- 1Department of Biochemistry, Faculty of Life Sciences, Aligarh Muslim University, Aligarh, India
- 2Department of Biochemistry, Faculty of Medicine & Applied Medical Sciences, Northern Border University, Arar, Saudi Arabia
- 3Department of Surgery, Loyola University Medical Center, Maywood, IL, United States
- 4King Fahd Medical Research Center, King Abdulaziz University, Jeddah, Saudi Arabia
- 5Department of Clinical Laboratory Sciences, College of Applied Medical Sciences, King Saud University, Riyadh, Saudi Arabia
- 6College of Medical Rehabilitation, Qassim University, Buraidah, Saudi Arabia
- 7Novel Global Community Educational Foundation, Hebersham, NSW, Australia
Psychological stress contributes to increased susceptibility to a number of diseases including cancer. The present study was designed to assess the effect of chronic unpredictable stress on N-nitrosodiethylamine induced liver toxicity in terms of in vivo antioxidant status and DNA damage in Swiss albino mice. The animals used in this study were randomized into different groups based on the treatment with N-nitrosodiethylamine or chronic unpredictable stress alone and post-stress administration of N-nitrosodiethylamine. The mice were sacrificed after 12 weeks of treatment, and the status of major enzymatic and non-enzymatic antioxidants, liver function markers, lipid peroxidation and the extent of DNA damage were determined in circulation and liver tissues of all the groups. The N-nitrosodiethylamine treated group showed significantly compromised levels of the antioxidant enzymes, lipid peroxidation, and the liver function markers with enhanced DNA damage as compared to chronic unpredictable stress or control groups. A similar but less typical pattern observed in the chronic unpredictable stress treated mice. All the measured biochemical parameters were significantly altered in the group treated with the combination of chronic unpredictable stress and N-nitrosodiethylamine when compared to controls, or chronic unpredictable stress alone and/or N-nitrosodiethylamine alone treated groups. Thus, exposure to continuous, unpredictable stress conditions even in general life may significantly enhance the hepatotoxic potential of N-nitrosodiethylamine through an increase in the oxidative stress and DNA damage.
Introduction
The behavioral processes have long been suspected to influence many health conditions including cancer. Both progression and to a lesser extent cancer onset, have been related to chronic stress, depression, lack of social support and various other psychological factors (Reiche et al., 2004; Antoni et al., 2006). Stress exposure induces a cluster of physiological and behavioral changes that have been linked to the response of the organism to maintain the homeostasis. Moreover, stress has been shown to markedly influence incidence, growth, metastasis, and the rejection of both the chemically induced and implanted tumors (Amkraut and Solomon, 1972; Zygmunt et al., 1985). Both the exacerbation and attenuation of tumor development have, however, been reported in response to stress (Pradhan and Prabhati, 1974; Justice, 1985).
N-nitrosodiethylamine (NDEA) causes oxidative stress and cellular injury by enhancing the formation of free radicals (Ramakrishnan et al., 2006; Valko et al., 2006). The metabolic activation of NDEA by cytochrome P450 enzymes converts it to ethyl-acetoxyethyl-nitrosamine, which can be conjugated by the phase II enzymes (Subramanian et al., 2007) to a non-toxic compound. Alternatively, it can form ethyldiazonium ion that directly ethylates cellular macromolecules (Muzio et al., 1999), and is responsible for its cytotoxic, mutagenic, and carcinogenic effects (Abdel-Hamid et al., 2013) through the production of ROS (Bansal et al., 2000).
Oxidative stress, a pervasive condition induced by psychological stress, has been implicated in and recognized to be a prominent feature associated with various diseases like cancer and their progression. An imbalance in the pro-oxidant/antioxidant status indicates the enhanced production of reactive oxygen species (ROS), such as peroxides, hydroxyl, and superoxide anion radicals (Muqbil et al., 2006), which induces cellular oxidative damage through DNA strand breaks and lipid peroxidation (Batcioglu et al., 2002). Thus, DNA damage induced by oxidative stress and/or deficient DNA-repair has an etiological or prognostic role in cancer (Mussarat et al., 1996). A previous study (Ip et al., 1996) has demonstrated the effect of restraint stress on the antioxidant status and DNA damage in rats. It was found that chronic restraint stress increased lipid peroxidation, compromised antioxidant status and enhanced DNA damage (Zaidi et al., 2005; Muqbil and Banu, 2006; Muqbil et al., 2006). Other studies have confirmed that stress is associated with low concentrations of O6 methyltransferase, which is an important DNA-repair enzyme (Glaser et al., 1985). Hence, it is important to correlate between alterations in the parameters of psychological stress with those of redox state, DNA-repair activity, and antioxidant defense so as to identify the possible relationship between chronic stress and cancer.
The chronic unpredictable stress (CUS) is a well-recognized model for inducing chronic physical and psychological stress. CUS has been used in the present study to assess the effects of various psychological factors and stressors on some biochemical parameters and DNA damage, also in combination with the known chemical carcinogen NDEA. This study may throw light on the effect of CUS on the early stages of liver cancer as evident from the measured biochemical markers, antioxidant status, and the DNA damage. This also suggests that the life changing unpredictable stresses may enhance the carcinogenic potential of environmental chemicals through compromised oxidative status and DNA damage.
Materials and Methods
Chemicals
N-nitrosodiethylamine, Histopaque 1077, HBSS, RPMI 1640 were purchased from Sigma, United States (St. Louis, MO), GSH, GSSG, NADPH, NADP, CDNB, DTNB, Pyrogallol were purchased from SRL, India (Muqbil et al., 2006). All other chemicals were of analytical grade purchased from commercial sources. The uric acid (SPN099) and glucose (SPN077) estimation kits were purchased from Span Diagnostics Limited, India.
Animals
Six-eight week old male Swiss albino mice weighing 35 ± 5 g used for this study were purchased from Jamia Hamdard University, New Delhi, India. The animals were acclimatized for 1 week (Umukoro et al., 2015) and maintained under standard housing conditions with 12 h light and dark cycle. The food in the form of dry pellets (Ashirwad Industries, Chandigarh, India) and water was available ad libitum. The Institutional Research Committee approved all animal procedures, and the study has been conducted according to the principles of the University Ethical Committee (UEC) of the Aligarh Muslim University, Aligarh, UP, India, for the Control and Prevention of Cruelty toward Experimental Animals (CPCEA), an Indian National body enacting the ethical rules for performing the research on experimental animals.
Experimental Design
The experimental mice were randomly divided into four groups of 12 animals each. Group I animals served as normal controls and received no treatments; Group II animals were exposed to CUS for 15 consecutive days just before termination of the experiment. Group III mice were given NDEA (100 mg/kg body weight in normal saline) p.o. once a week for 3 weeks; Group IV animals were treated with NDEA after stress exposure as in group III (post-stress NDEA).
CUS Procedure
The CUS procedure employed in this study was based on published reports (Katz et al., 1981; Willner et al., 1987; Lu et al., 2006). To maximize unpredictability, different types of stressors were applied in seemingly random order, two per day for 15 days and at various times during the light phase (0800–2000 h) as outlined in Table 1.
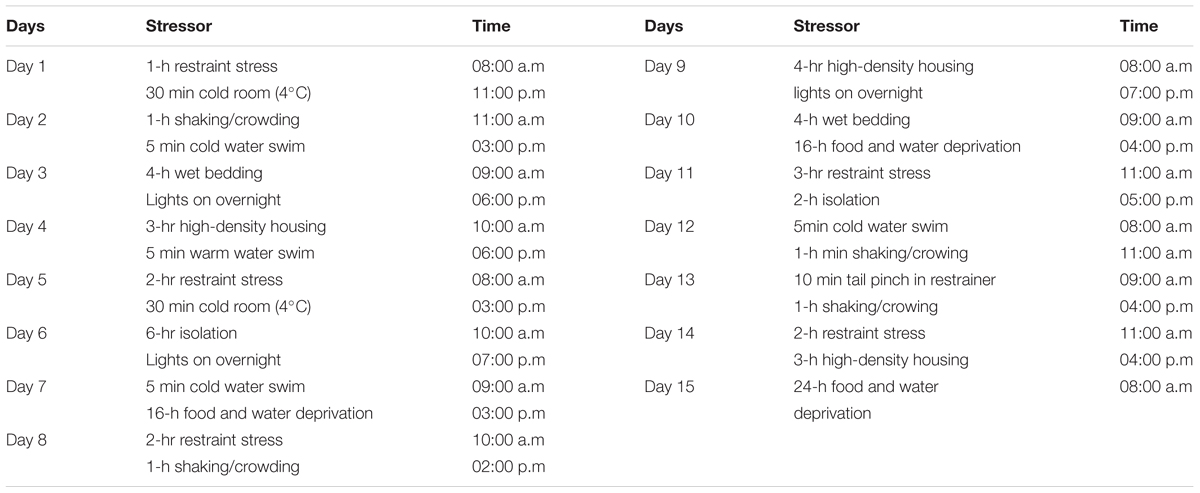
TABLE 1. Chronic unpredictable stress protocol (Suhail et al., 2011).
The specific details of the CUS procedure were as follows: for restraint stress, mice were placed individually in body sized wire mesh cages attached to wooden boards, with no movement allowed (Bondi et al., 2008). The shaking-crowding procedure was carried out by placing (Bondi et al., 2008) six mice in a cardboard box atop a lab shaker set to produce 220 back-and-forth movements (Bondi et al., 2008) per minute. Warm swim and cold (Bondi et al., 2008) swim were accomplished by placing the mice in a cylindrical tank (60 cm height-30 cm diameter) filled with water to a 30 cm depth at 25°C or 18°C, respectively. For wet bedding 300 ml tap water was poured in the home cage. The mice were subjected to a high-density housing by placing six mice in a restricted place for 4–6 h. The tail pinch involved placing the mice in the previously described restraining device and applying a clothespin 1 cm from the base of the tail (Suhail et al., 2011) for 10 min.
Assessment of DNA Damage in Lymphocytes and Liver Cells
Six mice from each group were sacrificed after a period of 12 weeks; their liver and blood tissues were subjected to DNA damage studies by single-cell gel electrophoresis (comet assay). The remaining six mice from each group were sacrificed, and their blood and liver samples were collected and subjected to biochemical estimations.
Immediately after each sacrifice, blood samples of mice were collected in heparinized tubes, and their livers were excised and washed with ice-cold saline. The heparinized blood was suitably diluted in PBS (Ca2+ and Mg2+ free) (Muqbil et al., 2006). Lymphocytes were isolated using Histopaque 1077 (Sigma), and the cells (≈2 × 105) were finally suspended in RPMI 1640. Excised livers were then filled with Hank’s balanced salt solution (HBSS) Ca2+ and Mg2+ free, containing proteinase K (17 units/mL) and incubated at 37°C for 30 min. After centrifugation cells were suspended in RPMI 1640. The viability of cells was assessed using trypan blue exclusion test (Pool-Zobel et al., 1993).
The DNA damage in the liver cells was assessed by single alkaline cell gel electrophoresis (comet assay) according to the method of Singh et al. (1988) with slight modification as described earlier (Muqbil and Banu, 2006). The cellular DNA damage was assessed by measuring tail length (migration of DNA from the nucleus in μm) which was automatically generated by Komet 5.5 image analysis system.
Biochemical Estimations
The biochemical estimations were performed on plasma and liver tissues of mice after 12 weeks of treatment. The plasma of six mice from each group was collected after centrifugation of heparinized blood for 10 min at 2500 × g at 4°C. The liver tissues were placed in ice-cold saline containers, and a 10% homogenate was prepared in 0.1 M sodium phosphate buffer, pH 7.4, centrifuged at 10,000 × g (4°C) for 15 min to remove cellular debris and the supernatant (Zafir and Banu, 2009) was used for analysis.
The Plasma and liver tissues were used for the estimation of the antioxidant enzymes; superoxide dismutase (SOD), catalase (CAT), glutathione S-transferase (GST), and glutathione reductase (GR) as described below. The levels of malondialdehyde (MDA), glutathione (GSH), and liver marker enzymes viz glutamate oxaloacetate transaminase (GOT), glutamate pyruvate transaminase (GPT), and alkaline phosphatase (ALP) were also determined according to the following methods.
SOD Activity
Superoxide dismutase activity was assayed by monitoring the inhibition of auto-oxidation of Pyrogallol (0.05 M tris succinate buffer, pH 8.2) at 420 nm (Marklund and Marklund, 1974). One enzyme unit is defined as the amount of enzyme required to cause 50% inhibition of the rate of Pyrogallol auto-oxidation.
CAT Activity
The CAT activity was measured in 0.05 M phosphate buffer (pH 7.0) by following the decrease in absorbance at 240 nm due to decomposition of 30 mM hydrogen peroxide (Aebi, 1984; Zafir and Banu, 2009). One enzyme unit is defined as the amount of enzyme (Suhail et al., 2015) decomposing 1 μM H2O2 per minute at 25°C.
GST Activity
The GST activity was assayed in 0.2 M phosphate buffer (pH 6.5) after adding 1 mM 1-chloro-2,4-dinitrobenzene (CDNB) and 1 mM GSH in the reaction mixture and following the increase in absorbance at 340 nm due to the formation of the CDNB–GSH conjugate (Habig et al., 1974). One unit of enzyme activity is defined as the amount of enzyme catalyzing the formation of 1 μM product per min under the specific assay conditions. Enzyme activity was expressed as units per mg of protein (molar extinction coefficient = 9.6 × 103 M/cm).
GR Activity
The GR activity was assayed by monitoring the oxidation of 0.1 mM NADPH as a decrease in absorbance at 340 nm (Zafir and Banu, 2009) due to an NADPH-dependent reduction of 1.0 mM oxidized glutathione disulphide to glutathione (0.1 M phosphate buffer, pH 7.6) by the catalytic action of GR (Carlberg and Mannervik, 1975; Zafir and Banu, 2009). One unit of enzyme activity is defined as the amount of enzyme catalyzing the 1 μM NADPH per min under assay conditions. Enzyme activity was calculated using a molar extinction coefficient of 6.22 × 103 M/cm and expressed as units per mg of protein.
Total Reduced Glutathione
The levels of GSH were determined using the classical thiol reagent 5,5′-dithiobis-2-nitrobenzoic acid (DTNB) (0.1 M phosphate buffer, pH 7.4). The yellow color developed by the reaction of GSH with DTNB was read at 412 nm (Jollow et al., 1974).
Uric Acid and Glucose
The levels of Uric Acid and Glucose in plasma were measured by using commercial kits (Span Diagnostics Ltd., India).
Lipid Peroxidation, Malondialdehyde (MDA)
The lipid peroxidation was measured by determining MDA (a thiobarbituric acid reactive species, TBARS) spectrophotometrically following the thiobarbituric acid (TBA) test for the formation of TBARS during an acid-heating (Bondi et al., 2008) reaction (Beuge and Aust, 1978). The pink chromogen formed by MDA–TBA complex was detected at 535 nm and quantified using an extinction coefficient of 1.56 × 103 M/cm (Zafir and Banu, 2009).
Liver Marker Enzymes
The commercial kits (Span Diagnostics Ltd., India) were used for the measurements of the GOT and GPT in the liver tissues and plasma. The ALP activity was measured in the circulation and liver tissues by using p-nitro phenylphosphate (pNPP) as substrate (Shah et al., 1979).
Protein Estimation
The protein content was determined by the method of Lowry et al. (1951) using bovine serum albumin as standard.
Statistical Analysis
The data were expressed as group mean ± SEM of six values and analyzed by one-way ANOVA for differences among controls and treatment groups. The ‘p’ values of 0.05 or less were considered statistically significant.
Results
Effect of CUS and NDEA on Lipid Peroxidation and GSH Levels
The plasma and liver tissue levels of MDA showed a significant (p < 0.05) increase when the mice were exposed to CUS or NDEA alone as compared to controls. The post-stress NDEA administration further significantly (P < 0.02) enhanced the levels as compared to all the other groups (Figures 1A,B). While the levels of GSH were significantly (P < 0.05) decreased in the plasma and liver tissues of CUS alone or NDEA alone treated mice, as compared to control groups, they were further decreased significantly (P < 0.02) in the post-NDEA stress treated mice as compared to either CUS or NDEA alone treated groups (Figures 1C,D).
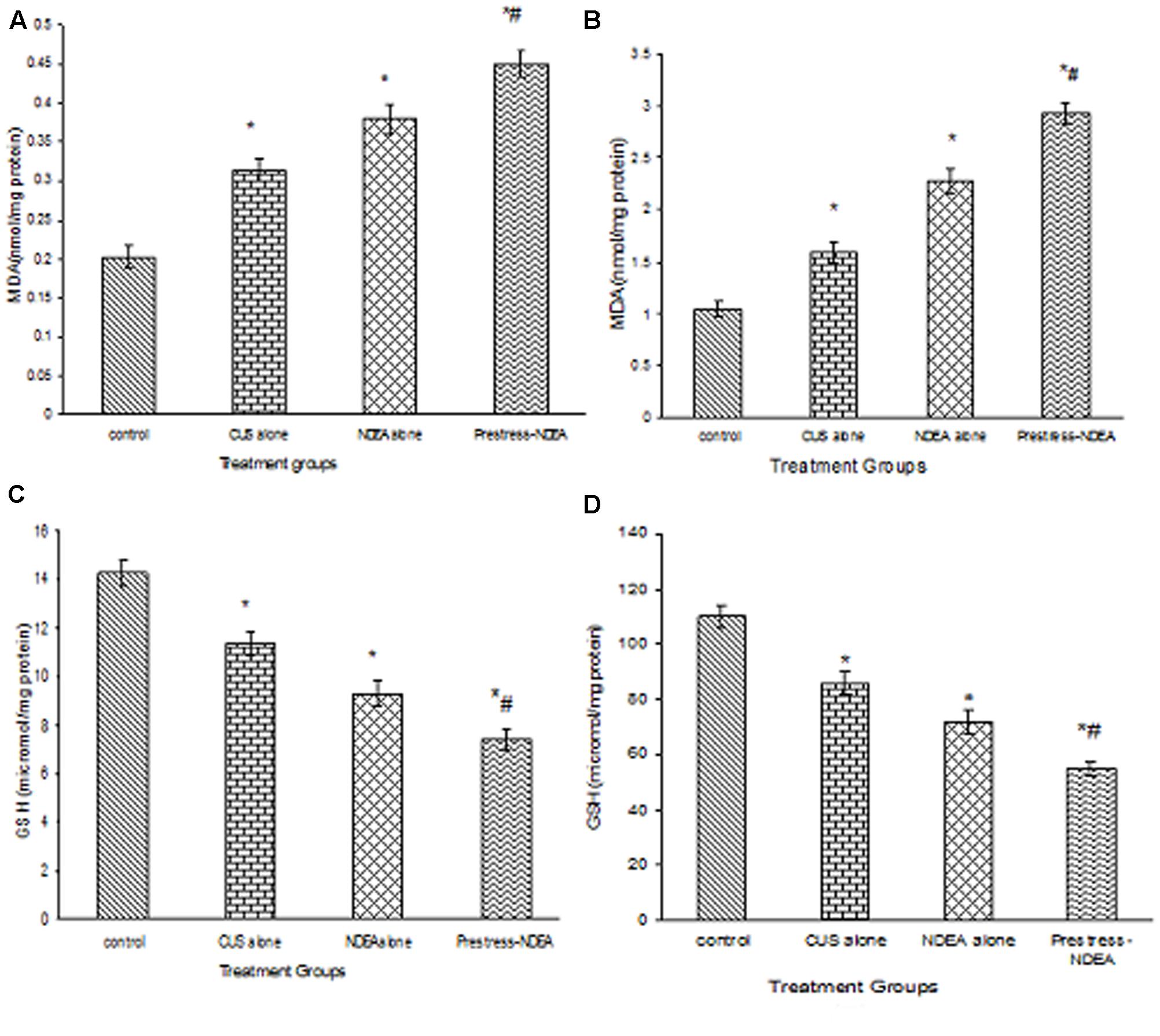
FIGURE 1. The effect of chronic unpredictable stress (CUS) and N-nitrosodiethylamine (NDEA), alone and in combination on the plasma (A), hepatic (B) levels of malondialdehyde (MDA); and plasma (C) and hepatic (D) levels of GSH in the experimental mice. ∗P < 0.05 when compared to control group. #P < 0.02 when compared to CUS alone and NDEA alone treated.
Effect of Various Treatments on the Antioxidant Status
The activities of antioxidant enzymes, SOD, CAT, GST, and GR in liver tissues were significantly (P < 0.05) decreased in CUS alone, and NDEA alone treated groups, as compared to the controls (Table 2). The activities were further significantly (P < 0.02) decreased in post-NDEA stress treated mice as compared to the other groups.

TABLE 2. The activities of free radical metabolizing enzymes in the liver tissues of mice exposed to chronic unpredictable stress (CUS) alone, N-nitrosodiethylamine (NDEA) alone, and post-stress NDEA treatments.
Alterations in the Functional Markers and Biochemical Parameters by CUS and NDEA Exposure Alone or Combination
The levels of various marker enzymes of liver function (Muqbil et al., 2006) were significantly (p < 0.05) increased in the liver tissues of stressed as well as NDEA alone treated mice (Table 3). The levels were further increased significantly (p < 0.02) in post-NDEA stress treated mice as compared to either control, stress alone or NDEA alone treated groups. The circulating levels of the biochemical liver markers of control and treated groups showed a similar pattern. The in vivo antioxidant levels were significantly (p < 0.05) decreased in CUS alone, and NDEA alone treated groups as compared to controls (Table 4). These activities were further significantly (p < 0.02) decreased in the post-NDEA stress treated mice as compared to other groups. In the CUS alone and NDEA alone treated groups the circulatory levels of uric acid and glucose were significantly decreased (p < 0.05) as compared to controls, however, these levels were further significantly decreased (p < 0.02) in post-NDEA stress treated group as compared to NDEA alone and/or CUS alone treatments (Table 4).
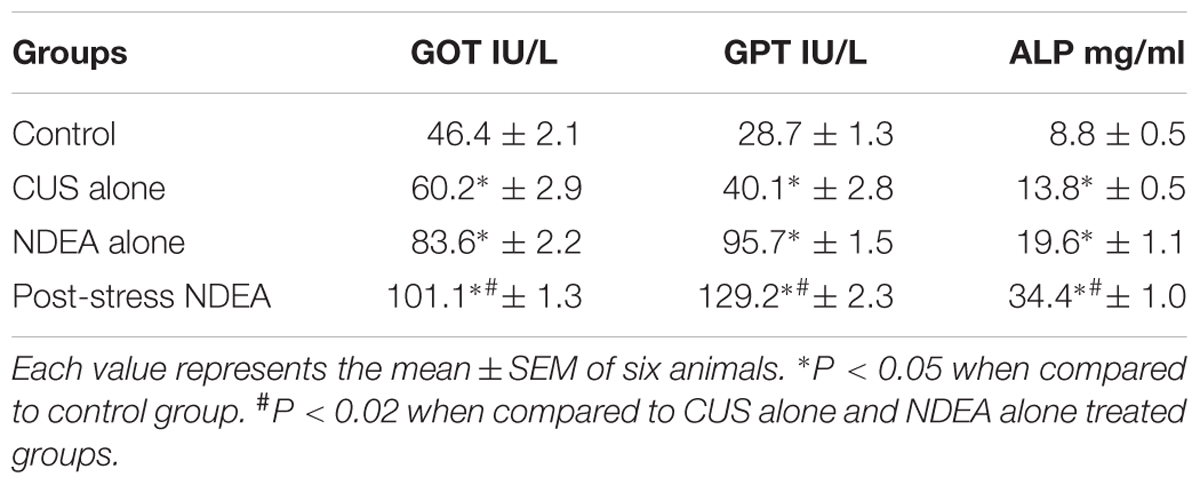
TABLE 3. The effect of CUS alone, NDEA alone, and post-stress NDEA treatments on various circulatory biochemical parameters.
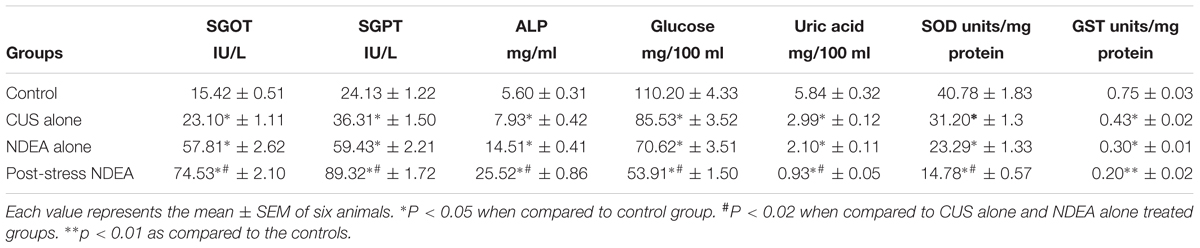
TABLE 4. The tissue levels of liver function enzymes GOT, GPT, and ALP in the mice exposed to CUS alone, NDEA alone, and post-stress NDEA treatments.
The Effect of Various Treatments on the Lymphocyte and Liver Cell DNA Damage
Both in the lymphocytes and liver cells, the DNA damage was increased significantly (p < 0.05) both in CUS or NDEA alone treated mice as compared to the control groups as depicted by comet tail lengths (Table 5). The DNA damage was further increased significantly (p < 0.02) by post-stress NDEA treatment as compared to the other groups (Table 5; liver cells Figure 2).
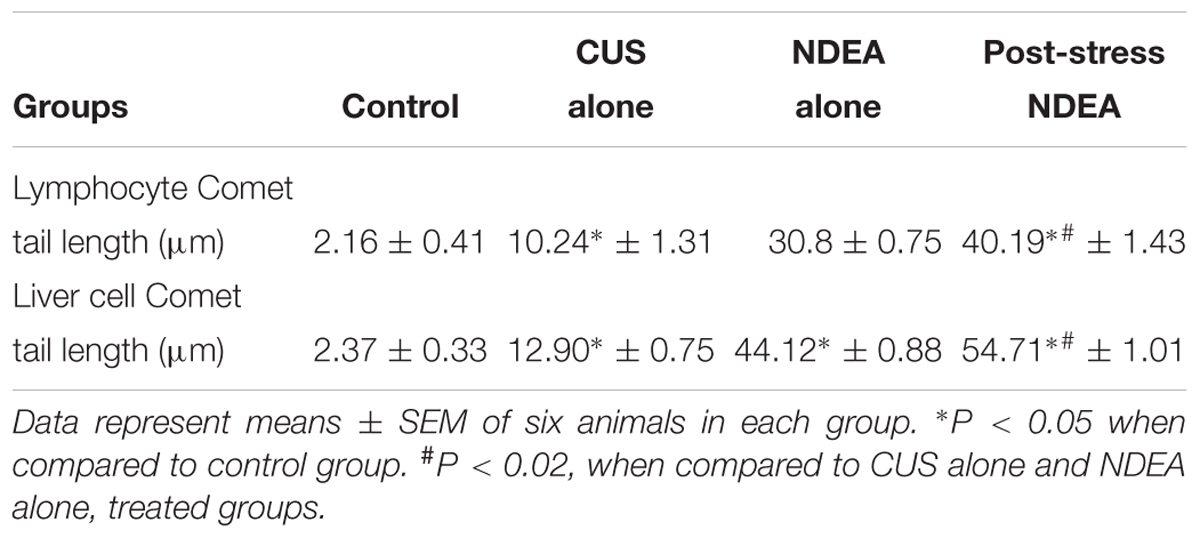
TABLE 5. The DNA damage caused by CUS, NDEA, and post-stress NDEA treatments on the peripheral blood lymphocytes and the liver cells of mice.
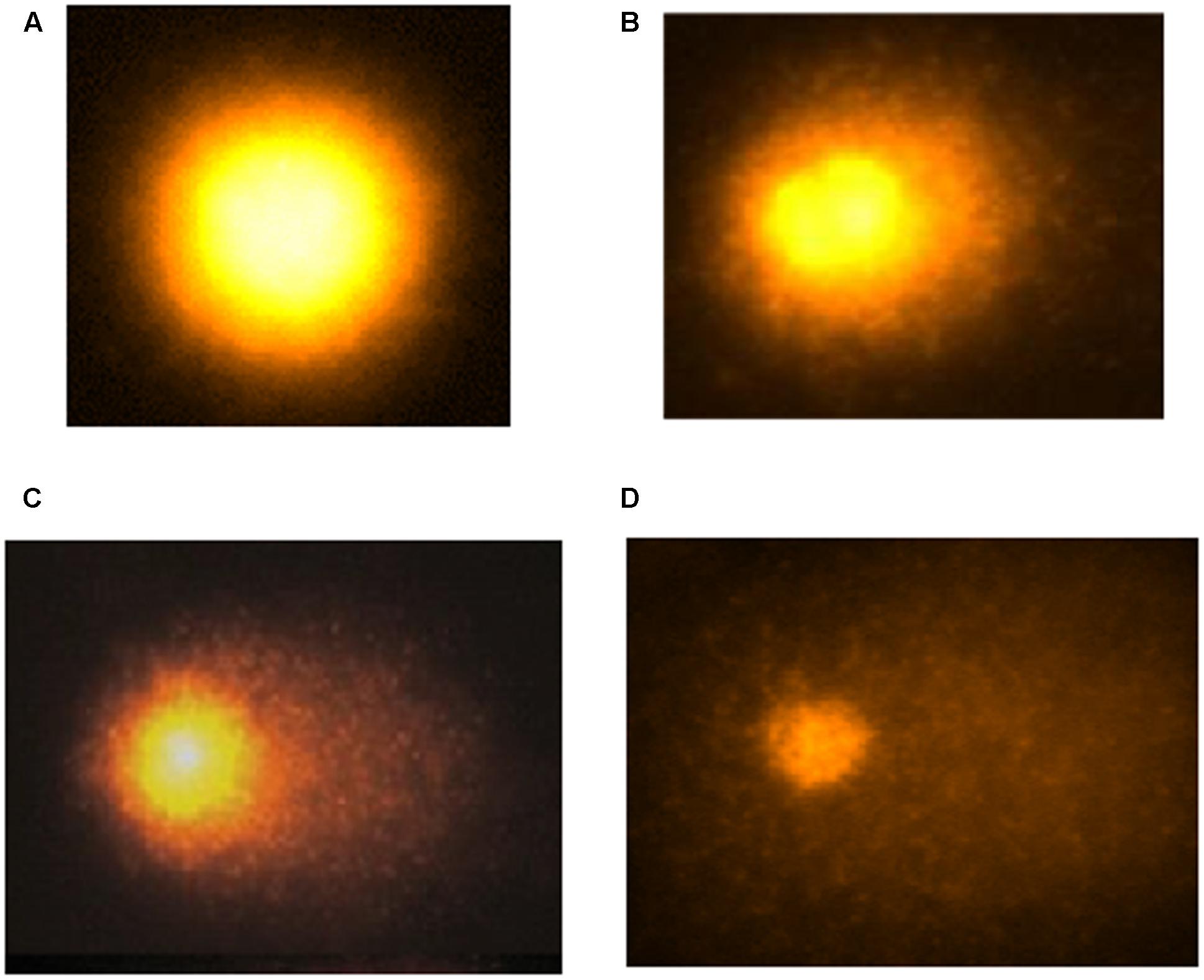
FIGURE 2. The hepatocyte DNA damage measured as comet tail lengths in control (A) and after CUS (B); NDEA (C); and post-CUS NDEA (D) treatments in the mice.
Thus, these results reveal that the CUS treatment aggravates the NDEA induced alterations of lipid peroxidation, antioxidant status, and biochemical markers, leading to an increase in carcinogen induced oxidative stress and DNA damage, which may play a significant role in enhancing the carcinogenic potential of NDEA in liver carcinogenesis.
Discussion
Exposure to stress situation stimulates numerous pathways leading to increased production of free radicals. Several human chronic diseases including cancer have been associated with the altered oxidative stress, produced either through an increased free radical generation and/or a compromised antioxidant level in the target cells and tissues (Trush and Kensler, 1991; Rice-Evans and Burdon, 1993). The free radicals thus generated induce strand breaks and cause oxidative modification of DNA bases (Cerutti, 1985). The participation of ROS in hepatocarcinogenesis is evident as initiation with low doses of NDEA induces liver DNA-8-hydroxydeoxy-guanosine adduct formation (Nakae et al., 1997) and causes mutations, which leads to carcinogenesis. The free radicals, mostly ROS cause cellular injury. The consequences of which are often exhibited and measured as lipid peroxidation product MDA, the major end product of this reaction. Malondialdehyde directly reacts with DNA to form oxidative DNA adducts (Chaudhary et al., 1994), thereby causing DNA damage and interfering with the DNA-repair mechanism.
The present study shows that exposure of mice to CUS result in a significant increase in the levels of MDA, and a significant decrease in the antioxidant enzyme activities and the levels of GSH. These results are supported by previous studies in which CUS showed a disturbed oxidant-antioxidant balance (Zhang et al., 2009). The significant increase of MDA in NDEA-administered mice has also been reported by others (Thirunavukkarasu and Sakthisekaran, 2001; Pradeep et al., 2007). However, the levels of MDA were further significantly increased in post-NDEA stress treated group, which could be due to either increased production or decreased destruction of ROS, as the exposure to stress already causes a compromised status of the antioxidant system and thus decrease in anti-oxygenic potential in vivo. Reduced GSH is the major cytosolic thiol compound which plays important cellular functions including the destruction of hydrogen peroxide, lipid peroxides, and free radicals. The administration of NDEA caused a significant decrease in the levels of GSH, which may be responsible for the increased lipid peroxidation. NDEA administration after stress exposure caused a further decrease in the levels of GSH. This enhanced decrease of GSH in post-stress NDEA treatment suggests that the cells which are already deficient in thiol (-SH) groups undergo fast lipid per oxidation, as GSH is one of the guarding factors against oxidative stress. Enhanced lipid peroxidation associated with depletion of antioxidants is a characteristic finding in a variety of malignancies (Anis et al., 2001). The primary antioxidant enzymes SOD and CAT are involved in the inactivation of environmental carcinogens and direct elimination of toxic free radicals and electrophiles in vivo, which helps in the amelioration of the oxidative damage. The activities of both these free radical scavenging enzymes were found to be decreased in rats treated with NDEA (Yadav and Bhatnagar, 2007). The results of our study also indicate that the NDEA administration increases the susceptibility of hepatocytes to carcinogenesis by reducing the activities of SOD and CAT. The oxidative stress through the generation of ROS causes denaturation of protein/enzyme by the formation of protein carbonyls which might have caused inhibition of enzymatic activities (Höhn and Grune, 2014). A further significant decrease in the activities of SOD and CAT by CUS in NDEA-treated mice could be due to over utilization/less production of these enzymatic antioxidants to scavenge the products of lipid peroxidation and oxidative stress.
A large number of studies have established a correlation between cancer incidence and various disorders of GSH-related enzyme functions, alterations of GSTs being most frequently reported. A significant decrease in the activity of GSH dependent enzymes, GST and GR was observed in NDEA treated mice. This may be due to the decreased expression of these antioxidants during hepatocellular damage as previously described (Kweon et al., 2003). The further decreased levels of GST and GR as observed in the post-stress NDEA treated mice might have facilitated the NDEA induced liver carcinogenesis by decreasing its clearance from the system. The already compromised state of antioxidant level due to stress exposure might have further contributed in aggravation of the oxidative stress and NDEA induced toxicity. The diagnostic biomarker enzymes of hepatic damage GOT, GPT, and ALP are released into the circulation after cellular damage (Naik and Panda, 2007) and a rise of these marker enzymes in the serum causes increased permeability and necrosis of the cells (Al-Athar, 2004). In this study, we demonstrated that the administration of NDEA to mice leads to a marked elevation in the levels of GOT, GPT, and ALP which is indicative of hepatocellular damage, as also previously reported (Sadik et al., 2008). Further elevations of these enzymes in post-stress NDEA treatment could potentially be attributed to the release of these enzymes from the cytoplasm into the blood (Ritter et al., 2004) circulation after rupture of the plasma membrane due to increased free radical induced cellular damage. Both uric acid and glucose are also considered to be the major antioxidants found in blood plasma. The uric acid can act as an antioxidant either by binding to the radicals or by directly scavenging oxidizing species. It, therefore, inhibits lipid peroxidation (Muqbil et al., 2006). Glucose also acts as a scavenger of hydroxyl radicals (Halliwel and Gutteridge, 1990). The levels of these antioxidants were found to be decreased with increase in lipid peroxidation in plasma of mice exposed either to CUS alone or NDEA alone. The levels were further decreased when NDEA was given to the mice after exposure to stress, which shows that stress itself alters the antioxidant status, thus facilitating (Muqbil et al., 2006) and enhancing the NDEA induced liver carcinogenesis. Earlier studies from our laboratory have shown that CUS enhanced both the nephrotoxic and hepatotoxic potential of 7,12-dimethylbenz(a) anthracene in Swiss albino mice (Suhail et al., 2011), by altering the oxidative stress and compromising the antioxidant system, thus stress may act as a promoter of carcinogenesis by enhancing the pro-oxidant potential of carcinogens. Moreover, CUS also aggravated the development of skin tumors (in terms of their incidence, tumor yield, and tumor burden) in mice which further strongly supported our studies on the effect of stress on cancer promotion through DNA damage and modulation of oxidant/antioxidant system in vivo (Suhail et al., 2015). Thus, irrespective of the carcinogen used or the mode of application topical (Suhail et al., 2011, 2015) or oral as in the present study, CUS increased the toxic potential of the carcinogens.
All the above findings of oxidative stress were also supported by studies which showed CUS and NDEA caused significant damage to the DNA of lymphocytes and the liver cells as compared to controls. Post-CUS NDEA treatment showed further higher DNA damage in comparison with control, CUS alone or NDEA alone groups. The explanation for this may be that the chronic stress caused DNA damage within the cell either by altering the ability of the cells to repair DNA due to compromised antioxidant defense system (Bondi et al., 2008), or by causing oxidative stress and inhibiting apoptosis as observed by others also (Glaser et al., 1985; Kiecolt-Glaser et al., 1985; Adachi et al., 1993). The mechanisms that play roles in NDEA carcinogenicity in hepatocytes include DNA adduct formation followed by a gene mutation (Dyroff et al., 1986; Deal et al., 1989; Travis et al., 1991). Administration of NDEA generates lipid peroxidation products in general (Hietanen et al., 1987) and enhances the formation of the activated oxygen species in the preneoplastic nodules (Scholz et al., 1990) in the liver. The increased formation of reactive oxygen substances both in the circulation and in the liver tissues by NDEA administration either alone or after stress exposure further confirms an enhanced DNA damage by the chronic stress. Thus, the chronic stress plays an important role in the initial stages of liver carcinogenesis by compromising the antioxidant status, inducing oxidative stress and enhancing the DNA damaging potential of a carcinogen. Human body remains in a compromised antioxidant and enhanced oxidative status due to physical, physiological or psychological stress, and under this condition, any insult due to environmental carcinogens can aggravate the situation and may pave the way for carcinogenesis by enhancing the carcinogenic potential of any potent carcinogen irrespective of the mode of exposure.
Conclusion
Our results suggest a strong correlation between the observed alterations in the biochemical parameters, notably decreased antioxidant status and increased oxidative stress, due to the chronic stress exposure and the toxicity of environmental chemicals. Exposure to NDEA caused induction of oxidative stress and DNA damage by the generation and accumulation of large amounts of free radicals. Chronic stress further exacerbated this condition, thus putting at an increased risk of developing cancer. This study may offer a sensitive and useful approach for assessment of the effects of psychological stress on the carcinogenic risks of environmental chemicals (Bondi et al., 2008). Further studies may be aimed to combine the parameters of psychological stress with those of redox state, DNA-repair activity, and antioxidant defense system, so as to identify the relationship between psychological/physical stress and carcinogenesis at the molecular level to give a clearer picture.
Ethics Statement
This study was carried out after the protocol was approved by the Aligarh Muslim University ethics committee in accordance with the recommendations of the Indian national committee for the ‘Control and Prevention of Cruelty towards Experimental Animals’.
Author Contributions
NB conceived and designed the study. NB, NS, GA, HK, and SH performed the experiments and wrote the manuscript. NB, AA, MA, and SF provided critical revision of the manuscript for intellectual content.
Conflict of Interest Statement
The authors declare that the research was conducted in the absence of any commercial or financial relationships that could be construed as a potential conflict of interest.
Acknowledgments
The authors acknowledge the facilities provided by the Aligarh Muslim University, Aligarh, India. Thanks are due to University grants commission (New Delhi), UGC-DRS and DST-(FIST) for providing lab facilities. NB thanks the support of Qassim University, Saudi Arabia. GA gratefully acknowledges the facilities provided by King Fahd Medical Research Center (KFMRC) and Deanship of Scientific Research (DSR), King Abdulaziz University, Jeddah, Saudi Arabia.
References
Abdel-Hamid, N., Ramadan, M., and Amgad, S. (2013). Glycoregulatory enzymes as early diagnostic markers during premalignant stage in hepatocellular carcinoma. Am. J. Cancer Prev. 1, 14–19. doi: 10.12691/ajcp-1-2-1
Adachi, S., Kawamura, K., and Takemoto, K. (1993). Oxidative damage of nuclear DNA in liver of rats exposed to psychological stress. Cancer Res. 53, 4153–4155.
Aebi, H. (1984). Catalase in vitro. Methods Enzymol. 105, 121–126. doi: 10.1016/S0076-6879(84)05016-3
Al-Athar, A. M. (2004). The influence of dietary grape seed oil on DMBA-induced liver enzymes disturbances in the frog, Rana ridibunda. Pak. J. Nutr. 5, 304–309.
Amkraut, A., and Solomon, G. F. (1972). Stress and murine-sarcoma virus induced tumors. Cancer Res. 32, 1428–1433
Anis, K. V., Rajesh Kumar, N. V., and Kuttan, R. (2001). Inhibition of chemical carcinogenesis by biberine in rats and mice. J. Pharm. Pharmacol. 53, 763–768. doi: 10.1211/0022357011775901
Antoni, M. H., Lutgendorf, S. K., Cole, S. W., Dhabhar, F. S., Sephton, S. E., McDonald, P. G., et al. (2006). The influence of bio-behavioural factors on tumour biology: pathways and mechanisms. Nat. Rev. Cancer 6, 240–248. doi: 10.1038/nrc1820
Bansal, A. K., Trivedi, R., Soni, G. L., and Bhatnagar, D. (2000). Hepatic and renal oxidative stress in acute toxicity of N-nitrosodiethylamine in rats. Indian J. Exp. Biol. 38, 916–920.
Batcioglu, K., Karagozler, A. A., Genc, M., and Celik, S. (2002). Comparison of the chemopreventive potentials of melatonin and vitamin E plus selenium on 7,12-dimethylbenz(a)anthraceneinduced inhibition of mouse liver antioxidant enzymes. Eur. Cancer. Prev. 11, 57–61. doi: 10.1097/00008469-200202000-00008
Beuge, J. A., and Aust, S. D. (1978). Microsomal lipid peroxidation. Methods Enzymol. 52, 302–310. doi: 10.1016/S0076-6879(78)52032-6
Bondi, C. O., Rodriguez, G., Gould, G. G., Frazer, A., and Morilak, D. A. (2008). Chronic unpredictable stress induces a cognitive deficit and anxiety-like behavior in rats that is prevented by chronic antidepressant drug treatment. Neuropsychopharmacology 33, 320–331. doi: 10.1038/sj.npp.1301410
Carlberg, I., and Mannervik, B. (1975). Purification and characterization of the flavoenzyme glutathione reductase from rat liver. J. Biol. Chem. 250, 5475–5480.
Cerutti, P. A. (1985). Pro oxidant states and tumor promotion. Science 227, 375–381. doi: 10.1126/science.2981433
Chaudhary, A. K., Nokubo, M., Marnett, L. J., and Blair, I. A. (1994). Analysis of the malondialdehyde-2’-deoxyguanosine adduct in rat liver DNA by gas chromatography/electron capture negative chemical ionization mass spectrometry. Biol. Mass Spectrom. 23, 457–464. doi: 10.1002/bms.1200230802
Deal, F. H., Richardson, F. C., and Swenberg, J. A. (1989). Dose response of hepatocyte replication in rats following continous exposure to diethylnitosamine. Cancer Res. 49, 6985–6988.
Dyroff, M. C., Richardson, F. C., Poppy, J. A., Bedell, M. A., and Wenberg, J. A. (1986). Correlation of O4-ethyldeoxythymidine accumulation, hepatic initiation and hepatocellular carcinoma induction in rats continuously administered diethylnitrosamine. Carcinogenesis 7, 241–246. doi: 10.1093/carcin/7.2.241
Glaser, R., Thorn, B. E., Tarr, K. L., Kiecolt-Glaser, J. K., and D’Ambrosio, S. M. (1985). Effects of stress on methyltransferase synthesis: an important DNA repair enzyme. Health Psychol. 4, 403–412. doi: 10.1037/0278-6133.4.5.403
Habig, W. H., Pabst, M. J., and Jakoby, W. B. (1974). Glutathione s-transferases: the first enzymatic step in mercapturic acid formation. J. Biol. Chem. 249, 7130–7139.
Halliwel, B., and Gutteridge, J. M. C., (1990). The antioxidants of human extracellular fluids. Arch. Biochem. Biophys. 280, 1–8. doi: 10.1016/0003-9861(90)90510-6
Hietanen, E., Ahotupa, M., Bereziat, J. C., Bussacchini, V., Camus, A. M., Bartsch, H., et al. (1987). “Lipid peroxidation and chemically-induced cancer in rats fed lipid rich diet,” in Carcinogenesis and Tumour Progression, Vol. 4, eds K. Lapis and S. Eckhardt (Budapest: Karger Publishers) 9–16.
Höhn, T. J., and Grune, T. (2014). The proteasome and the degradation of oxidized proteins: part III-Redox regulation of the proteasomal system. Redox Biol. 2, 388–394 doi: 10.1016/j.redox.2013.12.029
Ip, S. P., Poon, M. K., Che, C. T., Ng, K. H., Kong, Y. C., and Ko, K. M. (1996). Schisandrin B protects against carbon tetrachloride toxicity by enhancing the mitochondrial glutathione redox status in mouse liver, Free Radic. Biol. Med. 21, 709–712. doi: 10.1016/0891-5849(96)00179-7
Jollow, D. J., Mitchel, J. R., Zampaglione, N., and Gillete, J. R. (1974). Bromobenzene induced liver necrosis:protective role of glutathione and evidence for 3, 4 bromobenzene oxide as the hepatotoxic intermediate. Pharmacology 11, 151–169
Justice, A. (1985). Review of the effects of stress on cancer in laboratory animals: importance of time of stress application and type of tumour. Psychol. Bull. 98, 108–138. doi: 10.1037/0033-2909.98.1.108
Katz, R. J., Roth, K. A., and Carroll, B. J. (1981). Acute and chronic effects on open field activity in the rat: implications for a model of depression. Neurosci. Biobehav. Rev. 5, 247–251. doi: 10.1016/0149-7634(81)90005-1
Kiecolt-Glaser, J. K., Stephens, R. E., Lipetz, P. D., Speicher, C. E., and Glaser, R., (1985). Distress and DNA repair in human lymphocytes. J. Behav. Med. 8, 311–320. doi: 10.1007/BF00848366
Kweon, S., Park, K. A., and Choi, H. (2003). Chemopreventive effect of garlic powder diet in diethylnitrosamine-induced rat hepatocarcinogenesis. Life Sci. 73, 2515–2526. doi: 10.1016/S0024-3205(03)00660-X
Lowry, O. H., Rosebrough, N. J., Farr, A. L., and Randall, R. J. (1951). Protein measurement with the folin phenol reagent. J. Biol. Chem. 193, 265–275.
Lu, X. Y., Kim, C. S., Frazer, A., and Zhang, W. (2006). Leptin: a potential novel antidepressant. Proc. Natl. Acad. Sci. U.S.A. 103, 1593–1598. doi: 10.1073/pnas.0508901103
Marklund, S., and Marklund, G. (1974). Involvement of superoxide anion radical in the autooxidation of pyrogallol and a convenient assay for superoxide dismutase. Eur. J. Biochem. 47, 469–474. doi: 10.1111/j.1432-1033.1974.tb03714.x
Muqbil, I., and Banu, N. (2006). Enhancement of pro oxidant effect of Dimethylbenz(a)anthracene (DMBA) in rats by pre exposure to restraint stress. Cancer Lett. 240, 213–220. doi: 10.1016/j.canlet.2005.09.008
Muqbil, I., Asfar, S. A., and Banu, N. (2006) Prior exposure to restraint stress enhances7,12- dimethylbenz(a)anthracene (DMBA) induced DNA damage in rats. FEBS Lett. 580, 3995–3999. doi: 10.1016/j.febslet.2006.06.030
Mussarat, J., Arezine-Wilson, J., and Wani, A. A. (1996). Prognostic and aetiological relevance of 8-hydroxyguanosine in human breast carcinogenesis. Eur. J. Cancer 32 A, 1209–1214. doi: 10.1016/0959-8049(96)00031-7
Muzio, G., Marengo, B., and Salvo, R. (1999). Liver cancer is induced by a subnecrogenic dose of NDEA when associated with fasting/refeeding: role of glutathione-transferase and lipid peroxidation. Free Radic. Biol. Med. 26, 1314–1320. doi: 10.1016/S0891-5849(98)00329-3
Naik, S. R., and Panda, V. S. (2007). Antioxidant and hepatoprotective effects of Ginkgo biloba phytosomes in carbon tetrachloride-induced liver injury in rodents. Liver Int. 27, 393–399. doi: 10.1111/j.1478-3231.2007.01463.x
Nakae, D., Kobayashi, Y., Akai, H., Andoh, N., Satoh, H., Ohashi, K., et al. (1997). Involvement of 8-hydroxyguanine formation in the initiation of rat liver carcinogenesis by low dose levels of N-nitrosodiethylamine. Cancer Res. 57, 1281–1287.
Pool-Zobel, B. L., Guigas, C., Klein, R., Neudecker, C., Renner, H. W., and Schmezer, P. (1993). Assessment of genotoxic effect by lindane. Food. Chem. Toxicol. 31, 271–283. doi: 10.1016/0278-6915(93)90077-C
Pradeep, K., Mohan, C. V., Gobianand, K., and Karthikeyan, S. (2007). Silymarin modulates the oxidant-antioxidant imbalance during diethylnitrosamine induced oxidative stress in rats. Eur. J. Pharmacol. 560, 110–116. doi: 10.1016/j.ejphar.2006.12.023
Pradhan, S. N., and Prabhati, R. (1974). Effects of stress on growth of transplanted and 7,12-dimethlybenz(a)anthracene-induced tumors and their modification by psychotropic drugs. J. Natl. Inst. 53, 1241–1245. doi: 10.1093/jnci/53.5.1241
Ramakrishnan, G., Raghavendran, H. R., Vinodhkumar, R., and Devaki, T. (2006). Suppression of N-nitrosodiethylamine induced hepatocarcinogenesis by silymarin in rats. Chem. Biol. Interact. 161, 104–114. doi: 10.1016/j.cbi.2006.03.007
Reiche, E. M., Nunes, S. O., and Morimoto, H. K. (2004). Stress, depression, the immune system, and cancer. Lancet Oncol. 5, 617-625. doi: 10.1016/S1470-2045(04)01597-9
Rice-Evans, C., and Burdon, R. (1993). Free radical-interactions and their pathological consequences. Prog. Lipid Res. 32, 71–110 doi: 10.1016/0163-7827(93)90006-i
Ritter, R., Reinke, A., Andrades, M., Martins, M. R., Rocha, J., Menna-Barreto, S., et al. (2004). Protective effect of N-acetylcysteine and deferoxamine on carbon tetrachloride-induced acute hepatic failure in rats. Crit. Care Med. 32, 2079–2083. doi: 10.1097/01.CCM.0000142699.54266.D9
Sadik, A. H. N., EL-Maraghy, S. A., and Ismail, M. F. (2008). Diethylnitrosamine-induced hepatocarcinogenesis in rats: possible chemoprevention by blueberries. Afr. J. Biochem. Res. 2, 081–087.
Scholz, W., Schutze, K., Kunz, W., and Schwarz, M. (1990). Phenobarbital enhances the formation of reactive oxygen in neoplastic rat liver nodules. Cancer Res. 50, 7015–7022.
Shah, S. V., Kempson, S. A., Northrup, T. E., and Dousa, T. P. (1979). Renal adaptation to low phosphate diet in rats. J. Clin. Invest. 64, 955–966. doi: 10.1172/JCI109562
Singh, N. P., McCoy, M. T., Tice, R. R., and Schneider, E. L. (1988). A simple technique for quantitation of low levels of DNA damage in individual cells. Exp. Cell. Res. 175, 184–191. doi: 10.1016/0014-4827(88)90265-0
Subramanian, P., Mirunalini, S., Dakshayani, K. B., Pandi-Perumal, S. R., Trakht, I., and Cardinali, D. P. (2007). Prevention by melatonin of hepatocarcinogenesis in rats injected with N-nitrosodiethylamine. J. Pineal Res. 43, 305–312. doi: 10.1111/j.1600-079X.2007.00478.x
Suhail, N., Bilal, N., Hasan, S., Ahmad, A., Ashraf, G. M., and Banu, N. (2015). Chronic unpredictable stress (CUS) enhances the carcinogenic potential of 7,12-dimethylbenz (a) anthracene (DMBA) and accelerates the onset of tumor development in Swiss albino mice. Cell Stress Chaperones 20, 1023–1036. doi: 10.1007/s12192-015-0632-x
Suhail, N., Bilal, N., Hasan, S., and Banu, N. (2011). Chronic unpredictable stress exacerbates 7,12-dimethylbenz (a) anthracene induced hepatotoxicity and nephrotoxicity in Swiss albino mice. Mol. Cell. Biochem. 355, 117–126. doi: 10.1007/s11010-011-0845-y
Thirunavukkarasu, C., and Sakthisekaran, C. D. (2001). Effect of selenium on N-nitrosodiethylamine-induced multistage hepatocarcinogenesis with reference to lipid peroxidation and enzymic antioxidants. Cell Biochem. Funct. 19, 27–35. doi: 10.1002/cbf.895
Travis, C. C., McClain, T. W., and Birkner, P. D. (1991). Diethylnitrosamine- induced hepatocarcinogenesis in rats: a theroretical study. Toxicol. Appl. Pharmacol. 109, 289–304. doi: 10.1016/0041-008X(91)90176-F
Trush, M. A., and Kensler, T. W. (1991). An overview of the relationship between oxidative stress and chemical carcinogenesis. Free Radic. Biol. Med. 10, 201–209. doi: 10.1016/0891-5849(91)90077-G
Umukoro, S., Oluwole, G. O., Olamijowon, H. E., Omogbiya, A. I., and Eduviere, A. T. (2015). Effect of monosodium glutamate on behavioral phenotypes, biomarkers of oxidative stress in brain tissues and liver enzymes in mice. World J. Neurosci. 5, 339–349. doi: 10.4236/wjns.2015.55033
Valko, M., Rhodes, C. J., Moncol, J., Izakovic, M., and Mazur, M. (2006). Free radicals, metals and antioxidants in oxidative stress-induced cancer. Chem. Biol. Interact. 160, 1–40. doi: 10.1016/j.cbi.2005.12.009
Willner, P., Towell, A., Sampson, D., Sophokleous, S., and Muscat, R. (1987). Reduction of sucrose preference by chronic unpredictable mild stress, and its restoration by a tricyclic antidepressant. Psychopharmacology 93, 358–364. doi: 10.1007/BF00187257
Yadav, A. S., and Bhatnagar, D. (2007). Chemo-preventive effect of Star anise in N-nitrosodiethylamine initiated and phenobarbital promoted hepato-carcinogenesis. Chem. Biol. Interact. 169, 207–214. doi: 10.1016/j.cbi.2007.06.032
Zafir, A., and Banu, N. (2009). Induction of oxidative stress by restraint stress and corticosterone treatment in rats. Indian J. Biochem. Biophys. 46, 53–58.
Zaidi, S. M., Al-Qirim, T., and Banu, N. (2005). Effects of antioxidant vitamins on glutathione depletion and lipid peroxidation induced by restraint stress in the rat liver. Drugs R D 6, 157–165. doi: 10.2165/00126839-200506030-00004
Zhang, D., Wen, X. S., Wang, X. Y., Shi, M., and Zhao, Y. (2009). Antidepressant effect of Shudihuang on mice exposed to unpredictable chronic mild stress, J. Ethnopharmacol. 123, 55–60. doi: 10.1016/j.jep.2009.02.029
Keywords: N-nitrosodiethylamine, chronic unpredictable stress, hepatotoxicity, DNA damage, carcinogenesis
Citation: Bilal N, Suhail N, Hasan S, Ashraf GM, Fatima S, Khan HY, Alharbi MS, Alexiou A and Banu N (2017) Exacerbation of N-nitrosodiethylamine Induced Hepatotoxicity and DNA Damage in Mice Exposed to Chronic Unpredictable Stress. Front. Pharmacol. 8:360. doi: 10.3389/fphar.2017.00360
Received: 03 March 2017; Accepted: 26 May 2017;
Published: 15 June 2017.
Edited by:
Anna Rita Migliaccio, Icahn School of Medicine at Mount Sinai, United StatesReviewed by:
Subash Gupta, Banaras Hindu University, IndiaJian Lu, Johns Hopkins University, United States
Copyright © 2017 Bilal, Suhail, Hasan, Ashraf, Fatima, Khan, Alharbi, Alexiou and Banu. This is an open-access article distributed under the terms of the Creative Commons Attribution License (CC BY). The use, distribution or reproduction in other forums is permitted, provided the original author(s) or licensor are credited and that the original publication in this journal is cited, in accordance with accepted academic practice. No use, distribution or reproduction is permitted which does not comply with these terms.
*Correspondence: Naheed Banu, bmFoZWVkYmFudTdAeWFob28uY29t;, bmJhbnVhbXVAZ21haWwuY29t Athanasios Alexiou, YWxleGlvdUBuZ2NlZi5uZXQ=;, YWxleHRoYUB5YWhvby5ncg==