- 1Shenzhen Hospital, Beijing University of Chinese Medicine, Shenzhen, China
- 2School of Life Sciences, Beijing University of Chinese Medicine, Beijing, China
Rubus chingii Hu (R. chingii), referred to as “Fu-Pen-Zi” in Chinese, has great medicinal and dietary values since ancient times. The dried fruits of R. chingii have been widely used in traditional Chinese medicine (TCM) for the treatment of kidney enuresis and urinary frequency for centuries. According to current findings, R. chingii has been reported to contain a variety of chemical constituents, mostly triterpenoids, diterpenoids, flavonoids, and organic acids. These compounds have been demonstrated to be the major bioactive components responsible for pharmacological effects such as anticomplementary, anticancer, antioxidant, antimicrobial, and anti-inflammatory functions. Therefore, this review focused on the up-to-date published data of the literature about R. chingii and comprehensively summarized its phytochemistry, pharmacology, quality control, and toxicity to provide a beneficial support to its further investigations and applications in medicines and foods.
Introduction
The genus Rubus, belonging to the Rosaceae family, has edible and economically important fruits and is widely distributed throughout the Northern Hemisphere (Moreno-Medina et al., 2018). This genus consists of over 700 species, about 194 of which occur in China, including R. chingii, R. idaeus, R. rosifolius, R. parvifolius, and so on (Li et al., 2015). Among them, R. chingii is an important functional food with the fruits known as “Fu-Pen-Zi” in Chinese. It is mainly cultivated in East China, especially in Jiangxi province, Anhui province, Jiangsu province, Zhejiang province, and Fujian province. Due to its rich nutritional and medicinal value, R. chingii has been frequently used in traditional Chinese medicine (TCM) for centuries (Liu and Niu, 2014). The medical properties of R. chingii have been mentioned in many landmark Chinese medical monographs, such as “Compendium of Materia Medica,” “Bencao Mengquan,” “Leigong Paozhi Lun,” and “Qianjin Yi Fang.” According to the theory of traditional Chinese herbal medical science, R. chingii is commonly used as a tonic for the treatment of enuresis, kidney deficiency, impotence and prospermia, frequency of micturition, spermatorrhea, and other illnesses (Xie et al., 2013a).
Since the universal uses of R. chingii in folk medicines, a great deal of studies concerning the chemical constituents and pharmacological activities of this medicinal plant have been carried out, which gave rise to numerous interesting and attractive results. Many in vitro and in vivo investigations have indicated that the extracts and the ingredients isolated from R. chingii possess abundant pharmacological effects, such as anticomplementary, anticancer, antioxidant, antimicrobial, anti-aging and anti-inflammatory activities (Shi, 2017). These marvelous biological functions of this herb can be attributed to the presence of a broad spectrum of phytochemical constituents including triterpenoids, diterpenoids, flavonoids, organic acids, and many other compounds.
Although some brief reviews about the chemical constituents and biological activities have been conducted, these papers were written in Chinese and not studied in a systematic manner. This paper strives for a comprehensive overview of the latest information on the phytochemistry, biological activities, quality control, as well as the toxicity of this herb. More importantly, the correlation between the biological properties and the existence of the bioactive chemical components responsible for the actions has also been discussed based on the published literatures. Finally, the major achievements and shortcomings, together with the possible tendency and perspective for future food and pharmacological research of this herb, have been put forward, too. We believe that this review will highlight the significance of R. chingii and indicate new research directions of this species.
Phytochemical Constituents of R. chingii
So far, more than 235 chemical constituents have been isolated and identified from R. chingii (Table 1). These compounds include 15 triterpenoids, 15 diterpenoids, 18 flavonoids, 7 alkaloids, 95 volatile compounds, 5 coumarins, 9 steroids, 56 organic acids, and 15 other compounds. Among them, triterpenoids and diterpenoids have been identified as the characteristic components.
Triterpenoids
Triterpenoids are the major chemical compounds present in R. chingii. They are mainly pentacyclic triterpenoids or thereof derivatives, with oleanane-type and ursane-type skeletons (Figure 1). The first study of triterpenes identified in R. chingii dates back to the 1980s, when Masao et al. reported the isolation of a new diosphenol-type triterpene named fupenzic acid (1) (Hattori et al., 1988). In another work (Guo, 2005), the fruits of R. chingii were extracted with methanol. Further fractionation of the methanol extract led to the isolation of five oleanane-type triterpene acids [oleanic acid (2), maslinic acid (3), arjunic acid (4), 2α, 3α, 19α-trihydroxyolean-12-ene-28-oic-acid (5), and sericic acid (6)] together with four ursane-type triterpene acids [ursolic acid (7), 2α-hydroxyursolic acid (8), euscaphic acid (9), and hyptatic acid (10)]. Moreover, Cheng et al. found that the roots of this plant were rich in triterpenoids. They obtained three triterpene acids, namely, ursolic acid (7), euscaphic acid (9), and 11α-hydroxyeuscaphic acid (11) from this plant part (Cheng, 2008). In further studies, Chai et al. obtained 2α,19α,24-trihydroxyurs-12-ene-3-oxo-28-acid (12) and tormentic acid (13) from the 95% ethanol extract of R. chingii fruit (Chai, 2008). Lately, investigation of the 80% ethanol extract of the fruits of R. chingii yielded nigaichigoside F1 (14) and 2α,19α-dihydroxy-3-oxo-12-ursen-28-oic acid (15) (Xiao et al., 2011).
Diterpenoids
Diterpenoids are also characterized as the representative ingredients of R. chingii. Currently, 15 diterpenoids (Figure 2), including 2 kaurane-type diterpenoids and 13 labdane-type diterpenoids, have been identified in R. chingii. Rubusoside(16) was the first diterpenoid isolated from the methanol extract of the leaves of R. chingii in 1981 (Tanaka et al., 1981), and subsequent investigations have led to the isolation of five additional labdane-type diterpene glucosides (Goshonoside-F1-F5, 17–21) (Tanaka et al., 1984). Furthermore, another two labdane-type diterpene glucosides, namely, goshonoside-F6(22) and goshonoside-F7(23), were reported to be obtained from both the leaves and fruits of R. chingii (Wang, 1991). In 2013, a new ent-labdane diterpene saponin, named goshonoside-G(24), was separated from the 70% ethanol extract of R. chingii unripe fruit, and its structure was determined based on NMR spectroscopic studies and mass spectrometry data (Sun et al., 2013b). Later, from the ethyl acetate extract of R. chingii fruit, Guo (2015) isolated five labdane-type diterpene glycosides that were elucidated as ent-Labda-8(17),13E-diene-3β,15,18-triol(25), ent-Labda-8(17),13E-diene-3α,15,18-triol(26), 15,18-di-O-β-D-glucopyranosyl-13(E)-ent-labda-7(8),13(14)-diene-3β,15,18-triol(27), 15,18-di-O-β-D-glucopyranosyl-13(E)-ent-labda-8(9),13(14)-diene-3β,15,18-triol(28), and 15-O-β-D-apiofuranosyl-(1→2)β-D-glucopranosyl-18-O-β-D-glucopyranosyl-13(E)-ent-labda-8(9),13(14)-diene-3β,15,18-triol(29). More recently, Zhang et al. (2017b) found a kaurane-type diterpenoid called ent-16α,17-dihydroxy-kauran-19-oic acid(30) from fruits of R. chingii by bio-guided isolation.
Flavonoids
Flavonoids, occurring naturally in dietary and medicinal plants (Azietaku et al., 2017), are important polyphenol constituents with various pharmacological effects (Cai et al., 2018). The main types of flavonoids found in R. chingii were kaempferol, quercetin, and their derivatives. To date, a total of 18 flavonoids have been reported mainly from the fruits of R. chingii. Guo et al. isolated six compounds: kaempferol(31), quercetin(32), tiliroside(33), astragalin(34), quercetin-3-O-β-D-glucopyranoside(35), and kaempferol-3-O-β-D-glucuronic acid methyl ester(36) (Guo, 2005). In the same year, Liu (2005) obtained kaempferol-7-O-α-L-rhamnoside(37) and 2″-O-Galloyl-hyperin(38). Then, by using a series of chromatographic and spectrum technologies, Cheng (2008) isolated and identified aromadedrin(39), quercitrin(40), hyperoside(41), and cis-tiliroside(42) in 2008. Furthermore, investigation of the 80% ethanol extract of the dried fruits of R. chingii yielded phlorizin(43) (Xiao et al., 2011). Lately, kaempferol-3-O-hexoside(44), quercetin-3-O-glucuronide(45), and kaempferol-3-O-glucuronide(46) were identified in the fruits of R. chingii by high-performance liquid chromatography (HPLC) coupled with linear ion trap-OrbiTrap hybrid mass spectrometer (Li et al., 2018). In addition, kaempferol-3-O-β-D-rutinoside(47) (He et al., 2013) and rutin(48) (Zhang et al., 2017a) were also found in this plant. Their structures are shown in Figure 3.
Alkaloids
Alkaloids represent a relatively small class of compounds in R. chingii. Only seven of this class of compounds have been isolated from R. chingii (Figure 4), with skeletons of the quinoline, isoquinoline, and indole types. In 2008, Chai (2008) reported that from the 95% and 50% ethanol extract of the fruits of R. chingii, three alkaloids were isolated and identified as 4-hydroxy-2-oxo-1,2,3,4-terahydroquinoline-4-carboxylic acid(49), methyl 1-oxo-1, 2-dihydroisoquinoline-4-carboxylate(50), and 1-oxo-1, 2-dihydroisoquinoline-4-carboxylic acid(51). In 2011, guiding with 1,1-diphenyl-2-picrylhydrazyl (DPPH) free radical scavenging activity, another four alkaloids, including rubusine(52), methyl (3-hydroxy-2-oxo-2,3-dihydroindol-3-yl)-acetate(53), methyldioxindole-3-acetate(54), and 2-oxo-1,2-dihydroquinoline-4-carboxylic acid(55), were isolated from the ethanol extract of the same plant part (Ding, 2011).
Volatile Constituents
Volatile compounds (Figure 5) comprise an important part of R. chingii (Pi and Wu, 2003; Dian et al., 2005; Han et al., 2014; Zhang and Jiang, 2015). Han et al. (2014) investigated the volatile constituents from the leaves of R. chingii by employing head-space gas chromatography–mass spectrometry (GC/MS) and identified 37 constituents, mainly including hexadecanoic acid (44.97%), tetradecanoic acid (10.88%), and acetic acid (4.13%). In another study conducted in 2015, a total of 58 volatile compounds were identified from the unripe fruits of R. chingii using GC/MS (Zhang and Jiang, 2015). According to their structures, these volatile compounds could be divided into eight chemical groups: saturated hydrocarbons (9 compounds), unsaturated hydrocarbons (10 compounds), alcohols (9 compounds), carbonyl compounds (2 compounds), esters (11 compounds), organic acids (7 compounds), oxides and epoxides (8 compounds), and others (2 compounds).
Coumarins
Coumarins are phenolic compounds characterized by a benzene ring attached to a pyrone ring. They have a fragrant smell and exist throughout the plant kingdom (Azietaku et al., 2017). To date, limited studies have been performed to investigate the coumarins in R. chingii and only five coumarins have been isolated, including two simple coumarins and three furocoumarins (Figure 6). Liu (2005) isolated and identified esculetin(151), esculin(152), and imperatorin(153) from the 70% ethanol extract of the fruits of R. chingii by various chromatographic methods. You reported the isolation and structure elucidation of a new furocoumarins, 3,5,9-trihydroxy-7,8-dihydrocyclopenta[g]chromene-2,6-dione(154), which they named Fu-Pen-Zi-Su (You, 2009) or rubusin A (Sun et al., 2011), from the n-butanol extract of the fruits of R. chingii. Recently, phytochemical analysis of R. chingii afforded a new chromone called rubusin B(155), which was confirmed according to the 1D and 2D NMR data and MS data (Liang et al., 2015).
Steroids
Phytosterols are a class of physiologically active compounds extensively used in cosmetics, foods, and medicines. In R. chingii, steroids are relatively rare, and only nine steroidal metabolites have been reported and characterized (Figure 7). In 2005, three steroids, namely, β-sitosterol(156), daucosterol(157), and stigmast-4-ene-(3β,6α)-diol(158) (Guo, 2005), were found to exist in methanol extract of the fruits of R. chingii. Moreover, β-sitosterol(156) and daucosterol (157) were isolated from the roots of R. chingii by Cheng in 2008 (Cheng, 2008). In further studies, another steroid called stigmast-5-en-3-ol,oleate(159) was obtained from the methylene chloride extract of R. chingii fruit (You, 2009). Other steroidal compounds that were isolated from this plant were β-stigmasterol(160) (Xiao, 2011), 7α-hydroxy-β-sitosterol(161) (Du et al., 2014), and sitosterol palmitate (162) (Liu et al., 2014). In addition, campesterol(163) and γ-sitosterol(164) were tentatively elucidated by GC/MS (Zhang and Jiang, 2015).
Organic Acids
Organic acids are a class of carboxyl-group-containing compounds that could be found in numerous plants worldwide. R. chingii extracts contain a high percentage of organic acids. A total of 56 organic acids, including 23 phenolic acids (165–187), 20 fatty acids (188–207), 4 tannins (208–211), and 9 other compounds (212–220) have been reported mainly from the fruits of R. chingii (Pi and Wu, 2003; Lim et al., 2004; Dian et al., 2005; Guo, 2005; Liu, 2005; Xie et al., 2005; Chai, 2008; Cheng, 2008; You, 2009; You et al., 2009; Xiao et al., 2011; Han et al., 2013; Sun et al., 2013a; Xie et al., 2013b; Du et al., 2014; Han et al., 2014; Liu et al., 2014; Zhang, 2014; Guo, 2015; Zhang and Jiang, 2015; Chai et al., 2016; Li et al., 2018). Detailed information of these organic acid compounds is shown in Table 1 (165–220) and Figure 8.
Other Compounds
In addition to these compounds mentioned above, a range of other compounds have also been isolated from R. chingii. Detailed information of these compounds is shown in Table 1 (221–235) and Figure 9 (Guo, 2005; Guo et al., 2007; Chai, 2008; Cheng, 2008; You, 2009; Kong et al., 2011; Xiao et al., 2011; Sun et al., 2013a; Xie et al., 2013b; Du et al., 2014; Zhang and Jiang, 2015).
Pharmacological Activities of R. chingii
As a well-known medicinal plant in TCM, the fruits and leaves of R. chingii are widely used for the treatment of various diseases. The major pharmacological properties such as anticomplementary, anticancer, antioxidant, antimicrobial, anti-inflammatory, anti-hypotensive, anti-aging, antithrombotic, antidiabetic, neuroprotective, and anti-osteoporosis activities of this herbaceous medicine are summarized in Table 2, and the details will be further discussed below.
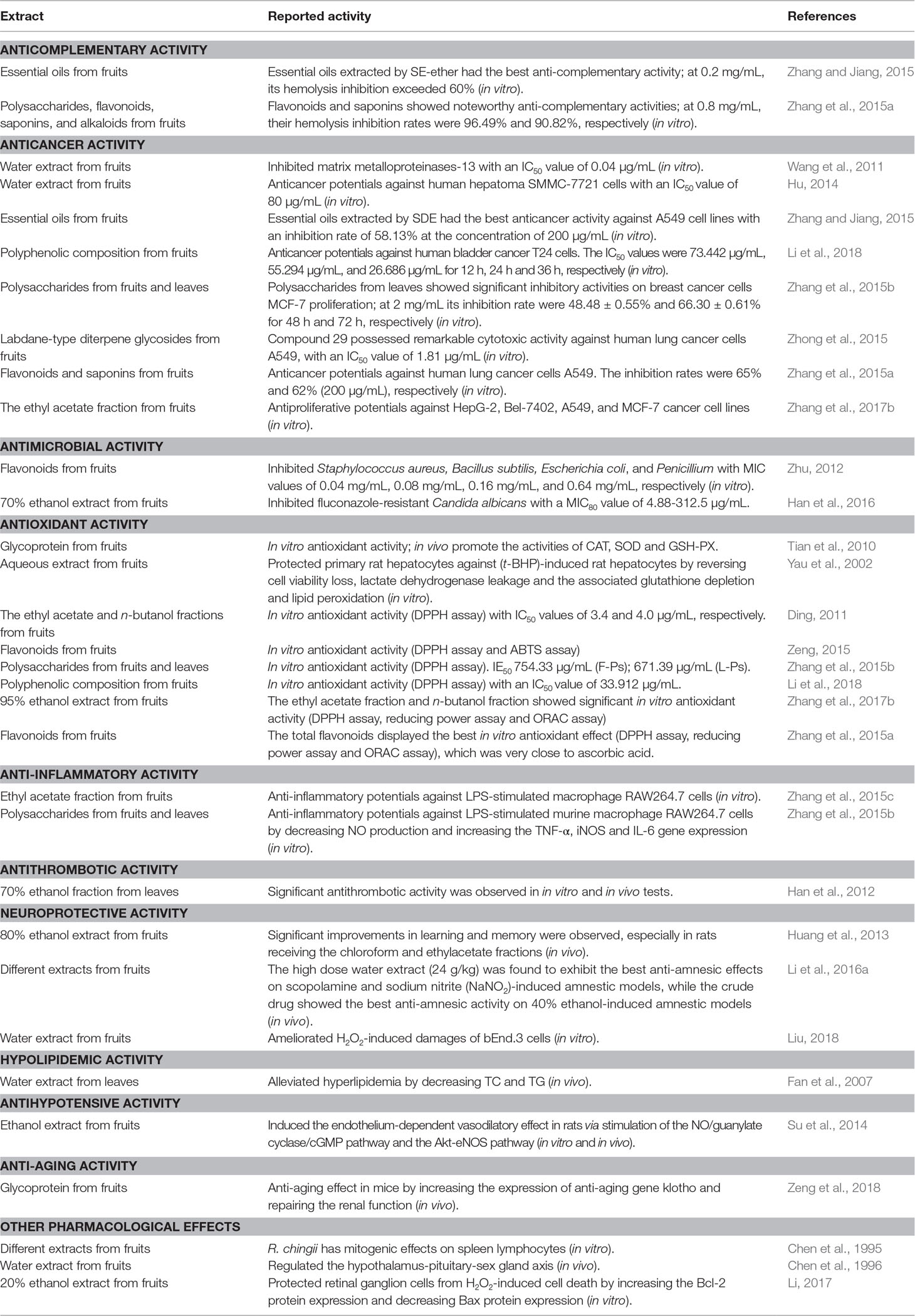
Table 2 Reported biological activities in vitro and in vivo of R. chingii crude extracts and fractions.
Anticomplementary Activity
Several studies demonstrated that the extracts of R. chingii possess anticomplementary activity. Zhang and Jiang employed a complement fixation test to assess the in vitro anticomplementary activity of the essential oils from fruits of R. chingii by three different extraction methods [steam distillation extraction (SDE), soxhlet extraction (SE) with ethanol, and SE with ether]. The results showed that the essential oils obtained by SE-ether had the strongest anticomplementary effect, even stronger than heparin (control) (Zhang and Jiang, 2015). The flavonoids and saponins extracted from R. chingii also showed noteworthy anticomplementary activities when compared to its polysaccharides and alkaloids. The hemolysis inhibition rates of the flavonoids and saponins were 96.49% and 90.82% (at the concentration of 0.8 mg/ml), respectively, which were even higher than heparin sodium (Zhang et al., 2015a).
Anticancer Activity
The antitumor effects of the various extracts of R. chingii have been extensively investigated through a large number of in vivo and in vitro experiments. Wang et al. (2011) found that the water extract of R. chingii could inhibit the activities of matrix metalloproteinases-13 with an IC50 value (half maximal inhibitory concentration) of 0.04 μg/ml. The results suggested that this herbal medicine may be used for the treatment of cancer. Another study showed that the water extract of R. chingii gave rise to a dose-dependent antiproliferative effect on hepatocellular carcinoma cells with an IC50 value of 80 μg/ml (Hu, 2014). Anticancer activity was also reported for the essential oils from the unripe fruits of R. chingii by in vitro MTT cytotoxicity assay against A549 cell lines. The results showed that the essential oils extracted by SDE exhibited stronger activity than SE-ethanol, which may be due to the extract obtained by SDE, which had a higher content of unsaturated fatty acids (Zhang and Jiang, 2015). An in vitro study showed that polyphenolic composition in the fruits of R. chingii could inhibit the proliferation and induce apoptosis of human bladder cancer T24 cells remarkably in a dose-dependent and time-response manner. The IC50 values were 73.442, 55.294, and 26.686 μg/ml for 12, 24, and 36 h, respectively (Li et al., 2018). In a similar study, Zhang et al. (2015b) evaluated the anticancer activity of the polysaccharides from R. chingii via MTT assay and found that inhibitory activities on breast cancer cells’ MCF-7 and liver cancer cells’ Bel-7402 proliferation were also concentration- and time-dependent. From 70% ethanol extract of the fruits of R. chingii, Zhong et al. (2015) isolated three new labdane-type diterpene glycosides and in vitro tests of these compounds for anticancer activity showed that compound 29 possessed remarkable cytotoxic activity against A549 (human lung cancer cell line), with an IC50 value of 1.81 μg/ml (2.32 μM). Furthermore, tiliroside, a representative flavonoid isolated from R. chingii, induced the apoptosis of A549 cells in a dose-dependent manner, with an IC50 value of 113.41 ± 1.89 μg/ml (190.76 ± 3.18 μM) (Zhang et al., 2015a). In 2017, Zhang et al. (2017b) investigated the antiproliferative ingredients in the fruits of R. chingii by using bio-assay guided isolation, and found that tormentic acid possessed notable cytotoxicity activities against HepG-2, Bel-7402, A549, and MCF-7 cancer cell lines with the IC50 values of 40.57, 54.22, 62.36, and 24.23 μg/ml, respectively. All these results described above suggest that R. chingii has an exact effect on prevention of cancer. However, a common mechanism about the exact cellular and molecular targets needs to be fully elucidated and the diversity of extracts makes data interpretation difficult.
Antimicrobial Activity
Antimicrobial activity, an important effect of R. chingii, had been comprehensively studied. A moderate antibacterial activity was evident for the flavonoids from R. chingii against Staphylococcus aureus, Bacillus subtilis, Escherichia coli, and Penicillium with MIC (minimum inhibitory concentration) values of 0.04, 0.08, 0.16, and 0.64 mg/ml, respectively. However, it could not inhibit the growth of Saccharomyces cerevisiae, Rhizopus, and Mucor (Zhu, 2012). In addition, R. chingii extract combined with fluconazole displayed synergistic antifungal activity on fluconazole-resistant Candida albicans with an MIC80 (the lowest concentration to inhibit 80% of fungal growth) value of 0.0625–16 μg/ml for fluconazole and 4.88–312.5 μg/ml for the 70% ethanol extract of R. chingii (Han et al., 2016).
Antioxidant Activity
Oxidative stress by free radicals is a significant event in the cell, which is associated with a wide range of human degenerative diseases (Bi et al., 2016). The glycoprotein from R. chingii showed significant in vitro antioxidant activity via free radical scavenging assay and reducing power assays. An in-depth in vivo study revealed that the glycoprotein could significantly increase the activities of catalase (CAT), superoxide dismutase (SOD), and glutathione peroxidase (GSH-PX) in serum, liver, and brain tissues of rats, which also confirmed the strong reducing power of the glycoprotein (Tian et al., 2010). The aqueous extract of R. chingii has also been reported to reverse tert-butyl hydroperoxide (t-BHP)-induced oxidative damage in rat hepatocytes by inhibiting lactate dehydrogenase leakage, lipid peroxidation, and the associated glutathione depletion (Yau et al., 2002). Moreover, among nine compounds isolated from the fruits of R. chingii, methyl (3-hydroxy-2-oxo-2,3-dihydroindol-3-yl)-acetate, vanillic acid, kaempferol, and tiliroside displayed antioxidative capacity. Their IC50 values were 45.2, 34.9, 78.5, and 13.7 μM, respectively (ascorbic acid, 131.8 μM) (Ding, 2011). Zeng et al. studied the in vitro antioxidant capacities of the total flavonoid contents of R. chingii by the 1,1-diphenyl-2-picrylhydrazyl (DPPH) and 2,2’-azino-bis 3-ethylbenzothiazoline-6-sulphonic acid (ABTS) methods. The results showed that the total flavonoid content exhibited a significant correlation with antioxidant activity in the DPPH assay (r 2 = 0.758, ρ = 0.004) and the ABTS assay (r 2 = 0.788, ρ = 0.002) (Zeng et al., 2015). Zhang et al. (2015b) studied the activities of polysaccharides from R. chingii fruit (F-Ps) and leaf (L-Ps) through DPPH scavenging assay and found that the scavenging activities of F-Ps and L-Ps had almost 10 folds lower antioxidant potential than the vitamin C with half inhibition effect (IE50) values of 754.33 and 671.39 μg/ml, respectively. Similarly, the polyphenolic composition in the fruits of R. chingii exhibited high DPPH scavenging effect with an IC50 value of 33.912 μg/ml, which was half of the standard ascorbic acid (Li et al., 2018). In 2017, an interesting study investigated the antioxidant effects of fruits of R. chingii by using the DPPH assay, reducing power assay and oxygen radical absorbance capacity (ORAC) assay, and the results revealed that the ethyl acetate fraction and n-butanol fraction were found to be the most potent (Zhang et al., 2017b). The polysaccharides, flavonoids, saponins, and alkaloids extracted from R. chingii were also assessed for their antioxidant activity through the same methods. The results indicated that total flavonoids displayed the best antioxidant effect, which was very close to ascorbic acid (Zhang et al., 2015a). From the results mentioned above, we can conclude that the strong antioxidant activity of R. chingii might be predominantly related to the presence of the glycoproteins and phenolic compounds, especially flavonoids. Additionally, it is worthy to note that the in vitro experiments used to test total antioxidant are not specific and prone to interferences, which may give unreliable results. Therefore, further in vivo studies are needed to validate these results.
Anti-Inflammatory Activity
Sun et al. (2013b) extracted a new compound called goshonoside-G from the fruits of R. chingii. This compound possessed notable inhibitory effect on NO production in LPS-stimulated macrophage RAW264.7 cells with an IC50 value of 54.98 μg/ml. In bio-assay guided fractionation of the ethanol extract of R. chingii, which provided the best anti-inflammatory effect, tiliroside, astragalin, hyperoside, quercitrin, and kaempferol 3-rutinoside were isolated. Among the flavonoid glycosides, tiliroside possessed the strongest inhibitory effect on NO production in LPS-stimulated macrophage RAW 264.7 cells with the inhibitory rate of 30.4% at a concentration of 100 μg/ml, which was very close to that of dexamethasone at a concentration of 50 μg/ml. Western blot and RT-PCR showed that the underlying mechanism of the suppression of inflammatory reactions by tiliroside may be due to its modulation of a signaling mitogen-activated protein kinase (MAPK) and pro-inflammatory cytokines activities (Zhang et al., 2015c). In addition, the polysaccharides from leaves and fruits induced a dose-dependent (2–400 μg/ml) inhibition of the nitric oxide (NO) production in murine macrophage RAW 264.7 cells through suppressing the TNF-α, iNOS, and IL-6 gene expression (Zhang et al., 2015b). Therefore, flavonoid glycosides and polysaccharides along with goshonoside-G of the plant could be considered as potential anti-inflammatory agents.
Antithrombotic Activity
The 70% ethanol fraction from an aqueous extract of R. chingii leaves was found to treat thrombosis through inhibiting the aggregation of blood platelets using activity tests carried out in vitro and in vivo. The bio-guided isolation of the extract yielded six compounds (salicylic acid, kaempferol, quercetin, tiliroside, quercetin 3-O-β-D-glucopyranoside, and kaempferol 3-O-β-D-glucopyranoside). Their anticoagulant activities were examined using plasma recalcification time (PRT) test. It is noteworthy that kaempferol, quercetin, and tiliroside obviously delayed PRT in blood at a concentration of 2 mg/ml, while salicylic acid, quercetin 3-O-β-D-glucopyranoside, and kaempferol 3-O-β-D-glucopyranoside demonstrated the weakest effect in the in vitro experiment (Han et al., 2012).
Neuroprotective Activity
Huang et al. investigated whether or not R. chingii was involved in attenuating learning and memory deficits on a classical model of Kidney Yang Deficiency Syndrome (KDS-Yang) in Alzheimer’s disease rats induced by D-galactose combined with hydrocortisone. Morris water maze tests demonstrated significant improvements in learning and memory, especially in rats receiving the chloroform and ethylacetate fractions of R. chingii (Huang et al., 2013). The major mechanism may be that R. chingii could protect neurons in rat hippocampal CA1 region by increasing choline acyltransferase (ChAT) activity but decreasing acetylcholinesterase (AChE) activity and Tau protein expression. The possible memory-enhancing effects of different extracts of R. chingii on amnesic rats induced by scopolamine, sodium nitrite, and 40% ethanol were also studied by assessing a Morris water maze test. The results showed that the high-dose water extract (24 g/kg) exhibited the best anti-amnesic effects on scopolamine and sodium nitrite (NaNO2)-induced amnestic models, while the crude drug showed the best anti-amnesic activity on 40% ethanol-induced amnestic models (Li et al., 2016a). Moreover, Liu et al. (2018) demonstrated that the water extract of R. chingii could ameliorate H2O2-induced damages of brain microvascular endothelial cells (bEnd.3 cells) via regulating the expression of apoptosis-related proteins. In addition, two flavonoids (kaempferol and quercetin) isolated from R. chingii were investigated for neuroprotective activity. It was observed that at 80 μmol/L concentration, both compounds significantly inhibited the decrease of cell viability (MTT reduction), prevented membrane damage (LDH release), scavenged ROS formation, and attenuated the decrease of malondialdehyde (MDA) in H2O2-induced PC12 cells (Zhao et al., 2018). These abovementioned results of preclinical investigations show that R. chingii may be a promising herbal medicine to combat nerve injury.
Antidiabetic Activity and Hypolipidemic Activity
Xie et al. reported antihyperglycemic effects of raspberry ketone in the alloxan-induced diabetic rat model, which were beneficial for the treatment of diabetes. The study showed that raspberry ketone reduced the level of the blood glucose, protected the normal physiological function of pancreatic β cells, and stimulated insulin secretion by effectively inhibiting the oxidative stress (Xie et al., 2012). Another study showed that raspberry ketone could significantly promote glucose uptake in HepG2 cells by increasing the IRS-1 protein expression and decreasing SHP-1 mRNA gene expression (Xie et al., 2014).
The hypolipidemic activity of the leaves from R. chingii was evaluated in the hyperlipidemia rats induced by a high-fat diet and adults with hyperlipidemia. The results revealed that treatment with raspberry leaves exhibited significant hypolipidemic effect, indicated by reduced level of serum total cholesterol (TC) and triacylglycerols (TGs). Therefore, it suggested that raspberry leaves could be further explored as a therapy for the treatment of hyperlipidemia diseases (Fan et al., 2007).
Anti-Osteoporotic Activity
Liang et al. (2015) isolated a novel compound, rubusin B, and six known compounds from the fruits of R. chingii, and an in vitro study showed that rubusin B, kaempferol, rubusin A, and quercetin exhibited anti-osteoporotic activities with different characteristics. Quercetin and kaempferol had a direct stimulatory effect on alkaline phosphatase (ALP) activity and bone formation, while rubusin A and B could effectively attenuate osteoclastic resorption even at a very low concentration (0.01 ppm).
Antihypotensive Activity
Recently, it was shown that the ethanol extract of R. chingii could induce the endothelium-dependent vasodilatory effect in rats, via stimulation of the NO/guanylate cyclase/cGMP pathway and the Akt-eNOS pathway (Su et al., 2014).
Anti-Aging Activity
A novel glycoprotein isolated from R. chingii exhibited notable anti-aging effect in the D-galactose-induced aging mice model by increasing the expression of anti-aging gene klotho and repairing the renal function (Zeng et al., 2018).
Other Pharmacological Effects
In addition to the bio-activities mentioned above, some other pharmacological effects of R. chingii and its constituents were also reported. Chen et al. (1995) demonstrated that R. chingii has mitogenic effects on spleen lymphocytes. They also found that R. chingii could regulate the hypothalamus–pituitary–sex gland axis (Chen et al., 1996). Li (2017) reported that R. chingii could protect retinal ganglion cells from H2O2-induced cell death by increasing the Bcl-2 protein expression and decreasing Bax protein expression.
Toxicity
Limited data are available concerning the safety assessments of R. chingii. In an acute toxicity test, the dose of the water extract of R. chingii leaves used in mice was 20 g/kg/day, and it did not induce any toxicity sign or death in 2 weeks (Tang et al., 2007). The potential adverse effects of R. chingii leaves were also determined by a repeated dose oral toxicity study, which was conducted on Wistar rats administered for 90 days at oral dosages of 2.5, 5, and 10 g/kg. The researchers found no significant differences between groups in body weights, food consumption, blood biochemistry, organ weights, gross pathology, and histopathology. Further study indicated that R. chingii leaves had no mutagenic or genotoxic effect using the Ames test, bone marrow micronucleus test, and sperm aberration test (Tang et al., 2007). Based on the results described above, we can conclude that R. chingii leaves are not toxic and hence reliably safe for use for pharmacological purposes. However, more in-depth investigations are still needed to explore the toxicity of the fruits of R. chingii to human health.
Quality Control
It is well known that the inherent quality of herb medicine may vary significantly in different geographical conditions and different harvest times (Zhang et al., 2018). In the Chinese Pharmacopoeia (2015), the contents of ellagic acid and kaempferol-3-O-rutinoside in R. chingii should not be less than 0.2% and 0.03%, respectively (Chinese Pharmacopoeia Commission, 2015). It is extensively accepted that the multiple components of TCM are responsible for their curative effects by exerting their synergistic effects on multiple targets and levels (Li et al., 2016b). Thus, relying only on the two components for quality control seems insufficient to determine the strengths and weaknesses of R. chingii. With the advancement of analytical tools, the multi-component determination has been extensively used for comprehensive quality assessment of R. chingii. A total of 21 compounds: tiliroside (Chai et al., 2009), kaempferol (Xie et al., 2015; Ping et al., 2016), gallic acid (Li and Tan, 2008), ellagic acid, quercetin-3-O-β-D-glucopyranoside, kaempferol-3-O-rutinoside, goshonoside-F5 (Han et al., 2013), rutin (Zhang et al., 2017a), hyperoside (Chen et al., 1996), astragalin (Zhong et al., 2014; Ma et al., 2017), quercetin (Cheng et al., 2012), maslinic acid, 2α-hydroxyursolic acid, oleanic acid (Cao et al., 2017), ursolic acid, arjunic acid, 2α,3α,19α-trihydroxy-12-oleanen-28-oic acid, euscaphic acid (Guo et al., 2005), adenosine, brevifolin carboxylic acid, and ethyl gallate (Chai et al., 2016), have been quantified by HPLC or CE by different research groups (Chen et al., 2006). The volatile constituents such as hexadecanoic acid, tetradecanoic acid, and acetic acid were detected by GC/MS (Han et al., 2014; Zhang and Jiang, 2015). In addition, a pharmacokinetic study was carried out to determine quercetin-3-O-β-D-glucopyranoside, kaempferol-3-O-rutinoside, and tiliroside in rat plasma after oral administration of R. chingii to rats (Zan et al., 2018). However, there is still no unified method for quality control and fingerprinting of R. chingii. The quantitative analysis of R. chingii is listed in Table 3.
Conclusion and Future Perspectives
R. chingii is a nutritive plant commonly used as a functional food and medicine in China. It has been applied in clinical practice successfully for centuries to tonify the kidney, control nocturnal emissions, and reduce urination (Han et al., 2012). Although chemical compositions and biological activities of this medical plant are well documented, more conclusive studies are still needed to fill certain specific gaps in R. chingii science.
Firstly, and particularly, it is noteworthy that most pharmacological studies on R. chingii have only been conducted in animal models, cell models, and other in vitro experiments. Therefore, comprehensive placebo-controlled and double-blind clinical trials should be undertaken in the future to provide remarkable evidence for these positive findings on the efficacy of R. chingii. Besides, some of the pharmacological studies were carried out at too high doses that could hardly be translated to clinical practice and more in-depth investigations are needed to standardize the best dosage for these claimed bioactivities of R. chingii in ethnomedicine. In addition, the exact mechanisms of many medicinal properties of this herb still remain vague to date; thus, additional studies to better identify the functions and molecular targets seem to be necessary.
Secondly, most pharmacological activities were measured using uncharacterized crude extracts of R. chingii, and this makes it hard to reproduce the results of these investigations and elucidate the link between activity and particular compounds. Additionally, most of these phytochemicals were isolated from the fruits, and the chemical composition of other parts of this plant was largely unknown. Therefore, in-depth phytochemical investigations of all parts of R. chingii based on bio-guided isolation strategies are still needed, which may lead to the expansion of existing therapeutic potential of this miracle herb.
Thirdly, toxicological studies are important to understand the safety profile of herbal drugs, but data on toxicological aspects of R. chingii remain unexplored. The only toxicological study about R. chingii was conducted in the leaf extract, which revealed its non-toxic nature. Hence, to ensure a full utilization of the medicinal resource, further relative systematic toxicity and safety evaluation studies were quite considerable and necessary, especially in fruit extract and other effective extracts, to meet the Western standards of evidence-based medicine.
Fourthly, pharmacokinetic studies involving R. chingii are very limited and only focus on a few biological active substances present in R. chingii, which do not fully reflect the pharmacokinetic properties of this herb medicine. Thus, further investigations should be carried out to assess the absorption, distribution, metabolism, and excretion of the crude extracts of this plant in vivo. Additionally, metabolic studies of single isolated compounds in R. chingii should be strengthened, which could provide a scientific basis for clarifying the major metabolic route and action mechanism and defining the bio-active components responsible for the curative effects. Meanwhile, the identification of unknown metabolites may contribute to the drug discovery and development process.
Lastly, and importantly, because of the complex composition of TCM, quality control of TCM is a great challenge and has become a key factor to restrict its modernization process. Thus, setting up an effective and standardized quality control method of R. chingii is indispensable and emergent, which is crucial for ensuring the safety and efficacy of this medicinal product. In addition, good plant practice ought to be enforced to fulfill quantity and quality requirements for R. chingii.
Author Contributions
GY and ZL searched the literature, collected the data, and drafted the manuscript. GY and WW contributed to analysis and manuscript preparation. YL and YZ helped check the chemical structures and formula. YS provided comments on the manuscript. All authors read and approved the final manuscript.
Funding
This study was supported by the Start-up fund from Beijing University of Chinese Medicine to YS (No. 1000061020044 and No. 1000041510052).
Conflict of Interest Statement
The authors declare that the research was conducted in the absence of any commercial or financial relationships that could be construed as a potential conflict of interest.
Acknowledgments
We acknowledge Beijing University of Chinese Medicine for providing support and assistance for this review article.
References
Azietaku, J. T., Ma, H., Yu, X. A., Li, J., Oppong, M. B., Cao, J., et al. (2017). A review of the ethnopharmacology, phytochemistry and pharmacology of Notopterygium incisum. J. Ethnopharmacol. 202, 241–255. doi: 10.1016/j.jep.2017.03.022
Bi, W., Gao, Y., Shen, J., He, C., Liu, H., Peng, Y., et al. (2016). Traditional uses, phytochemistry, and pharmacology of the genus Acer (maple): a review. J. Ethnopharmacol. 189, 31–60. doi: 10.1016/j.jep.2016.04.021
Cai, Y. Q., Hu, J. H., Qin, J., Sun, T., Li, X. L. (2018). Rhododendron Molle(Ericaceae): phytochemistry, pharmacology, and toxicology. Chin. J. Nat. Med. 16, 401–410. doi: 10.1016/S1875-5364(18)30073-6
Cao, F., Qiu, X. X., Zhang, L., Wang, Y. Y. (2017). Determination of three triterpene acids in Rubi Fructus by reverse-phase high-performance liquid chromatography. J. Anhui. Univ. Chin. Med. 36, 79–82. doi: 10.3969/j.issn.2095-7246.2017.04.023
Chai, W. (2008). Studies on the chemical constituents and quality standard of Rubus chingii Hu. Ph.D Thesis. Beijing, China: China Academy of Chinese Medical Science.
Chai, W., Wang, Z. J., Tang, L. Y., Fu, M. H. (2009). Quantitive determination of tiliroside from Rubus chingii Hu by HPLC. Chin. J. Chin. Mater. Med. 34, 2534–2535. doi: 10.3321/j.issn:1001-5302.2009.19.027
Chai, X., Du, L. F., Yang, J., Jiang, Z. Z., Yang, L., Wang, Y. F., et al. (2016). Simultaneous determination of eight constituents in fruits of Rubus chingii by UPLC. Chin. Herbal Med. 8, 280–285. doi: 10.1016/S1674-6384(16)60051-5
Chen, K. H., Fang, J., Gong, B., Mo, Q. Z., Quan, H. B., Sun, W. P. (1995). The enhancing effect of Rubus chingii Hu on the lymphocyte proliferation and its relationship with cyclic nucleotides. Shanghai J. Immunol. 15, 302–304.
Chen, K. H., Fang, J., Kuang, X. W., Mo, Q. Z. (1996). Effects of the fruit of Rubus chingii Hu on hypothalamus–pituitary–sex gland axis in rats. Chin. J. Chin. Mater. Med. 21, 560–562.
Chen, L. L., Pan, J., Zhao, L. H., Yang, L. Y. (2006). HPLC fingerprint of Rubus chingii Hu. Chin. Trad. Patent Med. 28, 937–940. doi: 10.3969/j.issn.1001-1528.2006.07.001
Cheng, K. J. (2008). Studies on the bioactive constituents of Rubus chingii II. Studies on the stability of stilbenoids from the roots of Caragana sinica. Ph.D Thesis. Shanghai China: Fudan University.
Cheng, L. Z., Zhang, L., Xie, X. M. (2012). Simultaneous determination of three flavonoids in fructus rubi by ultra performance liquid chromatography. J. Anhui TCM Coll. 31, 75–77. doi: 10.3969/j.issn.1000-2219.2012.05.028
Chinese Pharmacopoeia Commission. (2015). Pharmacopoeia of People’s Republic of China Part I. Beijing, China: People’s Medical Publishing House, 382.
Dian, L. H., Gong, X. L., Cai, C., Zhang, L. J. (2005). Analysis of volatile oils in Rubus chingii Hu by GC-MS. Tianjin Pharma. 17, 9–10.
Ding, H. Y. (2011). Extracts and constituents of Rubus chingii with 1,1-diphenyl-2-picrylhydrazyl (DPPH) free radical scavenging activity. Int. J. Mol. Sci. 12, 3941–3949. doi: 10.3390/ijms12063941
Du, L. F., Li, J., Yang, L., He, Y., Chai, X., Zhang, P., et al. (2014). Chemical constituents from the fruits of Rubus chingii Hu. Nat. Prod. Res. Dev. 26, 1957–1960. doi: 10.16333/j.1001-6880.2014.12.012
Fan, B. L., Gong, C. R., Sun, F. Z., Wang, H. M., Wang, Y. E., Tian, J., et al. (2007). Effects of Hubei Rubus chingii Hu leaf on serum lipid metabolism in hyperlipidemia rats and human adults. Food Sci. 28, 526–529. doi: 10.1016/S1872-2075(07)60055-7
Guo, Q. (2015). Studies on the chemical constituents and quality control standard of Rubus chingii Hu. Master’s Thesis. Nanchang, China: Jiangxi University of Chinese Medicine.
Guo, Q. L. (2005). Studies on the chemical constituents of Rubus chingii and inula cappa. Ph.D Thesis. Beijing, China: Peking Union Medical College.
Guo, Q. L., Gao, J. Y., Yang, J. S. (2005). Analysis of bioactive triterpenes from Rubus chingii by cyclodextrin-modified capillary electrophoresis. Chromatographia 62, 145–150. doi: 10.1365/s10337-005-0591-y
Guo, Q. L., Yang, J. S., Liu, J. X. (2007). Studies on chemical constituents in fruits of Rubus chingii. Chin. Pharm. J. 42, 1141–1143.
Han, B., Chen, J., Yu, Y. Q., Cao, Y. B., Jiang, Y. Y. (2016). Antifungal activity of Rubus chingii extract combined with fluconazole against fluconazole-resistant Candida albicans. Microbiol. Immunol. 60, 82–92. doi: 10.1111/1348-0421.12357
Han, N., Gu, Y. H., Ye, C., Cao, Y., Liu, Z., Yin, J. (2012). Antithrombotic activity of fractions and components obtained from raspberry leaves (Rubus chingii). Food Chem. 132, 181–185. doi: 10.1016/j.foodchem.2011.10.051
Han, Z., Chen, X. Y., Li, Y. H., Sun, H. J. (2014). Analysis of the volatile constituents from the leaves of Rubus chingii Hu by HS-GC/MS. Med. Plant 5, 31–33.
Han, Z., Yan, C. X., Li, Y. H., Sun, H. J. (2013). Analysis of volatile constituents of raspberry leaf using HS-GC/MS. Food Mach. 29, 11–13. doi: 10.3969/j.issn.1003-5788.2013.04.004
Hattori, M., Kuo, K. P., Shu, Y. Z., Tezuka, Y., Kikuchi, T., Namba, T. (1988). A triterpene from the fruits of Rubus chingii. Phytochemistry 27, 3975–3976. doi: 10.1016/0031-9422(88)83061-9
He, J. M., Sun, N., Wu, W. D., Fan, L. J., Guo, M. L. (2013). Determination of ellagic acid, flavonoids and goshonoside-F5 in Rubi Fructus by HPLC. Chin. J. Chin. Mater. Med. 38, 4351–4356. doi: 10.4268/cjcmm20132431
Hu, Y. L. (2014). The research of the inhibition on hepatoma cell line SMMC-7721 by raspberry extract. Master’s Thesis. Jinan, China: Shandong University of Chinese Medicine.
Huang, L. P., Xiong, Y. J., Zhao, M. L., Gong, J. H., Xie, Y. H. (2013). The impact of raspberry different extract parts on kidney-YANG deficiency AD rats’ learning and memory abilities. Pharmacol. Clin. Chin. Mater. Med. 29, 111–113. doi: 10.13412/j.cnki.zyyl.2013.04.018
Kong, D. P., Qian, D. W., Guo, S., Duan, J. A. (2011). Determination of nucleosides compounds in nine tonic traditional Chinese medicines of fruit and seeds. Chin. J. Exp. Trad. Med. Form. 17, 98–101. doi: 10.3969/j.issn.1005-9903.2011.04.030
Li, G. (2017). Study on the protective effect of raspberry on retinal ganglion cells (RGCs). Master’s Thesis. Shenyang, China: Liaoning University of Traditional Chinese Medicine.
Li, J., Du, L. F., He, Y., Yang, L., Li, Y. Y., Wang, Y. F., et al. (2015). Chemical constituents and biological activities of plants from the genus Rubus. Chem. Biodivers. 12, 1809–1847. doi: 10.1002/cbdv.201400307
Li, K., Xia, X. X., Ding, Y. H., Zhou, W. Y., Xie, B. S., Cai, J. B., et al. (2016a). Effect of different extract parts from Rubi Fructus on improving memory disorder in mice. Chin. J. Exp. Trad. Med. Form. 22, 142–147. doi: 10.13422/j.cnki.syfjx.2016020142
Li, K. Y., Zeng, M. L., Li, Q. L., Zhou, B. H. (2018). Identification of polyphenolic composition in the fruits of Rubus chingii Hu and its antioxidant and antiproliferative activity on human bladder cancer T24 cells. J. Food Meas. Charact. 13 (1), 51–60. doi: 10.1007/s11694-018-9918-x
Li, T. A., Tan, X. Y. (2008). Quantitative determination of gallic acid in Fructus Rubi by HPLC. Chin. J. Mod. Appl. Pharm. 25, 235–237. doi: 10.3969/j.issn.1007-7693.2008.03.023
Li, X., Zhao, J., Liu, J. X., Li, G., Zhao, Y., Zeng, X. (2016b). Systematic analysis of absorbed anti-inflammatory constituents and metabolites of sarcandra glabrain rat plasma using ultra-high-pressure liquid chromatography coupled with linear trap quadrupole orbitrap mass spectrometry. Plos One 11, e0150063. doi: 10.1371/journal.pone.0150063
Liang, W. Q., Xu, G. J., Weng, D., Gao, B., Zheng, X. F., Qian, Y. (2015). Anti-osteoporotic components of Rubus chingii. Chem. Nat. Comp. 51, 47–49. doi: 10.1007/s10600-015-1200-4
Lim, J. D., Yun, S. J., Lee, S. J., Chung, I. M., Kim, M. J., Heo, K., et al. (2004). Comparison of resveratrol contents in medicinal plants. Korean J. Med. Crop Sci. 12, 163–170.
Liu, J. (2005). Study on the chemical constituents of Rubus chingii Hu and its pharmacological effects on renal asthenia yang test of mouse. Master’s Thesis. Shenyang, China: Shenyang Pharmaceutical University.
Liu, M. X., Niu, J. (2014). Advance research on raspberry (Rubus chingii Hu) and resource utilization. Sci. Techn. Vis. 22, 26–27. doi: 10.3969/j.issn.2095-2457.2014.22.015
Liu, W. Q., Gong, J. H., Wang, Y. X., Lian, B., Huang, L. P., Xie, Y. H. (2014). Isolation and identification of chemical constituents from the active site of Rubus chingii Hu. Mod. Chin.Med. 16, 372–373. doi: 10.13313/j.issn.1673-4890.2014.05.006
Liu, X. H. (2018). The effects of raspberry decoction on apoptosis of bEnd.3 cells induced by H2O2 and related clinical application. Master’s Thesis. Nanjing, China: Nanjing University of Chinese Medicine.
Ma, Y. J., Bai, W. T., Zhu, X. F., Huang, L. P., Xie, Y. H. (2017). Simultaneous determination of four flavonoids in Rubus chingii by MIPs-HPLC. Chin. Trad. Patent Med. 39, 2097–2101. doi: 10.3969/j.issn.1001-1528.2017.10.023
Moreno-Medina, B. L., Casierra-Posada, F., Cutler, J. (2018). Phytochemical composition and potential use of Rubus species. Gesunde Pflanzen. 70, 65. doi: 10.1007/s10343-018-0416-1
Pi, H., Wu, J. (2003). Phytochemistry and pharmacology of Rubus chingii Hu: A review. Stud. J. Trad. Chin. Med. 21, 2169–2174. doi: 10.13193/j.archtcm.2003.12.182.pihf.108
Ping, Y. H., Li, C. L., Xie, Y. H. (2016). Determination of tiliroside and kaempferol in Rubus chingii Hu. Food Res. Dev. 37, 139–141. doi: 10.3969/j.issn.1005-6521.2016.07.034
Shi, Y. F. (2017). Raspberry research progress of nutritional ingredients and pharmacological effects. ShanDong. Chem. Ind. 46, 71–72. doi: 10.3969/j.issn.1008-021X.2017.06.025
Su, X. H., Duan, R., Sun, Y. Y., Wen, J. F., Kang, D. G., Lee, H. S., et al. (2014). Cardiovascular effects of ethanol extract of Rubus chingii Hu (Rosaceae) in rats: An in vivo and in vitro approach. J. Physiol. Pharmacol. 65, 417–424.
Sun, J. X., Zhu, H. X., Xiao, D. G. (2013a). Determination of the contents of organic acids of raspberry. Mod. Food Sci. Tech. 29, 1374–1376. doi: 10.13982/j.mfst.1673-9078.2013.06.040
Sun, N., Wang, Y., Liu, Y., Guo, M. L., Yin, J. (2013b). A new ent-labdane diterpene saponin from the fruits of Rubus chingii. Chem. Nat. Comp. 49, 49–53. doi: 10.1007/s10600-013-0503-6
Sun, Z. L., Zhang, Y., Wan, A. H., Zhang, X. L., Feng, J. (2011). A new active compound against kidney deficiency from the fruits of Rubus corchorifolius. J. Asian Nat. Prod. Res. 13, 68–74. doi: 10.1080/10286020.2010.541156
Tanaka, T., Kawamura, K., Kitahara, T., Kohda, H., Tanaka, O. (1984). Ent-labdane-type diterpene glucosides from leaves of Rubus chingii. Phytochemistry 23, 615–621. doi: 10.1016/S0031-9422(00)80393-3
Tanaka, T., Kohda, H., Tanaka, O., Chen, F. H., Chou, W. H., Leu, J. L. (1981). Rubusoside (β-D-glucosyl ester of 13-O-β-D-glucosyl-steviol), a sweet principle of Rubus chingii Hu (Rosaceae). Agric. Biol. Chem. 45, 2165–2166. doi: 10.1271/bbb1961.45.2165
Tang, X. Q., Liu, Y., Sun, F. Z., Fu, S. H., Tian, H., Yang, W. X., et al. (2007). Toxicological evaluation of Hubei R. chingi Hu. Carcin. Teratogen. Mut. 19, 395–398.
Tian, T., Duan, Y. F., Niu, F. G. (2010). Antioxidant effect of raspberry glycoprotein. Food Sci. 31, 357–360.
Wang, Y. C., Li, F., Tao, L. (2011). Inhibiting effect of Rubus chingii Hu extract on matrix metalloproteinase in vitro. Chin. J. Clin. Pharmacol. 27, 291–292. doi: 10.1007/s10008-010-1224-4
Xiao, H. M. (2011). Studies on the chemical constituents from fruits of Rubus chingii. Master’s Thesis. Hefei, China: Anhui University. doi: 10.1007/s10570-010-9464-0
Xiao, H. M., Zu, L. B., Li, S. P., Wang, K. J., Li, N. (2011). Chemical constituents from dried fruits of Rubus chingii. Chin. J. Med. Chem. 21, 220–226.
Xie, X. M., Pang, X. B., Li, X. T. (2012). Hypoglycemic effect and mechanism of raspberry ketone on diabetic model mice. Chin. Pharm. J. 47, 1899–1904.
Xie, X. M., Pang, X. B., Li, X. T. (2014). Effect of raspberry ketone on expression of SHP-1 and IRS-1 in insulin signaling pathway of HepG2 cells. Chin. Trad. Patent Med. 36, 1579–1583. doi: 10.3969/j.issn.1001-1528.2014.08.003
Xie, Y. H., Ding, Y. H., Lian, B., Bin, Y. U., Fan, H. (2015). Quantitative determination of active ingredient kaempferol in Rubus chingii Hu. J. Jiangxi Univ. Trad. Chin. Med. 27, 77–78.
Xie, Y. H., Lian, B., Gong, J. H., Tu, L. D., Zhang, Y. T., Huang, L. P. (2013a). Preparation of magnetic chitosan hyamine microspheres and separation of phenolic acids from Rubus chingii Hu. Adv. Mater. Res. 634–638, 1347–1351 doi: 10.4028/www.scientific.net/AMR.634-638.1347
Xie, Y. H., Miao, J. R., Liu, W. Q. (2005). Studies on the chemical constituents from Fructus Rubi. Zhong Yao Cai 28, 99–100. doi: 10.3321/j.issn:1001-4454.2005.02.012
Xie, Y. H., Zhou, L. J., Luo, J. L., Gong, J. H., Huang, L. P. (2013b). Isolation and structure identification of chemical constituents from Rubus chingii Hu. Lishizhen Med. Mater. Med. Res. 24, 786–787. doi: 10.3969/j.issn.1008-0805.2013.04.008
Yau, M. H., Che, C. T., Liang, S. M., Kong, Y. C., Fong, W. P. (2002). An aqueous extract of Rubus chingii fruits protects primary rat hepatocytes against tert-butyl hydroperoxide induced oxidative stress. Life Sci. 72, 329–338. doi: 10.1016/S0024-3205(02)02239-7
You, M. T. (2009). The active components on kidney-yang deficient mice from the fruits of Rubus chingii. Master’s Thesis. Shanghai, China: Second Military Medical University.
You, M. T., Li, Y. K., Guo, M. L. (2009). Study on chemical constituents of methylene chloride extract of Rubus chingii. Acad. J. Second Mil. Med. Univ. 29, 1199–1202. doi: 10.3724/SP.J.1008.2009.01199
Zan, T., Piao, L., Wei, Y., Gu, Y., Liu, B., Jiang, D. (2018). Simultaneous determination and pharmacokinetic study of three flavonoid glycosides in rat plasma by LC-MS/MS after oral administration of Rubus chingii Hu extract. Biomed. Chromatogra. 32, e4106. doi: 10.1002/bmc.4106
Zeng, H. J., Liu, Z., Wang, Y. P., Yang, D., Yang, R., Qu, L. B. (2018). Studies on the anti-aging activity of a glycoprotein isolated from Fupenzi (Rubus chingii Hu). and its regulation on klotho gene expression in mice kidney. Int. J. Biol. Macromol. 119, 470–476. doi: 10.1016/j.ijbiomac.2018.07.157
Zeng, H. J., Yang, R., Lei, L. F., Wang, Y. P. (2015). Total flavonoid content, the antioxidant capacity, fingerprinting and quantitative analysis of Fupenzi (Rubus chingii Hu). Chinese Med. 6, 204–213. doi: 10.4236/cm.2015.64023
Zhang, H. (2014). Research progress in chemical synthesis of raspberry ketone. Acetaldehyde Acetic Acid Chem. Ind. 16 (9), 12–19.
Zhang, L., Qiu, X. X., Yue, J. Y. (2017a). Simultaneous determination of ellagic acid and five flavonoids of Rubus chingii Hu by HPLC. J. Chin. Med. Mater. 40, 2625–2628. doi: 10.13863/j.issn1001-4454.2017.11.029
Zhang, T. T., Jiang, J. G. (2015). Analyses on essential oil components from the unripe fruits of Rubus chingii Hu by different methods and their comparative cytotoxic and anti-complement activities. Food Anal. Method 8, 937–944. doi: 10.1007/s12161-014-9973-9
Zhang, T. T., Li, Y., Jiang, J. G. (2015a). Bioactive comparison of main components from unripe fruits of Rubus chingii Hu and identification of the effective component. Food Funct. 6, 2205–2214. doi: 10.1039/C5FO00406C
Zhang, T. T., Liu, Y. J., Yang, L., Jiang, J. G., Zhao, J. W., Zhu, W. (2017b). Extraction of antioxidant and antiproliferative ingredients from fruits of Rubus chingii Hu by active tracking guidance. Medchemcomm 8, 1673–1680. doi: 10.1039/C7MD00240H
Zhang, T. T., Lu, C. L., Jiang, J. G., Wang, M., Wang, D. M., Zhu, W. (2015b). Bioactivities and extraction optimization of crude polysaccharides from the fruits and leaves of Rubus chingii Hu. Carbohydr. Polym. 130, 307–315. doi: 10.1016/j.carbpol.2015.05.012
Zhang, T. T., Wang, M., Yang, L., Jiang, J. G., Zhao, J. W., Zhu, W. (2015c). Flavonoid glycosides from Rubus chingii Hu fruits display anti-inflammatory activity through suppressing MAPKs activation in macrophages. J. Funct. Foods 18, 235–243. doi: 10.1016/j.jff.2015.07.006
Zhang, X., Zhan, G., Jin, M., Zhang, H., Dang, J., Zhang, Y., et al. (2018). Botany, traditional use, phytochemistry, pharmacology, quality control, and authentication of Radix Gentianae Macrophyllae—a traditional medicine: A review. Phytomedicine 46, 142–163. doi: 10.1016/j.phymed.2018.04.020
Zhao, M. L., Yan, B., Liu, C., Hou, M., Xie, Y. H., Huang, L. (2018). Different components of raspberry extracted by ethyl acetate could improve the impairments of PC12 induced by H2O2. Pharmacol. Clin. Chin. Mater. Med. 34, 58–62.
Zhong, R. J., Guo, Q., Zhou, G. P., Fu, H. Z. (2014). RP- HPLC simultaneous determination of two main flavonoid glycoside components in Rubus chingii Hu. Chin. J. Pharm. Anal. 34, 971–974. doi: 10.16155/j.0254-1793.2014.06.018
Zhong, R. J., Guo, Q., Zhou, G. P., Fu, H. Z., Wan, K. H. (2015). Three new labdane-type diterpene glycosides from fruits of Rubus chingii and their cytotoxic activities against five humor cell lines. Fitoterapia 102, 23–26. doi: 10.1016/j.fitote.2015.01.007
Keywords: Rubus chingii Hu, phytochemistry, pharmacology, toxicity, quality control
Citation: Yu G, Luo Z, Wang W, Li Y, Zhou Y and Shi Y (2019) Rubus chingii Hu: A Review of the Phytochemistry and Pharmacology. Front. Pharmacol. 10:799. doi: 10.3389/fphar.2019.00799
Received: 03 March 2019; Accepted: 20 June 2019;
Published: 16 July 2019.
Edited by:
Matthias F. Melzig, Freie Universität Berlin, GermanyReviewed by:
Carel Basson Oosthuizen, University of Pretoria, South AfricaHelen Skaltsa, National and Kapodistrian University of Athens, Greece
Monika E. Czerwińska, Medical University of Warsaw, Poland
Copyright © 2019 Yu, Luo, Wang, Li, Zhou and Shi. This is an open-access article distributed under the terms of the Creative Commons Attribution License (CC BY). The use, distribution or reproduction in other forums is permitted, provided the original author(s) and the copyright owner(s) are credited and that the original publication in this journal is cited, in accordance with accepted academic practice. No use, distribution or reproduction is permitted which does not comply with these terms.
*Correspondence: Yuanyuan Shi, eXNoaUBidWNtLmVkdS5jbg==