- Department of Pharmaceutics, Faculty of Pharmacy and Pharmaceutical Sciences, University of Karachi, Karachi, Pakistan
The 2019 novel coronavirus (2019-nCoV), commonly known as severe acute respiratory syndrome coronavirus 2 (SARS-CoV-2) or coronavirus disease 2019 (COVID-19), was first revealed in late 2019 in Wuhan city, Hubei province, China. It was subsequently spread globally and thereby declared as a pandemic by WHO in March 2020. The disease causes severe acute respiratory illness and is highly contagious due to the fast-onward transmission. As of the mid of November 2020, the disease has affected 220 countries with more than 16 million active cases and 1.3 million deaths worldwide. Males, pregnant women, the elderly, immunosuppressed patients, and those with underlying medical conditions are more vulnerable to the disease than the general healthy population. Unfortunately, no definite treatment is available. Although remdesivir as an antiviral had been approved for use in those above 12 years of age and 40 kg weight group, it has been observed to be ineffective in large-scale SOLIDARITY trials by WHO. Moreover, dexamethasone has been found to increase the recovery rate of ventilated patients; oxygen and inhaled nitric oxide as a vasodilator have been given emergency expanded access. In addition, more than 57 clinical trials are being conducted for the development of the vaccines on various platforms. Two vaccines were found to be significantly promising in phase III results. It is concluded that till the approval of a specific treatment or development of a vaccine against this deadly disease, the preventive measures should be followed strictly to reduce the spread of the disease.
Introduction
SARS-CoV-2 and COVID-19
Severe acute respiratory syndrome coronavirus 2, which is abbreviated as SARS-CoV-2, is a single-stranded RNA virus that belongs to the Coronaviridae family (subfamily: Coronavirinae) in the order Nidovirales. The consensus report after its phylogenetic analysis by Coronaviridae Study Group of International Committee on Taxonomy of Viruses has concluded that this virus belongs to a species group of similar coronaviruses called “Severe Acute Respiratory Syndrome-Related Viruses”. This particular virus has thus been recognized as “novel” in its phylogenetic character and is far more distinct than just strain and isolate of any previously known viruses (Gorbalenya et al., 2020). On January 3, 2020, the virus was first named 2019-nCoV (2019 novel coronavirus), and the disease was called novel coronavirus-infected pneumonia (NCIP) by the National Health Commission and China CDC after the revelation and analysis of the complete viral genome of the virus (Wenjie et al., 2020; Zhu et al., 2020). WHO has termed the infectious disease caused by SARS-CoV-2 as coronavirus disease 2019 (COVID-19) (WHO, 2020d). The collective symptoms due to the infection include a range of mild symptoms such as fever, dry cough, malaise, sore throat, fatigue, pain, and loss of taste or smell to a range of moderate symptoms predominantly including dyspnea, diarrhea, and pneumonia (Tay et al., 2020a; CDC, 2020). In critical situations, the patients were found to have been affected by dysfunctional immune response clinically identified as “cytokine release syndrome” and thrombosis, which often lead to fatal consequences (Merad and Martin, 2020). Since the initial report of the outbreak of the virus in Wuhan city, Hubei province, China, in December 2019, where a cluster of infections with pneumonia-like symptoms was reported, WHO declared COVID-19 a pandemic on March 11, 2020. Currently, it affects almost 220 countries across the world, with widely varying distribution of incidence and mortality among different geographies and countries. As of November 15, 2020, the total incidence of the infection stands at more than 57 million people diagnosed, with more than 1.3 million confirmed deaths reported globally (WHO, 2020a). A graphical presentation of the COVID 19 disease is presented in Figure 1.
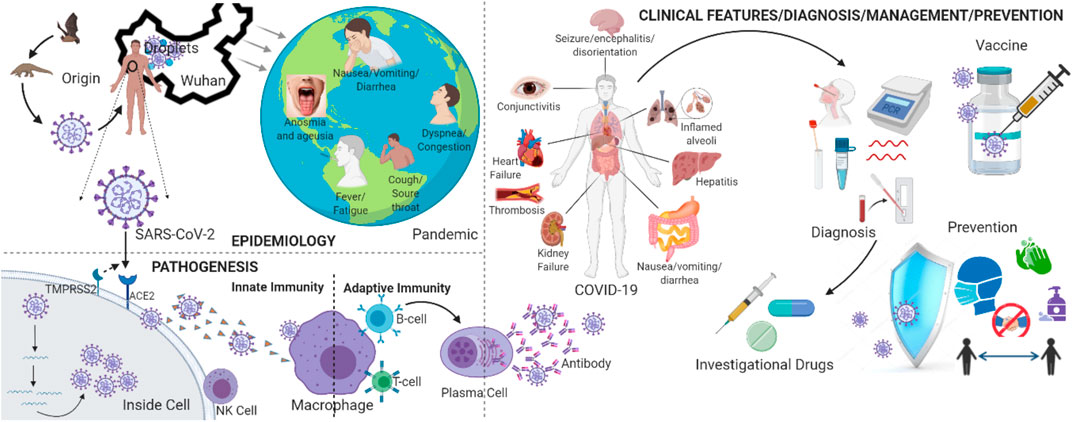
FIGURE 1. Schematic overview of SARS-CoV-2 epidemiology, pathogenesis and clinical features, diagnosis, management, and prevention [Figure created with BioRender, www.biorender.com].
Morphology and Genetic Composition
The SARS-CoV-2 is a spherical-shaped virus with irregular crown-like projections on its surface. These crowns are surrounded by several types of functional proteins submerged and protruding from them. It is enveloped with a positive-sense single-stranded RNA genome with an approximate size of 30 kilobases (Zhao et al., 2020). In terms of its size, it has an overall large diameter in a size range of 75–160 nm (Guobao et al., 2007). The genome of SARS-CoV-2 consists of 14 open reading frames (ORFs) that encode 27 proteins, 15 nonstructural proteins that are important for viral replication, and four structural proteins named spike (S), envelope (E), membrane (M), and nucleocapsid (N) along with accessory proteins (Malik et al., 2020; Wu A. et al., 2020) (see Figure 2). The studies have indicated its similarity to Bat-SARS-like coronavirus, SARS-CoV, and MERS-CoV (Zhou P. et al., 2020; Chen Y. et al., 2020).
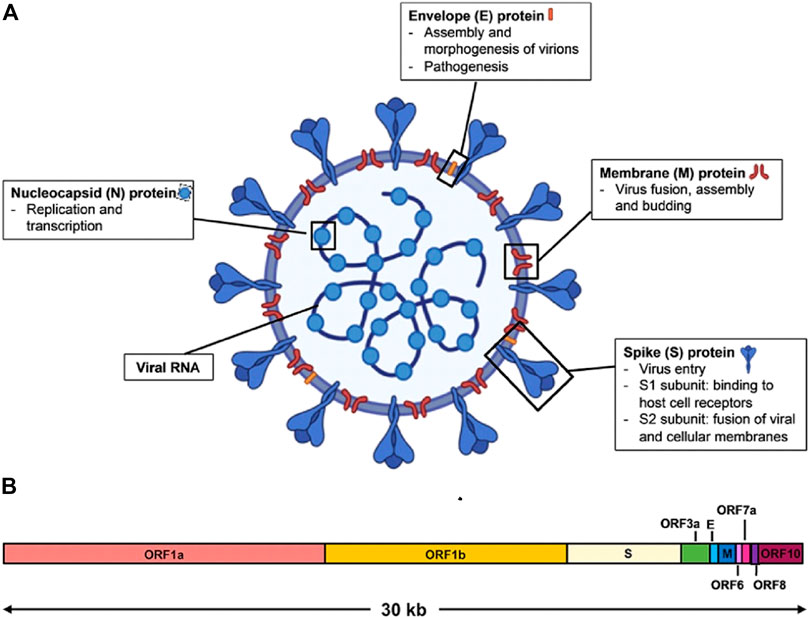
FIGURE 2. Schematic diagram of SARS-CoV-2 virus structure and genome organization. (A) The viral surface proteins, spike (S), envelope (E), and membrane (M) are embedded in a lipid bilayer. The single-stranded positive-sense viral RNA is associated with the nucleocapsid (N) protein. Diagram was created with BioRender. (B) The genome organization of SARS-CoV-2 viral RNA, which is adapted from GenBank accession number: MN908947, is characterized by sequence alignment against two representative members of the betacoronavirus genus. The entire genome sequence is ∼30 kb long [reproduced with permission] (Lee et al., 2020b).
Mechanism of Cell Entry and Life Cycle of the Virus
The virus enters the host’s cell through angiotensin-converting enzyme 2 (ACE2) receptors present on the cell membrane of the cells of several tissues, particularly of the lower respiratory tract (LRT), heart, kidneys, and gastrointestinal tract (GIT) (Imai et al., 2010; Hoffmann et al., 2020; Wan et al., 2020).
The entry is also facilitated by TMPRSS2 protease or endosomal cathepsin L present on host’s cells. The viral S protein consists of S1 and S2 subunits. The S1 binds ACE2 receptors through the RBD region, while S2 and TMPRSS2 or cathepsin L complex promote membrane fusion between the virus and the host cell. The entry is followed by the release of viral RNA, translation of ORF, production of nonstructured proteins, and formation of viral replication transcriptase complex. The complex initiates genome replication and subgenomic transcription. The viral structural proteins (S, E, M, and N) are encoded, including certain accessory proteins. Afterward, translation proteins are assembled at the endoplasmic-reticulum-Golgi intermediate compartment (ERGIC). Here, the S protein may also be modified by furin. The viral particles are thereby released from the host’s cell through exocytosis (Hoffmann et al., 2020; Tang T. et al., 2020; Shereen et al., 2020; Su and Wu, 2020).
This is the same mechanism as that observed previously for the SARS-CoV virus (Imai et al., 2010; Hoffmann et al., 2020; Wan et al., 2020). Some studies have found that the ACE2 receptor affinity of SARS-CoV-2 is more efficient than that of SARS-CoV(2003) but less efficient than its 2002 strain (Guobao et al., 2007; Wu A. et al., 2020) (see Figure 3). It is believed that any mutation on the receptor-binding domain (RBD) of S protein could make the virus more pathogenic. However, some mutations other than the receptor interaction sites in RBD of S protein have been discovered, but the role of such mutations in its pathogenicity is still not clear (Wu A. et al., 2020; Wan et al., 2020).
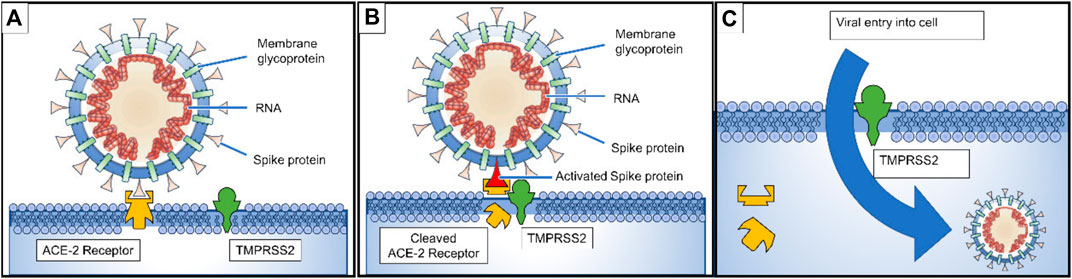
FIGURE 3. (A) Spike proteins on the surface of the coronavirus bind to angiotensin-converting enzyme 2 (ACE2) receptors on the surface of the target cell; (B) The type II transmembrane serine protease (TMPRSS2) binds to and cleaves the ACE2 receptor. In the process, the spike protein is activated; (C) cleaved ACE2 and activated spike protein facilitate viral entry. TMPRSS2 expression increases cellular uptake of the coronavirus (Lee et al., 2020).
Epidemiology
Origin
The novel coronavirus (nCoV), which has been later named SARS-CoV-2, was first reported to spread among contacts in the Huanan seafood wholesale market in Wuhan city, Hubei province, China (Wang et al., 2020b). The isolated agent was later identified as the seventh species of the coronavirus family to have caused infectious conditions in humans (Tang X. et al., 2020). It has been strongly believed that the species originated from Rhinolophus affinis (horseshoe bats, 96% identical genome with RaTG13 coronavirus species found in the host) with likely zoonotic spillover in Manis javanica intermediary host (Malayan pangolins, identification of strong similarity in six places of the RBDs of the virus with species in the hosts). This assessment substantiates the argument of its natural selection in humans either before or after zoonotic spillovers from intermediary hosts (Andersen et al., 2020).
Geographical Epidemiology, Ethnicity, and Culture
The SARS-CoV-2 virus has affected over 220 countries as of November 15, 2020. The epicenters of the pandemic are currently centered in the Americas (the United States and Central American and South American countries) and Europe, where the incidence is reported to be more than 24 million and 16 million cases, respectively (WHO, 2020a). In terms of mortality, United States, Mexico, Brazil, Columbia, and Peru are the worst affected countries in the Americas, whereas the United Kingdom, Spain, Italy, and France depict a similarly grim picture in Europe. India, Iran, and Russia are also among those countries that are badly affected globally (WHO, 2020a). In the United States, there have been approximately 11.5 million cases (highest incidence) reported with mortality of over 250,000. This is the highest mortality of any country reported so far. In terms of country-wise mortality figures, United States is followed by Brazil (∼168,000), Mexico (∼100,000), Argentina (∼37,000), Columbia (∼35,000), and Peru (∼34,000). Among the various European countries that have been hit badly by the pandemic, the highest mortality is documented in the United Kingdom (∼54,000), followed by Italy (∼48,000), France (∼47,000), and Spain (∼42,000). In Asia, India leads the mortality figures with more than ∼133,000 deaths attributed to COVID-19, followed by Iran with ∼44,000 deaths and Russia with ∼35,000 deaths (WHO, 2020e). Recently, many countries have seen a significant spike or surge in the number of new cases since the start of September 2020. This is especially true for many European countries where collectively ∼270,000 cases are now being reported each day in Europe against a modest value of ∼30,000 back in late August. Similarly, these figures are also being reported for the Americas against the lowest values ∼65,000 in August (WHO, 2020e). This is being referred to as the “second wave” of new infections, especially pertinent to European countries, where after significant depression in the number of new cases in recent 3–4 months (due to government measures), the recent lifting of lockdowns has led to a huge spike of new cases (Looi, 2020). Governments have therefore implemented strong measures equivalent to initial measures taken during the first wave of infections.
There is wide intercontinental variability in the spreading of COVID-19, and to assess the regional population data, individual expression of transmembrane protease serine 2 (TMPRSS2), which allows cellular uptake of the S protein, may be a determinant of SARS-CoV-2 regional infection susceptibility. Four variants of TMPRSS2 were evaluated in the local population of Africa, America, Europe, and Asia (China, Japan, and Taiwan), and the frequencies of variant alleles with high TMPRSS 2 expression in lungs were reported to be highest in the European and American population and lowest in the Asian population. Similarly, phylogenic analysis of time to the most recent common ancestor (TMRCA) was performed for SARS-CoV-2, dating to November 12, 2019, which also matched the epidemiological records of the disease. The non-Asian (Africa and Europe) outbreak of the disease has been associated with the reported reason of subhaplogroup A2 originated in Europe from Asian ancestor, where haplogroup A is regarded as an ancestral node (Gómez-Carballa et al., 2020). The emergence of COVID-19 was late in South America. The first case was reported on the 25th of February 2020 in Brazil, after which the entire country went into strict lockdown within few weeks. More than 65,000 cases were reported on the 14th of April, and Ecuador was the one found to be badly affected. The high incidence of COVID-19 was found in the state of Ceara, and most of the cases were originated from Fortaleza, the capital. The exponential rise in COVID-19 cases also led to the need for epidemiological surveillance and consistent analysis (Burki, 2020). A bibliometric study was also performed to evaluate the current trend in research on COVID-19 conducted in Latin America, and Aldeota, Cais do Porto, Centro, Edson Queiroz, and Cambeba were found to be the neighborhoods with the highest propensity for COVID-19 (Braga et al., 2020).
Ethnic factor is also a significant factor determining vulnerability among different racial groups. The susceptibility of COVID-19 among different races was reported in the following order: Black (Central and Western Africa) > Asian (Bangladesh > Indian ≥ Chinese ≥ Pakistani) > mixed ethnic groups (Epi cell, 2020). Similarly, culture plays a vital role in determining the attribution to disease, help-seeking behavior, and community acceptance to comply with measures and interventions to counter pandemic spread. Cultural norms greatly influence the failure or success of the strategies derived for the containment of the COVID-19 outbreak. They may augment the community response to volunteering efforts and making social distancing and lockdown an easier task (Mckee, 2020). For example, some Asian countries like Japan, Vietnam, and Taiwan implemented stringent lockdown at the beginning of the outbreak, overlooking their national economic damage. However, for the United States of America, the primary concern was their economy, and American authorities neglected the severity of the COVID-19 outbreak (Huynh, 2020). In addition, 1.76 million people might have been saved in the United States if strict social distancing was practiced. The Russian approach was more different, comprising penalties against the violation of the governmental instructions, whereas India and the Philippines were more stringent and were reported to arrest people not following social distancing practice (Greenstone and Nigam, 2020). Fetzer et al. (2020), studied the heterogeneous behavior of 51 countries for following social distancing and reported that Peru strictly followed the “staying at home” policy and ranked second among the countries with the highest percentage of residentials (Fetzer et al., 2020). The Asian countries applied a punitive approach to social distancing as their strict cultures, while the European countries are likely to be lenient in forcing people to stay at home. It has also been observed that countries with higher “Uncertainty Avoidance Index (UAI),” exhibited a lower proportion of public gathering. UAI shows that people in a society are in fear of unknown, uncertain, and unstructured situations; this can influence cultural perception and decision power (Huynh, 2020). The perceptions of different communities also affect the local medicinal practices; in a study performed in India, 48% of participants favored eating garlic in prophylaxis against COVID-19 (Vadivu and Annamuthu, 2014). But there can also be a sense of truculence and false hope that may drive some communities out of isolation due to a high degree of hubris. For example, in India, some organizations promoted people to take a bath in cow dung to defeat the SARS-CoV-2 (Theinterpreter, 2020).
Demographic Distribution
In terms of the distribution of incidence rates among different age and racial groups, the virus seems to be very discriminative. The data of 10 European countries on the distribution of COVID-19 cases by gender and age revealed that females of working age outnumber infected males. However, the rate in females declines at the retirement age of 60–69, which results in a crossover among males with COVID-19, so the vulnerable age group for a male is 70–79 and that of the female is 20–29, especially those bearing health and care–related occupation profiles (Sobotka et al., 2020). Moreover, it has been found that pregnant women are more vulnerable to COVID-19 infection, with a higher rate of death being associated with physiological changes during pregnancy, such as an increase in heart rate, a decrease in lung capacity, and a higher risk of thromboembolic disease (Centers for Disease Control and Prevention (CDC), 2020b). Across the globe, one pattern is very persistently observed; that is, infected males are more likely to die than females, despite all the uncertainties and dubitation (Zhou F. et al., 2020; Jordan et al., 2020; Lawton, 2020). Globally, in terms of the incidence of symptomatic incidence and mortality, elderly patients, especially with underlying conditions, are identified as the most vulnerable group (Kang and Jung, 2020). In New York state for the two oldest age groups, 65–74 years old and 75 and above, the weekly calculated infection fatality risk (IFR) was more significant than that of the younger age (0.0097% for <25 years and 0.12% for 25–44 years) group and was reported to be 6.7% and 19.1%, respectively (Yang W. et al., 2020). The cumulative infection rate across the globe is the lowest, i.e., below 1 per 1,000 among children and adolescents. The rates were found to be lowest in Spain, 0.6% (age, 0–14), and highest in Czechia, 9.2%. Comparatively stable infection rates were reported in Portugal at the ages of 20–59, but the irregular profile was exhibited in Czechia and Germany; however, a steeper rise in infection rate with age was observed in England (Sobotka et al., 2020).
Empirically, older people with comorbidities, residing in nursing homes, are at the highest risk of adverse outcomes and mortality during the running phases of a pandemic. Moreover, behavioral problems, cognitive disorders, and functional impairment may synergize the threat posed to nursing homes (Fallon et al., 2020). In Washington, about two-thirds of the residents were reported to be infected within a period of 3 weeks only at the death rate of 33%, along with 50 staff members and 16 visitors infected (Mcmichael et al., 2020). A significant number of deaths reported in Spain have been associated with nursing home residents. Many authorities are not including deaths at nursing homes from the total COVID-19 death toll (Wang et al., 2020a).
Mode of Transmission
SARS-CoV-2 has been found to transmit primarily through respiratory droplets (5–10 µm) and physical contact with contaminated matter. It is believed that the virus could also be transferred through airborne mechanisms (aerosols) where the virus gets trapped in particles (<5 µm) for an extended period of time and can be transmitted through distances of over 1 m (WHO, 2020c; Liu et al., 2020b; Morawska and Cao, 2020). Such instances are likely in closed spaces such as saturated ventilation systems or proximities where the patients with fluids of high virus loads are in contact with the susceptible individuals, such as exposure of healthcare workers and medics during intubation procedures and noninvasive positive pressure ventilation (Liu et al., 2020b). There have been reports that SARS-CoV-2 is also present in the fecal matter of the patients and can infect the gastrointestinal tract (Lamers et al., 2020). However, the scientific evidence for confirmed transmission through the fecal-oral route is missing (Xu Y. et al., 2020). Furthermore, there have been instances where the virus has been found to transfer through another intermediary host such as domesticated cats (human-cat-human), raising significant concerns of additional factors that could aid in the spread of the virus (Shi J. et al., 2020; Halfmann et al., 2020; Mallapaty, 2020).
In terms of epidemic modeling, the mean reported “reproduction number” (R0) for the current first wave of the pandemic has been estimated around 3.28 with a median value of 2.79, which is surprisingly very high compared to the estimates of 1.25–2.5 given by WHO at the beginning of the epidemic (Liu et al., 2020a). Moreover, this estimate is also higher than ∼2 reported for SARS-CoV-1. The surprising element behind the unprecedented spread of this coronavirus is its capability of transmission presymptomatically (∼48%, cases that show symptoms afterward) and asymptomatically (∼10%, cases where the person does not show any symptoms). The symptomatic cases are reported to be around 38% of the total transmissions (Ferretti et al., 2020). It must also be mentioned here that citing some recent studies, WHO, in its interim guidance and public press briefing, has made claims that asymptomatic transfer is unlikely with a wide level of interest in the announcement by governments around the world in favor of reopening the economies; however, due to significant criticism from public health experts around the world, WHO has since changed the stance and maintained that the matter is not yet close to a verdict and even if there is weak evidence of asymptomatic transfer, there is still a chance of its spread. As far as the current spread is concerned, WHO has categorized the transmission within countries as either “sporadic,” “cluster-based,” or “community-based” transmissions and thus with the current trend of data, Saudi Arabia, Somalia, Yemen, and Magnolia are classified as “sporadic transmission” areas, whereas countries such as China, Pakistan, India, Australia, Russia, Germany, Italy and Portugal are being classified as “cluster-based transmission” areas. The remaining areas are largely classified as “community-based transmission” areas, including the Americas, Africa, and remaining countries of Europe.
Precipitating Factors Influencing the Transmission
Wide varieties of factors have been identified to influence and affect the transmission rate of SARS-CoV-2 and the severity of COVID-19 among humans. Some of these factors are related to social behavior, while others are identified as physical and environmental conditions. According to a detailed study investigating the interrelationship of various factors influencing the virulence of the COVID-19, the primary factors to limit the spread of COVID-19 are social distancing and community sense of mitigation measures recommended by WHO such as personal hygiene and mandatory wearing of face masks, especially in closed spaces (Lakshmi Priyadarsini and Suresh, 2020). Conversely, in terms of physical conditions, lower air temperature (∼22°C) and lower relative humidity (40–60% RH), turbulent airflow patterns in packed areas, and closed-circuit ventilation have all been reported to participate in the spread of contaminated aerosols and thus are likely participants in the increased rate of transmission. Primary physical factors related to environmental conditions such as air temperature, relative humidity, and UV light exposure have been previously studied in detail for the SARS-CoV-1 virus, with a significant loss of virulence observed at a temperature of 38°C and >95% RH (Chan et al., 2011; Kowalski et al., 2020). Although there are few such studies on SARS-CoV-2, the same effects have been observed related to temperature and relative humidity for this virus, thereby impacting its spread (Bannister-Tyrrell et al., 2020). The virus has been found to be stable over a wide range of pH (3–10) at room temperature (Chin et al., 2020). The stability studies on different surfaces have identified that the viral titer was undetectable from printing or tissue paper after 3 h incubation and 2 h on wood and cloth but could last for 4–7 days on other surfaces. Furthermore, it has been found to be susceptible to typical disinfectants such as hydrogen peroxide, sodium hypochlorite, and hand soaps (Chin et al., 2020).
Another significant factor related to demographics is the age bracket of exposed individuals (higher age groups are more susceptible), as it is continuously reported as a significant predisposing factor for increased mortality and morbidity due to COVID-19 (Lakshmi Priyadarsini and Suresh, 2020). The other major precipitating factor that has been noted to contribute to the severity of COVID-19 is underlying medical conditions, such as hypertension, diabetes, asthma, renal disease, and other respiratory conditions such as COPD (Lakshmi Priyadarsini and Suresh, 2020). It has been suggested that the higher baseline levels of IL-6 and other inflammatory cytokines may be the probable reason for severe infection in these conditions (Tay et al., 2020b; Lakshmi Priyadarsini and Suresh, 2020). However, the anticipated response is not observed in inflammatory arthritis patients, even with raised levels of IL-6 (Schett et al., 2020). In addition, immune-compromised patients and patients on immunomodulators are also likely to exhibit a quite grim picture of COVID-19 (Monti et al., 2020). In a study, 58 patients with multiple myeloma (MM) receiving different immunomodulators diagnosed with COVID-19 showed a mortality rate of 24%. Therefore, early intervention in immunomodulatory therapies and strict adherence to the safety measures are recommended to encounter the future outbreak of COVID-19 (Wang B. et al., 2020).
Furthermore, one of the most significant socioenvironmental factors affecting the rate of spread is the population density. This factor alone can significantly contribute to the major wave of large-scale epidemics observed in localities like New York, New Jersey, and Indian Slums Metropolitan areas (Corburn et al., 2020; Gonzalez-Reiche et al., 2020; Mishra et al., 2020). Similarly, the transfer rate and infection reproduction number (R0) were calculated to be four times higher than those in the initial spread in Wuhan in Diamond Princess (a cruise ship affected by SARS-CoV-2 (Rocklöv and Sjödin, 2020).
Global Measures in Response to Pandemic
Since the first identification of the spread of this virus in the Wuhan city of Hubei province China, governments and policy advising agencies have been advocating for various containment and mitigation strategies to minimize the spread and flatten the pandemic curve to avoid crippling consequences on the healthcare systems due to out-of-capacity inflow of critical cases that may result in higher mortality. Four key response measures have been suggested by the OECD to the governments worldwide in light of the scientific evidence established from earlier and current pandemics. These include 1) large-scale surveillance, monitoring, and detection through centralized epidemiological and disaster management centers; 2) prevention of the spread in the community by means of social distancing measures and smart and complete lockdowns wherever necessary; 3) clinical management of cases by means of the best available scientific evidence and practices; 4) maintaining essential services to ensure the smooth running of the system and avoiding any other potential catastrophe (OECD, 2020). A mix of various containment and mitigation strategies well suited for the given country and region is advocated. In this regard, the United Nations had advised implementing contact tracing and hotspot mapping strategy to mitigate the spread for developing countries. This is especially true for developing countries such as Bangladesh, Myanmar, and India, where the economic consequences of complete lockdown are becoming disastrous (UN-DGC, 2020).
After a nearly complete global shutdown, economists are ringing alarms of the unprecedented recession of the 21st century, which is already devastating news for the developing economies, owing to their large informal sectors. The projected median decline in GDP is already threatening for many economies and may likely dip down further below the projections (Fernandes, 2020).
The measures to circumvent the spread of this virus based on manual contact tracing are insufficient and thus, along with the advocated measures of social distancing, hygiene, manual tracing of contacts, quarantine, and lockdowns, the use of digital applications, where the contacts are automatically alarmed of any potential transmission with a known case, is touted to be a major driving factor to control the spread. This strategy has been in various ways successful in countries such as South Korea if implemented with transparency and integrity to secure the public data (Ahn, 2020). Moreover, the strict implementation strategy and early timing of enforcing social distancing measures across many countries have resulted in a very contrasting and significant consequence in terms of reduction of “R” value of the spread and total fatalities and incidence of the infections (Ketchell, 2020).
Diagnostic and Monitoring Tools
SARS-CoV-2 genetic material is reported to be successfully detected through throat swabs and the upper and lower respiratory tract, blood, stool, or urine samples (Pan Y. et al., 2020; Chen W. et al., 2020; Yan et al., 2020). Several methods have been introduced for the detection of SARS-CoV-2. However, the collection of various samples from different sites and the utilization of multiple techniques is usually recommended to avoid false results related to the use of a single sample or method (Wang et al., 2020b). Furthermore, the application of positive, negative, and inhibition controls is also recommended to assure quality diagnosis (Yan et al., 2020).
Nucleic Acid Amplification Tests
PCR and Real-Time PCR
PCR and RT-PCR are considered important molecular biology techniques, first introduced in the 1980s. The techniques are based on the amplification and detection of a particular gene (Panawala, 2017). The amplification of genetic material is beneficial for obtaining the satisfying quantity of specimens required for a laboratory study. Both PCR and RT-PCR involve the utilization of certain enzymes. PCR uses a DNA template, whereas RT-PCR uses RNA (Panawala, 2017). Several RT-PCR-based test kits have also been developed (Li X. et al., 2020).
Sensitivity and Specificity
The techniques are considered highly sensitive, highly specific, and reliable for the detection of CoVs (Shen M. et al., 2020). However, these methods were observed to be commonly used for SARS-CoV-2 detection (Li X. et al., 2020). Yet, the procedures are claimed to be time-consuming and expensive that require costly reagents or equipment. The absence of safe and stable EPC (external positive controls), as available for SARS-CoV-1, is another severe problem in the detection of SARS-CoV-2 (Shen M.et al., 2020). Furthermore, adequate sampling, proper handling of the sample, and sufficient genetic material are recommended for a reliable PCR-based report (Lee et al., 2020). Additionally, RT-PCR is recommended over PCR due to its superior sensitivity (Shen M. et al., 2020).
Several rapid diagnosis kits that have been developed as per WHO standards are claimed to be 95% accurate against SARS-CoV-2. An RT-PCR-based test kit has also been introduced by the Centers for Disease Control and Prevention (CDC) CDC (2020b). Rapid nucleic acid diagnostic papers have also been invented, which provide a rapid detection facility of only 3 min with unaided eye observation (Jin Y. et al., 2020). Furthermore, the kits are only limited to upper and lower respiratory tract specimens. However, FDA has recently authorized the first RT-PCR-based LabCorp COVID-19 kit with a home collection option (FDA, 2020b).
Loop-Mediated Isothermal Amplification
LAMP is known as an ultrasensitive novel isothermal nucleic acid amplification–based method. It has been claimed to be capable of detecting even a small quantity of biomaterial within an hour and without the need for expensive reagents or equipment.
Sensitivity and Specificity
Its sensitivity and detection rate against coronaviruses have been found to be similar to those of PCR-based methods. However, the technique requires a high temperature, usually 65 °C, which restricts its application (Shen M. et al., 2020).
Microarray
The microarray technique has been widely used for the detection of coronaviruses. In this method, the virus RNA is used to produce cDNA, labeled with a specific probe through reverse transcriptase followed by subsequent detection of that specific probe. The method offers a low cost with sensitivity equal to that of RT-PCR. Moreover, portable microarray chips with adequate detection limits have also been introduced (Shen M. et al., 2020).
Specific High-Sensitivity Enzymatic Reporter Unlocking
This method is based on RNA-targeting CRISPR (Clustered Regularly Interspaced Short Palindromic Repeats) related enzyme Cas13. Cas13 has been combined with LAMP to detect DNA or RNA molecules.
Sensitivity and Specificity
The method has been shown to be quick, portable, and highly sensitive for nucleic acid detection (Shen M. et al., 2020; Udugama et al., 2020).
Radiological Examination
Although the nucleic acid amplification test has been widely recommended for the detection of SARS-CoV-2, its false reports could not be overlooked that may result in a false diagnosis and other severe consequences (Li X. et al., 2020). Consequently, the CT (chest radiography) scan has become a reliable method for the diagnosis of COVID-19 in clinical practice (Jin Y. et al., 2020). The scan images of almost all COVID-19 cases indicate the same features, particularly bilateral pulmonary parenchymal ground-glass opacification and consolidative pulmonary opacities, that have been observed in nearly 60 to 77 percent of cases (Forouzesh et al., 2020). At the same time, it has been observed that patients with negative nucleic acid amplification tests may show positive chest CT scan findings. However, a repeated nucleic acid amplification test is suggested for the final remarks (Forouzesh et al., 2020). Artificial Intelligence (AI) technology has also been used for accurate and instant interpretation of CT images (Jin Y. et al., 2020; Mak, 2020).
Limitations
Some disadvantages of CT imaging have also been reported, particularly nonselectivity and hysteresis of irregular imaging (Li X. et al., 2020). Moreover, prevention from frequent exposure to radiation, especially for pregnant women and children, is strongly recommended (Forouzesh et al., 2020).
Serological Tests
Acute serological responses have been identified in COVID-19 patients (Zhou P. et al., 2020). The serological tests are considered alternative to the nucleic acid test and CT imaging. For this purpose, several colloidal gold immunochromatography assays and other related techniques, kits, and detection methods have been applied and established (Jin Y. et al., 2020; Lee et al., 2020; Li X. et al., 2020; Rashid et al., 2020). The techniques generally target coronavirus immunogenic proteins (S, N, E, and M) and RBD to detect the presence of SARS-CoV-2 related antibodies (Mcintosh et al., 2020). The IgG levels are reported to be usually increased as the IgM levels start decreasing during viral infection (Rashid et al., 2020). IgM antibodies have been detected successfully during the early phases of infection, usually within 3 days, and are claimed to be present even after a month. Similarly, SARS-CoV-2 specific IgG antibodies have been reported to be detected after 4 days of infection period with a peak level after 2 weeks. It has been shown that their levels are related to disease severity; a higher level of both antibodies indicates greater severity of the infection. Researchers have also suggested IgA detection for SARS-CoV-2 diagnosis that is related to mucosal immunity usually activated in COVID-19 patients. However, it is considered less specific than IgM and IgG (Lee et al., 2020).
A list of other serological markers has been reported for the prediction of infection severity and prognosis of the disease in patients suffering from COVID-19. Some of these include an examination of interleukins (IL) levels, particularly IL-6, IL-10, and IL-2R, ESR (Erythrocyte Sedimentation Rate), CBC (Complete Blood Count), PT (Prothrombin Time), and levels of liver, kidney, heart, and other related enzymes (Forouzesh et al., 2020).
Limitations
Although serological tests are regarded as fast, powerful, and easy to conduct, it has been noted that the antibodies' response develops after several days of infection. The CDC does not recommend these tests for the diagnosis of current COVID-19 disease. Moreover, only 70% of their sensitivity is reported even after 4–6 days of infection (Centers for Disease Control and Prevention (CDC), 2020a; Wang et al., 2020b). The antibodies, IgM, and IgG, against SARS-CoV-2, have been observed to increase progressively with infection (Lee et al., 2020). Thereby, early diagnosis of infection is not possible and may lead to false-negative reports. It has also been reported that a large population has already been exposed to other human coronaviruses, and thus the false-positive response is commonly observed due to a high level of SARS-CoV-2 similarity to other coronaviruses. Therefore, the utilization of multiple serological approaches is recommended for a true report (Lee et al., 2020).
Coronavirus Disease 2019
Immune System
The immune system works as a defense system and plays a key role in the prevention of pathogenic attacks throughout the body; however, uncontrolled or impaired immune response may result in harmful tissue damage (Cao, 2020; Li G. et al., 2020). Overwhelming of the inflammatory response is considered to be initiated as a result of the antagonism effect of interferon by SARS-CoV-2 to promote its replication inside the cell (Tay et al., 2020a). Interferon (IFN) response is considered directly related to viral load. An increase in type 1 IFN response causes decreased viral load and vice versa. It has been observed that a decrease in total T cell count causes a declined function of these cells in COVID-19 patients (Diao et al., 2020). However, increased levels of cytokines such as interleukins (IL-6, IL-1β, IL-2, IL-8, and IL-17), granulocytes like granulocyte colony-stimulating factor (G-CSF), granulocyte-macrophage colony-stimulating factor (GM- CSF), interferon gamma-induced protein 10 (IP10), monocyte chemoattractant protein-1 (MCP1), macrophage inflammatory proteins-1 alpha (MIPα), and tumor necrosis factor (TNF) along with C-reactive proteins, D-dimers, and ferritin are reported in COVID-19 infection (Cao and Li, 2020; Xu Z. et al., 2020; Huang et al., 2020). Cytokines are responsible for shock and severe tissue damage to different organs, and slow healing of lungs is observed in patients with elevated IL-6 levels (Wang et al., 2020b). Another unique characteristic of hypercoagulation has also been commonly noticed in serious COVID-19 patients (Tang et al., 2020b; Merad and Martin, 2020). The cytokine storm and sepsis are considered the primary cause of death in about 28% of severe cases of COVID-19 (Zhang B. et al., 2020). But these immunological changes are often restored, particularly in mild to medium cases. Simultaneously, individuals with robust immunity and without comorbidities may successfully eliminate the virus before the exacerbation of immune overreaction (Cao and Li, 2020).
Organs Involvement
The organs that have been confirmed clinically to be involved in the COVID-19 infection include the eye, nervous, digestive, respiratory, circulatory, and urinary systems (Wang L.-S. et al., 2020). Although the lungs are the primary target of COVID-19 infection, it can attack or damage almost all body organs, particularly the heart, blood vessels, kidneys, intestines, and brain. The cells of these organs are rich in ACE2 receptors that are essentially required for the virus entry into the cells (Cao and Li, 2020; Cyranoski, 2020; Ky and Mann, 2020; Wadman et al., 2020; Chris Baraniuk, 2020, April 29).
Nasal Passage
The cells of the nose and throat are rich in ACE2 receptors providing an adequate environment for the virus where it starts replication. This is an asymptomatic phase, but a person could be the carrier of this deadly virus to another person (Peiris et al., 2003; Zou et al., 2020; Chris Baraniuk, 2020). The viral load of SARS-CoV-2 has been found higher in the nose than throat, unlike SARS-CoV-1, which is the probable reason for its rapid spread through respiratory droplets on close person-to-person contact (Chavez et al., 2020; Zou et al., 2020). The other sources of its spread could be the air contaminated with viral load from cough or sneeze of an infected person, touching or shaking hands, touching the mouth, nose, or eye after viral exposure, or less frequently through the fecal-oral route(Joseph and Ashkan, 2020).
It has been observed that in some cases, viruses may bypass the throat cells and enter into the lungs directly and may cause acute pneumonia without developing mild symptoms related to the throat, including cough and low-grade fever. (Cyranoski, 2020; Wadman et al., 2020).
Lungs
The lungs are considered as the main battle area. The alveoli of the lungs are rich in ACE2 receptors (Wadman et al., 2020; Chris Baraniuk, 2020, April 29). The virus attacks epithelial cells of the lungs and causes Diffuse Alveolar Damage (DAD), resulting in respiratory failure in some patients (Gu and Korteweg, 2007; Schaefer et al., 2020). The WBCs (White Blood Cells), dead cells, mucous, and pus or fluid together in alveoli after the virus attack causes ARDS (Acute Respiratory Distress Syndrome) with parallel symptoms of pneumonia-like fever, cough, and difficulty in breathing, resulting in hypoxemia (Tay et al., 2020a; Xu Z. et al., 2020; Huang et al., 2020). Fortunately, some cases are resolved by just oxygen supply, but many individuals could not survive or require intensive care and end up on ventilation commonly (Xu Z. et al., 2020; Huang et al., 2020; Wadman et al., 2020). Furthermore, the development of pulmonary lymphopenia and increased neutrophil-lymphocyte ratio in almost 80 percent of infected patients is considered due to immune cells' stimulation toward the infection site (Tay et al., 2020a; Jamilloux et al., 2020). Lymphopenia is described as a result of either T cells’ death due to direct viral attack, cytokine-induced apoptosis of T cells, or immune cell redistribution (Jamilloux et al., 2020).
The clinicians believe that releasing a high amount of chemical signaling molecules or cytokine storm by the immune system may overreact or start attacking healthy cells and responsible for severe infection (Tay et al., 2020a; Wang et al., 2020b).
Cardiovascular System
Heart and blood vessels are rich in ACE2 receptors (Zheng et al., 2020; Chris Baraniuk, 2020). The studies conducted in China reveal that almost 20 to 44 COVID-19 percent of patients develop cardiac symptoms. These symptoms include arrhythmia, cardiac muscle damage, cardiac swelling and scarring, decreased heart function, and heart attack. Moreover, cardiac symptoms may develop secondary to pneumonia (Zhou F. et al., 2020; Ky and Mann, 2020; Wadman et al., 2020; Zheng et al., 2020). The cardiac symptoms may result in clotting defects, which are the additive COVID-19 severity and mortality. The hypercoagulation state is characterized by extended prothrombin time, high D-dimer levels, and fibrinogen with almost satisfactory partial thromboplastin time (Tang et al., 2020b; Merad and Martin, 2020). It is considered that cytokines, particularly IL-6, and endothelial cell injury are responsible for the activation of the coagulation system and suppression of the fibrinolytic system (Merad and Martin, 2020; Tang et al., 2020b; Wang et al., 2020b). It has been reported that almost 71.4 percent of nonsurvivors developed disseminated intravascular coagulation (DIC) (Tang et al., 2020b). The clots may progress to thrombosis formation, which may cause pulmonary embolism or stroke. It is considered to be a major cause of death due to COVID-19 infection (Zheng et al., 2020; Merad and Martin, 2020; Wang et al., 2020b). The data from the United States show that almost one-third of hospitalized patients had preexisting cardiovascular symptoms or diabetes. The studies suggest that the lack of oxygen and cytokine storm after the viral attack on the lungs is also responsible for blood vessels and heart damage (Tay et al., 2020a; Ky and Mann, 2020).
Renal System
Like other organs, kidneys are also abundant in ACE2 receptors (Chris Baraniuk, 2020, April 29). The studies show that about 59 percent of hospitalized patients develop proteinuria, whereas hematuria, increased blood urea nitrogen, and high levels of creatinine have been observed in 44 percent, 14 percent, and 10 percent of patients, respectively. Acute kidney injury (AKI) and kidney failure are seen as common (Li Z. et al., 2020). Reduced blood flow to the kidney is observed due to cytokine storms, resulting in kidney injury (Cheng et al., 2020). AKI is considered to be serious organ damage caused by COVID-19. A critical high serum creatinine (SCr) level and low urine output are reported during kidney injury (Xu D. et al., 2020). On the contrary, a study conducted in China by Wang et al. indicated fewer associations between AKI and COVID-19 (Wang et al., 2020a). Moreover, ventilators and some antivirals suggested for the treatment of COVID-19 may damage kidneys extensively, particularly in patients with preexisting conditions like diabetes, hypertension, and kidney diseases, respectively (Wadman et al., 2020) (Li Z. et al., 2020).
Central Nervous System
A large number of ACE2 receptors are present in the neural cortex and brain stem (Wadman et al., 2020; Chris Baraniuk, 2020, April 29). The SARS-CoV-2 has been detected in the cerebrospinal fluid. Neurological symptoms are seen in almost 5–10 percent of hospitalized patients (Wadman et al., 2020). However, the brain and nervous system damage should not be underestimated in patients on ventilators (Stevens et al., 2008). Hyperactivity of the nervous system, unconsciousness, loss of sense of smell and taste, meningitis, encephalitis, stroke, brain injury and seizure have been reported. Moreover, it is revealed that the cytokine storm and thrombosis are also responsible for brain swelling, stroke, and severe brain injury (Wu Y. et al., 2020).
Gastrointestinal Tract
The SARS-CoV-2 attacks the lining of the lower digestive tract that is rich in ACE2 receptors (Wadman et al., 2020; Chris Baraniuk, 2020). The virus has been detected in the stool samples of almost 53 percent of patients suffering from COVID-19. Additionally, the viral protein shell is found in the intestines biopsy indicating its replication in the gut linings (Zhou F. et al., 2020). Likewise, viral RNA has been detected on rectal swabs even after negative nasopharyngeal testing (Wang W. et al., 2020). The gastrointestinal symptoms include diarrhea, vomiting, and abdominal pain and have been observed in almost 20 percent of infected patients (Huang et al., 2020).
Liver
The injury to the liver and bile is found common in hospitalized patients, but the direct invasion of SARS-CoV-2 to the liver is not confirmed (Gu et al., 2020; Wadman et al., 2020). However, multiple events during the infection and administration of several drugs are considered responsible for elevated levels of liver enzymes and liver damage (Bangash et al., 2020). In a study conducted in China, 58% of 148 COVID-19 patients had an abnormal liver function. Higher levels of procalcitonin and C-reactive proteins have also been observed in these patients (Fan et al., 2020). Liver dysfunction is observed dominantly in severe cases (Zhang C. et al., 2020).
Eyes
Symptoms like conjunctivitis or pink eyes and watery eyes have been observed in almost one-third of hospitalized patients (Wadman et al., 2020). Chemosis, conjunctival hyperemia, epiphora, and increased secretions are reported in patients in addition to conjunctivitis. Patients with ocular symptoms have demonstrated extraordinary WBCs, neutrophil counts, procalcitonin, C-reactive protein, and lactate dehydrogenase levels compared to those without any ocular symptoms. Furthermore, RT-PCR assay of 90% of infected ocular patients showed positive results for SARS-CoV-2 from a conjunctival swab in addition to a nasopharyngeal swab (Wu P. et al., 2020).
Skin
The skin-related symptoms associated with COVID-19 were first reported in China, followed by Italy and in Spain; when a study was conducted on 375 patients with skin lesions and positive SARS-CoV-2 test, a relationship between skin lesions and COVID-19 was established (Diotallevi et al., 2020). It has been observed that the viral attacks on the ACE2 receptors, present in arterial and venous endothelial cells and arterial smooth muscle cells, trigger the host’s inflammatory response, including activation of mast cells and basophils, which may cause multiple skin conditions like rashes, diffuse or disseminated erythema, urticaria, livedo racemosa, blue toe syndrome, retiform purpura, vesicle trunk, purpuric exanthema, atopic dermatitis, and neutrophilic dermatoses, as well as less frequent cases of chilblains affecting fingers or toes (acral rash). It has been suggested that the skin manifestations may be the result of minor thrombotic events or damage to the endothelial walls of small distal vessels. These conditions have been observed in COVID-19 patients of all ages, but the rashes may be paraviral due to cytokines or drug-related during the treatment of any disease (Criado et al., 2020). Although these conditions may not be accompanied by pain or itching or other systemic symptoms, identifying rashes is important in earlier COVID-19 cases, and therefore attention to skin involvement during COVID-19 is also suggested (Bataille et al., 2020).
Drugs Being Investigated for the Treatment of COVID-19 and Its Management
Supportive Therapy
Antipyretics or NSAIDs for reducing fever and pain (Wu A. et al., 2020), oxygen therapy to maintain oxygen saturation (Røsjø et al., 2011), antibiotics as an empiric therapy (Rhodes et al., 2017), intravenous fluid resuscitation or vasopressor for regulating persistent shock (Schultz et al., 2017), early blood purification for reduction of renal workload and renal function recovery (Wang D. et al., 2020), beta-agonists such as dobutamine for the management of cardiac shock or failure, and systemic steroids against COPD exacerbation are commonly suggested as supportive therapy in COVID-19 infection (Alhazzani et al., 2020; World Health Organization, 2020a; Mcintosh, 2020). Furthermore, the use of vitamins as an immunity booster and some Chinese medicines against inflammatory responses has also been reported for the management of COVID-19 (People’s daily of China, 2020; Runfeng et al., 2020; Wang L.-S. et al., 2020). A description of such medications is as follows.
Antibacterials
Azithromycin
Clinical trials are currently perceiving its effectiveness against SARS-CoV-2 (Akram et al., 2020; Hinks et al., 2020; Sivapalan et al., 2020). In a trial conducted in France, it revealed that the group of COVID-19 patients who were receiving hydroxychloroquine along with azithromycin showed significant response in comparison with the group receiving hydroxychloroquine alone. Azithromycin was used in a dose of 500 mg per day on day one, followed by 250 mg per day for 5 days along with 600 mg hydroxychloroquine per day, respectively (Gautret et al., 2020). Another study showed its combination with other drugs; especially, hydroxychloroquine was beneficial on laboratory-confirmed COVID-19 patients (Sekhavati et al., 2020). However, Molina et al. and Remo H M Furtado et al. observed contrary results in their trials and did not support this combination therapy especially in patients with severe COVID-19 infection (Furtado et al., 2020; Molina et al., 2020). Similarly, the cardiac toxicity related to these drugs is also considered a major weakness of the regimen (Juurlink, 2020).
Teicoplanin
Teicoplanin has been shown to be active against the Ebola virus, SARS-CoV-1, and MERS-CoV and suggested for the treatment of COVID-19. It targets viral S protein and has been observed to be useful during the early phases of COVID-19 infection (Zhang J. et al., 2020). The recommended dose is 100–400 mg twice daily for 10 days (Parente and Laplante, 2017).
Immunomodulators and Immunosuppressants
Interferon-1
Clinical trials are being conducted to investigate its effectiveness against COVID-19 infection (Alavi Darazam et al., 2020). It has also been found to be effective previously against SARS-CoV-1 and MERS-CoV and suggested presently for the treatment of SARS-CoV-2 (Arabi et al., 2020; Jamilloux et al., 2020; Sallard et al., 2020). Researchers believed that SARS-CoV-2 could be more sensitive to IFN (Sallard et al., 2020). However, it has been advised for the treatment of COVID-19 patients who are suffering from hyperinflammation and ARDS (Jamilloux et al., 2020). The recently published interim results of the WHO SOLIDARITY trial consortium has downplayed the role of interferon alone or in combination with lopinavir (initially) to reduce the overall mortality of hospitalized moderate and severe COVID-19 patients. A total of over 1,412 patients reportedly enrolled in the study were compared with 4,088 patients with no study drug. The study has thus concluded that no difference has been observed in the 28-day survival rate among hospitalized patients receiving 44 µg subcutaneous injection thrice weekly or 10 µg daily for 6 days in patients on high oxygen or ECMO (Pan H. et al., 2020).
Systemic Corticosteroids
Methylprednisolone has been studied on COVID-19 patients with COPD and a dose of 1–2 mg per kg per day intravenously for 5–7 days has been found effective in reducing the mortality rate reported (Wu C. et al., 2020). Other studies reported 40–80 mg dose for a period of 3–6 days for the treatment of COVID-19 (Wu R. et al., 2020). Similarly, reduction in the disease course and improvement in symptoms have been observed in patients while administering corticosteroids (Wang Y. et al., 2020). However, the IDSA (Infectious Disease Society of American) recommends using the therapy only in the treatment of ARDS (Bhimraj et al., 2020).
A comprehensive trial in the United Kingdom named “Randomized Evaluation of COVID-19 Therapy” (RECOVERY), which is studying lopinavir-ritonavir, low-dose dexamethasone, hydroxychloroquine, azithromycin, tocilizumab, and convalescent plasma as potential treatments for the ongoing pandemic patients, has announced that low-dose dexamethasone (6 mg once daily po/iv) is found to have substantially (41%, patients on ventilation; 25%, patients of oxygen; 13%, no respiratory intervention) reduced the 28-day mortality of patients. The trial includes over 2,104 patients receiving low-dose dexamethasone and 4,321 patients on randomized to usual care (Peter Horby, 2020). FDA has also included it in the list of drugs for temporary compounding by outsourcing facilities and pharmacy compounders (FDA, 2020a). Some clinical trials are also being conducted to evaluate its effectiveness against COVID-19 (Maskin et al., 2020; Tomazini et al., 2020).
Tocilizumab/Sarilumab
Clinical trials are being conducted using these antibodies against SARS-CoV-2 (ClinicalTrials.gov, 2020; Farias et al., 2020). A study conducted in China revealed recovery of 20 out of 21 patients while using tocilizumab (NIH - U.S. National Library of Medicine, 2020, April 28). It has been found to be more effective in COVID-19 patients without the need for ventilator support and almost no toxicity is reported (Perrone et al., 2020). Furthermore, its efficacy has also been studied in the combination of high-dose methylprednisolone in COVID-19 patients and a decrease in mortality rate and mechanical ventilation support and increase in recovery have been observed (Ramiro et al., 2020). Similar significant results against COVID-19 infection and a reduction in fever at the first dose have also been reported in studies (Xu X. et al., 2020; Fu et al., 2020).
Sirolimus
It has been used for the treatment of viral infections, including infections caused by coronaviruses (Wang et al., 2014; Kindrachuk et al., 2015), and has been proposed as a potential candidate for the treatment of COVID-19 (Zhou Y. et al., 2020). Clinical trials are scheduled to be conducted using sirolimus in COVID-19 patients (NIH - U.S. National Library of Medicine, 2020, May).
Miscellaneous
NSAIDs
NSAIDs, especially acetaminophen, are considered important agents for the suppression of fever during an infection. These drugs also play a key role in reducing severe immune responses and preventing viral shedding. However, the side-effects such as GI bleeding, fluid retention, and kidney dysfunction related to these agents are of great concern (Little, 2020). Moreover, it has been claimed that NSAIDs, especially ibuprofen, upregulate ACE2 receptors and could exacerbate the factors for the COVID-19 infection (Day, 2020). Studies reported that no sufficient evidence had been found against the use of ibuprofen in patients with COVID-19 (Sodhi and Etminan, 2020).
Thiazolidinediones, ARBs, and ACE2 Receptor Blocker
It is believed that thiazolidinediones might be responsible for increased ACE2 receptor expression and could result in severe or deadly COVID-19 infection (Bauer et al., 2010; Fang et al., 2020). Researchers hypothesized that ARB's (Angiotensin Receptor Blocker) long-term use and ACE2 receptor blockers could result in overexpression of their receptors. It has been further concluded that high mortality in patients with a history of preexisting cardiovascular diseases and diabetes might be related to the long-term use of these drugs (Fang et al., 2020). On the contrary, a group of researchers claimed that no such evidence is available against thiazolidinediones, ARB, ACE inhibitors, or other related drugs (Gracia-Ramos, 2020).
Vasodilators (Nitric Oxide and Epoprostenol)
Since hypoxemia is a major risk of death in severe cases of COVID-19, vasodilators are considered to be useful. Unfortunately, no major study is reported on the treatment of vasodilators against SARS-CoV-2. However, the agents were used effectively against SARS-CoV-1 and MERS-CoV (Chen et al., 2004; Åkerström et al., 2005), and trials are also being proceeded or planned for the prevention and treatment of COVID-19 (Begun et al., 2020). In addition, FDA granted emergency expanded access to a biotherapeutics company, Bellerophon Therapeutics, for its inhaled nitric oxide (iNO plus) (GlobeNewswire, 2020, March 20). The company recently announced the first successful treatment of the COVID-19 patients (Bellerophon Therapeutics, 2020).
Vitamins
It is observed that the vitamin D levels decrease in several healthy individuals, especially during winter and additionally who get less exposure to sunlight, housebound, or work at night (Enwemeka et al., 2020). An adequate vitamin D level during summer strengthens the immune system and thereby decreases viruses’ attack (News Scientist, 2020, April 1). Clinicians claimed that the low level of vitamin D in the body could be the cause of the COVID-19 outbreak during the winter season (Enwemeka et al., 2020). Vitamin C, along with vitamin D and vitamin E, is also recommended in some studies. These vitamins have been beneficial in preventing respiratory infections and enhancing body resistance toward nCoV (Wang L.-S. et al., 2020).
Anticoagulants (Low-Molecular-Weight Heparin)
A decrease in mortality has been observed in patients while administering anticoagulants (Tang et al., 2020a). Low-molecular-weight heparin is suggested for the treatment of hypercoagulation and thrombosis-associated vascular damage in COVID-19 patients (Tang et al., 2020b). In addition, heparin has also been claimed to have anti-inflammatory and antiviral activities against SARS-CoV-2 (Shi C. et al., 2020; Mycroft-West et al., 2020). The European Society of Cardiology has recently schemed an anticoagulation protocol for coagulopathy management in patients suffering from COVID-19. Patients with respiratory rate >24 bpm, dyspnea, oxygen saturation <90%, rising D-dimer levels, elevated C-reactive protein, and elevated fibrinogen levels are characterized in high thrombotic risk group and various anticoagulation strategies are therefore suggested for them. A target of 60–85 prothrombin time (aPTT) range is considered and parenteral drip of heparin for patients admitted to Intensive Care Unit (ICU) or subcutaneous enoxaparin at a dose of 1 mg/kg two times a day for patients who do not require intensive care is suggested. Furthermore, Point-of-Care Ultrasound (POCUS) is recommended for deciding the continuation of the anticoagulation therapy (Atallah et al., 2020).
Traditional Herbal Medicines
Traditional herbal medicines have been found useful for the treatment of epidemic outbreaks, including influenza and coronaviruses (Chen et al., 2011; Xiaoyan et al., 2018; Redeploying plant defences, 2020; Xiaoyan et al., 2020). Traditional medicine treatment guidelines have also been issued by China and Korea on the treatment of COVID-19 (Ang et al., 2020). Chinese medicines, particularly Shuanghuanglian oral liquid and LianhuaQingwen capsule, have been extensively used for the treatment of COVID-19 disease (People's daily of China, 2020; Runfeng et al., 2020). The other traditional herbal medicines commonly used for the treatment of COVID-19 include Astragalus membranaceus, Saposhnikoviae divaricata, Glycyrrhiza uralensis, Rhizoma atractylodis macrocephalae, Fructus forsythia, Lonicerae Japonicae Flos, Atractylodis Rhizoma, Radix platycodonis, and Agastache rugosa (Luo et al., 2020). It is believed that these medicines could play a key role in the reduction of inflammatory responses developed in the human body as a result of viruses and bacteria (People's daily of China, 2020; Runfeng et al., 2020)
Specific Therapy
It is believed that the drugs targeting the SARS-CoV-2 main protease (Mpro), an enzyme that is important to viral replication and transcription, could play a key role in COVID-19 treatment (Jin Z. et al., 2020). A list of drugs is described as follows.
Antiprotozoals
Chloroquine (CQ) and Hydroxychloroquine (HCQ)
Researchers analyzed that antiprotozoals reduce cytokine storm, the main cause of severe infection and death in COVID-19 (Cao, 2020). The therapy is claimed to be responsible for the inhibition of ACE2 receptors glycosylation. The drugs also bind with viral S protein, resulting in the prevention of SARS-CoV-2 entry into the cells (Savarino et al., 2006; Liu J. et al., 2020; Wang M. et al., 2020). In vitro studies have shown supportive results in reducing viral replication and, thus, symptoms duration (Gao et al., 2020; Singh et al., 2020). A study conducted on patients with positive SARS-CoV-2 indicated that the viral RNA became undetectable on day 6 after administering 200 mg HCQ three times a day (Frie and Gbinigie, 2020).
On March 28, both were granted EUA (Emergency Use Authorization) for the treatment of COVID-19 by FDA (FDA, 2020, March 28). Later FDA, on April 24, warned the use of these agents, alone or in combination with other drugs, particularly azithromycin, outside a hospital setting because of the reported evidence of abnormal heart rhythm in a clinical trial (FDA, 2020, April 24) (Borba et al., 2020). Furthermore, their use against COVID-19 was not successful and therefore not supported by WHO (WHO, 2020, March 11). HCQ is considered less toxic due to the hydroxyl group present in its structure, which helps in easy clearance from the body (Schultz and Gilman, 1997; Singh et al., 2020).
In rather recent developments, the large-scale trials have either stopped or paused the study on HCQ, citing that the treatment does not offer any improvement in mortality; moreover, initial data showed significant adverse effects associated. This is true for RECOVERY and SOLIDARITY trials as well, which are funded by UKRI and WHO, respectively. In the case of the RECOVERY trial, 1,542 patients treated with HCQ had higher (25.7%) fatality in 28 days compared to 3,132 patients (23.5%) with standard care. Similarly, SOLIDARITY trials by WHO were also suspended on May 24, 2020, owing to reports of toxicity and nonsuperiority of the treatment. The development has also led to the retraction of a major article published in Lancet where the journal could not verify the data due to confidentiality issues of the patients amid the widespread skepticism of the drug (Kupferschmidt, 2020). Similar results have also been observed recently by Lyngbakken et al., and no improvement was found for using 400 mg HCQ two times a day for 7 days in hospitalized patients suffering from COVID-19 infection (Lyngbakken et al., 2020).
Nitazoxanide
Nitazoxanide is suggested to have strong antiviral activity against SARS-CoV-2 (Srivatsan Padmanabhan and Tech, 2020). Additionally, it has also been proposed in combination with hydroxychloroquine for the treatment of COVID-19 infection and suggested to help eradicate viral load and control overwhelming the immune system, particularly in severe cases (Srivatsan Padmanabhan, 2020). The optimal doses against SARS-CoV-2 are predicted to be 1,200 mg four times, 1,600 mg three times, and 2,900 mg two times a day in a fasted state and 700 mg four times, 900 mg three times, and 1,400 mg two times a day in fed state, respectively (Rajoli et al., 2020). In a recent clinical trial conducted on ambulatory, hospitalized, and pregnant women suffering from COVID-19 infection, nitazoxanide has been observed to be a safe therapy and found effective against SARS-CoV-2 (Meneses Calderón et al., 2020).
Antivirals
Remdesivir
It has been widely used against the Ebola virus and has shown effective against other single-stranded RNA viruses such as Marburg virus, Nipah virus, parainfluenza type 3 virus, and human coronaviruses (Warren et al., 2016; Lo et al., 2017). Clinical trials are being carried out currently to evaluate its safety and efficacy against SARS-CoV-2 (Al-Tawfiq et al., 2020; NIH - U.S. National Library of Medicine, 2020a; NIH - U.S. National Library of Medicine, 2020b). Its intravenous administration to COVID-19 patients resulted in notable recovery from pneumonia (Holshue et al., 2020). A study in USA, Japan, and Europe or Canada showed clinical improvements in 36 out of the 53 hospitalized severe COVID-19 patients (68%) with remdesivir at a dose of 200 mg on day 1, followed by 100 mg per day for 9 days (total: 10 days of therapy). In terms of therapeutic goals, in a large randomized, double-blind, placebo-controlled clinical trial conducted in over 1,000 patients, remdesivir was found to have shortened the duration of therapy from 15 to 11 days and improvements in mortality rates have been observed (Beigel et al., 2020). In May 2020, FDA issued EUA to this drug for the treatment of severe COVID-19 cases in both adults and children. The FDA further defined severe conditions as individuals with low blood oxygen saturation levels or requiring mechanical oxygen support (FDA, 2020, May 1). Later, in October 2020, it became the first antiviral drug approved by the FDA for use in COVID-19 patients above 11 years of age and 40 kg individuals (FDA, 2020 ; U.S.F.D.A., 2020). However, very recently, the use of remdesivir has been rejected by WHO after the interim results of the SOLIDARITY trial are released. A total of 2750 COVID-19 patients were given 200 mg loading dose followed by 100 mg of drug once daily till the 9th day. The data showed that the treatment group did not show any improvement over the no-drug-of-study group comprising 4,088 patients. The drug failed to improve overall mortality or prolonged the initiation of ventilation of moderately ill patients. (Pan H. et al., 2020). Though the trial is a multicenter global study, its case-by-case recommendation remains largely weak against low-risk vs. high-risk patients as described in randomized double-blind placebo-controlled clinical study “adaptive COVID-19 treatment trial” (ACTT-1), which favors the treatment with the drug and was subsequently used by FDA before giving approval. The final results, however, are awaited before any conclusion is made on its (SOLIDARITY trial) effectiveness in any subgroups of patients (NIAID, 2020; Pan H. et al., 2020).
Lopinavir/Ritonavir
Clinical trials have been investigated using a combination of a dose of 400 mg for lopinavir and 100 mg for ritonavir two times a day for the treatment of COVID-19. The clinicians claimed that no remarkable benefits were observed. The studies further found nausea, diarrhea, and asthenia as common side-effects (Cao et al., 2020). However, a study conducted in China using 400 mg lopinavir per day with or without IFN- α2b has claimed that it is effective against COVID-19. But, the consideration of gastrointestinal side-effects and hypokalemia was also suggested (Liu F. et al., 2020). Similarly, improved COVID-19 related clinical symptoms such as fever and no reduction in SARS-CoV-2 titers have been reported in a study conducted in Korea (Lim et al., 2020). Another clinical trial has also been announced recently by Prasan Kumar Panda et al. for investigating the efficacy of lopinavir-ritonavir or hydroxychloroquine along with ribavirin in hospitalized COVID-19 patients (Panda et al., 2020). Similar to the case of remdesivir, lopinavir and ritonavir have also failed to demonstrate any considerable superiority to other treatment options used for patients with moderately ill patients in the SOLIDARITY trials (Pan H. et al., 2020).
Ribavirin
Ribavirin has been observed to be effective, particularly in combination with IFN, against viruses, including coronaviruses (Scott and Perry, 2002; Khalili et al., 2020). However, a decrease in hemoglobin concentration is reported in patients with COVID-19, while administering this drug (Khalili et al., 2020). Its efficacy in hospitalized COVID-19 patients has been recently investigated alone and in combination with sofosbuvir/daclatasvir and no significant improvement was observed. However, clinicians suggested further investigation on large clinical trials (Abbaspour Kasgari et al., 2020; Eslami et al., 2020).
Umifenovir
It has been reported effective against both SARS-CoV-1 and SARS-CoV-2 (Blaising et al., 2014; Dong et al., 2020). A retrospective study found that an increase in efficacy of lopinavir and ritonavir was observed against SARS-CoV-2 when augmented with umifenovir (Deng et al., 2020).
Favipiravir
It has been proposed as an experimental drug for the treatment of COVID-19 infection (Li and De Clercq, 2020). The clinical trial indicated that patients suffering from moderate COVID-19 infection when treated with favipiravir showed remarkable recovery within a week. It has also been found clinically superior to umifenovir against SARS-CoV-2. The former showed about 71% recovery rate, and the latter 55% only (Chen C. et al., 2020). Several clinical trials are being conducted to examine its safety and efficacy against SARS-CoV-2 (Seneviratne et al., 2020; WHO, 2020, April 11). It has been observed to be effective against SARS-CoV-2 at a dose of 1600 mg twice a day first, followed by 600 mg two times a day for 13 days (Cai et al., 2020). However, low blood concentration of this drug in severe COVID-19 patients compared to healthy individuals has been observed in a recent clinical trial and therefore, further investigation for the development of optimal treatment strategy in critically ill COVID-19 patients has been suggested (Irie et al., 2020).
Oseltamivir
Clinical trials are being conducted using this drug alone and in combination with other antivirals and antiprotozoals against COVID-19 infection (Rosa and Santos, 2020). However, scientists claim that no satisfactory results have been observed while administering oseltamivir to COVID-19 patients (Wu A. et al., 2020). Similarly, it has been suggested that as SARS-CoV-2 does not contain neuraminidase enzyme, oseltamivir and other related drugs are not expected to be effective against COVID-19 (Orders, 2020).
Convalescent Plasma Therapy
Convalescent plasma therapy has been successfully used previously against SARS-CoV and MERS-CoV coronaviruses (Zhang et al., 2005; Mair-Jenkins et al., 2015) and is now being explored against COVID-19 (Shen C. et al., 2020; Chen L. et al., 2020). The technique has also been recommended as an emergency investigational new drug application by the FDA for the treatment of fatal or deadly COVID-19 infections (Food and Administration, 2020). A number of clinical trials exploring the safety of the therapy have concluded that the use of convalescent plasma is safe with or without other treatment options used. However, the studies have largely failed to confirm its effectiveness in a variety of settings, especially in a large randomized clinical trial with over 100 enrolled patients; the effectiveness of the therapy has been largely inconclusive in improving the condition of moderately and severely ill patients (Agarwal et al., 2020; Li L. et al., 2020; Olivares-Gazca et al., 2020). Moreover, in one study, the convalescent plasma transfusion failed to increase the neutralizing antibody titers in recipients (Bradfute et al., 2020). The treatment and management option is also described by Yang et al in 2020 (see Figure 4)
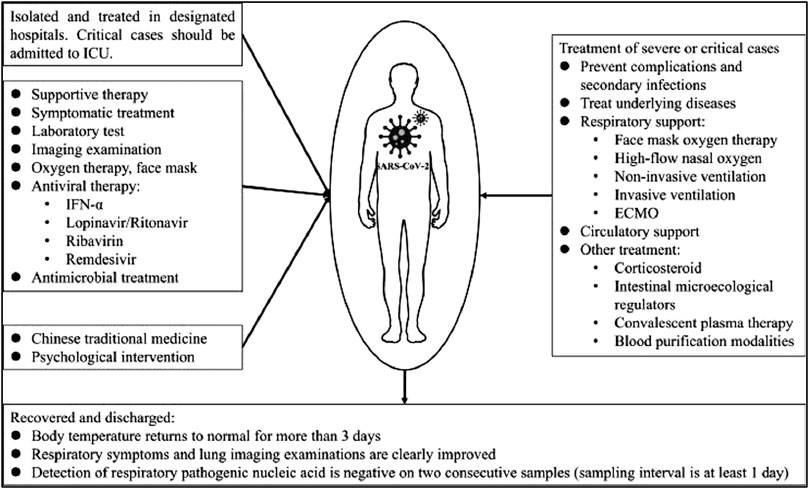
FIGURE 4. The treatment and management option for COVID-19 patients [reproduced with permission] (Yang Y. et al., 2020).
Landscape of Vaccine Development for SARS-CoV-2
In the wake of the current pandemic, an unprecedented response has been observed globally on the front for vaccine development against SARS-CoV-2 to protect the large masses of the global population from getting infected with long-term immunity against the virus. There are largely three ways due to which this development response is unprecedented in the history of pandemics ever. The first reason is that there are over 120 vaccine research groups and teams participating in the development, comprising majorly large and small commercial sector biotech companies followed by academic institutions and public sector research organizations (Shang et al., 2020; WHO, 2020b). A large fraction of these teams are working on already developed and tested platforms such as live-attenuated virus and chemically inactivated viruses that have been successful for a wide range of viral diseases (Shang et al., 2020). This also ensures that if one method becomes successful, the challenges to develop and scale things up would not be cumbersome. Moreover, novel strategies like DNA plasmids and mRNA-based vaccines are also being touted as a potential breakthrough in the field as these platforms offer high production and quality control during manufacturing and can be replicated to suffice the global demand (Callaway, 2020b). On the other hand, some leading researchers are skeptical of the timeline prediction of 18 months for the vaccine citing various technical challenges and regulatory oversight reasons (GeneScript, 2020). The fastest time for a vaccine development that has been recorded is of 7 months for the Ebola, Swine flu, and Zika virus. However, in the case of SARS-CoV-2, it took only 10 weeks for RNA-based Moderna vaccine candidate to enter clinical trials (Callaway, 2020a; GeneScript, 2020). Concise information about a variety of vaccine platforms currently under various stages of clinical development (regulatory approval for human use) is given in Table 1. Moreover, the detailed landscape of vaccines that successfully demonstrated a safety profile and immunogenicity in phase I and II studies has been given in Tables 2 and 3. A variety of platforms are being explored for the development of a vaccine. These include mRNA-based vaccines, DNA- and plasmid-based vaccines, nonreplicating viral vectors, and attenuated viral vaccines, protein subunits. Apart from that, some already existing vaccines are also being explored for likely cross-/nonspecific immunity against SARS-CoV-2 infection. These vaccines include BCG, polio, and MMR vaccines, which are also part of many national child-immunization programs in the developing countries. Among all the vaccines being developed, ten vaccines have entered phase III clinical trials and have shown promising results in terms of safety and immunogenicity (WHO, 2020b).
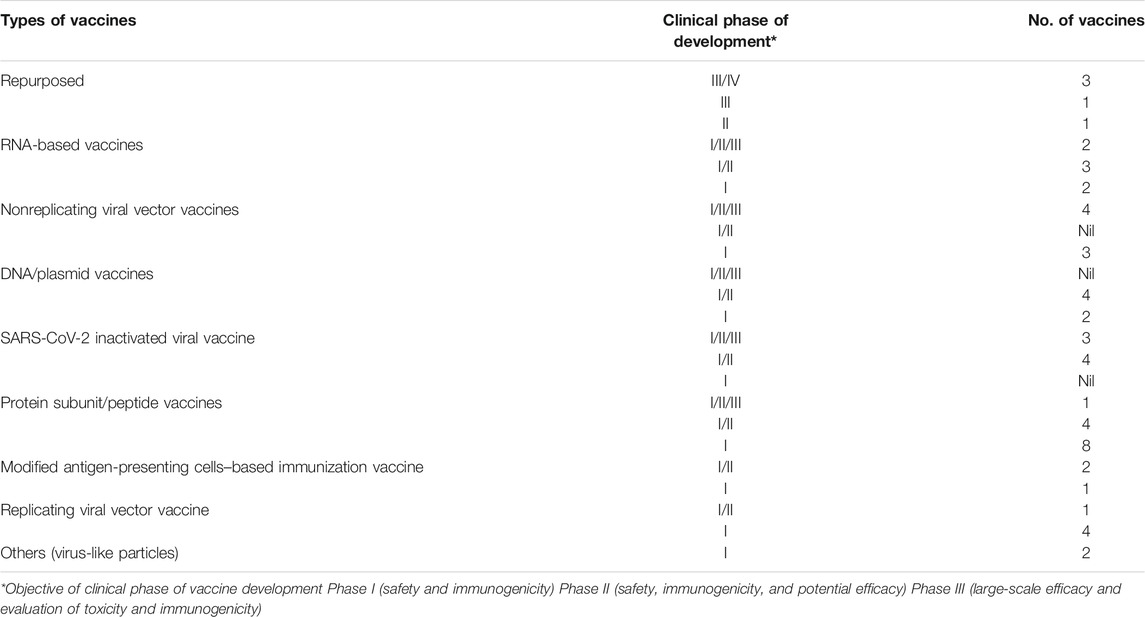
TABLE 1. Number and types of SARS-Cov-2 vaccines with respect to clinical development phase [sources: ClinicalTrials.gov, WHO COVID-19 (DRAFT landscape of COVID-19 candidate vaccines), and Biorender.com (COVID-19 vaccine tracker)].
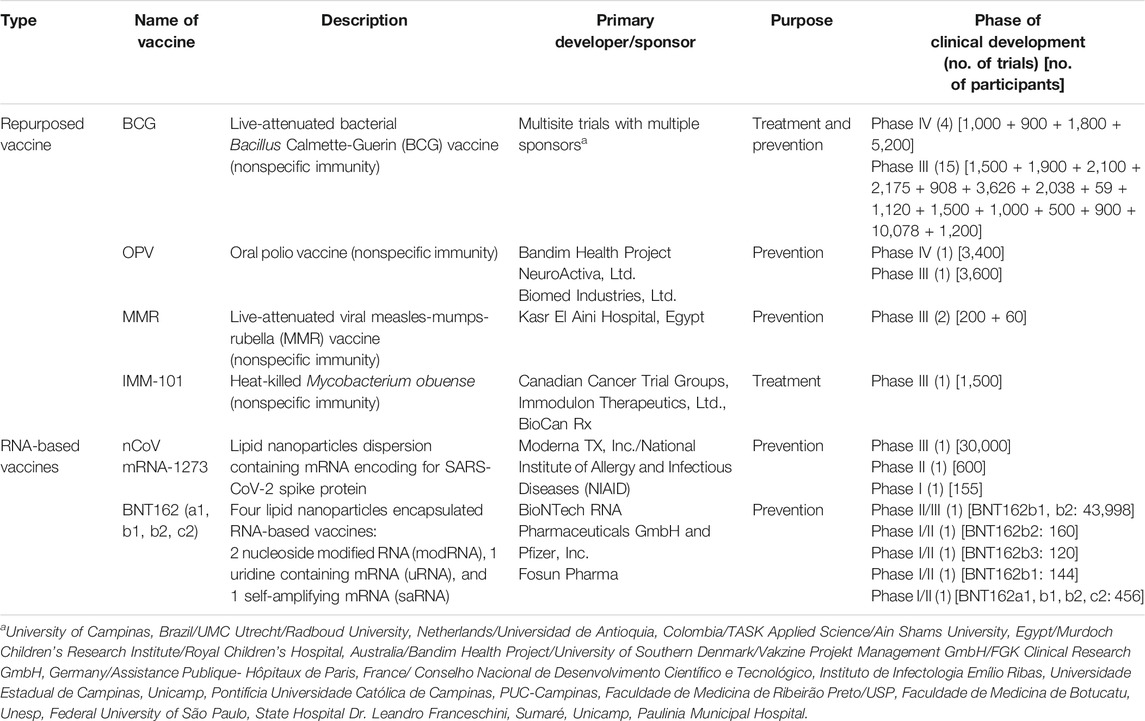
TABLE 2. Description of vaccines under the clinical phase of development for SARS-CoV-2 (repurposed and RNA vaccines) [sources: ClinicalTrials.gov, WHO COVID-19 (DRAFT landscape of COVID-19 candidate vaccines), and Biorender.com (COVID-19 vaccine tracker)].
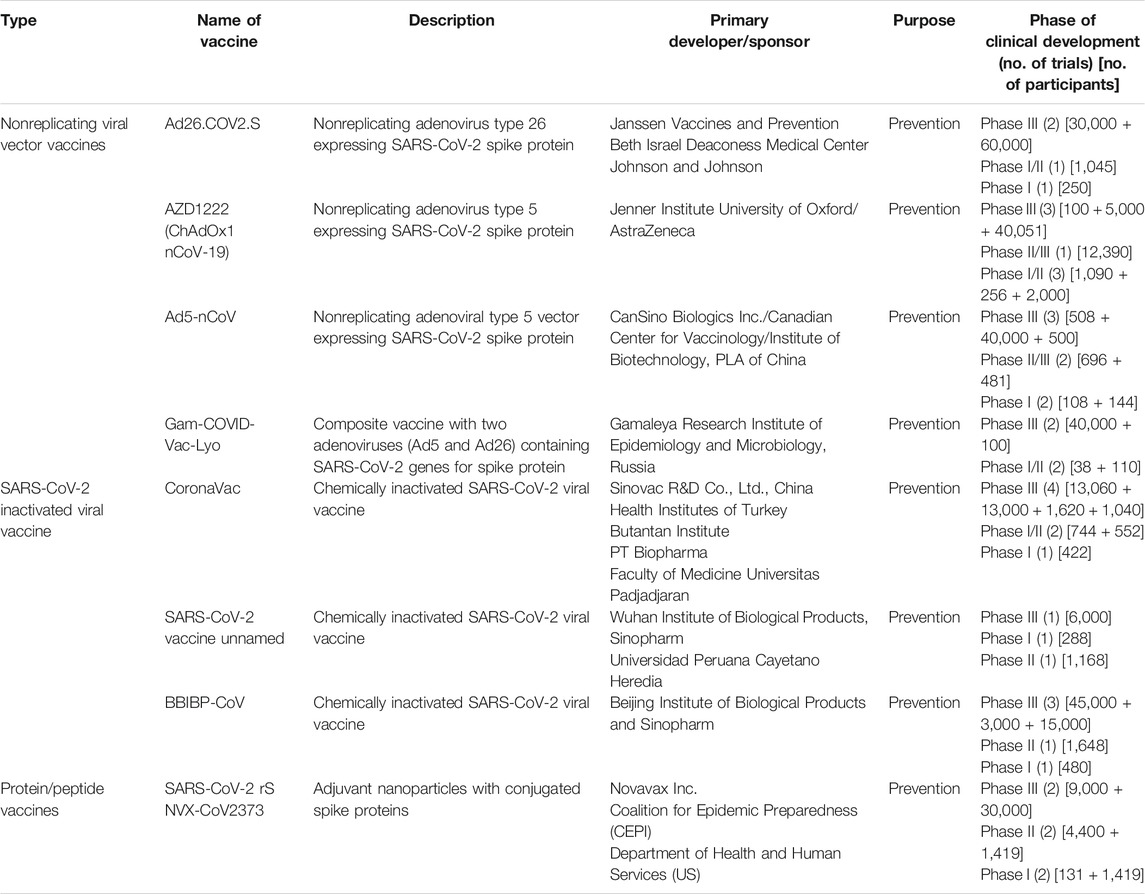
TABLE 3. Description of vaccines under clinical phase development for SARS-CoV-2 (viral vector, attenuated vaccines, and protein subunit vaccines) [sources: ClinicalTrials.gov, WHO COVID-19 (DRAFT landscape of COVID-19 candidate vaccines), and Biorender.com (COVID-19 vaccine tracker)].
Preventive Measures
As no specific treatment is available for this nCoV, it is recommended to obtain preventive measures and reduce the spread of the virus. It is advised to maintain personal hygiene, proper ventilation, a healthy lifestyle, and adequate nutritional consumption to boost immunity and enhance self-resistance (Yoshikawa and High, 2001; Simpson et al., 2015). Handwashing with soap and water or alcohol-based sanitizer after contact with any contaminated surface is directed (World Health Organization, 2020b). Protective equipment such as face mask, gown, gloves, face shield or goggles, and N95 respirator is suggested, especially in hospital settings (World Health Organization, 2020b). A study conducted in a hospital in Wuhan, China, on the association between the use of face masks and spread of COVID-19 has revealed that the infection rate in the departments using face masks, disinfectants, and handwashing was lower than that in the departments not using or frequently using the preventive measures (Wenjie et al., 2020). Close interaction is the cause of viral transmission (World Health Organization, 2020b). The infected people are recommended to be isolated or use airborne infection isolation (AIIR)/negative pressure isolation (NPI) room (Wax and Christian, 2020). Social distancing by reducing mass gatherings, social events, and group meetings is considered the best preventive measure (CDC, 2020, March 27).
Conclusion
COVID-19 disease is an enormous challenge to the global community. This once-in-a-century pandemic has catastrophically affected our daily lives and is an unprecedented threat to the economies of many leading nations across the globe. Besides plethoric technological advancement and awareness, the world was not prepared to face the sudden outbreak of this disease. The pace of disease progression in different regions of the world has made it imperative that quick and honest preventive measures should be implemented at macro and micro levels to limit the spread till the development and approval of effective vaccine and evidence-based management at a global scale. The virus demands respect, so keeping self-hygiene, wearing masks, sanitizing, and maintaining social distancing seem to be the only effective means of mitigating the disease. Cultural diversity among different regions should be considered in developing robust strategies and communication. Many vaccines are under various phases of clinical trials, their efficacy, safety, scale-up production, supply chain management, and cold chain maintenance are another set of challenges, especially for developing and underdeveloped nations.
Author Contributions
MSH conceptualized the theme of this review article and helped in the write-up and arranging the sequence. FA helped in the write-up, collection of data, and tables. MSI has done extensive literature study and helped in writing the epidemiology section. RY helped in writing therapeutic management and MSA helped in organizing figures and formatting work.
Conflict of Interest
The authors declare that the research was conducted in the absence of any commercial or financial relationships that could be construed as a potential conflict of interest.
References
Abbaspour Kasgari, H., Moradi, S., Shabani, A. M., Babamahmoodi, F., Davoudi Badabi, A. R., Davoudi, L., et al. (2020). Evaluation of the efficacy of sofosbuvir plus daclatasvir in combination with ribavirin for hospitalized COVID-19 patients with moderate disease compared with standard care: a single-centre, randomized controlled trial. J. Antimicrob. Chemother. 75, 3373–3378. doi:10.1093/jac/dkaa33 |
Agarwal, A., Mukherjee, A., Kumar, G., Chatterjee, P., Bhatnagar, T., and Malhotra, P. (2020). Convalescent plasma in the management of moderate COVID-19 in adults in India: open label phase II multicentre randomised controlled trial (PLACID Trial). BMJ 371, m3939. doi:10.1136/bmj.m3939 |
Ahn, M. (2020). How South Korea flattened the coronavirus curve with technology. Global Health NewsWireAvailable at: https://globalhealthnewswire.com/technology/2020/04/22/how-south-korea-flattened-the-coronavirus-curve-with-technology (Accessed June 17, 2020).
Åkerström, S., Mousavi-Jazi, M., Klingström, J., Leijon, M., Lundkvist, Å., and Mirazimi, A. (2005). Nitric oxide inhibits the replication cycle of severe acute respiratory syndrome coronavirus. J. Virol. 79, 1966–1969. doi:10.1128/JVI.79.3.1966 |
Akram, J., Azhar, S., Shahzad, M., Latif, W., and Khan, K. S. (2020). Pakistan randomized and observational trial to evaluate coronavirus treatment (PROTECT) of hydroxychloroquine, oseltamivir and azithromycin to treat newly diagnosed patients with COVID-19 infection who have no comorbidities like diabetes mellitus: a structured summary of a study protocol for a randomized controlled trial. Trials 21, 702. doi:10.1186/s13063-020-04616-4 |
Al-Tawfiq, J. A., Al-Homoud, A. H., and Memish, Z. A. (2020). Remdesivir as a possible therapeutic option for the COVID-19. Travel Med. Infect. Dis. 34, 101615. doi:10.1016/j.tmaid.2020.101615 |
Alavi Darazam, I., Hatami, F., Rabiei, M. M., Pourhoseingholi, M. A., Moradi, O., Shokouhi, S., et al. (2020). An investigation into the beneficial effects of high-dose interferon beta 1-a, compared to low-dose interferon beta 1-a (the base therapeutic regimen) in moderate to severe COVID-19: a structured summary of a study protocol for a randomized controlled l trial. Trials 21, 880. doi:10.1186/s13063-020-04812-2 |
Alhazzani, W., Møller, M. H., Arabi, Y. M., Loeb, M., Gong, M. N., Fan, E., et al. (2020). Surviving sepsis campaign: guidelines on the management of critically ill adults with coronavirus disease 2019 (COVID-19). Intensive Care Med. 48, 1–34. doi:10.1097/CCM.0000000000004363
Andersen, K. G., Rambaut, A., Lipkin, W. I., Holmes, E. C., and Garry, R. F. (2020). The proximal origin of SARS-CoV-2. Nat. Med. 26, 450–452. doi:10.1038/s41591-020-0820-9 |
Ang, L., Lee, H. W., Choi, J. Y., Zhang, J., and Lee, M. S. (2020). Herbal medicine and pattern identification for treating COVID-19: a rapid review of guidelines. Integr. Med. Res. 9, 100407. doi:10.1016/j.imr.2020.100407 |
Arabi, Y. M., Asiri, A. Y., Assiri, A. M., Balkhy, H. H., Al Bshabshe, A., Al Jeraisy, M., et al. (2020). Interferon beta-1b and lopinavir-ritonavir for Middle East respiratory syndrome. N. Engl. J. Med. 383, 1645–1656. doi:10.1056/NEJMoa2015294 |
Atallah, B., Mallah, S. I., and Almahmeed, W. (2020). Anticoagulation in COVID-19. Eur. Heart J. Cardiovasc. Pharmacother. 6, 260–261. doi:10.1093/ehjcvp/pvaa036 |
Bangash, M. N., Patel, J., and Parekh, D. (2020). COVID-19 and the liver: little cause for concern. Lancet Gastroenterol. Hepatol. 5, 529–530. doi:10.1016/S2468-1253(20)30084-4 |
Bannister-Tyrrell, M., Meyer, A., Faverjon, C., and Cameron, A. (2020). Preliminary evidence that higher temperatures are associated with lower incidence of COVID-19, for cases reported globally up to 29th February 2020. medRxiv medRxiv 20036731v1 [Preprint] doi:10.1101/2020.03.18.20036731
Bataille, V., Visconti, A., Murray, B., Bournot, A., Wolf, J., Ourselin, S., et al. (2020). Diagnostic value of skin manifestation of SARS-CoV-2 infection. medRxiv. [e-pub ahead of print], doi:10.1101/2020.07.10.20150656
Bauer, C. M., Zavitz, C. C., Botelho, F. M., Lambert, K. N., Brown, E. G., Mossman, K. L., et al. (2010). Treating viral exacerbations of chronic obstructive pulmonary disease: insights from a mouse model of cigarette smoke and H1N1 influenza infection. PLoS One 5, e13251. doi:10.1371/journal.pone.0013251 |
Begun, C., Heins, J., and Tyndall, M. (2020). Mallinckrodt evaluates the potential role for inhaled nitric oxide to treat COVID-19 associated lung complications, engages with scientific, governmental and regulatory agencies. Available at: https://www.biospace.com/article/releases/mallinckrodt-evaluates-the-potential-role-for-inhaled-nitric-oxide-to-treat-covid-19-associated-lung-complications-engages-with-scientific-governmental-and-regulatory-agencies/.
Beigel, J. H., Tomashek, K. M., Dodd, L. E., Mehta, A. K., Zingman, B. S., Kalil, A. C., et al. (2020). Remdesivir for the treatment of COVID-19 — preliminary report. N. Engl. J. Med. 383, 1813–1826. doi:10.1056/NEJMoa2007764 |
Bellerophon. Therapeutics (2020). Bellerophon therapeutics announces first patient treated in phase 3 clinical study of INOpulse® inhaled nitric oxide therapy for COVID-19, initiation of the COViNOX study follows the conclusion of the FDA emergency access program through which 180 patients with COVID-19 were treated in the United States. Available at: https://www.globenewswire.com/news-release/2020/07/13/2061229/0/en/Bellerophon-Therapeutics-Announces-First-Patient-Treated-in-Phase-3-Clinical-Study-of-INOpulse-Inhaled-Nitric-Oxide-Therapy-for-COVID-19.html.
Bhimraj, A., Morgan, R. L., Shumaker, A. H., Lavergne, V., Baden, L., Cheng, V. C.-C., et al. (2020). Infectious Diseases Society of America guidelines on the treatment and management of patients with COVID-19. Clin. Infect. Dis. ciaa478. doi:10.1093/cid/ciaa478 |
Blaising, J., Polyak, S. J., and Pécheur, E. I. (2014). Arbidol as a broad-spectrum antiviral: an update. Antiviral Res. 107, 84–94. doi:10.1016/j.antiviral.2014.04.006 |
Borba, M. G. S., Val, F. F. A., Sampaio, V. S., Alexandre, M. a. A., Melo, G. C., Brito, M., et al. (2020). Chloroquine diphosphate in two different dosages as adjunctive therapy of hospitalized patients with severe respiratory syndrome in the context of coronavirus (SARS-CoV-2) infection: preliminary safety results of a randomized, double-blinded, phase IIb clinical trial (CloroCOVID-19 study). medRxiv doi:10.1101/2020.04.07.20056424
Bradfute, S. B., Hurwitz, I., Yingling, A. V., Ye, C., Cheng, Q., Noonan, T. P., et al. (2020). Severe acute respiratory syndrome coronavirus 2 neutralizing antibody titers in convalescent plasma and recipients in New Mexico: an open treatment study in patients with coronavirus disease 2019. J. Infect. Dis. 222, 1620–1628. doi:10.1093/infdis/jiaa505 |
Braga, J. U., Ramos, A. N., Ferreira, A. F., Lacerda, V. M., Freire, R. M. C., and Bertoncini, B. V. (2020). Propensity for COVID-19 severe epidemic among the populations of the neighborhoods of Fortaleza, Brazil, in 2020. BMC Public Health 20, 1486. doi:10.1186/s12889-020-09558-9 |
Burki, T. (2020). COVID-19 in Latin America. Lancet Infect. Dis. 20, 547–548. doi:10.1016/S1473-3099(20)30303-0 |
Cai, Q., Yang, M., Liu, D., Chen, J., Shu, D., Xia, J., et al. (2020). Experimental treatment with favipiravir for COVID-19: an open-label control study. Engineering (Beijing) 6, 1192–1198. doi:10.1016/j.eng.2020.03.007 |
Callaway, E. (2020a). Coronavirus vaccines: five key questions as trials begin. NatureSpringerAvailable at: https://www.nature.com/articles/d41586-020-00798-8. (Accessed March 18, 2020)
Callaway, E. (2020b). “The race for coronavirus vaccines: a graphical guide,” in Nature. Berlin UK: SpringerNature.
Cao, B., Wang, Y., Wen, D., Liu, W., Wang, J., Fan, G., et al. (2020). A trial of lopinavir–ritonavir in adults hospitalized with severe COVID-19. N. Engl. J. Med. 382, 1787–1799. doi:10.1056/NEJMoa2001282 |
Cao, W., and Li, T. (2020). COVID-19: towards understanding of pathogenesis. Cell Res. 1–3. doi:10.1038/s41422-020-0327-4
Cao, X. (2020). COVID-19: immunopathology and its implications for therapy. Nat. Rev. Immunol. 20, 269–270. doi:10.1038/s41577-020-0308-3 |
CDC (2020a, March 27). Interim US guidance for risk assessment and public health management of persons with potential coronavirus disease 2019 (COVID-19) exposures: geographic risk and contacts of laboratory-confirmed cases. Available at: https://www.cdc.gov/coronavirus/2019-ncov/hcp/guidance-risk-assesment-hcp.html
CDC (2020b). Symptoms. Centers for Disease Control and Prevention (CDC) (2020a). CDC diagnostic tests for COVID-19, Available at: https://www.cdc.gov/coronavirus/2019-ncov/hcp/testing-overview.html.
Centers for Disease Control and Prevention (CDC) (2020a). Coronavirus disease 2019 (COVID-19), test for past infection. Centers for Disease Control and Prevention (CDC) (2020a). CDC diagnostic tests for COVID-19, https://www.cdc.gov/coronavirus/2019-ncov/hcp/testing-overview.html.
Centers for Disease Control and Prevention (CDC) (2020b). Update: characteristics of symptomatic women of reproductive age with laboratory-confirmed SARS-CoV-2 infection by pregnancy status — United States, January 22–October 3, 2020. MMWR Morb. Mortal. Wkly Rep. 69, 1641–1647. doi:10.15585/mmwr.mm6944e3
Chan, K. H., Peiris, J. S., Lam, S. Y., Poon, L. L., Yuen, K. Y., and Seto, W. H. (2011). The effects of temperature and relative humidity on the viability of the SARS coronavirus. Adv. Virol. 2011, 734690. doi:10.1155/2011/734690 |
Chavez, S., Long, B., Koyfman, A., and Liang, S. Y. (2020). Coronavirus disease (COVID-19): a primer for emergency physicians. Am. J. Emerg. Med. S0735-6757 (20), 30178-30179. 24 Mar. 2020, doi:10.1016/j.ajem.2020.03.036
Chen, C., Huang, J., Cheng, Z., Wu, J., Chen, S., Zhang, Y., et al. (2020). Favipiravir versus arbidol for COVID-19: a randomized clinical trial. medRxiv. [Preprint]. doi:10.1101/2020.03.17.20037432
Chen, L., Liu, P., Gao, H., Sun, B., Chao, D., Wang, F., et al. (2004). Inhalation of nitric oxide in the treatment of severe acute respiratory syndrome: a rescue trial in Beijing. Clin. Infect. Dis. 39, 1531–1535. doi:10.1086/425357 |
Chen, L., Xiong, J., Bao, L., and Shi, Y. (2020). Convalescent plasma as a potential therapy for COVID-19. Lancet Infect. Dis. 20, 398–400. doi:10.1016/S1473-3099(20)30141-9 |
Chen, W., Lim, C. E., Kang, H. J., and Liu, J. (2011). Chinese herbal medicines for the treatment of type A H1N1 influenza: a systematic review of randomized controlled trials. PLoS One 6, e28093. doi:10.1371/journal.pone.0028093 |
Chen, W., Lan, Y., Yuan, X., Deng, X., Li, Y., Cai, X., et al. (2020). Detectable 2019-nCoV viral RNA in blood is a strong indicator for the further clinical severity. Emerg. Microb. Infect. 9, 469–473. doi:10.1080/22221751.2020.1732837
Chen, Y., Liu, Q., and Guo, D. (2020). Emerging coronaviruses: genome structure, replication, and pathogenesis. J. Med. Virol. 92, 418–423. doi:10.1002/jmv.25681 |
Cheng, Y., Luo, R., Wang, K., Zhang, M., Wang, Z., Dong, L., et al. (2020). Kidney disease is associated with in-hospital death of patients with COVID-19. Kidney Int. 97, 829–838. doi:10.1016/j.kint.2020.03.005 |
Chin, A., Chu, J., Perera, M., Hui, K., Yen, H.-L., Chan, M., et al. (2020). Stability of SARS-CoV-2 in different environmental conditions. The Lancet 1, E10, doi:10.1016/S2666-5247(20)30003-3
Chris Baraniuk (2020). “Receptors for SARS-CoV-2 present in wide variety of human cells,” in The scientist exploring life. USA: Inspiring Innovation.
Clinicaltrials.Gov (2020). Evaluation of the efficacy and safety of sarilumab in hospitalized patients with COVID-19. Available at:https://clinicaltrials.gov/ct2/show/NCT04315298.
Corburn, J., Vlahov, D., Mberu, B., Riley, L., Caiaffa, W. T., Rashid, S. F., et al. 2020). Slum health: arresting COVID-19 and improving well-being in urban informal settlements. J. Urban Health, 97, 348–357. doi:10.1007/s11524-020-00438-6 |
Criado, P. R., Abdalla, B. M. Z., De Assis, I. C., Van Blarcum De Graaff Mello, C., Caputo, G. C., and Vieira, I. C. (2020). Are the cutaneous manifestations during or due to SARS-CoV-2 infection/COVID-19 frequent or not? Revision of possible pathophysiologic mechanisms. Inflamm. Res. 69, 745–756. doi:10.1007/s00011-020-01370-w |
Cyranoski, D. (2020). Profile of a killer: the complex biology powering the coronavirus pandemic. Nature 581, 22–26. doi:10.1038/d41586-020-01315-7 |
Day, M. (2020). COVID-19: ibuprofen should not be used for managing symptoms, say doctors and scientists. BMJ 368, m1086. doi:10.1136/bmj.m1086
Deng, L., Li, C., Zeng, Q., Liu, X., Li, X., Zhang, H., et al. (2020). Arbidol combined with LPV/r versus LPV/r alone against corona virus disease 2019: a retrospective cohort study. J. Infect. 81, el–e5. doi:10.1016/j.jinf.2020.03.002
Diao, B., Wang, C., Tan, Y., Chen, X., Liu, Y., Ning, L., et al. (2020). Reduction and functional exhaustion of T cells in patients with coronavirus disease 2019 (COVID-19). Frontiers Immunol. 2020;11, 827. doi:10.3389/fimmu.2020.00827
Diotallevi, F., Campanati, A., Bianchelli, T., Bobyr, I., Luchetti, M. M., Marconi, B., et al. (2020). Skin involvement in SARS-CoV-2 infection: case series. J. Med. Virol. 92, 2332–2334. doi:10.1002/jmv.26012 |
Dong, L., Hu, S., and Gao, J. (2020). Discovering drugs to treat coronavirus disease 2019 (COVID-19). Drug Discov. Ther. 14, 58–60. doi:10.5582/ddt.2020.01012 |
Enwemeka, C. S., Bumah, V. V., and Masson-Meyers, D. S. (2020). Light as a potential treatment for pandemic coronavirus infections: a perspective. J. Photochem. Photobiol. B Biol. 207, 111891. doi:10.1016/j.jphotobiol.2020.111891
Epi cell, Surveillance Cell and Health Intelligence Team (2020). Disparities in the risk and outcomes of COVID-19. Editor P. H. England (London: Public Health England).
Eslami, G., Mousaviasl, S., Radmanesh, E., Jelvay, S., Bitaraf, S., Simmons, B., et al. (2020). The impact of sofosbuvir/daclatasvir or ribavirin in patients with severe COVID-19. J. Antimicrob. Chemother. 75, 3366–3372. doi:10.1093/jac/dkaa331 |
Fallon, A., Dukelow, T., Kennelly, S. P., and O'neill, D. (2020). COVID-19 in nursing homes. QJM 113, 391–392. doi:10.1093/qjmed/hcaa136 |
Fan, Z., Chen, L., Li, J., Cheng, X., Yang, J., Tian, C., et al. (2020). Clinical features of COVID-19-related liver damage. Clin. Gastroenterol. Hepatol. 18, 1561–1566. doi:10.1016/j.cgh.2020.04.002 |
Fang, L., Karakiulakis, G., and Roth, M. (2020). Are patients with hypertension and diabetes mellitus at increased risk for COVID-19 infection? Lancet Respir. Med. 8, e21. doi:10.1016/S2213-2600(20)30116-8 |
Farias, D. L. C., Prats, J., Cavalcanti, A. B., Rosa, R. G., Machado, F. R., Berwanger, O., et al. (2020). Rationale and design of the “Tocilizumab in patients with moderate to severe COVID-19: an open-label multicentre randomized controlled” trial (TOCIBRAS). Rev. Bras. Ter Intensiva 32, 337–347. doi:10.5935/0103-507X.20200060 |
FDA (2020a).Coronavirus (COVID-19) update: Daily Roundup July 14, 2020. Available at: https://www.fda.gov/news-events/press-announcements/coronavirus-covid-19-update-daily-roundup-july-14-2020
FDA (2020b). Coronavirus (COVID-19) update: FDA authorizes first test for patient At-home sample collection. Available at: https://www.fda.gov/news-events/press-announcements/coronavirus-covid-19-update-fda-authorizes-first-test-patient-home-sample-collection#:∼:text=The%20U.S.%20Food%20and%20Drug,collection%20option%20for%20COVID%2D19.&text=Hahn%2C%20M.D.%20%E2%80%9CThe%20FDA's%20around,with%20over%20350%20test%20developers.
FDA (2020, May 1). Coronavirus (COVID-19) update: FDA issues emergency use authorization for potential COVID-19 treatment.
FDA (2020, April 24). FDA cautions against use of hydroxychloroquine or chloroquine for COVID-19 outside of the hospital setting or a clinical trial due to risk of heart rhythm problems.
FDA (2020, March 28). Request for emergency use authorization for use of chloroquine phosphate or hydroxychloroquine sulfate supplied from the strategic national stockpile for treatment of 2019 coronavirus disease
Fernandes, N. (2020). Economic effects of coronavirus outbreak (COVID-19) on the world economy. Available at SSRN: https://ssrn.com/abstract=3557504 (Accessed March 22, 2020).
Ferretti, L., Wymant, C., Kendall, M., Zhao, L., Nurtay, A., Abeler-Dörner, L., et al. (2020). Quantifying SARS-CoV-2 transmission suggests epidemic control with digital contact tracing. Science 368, eabb6936. doi:10.1126/science.abb6936 |
Fetzer, T., Witte, M., Hensel, L., Jachimowicz, J. M., Haushofer, J., Ivchenko, A., et al. (2020). Measuring worldwide COVID-19 attitudes and beliefs. NY, USA: Mimeo.
Food and Administration (2020). Recommendations for investigational COVID-19 convalescent plasma. USA
Forouzesh, M., Rahimi, A., Valizadeh, R., Dadashzadeh, N., and Mirzazadeh, A. (2020). Clinical display, diagnostics and genetic implication of novel coronavirus (COVID-19) epidemic. Eur. Rev. Med. Pharmacol. Sci. 24, 4607–4615. doi:10.26355/eurrev_202004_21047 |
Frie, K., and Gbinigie, K. (2020). Chloroquine and hydroxychloroquine: current evidence for their effectiveness in treating COVID-19. Centre for Evidence-Based Medicine, Nuffield Department of Primary Care Health Sciences University of Oxford–25. United Kingdom: Maart.
Fu, B., Xu, X., and Wei, H. (2020). Why tocilizumab could be an effective treatment for severe COVID-19? J. Transl. Med. 18, 164. doi:10.1186/s12967-020-02339-3 |
Furtado, R. H. M., Berwanger, O., Fonseca, H. A., Corrêa, T. D., Ferraz, L. R., Lapa, M. G., et al. (2020). Azithromycin in addition to standard of care versus standard of care alone in the treatment of patients admitted to the hospital with severe COVID-19 in Brazil (COALITION II): a randomised clinical trial. Lancet 396, 959–967. doi:10.1016/S0140-6736(20)31862-6 |
Gao, J., Tian, Z., and Yang, X. (2020). Breakthrough: chloroquine phosphate has shown apparent efficacy in treatment of COVID-19 associated pneumonia in clinical studies. Biosci. Trends 14, 72–73. doi:10.5582/bst.2020.01047 |
Gautret, P., Lagier, J.-C., Parola, P., Meddeb, L., Mailhe, M., Doudier, B., et al. (2020). Hydroxychloroquine and azithromycin as a treatment of COVID-19: results of an open-label non-randomized clinical trial. Int. J. Antimicrob. Agents 56, 105949. doi:10.1016/j.ijantimicag.2020.105949 |
Genescript (2020). A surprising player in the race for a SARS-CoV-2 vaccine. (Berlin UK:Nature news.).
Globenewswire (2020). FDA grants Bellerophon emergency expanded access for INOpulse® for the treatment of COVID-19 virus. USA
Gómez-Carballa, A., Bello, X., Pardo-Seco, J., Martinón-Torres, F., and Salas, A. (2020). Mapping genome variation of SARS-CoV-2 worldwide highlights the impact of COVID-19 super-spreaders. Genome Res. 30, 1434–1448. doi:10.1101/gr.266221.120 |
Gonzalez-Reiche, A. S., Hernandez, M. M., Sullivan, M. J., Ciferri, B., Alshammary, H., Obla, A., et al. (2020). Introductions and early spread of SARS-CoV-2 in the New York city area. Science 369, eabc1917. doi:10.1126/science.abc1917
Gorbalenya, A. E., Baker, S. C., Baric, R. S., De Groot, R. J., Drosten, C., Gulyaeva, A. A., et al. (2020). The species Severe acute respiratory syndrome-related coronavirus: classifying 2019-nCoV and naming it SARS-CoV-2. Nature Microbiology 5, 536–544. doi:10.1038/s41564-020-0695-z |
Gracia-Ramos, A. E. (2020). Is the ACE2 overexpression a risk factor for COVID-19 infection? Arch. Med. Res. 51, 345–346. doi:10.1016/j.arcmed.2020.03.011 |
Greenstone, M., and Nigam, V. (2020). Does social distancing matter? University of Chicago, Becker Friedman Institute for Economics. Working Paper No. 2020-26.
Gu, J., Han, B., and Wang, J. (2020). COVID-19: gastrointestinal manifestations and potential fecal-oral transmission. Gastroenterology 158, 1518–1519. doi:10.1053/j.gastro.2020.02.054 |
Gu, J., and Korteweg, C. (2007). Pathology and pathogenesis of severe acute respiratory syndrome. Am. J. Pathol. 170, 1136–1147. doi:10.2353/ajpath.2007.061088 |
Guobao, F., Lu, L., Wanzhao, C., and Fang, W. (2007). Electron beam irradiation on novel coronavirus (COVID-19): a Monte-Carlo simulation. Chin. Phys. B 29, 048703. doi:10.1088/1674-1056/ab7dac
Halfmann, P. J., Hatta, M., Chiba, S., Maemura, T., Fan, S., Takeda, M., et al. (2020). Transmission of SARS-CoV-2 in domestic cats. N. Engl. J. Med. 383, 592–594. doi:10.1056/NEJMc2013400 |
Hinks, T. S. C., Barber, V. S., Black, J., Dutton, S. J., Jabeen, M., Melhorn, J., et al. (2020). A multi-centre open-label two-arm randomised superiority clinical trial of azithromycin versus usual care in ambulatory COVID-19: study protocol for the ATOMIC2 trial. Trials 21, 718. doi:10.1186/s13063-020-04593-8 |
Hoffmann, M., Kleine-Weber, H., Schroeder, S., Krüger, N., Herrler, T., Erichsen, S., et al. (2020). SARS-CoV-2 cell entry depends on ACE2 and TMPRSS2 and is blocked by a clinically proven protease inhibitor. Cell 181, 271–280.e8.e278. doi:10.1016/j.cell.2020.02.052 |
Holshue, M. L., Debolt, C., Lindquist, S., Lofy, K. H., Wiesman, J., Bruce, H., et al. (2020). First case of 2019 novel coronavirus in the United States. N. Engl. J. Med. 382, 929–936. doi:10.1056/NEJMoa2001191 |
Huang, C., Wang, Y., Li, X., Ren, L., Zhao, J., Hu, Y., et al. (2020). Clinical features of patients infected with 2019 novel coronavirus in Wuhan, China. Lancet 395, 497–506. doi:10.1016/S0140-6736(20)30183-5 |
Huynh, T. L. D. (2020). Does culture matter social distancing under the COVID-19 pandemic? Saf. Sci. 130, 104872. doi:10.1016/j.ssci.2020.104872 |
Imai, Y., Kuba, K., Ohto-Nakanishi, T., and Penninger, J. M. (2010). Angiotensin-converting enzyme 2 (ACE2) in disease pathogenesis. Circ. J. 74, 405–410. doi:10.1253/circj.cj-10-0045 |
Irie, K., Nakagawa, A., Fujita, H., Tamura, R., Eto, M., Ikesue, H., et al. (2020). Pharmacokinetics of favipiravir in critically ill patients with COVID-19. Clin. Transl. Sci. 13, 880–885. doi:10.1111/cts.12827 |
Jamilloux, Y., Henry, T., Belot, A., Viel, S., Fauter, M., El Jammal, T., et al. (2020). Should we stimulate or suppress immune responses in COVID-19? Cytokine and anti-cytokine interventions. Autoimmun. Rev., 19, 102567. doi:10.1016/j.autrev.2020.102567 |
Jin, Y., Yang, H., Ji, W., Wu, W., Chen, S., Zhang, W., et al. (2020). Virology, epidemiology, pathogenesis, and control of COVID-19. Viruses 12, 372. doi:10.3390/v12040372
Jin, Z., Du, X., Xu, Y., Deng, Y., Liu, M., Zhao, Y., et al. (2020). Structure of Mpro from SARS-CoV-2 and discovery of its inhibitors. Nature. 582 (7811), 289–293. doi:10.1038/s41586-020-2223-y
Jordan, R. E., Adab, P., and Cheng, K. (2020). COVID-19: risk factors for severe disease and death. United Kingdom:British Medical Journal Publishing Group).
Joseph, T., and Ashkan, M. (2020). COVID-19. COVID-19 E-Book. “International Pulmonologist's consensus on COVID-19” - 2nd Edn, Available at: https://www.unah.edu.hn/dmsdocument/9674-consenso-internacional-de-neumologos-sobre-covid-19-version-ingles.
Juurlink, D. N. (2020). Safety considerations with chloroquine, hydroxychloroquine and azithromycin in the management of SARS-CoV-2 infection. CMAJ 192, E450–E453. doi:10.1503/cmaj.200528 |
Kang, S. J., and Jung, S. I. (2020). Age-related morbidity and mortality among patients with COVID-19. Infect. Chemother. 52, 154–164. doi:10.3947/ic.2020.52.2.154 |
Ketchell, M. (2020). 6 countries, 6 curves: how nations that moved fast against COVID-19 avoided disaster. The ConversationAvailable at: https://theconversation.com/6-countries-6-curves-how-nations-that-moved-fast-against-covid-19-avoided-disaster-137333 (Accessed June 16, 2020).
Khalili, J. S., Zhu, H., Mak, A., Yan, Y., and Zhu, Y. (2020). Novel coronavirus treatment with ribavirin: groundwork for evaluation concerning COVID‐19. J. Med. Virol. 92, 740–746. doi:10.1002/jmv.25798 |
Kindrachuk, J., Ork, B., Hart, B. J., Mazur, S., Holbrook, M. R., Frieman, M. B., et al. 2015). Antiviral potential of ERK/MAPK and PI3K/AKT/mTOR signaling modulation for Middle East respiratory syndrome coronavirus infection as identified by temporal kinome analysis. Antimicrob. Agents Chemother. 59, 1088–1099. doi:10.1128/AAC.03659-14 |
Kowalski, W., Walsh, T., and Petraitis, V. (2020).2020 COVID-19 coronavirus ultraviolet susceptibility. NY, USA: PurpleSun Inc
Kupferschmidt, K. (2020). Three big studies dim hopes that hydroxychloroquine can treat or prevent COVID-19. SciencemagAvailable: https://www.sciencemag.org/news/2020/06/three-big-studies-dim-hopes-hydroxychloroquine-can-treat-or-prevent-covid-19. (Accessed June 9, 2020)
Ky, B., and Mann, D. L. (2020). COVID-19 clinical trials: a primer for the cardiovascular and cardio-oncology communities. JACC: Basic Transl. Sci. 5, 501–517. doi:10.1016/j.jacbts.2020.04.003 |
Lakshmi Priyadarsini, S., and Suresh, M. (2020). Factors influencing the epidemiological characteristics of pandemic COVID 19: a TISM approach. Int. J. Healthc. Manag. 13, 89–98. doi:10.1080/20479700.2020.1755804
Lamers, M. M., Beumer, J., Van Der Vaart, J., Knoops, K., Puschhof, J., Breugem, T. I., et al. (2020). SARS-CoV-2 productively infects human gut enterocytes. Science 369, eabc1669. doi:10.1126/science.abc1669
Lawton, G. (2020). Why are men more likely to get worse symptoms and die from C 5. The New Scientist 16.
Lee, C. Y.-P., Lin, R. T. P., Renia, L., and Ng, L. F. P. (2020). Serological approaches for COVID-19: epidemiologic perspective on surveillance and control. Front. Immunol. 11, 879. doi:10.3389/fimmu.2020.00879 |
Li, G., Fan, Y., Lai, Y., Han, T., Li, Z., Zhou, P., et al. 2020). Coronavirus infections and immune responses. J. Med. Virol. 92, 424–432. doi:10.1002/jmv.25685 |
Li, G., and De Clercq, E. (2020). Therapeutic options for the 2019 novel coronavirus (2019-nCoV). Berlin United Kingdom: (Nature Publishing Group).
Li, L., Zhang, W., Hu, Y., Tong, X., Zheng, S., Yang, J., et al. (2020). Effect of convalescent plasma therapy on time to clinical improvement in patients with severe and life-threatening COVID-19: a randomized clinical trial. JAMA 324, 460–470. doi:10.1001/jama.2020.10044 |
Li, X., Geng, M., Peng, Y., Meng, L., and Lu, S. (2020). Molecular immune pathogenesis and diagnosis of COVID-19. J. Pharm. Anal. 10, 102–108. doi:10.1016/j.jpha.2020.03.001 |
Li, Z., Wu, M., Yao, J., Guo, J., Liao, X., Song, S., et al. (2020). Caution on kidney dysfunctions of COVID-19 patients. medRxiv [Preprint]. doi:10.1101/2020.02.08.20021212
Lim, J., Jeon, S., Shin, H.-Y., Kim, M. J., Seong, Y. M., Lee, W. J., et al. (2020). Case of the index patient who caused tertiary transmission of COVID-19 infection in Korea: the application of lopinavir/ritonavir for the treatment of COVID-19 infected pneumonia monitored by quantitative RT-PCR. J. Kor. Med. Sci. 35, e79. doi:10.3346/jkms.2020.35.e79
Little, P. (2020). Non-steroidal anti-inflammatory drugs and COVID-19. British Medical Journal Publishing Group).
Liu, F., Xu, A., Zhang, Y., Xuan, W., Yan, T., Pan, K., et al. (2020). Patients of COVID-19 may benefit from sustained lopinavir-combined regimen and the increase of eosinophil may predict the outcome of COVID-19 progression. Int. J. Infect. Dis. 95, 183–191. doi:10.1016/j.ijid.2020.03.013 |
Liu, J., Cao, R., Xu, M., Wang, X., Zhang, H., Hu, H., et al. (2020). Hydroxychloroquine, a less toxic derivative of chloroquine, is effective in inhibiting SARS-CoV-2 infection in vitro. Cell discovery 6, 1–4. doi:10.1038/s41421-020-0156-0
Liu, Y., Gayle, A. A., Wilder-Smith, A., and Rocklöv, J. (2020a). The reproductive number of COVID-19 is higher compared to SARS coronavirus. J. Travel Med. 27, taaa021. doi:10.1093/jtm/taaa021 |
Liu, Y., Ning, Z., Chen, Y., Guo, M., Liu, Y., Gali, N. K., et al. (2020b). Aerodynamic analysis of SARS-CoV-2 in two Wuhan hospitals. Nature 582, 557–560. doi:10.1038/s41586-020-2271-3 |
Lo, M. K., Jordan, R., Arvey, A., Sudhamsu, J., Shrivastava-Ranjan, P., Hotard, A. L., et al. (2017). GS-5734 and its parent nucleoside analog inhibit Filo-, Pneumo-, and paramyxoviruses. Sci. Rep. 7, 43395. doi:10.1038/srep43395 |
Looi, M.-K. (2020). COVID-19: is a second wave hitting Europe? BMJ 371, m4113. doi:10.1136/bmj.m4113 |
Luo, H., Tang, Q.-L., Shang, Y.-X., Liang, S.-B., Yang, M., Robinson, N., et al. (2020). Can Chinese medicine be used for prevention of corona virus disease 2019 (COVID-19)? A review of historical classics, research evidence and current prevention programs. Chin. J. Integr. Med., 1–8. doi:10.1007/s11655-020-3192-6
Lyngbakken, M. N., Berdal, J. E., Eskesen, A., Kvale, D., Olsen, I. C., Rueegg, C. S., et al. (2020). A pragmatic randomized controlled trial reports lack of efficacy of hydroxychloroquine on coronavirus disease 2019 viral kinetics. Nat. Commun. 11, 5284. doi:10.1038/s41467-020-19056-6 |
Mair-Jenkins, J., Saavedra-Campos, M., Baillie, J. K., Cleary, P., Khaw, F.-M., Lim, W. S., et al. (2015). The effectiveness of convalescent plasma and hyperimmune immunoglobulin for the treatment of severe acute respiratory infections of viral etiology: a systematic review and exploratory meta-analysis. J. Infect. Dis. 211, 80–90. doi:10.1093/infdis/jiu396 |
Malik, Y. S., Sircar, S., Bhat, S., Sharun, K., Dhama, K., Dadar, M., et al. (2020). Emerging novel coronavirus (2019-nCoV)—current scenario, evolutionary perspective based on genome analysis and recent developments. Vet. Q. 40, 68–76. doi:10.1080/01652176.2020.1727993 |
Maskin, L. P., Olarte, G. L., Palizas, F., Velo, A. E., Lurbet, M. F., Bonelli, I., et al. (2020). High dose dexamethasone treatment for acute respiratory distress syndrome secondary to COVID-19: a structured summary of a study protocol for a randomised controlled trial. Trials 21, 743. doi:10.1186/s13063-020-04646-y |
Mcintosh, K. (2020). “Coronavirus disease 2019 (COVID-19),” in UpToDate. Editors M. S. Hirsch, and A. Bloom). Available at: https://www.google.com/search?rlz=1C1SQJL_enPK914PK914&sxsrf=ALeKk03wfwq6PzABl_2TEGg3ttaHfTy7MQ:1612805854998&q=Waltham,+Massachusetts&stick=H4sIAAAAAAAAAOPgE-LSz9U3MDJLKy-rVOIAsTOqjE21tLKTrfTzi9IT8zKrEksy8_NQOFYZqYkphaWJRSWpRcWLWMXCE3NKMhJzdRR8E4uLE5MzSotTS0qKd7AyAgDpagSUYAAAAA&sa=X&ved=2ahUKEwj6ndCA6truAhWZFMAKHWrtArUQmxMoATAjegQIQRAD” Waltham, MA, USA.
Mcintosh, K., Bloom, A., and Hirsch, M. (2020). “Coronavirus disease 2019 (COVID-19): epidemiology, virology, clinical features, diagnosis, and prevention,” in UpToDate. Waltham, Mass.: UpToDate.
Mckee, M. (2020). A European roadmap out of the COVID-19 pandemic. (United Kingdom:British Medical Journal Publishing Group).
Mcmichael, T. M., Currie, D. W., Clark, S., Pogosjans, S., Kay, M., Schwartz, N. G., et al. (2020). Epidemiology of COVID-19 in a long-term care facility in King county, Washington. N. Engl. J. Med. 382, 2005–2011. doi:10.1056/NEJMoa2005412 |
Meneses Calderón, J., Figueroa Flores, M. D. R., Paniagua Coria, L., Briones Garduño, J. C., Meneses Figueroa, J., Vargas Contretas, M. J., et al. (2020). Nitazoxanide against COVID-19 in three explorative scenarios. J. Infect. Dev. Ctries 14, 982–986. doi:10.3855/jidc.13274 |
Merad, M., and Martin, J. C. (2020). Pathological inflammation in patients with COVID-19: a key role for monocytes and macrophages. Nat. Rev. Immunol. 20, 1–8. doi:10.1038/s41577-020-0331-4 |
Mishra, S. V., Gayen, A., and Haque, S. M. (2020). COVID-19 and urban vulnerability in India. Habitat International 103, 102230. doi:10.1016/j.habitatint.2020.102230 |
Molina, J. M., Delaugerre, C., Le Goff, J., Mela-Lima, B., Ponscarme, D., Goldwirt, L., et al. (2020). No evidence of rapid antiviral clearance or clinical benefit with the combination of hydroxychloroquine and azithromycin in patients with severe COVID-19 infection. Med. Maladies Infect. 50, 384. doi:10.1016/j.medmal.2020.03.006
Monti, S., Balduzzi, S., Delvino, P., Bellis, E., Quadrelli, V. S., and Montecucco, C. (2020). Clinical course of COVID-19 in a series of patients with chronic arthritis treated with immunosuppressive targeted therapies. Ann. Rheum. Dis. 79, 667. doi:10.1136/annrheumdis-2020-217424 |
Morawska, L., and Cao, J. (2020). Airborne transmission of SARS-CoV-2: the world should face the reality. Environ. Int. 139, 105730. doi:10.1016/j.envint.2020.105730 |
Mycroft-West, C., Su, D., Elli, S., Guimond, S., Miller, G., Turnbull, J., et al. (2020). The 2019 coronavirus (SARS-CoV-2) surface protein (Spike) S1 receptor binding domain undergoes conformational change upon heparin binding. bioRxiv [Preprint] doi:10.1101/2020.02.29.971093
NIAID (2020). Niaid “adaptive COVID-19 treatment trial (ACTT)”. National Institute of Allergy and Infectious Diseases.
NIH - U.S. National Library of Medicine (2020a). A trial of remdesivir in adults with mild and moderate COVID-19.
NIH - U.S. National Library of Medicine (2020b). A trial of remdesivir in adults with severe COVID-19.
NIH - U.S. National Library of Medicine (2020, April 28). Tocilizumab in the treatment of coronavirus induced disease (COVID-19) (CORON-ACT).
NIH - U.S. National Library of Medicine (2020, May). Sirolimus treatment in hospitalized patients with COVID-19 pneumonia (SCOPE).
OECD (2020). “Flattening the COVID-19 peak: containment and mitigation policies,” in OECD policy responses to coronavirus (COVID-19). Paris, France
Olivares-Gazca, J. C., Priesca-Marín, J. M., Ojeda-Laguna, M., Garces-Eisele, J., Soto-Olvera, S., Palacios-Alonso, A., et al. (2020). Infusion of convalescent plasma is associated with clinical improvement in critically ill patients with COVID-19: a pilot study. Rev. Invest. Clin. 72, 159–164. doi:10.24875/RIC.20000237 |
Pan, H., Peto, R., Karim, Q. A., Alejandria, M., Restrepo, A. M. H., Garcia, C. H., et al. (2020). Repurposed antiviral drugs for COVID-19- interim WHO SOLIDARITY trial results. medRxiv 1–17. doi:10.1056/NEJMoa2023184
Pan, Y., Zhang, D., Yang, P., Poon, L. L., and Wang, Q. (2020). Viral load of SARS-CoV-2 in clinical samples. Lancet Infect. Dis. 20, 411–412. doi:10.1016/S1473-3099(20)30113-4 |
Panda, P. K., Bandyopadhyay, A., Singh, B. C., Moirangthem, B., Chikara, G., Saha, S., et al. (2020). Safety and efficacy of antiviral combination therapy in symptomatic patients of COVID-19 infection - a randomised controlled trial (SEV-COVID Trial): a structured summary of a study protocol for a randomized controlled trial. Trials 21, 866. doi:10.1186/s13063-020-04774-5 |
Parente, D. M., and Laplante, K. L. (2017). “145 - glycopeptides,” in Infectious diseases. 4th Edn, Editors. J. Cohen, W. G. Powderly, and S. M. Opal (Oval Road, London: Elsevier), 1249–1255.e1242.
Peiris, J., Chu, C., Cheng, V., Chan, K., Hung, I., Poon, L., et al. (2003). Clinical progression and viral load in a community outbreak of coronavirus-associated SARS pneumonia: a prospective study. Lancet 361, 1767–1772. doi:10.1016/s0140-6736(03)13412-5 |
People's Daily of China (2020). The novel coronavirus can be inhibited by Chinese patent medicine Shuanghuanglian oral liquid. Beijing, China
Perrone, F., Piccirillo, M. C., Ascierto, P. A., Salvarani, C., Parrella, R., Marata, A. M., et al. (2020). Tocilizumab for patients with COVID-19 pneumonia. The single-arm TOCIVID-19 prospective trial. J. Transl. Med. 18, 405. doi:10.1186/s12967-020-02573-9 |
Peter Horby, M. L. (2020). Low-cost dexamethasone reduces death by up to one third in hospitalised patients with severe respiratory complications of COVID-19. Oxford UniversityAvailable at: http://www.ox.ac.uk/news/2020-06-16-low-cost-dexamethasone-reduces-death-one-third-hospitalised-patients-severe (Accessed June 18, 2020).
Rajoli, R. K., Pertinez, H., Arshad, U., Box, H., Tatham, L., Curley, P., et al. (2020). Dose prediction for repurposing nitazoxanide in SARS-CoV-2 treatment or chemoprophylaxis. medRxiv. [Preprint]. 2020 May 6. doi:10.1101/2020.05.01.20087130
Ramiro, S., Mostard, R. L. M., Magro-Checa, C., Van Dongen, C. M. P., Dormans, T., Buijs, J., et al. (2020). Historically controlled comparison of glucocorticoids with or without tocilizumab versus supportive care only in patients with COVID-19-associated cytokine storm syndrome: results of the CHIC study. Ann. Rheum. Dis. 79, 1143–1151. doi:10.1136/annrheumdis-2020-219479 |
Rashid, Z. Z., Othman, S. N., Samat, M. N. A., Ali, U. K., and Wong, K. K. (2020). Diagnostic performance of COVID-19 serology assays. Malays. J. Pathol. 42, 13–21.
Rhodes, A., Evans, L. E., Alhazzani, W., Levy, M. M., Antonelli, M., Ferrer, R., et al. (2017). Surviving sepsis campaign: international guidelines for management of sepsis and septic shock: 2016. Intensive Care Med. 43, 304–377. doi:10.1007/s00134-017-4683-6 |
Rocklöv, J., and Sjödin, H. (2020). High population densities catalyse the spread of COVID-19. J. Travel Med. 27, taaa038. doi:10.1093/jtm/taaa038 |
Rosa, S. G. V., and Santos, W. C. (2020). Clinical trials on drug repositioning for COVID-19 treatment. Rev. Panam. Salud Públic 44, e40. doi:10.1093/jtm/taaa038
Røsjø, H., Varpula, M., Hagve, T.-A., Karlsson, S., Ruokonen, E., Pettilä, V., et al. (2011). Circulating high sensitivity troponin T in severe sepsis and septic shock: distribution, associated factors, and relation to outcome. Intensive Care Med. 37, 77–85. doi:10.1007/s00134-010-2051-x |
Runfeng, L., Yunlong, H., Jicheng, H., Weiqi, P., Qinhai, M., Yongxia, S., et al. (2020). Lianhuaqingwen exerts anti-viral and anti-inflammatory activity against novel coronavirus (SARS-CoV-2). Pharm. Res. 156, 104761. doi:10.1016/j.phrs.2020.104761
Sallard, E., Lescure, F.-X., Yazdanpanah, Y., Mentre, F., and Peiffer-Smadja, N. (2020). Type 1 interferons as a potential treatment against COVID-19. Antiviral Res. 178, 104791. doi:10.1016/j.antiviral.2020.104791 |
Savarino, A., Di Trani, L., Donatelli, I., Cauda, R., and Cassone, A. (2006). New insights into the antiviral effects of chloroquine. Lancet Infect. Dis. 6, 67–69. doi:10.1016/S1473-3099(06)70361-9 |
Schaefer, I.-M., Padera, R. F., Solomon, I. H., Kanjilal, S., Hammer, M. M., Hornick, J. L., et al. (2020). In situ detection of SARS-CoV-2 in lungs and airways of patients with COVID-19. Mod. Pathol. 33, 2104–2114. doi:10.1038/s41379-020-0595-z |
Schett, G., Manger, B., Simon, D., and Caporali, R. (2020). COVID-19 revisiting inflammatory pathways of arthritis. Nat. Rev. Rheumatol. 16, 1–6. doi:10.1038/s41584-020-0451-z |
Schultz, K. R., and Gilman, A. L. (1997). The lysosomotropic amines, chloroquine and hydroxychloroquine: a potentially novel therapy for graft-versus-host disease. Leuk. Lymphoma 24, 201–210. doi:10.3109/10428199709039008 |
Schultz, M. J., Dunser, M. W., Dondorp, A. M., Adhikari, N. K., Iyer, S., Kwizera, A., et al. (2017). Current challenges in the management of sepsis in ICUs in resource-poor settings and suggestions for the future. Intensive Care Med. 43, 612–624. |
Scott, L. J., and Perry, C. M. (2002). Interferon-α-2b plus ribavirin. Drugs 62, 507–556. doi:10.2165/00003495-200262030-00009 |
Sekhavati, E., Jafari, F., Seyedalinaghi, S., Jamalimoghadamsiahkali, S., Sadr, S., Tabarestani, M., et al. (2020). Safety and effectiveness of azithromycin in patients with COVID-19: an open-label randomised trial. Int. J. Antimicrob. Agents 56, 106143. doi:10.1016/j.ijantimicag.2020.106143 |
Seneviratne, S. L., Abeysuriya, V., De Mel, S., De Zoysa, I., and Niloofa, R. (2020). Favipiravir in COVID-19.
Shang, W., Yang, Y., Rao, Y., and Rao, X. (2020). The outbreak of SARS-CoV-2 pneumonia calls for viral vaccines. NPJ Vaccines 5, 18. doi:10.1038/s41541-020-0170-0 |
Shen, C., Wang, Z., Zhao, F., Yang, Y., Li, J., Yuan, J., et al. (2020). Treatment of 5 critically ill patients with COVID-19 with convalescent plasma. JAMA 323, 1582–1589. doi:10.1001/jama.2020.4783 |
Shen, M., Zhou, Y., Ye, J., Al-Maskri, A. a. A., Kang, Y., Zeng, S., et al. (2020). Recent advances and perspectives of nucleic acid detection for coronavirus. J. Pharm. Anal. 10, 97–101. doi:10.1016/j.jpha.2020.02.010 |
Shereen, M. A., Khan, S., Kazmi, A., Bashir, N., and Siddique, R. (2020). COVID-19 infection: origin, transmission, and characteristics of human coronaviruses. J. Adv. Res. 24, 91–98. doi:10.1016/j.jare.2020.03.005 |
Shi, C., Wang, C., Wang, H., Yang, C., Cai, F., Zeng, F., et al. (2020). The potential of low molecular weight heparin to mitigate cytokine storm in severe COVID-19 patients: a retrospective Cohort Study. Sci. 13 (6), 1087-1095. doi:10.1111/cts.12880
Shi, J., Wen, Z., Zhong, G., Yang, H., Wang, C., Huang, B., et al. (2020). Susceptibility of ferrets, cats, dogs, and other domesticated animals to SARS–coronavirus 2. Science 368, 1016. doi:10.1126/science.abb7015 |
Simpson, R. J., Kunz, H., Agha, N., and Graff, R. (2015). “Exercise and the regulation of immune functions,” in Progress in molecular biology and translational science. (Oval Road, London:Elsevier), 355–380. |
Singh, A. K., Singh, A., Shaikh, A., Singh, R., and Misra, A. (2020). Chloroquine and hydroxychloroquine in the treatment of COVID-19 with or without diabetes: a systematic search and a narrative review with a special reference to India and other developing countries. Diabetes Metab. Syndr. 14, 241–246. doi:10.1016/j.dsx.2020.03.011 |
Sivapalan, P., Ulrik, C. S., Lappere, T. S., Eklöf, J. V., Shaker, S. B., Bødtger, U. C. S., et al. (2020). Proactive prophylaxis with azithromycin and hydroxychloroquine in hospitalized patients with COVID-19 (ProPAC-COVID): a statistical analysis plan. Trials 21, 867. doi:10.1186/s13063-020-04795-0 |
Sobotka, T., Brzozowska, Z., Muttarak, R., Zeman, K., and Di Lego, V. (2020). Age, gender and COVID-19 infections. medRxiv. [Preprint], doi:10.1101/2020.05.24.20111765
Sodhi, M., and Etminan, M. (2020). Safety of ibuprofen in patients with COVID-19: causal or confounded? Chest 158, 55–56. doi:10.1016/j.chest.2020.03.040 |
Srivatsan Padmanabhan, M. (2020). Potential dual therapeutic approach against SARS-CoV-2/COVID-19 with nitazoxanide and Hydroxychloroquine. doi:10.13140/RG.2.2.28124.74882
Srivatsan Padmanabhan, M., and Tech, K. P. M. (2020). Nitazoxanide–a potential ally in the treatment of COVID-19. Available at: https://www.researchgate.net/deref/http%3A%2F%2Fdx.doi.org%2F10.13140%2FRG.2.2.22854.83527”10.13140/RG.2.2.22854.83527
Stevens, R. D., Lazaridis, C., and Chalela, J. A. (2008). The role of mechanical ventilation in acute brain injury. Neurol. Clin. 26, 543–563. doi:10.1016/j.ncl.2008.03.014 |
Su, Z., and Wu, Y. (2020). A multiscale and comparative model for receptor binding of 2019 novel coronavirus and the implication of its life cycle in host cells. bioRxiv. [Preprint] doi:10.1101/2020.02.20.958272
Tang, N., Bai, H., Chen, X., Gong, J., Li, D., and Sun, Z. (2020a). Anticoagulant treatment is associated with decreased mortality in severe coronavirus disease 2019 patients with coagulopathy. J. Thromb. Haemostasis 18, 1094–1099. doi:10.1111/jth.14817
Tang, N., Li, D., Wang, X., and Sun, Z. (2020b). Abnormal coagulation parameters are associated with poor prognosis in patients with novel coronavirus pneumonia. J. Thromb. Haemostasis 18, 844–847. doi:10.1111/jth.14768
Tang, T., Bidon, M., Jaimes, J. A., Whittaker, G. R., and Daniel, S. (2020).Coronavirus membrane fusion mechanism offers as a potential target for antiviral development. Antiviral Res. 178, 104792. doi:10.1016/j.antiviral.2020.10479 |
Tang, X., Wu, C., Li, X., Song, Y., Yao, X., Wu, X., et al. (2020). On the origin and continuing evolution of SARS-CoV-2. Nat. Sci. Rev. 7, 1012–1023. doi:10.1093/nsr/nwaa036
Tay, M. Z., Poh, C. M., Rénia, L., Macary, P. A., and Ng, L. F. P. (2020b). The trinity of COVID-19: immunity, inflammation and intervention. Nat. Rev. Immunol. 20, 363–374. doi:10.1038/s41577-020-0311-8 |
Tay, M. Z., Poh, C. M., Rénia, L., Macary, P. A., and Ng, L. F. (2020a). The trinity of COVID-19: immunity, inflammation and intervention. Nat. Rev. Immunol. 20, 1–12. doi:10.1038/s41577-020-0311-8
Tomazini, B. M., Maia, I. S., Bueno, F. R., Silva, M., Baldassare, F. P., Costa, E. L. V., et al. (2020). COVID-19-associated ARDS treated with DEXamethasone (CoDEX): study design and rationale for a randomized trial. Rev Bras Ter Intensiva 32, 354–362. doi:10.5935/0103-507X.20200063 |
Udugama, B., Kadhiresan, P., Kozlowski, H. N., Malekjahani, A., Osborne, M., Li, V. Y., et al. (2020). Diagnosing COVID-19: the disease and tools for detection. ACS Nano. 14, 3822–3835. doi:10.1021/acsnano.0c02624 |
UN-DGC (2020). United nations working to flatten COVID-19 curve in southeast Asia. Department of Global Communications. (Geneva, Switzerland: United Nations).
Vadivu, T. S., and Annamuthu, P. (2014). An awareness and perception of COVID-19 among general public–A cross sectional analysis. Int. J. Mod. Trends Sci. Technol. 6, 49–5.
Wadman, M., Couzin-Frankel, J., Kaiser, J., and Matacic, C. (2020). A rampage through the body. (Washington, D.C., United States American Association for the Advancement of Science). Available at: https://www.google.com/search?rlz=1C1SQJL_enPK914PK914&sxsrf=ALeKk03QpRmQenKj2QfQsim3DPZgL2rfRw:1612806475389&q=Washington,+D.C.&stick=H4sIAAAAAAAAAOPgE-LUz9U3MMy2MC1W4gAxizLMsrW0spOt9POL0hPzMqsSSzLz81A4VhmpiSmFpYlFJalFxYtYBcITizMy89JL8vN0FFz0nPV2sDICAHSiv9NZAAAA&sa=X&ved=2ahUKEwjB5Lmo7NruAhWObsAKHQ9zAkYQmxMoATAjegQITBAD.
Wan, Y., Shang, J., Graham, R., Baric, R. S., and Li, F. (2020). Receptor recognition by the novel coronavirus from Wuhan: an analysis based on decade-long structural studies of SARS coronavirus. J. Virol. 94, e00127-20. doi:10.1128/JVI.00127-20 |
Wang, B., Van Oekelen, O., Mouhieddine, T., Del Valle, D. M., Richter, J., Cho, H. J., et al. (2020). A tertiary center experience of multiple myeloma patients with COVID-19: lessons learned and the path forward. medRxiv.
Wang, C.-H., Chung, F.-T., Lin, S.-M., Huang, S.-Y., Chou, C.-L., Lee, K.-Y., et al. (2014). Adjuvant treatment with a mammalian target of rapamycin inhibitor, sirolimus, and steroids improves outcomes in patients with severe H1N1 pneumonia and acute respiratory failure. Crit. Care Med. 42, 313–321. doi:10.1097/CCM.0b013e3182a2727d |
Wang, D., Hu, B., Hu, C., Zhu, F., Liu, X., Zhang, J., et al. (2020). Clinical characteristics of 138 hospitalized patients with 2019 novel coronavirus–infected pneumonia in Wuhan, China. JAMA 323, 1061–1069. doi:10.1001/jama.2020.1585 |
Wang, H., Li, T., Barbarino, P., Gauthier, S., Brodaty, H., Molinuevo, J. L., et al. (2020a). Dementia care during COVID-19. Lancet 395, 1190–1191. doi:10.1016/S0140-6736(20)30755-8 |
Wang, H., Li, X., Li, T., Zhang, S., Wang, L., Wu, X., et al. (2020b). The genetic sequence, origin, and diagnosis of SARS-CoV-2. Eur. J. Clin. Microbiol. Infect. Dis., 1, 1–7. doi:10.1007/s10096-020-03899-4
Wang, L.-S., Wang, Y.-R., Ye, D.-W., and Liu, Q.-Q. (2020). A review of the 2019 Novel Coronavirus (COVID-19) based on current evidence. Int. J. Antimicrob. Agents 56, 105948. doi:10.1016/j.ijantimicag.2020.106137 |
Wang, M., Cao, R., Zhang, L., Yang, X., Liu, J., Xu, M., et al. (2020). Remdesivir and chloroquine effectively inhibit the recently emerged novel coronavirus (2019-nCoV) in vitro. Cell Res. 30, 269–271. doi:10.1038/s41422-020-0282-0 |
Wang, W., Xu, Y., Gao, R., Lu, R., Han, K., Wu, G., et al. (2020). Detection of SARS-CoV-2 in different types of clinical specimens. JAMA 323, 1843–1844. doi:10.1001/jama.2020.3786 |
Wang, Y., Jiang, W., He, Q., Wang, C., Wang, B., Zhou, P., et al. (2020). Early, low-dose and short-term application of corticosteroid treatment in patients with severe COVID-19 pneumonia: single-center experience from Wuhan, China. medRxiv. [Preprint]. doi:10.1101/2020.03.06.20032342
Warren, T. K., Jordan, R., Lo, M. K., Ray, A. S., Mackman, R. L., Soloveva, V., et al. (2016). Therapeutic efficacy of the small molecule GS-5734 against Ebola virus in rhesus monkeys. Nature 531, 381–385. doi:10.1038/nature17180 |
Wax, R. S., and Christian, M. D. (2020). Practical recommendations for critical care and anesthesiology teams caring for novel coronavirus (2019-nCoV) patients. Can. J. Anaesth. 67, 568–576. doi:10.1007/s12630-020-01591-x |
Wenjie, T., Xiang, Z., Xuejun, M., Wenling, W., Peihua, N., Wenbo, X., et al. (2020). A novel coronavirus genome identified in a cluster of pneumonia cases — Wuhan, China 2019−2020. China CDC Weekly 2, 61–62. doi:10.1056/NEJMoa2001017
WHO (2020a). Coronavirus disease (COVID-19) weekly epidemiological and operational update-report. Geneva, Switzerland: Department of Communications, World Health Orgnaizations.
WHO (2020c). Modes of transmission of virus causing COVID-19: implications for IPC precaution recommendations. Geneva, Switzerland: Department of Communications, World Health Organization.
WHO (2020d). Naming the coronavirus disease (COVID-19) and the virus that causes it. Geneva, Switzerland: Department of Communications, World Health Organization.
WHO (2020, April 11). WHO R&D Blueprint COVID-19: informal consultation on the potential inclusion of Favipiravir in a clinical trial. Geneva, Switzerland: World Health Organization.
WHO (2020b). Draft landscape of COVID-19 candidate vaccines. Geneve, Switzerland: World Health Organization.
WHO (2020, March 11). Informal consultation on the potential role of chloroquine in the clinical management of COVID 19 infection. Geneve, Switzerland: World Health Organization
WHO (2020e). WHO coronavirus disease (COVID-19) dashboard. Geneva, Switzerland: Department of Communications, World Health Organization.
World Health Organization (2020a). Clinical management of severe acute respiratory infection when novel coronavirus (nCoV) infection is suspected: interim guidance.
World Health Organization (2020b). Rational use of personal protective equipment (PPE) for coronavirus disease (COVID-19): interim guidance, 19 March 2020. World Health Organization).
Wu, A., Peng, Y., Huang, B., Ding, X., Wang, X., Niu, P., et al. (2020). Genome composition and divergence of the novel coronavirus (2019-nCoV) originating in China. Cell Host Microbe 27, 325–328. doi:10.1016/j.chom.2020.02.001 |
Wu, C., Chen, X., Cai, Y., Zhou, X., Xu, S., Huang, H., et al. (2020). Risk factors associated with acute respiratory distress syndrome and death in patients with coronavirus disease 2019 pneumonia in Wuhan, China. JAMA Intern. Med. 180, 934–943. doi:10.1001/jamainternmed.2020.0994 |
Wu, P., Duan, F., Luo, C., Liu, Q., Qu, X., Liang, L., et al. (2020). Characteristics of ocular findings of patients with coronavirus disease 2019 (COVID-19) in Hubei Province, China. JAMA Ophthalmol. 138, 575–578. doi:10.1001/jamaophthalmol.2020.1291 |
Wu, R., Wang, L., Kuo, H.-C. D., Shannar, A., Peter, R., Chou, P. J., et al. (2020). An update on current therapeutic drugs treating COVID-19. Curr. Pharmacol. Rep. 1, 1–15. doi:10.1007/s40495-020-00216-7
Wu, Y., Xu, X., Chen, Z., Duan, J., Hashimoto, K., Yang, L., et al. (2020). Nervous system involvement after infection with COVID-19 and other coronaviruses. Brain Behav. Immun. 87, 18–22. doi:10.1016/j.bbi.2020.03.031 |
Xiaoyan, L., Lundborg, C. S., Banghan, D., Bojun, C., Hong, Z., Jiqiang, L., et al. (2018). Clinical outcomes of influenza-like illness treated with Chinese herbal medicine: an observational study. J. Tradit. Chin. Med. 38, 107–116. |
Xu, D., Zhang, H., Gong, H.-Y., Chen, J.-X., Ye, J.-Q., Meng, T., et al. (2020). Identification of a potential mechanism of acute kidney injury during the COVID-19 outbreak: a study based on single-cell transcriptome analysis. Intensive Care Med 46, 1114–1116. doi:10.1007/s00134-020-06026-1 |
Xu, X., Han, M., Li, T., Sun, W., Wang, D., Fu, B., et al. (2020). Effective treatment of severe COVID-19 patients with tocilizumab. Proc. Natl. Acad. Sci. U.S.A. 117, 202005615. doi:10.1073/pnas.2005615117
Xu, Y., Li, X., Zhu, B., Liang, H., Fang, C., Gong, Y., et al. (2020). Characteristics of pediatric SARS-CoV-2 infection and potential evidence for persistent fecal viral shedding. Nat. Med. 26, 502–505. doi:10.1038/s41591-020-0817-4 |
Xu, Z., Shi, L., Wang, Y., Zhang, J., Huang, L., Zhang, C., et al. (2020). Pathological findings of COVID-19 associated with acute respiratory distress syndrome. Lancet Respir. Med. 8, 420–422. doi:10.1016/S2213-2600(20)30076-X |
Yan, Y., Chang, L., and Wang, L. (2020). Laboratory testing of SARS‐CoV, MERS‐CoV, and SARS‐CoV‐2 (2019‐nCoV): current status, challenges, and countermeasures. Rev. Med. Virol. 30, e2106. doi:10.1002/rmv.2106 |
Yang, W., Kandula, S., Huynh, M., Greene, S. K., Van Wye, G., Li, W., et al. (2020). Estimating the infection fatality risk of COVID-19 in New York city during the spring 2020 pandemic wave. The Lancet. 21, 203-212. doi:10.1016/S1473-3099(20)30769-6
Yang, Y., Peng, F., Wang, R., Yange, M., Guan, K., Jiang, T., et al. (2020). The deadly coronaviruses: the 2003 SARS pandemic and the 2020 novel coronavirus epidemic in China. J. Autoimmun. 109, 3. doi:10.1016/j.jaut.2020.102434
Yoshikawa, T. T., and High, K. P. (2001). Nutritional strategies to boost immunity and prevent infection in elderly individuals. Clin. Infect. Dis. 33, 1892–1900. doi:10.1086/324509 |
Zhang, B., Zhou, X., Qiu, Y., Feng, F., Feng, J., Jia, Y., et al. (2020). Clinical characteristics of 82 death cases with COVID-19. PloS one. 15 (7), e0235458. doi:10.1371/journal.pone.0235458
Zhang, C., Shi, L., and Wang, F.-S. (2020). Liver injury in COVID-19: management and challenges. Lancet Gastroenterol. Hepatolo. 5, 428–430. doi:10.1016/S2468-1253(20)30057-1
Zhang, J., Ma, X., Yu, F., Liu, J., Zou, F., Pan, T., et al. (2020). Teicoplanin potently blocks the cell entry of 2019-nCoV. bioRxiv. [Preprint]. doi:10.1101/2020.02.05.935387
Zhang, J. S., Chen, J. T., Liu, Y. X., Zhang, Z. S., Gao, H., Liu, Y., et al. (2005). A serological survey on neutralizing antibody titer of SARS convalescent sera. J. Med. Virol. 77, 147–150. doi:10.1002/jmv.20431 |
Zhao, X., Liu, B., Yu, Y., Wang, X., Du, Y., Gu, J., et al. (2020). The characteristics and clinical value of chest CT images of novel coronavirus pneumonia. Clin. Radiol. 75, 335–340. doi:10.1016/j.crad.2020.03.002 |
Zheng, Y.-Y., Ma, Y.-T., Zhang, J.-Y., and Xie, X. (2020). COVID-19 and the cardiovascular system. Nat. Rev. Cardiol. 17, 259–260. doi:10.1038/s41569-020-0360-5 |
Zhou, F., Yu, T., Du, R., Fan, G., Liu, Y., Liu, Z., et al. (2020). Clinical course and risk factors for mortality of adult inpatients with COVID-19 in Wuhan, China: a retrospective cohort study. Lancet 395, 1054–1062. doi:10.1016/S0140-6736(20)30566-3 |
Zhou, P., Yang, X.-L., Wang, X.-G., Hu, B., Zhang, L., Zhang, W., et al. (2020). A pneumonia outbreak associated with a new coronavirus of probable bat origin. Nature 579, 270–273. doi:10.1038/s41586-020-2012-7 |
Zhou, Y., Hou, Y., Shen, J., Huang, Y., Martin, W., and Cheng, F. (2020). Network-based drug repurposing for novel coronavirus 2019-nCoV/SARS-CoV-2. Cell Discov. 6, 1–18. doi:10.1038/s41421-020-0153-3
Zhu, N., Zhang, D., Wang, W., Li, X., Yang, B., Song, J., et al. (2020). A novel coronavirus from patients with pneumonia in China, 2019. N. Engl. J. Med. 382, 727–733. doi:10.1056/NEJMoa2001017 |
Keywords: coronavirus, SARS-CoV-2, COVID-19, remdesivir, dexamethasone, hydroxychloroquine, azithromycin, vaccines
Citation: Shoaib MH, Ahmed FR, Sikandar M, Yousuf RI and Saleem MT (2021) A Journey From SARS-CoV-2 to COVID-19 and Beyond: A Comprehensive Insight of Epidemiology, Diagnosis, Pathogenesis, and Overview of the Progress into Its Therapeutic Management. Front. Pharmacol. 12:576448. doi: 10.3389/fphar.2021.576448
Received: 26 June 2020; Accepted: 11 January 2021;
Published: 26 February 2021.
Edited by:
Rafael Maldonado, Pompeu Fabra University, SpainReviewed by:
Marc Henri De Longueville, UCB Pharma, BelgiumFlorentina Ligia Furtunescu, Carol Davila University of Medicine and Pharmacy, Romania
Copyright © 2021 Shoaib, Ahmed, Sikandar, Yousuf and Saleem. This is an open-access article distributed under the terms of the Creative Commons Attribution License (CC BY). The use, distribution or reproduction in other forums is permitted, provided the original author(s) and the copyright owner(s) are credited and that the original publication in this journal is cited, in accordance with accepted academic practice. No use, distribution or reproduction is permitted which does not comply with these terms.
*Correspondence: Muhammad Harris Shoaib, aGFycmlzc2hvYWliMjAwMEB5YWhvby5jb20=