- 1Shanghai Mental Health Centre, School of Medicine, Shanghai Jiao Tong University, Shanghai, China
- 2First Affiliated Hospital of Guizhou University of Traditional Chinese Medicine, Guiyang, China
- 3School of Pharmacy, Guizhou University of Traditional Chinese Medicine, Guiyang, China
- 4Department of Anesthesiology, Renji Hospital, School of Medicine, Shanghai Jiao Tong University, Shanghai, China
Ischemic stroke is a leading cause of death and disability worldwide. Currently, only a limited number of drugs are available for treating ischemic stroke. Hence, studies aiming to explore and develop other potential strategies and agents for preventing and treating ischemic stroke are urgently needed. Ginseng Rb1 (GRb1), a saponin from natural active ingredients derived from traditional Chinese medicine (TCM), exerts neuroprotective effects on the central nervous system (CNS). We conducted this review to explore and summarize the protective effects and mechanisms of GRb1 on cerebral ischemic injury, providing a valuable reference and insights for developing new agents to treat ischemic stroke. Our summarized results indicate that GRb1 exerts significant neuroprotective effects on cerebral ischemic injury both in vivo and in vitro, and these network actions and underlying mechanisms are mediated by antioxidant, anti-inflammatory, and antiapoptotic activities and involve the inhibition of excitotoxicity and Ca2+ influx, preservation of blood–brain barrier (BBB) integrity, and maintenance of energy metabolism. These findings indicate the potential of GRb1 as a candidate drug for treating ischemic stroke. Further studies, in particular clinical trials, will be important to confirm its therapeutic value in a clinical setting.
Introduction
When the brain becomes blocked by a blood clot and blood is prevented from reaching the brain, an ischemic stroke occurs. Ischemic stroke, approximately accounting for 85% of all diagnosed strokes, has the characteristics of high morbidity, high mortality, high disability, and high recurrence rates and is mainly caused by cerebral ischemia and reperfusion (I/R) injury (CIRI) (Turley et al., 2005; Eltzschig and Eckle, 2011). I/R is a pathological condition characterized by an initial restriction of blood supply to an organ followed by the subsequent restoration of perfusion and concomitant reoxygenation (Turley et al., 2005; Eltzschig and Eckle, 2011), which is one of the leading causes of death worldwide (Turley et al., 2005; Woodruff et al., 2011; Feigin et al., 2014). I/R injury mainly includes ischemic stroke, acute kidney injury, and myocardial infarction. Due to the interrupted blood supply to the CNS, cerebral infarction causes ischemic stroke and other forms of CNS injury. As these mechanisms often involve complex combinations of necrosis, apoptosis, necroptosis, and autophagy, ischemic stroke–related pathogenesis is not totally clear (Turley et al., 2005; Eltzschig and Eckle, 2011). Based on accumulating evidence, once an ischemic stroke occurs, the insufficient blood supply directly stimulates neurons, causes the accumulation of glutamate, overactivates a plethora of downstream signaling pathways, and increases the intracellular calcium concentration, which triggers energy metabolism disorders (Bolaños et al., 2009), oxidative stress (Chamorro et al., 2016), Ca2+ overload, excitatory neurotransmitter release, the immune-mediated inflammatory response following acute ischemic stroke, and its related apoptosis and necrosis processes (Kamel and Iadecola, 2012; Chamorro et al., 2016), finally resulting in ischemic infarction of the brain. Hence, the main aim of acute stroke treatment is to salvage the ischemic penumbra or volume of hypoperfused, nonfunctional, yet still viable tissue surrounding the infarcted core.
Currently, tissue plasminogen activator (TPA) is regarded as the main effective pharmacological therapy and drug for ischemic stroke (Turley et al., 2005; Woodruff et al., 2011; Chamorro et al., 2016). Scholars have conducted extensive studies and developed some neuroprotective drugs and other interventions for treating ischemic injury (Tuttolomondo et al., 2011; Woodruff et al., 2011), ranging from pharmacologically blocking neurotransmitter receptors to intercepting cell death pathways, as well as the induction of hypothermia or hyperoxygenation. However, most of these studies failed to show the efficacy of any of these promising strategies. The current existing neuroprotective drugs remain limited and insufficient for the clinical treatment needs for ischemic stroke (Turley et al., 2005; Woodruff et al., 2011; Chamorro et al., 2016). Therefore, the development of novel therapeutic strategies and agents for preventing and treating ischemic stroke is urgently needed.
Panax ginseng C. A. Mey and P. notoginseng (Burk) F. H. Chen are commonly used as natural medicinal plants in TCM (Hui et al., 2010; Bao-Ying et al., 2014; Yang et al., 2014; Xie et al., 2018a; Xie et al., 2018b), the roots and medicinal ingredients of which have been in use for several hundred years. Pharmacological studies have shown that P. notoginseng, P. ginseng, and their extracts have many functions (Mancuso and Santangelo, 2017; Kim, 2018), such as anti-inflammatory activity (Allison and Ditor, 2014; Zheng et al., 2014; Zhang et al., 2015; Jeon and Kim, 2016), antioxidant activity, blood glucose regulation (Xie et al., 2016; Zhang et al., 2016), insulin resistance improvement (Zhang and Jiang, 2012; Zhai et al., 2018), inhibition of neuronal apoptosis (Li et al., 2014; Hou et al., 2017; Yang et al., 2017), and neuroprotection (Xie et al., 2018a; Xie et al., 2018b; Wang et al., 2016; Berge and Riise, 2015). Hence, one of the main tasks is to identify natural active substances and compounds that can be utilized for the prevention and treatment of ischemic stroke.
Ginseng Rb1 (GRb1) is a ginsenoside glycol (Liu et al., 2015; Ju et al., 2019) and one of the main active ingredients of P. notoginseng and P. ginseng (Figure 1). GRb1 has been proven to exert significant protective effects on the CNS, cardiovascular system (Xie et al., 2018a; Xie et al., 2018b), and immune system and possesses antitumor activities (Zhou et al., 2018; Zhou et al., 2019a; Zhou et al., 2019b). As shown in Figure 1, GRb1 possesses various pharmacological activities, including neuroprotective (Yang et al., 2008; Liu et al., 2013; Huang et al., 2015; Huang et al., 2015; Ye et al., 2019), acute renal injury-protective (Wang et al., 2008; Sun et al., 2012; Chen et al., 2019), cardiovascular-protective (Wang et al., 2008; Xia et al., 2011; Zheng et al., 2017; Li et al., 2020), lung injury-protective (Wang et al., 2013; Chen et al., 2014; Jiang et al., 2015; Li et al., 2015), and antiaging (Dong et al., 2017; Zhou et al., 2019a) effects, in many in vitro and in vivo models.
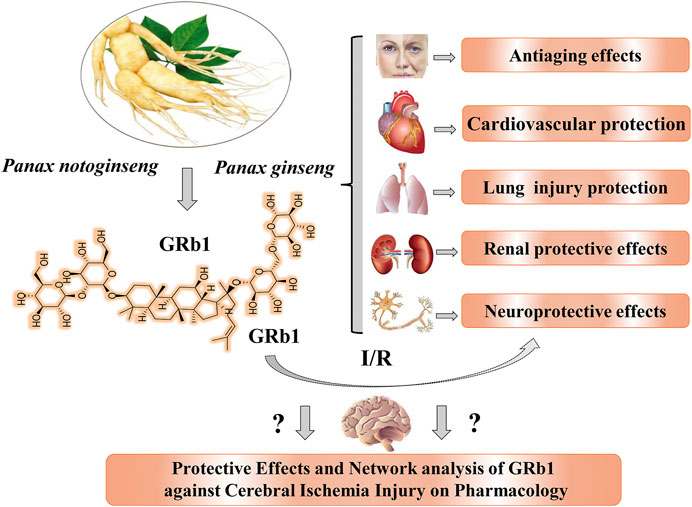
FIGURE 1. Natural sources, chemical structure, and main pharmacological activities of ginsenoside Rb1 obtained from P. notoginseng and P. ginseng. GRb1 exerts significant neuroprotective effects, but its efficiency and action network must be further explored and analyzed. GRb1, ginsenoside Rb1; I/R, indicates ischemia and reperfusion.
Currently, accumulated experiments and data suggest that GRb1 exerts neuroprotective effects both in vivo and in vitro and has a great potential as a novel candidate agent for ischemic stroke. To date, researchers have not clearly determined whether GRb1 can be used to treat ischemic stroke and cerebral I/R injury. No systematic review or analysis has been conducted to assess the protective effects and mechanisms by which GRb1 combats ischemic stroke and I/R injury (Figure 1). Hence, we conducted this analysis of preclinical studies of the effect of GRb1 on ischemic stroke. We searched the PubMed and China National Knowledge Infrastructure databases via using “Ginsenoside Rb1” and “Ischemia” as search terms. The PubMed database was comprehensively searched up to September 2020, and it showed 66 literatures; we excluded some irrelevant ones and then divided them into several aspects to further analyze the pharmacological effects and mechanisms of GRb1 in pre-treating and treating CIRI. Furthermore, we manually searched for other potential and relevant references, and there were no limitations in the language of all publications.
In this review, we found that GRb1 might alleviate cerebral/neural ischemia injury via its antiapoptotic, antioxidant, and anti-inflammatory activities, and effects on mitochondrial homeostasis, promoting neurogenesis, and improving brain functional connections and interactions, which provides more evidence for basic studies and further promotes the development of GRb1 as a candidate drug for the clinical treatment of ischemic stroke.
Antiapoptotic Effects and Neurons
Recent reports have suggested that GRb1, a natural saponin ingredient of TCM, exerts remarkable neuroprotective effects on the CNS (Ahmed et al., 2016), prevents and alleviates cerebral I/R injury via anti-inflammatory activity, antioxidant activity, enhanced neuroproliferation and neurodifferentiation, and improved energy metabolism (Chen et al., 2010; Zhu et al., 2012; Huang et al., 2015), confirming that Rb1 exerts antiapoptotic effects on neurons.
First, in vivo experiments showed that Rb1 significantly inhibited CA1 neuronal death caused by a 2-vessel occlusion (2-VO) model in rats (Luo et al., 2014) and delayed neuronal death in gerbils (Lim et al., 1997); noticeably reduced the infarct size and neuronal deficits, relieved pathological changes (Yuan et al., 2007), and decreased the number of neural apoptotic cells (Wen et al., 1996) in rats with middle cerebral artery occlusion (MCAO) (Lim et al., 1997; Yuan et al., 2007; Yang et al., 2008); and scavenged free radicals (Lim et al., 1997) and improved hippocampal blood flow at 5 min after transient forebrain ischemia (Lim et al., 1997). Rb1 significantly prolonged the response latency of ischemic gerbils and rescued a significant number of ischemic CA1 pyramidal neurons (Wen et al., 1996; Lim et al., 1997). Under abnormal ischemic microenvironmental conditions, Rb1 evidently decreased the concentrations of glutamic acid and Ca2+ (Wang et al., 2017), noticeably alleviated memory deficits in rats, and reduced pyramidal cellular necrosis and apoptosis in the hippocampus induced by glutamate (Glu) and Ca2+ (Wang et al., 2017; Guo et al., 2018). Additionally, Rb1 suppressed the loss of BBB integrity by suppressing the induction of neuroinflammation in a model of ischemic stroke (Chen et al., 2015). Based on these results, Rb1, an effective neuroprotective drug, plays key roles in cerebral I/R injury.
Second, in vitro experiments (Table 1) suggested that Rb1 might increase cell viability, inhibit oxygen and glucose deprivation (OGD)–induced neuronal death, and reduce autophagic vacuoles in SH-SY5Y cells (Luo et al., 2014), changes that were blocked by the inhibitor LY294002. GRb1 significantly decreased the levels of free radicals, protected hippocampal neurons from lethal damage caused by the hydroxyl radical–promoting agent FeSO4in vitro (Lim et al., 1997), and markedly suppressed the uptake of Glu and overload of Ca2+ in OGD-induced SH-SY5Y cells (Wang et al., 2017; Guo et al., 2018). GRb1 also significantly reduced the levels of lactate dehydrogenase (LDH) (Park et al., 2005; Li et al., 2016), nitric oxide (NO), and superoxide (O-) (Zhu et al., 2012; Huang et al., 2014). Thus, Rb1 might be regarded as an antiapoptotic agent with neuroprotective effects.
Furthermore, GRb1 significantly inhibited the expression of the proapoptotic genes Bax, Bad, and caspase-3 (Yang et al., 2008; Gao et al., 2010), upregulated Bcl-2 and the ratio of Bcl-2/Bax in vivo and in vitro (Yuan et al., 2007; Yang et al., 2008), increased the expression of glial-derived neurotrophic factor (GDNF) and brain-derived neurotrophic factor (BDNF) (Yuan et al., 2007; Gao et al., 2010), and thus prevented neuronal death induced by cerebral ischemia. GRb1 significantly increased the levels of Akt phosphorylated at Ser473 (P-Akt) and reduced the expression levels of LC3II and Beclin1, indicating that GRb1 might prevent ischemic neuronal death by modulating autophagy activation (Luo et al., 2014); moreover, GRb1 increased the levels of P-Akt and P-mTOR, reduced P-PTEN levels in vivo and in vitro, and ameliorated the abnormal microenvironment by activating the P-AKT/P-mTOR pathway and inhibiting P-PTEN (Guo et al., 2018). In contrast, the LY294002 treatment reversed these changes induced by GRb1. Furthermore, GRb1 inhibited the expression of NMDAR, increased the expression of glial glutamate transporter 1 (GLT-1), and downregulated the levels of cytochrome C (Cyt-C) in response to neuronal mitochondrial stress, which reduced the excessive Glu and Ca2+ levels (Wang et al., 2017; Guo et al., 2018).
In general, evidence from recent studies suggests that GRb1 may exert its antiapoptotic effects by regulating the phosphoinositide 3-kinase (PI3K)-protein kinase B (AKT)-TOR signaling pathways, inhibiting autophagy, alleviating mitochondrial stress and apoptosis pathways, modulating the expression of N-methyl-D-aspartate-receptor (NMDAR) and GLT-1, and improving neurogenesis and BDNF levels. Nonetheless, currently, the mechanisms by which GRb1 regulates ischemic neuronal apoptosis are not completely elaborated and summarized and should be further explored in the future.
Regulation of Neuroinflammation and Microglia
Although CIRI is a complex pathology caused by the interaction of numerous pathophysiological factors (Allison and Ditor, 2014; Anderson et al., 2014), accumulating evidence indicates that acute inflammation and subsequent apoptosis and necrosis are involved in the progression of a cerebral ischemic insult (Jianhua et al., 2008; Eltzschig and Eckle, 2011; Jiang et al., 2014; Tao et al., 2015). Recently, GRb1 was reported to exert beneficial effects on cerebral ischemic stroke and to inhibit inflammatory cascades in the acute phases of cerebral ischemia (Wang et al., 2008; Zhu et al., 2012; Jiang et al., 2015).
According to recent studies (Table 2), GRb1 noticeably reduces the infarct volume, significantly alleviates neurological deficits, decreases neurological severity scores (Zhu et al., 2012; Liu et al., 2013; Liu et al., 2018; Chen et al., 2020), preserves the neuronal morphology and structure (Ke et al., 2014), improves pathological changes (Liu et al., 2018), and inhibits the activation of microglia in MCAO/R model rats (Zhu et al., 2012) and N9 microglia in vitro (Ke et al., 2014). These neuroprotective effects may be involved in microglia-mediated CNS inflammation and related neuronal damage in the acute phases of cerebral ischemia/hypoxia.
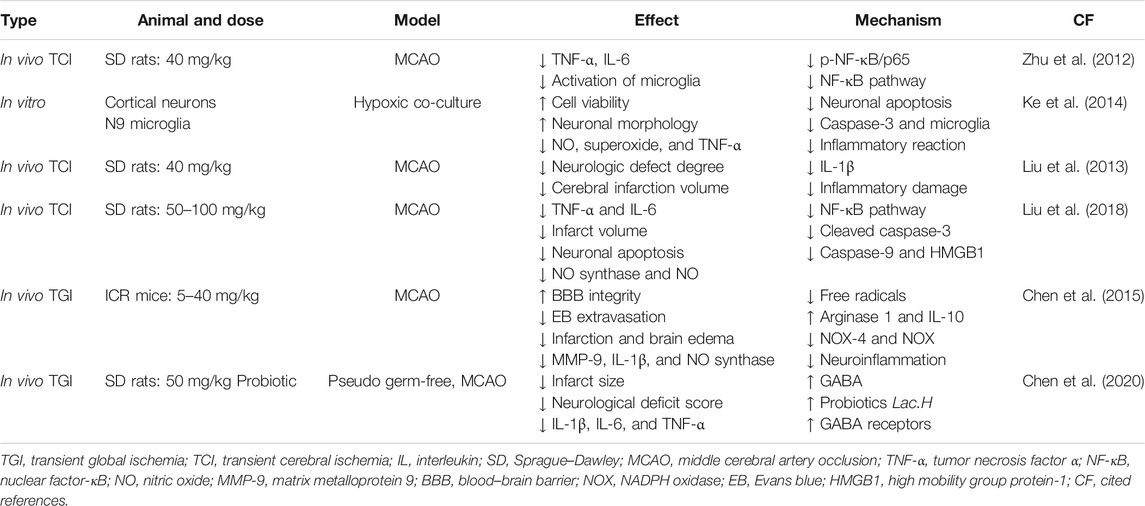
TABLE 2. Neuroprotective effects of GRb1 on cerebral ischemia injury are mediated by suppressing neuroinflammation and microglia-mediated inflammatory reactions, based on current reports and results.
On the one hand, ginsenoside GRb1 notably decreased the activation of microglia induced by I/R (Zhu et al., 2012) and a hypoxic coculture system (Ke et al., 2014) and significantly reduced the levels of the interleukin (IL)-1β (Zhu et al., 2012; Liu et al., 2013; Chen et al., 2015; Chen et al., 2020), tumor necrosis factor-α (TNF-α) (Zhu et al., 2012; Ke et al., 2014; Liu et al., 2018; Chen et al., 2020), IL-6 (Zhu et al., 2012; Liu et al., 2018; Chen et al., 2020), and high mobility group protein 1 (HMGB1) mRNAs and proteins in brain tissue and serum (Liu et al., 2018); on the other hand, GRb1 significantly decreased the levels of NO (Chen et al., 2015), superoxide (Ke et al., 2014; Liu et al., 2018), and nitric oxide synthase (Liu et al., 2018) produced by microglia in vitro (Ke et al., 2014) and in vivo (Zhu et al., 2012; Liu et al., 2018), reduced neuronal apoptosis (Ke et al., 2014; Liu et al., 2018), and reduced the levels of cleaved caspase-3 and caspase-9 (Ke et al., 2014; Liu et al., 2018).
Further studies revealed that GRb1 inhibited nuclear factor kappa B (NF-κB) signaling pathways by decreasing the levels of phosphorylated NF-κB/p65 and IB-kinase complex (IKK) and downregulating HMGB1 and its related local inflammation (Ke et al., 2014; Liu et al., 2018), which stimulate NF-κB translocation and mitogen-activated protein kinase (MAPK) phosphorylation triggered by HMGB-1/TLR4 (Lu et al., 2011; Procaccio et al., 2014; Sun and Nan, 2016; Xie et al., 2019). Moreover, GRb1 reduced the expression levels of proinflammatory factors, attenuated the activity of MMP-9 (Chen et al., 2015), upregulated the expression of γ-aminobutyric acid (GABA) receptors in I/R rats (Chen et al., 2020), protected BBB integrity in ischemic stroke by suppressing neuroinflammation, decreased the production of MMP-9 and NOX4-derived free radicals (Chen et al., 2015), and regulated the probiotic Lac.H and GABA receptor levels (Chen et al., 2020). Overall, GRb1 may exert a neuroprotective effect on I/R-induced injury by suppressing neuroinflammation and microglia-mediated inflammatory reactions.
Antioxidant Effects and Mitochondria
Oxidative stress plays an important role, and reactive oxygen species (ROS) is implicated in the tissue damage that occurs in cerebral ischemic pathogenesis, which results in the production of toxic molecules that alter cellular proteins, lipids, and ribonucleic acids, leading to cell dysfunction or death (Crack and Taylor, 2005; Chamorro et al., 2016). Excessive ROS severely impair mitochondria and their related energetic metabolism functions. GRb1 exhibits various pharmacological activities, including antioxidant (Dong et al., 2017; Li et al., 2019; Xu et al., 2019; Ye et al., 2019), antiapoptotic, and neuroprotective properties.
First, the results of in vivo experiments (Table 3) showed that GRb1 protected hippocampal CA1 neurons (Lim et al., 1997), reduced free radicals (Chen et al., 2015), and possessed potential for treating brain injuries (Yang et al., 2008); meanwhile, GRb1 increased superoxide dismutase (SOD) activity, decreased malondialdehyde (MDA) contents in the serum and spinal cord tissue (Ye et al., 2019), and inhibited oxidative stress and extracellular signal-regulated kinase (ERK) activation in aged mice exposed to cerebral ischemia (Dong et al., 2017). All of the antioxidant effects of GRb1 are beneficial for reducing neuronal death, mitochondrial damage, and astrocyte injury induced by I/R, indicating that GRb1 may reduce pathological changes and decrease neural cell apoptosis (Yang et al., 2008; Chen et al., 2015).
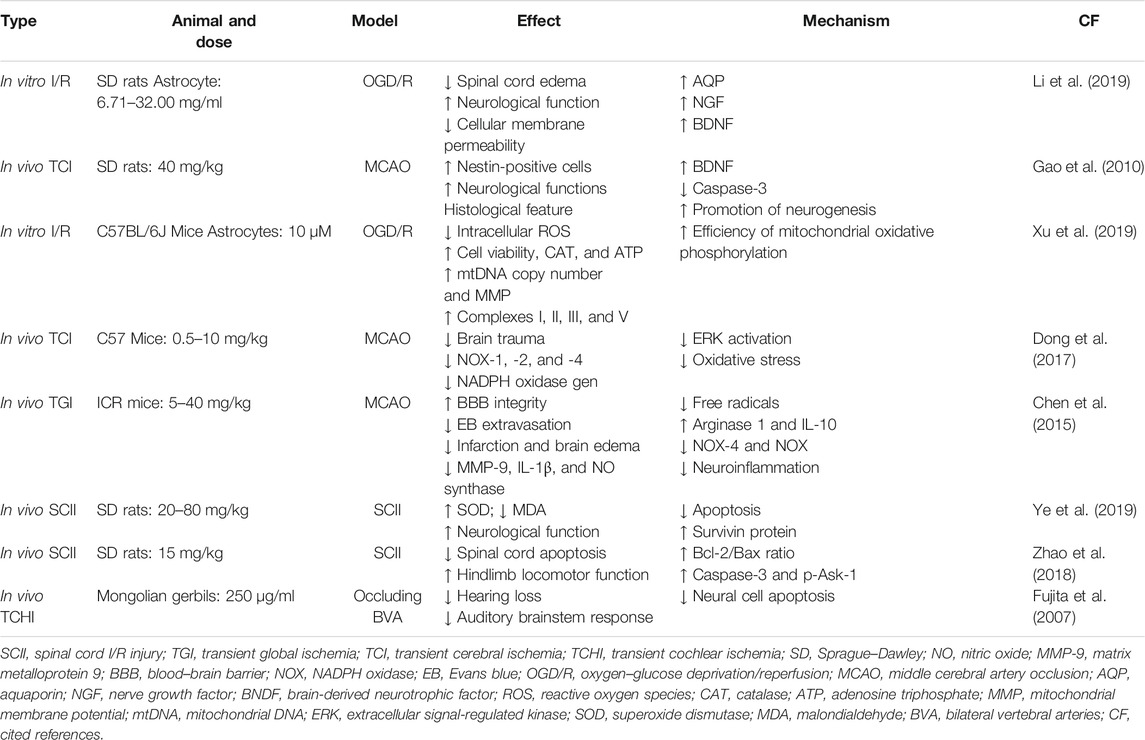
TABLE 3. Antioxidant effects of GRb1 on I/R neuronal injury mediated by the inhibition of oxidative stress and mitochondrial injury, increases in energy metabolism and protection of the BBB, based on recent reports and results.
Second, in vitro experiments (Table 3) confirmed that GRb1 significantly improved cell viability, decreased intracellular ROS production, and increased catalase (CAT) activity and the mtDNA copy number in OGD/R-induced astrocytes, thus inhibiting oxidative phosphorylation (OXPHOS) (Xu et al., 2019). GRb1 exerted obvious protective effects on the mitochondria by distinctly attenuating MMP depolarization, improving the efficiency of mitochondrial OXPHOS, increasing the activities of complexes I, II, III, and V, and increasing the level of adenosine triphosphate (ATP) following OGD/R induction (Xu et al., 2019).
Further studies (Table 3) revealed the antioxidant effects of GRb1. On the one hand, GRb1 significantly downregulated NOX-4 expression and NOX activities in ischemic rat brain tissues (Chen et al., 2015; Dong et al., 2017), inhibited ischemia-stimulated NADPH oxidase gene expression, including NOX-1, 2, and 4 (Dong et al., 2017), and prevented ERK activation in aged mice (Dong et al., 2017). On the other hand, GRb1 significantly suppressed mitochondrial damage, increased the mitochondrial membrane potential (MMP) in OGD/R-induced astrocytes (Xu et al., 2019), and thus improved the antioxidant activity (CAT and SOD). GRb1 remarkably increased aquaporin (AQP) 4 levels (Li et al., 2019), decreased Bax expression (Yang et al., 2008), and upregulated Bcl-2 (Yang et al., 2008), nerve growth factor (NGF), and BDNT expression (Li et al., 2019) in vivo and in vitro. Thus, GRb1 is potentially useful for treating brain injury, due to its antioxidant effects and mitochondrial protection.
Inhibition of Other Types of Neural Ischemic Injury
Related studies have shown that GRb1 significantly alleviates I/R injury of the kidney (Wang et al., 2008; Sun et al., 2012; Sun et al., 2013; Chen et al., 2019) and brain (Chen et al., 2010; Zhu et al., 2012; Huang et al., 2015). However, few reports have assessed spinal cord ischemia–reperfusion injury (SCII). According to recent studies, GRb1 reduces cell apoptosis induced by SCII by inhibiting oxidative stress (Ye et al., 2019). GRb1 noticeably improves hindlimb locomotor dysfunction in rats (Zhao et al., 2018), increases SOD activity, decreases the MDA content in serum and spinal cord tissue (Ye et al., 2019), and inhibits neuronal apoptosis. The potential mechanisms may be tightly associated with promoting the expression of survivin (Ye et al., 2019), downregulating the levels of caspase-3 and phosphorylated Ask-1 (p-Ask-1), and improving the Bcl-2/Bax ratio (Zhao et al., 2018) in SCII rats. After the exposure of spiral ganglion cells (SGCs)to transient cochlear ischemia, GRb1 significantly reduced the percentage of the auditory brainstem response threshold shift and prevented hearing loss caused by ischemic injury to SGCs in Mongolian gerbils (Fujita et al., 2007), indicating that GRb1 might protect SGCs from ischemic injury.
In addition, GRb1 clearly increased the expression of NGF in OGD/R-stimulated astrocytes (Li et al., 2019), upregulated BDNF expression, and downregulated caspase-3 expression in MCAO/R model rats (Yuan et al., 2007; Gao et al., 2010). Therefore, GRb1 may exert neuroprotective effects by promoting neurogenesis.
Analysis of Safety and Therapeutic Effectiveness
Based on the above summarized findings (Tables 1–3), Rb1 has a potential therapeutic value for ischemic stroke via immunological, antioxidant, and neuroprotective effects. However, the efficacy and safety of Rb1 for ischemic stroke in human have not been tested, and its effective dose is not known. Nevertheless, there is evidence that red ginseng extract, which contains 20–30% of GRb1, at 23, 25, 50, and 100 mg with repeated oral administration for 1–2 weeks, is safe and well tolerant in healthy subjects (Kim, 2013; Jin et al., 2019; Choi et al., 2020). In addition, randomized controlled studies showed that 200–400 mg of ginsenosides or its preparations significantly ameliorated neurological deficit (He et al., 2011) and improved working memory and daily activities of patients with ischemic stroke (Reay et al., 2010; He et al., 2011), indicating that a daily dose of 40–120 mg of GRb1 may be safe and effective for treating ischemic stroke. The effective dose of Rb1 tested in animal studies with notably attenuated ischemia-induced cerebral I/R injury ranges from 10 to 40 mg/kg (Tables 1–3), which is equivalent to 0.8–3.2 mg/kg in human (calculated from dose in mice) or 48–192 mg Rb1 for a 60 kg person. Thus, an effective concentration of Rb1 may be achievable in human.
Conclusion and Remarks
GRb1, a natural active ingredient, exerts neurotrophic and neuroprotective effects on the CNS. In the present review (Figure 2), we summarized the available reports on the therapeutic effects and the molecular mechanisms of GRb1 in cerebral I/R injury. The currently available data and our summarized results suggest that GRb1 may alleviate cerebral/neural ischemic injury via multiple pharmacological activities, such as its antiapoptotic, antioxidant, and anti-inflammatory properties, preservation of mitochondrial homeostasis, promotion of neurogenesis, and maintenance of the probiotic balance. Based on these results, GRb1, a special natural compound, has bright prospects for the prevention and treatment for ischemic stroke with a pharmacological network of multiple effects, targets, and molecular pathways.
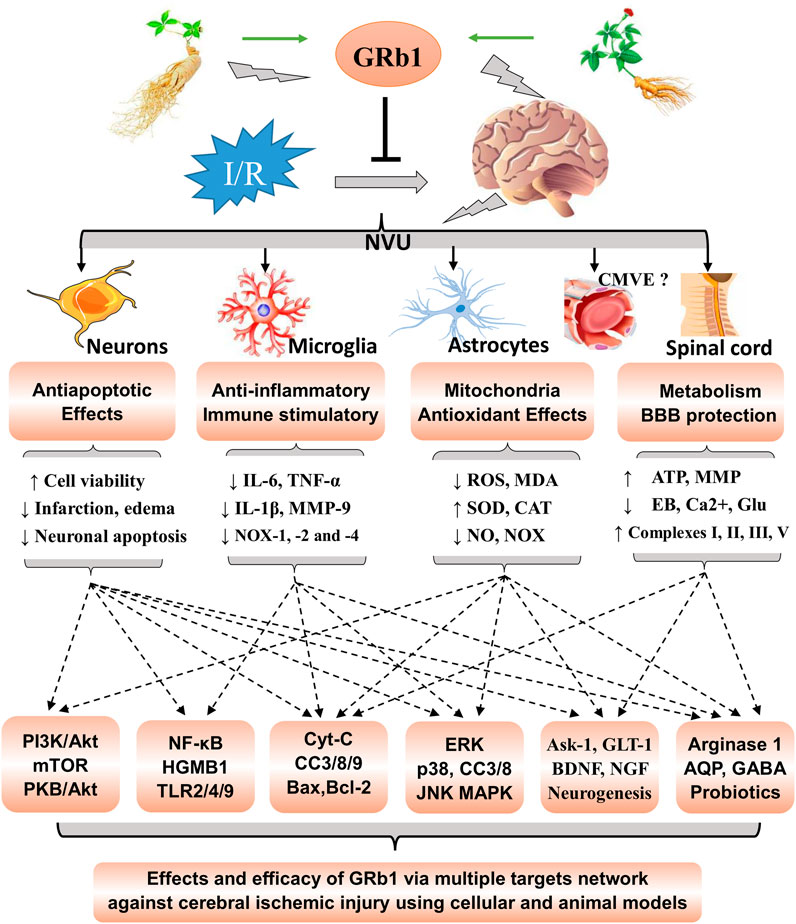
FIGURE 2. Summarized effects and molecular network analysis of GRb1 in cerebral ischemic injury. Rb1 exerts significant neuroprotective effects on the neurovascular unit (NVU) and other neural cells via the network actions of its antiapoptotic, antioxidant, and anti-inflammatory activities; mitochondrial homeostasis; neurogenesis promotion; and regulation of the probiotic balance. The molecular mechanisms involve multiple effects, multiple targets, and multiple pathways. NVU, neurovascular unit; CMVE, cerebral microvascular endothelium. GRb1, ginsenoside Rb1; I/R, indicates ischemia and reperfusion. CC, cleaved caspase.
Cerebral I/R injury is a complicated pathological process (Turley et al., 2005; Eltzschig and Eckle, 2011; Chamorro et al., 2016) that includes various types of I/R damage to the neurovascular unit (NVU), which is composed of nerve cells, BBB, microglia, and extracellular matrix (Lok et al., 2007; Guo and Lo, 2009; Lo, 2017). For many decades, neuronal injury has been considered the main cause of functional deficits after brain injury. Accordingly, almost all therapeutic strategies were targeted at rescuing neurons and repairing neuronal damage. However, emerging data from both experimental models and patients now clearly show that, in patients with stroke, saving neurons alone may also be insufficient for treating brain infarcts (Lok et al., 2015) as brain functional connections and interactions among the different components in the NVU are also important (Cai et al., 2017; Jiang et al., 2018). Coincidentally, as shown in Figure 2, GRb1 exerts significant antiapoptotic effects on neurons exposed to cerebral ischemia stroke, suppresses neuroinflammation and microglia-mediated inflammatory stress in the acute phase of I/R, and protects ischemia-exposed astrocyte cells. Meanwhile, GRb1 inhibits oxidative stress and mitochondrial damage in cells exposed to I/R, preserves the BBB integrity, improves energy metabolism, and promotes neurogenesis. Moreover, GRb1 may alleviate spinal cord ischemia–reperfusion injury (SCII) and regulate the probiotic balance (Figure 2). Hence, GRb1 obviously plays a vital role not only in neural protection but also via the inhibition of NVU damage caused by CIRI.
From the perspective of protecting the NVU, GRb1 prevents I/R-induced cell apoptosis and death, suppresses excessive Ca2+, glutamate, and RNS levels, alleviates mitochondrial stress, improves neurogenesis, and reduces the cerebral infarct volume in vitro and in vivo, indicating that GRb1 may exert antiapoptotic effects and protect the NVU from CIRI (Figure 2). Although the mechanisms have not been completely elaborated, our summarized results (Table 1) further reveal the molecular mechanisms and vital biomarkers of the effects of GRb1 on CIRI, which may be related to the PI3K-AKT-mTOR, autophagy, and mitochondrial apoptosis signaling pathways, NMDA receptor and GLT-1 targets, and neurogenesis.
Neuroinflammation underlies the etiology of multiple neurodegenerative diseases and stroke (Eldahshan et al., 2019). In the acute phase of ischemic stroke, resident microglia and recruited macrophages assume an M2 phenotype, and the immune-mediated inflammatory response is activated, resulting in the secretion of a number of inflammatory cytokines (IL-6, IL-1β, and TNF-α and the proteolytic enzymes MMP 3 and 9) (Jiang et al., 2018). Proinflammatory cytokines further trigger severe inflammatory stress, aggravate BBB dysfunction (Jiang et al., 2018), and induce NVU damage. All these events lead to the exacerbation of ischemic injury. Based on the summarized results listed in Table 2, we found that GRb1 inhibited the NF-KB, HMGB-1/TLR4, and HMGB1 signaling pathways, regulated the MAPK signaling pathway, increased the expression of GABA receptors, and reduced the levels of proinflammatory cytokines (IL-6, MMP-9, IL-1β, and TNF-α). By regulating these pathways, GRb1 suppresses neuroinflammation and microglia-mediated inflammatory reactions, reduces the damage caused by inflammatory factors, improves the integrity and normal functions of the BBB, and thus inhibits necrosis and apoptosis associated with anti-inflammatory activities at the early stages after stroke, which plays a fundamental role in the maintenance of CNS homeostasis, BBB structural integrity, and normal functions of the NVU.
The neutralization of oxidative and nitrosative stresses is a potential therapeutic strategy because the ischemic brain is highly susceptible to oxidative damage (Crack and Taylor, 2005; Xie et al., 2020). After CI/RI, the production of ROS increases, leading to lipid peroxidation, mitochondrial and DNA damage, protein nitration, and mitochondrial injury that evokes the mitochondrial release of apoptosis inducers (Bolaños et al., 2009; Chamorro et al., 2016). According to recent studies (Table 3), GRb1 decreases the production of ROS, MDA, and NO, improves antioxidant defenses (CAT, SOD, and GSH-px), and inhibits I/R-induced mitochondrial injury in vivo and in vitro. Moreover, it remarkably increases AQP4 levels (Li et al., 2019), decreases Bax expression (Yang et al., 2008), upregulates the expression of Bcl-2 (Yang et al., 2008), downregulates NOX-4 expression and NOX activities, and inhibits the cascade reactions of caspase-3 and caspase-9. Thus, GRb1 exerts significant neuroprotective effects due to its antioxidant activity and protects the mitochondria in the treatment of cerebral ischemic injury.
In summary, this review addresses the effects and efficacy of GRb1 by discussing research results obtained using cellular and animal models; the summarized results and analysis indicate that GRb1, a new candidate agent, has bright prospects for preventing and treating ischemic stroke.
However, unrecognized actions and limitations of GRb1 still exist. 1) Researchers have not clearly determined whether GRb1 alleviates ischemic injury of the cerebral microvascular endothelium (CMVE) of the NVU. 2) Because of the lack of clinical testing and validation of preclinical data, the use of GRb1 as a new drug for treating ischemic stroke remains a challenge. 3) The existing research and data only showed the effects of GRb1 on I/R in the early stages and acute phases. Therefore, currently available clinical trials and data collection are urgently needed, and future studies should focus on the effects and molecular mechanisms of GRb1 on the NVU system and the recovery phase of ischemic stroke.
Author Contributions
WX and XW designed the review; WX, XW, and YW wrote the manuscript; YC, DY, and TX helped map the figures and revise the manuscript. SZ was mainly responsible for the supervision of the works and the management of related projects. All authors discussed, edited and approved the final version.
Funding
This work was funded by the Natural Science Foundation of China (No. 81801096). We greatly expressed our sincere gratitude to the foundations.
Conflict of Interest
The authors declare that the research was conducted in the absence of any commercial or financial relationships that could be construed as a potential conflict of interest.
The reviewer MW declared a shared affiliation with several of the authors, KW, XW, SC, XZ, and TX, to the handling editor at time of review.
References
Ahmed, T., Raza, S. H., Maryam, A., Setzer, W. N., Braidy, N., Nabavi, S. F., et al. (2016). Ginsenoside Rb1 as a Neuroprotective Agent: A Review. Brain Res. Bull. 125, 30–43. doi:10.1016/j.brainresbull.2016.04.002
Allison, D. J., and Ditor, D. S. (2014). The Common Inflammatory Etiology of Depression and Cognitive Impairment: a Therapeutic Target. J. Neuroinflammation 11, 151. doi:10.1186/s12974-014-0151-1
Anderson, G., Berk, M., Dean, O., Moylan, S., and Maes, M. (2014). Role of Immune-Inflammatory and Oxidative and Nitrosative Stress Pathways in the Etiology of Depression: Therapeutic Implications. CNS Drugs 28 (1), 1–10. doi:10.1007/s40263-013-0119-1
Bao-Ying, H., Liu, X. J., Qiang, R., Jiang, Z. L., Xu, L. H., Wang, G. H., et al. (2014). Treatment with Ginseng Total Saponins Improves the Neurorestoration of Rat after Traumatic Brain Injury. J. Ethnopharmacol. 155 (2), 1243–1255. doi:10.1016/j.jep.2014.07.009
Berge, L. I., and Riise, T. (2015). Comorbidity between Type 2 Diabetes and Depression in the Adult Population: Directions of the Association and Its Possible Pathophysiological Mechanisms. Int. J. Endocrinol. 2015, 164760. doi:10.1155/2015/164760
Bolaños, J. P., Moro, M. A., Lizasoain, I., and Almeida, A. (2009). Mitochondria and Reactive Oxygen and Nitrogen Species in Neurological Disorders and Stroke: Therapeutic Implications☆. Adv. Drug Deliv. Rev. 61 (14), 1299–1315. doi:10.1016/j.addr.2009.05.009
Cai, W., Zhang, K., Li, P., Zhu, L., Xu, J., Yang, B., et al. (2017). Dysfunction of the Neurovascular Unit in Ischemic Stroke and Neurodegenerative Diseases: An Aging Effect. Ageing Res. Rev. 34, 77–87. doi:10.1016/j.arr.2016.09.006
Chamorro, Á., Dirnagl, U., Urra, X., and Planas, A. M. (2016). Neuroprotection in Acute Stroke: Targeting Excitotoxicity, Oxidative and Nitrosative Stress, and Inflammation. Lancet Neurol. 15 (8), 869–881. doi:10.1016/s1474-4422(16)00114-9
Chen, W., Dang, Y., and Zhu, C. (2010). Simultaneous Determination of Three Major Bioactive Saponins of Panax Notoginseng Using Liquid Chromatography-Tandem Mass Spectrometry and a Pharmacokinetic Study. Chin. Med. 5, 12. doi:10.1186/1749-8546-5-12
Chen, Y.-Q., Rong, L., and Qiao, J.-O. (2014). Anti-inflammatory Effects of Panax Notoginseng Saponins Ameliorate Acute Lung Injury Induced by Oleic Acid and Lipopolysaccharide in Rats. Mol. Med. Rep. 10 (3), 1400–1408. doi:10.3892/mmr.2014.2328
Chen, W., Guo, Y., Yang, W., Zheng, P., Zeng, J., and Tong, W. (2015). Protective Effect of Ginsenoside Rb1 on Integrity of Blood-Brain Barrier Following Cerebral Ischemia. Exp. Brain Res. 233 (10), 2823–2831. doi:10.1007/s00221-015-4352-3
Chen, S., Li, X., Wang, Y., Mu, P., Chen, C., Huang, P., et al. (2019). Ginsenoside Rb1 Attenuates Intestinal Ischemia/reperfusion induced Inflammation and Oxidative Stress via Activation of the PI3K/Akt/Nrf2 Signaling Pathway. Mol. Med. Rep. 19 (5), 3633–3641. doi:10.3892/mmr.2019.10018
Chen, H., Shen, J., Li, H., Zheng, X., Kang, D., Xu, Y., et al. (2020). Ginsenoside Rb1 Exerts Neuroprotective Effects through Regulation of Lactobacillus Helveticus Abundance and GABAA Receptor Expression. J. Ginseng Res. 44 (1), 86–95. doi:10.1016/j.jgr.2018.09.002
Choi, M.-K., Jin, S., Jeon, J.-H., Kang, W. Y., Seong, S. J., Yoon, Y.-R., et al. (2020). Tolerability and Pharmacokinetics of Ginsenosides Rb1, Rb2, Rc, Rd, and Compound K after Single or Multiple Administration of Red Ginseng Extract in Human Beings. J. Ginseng Res. 44 (2), 229–237. doi:10.1016/j.jgr.2018.10.006
Crack, P. J., and Taylor, J. M. (2005). Reactive Oxygen Species and the Modulation of Stroke☆. Free Radic. Biol. Med. 38 (11), 1433–1444. doi:10.1016/j.freeradbiomed.2005.01.019
Dong, X., Zheng, L., Lu, S., and Yang, Y. (2017). Neuroprotective Effects of Pretreatment of Ginsenoside Rb1 on Severe Cerebral Ischemia-Induced Injuries in Aged Mice: Involvement of Anti-oxidant Signaling. Geriatr. Gerontol. Int. 17 (2), 338–345. doi:10.1111/ggi.12699
Eldahshan, W., Fagan, S. C., and Ergul, A. (2019). Inflammation within the Neurovascular Unit: Focus on Microglia for Stroke Injury and Recovery. Pharmacol. Res. 147, 104349. doi:10.1016/j.phrs.2019.104349
Eltzschig, H. K., and Eckle, T. (2011). Ischemia and Reperfusion-From Mechanism to Translation. Nat. Med. 17 (11), 1391–1401. doi:10.1038/nm.2507
Feigin, V. L., Forouzanfar, M. H., Krishnamurthi, R., Mensah, G. A., Connor, M., Bennett, D. A., et al. (2014). Global and Regional burden of Stroke during 1990-2010: Findings from the Global Burden of Disease Study 2010. The Lancet 383 (9913), 245–255. doi:10.1016/s0140-6736(13)61953-4
Fujita, K., Hakuba, N., Hata, R., Morizane, I., Yoshida, T., Shudou, M., et al. (2007). Ginsenoside Rb1 Protects against Damage to the Spiral Ganglion Cells after Cochlear Ischemia. Neurosci. Lett. 415 (2), 113–117. doi:10.1016/j.neulet.2007.01.005
Gao, X.-Q., Yang, C.-X., Chen, G.-J., Wang, G.-Y., Chen, B., Tan, S.-K., et al. (2010). Ginsenoside Rb1 Regulates the Expressions of Brain-Derived Neurotrophic Factor and Caspase-3 and Induces Neurogenesis in Rats with Experimental Cerebral Ischemia. J. Ethnopharmacol. 132 (2), 393–399. doi:10.1016/j.jep.2010.07.033
Guo, S., and Lo, E. H. (2009). Dysfunctional Cell-Cell Signaling in the Neurovascular Unit as a Paradigm for Central Nervous System Disease. Stroke 40 (3Suppl. 1), 4–7. doi:10.1161/strokeaha.108.534388
Guo, Y., Wang, L.-P., Li, C., Xiong, Y.-X., Yan, Y.-T., Zhao, L.-Q., et al. (2018). Effects of Ginsenoside Rb1 on Expressions of Phosphorylation Akt/Phosphorylation mTOR/Phosphorylation PTEN in Artificial Abnormal Hippocampal Microenvironment in Rats. Neurochem. Res. 43 (10), 1927–1937. doi:10.1007/s11064-018-2612-x
He, L., Chen, X., Zhou, M., Zhang, D., Yang, J., Yang, M., et al. (2011). Radix/Rhizoma Notoginseng Extract (Sanchitongtshu) for Ischemic Stroke: A Randomized Controlled Study. Phytomedicine 18 (6), 437–442. doi:10.1016/j.phymed.2010.10.004
Hou, Q. L., Wang, Y., Li, Y. B., Hu, X. L., and Wang, S. L. (2017). Protective Effect of Notoginsenoside R1 on Neuron Injury Induced by OGD/R through ATF6/Akt Signaling Pathway. Zhongguo Zhong Yao Za Zhi. 42 (6), 1167–1174. doi:10.19540/j.cnki.cjcmm.20170121.014
Huang, X. P., Qiu, Y. Y., Wang, B., Ding, H., Tang, Y. H., Zeng, R., et al. (2014). Effects of Astragaloside IV Combined with the Active Components of Panax Notoginseng on Oxidative Stress Injury and Nuclear Factor-Erythroid 2-related Factor 2/heme Oxygenase-1 Signaling Pathway after Cerebral Ischemia-Reperfusion in Mice. Pharmacogn Mag. 10 (40), 402–409. doi:10.4103/0973-1296.141765
Huang, X. P., Ding, H., Wang, B., Qiu, Y. Y., Tang, Y. H., Zeng, R., et al. (2015). Effects of the Main Active Components Combinations of Astragalus and Panax Notoginseng on Energy Metabolism in Brain Tissues after Cerebral Ischemia-Reperfusion in Mice. Pharmacogn Mag. 11 (44), 732–739. doi:10.4103/0973-1296.165572
Huang, X.-P., Ding, H., Lu, J.-D., Tang, Y.-H., Deng, B.-X., and Deng, C.-Q. (2015). Effects of the Combination of the Main Active Components of Astragalus and Panax Notoginseng on Inflammation and Apoptosis of Nerve Cell after Cerebral Ischemia-Reperfusion. Am. J. Chin. Med. 43 (7), 1419–1438. doi:10.1142/s0192415x15500809
Hui, Q., Zhang, C., Shi, Z. B., Yang, H. Q., and Wang, K. Z. (2010). Protective Effects and Mechanism of Panax Notoginseng Saponins on Oxidative Stress-Induced Damage and Apoptosis of Rabbit Bone Marrow Stromal Cells. Chin. J. Integr. Med. 16 (6), 525–530. doi:10.1007/s11655-010-0566-1
Jeon, S. W., and Kim, Y. K. (2016). Neuroinflammation and Cytokine Abnormality in Major Depression: Cause or Consequence in that Illness? Wjp 6 (3), 283–293. doi:10.5498/wjp.v6.i3.283
Jiang, M., Li, J., Peng, Q., Liu, Y., Liu, W., Luo, C., et al. (2014). Neuroprotective Effects of Bilobalide on Cerebral Ischemia and Reperfusion Injury Are Associated with Inhibition of Pro-inflammatory Mediator Production and Down-Regulation of JNK1/2 and P38 MAPK Activation. J. Neuroinflammation 11 (1), 167. doi:10.1186/s12974-014-0167-6
Jiang, Y., Zhou, Z., Meng, Q. T., Sun, Q., Su, W., Lei, S., et al. (2015). Ginsenoside Rb1 Treatment Attenuates Pulmonary Inflammatory Cytokine Release and Tissue Injury Following Intestinal Ischemia Reperfusion Injury in Mice. Oxid Med. Cel Longev 2015, 843721. doi:10.1155/2015/843721
Jiang, X., Andjelkovic, A. V., Zhu, L., Yang, T., Bennett, M. V. L., Chen, J., et al. (2018). Blood-brain Barrier Dysfunction and Recovery after Ischemic Stroke. Prog. Neurobiol. 163-164, 144–171. doi:10.1016/j.pneurobio.2017.10.001
Jianhua, Q., Nishimura, M., Wang, Y., Sims, J. R., Qiu, S., Savitz, S. I., et al. (2008). Early Release of HMGB-1 from Neurons after the Onset of Brain Ischemia. J. Cereb. Blood Flow Metab. 28 (5), 927–938. doi:10.1038/sj.jcbfm.9600582
Jin, S., Jeon, J. H., Lee, S., Kang, W. Y., Seong, S. J., Yoon, Y. R., et al. (2019). Detection of 13 Ginsenosides (Rb1, Rb2, Rc, Rd, Re, Rf, Rg1, Rg3, Rh2, F1, Compound K, 20(S)-Protopanaxadiol, and 20(S)-Protopanaxatriol) in Human Plasma and Application of the Analytical Method to Human Pharmacokinetic Studies Following Two Week-Repeated Administration of Red Ginseng Extract. Molecules 24 (14), 2618. doi:10.3390/molecules24142618
Ju, Z., Li, J., Lu, Q., Yang, Y., Yang, L., and Wang, Z. (2019). Identification and Quantitative Investigation of the Effects of Intestinal Microflora on the Metabolism and Pharmacokinetics of Notoginsenoside Fc Assayed by Liquid Chromatography with Electrospray Ionization Tandem Mass Spectrometry. J. Sep. Sci. 42 (9), 1740–1749. doi:10.1002/jssc.201801237
Kamel, H., and Iadecola, C. (2012). Brain-Immune Interactions and Ischemic Stroke: Clinical Implications. Arch. Neurol. 69 (5), 576–581. doi:10.1001/archneurol.2011.3590
Ke, L., Guo, W., Xu, J., Zhang, G., Wang, W., and Huang, W. (2014). Ginsenoside Rb1 Attenuates Activated Microglia-Induced Neuronal Damage. Neural Regen. Res. 9 (3), 252–259. doi:10.4103/1673-5374.128217
Kim, H.-K. (2013). Pharmacokinetics of Ginsenoside Rb1 and its Metabolite Compound K after Oral Administration of Korean Red Ginseng Extract. J. Ginseng Res. 37 (4), 451–456. doi:10.5142/jgr.2013.37.451
Kim, J.-H. (2018). Pharmacological and Medical Applications of Panax Ginseng and Ginsenosides: a Review for Use in Cardiovascular Diseases. J. Ginseng Res. 42 (3), 264–269. doi:10.1016/j.jgr.2017.10.004
Li, W., Ling, S., Yang, Y., Hu, Z., Davies, H., and Fang, M. (2014). Systematic Hypothesis for post-stroke Depression Caused Inflammation and Neurotransmission and Resultant on Possible Treatments. Neuro Endocrinol. Lett. 35 (2), 104–109.
Li, T., Shu, Y.-J., Cheng, J.-Y., Liang, R.-C., Dian, S.-N., Lv, X.-X., et al. (2015). Pharmacokinetics and Efficiency of Brain Targeting of Ginsenosides Rg1 and Rb1 Given as Nao-Qing Microemulsion. Drug Develop. Ind. Pharm. 41 (2), 224–231. doi:10.3109/03639045.2013.858734
Li, F., Fan, X., Zhang, Y., Pang, L., Ma, X., and Song, M. (2016). Cardioprotection by Combination of Three Compounds from ShengMai Preparations in Mice with Myocardial Ischemia/reperfusion Injury through AMPK Activation-Mediated Mitochondrial Fission. Sci. Rep. 6, 37114. doi:10.1038/srep37114
Li, Y. N., Gao, Z. W., Li, R., Zhang, Y. F., Zhu, Q. S., and Huang, F. (2019). Aquaporin 4 Regulation by Ginsenoside Rb1 Intervenes with Oxygen-Glucose Deprivation/reoxygenation-Induced Astrocyte Injury. Medicine (Baltimore) 98 (42), e17591. doi:10.1097/md.0000000000017591
Li, C.-Y., Yang, P., Jiang, Y.-L., Lin, Z., Pu, Y.-W., Xie, L.-Q., et al. (2020). Ginsenoside Rb1 Attenuates Cardiomyocyte Apoptosis Induced by Myocardial Ischemia Reperfusion Injury through mTOR Signal Pathway. Biomed. Pharmacother. 125, 109913. doi:10.1016/j.biopha.2020.109913
Lim, J.-H., Wen, T.-C., Matsuda, S., Tanaka, J., Maeda, N., Peng, H., et al. (1997). Protection of Ischemic Hippocampal Neurons by Ginsenoside Rb1, a Main Ingredient of Ginseng Root. Neurosci. Res. 28 (3), 191–200. doi:10.1016/s0168-0102(97)00041-2
Liu, J. W., Ren, Y. L., Liu, X. L., Xia, H. L., Zhang, H. L., Jin, S. H., et al. (2013). Effect of Ginsenoside Rb1 on Cerebral Infarction Volume and IL-1 Beta in the Brain Tissue and Sera of Focal Cerebral Ischemia/reperfusion Injury Model Rats. Zhongguo Zhong Xi Yi Jie He Za Zhi. 33 (12), 1696–1700. doi:10.7661/CJIM.2013.12.1696
Liu, M. H., Yang, B. R., Cheung, W. F., Yang, K. Y., Zhou, H. F., Kwok, J. S., et al. (2015). Transcriptome Analysis of Leaves, Roots and Flowers of Panax Notoginseng Identifies Genes Involved in Ginsenoside and Alkaloid Biosynthesis. BMC Genomics 16, 265. doi:10.1186/s12864-015-1477-5
Liu, A., Zhu, W., Sun, L., Han, G., Liu, H., Chen, Z., et al. (2018). Ginsenoside Rb1 Administration Attenuates Focal Cerebral Ischemic Reperfusion Injury through Inhibition of HMGB1 and Inflammation Signals. Exp. Ther. Med. 16 (4), 3020–3026. doi:10.3892/etm.2018.6523
Lo, E. H. (2017). The Neurovascular Unit. Primer on Cerebrovascular Diseases. Editors L. R. Caplan, M. C. Leary, A. J. Thomas, J. H. Zhang, J. Biller, and E. H. Lo, Second Edition (London, United Kingdom: Elsevier), 226–229. doi:10.1016/b978-0-12-803058-5.00046-1
Lok, J., Gupta, P., Guo, S., Kim, W. J., Whalen, M. J., van Leyen, K., et al. (2007). Cell–cell Signaling in the Neurovascular Unit. Neurochem. Res. 32 (12), 2032–2045.
Lok, J., Wang, X.-S., Xing, C.-H., Maki, T.-K., Wu, L.-M., Guo, S.-Z., et al. (2015). Targeting the Neurovascular Unit in Brain Trauma. CNS Neurosci. Ther. 21 (4), 304–308. doi:10.1111/cns.12359
Lu, T.-H., Hsieh, S.-Y., Yen, C.-C., Wu, H.-C., Chen, K.-L., Hung, D.-Z., et al. (2011). Involvement of Oxidative Stress-Mediated ERK1/2 and P38 Activation Regulated Mitochondria-dependent Apoptotic Signals in Methylmercury-Induced Neuronal Cell Injury. Toxicol. Lett. 204 (1), 71–80. doi:10.1016/j.toxlet.2011.04.013
Luo, T., Liu, G., Ma, H., Lu, B., Xu, H., Wang, Y., et al. (2014). Inhibition of Autophagy via Activation of PI3K/Akt Pathway Contributes to the protection of Ginsenoside Rb1 against Neuronal Death Caused by Ischemic Insults. Int. J. Mol. Sci. 15 (9), 15426–15442. doi:10.3390/ijms150915426
Mancuso, C., and Santangelo, R. (2017). Panax Ginseng and Panax Quinquefolius : From Pharmacology to Toxicology. Food Chem. Toxicol. 107 (Pt), 362–372. doi:10.1016/j.fct.2017.07.019
Park, J. K., Namgung, U., Lee, C. J., Park, J. O., Jin, S.-H., Kwon, O.-B., et al. (2005). Calcium-independent CaMKII Activity Is Involved in Ginsenoside Rb1-Mediated Neuronal Recovery after Hypoxic Damage. Life Sci. 76 (9), 1013–1025. doi:10.1016/j.lfs.2004.10.011
Procaccio, V., Bris, C., Chao de la Barca, J. M., Oca, F., Chevrollier, A., Amati-Bonneau, P., et al. (2014). Perspectives of Drug-Based Neuroprotection Targeting Mitochondria. Revue Neurologique 170 (5), 390–400. doi:10.1016/j.neurol.2014.03.005
Reay, J. L., Scholey, A. B., and Kennedy, D. O. (2010). Panax Ginseng (G115) Improves Aspects of Working Memory Performance and Subjective Ratings of Calmness in Healthy Young Adults. Hum. Psychopharmacol. Clin. Exp. 25 (6), 462–471. doi:10.1002/hup.1138
Sun, J., and Nan, G. (2016). The Mitogen-Activated Protein Kinase (MAPK) Signaling Pathway as a Discovery Target in Stroke. J. Mol. Neurosci. 59 (1), 90–98. doi:10.1007/s12031-016-0717-8
Sun, Q., Meng, Q.-T., Jiang, Y., and Xia, Z.-Y. (2012). Ginsenoside Rb1 Attenuates Intestinal Ischemia Reperfusion Induced Renal Injury by Activating Nrf2/ARE Pathway. Molecules 17 (6), 7195–7205. doi:10.3390/molecules17067195
Sun, Q., Meng, Q. T., Jiang, Y., Liu, H. M., Lei, S. Q., Su, W. T., et al. (2013). Protective Effect of Ginsenoside Rb1 against Intestinal Ischemia-Reperfusion Induced Acute Renal Injury in Mice. PLoS One 8 (12), e80859. doi:10.1371/journal.pone.0080859
Tao, X., Sun, X., Yin, L., Han, X., Xu, L., Qi, Y., et al. (2015). Dioscin Ameliorates Cerebral Ischemia/reperfusion Injury through the Downregulation of TLR4 Signaling via HMGB-1 Inhibition. Free Radic. Biol. Med. 84, 103–115. doi:10.1016/j.freeradbiomed.2015.03.003
Turley, K. R., Toledo-Pereyra, L. H., and Kothari, R. U. (2005). Molecular Mechanisms in the Pathogenesis and Treatment of Acute Ischemic Stroke. J. Invest. Surg. 18 (4), 207–218. doi:10.1080/08941930591004449
Tuttolomondo, A., Di Sciacca, R., Di Raimondo, D., Pedone, C., La Placa, S., Pinto, A., et al. (2011). Effects of Clinical and Laboratory Variables and of Pretreatment with Cardiovascular Drugs in Acute Ischaemic Stroke: a Retrospective Chart Review from the GIFA Study. Int. J. Cardiol. 151 (3), 318–322. doi:10.1016/j.ijcard.2010.06.005
Wang, J., Qiao, L., Li, Y., and Yang, G. (2008). Ginsenoside Rb1 Attenuates Intestinal Ischemia-Reperfusion-Induced Liver Injury by Inhibiting NF-κB Activation. Exp. Mol. Med. 40 (6), 686–698. doi:10.3858/emm.2008.40.6.686
Wang, Z., Li, M., Wu, W.-k., Tan, H.-m., and Geng, D.-f. (2008). Ginsenoside Rb1 Preconditioning Protects against Myocardial Infarction after Regional Ischemia and Reperfusion by Activation of Phosphatidylinositol-3-Kinase Signal Transduction. Cardiovasc. Drugs Ther. 22 (6), 443–452. doi:10.1007/s10557-008-6129-4
Wang, J., Qiao, L., Li, S., and Yang, G. (2013). Protective Effect of Ginsenoside Rb1 against Lung Injury Induced by Intestinal Ischemia-Reperfusion in Rats. Molecules 18 (1), 1214–1226. doi:10.3390/molecules18011214
Wang, T., Guo, R., Zhou, G., Zhou, X., Kou, Z., Sui, F., et al. (2016). Traditional Uses, Botany, Phytochemistry, Pharmacology and Toxicology of Panax Notoginseng (Burk.) F.H. Chen: A Review. J. Ethnopharmacol. 188, 234–258. doi:10.1016/j.jep.2016.05.005
Wang, S., Li, M., Guo, Y., Li, C., Wu, L., Zhou, X.-F., et al. (2017). Effects of Panax Notoginseng Ginsenoside Rb1 on Abnormal Hippocampal Microenvironment in Rats. J. Ethnopharmacol. 202, 138–146. doi:10.1016/j.jep.2017.01.005
Wen, T. C., Yoshimura, H., Matsuda, S., Lim, J. H., and Sakanaka, M. (1996). Ginseng Root Prevents Learning Disability and Neuronal Loss in Gerbils with 5-minute Forebrain Ischemia. Acta Neuropathol. 91 (1), 15–22. doi:10.1007/s004010050387
Woodruff, T. M., Thundyil, J., Tang, S.-C., Sobey, C. G., Taylor, S. M., and Arumugam, T. V. (2011). Pathophysiology, Treatment, and Animal and Cellular Models of Human Ischemic Stroke. Mol. Neurodegeneration 6 (1), 11. doi:10.1186/1750-1326-6-11
Xia, R., Zhao, B., Wu, Y., Hou, J. B., Zhang, L., Xu, J. J., et al. (2011). Ginsenoside Rb1 Preconditioning Enhances eNOS Expression and Attenuates Myocardial Ischemia/reperfusion Injury in Diabetic Rats. J. Biomed. Biotechnol. 2011, 767930. doi:10.1155/2011/767930
Xie, T., Zhou, X. P., Lin, L. L., Xu, J. Y., Shen, C. S., Feng, Z., et al. (2016). Metabolomics Analysis of Tripterygium Wilfordii Formulation Based on Theory of Detoxicity Compatibility. Zhongguo Zhong Yao Za Zhi. 41 (6), 1124–1129. doi:10.4268/cjcmm20160625
Xie, W., Meng, X., Zhai, Y., Zhou, P., Ye, T., Wang, Z., et al. (2018a). Panax Notoginseng Saponins: A Review of Its Mechanisms of Antidepressant or Anxiolytic Effects and Network Analysis on Phytochemistry and Pharmacology. Molecules 23 (4), 940. doi:10.3390/molecules23040940
Xie, W., Zhou, P., Sun, Y., Meng, X., Dai, Z., Sun, G., et al. (2018b). Protective Effects and Target Network Analysis of Ginsenoside Rg1 in Cerebral Ischemia and Reperfusion Injury: A Comprehensive Overview of Experimental Studies. Cells 7 (12), 270. doi:10.3390/cells7120270
Xie, W., Zhu, T., Dong, X., Nan, F., Meng, X., Zhou, P., et al. (2019). HMGB1-triggered Inflammation Inhibition of Notoginseng Leaf Triterpenes against Cerebral Ischemia and Reperfusion Injury via MAPK and NF-κB Signaling Pathways. Biomolecules 9 (10), 512. doi:10.3390/biom9100512
Xie, W., Zhu, T., Zhou, P., Xu, H., Meng, X., Ding, T., et al. (2020). Notoginseng Leaf Triterpenes Ameliorates OGD/R-Induced Neuronal Injury via SIRT1/2/3-Foxo3a-MnSOD/PGC-1α Signaling Pathways Mediated by the NAMPT-NAD Pathway. Oxid Med. Cel Longev. 2020, 7308386. doi:10.1155/2020/7308386
Xu, M., Ma, Q., Fan, C., Chen, X., Zhang, H., and Tang, M. (2019). Ginsenosides Rb1 and Rg1 Protect Primary Cultured Astrocytes against Oxygen-Glucose Deprivation/Reoxygenation-Induced Injury via Improving Mitochondrial Function. Int. J. Mol. Sci. 20 (23), 6086. doi:10.3390/ijms20236086
Yang, C. X., Liu, J. X., Sun, Z. L., Gao, X. Q., Deng, L., and Yuan, Q. L. (2008). Effects of Ginsenoside RB1 on Neural Cell Apoptosis and Expressions of Bcl-2 and Bax in Rats Following Subjected to Cerebral Ischemia-Reperfusion. Sichuan Da Xue Xue Bao Yi Xue Ban 39 (2), 214–217. doi:10.1016/S1872-2075(08)60026-6
Yang, X., Xiong, X., Wang, H., and Wang, J. (2014). Protective Effects of Panax Notoginseng Saponins on Cardiovascular Diseases: a Comprehensive Overview of Experimental Studies. Evidence-Based Complementray Altern. Med. 2014 (12), 204840. doi:10.1155/2014/204840
Yang, X., Yang, S., Hong, C., Yu, W., and Guonian, W. (2017). Panax Notoginseng Saponins Attenuates Sevoflurane-Induced Nerve Cell Injury by Modulating AKT Signaling Pathway. Mol. Med. Rep. 16 (5), 7829–7834. doi:10.3892/mmr.2017.7519
Ye, J. T., Li, F. T., Huang, S. L., Xue, J. L., Aihaiti, Y., Wu, H., et al. (2019). Effects of Ginsenoside Rb1 on Spinal Cord Ischemia-Reperfusion Injury in Rats. J. Orthop. Surg. Res. 14 (1), 259. doi:10.1186/s13018-019-1299-2
Yuan, Q.-L., Yang, C.-X., Xu, P., Gao, X.-Q., Deng, L., Chen, P., et al. (2007). Neuroprotective Effects of Ginsenoside Rb1 on Transient Cerebral Ischemia in Rats. Brain Res. 1167, 1–12. doi:10.1016/j.brainres.2007.06.024
Zhai, Y., Meng, X., Luo, Y., Wu, Y., Ye, T., Zhou, P., et al. (2018). Notoginsenoside R1 Ameliorates Diabetic Encephalopathy by Activating the Nrf2 Pathway and Inhibiting NLRP3 Inflammasome Activation. Oncotarget 9 (10), 9344–9363. doi:10.18632/oncotarget.24295
Zhang, T.-T., and Jiang, J.-G. (2012). Active Ingredients of Traditional Chinese Medicine in the Treatment of Diabetes and Diabetic Complications. Expert Opin. Investig. Drugs 21 (11), 1625–1642. doi:10.1517/13543784.2012.713937
Zhang, J., Ding, L., Wang, B., Ren, G., Sun, A., Deng, C., et al. (2015). Notoginsenoside R1 Attenuates Experimental Inflammatory Bowel Disease via Pregnane X Receptor Activation. J. Pharmacol. Exp. Ther. 352 (2), 315–324. doi:10.1124/jpet.114.218750
Zhang, H., Li, Z., Zhou, Z., Yang, H., Zhong, Z., and Lou, C. (2016). Antidepressant-like Effects of Ginsenosides: A Comparison of Ginsenoside Rb3 and its Four Deglycosylated Derivatives, Rg3, Rh2, Compound K, and 20(S)-protopanaxadiol in Mice Models of Despair. Pharmacol. Biochem. Behav. 140, 17–26. doi:10.1016/j.pbb.2015.10.018
Zhao, D., Zhang, M., Yuan, H., Meng, C., Zhang, B., and Wu, H. (2018). Ginsenoside Rb1 Protects against Spinal Cord Ischemia-Reperfusion Injury in Rats by Downregulating the Bax/Bcl-2 Ratio and Caspase-3 and P-Ask-1 Levels. Exp. Mol. Pathol. 105 (3), 229–235. doi:10.1016/j.yexmp.2018.09.001
Zheng, X., Liang, Y., Kang, A., Ma, S.-J., Xing, L., Zhou, Y.-Y., et al. (2014). Peripheral Immunomodulation with Ginsenoside Rg1 Ameliorates Neuroinflammation-Induced Behavioral Deficits in Rats. Neuroscience 256, 210–222. doi:10.1016/j.neuroscience.2013.10.023
Zheng, Q., Bao, X. Y., Zhu, P. C., Tong, Q., Zheng, G. Q., Wang, Y., et al. (2017). Ginsenoside Rb1 for Myocardial Ischemia/Reperfusion Injury: Preclinical Evidence and Possible Mechanisms. Oxid Med. Cel Longev. 2017, 6313625. doi:10.1155/2017/6313625
Zhou, P., Xie, W., Luo, Y., Lu, S., Dai, Z., Wang, R., et al. (2018). Inhibitory Effects of Ginsenoside Rb1 on Early Atherosclerosis in ApoE-/- Mice via Inhibition of Apoptosis and Enhancing Autophagy. Molecules 23 (11). doi:10.3390/molecules23112912
Zhou, P., Xie, W., He, S., Sun, Y., Meng, X., Sun, G., et al. (2019a). Ginsenoside Rb1 as an Anti-Diabetic Agent and Its Underlying Mechanism Analysis. Cells 8 (3), 204. doi:10.3390/cells8030204
Zhou, P., Xie, W., Sun, Y., Dai, Z., Li, G., Sun, G., et al. (2019b). Ginsenoside Rb1 and Mitochondria: A Short Review of the Literature. Mol. Cell Probes. 43, 1–5. doi:10.1016/j.mcp.2018.12.001
Keywords: ginsenoside Rb1, ischemia and reperfusion injury, ischemia stroke, anti-inflammatory, antioxidant, antiapoptosis
Citation: Xie W, Wang X, Xiao T, Cao Y, Wu Y, Yang D and Zhang S (2021) Protective Effects and Network Analysis of Ginsenoside Rb1 Against Cerebral Ischemia Injury: A Pharmacological Review. Front. Pharmacol. 12:604811. doi: 10.3389/fphar.2021.604811
Received: 10 September 2020; Accepted: 13 May 2021;
Published: 02 July 2021.
Edited by:
Chun Guang Li, Western Sydney University, AustraliaReviewed by:
Min Wu, China Academy of Chinese Medical Sciences, ChinaPing Zhou, Chinese Academy of Medical Sciences and Peking Union Medical College, China
Copyright © 2021 Xie, Wang, Xiao, Cao, Wu, Yang and Zhang. This is an open-access article distributed under the terms of the Creative Commons Attribution License (CC BY). The use, distribution or reproduction in other forums is permitted, provided the original author(s) and the copyright owner(s) are credited and that the original publication in this journal is cited, in accordance with accepted academic practice. No use, distribution or reproduction is permitted which does not comply with these terms.
*Correspondence: Song Zhang, emhhbmdzb25nMTAzMUAxNjMuY29t
†These authors have contributed equally to this work