- 1State Key Laboratory of Toxicology and Medical Countermeasures, Department of Neuroimmunopharmacology, Beijing Institute of Pharmacology and Toxicology, Beijing, China
- 2Department of Pharmacy, 302 Hospital of People's Liberation Army, Beijing, China
It is highly valuable to study the pharmacokinetics of herbal components under the pathological condition of liver dysfunction for safe and rational use of herbal medicines. In this study, the pharmacokinetic profiles of four effective lignans from Schisandra chinensis (SC), schisandrin, schisantherin A, deoxyshisandrin and γ-schisandrin, were investigated in carbon tetrachloride (CCl4)-intoxicated rats. The metabolism of the four lignans was also studied using microsomes from patients with advanced hepatocellular carcinoma. In situ intestinal and hepatic perfusions were conducted to clarify the contributions from impairments of gut and liver on the pharmacokinetics of the four schisandra lignans in CCl4-intoxicated rats. The metabolism in rat and human liver microsomes and transport in Caco-2 monolayer cell model were studied to reveal the key factors for the in vivo disposition of the four lignans. When SC alcoholic extract was orally administrated to CCl4-intoxicated rat for a short term (4 days), the pharmacokinetics of four active SC lignans was significantly changed while its hepatotherapeutic effect was not obviously observed. The plasma concentrations of the four schisandra lignans were dramatically elevated compared with the control. The Cmax, AUC and MRT were all increased or prolonged significantly while parameter CLz/F was obviously reduced in rat pretreated with CCl4. In hepatic perfusion study and liver microsomes incubation, it was found that the hepatic metabolism of the four lignans was markedly decreased mainly due to the activity reduction of multiple CYP450 isoenzymes involved the metabolism, which, eventually, might lead to the alternation of their pharmacokinetic profiles in CCl4-intoxicated rats or patients with advanced hepatocellular carcinoma. The pharmacokinetic studies of SC components in pathological situation of liver dysfunction are expected to provide useful data for rational and safe application of SC preparations in clinic or further pharmacological and toxicological research.
Introduction
Investigations revealed that the use of herbal medicines, usually as the complementary and alternative treatment, increased dramatically and became popular around the world (Ramos-Remus and Raut, 2008; Harris et al., 2012; Putthapiban et al., 2017). Meanwhile, the safety of herbal medicine and herbal-drug interactions have been highlighted and drawn extensive attention from the medical community (Brazier and Levine, 2003; Samuels et al., 2017; Singh and Zhao, 2017).
Liver is the most important organ for in vivo metabolism and biotransformation of xenobiotics irrespective of their origins. The pharmacokinetic profiles of drugs could be altered by the dysfunctions of liver in pathological conditions, which exerts a negative effect on drug safety and therapy (Tamura et al., 2004). Therefore, it is highly valuable to conduct the pharmacokinetic study of herbal components under the pathological condition of liver dysfunction, which could provide reliable and referential data for safe and rational clinical application of herbal drugs (McLean and Morgan, 1991; Yokogawa et al., 2004).
Schisandra chinensis (SC), subjected to Magnoliaceae, comes from the dry mature fruit of S. chinensis (Turcz.) Baill or S. Sphenanchera Rehd. etWils. It has been used widely as a restorative or tonic in asthenic diseases in Asia and as a common constituent in many prescriptions in Traditional Chinese Medicines (TCM). Moreover, Wuweizi tablet (a preparation of an ethanol extract of SC) has been officially applied in clinic for treatment of liver diseases. Many pharmacological studies revealed that SC and schisandra lignans, the major effective components, showed various beneficial biological activities including hepatoprotection against viral and various hepatotoxins, tranquilization, hypnogenesis, anticonvulsive and neuro-protective effects, and so on (Lu and Liu, 1992; Fujihashi et al., 1995; Zhu et al., 2016; Szopa et al., 2017). However, it was recently reported that schisandrin B and schisandrae fructus oil could elevate hepatic and serum triglyceride levels, heighten serum alanine aminotransferase (ALT) activity, and eventually induce hepatotoxicity (hypertriglyceridemia, hepatomegaly and liver damage) in mice (Zhang et al., 2014). SC extracts exhibited inhibitive or inductive effects on rat hepatic cytochrome P450 (CYP450) enzymes and caused herb-drug interaction mediated by CYP450s (Wang et al., 2014). The pharmacokinetics of the SC lignans was correlated well with CYP3A, ALT and AST (Xie et al., 2010). Based on these former results, the pharmacokinetics of SC ingredients under conditions of liver dysfunctions should be further studied concerning that SC ingredients were often applied for treatment of various liver diseases.
In our present study, we selected four effective schisandra lignans with high abundance in SC alcoholic extract, schisandrin, schisantherin A, deoxyshisandrin and γ-schisandrin (Figure 1), to describe their pharmacokinetics in rat pretreated with carbon tetrachloride (CCl4), a classic hepatotoxin, using HPLC-MS method. Moreover, intestinal and hepatic perfusions were conducted to clarify the contributions from impairments of gut and liver on the pharmacokinetics of the four schisandra lignans in CCl4-intoxicated rats. The metabolism in rat and human liver microsomal incubations and transport in Caco-2 monolayer cell model were also studied to reveal the key factors for the in vivo disposition of the four lignans. The results of the study are expected to provide useful data for rational and safe application of SC preparations in clinic.
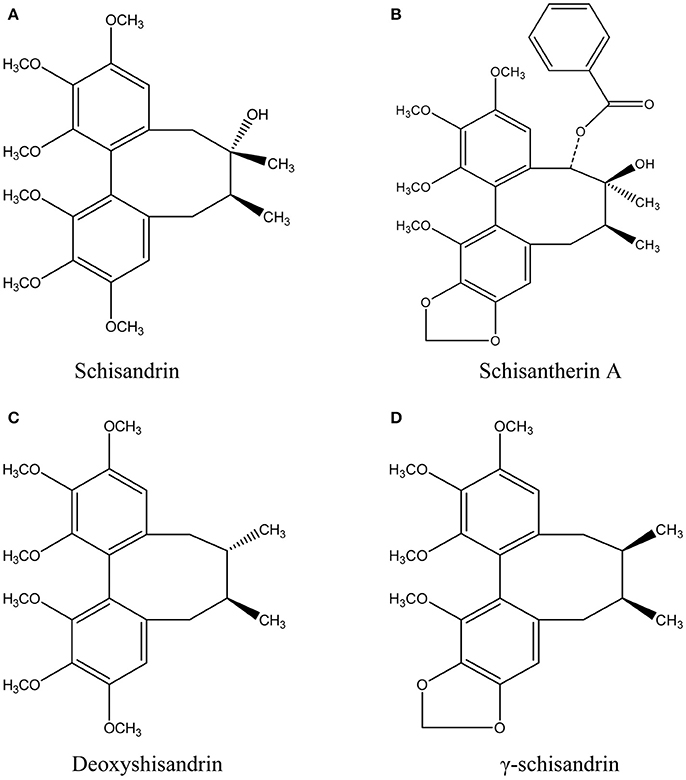
Figure 1. Chemical structures of schisandrin (A), schisantherin A (B), deoxyshisandrin (C), and γ-schisandrin (D) from Schisandra chinensis.
Materials and Methods
Materials
Dried alcoholic extract of SC containing schisandrin (1.83%), schisantherin A (1.52%), deoxyshisandrin (2.41%) and γ-schisandrin (1.22%), was purchased from Nantong Sihai Plant Extracts Co., Ltd (Jiangsu, China). Schisandrin, schisantherin A, deoxyshisandrin, γ-schisandrin (>99% purity) and midazolam were purchased from the National Institute for the Control of Pharmaceutical and Biological Products (Beijing, China). Phenacetin, acetaminophen, dextromethorphan, dextrophan, diclofenac, 4-hydroxydiclofena, mephenytoin, 4-hydroxymephenytoin, chlorzoxazone, 6-hydroxychlorzoxazone, 1-hydroxymidazolam, ketoconazole, ticlopidine, sulfaphenazole, quinidine, furafylline, disulfiram were obtained from Sigma-Aldrich Corporation (MO, US). Bicyclol (>99% purity) was provided by Beijing Union Pharmaceutical Plant. CCl4 and glycerin were purchased from Beijing Chemical Reagents Company. Methanol and acetonitrile were HPLC grade (Fisher, USA), other reagents were analytical reagent grade. The pooled male human microsomes were purchased from BD corporation (MA, US). Ultrapure water was prepared using Milli-Q Reagent System (Millipore, MA, USA); AST, ALT, ALP, LDH, TB, BUN, and CRE kits were purchased from Nanjing Jiancheng Reagents Company.
Animal Treatment
Male Sprague-Dawley rats, weighing 180–220 g, were obtained from Beijing Vital River Experimental Animal Co., Ltd. The animals were quarantined for 1 week prior to study and maintained on a 12-h light/12-h dark cycle at 22 ± 1°C and at 50–55% relative humidity with free access to solid pellet diet and water ad libitum throughout the study. All rats were assigned randomly to three groups, including acute liver injury (ALI) rat, SC-treated ALI rat and the control, 5 in each group. The ALI and SC-treated ALI rats were intraperitoneal injected two doses of the mixture of CCl4 and olive oil (1:1, V/V) at 2 mL/kg in a 24 h time interval (Huang et al., 2001), while the control group was given the same volume of olive oil. Then, the SC-treated ALI rat was orally dosed with SC alcoholic extract suspended in 0.5% sodium carboxymethyl cellulose (CMC) at 1.5 g/kg/day for 4 days after CCl4 injection. The dosages were set according to the amounts of the four active lignans used previously for pharmacological studies and their contents in the extract (Chiu et al., 2002, 2007; Cheng et al., 2013). All animal protocols were approved by the Animal Care and Welfare Committee of the Academy of Military Medical Sciences. All animal procedures complied with the Guide for the Care and Use of Laboratory Animals issued by Beijing Association on Laboratory Animal Care (BALAC).
Assays for Biochemical Indexes
Serum aspartate transaminase (AST), alanine transaminase (ALT), alkaline phosphatase (ALP), lactate dehydrogenase (LDH), total bilirubin, blood urea nitrogen (BUN), and creatinine (CRE) levels were determined with commercial kits (Nanjing Jiancheng Bioengineering Institute, China).
Pharmacokinetic Studies of Four Effective Lignans in ALI Rat
The pharmacokinetic profiles of four lignans were studied in ALI rat after multiple oral administrations of SC alcoholic extract at dose of 1.5 g/kg/day. After the ALI rat was established, SC alcoholic extract suspended in 0.5% sodium carboxymethyl cellulose was given to ALI rats and the control via oral gavage once a day for 4 consecutive days. Before the last dosing, rats were fasted for 12 h while water was taken freely. Blood samples were collected at 0, 0.25, 0.5, 1, 2, 3, 4, 6, 8, 12, 24, 36, and 48 h after the last dosing. A cannula was surgically placed into the jugular vein for collection of blood samples (about 0.1 mL) and blood loss compensation using sterile isotonic saline in the pharmacokinetic study. Plasma was separated by centrifugation and stored at −20°C until analysis. Plasma concentrations of the four lignans were quantified using HPLC-MS analysis developed according to the reported method (Wang et al., 2008).
In Situ Intestinal Perfusion of Four Effective Lignans
The surgical procedures of intestinal perfusion were conducted according to those published reports (Savina et al., 1981). Hank's balanced salt solution (HBSS, pH 7.4) with four schisandra lignans (50 μM) at 37°C was infused into the jejunum segment at rate of 0.3 mL/min. Phenol Red (50 μM) was added to serve as a nonabsorbable marker for measuring water flux. Blood samples from mesenteric vein and perfusate were collected at 5-min interval. The plasma separated by centrifugation and perfusate was stored at −20°C until HPLC-MS analysis.
In Situ Hepatic Perfusion Studies of Four Effective Lignans
In situ liver perfusion was performed similarly as the method described previously (Uraki et al., 2016). Rats were cannulated in the bile duct, hepatic portal vein, and inferior vena cava. The perfusate consisted of Krebs–Hensleit buffer (pH 7.4) containing sodium taurocholate (4.75 mg/L), 1% bovine serum albumin, and glucose (1.2 g/L) was oxygenated using carbogen, 95% O2/5% CO2. The perfusate at 37°C was infused into the portal vein at a flow rate of 30 mL/min in a recirculatory manner. After 10-min equilibration, four schisandra lignans were added to the reservoir (final concentration of 10 μM). Perfusate (0.5 mL) and bile samples were collected at 0, 10, 20, 30, 40, 50, and 60 min after the addition of lignans. The same volume of blank perfusion buffer was immediately added to the reservoir after collection of the perfusate samples. After hepatic perfusion, rat was sacrificed and liver was removed, blotted dry, and weighed.
Measurement of Cytochrome P450 Activities With Probe Substrates
Liver microsomes of ALI rats and patient with advanced hepatocellular carcinoma (AHC) were prepared by differential centrifugation as described previously (Eriksson, 1978). The study protocol preparing liver microsomes from patients was approved by the Ethics Committee of 302 Hospital of People's Liberation Army. The pellet was resuspended in homogenization medium and stored at −80°C until use. The CYP450 isozymes activities were determined by probe substrate method reported previously (He et al., 2007; Li et al., 2007). The activities of CYP isoforms were indicated by the formation rates of the specific metabolites of six probe substrates (phenacetin for rat and human CYP1A2, diclofenac for rat CYP2C6 and human CYP2C9, S-mephenytoin for rat CYP2C11 and human CYP2C19, dextromethorphan for rat CYP2D2 and human CYP2D6, chlorzoxazone for rat and human CYP2E1, and midazolam for rat CYP3A1/2 and human CYP3A4/5) incubated in rat and human liver microsomes. All metabolites were determined using validated LC-MS/MS methods.
Metabolism of Four Schisandra Lignans in Rat or Human Liver Microsomes Incubation
The metabolism of four schisandra lignans was investigated in liver microsomes prepared from CCl4-intoxicated rat or AHC patient. The incubation system (200 μL, ≤ 1% of organic solvent) included rat (final protein concentration: 1 mg/mL) or human (final protein concentration: 0.5 mg/mL) liver microsomes, NADPH (1.2 mM), Tris-Hcl buffer (50 mM, pH = 7.4) and four lignans (final concentration was 5 μM). After 5 min preincubation in water bath at 37°C, NADPH was added to start the reaction, after a series of given time incubation (0, 5, 10, 20, 30, 45, 60 min), 400 μL ice acetonitrile was added to stop the reaction followed by centrifugation (14,000 rpm × 5 min). The four lignans in the supernate were analyzed by the similar HPLC-MS method in the pharmacokinetic study.
Identification of CYP450 Isozymes Involved in the Metabolism of Four Schisandra Lignans in Rat or Human Liver Microsomes (Rendic and Di Carlo, 1997)
The CYP450s involved in the metabolism of schisandrin, schisantherin A, deoxyshisandrin and γ-schisandrin in rat or human liver microsomes were confirmed by comparison of the metabolism ratio before and after the addition of selective inhibitors to CYP450 isozymes. The incubation system includes rat liver microsomes (1 mg/mL) or human liver microsomes (0.5 mg/mL), NADPH (1.2 mM), Tris-Hcl buffer (50 mM, pH = 7.4), four lignans (final concentration was 5 μM), and CYP450 isozymes inhibitors (CYP3A/ ketoconazole 100 μM; CYP2C11/ticlopidine 50 μM; CYP2C6/ sulfaphenazole 50 μM; CYP2D2/ quinidine 50 μM; CYP1A2/ furafylline 50 μM; CYP2E1/disulfiram 250 μM), while the organic solvent volume was not more than 1% of total volume (500 μL). After 5 min preincubation in water bath at 37°C, NADPH was added to start the reaction (furafylline and disulfiram whose metabolites actually inhibited the activities of CYP450s were preincubated 15 min in the present of NADPH before substrate addition, other steps were similar), after a given time incubation (15 min for schisandrin, 40 min for schisantherin A, deoxyshisandrin and γ-schisandrin, the time was set by a pre-experiment), 500 μL ice acetonitrile was added to stop the reaction followed by centrifugation. The supernate was injected to HPLC-MS for analysis of the four lignans.
Transport Studies in Caco-2 Cell Monolayer Model
Caco-2 cell (passage 38) was used in the transport experiments. Cells were seeded on polycarbonate filters (12 mm diameter, pore size 0.4 mm) at 1 × 105/cm2. The cell monolayer was formed 21 days post seeding when the transepithelial electrical resistance (TEER) was above 1,000 Ω• cm2. Three concentration levels (5, 10, and 50 μM) were set for the four schisandra lignans in the study. After preincubation for 1 h at 37°C, the solution of the four lignans was respectively added to the apical (0.4 mL) or basolateral (0.6 mL) chamber for absorptive (AP → BL) and secretive (BL → AP) permeability test. At the specified timepoints (0.5, 1, 1.5 and 2 h), 50 μL samples (n = 3) were withdrawn from receiver chamber, and meanwhile, 50 μL fresh buffer was added. The four lignans were determined by HPLC-MS method.
Data Analysis
The permeability of four lignans across the rat intestine was calculated based on the disappearance of parent drug from the lumen (Plumen) as well as the appearance of the drug in blood (Pblood) using the following equations (Singhal et al., 1998):
where r is the radius of the perfused intestinal lumen; l is the length of the segment; Q is the flow rate of perfusate; Cin is the concentration of drug at the start of perfusion; and Cout is the steady-state concentration of drug exiting the lumen. ΔMB/Δt is the rate of drug appearance in mesenteric blood; and {C} is the logarithmic mean concentration of drug in the lumen.
The apparent permeability coefficient (Papp) in the Caco-2 cell was calculated by the formula Papp = (ΔQ/Δt)/AC0, where ΔQ/Δt is the transport rate (nmol/s), which remained approximately constant in time, A is the well membrane area (0.6 cm2), and C0 is the initial concentration of the four lignans (μM). The efflux rate (ER) was calculated by the formula ER = Papp (BL → AP)/Papp(AP → BL).
Pharmacokinetics Analysis
Data fitting and pharmacokinetic parameter calculations were carried out using the Winnolin software. Non-compartmental analysis was used to calculate the pharmacokinetic parameters. Parameters, such as AUC and MRT, were calculated using statistical moment method.
Statistical Analysis
Statistically significant difference between two groups was evaluated by Student's t-test. Non-parametric tests was used to analyze Tmax or the data failed in homogeneity of variance test. The prior level of significance was set at P < 0.05. All results are expressed as mean ± standard deviation (SD).
Results
The Evaluation of Liver and Renal Functions in Rat Intoxicated With CCl4
The functions of liver and kidney in rat intoxicated with CCl4 were evaluated by determining the related biochemical indexes (Table 1). The results showed that the values of liver index, serum ALT and AST, and BUN was increased by 40, 720, 30, and 30% in ALI rat intoxicated with CCl4, while the content of liver microsomal CYP450 enzyme was decreased by 56% compared with control rats. In addition, it was found that the 4-day administration of SC alcoholic extract (1.5 g/kg/day) did not show positive effect on the liver dysfunction in ALI rats indicated by elevated serum ALT and AST or decreased CYP450 content.
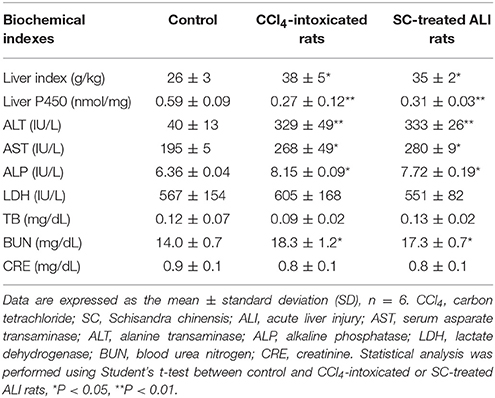
Table 1. Indexes of liver and renal functions in acute liver injury (ALI) rats intoxicated by carbon tetrachloride.
These data suggested that the CCl4 injection caused significant liver injury and little alteration in renal function, which is mostly consistent with the previous study. Short-term administration of SC alcoholic extract may not exhibit hepatotherapeutic effect.
Pharmacokinetics of the Four Lignans in CCl4-Intoxicated Rats After Orally Dosing of SC Alcoholic Extract
The pharmacokinetic profiles of the four lignans were investigated in CCl4 intoxicated rat after orally multiple dosing of SC alcoholic extract at 1.5 g/kg. The results showed that most of the pharmacokinetic parameters of the four lignans including Cmax, AUC and MRT were significantly increased in CCl4-treated group, compared with control rats that received vehicle alone (Figure 2, Table 2). In addition, the systemic clearance (CLz/F) in CCl4 intoxicated rats was reduced by about 83% for schisandrin, 89% for schisantherin A, 56% for deoxyshisandrin and 54% for γ-schisandrin, respectively. These results suggested that the in vivo disposition of the lignans was dramatically altered in liver-injured rats pretreated with CCl4.
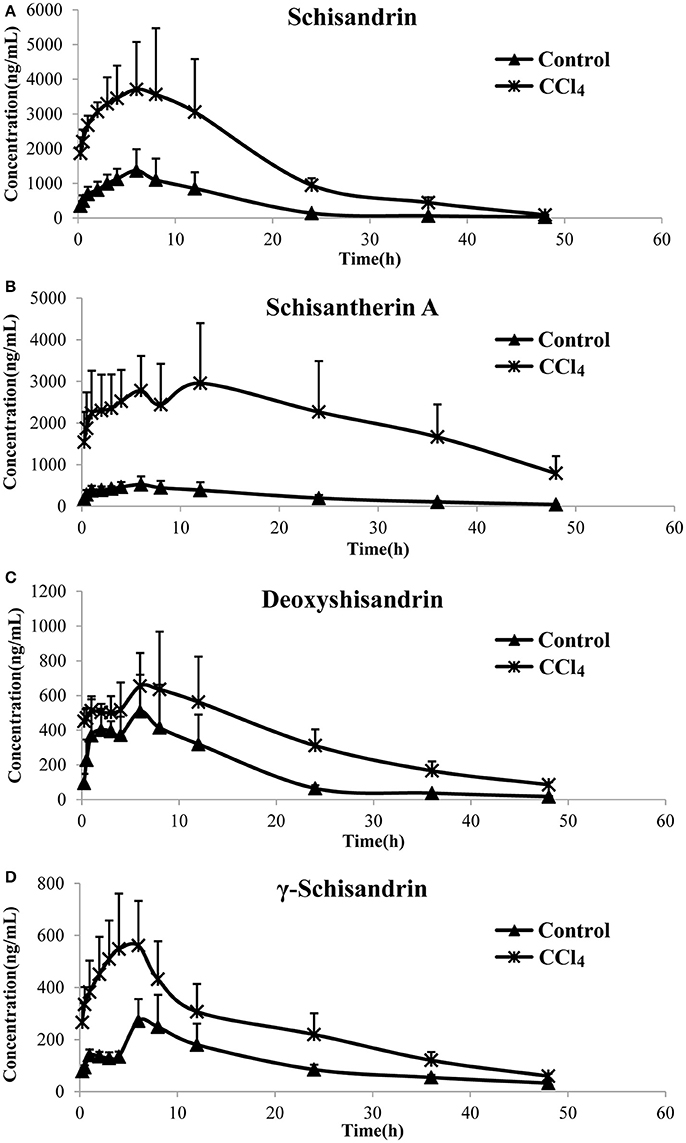
Figure 2. Plasma concentration–time curves of the four lignans in carbon tetrachloride (CCl4)-intoxicated rats after oral administration of alcoholic extract of Schisandra chinensis (1.5 g/kg, n = 5). Control, group of normal rats; CCl4, group of CCl4-intoxicated rats; (A) schisandrin; (B) schisantherin A; (C) deoxyshisandrin; (D) γ-schisandrin.
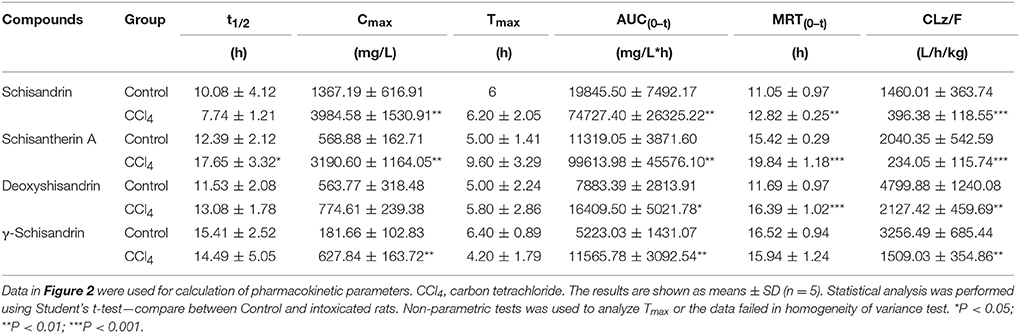
Table 2. Pharmacokinetic parameters of four lignans in carbon tetrachloride -intoxicated rats after oral administration of alcoholic extract of Schisandra chinensis at 1.5 g/kg (n = 5).
In Situ Intestinal and Hepatic Perfusion of Four Lignans
In situ intestinal and hepatic perfusion was conducted in our study to evaluate the effect of intestinal absorption and hepatic metabolism on the pharmacokinetic profiles of four schisandra lignans in CCl4-intoxicated rats.
As shown in Table 3, the remaining amounts of schisandrin and deoxyshisandrin in perfusate were both slightly increased about 10% in intestinal autoperfusion (60 min) of CCl4-treated rats, while those of schisantherin A and γ-schisandrin were not changed compared with the control. Besides, similar results were also found in the cumulative plasma amounts of schisandrin and deoxyshisandrin which raised about 91% and 63% in CCl4-intoxicated rats. The other parameters including cumulative intestinal amount, Pblood and Plumen were not obviously altered in CCl4-treated rats.
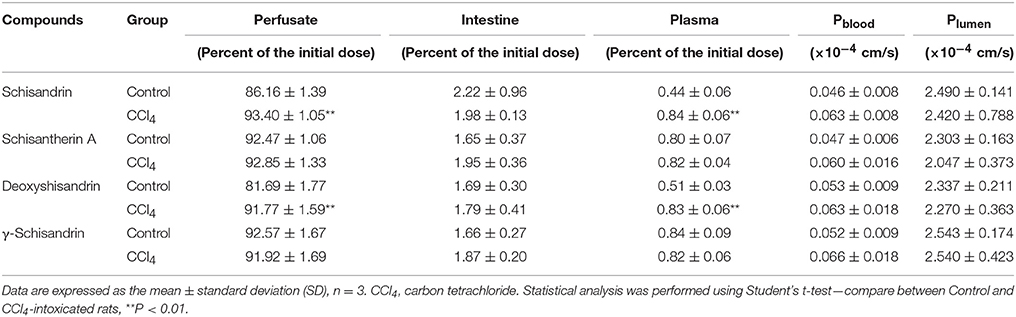
Table 3. Determination of the four lignans in perfusate, intestinal tissue, and plasma after intestinal perfusion in situ.
In hepatic perfusion study, the results showed that the AUC(0−1h) of four schisandra lignans in perfusate and their cumulative amounts in liver were significantly increased. The total amount of deoxyshisandrin in bile was reduced in CCl4-treated rats (Table 4). The above data demonstrated that the hepatic metabolism of the four lignans was markedly reduced in rats pretreated with CCl4.
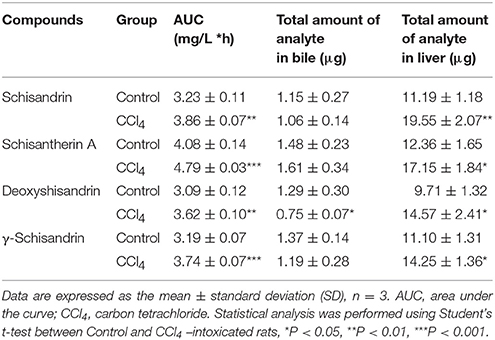
Table 4. Determination of four lignans in perfusate, liver tissue, and bile after hepatic perfusion in situ.
Determination of Liver Microsomal CYP450 Isozymes Activities
The activities of six liver CYP450 isozymes in CCl4-intoxicated rat or patient with advanced hepatocellular carcinoma (AHC) were subsequently determined by method of probe substrates incubation in liver microsomes. The results, shown in Figure 3, demonstrated that the metabolic production rates of the six probe substrates in liver microsomes of CCl4-intoxicated rats were significantly decreased by 43% for acetaminophen (CYP1A2), 77% for 4-hydroxydiclofenac (CYP2C6), 63% for 4-hydroxymephenytoin (CYP2C11), 79% for dextrophan (CYP2D2), 43% for 6-hydroxychlorzoxazone (CYP2E1), and 46% for 1-hydroxymidazolam (CYP3A), respectively. Likewise, the activities of six liver CYP450s from AHC patient were reduced by about 50% for CYP1A2, 87% for CYP2C9, 80% for CYP2C19, 66% for CYP2D6, 68% for CYP2E1, and 76% for CYP3A4/5.
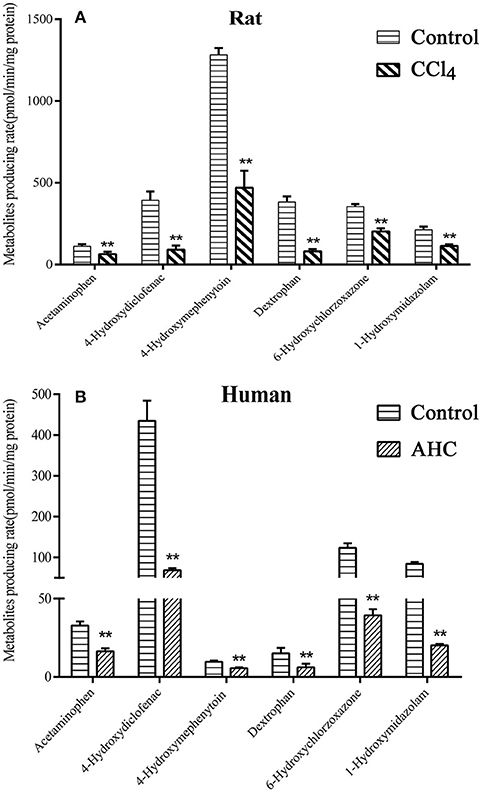
Figure 3. In vitro metabolites producing rate of CYP450s probe substrates in liver microsomal incubation of carbon tetrachloride (CCl4)-intoxicated rats (A) and patients (B) with advanced hepatocellular carcinoma (AHC). (A) Control, liver microsomes from normal rats; CCl4, liver microsomes from CCl4-intoxicated rats; (B) Control, pooled male human liver microsomes purchased from BD corporation; AHC, liver microsomes prepared from patients with advanced hepatocellular carcinoma. Acetaminophen-rat and human CYP1A2; 4-Hydroxydiclofenac-rat CYP2C6 and human CYP2C9; 4-Hydroxymephenytoin-rat CYP2C11 and human CYP2C19; Dextrophan-rat CYP2D2 and human CYP2D6; 6-Hydroxychlorzoxazone- rat and human CYP2E1; 1-Hydroxymidazolam- rat CYP3A1/2 and human CYP3A4/5. Statistical analysis was performed using Student's t-test, **P < 0.01, vs. CCl4-intoxicated rat or AHC patient, n = 5.
Metabolism of Four Schisandra Lignans in Rat or Human Liver Microsomes Incubation
It was investigated the metabolism of four schisandra lignans in vitro using rat or human liver microsomes from CCl4 intoxicated rat or patient with advanced hepatocellular carcinoma (AHC). The metabolism of the four lignans was indicated by the percentage of the substrate reduced after incubation compared with the initial concentration. As shown in Figures 4, 5, the substrate left percentage of four lignans was 30.2–59.9% in CCl4-treated rat liver microsomes incubation, while that was 1.92–13.74% in control samples after incubation for 60 min. Likely, the substrate left percentage was 24.6–70.4% for four schisandra lignans in AHC patient liver microsomes incubation compared with 9.7–18.3% of four lignans left in control group. All these results indicated that the in vitro metabolism of four schisandra lignans was reduced in liver microsomes from CCl4-treated rat and AHC patient.
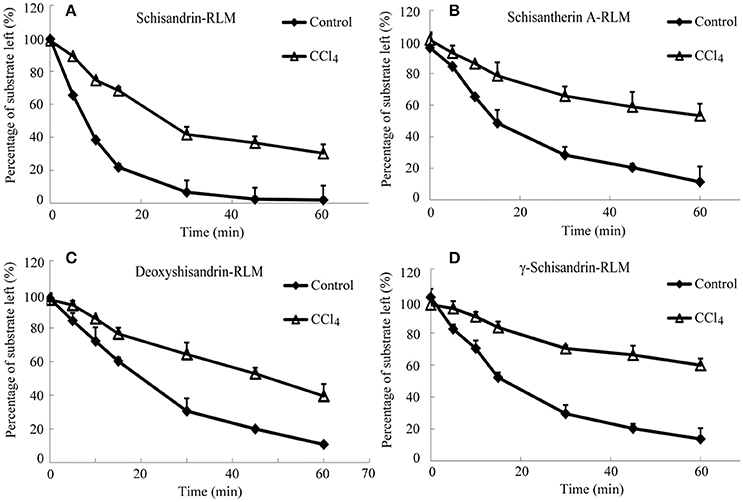
Figure 4. Substrate left-incubation time curve of schisandrin (A), schisantherin A (B), deoxyshisandrin (C), and γ-schisandrin (D) in carbon tetrachloride (CCl4)-intoxicated rat liver microsomes (n = 3). Control, liver microsomes from normal rats; CCl4, liver microsomes from CCl4-intoxicated rats.
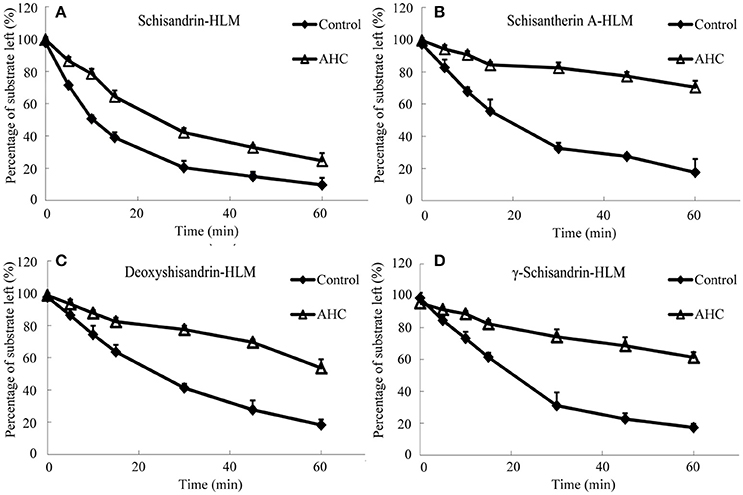
Figure 5. Substrate left-incubation time curve of schisandrin (A), schisantherin A (B), deoxyshisandrin (C), and γ-schisandrin (D) in liver microsomes from patient with advanced hepatocellular carcinoma (AHC) (n = 3). Control, pooled male human liver microsomes purchased from BD corporation; AHC, liver microsomes prepared from patients with advanced hepatocellular carcinoma.
Identification of CYP450 Isozymes Involved in the Metabolism of Four Schisandra Lignans in Rat and Human Liver Microsomes
The CYP450 isozymes involved in the metabolism of the four effective schisandra lignans were identified in rat and human liver microsomes (Tables 5, 6). In the rat liver microsomes incubation, it was found that multiple CYP450 isozymes participated in the metabolism of the four lignans (≥20% inhibition), i.e., CYP2C6/11, CYP2E1, and CYP3A for schisandrin, CYP1A2, CYP2C6/11, and CYP2E1 for schisantherin A, CYP2C11, CYP2D2, CYP2E1, and CYP3A for deoxyshisandrin, and CYP1A2, CYP2D2 and CYP2E1 for γ-schisandrin. Likewise, multiple CYP450s were found involved in the metabolism of the four compounds in human liver microsome incubation. These results proved CYP450s played a key role in the metabolism of four schisandra lignans.
Transport Studies of Four Lignans Across Caco-2 Cell Monolayer
The transport across Caco-2 cell monolayer was studied to observe the role of multi-drug resistance (MDR) proteins on the in vivo disposition process of the four lignans. The results (Table 7) showed that there was no obvious dose-response relationship between the permeability coefficients Papp(AP → BL) or Papp (BL → AP) and the escalating concentrations of the four lignans (5, 10, and 50 μm). According the values of Papp and ER, the four lignans could be classified as moderately absorbed compounds, but no substrates of P-glycoprotein (P-gp), although there was some efflux trend in transport of deoxyschizandrin. The results of digoxin (P-gp substrate), atenolol (poor permeability) and propranolol (high permeability) in Caco-2 cell were consistent with the previously reported data, indicating good reliability and integrity of the Caco-2 cell monolayer model in our study.
Discussion
Herbal medicines have been used for treatments of many kinds of diseases for a long time around the world. A general misconception prevails among the general public that herbal medicines are safe and free from adverse effects because of the natural origin. Moreover, most users of herbal products often self-administered along with therapeutic drugs without informing their allopathic doctors (Putthapiban et al., 2017). Actually, “all natural” herbal products are mixtures of potentially biologically active ingredients without clear quantities. These ingredients may possess inherent pharmacological activities, cause unexpected herb-drug interactions and even have direct toxicities (Baig et al., 2014; Banumathi et al., 2017). Meanwhile, it is known that expressions of proteins, activities of enzymes and functions of organs would be altered in pathological conditions. These alternations might exert an important influence on the in vivo pharmacokinetics of drugs, and furthermore, affect the therapy, or safety (Yokogawa et al., 2004; Tan et al., 2009). Therefore, it is important and meaningful to reveal the pharmacokinetics of herbal ingredients in pathological conditions for safe and rational application of herbal drugs in clinic.
Most of the previous studies reported that the SC active lignans had hepatoprotective effect against many kinds of liver diseases. However, it was recently revealed that schisandrin B and schisandrae fructus oil could induce hepatotoxicity associated with elevations in hepatic and serum triglyceride levels in mice (Zhang et al., 2014). In addition, it was found that SC lignans exhibited inhibition or induction effects on CYP450s, the most important drug metabolism enzymes, causing herbal-drug interaction which might affect the drug therapy or safety (Wang et al., 2014). Therefore, we conducted the pharmacokinetic study of SC effective components under pathological condition, especially considering the common use of SC preparations for different liver diseases and the key role of liver in disposition of xenobiotics.
CCl4 is an often used toxicant for establishment of Acute Liver Injury (ALI) animal model. This agent could be transformed into reactive toxic metabolites by the CYP450 system to cause liver injury, characterized by the elevation of serum ALT and AST, and morphological changes including hepatocyte inflammation, necrosis and steatosis, etc. (Li et al., 2014; Abdel-Bakky et al., 2015; Liang et al., 2015). In our present study, the serum ALT and AST increased by 8.2 and 1.3-folds, respectively. Moreover, BUN, an index of kidney function, was mildly increased in CCl4-intoxicated rats. These results was consistent with the data published, suggesting successful establishment of ALI rat model. However, the short-term (4 day) SC treatment to ALI rat did not show hepatotherapeutic effect on liver injury, which was different from the results of SC pretreatment before CCl4 injection to rats or long-term application of SC extract to mice (Xie et al., 2010; Cheng et al., 2013; Chen et al., 2017). Meanwhile, after dosing of SC extract to ALI rat, it was found that the phamacokinetic profiles of the four schisandra lignans were obviously different from those in control group, exhibited by elevated Cmax and AUC, prolonged MRT and decreased clearance, all of which might increase the possibility of adverse effects or safety concern. To elucidate the mechanism of the pharmacokinetic alternation of the four lignans in ALI rats, the in situ intestinal and hepatic perfusion models were applied for investigation of the respective contributions of intestinal absorption and hepatic disposition. The results suggested that the obviously reduced metabolism of the four lignans in intestine or liver might the main reason for the alternation of the pharmacokinetic profiles.
It has been known that liver CYP450s were main enzymes involved in the in vivo metabolism of xenobiotics, including drugs. Therefore, it was very possible that the change in the metabolism of four active lignans was associated with CYP450s. It was reported that the mRNA expressions of liver CYP450s, including CYP2C11, CYP2E1, CYP3A2, and CYP1A2, were depressed in ALI model rat, indicating the reduction of the protein expression and function of liver CYP450 enzymes (Jia et al., 2007). Hence, the activities of liver CYP450s in ALI rat were further determined in our study. The results revealed that the content of liver CYP450 was decreased by 54% in CCl4-treated rats, while the activities of six major CYP450s isozymes were also depressed significantly. Similarly, the activities of liver CYP450s from AHC patient were also significantly reduced. Moreover, the in vitro metabolism of four schisandra lignans was markly decreased in liver microsomes from CCl4-treated rat and AHC patient. The decreased activity of the cytochrome P450 enzymes indicated by the change in the isozyme spectrum after CCL4-intoxication was basically coincident with the reduced metabolism of the four lignans in liver microsomes following the application of selective inhibitors of CYP450s. Multiple CYP450s, including CYP1A, CYP2C, CYP2D, CYP2E, and CYP3A, were proved to involve the metabolism of the four schisandra lignans in rat and human liver microsomes. Of course, it was better to confirm the results using recombinant human CYP450 isozymes while the inhibition of CYP450s by specific drug inhibitors only gave a relative answer. It was also observed that four lignans were not the substrates of the drug resistant protein P-gp, suggesting little possibility of involvement of P-gp into the disposition of the four active lignans in CCl4-treated rat. Based on all the data in our study, it could be concluded that the depression of the liver CYP450s activities in ALI rat resulted in the pharmacokinetic alternation of the four SC active lignans. When SC preparations were used in clinic, it is better to adjust the dosage according to the liver function and CYP450s activities of patients to avoid toxicity or adverse effects, considering decreased metabolism of the active SC lignans in liver microsomes from AHC patients.
In summary, the pharmacokinetics of four active SC lignans after oral administration was significantly changed when its hepatoprotective effect was not obviously observed after a short-term treatment of SC alcoholic extract to ALI model rat intoxicated with CCl4. The decreased metabolism of the lignans in liver or intestine due to the activity reduction of multiple CYP450s involved was believed to contribute the most to the increased exposure of the four SC components in ALI rats. The pharmacokinetic study of the effective SC lignans under the pathological condition of liver dysfunction provided reliable experimental data for rational and safe clinical application of SC preparations as well as the related pharmacological or toxicological research.
Author Contributions
RW: Performed the experiments, analyzed the data, and wrote the manuscript; ZX, XZ, and FL: Performed concentration detection; WZ and YZ: Participated in research design and amended the paper.
Funding
Funding for this study was provided in part by research grants from the supported by National Science and Technology Major Project (2016ZX09J16104, 2012ZX09301003-002-001).
Conflict of Interest Statement
The authors declare that the research was conducted in the absence of any commercial or financial relationships that could be construed as a potential conflict of interest.
References
Abdel-Bakky, M. S., Helal, G. K., El-Sayed, E. M., and Saad, A. S. (2015). Carbon tetrachloride-induced liver injury in mice is tissue factor dependent. Environ. Toxicol. Pharmacol. 39, 1199–1205. doi: 10.1016/j.etap.2015.02.012
Baig, I. A., Mehjabeen., Muhammed, S., Jahan, N., and Haq, N. (2014). Toxicity studies on herbal formulation used in diabetes mellitus. Pak. J. Pharm. Sci. 27, 2213–2220.
Banumathi, B., Vaseeharan, B., Ishwarya, R., Govindarajan, M., Alharbi, N. S., Kadaikunnan, S., et al. (2017). Toxicity of herbal extracts used in ethno-veterinary medicine and green-encapsulated ZnO nanoparticles against Aedes aegypti and microbial pathogens. Parasitol. Res. 116, 1637–1651. doi: 10.1007/s00436-017-5438-6
Brazier, N. C., and Levine, M. A. (2003). Understanding drug-herb interactions. Pharmacoepidemiol. Drug Saf. 12, 427–430. doi: 10.1002/pds.870
Chen, Q., Zhan, Q., Li, Y., Sun, S., Zhao, L., Zhang, H., et al. (2017). Schisandra lignan extract protects against carbon tetrachloride-induced liver injury in mice by inhibiting oxidative stress and regulating the NF-κB and JNK signaling pathways. Evid. Based Complement. Alternat. Med. 2017:5140297. doi: 10.1155/2017/5140297
Cheng, N., Ren, N., Gao, H., Lei, X., Zheng, J., and Cao, W. (2013). Antioxidant and hepatoprotective effects of Schisandra chinensis pollen extract on CCl4-induced acute liver damage in mice. Food Chem. Toxicol. 55, 234–240. doi: 10.1016/j.fct.2012.11.022
Chiu, P. Y., Leung, H. Y., Siu, A. H., Poon, M. K., and Ko, K. M. (2007). Schisandrin B decreases the sensitivity of mitochondria to calcium ion-induced permeability transition and protects against carbon tetrachloride toxicity in mouse livers. Biol. Pharm. Bull. 30, 1108–1112. doi: 10.1248/bpb.30.1108
Chiu, P. Y., Mak, D. H., Poon, M. K., and Ko, K. M. (2002). In vivo antioxidant action of a lignan-enriched extract of Schisandra fruit and an anthraquinone-containing extract of Polygonum root in comparison with schisandrin B and emodin. Planta Med. 68, 951–956. doi: 10.1055/s-2002-35661
Eriksson, L. C. (1978). Preparation of liver microsomes with high recovery of endoplasmic reticulum and a low grade of contamination. Biochim. Biophys. Acta 508, 155–164. doi: 10.1016/0005-2736(78)90197-9
Fujihashi, T., Hara, H., Sakata, T., Mori, K., Higuchi, H., Tanaka, A., et al. (1995). Anti-Human-Immunodeficiency-Virus (HIV) activities of halogenated gomisin-J derivatives, new nonnucleoside inhibitors of HIV type-1 reverse-transcriptase. Antimicr. Agents Chemother. 39, 2000–2007. doi: 10.1128/AAC.39.9.2000
Harris, P. E., Cooper, K. L., Relton, C., and Thomas, K. J. (2012). Prevalence of complementary and alternative medicine (CAM) use by the general population: a systematic review and update. Int. J. Clin. Pract. 66, 924–939. doi: 10.1111/j.1742-1241.2012.02945.x
He, F., Bi, H. C., Xie, Z. Y., Zuo, Z., Li, J. K., Li, X., et al. (2007). Rapid determination of six metabolites from multiple cytochrome P450 probe substrates in human liver microsome by liquid chromatography/mass spectrometry: application to high-throughput inhibition screening of terpenoids. Rapid Commun. Mass Spectr. 21, 635–643. doi: 10.1002/rcm.2881
Huang, Z. H., Murakami, T., Okochi, A., Yumoyo, R., Nagai, J., and Takano, M. (2001). Expression and function of P-glycoprotein in rats with carbon tetrachloride-induced acute hepatic failure. J. Pharm. Pharmacol. 53, 873–881. doi: 10.1211/0022357011776036
Jia, N., Liu, X., Wen, J., Qian, L., Qian, X., Wu, Y., et al. (2007). A proteomic method for analysis of CYP450s protein expression changes in carbon tetrachloride induced male rat liver microsomes. Toxicology 237, 1–11. doi: 10.1016/j.tox.2007.04.015
Li, G., Wang, X. Y., Suo, Y. R., and Wang, H. L. (2014). Protective effect of seed oil of Herpetospermum pedunculosum against carbon tetrachloride-induced liver injury in rats. Saudi Med. J. 35, 981–987.
Li, X., Chen, X., Li, Q., Wang, L., and Zhong, D. (2007). Validated method for rapid inhibition screening of six cytochrome P450 enzymes by liquid chromatography-tandem mass spectrometry. J. Chromatogr. B Anal. Technol. Biomed. Life Sci. 852, 128–137. doi: 10.1016/j.jchromb.2007.01.006
Liang, B., Guo, X. L., Jin, J., Ma, Y. C., and Feng, Z. Q. (2015). Glycyrrhizic acid inhibits apoptosis and fibrosis in carbon-tetrachloride-induced rat liver injury. World J. Gastroenterol. 21, 5271–5280. doi: 10.3748/wjg.v21.i17.5271
Lu, H., and Liu, G. T. (1992). Antioxidant activity of dibenzocyclooctene lignans isolated from Schisandraceae. Planta Med. 58, 311–313. doi: 10.1055/s-2006-961473
McLean, A. J., and Morgan, D. J. (1991). Clinical pharmacokinetics in patients with liver disease. Clin. Pharmacokinet. 21, 42–69. doi: 10.2165/00003088-199121010-00004
Putthapiban, P., Sukhumthammarat, W., and Sriphrapradang, C. (2017). Concealed use of herbal and dietary supplements among Thai patients with type 2 diabetes mellitus. J. Diabetes Metab. Disord. 16:36. doi: 10.1186/s40200-017-0317-3
Ramos-Remus, C., and Raut, A. (2008). Complementary and alternative practices in rheumatology. Best Pract. Res. Clin. Rheumatol. 22, 741–757. doi: 10.1016/j.berh.2008.05.001
Rendic, S., and Di Carlo, F. J. (1997). Human cytochrome P450 enzymes: a status report summarizing their reactions, substrates, inducers, and inhibitors. Drug Metab. Rev. 29, 413–580. doi: 10.3109/03602539709037591
Samuels, N., Ben-Arye, E., Maimon, Y., and Berger, R. (2017). Unmonitored use of herbal medicine by patients with breast cancer: reframing expectations. J. Cancer Res. Clin. Oncol. 143, 2267–2273. doi: 10.1007/s00432-017-2471-x
Savina, P. M., Staubus, A. E., Gaginella, T. S., and Smith, D. F. (1981). Optimal perfusion rate determined for in situ intestinal absorption studies in rats. J. Pharm. Sci. 70, 239–243. doi: 10.1002/jps.2600700303
Singh, A., and Zhao, K. (2017). Herb-drug interactions of commonly used Chinese medicinal herbs. Int. Rev. Neurobiol. 135, 197–232. doi: 10.1016/bs.irn.2017.02.010
Singhal, D., Ho, N. F., and Anderson, B. D. (1998). Absorption and intestinal metabolism of purine dideoxynucleosides and an adenosine deaminase-activated prodrug of 2′,3′-dideoxyinosine in the mesenteric vein cannulated rat ileum. J. Pharm. Sci. 87, 569–577. doi: 10.1021/js9703582
Szopa, A., Ekiert, R., and Ekiert, H. (2017). Current knowledge of Schisandra chinensis (Turcz.) Baill. (Chinese magnolia vine) as a medicinal plant species: a review on the bioactive components, pharmacological properties, analytical and biotechnological studies. Phytochem. Rev. 16, 195–218. doi: 10.1007/s11101-016-9470-4
Tamura, S., Ohike, A., Tokunaga, Y., Ibuki, R., Amidon, G. L., Sezaki, H., et al. (2004). Effect of experimental acute renal and hepatic failure on absorption of tacrolimus in rat small intestine. Drug Metab. Pharmacokinet. 19, 190–197. doi: 10.2133/dmpk.19.190
Tan, W., Wang, B. L., Hu, J. P., Sheng, L., and Li, Y. (2009). Establishment of liquid chromatography/mass spectrometry for determination of bicyclol in rat single-pass intestinal perfusion. Biomed. Chromatogr. 23, 1059–1063. doi: 10.1002/bmc.1223
Uraki, M., Kawase, A., Matsushima, Y., and Iwaki, M. (2016). Effects of dose, flow rate, and bile acid on diclofenac disposition in the perfused rat liver. Eur. J. Drug Metab. Pharmacokinet. 41, 301–307. doi: 10.1007/s13318-015-0259-4
Wang, B. L., Hu, J. P., Tan, W., Sheng, L., Chen, H., and Li, Y. (2008). Simultaneous quantification of four active schisandra lignans from a traditional Chinese medicine Schisandra chinensis (Wuweizi) in rat plasma using liquid chromatography/mass spectrometry. J. Chromatogr. B Analyt. Technol. Biomed. Life Sci. 865, 114–120. doi: 10.1016/j.jchromb.2008.02.016
Wang, B., Yang, S., Hu, J., and Li, Y. (2014). Multifaceted interaction of the traditional Chinese medicinal herb Schisandra chinensis with cytochrome P450-mediated drug metabolism in rats. J. Ethnopharmacol. 155, 1473–1482. doi: 10.1016/j.jep.2014.07.026
Xie, Y., Hao, H., Kang, A., Liang, Y., Xie, T., Sun, S., et al. (2010). Integral pharmacokinetics of multiple lignan components in normal, CCl4-induced hepatic injury and hepatoprotective agents pretreated rats and correlations with hepatic injury biomarkers. J. Ethnopharmacol. 131, 290–299. doi: 10.1016/j.jep.2010.06.038
Yokogawa, K., Watanabe, M., Takeshita, H., Nomura, M., Mano, Y., and Miyamoto, K. (2004). Serum aminotransferase activity as a predictor of clearance of drugs metabolized by CYP isoforms in rats with acute hepatic failure induced by carbon tetrachloride. Int. J. Pharm. 269, 479–489. doi: 10.1016/j.ijpharm.2003.09.045
Zhang, Y., Pan, S. Y., Zhou, S. F., Wang, X. Y., Sun, N., Zhu, P. L., et al. (2014). Time and dose relationships between schisandrin B- and schisandrae fructus oil-induced hepatotoxicity and the associated elevations in hepatic and serum triglyceride levels in mice. Drug Des. Devel. Ther. 8, 1429–1439. doi: 10.2147/DDDT.S67518
Keywords: Schisandra chinensis (SC), lignans, pharmacokinetics, liver injury, cytochrome P450(CYP450)
Citation: Wu R, Xiao Z, Zhang X, Liu F, Zhou W and Zhang Y (2018) The Cytochrome P450-Mediated Metabolism Alternation of Four Effective Lignans From Schisandra chinensis in Carbon Tetrachloride-Intoxicated Rats and Patients With Advanced Hepatocellular Carcinoma. Front. Pharmacol. 9:229. doi: 10.3389/fphar.2018.00229
Received: 11 January 2018; Accepted: 28 February 2018;
Published: 14 March 2018.
Edited by:
Yurong Lai, Gilead (United States), United StatesReviewed by:
Xufeng Tao, Dalian Medical University, ChinaStanislav Yanev, Institute of Neurobiology (BAS), Bulgaria
Copyright © 2018 Wu, Xiao, Zhang, Liu, Zhou and Zhang. This is an open-access article distributed under the terms of the Creative Commons Attribution License (CC BY). The use, distribution or reproduction in other forums is permitted, provided the original author(s) and the copyright owner are credited and that the original publication in this journal is cited, in accordance with accepted academic practice. No use, distribution or reproduction is permitted which does not comply with these terms.
*Correspondence: Wenxia Zhou, zhouwx@bmi.ac.cn
Yongxiang Zhang, zhangyx@bmi.ac.cn