- 1Department of Pharmacology, Faculty of Pharmacy and Health Sciences, University of Balochistan, Quetta, Pakistan
- 2Department of Drug Design and Pharmacology, Faculty of Health and Medical Sciences, University of Copenhagen, Copenhagen, Denmark
This work describes an analytical platform based on semi-high-resolution antileishmanial profiling combined with hyphenation of high-performance liquid chromatography – high-resolution mass spectrometry – solid-phase extraction – nuclear magnetic resonance spectroscopy, i.e., semiHR-antileishmanial assay/HPLC-HRMS-SPE-NMR. The platform enables fast pinpointing of HPLC peaks representing Leishmania tropica inhibitors in complex matrices, with subsequent structural identification of targeted inhibitors. Active analytes were cumulatively trapped on SPE cartridges and the structures elucidated by analysis of NMR spectra obtained in the HPLC-HRMS-SPE-NMR mode. This led to the identification of six known compounds 2,4,6-trihydroxyacetophenone-2-O-β-D-glucopyranoside (1), lalioside (2), luteolin-4′-O-β-D-glucopyranoside (3), apigenin-4′-O-β-D-glucopyranoside (4), luteolin (5), and apigenin (6). IC50 of the active compounds were determined with luteolin being the most potent inhibitor with an IC50 value of 4.15 μg/ml. The platform proved to be an efficient method for the identification of L. tropica inhibitors.
Introduction
Leishmaniasis, caused by parasites belonging to the genus Leishmania (Family Trypanosomatidae), is a major public health problem in tropical and sub-tropical regions. The parasite is transmitted by the sand fly vector, with dogs, sheep, rats, horses, and cats being common animal hosts of leishmaniasis (Pearson and Sousa, 1996; Markle and Makboul, 2004). WHO has reported that people from 98 countries – covering five continents – are at high risk of contracting leishmaniasis, and it is estimated that approximately 12 million people are currently infected (Alvar et al., 2012). Cutaneous leishmaniasis is caused by different Leishmania species, e.g., Leishmania tropica, Leishmania major, Leishmania amazonensis, and Leishmania braziliensis. In Pakistan, L. tropica is the main cause of cutaneous leishmaniasis (Rowland et al., 1999; Brooker et al., 2004). First line therapy for cutaneous leishmaniasis in Europe, Asia, and Africa is pentavalent antimonials, i.e., sodium stibogluconate and meglumine antimoniate (Markle and Makboul, 2004). However, antimonials have severe side effects like myalgia, pancreatitis, cardiac arrhythmia, hepatitis, and accumulation of the drug in liver and spleen. Thus, there is an urgent need for new chemical entities for non-toxic and effective treatment of leishmaniasis (Brooker et al., 2004; Markle and Makboul, 2004).
Lawsonia inermis L. is commonly known as Henna or Mehndi (Family Lythraceae) (Kumar et al., 2005). It is native to Northern Africa and South-western Asia, and is cultivated in many tropical and sub-tropical regions (Cartwright-Jones, 2006). In Pakistan, it is widely found in the Dera Ismail khan and Bannu districts of the Khyber Pakhtunkhwa province. L. inermis is extensively used for different medicinal purposes, and possess a variety of biological and pharmacological activities, including antioxidant (Dasgupta et al., 2003), antibacterial (Ali et al., 2001), antifungal (Singh and Pandey, 1989), antiviral (Khan et al., 1991), antiparasitic (Okpeton et al., 2004), analgesic (Mohsin et al., 1989), cytotoxic (Ali and Grever, 1998), antidiabetic (Arayne et al., 2007), antileishmanial (Iqbal et al., 2016a,b) and protein glycation inhibitory activity (Sultana et al., 2009). L. inermis is chemically well investigated (Semwal et al., 2014), and more than 135 compounds have been reported from the genus. This includes phenolic compounds [flavonoids (Liou et al., 2013), naphthalenes (Hsouna et al., 2011), naphthoquinones (Almeida et al., 2012), coumarins (Chaudhary et al., 2010), alkylphenones (Hsouna et al., 2011)], terpenes [volatile terpenes (Hema et al., 2010), non-volatile terpenes (Liou et al., 2013)], aliphatic hydrocarbons, and alkaloids (Iqbal et al., 2016b).
A major bottleneck in our ongoing search for antiparasitic constituents from plants (Sairafianpour et al., 2002; Ziegler et al., 2002; Pedersen et al., 2009) has been the traditional time-consuming bioassay-guided isolation of the antileishmanial compounds. This urged us to implement new bioanalytical technologies for faster analyses targeting the bioactive constituents. Hyphenation of separation techniques, spectroscopic methods, and bioassays has in recent years proven to be an efficient strategy for this purpose (Van Beek et al., 2009). While the commonly used hyphenation of high-performance liquid chromatography coupled with high-resolution mass spectrometry (HPLC-HRMS) is a fast and sensitive technique, it has several limitations when it comes to full structural elucidation of complex natural products. Especially promising is therefore the additional hyphenation of HPLC and HPLC-HRMS with solid-phase extraction and nuclear magnetic resonance spectroscopy, i.e., HPLC-(HRMS)-SPE-NMR (Lambert et al., 2005; Johansen et al., 2011), which allows full structural identification of complex natural products directly from crude extracts. As HPLC-HRMS-SPE-NMR only allows for chemical analysis, the recent combination with semi-high-resolution and/or high-resolution bioactivity profiling, i.e., microfractionation in microplates followed by bioassaying to yield a semi-high or high-resolution inhibition profile, is one of the most promising technological developments within bioanalytical plant research. The resulting HR-bioassay/HPLC-HRMS-SPE-NMR technology platform has already proven effective for fast identification of α-glucosidase inhibitors (Schmidt et al., 2012, 2014; Kongstad et al., 2015; Wubshet et al., 2015), aldose reductase inhibitors (Tahtah et al., 2015), α-amylase inhibitors (Okutan et al., 2014), and radical scavengers (Wiese et al., 2013; Wubshet et al., 2013; Liu et al., 2015) directly from crude plant extracts. However, the current work is the first example of semi-high-resolution antileishmanial inhibition profiling coupled with HPLC-HRMS-SPE-NMR.
Materials and Methods
Chemicals
Fetal bovine serum (FBS), dimethyl sulfoxide (DMSO), RPMI-1640 medium, Amphotericin B, penicillin, streptomycin, formic acid, analytical grade HPLC solvents (chloroform, methanol, ethyl acetate, n-hexane, acetone), and methanol-d4 (99.8 atom % of deuterium) were purchased from Sigma-Aldrich (St. Louis, MO, United States), whereas silica gel 60 0.063–0.200 mm (70–230 mesh ASTM) was purchased from MERCK (Darmstadt, Germany). Water used for HPLC was purified by deionization and 0.22 μm membrane filtration (Millipore, Billerica, MA, United States).
Collection of Plant Material and Preparation of Crude Extract
Leaves of Lawsonia inermis L. were collected from the territory of Dera Ismail Khan, Khyber Pakhtunkhwa (KPK), Pakistan in August and September 2014. Identification was performed by Dr. Siraj-ud-Din, Department of Botany, and a voucher specimen [accession number: Bot, 200101 (pup)] was deposited at Department of Botany, University of Peshawar (UOP), KPK. The leaves were washed with distilled water before drying in the shade at temperatures below 35°C. The leaves were stored in a cool dark place until use. Ground material of L. inermis (leaves, 1 kg) was extracted with methanol (2 L) for 1 week with regular stirring. The extract was filtered and concentrated in vacuo to afford 500 g of an oily extract.
Preparative-Scale Fractionation
The crude leaves extract (250 g) was fractionated by means of silica gel column chromatography (100 cm × 50 mm i.d column, silica gel 60 0.063–0.200 mm from MERCK (Darmstadt), Germany). A gradient elution was used starting with n-hexane-chloroform (99:1) with 20% step-wise increments to 100% chloroform, followed by 20% increments of ethyl acetate, and subsequent 20% increments of methanol to 100% methanol. Each of the increments constituted 500 mL solvent, which yielded 89 fractions. Based on TLC, fractions 1–5 were pooled (fraction F1, 6 g), fractions 6–25 (F2, 9.5 g), fractions 26–40 (F3, 3.5 g), fractions 41–45 (F4, 5 g), fractions 46–65 (F5, 10 g), fractions 66–80 (F6, 6 g), and fractions 81–89 (F7, 8.5 g). Fraction F1–F7 was subjected for in vitro antileishmanial activity, vide infra.
In Vitro Antileishmanial Activity
In vitro antileishmanial activity of L. inermis fractions were performed with clinically isolated L. tropica promastigotes (KWH23, Recently Pakistani clinically isolated strain, UOP, Pakistan). The in vitro antileishmanial growth inhibition assay was adopted from Iqbal et al. (2016b). Promastigotes of L. tropica were cultured in RPMI-1640 medium containing 10% FBS, 200 U/mL of penicillin, and 0.2 mg/mL of streptomycin. The parasites were cultured at 26°C for 4 days in an incubator (Gallenkamp, Size 1, United Kingdom), where after promastigotes were harvested in sterile tubes. The number of promastigotes was measured by transferring 5–10 μL to a haemocytometer (Reichert Technologies, Depew, NY, United States), and counting the number of promastigotes under upright microscope (CX31, Olympus, Tokyo, Japan). The viable cell count was calculated using the formula:
The harvested promastigotes were subsequently centrifuge at 4°C at 2000 rpm for 10 min, the supernatant removed, and the pellet reconstituted in fresh RPMI-1640 medium with 10% FBS to obtain a concentration of 1.4 × 106 promastigotes/mL which was distributed in a 96 well culture plate (180 μL each) and incubated for 2 days at 26°C with fractions of L. inermis.
The percentage inhibition of parasite growth was calculated as the mean of three replicate measurements with standard deviation using the equation:
IC50 values were determined by non-linear regression analysis using Graph Pad Prism 6 software.
Preparative-Scale Fractionation of F5
An injection solution of 0.1 g/mL of fraction F5 was subjected to preparative-scale RP-HPLC using an Agilent 1100 system equipped with two preparative solvent delivery units, a multiple wavelength detector, an autosampler, and a fraction collector. Separation was performed using a 250 mm × 21.2 mm i.d. Phenomenex Luna C18 column with 5 μm particle size (Phenomenex, Torrance, CA, United States) operated at room temperature. The aqueous eluent (A) consisted of water–acetonitrile (95:5), and the organic eluent (B) consisted of water–acetonitrile (5:95) both acidified with 0.1% formic acid. The eluent flow rate was maintained at 20 mL/min. Repeated injection (3 × 900 μL) of the above solution was followed by a gradient elution profile as follows: 0 min, 10% B; 40 min, 40% B; 45 min, 100% B; 55 min, 100% B; 56 min, 10% B. This afforded 27.1 mg of subfraction 1, 10.9 mg of subfraction 2, 9.3 mg of subfraction 3, 8.3 mg of subfraction 4, 15.2 mg of subfraction 5, 10.0 mg of subfraction 6, 10.3 mg of subfraction 7, 6.8 mg of subfraction 8, 21.1 mg of subfraction 9, 5.3 mg of subfraction 10, 6.9 mg of subfraction 11, 5.3 mg of subfraction 12, 3.4 mg of subfraction 13, 2.5 mg of subfraction 14, 4.4 mg of subfraction 15, 96.6 mg of subfraction 16, 35.1 mg of subfraction 17, 3.9 mg of subfraction 18, 6.3 mg of subfraction 19, and 3.7 mg of subfraction 20. All collected subfractions were concentrated under reduced pressure at 45°C in rotary evaporator. Initially, the subfractions were reconstituted in 0.1% DMSO in order to assess the IC50 values in the in vitro antileishmanial growth inhibition assay, vide supra.
HPLC-HRMS-SPE-NMR
The HPLC-HRMS-SPE-NMR system consisted of an Agilent 1200 chromatograph comprising of a quaternary pump, degasser, thermostated column compartment, auto sampler, and photodiode array detector (Santa Clara, CA, United States), a Bruker microOTOF-Q II mass spectrometer (Bruker Daltonik, Bremen, Germany) equipped with an electrospray ionization source and operated via a 1:99 flow splitter, a Knauer Smartline K120 pump for post-column dilution (Knauer, Berlin, Germany), a Spark Holland Prospekt2 SPE unit (Spark Holland, Emmen, Netherlands), a Gilson 215 liquid handler equipped with a 1 mm needle for automated filling of 1.7 mm NMR tubes, and a Bruker Avance III 600 MHz NMR spectrometer (1H operating frequency 600.13 MHz) equipped with a Bruker Sample Jet sample changer and a cryogenically cooled gradient inverse triple-resonance 1.7 mm TCI probe-head (Bruker Biospin, Rheinstetten, Germany). Mass spectra were acquired in positive and negative ion modes, using drying temperature of 200°C, capillary voltage of -4100 and +4000 V for positive and negative ion modes, respectively, nebulizer pressure of 2.0 bar, and drying gas flow of 7 L/min. The negative ion mode HPLC-HRMS analysis was performed in a different experiment using identical chromatographic condition. A solution of sodium formate clusters was injected in the beginning of each run to enable internal mass calibration. Chromatographic separation was acquired on a Phenomenex Luna C18(2) column (150 mm × 4.6 mm, 3 μm, 100 Å; Phenomenex, Torrance, CA, United States) maintained at 40°C, using water–acetonitrile (95:5) (eluent A) and acetonitrile–water (95:5) (eluent B), both acidified with 0.1% formic acid. At a flow rate of 0.5 mL/min the following gradient elution profile was used: 0 min, 10% B; 40 min, 40% B; 45 min, 100% B; 55 min, 100% B; 56 min, 10% B. Cumulative SPE trapping of peaks 1–9 of L. inermis fraction F5 was performed after 10 consecutive separations. The HPLC eluate was diluted with Milli-Q water at a flow rate of 1.5 mL/min prior to trapping on 10 mm × 2 mm i.d. resin GP (general purpose, 5–15 μm, spherical shape, polydivinyl-benzene phase) SPE cartridges from Spark Holland (Emmen, Netherlands), and analytes were trapped using absorption thresholds (280 and 330 nm). SPE cartridges were conditioned with 1000 μL of acetonitrile at 6 mL/min and equilibrated with 500 μL of Milli-Q water at 1 mL/min prior to trapping. Loaded cartridges were dried with pressurized nitrogen gas for 45 min each prior to elution with methanol-d4. Separations were controlled by Bruker HyStar version 3.2 software, automated filling of NMR tubes was controlled by Prep Gilson ST version 1.2 software, and automated NMR acquisition was controlled by Bruker IconNMR version 4.2 software. NMR data processing was performed using Bruker Topspin version 3.2 software.
NMR Experiments
All NMR spectra were recorded in methanol-d4 at 300 K. 1H and 13C chemical shifts were referenced to the residual solvent signal (δ 3.31 and 49.00, respectively). One dimensional 1H NMR spectra were with 30° pulses, 3.66 s interpulse intervals, 64k data points, and multiplied with an exponential function corresponding to line-broadening of 0.3 Hz prior to Fourier transform. Phase-sensitive DQF-COSY spectra were recorded using a gradient-based pulse sequence with a 20 ppm spectral width and 2k × 256 data points (processed with forward linear prediction to 1k data points). Multiplicity-edited HSQC spectra were acquired with the following parameters: spectral width 20 ppm for 1H and 165 ppm for 13C, 2k × 256 data points (processed with forward linear prediction to 1k data points), and 2.0 s relaxation delay. HMBC spectra were optimized for nJC,H = 10 Hz and acquired using the following parameters: spectral width 20 ppm for 1H and 222 ppm for 13C, 2k × 128 data points (processed with forward linear prediction to 1k data points), and 1.5 s relaxation delay.
Results
The current work describes the use of HPLC-HRMS-SPE-NMR for dereplication of metabolites in extract of Lawsonia inermis leaves – in combination with semi-high-resolution antileishmanial profiling. Initially, crude methanol extract was tested for its ability to inhibit extracellular promastigotes of L. tropica at concentrations of 100, 50, 25, and 10 mg/ml, which resulted in 100% inhibition at all concentrations. The crude methanol extract was subsequently subjected to preparative-scale column chromatography for separation into seven fractions (F1–F7). These fractions were tested for their ability to inhibit extracellular promastigotes, and as seen in Table 1, Fraction 5 showed the strongest antileishmanial activity.
Fraction 5 was selected for semi-high-resolution antileishmanial screening with the eluate from 10 to 40 min being separated into 20 sub-fractions in 96-well microplates. The eluate was evaporated, and the dried material in each well was redissolved in DMSO to make stock and working solutions. These solutions were assayed for their in vitro antileishmanial activity toward extracellular promastigotes. The active major constituents of subfractions 5, 7, 10, and 13 were identified by HPLC-HRMS-SPE-NMR directly from the crude extract and the IC50 values of the identified, active metabolites were determined.
Discussion
HPLC-HRMS-SPE-NMR
The major metabolites of the active fractions (peaks 2, 4, 5, 7, and 9), together with additional four peaks, were subjected to HPLC-HRMS-SPE-NMR analysis (Figure 1). Detailed HRMS and NMR analysis resulted in identification of six known compounds 1–6 (Figure 2) corresponding to peaks 1, 2, 4, 5, 7, and 9, while for peaks 3, 6, and 8, the quality of the NMR data did not allow for full structure elucidation. 1H NMR and HRMS data of 1–6 obtained in the HPLC-HRMS-SPE-NMR mode is provided in the compound summary paragraph.
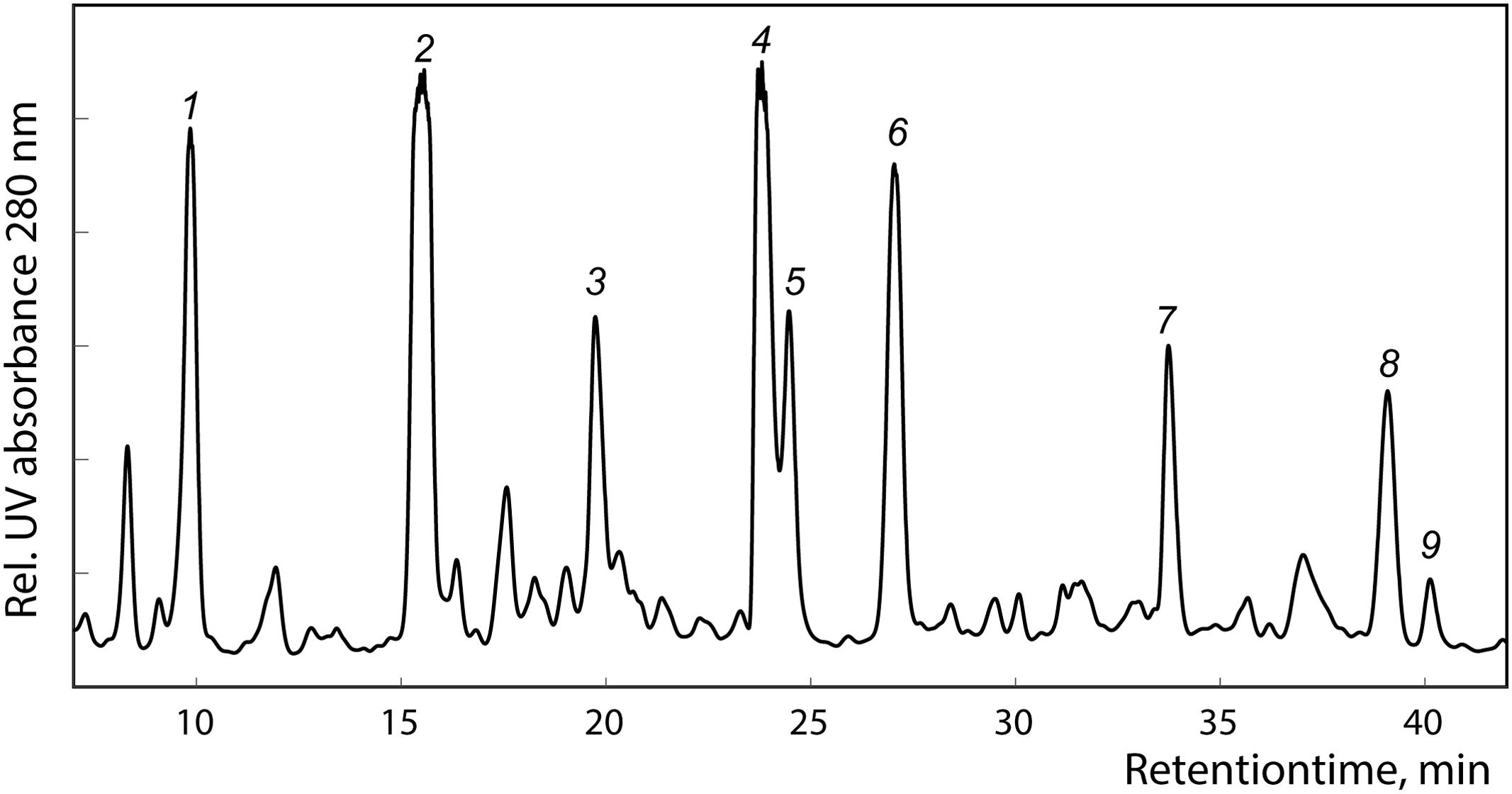
FIGURE 1. HPLC chromatogram of Lawsonia inermis L. leaves F5 extract at 280 nm acquired in the HPLC-HRMS-SPE-NMR mode.
The known compounds were identified by comparison of their spectral data with those reported in the literature as 2,4,6-trihydroxyacetophenone-2-O-β-D-glucopyranoside (1) (Lee et al., 1996), lalioside (2) (Takeda and Fatope, 1988), luteolin-4′-O-β-D-glucopyranoside (3) (Lee et al., 2002), apigenin 4′-O-β-D-glucopyranoside (4) (Ding et al., 2004), luteolin (5) (Scognamiglio et al., 2014), and apigenin (6) (Miyazawa and Hisama, 2003) and confirmed by 2D NMR analysis.
Antileishmanial Activity
In our work, F5 was subjected to prep HPLC for time slice based fractionation. It results in 20 sub-fractions which were further analyzed by extracellular promastigotes in which four sub-fractions showed promising inhibitory activity as shown in Table 2. After observation of their significant antileishmanial activity in the sub-fraction screening, lalioside (2), luteolin-4′-O-β-D-glucopyranoside (3), apigenin 4′-O-β-D-glucopyranoside (4), luteolin (5), and apigenin (6) were isolated by preparative- and analytical-scale HPLC, and the materials were used for assessing IC50 values in antileishmanial activity assay.
This is in agreement with previously observed inhibition of L. tropica by crude extract of different L. inermis plant parts (Iqbal et al., 2016b). Tasdemir et al. (2006) have previously investigated the structure-activity relationship of flavonoids, including luteolin (5) and apigenin (6). It was found that a minimum of two hydroxy substituents on the A ring, preferably on position 5, 7, and 8, greatly increased the antileishmanial potential. Furthermore, the double bond between C-2 and C-3 proved to be important for the activity. Both luteolin (5) and apigenin (6) are di-substituted on the ‘A’ ring on positions 5 and 7 and both having the important double bond Δ2,3 accounting for the IC50 values of 4.15 and 8.30 μg/mL, respectively, with the differences in the antileishmanial potential attributes to the different substitution pattern on the B ring. Interestingly, the 4′-O-β-D-glucosides of 5 and 6 (10.27 and 9.51 μg/mL, 3 and 4, respectively) showed a markedly decreased inhibitory effect against extracellular promastigotes. This is in line with previous reports that glucosylation on the A ring have similar effects (Tasdemir et al., 2006). Lalioside (2), a tetra-substituted acetophenone glucoside, showed promising inhibitory effects with an IC50 value of 5.02 μg/mL while the tri-substituted acetophenone glucoside (1) did not show noteworthy antileishmanial potential in the semi-high-resolution screening, indicating the importance of the C-3 hydroxyl substituent. While both luteolin (5) and apigenin (6) have previously been shown to possess activity against L. donovani (0.8 and 1.8 μg/mL, respectively) (Tasdemir et al., 2006) this is the first report of their in vitro activity against L. tropica and the first time lalioside (2), luteolin-4′-O-β-D-glucopyranoside (3), and apigenin-4′-O-β-D-glucopyranoside (4) are reported against any Leishmania species.
Conclusion
In conclusion, luteolin and lalioside are potent inhibitors while apigenin, apigenin-4′-O-β-D-glucopyranoside, and luteolin-4′-O-β-D-glucopyranoside have moderate inhibitory effect on extracellular promastigotes of L. tropica. Further studies on identified compounds of Lawsonia Inermis are planned to investigate specificity and structure-activity relationship.
Author Contributions
KI: Performed assay and chemical investigation. Participated in writing the manuscript. JI: Supervised and assisted KI on the antileishmanial work and participated in writing the manuscript. DS: Supervised and assisted KI on the chemical investigation and participated in writing the manuscript. KK: Took part in the chemical investigation and participated in writing the manuscript.
Conflict of Interest Statement
The authors declare that the research was conducted in the absence of any commercial or financial relationships that could be construed as a potential conflict of interest.
Acknowledgments
HPLC equipment used for semi-high resolution bioassay profiles was obtained via a grant from The Carlsberg Foundation. The 600 MHz HPLC-HRMS-SPE-NMR system used in this work was acquired through a grant from “Apotekerfonden af 1991”, The Carlsberg Foundation, and the Danish Agency for Science, Technology and Innovation via the National Research Infrastructure funds. The research scholarship to KI is provided by the International Research Support Initiative Program by the Higher Education Commission of Pakistan. Laboratory technician Arife Önder is gratefully acknowledged for assistance in preparative isolation of pure compounds.
2,4,6-Trihydroxyacetophenone-2-O-β-D-glucopyranoside (1): 1H NMR (CD3OD, 600 MHz) δ 6.18 (1H, d, J = 1.8 Hz, H-3), 5.95 (1H, t, J = 1.8 Hz, H-5), 5.02 (1H, d, J = 7.8 Hz, H-1′), 3.93, d, J = 12.1 Hz H-6′A), 3.73 (dd, J = 12.1, 5.5 Hz, H-6′B), 3.53 (1H, dd, J = 9.2, 7.8 Hz, H-2′), 3.48 (2H, m, H-3′, H-5′), 3.41 (1H, t, J = 9.5 Hz, H-3′), 2.69 (3H, s, Me); (+) HRESIMS m/z 331.1022 [M+H]+ (calcd for C14H19O9+, ΔM 0.4 ppm).
Lalioside (2): 1H NMR (CD3OD, 600 MHz) δ 5.90 (1H, s, H-5), 4.53 (1H, d, J = 7.8 Hz, H-1′), 3.84, dd, J = 12.1, 2.3 Hz, H-6′A), 3.76 (dd, J = 12.1, 4.4 Hz, H-6′B), 3.4-3.51 (3H, m, H-3′, H-4′, H-5′), 3.45 (1H, m, H-2′), 2.63 (3H, s, Me); (+) HRESIMS m/z 347.0976 [M+H]+ (calcd for C14H19O10+, ΔM -0.8 ppm).
Luteolin-4′-O-β-D-glucopyranoside (3): 1H NMR (CD3OD, 600 MHz) δ 7.43 (2H, m, H-2′, H-6′), 7.30 (1H, d, J = 8.3 Hz, H-5′), 6.59 (1H, s, H-3), 6.44 (1H, brs, H-8), 6.20 (1H, brs, H-6), 4.94 (1H, d, J = 7.6 Hz, H-1″), 3.93 (1H, dd, J = 12.2, 1.9 Hz, H-6″A), 3.74 (1H, dd, J = 12.2, 5.5 Hz, H-6″B), 3.54 (1H, m, H-2″), 3.50 (2H, m, H-3″, H-5″), 3.43 (1H, m, H-4″); (+) HRESIMS m/z 449.1084 [M+H]+ (calcd for C21H21O11+, ΔM -1.3 ppm).
Apigenin-4′-O-β-D-glucopyranoside (4): 1H NMR (CD3OD, 600 MHz) δ 7.95 (2H, d, J = 8.9 Hz, H-2′/H-6′), 7.25 (2H, d, J = 8.9 Hz, H-3′/H-5′), 6.66 (1H, s, H-3), 6.48 (1H, d, J = 2.1 Hz, H-8), 6.26 (1H, d, J = 2.1 Hz, H-6), 5.04 (1H, d, J = 7.6 Hz, H-1″), 3.92 (1H, dd, J = 12.1, 2.1 Hz, H-6″A), 3.72 (1H, dd, J = 12.1, 5.6 Hz, H-6″B), 3.50 (3H, m, H-2″, H-3″, H-5″), 3.43 (1H, m, H-4″); (+) HRESIMS m/z 433.1128 [M+H]+ (calcd for C20H21O10+, ΔM 0.2 ppm).
Luteolin (5) : 1H NMR (CD3OD, 600 MHz) δ 7.39 (1H, dd, J = 8.6, 2.3 Hz, H-6′), 7.38 (1H, d, J = 2.3 Hz, H-2′), 6.90 (1H, d, J = 8.6 Hz, H-5′), 6.55 (1H, s, H-3), 6.45 (1H, d, J = 2.1 Hz, H-8), 6.22 (1H, d, J = 2.1 Hz, H-6); (+) HRESIMS m/z 287.0552 [M+H]+ (calcd for C15H11O6+, ΔM -0.8 ppm).
Apigenin (6) : 1H NMR (CD3OD, 600 MHz) δ 7.86 (2H, d, J = 8.9 Hz, H-2′/H-6′), 6.94 (2H, d, J = 8.9 Hz, H-3′/H-5′), 6.60 (1H, s, H-3), 6.47 (1H, d, J = 2.1 Hz, H-8), 6.22 (1H, d, J = 2.1 Hz, H-6); (+) HRESIMS m/z 271.0606 [M+H]+ (calcd for C15H11O5+, ΔM -1.9 ppm).
References
Ali, M., and Grever, M. R. (1998). A cytotoxic napthoquinone from Lawsonia inermis. Fitoterapia 69, 181–183.
Ali, N. A., Julich, W. D., Kusnick, C., and Lindequist, U. (2001). Screening of Yemeni medicinal plants for antibacterial and cytotoxic activities. J. Ethnopharmacol. 74, 173–179. doi: 10.1016/S0378-8741(00)00364-0
Almeida, P. J., Borrego, L., Pulido-Melian, E., and Gonzalez-Diaz, O. (2012). Quantification of p-phenylenediamine and 2-hydroxy-1,4-naphthoquinone in henna tattoos. Contact. Dermatitis 66, 33–37. doi: 10.1111/j.1600-0536.2011.01992.x
Alvar, J., Velez, I. D., Bern, C., Herrero, M., Desjeux, P., Cano, J., et al. (2012). Leishmaniasis worldwide and global estimates of its incidence. PLoS ONE 7:e35671. doi: 10.1371/journal.pone.0035671
Arayne, M. S., Sultana, N., Mirza, A. Z., Zuberi, M. H., and Siddiqui, F. A. (2007). In vitro hypoglycemic activity of methanolic extract of some indigenous plants. Pak. J. Pharm. Sci. 20, 268–273.
Brooker, S., Mohammed, N., Adil, K., Agha, S., Reithinger, R., Rowland, M., et al. (2004). Leishmaniasis in refugee and local Pakistan populations. Emerg. Infect. Dis. 10, 1681–1684. doi: 10.3201/eid1009.040179
Cartwright-Jones, C. (2006). Developing Guidelineson Henna: A Geographical Approach. Masters’ dissertation, Kent State University, Kent, OH.
Chaudhary, G., Goyal, S., and Poonia, P. (2010). Lawsonia inermis Linnaeus: a phytopharmacological review. Int. J. Pharm. Sci. Drug. Res. 2, 91–98.
Dasgupta, T., Rao, A. R., and Yadava, P. K. (2003). Modulatory effect of henna leaf (Lawsonia inermis) on drug metabolizing phase I and Phase II enzymes, antioxidant enzymes, lipid peroxidation and chemically induced skin and forestomach papillomagenesis in mice. Mol. Cell. Biochem. 245, 11–22. doi: 10.1023/A:1022853007710
Ding, H. Y., Chan, Y. Y., Chang, W. L., and Lin, H. C. (2004). Flavonoids from the flowers of Pueraria lobata. J. Chin. Chem. Soc. 51, 1425–1428. doi: 10.1002/jccs.200400210
Hema, R., Kumaravel, S., Gomathi, S., and Sivasubramaniam, C. (2010). Gas chromatography-Mass spectroscopic analysis of Lasonia inermis leaves. Life Sci. J. 7, 48–50.
Hsouna, A. B., Trigui, M., Culioli, G., Blache, Y., and Jaoua, S. (2011). Antioxidants constituents from Lawsonia inermis leaves: Isolation, structure elucidation and antioxidative capacity. Food Chem. 125, 193–200. doi: 10.1016/j.foodchem.2010.08.060
Iqbal, K., Iqbal, J., and Afreen, M. S. (2016a). Comparative study on antileishmanial and cytotoxic activity of Lawsonia inermis bark and Aloe Vera leaves. Int. J. Biol. Pharm. Allied. Sci. 5, 1490–1500.
Iqbal, K., Iqbal, J., Umair, M., Farooq, U., Iqbal, M. M., Qamar, S., et al. (2016b). Anti-leishmanial and cytotoxic activities of extracts from three Pakistani Plants. Trop. J. Pharm. Res. 15, 2113–2119. doi: 10.4314/tjpr.v15i10.9
Johansen, K. T., Wubshet, S. G., Nyberg, N. T., and Jaroszewski, J. W. (2011). From retrospective assessment to prospective decisions in natural product isolation: HPLC-SPE-NMR analysis of Carthamus oxyacantha. J. Nat. Prod. 74, 2454–2461. doi: 10.1021/np200780m
Khan, M. M., Ali, A., Jain, D. C., Bhakuni, R. S., Zaim, M., and Thakur, R. S. (1991). Occurrence of some antiviral sterols in Artemisia annua. Plant Sci. 75, 161–165. doi: 10.1016/0168-9452(91)90230-6
Kongstad, K. T., Özdemir, C., Barzak, A., Wubshet, S. G., and Staerk, D. (2015). Combined use of high-resolution α-glucosidase inhibition profiling and high-performance liquid chromatography-high-resolution mass spectrometry-solid-phase extraction-nuclear magnetic resonance spectroscopy for investigation of antidiabetic principles in crude plant extracts. J. Agric. Food. Chem. 63, 2257–2263. doi: 10.1021/jf506297k
Kumar, S., Singh, Y. V., and Singh, M. (2005). Agro-History, Uses, Ecology and Distribution of Henna (Lawsonia inermis L. syn. Alba Lam). Henna: Cultivation, Improvement, and Trade. Jodhpur: Central Arid Zone Research Institute, 11–12.
Lambert, M., Staerk, D., Hansen, S. H., and Jaroszewski, J. W. (2005). HPLC-SPE-NMR hyphenation in natural products research: optimization of analysis of Croton membranaceus extract. Magn. Reson. Chem. 43, 771–775. doi: 10.1002/mrc.1613
Lee, K. R., Hong, S. W., Kwak, J. H., Pyo, S. P., and Jee, O. P. (1996). Phenolic constituents from the Aerial parts of Artemisia stolonifera. Arch. Pharm. Res. 19, 231–234. doi: 10.1007/BF02976896
Lee, M. H., Son, Y. K., and Han, Y. N. (2002). Tissue factor inhibitory flavonoids from the fruits of Chaenomeles sinensis. Arch. Pharm. Res. 25, 842–850. doi: 10.1007/BF02977002
Liou, J. R., El-Shazly, M., Du, Y. C., Tseng, C. N., Hwang, T. L., Chuang, Y. L., et al. (2013). 1,5-Diphenylpent-3-en-1-ynes and methyl naphthalene carboxylates from Lawsonia inermis and their anti-inflammatory activity. Phytochemistry 88, 67–73. doi: 10.1016/j.phytochem.2012.11.010
Liu, B., Kongstad, K. T., Qinglei, S., Nyberg, N. T., Jäger, A. K., and Staerk, D. (2015). Dual high-resolution α-glucosidase and radical scavenging profiling combined with HPLC-HRMS-SPE-NMR for identification of minor and major constituents directly from the crude extract of Pueraria lobata. J. Nat. Prod. 78, 294–300. doi: 10.1021/np5009416
Markle, W. H., and Makboul, K. (2004). Cutaneous leishmaniasis recognition and treatment. Am. Fam. Physician 69, 455–460.
Miyazawa, M., and Hisama, M. (2003). Antimutagenic activity of flavonoids from Chrysanthemum morifolium. Biosci. Biotechnol. Biochem. 67, 2091–2099. doi: 10.1271/bbb.67.2091
Mohsin, A., Shah, A. H., Al-Yahya, M. A., Tariq, M., Tanira, M. O., and Ageel, A. A. (1989). Analgesic, antipyretic activity and phytochemical screening of some plants used in traditional Arab system of medicine. Fitoterapia 60, 174–177.
Okpeton, T., Yolou, S., Gleye, C., Roblot, F., Loiseau, P., Bories, C., et al. (2004). Antiparasitic activities of medicinal plants used in ivory Coast. J. Ethnopharmacol. 90, 91–97. doi: 10.1016/j.jep.2003.09.029
Okutan, L., Kongstad, K. T., Jäger, A. K., and Staerk, D. (2014). High-resolution α-amylase assay combined with high-performance liquid chromatography-solid-phase extraction-nuclear magnetic resonance spectroscopy for expedited identification of α-amylase inhibitors: proof of concept and α-amylase inhibitor in cinnamon. J. Agric. Food Chem. 62, 11465–11471. doi: 10.1021/jf5047283
Pearson, R. D., and Sousa, A. D. Q. (1996). Clinical spectrum of leishmaniasis. Clin. Infect. Dis. 22, 1–13. doi: 10.1093/clinids/22.1.1
Pedersen, M. M., Chukwujekwu, J. C., Lategan, C. A., Staden, J. V., Smith, P. J., and Staerk, D. (2009). Antimalarial sesquiterpene lactones from Distephanus angulifolius. Phytochemistry 70, 601–607. doi: 10.1016/j.phytochem.2009.02.005
Rowland, M., Munir, A., Durrani, N., Noyes, H., and Reyburn, H. (1999). An outbreak of cutaneous leishmaniasis in an Afghan refugee settlement in north-west Pakistan. Trans. R. Soc. Trop. Med. Hyg. 93, 133–136. doi: 10.1016/S0035-9203(99)90285-7
Sairafianpour, M., Kayser, O., Christensen, J., Asfa, M., Witt, M., Staerk, D., et al. (2002). Leishmanicidal and antiplasmodial activity of constituents of Smirnowia iranica. J. Nat. Prod. 65, 1754–1758. doi: 10.1021/np020244s
Schmidt, J. S., Lauridsen, M. B., Dragsted, L. O., Nielsen, J., and Staerk, D. (2012). Development of a bioassay-coupled HPLC-SPE-ttNMR platform for identification of α-glucosidase inhibitors in apple peel (Malus × domestica Borkh.). Food Chem. 135, 1692–1699. doi: 10.1016/j.foodchem.2012.05.075
Schmidt, J. S., Nyberg, N. T., and Staerk, D. (2014). Assessment of constituents in Allium by multivariate data analysis, high-resolution α-glucosidase inhibition assay and HPLC-SPE-NMR. Food. Chem. 161, 192–198. doi: 10.1016/j.foodchem.2014.03.062
Scognamiglio, M., Fiumano, V., Abrosca, B. D., Esposito, A., Choi, Y. H., Verpoortw, R., et al. (2014). Chemical interactions between plants in Mediterranean vegetation: the influence of selected plant extracts on Aegilops geniculata metabolome. Phytochemistry 106, 69–85. doi: 10.1016/j.phytochem.2014.07.006
Semwal, R. B., Semwal, D. K., Combrinck, S., Cartwright-Jones, C., and Viljoen, A. (2014). Lawsonia inermis L. (Henna): Ethnobotanical, phytochemical and pharmacological aspects. J. Ethnopharmacol. 155, 80–103. doi: 10.1016/j.jep.2014.05.042
Singh, V. K., and Pandey, D. K. (1989). Fungitoxic studies on bark extract of Lawsonia inermis against ringworm fungi. Hindustan Antibiot. Bull. 31, 32–35.
Sultana, N., Chaudhary, M. I., and Khan, A. J. (2009). Protein glycation inhibitory activities of Lawsonia inermis and its active principles. J. Enzym. Inhib. Med. Chem. 24, 257–261. doi: 10.1080/14756360802057500
Tahtah, Y., Kongstad, K. T., Wubshet, S. G., Nyberg, N. T., Jønsson, L. H., Jäger, A. K., et al. (2015). Triple aldose reductase/α-glucosidase/radical scavenging high-resolution profiling combined with high-performance liquid chromatography-high-resolution mass spectrometry-solid-phase extraction-nuclear magnetic resonance spectroscopy for identification of antidiabetic constituents in crude extract of Radix Scutellariae. J. Chromatogr. A 1408, 125–132. doi: 10.1016/j.chroma.2015.07.010
Takeda, Y., and Fatope, M. O. (1988). New phenolic glucosides from Lawsonia inermis. J. Nat. Prod. 51, 725–729. doi: 10.1021/np50058a010
Tasdemir, D., Kaiser, M., Brun, R., Yardley, V., Schmidt, T. J., Tosun, F., et al. (2006). Antitrypanosomal and antileishmanial activities of flavonoids and their analogues: in vitro, in vivo, structure-activity relationship, and quantitative structure-activity relationship studies. Antimicrob. Agents Chemother. 50, 1352–1364. doi: 10.1128/AAC.50.4.1352-1364.2006
Van Beek, T., Tetala, K., Koleva, I., Dapkevicius, A., Exarchou, V., Jeurissen, S., et al. (2009). Recent developments in the rapid analysis of plants and tracking their bioactive constituents. Phytochemistry Rev. 8, 387–399. doi: 10.1007/s11101-009-9125-9
Wiese, S., Wubshet, S. G., Nielsen, J., and Staerk, D. (2013). Coupling HPLC-SPE-NMR with a microplate-based high-resolution antioxidant assay for efficient analysis of antioxidants in food – Validation and proof-of-concept study with caper buds. Food Chem. 141, 4010–4018. doi: 10.1016/j.foodchem.2013.06.115
Wubshet, S. G., Moresco, H. H., Tahtah, Y., Brighente, I. M., and Staerk, D. (2015). High-resolution bioactivity profiling combined with HPLC-HRMS-SPE-NMR: α-Glucosidase inhibitors and acetylated ellagic acid rhamnosides from Myrcia palustris DC. (Myrtaceae). Phytochemistry 116, 246–252. doi: 10.1016/j.phytochem.2015.04.004
Wubshet, S. G., Schmidt, J. S., Wiese, S., and Staerk, D. (2013). High-resolution screening combined with HPLC-HRMS-SPE-NMR for identification of potential health-promoting constituents in sea aster and searocket–new Nordic food ingredients. J. Agric. Food Chem. 61, 8616–8623. doi: 10.1021/jf402949y
Ziegler, H. L., Jensen, T. H., Christensen, J., Staerk, D., Hägerstrand, H., Sittie, A. A., et al. (2002). Possible artefacts in the in vitro determination of antimalarial activity of natural products that incorporate into lipid bilayer: apparent antiplasmodial activity of dehydroabietinol, a constituent of Hyptis suaveolens. Planta Med. 68, 547–549. doi: 10.1055/s-2002-32548
Keywords: Leishmania tropica, Lawsonia inermis, leishmaniasis, semi-high-resolution inhibition profile, HPLC-HRMS-SPE-NMR
Citation: Iqbal K, Iqbal J, Staerk D and Kongstad KT (2017) Characterization of Antileishmanial Compounds from Lawsonia inermis L. Leaves Using Semi-High Resolution Antileishmanial Profiling Combined with HPLC-HRMS-SPE-NMR. Front. Pharmacol. 8:337. doi: 10.3389/fphar.2017.00337
Received: 21 December 2016; Accepted: 18 May 2017;
Published: 31 May 2017.
Edited by:
Marco Leonti, University of Cagliari, ItalyReviewed by:
Kannan R. R. Rengasamy, China Agricultural University, ChinaBanasri Hazra, Jadavpur University, India
Copyright © 2017 Iqbal, Iqbal, Staerk and Kongstad. This is an open-access article distributed under the terms of the Creative Commons Attribution License (CC BY). The use, distribution or reproduction in other forums is permitted, provided the original author(s) or licensor are credited and that the original publication in this journal is cited, in accordance with accepted academic practice. No use, distribution or reproduction is permitted which does not comply with these terms.
*Correspondence: Kenneth T. Kongstad, a2VubmV0aC5rb25nc3RhZEBzdW5kLmt1LmRr