- 1Phytochemistry Research Center, Shahid Beheshti University of Medical Sciences, Tehran, Iran
- 2Facultad de Medicina, Universidad del Azuay, Cuenca, Ecuador
- 3Department of Life Sciences, Presidency University, Kolkata, India
- 4Department of Natural Products Drugs Discovery, Dr. Koirala Research Institute for Biotechnology and Biodiversity, Kathmandu, Nepal
- 5Department of Botany, Lahore College for Women University, Lahore, Pakistan
- 6Laboratory of Histology, Embryology and Cytogenetic, Faculty of Medicine and Pharmacy, Mohammed V University in Rabat, Rabat, Morocco
- 7Medical Ethics and Law Research Center, Shahid Beheshti University of Medical Sciences, Tehran, Iran
- 8Institute of Botany, Plant Physiology and Genetic Resources, Ilia State University, Tbilisi, Georgia
- 9Department of Food Science, Faculty of Food Engineering (FEA), University of Campinas (UNICAMP), Campinas, Brazil
- 10Laboratory of Human Pathology Biology, Faculty of Sciences, Genomic Center of Human Pathology, Faculty of Medicine and Pharmacy, Mohammed V University of Rabat, Rabat, Morocco
- 11Department of Agricultural and Environmental Sciences, Milan State University, Milan, Italy
- 12Department of Biomedical, Surgical and Dental Sciences, Milan State University, Milan, Italy
- 13Department of Nutrition and Dietetics, Faculty of Pharmacy, University of Concepcion, Concepcion, Chile
- 14Universidad de Concepción, Unidad de Desarrollo Tecnológico, UDT, Concepcion, Chile
- 15Food Safety Research Center (salt), Semnan University of Medical Sciences, Semnan, Iran
- 16Department of Toxicology, University of Medicine and Pharmacy of Craiova, Craiova, Romania
- 17Department of Clinical Pharmacy, University of Medicine and Pharmacy of Craiova, Craiova, Romania
- 18Department of Pharmacy, Faculty of Health Sciences, Universidad San Jorge, Zaragoza, Spain
- 19Instituto Agroalimentario de Aragón-IA2 (CITA-Universidad de Zaragoza), Zaragoza, Spain
- 20Department of Physiology, "Carol Davila" University of Medicine and Pharmacy, Bucharest, Romania
- 21Department of Dermatology, "Prof. N.C. Paulescu" National Institute of Diabetes, Nutrition and Metabolic Diseases, Bucharest, Romania
The genus Cinnamomum includes a number of plant species largely used as food, food additives and spices for a long time. Different traditional healing systems have used these plants as herbal remedies to cure diverse ailments. The aim of this comprehensive and updated review is to summarize the biodiversity of the genus Cinnamomum, its bioactive compounds, the mechanisms that underlie the pharmacological activities and molecular targets and toxicological safety. All the data in this review have been collected from databases and recent scientific literature including Web of Science, PubMed, ScienceDirect etc. The results showed that the bioactive compounds of Cinnamomum species possess antimicrobial, antidiabetic, antioxidant, anti-inflammatory, anticancer and neuroprotective effects. The preclinical (in vitro/in vivo) studies provided the possible molecular mechanisms of these action. As a novelty, recent clinical studies and toxicological data described in this paper support and confirm the pharmacological importance of the genus Cinnamomum. In conclusion, the obtained results from preclinical studies and clinical trials, as well as reduced side effects provide insights into future research of new drugs based on extracts and bioactive compounds from Cinnamomum plants.
Introduction
The Cinnamomum plants have been studied for its phyto-constituents and pharmacological properties as well as traditional medicinal significance. Cinnamomum verum, known as the “true cinnamon tree” and “Ceylon cinnamon tree” is an evergreen small, tree that belongs to the Lauraceae family. Along with other cinnamon species, such as Cinnamomum cassia, Cinnamomum verum etc., the tree bark is used to obtain cinnamon (Ribeiro-Santos et al., 2017). The ancient botanical name of this tree – Cinnamomum zeylanicum-derives from Ceylon the old name for Sri Lanka (Ribeiro-Santos et al., 2017). Cinnamomum cassia, also called “Chinese cinnamon,” is an evergreen tree, native to South China; Chinese cinnamon being produced mainly in the southern regions and is also widely grown in the other areas of the South and East Asia (Bedigian, 2005). People used cinnamon obtained from a variety of Cinnamomum plants as a spice from ancient times. It is mentioned in the texts written in Sanskrit and in the Bible, as well as in the works of Herodotus and Pliny (Lu et al., 2011). In Egypt, Cinnamomum zeylanicum was used in the embalming process. It was also added to foods for preservation. Both in India and Europe, Cinnamomum species have been traditionally used in the treatment of respiratory viruses, especially combined with ginger (Zingiber officinale). Ginger stimulates blood circulation in the extremities (toes, fingers), and Cinnamomum plants are an alternative natural medicine for reducing muscle pain and other signs and symptoms of colds and flu. Other traditional uses include urinary tract infections, relieve the abdominal discomfort, and improves digestion, antidiabetic, analgesic, and neuroprotective effects (Ravindran et al., 2003).
All types of cinnamon contain the active ingredient cinnamaldehyde, which accounts for between 65 and 80% of the essential natural oil. Cinnamon is used in case of dyspepsia, flatulence, nausea, intestinal colic, slow digestion, diarrhea, and digestive atony. This antispastic effect is attributed to the natural chemical compound catechin that contributes to the reduction of nausea and vomiting. Also, its volatile oil can help better food processing by breaking down fat during digestion. The studies showed that cinnamon helps diabetic patients to metabolize sugar more easily. In the case of people with type II diabetes, the pancreas produces insulin, but their body cannot use it effectively for decreasing blood sugar concentration (Chen et al., 2012). The researchers have found in recent studies that cinnamon improves insulin’s ability to metabolize glucose, helping to control blood sugar levels. It contains the antioxidant glutathione and a type of flavonoid called methyl hydroxychalcone polymer (MHCP) (Qin et al., 2010). The potential antidiabetic mechanism of cinnamon is associated with increasing the receptivity of adipose cells to the hormone insulin that regulates the metabolism of glucose and controls the level of sugar in the blood.
Cinnamon helps reduce pain due to its action of inhibiting prostaglandin. Cinnamaldehyde acts against the coagulation of platelets in the blood, which can hinder blood flow. It inhibits arachidonic acid’s release (a trigger for the inflammatory response) from cell membranes (Vallianou et al., 2019). Therefore, cinnamon is useful for the pharmacotherapy of inflammatory diseases, such as rheumatoid arthritis (Rogoveanu et al., 2018; Shishehbor et al., 2018). Recent studies showed that the scent and aroma of cinnamon act as cognitive stimuli, which could improve memory, visual-motor capacity and virtual memory (Nussbaum et al., 2017), due to its compound cinnamic aldehyde. Experimental researches on rodents receiving cinnamic aldehyde have reported improvements in stress-induced depressive behaviors. Cinnamic aldehyde is administered orally in the treatment of behavioral and mental disorders. Recent findings in this regard may also be helpful in treating depression (Panickar et al., 2009).
Starting from the ethnopharmacological premises of the therapeutic beneficial effects of Cinnamomum genus, in this manuscript were highlighted, based on scientific evidence the potential current pharmacological mechanisms and human clinical studies for the benefits of human health. Therefore, this current work reviews the comprehensive and current knowledge on the phyto-constituents, potential mechanisms of the main pharmacological activities evidenced by preclinical studies (in vivo, in vitro), recent clinical trials and toxicological data regarding safety of Cinnamomum plants.
Review Methodology
Scientific search engines Medline, PubMed, ScienceDirect and Scopus were searched to retrieve literature and cross-references using key words: “Cinnamomum,” “phytochemistry,” “pharmacology”; “mechanisms of action”; “toxicology.” We included literature in relation to the bioactive compounds, pharmacological activities and underlying mechanism of action, clinical studies, toxicological and safety considerations of different Cinnamomum species. Plants’ taxonomy was validated using The Plant List (http://www.theplantlist.org/), and chemical formulas were validated by consulting the PubChem chemical base data (http://pubchem.ncbi.nlm.nih.gov/search/#collection=compounds). Inclusion criteria: in vitro/in vivo pharmacological studies using cell lines and laboratory animals, studies involving extracts of the genus Cinnamomum, studies with obvious mechanisms of action. Exclusion criteria: studies that included homeopathic preparations and other associated nutritional supplements, studies without explaining the mechanism of action.
Phytochemistry of Cinnamomum Genus
Chemical Composition
The chemical composition of cinnamon EOs (essential oils) varies depending on several factors that include the part of the plant used, growing season, age of trees, location, and extraction methods (Kaul et al., 2003; Barceloux, 2009; Wang et al., 2009; Chakraborty et al., 2015). Cinnamaldehyde and its analogs, butanolides, diterpenoids, lignans and several other compounds, are present in this genus. From the genus Cinnamomum, a total of 127 chemical compounds have been identified (Zhao and Ma, 2016).
Cinnamon presents a diversity of resinous compounds, including cinnamaldehyde, cinnamates, cinnamic acid and natural EOs (Senanayake et al., 1978) (Figure 1; Table 1). Over time, cinnamaldehyde changes color, absorbs oxygen and thus explains the appearance of the perfume and spicy taste (Singh et al., 2007). EOs contain a great variety of volatile natural compounds, such as trans-cinnamaldehyde, eugenol, cinnamyl acetate, L-borneol, ß-caryophyllene, caryophyllene oxide, L-bornyl acetate, a-thujene, a-terpineol, a-cubebene, terpinolene and E-nerolidol (Jantan and Goh, 1992; Jantan et al., 2003; Jantan et al., 2005; Chua et al., 2008; Abdelwahab et al., 2010; Tung et al., 2010; Geng et al., 2011). Spathulenol was reported as the major compound in leaf oil of Cinnamomum altissimum Kosterm (Jantan et al., 2003).
According to (Wang et al., 2009), eugenol (80%) is the main volatile compound in the EO of Cinnamomum verum J. Presl (synonym: Cinnamomum zeylanicum Blume) instead of trans-cinnamaldehyde (16.25%) and the other constituents such as: alcohols, aldehydes, ketones, alkanes, sulfides, and ethers. The chemical composition in the bark and leaf EOs of C. verum consists of high levels of eugenol (90.2%) and cinnamaldehyde (44.2%). The chemical constituents of C. verum bark EO include three major compounds and six minor chemical derivatives (Yang et al., 2012). Cinnamaldehyde (59%), benzaldehyde (12%) and eugenol (5%) are the major compounds, while the six minor constituents are α-phellandrene (1.1%), linalool (1.1%), benzoic acid (0.8%), β-caryophyllene (0.7%), linalyl acetate (0.6%) and benzyl cinnamate (0.6%). The most important compounds identified in the leaf oil of C. verum grown are eugenol (75%), linalool (8%) and piperitone (2.5%) (Raina et al., 2001).
Chemical constituents in the leaf EO of Cinnamomum burmanni (Nees & T. Nees) Blume are trans-cinnamaldehyde (60%), eugenol (18%) and coumarin (14%). Other constituents identified in the oils are alcohols, aldehydes, and ketones. The major components in the stem bark oil of Cinnamomum iners (Reinw. ex Nees & T. Nees) Blume are 1,8-cineole (41%), α-terpineol (15%) and terpinen-4-ol (14%). The other components identified are β-pinene (4.75%), γ-terpinolene (1.61%) and caryophyllene oxide (4.37%) (Wang et al., 2009).
Other minor constituents reported in cinnamon EO include: cinnamic acid, phenolic acids, oligopolymeric procyanidins, pentacyclic diterpenes, cinnzeylanol, and its acetyl derivative cinnzeylanine, mannitol, xylose, arabinose, xylanose, glucose, mucilage polysaccharides (European Scientific Cooperative on Phytotherapy, 2003). Several nonvolatile compounds have been found in cinnamon EOs such as cinncassiols, cinnzeylanol, cinnzeylanin, anhydrocinnzeylanol, anhydrocinnzeylanin, several benzyl isoquinoline alkaloids, cinnamic acid, β-sitosterol, flavanol glucosides, coumarin, protocatechuic acid, vanillic acid and syringic acid (Leela, 2008).
Cinnamomum’s Natural Compounds: Pharmacokinetics, Bioavailability, Bioactivity and Metabolism
Considering the pharmacokinetics, bioavailability and metabolism of Cinnamomum active ingredients, very little works have been performed. Many studies described the role of bioactive compounds from the plants in enhancing bioavailability of some standard drugs (Salehi et al., 2020b).
Pharmacokinetics of cinnamic acid (CA) indicated the CA was readily absorbed and then metabolized quickly into hippuric acid (HA) when a decoction of Ramulus Cinnamomi (RC) [containing CA 7.62 × 10–5 mol/kg and cinnamaldehyde (CNMA) 1.77 × 10–5 mol/kg] was administered in rats via oral route. CNMA was found to be metabolized partially in stomach and small intestine into CA and almost fully metabolized in liver into CA before being absorbed into rat blood. The results indicated that plasma CA in RC group probably came from CNMA transformation in RC (Chen et al., 2009). Cinnamon also increased pioglitazone bioavailability via CYP3A4 enzyme inhibition in rat following oral administration owing to its possible use in combination with pioglitazone against diabetes (Mamindla et al., 2017). CA significantly inhibited rosuvastatin (RSV) (a specific breast cancer resistance protein) transport into rat bile thus enhanced the plasma exposure of the same (Basu et al., 2013).
In a recent study, comparative pharmacokinetic analysis of Cinnamomum cassia twigs’ standard decoction and dispensing granules containing three phenolics such as cinnamic acid, vanillic acid and protocatechuic acid in rats revealed the AUC0–t values of the compounds by LC–MS/MS (Tao et al., 2019).
Preclinical Studies Related to Pharmacological Activities and Potential Mechanisms of Cinnamomum’s Phyto-Constituents
Various traditional uses of Cinnamomum plants motivated a series of experimental investigations of the plant’s pharmacological properties (Wang et al., 2020). Those experimental approaches tempted to validate the potential uses of these plants as therapeutic remedies. Studies have been reported on extracts and isolated compounds of Cinnamomum plants, investigating antibacterial, anti-diabetes, anti-inflammatory, antioxidant, antitumor, and neuroprotective properties.
Antibacterial Activity
In order to inhibit the growth and proliferation of pathogenic microorganisms, synthetic drugs have been commonly used for the treatment of microbial infections. The overuse of conventional antibacterial drugs could lead to serious side effects, including the selection of resistant bacterial strains and the development of antibiotic resistance during treatment, posing a real threat to global public health (Russell, 2002; Goyal et al., 2008; Călina et al., 2017; Ungureanu et al., 2017; Zlatian et al., 2018). Therefore, it is necessary to discover new sources of antibiotics such us natural antimicrobial compounds that could be an effective and cheaper alternative (Mbwambo et al., 2007; Salehi et al., 2019a).
The mechanisms underlying the antibacterial effects of natural derivatives of Cinnamomum plants are complex. Cinnamaldehyde, as well as eugenol, inhibited β-lactamase’s production by the bacterium and destroyed its cell wall (Helander et al., 1998; Di Pasqua et al., 2007). Phenolic compounds such as carvacrol can also cause destruction of the cell cytoplasmic membrane (Ultee et al., 1999), and terpenes interact with the bacterial membrane by modifying its permeability (Lambert et al., 2001) and increasing the penetration of antibacterial agents. Essential oils of Cinnamomum contain a wide range of different groups of chemical compounds, suggesting that their antibacterial activity might have several mechanisms (Skandamis and Nychas, 2001; Carson et al., 2002). (Figure 2).
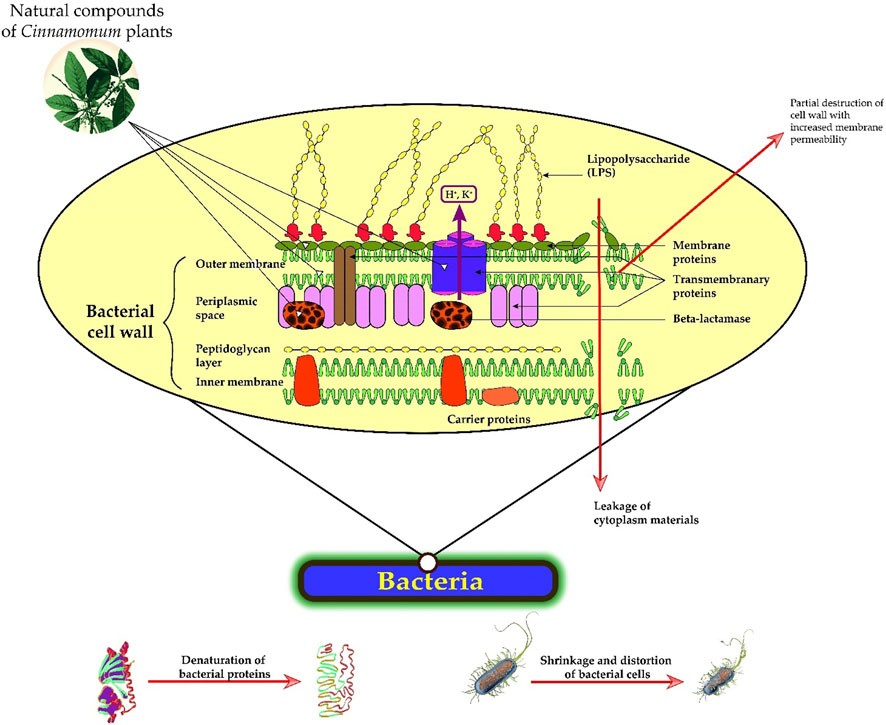
FIGURE 2. Antibacterial properties of Cinnamomum plants’ derivatives. The main potential mechanisms of antibacterial action are related to: 1) the partial degradation of the bacterial cell wall, 2) the increase of membrane permeability, 3) the leakage of cytoplasm materials, 4) the shrinkage of bacterial cells and prominent distortion, and 5) the alteration of secondary, tertiary structures and bacterial protein.
A recent study highlighted that MBC (minimum bactericidal concentration) and MIC (minimum inhibitory concentration) of the methanol extract of C. burmanni leaves were 625 and 2,500 μg/ml, against Bacillus cereus. For other bacterial strains (Staphilococcus aureus, Listeria monocytogenes, Escherichia coli, and Salmonella anatum), the values are >2,500 μg/ml for both concentrations (Shan et al., 2007).
The methanol extract of Cinnamomum tamala (Buch.-Ham.) T. Nees & Eberm. stem bark revealed MIC values of 256, 4,096, 4,096, and 2048 μg/ml against Staphilococcus aureus, Streptococcus pyogenes, B. cereus and Bacillus subtilis, respectively, with inhibition diameters ranging from 14.0 to 20.8 mm (Goyal et al., 2009). The aqueous extract of C. verum bark demonstrated antibacterial activity against Moraxella cattarhalis with MIC and MBC values of 120 and 240 mg/ml, respectively, and an inhibition zone of 11 mm (Rasheed and Thajuddin, 2011). (Table 2).
Regarding the EO of C. osmophloeum leaves, the MIC values were 200 and 500 μg/ml for the 9 bacteria tested (Chang et al., 2001), and the chemical components of this oil were similar to those of Cinnamomum cassia (L.) J. Presl (Hu et al., 1985). In addition, (Oussalah et al., 2006) evaluated the antibacterial efficacy of essential oils of two Cinnamomum species: C. cassi and C. verum against Pseudomonas putida and found MIC values of 0.05 and 0.1 % wt/vol, respectively. In another study, the MIC values for the EO of C. cassia leaves were 500 ppm for E. coli, B. cereus, Lactobacillus sakei and 750 mg/ml for Salmonella typhimurium (Turgis et al., 2012).
The antibacterial potency of the methanol extract of C. cassia was also tested against different species of E. coli by the disk diffusion method and the microdilution (Kim et al., 2004). The C. cassia bark EO was tested on L. monocytogenes and EPEC with a zone of inhibition of 22.42 and 13.72 mm, respectively, and a MIC of 0.03 and 0.06 μg/ml respectively (de Oliveira et al., 2012). Another study on the same part of C. cassia investigated the permeability of the bacterial membrane by measuring the relative electrical conductivity, which increased with the increased concentration of the EO (Huang et al., 2014).
The antimicrobial property of C. verum EO was assayed against 21 bacteria strains, using MIC methods and disc diffusion. The EO highlighted the significant antibacterial activity against the tested strains. It showed higher activity against Gram-positive (Enterococcus, Streptococcus, Staphylococcus) and Gram-negative (Pseudomonas aeruginosa) bacteria strains. These results are consistent with those of Chao et al. (2000) revealing that bark oil of cinnamon completely inhibited the growth of selected Gram-negative and Gram-positive bacteria.
In vitro antibacterial efficacy of different EOs (Al-Bayati and Mohammed, 2009; Noudeh et al., 2010; Guerra et al., 2012; Utchariyakiat et al., 2016) and methanol extracts (Hameed et al., 2016) of C. verum bark was evaluated against Gram-positive and Gram-negative bacteria using broth dilution and diffusion methods. The obtained results showed that C. verum was able to inhibit all of the tested strains. Furthermore, the main chemical constituents of C. cassia and C. verum natural oils were eugenol cinnamaldehyde (Ross, 1976; Hu et al., 1985) which could inhibit microbial growth (Lee and Ahn, 1998; Ooi et al., 2006).
EOs of Cinnamomum micranthum f. kanehirae (Hayata) S. S. Ying (leaves and twigs) were effective against Vibrio parahemolyticus, V. alginolyticus, V. vulnificus, Lactococcus garvieae, Debaryomyces hansenii, Photobacteria damsel, Streptococcus sp. and Aeromonas hydrophila, with diameters reduction from 0.1 to 1 cm (Yeh et al., 2009). The observed difference may be due to the difference of antibacterial constituents in leaves and twigs. The antibacterial screening of EO samples extracted from Cinnamomum bejolghota (Buch.-Ham). Sweet was evaluated using disc diffusion assay against zoonotic enteropathogens and showed promising antibacterial activity (Wannissorn et al., 2005). Indeed, EOs are complexes of antibacterial agents including natural aromatic and volatile compounds (Loy et al., 2001).
The volatile oils and extracts from the stem bark of Cinnamomum impressicostatum Kosterm., C. altissimum and C. porrectum (Roxb.) Kosterm showed high antibacterial activity against Gram-positive and Gram-negative bacteria, including methicillin-resistant Staphylococcus aureus (MRSA). Some tested extracts exhibited relevant activity against MRSA compared with methicillin-sensitive S. aureus (MSSA). The highest inhibition zone (21.0 mm) was measured for C. impressicostatum stem-bark water extract against MRSA with MIC and MBC values of 19.5 and 39.0 μg/ml, respectively (Buru et al., 2014). The leaf oil of Cinnamomum chemungianum M. Mohanan and A. N. Henry enhanced a moderate activity against the tested bacteria (Rameshkumar et al., 2007).
Agarwal et al. (2019) used C. tamala leaf extract to synthesize eco-friendly zinc oxide nanoparticles with important antibacterial effects against S. aureus. Authors showed that these nanoparticles induced reduction in bacterial growth, in a time and concentration-dependent pattern, due to membrane damage that leads to leakage of intracellular proteins and cellular contents. On the other hand, C. verum EOs exhibited remarkable inhibitory effects of Staphylococcus aureus multi-drug resistant (MDR). Indeed, cinnamon oil at concentrations of 3.12% strongly inhibited all the Streptococcus mutans isolated with the MIC values of 12.8–51.2 and 64–256 mg/ml, respectively (Fani and Kohanteb, 2011). (Rossi et al., 2019) showed that C. verum EOs inhibited the adhesion of Salmonella enterica which involved the reduction of polyphenol oxidase activity. (Wu et al., 2019) showed that C. camphora EOs had an important antibacterial potency against E. coli with a MIC and MBC of 200 μg/L.
(Yap et al., 2015) have studied the EO of C. verum bark against E. coli by testing the permeability of its outer membrane, and they found that the observed membrane damage was determined by denaturation and acidification of the cell membrane (Borges et al., 2013). This suggested that the EO of C. verum bark disrupts the cell membrane at lethal and sub-lethal concentrations by increasing the availability of the antibiotic in the bacterial cell (Eumkeb et al., 2012). Indeed, EOs are complexes of antibacterial agents including natural aromatic and volatile compounds (Loy et al., 2001).
Antidiabetic Activities
Diabetes mellitus is a chronic disease that affects about 7% of the world's people (Zimmet et al., 2001) and it is expected to increase by 5.5% in 2025 (King et al., 1998). Diabetes mellitus type 2 (T2DM) accounts for 85–90% of all diagnosed diabetic patients (Wild et al., 2004) with high medical and social costs. Diabetes mellitus is characterized by an altered metabolism (of carbohydrates, lipids, and lipoproteins) and chronic hyperglycemia resulting from pancreatic β-cell dysfunction (Wild et al., 2004; Qin et al., 2010), insulin production deficiency, insulin resistance in key target tissues and impaired glycemic index control (Leiter and Lewanczuk, 2005), (DeFronzo et al., 1992). These alterations cause severe complications in the functioning of the cardiovascular system, as well as hypertension and dyslipidemia that are risk factors for stroke and myocardial infarction (Luscher and Steffel, 2008). Postprandial glucose control is essential for treating diabetes mellitus and avoiding its complications (Ortiz-Andrade et al., 2007). Moreover, correct diet and sport are necessary for the prevention of T2DM (Kahn et al., 2006; Hu, 2011).
The antidiabetic mechanisms of natural compounds derived from Cinnamomum spp. are explained as follow: 1) stimulation of insulin secretion by pancreatic β-cells of the islets of Langerhans, 2) increasing the muscle and hepatic glycogen content, 3) inhibition of α-amylase and α-glucosidase activities (key enzymes of carbohydrate metabolism) (Subash Babu et al., 2007; Zhang et al., 2008) 4) stimulation of glycogen synthesis and cellular glucose uptake by membrane translocation of glucose transporter (GLUT 4); stimulation of the glucose metabolism, 5) reduction of the gluconeogenesis by its actions on the most important regulatory enzymes, 6) potentiation of insulin release and increasing insulin receptor activity (Bandara et al., 2012; Ranasinghe et al., 2012). (Figure 3) and Table 2.
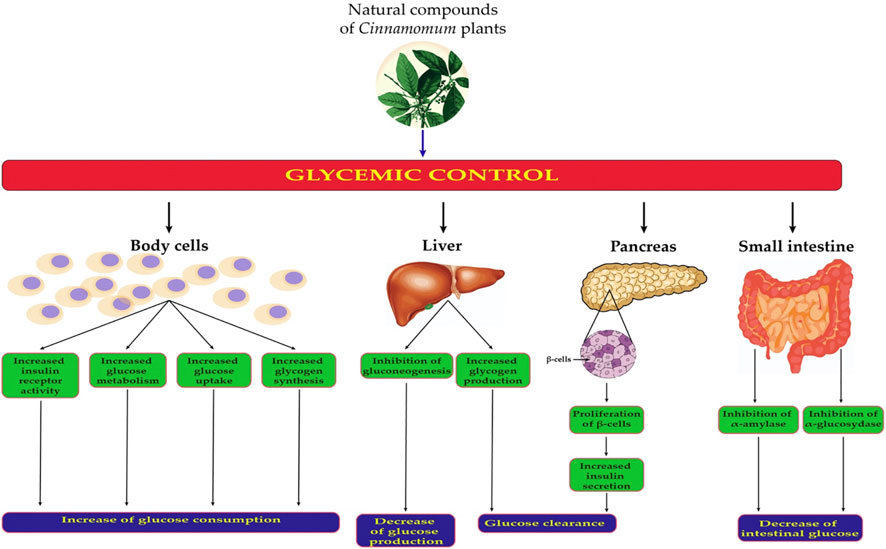
FIGURE 3. Potential mechanisms of antidiabetic effects of chemical compounds of Cinnamomum plants. Body cells mean all the human cells with receptors for insulin.
The antihyperglycemic action of the soluble polyphenols of C. verum bark was verified in SZT-induced diabetic rats at the dose of 200 mg/body weight (Krishnakumar et al., 2014). Cinnamaldehyde isolated from the volatile oil of C. verum showed a highly significant effect on plasma glucose levels using a rat model of SZT-induced diabetes (Subash Babu et al., 2007). This major component has a wide variety of pharmacological properties, including antihyperglycaemic activity in diabetic rats (Subash Babu et al., 2007; Zhang et al., 2008). Al-LogmaniI and Zari (2009) have also tested the EO of C. verum and showed an important reduction in blood sugar levels.
The chloroform extract of C. verum bark stem exhibited antidiabetic activity in vivo at 20 mg/body weight using a rat model of SZT-induced diabetes (Anand et al., 2010). The authors demonstrated that the chemical compounds of extract increased the muscle and liver glycogen content. By using alloxan-induced diabetes in rats, other studies showed that the EO of C. verum bark decreased fasting blood sugar in a dose-dependent manner and reduced total cholesterol level, blood urea, urinary protein, thiobarbituric acid reactive substances (TBARS), and catalase levels in diabetic rats (Rajbir et al., 2009; Mishra et al., 2010). A few studies have reported the antidiabetic effect of C. verum aqueous extracts (Verspohl et al., 2005; Kannappan et al., 2006; Ranilla et al., 2010; Shen et al., 2010; Chen et al., 2013).
The antihyperglycemic activity can also be evaluated by the inhibition test of α-glucosidase and α-amylase. Inhibitors of these enzymes are intended to maintain glucose homeostasis in diabetics by decreasing the rate of glucose uptake (Bösenberg and van Zyl, 2008; Hanhineva et al., 2010). (Ranilla et al., 2010) showed an important inhibition of α-glucosidase and α-amylase actions by C. verum bark aqueous extract. The same extract attenuated hyperglycemia depending on the dose in SZT diabetic rats (Shen et al., 2010; Chen et al., 2013). The antidiabetic effect of this extract was confirmed by the decrease in blood glucose using oral glucose tolerance test in rats (Kannappan et al., 2006; Chen et al., 2013).
In an in vitro study, C. verum showed a potential antidiabetic effect by reducing post-prandial intestinal glucose absorption via enzymatic reduction of pancreatic α–amylase and intestinal α–glucosidase. In vivo studies also confirmed the anti-hyperglycemic effects of C. verum (Bandara et al., 2012; Ranasinghe et al., 2012) through the decrease in LDL cholesterol, total cholesterol and triglycerides with increasing HDL in hyper-lipidaemic albino rabbits. (Javed et al., 2012).
Antioxidant Activity
Some clinical investigations showed that long term consumption of cinnamon extracts improved the levels of blood markers of oxidative stress, such as the antioxidant capacity. The extracts also reduced the transaminase and lipid peroxidase activities (Ranjbar et al., 2007; Rashidi et al., 2014). Diverse tests were used to evaluate potential antioxidant action of Cinnamomum plant extracts and secondary metabolites. These include 2,2′-azinobis (3-ethyl-benzothiazoline-6-sulphonic acid (ABTS), 2,2-diphenyl-1-picrylhydrazyl (DPPH), ferric reducing antioxidant power (FRAP), oxygen radical absorbance capacity (ORAC), Folin Ciocalteau reduction assay (FCR), β carotene linoleic acid bleaching (BCLB), and enzyme inhibition assays.
The antioxidant activity of C. osmophloeum leaf EOs was assessed using DPPH radical scavenging assay, with IC50 = 29.7 μg/ml (Lin et al., 2007). The ethanol extract of the same species was tested using DPPH and FRAP assays by (Lee et al., 2015). The results showed inhibitory values of 38.97 and 0.48% by DPPH and FRAP test, respectively.
(Prasad et al., 2009) tested the antioxidant activity of ethanolic extract of Cinnamomum curvifolium (Lam.) Nees, C. burmanni, C. cassia, C. verum, and C. tamala using DPPH, FRAP and ORAC assays. All tested species showed important antioxidant activities with some variability depending on the species and the used method (Prasad et al., 2009). C. cassia was also reported as an antioxidant species by several studies (Lin et al., 2003; Kim et al., 2006; Jang et al., 2007). C. cassia water extract showed important capacities to inhibit the key enzymes involved in ROS generation such as catalase, superoxide dismutase and glutathione peroxidase (Kim et al., 2006). Methanolic extract of C. verum revealed remarkable antioxidant activities particularly by chelating metal and inhibition of lipid peroxidation (Mathew and Abraham, 2006). Moreover, the inhibition of lipid peroxidation was also demonstrated by EOs of C. verum (Jayaprakasha et al., 2003; Jayaprakasha et al., 2006). The research that highlighted the antioxidative activity of Cinnamomum plants is summarized in Table 2.
Anti-inflammatory Activities
Inflammation is an important pathophysiological process of the organism that maintains homeostasis, fighting pathogens and repairing damaged tissues caused by various injuries such as trauma, infection or immune response (Salehi et al., 2019b), (Stalnikowitz and Weissbrod, 2003). The same inflammatory process is involved in the maintenance of several disorders and is characterized by the production of diverse pro-inflammatory mediators (Salehi et al., 2020a). The current common class of medications against inflammation disorders still relies on non-steroidal anti-inflammatory drugs (Padureanu et al., 2019). However, in spite of having important anti-inflammatory potential, these drug class can cause several side effects such as bleeding, kidney failure and gastrointestinal ulceration. Therefore, increasing attention has been directed towards natural and health-friendly alternatives (Salehi et al., 2019c; Salehi et al., 2019d). The use of natural compounds constitutes an attractive approach for the treatment of several inflammatory disorders.
Inflammation involves reactive oxygen species (ROS) generation, NO production, phospholipase A2 activation and histamine release in neutrophils, macrophages and mast cells. NO has an important role in lipopolysaccharide (LPS), TNF or IL-1 mediated inflammatory process. It is also essential in the cell function maintenance (Stalnikowitz and Weissbrod, 2003) though, on the other hand, NO is able to induce cell injury as a reactive radical. The activator protein-1 (AP-1) and nuclear factor kappa B (NF-kB) are important modulators of inflammation.
The main mechanism of the anti-inflammatory activity of the major chemical compound of Cinnamomum plants, cinnamaldehyde is the effective inhibitor of the expression of NF-kB mediated by its antioxidant activity (Kim et al., 2007). In addition, cinnamaldehyde inhibits pro-inflammatory mediators such as chemokines, interferons, interleukins, lymphokines, eicosanoids (prostaglandins and leukotrienes) and ROS involved in Alzheimer’s disease (Latta et al., 2015). Preclinical studies showed a reasonably good anti-inflammatory effect of Cinnamomum constituents. Diverse extracts and isolated compounds of Cinnamomum plants have been studied for possible anti-inflammatory activity in various animal models. (Liao et al., 2012b) showed an anti-inflammatory effect of cinnamaldehyde on lipopolysaccharide (LPS) stimulated mouse macrophage (RAW 264.7) at 50 µM.
The in vivo investigations confirmed the anti-inflammatory effect using the carrageenan-induced mouse paw edema (Liao et al., 2012b). Cinnamic alcohol (another volatile compound from Cinnamomum plants) also exerted anti-inflammatory activity using the same model (Liao et al., 2012b). In another study, cinnamyl acetate revealed a significant anti-inflammatory action on LPS-stimulated mouse macrophages (RAW264.7) (Chua et al., 2008). The research highlighting the anti-inflammatory activity of Cinnamomum plants is displayed in Table 2.
Anticancer Activities
Recent researches showed that the in vivo anticancer activity of the cinnamon extract was mediated by the induction of tumor apoptosis through the inhibition of NF-κB levels (Kwon et al., 2010). On the other hand, cinnamon showed important anti-cancer effects via affecting on numerous cancer-related pathways such as apoptosis (Kwon et al., 2010). This apoptotic action is mediated by targeting Fas/Fas/CD95, caspase-3, and Bcl-XL (B-cell lymphoma-extra-large) pathways (Sadeghi et al., 2019).
C. burmanni stem bark methanolic extract was tested on human cell lines (NPC/HK1 and C666-1) by Daker et al. (2013). The results showed important cytotoxic effect against HK1 (IC50 = 224.3 μg/ml) and C666-1 (IC50 = 6.30 μg/ml) Daker et al. (2013). Koppikar et al. (2010) tested the aqueous bark extract of C. cassia on human cervical carcinoma (SiHa) cell lines. This extract decreased the growth of cancer up to 2-fold compared to the untreated control cells at the concentration of 80 μg/ml Koppikar et al. (2010).
In another study, the ethanolic extract of the same species tested by Wondrak et al. (2010) on human colorectal carcinoma (HCT 116 and HT 29) cell lines showed anticancer properties. The C. cassia bark aqueous extract was evaluated on cancer cell lines of lymphoma, melanoma and cervix as well as in a melanoma mouse model (Kwon et al., 2010). The cinnamon extract inhibited tumor cell growth in vitro at 0.5 mg/ml.
Cinnamaldehyde showed an important antitumor effect as well (Ng and Wu, 2011). It inhibited the growth of hepatoma Hep G2 cells line at IC50 = 9.8 μM. Some bioactive compounds isolated from Cinnamomum subavenium Miq. such as subamolide D and E showed remarkable anticancer effects on human colon adenocarcinoma (SW 480) cell lines. The cytotoxic effect was mediated by the capacity of these compounds to cause DNA damage in a dose- and time-dependent manner (Kuo et al., 2008). Moreover, subamolide B isolated from the stem of the same plant showed significant cytotoxic effects on human SCC12 (IC50 = 9.12 μg/ml), epidermoid carcinoma A431 (IC50 = 13.30 μg/ml), and human melanoma A375 (IC50 = 17.59 μg/ml) cell lines (Yang et al., 2013).
In another study, subamolide B and its isomer subamolide A induced apoptosis in human colon adenocarcinoma cell line SW480 and human urothelial carcinoma cell line NTUB1(Chen et al., 2007; Wang et al., 2011). Furthermore, subamolide E, isolated from C. subavenium, exhibited an important in vitro anti-melanoma activity (Kuo et al., 2008; Wang et al., 2011). (Lin et al., 2009) reported that butanolides isolated from the stem of Cinnamomum tenuifolium (Makino) Sugim showed anticancer activity on human prostate cancer (DU145) cell line. (Lin et al., 2009). In addition, the extracts of C. kotoense was found cytotoxic against HeLa cells (Chen et al., 2008). Butanolides isolated from the C. kotoense leaves reported genotoxic and cytotoxic effects on various cell lines, such as human laryngeal carcinoma cells Hep-2, Chinese hamster ovarian cells CHO-K1 and rat hepatoma tissue cultures (Garcez et al., 2005) and mouse lymphoid leukemia P-388 cells (Tsai et al., 2002). In vitro antineoplastic activities of Cinnamomum species are summarized in Table 2.
Neuroprotective Activities: Potential Mechanisms and Molecular Targets in Neurodegenerative Diseases
Parkinson's and Alzheimer’s diseases are common neurodegenerative diseases, accompanied by cognitive and memory impairments, sometimes difficult to differentiate from real psychosis or other neurological diseases (Nussbaum et al., 2017; Buga et al., 2019; Tsatsakis et al., 2019). The mechanisms of neuroprotective effects of Cinnamomum plants and their derivatives have been reported by several studies (Liao et al., 2008; Peng et al., 2008; Peterson et al., 2009; Panickar et al., 2012; Jiao et al., 2013). (Figure 4).
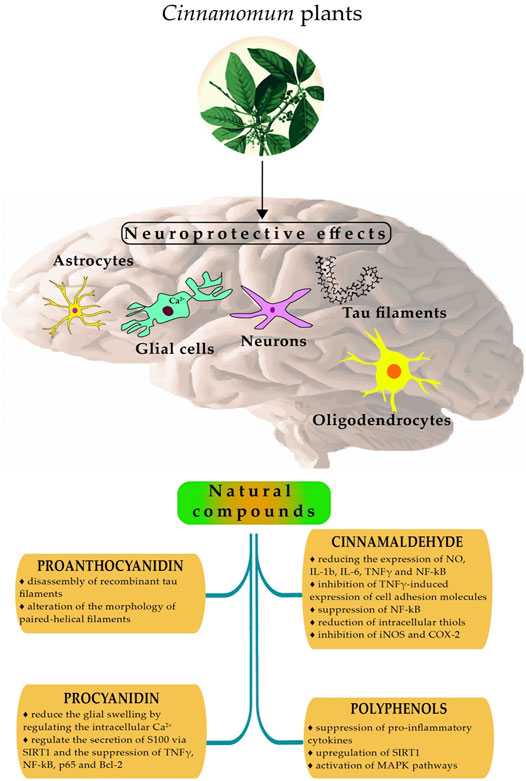
FIGURE 4. Summarized mechanisms of neuroprotective effects of Cinnamomum plants. Abbreviations: SIRT1, Sirtuin 1; MAPK, mitogen-activated protein kinase; iNOS, inducible Nitric Oxide synthase; TNF-γ, tumor necrosis factor; COX-2, Cyclooxygenase-2; NF-kB, Nuclear Factor- Kappa B; p65 (RelA subunit of NF-κB family of transcription factors); Bcl-2, B-cell lymphoma 2; IL-1b, Interleukin-1beta; IL-6, Interleukin 6.
(Ho et al., 2013) showed that procyanidinA trimer 1 (a bioactive compound isolated from C. burmanni) had an essential neuroprotective effect which reduced the glial swelling by regulating the intracellular calcium concentration in glial neuronal cells (Peng et al., 2008). Procyanidin exhibited neuroprotective activity by its capacity to regulate the secretion of S100 via the regulation of SIRT1 and the suppression of TNF-γ, NF-kB p65 (RelA subunit of NF-κB family of transcription factors) and B-cell lymphoma 2 (Bcl-2) on glioma cells (Jiao et al., 2013).
(Panickar et al., 2012) studied the neuroprotection of procyanidin B2 isolated from C. verum. The results showed that this molecule inhibited advanced glycation end-product production in the bovine serum albumin-glucose model (Panickar et al., 2012).
Cinnamaldehyde is a volatile compound found in C. cassia extracts. (Ho et al., 2013) showed that this compound possessed an important neuroprotective effect by reducing the expression of NO, tumor necrosis factor (NF-γ), interleukin-1beta (IL-1b), interleukin 6 (IL-6), and nuclear factor-kappa B (NF-kB) in LPS induced BV2 microglia cells.
In a recent study, (Liao et al., 2008) revealed that cinnamaldehyde from C. cassia exhibited remarkable neuroprotection by the reduction of TNFγ-induced expression of cell adhesion molecules, the suppression of nuclear factor-kappa B (NF-kB) and the reduction of intracellular thiols in endothelial cells (Liao et al., 2008).
Cinnamaldehyde isolated from C. ramulus exhibited anti-neuro-inflammatory properties by the inhibition of inducible nitric oxide synthase (iNOS) and cyclooxygenase-2 (COX-2) in LPS-induced BV2 microglial cells (Pyo et al., 2013).
In addition, cinnamon bioactive compounds exhibited neuroprotective effects in in vivo models of Alzheimer’s disease and extended the lifespan via regulation of key antioxidant pathways (Crews et al., 2016). C. verum extracts provided an important protection against Alzheimer’s disease and dementia in the scopolamine-induced memory impairment in experimental rat model (Jain et al., 2015).
In the same way, a different mixture of cinnamon rich in polyphenols exhibited important neuroprotective activities in rat glioma cells by suppression of pro-inflammatory cytokines, upregulation of sirtuin 1 (SIRT1) and activation of mitogen-activated protein kinase (MAPK) pathways (Qin et al., 2014). Neuroprotective activities of Cinnamomum plants are tabulated in Table 2.
Other Pharmacological Activities
Cinnamomum plants have also shown an array of other biological activities. (Nyadjeu et al., 2011) reported that C. verum extracts decreased arterial blood pressure in rats with normal arterial hypertension and various models of hypertensive rats (salt-loaded and spontaneously) (Nyadjeu et al., 2011). In another study, (Wansi et al., 2007) reported that C. verum extracts showed similar effects in arterial blood pressure of normotensive rats and salt-loaded hypertensive rats. Moreover, they showed that C. verum had a vasorelaxant action on the thoracic aortic ring segments, which suggests that C. verum might inhibit extracellular calcium through L-type voltage-sensitive channels (Wansi et al., 2007).
The antiparasitic effects of EOs from bark and leaves of C. verum were investigated by (Samarasekera et al., 2005) against Anopheles tessellates, Culex quinquefasciatus, and Aedes aegypti. These oils showed knock-down and mortality against A. tessellatus (LD50 = 0.33 μg/ml) and C. quinquefasciatus (LD50 = 0.66 μg/ml). (Yang et al., 2005) showed fewer effect of C. verum bark EO against Pediculus humanus capitis (eggs, adult females of human head louse) than either phenothrin or pyrethrum using direct contact bioassay (Yang et al., 2005).
Collagen synthesis was stimulated in human dermal fibroblasts by C. verum extracts (Takasao et al., 2012). C. verum extract enhanced both mRNA and protein expression levels of type I collagen without cytotoxicity, and cinnamaldehyde was the most active component stimulating the expression of collagen, suggesting that C. verum extracts might be useful in skin anti-aging treatments (Takasao et al., 2012). Moreover, C. verum extracts inhibited osteoclastogenesis (Tsuji-Naito, 2008). At concentrations of 12.5–50 μg/ml, C. verum inhibited osteoclast-like cell formation in a dose-dependent manner without affecting cell viability. This finding suggests its potential use in the treatment of osteopenia diseases (Tsuji-Naito, 2008). In addition, C. verum exhibited in vivo anti-secretagogue and anti-gastric ulcer effects (Alqasoumi, 2012). In pylorus-ligated rats, C. verum extract pre-treatment reduced the basal gastric acid secretion and inhibited gastric hemorrhagic lesions (Alqasoumi, 2012). Furthermore, C. verum extracts at 100 and 200 mg/b. w. effectively diminished the extent of diarrhea (71.7 and 80.4%, respectively) in test animals (RAO, 2012).
In another research performed in two rat models, the evaluation of anti-nociceptive effects of C. verum and selected plants showed that C. verum produced a dose-dependent analgesic protective effect against thermal stimuli. Moreover, C. verum enhanced an anti-inflammatory activity against chronic inflammation of cotton pellet granuloma (Atta and Alkofahi, 1998; RAO, 2012). C. verumhas also demonstrated wound healing properties (Kamath et al., 2003; Farahpour and Habibi, 2012). C. verum (0.01, 0.05, and 0.1 g/b. w. for 28 days) displayed hepatoprotective effects in a study where the liver injury was induced in rats by CCl4 (carbon tetrachloride) (Eidi et al., 2012). Other pharmacological properties of Cinnamomum plants are summarized in Table 2.
Clinical Trials Related to Efficacy of Bioactive Compounds Derived From Cinnamomum
Type 2 Diabetes Mellitus, Obesity and Metabolic Syndrome
Cinnamon consumption was associated with reduction in the levels of fasting plasma glucose (FPG), total cholesterol (TC), triglyceride levels (TG), LDL cholesterol and hemoglobin A1c (HbA1c). In one study, cinnamon consumption resulted in significantly increased FPG levels and HbA1c (Blevins et al., 2007) or no significant change in HDL cholesterol levels. However, the most preferred and effective doses with fewer side effects are still not clear. Doses applied in randomized clinical trials showed conflicting results as consumption of 1 g/day (Khan et al., 2003; Blevins et al., 2007; Crawford, 2009), 1.5 g/day (Vanschoonbeek et al., 2006; Radhia et al., 2010), 2 g/day (Akilen et al., 2010), 3 g/day (Khan et al., 2003; Mang et al., 2006; Vafa et al., 2012), or 5 g/day (Gutierrez et al., 2016), 6 g/day (Khan et al., 2003) showed different effects in glycemic and lipid parameters. FPG and HbA1c are commonly used parameters for diabetes diagnosis. HbA1c values of more than 6.5% can be related to diabetes. Vafa et al. showed FPG and HbA1c at baseline modestly elevated (7.02 mmol/L to 8 mmol/L) and 6.9–8.0%, respectively (Vafa et al., 2012). Both the studies by Akilen et al. and Crowfrod reported a drop of 0.36 and 0.83% of HbA1c values at low dose cinnamon supplementation which were safe and well tolerated for 3 months in patients using simultaneous hypoglycemic medications (Crawford, 2009; Akilen et al., 2010). Mang et al. observed that aqueous extract of cassia cinnamon significantly reduced glucose levels like 10.3% in the cinnamon group and 3.4% in the placebo group, however, no considerable difference in HbA1c and cholesterol (LDL, HDL, TC) levels were observed (Mang et al., 2006). Suppapitiporn et al. observed no significant differences in cinnamon and placebo group for FPG and lipid profiles but HbA1c level decreased in both groups by 0.38 and 0.19% respectively (Suppapitiporn and Kanpaksi, 2006).
Khan et al. reported that intake of 1, 3, or 6 g of cinnamon reduced fasting serum glucose by 18–29%, LDL by 7–27%, triacylglycerol by 23–30%, and total cholesterol by 12 26% after 40 days treatment. Nevertheless, they did not investigate the HbA1c value (Khan et al., 2003). One study showed that, consumption of Cinnamomum cassia powder 1.5 g/day for 6 weeks, did not improve plasma glucose levels, insulin, and cholesterol levels and no reduction in HbA1c level was noted (Vanschoonbeek et al., 2006). Only one trial by Blevins et al. observed significant increases in HbA1c and FPG levels (Blevins et al., 2007). In healthy, sedentary and obese women with Cassia supplementation a statistically significant reduction in glucose level was noted suggesting that the bark of Cassiacan improve glucose tolerance. However, this study did not mention the acute dose of Cassia to maintain insulin resistance or sensitivity (Gutierrez et al., 2016). Some studies proposed that cinnamon also improved lipid profiles in clinical trials. Vafa et al. showed that in type 2 diabetes treated with cinnamon increased LDL levels but decreased TC, insulin and triglyceride levels with the improved glycemic index (Vafa et al., 2012). In addition, intercellular adhesion molecule-1 (ICAM-1) levels are increased in serum associated with increasing type-2 diabetes. Azimi et al. studied that consumption of Cinnamomum verum extract for 8 weeks decreased serum ICAM-1 level in patients with type-2 diabetes (Azimi et al., 2016).
26 clinical trial studies on cinnamon are present on Clinical Trials Govt. Database, among them most of the studies are under process and 14 studies are completed. Clinical trial numbers NCT03219411 and NCT01301521 showed effects of cinnamon supplementation in pre-diabetic patients. Clinical trials NCT03711682 and NCT00237640 observed plasma glucose and lipid levels reduction mediated by cinnamon in type 2 diabetic patients and in noninsulin dependent type 2 diabetes mellitus patients respectively. NCT01302743 trial demonstrated the application of water-soluble cinnamon bark extract and metformin for the treatment of type 2 diabetes mellitus patients. Table 3 displayed the clinical studies on Cinnamon plants in relation to diabetes.
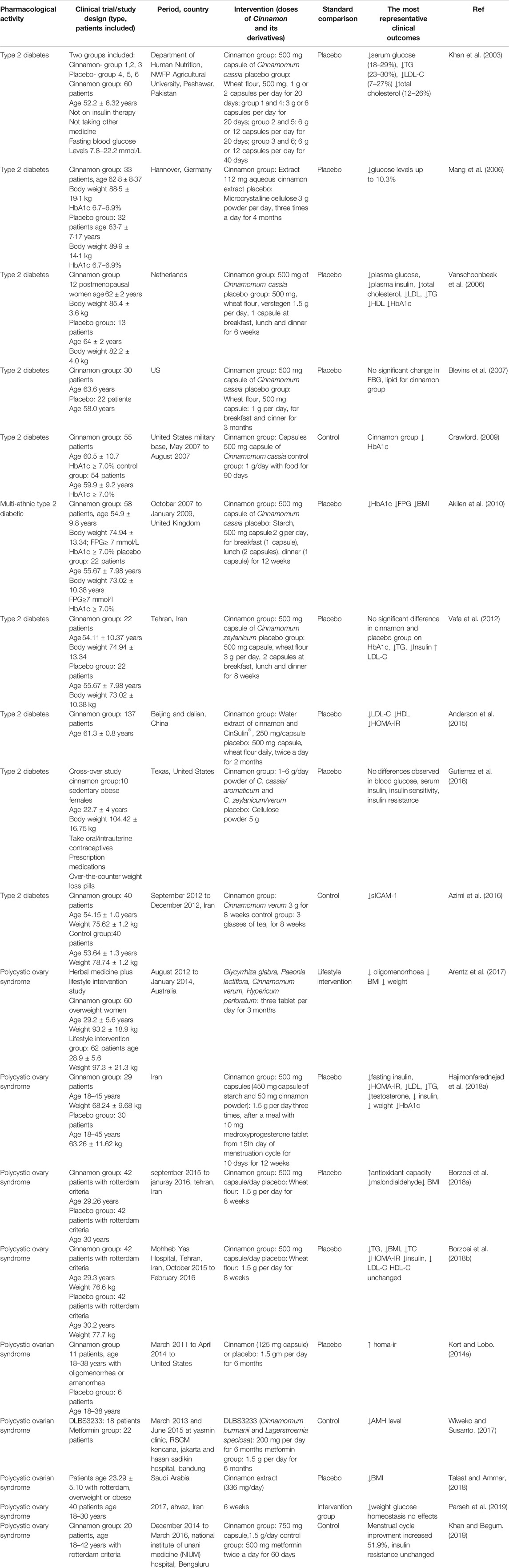
TABLE 3. Description of recent clinical studies related to pharmacological activity of natural compounds from Cinnamomum species.
Neurodegenerative Disorders
Neurodegenerative disorders (ND) include disorders like Alzheimer’s disease, amyotrophic lateral sclerosis, Parkinson's disease, Huntington’s disease, motor neuron disorder, and frontotemporal dementia that result from slow progressive and unalterable drop of certain areas of the nervous system, leading to disruption in nervous system working or death (Nussbaum et al., 2017).
Various Cinnamomum species displayed efficacy in the management of neurodegenerative diseases. Several in vitro studies are present for cinnamon that can regulate factors that trigger neurodegenerative diseases. Cinnamon extract inhibited Tau aggregation in vitro attenuating Alzheimer’s diseases (Peterson et al., 2009). C. cassia bark extracted in ethanol demonstrated in vitro efficacy against Huntington disease Kaur and Shri (2018). Therefore, cinnamon is necessary to put into clinical trials that develop drugs for neurodegenerative disorders. One clinical trial (NCT03225144) for patients with motor neuron disorder, frontotemporal dementia, or related adult-onset neurodegenerative disorder is ongoing involving 200 participants (Table 3).
Polycystic Ovary Syndrome: A Possible Control on Its Metabolic Parameters
Polycystic ovary syndrome (PCOS) is caused by an associated endocrine dysfunction and is associated with increased risk of developing insulin resistance, type 2 diabetes, high blood pressure, hypercholesteromy and heart disease.
Several clinical trials reported conflicts in results for cinnamon efficiency on the improvement of BMI, body weight, oxidative stress, and fertility (Hajimonfarednejad et al., 2018a; Borzoei et al., 2018b; Khan and Begum, 2019). Consumption of daily 1.5 g cinnamon did not show any significant effect on BMI and body weight but improved glucose balance in patients with PCOS (Hajimonfarednejad et al., 2018a). However, Borzoei et al. decreased BMI and improved glucose balance treated with the same concentration as applied by Hajimonfarednejad et al. (Hajimonfarednejad et al., 2018a; Borzoei et al., 2018b). Oral supplementation of cinnamon also showed weight loss. This study further exhibited decreased BMI in comparison to the placebo group in PCOS patients (Talaat and Ammar, 2018; Parseh et al., 2019). In contrast to the report by Hajimonfarednejad et al. and Kort and Lobo observed that oral cinnamon consumption did not show any effect on serum glucose balance in PCOS patients (Kort and Lobo, 2014a; Hajimonfarednejad et al., 2018a). This study also supported by Parseh et al., who also did not report any changes in glucose homeostasis (Parseh et al., 2019). All of the studies discussed here showed an effective dose of cinnamon as 1.5 g per day, three times after meal for 1.5–6 months except Talaat and Ammar reported effective dose of 336 mg cinnamon extract per day whereas Wiweko and Susanto (2017) treated with 200 mg of Cinnamomum burmanii and Lagerstroemia speciosa combination extract (Talaat and Ammar, 2018).
Serum LDL-C, TG and TC levels were also found to be improved in PCOS patients in comparison to placebo (Hajimonfarednejad et al., 2018a; Borzoei et al., 2018b). However, HDL-C level did not show any significant improvement (Borzoei et al., 2018b). Khan and Begum observed no changes in insulin resistance and did not found any significant improvement in patients’ life in comparison with metformin treatment as a control (Khan and Begum, 2019).
Herbal medicine combination extract of Glycyrrhiza glabra, Paeonia lactiflora, Cinnamomum verum and Hypericum perforatum (supplemented with 1.5 g tablet per day for 3 months) with lifestyle intervention positively improved menstrual regulation and reduction in oligomenorrhoea of 32.9% in patients in comparison with only lifestyle intervention in overweight women with PCOS (Arentz et al., 2017).
Oxidative stress is one of the main causes for increasing lipogenes, BMI in PCOS due to molecular damage, and reduction of serum antioxidants. Borzoei et al. demonstrated that cinnamon extract has antioxidant activity that can improve oxidative stress in women with PCOS (Borzoei et al., 2018a). Table 3 summarized the clinical studies on cinnamon plants in relation to PCOS.
Toxicological Safety and Adverse Effects of Natural Derivatives of Cinnamomum Species
Besides the numerous health benefits, all phyto-therapeutics are not always safe and might result in adverse effects such as allergic dermatitis, the toxicity of organs and interactions with foods and pharmaceuticals (Calixto, 2000). The usual dose for dietary supplements has been suggested to be between 1 and 4 g per day (NIHU, 2015). The usual doses for the administration of cinnamon oil, which is stronger, vary between 50 and 200 mg per day. For doses up to 6 g per day, no adverse reactions were reported (Yun et al., 2018). The common use of cinnamon in food as a spice, food additives and flavoring agent would suggest that it is likely to be safe (Gowder, 2014). However, when consumed in excess, cinnamon can cause respiratory distress, increase pulse rate and increase the sweating process, followed by depressive and drowsy states. This may aggravate the symptoms of rosacea and may increase the risk of developing oral cancer. Coumarin, naturally found in cinnamon, can have a negative influence on the liver, so people with liver disorders should avoid excessive consumption (Organization, 2001).
Cinnamon extract at different doses didn’t produce any toxicity or mortality on rats, as well as no adverse effect was observed (Ahmad et al., 2013) though trans-cinnamaldehyde and coumarins are toxic components of cinnamon (Woehrlin et al., 2010; Zhang et al., 2010). High levels of coumarin and cinnamaldehyde might be correlated to liver damage (Deng, 2012), risk of cancer (Abraham et al., 2010), mouth sores (Vivas and Migliari, 2015), low blood sugar (Adisakwattana et al., 2011; Deng, 2012) breathing problems and interaction with certain medications (Abraham et al., 2010). Therefore, the long-term use of a high amount of cinnamon should be continuously monitored. The tolerable daily intake for coumarin (0.1 mg/b. w.) is to be regarded as safe in terms of daily cinnamon intake without the risk of adverse effects (Abraham et al., 2010). When used in large quantities Cinnamomum may interact with certain drugs, which could damage the liver.
Used externally, the cinnamon essential oil can produce skin redness, irritation and burning sensation in the epidermis (Markman, 2002). In addition, negative interactions with the drugs such as statins, acetaminophen, amiodarone (used to treat heart conditions), carbamazepine (given in the treatment of seizures), medicines for treating fungal infections, methotrexate (used in antitumor treatments) and methyldopa (used in hypertension) may also occur.
Because cinnamon can reduce the concentration of blood sugar, consumed in large quantities, it can interact with diabetes medicines, leading to a very low level of sugar. It is also possible to interfere negatively with tetracycline. Noteworthy, the safety of cinnamon is dependent on supplier quality assurance (SQA), good manufacturing practice (GMP) and good agricultural practice (GAP). It is necessary to control the cultivation, harvesting, plant identification, contamination, adulteration, preparation, packaging, transportation, storage etc. In order to guarantee the safety of the cinnamon products, it is required to assess the quality control of identity/authenticity and purity, stability and shelf life, toxicity physical/chemical/biological/microbial properties and finally standardization of the plant material as well as the products (Kumari and Kotecha, 2016).
Discussion
One of the strengths of this updated review is that the pharmacological effects highlighted in preclinical in vitro studies and in vitvo models could be translated into recent clinical trials in human subjects at well-defined doses, providing strong scientific evidence-based support for therapeutic effects. ethnopharmacological features of cinamomum species.
In addition, current toxicological data show that unwanted side effects may occur at higher doses of cinnamon than shown in pharmacological studies.
Another strength of this comprehensive review is the summarized presentation of the pharmacological mechanisms and molecular targets of action resulting from the meta-analyzes included in the study in order to open new clinical pharmacotherapeutic perspectives.
Starting from traditional uses, pharmacognostic research on Cinnamomum plants have identified chemical compounds with biological activities. Three major components found in cinnamon oil and powder: are cinnamaldehyde, acetate, and cinnamyl alcohol, give it beneficial properties. Also, cinnamon is a good source of iron, calcium, manganese and dietary fiber (Vallverdú-Queralt et al., 2014).
Plant organic extracts are a rich source of antimicrobial agents and methanol extracts are known for their antimicrobial properties. Indeed, organic solvents have a good ability to solubilize the active components (de Boer et al., 2005) and their use does not influence the bioactivity of these components (Goyal et al., 2009). (Thongson et al., 2004) also suggested that organic solvents are better solvents of antimicrobial substances. Therefore, a number of preclinical experimental studies showed the antibacterial effects of Cinnamomum plants. Secondary metabolites of Cinnamon plants have the ability to adhere to the microbial cell surface by crossing the lipid layer of the cytoplasmic wall, thus accumulating in the bacterial cell wall. Therefore, phytochemicals alter the structure of the membrane and increase its permeability, causing leakage of vital intracellular metabolites and, finally, cell death (Rhayour, 2003; Wu et al., 2009).
The bacterial cytoplasmic membrane plays a role in selective permeability by ensuring the crossing of H+ and K ions+. The maintenance and regulation of this barrier are provided by the structural, chemical composition of the cell wall. Increased ion leakage means a disruption of the permeability barrier. Cell metabolism can be influenced by changes in the structural integrity of the cell membrane that, in turn, can lead to the death of cells. (Cox et al., 2001).
Several preclinical experimental studies have been developed to highlight the antidiabetic effects of natural molecules from Cinnamomum species. Current conventional therapies used in the treatment of diabetes have many limitations, such as short- and long-term undesirable effects and high rates of failure (Sharifi-Rad et al., 2020a). Therefore, it is necessary to develop more effective drugs to treat diabetes. Presently, complementary herbal remedies are expected to have hypoglycemic properties similar to those of conventional medications without troublesome side effects (Sharifi-Rad et al., 2020b). Traditional herbs and spices can also delay the onset of diabetic complications, control blood sugar levels and correct metabolic abnormalities. The in vitro methods are essentially based on the inhibition of enzymes involved in the carbohydrate catabolism and therefore sugar absorption. However, the determination of the in vivo antidiabetic activity requires the development of approaches such as oral glucose tolerance test, streptozotocin (SZT)-induced diabetes and alloxan-induced animal models.
The potential mechanisms of antidiabetic effects of Cinnamomum species were highlighted by recent studies. These have shown that secondary plant metabolites, such as terpenoids and flavonoids, have a significant hypoglycemic effect (Marles and Farnsworth, 1995). Terpenoids stimulate insulin secretion from pancreatic β-cells (Marles and Farnsworth, 1995) whereas cinnamaldehyde has a significantly anti-hyperglycemic effect (Subash Babu et al., 2007).
The antioxidant properties of Cinnamomum plants have extensively reported. Polar extracts of the leaves, fruits and seeds, as well as several pure compounds, exhibited relevant activities in a variety of antioxidant assays when compared to positive controls. Cinnamon extracts and its phenolic compounds showed important free radical scavenging properties by the modulation of key enzymes implicated in oxidative stress or by modulation of oxidative pathways that influence the maintaining of redox homeostasis. Cinnamon extracts enhanced ferric reducing antioxidant power (FRAP) and plasma thiol (P-SH), and decreased MDA levels. In addition, they also increased antioxidant enzyme properties such as SOD and catalase (Moselhy and Ali, 2009; Roussel et al., 2009). Our study also showed that Cinnamomum derivatives have a good anti-inflammatory properties.
The antitumor effects of Cinnamomum species have been reported by several studies (Koppikar et al., 2010; Kwon et al., 2010; Wondrak et al., 2010; Daker et al., 2013). These antineoplastic activities were tested in vitro on several types of human cancer cell lines. These studies highlighted the next possible anticancer mechanisms of Cinnamomum plants: 1) inhibition of tumor cells growth (Koppikar et al., 2010), 2) the cinnamon extract is a potent activator of the transcription factor NRF2 (nuclear factor erythroid 2-related factor 2) orchestrated antioxidant response in human cells (Wondrak et al., 2010), 3) cinnamaldehyde involves apoptosis mediated by the increase of p53 and APO-19 (Fas/CD95) protein signaling pathways (Ng and Wu, 2011), 4) DNA (deoxyribonucleic acid) damage (Ng and Wu, 2011), v)apoptosis mediated by the tyrosinase inhibition (Chen et al., 2007; Wang et al., 2011), 6) reducing the mitochondrial transmembrane potential, increasing the ratio of cytochrome C concentration and subsequently activated caspase-9/caspase-3 (Lin et al., 2009).
Recent studies showed neuroprotective effects of Cinnamomum plants and their derivatives. The aqueous extract of C. verum reduced tau aggregation and filament formation, the markers of Alzheimer’s disease (Peterson et al., 2009). From the brains of patients diagnosed with Alzheimer’s disease, the C. verum extract produced the complete disassembly of recombinant tau filaments and caused important alteration of the histology of paired helical filaments isolated, even though it was not deleterious to the normal cellular function of tau. (Peterson et al., 2009). Specifically, the proanthocyanidin, a chemical constituent isolated from the C. verum extract was observed to be mainly responsible for this inhibitory activity (Peterson et al., 2009).
Recent clinical studies have found that complementary herbal treatments with cinnamon regulate the frequency of menstrual cycles and may reduce insulin resistance in women with polycystic ovaries.
Regarding the toxicity data, some clinical studies reported that the most common adverse events after cinnamon consumption were gastrointestinal problems in patients with diabetes (Altschuler et al., 2007; Crawford, 2009), Helicobacter infection (Nir et al., 2000), polycystic ovarian syndrome (Kort and Lobo, 2014b) and seasonal allergies (Walanj et al., 2014). Dermatitis (Calnan, 1976; Ackermann et al., 2009; Isaac-Renton et al., 2015) and steatites (Miller et al., 1992; Endo and Rees, 2007) were also reported in some case reports. A systematic review of clinical trials and case reports/series on side effects associated with the use of cinnamon in humans indicated that no important difference obtained between cinnamon treated and control group in most cases (Food and Administration, 2005; Hajimonfarednejad et al., 2018b).
Further efforts should be made to investigate future applications of standardized Cinnamomum plants using well-designed research.
Overall Conclusions
Cinnamomum plants, its extracts and chemical constituents have several activities promoting human health. Antibacterial, antidiabetic, antioxidant, anti-inflammatory, antitumor and neuroprotective activities are the most studied biological properties of Cinnamomum plants. Different from conventional therapeutic medicines, Cinnamomum species can be daily consumed in our diet without harmful effects and maybe used as a disease-preventive agent.
In general, chemical constituents of Cinnamomum plants such as cinnamon, cinnamaldehyde, cinnamophilin and others, have both direct and indirect activities, i.e. antioxidant and antibacterial activities occur by direct action on oxidant species or bacterial, whereas the antidiabetic, anticancer and anti-inflammatory activities occur indirectly via some yet undefined receptor-mediated mechanisms. In this present review, we have retrieved and summarized the wide applications and recent literature on the phytochemistry, bioactivity and pre-clinical and clinical investigations performed on many species of the genus. Besides, a detailed account on their toxicological considerations also indicate their safety and efficacy for human consumption. However, since a plethora of reports exist on the plant species, details on ethnobotany, biotechnological interventions and detailing structure-activity studies are beyond the scope and coverage of the present attempt.
Botanical research advances can improve the obtaining of Cinnamomum plants with the best phytochemical compounds. Moreover, the antimicrobial effects of Cinnamomum spp. can be potentiated in the food industry as the development of green foods.
Author Contributions
JS-R, AD, and DC conceptualization. MM, AD, NK, SS, NE, and BH validation investigation. NC, CS, AB, and SV resources. BS, MM, AD, EV, and FL. VL, TG, AA, and CC. data curation. JS-R, AD, FL, BS, VL, CC, and DC review and editing. DC supervision. All authors: writing. All authors read and approved the final version and contributed equally to the article.
Funding
This research and article processing charges were funded by a grant of Romanian Ministry of Research and Innovation, CCCDI-UEFISCDI, (project number 61PCCDI/2018PN-III-P1-1.2-PCCDI-2017-0341), within PNCDI-III; CONICYT PIA/APOYO CCTE AFB170007.
Conflict of Interest
The authors declare that the research was conducted in the absence of any commercial or financial relationships that could be construed as a potential conflict of interest.
References
Abdelwahab, S. I., Zaman, F. Q., Mariod, A. A., Yaacob, M., Ahmed Abdelmageed, A. H., and Khamis, S. (2010). Chemical Composition, Antioxidant and Antibacterial Properties of the Essential Oils of Etlingera Elatior and Cinnamomum Pubescens Kochummen. J. Sci. Food Agric. 90, 2682–2688. doi:10.1002/jsfa.4140
Abraham, K., Wöhrlin, F., Lindtner, O., Heinemeyer, G., and Lampen, A. (2010). Toxicology and Risk Assessment of Coumarin: Focus on Human Data. Mol. Nutr. Food Res. 54, 228–239. doi:10.1002/mnfr.200900281
Ackermann, L., Aalto-Korte, K., Jolanki, R., and Alanko, K. (2009). Occupational Allergic Contact Dermatitis from Cinnamon Including One Case from Airborne Exposure. Contact Dermatitis 60, 96–99. doi:10.1111/j.1600-0536.2008.01486.x
Adisakwattana, S., Lerdsuwankij, O., Poputtachai, U., Minipun, A., and Suparpprom, C. (2011). Inhibitory Activity of Cinnamon Bark Species and Their Combination Effect with Acarbose against Intestinal α-glucosidase and Pancreatic α-amylase. Plant Foods Hum. Nutr. 66, 143–148. doi:10.1007/s11130-011-0226-4
Agarwal, H., Nakara, A., Menon, S., and Shanmugam, V. (2019). Eco-friendly Synthesis of Zinc Oxide Nanoparticles Using Cinnamomum Tamala Leaf Extract and its Promising Effect towards the Antibacterial Activity. J. Drug Deliv. Sci. Tech. 53, 101212. doi:10.1016/j.jddst.2019.101212
Ahmad, M., Lim, C. P., Akowuah, G. A., Ismail, N. N., Hashim, M. A., Hor, S. Y., et al. (2013). Safety Assessment of Standardised Methanol Extract of Cinnamomum Burmannii. Phytomedicine 20, 1124–1130. doi:10.1016/j.phymed.2013.05.005
Akilen, R., Tsiami, A., Devendra, D., and Robinson, N. (2010). Glycated Haemoglobin and Blood Pressure-Lowering Effect of Cinnamon in Multi-Ethnic Type 2 Diabetic Patients in the UK: a Randomized, Placebo-Controlled, Double-Blind Clinical Trial. Diabetic Med. 27, 1159–1167. doi:10.1111/j.1464-5491.2010.03079.x
Al-Bayati, F. A., and Mohammed, M. J. (2009). Isolation, Identification, and Purification of Cinnamaldehyde fromCinnamomum Zeylanicumbark Oil. An Antibacterial Study. Pharm. Biol. 47, 61–66. doi:10.1080/13880200802430607
Al-Logmanii, A. S., and Zari, T. A. (2009). Effects of Nigella Sativa L. And Cinnamomum Zeylanicum Blume Oils on Some Physiological Parameters in Streptozotocin-Induced Diabetic Rats. Boletínl Atino Americano y Del. Caribe de Plantas Medicinales y Aromáticas 8, 159.
Alqasoumi, S. (2012). Anti-secretagogue and Antiulcer Effects of Cinnamon Cinnamomum Zeylanicum in Rats. J. Pharmacognosy Phytother. 4, 53–61. doi:10.5897/jpp12.023
Altschuler, J. A., Casella, S. J., Mackenzie, T. A., and Curtis, K. M. (2007). The Effect of Cinnamon on A1C Among Adolescents with Type 1 Diabetes. Diabetes care 30, 813–816. doi:10.2337/dc06-1871
Anand, P., Murali, K. Y., Tandon, V., Murthy, P. S., and Chandra, R. (2010). Insulinotropic Effect of Cinnamaldehyde on Transcriptional Regulation of Pyruvate Kinase, Phosphoenolpyruvate Carboxykinase, and GLUT4 Translocation in Experimental Diabetic Rats. Chemico-Biological Interactions 186, 72–81. doi:10.1016/j.cbi.2010.03.044
Anderson, D., Cordell, H. J., Fakiola, M., Francis, R. W., Syn, G., Scaman, E. S. H., et al. (2015). First Genome-wide Association Study in an Australian Aboriginal Population Provides Insights into Genetic Risk Factors for Body Mass Index and Type 2 Diabetes. PloS one 10, e0119333. doi:10.1371/journal.pone.0119333
Arentz, S., Smith, C. A., Abbott, J., Fahey, P., Cheema, B. S., and Bensoussan, A. (2017). Combined Lifestyle and Herbal Medicine in Overweight Women with Polycystic Ovary Syndrome (PCOS): A Randomized Controlled Trial. Phytother. Res. 31, 1330–1340. doi:10.1002/ptr.5858
Atta, A. H., and Alkofahi, A. (1998). Anti-nociceptive and Anti-inflammatory Effects of Some Jordanian Medicinal Plant Extracts. J. Ethnopharmacology 60, 117–124. doi:10.1016/s0378-8741(97)00137-2
Azimi, P., Ghiasvand, R., Feizi, A., Hosseinzadeh, J., Bahreynian, M., Hariri, M., et al. (2016). Effect of Cinnamon, Cardamom, Saffron and Ginger Consumption on Blood Pressure and a Marker of Endothelial Function in Patients with Type 2 Diabetes Mellitus: A Randomized Controlled Clinical Trial. Blood Press. 25, 133–140. doi:10.3109/08037051.2015.1111020
Bakar, A., Yao, P. C., Ningrum, V., Liu, C. T., and Lee, S. C. (2020). Beneficial Biological Activities of Cinnamomum Osmophloeum and its Potential Use in the Alleviation of Oral Mucositis: A Systematic Review. Biomedicines 8. doi:10.3390/biomedicines8010003
Bandara, T., Uluwaduge, I., and Jansz, E. R. (2012). Bioactivity of Cinnamon with Special Emphasis on Diabetes Mellitus: a Review. Int. J. Food Sci. Nutr. 63, 380–386. doi:10.3109/09637486.2011.627849
Barceloux, D. G. (2009). Cinnamon (Cinnamomum Species). Disease-a-Month 55, 327–335. doi:10.1016/j.disamonth.2009.03.003
Basu, S., Jana, S., Patel, V. B., and Patel, H. (2013). Effects of Piperine, Cinnamic Acid and Gallic Acid on Rosuvastatin Pharmacokinetics in Rats. Phytother Res. 27, 1548–1556. doi:10.1002/ptr.4894
Bedigian, D. (2005). Cinnamon and Cassia. The Genus Cinnamomum. Medicinal and Aromatic Plants-Industrial Profiles, Vol. 36. Econ. Bot. 3659, 93–94. doi:10.1663/0013-0001(2005)059
Blevins, S. M., Leyva, M. J., Brown, J., Wright, J., Scofield, R. H., and Aston, C. E. (2007). Effect of Cinnamon on Glucose and Lipid Levels in Non Insulin-dependent Type 2 Diabetes. Diabetes care 30, 2236–2237. doi:10.2337/dc07-0098
Borges, A., Ferreira, C., Saavedra, M. J., and Simões, M. (2013). Antibacterial Activity and Mode of Action of Ferulic and Gallic Acids against Pathogenic Bacteria. Microb. Drug Resist. 19, 256–265. doi:10.1089/mdr.2012.0244
Borzoei, A., Rafraf, M., and Asghari-Jafarabadi, M. (2018a). Cinnamon Improves Metabolic Factors without Detectable Effects on Adiponectin in Women with Polycystic Ovary Syndrome. Asia Pac. J. Clin. Nutr. 27, 556–563. doi:10.6133/apjcn.062017.13
Borzoei, A., Rafraf, M., Niromanesh, S., Farzadi, L., Narimani, F., and Doostan, F. (2018b). Effects of Cinnamon Supplementation on Antioxidant Status and Serum Lipids in Women with Polycystic Ovary Syndrome. J. traditional Complement. Med. 8, 128–133. doi:10.1016/j.jtcme.2017.04.008
Bösenberg, L. H., and Van Zyl, D. G. (2008). The Mechanism of Action of Oral Antidiabetic Drugs: A Review of Recent Literature. J. Endocrinol. Metab. Diabetes South Africa 13, 80–88. doi:10.1080/22201009.2008.10872177
Buga, A.-M., Docea, A. O., Albu, C., Malin, R. D., Branisteanu, D. E., Ianosi, G., et al. (2019). Molecular and Cellular Stratagem of Brain Metastases Associated with Melanoma. Oncol. Lett. 17, 4170–4175. doi:10.3892/ol.2019.9933
Buru, A. S., Pichika, M. R., Neela, V., and Mohandas, K. (2014). In vitro antibacterial Effects of Cinnamomum Extracts on Common Bacteria Found in Wound Infections with Emphasis on Methicillin-Resistant Staphylococcus aureus. J. Ethnopharmacology 153, 587–595. doi:10.1016/j.jep.2014.02.044
Călina, D., Docea, A. O., Rosu, L., Zlatian, O., Rosu, A. F., Anghelina, F., et al. (2017). Antimicrobial Resistance Development Following Surgical Site Infections. Mol. Med. Rep. 15, 681–688. doi:10.3892/mmr.2016.6034
Calixto, J. B. (2000). Efficacy, Safety, Quality Control, Marketing and Regulatory Guidelines for Herbal Medicines (Phytotherapeutic Agents). Braz. J. Med. Biol. Res. 33, 179–189. doi:10.1590/s0100-879x2000000200004
Calnan, C. D. (1976). Cinnamon Dermatitis from an Ointment. Contact dermatitis 2, 167–170. doi:10.1111/j.1600-0536.1976.tb03018.x
Carson, C. F., Mee, B. J., and Riley, T. V. (2002). Mechanism of Action of Melaleuca Alternifolia (Tea Tree) Oil on Staphylococcus aureus Determined by Time-Kill, Lysis, Leakage, and Salt Tolerance Assays and Electron Microscopy. Aac 46, 1914–1920. doi:10.1128/aac.46.6.1914-1920.2002
Chakraborty, A., Sankaran, V., Sankaran, M. R., and Chellappan, D. R. (2015). Chemical Analysis of Leaf Essential Oil of Cinnamomum Verum from Palni Hills, Tamil Nadu. J. Chem. Pharm. Sci. 8, 476–479.
Chang, C.-W., Chang, W.-L., Chang, S.-T., and Cheng, S.-S. (2008). Antibacterial Activities of Plant Essential Oils against Legionella pneumophila. Water Res. 42, 278–286. doi:10.1016/j.watres.2007.07.008
Chang, S.-T., Chen, P.-F., and Chang, S.-C. (2001). Antibacterial Activity of Leaf Essential Oils and Their Constituents from Cinnamomum Osmophloeum. J. Ethnopharmacology 77, 123–127. doi:10.1016/s0378-8741(01)00273-2
Chao, S. C., Young, D. G., and Oberg, C. (2000). Screening for Inhibitory Activity of Essential Oils on Selected Bacteria, Fungi and Viruses. J. Essential Oil Res. 12 (5), 639–649. doi:10.1080/10412905.2000.9712177
Chen, C.-Y., Chen, C.-H., Lo, Y.-C., Wu, B.-N., Wang, H.-M., Lo, W.-L., et al. (2008). Anticancer Activity of Isoobtusilactone A fromCinnamomum Kotoense: Involvement of Apoptosis, Cell-Cycle Dysregulation, Mitochondria Regulation, and Reactive Oxygen Species. J. Nat. Prod. 71, 933–940. doi:10.1021/np070620e
Chen, C.-Y., Chen, C.-H., Wong, C.-H., Liu, Y.-W., Lin, Y.-S., Wang, Y.-D., et al. (2007). Cytotoxic Constituents of the Stems of Cinnamomum Subavenium. J. Nat. Prod. 70, 103–106. doi:10.1021/np060425k
Chen, G., Lu, F., Xu, L., Dong, H., Yi, P., Wang, F., et al. (2013). The Anti-diabetic Effects and Pharmacokinetic Profiles of Berberine in Mice Treated with Jiao-Tai-Wan and its Compatibility. Phytomedicine 20, 780–786. doi:10.1016/j.phymed.2013.03.004
Chen, L., Sun, P., Wang, T., Chen, K., Jia, Q., Wang, H., et al. (2012). Diverse Mechanisms of Antidiabetic Effects of the Different Procyanidin Oligomer Types of Two Different Cinnamon Species ondb/dbMice. J. Agric. Food Chem. 60, 9144–9150. doi:10.1021/jf3024535
Chen, Y., Ma, Y., and Ma, W. (2009). Pharmacokinetics and Bioavailability of Cinnamic Acid after Oral Administration of Ramulus Cinnamomi in Rats. Eur. J. Drug Metabol. Pharmacokinet. 34, 51–56. doi:10.1007/bf03191384
Cheng, D. M., Kuhn, P., Poulev, A., Rojo, L. E., Lila, M. A., and Raskin, I. (2012). In vivo and In Vitro Antidiabetic Effects of Aqueous Cinnamon Extract and Cinnamon Polyphenol-Enhanced Food Matrix. Food Chem. 135, 2994–3002. doi:10.1016/j.foodchem.2012.06.117
Chua, M.-T., Tung, Y.-T., and Chang, S.-T. (2008). Antioxidant Activities of Ethanolic Extracts from the Twigs of Cinnamomum Osmophloeum. Bioresour. Tech. 99, 1918–1925. doi:10.1016/j.biortech.2007.03.020
Cox, S., Mann, C., Markham, J., Gustafson, J., Warmington, J., and Wyllie, S. (2001). Determining the Antimicrobial Actions of Tea Tree Oil. Molecules 6, 87–91. doi:10.3390/60100087
Crawford, P. (2009). Effectiveness of Cinnamon for Lowering Hemoglobin A1C in Patients with Type 2 Diabetes: a Randomized, Controlled Trial. J. Am. Board Fam. Med. 22, 507–512. doi:10.3122/jabfm.2009.05.080093
Crews, R., Gomada, Y., Jamison, B., and Vattem, D. (2016). Molecular Effects of Cinnamon Bioactive Compounds for Neuroprotection in D. Melanogaster. FASEB J. 30, 692.
Daker, M., Lin, V. Y., Akowuah, G. A., Yam, M. F., and Ahmad, M. (2013). Inhibitory Effects of Cinnamomum Burmannii Blume Stem Bark Extract and Trans-cinnamaldehyde on Nasopharyngeal Carcinoma Cells; Synergism with Cisplatin. Exp. Ther. Med. 5, 1701–1709. doi:10.3892/etm.2013.1041
De Boer, H. J., Kool, A., Broberg, A., Mziray, W. R., Hedberg, I., and Levenfors, J. J. (2005). Anti-fungal and Anti-bacterial Activity of Some Herbal Remedies from Tanzania. J. Ethnopharmacology 96, 461–469. doi:10.1016/j.jep.2004.09.035
De Oliveira, M. M. M., Brugnera, D. F., Do Nascimento, J. A., and Piccoli, R. H. (2012). Control of Planktonic and Sessile Bacterial Cells by Essential Oils. Food Bioproducts Process. 90, 809–818. doi:10.1016/j.fbp.2012.03.002
Defronzo, R. A., Bonadonna, R. C., and Ferrannini, E. (1992). Pathogenesis of NIDDM: A Balanced Overview. Diabetes Care 15, 318–368. doi:10.2337/diacare.15.3.318
Deng, R. (2012). A Review of the Hypoglycemic Effects of Five Commonly Used Herbal Food Supplements. Fna 4, 50–60. doi:10.2174/1876142911204010050
Di Pasqua, R., Betts, G., Hoskins, N., Edwards, M., Ercolini, D., and Mauriello, G. (2007). Membrane Toxicity of Antimicrobial Compounds from Essential Oils. J. Agric. Food Chem. 55, 4863–4870. doi:10.1021/jf0636465
Eidi, A., Mortazavi, P., Bazargan, M., and Zaringhalam, J. (2012). Hepatoprotective Activity of Cinnamon Ethanolic Extract against CCI4-Induced Liver Injury in Rats. Excli j 11, 495–507.
Endo, H., and Rees, T. D. (2007). Cinnamon Products as a Possible Etiologic Factor in Orofacial Granulomatosis. Med. Oral Patol Oral Cir Bucal 12, E440–E444.
Eumkeb, G., Siriwong, S., and Thumanu, K. (2012). Synergistic Activity of Luteolin and Amoxicillin Combination against Amoxicillin-Resistant Escherichia coli and Mode of Action. J. Photochem. Photobiol. B: Biol. 117, 247–253. doi:10.1016/j.jphotobiol.2012.10.006
EUROPEAN SCIENTIFIC COOPERATIVE ON PHYTOTHERAPY (2003). ESCOP Monographs. 2nd ed. Amsterdam, Netherlands: World Press.
Fani, M. M., and Kohanteb, J. (2011). Inhibitory Activity of Cinnamon Zeylanicum and Eucalyptus Globulus Oils on Streptococcus Mutans, Staphylococcus Aureus, and Candida Species Isolated from Patients with Oral Infections. Shiraz Univ. Dent J. 11, 14–22.
Farahpour, M., and Habibi, M. (2012). Evaluation of the Wound Healing Activity of an Ethanolic Extract of Ceylon Cinnamon in Mice. Veterinarni Medicina 57, 53–57. doi:10.17221/4972-vetmed
Food and Administration 2005. Guidance for Industry: Estimating the Maximum Safe Dose in Initial Clinical Trials for Therapeutics in Adult Healthy Volunteers. Mitochondrion 9. 9–16. doi:10.1016/j.mito.2008.09.002
Garcez, F., Garcez, W., Martins, M., Matos, M., Guterres, Z., Mantovani, M., et al. (2005). Cytotoxic and Genotoxic Butanolides and Lignans fromAiouea Trinervis. Planta Med. 71, 923–927. doi:10.1055/s-2005-871251
Geng, S., Cui, Z., Huang, X., Chen, Y., Xu, D., and Xiong, P. (2011). Variations in Essential Oil Yield and Composition during Cinnamomum cassia Bark Growth. Ind. Crops Prod. 33, 248–252. doi:10.1016/j.indcrop.2010.10.018
Goyal, P., Chauhan, A., and Kaushik, P. (2009). Laboratory Evaluation of Crude Extracts of Cinnamomumtamala for Potential Antibacterial Activity. Electron. J. Biol. 5, 75–79.
Goyal, P., Khanna, A., Chauhan, A., Chauhan, G., and Kaushik, P. (2008). In vitro evaluation of Crude Extracts of Catharanthus Roseus for Potential Antibacterial Activity. Int. J. Green. Pharm. 2, 176–181. doi:10.4103/0973-8258.42739
Guerra, F. Q. S., Mendes, J. M., Sousa, J. P. d., Morais-Braga, M. F. B., Santos, B. H. C., Melo Coutinho, H. D., et al. (2012). Increasing Antibiotic Activity against a Multidrug-resistantAcinetobacterspp by Essential Oils ofCitrus limonandCinnamomum Zeylanicum. Nat. Product. Res. 26, 2235–2238. doi:10.1080/14786419.2011.647019
Gutierrez, J. L., Bowden, R. G., and Willoughby, D. S. (2016). CassiaCinnamon Supplementation Reduces Peak Blood Glucose Responses but Does Not Improve Insulin Resistance and Sensitivity in Young, Sedentary, Obese Women. J. Dietary Supplements 13, 461–471. doi:10.3109/19390211.2015.1110222
Hajimonfarednejad, M., Nimrouzi, M., Heydari, M., Zarshenas, M. M., Raee, M. J., and Jahromi, B. N. (2018a). Insulin Resistance Improvement by Cinnamon Powder in Polycystic Ovary Syndrome: A Randomized Double-Blind Placebo Controlled Clinical Trial. Phytotherapy Res. 32, 276–283. doi:10.1002/ptr.5970
Hajimonfarednejad, M., Ostovar, M., Raee, M. J., Hashempur, M. H., Mayer, J. G., and Heydari, M. (2018b). Cinnamon: A Systematic Review of Adverse Events. Clin. Nutr. 38. 594–602. doi:10.1016/j.clnu.2018.03.013
Hameed, I., Altameme, H., and Mohammed, G. (2016). Evaluation of Antifungal and Antibacterial Activity and Analysis of Bioactive Phytochemical Compounds of Cinnamomum Zeylanicum (Cinnamon Bark) Using Gas Chromatography-Mass Spectrometry. Orient. J. Chem. 32, 1769–1788. doi:10.13005/ojc/320406
Hanhineva, K., Törrönen, R., Bondia-Pons, I., Pekkinen, J., Kolehmainen, M., Mykkänen, H., et al. (2010). Impact of Dietary Polyphenols on Carbohydrate Metabolism. Ijms 11, 1365–1402. doi:10.3390/ijms11041365
Helander, I. M., Alakomi, H.-L., Latva-Kala, K., Mattila-Sandholm, T., Pol, I., Smid, E. J., et al. (1998). Characterization of the Action of Selected Essential Oil Components on Gram-Negative Bacteria. J. Agric. Food Chem. 46, 3590–3595. doi:10.1021/jf980154m
Ho, S.-C., Chang, K.-S., and Chang, P.-W. (2013). Inhibition of Neuroinflammation by Cinnamon and its Main Components. Food Chem. 138, 2275–2282. doi:10.1016/j.foodchem.2012.12.020
Hu, F. B. (2011). Globalization of Diabetes: the Role of Diet, Lifestyle, and Genes. Diabetes Care 34, 1249–1257. doi:10.2337/dc11-0442
Hu, T. W., Lin, Y. T., and Ho, C. K. (1985). Natural Variation of Chemicalcomponents of the Leaf Oil of Cinnamomum Osmophloeum Kaneh. Bull. Taiwan For. Res. Instustry New Ser. 78, 18.
Huang, D. F., Xu, J.-G., Liu, J.-X., Zhang, H., and Hu, Q. P. (2014). Chemical Constituents, Antibacterial Activity and Mechanism of Action of the Essential Oil from Cinnamomum cassia Bark against Four Food-Related Bacteria. Microbiology 83, 357–365. doi:10.1134/s0026261714040067
Isaac-Renton, M., Li, M. K., and Parsons, L. M. (2015). Cinnamon Spice and Everything Not Nice. Dermatitis 26, 116–121. doi:10.1097/der.0000000000000112
Jain, S., Sangma, T., Shukla, S. K., and Mediratta, P. K. (2015). Effect ofCinnamomum Zeylanicumextract on Scopolamine-Induced Cognitive Impairment and Oxidative Stress in Rats. Nutr. Neurosci. 18, 210–216. doi:10.1179/1476830514y.0000000113
Jang, H.-D., Chang, K.-S., Huang, Y.-S., Hsu, C.-L., Lee, S.-H., and Su, M.-S. (2007). Principal Phenolic Phytochemicals and Antioxidant Activities of Three Chinese Medicinal Plants. Food Chem. 103, 749–756. doi:10.1016/j.foodchem.2006.09.026
Jantan, I. B., and Goh, S. H. (1992). Essential Oils ofCinnamomumSpecies from Peninsular Malaysia. J. Essent. Oil Res. 4, 161–171. doi:10.1080/10412905.1992.9698038
Jantan, I. B., Yalvema, M. F., Ayop, N., and Ahmad, A. S. (2005). Constituents of the Essential Oils ofCinnamomum Sintoc Blume from a Mountain Forest of Peninsular Malaysia. Flavour Fragr. J. 20, 601–604. doi:10.1002/ffj.1495
Jantan, I., Ling, Y. E., Romli, S., Ayop, N., and Ahmad, A. S. (2003). A Comparative Study of the Constituents of the Essential Oils of ThreeCinnamomumSpecies from Malaysia. J. Essent. Oil Res. 15, 387–391. doi:10.1080/10412905.2003.9698618
Javed, I., Faisal, I., Rahman, Z., Khan, M. Z., Muhammad, F., Aslam, B., et al. (2012). Lipid Lowering Effect of Cinnamomum Zeylanicum in Hyperlipidaemic Albino Rabbits. Pak J. Pharm. Sci. 25, 141–147.
Jayaprakasha, G. K., Jagan Mohan Rao, L., and Sakariah, K. K. (2003). Volatile Constituents fromCinnamomum zeylanicumFruit Stalks and Their Antioxidant Activities. J. Agric. Food Chem. 51, 4344–4348. doi:10.1021/jf034169i
Jayaprakasha, G. K., Ohnishi-Kameyama, M., Ono, H., Yoshida, M., and Jaganmohan Rao, L. (2006). Phenolic Constituents in the Fruits ofCinnamomum Zeylanicumand Their Antioxidant Activity. J. Agric. Food Chem. 54, 1672–1679. doi:10.1021/jf052736r
Jia, Q., Liu, X., Wu, X., Wang, R., Hu, X., Li, Y., et al. (2009). Hypoglycemic Activity of a Polyphenolic Oligomer-Rich Extract of Cinnamomum Parthenoxylon Bark in Normal and Streptozotocin-Induced Diabetic Rats. Phytomedicine 16, 744–750. doi:10.1016/j.phymed.2008.12.012
Jiao, L., Zhang, X., Huang, L., Gong, H., Cheng, B., Sun, Y., et al. (2013). Proanthocyanidins Are the Major Anti-diabetic Components of Cinnamon Water Extract. Food Chem. Toxicol. 56, 398–405. doi:10.1016/j.fct.2013.02.049
Kahn, S. E., Hull, R. L., and Utzschneider, K. M. (2006). Mechanisms Linking Obesity to Insulin Resistance and Type 2 Diabetes. Nature 444, 840–846. doi:10.1038/nature05482
Kamath, J. V., Rana, A. C., and Roy Chowdhury, A. (2003). Pro-healing Effect ofCinnamomum Zeylanicum Bark. Phytother. Res. 17, 970–972. doi:10.1002/ptr.1293
Kamble, S., and Rambhimaiah, S. (2015). Antidiabetic Activity of Aqueous Extract of Cinnamomum cassia in Alloxan-Induced Diabetic Rats. Biomed. Pharmacol. J. 6, 83–88.
Kang, B.-H., Racicot, K., Pilkenton, S. J., and Apostolidis, E. (2014). Evaluation of the In Vitro Anti-hyperglycemic Effect of Cinnamomum cassia Derived Phenolic Phytochemicals, via Carbohydrate Hydrolyzing Enzyme Inhibition. Plant Foods Hum. Nutr. 69, 155–160. doi:10.1007/s11130-014-0415-z
Kannappan, S., Jayaraman, T., Rajasekar, P., Ravichandran, M. K., and Anuradha, C. V. (2006). Cinnamon Bark Extract Improves Glucose Metabolism and Lipid Profile in the Fructose-Fed Rat. Singapore Med. J. 47, 858–863.
Kar, A., Choudhary, B. K., and Bandyopadhyay, N. G. (2003). Comparative Evaluation of Hypoglycaemic Activity of Some Indian Medicinal Plants in Alloxan Diabetic Rats. J. Ethnopharmacology 84, 105–108. doi:10.1016/s0378-8741(02)00144-7
Kaul, P. N., Bhattacharya, A. K., Rajeswara Rao, B. R., Syamasundar, K. V., and Ramesh, S. (2003). Volatile Constituents of Essential Oils Isolated from Different Parts of Cinnamon (Cinnamomum Zeylanicum Blume). J. Sci. Food Agric. 83, 53–55. doi:10.1002/jsfa.1277
Kaur, R., and Shri, R. (2018). Role of the Genus Cinnamomum in the Management of Neurodegenerative Diseases: Outcomes and Shortcomings. Indian J. Pharm. Sci. 80 (6), 984–995. doi:10.4172/pharmaceutical-sciences.1000448
Khan, A. A., and Begum, W. (2019). Efficacy of Darchini in the Management of Polycystic Ovarian Syndrome: A Randomized Clinical Study. J. Herbal Med. 15, 100249. doi:10.1016/j.hermed.2018.11.005
Khan, A., Safdar, M., Ali Khan, M. M., Khattak, K. N., and Anderson, R. A. (2003). Cinnamon Improves Glucose and Lipids of People with Type 2 Diabetes. Diabetes care 26, 3215–3218. doi:10.2337/diacare.26.12.3215
Kim, D. H., Kim, C. H., Kim, M.-S., Kim, J. Y., Jung, K. J., Chung, J. H., et al. (2007). Suppression of Age-Related Inflammatory NF-Κb Activation by Cinnamaldehyde. Biogerontology 8, 545–554. doi:10.1007/s10522-007-9098-2
Kim, H.-O., Park, S.-W., and Park, H.-D. (2004). Inactivation of Escherichia coli O157:H7 by Cinnamic Aldehyde Purified from Cinnamomum cassia Shoot. Food Microbiol. 21, 105–110. doi:10.1016/s0740-0020(03)00010-8
Kim, S. H., Hyun, S. H., and Choung, S. Y. (2006). Antioxidative Effects ofCinnamomi cassiaeandRhodiola Roseaextracts in Liver of Diabetic Mice. Biofactors 26, 209–219. doi:10.1002/biof.5520260306
King, H., Aubert, R. E., and Herman, W. H. (1998). Global Burden of Diabetes, 1995-2025: Prevalence, Numerical Estimates, and Projections. Diabetes Care 21, 1414–1431. doi:10.2337/diacare.21.9.1414
Koppikar, S. J., Choudhari, A. S., Suryavanshi, S. A., Kumari, S., Chattopadhyay, S., and Kaul-Ghanekar, R. (2010). Aqueous Cinnamon Extract (ACE-C) from the Bark of Cinnamomum cassia Causes Apoptosis in Human Cervical Cancer Cell Line (SiHa) through Loss of Mitochondrial Membrane Potential. BMC Cancer 10, 210. doi:10.1186/1471-2407-10-210
Kort, D. H., and Lobo, R. A. (2014a). Preliminary Evidence that Cinnamon Improves Menstrual Cyclicity in Women with Polycystic Ovary Syndrome: a Randomized Controlled Trial. Am. J. Obstet. Gynecol. 211, 487–496. doi:10.1016/j.ajog.2014.05.009e1
Krishnakumar, I. M., Abin, I., Johannah, N. M., Eapen, N., Balu, M., and Ramadassan, K. (2014). Effects of the Polyphenol Content on the Anti-diabetic Activity of Cinnamomum Zeylanicum Extracts. Food Funct. 5, 2208–2220.
Kumar, S., Vasudeva, N., and Sharma, S. (2012). GC-MS Analysis and Screening of Antidiabetic, Antioxidant and Hypolipidemic Potential of Cinnamomum Tamala Oil in Streptozotocin Induced Diabetes Mellitus in Rats. Cardiovasc. Diabetol. 11, 95. doi:10.1186/1475-2840-11-95
Kumari, R., and Kotecha, M. (2016). A Review on the Standardization of Herbal Medicines. Int. J. Pharm. Sci. Res. 7, 97–106. doi:10.7897/2230-8407.07319
Kuo, S.-Y., Hsieh, T.-J., Wang, Y.-D., Lo, W.-L., Hsui, Y.-R., and Chen, C.-Y. (2008). Cytotoxic Constituents from the Leaves of Cinnamomum Subavenium. Chem. Pharm. Bull. 56, 97–101. doi:10.1248/cpb.56.97
Kwon, H.-K., Hwang, J.-S., So, J.-S., Lee, C.-G., Sahoo, A., Ryu, J.-H., et al. (2010). Cinnamon Extract Induces Tumor Cell Death through Inhibition of NFκB and AP1. BMC cancer 10, 392. doi:10.1186/1471-2407-10-392
Lambert, R. J. W., Skandamis, P. N., Coote, P. J., and Nychas, G.-J. E. (2001). A Study of the Minimum Inhibitory Concentration and Mode of Action of Oregano Essential Oil, Thymol and Carvacrol. J. Appl. Microbiol. 91, 453–462. doi:10.1046/j.1365-2672.2001.01428.x
Latta, C. H., Brothers, H. M., and Wilcock, D. M. (2015). Neuroinflammation in Alzheimer's Disease; A Source of Heterogeneity and Target for Personalized Therapy. Neuroscience 302, 103–111. doi:10.1016/j.neuroscience.2014.09.061
Lee, H.-S., and Ahn, Y.-J. (1998). Growth-Inhibiting Effects ofCinnamomum cassiaBark-Derived Materials on Human Intestinal Bacteria. J. Agric. Food Chem. 46, 8–12. doi:10.1021/jf970548y
Lee, H. J., Hyun, E.-A., Yoon, W. J., Kim, B. H., Rhee, M. H., Kang, H. K., et al. (2006). In vitro anti-inflammatory and Anti-oxidative Effects of Cinnamomum Camphora Extracts. J. Ethnopharmacology 103, 208–216. doi:10.1016/j.jep.2005.08.009
Lee, M.-G., Kuo, S.-Y., Yen, S.-Y., Hsu, H.-F., Leung, C.-H., Ma, D.-L., et al. (2015). Evaluation of Cinnamomum Osmophloeum Kanehira Extracts on Tyrosinase Suppressor, Wound Repair Promoter, and Antioxidant. Scientific World J. 7. 303415. doi:10.1155/2015/303415
Leela, N. K. (2008). “Cinnamon and cassia,” in Chemistry of Spices. Editors V. A. PARTHASARATHY, B. CHEMPAKAM, and T. J. ZACHARIAH (Wallingford, Oxfordshire, UK: CAB International).
Leiter, L., and Lewanczuk, R. (2005). Of the Renin-Angiotensin System and Reactive Oxygen speciesType 2 Diabetes and Angiotensin II Inhibition. Am. J. Hypertens. 18, 121–128. doi:10.1016/j.amjhyper.2004.07.001
Li, L., Li, Z. W., Yin, Z. Q., Wei, Q., Jia, R. Y., Zhou, L. J., et al. (2014). Antibacterial Activity of Leaf Essential Oil and its Constituents from Cinnamomum Longepaniculatum. Int. J. Clin. Exp. Med. 7, 1721–1727.
Liao, B.-C., Hsieh, C.-W., Liu, Y.-C., Tzeng, T.-T., Sun, Y.-W., and Wung, B.-S. (2008). Cinnamaldehyde Inhibits the Tumor Necrosis Factor-α-Induced Expression of Cell Adhesion Molecules in Endothelial Cells by Suppressing NF-Κb Activation: Effects upon IκB and Nrf2. Toxicol. Appl. Pharmacol. 229, 161–171. doi:10.1016/j.taap.2008.01.021
Liao, J.-C., Deng, J.-S., Chiu, C.-S., Hou, W.-C., Huang, S.-S., Shie, P.-H., et al. (2012a). Anti-inflammatory Activities of Cinnamomum cassia Constituents In Vitro and In Vivo. Evidence-Based Complement. Altern. Med. 2012, 429320. doi:10.1155/2012/429320
Liao, J. C., Deng, J. S., Chiu, C. S., Hou, W. C., Huang, S. S., Shie, P. H., et al. (2012b). Anti-Inflammatory Activities of Cinnamomum cassia Constituents In Vitro and In Vivo. Evid. Based Complement. Alternat Med. 2012, 429320. doi:10.1155/2012/429320
Lin, C.-C., Wu, S.-J., Chang, C.-H., and Ng, L.-T. (2003). Antioxidant Activity ofCinnamomum cassia. Phytother. Res. 17, 726–730. doi:10.1002/ptr.1190
Lin, G.-M., Chen, Y.-H., Yen, P.-L., and Chang, S.-T. (2016). Antihyperglycemic and Antioxidant Activities of Twig Extract from Cinnamomum Osmophloeum. J. Traditional Complement. Med. 6, 281–288. doi:10.1016/j.jtcme.2015.08.005
Lin, K., Yeh, S., Lin, M., Shih, M., Yang, K., and Hwang, S. (2007). Major Chemotypes and Antioxidative Activity of the Leaf Essential Oils of Cinnamomum Osmophloeum Kaneh. From a Clonal Orchard. Food Chem. 105, 133–139. doi:10.1016/j.foodchem.2007.03.051
Lin, R.-J., Cheng, M.-J., Huang, J.-C., Lo, W.-L., Yeh, Y.-T., Yen, C.-M., et al. (2009). Cytotoxic Compounds from the Stems ofCinnamomum Tenuifolium. J. Nat. Prod. 72, 1816–1824. doi:10.1021/np900225p
Loy, G., Cottiglia, F., Garau, D., Deidda, D., Pompei, R., and Bonsignore, L. (2001). Chemical Composition and Cytotoxic and Antimicrobial Activity of Calycotome Villosa (Poiret) Link Leaves. Il Farmaco 56, 433–436. doi:10.1016/s0014-827x(01)01056-4
Lu, Z., Jia, Q., Wang, R., Wu, X., Wu, Y., Huang, C., et al. (2011). Hypoglycemic Activities of A- and B-type Procyanidin Oligomer-Rich Extracts from Different Cinnamon Barks. Phytomedicine 18, 298–302. doi:10.1016/j.phymed.2010.08.008
Luscher, T. F., and Steffel, J. (2008). Sweet and Sour. Circ. Res. 102, 9–11. doi:10.1161/01.res.0000303937.73170.31
Mamindla, S., Srgp Koganti, V., Ravouru, N., and Koganti, B. (2017). Effect of Cinnamomum cassia on the Pharmacokinetics and Pharmacodynamics of Pioglitazone. Curr. Clin. Pharmacol. 12, 41–49. doi:10.2174/1574884712666170207152020
Mang, B., Wolters, M., Schmitt, B., Kelb, K., Lichtinghagen, R., Stichtenoth, D. O., et al. (2006). Effects of a Cinnamon Extract on Plasma Glucose, HbA1c, and Serum Lipids in Diabetes Mellitus Type 2. Eur. J. Clin. Invest. 36, 340–344. doi:10.1111/j.1365-2362.2006.01629.x
Markman, M. (2002). Safety Issues in Using Complementary and Alternative Medicine. J. Clin. Oncol. 20, 39S–41S.
Marles, R. J., and Farnsworth, N. R. (1995). Antidiabetic Plants and Their Active Constituents. Phytomedicine 2, 137–189. doi:10.1016/s0944-7113(11)80059-0
Mathew, S., and Abraham, T. E. (2006). Studies on the Antioxidant Activities of Cinnamon (Cinnamomum Verum) Bark Extracts, through Various In Vitro Models. Food Chem. 94, 520–528. doi:10.1016/j.foodchem.2004.11.043
Mbwambo, Z. H., Moshi, M. J., Masimba, P. J., Kapingu, M. C., and Nondo, R. S. (2007). Antimicrobial Activity and Brine Shrimp Toxicity of Extracts of Terminalia Brownii Roots and Stem. BMC Complement. Altern. Med. 7, 9. doi:10.1186/1472-6882-7-9
Miller, R. L., Gould, A. R., and Bernstein, M. L. (1992). Cinnamon-induced Stomatitis Venenata. Oral Surg. Oral Med. Oral Pathol. 73, 708–716. doi:10.1016/0030-4220(92)90016-j
Mishra, A., Bhatti, R., Singh, A., and Singh Ishar, M. (2010). Ameliorative Effect of the Cinnamon Oil fromCinnamomum Zeylanicumupon Early Stage Diabetic Nephropathy. Planta Med. 76, 412–417. doi:10.1055/s-0029-1186237
Moselhy, S. S., and Ali, H. K. (2009). Hepatoprotective Effect of Cinnamon Extracts against Carbon Tetrachloride Induced Oxidative Stress and Liver Injury in Rats. Biol. Res. 42, 93–98. doi:10.4067/s0716-97602009000100009
Ng, L. T., and Wu, S. J. (2011). Antiproliferative Activity of Cinnamomum cassia Constituents and Effects of Pifithrin-Alpha on Their Apoptotic Signaling Pathways in Hep G2 Cells. Evid. Based Complement. Alternat Med. 2011, 492148. doi:10.1093/ecam/nep220
Nir, Y., Potasman, I., Stermer, E., Tabak, M., and Neeman, I. (2000). Controlled Trial of the Effect of Cinnamon Extract on Helicobacter pylori. Helicobacter 5, 94–97. doi:10.1046/j.1523-5378.2000.00014.x
Noudeh, G. D., Sharififar, F., Noodeh, A. D., Moshafi, M. H., Afzadi, M. A., Behravan, E., et al. (2010). Antitumor and Antibacterial Activity of Four Fractions from Heracleumpersicum Desf. And Cinnamomum Zeylanicum Blume. J. Med. Plants Res. 4, 2176–2180.
Nussbaum, L., Hogea, L. M., Călina, D., Andreescu, N., Grădinaru, R., S,tefănescu, R., et al. (2017). Modern Treatment Approaches in Psychoses. Pharmacogenetic, Neuroimagistic and Clinical Implications. Farmacia 65, 75–81.
Nyadjeu, P., Dongmo, A., Nguelefack, T. B., and Kamanyi, A. (2011). Antihypertensive and Vasorelaxant Effects of Cinnamomum Zeylanicum Stem Bark Aqueous Extract in Rats. J. Complement. Integr. Med. 8. doi:10.2202/1553-3840.1490
Ooi, L. S. M., Li, Y., Kam, S.-L., Wang, H., Wong, E. Y. L., and Ooi, V. E. C. (2006). Antimicrobial Activities of Cinnamon Oil and Cinnamaldehyde from the Chinese Medicinal HerbCinnamomum cassiaBlume. Am. J. Chin. Med. 34, 511–522. doi:10.1142/s0192415x06004041
ORGANIZATION (2001). Joint FAO/WHO Expert Committee on Food Additives. Safety evaluation of certain food additives and contaminants in food: Fumonisins. Proceedings of the 56th Meeting of the Joint FAO/WHO Expert Committee on Food Additives, 103–279.
Ortiz-Andrade, R. R., García-Jiménez, S., Castillo-España, P., Ramírez-Ávila, G., Villalobos-Molina, R., and Estrada-Soto, S. (2007). α-Glucosidase Inhibitory Activity of the Methanolic Extract from Tournefortia Hartwegiana: An Anti-hyperglycemic Agent. J. Ethnopharmacology 109, 48–53. doi:10.1016/j.jep.2006.07.002
Oussalah, M., Caillet, S., Saucier, L., and Lacroix, M. (2006). Antimicrobial Effects of Selected Plant Essential Oils on the Growth of a Pseudomonas Putida Strain Isolated from Meat. Meat Sci. 73, 236–244. doi:10.1016/j.meatsci.2005.11.019
Padureanu, R., Albu, C. V., Mititelu, R. R., Bacanoiu, M. V., Docea, A. O., Calina, D., et al. (2019). Oxidative Stress and Inflammation Interdependence in Multiple Sclerosis. Jcm 8, 1815. doi:10.3390/jcm8111815
Panickar, K. S., Polansky, M. M., and Anderson, R. A. (2009). Cinnamon Polyphenols Attenuate Cell Swelling and Mitochondrial Dysfunction Following Oxygen-Glucose Deprivation in Glial Cells. Exp. Neurol. 216, 420–427. doi:10.1016/j.expneurol.2008.12.024
Panickar, K. S., Polansky, M. M., Graves, D. J., Urban, J. F., and Anderson, R. A. (2012). A Procyanidin Type A Trimer from Cinnamon Extract Attenuates Glial Cell Swelling and the Reduction in Glutamate Uptake Following Ischemia-like Injury In Vitro. Neuroscience 202, 87–98. doi:10.1016/j.neuroscience.2011.11.051
Parseh, S., Shakerian, S., and Alizadeh, A. A. (2019). Effect of Chronic Aerobic/Resistive Exercises with Supplementation of Cinnamon on Insulin Resistance in Women with Polycystic Ovary Syndrome in Ahvaz City in 2017. J. Arak Univ. Med. Sci. 22, 15–26.
Peng, X., Cheng, K.-W., Ma, J., Chen, B., Ho, C.-T., Lo, C., et al. (2008). Cinnamon Bark Proanthocyanidins as Reactive Carbonyl Scavengers to Prevent the Formation of Advanced Glycation Endproducts. J. Agric. Food Chem. 56, 1907–1911. doi:10.1021/jf073065v
Peterson, D. W., George, R. C., Scaramozzino, F., Lapointe, N. E., Anderson, R. A., Graves, D. J., et al. (2009). Cinnamon Extract Inhibits Tau Aggregation Associated with Alzheimer's Disease In Vitro. Jad 17, 585–597. doi:10.3233/jad-2009-1083
Prasad, K. N., Yang, B., Dong, X., Jiang, G., Zhang, H., Xie, H., et al. (2009). Flavonoid Contents and Antioxidant Activities from Cinnamomum Species. Innovative Food Sci. Emerging Tech. 10, 627–632. doi:10.1016/j.ifset.2009.05.009
Pyo, J.-H., Jeong, Y.-K., Yeo, S., Lee, J.-H., Jeong, M.-Y., Kim, S.-H., et al. (2013). Neuroprotective Effect of Trans-cinnamaldehyde on the 6-Hydroxydopamine-Induced Dopaminergic Injury. Biol. Pharm. Bull. 36, 1928–1935. doi:10.1248/bpb.b13-00537
Qin, B., Panickar, K. S., and Anderson, R. A. (2014). Cinnamon Polyphenols Regulate S100β, Sirtuins, and Neuroactive Proteins in Rat C6 Glioma Cells. Nutrition 30, 210–217. doi:10.1016/j.nut.2013.07.001
Qin, B., Panickar, K. S., and Anderson, R. A. (2010). Cinnamon: Potential Role in the Prevention of Insulin Resistance, Metabolic Syndrome, and Type 2 Diabetes. J. Diabetes Sci. Technol. 4, 685–693. doi:10.1177/193229681000400324
Radhia, K., Zakkia, K., and Shah, S. H. (2010). Cinnamon May Reduce Glucose, Lipid and Cholesterol Level in Type 2 Diabetic Individuals. Pakistan J. Nutr. 9, 430–433.
Raina, V. K., Srivastava, S. K., Aggarwal, K. K., Ramesh, S., and Kumar, S. (2001). Essential Oil Composition ofCinnamomum Zeylanicum Blume Leaves from Little Andaman, India. Flavour Fragr. J. 16, 374–376. doi:10.1002/ffj.1016
Rajbir, B., Kaur, S., and Singh, J.ISHARMPS (2009). Ameliorative Effect of Volatile Oil from Cinnamomum Zeylanicum on Hyperalgesia in Alloxan Diabetic Rats. Can. J. Pure Applsci 3, 887–895.
Rameshkumar, K. B., George, V., and Shiburaj, S. (2007). Chemical Constituents and Antibacterial Activity of the Leaf Oil ofCinnamomum chemungianumMohan et Henry. J. Essent. Oil Res. 19, 98–100. doi:10.1080/10412905.2007.9699238
Ranasinghe, P., Jayawardana, R., Galappaththy, P., Constantine, G. R., De Vas Gunawardana, N., and Katulanda, P. (2012). Efficacy and Safety of 'true' cinnamon(Cinnamomum Zeylanicum)as a Pharmaceutical Agent in Diabetes: a Systematic Review and Meta-Analysis. Diabet Med. 29, 1480–1492. doi:10.1111/j.1464-5491.2012.03718.x
Ranilla, L. G., Kwon, Y.-I., Apostolidis, E., and Shetty, K. (2010). Phenolic Compounds, Antioxidant Activity and In Vitro Inhibitory Potential against Key Enzymes Relevant for Hyperglycemia and Hypertension of Commonly Used Medicinal Plants, Herbs and Spices in Latin America. Bioresour. Tech. 101, 4676–4689. doi:10.1016/j.biortech.2010.01.093
Ranjbar, A., Ghaseminejhad, S., Takalu, H., Baiaty, A., Rahimi, F., and Abdollahi, M. (2007). Anti Oxidative Stress Potential of Cinnamon (Cinnamomum Zeylanicum) in Operating Room Personnel; A Before/After Cross Sectional Clinical Trial. Int. J. Pharmacol. 3, 482–486.
Rao, H. J.LAKSHMI (2012). Anti-diarrhoeal Activity of the Aqueous Extract of the Bark of Cinnamomum Zeylanicum Linn in Mice. J. Clin. Diag Res. 6, 215–219.
Rao, P. V., and Gan, S. H. (2014). Cinnamon: A Multifaceted Medicinal Plant. Evidence-Based Complement. Altern. Med. 2014, 642942. doi:10.1155/2014/642942
Rasheed, M. U., and Thajuddin, N. (2011). Effect of Medicinal Plants on Moraxella Cattarhalis. Asian Pac. J. Trop. Med. 4, 133–136. doi:10.1016/S1995-7645(11)60053-9
Rashidi, M., Malekirad, A. A., Abdollahi, M., Habibollahi, S., Dolatyari, N., and Narimani, M. (2014). The Effect of Tea-Cinnamon and Melissa Officinalis L. Aqueous Extraction, on Neuropsychology Distress, Biochemical and Oxidative Stress Biomarkers in Glass Production Workers. Health 06, 2592–2601. doi:10.4236/health.2014.619298
Ravindran, P., Nirmal-Babu, K., and Shylaja, M. (2003). Cinnamon and cassia: The Genus Cinnamomum. CRC Press.
Rhayour, K., Bouchikhi, T., Tantaoui-Elaraki, A., Sendide, K., and Remmal, A. (2003). The Mechanism of Bactericidal Action of Oregano and Clove Essential Oils and of Their Phenolic Major Components onEscherichia coliandBacillus Subtilis. J. Essent. Oil Res. 15 (4), 286–292. doi:10.1080/10412905.2003.9712144
Ribeiro-Santos, R., Andrade, M., Madella, D., Martinazzo, A. P., de Aquino Garcia Moura, L., De Melo, N. R., et al. (2017). Revisiting an Ancient Spice with Medicinal Purposes: Cinnamon. Trends Food Sci. Tech. 62, 154–169. doi:10.1016/j.tifs.2017.02.011
Rogoveanu, O. C., Calina, D., Cucu, M. G., Burada, F., Docea, A. O., Sosoi, S., et al. (2018). Association of Cytokine Gene Polymorphisms with Osteoarthritis Susceptibility. Exp. Ther. Med. 16, 2659–2664. doi:10.3892/etm.2018.6477
Ross, M. S. F. (1976). Analysis of Cinnamon Oils by High-Pressure Liquid Chromatography. J. Chromatogr. A 118, 273–275. doi:10.1016/s0021-9673(00)81222-4
Rossi, C., Chaves-López, C., Možina, S. S., Di Mattia, C., Scuota, S., Luzzi, I., et al. (2019). Salmonella enterica Adhesion: Effect of Cinnamomum Zeylanicum Essential Oil on Lettuce. LWT 111, 16–22. doi:10.1016/j.lwt.2019.05.026
Roussel, A.-M., Hininger, I., Benaraba, R., Ziegenfuss, T. N., and Anderson, R. A. (2009). Antioxidant Effects of a Cinnamon Extract in People with Impaired Fasting Glucose that Are Overweight or Obese. J. Am. Coll. Nutr. 28, 16–21. doi:10.1080/07315724.2009.10719756
Russell, A. D. (2002). Antibiotic and Biocide Resistance in Bacteria: Introduction. J. Appl. Microbiol. 92 (Suppl. l), 1s–3s. doi:10.1046/j.1365-2672.92.5s1.14.x
Sadeghi, S., Davoodvandi, A., Pourhanifeh, M. H., Sharifi, N., Arefnezhad, R., Sahebnasagh, R., et al. (2019). Anti-cancer Effects of Cinnamon: Insights into its Apoptosis Effects. Eur. J. Med. Chem. 178, 131–140. doi:10.1016/j.ejmech.2019.05.067
Salehi, B., Capanoglu, E., Adrar, N., Catalkaya, G., Shaheen, S., Jaffer, M., et al. (2019a). Cucurbits Plants: A Key Emphasis to its Pharmacological Potential. Molecules 24, 1854. doi:10.3390/molecules24101854
Salehi, B., Jornet, P. L., Lopez, E. P. F., Calina, D., Sharifi-Rad, M., Ramirez-Alarcon, K., et al. (2019b). Plant-Derived Bioactives in Oral Mucosal Lesions: A Key Emphasis to Curcumin, Lycopene, Chamomile, Aloe Vera, Green Tea and Coffee Properties. Biomolecules 9, 23. doi:10.3390/biom9030106
Salehi, B., Rescigno, A., Dettori, T., Calina, D., Docea, A. O., Singh, L., et al. (2020a). Avocado-Soybean Unsaponifiables: A Panoply of Potentialities to Be Exploited. Biomolecules 10, 130. doi:10.3390/biom10010130
Salehi, B., Sharifi-Rad, J., Capanoglu, E., Adrar, N., Catalkaya, G., Shaheen, S., et al. (2019c). Cucurbita Plants: From Farm to Industry. Appl. Sci. 9, 3387. doi:10.3390/app9163387
Salehi, B., Sharifi-Rad, J., Cappellini, F., Reiner, A., Zorzan, D., Imran, M., et al. (2020b). The Therapeutic Potential of Anthocyanins: Current Approaches Based on Their Molecular Mechanism of Action. Front. Pharmacol. 11, 20. doi:10.3389/fphar.2020.01300
Salehi, B., Shivaprasad Shetty, M., V. Anil Kumar, N., Živković, J., Calina, D., Oana Docea, A., et al. (2019d). Veronica Plants-Drifting from Farm to Traditional Healing, Food Application, and Phytopharmacology. Molecules 24, 2454. doi:10.3390/molecules24132454
Samarasekera, R., Kalhari, K. S., and Weerasinghe, I. S. (2005). Mosquitocidal Activity of Leaf and Bark Essential Oils of CeylonCinnamomum Zeylanicum. J. Essent. Oil Res. 17, 301–303. doi:10.1080/10412905.2005.9698909
Senanayake, U. M., Lee, T. H., and Wills, R. B. H. (1978). Volatile Constituents of Cinnamon (Cinnamomum Zeylanicum) Oils. J. Agric. Food Chem. 26, 822–824. doi:10.1021/jf60218a031
Shan, B., Cai, Y.-Z., Brooks, J. D., and Corke, H. (2007). Antibacterial Properties and Major Bioactive Components of Cinnamon Stick (Cinnamomum Burmannii): Activity against Foodborne Pathogenic Bacteria. J. Agric. Food Chem. 55, 5484–5490. doi:10.1021/jf070424d
Sharifi-Rad, J., Rodrigues, C. F., Sharopov, F., Docea, A. O., Karaca, A. C., Sharifi-Rad, M., et al. (2020a). Diet, Lifestyle and Cardiovascular Diseases: Linking Pathophysiology to Cardioprotective Effects of Natural Bioactive Compounds. Int. J. Environ. Res. Public Health 17, 31. doi:10.3390/ijerph17072326
Sharifi-Rad, M., Kumar, N. V. A., Zucca, P., Varoni, E. M., Dini, L., Panzarini, E., et al. (2020b). Lifestyle, Oxidative Stress, and Antioxidants: Back and Forth in the Pathophysiology of Chronic Diseases. Front. Physiol. 11, 21. doi:10.3389/fphys.2020.00694
Shen, Y., Fukushima, M., Ito, Y., Muraki, E., Hosono, T., Seki, T., et al. (2010). Verification of the Antidiabetic Effects of Cinnamon (Cinnamomum Zeylanicum) Using Insulin-Uncontrolled Type 1 Diabetic Rats and Cultured Adipocytes. Biosci. Biotechnol. Biochem. 74, 2418–2425. doi:10.1271/bbb.100453
Shihabudeen, H. M. S., Priscilla, D. H., and Thirumurugan, K. (2011). Cinnamon Extract Inhibits Alpha-Glucosidase Activity and Dampens Postprandial Glucose Excursion in Diabetic Rats. Nutr. Metab. (Lond) 8, 46.
Shishehbor, F., Rezaeyan Safar, M., Rajaei, E., and Haghighizadeh, M. H. (2018). Cinnamon Consumption Improves Clinical Symptoms and Inflammatory Markers in Women with Rheumatoid Arthritis. J. Am. Coll. Nutr. 37, 685–690. doi:10.1080/07315724.2018.1460733
Singh, G., Maurya, S., Delampasona, M. P., and Catalan, C. A. N. (2007). A Comparison of Chemical, Antioxidant and Antimicrobial Studies of Cinnamon Leaf and Bark Volatile Oils, Oleoresins and Their Constituents. Food Chem. Toxicol. 45, 1650–1661. doi:10.1016/j.fct.2007.02.031
Skandamis, P. N., and Nychas, G.-J. E. (2001). Effect of Oregano Essential Oil on Microbiological and Physico-Chemical Attributes of Minced Meat Stored in Air and Modified Atmospheres. J. Appl. Microbiol. 91, 1011–1022. doi:10.1046/j.1365-2672.2001.01467.x
Stalnikowitz, D. K., and Weissbrod, A. B. (2003). Liver Fibrosis and Inflammation. A Review. Ann. Hepatol. 2, 159–163. doi:10.1016/s1665-2681(19)32127-1
Subash Babu, P., Prabuseenivasan, S., and Ignacimuthu, S. (2007). Cinnamaldehyde-A Potential Antidiabetic Agent. Phytomedicine 14, 15–22. doi:10.1016/j.phymed.2006.11.005
Suppapitiporn, S., and Kanpaksi, N. (2006). The Effect of Cinnamon cassia Powder in Type 2 Diabetes Mellitus. J. Med. Assoc. Thai 89 Suppl 3, S200–S205.
Takasao, N., Tsuji-Naito, K., Ishikura, S., Tamura, A., and Akagawa, M. (2012). Cinnamon Extract Promotes Type I Collagen Biosynthesis via Activation of IGF-I Signaling in Human Dermal Fibroblasts. J. Agric. Food Chem. 60, 1193–1200. doi:10.1021/jf2043357
Talaat, B., and Ammar, I. M. M. (2018). The Added Value of Cinnamon to Metformin in Controlling Symptoms of Polycystic Ovary Syndrome, a Randomized Controlled Trial. Middle East Fertil. Soc. J. 23, 440–445. doi:10.1016/j.mefs.2018.03.005
Tao, Y., Xu, X., Yan, J., and Cai, B. (2019). A Sensitive UPLC–MS/MS Method for Simultaneous Determination of Polyphenols in Rat Plasma: Application to a Pharmacokinetic Study of Dispensing Granules and Standard Decoction of Cinnamomum cassia Twigs. Biomed. Chromatogr. 33, e4534. doi:10.1002/bmc.4534
Thongson, C., Davidson, P. M., Mahakarnchanakul, W., and Weiss, J. (2004). Antimicrobial Activity of Ultrasound-Assisted Solvent-Extracted Spices. Lett. Appl. Microbiol. 39, 401–406. doi:10.1111/j.1472-765x.2004.01605.x
Tsai, I.-L., Hung, C.-H., Duh, C.-Y., and Chen, I.-S. (2002). Cytotoxic Butanolides and Secobutanolides from the Stem Wood of Formosan Lindera Communis. Planta Med. 68, 142–145. doi:10.1055/s-2002-20260
Tsatsakis, A., Docea, A. O., Calina, D., Tsarouhas, K., Zamfira, L.-M., Mitrut, R., et al. (2019). A Mechanistic and Pathophysiological Approach for Stroke Associated with Drugs of Abuse. Jcm 8, 1295. doi:10.3390/jcm8091295
Tsuji-Naito, K. (2008). Aldehydic Components of Cinnamon Bark Extract Suppresses RANKL-Induced Osteoclastogenesis through NFATc1 Downregulation. Bioorg. Med. Chem. 16, 9176–9183. doi:10.1016/j.bmc.2008.09.036
Tung, Y.-T., Yen, P.-L., Lin, C.-Y., and Chang, S.-T. (2010). Anti-inflammatory Activities of Essential Oils and Their Constituents from Different Provenances of Indigenous Cinnamon (Cinnamomum Osmophloeum) Leaves. Pharm. Biol. 48, 1130–1136. doi:10.3109/13880200903527728
Turgis, M., Vu, K. D., Dupont, C., and Lacroix, M. (2012). Combined Antimicrobial Effect of Essential Oils and Bacteriocins against Foodborne Pathogens and Food Spoilage Bacteria. Food Res. Int. 48, 696–702. doi:10.1016/j.foodres.2012.06.016
Ultee, A., Kets, E. P. W., and Smid, E. J. (1999). Mechanisms of Action of Carvacrol on the Food-Borne Pathogen Bacillus Cereus. Appl. Environ. Microbiol. 65, 4606–4610. doi:10.1128/aem.65.10.4606-4610.1999
Ungureanu, A., Zlatian, O., Mitroi, G., Drocaş, A., Ţîrcă, T., Călina, D., et al. (2017). Staphylococcus aureus Colonisation in Patients from a Primary Regional Hospital. Mol. Med. Rep. 16, 8771–8780. doi:10.3892/mmr.2017.7746
Unlu, M., Ergene, E., Unlu, G. V., Zeytinoglu, H. S., and Vural, N. (2010). Composition, Antimicrobial Activity and In Vitro Cytotoxicity of Essential Oil from Cinnamomum Zeylanicum Blume (Lauraceae). Food Chem. Toxicol. 48, 3274–3280. doi:10.1016/j.fct.2010.09.001
Utchariyakiat, I., Surassmo, S., Jaturanpinyo, M., Khuntayaporn, P., and Chomnawang, M. T. (2016). Efficacy of Cinnamon Bark Oil and Cinnamaldehyde on Anti-multidrug Resistant Pseudomonas aeruginosa and the Synergistic Effects in Combination with Other Antimicrobial Agents. BMC Complement. Altern. Med. 16, 158. doi:10.1186/s12906-016-1134-9
Vafa, M., Mohammadi, F., Shidfar, F., Sormaghi, M. S., Heidari, I., Golestan, B., et al. (2012). Effects of Cinnamon Consumption on Glycemic Status, Lipid Profile and Body Composition in Type 2 Diabetic Patients. Int. J. Prev. Med. 3, 531–536.
Vallianou, N., Tsang, C., Taghizadeh, M., Davoodvandi, A., and Jafarnejad, S. (2019). Effect of Cinnamon (Cinnamomum Zeylanicum) Supplementation on Serum C-Reactive Protein Concentrations: A Meta-Analysis and Systematic Review. Complement. therapies Med. 42, 271–278. doi:10.1016/j.ctim.2018.12.005
Vallverdú-Queralt, A., Regueiro, J., Martínez-Huélamo, M., Rinaldi Alvarenga, J. F., Leal, L. N., and Lamuela-Raventos, R. M. (2014). A Comprehensive Study on the Phenolic Profile of Widely Used Culinary Herbs and Spices: Rosemary, Thyme, Oregano, Cinnamon, Cumin and Bay. Food Chem. 154, 299–307. doi:10.1016/j.foodchem.2013.12.106
Vanschoonbeek, K., Thomassen, B. J. W., Senden, J. M., Wodzig, W. K. W. H., and Van Loon, L. J. C. (2006). Cinnamon Supplementation Does Not Improve Glycemic Control in Postmenopausal Type 2 Diabetes Patients. J. Nutr. 136, 977–980. doi:10.1093/jn/136.4.977
Verspohl, E. J., Bauer, K., and Neddermann, E. (2005). Antidiabetic Effect ofCinnamomum cassia andCinnamomum Zeylanicum In vivo andIn Vitro. Phytother. Res. 19, 203–206. doi:10.1002/ptr.1643
Vivas, A. P. M., and Migliari, D. A. (2015). Cinnamon-induced Oral Mucosal Contact Reaction. Todentj 9, 257–259. doi:10.2174/1874210601509010257
Walanj, S., Walanj, A., Mohan, V., and Thakurdesai, P. A. (2014). Efficacy and Safety of the Topical Use of Intranasal Cinnamon Bark Extract in Seasonal Allergic Rhinitis Patients: A Double-Blind Placebo-Controlled Pilot Study. J. Herbal Med. 4, 37–47. doi:10.1016/j.hermed.2013.12.002
Wang, H.-M., Chen, C.-Y., and Wen, Z.-H. (2011). Identifying Melanogenesis Inhibitors from Cinnamomum Subavenium with In Vitro and In Vivo Screening Systems by Targeting the Human Tyrosinase. Exp. Dermatol. 20, 242–248. doi:10.1111/j.1600-0625.2010.01161.x
Wang, J., Su, B., Jiang, H., Cui, N., Yu, Z., Yang, Y., et al. (2020). Traditional Uses, Phytochemistry and Pharmacological Activities of the Genus Cinnamomum (Lauraceae): A Review. Fitoterapia 146, 104675. doi:10.1016/j.fitote.2020.104675
Wang, R., Wang, R., and Yang, B. (2009). Extraction of Essential Oils from Five Cinnamon Leaves and Identification of Their Volatile Compound Compositions. Innovative Food Sci. Emerging Tech. 10, 289–292. doi:10.1016/j.ifset.2008.12.002
Wannissorn, B., Jarikasem, S., Siriwangchai, T., and Thubthimthed, S. (2005). Antibacterial Properties of Essential Oils from Thai Medicinal Plants. Fitoterapia 76, 233–236. doi:10.1016/j.fitote.2004.12.009
Wansi, S. L., Nyadjeu, P., Ngamga, D., Mbuyo, E. P. N., Nguelefack, T. B., and Kamanyi, A. (2007). Blood Pressure Lowering Effect of the Ethanol Extract from the Stembark of Cinnamomum Zeylanicum (Lauraceae) in Rats. Pharmacol. Online 3, 166–176.
Wild, S., Roglic, G., Green, A., Sicree, R., and King, H. (2004). Global Prevalence of Diabetes: Estimates for the Year 2000 and Projections for 2030. Diabetes Care 27, 1047–1053. doi:10.2337/diacare.27.5.1047
Wiweko, B., and Susanto, C. A. (2017). The Effect of Metformin and Cinnamon on Serum Anti-mullerian Hormone in Women Having PCOS: A Double-Blind, Randomized, Controlled Trial. J. Hum. Reprod. Sci. 10, 31–36. doi:10.4103/jhrs.JHRS_90_16
Woehrlin, F., Fry, H., Abraham, K., and Preiss-Weigert, A. (2010). Quantification of Flavoring Constituents in Cinnamon: High Variation of Coumarin in cassia Bark from the German Retail Market and in Authentic Samples from Indonesia. J. Agric. Food Chem. 58, 10568–10575. doi:10.1021/jf102112p
Wondrak, G., Villeneuve, N. F., Lamore, S. D., Bause, A. S., Jiang, T., and Zhang, D. D. (2010). The Cinnamon-Derived Dietary Factor Cinnamic Aldehyde Activates the Nrf2-dependent Antioxidant Response in Human Epithelial Colon Cells. Molecules 15, 3338–3355. doi:10.3390/molecules15053338
Wu, K., Lin, Y., Chai, X., Duan, X., Zhao, X., and Chun, C. (2019). Mechanisms of Vapor-phase Antibacterial Action of Essential Oil from Cinnamomum Camphora Var. Linaloofera Fujita against Escherichia coli. Food Sci. Nutr. 7, 2546–2555. doi:10.1002/fsn3.1104
Wu, V., Qiu, X., Delosreyes, B., Lin, C., and Pan, Y. (2009). Application of Cranberry Concentrate (Vaccinium Macrocarpon) to Control Escherichia coli O157:H7 in Ground Beef and its Antimicrobial Mechanism Related to the Downregulated Slp, hdeA and Cfa. Food Microbiol. 26, 32–38. doi:10.1016/j.fm.2008.07.014
Yanakiev, S. (2020). Effects of Cinnamon (Cinnamomum spp.) in Dentistry: A Review. Molecules 25. doi:10.3390/molecules25184184
Yang, C.-H., Li, R.-X., and Chuang, L.-Y. (2012). Antioxidant Activity of Various Parts of Cinnamomum cassia Extracted with Different Extraction Methods. Molecules 17, 7294–7304. doi:10.3390/molecules17067294
Yang, S. Y., Wang, H. M., Wu, T. W., Chen, Y. J., Shieh, J. J., Lin, J. H., et al. (2013). Subamolide B Isolated from Medicinal Plant Cinnamomum Subavenium Induces Cytotoxicity in Human Cutaneous Squamous Cell Carcinoma Cells through Mitochondrial and CHOP-dependent Cell Death Pathways. Evid. Based Complement. Alternat Med. 2013, 630415. doi:10.1155/2013/630415
Yang, Y.-C., Lee, H.-S., Lee, S. H., Clark, J. M., and Ahn, Y.-J. (2005). Ovicidal and Adulticidal Activities of Cinnamomum Zeylanicum Bark Essential Oil Compounds and Related Compounds against Pediculus Humanus Capitis (Anoplura: Pediculicidae). Int. J. Parasitol. 35, 1595–1600. doi:10.1016/j.ijpara.2005.08.005
Yap, P. S. X., Krishnan, T., Chan, K.-G., and Lim, S. H. E. (2015). Antibacterial Mode of Action of Cinnamomum Verum Bark Essential Oil, Alone and in Combination with Piperacillin, against a Multi-Drug-Resistant Escherichia coli Strain. J. Microbiol. Biotechnol. 25, 1299–1306. doi:10.4014/jmb.1407.07054
Yeh, R.-Y., Shiu, Y.-L., Shei, S.-C., Cheng, S.-C., Huang, S.-Y., Lin, J.-C., et al. (2009). Evaluation of the Antibacterial Activity of Leaf and Twig Extracts of Stout Camphor Tree, Cinnamomum Kanehirae, and the Effects on Immunity and Disease Resistance of White Shrimp, Litopenaeus Vannamei. Fish Shellfish Immunol. 27, 26–32. doi:10.1016/j.fsi.2008.11.008
Yun, J.-W., You, J.-R., Kim, Y.-S., Kim, S.-H., Cho, E.-Y., Yoon, J.-H., et al. (2018). In vitro and In Vivo Safety Studies of Cinnamon Extract ( Cinnamomum cassia ) on General and Genetic Toxicology. Regul. Toxicol. Pharmacol. 95, 115–123. doi:10.1016/j.yrtph.2018.02.017
Zhang, J.-H., Liu, L.-Q., He, Y.-L., Kong, W.-J., and Huang, S.-A. (2010). Cytotoxic Effect of Trans-cinnamaldehyde on Human Leukemia K562 Cells. Acta Pharmacol. Sin 31, 861–866. doi:10.1038/aps.2010.76
Zhang, W., Xu, Y.-c., Guo, F.-j., Meng, Y., and Li, M.-l. (2008). Anti-diabetic Effects of Cinnamaldehyde and Berberine and Their Impacts on Retinol-Binding Protein 4 Expression in Rats with Type 2 Diabetes Mellitus. Chin. Med. J. 121, 2124–2128. doi:10.1097/00029330-200811010-00003
Zhao, J., and Ma, J.-S. (2016). Phytochemicals and Biological Activities of Genus Cinnamomum. Res. Rev. J. Pharmacognosy Phytochemistry 4, 27–34.
Zimmet, P., Alberti, K. G. M. M., and Shaw, J. (2001). Global and Societal Implications of the Diabetes Epidemic. Nature 414, 782–787. doi:10.1038/414782a
Keywords: Ciannamomum spp., phytochemistry, Pharmacology, mechanisms of action, clinical trials, Toxicological data
Citation: Sharifi-Rad J, Dey A, Koirala N, Shaheen S, El Omari N, Salehi B, Goloshvili T, Cirone Silva NC, Bouyahya A, Vitalini S, Varoni EM, Martorell M, Abdolshahi A, Docea AO, Iriti M, Calina D, Les F, López V and Caruntu C (2021) Cinnamomum Species: Bridging Phytochemistry Knowledge, Pharmacological Properties and Toxicological Safety for Health Benefits. Front. Pharmacol. 12:600139. doi: 10.3389/fphar.2021.600139
Received: 28 August 2020; Accepted: 06 April 2021;
Published: 11 May 2021.
Edited by:
Thomas Efferth, Johannes Gutenberg University Mainz, GermanyReviewed by:
Wannee Jiraungkoorskul, Mahidol University, ThailandDeepak Kumar Semwal, Uttarakhand Ayurved University, India
Copyright © 2021 Sharifi-Rad, Dey, Koirala, Shaheen, El Omari, Salehi, Goloshvili, Cirone Silva, Bouyahya, Vitalini, Varoni, Martorell, Abdolshahi, Docea, Iriti, Calina, Les, López and Caruntu. This is an open-access article distributed under the terms of the Creative Commons Attribution License (CC BY). The use, distribution or reproduction in other forums is permitted, provided the original author(s) and the copyright owner(s) are credited and that the original publication in this journal is cited, in accordance with accepted academic practice. No use, distribution or reproduction is permitted which does not comply with these terms.
*Correspondence: Javad Sharifi-Rad, javad.sharifirad@gmail.com; Bahare Salehi, bahar.salehi007@gmail.com; Abdelhakim Bouyahya, boyahyaa-90@hotmail.fr; Miquel Martorell, martorellpons@gmail.com; Daniela Calina, calinadaniela@gmail.com; Francisco Les, fles@usj.es; Constantin Caruntu, costin.caruntu@gmail.com