- 1Department of Cardiology, West China Hospital, Sichuan University, Chengdu, China
- 2Division of Cardiology, Department of Medicine, Karolinska University Hospital, Karolinska Institutet, Stockholm, Sweden
- 3Laboratory of Cardiovascular Diseases, Regenerative Medicine Research Center, West China Hospital, Sichuan University, Chengdu, China
- 4School of Acupuncture and Tuina and International Collaborative Centre on Big Science Plan for Purinergic Signalling, Chengdu University of Traditional Chinese Medicine, Chengdu, China
- 5Acupuncture and Chronobiology Key Laboratory of Sichuan Province, Chengdu, China
Purinergic P2X7 receptor, a nonselective cation channel, is highly expressed in immune cells as well as cardiac smooth muscle cells and endothelial cells. Its activation exhibits to mediate nucleotide-binding domain (NOD)-like receptor protein 3 (NLRP3) inflammasome activation, resulting in the release of interleukin-1 beta (IL-1β) and interleukin-18 (IL-18), and pyroptosis, thus triggering inflammatory response. These pathological mechanisms lead to the deterioration of various cardiovascular diseases, including atherosclerosis, arrhythmia, myocardial infarction, pulmonary vascular remodeling, and cardiac fibrosis. All these worsening cardiac phenotypes are proven to be attenuated after the P2X7 receptor inhibition in experimental studies. The present review aimed to summarize key aspects of P2X7 receptor–mediated inflammation and pyroptosis in cardiovascular diseases. The main focus is on the evidence addressing the involvement of the P2X7 receptor in the inflammatory responses to the occurrence and development of cardiovascular disease and therapeutic interventions.
Introduction
Cardiovascular disease is a major cause of morbidity and mortality worldwide. Chronic inflammation is an important player in the pathogenesis of cardiovascular disease (Golia et al., 2014), which simultaneously impairs cardiac function, and continuously aggravates the symptoms of patients (Westermann et al., 2011).
Recent studies demonstrated that purinergic P2X7 receptors played an important role in a variety of cardiovascular diseases, including atherosclerosis (Piscopiello et al., 2013; Wernly and Zhou, 2020), arrhythmia after myocardial infarction (Gao et al., 2017), vascular remodeling (Mahdi et al., 2018; Hansen et al., 2020), and cardiac fibrosis (Zhou J. et al., 2020). P2X7 receptors are highly expressed in immune cells, including dendritic cells, mast cells, macrophages, and monocytes. As an ATP-gated ion channel, activation of the P2X7 receptor causes the release of interleukin-1 beta (IL-1β) and interleukin-18 (IL-18) recruitment by NLRP3, resulting in inflammatory response (Bracey et al., 2013; Stachon et al., 2017). A recent review made a summary on the role of P2X7 receptor–mediated endothelial dysfunction in hypertension, atherosclerosis, renal dysfunction, and cardiac and cerebral ischemia by promoting inflammatory responses (Shokoples et al., 2020). Apart from endothelial dysfunction, pyroptosis in cardiomyocytes was also demonstrated to be associated with the P2X7 receptor in heart injury (Liu et al., 2016). In our review, we will focus on key aspects of P2X7 receptor–mediated inflammation and pyroptosis in cardiovascular diseases, including atherosclerosis, arrhythmia, myocardial infarction, pulmonary vascular remodeling, and cardiac fibrosis, and the therapeutic value of targeting the P2X7 receptor.
Relationship Among Adenosine Triphosphate, P2X7 Receptor, and Inflammation
ATP is a selective endogenous ligand and exerts as a natural agonist for P2X7 receptors. Under physiological conditions, the extracellular ATP concentration is about 30 nM in circulation. In pathological conditions, when the tissue is subjected to hypoxia or inflammation, a large amount of ATP is released, accumulated at the injured site or entered into circulation to activate P2X7 receptors and subsequent signaling (Bours et al., 2006).
A large and negatively charged ATP molecule is unable to directly diffuse across the lipid bilayer of the plasma membrane but can pass through the cell membrane via other regulatory or nonregulatory channels, such as connexins and pannexins (Novitskaya et al., 2016). During myocardial injury, ATP released from ischemic cardiomyocytes can bind to P2X7 receptors and activate platelets and inflammatory cells (Erlinge and Burnstock, 2008; Nishida et al., 2008; Burnstock and Pelleg, 2015). Activation of P2X7 receptors by ATP opens cation channels that are permeable to several cations, such as K+, Na+, and Ca2+, triggering a series of inflammatory responses (Baroja-Mazo et al., 2013; Sluyter, 2017). Moreover, continuous activation of P2X7 receptors forms nonselective membrane pores that allow molecules up to 900 kDa to pass, leading to cell membrane perforation and cell apoptosis (Hechler and Gachet, 2015). In addition, extracellular ATP opens K+ channels through ATP-gated P2X7 receptors, accelerating K+ outflow and thereby triggering NLRP3 inflammasome activation (He et al., 2017). The inflammasome is a multi-protein complex involved in the assembly and formation of cytoplasm by the pattern recognition receptor, mainly composed of receptor proteins (NLR or ALR family), apoptosis-related speck-like protein (ASC, apoptosis-associated speck-like protein containing CARD), and procaspase-1 (Atianand et al., 2013). It regulates the maturation and secretion of IL-1β and IL-18, as well as pyroptosis, playing an important role in the development of chronic inflammatory conditions, including cardiovascular disease (Dinarello, 2009; He et al., 2013; Mangan et al., 2018). The pro-inflammatory cytokines IL-1β and IL-18 are secreted by many cell types, and their gene expressions are regulated at both transcriptional and posttranslational levels. IL-1β precursors (pro–IL-1β) are inactive in vivo, and serine protease or caspase-1 processes them to the bioactive form (Afonina et al., 2015). Pattern recognition receptor (first signal, Signal 1), such as Toll-like receptors (TLRs) or cytokines receptors, leads to synthesis of the cytokine precursor pro–IL-1β and pro–IL-18 via NF-κB (Bauernfeind et al., 2009), whereas inflammasome (second signal, Signal 2) converts procaspase-1 into an enzyme-active form of caspase-1 (Franchi et al., 2009). Finally, caspase-1 processes pro–IL-1β and pro–IL-18 into their active forms, that is, IL-1β and IL-18, respectively, thus triggering inflammation (Kelley et al., 2019). Another study has shown that activation of P2X7 receptors resulted in a large influx of calcium ions, thus activating calmodulin-dependent protein kinase (CaMK) II and Ca2+-dependent phospholipase A2, and inducing the release of IL-1β (Xu and Liang, 2013).
Pyroptosis is a type of programmed cell death characterized by cellular swelling, rupture of membrane, release of cellular contents, and remarkable inflammatory response (Shi et al., 2015). Pyroptosis can be divided into caspase-1–dependent and caspase-independent pathways as follows: 1) under the stimulation of pathogens and bacteria, intracellular NLR recognizes these signals and activates caspase-1 by connecting ASC to pro–caspase-1. Gasdermin-D (GSDM-D), a pore-forming protein, cleaved by caspase-1, induces pyroptosis (Kayagaki et al., 2015; Shi et al., 2017). 2) Caspase-4/5/11 binds to LPS through the CARD domain inside the cell and triggers pyroptosis (Zhaolin et al., 2019). When P2X7 receptors activate the NLRP3 inflammasome in response to ATP, a circular platform is formed for the aggregation of ASC and caspase-1. Caspase-1 and other inflammatory caspases (caspase-4/5/11) cut GSDM-D into two fragments, resulting in the destruction of cell membranes through their pore-forming activity and promoting pyroptosis, as well as the releasing of IL-1β (Liu et al., 2016). IL-1β prolongs myocardial action potential duration (APD), decreases potassium current, and increases calcium sparks, oxidation, and phosphorylation of CaMK II, which facilitate the susceptibility of spontaneous systolic events and arrhythmias in cardiomyocytes (Monnerat et al., 2016).
Collectively, P2X7 receptors activated NLRP3 inflammasome in response to extracellular ATP, resulting in the release of IL-1β and IL-18, and play an important role in regulating inflammation and pyroptosis. This inflammatory response contributed to the pathology of cardiovascular diseases (Figure 1).
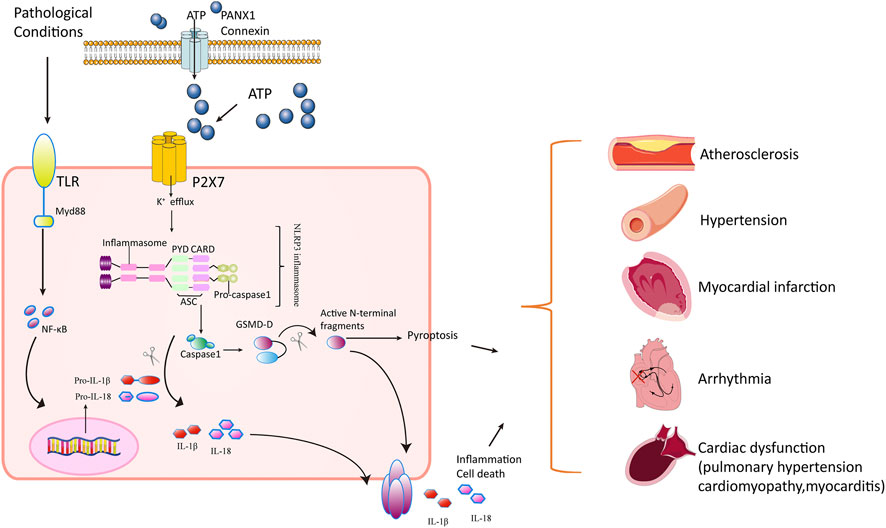
FIGURE 1. Mechanisms of P2X7 receptor’s action in cardiovascular disorders. In pathological stress including hypoxia/ischemia/hyperglycemia, the Toll-like receptors (TLRs) are activated, producing progenitors of inflammatory cytokines such as pro-IL-1β and pro-IL-18. Meanwhile, the P2X7 receptor is activated in response to ATP released through PANX1 and connexins in cardiomyocytes. The openness of P2X7 receptor leads to K+ efflux and Ca2+ influx, triggering NLRP3 inflammasome assembly (a circular platform consisting of NLR, ASC, and pro–caspase-1). NLRP3 inflammasomes convert pro–caspase-1 into active caspase-1. Caspase-1 cleaves the inactive pro–IL-1β and pro–IL-18 cytokines into active cytokines IL-1β and IL-18, respectively, and cuts GSDM-D into active N-terminal fragment. The active form of GSDM-D induces cell membrane disruption through their pore-forming activity and by promoting pyroptosis and releasing IL-1β and IL-18. The inflammatory response mediated by cytokines and pyroptosis contributes to pathology in atherosclerosis, hypertension, pulmonary hypertension, myocardial infarction, arrhythmia, cardiomyopathy, and autoimmune myocarditis.
Role of the P2X7 Receptor in Cardiovascular Diseases
The P2X7 Receptor and Atherosclerosis
Atherosclerosis, characterized by lipid deposition and inflammatory response, is the major cause of coronary heart disease, cerebral infarction, and peripheral vascular disease. Once the lesions of atherosclerosis block the arterial lumen, tissues or organs supplied by the artery will show manifestations of ischemia or necrosis (Libby, 2013). One of the key features of atherosclerosis was the formation of atherosclerosis plaque that usually involves endothelial dysfunction followed by a series of complex pathological processes, including platelet activation and inflammatory cell aggregation. However, the specific mechanism underlying the formation of atherosclerosis plaque was not fully understood (Yang et al., 2017). Of importance, activation of P2X7 receptors has been shown to play a significant role in that process. Thus, expression of P2X7 receptors markedly increased in human carotid artery plaques and was correlated to the degree of coronary artery stenosis (Piscopiello et al., 2013; Shi et al., 2020). Gidlof et al.suggest that a common loss-of-function missense variant of the P2RX7 gene may be associated with reduced ischemic heart disease in smokers (Gidlof et al., 2012). Therefore, it is reasonable to specify its involvement in plaque formation and rupture in atherosclerotic regions.
The P2X7 receptor is widely expressed in smooth muscle cells (SMCs) and endothelial cells, and the activation of the receptor in those cells plays a major role in the initiation and development of atherosclerosis (North, 2002; Franco et al., 2015). In atherosclerosis, turbulent blood flows at the lesion cause a significant increase in ATP to activate P2X7 receptors and their downstream signaling, such as p38 activation (Milner et al., 1990; Green et al., 2018). Impaired vascular regulation, oxidative stress, and inflammation can cause endothelial dysfunction in hyperglycemia and diabetes, which are risk factors for atherosclerosis (Zhou R. et al., 2020). Under the stimulation by high glucose and palmitic acid, the release of ATP activated endothelial P2X7 receptors, contributing to the production of pro-inflammatory factors and ROS, increase in cell permeability and cell adhesion molecules, and reduction of bioavailability of nitric oxide (NO) (Sathanoori et al., 2015; Lombardi et al., 2017; Stachon et al., 2017). In addition to endothelial cells and smooth muscle cells, increased expression of P2X7 receptors was also found in macrophages in the plaque of coronary atherosclerosis, suggesting the immune activity of P2X7 receptors. Knockout of the P2X7 receptor can reduce the infiltration of macrophages, caspase-1 activity, and the adhesion molecules, leading to small atherosclerotic degeneration (Lombardi et al., 2017; Stachon et al., 2017). Besides, lipid oxidation can activate NLRP3, and thus releases IL-1β and IL-18, accelerating the progression of atherosclerotic lesions, and reducing lipid oxidation can delay this progression (Leonarduzzi et al., 2012; Escalante et al., 2017). Studies have confirmed that activation of P2X7 receptors was involved in lipid metabolism of epithelial cells and immune cells, resulting in lipid oxidation and phagocytic clearance dysfunction (Costa-Junior et al., 2011; Guha et al., 2013). The P2X7 receptor knockout mice exhibited a lower cholesterol level and smaller atherosclerotic lesions than wide-type mice, suggesting the role of the P2X7 receptor in inflammation that is mediated by lipid oxidation (Duewell et al., 2010; Peng et al., 2015; Stachon et al., 2017). Interestingly, in Canakinumab Anti-Inflammatory Thrombosis Outcome Study (CANTOS), although IL-1 antagonist was associated with a reduction in cardiovascular events, cholesterol levels hardly altered, raising new challenges that whether the P2X7 receptor antagonists can reduce cholesterol levels (Ridker et al., 2017). It is also debatable whether atorvastatin played an anti-inflammatory role and reduced atherosclerosis by activating P2X7 receptors in endothelial cells, which is contradictory to the results of inflammation caused by endothelial P2X7 receptor activation (Mistafa et al., 2008; Lombardi et al., 2017). Therefore, the relationship between the P2X7 receptor and atherosclerosis warrants further study in the future.
The P2X7 Receptor and Hypertension
Although the causes of hypertension are not fully understood, growing evidence has indicated that inflammation is not only associated with high blood pressure but also with the progression of this disease in recent years (Guzik et al., 2007; Kirabo et al., 2014). Studies have shown that activation of P2X7 receptors by uridine adenosine tetraphosphate led to vascular contraction (Zhou et al., 2015). Moreover, a single-nucleotide polymorphism (SNP) for the P2RX7 gene was associated with blood pressure, especially for rs591874, which was related to nocturnal diastolic blood pressure (Palomino-Doza et al., 2008). Among Chinese postmenopausal women, single-nucleotide polymorphism (rs3751143-C) of the P2RX7 gene was linked to a reduced risk of essential hypertension (Gong et al., 2019). Of note, plasma ATP levels in hypertensive mice began to increase from the third day after the induction of hypertension, which was consistent with the higher plasma ATP levels in hypertensive patients than in normal subjects (Zhao et al., 2019). Also, sustained shear stress can induce the release of ATP in endothelial cells, which may lead to the activation of P2X7 receptors (Yamamoto et al., 2000). These results indicate that the P2X7 receptor is involved in the occurrence and development of hypertension.
Hypertensive rats on a high-salt diet exhibited increased expression of P2X7 receptors, whereas the P2X7 receptor knockout mice showed decreased systolic and diastolic blood pressure (Ji et al., 2012). Likewise, IL-1β in cultured macrophages significantly increased after treatment with the P2X7 receptor agonist BzATP in salt-sensitive rats when compared with salt-resistant rats (Ji et al., 2012). In addition to blood pressure, the P2X7 receptor was also closely related to hypertension-related renal injury. Chronic activation of the renin–angiotensin system promoted the occurrence of hypertension, renal microvascular dysfunction, hypoxia, and inflammation. In this process, activation of P2X7 receptors can intensify microcirculatory obstruction, regional ischemia, and hypoxia, accelerating the progression of renal injury induced by angiotensin II (Menzies et al., 2015). Blocking P2X7 receptors may benefit renal function, which can reduce renal vascular resistance, mainly in the anterior glomerular artery and arteriole. The possible mechanisms might be as follows: first, the antagonistic effect of P2X7 receptors increased myelin perfusion, oxygenation, and attenuated renal inflammation; second, the P2X7 receptor blockade enhanced the pressure–urinary sodium excretion reaction (Arulkumaran et al., 2011; Solini et al., 2013); last, in an angiotensin II–dependent hypertension rat model, the P2X7 receptor antagonist A438079 reduced efferent and afferent arteriole resistance, suggesting the role of the P2X7 receptor in glomerular hemodynamics (Franco et al., 2017).
It is worth noting that hypertension was prevalent in patients with autoimmune diseases, in which both antigen-presenting cells (APCs) and T cells caused higher blood pressure (Madhur et al., 2010; Wilck et al., 2017). The elevated concentration of extracellular ATP acted as a “danger signal” to mobilize immune cells, especially through the P2X7 receptor that upregulated CD86 expression in APCs. Of note, hydrolyzing ATP or blocking the P2X7 receptor can effectively block the upregulation of CD86 and decrease the reactivity of T cells under hypertension (Zhao et al., 2019). Interestingly, IL-1β antagonist significantly reduced blood pressure in one kidney/deoxycorticosterone acetate (DOCA)/salt-induced hypertension in rats, despite the fact that monoclonal antibody against IL-1β did not reduce blood pressure or incident hypertension during follow-up in CANTOS (Ling et al., 2017; Ridker et al., 2017; Rothman et al., 2020). Therefore, the P2X7 receptor, as the upstream of IL-1β, may become a promising target for the treatment of hypertension in the future.
The P2X7 Receptor and Pulmonary Arterial Hypertension
PAH is a disease with poor prognosis and high mortality, which is accompanied by a progressive increase of pulmonary artery resistance and eventually right heart failure (Simonneau et al., 2019). This disease is characterized by the abnormal structure of pulmonary artery endothelial cells (PAECs), secretion of inflammatory factors, proliferation of pulmonary artery smooth muscle cells (PASMCs), thickening of the pulmonary artery wall, and narrowing of its lumen, leading to the remodeling of pulmonary artery structure (Vonk et al., 2019). Currently, the five-year mortality rate is as high as 59% for the lack of specific treatment for PAH (Boucly et al., 2017). Therefore, it is urgent to explore the pathogenesis and new therapeutic targets for PAH.
Inflammation is involved in the development and progression of PAH. Pulmonary arterial lesions are infiltrated by macrophages, T cells, B cells, and other inflammatory cells in the damaged artery of PAH patients (Price et al., 2012). Several other studies found that the levels of pro-inflammatory cytokines, such as C-reactive protein (CRP), IL-1β, IL-6, IL-8, IL-10, monocyte chemotactic protein 1 (MCP-1), tumor necrosis factor α, and interferon, significantly increased in the plasma of patients with idiopathic PAH (Rabinovitch et al., 2014; Pezzuto et al., 2015). Despite the heterogeneity among species, the P2X7 receptor is expressed in isolated PAECs and intact pulmonary vascular systems from the lungs of mice and humans (Cai et al., 2020). In animal models of pulmonary hypertension induced by monocrotaline (MCT), the activation of P2X7 receptors is accompanied by an increase in NLRP3 inflammasome and IL-1β. At the same time, activated P2X7 receptors colocate with myeloid cells and vascular cells (Yin et al., 2017b;Duan et al., 2018). Additionally, studies have confirmed the role of ATP–P2X7 receptor axis in the development of pulmonary inflammation, and the proliferation and migration of vascular smooth muscle cells (Robinson et al., 2006; Lucattelli et al., 2011). The above evidence implies that P2X7 receptors’ activation may be associated with PAH by increasing a strong inflammation response.
Blockade of the P2X7 receptor ameliorated PAH to some extent. In a rat model of PAH induced by MCT, administration of P2X7 receptor antagonist A74003 for 2 weeks reversed pulmonary vascular remodeling and significantly reduced NLRP3 inflammasome, TNF-α, and IL-1β levels (Yin et al., 2017b). In another study using the same model, Brilliant Blue G (BBG), a P2X7 receptor antagonist, not only alleviated the remodeling of pulmonary vessels and function but also reduced the levels of pro-inflammatory cytokine IL-1β and tumor necrosis factor α through the P38/MAPK signaling pathway (Duan et al., 2018). Interestingly, a novel inhibitor of P2X7 receptor PKT100 improved the right ventricle (RV) contractility and systolic function and survival in the PAH mice model induced by bleomycin, with no effect on pulmonary arterial pressure and pulmonary vascular remodeling (Hansen et al., 2020). This provides a new perspective for current treatment strategies that target both the RV function and pulmonary vessels, which may improve the prognosis of PAH patients. To sum up, the inhibition of the P2X7 receptor exerted anti-inflammatory and anti-remodeling effects on PAH, suggesting that the P2X7 receptor may be a potential therapeutic target. Although studies on the role of P2X7 receptor in PAHs are not completely understood, further exploration on novel disease mechanisms and validation of this receptor in clinical trials are requested.
The P2X7 Receptor and Myocardial Infarction
The main cause of acute myocardial infarction (AMI) is coronary thrombosis and continuous blood flow obstruction, secondary to rupture of unstable atherosclerotic plaque. Large amounts of ATP and oxidative stress products (reactive oxygen species) are released from cardiomyocytes and endothelial cells, leading to P2X7-mediated NLRP3 inflammasome formation and activation around the border area of infarcted tissues (Cheung et al., 2015; Hesse et al., 2017). It should be noted that activation of NLRP3 inflammasome in cardiomyocytes induces caspase-dependent pyroptosis rather than producing IL-1β (Toldo et al., 2018; Zeng et al., 2019; Wang et al., 2020). This is supported by the fact that fibroblasts and endothelial cells produce IL-1β in ischemia/reperfusion injury, but not cardiomyocytes (Heid et al., 2013; Sandanger et al., 2013; Liu et al., 2014). Additionally, epicardial-derived cells (EPDCs) also play an important role in the P2X7–NLRP3–IL-1β axis. After MI in the adult heart, EPDCs are activated, proliferated, and migrated into the damaged myocardial layer. They differentiate into fibroblasts, vascular smooth muscle cells, pericytes, and adipocytes, and secrete many signaling molecules that affect myocardial regeneration (Cai et al., 2008; Dube et al., 2017; Quijada et al., 2019). CD73 on EPDCs degrades extracellular ATP and nicotinamide adenine dinucleotide (NAD) to adenosine, thereby activating the A2B receptor. In turn, in addition to IL-6 formation, activation of the A2B receptor also enables intracellular vesicles to release ATP and NAD, forming a positive feedback. A large amount of ATP/NAD activates P2X7 receptors, leading to the formation of the NLRP3 inflammasome and release of IL-1β (Hesse et al., 2017). Also, the NAD from EPDCs can bind to the enzyme ARTC2 expressed on the regulatory T (Treg) cell, activating P2X7 receptors by transferring ADP-ribose to cell surface. The downstream signaling pathway, L-selectin, and CD27 will be cleaved, causing phosphatidylserine to flip outward and leading to Treg cell apoptosis (Seman et al., 2003; Aswad et al., 2005; Adriouch et al., 2008; Scheuplein et al., 2009). Treg can protect cardiomyocytes from apoptosis, reduce inflammation and adverse ventricular remodeling after infarction, and promote cardiac healing (Matsumoto et al., 2011; Meng et al., 2016).
It is also worth noting that SNPs for the P2RX7 gene played a role in hypertension but not in myocardial infraction. None of the SNPs (A1513C, rs208294, and rs3751143) for the P2RX7 gene was associated with mortality in patients with heart failure, a common consequence of myocardial infarction (Eslick et al., 2009; Pasqualetti et al., 2017).
Accordingly, inhibition of P2X7 receptors may reduce infarcted areas and improve cardiac function and survival by suppressing inflammation. After MI, treatment of small interfering RNA (siRNA) or P2X7 receptors inhibitor can prevent the formation of NLRP3 inflammasome and limit the infarcted area (Barth et al., 2010). Moreover, Mezzaroma et al. demonstrated that inhibition of cryopyrin or P2X7 receptors could blunt caspase-1 activation and assembly of inflammasomes, subsequently reducing cell death and reversing ventricular remodeling (Mezzaroma et al., 2011). Canakinumab, a monoclonal antibody targeting IL-1β, has been shown to reduce the adverse cardiac event (myocardial infarction and/or stroke) by 15% in patients with a prior MI (Ridker et al., 2017). Therefore, despite the limited clinical data, targeting P2X7 receptor to reduce inflammation may provide a new direction for future studies.
The P2X7 Receptor and Arrhythmia
After ischemia-reperfusion injury or MI, arrhythmia is one of the most common complications, putting patients at risk for deterioration of cardiac function and sudden cardiac death (Arevalo et al., 2016). The release of various inflammatory cytokines induced a continuous inflammatory response in cardiac tissue, and thus generated pro-arrhythmic substrates of tissue fibrosis and electrical remodeling (Eisenhut and Wallace, 2011). NLRP3 and IL-1β played an important role throughout the whole process. After cardiac injury, IL-1β was elevated by TLR and NLRP3 inflammasomes, and it prolonged APD, decreased outward potassium current, and increased calcium spark, oxidation, and phosphorylation of CaMK II (Cerrone et al., 2012; Anumonwo and Pandit, 2015). These changes resulted in impaired contractility and the propensity of arrhythmia for cardiomyocytes. In addition, inflammatory responses were also involved in sympathetic nerve regeneration by increasing sympathetic nerve density and tone. Sympathetic hyperinnervation promoted cell apoptosis and deteriorated cardiac function (Lindholm et al., 1987; Hoffmann et al., 2013). The sympathetic nerve of the heart branches from stellate ganglia. After MI, the expression of P2X7 receptor increased in stellate ganglia, indicating that the P2X7 receptor was involved in neural remodeling (Kong et al., 2013; Tu et al., 2016). These observations suggest that the P2X7 receptor–NLRP3–IL-1β axis is associated with arrhythmia.
In animal studies, blockade of the P2X7 receptor or its downstream regulators has been proven to be effective for arrhythmias. The P2X7 receptor inhibitor A740003 or IL-1β antagonist Anakinra can blunt sympathetic hyperinnervation and sympathetic sprouting by suppressing the infiltration of macrophages and production of IL-1β and nerve growth factor (NGF) (Yin et al., 2017a). Inhibiting P2X7 receptors with short hairpin RNA (shRNA) improved sympathetic hyperinnervation and cardiac remodeling by inhibiting the Akt and ERK1/2 pathways and NF-κB activation, which in turn promoted IL-1β production (Gao et al., 2017). In addition, NONRATT021972 siRNA targeting the P2X7 receptor restored the abnormal distribution of sympathetic nerve fibers around the ischemic myocardium and sympathetic overexcitation, and increased norepinephrine and epinephrine concentrations and blood pressure (Tu et al., 2016). Besides, De Jesus et al. demonstrated that IL-1β antagonist Anakinra significantly improved cardiac conduction function and intracellular Ca2+ concentration, decreased transmembrane potential and Ca2+ alternating amplitude, and reduced the incidence of spontaneous ventricular arrhythmia. The mechanism underlying the myocardial infarction–induced arrhythmia may be related to increased expression levels of connexin 43 and Ca2+-ATPase in the sarcoplasmic reticulum (De Jesus et al., 2017). Together, therapeutic interventions targeting P2X7 receptor may protect against cardiac arrhythmia.
The P2X7 Receptor and Cardiomyopathy
Cardiomyopathy is an anatomic and pathologic diagnosis associated with a high incidence of morbidity and mortality. Cardiomyopathy may be secondary (e.g., infiltrative, toxic, and inflammatory) (Wexler et al., 2009). The pathophysiological mechanism of cardiomyopathy is still unclear. However, cardiac fibrosis has been successively confirmed to be involved in its pathophysiological process, among which inflammatory reaction plays a key role (Nishida and Otsu, 2017). IL-1β and IL-18 lead to the initiation of pyroptosis and fibrosis in diabetic cardiomyopathy (Vandanmagsar et al., 2011; Luo et al., 2014). In a calcineurin-induced structural heart disease mouse model, elevated NLRP3 mRNA levels, cardiac hypertrophy, inflammation, and ventricular dilation were observed (Bracey et al., 2013). Meanwhile, in transverse aortic constriction (TAC)-induced dilated cardiomyopathy, the expression of P2X7 receptor, NLRP3 inflammasome, and its downstream effectors significantly increased, accompanied by impaired cardiac function and collagen deposition (Zhou J. et al., 2020). Likewise, SNP rs28360451-A for the P2RX7 gene is involved in hypertrophic cardiomyopathy, and loss of function mutation will cause a defective phenotype (Biswas et al., 2019).
The P2X7 receptor–NLRP3–IL-1β axis is involved in the pathophysiological process of cardiac fibrosis. In pathological conditions, plenty of collagen I and III are produced by activated fibroblasts under the long-term stimulation of IL-18 and IL-1β, leading to the excessive extracellular matrix, cardiac fibrosis, and remodeling (Turner, 2016). Additionally, under TGF-β stimulation, NLRP3 inflammasomes increase in cardiac fibroblasts, which promote the activation of receptor-associated Smad (R-SMad) in myofibroblasts. Activated R-Smads (RSmad 2/3) bind to co-SMAD (Smad 4) to form a transcription complex to promote the expression of fibrogenic genes (Bracey et al., 2014). Moreover, caspase-1, the product of NLRP3, and other caspases (caspase-4/5/11) cut GSDMD into two fragments, in which the amino-terminal one damages cell membranes through its pore-forming activity, thereby promoting pyroptosis and the release of IL-1β (Liu et al., 2016).
Targeting P2X7 receptor directly alleviated structural and functional abnormalities in cardiomyopathy. H3 relaxin, a member of the insulin-like growth factor superfamily, can inhibit collagen synthesis of cardiac fibroblasts in the high-glucose environment by attenuating the activation of the ROS and P2X7 receptor–mediated NLRP3 inflammasome (Zhang et al., 2017; Zhang et al., 2018). In addition, in the TAC mouse model, treatment with the P2X7 receptor antagonist BBG reduced cardiac fibrosis and improved systolic function by inhibiting the expression of NLRP3 and IL-1β. Inhibition of downstream regulators of NLRP3 inflammasome also prevents the progression of cardiomyopathy. It has been proven that the knockout of NLRP3 or IL-1β receptor antagonists improved the mitochondrial structure of muscle fibers and ultimately restored cardiac function of cardiomyopathy via reducing the extracellular matrix (Bracey et al., 2013; Luo et al., 2014). Overall, the blockade of P2X7 receptor may ameliorate the progression of cardiomyopathy by suppressing inflammation and fibrosis, especially in cardiac fibroblasts.
The P2X7 Receptor and Autoimmune Myocarditis
Autoimmune myocarditis is characterized by a series of inflammatory responses accompanied by the necrosis of cardiomyocytes and infiltration of monocytes. No specific treatment is available until now (Suzuki et al., 2007; Fung et al., 2016). Heart biopsy from patients with acute myocarditis showed that inflammasomes were only observed in the patient group when compared with control subjects who died without cardiac conditions and were higher in patients with New York Heart Association (NYHA) III-IV than in those with NYHA I-II (Toldo et al., 2014). In the primary mouse submandibular gland, P2X7 receptors activated NLRP3 inflammasome and released IL-1β, contributing to autoimmunity (Khalafalla et al., 2017). These data suggest that P2X7 receptor may be involved in autoimmune myocarditis through pro-inflammatory cascades.
In a murine experimental autoimmune myocarditis (EAM) model, P2X7 receptors were activated and the EAM model showed impairment of cardiac systolic function and infiltration of inflammatory cells 21 days after EAM induction. The application of P2X7 receptor antagonist A74003 restored the systolic function by suppressing the CD4+ T cells and macrophage infiltration, as well as the IL-1β mRNA expression (Zempo et al., 2015). It is worth mentioning that the P2X7 receptor knockout mice exhibited the phenotype of dilated cardiomyopathy despite lower IL-1β and IL-17 levels than its wide-type ones in an anti-M2 muscarinic receptor–induced autoimmune cardiomyopathy model (Martinez et al., 2015). Thus, further studies are needed to better understand the safety and efficacy of the P2X7 receptor in autoimmune myocarditis.
Conclusions and Perspectives
Numerous studies suggest that activation of P2X7 receptors plays a crucial role in cardiovascular disease, and targeting P2X7 receptor is an effective tool to alleviate the progression of cardiovascular diseases such as atherosclerosis, hypertension, pulmonary hypertension, myocardial infarction, arrhythmia, cardiomyopathy, and autoimmune myocarditis. Further attention should be paid to potential roles for P2X7 receptor activation in cardiac allograft rejection (Wu et al., 2016; Vergani et al., 2013; D’Addio et al., 2018). The mechanisms mainly involve the P2X7 receptor–mediated inflammatory response induced by cytokines and pyroptosis. However, P2X7 inhibitors showed limited efficacy in clinical trials of other inflammatory diseases. In a clinical trial of rheumatoid arthritis, the P2X7 inhibitors AZD9056 (AstraZeneca) and CE-224535 (Pfizer) did not significantly improve symptoms (Keystone et al., 2012; Stock et al., 2012). Similarly, in Crohn’s disease, AZD9056 hardly lowers the inflammatory markers, although it improved the patients’ activity index scores (Eser et al., 2015). Given the good tolerance of P2X7 inhibitors in clinical trials and the favorable efficacy obtained in various animal models, P2X7 receptor inhibition could represent a novel approach to the treatment of cardiovascular disease in humans. However, clinical trials by targeting the P2X7 receptor to alleviate cardiovascular disease have not been conducted. Therefore, it is necessary to conduct in-depth research on the role of P2X7 receptor in cardiovascular disease and translate these findings from animal models into clinical studies.
Author Contributions
All authors equally contributed to this research with conception and design of the study; literature and analysis; drafting, critical revision, and editing; and final approval of the final version.
Funding
This work was supported by grants from the National Natural Science Foundation of China (81904306, 12072215, and 81774437), Sichuan Science and Technology Program (2019YFH0108 and 2020YFH0115), the Swedish Heart and Lung Foundation (20190341 and 20200326), the Karolinska Institutet Grant (2018 and 2020), the Loo and Hans Ostermans Stiftelse (2018-01213 and 2020-01209), the Lars Hiertas Minne Foundation (FO2018-0156), the National Key R&D Program of China (2019YFC1709101), and the Project First-Class Disciplines Development of Chengdu University of Traditional Chinese Medicine (CZYHW 1901).
Conflict of Interest
The authors declare that the research was conducted in the absence of any commercial or financial relationships that could be construed as a potential conflict of interest.
References
Adriouch, S., Bannas, P., Schwarz, N., Fliegert, R., Guse, A. H., Seman, M., et al. (2008). ADP‐ribosylation at R125 Gates the P2X7 Ion Channel by Presenting a Covalent Ligand to its Nucleotide Binding Site. FASEB j. 22 (3), 861–869. doi:10.1096/fj.07-9294com
Afonina, I. S., Müller, C., Martin, S. J., and Beyaert, R. (2015). Proteolytic Processing of Interleukin-1 Family Cytokines: Variations on a Common Theme. Immunity 42 (6), 991–1004. doi:10.1016/j.immuni.2015.06.003
Anumonwo, J. M., and Pandit, S. V. (2015). Ionic Mechanisms of Arrhythmogenesis. Trends Cardiovasc. Med. 25 (6), 487–496. doi:10.1016/j.tcm.2015.01.005
Arevalo, H. J., Vadakkumpadan, F., Guallar, E., Jebb, A., Malamas, P., Wu, K. C., et al. (2016). Arrhythmia Risk Stratification of Patients after Myocardial Infarction Using Personalized Heart Models. Nat. Commun. 7, 11437. doi:10.1038/ncomms11437
Arulkumaran, N., Unwin, R. J., and Tam, F. W. (2011). A Potential Therapeutic Role for P2X7 Receptor (P2X7R) Antagonists in the Treatment of Inflammatory Diseases. Expert Opin. Investig. Drugs 20 (7), 897–915. doi:10.1517/13543784.2011.578068
Aswad, F., Kawamura, H., and Dennert, G. (2005). High Sensitivity of CD4+CD25+Regulatory T Cells to Extracellular Metabolites Nicotinamide Adenine Dinucleotide and ATP: A Role for P2X7Receptors. J. Immunol. 175 (5), 3075–3083. doi:10.4049/jimmunol.175.5.3075
Atianand, M. K., Rathinam, V. A., and Fitzgerald, K. A. (2013). SnapShot: Inflammasomes. Cell 153 (1), 272. doi:10.1016/j.cell.2013.03.009
Baroja-Mazo, A., Barberà-Cremades, M., and Pelegrín, P. (2013). The Participation of Plasma Membrane Hemichannels to Purinergic Signaling. Biochim. Biophys. Acta (Bba) - Biomembranes 1828 (1), 79–93. doi:10.1016/j.bbamem.2012.01.002
Barth, K., Pfleger, C., Linge, A., Sim, J. A., Surprenant, A., Steinbronn, N., et al. (2010). Increased P2X7R Expression in Atrial Cardiomyocytes of Caveolin-1 Deficient Mice. Histochem. Cel Biol. 134 (1), 31–38. doi:10.1007/s00418-010-0716-8
Bauernfeind, F. G., Horvath, G., Stutz, A., Alnemri, E. S., Macdonald, K., Speert, D., et al. (2009). Cutting Edge: NF-Κb Activating Pattern Recognition and Cytokine Receptors License NLRP3 Inflammasome Activation by Regulating NLRP3 Expression. J. Immunol. 183 (2), 787–791. doi:10.4049/jimmunol.0901363
Biswas, A., Raza, A., Das, S., Kapoor, M., Jayarajan, R., Verma, A., et al. (2019). Loss of Function Mutation in the P2X7, a Ligand-Gated Ion Channel Gene Associated with Hypertrophic Cardiomyopathy. Purinergic Signal. 15 (2), 205–210. doi:10.1007/s11302-019-09660-7
Boucly, A., Weatherald, J., Savale, L., Jaïs, X., Cottin, V., Prevot, G., et al. (2017). Risk Assessment, Prognosis and Guideline Implementation in Pulmonary Arterial Hypertension. Eur. Respir. J. 50 (2), 1700889. doi:10.1183/13993003.00889-2017
Bours, M. J. L., Swennen, E. L. R., Di Virgilio, F., Cronstein, B. N., and Dagnelie, P. C. (2006). Adenosine 5′-triphosphate and Adenosine as Endogenous Signaling Molecules in Immunity and Inflammation. Pharmacol. Ther. 112 (2), 358–404. doi:10.1016/j.pharmthera.2005.04.013
Bracey, N. A., Beck, P. L., Muruve, D. A., Hirota, S. A., Guo, J., Jabagi, H., et al. (2013). The Nlrp3 Inflammasome Promotes Myocardial Dysfunction in Structural Cardiomyopathy through Interleukin-1β. Exp. Physiol. 98 (2), 462–472. doi:10.1113/expphysiol.2012.068338
Bracey, N. A., Gershkovich, B., Chun, J., Vilaysane, A., Meijndert, H. C., Wright, J. R., et al. (2014). Mitochondrial NLRP3 Protein Induces Reactive Oxygen Species to Promote Smad Protein Signaling and Fibrosis Independent from the Inflammasome. J. Biol. Chem. 289 (28), 19571–19584. doi:10.1074/jbc.M114.550624
Burnstock, G., and Pelleg, A. (2015). Cardiac Purinergic Signalling in Health and Disease. Purinergic Signal. 11 (1), 1–46. doi:10.1007/s11302-014-9436-1
Cai, C.-L., Martin, J. C., Sun, Y., Cui, L., Wang, L., Ouyang, K., et al. (2008). A Myocardial Lineage Derives from Tbx18 Epicardial Cells. Nature 454 (7200), 104–108. doi:10.1038/nature06969
Cai, Z., Tu, L., Guignabert, C., Merkus, D., and Zhou, Z. (2020). Purinergic Dysfunction in Pulmonary Arterial Hypertension. Jaha 9 (18). doi:10.1161/JAHA.120.017404
Cerrone, M., Cummings, S., Alansari, T., and Priori, S. G. (2012). A Clinical Approach to Inherited Arrhythmias. Circ. Cardiovasc. Genet. 5 (5), 581–590. doi:10.1161/CIRCGENETICS.110.959429
Cheung, K. K., Marques-Da-Silva, C., Vairo, L., dos Santos, D. S., Goldenberg, R., Coutinho-Silva, R., et al. (2015). Pharmacological and Molecular Characterization of Functional P2 Receptors in Rat Embryonic Cardiomyocytes. Purinergic Signal. 11 (1), 127–138. doi:10.1007/s11302-014-9441-4
Costa-Junior, H. M., Marques-Da-Silva, C., Vieira, F. S., Monção-Ribeiro, L. C., and Coutinho-Silva, R. (2011). Lipid Metabolism Modulation by the P2X7 Receptor in the Immune System and during the Course of Infection: New Insights into the Old View. Purinergic Signal. 7 (4), 381–392. doi:10.1007/s11302-011-9255-6
D’Addio, F., Vergani, A., Potena, L., Maestroni, A., Usuelli, V., Ben Nasr, M., et al. (2018). P2X7R Mutation Disrupts the NLRP3-Mediated Th Program and Predicts Poor Cardiac Allograft Outcomes. J. Clin. Invest. 128 (8), 3490–3503. doi:10.1172/JCI94524
De Jesus, N. M., Wang, L., Lai, J., Rigor, R. R., Francis Stuart, S. D., Bers, D. M., et al. (2017). Antiarrhythmic Effects of Interleukin 1 Inhibition after Myocardial Infarction. Heart Rhythm 14 (5), 727–736. doi:10.1016/j.hrthm.2017.01.027
Dinarello, C. A. (2009). Immunological and Inflammatory Functions of the Interleukin-1 Family. Annu. Rev. Immunol. 27, 519–550. doi:10.1146/annurev.immunol.021908.132612
Duan, L., Hu, G.-h., Li, Y.-j., Zhang, C.-l., and Jiang, M. (2018). P2X7 Receptor Is Involved in Lung Injuries Induced by Ischemia-Reperfusion in Pulmonary Arterial Hypertension Rats. Mol. Immunol. 101, 409–418. doi:10.1016/j.molimm.2018.07.027
Dubé, K. N., Thomas, T. M., Munshaw, S., Rohling, M., Riley, P. R., and Smart, N. (2017). Recapitulation of Developmental Mechanisms to Revascularize the Ischemic Heart. JCI Insight 2 (22). doi:10.1172/jci.insight.96800
Duewell, P., Kono, H., Rayner, K. J., Sirois, C. M., Vladimer, G., Bauernfeind, F. G., et al. (2010). NLRP3 Inflammasomes Are Required for Atherogenesis and Activated by Cholesterol Crystals. Nature 464 (7293), 1357–1361. doi:10.1038/nature08938
Eisenhut, M., and Wallace, H. (2011). Ion Channels in Inflammation. Pflugers Arch. - Eur. J. Physiol. 461 (4), 401–421. doi:10.1007/s00424-010-0917-y
Erlinge, D., and Burnstock, G. (2008). P2 Receptors in Cardiovascular Regulation and Disease. Purinergic Signal. 4 (1), 1–20. doi:10.1007/s11302-007-9078-7
Escalante, C., Mora, S., and Bolaños, L. (2017). Hormone Replacement Therapy Reduces Lipid Oxidation Directly at the Arterial Wall: A Possible Link to Estrogens’ Cardioprotective Effect through Atherosclerosis Prevention. J. Mid-life Health 8 (1), 11–16. doi:10.4103/0976-7800.201967
Eser, A., Colombel, J.-F., Rutgeerts, P., Vermeire, S., Vogelsang, H., Braddock, M., et al. (2015). Safety and Efficacy of an Oral Inhibitor of the Purinergic Receptor P2X7 in Adult Patients with Moderately to Severely Active Crohnʼs Disease. Inflamm. Bowel Dis. 21 (10), 1–53. doi:10.1097/MIB.0000000000000514
Eslick, G. D., Thampan, B. V., Nalos, M., Mclean, A. S., and Sluyter, R. (2009). Circulating Interleukin-18 Concentrations and a Loss-Of-Function P2X7 Polymorphism in Heart Failure. Int. J. Cardiol. 137 (1), 81–83. doi:10.1016/j.ijcard.2008.05.017
Franchi, L., Eigenbrod, T., Muñoz-Planillo, R., and Nuñez, G. (2009). The Inflammasome: a Caspase-1-Activation Platform that Regulates Immune Responses and Disease Pathogenesis. Nat. Immunol. 10 (3), 241–247. doi:10.1038/ni.1703
Franco, M., Bautista-Pérez, R., Cano-Martínez, A., Pacheco, U., Santamaría, J., del Valle Mondragón, L., et al. (2017). Physiopathological Implications of P2X1and P2X7receptors in Regulation of Glomerular Hemodynamics in Angiotensin II-Induced Hypertension. Am. J. Physiology-Renal Physiol. 313 (1), F9–F19. doi:10.1152/ajprenal.00663.2016
Franco, M., Bautista-Pérez, R., and Pérez-Méndez, O. (2015). Purinergic Receptors in Tubulointerstitial Inflammatory Cells: a Pathophysiological Mechanism of Salt-Sensitive Hypertension. Acta Physiol. 214 (1), 75–87. doi:10.1111/apha.12471
Fung, G., Luo, H., Qiu, Y., Yang, D., and Mcmanus, B. (2016). Myocarditis. Circ. Res. 118 (3), 496–514. doi:10.1161/CIRCRESAHA.115.306573
Gao, H., Yin, J., Shi, Y., Hu, H., Li, X., Xue, M., et al. (2017). Targeted P2X7R shRNA Delivery Attenuates Sympathetic Nerve Sprouting and Ameliorates Cardiac Dysfunction in Rats with Myocardial Infarction. Cardiovasc. Ther. 35 (2), e12245. doi:10.1111/1755-5922.12245
Gidlöf, O., Smith, J. G., Melander, O., Lövkvist, H., Hedblad, B., Engström, G., et al. (2012). A Common Missense Variant in the ATP Receptor P2X7 Is Associated with Reduced Risk of Cardiovascular Events. PLoS One 7 (5), e37491. doi:10.1371/journal.pone.0037491
Golia, E., Limongelli, G., Natale, F., Fimiani, F., Maddaloni, V., Russo, P. E., et al. (2014). Adipose tissue and vascular inflammation in coronary artery disease. World J. Cardiol. 6 (7), 539–554. doi:10.4330/wjc.v6.i7.539
Gong, C., Liu, X., Ding, L., Liu, Y., Li, T., Wang, S., et al. (2019). A Non-synonymous Polymorphism in Purinergic P2X7 Receptor Gene Confers Reduced Susceptibility to Essential Hypertension in Chinese Postmenopausal Women. Clin. Exp. Hypertens. 41 (6), 558–563. doi:10.1080/10641963.2018.1523914
Green, J. P., Souilhol, C., Xanthis, I., Martinez-Campesino, L., Bowden, N. P., Evans, P. C., et al. (2018). Atheroprone Flow Activates Inflammation via Endothelial ATP-dependent P2X7-P38 Signalling. Cardiovasc. Res. 114 (2), 324–335. doi:10.1093/cvr/cvx213
Guha, S., Baltazar, G. C., Coffey, E. E., Tu, L. A., Lim, J. C., Beckel, J. M., et al. (2013). Lysosomal Alkalinization, Lipid Oxidation, and Reduced Phagosome Clearance Triggered by Activation of the P2X7 Receptor. FASEB j. 27 (11), 4500–4509. doi:10.1096/fj.13-236166
Guzik, T. J., Hoch, N. E., Brown, K. A., Mccann, L. A., Rahman, A., Dikalov, S., et al. (2007). Role of the T Cell in the Genesis of Angiotensin II-Induced Hypertension and Vascular Dysfunction. J. Exp. Med. 204 (10), 2449–2460. doi:10.1084/jem.20070657
Hansen, T., Karimi Galougahi, K., Besnier, M., Genetzakis, E., Tsang, M., Finemore, M., et al. (2020). The Novel P2X7 Receptor Antagonist PKT100 Improves Cardiac Function and Survival in Pulmonary Hypertension by Direct Targeting of the Right Ventricle. Am. J. Physiology-Heart Circulatory Physiol. 319 (1), H183–H191. doi:10.1152/ajpheart.00580.2019
He, Y., Franchi, L., and Núñez, G. (2013). TLR Agonists Stimulate Nlrp3-dependent IL-1β Production Independently of the Purinergic P2X7 Receptor in Dendritic Cells and In Vivo. J.I. 190 (1), 334–339. doi:10.4049/jimmunol.1202737
He, Y., Taylor, N., Fourgeaud, L., and Bhattacharya, A. (2017). The Role of Microglial P2X7: Modulation of Cell Death and Cytokine Release. J. Neuroinflammation 14 (1), 135. doi:10.1186/s12974-017-0904-8
Hechler, B., and Gachet, C. (2015). Purinergic Receptors in Thrombosis and Inflammation. Arterioscler Thromb. Vasc. Biol. 35 (11), 2307–2315. doi:10.1161/ATVBAHA.115.303395
Heid, M. E., Keyel, P. A., Kamga, C., Shiva, S., Watkins, S. C., and Salter, R. D. (2013). Mitochondrial Reactive Oxygen Species Induces NLRP3-dependent Lysosomal Damage and Inflammasome Activation. J.I. 191 (10), 5230–5238. doi:10.4049/jimmunol.1301490
Hesse, J., Leberling, S., Boden, E., Friebe, D., Schmidt, T., Ding, Z., et al. (2017). CD73‐derived Adenosine and tenascin‐C Control Cytokine Production by Epicardium‐derived Cells Formed after Myocardial Infarction. FASEB j. 31 (7), 3040–3053. doi:10.1096/fj.201601307R
Hoffmann, B. A., Steven, D., Willems, S., and Sydow, K. (2013). Renal Sympathetic Denervation as an Adjunct to Catheter Ablation for the Treatment of Ventricular Electrical Storm in the Setting of Acute Myocardial Infarction. J. Cardiovasc. Electrophysiol. 24 (12), E21. doi:10.1111/jce.12282
Ji, X., Naito, Y., Weng, H., Endo, K., Ma, X., and Iwai, N. (2012). P2X7 Deficiency Attenuates Hypertension and Renal Injury in Deoxycorticosterone Acetate-Salt Hypertension. Am. J. Physiology-Renal Physiol. 303 (8), F1207–F1215. doi:10.1152/ajprenal.00051.2012
Kayagaki, N., Stowe, I. B., Lee, B. L., O’Rourke, K., Anderson, K., Warming, S., et al. (2015). Caspase-11 Cleaves Gasdermin D for Non-canonical Inflammasome Signalling. Nature 526 (7575), 666–671. doi:10.1038/nature15541
Kelley, N., Jeltema, D., Duan, Y., and He, Y. (2019). The NLRP3 Inflammasome: An Overview of Mechanisms of Activation and Regulation. Ijms 20 (13), 3328. doi:10.3390/ijms20133328
Keystone, E. C., Wang, M. M., Layton, M., Hollis, S., and Mcinnes, I. B. (2012). Clinical Evaluation of the Efficacy of the P2X7purinergic Receptor Antagonist AZD9056 on the Signs and Symptoms of Rheumatoid Arthritis in Patients with Active Disease Despite Treatment with Methotrexate or Sulphasalazine. Ann. Rheum. Dis. 71 (10), 1630–1635. doi:10.1136/annrheumdis-2011-143578
Khalafalla, M. G., Woods, L. T., Camden, J. M., Khan, A. A., Limesand, K. H., Petris, M. J., et al. (2017). P2X7 Receptor Antagonism Prevents IL-1β Release from Salivary Epithelial Cells and Reduces Inflammation in a Mouse Model of Autoimmune Exocrinopathy. J. Biol. Chem. 292 (40), 16626–16637. doi:10.1074/jbc.M117.790741
Kirabo, A., Fontana, V., de Faria, A. P. C., Loperena, R., Galindo, C. L., Wu, J., et al. (2014). DC Isoketal-Modified Proteins Activate T Cells and Promote Hypertension. J. Clin. Invest. 124 (10), 4642–4656. doi:10.1172/JCI74084
Kong, F., Liu, S., Xu, C., Liu, J., Li, G., Li, G., et al. (2013). Electrophysiological Studies of Upregulated P2X7 Receptors in Rat Superior Cervical Ganglia after Myocardial Ischemic Injury. Neurochem. Int. 63 (3), 230–237. doi:10.1016/j.neuint.2013.06.003
Leonarduzzi, G., Gamba, P., Gargiulo, S., Biasi, F., and Poli, G. (2012). Inflammation-related Gene Expression by Lipid Oxidation-Derived Products in the Progression of Atherosclerosis. Free Radic. Biol. Med. 52 (1), 19–34. doi:10.1016/j.freeradbiomed.2011.09.031
Libby, P. (2013). Mechanisms of Acute Coronary Syndromes and Their Implications for Therapy. N. Engl. J. Med. 368 (21), 2004–2013. doi:10.1056/NEJMra1216063
Lindholm, D., Heumann, R., Meyer, M., and Thoenen, H. (1987). Interleukin-1 Regulates Synthesis of Nerve Growth Factor in Non-neuronal Cells of Rat Sciatic Nerve. Nature 330 (6149), 658–659. doi:10.1038/330658a0
Ling, Y. H., Krishnan, S. M., Chan, C. T., Diep, H., Ferens, D., Chin-Dusting, J., et al. (2017). Anakinra Reduces Blood Pressure and Renal Fibrosis in One kidney/DOCA/salt-induced Hypertension. Pharmacol. Res. 116, 77–86. doi:10.1016/j.phrs.2016.12.015
Liu, Y., Lian, K., Zhang, L., Wang, R., Yi, F., Gao, C., et al. (2014). TXNIP Mediates NLRP3 Inflammasome Activation in Cardiac Microvascular Endothelial Cells as a Novel Mechanism in Myocardial Ischemia/reperfusion Injury. Basic Res. Cardiol. 109 (5), 415. doi:10.1007/s00395-014-0415-z
Liu, X., Zhang, Z., Ruan, J., Pan, Y., Magupalli, V. G., Wu, H., et al. (2016). Inflammasome-activated Gasdermin D Causes Pyroptosis by Forming Membrane Pores. Nature 535 (7610), 153–158. doi:10.1038/nature18629
Lombardi, M., Mantione, M. E., Baccellieri, D., Ferrara, D., Castellano, R., Chiesa, R., et al. (2017). P2X7 Receptor Antagonism Modulates IL-1β and MMP9 in Human Atherosclerotic Vessels. Sci. Rep. 7 (1), 4872. doi:10.1038/s41598-017-05137-y
Lucattelli, M., Cicko, S., Müller, T., Lommatzsch, M., Cunto, G. D., Cardini, S., et al. (2011). P2X7Receptor Signaling in the Pathogenesis of Smoke-Induced Lung Inflammation and Emphysema. Am. J. Respir. Cel Mol Biol 44 (3), 423–429. doi:10.1165/rcmb.2010-0038OC
Luo, B., Li, B., Wang, W., Liu, X., Xia, Y., Zhang, C., et al. (2014). NLRP3 Gene Silencing Ameliorates Diabetic Cardiomyopathy in a Type 2 Diabetes Rat Model. PLoS One 9 (8), e104771. doi:10.1371/journal.pone.0104771
Madhur, M. S., Lob, H. E., Mccann, L. A., Iwakura, Y., Blinder, Y., Guzik, T. J., et al. (2010). Interleukin 17 Promotes Angiotensin II-Induced Hypertension and Vascular Dysfunction. Hypertension 55 (2), 500–507. doi:10.1161/HYPERTENSIONAHA.109.145094
Mahdi, A., Jiao, T., Tratsiakovich, Y., Yang, J., Östenson, C.-G., Pernow, J., et al. (2018). Altered Purinergic Receptor Sensitivity in Type 2 Diabetes-Associated Endothelial Dysfunction and Up4A-Mediated Vascular Contraction. Ijms 19 (12), 3942. doi:10.3390/ijms19123942
Mangan, M. S. J., Olhava, E. J., Roush, W. R., Seidel, H. M., Glick, G. D., and Latz, E. (2018). Erratum: Targeting the NLRP3 Inflammasome in Inflammatory Diseases. Nat. Rev. Drug Discov. 17 (9), 688. doi:10.1038/nrd.2018.149
Martinez, C. G., Zamith-Miranda, D., Da Silva, M. G., Ribeiro, K. C., Brandão, I. T., Silva, C. L., et al. (2015). P2×7 Purinergic Signaling in Dilated Cardiomyopathy Induced by Auto-Immunity against Muscarinic M2 Receptors: Autoantibody Levels, Heart Functionality and Cytokine Expression. Sci. Rep. 5 (1). doi:10.1038/srep16940
Matsumoto, K., Ogawa, M., Suzuki, J.-i., Hirata, Y., Nagai, R., and Isobe, M. (2011). Regulatory T Lymphocytes Attenuate Myocardial Infarction-Induced Ventricular Remodeling in Mice. Int. Heart J. 52 (6), 382–387. doi:10.1536/ihj.52.382
Meng, X., Yang, J., Dong, M., Zhang, K., Tu, E., Gao, Q., et al. (2016). Regulatory T Cells in Cardiovascular Diseases. Nat. Rev. Cardiol. 13 (3), 167–179. doi:10.1038/nrcardio.2015.169
Menzies, R. I., Howarth, A. R., Unwin, R. J., Tam, F. W. K., Mullins, J. J., and Bailey, M. A. (2015). Inhibition of the Purinergic P2X7 Receptor Improves Renal Perfusion in Angiotensin-II-Infused Rats. Kidney Int. 88 (5), 1079–1087. doi:10.1038/ki.2015.182
Mezzaroma, E., Toldo, S., Farkas, D., Seropian, I. M., Van Tassell, B. W., Salloum, F. N., et al. (2011). The Inflammasome Promotes Adverse Cardiac Remodeling Following Acute Myocardial Infarction in the Mouse. Proc. Natl. Acad. Sci. 108 (49), 19725–19730. doi:10.1073/pnas.1108586108
Milner, P., Bodin, P., Loesch, A., and Burnstock, G. (1990). Rapid Release of Endothelin and ATP from Isolated Aortic Endothelial Cells Exposed to Increased Flow. Biochem. Biophysical Res. Commun. 170 (2), 649–656. doi:10.1016/0006-291x(90)92141-l
Mistafa, O., Högberg, J., and Stenius, U. (2008). Statins and ATP Regulate Nuclear pAkt via the P2X7 Purinergic Receptor in Epithelial Cells. Biochem. Biophysical Res. Commun. 365 (1), 131–136. doi:10.1016/j.bbrc.2007.10.148
Monnerat, G., Alarcón, M. L., Vasconcellos, L. R., Hochman-Mendez, C., Brasil, G., Bassani, R. A., et al. (2016). Macrophage-dependent IL-1β Production Induces Cardiac Arrhythmias in Diabetic Mice. Nat. Commun. 7, 13344. doi:10.1038/ncomms13344
Nishida, K., and Otsu, K. (2017). Inflammation and Metabolic Cardiomyopathy. Cardiovasc. Res. 113 (4), 389–398. doi:10.1093/cvr/cvx012
Nishida, M., Sato, Y., Uemura, A., Narita, Y., Tozaki-Saitoh, H., Nakaya, M., et al. (2008). P2Y6 Receptor-Gα12/13 Signalling in Cardiomyocytes Triggers Pressure Overload-Induced Cardiac Fibrosis. EMBO J. 27 (23), 3104–3115. doi:10.1038/emboj.2008.237
North, R. A. (2002). Molecular Physiology of P2X Receptors. Physiol. Rev. 82 (4), 1013–1067. doi:10.1152/physrev.00015.2002
Novitskaya, T., Chepurko, E., Covarrubias, R., Novitskiy, S., Ryzhov, S. V., Feoktistov, I., et al. (2016). Extracellular Nucleotide Regulation and Signaling in Cardiac Fibrosis. J. Mol. Cell Cardiol. 93, 47–56. doi:10.1016/j.yjmcc.2016.02.010
Palomino-Doza, J., Rahman, T. J., Avery, P. J., Mayosi, B. M., Farrall, M., Watkins, H., et al. (2008). Ambulatory Blood Pressure Is Associated with Polymorphic Variation in P2X Receptor Genes. Hypertension 52 (5), 980–985. doi:10.1161/HYPERTENSIONAHA.108.113282
Pasqualetti, G., Seghieri, M., Santini, E., Rossi, C., Vitolo, E., Giannini, L., et al. (2017). P2X7 Receptor and APOE Polymorphisms and Survival from Heart Failure: A Prospective Study in Frail Patients in a Geriatric Unit. Aging Dis. 8 (4), 434–441. doi:10.14336/AD.2016.1202
Peng, K., Liu, L., Wei, D., Lv, Y., Wang, G., Xiong, W., et al. (2015). P2X7R Is Involved in the Progression of Atherosclerosis by Promoting NLRP3 Inflammasome Activation. Int. J. Mol. Med. 35 (5), 1179–1188. doi:10.3892/ijmm.2015.2129
Pezzuto, B., Badagliacca, R., Poscia, R., Ghio, S., D’Alto, M., Vitulo, P., et al. (2015). Circulating Biomarkers in Pulmonary Arterial Hypertension: Update and Future Direction. J. Heart Lung Transplant. 34 (3), 282–305. doi:10.1016/j.healun.2014.12.005
Piscopiello, M., Sessa, M., Anzalone, N., Castellano, R., Maisano, F., Ferrero, E., et al. (2013). P2X7 Receptor Is Expressed in Human Vessels and Might Play a Role in Atherosclerosis. Int. J. Cardiol. 168 (3), 2863–2866. doi:10.1016/j.ijcard.2013.03.084
Price, L. C., Wort, S. J., Perros, F., Dorfmüller, P., Huertas, A., Montani, D., et al. (2012). Inflammation in Pulmonary Arterial Hypertension. Chest 141 (1), 210–221. doi:10.1378/chest.11-0793
Quijada, P., Misra, A., Velasquez, L. S., Burke, R. M., Lighthouse, J. K., Mickelsen, D. M., et al. (2019). Pre-existing Fibroblasts of Epicardial Origin Are the Primary Source of Pathological Fibrosis in Cardiac Ischemia and Aging. J. Mol. Cell Cardiol. 129, 92–104. doi:10.1016/j.yjmcc.2019.01.015
Rabinovitch, M., Guignabert, C., Humbert, M., and Nicolls, M. R. (2014). Inflammation and Immunity in the Pathogenesis of Pulmonary Arterial Hypertension. Circ. Res. 115 (1), 165–175. doi:10.1161/CIRCRESAHA.113.301141
Ridker, P. M., Everett, B. M., Thuren, T., Macfadyen, J. G., Chang, W. H., Ballantyne, C., et al. (2017). Antiinflammatory Therapy with Canakinumab for Atherosclerotic Disease. N. Engl. J. Med. 377 (12), 1119–1131. doi:10.1056/NEJMoa1707914
Robinson, W. P., Douillet, C. D., Milano, P. M., Boucher, R. C., Patterson, C., and Rich, P. B. (2006). ATP Stimulates MMP-2 Release from Human Aortic Smooth Muscle Cells via JNK Signaling Pathway. Am. J. Physiology-Heart Circulatory Physiol. 290 (5), H1988–H1996. doi:10.1152/ajpheart.00344.2005
Rothman, A. M., Macfadyen, J., Thuren, T., Webb, A., Harrison, D. G., Guzik, T. J., et al. (2020). Effects of Interleukin-1β Inhibition on Blood Pressure, Incident Hypertension, and Residual Inflammatory Risk. Hypertension 75 (2), 477–482. doi:10.1161/HYPERTENSIONAHA.119.13642
Sandanger, Ø., Ranheim, T., Vinge, L. E., Bliksøen, M., Alfsnes, K., Finsen, A. V., et al. (2013). The NLRP3 Inflammasome Is Up-Regulated in Cardiac Fibroblasts and Mediates Myocardial Ischaemia-Reperfusion Injury. Cardiovasc. Res. 99 (1), 164–174. doi:10.1093/cvr/cvt091
Sathanoori, R., Swärd, K., Olde, B., and Erlinge, D. (2015). The ATP Receptors P2X7 and P2X4 Modulate High Glucose and Palmitate-Induced Inflammatory Responses in Endothelial Cells. PLoS One 10 (5), e0125111. doi:10.1371/journal.pone.0125111
Scheuplein, F., Schwarz, N., Adriouch, S., Krebs, C., Bannas, P., Rissiek, B., et al. (2009). NAD+and ATP Released from Injured Cells Induce P2X7-dependent Shedding of CD62L and Externalization of Phosphatidylserine by Murine T Cells. J. Immunol. 182 (5), 2898–2908. doi:10.4049/jimmunol.0801711
Seman, M., Adriouch, S., Scheuplein, F., Krebs, C., Freese, D., Glowacki, G., et al. (2003). NAD-induced T Cell Death. Immunity 19 (4), 571–582. doi:10.1016/s1074-7613(03)00266-8
Shi, J., Gao, W., and Shao, F. (2017). Pyroptosis: Gasdermin-Mediated Programmed Necrotic Cell Death. Trends Biochem. Sci. 42 (4), 245–254. doi:10.1016/j.tibs.2016.10.004
Shi, J., Zhao, Y., Wang, K., Shi, X., Wang, Y., Huang, H., et al. (2015). Cleavage of GSDMD by Inflammatory Caspases Determines Pyroptotic Cell Death. Nature 526 (7575), 660–665. doi:10.1038/nature15514
Shi, X., Zheng, K., Shan, P., Zhang, L., Wu, S., and Huang, Z. (2020). Elevated Circulating Level of P2X7 Receptor Is Related to Severity of Coronary Artery Stenosis and Prognosis of Acute Myocardial Infarction. Cardiol. J. doi:10.5603/CJ.a2020.0074
Shokoples, B. G., Paradis, P., and Schiffrin, E. L. (2020). P2X7. Atvb, 41. ATVBAHA120315116. doi:10.1161/ATVBAHA.120.315116
Simonneau, G., Montani, D., Celermajer, D. S., Denton, C. P., Gatzoulis, M. A., Krowka, M., et al. (2019). Haemodynamic Definitions and Updated Clinical Classification of Pulmonary Hypertension. Eur. Respir. J. 53 (1), 1801913. doi:10.1183/13993003.01913-2018
Solini, A., Menini, S., Rossi, C., Ricci, C., Santini, E., Blasetti Fantauzzi, C., et al. (2013). The Purinergic 2X7receptor Participates in Renal Inflammation and Injury Induced by High-Fat Diet: Possible Role of NLRP3 Inflammasome Activation. J. Pathol. 231 (3), 342–353. doi:10.1002/path.4237
Stachon, P., Heidenreich, A., Merz, J., Hilgendorf, I., Wolf, D., Willecke, F., et al. (2017). P2X 7 Deficiency Blocks Lesional Inflammasome Activity and Ameliorates Atherosclerosis in Mice. Circulation 135 (25), 2524–2533. doi:10.1161/CIRCULATIONAHA.117.027400
Stock, T. C., Bloom, B. J., Wei, N., Ishaq, S., Park, W., Wang, X., et al. (2012). Efficacy and Safety of CE-224,535, an Antagonist of P2X7Receptor, in Treatment of Patients with Rheumatoid Arthritis Inadequately Controlled by Methotrexate. J. Rheumatol. 39 (4), 720–727. doi:10.3899/jrheum.110874
Suzuki, J.-i., Ogawa, M., Futamatsu, H., Kosuge, H., Sagesaka, Y. M., and Isobe, M. (2007). Tea Catechins Improve Left Ventricular Dysfunction, Suppress Myocardial Inflammation and Fibrosis, and Alter Cytokine Expression in Rat Autoimmune Myocarditis. Eur. J. Heart Fail. 9 (2), 152–159. doi:10.1016/j.ejheart.2006.05.007
Toldo, S., Kannan, H., Bussani, R., Anzini, M., Sonnino, C., Sinagra, G., et al. (2014). Formation of the Inflammasome in Acute Myocarditis. Int. J. Cardiol. 171 (3), e119–e121. doi:10.1016/j.ijcard.2013.12.137
Toldo, S., Mauro, A. G., Cutter, Z., and Abbate, A. (2018). Inflammasome, Pyroptosis, and Cytokines in Myocardial Ischemia-Reperfusion Injury. Am. J. Physiology-Heart Circulatory Physiol. 315 (6), H1553–H1568. doi:10.1152/ajpheart.00158.2018
Tu, G., Zou, L., Liu, S., Wu, B., Lv, Q., Wang, S., et al. (2016). Long Noncoding NONRATT021972 siRNA Normalized Abnormal Sympathetic Activity Mediated by the Upregulation of P2X7 Receptor in Superior Cervical Ganglia after Myocardial Ischemia. Purinergic Signal. 12 (3), 521–535. doi:10.1007/s11302-016-9518-3
Turner, N. A. (2016). Inflammatory and Fibrotic Responses of Cardiac Fibroblasts to Myocardial Damage Associated Molecular Patterns (DAMPs). J. Mol. Cell Cardiol. 94, 189–200. doi:10.1016/j.yjmcc.2015.11.002
Vandanmagsar, B., Youm, Y.-H., Ravussin, A., Galgani, J. E., Stadler, K., Mynatt, R. L., et al. (2011). The NLRP3 Inflammasome Instigates Obesity-Induced Inflammation and Insulin Resistance. Nat. Med. 17 (2), 179–188. doi:10.1038/nm.2279
Vergani, A., Tezza, S., D’Addio, F., Fotino, C., Liu, K., Niewczas, M., et al. (2013). Long-term Heart Transplant Survival by Targeting the Ionotropic Purinergic Receptor P2X7. Circulation 127 (4), 463–475. doi:10.1161/CIRCULATIONAHA.112.123653
Vonk Noordegraaf, A., Chin, K. M., Haddad, F., Hassoun, P. M., Hemnes, A. R., Hopkins, S. R., et al. (2019). Pathophysiology of the Right Ventricle and of the Pulmonary Circulation in Pulmonary Hypertension: an Update. Eur. Respir. J. 53 (1). doi:10.1183/13993003.01900-2018
Wang, Y., Liu, X., Shi, H., Yu, Y., Yu, Y., Li, M., et al. (2020). NLRP3 Inflammasome, an Immune‐inflammatory Target in Pathogenesis and Treatment of Cardiovascular Diseases. Clin. Translational Med. 10 (1), 91–106. doi:10.1002/ctm2.13
Wernly, B., and Zhou, Z. (2020). More Purinergic Receptors Deserve Attention as Therapeutic Targets for the Treatment of Cardiovascular Disease. Am. J. Physiology-Heart Circulatory Physiol. 319 (4), H723–H729. doi:10.1152/ajpheart.00417.2020
Westermann, D., Lindner, D., Kasner, M., Zietsch, C., Savvatis, K., Escher, F., et al. (2011). Cardiac Inflammation Contributes to Changes in the Extracellular Matrix in Patients with Heart Failure and Normal Ejection Fraction. Circ. Heart Fail. 4 (1), 44–52. doi:10.1161/CIRCHEARTFAILURE.109.931451
Wexler, R. K., Elton, T., Pleister, A., and Feldman, D. (2009). Cardiomyopathy: an Overview. Am. Fam. Physician 79 (9), 778–784.
Wilck, N., Matus, M. G., Kearney, S. M., Olesen, S. W., Forslund, K., Bartolomaeus, H., et al. (2017). Salt-responsive Gut Commensal Modulates TH17 axis and Disease. Nature 551 (7682), 585–589. doi:10.1038/nature24628
Wu, C., Zhao, Y., Xiao, X., Fan, Y., Kloc, M., Liu, W., et al. (2016). Graft‐Infiltrating Macrophages Adopt an M2 Phenotype and Are Inhibited by Purinergic Receptor P2X7 Antagonist in Chronic Rejection. Am. J. Transpl. 16 (9), 2563–2573. doi:10.1111/ajt.13808
Xu, H., and Liang, S. D. (2013). [Effect of P2X7 Receptor on Inflammatory Diseases and its Mechanism]. Sheng Li Xue Bao 65 (2), 244–252.
Yamamoto, K., Korenaga, R., Kamiya, A., and Ando, J. (2000). Fluid Shear Stress Activates Ca 2+ Influx into Human Endothelial Cells via P2X4 Purinoceptors. Circ. Res. 87 (5), 385–391. doi:10.1161/01.res.87.5.385
Yang, X., Li, Y., Li, Y., Ren, X., Zhang, X., Hu, D., et al. (2017). Oxidative Stress-Mediated Atherosclerosis: Mechanisms and Therapies. Front. Physiol. 8, 600. doi:10.3389/fphys.2017.00600
Yin, J., Wang, Y., Hu, H., Li, X., Xue, M., Cheng, W., et al. (2017a). P2X7receptor Inhibition Attenuated Sympathetic Nerve Sprouting after Myocardial Infarctionviathe NLRP3/IL-1β Pathway. J. Cel. Mol. Med. 21 (11), 2695–2710. doi:10.1111/jcmm.13185
Yin, J., You, S., Liu, H., Chen, L., Zhang, C., Hu, H., et al. (2017b). Role of P2X7R in the Development and Progression of Pulmonary Hypertension. Respir. Res. 18 (1), 127. doi:10.1186/s12931-017-0603-0
Zempo, H., Sugita, Y., Ogawa, M., Watanabe, R., Suzuki, J.-i., and Isobe, M. (2015). A P2X7 Receptor Antagonist Attenuates Experimental Autoimmune Myocarditis via Suppressed Myocardial CD4+ T and Macrophage Infiltration and NADPH Oxidase 2/4 Expression in Mice. Heart Vessels 30 (4), 527–533. doi:10.1007/s00380-014-0527-2
Zeng, C., Wang, R., and Tan, H. (2019). Role of Pyroptosis in Cardiovascular Diseases and its Therapeutic Implications. Int. J. Biol. Sci. 15 (7), 1345–1357. doi:10.7150/ijbs.33568
Zhang, X., Fu, Y., Li, H., Shen, L., Chang, Q., Pan, L., et al. (2018). H3 Relaxin Inhibits the Collagen Synthesis via ROS- and P2X7R-Mediated NLRP3 Inflammasome Activation in Cardiac Fibroblasts under High Glucose. J. Cel. Mol. Med. 22 (3), 1816–1825. doi:10.1111/jcmm.13464
Zhang, X., Pan, L., Yang, K., Fu, Y., Liu, Y., Chi, J., et al. (2017). H3 Relaxin Protects against Myocardial Injury in Experimental Diabetic Cardiomyopathy by Inhibiting Myocardial Apoptosis, Fibrosis and Inflammation. Cell. Physiol. Biochem. 43 (4), 1311–1324. doi:10.1159/000481843
Zhao, T. V., Li, Y., Liu, X., Xia, S., Shi, P., Li, L., et al. (2019). ATP Release Drives Heightened Immune Responses Associated with Hypertension. Sci. Immunol. 4 (36), eaau6426. doi:10.1126/sciimmunol.aau6426
Zhaolin, Z., Guohua, L., Shiyuan, W., and Zuo, W. (2019). Role of Pyroptosis in Cardiovascular Disease. Cell Prolif 52 (2), e12563. doi:10.1111/cpr.12563
Zhou, J., Tian, G., Quan, Y., Li, J., Wang, X., Wu, W., et al. (2020). Inhibition of P2X7 Purinergic Receptor Ameliorates Cardiac Fibrosis by Suppressing NLRP3/IL-1β Pathway. Oxidative Med. Cell Longevity 2020, 1. doi:10.1155/2020/7956274
Zhou, R., Dang, X., Sprague, R. S., Mustafa, S. J., and Zhou, Z. (2020). Alteration of Purinergic Signaling in Diabetes: Focus on Vascular Function. J. Mol. Cell Cardiol. 140, 1–9. doi:10.1016/j.yjmcc.2020.02.004
Keywords: P2X7 receptor, inflammation, atherosclerosis, arterial hypertension, myocardial infarction
Citation: Zhou J, Zhou Z, Liu X, Yin H-Y, Tang Y and Cao X (2021) P2X7 Receptor–Mediated Inflammation in Cardiovascular Disease. Front. Pharmacol. 12:654425. doi: 10.3389/fphar.2021.654425
Received: 18 January 2021; Accepted: 09 April 2021;
Published: 29 April 2021.
Edited by:
Peter Illes, Leipzig University, GermanyReviewed by:
Ronald Sluyter, University of Wollongong, AustraliaShangdong Liang, Nanchang University, China
Copyright © 2021 Zhou, Zhou, Liu, Yin, Tang and Cao. This is an open-access article distributed under the terms of the Creative Commons Attribution License (CC BY). The use, distribution or reproduction in other forums is permitted, provided the original author(s) and the copyright owner(s) are credited and that the original publication in this journal is cited, in accordance with accepted academic practice. No use, distribution or reproduction is permitted which does not comply with these terms.
*Correspondence: Xin Cao, Y2FveGluQGNkdXRjbS5lZHUuY24=