- 1Graduate Institute of Clinical Medical Sciences, College of Medicine, Chang Gung University, Taoyuan, Taiwan
- 2Puxin Fengze Chinese Medicine Clinic, Taoyuan, Taiwan
- 3Graduate Institute of Natural Products, College of Medicine, Chang Gung University, Taoyuan, Taiwan
- 4Research Center for Chinese Herbal Medicine, Research Center for Food and Cosmetic Safety, and Graduate Institute of Health Industry Technology, Chang Gung University of Science and Technology, Taoyuan, Taiwan
- 5Department of Biotechnology, College of Life Science, Kaohsiung Medical University, Kaohsiung, Taiwan
- 6School of Traditional Chinese Medicine, College of Medicine, Chang Gung University, Taoyuan, Taiwan
- 7Center for Traditional Chinese Medicine, Chang Gung Memorial Hospital, Taoyuan, Taiwan
- 8Department of Anesthesiology, Chang Gung Memorial Hospital, Taoyuan, Taiwan
- 9Department of Chemical Engineering, Ming Chi University of Technology, New Taipei City, Taiwan
This review describes targeting neutrophils as a potential therapeutic strategy for acute respiratory distress syndrome (ARDS) associated with coronavirus disease 2019 (COVID-19), a pandemic caused by severe acute respiratory syndrome coronavirus 2 (SARS-CoV-2). Neutrophil counts are significantly elevated in patients with COVID-19 and significantly correlated with disease severity. The neutrophil-to-lymphocyte ratio can serve as a clinical marker for predicting fatal complications related to ARDS in patients with COVID-19. Neutrophil-associated inflammation plays a critical pathogenic role in ARDS. The effector functions of neutrophils, acting as respiratory burst oxidants, granule proteases, and neutrophil extracellular traps, are linked to the pathogenesis of ARDS. Hence, neutrophils can not only be used as pathogenic markers but also as candidate drug targets for COVID-19 associated ARDS.
Introduction
Severe acute respiratory syndrome coronavirus 2 (SARS-CoV-2), an enveloped, nonsegmented, positive-sense RNA β-coronavirus, is the cause of the ongoing coronavirus disease 2019 (COVID-19) pandemic (Guo et al., 2020). SARS-CoV-2 is primarily transmitted by respiratory droplets and airway secretions through close contact with infected individuals (Lee and Hsueh, 2020). The main manifestations of COVID-19 are fever, cough, dyspnea, sore throat, fatigue, diarrhea (Guan et al., 2020), headache, nausea, vomiting (Li Y. C. et al., 2020), anosmia (loss of smell), and ageusia (loss of taste) (Vaira et al., 2020). Severe complications include acute respiratory distress syndrome (ARDS), septic shock, coagulation dysfunction, and multiple organ failure (Wang et al., 2020). The elderly (>65 years of age) and individuals with underlying secondary diseases, such as chronic obstructive pulmonary disease (COPD), cardiovascular disease, hypertension, and diabetes mellitus, tend to have severe complications and higher mortality rates (Yang et al., 2020). An effective therapy for COVID-19 remains under investigation (Lu, 2020).
Neutrophils are pivotal effector cells in the innate immune defense against infections in humans. Neutrophils migrate to infected tissues in multiple ways including rolling, adhesion, crawling, and transmigration. Subsequently, they are activated and exert inflammatory responses, such as phagocytosis, respiratory burst with superoxide anion production, degranulation with protease release, and NETosis with neutrophil extracellular trap (NET) formation (Liew and Kubes, 2019). Neutrophil inflammatory responses may be considered a double-edged sword; although they protect against infection, they also cause severe tissue damage. Activated neutrophils are involved in many acute and chronic inflammatory diseases as well as autoimmune disorders, such as respiratory diseases (ARDS, COPD, and asthma), cardiovascular diseases (atherosclerosis and thrombosis) (Németh et al., 2020), gastrointestinal diseases (inflammatory bowel disease and autoimmune hepatitis) (Honda and Kubes, 2018), neurological diseases (multiple sclerosis and Alzheimer’s disease) (Dong et al., 2018; Woodberry et al., 2018), skin diseases (psoriasis and Behçet’s disease) (Safi et al., 2018; Chiang et al., 2019), and metabolic diseases (diabetes mellitus and obesity) (Talukdar et al., 2012; Brotfain et al., 2015).
During the incubation period and nonsevere stage of COVID-19, the host immune system successfully destroys the virus and protects against disease progression. However, in the severe stage, SARS-CoV-2 replicates rapidly and causes massive tissue damage, particularly in the lungs. Thereafter, the destroyed cells cause a dysregulated inflammatory response and cytokine storm, leading to ARDS and other severe complications (Shi H. et al., 2020). Therefore, therapeutic strategies targeting hyperactivated neutrophils may be useful for treating COVID-19 associated ARDS. It has been suggested that a combination of antiviral and anti-inflammatory therapies effectively inhibit SARS-CoV-2 activity and reduce dysregulated immune reactions in COVID-19 (Stebbing et al., 2020).
In this review, we describe the roles of neutrophils in COVID-19 associated ARDS and provide an overview of suitable therapeutic strategies for targeting neutrophils. The particular focus is on clinical drugs and clinical trial drugs shown to affect neutrophil function (Table 1).
General Characteristics of COVID-19 Associated ARDS
ARDS is a critical noncardiogenic pulmonary edema caused by alveolar infection or inflammation. Patients who develop ARDS suffer from a series of nonspecific manifestations, such as cough, shortness of breath, dyspnea, tachycardia, or cyanosis of the nail bed (Sweeney and Mcauley, 2016). If respiratory failure occurs, patients require endotracheal intubation and mechanical ventilation. The mortality rate is approximately 30%–40% (Stevens et al., 2018). ARDS is diagnosed using the Berlin criteria, i.e., acute onset or worsening within one week, bilateral lung infiltrates upon chest X-ray or computed tomography scan, origin exclusive of heart failure or volume overload, disease severity based on desaturation values (severe: arterial oxygen tension/inspired oxygen fraction (PaO2/FiO2) ≤ 100 mmHg, moderate: PaO2/FiO2 100 to ≤ 200 mmHg, and mild: PaO2/FiO2 200 to ≤ 300 mmHg), and minimum positive end-expiratory pressure (PEEP) of 5 cm H2O for mechanical ventilation (Figure 1) (Ranieri et al., 2012). Patients with pneumonia, sepsis, gastric aspiration, or chest trauma may readily develop ARDS. Respiratory viruses, such as influenza virus, Middle East respiratory syndrome-related coronavirus (MERS), SARS-CoV, rhinovirus, respiratory syncytial virus, parainfluenza virus, human metapneumovirus, and adenoviruses may cause viral pneumonia and severe ARDS (Shah and Wunderink, 2017). SARS-CoV-2 emerged in 2019 and caused the COVID-19 outbreak. Patients with COVID-19 may experience lethal pneumonia and ARDS (Badraoui et al., 2020; Zhou P. et al., 2020). Matthay et al. provided a list of recommended treatments for patients with ARDS caused by COVID-19 including adjustment of the tidal volume to 6 ml/kg predicted weight, maintenance of the plateau airway pressure at <30 cm H2O, neuromuscular blockade for patient-ventilator dyssynchrony, maintenance of a prone position during ventilation for severe ARDS, maintenance of a negative fluid balance of 0.5–1.0 L/day, and antibiotic administration for secondary bacterial and fungal infections (Matthay et al., 2020). Extracorporeal membrane oxygenation (ECMO) for ARDS related to COVID-19 requires careful patient selection, intensive care, and secondary infection prevention to rescue lung injury in severe cases of ARDS (Mi et al., 2018; Ramanathan et al., 2020).
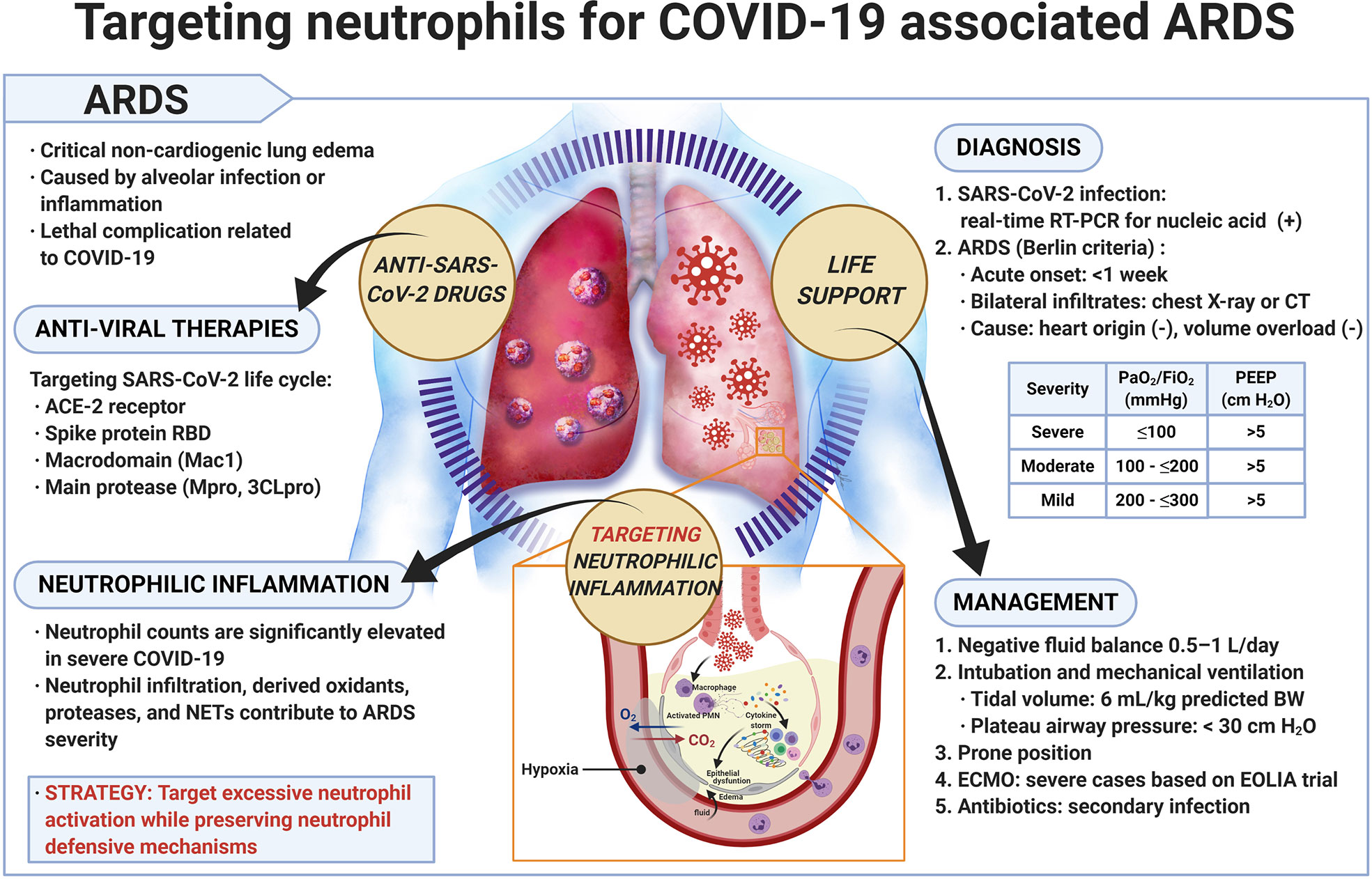
Figure 1 Targeting neutrophils for COVID-19 associated ARDS. Precise diagnosis of SARS-CoV-2 infection and effective management of COVID-19 are important. Application of antiviral therapy together with suppression of overly active neutrophilic inflammation appears to be a promising strategy for treating patients with COVID-19 associated ARDS. ARDS, acute respiratory distress syndrome; BW, body weight; COVID-19, coronavirus disease 2019; CT, computed tomography; ECMO, extracorporeal membrane oxygenation; EOLIA, ECMO to rescue lung injury in severe ARDS; FiO2, inspired oxygen fraction; ACE2, angiotensin-converting enzyme 2; NET, neutrophil extracellular trap; PaO2, arterial oxygen tension; PEEP, positive end-expiratory pressure; PMN, polymorphonuclear leukocyte; RBD, receptor binding domain; RT-PCR, reverse transcription polymerase chain reaction; SARS-CoV-2, severe acute respiratory syndrome coronavirus 2.
Contribution of Neutrophils to COVID-19 Associated ARDS
SARS-CoV-2 employs human angiotensin-converting enzyme 2 (hACE2) as an entry receptor for invading host (Zhou P. et al., 2020). The hACE2 receptor is abundant in the respiratory airway, blood vessels, kidney, and intestine (Li Y. C. et al., 2020). Viral RNAs serve as pathogen-associated molecular patterns (PAMPs) and are sensed by Toll-like receptors (TLRs) such as TLR3, TLR7, TLR8, and TLR9. This results in the production of interferon α and β, along with various proinflammatory cytokines (Kawai and Akira, 2010). Lung inflammation initiated by proinflammatory macrophages and neutrophils causes ARDS, a critical issue in the severe form of COVID-19 (Shi H. et al., 2020). Patients with severe COVID-19 exhibit dysregulated immune responses, such as decreased lymphocyte levels, but increased neutrophil levels (Qin et al., 2020). The neutrophil count in patients with pneumonia was found higher than in patients with only mild acute respiratory disease related to COVID-19 (Lai et al., 2020). The remarkably elevated neutrophil count was found to serve as a marker for poor prognosis in a retrospective review of 25 deaths related to SARS-CoV-2 (Li X. et al., 2020). In another retrospective analysis of 95 patients with COVID-19, an increased neutrophil count was related to disease severity and reflected an overt inflammatory response causing complications (Zhang et al., 2020). The neutrophil-to-lymphocyte ratio was significantly elevated in patients with severe COVID-19 based on a meta-analysis. Furthermore, this ratio could be used as a marker for predicting whether more severe complications such as ARDS would arise (Lagunas-Rangel, 2020). Finally, neutrophils are suggested as a target for immunopathologic complications in severe COVID-19 patients (Tomar et al., 2020). The elevated neutrophil count in COVID-19 patients and its significant correlation with disease severity indicates the importance of neutrophils in the management of COVID-19. The elevated neutrophil count is not only an abnormal laboratory finding but also a characteristic feature that should be further evaluated to develop treatments for patients infected with SARS-CoV-2.
Cellular invasion of SARS-CoV-2 reduces hACE2 expression, thereby promoting the recruitment of neutrophils (Tomar et al., 2020). Possible strategies for developing anti-SARS-CoV-2 drugs include targeting the ACE2 receptor, the spike (S) protein receptor binding domain, the macrodomain (Mac1), and the main protease (Mpro, 3CLpro) (Alhammad et al., 2020; Ton et al., 2020; Zhu et al., 2020). Neutrophils were widespread in the alveoli in COVID-19 patients (Zuo et al., 2020). In rats, excessive neutrophil migration to the lungs caused severe lung hemorrhage and increased microvascular permeability. Therefore, neutrophils participate in viral clearance while contributing to pathological symptoms in rat respiratory coronavirus infection (Haick et al., 2014). Moreover, neutrophils play a pivotal role in the cytokine storm (Tisoncik et al., 2012). In COVID-19 patients, neutrophils secrete interleukin (IL)-6 via a TLR8-mediated mechanism leading to a cytokine storm and subsequent lung damage (Mohamed et al., 2020). IL-1β and NETs form a feedback loop, which contribute to the pathogenesis of ARDS in COVID-19 patients (Yaqinuddin and Kashir, 2020). SARS-CoV-2 may invade nerves and aggravate respiratory failure (Li Y.C. et al., 2020). Neutrophil reactive oxygen species (ROS) and NETs participate in demyelination of the central neural system in mice with neurological diseases and coronavirus infection (Cheng et al., 2019). Therefore, treatments targeting excessive neutrophil activation may improve pathological neutrophilic inflammation during COVID-19 infection complicated by ARDS and nerve invasion.
Targeting Neutrophils May Improve the Treatment of ARDS Caused by SARS-COV-2 Infection
Neutrophil infiltration is the defining hallmark of ARDS (Zemans and Matthay, 2017). Elevated neutrophils and neutrophil-derived microparticles are found in bronchoalveolar lavage fluid from patients with ARDS (Nakos et al., 1998; Guervilly et al., 2011). In ARDS patients, macrophages secrete CXCL8 (IL-8) to activate a circulating neutrophil recruitment cascade via C-X-C chemokine receptor 1 (CXCR1) receptors. CXCL8 levels are also correlated with the severity and outcome of ARDS (Miller et al., 1992; Groeneveld et al., 1995). CXC chemokines including CXCL1/2, CXCL8, CXCL5, CXCL12, and CXCL15 are responsible for neutrophil recruitment to the lungs during lung injury. However, their blockade would not completely prevent neutrophil recruitment, which indicates a rather complicated mechanism operating during immune activation (Zemans and Matthay, 2017). The SARS-CoV S protein stimulates lung epithelial cells to release IL-8 via activation of MAPK and AP-1, the IL-8 promoter (Chang et al., 2004). Epithelial membrane protein 2 (Emp2) of alveolar epithelial type 1 cells is important for the regulation of neutrophil migration in ARDS. Emp2 knock-out mice displayed decreased neutrophil influx to the lungs and an improved survival rate of bacterial pneumonia (Lin et al., 2020). Therefore, anti-EMP2 diabodies may be helpful in treating ARDS by mitigating neutrophil infiltration. Moreover, CCL2 (monocyte chemoattractant protein-1, MCP-1) and CCL7 are increased in the lungs of patients with ARDS (Bhatia et al., 2012; Mercer et al., 2014). Extravasated neutrophils exhibit elevated CCL2 and CCL7 binding to CCR2 receptors. Proteinase-activated receptors (PARs) are present on epithelial cells, monocytes, macrophages, and vascular endothelial cells, and their activation leads to the release of proinflammatory mediators including the cytokines TNF, IL-1β, IL-2, and IL-6, and the chemokines CXCL8 (IL-8) and CCL2, all of which are associated with ARDS pathogenesis. Modulating the CC chemokine response via antagonism of PAR1 signaling or by blocking these chemokines directly, may represent a treatment model for excessive neutrophilia and tissue damage associated with ARDS (Mercer et al., 2014). The level of neutrophil-derived calprotectin, along with other acute inflammatory markers, is correlated with pulmonary severity caused by SARS-CoV-2, which indicates that neutrophils are drivers of the thrombo-inflammatory storm, not just bystanders (Shi Y. et al., 2020). Mitochondrial formyl peptides are elevated in ARDS patients (Dorward et al., 2017) and formyl peptides are known to drive neutrophils in ARDS. Formyl peptide receptors (FPRs) play an important role in the activation of neutrophils (Yang and Hwang, 2016; Chen et al., 2017), and FPR-1 expression is elevated in lung injury and fibrosis (Leslie et al., 2020). Several FPR1 antagonists were discovered previously in our lab including the clinical drug propofol (Yang et al., 2013; Liu et al., 2017; Yang et al., 2017; Chen et al., 2018) that may have potential in the development of the treatment for COVID-19 associated ARDS.
NETs are composed of sticky chromatin decorated with various granular components (Zawrotniak and Rapala-Kozik, 2013). Interestingly, sputum viscosity is correlated with the level of NETs (Papayannopoulos et al., 2011; Manzenreiter et al., 2012). Mucokinetic drugs that preserve viscoelasticity, not mucolytics, were recommended for the management of cystic fibrosis (Henke and Ratjen, 2007). This could also be applied to ARDS in COVID-19 patients. NET level was found related to the polarization of proinflammatory M1 macrophages in ARDS patients; furthermore, NET inhibitors repressed NET formation and reduced M1 macrophage markers in a mouse model of acute lung injury (Song et al., 2019). Phagocytosis of NETs by macrophages is impaired in ARDS (Grégoire et al., 2018). Activation of the AMP-activated protein kinase (AMPK) pathway stimulates macrophage efferocytosis (Bae et al., 2011). Therefore, drugs interacting with AMPK, such as metformin, may reduce ARDS severity (Grégoire et al., 2018). Moreover, NET levels in the plasma are known to be correlated with ARDS mortality (Lefrançais et al., 2018). NETs consist of neutrophil-extruded DNA coated with histones, neutrophil elastase (NE), and myeloperoxidase (MPO). Peptidylarginine deiminase 4 (PAD4), NE, and gasdermin D along with free DNA, all participate in the NET formation (Nathan, 2020). NETs are prevalent in blood, trachea, and lung specimens of COVID-19 patients (Veras et al., 2020). Moreover, SARS-CoV-2 stimulates neutrophils from healthy donors into forming NETs, which can cause apoptosis in respiratory epithelial cells in vitro (Oubihi and Wang, 2020). High levels of plasma MPO-DNA complex and aberrant NET formation are correlated with severe ARDS in COVID-19 (Middleton et al., 2020; Zuo et al., 2020). Also, elevated levels of cell-free DNA were observed in COVID-19 patients (n = 50), as well as highly specific markers of NETs, such as MPO-DNA and citrullinated histone H3 (Cit-H3), along with other typical markers (C-reactive protein, D-dimer, neutrophil count, etc.) (Zuo et al., 2020). NETs contributed to microthrombi through platelet-neutrophil interactions in COVID-19 associated ARDS, and neonatal NET-Inhibitory Factor (nNIF) could block NET formation induced by COVID-19 plasma. This represents a potential therapeutic intervention for COVID-19 (Middleton et al., 2020). PAD4 is a predominant driver of histone citrullination in NETs (Wong and Wagner, 2018). Currently, several PAD4 inhibitors were demonstrated to inhibit NET formation in vitro. Among them, Cl-amidine (Kusunoki et al., 2016), GSK484 (Lewis et al., 2015), and BMS-P5 (Li M. et al., 2020) may have future development potential. Therefore, inhibition of neutrophil activation and NET formation may be beneficial in COVID-19-associated ARDS.
Drugs Targeting Neutrophils for COVID-19 ASSOCIATED ARDS
Currently, there are several clinical drugs indicated for use in respiratory diseases that affect neutrophil function, but other drugs should also be considered for the treatment of ARDS in COVID-19. A summary of commercially available approved drugs or those in clinical trials, allocated to different groups based on their specific target in neutrophils, is provided (Figure 2, Table 1).
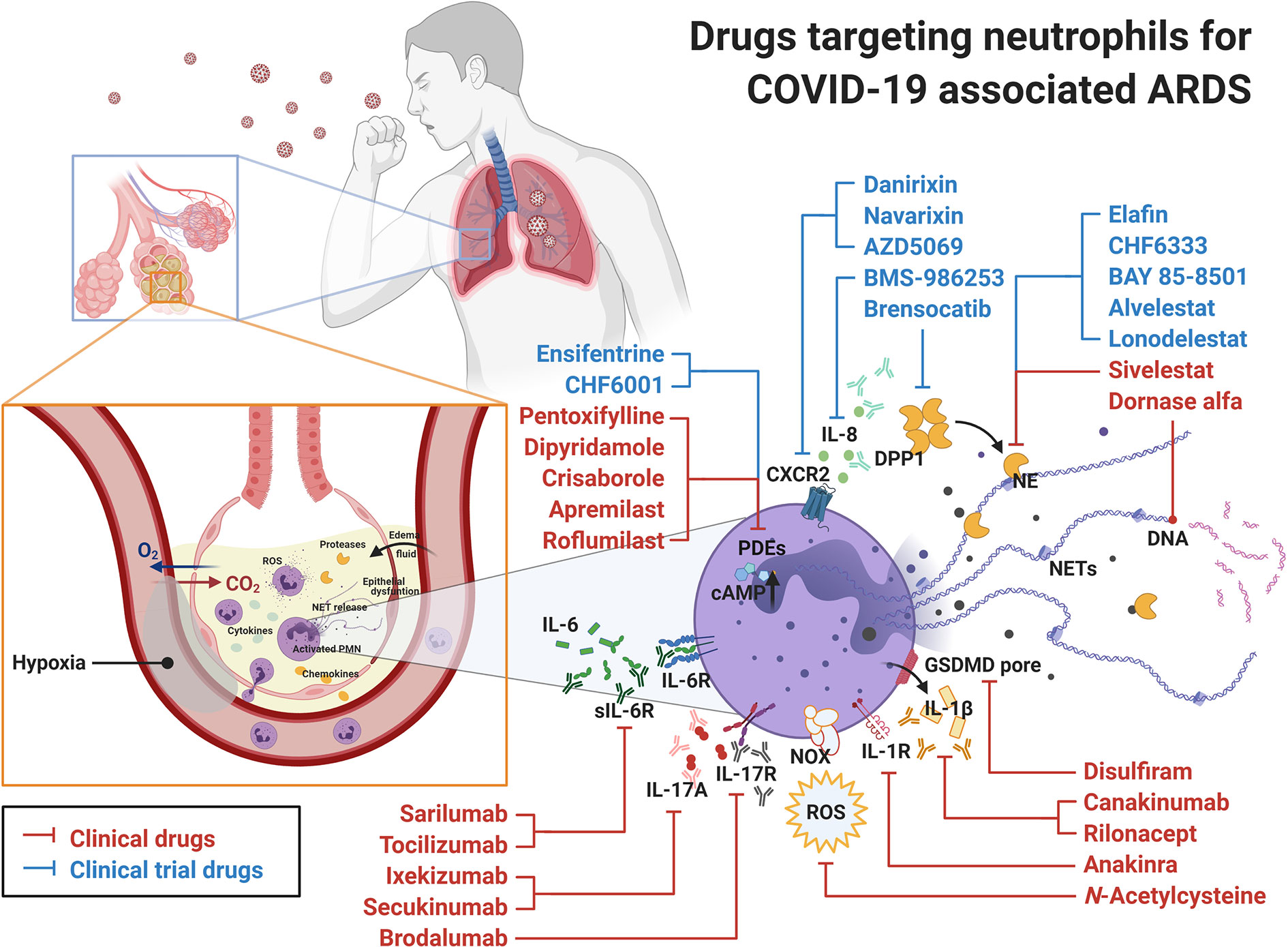
Figure 2 Drugs targeting neutrophils for COVID-19 associated ARDS. Clinical drugs (in red) and clinical trial drugs (in blue) are displayed with their targets in neutrophils. In COVID-19 infection, the release of SARS-CoV-2 RNA in lungs serves as PAMP and induces complicated immune reactions leading to ARDS and respiratory failure. Under these circumstances, neutrophils recruited to the site of infection participate in the elimination of SARS-CoV-2, but they also contribute to the pathogenesis of ARDS. Therefore, the commercial drugs targeting neutrophils might be considered as novel candidates to treat COVID-19 associated ARDS. ARDS, acute respiratory distress syndrome; CXCR, C-X-C chemokine receptor; DPP1, dipeptidyl peptidase 1; GSDMD, gasdermin D; IL, interleukin; NE, neutrophil elastase; NET, neutrophil extracellular trap; NOX, NADPH oxidase; PDE, phosphodiesterase; PMN, polymorphonuclear leukocyte; ROS, reactive oxygen species.
Neutrophil Elastase Inhibitors
Neutrophil elastase (NE) contributes to the invasion of SARS-CoV-2 into host cells and can also damage lung tissue directly, thus participating in the pathogenesis of COVID-19 associated ARDS (Thierry, 2020). Moreover, NE is an important component and plays an important role in NETosis. For instance, the administration of elastase inhibitors such as sivelestat (Kim et al., 2014) and BAY 85-8501 (Von Nussbaum et al., 2015) ameliorated acute lung injury in mice. Currently, sivelestat is the only approved NE inhibitor for the treatment of ARDS in Korea and Japan (Aikawa and Kawasaki, 2014). There are several other NE inhibitors in different stages of clinical trials including alvelestat (AZD9668, COPD, phase 2, US national clinical trial number NCT03636347) (Stockley et al., 2013), BAY 85-8501 (noncystic fibrosis bronchiectasis, phase 2, NCT01818544), (Watz et al., 2019), lonodelestat (POL6014, cystic fibrosis, phase 1, NCT03748199) (Barth et al., 2020), CHF6333 (noncystic fibrosis bronchiectasis, phase 1, NCT03056326, NCT04010799) (Gramegna et al., 2017), and elafin (ARDS, phase 1, NCT02944279) (Barnes et al., 2020). In a meta-analysis study, sivelestat failed to improve the survival of patients with ARDS (Tagami et al., 2014). However, a retrospective cohort study with 66 ARDS patients demonstrated that sivelestat treatment yields positive outcomes (Maki et al., 2020). In particular, aerosol- or nebulizer-dosed NE inhibitors significantly improved their efficacy and lowered adverse effects (Barth et al., 2020). Thus, prompt administration of NE inhibitors may be helpful in severe COVID-19 patients with ARDS (Mohamed et al., 2020).
Respiratory Burst Inhibitor
N-Acetylcysteine is a potential therapeutic in the treatment of COVID-19 (Andreou et al., 2020). It is a mucolytic drug with antioxidant activity that is used in respiratory diseases (Mokhtari et al., 2017) and skin diseases (Adil et al., 2018), as well as an antidote in acetaminophen overdose (Mokhtari et al., 2017). Moreover, N-acetylcysteine inhibited the respiratory burst in activated neutrophils in vitro (Allegra et al., 2002) and in patients in the intensive care unit (Heller et al., 2001). Furthermore, N-acetylcysteine alleviated acute lung injury in vivo under various conditions (Alkan et al., 2006; Chuang et al., 2007; Liu et al., 2008; Yubero et al., 2012; Guo et al., 2019), and inhibited lung fibrosis in vivo (Kulshrestha et al., 2020) with limited patient outcomes, according to a meta-analysis study (Sun et al., 2016). In another meta-analysis study, N-acetylcysteine treatment of ARDS patients shortened their stay in the intensive care unit (Zhang et al., 2017). Finally, it has also been suggested that N-acetylcysteine should be used in combination with other drugs to manage ARDS (Guo et al., 2019; Andreou et al., 2020; Horowitz and Freeman, 2020). Currently, several clinical trials with N-acetylcysteine to treat COVID-19 (NCT04419025, NCT04455243, NCT04374461, etc.) are ongoing. Based on the above, we believe that N-acetylcysteine may be helpful in treating ARDS caused by SARS-CoV-2 via its antioxidant and anti-respiratory burst activity.
Dipeptidyl Peptidase 1 Inhibitor
Dipeptidyl peptidase 1 (DPP1), also known as cathepsin C, is a cysteine dipeptidyl aminopeptidase that activates serine proteases such as NE during maturation of neutrophils. Excessive serine protease activity causes various inflammatory lung diseases such as ARDS and contributes to COPD and asthma. Brensocatib (also called INS1007 or AZD7986), a DPP1 inhibitor, was found to reduce NE activity in healthy humans (Palmér et al., 2018) and is now in clinical trials for bronchiectasis (NCT03218917) and COVID-19 (EU clinical trial number EudraCT 2020-001643-13). The administration of DPP1 inhibitors may prevent ARDS progression caused by SARS-CoV-2 (Korkmaz et al., 2020). Thus, DPP1 inhibitors are of interest in treating COVID-19 associated ARDS.
PDE4 Inhibitors
Phosphodiesterases (PDEs) belong to the class of enzymes that metabolize the intracellular second messenger cyclic adenosine monophosphate (cAMP) and cyclic guanosine monophosphate (cGMP). In particular, cAMP-specific PDE4 type is widely present in immune cells including neutrophils and contributes to neutrophil-mediated lung inflammation (Baillie et al., 2019). There are currently three FDA-approved PDE4 inhibitors: roflumilast for COPD (Phillips, 2020), apremilast for psoriasis (Queiro Silva et al., 2020), and crisaborole for atopic dermatitis (Hashim et al., 2020). Other drugs such as CHF6001 (NCT02986321 for COPD and NCT01689571 for asthma) (Singh et al., 2019), and ensifentrine (RPL554, NCT04027439 for COPD) (Cazzola et al., 2019) are awaiting phase 3 clinical trials. However, many of the experimental drugs were discontinued in clinical trials due to side effects (Phillips, 2020). Several PDE inhibitors have been proposed to be suitable drugs for COVID-19 treatment (Giorgi et al., 2020). Thus, we suggest their clinical consideration in COVID-19 associated ARDS. In light of the above, clinical trials targeting COVID-19 using apremilast (NCT04488081) or ensifentrine (NCT04527471) have been initiated.
Dipyridamole is an FDA-approved nonspecific PDE inhibitor used for thrombosis and was discovered to inhibit NET formation (Ali et al., 2019). Dipyridamole acts by increasing intracellular cAMP levels and blocking adenosine reuptake in cells, thereby leading to its antiplatelet and vasodilatory effects (Tan et al., 2019). In a trial including 31 COVID-19 patients, dipyridamole showed improvement in severe cases with significantly reduced D-dimer levels (Liu et al., 2020). Currently, several COVID-related trials are ongoing for dipyridamole (NCT04410328, NCT04424901, NCT04391179). Another FDA-approved nonspecific PDE inhibitor, pentoxifylline, is a derivative of caffeine. Pentoxifylline stimulates blood flow, inhibits platelets, and has immunomodulatory and anti-inflammatory properties. Pentoxifylline also inhibits neutrophil adhesion (Bone, 1992). It is currently in ongoing clinical trials for COVID-19 (NCT04433988). Along with specific PDE4 inhibitors, dipyridamole and pentoxifylline may represent suitable candidates for further anti-COVID-19 development (Hendry et al., 2020).
Gasdermin D Inhibitor
Disulfiram, an FDA-approved gasdermin D inhibitor, blocks SARS-CoV-2 replication in silico (Lobo-Galo et al., 2020). Moreover, disulfiram was shown to abrogate gasdermin D pore formation by covalent bonding to Cys191/Cys192 (Hu et al., 2020). Gasdermin D is important in the formation of NETs (Sollberger et al., 2018). Therefore, disulfiram has the potential to reduce NET-related pathogenesis in ARDS caused by SARS-CoV-2. Currently, a clinical trial is ongoing on the potential use of disulfiram in COVID-19 (NCT04485130).
DNase Inhibitors
Application of DNase I to mice with severe bacterial pneumonia and acute lung injury reduced NET formation and improved their survival rate (Lefrançais et al., 2018). Administration of dornase alfa, an FDA-approved recombinant human DNAse I, using a nebulizer in severe COVID-19 associated ARDS patients may help lyse the sputum and improve disease progression (Barnes et al., 2020; Weber et al., 2020). Currently, there are several COVID-19 clinical trials using dornase alfa (NCT04402970, NCT04359654, NCT04355364, EudraCT 2020-001492-33, etc.) (Desilles et al., 2020). Another promising DNase inhibitor, AIR DNAse™, is currently undergoing phase 2 clinical trials in cystic fibrosis patients (NCT02605590, NCT02722122).
Chemokine-Related Drugs (IL-8, IL-17, IL-1β and IL-6)
IL-8 secreted by macrophages and lung epithelial cells is a neutrophil chemoattractant. Moreover, IL-8 contributes to neutrophil activation and NET formation after binding to CXCR2 on neutrophils, thereby causing hyperinflammation. Interestingly, anti-IL-8 monoclonal antibody BMS-986253 (Humax IL-8), developed as an anti-tumor treatment (Bilusic et al., 2019), is currently in clinical trial for COVID-19 (NCT04347226). AZD5069 (Nicholls et al., 2015; Cullberg et al., 2018), danirixin (Madan et al., 2019; Lazaar et al., 2020), and navarixin (SCH527123) (Holz et al., 2010; Todd et al., 2016) are available CXCR2 inhibitors that ameliorate neutrophil activation in pulmonary diseases including bronchiectasis, virus-associated lung infection, COPD and asthma. Therefore, they may represent valuable drugs for the treatment of ARDS in COVID-19 patients (Narasaraju et al., 2020). However, the development of the CXCR2 antagonist QBM076 was terminated for safety reasons (NCT01972776).
IL-17A is a proinflammatory cytokine involved in inflammation and immune responses; thus, blocking its effect is beneficial in treating neutrophilic inflammatory diseases. Ixekizumab (NCT03099538) and secukinumab (NCT03099980) are monoclonal antibodies (mAb) against IL-17A that prevent its interaction with the IL-17A receptor. In particular, IL-17A antagonists have been used for the treatment of rheumatoid arthritis and psoriasis (Chiang et al., 2019). Brodalumab (NCT04249713) binds with a high affinity to interleukin IL-17 receptor A, thereby inhibiting IL-17 proinflammatory cytokines (Bulat et al., 2020). Secukinumab is currently tested in coronavirus trials (NCT04403243, EudraCT 2020-001246-18).
Anakinra is a IL-1 receptor inhibitor, while canakinumab and rilonacept are IL-1β inhibitors (Prieto-Peña and Dasgupta, 2020). Anakinra was reported in the treatment of COVID-19 (Van De Veerdonk and Netea, 2020). Subcutaneous administration of anakinra reduced mortality and lowered the need for advanced respiratory support in severe COVID-19 patients (Huet et al., 2020); however, several limitations were observed (Khan et al., 2020). There are currently several clinical trials in relation to COVID-19 on anakinra (NCT04330638, NCT04364009, etc.) and canakinumab (NCT04362813, etc.).
Tocilizumab, an IL-6 receptor inhibitor, reduced the level of serum C-reactive protein and ameliorated pulmonary computed tomography appearances in patients with COVID-19 (Guaraldi et al., 2020; Soy et al., 2020; Xu et al., 2020). A plethora of clinical trials using tocilizumab is currently ongoing in relation to COVID-19 (Actemra, Roche, NCT04317092, NCT04320615, etc.). Similarly, several clinical trials for another IL-6 receptor inhibitor, sarilumab (Kevzara, Regeneron, NCT04315298, etc.), have been initiated (Lu et al., 2020).
Other Drugs
Corticosteroids are recommended by the US National Institutes of Health (NIH) for use in COVID-19 patients who are being mechanically ventilated or require oxygen supplementation (https://www.covid19treatmentguidelines.nih.gov). However, there are several concerns, and corticoids were previously not recommended in patients with ARDS related to viral pneumonia (Ni et al., 2019). Corticosteroids also reduce the level of neutrophil-derived secreted IL-1 receptor antagonist (sIL-1Ra), which leads to increased IL-1β expression (Langereis et al., 2011). Hence, corticosteroids have proinflammatory effects in neutrophils. Corticosteroids are generally thought to weakly suppress neutrophilic inflammation, although several research groups reported them to inhibit the neutrophil respiratory burst and interfere with neutrophil recruitment (Strausbaugh and Rosen, 2001; Ronchetti et al., 2018; Ricci et al., 2019). For instance, corticosteroids do not improve neutrophilic inflammation in corticosteroid-resistant asthma or COPD (Yang et al., 2012; Ronchetti et al., 2018).
Dapsone, colchicine, and olanzapine were suggested as potential adjuvant therapies in ARDS caused by SARS-CoV-2 (Altschuler and Kast, 2020). Dapsone has anti-neutrophilic activity through its inhibition of IL-8 mediated chemotaxis and thus may be therapeutic in COVID-19 associated ARDS (Farouk and Salman, 2020; Kast, 2020). Colchicine, a neutrophil microtubule polymerization inhibitor, reduces IL-1 production and is undergoing several clinical trials for COVID-19 (NCT04322682 and 18 more) (Soy et al., 2020). Olanzapine is an antipsychotic drug targeting the histamine 1 receptor, thereby reducing IL-6 generation (Altschuler and Kast, 2020).
Hydroxychloroquine and azithromycin were reported as effective anti-SARS-CoV-2 agents (Ohe et al., 2020; Zhou D. et al., 2020). Fujita et al. demonstrated that hydroxychloroquine significantly inhibited IL-1β production in activated human neutrophils in vitro (Fujita et al., 2019). Hydroxychloroquine inhibited NET formation and ameliorated the hypercoagulation state in mice with orthotopic pancreatic adenocarcinoma. A chemotherapy regimen with hydroxychloroquine prior to surgery improved the rate of perioperative venous thromboembolism in a clinical trial (Boone et al., 2018). With respect to the effect of azithromycin on neutrophils, Bystrzycka et al. revealed that azithromycin decreased NET release in activated neutrophils in vitro (Bystrzycka et al., 2017). Anderson et al. demonstrated that azithromycin inhibited neutrophil migration in dextran sulfate sodium-induced mice (Anderson et al., 2019). However, Cavalcanti et al. showed that hydroxychloroquine and azithromycin did not alter disease progression in patients with mild-to-moderate COVID-19 (Cavalcanti et al., 2020). The World Health Organization does not recommend the use of hydroxychloroquine and azithromycin due to the controversial outcomes of several trials.
Conclusion
ARDS is the most lethal complication of COVID-19. Neutrophils, although involved in the elimination of SARS-CoV-2, also participate in the pathogenesis of COVID-19 associated ARDS. Suppression of aberrant neutrophil activation may provide an effective strategy for treating COVID-19 associated ARDS. Several clinical drugs that target neutrophils can be chosen for further therapeutic use in ARDS associated with SARS-CoV-2 infection.
Author Contributions
C-CC, MK, and W-JC wrote the manuscript. T-LH conceived the study and edited the manuscript. All authors contributed to the article and approved the submitted version.
Funding
This work was supported by grants from the Ministry of Science and Technology (MOST 108-2320-B-255-003-MY3, MOST 109-2327-B-255-001, and MOST 109-2327-B-182-002) and Chang Gung Memorial Hospital (BMRP450, and CORPG5K0031). The funding sources had no role in the study design; in the collection, analysis and interpretation of data; in the writing of the report; or in the decision to submit the article for publication.
Conflict of Interest
The authors declare that the research was conducted in the absence of any commercial or financial relationships that could be construed as a potential conflict of interest.
Acknowledgments
This manuscript was edited by Editage. The figures were supported by BioRender.
References
Adil, M., Amin, S. S., Mohtashim, M. (2018). N-acetylcysteine in dermatology. Indian J. Dermatol. Venereol. Leprol. 84, 652–659. doi: 10.4103/ijdvl.IJDVL_33_18
Aikawa, N., Kawasaki, Y. (2014). Clinical utility of the neutrophil elastase inhibitor sivelestat for the treatment of acute respiratory distress syndrome. Ther. Clin. Risk Manage. 10, 621–629. doi: 10.2147/TCRM.S65066
Alhammad, Y. M. O., Kashipathy, M. M., Roy, A., Johnson, D. K., Mcdonald, P., Battaile, K. P., et al. (2020). The SARS-CoV-2 conserved macrodomain is a highly efficient ADP-ribosylhydrolase enzyme. bioRxiv 2020.05.11.089375. doi: 10.1101/2020.05.11.0893755
Ali, R. A., Gandhi, A. A., Meng, H., Yalavarthi, S., Vreede, A. P., Estes, S. K., et al. (2019). Adenosine receptor agonism protects against NETosis and thrombosis in antiphospholipid syndrome. Nat. Commun. 10, 1916. doi: 10.1038/s41467-019-09801-x
Alkan, A., Eroğlu, F., Eroğlu, E., Ergin, C., Cerçi, C., Alsancak, G. (2006). Protective effects of N-acetylcysteine and erdosteine on hemorrhagic shock-induced acute lung injury. Eur. J. Emerg. Med. 13, 281–285. doi: 10.1097/00063110-200610000-00007
Allegra, L., Dal Sasso, M., Bovio, C., Massoni, C., Fonti, E., Braga, P. C. (2002). Human neutrophil oxidative bursts and their in vitro modulation by different N-acetylcysteine concentrations. Arzneimittelforschung 52, 669–676. doi: 10.1055/s-0031-1299949
Altschuler, E. L., Kast, R. E. (2020). Dapsone, colchicine and olanzapine as treatment adjuncts to prevent COVID-19 associated adult respiratory distress syndrome (ARDS). Med. Hypotheses 141, 109774. doi: 10.1016/j.mehy.2020.109774
Anderson, S. J., Lockhart, J. S., Estaki, M., Quin, C., Hirota, S. A., Alston, L., et al. (2019). Effects of azithromycin on behavior, pathologic signs, and changes in cytokines, chemokines, and neutrophil migration in C57BL/6 mice exposed to dextran sulfate sodium. Comp. Med. 69, 4–15. doi: 10.30802/AALAS-CM-18-000001
Andreou, A., Trantza, S., Filippou, D., Sipsas, N., Tsiodras, S. (2020). COVID-19: The potential role of copper and N-acetylcysteine (NAC) in a combination of candidate antiviral treatments against SARS-CoV-2. In Vivo 34, 1567–1588. doi: 10.21873/invivo.11946
Badraoui, R., Alrashedi, M. M., El-May, M. V., Bardakci, F. (2020). Acute respiratory distress syndrome: a life threatening associated complication of SARS-CoV-2 infection inducing COVID-19. J. Biomol. Struct. Dyn., 1–10. doi: 10.1080/07391102.2020.1803139 Online ahead of print.
Bae, H. B., Zmijewski, J. W., Deshane, J. S., Tadie, J. M., Chaplin, D. D., Takashima, S., et al. (2011). AMP-activated protein kinase enhances the phagocytic ability of macrophages and neutrophils. FASEB J. 25, 4358–4368. doi: 10.1096/fj.11-190587
Baillie, G. S., Tejeda, G. S., Kelly, M. P. (2019). Therapeutic targeting of 3’,5’-cyclic nucleotide phosphodiesterases: inhibition and beyond. Nat. Rev. Drug Discovery 18, 770–796. doi: 10.1038/s41573-019-0033-4
Barnes, B. J., Adrover, J. M., Baxter-Stoltzfus, A., Borczuk, A., Cools-Lartigue, J., Crawford, J. M., et al. (2020). Targeting potential drivers of COVID-19: Neutrophil extracellular traps. J. Exp. Med. 217 (6), e20200652. doi: 10.1084/jem.20200652
Barth, P., Bruijnzeel, P., Wach, A., Sellier Kessler, O., Hooftman, L., Zimmermann, J., et al. (2020). Single dose escalation studies with inhaled POL6014, a potent novel selective reversible inhibitor of human neutrophil elastase, in healthy volunteers and subjects with cystic fibrosis. J. Cyst. Fibros. 19, 299–304. doi: 10.1016/j.jcf.2019.08.020
Bhatia, M., Zemans, R. L., Jeyaseelan, S. (2012). Role of chemokines in the pathogenesis of acute lung injury. Am. J. Respir. Cell Mol. Biol. 46, 566–572. doi: 10.1165/rcmb.2011-0392TR
Bilusic, M., Heery, C. R., Collins, J. M., Donahue, R. N., Palena, C., Madan, R. A., et al. (2019). Phase I trial of HuMax-IL8 (BMS-986253), an anti-IL-8 monoclonal antibody, in patients with metastatic or unresectable solid tumors. J. Immunother. Cancer 7, 240. doi: 10.1186/s40425-019-0706-x
Bone, R. C. (1992). Inhibitors of complement and neutrophils: a critical evaluation of their role in the treatment of sepsis. Crit. Care Med. 20, 891–898. doi: 10.1097/00003246-199206000-00029
Boone, B. A., Murthy, P., Miller-Ocuin, J., Doerfler, W. R., Ellis, J. T., Liang, X., et al. (2018). Chloroquine reduces hypercoagulability in pancreatic cancer through inhibition of neutrophil extracellular traps. BMC Cancer 18, 678. doi: 10.1186/s12885-018-4584-2
Brotfain, E., Hadad, N., Shapira, Y., Avinoah, E., Zlotnik, A., Raichel, L., et al. (2015). Neutrophil functions in morbidly obese subjects. Clin. Exp. Immunol. 181, 156–163. doi: 10.1111/cei.12631
Bulat, V., Situm, M., Azdajic, M. D., Likic, R. (2020). Potential role of IL-17 blocking agents in the treatment of severe COVID-19? Br. J. Clin. Pharmacol. doi: 10.1111/bcp.14437 Online ahead of print.
Bystrzycka, W., Manda-Handzlik, A., Sieczkowska, S., Moskalik, A., Demkow, U., Ciepiela, O. (2017). Azithromycin and chloramphenicol diminish neutrophil extracellular traps (NETs) release. Int. J. Mol. Sci. 18 (12), 2666. doi: 10.3390/ijms18122666 Online ahead of print.
Cavalcanti, A. B., Zampieri, F. G., Rosa, R. G., Azevedo, L. C. P., Veiga, V. C., Avezum, A., et al. (2020). Hydroxychloroquine with or without azithromycin in mild-to-moderate Covid-19. N. Engl. J. Med. doi: 10.1056/NEJMoa2019014
Cazzola, M., Calzetta, L., Rogliani, P., Matera, M. G. (2019). Ensifentrine (RPL554): an investigational PDE3/4 inhibitor for the treatment of COPD. Expert Opin. Investig. Drugs 28, 827–833. doi: 10.1080/13543784.2019.1661990
Chang, Y. J., Liu, C. Y., Chiang, B. L., Chao, Y. C., Chen, C. C. (2004). Induction of IL-8 release in lung cells via activator protein-1 by recombinant baculovirus displaying severe acute respiratory syndrome-coronavirus spike proteins: identification of two functional regions. J. Immunol. 173, 7602–7614. doi: 10.4049/jimmunol.173.12.7602
Chen, K., Bao, Z., Gong, W., Tang, P., Yoshimura, T., Wang, J. M. (2017). Regulation of inflammation by members of the formyl-peptide receptor family. J. Autoimmun. 85, 64–77. doi: 10.1016/j.jaut.2017.06.012
Chen, C. Y., Tsai, Y. F., Huang, W. J., Chang, S. H., Hwang, T. L. (2018). Propofol inhibits endogenous formyl peptide-induced neutrophil activation and alleviates lung injury. Free Radic. Biol. Med. 129, 372–382. doi: 10.1016/j.freeradbiomed.2018.09.048
Cheng, Y., Skinner, D. D., Lane, T. E. (2019). Innate immune responses and viral-induced neurologic disease. J. Clin. Med. 8 (1), 3. doi: 10.3390/jcm8010003
Chiang, C. C., Cheng, W. J., Korinek, M., Lin, C. Y., Hwang, T. L. (2019). Neutrophils in psoriasis. Front. Immunol. 10, 2376. doi: 10.3389/fimmu.2019.02376
Chuang, I. C., Liu, D. D., Kao, S. J., Chen, H. I. (2007). N-acetylcysteine attenuates the acute lung injury caused by phorbol myristate acetate in isolated rat lungs. Pulm. Pharmacol. Ther. 20, 726–733. doi: 10.1016/j.pupt.2006.08.010
Cullberg, M., Arfvidsson, C., Larsson, B., Malmgren, A., Mitchell, P., Wahlby Hamren, U., et al. (2018). Pharmacokinetics of the oral selective CXCR2 antagonist AZD5069: a summary of eight phase I studies in healthy volunteers. Drugs R. D. 18, 149–159. doi: 10.1007/s40268-018-0236-x
Desilles, J. P., Gregoire, C., Le Cossec, C., Lambert, J., Mophawe, O., Losser, M. R., et al. (2020). Efficacy and safety of aerosolized intra-tracheal dornase alfa administration in patients with SARS-CoV-2-induced acute respiratory distress syndrome (ARDS): a structured summary of a study protocol for a randomised controlled trial. Trials 21, 548. doi: 10.1186/s13063-020-04488-8
Dong, Y., Lagarde, J., Xicota, L., Corne, H., Chantran, Y., Chaigneau, T., et al. (2018). Neutrophil hyperactivation correlates with Alzheimer’s disease progression. Ann. Neurol. 83, 387–405. doi: 10.1002/ana.25159
Dorward, D. A., Lucas, C. D., Doherty, M. K., Chapman, G. B., Scholefield, E. J., Conway Morris, A., et al. (2017). Novel role for endogenous mitochondrial formylated peptide-driven formyl peptide receptor 1 signalling in acute respiratory distress syndrome. Thorax 72, 928–936. doi: 10.1136/thoraxjnl-2017-210030
Farouk, A., Salman, S. (2020). Dapsone and doxycycline could be potential treatment modalities for COVID-19. Med. Hypotheses 140, 109768. doi: 10.1016/j.mehy.2020.109768
Fujita, Y., Matsuoka, N., Temmoku, J., Furuya, M. Y., Asano, T., Sato, S., et al. (2019). Hydroxychloroquine inhibits IL-1β production from amyloid-stimulated human neutrophils. Arthritis Res. Ther. 21, 250. doi: 10.1186/s13075-019-2040-6
Giorgi, M., Cardarelli, S., Ragusa, F., Saliola, M., Biagioni, S., Poiana, G., et al. (2020). Phosphodiesterase inhibitors: could they be beneficial for the treatment of COVID-19? Int. J. Mol. Sci. 21, 5338. doi: 10.3390/ijms21155338
Gramegna, A., Amati, F., Terranova, L., Sotgiu, G., Tarsia, P., Miglietta, D., et al. (2017). Neutrophil elastase in bronchiectasis. Respir. Res. 18, 211–211. doi: 10.1186/s12931-017-0691-x
Grégoire, M., Uhel, F., Lesouhaitier, M., Gacouin, A., Guirriec, M., Mourcin, F., et al. (2018). Impaired efferocytosis and neutrophil extracellular trap clearance by macrophages in ARDS. Eur. Respir. J. 52, 1702590. doi: 10.1183/13993003.02590-2017
Groeneveld, A. B., Raijmakers, P. G., Hack, C. E., Thijs, L. G. (1995). Interleukin 8-related neutrophil elastase and the severity of the adult respiratory distress syndrome. Cytokine 7, 746–752. doi: 10.1006/cyto.1995.0089
Guan, W. J., Ni, Z. Y., Hu, Y., Liang, W. H., Ou, C. Q., He, J. X., et al. (2020). Clinical characteristics of coronavirus cisease 2019 in China. N. Engl. J. Med. 382, 1708–1720. doi: 10.1056/NEJMoa2002032
Guaraldi, G., Meschiari, M., Cozzi-Lepri, A., Milic, J., Tonelli, R., Menozzi, M., et al. (2020). Tocilizumab in patients with severe COVID-19: a retrospective cohort study. Lancet Rheumatol. 2, e474–e484. doi: 10.1016/S2665-9913(20)30173-9
Guervilly, C., Lacroix, R., Forel, J. M., Roch, A., Camoin-Jau, L., Papazian, L., et al. (2011). High levels of circulating leukocyte microparticles are associated with better outcome in acute respiratory distress syndrome. Crit. Care 15, R31. doi: 10.1186/cc9978
Guo, D. W., Wang, C. Y., Shih, H. C. (2019). N-acetylcysteine and atorvastatin alleviates lung injury due to ischemia-reperfusion injury in rats. J. Chin. Med. Assoc. 82, 909–914. doi: 10.1097/JCMA.0000000000000193
Guo, Y. R., Cao, Q. D., Hong, Z. S., Tan, Y. Y., Chen, S. D., Jin, H. J., et al. (2020). The origin, transmission and clinical therapies on coronavirus disease 2019 (COVID-19) outbreak - an update on the status. Mil. Med. Res. 7, 11. doi: 10.1186/s40779-020-00240-0
Haick, A. K., Rzepka, J. P., Brandon, E., Balemba, O. B., Miura, T. A. (2014). Neutrophils are needed for an effective immune response against pulmonary rat coronavirus infection, but also contribute to pathology. J. Gen. Virol. 95, 578–590. doi: 10.1099/vir.0.061986-0
Hashim, P. W., Chima, M., Kim, H. J., Bares, J., Yao, C. J., Singer, G., et al. (2020). Crisaborole 2% ointment for the treatment of intertriginous, anogenital, and facial psoriasis: A double-blind, randomized, vehicle-controlled trial. J. Am. Acad. Dermatol. 82, 360–365. doi: 10.1016/j.jaad.2019.06.1288
Heller, A. R., Groth, G., Heller, S. C., Breitkreutz, R., Nebe, T., Quintel, M., et al. (2001). N-acetylcysteine reduces respiratory burst but augments neutrophil phagocytosis in intensive care unit patients. Crit. Care Med. 29, 272–276. doi: 10.1097/00003246-200102000-00009.
Hendry, B. M., Stafford, N., Arnold, A. D., Sangwaiya, A., Manglam, V., Rosen, S. D., et al. (2020). Hypothesis: pentoxifylline is a potential cytokine modulator therapeutic in COVID-19 patients. Pharmacol. Res. Perspect. 8, e00631. doi: 10.1002/prp2.631
Henke, M. O., Ratjen, F. (2007). Mucolytics in cystic fibrosis. Paediatr. Respir. Rev. 8, 24–29. doi: 10.1016/j.prrv.2007.02.009
Holz, O., Khalilieh, S., Ludwig-Sengpiel, A., Watz, H., Stryszak, P., Soni, P., et al. (2010). SCH527123, a novel CXCR2 antagonist, inhibits ozone-induced neutrophilia in healthy subjects. Eur. Respir. J. 35, 564–570. doi: 10.1183/09031936.00048509
Honda, M., Kubes, P. (2018). Neutrophils and neutrophil extracellular traps in the liver and gastrointestinal system. Nat. Rev. Gastroenterol. Hepatol. 15, 206–221. doi: 10.1038/nrgastro.2017.183
Horowitz, R. I., Freeman, P. R. (2020). Three novel prevention, diagnostic, and treatment options for COVID-19 urgently necessitating controlled randomized trials. Med. Hypotheses 143, 109851. doi: 10.1016/j.mehy.2020.109851
Hu, J. J., Liu, X., Xia, S., Zhang, Z., Zhang, Y., Zhao, J., et al. (2020). FDA-approved disulfiram inhibits pyroptosis by blocking gasdermin D pore formation. Nat. Immunol. 21, 736–745. doi: 10.1038/s41590-020-0669-6
Huet, T., Beaussier, H., Voisin, O., Jouveshomme, S., Dauriat, G., Lazareth, I., et al. (2020). Anakinra for severe forms of COVID-19: a cohort study. Lancet Rheumatol. 2, e393–e400. doi: 10.1016/S2665-9913(20)30164-8
Kast, R. E. (2020). Dapsone as treatment adjunct in ARDS. Exp. Lung Res. 46, 157–161. doi: 10.1080/01902148.2020.1753266
Kawai, T., Akira, S. (2010). The role of pattern-recognition receptors in innate immunity: update on Toll-like receptors. Nat. Immunol. 11, 373–384. doi: 10.1038/ni.1863
Khan, A. R., Soneja, M., George, N., Wig, N. (2020). Anakinra for severe forms of COVID-19. Lancet Rheumatol. doi: 10.1016/S2665-9913(20)30271-X
Kim, D. H., Chung, J. H., Son, B. S., Kim, Y. J., Lee, S. G. (2014). Effect of a neutrophil elastase inhibitor on ventilator-induced lung injury in rats. J. Thorac. Dis. 6, 1681–1689. doi: 10.3978/j.issn.2072-1439.2014.11.10
Korkmaz, B., Lesner, A., Marchand-Adam, S., Moss, C., Jenne, D. E. (2020). Lung protection by cathepsin C inhibition: a new hope for COVID-19 and ARDS? J. Med. Chem. 7, 2020. doi: 10.1021/acs.jmedchem.0c00776
Kulshrestha, R., Pandey, A., Jaggi, A., Bansal, S. (2020). Beneficial effects of N-acetylcysteine on protease-antiprotease balance in attenuating bleomycin-induced pulmonary fibrosis in rats. Iran. J. Basic Med. Sci. 23, 396–405. doi: 10.22038/IJBMS.2020.39031.9261
Kusunoki, Y., Nakazawa, D., Shida, H., Hattanda, F., Miyoshi, A., Masuda, S., et al. (2016). Peptidylarginine deiminase inhibitor suppresses neutrophil extracellular trap formation and MPO-ANCA production. Front. Immunol. 7:227. doi: 10.3389/fimmu.2016.00227
Lagunas-Rangel, F. A. (2020). Neutrophil-to-lymphocyte ratio and lymphocyte-to-C-reactive protein ratio in patients with severe coronavirus disease 2019 (COVID-19): A meta-analysis. J. Med. Virol. doi: 10.1002/jmv.25819
Lai, C. C., Liu, Y. H., Wang, C. Y., Wang, Y. H., Hsueh, S. C., Yen, M. Y., et al. (2020). Asymptomatic carrier state, acute respiratory disease, and pneumonia due to severe acute respiratory syndrome coronavirus 2 (SARS-CoV-2): facts and myths. J. Microbiol. Immunol. Infect. 53, 404–412. doi: 10.1016/j.jmii.2020.02.012
Langereis, J. D., Oudijk, E. J., Schweizer, R. C., Lammers, J. W., Koenderman, L., Ulfman, L. H. (2011). Steroids induce a disequilibrium of secreted interleukin-1 receptor antagonist and interleukin-1β synthesis by human neutrophils. Eur. Respir. J. 37, 406–415. doi: 10.1183/09031936.00170409
Lazaar, A. L., Miller, B. E., Donald, A. C., Keeley, T., Ambery, C., Russell, J., et al. (2020). CXCR2 antagonist for patients with chronic obstructive pulmonary disease with chronic mucus hypersecretion: a phase 2b trial. Respir. Res. 21, 149. doi: 10.1186/s12931-020-01401-4
Lee, P. I., Hsueh, P. R. (2020). Emerging threats from zoonotic coronaviruses-from SARS and MERS to 2019-nCoV. J. Microbiol. Immunol. Infect. 53, 365–367. doi: 10.1016/j.jmii.2020.02.001
Lefrançais, E., Mallavia, B., Zhuo, H., Calfee, C. S., Looney, M. R. (2018). Maladaptive role of neutrophil extracellular traps in pathogen-induced lung injury. JCI Insight 3 (3), e98178. doi: 10.1172/jci.insight.98178
Leslie, J., Millar, B. J., Del Carpio Pons, A., Burgoyne, R. A., Frost, J. D., Barksby, B. S., et al. (2020). FPR-1 is an important regulator of neutrophil recruitment and a tissue-specific driver of pulmonary fibrosis. JCI Insight 5 (4), e125937. doi: 10.1172/jci.insight.125937
Lewis, H. D., Liddle, J., Coote, J. E., Atkinson, S. J., Barker, M. D., Bax, B. D., et al. (2015). Inhibition of PAD4 activity is sufficient to disrupt mouse and human NET formation. Nat. Chem. Biol. 11, 189–191. doi: 10.1038/nchembio.1735
Li, M., Lin, C., Deng, H., Strnad, J., Bernabei, L., Vogl, D. T., et al. (2020). A novel peptidylarginine deiminase 4 (PAD4) inhibitor BMS-P5 blocks formation of neutrophil extracellular traps and delays progression of multiple myeloma. Mol. Cancer Ther. 19, 1530–1538. doi: 10.1158/1535-7163.MCT-19-1020
Li, X., Wang, L., Yan, S., Yang, F., Xiang, L., Zhu, J., et al. (2020). Clinical characteristics of 25 death cases infected with COVID-19 pneumonia: a retrospective review of medical records in a single medical center, Wuhan, China. Int. J. Infect. Dis. 94, 128–132. doi: 10.1016/j.ijid.2020.03.053
Li, Y. C., Bai, W. Z., Hashikawa, T. (2020). The neuroinvasive potential of SARS-CoV2 may play a role in the respiratory failure of COVID-19 patients. J. Med. Virol. 92, 552–555. doi: 10.1002/jmv.25728
Liew, P. X., Kubes, P. (2019). The neutrophil’s role during health and disease. Physiol. Rev. 99, 1223–1248. doi: 10.1152/physrev.00012.2018
Lin, W. C., Gowdy, K. M., Madenspacher, J. H., Zemans, R. L., Yamamoto, K., Lyons-Cohen, M., et al. (2020). Epithelial membrane protein 2 governs transepithelial migration of neutrophils into the airspace. J. Clin. Invest. 130, 157–170. doi: 10.1172/JCI127144
Liu, D. D., Kao, S. J., Chen, H. I. (2008). N-acetylcysteine attenuates acute lung injury induced by fat embolism. Crit. Care Med. 36, 565–571. doi: 10.1097/01.CCM.0000299737.24338.5C
Liu, F. C., Yu, H. P., Syu, Y. T., Fang, J. Y., Lin, C. F., Chang, S. H., et al. (2017). Honokiol suppresses formyl peptide-induced human neutrophil activation by blocking formyl peptide receptor 1. Sci. Rep. 7, 6718. doi: 10.1038/s41598-017-07131-w
Liu, X., Li, Z., Liu, S., Sun, J., Chen, Z., Jiang, M., et al. (2020). Potential therapeutic effects of dipyridamole in the severely ill patients with COVID-19. Acta Pharm. Sin. B 10 (7), 1205–1215. doi: 10.1016/j.apsb.2020.04.008
Lobo-Galo, N., Terrazas-López, M., Martínez-Martínez, A., Díaz-Sánchez, A. G. (2020). FDA-approved thiol-reacting drugs that potentially bind into the SARS-CoV-2 main protease, essential for viral replication. J. Biomol. Struct. Dyn. 1–9. doi: 10.1080/07391102.2020.1764393
Lu, C. C., Chen, M. Y., Lee, W. S., Chang, Y. L. (2020). Potential therapeutic agents against COVID-19: What we know so far. J. Chin. Med. Assoc. 83, 534–536. doi: 10.1097/JCMA.0000000000000318
Lu, H. (2020). Drug treatment options for the 2019-new coronavirus, (2019-nCoV). Biosci. Trends 14, 69–71. doi: 10.5582/bst.2020.01020
Madan, A., Chen, S., Yates, P., Washburn, M. L., Roberts, G., Peat, A. J., et al. (2019). Efficacy and safety of danirixin (GSK1325756) co-administered with standard-of-care antiviral (oseltamivir): a phase 2b, global, randomized study of adults hospitalized with influenza. Open Forum Infect. Dis. 6, ofz163. doi: 10.1093/ofid/ofz163
Maki, C., Inoue, Y., Ishihara, T., Hirano, Y., Kondo, Y., Sueyoshi, K., et al. (2020). Evaluation of appropriate indications for the use of sivelestat sodium in acute respiratory distress syndrome: a retrospective cohort study. Acute Med. Surg. 7, e471. doi: 10.1002/ams2.471
Manzenreiter, R., Kienberger, F., Marcos, V., Schilcher, K., Krautgartner, W. D., Obermayer, A., et al. (2012). Ultrastructural characterization of cystic fibrosis sputum using atomic force and scanning electron microscopy. J. Cyst. Fibros. 11, 84–92. doi: 10.1016/j.jcf.2011.09.008
Matthay, M. A., Aldrich, J. M., Gotts, J. E. (2020). Treatment for severe acute respiratory distress syndrome from COVID-19. Lancet Respir. Med. 8, 433–434. doi: 10.1016/S2213-2600(20)30127-2
Mercer, P. F., Williams, A. E., Scotton, C. J., José, R. J., Sulikowski, M., Moffatt, J. D., et al. (2014). Proteinase-activated receptor-1, CCL2, and CCL7 regulate acute neutrophilic lung inflammation. Am. J. Respir. Cell Mol. Biol. 50, 144–157. doi: 10.1165/rcmb.2013-0142OC
Mi, M. Y., Matthay, M. A., Morris, A. H. (2018). Extracorporeal membrane oxygenation for severe acute respiratory distress syndrome. N. Engl. J. Med. 379, 884–887. doi: 10.1056/NEJMclde1804601
Middleton, E. A., He, X. Y., Denorme, F., Campbell, R. A., Ng, D., Salvatore, S. P., et al. (2020). Neutrophil extracellular traps contribute to immunothrombosis in COVID-19 acute respiratory distress syndrome. Blood 136 (10), 1169–1179. doi: 10.1182/blood.2020007008
Miller, E. J., Cohen, A. B., Nagao, S., Griffith, D., Maunder, R. J., Martin, T. R., et al. (1992). Elevated levels of NAP-1/interleukin-8 are present in the airspaces of patients with the adult respiratory distress syndrome and are associated with increased mortality. Am. Rev. Respir. Dis. 146, 427–432. doi: 10.1164/ajrccm/146.2.427
Mohamed, M. M. A., El-Shimy, I. A., Hadi, M. A. (2020). Neutrophil elastase inhibitors: a potential prophylactic treatment option for SARS-CoV-2-induced respiratory complications? Crit. Care 24, 311. doi: 10.1186/s13054-020-03023-0
Mokhtari, V., Afsharian, P., Shahhoseini, M., Kalantar, S. M., Moini, A. (2017). A review on various uses of N-acetyl cysteine. Cell J. 19, 11–17. doi: 10.22074/cellj.2016.4872
Nakos, G., Kitsiouli, E. I., Tsangaris, I., Lekka, M. E. (1998). Bronchoalveolar lavage fluid characteristics of early intermediate and late phases of ARDS. Alterations in leukocytes, proteins, PAF and surfactant components. Intensive Care Med. 24, 296–303. doi: 10.1007/s001340050571
Narasaraju, T., Tang, B. M., Herrmann, M., Muller, S., Chow, V. T. K., Radic, M. (2020). Neutrophilia and NETopathy as key pathologic drivers of progressive lung impairment in patients with COVID-19. Front. Pharmacol. 11:870. doi: 10.3389/fphar.2020.00870
Nathan, C. (2020). Neutrophils and COVID-19: Nots, NETs, and knots. J. Exp. Med. 21 (9), e20201439.7. doi: 10.1084/jem.20201439
Németh, T., Sperandio, M., Mócsai, A. (2020). Neutrophils as emerging therapeutic targets. Nat. Rev. Drug Discovery 19, 253–275. doi: 10.1038/s41573-019-0054-z
Ni, Y. N., Chen, G., Sun, J., Liang, B. M., Liang, Z. A. (2019). The effect of corticosteroids on mortality of patients with influenza pneumonia: a systematic review and meta-analysis. Crit. Care 23, 99. doi: 10.1186/s13054-019-2395-8
Nicholls, D. J., Wiley, K., Dainty, I., Macintosh, F., Phillips, C., Gaw, A., et al. (2015). Pharmacological characterization of AZD5069, a slowly reversible CXC chemokine receptor 2 antagonist. J. Pharmacol. Exp. Ther. 353, 340–350. doi: 10.1124/jpet.114.221358
Ohe, M., Shida, H., Jodo, S., Kusunoki, Y., Seki, M., Furuya, K., et al. (2020). Macrolide treatment for COVID-19: will this be the way forward? Biosci. Trends 14, 159–160. doi: 10.5582/bst.2020.03058
Oubihi, Z., Wang, L. (2020). Caught by NETs. Nat. Rev. Immunol. 20, 462. doi: 10.1038/s41577-020-0384-4
Palmér, R., Mäenpää, J., Jauhiainen, A., Larsson, B., Mo, J., Russell, M., et al. (2018). Dipeptidyl Peptidase 1 inhibitor AZD7986 induces a sustained, exposure-dependent reduction in neutrophil elastase activity in healthy subjects. Clin. Pharmacol. Ther. 104, 1155–1164. doi: 10.1002/cpt.1053
Papayannopoulos, V., Staab, D., Zychlinsky, A. (2011). Neutrophil elastase enhances sputum solubilization in cystic fibrosis patients receiving DNase therapy. PloS One 6, e28526. doi: 10.1371/journal.pone.0028526
Phillips, J. E. (2020). Inhaled phosphodiesterase 4 (PDE4) inhibitors for inflammatory respiratory diseases. Front. Pharmacol. 11, 259. doi: 10.3389/fphar.2020.00259
Prieto-Peña, D., Dasgupta, B. (2020). Biologic agents and small-molecule inhibitors in systemic autoimmune conditions: an update. Pol. Arch. Intern. Med. doi: 10.20452/pamw.15438
Qin, C., Zhou, L., Hu, Z., Zhang, S., Yang, S., Tao, Y., et al. (2020). Dysregulation of immune response in patients with COVID-19 in Wuhan, China. Clin. Infect. Dis. 71, 762–768. doi: 10.1093/cid/ciaa248
Queiro Silva, R., Armesto, S., González Vela, C., Naharro Fernández, C., González-Gay, M. A. (2020). COVID-19 patients with psoriasis and psoriatic arthritis on biologic immunosuppressant therapy vs apremilast in North Spain. Dermatol. Ther. e13961. doi: 10.1111/dth.13961
Ramanathan, K., Antognini, D., Combes, A., Paden, M., Zakhary, B., Ogino, M., et al. (2020). Planning and provision of ECMO services for severe ARDS during the COVID-19 pandemic and other outbreaks of emerging infectious diseases. Lancet Respir. Med. 8, 518–526. doi: 10.1016/S2213-2600(20)30121-1
Ranieri, V. M., Rubenfeld, G. D., Thompson, B. T., Ferguson, N. D., Caldwell, E., Fan, E., et al. (2012). Acute respiratory distress syndrome: the Berlin Definition. JAMA 307, 2526–2533. doi: 10.1001/jama.2012.5669
Ricci, E., Ronchetti, S., Gabrielli, E., Pericolini, E., Gentili, M., Roselletti, E., et al. (2019). GILZ restrains neutrophil activation by inhibiting the MAPK pathway. J. Leukoc. Biol. 105, 187–194. doi: 10.1002/JLB.3AB0718-255R
Ronchetti, S., Ricci, E., Migliorati, G., Gentili, M., Riccardi, C. (2018). How glucocorticoids affect the neutrophil life. Int. J. Mol. Sci. 19 (12), 4090. doi: 10.3390/ijms19124090
Safi, R., Kallas, R., Bardawil, T., Mehanna, C. J., Abbas, O., Hamam, R., et al. (2018). Neutrophils contribute to vasculitis by increased release of neutrophil extracellular traps in Behçet’s disease. J. Dermatol. Sci. 92, 143–150. doi: 10.1016/j.jdermsci.2018.08.010
Shah, R. D., Wunderink, R. G. (2017). Viral pneumonia and acute respiratory distress syndrome. Clin. Chest Med. 38, 113–125. doi: 10.1016/j.ccm.2016.11.013
Shi, H., Zuo, Y., Yalavarthi, S., Gockman, K., Zuo, M., Madison, J. A., et al. (2020). Neutrophil calprotectin identifies severe pulmonary disease in COVID-19. Medrxiv Preprint Server Health Sci. 2020. doi: 10.1101/2020.05.06.20093070
Shi, Y., Wang, Y., Shao, C., Huang, J., Gan, J., Huang, X., et al. (2020). COVID-19 infection: the perspectives on immune responses. Cell Death Differ. 27 (5), 1451–1454. doi: 10.1038/s41418-020-0530-3
Singh, D., Beeh, K. M., Colgan, B., Kornmann, O., Leaker, B., Watz, H., et al. (2019). Effect of the inhaled PDE4 inhibitor CHF6001 on biomarkers of inflammation in COPD. Respir. Res. 20, 180. doi: 10.1186/s12931-019-1142-7
Sollberger, G., Choidas, A., Burn, G. L., Habenberger, P., Di Lucrezia, R., Kordes, S., et al. (2018). Gasdermin D plays a vital role in the generation of neutrophil extracellular traps. Sci. Immunol. 3 (26), eaar6689. doi: 10.1126/sciimmunol.aar6689
Song, C., Li, H., Li, Y., Dai, M., Zhang, L., Liu, S., et al. (2019). NETs promote ALI/ARDS inflammation by regulating alveolar macrophage polarization. Exp. Cell Res. 382, 111486. doi: 10.1016/j.yexcr.2019.06.031
Soy, M., Keser, G., Atagündüz, P., Tabak, F., Atagündüz, I., Kayhan, S. (2020). Cytokine storm in COVID-19: pathogenesis and overview of anti-inflammatory agents used in treatment. Clin. Rheumatol. 39, 2085–2094. doi: 10.1007/s10067-020-05190-5
Stebbing, J., Phelan, A., Griffin, I., Tucker, C., Oechsle, O., Smith, D., et al. (2020). COVID-19: combining antiviral and anti-inflammatory treatments. Lancet Infect. Dis. 20, 400–402. doi: 10.1016/S1473-3099(20)30132-8
Stevens, J. P., Law, A., Giannakoulis, J. (2018). Acute respiratory distress syndrome. JAMA 319, 732. doi: 10.1001/jama.2018.0483
Stockley, R., De Soyza, A., Gunawardena, K., Perrett, J., Forsman-Semb, K., Entwistle, N., et al. (2013). Phase II study of a neutrophil elastase inhibitor (AZD9668) in patients with bronchiectasis. Respir. Med. 107, 524–533. doi: 10.1016/j.rmed.2012.12.009
Strausbaugh, H. J., Rosen, S. D. (2001). A potential role for annexin 1 as a physiologic mediator of glucocorticoid-induced L-selectin shedding from myeloid cells. J. Immunol. 166, 6294–6300. doi: 10.4049/jimmunol.166.10.6294
Sun, T., Liu, J., Zhao De, W. (2016). Efficacy of N-acetylcysteine in idiopathic pulmonary fibrosis: a systematic review and meta-analysis. Med. (Baltimore) 95, e3629. doi: 10.1097/MD.0000000000003629
Sweeney, R. M., Mcauley, D. F. (2016). Acute respiratory distress syndrome. Lancet 388, 2416–2430. doi: 10.1016/S0140-6736(16)00578-X
Tagami, T., Tosa, R., Omura, M., Fukushima, H., Kaneko, T., Endo, T., et al. (2014). Effect of a selective neutrophil elastase inhibitor on mortality and ventilator-free days in patients with increased extravascular lung water: a post hoc analysis of the PiCCO Pulmonary Edema Study. J. Intensive Care 2, 67. doi: 10.1186/s40560-014-0067-y
Talukdar, S., Oh, D. Y., Bandyopadhyay, G., Li, D., Xu, J., Mcnelis, J., et al. (2012). Neutrophils mediate insulin resistance in mice fed a high-fat diet through secreted elastase. Nat. Med. 18, 1407–1412. doi: 10.1038/nm.2885
Tan, M. K. X., Heng, T. Y. J., Mak, A. (2019). The potential use of metformin, dipyridamole, N-acetylcysteine and statins as adjunctive therapy for systemic lupus erythematosus. Cells 8 (4), 323. doi: 10.3390/cells8040323
Thierry, A. R. (2020). Anti-protease treatments targeting plasmin(ogen) and neutrophil elastase may be beneficial in fighting COVID-19. Physiol. Rev. 100, 1597–1598. doi: 10.1152/physrev.00019.2020
Tisoncik, J. R., Korth, M. J., Simmons, C. P., Farrar, J., Martin, T. R., Katze, M. G. (2012). Into the eye of the cytokine storm. Microbiol. Mol. Biol. Rev. 76, 16–32. doi: 10.1128/MMBR.05015-11
Todd, C. M., Salter, B. M., Murphy, D. M., Watson, R. M., Howie, K. J., Milot, J., et al. (2016). The effects of a CXCR1/CXCR2 antagonist on neutrophil migration in mild atopic asthmatic subjects. Pulm. Pharmacol. Ther. 41, 34–39. doi: 10.1016/j.pupt.2016.09.005
Tomar, B., Anders, H. J., Desai, J., Mulay, S. R. (2020). Neutrophils and neutrophil extracellular traps drive necroinflammation in COVID-19. Cells 9 (6), 1383. doi: 10.3390/cells9061383
Ton, A. T., Gentile, F., Hsing, M., Ban, F., Cherkasov, A. (2020). Rapid Identification of Potential Inhibitors of SARS-CoV-2 Main Protease by Deep Docking of 1.3 Billion Compounds. Mol. Inform. 39 (8), e2000028. doi: 10.1002/minf.202000028
Vaira, L. A., Salzano, G., Deiana, G., De Riu, G. (2020). Anosmia and ageusia: common findings in COVID-19 patients. Laryngoscope 130, 1787. doi: 10.1002/lary.28692
Van De Veerdonk, F. L., Netea, M. G. (2020). Blocking IL-1 to prevent respiratory failure in COVID-19. Crit. Care 24, 445. doi: 10.1186/s13054-020-03166-0
Veras, F. P., Pontelli, M. C, Silva, C. M., Toller-Kawahisa, J. E., De Lima, M., Nascimento, D. C., et al. (2020). SARS-CoV-2-triggered neutrophil extracellular traps mediate COVID-19 pathology. J. Exp. Med. 217 (12), e20201129. doi: 10.1084/jem.20201129
Von Nussbaum, F., Li, V. M., Allerheiligen, S., Anlauf, S., Bärfacker, L., Bechem, M., et al. (2015). Freezing the Bioactive Conformation to Boost Potency: The Identification of BAY 85-8501, a Selective and Potent Inhibitor of Human Neutrophil Elastase for Pulmonary Diseases. ChemMedChem 10, 1163–1173. doi: 10.1002/cmdc.201500131
Wang, D., Hu, B., Hu, C., Zhu, F., Liu, X., Zhang, J., et al. (2020). Clinical characteristics of 138 hospitalized patients with 2019 novel coronavirus-infected pneumonia in Wuhan, China. JAMA 323 (11), 1061–1069. doi: 10.1001/jama.2020.1585
Watz, H., Nagelschmitz, J., Kirsten, A., Pedersen, F., Van Der Mey, D., Schwers, S., et al. (2019). Safety and efficacy of the human neutrophil elastase inhibitor BAY 85-8501 for the treatment of non-cystic fibrosis bronchiectasis: a randomized controlled trial. Pulm. Pharmacol. Ther. 56, 86–93. doi: 10.1016/j.pupt.2019.03.009
Weber, A. G., Chau, A. S., Egeblad, M., Barnes, B. J., Janowitz, T. (2020). Nebulized in-line endotracheal dornase alfa and albuterol administered to mechanically ventilated COVID-19 patients: a case series. medRxiv 2020.05.13.20087734. doi: 10.1101/2020.05.13.20087734
Wong, S. L., Wagner, D. D. (2018). Peptidylarginine deiminase 4: a nuclear button triggering neutrophil extracellular traps in inflammatory diseases and aging. FASEB J. 32 (12), 6358 –6370. doi: 10.1096/fj.201800691R
Woodberry, T., Bouffler, S. E., Wilson, A. S., Buckland, R. L., Brüstle, A. (2018). The emerging role of neutrophil granulocytes in multiple sclerosis. J. Clin. Med. 7 (12):511. doi: 10.3390/jcm7120511
Xu, X., Han, M., Li, T., Sun, W., Wang, D., Fu, B., et al. (2020). Effective treatment of severe COVID-19 patients with tocilizumab. Proc. Natl. Acad. Sci. U.S.A. 117, 10970–10975. doi: 10.1073/pnas.2005615117
Yang, S. C., Hwang, T. L. (2016). The potential impacts of formyl peptide receptor 1 in inflammatory diseases. Front. Biosci. (Elite Ed.) 8, 436–449.
Yang, I. A., Clarke, M. S., Sim, E. H., Fong, K. M. (2012). Inhaled corticosteroids for stable chronic obstructive pulmonary disease. Cochrane Database Syst. Rev. (7), Cd002991. doi: 10.1002/14651858.CD002991.pub3
Yang, S. C., Chung, P. J., Ho, C. M., Kuo, C. Y., Hung, M. F., Huang, Y. T., et al. (2013). Propofol inhibits superoxide production, elastase release, and chemotaxis in formyl peptide-activated human neutrophils by blocking formyl peptide receptor 1. J. Immunol. 190, 6511–6519. doi: 10.4049/jimmunol.1202215
Yang, S. C., Chang, S. H., Hsieh, P. W., Huang, Y. T., Ho, C. M., Tsai, Y. F., et al. (2017). Dipeptide HCH6-1 inhibits neutrophil activation and protects against acute lung injury by blocking FPR1. Free Radic. Biol. Med. 106, 254–269. doi: 10.1016/j.freeradbiomed.2017.02.038
Yang, X., Yu, Y., Xu, J., Shu, H., Xia, J., Liu, H., et al. (2020). Clinical course and outcomes of critically ill patients with SARS-CoV-2 pneumonia in Wuhan, China: a single-centered, retrospective, observational study. Lancet Respir. Med. 8, 475–481. doi: 10.1016/S2213-2600(20)30079-5
Yaqinuddin, A., Kashir, J. (2020). Novel therapeutic targets for SARS-CoV-2-induced acute lung injury: targeting a potential IL-1beta/neutrophil extracellular traps feedback loop. Med. Hypotheses 143, 109906. doi: 10.1016/j.mehy.2020.109906
Yubero, S., Ramudo, L., Manso, M. A., Collía, F., De Dios, I. (2012). Evaluation of N-acetylcysteine treatment in acute pancreatitis-induced lung injury. Inflamm. Res. 61, 699–705. doi: 10.1007/s00011-012-0462-6
Zawrotniak, M., Rapala-Kozik, M. (2013). Neutrophil extracellular traps (NETs) - formation and implications. Acta Biochim. Pol. 60, 277–284. doi: 10.18388/abp.2013_1983
Zemans, R. L., Matthay, M. A. (2017). What drives neutrophils to the alveoli in ARDS? Thorax 72, 1–3. doi: 10.1136/thoraxjnl-2016-209170
Zhang, Y., Ding, S., Li, C., Wang, Y., Chen, Z., Wang, Z. (2017). Effects of N-acetylcysteine treatment in acute respiratory distress syndrome: A meta-analysis. Exp. Ther. Med. 14, 2863–2868. doi: 10.3892/etm.2017.4891
Zhang, G., Zhang, J., Wang, B., Zhu, X., Wang, Q., Qiu, S. (2020). Analysis of clinical characteristics and laboratory findings of 95 cases of 2019 novel coronavirus pneumonia in Wuhan, China: a retrospective analysis. Respir. Res. 21, 74. doi: 10.1186/s12931-020-01338-8
Zhou, D., Dai, S. M., Tong, Q. (2020). COVID-19: a recommendation to examine the effect of hydroxychloroquine in preventing infection and progression. J. Antimicrob. Chemother. 75, 1667–1670. doi: 10.1093/jac/dkaa114
Zhou, P., Yang, X. L., Wang, X. G., Hu, B., Zhang, L., Zhang, W., et al. (2020). A pneumonia outbreak associated with a new coronavirus of probable bat origin. Nature 579, 270–273. doi: 10.1038/s41586-020-2012-7
Zhu, Y., Li, J., Pang, Z. (2020). Recent insights for the emerging COVID-19: drug discovery, therapeutic options and vaccine development. Asian J. Pharm. Sci. doi: 10.1016/j.ajps.2020.06.001
Keywords: coronavirus disease 2019, severe acute respiratory syndrome coronavirus 2, acute respiratory distress syndrome, neutrophils, neutrophil extracellular trap
Citation: Chiang C-C, Korinek M, Cheng W-J and Hwang T-L (2020) Targeting Neutrophils to Treat Acute Respiratory Distress Syndrome in Coronavirus Disease. Front. Pharmacol. 11:572009. doi: 10.3389/fphar.2020.572009
Received: 12 June 2020; Accepted: 11 September 2020;
Published: 09 October 2020.
Edited by:
Siddappa N. Byrareddy, University of Nebraska Omaha, United StatesReviewed by:
Betsy J. Barnes, Feinstein Institute for Medical Research, United StatesHitendra S. Chand, Florida International University, United States
Copyright © 2020 Chiang, Korinek, Cheng and Hwang. This is an open-access article distributed under the terms of the Creative Commons Attribution License (CC BY). The use, distribution or reproduction in other forums is permitted, provided the original author(s) and the copyright owner(s) are credited and that the original publication in this journal is cited, in accordance with accepted academic practice. No use, distribution or reproduction is permitted which does not comply with these terms.
*Correspondence: Tsong-Long Hwang, aHRsQG1haWwuY2d1LmVkdS50dw==
†These authors have contributed equally to this work