- Key Laboratory of Shaanxi Administration of Traditional Chinese Medicine for TCM Compatibility, and State Key Laboratory of Research & Development of Characteristic Qin Medicine Resources (Cultivation), and Shaanxi Key Laboratory of Chinese Medicine Fundamentals and New Drugs Research, and Shaanxi Collaborative Innovation Center of Chinese Medicinal Resources Industrialization, Shaanxi University of Chinese Medicine, Xi’an, China
The incidence rate of cardio-cerebrovascular diseases (CCVDs) is increasing worldwide, causing an increasingly serious public health burden. The pursuit of new promising treatment options is thus becoming a pressing issue. Hydroxysafflor yellow A (HSYA) is one of the main active quinochalcone C-glycosides in the florets of Carthamus tinctorius L., a medical and edible dual-purpose plant. HSYA has attracted much interest for its pharmacological actions in treating and/or managing CCVDs, such as myocardial and cerebral ischemia, hypertension, atherosclerosis, vascular dementia, and traumatic brain injury, in massive preclinical studies. In this review, we briefly summarized the mode and mechanism of action of HSYA on CCVDs based on these preclinical studies. The therapeutic effects of HSYA against CCVDs were presumed to reside mostly in its antioxidant, anti-inflammatory, and neuroprotective roles by acting on complex signaling pathways.
Introduction
Cardio-cerebrovascular diseases (CCVDs) are characterized by ischemic or hemorrhagic lesions of the heart, brain, and peripheral circulatory tissues (Liu Z. et al., 2018). It is the high incidence, recurrence, and disability rates of CCVDs that directly aggravate the global burden of public health and hinder socio-economic development (Minno et al., 2019). Although much progress has been made in understanding the pathological mechanisms of CCVDs, there is still no effective therapy to prevent or stop the epidemic trend of CCVDs, resulting in the urgent need to identify novel therapeutic options (Kazantsev and Outeiro, 2010; Upadhyay, 2014).
Traditional Chinese medicine (TCM), a cost-effective and safe remedy, has been widely used in China and surrounding countries (including Japan and Korea) for the treatment and management of CCVDs with exact and prominent efficacy. Carthamus tinctorius L. (Compositae) (Figure 1A) seeds are known to be rich in α-linoleic acid and have been used since ancient times as a source of cooking oil. Meanwhile, its flowers are widely used for coloring and flavoring foods and manufacturing dyes (Hu et al., 2018; Guo et al., 2019). Notably, the medical use of Carthami Flos (Figure 1B, the dried florets of C. tinctorius) was first documented in the Golden Chamber Synopsis (Han Dynasty, ~2000 years ago) (Ma et al., 2014), and also described in the Compendium of Materia Medica (Ming Dynasty, ~500 years ago) as being able to “invigorate the blood circulation”, suggestive of its potential uses against circulatory system diseases. In modern Chinese clinic, Honghua injection (made from the water extract of Carthami Flos) and Danhong injection (extracted and refined from Salviae miltiorrhizae Radix et Rhizoma and Carthami Flos herb pair) are widely used for the treatment of coronary heart disease, angina pectoris, myocardial infarction, ischemic encephalopathy, and cerebral thrombosis (Fan et al., 2019; Zhang et al., 2019).
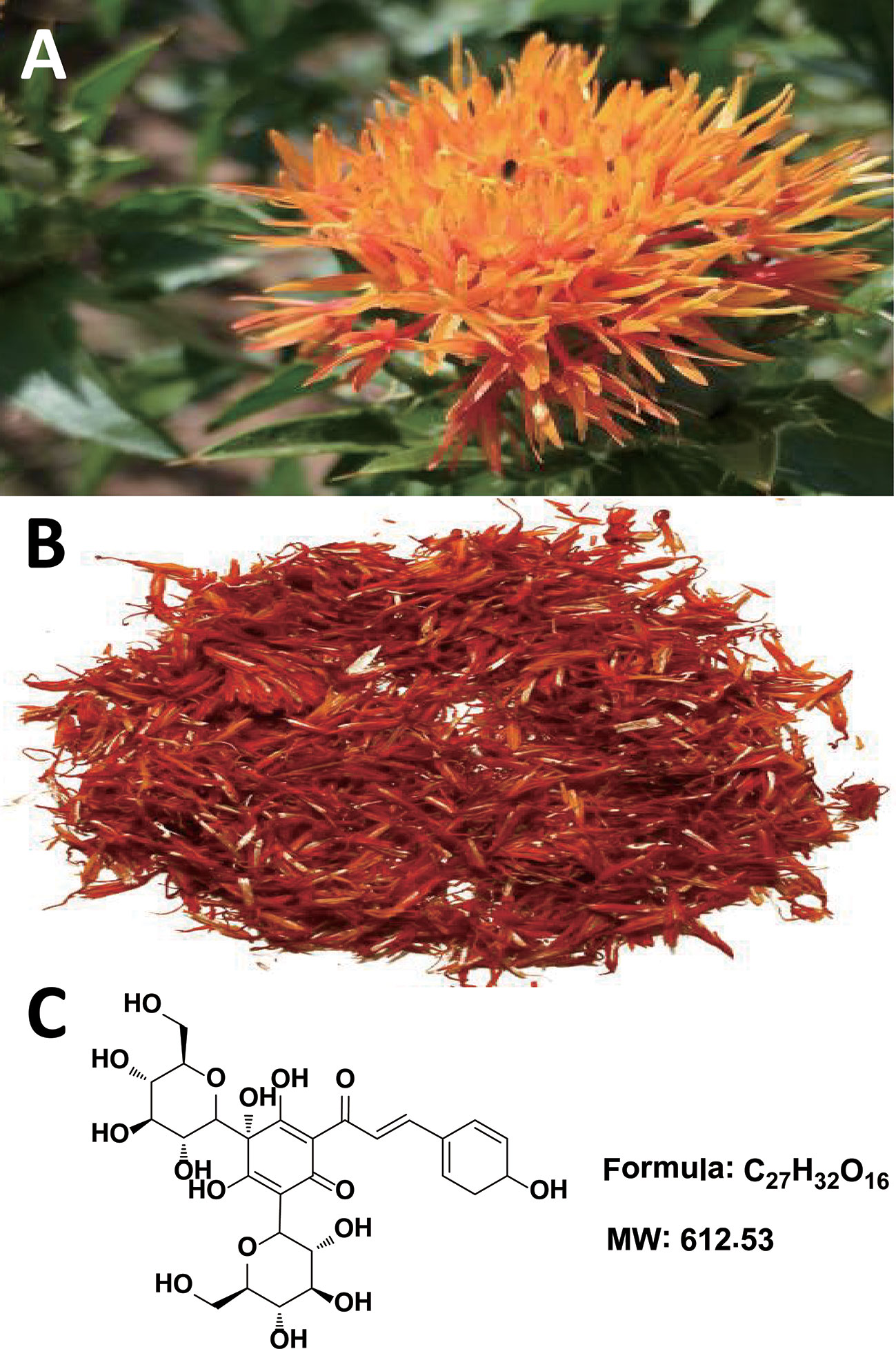
Figure 1 Carthamus tinctorius L. (A), Carthami Flos (the dried florets of C. tinctorius) (B), and hydroxysafflor yellow A (C).
The chemical constituents of Carthami Flos are plentiful, and include flavonoids (e.g. quinochalcone C-glycosides), alkaloids, phenolic acids, fatty acids, and more (Yue et al., 2013). Among them, hydroxysafflor yellow A (HSYA, Figure 1C) is both a representative water-soluble quinochalcone C-glycoside pigment and the quality marker of Carthami Flos. It produces remarkable pharmacological activities in CCVDs that have aroused great interest worldwide (Zhang et al., 2019), and massive preclinical studies have aimed to prove the pharmacological effects and dissect the mechanisms of actions of HSYA in treating CCVDs. Safflower yellow injection, a purified yellow pigment extract from Carthami Flos containing no less than 70% of HSYA, is commercially available for stable exertional angina pectoris of coronary heart disease with a marked curative effect in Chinese clinic (Liu et al., 2014; Xuan et al., 2018). Here, we briefly summarize the existing evidence to provide valuable references and implications for the clinical uses of HSYA.
Therapeutic Potential of HSYA on CCVDs
Effects on Myocardial Ischemia (MI)
It is acknowledged that MI results from insufficient blood-oxygen supply (Thiagarajan et al., 2017) and the improved vasomotor and circulatory functions exert beneficial effects on MI (Ribeiro et al., 2019). The vasoconstrictor endothelin and the vasodilator nitric oxide (NO) are known as two common regulators modulating vasomotor function. HSYA can reverse the circulating levels of both in acute MI animals (e.g., dogs and rats), thereby elevating myocardial blood-oxygen supply and reducing myocardial injury and apoptosis (Li et al., 2006; Wang et al., 2007). Other vasomotor function-related factors, such as 6-keto-prostaglandin F1α, thromboxane B2, and angiotensin II (Ang II), were also of great importance for HSYA (Wang et al., 2007). Angiogenesis participates in the circulatory function recovery from MI, and HSYA exerts the pro-angiogenic effects in two main ways: (1) nucleolin-mediated post-transcriptional regulation of vascular endothelial growth factor-A (VEGF-A) and matrix metalloproteinase (MMP) -9 expressions (Zou et al., 2018); and (2) the up-regulation of heme oxygenase-1 (HO-1)/VEGF-A/stromal cell-derived factor-1α cascade (Wei et al., 2017).
A specialized piece of in vivo research demonstrated that the antioxidant effect of HSYA was involved in the prevention of Ang II-induced myocardial hypertrophy (a compensatory response to MI), which may act through the activation of the nuclear factor erythroid-2-related factor 2 (Nrf2)/NAD(P)H: quinone oxidoreductase 1/HO-1 signaling pathway (Ni et al., 2018). Nrf-2, as the main regulator of the antioxidant system present on the cardiovascular system, is becoming a very promising pharmacological target for cardiovascular diseases (McSweeney et al., 2016). Our research group has found that HSYA possessed significant antioxidant activity in vitro (Yue et al., 2014). Thus, the antioxidant effect of HSYA may be essential to improve the outcomes of cardiovascular diseases.
Effects on Myocardial Ischemia/Reperfusion (MI/R) Injury
Thrombolytic or percutaneous coronary intervention reperfusion for acute myocardial infarction is favorable in most cases, but can also cause MI/R injury, resulting in excessive pro-inflammatory cytokines in myocardial tissue (Xie et al., 2018). HSYA has been reported to possess significant anti-inflammatory activity in vitro (Yue et al., 2016). In neonatal rat ventricular myocytes induced by hypoxia/reoxygenation (H/R) and lipopolysaccharide (LPS), HSYA not only inhibited the excessive secretion of pro-inflammatory cytokines but also suppressed the over-expression of toll like receptor (TLR) 4 and nuclear factor kappa beta (NF-κB). Importantly, HSYA was found to alleviate cardiac damage caused by MI/R in normal rats instead of TLR4-knockout mice (Han et al., 2016). It is important to note that TLR4 is the receptor of LPS, the component of the outer membrane of Gram-negative bacteria. There is evidence that NOD-like receptor 3 (NLRP3) inflammasome activation promotes myocardial injury and apoptosis via inducing the production of pro-inflammatory cytokines. HSYA improved H/R-induced H9c2 cell viability, maintained mitochondrial membrane potential, and inhibited NLRP3 inflammasome activation, while the adenosine 5’-monophosphate-activated protein kinase (AMPK) inhibitor partially abolished these observed effects of HSYA, which suggests that HSYA suppresses NLRP3 inflammasome activation via the AMPK pathway (Ye et al., 2020). Together, the TLR4 signaling pathway and NLRP3 inflammasome impact on the anti-inflammatory action of HSYA in MI/R.
Apoptosis is initiated shortly after the onset of myocardial infarction and is enhanced markedly during reperfusion. In H/R-induced H9c2 cells, the anti-apoptotic effect of HSYA not only depends on the up-regulation of HO-1 expression through the phosphoinositide 3-kinase (PI3K)/the protein kinase B (Akt)/Nrf2 signaling pathway, a compensatory mechanism limiting the apoptotic events in the presence of aggressive factors (Liu et al., 2012), but also targets the Akt/hexokinase II pathway to activate the hexokinase II protein and restore mitochondrial energy to reduce intracellular reactive oxygen species (ROS) generation (Min and Wei, 2017). Additionally, Zhou et al. reported that the anti-apoptotic effect of HSYA might be largely dependent on the Janus kinase 2/signal transducer (JAK2) and activation of the transcription 1 pathway (Zhou et al., 2019).
Beside inflammation and apoptosis, MI/R damages cardiomyocytes in part via the opening of the mitochondrial permeability transition pore (mtPTP), a non-selective pore that penetrates the inner and outer mitochondrial membranes (Bhosale and Duchen, 2019). HSYA has the capability to enter the cardiomyocytes and then inhibit mtPTP opening to alleviate H/R-induced myocardial injury through the enhanced endothelial nitric oxide synthase (eNOS)-produced NO (Liu et al., 2008; Huber et al., 2018).
Effects on Hypertension
Hypertension is a major global health challenge and an important risk factor of CCVDs. The blood pressure control rate of hypertensive patients in developing countries remains at unacceptably low levels (Mills et al., 2016). There is evidence that the conspicuous antihypertensive effect of HSYA is attributed to the inhibition of voltage-gated channels, the renin-angiotensin-aldosterone system, and the sympathetic nervous system. Specifically, HSYA inhibited the endotehlin-independent contraction of the thoracic aorta rings of rats through the blockade of inositol 1,4,5-triphosphate receptor in vascular smooth muscle cells (VSMCs), leading to the decrease of extracellular Ca2+ influx (Zhang et al., 2011). Beside VSMCs, endothelial cells participate in vasoconstriction and relaxation. Yang et al. found that oral HSYA has a concentration-dependent antihypertensive effect. It reversed the constriction of mesenteric arteries induced by a thromboxane A2 mimetic agent, the potential mechanism of which might be associated with the TRPV4 channel-dependent Ca2+ influx, protein kinase A-dependent eNOS phosphorylation, and NO production (Yang et al., 2020). A further study disclosed that HSYA could normalize blood pressure and heart rate dose-dependently in spontaneously hypertensive rats, which might be related to activating KATP and BKCa channels, inhibiting L-type Ca channels, decreasing Ca2+ influx, and subsequently inhibiting cardiac contractility (Nie et al., 2012; Wang et al., 2020). However, HSYA reduced blood pressure and heart rate in normotensive rats, on which more focus needs to be placed in the future. Moreover, HSYA can increase the reduced diastolic response of the thoracic aortic to acetylcholine and sodium nitroprusside, and thus attenuate the vascular contractile effect of phenylephrine (Jin et al., 2013). HSYA can also inhibit proliferative activity and collagen synthesis of Ang II-induced adventitial fibroblasts, reduce the expressions of MMP-1, transforming growth factor-β1, α-smooth muscle actin, and NF-κB p65, and thereby decreasing vascular adventitia proliferation and hyperplasia during vascular remodeling (Yuan et al., 2014). Obviously, it could be drawn that HSYA might be potentially useful as an antihypertensive drug via multiple mechanisms mainly involved in both cardiac output and peripheral resistance.
Hypertension may cause ventricular hypertrophy, which produces mechanical stimulation to the heart, further causing arrhythmia, heart failure, coronary occlusion, and sudden death (Pearson et al., 1991). A study by Wang et al. indicated that oral HSYA exhibited anti-apoptotic effects on hypertensive ventricular hypertrophy in rats by increasing the B-cell lymphoma-2 (Bcl-2)/Bcl-2 associated X protein (Bax) ratio and blocking serum MMP-2 and MMP-9 levels (Wang et al., 2014). Moreover, pulmonary arterial hypertension is a common combination of congenital heart disease with systemic-to-pulmonary artery shunt diseases, leading to right ventricular heart failure and premature death (Huang et al., 2018). Voltage-gated K+ channel (KV channel) is an important channel for maintaining normal membrane potential and muscle tension of VSMCs, while HSYA could activate the Kv channel of pulmonary artery VSMCs to reduce vascular tension, suggesting that HSYA may be a potential medication for pulmonary arterial hypertension (Bai et al., 2012).
Effects on Atherosclerosis (AS)
AS could precipitate the onset of myocardial infarction and inflammation and is being increasingly recognized as the main pathogenic mechanism through the narrowing and blockage of arteries and the increased risk of blood vessel rupture (Kotla et al., 2017). HSYA could inhibit ROS-induced inflammation in THP-1 macrophages (Jiang et al., 2017), but could also suppress tumor necrosis factor-α (TNF-α)-induced inflammatory responses through inhibiting the TNF-α receptor type 1-mediated classical NF-κB pathway in arterial endothelial cells (Wang H. F. et al., 2016). Jiang et al. discovered that HSYA-mediated sonodynamic therapy induced an autophagic response to inhibit inflammation via the PI3K/Akt/mammalian target of rapamycin (mTOR) signaling pathway in THP-1 macrophages (Jiang et al., 2017). Nevertheless, it is very interesting to justify the potential biphasic effect of HSYA under excessive macrophage autophagy, which can drive the instability of atherosclerotic plaque (Petrovski et al., 2011).
In fact, the oxidation of low-density lipoprotein (LDL) and oxidized LDL (ox-LDL)-induced vascular damage are key events in early AS. Although its LDL-lowering effect remains unknown, HSYA was able to reduce the susceptibility of LDL to copper-induced lipid peroxidation in vitro (Bacchetti et al., 2020). It is interesting to identify the effect of HSYA against ox-LDL formation in vivo. In ox-LDL-induced foamy macrophages, HSYA displayed obvious repairing effects on the de novo fatty acid biosynthesis pathway, among which oleoyl-(acyl-carrier-protein) hydrolase was postulated to be a target of HSYA (Wei et al., 2018). HSYA also exerted protective effects against ox-LDL-induced VSMCs proliferation via increasing mitogen-activated protein kinase phospholipase-1 expression and the proportion of cells in the G0/G1 phase, followed by reducing p-extracellular regulated protein kinases activity (Sheng et al., 2012). Moreover, HSYA has been shown to significantly improve ox-LDL-induced human endothelial injury, partially via the anti-apoptotic effect of the mitochondrial membranous voltage-dependent anion-selective channel protein 2 (Ye et al., 2017). Recently, Miao et al. revealed that HSYA could inhibit the high ox-LDL-induced human coronary artery endothelial cell injury, possibly via increasing eNOS expression and NO release, while inhibiting LDL receptor-1 expression and lactate dehydrogenase release (Miao et al., 2019).
During the development of AS, platelets can accelerate activation and release a variety of active substances, such as platelet-activating factor (PAF) and thromboxane B2, conversely promoting platelet adhesion and aggregation, and even damaging vascular endothelial cells (Wang et al., 2008). HSYA was able to inhibit PAF-induced platelet aggregation in rabbits by blocking PAF-mediated washed rabbit platelets (WRPs) and polymorphonuclear leukocyte aggregation (Zang et al., 2002).
Collectively, the above in vitro studies have manifested the potential anti-AS effects of HSYA, which should go through additional in vivo studies to determine its clinical implications.
Effects on Vascular Injury and Remodeling Diseases
The vascular endothelium plays an important role in modulating numerous aspects of vascular homeostasis (Scarabelli et al., 2002). HSYA was capable of promoting the survival and proliferation of vascular endothelial cells under both normoxic and hypoxic conditions, and its effect was stronger under hypoxia via up-regulating the Bcl-2/Bax ratio and accumulating hypoxia-inducible factor-1 (HIF-1) α, which was related to VEGF and its receptor system (Song et al., 2005; Ji et al., 2008). Also, HSYA could protect human umbilical vein endothelial cells (HUVECs) from hypoxia-induced injury by reducing p53 expression in the cell nucleus and up-regulating eNOS expression to produce NO in cell supernatant (Ji et al., 2009).
Abnormal proliferation of VSMCs is a crucial cytopathological basis for the development and progression of vascular remodeling diseases (Ivey et al., 2008). HSYA could inhibit platelet-derived growth factor-BB induced VSMCs proliferation by decreasing proliferating cell nuclear antigen expression and blocking mitogen-activated protein kinase/extracellular regulated protein kinases and Akt signaling pathways (Song et al., 2014; Zhao et al., 2015). In the LPS-induced VSMCs proliferation and migration model, HSYA inhibits the up-regulation of TLR4 expression and the activation of Ras-related C3 botulinum toxin substrate 1/Akt pathway (Yang et al., 2015).
Effects on Cerebral Ischemia (CI)
CI is one of the leading causes of death worldwide, and patients who survive CI often experience paralysis, impaired speech, or loss of vision (Moskowitz et al., 2010). HSYA appears to treat focal CI injury in rats through its anti-coagulation effects on thrombosis formation and platelet aggregation, as well as beneficial regulation on prostacyclin/thromboxane and blood rheological changes (Zhu et al., 2005). HSYA could also preserve cortex mitochondrial function of CI rats via scavenging free radicals, reducing lipid peroxides, and antagonizing Ca2+ (Tian et al., 2004). Importantly, HSYA possessed a better effect on cerebrovascular vasodilatation than on cardiovascular vasodilatation (Sun Y. et al., 2018), but the differential molecular mechanism remains to be discovered.
The blood-brain barrier (BBB) essentially maintains a stable cerebral homeostasis, while the destruction or increased permeability of BBB are common pathological processes during many serious cerebrovascular diseases (Chen Z. X. et al., 2019). A study by Lv et al. revealed that HSYA significantly attenuated BBB dysfunction in anti-inflammatory patterns in ischemia stroke via the tight junction pathway, especially the NMMHC IIA, TLR4/PI3K/Akt/Jun N-terminal kinase 1/2/14-3-3ϵ pathway while inhibiting the expressions of occludin, claudin-5, and zonula occludens-1 (Lv and Fu, 2018). Since MMPs are the main endoproteinases involved in BBB destruction (Romanic et al., 1998), the prominent inhibitory effects of HSYA on MMP-2 and MMP-9 mentioned in cardiovascular diseases may also contribute to BBB improvement.
It is worth mentioning that CI plays a causal role in facilitating neuronal death (Martin and Wang, 2010). A metabonomic study revealed that HSYA could attenuate excitatory amino acid-induced neurotoxicity, at least partially, through inhibiting the NF-κB pathway in the cerebral tissues of the middle cerebral artery occlusion (MCAO) model rats (Liu et al., 2013). Other protective mechanisms of HSYA against excitotoxic neuronal death include the inhibition of NR2B-containing N-methyl-d-aspartate receptors (NMDARs) expression and the Bcl-2 family regulation in cortical cultures, and the inhibition of the N-methyl-d-aspartate-induced and NMDARs-mediated intracellular Ca2+ increase in hippocampal cultures (Yang et al., 2010; Wang X. T. et al., 2016). In oxygen-glucose deprivation (OGD)-induced BV2 microglia, the neuroprotective action of HSYA involves the decreased expressions of pro-inflammatory cytokines, including interleukin (IL) -1β, TNF-α, inducible nitric oxide synthase, cyclooxygenase-2, and monocyte chemotactic protein-1, as well as the reserved phosphorylation of p38 and nuclear translocation of p65 (Li et al., 2013). Peroxynitrite-mediated protein tyrosine nitration and nitrosative stress represent the crucial pathogenic mechanisms of CI, while the anti-nitrative pathway might contribute to the neuroprotective efficacy of HSYA. Specifically, Sun et al. discovered that HSYA blocked authentic peroxynitrite-induced tyrosine nitration in primary cortical neurons by the reduction of inducible nitric oxide synthase expression and NO content, suggestive of its peroxynitrite scavenging abilities (Sun et al., 2013). They further reported that HSYA prevented peroxisome proliferator-activated receptor γ nitrative modification in primary neurons and resumed eroxisome proliferator-activated receptor γ activity stimulated by either 15-deoxy-delta prostaglandin J2 or rosiglitazone (Sun L. et al., 2018).
Effects on Cerebral Ischemia/Reperfusion (CI/R) Injury
A growing body of research has evidenced that oxidative stress is implicated in the pathogenesis of CI/R injury. Wei et al. showed that HSYA might oppose CI/R injury of MCAO rats through attenuating the elevation of malondialdehyde (MDA) level and decreasing superoxide dismutase (SOD) activity in the ipsilateral hemisphere and serum (Wei et al., 2005). HSYA could also reduce CI/R-induced protein oxidation and nitration, attenuate BBB destruction, and importantly inhibit the up-regulation of 12/15-lipoxygenase, which is implicated in the oxidative stress of CI/R (Sun et al., 2012). In an in vitro assay, HSYA was shown to block OGD/reoxygenation (OGD/R)-induced PC12 cells apoptosis through the suppression of intracellular oxidative stress (Fan et al., 2011).
An inflammatory reaction is a recognized player in CI/R damage. Through suppressing TLR4 pathway-mediated signaling responses, HSYA could up-regulate brain-derived neurotrophic factor (BDNF) in MCAO mice at post-ischemia/reperfusion (Lv et al., 2015), but also exert neurotrophic and anti-inflammatory functions in LPS-activated co-existence systems for microglia and neurons (Lv et al., 2016). In the microglia of the ischemic cortex after acute CI/R, HSYA exerted anti-inflammatory effects by activating the TLR9 signaling pathway and suppressing the NF-κB pathway (Gong et al., 2018). Further studies demonstrated that HSYA significantly inhibited NF-κB p65 nuclear translation and p65 binding activity, both mRNA and protein levels of intercellular adhesion molecule 1, and the infiltration of neutrophils (Sun et al., 2010).
Cognitive impairment is becoming a serious mental deficit that severely affects the life quality of patients following CI/R (Jokinen et al., 2006). HSYA has the capacity to improve neurological deficit scores and increase the surviving hippocampal CA1 pyramidal cells in focal CI/R rats (Sun et al., 2010). HSYA injected via the common carotid artery significantly rescued the neurological and cognitive functional deficits of MCAO rats against CI/R injury. Meanwhile, HSYA could markedly down-regulate JAK2-mediated signaling, while promoting the expression of the suppressor of cytokine signaling protein 3 (SOCS3) (Yu et al., 2018; Yu et al., 2020). Furthermore, the neuroprotective effect of HSYA against CI/R injury might be conferred through activating the Akt-dependent autophagy pathway (Qi et al., 2014). In both OGD/R-induced primary mouse neurons and PC12 cells, HSYA inhibited phenylalanine biosynthesis to enhance mitochondrial function and biogenesis for neuroprotection (Chen S. N. et al., 2019).
PI3K-mediated signaling pathways are also involved in the protective effects of HSYA against apoptosis and autophagy during CI/R. Chen et al. reported that HSYA critically reduced CI/R-mediated apoptosis through the PI3K/Akt/glycogen synthase kinase 3β signaling pathway (Chen et al., 2013). HSYA protected the cerebral microvascular endothelial cells against OGD/R-induced injury by inhibiting autophagy via the Class I PI3K/Akt/mTOR signaling pathway (Yang et al., 2018). Proteomic analysis showed that mTOR, Eftud2, Rab11, Ppp2r5e, and HIF-1 signaling pathways were the key hub proteins and pathways in HSYA against CI/R injury (Xu et al., 2019). Therefore, the PI3K/Akt/mTOR signaling pathway needs to be further studied to clarify the mechanisms of actions of HSYA against CI/R injury.
Similar to MI/R, CI/R also results in mtPTP opening. Mechanically, HSYA could inhibit mtPTP opening by inhibiting Ca2+-induced ROS generation and H2O2-induced swelling of mitochondria isolated from rat brains, improving mitochondrial energy metabolism and enhancing ATP levels and the respiratory control ratio in the ischemia brain (Tian et al., 2008).
Effects on Vascular Dementia (VaD)
VaD is characterized by pathological damage and a decline in intelligence resulting from hypoxic-ischemic or hemorrhagic brain injury (Zhao X. X. et al., 2018). As mentioned before, HSYA has the capacity to improve cognitive impairment. In VaD rats, Zhang et al. revealed that HSYA promoted angiogenesis and increased synaptic plasticity via up-regulating the hippocampal expressions of VEGF-A, NMDAR type-1, BDNF, and GluN2B (a subunit of NMDAR), thus improving spatial learning and memory (Zhang et al., 2014; Xing et al., 2016). Although no drug is approved, the above findings may shed light on the therapeutic potential of HSYA for managing the progress of VaD.
Effects on Traumatic Brain Injury (TBI)
TBI refers to the injury of cerebral tissue structure/function caused by various kinds of mechanical violence in the outside world (Xiu et al., 2018). HSYA has the potential to be utilized as a neuroprotective agent in cases of TBI. Firstly, TBI enables HSYA to distribute in the cerebral tissues of rats (Bie et al., 2010). Then, the antioxidant effect of HSYA in the brain of the TBI rats could explain the TBI improvement via increasing SOD, catalase and glutathione levels, while reducing MDA and oxidized glutathione (GSSG) levels (Bie et al., 2010; Wang Y. et al., 2016). Lastly, HSYA could increase mitochondrial ATPase (i.e., Na+, K+-ATPase, Ca2+-ATPase, and Mg2+-ATPase) and tissue plasminogen activator activities, while decreasing plasma plasminogen activator inhibitor-1 activity and MMP-9 expression in the hippocampus of TBI rats (Wang Y. et al., 2016).
In summary, the above findings buttress the assertion that HSYA exerts cardio-cerebrovascular protective activities through complex pathways and exhibits a definite curative effect in the application of CCVDs (Table 1 and Figure 2).
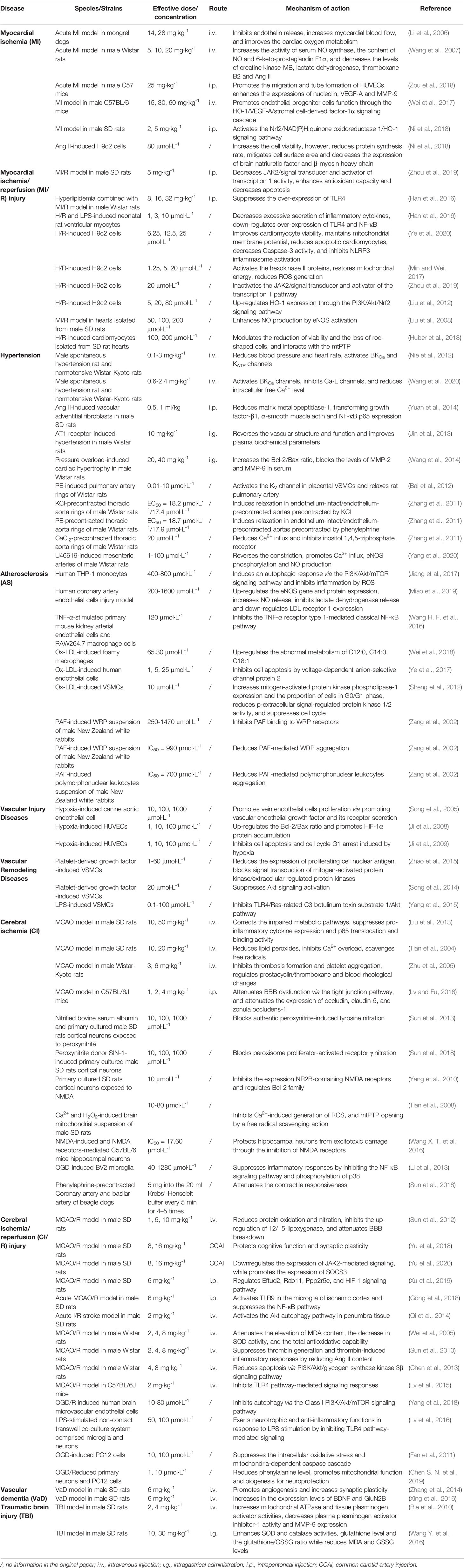
Table 1 Summary of pharmacological effects and mechanisms of HSYA on cardio-cerebrovascular diseases.
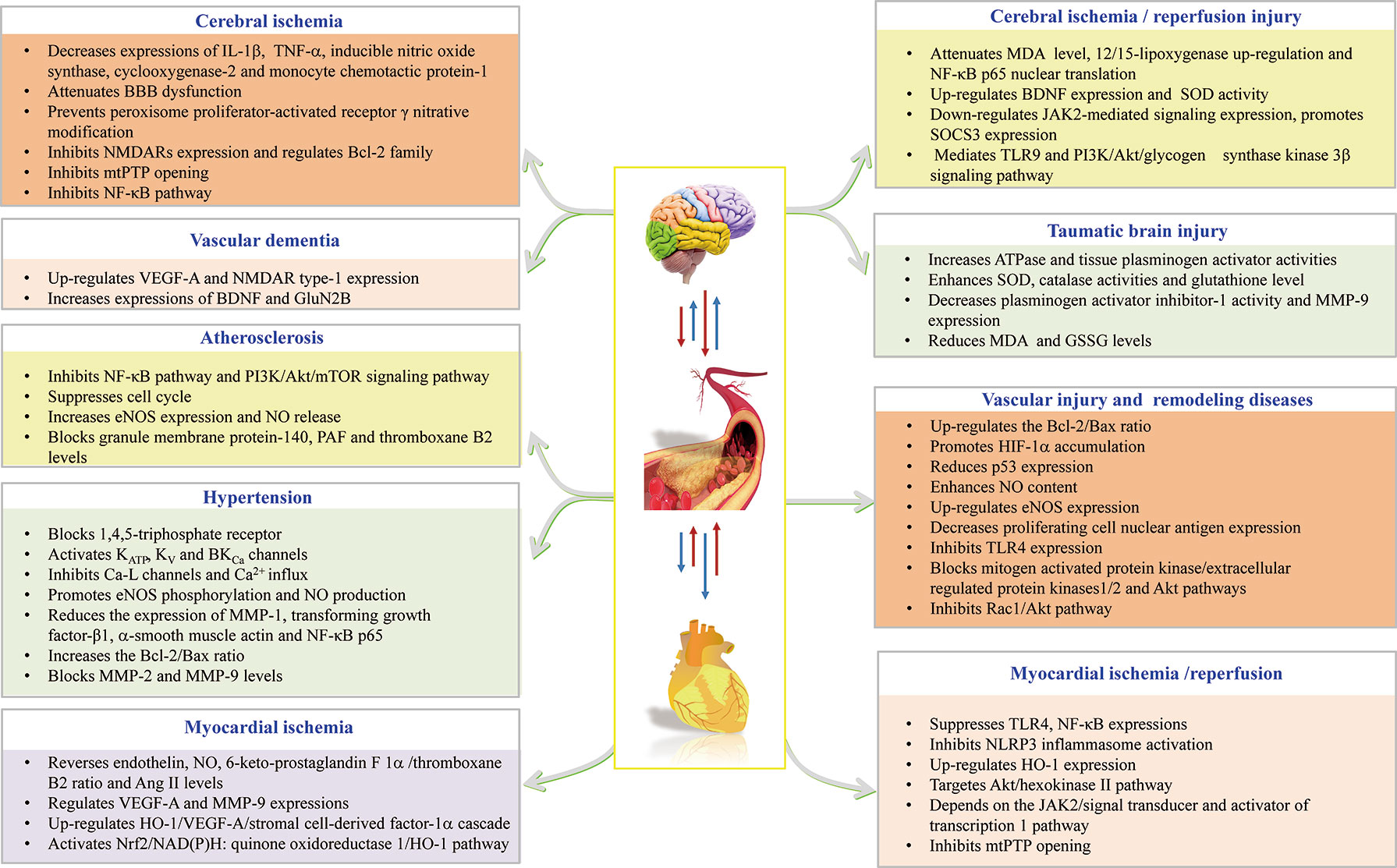
Figure 2 HSYA acts on the functional targets and signaling pathways of cardio-cerebrovascular diseases.
Conclusions and Prospects
It is becoming clear that the important mechanisms by which HSYA exerts extensive biological activities in CCVDs are through its antioxidant, anti-inflammatory, and neuroprotective effects. And there is no doubt that HSYA is a promising lead drug candidate in designing new multi-targeted therapeutic agents against CCVDs. The other analogues of HSYA, safflor yellow A (Duan et al., 2013) and safflor yellow B (Wang et al., 2009; Wang et al., 2013), showed similar protective effects against CCVDs. Further structural modification of HSYA should be extensively made and coupled with quantitative structure-activity relationship studies to develop more selective and safe drugs.
The oral bioavailability of HSYA is extremely low (~1.2%) (Ekin, 2005) and oral administration of HSYA accounts for about 0.9% of all in vivo experiments from Table 1. However, among many administration routes, oral administration is of great significance in drug formation because of its convenience and safety. Thus, the microemulsion, nanoemulsion, and nanoparticles of HSYA have been developed to overcome the low oral bioavailability (Li et al., 2010; Qi et al., 2011; Lv et al., 2012; Shi et al., 2018; Zhao B. X. et al., 2018). Considering the weak ability of HSYA to penetrate the BBB in physiologic condition (He et al., 2008), Borneolum Syntheticum and Acori Talarinowii Rhizoma were used to enhance its BBB permeability (Wu et al., 2011). Nevertheless, more attention should be payed to the overall efficacy and safety evaluation of HSYA before and after improving oral bioavailability and BBB permeability.
In the past decade, cohesive evidence showed that gut microbiota may serve as a therapeutic target of natural compounds derived from TCM (Yue et al., 2019a; Yue et al., 2019b). Oral HSYA is mostly retained in the intestinal tract as its prototype, which inevitably interacts with gut microbiota. Obesity is considered to be one of the most important risk factors of CCVDs. Our research group reported that HSYA mediated its anti-obesity effects by reversing gut microbiota dysbiosis in obese mice, followed by increasing short-chain fatty acid (SCFA)-producing bacteria (Liu J. et al., 2018). For SCFAs, it has a well-established role in maintaining host immune function after MI (Tang et al., 2019). The potential for microbial synthesis of SCFAs, including propionate and butyrate, was low in patients with atherosclerotic cardiovascular disease (Jie et al., 2017). Recently, the microbiota-gut-brain axis has shown to influence BBB permeability and the pathological process of TBI (Braniste et al., 2014; Ma et al., 2017). Thus, it is feasible to unveil the underlying mechanisms of oral HSYA on CCVDs from the new perspective of gut microbiota modulation.
Carthami Flos is a common part of preparations used in TCM and other traditional medicinal systems. It is necessary to strengthen the compatibility research of HSYA and other TCM-derived components. As an example, HSYA and Danshensu synergistically enhanced the antioxidant defense system and anti-apoptotic effects on MI/R injury through the Akt/Nrf2/HO-1 signaling pathway (Hu et al., 2016). Their combination further achieved enhanced neuroprotective effects on CI/R injury by alleviating pro-inflammatory and oxidative stress reactions via the TLR4/NF-κB and Nrf2/HO-1 pathways (Xu et al., 2017). On the other hand, combinations with existing western medications may also provide new therapy options for CCVDs patients. For example, HSYA as an add-on therapy to acetylglutamine could synergistically modulate the neuronal apoptosis and inflammation process during CI/R (Deng et al., 2018). However, it is important to note that HSYA is able to inhibit cytochrome P450 (CYP) enzymes’ (i.e. CYP1A2 and CYP2C11) activities but induces CYP3A1 activity (Xu et al., 2014). Hence, more detailed and advanced research should be done in the future to develop new compound formulas with HSYA, which may bring about important benefits for CCVDs patients and TCM modernization.
The randomized controlled clinical trial (RCT) is an essential step in confirming the efficacy and safety of drugs. In contrast with the large numbers of preclinical experiments, only a few completed RCTs of HSYA were reported, which were mainly reflected in evaluating the efficacy and safety of HSYA injection in the treatment of acute ischemic stroke with blood stasis syndrome (Qin et al., 2016; Hu et al., 2020), followed by a currently ongoing phase III RCT (No. CTR20150839, http://www.chinadrugtrials.org.cn/). However, none of the existing RCTs were of high methodological quality, and the conclusions need to be further verified by large sample, multicenter, and double-blind RCTs (as compared to traditional treatment regimens). In addition, clinical evidence supporting the application of HSYA for the management of CCVDs other than acute ischemic stroke with blood stasis syndrome is still lacking.
Author Contributions
S-JY and Y-PT conceived and designed the review. XB searched the literature and drafted the manuscript. W-XW and R-JF examined the literature and made the figures. HG edited the manuscript. S-JY, Y-YC, and Y-PT made a critical revision of the review. All authors contributed to the article and approved the submitted version.
Funding
This work was supported by the National Natural Science Foundation of China (81903786, 81773882), the Natural Science Foundation of Shaanxi Province (2019JQ-054), the Young Talent Support Program from the Association for Science and Technology of Colleges in Shaanxi Province (20190306), grants from the Key Research and Development Program of Shaanxi (2019ZDLSF04-05), Shaanxi Administration of Traditional Chinese Medicine (2019-ZZ-JC018), and Subject Innovation Team of Shaanxi University of Chinese Medicine (2019-YL10).
Conflict of Interest
The authors declare that the research was conducted in the absence of any commercial or financial relationships that could be construed as a potential conflict of interest.
Abbreviations
Akt, the protein kinase B; AMPK, 5’-monophosphate -activated protein kinase; Ang II, angiotensin II; AS, atherosclerosis; Bax, Bcl-2 associated X protein; BBB, blood-brain barrier; Bcl-2, B-cell lymphoma-2; BDNF, brain-derived neurotrophic factor; CCVDs, cardio-cerebrovascular diseases; CI, cerebral ischemia; CI/R, cerebral ischemia/reperfusion; CYP, cytochrome P450; eNOS, endothelial nitric oxide synthase; GSSG, oxidized glutathione; H/R, hypoxia/reoxygenation; HIF-1, hypoxia inducible factor-1; HO-1, heme oxygenase-1; HSYA, hydroxysafflor yellow A; HUVECs, human umbilical vein endothelial cells; IL, interleukin; JAK2, janus kinase 2/signal transducer; LDL, low-density lipoprotein; LPS, lipopolysaccharide; MCAO, middle cerebral artery occlusion; MDA, malondialdehyde; MI, myocardial ischemia; MI/R, myocardial ischemia/reperfusion; MMP, matrix metalloproteinase; mTOR, mammalian target of rapamycin; mtPTP, mitochondrial permeability transition pore; NF-κB, nuclear factor kappa beta; NLRP3, NOD-like receptor 3; NMDARs, NR2B-containing N-methyl-d-aspartate receptors; NO, nitric oxide; Nrf2, nuclear factor erythroid-2-related factor 2; OGD, oxygen-glucose deprivation; OGD/R, OGD/reoxygenation; ox-LDL, oxidized low-density lipoprotein; PAF, platelet activating factor; PI3K, phosphoinositide 3-kinase; RCT, randomized controlled clinical trial; ROS, reactive oxygen species; SCFA, short-chain fatty acid; SD, Sprague-Dawley; SOCS3, suppressor of cytokine signaling protein 3; SOD, superoxide dismutase; TBI, traumatic brain injury; TCM, traditional Chinese medicine; TLR, toll like receptor; TNF-α, tumor necrosis factor-α; VaD, vascular dementia; VEGF, vascular endothelial growth factor; VSMCs, vascular smooth muscle cells; WRP, washed rabbits platelet.
References
Bacchetti, T., Morresi, C., Bellachioma, L., Ferretti, G. (2020). Antioxidant and pro-oxidant properties of Carthamus tinctorius, hydroxy safflor yellow A, and safflor yellow A. Antioxidants 9, 119. doi: 10.3390/antiox9020119
Bai, Y. H., Lu, P., Han, C. H., Yu, C. Y., Chen, M. G., He, F., et al. (2012). Hydroxysafflor yellow A (HSYA) from flowers of Carthamus tinctorius L. and its vasodilatation effects on pulmonary artery. Molecules 17, 14918–14927. doi: 10.3390/molecules171214918
Bhosale, G., Duchen, M. R. (2019). Investigating the mitochondrial permeability transition pore in disease phenotypes and drug screening. Curr. Protoc. Pharmacol. 85, e59. doi: 10.1002/cpph.59
Bie, X. D., Han, J., Dai, H. B. (2010). Effects of hydroxysafflor yellow A on the experimental traumatic brain injury in rats. J. Asian Nat. Prod. Res. 12, 239–247. doi: 10.1080/10286020903510636
Braniste, V., AlAsmakh, M., Kowal, C., Anuar, F., Abbaspour, A., Toth, M., et al. (2014). The gut microbiota influences blood-brain barrier permeability in mice. Sci. Transl. Med. 6, 263ra158. doi: 10.1126/scitranslmed.3009759
Chen, L., Xiang, Y. X., Kong, L. J., Zhang, X. M., Sun, B. Z., Wei, X. B. (2013). Hydroxysafflor yellow A protects against cerebral ischemia-reperfusion injury by antiapoptotic effect through PI3K/Akt/GSK3β pathway in rat. Neurochem. Res. 38, 2268–2275. doi: 10.1007/s11064-013-1135-8
Chen, S. N., Sun, M., Zhao, X. H., Yang, Z. F., Liu, W. X., Cao, J. Y., et al. (2019). Neuroprotection of hydroxysafflor yellow A in experimental cerebral ischemia/reperfusion injury via metabolic inhibition of phenylalanine and mitochondrial biogenesis. Mol. Med. Rep. 19, 3009–3020. doi: 10.3892/mmr.2019.9959
Chen, Z. X., Xu, Q. Q., Shan, C. S., Shi, Y. H., Wang, Y., Chang, R. C. C., et al. (2019). Borneol for regulating the permeability of the blood-brain barrier in experimental ischemic stroke: preclinical evidence and possible mechanism. Oxid. Med. Cell Longev. 2019:2936737. doi: 10.1155/2019/2936737
Deng, L., Wan, H. T., Zhou, H. F., Yu, L., He, Y. (2018). Protective effect of hydroxysafflor yellow A alone or in combination with acetylglutamine on cerebral ischemia reperfusion injury in rat: A PET study using 18F-fuorodeoxyglucose. Eur. J. Pharmacol. 825, 119–132. doi: 10.1016/j.ejphar.2018.02.011
Duan, J. L., Wang, J. W., Guan, Y., Yin, Y., Wei, G., Cui, J., et al. (2013). Safflor yellow A protects neonatal rat cardiomyocytes against anoxia/reoxygenation injury in vitro. Acta Pharmacol. Sin. 34, 487–495. doi: 10.1038/aps.2012.185
Ekin, Z. (2005). Resurgence of safflower (Carthamus tinctorius L.) utilization: a global view. J. Agron. 4, 83–87. doi: 10.3923/ja.2005.83.87
Fan, L. H., Dang, X. Q., Shi, Z. B., Zhang, C., Wang, K. Z. (2011). Hydroxysafflor yellow A protects PC12 cells against the apoptosis induced by oxygen and glucose deprivation. Cell. Mol. Neurobiol. 31, 1187–1194. doi: 10.1007/s10571-011-9720-3
Fan, J. X., Qin, X. M., Li, Z. Y. (2019). Molecular docking and multivariate analysis studies of active compounds in the safflower injection. J. Liq. Chromatogr. R. T. 42, 673–680. doi: 10.1080/10826076.2019.1665540
Gong, Z., Pan, J. R., Li, X. P., Wang, H. X., He, L., Peng, Y. (2018). Hydroxysafflor yellow A reprograms TLR9 signalling pathway in ischaemic cortex after cerebral ischaemia and reperfusion. CNS Neurol. Disord-DR. 17, 370–382. doi: 10.2174/1871527317666180502110205
Guo, X. J., Zheng, M., Pan, R. Y., Zang, B. X., Gao, J. W., Ma, H. Y., et al. (2019). Hydroxysafflor yellow A (HSYA) targets the platelet-activating factor (PAF) receptor and inhibits human bronchial smooth muscle activation induced by PAF. Food Funct. 10, 4661–4673. doi: 10.1039/c9fo00896a
Han, D., Wei, J., Zhang, R., Ma, W., Shen, C., Feng, Y. D., et al. (2016). Hydroxysafflor yellow A alleviates myocardial ischemia/reperfusion in hyperlipidemic animals through the suppression of TLR4 signaling. Sci. Rep. 6:35319. doi: 10.1038/srep35319
He, P. P., Fu, F. H., Wang, T., Li, C. K., Xin, W. Y., Zhang, X. M. (2008). Effect of cerebral ischemia/reperfusion injury on hydroxysafflor yellow A penetrating across the blood-brain barrier. Sci. Pharm. 76, 713–724. doi: 10.3797/scipharm.0811-01
Hu, T. X., Wei, G., Xi, M. M., Yan, J. J., Wu, X. X., Wang, Y. H., et al. (2016). Synergistic cardioprotective effects of Danshensu and hydroxysafflor yellow A against myocardial ischemia-reperfusion injury are mediated through the Akt/Nrf2/HO-1 pathway. Int. J. Mol. Med. 38, 83–94. doi: 10.3892/ijmm.2016.2584
Hu, Z. C., Xie, Z. J., Tang, Q., Li, X. B., Fu, X., Feng, Z. H., et al. (2018). Hydroxysafflor yellow A (HSYA) targets the NF-κB and MAPK pathways and ameliorates the development of osteoarthritis. Food Funct. 9, 4443–4456. doi: 10.1039/C8FO00732B
Hu, M. Z., Zhou, Z. Y., Zhou, Z. Y., Lu, H., Gao, M., Liu, L. M., et al. (2020). Effect and safety of hydroxysafflor yellow A for injection in patients with acute ischemic stroke of blood stasis syndrome: A phase II, multicenter, randomized, double-blind, multiple-dose, active-controlled clinical trial. Chin. J. Integr. Med. 26, 420–427. doi: 10.1007/s11655-020-3094-7
Huang, L., Li, L., Hu, E. C., Chen, G., Meng, X. M., Xiong, C. M., et al. (2018). Potential biomarkers and targets in reversibility of pulmonary arterial hypertension secondary to congenital heart disease: an explorative study. Pulm. Circ. 8:204589321875598. doi: 10.1177/2045893218755987
Huber, G. A., Priest, S. M., Geisbuhler, T. P. (2018). Cardioprotective effect of hydroxysafflor yellow A via the cardiac permeability transition pore. Planta Med. 84, 507–518. doi: 10.1055/s-0043-122501
Ivey, M. E., Osman, N., Little, P. J. (2008). Endothelin-1 signalling in vascular smooth muscle: Pathways controlling cellular functions associated with atherosclerosis. Atherosclerosis 199, 237–247. doi: 10.1016/j.atherosclerosis.2008.03.006
Ji, D. B., Zhu, M. C., Zhu, B., Zhu, Y. Z., Li, C. L., Ye, J., et al. (2008). Hydroxysafflor yellow A enhances survival of vascular endothelial cells under hypoxia via upregulation of the HIF-1α-VEGF pathway and regulation of Bcl-2/Bax. J. Cardiovasc. Pharm. 52, 191–202. doi: 10.1097/FJC.0b013e318181fb02
Ji, D. B., Zhang, L. Y., Li, C. L., Ye, J., Zhu, H. B. (2009). Effect of hydroxysafflor yellow A on human umbilical vein endothelial cells under hypoxia. Vasc. Pharmacol. 50, 137–145. doi: 10.1016/j.vph.2008.11.009
Jiang, Y. Q., Kou, J. Y., Han, X. B., Li, X. S., Zhong, Z. Y., Liu, Z. N., et al. (2017). ROS-dependent activation of autophagy through the PI3K/Akt/mTOR pathway is induced by hydroxysafflor yellow A-sonodynamic therapy in THP-1 macrophages. Oxid. Med. Cell. Longev. 2017, 8519169. doi: 10.1155/2017/8519169
Jie, Z. Y., Xia, H. H., Zhong, S. L., Feng, Q., Li, S. H., Liang, S. S., et al. (2017). The gut microbiome in atherosclerotic cardiovascular disease. Nat. Commun. 8, 845. doi: 10.1038/s41467-017-00900-1
Jin, Z., Zhang, W. H., Chai, W. R., Zheng, Y. Q., Zhi, J. M. (2013). Antibodies against AT1 receptors are associated with vascular endothelial and smooth muscle function impairment: Protective effects of hydroxysafflor yellow A. PloS One 8, e67020. doi: 10.1371/journal.pone.0067020
Jokinen, H., Kalska, H., Mäntylä, R., Pohjasvaara, T., Ylikoski, R., Hietanen, M., et al. (2006). Cognitive profile of subcortical ischaemic vascular disease. J. Neurol. Neurosurg. Psychiatry 77, 28–33. doi: 10.1136/jnnp.2005.069120
Kazantsev, A. G., Outeiro, T. F. (2010). Drug discovery for CNS disorders: from bench to bedside. CNS Neurol. Disord-DR. 9, 668. doi: 10.2174/187152710793237395
Kotla, S., Singh, N. K., Rao, G. N. (2017). ROS via BTK-p300-STAT1-PPARγ signaling activation mediates cholesterol crystals-induced CD36 expression and foam cell formation. Redox Biol. 11, 350–364. doi: 10.1016/j.redox.2016.12.005
Li, X. Z., Liu, J. X., Shang, X. H., Fu, J. H. (2006). Protective effects of hydroxysafflor yellow A on acute myocardial ischemia in dogs. Chin. Pharm. Bull. 22, 533–537.
Li, J. R., Sun, M. J., Ping, Q. N., Chen, X. J., Qi, J. P., Han, D. E. (2010). Metabolism, excretion and bioavailability of hydroxysafflor yellow A after oral administration of its lipid-based formulation and aqueous solution in rats. Chin. J. Nat. Med. 8, 233–240. doi: 10.3724/SP.J.1009.2010.00223
Li, J., Zhang, S. Y., Lu, M. R., Chen, Z. B., Chen, C., Han, L. J., et al. (2013). Hydroxysafflor yellow A suppresses inflammatory responses of BV2 microglia after oxygen-glucose deprivation. Neurosci. Lett. 535, 51–56. doi: 10.1016/j.neulet.2012.12.056
Liu, Y. N., Zhou, Z. M., Chen, P. (2008). Evidence that hydroxysafflor yellow A protects the heart against ischaemia-reperfusion injury by inhibiting mitochondrial permeability transition pore opening. Clin. Exp. Pharmacol. P. 35, 211–216. doi: 10.1111/j.1440-1681.2007.04814.x
Liu, S. X., Zhang, Y., Wang, Y. F., Li, X. C., Xiang, M. X., Bian, C., et al. (2012). Upregulation of heme oxygenase-1 expression by hydroxysafflor yellow A conferring protection from anoxia/reoxygenation-induced apoptosis in H9c2 cardiomyocytes. Int. J. Cardiol. 160, 95–101. doi: 10.1016/j.ijcard.2011.03.033
Liu, Y. Y., Lian, Z. Q., Zhu, H. B., Wang, Y. H., Yu, S. S., Chen, T. T., et al. (2013). A systematic, integrated study on the neuroprotective effects of hydroxysafflor yellow A revealed by 1H NMR-based metabonomics and the NF-κB pathway. Evid. Based. Compl. Alt. 2013, 147362. doi: 10.1155/2013/147362
Liu, Y. Q., Tian, X. F., Cui, M. Z., Zhao, S. Z. (2014). Safflower yellow inhibits angiotensin II-induced adventitial fibroblast proliferation and migration. J. Pharmacol. Sci. 126, 107–114. doi: 10.1254/jphs.14055FP
Liu, J., Yue, S. J., Yang, Z. R., Feng, W. W., Meng, X. T., Wang, A. T., et al. (2018). Oral hydroxysafflor yellow A reduces obesity in mice by modulating the gut microbiota and serum metabolism. Pharmacol. Res. 134, 40–50. doi: 10.1016/j.phrs.2018.05.012
Liu, Z., Xu, Y. Q., Ji, X. M. (2018). Progress in clinical diagnosis and treatment of cardio-cerebrovascular diseases. Chin. J. Geriatr. Heart Brain Vessel Dis. 20, 1219–1220. doi: 10.3969/j.issn.1009-0126.2018.11.025
Lv, Y. N., Fu, L. S. (2018). The potential mechanism for hydroxysafflor yellow A attenuating blood-brain barrier dysfunction via tight junction signaling pathways excavated by an integrated serial affinity chromatography and shotgun proteomics analysis approach. Neurochem. Int. 112, 38–48. doi: 10.1016/j.neuint.2017.10.012
Lv, L. Z., Tong, C. Q., Lv, Q., Tao, X. J., Li, L. M., Fang, Q. X., et al. (2012). Enhanced absorption of hydroxysafflor yellow A using a self-double-emulsifying drug delivery system: In vitro and in vivo studies. Int. J. Nanomed. 7, 4099–4107. doi: 10.2147/IJN.S33398
Lv, Y. N., Qian, Y. S., Fu, L. S., Chen, X. Y., Zhong, H. L., Wei, X. H. (2015). Hydroxysafflor yellow A exerts neuroprotective effects in cerebral ischemia reperfusion-injured mice by suppressing the innate immune TLR4-inducing pathway. Eur. J. Pharmacol. 769, 324–332. doi: 10.1016/j.ejphar.2015.11.036
Lv, Y. N., Qian, Y. S., Ouyang, A. J., Fu, L. S. (2016). Hydroxysafflor yellow A attenuates neuron damage by suppressing the lipopolysaccharide-induced TLR4 pathway in activated microglial cells. Cell. Mol. Neurobiol. 36, 1241–1256. doi: 10.1007/s10571-015-0322-3
Ma, G. N., Yu, F. L., Zhang, H., Li, Z. P., Mei, X. G. (2014). Pharmacokinetics and biopharmaceutics of hydroxysafflor yellow A: research advances. J. Int. Pharm. Res. 41, 195–200. doi: 10.13220/j.cnki.jipr.2014.02.012
Ma, E. L., Smith, A., Desai, N., Cheung, L., Hanscom, M., Stoica, B. A., et al. (2017). Bidirectional brain-gut interactions and chronic pathological changes after traumatic brain injury in mice. Brain Behav. Immun. 66, 56–69. doi: 10.1016/j.bbi.2017.06.018
Martin, H. G. S., Wang, Y. T. (2010). Blocking the deadly effects of the NMDA receptor in stroke. Cell 140, 174–176. doi: 10.1016/j.cell.2010.01.014
McSweeney, S. R., Warabi, E., Siow, R. C. M. (2016). Nrf2 as an endothelial mechanosensitive transcription factor. Hypertension 67, 20–29. doi: 10.1161/HYPERTENSIONAHA.115.06146
Miao, T. J., Qian, L., Yu, F., Hu, L. G., Han, J. Q., An, Y. (2019). Protective effects of hydroxysafflor yellow A on high oxidized low-density lipoprotein induced human coronary artery endothelial cells injuries. Cancer Cell Res. 22, 581–589.
Mills, K. T., Bundy, J. D., Kelly, T. N., Reed, J. E., Kearney, P. M., Reynolds, K., et al. (2016). Global disparities of hypertension prevalence and control: a systematic analysis of population-based studies from 90 countries. Circulation 134, 441–450. doi: 10.1161/CIRCULATIONAHA.115.018912
Min, J., Wei, C. (2017). Hydroxysafflor yellow A cardioprotection in ischemia-reperfusion (I/R) injury mainly via Akt/hexokinase II independent of ERK/GSK-3β pathway. Biomed. Pharmacother. 87, 419–426. doi: 10.1016/j.biopha.2016.12.113
Minno, A. D., Stornaiuolo, M., Novellino, E. (2019). Molecular scavengers, oxidative stress and cardiovascular disease. J. Clin. Med. 8, 1895. doi: 10.3390/jcm8111895
Moskowitz, M. A., Lo, E. H., Iadecola, C. (2010). The science of stroke: mechanisms in search of treatments. Neuron 67, 181–198. doi: 10.1016/j.neuron.2010.07.002
Ni, B., Zhou, D. L., Jing, Y. Y., Liu, S. X. (2018). Hydroxysafflor yellow A protects against angiotensin II-induced hypertrophy. Mol. Med. Rep. 18, 3649–3656. doi: 10.3892/mmr.2018.9399
Nie, P. H., Zhang, L., Zhang, W. H., Rong, W. F., Zhi, J. M. (2012). The effects of hydroxysafflor yellow A on blood pressure and cardiac function. J. Ethnopharmacol. 139, 746–750. doi: 10.1016/j.jep.2011.11.054
Pearson, A. C., Pasierski, T., Labovitz, A. J. (1991). Left ventricular hypertrophy: Diagnosis, prognosis and management. Am. Heart J. 121, 148–157. doi: 10.1016/0002-8703(91)90968-N
Petrovski, G., Ayna, G., Májai, G., Hodrea, J., Benkő, S., Mádi, A., et al. (2011). Phagocytosis of cells dying through autophagy induces inflammasome activation and IL-1β release in human macrophages. Autophagy 7, 321–330. doi: 10.4161/auto.7.3.14583
Qi, J. P., Zhuang, J., Wu, W., Lu, Y., Song, Y. M., Zhang, Z. T., et al. (2011). Enhanced effect and mechanism of water-in-oil microemulsion as an oral delivery system of hydroxysafflor yellow A. Int. J. Nanomed. 6, 985–991. doi: 10.2147/IJN.S18821
Qi, Z. F., Yan, F., Shi, W. J., Zhang, C. C., Dong, W., Zhao, Y. M., et al. (2014). AKT-related autophagy contributes to the neuroprotective efficacy of hydroxysafflor yellow A against ischemic stroke in rats. Transl. Stroke Res. 5, 501–509. doi: 10.1007/s12975-014-0346-x
Qin, S. C., Liang, W. X., Wen, X. W., Deng, M. Z. (2016). Clinical efficacy of hydroxysafflor yellow A injection for acute ischemic stroke with blood stasis syndrome. Chin. J. Exp. Tradit. Med. Form. 9, 40–41. doi: 10.13422/j.cnki.syfjx.2016210157
Ribeiro, R., Badiwala, M. V., Ramzy, D., Tumiati, L. C., Rao, V. (2019). Recipient hypertonic saline infusion prevents cardiac allograft dysfunction. J. Thorac. Cardiov. Surg. 157, 615–625. doi: 10.1016/j.jtcvs.2018.07.018
Romanic, A. M., White, R. F., Arleth, A. J., Ohlstein, E. H., Barone, F. C. (1998). Matrix metalloproteinase expression increases after cerebral focal ischemia in rats: inhibition of matrix metalloproteinase-9 reduces infarct size. Stroke 29, 1020–1030. doi: 10.1161/01.str.29.5.1020
Scarabelli, T. M., Stephanou, A., Pasini, E. (2002). Different signaling pathways induce apoptosis in endothelial cells and cardiac myocytes during ischemia/reperfusion injury. Circ. Res. 90, 745–748. doi: 10.1161/01.RES.0000015224.07870.9A
Sheng, L., Bi, S. J., Cheng, C., Zhang, J. B. (2012). Hydroxysafflor yellow A suppresses oxidized low density lipoprotein induced proliferation of vascular smooth muscle cells. Bangl. J. Pharmacol. 7, 87–93. doi: 10.3329/bjp.v7i2.10499
Shi, M. X., Guo, Q., Huang, Y. R., Lu, W. J., Zhang, J. Q. (2018). Pharmacokinetics of hydroxysafflor yellow A water-in-oil nanoemulsion. Chin. Pharm. J. 53, 2108–2112. doi: 10.11669/cpj.2018.24.007
Song, Y., Zhang, L., Qu, K., Li, C. L., Zhu, H. B. (2005). Hydroxysafflor yellow A promotes vascular endothelial cell proliferation via VEGF/VEGF receptor. J. Chin. Pharm. Sci. 14, 181–185.
Song, Y. M., Long, L. L., Zhang, N., Liu, Y. H. (2014). Inhibitory effects of hydroxysafflor yellow A on PDGF-BB-induced proliferation and migration of vascular smooth muscle cells via mediating Akt signaling. Mol. Med. Rep. 10, 1555–1560. doi: 10.3892/mmr.2014.2336
Sun, X., Wei, X. B., Qu, S. F., Zhao, Y. X., Zhang, X. M. (2010). Hydroxysafflor yellow A suppresses thrombin generation and inflammatory responses following focal cerebral ischemia-reperfusion in rats. Bioorg. Med. Chem. Lett. 20, 4120–4124. doi: 10.1016/j.bmcl.2010.05.076
Sun, L., Yang, L., Xu, Y. W., Liang, H., Han, J., Zhao, R. J., et al. (2012). Neuroprotection of hydroxysafflor yellow A in the transient focal ischemia: inhibition of protein oxidation/nitration, 12/15-lipoxygenase and blood-brain barrier disruption. Brain Res. 1473, 227–235. doi: 10.1016/j.brainres.2012.07.047
Sun, L., Yang, L., Fu, Y., Han, J., Xu, Y. W., Liang, H., et al. (2013). Capacity of HSYA to inhibit nitrotyrosine formation induced by focal ischemic brain injury. Nitric. Oxide 35, 144–151. doi: 10.1016/j.niox.2013.10.002
Sun, L., Xu, Y. W., Han, J., Xiao, C., Cao, S. S., Liang, H., et al. (2018). Hydroxysafflor yellow A shows protection against PPARγ inactivation in nitrosative neurons. Oxid. Med. Cell Longev. 2018:9101740. doi: 10.1155/2018/9101740
Sun, Y., Xu, D. P., Qin, Z., Wang, P. Y., Hu, B. H., Yu, J. G., et al. (2018). Protective cerebrovascular effects of hydroxysafflor yellow A (HSYA) on ischemic stroke. Eur. J. Pharmacol. 818, 604–609. doi: 10.1016/j.ejphar.2017.11.033
Tang, T. W. H., Chen, H. C., Chen, C. Y., Yen, C. Y. T., Lin, C. J., Prajnamitra, R. P., et al. (2019). Loss of gut microbiota alters immune system composition and cripples postinfarction cardiac repair. Circulation 139, 647–659. doi: 10.1161/CIRCULATIONAHA.118.035235
Thiagarajan, H., Thiyagamoorthy, U., Shanmugham, I., Nandagopal, G. D., Kaliyaperumal, A. (2017). Angiogenic growth factors in myocardial infarction: A critical appraisal. Heart Fail. Rev. 22, 665–683. doi: 10.1007/s10741-017-9630-7
Tian, J. W., Fu, F. H., Jiang, W. L., Wang, C. Y., Sun, F., Zhang, T. P. (2004). Protective effect of hydroxysaflor yellow A against rat cortex mitochondrial injuries induced by cerebral ischemia. Acta Pharm. Sin. 39, 774–777. doi: 10.16438/j.0513-4870.2004.10.002
Tian, J. W., Li, G. S., Liu, Z. F., Fu, F. H. (2008). Hydroxysafflor yellow A inhibits rat brain mitochondrial permeability transition pores by a free radical scavenging action. Pharmacology 82, 121–126. doi: 10.1159/000141653
Upadhyay, R. K. (2014). Drug delivery systems, CNS protection, and the blood brain barrier. Biomed. Res. Int. 2014, 869269. doi: 10.1155/2014/869269
Wang, T., Fu, F. H., Han, B., Zhu, M., Li, G. S., Liu, K. (2007). Protection and mechanisms of hydroxysafflor yellow A on experimental myocardial infarction in rats. Chin. Tradit. Herb. Drugs 38, 1853–1856.
Wang, Z. J., Ke, Y. N., Chen, W. L., Yu, C. A. (2008). Analysis of the platelet activated function of hyperlipemia rats. Chin. J. Compar. Med. 18, 10–13.
Wang, C. Y., Ma, H. M., Zhang, S. P., Wang, Y. F., Liu, J. T., Xiao, X. H. (2009). Safflor yellow B suppresses pheochromocytoma cell (PC12) injury induced by oxidative stress via antioxidant systemand Bcl-2/Bax pathway. N. S. Arch. Pharmacol. 380, 135–142. doi: 10.1007/s00210-009-0424-x
Wang, C. Y., He, Y. H., Yang, M., Sun, H. L., Zhang, S. P., Wang, C. H. (2013). Safflor yellow B suppresses angiotensin II-mediated human umbilical vein cell injury via regulation of Bcl-2/p22phox expression. Toxicol. Appl. Pharm. 273, 59–67. doi: 10.1016/j.taap.2013.08.018
Wang, J. P., Zhang, Q., Mei, X. H., Zhang, X. Z. (2014). Hydroxysafflor yellow A attenuates left ventricular remodeling after pressure overload-induced cardiac hypertrophy in rats. Pharm. Biol. 52, 31–35. doi: 10.3109/13880209.2013.805791
Wang, H. F., Liu, J. L., Yang, Y. J., Cao, Q. W., Huo, X. P., Ma, S. H., et al. (2016). Hydroxy-safflower yellow A inhibits the TNFR1-mediated classical NF-кB pathway by inducing shedding of TNFR1. Phytother. Res. 30, 790–796. doi: 10.1002/ptr.5579
Wang, X. T., Ma, Z. Y., Fu, Z. X., Gao, S., Yang, L., Jin, Y., et al. (2016). Hydroxysafflor yellow A protects neurons from excitotoxic death through inhibition of NMDARs. ASN Neuro 8, 1759091416642345. doi: 10.1177/1759091416642345
Wang, Y., Zhang, C. H., Peng, W. J., Xia, Z. A., Gan, P. P., Huang, W., et al. (2016). Hydroxysafflor yellow A exerts antioxidant effects in a rat model of traumatic brain injury. Mol. Med. Rep. 14, 3690–3696. doi: 10.3892/mmr.2016.5720
Wang, N., He, D. M., Zhou, Y. Q., Wen, J., Liu, X. Q., Li, P. Y., et al. (2020). Hydroxysafflor yellow A actives BKCa channels and inhibits L-type Ca channels to induce vascular relaxation. Eur. J. Pharmacol. 870, 172873. doi: 10.1016/j.ejphar.2019.172873
Wei, X. B., Liu, H. Q., Sun, X., Fu, F. H., Zhang, X. M., Wang, J., et al. (2005). Hydroxysafflor yellow A protects rat brains against ischemia-reperfusion injury by antioxidant action. Neurosci. Lett. 386, 58–62. doi: 10.1016/j.neulet.2005.05.069
Wei, G., Yin, Y., Duan, J. L., Guo, C., Zhu, Y. R., Wang, Y. H., et al. (2017). Hydroxysafflor yellow A promotes neovascularization and cardiac function recovery through HO-1/VEGF-A/SDF-1α cascade. Biomed. Pharmacother. 88, 409–420. doi: 10.1016/j.biopha.2017.01.074
Wei, Z. Y., Xu, W. J., Dong, J. J., Liu, J., Jia, Z. X., Chen, Y. J., et al. (2018). Hydroxysafflor yellow A repairing the metabolic disturbances of early atherosclerosis based on fatty acid profiling. Acta Pharm. Sin. 53, 1680–1688. doi: 10.16438/j.0513-4870.2018-0409
Wu, X., Ouyang, L. N., Xiang, D. W., Xiang, D. X. (2011). Enhancing effect of Borneolum Syntheticum and Acori Talarinowii Rhizoma on penetrating blood-brain barrier of hydroxysafflor yellow A. Chin. Tradit. Herb. Drugs 42, 734–737.
Xie, Y. Y., Zhang, J. P., Fang, Z. H., Li, Y. F., Wang, M. Y. (2018). Research progress of different sources exosomes and myocardial ischemia reperfusion injury. J. Clin. Cardiol. 34, 841–845. doi: 10.13201/j.issn.1001-1439.2018.09.002
Xing, M. Y., Sun, Q. N., Wang, Y. Y., Cheng, Y., Zhang, N. (2016). Hydroxysafflor yellow A increases BDNF and NMDARs in the hippocampus in a vascular dementia rat model. Brain Res. 1642, 419–425. doi: 10.1016/j.brainres.2016.04.030
Xiu, G. H., Sun, J., Li, X. L., Yin, Y. Y., Li, B. Q., Lin, B. (2018). Effects of hyperbaric oxygen treatment on neurological functional and expression of CyclinD1 in rats with traumatic brain injury. J. Clin. Neurosurg. 15, 46–54. doi: 10.3969/j.issn.1672-7770.2018.01.011
Xu, R. A., Xu, Z. S., Ge, R. S. (2014). Effects of hydroxysafflor yellow A on the activity and mRNA expression of four CYP isozymes in rats. J. Ethnopharmacol. 151, 1141–1146. doi: 10.1016/j.jep.2013.12.025
Xu, H., Liu, W. X., Liu, T. L., Su, N., Guo, C., Feng, X. N., et al. (2017). Synergistic neuroprotective effects of Danshensu and hydroxysafflor yellow A on cerebral ischemia-reperfusion injury in rats. Oncotarget 8, 115434–115443. doi: 10.18632/oncotarget.23272
Xu, H., Liu, T. L., Wang, W. J., Su, N., Yang, L. D., Yang, Z. F., et al. (2019). Proteomic analysis of hydroxysafflor yellow A against cerebral ischemia-reperfusion injury in rats. Rejuv. Res. 22, 503–512. doi: 10.1089/rej.2018.2145
Xuan, J. W., Huang, M., Lu, Y. J., Tao, L. B. (2018). Economic evaluation of safflower yellow injection for the treatment of patients with stable angina pectoris in China: A cost-effectiveness analysis. J. Altern. Complem. Med. 24, 564–569. doi: 10.1089/acm.2017.0284
Yang, Q., Yang, Z. F., Liu, S. B., Zhang, X. N., Hou, Y., Li, X. Q., et al. (2010). Neuroprotective effects of hydroxysafflor yellow A against excitotoxic neuronal death partially through down-regulation of NR2B-containing NMDA receptors. Neurochem. Res. 35, 1353–1360. doi: 10.1007/s11064-010-0191-6
Yang, G. S., Zhou, X. Y., Chen, T., Deng, Y. D., Yu, D., Pan, S. Y., et al. (2015). Hydroxysafflor yellow A inhibits lipopolysaccharide-induced proliferation and migration of vascular smooth muscle cells via Toll-like receptor-4 pathway. Int. J. Clin. Exp. Med. 8, 5295–5302.
Yang, G., Wang, N., Seto, S. W., Chang, D., Liang, H. Z. (2018). Hydroxysafflor yellow A protects brain microvascular endothelial cells against oxygen glucose deprivation/reoxygenation injury: Involvement of inhibiting autophagy via class I PI3K/Akt/mTOR signaling pathway. Brain Res. Bull. 140, 243–257. doi: 10.1016/j.brainresbull.2018.05.011
Yang, J. F., Wang, R., Cheng, X. H., Qu, H. C., Qi, J., Li, D., et al. (2020). The vascular dilatation induced by hydroxysafflor yellow A (HSYA) on rat mesenteric artery through TRPV4-dependent calcium influx in endothelial cells. J. Ethnopharmacol. 256, 112790. doi: 10.1016/j.jep.2020.112790
Ye, F., Wang, J. H., Meng, W., Qian, J. R., Jin, M. (2017). Proteomic investigation of effects of hydroxysafflor yellow A in oxidized low-density lipoprotein-induced endothelial injury. Sci. Rep. 7, 17981. doi: 10.1038/s41598-017-18069-4
Ye, J. X., Wang, M., Wang, R. Y., Liu, H. T., Qi, Y. D., Fu, J. H., et al. (2020). Hydroxysafflor yellow A inhibits hypoxia/reoxygenation-induced cardiomyocyte injury via regulating the AMPK/NLRP3 inflammasome pathway. Int. Immunopharmacol. 82, 106316. doi: 10.1016/j.intimp.2020.106316
Yu, L., Duan, Y. H., Zhao, Z., He, W. D., Xia, M., Zhang, Q. J., et al. (2018). Hydroxysafflor yellow A (HSYA) improves learning and memory in cerebral ischemia reperfusion-injured rats via recovering synaptic plasticity in the hippocampus. Front. Cell. Neurosci. 12, 371. doi: 10.3389/fncel.2018.00371
Yu, L., Liu, Z. L., He, W. D., Chen, H. F., Lai, Z. L., Duan, Y. H., et al. (2020). Hydroxysafflor Yellow A Confers Neuroprotection from focal cerebral ischemia by modulating the crosstalk between JAK2/STAT3 and SOCS3 signaling pathways. Cell. Mol. Neurobiol. 40, 1271–1281. doi: 10.1007/s10571-020-00812-7
Yuan, W. D., Yang, D. X., Sun, X. H., Liu, W., Wang, L., Li, X. Y., et al. (2014). Effects of hydroxysafflor yellow A on proliferation and collagen synthesis of rat vascular adventitial fibroblasts induced by angiotensin II. Int. J. Clin. Exp. Patho. 7, 5772–5781.
Yue, S. J., Tang, Y. P., Li, S. J., Duan, J. A. (2013). Chemical and biological properties of quinochalcone C-glycosides from the florets of Carthamus Tinctorius. Molecules 18, 15220–15254. doi: 10.3390/molecules181215220
Yue, S. J., Tang, Y. P., Wang, L. Y., Tang, H., Li, S. J., Liu, P., et al. (2014). Separation and evaluation of antioxidant constituents from Carthamus tinctorius. China J. Chin. Mater. Med. 39, 3295–3300.
Yue, S. J., Qu, C., Zhang, P. X., Tang, Y. P., Jin, Y., Jiang, J. S., et al. (2016). Carthorquinosides A and B, quinochalcone C−glycosides with diverse dimeric skeletons from Carthamus tinctorius. J. Nat. Prod. 79, 2644–2651. doi: 10.1021/acs.jnatprod.6b00561
Yue, S. J., Wang, W. X., Yu, J. G., Chen, Y. Y., Shi, X. Q., Yan, D., et al. (2019a). Gut microbiota modulation with traditional Chinese medicine: A system biology-driven approach. Pharmacol. Res. 148, 104453. doi: 10.1016/j.phrs.2019.104453
Yue, S. J., Liu, J., Wang, A. T., Meng, X. T., Yang, Z. R., Peng, C., et al. (2019b). Berberine alleviates insulin resistance by reducing peripheral branched-chain amino acids. Am. J. Physiol. Endoc. M. 316, E73–E85. doi: 10.1152/ajpendo.00256.2018
Zang, B. X., Jin, M., Si, N., Zhang, Y., Wu, W., Piao, Y. Z. (2002). Antagonistic effect of hydroxysafflor yellow A on the platelet activating factor receptor. Acta Pharm. Sin. 37, 696–699. doi: 10.16438/j.0513-4870.2002.09.006
Zhang, L., Nie, P. H., Zhang, G. H., Rong, W. F., Zhi, J. M. (2011). Endothelium-independent vasodilation effect of hydroxysafflor yellow A in thoracic aorta of wistar rats. J. Med. Plant Res. 5, 2187–2191. doi: 10.1002/cmdc.201100121
Zhang, N., Xing, M. Y., Wang, Y. Y., Liang, H., Yang, Z., Shi, F. D., et al. (2014). Hydroxysafflor yellow A improves learning and memory in a rat model of vascular dementia by increasing VEGF and NR1 in the hippocampus. Neurosci. Bull. 30, 417–424. doi: 10.1007/s12264-013-1375-2
Zhang, J. X., Qi, M. J., Shi, M. Z., Chen, J. J., Zhang, X. Q., Yang, J., et al. (2019). Effects of Danhong injection, a traditional Chinese medicine, on nine cytochrome P450 isoforms in vitro. Biomed. Chromatogr. 33, e4454. doi: 10.1002/bmc.4454
Zhao, J. S., Fang, M. X., Guo, Q. S., Li, Y. F., Xu, B. Y., Lai, S. H., et al. (2015). Hydroxysafflor yellow A inhibited rat vascular smooth muscle cells proliferation induced by PDGF. Chin. J. Cell Biol. 37, 827–831. doi: 10.11844/cjcb.2015.06.0032
Zhao, B. X., Gu, S. F., Du, Y., Shen, M. J., Liu, X. R., Shen, Y. Q. (2018). Solid lipid nanoparticles as carriers for oral delivery of hydroxysafflor yellow A. Int. J. Pharmacol. 535, 164–171. doi: 10.1016/j.ijpharm.2017.10.040
Zhao, X. X., Li, H. S., Wang, H. Y. (2018). Research progress on risk factors of vascular dementia. Adv. Cardiovasc. Dis. 39, 328–331. doi: 10.16806/j.cnki.issn.1004-3934.2018.03.007
Zhou, D. L., Ding, T. T., Ni, B., Jing, Y. Y., Liu, S. X. (2019). Hydroxysafflor yellow A mitigated myocardial ischemia/reperfusion injury by inhibiting the activation of the JAK2/STAT1 pathway. Int. J. Mol. Med. 44, 405–416. doi: 10.3892/ijmm.2019.4230
Zhu, H. B., Zhang, L., Wang, Z. H., Tian, J. W., Fu, F. H., Liu, K., et al. (2005). Therapeutic effects of hydroxysafflor yellow A on focal cerebral ischemic injury in rats and its primary mechanisms. J. Asian Nat. Prod. Res. 7, 607–613. doi: 10.1080/10286020310001625120
Keywords: hydroxysafflor yellow A, quinochalcone C-glycoside, cardio-cerebrovascular diseases, Carthami Flos, ischemia
Citation: Bai X, Wang W-X, Fu R-J, Yue S-J, Gao H, Chen Y-Y and Tang Y-P (2020) Therapeutic Potential of Hydroxysafflor Yellow A on Cardio-Cerebrovascular Diseases. Front. Pharmacol. 11:01265. doi: 10.3389/fphar.2020.01265
Received: 06 June 2020; Accepted: 30 July 2020;
Published: 29 September 2020.
Edited by:
M. Carmen González-Mas, University of Valencia, SpainReviewed by:
Li Yu, Zhejiang Chinese Medical University, ChinaYa-nan Yang, Chinese Academy of Medical Sciences and Peking Union Medical College, China
Copyright © 2020 Bai, Wang, Fu, Yue, Gao, Chen and Tang. This is an open-access article distributed under the terms of the Creative Commons Attribution License (CC BY). The use, distribution or reproduction in other forums is permitted, provided the original author(s) and the copyright owner(s) are credited and that the original publication in this journal is cited, in accordance with accepted academic practice. No use, distribution or reproduction is permitted which does not comply with these terms.
*Correspondence: Shi-Jun Yue, c2hpanVuX3l1ZUAxNjMuY29t; Yu-Ping Tang, eXVwaW5ndGFuZ0BzbnRjbS5lZHUuY24=