- 1Department of Pharmacy, Nanfang Hospital, Southern Medical University, Guangzhou, China
- 2Rational Medication Evaluation and Drug Delivery Technology Lab, Nanfang Hospital, Southern Medical University, Guangzhou, China
- 3General Hospital of Guangzhou Military Command of PLA, Guangzhou, China
- 4Department of Pharmacy, the Third Affiliated Hospital of Guangzhou Medical University, Guangzhou, China
- 5Guangdong Provincial Key Laboratory of New Drug Screening, Southern Medical University, Guangzhou, China
Background and Purpose: Novobiocin (NOVO), an ABC transporter inhibitor, decreases intestinal wall permeability of capsaicin (CAP), an ABC transporter substrate. However, the mechanism of this effect is not consistent with the action of NOVO as an ABC transporter inhibitor. We previously found that CAP can also be transported via TRPV1, which was site-specific in the permeability of CAP across the intestine. We explored the regulation by NOVO of TRPV1 in the present study.
Methods: Rats and transfected IEC-6 cells were used as the models to assess intestinal permeability and expression of TRPV1. Ussing chamber and intracellular accumulation were used to evaluate the influence of NOVO on the transport of CAP in vitro. The expression of TRPV1 was detected after administration of NOVO by qRT-PCR, western blot and immunofluorescent imaging. In addition, MTT and lactate dehydrogenase (LDH) were used to evaluate the cytotoxicity of NOVO in both rat and cell models. Finally, the effect of NOVO on the absorption of CAP in vivo was studied by LC-MS/MS.
Results: In vitro data showed that there existed a dose-dependent relationship in the range of concentration between 5 and 50 μM, and even 5 μM NOVO could decrease intestinal permeability of CAP across the intestine. Meanwhile, cytosolic accumulation of CAP decreased when NOVO was used simultaneously or 24 h in advance. NOVO exhibited an inhibition level similar to that of ruthenium red (RR) or SB-705498, a TRPV1-specific inhibitor. NOVO down-regulated TRPV1 expression in the intestine and in transfected cells in a concentration-dependent fashion, hinting that its inhibition of the permeability of CAP is due to its inhibition of TRPV1 expression. Immunofluorescent imaging data showed that the fluorescence intensity of TRPV1 was reduced after pre-treatment with NOVO and SB-705498. In vivo data further demonstrated that oral co-administration of NOVO decreased Cmax and AUC of CAP in dosage-dependent ways, consistent with its role as a TRPV1 inhibitor.
Conclusion: NOVO could be a potential TRPV1 inhibitor by attenuating the expression of TRPV1 and may be used to attenuate permeability of TRPV1 substrates.
Introduction
Transient receptor potential vanilloid member 1 (TRPV1) is a ligand-gated cation channel, which activates a series of cellular signals (Tominaga et al., 1998). Because TRPV1 is activated by CAP, TRPV1 has also been called the CAP receptor (Caterina et al., 1997). In addition to allowing influx of large cation such as FM1-43, tetraethylammonium (Meyers et al., 2003; Hellwig et al., 2004; Munns et al., 2015), TRPV1 has also been reported to allow permeability of cationic aminoglycoside antibiotics, including gentamicin (Ocana and Baeyens, 1991; Prado and Machado Filho, 2002). Inhibiting TRPV1 would attenuate the permeation of gentamicin across kidney epithelial and inner ear sensory cells. Hence it might protect against serious kidney and ear side effects induced by the administration of gentamicin (Myrdal and Steyger, 2005; Ishibashi et al., 2009). Furthermore, data provide evidence that gentamicin entered cell via cation channels and that this penetration was mediated by TRPV1 (Lee et al., 2013). Thus, TRPV1 may not only mediate the transport of cellular ions, but also constitute one of the transporting pathways for some drugs.
Our previous study revealed that the transmembrane permeability of CAP across the colon was remarkably higher than that across the other regions of the gastrointestinal mucous membranes of rats. This observation may be explained by the distribution of TRPV1 in the intestine, which has distinct differences across different parts of the intestine and was most expressed in the colon (Funakoshi et al., 2006). Moreover, colon-specific transport of CAP vanished in presence of RR, a non-specific antagonist of TRPV1. This observation suggested that TRPV1 is involved in transmembrane permeability of CAP (Duan et al., 2013). Coincidentally, our previous data showed that NOVO, a BCRP inhibitor, could also decrease permeability of CAP.
NOVO is an aminocoumarin natural antibiotic used to treat bacterial infections caused by staphylococci (such as methicillin-resistant Staphylococcus aureus), pneumococci, enterococci and many other Gram-positive organisms (Gombert and Aulicino, 1984; Jones, 1989; Schmutz et al., 2003). NOVO was demonstrated to have a specific inhibitory effect on BCRP and has been used with some antitumor drugs to block BCRP-mediated drug efflux, potentially reversing multidrug resistance (Su et al., 2007). ABC transporters, including “BCRP, P-gp and multidrug resistance protein 2 (MRP2),” were usually located in the serosa of intestine. ABC transporters significantly restrict oral absorption and efflux of substrates from the serosal side to the mucosal side of the intestinal wall (Sinko et al., 2004; Su et al., 2006; You et al., 2013). Therefore, ABC transporter inhibitors would increase the intracellular accumulation of drugs. However, NOVO did not exhibit these expected results. In fact, our data showed that NOVO decreased the absorption of CAP. These conflicting results suggested the inhibition of CAP permeability was not associated with BCRP. These findings hinted that there might exist other mechanisms which are not related to BCRP-mediated transport.
Some researchers have advised that some antibiotics should not be taken with spicy food (chili) because it might affect pharmacokinetics of these antibiotics. Sumano-Lopez et al. (2007) found the oral bioavailability of ciprofloxacin would increase by 70% when administrated in combination with 0.01–0.5% CAP. It was also reported that CAP affected the oral absorption rate of cephalexin and cefazolin. Importantly, the absorption rate returned to a normal level after co-administration of RR (Komori et al., 2007a,b), suggesting that the absorption of cephalexin and cefazolin was affected by intestinal TRPV1. Based on these findings, we speculated that the interaction between spicy foods containing CAP and these antibiotics may be induced by the TRPV1 pathway.
Until now, the mechanism by which NOVO decreases the transport of CAP across intestinal membranes has remained unclear. Considering CAP’s role as a TRPV1 substrate, we hypothesized that NOVO may play a novel role, as a TRPV1 inhibitor, and decrease the permeability of CAP. Therefore, in the present study, intestinal cells (IEC-6) and rats were chosen as test models. CAP was used as an indicator of TRPV1 transport, while RR (a non-specific TRPV1 inhibitor) and SB-705498 (a specific TRPV1 inhibitor) were used as positive controls to explore the influence of NOVO in regulating TRPV1 in vitro and in vivo.
Materials and Methods
Reagents and Materials
CAP (lot number: 048K5058V), HEPES (lot number: 016K54331), and Trizma base (lot number: 103K5411) were obtained from Sigma–Aldrich Chemical Co. (St. Louis, MO, United States). RR and NOVO were provided from Guangzhou Institute for Drug Control, China. HPLC-grade methanol and acetonitrile were purchased from Merck (Darmstach, Germany). SB-705498 was purchased from Skelleck Chemicals LCC (Houston, TX, United States).
Animals
All of the animal experiments were performed according to the guideline of Experimental Animal Ethics Committee of Southern Medical University, and animal studies were reported in compliance with the ARRIVE guidelines (Kilkenny et al., 2010; McGrath and Lilley, 2015). We used 5–7-week-old male SD rats (200–250 g) which were purchased from the Laboratory Animal Center, Southern Medical University (Guangzhou, China; Permit number for rats: 44002100008791). Animal study was performed according to National Institutes of Health guide for the care and use of laboratory animals. The rats were fed with standard food and water and maintained on 12/12-h light/dark cycle in a temperature (20 ± 2°C) and were given 1 week to acclimate before experimental use.
Ussing Chamber Experiments
The intestinal permeability of CAP in vitro was performed using Ussing chamber. For the in vitro permeability studies, CAP was prepared in 1% ethanol in oxygenated (O2/CO2, 95/5) HEPES buffer (3 M KCl, 1 M CaCl2, 1 M MgSO4, 8.18 g NaCl, pH 7.4), which was prepared daily, to yield final concentration of 100 μM. NOVO was also prepared in HEPES buffer to yield final concentration at 5, 10, 25, 50, 100, and 200 μM. Animal intestinal segments for the permeability study were prepared in accordance with the experimental method as described previously (Yodoya et al., 1994; Wallon et al., 2005; Duan et al., 2013). Briefly, male SD rats, weighting 240–260 g, were fasted for 18 h before each experiment and anesthetized by injecting 10 % chloral hydrate anesthesia (i.p.). Different portions of the rat intestine were excised and flushed with HEPES buffer, including jejunum (after the first 5 cm of the top of small intestine), ileum (the distal part of small intestine) and colon (proximal to cecal-colonic junction), and incubated in the ice-cold HEPES buffer. Next, 3–4 cm of the intestine was clipped, and the serosa was removed rapidly on an ice-cold glass. The intestinal segments were fixed in the Ussing chamber. Finally, 7 mL of HEPES buffer was added to the receiving side while an equal volume of drug solution to the dosing side. All the chambers were maintained at 37°C by using a warm water-circulating pump and a mixture of 95% O2 and 5% CO2 aerated to ensure the activity of the membrane. 0.5 mL of the sample was collected from the receiving side at 30, 60, 75, 90, and 120 min and a 0.5 mL aliquot of HEPES was added at the same side after each sampling point. All the samples were kept at -20°C till HPLC analysis.
Preparation of Tissue Extract
Forty male SD rats (200–250 g) were used for orally administered experiment. The animals were arbitrarily distributed in eight different groups and each group was treated with its respective dose of calculated amount. Group I was orally administered 0.9% normal saline (5 mL⋅kg-1). Group II labeled as positive control was orally administered with 10 μM RR (5 mL⋅kg-1). Rats of Group III–VIII were treated with 5 mL⋅kg-1 of NOVO dissolved in 0.9% normal saline (5, 10, 25, and 50 μM, respectively). The animals were orally administered twice a day for 2 weeks. After another 14 days, animals were sacrificed and then the jejunum, ileum and colon tissue were excised. The intestinal tissues were frozen in liquid nitrogen, and then stored at -80°C for protein or ribonucleic acid (RNA) isolation.
Cells Culture and Plasmid Transfection
The rat intestinal epithelial cell line IEC-6, purchased from Kunming Institute of Zoology. CAS, was cultured in Dulbecco’s Modified Eagle’s Medium (DMEM) (Gibco, Grand Island, NY, United States) supplemented with 10% fetal bovine serum (FBS; Gibco) at 37°C in a humidified atmosphere of 5% CO2.
The plasmid pENTER containing the TRPV1 coding sequence NM_080704 was used as template in a PCR with the following primers: 5′-CGCAAATGGGCGGTAGGCGTG-3′ (forward) and 5′-CCTCTACAAATGTGGTATGGC (reverse). And synthetic small interfering RNA (siRNA) specific for rat TRPV1 (TRPV1-siRNA) was purchased from Vigene Bioscience (Rockville, MD, United States) with the following sequences: 5′-AGCGCAUCUUCUACUUCAACU-3′ (forward) and 5′-UUGAAGUAGAAGAUGCGCUUG-3′ (reverse) for TRPV1-siRNA. The transfecting process was conducted by using the lipofectamin® 3000 transfection reagent (InvitrogenTM, Carlsbad, CA, United States), according to the manufacturer’s protocol.
Measurement of Cell Viability and Cytotoxicity
This experiment focused on evaluating the cyctoxicity of NOVO, CAP and RR in IEC-6/TRPV1+ and IEC-TRPV1- cells and finding their dosing with non-cytotoxicity on the test cells separately. The MTT assay was used to identify non-toxic doses of NOVO, CAP and RR in IEC6/TRPV1+ and IEC6/TRPV1- cells. The transfected cells were plated in 96-well plates. NOVO, CAP or RR was added to the wells, and the plates were incubated for 12 or 24 h. Optical density at 490 nm was used to evaluate cell viability in 96-well plates.
After determination of the non-cytotoxicity concentration, LDH Release Assay Kit (Nanjing Jiancheng Bioengineering Inc., Nanjing, China) was further used to check the influence of NOVO, CAP or RR at non-cytotoxic concentration on the plasma membrane of the transfected cells. The transfected cells were plated in 12-well plates. NOVO, CAP and RR at non-cytotoxic concentration from the MTT assay were added to wells after cells were plated, and the plates were incubated for 12 or 24 h. The cells were centrifuged at 1000 g for 5 min. Next, 50 μL of supernatants were aspirated and the rest of supernatants were discarded. The cells were washed twice with deionized water and then lysed by ultrasonic wave cracker. And then cell lysate and supernatants were analyzed by using LDH Release Assay Kit, according to the manufacturer’s instructions.
CAP Accumulation Assay
The transfected cells were grown to 80% confluence in 12-well plates. The experiment was divided into three parts: (1) Cells were incubated with CAP (25 μM) alone, or in combination with SB-705498 (0.1 μM), or in combination with RR (10 μM), or in combination with NOVO (1, 5, and 10 μM), for 5, 15, 30, and 60 min; (2) Cells were incubated with CAP (25 μM) for 5, 15, 30, and 60 min at the beginning. SB-705498, RR or NOVO was then added to the medium for another 15, 30, and 60 min. (3) After pretreatment with SB-705498, RR or NOVO for 24 h, cells were incubated with CAP (25 μM) in culture medium at 37°C in 5% CO2 for 5, 15, 30, and 60 min, respectively. After incubation, the medium was removed, and cell layers were washed three times with ice-cold PBS and then extracted with Membrane Protein Extraction Kit (Thermo Fisher Scientific Inc, Rockford, IL, United States), according to the manufacturer’s protocol. The cytosolic protein extracts were stored at -80°C until LC-MS/MS analysis.
Immunofluorescent Staining
The IEC-6/TRPV1+ cells were cultured on coverslips in 24-well plates overnight. Briefly, cells were stimulated with NOVO (10 μM), SB-705498 (0.1 μM) for 24 h. At the end of incubation, cells were fixed with 4% paraformaldehyde in 0.1 M PBS for 20 min. The cells were then treated by 0.25% (v/v) TritonX-100 for 5 min, and washed again. Followed by blocking in 5% (v/v) horse serum for 60 min, cells were then incubated with primary antibodies for the anti-TRPV1 receptor antibody (1:1000, NB100-1617, Novus) overnight in a 4°C refrigerator. Alexa Flour® 488-conjugated goat anti-rabbit IgG (Zhongshan Goldenbridge Biotechnology) at 1:100 dilution was used as the secondary antibody that incubated cells for 60 min. Thereafter, the coverslips were mounted face down onto slides with mounting medium containing DAPI and stored at 4°C before microscopy. Fluorescence images were captured using a fluorescence microscope (Olympus, Japan).
Quantitative Real Time (qRT)-PCR
qRT-PCR was performed to evaluate TRPV1 mRNA expression after NOVO treatment. The total RNA of the cells or tissues was isolated with an RNA isolation plus kit (Takara Shuzo, Kyoto, Japan), according to the manufacturer’s protocol. The quality of RNA was confirmed by agarose gel electrophoresis and an optical density measurement of A260/A280. The cDNAs were prepared using SYBR® Premix Ex TaqTMII (Takara Shuzo, Kyoto, Japan) and the following primers: 5′-CACAGAGTGGACCCAGATAACG-3′ and 5′-CACTCGAGATAGACATGCCACC-3′ for TRPV1, and 5′-AGAAGGCTGGGGCTCATTTG-3′, 5′-AGGGGCCATCCACAGTCTTC-3′ for GAPDH were carried out by using the LightCycler480 System. The relative expression was calculated by the 2-ΔΔC(t) method (Livak and Schmittgen, 2001).
Protein Extract and Western Blot
After treatment, the cells or tissues were collected and washed with PBS. Membrane protein extracts were obtained with Membrane Protein Extraction Kit (Thermo Fisher Scientific Inc, United States), according to the manufacturer’s protocol. Protein concentrations were quantified by using the BCA Protein Assay kit (Beyotime, Shanghai, China). Equal amounts of protein were resolved by SDS-PAGE on a 10% polyacrylamide gel and transferred onto a polyvinylidene fluoride membrane. The membrane was incubated with antibodies against TRPV1 (Novus Biologicals, LLC. Catalog: NB100-1617) and GAPDH (Bioworld Technology, Inc., Catalog: BS6007M), followed by incubation with anti-rabbit secondary antibodies (Cell Signaling Technology, Inc., Catalog: 7074).
In vivo Pharmacokinetics Study
SD rats, weighing 240–260 g, were fasted for 18 h before the study and orally administered CAP (10 mg⋅kg-1) in combination with different doses of NOVO (12.5 mg⋅kg-1, 25 mg⋅kg-1, 50 mg⋅kg-1, and 100 mg⋅kg-1) or 0.9% saline. Blood samples were collected into heparinized tubes before and at 30, 60, 120, 180, 210, 240, 270, 330, 390, and 720 min after administration. Blood samples were immediately centrifuged at 4000 g (4°C) for 10 min to obtain plasma. The plasma samples were stored at -80°C until LC-MS/MS analysis.
Plasma Sample and Protein Sample Preparation
The plasma samples and protein sample with 100 μL were extracted with 400 μL of methanol involving IS (verapamil 50 ng⋅mL-1) by vortex-mix for 1 min, ultrasonic extraction for 1 h, and then centrifugation for 30 min at 15000 g. The supernatant was transferred into a borosilicate glass and 2 μL was subjected to LC-MS/MS analysis.
Analytical System
HPLC
The concentrations of CAP and NOVO in vitro permeability studies were determined by HPLC system and the HPLC were respectively performed as described (Guo et al., 2014) and in Zuhowski et al. (1994). In brief, samples were injected into a C18 column (3.5 μm, 2.1 mm × 100 mm). An aliquot of 20 μL of sample solutions were injected. Elution solvents were waster- methanol (30–70, v/v) for CAP and water with 0.1% phosphoric acid-methanol (10–90, v/v) for NOVO. The wavelength were set at 280 nm (CAP) and 340 nm (NOVO), respectively.
LC-MS/MS
The LC-MS/MS system consisted of Agilent 6460 Triple Quadrupole LC/MS systems via an ESI interface. The samples were separated on an Agilent ZORBAX SB-C18 column (3.5 μm, 2.1 mm × 150 mm) and the column temperature was maintained at 30°C. The mobile phase consisted of acetonitrile and water contains 0.1% formic acid (70:30, v/v). The flow rate was 0.2 mL⋅min-1. The ion source was an electrospray ionization interface in the positive mode. The precursor/product ion transitions were monitored at m/z 306→137 for CAP and m/z 455→165 for verapamil. Other parameters were as follows: spray voltage, 4.0 kV; capillary temperature, 350°C; gas flow, 10 L⋅min-1.
Statistical Analysis
The data and statistical analysis comply with the recommendations on experimental design and analysis in pharmacology (Curtis et al., 2015). Data in all experiments were presented as the mean ± SD. Significant differences were tested by one-way ANOVA, Student’s t-test and Fisher’s Least Significant Difference (LSD) test using SPSS 13.0 software. For all tests, p < 0.05 was considered as statistically significant.
Results
NOVO Exhibits Dose-Dependent Inhibition of Intestinal Permeability of CAP
RR is a non-specific antagonist of TRPV1 which significantly reduced permeability of CAP. The TRPV1 inhibition mediated by RR at 10 μM was significantly different in the ileum (1.46-fold) and colon (1.64-fold) when compared with the CAP-only control. Hence, 10 μM RR was used as the positive control in order to compare the effects of NOVO and RR on the transport of CAP across the intestine. As shown in Table 1, NOVO greatly inhibited the permeability of CAP across the intestine. 5–25 μM NOVO could significantly decrease the permeability across the jejunum, ileum and colon in a dose-dependent way. Meanwhile, 50 μM NOVO could also reduce the transport of CAP in the jejunum and colon. More interestingly, 50 μM NOVO displayed an effect similar to 10 μM RR on the transport of CAP. However, NOVO at lower doses, 10 and 25 μM exhibited a stronger inhibitory effect than did 10 μM RR.
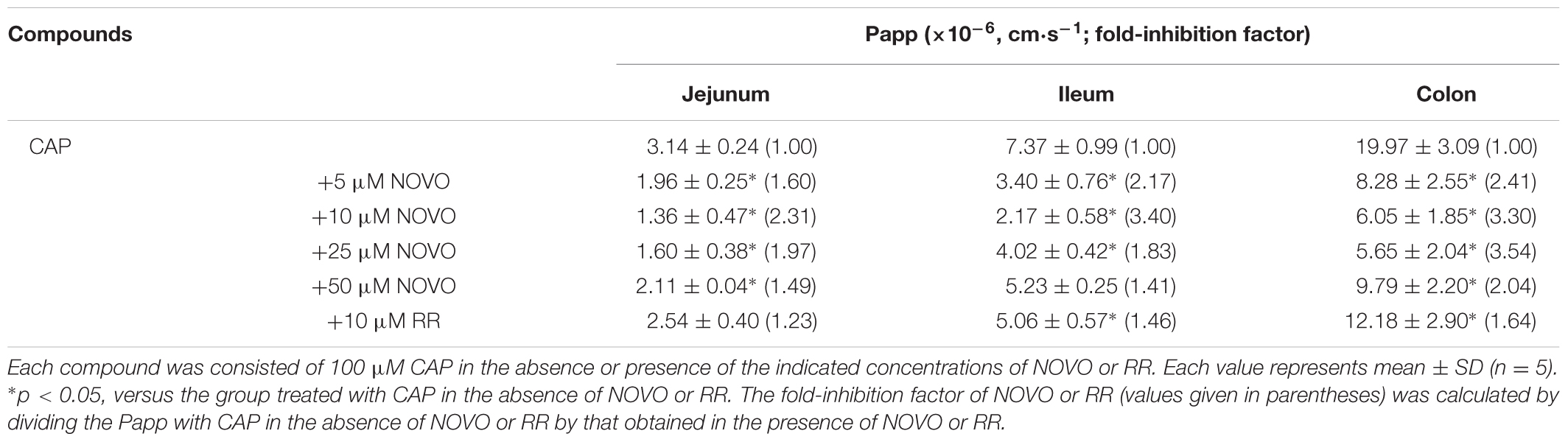
TABLE 1. The contribution of NOVO and RR in the transport of CAP across the jejunum, ileum, and colon.
The Contribution of TRPV1 Inhibitor in the Transport of NOVO
In order to determine whether the transport of NOVO across the intestine was related to TRPV1, we evaluated how RR affected the transport of NOVO. As shown in Figure 1, permeability of NOVO was much greater in the jejunum than in the ileum and colon. Additionally, there was no change in permeability of NOVO across these intestinal segments compared to the case when NOVO was administered in combination with RR.
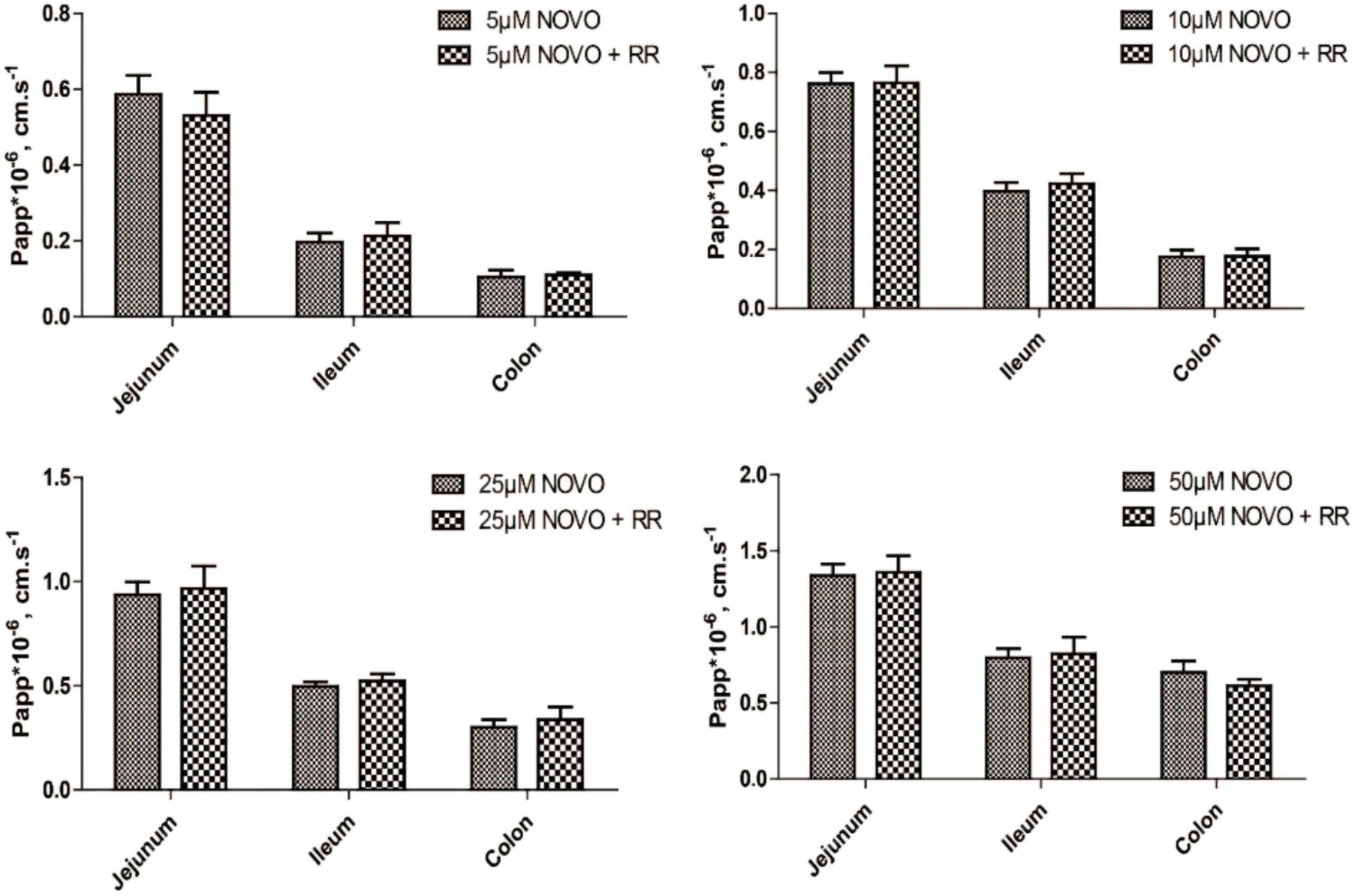
FIGURE 1. The effect of RR on the permeability of NOVO across the jejunum, ileum and colon. The samples contained the indicated concentrations of NOVO in the absence or presence of 10 μM RR. Each value represents mean ± SD (n = 5).
Characterization of TRPV1-Overexpressing (IEC-6/TRPV1+) and TRPV1-Knockdown (IEC-6/TRPV1-) Intestinal Epithelium Cell Lines 6
To elucidate the regulation by NOVO of TRPV1 activity, intestinal IEC-6 cells were transiently transfected with a plasmid containing the TRPV1 coding sequence. Successful overexpression (IEC-6/TRPV1+) and knockdown (IEC-6/TRPV1-) compared with untreated controls were verified by qRT-PCR (Figures 2A,B) and western blot (Figure 2C). Then we examined the individual effects of NOVO, CAP, RR, and SB-705498 on the survival of IEC-6/TRPV1+ and IEC-6/TRPV1- cells by using a MTT assay. As shown in Table 2, more than 80% of the cells were viable up to a concentration of 10 μM NOVO, 25 μM CAP, and 10 μM RR for both IEC-6/TRPV1+ and IEC-6/TRPV1- which had been incubated for 24 h with these inhibitors. It is known that SB-705498 has low cytotoxicity in transfected cells at doses ranging from 1 to 5000 nM. Hence, NOVO at concentrations of 2.5, 5, and 10 μM were used as low, medium, and high concentrations in assessing its mode of action. Similarly, 25 μM CAP, 10 μM RR, or 0.1 μM SB-705498 was used as the non-cytotoxic concentration in the experiments.
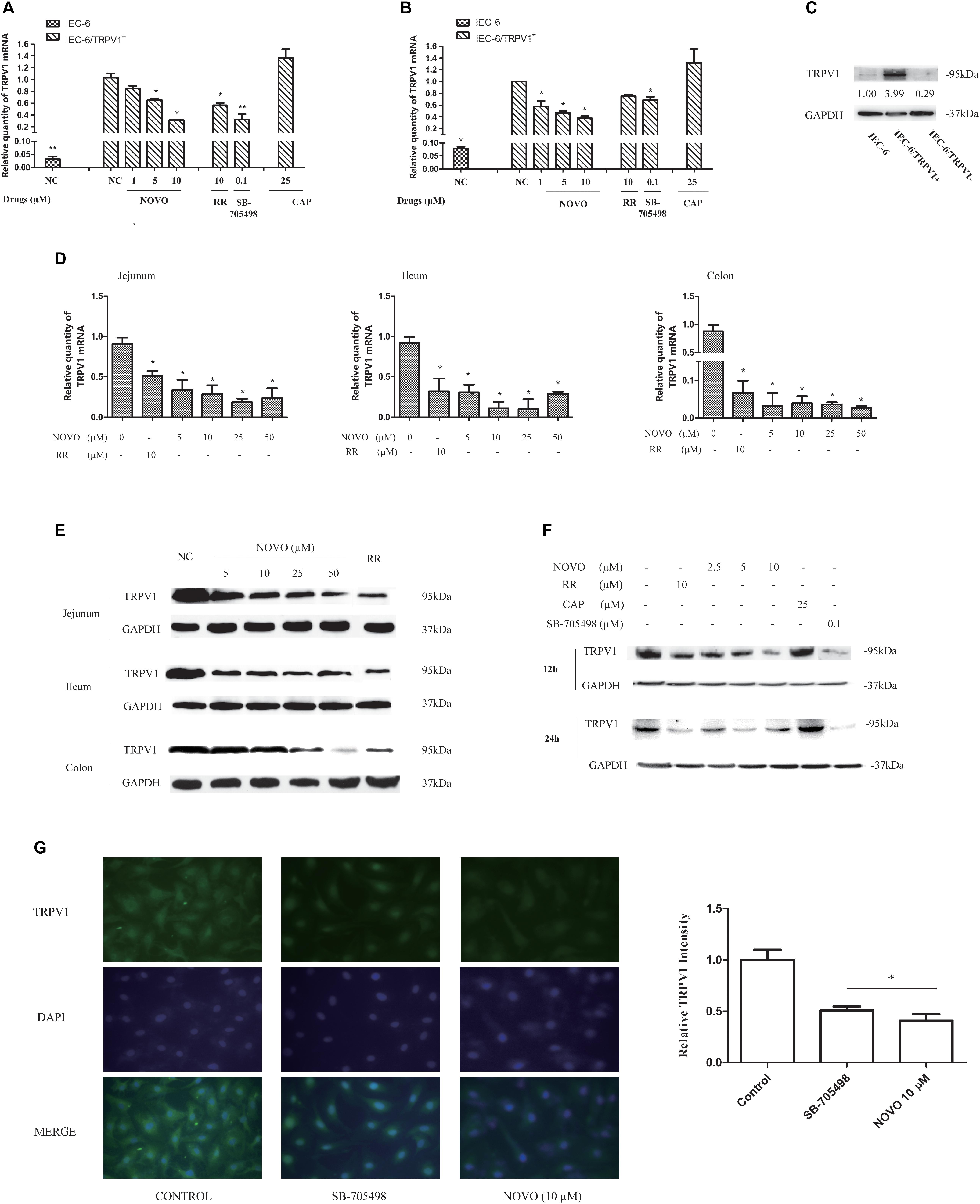
FIGURE 2. NOVO attenuated the expression of TRPV1. (A,B) Effects of NOVO on the expression of TRPV1 mRNA in IEC-6/TRPV1+ for 12 h (A) and 24 h (B). IEC-6/TRPV1+ cells were treated with 2.5–10 μM NOVO for 12 or 24 h, or 10 μM RR for 12 or 24 h, or 0.1 μM SB-705498 for 12 or 24 h, 25 μM CAP for 12 or 24 h, or 0.1% DMSO for 12 or 24 h. Shown are the mean relative mRNA expression levels normalized to untreated cells from three independent experiments performed in triplicate with standard deviations. ∗p < 0.05 vs. control group. (C) The expression of TRPV1 protein in non-transfected and transfected cells. (D) Effects of NOVO on mRNA expression of TRPV1 in jejunum, ileum and colon. Shown are the mean relative mRNA expression levels normalized to NC from three independent experiments performed in triplicate with standard deviations. ∗p < 0.05, statistic versus NC. Each value represents mean ± SD (n = 5). Rats were exposed to different concentrations of NOVO (5, 10, 25, and 50 μM), RR (10 μM) or 0.9% saline (NC) through oral administration (p.o.) twice per day for 14 days. Total RNA was obtained from the intestinal tissues extracted from treated rats. (E) Effects of NOVO on the protein expression of TRPV1 in jejunum, ileum and colon. Total membrane proteins were obtained from the intestinal tissues extracted from treated rats. (F) Effects of NOVO on the expression of TRPV1 protein in IEC-6/TRPV1+. (G) Subcellular distribution of TRPV1 in the transfected cell lines. IEC-6/TRPV1+ cells were fixed for immunofluorescence testing followed by pre-treatment with NOVO (10 μM), SB-705498 (0.1 μM), or 0.1% DMSO for 24 h and then labeled with anti-TRPV1 antibody (green) and DAPI (blue). Original magnification × 400. ∗p < 0.05, statistic versus control. Each value represents mean ± SD (n = 5).
Analysis of cell membrane integrity was carried out using the LDH release assay. This assay was used to re-evaluate whether there was any damage to the cell membranes in the presence of NOVO, CAP, RR, and SB-705498 at the non-cytotoxic concentrations determined by the MTT assay. As shown in Table 3, there was not any significant difference in LDH release after cells were treated with NOVO (2.5, 5, and 10 μM), CAP (25 μM), RR (10 μM), and SB-705498 (0.1 μM) for 12 and 24 h, compared with the control. This meant no damage was induced by NOVO, CAP, RR, and SB-705498.
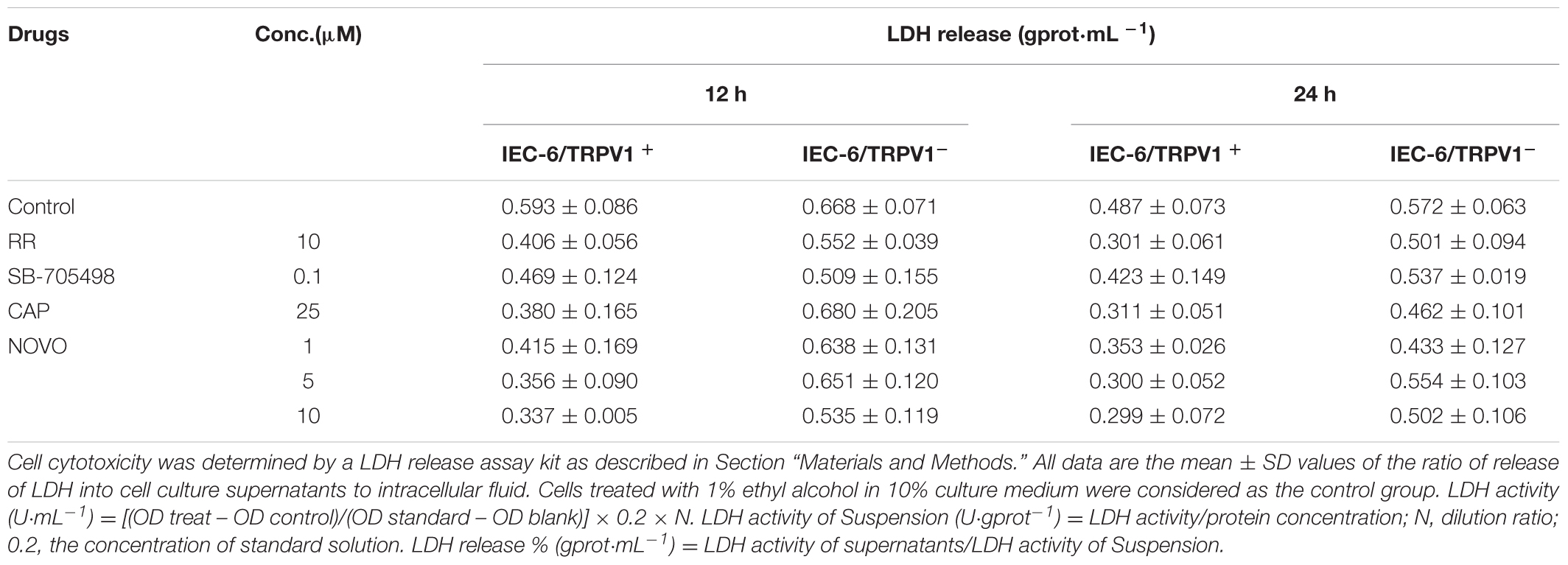
TABLE 3. Effects of NOVO, CAP, and the TRPV1 inhibitors on cell membrane integrity of IEC-6/TRPV1+ cells (percentage of LDH release was used as an index).
NOVO Attenuated the Expression of TRPV1 in the Intestine
qRT-PCR and western blot analysis were performed to detect any change in mRNA and protein levels of TRPV1 upon pretreatment with NOVO, respectively. Figure 2D shows the influence of NOVO on the expression of TRPV1 mRNA in the rat intestine after 14 days of p.o. administration of NOVO. The data demonstrate that the TRPV1 mRNA level decreased markedly in a NOVO-dose-dependent manner. The mRNA expression analysis showed less mRNA in the jejunum than in the ileum, and less mRNA in the ileum than in the colon. Similarly, NOVO also decreased the TRPV1 protein level in a dose-dependent manner (Figure 2E). However, 5 μM NOVO was not sufficient to attenuate TRPV1 protein expression.
NOVO Down-Regulated TRPV1 mRNA and Protein Expression in IEC-6/TRPV1+ Cells
NOVO could also dramatically attenuate the expression of TRPV1 mRNA (Figures 2A,B) and protein (Figure 2F) in a concentration-dependent manner over 24 h in IEC-6/TRPV1+ cells. Moreover, NOVO displayed a similar suppression effect on the expression of TRPV1 as did RR and SB-705498.
To further validate this change in TRPV1 expression, we investigated the subcellular localization of TRPV1 in IEC-6/TRPV1+ cells (Figure 2G). Immunofluorescent imaging data showed that TRPV1 was distributed diffusely throughout the cell with detectable cell surface expression in the untreated group, which corresponded with the protein expression levels identified with western blot analysis. The fluorescence intensity of TRPV1 was reduced after pre-treatment with NOVO (10 μM) and SB-705498.
NOVO Inhibited the Accumulation of CAP in Cells
To validate the relationship between TRPV1 expression and permeability of CAP, the intracellular accumulation of CAP through cell membranes was examined. We found first that the accumulation of CAP in IEC-6/TRPV1+ cells surpassed that in IEC-6 cells. However, IEC-6 cells with TRPV1 knockdown significantly decreased the accumulation of CAP (Figure 3A). Second, IEC-6/TRPV1+ cells were exposed to 2.5–10 μM NOVO, 10 μM RR, or 0.1 μM SB-705498 for 24 h prior to incubation with CAP for 60 min. Figure 3B shows that NOVO concentrations (2.5–10 μM) exhibited a positive correlation with a decrease in the accumulation of CAP in the cells. Additionally, 10 μM NOVO displayed similar effects as SB-705498 and RR did in the transport of CAP. Third, after successful construction, transfected cells were exposed to CAP in the presence of NOVO, RR or SB-705498. Fourth, transfected cells were exposed to 2.5–10 μM NOVO, 10 μM RR, or 0.1 μM SB-705498 for 24 h in the presence of CAP for 60 min. Compared to the control, NOVO exhibited strong inhibition of the accumulation of CAP in IEC-6/TRPV1+ cells (Figure 3C). Furthermore, 10 μM NOVO displayed a similar effect as SB-705498 did in the transport of CAP. However, in IEC-6/TRPV1-, the intracellular accumulation of CAP was far less than that in IEC-6. Moreover, the accumulation of CAP in IEC-6/TRPV1- did not change when the cells were treated with RR, SB-705498, or NOVO (Figure 3D). Finally, IEC-6/TRPV1+ cells were exposed to CAP alone for 60 min prior to administration of NOVO, RR, or SB-705498. The plot indicates that the accumulation of CAP decreased immediately at 75 min after cells were treated with SB-705498 and NOVO (5 and 10 μM) (Figure 3E). SB-705498 and 10 μM NOVO exhibited sustained inhibition of the accumulation of CAP. Compared to the control, NOVO at 2.5 and 5 μM decreased the permeation of CAP.
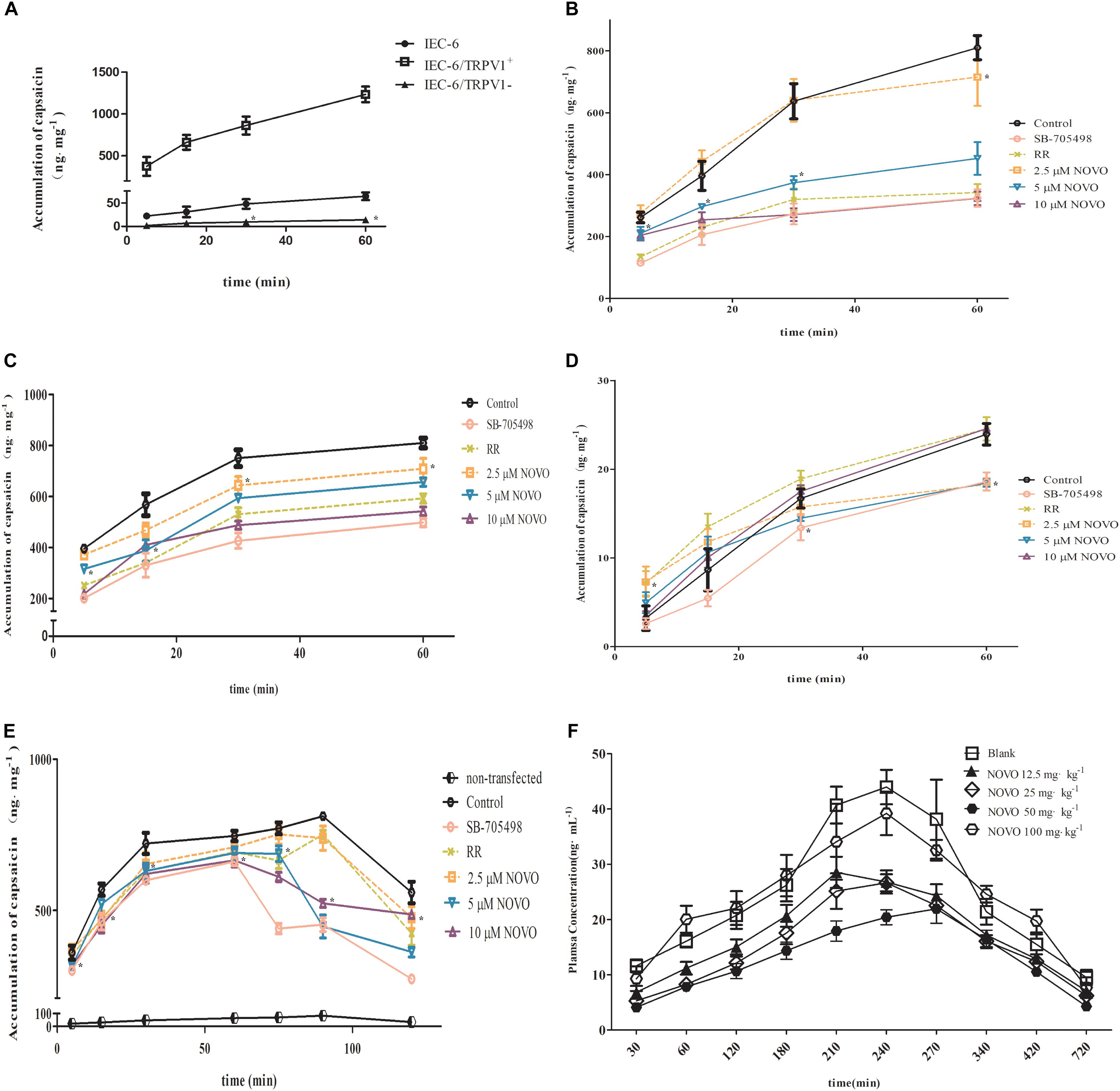
FIGURE 3. Effects of NOVO on the intracellular accumulation of CAP in cells and on the pharmacokinetics of CAP in vivo. (A) The accumulation of CAP in non-transfected and transfected cells. Cells were treated with 25 μM CAP for 5, 15, 30, and 60 min. ∗p < 0.05, statistic versus control. (B) The accumulation of CAP after different pre-treatments in TRPV1-overexpressing IEC-6 cells. Pre-treatment (24 h) of IEC-6/TRPV1+ with 2.5–10 μM NOVO, RR (non-specific inhibitor), SB-705498 (specific inhibitor) or 0.1% DMSO. Subsequently, IEC-6/TRPV1+ cells were incubated with 25 μM CAP for 5, 15, 30, and 60 min. ∗p < 0.05, statistic versus control. (C) The accumulation of CAP after the administration of CAP in combination with 2.5–10 μM NOVO, RR (non-specific inhibitor), SB-705498 (specific inhibitor) or 0.1% dimethyl sulfoxide (DMSO) in TRPV1-overexpressing IEC-6 cells, respectively. ∗p < 0.05, statistic versus control. (D) The accumulation of CAP after the administration of CAP in combination with 2.5–10 μM NOVO, RR (non-specific inhibitor), SB-705498 (specific inhibitor) or 0.1% DMSO in TRPV1-knockdown IEC-6 cells. ∗p < 0.05, statistic versus control. (E) The effect of NOVO, RR and SB-705498 on the accumulation of CAP in IEC-6/TRPV1+. Cells were treated with 25 μM CAP and then treated with NOVO, RR, or SB-705498 at 60 min. ∗p < 0.05, statistic versus control. (F) Mean plasma concentration versus time profiles of CAP after the oral administration of CAP (10 mg⋅kg-1) in combination with NOVO (12.5–100 mg⋅kg-1) to rats. In vivo data are represented as the mean ± SD values from six independent experiments. Cell data were represented as the mean ± SD values from three independent experiments performed in triplicate.
NOVO Decreased the Absorption of CAP After Oral Co-administration
Plasma samples for analysis of CAP after oral administration of different doses of NOVO were taken from anesthetized rats in the in vivo study. The peak time (Tmax) of CAP was about 240 min and it did not change with dosage. Cmax and AUC0-720min were gradually attenuated as the dose of NOVO increased in the 12.5-50 mg⋅kg-1 range (Table 4), indicating that NOVO could inhibit the absorption and affect the pharmacokinetic properties of CAP. However, 100 mg⋅kg-1 NOVO had no impact on the pharmacokinetic properties of CAP compared with the CAP-only group. As shown in Figure 3F, the variation of plasma concentration versus time profiles of CAP was related to the dose of NOVO after oral administration of NOVO (12.5-50 mg⋅kg-1).

TABLE 4. Pharmacokinetic parameters of CAP after the oral administration of CAP (10 mg⋅kg-1) in combination with NOVO (12.5–100 mg⋅kg-1) in rats.
Discussion
Few studies have shown how CAP permeates across the membranes. In theory, CAP is a lipophilic compound (Georgescu et al., 2017) and its logP is 4 (Iida et al., 2003), which means it might permeate lipid bilayers. Several groups have proposed CAP may interact with lipids within cell membranes. Hanson et al. indicated that CAP could travel through lipid bilayers by interacting with TRPV1 in molecular dynamics simulations (Hanson et al., 2015). However, most drugs/compounds do not have only way to access the cytoplasm. Many parameters, including pKa and solubility of drugs/compounds, can also play a role in determination of their transport pathways. Furthermore, our previous study demonstrated that the highest permeation of CAP was colon-specific and therefore could be a consequence of the colon-specific distribution of TRPV1 (Duan et al., 2013). In addition, some researchers indicated that TRPV1 could be a transport pathway to regulate the absorption of certain drugs, including CAP (Myrdal and Steyger, 2005; Duan et al., 2013; Lee et al., 2013). Therefore, we hypothesized that CAP may not only be an agonist of TRPV1 (Vriens et al., 2009), but also a transporter substrate of TRPV1.
We used the intestinal cell line IEC-6 to explore whether CAP permeates intestinal cells via TRPV1. IEC-6 is a common model for in vitro experiments and has characteristics similar to intestinal basement membrane. These cells have microvilli, centrioles, Golgi complexes, and junctional complexes according to an ultrastructural study (Quaroni et al., 1979). Due to the characteristics of IEC-6 cells, this cell line has also been used in studies of hepatotoxicity (Zhou et al., 2017), tight junctions (Yu et al., 2016) and TRP channels (Kono et al., 2013; Yamawaki et al., 2014). Cells were transiently transfected with plasmid to produce TRPV1- overexpressing and TRPV1-knockdown cells. The data showed that knockdown significantly suppressed the permeability of CAP in IEC-6/TRPV1- compared to non-transfected cells. Moreover, the permeability of CAP was greatly increased in IEC-6/TRPV1+. The above results indicated that overexpression of TRPV1 could accelerate the permeation of CAP. TRPV1 knockdown suppressed the intracellular accumulation of CAP. Together, these findings suggested that TRPV1 plays an important role in CAP transport through membranes as we had hypothesized.
Since we had demonstrated that TRPV1 affected CAP traveling through membranes, we used rat intestinal tissues to evaluate the effect of NOVO on the permeability of CAP across the intestine with an Ussing chamber system. First, intestinal samples were treated with concentrations of NOVO ranging from 5 to 50 μM to evaluate the contribution of NOVO to the transport of CAP in vitro. The data showed that NOVO at 5–25 μM significantly decreased the permeability of CAP, in a concentration-dependent manner. RR, a non-specific blocker of TRPV1, interferes with the opening of TRPV1 channels, thereby decreasing calcium entry and subsequent peptide release (Dray et al., 1990; Lou et al., 1991). A previous study showed that RR produced long-lasting, selective, and reversible inhibition of CAP-induced plasma extravasation in rat tracheas (Brokaw and White, 1995). Loris et al. found that pretreatment with RR of the ileum abolished the response to CAP and blocked TRPV1 activation (Chahl, 1989). Therefore, we used RR (10 μM) as a positive control in order to compare the effect of NOVO and RR in the transport of CAP across the intestine. Table 1 shows that RR decreased the permeability of CAP across the jejunum, ileum, and colon. Furthermore, NOVO showed an even stronger (5–25 μM) effect on inhibiting permeability than RR did. However, this led to a question: was the inhibitory contribution of NOVO a result of its role as a TRPV1 inhibitor or a result of its role as a competitive substrate? As shown in Figure 1, RR did not affect the transport of NOVO. In addition, we had found that the TRPV1-mediated permeability of CAP across the colonic mucosa was remarkably higher than that across the jejunal or ileac mucosa. NOVO itself did not show transport permeability similar to that of CAP, suggesting that NOVO was not a competitive substrate of TRPV1. This excluded the possibility that NOVO decreased intestinal permeability of CAP through its competitive binding to the binding site of TRPV1. Preliminary results suggested NOVO might inhibit the transport function of TRPV1.
RR is a non-specific antagonist of TRPV1. RR inhibits a large number of TRP channels, ryanodine receptors and many other membrane proteins (Gustafsson et al., 2005; Maqboul and Elsadek, 2018). In addition, RR is used as a cell surface stain in histology suggesting that it may interact with cell membranes. Therefore, we chose a specific TRPV1 antagonist, SB-705498 (Gunthorpe et al., 2007; Bareille et al., 2013; Holland et al., 2014), as another positive control in our intestinal cell models in order to compare the effect of the inhibition of TRPV1 and the accumulation of CAP in the presence or absence of NOVO.
Next, we used the TRPV1-overexpression and TRPV1-knockdown cell models to evaluate how NOVO affected cell permeability of CAP with different levels of expression of TRPV1. Due to the apparent binding affinity of CAP to TRPV1, the accumulation of CAP may increase in the presence of membrane protein. Furthermore, the protein binding ratio of CAP is higher than 90% (data not shown). Hence, the cells were separated into cytosolic and membrane fractions. The concentrations of CAP in cytosolic fractions were used to estimate intracellular accumulation. These samples excluded the membrane proteins, including TRPV1, to which CAP may bind in the membrane. First, we explored the intracellular accumulation of CAP in the presence of NOVO or SB-705498 in IEC-6/TRPV1+. The data showed that both NOVO and SB-705498 decreased the accumulation of CAP in the cells. Second, we evaluated the effect of SB-705498 and NOVO on the intracellular accumulation of CAP in IEC-6/TRPV1-. The data showed that the accumulation of CAP did not change in IEC-6/TRPV1- cells in the presence of NOVO or SB-705498. These results indicated that TRPV1 played a key role in the effect of NOVO on the accumulation of CAP. Third, IEC-6/TRPV1+ cells were exposed to CAP alone for 60 min, and then NOVO or SB-705498 was added in the culture medium at 60 min. The accumulation of CAP decreased immediately after cells were exposed to SB-705498 or NOVO. Both 5 and 10 μM NOVO showed similar inhibition as SB-705498 did in the accumulation of CAP. These data demonstrated that the increasing accumulation of CAP in the TRPV1-overexpressing cells would decrease rapidly if followed with the specific TRPV1 inhibitor. This observation verified the effect of NOVO on the TRPV1 pathway.
After verifying the effect of NOVO on cellular permeability of CAP, we tried to explore the effects of NOVO on the expression of TRPV1. Rats and TRPV1-overexpressing cells were used as a model to evaluate any correlation between NOVO treatment and TRPV1 expression. TRP channels are integral membrane proteins and require a lipid membrane environment for proper function (Huynh et al., 2014). Several studies have determined the structures of the TRPV1 and revealed its mechanisms of ligand and lipid action (Cao et al., 2013; Liao et al., 2013; Gao et al., 2016), which verified TRPV1 stable expression in the cell membrane (Melnick and Kaviany, 2018). And Salvador et al. indicated that TRPV1 was located on the cell surface in both primary DRG neurons and transfected HEK293 (Sanz-Salvador et al., 2012). Photomicrographs shown in Figure 2G also illustrate that TRPV1 was distributed throughout the transfected cells. Moreover, both the specific TRPV1 inhibitor and NOVO could reduce the fluorescence intensity of TRPV1, compared with the untreated control. TRPV1, known as integral membrane protein, might exhibit the effect as a transporter when it located on the cell surface. Hence, we used Membrane Protein Extraction Kit for enrichment of integral membrane protein for better analysis. The results showed that increasing the concentration of NOVO dose-dependently suppressed the expression of TRPV1 mRNA and protein. It further suggested that NOVO might inhibit TRPV1 by affecting its expression in the intestine and inhibiting the permeation of CAP through membrane. Based on these findings, IEC-6/TRPV1+ cells were pre-incubated with RR, SB-705498, and NOVO for 24 h in order to evaluate any effects of these changes on CAP permeation. The results indicated that NOVO exhibited similar effects as the TRPV1 specific inhibitor SB-705498 in inhibiting the accumulation of CAP after pre-incubation for 24 h. Thus, the reason why the permeability of CAP decreased was that NOVO inhibited the expression of TRPV1. It is known that NOVO is a DNA gyrase inhibitor, and it has been shown that NOVO can affect the regulation of the transcription and translation of many genes (Gmuender et al., 2001; Chatt et al., 2014; Byrd et al., 2016). Therefore, decreased TRPV1 expression may be regulated by one of the genes that are affected by NOVO. However, it is unclear how the signaling pathway works, and more studies should be undertaken.
On the basis of the in vitro study, LC-MS/MS was used to detect the plasma concentration of CAP after oral administration of NOVO and to verify the impact of NOVO on the absorption of CAP in vivo. The in vivo pharmacokinetic study found that NOVO affected the pharmacokinetic properties of CAP in a dose-dependent fashion. This study showed that 12.5–50 mg⋅kg-1 NOVO significantly attenuated Cmax and AUC0-720 min of CAP in a dosage-dependent manner. However, NOVO at 100 mg⋅kg-1 did not affect Cmax and AUC0-720 min of CAP when compared with the CAP-alone group. In addition, we did find that 50 μM NOVO did not affect the permeability of CAP (Table 1). We found that the permeability of CAP increased when the concentration was higher than 100 μM in the Ussing chamber experiment (data not shown). Based on these results, we hypothesized that NOVO would inhibit TRPV1 only at certain dosage. Once the dose of NOVO was out of range, such as the 100 mg⋅kg-1 in our in vivo experiment, the weakening inhibition may be due to saturation or some other unknown effects that lead to an enhancement of the permeation of CAP across the intestine. It is well known that TRPV1 can also affect TJ and regulate the expression of TJ -related proteins, such as occludin, ZO-1, and ZO-2 (Li et al., 2015). In addition, the paracellular pathway, an important pathway for intercellular permeation, is mainly restricted by TJ located on the apical side of epithelial cells (Gunzel and Fromm, 2012). The controlled opening of TJ is a way to increase the absorption of drugs across the epithelium. Hence there might be a possibility, based on the above results, that high concentrations of NOVO would increase the opening of TJ in the intestine, and even damage the structure of TJ, which may have resulted in increasing the permeation of CAP across the intestine. Further investigations are yet to be done to verify this hypothesis. Additional work should also be done using fluorescent CAP analogs (Li et al., 2011) to examine the intracellular accumulation of CAP and the effect of NOVO on CAP internalization (Sanz-Salvador et al., 2012), which would define whether the above findings are specific to CAP or can be generalized to all TRPV1 substrates.
In this study, we demonstrate for the first time that NOVO can regulate the expression of TRPV1 and that this regulation is concentration-dependent. Moreover, we have shown that inhibition of TRPV1 expression might affect the transport of CAP across the intestine and its accumulation in cells. As far as human tissues are concerned, altered TRPV1 expression in nerve fibers has been demonstrated in biopsies from patients with rectal hypersensitivity or inflammatory bowel disease (IBD). Moreover, TRPV1 expression correlated with the degree of abdominal pain in IBD (Yiangou et al., 2001; Chan et al., 2003; Akbar et al., 2008). In rats, stress-induced visceral hypersensitivity, which is dependent on mast cell degranulation and subsequent TRPV1 activation, occurs in the absence of overt inflammation (Kihara et al., 2003; Miranda et al., 2007; van den Wijngaard et al., 2009). NOVO has been clinically used for decades and the therapeutic dose in human is 2 g per day (Kennedy et al., 1995). NOVO can exert its antibiotic effects and at the same time have a significant inhibitory effect on TRPV1 at equivalent doses in rats. The potency of NOVO suggests that it might be a useful therapeutic agent for rectal hypersensitivity or IBD due to its inhibition of TRPV1 along with its antimicrobial actions. It will be interesting to explore whether the inhibition of TRPV1 is specific to aminocoumarin antibiotics or other groups of antibiotics sharing the same properties.
Conclusion
This study demonstrates that NOVO may be a potential TRPV1 inhibitor. In our knowledge, this study is the first of its kind to report that NOVO attenuates the expression of TRPV1 in cells and tissues in vitro and in vivo. Therefore, NOVO can be used to inhibit the transport of TRPV1 substrates and regulate TRPV1 expression.
Author Contributions
QL, GL, and BZ designed the experiment and interpreted the results. QL and QC analyzed the data and wrote the manuscript. QL, XL, and YC performed the experiments. All the authors approved the final manuscript.
Funding
This work was supported by grants from the National Natural Science Foundations of China (No. 81173014), the Natural Science Foundations of Guangdong Province (No. 2015A030313281), and Guangzhou City Science and Technology Plan Projects (No. 2018o4010065).
Conflict of Interest Statement
The authors declare that the research was conducted in the absence of any commercial or financial relationships that could be construed as a potential conflict of interest.
Acknowledgments
We thank Dr. Lv Lisong and LetPub (www.letpub.com) for his linguistic assistance during the preparation of this manuscript.
Abbreviations
ABC, ATP-binding cassette protein family; AUC, Area Under Curve; BCRP, breast cancer resistant protein; CAP, capsaicin; Cmax, maximum plasma concentration; h, hour; IEC-6/TRPV1+, overexpression-TRPV1 IEC-6 cells; IEC-6/TRPV1-, TRPV1-knockdown IEC-6 cells; LDH, lactate dehydrogenase; logP, octanol/water partition coefficients; min, minute; MTT, 3-(4,5-dimethyl-2-thiazolyl)-2,5-diphenyl-2-H-tetrazolium bromide; NOVO, novobiocin; Papp, Apparent Permeability; qRT-PCR, quantitative real time PCR; RR, ruthenium red; SD, Sprague Dawley; TJ, tight junction; Tmax, time to peak; TRPV1, Transient receptor potential cation channel subfamily V member 1.
References
Akbar, A., Yiangou, Y., Facer, P., Walters, J. R., Anand, P., and Ghosh, S. (2008). Increased capsaicin receptor TRPV1-expressing sensory fibres in irritable bowel syndrome and their correlation with abdominal pain. Gut 57, 923–929. doi: 10.1136/gut.2007.138982
Bareille, P., Murdoch, R. D., Denyer, J., Bentley, J., Smart, K., Yarnall, K., et al. (2013). The effects of a TRPV1 antagonist, SB-705498, in the treatment of seasonal allergic rhinitis. Int. J. Clin. Pharmacol. Ther. 51, 576–584. doi: 10.5414/CP201890
Brokaw, J. J., and White, G. W. (1995). Characterization of ruthenium red as an inhibitor of neurogenic inflammation in the rat trachea. Gen. Pharmacol. 26, 327–331. doi: 10.1016/0306-3623(94)00202-X
Byrd, K. M., Subramanian, C., Sanchez, J., Motiwala, H. F., Liu, W., Cohen, M. S., et al. (2016). Synthesis and biological evaluation of novobiocin core analogues as Hsp90 inhibitors. Chemistry 22, 6921–6931. doi: 10.1002/chem.201504955
Cao, E., Liao, M., Cheng, Y., and Julius, D. (2013). TRPV1 structures in distinct conformations reveal activation mechanisms. Nature 504, 113–118. doi: 10.1038/nature12823
Caterina, M. J., Schumacher, M. A., Tominaga, M., Rosen, T. A., Levine, J. D., and Julius, D. (1997). The capsaicin receptor: a heat-activated ion channel in the pain pathway. Nature 389, 816–824. doi: 10.1038/39807
Chahl, L. A. (1989). The effects of ruthenium red on the response of guinea-pig ileum to capsaicin. Eur. J. Pharmacol. 169, 241–247. doi: 10.1016/0014-2999(89)90021-6
Chan, C. L., Facer, P., Davis, J. B., Smith, G. D., Egerton, J., Bountra, C., et al. (2003). Sensory fibres expressing capsaicin receptor TRPV1 in patients with rectal hypersensitivity and faecal urgency. Lancet 361, 385–391. doi: 10.1016/S0140-6736(03)12392-6
Chatt, A., Aloui, M., Tagourti, J., Mihoub, M., and Landoulsi, A. (2014). Novobiocin sensitivity of Salmonella typhimurium dam and/or seqA mutants. Pol. J. Microbiol. 63, 51–56.
Curtis, M. J., Bond, R. A., Spina, D., Ahluwalia, A., Alexander, S. P., Giembycz, M. A., et al. (2015). Experimental design and analysis and their reporting: new guidance for publication in BJP. Br. J. Pharmacol. 172, 3461–3471. doi: 10.1111/bph.12856
Dray, A., Forbes, C. A., and Burgess, G. M. (1990). Ruthenium red blocks the capsaicin-induced increase in intracellular calcium and activation of membrane currents in sensory neurones as well as the activation of peripheral nociceptors in vitro. Neurosci. Lett. 110, 52–59. doi: 10.1016/0304-3940(90)90786-9
Duan, L., Yan, Y., Sun, Y., Zhao, B., Hu, W., and Li, G. (2013). Contribution of TRPV1 and multidrug resistance proteins in the permeation of capsaicin across different intestinal regions. Int. J. Pharm. 445, 134–140. doi: 10.1016/j.ijpharm.2013.02.007
Funakoshi, K., Nakano, M., Atobe, Y., Goris, R. C., Kadota, T., and Yazama, F. (2006). Differential development of TRPV1-expressing sensory nerves in peripheral organs. Cell Tissue Res. 323, 27–41. doi: 10.1007/s00441-005-0013-3
Gao, Y., Cao, E., Julius, D., and Cheng, Y. (2016). TRPV1 structures in nanodiscs reveal mechanisms of ligand and lipid action. Nature 534, 347–351. doi: 10.1038/nature17964
Georgescu, S. R., Sarbu, M. I., Matei, C., Ilie, M. A., Caruntu, C., Constantin, C., et al. (2017). Capsaicin: friend or foe in skin cancer and other related malignancies? Nutrients 9:E1365. doi: 10.3390/nu9121365
Gmuender, H., Kuratli, K., Di Padova, K., Gray, C. P., Keck, W., and Evers, S. (2001). Gene expression changes triggered by exposure of Haemophilus influenzae to novobiocin or ciprofloxacin: combined transcription and translation analysis. Genome Res. 11, 28–42. doi: 10.1101/gr.157701
Gombert, M. E., and Aulicino, T. M. (1984). Susceptibility of multiply antibiotic-resistant pneumococci to the new quinoline antibiotics, nalidixic acid, coumermycin, and novobiocin. Antimicrob. Agents Chemother. 26, 933–934. doi: 10.1128/AAC.26.6.933
Gunthorpe, M. J., Hannan, S. L., Smart, D., Jerman, J. C., Arpino, S., Smith, G. D., et al. (2007). Characterization of SB-705498, a potent and selective vanilloid receptor-1 (VR1/TRPV1) antagonist that inhibits the capsaicin-, acid-, and heat-mediated activation of the receptor. J. Pharmacol. Exp. Ther. 321, 1183–1192. doi: 10.1124/jpet.106.116657
Gunzel, D., and Fromm, M. (2012). Claudins and other tight junction proteins. Compr. Physiol. 2, 1819–1852. doi: 10.1002/cphy.c110045
Guo, L., Duan, L., Dong, X., Dou, L. L., Zhou, P., Liu, E. H., et al. (2014). A simple and sensitive LC-MS/MS method for determination of miltirone in rat plasma and its application to pharmacokinetic studies. J. Chromatogr. B Analyt. Technol. Biomed. Life Sci. 973C, 33–38. doi: 10.1016/j.jchromb.2014.10.008
Gustafsson, A. J., Ingelman-Sundberg, H., Dzabic, M., Awasum, J., Nguyen, K. H., Ostenson, C. G., et al. (2005). Ryanodine receptor-operated activation of TRP-like channels can trigger critical Ca2 + signaling events in pancreatic beta-cells. FASEB J. 19, 301–303. doi: 10.1096/fj.04-2621fje
Hanson, S. M., Newstead, S., Swartz, K. J., and Sansom, M. S. P. (2015). Capsaicin interaction with TRPV1 channels in a lipid bilayer: molecular dynamics simulation. Biophys. J. 108, 1425–1434. doi: 10.1016/j.bpj.2015.02.013
Hellwig, N., Plant, T. D., Janson, W., Schafer, M., Schultz, G., and Schaefer, M. (2004). TRPV1 acts as proton channel to induce acidification in nociceptive neurons. J. Biol. Chem. 279, 34553–34561. doi: 10.1074/jbc.M402966200
Holland, C., van Drunen, C., Denyer, J., Smart, K., Segboer, C., Terreehorst, I., et al. (2014). Inhibition of capsaicin-driven nasal hyper-reactivity by SB-705498, a TRPV1 antagonist. Br. J. Clin. Pharmacol. 77, 777–788. doi: 10.1111/bcp.12219
Huynh, K. W., Cohen, M. R., and Moiseenkova-Bell, V. Y. (2014). Application of amphipols for structure-functional analysis of TRP channels. J. Membr. Biol. 247, 843–851. doi: 10.1007/s00232-014-9684-6
Iida, T., Moriyama, T., Kobata, K., Morita, A., Murayama, N., Hashizume, S., et al. (2003). TRPV1 activation and induction of nociceptive response by a non-pungent capsaicin-like compound, capsiate. Neuropharmacology 44, 958–967. doi: 10.1016/S0028-3908(03)00100-X
Ishibashi, T., Takumida, M., Akagi, N., Hirakawa, K., and Anniko, M. (2009). Changes in transient receptor potential vanilloid (TRPV) 1, 2, 3 and 4 expression in mouse inner ear following gentamicin challenge. Acta Otolaryngol. 129, 116–126. doi: 10.1080/00016480802032835
Jones, R. N. (1989). Should novobiocin be clinically re-evaluated? Diagn. Microbiol. Infect. Dis. 12, 363–365. doi: 10.1016/0732-8893(89)90105-3
Kennedy, M. J., Armstrong, D. K., Huelskamp, A. M., Ohly, K., Clarke, B. V., Colvin, O. M., et al. (1995). Phase I and pharmacologic study of the alkylating agent modulator novobiocin in combination with high-dose chemotherapy for the treatment of metastatic breast cancer. J. Clin. Oncol. 13, 1136–1143. doi: 10.1200/JCO.1995.13.5.1136
Kihara, N., de la Fuente, S. G., Fujino, K., Takahashi, T., Pappas, T. N., and Mantyh, C. R. (2003). Vanilloid receptor-1 containing primary sensory neurones mediate dextran sulphate sodium induced colitis in rats. Gut 52, 713–719. doi: 10.1136/gut.52.5.713
Kilkenny, C., Browne, W., Cuthill, I. C., Emerson, M., Altman, D. G., and NC3Rs Reporting Guidelines Working Group. (2010). Animal research: reporting in vivo experiments: the ARRIVE guidelines. Br. J. Pharmacol. 160, 1577–1579. doi: 10.1111/j.1476-5381.2010.00872.x
Komori, Y., Aiba, T., Nakai, C., Sugiyama, R., Kawasaki, H., and Kurosaki, Y. (2007a). Capsaicin-induced increase of intestinal cefazolin absorption in rats. Drug Metab. Pharmacokinet. 22, 445–449.
Komori, Y., Aiba, T., Sugiyama, R., Nakai, C., Kawasaki, H., and Kurosaki, Y. (2007b). Effects of capsaicin on intestinal cephalexin absorption in rats. Biol. Pharm. Bull. 30, 547–551.
Kono, T., Kaneko, A., Omiya, Y., Ohbuchi, K., Ohno, N., and Yamamoto, M. (2013). Epithelial transient receptor potential ankyrin 1 (TRPA1)-dependent adrenomedullin upregulates blood flow in rat small intestine. Am. J. Physiol. Gastrointest. Liver Physiol. 304, G428–G436. doi: 10.1152/ajpgi.00356.2012
Lee, J. H., Park, C., Kim, S. J., Kim, H. J., Oh, G. S., Shen, A., et al. (2013). Different uptake of gentamicin through TRPV1 and TRPV4 channels determines cochlear hair cell vulnerability. Exp. Mol. Med. 45:e12. doi: 10.1038/emm.2013.25
Li, H., Wang, S., Chuang, A. Y., Cohen, B. E., and Chuang, H. H. (2011). Activity-dependent targeting of TRPV1 with a pore-permeating capsaicin analog. Proc. Natl. Acad. Sci. U.S.A. 108, 8497–8502. doi: 10.1073/pnas.1018550108
Li, J., Cong, X., Zhang, Y., Xiang, R. L., Mei, M., Yang, N. Y., et al. (2015). ZO-1 and -2 are required for TRPV1-modulated paracellular permeability. J. Dent. Res. 94, 1748–1756. doi: 10.1177/0022034515609268
Liao, M., Cao, E., Julius, D., and Cheng, Y. (2013). Structure of the TRPV1 ion channel determined by electron cryo-microscopy. Nature 504, 107–112. doi: 10.1038/nature12822
Livak, K. J., and Schmittgen, T. D. (2001). Analysis of relative gene expression data using real-time quantitative PCR and the 2(-Delta Delta C(T)) Method. Methods 25, 402–408. doi: 10.1006/meth.2001.1262
Lou, Y. P., Karlsson, J. A., Franco-Cereceda, A., and Lundberg, J. M. (1991). Selectivity of ruthenium red in inhibiting bronchoconstriction and CGRP release induced by afferent C-fibre activation in the guinea-pig lung. Acta Physiol. Scand. 142, 191–199. doi: 10.1111/j.1748-1716.1991.tb09147.x
Maqboul, A., and Elsadek, B. (2018). Expression profiles of TRPV1, TRPV4, TLR4 and ERK1/2 in the dorsal root ganglionic neurons of a cancer-induced neuropathy rat model. PeerJ 6:e4622. doi: 10.7717/peerj.4622
McGrath, J. C., and Lilley, E. (2015). Implementing guidelines on reporting research using animals (ARRIVE etc.): new requirements for publication in BJP. Br. J. Pharmacol. 172, 3189–3193. doi: 10.1111/bph.12955
Melnick, C., and Kaviany, M. (2018). Thermal actuation in TRPV1: role of embedded lipids and intracellular domains. J. Theor. Biol. 444, 38–49. doi: 10.1016/j.jtbi.2018.02.004
Meyers, J. R., MacDonald, R. B., Duggan, A., Lenzi, D., Standaert, D. G., Corwin, J. T., et al. (2003). Lighting up the senses: FM1-43 loading of sensory cells through nonselective ion channels. J. Neurosci. 23, 4054–4065. doi: 10.1523/JNEUROSCI.23-10-04054.2003
Miranda, A., Nordstrom, E., Mannem, A., Smith, C., Banerjee, B., and Sengupta, J. N. (2007). The role of transient receptor potential vanilloid 1 in mechanical and chemical visceral hyperalgesia following experimental colitis. Neuroscience 148, 1021–1032. doi: 10.1016/j.neuroscience.2007.05.034
Munns, C. H., Chung, M. K., Sanchez, Y. E., Amzel, L. M., and Caterina, M. J. (2015). Role of the outer pore domain in transient receptor potential vanilloid 1 dynamic permeability to large cations. J. Biol. Chem. 290, 5707–5724. doi: 10.1074/jbc.M114.597435
Myrdal, S. E., and Steyger, P. S. (2005). TRPV1 regulators mediate gentamicin penetration of cultured kidney cells. Hear. Res. 204, 170–182. doi: 10.1016/j.heares.2005.02.005
Ocana, M., and Baeyens, J. M. (1991). Analgesic effects of centrally administered aminoglycoside antibiotics in mice. Neurosci. Lett. 126, 67–70. doi: 10.1016/0304-3940(91)90373-2
Prado, W. A., and Machado Filho, E. B. (2002). Antinociceptive potency of aminoglycoside antibiotics and magnesium chloride: a comparative study on models of phasic and incisional pain in rats. Braz. J. Med. Biol. Res. 35, 395–403. doi: 10.1590/S0100-879X2002000300017
Quaroni, A., Wands, J., Trelstad, R. L., and Isselbacher, K. J. (1979). Epithelioid cell cultures from rat small intestine. Characterization by morphologic and immunologic criteria. J. Cell Biol. 80, 248–265. doi: 10.1083/jcb.80.2.248
Sanz-Salvador, L., Andres-Borderia, A., Ferrer-Montiel, A., and Planells-Cases, R. (2012). Agonist- and Ca2 + -dependent desensitization of TRPV1 channel targets the receptor to lysosomes for degradation. J. Biol. Chem. 287, 19462–19471. doi: 10.1074/jbc.M111.289751
Schmutz, E., Muhlenweg, A., Li, S. M., and Heide, L. (2003). Resistance genes of aminocoumarin producers: two type II topoisomerase genes confer resistance against coumermycin A1 and clorobiocin. Antimicrob. Agents Chemother. 47, 869–877. doi: 10.1128/AAC.47.3.869-877.2003
Sinko, P. J., Kunta, J. R., Usansky, H. H., and Perry, B. A. (2004). Differentiation of gut and hepatic first pass metabolism and secretion of saquinavir in ported rabbits. J. Pharmacol. Exp. Ther. 310, 359–366. doi: 10.1124/jpet.103.064394
Su, Y., Hu, P., Lee, S. H., and Sinko, P. J. (2007). Using novobiocin as a specific inhibitor of breast cancer resistant protein to assess the role of transporter in the absorption and disposition of topotecan. J. Pharm. Pharm. Sci. 10, 519–536. doi: 10.18433/J3QP4W
Su, Y., Lee, S. H., and Sinko, P. J. (2006). Inhibition of efflux transporter ABCG2/BCRP does not restore mitoxantrone sensitivity in irinotecan-selected human leukemia CPT-K5 cells: evidence for multifactorial multidrug resistance. Eur. J. Pharm. Sci. 29, 102–110. doi: 10.1016/j.ejps.2006.06.001
Sumano-Lopez, H., Gutierrez-Olvera, L., Aguilera-Jimenez, R., Gutierrez-Olvera, C., and Jimenez-Gomez, F. (2007). Administration of ciprofloxacin and capsaicin in rats to achieve higher maximal serum concentrations. Arzneimittelforschung 57, 286–290. doi: 10.1055/s-0031-1296620
Tominaga, M., Caterina, M. J., Malmberg, A. B., Rosen, T. A., Gilbert, H., Skinner, K., et al. (1998). The cloned capsaicin receptor integrates multiple pain-producing stimuli. Neuron 21, 531–543. doi: 10.1016/S0896-6273(00)80564-4
van den Wijngaard, R. M., Klooker, T. K., Welting, O., Stanisor, O. I., Wouters, M. M., van der Coelen, D., et al. (2009). Essential role for TRPV1 in stress-induced (mast cell-dependent) colonic hypersensitivity in maternally separated rats. Neurogastroenterol. Motil. 21:1107-e94. doi: 10.1111/j.1365-2982.2009.01339.x
Vriens, J., Appendino, G., and Nilius, B. (2009). Pharmacology of vanilloid transient receptor potential cation channels. Mol. Pharmacol. 75, 1262–1279. doi: 10.1124/mol.109.055624
Wallon, C., Braaf, Y., Wolving, M., Olaison, G., and Soderholm, J. D. (2005). Endoscopic biopsies in Ussing chambers evaluated for studies of macromolecular permeability in the human colon. Scand. J. Gastroenterol. 40, 586–595. doi: 10.1080/00365520510012235
Yamawaki, H., Mihara, H., Suzuki, N., Nishizono, H., Uchida, K., Watanabe, S., et al. (2014). Role of transient receptor potential vanilloid 4 activation in indomethacin-induced intestinal damage. Am. J. Physiol. Gastrointest. Liver Physiol. 307, G33–G40. doi: 10.1152/ajpgi.00105.2013
Yiangou, Y., Facer, P., Ford, A., Brady, C., Wiseman, O., Fowler, C. J., et al. (2001). Capsaicin receptor VR1 and ATP-gated ion channel P2X3 in human urinary bladder. BJU Int. 87, 774–779. doi: 10.1046/j.1464-410x.2001.02190.x
Yodoya, E., Uemura, K., Tenma, T., Fujita, T., Murakami, M., Yamamoto, A., et al. (1994). Enhanced permeability of tetragastrin across the rat intestinal membrane and its reduced degradation by acylation with various fatty acids. J. Pharmacol. Exp. Ther. 271, 1509–1513.
You, F., Hu, J., Li, X., and Li, Y. (2013). The elimination of MTC-220, a novel anti-tumor agent of conjugate of paclitaxel and muramyl dipeptide analogue, in rats. Cancer Chemother. Pharmacol. 71, 1453–1462. doi: 10.1007/s00280-013-2144-7
Yu, T., Lu, X. J., Li, J. Y., Shan, T. D., Huang, C. Z., Ouyang, H., et al. (2016). Overexpression of miR-429 impairs intestinal barrier function in diabetic mice by down-regulating occludin expression. Cell Tissue Res. 366, 341–352. doi: 10.1007/s00441-016-2435-5
Zhou, Y., Xu, X., Yu, B., and Yu, G. (2017). Characterization of in vitro effects of microcystin-LR on intestinal epithelial cells. Environ. Toxicol. 32, 1539–1547. doi: 10.1002/tox.22375
Keywords: novobiocin, capsaicin, TRPV1, inhibitor, expression
Citation: Liang Q, Lv X, Cai Q, Cai Y, Zhao B and Li G (2018) Novobiocin, a Newly Found TRPV1 Inhibitor, Attenuates the Expression of TRPV1 in Rat Intestine and Intestinal Epithelial Cell Line IEC-6. Front. Pharmacol. 9:1171. doi: 10.3389/fphar.2018.01171
Received: 08 June 2018; Accepted: 26 September 2018;
Published: 15 October 2018.
Edited by:
Salvatore Salomone, Università degli Studi di Catania, ItalyReviewed by:
Bidhan C. Bandyopadhyay, Washington DC VA Medical Center, United StatesPeter S. Reinach, Wenzhou Medical University, China
Copyright © 2018 Liang, Lv, Cai, Cai, Zhao and Li. This is an open-access article distributed under the terms of the Creative Commons Attribution License (CC BY). The use, distribution or reproduction in other forums is permitted, provided the original author(s) and the copyright owner(s) are credited and that the original publication in this journal is cited, in accordance with accepted academic practice. No use, distribution or reproduction is permitted which does not comply with these terms.
*Correspondence: Boxin Zhao, Ym94aW5femhhb0AxMjYuY29t Guofeng Li, bGlndW9mZW5nX2dkQDEyNi5jb20=