- 1National Centre for Drug Research and Evaluation, Istituto Superiore di Sanitá, Rome, Italy
- 2Department of Neuroscience, Istituto Superiore di Sanità, Rome, Italy
The STriatal-Enriched protein tyrosine phosphatase STEP is a brain-specific tyrosine phosphatase that plays a pivotal role in the mechanisms of learning and memory, and it has been demonstrated to be involved in several neuropsychiatric diseases. Recently, we found a functional interaction between STEP and adenosine A2A receptor (A2AR), a subtype of the adenosine receptor family widely expressed in the central nervous system, where it regulates motor behavior and cognition, and plays a role in cell survival and neurodegeneration. Specifically, we demonstrated the involvement of STEP in A2AR-mediated cocaine effects in the striatum and, more recently, we found that in the rat striatum and hippocampus, as well as in a neuroblastoma cell line, the overexpression of the A2AR, or its stimulation, results in an increase in STEP activity. In the present article we will discuss the functional implication of this interaction, trying to examine the possible mechanisms involved in this relation between STEP and A2ARs.
Introduction
P1 adenosine receptors are the most investigated purinergic receptors within the central nervous system (CNS). Since their identification in the late 70s, they have been the subject of numerous studies that established their widespread distribution in the brain and their pivotal role in the functioning of the CNS. The adenosine A2A receptor (A2AR) is one of the four G protein coupled receptor subtypes (A1, A2A, A2B, and A3), it is coupled with Gs protein and its stimulation activates adenylate cyclase causing an increase in intracellular cAMP levels (Borea et al., 2018). With the exception of the dorsal and ventral striatum, where A2AR is present at remarkably high levels, in the rest of the brain the expression of the receptor is quite low (Rosin et al., 2003). Despite this, the huge importance of A2AR in the CNS is witnessed by its role in the regulation of fundamental functions such as movement, cognition and emotions and, for this reason, it has attracted the interest of researchers as a potential therapeutic target (Borah et al., 2019). Indeed, the A2AR antagonist istradefylline (Nourianz®) has recently been approved in the United States, after its first registration in Japan, for the treatment of Parkinson’s disease, as an add-on to levodopa (Chen and Cunha, 2020).
One of the peculiarities of A2AR is the ability to modulate the activation and function of several other receptors, such as dopamine D2, cannabinoid CB1, metabotropic glutamate 5 receptor (mGlu5R), as well as adenosine A1 receptors, by forming heteroreceptor complexes (Cabello et al., 2009; Tebano et al., 2012; Moreno et al., 2018; Ferré and Ciruela, 2019). Recently, we identified a novel role of A2ARs in the rodent brain and in neuronal cells. Specifically, we demonstrated that the stimulation of A2ARs results in the activation of the STriatal-Enriched protein tyrosine phosphatase STEP, a brain-specific tyrosine phosphatase involved in several functions, including learning and memory (Goebel-Goody et al., 2012; Chiodi et al., 2014; Mallozzi et al., 2020).
In this article we will present some recent results on the A2ARs/STEP interaction and on the possible mechanisms involved. The physiological implication of this new receptor function will be discussed.
STriatal-Enriched Protein Tyrosine Phosphatase
In the early 90s, Paul J. Lombroso and collaborators isolated a new protein tyrosine phosphatase in the brain, particularly enriched in the striatum, that strongly colocalized with DARPP32 and tyrosine hydroxylase-positive neurons, which was denominated STEP (Lombroso et al., 1991, 1993). STEP exists in several isoforms that differ in intracellular localization and functions, and all originate by alternative splicing of a single Ptpn5 gene (Boulanger et al., 1995). The two major isoforms are STEP61, associated with membrane compartments, and the cytosolic protein STEP46, and both carry the consensus sequence required for the phosphatase catalytic activity and a kinase-interacting motif (KIM), that allows the interaction with the substrates. When phosphorylated at the specific Ser residues (221 for STEP61 and 49 for STEP46) within the KIM domains, STEP61 and STEP46 become inactive since they lose their ability to bind to the substrates (Bult et al., 1996; Pulido et al., 1998; Kamceva et al., 2016). STEP activity is regulated by quite complex phosphorylation/dephosphorylation mechanisms, in which calcineurin (a calcium/calmodulin-activated serine/threonine phosphatase, also known as PP2B) and protein kinase A (PKA) play a major role (Figure 1). Calcineurin activates STEP through protein phosphatase 1 (PP1), which dephosphorylates the regulatory serine residue and activates STEP (Paul et al., 2000). The activation of PKA results in the inhibition of STEP activity either through the direct phosphorylation of STEP61 and STEP46 at the specific serine residues and, indirectly, through the phosphorylation of DARPP-32 and the inhibition of PP1(Paul et al., 2000; Valjent et al., 2005; Giralt et al., 2011). Several neurotransmitter receptors, such as dopamine D1 receptor and nicotinic α7 nAChR, are able to modulate STEP activity (Paul et al., 2000; Zhang et al., 2013). Moreover, mGlu5R has been shown to increase STEP translation at dendritic levels that mediates AMPA receptor endocytosis, a mechanism that could be involved in DHPG-induced LTD. (Moult et al., 2002; Zhang et al., 2008; Goebel-Goody et al., 2012; Chen et al., 2013). As already mentioned, and as we will discuss later, STEP activity is also modulated by A2AR (Chiodi et al., 2014; Mallozzi et al., 2020).
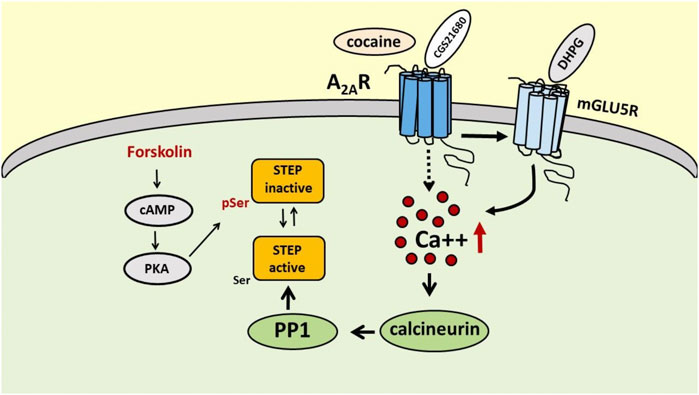
FIGURE 1. Schematic representation of the possible mechanisms involved in the regulation of STEP activity by A2ARs. Activation of A2ARs, directly with the selective A2AR agonist CGS 21680 or indirectly with cocaine, increases STEP activity through a peculiar mechanism involving mGlu5R, intracellular Ca++ increase and calcineurin recruitment. On the contrary, activation of PKA by forkolin promotes STEP inactivation through phosphorylation of Ser residue.
Several substrates of STEP have been identified. The glutamate receptor subunits GluN2B and GluA1/GluA2 of the NMDA and AMPA receptors, respectively, are important STEP substrates whose dephosphorylation at specific tyrosine residues promotes receptor internalization and reduces NMDA- and AMPA-mediated synaptic transmission, having a strong impact on synaptic plasticity (Won and Roche, 2021). Pyk2 and Fyn, two other STEP substrates, are also involved in the modulation of synaptic transmission and synaptic plasticity by influencing several mechanisms, including the direct or indirect phosphorylation of glutamate receptor subunits (Huang et al., 2001; Giralt et al., 2017; Matrone et al., 2020). Moreover, STEP shows a role at presynaptic level, modulating glutamate neurotransmitter release (Bosco et al., 2018). Additional STEP substrates are the extracellular signal-regulated kinases 1 and 2 (ERK1/2), involved in memory processes and in synaptic plasticity, and p38, implicated in cell death and survival, and both are inactivated by dephosphorylation of specific tyrosine residues upon STEP activation (Goebel-Goody et al., 2012).
STEP appears to be strongly involved in neurological disorders where synaptic dysfunctions have been identified, as well as in diseases where excitotoxicity play a major role.
Indeed, in the last years a dysregulation of STEP has been found in several neuropsychiatric diseases and its modulation, by genetic or pharmacological tools, was accompanied by the attenuation of the symptoms in animal models of diseases (Karasawa and Lombroso, 2014; Kulikova and Kulikov, 2017). The general idea is that elevated STEP levels or activity have detrimental effects on cognition by negatively influencing synaptic strengthening through the dephosphorylation of substrates regulating synaptic plasticity (Pelkey et al., 2002; Fitzpatrick and Lombroso, 2011). Indeed, high levels of STEP expression have been found in animal models of Alzheimer’s and Parkinson’s diseases (neurodegenerative diseases characterized by cognitive impairment), and in the hippocampus of aged mice, rats and rhesus monkeys and in the brain of individuals with mild cognitive impairment (Xu et al., 2012; Kurup et al., 2015; Castonguay et al., 2018). Furthermore, STEP over-expression induced memory deficits in mice, and its inhibition ameliorates memory performances in aged rats and in animal models of neuropsychiatric diseases (Castonguay et al., 2018). However, during aging reduced STEP activity and expression have also been reported (Rajagopal et al., 2016; Cases et al., 2018).
Beside its role in modulating synaptic plasticity and cognition, STEP is emerging as a key regulator of neuronal survival and death. As demonstrated by Choi et al. (2007), STEP increases neuronal vulnerability to excitotoxic cell death in primary hippocampal cultures and the sensitivity of neurons to excitotoxicity induced by Status Epilepticus in mice. These effects were due to the blockade of neuroprotective responses initiated by the ERK/MAPK signaling pathway. On the other hand, in an in vivo model of cerebral ischemia, where excitotoxic cell death plays a major role, STEP exerts a neuroprotective effect by inhibiting the p38 MAPK signaling pathway. In fact, administration of the STEP-derived peptide prevents p38 MAPK activation and reduces ischemic brain damage in STEP KO mice (Deb et al., 2013). In order to reconcile those apparently conflicting results, it should be considered that, depending on the level of calcium increase following NMDA receptors stimulation, STEP activity can be increased and promote neuroprotection by reducing p38 activation or, in case of a prolonged insult, the resulting STEP degradation will facilitate cell death pathways by increasing the phosphorylation of p38 MAPK (Poddar et al., 2010). In addition, the stimulation of synaptic or extrasynaptic NMDA receptors differently impacts on STEP expression, resulting in the activation of ERK1/2 or p38 MAPK, respectively, and promoting cell survival or death (Xu et al., 2009).
Another well identified role for STEP is the modulation of the effects of psychostimulant drugs such as cocaine and amphetamine (Valjent et al., 2005; Hopf and Bonci, 2009; Sun et al., 2013; Siemsen et al., 2018). As for cocaine effects, initial studies demonstrated that following acute cocaine treatment in mice, the increase in ERK1/2 phosphorylation (pERK1/2) in a subpopulation of dopamine D1R-containing striatal neurons was mediated, at least in part, by D1R-mediated STEP inactivation (Valjent et al., 2005). However, in condition of chronic cocaine consumption, such as in models of cocaine self-administration, a decrease in STEP phosphorylation and pERK1/2 are observed in the rat prefrontal cortex, that could represent early events in withdrawal mechanisms (Sun et al., 2013). More recently, cocaine-induced STEP activation has been demonstrated in the early phase of abstinence, which mediates the decrease in p-ERK observed in the pre-limbic cortex of cocaine-seeking rats (Siemsen et al., 2018). These studies demonstrate an active role of STEP in cocaine-mediated effects. In line with this, as we will describe below, we found that the synaptic depression exerted by cocaine in the striatum involved STEP activation through the stimulation of A2ARs (Chiodi et al., 2014), suggesting an interaction between the receptor and the phosphatase.
Evidence of a Functional Interaction Between A2AR and STriatal-Enriched Protein Tyrosine Phosphatase
The first evidence of an involvement of STEP in A2AR-mediated effects came from our study investigating the synaptic effects of cocaine in the striatum (Chiodi et al., 2014). We found that cocaine reduced striatal synaptic transmission, evaluated by recording extracellular field potentials and AMPA- and NMDA-mediated currents in whole cell patch-clamp experiments in corticostriatal slices. Cocaine effects were reduced by A2AR antagonist, by inhibitor of protein tyrosine phosphatases, by a calcineurin inhibitor and by TAT-STEP, a substrate trapping mutant peptide that makes STEP enzymatically inactive. In addition, the effect of cocaine was strongly reduced in A2AR knock-out mice. In order to understand the relationship among cocaine, A2ARs and tyrosine phosphatases (and STEP in particular), we evaluated the enzimatic activity of the total tyrosine phosphatases, and of STEP in particular, in mice striatal tissue after cocaine stimulation. We could show that cocaine increased tyrosine phosphatase activity, and in particular STEP activity, in A2AR-dependent manner. In fact, cocaine failed to activate STEP in the presence of the A2AR antagonist or in A2AR knock-out mice. These results suggested that a possible mechanism through which cocaine reduced synaptic transmission is the recruitment of A2AR and STEP activation. Indeed, STEP activation results in the dephosphorylation and internalization of NMDA and AMPA receptor subunits causing depression of excitatory synaptic transmission (Zhang et al., 2008; Zhang et al., 2010; Zhang et al., 2011; Kurup et al., 2010). Moreover, the involvement of calcineurin suggests the need of intracellular calcium increase. These mechanisms have been very nicely examined and depicted by Robert Yasuda (Yasuda, 2020) who recapitulated the way through which A2AR modulates cocaine-induced synaptic depression and, possibly, cocaine self-administration, via STEP activation.
In a recent paper, in order to confirm and further investigate the relationship between A2AR and STEP, we used cellular, genetic, and pharmacological approaches to evaluated STEP activity in different condition of A2AR stimulation and in different brain areas (Mallozzi et al., 2020). We took advantage of a transgenic rat strain overexpressing A2AR in the brain (Chiodi et al., 2016) in which we evaluated STEP activity in the striatum and hippocampus. In basal conditions, we found a significant increase in STEP activity in the striatum and hippocampus of A2AR overexpressing rats with respect to wild type. Moreover, in the striatum the selective A2AR agonist CGS21680 increased STEP activity in wild type but not in A2AR overexpressing rats (where STEP activity was already high), while ZM241385, the A2AR antagonist, reduced STEP activity in overexpressing rats (up to wild type levels), without any effects in wild type animals. In addition, in A2AR overexpressing rats we found a decrease in the phosphorylation levels of GluN2B and Pyk2, two well-known STEP substrates, consistent with an increased phosphatase activity (Mallozzi et al., 2020).
Similar results have been obtained in the neuroblastoma cell line SH-SY5Y, which expresses both STEP and A2ARs, where we confirmed that the stimulation of A2AR with CGS21680 causes an increase in STEP activity, evaluated also by western blotting analysis as a decrease in STEP phosphorylation status.
An interesting point to address is by which mechanism the stimulation of A2ARs results in STEP activation. It is demonstrated, in fact, that the activation of the cAMP/PKA pathway, as it occurs with the activation of Gs-coupled receptors (and the A2AR belongs indeed to the family of Gs-coupled receptors), rather results in the phosphorylation and inactivation of STEP (Paul et al., 2000). Actually, also in our hands the treatment of SH-SY5Y cells with forskolin (Mallozzi et al., 2020), which induces activation of the cAMP/PKA pathway, causes an up-regulation of phosphoSTEP, consistent with the inactivation of the phosphatase. Thus, a different mechanism must be hypothesized to explain A2AR-mediated STEP activation.
To assess if a physical interaction between A2AR and STEP could be necessary, we performed Bioluminescence Resonance Energy Transfer (BRET) assays (Molinari et al., 2008; Casella et al., 2011) in SH-SY5Y cell populations co-expressing a green fluorescent version of STEP61 with either luminescent-A2AR (a kind gift from Francisco Ciruela) or luminescent-β-arrestin 2 protein (a well recognized G-protein independent signal transducer) (Sachs et al., 2005). In our experiments, exposure of these cells to the A2AR agonist CGS21680 failed to enhance the BRET signal over the level of unstimulated samples, suggesting that STEP61 is probably not an A2AR interacting partner (unpublished data) and that the signaling route of A2AR to STEP61 probably does not depend on their direct interaction. However, to definitively exclude a direct interaction between A2AR and STEP, BRET experiments should be performed also by using other STEP isoforms (i.e., STEP46).
In a recent paper Won and collaborators used mass spectrometry to study STEP binding proteins and identified 315 candidate proteins and, among them, the authors recognized mGlu5R as an interactor of STEP (Won et al., 2019). This finding is particularly interesting since it is well known that A2AR and mGlu5R physically and functionally interact in several brain areas, that activation of A2ARs exerts a permissive role on mGluR5R-mediated effects (Ferre et al., 2002; Domenici et al., 2004; Tebano et al., 2005; Krania et al., 2018) and, most importantly, that mGlu5R stimulation results in an increase in STEP translation and, presumably, activation (Zhang et al., 2008). Moreover, mGlu5R interacts with Gq proteins and its stimulation enables the activation of PLC signaling and intracellular calcium increase (Conn and Pin, 1997). Interestingly, in our recent paper we found that A2AR-induced STEP activation is calcium-dependent since in SH-SY5Y cells it is prevented by the calcium chelator BAPTA-AM and by the calcineurin inhibitor FK506 (Mallozzi et al., 2020). Thus, on the basis of this calcium dependence, the mGlu5R could be a good candidate to mediate A2AR effects on STEP activity. Therefore, in preliminary experiments we verified in the SH-SY5Y cell line the effect of the selective A2AR agonist CGS 21680 on STEP activity in the presence of the mGlu5R antagonist MPEP, and we found that by blocking mGlu5R, CGS 21680 was no longer able to increase STEP activity (unpublished results). Even though additional experiments are needed, these results clearly suggest that A2ARs modulate STEP activity through the involvement of mGlu5R (Figure 1).
Discussion and Conclusion
The studies presented above provide a clear demonstration of a functional interaction between A2ARs and STEP in the striatum and hippocampus of the rat and mouse brain, which has been confirmed in the SH-SY5Y neuroblastoma cell line, suggesting that this interaction can occur in different cell types. The mechanism through which A2AR and STEP interact is still not clearly identified, but the calcium dependence and the involvement of mGlu5R are both very likely. Even though a strong evidence that this interaction occurs also in vivo is still lacking, a review of the scientific literature shows that in some neuropathologic conditions STEP and the A2AR are dysregulated in a similar way. For example, STEP levels are elevated in rodent models of Alzheimer’s disease, in postmortem brains of patients with Alzheimer’s disease and in the brain of individuals with mild cognitive impairment (Zhang et al., 2011; Xu et al., 2012; Castonguay et al., 2018). In the same way, A2ARs are upregulated in Alzheimer’s disease, both in animal models and in the brain of patients (Arendash et al., 2006; Albasanz et al., 2008; Orr et al., 2015; Temido-Ferreira et al., 2020). More interestingly, during aging both STEP and A2ARs are upregulated and show an enhanced activity in animal models and in the human aged brain, and inhibition of STEP activity or the blockade of A2ARs improved memory performances (Castonguay et al., 2018; Orr et al., 2018; Ferré and Ciruela, 2019; Temido-Ferreira et al., 2019; Temido-Ferreira et al., 2020). Finally, STEP over-expression induced memory impairment in adult mice (Castonguay et al., 2018), and the same occurs in conditions of increased A2ARs activation (Gimenez-Llort et al., 2007; Li et al., 2015; Pagnussat et al., 2015). Accordingly, in A2AR overexpressing rats, in which we demonstrated an increased basal STEP activity in the striatum and hippocampus, working memory deficits have been reported (Gimenez-Llort et al., 2007; Mallozzi et al., 2020). Very recently, Ferrante et al. (2021) demonstrated that STEP protein expression and activity were increased in Fragile X mice and normalized by the A2AR antagonist KW6002 treatment, which improved the behavioral phenotype as well.
Thus, one important conclusion is that the modulation of STEP activity could contribute to the effects of A2ARs on cognitive functions (Chen, 2014; Uchida et al., 2014; Temido-Ferreira et al., 2019). As for Parkinson’s disease, an interesting consideration is that long-term treatment of patients with istradefylline could result not only in the improvement of motor deficits but also in beneficial effects on cognitive dysfunction, and that the inhibition of STEP could play a major role in this effect. In fact, STEP levels are increased in human brains and in animal models of Parkinson’s disease, which may contribute to the cognitive impairment that occurs in the disease (Kurup et al., 2015).
In conclusion, the interaction between A2AR and STEP (possibly through the involvement of mGlu5R) could have clinical relevance and its possible consequences should be contemplated when proposing drugs targeting the A2ARs. Notably, particular attention should be payed when considering A2AR agonists as potential treatment for human pathologies (Borea et al., 2018; Borah et al., 2019), given their potential to impair cognitive performance by increasing STEP activity.
Data Availability Statement
The original contributions presented in the study are included in the article/Supplementary Material, further inquiries can be directed to the corresponding author.
Author Contributions
MRD and CM conceived the work; MRD drafted the manuscript; RP composed the figure; IC, CM, and RP, produced the original data, VC, AF, and PP contributed in writing and editing and all read and approved the present version of the manuscript for publication.
Conflict of Interest
The authors declare that the research was conducted in the absence of any commercial or financial relationships that could be construed as a potential conflict of interest.
References
Albasanz, J. L., Perez, S., Barrachina, M., Ferrer, I., and Martín, M. (2008). Research article: up-regulation of adenosine receptors in the frontal cortex in alzheimer's disease. Brain Pathol. 18 (2), 211–219. doi:10.1111/j.1750-3639.2007.00112.x
Arendash, G. W., Schleif, W., Rezai-Zadeh, K., Jackson, E. K., Zacharia, L. C., Cracchiolo, J. R., et al. (2006). Caffeine protects Alzheimer's mice against cognitive impairment and reduces brain β-amyloid production. Neuroscience 142 (2), 941–952. doi:10.1016/j.neuroscience.2006.07.021
Borah, P., Deka, S., Mailavaram, R. P., and Deb, P. K. (2019). P1 receptor agonists/antagonists in clinical trials - potential drug candidates of the future. Curr. Pharm. Des. 25 (26), 2792–2807. doi:10.2174/1381612825666190716111245
Borea, P. A., Gessi, S., Merighi, S., Vincenzi, F., and Varani, K. (2018). Pharmacology of adenosine receptors: the state of the art. Physiol. Rev. 98 (3), 1591–1625. doi:10.1152/physrev.00049.2017
Bosco, F., Valente, P., Milanese, M., Piccini, A., Messa, M., Bonanno, G., et al. (2018). Altered intracellular calcium homeostasis underlying enhanced glutamatergic transmission in striatal-enriched tyrosine phosphatase (STEP) knockout mice. Mol. Neurobiol. 55 (10), 8084–8102. doi:10.1007/s12035-018-0980-5
Boulanger, L., Lombroso, P., Raghunathan, A., During, M., Wahle, P., and Naegele, J. (1995). Cellular and molecular characterization of a brain-enriched protein tyrosine phosphatase. J. Neurosci. 15 (12), 1532–1544. doi:10.1523/jneurosci.15-02-01532.1995
Bult, A., Zhao, F., Dirkx Jr., R., Sharma, E., Lukacsi, E., Solimena, M., et al. (1996). Step61: a member of a family of brain-enriched PTPs is localized to the endoplasmic reticulum. J. Neurosci. 16 (24), 7821–7831. doi:10.1523/jneurosci.16-24-07821.1996
Cabello, N., Gandía, J., Bertarelli, D. C. G., Watanabe, M., Lluís, C., Franco, R., et al. (2009). Metabotropic glutamate type 5, dopamine D2and adenosine A2areceptors form higher-order oligomers in living cells. J. Neurochem. 109 (5), 1497–1507. doi:10.1111/j.1471-4159.2009.06078.x
Casella, I., Ambrosio, C., Grò, M. C., Molinari, P., and Costa, T. (2011). Divergent agonist selectivity in activating β1- and β2-adrenoceptors for G-protein and arrestin coupling. Biochem. J. 438 (1), 191–202. doi:10.1042/BJ20110374
Cases, S., Saavedra, A., Tyebji, S., Giralt, A., Alberch, J., and Pérez-Navarro, E. (2018). Age-related changes in STriatal-Enriched protein tyrosine Phosphatase levels: regulation by BDNF. Mol. Cell Neurosci. 86, 41–49. doi:10.1016/j.mcn.2017.11.003
Castonguay, D., Dufort-Gervais, J., Ménard, C., Chatterjee, M., Quirion, R., Bontempi, B., et al. (2018). The tyrosine phosphatase STEP is involved in age-related memory decline. Curr. Biol. 28 (7), 1079–1089.e4. doi:10.1016/j.cub.2018.02.047
Chen, J.-F. (2014). Adenosine receptor control of cognition in normal and disease. Int. Rev. Neurobiol. 119, 257–307. doi:10.1016/B978-0-12-801022-8.00012-X
Chen, J.-F., and Cunha, R. A. (2020). The belated US FDA approval of the adenosine A2A receptor antagonist istradefylline for treatment of Parkinson's disease. Purinergic Signal. 16 (2), 167–174. doi:10.1007/s11302-020-09694-2
Chen, X., Lin, R., Chang, L., Xu, S., Wei, X., Zhang, J., et al. (2013). Enhancement of long-term depression by soluble amyloid β protein in rat hippocampus is mediated by metabotropic glutamate receptor and involves activation of p38MAPK, STEP and caspase-3. Neuroscience 253, 435–443. doi:10.1016/j.neuroscience.2013.08.054
Chiodi, V., Ferrante, A., Ferraro, L., Potenza, R. L., Armida, M., Beggiato, S., et al. (2016). Striatal adenosine-cannabinoid receptor interactions in rats over-expressing adenosine A2Areceptors. J. Neurochem. 136 (5), 907–917. doi:10.1111/jnc.13421
Chiodi, V., Mallozzi, C., Ferrante, A., Chen, J. F., Lombroso, P. J., Di Stasi, A. M. M., et al. (2014). Cocaine-induced changes of synaptic transmission in the striatum are modulated by adenosine A2A receptors and involve the tyrosine phosphatase STEP. Neuropsychopharmacology 39 (3), 569–578. doi:10.1038/npp.2013.229
Choi, Y. S., Lin, S. L., Lee, B., Kurup, P., Cho, H. Y., Naegele, J. R., et al. (2007). Status epilepticus-induced somatostatinergic hilar interneuron degeneration is regulated by striatal enriched protein tyrosine phosphatase. J. Neurosci. 27 (11), 2999–3009. doi:10.1523/JNEUROSCI.4913-06.2007
Conn, P. J., and Pin, J.-P. (1997). Pharmacology and functions of metabotropic glutamate receptors. Annu Rev Pharmacol Toxicol. 37, 205–237. doi:10.1146/annurev.pharmtox.37.1.205
Deb, I., Manhas, N., Poddar, R., Rajagopal, S., Allan, A. M., Lombroso, P. J., et al. (2013). Neuroprotective role of a brain-enriched tyrosine phosphatase, STEP, in focal cerebral ischemia. J. Neurosci. 33 (45), 17814–17826. doi:10.1523/JNEUROSCI.2346-12.2013
Domenici, M. R., Pepponi, R., Martire, A., Tebano, M. T., Potenza, R. L., and Popoli, P. (2004). Permissive role of adenosine A2A receptors on metabotropic glutamate receptor 5 (mGluR5)-mediated effects in the striatum. J. Neurochem. 90 (5), 1276–1279. doi:10.1111/j.1471-4159.2004.02607.x
Ferrante, A., Boussadia, Z., Borreca, A., Mallozzi, C., Pedini, G., Pacini, L., et al. (2021). Adenosine A2A receptor inhibition reduces synaptic and cognitive hippocampal alterations in Fmr1 KO mice. Transl. Psychiatry 11 (1), 112. doi:10.1038/s41398-021-01238-5
Ferré, S., and Ciruela, F. (2019). Functional and neuroprotective role of striatal adenosine A2AReceptor heterotetramers. J. Caffeine Adenosine Res. 9 (3), 89–97. doi:10.1089/caff.2019.0008
Ferre, S., Karcz-Kubicha, M., Hope, B. T., Popoli, P., Burgueno, J., Gutierrez, M. A., et al. (2002). Synergistic interaction between adenosine A2A and glutamate mGlu5 receptors: implications for striatal neuronal function. Proc. Natl. Acad. Sci. 99 (18), 11940–11945. doi:10.1073/pnas.172393799
Fitzpatrick, C. J., and Lombroso, P. J. (2011). The role of striatal-enriched protein tyrosine phosphatase (STEP) in cognition. Front. Neuroanat. 5, 47. doi:10.3389/fnana.2011.00047
Giménez-Llort, L., Schiffmann, S. N., Shmidt, T., Canela, L., Camón, L., Wassholm, M., et al. (2007). Working memory deficits in transgenic rats overexpressing human adenosine A2A receptors in the brain. Neurobiol. Learn. Mem. 87 (1), 42–56. doi:10.1016/j.nlm.2006.05.004
Giralt, A., Brito, V., Chevy, Q., Simonnet, C., Otsu, Y., Cifuentes-Díaz, C., et al. (2017). Pyk2 modulates hippocampal excitatory synapses and contributes to cognitive deficits in a Huntington's disease model. Nat. Commun. 8 (1), 15592. doi:10.1038/ncomms15592
Giralt, A., Saavedra, A., Carretón, O., Xifró, X., Alberch, J., and Pérez-Navarro, E. (2011). Increased PKA signaling disrupts recognition memory and spatial memory: role in Huntington's disease. Hum. Mol. Genet. 20 (21), 4232–4247. doi:10.1093/hmg/ddr351
Goebel-Goody, S. M., Baum, M., Paspalas, C. D., Fernandez, S. M., Carty, N. C., Kurup, P., et al. (2012). Therapeutic implications for striatal-enriched protein tyrosine phosphatase (STEP) in neuropsychiatric disorders. Pharmacol. Rev. 64 (1), 65–87. doi:10.1124/pr.110.003053
Hopf, F. W., and Bonci, A. (2009). Striatal-enriched protein-tyrosine-phosphatase, synaptic plasticity, and psychostimulant-induced stereotypies. Biol. Psychiatry 65 (8), 635–636. doi:10.1016/j.biopsych.2009.02.008
Huang, Y.-Q., Lu, W.-Y., Ali, D. W., Pelkey, K. A., Pitcher, G. M., Lu, Y. M., et al. (2001). CAKβ/Pyk2 kinase is a signaling link for induction of long-term potentiation in CA1 Hippocampus. Neuron 29 (2), 485–496. doi:10.1016/s0896-6273(01)00220-3
Kamceva, M., Benedict, J., Nairn, A. C., and Lombroso, P. J. (2016). Role of striatal-enriched tyrosine phosphatase in neuronal function. Neural Plasticity 2016, 1–9. doi:10.1155/2016/8136925
Karasawa, T., and Lombroso, P. J. (2014). Disruption of striatal-enriched protein tyrosine phosphatase (STEP) function in neuropsychiatric disorders. Neurosci. Res. 89, 1–9. doi:10.1016/j.neures.2014.08.018
Krania, P., Dimou, E., Bantouna, M., Kouvaros, S., Tsiamaki, E., Papatheodoropoulos, C., et al. (2018). Adenosine A2A receptors are required for glutamate mGluR5- and dopamine D1 receptor-evoked ERK1/2 phosphorylation in rat hippocampus: involvement of NMDA receptor. J. Neurochem. 145 (3), 217–231. doi:10.1111/jnc.14268
Kulikova, E., and Kulikov, A. (2017). Striatal-enriched tyrosine protein phosphatase (STEP) in the mechanisms of depressive disorders. Curr. Protein Pept. Sci. 18 (11), 1152–1162. doi:10.2174/1389203718666170710121532
Kurup, P. K., Xu, J., Videira, R. A., Ononenyi, C., Baltazar, G., Lombroso, P. J., et al. (2015). STEP61 is a substrate of the E3 ligase parkin and is upregulated in Parkinson's disease. Proc. Natl. Acad. Sci. 112 (4), 1202–1207. doi:10.1073/pnas.1417423112
Kurup, P., Zhang, Y., Xu, J., Venkitaramani, D. V., Haroutunian, V., Greengard, P., et al. (2010). A -mediated NMDA receptor endocytosis in alzheimer's disease involves ubiquitination of the tyrosine phosphatase STEP61. J. Neurosci. 30 (17), 5948–5957. doi:10.1523/JNEUROSCI.0157-10.2010
Li, W., Silva, H. B., Real, J., Wang, Y.-M., Rial, D., Li, P., et al. (2015). Inactivation of adenosine A2A receptors reverses working memory deficits at early stages of Huntington's disease models. Neurobiol. Dis. 79, 70–80. doi:10.1016/j.nbd.2015.03.030
Lombroso, P. J., Murdoch, G., and Lerner, M. (1991). Molecular characterization of a protein-tyrosine-phosphatase enriched in striatum. Proc. Natl. Acad. Sci. 88 (16), 7242–7246. doi:10.1073/pnas.88.16.7242
Lombroso, P., Naegele, J., Sharma, E., and Lerner, M. (1993). A protein tyrosine phosphatase expressed within dopaminoceptive neurons of the basal ganglia and related structures. J. Neurosci. 13 (7), 3064–3074. doi:10.1523/jneurosci.13-07-03064.1993
Mallozzi, C., Pepponi, R., Visentin, S., Chiodi, V., Lombroso, P. J., Bader, M., et al. (2020). The activity of the Striatal‐enriched protein tyrosine phosphatase in neuronal cells is modulated by adenosine A 2A receptor. J. Neurochem. 152 (3), 284–298. doi:10.1111/jnc.14866
Matrone, C., Petrillo, F., Nasso, R., and Ferretti, G. (2020). Fyn tyrosine kinase as harmonizing factor in neuronal functions and dysfunctions. Int. J. Mol. Sci. 21 (12), 4444. doi:10.3390/ijms21124444
Molinari, P., Casella, I., and Costa, T. (2008). Functional complementation of high-efficiency resonance energy transfer: a new tool for the study of protein binding interactions in living cells. Biochem. J. 409 (1), 251–261. doi:10.1042/bj20070803
Moreno, E., Chiarlone, A., Medrano, M., Puigdellívol, M., Bibic, L., Howell, L. A., et al. (2018). Singular location and signaling profile of adenosine a2a-cannabinoid CB1 receptor heteromers in the dorsal striatum. Neuropsychopharmacol. 43 (5), 964–977. doi:10.1038/npp.2017.12
Moult, P. R., Schnabel, R., Kilpatrick, I. C., Bashir, Z. I., and Collingridge, G. L. (2002). Tyrosine dephosphorylation underlies DHPG-induced LTD. Neuropharmacology 43 (2), 175–180. doi:10.1016/s0028-3908(02)00110-7
Orr, A. G., Hsiao, E. C., Wang, M. M., Ho, K., Kim, D. H., Wang, X., et al. (2015). Astrocytic adenosine receptor A2A and Gs-coupled signaling regulate memory. Nat. Neurosci. 18 (3), 423–434. doi:10.1038/nn.3930
Orr, A. G., Lo, I., Schumacher, H., Ho, K., Gill, M., Guo, W., et al. (2018). Istradefylline reduces memory deficits in aging mice with amyloid pathology. Neurobiol. Dis. 110 (17), 29–36. doi:10.1016/j.nbd.2017.10.014
Pagnussat, N., Almeida, A. S., Marques, D. M., Nunes, F., Chenet, G. C., Botton, P. H. S., et al. (2015). Adenosine A2Areceptors are necessary and sufficient to trigger memory impairment in adult mice. Br. J. Pharmacol. 172 (15), 3831–3845. doi:10.1111/bph.13180
Paul, S., Snyder, G. L., Yokakura, H., Picciotto, M. R., Nairn, A. C., and Lombroso, P. J. (2000). The Dopamine/D1 receptor mediates the phosphorylation and inactivation of the protein tyrosine phosphatase STEP via a PKA-dependent pathway. J. Neurosci. 20 (15), 5630–5638. doi:10.1523/jneurosci.20-15-05630.2000
Pelkey, K. A., Askalan, R., Paul, S., Kalia, L. V., Nguyen, T.-H., Pitcher, G. M., et al. (2002). Tyrosine phosphatase STEP is a tonic brake on induction of long-term potentiation. Neuron 34 (1), 127–138. doi:10.1016/s0896-6273(02)00633-5
Poddar, R., Deb, I., Mukherjee, S., and Paul, S. (2010). NR2B-NMDA receptor mediated modulation of the tyrosine phosphatase STEP regulates glutamate induced neuronal cell death. J. Neurochem. 115 (6), 1350–1362. doi:10.1111/j.1471-4159.2010.07035.x
Pulido, R., Zuniga, A., and Ullrich, A. (1998). PTP-SL and STEP protein tyrosine phosphatases regulate the activation of the extracellular signal-regulated kinases ERK1 and ERK2 by association through a kinase interaction motif. EMBO J. 17 (24), 7337–7350. doi:10.1093/emboj/17.24.7337
Rajagopal, S., Deb, I., Poddar, R., and Paul, S. (2016). Aging is associated with dimerization and inactivation of the brain-enriched tyrosine phosphatase STEP. Neurobiol. Aging 41, 25–38. doi:10.1016/j.neurobiolaging.2016.02.004
Rosin, D. L., Hettinger, B. D., Lee, A., and Linden, J. (2003). Anatomy of adenosine A2A receptors in brain: morphological substrates for integration of striatal function. Neurology 61 (Issue 11, Supplement 6), S12–S18. doi:10.1212/01.wnl.0000095205.33940.99
Sachs, K., Perez, O., Pe’er, D., Lauffenburger, D. A., and Nolan, G. P. (2005). Causal protein-signaling networks derived from multiparameter single-cell data. Science 308 (5721), 523–529. doi:10.1126/science.1105809
Siemsen, B. M., Lombroso, P. J., and McGinty, J. F. (2018). Intra-prelimbic cortical inhibition of striatal-enriched tyrosine phosphatase suppresses cocaine seeking in rats. Addict. Biol. 23 (1), 219–229. doi:10.1111/adb.12504
Sun, W.-L., Zelek-Molik, A., and McGinty, J. F. (2013). Short and long access to cocaine self-administration activates tyrosine phosphatase STEP and attenuates GluN expression but differentially regulates GluA expression in the prefrontal cortex. Psychopharmacology 229 (4), 603–613. doi:10.1007/s00213-013-3118-5
Tebano, M. T., Martire, A., and Popoli, P. (2012). Adenosine A2A-cannabinoid CB1 receptor interaction: an integrative mechanism in striatal glutamatergic neurotransmission. Brain Res. 1476, 108–118. doi:10.1016/j.brainres.2012.04.051
Tebano, M. T., Martire, A., Rebola, N., Pepponi, R., Domenici, M. R., Gro, M. C., et al. (2005). Adenosine A2A receptors and metabotropic glutamate 5 receptors are co-localized and functionally interact in the hippocampus: a possible key mechanism in the modulation of N-methyl-D-aspartate effects. J. Neurochem. 95 (4), 1188–1200. doi:10.1111/j.1471-4159.2005.03455.x
Temido-Ferreira, M., Coelho, J. E., Pousinha, P. A., and Lopes, L. V. (2019). Novel players in the aging synapse: impact on cognition. J. Caffeine Adenosine Res. 9 (3), 104–127. doi:10.1089/caff.2019.0013
Temido-Ferreira, M., Ferreira, D. G., Batalha, V. L., Marques-Morgado, I., Coelho, J. E., Pereira, P., et al. (2020). Age-related shift in LTD is dependent on neuronal adenosine A2A receptors interplay with mGluR5 and NMDA receptors. Mol. Psychiatry 25 (8), 1876–1900. doi:10.1038/s41380-018-0110-9
Uchida, S.-i., Kadowaki-Horita, T., and Kanda, T. (2014). Effects of the adenosine A2A receptor antagonist on cognitive dysfunction in Parkinson's disease. Int. Rev. Neurobiol. 119, 169–189. doi:10.1016/B978-0-12-801022-8.00008-8
Valjent, E., Pascoli, V., Svenningsson, P., Paul, S., Enslen, H., Corvol, J.-C., et al. (2005). From the Cover: regulation of a protein phosphatase cascade allows convergent dopamine and glutamate signals to activate ERK in the striatum. Proc. Natl. Acad. Sci. 102 (2), 491–496. doi:10.1073/pnas.0408305102
Won, S., Incontro, S., Li, Y., Nicoll, R. A., and Roche, K. W. (2019). The STEP61 interactome reveals subunit-specific AMPA receptor binding and synaptic regulation. Proc. Natl. Acad. Sci. U.S.A. 116 (16), 8028–8037. doi:10.1073/pnas.1900878116
Won, S., and Roche, K. W. (2021). Regulation of glutamate receptors by striatal‐enriched tyrosine phosphatase 61 (STEP 61 ). J. Physiol. 599 (2), 443–451. doi:10.1113/JP278703
Xu, J., Kurup, P., Nairn, A. C., and Lombroso, P. J. (2012). Striatal-enriched protein tyrosine phosphatase in alzheimer's disease. Adv. Pharmacol. 64, 303–325. doi:10.1016/B978-0-12-394816-8.00009-X
Xu, J., Kurup, P., Zhang, Y., Goebel-Goody, S. M., Wu, P. H., Hawasli, A. H., et al. (2009). Extrasynaptic NMDA receptors couple preferentially to excitotoxicity via calpain-mediated cleavage of STEP. J. Neurosci. 29 (29), 9330–9343. doi:10.1523/JNEUROSCI.2212-09.2009
Yasuda, R. P. (2020). Adenosine STEPs on synaptic function. J. Neurochem. 152 (3), 270–272. doi:10.1111/jnc.14901
Zhang, L., Xie, J.-W., Yang, J., and Cao, Y.-P. (2013). Tyrosine phosphatase STEP61negatively regulates amyloid β-mediated ERK/CREB signaling pathways via α7 nicotinic acetylcholine receptors. J. Neurosci. Res. 91 (12), 1581–1590. doi:10.1002/jnr.23263
Zhang, Y., Kurup, P., Xu, J., Anderson, G. M., Greengard, P., Nairn, A. C., et al. (2011). Reduced levels of the tyrosine phosphatase STEP block beta amyloid-mediated GluA1/GluA2 receptor internalization. J. Neurochem. 119 (3), 664–672. doi:10.1111/j.1471-4159.2011.07450.x
Zhang, Y., Kurup, P., Xu, J., Carty, N., Fernandez, S. M., Nygaard, H. B., et al. (2010). Genetic reduction of striatal-enriched tyrosine phosphatase (STEP) reverses cognitive and cellular deficits in an Alzheimer's disease mouse model. Proc. Natl. Acad. Sci. 107 (44), 19014–19019. doi:10.1073/pnas.1013543107
Keywords: adenosine A2A receptor, striatal-enriched protein tyrosine phosphatase, brain, SH-SY5Y neuroblastoma cell lines, functional interaction
Citation: Domenici MR, Mallozzi C, Pepponi R, Casella I, Chiodi V, Ferrante A and Popoli P (2021) Insight into the Role of the STriatal-Enriched Protein Tyrosine Phosphatase (STEP) in A2A Receptor-Mediated Effects in the Central Nervous System. Front. Pharmacol. 12:647742. doi: 10.3389/fphar.2021.647742
Received: 05 January 2021; Accepted: 26 February 2021;
Published: 19 April 2021.
Edited by:
Francisco Ciruela, University of Barcelona, SpainReviewed by:
Hyung Nam, Louisiana State University Health Shreveport, United StatesMarco Milanese, University of Genoa, Italy
Copyright © 2021 Domenici, Mallozzi, Pepponi, Casella, Chiodi, Ferrante and Popoli. This is an open-access article distributed under the terms of the Creative Commons Attribution License (CC BY). The use, distribution or reproduction in other forums is permitted, provided the original author(s) and the copyright owner(s) are credited and that the original publication in this journal is cited, in accordance with accepted academic practice. No use, distribution or reproduction is permitted which does not comply with these terms.
*Correspondence: Maria Rosaria Domenici, bWFyaWFyb3NhcmlhLmRvbWVuaWNpQGlzcy5pdA==