- Department of General Biochemistry, Faculty of Biology and Environmental Protection, University of Lodz, Lodz, Poland
Antioxidants present in the diet may have a significant effect on the prophylaxis and progression of various diseases associated with oxidative stress. Berries contain a range of chemical compounds with antioxidant properties, including phenolic compounds. The aim of this review article is to provide an overview of the current knowledge of such phenolic antioxidants, and to discuss whether these compounds may always be natural gifts for human health, based on both in vitro and in vivo studies. It describes the antioxidant properties of fresh berries (including aronia berries, grapes, blueberries, sea buckthorn berries, strawberries and other berries) and their various products, especially juices and wines. Some papers report that these phenolic compounds may sometimes behave like prooxidants, and sometimes demonstrate both antioxidant and prooxidant activity, while others note they do not behave the same way in vitro and in vivo. However, no unwanted or toxic effects (i.e., chemical, hematological or urinary effect) have been associated with the consumption of berries or berry juices or other extracts, especially aronia berries and aronia products in vivo, and in vitro, which may suggest that the phenolic antioxidants found in berries are natural gifts for human health. However, the phenolic compound content of berries and berry products is not always well described, and further studies are required to determine the therapeutic doses of different berry products for use in future clinical studies. Moreover, further experiments are needed to understand the beneficial effects reported so far from the mechanistic point of view. Therefore, greater attention should be paid to the development of well-controlled and high-quality clinical studies in this area.
Introduction
Natural phenolic compounds are found in many foods, including vegetables, fruits, tea, coffee, chocolate, wine, honey, and oil (Kulling and Rawel, 2008; Szajdek and Borowska, 2008; Chong et al., 2010; Chrubasik et al., 2010; Christaki, 2012; Kutlesa and Mrsic, 2016; Gomes-Rochette et al., 2016).
Recent years have seen increased consumption of berries, and fruit in general. Research suggests that this increased intake of fruits and berries may be associated with a reduced incidence of disorders induced by reactive oxygen species (ROS), including cardiovascular disorders, cancer and inflammatory processes (Gomes-Rochette et al., 2016). Berries and their products (i.e., berry juice and jam) are very often recognized as “superfoods.” They possess high concentrations of phenolic compounds, which have been found in in vitro and in vivo studies to possess a range of biological activities, including anticancer and antiplatelet activities, as well as antioxidant properties (Valcheva-Kuzmanova et al., 2006; Erlund et al., 2008; Kulling and Rawel, 2008; Szajdek and Borowska, 2008; Chong et al., 2010; Chrubasik et al., 2010; Christaki, 2012; Giampieri et al., 2012, 2015; McEwen, 2014; Nile and Park, 2014; Del Bo et al., 2015; Skrovankova et al., 2015; Wightman and Henberger, 2015; Kristo et al., 2016; Olas, 2016, 2017). However, these compounds may not influence the levels of oxidative stress biomarkers, and may even have prooxidative effects. In addition, the precise biological activities of berry phenolics are dependent on a range of factors including the class of phenolics, their concentration, the type of berry and even the form consumed, be it fresh berries, juice, wine, jam, oil or medicinal products. This review article summarizes the current knowledge concerning whether the phenolic compounds within berries may always have a beneficial influence on human health as antioxidants, and to what extent these compounds may sometimes act as prooxidants. The source information for this paper is derived not only from in vitro models, but also in vivo models.
The Botanical Classification of Berries
Although, according to botanical terminology, a berry is a simple fruit with seeds and pulp produced from the ovary of a single flower with a fleshy pericarp, the term “berry” is also commonly used to refer in general to a small, pulpy and often edible fruit. Blueberries may be categorized as berries under both definitions, but grapes are berries only according to the botanical definition. Moreover, while strawberries and blackberries are typically referred to as berries, they are not officially categorized as such (Hickey and King, 2001).
Berries belong to several families, although the two key examples are the Rosaceae, including black chokeberry (Aronia melanocarpa), strawberry (Fragaria ananassa), red raspberry (Rubus ideaus), black raspberry (Rubus occidentalis), blackberry (Rubus fruticosus) and cloudberry (Rubus chamaemorus), and the Ericaceae, including cranberry (Vaccinium macrocarpon), bilberry (Vaccinium myritillus), lowbush blueberry (Vaccinium angustifolium), highbush blueberry (Vaccinium corymbosum). Examples of berries from other families include blackcurrants (Ribes nigrum; family: Grossulariaceae), sea buckthorn (Elaaagnus rhamnoides (L.); family: Elaeagnaceae) and grapes (Vitis; family: Vitaceae).
The Chemical Composition of Berries
A huge variety of phenolic compounds are produced by plants, with 1000s recognized throughout the plant kingdom. They can be found in various parts of the plant, but particularly the fruits, leaves and seeds, where they are typically involved in the defense against ultraviolet radiation and pathogens. Phenolics possess one or more aromatic rings bearing one or more hydroxyl groups. They occur in free and conjugated forms with acids, sugars, or other water-soluble or fat-soluble compounds (Szajdek and Borowska, 2008; Nile and Park, 2014; Del Bo et al., 2015; Skrovankova et al., 2015).
For years, phenolic compounds were regarded as anti-nutritional compounds, and in some cases as toxic and mutagenic. Their anti-nutritional activities result from their interactions with proteins, which reduce nutrient assimilation by the inhibition of proteolytic, lipolytic and glycolytic enzymes. Moreover, metal cations are often made unobtainable by complexing with phenolic compounds in humans consuming a plant-based diet. It is important to note that the toxicity of phenolic compounds has not yet been fully recognized and was ignored for years (Bisson et al., 2015).
Berries are not only a source of non-nutritive compounds, including phenolics (Singh and Basu, 2102), but are also a rich source of wide variety of nutritive compounds, including sugars (glucose, fructose) and minerals (phosphorus, calcium, iron, potassium, magnesium, manganese, sodium and copper) (Kulling and Rawel, 2008; Szajdek and Borowska, 2008; Giampieri et al., 2012; Del Bo et al., 2015; Malinowska and Olas, 2016). In addition, iron and manganese are important components of antioxidant enzymes. Berries contain a large amount of the vitamins A, C and E, which act as antioxidants and may reduce the inflammation process (Skrovankova et al., 2015). Blackcurrants and sea buckthorn berries have particularly high concentrations of vitamin C, ranging from 120 to 215 mg per 100 g fruit for blackcurrants, and as high as 600 mg per 100 g fruit for sea buckthorn berries (Olas, 2016; Malinowska and Olas, 2016). Furthermore, berries contain low concentrations of lipids but high concentrations of dietary fiber, which has a nutritional function and reduces the level of low density lipoprotein (LDL) in serum. In addition, it is notable that sea buckthorn oil (extracted from seeds and fruits) and grape seed oil are rich source of fatty acids, unsaturated fatty acids in particular, which have beneficial effects on cardiovascular diseases, neurodegenerative diseases and cancer (Olas, 2016). All these compounds together have a synergistic and multifunctional effect on human health. The chemical composition of a particular berry depends on a range of factors, such as cultivar and variety, plant nutrition, time of harvest, growing location and environmental conditions (Skrovankova et al., 2015).
The Chemical Structure of Phenolic Compounds With Antioxidant Properties
Anthocyanins
Anthocyanins confer the blue, purple and red color of many fruits, including berries. However, berry anthocyanins are not only responsible for fruit color, but also may be used as natural pigments for the food industry (He and Giusti, 2009; Lee et al., 2015). In addition, anthocyanins are known to be one of the most powerful natural antioxidants. Berries are one of the richest sources of anthocyanins among all the fruits (He and Giusti, 2009; You et al., 2011; Lee et al., 2015; Olivas-Aguirre et al., 2016) and are found at the highest concentrations in the skins of berries. Anthocyanins consist of an aromatic ring bonded to a heterocyclic ring containing oxygen, which is also bonded by a carbon-carbon bond to a third aromatic ring. They can be classified into six forms based on the presence of hydroxyl and methoxyl substitutions on the B-ring: cyanidin, malvidin, peonidin, petunidin, pelargonidin and delphinidin (He and Giusti, 2009). The most common types of anthocyanins present in various berries are given in Table 1.
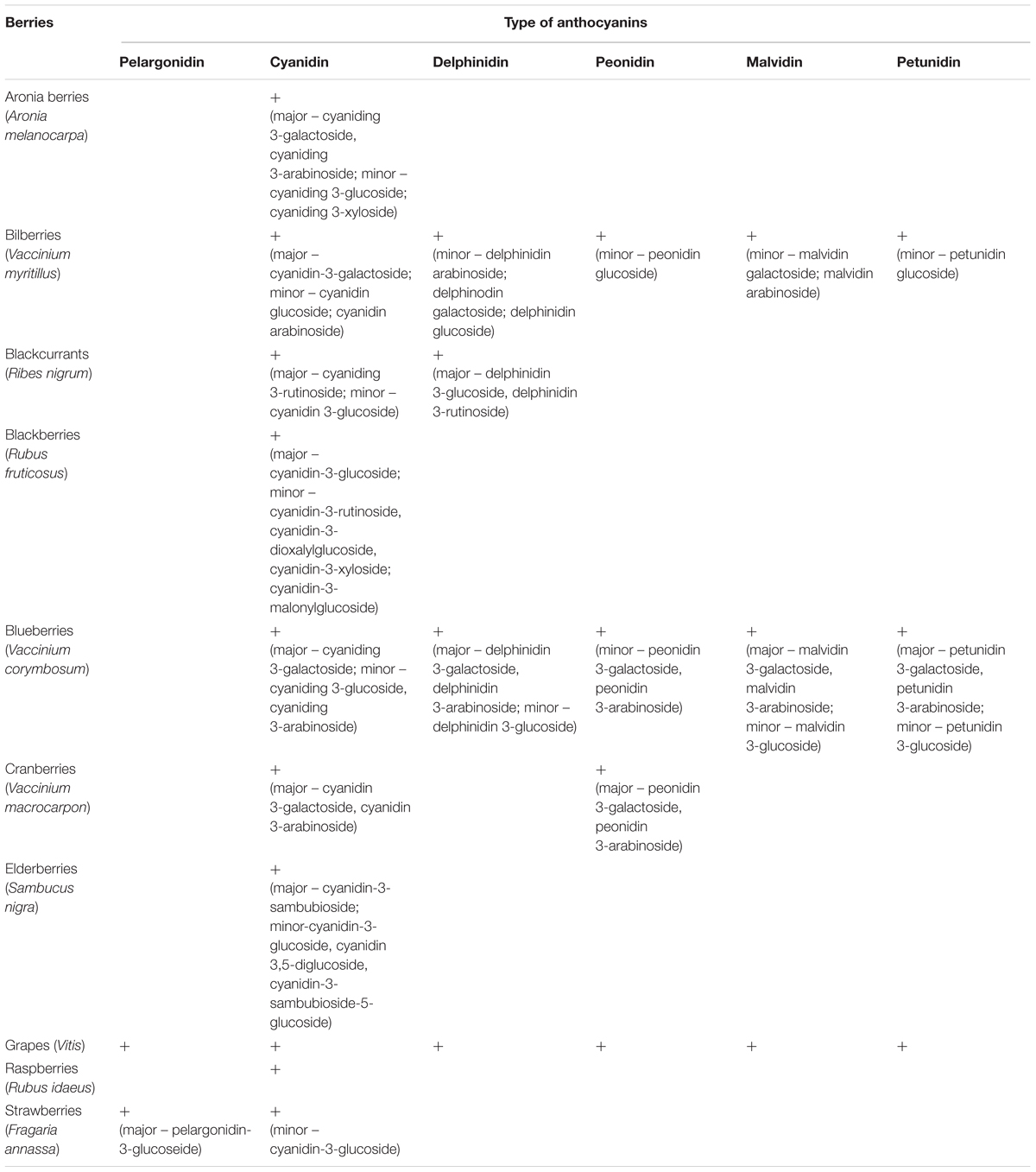
TABLE 1. Major types of anthocyanins, which are presented in various berries (Lee et al., 2015; Nayak et al., 2015; Wang et al., 2015; Kristo et al., 2016; Kšonžeková et al., 2016; Samoticha et al., 2017; modified).
An important property of anthocyanins is that they are able to cross the blood-brain barrier (Andres-Lacueva et al., 2005; Kalt et al., 2008). However, they also have low bioavailability compared with other phenolic compounds (Manach et al., 2004, 2005; Talavera et al., 2006).
Lee et al. (2015) note that the total antioxidant capacity of berries rich in distinct anthocyanins is derived from both anthocyanin composition and the antioxidant capacity of individual anthocyanins.
Other Phenolic Compounds
A wide range of other secondary compounds are also available in different types of berries. Strawberries, blueberries and chokeberries are rich sources of flavon-3-ols, while red raspberries and cloudberries provide high levels of such tannins as ellagitannins. In addition, berries are good sources of such phenolic acids as ellagic acid, chlorogenic acid and gallic acid: blueberry, for example, contains up to 2 g/kg FW of chlorogenic acid (Romani et al., 2016). The absorbance rate varies depending on the type of acid, with chlorogenic acid being poorly absorbed, and gallic acid rapidly absorbed. Ellagic acid represents about 50% of the total phenolic compounds in cranberries and raspberries (Nile and Park, 2014; Skrovankova et al., 2015). In addition, both grapes and red currants are rich in resveratrol, which belongs to the group of stilbenes.
Table 2 presents the total concentrations of phenolic compounds, including anthocyanins, in various berries and berry products. For example, the concentration of phenolic compounds in aronia is about 2080 mg/100 g fruits, which is higher than other berries (for blackberries is about 248 mg/100 g fruits and for blueberries is about 525 mg/100 g fruits) (Lee et al., 2015). Industrial berry products such as aronia berry juice have also a high concentration of phenolic compounds (Table 2). However, only a few commercial products derived from berries (e.g., Aronox® aronia berry extract by Agropharm, Poland), have well-documented chemical compositions and biological activities, including antioxidant properties (Olas et al., 2008; Lee et al., 2015; Daskalova et al., 2015) (Table 2). Aronia berries and aronia juice are believed to possess the highest antioxidant capacity of all studied berries and their juices (Table 3).
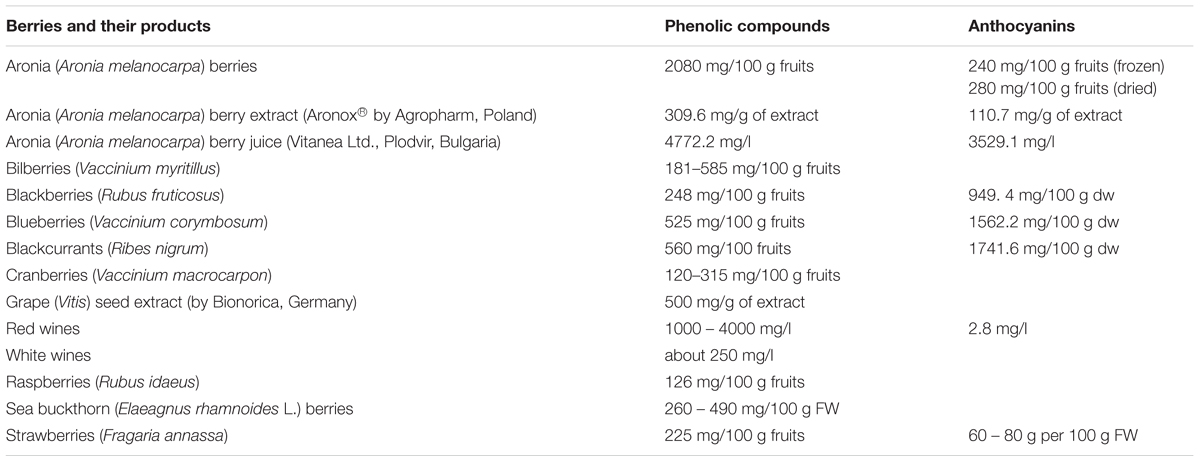
TABLE 2. The concentration of total phenolic compounds and anthocyanins in different berries and their products (Olas et al., 2008; Daskalova et al., 2015; Lee et al., 2015).
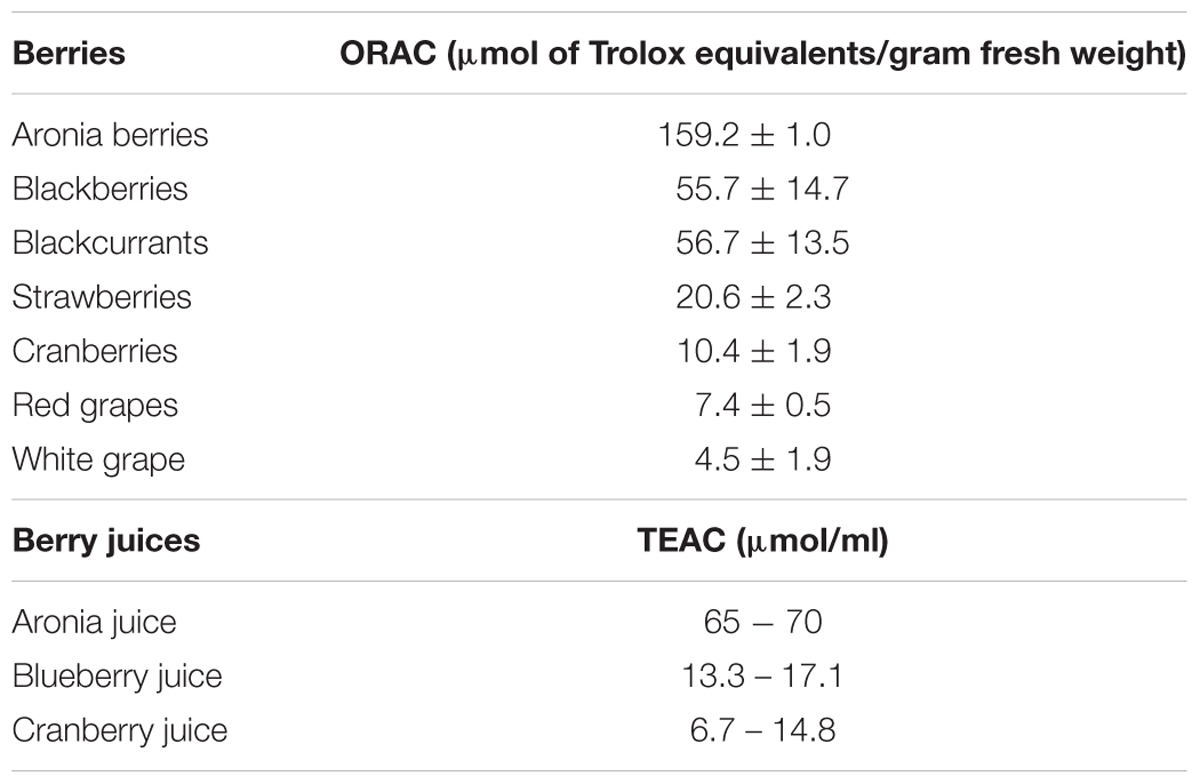
TABLE 3. Antioxidant capacity [measured by oxygen radical absorbing capacity (ORAC) or by Trolox equivalent capacity (TEAC)] of various fresh berries and berry juice [Kulling and Rawel, 2008; modified].
Other authors have reported that berry seeds may be a source of phenolic compounds: grape seeds were found to contain various phenolic acids including gallic acid, p-qumaric acid and ferulic acid (Nassiri-Asl and Hosseinzadeh, 2016). Duba and Fiori (2015) and Garavaglia et al. (2016) have also reported a large amount of phenolic acids, flavonoids, tannins and stilbenes in grape seed oil, with the main phenolic components being epicatechins, catechins, procyanidins and resveratrol (Garavaglia et al., 2016). The total amounts of phenolic compounds extracted from grape seed oil by cold-pressing is about 2.9 mg/kg; this amount includes small amounts of resveratrol (0.3 mg/kg), catechin and epicatechin (1.3 mg/kg each) (Garavaglia et al., 2016). Another source of phenolic compounds, including the flavonoids rutin and quercetin, is sea buckthorn oil extracted from the berry pulp and seeds (Olas, 2016). Many phenolic compounds are found in the small seeds on the outside of strawberries; their antioxidant value is about 14% of the entire value of the fruit.
Similar bioactive compounds, including phenolic compounds, are found in berries and berry leaves, i.e., fresh and dried leaves of sea buckthorn have different anthocyanins and flavonoids, such as gallocatechin and epicatechin (Christaki, 2012; Olas, 2016; Ferlemi and Lamari, 2016). Berry leaves are one of the richest sources of chlorogenic acid (Ferlemi and Lamari, 2016).
Metabolism and Bioavailability of Phenolic Compounds
Berries are an integral part of the human diet, both as fresh berries and as various products, such as jams, juices, wines and berry extracts, which may act as functional foods. They also have a pleasant taste and little calorific content. In addition, both fresh berries and their products have high concentrations of phenolic compounds: flavonoids such as anthocyanins, and non-flavonoids such as stilbenes and phenolic acids. As berries are very often consumed raw, these compounds are not deactivated by cooking. About 8000 phenolic compounds are known to be present in the modern human diet (Ogah et al., 2014; Lall et al., 2015; Del Bo et al., 2015; Terahara, 2015; Kristo et al., 2016).
From the nutritional point of view, phenolic compounds are xenobiotics, which are metabolized in the digestive system as in a “normal dietary situation” (Gheribi, 2011; Bisson et al., 2015). Phenolic compounds are metabolized to sulfated compounds and methylated compounds, and are glucuronidated in the liver. An important metabolite formed from phenolic compounds following the consumption of fruits such as berries is hippuric acid (Toromanovic et al., 2008; Del Bo et al., 2015; Santhakumar et al., 2015).
The Recommended Daily Intake for phenolic compounds remains unknown and given the range of various biological effects occurring at different concentrations, it may well be impossible to determine a uniform value (Gheribi, 2011).
Recently, various in vitro and in vivo experiments have demonstrated that phenolic compounds have a range of beneficial properties including anticancer, anti-platelet, anti-inflammatory and antioxidant effects (Valcheva-Kuzmanova et al., 2006; Erlund et al., 2008; Kulling and Rawel, 2008; Szajdek and Borowska, 2008; Chong et al., 2010; Chrubasik et al., 2010; Christaki, 2012; Giampieri et al., 2012, 2015; McEwen, 2014; Nile and Park, 2014; Del Bo et al., 2015; Skrovankova et al., 2015; Wightman and Henberger, 2015; Kristo et al., 2016; Olas, 2016, 2017; Umeno et al., 2016). Not only does the concentration of phenolic compounds have an effect on human health, but also their metabolism and bioavailability (Yang et al., 2011; Wilczak et al., 2013).
Regular consumption of darker-colored berries, such as blackberries, blueberries, strawberries, raspberries and aronia berries, may provide a high intake of anthocyanin. For example, anthocyanins constitute about 30% of all phenolic compounds in blackcurrants and about 70% in blueberries. However, plasma concentrations of anthocyanins are typically quite low due to their poor absorbance profile (<1%) (Fang, 2014a,b, 2015). The average total intake of these compounds is about 200 mg/day and their concentration ranges from 10 to 50 nM in plasma following the consumption of berries. In addition, human experiments have found 0.1% of anthocyanin intake to be excreted in urine. Fang (2014a,b) suggest that the apparent low bioavailability of some anthocyanins may be due to extensive presystemic metabolism rather than poor absorption. Xie et al. (2016) also indicate that the anthocyanins in aronia extract, constituting 34% of the total phenolic content, are extensively metabolized.
Studies have shown that the bioavailability of phenolic compounds differs from berry to berry, and this can also be affected by the method of processing (Scalbert and Williamson, 2000; McGhie and Alton, 2007; Del Bo et al., 2012; Kuntz et al., 2015). Food processing procedures, such as high-temperature treatments, are recognized as one of the major factors responsible for the destruction or modification of natural phytochemicals, which may in turn affect the antioxidant properties of foods (Nicoli et al., 1999; Nayak et al., 2015). However, this reduction could be compensated for by the degradation of higher molecular weight phenolic compounds to smaller ones with greater antioxidant properties (Nayak et al., 2015).
In a study of the phenolic profiles of 26 berry samples and their antioxidant activity, Kahkonen et al. (2001) report that the choice of extraction method significantly affected both phenolic composition and antioxidant property of the resulting product. However, statistical analysis found no significant relationship between the observed activity and the contents of individual phenolic compounds.
Several factors, including the technological procedures used in winemaking, can also qualitatively and quantitatively affect the phenolic compound composition of wine (Garrido and Borges, 2013; Lingua et al., 2016). Phenolic compounds are transferred from the grape into the wine during crushing, maceration and fermentation. The majority of phenolic compounds in grapes are present in the skin and seeds (Lingua et al., 2016). Lingua et al. (2016) report a high correlation between phenolic composition and antioxidant capacity, with anthocyanins offering the greatest contribution to antioxidant capacity.
Berries are often consumed as fresh fruit, and in this form, their antioxidant capacity is not reduced by any factors such as heat or oxidation during processing (Patras et al., 2010; Skrovankova et al., 2015). It is very important to retain the beneficial properties of antioxidants in processed food products (Nayak et al., 2015). In the last decade, some papers have examined the influence of processing operations, such as drying or dehydration, on phytochemicals in fruit, including those of berries: for example, the flavonoid content of frozen aronia berries is 12.2 mg/100 g fruit, and of dried aronia berries is 107 mg/100 g fruit. Recently, Oszmianski and Lachowicz (2016) found the phenolics in dried aronia berry pomace and in juice obtained from crushed berries to have higher activity than those from the whole foods.
Freshly produced strawberry juices have higher anthocyanin concentrations than those stored for 6 months at 4°C and 30°C (Oszmianski and Wojdylo, 2009; Skrovankova et al., 2015). Moreover, a number of phenolic compounds in clear strawberry juices were found to be have been lost during processing (Skrovankova et al., 2015). After heat processing and drying, the total phenolic compound concentration is also less than 70% (Rudy et al., 2015; Skrovankova et al., 2015). Interestingly, the concentration of anthocyanins in raspberry juice may increase about 2.5-fold after a week of storage at 20°C (Kalt et al., 1999; Skrovankova et al., 2015); however, in canned blackberries, significant amounts of anthocyanins are leached into the brine during processing and storage (Hager et al., 2008; Skrovankova et al., 2015). Elsewhere, anthocyanin concentration was found to decrease by about 30% in blueberries following thermal treatment (Giovanelli et al., 2013; Skrovankova et al., 2015). However, a 7% increase in anthocyanin concentration was found in blueberries following blanching at 85°C for 3 min (Giovanelli et al., 2012; Skrovankova et al., 2015); in addition, a study of anthocyanin absorption following the consumption of one portion (300 g) of minimally processed blueberry puree obtained from blanched or unblanched berries by Del Bo et al. (2012) found blanching to have no significant effect on total anthocyanin content, but in fact enhanced their absorption from minimally processed purees.
In addition, the concentration of berry phenolic compounds may change during berry development. The phenolics content of strawberries is known to decrease significantly by about 90% during ripening from green to red berries (Regonold et al., 2010; Crecente-Campo et al., 2012; Skrovankova et al., 2015).
The Oxidative Stress and Its Biomarkers; the Role of Berry Phenolic Compounds in the Oxidative Stress
In a healthy organism, the generation of reactive oxygen species is balanced by the activities of antioxidants (Bartosz and Sadowska-Bartosz, 2015). Increased ROS generation or diminished antioxidant defense is referred to as oxidative stress, which may participate in the development of various diseases, including cancer, cardiovascular diseases and neurodegenerative disorders (Bartosz and Sadowska-Bartosz, 2015). Oxidative stress is usually a local event, one which may be indicated by different biomarkers, including such markers of lipid oxidative modification as malondialdehyde (MDA), conjugated dienes or F2-isoprostanes, markers of protein modification including carbonylated proteins, oxidation of thiol groups, protein fragmentation and nitrated proteins, or markers of oxidative damage of nucleic acids (Bartosz and Sadowska-Bartosz, 2015). These biomarkers not only have diagnostic value, but they may be also useful indicators of the need for antioxidant supplementation.
Various medicinal effects of berries against diseases associated with oxidative stress have been attributed to their high phenolic antioxidant content, especially anthocyanins and phenolic acids. In addition, berries are recognized to have high levels of vitamins A, C and E, which may act as antioxidants (Skrovankova et al., 2015; Olas, 2016). Various authors have attributed the health benefits of whole foods to complex mixtures of phytochemicals (i.e., phenolics). Moreover, greater beneficial effects have been associated with the antioxidants obtained from whole foods than those obtained singly (Eberhardt et al., 2000; Nayak et al., 2015).
A number of in vitro and in vivo studies have examined the antioxidant activities of berries and their products, especially berry juices (Table 4). They have examined inter alia the inhibition of lipid peroxidation, inhibition of protein carbonylation, inhibition of ROS generation, increase of total antioxidant status and the increase of antioxidant enzyme activity. The results of these studies are given in Table 4. It is important to note that antioxidant effects were not only found in in vitro models or in animals, but also in humans, where dietary supplementation with a range of berry products, including berry juices, reduces the levels of a number of biomarkers of oxidative stress.
Previous studies have demonstrated that the consumption of berries rich in antioxidant phenolic compounds results in an increase in plasma total antioxidant status in humans (Wilson and Bauer, 2009; Negi et al., 2013; Kardum et al., 2014; Del Bo et al., 2015). The modulation of various antioxidant/prooxidant status markers observed in healthy subjects demonstrates the potential prophylactic actions of fresh berries and their products, and underlines their importance as part of an optimal diet. These benefits have also been observed in subjects with poor health, including patients with diseases which are very often correlated with oxidative stress, i.e., patients with cancer, metabolic syndrome or cardiovascular diseases (Table 4). Zafra-Stone et al. (2007) note that a combination of six berry extracts (wild blueberry, wild bilberry, cranberry, elderberry, raspberry seed and strawberry) exhibited significantly superior antioxidant potential than the consumption of individual berries.
However, some papers note that phenolic compounds may behave like prooxidants under conditions that favor autooxidation, such as high pH and in the presence of high concentrations of transition metal ions and oxygen molecules (Cotoras et al., 2014). Moreover, while small phenolic compounds (i.e., quercetin and gallic acid) are easily oxidized and possess prooxidant properties, phenolic compounds of high molecular weights (i.e., condensed and hydrolysable tannins) have little or no prooxidant properties (Hagerman et al., 1998; Cotoras et al., 2014). In addition, phenolic compounds such as vanillic acid, ellagic acid, gallic acid and rutin have been reported to possess dual antioxidant and prooxidant properties (Fukumoto and Mazza, 2000). Cotoras et al. (2014) found that grape extracts demonstrated antioxidant or prooxidant properties depending on the method of extraction and the variety of the grape. It is very important that the potential antioxidant function of a plant extract with phenolic compounds in vivo cannot be safely correlated from in vitro experiments, because they do not take into account the metabolic transformations and interactions that are known to affect the bioavailability and biological properties of phenolic compounds (Veskouis et al., 2012).
Veskouis et al. (2012) report the presence of such dual effects of a phenol-rich extract of grape pomace in in vitro and in vivo models. This extract inhibited ROS production and DNA damage stimulated by peroxyl and hydroxyl radicals in vitro, but induced protein carbonylation, and lipid peroxidation, and decreased the level of glutathione in vivo (Table 4). Practical recommendations for the use of phenolic antioxidant should involve the use of both in vitro and in vivo experiments.
Conclusion
In recent years, a number of studies have examined the role of phenolic compounds in berries as antioxidants protecting against the most common diseases related to oxidative stress-driven pathologies, such as cardiovascular diseases, inflammation, cancer and neurodegenerative diseases. Berries and their products have been shown to play a beneficial role as antioxidants in humans in both in vitro and in vivo models using dietary supplementation with various berries (Del Bo et al., 2015), and the most potent antioxidants commonly found in berries may well be the anthocyanins. In contrast, a few papers have demonstrated that the phenolic compounds also have prooxidative activity, and berry extracts rich in phenolic compounds do not behave the same way in in vitro and in vivo models (Table 4).
However, no unwanted or toxic effects (i.e., chemical, hematological or urinary effect) have been associated with the consumption of berries or berry juices or other extracts, especially aronia berries and aronia products in vivo, and in vitro (Kulling and Rawel, 2008), which may suggest that the phenolic antioxidants found in berries are natural gifts for human health. However, the phenolic compound content of berries and berry products is not always well described, and further studies are required to determine the therapeutic doses of different berry products for use in future clinical studies. Moreover, further experiments are needed to understand the beneficial effects reported so far from the mechanistic point of view. Therefore, greater attention should be paid to the development of well-controlled and high-quality clinical studies in this area.
Author Contributions
The author confirms being the sole contributor of this work and approved it for publication.
Conflict of Interest Statement
The author declares that the research was conducted in the absence of any commercial or financial relationships that could be construed as a potential conflict of interest.
The reviewer CT and handling Editor declared their shared affiliation.
Acknowledgments
This work was supported grant 506/1136 from the University of Lodz.
References
Alavrez-Suarez, J. M., Dekanski, D., Ristic, S., Radonjic, N. V., Petronijevic, N. D., GIampieri, F., et al. (2011). Strawberry polyphenols attenuate ethanol-induced gastric lesions in rats by activation of antioxidant enzymes and attenuation of MDA increase. PLoS One 6:e25878. doi: 10.1371/journal.pone.0025878
Andres-Lacueva, C., Shukitt-Hale, B., Galli, R. L., Jauregui, O., Lamuela-Raventos, R. M., and Joseph, J. A. (2005). Anthocyanins in aged blueberry-fed rats are found centrally and may enhance memory. Nutr. Neurosci. 8, 111–120. doi: 10.1080/10284150500078117
Bartosz, G., and Sadowska-Bartosz, I. (2015). “Oxidative, nitrosative, and chlorinative stress: biomarkers,” in Studies on Psychiatric Disorders, Oxidative Stress in Applied Basic Research and Clinical Practice, eds A. Dietrich-Muszalska, V. Chauhan, and S. Grignon (New York, NY: Humana Press), 1–39.
Basu, A., Betts, N. M., Nguyen, A., Newman, E. D., Fu, D., and Lyons, T. J. (2014). Freeze-dried strawberries lower serum cholesterol and lipid peroxidation in adults with abdominal adiposity and elevated serum lipids. J. Nutr. 144, 830–837. doi: 10.3945/jn.113.188169
Basu, A., Du, M., Leyva, M. J., Sanchez, K., Betts, N. M., Wu, M., et al. (2010). Blueberries decrease cardiovascular risk factors in obese men and women with metabolic syndrome. J. Nutr. 140, 1582–1587. doi: 10.3945/jn.110.124701
Basu, A., Wilkinson, M., Penugonda, K., Simmons, B., Betts, N. M., and Lyons, T. J. (2009). Freeze-dried strawberry powder improves lipid profile and lipid peroxidation in women with metabolic syndrome: baseline and post intervention effects. Nutr. J. 8, 1–7. doi: 10.1186/1475-2891-8-43
Bisson, J., McAlpine, J. B., Friesen, J. B., Chen, S. N., Graham, J., and Pauli, G. F. (2015). Can invalid bioactive undermine natural product-based drug discover? J. Med. Chem. 59, 1671–1690. doi: 10.1021/acs.jmedchem.5b01009
Cetin, A., Kaynar, L., Kocyigit, I., Hocioglu, S. K., Saraymen, R., Ozturk, A., et al. (2008). The effect of grape seed extract on radiation-induced oxidative stress in the rat liver. Turk. J. Gastroenetrol. 19, 92–98.
Chong, M. F. F., Macdonald, R., and Lovegrove, J. A. (2010). Fruit polyphenols and CDV risk: a review of human intervention studies. Br. J. Nutr. 104, S28–S39. doi: 10.1017/S0007114510003922
Christaki, E. (2012). Hippophae rhamnoides L. (Sea Buckthorn): a potential source of nutraceuticals. Food Public Health 2, 69–72. doi: 10.1002/pca.1353
Chrubasik, C., Li, G., and Chrubasik, S. (2010). The clinical effectiveness of chokeberry: a systematic review. Phytother. Res. 24, 1107–1114. doi: 10.1002/ptr.3226
Cotoras, M., Vinaco, H., Melo, R., Aguirre, M., Silva, E., and Mendoza, L. (2014). In vitro and in vivo evaluation of the antioxidant and prooxidant activity of phenolic compounds obtained from grape (Vitis vinifera) pomace. Molecules 19, 21154–21167. doi: 10.3390/molecules191221154
Crecente-Campo, J., Nunes-Damaceno, M., Romero-Rodriguez, M. A., and Vazquez-Oderiz, M. L. (2012). Color, anthocyanin pigment, ascorbic acid and total phenolic compound determination in organic versus conventional strawberries (Fragaria ananassa Duch, cv Selva). J. Food Comp. Anal. 28, 23–30. doi: 10.1016/j.jfca.2012.07.004
Daskalova, E., Delchev, S., Peeva, Y., Vladimirova-Kitova, L., Kratchanova, M., Kratchanov, C., et al. (2015). Antiatherogenic and cardioprotective effects of black chokeberry (Aronia melanocarpa) juice in aging rats. Evid. Based Complement. Alternat. Med. 2015:10. doi: 10.1155/2015/717439
Del Bo, C., Martini, D., Porrini, M., Klimis-Zacas, D., and Riso, P. (2015). Berries and oxidative stress markers: an overview of human intervention studies. Food Funct. 6, 2890–2917. doi: 10.1039/c5fo00657k
Del Bo, C., Martini, D., Vendrame, S., Riso, P., Ciappellano, S., Klimis-Zacas, D., et al. (2010). Improvement of lymphocyte resistance against H2O2-induced DNA damage in Sprague–Dawley rats after eight weeks of w wild blueberry (Vaccinium angustifolium)-enriched diet. Mutat. Res. 703, 158–162. doi: 10.1016/j.mrgentox.2010.08.013
Del Bo, C., Porrini, M., Campolo, J., Parolini, M., Lanti, C., Klimis-Zacaz, D., et al. (2016). A single blueberry (Vaccinium corymbosum) portion does not affect markers of antioxidant defence and oxidative stress in healthy volunteers following cigarette smoking. Mutagenesis 31, 215–224. doi: 10.1093/mutage/gev079
Del Bo, C., Riso, P., Brambill, A., Gardana, C., Rizzolo, A., Simonetti, P., et al. (2012). Blanching improves anthocyanin absorption from highbush blueberry (Vaccinium corymbosum L.) puree in healthy human volunteers: a pilot study. J. Agric. Food Chem. 60, 9298–9304. doi: 10.1021/jf3021333
Duba, K. S., and Fiori, L. (2015). Supercritical CO2 extraction of grape seed oil: effect of process parameters on the extraction kinetics. J. Supercrit. Fluids 98, 33–43. doi: 10.1016/j.supflu.2014.12.021
Duthie, S. J., McE Jenkinson, A., Crozier, A., Mullen, W., Pirie, L., Kyle, J., et al. (2006). The effects of cranberry juice consumption on antioxidant status and biomarkers relating to heart disease and cancer in healthy human volunteers. Eur. J. Nutr. 45, 113–122. doi: 10.1007/s00394-005-0572-9
Eberhardt, M. V., Lee, C. Y., and Liu, R. H. (2000). Nutrition – antioxidant activity of fresh apples. Nature 405, 903–904. doi: 10.1038/35016151
Eccleston, C., Baoru, Y., Tahvonem, R., Kallio, H., Rimbach, G. H., and Minihane, A. M. (2002). Effects of an antioxidant-rich juice (sea buckthorn) on risk factors for coronary heart disease in humans. J. Nutr. Biochem. 13, 346–354. doi: 10.1016/S0955-2863(02)00179-1
Erlund, I., Koli, R., Alfthan, G., Marniemi, J., Puukka, P., Mustonen, P., et al. (2008). Favorable effects of berry consumption of platelet function, blood pressure, and HDL cholesterol. Am. J. Clin. Nutr. 87, 323–331.
Fang, J. (2014a). Bioavailability of anthocyanins. Drug Metab. Rev. 46, 508–520. doi: 10.3109/03602532.2014.978080
Fang, J. (2014b). Some anthocyanins could be efficiently absorbed across the gastrointestinal mucosa: extensive presystemic metabolism reduces apparent bioavailability. J. Agric. Food Chem. 62, 3904–3911. doi: 10.1021/jf405356b
Fang, J. (2015). Classification of fruits based on anthocyanin types and relevance to their health effects. Nutrition 31, 1301–1306. doi: 10.1016/j.nut.2015.04.015
Ferlemi, A. V., and Lamari, F. N. (2016). Berry leaves: an alternative source of bioactive natural products of nutritional and medicinal value. Antioxidants 5, 1–20. doi: 10.3390/antiox5020017
Fukumoto, L. R., and Mazza, G. (2000). Assessing antioxidant and prooxidant activities of phenoic compounds. J. Agric. Food Chem. 48, 3597–3604. doi: 10.1021/jf000220w
Garavaglia, J., Markoski, M. N., Oliviers, A., and Marcadenti, A. (2016). Grape seed oil compounds: biological and chemical actions for health. Nutr. Metab. Insights 9, 59–64. doi: 10.4137/NMI.S32910
Garrido, J., and Borges, F. (2013). Wine and grape polyphenols – a chemical perspective. Food Res. Int. 54, 1844–1858. doi: 10.1002/jsfa.7051
Giampieri, F., Alvarez-Suarez, J. M., Gasparrini, M., Forbes-Hernandez, T. Y., Afrin, S., Bompadre, S., et al. (2016). Strawberry consumption alleviates doxorubicin-induced toxicity by suppressing oxidative stress. Food Chem. Toxicol. 94, 128–137. doi: 10.1016/j.fct.2016.06.003
Giampieri, F., Forbes-Hernandez, T. Y., Gasparrini, M., Avarez-Suarez, J. M., Afrin, S., Bompadre, S., et al. (2015). Strawberry as a health: an evidence based review. Food Funct. 6, 1386–1398. doi: 10.1039/c5fo00147a
Giampieri, F., Tulipani, S., Alvarez-Suarez, J. M., Quiles, J. L., Mezzetti, B., and Battino, M. (2012). The strawberry: composition, nutritional quality, and impact on human health. Nutrition 28, 9–19. doi: 10.1016/j.nut.2011.08.009
Giovanelli, G., Brambilla, A., Rizzolo, A., and Sinelli, N. (2012). Effects ofblanching pre-treatment and sugar composition of the osmotic solution on physico-chemical, morphological and antioxidant characteristics of osmodehydrated blueberries (Vaccinium corymbosum L.). Food Res. Int. 49, 263–271. doi: 10.1016/j.foodres.2012.08.015
Giovanelli, G., Brambilla, A., and Sinelli, N. (2013). Effects of osmo-air dehydration treatments on chemical, antioxidant and morphological characteristics of blueberries. LWT Food Sci. Technol. 54, 577–584. doi: 10.1016/j.lwt.2013.06.008
Gomes-Rochette, N. F., Da Silveira Vasconcelos, M., Nabavi, S. M., Mota, E. F., Nunes-Pinheiro, D. C., Daglia, M., et al. (2016). Fruit as potent natural antioxidants and their biological effects. Curr. Pharm. Biotechnol. 17, 986–993. doi: 10.2174/1389201017666160425115401
Guo, H., Zhong, R., Liu, Y., Jiang, X., Tang, X., Li, Z., et al. (2014). Effects of bayberry juice on inflammatory and apoptotic markers in young adults with features of non-alcoholic fatty liver disease. Nutrition 30, 198–203. doi: 10.1016/j.nut.2013.07.023
Hager, T. J., Howard, L. R., and Prior, R. L. (2008). Processing and storage effects on monomeric anthocyanins, percent polymeric color, and antioxidant capacity of processed blackberry products. J. Agric. Food Chem. 56, 689–695. doi: 10.1021/jf071994g
Hagerman, A. E., Riedl, K. M., Jones, G. A., Sovik, K. N., Ritchared, N. T., Hartzfeld, P. W., et al. (1998). High molecular weight plant polyphenolics (tannins) as biological antioxidants. J. Agric. Food Chem. 46, 1887–1892. doi: 10.1021/jf970975b
He, J., and Giusti, M. M. (2009). Anthocyanins: natural colorants with health-promoting properties. Annu. Rev. Food Sci. Technol. 1, 163–187. doi: 10.1146/annurev.food.080708.100754
Hickey, M., and King, C. (2001). The Cambridge Illustrated Glossary of Botanical Terms, 1st. Edn. Cambridge: Cambridge University Press.
Jenkins, D. J., Nguyen, T. H., Kendall, C. W., Faulkner, D. A., Bashyam, B., Kim, I. J., et al. (2008). The effect of strawberries ina cholesterol-lowering dietary portfolio. Met. Clin. Exp. 57, 1636–1644. doi: 10.1016/j.metabol.2008.07.018
Johnson, S. A., Figueroa, A., Navaei, N., Wong, A., Kalfon, R., Ormesbee, L. T., et al. (2015). Daily blueberry consumption improves blood pressure and arterial stiffness in postmenopausal women with pre- and stage 1-hypertension: a randomized, double-blind, placebo-controlled clinical trial. J. Acad. Nutr. Diet. 115, 369–377. doi: 10.1016/j.jand.2014.11.001
Kahkonen, M. P., Hopia, A. I., and Heinonen, M. (2001). Berry phenolics and their antioxidant activity. J. Agric. Food Chem. 49, 4076–4082. doi: 10.1021/jf010152t
Kalt, W., Blumberg, J. B., McDonald, J. E., Vinqvist-Tymchuk, M. R., Fillmore, S. A., Graf, B. A., et al. (2008). Identification of anthocyanins in the liver, eye, and brain of blueberry-fed pigs. J. Agric. Food Chem. 56, 705–712. doi: 10.1021/jf071998l
Kalt, W., Forney, C. F., Martin, A., and Prior, R. L. (1999). Antioxidant capacity, vitamin C, phenolics, and anthocyanins after fresh storage of small fruits. J. Agric. Food Chem. 47, 4638–4644. doi: 10.1021/jf990266t
Kardum, N., Konic-Ristic, A., Savikin, K., Spasic, S., Stefanovic, A., Ivanisevic, J., et al. (2014). Effects of polyphenol-rich chokeberry juice on antioxidant/prooxidant status in healthy subjects. J. Med. Food 17, 869–874. doi: 10.1089/jmf.2013.0135
Karlsen, A., Paur, I., Bohn, S. K., Sakhi, A. K., Borge, G. I., Serafini, M., et al. (2010). Bilberry juice modulates plasma concentration of NF-(B related inflammatory markers in subjects at increased risk of CVD. Eur. J. Nutr. 49, 345–355. doi: 10.1007/s00394-010-0092-0
Kay, C. D., and Holub, B. J. (2002). The effect of wild blueberry (Vaccinium angustifolium) consumption on postprandial serum antioxidant status in human subjects. Br. J. Nutr. 88, 389–397. doi: 10.1079/BJN2002665
Kȩdzierska, M., Głowacki, R., Czernek, U., Szydłowska-Pazera, K., Potemski, P., Piekarski, J., et al. (2013a). Changes in plasma thiol levels induced by different phases of treatment in breast cancer; the role of commercial extract from black chokeberry. Mol. Cell. Biochem. 372, 47–55. doi: 10.1007/s11010-012-1444-2
Kȩdzierska, M., Malinowska, J., Kontek, B., Kołodziejczyk-Czepas, J., Czernek, U., Potemski, P., et al. (2013b). Chemotherapy modulates the biological activity of breast cancer patients plasma; the protective properties of black chokeberry extract. Food Chem. Toxicol. 53, 126–132. doi: 10.1016/j.fct.2012.11.042
Kedzierska, M., Olas, B., Wachowicz, B., Glowacki, R., Bald, E., Czernek, U., et al. (2012). Effects of the commercial extract of aronia on oxidative stress in blood platelets isolated from breast cancer patients after the surgery and various phases of the chemotherapy. Fitoterapia 83, 310–317. doi: 10.1016/j.fitote.2011.11.007
Kȩdzierska, M., Olas, B., Wachowicz, B., Stochmal, A., Oleszek, W., and Erler, J. (2011). Changes of platelet antioxidative enzymes during oxidative stress; the protective effect of polyphenol-rich extract from berries of Aronia melanocarpa and grape seeds. Platelets 22, 385–389. doi: 10.3109/09537104.2010.545151
Kedzierska, M., Olas, B., Wachowicz, B., Stochmal, A., Oleszek, W., Jeziorski, A., et al. (2009). An extract from berries of Aronia melanocarpa modulates the generation of superoxide anion radicals in blood platelets from breast cancer patients. Planta Med. 75, 1405–1409. doi: 10.1055/s-0029-1185718
Kȩdzierska, M., Olas, B., Wachowicz, B., Stochmal, A., Oleszek, W., Jeziorski, A., et al. (2010). The nitrative and oxidative stress in blood platelets isolated from breast cancer patients; the protectory action of Aronia melanocarpa extract. Platelets 21, 541–548. doi: 10.3109/09537104.2010.492534
Khan, F., Ray, S., Craigie, A. M., Kennedy, G., Hill, A., Barton, K. L., et al. (2014). Lowering of oxidative stress improves endothelial function in healthy subjects with habitually low intake of fruit and vegetables: a randomized controlled trial of antioxidant- and polyphenol-rich blackcurrant juice. Free Radic. Biol. Med. 72, 232–237. doi: 10.1016/j.freeradbiomed.2014.04.006
Kondeva-Burdina, M., Valcheva-Kuzmanova, S., Markova, T., Mitcheva, M., and Belcheva, A. (2015). Effects of Aronia melanocarpa fruit juice on isolated rat hepatocytes. Pharmacogn. Mag. 11, S592–S597. doi: 10.4103/0973-1296.172967
Kowalczyk, E., Fijalkowski, P., Kura, M., Krzesinski, P., Blaszczyk, J., Kowalski, J., et al. (2005). The influence of anthocyanins from Aronia melanocarpa on selected parameters of oxidative stress and microelements contents in men with hypercholesterolemia. Pol. Merkur. Lekarski 19, 651–653.
Kresty, L. A., Frankel, W. L., Hammond, C. D., Baird, M. E., Mele, J. M., Stoner, G. D., et al. (2006). Transitioning from preclinical to clinical chemopreventive assessments of lyophilized black raspberries: interim results show berries modulate markers of oxidative stress in Barrett’s esophagus patients. Nutr. Cancer 54, 158–156. doi: 10.1207/s15327914nc5401_15
Kristo, A. S., Klimis-Zacas, D., and Sikalidis, A. K. (2016). Protective role of dietary berries in cancer. Antioxidants 5, 1–23. doi: 10.3390/antiox5040037
Kšonžeková, P., Mariychuk, R., Eliašová, A., Mudroòová, D., Csank, T., Király, J., et al. (2016). In vitro study of biological activities of anthocyanin-rich berry extracts on porcine intestinal epithelial cells. J. Sci. Food Agric. 15, 1093–1100. doi: 10.1002/jsfa.7181
Kujawska, M., Ignatowicz, E., Ewertowska, M., Oszmianski, J., and Jodynis-Libert, J. (2011). Protective effect of chokeberry on chemical-induced oxidative stress in rat. Hum. Exp. Toxicol. 30, 199–208. doi: 10.1177/0960327110371697
Kulling, S. E., and Rawel, H. M. (2008). Chokeberry (Aronia melanocarpa) – A review on the characteristic components and potential health effects. Planta Med. 74, 1625–1634. doi: 10.1055/s-0028-1088306
Kuntz, S., Kunz, C., Herrmann, J., Borsch, C. H., Abel, G., Frohling, B., et al. (2014). Anthocyanins from fruit juices improve the antioxidant status of healthy young female volunteers without affecting anti-inflammatory parameters: results from the randomized, double-blind, placebo-controlled, cross-over ANTHONIA (ANTHOcyanins in Nutrition Investigation Allliance) study. Br. J. Nutr. 112, 925–936. doi: 10.1017/S0007114514001482
Kuntz, S., Rudloff, S., Asseburg, H., Brsch, C., Frohling, B., Unger, F., et al. (2015). Uptake and biovailability of anthocyanins and phenolic acids from grape/blueberry juice and smoothie in vitro and in vivo. Br. J. Nutr. 113, 1044–1055. doi: 10.1017/S0007114515000161
Kutlesa, Z., and Mrsic, D. B. (2016). Wine and bone health: a review. J. Bone. Miner. Metab. 34, 11–22. doi: 10.1007/s00774-015-0660-8
Lakshmi, B. V., Sudhakar, M., and Aparma, M. (2013). Protective potential of black grapes against lead induced oxidative stress in rats. Environ. Toxicol. Pharmacol. 35, 361–368. doi: 10.1016/j.etap.2013.01.008
Lall, R. K., Syed, D. N., Adhami, V. M., Khan, M. I., and Mukhtar, H. (2015). Dietary polyphenols in prevention and treatment of prostate cancer. Int. J. Mol. Sci. 16, 3350–3376. doi: 10.3390/ijms16023350
Lee, J. E., Park, E., Lee, J. E., Auh, J. H., Choi, H. K., Lee, J., et al. (2011). Effects of a Rubus coreanus Miquel supplement on plasma antioxidant capacity in healthy Korean men. Nutr. Res. Pract. 5, 429–434. doi: 10.4162/nrp.2011.5.5.429
Lee, S. G., Vance, T. M., Nam, T. G., Kim, D. O., Koo, S. I., and Chun, O. K. (2015). Contribution of anthocyanin composition to total antioxidant capacity of berries. Plant Foods Hum. Nutr. 70, 427–432. doi: 10.1007/s11130-015-0514-5
Lingua, M. S., Fabani, M. P., Wunderlin, D. A., and Baroni, M. V. (2016). From grape to wine: changes in phenolic composition and its influence on antioxidant activity. Food Chem. 208, 228–238. doi: 10.1016/j.foodchem.2016.04.009
Malinowska, J., Oleszek, W., Stochmal, A., and Olas, B. (2013). The polyphenol-rich extracts from black chokeberry and grape seeds impair changes in the platelet adhesion and aggregation induced by a model of hyperhomocysteinemia. Eur. J. Nutr. 52, 1049–1057. doi: 10.1007/s00394-012-0411-8
Manach, C., Scalbert, A., Morand, C., Remesy, C., and Jimenez, L. (2004). Polyphenols: food sources and bioavailability. Am. J. Clin. Nutr. 79, 727–747. doi: 10.1093/ajcn/79.5.727
Manach, C., Williamson, G., Morand, C., Scalbert, A., and Remesy, C. (2005). Bioavailability and bioefficacy of polyphenols in humans. Review of 97 bioavailability studies. Am. J. Clin. Nutr. 81, 230S–242S.
Marniemi, J., Hakala, P., Maki, J., and Ahotupa, M. (2000). Partial resistance of low density lipoprotein to oxidation in vivo after increased intake of berries. Nutr. Metab. Cardiovasc. Dis. 10, 331–337.
Mazza, G., Kay, C. D., Cottrell, T., and Holub, B. J. (2002). Absorption of anthocyanins from blueberries and serum antioxidant status in human subjects. J. Agric. Food Chem. 50, 7731–7737. doi: 10.1021/jf020690l
McAnulty, S. R., McAnulty, L. S., Morrow, J. D., Khardouni, D., Shooter, I., and Monk, J. (2015). Effect of daily fruit ingestion on angiotensin converting enzyme activity, blood pressure, and oxidative stress in chronic smokers. Free Radic. Res. 39, 1241–1248. doi: 10.1080/10715760500306836
McEwen, B. J. (2014). The influence of diet and nutrients on platelet function. Semin. Thromb. Hemost. 40, 214–226. doi: 10.1055/s-0034-1365839
McGhie, T. K., and Alton, M. C. (2007). The bioavailability and absorption of anthocyanins: towards a better understanding. Mol. Nutr. Food Res. 51, 702–713. doi: 10.1002/mnfr.200700092
Moazen, S., Amani, R., Homoyouni, R. A., Shahbazian, H., Ahmadi, K., and Taha Jaliali, M. (2013). Effects of freeze-dried strawberry supplementation on metabolic biomarkers of atherosclerosis in subjects with type 2 diabetes: a randomized double-blind controlled trial. Ann. Nutr. Metab. 63, 256–264. doi: 10.1159/000356053
Muresan, A., Alb, C., Suciu, S., Clichici, S., Filip, A., Login, C., et al. (2010). Studies on antioxidant effects of red grapes seed extract from Vitis vinifera, Burgund Mare, Recas in pregnant rats. Acta Physiol. Hung. 97, 240–246. doi: 10.1556/APhysiol.97.2010.2.11
Murkovic, M., Abuja, P. M., Bergmann, A. R., Zirngast, A., Adam, U., Winklhofer-Roob, B. M., et al. (2004). Effects of elderberry juice on fasting and postprandial serum lipids and low-density lipoprotein oxidation in healthy volunteers: a randomized, double-blind, placebo-controlled study. Eur. J. Clin. Nutr. 58, 244–249. doi: 10.1038/sj.ejcn.1601773
Nassiri-Asl, M., and Hosseinzadeh, H. (2016). Review of the pharmacological effects of Vitis vinifera (Grape) and its bioactive constituents: an update. Phytother. Res. 30, 1392–1403. doi: 10.1002/ptr.5644
Nayak, B., Liu, R. H., and Tang, J. (2015). Effect of processing on phenolic antioxidnats of fruits, vegetables, and grains – a review. Crit. Rev. Food Sci. Nutr. 55, 887–919. doi: 10.1080/10408398.2011.654142
Negi, B., Kaur, R., and Dey, G. (2013). Protective effects of a novel sea buckthorn wine on oxidative stress and hipercholesterolemia. Food Funct. 4, 240–248. doi: 10.1039/c2fo30125c
Netzel, M., Strass, G., Herbst, M., Dietrich, H., Bitsch, R., Bitsch, I., et al. (2005). The extraction and biological antioxidant activity of elderberry antioxidants in healthy humans. Food Res. Int. 38, 905–910. doi: 10.1016/j.foodres.2005.03.010
Nicoli, M. C., Anese, M., and Parpinel, M. (1999). Influence of processing on the antioxidant properties of fruit and vegetables. Trends Food Sci. Technol. 10, 94–100. doi: 10.1016/S0924-2244(99)00023-0
Nile, S. H., and Park, S. W. (2014). Edible berries: bioactive components and their effect of human health. Nutrition 30, 134–144. doi: 10.1016/j.nut.2013.04.007
Ogah, O., Watkins, C. S., Ubi, B. E., and Oraguzie, N. C. (2014). Phenolic compounds in Rosaceae fruit and nut crops. J. Agric. Food Chem. 62, 9369–9386. doi: 10.1021/jf501574q
Olas, B. (2016). Sea buckthorn as a source of important bioactive compounds in cardiovascular diseases. Food Chem. Toxicol. 97, 199–204. doi: 10.1016/j.fct.2016.09.008
Olas, B. (2017). The multifunctionality of berries toward blood platelets and the role of berry phenolics in cardiovascular disorders. Platelets 28, 540–549. doi: 10.1080/09537104.2016.1235689
Olas, B., Kedzierska, M., Wachowicz, B., Stochmal, A., Oleszek, W., Jeziorski, A., et al. (2010). Effect of aronia on thiol levels in plasma of breast cancer patients. Cent. Eur. J. Biol. 5, 38–46.
Olas, B., Kontek, B., Malinowska, P., Żuchowski, J., and Stochmal, A. (2016). Hippophae rhamnoides L. fruits reduce the oxidative stress in human blood platelets and plasma. Oxid. Med. Cell. Longev. 2016:4692486. doi: 10.1155/2016/4692486
Olas, B., Wachowicz, B., Stochmal, A., and Oleszek, W. (2012). The polyphenol-rich extract from grape seeds inhibits platelet signaling pathways triggered by both proteolytic and non-proteolytic agonists. Platelets 23, 282–289. doi: 10.3109/09537104.2011.618562
Olas, B., Wachowicz, B., Tomczak, A., Erler, J., Stochmal, A., and Oleszek, W. (2008). Comparative anti-platelet and antioxidant properties of polyphenol-rich extracts from: berries of Aronia melanocarpa, seeds of grape, bark of Yucca schidigera in vitro. Platelets 19, 70–77. doi: 10.1080/09537100701708506
Olchowik, E., Lotkowski, K., Mavlyanov, S., Abdullajanova, N., Ionov, M., Bryszewska, M., et al. (2012). Stabilization of erythrocytes against oxidative and hypotonic stress by tannins isolated from sumac leaves (Rhus typhina L.) and grape seeds (Vitis vinifera L.). Cell. Mol. Biol. Let. 17, 333–348. doi: 10.2478/s11658-012-0014-7
Olivas-Aguirre, F. J., Rodrigo-Garcia, J., del Martines-Ruiz, N. R., Cardenas-Robles, A. I., Mendoza-Diaz, S. O., Alvarez-Parrilla, E., et al. (2016). Cyanidin-3-O-glucoside: physical-chemistry, foodomics and health effects. Molecules 21, 1–30. doi: 10.3390/molecules21091264
Oszmianski, J., and Lachowicz, S. (2016). Effect of the production of dried fruits and juice from chokeberry (Aronia melanocarpa L.) on the content and antioxidative activity of bioactive compounds. Molecules 21, 1–14. doi: 10.3390/molecules21081098
Oszmianski, J., and Wojdylo, A. (2009). Comparative study of phenolic content and antioxidant activity of strawberry puree, clear, and cloudy juices. Eur. Food Res. Technol. 228, 623–631. doi: 10.1007/s00217-008-0971-2
Patras, A., Brunton, N. P., O’Donnell, C., and Tiwari, B. K. (2010). Effect of thermal processing on anthocyanin stability in foods; mechanisms and kinetics of degradation. Trends Food Sci. Technol. 21, 3–11. doi: 10.1016/j.tifs.2009.07.004
Regonold, J. P., Andrews, P. K., Reeve, J. R., Carpenter-Boggs, L., and Schadt, C. W. (2010). Fruit and soil quality of organic and conventional strawberry agroecosystems. PLoS One 5:e12346. doi: 10.1371/journal.pone.0012346
Reul, G., Pomerleau, S., Couture, P., Lamarche, B., and Couillard, C. (2005). Changes in plasma antioxidant capacity and oxidized low-density lipoprotein levels in men after short-term cranberry juice consumption. Metabolism 54, 856–861. doi: 10.1016/j.metabol.2005.01.031
Riso, P., Klimis-Zacas, D., Del Bo, C., Martini, D., Campolo, J., Vendrame, S., et al. (2013). Effect of a wild blueberry (Vaccinium angustifolium) drink intervention on markers of oxidative stress, inflammation and endothelial function in humans with cardiovascular risk factors. Eur. J. Nutr. 52, 949–961. doi: 10.1007/s00394-012-0402-9
Romani, A., Vignolini, P., Ieri, F., and Heimler, D. (2016). Polyphenols and volatile compounds in commercial chokebery (Aronia melanocarpa) products. Nat. Prod. Commun. 11, 99–102.
Rudy, S., Dziki, D., Krzykowski, A., Gawlik-Dziki, U., Polak, R., Rozilo, R., et al. (2015). Influence of pre-treatments and freeze-drying temperature on the process kinetics and selected physico-chemical properties of cranberries (Vaccinium macrocarpon Ait.). LWT Food Sci. Technol. 63, 497–503. doi: 10.1016/j.lwt.2015.03.067
Ryszawa, N., Kawczynska-Drodz, A., Pryjma, J., Czesnikiewicz-Guzik, M., Adamek-Guzik, T., Naruszewicz, M., et al. (2006). Effects of novel plant antioxidants on platelet superoxide production and aggregation in atherosclerosis. J. Physiol. Pharmacol. 57, 611–626.
Saada, H. N., Said, U. Z., Meky, N. H., and Abd El Azime, A. S. (2009). Grape seed extract Vitis vinifera protects against radiation-induced oxidative damage and metabolic disorders in rat. Phytother. Res. 23, 434–438. doi: 10.1002/ptr.2684
Samoticha, J., Wojdylo, A., and Golis, T. (2017). Phenolic composition, physicochemical properties and antioxidant activity of interspecific hybrids of grapes growing in Poland. Food Chem. 215, 263–273. doi: 10.1016/j.foodchem.2016.07.147
Santhakumar, A. B., Stanley, R., and Singh, I. (2015). The ex vivo antiplatelet activation potential of fruit phenolic metabolite hippuric acid. Food Funct. 6, 2679–2683. doi: 10.1039/c5fo00715a
Saponidou, V. S., Margaritis, I., Siahos, N., Arsenopoulos, K., Dragatidou, E., Taitzoglou, I. A., et al. (2014). Antioxidant effect of a polyphenol-rich grape pomace extract on motility, viability and lipid peroxidation of thawed bovine spermatozoa. J. Biol. Res. 21, 1–6. doi: 10.1186/2241-5793-21-19
Scalbert, A., and Williamson, G. (2000). Dietary intake and bioavailability of polyphenols. J. Nutr. 130, 2073–2085. doi: 10.1093/jn/130.8.2073S
Simao, T. N., Lozovoy, M. A., SIamo, A. N., Oliveira, S. R., Venturini, D., Morimoto, H. K., et al. (2013). Reduced-energy cranberry juice increases folic acid and adiponectin and reduces homocysteine and oxidative stress in patients with the metabolic syndrome. Br. J. Nutr. 110, 1885–1894. doi: 10.1017/S0007114513001207
Singh, J., and Basu, P. S. (2102). Non-nutritive bioactive compounds in pulses and their impact on human health: an overview. Food Nutr. Sci. 3, 1664–1672. doi: 10.4236/fns.2012.312218
Skrovankova, S., Sumczynski, D., Mlcek, J., Jurikova, T., and Sochor, J. (2015). Bioactive compounds and antioxidant activity in different types of berries. Int. J. Mol. Sci. 16, 24673–24706. doi: 10.3390/ijms161024673
Szajdek, A., and Borowska, E. J. (2008). Bioactive compounds and health-promoting properties of berry fruits: a review. Plant Foods Hum. Nutr. 63, 147–156. doi: 10.1007/s11130-008-0097-5
Talavera, S., Felgines, C., Texier, O., Besson, C., Mazur, A., Lamaison, J. L., et al. (2006). Bioavailability of a bilberry anthocyanin extract and its impact on plasma antioxidant capacity in rats. J. Sci. Food Agric. 86, 90–97. doi: 10.1002/jsfa.2327
Toromanovic, J., Kovac-Besovic, E., Sapcainin, A., Tahirovic, I., Rimpapa, Z., Kroyer, G., et al. (2008). Urinary hippuric acid after ingestion of edible fruits Bosn. J. Basic. Med. Sci. 8, 38–43.
Tulipani, S., Armeni, T., GIampieri, F., Alvarez-Saurez, J. M., Gonzalez-Paramas, A. M., Santos-Buelga, C., et al. (2014). Strawberry intake increases blood fluid, erythrocyte and mononuclear cell defenses against oxidative challenge. Food Chem. 156, 87–93. doi: 10.1016/j.foodchem.2014.01.098
Umeno, A., Horie, M., Murotami, K., Nakajima, Y., and Yoshida, Y. (2016). Antioxidative and antidiabetic effects of natural polyphenols and isoflavones. Molecules 21, 1–15. doi: 10.3390/molecules21060708
Valcheva-Kuzmanova, S., Borisova, P., Galunska, B., Krasnaliev, I., and Belcheva, A. (2004). Hepatoprotective effect of the natural fruit from Aronia melanocarpa on carbon tetrachloride-induced acute liver damage in rats. Exp. Toxicol. Pathol. 56, 195–201. doi: 10.1016/j.etp.2004.04.012
Valcheva-Kuzmanova, S. V., Popova, P. B., Galunska, B. T., and Belcheva, A. (2006). Protective effect of Aronia melanocarpa fruit juice pretreatment in a model of carbon tetrachloride-induced hepatotoxicity in rats. Folia Med. (Plovdiv) 48, 57–62.
Valls-Belles, V., Torres, M. C., Muniz, P., Beltran, S., Martinez-Alvarez, J. R., and Codoner-Franch, P. (2006). Defatted milled grape seed protects Adriamycin-treated hepatocytes against oxidative damage. Eur. J. Nutr. 45, 251–258. doi: 10.1007/s00394-006-0591-1
Veskouis, A. S., Kyparos, A., Nikolaidis, M. G., Stagos, D., Aligiannis, N., Halabalaki, M., et al. (2012). The antioxidant effects of a polyphenol-rich grape pomace extract in vitro do not correspond in vivo using exercise as an oxidant stimulus. Oxid. Med. Cell. Longev. 2012:185867. doi: 10.1155/2012/185867
Vinson, J. A., Bose, P., Proch, J., Al Kharrat, H., and Samman, N. (2008). Cranberries and cranberry products: powerful in vitro, ex vivo, and in vivo sources of antioxidants. J. Agric. Food Chem. 56, 5884–5891. doi: 10.1021/jf073309b
Wang, L., Li, Y. M., Lei, L., Wang, X., Ma, K. Y., and Chen, Z. Y. (2015). Cranberry anthocyanin extract prolongs lifespan of fruit flies. Exp. Gerantol. 69, 189–195. doi: 10.1016/j.exger.2015.06.021
Weisel, T., Baum, M., Eisenbrand, G., Dietrich, H., Will, F., Stockis, J. P., et al. (2006). An anthocyanin/polyphenolic-rich fruit juice reduces oxidative DNA damage and increases glutathione level in healthy probands. Biotechnol. J. 1, 388–397. doi: 10.1002/biot.200600004
Wightman, J. L. D., and Henberger, R. A. (2015). Effect of grape and other berries on cardiovascular health. J. Sci. Food Agric. 95, 1584–1597. doi: 10.1002/jsfa.6890
Wilczak, J., Kamola, D., and Jank, M. (2013). Bioavailability of polyphenolic compounds and their influence on antioxidant potential of rat plasma. Probl. Hig. Epidemiol. 94, 532–535.
Wilson, T., and Bauer, B. A. (2009). Advising consumers about dietary supplements: lessons from cranberry products. J. Diet. Suppl. 6, 377–384. doi: 10.3109/19390210903280298
Xie, L., Lee, S. G., Vance, T. M., Wang, Y., Kim, B., Lee, J. Y., et al. (2016). Bioavailability of anthocyanins and colonic polyphenol metabolites following consumption of aronia berry extract. Food Chem. 211, 860–868. doi: 10.1016/j.foodchem.2016.05.122
Yang, M., Koo, S. I., Song, W. O., and Chun, O. K. (2011). Food matrix affecting anthocynanin bioavailability: review. Curr. Med. Chem. 18, 291–300. doi: 10.2174/092986711794088380
You, Q., Wang, B., Chen, F., Huang, Z., Wang, X., and Luo, P. G. (2011). Comparison of anthocyanins and phenolics in organically and conventionally grown blueberries in selected cultivars. Food Chem. 125, 201–208. doi: 10.1016/j.foodchem.2010.08.063
Keywords: berries, phenolic compounds, antioxidants, health, oxidative stress
Citation: Olas B (2018) Berry Phenolic Antioxidants – Implications for Human Health? Front. Pharmacol. 9:78. doi: 10.3389/fphar.2018.00078
Received: 14 October 2017; Accepted: 23 January 2018;
Published: 26 March 2018.
Edited by:
Claudio Bucolo, Università degli Studi di Catania, ItalyReviewed by:
Corrado Tringali, Università degli Studi di Catania, ItalyDavid Auñón-Calles, SEPROX BIOTECH, Spain
Copyright © 2018 Olas. This is an open-access article distributed under the terms of the Creative Commons Attribution License (CC BY). The use, distribution or reproduction in other forums is permitted, provided the original author(s) and the copyright owner are credited and that the original publication in this journal is cited, in accordance with accepted academic practice. No use, distribution or reproduction is permitted which does not comply with these terms.
*Correspondence: Beata Olas, YmVhdGEub2xhc0BiaW9sLnVuaS5sb2R6LnBs