- 1School of Pharmaceutical Sciences, Guangzhou University of Chinese Medicine, Guangzhou, China
- 2Department of Pharmacy, Affiliated Dongguan Hospital, Southern Medical University, Dongguan, China
- 3Department of Systems Biomedical Sciences, School of Medicine, Jinan University, Guangzhou, China
- 4NHC Key Laboratory of Male Reproduction and Genetics, Guangzhou, China
- 5Department of Central Laboratory, Family Planning Research Institute of Guangdong Province, Guangzhou, China
- 6Shaoguan Institute of Danxia Dendrobium Officinale, Shaoguan, China
We investigated the antitumor effects of four fractions of Dendrobium officinale Kimura & Migo (D. officinale) polysaccharides with different molecular weights (Mw), Astragalus membranaceus polysaccharides (APS) and Lentinus edodes polysaccharides (LNT) on colorectal cancer (CRC) using a zebrafish xenograft model. Transcriptome sequencing was performed to further explore the possible antitumor mechanisms of D. officinale polysaccharides. Fractions of D. officinale polysaccharides, LNT, and APS could significantly inhibit the growth of HT-29 cells in a zebrafish xenograft model. One fraction of D. officinale polysaccharides called DOPW-1 (Mw of 389.98 kDa) exhibited the strongest tumor inhibition. Compared with the control group, RNA-seq revealed that the DOPW-1–treated experimental group had 119 differentially expressed genes (DEGs), of which 45 had upregulated expression and 74 had downregulated expression. Analyses using Gene Ontology and Kyoto Encyclopedia of Genes and Genomes suggested that the pathway “apoptosis-multiple species” was the most significantly enriched. Our data indicated that 1) fractions of D. officinale polysaccharides of Mw 389.98 kDa were most suitable against CRC; 2) DOPW-1 could be developed into a clinical agent against CRC; and 3) an apoptosis pathway is important for DOPW-1 to inhibit the proliferation of HT-29 cells.
Introduction
Dendrobium officinale Kimura & Migo (D. officinale) is a perennial herb in the Orchidaceae family. It possesses high pharmacological value and nutritional value. D. officinale has been used in traditional Chinese medicine formulations and a functional food in China for more than 1,000 years (Ng et al., 2012).
D. officinale polysaccharides possess anticancer (Yu et al., 2018a; Zhang et al., 2019; Zhang et al., 2020; Tao et al., 2021), immunomodulation (Huang et al., 2016; Tao et al., 2019), antioxidant (Luo et al., 2016), hypoglycemic (Wang et al., 2018; Kuang et al., 2020) and antifatigue (Wei et al., 2017) activities. However, few studies have focused on the activity of D. officinale polysaccharides against colorectal cancer (CRC).
The molecular weight (Mw) of polysaccharides has been considered to have a close relationship with the biological activities of polysaccharides (Wasser, 2002). Wang et al. (2014) showed that high-Mw polysaccharides (524 kDa) from Lentinus edodes displayed robust antitumor effects. Ren et al. (2016) revealed that a high-Mw polysaccharide (463 kDa) from Pleurotus eryngii had antitumor effects against HepG2 cells. However, Xu et al. (2016) revealed that medium-Mw polysaccharides (rather than high-Mw polysaccharides) from Camellia-species seed cake displayed excellent antioxidant activity. Bhadja et al. (2016) revealed that polysaccharides from seaweed with low Mw had a repairing effect on damaged HK-2 cells. Unfortunately, the relationship between the Mw and biological activity of D. officinale polysaccharides has not been investigated deeply. Zhang et al. (2019) explored the suitable Mw range of polysaccharides from D. officinale on inducing the apoptosis of HeLa cells, but was studied in vitro only. Therefore, for CRC, the Mw of D. officinale polysaccharides that are most suitable in vivo is still unknown.
In China and Japan, lentinan injection (which contains the main active ingredients of Lentinus edodes polysaccharides (LNT)) has been approved as adjuvant therapy for malignant tumors (Sun et al., 2020). Astragalus membranaceus polysaccharides (APS) have been applied widely in the clinic due to their immunomodulatory and antitumor activities (Zhou et al., 2017). Therefore, comparing the anticancer effects of D. officinale polysaccharides with those of APS and LNT could help provide a scientific basis for developing the polysaccharides of D. officinale as pharmacological agents.
Zebrafish (Danio rerio) is regarded as an “ideal” model organism for assessing human diseases because it possesses several orthologs with human genes and drug targets (Gunnarsson et al., 2008; Howe et al., 2013; Kim et al., 2013; Dai et al., 2014). Hence, zebrafish could be used to test the effects of D. officinale polysaccharides.
Transcriptome studies provide a promising perspective for exploring the molecular mechanisms of drug functions and investigating the underlying pathogenesis of diseases and modes of drug action (Wang et al., 2008; Li et al., 2011; Chen et al., 2012). Transcriptome abundance is measured by RNA sequencing (RNA-seq) (Hao et al., 2018). Some transcriptome analyses that aimed at elucidating the molecular mechanisms related to the antitumor effects of polysaccharides have been undertaken. For example, Kang et al. (2016) revealed the underlying antitumor mechanism of marine algae (Gracilariopsis lemaneiformis) polysaccharides by transcriptome profiling. Ren et al. (2019) uncovered the underlying antitumor mechanism of the action of polysaccharides from dandelions in inhibiting a specific pathway: phosphoinositide 3-kinase/protein kinase B/mammalian target of rapamycin. Qi et al. (2020) exposed the underlying anti-CRC mechanism of Cordyceps sinensis polysaccharides. Wang et al. (2019) revealed the underlying antitumor mechanism of the polysaccharides of Taishan Pinus massoniana pollen. All these research teams used RNA-seq to provide these observations.
We compared the effects of D. officinale polysaccharides of different Mw, as well as LNT and APS, on the growth of HT-29 cells in a zebrafish xenograft model. Also, we evaluated the changes in the gene-expression profile induced by treatment with D. officinale polysaccharides on HT-29 cells by RNA-seq to explore the underlying anti-CRC mechanism.
Materials and Methods
Ethical Approval of the Study Protocol
Animal care and experimental protocols were approved (IACUC-2019-194, IACUC-2020-097) by the Animal Ethics Committee at Hunter Biotechnology (Hangzhou, China) and were conducted in accordance with the Chinese Association for Laboratory Animal Sciences guidelines.
Materials
5-Fluorouracil (5-Fu) was purchased from Sigma-Aldrich (Saint Louis, MO, United States). APS was obtained from Tianjin Cinorch Pharmaceuticals (Tianjin, China). LNT was purchased from Jinling Pharmaceuticals (Jinling, China). Also, 5× All-In-One RT MasterMix (with AccuRT Genomic DNA Removal Kit) and EvaGreen 2× quantitative polymerase chain reaction (qPCR) MasterMix-LOW ROX were purchased from Applied Biological Materials (Vancouver, Canada). The TRIzol® reagent was obtained from CoWin Biosciences (Beijing, China).
Cell Lines
A human CRC cell line (HT-29) was purchased from the Cell Line Bank of the Chinese Academy of Sciences (Shanghai, China). HT-29 cells were cultured in McCoy’s 5A medium (Gibco, Grand Island, NY, United States) containing 10% fetal bovine serum (Gibco), penicillin (100 U·ml−1), and streptomycin (100 μg·ml−1) at 37°C in a humidified atmosphere with 5% CO2.
Zebrafish
Wild-type AB zebrafish maintained at Hunter Biotechnology was incubated at 28°C with E3 medium (pH 7.2) under a 14 h: 10 h light-dark cycle according to a standard zebrafish breeding protocol (Kimmel et al., 1995). The embryos were obtained by natural spawning.
Polysaccharides From Dendrobium officinale Kimura & Migo
Three polysaccharide fractions, DOP2 (D2), DOP8 (D8), and DOP122 (D122), with a Mw (in kDa) of 24.89, 80.32, and 1,224.54, respectively, were obtained from the stems of D. officinale, as described previously (Zhang et al., 2020). In brief, after hot water extraction, a D. officinale–extracting solution was precipitated with ethanol followed by oxidative degradation with a Vc–H2O2–FeCl2·4H2O system. Then, D2, D8, and D122 were obtained by freeze-drying.
Another polysaccharide, DOPW-1, of Mw 389.98 kDa, was isolated from the stems of D. officinale according to a method described previously (Tao et al., 2019). In brief, after hot water extraction, a D. officinale–extracting solution was precipitated with ethanol followed by purification using a DEAE cellulose-52 column and Sephadex G-300 HR column. Then, DOPW-1 was obtained by freeze-drying.
Effects of Polysaccharides of Different Molecular Weight in a Zebrafish Xenograft Model
A zebrafish xenograft model was established according to the method described by Hamilton et al. (2016). In brief, zebrafish xenografts were generated by microinjection of ∼200 chloromethylbenz-amino derivatives of 1,1′-dioctadecyl-3,3,3′,3′-tetramethylindocarbocyanine perchlorate (CM-Dil)–labeled HT-29 cells into the yolk sac of zebrafish embryos 2 days post fertilization (dpf).
After establishing the zebrafish xenograft model, the embryos (3 dpf) were placed into six groups randomly: model; 5-Fu (50 ng/zebrafish); D2 (250 μg·ml−1); D8 (250 μg·ml−1); DOPW-1 (250 μg·ml−1); D122 (250 μg·ml−1). The embryos were transferred into six-well plates (30 embryos/well). The dose of 5-Fu was selected based on the study by Xu et al. (2019), and the dose of experimental groups was selected based on our previous study (Tao et al., 2021), where we explored the maximum tolerance concentration (MTC) of polysaccharides from D. officinale and determined the effect of polysaccharides from D. officinale (27.8, 83.3, 250 μg·ml−1) in the zebrafish xenograft model. The results indicated that the concentration of 250 μg·ml−1 is determined as MTC and it has the most significant inhibitory effect on CRC. Subsequently, 5-Fu was administered through caudal vein microinjection. D2, D8, DOPW-1, and D122 were dissolved in the embryo medium. 2 days after administration, fluorescence images were captured using a fluorescence microscope (AZ100; Nikon, Japan). The fluorescence intensity was quantified with NIS-Elements D 3.10 (Nikon). The inhibition of tumor growth was calculated according to the following formula:
Effects of Astragalus membranaceus Polysaccharides, Dendrobium officinale Kimura & Migo Polysaccharide-1, and Lentinus edodes Polysaccharides in a Zebrafish Xenograft Model
After establishing the zebrafish xenograft model, the embryos (3 dpf) were divided randomly into five groups: model; 5-Fu (50 ng/zebrafish); APS (250 μg·ml−1); DOPW-1 (250 μg·ml−1); LNT (250 μg·ml−1). The embryos were transferred into six-well plates (30 embryos/well). Subsequently, 5-Fu was administered through caudal vein microinjection. APS, DOPW-1, and LNT were dissolved in the embryo medium. 2 days after administration, and fluorescence images were captured using a fluorescence microscope (AZ100). The fluorescence intensity was quantified with NIS-Elements D 3.10 (Nikon). Inhibition of tumor growth was calculated as described in the previous section.
RNA Extraction, Library Preparation, and Transcriptome Sequencing
In our previous study, cell proliferation assay was conducted (Tao et al., 2021), and the results demonstrated that the concentration of 400 μg·ml−1 possess the most significant inhibitory effect on HT-29 cells. Therefore, 400 μg·ml−1 was selected as the intervention dosage in this experiment. In brief, HT-29 cells were seeded in six-well plates (1×106 cells/well) for 24 h, and treated/ untreated with DOPW-1 (400 μg·ml−1) for an additional 48 h. After cell harvesting, total RNA was extracted using the TRIzol reagent at 4°C, and the concentration and purity (optical density at 260 nm (OD260)/OD280 ≥ 1.8, OD260/OD230 ≥ 1.5) were tested using a spectrophotometer (NanoDrop™ 2000; Thermo Fisher Scientific, Wilmington, DE, United States). The RNA Nano 6000 Assay Kit of the Bioanalyzer 2,100 system (Agilent Technologies, Santa Clara, CA, United States) was used to evaluate RNA integrity to ensure that the RNA integrity number ≥8.0. Finally, extracted RNA samples were frozen immediately at −80°C until use.
According to the manufacturer’s recommendations, the NEBNext® Ultra™ RNA Library Prep Kit for Illumina® (New England Biolabs, Ipswich, MA, United States) was used to construct the sequencing libraries. This was achieved using 1 μg of total RNA from each sample, and then index codes were added to attribute sequences to each sample. Clustering of the index-coded samples was performed using the TruSeq PE Cluster Kit v4-cBot-HS (Illumina, San Diego, CA, United States) on a cBot Cluster Generation System in accordance with the manufacturer’s (Illumina) directions. After cluster generation, sequencing of libraries was performed on an Illumina platform, and paired-end reads were generated (Chao et al., 2019).
Bioinformatics Analysis
Raw data with the “fastq” format were analyzed using in-house Perl scripts. In this step, the adapter sequence, poly-N-containing reads, and low-quality reads in raw data were discarded to obtain clean data. Meanwhile, Q20, Q30, GC-content, and sequence duplication in clean data were measured for quality control. Then, the filtered clean data of high quality were used for further analyses.
After the raw sequences had been processed and converted to clean reads, the latter were mapped to the reference genome sequence using HISAT2. Only reads with perfect matches or single mismatches were utilized further for this analysis based on the reference genome.
Several databases were used to annotate gene function: Nr (National Center for Biotechnology Information non-redundant protein sequences, ftp://ftp.ncbi.nih.gov/blast/db/); Pfam (Protein Family, http://pfam.xfam.org/); COG (Clusters of Orthologous Groups of Proteins, www.ncbi.nlm.nih.gov/COG/); KOG (Clusters of Protein Homology, www.ncbi.nlm.nih.gov/KOG/); SWISS-PROT (manually annotated and reviewed protein sequences, http://www.expasy.ch/sprot); KO (Kyoto Encyclopedia of Genes and Genomes (KEGG) Ortholog, http://www.genome.jp/kegg/); GO (Gene Ontology, www.geneontology.org/).
Fragments per kilobase of transcript per million mapped (FPKM) values were used to estimate gene expression. FPKM was calculated using the following formula:
Analysis of differential expression of the two groups was executed using edgeR (Robinson et al., 2010). Differentially expressed genes (DEGs) with P < 0.05 and fold change ≥2 were screened for further analysis. Analysis of the functional enrichment of DEGs using the GO database was performed using GOseq within R (R Institute for Statistical Computing, Vienna, Austria) based on Wallenius’ noncentral hyper-geometric distribution (Young et al., 2010), which can adjust for bias in gene length in DEGs. KOBAS (Mao et al., 2005) was applied for the analysis of pathway enrichment of DEGs using the KEGG database.
Analysis of Gene Expression by Real-Time Reverse Transcription-Quantitative Polymerase Chain Reaction
HT-29 cells were seeded in six-well plates at 1×106 cells/well for 24 h, and then treated or not treated with DOPW-1 (400 μg·ml−1) for an additional 48 h. After cell harvesting, total RNA was extracted using the TRIzol reagent. The quantity and quality of cells were evaluated using a spectrophotometer (NanoDrop 2000). RNA was transcribed into complementary DNA using the 5× All-In-One RT MasterMix (with AccuRT Genomic DNA Removal Kit) according to the manufacturer’s instructions. Expression of apoptosis-related genes (Bax, B-cell lymphoma (Bcl)-2, P53, caspase-3, cytochrome C) was detected using a real-time PCR instrument (ABI 7500; Applied Biosystems, Foster City, CA, United States) with EvaGreen 2× qPCR MasterMix-LOW ROX™ following the manufacturer’s instructions. β-actin was used as an internal standard to normalize gene targets. Relative RNA expression was calculated using the 2−∆∆CT method (Rieu and Powers 2009). The primers used were designed by Takara Biotechnology (Shiga, Japan) and are listed in Table 1. RT-qPCR experiments were carried out in triplicate.
Statistical Analyses
Statistical analyses were undertaken using SPSS 19.0 (IBM, Armonk, NY, United States) applying a two-tailed independent Student’s t-test or one-way ANOVA followed by Dunnett’s t-test. Data are expressed as the mean ± standard error of the mean (SEM). P < 0.05 was considered statistically significant.
Results
Effects of Polysaccharides of Different Molecular Weights in a Zebrafish Xenograft Model
To determine the antitumor effects of D. officinale polysaccharides of different Mw on tumorigenesis in vivo, we established a novel zebrafish xenograft model by transplanting HT-29 cells. Zebrafish embryos (3 dpf) were treated with 5-Fu, D2, D8, DOPW-1, or D122.
The fluorescence intensity reflected the tumor volume. The inhibitory effect was revealed by reduced fluorescence intensity (Figure 1A). Notably, all treatments exhibited antitumor effects in the HT-29 xenograft model (Figures 1B,C). Compared with the model group, the inhibition of the tumor xenograft treated with 5-Fu, D2, D8, DOPW-1, or D122 was 28.77 ± 4.96%, 64.41 ± 1.90%, 65.49 ± 2.23%, 67.91 ± 1.69%, and 48.64 ± 2.23%, respectively (Table 2). Tumor growth was inhibited potently and significantly by 5-Fu, D2, D8, DOPW-1, and D122 (Figure 1). DOPW-1, with a Mw of 389.98 kDa, exhibited the strongest tumor inhibition.
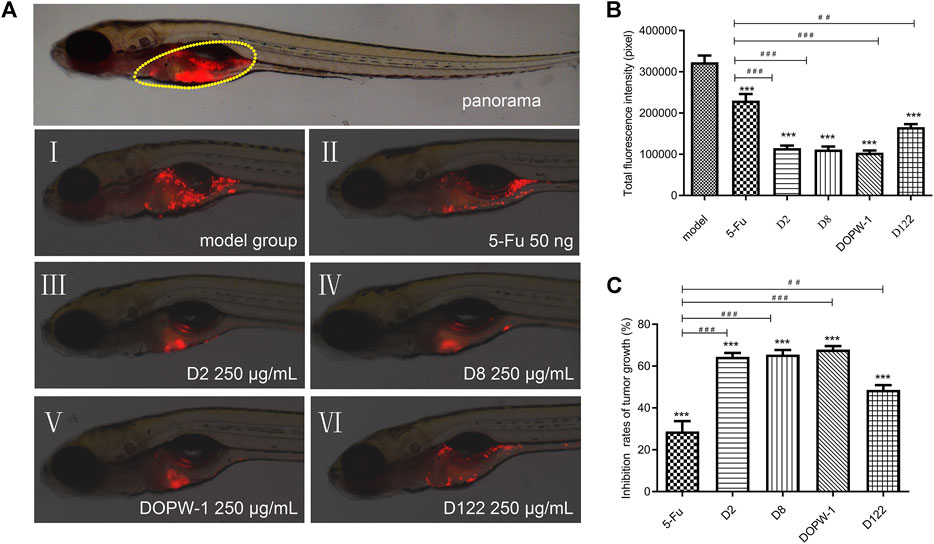
FIGURE 1. Inhibitory effect of polysaccharides of different Mw derived from D. officinale stems in a zebrafish xenograft model. (A) Red fluorescence represents a tumor area. (B) Fluorescence intensity of the tumor xenograft model. (C) Quantification of the inhibition of tumor growth. Data are expressed as the mean ± SEM. ***P < 0.001 for each group vs. the model group; ##P < 0.01 and ###P < 0.001 for each group vs. the 5-Fu group.
Effects of Astragalus membranaceus Polysaccharides, Dendrobium officinale Kimura & Migo Polysaccharide-1, and Lentinus edodes Polysaccharides in a Zebrafish Xenograft Model
The zebrafish model (created by implanting HT-29 cells) was also applied to determine the antitumor effects of APS, DOPW-1, and LNT on tumorigenesis in vivo. Zebrafish embryos (3 dpf) were treated with 5-Fu, APS, DOPW-1, or LNT. Growth inhibition was determined by capturing the fluorescence images using a fluorescence microscope. Figure 2A shows the tumor-inhibitory effect, which was indicated by reduced fluorescence intensity. Figures 2B,C show that all treatments exhibited antitumor effects in the zebrafish xenograft model. Compared with the model group, the inhibition of the tumor xenograft model treated with 5-Fu, APS, DOPW-1, or LNT was 34.03 ± 6.09%, 43.08 ± 5.19%, 55.13 ± 3.16%, and 22.48 ± 3.65%, respectively (Table 3). 5-Fu, APS, DOPW-1, and LNT inhibited tumor growth potently and significantly when compared with that in the model group (Figure 2). The inhibition of tumor growth elicited by DOPW-1 was significantly greater than that in the 5-Fu group. Moreover, compared with the APS and LNT groups, the DOPW-1 group exhibited the strongest tumor inhibition.
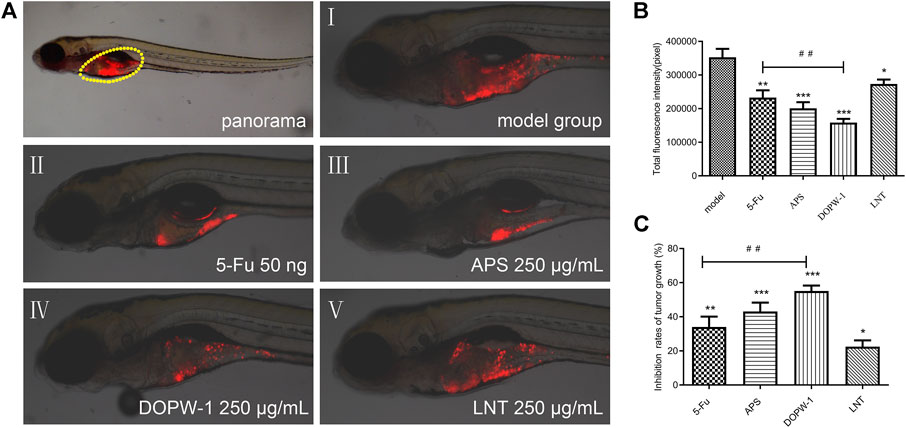
FIGURE 2. Tumor inhibition elicited by APS, DOPW-1, and LNT in a zebrafish xenograft model. (A) HT-29 cells (red) after xenotransplantation. (B) Fluorescence intensity of the tumor xenograft. (C) Quantification of the inhibition of tumor growth. Data are expressed as the mean ± SEM. *P < 0.05, **P < 0.01, and ***P < 0.001 for each group vs. the model group; ##P < 0.01 for the DOPW-1 group vs. the 5-Fu group.
Mapping of Reads
The strongest inhibitory effect on the growth of HT-29 cells was elicited by DOPW-1. Hence, DOPW-1 was selected as the experimental group to explore the difference in transcriptomes between it and the control group. All six constructed libraries (named control-1, control-2, control-3, DOPW-1-1, DOPW-1-2, and DOPW-1-3) were sequenced with raw reads.
An average of 52.39 (range, 46.14–63.26) million clean reads was obtained after discarding the adaptor sequence, poly-N–containing reads, and low-quality raw reads. HISAT2 was used to map clean reads to the human reference genome. A summary of the detailed mapping output is listed in Table 4. The ratio of mapping for the control and DOPW-1 groups was 94.69 and 94.71%, respectively, which demonstrated high gene expression in both groups.
Functional Annotation and Differentially Expressed Genes Analysis
Analysis of gene function was performed using gene annotation. The genes were aligned against the publicly available protein databases KEGG, KOG, and GO. In total, 22,478 (96.88%) genes were annotated (Table 5). Most of the genes were annotated by gene functions using KOG (59.26%), KEGG (58.88%), and GO (51.75%) databases.
Hierarchical clustering was undertaken, and volcano plots were created to visualize the differential expression of genes (Figure 3A). We identified 119 genes with significant differential expression in the DOPW-1 group compared with that in the control group. Forty-five genes had upregulated expression and 74 genes had downregulated expression, which was likely due to activation of HT-29 cells after DOPW-1 treatment. Agglomerative hierarchical clustering was used to identify the number of clusters from the cluster dendrogram (Figure 3B).
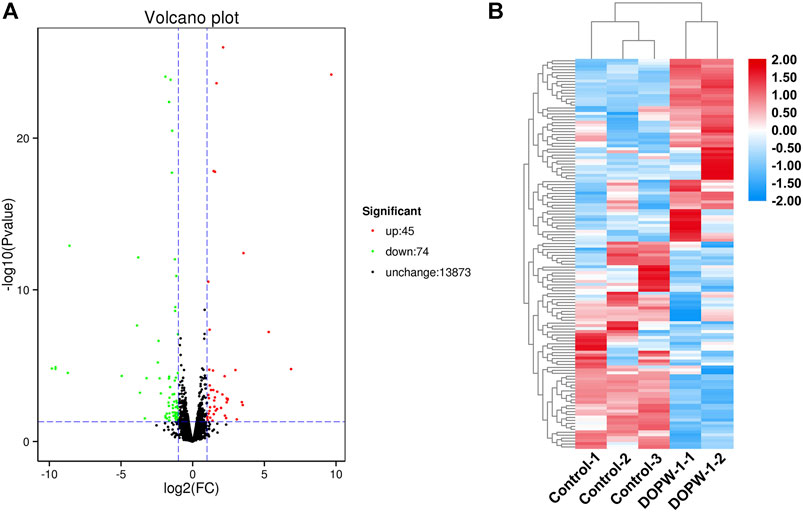
FIGURE 3. (A) Volcano map of DEGs between the DOPW-1 and control groups. Black, red, and green dots represent genes with no significant change in expression, significantly upregulated expression, and significantly downregulated expression in DEGs, respectively. (B) Hierarchical clustering of DEGs for the DOPW-1 group vs. the control group. Different colors represent different gene expressions in the DOPW-1 and control groups.
Functional Analysis of Genes Using Clusters of Protein Homology and Gene Ontology Databases
To evaluate and categorize the possible functions of genes, all unigenes were aligned to the KOG database. Orthologous genes were classified in the KOG database.
According to the KOG database, the genes were distributed in 17 orthologous clusters (Figure 4). Most of the genes were assigned to “general function prediction only” (16–20%), followed by “signal transduction mechanisms” (15–18.75%), “posttranslational modification, protein turnover, and chaperones” (8–10%), “replication, recombination, and repair” (6–7.5%), and “intracellular trafficking, secretion, and vesicular transport” (5–6.25%).
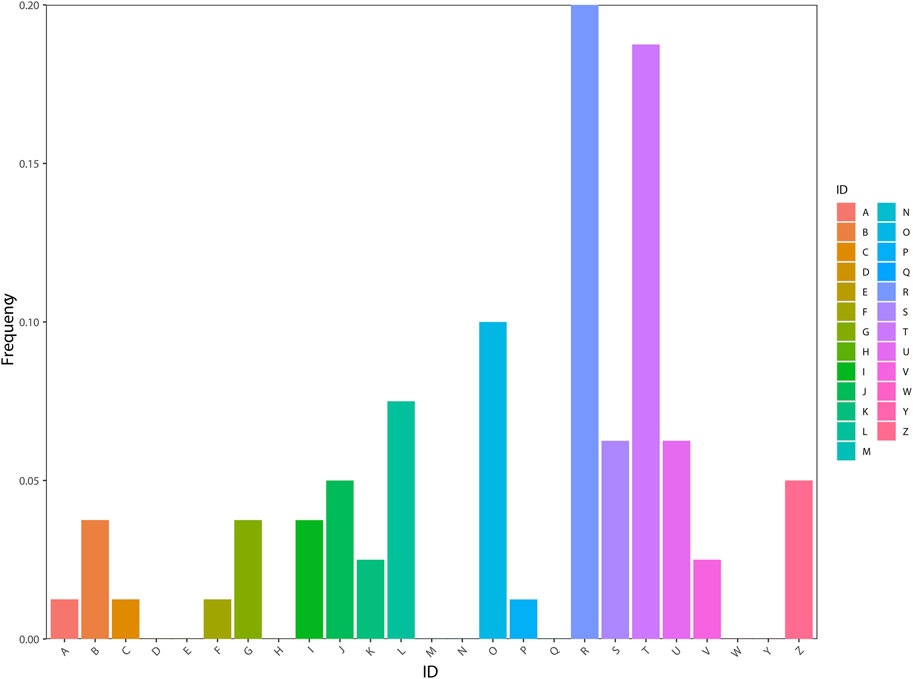
FIGURE 4. Functional classification of genes using the KOG database. (A) RNA processing and modification [1-1.25%]; (B) Chromatin structure and dynamics [3-3.75%]; (C) Energy production and conversion [1-1.25%]; (D) Cell cycle control, cell division, and chromosome partitioning [0-0%]; (E) Amino acid transport and metabolism [0-0%]; (F) Nucleotide transport and metabolism [1-1.25%]; (G) Carbohydrate transport and metabolism [3-3.75%]; (H) Coenzyme transport and metabolism [0-0%]; (I) Lipid transport and metabolism [3-3.75%]; (J) Translation, ribosomal structure, and biogenesis [4-5%]; (K) Transcription [2-2.5%]; (L) Replication, recombination, and repair [6-7.5%]; (M) Cell wall/membrane/envelope biogenesis [0-0%]; (N) Cell motility [0-0%]; (O) Posttranslational modification, protein turnover, and chaperones [8-10%]; (P) Inorganic ion transport and metabolism [1-1.25%]; (Q) Secondary metabolite biosynthesis, transport, and catabolism [0-0%]; (R) General function prediction only [16-20%]; (S) Function unknown [5-6.25%]; (T) Signal transduction mechanisms [15-18.75%]; (U) Intracellular trafficking, secretion, and vesicular transport [5-6.25%]; (V) Defense mechanisms [2-2.5%]; (W) Extracellular structures [0-0%]; (Y) Nuclear structure [0-0%]; (Z) Cytoskeleton [4-5%].
Further analysis of gene function was performed using the GO database employing the categories of biological process, cell component, and molecular function. A total of 12,006 genes were mapped using the GO database (Figure 5). Specifically, 10,434, 11,381, and 10,623 genes were assigned to biological process, cell component, and molecular function, respectively. Importantly, several different GO terms may be assigned to the same gene.
Analysis of Pathway Enrichment Using the Kyoto Encyclopedia of Genes and Genomes Database
Analysis of pathway enrichment revealed most genes to be assigned to “apoptosis and apoptosis-multiple species” (12.5%), “focal adhesion” (12.5%), and “ras signaling pathway” (12.5%) followed by “regulation of actin cytoskeleton” (9.38%) and “ErbB signaling pathway” (9.38%) (Figure 6).
To further investigate the anticancer signaling pathways of HT-29 cells treated with DOPW-1, we conducted enrichment analysis of DEGs using the KEGG database. The top 20 enriched pathways were selected (Figure 7). “Apoptosis-multiple species,” “ErbB signaling pathway,” “focal adhesion,” “ras signaling pathway,” “renal cell carcinoma,” and “steroid hormone biosynthesis” were the top six enriched pathways. Among them, “apoptosis-multiple species” was the most significantly enriched pathway. Hence, an apoptosis pathway is one of the most important pathways to consider if DOPW-1 inhibits the proliferation of HT-29 cells. This finding is consistent with an observation in our previous report (Tao et al., 2021).
Transcriptome Analysis of “Apoptosis-Multiple Species”
We discovered a close relationship among Bcl-2 family proteins, P53 protein, cytochrome C protein, caspase-3 protein, and apoptosis (Figure 8). In addition, the intrinsic pathway of apoptosis analyzed using RT-qPCR has been further verified by our previous study using Western blotting (Tao et al., 2021).
The apoptosis-related gene expression of P53, Bax, Bcl-2, cytochrome C, and caspase-3 was evaluated using RT-qPCR. Treatment with DOPW-1 (400 μg·ml−1) significantly increased the corresponding gene expression of P53, cytochrome C, and caspase-3 (Figure 9). Interestingly, the ratio of the expression of Bax/Bcl-2 in the DOPW-1 group was not significantly higher than that of the control group (P > 0.05). In our previous study, we calculated the ratio of the expression of Bak/Mcl-1 (anti- and pro-apoptotic factors, respectively) using Western blotting (Tao et al., 2021). Those results showed that the expression of Bak increased and that of Mcl-1 decreased after DOPW-1 treatment, which led to an increase in the Bak/Mcl-1 ratio when compared with that in the control group.
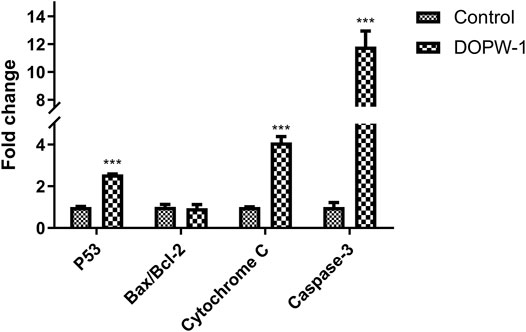
FIGURE 9. Effect of different treatments on gene expression. ***P < 0.001 for the DOPW-1 group vs. the control group.
Discussion
D. officinale polysaccharides possess anti-CRC activities (Tao et al., 2021). The Mw of polysaccharides is closely related to their antitumor activities (Huang et al., 2020). The side effects of chemotherapeutics warrant the development of nature-derived drugs based on polysaccharides. We investigated the effect of D. officinale polysaccharides of different Mw and currently used medicines based on polysaccharides (APS and LNT) in inhibiting tumor growth in a zebrafish xenograft model. We also studied the mechanism of action of D. officinale polysaccharides on the growth of HT-29 cells by RNA-seq.
In the study, the dose of D. officinale polysaccharides were selected based on our previous study (Tao et al., 2021), where we explored the MTC of polysaccharides from D. officinale and determined the effect of D. officinale polysaccharides (27.8, 83.3, 250 μg·ml−1) in a zebrafish xenograft model. The results indicated that the concentration of 250 μg·ml−1 is determined as the MTC and has the most significant inhibitory effect on CRC. D. officinale polysaccharides with a Mw ranging between 24.89 and 1,224.54 kDa exhibited significant antitumor activity, among which D. officinale polysaccharides of Mw 389.98 kDa exhibited the strongest tumor inhibition. This finding is in accordance with that of scholars who reported that lentinan of Mw 400–600 kDa exhibits strong antitumor activity (Wasser, 2002; Zhang et al., 2011). Also, Chen et al. (2009) postulated that polysaccharides of relatively high Mw may have more opportunity to “collide effectively”. Furthermore, long-chain polysaccharides have more repeating units and higher valency to bind to more receptors than that of short-chain polysaccharides. In addition, Zhang et al. (2016) reported that most polysaccharides of Mw from 10 kDa to 103 kDa exhibited a marked antitumor effect. In the present study, D2 (24.89 kDa) and D8 (80.32 kDa) showed a strong antitumor effect, whereas D122 (1,224.54 kDa) showed a relatively weaker effect. One reason may be that polysaccharides of low Mw could enter tumor cells more readily than polysaccharides of high Mw. Yu et al. (2015) showed that high-Mw polysaccharides from Porphyra yezoensis were not conducive to inhibiting gastric cancer cells. Huang et al. (2020) revealed that high-Mw polysaccharides from Dendrobium densiflorum could not exert biological effects by breaking through membranes to enter cells. In general, the degradation of high-Mw polysaccharides to low-Mw polysaccharides can improve their biological activity significantly. Nevertheless, a too low Mw of polysaccharides will inhibit the formation of an active polymer structure.
Naturally derived polysaccharides have been widely used as medicines in China, thanks to their low toxicity and efficacy. For example, polysaccharides from Astragalus species, Poria cocos, Ginseng species, and L. edodes have been approved for clinical use by the National Medical Products Administration of China (Yu et al., 2018b). In the present study, the effect of DOPW-1 on CRC was more robust than that of the medicinal polysaccharides APS and LNT. Hence, DOPW-1 could be a promising potential agent against CRC. The effect of LNT on CRC was weaker than that of APS and DOPW-1, and the dose plays an important role in this result. Jeff et al. (2013) reported that the efficacious dose of LNT against the proliferation of HCT-116 cells and HT-29 cell was 5 mg·ml−1, whereas we employed an LNT dose of 250 μg·ml−1. Therefore, the anti-CRC activity of DOPW-1 was better than that of LNT at the same dose (250 μg·ml−1).
Transcriptome studies are used to explore the underlying molecular mechanisms of drug actions (Zhang et al., 2017). RNA-seq was undertaken to investigate how DOPW-1 inhibited the growth of HT-29 cells. Use of the KEGG database showed that the DEGs mainly encoded proteins involved in “apoptosis-multiple species,” “ErbB signaling pathway,” “focal adhesion,” “ras signaling pathway,” “renal cell carcinoma,” and “steroid hormone biosynthesis”. Among them, “apoptosis-multiple species” was the most significantly enriched. It is known that polysaccharides of D. officinale possess pharmacological activities of anticancerous nature (Yu et al., 2018a; Liang et al., 2019; Zhang et al., 2019), and this study might supply valuable information for DOPW-1 anti-CRC.
Apoptosis as an important molecular mechanism for polysaccharides in inhibiting colon cancer has been reported (Feregrino-Pérez et al., 2008; Di et al., 2017). For example, Di et al. (2017) reported that exopolysaccharides produced by Lactobacillus casei SB27 from yak milk induced the apoptosis of HT-29 cells. Feregrino-Pérez et al. (2008) revealed that a polysaccharide extract from common beans (Phaseolus vulgaris L.) could induce apoptosis via mitochondria-based mechanisms. In the present study, RT-qPCR demonstrated that gene expression of P53, cytochrome C, and caspase-3 was increased significantly after DOPW-1 treatment. However, the ratio of the expression of Bax/Bcl-2 in the DOPW-1 group was not significantly higher than that in the control group. Western blotting in our previous study showed that the expression of Bak increased and that of Mcl-1 decreased after DOPW-1 treatment, which led to an increase in the Bak/Mcl-1 ratio compared with that in the control group (Tao et al., 2021). Taken together, these results suggested that DOPW-1–triggered apoptosis of HT-29 cells was associated with the upregulation of the intrinsic p53 signaling pathway through caspase activation and, finally, led to apoptosis through the mitochondrial pathway (intrinsic pathway of apoptosis).
Conclusion
Fractions of D. officinale polysaccharides with a Mw of 389.98 kDa may be the most suitable for antitumor effects against CRC. The antitumor effect of DOPW-1 on CRC was stronger than that of currently available alternatives: APS and LNT. RNA-seq revealed that an apoptosis pathway was important for DOPW-1 to inhibit the proliferation of HT-29 cells. We revealed that the pathway DOPW-1 induces the apoptosis of HT-29 cells and provided a scientific basis for use of DOPW-1 as a naturally occurring medicine against CRC. However, there are some limitations in this study. We merely performed the experiments in a zebrafish xenograft model, and clinical trials are needed to verify our data. On the other hand, a deeper understanding about the transcellular transport and subcellular location of D. officinale polysaccharides in CRC cells is yet to be gained. In the following studies, we will conduct further research to explore the transport and absorption of D. officinale polysaccharides in CRC cells by using labeling technology.
Data Availability Statement
The datasets presented in this study can be found in online repositories. The names of the repository/repositories and accession number(s) can be found below: https://www.ncbi.nlm.nih.gov/bioproject/PRJNA727088 PRJNA727088.
Ethics Statement
All animal care and experimental protocols were approved by the Animal Ethics Committee at Hunter Biotechnology, Inc. (No. IACUC-2019-194, No. IACUC-2020-097) and were conducted in accordance with the Chinese Association for Laboratory Animal Sciences guidelines.
Author Contributions
Conceptualization: GW and ST. Methodology: ST, ZR, ZW and JH. Software: ST and ZY. Validation: ST and SD. Data analysis: ST, ZR and CL. Resources: GW. Data curation: ST. Writing (preparation of original draft and revised the manuscript): ST. Supervision: GW. Project administration: GW. Funding acquisition: GW.
Funding
This work was supported by the 2018 Shaoguan City Science and Technology Plan Project: Special Project of Industry-University-Research Cooperation (2018CS11919) and 2019 Guangdong Province Special Fund for Science and Technology (‘Big project + task list’) Project: Ecological Cultivation and Sustainable Utilization of Danxia Dendrobium officinale, a rare Southern Medicine in Guangdong Province (no. 2019gdskjzxzj-zt3-2).
Conflict of Interest
The authors declare that the research was conducted in the absence of any commercial or financial relationships that could be construed as a potential conflict of interest.
Publisher’s Note
All claims expressed in this article are solely those of the authors and do not necessarily represent those of their affiliated organizations, or those of the publisher, the editors, and the reviewers. Any product that may be evaluated in this article, or claim that may be made by its manufacturer, is not guaranteed or endorsed by the publisher.
References
Bhadja, P., Tan, C. Y., Ouyang, J. M., and Yu, K. (2016). Repair Effect of Seaweed Polysaccharides with Different Contents of Sulfate Group and Molecular Weights on Damaged HK-2 Cells. Polymers (Basel). 8:188. doi:10.3390/polym8050188
Chao, Q., Gao, Z. F., Zhang, D., Zhao, B. G., Dong, F. Q., Fu, C. X., et al. (2019). The Developmental Dynamics of the Populus Stem Transcriptome. Plant Biotechnol. J. 17:206–219. doi:10.1111/pbi.12958
Chen, R., Mias, G. I., Li-Pook-Than, J., Jiang, L., Lam, H. Y., Chen, R., et al. (2012). Personal Omics Profiling Reveals Dynamic Molecular and Medical Phenotypes. Cell. 148:1293–1307. doi:10.1016/j.cell.2012.02.009
Chen, X., Xu, X., Zhang, L., and Zeng, F. (2009). Chain Conformation and Anti-tumor Activities of Phosphorylated (1→3)-β-D-Glucan from Poria Cocos. Carbohydr. Polym. 78:581–587. doi:10.1016/j.carbpol.2009.05.019
Dai, Y. J., Jia, Y. F., Chen, N., Bian, W. P., Li, Q. K., Ma, Y. B., et al. (2014). Zebrafish as a Model System to Study Toxicology. Environ. Toxicol. Chem. 33:11–17. doi:10.1002/etc.2406
Di, W., Zhang, L., Wang, S., Yi, H., Han, X., Fan, R., et al. (2017). Physicochemical Characterization and Antitumour Activity of Exopolysaccharides Produced by Lactobacillus Casei SB27 from Yak Milk. Carbohydr. Polym. 171:307–315. doi:10.1016/j.carbpol.2017.03.018
Feregrino-Pérez, A. A., Berumen, L. C., García-Alcocer, G., Guevara-Gonzalez, R. G., Ramos-Gomez, M., Reynoso-Camacho, R., et al. (2008). Composition and Chemopreventive Effect of Polysaccharides from Common Beans (Phaseolus vulgaris L.) on Azoxymethane-Induced colon Cancer. J. Agric. Food Chem. 56:8737–8744. doi:10.1021/jf8007162
Gunnarsson, L., Jauhiainen, A., Kristiansson, E., Nerman, O., and Larsson, D. G. (2008). Evolutionary Conservation of Human Drug Targets in Organisms Used for Environmental Risk Assessments. Environ. Sci. Technol. 42, 5807–5813. doi:10.1021/es8005173
Hamilton, L., Astell, K. R., Velikova, G., and Sieger, D. (2016). A Zebrafish Live Imaging Model Reveals Differential Responses of Microglia toward Glioblastoma Cells In Vivo. Zebrafish. 13:523–534. doi:10.1089/zeb.2016.1339
Hao, S., Li, S., Wang, J., Zhao, L., Yan, Y., Cao, Q., et al. (2018). Transcriptome Analysis of Phycocyanin-Mediated Inhibitory Functions on Non-small Cell Lung Cancer A549 Cell Growth. Mar. Drugs. 16:511. doi:10.3390/md16120511
Howe, K., Clark, M. D., Torroja, C. F., Torrance, J., Berthelot, C., Muffato, M., et al. 2013). The Zebrafish Reference Genome Sequence and its Relationship to the Human Genome. Nature. 496:498–503. doi:10.1038/nature12111
Huang, K., Li, Y., Tao, S., Wei, G., Huang, Y., Chen, D., et al. (2016). Purification, Characterization and Biological Activity of Polysaccharides from Dendrobium officinale. Molecules. 21:701. doi:10.3390/molecules21060701
Huang, S., Chen, F., Cheng, H., and Huang, G. (2020). Modification and Application of Polysaccharide from Traditional Chinese Medicine Such as Dendrobium officinale. Int. J. Biol. Macromol. 157:385–393. doi:10.1016/j.ijbiomac.2020.04.141
Jeff, I. B., Yuan, X., Sun, L., Kassim, R. M., Foday, A. D., and Zhou, Y. (2013). Purification and In Vitro Anti-proliferative Effect of Novel Neutral Polysaccharides from Lentinus Edodes. Int. J. Biol. Macromol. 52:99–106. doi:10.1016/j.ijbiomac.2012.10.007
Kang, Y., Li, H., Wu, J., Xu, X., Sun, X., Zhao, X., et al. (2016). Transcriptome Profiling Reveals the Antitumor Mechanism of Polysaccharide from Marine Algae Gracilariopsis lemaneiformis. Plos One. 11:e0158279. doi:10.1371/journal.pone.0158279
Kim, J. Y., Kim, H. H., and Cho, K. H. (2013). Acute Cardiovascular Toxicity of Sterilizers, PHMG, and PGH: Severe Inflammation in Human Cells and Heart Failure in Zebrafish. Cardiovasc. Toxicol. 13:148–160. doi:10.1007/s12012-012-9193-8
Kimmel, C. B., Ballard, W. W., Kimmel, S. R., Ullmann, B., and Schilling, T. F. (1995). Stages of Embryonic Development of the Zebrafish. Dev. Dyn. 203:253–310. doi:10.1002/aja.1002030302
Kuang, M. T., Li, J. Y., Yang, X. B., Yang, L., Xu, J. Y., Yan, S., et al. (2020). Structural Characterization and Hypoglycemic Effect via Stimulating Glucagon-like Peptide-1 Secretion of Two Polysaccharides from Dendrobium officinale. Carbohydr. Polym. 241: 116326. doi:10.1016/j.carbpol.2020.116326
Li, M., Wang, I. X., Li, Y., Bruzel, A., Richards, A. L., Toung, J. M., et al. (2011). Widespread RNA and DNA Sequence Differences in the Human Transcriptome. Science. 333:53–58. doi:10.1126/science.1207018
Liang, J., Li, H., Chen, J., He, L., Du, X., Zhou, L., et al. (2019). Dendrobium Officinale Polysaccharides Alleviate colon Tumorigenesis via Restoring Intestinal Barrier Function and Enhancing Anti-tumor Immune Response. Pharmacol. Res. 148:104417. doi:10.1016/j.phrs.2019.104417
Luo, Q. L., Tang, Z. H., Zhang, X. F., Zhong, Y. H., Yao, S. Z., Wang, L. S., et al. (2016). Chemical Properties and Antioxidant Activity of a Water-Soluble Polysaccharide from Dendrobium officinale. Int. J. Biol. Macromol. 89:219–227. doi:10.1016/j.ijbiomac.2016.04.067
Mao, X., Cai, T., Olyarchuk, J. G., and Wei, L. (2005). Automated Genome Annotation and Pathway Identification Using the KEGG Orthology (KO) as a Controlled Vocabulary. Bioinformatics. 21:3787–3793. doi:10.1093/bioinformatics/bti430
Ng, T. B., Liu, J., Wong, J. H., Ye, X., Wing Sze, S. C., Tong, Y., et al. (2012). Review of Research on Dendrobium, a Prized Folk Medicine. Appl. Microbiol. Biotechnol. 93:1795–1803. doi:10.1007/s00253-011-3829-7
Qi, W., Zhou, X., Wang, J., Zhang, K., Zhou, Y., Chen, S., et al. (2020). Cordyceps Sinensis Polysaccharide Inhibits colon Cancer Cells Growth by Inducing Apoptosis and Autophagy Flux Blockage via mTOR Signaling. Carbohydr. Polym. 237:116113. doi:10.1016/j.carbpol.2020.116113
Ren, D., Wang, N., Guo, J., Yuan, L., and Yang, X. (2016). Chemical Characterization of Pleurotus Eryngii Polysaccharide and its Tumor-Inhibitory Effects against Human Hepatoblastoma HepG-2 Cells. Carbohydr. Polym. 138:123–133. doi:10.1016/j.carbpol.2015.11.051
Ren, F., Li, J., Yuan, X., Wang, Y., Wu, K., Kang, L., et al. (2019). Dandelion Polysaccharides Exert Anticancer Effect on Hepatocellular Carcinoma by Inhibiting PI3K/AKT/mTOR Pathway and Enhancing Immune Response. J. Funct. Foods. 55:263–274. doi:10.1016/j.jff.2019.02.034
Rieu, I., and Powers, S. J. (2009). Real-time Quantitative RT-PCR: Design, Calculations, and Statistics. Plant Cell. 21:1031–1033. doi:10.1105/tpc.109.066001
Robinson, M. D., McCarthy, D. J., and Smyth, G. K. (2010). edgeR: a Bioconductor Package for Differential Expression Analysis of Digital Gene Expression Data. Bioinformatics. 26:139–140. doi:10.1093/bioinformatics/btp616
Sun, M., Bu, R., Zhang, B., Cao, Y., Liu, C., and Zhao, W. (2020). Lentinan Inhibits Tumor Progression by Immunomodulation in a Mouse Model of Bladder Cancer. Integr. Cancer Ther. 19, 1–7. doi:10.1177/1534735420946823
Tao, S., Huang, C., Tan, Z., Duan, S., Zhang, X., Ren, Z., et al. (2021). Effect of the Polysaccharides Derived from Dendrobium Officinale Stems on Human HT-29 Colorectal Cancer Cells and a Zebrafish Model. Food Biosci. 41:100995. doi:10.1016/j.fbio.2021.100995
Tao, S., Lei, Z., Huang, K., Li, Y., Ren, Z., Zhang, X., et al. (2019). Structural characterization and immunomodulatory activity of two novel polysaccharides derived from the stem of Dendrobium officinale Kimura et Migo. J. Funct. Foods. 57:121–134. doi:10.1016/j.jff.2019.04.013
Wang, E. T., Sandberg, R., Luo, S., Khrebtukova, I., Zhang, L., Mayr, C., et al. (2008). Alternative Isoform Regulation in Human Tissue Transcriptomes. Nature. 456:470–476. doi:10.1038/nature07509
Wang, K.-p., Wang, J., Li, Q., Zhang, Q.-l., You, R.-x., Cheng, Y., et al. (2014). Structural Differences and Conformational Characterization of Five Bioactive Polysaccharides from Lentinus edodes. Food Res. Int. 62:223–232. doi:10.1016/j.foodres.2014.02.047
Wang, K., Wang, H., Liu, Y., Shui, W., Wang, J., Cao, P., et al. (2018). Dendrobium Officinale Polysaccharide Attenuates Type 2 Diabetes Mellitus via the Regulation of PI3K/Akt-Mediated Glycogen Synthesis and Glucose Metabolism. J. Funct. Foods. 40:261–271. doi:10.1016/j.jff.2017.11.004
Wang, Q., Miao, Y., Xu, Y., Meng, X., Cui, W., Wang, Y., et al. (2019). Taishan Pinus Massoniana Pollen Polysaccharide Inhibits the Replication of Acute Tumorigenic ALV-J and its Associated Tumor Growth. Vet. Microbiol. 236:108376. doi:10.1016/j.vetmic.2019.07.028
Wasser, S. P. (2002). Medicinal Mushrooms as a Source of Antitumor and Immunomodulating Polysaccharides. Appl. Microbiol. Biotechnol. 60:258–274. doi:10.1007/s00253-002-1076-7
Wei, W., Li, Z. P., Zhu, T., Fung, H. Y., Wong, T. L., Wen, X., et al. (2017). Anti-Fatigue Effects of the Unique Polysaccharide Marker of Dendrobium Officinale on BALB/c Mice. Molecules. 22:155. doi:10.3390/molecules22010155
Xu, Z., Hu, C., Chen, S., Zhang, C., Yu, J., Wang, X., et al. (2019). Apatinib Enhances Chemosensitivity of Gastric Cancer to Paclitaxel and 5-fluorouracil. Cancer Manag. Res. 11:4905–4915. doi:10.2147/CMAR.S196372
Xu, Z., Li, X., Feng, S., Liu, J., Zhou, L., Yuan, M., et al. (2016). Characteristics and Bioactivities of Different Molecular Weight Polysaccharides from Camellia Seed Cake. Int. J. Biol. Macromol. 91:1025–1032. doi:10.1016/j.ijbiomac.2016.06.067
Young, M. D., Wakefield, M. J., Smyth, G. K., and Oshlack, A. (2010). Gene Ontology Analysis for RNA-Seq: Accounting for Selection Bias. Genome Biol. 11:R14. doi:10.1186/gb-2010-11-2-r14
Yu, W., Ren, Z., Zhang, X., Xing, S., Tao, S., Liu, C., et al. (2018a). Structural Characterization of Polysaccharides from Dendrobium Officinale and Their Effects on Apoptosis of HeLa Cell Line. Molecules. 23:2484. doi:10.3390/molecules23102484
Yu, X., Zhou, C., Yang, H., Huang, X., Ma, H., Qin, X., et al. (2015). Effect of Ultrasonic Treatment on the Degradation and Inhibition Cancer Cell Lines of Polysaccharides from Porphyra yezoensis. Carbohydr. Polym. 117:650–656. doi:10.1016/j.carbpol.2014.09.086
Yu, Y., Shen, M., Song, Q., and Xie, J. (2018b). Biological Activities and Pharmaceutical Applications of Polysaccharide from Natural Resources: A Review. Carbohydr. Polym. 183:91–101. doi:10.1016/j.carbpol.2017.12.009
Zhang, X., Luo, Y., Wei, G., Li, Y., Huang, Y., Huang, J., et al. (2019). Physicochemical and Antioxidant Properties of the Degradations of Polysaccharides from Dendrobium Officinale and Their Suitable Molecular Weight Range on Inducing HeLa Cell Apoptosis. Evid. Based Complement. Alternat. Med. 2019, 1–11. doi:10.1155/2019/4127360
Zhang, X., Qi, C., Guo, Y., Zhou, W., and Zhang, Y. (2016). Toll-like Receptor 4-related Immunostimulatory Polysaccharides: Primary Structure, Activity Relationships, and Possible Interaction Models. Carbohydr. Polym. 149:186–206. doi:10.1016/j.carbpol.2016.04.097
Zhang, X., Ruan, Z., You, X., Wang, J., Chen, J., Peng, C., et al. (2017). De Novo assembly and Comparative Transcriptome Analysis of the Foot from Chinese green Mussel (Perna Viridis) in Response to Cadmium Stimulation. Plos One. 12:e0176677. doi:10.1371/journal.pone.0176677
Zhang, X., Duan, S., Tao, S., Huang, J., Liu, C., Xing, S., et al. (2020). Polysaccharides from Dendrobium Officinale Inhibit Proliferation of Osteosarcoma Cells and Enhance Cisplatin-Induced Apoptosis. J. Funct. Foods. 73:104143. doi:10.1016/j.jff.2020.104143
Zhang, Y., Li, S., Wang, X., Zhang, L., and Cheung, P. C. K. (2011). Advances in Lentinan: Isolation, Structure, Chain Conformation and Bioactivities. Food Hydrocolloids. 25:196–206. doi:10.1016/j.foodhyd.2010.02.001
Zhou, L., Liu, Z., Wang, Z., Yu, S., Long, T., Zhou, X., et al. (2017). Astragalus Polysaccharides Exerts Immunomodulatory Effects via TLR4-Mediated MyD88-dependent Signaling Pathway In Vitro and In Vivo. Sci. Rep. 7:44822. doi:10.1038/srep44822
Glossary
APS Astragalus membranaceus polysaccharides
CRC Colorectal cancer
DEGs Differentially expressed genes
D. officinale Dendrobium officinale Kimura & Migo
DOPW-1 Dendrobium officinale Kimura & Migo polysaccharide
FPKM Fragments per kilobase of transcript per million mapped
GO Gene Ontology
KOG Clusters of protein homology
KEGG Kyoto Encyclopedia of Genes and Genomes
LNT Lentinus edodes polysaccharides
MTC maximum tolerance concentration
Mw molecular weight
RNA-seq RNA sequencing
Keywords: Dendrobium officinale Kimura & Migo, polysaccharide, molecular weight, colorectal cancer, Astragalus membranaceus polysaccharides, Lentinus edodes polysaccharides, RNA sequencing
Citation: Tao S, Ren Z, Yang Z, Duan S, Wan Z, Huang J, Liu C and Wei G (2021) Effects of Different Molecular Weight Polysaccharides From Dendrobium officinale Kimura & Migo on Human Colorectal Cancer and Transcriptome Analysis of Differentially Expressed Genes. Front. Pharmacol. 12:704486. doi: 10.3389/fphar.2021.704486
Received: 03 May 2021; Accepted: 27 October 2021;
Published: 03 December 2021.
Edited by:
Sp Li, University of Macau, ChinaReviewed by:
Prashant Kaushik, University of Valencia, SpainXiuping Chen, University of Macau, China
Copyright © 2021 Tao, Ren, Yang, Duan, Wan, Huang, Liu and Wei. This is an open-access article distributed under the terms of the Creative Commons Attribution License (CC BY). The use, distribution or reproduction in other forums is permitted, provided the original author(s) and the copyright owner(s) are credited and that the original publication in this journal is cited, in accordance with accepted academic practice. No use, distribution or reproduction is permitted which does not comply with these terms.
*Correspondence: Gang Wei, weigang021@outlook.com