- 1Department of Basic and Applied Medical Sciences, Heymans Institute of Pharmacology, Faculty of Medicine and Health Sciences, Ghent University, Ghent, Belgium
- 2Department of Human Structure and Repair, Faculty of Medicine and Health Sciences, Ghent University, Ghent, Belgium
Objective:
Intestinal inflammation triggers postoperative ileus (POI), commonly seen after abdominal surgery and characterized by impaired gastrointestinal transit; when prolonged, this leads to increased morbidity. Hydrogen sulfide (H2S) is recognized as an important mediator of many (patho)physiological processes, including inflammation, and is now investigated for anti-inflammatory application. Therefore, the aim of this study was to investigate the effect of the H2S-releasing naproxen derivative ATB-346, developed to reduce gastrointestinal injury by naproxen, and the slow-release H2S donor GYY4137 on intestinal inflammation and delayed gastrointestinal transit in murine POI.
Methods:
C57Bl6J mice were fasted for 6 h, anesthetized and after laparotomy, POI was induced by compressing the small intestine with two cotton applicators for 5 min (intestinal manipulation; IM). GYY4137 (50 mg/kg, intraperitoneally), ATB-346 (16 mg/kg, intragastrically) or naproxen (10 mg/kg, intragastrically) were administered 1 h before IM. At 24 h postoperatively, gastrointestinal transit was assessed via fluorescent imaging, and mucosa-free muscularis segments were prepared for later analysis. Inflammatory parameters and activity of inducible nitric oxide synthase (iNOS) and cyclo-oxygenase (COX)-2 were measured. Histological examination of whole tissue sections was done on hematoxylin-eosin stained slides.
Results:
Pre-treatment with GYY4137 (geometric center; GC: 7.6 ± 0.5) and ATB-346 (GC: 8.4 ± 0.3) prevented the delayed transit induced by IM (GC: 3.6 ± 0.5 vs. 9.0 ± 0.4 in non-operated controls) while naproxen only partially did (GC: 5.9 ± 0.5; n = 8 for all groups). GYY4137 and ATB-346 significantly reduced the IM-induced increase in muscular myeloperoxidase (MPO) activity and protein levels of interleukin (IL)-6, IL-1β and monocyte chemotactic protein 1; the reduction by naproxen was less pronounced and only reached significance for MPO activity and IL-6 levels. All treatments significantly reduced the increase in COX-2 activity caused by IM, whereas only GYY4137 significantly reduced the increase in iNOS activity. Naproxen treatment caused significant histological damage of intestinal villi.
Conclusion:
The study shows that naproxen partially prevents POI, probably through its inhibitory effect on COX-2 activity. Both ATB-346 and GYY4137 were more effective, the result with GYY4137 showing that H2S per se can prevent POI.
Introduction
Postoperative ileus (POI) refers to the transient impairment of gastrointestinal motility, which commonly occurs after abdominal surgery. It presents clinically as abdominal distension, inability to tolerate an oral diet, absence of bowel sounds and lack of flatus and defecation. Normally it resolves within 3 days, but when prolonged, nausea and vomiting will further contribute to increased morbidity, length of hospital stay and increased healthcare costs (Boeckxstaens and De Jonge, 2009). In the United States alone, the annual socio-economic impact of prolonged POI is estimated up to 1.46 billion US$ (Goldstein et al., 2007). POI upon intra-abdominal manipulation is induced by an acute neurogenic reaction with activation of inhibitory neural pathways and more importantly by a following prolonged inflammatory reaction triggered in the muscularis externa. Activated resident macrophages in the muscular layer play a critical role as they release inflammatory cytokines such as interleukin (IL)-6 and chemokines such as monocyte chemoattractant protein-1 (MCP-1), resulting in an increased endothelial expression of adhesion molecules such as intercellular adhesion molecule-1 and recruitment of circulatory leukocytes. Nitric oxide and prostaglandins released from these inflammatory cells will directly impair the smooth muscle contractility causing the delay in gastrointestinal transit (Boeckxstaens and De Jonge, 2009; Wehner et al., 2012). Laparoscopic procedures, enhanced recovery pathways and pharmacological treatment options such as prokinetics and non-steroidal anti-inflammatory drugs (NSAIDs) are advised to prevent prolonged POI but no full protection is obtained (Person and Wexner, 2006; Augestad, 2010; Bragg et al., 2015). Therefore, there is need for new treatment options, especially those targeting the intestinal inflammation (Van Bree et al., 2012).
Hydrogen sulfide (H2S), primarily known as a toxic environmental gas, is endogenously produced in mammalian tissue from cysteine, largely via three enzymes: cystathionine γ-lyase, cystathionine β-synthetase and 3-mercaptosulfurtransferase (Rose et al., 2017). H2S biosynthesis has been identified in a variety of mammalian tissues including lung, liver, heart and intestine and it has become clear that H2S, next to other endogenous gases like nitric oxide and carbon monoxide, plays an important role in both physiological and pathophysiological processes (Chen et al., 2007; Olas, 2015). Its role in inflammation is possibly one of the more controversial areas of the H2S biology as there are a lot of conflicting data concerning the pro- and/or anti-inflammatory properties of exogenous H2S. For example, administration of sodium hydrosulfide (NaHS), an H2S donor, was shown to inhibit aspirin-induced leukocyte adherence in mesenteric venules and reduced the leukocyte infiltration in an air pouch model in rats (Zanardo et al., 2006). In contrast, pre-treatment of mice with NaHS was shown to significantly enhance the lipopolysaccharide (LPS)-induced leukocyte adhesion, neutrophil migration and expression of adhesion molecules like P-selectin and intercellular adhesion molecule-1 in venular endothelium (Dal-Secco et al., 2008). One reason for the lack of clarity is the reliance on NaHS as an H2S donor in many studies; sulfide salts like NaHS, dissolved in aqueous solutions, will release large amounts of H2S within seconds (Li et al., 2008). Although the precise kinetic profile of endogenous H2S release within individual tissues has yet to be evaluated, it is likely that the controlled enzymatic H2S synthesis occurs at a much slower rate and in lesser amounts. Therefore, NaHS may not mimic the biological effects of endogenously produced H2S and, depending on the used dosage, may even exert toxic effects (Rose et al., 2015). In contrast to sulfide salts, GYY4137 [morpholin-4-ium 4 methoxyphenyl(morpholino) phosphinodithioate] releases H2S slowly both in vitro and in vivo for several hours, better mimicking the time course of naturally produced H2S, making this H2S donor more suitable to investigate the effect of exogenous H2S (Li et al., 2008; Lee et al., 2011). The anti-inflammatory effects of GYY4137 have already been shown in a variety of animal models including myocardial (Meng et al., 2015; Karwi et al., 2016; Qiu et al., 2018) and intestinal (Jensen et al., 2018) ischemia/reperfusion injury, LPS-induced endotoxemia (Li et al., 2009; Chen et al., 2016), atherosclerosis (Liu et al., 2013; Xie et al., 2016) and cisplatin-induced nephrotoxicity (Cao et al., 2018), whereby GYY4137 was able to reduce myeloperoxidase (MPO) activity and the level of pro-inflammatory cytokines such as IL-1β, IL-6, tumor necrosis factor-α and interferon (IFN)γ.
As mentioned earlier, NSAIDs are already used to treat pain and inflammation during prolonged POI allowing to spare opioids (Person and Wexner, 2006). However, NSAIDs, which suppress synthesis of prostaglandins by inhibiting cyclooxygenase (COX), are a major cause of gastric and duodenal ulceration and have been shown to also injure more distal parts of the small intestine, where the damage is more difficult to detect and treat (Wallace et al., 2011; Takeuchi and Satoh, 2015). It has been shown that H2S-releasing NSAIDs like ATB-346 [2-(6-methoxy-napthalen-2-yl)-propionic acid 4-thiocarbamoyl-phenyl ester], being the H2S-releasing derivative of naproxen, reduce the NSAID stimulated gastric leukocyte adhesion and protect the mucosa from ulceration (Wallace, 2007; Ekundi-Valentim et al., 2013). Moreover, released H2S will contribute to the overall anti-inflammatory effect of ATB-346; multiple studies have demonstrated a superior anti-inflammatory effect of ATB-346 when compared to naproxen as ATB-346 was able to reduce several inflammatory parameters like leukocyte infiltration, COX-2 activity and expression of IL-1β and tumor necrosis factor-α more effectively (Wallace et al., 2010; Campolo et al., 2013; Dief et al., 2015; Magierowski et al., 2017).
In the present study, we investigate the effect of a single dose of GYY4137 and ATB-346 on the delay in transit and inflammation during POI in mice and compare their effect with that of naproxen.
Materials and Methods
Materials
GYY4137 (Tocris Bioscience, Bristol, UK) was dissolved in saline (6.25 mg/ml) and ATB-346 (2 mg/ml; Axon Medchem, Groningen, Netherlands) and naproxen (1.25 mg/ml; Sigma-Aldrich, Diegem, Belgium) were suspended in 0.5% (m/v) methocel (methylcellulose, Sigma-Aldrich)/H2O. Isoflurane (IsoFlo®, Abbott Laboratories Ltd, Maidenhead, Berkshire, UK) was used to anaesthetize the mice when inducing POI. Fluorescein-labeled dextran (70 kDa; Invitrogen, Merelbeke, Belgium) was used to measure postoperative gastrointestinal transit.
Animals
Seven weeks old male C57BL/6J mice were purchased from Janvier, Le Genest St-Isle, France and were used between 8 and 12 weeks of age (22–25 g). Mice were housed in an animal care facility with a 12 h light/dark cycle and had free access to water and commercially available chow. Animal care and experimental procedures were approved by the Ethical Committee for Animal Experiments from the Faculty of Medicine and Health Sciences at Ghent University (ECD 16–48).
Surgical Procedure
POI was induced by surgical manipulation of the small intestine (intestinal manipulation, IM) as previously applied by several groups (Kalff et al., 2000; De Jonge et al., 2003; Cosyns et al., 2015). Mice were anaesthetized with inhaled isoflurane (induction, 5%; maintenance, 2%) and the abdomen was opened by midline laparotomy. The small intestine was exteriorized and then compressed for 5 min along its entire length by using sterile moist cotton swabs. The bowel was repositioned in the abdominal cavity and the incision was closed by two layers of continuous sutures. The total duration of the procedure was approximately 20–25 min. After the operation, mice were sacrificed at 24 h after induction of POI. The gastrointestinal tract from stomach until colon was then isolated. After measuring intestinal transit (see Section “Measurement of Intestinal Transit”), the gastrointestinal tract was flushed with aerated (5% CO2 in O2) ice-cold Krebs solution containing 1 mM phenylmethylsulfonyl fluoride. The small intestine was divided in 6 segments of equal length. Whole tissue sections of segment 3 were fixed in formalin for further histological examination. The mucosa was removed in the other segments using a glass slide and the muscular layer was stored at −80°C until further analysis.
Experimental Protocol
Mice were randomly assigned to five experimental groups (n = 8 per group). Group I served as control (non-treated, non-operated). Group II underwent surgical manipulation of the small intestine (IM). Group III to V received respectively GYY4137 intraperitoneally (i.p., 50 mg/kg), naproxen intragastrically (i.g., 10 mg/kg) and ATB-346 i.g. (16 mg/kg) at 1 h before the surgical procedure. All animals were deprived of food from 6 h before IM but had again access to food after IM. At 21 h after surgery, mice were again deprived of food to allow measurement of intestinal transit (see Section “Measurement of Intestinal Transit”). Mice were sacrificed at 24 h after surgery. Figure 1 shows a schematic overview of the protocol.
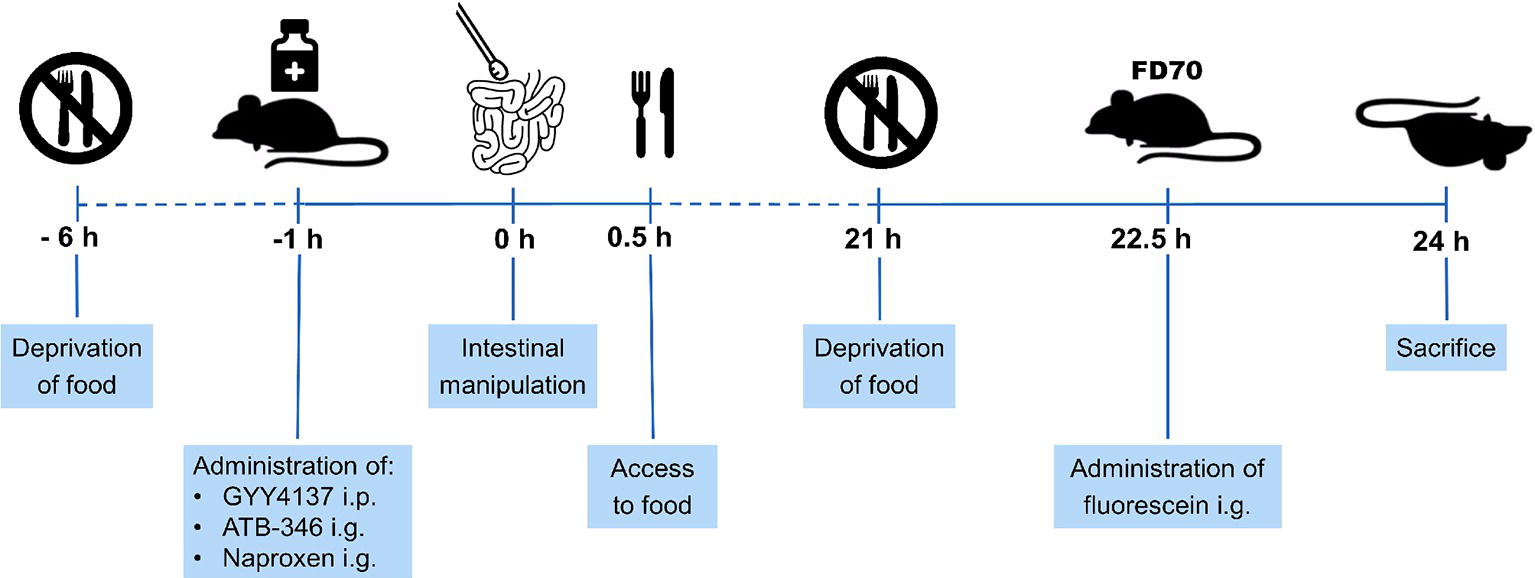
Figure 1. Schematic overview of experimental protocol. Mice were deprived of food from 6 h before intestinal manipulation (IM) and given GYY4137, ATB-346 or naproxen 1 h before IM. When mice recovered from surgery, they had again access to food. Mice were deprived of food at 21 h after IM and received 200 μl of fluorescein-labeled dextran (FD70) at 22.5 h after IM. 1.5 h later, mice were sacrificed followed by measurement of the gastrointestinal transit and collection of tissue samples of the small intestine.
Measurement of Intestinal Transit
Intestinal transit was measured by evaluating the distribution of non-absorbable fluorescein-labeled dextran (70 kDa; FD70) along the gastrointestinal tract as previously described (De Backer et al., 2008). 21 h after IM, the mice were deprived of food and 1.5 h hereafter a liquid fluorescein-labeled dextran meal (200 μl of a 25 mg/ml solution) was gavaged. 1.5 h later, i.e. 24 h after surgery, the animals were sacrificed. After excision of the gastrointestinal tract, two full-field images (one in normal illumination mode and another in fluorescent mode) were taken with a CCD camera and subsequently matched for calculation of fluorescence distribution along the gastrointestinal tract. The fluorescent intensity throughout the entire gastrointestinal tract was analyzed and calculated. Data were expressed as the percentage of fluorescence intensity per segment (stom, stomach; sb, small bowel segments 1–10; caec, caecum; col, colon segments 1–2). The geometric center (GC) of the fluorescence distribution, which can be described as the center of gravity for the distribution of fluorescein, was calculated by the formula: ∑(% FD70 per segment*segment number)/100). It can range from 1, if all fluorescein were found in the stomach, to 14, if all fluorescein were found in the second segment of the colon.
Biochemical Analyses
MPO Activity
MPO activity in mucosa-free segments of the small intestine was measured as an index of neutrophil infiltration, based on a previously described protocol (De Jonge et al., 2003).
Frozen tissue samples were homogenized with a Mikro-Dismembrator and dissolved in 10 volumes of 50 mM potassium phosphate buffer (pH 6.0) containing 0.5% hexadecyl-trimethylammonium bromide (HETAB). The homogenate was sonicated on ice (15 pulses of 0.7 s at full power) and subsequently subjected to freeze/thaw. The suspension was centrifuged (14,000 g, 20 min, 4°C) and 10 μl of the supernatant was added to 200 μl of assay mixture, containing ready-to-use 3,3′,5,5′-tetramethylbenzidine substrate, 0.5% HETAB, and 10 mM EDTA (on ice). The optical density was immediately read at 620 nm (Biotrak II). The reaction was then allowed to proceed for 3 min at 37°C. The reaction was stopped by placing the 96-well plate on ice, and the optical density was measured again. One unit of MPO activity was defined as the amount of enzyme that produces a change in optical density of 1.0 per minute at 37°C. Results were normalized to total protein content (Pierce BCA Protein Assay Kit) and expressed as U/mg protein.
IL-1β, IL-6, IL-10, IFNγ and MCP-1 Protein Levels
Protein expression levels of IL-1β, IL-6, IL-10, IFNγ and MCP-1 in mucosa-free segments of the small intestine were assessed by a Luminex magnetic bead assay according to the manufacturer’s guidelines (Bio-Rad, Temse, Belgium); the samples were processed using the BioPlex Pro Reagent Kit (Bio-Rad).
Frozen tissue samples were homogenized in PBS, containing protease and phosphatase inhibitors. The homogenate was sonicated on ice (5 pulses of 0.7 s) and after centrifugation (15,000 rpm, 15 min, 4°C), 50 μl of the supernatant was added to the corresponding well of a 96-well plate loaded with coupled beats for IL-1β, IL-6, IL-10, IFNγ and MCP-1. After placing the plate for 30 min on a shaking platform at 850 rpm protected from light, wells were washed and 25 μl of the mixture of detection antibodies for IL-1β, IL-6, IL-10, IFNγ and MCP-1 was added to each well. After placing the plate for another 30 min on the shaking platform at 850 rpm protected from light, wells were washed and 50 μl of Streptavidin Phycoerythrin solution was added to each well. After placing the plate for 10 min on the shaking platform at 850 rpm protected from light, wells were washed and the beads in each well were re-suspended in 125 μl assay buffer. After a last incubation step of 30 s, the wells were analyzed for IL-1β, IL-6, IL-10, IFNγ and MCP-1 protein concentration using Bio-Plex Manager Software (Bio-Rad). Data from tissue samples were normalized to the total protein content (Pierce BCA Protein Assay Kit).
COX-2 Activity
COX-2 enzyme activity was measured in mucosa-free segments by COX activity assay (Cayman Chemical) using arachidonic acid as a substrate and 10-acetyl-3,7-dihydroxy-phenoxazine (ADHP) as a fluorometric co-substrate. The reaction between COX-derived prostaglandin G2 and ADHP produces the highly fluorescent compound resorufin, which was measured.
Frozen tissue samples were homogenized with a Mikro-Dismembrator, dissolved in 5 volumes of 100 mM Tris-HCl buffer (pH 7.5), containing protease inhibitors, and centrifuged at 10,000 g for 15 min at 4°C. 10 μl of the supernatant was transferred to the appropriate well containing 140 μl of 1X Assay Buffer (100 mM Tris-HCl, pH 8.0), 10 μl of Heme in DMSO and 10 μl of the COX-1 inhibitor SC-560 (66 μM). After an incubation period of 5 min, 10 μl of ADHP Assay Reagent was added to each well. Administration of 10 μl of a 2 mM arachidonic acid solution to each well initiated the reaction; 1 min later the plate was measured using a spectrofluorometer (excitation wavelength: 530–540 nm, emission wavelength: 585–595 nm; Victor Wallac, PerkinElmer Life and Analytical Sciences). Background values were measured by not adding 10 μl of the arachidonic acid solution to the assigned wells and were deducted from the corresponding sample values. Results were normalized to total protein content (Pierce BCA Protein Assay Kit) and COX-2 activity was expressed as % of mean fluorescence in tissue from non-manipulated control mice.
iNOS Activity
Inducible NO synthase (iNOS) enzyme activity in intestinal muscularis samples was assayed by measuring the conversion of [3H]-arginine to [3H]-citrulline by use of a NOS activity assay (Cayman Chemical), according to the manufacturer’s protocol. The assay was conducted in calcium-free conditions to measure only iNOS.
Frozen tissue samples were homogenized with a Mikro-Dismembrator, dissolved in five volumes of 1X Homogenization Buffer and centrifuged at 10,000 g for 15 min at 4°C. 10 μl of the supernatant was added to 40 μl reaction mix (22.72 μl of Reaction Buffer [50 mM Tris-HCl (pH 7.4), 6 μM tetrahydrobiopterin, 2 μM flavin adenine dinucleotide, 2 μM flavin adenine mononucleotide], 4.55 μl of 10 mM NADPH (prepared in 10 mM Tris-HCl), 0.90 μl of [3H]-arginine (1 μCI/μl), 4.55 μl 8 mM Mg acetate, 3.64 μl calmodulin and 3.64 μl ddH2O). The reaction samples were incubated for 1 h at room temperature, and the reaction was stopped by adding 400 μl of Stop Buffer to the reaction sample. 100 μl of the equilibrated resin was added into each reaction sample and the reaction samples were then transferred in the provided spin cups, which were centrifuged for 30 s in a microcentrifuge at full speed. The eluate was then transferred to scintillation vials, and, after adding 2 ml scintillation solution (Ultima Gold, Canberra Packard, USA) to each vial, the radioactivity was quantified in a liquid scintillation counter (Packard Tri-Carb 2,100 TR, Canberra Packard, USA). Results were normalized to total protein content (Pierce BCA Protein Assay Kit) and iNOS activity was expressed as % of [3H]-citrulline in tissue from non-manipulated control mice.
Intestinal Histological Evaluation
Intestinal samples were fixed in formalin, dehydrated in a graded ethanol series and embedded in paraffin. Tissue sections of 5 μM were cut with a microtome and then stained with hematoxylin-eosin. Jonas Van Dingenen and Leen Pieters independently scored the slides to evaluate intestinal mucosal damage using the Chiu score (Chiu et al., 1970): grade 0, normal mucosa villi; grade 1, development of sub-epithelial Gruenhagen’s space at the apex of the villi; grade 2, extension of the sub-epithelial space with moderate epithelial lifting; grade 3, massive epithelial lifting, possibly with a few denuded villi; grade 4, denuded villi with lamina propria and exposed capillaries; and grade 5, disintegration of the lamina propria, ulceration and hemorrhage. For each sample, 100 villi were scored by both evaluators and the mean score per sample was calculated. Good correlation between the scores of the two evaluators is illustrated by a Pearson’s r-value of 0.8324.
Statistical Analysis
Data are given as mean ± s.e.m. as indicated; n refers to tissues obtained from different animals. The results were compared by one-way analysis of variance (ANOVA), followed by Bonferroni multiple comparison t-test for all manipulated groups vs. the non-operated control group and for the treated manipulated groups vs. the non-treated manipulated group. Results were considered different from a p less than 0.05 on (GraphPad version 5.03, San Diego, CA, USA). The Grubbs’ test was used to determine significant outliers (p < 0.05), that were excluded for further statistical analysis (Grubbs, 1969). The Pearson correlation test was used to investigate the correlation between the scores of the two evaluators performing the intestinal histological evaluation.
Results
Effect of GYY4137, Naproxen and ATB-346 on Manipulation-Induced Intestinal Dysmotility
In non-manipulated control mice, fluorescein-labeled dextran had mainly moved to the distal part of the small bowel (Figure 2A). In mice that underwent intestinal manipulation, fluorescein-labeled dextran was mostly present in the proximal part of the small bowel, pointing out a delay in transit (Figure 2B), which was confirmed by a significant reduction in GC (Figure 2F). Pre-treatment with 50 mg/kg GYY4137 i.p. or 16 mg/kg ATB-346 i.g. reduced the delay in transit caused by IM, as indicated by the shift of fluorescein-labeled dextran to the distal part of the small bowel (Figures 2C–E); the GC was significantly increased vs. that in untreated manipulated animals and no longer significantly different from that in non-manipulated control mice (Figure 2F). Pre-treatment with naproxen did not completely prevent the delay in transit caused by IM as the GC-value was still significantly different from that in non-manipulated control mice (Figure 2F).
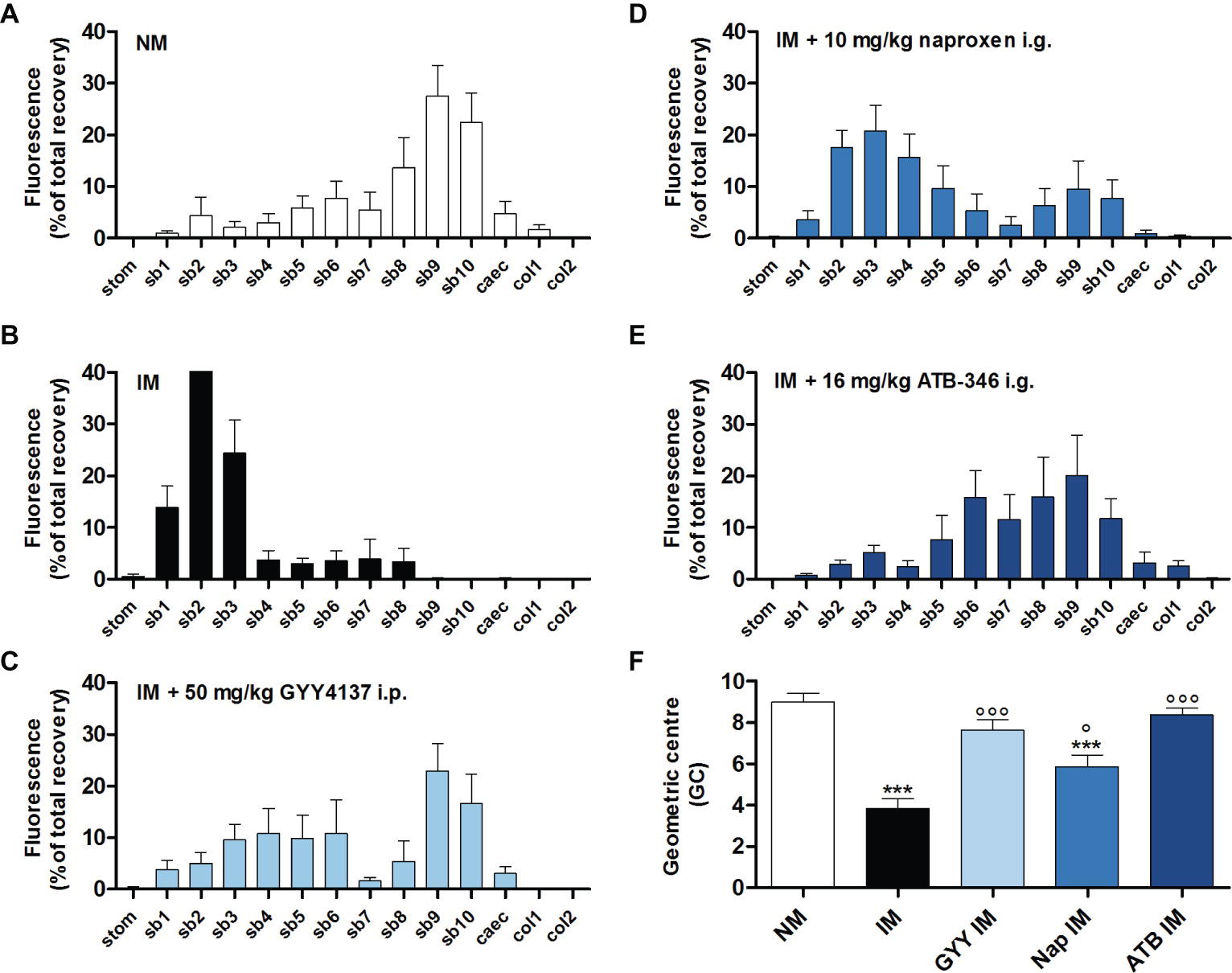
Figure 2. Influence of GYY4137, naproxen and ATB-346 on the delay in gastrointestinal transit caused by intestinal manipulation. Transit histograms (A–E) and geometric center (F) for the distribution of fluorescein-labeled dextran (70 kDa) along the gastrointestinal tract (stom, stomach; sb, small bowel segments; caec, caecum; col, colon segments), measured 24 h after intestinal manipulation (IM). Data represent the means ± S.E.M. of n = 8. ***p < 0.001 for comparison with non-manipulated (NM) control mice; °p < 0.05, °°°p < 0.001 for comparison with non-treated IM mice: one-way ANOVA followed by a Bonferroni multiple comparison test.
Effect of GYY4137, Naproxen and ATB-346 on Manipulation-Induced Inflammation
The leukocyte infiltration into the muscularis, measured as MPO activity, was increased 24 h after IM. This IM-induced accumulation of leukocytes in the muscular layer was significantly reduced when mice were pre-treated with 50 mg/kg GYY4137 i.p., 10 mg/kg naproxen i.g. or 16 mg/kg ATB-346 i.g. (Figure 3A). However, the MPO activity in the naproxen treated group was still significantly increased compared to the non-manipulated control group.
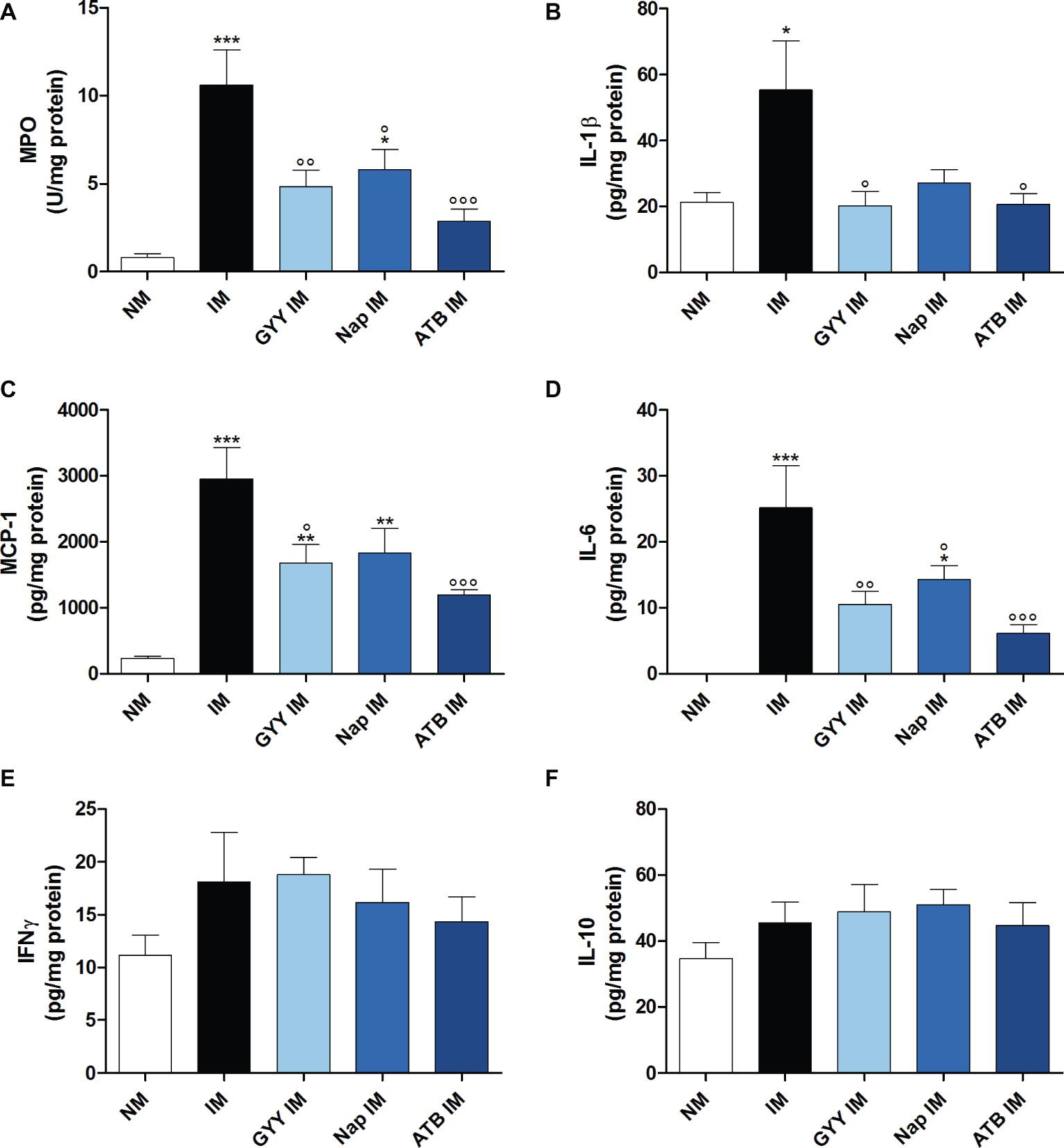
Figure 3. Influence of GYY4137, naproxen and ATB-346 on intestinal manipulation induced inflammation. Leukocyte infiltration ((A); myeloperoxidase activity, MPO) and IL-1β (B), MCP-1 (C), IL-6 (D), IFNγ (E) and IL-10 (F) protein levels in the muscular layer, measured 24 h after intestinal manipulation (IM). Data represent the means ± S.E.M. of n = 7–8. *p < 0.05, **p < 0.01, ***p < 0.001 for comparison with non-manipulated (NM) control mice; °p < 0.05, °°p < 0.01, °°°p < 0.001 for comparison with non-treated IM mice: one-way ANOVA followed by a Bonferroni multiple comparison test.
As shown in Figures 3B–D, surgical manipulation of the small intestine markedly increased IL-1β, MCP-1 and IL-6 protein expression in the intestinal muscular layer at 24 h after IM. Pre-treatment with all three compounds resulted in a significant reduction of the elevated IL-6 levels but the level of IL-6 in the group treated with naproxen remained significantly different from that in non-manipulated control animals (Figure 3D). Only GYY4137 and ATB-346 were able to significantly reduce the increase in IL-1β and MCP-1 levels caused by IM (Figures 3B,C). No significant increase in protein expression of IL-10 and IFNγ was observed in the muscularis of manipulated mice with the Luminex magnetic bead assay (Figures 3E,F).
Effect of GYY4137, Naproxen and ATB-346 on Intestinal iNOS and COX-2 Activity
Surgical manipulation of the small intestine caused a significant increase in iNOS enzyme activity in the muscularis. Only GYY4137 significantly reduced this increase in iNOS activity. Treatment with ATB-346 also tended to reduce the elevated iNOS activity, but this effect was not significant (Figure 4A). Surgery also caused a pronounced increase in COX-2 activity. All three compounds were able to significantly reduce the increased COX-2 activity caused by IM (Figure 4B).
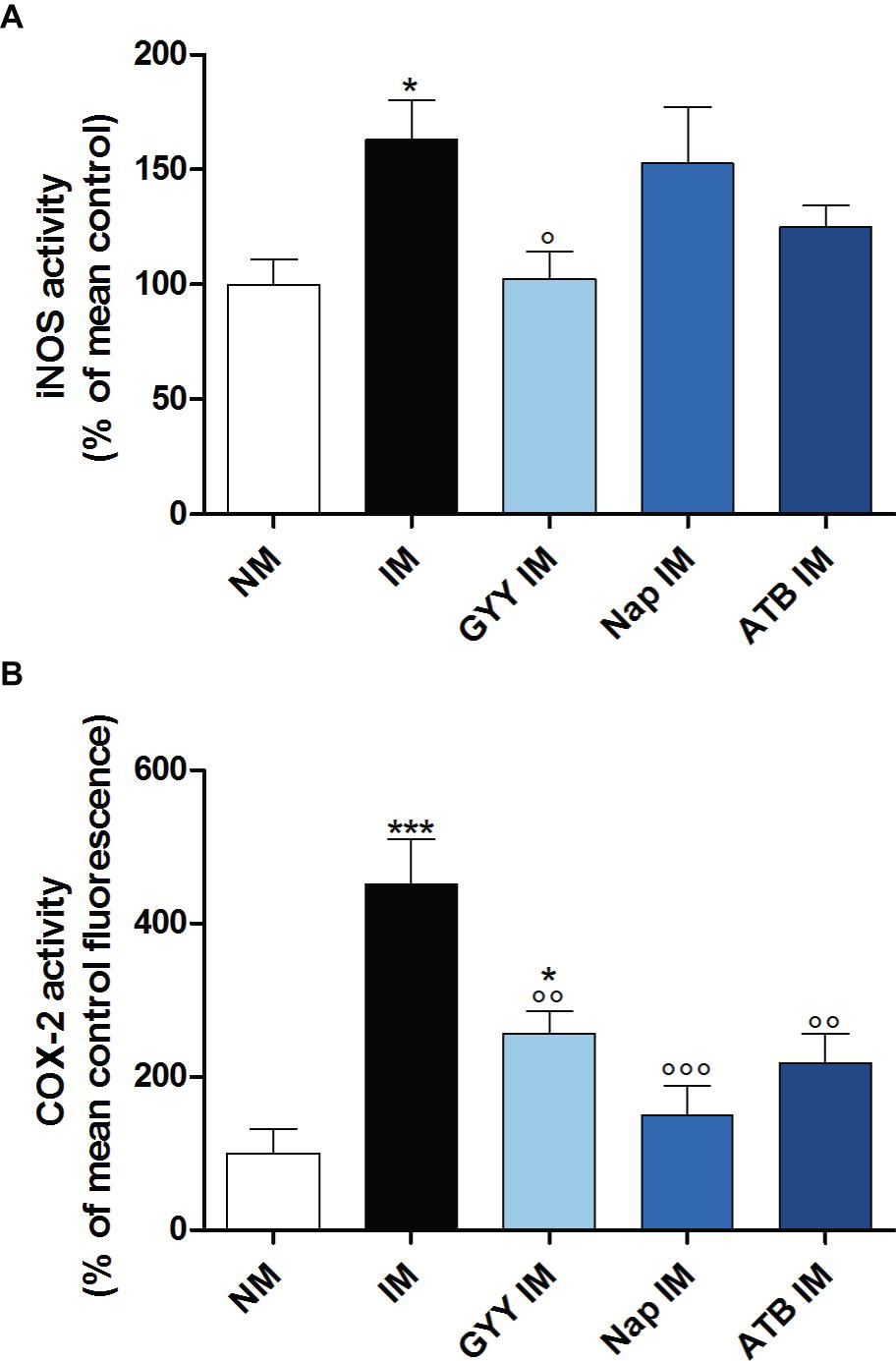
Figure 4. Influence of GYY4137, naproxen and ATB-346 on intestinal iNOS and COX-2 activity. iNOS (A) and COX-2 (B) activity in the muscular layer, measured 24 h after intestinal manipulation (IM). Data represent the means ± S.E.M. of n = 8. *p < 0.05, ***p < 0.001 for comparison with non-manipulated (NM) control mice; °p < 0.05, °°p < 0.01, °°°p < 0.001 for comparison with non-treated IM mice: one-way ANOVA followed by a Bonferroni multiple comparison test.
Effect of GYY4137, Naproxen and ATB-346 on Morphology of Intestinal Villi
No injury was seen in the non-manipulated control group, which demonstrated normal mucosal villi (Figure 5A). However, in the non-treated (Figure 5B) and GYY4137 treated (Figure 5C) manipulated mice, development of sub-epithelial Gruenhagen’s space in the tip of the villi was observed although the increase in Chiu score did not reach significance. The intestinal structure was damaged most severely in the naproxen treated IM group (Figure 5D), regularly showing moderate to massive epithelial lifting down the sides of the villi leading to a significant increase in Chiu score. Compared to the naproxen treated IM group, villi were less damaged when animals were treated with ATB-346 (Figure 5E), showing Gruenhagen’s space at the apex of the villi and moderate epithelial lifting.
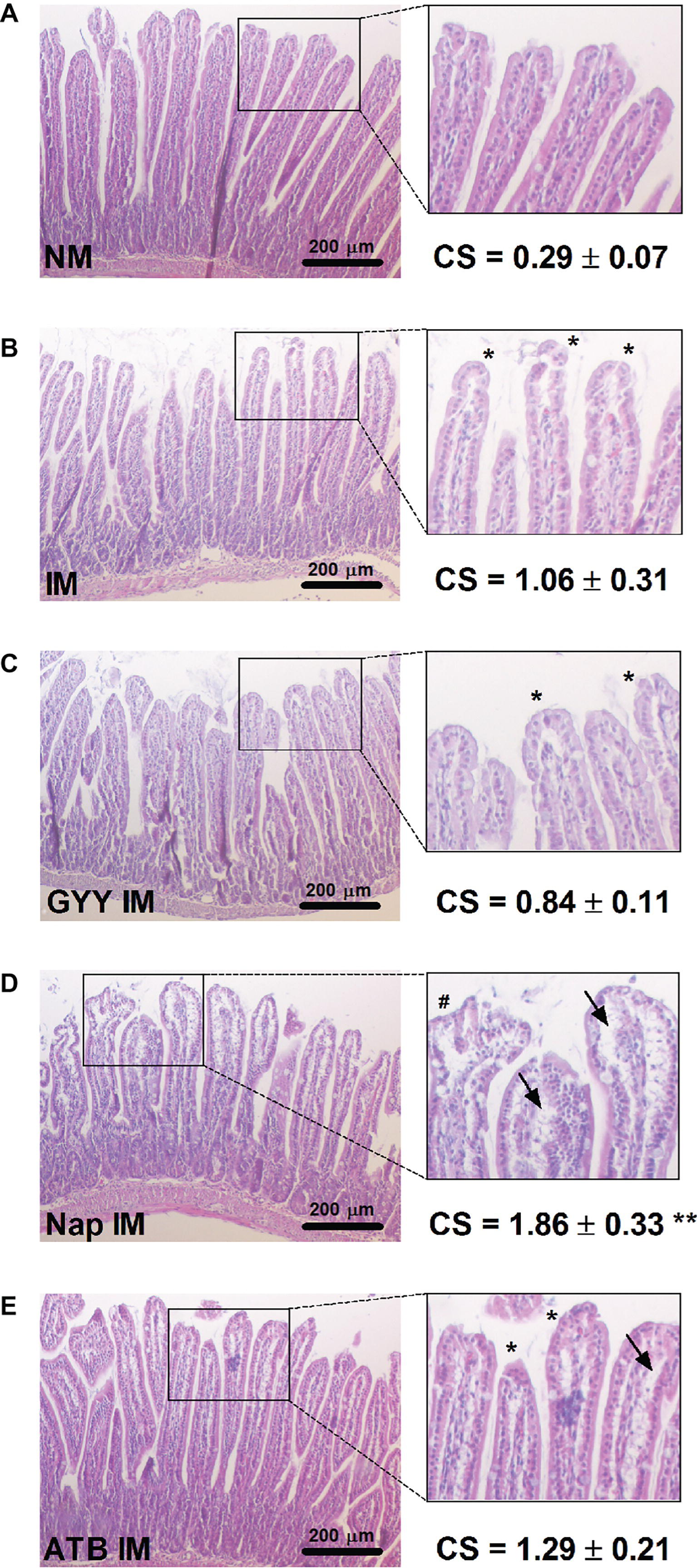
Figure 5. Influence of GYY4137, naproxen and ATB-346 on morphology of intestinal villi. Histological analysis and Chiu score (CS;A–E) of small intestinal mucosa following hematoxylin-eosin staining, analyzed 24 h after intestinal manipulation (IM). Asterisks (*) mark the development of sub-epithelial Gruenhagen’s space at villus apex; arrows (→) indicate further epithelial lifting down the sides of the villi and the number signs (#) mark massive epithelial lifting. Data represent the means ± S.E.M. of n = 4. **p < 0.01 for comparison with non-manipulated (NM) control mice: one-way ANOVA followed by a Bonferroni multiple comparison test.
Discussion
Hydrogen sulfide therapy has attracted significant attention as a potent biological mediator. Although its benefits have been appreciated in different gastrointestinal disease models like colitis (Fiorucci et al., 2007; Chen and Liu, 2016) and intestinal ischemia/reperfusion injury (Henderson et al., 2010; Jensen et al., 2018), its potential effect on POI has not yet been investigated. POI is a serious complication seen after abdominal surgery, leading to impairment of the gastrointestinal transit that traditionally was thought to result from neuronal dysfunction. Presently, it is clear that the immune response in the intestinal muscular layer upon abdominal surgery, characterized by the activation of resident macrophages and upregulation of inflammatory cytokines, adhesion molecules and chemokines, is the main mechanism of POI (Boeckxstaens and de Jonge, 2009; Van Bree et al., 2012; Bragg et al., 2015). Many of the data leading to the pathophysiological explanation of POI were obtained in the murine model of POI with manipulation of the small intestine. Throughout the years, this model yielded consistent results for transit and inflammation in our and other laboratories (Moore et al., 2003; Nakao et al., 2006; De Backer et al., 2009; Engel et al., 2010; Snoek et al., 2012; Cosyns et al., 2015). Also in the present study an inflammatory response is observed after manipulation of the murine small intestine, as shown by the increased leukocyte infiltration and elevated protein levels of the pro-inflammatory cytokines IL-6 and IL-1β and the chemokine MCP-1 in the intestinal muscularis externa at 24 h after induction of POI. Although previous reports (De Backer et al., 2009; Engel et al., 2010) show elevated IL-10 protein and IFNγ mRNA levels at 24 h after IM, we could not measure a significant increase in protein expression for these cytokines with the Luminex magnetic bead assay used in this study.
GYY4137 is an H2S-releasing molecule that was shown to have a pronounced anti-inflammatory effect in rodent models of myocardial (Qiu et al., 2018) and intestinal (Jensen et al., 2018) ischemia/reperfusion injury, endotoxemia (Chen et al., 2016) and atherosclerosis (Liu et al., 2013). The usual dose applied is 50 mg/kg by i.p. route (Liu et al., 2013; Chen et al., 2016; Jensen et al., 2018; Qiu et al., 2018). I.g. administration of GYY4137 has to be avoided since the release of H2S from GYY4137 will be enhanced under acidic conditions (Li et al., 2008), possibly leading to toxic H2S levels as seen with fast-release H2S donors such as NaHS. H2S-releasing naproxen ATB-346 showed beneficial anti-inflammatory effects in rodent models of colorectal cancer (Elsheikh et al., 2014), melanoma (De Cicco et al., 2016), zymosan-induced arthritis (Dief et al., 2015), spinal cord injury (Campolo et al., 2013) and carrageenan-induced synovitis (Ekundi-Valentim et al., 2013); the usual dose is 16 mg/kg by i.g. route (Ekundi-Valentim et al., 2013; Elsheikh et al., 2014; Dief et al., 2015). The studied route of administration for ATB-346 in rodents is indeed the i.g. one, as the compound is developed to improve the efficacy and decrease the side effects of the NSAID naproxen, used perorally in clinical practice. As neither GYY4137 nor ATB-346 has been tested before for POI, this was done in the actual study. I.g. naproxen was studied in comparison with ATB-346; the dose used (10 mg/kg) is the molar equivalent of the amount of naproxen contained within 16 mg/kg ATB-346 and has already been shown to exert anti-inflammatory effects in a rat model of carrageenan-induced synovitis (Ekundi-Valentim et al., 2013).
In the present study we show that both GYY4137 and ATB-346 were able to completely reverse the delay in gastrointestinal transit seen after IM, whereas naproxen was only able to partially restore the delayed transit. The findings for the NSAID naproxen are similar to previous work, in which the authors show the beneficial effects of pre-treatment with the non-selective COX inhibitors indomethacin and ketorolac and the selective COX-2 inhibitor 5,5-dimethyl-3-(3-fluorophenyl)-4-(4-methylsulphonyl) phenyl-2(5H)-furan on the impaired transit in a rat model of POI (De Winter et al., 1998; Schwarz et al., 2001). Treatment with GYY4137 and ATB-346 was also found to reduce the IM-induced inflammatory response, as evidenced by a marked reduction of the inflammatory mediators IL-1β, IL-6 and MCP-1 and leukocyte infiltration; whereas the reduction caused by naproxen only reached significance for IL-6 protein levels and leukocyte infiltration. These data are consistent with previous evidence showing that the H2S-releasing moiety of ATB-346 adds complimentary anti-inflammatory effects to the COX inhibiting effect of naproxen (Wallace et al., 2010; Campolo et al., 2013; Dief et al., 2015; Magierowski et al., 2017). The functional importance of the kinetically active substances NO and prostaglandins, that directly inhibit smooth muscle contractility, in the pathogenesis of POI has been demonstrated in earlier studies (Kalff et al., 2000; Schwarz et al., 2001). Therefore, we further investigated whether GYY4137, naproxen or ATB-346 could alter iNOS and COX-2 enzyme activity levels. Only GYY4137 was able to significantly reduce both the increased iNOS and COX-2 activity. Similarly, in an in vitro study in human synoviocytes and articular chondrocytes GYY4137 was able to dose-dependently reduce the LPS-induced increase in both iNOS and COX-2 expression and activity (Li et al., 2013). ATB-346 and naproxen did only cause a significant reduction in COX-2 activity, although ATB-346 tended to reduce iNOS activity. These findings are in line with the study of Campolo et al. in which ATB-346, but not naproxen, was able to attenuate iNOS expression in a murine model of spinal cord injury; it has to be noted that naproxen did also not affect COX-2 expression in this model (Campolo et al., 2013). However, the same research group also reported that in a model of traumatic brain injury in mice, administration of both ATB-346 and naproxen led to a significant reduction of COX-2 and iNOS expression (Campolo et al., 2014). The absence of any effect with naproxen on iNOS activity in the actual study might contribute to the less pronounced effect of naproxen on retarded transit.
As mentioned earlier, NSAIDs are a major cause of gastric and duodenal ulceration and have been shown to also injure more distal parts of the small intestine. The protective effect of H2S-releasing NSAIDs like ATB-346 on gastric and intestinal lesions has been well described in the literature and a double-blind, controlled clinical study to compare the gastrointestinal safety of ATB-346 vs. naproxen in healthy subjects is ongoing (ClinicalTrials.gov1; Wallace, 2007; Wallace et al., 2010; Magierowski et al., 2017). In this study intestinal manipulation per se tended to induce moderate mucosal damage although not reaching significance; a similar Chiu score was seen in manipulated mice pretreated with GYY4137. In manipulated mice, pretreated with naproxen, a significant increase in Chiu score was seen, confirming the well-known intestinal mucosal injury by NSAIDs. However in manipulated mice pretreated with ATB-346, the Chiu score was similar to that in non-treated manipulated mice showing that the H2S-release from this molecule was able to counteract mucosal injury of the naproxen moiety.
Follow-up studies can be performed to unravel the underlying mechanisms by which these H2S-releasing compounds exert their beneficial effects in the murine model of POI. The most frequently described mechanisms by which exogenous H2S exerts its anti-inflammatory effect are inhibition of the pro-inflammatory nuclear transcription factor-κB (Oh et al., 2006; Li et al., 2013; Chen and Liu, 2016), activation of the anti-oxidant nuclear factor erythroid 2–related factor 2 (Shimada et al., 2015; Xie et al., 2016; Qiu et al., 2018) and inhibition of the mitogen-activated protein kinase signaling pathways like p38 and extracellular signal-regulated kinase 1/2 (Hu et al., 2007; Xu et al., 2013). Furthermore, it can be investigated whether the endogenous H2S pathway is upregulated during POI as a protective mechanism, whereby exogenous H2S donors then strengthen this reaction; enhanced H2S synthesis with increased expression of H2S synthetizing enzymes at sites of mucosal ulceration was indeed demonstrated in rat colitis (Wallace et al., 2009; Flannigan et al., 2013).
This study demonstrates that pre-treatment with GYY4137, ATB-346 or naproxen reduces the impaired transit and suppresses the IM-induced inflammatory response in the muscularis seen during POI in mice, with naproxen being the least effective compound. Furthermore, the intestinal mucosal damage with naproxen alone was prevented by administering it as a H2S-releasing compound. The data suggest that delivery of H2S may represent a new pharmacological approach to prevent POI or to improve the effect of NSAIDs in POI. NSAIDs are indeed already studied for postoperative ileus with some improvement of time to gut recovery (Milne et al., 2018). The current data suggest that ATB-346 is preferable over naproxen per se for application in POI. The step to a clinical trial with ATB-346 to prevent POI in patients undergoing colorectal surgery seems easily feasible as safety of ATB-346 was already shown in a phase 1 study in healthy subjects and a phase 2 clinical trial demonstrated pain relief of ATB-346 in patients with osteoarthritis; in both studies whole blood COX activity was profoundly suppressed (Wallace et al., 2018).
Data Availability
The datasets generated for this study are available on request to the corresponding author.
Ethics Statement
This study was carried out in accordance with the recommendations of the most recent national legislation (Belgian Royal Decree, 29/05/2013) and European Directive (Directive 2010/63/EU). The protocol was approved by the Ethical Committee for Animal Experiments from the Faculty of Medicine and Health Sciences at Ghent University (ECD 16-48).
Author Contributions
RL designed the study. JD performed the experiments and data analysis. Intestinal histological evaluation was done by LP and JD under supervision of AV. RL and JD interpreted the findings and prepared and completed the manuscript. All authors approved the final version of the manuscript.
Conflict of Interest Statement
The authors declare that the research was conducted in the absence of any commercial or financial relationships that could be construed as a potential conflict of interest.
Acknowledgments
The authors thank Mrs. Els Van Deynse for technical support. Preliminary accounts of the study were presented at the Belgian Week of Gastroenterology, February 22th 2018, Antwerp, Belgium (Van Dingenen and Lefebvre, 2018a) and at the Meeting of the Federation of Neurogastroenterology and Motility, August 31st 2018, Amsterdam, The Netherlands (Van Dingenen and Lefebvre, 2018b).
Abbreviations
ADHP, 10-acetyl-3,7-dihydroxy-phenoxazine; ANOVA, One-way analysis of variance; COX, Cyclo-oxygenase; FD70, Fluorescein-labeled dextran (70 kDa); GC, Geometric center; HETAB, Hexadecyl-trimethylammonium bromide; H2S, Hydrogen sulfide; IFN, Interferon; i.g., Intragastrically; IL, Interleukin; IM, Intestinal manipulation; i.p., Intraperitoneally; MCP-1, Monocyte chemoattractant protein-1; MPO, Myeloperoxidase; NaHS, Sodium hydrosulfide; NSAID, Non-steroidal anti-inflammatory drug; POI, Postoperative ileus.
Footnotes
1. Clinicaltrials.gov. To Compare the Gastrointestinal Safety of a 14-Day Oral Dosing Regimen of ATB-346 to Sodium Naproxen in Healthy Subjects (2018). Retrieved October 10, 2018, from https://ClinicalTrials.gov/show/NCT03291418
References
Augestad, K. M. (2010). Postoperative ileus: impact of pharmacological treatment, laparoscopic surgery and enhanced recovery pathways. World J. Gastroenterol. 16, 2067–2074. doi: 10.3748/wjg.v16.i17.2067
Boeckxstaens, G. E., and De Jonge, W. J. (2009). Neuroimmune mechanisms in postoperative ileus. Gut 58, 1300–1311. doi: 10.1136/gut.2008.169250
Bragg, D., El-Sharkawy, A. M., Psaltis, E., Maxwell-Armstrong, C. A., and Lobo, D. N. (2015). Postoperative ileus: recent developments in pathophysiology and management. Clin. Nutr. 34, 367–376. doi: 10.1016/j.clnu.2015.01.016
Campolo, M., Esposito, E., Ahmad, A., Di Paola, R., Paterniti, I., Cordaro, M., et al. (2014). Hydrogen sulfide-releasing cyclooxygenase inhibitor ATB-346 enhances motor function and reduces cortical lesion volume following traumatic brain injury in mice. J. Neuroinflammation 11:196 doi: 10.1186/s12974-014-0196-1
Campolo, M., Esposito, E., Ahmad, A., Di Paola, R., Wallace, J. L., and Cuzzocrea, S. (2013). A hydrogen sulfide-releasing cyclooxygenase inhibitor markedly accelerates recovery from experimental spinal cord injury. FASEB J. 27, 4489–4499. doi: 10.1096/fj.13-234716
Cao, X., Xiong, S., Zhou, Y., Wu, Z., Ding, L., Zhu, Y., et al. (2018). Renal protective effect of hydrogen sulfide in cisplatin-induced nephrotoxicity. Antioxid. Redox Signal. 29, 455–470. doi: 10.1089/ars.2017.7157
Chen, C. Q., Xin, H., and Zhu, Y. Z. (2007). Hydrogen sulfide: third gaseous transmitter, but with great pharmacological potential. Acta Pharmacol. Sin. 28, 1709–1716. doi: 10.1111/j.1745-7254.2007.00629.x
Chen, S., Bu, D., Ma, Y., Zhu, J., Sun, L., Zuo, S., et al. (2016). GYY4137 ameliorates intestinal barrier injury in a mouse model of endotoxemia. Biochem. Pharmacol. 118, 59–67. doi: 10.1016/j.bcp.2016.08.016
Chen, X., and Liu, X. S. (2016). Hydrogen sulfide from a NaHS source attenuates dextran sulfate sodium (DSS)-induced inflammation via inhibiting nuclear factor-kappaB. J. Zhejiang Univ. Sci. B 17, 209–217. doi: 10.1631/jzus.B1500248
Chiu, C.-J., Mcardle, A. H., Brown, R., Scott, H. J., and Gurd, F. N. (1970). Intestinal mucosal lesion in low-flow states: I. A morphological, hemodynamic, and metabolic reappraisal. Arch. Surg. 101, 478–483. doi: 10.1001/archsurg.1970.01340280030009
Cosyns, S., Shiva, S., and Lefebvre, R. A. (2015). Protective effect of exogenous nitrite in postoperative ileus. Br. J. Pharmacol. 172, 4864–4874. doi: 10.1111/bph.13255
Dal-Secco, D., Cunha, T. M., Freitas, A., Alves-Filho, J. C., Souto, F. O., Fukada, S. Y., et al. (2008). Hydrogen sulfide augments neutrophil migration through enhancement of adhesion molecule expression and prevention of CXCR2 internalization: role of ATP-sensitive potassium channels. J. Immunol. 181, 4287–4298. doi: 10.4049/jimmunol.181.6.4287
De Backer, O., Blanckaert, B., Leybaert, L., and Lefebvre, R. A. (2008). A novel method for the evaluation of intestinal transit and contractility in mice using fluorescence imaging and spatiotemporal motility mapping. Neurogastroenterol. Motil. 20, 700–707. doi: 10.1111/j.1365-2982.2007.01073.x
De Backer, O., Elinck, E., Blanckaert, B., Leybaert, L., Motterlini, R., and Lefebvre, R. A. (2009). Water-soluble CO-releasing molecules reduce the development of postoperative ileus via modulation of MAPK/HO-1 signalling and reduction of oxidative stress. Gut 58, 347–356. doi: 10.1136/gut.2008.155481
De Cicco, P., Panza, E., Ercolano, G., Armogida, C., Sessa, G., Pirozzi, G., et al. (2016). ATB-346, a novel hydrogen sulfide-releasing anti-inflammatory drug, induces apoptosis of human melanoma cells and inhibits melanoma development in vivo. Pharmacol. Res. 114, 67–73. doi: 10.1016/j.phrs.2016.10.019
De Jonge, W. J., Van Den Wijngaard, R. M., The, F. O., Ter Beek, M.-L., Bennink, R. J., Tytgat, G. N. J., et al. (2003). Postoperative ileus is maintained by intestinal immune infiltrates that activate inhibitory neural pathways in mice. Gastroenterology 125, 1137–1147. doi: 10.1016/S0016-5085(03)01197-1
De Winter, B. Y., Boeckxstaens, G. E., De Man, J. G., Moreels, T. G., Herman, A. G., and Pelckmans, P. A. (1998). Differential effect of indomethacin and ketorolac on postoperative ileus in rats. Eur. J. Pharmacol. 344, 71–76. doi: 10.1016/S0014-2999(97)01563-X
Dief, A., Mostafa, D., Sharara, G., and Zeitoun, T. (2015). Hydrogen sulfide releasing naproxen offers better anti-inflammatory and chondroprotective effect relative to naproxen in a rat model of zymosan induced arthritis. Eur. Rev. Med. Pharmacol. Sci. 19, 1537–1546. https://www.europeanreview.org/
Ekundi-Valentim, E., Mesquita, P., Santos, K., Vieira De Paula, M., Florenzano, J., Zanoni, C., et al. (2013). A comparative study on the anti-inflammatory effects of single oral doses of naproxen and its hydrogen sulfide (H2S)-releasing derivative ATB-346 in rats with carrageenan-induced synovitis. Med. Gas Res. 3:24. doi: 10.1186/2045-9912-3-24
Elsheikh, W., Blackler, R. W., Flannigan, K. L., and Wallace, J. L. (2014). Enhanced chemopreventive effects of a hydrogen sulfide-releasing anti-inflammatory drug (ATB-346) in experimental colorectal cancer. Nitric Oxide 41, 131–137. doi: 10.1016/j.niox.2014.04.006
Engel, D. R., Koscielny, A., Wehner, S., Maurer, J., Schiwon, M., Franken, L., et al. (2010). T helper type 1 memory cells disseminate postoperative ileus over the entire intestinal tract. Nat. Med. 16, 1407–1413. doi: 10.1038/nm.2255
Fiorucci, S., Orlandi, S., Mencarelli, A., Caliendo, G., Santagada, V., Distrutti, E., et al. (2007). Enhanced activity of a hydrogen sulphide-releasing derivative of mesalamine (ATB-429) in a mouse model of colitis. Br. J. Pharmacol. 150, 996–1002. doi: 10.1038/sj.bjp.0707193
Flannigan, K. L., Ferraz, J. G. P., Wang, R., and Wallace, J. L. (2013). Enhanced synthesis and diminished degradation of hydrogen sulfide in experimental colitis: a site-specific, pro-resolution mechanism. PLoS One 8:e71962. doi: 10.1371/journal.pone.0071962
Goldstein, J. L., Matuszewski, K. A., Delaney, C., Senagore, A. J., Chiao, E. F., Shah, M., et al. (2007). Inpatient economic burden of postoperative ileus associated with abdominal surgery in the United States. Pharmacol. Ther. 32, 82–90. https://www.ptcommunity.com/
Grubbs, F. E. (1969). Procedures for detecting outlying observations in samples. Technometrics 11, 1–21. doi: 10.1080/00401706.1969.10490657
Henderson, P. W., Weinstein, A. L., Sohn, A. M., Jimenez, N., Krijgh, D. D., and Spector, J. A. (2010). Hydrogen sulfide attenuates intestinal ischemia-reperfusion injury when delivered in the post-ischemic period. J. Gastroenterol. Hepatol. 25, 1642–1647. doi: 10.1111/j.1440-1746.2010.06380.x
Hu, L. F., Wong, P. T., Moore, P. K., and Bian, J. S. (2007). Hydrogen sulfide attenuates lipopolysaccharide-induced inflammation by inhibition of p38 mitogen-activated protein kinase in microglia. J. Neurochem. 100, 1121–1128. doi: 10.1111/j.1471-4159.2006.04283.x
Jensen, A. R., Drucker, N. A., Te Winkel, J. P., Ferkowicz, M. J., and Markel, T. A. (2018). The route and timing of hydrogen sulfide therapy critically impacts intestinal recovery following ischemia and reperfusion injury. J. Pediatr. Surg. 53, 1111–1117. doi: 10.1016/j.jpedsurg.2018.02.072
Kalff, J. C., Schraut, W. H., Billiar, T. R., Simmons, R. L., and Bauer, A. J. (2000). Role of inducible nitric oxide synthase in postoperative intestinal smooth muscle dysfunction in rodents. Gastroenterology 118, 316–327. doi: 10.1016/S0016-5085(00)70214-9
Karwi, Q. G., Whiteman, M., Wood, M. E., Torregrossa, R., and Baxter, G. F. (2016). Pharmacological postconditioning against myocardial infarction with a slow-releasing hydrogen sulfide donor, GYY4137. Pharmacol. Res. 111, 442–451. doi: 10.1016/j.phrs.2016.06.028
Lee, Z. W., Zhou, J., Chen, C. S., Zhao, Y., Tan, C. H., Li, L., et al. (2011). The slow-releasing hydrogen sulfide donor, GYY4137, exhibits novel anti-cancer effects in vitro and in vivo. PLoS One 6:e21077. doi: 10.1371/journal.pone.0029758
Li, L., Fox, B., Keeble, J., Salto-Tellez, M., Winyard, P. G., Wood, M. E., et al. (2013). The complex effects of the slow-releasing hydrogen sulfide donor GYY4137 in a model of acute joint inflammation and in human cartilage cells. J. Cell. Mol. Med. 17, 365–376. doi: 10.1111/jcmm.12016
Li, L., Salto-Tellez, M., Tan, C. H., Whiteman, M., and Moore, P. K. (2009). GYY4137, a novel hydrogen sulfide-releasing molecule, protects against endotoxic shock in the rat. Free Radic. Biol. Med. 47, 103–113. doi: 10.1016/j.freeradbiomed.2009.04.014
Li, L., Whiteman, M., Guan, Y. Y., Neo, K. L., Cheng, Y., Lee, S. W., et al. (2008). Characterization of a novel, water-soluble hydrogen sulfide-releasing molecule (GYY4137): new insights into the biology of hydrogen sulfide. Circulation 117, 2351–2360. doi: 10.1161/CIRCULATIONAHA.107.753467
Liu, Z., Han, Y., Li, L., Lu, H., Meng, G., Li, X., et al. (2013). The hydrogen sulfide donor, GYY4137, exhibits anti-atherosclerotic activity in high fat fed apolipoprotein E(−/−) mice. Br. J. Pharmacol. 169, 1795–1809. doi: 10.1111/bph.12246
Magierowski, M., Magierowski, K., Surmiak, M., Hubalewska-Mazgaj, M., Kwiecien, S., Wallace, J. L., et al. (2017). The effect of hydrogen sulfide-releasing naproxen (ATB-346) versus naproxen on formation of stress-induced gastric lesions, the regulation of systemic inflammation, hypoxia and alterations in gastric microcirculation. J. Physiol. Pharmacol. 68, 749–756. http://www.jpp.krakow.pl/
Meng, G., Wang, J., Xiao, Y., Bai, W., Xie, L., Shan, L., et al. (2015). GYY4137 protects against myocardial ischemia and reperfusion injury by attenuating oxidative stress and apoptosis in rats. J. Biomed. Res. 29, 203–213. doi: 10.7555/JBR.28.20140037
Milne, T. G. E., Jaung, R., O’grady, G., and Bissett, I. P. (2018). Nonsteroidal anti-inflammatory drugs reduce the time to recovery of gut function after elective colorectal surgery: a systematic review and meta-analysis. Color. Dis. 20, O190–O198. doi: 10.1111/codi.14268
Moore, B. A., Otterbein, L. E., Türler, A., Choi, A. M. K., and Bauer, A. J. (2003). Inhaled carbon monoxide suppresses the development of postoperative ileus in the murine small intestine. Gastroenterology 124, 377–391. doi: 10.1053/gast.2003.50060
Nakao, A., Schmidt, J., Harada, T., Tsung, A., Stoffels, B., Cruz, R. J., et al. (2006). A single intraperitoneal dose of carbon monoxide-saturated ringer’s lactate solution ameliorates postoperative ileus in mice. J. Pharmacol. Exp. Ther. 319, 1265–1275. doi: 10.1124/jpet.106.108654
Oh, G. S., Pae, H. O., Lee, B. S., Kim, B. N., Kim, J. M., Kim, H. R., et al. (2006). Hydrogen sulfide inhibits nitric oxide production and nuclear factor-kappaB via heme oxygenase-1 expression in RAW264.7 macrophages stimulated with lipopolysaccharide. Free Radic. Biol. Med. 41, 106–119. doi: 10.1016/j.freeradbiomed.2006.03.021
Olas, B. (2015). Hydrogen sulfide in signaling pathways. Clin. Chim. Acta 439, 212–218. doi: 10.1016/j.cca.2014.10.037
Person, B., and Wexner, S. D. (2006). The management of postoperative ileus. Curr. Probl. Surg. 43, 6–65. doi: 10.1067/j.cpsurg.2005.10.003
Qiu, Y., Wu, Y., Meng, M., Luo, M., Zhao, H., Sun, H., et al. (2018). GYY4137 protects against myocardial ischemia/reperfusion injury via activation of the PHLPP-1/Akt/Nrf2 signaling pathway in diabetic mice. J. Surg. Res. 225, 29–39. doi: 10.1016/j.jss.2017.12.030
Rose, P., Dymock, B. W., and Moore, P. K. (2015). GYY4137, a novel water-soluble, H2S-releasing molecule. Methods Enzymol. 554, 143–167. doi: 10.1016/bs.mie.2014.11.014
Rose, P., Moore, P. K., and Zhu, Y. Z. (2017). H2S biosynthesis and catabolism: new insights from molecular studies. Cell. Mol. Life Sci. 74, 1391–1412. doi: 10.1007/s00018-016-2406-8
Schwarz, N. T., Kalff, J. C., Türler, A., Engel, B. M., Watkins, S. C., Billiar, T. R., et al. (2001). Prostanoid production via COX-2 as a causative mechanism of rodent postoperative ileus. Gastroenterology 121, 1354–1371. doi: 10.1053/gast.2001.29605
Shimada, S., Fukai, M., Wakayama, K., Ishikawa, T., Kobayashi, N., Kimura, T., et al. (2015). Hydrogen sulfide augments survival signals in warm ischemia and reperfusion of the mouse liver. Surg. Today 45, 892–903. doi: 10.1007/s00595-014-1064-4
Snoek, S. A., Dhawan, S., Van Bree, S. H., Cailotto, C., Van Diest, S. A., Duarte, J. M., et al. (2012). Mast cells trigger epithelial barrier dysfunction, bacterial translocation and postoperative ileus in a mouse model. Neurogastroenterol. Motil. 24, 172–184:e191. doi: 10.1111/j.1365-2982.2011.01820.x
Takeuchi, K., and Satoh, H. (2015). NSAID-induced small intestinal damage–roles of various pathogenic factors. Digestion 91, 218–232. doi: 10.1159/000374106
Van Bree, S. H. W., Nemethova, A., Cailotto, C., Gomez-Pinilla, P. J., Matteoli, G., and Boeckxstaens, G. E. (2012). New therapeutic strategies for postoperative ileus. Nat. Rev. Gastroenterol. Hepatol. 9, 675–683. doi: 10.1038/nrgastro.2012.134
Van Dingenen, J., and Lefebvre, R. A. (2018a). Influence of the slow-release H2S donor GYY4137 and the H2S-releasing naproxen derivative ATB-346 on postoperative ileus. Acta Gastroenterol. Belg. 81, Abstracts XXXth Belgian Week of Gastroenterology, B13.
Van Dingenen, J., and Lefebvre, R. A (2018b). Influence of the slow- release H2S donor GYY4137 and the H2S- releasing naproxen derivative ATB-346 on postoperative ileus. Neurogastroenterol. Motil. 30:e13422, Abstracts 3rd Meeting of the Federation of Neurogastroenterology and Motility, 043. doi: 10.1007/s11103-018-0803-0
Wallace, J. L. (2007). Hydrogen sulfide-releasing anti-inflammatory drugs. Trends Pharmacol. Sci. 28, 501–505. doi: 10.1016/j.tips.2007.09.003
Wallace, J. L., Caliendo, G., Santagada, V., and Cirino, G. (2010). Markedly reduced toxicity of a hydrogen sulphide-releasing derivative of naproxen (ATB-346). Br. J. Pharmacol. 159, 1236–1246. doi: 10.1111/j.1476-5381.2009.00611.x
Wallace, J. L., Syer, S., Denou, E., De Palma, G., Vong, L., Mcknight, W., et al. (2011). Proton pump inhibitors exacerbate NSAID-induced small intestinal injury by inducing dysbiosis. Gastroenterology 141, 1314–1322:1322 e1311–1315. doi: 10.1053/j.gastro.2011.06.075
Wallace, J. L., Vaughan, D., Dicay, M., Macnaughton, W. K., and De Nucci, G. (2018). Hydrogen sulfide-releasing therapeutics: translation to the clinic. Antioxid. Redox Signal. 28, 1533–1540. doi: 10.1089/ars.2017.7068
Wallace, J. L., Vong, L., McKnight, W., Dicay, M., and Martin, G. R. (2009). Endogenous and exogenous hydrogen sulfide promotes resolution of colitis in rats. Gastroenterology 137, 569–578:e561. doi: 10.1053/j.gastro.2009.04.012
Wehner, S., Vilz, T. O., Stoffels, B., and Kalff, J. C. (2012). Immune mediators of postoperative ileus. Langenbeck’s Arch. Surg. 397, 591–601. doi: 10.1007/s00423-012-0915-y
Xie, L., Gu, Y., Wen, M., Zhao, S., Wang, W., Ma, Y., et al. (2016). Hydrogen sulfide induces keap1 S-sulfhydration and suppresses diabetes-accelerated atherosclerosis via Nrf2 activation. Diabetes 65, 3171–3184. doi: 10.2337/db16-0020
Xu, W., Wu, W., Chen, J., Guo, R., Lin, J., Liao, X., et al. (2013). Exogenous hydrogen sulfide protects H9c2 cardiac cells against high glucose-induced injury by inhibiting the activities of the p38 MAPK and ERK1/2 pathways. Int. J. Mol. Med. 32, 917–925. doi: 10.3892/ijmm.2013.1462
Keywords: ATB-346, GYY4137, hydrogen sulfide, naproxen, postoperative ileus
Citation: Van Dingenen J, Pieters L, Vral A and Lefebvre RA (2019) The H2S-Releasing Naproxen Derivative ATB-346 and the Slow-Release H2S Donor GYY4137 Reduce Intestinal Inflammation and Restore Transit in Postoperative Ileus. Front. Pharmacol. 10:116. doi: 10.3389/fphar.2019.00116
Edited by:
Gabriella Aviello, University of Aberdeen,United KingdomReviewed by:
Jose A. Uranga, Universidad Rey Juan Carlos, SpainElisabetta Panza, University of Naples Federico II, Italy
Copyright © 2019 Van Dingenen, Pieters, Vral and Lefebvre. This is an open-access article distributed under the terms of the Creative Commons Attribution License (CC BY). The use, distribution or reproduction in other forums is permitted, provided the original author(s) and the copyright owner(s) are credited and that the original publication in this journal is cited, in accordance with accepted academic practice. No use, distribution or reproduction is permitted which does not comply with these terms.
*Correspondence: Romain A. Lefebvre, romain.lefebvre@ugent.be