- 1Faculty of Medicine, University of Limoges, Limoges, France
- 2Department of Internal Medicine, University Hospital Limoges Dupuytren Hospital, Limoges, France
- 3Department of Immunology, University Hospital Limoges Dupuytren Hospital, Limoges, France
Sortilin, also known as Neurotensin Receptor-3, and the sorting-related receptor with type-A repeats (SorLA) are both members of the Vps10p domain receptor family. Initially identified in CNS cells, they are expressed in various other cell types where they exert multiple functions. Although mostly studied for its involvement in Alzheimer’s disease, SorLA has recently been shown to be implicated in immune response by regulating IL-6-mediated signaling, as well as driving monocyte migration. Sortilin has been shown to act as a receptor, as a co-receptor and as an intra- and extracellular trafficking regulator. In the last two decades, deregulation of sortilin has been demonstrated to be involved in many human pathophysiologies, including neurodegenerative disorders (Alzheimer and Parkinson diseases), type 2 diabetes and obesity, cancer, and cardiovascular pathologies such as atherosclerosis. Several studies highlighted different functions of sortilin in the immune system, notably in microglia, pro-inflammatory cytokine regulation, phagosome fusion and pathogen clearance. In this review, we will analyze the multiple roles of sortilin and SorLA in the human immune system and how their deregulation may be involved in disease development.
Introduction
The vacuolar protein sorting 10 protein (Vps10p) domain receptors family is composed of five members: sortilin, SorLA, sorCS1, sorCS2, and sorCS3. In adult human, Vps10p receptors expression was primarily shown in the brain but has also been detected in various other tissues and organs (Hermey, 2009). They also have a dynamic and transient expression during embryonic and post-natal development in rodents, mainly in the nervous system (Hermans-Borgmeyer et al., 1999). Moreover, sortilin is highly expressed in embryonic lung, and SorLA in embryonic lung, kidney, and developing glands (Hermey, 2009). These observations suggest specific functions of sortilin and SorLA in the developing organs.
Since the discovery in the 1990s of sortilin (Petersen et al., 1997; Mazella et al., 1998) and SorLA (Jacobsen et al., 1996), these two proteins have been extensively studied for their functions as regulators of intracellular trafficking through their Vps10p domain. Vps10p is an extracellular ligand binding domain of 700 amino acids folded in a ten-bladed β-propeller (Quistgaard et al., 2009; Kitago et al., 2015). Vps10 domain proteins (Figure 1A) are subjected to various trafficking paths (Figure 1B): (i) transport from the Trans-Golgi Network (TGN) to the plasma membrane where they can act as receptors, or be shedded by metalloproteases and γ-secretases to finally be released as soluble proteins (Hermey et al., 2006; Nyborg et al., 2006; Ohwaki et al., 2007; Evans et al., 2011); (ii) clathrin-dependent internalization from the plasma membrane to endosomes (Nielsen et al., 2001; Morinville et al., 2004) and then either to the TGN through the retromer complex (Mari et al., 2007; Seaman, 2007) or to lysosomes for degradation (Dumanis et al., 2015; Tanimoto et al., 2017); (iii) finally they can be exported from the TGN to the extracellular medium through secretory granules (Yang et al., 2011) or extracellular vesicles such as exosomes (Wilson et al., 2014b).
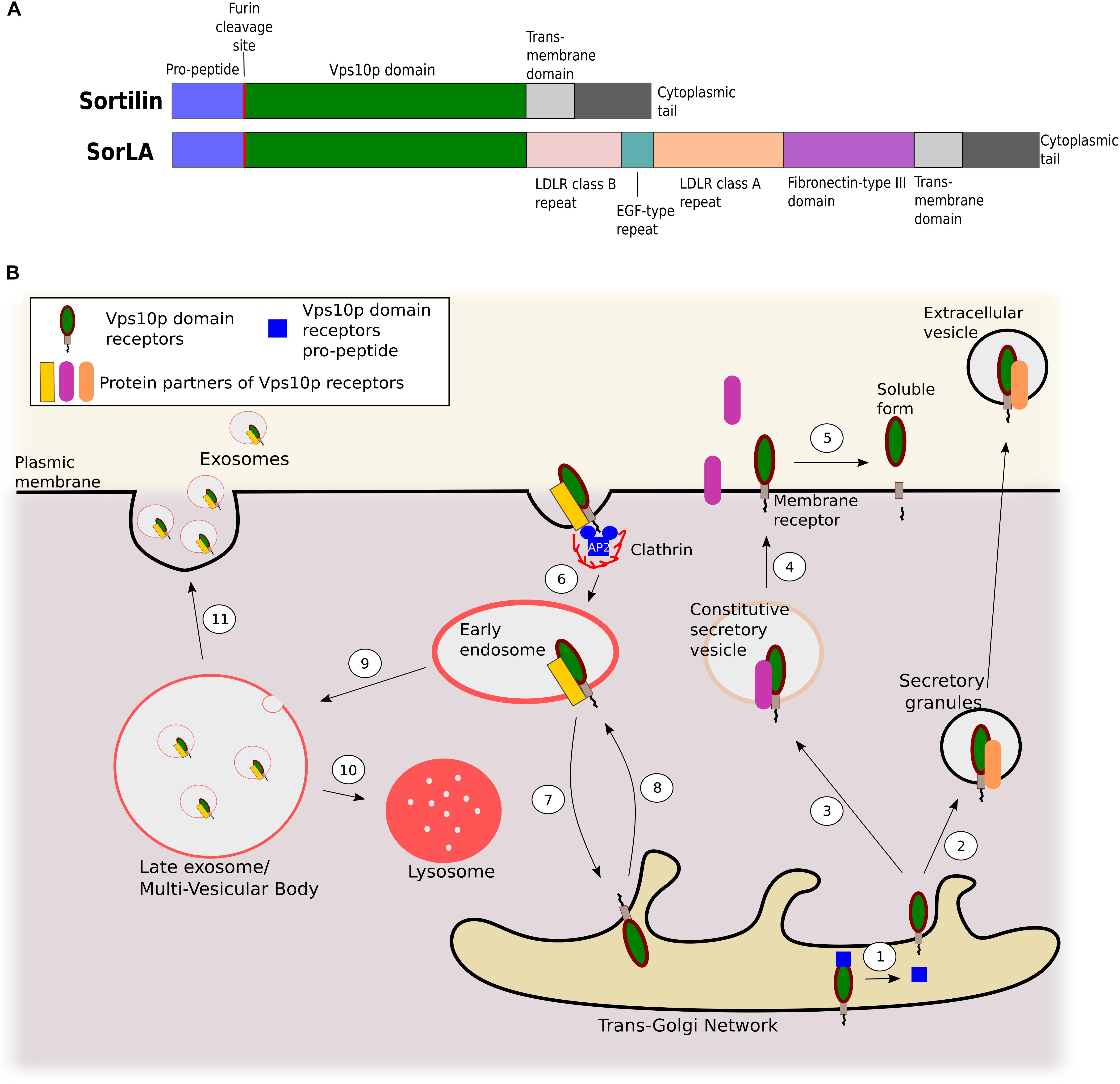
Figure 1. Role of Vps10p domain receptors in intracellular protein trafficking. (A) Schematic representation of sortilin and SorLA protein domains. (B) Trafficking pathways of Vps10p domain receptors. The propeptide is cleaved by pro-protein convertases in the trans Golgi network (step 1). Vps10p domain receptors associated with a protein partner can then be secreted from the TGN to extracellular vesicles through secretory granules (step 2) or addressed to the plasma membrane via constitutive secretory vesicles. Partners can either be anchored to the membrane or secreted as soluble proteins (steps 3–4). Once anchored to the membrane, Vps10p domain receptors can undergo proteolytic cleavage by a disintegrin and metalloprotease (ADAM) 10 or ADAM17, followed by γ-secretase, and be released under a soluble form (step 5). They can mediate internalization of partners from the plasma membrane through AP-2/clathrin-dependent endocytosis (step 6). They can navigate, alone or with partners, between early endosomes and the TGN via the retromer complex (step 7) or anterograde transport via interaction with GCAs/AP-1 (step 8). Early endosomes mature into late endosomes or multi-vesicular bodies (step 9). From there, Vps10p domain receptors and their partners can either be addressed to the lysosome for degradation (step 10) or exocytized in exosomes (step 11).
Both sortilin and SorLA have a wide variety of ligands and can either act as sorting regulators or receptors/co-receptors for cell signaling. Therefore, they are involved in many processes depending on cell type, as well as in many associated cellular disorders. Sortilin and SorLA are both involved in neurotensin (NTS) (Mazella et al., 1998; Jacobsen et al., 2001; Mazella, 2001) and neurotrophins signaling (Nykjaer et al., 2004; Teng et al., 2005; Rohe et al., 2009, 2013; Larsen et al., 2010, 2016). They are implicated in Alzheimer’s disease development since sortilin interacts with apoE (Carlo et al., 2013) and SorLA regulates amyloid precursor protein and amyloid-β peptides sorting (Andersen et al., 2005; Offe et al., 2006). Sortilin also regulates glucose transporter (Glut-4) sorting (Pan et al., 2017). Moreover, SorLA has been described as a risk factor for obesity (Whittle et al., 2015; Schmidt et al., 2016). Both proteins are involved in cardiovascular and metabolic diseases, such as atherosclerosis and type-2 diabetes, because of their lipoproteins regulatory functions reviewed in (Schmidt and Willnow, 2016). Finally, sortilin has been shown to be implicated in the development of different cancers (Wilson et al., 2016).
Few studies showed the expression of sortilin and SorLA and their potential functions in lymphoid tissues and bone marrow (BM). Sortilin is expressed in T and B lymphocytes, dendritic cells, NK cells, macrophages and microglia (Martin et al., 2003; Fauchais et al., 2008; Herda et al., 2012; Yabe-Wada et al., 2016) and SorLA expression was detected in monocytes, T and B cells and hematopoietic precursors (Zhang et al., 2000; Sakai et al., 2012). Here, we reviewed their involvement in immune-related processes, their known protein partners during these processes, and the pathologies associated with their deregulation.
Sortilin, SorLA, and Inflammation
Inflammation is the organism’s response to harmful stimuli. Upon exposure of tissues and organs to pathogens or toxic products, both innate and adaptive immune responses are activated at the inflammatory site. Main inflammation actors are innate and adaptive immune cells (macrophages, monocytes, dendritic cells, T-cells, B-cells…) which are recruited to the inflammatory site via pro-inflammatory chemokines. Inflammation mechanisms are controlled by pro-inflammatory cytokines, inducing complex intracellular signaling pathways. These cytokines are mainly interleukin (IL)-1, IL-6, IL-17, TNF-α and types I, II, and III interferon (IFN). Disruption of these factors may lead to auto-inflammatory and pro-inflammatory disorders (Turner et al., 2014).
Sortilin, SorLA, and Pro-inflammatory Cytokines
Sortilin Regulates Pro-inflammatory Cytokines Exocytosis and Signaling
Recent studies have highlighted the involvement of sortilin in the regulation of cytokines secretion during different immune functions, related to cell cytotoxicity and inflammation, through the control of IFN-γ and IL-6 exocytosis.
These functions were deduced from experimental models of Sort1 knock-out (sort1-/-) C57BL/6 mice. In natural killer cells (NK) and cytotoxic T lymphocytes (CTL), sortilin deficiency impaired the endosomal trafficking of cytolytic vesicles containing granzyme A. An upregulation of vesicle-associated membrane protein 7 (VAMP7), a late endosomal trafficking regulator, was observed due to its diminished lysosomal degradation. This was associated with the increase of granzyme A release and cytotoxic activity of CTL and NK cells (Herda et al., 2012). In this model, IFN-γ retention was detected in the Golgi network of CTLs, NKs, and Th1 cells. Indeed, sortilin interacts with IFN-γ in early endosomes sorting platforms at the TGN–toward different vesicle compartments: recycling endosomes, late endosomes or secretory lysosomes. The consequences of sortilin inactivation are demonstrated both during bacterial infection leading to a decreased IFN-γ release and in experimental autoimmune colitis reducing inflammatory lesions. In these models, TNF-α secretion was not modified and remains independent from sortilin binding and trafficking. Hence, through the regulation of both granzyme A and IFN-γ release, sortilin regulates the immune functions of T lymphocytes and NK cells during adaptive immune responses and target cell killing (Herda et al., 2012).
In addition to INF-γ, sortilin binding to IL-6 was demonstrated in LPS-activated type 1 macrophages (M1). This interaction depends on its extracellular domain and occurs intracellularly to regulate their secretion (Mortensen et al., 2014). IL-6 and IFN-γ effects are synergistic since IL-6 stimulates Th1 activation and IFN-γ secretion, and IFN-γ promotes IL-6 production by macrophages. In a C57BL/6 mouse model of atherosclerosis, the absence of sortilin induced a defect of IL-6 and IFN-γ secretion in activated macrophages and Th1 cells, reducing the inflammatory component of vascular lesions and atherosclerosis, independently of sortilin effect on lipid metabolism. Hence, by directly regulating IFN-γ and IL-6 secretion, sortilin could be a key regulator of inflammatory response increasing inflammatory component of atherosclerosis (Mortensen et al., 2014). Its deregulation in other inflammatory diseases needs to be investigated.
Beside IFN-γ and IL-6, surface plasmon resonance analysis demonstrated that sortilin also binds to other cytokines IFN-α, IL-17A, IL-10, and IL-12 (Yabe-Wada et al., 2016). The functions of sortilin in the exocytic trafficking of IFN-α was demonstrated in plasmacytoid dendritic cells (pDCs), known to secrete IFN-α (Yabe-Wada et al., 2016). This IFN trafficking depends on the dimerization of the sortilin ectodomain in acidic pH conditions encountered in the RE and TGN (Yabe-Wada et al., 2018). Through TLR9 activation, IFN-α release depends on sortilin and is diminished in sortilin-inactivated pDCs, without affecting its RNA transcription. However, a sustained TLR9 activation induces a subsequent negative control of IFN-α release depending on a post transcriptional degradation of sortilin, induced by TLR activation. Given the importance of IFN-α in antiviral immunity or in certain autoimmune diseases such as systemic lupus erythematous, it would be interesting to further study the role of sortilin in the regulation of type I IFN secretion in these related disorders.
In addition to the regulation of cytokine exocytosis, sortilin also regulates their signaling as reported for ciliary neurotrophic factor (CNTF) belonging to IL-6 family of cytokines sharing a common gp130 subunit receptor. This family includes IL-6, IL-11, IL-27, leukemia inhibitory factor (LIF), oncostatin M (OSM), ciliary neurotrophic factor (CNTF), cardiotrophin-1 (CT-1), cardiotrophin-like cytokine (CLC), neuropoietin (NP), and IL-31 (Scheller et al., 2011). CNTF activation depends on its binding to the receptor CNTFRα, followed by the recruitment of the heterodimeric complex gp130/LIFRβ receptor, leading to Janus Kinase (JAK) and STAT3 activation/phosphorylation. Sortilin binds the C-terminus tail of CNTF with a high affinity, at a distinct site of CNTFRβ binding, and mediates rapid uptake and clearance of extracellular CNTF. Sortilin also interacts with LIFRβ, and thus facilitates CNTF signaling through the heterodimer gp130/LIFRβ regardless of CNTF or CNTFRα binding to sortilin (Figure 2A). Similar interactions of CLC:CLF-1 with sortilin have been observed. However, its implication in endocytosis and/or secretion process of the cytokine remains unknown. According to the direct interaction of sortilin with LIFRβ subunit, it is worth noting that sortilin facilitates cytokine signaling depending on the LIFRβ subunit receptor. This concerns cytokines CT-1, LIF, OSM that engage the gp130/LIFRβ complex, but not IL-6 which engages the homodimer gp130 (Larsen et al., 2010). Thus sortilin is a key regulator of gp130/LIFRβ-IL-6 family in both physiological and pathophysiological processes, such as B-cell stimulation, the control of regulatory and effector T cells balance, metabolic functions, neural functions or autoimmune diseases (extensively reviewed in Rose-John, 2018). Further studies may highlight an important role of sortilin in the modulation of cell signaling induced by this pleiotropic cytokine family.
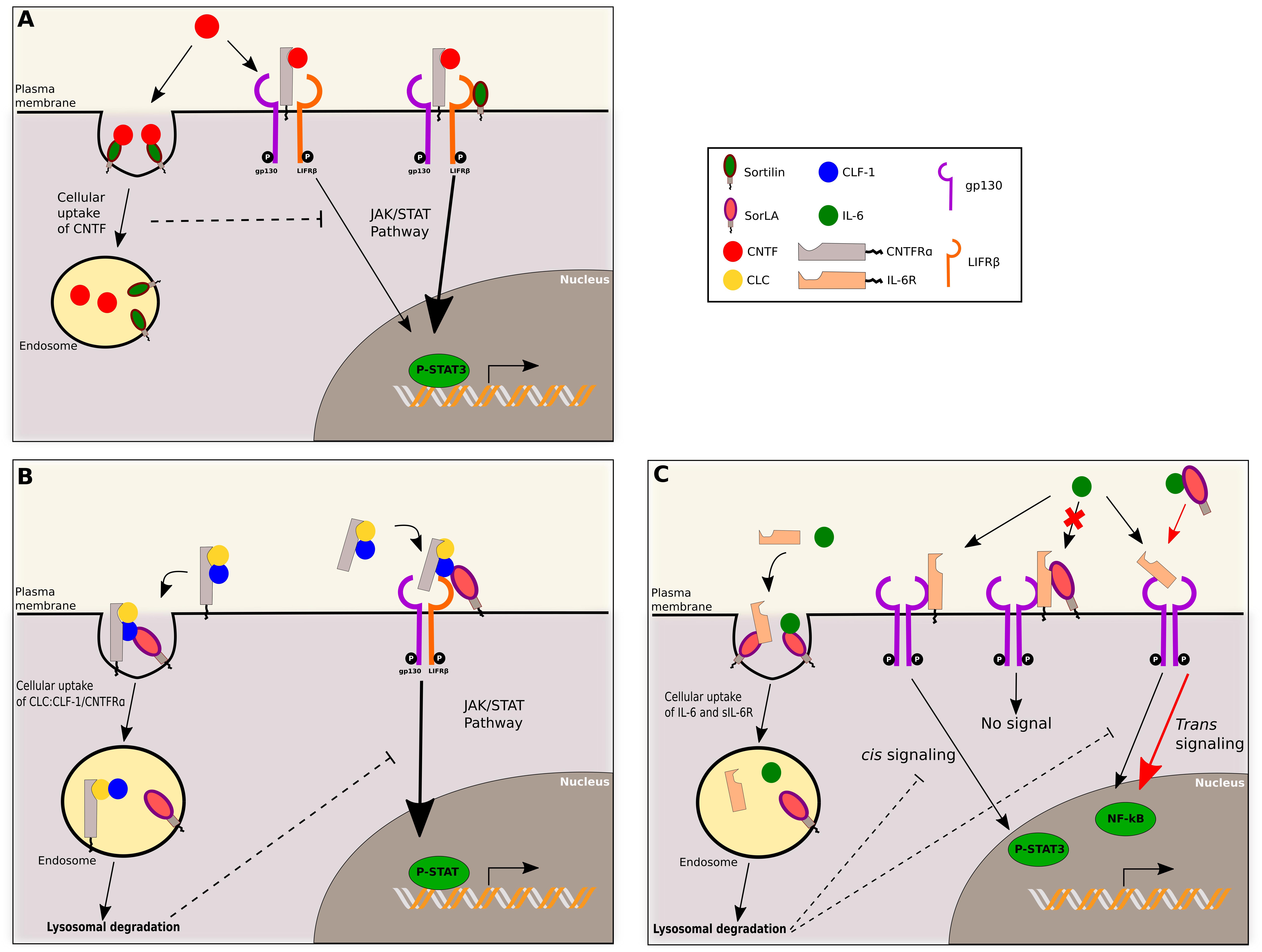
Figure 2. Sortilin and SorLA regulate IL-6 family signaling. (A) CNTF binds to CNTFRα, this complex engages the heterodimer gp130/LIFRβ to induce JAK/STAT pathway signaling. Sortilin can bind to LIFRβ and enhance this JAK/STAT signaling. Sortilin also mediates soluble CNTF cellular uptake, and decreases associated signaling. (B) SorLA interacts with CLF-1. CLF-1 can form a complex with CLC and CNTFRα. If this complex is soluble, SorLA binds to CLF-1 and concentrates the complex on the membrane, enhancing interaction with gp130/LIFRβ and JAK/STAT signaling. If this complex is anchored to the membrane, SorLA binds to CLF-1 and mediates endocytosis of the complex, followed by its degradation, decreasing associated signaling. (C) SorLA can bind to IL-6 or IL-6R. Binding of IL-6 to membrane anchored IL-6R, in association with gp130 homodimer, induces cis signaling (JAK/STAT pathway). SorLA can bind IL-6R and inhibits IL-6 binding and subsequent signaling. IL-6 can also engage soluble IL-6R, associated with gp130 homodimer, and induce trans signaling (NF-kB pathway). SorLA may bind to soluble IL-6 and act as a stabilizer, enhancing IL-6 half-life, and thus trans signaling. Additionally, SorLA negatively controls IL-6 turnover by binding to IL-6 or sIL-6R and mediating their endocytosis, followed by lysosomal degradation.
SorLA Regulates CLF-1 and IL-6 Signaling
Like sortilin, SorLA is implicated into the regulation of IL-6 family signaling as reported in a pro-B lymphocytes murine cell line. Whereas CLC and CLF-1 have a binding site for CNTFRα, only CLF-1 displays a binding site for SorLA. Thus, SorLA interacts either with CLF-1 alone, or with the cytokine CLC:CLF-1 complex via CLF-1 binding as well as with the heterotrimer of CLC:CLF-1 associated with CNTFRα. According to its partners, different functions of SorLA were described (Figure 2B). If the trimer is anchored to the membrane through membranous CNTFRα, SorLA mediates the internalization and lysosomal degradation of the complex and thus downregulates CLC:CLF-1/CNTFRα signaling. On the other hand, if the formed complex includes the soluble form of CNTFRα, SorLA concentrates the complex at the membrane, enhances its interaction with gp130/LIFRβ and thus increases JAK/STAT signaling. So, through interaction with CLF-1 only, SorLA regulates CLC/CNTFRα signaling and turnover (Larsen et al., 2016). SorLA additionally regulates IL-6 signaling (Figure 2C). IL-6 can induce signaling pathways, namely cis signaling when it binds to membrane-bound IL-6 Receptor (IL-6R) and trans signaling when binding the soluble form of IL-6R (sIL-6R). The complex formed then engages homodimeric gp130 to induce signaling via the JAK/STAT pathway. SorLA binds by its Vps10p domain both IL-6 and IL-6R independently or in complex. When interacting with IL-6 or sIL-6R, SorLA mediates cellular uptake and endocytosis of both proteins. Membrane-bound IL-6R has also been found to interact with SorLA. In such cases, SorLA downregulates cis signaling, probably by inhibiting IL-6 binding on IL-6R. The soluble form of SorLA can interact with circulating IL-6, in this complex SorLA acts as a stabilizing carrier protein, increasing IL-6 functional half-life and thus enhancing IL-6 trans signaling (Larsen and Petersen, 2017).
Larsen and colleagues highlight in this study a potentially crucial role of SorLA in regulation of IL-6 cytokine family signaling. Once again, the IL-6 family of cytokines is involved in many physiological and pathophysiological processes including, but not limited to, immune response and hepatic acute phase reaction (Rose-John, 2018). Uncovering the role of SorLA in modulation of IL-6 family signaling, could further deepen our understanding of these processes. For example, CLF-1 can interact with p28, a subunit of IL-27, to form a cytokine complex activating NK and T cells in the presence of IL-6Rα (Crabé et al., 2009) and a potential involvement of sortilin or SorLA in this pathway should be investigated given their role in CLF-1 signaling (Larsen et al., 2010, 2016).
Inflammatory Conditions Regulate Sortilin Expression
We mentioned previously that sortilin might be a key regulator of pro-inflammatory cytokines. Upon viral infection, viruses are sensed by Toll-like receptors (TLRs) 7 and 9 on pDCs, leading to massive secretion of IFN-α, under the control of sortilin (Yabe-Wada et al., 2016). In the same time, TLR signaling, in response to inflammatory conditions, negatively regulates sortilin expression which impairs IFN-α secretion. Similar findings were observed in adipocytes. Under inflammatory conditions and increase of TNF-α levels, a diminution of both sortilin mRNA and protein were detected (Kaddai et al., 2009; Hivelin et al., 2017), by an unknown mechanism. Recently, several mechanisms are described and correlated with the regulation of sortilin expression, depending on different transcriptional and post transcriptional regulating factors (Sung et al., 2018).
Some mechanisms regulating sortilin expression are linked to transcriptional regulation of SORT1 gene by different transcriptional factors. In an anti-inflammatory mice model depleted of regulatory T cells (Treg), IFN-γ and TNF-α levels are increased, notably in the liver, in parallel to a local inflammation (Klingenberg et al., 2013). IFN-γ activation decreased SORT1 transcription and sortilin expression in hepatocytes, through the activation of the JAK/STAT1 pathway and the binding of P-STAT1 to the promoter of sortilin (Pirault et al., 2017).
Another transcriptional regulation of SORT1 implicates the activating transcription factor 3 (ATF3), a transcriptional repressor and regulator of inflammation pathway. ATF3 is a member of the cyclic adenosine monophosphate (cAMP) response element binding protein (CREB), containing a DNA binding region and a bZIP domain (Chen et al., 1994; Liang et al., 1996; Hai and Hartman, 2001) ATF3 homodimerization represses transcription, but conversely, its heterodimerization with Jun proteins activates transcription (Liang et al., 1996). ATF3 promoter possesses many binding sites of transcription factors such as ATF/CRE (C-rich element), activator protein 1 (AP1) and NF-κB, suggesting that its expression is regulated by stress conditions like pro-inflammatory environment (Hai et al., 1999; Hashimoto et al., 2002). Under stress or pathogenic conditions, TLRs stimulation induces NF-κB activation and ATF3 transcription. ATF3 has been shown to downregulate pro-inflammatory cytokines transcription, notably ofIL-4, IL-5, IL-6, IL-12, IL-13, IFN-γ, IFN-β, TNF-α as well as chemokines CCL1, CCL2, CCL4, CCL5, CCL7, CCL8, and CCL11 (Gilchrist et al., 2006; Khuu et al., 2007; Rosenberger et al., 2008; Hoetzenecker et al., 2012; Lai et al., 2013; Zheng and Abraham, 2013; Boespflug et al., 2014). On the other hand, ATF3 expression is also induced by feedback regulation of some of these cytokines, including IL-6, IFN-γ, and IFN-β (Hai et al., 1999; Ho et al., 2008; Labzin et al., 2015). These results highlight ATF3 role in the modulation of host immune response under stress or physiopathological conditions (Jadhav and Zhang, 2017).
ATF3 binding to the proximal promoter region of SORT1 represses SORT1 transcription (Ai et al., 2012). In hepatocytes, endoplasmic reticulum stress induces an increase of ATF3 and thus a reduction of sortilin expression, leading to reduced VLDL clearance and promoting atherosclerosis (Ai et al., 2012; Klingenberg et al., 2013). In parallel, the IFN-γ and TNF-α – induced drop of sortilin expression are both associated with an increase in ATF3 expression.
We hypothesize that regulation of sortilin expression by ATF3 might be of major importance in immunomodulation. Indeed, ATF3 regulates the expression of various pro-inflammatory cytokines and chemokines, and is itself regulated by them. On the other hand, sortilin can modulate the production and secretion of some of those cytokines and is also regulated by them. We believe that a balance between ATF3 and sortilin expression might be of great importance in the modulation of inflammation and immune response. Sortilin promotes inflammation and consequently activates an autoregulation loop by ATF3 activation, although only few studies, all focused on atherosclerosis, confirmed the important role of sortilin/ATF3 axis in innate immunity. These observations should be more deeply investigated especially its potential deregulation in chronic inflammatory diseases.
Moreover, another post transcriptional mechanism controlling sortilin expression was detected under TLR activation. It concerns its mRNA stability depending on CRE, identified in the 3′ UTR of sortilin mRNA. Under physiological conditions, poly-rC binding protein 1 (PCBP1), a protein involved in RNA processing, translation and stability (Makeyev and Liebhaber, 2002), binds to CRE and improves sortilin mRNA stability. TLR signaling activation by viral infection induces an increase in intracellular metal zinc, which in turn leads to PCBP1/CRE dissociation in the 3′ UTR of sortilin mRNA, and finally its degradation. This post transcriptional regulation of sortilin by TLR results in decrease of sortilin protein level and thus activity, and consequently a decrease in pro-inflammatory cytokines production (Yabe-Wada et al., 2016).
Sortilin, SorLA, and Atherosclerosis
Sortilin Regulates Inflammation in Atherosclerosis
Sortilin has been identified as an important regulator of cardiovascular and metabolic disorders. Among them, sortilin is implicated in atherosclerosis and atherosclerotic plaques development through multiple processes, including regulation of calcification (Goettsch et al., 2016), lipoprotein metabolism (Kjolby et al., 2010; Strong et al., 2012), Glut4 biogenesis and glucose uptake in type-2 diabetes (Shi and Kandror, 2005) and arterial wall inflammation (Mortensen et al., 2014; Patel et al., 2015). In this review we will only discuss the relationship between sortilin and the immune system in atherosclerosis (more details on the implication of sortilin in atherosclerosis can be found in recent reviews) (Schmidt and Willnow, 2016; Zhong et al., 2016; Goettsch et al., 2018).
Sortilin is expressed by immune cells, notably macrophages and Th1 cells. In macrophages, sortilin promotes native LDL uptake, leading to the formation of cholesterol-loaded macrophages called foam cells (Patel et al., 2015), implicated in atherosclerosis development (Yu et al., 2013). As described above, sortilin plays an important role in pro-inflammatory cytokines secretion, such as IL-6 in macrophages and IFN-γ in Th1 cells (Mortensen et al., 2014). IL-6 facilitates the secretion of IFN-γ by T cells (Nurieva et al., 2007). Both cytokines have been associated to atherosclerosis promotion (Koga et al., 2007; Schuett et al., 2012). Surprisingly, pro-inflammatory cytokine regulation in macrophages described by Mortensen et al. (2014) in their sort1-/- mouse model was not confirmed by Patel et al. (2015) despite a reduction in atherosclerosis lesions.
Correlating with previously discussed results on T Reg (FOXP3+)-depleted mice (mimicking hyperinflammation), the increase of IFN-γ and TNF-α downregulates sortilin expression in the liver. This model was marked by extensive atherosclerosis lesions with vascular inflammation. These results are associated with reduced VLDL and chylomicron clearance and augmented plasmatic cholesterol levels, which promote atherosclerosis. They demonstrate that immunity could regulate sortilin-mediated metabolic processes and that chronic inflammation might also promote metabolic disorders and cardiovascular diseases (Klingenberg et al., 2013).
Although very few studies investigated the immunomodulatory role of sortilin in atherosclerosis, we can hypothesize that sortilin promotes formation of foam cells and chronic inflammation in blood vessels inducing atherosclerosis development. This chronic inflammation might in turn downregulate sortilin in the liver and disrupt lipoprotein metabolism, further enhancing atherosclerosis and cardiovascular diseases. Given the small number of studies, some results remain conflicting and need further investigation and clarification.
SorLA Modulates Monocyte Migration to Atherosclerotic Lesions
Following vascular injury, circulating monocytes infiltrate the intima and differentiate into macrophages. Those macrophages secrete chemoattractant molecules enhancing inflammatory cells recruitment. One of them, MCP-1 (CCL-2) plays a crucial role in monocyte recruitment and activation. Moreover, an increase of MCP-1 expression has been detected in atheromatous plaques (Lusis, 2000; Charo and Taubman, 2004; McCarthy et al., 2010; Schmidt et al., 2017). Conjugated Linoleic Acids (CLA) were identified as atheroprotective by inhibiting inflammatory cytokines (Yu et al., 2002; Changhua et al., 2005), including MCP-1 secretion and thus monocyte migration, as well as inflammatory phenotypes of activated macrophages (McClelland et al., 2010).
In atherosclerotic tissues, SorLA is overexpressed in monocytes/macrophages, and CLA inhibits its expression through a PPAR-γ-dependent pathway and a decrease of SorLA, reducing migration of monocytes to MCP-1 stimuli. Furthermore, an increase in SorLA was followed by an increase in urokinase-type Plasminogen Activator Receptor (uPAR) known to be involved in the infiltration of intima by monocytes cells and in foam cells formation (May et al., 2002; Gu et al., 2005; McCarthy et al., 2010). Soluble form of SorLA, highly increased in atheromatous plaques, activates uPAR expressed at the cell surface, promoting migration of macrophages and lipids accumulation (Ohwaki et al., 2007).
In atherosclerosis, SorLA exerts a dual role: it is implicated in the regulation of lipolysis (Nilsson et al., 2007, 2008; Klinger et al., 2011; Mendoza-Barberá et al., 2013; Schmidt et al., 2017) and in the regulation of monocyte migration to MCP-1 stimuli. Although these processes were only described in atherosclerosis cases, it would be interesting to study SorLA implication in monocyte/macrophage migration in physiological immune process such as inflammation following antigens stimuli. Hence, uPAR, known as an important player in tissue repair process (Blasi and Carmeliet, 2002), may also implicate SorLA.
Sortilin, Microglia, and Neuroinflammation
Microglia cells, tissue-based macrophages of the Central Nervous System (CNS), are considered as the key innate immunity cells in the CNS (Gehrmann et al., 1995; Kofler and Wiley, 2011). They have a sentinel immune function, constantly surveying their environment to detect tissue damage and pathogens. As most tissue macrophages, they recognize damage-associated molecular patterns (DAMPs) and pathogen-associated molecular patterns (PAMPs) by high expression of surface receptors such as TLRs, CD68, or CD206, as well as cytoplasmic receptors such as retinoic acid-inducible gene-1-like (RLR) and nuclear oligomerization domain-like receptors (NLR). TLRs signaling results in activation of pro-inflammatory cytokines, such as IL-1β and IL-18, gene transcription and pro-protein synthesis, while RLR or NLR induces formation of an inflammasome (Aravalli et al., 2007; Lehnardt, 2010; Ross, 2010).
Murine and human microglia cells express sortilin but not the two other NTS receptors NTSR1 and NTSR2 (Martin et al., 2003; Patel et al., 2016). Upon stimulation by NTS, sortilin activates both PI3K/AKT and MAPK/ERK1-2 pathways. These signaling pathways induce an increase in pro-inflammatory cytokines and chemokines transcription, including Monocyte Chemoattractant Protein-1 (MCP-1), MIP-2 (or CXCL2), IL-1β, and TNF-α, responsible for inflammatory processes and leucocytes recruitment (Dicou et al., 2004). Upon stimulation by NTS, sortilin induces the migration of murine microglial cells through chemoattractive effects and induces their maturation into pro-inflammatory cytokines producing phagocytes which might contribute to neuroinflammation and development of neurodegenerative disorders (Martin et al., 2003, 2005; Dicou et al., 2004). In human microglia, a similar activation is obtained in vitro by NTS, inducing production of pro-inflammatory cytokines IL-1β, CXCL8, CCL2, and CCL5 through PI3K/mTOR activation. These signaling effects were inhibited by mTOR inhibitors such as methoxyluteolin (Patel et al., 2016). A relationship with autism is hypothesized based on the increase in plasmatic NTS levels, evidenced in children with autism spectrum disorders, notably with the accompanying gastrointestinal dysfunction. There is an hypothesized link between such results, neuroinflammation, and the interaction of microglia and microbiome disorders (recently reviewed in Lebovitz et al., 2018), but the mechanisms have yet to be determined.
Sortilin can also regulate brain inflammation by mediating progranulin (PGRN) uptake by microglial cells. PGRN is a growth factor implicated in the regulation of various processes, including wound healing, tumorigenesis and inflammation (recently reviewed in Paushter et al., 2018). PGRN is produced and secreted at high levels by microglia, especially by trauma activated cells, and associated to anti-inflammatory effects by controlling microglial activation, migration, phagocytosis and synapse pruning (Paushter et al., 2018). PGRN could potentially bind to TNFR, inhibiting inflammatory effects by competing with TNF-α (Tang et al., 2011). Sortilin in microglia is able to bind extracellular PGRN and mediate its endocytosis and clearance, thus reducing anti-inflammatory properties (Kumar-Singh, 2011; Chen et al., 2013; Jian et al., 2013). PGRN deficiency causes several neurodegenerative pathologies; PGNR haploinsufficiency is associated with frontotemporal dementia and a total deficiency can cause neuronal ceroid lipofuscinosis. Gene therapies recovering PGRN expression have beneficial effects on the patients affected with frontotemporal dementia or neuronal ceroid lipofuscinosis, and a combined inhibition of sortilin in microglia could reduce PGRN clearance, increasing extracellular PGRN concentration and potentially enhancing its beneficial effects (Arrant et al., 2018).
As microglia are activated via sortilin, its involvement in the development of Multiple Sclerosis has been studied through a model of experimental-induced encephalomyelitis in sort1-/- mice. Whereas sortilin is expressed in mouse microglia and dendritic cells, its loss reduced the antigen-processing ability in dendritic cells but did not affect the development and progression of brain lesions, suggesting that sortilin did not control this autoimmune encephalitis (Reuter et al., 2015). This discrepancy with the models previously described may be related to the acute experimental model and that sortilin regulatory functions are more relevant in chronic inflammatory models.
Sortilin, Inflammation, and Cancer?
To further expand on the implication of sortilin in inflammatory context, we open perspectives on cancer-mediated inflammation. As previously discussed, while sortilin deregulation were well characterized in neurodegenerative and cardiovascular diseases (Wilson et al., 2014a; Schmidt and Willnow, 2016), the involvement of sortilin in cancer cells homeostasis remains misunderstood and subject to controversies. Indeed, sortilin participates actively in the release of growth factors promoting thereby autocrine survival loops through “sustained proliferative signaling,” a central hallmark of cancer. Inversely, a cell suicide may triggers following the association of the sortilin with the death domain receptor p75, alimenting thus controversies toward sortilin. Surprisingly, while sortilin regulates the immune functions of T lymphocytes and NK cells, no data are stated whether sort1-/- mice develop spontaneous tumors. Likewise, no clinical data stated about the expression of sortilin in tumor-infiltrating immune cells. Intriguingly, while immune surveillance remains a crucial barrier against transformed cells, immune cells play an important role in tumor initiation, progression, and invasion. Indeed, pre-neoplastic lesions trigger pro-inflammatory cells recruitment and infiltration. For example, gastric cancer cells express both IL-6 and its receptor and may illustrate an indirect role of sortilin mediated pre-metastasis niche formation. Indeed, infiltrated-inflammatory cells release IL-6 upon the dependence of sortilin. Exogenous IL-6 promotes the proliferation and the invasion of gastric cells as well as the secretion of the vascular endothelial growth factor C (VEGFR-C) promoting lymphatic drainage. Thus, the release of IL-6 toward neoplastic lesion promotes lymphangiogenesis, a first step before lymph node invasion (Zhao et al., 2016). Interestingly, sortilin expression is correlated with breast cancer aggressiveness and lymph node metastasis (Roselli et al., 2015). Interestingly, sortilin is also involved in the release of progranulin by breast cancer cells, inducing migration and cancer stem cell expansion (Rhost et al., 2018). However, no data stated about the expression of sortilin in infiltrated-inflammatory cells as well as the implication of sortilin in the release of IL-6 from cancer cells.
In conjunction with IL-6, IFNγ plays a major role in modulation of the cancer immune network to inhibit or promote tumor progression (Landskron et al., 2014; Shalapour and Karin, 2015; Mojic et al., 2017). Through regulation of IFN-γ and IL-6 cytokine family, we speculate that sortilin could be involved in tumor microenvironment inflammation and promote tumor progression.
Sortilin and Phagocytosis/Scavenging
Sortilin Mediates Macrophage Antigen Scavenging
Alternatively activated macrophages (AAM), M2-type macrophages, are obtained by stimulation of macrophages by Th2 lymphocytes producing anti-inflammatory cytokines such as TGF-β, IL-4, IL-10, IL-13. These macrophages are characterized by an increase of innate immunity markers, class II MHC and CD14 expression, an increased phagocytic activity and a decreased antigen processing ability (Tzachanis et al., 2002; Gordon, 2003; Martinez et al., 2008; Mosser and Edwards, 2008). AAM showed augmented levels of known scavenger receptors Macrophage Mannose Receptor (or CD206) and sortilin expression. CD206 and sortilin might participate to the increased extracellular antigen scavenging. Associated with AAM inability to process scavenged antigens, this process participates in T cell response inhibition, which is essential for transplantation tolerance (Tzachanis et al., 2002). By regulating antigen clearance by macrophages, sortilin might be indirectly implicated in modulation of T cell mediated immunity and transplant rejection (Figure 3A).
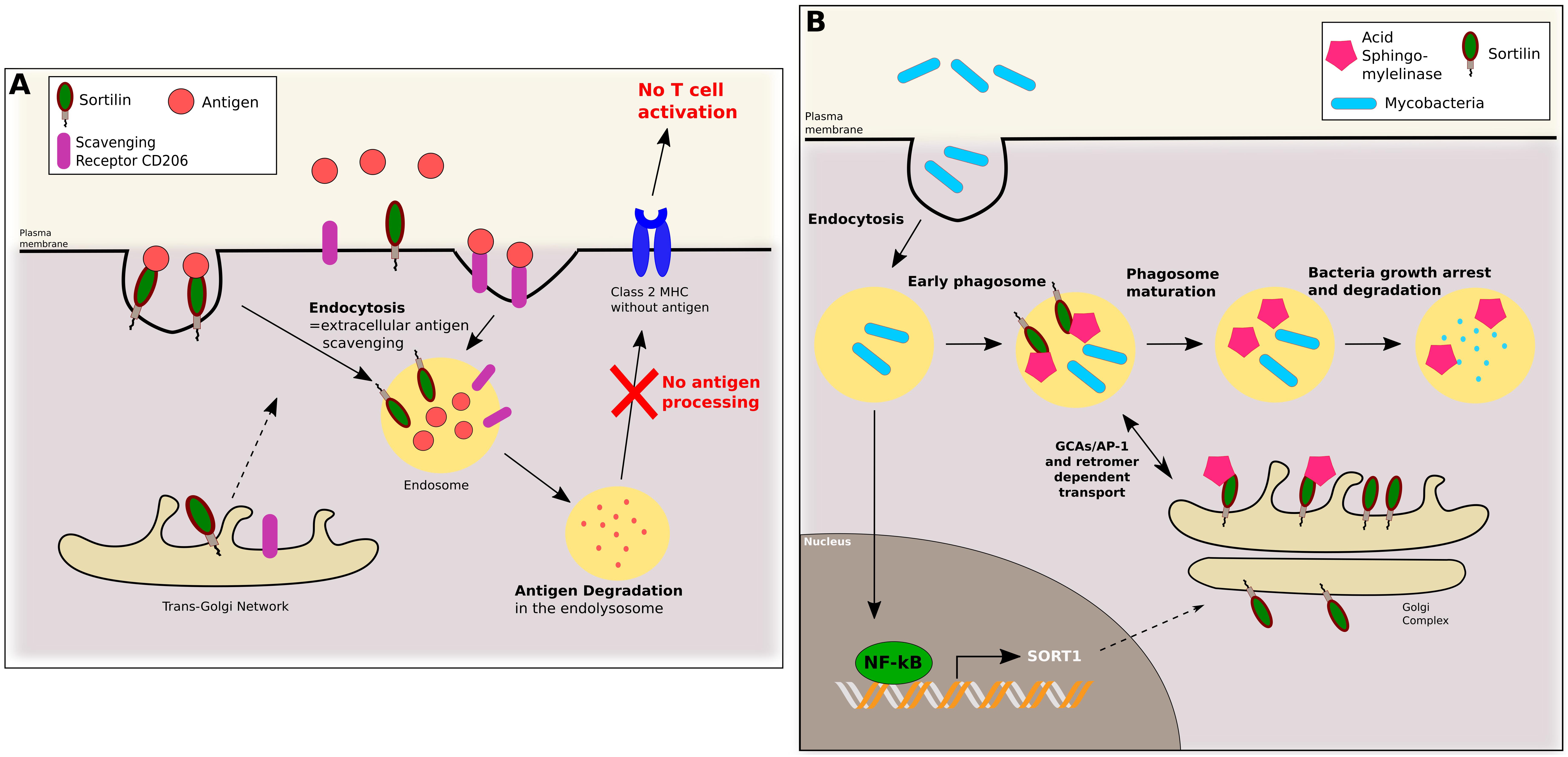
Figure 3. In macrophages, sortilin is implicated in antigen scavenging and phagosome maturation. (A) Sortilin and scavenger receptor CD206 are addressed from the TGN to the plasma membrane. In Alternatively Associated Macrophages (AAMs), sortilin can bind extracellular antigens and mediate their endocytosis. Antigens are then degraded in the lysosome, and AAMs being incapable of antigen processing, antigen peptides are not presented to T cells by class 2 MHC. (B) Upon phagocytosis of mycobacteria by macrophages, NF-kB pathway is activated, inducing SORT1 transcription. Sortilin is then transported and vehicles Acid Sphingomyelinase, to early phagosomes. This step is crucial to phagosome maturation and mycobacteria growth arrest and degradation.
Sortilin Regulates Phagosome Maturation and Lysosomal Enzyme Delivery
Fusion between the phagosome and the lysosome is a key event in mycobacteria elimination by macrophages. It allows phagolysosome formation, able to efficiently eradicate the infection. Upon infection by the non-pathogenic mycobacteria Mycobacterium smegmatis, macrophages activate the NF-κB pathway, inducing phagolysosome fusion and mycobacteria killing. NF-κB activation results in a de novo synthesis of both lysosomal enzymatic components and proteins involved in intracellular trafficking, including sortilin (Gutierrez et al., 2008). In macrophages, sortilin is located toward the Golgi apparatus and acquired by phagosomes after their internalization. Sortilin interacts with two lysosomal proteins, Acid Sphingomyelinase (ASM) and Prosaposin (PS), and is required for their delivery from the Golgi to the intracellular phagosomes containing bacteria (Wahe et al., 2010). Likewise, sortilin is required for the targeting of the lysosomal proteases cathepsin D and H (Canuel et al., 2008). Sortilin and ASM are acquired in phagosomes at the early stages of mycobacteria infection. Sortilin and phagosome interaction (and subsequent delivery of ASM) is dependent of both anterograde transport and the retromer complex. Anterograde transport is dependent on interaction of sortilin with GGA/AP-1 complex through the dileucine motif 829LL in the sortilin cytoplasmic tail, and retromer complex interaction is dependent on sortilin residues 787 to 792 (FLVHRY) and cysteine 783 palmitoylation, also in the sortilin cytoplasmic tail. Upon infection by Mycobacterium bovis or tuberculosis, lack of sortilin elicits an increase of bacteria replication in macrophages, increasing neutrophils lung infiltration and pathogenesis (Vázquez et al., 2016).
In conclusion, macrophages infection by mycobacteria triggers the NF-κB pathway stimulating sortilin and ASM de novo synthesis. Then, sortilin transports ASM from the TGN to phagosomes through interaction of its cytoplasmic tail with GGA/AP-1 and the retromer complex essential for anterograde and retrograde transport. Hence, in concert, both sortilin and ASM delivery are required for phagosome maturation, bacteria growth restriction and efficient elimination (Figure 3B).
SorLA May Be Implicated in Maintaining the Hematological Pool
Hematopoietic Stem and Progenitor Cells (HSPCs) differentiation from immature quiescent self-renewable cells into mature and proliferating cells, is planned by crucial events such as their adhesion to bone marrow cells. The BM pool of HSPCs is regulated by modulation of various molecules under hypoxic conditions (Chow et al., 2001; Lévesque et al., 2010; Trumpp et al., 2010; Suda et al., 2011). Among them, uPAR has been identified as a regulator of HSPCs adhesion in osteoblastic niches as well as a regulator of HSPCs proliferation and marrow pool size (Tjwa et al., 2009).
High levels of SorLA have been observed in immature hematopoietic precursors both at the plasma membrane and in soluble form following proteolytic shedding (Zhang et al., 2000; Hermey et al., 2006). Under hypoxic conditions, intracellular and soluble SorLA levels are increased after HIF-1α binding on SorLA promoter. Both soluble SorLA and uPAR are upregulated by hypoxia in the BM niche. Soluble SorLA interacts with membrane bound uPAR in immature hematological cells and modulates its activity, enhancing HSPCs adhesion to BM stromal cells, maintaining thus a normal hematological cell pool size (Nishii et al., 2013).
Conclusion and Remarks
Sortilin and SorLA play incredibly versatile functions depending on their “interactome.” Studies to date emphasize their roles in NTS and neurotrophin signaling pathways in cancer progression, and in cardiovascular, metabolic and neurodegenerative disease. In the present review, we highlighted some of their roles in the immune homeostasis (summarized in Figure 4). Sortilin regulates the production and exocytosis of pro-inflammatory cytokines and is in turn downregulated by them. Both sortilin and SorLA are implicated in the modulation of IL-6 family cytokines signaling and turnover, which could potentially be of major significance in IL-6-dependent immune processes. Sortilin also stimulates neuroinflammation through both pro-inflammatory cytokines release and PGRN clearance by microglia. Furthermore, ATF3 identified as a sortilin expression repressor, may thus have a key immunomodulatory effect by inhibiting inflammation. However, sortilin and SorLA are not only involved in inflammation. Indeed, sortilin is expressed by macrophages and is implicated in antigen clearance, probably impacting T cell immunity and graft tolerance, and is also required during the maturation stages of phagolysosomes formation in cases of infection by mycobacteria. Interestingly, SorLA seems to be important for the differentiation of hematological stem cells in the BM and the maintenance of a normal hematological pool size.
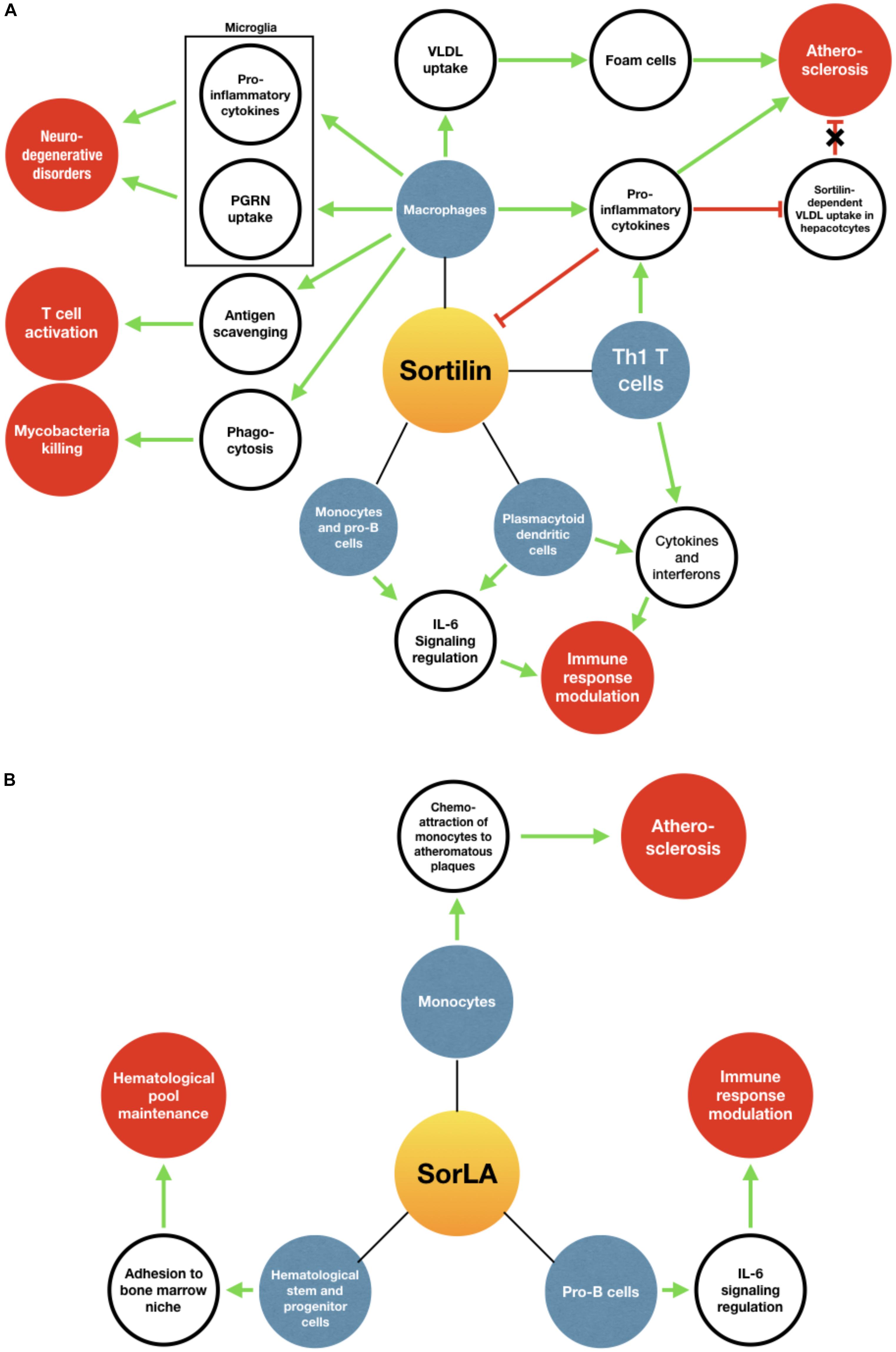
Figure 4. Implications of sortilin (A) and SorLA (B) in immune-related processes. Blue circles represent cellular models expressing sortilin or SorLA. Blank circles represent pathways/processes/proteins modified by sortilin or SorLA. Red circles represent physiological or pathological processes induced. Green arrows mean a stimulating role, while red arrows mean an inhibition. The red arrow with a black cross means that the inhibition is lifted.
Taken together these observations suggest that sortilin and SorLA may be involved in innate immunity, through regulation of inflammation and phagocytosis, as well as in adaptive immunity by controlling immune cells maturation and modulating T and NK cells activation. For now, only few studies high lighted a role for sortilin and SorLA in normal and/or pathological immunity, however, deeper investigations might identify unknown news functions in immunomodulatory pathways.
Author Contributions
HT wrote the original draft of the manuscript and created the figures. SS, TN, P-FG, A-LF, and M-OJ reviewed and edited the manuscript and supervised this work.
Funding
This work was supported and funded by grants from FEDER (Fonds Européen de Développement Régional), the Limousin Regional Council, La Ligue Nationale Contre le Cancer (Comiteì Deìpartemental de La Haute-Vienne), and CORC (Comiteì d’Orientation de la Recherche en Canceìrologie) from Limoges.
Conflict of Interest Statement
The authors declare that the research was conducted in the absence of any commercial or financial relationships that could be construed as a potential conflict of interest.
References
Ai, D., Baez, J. M., Jiang, H., Conlon, D. M., Hernandez-Ono, A., Frank-Kamenetsky, M., et al. (2012). Activation of ER stress and mTORC1 suppresses hepatic sortilin-1 levels in obese mice. J. Clin. Invest. 122, 1677–1687. doi: 10.1172/JCI61248
Andersen, O. M., Reiche, J., Schmidt, V., Gotthardt, M., Spoelgen, R., Behlke, J., et al. (2005). Neuronal sorting protein-related receptor sorLA/LR11 regulates processing of the amyloid precursor protein. Proc. Natl. Acad. Sci. U.S.A. 102, 13461–13466. doi: 10.1073/pnas.0503689102
Aravalli, R. N., Peterson, P. K., and Lokensgard, J. R. (2007). Toll-like receptors in defense and damage of the central nervous system. J. Neuroimmune Pharmacol. 2, 297–312. doi: 10.1007/s11481-007-9071-5
Arrant, A. E., Onyilo, V. C., Unger, D. E., and Roberson, E. D. (2018). Progranulin gene therapy improves lysosomal dysfunction and microglial pathology associated with frontotemporal dementia and neuronal ceroid lipofuscinosis. J. Neurosci. 38, 2341–2358. doi: 10.1523/JNEUROSCI.3081-17.2018
Blasi, F., and Carmeliet, P. (2002). uPAR: a versatile signalling orchestrator. Nat. Rev. Mol. Cell Biol. 3, 932–943. doi: 10.1038/nrm977
Boespflug, N. D., Kumar, S., McAlees, J. W., Phelan, J. D., Grimes, H. L., Hoebe, K., et al. (2014). ATF3 is a novel regulator of mouse neutrophil migration. Blood 123, 2084–2093. doi: 10.1182/blood-2013-06-510909
Canuel, M., Korkidakis, A., Konnyu, K., and Morales, C. R. (2008). Sortilin mediates the lysosomal targeting of cathepsins D and H. Biochem. Biophys. Res. Commun. 373, 292–297. doi: 10.1016/j.bbrc.2008.06.021
Carlo, A.-S., Gustafsen, C., Mastrobuoni, G., Nielsen, M. S., Burgert, T., Hartl, D., et al. (2013). The pro-neurotrophin receptor sortilin is a major neuronal apolipoprotein E receptor for catabolism of amyloid-β peptide in the brain. J. Neurosci. 33, 358–370. doi: 10.1523/JNEUROSCI.2425-12.2013
Changhua, L., Jindong, Y., Defa, L., Lidan, Z., Shiyan, Q., and Jianjun, X. (2005). Conjugated linoleic acid attenuates the production and gene expression of proinflammatory cytokines in weaned pigs challenged with lipopolysaccharide. J. Nutr. 135, 239–244. doi: 10.1093/jn/135.2.239
Charo, I. F., and Taubman, M. B. (2004). Chemokines in the pathogenesis of vascular disease. Circ. Res. 95, 858–866. doi: 10.1161/01.RES.0000146672.10582.17
Chen, B. P., Liang, G., Whelan, J., and Hai, T. (1994). ATF3 and ATF3 delta Zip. Transcriptional repression versus activation by alternatively spliced isoforms. J. Biol. Chem. 269, 15819–15826.
Chen, X., Chang, J., Deng, Q., Xu, J., Nguyen, T. A., Martens, L. H., et al. (2013). Progranulin does not bind tumor necrosis factor (TNF) receptors and is not a direct regulator of TNF-dependent signaling or bioactivity in immune or neuronal cells. J. Neurosci. 33, 9202–9213. doi: 10.1523/JNEUROSCI.5336-12.2013
Chow, D. C., Wenning, L. A., Miller, W. M., and Papoutsakis, E. T. (2001). Modeling pO2 distributions in the bone marrow hematopoietic compartment. II. Modified kroghian models. Biophys. J. 81, 685–696. doi: 10.1016/S0006-3495(01)75733-5
Crabé, S., Guay-Giroux, A., Tormo, A. J., Duluc, D., Lissilaa, R., Guilhot, F., et al. (2009). The IL-27 p28 subunit binds cytokine-like factor 1 to form a cytokine regulating NK and T cell activities requiring IL-6R for signaling. J. Immunol. 183, 7692–7702. doi: 10.4049/jimmunol.0901464
Dicou, E., Vincent, J.-P., and Mazella, J. (2004). Neurotensin receptor-3/sortilin mediates neurotensin-induced cytokine/chemokine expression in a murine microglial cell line. J. Neurosci. Res. 78, 92–99. doi: 10.1002/jnr.20231
Dumanis, S. B., Burgert, T., Caglayan, S., Füchtbauer, A., Füchtbauer, E.-M., Schmidt, V., et al. (2015). Distinct functions for anterograde and retrograde sorting of SORLA in amyloidogenic processes in the brain. J. Neurosci. 35, 12703–12713. doi: 10.1523/JNEUROSCI.0427-15.2015
Evans, S. F., Irmady, K., Ostrow, K., Kim, T., Nykjaer, A., Saftig, P., et al. (2011). Neuronal brain-derived neurotrophic factor is synthesized in excess, with levels regulated by sortilin-mediated trafficking and lysosomal degradation. J. Biol. Chem. 286, 29556–29567. doi: 10.1074/jbc.M111.219675
Fauchais, A.-L., Lalloue, F., Lise, M.-C., Boumediene, A., Preud’homme, J.-L., Vidal, E., et al. (2008). Role of endogenous brain-derived neurotrophic factor and sortilin in B cell survival. J. Immunol. 181, 3027–3038. doi: 10.4049/jimmunol.181.5.3027
Gehrmann, J., Matsumoto, Y., and Kreutzberg, G. W. (1995). Microglia: intrinsic immuneffector cell of the brain. Brain Res. Brain Res. Rev. 20, 269–287. doi: 10.1016/0165-0173(94)00015-H
Gilchrist, M., Thorsson, V., Li, B., Rust, A. G., Korb, M., Kennedy, K., et al. (2006). Systems biology approaches identify ATF3 as a negative regulator of Toll-like receptor 4. Nature 441, 173–178. doi: 10.1038/nature04768
Goettsch, C., Hutcheson, J. D., Aikawa, M., Iwata, H., Pham, T., Nykjaer, A., et al. (2016). Sortilin mediates vascular calcification via its recruitment into extracellular vesicles. J. Clin. Invest. 126, 1323–1336. doi: 10.1172/JCI80851
Goettsch, C., Kjolby, M., and Aikawa, E. (2018). Sortilin and its multiple roles in cardiovascular and metabolic diseases. Arterioscler. Thromb. Vasc. Biol. 38, 19–25. doi: 10.1161/ATVBAHA.117.310292
Gordon, S. (2003). Alternative activation of macrophages. Nat. Rev. Immunol. 3, 23–35. doi: 10.1038/nri978
Gu, J.-M., Johns, A., Morser, J., Dole, W. P., Greaves, D. R., and Deng, G. G. (2005). Urokinase plasminogen activator receptor promotes macrophage infiltration into the vascular wall of ApoE deficient mice. J. Cell. Physiol. 204, 73–82. doi: 10.1002/jcp.20262
Gutierrez, M. G., Mishra, B. B., Jordao, L., Elliott, E., Anes, E., and Griffiths, G. (2008). NF- B activation controls phagolysosome fusion-mediated killing of mycobacteria by macrophages. J. Immunol. 181, 2651–2663. doi: 10.4049/jimmunol.181.4.2651
Hai, T., and Hartman, M. G. (2001). The molecular biology and nomenclature of the activating transcription factor/cAMP responsive element binding family of transcription factors: activating transcription factor proteins and homeostasis. Gene 273, 1–11. doi: 10.1016/S0378-1119(01)00551-0
Hai, T., Wolfgang, C. D., Marsee, D. K., Allen, A. E., and Sivaprasad, U. (1999). ATF3 and stress responses. Gene Expr. 7, 321–335.
Hashimoto, Y., Zhang, C., Kawauchi, J., Imoto, I., Adachi, M. T., Inazawa, J., et al. (2002). An alternatively spliced isoform of transcriptional repressor ATF3 and its induction by stress stimuli. Nucleic Acids Res. 30, 2398–2406. doi: 10.1093/nar/30.11.2398
Herda, S., Raczkowski, F., Mittrücker, H.-W., Willimsky, G., Gerlach, K., Kühl, A. A., et al. (2012). The sorting receptor sortilin exhibits a dual function in exocytic trafficking of interferon-γ and granzyme A in T cells. Immunity 37, 854–866. doi: 10.1016/j.immuni.2012.07.012
Hermans-Borgmeyer, I., Hermey, G., Nykjaer, A., and Schaller, C. (1999). Expression of the 100-kDa neurotensin receptor sortilin during mouse embryonal development. Brain Res. Mol. Brain Res. 65, 216–219. doi: 10.1016/S0169-328X(99)00022-4
Hermey, G. (2009). The Vps10p-domain receptor family. Cell. Mol. Life Sci. 66, 2677–2689. doi: 10.1007/s00018-009-0043-1
Hermey, G., Sjøgaard, S. S., Petersen, C. M., Nykjær, A., and Gliemann, J. (2006). Tumour necrosis factor α-converting enzyme mediates ectodomain shedding of Vps10p-domain receptor family members. Biochem. J. 395, 285–293. doi: 10.1042/BJ20051364
Hivelin, C., Mazella, J., and Coppola, T. (2017). Sortilin derived propeptide regulation during adipocyte differentiation and inflammation. Biochem. Biophys. Res. Commun. 482, 87–92. doi: 10.1016/j.bbrc.2016.10.139
Ho, H. H., Antoniv, T. T., Ji, J.-D., and Ivashkiv, L. B. (2008). Lipopolysaccharide-induced expression of matrix metalloproteinases in human monocytes is suppressed by IFN-γ via superinduction of ATF-3 and suppression of AP-1. J. Immunol. 181, 5089–5097. doi: 10.4049/jimmunol.181.7.5089
Hoetzenecker, W., Echtenacher, B., Guenova, E., Hoetzenecker, K., Woelbing, F., Brück, J., et al. (2012). ROS-induced ATF3 causes susceptibility to secondary infections during sepsis-associated immunosuppression. Nat. Med. 18, 128–134. doi: 10.1038/nm.2557
Jacobsen, L., Madsen, P., Jacobsen, C., Nielsen, M. S., Gliemann, J., and Petersen, C. M. (2001). Activation and functional characterization of the mosaic receptor SorLA/LR11. J. Biol. Chem. 276, 22788–22796. doi: 10.1074/jbc.M100857200
Jacobsen, L., Madsen, P., Moestrup, S. K., Lund, A. H., Tommerup, N., Nykjaer, A., et al. (1996). Molecular characterization of a novel human hybrid-type receptor that binds the alpha2-macroglobulin receptor-associated protein. J. Biol. Chem. 271, 31379–31383. doi: 10.1074/jbc.271.49.31379
Jadhav, K., and Zhang, Y. (2017). Activating transcription factor 3 in immune response and metabolic regulation. Liver Res. 1, 96–102. doi: 10.1016/j.livres.2017.08.001
Jian, J., Zhao, S., Tian, Q., Gonzalez-Gugel, E., Mundra, J. J., Uddin, S. M., et al. (2013). Progranulin directly binds to the CRD2 and CRD3 of TNFR extracellular domains. FEBS Lett. 587, 3428–3436. doi: 10.1016/j.febslet.2013.09.024
Kaddai, V., Jager, J., Gonzalez, T., Najem-Lendom, R., Bonnafous, S., Tran, A., et al. (2009). Involvement of TNF-α in abnormal adipocyte and muscle sortilin expression in obese mice and humans. Diabetologia 52, 932–940. doi: 10.1007/s00125-009-1273-3
Khuu, C. H., Barrozo, R. M., Hai, T., and Weinstein, S. L. (2007). Activating transcription factor 3 (ATF3) represses the expression of CCL4 in murine macrophages. Mol. Immunol. 44, 1598–1605. doi: 10.1016/j.molimm.2006.08.006
Kitago, Y., Nagae, M., Nakata, Z., Yagi-Utsumi, M., Takagi-Niidome, S., Mihara, E., et al. (2015). Structural basis for amyloidogenic peptide recognition by sorLA. Nat. Struct. Mol. Biol. 22, 199–206. doi: 10.1038/nsmb.2954
Kjolby, M., Andersen, O. M., Breiderhoff, T., Fjorback, A. W., Pedersen, K. M., Madsen, P., et al. (2010). Sort1, encoded by the cardiovascular risk locus 1p13.3, is a regulator of hepatic lipoprotein export. Cell Metab. 12, 213–223. doi: 10.1016/j.cmet.2010.08.006
Klingenberg, R., Gerdes, N., Badeau, R. M., Gisterå, A., Strodthoff, D., Ketelhuth, D. F. J., et al. (2013). Depletion of FOXP3+ regulatory T cells promotes hypercholesterolemia and atherosclerosis. J. Clin. Invest. 123, 1323–1334. doi: 10.1172/JCI63891
Klinger, S. C., Glerup, S., Raarup, M. K., Mari, M. C., Nyegaard, M., Koster, G., et al. (2011). SorLA regulates the activity of lipoprotein lipase by intracellular trafficking. J. Cell Sci. 124, 1095–1105. doi: 10.1242/jcs.072538
Kofler, J., and Wiley, C. A. (2011). Microglia: key innate immune cells of the brain. Toxicol. Pathol. 39, 103–114. doi: 10.1177/0192623310387619
Koga, M., Kai, H., Yasukawa, H., Yamamoto, T., Kawai, Y., Kato, S., et al. (2007). Inhibition of progression and stabilization of plaques by postnatal interferon-γ function blocking in apoe-knockout mice. Circ. Res. 101, 348–356. doi: 10.1161/CIRCRESAHA.106.147256
Kumar-Singh, S. (2011). Progranulin and TDP-43: mechanistic links and future directions. J. Mol. Neurosci. 45, 561–573. doi: 10.1007/s12031-011-9625-0
Labzin, L. I., Schmidt, S. V., Masters, S. L., Beyer, M., Krebs, W., Klee, K., et al. (2015). ATF3 is a key regulator of macrophage IFN responses. J. Immunol. 195, 4446–4455. doi: 10.4049/jimmunol.1500204
Lai, P. F., Cheng, C. F., Lin, H., Tseng, T. L., Chen, H. H., and Chen, S. H. (2013). ATF3 protects against LPS-induced inflammation in mice via inhibiting HMGB1 expression. Evid. Based Complement. Alternat. Med. 2013:716481. doi: 10.1155/2013/716481
Landskron, G., De la Fuente, M., Thuwajit, P., Thuwajit, C., and Hermoso, M. A. (2014). Chronic inflammation and cytokines in the tumor microenvironment. J. Immunol. Res. 2014:149185. doi: 10.1155/2014/149185
Larsen, J. V., Hansen, M., Moller, B., Madsen, P., Scheller, J., Nielsen, M., et al. (2010). Sortilin facilitates signaling of ciliary neurotrophic factor and related helical type 1 cytokines targeting the gp130/leukemia inhibitory factor receptor heterodimer. Mol. Cell. Biol. 30, 4175–4187. doi: 10.1128/MCB.00274-10
Larsen, J. V., Kristensen, A. M., Pallesen, L. T., Bauer, J., Vægter, C. B., Nielsen, M. S., et al. (2016). Cytokine-like factor 1, an essential facilitator of cardiotrophin-like cytokine:ciliary neurotrophic factor receptor α signaling and sorLA-mediated turnover. Mol. Cell. Biol. 36, 1272–1286. doi: 10.1128/MCB.00917-15
Larsen, J. V., and Petersen, C. M. (2017). SorLA in interleukin-6 signaling and turnover. Mol. Cell. Biol. 37:e00641-16. doi: 10.1128/MCB.00641-16
Lebovitz, Y., Ringel-Scaia, V. M., Allen, I. C., and Theus, M. H. (2018). Emerging developments in microbiome and microglia research: implications for neurodevelopmental disorders. Front. Immunol. 9:1993. doi: 10.3389/fimmu.2018.01993
Lehnardt, S. (2010). Innate immunity and neuroinflammation in the CNS: the role of microglia in Toll-like receptor-mediated neuronal injury. Glia 58, 253–263. doi: 10.1002/glia.20928
Lévesque, J.-P., Helwani, F. M., and Winkler, I. G. (2010). The endosteal “osteoblastic” niche and its role in hematopoietic stem cell homing and mobilization. Leukemia 24, 1979–1992. doi: 10.1038/leu.2010.214
Liang, G., Wolfgang, C. D., Chen, B. P. C., Chen, T.-H., and Hai, T. (1996). ATF3 gene genomic organization, promoter, and regulation. J. Biol. Chem. 271, 1695–1701. doi: 10.1074/jbc.271.3.1695
Makeyev, A. V., and Liebhaber, S. A. (2002). The poly(C)-binding proteins: a multiplicity of functions and a search for mechanisms. RNA 8, 265–278. doi: 10.1017/S1355838202024627
Mari, M., Bujny, M. V., Zeuschner, D., Geerts, W. J., Griffith, J., Petersen, C. M., et al. (2007). SNX1 defines an early endosomal recycling exit for sortilin and mannose 6-phosphate receptors. Traffic 9, 380–393. doi: 10.1111/j.1600-0854.2007.00686.x
Martin, S., Dicou, E., Vincent, J.-P., and Mazella, J. (2005). Neurotensin and the neurotensin receptor-3 in microglial cells. J. Neurosci. Res. 81, 322–326. doi: 10.1002/jnr.20477
Martin, S., Vincent, J.-P., and Mazella, J. (2003). Involvement of the neurotensin receptor-3 in the neurotensin-induced migration of human microglia. J. Neurosci. 23, 1198–1205. doi: 10.1523/JNEUROSCI.23-04-01198.2003
Martinez, F. O., Sica, A., Mantovani, A., and Locati, M. (2008). Macrophage activation and polarization. Front. Biosci. J. Virtual Libr. 13:453–461. doi: 10.2741/2692
May, A. E., Schmidt, R., Kanse, S. M., Chavakis, T., Stephens, R. W., Schömig, A., et al. (2002). Urokinase receptor surface expression regulates monocyte adhesion in acute myocardial infarction. Blood 100, 3611–3617. doi: 10.1182/blood-2002-03-0778
Mazella, J. (2001). Sortilin/neurotensin receptor-3: a new tool to investigate neurotensin signaling and cellular trafficking? Cell. Signal. 13, 1–6. doi: 10.1016/S0898-6568(00)00130-3
Mazella, J., Zsürger, N., Navarro, V., Chabry, J., Kaghad, M., Caput, D., et al. (1998). The 100-kDa neurotensin receptor is gp95/sortilin, a non-G-protein-coupled receptor. J. Biol. Chem. 273, 26273–26276. doi: 10.1074/jbc.273.41.26273
McCarthy, C., O’Gaora, P., James, W. G., McClelland, S., Fitzgerald, D. J., Belton, O., et al. (2010). SorLA modulates atheroprotective properties of CLA by regulating monocyte migration. Atherosclerosis 213, 400–407. doi: 10.1016/j.atherosclerosis.2010.09.025
McClelland, S., Cox, C., O’Connor, R., de Gaetano, M., McCarthy, C., Cryan, L., et al. (2010). Conjugated linoleic acid suppresses the migratory and inflammatory phenotype of the monocyte/macrophage cell. Atherosclerosis 211, 96–102. doi: 10.1016/j.atherosclerosis.2010.02.003
Mendoza-Barberá, E., Julve, J., Nilsson, S. K., Lookene, A., Martín-Campos, J. M., Roig, R., et al. (2013). Structural and functional analysis of APOA5 mutations identified in patients with severe hypertriglyceridemia. J. Lipid Res. 54, 649–661. doi: 10.1194/jlr.M031195
Mojic, M., Takeda, K., and Hayakawa, Y. (2017). The dark side of IFN-γ: its role in promoting cancer immunoevasion. Int. J. Mol. Sci. 19:E89. doi: 10.3390/ijms19010089
Morinville, A., Martin, S., Lavallée, M., Vincent, J.-P., Beaudet, A., and Mazella, J. (2004). Internalization and trafficking of neurotensin via NTS3 receptors in HT29 cells. Int. J. Biochem. Cell Biol. 36, 2153–2168. doi: 10.1016/j.biocel.2004.04.013
Mortensen, M. B., Kjolby, M., Gunnersen, S., Larsen, J. V., Palmfeldt, J., Falk, E., et al. (2014). Targeting sortilin in immune cells reduces proinflammatory cytokines and atherosclerosis. J. Clin. Invest. 124:5317. doi: 10.1172/JCI76002
Mosser, D. M., and Edwards, J. P. (2008). Exploring the full spectrum of macrophage activation. Nat. Rev. Immunol. 8, 958–969. doi: 10.1038/nri2448
Nielsen, M. S., Madsen, P., Christensen, E. I., Nykjaer, A., Gliemann, J., Kasper, D., et al. (2001). The sortilin cytoplasmic tail conveys Golgi-endosome transport and binds the VHS domain of the GGA2 sorting protein. EMBO J. 20, 2180–2190. doi: 10.1093/emboj/20.9.2180
Nilsson, S. K., Christensen, S., Raarup, M. K., Ryan, R. O., Nielsen, M. S., and Olivecrona, G. (2008). Endocytosis of apolipoprotein A-V by members of the low density lipoprotein receptor and the VPS10p domain receptor families. J. Biol. Chem. 283, 25920–25927. doi: 10.1074/jbc.M802721200
Nilsson, S. K., Lookene, A., Beckstead, J. A., Gliemann, J., Ryan, R. O., and Olivecrona, G. (2007). Apolipoprotein A-V interaction with members of the low density lipoprotein receptor gene family. Biochemistry 46, 3896–3904. doi: 10.1021/bi7000533
Nishii, K., Nakaseko, C., Jiang, M., Shimizu, N., Takeuchi, M., Schneider, W. J., et al. (2013). The soluble form of LR11 protein is a regulator of hypoxia-induced, urokinase-type plasminogen activator receptor (uPAR)-mediated adhesion of immature hematological cells. J. Biol. Chem. 288, 11877–11886. doi: 10.1074/jbc.M112.442491
Nurieva, R., Yang, X. O., Martinez, G., Zhang, Y., Panopoulos, A. D., Ma, L., et al. (2007). Essential autocrine regulation by IL-21 in the generation of inflammatory T cells. Nature 448, 480–483. doi: 10.1038/nature05969
Nyborg, A. C., Ladd, T. B., Zwizinski, C. W., Lah, J. J., and Golde, T. E. (2006). Sortilin, SorCS1b, and SorLA Vps10p sorting receptors, are novel γ-secretase substrates. Mol. Neurodegener. 1:3.
Nykjaer, A., Lee, R., Teng, K. K., Jansen, P., Madsen, P., Nielsen, M. S., et al. (2004). Sortilin is essential for proNGF-induced neuronal cell death. Nature 427, 843–848. doi: 10.1038/nature02319
Offe, K., Dodson, S. E., Shoemaker, J. T., Fritz, J. J., Gearing, M., Levey, A. I., et al. (2006). The lipoprotein receptor LR11 regulates amyloid beta production and amyloid precursor protein traffic in endosomal compartments. J. Neurosci. 26, 1596–1603. doi: 10.1523/JNEUROSCI.4946-05.2006
Ohwaki, K., Bujo, H., Jiang, M., Yamazaki, H., Schneider, W. J., and Saito, Y. (2007). A secreted soluble form of LR11, specifically expressed in intimal smooth muscle cells, accelerates formation of lipid-laden macrophages. Arterioscler. Thromb. Vasc. Biol. 27, 1050–1056. doi: 10.1161/ATVBAHA.106.137091
Pan, X., Zaarur, N., Singh, M., Morin, P., and Kandror, K. V. (2017). Sortilin and retromer mediate retrograde transport of Glut4 in 3T3-L1 adipocytes. Mol. Biol. Cell 28, 1667–1675. doi: 10.1091/mbc.E16-11-0777
Patel, A. B., Tsilioni, I., Leeman, S. E., and Theoharides, T. C. (2016). Neurotensin stimulates sortilin and mTOR in human microglia inhibitable by methoxyluteolin, a potential therapeutic target for autism. Proc. Natl. Acad. Sci. U.S.A. 113, E7049–E7058. doi: 10.1073/pnas.1604992113
Patel, K. M., Strong, A., Tohyama, J., Jin, X., Morales, C. R., Billheimer, J., et al. (2015). Macrophage sortilin promotes LDL uptake, foam cell formation, and atherosclerosis. Circ. Res. 116, 789–796. doi: 10.1161/CIRCRESAHA.116.305811
Paushter, D. H., Du, H., Feng, T., and Hu, F. (2018). The lysosomal function of progranulin, a guardian against neurodegeneration. Acta Neuropathol. 136, 1–17. doi: 10.1007/s00401-018-1861-8
Petersen, C. M., Nielsen, M. S., Nykjaer, A., Jacobsen, L., Tommerup, N., Rasmussen, H. H., et al. (1997). Molecular identification of a novel candidate sorting receptor purified from human brain by receptor-associated protein affinity chromatography. J. Biol. Chem. 272, 3599–3605. doi: 10.1074/jbc.272.6.3599
Pirault, J., Polyzos, K. A., Petri, M. H., Ketelhuth, D. F. J., Bäck, M., and Hansson, G. K. (2017). The inflammatory cytokine interferon-gamma inhibits sortilin-1 expression in hepatocytes via the JAK/STAT pathway. Eur. J. Immunol. 47, 1918–1924. doi: 10.1002/eji.201646768
Quistgaard, E. M., Madsen, P., Grøftehauge, M. K., Nissen, P., Petersen, C. M., and Thirup, S. S. (2009). Ligands bind to sortilin in the tunnel of a ten-bladed β-propeller domain. Nat. Struct. Mol. Biol. 16, 96–98. doi: 10.1038/nsmb.1543
Reuter, E., Weber, J., Paterka, M., Ploen, R., Breiderhoff, T., van Horssen, J., et al. (2015). Role of sortilin in models of autoimmune neuroinflammation. J. Immunol. 195, 5762–5769. doi: 10.4049/jimmunol.1403156
Rhost, S., Hughes,É., Harrison, H., Rafnsdottir, S., Jacobsson, H., Gregersson, P., et al. (2018). Sortilin inhibition limits secretion-induced progranulin-dependent breast cancer progression and cancer stem cell expansion. Breast Cancer Res. 20:137. doi: 10.1186/s13058-018-1060-5
Rohe, M., Hartl, D., Fjorback, A. N., Klose, J., and Willnow, T. E. (2013). SORLA-mediated trafficking of TrkB enhances the response of neurons to BDNF. PLoS One 8:e72164. doi: 10.1371/journal.pone.0072164
Rohe, M., Synowitz, M., Glass, R., Paul, S. M., Nykjaer, A., and Willnow, T. E. (2009). Brain-derived neurotrophic factor reduces amyloidogenic processing through control of SORLA gene expression. J. Neurosci. 29, 15472–15478. doi: 10.1523/JNEUROSCI.3960-09.2009
Rose-John, S. (2018). Interleukin-6 family cytokines. Cold Spring Harb. Perspect. Biol. 10:a028415. doi: 10.1101/cshperspect.a028415
Roselli, S., Pundavela, J., Demont, Y., Faulkner, S., Keene, S., Attia, J., et al. (2015). Sortilin is associated with breast cancer aggressiveness and contributes to tumor cell adhesion and invasion. Oncotarget 6, 10473–10486. doi: 10.18632/oncotarget.3401
Rosenberger, C. M., Clark, A. E., Treuting, P. M., Johnson, C. D., and Aderem, A. (2008). ATF3 regulates MCMV infection in mice by modulating IFN-γ expression in natural killer cells. Proc. Natl. Acad. Sci. 105, 2544–2549. doi: 10.1073/pnas.0712182105
Ross, I. (2010). “A bird’s-eye view of macrophage biology,” in Lentiviruses and Macrophages: Molecular and Cellular Interactions, ed. M. Desport (Norfolk, VA: Caister Academic Press), 25–80.
Sakai, S., Nakaseko, C., Takeuchi, M., Ohwada, C., Shimizu, N., Tsukamoto, S., et al. (2012). Circulating soluble LR11/SorLA levels are highly increased and ameliorated by chemotherapy in acute leukemias. Clin. Chim. Acta 413, 1542–1548. doi: 10.1016/j.cca.2012.06.025
Scheller, J., Chalaris, A., Schmidt-Arras, D., and Rose-John, S. (2011). The pro- and anti-inflammatory properties of the cytokine interleukin-6. Biochim. Biophys. Acta 1813, 878–888. doi: 10.1016/j.bbamcr.2011.01.034
Schmidt, V., Schulz, N., Yan, X., Schürmann, A., Kempa, S., Kern, M., et al. (2016). SORLA facilitates insulin receptor signaling in adipocytes and exacerbates obesity. J. Clin. Invest. 126, 2706–2720. doi: 10.1172/JCI84708
Schmidt, V., Subkhangulova, A., and Willnow, T. E. (2017). Sorting receptor SORLA: cellular mechanisms and implications for disease. Cell. Mol. Life Sci. 74, 1475–1483. doi: 10.1007/s00018-016-2410-z
Schmidt, V., and Willnow, T. E. (2016). Protein sorting gone wrong – VPS10P domain receptors in cardiovascular and metabolic diseases. Atherosclerosis 245, 194–199. doi: 10.1016/j.atherosclerosis.2015.11.027
Schuett, H., Oestreich, R., Waetzig, G. H., Annema, W., Luchtefeld, M., Hillmer, A., et al. (2012). Transsignaling of interleukin-6 crucially contributes to atherosclerosis in mice. Arterioscler. Thromb. Vasc. Biol. 32, 281–290. doi: 10.1161/ATVBAHA.111.229435
Seaman, M. N. J. (2007). Identification of a novel conserved sorting motif required for retromer-mediated endosome-to-TGN retrieval. J. Cell Sci. 120, 2378–2389. doi: 10.1242/jcs.009654
Shalapour, S., and Karin, M. (2015). Immunity, inflammation, and cancer: an eternal fight between good and evil. J. Clin. Invest. 125, 3347–3355. doi: 10.1172/JCI80007
Shi, J., and Kandror, K. V. (2005). Sortilin is essential and sufficient for the formation of Glut4 storage vesicles in 3T3-L1 adipocytes. Dev. Cell 9, 99–108. doi: 10.1016/j.devcel.2005.04.004
Strong, A., Ding, Q., Edmondson, A. C., Millar, J. S., Sachs, K. V., Li, X., et al. (2012). Hepatic sortilin regulates both apolipoprotein B secretion and LDL catabolism. J. Clin. Invest. 122, 2807–2816. doi: 10.1172/JCI63563
Suda, T., Takubo, K., and Semenza, G. L. (2011). Metabolic regulation of hematopoietic stem cells in the hypoxic niche. Cell Stem Cell 9, 298–310. doi: 10.1016/j.stem.2011.09.010
Sung, H. Y., Lee, J. Y., Park, A. K., Moon, Y. J., Jo, I., Park, E.-M., et al. (2018). Aberrant promoter hypomethylation of sortilin 1: a moyamoya disease biomarker, aberrant promoter hypomethylation of sortilin 1: a moyamoya disease biomarker. J. Stroke 20, 350–361. doi: 10.5853/jos.2018.00962
Tang, W., Lu, Y., Tian, Q.-Y., Zhang, Y., Guo, F.-J., Liu, G.-Y., et al. (2011). The growth factor progranulin binds to TNF receptors and is therapeutic against inflammatory arthritis in mice. Science 332, 478–484. doi: 10.1126/science.1199214
Tanimoto, R., Palladino, C., Xu, S.-Q., Buraschi, S., Neill, T., Gomella, L. G., et al. (2017). The perlecan-interacting growth factor progranulin regulates ubiquitination, sorting, and lysosomal degradation of sortilin. Matrix Biol. 64, 27–39. doi: 10.1016/j.matbio.2017.04.001
Teng, H. K., Teng, K. K., Lee, R., Wright, S., Tevar, S., Almeida, R. D., et al. (2005). ProBDNF induces neuronal apoptosis via activation of a receptor complex of p75NTR and sortilin. J. Neurosci. 25, 5455–5463. doi: 10.1523/JNEUROSCI.5123-04.2005
Tjwa, M., Sidenius, N., Moura, R., Jansen, S., Theunissen, K., Andolfo, A., et al. (2009). Membrane-anchored uPAR regulates the proliferation, marrow pool size, engraftment, and mobilization of mouse hematopoietic stem/progenitor cells. J. Clin. Invest. 119, 1008–1018. doi: 10.1172/JCI36010
Trumpp, A., Essers, M., and Wilson, A. (2010). Awakening dormant haematopoietic stem cells. Nat. Rev. Immunol. 10, 201–209. doi: 10.1038/nri2726
Turner, M. D., Nedjai, B., Hurst, T., and Pennington, D. J. (2014). Cytokines and chemokines: at the crossroads of cell signalling and inflammatory disease. Biochim. Biophys. Acta 1843, 2563–2582. doi: 10.1016/j.bbamcr.2014.05.014
Tzachanis, D., Berezovskaya, A., Nadler, L. M., and Boussiotis, V. A. (2002). Blockade of B7/CD28 in mixed lymphocyte reaction cultures results in the generation of alternatively activated macrophages, which suppress T-cell responses. Blood 99, 1465–1473. doi: 10.1182/blood.V99.4.1465
Vázquez, C. L., Rodgers, A., Herbst, S., Coade, S., Gronow, A., Guzman, C. A., et al. (2016). The proneurotrophin receptor sortilin is required for Mycobacterium tuberculosis control by macrophages. Sci. Rep. 6:29332. doi: 10.1038/srep29332
Wahe, A., Kasmapour, B., Schmaderer, C., Liebl, D., Sandhoff, K., Nykjaer, A., et al. (2010). Golgi-to-phagosome transport of acid sphingomyelinase and prosaposin is mediated by sortilin. J. Cell Sci. 123, 2502–2511. doi: 10.1242/jcs.067686
Whittle, A. J., Jiang, M., Peirce, V., Relat, J., Virtue, S., Ebinuma, H., et al. (2015). Soluble LR11/SorLA represses thermogenesis in adipose tissue and correlates with BMI in humans. Nat. Commun. 6:8951. doi: 10.1038/ncomms9951
Wilson, C. M., Naves, T., Al Akhrass, H., Vincent, F., Melloni, B., Bonnaud, F., et al. (2016). A new role under sortilin’s belt in cancer. Commun. Integr. Biol. 9:e1130192. doi: 10.1080/19420889.2015.1130192
Wilson, C. M., Naves, T., Vincent, F., Melloni, B., Bonnaud, F., Lalloue, F., et al. (2014b). Sortilin mediates the release and transfer of exosomes in concert with two tyrosine kinase receptors. J. Cell Sci. 127, 3983–3997. doi: 10.1242/jcs.149336
Wilson, C. M., Naves, T., Saada, S., Pinet, S., Vincent, F., Lalloué, F., et al. (2014a). The implications of sortilin/vps10p domain receptors in neurological and human diseases. CNS Neurol. Disord. Drug Targets 13, 1354–1365.
Yabe-Wada, T., Matsuba, S., Takeda, K., Sato, T., Suyama, M., Ohkawa, Y., et al. (2016). TLR signals posttranscriptionally regulate the cytokine trafficking mediator sortilin. Sci. Rep. 6:26566. doi: 10.1038/srep26566
Yabe-Wada, T., Matsuba, S., Unno, M., and Onai, N. (2018). Crystal structure of the ligand-free form of the Vps10 ectodomain of dimerized sortilin at acidic pH. FEBS Lett. 592, 2647–2657. doi: 10.1002/1873-3468.13181
Yang, M., Lim, Y., Li, X., Zhong, J.-H., and Zhou, X.-F. (2011). Precursor of brain-derived neurotrophic factor (proBDNF) forms a complex with huntingtin-associated protein-1 (HAP1) and sortilin that modulates proBDNF trafficking, degradation, and processing. J. Biol. Chem. 286, 16272–16284. doi: 10.1074/jbc.M110.195347
Yu, X.-H., Fu, Y.-C., Zhang, D.-W., Yin, K., and Tang, C.-K. (2013). Foam cells in atherosclerosis. Clin. Chim. Acta 424, 245–252. doi: 10.1016/j.cca.2013.06.006
Yu, Y., Correll, P. H., and Heuvel, J. P. V. (2002). Conjugated linoleic acid decreases production of pro-inf lammatory(products )in macrophages: evidence for a PPARg-dependent mechanism. Biochim. Biophys. Acta 1581, 89–99. doi: 10.1016/S1388-1981(02)00126-9
Zhang, X.-M., Dormady, S. P., and Basch, R. S. (2000). Identification of four human cDNAs that are differentially expressed by early hematopoietic progenitors. Exp. Hematol. 28, 1286–1296. doi: 10.1016/S0301-472X(00)00539-7
Zhao, G., Zhu, G., Huang, Y., Zheng, W., Hua, J., Yang, S., et al. (2016). IL-6mediates the signal pathway of JAK-STAT3-VEGF-C promoting growth, invasion and lymphangiogenesis in gastric cancer. Oncol. Rep. 35, 1787–1795. doi: 10.3892/or.2016.4544
Zheng, S., and Abraham, C. (2013). NF-κB1 inhibits NOD2-induced cytokine secretion through ATF3-dependent mechanisms. Mol. Cell. Biol. 33, 4857–4871. doi: 10.1128/MCB.00797-13
Keywords: sortilin, SorLA, immune system, cytokines, trafficking, signaling, inflammation, phagocytosis
Citation: Talbot H, Saada S, Naves T, Gallet P-F, Fauchais A-L and Jauberteau M-O (2019) Regulatory Roles of Sortilin and SorLA in Immune-Related Processes. Front. Pharmacol. 9:1507. doi: 10.3389/fphar.2018.01507
Received: 12 October 2018; Accepted: 10 December 2018;
Published: 07 January 2019.
Edited by:
Jean Mazella, UMR7275 Institut de Pharmacologie Moléculaire et Cellulaire (IPMC), FranceReviewed by:
Gina Leinninger, Michigan State University, United StatesAlain Couvineau, Institut National de la Santé et de la Recherche Médicale (INSERM), France
Copyright © 2019 Talbot, Saada, Naves, Gallet, Fauchais and Jauberteau. This is an open-access article distributed under the terms of the Creative Commons Attribution License (CC BY). The use, distribution or reproduction in other forums is permitted, provided the original author(s) and the copyright owner(s) are credited and that the original publication in this journal is cited, in accordance with accepted academic practice. No use, distribution or reproduction is permitted which does not comply with these terms.
*Correspondence: Hugo Talbot, hugo.talbot@unilim.fr Marie-Odile Jauberteau, m-o.jauberteau-marchan@unilim.fr