- 1Key Laboratory of Liver and Kidney Diseases (Ministry of Education), Institute of Liver Diseases, Shuguang Hospital, Shanghai University of Traditional Chinese Medicine, Shanghai, China
- 2Central Research Institute, Shanghai Pharmaceuticals Holding Co., Ltd., Shanghai, China
- 3The MOE Key Laboratory for Standardization of Chinese Medicines and the SATCM Key Laboratory for New Resources and Quality Evaluation of Chinese Medicines, Institute of Chinese Materia Medica, Shanghai University of Traditional Chinese Medicine, Shanghai, China
- 4Shanghai Zhonghua Pharmaceutical Co., Ltd., Shanghai, China
- 5Huqingyutang Chinese Medicine Modernization Research Institute of Zhejiang Province, Hangzhou, China
Wei-Fu-Chun (WFC) tablet is a commercial medicinal product approved by China Food and Drug Administration, which is made of Panax ginseng C.A.Mey., Citrus aurantium L., and Isodon amethystoides (Benth.). WFC has been popularly used for the treatment of precancerous lesions of gastric cancer (PLGC) in clinical practice. In this study, a UHPLC-ESI-Q-TOF/MS method in both positive and negative ion mode was employed to rapidly survey the major constituents of WFC. 178 compounds including diterpenoids, triterpenes, sesquiterpenes, flavonoids, saponins, phenylpropanoids, lignans, coumarins, organic acids, fatty acids, quinones, and sterols, were identified by comparing their retention times, accurate mass within 5 ppm error, and MS fragmentation ions. In addition, 77 absorbed parent molecules and nine metabolites in rat serum were rapidly characterized by UHPLC-ESI-Q-TOF/MS. The network pharmacology method was used to predict the active components, corresponding therapeutic targets, and related pathways of WFC in the treatment of PLGC. Based on the main compounds in WFC and their metabolites in rat plasma and existing databases, 13 active components, 48 therapeutic targets, and 61 pathways were found to treat PLGC. The results of PLGC experiment in rats showed that WFC could improve the weight of PLGC rats and the histopathological changes of gastric mucosa partly by inhibiting Mitogen-activated protein kinase (MAPK) signaling pathway to increase pepsin secretion. This study offers an applicable approach to identify chemical components, absorbed compounds, and metabolic compounds in WFC, and provides a method to explore bioactive ingredients and action mechanisms of WFC.
Introduction
Traditional Chinese medicine (TCM) is based on the principle of holism, that is, all the body systems are interconnected. Thus, TCM treatments can be multi-target, multi-link, and with minimal side-effects in the prevention and treatment of precancerous lesions of gastric cancer (PLGC), among other diseases. The Wei-Fu-Chun (WFC) tablet, a well-known Chinese herbal preparation, is composed of three herbs: P. ginseng (HS: 131 g), C. aurantium (ZQ: 250 g), and I. amethystoides (XCC: 2,500 g). Dosage of these herbs was derived from Chinese Pharmacopoeia, 2020 edition. In the first part of this edition, WFC was mentioned to tonify spleen qi, promote blood circulation and detoxification, and to have been employed to treat PLGC in clinic for many years (Jin et al., 2015). PLGC is a group of diseases with pathological features of intestinal metaplasia or dysplasia based on chronic atrophic gastritis (Correa et al., 1975). PLGC is an important stage in the development of superficial gastritis to gastric cancer. According to the Chinese Cancer Statistics released by the Chinese Cancer Registry, the mortality rate of gastric cancer ranked second in China, following lung cancer. (Chen et al., 2016). Therefore, clinically halting the progression of PLGC to gastric cancer or reversing it has been the focus of current academic research. Some studies investigated the mechanisms of WFC to treat gastritis in vitro or in vivo (Zhao et al., 2012; Huang et al., 2014). However, no studies on the action mechanism of WFC in the treatment of PLGC have been conducted so far. With the prominence of network pharmacology in system biology, this distinct and novel approach to the study of complex analytical systems is becoming more widely known and more frequently used in the field of drug research. The role of network pharmacology includes uncovering the functions of TCM, providing scientific evidence for TCM, and establishing TCM as a scientifically-proven field (Ma et al., 2016). Here, we attempted to explore the action mechanisms of WFC in the treatment of PLGC using network pharmacology and experimental studies.
In this paper, a reliable and rapid ultra-high performance liquid chromatography coupled with electrospray ionization quadrupole time-of-flight mass spectrometry (UHPLC-ESI-Q-TOF/MS) method was established to profile complex compounds in WFC and describe their absorption behavior and metabolites in plasma samples after oral administration of WFC. The network pharmacology method was used to construct the pharmacology-network of the “origins-components-targets-pathways” of WFC to systematically predict the active components, targets, and signaling pathways that may treat PLGC. In addition, animal experiment was used to verify the prediction and clarify the mechanisms of action. This study provides a reference for further research and exploration of pharmacodynamics material basis and mechanisms of WFC.
Experimental Materials and Methods
Chemicals and Reagents
Drug samples of WFC were obtained from Huqingyutang Pharmaceutical Co., Ltd (Hangzhou, China, batch No. 17066174). HPLC-grade acetonitrile, methanol, and formic acid were purchased from Fisher Scientific (Santa Clara, United States). Ultrapure water was prepared using a Millipore Alpha-Q water system (Millipore, United States). All other reagents were of analytical grade. For animal experiments, the drugs were suspended in sterilized 0.5% carboxymethylcellulose sodium. Vitacoenzyme tablets (0.8 g) was purchased from Beihai Sunshine Pharmaceutical Co., Ltd (Guangxi, China, Lot no. 102029), and Pepsin kit from Nanjing Jiancheng Bioengineering Research Institute (Nanjing, China). MNNG was purchased from TOKYO Chemical Industry Co., Ltd (Tokyo, Japan). Ranitidine hydrochloride capsule was brought from Zhejiang Kangenbei Pharmaceutical Co., Ltd (Zhenjiang, China). TAKARA RNA reverse transcription kits were from Takara Biomedical Technology (Beijing) Co., Ltd (Beijing, China); TOYOBO amplification kit was purchased from toyo textile (Shanghai) biotechnology co., Ltd (Shanghai, China). Primers to amplify the genes ß-actin, Pi3k, Akt1, Mapk11, Fas, Mapk8, caspase3, Mapk14, Tp53, and Vegfα were designed by Shanghai Guanchun Biotechnology Co., Ltd (bioTNT) (Shanghai, China) (Table 1).
Ethics Statement
All feeding conditions were in compliance with the Chinese Animal Welfare Law and the relevant regulations of Fudan University and Shanghai University of Traditional Chinese Medicine Experimental Animal Ethics Committee. Wistar rats from the Experimental Animal Center of Shanghai University of Traditional Chinese Medicine were recruited to establish the model of gastric precancerous lesions. Animal license Code: SYXK Shanghai 2014-008. Ethics No.: PZSHUTCM19039006.
Animal Handling
Animal Model for Drug Metabolism Experiment in Rats
Ten specific pathogen-free male Sprague-Dawley rats (200 g) were provided by the Experimental Animal Center of Shanghai University of Traditional Chinese Medicine. Rats were housed in an animal room (24 ± 2°C, 60 ± 5% relative humidity) with the setting of a 12 h dark/12 h light cycle. Before the experiment, rats were given water and fed standard laboratory food for acclimatization for a week. Rats were fasted for 12 h before the sample collection but still had access to water throughout the experiment. WFC (dissolved in purified water) was orally administered to six rats at a dose of 4.0 g/kg (capsule powder/body weight) twice a day consecutively for 7 days to accumulate as many absorbed components as possible. Four rats were assigned to the blank control group. Whole blood samples (0.5 ml) were collected from the sub-orbital vein and placed in heparinized polythene tubes at 15, 30, 60, 90, and 120 min after the last drug administration. Plasma was separated immediately by centrifugation at 12,000 rpm for 10 min at 4°C. All samples were immediately stored at −80°C until further analysis.
Pharmacological Experiment of Precancerous Lesions of Gastric Cancer Model in Rats
Fifty 7-week-old male Wistar rats (171 ± 10 g, SPF Grade) were obtained from the Experimental Animal Center of Shanghai University of Traditional Chinese Medicine. All rats were supported and observed in research facility under alternating light and dark conditions (12 h:12 h). After 3 days of adaptive feeding, all rats were divided into five groups, normal group (N group), model group (M group), high-dose group (WH group), low-dose group (WL group), and control group (VM group), stratified randomly according to the body weight of 10 rats in each group. Based on literature modeling methods (Lin et al., 2019) and with the exception of the N group rats, all rats were given 100 mg/L methyl nitroguanidine aqueous solution drink and 0.3 g/L ranitidine fodder each day, and alternatively gavage fed 150 g/L sodium chloride solution at 56°C and 30% ethanol at 10 ml/kg. All model rats were starved and had satiation disorder. After 4 weeks, drugs were administered by gavage. Drug dosage was as follows: Rats in WH group and WL group were given WFC at 1.44 g/kg and 0.72 g/kg, respectively, and VM group was given vatacoenayme at 0.6 g/kg each day. Rats in N group were given gastric gavage of 10 ml/kg of normal saline. After fasting for 12 h at the end of the 16th week, all rats were sacrificed for sample collection.
Plasma Sample Preparation
All plasma samples from the experimental rats were combined into one sample so as to eliminate the individual variability in each experiment. To get the whole information of absorbed components and metabolites, equivalent plasma was taken from the five time points to form mixture as the analysis plasma. An aliquot of 3 ml of methanol was added to 1 ml of plasma and vortex-mixed for 3 min to precipitate proteins (PPT). After centrifugation at 12,000 rpm for 10 min, the supernatant was transferred into a clean tube and evaporated to dryness under a gentle stream of nitrogen at room temperature. The residue was re-dissolved in 200 μl initial mobile phase through vortex-mixing and ultrasonic processing. The resulting solution was centrifuged at 12,000 rpm for 10 min, and 5 μl of the supernatant was injected into the LC-MS system for analysis. The blank plasma sample was prepared as the drug-containing sample.
Preparation and Observation of Animal Samples of Precancerous Lesions of Gastric Cancer Model
Pepsin Detection and Histopathology Observation
Body weight and autonomic activity of rats were observed. Detection of pepsin was conducted in accordance with the kit instructions of Nanjing Jiancheng biological engineering institute. The gastric tissues of rats were subjected to tissue dehydration and paraffin discharge operations after being fully fixed with formaldehyde. The embedded tissues were cut into 0.04 μm blank tissue slices for HE staining and histomorphologic study of gastric mucosa under microscope (100×). Inflammatory cell infiltration, ulcer formation, glandular atrophy, basement membrane thickening, and dysplasia were observed.
Real-Time Polymerase Chain Reaction
According to operating manual, gastric tissues were extracted by “one-step” Trizol method and concentrations were calculated. Obtained RNA templates were reverse-transcribed into c-DNA using TAKARA RNA Reverse Transcription Kit. Next, RT-qPCR was performed following TOYOBO Amplification Kit instructions. Here, each well contained a PCR reaction volume of 10 μl (SYBR Green master mix 5 μl, forward primer 1 μl, reverse primer 1 μl, c-DNA sample 1 μl, and diethylpyrocarbonate 2 μl). ß-Actin was used as an endogenous control gene. PCR reaction consisted of 45 cycles as follows: 50°C for 2 min → 95°C for 1 min → 95°C for 15 s → 60°C for 1 min → 95°C for 15 s → 60°C for 1 min. 2−∆Ct represents the expression level of the tested genes relative to the normal group of genes.
Ultra-High Performance Liquid Chromatography and Mass Conditions
Chromatographic Conditions
UHPLC-MS conditions and data processing were described as previously reported (Wang et al., 2019). Chromatographic separations were performed on an Acquity UHPLC system (Waters, United States) equipped with a binary solvent delivery system and an autosampler. The extracts were separated using an Acquity UHPLC BEH C18 RP column (1.7 μm, 100 mm × 2.1 mm i. d.; Waters, United States) in which the column temperature was maintained at 45°C to avoid excessive column pressure. The autosampler temperature was fixed at 4°C. The mobile phase consisted of 0.1% formic acid in deionized water (mobile phase A) and acetonitrile (mobile phase B). Separation was conducted with the following gradient elution: 0–3.5 min, 5–20% B; 3.5–8.0 min, 20% B; 8.0–15.0 min, 20–30% B; 15.0–21.0 min, 30–50% B; 21.0–26.0 min, 50–95% B; 26.0–28.0 min, 95% B; 28.0–28.1 min, 95–5% B; and 28.1–32.0 min, 5% B for equilibration of the column. The flow rate was set at 0.3 ml/min, and an aliquot of 5 μl was set as injection volume.
Mass Spectrometric Conditions
MS detection was performed using Acquity Synapt G2 Q-TOF tandem mass spectrometer (Waters, United Kingdom) connected to the UHPLC system by an ESI interface and controlled by MassLynx version 4.1 (Waters, United Kingdom). The ESI source was operated in both positive (ESI+) and negative (ESI−) ionization modes. The optimized conditions to trigger maximum response of metabolites were listed as follows: capillary voltage, −2.5 kV (ESI−) or +3 kV (ESI+); sample cone, −25 V (ESI−) or +30 V (ESI+); extraction cone, −4.0 V (ESI−) or +4.0 V (ESI+); source temperature, 120°C; desolation temperature, 350°C; cone gas (nitrogen) flow, 50 L/h; and desolvation gas (nitrogen) flow, 600 L/h. Argon was used as collision gas. Leucine-enkephalin (2 ng/ml) was used as the lock mass generating a reference ion at m/z of 554.2615 (ESI−) or 556.2771 (ESI+) by a lock spray at 5 μl/min to acquire accurate mass during analysis.
Data were collected in a centroid mode. MSE approach was conducted with two scan functions. In function 1, the following parameters were set: m/z 50–1,500; scan duration, 0.3 s; interscan delay, 0.024 s; and collision energy ramp, 4 V. In function 2, the following parameters were set: m/z 50–1,500; scan duration, 0.3 s; interscan delay, 0.024 s; and collision energy ramp, 10–30 V. In MSE, MS, and MS/MS data can be acquired almost simultaneously in a single analytical run. Data acquisition and processing were conducted using Waters MassLynx version 4.1.
Data Processing
Construction of In-House Library
By comprehensive document retrieval, information about compounds in the three crude medicinal materials and WFC prescription was collected to form an in-house library of WFC. The in-house library content was described with respect to the known global phytochemical constituents and the metabolites database, including the English name, structure, molecular formula, characteristic fragment ions, all accurate monoisotopic mass values of the related chemical formula, and original source.
MassLynx Processing Approach for Analysis of Wei-Fu-Chun Phytochemical Constituents, Absorbed Compounds and Metabolites
Mass data processing was carried out using MassLynx 4.1, including extracted ion chromatograms (EIC) using a narrow mass window of 0.01 Da, calculation of EIC with mass errors within 5 ppm, and fraction isotope abundance value of 1.0. In particular, EIC were also applied to distinguish mixed peaks eluted almost at the same retention time. Target chemical structures were analyzed and confirmed based on EIC, accurately measured mass value, fragment behavior, and elution order, which were all compared with the in-house library data. Furthermore, WFC components in drug-containing samples were also determined by comparing the retention time and mass data with blank samples. By comparing postdose rat serum, pre-dose rat serum, and the extracts of WFC by UHPLC-ESI-Q-TOF/MS, absorbed compound profile of rat serum was obtained and analyzed. During the same retention time, peaks appeared in the drug-containing sample and the extracts sample of WFC, but were absent in the control sample or the peaks in the drug-containing sample were five folds greater than that in the control sample, which was extracted as absorption compound. These candidates were further collated and culled by serious MS spectra analysis to conform the true absorbed compounds.
Active Chemical Compositions of Wei-Fu-Chun and Potential Targets
According to the results of chemical profiling and metabolic study of WFC, we selected absorbed parent molecules and metabolites in rat serum as candidate compounds. The candidate compounds were paired one-to-one with protein targets using Stitch 5.0 database (http://stitch.embl.de/). We set the parameter to “Homo sapiens” with confidence greater than 0.4, and deleted candidate compounds without corresponding targets. Active components and corresponding potential targets were obtained.
Identifying Precancerous Lesion of Gastric Cancer Related Targets in Wei-Fu-Chun
“Precancerous lesion of gastric cancer” was used as a keyword to search Genecards (https://www.genecards.org/) and OMIM database (http://www.omim.org/) separately and to retrieve therapeutic targets for PLGC. Precancerous lesions of gastric cancer -associated target proteins were collected.
Targets GO-Enrichment and Pathways Enrichment Analysis
The targets of WFC in the treatment of PLGC corresponding to the selected active components were input into DAVID 6.8 database (https://david.ncifcrf.gov/) for GO enrichment analysis, and in KEGG database (http://www.genome.jp/kegg) for pathway enrichment analysis. The parameters were set to p < 0.5 and FDR < 0.05.
Network Construction and Its Features
We input the active component-target pair obtained from String database (http://string-db.org/cgi/input.pl) into Cytoscape 3.1, set the properties of the active components and targets, selected the most suitable node distribution form, and generated the active component-target interaction map. The primary pathway, biological processes, cellular components and molecular functions of p < 0.05 were selected. Visualize the network of “origins-components-targets-pathways” with the Merge feature of Cytoscape 3.1.
Statistical Analysis
SPSS 21.0 statistical software was used for data statistics and analysis. Data measurement was expressed as mean ± standard errors (±SEM). One-way ANOVA was used for comparison among groups, and LSD-t test for further two-paired comparison. A p-value <0.05 indicated a significant statistical difference between two groups.
Results
Sample Acquisition and LC-MS Conditions of Developed Method
Nowadays, UHPLC-ESI-Q-TOF/MS capable of high resolution, high sensitivity and high accuracy have been proven to be an excellent technique for quantitative and qualitative analysis of multi-components and metabolites in complex mixture especially in TCM formulas. Here, UHPLC-ESI-Q-TOF/MS has shown superior performance, in terms of high mass resolution and accurate mass measurement, fast scan speed and wide dynamic range of mass analyzer, as well as efficient separation capability and speed of UHPLC. The acquisition of dependable biological samples for UHPLC-ESI-Q-TOF/MS analysis played a crucial part in the comprehensive in vivo screening of WFC compounds. Primarily, all the possible components should be contained in the collected crude samples and have adequate levels to be detected, taken into account that drug administration method and collection time influence sample content (Zhang et al., 2017).
LC-MS conditions were optimized to enable samples to obtain the best instrumental performance. Both positive and negative ion modes were employed to screen as many potential compounds as possible, but the negative ion mode provided higher signal intensity and the ability to detect more peak signals. Different mobile phase systems and gradient programs were emphasized and investigated to achieve good ionization and separation behavior. A mixture of 0.1% aqueous formic acid and acetonitrile was nally chosen as the preferred mobile phase. This was because acetonitrile had stronger eluting power and the retention behavior of flavonoids on the reversed-phase column was easily affected by pH (increasing pH enhanced the ionization of flavonoids and could reduce the retention in a reversed-phase separation). Thus, small amounts of formic acids were normally included in the solvent to suppress ionization of phenolic or carboxylic groups, hence improving the resolution and reproducibility of each separation. Formic acid buffer have been used as part of the mobile phase for optimizing the analysis time and enhancing separation (Wang et al., 2019). Many components, especially flavonoids and ginsenosides, gave prominent molecular adductive ions when formic acid was used. The results indicated that the proposed method was acceptable and adequate for the comprehensive study of WFC.
Analysis of Wei-Fu-Chun Phytochemistry Components, Absorbed Components, and Metabolites by UHPLC-ESI-Q-TOF/MS
The base peak intensity (BPI) chromatograms of WFC extract at negative and positive ion modes are shown in Figure 1 and Table 2. A total of 178 compounds were identified or tentatively characterized, including 70 terpenes (56 diterpenoids, 12 triterpene, and 2 sesquiterpenes), 51 flavonoids, 33 saponin, six phenylpropanoids, four lignans, three coumarins, three organic acids, two fatty acid, one quinones, one sterol, and four unknown compounds. Structurally related compounds shared analogous MS response and fragment behavior. All compounds were characterized based on their ECs, MS data, and retention behavior with the help of the constructed in-house library and additional literature. The library made the workflow significantly more effective when one was familiar with the detailed information of these extensively investigated molecules and allowed the characterization of these compounds even when reference standards were not available. The calculated correct monoisotopic mass values of quasimolecular ions and rational fragment ions were especially critical to screen the various compounds fast and firmly. There were many isomers inevitably existing in the complex natural compounds and the tiny difference of the mass spectra made them difficult to distinguish. In this case, the electronic effect and their hydrophilicity should be considered (Li et al., 2015). The detailed identified information, including chemical formula, observed mass values of quasi-molecular ions, mass error, and botanical source, is listed in Table 2. A chemical library, including 569 compounds in WFC, was established. By matching against the chemical library, 178 ingredients in WFC were identified. Among them, 93 compounds belonged to XCC, 51 to ZQ, 31 to HS. Three compounds were unknown, meaning that the herb(s) from which they came remained undetermined. In addition, 77 absorbed parent molecules and nine metabolites in rat serum were characterized by UHPLC-ESI-Q-TOF/MS.
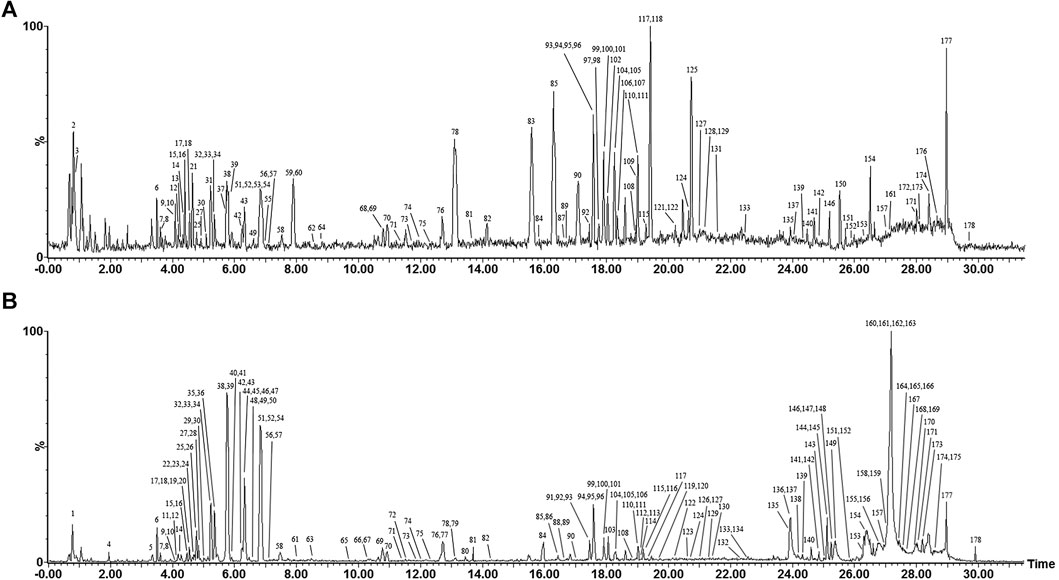
FIGURE 1. Representative base peak intensity (BPI) chromatograms of WFC in positive mode (A) and in negative mode (B) and total ions chromatogram (TIC) in positive mode (C) and in negative mode (D) by UHPLC-ESI-Q-TOF/MS.
Identification of Diterpenoids, Triterpene, and Sesquiterpenes
Diterpenoids are the major bioactive constituents of XCC. About 500 new diterpenoids (mainly ent-kauranoids) with different oxygenations and cleavage patterns have been isolated and characterized from plants of the genus Isodon. In our study, 70 terpenes, including 56 diterpenoids, 12 triterpene, and two sesquiterpenes, were detected and identified in WFC, by means of UHPLC-ESI-Q-TOF/MS in both negative and positive mode according to the literatures (Zhou et al., 2009; Jin et al., 2010). The accurate mass measurements and elemental compositions of molecular ions and main product ion were shown in Table 2. In this study, all terpenes from XCC were ionized as deprotonated molecules [M − H]− in negative mode, however, only part of the terpenes were detected in positive mode.
Identification of Flavonoids
Fifty-one flavonoids and their glycosides in WFC (39 from ZQ, 12 from XCC) were detected according to the literature (Fabre et al., 2001; Shi et al., 2007; Zhang et al., 2012), most of them having a common structure of C6-C3-C6. Their cleavage regularity in extracts has been well described. Simply, for aglycones, the main MS/MS behavior involved RDA fragmentation pathway and losses of small neutral molecules and radicals from [M − H]−, CO (28 Da), CO2 (44 Da), H2O (18 Da), and CH3 (15 Da) that are useful for determining the presence of specific functional groups; for flavonoid glycosides, the cleavage at the glycosideic linkages in positive and negative ion mode both could happen and produce the same fragmentations with low m/z as the fragmentations obtained in their aglycone. For example, flavone glycosides were characterized by the successive losses of an apiose residue C5H8O4 (132 Da), pentose residue (146 Da), hexose residue (162 Da), glucuronic acid (176 Da), rutinoside or glycoside neohesperidin (308 Da).
Compound 15 showed [M − H]− ion at m/z 741, and identical fragmentations at m/z 609, which lost an apiose residue (132 Da). Here, compound 15 was identified as Glucosyl-naringin (Table 2). Compound 18 showed [M − H]− ion at m/z 427, and identical fragmentations at m/z 265, which lost a hexose residue (162 Da). Compound 18 was identified as hyuganoside II or V. Compounds 19, 32, and 38 showed [M − H]− ion at m/z 595, 579, 579, and identical fragmentations at m/z 287, 271, 271, which lost a hexose residue (308 Da). Compound 19 was identified as eriocitrin. Both compounds 32 and 38 showed [M − H]− ion at m/z 579 [M + H]+ ion at m/z 581, and identical fragmentations at m/z 271 (negative) and 273 (positive), which lost a hexose residue (308 Da), as well as identical fragmentations at m/z 435 (positive), which lost a pentose residue (146 Da) of 38. Thus, 32 and 38 were deemed narirutin and naringin based on the fragmentations and appearance time. Compounds 42 and 52 were identified as hesperidin and neohesperidin, as both lost a hexose residue (308 Da) at negative and positive conditions (Li et al., 2004). Compound 57 showed identical [M − H]− ion at m/z 463, and fragmentation at m/z 301 and 286, suggesting that compound 57 had a hexose residue (162 Da), and CH3 (15 Da) in its aglycone. Compound 57 was identified as hesperetin-7-O-glucoside. Other flavonoids were tentatively identified based on the positive and negative parent ion and previous literature report.
Identification of Saponin
Thirty-two saponins (31 from HS) were detected and identified in WFC, by matching the empirical molecular formula and fragment ions with reported MS data of saponins in HS, ZQ, and XCC (Liu et al., 2006; Dan et al., 2009; Zhang et al., 2012). The detailed information was shown in Table 2. Most ginsenosides were liable to form [M − H]− ion and [M + HCOO]− ion in the negative mode and [M + H]+ ion in positive mode. The main pathway for mass spectrometry cleavage of ginsenosides to lose the glycosyl groups to form the parent ion of m/z 459 (diol type) and m/z 475 (triol type). For example, in the case of compounds 127 and 135, m/z 459 was detected in negative mode. The second point is the characteristic fragment ion formed by glycosylation. For compounds 96, 132, and 112, the same fragment m/z 621 lost one or two glucoses from parent ion. Fragment m/z 603 of compounds 129 and 137 lost one glucose from parent ion.
Identification of Phenylpropanoids
Six phenylpropanoids were detected in WFC. They were methyl rosmarinate (11), hyuganoside II/hyuganoside V (18) (+)-rabdosiin or (−)-rabdosiin (30, 34), rosmarinic acid (45), danshensu (47) (Zhang et al., 2012). Compound 18 showed [M − H]− ion at m/z 427.1615, and identical fragmentations at m/z 265, which lost a glucose residue (162 Da).
Identification of Lignans
Four lignans were detected in WFC, which were (+)-1-hydroxypinoresinol (50), Lariciresinol (51), sesamin (66), and Epipinoresinol (162). All of the four compounds were from XCC. They were liable to form [M − H]− ion in the negative mode and [M + H]+ ion in positive mode.
Identification of Coumarins
Meranzin (60) and Epoxybergamottin (87) were identified from ZQ, and Esculetin (86) was identified from XCC. Meranzin (60) showed a protonated molecule at m/z 261.1139 [M + H]+, with a molecular formula C15H16O4. MS/MS fragmentation appeared in m/z 189.0572 [M + H-C4H7O]+ (Tsujimoto et al., 2019). The MS fragmentation was typical for the fragmentation pattern of meranzin.
Identification of Organic Acids, Fatty Acid, Quinones, Sterol, and Unknown Compounds
Three organic acid, two fatty acid, one quinones, one sterol, and four unknown compounds were identified.
UHPLC-ESI-Q-TOF/MS Analysis of Compounds in Wei-Fu-Chun Absorbed into Blood Circulation
The absorbed compounds were explored based on the hypothesis that the active constituents were those absorbed into tissue (Zhao et al., 2010). By comparing post-dose rat serum, pre-dose rat serum, and the extracts of WFC by UHPLC-ESI-Q-TOF/MS, an absorbed compounds profile of rat serum was obtained and analyzed. The 77 parent molecules in the positive- and negative-ion mode were summarized in Figure 2 and Table 2 and marked with an asterisk (*). Of these 77 parent molecules, there were 24 diterpenoids (23 from XCC, one from ZQ), 20 flavonoids (15 from ZQ, five from XCC), 17 saponins (all are ginsenoside from HS), five triterpenes (three from XCC, two from ZQ), two phenylpropanoids (both are from XCC), two organic acids (both are from XCC), one sesquiterpenes (from ZQ), one sterol (from XCC), one fatty acid (from XCC), and two unknown compounds. Taken together, the most absorbed compounds were terpene from XCC, flavonoids from ZQ, and saponins from HS.
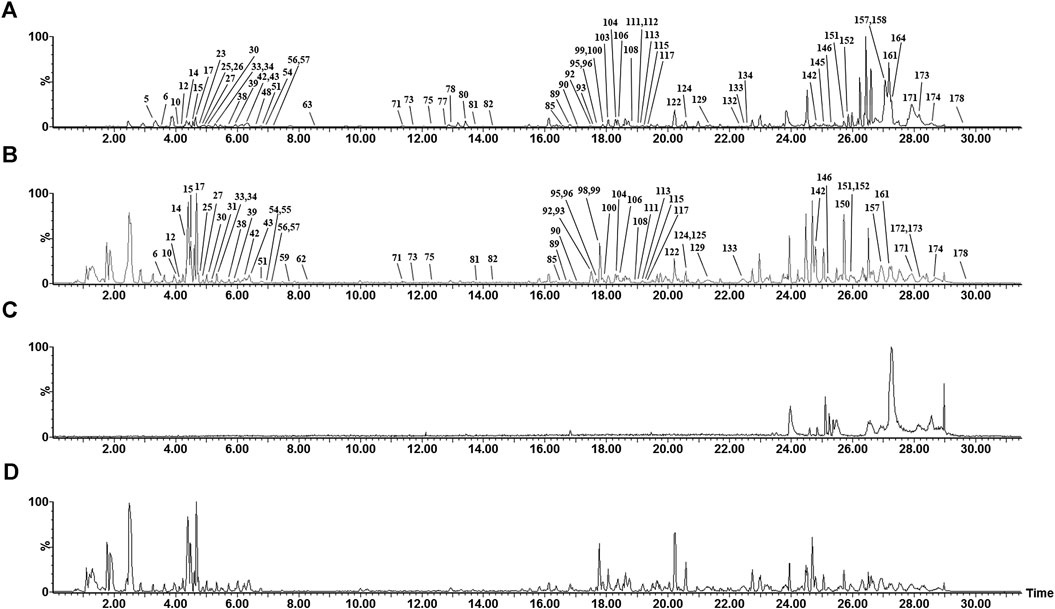
FIGURE 2. Representative base peak intensity (BPI) chromatograms of absorbed compounds after oral administration of WFC in negative mode (A) and in positive mode (B) by UHPLC-ESI-Q-TOF/MS; Representative base peak intensity (BPI) chromatograms of blank serum in negative mode (C) and in positive mode (D) by UHPLC-ESI-Q-TOF/MS.
Tentative Identification of the Wei-Fu-Chun Metabolites in Rats
Some drug components could be further metabolized by a variety of metabolic enzymes in the body and form phase I or phase II metabolites, which are highly related to the curative effect and drug elimination. Drug metabolites usually kept the core structure of the parent drug after biotransformation, hence the obtained candidate metabolites were further identified by comparing the change in molecular mass (△M), the retention time, and MS2 spectral with their parent drugs. Metabolites of all 178 compounds were tentatively predicted in rat plasma according to their molecular weights and literature reports; however, only the metabolites of limonin, ginsenoside Rg1, ginsenoside Rb3, oridonin were found in our study. M1 eluted at 1.07 min was observed at m/z 455.1891, which is 16 Da (−O) lower than limonin. M2 eluted at 1.08 min and the positive ion was observed at m/z 485.1989, which was 14 Da (+CH2) higher than limonin. The peak elution of M4 was at 4.34 min and the positive ion was observed at m/z 489.2314, which was 18 Da (A-ring lactone) higher than that of the parent drug limonin. The two metabolites (M3 and M5) of ginsenoside Rg1 were observed at m/z 859.4590 and 861.4748 in negative mode, which were 14 Da (+O-2H) and 16 Da (+O) higher than limonin (Wang et al., 2016). Oridonin + OH (M9) and +2OH (M8) compounds were observed at m/z 379.1540 and 395.1656.
Network Pharmacology Approach to Predict the Compounds and Action Mechanisms of Wei-Fu-Chun Against Precancerous Lesions of Gastric Cancer
Identification of Active Chemical Compositions, Candidate Targets and Biological Processes for Wei-Fu-Chun Against Precancerous Lesions of Gastric Cancer
The efficacy of TCM is based on the overall regulation of multiple active ingredients acting on different disease-related targets through multiple pathways (Li and Zhang, 2013). Therefore, we collected 86 candidate compounds (Tables 2, 3) and 105 potential targets. 13 active components and 48 protein targets to treat PLGC were then obtained. Detailed information of these therapeutic targets was described in Tables 4, 5 separately.
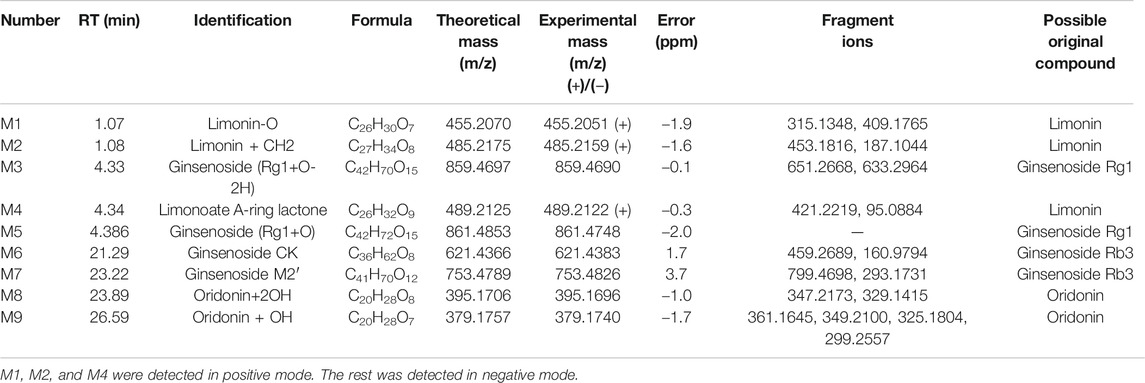
TABLE 3. UHPLC-ESI-Q-TOF/MS data obtained in negative and positive ion detection mode for identification of WFC metabolites.
Enrichment Analysis of Candidate Targets for Wei-Fu-Chun Against Precancerous Lesions of Gastric Cancer
To further explore the possible functions of the 48 therapeutic targets and reveal the relationship between active components and their underlying targets in PLGC, we performed pathway enrichment analysis on PLGC-related protein targets and obtained the main related signaling pathways of target proteins (p-value < 0.01). The 61 anti-PLGC pathways of WFC were listed in Table 6. These therapeutic targets were mainly distributed in PI3K/Akt pathway, MAPK pathway, vascular endothelial growth factor (VEGF) pathway, hypoxia-inducible factor-1 (HIF-1) pathway, and tumor necrosis factor (TNF) pathway, and FoxO pathway. In addition, According to the GO enrichment analysis results, we found that the functions of therapeutic targets were involved in multiple biological processes such as peptide chain serine phosphorylation, RNA polymerase II promoter transcription, cellular hypoxia, apoptosis, vascular endothelial cell migration, macrophage differentiation, vascular endothelial growth factor receptor, chemokine biosynthesis, cell proliferation, and nitric oxide biosynthesis (Figure 3).
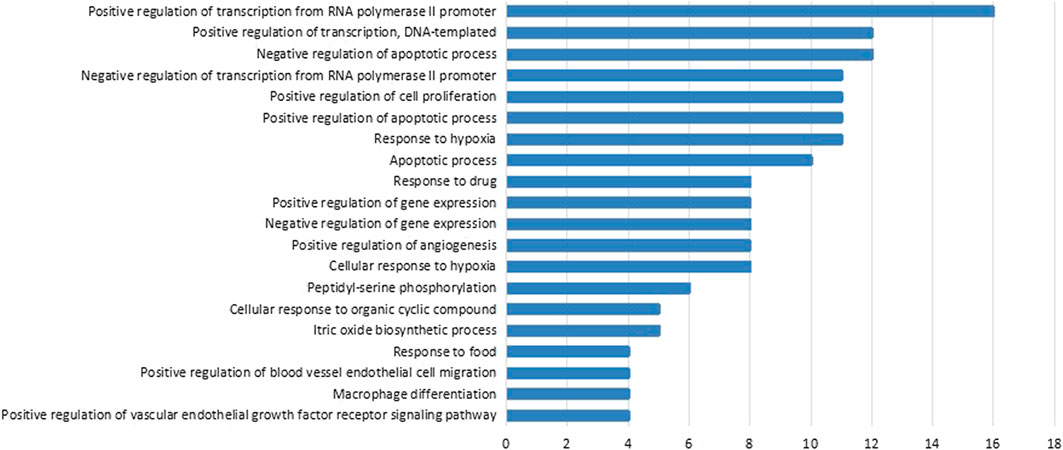
FIGURE 3. Biological processes regulated by WFC to treat PLGC. The count of each biological process was shown on the right side of the bar. The p-value of each biological process was less than 0.05. FDR of each biological process was less than 0.05.
Construction of Pharmacology-Network for Wei-Fu-Chun Against Precancerous Lesions of Gastric Cancer
Using the Cytoscape 3.1 software, we constructed an “origins-components-targets-pathways” pharmacology network of WFC (Figure 4). This network depicted the relationship between 61 pathways, 48 therapeutic targets, 13 active components and corresponding plant materials. The network also consisted of 64 nodes and 75 edges, of which Ginsenoside Rb1 (C3, degree = 7), Naringin (C7, degree = 9), Hesperidin (C8, degree = 8), Hesperetin (C9, degree = 7), and Oridonin (C11, degree = 8) had high degrees and were centrally located in the network, suggesting that these active compounds may be the key components of WFC in the treatment of PLGC.
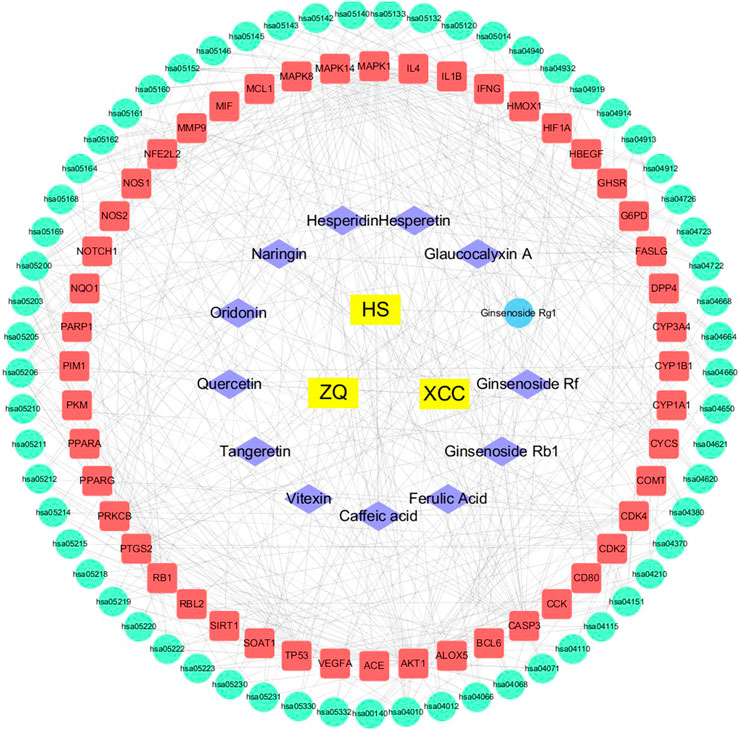
FIGURE 4. Pharmacology-network of the “origins-components-targets-pathways” regulated by WFC. The yellow rectangles represent Chinese herbal medicines, while the purple rhombuses represent active components of WFC. The blue ellipse represents metabolites of ginsenoside Rg1. The red rectangles represent target proteins, and the green ellipses represent pathways in Table 6.
Model Test Results of Precancerous Lesions of Gastric Cancer
The speed of weight gain in the model group was much lower than that in the normal group. At the end of the fourth week, the weight of the high dose group, the low dose group, and the Vitacoenzyme group showed a rising trend after the simultaneous modeling and administration of the high dose group, the low dose group, and the vitamin enzyme group (Figure 5A). The increase rate of the high dose group was higher than that of the other two groups. The weight of the model group increased and then decreased until the end of the model. Compared with the normal group, there was a significant statistical difference in the activity value of pepsin in the normal group and the model group (p < 0.05). Compared with the model group, the activity value of pepsin in the high-dose group was significantly different (p < 0.05). There was no significant difference in protease activity between the low dose group and the model group. There was also no significant difference in pepsin activity between the high-dose group and the normal group (p > 0.05) (Figure 5B). The histopathology of gastric mucosa was demonstrated by hematoxylin eosin staining. Atrophic glands, intestinal metaplasia and lymphocyte infiltration were shown in the model group, and compared with the model group, the above pathological manifestations were alleviated in varying degrees (Figure 5C). RT-PCR results showed that mRNA expression of VEGF, FOXO4, AKT, TP53, FAS, MAPK8, MAPK11, and MAPK14 in the model group was significantly up-regulated compared with that in the N group (p < 0.05)., while mRNA expression of interleukin-10 (interleukin-10, il-10) was down-regulated (p < 0.01). mRNA expressions of VEGF, FOXO4, AKT, TP53, FAS, MAPK8, MAPK11, and MAPK14 were all significantly down-regulated in the WFC high dose group (p < 0.05) (Figure 6).
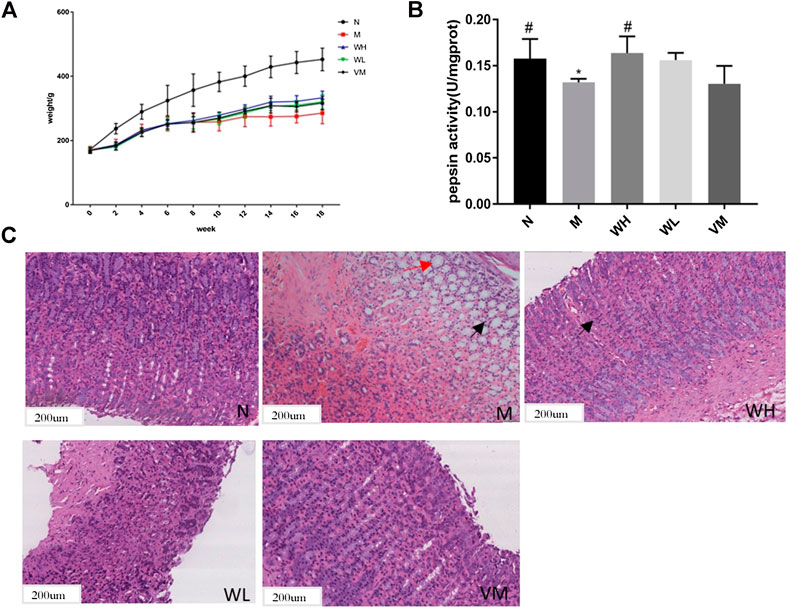
FIGURE 5. Pharmacodynamic effect of WFC on PLGC models. (A) The effect of WFC on the weight change of rats with PLGC. (B) Pepsin activity changes in gastric tissue of rats in different groups. (C) Pathological changes of gastric tissue of in different groups of rats (H&E: 100×). *p < 0.05 compared with the normal group; #p < 0.05 compared with the model group.
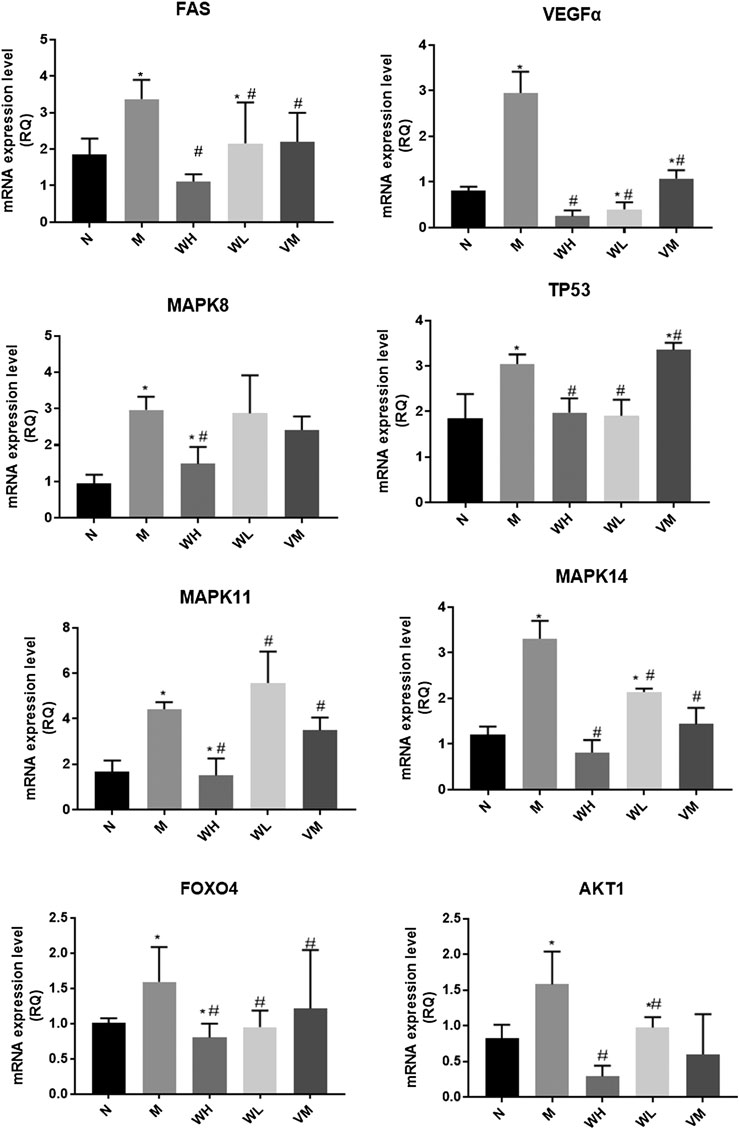
FIGURE 6. Gene expression related to MAPK pathways in gastric tissues of different groups of rats. *p < 0.05 compared with the normal group; #p < 0.05 compared with the model group.
Discussion
WFC, an herbal prescription with three medicines, may contain thousands of compounds, however, naringin is the only maker compound of WFC in 2015 edition of “Chinese Pharmacopoeia.” In this study, we hypothesized that unilateral factors and single targets are insufficient to demonstrate the complex mechanisms of WFC. The network pharmacology method used in this study is a novel methodology based on the construction of multilayer networks of disease phenotype-gene-drug to predict drug targets in a holistic manner and promote efficient drug discovery (Liu and Sun, 2012). This method represents a breakthrough in comparison with the traditional herbal medicine research pattern “gene target-disease” and initiates the new pattern of “multiple genes multiple targets-complex diseases” (Hopkins, 2008; Ma et al., 2016). With this method, we proved that XCC was the critical ingredient involved in the treatment of PLGC, which corresponded to the constituents of WFC with 86.8% weight of XCC in WFC. HS was also a major herb that regulated PLGC. Moreover, WFC is a multiple-component complex system. (Zhang et al., 2012) unambiguously identified or tentatively characterized 46 components in WFC tablet, including 26 saponins, 10 flavonoids, and 10 other compounds. However, it is not enough to understand the chemical components of WFC, which makes it rather difficult to define the functions of this herbal medicine from material basis and chemical properties. Therefore, a chemical fingerprint analysis of WFC was necessary. Compared with the material basis of the three herbs in WFC, a chemical fingerprint analysis of WFC was fully carried out by means of UHPLC-ESI-Q-TOF/MS. Finally, a total of 178 compounds were identified in WFC, including 93 compounds (70 terpene) originally from I. amethystoides, 51 compounds from Fructus aurantii, 31 compounds from red ginseng, which basically demonstrated the material basis in WFC. However, the main components’ content and dose-effect relationship should be further investigated.
In network pharmacology studies, there were 86 candidate compounds (77 absorbed components and nine metabolites) and 105 potential targets. Thirteen active components and 48 protein targets were selected to explore the effect of WFC against PLGC. The results showed that PI3K/Akt pathway, MAPK pathway, VEGF pathway, HIF-1 pathway, TNF pathway, and FOXO pathway may be involved as the anti-PLGC pathways of WFC. In addition, ginsenoside Rb1, naringin, hesperidin, hesperetin, and oridonin may be the key components of WFC in the treatment of PLGC. Ginsenoside Rb1was shown to exert anti-inflammatory effects in Modulating TLR-4 dimerization and NF-kB/MAPKs signaling pathways (Gao et al., 2020). Ginsenoside Rb1 also enhanced the phagocytic capacity of macrophages for bacteria via activation of the p38/Akt pathway, which may be a useful pharmacological adjuvant for the treatment of bacterial infections in clinically relevant conditions (Xin et al., 2019). Moreover, naringin induced autophagy-mediated growth inhibition by downregulating the PI3K/Akt/mTOR cascade via activation of MAPK pathways in AGS cancer cells (Raha et al., 2015). As for hesperetin, it induced apoptosis in human glioblastoma cells via p38 MAPK activation (Li et al., 2020). Finally, oridonin’s anticancer effects on colon cancer were mediated via BMP7/p38 MAPK/p53 signaling (Liu et al., 2018). Overall, this suggested that MAPK pathway, PI3K/Akt pathway, and p38/Akt pathway may be the key pathways of WFC treating PLGC.
To verify the network pharmacology prediction results, in vivo rat experiments were carried out. Pepsin is formed by pepsinogen stimulated by gastric acid. Pepsin is mainly secreted by the main cells. Atrophy of gastric glands and metaplasia of intestinal epithelium reduce the main cells. From superficial gastritis, atrophic gastritis/dysplasia to gastric cancer, pepsinogen in pathological tissues decreases and then pepsin activity decreases. In chronic atrophic gastritis patients with or without intestinal metaplasia, pepsinogen I often decreased (Terasawa et al., 2014). In this experiment, WFC restored pepsin activity in the rat model of PLGC.
MAPK is the main transmitter of intracellular and extracellular signals. P38 and JNK are the important parts of its four major subgroups. JNK pathway and p38 pathway mediate the transmission of cytokines and inflammatory mediators and participate in cell cycle, apoptosis, and migration. This process plays an important role in the development of precancerous lesions to gastric cancer. JNK mainly exists in the cytoplasm and accumulates rapidly and significantly in the nucleus after being activated by the superior kinase. The activation of transcription factors in the nucleus includes TP53, c-Jun and so on (Liu and Lin, 2005), and then produces biological effects. The positive rate of JNK expression in gastric cancer was 75%, and it positively correlated with the size of the tumor and the early and late stage of the tumor (Wang, 2009). Helicobacter pylori can stimulate the invasion of AGS gastric cancer cells through JNK signaling pathway (Díaz-Serrano et al., 2018). Inhibition of JNK pathway can enhance the antitumor effect of trail on MGC803 gastric cancer cells (Liu et al., 2011). P38 has five isomers, p38 α (p38), p38 ß 1, p38 ß 2, p38 γ, p38 δ. 38 α, and p38 ß tissues are widespread. P38 pathway plays an important role in cell cycle regulation and can induce cell cycle. The inhibition of p38 pathway may be one of the mechanisms of synergistic antitumor effect of Adriamycin and monomer PA-2 (Liang et al., 2020). In gastric cancer patients with high expression of lncRNA-aoc4p, inhibiting the expression of lncRNA-aoc4p could reduce the expression level of JNK and p38 protein and inhibit cell proliferation, migration, and invasion (Qu et al., 2019). FOXO4, as a member of the FOXO family of transcription factors, is regulated by microRNA in a variety of cancer cells, and its abnormal expression is closely related to gastrointestinal tumors (Gross et al., 2008; Liu et al., 2020). The current study further proved the therapeutic effect of WFC on PLGC in rats, and preliminarily explored and verified the results of network pharmacology. The detection results of MAPK pathway genes p38 α, p38 β, JNK and its upstream and downstream factors TP53 and VEGF α, as well as FOXO4 and AKT genes showed consistency. We believe that MAPK pathway is involved in the mechanism of action of WFC on PLGC, which needs to be further explored.
Conclusion
In conclusion, a simple and reliable UHPLC–ESI-Q-TOF/MS technique was established to profile complex compounds in WFC and describe their absorption behavior in plasma samples after oral administration of WFC. A total of 178 compounds were identified. In addition, 77 absorbed compounds of parent molecules in WFC were detected. Among these compounds, the most absorbed ones were terpenes from XCC, flavonoids from ZQ and saponins from HS. The current study supplemented previous WFC research, and MS data contributed to the identification of allied natural compounds. The screening of in vivo absorbed and metabolic compounds provided constructive material basis for further research on the pharmacology and curative mechanisms of WFC. Moreover, the network pharmacology method was used to predict the active components, corresponding therapeutic targets, and related pathways of WFC in the treatment of PLGC. Finally, based on the major compounds of WFC and their metabolites in rat plasma and existing databases, 13 active components, 48 therapeutic targets, and 61 pathways were found to act against PLGC. The results from rat experiment showed that WFC could improve the weight of PLGC rats and the gastric mucosa histopathological changes partly by inhibiting MAPK signaling pathway to increase pepsin secretion.
This study offered an applicable approach for the identification of chemical components, absorbed compounds, and metabolic compounds of WFC, and provided a method to explore bioactive ingredients and action mechanisms of WFC.
Data Availability Statement
The raw data supporting the conclusions of this manuscript will be made available by the authors, without undue reservation, to any qualified researcher.
Author Contributions
HW, RW, and DX contributed equally. HW conducted the experimental part of the main component analysis of WFC. Network pharmacology was mainly analyzed by RW, DX performed most of the pharmacological experiments, analyzed the data, and participated in the manuscript draft. LD and XL helped complete animal feeding and analysis of the main components in plasma of Weifuchun tablets. YB, XC, and BN polished the manuscript. SW, KL, and WC monitored the drug quality and helped complete the data collation. GY and MS directed the study, and drafted and finalized the manuscript. All authors read and approved the final manuscript.
Funding
This work was supported by Science and Technology Commission of Shanghai Municipality (Nos. 15DZ1900104 and 15DZ1900100 to MS and GY); the fourth batch of Chinese medicine (basic) talents of the State Administration of TCM (No. 2017-124 to MS); Construction of Postgraduate Innovation Course in Shanghai University of Traditional Chinese Medicine (No. 2017 to MS); and Natural Science Foundation of Shanghai (No. 20ZR1458100 to HW).
Conflict of Interest
HW, RW, XL, KL and GY were employed by Shangai Pharmaceuticals Holding Co., Ltd.. LD was employed by Shanghai Zhonghua Pharmaceuticals Co., Ltd. WC were employed by Huqingyutang Chinese Medicine Medernization Research Institute of Zhejiang Province. The remaining authors declare that the research was conducted in the absence of any commercial or financial relationships that could be constructed as a potential conflict of interest.
References
Chen, W., Zheng, R., Baade, P. D., Zhang, S., Zeng, H., Bray, F., et al. (2016). Cancer statistics in China, 2015. CA A Cancer J. Clin. 66 (2), 115–132. doi:10.3322/caac.21338
Correa, P., Haenszel, W., Cuello, C., Tannenbaum, S., and Archer, M. (1975). A model for gastric cancer epidemiology. Lancet 306 (7924), 58–60. doi:10.1016/s0140-6736(75)90498-5
Dan, M., Xie, G., Gao, X., Long, X., Su, M., Zhao, A., et al. (2009). A rapid ultra-performance liquid chromatography-electrospray Ionisation mass spectrometric method for the analysis of saponins in the adventitious roots of Panax notoginseng. Phytochem. Anal. 20 (1), 68–76. doi:10.1002/pca.1099
Díaz-Serrano, A., Angulo, B., Dominguez, C., Pazo-Cid, R., Salud, A., Jiménez-Fonseca, P., et al. (2018). Genomic profiling of HER2-positive gastric cancer: PI3K/Akt/mTOR pathway as predictor of outcomes in HER2-positive advanced gastric cancer treated with trastuzumab. Oncologist 23 (9), 1092–1102. doi:10.1634/theoncologist.2017-0379
Fabre, N., Rustan, I., Hoffmann, E., and Quetin-Leclercq, J. (2001). Determination of flavone, flavonol, and flavanone aglycones by negative ion liquid chromatography electrospray ion trap mass spectrometry. J. Am. Soc. Mass Spectrom. 12 (6), 707–715. doi:10.1016/s1044-0305(1)00226-4
Gao, H., Kang, N., Hu, C., Zhang, Z., Xu, Q., Liu, Y., et al. (2020). Ginsenoside Rb1 exerts anti-inflammatory effects in vitro and in vivo by modulating toll-like receptor 4 dimerization and NF-kB/MAPKs signaling pathways. Phytomedicine 69, 153197. doi:10.1016/j.phymed.2020.153197
Gross, D. N., van den Heuvel, A. P. J., and Birnbaum, M. J. (2008). The role of FoxO in the regulation of metabolism. Oncogene 27 (16), 2320–2336. doi:10.1038/onc.2008.25
Hopkins, L. A. (2008). Network pharmacology: the next paradigm in drug discovery. Nat. Chem. Biol. 4 (11), 682–690. doi:10.1038/nchembio.118
Huang, X., Lu, B., Zhang, S., Meng, L. N., and Zhao, M. (2014). Effect of Weifuchun on inhibiting inflammation of Helicobacter pylori-infected GES-1 cells and NF-kappaB signaling pathway. Zhongguo Zhong Xi Yi Jie He Za Zhi 34 (4), 450–454
Jin, Y., Du, Y., Shi, X., and Liu, P. (2010). Simultaneous quantification of 19 diterpenoids in Isodon amethystoides by high-performance liquid chromatography-electrospray ionization tandem mass spectrometry. J. Pharmaceut. Biomed. Anal. 53 (3), 403–411. doi:10.1016/j.jpba.2010.04.030
Jin, Y., Tian, T., Ma, Y., Xu, H., and Du, Y. (2015). Simultaneous determination of ginsenoside Rb1, naringin, ginsenoside Rb2 and oridonin in rat plasma by LC-MS/MS and its application to a pharmacokinetic study after oral administration of Weifuchun tablet. J. Chromatogr. B 1000, 112–119. doi:10.1016/j.jchromb.2015.06.027
Li, Q., Miao, Z., Wang, R., Yang, J., and Zhang, D. (2020). Hesperetin induces apoptosis in human glioblastoma cells via p38 MAPK activation. Nutr. Cancer 72 (3), 538–545. doi:10.1080/01635581.2019.1638424
Li, S., and Zhang, B. (2013). Traditional Chinese medicine network pharmacology: theory, methodology and application. Chin. J. Nat. Med. 11 (2), 110–120. doi:10.1016/s1875-5364(13)60037-0
Li, S., Zhang, M., Hou, P., Zhang, R., Hou, C., Bi, K., et al. (2015). Identification of the toxic components in Semen Strychni and their metabolites in rat serum by high performance liquid chromatography coupled with a Q Exactive high-resolution benchtop quadrupole Orbitrap mass spectrometer. RSC Adv. 5 (95), 77689–77698. doi:10.1039/c5ra14678j
Li, X., Xiao, H., Liang, X., Shi, D., and Liu, J. (2004). LC-MS/MS determination of naringin, hesperidin and neohesperidin in rat serum after orally administrating the decoction of Bulpleurum falcatum L. and Fractus aurantii. J. Pharmaceut. Biomed. Anal. 34 (1), 159–166. doi:10.1016/j.japna.2003.08.002
Liang, L., Amin, A., Cheung, W.-Y., Xu, R., Yu, R., Tang, J., et al. (2020). Parameritannin A-2 from Urceola huaitingii enhances doxorubicin-induced mitochondria-dependent apoptosis by inhibiting the PI3K/Akt, ERK1/2 and p38 pathways in gastric cancer cells. Chem. Biol. Interact. 316, 108924. doi:10.1016/j.cbi.2019.108924
Lin, L., Han, T., Lu, L., Sun, Q., and Sun, M. (2019). Summary and review of animal models for chronic atrophic gastritis and precancerous lesions of gastric cancer. Chin. J. Exp. Tradit. Med. Formulae 25 (2), 220–227.
Liu, J., and Lin, A. (2005). Role of JNK activation in apoptosis: a double-edged sword. Cell Res. 15 (1), 36–42. doi:10.1038/sj.cr.7290262
Liu, J., Qu, X. J., Liu, Y. P., Xu, L., and Zhang, Y. (2011). Effect of JNK signaling pathway inhibition on TRAIL-induced apoptosis in human gastric cancer cells. Chin. J. Cancer Prev. Treat. 18 (7), 481–484.
Liu, R.-X., Ma, Y., Hu, X.-L., Ren, W.-Y., Liao, Y.-P., Wang, H., et al. (2018). Anticancer effects of oridonin on colon cancer are mediated via BMP7/p38 MAPK/p53 signaling. Int. J. Oncol. 53 (5), 2091–2101. doi:10.3892/ijo.2018.4527
Liu, W., Li, Y., and Luo, B. (2020). Current perspective on the regulation of FOXO4 and its role in disease progression. Cell. Mol. Life Sci. 77 (4), 651–663. doi:10.1007/s00018-019-03297-w
Liu, Y., Yang, J., and Cai, Z. (2006). Chemical investigation on Sijunzi decoction and its two major herbs Panax ginseng and Glycyrrhiza uralensis by LC/MS/MS. J. Pharmaceut. Biomed. Anal. 41 (5), 1642–1647. doi:10.1016/j.jpba.2006.02.033
Liu, Z.-H., and Sun, X.-B. (2012). Network pharmacology: new opportunity for the modernization of traditional Chinese medicine. Yao Xue Xue Bao 47 (6), 696–703.
Ma, X., Lv, B., Li, P., Jiang, X., Zhou, Q., Wang, X., et al. (2016). Identification of “multiple components-multiple targets-multiple pathways” associated with naoxintong capsule in the treatment of heart diseases using UPLC/Q-TOF-MS and network pharmacology. Evid. Based Complement. Alternat. Med. 2016 (12), 9468087. doi:10.1155/2016/9468087
Qu, C. X., Shi, X. C., Bi, H., Zhai, L. Q., and Yang, Q. (2019). LncRNA AOC4P affects biological behavior of gastric cancer cells through MAPK signaling pathway. Eur. Rev. Med. Pharmacol. Sci. 23 (20), 8852–8860. doi:10.26355/eurrev_201910_19280
Raha, S., Yumnam, S., Hong, G. E., Lee, H. J., Saralamma, V. V. G., Park, H.-S., et al. (2015). Naringin induces autophagy-mediated growth inhibition by downregulating the PI3K/Akt/mTOR cascade via activation of MAPK pathways in AGS cancer cells. Int. J. Oncol. 47 (3), 1061–1069. doi:10.3892/ijo.2015.3095
Shi, P., He, Q., Song, Y., Qu, H., and Cheng, Y. (2007). Characterization and identification of isomeric flavonoid O-diglycosides from genus Citrus in negative electrospray ionization by ion trap mass spectrometry and time-of-flight mass spectrometry. Anal. Chim. Acta 598 (1), 110–118. doi:10.1016/j.aca.2007.07.027
Terasawa, T., Nishida, H., Kato, K., Miyashiro, I., Yoshikawa, T., Takaku, R., et al. (2014). Prediction of gastric cancer development by serum pepsinogen test and Helicobacter pylori seropositivity in Eastern Asians: a systematic review and meta-analysis. PloS One 9 (10), e109783. doi:10.1371/journal.pone.0109783
Tsujimoto, T., Yoshitomi, T., Maruyama, T., Yamamoto, Y., Hakamatsuka, T., and Uchiyama, N. (2019). High-resolution liquid chromatography-mass spectrometry-based metabolomic discrimination of citrus-type crude drugs and comparison with nuclear magnetic resonance spectroscopy-based metabolomics. J. Nat. Prod. 82 (8), 2116–2123. doi:10.1021/acs.jnatprod.8b00977
Wang, H., Guan, Y., Wu, R., Lv, X., Shen, X., and Ye, G. (2019). UPLC-Q‐TOF/MS characterization of efficacy substances on osteoblasts differentiation and function in rat serum after administration of Wang‐Bi tablet. Biomed. Chromatogr. 33 (10), e4628. doi:10.1002/bmc.4628
Wang, J.-R., Tong, T.-T., Yau, L.-F., Chen, C.-y., Bai, L.-P., Ma, J., et al. (2016). Characterization of oxygenated metabolites of ginsenoside Rg 1 in plasma and urine of rat. J. Chromatogr. B 1026 (10), 75–86. doi:10.1016/j.jchromb.2015.12.028
Wang, S. (2009). Expression and clinical significance of CD44V6, vegf-c and JNK in gastric cancer tissues. Dalian, China: Dalian Medical University.
Xin, C., Quan, H., Kim, J.-M., Hur, Y.-H., Shin, J.-Y., Bae, H.-B., et al. (2019). Ginsenoside Rb1 increases macrophage phagocytosis through p38 mitogen-activated protein kinase/Akt pathway. J. Ginseng. Res. 43 (3), 394–401. doi:10.1016/j.jgr.2018.05.003
Zhang, Y., Cheng, Y., Liu, Z., Ding, L., Qiu, T., Chai, L., et al. (2017). Systematic screening and characterization of multiple constituents in Guizhi Fuling capsule and metabolic profiling of bioactive components in rats using ultra-high-performance liquid chromatography/quadrupole-time-of-flight mass spectrometry. J. Chromatogr. B 1061–1062, 474–486. doi:10.1016/j.jchromb.2017.07.021
Zhang, Y., Pan, J., Zhong, J., Wang, Y., Fan, X., and Cheng, Y. (2012). Virtual separation of phytochemical constituents by their adduct-ion patterns in full mass spectra. J. Chromatogr. A 1227, 181–193. doi:10.1016/j.chroma.2012.01.006
Zhao, M., Bin, L. V., Huang, X., Chen, B. B., and Jin, H. F. (2012). Effects of Weifuchun on TNF-α-induced inflammation and intestinal metaplasia in GES-1 cells. Chin. J. Integr. Tradit. West. Med. Dig. 20 (9), 394–397. doi:10.11569/wcjd.v20.i34.3310
Zhao, X., Zhang, X., Di, L., Wang, S., Cai, B., Du, Q., et al. (2010). Exploration of thoughts and methods in study on material base of traditional Chinese medicinal herbs prescriptions. World Sci. Technol. 11 (4), 488–492. doi:10.1016/s1876-3553(10)60021-x
Keywords: Wei-Fu-Chun tablet, precancerous lesions of gastric cancer, network pharmacology, effective substances and mechanism, UHPLC-ESI-Q-TOF/MS
Citation: Wang H, Wu R, Xie D, Ding L, Lv X, Bian Y, Chen X, Nisma Lena BA, Wang S, Li K, Chen W, Ye G and Sun M (2020) A Combined Phytochemistry and Network Pharmacology Approach to Reveal the Effective Substances and Mechanisms of Wei-Fu-Chun Tablet in the Treatment of Precancerous Lesions of Gastric Cancer. Front. Pharmacol. 11:558471. doi: 10.3389/fphar.2020.558471
Received: 26 May 2020; Accepted: 21 September 2020;
Published: 18 November 2020.
Edited by:
Raffaele Capasso, University of Naples Federico II, ItalyReviewed by:
Xiao Liu, Nanjing University of Chinese Medicine, ChinaXi rui He, Zunyi Medical University, China
Copyright © 2020 Wang, Wu, Xie, Ding, Lv, Bian, Chen, Nisma Lena, Wang, Ye and Sun. This is an open-access article distributed under the terms of the Creative Commons Attribution License (CC BY). The use, distribution or reproduction in other forums is permitted, provided the original author(s) and the copyright owner(s) are credited and that the original publication in this journal is cited, in accordance with accepted academic practice. No use, distribution or reproduction is permitted which does not comply with these terms.
*Correspondence: Mingyu Sun, bXlzdW4yNDhAaG90bWFpbC5jb20=, Guan Ye, eWVnQHNwaGNoaW5hLmNvbQ==
†These authors have contributed equally to this work