- 1Lung Cancer Center of West China Hospital, Sichuan University, Chengdu, China
- 2The Medical Department, 3D Medicines Inc., Shanghai, China
The essence of precision medicine is to achieve the goal of “individualized treatment” through genotyping of patients and targeted therapy. At present, the pathogenic genes of non-small cell lung cancer (NSCLC) have been studied most thoroughly and targeted therapy based on genotyping has been the most successful. This paper focuses on the precision treatment of NSCLC based on genotyping, comparing gene detection methods and summarize the latest progress of NSCLC immunotherapy.
Introduction
Fundamentally, cancer is a genetic disease caused by gene variations or epigenetic alterations. However, even the same tumor can be caused by different genetic variations, which is the heterogeneity of the tumor. Even patients with the same pathological type of tumor may respond differently to the treatment because of heterogeneity. Precision medicine is an individualized medical model based on the rapid development of genome sequencing technology and the vigorous rise of biological information and big data science. According to the different molecular types of patients, different treatment regimens are the most reliable methods to improve response and reduce adverse reactions. With the continuous progress of biological detection technology, the cost of human gene molecular typing is becoming lower while the accuracy is getting higher, and individualized treatment is gradually becoming reality.
In recent years, the incidence and mortality of lung cancer have shown a sharp rise in the world. What’s more, lung cancer is the most common cause of cancer death worldwide, with 1.38 million people dying every year, accounting for 18.2% of the total cancer deaths (Jemal et al., 2011). It is also the cancer with the highest morbidity and mortality in China, approximately 781,000 new cases and 626,000 deaths had been reported in 2014 (Chen et al., 2014). Based on the data from the Global cancer statistics 2018, it shows that among the males, incidence rate of the NSCLC is 223.0 per 100,000 and mortality rate is 166.6 per 100,000. Besides, in the female, the incidence rate is 182.6 per 100,000 (Bray et al., 2018). Non-small cell lung cancer (NSCLC) accounts for about 80–85% of lung cancer, and its clinical manifestations are complex and diverse. There are many risks associated with surgical management of advanced NSCLC (Zhang et al., 2017a), drug therapy for advanced NSCLC is safer than surgical treatment (Nie et al., 2012). Patients with the same pathological type of NSCLC may have different responses to the same anticancer drug. At present, the pathogenic genes of NSCLC have been studied most thoroughly and targeted therapy based on genotyping has been the most successful. The purpose of this article is to review the accurate treatment of advanced NSCLC based on genotyping, including targeted therapy, immunotherapy, and comparison of several common detection methods.
Overview of Targeted Therapy of NSCLC
Chemotherapy was the most important treatment for stage III and IV NSCLC patients until targeted therapy was well developed. The generally acknowledged third-generation new chemotherapy drugs combined with platinum regimen have an overall effect resulted in a significant improvement in survival (HR, 0.79; 95% CI, 0.62–1.00; p = 0.05) and a 5 years survival improvement of 11% (67% with chemotherapy vs. 56% with observation) (Nagasaka and Gadgeel, 2018), however, the median survival period is only 8–10 months (Li and Liu, 2018). Meanwhile, chemotherapy drugs cannot differentiate tumor cells and normal cells while working, the treatment related adverse reactions are dramatically strong therefore being feared by patients.
It was not until the emergence of targeted therapy based on molecular typing that the survival period of patients with advanced NSCLC was improved to several years, such as the second generation ALK-TKI alectinib (Alecensa) achieved the PFS of first-line NSCLC patients with ALK fusion up to 34.8 months (Peters et al., 2017), and the adverse reactions were greatly reduced, such as the adverse events of grade 3 or higher was lower with the third generation EGFR-TKI osimertinib (Tagrisso, 23%) than with platinum-pemetrexed (47%) (Mok et al., 2017; Peters et al., 2017).
The discovery of NSCLC targeted therapy is an event of necessity in contingency. At the end of 2003, researchers from Dana-Farber and Massachusetts general hospital in the United States simultaneously found high remission rates in some NSCLC patients using tyrosine kinase inhibitors (TKIs), and these patients’ high remission rates were confirmed to be the result of EGFR gene mutation (Kris et al., 2003). The first drug, bevacizumab, was approved by the FDA in 2004 for the treatment of advanced colorectal cancer (Herbst et al., 2018). By 2009, the first large randomized controlled study, IPASS, demonstrated that gefitinib significantly prolongs PFS in lung cancer patients with EGFR mutations related to carboplatin-paclitaxel (hazard ratio for progression or death, 0.48; 95% CI, 0.36–0.64; P < 0.001) (Mok et al., 2009). An important advance in the management of advanced stage NSCLC occurred in 2015, when the US FDA approved the ICB nivolumab for the treatment of patients whose disease progressed during or after platinum-based therapy, heralding a new era in the management of lung cancer (Herbst et al., 2018). Since then, a series of genes related to the pathogenesis and treatment of NSCLC have been discovered, and a variety of targeted drugs and detection methods have been developed, changing the patterns of advanced NSCLC treatment thoroughly. The latest NSCLC guideline, 2019 v3, published by national comprehensive cancer network (NCCN) suggests that 9 genes related to targeted therapy should be detected, including EGFR, KRAS, HER2, ALK, ROS1, MET, BRAF, RET, and NTRK. Here we use the timeline to show the development of targeted therapies and immunotherapies for the treatment of NSCLC over two decades Figure 1 (Herbst et al., 2018).
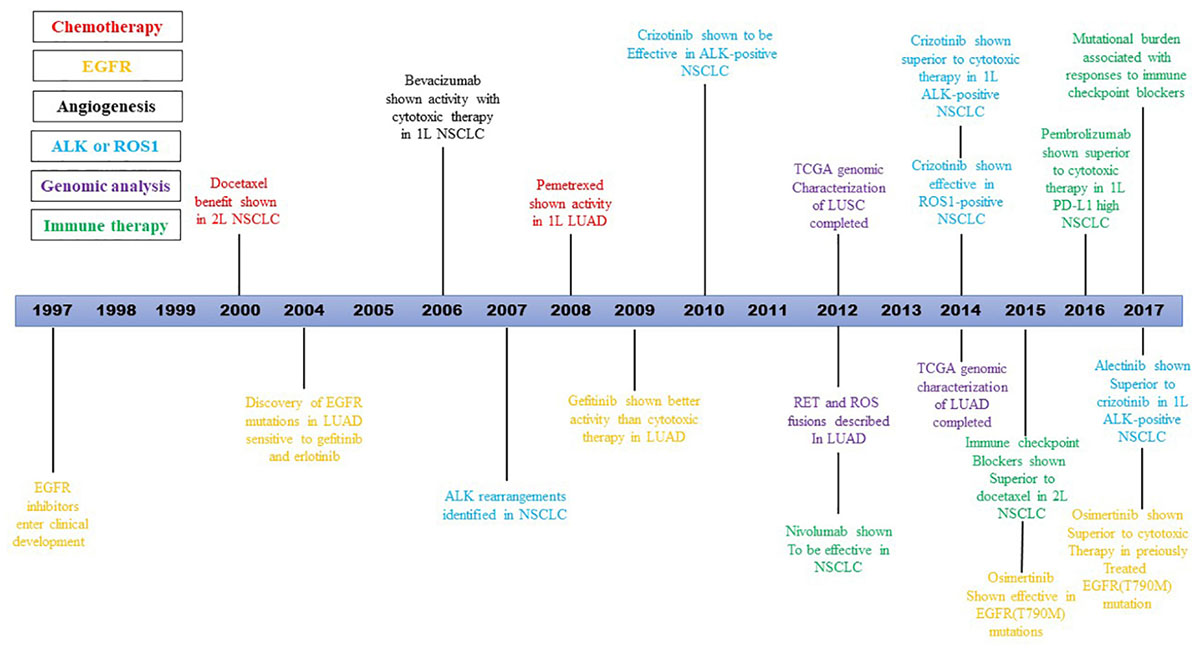
Figure 1. Timeline illustrating the development of targeted therapies and immunotherapies for the treatment of NSCLC over two decades.
EGFR
As the first therapeutic target discovered, EGFR has been the most thoroughly studied and the most successful. Based on recent studies, EGFR is the most common driving gene in NSCLC in Asia-Pacific and Russian, with an incidence of 49.3% (Han et al., 2017). Mutation types mainly include single nucleotide variation (SNV), insertion, deletion and copy number variation (CNV). The variations were mostly concentrated in exons 18–21, and the responses of exon 19 and 21 to EGFR-tyrosine kinase inhibitor (EGFR-TKI) were generally better than exons 18 and 20. The most common sensitive mutations are the deletion of amino acids at 747–750 of exon 19 (19Del) and the L858R mutation of exon 21, so the use of first-generation EGFR-TKI, namely gefitinib (Iresa), erlotinib (Trockai) and ecotinib (Kemet sodium), can be considered. Afatinib (Giotrif), the second generation of EGFR-TKI, is an irreversible inhibitor with two targets, EGFR and HER2. It is especially applicable for patients with EGFR-TKI resistance caused by HER2 mutation. Afatinib is effective for certain types of rare EGFR mutations and has been approved by FDA for use with rare EGFR mutations: G719X, L861Q, and S768I (Yang et al., 2015).
Drug resistance is almost inevitable after 8–14 months of the first or second-generation EGFR-TKI treatment (Maemondo et al., 2010; Mitsudomi et al., 2010; Tan et al., 2016). The reasons for drug resistance are varied. The mutation of T790M of EGFR exon 20 is the most common cause of drug resistance, accounting for about 50–60% (Kobayashi et al., 2005; Oxnard et al., 2011; Sequist et al., 2011; Yu et al., 2013). In addition, downstream KRAS, BRAF and other activation mutations, HER2 mutation, MET amplification lead to bypass activation, PTEN lost, and transformation to small-cell lung cancer (SCLC), which are also the mechanisms of acquired drug resistance.
If T790M mutation is detected, we can switch to the third-generation EGFR-TKI osimertinib. Studies have shown that after the first or second -generation EGFR-TKI resistance caused by T790M mutation, the survival period of 7.6m can still be obtained by using osimertinib. AURA3 studies in NSCLC patients with EGFR-T790M mutations showed significantly longer PFS with osimertinib compared with permetrexine (Mok et al., 2017). The FLAURA study showed that regardless of whether T790M mutations were detected, the PFS of the first-line treatment group with osimertinib reached 18.9 months, while the median PFS of the first-line standard treatment of the first-generation EGFR-TKI was only 10.2 months, and patients with osimertinib had a high safety (Soria et al., 2018). Thus, first-line use of osimertinib may have a longer FPS than switching to osimertinib after the resistance of first-generation EGFR-TKI.
Taking targeted drug osimertinib as an example, in NSCLC patients with epidermal growth factor receptor (EGFR) T790M mutation, the median duration of progression-free survival (PFS) was significantly longer with osimertinib than with platinum-pemetrexed (10.1 vs. 4.4 months; HR: 0.30; 95% CI, 0.23–0.41; P < 0.001); the objective response rate (ORR) were 71% vs. 31% (odds ratio, 5.39; 95% CI, 3.47–8.48; P < 0.001) (Mok et al., 2017).
Unfortunately, even if the initial treatment is very effective, patients still have to face drug resistance after months of using osimertinib. The mutation of C797S of EGFR exon 20 is the most common cause of acquired drug-resistance of osimertinib. Geoffrey and his team found out that non-invasive genotyping of cell-free plasma DNA (cfDNA) is a useful biomarker for prediction of outcome from osimertinib (Oxnard et al., 2016). If C797S and T790M are on different chromosomes (trans-configuration), it will still be sensitive to the combination of the first and third-generation EGFR-TKI (Arulananda et al., 2017). On the other hand, if these two mutations are in the same chromosomes (cis-configuration), EGFR-TKI should no longer be used (Goldberg et al., 2018). Interestingly, NSCLC patients with C797S/T790M/19Del or L858R (triple-mutation) are resistance to EGFR-TKIs, but sensitive to ALK-TKI brigatinib (Alunbrig) (Uchibori et al., 2017).
It has been reported that EGFR L718Q, G796D, L844V, et al. mutations are also an acquired drug-resistance site to osimertinib and first-generation EGFR-TKI, but is sensitive to afatinib (Liu et al., 2017; Zheng et al., 2017). Moreover, EGFR is expressed in many normal epithelial tissues and in many human cancers, including those of colon and rectal. For instance, cetuximab also received approval by the FDA for the treatment of head and neck cancer (Altaha and Abraham, 2007).
ALK
Anaplastic lymphoma kinase (ALK) fusion accounts for 3–5% of NSCLC, which is the second largest mutant gene found after EGFR gene. ALK is most common fused with echinoderm microtubule-associated protein-like 4 (EML4). EML4 is located at P21 of human chromosome 2, while ALK is located at P23. These two genes form a new gene EML4-ALK through inversion fusion. EML4-ALKfusion has more than 21 different forms according to the location of fracture. The sensitivity of different fusion forms to ALK-TKI was also different. The response rate and PFS of patients fused with exon 13 of EML4 gene and exon 20 of ALK gene to crizotinib (Xalkori) were significantly higher than those of other fusion types (Yoshida et al., 2016).
It was once thought that there was exclusion between ALK fusion and EGFR activating mutations (Crystal and Shaw, 2011), which was actually a misunderstanding. Both EGFR and ALK are strong driving genes, as a result, the presence of either of them is sufficient to form tumors, so the presence of them has been rarely observed before. As the data accumulated, some scientists felt obliged to investigate for the patients’ samples did show these two genetic variants coexist (Wen et al., 2016).
PROFILE series of clinical research evidence all confirmed that compared with chemotherapy, both the response rate and disease control time of crizotinib were significantly better (Blackhall et al., 2017). The response rate of crizotinib for ALK fusion positive patients could reach 60∼80%, while that of chemotherapy drugs was only about 30∼40%. Crizotinib is one of the most significant clinical benefits drugs in targeted therapy of lung cancer.
Unfortunately, after initial response to crizotinib, tumors inevitably relapse and end up with drug-resistant symptoms like brain metastasis. In order to overcome crizotinib resistance, alectinib, ceritinib (Zykadia), brigatinib and other second-generation ALK-TKIs were developed, which are more potent and brain-penetrable (Duruisseaux et al., 2017). Surprisingly, all three second-generation ALK inhibitors achieved significant improvements in the first line NSCLC patients with ALK positive. Next generation agents (alectinib and brigatinib) revealed significant improvement in PFS (HR 0.50 [0.43, 0.57; p < 0.00001]), ORR (OR 1.57 [1.21, 2.04; p = 0.0006]) in comparison to crizotinib and yielded better response intracranially than crizotinib in terms of objective response rate (OR 5.87 [3.49, 9.87; p < 0.00001]) and time to CNS progression (HR 0.25 [0.13, 0.46; p < 0.0001]) (Khan et al., 2018; Paik and Dhillon, 2018). What’s more, brigatinib received granted accelerated approval by the United States Food and Drug Administration. In ASCEND-4 study (Soria et al., 2017), the mPFS of ceritinib is 16.6 months. In ALTA-1L study (Camidge et al., 2018), the estimated 12 months PFS rate, brigatinib and crizotinib achieved 67 and 43%, respectively. Especially in ALEX study, alectinib was head-to-head compared with crizotinib in first-line advanced ALK-positive NSCLC (Peters et al., 2017). The updated follow-up results was reported in 2018 ASCO conference. The mPFS of first-line alectinib was 34.8 months, compared with 10.9 months for crizotinib, which was amazing 3 times longer. An mPFS of nearly 3 years is enough to allow patients to live for 4–5 years or more, making lung cancer turn into a chronic disease. In addition, significant differences were observed between the two treatment groups regardless of whether the patient’s baseline was associated with brain metastasis. Alectinib was also significantly superior to crizotinib in efficacy duration analysis. Safety data of alectinib is also excellent, and the incidence of serious adverse events remains low even after long-term use. The excellent efficacy and safety of alectinib may be related to its special molecular structure (Kinoshita et al., 2012). The drug structure determines the selectivity and affinity with the target. The higher the selectivity, the higher the safety and efficacy. Alectinib also has excellent blood-brain barrier permeability, which means better treatment and even prevention of brain metastases, and therefore longer PFS. Overall, ceritinib, brigatinib and alectinib have become the first-line treatment scheme recommended in NCCN guidelines for ALK fusion positive patients.
However, very similar to the case of EGFR-TKI, the second-generation ALK-TKIs also face resistance problems. Mutations in the ALK tyrosine kinase domain, such as G1202R, may affect the binding of ALK-TKIs, leading to drug resistance (Gainor et al., 2016). Fortunately, for patients with alectinib resistance, we can also choose the third-generation ALK-TKI lorlatinib (Lorbrena), which is specifically designed for drug resistance mutation sites and has a high blood-brain barrier permeability. By virtue of data already disclosed in the phase I/II studies (Basit et al., 2017; Shaw et al., 2017), lorlatinib has been approved by FDA as a breakthrough drug, and the phase III CROWN study is ongoing. Entrectinib was shown to be well tolerated and active against those gene fusions in solid tumors, including in patients with primary or secondary CNS disease (Drilon et al., 2017).
ROS1
Human ROS1 gene is a transmembrane tyrosine kinase gene of insulin receptor family, located at q21 of chromosome 6. ROS1 rearrangement represents a new and unique molecules subtype of NSCLC, whose frequency is 1–2%, and is common in young non-smoking female lung adenocarcinoma patients. However, ROS1 fusion occurs in up to 5% of patients with negative EGFR and ALK. Due to the low occurrence probability of ROS1 fusion in lung cancer patients, this molecular subtype was often neglected in the past in clinical practice, and patients with ROS1 fusion were often treated with standard regimens such as chemotherapy for first-line. With the development of gene sequencing technology, ROS1 fusion has been truly demonstrated to clinicians.
ROS1 rearrangement is mainly concentrated in exon 32–36, and at least 9 different fusion types have been found in NSCLC, among which CD74-ROS1 and SLC34A2-ROS1 are common (Jun et al., 2012).
Crizotinib and ceritinib have been shown to be effective in ROS1-positive NSCLC patients. A study published in 2014 showed that in NSCLC patients with ROS1 fusion using crizotinib showed amazing anti-tumor activity, with the objective response rate (ORR) being 72% and median duration of response (mDOR) being 17.6 months (Shaw et al., 2014). But for crizotinib, the first-generation ALK inhibitor, which can cause relapse of CNS metastases can be overcome by newer ROS1 inhibitors (Dong et al., 2016).
Similarly, NSCLC patients with ROS1 fusion who are treated with crizotinib may develop drug resistance, especially CNS metastases. Common drug resistance mutations include G2032R and D2033N of ROS1, which can be overcome by cabozantinib and lorlatinib (Katayama et al., 2015; Zou et al., 2015). A newer inhibitors, entrectinib (RXDX-101), is a ROS1, Pan-TRK, and ALK inhibitor with activity in multiple molecularly defined cancer indications. Entrectinib has extremely high anti-tumor activity against cell lines dependent on the pharmacological targets of this drug in vitro, which has shown great promise in phase I/II clinical trials (Ardini et al., 2016). One of the major differences between entrectinib and crizotinib is that crizotinib cannot normally penetrate the brain and CNS, and entrectinib has shown effective CNS activity.
BRAF
BRAF gene mutation is not only one of the mechanisms of EGFR-TKI resistance, but also an important driver gene and target of targeted therapy. BRAF mutations are found in 1.5–3.5% of NSCLC and cause downstream activation of the MAPK signaling pathway (Leonetti et al., 2018). V600E is the most common mutation. Selective BRAF inhibitors, such as dabrafenib (Tafinlar) and trametinib (Mekinist) has been recommended for the first-line and second-line treatment of advanced NSCLC in the NCCN guidelines.
The ORR of dabrafenib monotherapy for NSCLC patients with BRAF-V600E is 33% (95% CI, 23–45%) (Planchard et al., 2016b). In previously untreated metastatic NSCLC patients with BRAF-V600E, dabrafenib combined with trametinib achieved an ORR of 64% (95% CI, 46–79%), with 2 (6%) patients achieving a CR and 21 (58%) a PR (Planchard et al., 2017). Analogously, in pretreated patients the ORR was 63.2% (95% CI, 49.3–75.6%) (Planchard et al., 2016a).
Vemurafenib (Zelboraf) was approved by the FDA for the treatment of unrespectable and metastatic malignant melanoma with BRAF-V600E mutation in 2011. In the VE-BASKET study, vemurafenib was used for NSCLC patients with BRAF-mutant, the ORR was 42% (95% CI, 20–67%). The median PFS was 7.3 months (95% CI, 3.5–10.8%). The 12 months rate of PFS and OS were 23% (95% CI, 6–46%) and 66% (95% CI, 36–85%), respectively (Hyman et al., 2015).
MET
c-MET is a kind of transmembrane receptors with independent phosphorylation activity, encoded by mesenchymal epithelial transition (MET) gene (Gherardi et al., 2012). The hepatocyte growth factor (HGF) is the specificity ligand of c-MET (Naldini et al., 1991). MET gene amplification, or c-MET protein overexpression, is one of the causes of resistance in NSCLC patients with the first or second-generation EGFR-TKI, accounting for about 5% of resistances (Engelman et al., 2007; Cappuzzo et al., 2009; Yu et al., 2013). Therefore, some researchers believe that the combination of c-MET inhibitor and EGFR-TKI will be a new idea to overcome drug resistance.
There is also a special mutation form of MET genes: exon 14-skipping (Awad et al., 2016). The Cancer Genome Atlas (TCGA) showed that MET exon 14-skipping were present in about 4% (10/230) of lung adenocarcinoma, leading to partial or complete skipping deletion of MET exon14 at mRNA level (Cancer Genome Atlas Research Network, 2014). The NCCN guidelines recommended crizotinib for patients with c-MET protein overexpression, MET gene amplification, and MET exon 14-skipping mutation in NSCLC patients.
NTRK
Rearrangements including NTRK1, NTRK2, and NTRK3 are in approximately 2–3% NSCLC patients without other driving genes, such as EGFR, KRAS, EML4-ALK, and ROS1 (Ricciuti et al., 2017).
On November 26, 2018, the US FDA accelerated the approval of larotrectinib (LOXO-101, Vitrakvi) for the treatment of locally advanced or metastatic solid tumors in adults and children with neurotropic tyrosine kinase receptor (NTRK) gene fusion, regardless of the region of cancer occurrence.
It is the first broad-spectrum cancer targeting drug that has been approved by the FDA, which target at NTRK fusion mutations and regardless of cancer type. It have been reported in almost every cancer, and shown to be effective in 17 childhood and adult tumors (Berger et al., 2018).
According to the latest data released at the annual meeting of the European society of oncology (ESMO) in October 2018, larotrectinib was able to achieve 80% objective response rate (ORR) in 55 patients with NTRK fusion cancer that could be measured by RECIST 1.1 criteria. It is noteworthy that larotrectinib showed very consistent results in various cancer types.
Entrectinib was also effective for NTRK fusion. In the studies of ALKA-372-001 and STARTRK-1, the ORR of 5 cases with NTRK fusion was 100%, including 3 cases of brain metastasis (Drilon et al., 2017). Results of phase II study STARTRK-2 have been published on 2018 ESMO congress. The result shows that, regardless of whether brain metastases, 54 NTRK fusion patients (sarcoma 24%, NSCLC 19%, sample secretory breast carcinoma 13%, breast cancer 11%, colorectal cancer 7%, bile duct carcinoma and other gynecological tumor, neuroendocrine carcinoma and salivary gland carcinoma and pancreatic cancer) treated by entrectinib all have gain benefit: ORR 57.4%, mDOR 10.4 months, mPFS 11.2 months, and mOS 20.9 months.
Others
In recent years, due to the progress of molecular subtyping technology, people have gained a new understanding of the biological mechanism of the occurrence and development of NSCLC. Several specific subtypes of driving genes, such as HER2, RET, KRAS, have been discovered, and corresponding targeted drugs have been developed, thus paving the way for the era of personalized medicine of NSCLC.
The incidence of RET fusion in lung adenocarcinoma is unknown and may be anywhere between 0.4 and 2% (Smit, 2017). The drugs target at RET fusion are much less effective than EGFR-TKI or ALK-TKI, and combination therapies may be the key to improving response in the future.
Human epidermal growth factor receptor 2 (HER2) is expressed in many cancers, including NSCLC. HER2 amplification has been reported to occur in up to 13–22.8% of NSCLC (Yu et al., 2013; Ko et al., 2018). Afatinib could be a useful therapeutic agent as HER2-targeted therapy for patients with NSCLC harboring HER2 alterations (Torigoe et al., 2018).
KRAS is a driving gene with very high mutation frequency in NSCLC and colorectal cancer, accounting for 3% in western population and 6% in Asian population (Domagala et al., 2012). KRAS has a high mutation frequency in many other tumor species. However, unfortunately, there are currently no targeted drugs specifically targeting KRAS mutations approved in list. KRAS mutation may affect the efficacy of EGFR-TKI, but increase the efficacy of immunotherapy.
Overview of Detection Method
With the continuous progress of biological detection technology, the acquisition of human gene molecular typing is becoming more convenient and easier, and accurate treatment is gradually becoming the reality.
According to the different detection objects, there are many detection methods: ARMS, NGS, ddPCR, and FISH are targeted at DNA; RNA was targeted by RNA-Seq and RT-PCR, and IHC for protein. If the amount of tumor tissue is too small or unable to obtain tissue, we can supplement with liquid biopsy through blood, hydrothorax or ascites, pericardial effusion, cerebrospinal fluid and other specimens (Zhang et al., 2017b).
ARMS
The amplification refractory mutation system (ARMS) based on fluorescence quantitative PCR technology has become the mainstream technology of gene mutation detection due to its simple operation, high specificity, high sensitivity and good repeatability, which is suitable for hospital laboratory to carry out test by itself. ARMS can be used to detect gene mutations in tumor tissues and peripheral blood, but the prerequisite is to specify the specific site to be detected in advance and design the corresponding PCR primer. Therefore, ARMS is only applicable to the detection of known mutation sites and cannot be used for the detection of unknown sites.
NGS
High-throughput sequencing, or next-generation sequencing (NGS), because of its low cost, large throughput, high accuracy and rich information content, has become a very important role in the study of genome, transcriptome and epigenetics. NGS mainly includes the whole genome sequencing (WGS), whole exome sequencing (WES), as well as the targeted region sequencing (TRS) or cancer gene panel (CGP). Recently, NGS is used to sequence circulating tumor DNA (ctDNA), as a kind of liquid biopsy. ctDNA is derived from necrotic tumor cells, apoptotic tumor cells, circulating tumor cells and exosomes produced by tumor cells. Although ctDNA samples are relatively simple to obtain, they are extremely low in content, accounting for 0.01∼1.00% of cell free DNA (cfDNA) in plasma. It was not until the emergence of NGS technology that the difficulty of detecting mutations from extremely low abundance samples was solved.
The types of mutations detected by NGS mainly include SNV, insertion, deletion, CNV, etc., including known and unknown mutation forms, which can provide much more information than ARMS. However, in terms of laboratory hardware setting and personnel qualification, it has higher requirements than conventional PCR laboratories. Therefore, it cannot be widely used in more hospitals at present, and third-party testing institutions are more likely to provide testing services.
FISH
Fluorescence in situ hybridization (FISH), can be used for the detection of CNV and gene fusion frequently. The number and location of the corresponding genes can be clearly displayed under the microscope by fluorescent probe labeling, and the CNV and fusion can be determined by this method. FISH is the gold standard for the detection of gene fusion. Taking ALK fusion as an example: two probes, red and green, are designed to mark the two ends of ALK gene, respectively, once ALK gene is broken and rearranged or inverted, red and green signals will be separated under the microscope; while those that are not broken will show yellow fluorescence signals. FISH can detect whether a gene has been rearranged or reversed, but it cannot determine where it has been broken or fused with which gene. NGS detects gene fusion to identify the site of rupture and to identify which genes are fused. However, about 10% of fusion fracture sites were located in introns or not contained by probes, so NGS could not detect them. Therefore, NGS combined with FISH will help to determine the final result.
ddPCR
Droplet digital PCR (ddPCR), as the third generation of PCR technique usually used in ctDNA liquid biopsy, gain a great of attention in the field of clinical diagnosis because of its high sensitivity. By using the micro-PCR amplification system, absolute quantification can be achieved, which overcomes the difficulty of ctDNA not easy to be amplified due to its low content. Same as ARMS, ddPCR can only detect known sites, but it is fast and sensitive, with almost no detection threshold. ddPCR combined with NGS cannot only quickly check whether there are common mutations, but also examine whether there are rare mutations in a wide range.
Due to its high sensitivity, relatively non-invasive and absolute quantification, ddPCR has been used for dynamic monitoring of mutant copy number, which can be used for real-time monitoring of the relationship between tumor progression, drug resistance and gene evolution.
IHC
Genes ultimately function through proteins, so detection of proteins is of more concern. Immunohistochemistry (IHC) is the detection of protein level, which can detect protein deficiency, overexpression, fusion and so on. Surgical tissue, fine needle aspiration biopsy tissue, pleural or ascites centrifugal precipitates can all be used for the detection of immunohistochemistry.
The test results of DNA level and protein level sometimes do not match. Amplification of a gene usually implies overexpression of the corresponding protein, such as androgen receptor (AR). On the contrary, when the AR protein is overexpressed, but the AR gene is not necessarily amplified, because there are many regulatory mechanisms from DNA to protein. Similarly, if the promoter is methylated, then even if the DNA sequencing is normal, the corresponding protein may be missing. There is also a weak relationship between some genes and protein expression, such as CD274 gene and PD-L1 protein, and there is almost no relationship between the expression level of PD-L1 and the copy number of CD274.
Overview of Immune Checkpoint Inhibitors
The cause of the tumor is related to immune system oversight. All malignancies originate from genetic mutations, including germline and somatic mutations. According to the genetic central dogma, the changes in DNA ultimately cause changes in proteins. The altered proteins (tumor proteins) are immunogenic (neoantigens). A large number of neoantigens, caused by genetic and epigenetic changes, can induce an immune response, but tumors can induce tolerance between tumors and specific T cells by up-regulating ligands of inhibit receptors, ultimately resisting immune attacks (Mellman et al., 2011). According to the Cancer immunoediting theory first proposed by Dunn et al. (2002), the immune response has three stages of elimination, equilibrium and escape, and it is regulated by various stimulant and inhibitory factors (Dunn et al., 2002). Tumor immunotherapy is to activate the body’s auto defense mechanism or give exogenous substances to regulate the immune response to tumor, and stimulate the immune cells to identify, inhibit and kill tumor cells (Dong et al., 2018). The immune escape mechanism of tumors plays an important role in the occurrence and development of tumors (Siegel et al., 2017).
Currently, inhibitors of two checkpoints, cytotoxic T lymphocyte antigen – 4, (CTLA-4), programmed cell death protein – 1 (PD-1)/programmed cell death ligand 1 (PD-L1), have been approved to be listed. PD-1 inhibitors include nivolumab, pembrolizumab, and PD-L1 inhibitors include atezolizumab, durvalumab, avelumab, etc.
Immunotherapy often produces some magical results and has aroused great interest among oncologists worldwide. However, not every patient can achieve this effect, and some patients may even progress faster after receiving immunotherapy. MDM2/4 amplification, chromosome 11q13 (CCND1, FGF3, FGF4, and FGF19) amplification and EGFR amplification may be associated with hyperprogression in immunotherapy (Kato et al., 2017). Molecular typing is also needed to determine which patients are suitable for use, and biomarkers are used to screen out which patients can use immunotherapy.
PD-L1
PD-1 is expressed in T cells, natural killer cells (NK), monocytes and B cells. PD-L1 is mainly expressed on the surface of tumor cells and in the tumor microenvironment. When PD-1 binds to PD-L1, T cells are inhibited. PD-L1 on the tumor cell membrane can be abnormally up-regulated and inhibit the activation of T lymphocytes, leading to tumor immune escape (Haanen and Robert, 2015). The PD-L1 expression level is the first biomarker approved by FDA. Although there were some differences reported in the results of clinical trials in which different PD-1/PD-L1 inhibitors were involved, the benefit of immunotherapy was significantly higher in those with high expression of PD-L1 than in those with low expression (Dong et al., 2018).
IHC is the gold standard for detecting the expression rate of PD-L1 protein. There are two platforms (Dako, Ventana) and four testing kits (28-8, 22C3, SP263, SP142) used for PD-L1 detecting.
TMB
Tumor mutation burden (TMB) is also able to identify candidate patients who will benefit from immunotherapy. TMB is defined as the number of non-synonymous somatic mutations per million bases, excluding germline mutations. Therefore, TMB describes the stability of the genome. The higher the TMB is, the more mutations will be, as a result there will be more new antigens, more immunogenicity, and better effect of immunotherapy.
NGS is the only method for TMB detection. TMB measured by WES is the gold standard, but it is costly, time-consuming and inconvenient for clinical application. The TMB measured by TRS (CGP) avoids these problems successfully and has been widely carried out in clinical practice. The question then becomes how to define “TMB-H.” When different regions were selected for sequencing, the TMB value obtained was inconsistent and an accepted cutoff value could not be determined. A smart solution is to compare the ordering of the absolute value of CGP-TMB instead of comparing the absolute value directly. The CGP-TMB values of the same tumor species detected by the same detection institution can be sorted, with the highest 25% defined as “high,” the middle 50% defined as “medium,” and the lowest 25% defined as “low,” which can also be divided into three equal parts. Blood-based TMB (bTMB) has also been shown to be useful in screening people for immunotherapy (Gandara et al., 2018).
In October 2018, based on the results of Checkmate-227 (Hellmann et al., 2018) and Checkmate-026 (Carbone et al., 2017), the NCCN issued 2019 v1.0 guidelines for NSCLC, recommending TMB for the first time to identify lung cancer patients who are suitable for the combination of “nivolumab + ipilimumab” and “nivolumab.” But it also points out that there is no consensus on how to measure TMB.
MMR and MSI
Mismatch-repair (MMR) deficiency and microsatellite-instability (MSI) can predict the therapeutic effect of tumor immunotherapy (Le et al., 2015). MSI is major caused by MMR- deficient (dMMR), and we detect MSI to determine whether the function of MMR is proficient (pMMR). Therefore, like TMB, MSI, and MMR are indicators of genomic stability. dMMR enables tumors to synthesize new antigens with potential immunogenicity, thus causing an immune response (Colle et al., 2017).
The gold standard for MMR detection is IHC to detect whether four MMR proteins (MLH1, MSH2, MSH6, PMS2) are expressed. There are two possible reasons for the non-expression of MMR protein. 1. MMR gene variation; 2. Methylation of MMR gene promoter. The first cause can be also detected by NGS, but the second can only be confirmed by detecting promoter methylation (McCarthy et al., 2018).
MSI can be measured by PCR or NGS. PCR-MSI is easy to operate, and has been carried out in many hospitals, but only five sites have been detected so far. Compared with PCR, NGS detection has a wider range and can avoid detection omission effectively (Hempelmann et al., 2018). The sensitivity and specificity of CGP-MSI based on NGS can reach 93.1–96.6% and 97.2–100%, respectively (Salipante et al., 2014; Hempelmann et al., 2018). In addition to improved sensitivity, NGS-MSI testing offers several advantages over PCR-MSI methods. (1) They do not require matched non-tumor tissue; (2) Interpretation is streamlined and semiautomated (Hempelmann et al., 2018).
However, dMMR or MSI is a low-probability event in NSCLC.
Summary
Tumor-targeted therapy is to determine the treatment method for specific driving gene mutations by detecting whether there are gene mutations or gene spectrum changes in tumors that lead to tumor growth. In order to determine whether patients can use targeted therapeutic drugs, genetic molecular typing is needed first.
In the selection of gene detection technology, it is necessary to select appropriate detection technology according to its corresponding target to ensure the accuracy and reliability of detection results.
NSCLC has the most thorough research and the most sufficient evidence in driving gene molecular typing. Accurate treatment of NSCLC patients can only be achieved by using the technology and accurate detection. We hope that in the future, more detection technologies, more driving genes and more effective targeted drugs will emerge, so that NSCLC will become a controllable chronic disease.
Author Contributions
QZ put forward the content of the paper. JD wrote the manuscript. BL, DL, and DH literature and clinical data were reviewed.
Funding
This work was supported by the Department of Science and Technology of Sichuan Province (2018SZ0152) and Health Commission of Sichuan Province (18PJ432).
Conflict of Interest Statement
DH was employed by company 3D Medicines Inc.
The remaining authors declare that the research was conducted in the absence of any commercial or financial relationships that could be construed as a potential conflict of interest.
References
Altaha, R., and Abraham, J. (2007). Epidermal growth factor receptor as a target for cancer therapy. Expert Rev. Anticancer Ther. 7, 1–3. doi: 10.1586/14737140.7.1.1
Ardini, E., Menichincheri, M., Banfi, P., Bosotti, R., De Ponti, C., Pulci, R., et al. (2016). Entrectinib, a Pan-TRK. Mol. Cancer Ther. 15, 628–639. doi: 10.1158/1535-7163.MCT-15-0758
Arulananda, S., Do, H., Musafer, A., Mitchell, P., Dobrovic, A., and John, T. (2017). Combination osimertinib and gefitinib in C797S and T790M EGFR-mutated non-small cell lung cancer. J. Thorac. Oncol. 12, 1728–1732. doi: 10.1016/j.jtho.2017.08.006
Awad, M. M., Oxnard, G. R., Jackman, D. M., Savukoski, D. O., Hall, D., Shivdasani, P., et al. (2016). MET exon 14 mutations in non-small-cell lung cancer are associated with advanced age and stage-dependent MET genomic amplification and c-Met overexpression. J. Clin. Oncol. 34, 721–730. doi: 10.1200/JCO.2015.63.4600
Basit, S., Ashraf, Z., Lee, K., and Latif, M. (2017). First macrocyclic 3(rd)-generation ALK inhibitor for treatment of ALK/ROS1 cancer: clinical and designing strategy update of lorlatinib. Eur. J. Med. Chem. 134, 348–356. doi: 10.1016/j.ejmech.2017.04.032
Berger, S., Martens, U. M., and Bochum, S. (2018). Larotrectinib (LOXO-101). Recent Results Cancer Res. 211, 141–151. doi: 10.1007/978-3-319-91442-8_10
Blackhall, F., Ross Camidge, D., Shaw, A. T., Soria, J. C., Solomon, B. J., Mok, T., et al. (2017). Final results of the large-scale multinational trial PROFILE 1005: efficacy and safety of crizotinib in previously treated patients with advanced/metastatic ALK-positive non-small-cell lung cancer. ESMO Open 2:e000219. doi: 10.1136/esmoopen-2017-000219
Bray, F., Ferlay, J., Soerjomataram, I., Siegel, R. L., Torre, L. A., and Jemal, A. (2018). Global cancer statistics 2018: GLOBOCAN estimates of incidence and mortality worldwide for 36 cancers in 185 countries. CA Cancer J. Clin. 68, 394–424. doi: 10.3322/caac.21492
Camidge, D. R., Kim, H. R., Ahn, M. J., Yang, J. C.-H., Han, J. Y., Lee, J.-S., et al. (2018). Brigatinib versus crizotinib in ALK-positive non-small-cell lung cancer. N. Engl. J. Med. 379, 2027–2039. doi: 10.1056/NEJMoa1810171
Cancer Genome Atlas Research Network (2014). Comprehensive molecular profiling of lung adenocarcinoma. Nature 511, 543–550. doi: 10.1038/nature13385
Cappuzzo, F., Janne, P. A., Skokan, M., Finocchiaro, G., Rossi, E., Ligorio, C., et al. (2009). MET increased gene copy number and primary resistance to gefitinib therapy in non-small-cell lung cancer patients. Ann. Oncol. 20, 298–304. doi: 10.1093/annonc/mdn635
Carbone, D. P., Reck, M., Paz-Ares, L., Creelan, B., Horn, L., Steins, M., et al. (2017). First-line nivolumab in stage IV or recurrent non-small-cell lung cancer. N. Engl. J. Med. 376, 2415–2426. doi: 10.1056/NEJMoa1613493
Crystal, A. S., and Shaw, A. T. (2011). New targets in advanced NSCLC: EML4-ALK. Clin. Adv. Hematol. Oncol. 9, 207–214.
Chen, W. Q., Li, H., Sun, K. X., Zheng, R. S., Zhang, S. W., Zeng, H. M., et al. (2014). Report of cancer incidence and Mortality in China. Zhonghua Zhong Liu Za Zhi 40, 5–13.
Colle, R., Cohen, R., Cochereau, D., Duval, A., Lascols, O., Lopez-Trabada, D., et al. (2017). Immunotherapy and patients treated for cancer with microsatellite instability. Bull. Cancer 104, 42–51. doi: 10.1016/j.bulcan.2016.11.006
Domagala, P., Hybiak, J., Sulzyc-Bielicka, V., Cybulski, C., Ryś, J., and Domagała, W. (2012). KRAS mutation testing in colorectal cancer as an example of the pathologist’s role in personalized targeted therapy: a practical approach. Pol. J. Pathol. 63, 145–164. doi: 10.5114/pjp.2012.31499
Dong, J., Li, B., Zhou, Q., and Huang, D. (2018). Advances in evidence-based medicine for immunotherapy of non-small cell lung cancer. J. Evid. Based Med. 11, 278–287. doi: 10.1111/jebm.12322
Dong, X., Fernandez-Salas, E., Li, E., and Wang, S. (2016). Elucidation of resistance mechanisms to second-generation ALK inhibitors alectinib and ceritinib in non-small cell lung cancer cells. Neoplasia 18, 162–171. doi: 10.1016/j.neo.2016.02.001
Drilon, A., Siena, S., Ou, S. I., Patel, M., Ahn, M. J., Lee, J., et al. (2017). Safety and antitumor activity of the multitargeted pan-TRK, ROS1, and ALK inhibitor entrectinib: combined results from two phase I trials (ALKA-372-001 and STARTRK-1). Cancer Discov. 7, 400–409. doi: 10.1158/2159-8290.CD-16-1237
Dunn, G. P., Bruce, A. T., Ikeda, H., Old, L. J., and Schreiber, R. D. (2002). Cancer immunoediting: from immunosurveillance to tumor escape. Nat. Immunol. 3, 991–998. doi: 10.1038/ni1102-991
Duruisseaux, M., Mc Leer-Florin, A., Moro-Sibilot, D., and Cadranel, J. (2017). Are ALK rearrangement variants promising predictive biomarker of ALK tyrosine kinase inhibitors efficacy? Ann. Oncol. 28:1401. doi: 10.1093/annonc/mdx116
Engelman, J. A., Zejnullahu, K., Mitsudomi, T., Song, Y., Hyland, C., Park, J. O., et al. (2007). MET amplification leads to gefitinib resistance in lung cancer by activating ERBB3 signaling. Science 316, 1039–1043. doi: 10.1126/science.1141478
Gainor, J. F., Dardaei, L., Yoda, S., Friboulet, L., Leshchiner, I., Katayama, R., et al. (2016). Molecular mechanisms of resistance to first- and second-generation ALK inhibitors in ALK-rearranged lung cancer. Cancer Discov. 6, 1118–1133. doi: 10.1158/2159-8290.CD-16-0596
Gandara, D. R., Paul, S. M., Kowanetz, M., Schleifman, E., Zou, W., Li, Y., et al. (2018). Blood-based tumor mutational burden as a predictor of clinical benefit in non-small-cell lung cancer patients treated with atezolizumab. Nat. Med. 24, 1441–1448. doi: 10.1038/s41591-018-0134-3
Gherardi, E., Birchmeier, W., Birchmeier, C., and Vande Woude, G. (2012). Targeting MET in cancer: rationale and progress. Nat. Rev. Cancer 12, 89–103. doi: 10.1038/nrc3205
Goldberg, M. E., Montesion, M., Young, L., Suh, J., Greenbowe, J., Kennedy, M., et al. (2018). Multiple configurations of EGFR exon 20 resistance mutations after first- and third-generation EGFR TKI treatment affect treatment options in NSCLC. PLoS One 13:e0208097. doi: 10.1371/journal.pone.0208097
Haanen, J. B., and Robert, C. (2015). Immune checkpoint inhibitors. Prog. Tumor Res. 42, 55–66. doi: 10.1159/000437178
Han, B., Tjulandin, S., Hagiwara, K., Normanno, N., Wulandari, L., Laktionov, K., et al. (2017). EGFR mutation prevalence in Asia-Pacific and Russian patients with advanced NSCLC of adenocarcinoma and non-adenocarcinoma histology: the IGNITE study. Lung Cancer 113, 37–44. doi: 10.1016/j.lungcan.2017.08.021
Hellmann, M. D., Ciuleanu, T. E., Pluzanski, A., Lee, J. S., Otterson, G. A., Valette, C. A., et al. (2018). Nivolumab plus ipilimumab in lung cancer with a high tumor mutational burden. N. Engl. J. Med. 378, 2093–2104. doi: 10.1056/NEJMoa1801946
Hempelmann, J. A., Lockwood, C. M., Konnick, E. Q., Schweizer, M. T., Antonarakis, E. S., Lotan, T. L., et al. (2018). Microsatellite instability in prostate cancer by PCR or next-generation sequencing. J. Immunother. Cancer 6:29. doi: 10.1186/s40425-018-0341-y
Herbst, R. S., Morgensztern, D., and Boshoff, C. (2018). The biology and management of non-small cell lung cancer. Nature 553, 446–454.
Hyman, D. M., Puzanov, I., Subbiah, V., Faris, J. E., Chau, I., Blay, J.-Y., et al. (2015). Vemurafenib in multiple nonmelanoma cancers with BRAF V600 mutations. N. Engl. J. Med. 373, 726–736. doi: 10.1056/NEJMoa1502309
Jemal, A., Bray, F., Center, M. M., Ferlay, J., Ward, E., and Forman, D. (2011). Global cancer statistics. CA Cancer J. Clin. 61, 69–90. doi: 10.3322/caac.20107
Jun, H. J., Johnson, H., Bronson, R. T., de Feraudy, S., White, F., and Charest, A. (2012). The oncogenic lung cancer fusion kinase CD74-ROS activates a novel invasiveness pathway through E-Syt1 phosphorylation. Cancer Res. 72, 3764–3774. doi: 10.1158/0008-5472.CAN-11-3990
Katayama, R., Kobayashi, Y., Friboulet, L., Lockerman, E. L., Koike, S., Shaw, A. T., et al. (2015). Cabozantinib overcomes crizotinib resistance in ROS1 fusion-positive cancer. Clin. Cancer Res. 21, 166–174. doi: 10.1158/1078-0432.CCR-14-1385
Kato, S., Goodman, A., Walavalkar, V., Barkauskas, D. A., Sharabi, A., and Kurzrock, R. (2017). Hyperprogressors after immunotherapy: analysis of genomic alterations associated with accelerated growth rate. Clin. Cancer Res. 23, 4242–4250. doi: 10.1158/1078-0432.CCR-16-3133
Khan, M., Lin, J., Liao, G., Tian, Y., Liang, Y., Li, R., et al. (2018). ALK Inhibitors in the Treatment of ALK Positive NSCLC. Front. Oncol. 8:557. doi: 10.3389/fonc.2018.00557
Kinoshita, K., Asoh, K., Furuichi, N., Ito, T., Kawada, H., Hara, S., et al. (2012). Design and synthesis of a highly selective, orally active and potent anaplastic lymphoma kinase inhibitor (CH5424802). Bioorg. Med. Chem. 20, 1271–1280. doi: 10.1016/j.bmc.2011.12.021
Ko, Y. S., Kim, N. Y., and Pyo, J. S. (2018). Concordance analysis between HER2 immunohistochemistry and in situ hybridization in non-small cell lung cancer. Int. J. Biol. Markers 33, 49–54. doi: 10.5301/ijbm.5000271
Kobayashi, S., Boggon, T. J., Dayaram, T., Jänne, P. A., Kocher, O., Meyerson, M., et al. (2005). EGFR mutation and resistance of non-small-cell lung cancer to gefitinib. N. Engl. J. Med. 352, 786–792. doi: 10.1056/NEJMoa044238
Kris, M. G., Natale, R. B., Herbst, R. S., Lynch, T. J. Jr., Prager, D., Belani, C. P., et al. (2003). Efficacy of gefitinib, an inhibitor of the epidermal growth factor receptor tyrosine kinase, in symptomatic patients with non-small cell lung cancer: a randomized trial. JAMA 290, 2149–2158. doi: 10.1001/jama.290.16.2149
Le, D. T., Uram, J. N., Wang, H., Bartlett, B. R., Kemberling, H., Eyring, A. D., et al. (2015). PD-1 blockade in tumors with mismatch-repair deficiency. N. Engl. J. Med. 372, 2509–2520. doi: 10.1056/NEJMoa1500596
Leonetti, A., Facchinetti, F., Rossi, G., Minari, R., Conti, A., Friboulet, L., et al. (2018). BRAF in non-small cell lung cancer (NSCLC): pickaxing another brick in the wall. Cancer Treat. Rev. 66, 82–94. doi: 10.1016/j.ctrv.2018.04.006
Li, H. D., and Liu, S. L. (2018). Molecular targeted therapy for non-small cell lung cancer: the reality in China and coping strategy. Prac. J. Med. Pharm. 35, 373–379. doi: 10.14172/j.issn1671-4008.2018.04.030
Liu, Y., Li, Y., Qu, Q., Wu, X., Wang, X., Shao, Y. W., et al. (2017). Acquired EGFR L718V mutation mediates resistance to osimertinib in non-small cell lung cancer but retains sensitivity to afatinib. Lung Cancer 118, 1–5. doi: 10.1016/j.lungcan.2018.01.015
Maemondo, M., Inoue, A., Kobayashi, K., Sugawara, S., Oizumi, S., Isobe, H., et al. (2010). Gefitinib or chemotherapy for non-small-cell lung cancer with mutated EGFR. N. Engl. J. Med. 362, 2380–2388. doi: 10.1056/NEJMoa0909530
McCarthy, A. J., Capo-Chichi, J. M., Spence, T., Grenier, S., Stockley, T., Kamel-Reid, S., et al. (2018). Heterogenous loss of mismatch repair (MMR) protein expression: a challenge for immunohistochemical interpretation and microsatellite instability (MSI) evaluation. J. Pathol. Clin. Res. doi: 10.1002/cjp2.120 [Epub ahead of print].
Mellman, I., Coukos, G., and Dranoff, G. (2011). Cancer immunotherapy comes of age. Nature 480, 480–489. doi: 10.1038/nature10673
Mitsudomi, T., Morita, S., Yatabe, Y., Negoro, S., Okamoto, I., Tsurutani, J., et al. (2010). Gefitinib versus cisplatin plus docetaxel in patients with non-small-cell lung cancer harbouring mutations of the epidermal growth factor receptor (WJTOG3405): an open label, randomised phase 3 trial. Lancet Oncol. 11, 121–128. doi: 10.1016/S1470-2045(09)70364-X
Mok, T. S., Wu, Y. L., Ahn, M. J., Garassino, M. C., Kim, H. R., Ramalingam, S. R., et al. (2017). Osimertinib or platinum-pemetrexed in EGFR T790M-positive lung cancer. N. Engl. J. Med. 376, 629–640. doi: 10.1056/NEJMoa1612674
Mok, T. S., Wu, Y. L., Thongprasert, S., Yang, C. H., Chu, D. T., Saijo, N., et al. (2009). Gefitinib or carboplatin-paclitaxel in pulmonary adenocarcinoma. N. Engl. J. Med. 361, 947–957. doi: 10.1056/NEJMoa0810699
Nagasaka, M., and Gadgeel, S. M. (2018). Role of chemotherapy and targeted therapy in early-stage non-small cell lung cancer. Expert Rev. Anticancer Ther. 18, 63–70. doi: 10.1080/14737140.2018.1409624
Naldini, L., Vigna, E., Narsimhan, R. P., Gaudino, G., Zarnegar, R., Michalopoulos, G. K., et al. (1991). Hepatocyte growth factor (HGF) stimulates the tyrosine kinase activity of the receptor encoded by the proto-oncogene c-MET. Oncogene 6, 501–504.
Nie, Y. L., Liu, K. X., Mao, X. Y., Li, Y. L., Li, J., and Zhang, M. M. (2012). Effect of injection of brucea javanica oil emulsion plus chemoradiotherapy for lung cancer: a review of clinical evidence. J. Evid. Based Med. 5, 216–225. doi: 10.1111/jebm.12001
Oxnard, G. R., Arcila, M. E., Sima, C. S., Riely, G. J., Chmielecki, J., Kris, M. G., et al. (2011). Acquired resistance to EGFR tyrosine kinase inhibitors in EGFR-mutant lung cancer: distinct natural history of patients with tumors harboring the T790M mutation. Clin. Cancer Res. 17, 1616–1622. doi: 10.1158/1078-0432.CCR-10-2692
Oxnard, G. R., Thress, K. S., Alden, R. S., Lawrance, R., Paweletz, C. P., Cantarini, M., et al. (2016). Association between plasma genotyping and outcomes of treatment with osimertinib (AZD9291) in advanced non-small-cell lung cancer. J. Clin. Oncol. 34, 3375–3382. doi: 10.1200/JCO.2016.66.7162
Paik, J., and Dhillon, S. (2018). Alectinib: a review in advanced, ALK-Positive NSCLC. Drugs 78, 1247–1257. doi: 10.1007/s40265-018-0952-0
Peters, S., Camidge, D. R., Shaw, A. T., Gadgeel, S., Ahn, J. S., Kim, D. W., et al. (2017). Alectinib versus crizotinib in untreated ALK-positive non-small-cell lung cancer. N. Engl. J. Med. 377, 829–838. doi: 10.1056/NEJMoa1704795
Planchard, D., Besse, B., Groen, H. J. M., Mazieres, J., Besse, B., Helland, Å., et al. (2016a). Dabrafenib plus trametinib in patients with previously treated BRAF(V600E)-mutant metastatic non-small cell lung cancer: an open-label, multicentre phase 2 trial. Lancet Oncol. 17, 984–993. doi: 10.1016/S1470-2045(16)30146-2
Planchard, D., Kim, T. M., Mazieres, J., Quoix, E., Riely, G., Barlesi, F., et al. (2016b). Dabrafenibin patients with BRAF(V600E)-positive advanced non-small-cell lung cancer: a single-arm, multicentre, open-label, phase 2 trial. Lancet Oncol. 17, 642–650. doi: 10.1016/S1470-2045(16)00077-2
Planchard, D., Smit, E. F., Groen, H. J. M., Mazieres, J., Besse, B., Helland, Å., et al. (2017). Dabrafenib plus trametinib in patients with previously untreated BRAF(V600E)-mutant metastatic non-small-cell lung cancer: an open-label, phase 2 trial. Lancet Oncol. 18, 1307–1316. doi: 10.1016/S1470-2045(17)30679-4
Ricciuti, B., Brambilla, M., Metro, G., Baglivo, S., Matocci, R., Pirro, M., et al. (2017). Targeting NTRK fusion in non-small cell lung cancer: rationale and clinical evidence. Med. Oncol. 34:105. doi: 10.1007/s12032-017-0967-5
Salipante, S. J., Scroggins, S. M., Hampel, H. L., Turner, E. H., and Pritchard, C. C. (2014). Microsatellite instability detection by next generation sequencing. Clin. Chem. 60, 1192–1199. doi: 10.1373/clinchem.2014.223677
Sequist, L. V., Waltman, B. A., Dias-Santagata, D., Digumarthy, S., Turke, A. B., Fidias, P., et al. (2011). Genotypic and histological evolution of lung cancers acquiring resistance to EGFR inhibitors. Sci. Transl. Med. 3:75ra26. doi: 10.1126/scitranslmed.3002003
Shaw, A. T., Felip, E., Bauer, T. M., Besse, B., Navarro, A., Postel-Vinay, S., et al. (2017). Lorlatinib in non-small-cell lung cancer with ALK or ROS1 rearrangement: an international, multicentre, open-label, single-arm first-in-man phase 1 trial. Lancet Oncol. 18, 1590–1599. doi: 10.1016/S1470-2045(17)30680-0
Shaw, A. T., Ou, S. H., Bang, Y. J., Camidge, R., Solomon, B. J., Salgia, R., et al. (2014). Crizotinib in ROS1-rearranged non-small-cell lung cancer. N. Engl. J. Med. 371, 1963–1971. doi: 10.1056/NEJMoa1406766
Siegel, R. L., Miller, K. D., and Jemal, A. (2017). Cancer Statistics, 2017. CA Cancer J. Clin. 67, 7–30. doi: 10.3322/caac.21387
Smit, E. F. (2017). How should “RET positive” NSCLC be treated? Lung Cancer 108, 250–251. doi: 10.1016/j.lungcan.2017.02.006
Soria, J. C., Ohe, Y., Vansteenkiste, J., Reungwetwattana, T., Chewaskulyong, B., Lee, K. H., et al. (2018). Osimertinib in untreated EGFR-mutated advanced non-small-cell lung cancer. N. Engl. J. Med. 378, 113–125. doi: 10.1056/NEJMoa1713137
Soria, J. C., Tan, D. S. W., Chiari, R., Wu, Y. L., Paz-Ares, L., Wolf, J., et al. (2017). First-line ceritinib versus platinum-based chemotherapy in advanced ALK-rearranged non-small-cell lung cancer (ASCEND-4): a randomised, open-label, phase 3 study. Lancet 389, 917–929. doi: 10.1016/S0140-6736(17)30123-X
Tan, D. S., Yom, S. S., Tsao, M. S., Pass, H. I., Kelly, K., Peled, N., et al. (2016). The international association for the study of lung cancer consensus statement on optimizing management of EGFR mutation-positive non-small cell lung cancer: status in 2016. J. Thorac. Oncol. 11, 946–963. doi: 10.1016/j.jtho.2016.05.008
Torigoe, H., Shien, K., Takeda, T., Yoshioka, T., Namba, K., Sato, H., et al. (2018). Therapeutic strategies for afatinib-resistant lung cancer harboring HER2 alterations. Cancer Sci. 109, 1493–1502. doi: 10.1111/cas.13571
Uchibori, K., Inase, N., Araki, M., Kamada, M., Sato, S., Okuno, Y., et al. (2017). Brigatinib combined with anti-EGFR antibody overcomes osimertinib resistance in EGFR-mutated non-small-cell lung cancer. Nat. Commun. 8:14768. doi: 10.1038/ncomms14768
Wen, M., Wang, X., Sun, Y., Xia, J., Fan, L., Xing, H., et al. (2016). Detection of EML4-ALK fusion gene and features associated with EGFR mutations in Chinese patients with non-small-cell lung cancer. Onco Targets Ther. 9, 1989–1995. doi: 10.2147/OTT.S100303
Yang, J. C., Sequist, L. V., Geater, S. L., Tsai, C. M., Mok, T. S., Schuler, M., et al. (2015). Clinical activity of afatinib in patients with advanced non-small-cell lung cancer harbouring uncommon EGFR mutations: a combined post-hoc analysis of LUX-Lung 2, LUX-Lung 3, and LUX-Lung 6. Lancet Oncol. 16, 830–838. doi: 10.1016/S1470-2045(15)00026-1
Yoshida, T., Oya, Y., Tanaka, K., Shimizu, J., Horio, Y., Kuroda, H., et al. (2016). Differential crizotinib response duration among ALK fusion variants in ALK-positive non-small-cell lung cancer. J. Clin. Oncol. 34, 3383–3389. doi: 10.1200/JCO.2015.65.8732
Yu, H. A., Arcila, M. E., Rekhtman, N., Sima, C. S., Zakowski, M. F., Pao, W., et al. (2013). Analysis of tumor specimens at the time of acquired resistance to EGFR-TKI therapy in 155 patients with EGFR-mutant lung cancers. Clin. Cancer Res. 19, 2240–2247. doi: 10.1158/1078-0432.CCR-12-2246
Zhang, J., Zhao, T., Long, S., Liu, X., and Yu, H. (2017a). Risk factors for postoperative infection in Chinese lung cancer patients: a meta-analysis. J. Evid. Based Med. 10, 255–262. doi: 10.1111/jebm.12276
Zhang, J., Zhao, T., Xu, C., Huang, J., and Yu, H. (2017b). Genetic susceptibility of lung cancer in Chinese population: an overview of systematic reviews and meta-analyses. J. Evid. Based Med. 10, 207–211. doi: 10.1111/jebm.12269
Zheng, D., Hu, M., Bai, Y., Zhu, X., Lu, X., Wu, C., et al. (2017). EGFR G796D mutation mediates resistance to osimertinib. Oncotarget 8, 49671–49679. doi: 10.18632/oncotarget.17913
Keywords: NSCLC, targeted therapy, immunotherapy, EGFR, PD-L1, ALK, ROS1
Citation: Dong J, Li B, Lin D, Zhou Q and Huang D (2019) Advances in Targeted Therapy and Immunotherapy for Non-small Cell Lung Cancer Based on Accurate Molecular Typing. Front. Pharmacol. 10:230. doi: 10.3389/fphar.2019.00230
Received: 28 December 2018; Accepted: 22 February 2019;
Published: 12 March 2019.
Edited by:
Shuang Zhou, University of Houston, United StatesReviewed by:
Pooja Dhupkar, University of Texas MD Anderson Cancer Center, United StatesLu Zhao, Zhejiang University, China
Copyright © 2019 Dong, Li, Lin, Zhou and Huang. This is an open-access article distributed under the terms of the Creative Commons Attribution License (CC BY). The use, distribution or reproduction in other forums is permitted, provided the original author(s) and the copyright owner(s) are credited and that the original publication in this journal is cited, in accordance with accepted academic practice. No use, distribution or reproduction is permitted which does not comply with these terms.
*Correspondence: Qinghua Zhou, emhvdXFoMTM1QDE2My5jb20=