- Hepatic Surgery Center, Tongji Hospital, Tongji Medical College, Huazhong University of Science and Technology, Clinical Medicine Research Center for Hepatic Surgery of Hubei Province, Key Laboratory of Organ Transplantation, Ministry of Education and Ministry of Public Health, Wuhan, China
Doxorubicin (Dox), an antitumor antibiotic, has therapeutic effects on many kinds of tumors. However, Dox can produce some serious side effects that limit its clinical application. Thus, exploration of effective drug targets or active lead compounds against Dox-induced organ damage is necessary. Dioscin, one natural product, has potent effects against Dox-induced renal injury and cardiotoxicity. However, the effects of dioscin on Dox-induced hepatotoxicity have not been reported. In this study, the results showed that dioscin significantly ameliorated Dox-induced cell injury, reduced reactive oxygen species (ROS) level, and suppressed cell apoptosis in alpha mouse liver 12 (AML-12) cells caused by Dox. In vivo, dioscin evidently decreased the levels of alanine transaminase (ALT), aspartate transaminase (AST), malondialdehyde (MDA); increased the levels of superoxide dismutase (SOD), glutathione (GSH), and glutathione peroxidase (GSH-Px); and alleviated liver injury. Mechanism study showed that dioscin remarkably up-regulated the expression levels of silent information regulator 1 (Sirt1) and heme oxygenase-1 (HO-1) via increase of the nuclear translocation of NF-E2-related factor 2 (Nrf2) and suppressed the expression levels of forkhead box protein O1 (FOXO1) and kelch-like ECH-associated protein-1 (Keap1) to inhibit oxidative stress. Furthermore, dioscin obviously decreased the nuclear translocation of nuclear factor κB (NF-κB) and the mRNA levels of tumor necrosis factor alpha (TNF-α), interleukin 1β (IL-1β), and interleukin 6 (IL-6) to suppress inflammation. Meanwhile, dioscin significantly regulated tumor suppressor P53 (P53) expression level and BCL-2-associated X (BAX)/BCL-2 apoptosis regulator (BCL-2) ratio to inhibit cell apoptosis. These results were further validated by knockdown of Sirt1 using siRNA silencing in AML-12 cells, which confirmed that the target of dioscin against Dox-induced hepatotoxicity was Sirt1/FOXO1/NF-κB signal. In short, our findings showed that dioscin exhibited protective effects against Dox-induced liver damage via suppression of oxidative stress, inflammation, and apoptosis, which should be developed as one new candidate for the prevention of Dox-induced liver injury in the future.
Introduction
Doxorubicin (Dox) is one of the most effective chemotherapeutic drugs for the treatment of lung, gastric, ovarian, breast, thyroid, sarcoma, and pediatric cancers (Cristina et al., 2009; Thorn et al., 2011; Dragojevic-Simic et al., 2013). However, long-term use of Dox can produce serious side effects on non-tumor tissues, and thus, its clinical application is limited (Reddy et al., 2012). There are numerous side effects including hepatotoxicity, nephrotoxicity, and cardiotoxicity, and the toxic effects on the reproductive system and nervous system produced by Dox cannot be reversed (Okunewick et al., 1985; Eugene et al., 2000; Saad et al., 2001; Mihailovic-Stanojevic et al., 2008; Dragojevic-Simic et al., 2013; Roomi et al., 2014; Pugazhendhi et al., 2018). In addition, the metabolites of Dox by hepatic microsomal enzymes and cytoplasmic reductase are adriamycin and hepatotoxic glycosides (Camaggi et al., 1988; Ganey et al., 1988). As the largest metabolic organ in the body, the liver plays critical roles in metabolism, which can also be easily damaged by Dox. Hence, exploration of active lead compounds against Dox-induced liver damage is necessary.
Drug-induced hepatotoxicity may occur through the formation of free radicals and the production of reactive oxygen species (ROS), which can cause oxidative damage to organs (Minotti et al., 2004; Qin et al., 2008; Sassocerri et al., 2010; Aryal et al., 2014). In addition, the activation of inflammatory response has been found in Dox-induced cardiotoxicity, which can be reduced by suppressing nuclear factor κB (NF-κB) (Ashikawa et al., 2004; Austin et al., 2016; Zhang et al., 2019). Some studies have confirmed that Dox-derived ROS can adjust tumor suppressor P53 (P53) signal, release cytochrome c, and activate caspase-3 to promote apoptosis (Mukherjee et al., 2004; Morsi et al., 2006; Paweorn et al., 2015). Therefore, simultaneous inhibition of oxidative stress, inflammation, and apoptosis should be one effective method to treat Dox-induced hepatotoxicity.
Silent information regulator 1 (Sirt1), a NAD+-dependent class III histone deacetylase, has the ability to deacetylate key metabolic players including peroxisome coactivator 1 alpha, which is also a master regulator of oxidative metabolism and one inducer of oxidative stress protection systems such as manganese superoxide dismutase (SOD) and catalase (Danz et al., 2009). Sirt1 can increase cell resistance and survival from stress to protect against Dox-induced oxidative damage and cell death. Overexpression of Sirt1 can protect the heart from oxidative stress through up-regulating the actions of antioxidants (Danz et al., 2009; Yang et al., 2015). Furthermore, previous studies have demonstrated that Sirt1 can suppress NF-κB and adjust P53 signal, culminating in regulating cardiomyocyte inflammation and apoptosis (Han et al., 2008; Yang et al., 2015). Hence, Sirt1 plays critical roles in regulating inflammation, oxidative stress, and apoptosis. However, to the best of our knowledge, there are no mechanistic studies on the roles of Sirt1 in Dox-induced hepatotoxicity.
With the development of modern pharmacology and molecular biology, the potential of natural products and Chinese herbal compounds for the prevention and treatment of human diseases has attracted more and more attention in recent years. Dioscin (shown in Figure 1A), a naturally derived triterpenoid saponin, has potent effects against various cancers (Qi Y. et al., 2016; Si et al., 2016; Zhao et al., 2016; Han et al., 2017; Tao et al., 2017; Xu L. et al., 2019) and protective activities against organ damage (Qi et al., 2015; Qi M. et al., 2016;Qiao et al., 2017; Zheng et al., 2017; Zhu et al., 2017; Han et al., 2018; Hu et al., 2018; Qi et al., 2019), which also has active actions against metabolic diseases including diabetes, hyperuricemia, obesity, and osteoporosis (Yin et al., 2015; Tao et al., 2016; Xu et al., 2017; Tao et al., 2018). Actually, beneficial therapeutic effects of dioscin on acute liver injury through alleviation of oxidative stress and suppression of inflammation have been studied, which can also promote liver regeneration (Gu et al., 2015; Liu et al., 2015; Xu et al., 2015; Zhang et al., 2015; Yao et al., 2016; Zhang et al., 2016; Qi et al., 2017). Also, dioscin shows protective effects against Dox-induced nephrotoxicity and cardiotoxicity (Injac et al., 2008; Qi et al., 2018). Based on these prospective findings, we speculated that dioscin might exert protective effects against Dox-induced hepatotoxicity, and we designed in vivo and in vitro experiments to verify our hypothesis.
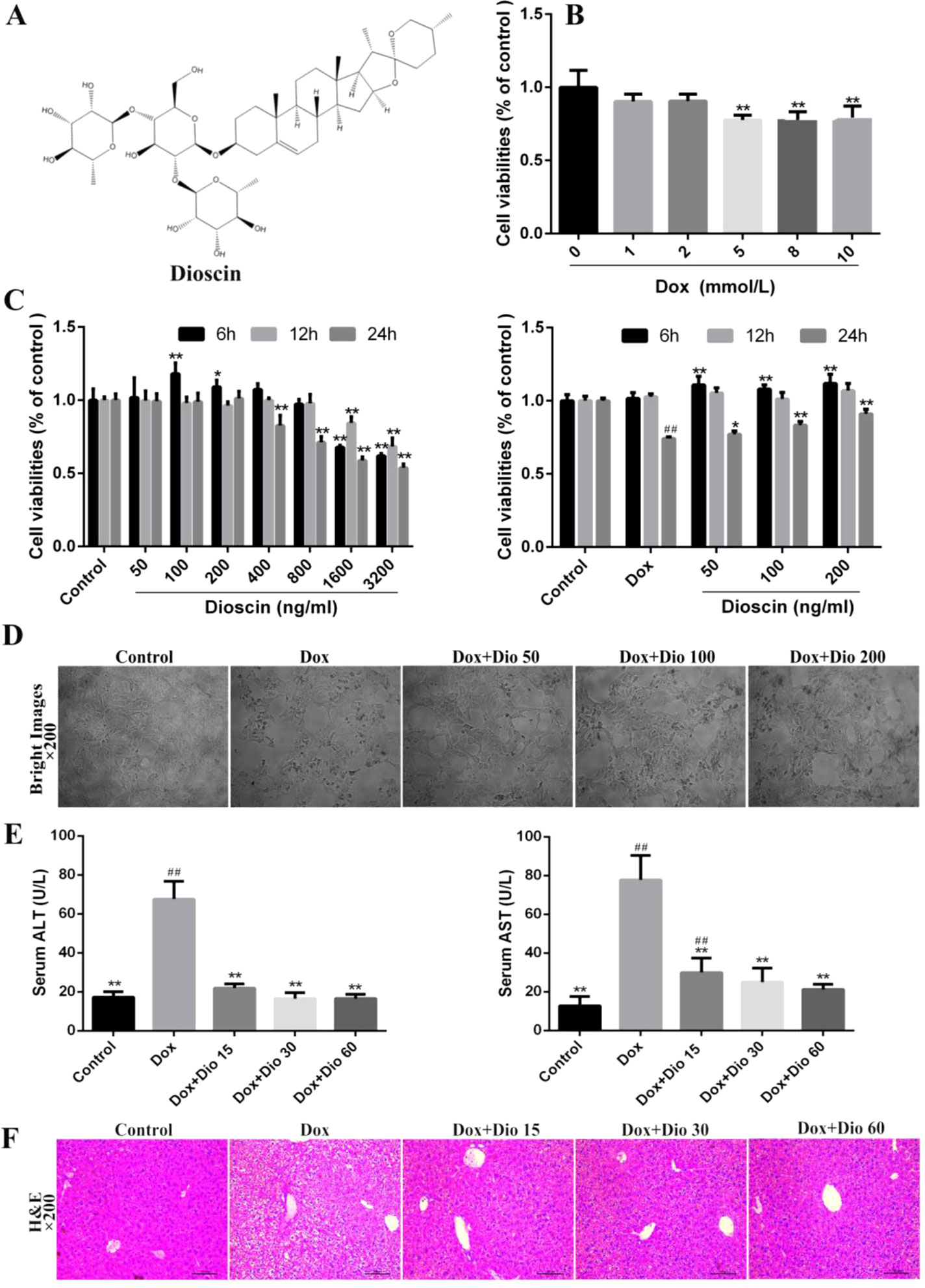
Figure 1 Dioscin inhibits Dox-induced AML-12 cell damage and liver injury in mice. (A) Chemical structure of dioscin. (B) Dox-induced nephrotoxicity on AML-12 cell. (C) Cytotoxicity of dioscin on AML-12 cells and the effects of dioscin on cell viability induced by Dox. (D) Effects of dioscin (50, 100, and 200 ng/ml) for 24-h pretreatment on the cellular morphology and structure of AML-12 cells by bright image (200× magnification) investigation. (E) Effects of dioscin on AST and ALT levels in mice. (F) H&E staining (200× original magnification) of the liver tissue in mice. All data are expressed as the mean ± SD (n = 5 for in vitro test and n = 8 for in vivo test). *p < 0.05, **p < 0.01 compared with the model group. ##p < 0.01 compared with the control group. ALT, alanine transaminase; AML-12, alpha mouse liver 12; AST, aspartate transaminase; Dox, doxorubicin; H&E, hematoxylin and eosin.
Materials and Methods
Chemicals and Materials
Dioscin (purity > 98%) was obtained from Dioscorea nipponica Makino (Yin et al., 2010), which was dissolved in 0.5% carboxymethyl cellulose sodium (CMC-Na) for in vivo experiments and in 0.1% dimethyl sulfoxide (DMSO) for in vitro tests. The alanine transaminase (ALT), aspartate transaminase (AST), malondialdehyde (MDA), superoxide dismutase (SOD), glutathione (GSH), and glutathione peroxidase (GSH-Px) kits were from Nanjing Jiancheng Institute of Biotechnology (Nanjing, China). 3-(4,5-Dimethylthiazol-2-yl)-2,5-diphenyltetrazolium bromide (MTT) was provided by Roche Diagnostics (Basel, Switzerland). The bicinchoninic acid (BCA) protein assay kit, cell lysis buffer, and phenylmethanesulfonyl fluoride (PMSF) were obtained from Beyotime Institute of Biotechnology (Jiangsu, China). Dox and were purchased from Sigma-Aldrich (St. Louis, MO, USA).
Cell Culture
Alpha mouse liver 12 (AML-12) cells (Shanghai Institute of Biochemistry and Cell Biology, China) were cultured in Dulbecco’s modified Eagle medium (DMEM) and Ham’s F12 medium with 5 μg/ml of insulin, 5 μg/ml of transferrin, 5 ng/ml of selenium, 40 ng/ml of dexamethasone, and 10% fetal bovine serum, which were maintained in a humidified atmosphere of 5% CO2 and 95% O2 at 37°C.
Dox-Induced Cell Injury
Dox was prepared to make a series of working dilutions in serum-free DMEM. The AML-12 cells were plated in 96-well plates at a density of 8 × 104 cells/ml per well for 24 h. Then, the medium was removed, and 100 μL of sample solution with various concentrations of Dox (0, 1, 2, 5, 8, and 10 mM) was added under different treatment times for 24 h. After 10 μL of MTT stock solution (5 mg/ml) was added, the plates were incubated for another 4 h at 37°C, and DMSO (100 ml/well) was added to dissolve formazan crystals. The absorbance was measured with a microplate reader (Thermo, USA) at 490 nm, the results were normalized to control unwanted sources of variation, and the cell morphology was imaged with a phase contrast microscope (Nikon, Japan). Then a suitable concentration of Dox on cell injury was optimized.
Dioscin Toxicity Assay
AML-12 cells were plated in 96-well plates at a density of 8 × 104 cells/ml for 24 h and treated with various concentrations of dioscin (0, 50, 100, 200 400, 800, 1,600, and 3,200 ng/ml) for 6, 12, and 24 h at 37°C, and the cells were then analyzed according to MTT method. The absorbance of the samples was quantified at 490 nm using a phase contrast microscope (Nikon, Japan).
Cell Viability Assay
AML-12 cells were seeded in 96-well plates at a density of 8 × 104 cells/ml for 24 h and then pretreated with various concentrations of dioscin (50, 100, or 200 ng/ml) for 6, 12, and 24 h before being challenged with Dox (5 μg/ml) for 24 h. The cells in the model group were cultured without dioscin pretreatment, and the cells in the control group were cultured in serum-free DMEM under normal conditions during the entire experiment. The MTT assay was used to assess cell viability. The cell morphology was observed by an inverted microscope (Olympus BX-51, Tokyo, Japan).
Measurement of Intracellular ROS Level
AML-12 cells were plated in 6-well culture plates at a density of 8 × 104 cells/ml and treated with dioscin at concentrations of 50, 100, and 200 ng/ml for 12 h before treatment with Dox. The cells were harvested and then re-suspended in 500 ml of DCFH-DA (10 mM) into each well for 20 min at 37°C. After that, the cells were washed three times with serum-free DMEM, and the images were captured by fluorescent microscopy (Olympus, Japan) with 200× magnification.
Dox-Induced Hepatotoxicity In Vivo
Male C57BL/6J mice weighing 18–22 g were obtained from the Experimental Animal Center of Dalian Medical University (Dalian, China) (SCXK: 2013-0003). All animal experiments were performed according to the guidelines of the Institutional Animal Ethical Committee. Animals were housed in a room under the conditions of constant temperature (22 ± 3°C) and humidity (60%), a 12-h light/dark schedule, and free access to food and water.
After adapting for 1 week, the animals were randomly divided into five groups (n = 8): control group, Dox model group, Dio 60 mg/kg + Dox group, Dio 30 mg/kg + Dox group, and Dio 15 mg/kg + Dox group. Dioscin was administered intragastrically at doses of 15, 30, and 60 mg/kg once daily for 14 consecutive days. The mice in the control and model groups were administered with vehicle (0.5% CMC-Na). One hour after dioscin administration on the seventh day, the animals in the model and dioscin-treated groups were injected intraperitoneally with Dox (15 mg/kg) in 0.9% saline (10 ml/kg). After the last dose on the 14th day, the animals were sacrificed, and blood and liver tissues were collected and stored for the test.
Assessment of Biochemical Parameters
The serum levels of ALS and AST were measured using the commercial kits according to the manufacturer’s instructions. The levels of MDA, SOD, GSH, and GSH-Px in liver tissues were detected according to the manufacturer’s instructions.
Histological Examination
The liver tissues were fixed in 10% formalin, embedded in paraffin, and sectioned into 5-mm slices. The slices were stained with hematoxylin and eosin (H&E) and photographed by a light microscope (Nikon Eclipse TE2000-U, Nikon, Japan).
Terminal Deoxynucleotidyl Transferase (TdT)-Mediated dUTP Nick-End Labeling (TUNEL) Assay In Vivo and In Vitro
Apoptosis detection was executed using the assay kit (Beijing TransGen Biotech Co., Ltd., China) following the manufacturer’s instructions. The cells and tissues were treated with or without dioscin before the fluorescein (green)-labeled dUTP solution was added. Finally, the images were captured by a fluorescent microscope (Olympus, Japan) with 200× magnification and analyzed by the Image J software. The experiment was carried out by the researchers blinded to each group of animals.
Immunofluorescence Assay
For the immunofluorescence staining of Sirt1, the formalin-fixed and deparaffinized cells and liver tissue sections of mice were incubated with rabbit anti-Sirt1 in a humidified chamber overnight at 4°C. Then, the fluorescein-labeled secondary antibody followed by DAPI (5 μg/ml) was incubated according to the manufacturer’s instructions. TUNEL staining was measured with an In Situ Cell Death Detection Kit (TMR Red; Roche, NJ, USA) according to the manufacturer’s instructions. The samples were imaged using fluorescence microscopy (Olympus, Japan).
Western Blotting Assay
The total protein samples of liver tissues and cells were extracted using cold lysis buffer containing 1 mM of PMSF based on the manufacturer’s protocol, and the protein contents were determined using the BCA Protein Assay Kit. Then the proteins were separated by sodium dodecyl sulfate–polyacrylamide gel electrophoresis (SDS–PAGE) (10–15%), transferred to poly(vinylidene difluoride) (PVDF) membranes (Millipore, USA), blocked, and incubated overnight at 4°C with the primary antibodies listed in Table 1. After blocking with 5% dried skim milk for 3 h at room temperature, the membranes were incubated with horseradish peroxidase-conjugated antibody at room temperature for 2 h. Finally, the proteins were detected using an enhanced chemiluminescence (ECL) method and imaged by a BioSpectrum Gel Imaging System (UVP, Upland, CA, USA). Finally, the expression levels of the proteins normalized to GAPDH were detected using the ECL method and ChemiDoc XRS (Bio-Rad, USA).
Quantitative Real-Time Polymerase Chain Reaction (PCR) Assay
The total RNA samples were extracted from the cells and liver tissues using TRIzol reagent (TaKaRa Biotechnology Co., Ltd., China) according to the manufacturer’s protocol. Then, the purity of the extracted RNA was determined, and cDNA was synthesized using a PrimeScript® RT reagent kit according to the manufacturer’s instructions (TransGen Biotech, Beijing, China). Relative quantitation was performed using the Ct method for recurrent versus primary expression, with GAPDH as an endogenous control, and the fold changes were calculated for each gene. The primers used in the present work are listed in Table 2.
Sirt1 siRNA Transfection Experiment In Vitro
Transfection experiment was performed on AML-12 cells. The Sirt1 siRNA and control siRNA were dissolved in Opti-MEM and then equilibrated for 5 min at room temperature. The cells were transfected with Sirt1 siRNA or non-binding control siRNA using Lipofectamine 2000 reagent according to the manufacturer’s protocol. Then, the intracellular ROS level, cell apoptosis, and the protein levels of Sirt1, forkhead box protein O1 (FOXO1), kelch-like ECH-associated protein-1 (keap1), NF-E2-related factor 2 (Nrf2), heme oxygenase-1 (HO-1), NF-κB, BCL-2-associated X (BAX), and BCL-2 were measured after 24 h of transfection.
Statistical Analysis
All data were performed with GraphPad Prism 5.0 software (San Diego, CA, USA) and presented as the mean and standard deviation (SD). Statistically significant differences were determined using one-way analysis of variance (ANOVA) followed by the Newman–Keuls test. Comparisons between two groups were performed using an unpaired Student t-test. The results were considered to be statistically significant at p < 0.05.
Results
Dioscin Rehabilitates Dox-Induced Injury in AML-12 Cells and Mice
As shown in Figure 1B, the viability of AML-12 cells treated with 5.0 μM of Dox, compared with the control group, for 24 h was decreased at 72.1%, Thus, Dox at the concentration of 5.0 μM was used to treat the cells. As shown in Figure 1C, dioscin at the concentrations of 50, 100, and 200 ng/ml significantly protected cells against Dox-induced injury in a dose- and time-dependent manner. Compared with the Dox group, dioscin on 24-h treatment significantly increased the viability of AML-12 cells. Importantly, dioscin at the concentrations of 50, 100, and 200 ng/ml showed no toxicity to the cells. As shown in Figure 1D, the morphological changes of the cells including cell shrinkage and structure caused by Dox were all markedly reversed by dioscin. As shown in Figure 1E, dioscin significantly reduced the levels of ALT and AST in mice compared with the Dox group. In addition, H&E staining (Figure 1F) revealed that the liver in the control group showed normal architecture, and apparent injuries were found in Dox-treated groups, which were all restored by dioscin.
Dioscin Inhibits Dox-Induced Oxidative Stress
Based on immunofluorescence staining shown in Figure 2A, after treatment with dioscin at the concentrations of 100 and 200 ng/ml for 12 h, the ROS levels in cells were significantly decreased than those in the Dox group. As shown in Figure 2B, dioscin significantly reduced the level of MDA and increased the levels of GSH, GSH-Px, and SOD in mice compared with the model group.
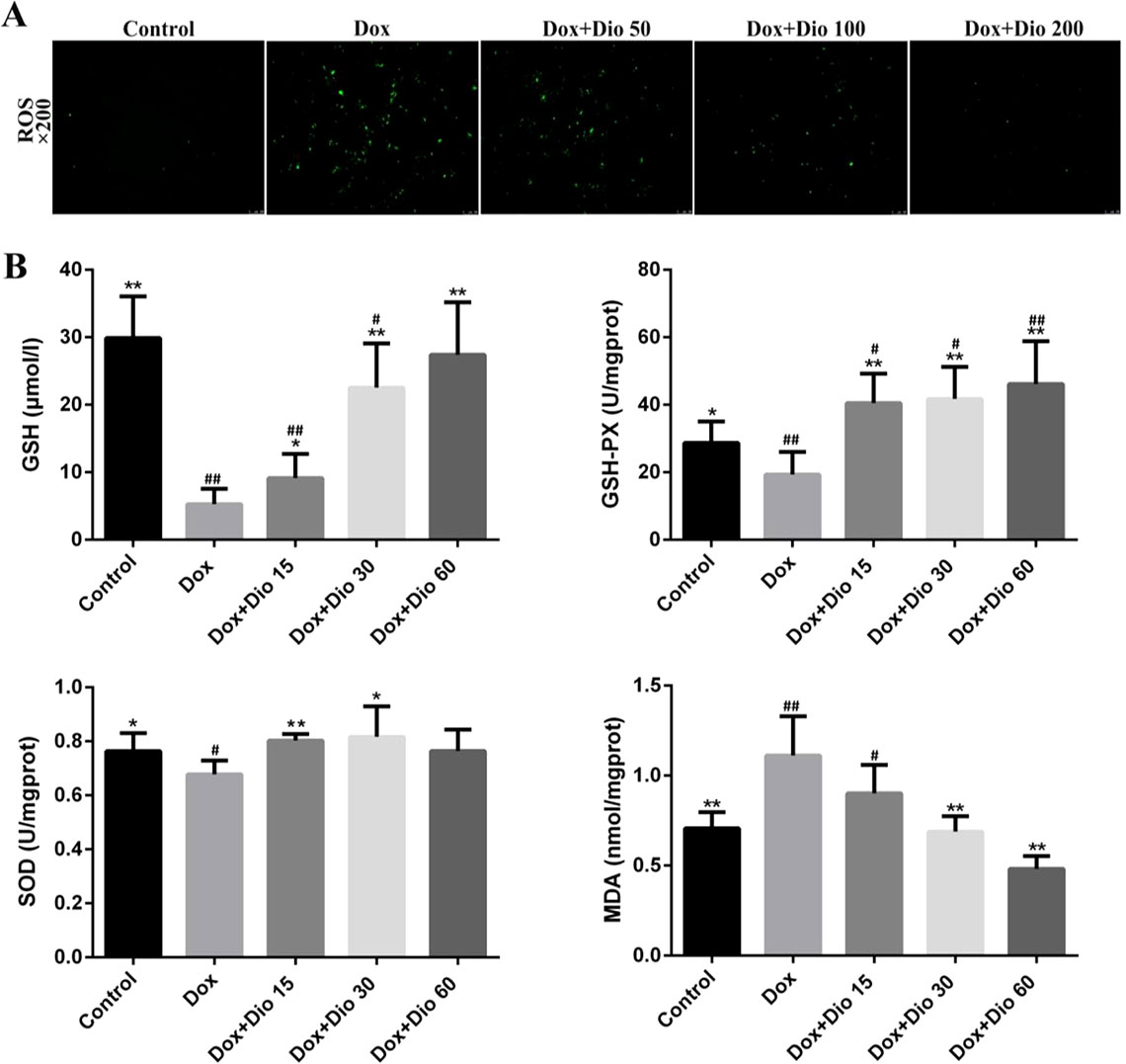
Figure 2 Dioscin attenuates oxidative damage in AML-12 cells and mice. (A) Effects of dioscin on intracellular ROS level in AML-12 cells treated with Dox. (B) Effects of dioscin on MDA, SOD, GSH, and GSH-Px levels in mice. Data are presented as the mean ± SD (n = 3 for in vitro test and n = 8 for in vivo test). *p < 0.05 and **p < 0.01 compared with the Dox group. #p < 0.05, ##p < 0.01 compared with the control group. AML-12, alpha mouse liver 12; Dox, doxorubicin; GSH, glutathione; GSH-Px, glutathione peroxidase; MDA, malondialdehyde; ROS, reactive oxygen species; SOD, superoxide dismutase.
Dioscin Attenuates Dox-Induced Apoptosis in AML-12 Cells and Mice
As shown in Figures 3A, B, more TUNEL-positive cells with green fluorescence were observed in Dox-treated groups than in dioscin-treated groups in AML-12 cells and mice.
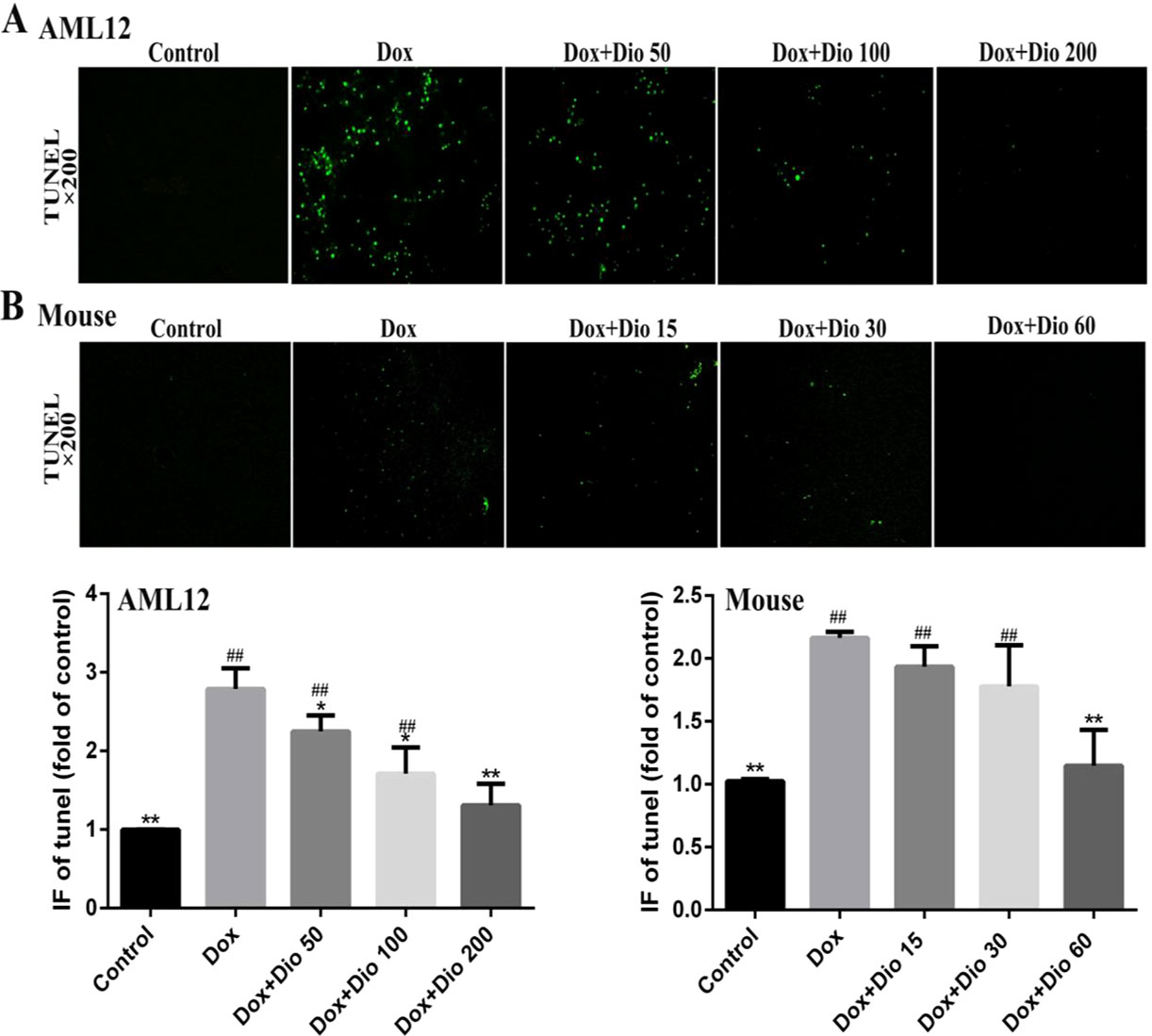
Figure 3 Effects of dioscin on the apoptosis of AML-12 cells and mice using TUNEL assay. (A) Dioscin attenuates Dox-induced apoptosis in AML-12 cells. (B) Dioscin inhibits Dox-induced liver cell apoptosis in mice. Data are presented as the mean ± SD (n = 5 for in vitro test and n = 8 for in vivo test). *p < 0.05 and **p < 0.01 compared with the model group. ##p < 0.01 compared with the control group. AML-12, alpha mouse liver 12; Dox, doxorubicin; TUNEL, terminal deoxynucleotidyl transferase-mediated dUTP nick-end labeling.
Dioscin Increases the Levels of Sirt1 in AML-12 Cells and Mice
As shown in Figures 4A, D, the expression levels of Sirt1 in the model groups were markedly decreased than in the control groups in vivo and in vitro, which were significantly up-regulated by dioscin based on western blotting assay. In addition, the results of immunofluorescence staining (Figures 4E, F) confirmed that dioscin markedly increased the expression levels of Sirt1 in AML-12 cells and mice.
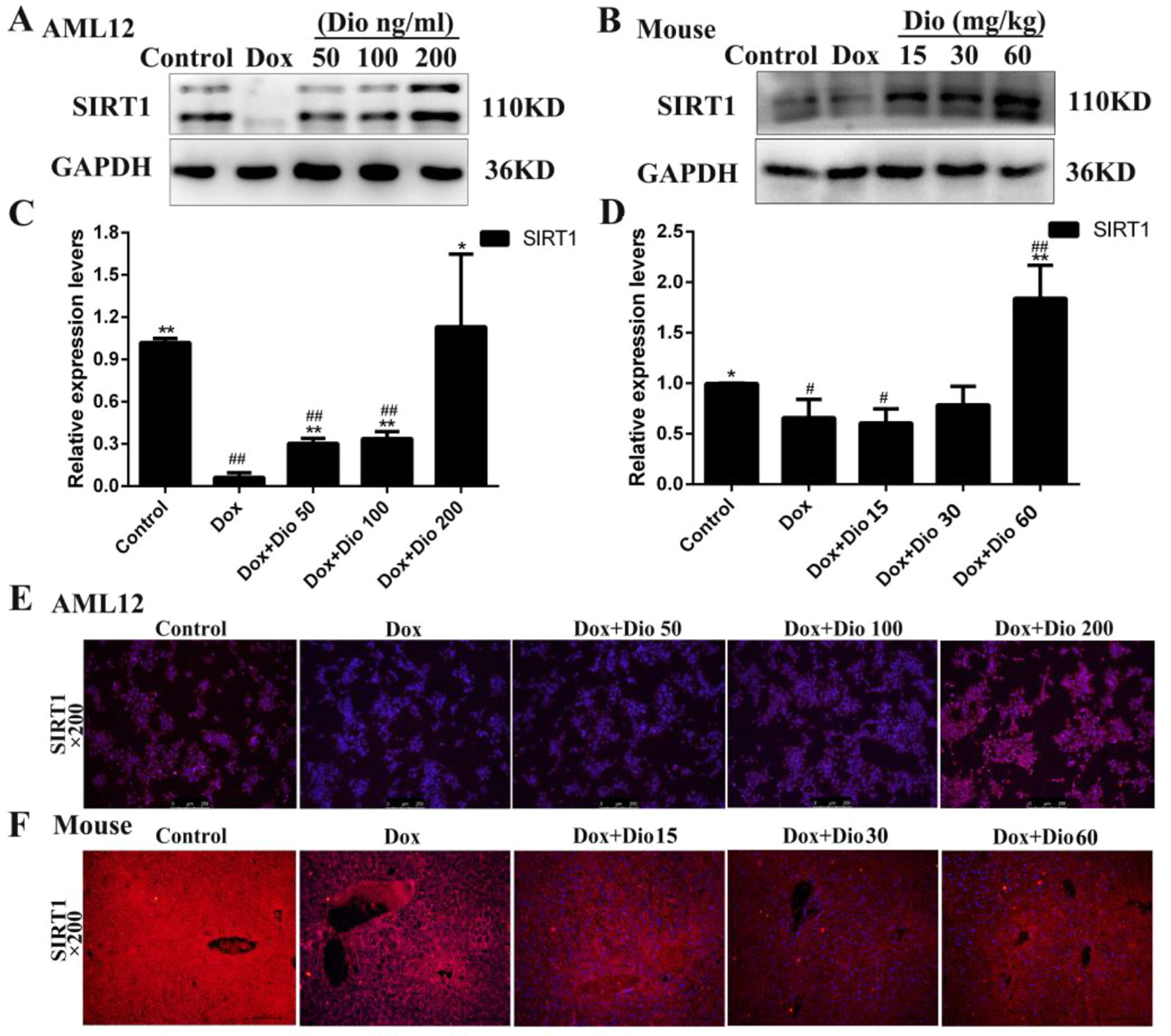
Figure 4 Sirt1 levels are activated in AML-12 cells and mice by dioscin. (A–D) Effects of dioscin on the protein levels of Sirt1 in AML-12 cells and mice. (E–F) Effects of dioscin on the expression levels of Sirt1 based on immunofluorescence staining in AML-12 cells and mice (200× magnification). Data are presented as the mean ± SD (n = 3 for in vitro test and n = 8 for in vivo test). *p < 0.05 and **p < 0.01 compared with the model group. #p < 0.05, ##p < 0.01 compared with the control group. AML-12, alpha mouse liver 12; Sirt1, silent information regulator 1.
Dioscin Up-Regulates FOXO1/Keap1/Nrf2 Signal in AML-12 Cells and Mice
As shown in Figures 5A, B, the protein levels of FOXO1 and Keap1 were significantly decreased, while the protein levels of Nrf2 and HO-1 were increased by dioscin than in the model groups in AML-12 cells and mice.
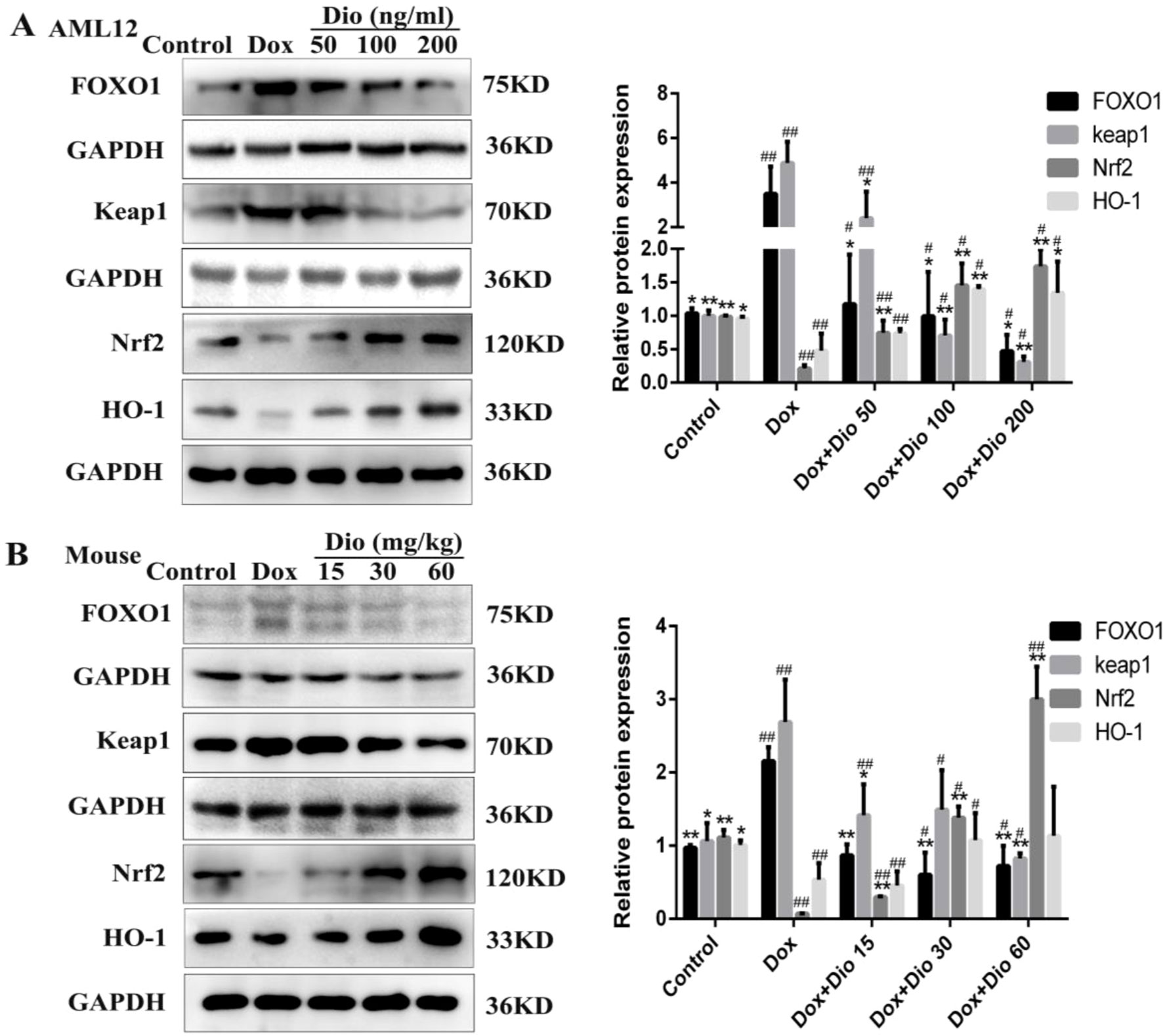
Figure 5 Dioscin suppresses the levels of protein in oxidative stress caused by Dox. (A) Effects of dioscin on the protein levels of FOXO1, Keap1, Nrf2, and HO-1 in AML-12 cells. (B) Effects of dioscin on the protein levels of FOXO1, Keap1, Nrf2, and HO-1 in mice. Data are presented as the mean ± SD (n = 3 for in vitro test and n = 8 for in vivo test). *p < 0.05 and **p < 0.01 compared with the model group. #p < 0.05, ##p < 0.01 compared with the control group. AML-12, alpha mouse liver 12; Dox, doxorubicin; FOXO1, forkhead box protein O1; HO-1, heme oxygenase-1; Keap1, kelch-like ECH-associated protein-1; Nrf2, NF-E2-related factor 2.
Dioscin Inhibits Dox-Induced Inflammation in AML-12 Cells and Mice
As shown in Figures 6A–D, the expression levels of NF-κB were significantly decreased by dioscin in AML-12 cells and mice than in the model groups. As shown in Figures 6E, F, the mRNA levels of interleukin 1β (IL-1β), interleukin 6 (IL-6), and tumor necrosis factor alpha (TNF-α) were all significantly decreased by dioscin.
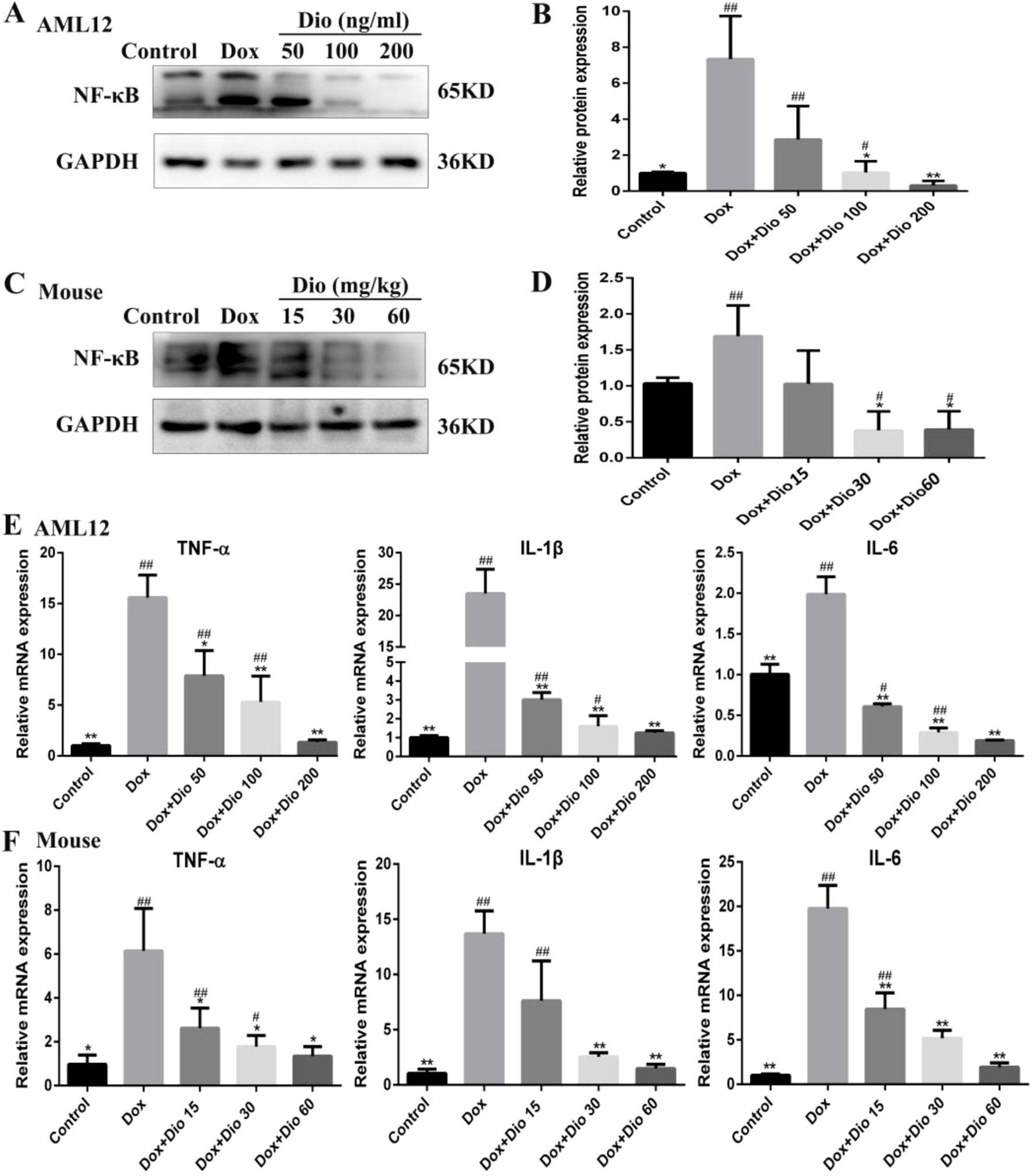
Figure 6 Dioscin ameliorates inflammation in vitro and in vivo. (A–D) Effects of dioscin on the expression levels of NF-κB in AML-12 and mice. (E–F) Effects of dioscin on the mRNA levels of IL-1β, IL-6, and TNF-α in AML-12 and mice. Data are presented as the mean ± SD (n = 3 for in vitro test and n = 8 for in vivo test). *p < 0.05 and **p < 0.01 compared with the model group. #p < 0.05, ##p < 0.01 compared with the control group. AML-12, alpha mouse liver 12; IL-1β, interleukin 1β; IL-6, interleukin 6; NF-κB, nuclear factor κB; TNF-α, tumor necrosis factor alpha.
Dioscin Affects the Protein Levels Related to Apoptosis in AML-12 Cells and Mice
As shown in Figures 7A–D, the expression levels of tumor suppressor P53 (P53) and BAX were significantly decreased while the expression levels of BCL-2 were increased by dioscin in a dose-dependent manner than in the model groups.
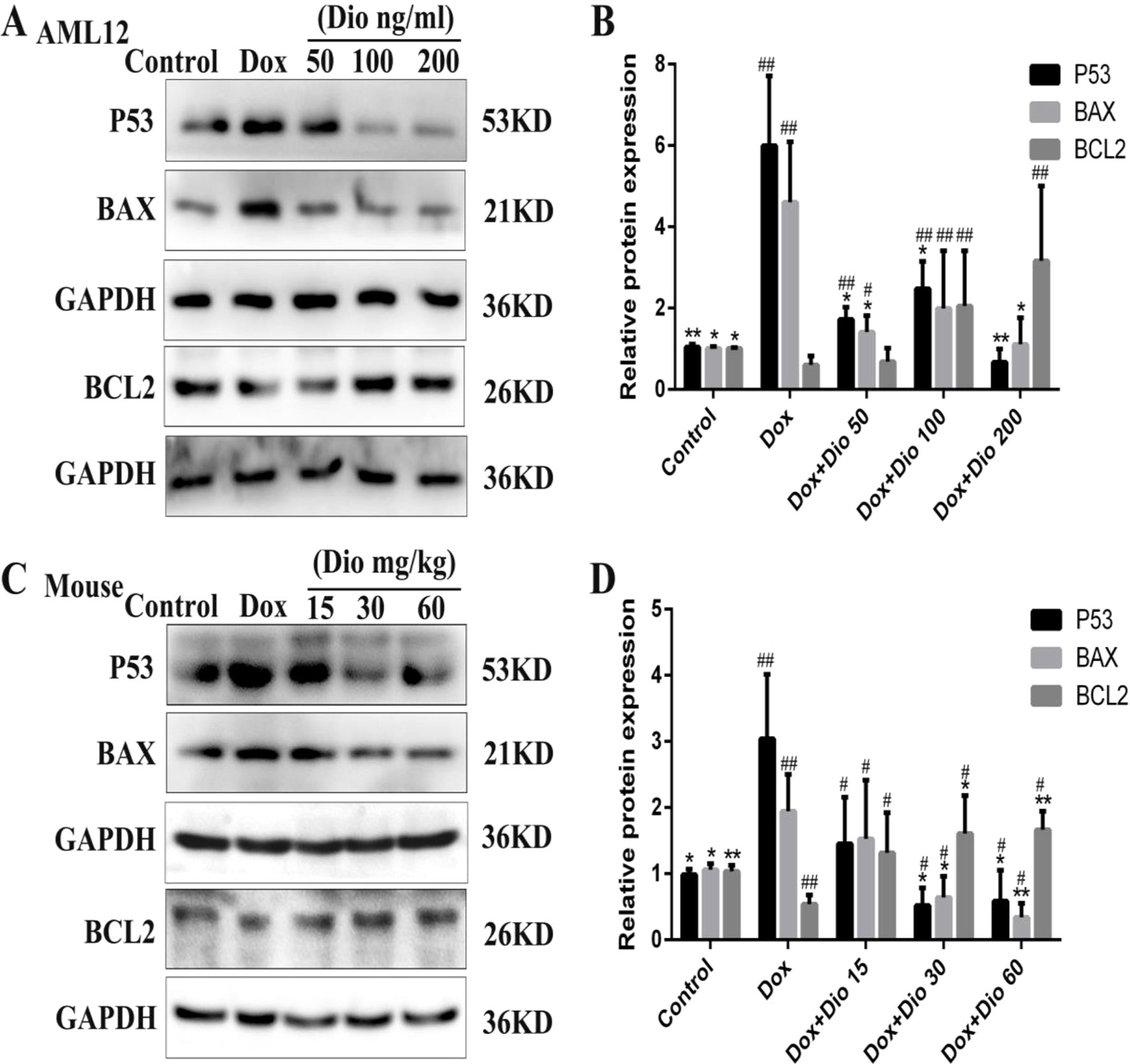
Figure 7 Dioscin regulates P53-mediated apoptosis pathway in vivo and in vitro. (A–B) Effects of dioscin on the expression levels of BAX and BCL-2 in AML-12 cells. (C–D) Effects of dioscin on the expression levels of P53, BAX, BCL-2 in mice. All data are presented as the mean ± SD (n = 3 for in vitro test and n = 8 for in vivo test). *p < 0.05 and **p < 0.01 compared with the model group. #p < 0.05, ##p < 0.01 compared with the control group. AML-12, alpha mouse liver 12; BAX, BCL-2-associated X; P53, tumor suppressor P53.
Sirt1 siRNA Reverses the Protective Effects of Dioscin on Dox-Induced Cell Injury
To investigate the roles of Sirt1 on the effects of dioscin against Dox-induced liver damage, a transfection approach using Sirt1 siRNA was carried out. As shown in Figure 8A, Sirt1 siRNA weakened the dioscin-induced up-regulation of Sirt1 based on immunofluorescence assay and increased the ROS level and the numbers of TUNEL-positive cells. However, dioscin still up-regulated the expression level of Sirt1, inhibited ROS level, and decreased the numbers of TUNEL-positive cells after transfection. Similar results were also found in the expression levels of the proteins related to oxidative stress, inflammation, and apoptosis in Sirt1 signal (Figure 8B). These findings confirmed that dioscin suppressed the signaling of oxidative stress, inflammation, and apoptosis via up-regulation Sirt1 signal.
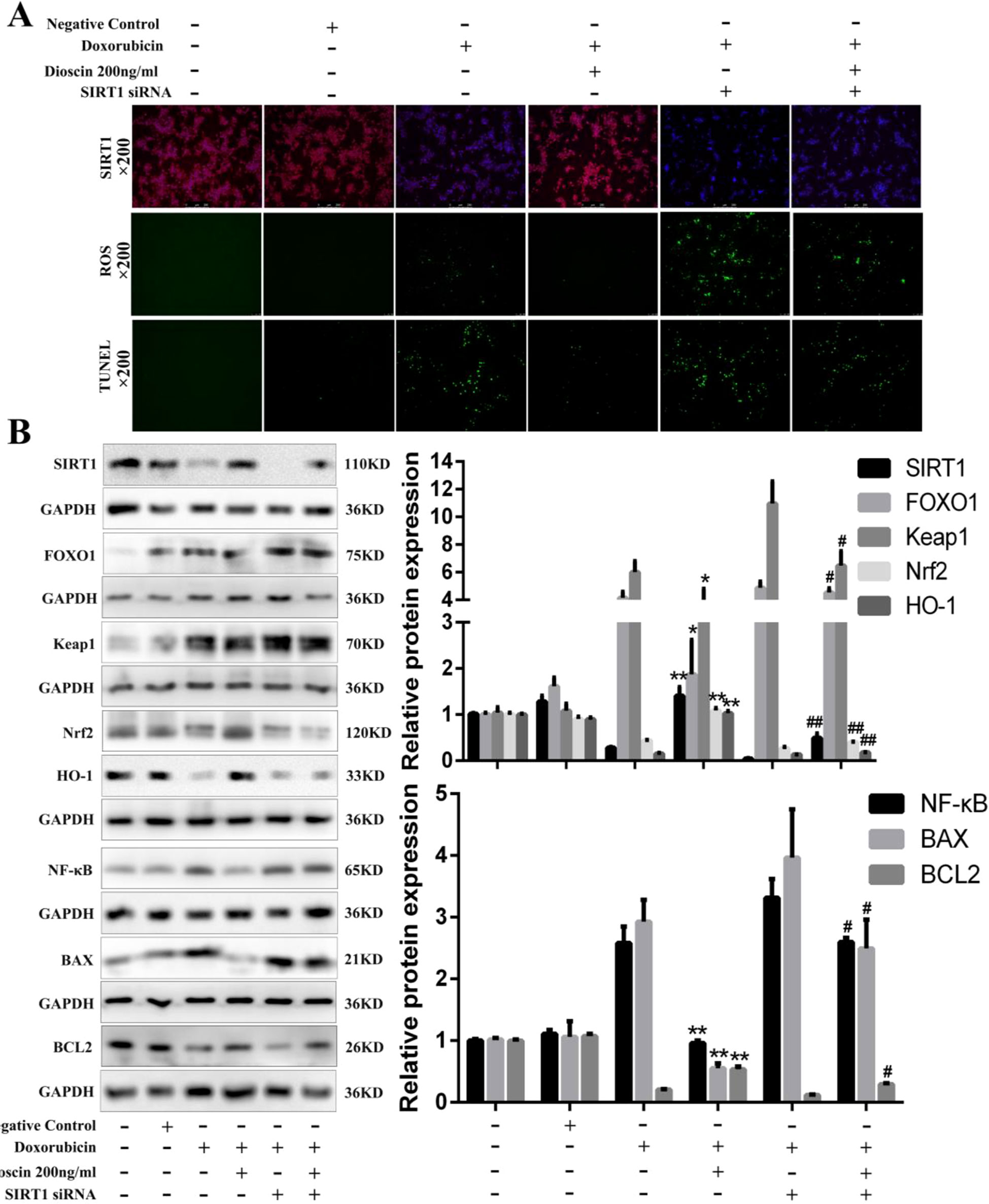
Figure 8 Sirt1 siRNA reverses the effects of dioscin on Dox-induced cell damage. (A) Effects of dioscin on Sirt1 expression, ROS level, and cell apoptosis with or without transfection of Sirt1 siRNA in vitro. (B) Effects of dioscin on the expression levels of Sirt1, FOXO1, Keap1, Nrf2, HO-1, NF-κB, BAX, and BCL-2 after transfection with Sirt1 in AML-12 cells. Data are presented as the mean ± SD (n = 5 for in vitro test and n = 8 for in vivo test). #p < 0.05 and ##p < 0.01 versus the Dox group; *p < 0.05 and **p < 0.01 versus the Dox group transfected with Sirt1 siRNA. AML-12, alpha mouse liver 12; BAX, BCL-2-associated X; Dox, doxorubicin; FOXO1, forkhead box protein O1; HO-1, heme oxygenase-1; Keap1, kelch-like ECH-associated protein-1; NF-κB, nuclear factor κB; Nrf2, NF-E2-related factor 2; ROS, reactive oxygen species; Sirt1, silent information regulator 1.
Discussion
Dox, one of the most widely used anticancer drugs, has therapeutic effects on a wide assortment of tumors (Rada et al., 2018). However, Dox can cause a range of significant side effects in normal tissues. Hepatotoxicity, a frequent side effect produced by Dox is of particular relevance under situation of preexisting abnormalities on liver function. Several studies have reported the protective effect of antioxidant agents against Dox-induced hepatotoxicity via adjustment of inflammation, oxidative stress, and apoptosis (Jeon et al., 2014; Wang et al., 2019). Dioscin, a naturally derived triterpenoid saponin, displayed the activity on fructose-induced renal damage via adjustment of Sirt3-mediated oxidative stress, renal fibrosis, lipid metabolism, and inflammation in rats (Qiao et al., 2017). In addition, dioscin inhibited Dox-induced nephrotoxicity by activating farnesoid X receptor (FXR) to suppress inflammation and oxidative stress, which was proved in NRK-52E cells and rats (Qi et al., 2017). In the present paper, dioscin exerted protective effects against Dox-induced damage on AML-12 cells and protected against Dox-induced liver injury in mice via suppression of ROS level, decreasing serum levels of ALT and AST and alleviating histopathological changes. These data implied that dioscin had protective effects by inhibiting Dox-induced hepatotoxicity.
The mechanisms responsible for Dox-induced hepatotoxicity are complex. In recent studies, oxidative stress has been considered as one major mechanism of oxidation-induced hepatotoxicity. It is reported that Dox can induce oxidative stress, which is characterized by ROS accumulation and the decrease of antioxidant defense on oxygen imbalance, culminating in attacking and oxidizing DNA and then inducing liver cell apoptosis (Wang et al., 2019). In the present study, Dox-induced hepatotoxicity produced oxidative stress as evidenced by the high ROS level in cells; high levels of GSH, GSH-Px, and SOD; and a low level of MDA in mice, which were ameliorated by dioscin. Herein, suppression of oxidative stress may be one potent strategy of dioscin against Dox-induced hepatotoxicity.
Many studies have shown that Sirt1 has critical roles against hepatotoxicity, which is a key player in pro-inflammatory context and oxidative stress during acetaminophen-mediated hepatotoxicity (Jeon et al., 2014). Sirt1 is also involved in controlling inflammatory responses, which can adjust NF-κB, a key mediator of pro-inflammatory signaling pathway triggered by cytokines. The latest work has confirmed that activating Sirt1/NF-κB pathway can cause the release of pro-inflammatory cytokines in cisplatin-induced nephrotoxicity (Jung et al., 2012). In the present work, dioscin exerted anti-inflammatory capability that resulted from the decreased levels of NF-κB, TNF-α, IL-1β, and IL-6 via increase of Sirt1 expression level. Therefore, inhibiting inflammatory response through adjusting Sirt1/NF-κB signal may be one powerful strategy of dioscin to treat Dox-induced hepatotoxicity.
FOXO1, one of the isoforms in the FOXO family, plays critical roles in cell proliferation and differentiation (Giannakou and Partridge, 2004). Activating Sirt1 can promote FOXO1 expression and transcription from cytoplasm to nucleus (Jung et al., 2012). Moreover, recent studies have shown that resveratrol can reduce Dox-induced cardiomyocytes cell apoptosis through Sirt1-mediated P53 deacetylation, suggesting that Sirt1 activation plays a critical role in apoptosis associated with P53 (Xu R. et al., 2019). The results of this study revealed that dioscin significantly decreased the expression levels of FOXO1, Nrf2, HO-1, P53, and BAX and dramatically up-regulated the expression levels of Keap1 and BCL-2. Taken together, the protective effect of dioscin against Dox-induced liver damage might be attributable to its antioxidative and anti-apoptosis properties through increasing Sirt1 level and activating FOXO1 and P53 pathways. In addition, Sirt1-siRNA transfection tests showed that dioscin showed potent effects against Dox-induced hepatotoxicity via regulation of Sirt1/FOXO1/NF-κB signal.
In conclusion, our results indicated that dioscin showed a protective effect against Dox-induced hepatotoxicity through adjusting Sirt1/FOXO1/NF-κB signal (Figure 9), which could be developed as a new potential candidate for clinical therapy to treat the disease. However, the deep mechanism of dioscin on Dox-induced hepatotoxicity and its clinical application remains to be further studied
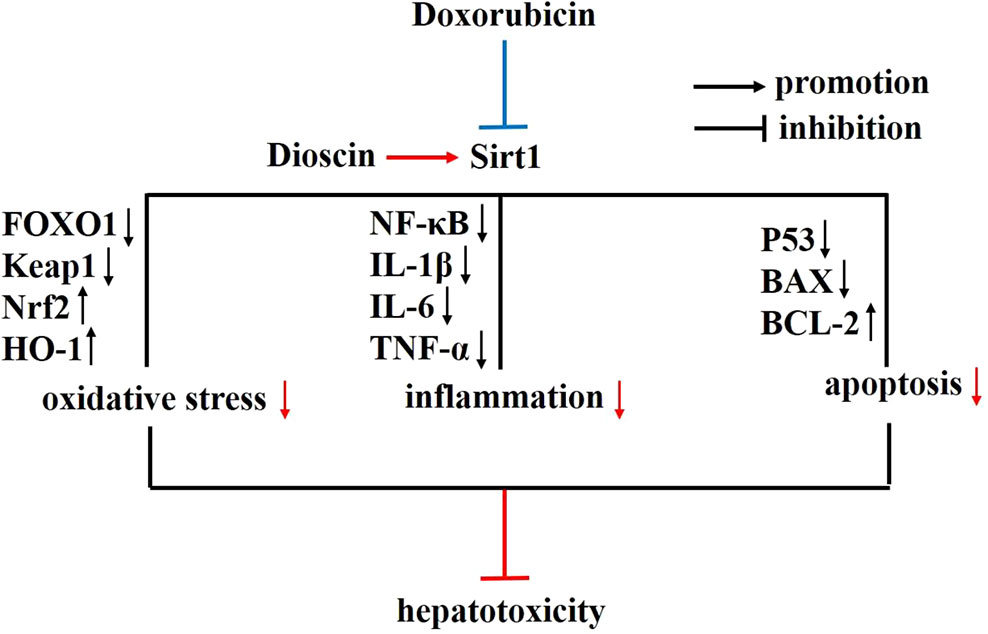
Figure 9 Diagram of the possible mechanism in which dioscin inhibits Dox-induced hepatotoxicity. Dox inhibits the expression of Sirt1, which could trigger cell oxidative stress, inflammation, and apoptosis via Sirt1/FOXO1/NF-κB signal pathway to induce hepatotoxicity. However, dioscin promotes the expression of Sirt1 and inhibits the oxidative stress, inflammation, and apoptosis to rescue Dox-induced hepatotoxicity through the Sirt1/FOXO1/NF-κB signal. Blue color indicates the function of Dox, and red color indicates the function and effect of dioscin. Dox, doxorubicin; FOXO1, forkhead box protein O1; NF-κB, nuclear factor κB; Sirt1, silent information regulator 1.
Data Availability
All datasets generated for this study are included in the manuscript.
Ethics Statement
The animal study was reviewed and approved by Institutional Animal Ethical Committee. Written informed consent was obtained from the owners for the participation of their animals in this study.
Author Contributions
SS was responsible for the planning, execution of all experiments, and preparation of the manuscript. LC and HL were responsible for the preparation, isolation, and bioavailability study of dioscin. JC, JL, and ZH were responsible for the immunofluorescence assay, Western blotting, and real-time PCR assays. BZ and XC were responsible for the conceptualization, planning, execution, and troubleshooting of the experiments; preparation of the manuscript; and the financial support.
Funding
This work was supported by National Natural Science Foundation of China (No. 81700056) and Postdoctoral Science Foundation of China (No. 2018M632878).
Conflict of Interest Statement
The authors declare that the research was conducted in the absence of any commercial or financial relationships that could be construed as a potential conflict of interest.
References
Aryal, B., Jeong, J., Rao, V. A. (2014). Doxorubicin-induced carbonylation and degradation of cardiac myosin binding protein c promote cardiotoxicity. Proc. Natl. Acad. Sci. U. S. A. 111, 2011–2016. doi: 10.1073/pnas.1321783111
Ashikawa, K., Shishodia, S., Fokt, I., Priebe, W., Aggarwal, B. B. (2004). Evidence that activation of nuclear factor-κb is essential for the cytotoxic effects of DOX and its analogues. Biochem. Pharmacol. 67, 353–364. doi: 10.1016/j.bcp.2003.08.039
Austin, R., Lee, S., Park, S. (2016). Rapid emergence and mechanisms of resistance by U87 glioblastoma cells to doxorubicin in an in vitro tumor microfluidic ecology. Proc. Natl. Acad. Sci. U. S. A. 113, 14283–14288. doi: 10.1073/pnas.1614898113
Camaggi, C. M., Comparsi, R., Strocchi, E., Testoni, F., Pannuti, F. (1988). Epirubicin and doxorubicin comparative metabolism and pharmacokinetics. Cancer Chemoth. Pharm. 21, 221–228. doi: 10.1007/BF00262774
Cristina, C., Santos, R. X., Susana, C., Sónia, C., Oliveira, P. J., Santos, M. S., et al. (2009). Doxorubicin: the good, the bad and the ugly effect. Curr. Med. Chem. 16, 3267–3285. doi: 10.2174/092986709788803312
Danz, E. D. B., Skramsted, J., Henry, N., Bennett, J. A., Keller, R. S. (2009). Resveratrol prevents doxorubicin cardiotoxicity through mitochondrial stabilization and the Sirt1 pathway. Free Radic. Biol. Med. 46, 1589–1597. doi: 10.1016/j.freeradbiomed.2009.03.011
Dragojevic-Simic, V., Dobrić, S., Jacević, V., Bokonjić, D., Milosavljević, I., Kovacević, A. (2013). Efficacy of amifostine in protection against doxorubicin-induced acute cardiotoxic effects in rats. Vojnosanit Pregl. 70, 38–45. doi: 10.2298/VSP110905041D
Eugene, H. H., Jun, Z., Douglas, P. C., Victor, J. F. (2000). Comparison of the protective effects of amifostine and dexrazoxane against the toxicity of doxorubicin in spontaneously hypertensive rats. Cancer Chemoth. Pharm. 45, 329–334. doi: 10.1007/s002800050048
Ganey, P. E., Kauffman, F. C., Thurman, R. G. (1988). Oxygen-dependent hepatotoxicity due to doxorubicin: role of reducing equivalent supply in perfused rat liver. Mol. Pharmacol. 34, 695–701.
Giannakou, M. E., Partridge, L. (2004). The interaction between FOXO and SIRT1: tipping the balance towards survival. Trends Cell Biol. 14, 408–412. doi: 10.1016/j.tcb.2004.07.006
Gu, L., Tao, X., Xu, Y., Han, X., Qi, Y., Xu, L., et al. (2015). Dioscin alleviates BDL- and DMN-induced hepatic fibrosis via Sirt1/Nrf2-mediated inhibition of p38 MAPK pathway. Toxicol. Appl. Pharmacol. 292, 19–29. doi: 10.1016/j.taap.2015.12.024
Han, M. K., Song, E. K., Guo, Y., Ou, X., Mantel, C., Broxmeyer, H. E. (2008). Sirt1 regulates apoptosis and Nanog expression in mice embryonic stem cells by controlling p53 subcellular localization. Cell Stem Cell. 2, 241–251. doi: 10.1016/j.stem.2008.01.002
Han, X., Hu, Y., Yin, L., Zheng, L., Zhao, X., Xu, L., et al. (2018). Dioscin ameliorates intestinal ischemia/reperfusion injury via adjusting miR-351-5p/MAPK13-mediated inflammation and apoptosis. Pharmacol. Res. 139, 431–439. doi: 10.1016/j.phrs.2018.11.040
Han, X., Xu, L., Yin, L., Qi, Y., Si, L., Xu, Y., et al. (2017). Potent effects of dioscin against pancreatic cancer via miR-149-3P-mediated inhibition of the AKT1 signaling pathway: dioscin against pancreatic cancer via miR-149-3P. Br. J. Pharmacol. 174, 553–568. doi: 10.1111/bph.13718
Hu, Y., Yin, L., Mao, Z., Xu, L., Tao, X., Tang, Z., et al. (2018). Protective effect of dioscin against intestinal ischemia/reperfusion injury via adjusting miR-351-5p-mediated oxidative stress. Pharmacol. Res. 137, 56–63. doi: 10.1016/j.phrs.2018.09.016
Injac, R., Perše, M., Djordjevic-Milic, V., Obermajer, N., Prijatelj, M., Djordjevic, A., et al. (2008). Potential hepatoprotective effects of fullerenol C60 (OH)24 in doxorubicin-induced hepatotoxicity in rats with mammary carcinomas. Biomaterial 29, 3451–3460. doi: 10.1016/j.biomaterials.2008.04.048
Jeon, B. T., Kim, K. E., Heo, R. W., Shin, H. J., Yi, C. O., Hah, Y. S., et al. (2014). Myeloid-specific deletion of SIRT1 increases hepatic steatosis and hypothalamic inflammation in mice fed a high-fat diet. Metab. Brain Dis. 29, 635–643. doi: 10.1007/s11011-014-9542-3
Jung, Y. J., Lee, J. E., Lee, A. S., Kang, K. P., Lee, S., Park, S. K., et al. (2012). SIRT1 overexpression decreases cisplatin-induced acetylation of NF-κB p65 subunit and cytotoxicity in renal proximal tubule cells. Biochem. Biophys. Res. Commun. 419, 206–210. doi: 10.1016/j.bbrc.2012.01.148
Liu, M., Xu, Y., Han, X., Yin, L., Xu, L., Qi, Y., et al. (2015). Dioscin alleviates alcoholic liver fibrosis by attenuating hepatic stellate cell activation via the TLR/MyD88/NF-κb signaling pathway. Sci. Rep. 5, 18038. doi: 10.1038/srep18038
Mihailovic-Stanojevic, N., Jovovic, D., Miloradovic, Z., Grujic-Milanovic, J., Jerkic, M., Markovic-Lipkovski, J. (2008). Reduced progression of adriamycin nephropathy in spontaneously hypertensive rats treated by losartan. Nephrol. Dial. Transpl. 24, 1142–1150. doi: 10.1093/ndt/gfn596
Minotti, G., Menna, P., Salvatorelli, E., Cario, G., Gianni, L. (2004). Anthracyclines: molecular advances and pharmacologic developments in antitumor activity and cardiotoxicity. Pharmacol. Rev. 56, 185–229. doi: 10.1124/pr.56.2.6
Morsi, M. I., Hussein, A. E., Mostafa, M., El-Abd, E., Abd El-Moneim, N. A. (2006). Evaluation of tumour necrosis factor-α, soluble p-selectin, γ-glutamyl transferase, glutathione s-transferase-π and α-fetoprotein in patients with hepatocellular carcinoma before and during chemotherapy. Br. J. Biomed. Sci. 63, 74–78. doi: 10.1080/09674845.2006.11732724
Mukherjee, S., Banerjee, S. K., Maulik, M., Dinda, A. K., Talwar, K. K. (2004). Protection against acute adriamycin-induced cardiotoxicity by garlic: role of endogenous antioxidants and inhibition of TNF-alpha expression. BMC Pharmacol. 3, 16–16. doi: 10.1186/1471-2210-3-16
Okunewick, J. P., Buffo, M., Kociban, D. L. (1985). Comparative toxicity of mitoxantrone and doxorubicin on hematopoietic stem cells. Exp. Hematol. 16, 23–30.
Paweorn, A., Sudjit, L., Surapol, I. (2015). Chemotherapy-induced cardiotoxicity: overview of the roles of oxidative stress. Oxid. Med. Cell Longev. 11, 1–13. doi: 10.1155/2015/795602
Pugazhendhi, A., Edison, T. N. J. I., Velmurugan, B. K., Jacob, J. A., Karuppusamy, I. (2018). Toxicity of doxorubicin (Dox) to different experimental organ systems. Life Sci. 200, 26–30. doi: 10.1016/j.lfs.2018.03.023
Qi, M., Yin, L., Xu, L., Tao, X., Qi, Y., Han, X., et al. (2016). Dioscin alleviates lipopolysaccharide-induced inflammatory kidney injury via the microRNA let-7i/TLR4/MyD88 signaling pathway. Pharmacol. Res. 111, 509–522. doi: 10.1016/j.phrs.2016.07.016
Qi, M., Zheng, L., Qi, Y., Han, X., Xu, Y., Xu, L., et al. (2015). Dioscin attenuates renal ischemia/reperfusion injury by inhibiting the TLR4/MyD88 signaling pathway via up-regulation of HSP70. Pharmacol. Res. 100, 341–352. doi: 10.1016/j.phrs.2015.08.025
Qi, Y., Li, R., Xu, L., Yin, L., Xu, Y., Han, X., et al. (2019). Neuroprotective effect of dioscin on the aging brain. Molecules 24, 1247. doi: 10.3390/molecules24071247
Qi, Y., Xu, L., Yin, L., Zhao, X., Zheng, L., Han, X., et al. (2016). Potent effects of dioscin against gastric cancer in vitro and in vivo. Phytomedicine 23, 274–282. doi: 10.1016/j.phymed.2016.01.012
Qi, Y., Xu, L., Zhao, L., Tao, X., Yin, L., Peng, J. (2018). Protective effect of dioscin against doxorubicin-induced cardiotoxicity via adjusting microRNA-140-5p-mediated myocardial oxidative stress. Redox Biol. 16, 189–198. doi: 10.1016/j.redox.2018.02.026
Qi, Y., Xu, L., Zhang, Y., Xu, Y., Song, S., Yin, L. (2017). Protective effects of dioscin against doxorubicin-induced nephrotoxicity via adjusting FXR-mediated oxidative stress and inflammation. Toxicology 378, 53–64. doi: 10.1016/j.tox.2017.01.007
Qiao, Y., Yin, L., Xu, L., Tao, X., Qi, Y., Xu, Y., et al. (2017). Protective effects of dioscin against fructose-induced renal damage via adjusting sirt3-mediated oxidative stress, fibrosis, lipid metabolism and inflammation. Toxicol. Lett. 284, 37–45. doi: 10.1016/j.toxlet.2017.11.031
Qin, X., He, W., Hai, C., Liang, X., Liu, R. (2008). Protection of multiple antioxidants Chinese herbal medicine on the oxidative stress induced by Adriamycin chemotherapy. J. Appl. Toxicol. 28, 271–282. doi: 10.1002/jat.1276
Rada, P., Pardo, V., Mobasher, M. A., Garcia, I., Ruiz, L., Ramos, C. S., et al. (2018). SIRT1 controls acetaminophen hepatotoxicity by modulating inflammation and oxidative stress. Antioxid. Redox Sign. 28, 1187–1208. doi: 10.1089/ars.2017.7373
Reddy, A., Anjaneyulu, Y., Shivakumar, P., Rani, M. (2012). A study on the toxic effects of doxorubicin on the histology of certain organs. Toxicol. Int. 19, 241. doi: 10.4103/0971-6580.103656
Roomi, M. W., Kalinovsky, T., Roomi, N. W., Rath, M. (2014). Prevention of Adriamycin-induced hepatic and renal toxicity in male balb/c mice by a nutrient mixture. Exp. Ther. Med. 7, 1040–1044. doi: 10.3892/etm.2014.1535
Saad, S. Y., Najjar, T. A., Al-Rikabi, A. C. (2001). The preventive role of deferoxamine against acute doxorubicin-induced cardiac, renal and hepatic toxicity in rats. Pharmacol. Res. 43, 211–218. doi: 10.1006/phrs.2000.0769
Sassocerri, E., Vendramini, V., Miraglia, S. M. (2010). Amifostine reduces the seminiferous epithelium damage in doxorubicin-treated prepubertal rats without improving the fertility status. Reprod. Biol. Endocrin. 8, 3. doi: 10.1186/1477-7827-8-3
Si, L., Zheng, L., Xu, L., Yin, L., Han, X., Qi, Y., et al. (2016). Dioscin suppresses human laryngeal cancer cells growth via induction of cell-cycle arrest and MAPK-mediated mitochondrial derived apoptosis and inhibition of tumor invasion. Eur. J. Pharmacol. 774, 105–117. doi: 10.1016/j.ejphar.2016.02.009
Tao, X., Qi, Y., Xu, L., Yin, L., Han, X., Xu, Y., et al. (2016). Dioscin reduces ovariectomy-induced bone loss by enhancing osteoblastogenesis and inhibiting osteoclastogenesis. Pharmacol. Res. 108, 90–101. doi: 10.1016/j.phrs.2016.05.003
Tao, X., Xu, L., Yin, L., Han, X., Qi, Y., Xu, Y., et al. (2017). Dioscin induces prostate cancer cell apoptosis through activation of estrogen receptor-β. Cell Death Dis. 8, e2989. doi: 10.1038/cddis.2017.391
Tao, X., Yin, L., Xu, L., Peng, J. (2018). Dioscin: a diverse acting natural compound with therapeutic potential in metabolic diseases, cancer, inflammation and infections. Pharmacol. Res. 137, 259–269. doi: 10.1016/j.phrs.2018.09.022
Thorn, C., Oshiro, C., Marsh, S., Hernandezboussard, T., Mcleod, H., Klein, T. (2011). Doxorubicin pathways: pharmacodynamics and adverse effects. Pharmacogenet. Genom. 21, 440. doi: 10.1097/FPC.0b013e32833ffb56
Wang, R., Dong, Z., Lan, X., Liao, Z., Chen, M. (2019). Sweroside alleviated LPS-induced inflammation via SIRT1 mediating NF-kappaB and FoxO1 signaling pathways in RAW264.7 Cells. Molecules 24, 872. doi: 10.3390/molecules24050872
Xu, L., Gu, L., Tao, X., Xu, Y., Qi, Y., Yin, L., et al. (2017). Effect of dioscin on promoting liver regeneration via activating Notch1/Jagged1 signal pathway. Phytomedicine 38, 107–117. doi: 10.1016/j.phymed.2017.11.006
Xu, L., Xu, Y., Han, X., Zhang, M., Chen, D., Yin, L., et al. (2019). Potent effects of dioscin against hepatocellular carcinoma through regulating TIGAR-mediated apoptosis, autophagy and DNA damage. Br. J. Pharmacol. 176, 919–937. doi: 10.1111/bph.14594
Xu, L., Yin, L., Qi, Y., Zhang, X., Han, X., Xu, Y. (2015). Potent effects of dioscin against liver fibrosis. Sci. Rep. 5, 9713. doi: 10.1038/srep09713
Xu, R., Xu, X., Deng, Y., Ma, Z., Li, X., Zhao, L., et al. (2019). Resveratrol attenuates myocardial hypoxia/reoxygenation-induced cell apoptosis through DJ-1-mediated SIRT1-p53 pathway. Biochem. Biophys. Res. Commun. 514, 401–406. doi: 10.1016/j.bbrc.2019.04.165
Yang, Y., Zhao, L., An, R., Yang, X., Liu, H. (2015). Melatonin alleviates brain injury in mice subjected to cecal ligation and puncture via attenuating inflammation, apoptosis, and oxidative stress: the role of Sirt1 signaling. J. Pineal. Res. 59, 230–239. doi: 10.1111/jpi.12254
Yao, H., Hu, C., Yin, L., Tao, X., Xu, L., Qi, Y., et al. (2016). Dioscin reduces lipopolysaccharide-induced inflammatory liver injury via regulating TLR4/MyD88 signal pathway. Int. Immunopharmacol. 36, 132–141. doi: 10.1016/j.intimp.2016.04.023
Yin, L., Xu, L., Qi, Y., Xu, Y., Liu, M., Han, X., et al. (2015). Potent effects of dioscin against obesity in mice. Sci. Rep. 5, 7973. doi: 10.1038/srep12183
Yin, L., Xu, Y., Qi, Y., Han, X., Xu, L., Peng, J. (2010). A green and efficient protocol for industrial-scale preparation of dioscin from Dioscorea nipponica Makino by two-step macroporous resin column chromatography. Chem. Eng. J. 165, 281–289. doi: 10.1016/j.cej.2010.09.045
Zhang, Q., Yang, J., Zhang, H. (2019). Carvedilol (CAR) combined with carnosic acid (CAA) attenuates doxorubicin-induced cardiotoxicity by suppressing excessive oxidative stress, inflammation, apoptosis and autophagy. Biomed. Pharmacother. 109, 71–83. doi: 10.1016/j.biopha.2018.07.037
Zhang, W., Yin, L., Tao, X., Xu, L., Zheng, L., Han, X., et al. (2016). Dioscin alleviates dimethylnitrosamine-induced acute liver injury through regulating apoptosis, oxidative stress and inflammation. Environ. Toxicol. Pharmacol. 45, 193–201. doi: 10.1016/j.etap.2016.06.002
Zhang, X., Xu, Y., Qi, Y., Yin, L., Xu, L., Han, X., et al. (2015). Quantitative chemical proteomics for investigating the biomarkers of dioscin against liver fibrosis caused by CCI4 in rats. Chem. Commun. 51, 11604–11607. doi: 10.1039/C4CC09160D
Zhao, X., Tao, X., Xu, L., Yin, L., Qi, Y., Xu, Y., et al. (2016). Dioscin induces apoptosis in human cervical carcinoma HeLa and SiHa cells through ROS-mediated DNA damage and the mitochondrial signaling pathways. Molecules 21, 730. doi: 10.3390/molecules21060730
Zheng, L., Yin, L., Xu, L., Qi, Y., Li, H., Xu, Y., et al. (2017). Protective effect of dioscin against thioacetamide-induced acute liver injury via FXR/AMPK signaling pathway in vivo. Biomed. Pharmacother. 97, 481–488. doi: 10.1016/j.biopha.2017.10.153
Keywords: dioscin, doxorubicin, liver injury, Sirt1/FOXO1/NF-κB signal, oxidative stress, inflammation, apoptosis
Citation: Song S, Chu L, Liang H, Chen J, Liang J, Huang Z, Zhang B and Chen X (2019) Protective Effects of Dioscin Against Doxorubicin-Induced Hepatotoxicity Via Regulation of Sirt1/FOXO1/NF-κb Signal. Front. Pharmacol. 10:1030. doi: 10.3389/fphar.2019.01030
Received: 14 July 2019; Accepted: 12 August 2019;
Published: 13 September 2019.
Edited by:
Jinyong Peng, Dalian Medical University, ChinaCopyright © 2019 Song, Chu, Liang, Chen, Liang, Huang, Zhang and Chen. This is an open-access article distributed under the terms of the Creative Commons Attribution License (CC BY). The use, distribution or reproduction in other forums is permitted, provided the original author(s) and the copyright owner(s) are credited and that the original publication in this journal is cited, in accordance with accepted academic practice. No use, distribution or reproduction is permitted which does not comply with these terms.
*Correspondence: Bixiang Zhang, Yml4aWFuZ3poYW5nQDE2My5jb20=; Xiaoping Chen, Y2hlbnhwY2hlbnhwQDE2My5jb20=