- 1Research and Development Laboratory for Biochemical, Molecular and Cellular Applications in Ophthalmological Sciences, IRCCS - Fondazione Bietti, Rome, Italy
- 2Ophthalmology Operative Complex Unit, University Campus Bio-Medico, Rome, Italy
Vision-threatening retinal diseases affect millions of people worldwide, representing an important public health issue (high social cost) for both technologically advanced and new-industrialized countries. Overall RD group comprises the retinitis pigmentosa, the age-related macular degeneration (AMD), the diabetic retinopathy (DR), and idiopathic epiretinal membrane formation. Endocrine, metabolic, and even lifestyles risk factors have been reported for these age-linked conditions that represent a “public priority” also in this COVID-19 emergency. Chronic inflammation and neurodegeneration characterize the disease evolution, with a consistent vitreoretinal interface impairment. As the vitreous chamber is significantly involved, the latest diagnostic technologies of imaging (retina) and biomarker detection (vitreous) have provided a huge input at both medical and surgical levels. Complement activation and immune cell recruitment/infiltration as well as detrimental intra/extracellular deposits occur in association with a reactive gliosis. The cell/tissue aging route shows a specific signal path and biomolecular profile characterized by the increased expression of several glial-derived mediators, including angiogenic/angiostatic, neurogenic, and stress-related factors (oxidative stress metabolites, inflammation, and even amyloid formation). The possibility to access vitreous chamber by collecting vitreous reflux during intravitreal injection or obtaining vitreous biopsy during a vitrectomy represents a step forward for an individualized therapy. As drug response and protein signature appear unique in each single patient, therapies should be individualized. This review addresses the current knowledge about biomarkers and pharmacological targets in these vitreoretinal diseases. As vitreous fluids might reflect the early stages of retinal sufferance and/or late stages of neurodegeneration, the possibility to modulate intravitreal levels of growth factors, in combination to anti-VEGF therapy, would open to a personalized therapy of retinal diseases.
Introduction
A recent evaluation of the current prevalence and pattern of retinal diseases (RD) in industrialized and nonindustrialized countries highlights that RD are increasing worldwide representing a serious problem even under COVID-19 emergency.
RD group includes the retinitis pigmentosa, the age-related macular degeneration, the diabetic retinopathy, and the idiopathic epiretinal membrane formations. Other than genetic background, several risk factors (endocrine, metabolic, and even lifestyles influencers) have been reported for these age-linked conditions that represent a “public priority” as worldwide population is more long-lived and its life expectancy is increasing. The chronic inflammation and neurodegeneration affect the neuronal network, with different vitreoretinal interface impairment. As the vitreous chamber is significantly affected due to release of a plethora of soluble mediators, the latest diagnostic technologies of imaging (retina) and biomarker detection (vitreous) have provided a huge input at both medical and surgical levels. As per consecutive increase, the early complement activation, the immune cell recruitment/infiltration with local release of soluble mediators, and the formation of intra/extracellular deposits lead to impairment of the neuronal network, sustained by reactive gliosis. Tissue/cell aging shows a specific signal path and biomolecular profile characterized by the increased expression of several glial-derived mediators, including angiogenic/angiostatic, neurogenic, and stress-related factors (oxidative stress metabolites, inflammation, and even amyloid formation).
This review addresses the current knowledge about potential candidate biomarkers and pharmacological targets in these vitreoretinal diseases. As vitreous fluids might reflect the early stages of retinal sufferance and/or late stages of degeneration, the possibility to modulate intravitreal levels of growth factors will be also discussed as an additional way to improve treatment approaches, most of them in combination to anti-VEGF therapy.
Precision Therapy for Retinal Diseases: State of the Art
Over the last years, numerous changes have been introduced to improve vitreoretinal surgery, such as the development of transconjunctival sutureless vitrectomy, ameliorated cutters, and new surgical approaches (Fujii et al., 2002). The possibility to collect vitreous biopsies represents a great opportunity of analysis for individualized medicine in retinal diseases (Oh and Oshima, 2014; Kasi et al., 2017; Coassin et al., 2020).
Globally, precision medicine approach represents a new way of thinking at prevention and treatment of multifactorial diseases, taking into consideration the individual variability in terms of genetic background, environment, and lifestyle. Although relatively new, the concept of precision medicine has represented for many years a fundamental aspect of healthcare outside ophthalmology. The possibility to predict and treat the disease depending on the specific local condition represents a crucial aspect of cancer therapy, and it is now extended to several other healthcare areas (Blix, 2014; Velez et al., 2018). In ophthalmology, precision medicine represents an effective approach for treating ocular tumors (Straatsma, 2018). In addition, this strategy is being applied successfully in the management of the inherited diseases (Ong et al., 2013). In the recent years, precision therapy has gained increasing interest for the management of retinal diseases. In particular, it has been recently proposed for intravitreal treatments in association with the biochemical analysis of vitreal reflux (Cacciamani et al., 2016). Collecting vitreous samples at the time of anti-VEGF intravitreal injection for wet AMD may be an effective method to elaborate further the precise clinical condition of the specific patient under treatment.
One aspect of precision medicine is the necessity of noninvasive indicators to drive the decision of the specialist. The contribution of computerized imaging, particularly the Optic Coherence Tomography (OCT) for examining the retinal layers in a noninvasive way, has improved drastically the posterior segment therapeutic approaches and reduced significantly healthcare costs, although a high percentage of senior population in industrialized countries is affected (Spaide et al., 2018). Diagnostic Imaging applied to retina and fundus has gained success worldwide, contributing to a better management of retinal diseases (Li et al., 2018). This computer-assisted technology has been recently associated with a considerable development of biomarkers coming from proteomic, metabolomic, and genetic basic and applied research investigation (Ristori et al., 2020).
Retinal Diseases: The Mechanisms Behind Clinical Manifestations
Many RD have a multifactorial etiology, most probably driven by a combination of genetic and environmental factors, interacting to produce a wide range of phenotypes (Hull et al., 2014). Environmental stressors allow epigenetic modifications by influencing cellular activity and tissue response (Crick, 1970; Jafari et al., 2017). Some morphological and biostrumental biomarkers (subretinal fluid, intraretinal fluid, intraretinal cysts, hyperreflective foci, drusen/pseudodrusen, epiretinal/limiting membranes, geographic/outer retinal atrophy, and fibrovascular pigment epithelial detachment) are currently of great utility in addition to the biostrumental biomarkers provided by OCT scansion (Cacciamani et al., 2019). For sustainment, some health-recognized biomarkers are also used to discriminate between absence of, association of, or defined pathological states (Ahmed et al., 2014). The possibility to have inflammatory biomarkers, as quantified in vitreal or vitreal reflux samples or even biopsies whenever accessible, represents a step forward in grading pathological manifestation as well as in guiding the surgical decision (Figure 1). RD forms will be discussed stepwise with respect to biomarkers’ association. RD group (RP, AMD, DR, and ERMs) will be discussed below with respect to clinical manifestations and biomarker association as quantified in vitreal fluids.
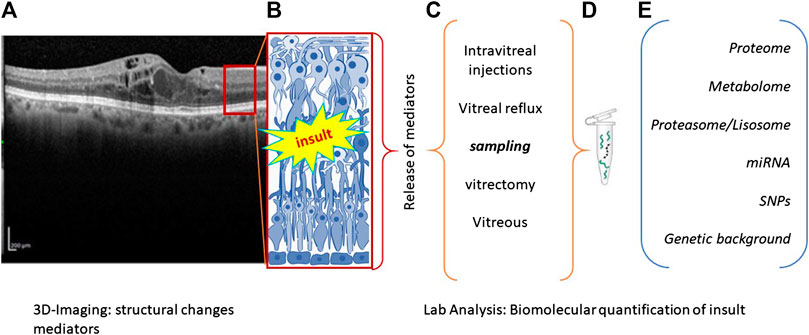
FIGURE 1. Flowchart summarizing the main steps of precision medicine. (A), (B) Biostrumental parameters’ acquisition by computerized tomography. Representative image showing retinal layers (A) with a red square schematized in (B). Retinal insult is followed by the release of a plethora of inflammatory mediators that can be quantified according to different sampling route (C). Samples can be appropriately processed to release RNA, DNA, or proteins (D), according to different new and old generation techniques (E). The main biomarkers used as outcome indicator are listed in (E).
Retinitis Pigmentosa (RP)
RP is a neurodegenerative eye disease characterized by degeneration of rod/cone photoreceptors leading to night blindness, followed by a progressive mid-peripheral vision loss, and culminating in complete blindness (around mid-40s) (Hamel, 2006). RP is genetically and phenotypically heterogeneous: mutations occur between 0.025 and 0.04% of worldwide population (Van Soest et al., 1999; Dias et al., 2018). RP outcome, disease onset (age), progression rate, and secondary clinical signs are variable, even in parents and relatives (Guadagni et al., 2015). Mutant proteins implicated in some functional process of retina may trigger nonsyndromic RP, while mutations in genes working in cells/tissues result in systemic manifestations (syndromic RP) (Waters and Beales, 2011; Wheway et al., 2014; Dias et al., 2018). Mutations in proteins associated with the classical components of the phototransduction cascade (rhodopsin and phosphodiesterase), cell signaling factors (such as Crx, Nrl), disc membrane-associated glycoproteins (peripherin), ion channels (such as the light sensitive cationic ones), and the structural proteins of the cilium can contribute to disease heterogeneity (Roesch et al., 2012; Daiger et al., 2013). Genetic and environmental factors (light) are known to modulate the entire disease: faster disease progression occurring at the inferior retina may result from a modifying effect caused by light. It has been hypothesized that light sources privilege the superior visual field, implying that a greater exposure occurs at the inferior retina, accelerating whole retinal degeneration (Paskowitz et al., 2006). This aspect was particularly evident when rd10 mice were exposed to increasing light intensity: particularly, both retinal function and photoreceptors’ number decreased as a function of light-dependent exposure, with morphological alterations and loss of synaptic connectivity inside the retinal network (Kutsyr et al., 2020).
Biomarkers in RP
Although genetic defects in photoreceptors or retinal pigment epithelium represent the primary causes of RP development, recent studies looked at the inflammatory response as a possible contributor for RP pathogenesis (Yoshida et al., 2013). In previous studies, Yoshida and coworkers showed that: i) inflammatory cells accumulate in the vitreous cavity of RP patients, mainly younger ones; ii) RP patients with increased inflammatory cells into vitreal cavity show decreased visual function; iii) increased proinflammatory cytokines and chemokines (IL-1α, IL-1β, IL-2, IL-4, IL-6, IL-8, IL-10, IFN-γ, GRO-α, I-309, IP-10, MCP-1, MCP-2, and TARC) typify both aqueous and vitreous from RP patients (Yoshida et al., 2013). All together, these aspects suggest the presence of a “strong” chronic inflammatory reaction depending on RP pathogenesis (Ohnaka et al., 2012). Similarly, proinflammatory cytokines and chemokines were quantified in retinas of rd10 experimental model (Ikeda et al., 2002; Ohnaka et al., 2012; Semba et al., 2013; Yoshida et al., 2013; Jové et al., 2014; Zhang et al., 2015; Kohler et al., 2016). Of those factors, MCP-1 levels were significantly increased in human RP aqueous and vitreous (Ikeda et al., 2002; Nakazawa et al., 2007; Ohnaka et al., 2012; Semba et al., 2013; Yoshida et al., 2013; Jové et al., 2014; Zhang et al., 2015; Kohler et al., 2016). As known from other diseases and experimental studies, the ability of MCP-1 is to recruit monocytes, activate dendritic cells, and stimulate memory T cells at injured sites (Ikeda et al., 2002; Nakazawa et al., 2007). More interestingly, MCP-1 activates microglia, which contributes to neuronal inflammation and subsequent neuronal apoptosis (Gupta et al., 2003; Minghetti et al., 2005). Nakazawa and coworkers demonstrated that MCP-1 contributes to photoreceptor apoptosis following retinal detachment, through a microglia/macrophage activation pathway (Nakazawa et al., 2007). In human RP retinas, another study demonstrated that an activated microglia phenotype was present in the outer nuclear layer of regions populated by death committed rods (Gupta et al., 2003). These data suggest that MCP-1, in concert with other soluble mediators, may play an important role in the inflammatory reactions and degenerative process of RP (Gupta et al., 2003; Nakazawa et al., 2007).
Age-Related Macular Degeneration (AMD)
AMD, a neurodegenerative late-onset retinal disease showing clinical and pathological aspects close to Alzheimer Diseases, is a retinal disease leading to central vision loss: it is characterized by neurodegeneration of the central region of retina and choroid (Haddad et al., 2006). Two major forms have been identified: atrophic (dry) and exudative (wet) AMD. While dry AMD (about 85% of cases) is characterized by yellow subretinal deposits (drusen) and/or retinal pigment epithelial (RPE) irregularities (hyperpigmentation/hypopigmentary changes), the wet form (around 15% of cases) is characterized by choroidal neovascularization and fibrovascular RPE detachments (Haddad et al., 2006). Both environment and genetic factors can contribute to developing and/or exacerbation of AMD (Haddad et al., 2006). The observation of 52 common and rare variants at 34 genetic loci, independently associated with late AMD, implies that genetic factors might represent a crucial aspect in the management of disease (Haddad et al., 2006). A strong genetic association in AMD pathogenesis was found for complement factor H (CFH) and complement factor I (CFI), as well as in the metalloproteinases tissue inhibitor 3 (TIMP3), due to the presence of rare coding variants (frequency < 0·1%) (Mitchell et al., 2018). Gene–environment interaction and factors such as age and race may lead to oxidative damage and inflammation (Sacca et al., 2009; Gemenetzi and Lotery, 2020). Strong association between several environmental factors and AMD has been reported and particularly the main demographic/environmental risk factors are aging, smoking, diet, fat intake, and obesity (Klein et al., 1992; Christen et al., 1996; Seddon et al., 1996; Cho et al., 2001; Seddon et al., 2003; Friedman et al., 2004). In the neovascular form of AMD, a higher risk of AMD development was associated with white populations, with respect to Hispanic and Black ones (Sommer et al., 1991; Cruickshanks et al., 1997). Protective effects came from diet (antioxidants, nuts, fish, and omega-3 fatty acids) (Age-Related Eye Disease Study Research Group, 2001). Other potential risk factors (hypertension, high cholesterol levels, and sunlight exposure) have been prospected although with no specific involvement (Seddon and Chen, 2004).
Biomarkers in AMD
Analytical procedures and related immunoassay for biomarker detection (screening and monitoring) would contribute significantly to the management of AMD, at both early and late disease (Nath et al., 2017). A strong biological correlation was observed between endothelial dysfunction and biomarkers of inflammation/oxidative stress. As anti-neovascular therapy is a popular strategy for AMD, the development of predictable biomarker would be of immense importance for strategizing therapeutic modalities based on the underlying pathology. Chau and coworkers determined matrix metalloproteinase- (MMP-) 2 and MMP-9 levels in the plasma collected from AMD suffering patients, highlighting that plasmatic MMP-9 levels were significantly higher in age-related maculopathy and choroidal neovascularization (CNV) groups, as compared to control groups (Chau et al., 2008). Machalinska and colleagues showed the presence of increased circulating endothelial cells (EndCs) and endothelial progenitor cells (EPCs) in AMD patients, as compared to healthy individuals. Authors highlighted that this specific increase would reflect a severe vascular disturbance (Machalińska et al., 2011). With respect to oxidative stress, Totan and coworkers showed the presence of increased Endothelin-1 (ET-1) and reduced Nitric Oxide (NO) plasmatic levels in AMD, suggesting an imbalance between vasoconstrictor and vasodilator agents, possibly reflecting either an endothelial dysfunction in AMD pathogenesis or a role of vasoconstriction in exudative AMD (Totan et al., 2015). Another study showed significant elevation of serum concentrations of IL-1α, IL-1β, IL-4, IL-5, IL-10, IL-13, and IL-17 in AMD patients compared to control subjects (Nassar et al., 2015). IL-1 is a macrophage-derived major proinflammatory cytokine acting mainly through the induction of a network of inflammatory cytokines, chemokines, and other small soluble mediators (Dinarello, 2009). IL-1 early release might elucidate, at least in part, the increased vascular exudation resulting in larger and persisting macular edema in these patients (Dinarello, 2009). Guymer et al. also demonstrated an association between elevated urinary cytokines, transforming growth factor- (TGF-) β1 and monocyte chemoattractant protein-1 (MCP-1), and AMD (Guymer et al., 2011). Therefore, the possibility to have a “urinary panel” (biomarkers) would facilitate the monitoring of disease progression or predicting vision-threatening complications or even measuring the response to treatments (Guymer et al., 2011). Some soluble receptors, taking actively part in the recognition of major proinflammatory cytokines, have gained increasing interest in the last years. For instance, the observation of increased plasma level of soluble Tumor Necrosis Factor Receptor-II (TNFR-II) in AMD supported the hypothesis of low-grade systemic inflammation in patients with AMD (Faber et al., 2015). Of crucial interest was the use of Paraoxonase-1 (PON1), an antioxidant agent used as an indicator of lipid peroxidation, to evaluate oxidative stress in patients with AMD. Authors reported a negative correlation between PON1 activity and Malondialdehyde (MDA) levels in patients with AMD. This may suggest some efficacy of antioxidant therapy to inhibit lipid peroxidation and that hypothetical agents able to increase PON1 activity could be a therapeutic option in AMD (Baskol et al., 2006).
Diabetic Retinopathy (DR)
DR, the result of diabetes causing damage to retinal blood vessels, is an ocular disease that may have different clinical characteristics but may lead to severe visual loss (Hayreh, 2014). Disease is associated with impaired glucose metabolism and after 20 years of type-1 diabetes, 80% of patients with insulin treated type-1 diabetes and 50% of patients with type-2 diabetes will have some degree of DR (Stitt et al., 2016). According to clinical manifestation, DR is currently categorized as mild, moderate, and severe nonproliferative diabetic retinopathy (NPDR) and proliferative diabetic retinopathy (PDR) in which retinal neovascularization is present (Cehofski et al., 2017). Diet and obesity have been reported to contribute massively to the development of type 2 diabetes. By the way, heritability can account for more than 52% of the advanced PDR, showing retinal neovascularization (Seddon, 2013).
Biomarkers in DR
DR is a common and often severe complication of diabetes. Nevertheless, despite the wide array of treatments available for DR management, vision restoration in advanced stage of disease is difficult. Although it is well known that tight glycemic control may protect from end-organ damage, the risk of developing diabetic complications despite adequate blood sugar control may be demonstrated by validated biomarkers. Fasching et al. demonstrated that, irrespective of metabolic control, serum concentrations of intercellular adhesion molecule-1 (ICAM-1) and vascular cell adhesion molecule-1 (VCAM-1) were elevated in patients with insulin-dependent diabetes mellitus (IDDM), reflecting ongoing endothelial cell stimulation and leukocyte activation (Fasching et al., 1996). No difference in serum E-selectin concentration was detected between diabetic patients (with or without macroangiopathy) and normal subjects, suggesting the contribution of adhesion molecules in the development of atherosclerosis occurring in diabetes (Kado and Nagata, 1999). Sharma et al. reported that circulating markers of inflammation, endothelial injury, and TNF signaling were significantly associated with DR in patients with type-1 diabetes (T1Ds). TNF receptor-I (TNFR-I) and TNFR-II receptors were highly correlated, but DR are associated more strongly with TNFR-I (Sharma et al., 2015). Studies showed the importance of TNF-α system in diabetic retinal microvascular damage (Behl et al., 2008). TNF-α binding to two specific membrane receptors (TNFR-I and TNFR-II) starts a signal pathway leading to the activation of transcription factors (NF-κB and Bax) involved in the proinflammatory and apoptotic cascade (Aderka, 1996). The diabetes-related vascular complications have been correlated with C-reactive protein (CRP), TNF-α, and IL-6 in the EURODIAB Prospective Complication Study (Schram et al., 2005). Indeed, a positive correlation was reported for DR and cardiovascular inflammatory factors, highlighting that strategies focused to decrease inflammatory activity may prevent the development of vascular complications in type 1 diabetes (Schram et al., 2005). Of interest, increased plasma levels of Endothelin (ET-1), a potent endothelium-derived vasoconstrictive peptide, have been found in non-insulin-dependent diabetic (NIDDM) patients, prospecting ET-1 as new marker of vascular damage in diabetic subjects (Laurenti et al., 1997). Jacqueminet and coworkers proposed the peripheral blood MMP-9 levels as “a suitable substitute biomarker” of retinopathy in type-1 diabetes not associated with vascular complications (Jacqueminet et al., 2006). Transforming growth factor-β (TGF-β), the main inducer of extracellular matrix remodeling and associated with collagen production and fibrogenesis, was found overexpressed in NIDDM patients (Pfeiffer et al., 1996). Lee et al. showed elevated levels of circulating endothelial progenitor cells (EPCs) and serum Erythropoietin (Epo), VEGF, and Substance P (SP) which may be involved in the progression of DR, sustaining a systemic vasculogenesis rather than a local angiogenesis (Lee et al., 2006). Both level and type of serum oxidative stress products–Malondialdehyde (MDA), Conjugated Diene (CD), Advanced Oxidation Protein Products (AOPPs), protein carbonyl, and 8-hydroxydeoxyguanosine (8-OHdG)– have been reported to have a predictive role in the development and progression of DR (Pan et al., 2008).
Idiopathic Epiretinal Membrane Formations (ERMs)
ERMs are thin and avascular sheet of fibrous tissue developing over the retinal layer, at the vitreoretinal interphase, merely at the macular area of retina, causing changes in architectonics and functioning with consequent reduced vision. ERMs etiology is idiopathic in many cases (80%) or secondary to different situations, including retinal detachment or vascular or inflammatory retinal diseases (Bottós et al., 2012). Prognostic and therapeutic decisions occur principally by OCT evaluation (Unsal et al., 2019). Vitrectomy followed by ERM peel-off is the routine surgical approach. Nevertheless, ERMs and even the associated internal limiting membrane can reform due to genetic/epigenetic influences or particular conditions (Gemenetzi and Lotery, 2020).
Biomarkers in ERMs
A recent study investigated the possibility to use an interplay of OCT and biochemical markers to allow a grading of ERM formation, severity, and traction entity, an indirect marker of retinal status or detach (Stevenson et al., 2016). Other than cytokine release, some additional biomarkers have been recently identified and quantified in both vitreous and tissues (Ahmad et al., 2018). Some of those inflammatory mediators were recognized as tissue remodeling actors (IL6, IL33, and IL8), implying the possibility of a direct modulation of cell migration and collagen metabolism at the vitreoretinal interface (Dinice et al., 2020). A special note should be devoted to Osteopontin, a tissue remodeling biomarker of recent attention (Dinice et al., 2020). In addition to the cell phenotyping markers (GFAP, Iba1, and CD56), other molecular targets have been observed and some oxidative ones are highlighted at both molecular and biochemical level (Marrocco et al., 2017). None of these biomarkers can be tissue or disease specific, although their increased expression as well as just their presence can be of great diagnostic and prognostic value (Strimbu and Tavel, 2010). Last, it is important to mention that some OCT parameters could be used to predict postoperative visual outcomes in patients with iERM treated with PPV (Minami et al., 2019).
DR and Novel Potential Players for Personalized Medicine
The possibility to select some biomarkers for predictive purposes will help to define patients suitable for therapy, according to the concept of precision medicine devoted to tailored individual needs. In the last decade, RNA and protein array approaches have been implemented by the metabolomic analysis, the proteasome/lysosome analysis, and the next generation sequencing coupled to the pharmacogenomics (PGx), as additional supports for individualized therapy (Laíns et al., 2019). Metabolome and associated pathways have been tested for improving our understanding of disease pathophysiology and associated mechanisms, as recently prospected for clarifying some aspects of a cicatricial disease of the ocular surface (Laíns et al., 2019; Di Zazzo et al., 2020). All the proteome and metabolome information should be verified with conventional approaches, bypassing some limits of these multiparametric techniques (Miteva et al., 2013). As known, metabolomics is strictly dependent on the influence of external factors (external environment, nutrition habits, age, and microbiome, among others) (Patti et al., 2012). The study of metabolomic variations allows the possibility to: i) increase the understanding of disease pathophysiology at the molecular level, generating new hypotheses for disease mechanisms; ii) identify those predictive/diagnostic biomarkers; iii) assess disease progression/exacerbation; iv) elucidate the influence of environment/lifestyle exposures in disease; and finally, v) assess drug efficacy and/or toxicity as well as eventual adverse-drug reactions (Nicholson et al., 2012; Jové et al., 2014; Kohler et al., 2016).
Epigenetic Factors
In the recent years, the epigenetic mechanisms gained attention for their promising ability to reduce the gap between environmental factors and disease development/exacerbation, by means of gene modulation (Lanza et al., 2019). Although many efforts have been carried out to elucidate genetic and environmental risk factors for retinal diseases, little is still unknown about their molecular mechanisms. Recent studies highlighted the association between epigenetic changes and incidence/progression of retinal diseases associated with visual loss (Lanza et al., 2019). While several gene mutations have been reported for RP, the mechanisms underlying photoreceptor death remain to be elucidated (He et al., 2013). A recent study carried out in an experimental model of RP (rd1 mouse) showed an increased histone deacetylase (HDAC) activity just before photoreceptor degeneration, an effect significantly reduced in the presence of HDAC inhibitors (Sancho-Pelluz et al., 2010). A reduced electroretinography response was observed in degenerated retinal cells when the retinal endoribonuclease Dicer (a helicase with rnase motif enzyme) is specifically knocked down (Damiani et al., 2008). As well, epigenetic mechanisms, including chromatin modifications, have been implicated in AMD pathogenesis (Liu et al., 2012). Merely, Suuronen and coworkers demonstrated that the addition of HDAC inhibitors resulted in expression/secretion of clusterin (a major component of drusen) by human RPE cell line, suggesting that the management of HDAC activity is important to limit or even counteract drusen formation (Suuronen et al., 2007). By using the DNA methylation microarray and bisulfite pyrosequencing applied to frozen human RPE/choroid samples (donors), Hunter and coworkers observed the hypermethylation of glutathione S-transferase isoforms mu1 and mu5 (GSTM1 and GSTM5) in AMD compared with age-matched control tissues (Hunter et al., 2012). The ability of GSTM1 and GSTM5 to reduce oxidative stress appears of great interest, as oxidative stress was hypothesized to contribute to AMD pathophysiology (Hunter et al., 2012).
In diabetic complications, the epigenetic contribution has been proposed to explain the exacerbation of retinal damage in the presence of poor glycemic control (Villeneuve and Natarajan, 2010). As observed in streptozotocin- (STZ-) treated rats (an experimental model of diabetes) with poor glycemic control, retinas and related retinal endothelial cells showed an overexpression of the histone modifiers HADC1, HADC2, and HADC8 and a reduced activity of histone H3-specific acetyltransferase (Zhong and Kowluru, 2010). Since histone-associated impairments did not reverse upon glycemia stabilization, an epigenetic-driven metabolic memory was hypothesized as major reason for DR endurance even in the case of restoration of normal circulating glucose levels (Zhong and Kowluru, 2010). Other epigenetic modifications include the expression of histone H3K4me2 associated with the transcriptional activation and decrease of superoxide dismutase gene (SOD-2), as observed in human DR retinas (donors) (Zhong and Kowluru, 2013).
microRNAs and Pharmacogenetic Biomarkers
Several microRNAs (miRNAs) have been implicated in photoreceptor degeneration in RP, DR, and ERMs (Wang et al., 2012; Russo et al., 2017; Anasagasti et al., 2018). Briefly, miRNAs are small nucleotide non-coding RNA sequences (≈25mers) interfering negatively with gene expression, at the RNA-induced silencing complex (RIS) level, through a binding/degrading activity (nucleases associated pattern) of specific transcripts in the cytosol. Either degrading or reducing transcript activity/translation inside the ribosomal machinery, miRNAs represent key regulators for tissue development and more properly cell growth, development, and differentiation (Jeker and Marone, 2015). One miRNA can target more than one mRNA and its ability to buffer variations in gene expression due to environmental/microenvironmental changes, and not the basic cell functions, highlights their important contribution to maintaining cellular homeostasis and para-inflammation (Lee et al., 2019). Impaired or even a complete loss of miRNA activity can result in several defects and malfunctioning at both tissue and cell level (Gurtner et al., 2016). Pathological implications of miRNA dysregulation have been described for retinal tissues, under either normal or pathological states (diabetes, neovascularization) (Liu et al., 2020). The usefulness of miRNA, as diagnostic tool, has been prospected from experimental models of diabetic retinopathy. Merely, both serum and retina of diabetic mice were found to express dysregulated miRNAs implicated in the regulation of VEGF, BDNF, PPAR-α, and CREB1 expression before the retinal vasculopathy occurs (Platania et al., 2019). Recently, a strong association of specific miRNAs with the progression and severity of retinal as well as vitreoretinal impairments has been observed (Russo et al., 2017; Martins et al., 2020). Their quantification in ocular fluids has been correlated with disease staging and severity, with promising diagnostic and/or prognostic outcomes.
Neuroprotective Factors
A unique attention has been devoted for years to the role of growth factors, including neurotrophic and angiogenic/angiostatic mediators, whose positive role is undoubted in RD. Machalinska et al. reported a marked decrease in the Pigment Epithelium-Derived Factor (PEDF) plasma levels in patients with dry AMD, whereas a significant higher level of PEDF and Vascular Endothelial Growth Factor (VEGF) was observed in the wet form, suggesting that different manifestations of AMD may be the result of altered concentrations of counterbalancing stimulators/inhibitors of angiogenesis (Machalińska et al., 2012). In the last decades, NGF has displayed interesting abilities in the visual system, working at both ocular surface and retina/optic nerve levels. The pleiotropic NGF effects have been described either in vitro (cell culture models) or in vivo (experimental models and humans), leading to the development of clinical trials devoted to demonstrating the useful NGF administration in neurodegenerative eye diseases. Other than in neurotrophic ulcer application, this neurotrophic route was particularly evident in the treatment of impaired retinal signal in experimental retinitis pigmentosa and in retinal cell death/optic nerve degeneration in response to abnormal elevated intraocular pressure (Sposato et al., 2008; Sivilia et al., 2009). Our recent observation in experimental models supports the neuroprotective effect of NGF in insulted eye, chiefly for retina and optic nerve degeneration (Rocco et al., 2017). Based on our results, NGF protective effects might be related to an increased survival of retinal ganglion cells and nerves in the optic nerve. The cell survival and neuritis outgrowth in NGF exposed were confirmed by further results, by administration of NGF/αVEGF combination implying an additional effect of the single NGF treatment (Rocco et al., 2017). With respect to NGF alone, the NGF/αVEGF combination significantly delayed and/or protected photoreceptors as well as retinal cells from degeneration. The concept of angiogenic depletion coupled to drugs and/or growth factors has been tested recently (Heier et al., 2020).
All the above reported biomarkers represent a concrete support to complete the imaging information. Essentially, genomics, proteomics, metabolomics, proteasome, and pharmacogenomics can represent valid tools for precision therapy applied to retinal healthcare (Figure 2).
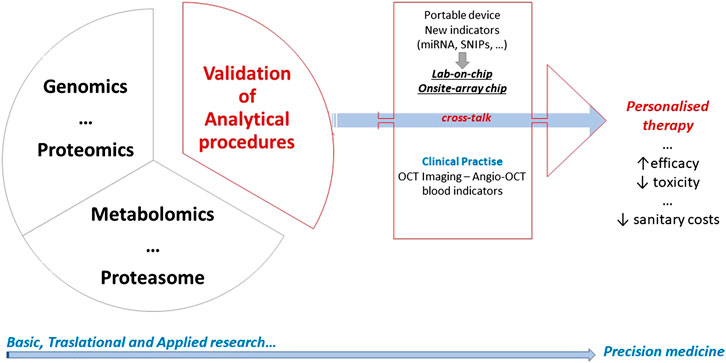
FIGURE 2. A graphical abstract summarizing the long-lasting and difficult process leading to personalized therapy. Basic and translational research crosstalk to clinical practice, and all convey to precision medicine. Overall steps in research field inform each other with the goal of improving the efficiency and effectiveness of disease prevention, diagnosis, and treatment.
Conclusion and Future Directions
As vitreous fluids might reflect the early stages of retinal sufferance and/or late stages of degeneration, the possibility to modulate intravitreal levels of growth factors, in combination to anti-VEGF therapy, would open to a new and more appropriate therapy to counteract retinal neurodegeneration. The pathways involved in this new way of thinking will be disclosed in the near future, opening to new alternative strategies of personalized therapies for a better management of retinal disorders through neuroprotection. The possibility to perform a grading of disease severity and finalize the surgical decisions is an adding value in personalized medicine.
Taken together, the above summarized findings sustain the great value of searching new research strategies for preserving the retina from neurodegeneration. The possibility to access vitreous chamber by collecting vitreous reflux during intravitreal injection or collecting vitreous biopsy at the time of vitrectomy represents a step forward for an individualized therapy. Drug response and protein signature appear to be unique in the single patient: therefore, therapies should be much as tailored as possible.
Author Contributions
All authors have made a substantial, direct, and intellectual contribution to the work and approved it for publication.
Funding
The study was partially supported by the Italian Ministry of Health and 5x1000 (2016) project to IRCCS-Fondazione Bietti.
Conflict of Interest
The authors declare that the research was conducted in the absence of any commercial or financial relationships that could be construed as a potential conflict of interest.
Acknowledgments
Many thanks are due to Fondazione Roma (Italy) for continuous support. The study was partially supported by the Italian Ministry of Health and 5x1000 (2016) project to IRCCS-Fondazione Bietti. The authors apologize for undesired missing of information or papers related to this topic.
References
Aderka, D. (1996). The potential biological and clinical significance of the soluble tumor necrosis factor receptors. Cytokine Growth Factor Rev. 7 (3), 231–240. doi:10.1016/s1359-6101(96)00026-3
Age-Related Eye Disease Study Research Group (2001). A randomized, placebo-controlled, clinical trial of high-dose supplementation with vitamins C and E, beta carotene, and zinc for age-related macular degeneration and vision loss: AREDS report no. 8. Arch. Ophthalmol. 119 (10), 1417–1436. doi:10.1001/archopht.119.10.1417
Ahmad, M. T., Zhang, P., Dufresne, C., Ferrucci, L., and Semba, R. D. (2018). The human eye proteome project: updates on an emerging proteome. Proteomics 18 (5-6), e1700394. doi:10.1002/pmic.201700394
Ahmed, R. M., Paterson, R. W., Warren, J. D., Zetterberg, H., O'Brien, J. T., Fox, N. C., et al. (2014). Biomarkers in dementia: clinical utility and new directions. J. Neurol. Neurosurg. Psychiatry 85 (12), 1426–1434. doi:10.1136/jnnp-2014-307662
Anasagasti, A., Ezquerra-Inchausti, M., Barandika, O., Muñoz-Culla, M., Caffarel, M. M., Otaegui, D., et al. (2018). Expression profiling analysis reveals key MicroRNA-mRNA interactions in early retinal degeneration in retinitis pigmentosa. Invest. Ophthalmol. Vis. Sci. 59 (6), 2381–2392. doi:10.1167/iovs.18-24091
Baskol, G., Karakucuk, S., Oner, A. O., Baskol, M., Kocer, D., Mirza, E., et al. (2006). Serum paraoxonase 1 activity and lipid peroxidation levels in patients with age-related macular degeneration. Ophthalmologica 220 (1), 12–16. doi:10.1159/000089269
Behl, Y., Krothapalli, P., Desta, T., DiPiazza, A., Roy, S., and Graves, D. T. (2008). Diabetes-enhanced tumor necrosis factor-alpha production promotes apoptosis and the loss of retinal microvascular cells in type 1 and type 2 models of diabetic retinopathy. Am. J. Pathol. 172 (5), 1411–1418. doi:10.2353/ajpath.2008.071070
Blix, A. (2014). Personalized medicine, genomics, and pharmacogenomics: a primer for nurses. Clin. J. Oncol. Nurs. 18 (4), 437–441. doi:10.1188/14.CJON.437-441
Bottós, J., Elizalde, J., Arevalo, J. F., Rodrigues, E. B., and Maia, M. (2012). Vitreomacular traction syndrome. J. Ophthalmic Vis. Res. 7 (2), 148–161.
Cacciamani, A., Esposito, G., Scarinci, F., Parravano, M., Dinice, L., Di Nicola, M., et al. (2019). Inflammatory mediators in the vitreal reflux of patients with diabetic macular edema. Graefes Arch. Clin. Exp. Ophthalmol. 257 (1), 187–197. doi:10.1007/s00417-018-4169-4
Cacciamani, A., Parravano, M., Scarinci, F., Esposito, G., Varano, M., and Micera, A. (2016). A simple spontaneous vitreal reflux collecting procedure during intravitreal injection: set-up and validation studies. Curr. Eye Res. 41 (7), 971–976. doi:10.3109/02713683.2015.1080282
Cehofski, L. J., Honoré, B., and Vorum, H. (2017). A review: proteomics in retinal artery occlusion, retinal vein occlusion, diabetic retinopathy and acquired macular disorders. Int. J. Mol. Sci. 18 (5), 907. doi:10.3390/ijms18050907
Chau, K. Y., Sivaprasad, S., Patel, N., Donaldson, T. A., Luthert, P. J., and Chong, N. V. (2008). Plasma levels of matrix metalloproteinase-2 and -9 (MMP-2 and MMP-9) in age-related macular degeneration. Eye 22 (6), 855–859.
Cho, E., Hung, S., Willett, W. C., Spiegelman, D., Rimm, E. B., Seddon, J. M., et al. (2001). Prospective study of dietary fat and the risk of age-related macular degeneration. Am. J. Clin. Nutr. 73, 209–218. doi:10.1093/ajcn/73.2.209
Christen, W. G., Glynn, R. J., Manson, J. E., Ajani, U. A., and Buring, J. E. (1996). A prospective study of cigarette smoking and risk of age-related macular degeneration in men. J. Am. Med. Assoc. 276 (14), 1147–1151. doi:10.1001/jama.1996.03540140035023
Coassin, M., Braconi, L., Sborgia, G., Mangano, G., Mastrofilippo, V., Di Zazzo, A., et al. (2020). One-port vs. three-port diagnostic vitrectomy for posterior segment diseases of unknown origin. Int. Ophthalmol. 40, 3217–3222. doi:10.1007/s10792-020-01504-3
Cruickshanks, K. J., Hamman, R. F., Klein, R., Nondahl, D. M., and Shetterly, S. M. (1997). The prevalence of age-related maculopathy by geographic region and ethnicity. The Colorado-Wisconsin Study of Age-Related Maculopathy. Arch. Ophthalmol. 115 (2), 242–250. doi:10.1001/archopht.1997.01100150244015
Daiger, S. P., Sullivan, L. S., and Bowne, S. J. (2013). Genes and mutations causing retinitis pigmentosa. Clin. Genet. 84, 132. doi:10.1111/cge.12203
Damiani, D., Alexander, J. J., O’Rourke, J. R., McManus, M., Jadhav, A. P., Cepko, C. L., et al. (2008). Dicer inactivation leads to progressive functional and structural degeneration of the mouse retina. J. Neurosci. 28 (19), 4878–4887. doi:10.1523/JNEUROSCI.0828-08.2008
Dinice, L., Cacciamani, A., Esposito, G., Taurone, S., Carletti, R., Ripandelli, G., et al. (2020). Osteopontin in vitreous and idiopathic epiretinal membranes. Graefes. Arch. Clin. Exp. Ophthalmol, 258 (7), 1503–1513. doi:10.1007/s00417-020-04685-w
Di Zazzo, A., Yang, W., Coassin, M., Micera, A., Antonini, M., Piccinni, F., et al. (2020). Signaling lipids as diagnostic biomarkers for ocular surface cicatrizing conjunctivitis. J. Mol. Med. 98 (5), 751–760. doi:10.1007/s00109-020-01907-w
Dias, M. F., Joo, K., Kemp, J. A., Ligório Fialho, S., da Silva Cunha, A., Woo, S. J., et al. (2018). Molecular genetics and emerging therapies for retinitis pigmentosa: basic research and clinical perspectives. Prog. Retin. Eye Res. 63, 107–131. doi:10.1016/j.preteyeres.2017.10.004
Dinarello, C. A. (2009). Immunological and inflammatory functions of the interleukin-1 family. Annu. Rev. Immunol. 27, 519–550. doi:10.1146/annurev.immunol.021908.132612
Faber, C., Jehs, T., Juel, H. B., Singh, A., Falk, M. K., Sørensen, T. L., et al. (2015). Early and exudative age-related macular degeneration is associated with increased plasma levels of soluble TNF receptor II. Acta Ophthalmol. 93 (3), 242–247. doi:10.1111/aos.12581
Fasching, P., Veitl, M., Rohac, M., Streli, C., Schneider, B., Waldhäusl, W., et al. (1996). Elevated concentrations of circulating adhesion molecules and their association with microvascular complications in insulin-dependent diabetes mellitus. J. Clin. Endocrinol. Metab. 81 (12), 4313–4317. doi:10.1210/jcem.81.12.8954033
Friedman, D. S., O'Colmain, B. J., Muñoz, B., Tomany, S. C., McCarty, C., de Jong, P. T., et al. (2004). Prevalence of age-related macular degeneration in the United States. Arch. Ophthalmol. 122, 564–572. doi:10.1001/archopht.122.4.564
Fujii, G. Y., De Juan, E., Humayun, M. S., Pieramici, D. J., Chang, T. S., Awh, C., et al. (2002). A new 25-gauge instrument system for transconjunctival sutureless vitrectomy surgery. Ophthalmology 109 (10), 1807–1813. doi:10.1016/s0161-6420(02)01179-x
Gemenetzi, M., and Lotery, A. J. (2020). Epigenetics in age-related macular degeneration: new discoveries and future perspectives. Cell. Mol. Life Sci. 77, 807–818. doi:10.1007/s00018-019-03421-w
Guadagni, V., Novelli, E., Piano, I., Gargini, C., and Strettoi, E. (2015). Pharmacological approaches to retinitis pigmentosa: a laboratory perspective. Prog. Retin. Eye Res. 48, 62–81. doi:10.1016/j.preteyeres.2015.06.005
Gupta, N., Brown, K. E., and Milam, A. H. (2003). Activated microglia in human retinitis pigmentosa, late-onset retinal degeneration, and age-related macular degeneration. Exp. Eye Res. 76 (4), 463–471. doi:10.1016/s0014-4835(02)00332-9
Gurtner, A., Falcone, E., Garibaldi, F., and Piaggio, G. (2016). Dysregulation of microRNA biogenesis in cancer: the impact of mutant p53 on Drosha complex activity. J. Exp. Clin. Canc. Res. 35, 45. doi:10.1186/s13046-016-0319-x
Guymer, R. H., Tao, L. W., Goh, J. K., Liew, D., Ischenko, O., Robman, L. D., et al. (2011). Identification of urinary biomarkers for age-related macular degeneration. Invest. Ophthalmol. Vis. Sci. 52, 4639–4644. doi:10.1167/iovs.10-7120
Haddad, S., Chen, C. A., Santangelo, S. L., and Seddon, J. M. (2006). The genetics of age-related macular degeneration: a review of progress to date. Surv. Ophthalmol. 51 (4), 316–363. doi:10.1016/j.survophthal.2006.05.001
Hayreh, S. S. (2014). Ocular vascular occlusive disorders: natural history of visual outcome. Prog. Retin. Eye Res. 41, 1–25. doi:10.1016/j.preteyeres.2014.04.001
He, S., Li, X., Chan, N., and Hinton, D. R. (2013). Review: epigenetic mechanisms in ocular disease. Mol. Vis. 19, 665–674.
Heier, J. S., Wykoff, C. C., Waheed, N. K., Kitchens, J. W., Patel, S. S., Vitti, R., et al. (2020). Intravitreal combined aflibercept + anti-platelet-derived growth factor receptor β for neovascular age-related macular degeneration: results of the phase 2 CAPELLA trial. Ophthalmology 127 (2), 211–220. doi:10.1016/j.ophtha.2019.09.021
Hull, S., Arno, G., Plagnol, V., Chamney, S., Russell-Eggitt, I., Thompson, D., et al. (2014). The phenotypic variability of retinal dystrophies associated with mutations in CRX, with report of a novel macular dystrophy phenotype. Invest. Ophthalmol. Vis. Sci. 55, 6934–6944. doi:10.1167/iovs.14-14715
Hunter, A., Spechler, P. A., Cwanger, A., Song, Y., Zhang, Z., Ying, G. S., et al. (2012). DNA methylation is associated with altered gene expression in AMD. Invest. Ophthalmol. Vis. Sci. 53 (4), 2089–2105. doi:10.1167/iovs.11-8449
Ikeda, Y., Yonemitsu, Y., Kataoka, C., Kitamoto, S., Yamaoka, T., Nishida, K., et al. (2002). Anti-monocyte chemoattractant protein-1 gene therapy attenuates pulmonary hypertension in rats. Am. J. Physiol. Heart Circ. Physiol. 283 (5), H2021–H2028. doi:10.1152/ajpheart.00919.2001
Jacqueminet, S., Ben Abdesselam, O., Chapman, M. J., Nicolay, N., Foglietti, M. J., Grimaldi, A., et al. (2006). Elevated circulating levels of matrix metalloproteinase-9 in type 1 diabetic patients with and without retinopathy. Clin. Chim. Acta 367 (1-2), 103–107. doi:10.1016/j.cca.2005.11.029
Jafari, M., Ansari-Pour, N., Azimzadeh, S., and Mirzaie, M. (2017). A logic-based dynamic modeling approach to explicate the evolution of the central dogma of molecular biology. PloS One 12, e0189922. doi:10.1371/journal.pone.0189922
Jeker, L. T., and Marone, R. (2015). Targeting microRNAs for immunomodulation. Curr. Opin. Pharmacol. 23, 25–31. doi:10.1016/j.coph.2015.05.004
Jové, M., Portero-Otín, M., Naudí, A., Ferrer, I., and Pamplona, R. (2014). Metabolomics of human brain aging and age-related neurodegenerative diseases. J. Neuropathol. Exp. Neurol. 73 (7), 640–657. doi:10.1097/NEN.0000000000000091
Kado, S., and Nagata, N. (1999). Circulating intercellular adhesion molecule-1, vascular cell adhesion molecule-1, and E-selectin in patients with type 2 diabetes mellitus. Diabetes Res. Clin. Pract. 46 (2), 143–148. doi:10.1016/s0168-8227(99)00083-2
Kasi, S. K., Hariprasad, S. M., and Hsu, J. (2017). Making the jump to 27-gauge vitrectomy: perspectives. Ophthalmic Surg. Lasers Imaging Retina 48 (6), 450–456. doi:10.3928/23258160-20170601-02
Klein, R., Klein, B. E., and Linton, K. L. (1992). Prevalence of age-related maculopathy. The beaver dam eye study. Ophthalmology 99, 933–943. doi:10.1016/s0161-6420(92)31871-8
Kohler, I., Verhoeven, A., Derks, R. J., and Giera, M. (2016). Analytical pitfalls and challenges in clinical metabolomics. Bioanalysis 8 (14), 1509–1532. doi:10.4155/bio-2016-0090
Kutsyr, O., Sánchez-Sáez, X., Martínez-Gil, N., de Juan, E., Lax, P., Maneu, V., et al. (2020). Gradual increase in environmental light intensity induces oxidative stress and inflammation and accelerates retinal neurodegeneration. Invest. Ophthalmol. Vis. Sci. 61 (10), 1. doi:10.1167/iovs.61.10.1
Laíns, I., Gantner, M., Murinello, S., Lasky-Su, J. A., Miller, J. W., Friedlander, M., et al. (2019). Metabolomics in the study of retinal health and disease. Prog. Retin. Eye Res. 69, 57–79. doi:10.1016/j.preteyeres.2018.11.002
Lanza, M., Benincasa, G., Costa, D., and Napoli, C. (2019). Clinical role of epigenetics and network analysis in eye diseases: a translational science review. J. Ophthalmol. 2019, 2424956. doi:10.1155/2019/2424956
Laurenti, O., Vingolo, E. M., Desideri, G. B., Ferri, C., Bellini, C., Cassone-Faldetta, M., et al. (1997). Increased levels of plasma endothelin-1 in non-insulin dependent diabetic patients with retinopathy but without other diabetes-related organ damage. Exp. Clin. Endocrinol. Diabetes 105 (Suppl. 2), 40–42. doi:10.1055/s-0029-1211795
Lee, I. G., Chae, S. L., and Kim, J. C. (2006). Involvement of circulating endothelial progenitor cells and vasculogenic factors in the pathogenesis of diabetic retinopathy. Eye 20 (59), 546–552. doi:10.1038/sj.eye.6701920
Lee, S. W. L., Paoletti, C., Campisi, M., Osaki, T., Adriani, G., Kamm, R. D., et al. (2019). MicroRNA delivery through nanoparticles. J. Contr. Release 313, 80–95. doi:10.1016/j.jconrel.2019.10.007
Li, Y., Xia, X., and Paulus, Y. M. (2018). Advances in retinal optical imaging. Photonics 5 (2), 9. doi:10.3390/photonics5020009
Liu, C. H., Huang, S., Britton, W. R., and Chen, J. (2020). MicroRNAs in vascular eye diseases. Int. J. Mol. Sci. 21 (2), 649. doi:10.3390/ijms21020649 | .
Liu, M. M., Chan, C. C., and Tuo, J. (2012). Genetic mechanisms and age-related macular degeneration: common variants, rare variants, copy number variations, epigenetics, and mitochondrial genetics. Hum. Genom. 6 (1), 13. doi:10.1186/1479-7364-6-13
Machalińska, A., Safranow, K., Dziedziejko, V., Mozolewska-Piotrowska, K., Paczkowska, E., Klos, P., et al. (2011). Different populations of circulating endothelial cells in patients with age-related macular degeneration: a novel insight into pathogenesis. Invest. Ophthalmol. Vis. Sci. 52 (1), 93–100. doi:10.1167/iovs.10-5756
Machalińska, A., Safranow, K., Mozolewska-Piotrowska, K., Dziedziejko, V., and Karczewicz, D. (2012). PEDF and VEGF plasma level alterations in patients with dry form of age-related degeneration--A possible link to the development of the disease. Klin. Oczna. 114 (2), 115–120.
Marrocco, I., Altieri, F., and Peluso, I. (2017). Measurement and clinical significance of biomarkers of oxidative stress in humans. Oxid. Med. Cell Longev. 2017, 6501046. doi:10.1155/2017/6501046
Martins, B., Amorim, M., Reis, F., Ambrósio, A. F., and Fernandes, R. (2020). Extracellular vesicles and MicroRNA: putative role in diagnosis and treatment of diabetic retinopathy. Antioxidants 9 (8), 705. doi:10.3390/antiox9080705
Minami, S., Shinoda, H., Shigeno, Y., Nagai, N., Kurihara, T., Watanabe, K., et al. (2019). Effect of axial length and age on the visual outcome of patients with idiopathic epiretinal membrane after pars plana vitrectomy. Sci. Rep. 9 (1), 19056. doi:10.1038/s41598-019-55544-6
Minghetti, L., Ajmone-Cat, M. A., De Berardinis, M. A., and De Simone, R. (2005). Microglial activation in chronic neurodegenerative diseases: roles of apoptotic neurons and chronic stimulation. Brain Res. Brain Res. Rev. 48 (2), 251–256. doi:10.1016/j.brainresrev.2004.12.015
Mitchell, P., Liew, G., Gopinath, B., and Wong, T. Y. (2018). Age-related macular degeneration. Lancet 392 (10153), 1147–1159. doi:10.1016/S0140-6736(18)31550-2
Miteva, Y. V., Budayeva, H. G., and Cristea, I. M. (2013). Proteomics-based methods for discovery, quantification, and validation of protein-protein interactions. Anal. Chem. 85 (2), 749–768. doi:10.1021/ac3033257
Nakazawa, T., Hisatomi, T., Nakazawa, C., Noda, K., Maruyama, K., She, H., et al. (2007). Monocyte chemoattractant protein 1 mediates retinal detachment-induced photoreceptor apoptosis. Proc. Natl. Acad. Sci. U.S.A. 104 (7), 2425–2430. doi:10.1073/pnas.0608167104
Nassar, K., Grisanti, S., Elfar, E., Lüke, J., Lüke, M., and Grisanti, S. (2015). Serum cytokines as biomarkers for age-related macular degeneration. Graefes Arch. Clin. Exp. Ophthalmol. 253 (5), 699–704. doi:10.1007/s00417-014-2738-8
Nath, M., Halder, N., and Velpandian, T. (2017). Circulating biomarkers in glaucoma, age-related macular degeneration, and diabetic retinopathy. Indian J. Ophthalmol. 65 (3), 191–197. doi:10.4103/ijo.IJO_866_16
Nicholson, J., Holmes, E., Kinross, J. M., Darzi, A. W., Takats, Z., and Lindon, J. C. (2012). Metabolic phenotyping in clinical and surgical environments. Nature 491 (7424), 384–392. doi:10.1038/nature11708
Oh, H., and Oshima, Y. (2014). Microincision vitrectomy surgery. Emerging techniques and technology. Dev. Ophthalmol. 54, 54–62. doi:10.1159/isbn.978-3-318-02661-0
Ohnaka, M., Miki, K., Gong, Y. Y., Stevens, R., Iwase, T., Hackett, S. F., et al. (2012). Long-term expression of glial cell line-derived neurotrophic factor slows, but does not stop retinal degeneration in a model of retinitis pigmentosa. J. Neurochem. 122 (5), 1047–1053. doi:10.1111/j.1471-4159.2012.07842.x
Ong, F. S., Kuo, J. Z., Wu, W. C., Cheng, C. Y., Blackwell, W. L., Taylor, B. L., et al. (2013). Personalized medicine in ophthalmology: from pharmacogenetic biomarkers to therapeutic and dosage optimization. J. Personalized Med. 3 (1), 40–69. doi:10.3390/jpm3010040
Pan, H. Z., Zhang, H., Chang, D., Li, H., and Sui, H. (2008). The change of oxidative stress products in diabetes mellitus and diabetic retinopathy. Br. J. Ophthalmol. 92 (4), 548–551. doi:10.1136/bjo.2007.130542
Paskowitz, D. M., LaVail, M. M., and Duncan, J. L. (2006). Light and inherited retinal degeneration. Br. J. Ophthalmol. 90, 1060. doi:10.1136/bjo.2006.097436
Patti, G. J., Yanes, O., and Siuzdak, G. (2012). Innovation: metabolomics: the apogee of the omics trilogy. Nat. Rev. Mol. Cell Biol. 13 (4), 263–269. doi:10.1038/nrm3314
Pfeiffer, A., Middelberg-Bisping, K., Drewes, C., and Schatz, H. (1996). Elevated plasma levels of transforming growth factor-beta 1 in NIDDM. Diabetes Care 19 (10), 1113–1117. doi:10.2337/diacare.19.10.1113
Platania, C. B. M., Maisto, R., Trotta, M., D’Amico, M., Rossi, S., Gesualdo, C., et al. (2019). Retinal and circulating miRNA expression patterns in diabetic retinopathy: an in silico and in vivo approach. Br. J. Pharmacol. 176 (13), 2179–2194. doi:10.1111/bph.14665
Ristori, M. V., Mortera, S. L., Marzano, V., Guerrera, S., Vernocchi, P., Ianiro, G., et al. (2020). Proteomics and metabolomics approaches towards a functional insight onto AUTISM spectrum disorders: phenotype stratification and biomarker discovery. Int. J. Mol. Sci. 21 (17), 6274. doi:10.3390/ijms21176274
Rocco, M. L., Balzamino, B. O., Esposito, G., Petrella, C., Aloe, L., and Micera, A. (2017). NGF/anti-VEGF combined exposure protects RCS retinal cells and photoreceptors that underwent a local worsening of inflammation. Graefes Arch. Clin. Exp. Ophthalmol. 255 (3), 567–574. doi:10.1007/s00417-016-3567-8
Roesch, K., Stadler, M. B., and Cepko, C. L. (2012). Gene expression changes within Müller glial cells in retinitis pigmentosa. Mol. Vis. 18, 1197.
Russo, A., Ragusa, M., Barbagallo, C., Longo, A., Avitabile, T., Uva, M. G., et al. (2017). miRNAs in the vitreous humor of patients affected by idiopathic epiretinal membrane and macular hole. PloS One 12 (3), e0174297. doi:10.1371/journal.pone.0174297
Sacca, S. C., Bolognesi, C., Battistella, A., Bagnis, A., and Izzotti, A. (2009). Gene-environment interactions in ocular diseases. Mutat. Res. 667 (1-2), 98–117. doi:10.1016/j.mrfmmm.2008.11.002
Sancho-Pelluz, J., Alavi, M. V., Sahaboglu, A., Kustermann, S., Farinelli, P., Azadi, S., et al. (2010). Excessive HDAC activation is critical for neurodegeneration in the rd1 mouse. Cell Death Dis. 1 (2), e24. doi:10.1038/cddis.2010.4
Schram, M. T., Chaturvedi, N., Schalkwijk, C. G., Fuller, J. H., and Stehouwer, C. D. (2005). EURODIAB prospective complications study groupmarkers of inflammation are cross-sectionally associated with microvascular complications and cardiovascular disease in type 1 diabetes–the EURODIAB prospective complications study. Diabetologia 48, 370–378. doi:10.1007/s00125-004-1628-8
Seddon, J. M., and Chen, C. (2004). The Epidemiology of age-related macular degeneration. Int. Ophthalmol. Clin. 44 (4), 17–39. doi:10.1097/00004397-200404440-00004
Seddon, J. M., Cote, J., Davis, N., and Rosner, B. (2003). Progression of age-related macular degeneration: association with body mass index, waist circumference, and waist-hip ratio. Arch. Ophthalmol. 121 (6), 785–792. doi:10.1001/archopht.121.6.785
Seddon, J. M., Willett, W. C., Speizer, F. E., and Hankinson, S. E. (1996). A prospective study of cigarette smoking and age-related macular degeneration in women. J. Am. Med. Assoc. 276 (14), 1141–1146.
Seddon, J. M. (2013). Genetic and environmental underpinnings to age-related ocular diseases. Invest. Ophthalmol. Vis. Sci. 54 (14), 28–30. doi:10.1167/iovs.13-13234
Semba, R. D., Enghild, J. J., Venkatraman, V., Dyrlund, T. F., and Van Eyk, J. E. (2013). The human eye proteome project: perspectives on an emerging proteome. Proteomics 13 (16), 2500–2511. doi:10.1002/pmic.201300075
Sharma, S., Purohit, S., Sharma, A., Hopkins, D., Steed, L., Bode, B., et al. (2015). Elevated serum levels of soluble TNF receptors and adhesion molecules are associated with diabetic retinopathy in patients with type-1 diabetes. Mediat. Inflamm. 2015, 279393. doi:10.1155/2015/279393
Sivilia, S., Giuliani, A., Fernández, M., Turba, M. E., Forni, M., Massella, A., et al. (2009). Intravitreal NGF administration counteracts retina degeneration after permanent carotid artery occlusion in rat. BMC Neurosci. 10, 52. doi:10.1186/1471-2202-10-52
Sommer, A., Tielsch, J. M., Katz, J., Quigley, H. A., Gottsch, J. D., Javitt, J. C., et al. (1991). Racial differences in the cause-specific prevalence of blindness in east Baltimore. N. Engl. J. Med. 325 (20), 1412–1417. doi:10.1056/NEJM199111143252004
Spaide, R. F., Fujimoto, J. G., Waheed, N. K., Sadda, S. R., and Staurenghi, G. (2018). Optical coherence tomography angiography. Prog. Retin. Eye Res. 64, 1–55. doi:10.1016/j.preteyeres.2017.11.003
Sposato, V., Bucci, M. G., Coassin, M., Russo, M. A., Lambiase, A., and Aloe, L. (2008). Reduced NGF level and TrkA protein and TrkA gene expression in the optic nerve of rats with experimentally induced glaucoma. Neurosci. Lett. 446 (1), 20–24. doi:10.1016/j.neulet.2008.09.024
Stevenson, W., Prospero Ponce, C. M., Agarwal, D. R., Gelman, R., and Christoforidis, J. B. (2016). Epiretinal membrane: optical coherence tomography-based diagnosis and classification. Clin. Ophthalmol. 10, 527–534. doi:10.2147/OPTH.S97722
Stitt, A. W., Curtis, T. M., Chen, M., Medina, R. J., McKay, G. J., Jenkins, A., et al. (2016). The progress in understanding and treatment of diabetic retinopathy. Prog. Retin. Eye Res. 51, 156–186. doi:10.1016/j.preteyeres.2015.08.001
Straatsma, B. R. (2018). Precision medicine and clinical ophthalmology. Indian J. Ophthalmol. 66 (10), 1389–1390. doi:10.4103/ijo.IJO_1459_18
Strimbu, K., and Tavel, J. A. (2010). What are biomarkers? Curr. Opin. HIV AIDS. 5 (6), 463–466. doi:10.1097/COH.0b013e32833ed177
Suuronen, T., Nuutinen, T., Ryhänen, T., Kaarniranta, K., and Salminen, A. (2007). Epigenetic regulation of clusterin/apolipoprotein J expression in retinal pigment epithelial cells. Biochem. Biophys. Res. Commun. 357 (2), 397–401. doi:10.1016/j.bbrc.2007.03.135
Totan, Y., Koca, C., Erdurmuş, M., Keskin, U., and Yiğitoğlu, R. (2015). Endothelin-1 and nitric Oxide levels in exudative age-related macular degeneration. J. Ophthalmic Vis. Res. 10 (2), 151–154. doi:10.4103/2008-322X.163765
Unsal, E., Cubuk, M. O., and Ciftci, F. (2019). Preoperative prognostic factors for macular hole surgery: which is better? Oman J. Ophthalmol. 12 (1), 20–24. doi:10.4103/ojo.OJO_247_2017
van Soest, S., Westerveld, A., de Jong, P. T., Bleeker-Wagemakers, E. M., and Bergen, A. A. (1999). Retinitis pigmentosa: defined from a molecular point of view. Surv. Ophthalmol. 43, 321–334. doi:10.1016/s0039-6257(98)00046-0
Velez, G., Tang, P. H., Cabral, T., Cho, G. Y., Machlab, D. A., Tsang, S. H., et al. (2018). Personalized proteomics for precision health: identifying biomarkers of vitreoretinal disease. Transl. Vis. Sci. Tech. 7, 12. doi:10.1167/tvst.7.5.12
Villeneuve, L. M., and Natarajan, R. (2010). The role of epigenetics in the pathology of diabetic complications. Am. J. Physiol. Ren. Physiol. 299 (1), F14–F25. doi:10.1152/ajprenal.00200.2010
Wang, S., Koster, K. M., He, Y., and Zhou, Q. (2012). miRNAs as potential therapeutic targets for age-related macular degeneration. Future Med. Chem. 4 (3), 277–287. doi:10.4155/fmc.11.176
Waters, A. M., and Beales, P. L. (2011). Ciliopathies: an expanding disease spectrum. Pediatr. Nephrol. 26, 1039–1056. doi:10.1007/s00467-010-1731-7
Wheway, G., Parry, D. A., and Johnson, C. A. (2014). The role of primary cilia in the development and disease of the retina. Organogenesis 10, 69–85. doi:10.4161/org.26710
Yoshida, N., Ikeda, Y., Notomi, S., Ishikawa, K., Murakami, Y., Hisatomi, T., et al. (2013). Laboratory evidence of sustained chronic inflammatory reaction in retinitis pigmentosa. Ophthalmology 120 (1), 5–12. doi:10.1016/j.ophtha.2012.07.008
Zhang, P., Dufresne, C., Turner, R., Ferri, S., Venkatraman, V., Karani, R., et al. (2015). The proteome of human retina. Proteomics 15 (4), 836–840. doi:10.1002/pmic.201400397
Zhong, Q., and Kowluru, R. A. (2013). Epigenetic modification of Sod2 in the development of diabetic retinopathy and in the metabolic memory: role of histone methylation. Invest. Ophthalmol. Vis. Sci. 54 (1), 244–250. doi:10.1167/iovs.12-10854
Keywords: retinal diseases, chronic inflammation, growth factors, angiogenesis, precision medicine, neurodegeneration, pharmacological targets
Citation: Micera A, Balzamino BO, Di Zazzo A, Dinice L, Bonini S and Coassin M (2021) Biomarkers of Neurodegeneration and Precision Therapy in Retinal Disease. Front. Pharmacol. 11:601647. doi: 10.3389/fphar.2020.601647
Received: 01 September 2020; Accepted: 09 November 2020;
Published: 18 January 2021.
Edited by:
Settimio Rossi, Second University of Naples, ItalyReviewed by:
Anca Hermenean, Vasile Goldiş Western University of Arad, RomaniaChiara Bianca Maria Platania, University of Catania, Italy
Copyright © 2021 Micera, Balzamino, Di Zazzo, Dinice, Bonini and Coassin. This is an open-access article distributed under the terms of the Creative Commons Attribution License (CC BY). The use, distribution or reproduction in other forums is permitted, provided the original author(s) and the copyright owner(s) are credited and that the original publication in this journal is cited, in accordance with accepted academic practice. No use, distribution or reproduction is permitted which does not comply with these terms.
*Correspondence: Alessandra Micera, YWxlc3NhbmRyYS5taWNlcmFAZm9uZGF6aW9uZWJpZXR0aS5pdA==