- 1College of Pharmacy, Chengdu University of Traditional Chinese Medicine, Chengdu, China
- 2School of Chinese Medicine, Li Ka Shing Faculty of Medicine, The University of Hong Kong, Hong Kong, China
Background and Purpose: Magnolol, as the main active ingredient of Traditional Chinese Medicine, can significantly improve gastrointestinal motility disorders (GMD). In the present study, metabolomics was used to investigate the mechanism of magnolol improving L-arginine induced GMD in rats.
Experimental Approach: SD rats were randomly divided into control group, model group and magnolol treated group. L-arginine was injected intraperitoneally in model and magnolol groups to induce GMD model. All intervention regimens were administered by oral gavage, once a day for five consecutive days. Relative gastric emptying rate and propulsive intestinal rate were measured. Metabolites in serum were analyzed based on UPLC-MS metabolomics technique.
Results: Magnolol significantly promoted gastric emptying and small intestinal propulsion. Compared with the model group, the level of serotonin and L-tryptophan significantly reversed (P < 0.05) and 22 metabolites reversed in the magnolol group. According to MetPA database analysis, magnolol has mainly affected 10 major metabolic pathways which were related to each other, Tryptophan metabolism is the most critical metabolic pathway associated with gastrointestinal tract.
Conclusion: These findings suggest that magnolol has a significantly promoting effect on L-arginine induced gastrointestinal motility disorder in rats, the mechanism is to reduce the production of nitric oxide to weaken the function of nitric oxide relaxing the gastrointestinal smooth muscle and increase the content of serotonin to promote gastrointestinal peristalsis and motility, secretion, absorption of nutrients.
Introduction
Gastrointestinal motility disorders (GMD) are a group of diseases, characterized by non-specific symptoms including nausea, vomiting, bloating, abdominal discomfort or pain, constipation or diarrhea. It includes gastrointestinal dysfunction, screw weakening, and delays gastric emptying and intestinal propulsion (Beyder and Farrugia, 2012; Valentin et al., 2015). Moreover, GMD mainly consists of irritable bowel syndrome and other functional gastrointestinal disorders (Manabe et al., 2010), has been a common public health issue worldwide. Normal peristalsis and homeostatic sensory and motor mechanisms along the gastrointestinal tract result from a series of control mechanisms involving extrinsic parasympathetic and sympathetic pathways, intrinsic nervous system and the electrical and contractile properties of the smooth muscle cells (Valentin et al., 2015). Abnormalities in either pathway can cause GMD. The most commonly used effective drugs for the treatment of GMD are cisapride and domperidone. However, both of them were associated with adverse cardiovascular events (Chen et al., 2010; Arana et al., 2015).
Magnolia Officinalis Rehder and EH Wilson (M. offcinalis) are traditional Chinese medicines widely used for gastrointestinal tract motility disorder in Asian countries (Kim et al., 2017). Magnolol (5,5′-diallyl-2,2′-dihydroxybipheny) is a lignan exacted from the bark of M. officinalis, the compounds of which demonstrating anti-cancer, anti-stress, anti-anxiety, anti-depressant, anti-oxidant and anti-inflammatory effects (Lee et al., 2011; Zhao et al., 2017). Several studies showed that magnolol could improve the gastric emptying of a semi-solid meal and intestinal propulsive activity in mice (Wei-Wei et al., 2005). It also can prevent sepsis-induced suppression of intestinal motility in rats by modulating self-amplified inflammatory events and block oxidative stress in the intestine (Yang et al., 2008). It was also found that it can prevent lipopolysaccharide-induced septic dysmotility in mice by regulating stem cell factor (SCF)/c-kit and nitric oxide (NO) signaling to maintain functional interstitial cells of Cajal (ICCs) (Miao et al., 2013). To some extent, these reports indicate that magnolol can regulate gastrointestinal motility disorder. However, its metabolism in vivo is unclear, and its regulatory mechanism cannot be further explained.
Metabolomics is the science that studies the metabolic content of a sample with the aim to associate the metabolite concentrations found in the physiology of the organism or the properties of the product (Gika and Theodoridis, 2011). It adopted metabolic profiling methods for the comprehensive analysis of biological fluids, and has a great impact on the investigation of discovering biomarkers, and identifying perturbed pathways due to disease or drug treatment. Recently, some reports have described metabolomic studies using various techniques, among the analytical techniques in metabonomic research, UPLC-MS has demonstrated its great potential essentially due to the high reproducibility of measurements and sensitivity of analysis (Ramirez et al., 2013; Lee et al., 2015; Xu et al., 2016). In recent years, metabolomics technology has also contributed to the study of the mechanism of drug action. For example, Metabolomics study showed that the anti-osteoporosis effect of Achyranthes bidentata polysaccharide in ovariectomized rats was related to lipid metabolism (Zhang M. et al., 2018); It is also found that Ilex pubescens extract can prevent blood stasis in rats through arachidonic acid metabolism and glycerol phospholipid metabolism by metabolomics (Zhang Y. et al., 2018). Therefore, this study aimed to study metabolomics platform based on UPLC-MS technique to investigate the effects and mechanisms of magnolol on GMD in rats.
Materials and Methods
Chemicals and Reagents
Methanol, was purchased from Ourchem (Shanghai, China). Acetonitrile, was purchased from Merck (Darmstadt, Germany). Formic acid, was purchased from TCI (Shanghai, China). Double distilled water was obtained from Arium® mini. Magnolol (Purity > 98%) was purchased from Chengdu Push Bio-technology Co., Ltd. (Sichuan, China). L-arginine was purchased from Biotal (United Kingdom). Dextran Blue 2000 (DB-2000) was purchased from Sigma-Aldrich (United States). Sodium Chloride Injection was obtained from Sichuan Kelun Pharmaceutical Co., Ltd. (Sichuan, China). Rat NOS2/iNOS(Nitric Oxide Synthase 2, Inducible) ELISA Kit was purchased from Elabscience Biotechnology Co., Ltd. (Wuhan, China). NO (Nitric Oxide) Assay Kit was purchased from Beyotime Biotechnology (Shanghai, China).
Animals
Male Sprague-Dawley rats weighing 180–200 g (Chengdu Dossy Biological Technology Co., Ltd.) were under the conditions of room temperature (20–23°C), humidity (50 ± 10%), light (12 h light/dark cycle) and were free access to diet. They acclimatized for 5 days before to the start of the experiment. All experiments and procedures performed according to the Regulations of Experimental Animal Administration issued by the State Committee of Science and Technology of China and the protocol (2014DL-023) approved by the Committee on the Ethics of Animal Experiments of Chengdu University of Traditional Chinese Medicine.
Animal Experiments and Sample Collection
After acclimatization, GMD model was induced by injected intraperitoneally with L-arginine at a dose of 5.2 g/kg on the first day and 2.6 g/kg on the second to the fifth day (Aiwu et al., 2012). Saline was injected intraperitoneally to rats in the control group for the same period. All the rats with comparable disease index were then randomly divided into two groups (n = 8 per group): model group: intragastric administrated with saline; magnolol group: intragastric administrated with magnolol (40 mg/kg). The rats were intragastric administrated with saline in control group. All the rats were administrated via gastric irrigation once daily for 5 days, then prohibited any food for 12 h before the experiments, but were allowed access to water freely.
On the fifth day, each group was administration 2% DB-2000 (0.4 mL) at the same time. After 30 min, the blood was collected from femoral artery. After clotting at 4°C for 2 h, the blood centrifuged at 3500 rpm for 10 min. The supernatant samples were transferred to Eppendorf tubes and immediately stored at -80°C until analysis (Tien et al., 2016).
Gastric Emptying and Small Intestinal Transit Experiments
After the rats were bled, pylorus and cardia ligated, the stomach was taken out, the greater curvature of the stomach was cut off. The gastric residual pigment fully washed in 4 ml deionized water, centrifuged at 3500 rpm for 15 min, taken the supernatant filtrate and measure the absorbance at 620 nm with a UV756CRT spectrophotometer, the percentage of the absorbance and the mean value of the blank group is the relative gastric retention rate. Meanwhile, the distance between the pyloric sphincter to the most front of the pigments and the cecum was measured, and the ratio of the two was used as small intestinal propulsive rates.
Where An is absorbance of DB-2000 recovered from the stomach of rat, A0 is mean absorbance of recovered from the stomachs of control rats.
Metabolic Analysis of Serum Samples
Sample Preparation
Prior to analysis, serum samples were thawed at 4°C, transferred 100 μL of each sample into 1.5 mL centrifuge tubes, add 400 μL of methanol (precooled at -20°C) to each tube and vortex for 60 s, centrifuged for 10 min at 12000 rpm 4°C and transferred all supernatant in each tube into another 1.5 mL centrifuge tube, filtered through 0.22 μm membrane and obtain the prepared sample extracts for UPLC-MS. For the quality control (QC) samples, taken 20 μL from each prepare sample extract and mix (These QC samples were used to monitor deviations of the analytical results from these pool mixtures and compare them to the errors caused by the analytical instrument itself), used the rest of the samples for UPLC-MS test (Zelena et al., 2009; Dunn et al., 2011).
Analysis of Serum Samples
Chromatographic separation was accomplished in an Acquity UPLC system equipped with an ACQUITY UPLC® BEH C18 (100 × 2.1 mm, 1.7 μm, Waters) column maintained at 40°C. The temperature of the autosampler was 4°C. Gradient elution of analytes was carried out with 0.1% formic acid in water (A) and 0.1% formic acid in acetonitrile (B) at a flow rate of 0.25 mL/min. Injection of 10 μL of each sample was done after equilibration. An increasing linear gradient of solvent B (v/v) was used as follows: 0∼1 min, 2% B; 1∼9.5 min, 2∼50% B; 9.5∼14 min, 50∼98% B; 14∼15 min, 98% B; 15∼15.5 min, 98∼2% B; 15.5∼17 min, 2%B (Sangster et al., 2006). The separated components were subsequently fragmented and analyzed using a mass spectrometer.
The ESI-MSn experiments were executed on the Thermo LTQ Orbitrap XL mass spectrometer with the spray voltage of 4.8 and -4.5 kV in positive and negative modes, respectively. Sheath gas and auxiliary gas were set at 45 and 15 arbitrary units, respectively. The capillary temperature was 325°C. The voltages of capillary and tube were 35 and 50, -15 and -50 V in positive and negative modes, respectively. The Orbitrap analyzer scanned over a mass range of m/z 89∼1000 for a full scan at a mass resolution of 60000. Data-dependent acquisition MS/MS experiments were performed with CID scan. The normalized collision energy was 30 eV. Dynamic exclusion was implemented with a repeat count of 2, and exclusion duration of 15 s (Want et al., 2013).
Data Processing and Analysis
Converting the original data obtained by Proteowizard software (v3.0.8789) was converted into mzXML format (Smith et al., 2006). R (v3.3.2) XCMS package was used to carry out peaks identification, peaks filtration and peaks alignment (The main parameters are bw = 5, ppm = 15, and peakwidth = c (10,120), mzwid = 0.015, mzdiff = 0.01. method = “centWave”). The data matrix including information of mass to charge ratio (m/z), retention time, and intensity were obtained. In addition, the data was exported to excel for subsequent analysis. In order to allow different magnitudes of data to be compared, the intensity had been batch normalization.
According to the characteristics of metabolomics, UV (Autoscalin, mean-centering and scaled to unit variance (Thevenot et al., 2015) was used to convert the data. The multivariate statistical analysis (software SIMCA-P (v13.0) and R language ropes package) was used to analyze the data, including Principal Component Analysis (PCA), Partial Least Squares-Discriminant Analysis (PLS-DA), and Orthogonal-Partial Least Squares-Discriminant Analysis (OPLS-DA) methods.
Metabolite Identifications and Pathway Analysis
By screening the metabolites, the biomarkers were found. Related differential metabolite screening conditions: p-value ≤ 0.05+VIP ≥ 1 (Trygg, 2010), one-way ANOVA p-value ≤ 0.05 (Haspel et al., 2014). The identification of metabolites is first confirmed according to the exact molecular weight (molecular weight error is < 20 ppm), followed by the MS/MS fragmentation pattern to Human Metabolome Database (HMDB)1, Metlin2, massbank3, LipidMaps4, mzclound (LipidMaps) database confirm the annotation to get metabolites.
Encyclopedia of Genes and Genomes (KEGG) is a systematic analysis of gene function and genome information database (Kanehisa and Goto, 2000). MetPA is a part of metaboanalyst5, which is mainly based on the KEGG metabolic pathway. MetPA database can be used to analyze metabolic pathways related to metabolites.
Detection of iNOS and NO Concentration
The concentration of iNOS was measured by ELISA using commercially available kits according to the manufacturer’s protocols. The serum level of NO was measured using a commercially available kit according to the manufacturer’s instructions.
Results
Effect of Magnolol Gastric Emptying and Small Intestinal Propulsion
The results of gastric emptying and small intestinal transit were shown in Figure 1. Compared with the control group, the relative gastric remnant rate had significantly increased and intestinal propulsive rate had significantly reduced in model group (P < 0.05). The pathogenic factors of gastrointestinal motility disorder are complex. At present, there is no objective criterion for gastrointestinal motility disorder model, but atropine and L-arginine are commonly selected drugs for this model (Ren et al., 2017; Wang et al., 2018). The results of gastric emptying and intestinal propulsion also indicate that L-arginine had inhibited gastric emptying and intestinal propulsion, which indicated that the model of gastrointestinal motility disorder was successful. Compared with model group, the relative gastric remnant rate had significantly reduced and the intestinal propulsive rate had a significant increase in magnolol group. The results showed that magnolol could promote gastric emptying and small intestinal propulsion, it can effectively improve the gastrointestinal motility disorder in rats.
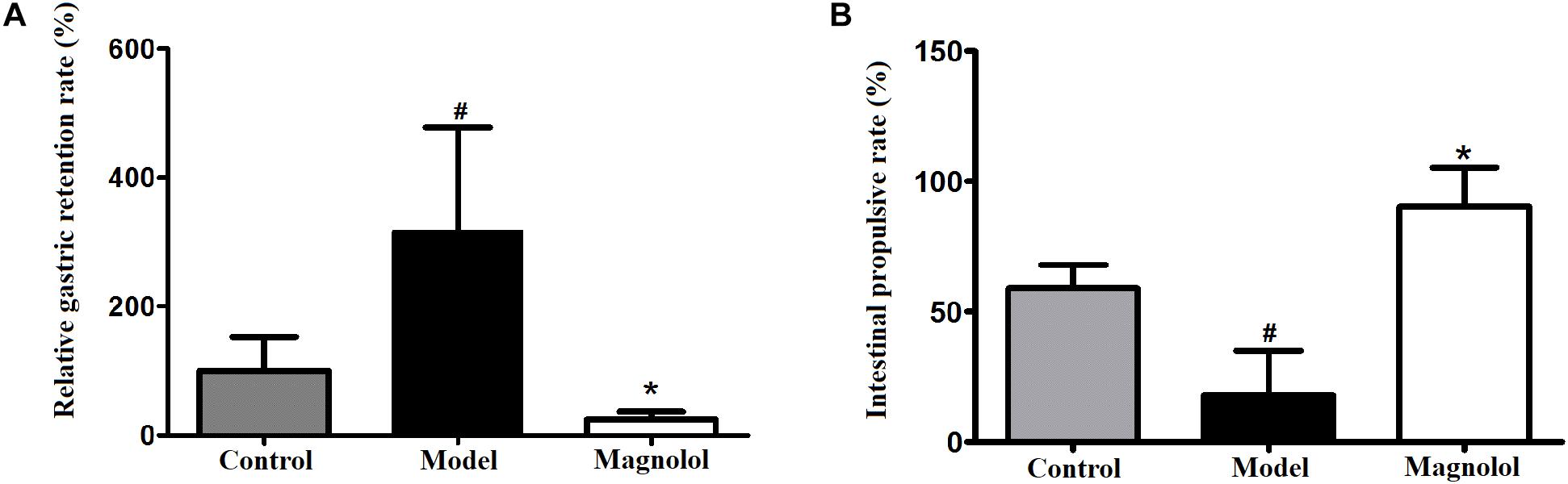
Figure 1. Effects on gastric emptying and intestinal propulsion. (A) Relative gastric remnant rate. (B) Intestinal propulsive rate. #Control group compared with model group P<0.05, ∗Magnolol group compared with model group P<0.05.
Metabolomic Analysis
Chromatographic Peak Separated
The base peak chromatograms of typical samples in each group are shown in Figures 2A,B. The peaks of the three groups are approximately the same in both positive and negative ion modes. In total, 4535 precursor molecules were obtained in positive ion mode, and 5654 precursor molecules were obtained from negative ion mode. After analysis, the number of metabolites in each group was shown in Figures 2C,D. Under the positive ion mode, there were 924 metabolites in the control group, 1116 metabolites in the model group, and 339 metabolites in the magnolol group. And there were 603 different metabolites between the control group and the model group, 359 different metabolites between the control group and the magnolol group, 436 different metabolites between model group and the magnolol group. Under the negative ion mode, there were 935 metabolites in the control group, 1064 metabolites in the model group and 498 metabolites in magnolol group. And there were 724 different metabolites between the control group and the model group, 459 different metabolites between the control group and the magnolol group, 515 different metabolites between model group and the magnolol group. Regarding the number of differential metabolites, the difference between the control group and the magnolol group was less than that between the control group and the model group, indicated that the serum composition of the magnolol was closer to the healthy rats.
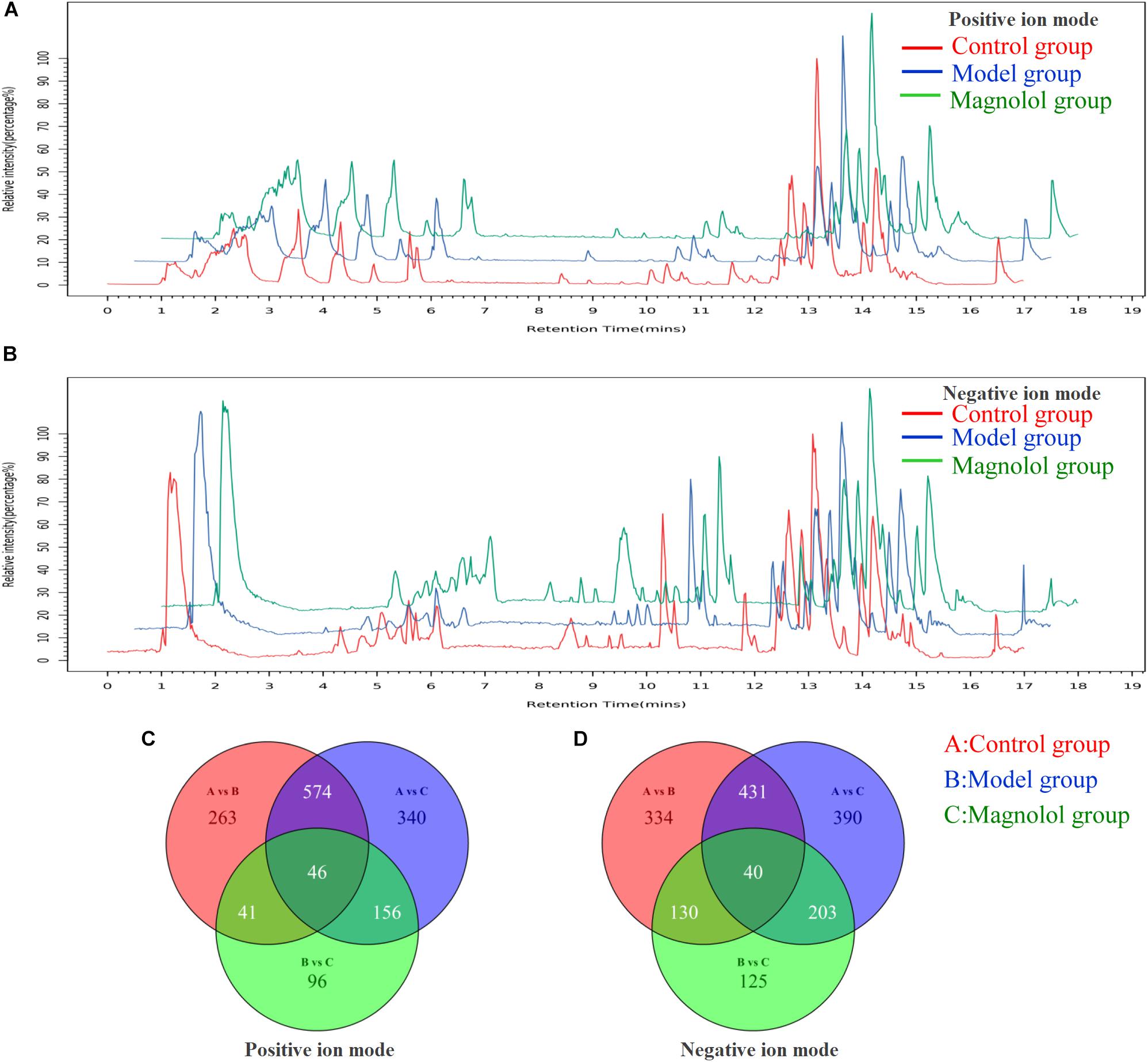
Figure 2. Typical sample base peak chromatogram and Venn diagrams. (A) Base peak chromatogram in positive ion mode. (B) Base peak chromatogram in negative ion mode. (C) Venn diagram in positive ion mode. (D) Venn diagram in negative ion mode.
Quality Control and Quality Assurance
Quality control (QC) can obtain whether the system error of the whole experiment is within the controllable range. Quality assurance (QA) can eliminate unreliable variables and control analysis errors within the scope of not affecting the results of multivariate statistical analysis. Generally, if the error of QC is less than 2SD, the QC sample aggregation system is reliable. For QA, it is acceptable that RSD (%) less than 30% is recommended for biomarker analysis. Therefore, variables with RSD (%) more than 30% are eliminated in the subsequent biomarker discovery process, and thus the RSD (%) LC-MS of QA is controlled within 30% (Dunn et al., 2011). As shown in Figure 3, the QC samples are clustered relative to the experimental samples, and the QC error is less than 2 times SD. The system error in the positive ion mode is smaller than that in the negative ion mode. After quality assurance, as shown in Figure 4, At positive ion mode, RSD is less than 30% of the variables, accounting for 75.24% of the total variables; at negative ion mode, RSD is less than 30% of the variables, accounting for 80.19% of the total variables, The total proportion is more than 70% proves to be a good data set, so the experimental data are reliable.
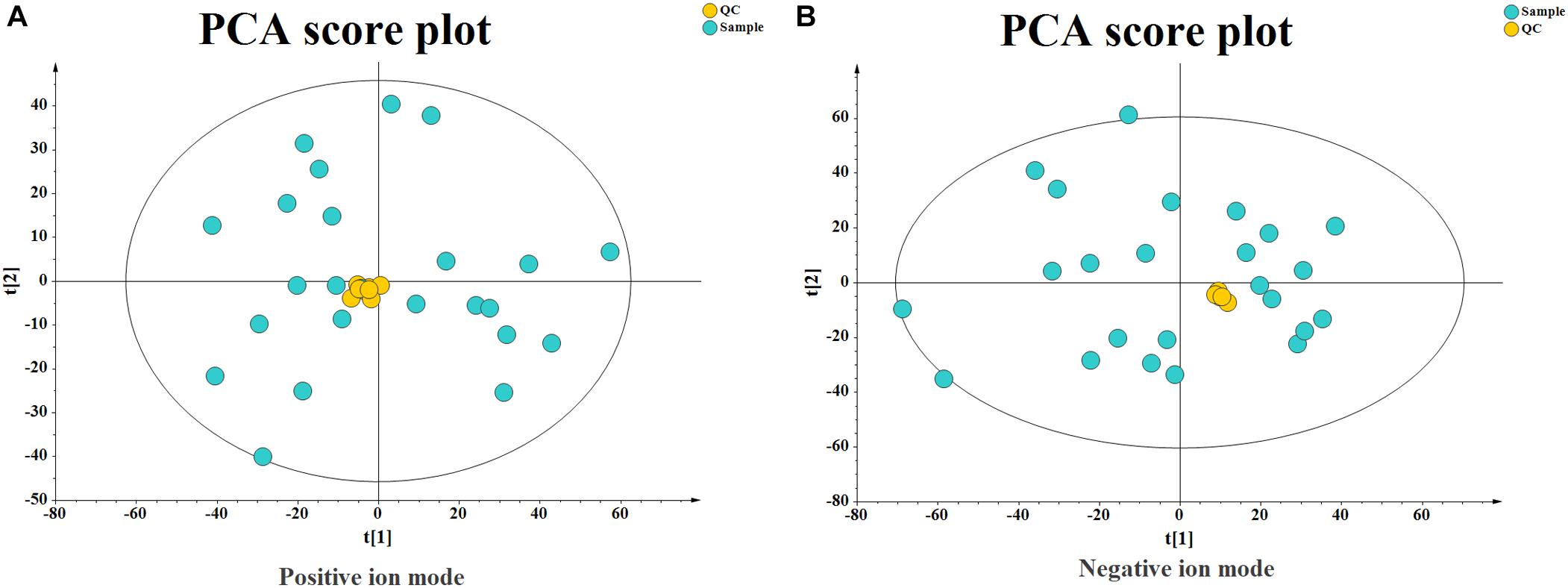
Figure 3. Principal Component Analysis (PCA) Score plot of quality control (QC) Samples. (A) At positive ion mode. (B) At negative ion mode.
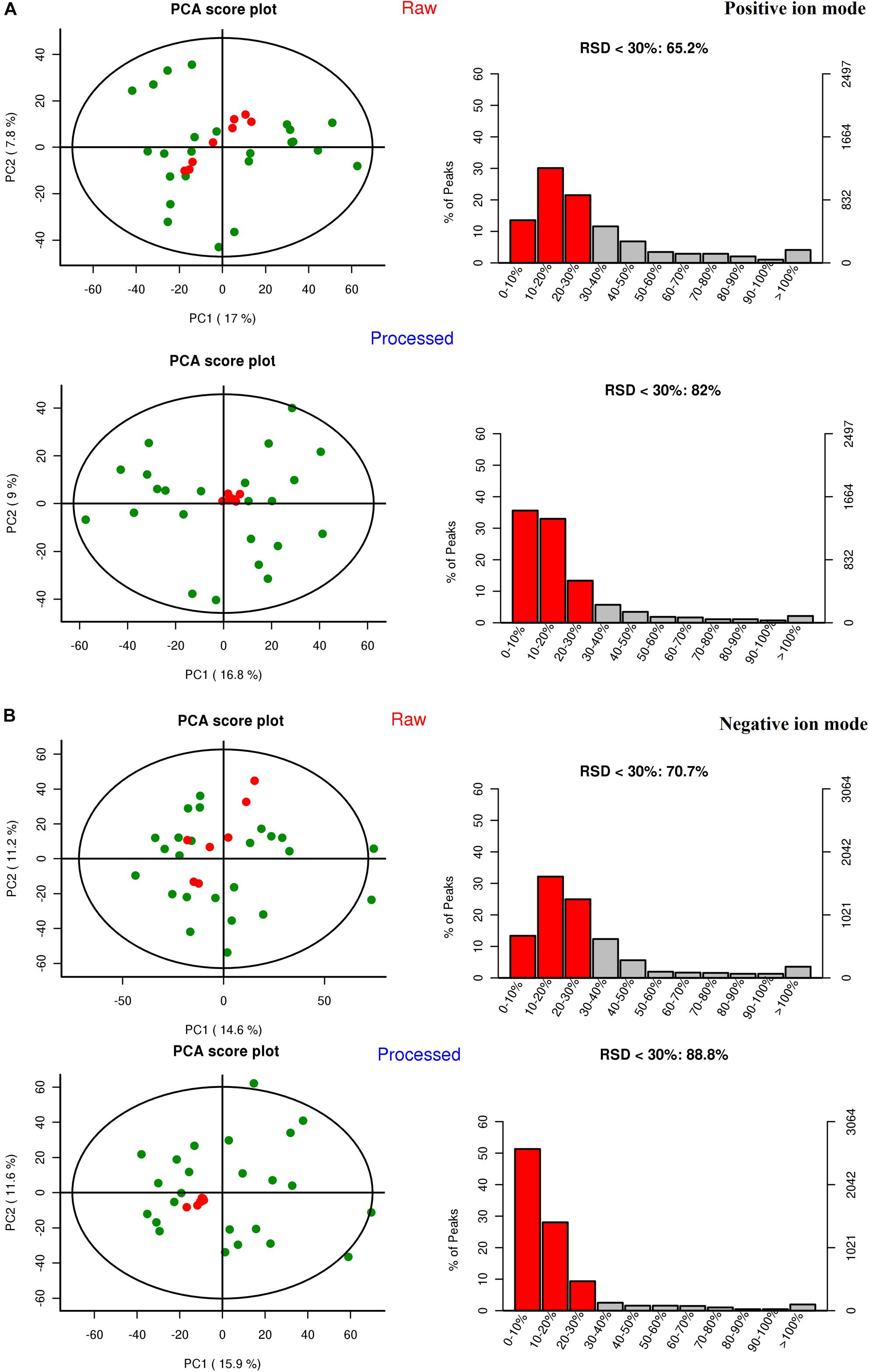
Figure 4. The results of the quality assurance (QA). (A) At positive ion mode. (B) At negative ion mode.
Multivariate Statistical Analysis
Good separation among the three groups was obtained using multivariate statistical analysis. PCA score plot showed the obvious separation trend between control and model groups (Figures 5A,B), which indicated that the model of gastrointestinal motility disorder was successfully established (ESI+: R2X = 0.423, Q2 = 0.0254; ESI-: R2X = 0.456, Q2 = 0.0966). To find the different compounds between groups, the PLS-DA is used to extract the information of variation between groups (Figures 5C,D). PLS-DA score plot showed that the classification effect was significant that each group separated from each other (ESI+: R2X = 0.363, R2Y = 0.986, Q2 = 0.659; ESI-: R2X = 0.343, R2Y = 0.922, Q2 = 0.650). PLS-DA not only tests the quality of the model by cross-validation, but also uses permutation test to evaluate whether the model is over-fitting (Figures 5E,F). In the positive ion mode, R2 = (0.0, 0.921), Q2 = (0.0, 0.00762); In negative ion mode, R2 = (0.0, 0.791), Q2 = (0.0, -0.225). In either mode, all blue Q2 points are lower from left to right than the original blue Q2 points, indicating that PLS-DA model has not been over-fitted. OPLS-DA was used to reduce the complexity of the model and enhance the explanatory power of the model (Figures 5G,H). The results illustrated that the metabolites in each group had been completely separated either the positive ion mode or the negative ion mode, and their VIP value was also one of the important conditions to identify the differential metabolites (ESI+: R2X = 0.365, R2Y = 0.983, Q2 = 0.632; ESI-: R2X = 0.457, R2Y = 0.989, Q2 = 0.659).
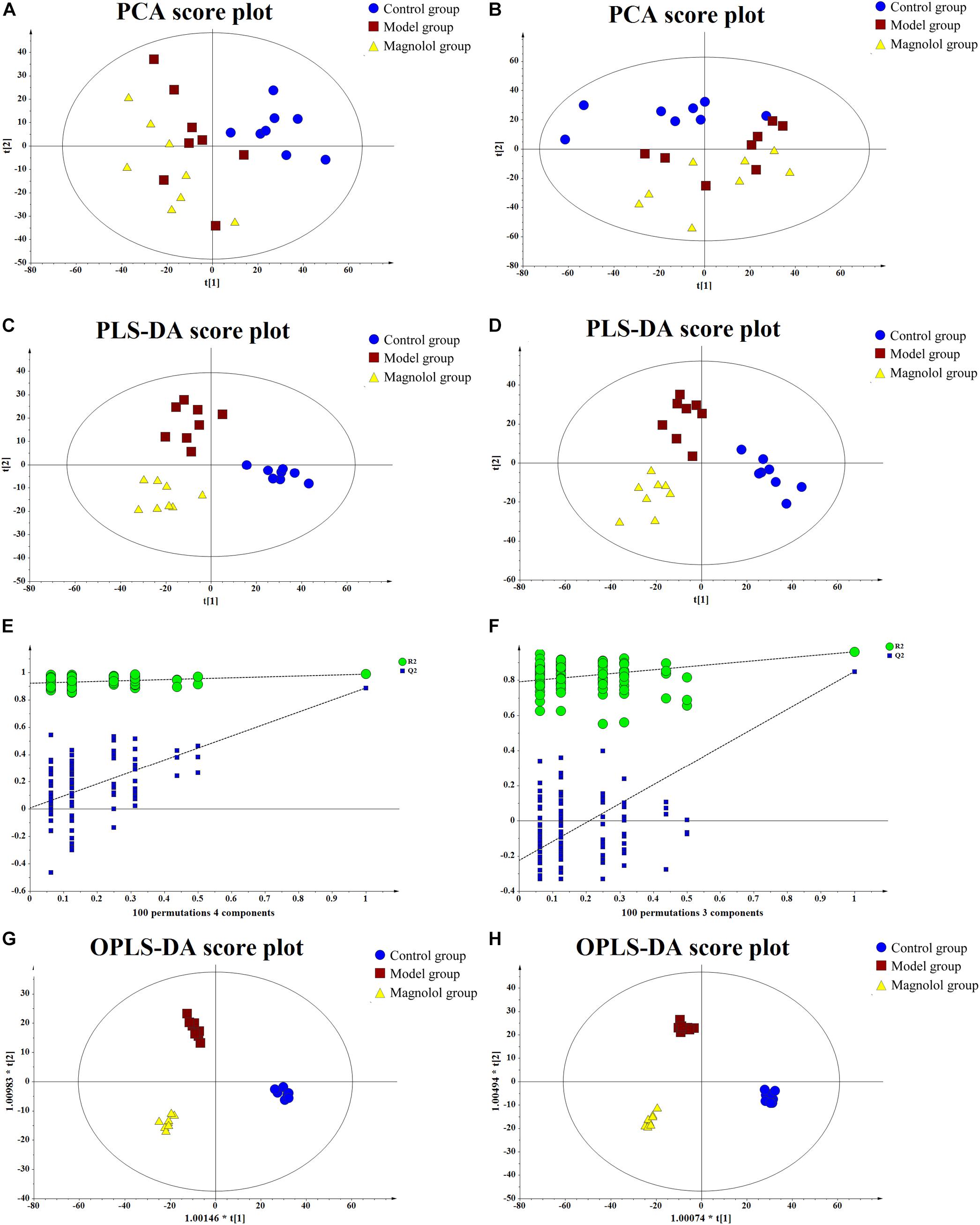
Figure 5. The results of multivariate statistical analysis. (A) The PCA score plot at positive ion mode. (B) The PCA score plot at negative ion mode. (C) The PLS-DA score plot at positive ion mode. (D) PLS-DA score plot at negative ion mode. (E) Permutations plot at positive ion mode. (F) Permutations plot at negative ion mode. (G) The OPLS-DA score plot at positive ion mode. (H) The OPLS-DA score plot at negative ion mode.
Screening and Identification of 30 Differential Metabolites
Potential biomarkers were screened according to p-value < 0.05 and VIP > 1. p-value was derived from Student’s t-test of metabolites. VIP was projected as the importance value of OPLS-DA first principal component variable. In total, 30 metabolic features majorly contributing to serum phenotype of model rats were identified (Table 1), and the majority of these metabolites were reversed by magnolol. Compared with the control group, the level of 6 metabolites significantly increased in the model group, including L-arginine, catechol, adenosine, N,N-dimethylglycine, L-valine, 4-hydroxy-L-proline, D-galactopyranose. Additionally, 24 metabolites significantly decreased. Compared with the model group, the level of 2 metabolites (serotonin and L-tryptophan) significantly reversed (P < 0.05) and 22 metabolites reversed in the magnolol group (Table 1).
Pearson correlation coefficient analysis was used to analyze the metabolite-metabolite correlation among identified metabolites in control group and model group. The consistency of metabolite and metabolite trends was examined to analyze the correlation among metabolites. Its calculation method is the core function in R(v3.1.3). Meanwhile, metabolite correlation analysis was performed for significant statistical tests. The statistical test method was the cor.test function in the R language package, and a false positive check was performed on the p-value. Using the FDR p-value ≤ 0.05 was a significant correlation (Rao et al., 2016). Metabolite-metabolite correlations between the serum of control group and model group showed in Figure 6. There were 326 significant correlations, of which 228 were positively correlated and 98 were negatively correlated. Notably, amino acids dominated the significant metabolite correlations, followed by organic acids. With the intake of L-arginine, the levels of multiple metabolites in the model group changed significantly. After the administration of magnolol, the reversal of serotonin and L-tryptophan was the most obvious, and L-arginine was found to be negatively correlated with serotonin. L-tryptophan is the precursor compound of serotonin. Therefore, L-arginine is indirectly associated with L-tryptophan. Through metabolite correlation analysis, each individual metabolite is interrelated and can know the changes of the quantity of each other.
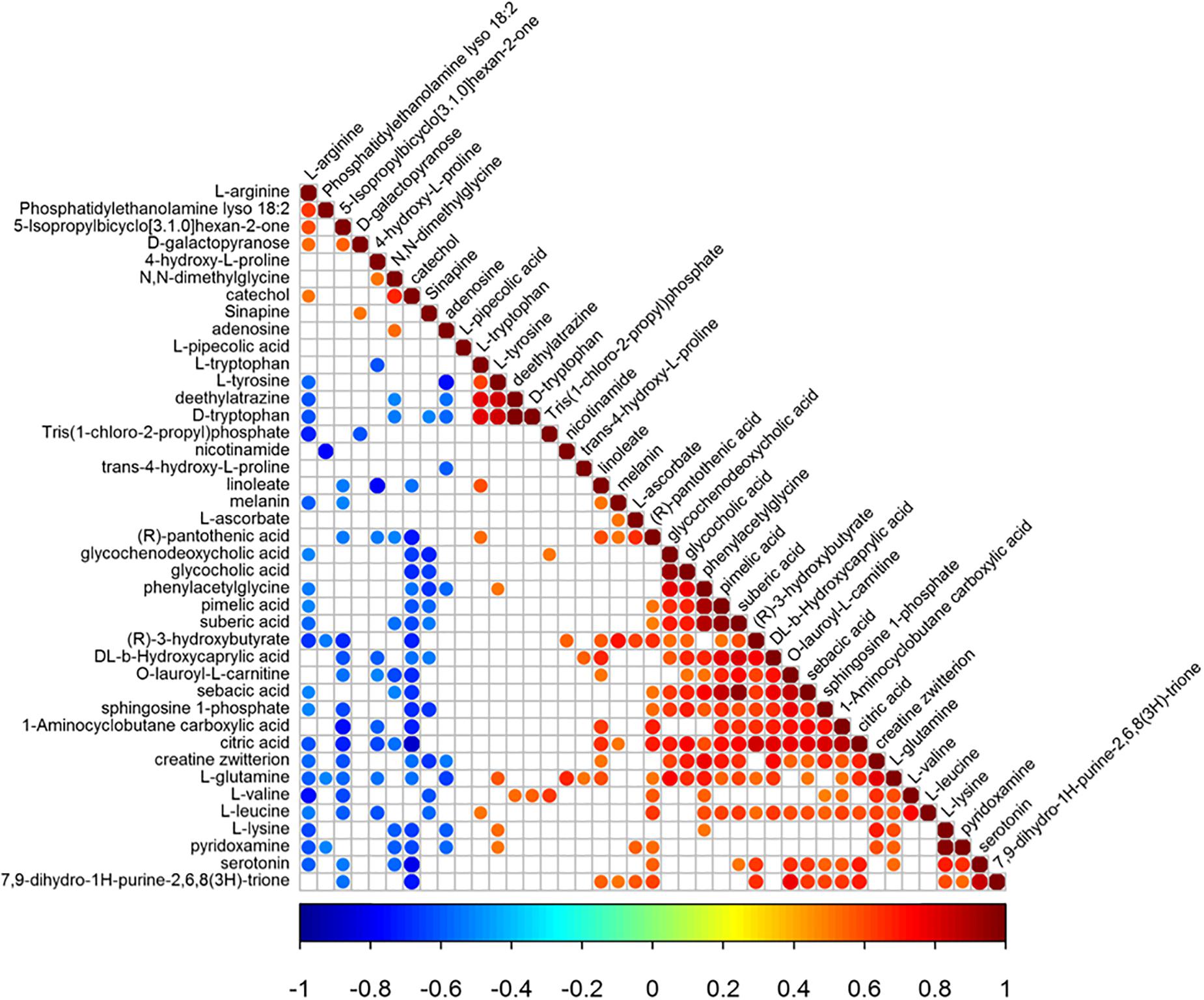
Figure 6. The results of metabolite-metabolite correlation analysis (Positive correlations are shown in red; negative correlations are shown in blue. The color-coded part is p-value < 0.05).
Metabolic Pathway Analysis
According to MetPA database analysis, there were 10 main metabolic pathways in the three groups, which were Linoleic acid metabolism; Valine, leucine and isoleucine biosynthesis; Glyoxylate and dicarboxylate metabolism; Alanine, aspartate and glutamate metabolism; Arginine and proline metabolism; Tryptophan metabolism; Citrate cycle (TCA cycle); Primary bile acid biosynthesis; Sphingolipid metabolism; Pantothenate and CoA biosynthesis (Figure 7A). Magnolol mainly affects four of these pathways, namely Tryptophan metabolism, Alanine, aspartate and glutamate metabolism, Arginine and proline metabolism and Citrate cycle (TCA cycle), among them, tryptophan metabolic pathway has the greatest impact. (Figure 7B). The enrichment analysis of KEGG metabolic pathway result was shown in Table 2. By consulting the KEGG database, the metabolic pathways were linked through the differential metabolites to form a metabolic pathway network (Figure 8). It can be seen that magnolol not only affected a single metabolic pathway but also formed a complex network of metabolic pathways through some interrelated metabolic pathways to achieve the purpose of treating GMD. Tryptophan metabolism is the core pathway to achieve a therapeutic effect, and other metabolic pathways play a supplementary role. Magnolol significantly affected L-tryptophan and serotonin. These two substances were both in the tryptophan metabolism pathway, and tryptophan metabolism pathway was directly related to gastrointestinal motility. From the metabolism pathway network, it could be seen that L-arginine has connected with tryptophan pathway through arginine and proline metabolism and alanine, aspartate and glutamate metabolism, and ultimately affects serotonin production. The content of L-arginine in the serum of model group increased, serotonin production was inhibited, magnolol significantly increased serotonin level, and made gastrointestinal tract movement normal in rats.
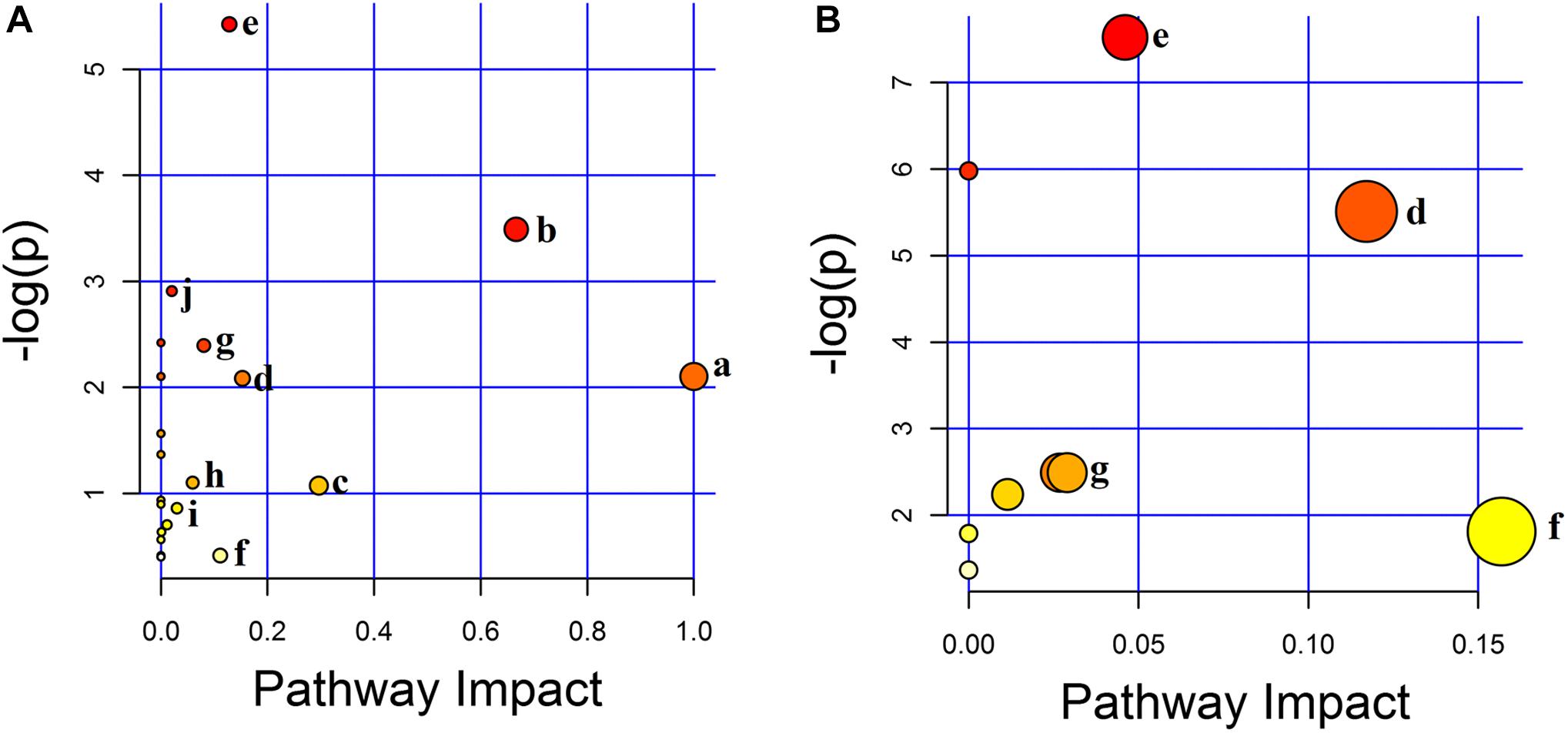
Figure 7. The result of metabolic pathway analysis. (A) The main metabolic pathways in the three groups. (B) The main metabolic pathways affected by magnolol (a–j represents the metabolic pathway details shown in Table 2).
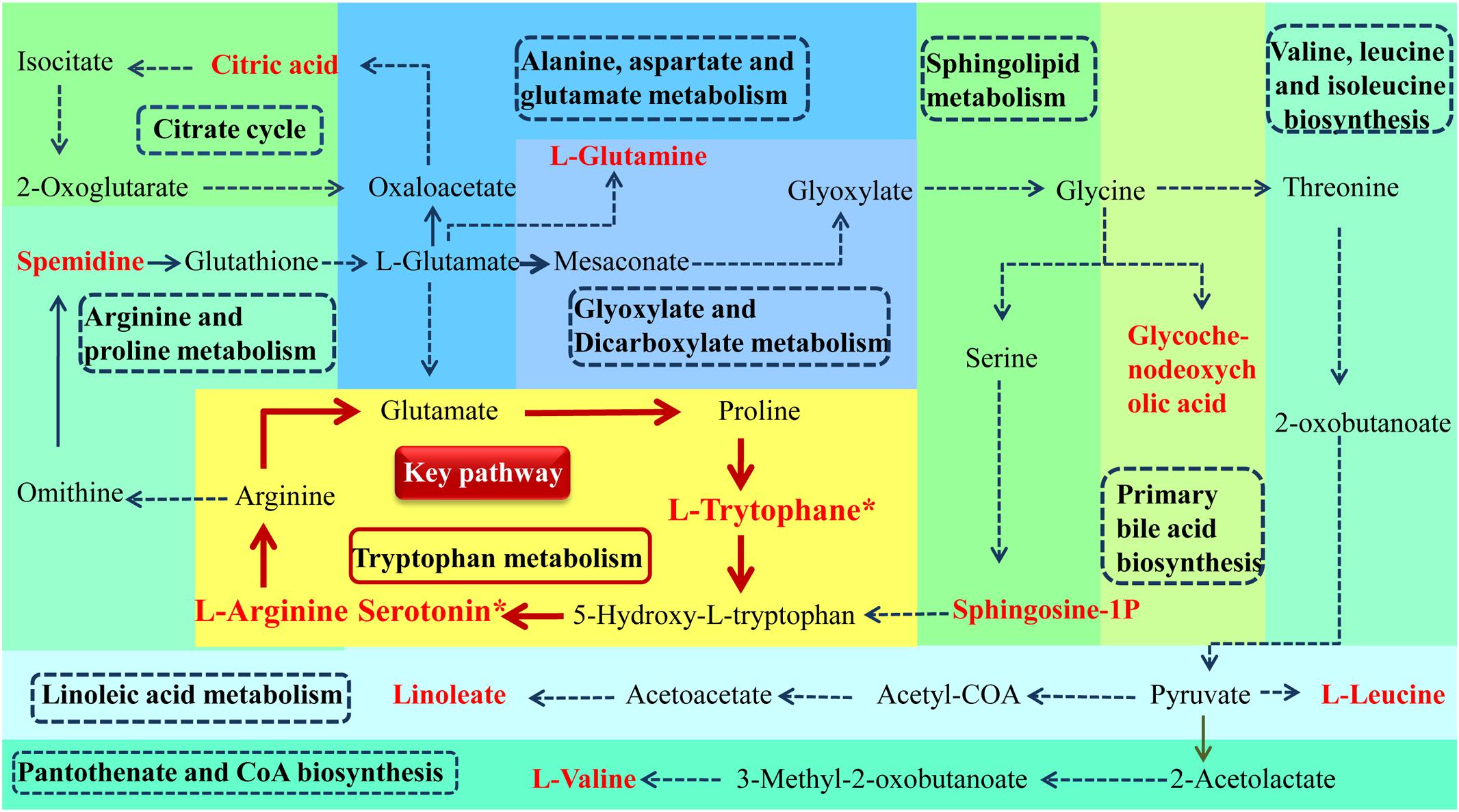
Figure 8. Metabolism pathway networks. (Different color plates represent different metabolic pathways. the name of pathway is in the dotted line frame. Metabolites name with red bold represent the differential metabolites which was identified (∗P < 0.05, magnolol group compared with model group).
Magnolol Decreases iNOS Expression and Suppresses NO Content in GMD Rats
We examined whether magnolol treatment affects the expression of iNOS using ELISA (Figure 9A). iNOS expression was significantly upregulated in the model group compared with the control group. Magnolol significantly suppressed the expression of iNOS in GMD rats. The slight increase in iNOS expression seen in magnolol group compared to control group was not statistically significant.
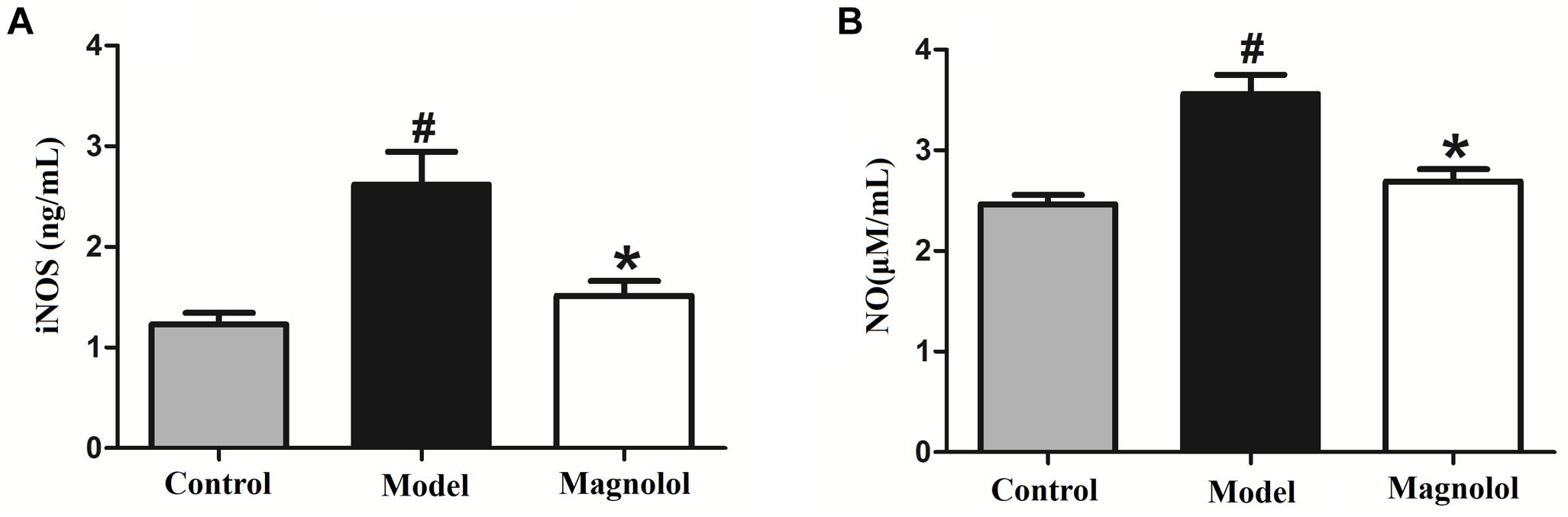
Figure 9. Magnolol prevents the L-arginine induced increase in iNOS and NO. (A) Concentrations of NO in serum in three groups. (B) Concentrations of iNOS in serum in three groups. #Control group compared with model group P<0.05, ∗Magnolol group compared with model group P<0.05.
We also tested whether magnolol affects the NO content in the serum of GMD rats (Figure 9B). NO concentration was significantly increased in L-arginine treated animals compared with control animals. Magnolol significantly reduced the increase in NO content in GMD rats. The slight increase in NO content seen in magnolol group compared to control group was not statistically significant. The results showed that magnolol has prevented the L-arginine induced increase in iNOS and NO.
Discussion
Magnolol has a good therapeutic effect on L-arginine induced gastrointestinal motility disorder in rats, which is supported by the following findings. (1)magnolol promoted gastric emptying; (2)magnolol promoted small intestinal propulsion; (3)magnolol significantly increased the level of serotonin and L-tryptophan in GMD rats; and (4) magnolol affected multiple metabolic pathways associated with the gastrointestinal tract in vivo, and tryptophan metabolism pathway played a major role in treatment. A recent study has shown that magnolol effectively improved the symptoms of Dextran Sulfate Sodium-Induced colitis in mice and its underlying mechanisms are associated with the restoring of tryptophan metabolites that inhibit the colonic inflammation (Zhao et al., 2017). It also found that magnolol acted on the tryptophan metabolism pathway, but it focused on the analysis of the kynurenine synthesis associated with anti-inflammatory effects and did not find the importance of serotonin for the gastrointestinal tract. Thus, this study used the metabolomics to study the mechanism of magnolol in the treatment of L-arginine induced GMD rats. According to the author’s knowledge, this study was the first time that a network of its metabolic pathway in vivo pathways was successfully constructed.
Rats were injected with L-arginine to cause GMD successfully. Yu et al. (2017) demonstrated L-arginine induced obvious functional disorders of the gastrointestinal tract, represented by significantly delayed gastric emptying and intestinal propulsion rates of mice. L-arginine is a multipurpose amino acid that also serves as a precursor for multiple metabolites, including polyamines and NO (Kim et al., 2018). L-arginine is the substrate for four enzymes, several of which exist as multiple isoforms: iNOS, arginases, glycine aminotransferase, and L-arginine decarboxylase. After arginine enters mammalian cells through the membrane-bound transporters CAT1 and CAT2B, it is metabolized by one of the iNOS to produce NO, which is a non-cholinergic and non-adrenergic neurotransmitter, can relax the smooth muscle and cause gastrointestinal motility to weaken. It plays an inhibitory role in gastrointestinal motility (Yu et al., 2017). The content of L-arginine in the model group increased significantly, magnolol reduced the amount of L-arginine in the model rats, reduced the production of NO, and alleviated the inhibition of gastrointestinal tract.
Through the metabolic pathway network, 11 red differential metabolites play an important role in linking, so that the metabolic pathways no longer exist alone, but constitute a whole. The 11 red differential metabolites including L-Arginine, Spermidine, L-Glutamine, Citric acid, Glycochenodeoxycholic acid, L-Leucine, L-Valine, Linoleate, Sphingosine-1P, L-Trytophane∗, Serotonin∗. L-arginine and Spermidine regulated gastrointestinal motility by affecting iNOS in the same metabolic pathway (Rodriguez-Gomez et al., 2016). L-arginine and L-glutamine are linked through two metabolic pathways. L-glutamine has protective effects on enteric neurons (Vicentini et al., 2016), most of the effects of L-glutamine on nerve cells are due to its conversion into glutathione which exerts an antioxidant effect through various mechanisms (Aoyama et al., 2008; Hermes-Uliana et al., 2014), comprising modulation in calcium homeostasis, apoptosis, and the intestinal immune system (Kuhn et al., 2010; Michalak et al., 2015). L-glutamine also has been shown to have antioxidant effects through influencing mechanisms of glutathione (GSH) synthesis and recycling (Chen and Herrup, 2012). The change of L-glutamine is not only related to the gastrointestinal function of magnolol, but also related to the antioxidant effect of magnolol. In addition, TCA cycle is an important energy metabolic pathway, and citric acid is one of its metabolites. Glycochenodeoxycholic acid is a metabolite of primary bile acid biosynthesis, which has a certain connection with the metabolism of bile acids. L-Leucine and L-Valine are branched-chain amino acids, have a protective effect on muscle (Maki et al., 2012).
The direct correlation of magnolol to the gastrointestinal tract is a tryptophan metabolic pathway. Sphingosine-1P, L-Trytophane∗ are associated with the pathway and play a role in regulating gastrointestinal motility by producing serotonin. Serotonin (5-HT), a monoamine hormone and neurotransmitter, is an essential gastrointestinal modulator whose effects regulate the intestinal physiology (Layunta et al., 2018), such as peristalsis and motility, secretion, absorption of nutrients (Mawe and Hoffman, 2013). Serotonin is synthesized in the central nervous system and the gastrointestinal tract where it is secreted from enteroendocrine cells. Its biosynthesis is regulated by two isoforms of the enzyme tryptophan hydroxylase (TPH) of which TPH1 is localized predominantly in gastrointestinal enteroendocrine cells. Serotonin activates the peristaltic reflexes, regulates gastrointestinal motility, and has a role in intestinal inflammation. Gastrointestinal 5-HT receptors are targets for the pharmacological management of gastrointestinal disorders that present with motility disturbances (Swami and Weber, 2018). The 5-HT4 receptor is expressed in various cell types in the colon and it possesses mainly prokinetic actions. 5-HT7 receptors are usually located near the 5-HT4 receptors where they help to augment their effects. Additionally, 5-HT3 receptors are localized throughout the gastrointestinal tract including enterocytes and the nerves of the myenteric plexus (Yaakob et al., 2015). Moreover, serotonin plays an important role in regulating the gastrointestinal tract by combining with various receptors. One of the mechanisms of magnolol in the treatment of gastrointestinal motility disorder is to increase serotonin content, promote gastrointestinal peristalsis and motility, secretion, absorption of nutrients and restore gastrointestinal motility to normal.
Conclusion
Magnolol can significantly improve gastric emptying and intestinal propulsion in rats with GMD. A variety of metabolic pathways were involved to achieve this effect. Among them, arginine and proline metabolism pathway would be the key metabolic pathway to play a therapeutic effect by reducing the production of L-arginine and NO, and Tryptophan metabolism would be the key metabolic pathway to play a therapeutic effect by increasing the content of serotonin. We propose the magnolol may rely on the role of relaxing gastrointestinal smooth muscle by NO and promoting gastrointestinal peristalsis and motility by serotonin to improve L-Arginine induced GMD in rats.
Author Contributions
XW contributed to the animal experiments, prepared and revised the manuscript. JZ and FL conceived and designed the experiments. CZ, MZ, and FG analyzed the data and wrote the manuscript.
Funding
This work was supported by National Natural Science Foundation of China (Grant No. 81603300).
Conflict of Interest Statement
The authors declare that the research was conducted in the absence of any commercial or financial relationships that could be construed as a potential conflict of interest.
Footnotes
- ^ http://www.hmdb.ca
- ^ http://metlin.scripps.edu
- ^ http://www.massbank.jp/
- ^ http://www.lipidmaps.org
- ^ www.metaboanalyst.ca
References
Aiwu, Y., Run, T., Guowen, H., and Miao, O. (2012). Effect of magnoliae officinalis cortex on stomach dynamic and ulcer in rat before and after its diaphoretic processing. Chin. J. Exp. Trad. Med. Form. 18, 212–215.
Aoyama, K., Watabe, M., and Nakaki, T. (2008). Regulation of neuronal glutathione synthesis. J. Pharmacol. Sci. 108, 227–238. doi: 10.1254/jphs.08R01CR
Arana, A., Johannes, C. B., Mcquay, L. J., Varas-Lorenzo, C., Fife, D., and Rothman, K. J. (2015). Risk of out-of-hospital sudden cardiac death in users of domperidone, proton pump inhibitors, or metoclopramide: a population-based nested case-control study. Drug Saf. 38, 1187–1199. doi: 10.1007/s40264-015-0338-0
Beyder, A., and Farrugia, G. (2012). Targeting ion channels for the treatment of gastrointestinal motility disorders. Therap. Adv. Gastroenterol. 5, 5–21. doi: 10.1177/1756283X11415892
Chen, J. H., and Herrup, K. (2012). Glutamine acts as a neuroprotectant against DNA damage, beta-amyloid and H2O2-induced stress. PLoS One 7:e33177. doi: 10.1371/journal.pone.0033177
Chen, S., Ji, J. R., Xu, P., Cao, Z. J., Mo, J. Z., Fang, J. Y., et al. (2010). Effect of domperidone therapy on nocturnal dyspeptic symptoms of functional dyspepsia patients. World J. Gastroenterol. 16, 613–617. doi: 10.3748/wjg.v16.i5.613
Dunn, W. B., Broadhurst, D., Begley, P., Zelena, E., Francis-Mcintyre, S., Anderson, N., et al. (2011). Procedures for large-scale metabolic profiling of serum and plasma using gas chromatography and liquid chromatography coupled to mass spectrometry. Nat. Protoc. 6, 1060–1083. doi: 10.1038/nprot.2011.335
Gika, H., and Theodoridis, G. (2011). Sample preparation prior to the LC-MS-based metabolomics_metabonomics of blood-derived samples. Bioanalysis 3, 1647–1661. doi: 10.4155/bio.11.122
Haspel, J. A., Chettimada, S., Shaik, R. S., Chu, J. H., Raby, B. A., Cernadas, M., et al. (2014). Circadian rhythm reprogramming during lung inflammation. Nat. Commun. 5:4753. doi: 10.1038/ncomms5753
Hermes-Uliana, C., Panizzon, C. P., Trevizan, A. R., Sehaber, C. C., Ramalho, F. V., Martins, H. A., et al. (2014). Is L-glutathione more effective than L-glutamine in preventing enteric diabetic neuropathy? Digest. Dis. Sci. 59, 937–948. doi: 10.1007/s10620-013-2993-2
Kanehisa, M., and Goto, S. (2000). KEGG_ kyoto encyclopedia of genes and genomes. Nucleic Acids Res. 28, 27–30. doi: 10.1093/nar/28.1.27
Kim, H. J., Han, T., Kim, Y. T., So, I., and Kim, B. J. (2017). Magnolia officinalis bark extract induces depolarization of pacemaker potentials through M2 and M3 muscarinic receptors in cultured murine small intestine interstitial cells of cajal. Cell Physiol. Biochem. 43, 1790–1802. doi: 10.1159/000484065
Kim, S.-H., Jason, R., Elizabeth, A. G., and Suhendan, E. (2018). Impact of L-arginine metabolism on immune response and anticancer immunotherapy. Front. Oncol. 8:67. doi: 10.3389/fonc.2018.00067
Kuhn, K. S., Muscaritoli, M., Wischmeyer, P., and Stehle, P. (2010). Glutamine as indispensable nutrient in oncology: experimental and clinical evidence. Eur. J. Nutr. 49, 197–210. doi: 10.1007/s00394-009-0082-2
Layunta, E., Latorre, E., Forcen, R., Grasa, L., Plaza, M. A., Arias, M., et al. (2018). NOD1 downregulates intestinal serotonin transporter and interacts with other pattern recognition receptors. J. Cell Physiol. 233, 4183–4193. doi: 10.1002/jcp.26229
Lee, J., Park, J. Y., Lee, S. H., Ryu, D. H., and Hwang, G. S. (2015). LC/MS-based polar metabolite profiling reveals gender differences in serum from patients with myocardial infarction. J. Pharm. Biomed. Anal. 115, 475–486. doi: 10.1016/j.jpba.2015.08.009
Lee, Y. J., Lee, Y. M., Lee, C. K., Jung, J. K., Han, S. B., and Hong, J. T. (2011). Therapeutic applications of compounds in the Magnolia family. Pharmacol. Ther. 130, 157–176. doi: 10.1016/j.pharmthera.2011.01.010
Maki, T., Yamamoto, D., Nakanishi, S., Iida, K., and Iguchi, G. (2012). Branched-chain amino acids reduce hindlimb suspension-induced muscle atrophy and protein levels of atrogin-1 and MuRF1 in rats. Nutr. Res. 32, 676–683. doi: 10.1016/j.nutres.2012.07.005
Manabe, N., Wong, B. S., and Camilleri, M. (2010). New-generation 5-HT4 receptor agonists_ potential for treatment of gastrointestinal motility disorders. Exp. Opin. Investig. Drugs 19, 765–775. doi: 10.1517/13543784.2010.482927
Mawe, G. M., and Hoffman, J. M. (2013). Serotonin signalling in the gut–functions, dysfunctions and therapeutic targets. Nat. Rev. Gastroenterol. Hepatol. 10, 473–486. doi: 10.1038/nrgastro.2013.105
Miao, B., Zhang, S., Wang, H., Yang, T., Zhou, D., and Wang, B. E. (2013). Magnolol pretreatment prevents sepsis-induced intestinal dysmotility by maintaining functional interstitial cells of Cajal. Inflammation 36, 897–906. doi: 10.1007/s10753-013-9617-z
Michalak, K. P., Maćkowska-Kȩdziora, A., Sobolewski, B., and Wozniak, P. (2015). Key roles of glutamine pathways in reprogramming the cancer metabolism. Oxid. Med. Cell. Longev. 2015:964321. doi: 10.1155/2015/964321
Ramirez, T., Daneshian, M., Kamp, H., Bois, F. Y., Clench, M. R., Coen, M., et al. (2013). Metabolomics in toxicology and preclinical research. ALTEX 30, 209–225. doi: 10.14573/altex.2013.2.209
Rao, G., Sui, J., and Zhang, J. (2016). Metabolomics reveals significant variations in metabolites and correlations regarding the maturation of walnuts (Juglans regia L.). Biol. Open 5, 829–836. doi: 10.1242/bio.017863
Ren, Y., Zhao, W., Zhao, J., Chen, X., Yu, C., and Liu, M. (2017). A comparative pharmacokinetic study of three flavonoids and three anthraquinones in normal and gastrointestinal motility disorders rat plasma after the oral administration of Wei-Chang-Shu tablet using high-performance liquid chromatography-tandem mass spectrometry. Biomed. Chromatogr. 31:e3997. doi: 10.1002/bmc.3997
Rodriguez-Gomez, I., Juan, N. M., Andres, Q., and Montoro-Molina, S. (2016). L-Arginine metabolism in cardiovascular and renal tissue from hyper- and hypothyroid rats. Exp. Biol. Med. 241, 550–556. doi: 10.1177/1535370215619042
Sangster, T., Plumb, R., Wilson, A. J., and Wilson, I. D. (2006). A pragmatic and readily implemented quality control strategy for HPLC-MS and GC-MS-based metabonomic analysis. Analyst 131, 1075–1078. doi: 10.1039/b604498k
Smith, C. A., Want, E. J., O’maille, G., Abagyan, R., and Siuzdak, G. (2006). XCMS_ processing mass spectrometry data for metabolite profiling using nonlinear peak alignment, matching, and identification. Anal. Chem. 78, 779–787. doi: 10.1021/ac051437y
Swami, T., and Weber, H. C. (2018). Updates on the biology of serotonin and tryptophan hydroxylase. Curr. Opin. Endocrinol. Diabetes Obes. 25, 12–21. doi: 10.1097/MED.0000000000000383
Thevenot, E. A., Roux, A., Xu, Y., Ezan, E., and Junot, C. (2015). Analysis of the human adult urinary metabolome variations with age, body mass index, and gender by implementing a comprehensive workflow for univariate and OPLS statistical analyses. J. Proteome Res. 14, 3322–3335. doi: 10.1021/acs.jproteome.5b00354
Tien, C. C., Peng, Y. C., Yang, F. L., Subeq, Y. M., and Lee, R. P. (2016). Slow infusion rate of doxorubicin induces higher pro-inflammatory cytokine production. Regul. Toxicol. Pharmacol. 81, 69–76. doi: 10.1016/j.yrtph.2016.08.002
Trygg, J. (2010). Orthogonal projections to latent structures (O-PLS). J. Chemometr. 16, 119–128. doi: 10.1002/cem.695
Valentin, N., Acosta, A., and Camilleri, M. (2015). Early investigational therapeutics for gastrointestinal motility disorders_ from animal studies to phase II trials. Exp. Opin. Investig. Drugs 24, 769–779. doi: 10.1517/13543784.2015.1025132
Vicentini, G. E., Fracaro, L., de Souza, S. R., Martins, H. A., Guarnier, F. A., and Zanoni, J. N. (2016). Experimental cancer cachexia changes neuron numbers and peptide levels in the intestine: partial protective effects after dietary supplementation with L-glutamine. PLoS One 11:e0162998. doi: 10.1371/journal.pone.0162998
Wang, B., Sun, X., Wang, S., Guo, P., Li, S., Zhang, M., et al. (2018). Comparative pharmacokinetics of (S)-MP3950, a novel 5-HT4 receptor agonist, in normal and atropine-induced gastrointestinal motility disorders rats. Xenobiotica 48, 824–830. doi: 10.1080/00498254.2017.1365974
Want, E. J., Masson, P., Michopoulos, F., Wilson, I. D., Theodoridis, G., Plumb, R. S., et al. (2013). Global metabolic profiling of animal and human tissues via UPLC-MS. Nat. Protoc. 8, 17–32. doi: 10.1038/nprot.2012.135
Wei-Wei, Z., Li, Y., Wang, X. Q., Tian, F., and Cao, H. (2005). Effects of magnolol and honokiol derived from traditional Chinese herbal remedies on gastrointestinal movement. World J. Gastroenterol. 11, 4414–4418. doi: 10.3748/wjg.v11.i28.4414
Xu, H., Zhang, L., Kang, H., Zhang, J., and Liu, J. (2016). Serum metabonomics of mild acute pancreatitis. J. Clin. Lab. Anal. 30, 990–998. doi: 10.1002/jcla.21969
Yaakob, N. S., Chinkwo, K. A., Chetty, N., Coupar, I. M., and Irving, H. R. (2015). Distribution of 5-HT3, 5-HT4, and 5-HT7 receptors along the human colon. J. Neurogastroenterol. Motil. 21, 361–369. doi: 10.5056/jnm14157
Yang, T. C., Zhang, S. W., Sun, L. N., Wang, H., and Ren, A. M. (2008). Magnolol attenuates sepsis-induced gastrointestinal dysmotility in rats by modulating inflammatory mediators. World J. Gastroenterol. 14, 7353–7360. doi: 10.3748/wjg.14.7353
Yu, J., Xu, W. H., Sun, W., Sun, Y., Guo, Z. L., and Yu, X. L. (2017). Curcumin alleviates the functional gastrointestinal disorders of mice in vivo. J. Med. Food 20, 1176–1183. doi: 10.1089/jmf.2017.3964
Zelena, E., Dunn, W. B., Broadhurst, D., Francis-McIntyre, S., Carroll, K. M., Begley, P., et al. (2009). Development of a robust and repeatable UPLC-MS method for the long-term metabolomic study of human serum. Anal. Chem. 81:1357. doi: 10.1021/ac8019366
Zhang, M., Wang, Y., Zhang, Q., Wang, C., Zhang, D., Wan, J. B., et al. (2018). UPLC/Q-TOF-MS-based metabolomics study of the anti-osteoporosis effects of Achyranthes bidentata polysaccharides in ovariectomized rats. Int. J. Biol. Macromol. 112, 433–441. doi: 10.1016/j.ijbiomac.2018.01.204
Zhang, Y., Fu, L. T., and Tang, F. (2018). The protective effects of magnolol on acute trinitrobenzene sulfonic acidinduced colitis in rats. Mol. Med. Rep. 17, 3455–3464. doi: 10.3892/mmr.2017.8321
Keywords: magnolol, gastrointestinal motility disorders, metabolomics, L-arginine, serotonin
Citation: Wang X, Zhang C, Zheng M, Gao F, Zhang J and Liu F (2019) Metabolomics Analysis of L-Arginine Induced Gastrointestinal Motility Disorder in Rats Using UPLC-MS After Magnolol Treatment. Front. Pharmacol. 10:183. doi: 10.3389/fphar.2019.00183
Received: 20 June 2018; Accepted: 14 February 2019;
Published: 01 March 2019.
Edited by:
Haitao Lu, Shanghai Jiao Tong University, ChinaReviewed by:
Aihua Zhang, Heilongjiang University of Chinese Medicine, ChinaXiaoyan Gao, Beijing University of Chinese Medicine, China
Dan Gao, Tsinghua University, China
Copyright © 2019 Wang, Zhang, Zheng, Gao, Zhang and Liu. This is an open-access article distributed under the terms of the Creative Commons Attribution License (CC BY). The use, distribution or reproduction in other forums is permitted, provided the original author(s) and the copyright owner(s) are credited and that the original publication in this journal is cited, in accordance with accepted academic practice. No use, distribution or reproduction is permitted which does not comply with these terms.
*Correspondence: Jinming Zhang, emhhbmdqaW5taW5nMTk4N0AxMjYuY29t Fang Liu, Yml0dGVyY2FmZmUxQDEyNi5jb20=