- 1EA 6309 - Myelin Maintenance and Peripheral Neuropathy, Faculties of Medicine and Pharmacy, University of Limoges, Limoges, France
- 2Department of Neurology, Reference Center for Rare Peripheral Neuropathies, University Hospital of Limoges, Limoges, France
- 3Neuro-Dol, UMR1107 INSERM, University of Clermont Auvergne, CHU Clermont-Ferrand, Clermont-Ferrand, France
Oxaliplatin is a key drug for colorectal cancer that causes OXP-induced peripheral neuropathy, a dose-limiting effect characterized by cold and tactile hyperesthesia. The relationship between the sensory nervous system and modulation of the renin-angiotensin system has been described, focusing on pain and neurodegeneration in several animal models. We assessed the effect of the RAS modulator, ramipril, an angiotensin converting-enzyme inhibitor in a mouse model of OXP-induced acute pain syndrome. OXP was administered in Swiss mice at a cumulative dose of 15 mg/kg (3 x 5 mg/kg/3 days, i.p.). RAM was administered i.p. every day from 24 h before the first OXP injection until the end of the experiments. We evaluated OIAS development and treatment effects by sensorimotor tests, intraepidermal nerve fiber and dorsal root ganglia-neuron immunohistochemical analyses, and sciatic nerve ultrastructural analysis. OXP-treated mice showed tactile allodynia and cold hypersensitivity, without motor impairment and evidence of nerve degeneration. RAM prevented cold sensitivity and improved recovery of normal tactile sensitivity in OXP-treated mice. Our finding that RAM alleviates OXP-induced pain is a step towards evaluating its therapeutic potential in patients receiving OXP treatment.
Introduction
Oxaliplatin, a platinum-based agent mostly used to treat colorectal cancer, frequently induces peripheral neuropathy, decreasing patients’ quality of life (Selvy et al., 2020). Development of oxaliplatin-induced peripheral neuropathy often leads to lower doses or disrupt treatment, thus limiting treatment efficacy and decreasing survival rate (Gewandter et al., 2017). OXP induces chronic cumulative sensory neuropathy (Pachman et al., 2015), probably caused by an accumulation of platinum in the dorsal root ganglia (Sprowl et al., 2013). This is often preceded by a specific acute syndrome, appearing during or within hours after each infusion (Pasetto et al., 2006), mainly consisting of numbness, paresthesia, dysesthesia, and pain, with cold allodynia (Pachman et al., 2015). Although not all patients with an OXP-induced acute syndrome will develop a long term disabling chronic sensory neuropathy, this OIAS probably represents the first step towards long term neurodegeneration (Park et al., 2009). Therefore, examining how different drugs could counteract these acute symptoms might be meaningful to develop strategies to prevent chronic OIPN. Because no treatment can be recommended for the prevention of chemotherapy-induced peripheral neuropathies and only duloxetine is moderately recommended for its treatment (Jordan et al., 2020; Loprinzi et al., 2020), preventing and limiting its progression by an appropriate therapeutic approach is a key priority. The renin angiotensin system is known for its involvement in blood pressure regulation and ion homeostasis (Rettig et al., 1986). Briefly, angiotensin-converting enzyme converts angiotensin I to angiotensin II, which mainly interacts with Ang II type 1 receptor and Ang II type 2 receptor. Most of the RAS components are known to be expressed into the sensory nervous system, tending to demonstrate the presence of a local RAS in the peripheral nervous system (Bessaguet et al., 2016). Various preclinical studies have highlighted the involvement of RAS modulation by the use of ACE inhibitors or angiotensin receptor blockers, in neuroprotection and pain control (Gallinat et al., 1998; Lucius et al., 1998; Patil et al., 2010; Pavel et al., 2013; Kaur et al., 2015; Yuksel et al., 2015; Bessaguet et al., 2017; Danigo et al., 2021). Modulation of RAS was neuroprotective in rodent models of traumatic nerve injury like chronic constriction injury and sciatic nerve transection, as well as in rodent models of diabetic neuropathy or toxic neuropathy (CIPN) (Oltman et al., 2008; Kaur et al., 2015; Yuksel et al., 2015; Bessaguet et al., 2017; Kim et al., 2019). Previously, we and others showed that ARBs were able to restore normal sensitivity in models of vincristine- and paclitaxel-associated CIPN (Bessaguet et al., 2018; Kim et al., 2019). Antitumoral activity of both paclitaxel and vincristine are based on the disruption of microtubule dynamics, which may probably cause an impairment of axoplasmic transport leading to neuropathic disorders. Among the other “traditional” chemotherapies, platinum agents, as OXP, act differentially by forming platinum-DNA adduct, and therefore lead to a different neurotoxicity. Neuroprotective effects of RAS modulation on platinum compound-induced neurotoxicity have not yet been evaluated. Although, a retrospective observational study showed that patients with a long lasting ACEIs treatment seemed to be less affected by OIPN symptoms (Roldan et al., 2017; Uchida et al., 2018). Hence, we evaluated the preventive effect of ramipril in a new mouse model of OIAS. This model is characterized by functional changes without evidence of sensory nerve fiber degeneration at the peripheral nerve and DRG level.
Materials and Methods
This study was conducted in accordance with the guidelines for the ethical care of experimental animals of the European Community (2010/63/EU) and was submitted to the French Ministry of Higher Education and Research and approved (number 11280#2017091510483336). Animal experiments are reported in compliance with ARRIVE guidelines (Percie du Sert et al., 2020). All effort was made to limit suffering and the number of animals used in the following experiments.
Animals
Swiss male mice (6–7 weeks old, 25–30 g) from Janvier labs (France) were housed in plastic cages and maintained on a 12 h light/dark cycle with food and water available ad libitum (BISCEm-animal care and facility center). Mice were randomly assigned to OXP or its vehicle (control group: CTRL), thereafter mice were randomly assigned to ramipril treatment or its vehicle, defining the following four treatment groups: control-vehicle (CTRL-VEH), oxaliplatin-vehicle (OXP-VEH), control-ramipril (CTRL-RAM) and oxaliplatin-ramipril (OXP-RAM).
Behavioral tests were performed on day 1 (D1), D3, and D5 following the last OXP injection, except for the jump test, which was performed on D1 and D3 only to avoid acclimation of the mice to the test. All animals were submitted to the functional tests, except for the jump test, the day before the start of experiment (reference day: RD) to obtain the baseline for each animal. Immunohistochemistry and morphological analyses were performed one day after the last OXP injection, the time corresponding to the maximum of sensory impairment (Figure 1).
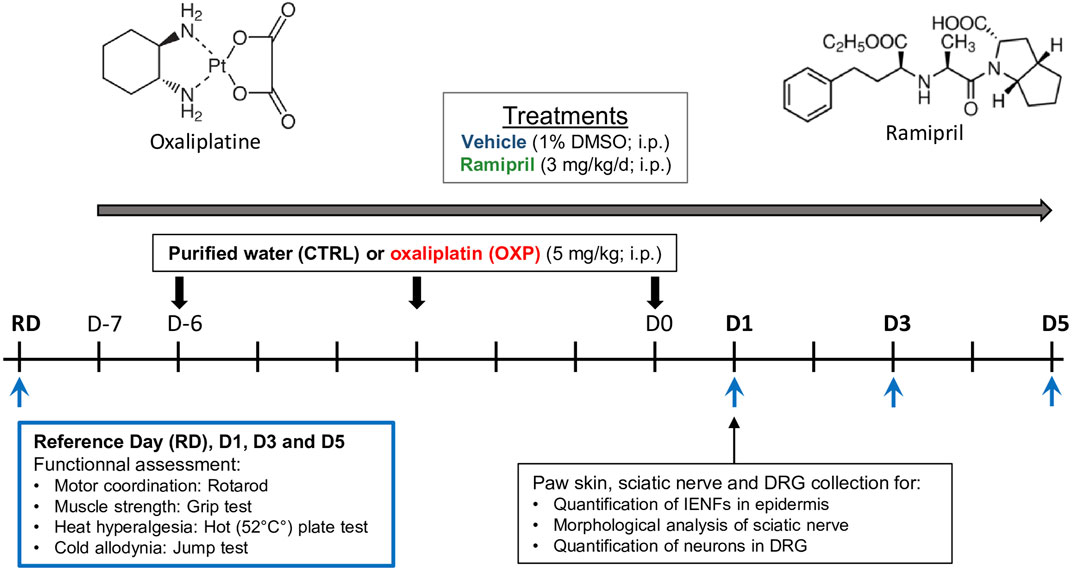
FIGURE 1. Schematic representation of the study design. CTRL, control; D, day; DMSO, diméthylsulfoxyde, DRG, dorsal root ganglion; IENFs, intraepidermal nerve fibers; i.p., intraperitoneal; RD, reference day.
Treatments
CIPN was induced by three injections of OXP (1 injection every 3 days) (5 mg/kg/3 days, intraperitoneally [i.p.]; Hospira, France). Control mice received an equivalent volume of the OXP diluent (purified water, i.p.). Treatments with ramipril (3 mg/kg/day, i.p.; Sigma, France) were started one day before the first OXP injection and administered each day for the next 10 days (Figure 1). The dose of 3 mg/kg/i.p. of ramipril in mice is equivalent to a dose of 30 mg/day per os in human, corresponding to a high dose (Alhusban et al., 2014). Although, high dose of ramipril had already shown neuroprotective effect in a rodent model of peripheral neuropathy (Kaur et al., 2015). Ramipril was diluted in a final vehicle solution of 1% dimethyl sulfoxide (DMSO) in saline solution (0.9% NaCl). VEH mice received injections of an equivalent volume of the vehicle used for ramipril. The volume of i.p injections was 100 µl/10 g of body weight.
Behavioral Tests
Motor Coordination
Motor coordination was assessed using the rotarod test (Bioseb, France) (Callizot et al., 2008). Three days before the start of the experiment (RD), mice were trained to walk against the motion of a rotating rod at a speed of 4 rpm. On the days of test, mice were placed on the rotarod and gradual 1 rpm/s acceleration was applied for 30 s, after which the speed was constant (4 rpm/s). The holding time (seconds) was recorded. The cut-off time was set on 60 s. Each test session consisted of three trials, separated by 5 min, and the mean value was calculated.
Muscle Strength
Muscle function was assessed with a grip strength meter (Bioseb, France) (Caillaud et al., 2020). Mice were held by the tail over the mesh of the meter, and once the forepaws, but neither hind paw, were both firmly grasping the grid, the mice were pulled along the axis of the force sensor until they were unable to retain their grip. The grip strength (grams) was recorded. Each test consisted of three trials, separated by 5 min, and the mean value was calculated.
Heat Hyperalgesia
Thermal nociception was assessed using the hot-plate test (Bioseb, France) (Bessaguet et al., 2017). Mice were placed on a 52°C hotplate for less than 30 s to avoid potential tissue damage. The latency (s) before first withdrawal criterion was recorded. The criteria of withdrawal included shaking, licking, or jumping on the hot plate. Mean latency was expressed as the threshold of an individual animal to thermal stimulation. Each test session consisted of three records separated by 5 min.
Tactile Allodynia
Tactile allodynia was assessed using von Frey filaments (Bioseb, France). Mice were placed in a plastic cage with a wire mesh floor which allowed access to the paws. The plastic cage was covered with an opacity cup to avoid visual stimulation. The area tested was the mid-plantar left hind paw. Mechanical threshold was tested using a modification of the simplified up-down method (Bonin et al., 2014). A test round started with filament #6 (0.40 g) and progressed to higher or lower filament value depending on the animal’s response. Each animal went through three test rounds for each paw at each experimental condition. Mechanical threshold is expressed as percentage according to baseline (%).
Cold Hypersensitivity
Thermal nociception was assessed using the jump to escape test (Descoeur et al., 2011). Noxious cold tolerance was assessed using a dynamic cold plate (Bioseb, France). Animals were placed on the test arena with the floor temperature progressively cooled from 22 to 3°C at a rate of 5°C/min. This procedure allows the paw surfaces to be cooled at the same rate as the floor arena. Nocifensive behavior (jumps) was noted as a function of cooling. Thus, we obtained a curve representing the number of jumps according to temperature for each mouse. Then, the area under the curve (AUC) was calculated for each animal and pooled in a histogram. Statistical analyses were performed on the AUC.
Immunohistochemistry of Footpad Skin and Dorsal Root Ganglia Neurons
To assess sensory innervation, animals (n = 6) were euthanized by transcardiac perfusion with phosphate buffer saline (PBS), followed by buffered 4% paraformaldehyde (PFA) solution. Then, footpads were removed by punch biopsy (diameter of 3 mm), post-fixed overnight in 4% PFA, cryoprotected (30% sucrose) and frozen at −20°C. Sections were cut on a cryostat set to 20 mm and incubated overnight at room temperature with primary antibody to protein gene product 9.5 (PGP9.5, 1:50, Abcam, Paris, France). Sections were then incubated with appropriate secondary antibody AF594-conjugated (1:500; Life Technologies, Saint-Aubin, France) during 2 h at room temperature. Epidermal nerve fibers were blindly counted under 400× magnification (Eclipse 50i, Nikon Instruments), according to established guidelines for Human (Lauria et al., 2005). The length of the dermo-epidermal junction was determined with NIS-Elements BR 2.30 software (Nikon) and was defined as the epidermal length. Epidermal nerve density was defined as the number of intact epidermal nerves crossing the dermo-epidermal junction divided by the epidermal length. Three slides per mouse were counted.
Two lumbar (L4 and L5) DRG per mouse were collected to assess the DRG neuron density by counting neuron cellular bodies visualized by PGP9.5, calcitonin gene-related peptide (CGRP), or substance P (SP) immunohistochemistry (Table 1). Each DRG section was systematically photographed at 200x under a fluorescence microscope (Eclipse 50i; Nikon Instruments, France). Immunoreactive DRG neurons were counted and the area containing neurons measured using NIS-Elements BR2.30 software (Nikon). The density of neurons positive for PGP9.5 is expressed as neurons/mm2. The density of peptidergic neurons was expressed as CGRP+ or SP+ neurons/PGP 9.5+ neurons. Three sections per DRG were counted.
Morphological Analysis of Sciatic Nerves
To assess the presence and morphology of unmyelinated nerve fibers, sciatic nerves were dissected after transcardiac perfusion with 2.5% glutaraldehyde diluted in Sorensen buffer, dehydrated, and embedded in Epon 812 resin (Euromedex, France). Semi-thin sections were stained with toluidine blue. Ultrathin sections were stained with uranyl acetate and lead citrate and observed under an electron microscope (Jeol 1011, Jeol, United States). Four images per animal (n = 3/group) were captured at 3000x magnification and the number of myelinated fibers per mm2 counted to calculate the density.
Data Analysis
Data were analyzed using GraphPad Prism 8 and expressed as the mean ± standard error of the mean (SEM). When statistical significance was identified by mixed-effects model statistical methods, individual comparisons were subsequently tested by Tukey’s multiple comparison test. Degree of significance was represented as follow: *p-value < 0.05, **p < 0.01, ***p < 0.001, ****p < 0.0001.
Results
Characterization of Oxaliplatin-Induced Acute Pain Syndrome
Short Course of Oxaliplatin Does Not Affect Motor Performance or Heat Sensitivity
The motor coordination of CTRL-VEH and OXP-VEH mice was evaluated using the rotarod test. There was no difference in the holding-time between OXP-VEH and CTRL-VEH mice on D1, D3, or D5 (Table 1). Muscular strength was assessed using the grip test. There was also no difference in the grip strength between OXP-VEH and CTRL-VEH mice on D1, D3, or D5 (Table 1). Thermal nociception, evaluated with the 52°C hotplate test, was not affected by OXP treatment on D1, D3, or D5 (Table 1).
Short Course of Oxaliplatin Does Not Affect the Morphology of Sensory Nerve Fibers
We quantified and examined morphological aspect of sensory nerve fibers at the cell body (DRG) and the terminal level (IENF). There was no significant difference in the densities of IENF (p = 0.5111, Figure 2A) and DRG neurons (p = 0.9787, Figure 3A) between OXP-VEH and CTRL-VEH mice. There was no visible morphological effect of OXP on DRG neurons under our conditions. Moreover, we quantified the SP+ and CGRP+ DRG neurons to explore the effect of OXP on neuropeptide depletion. There was no visible effect of OXP on peptidergic neuron population under our conditions (Figure 3B).
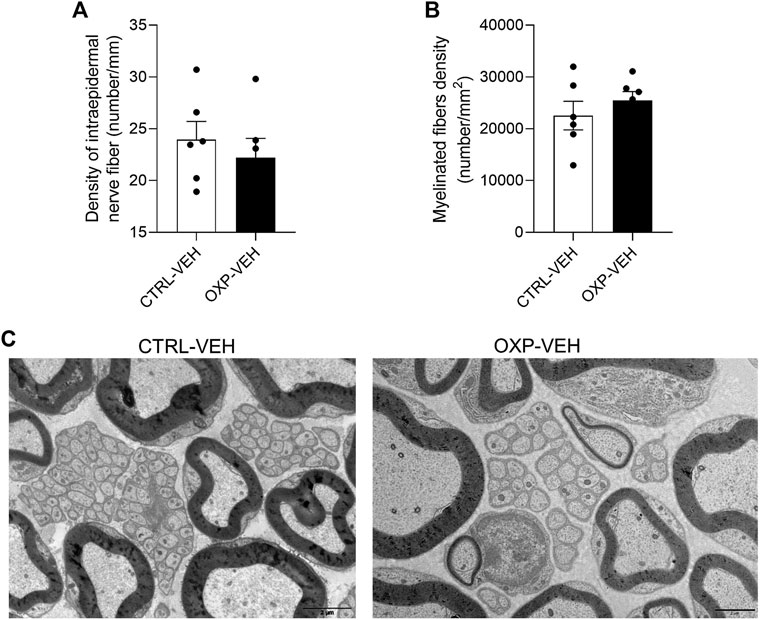
FIGURE 2. Effect of OXP on peripheral nerve fiber morphology. Footpad skin of CTRL-VEH and OXP-VEH mice were immunostained with PGP9.5 (A) Quantification of IENF positive for PGP9.5. Sciatic nerve fibers were examined by electron microscopy. (B) Quantification of myelinated fiber density. Analysis of three nerves per group and four fields of view per nerve (C) Visualization of myelinated and unmyelinated nerve fibers in the sciatic nerve. Scale bar = 2 µm n = 6 mice per group. OXP-treated mice received OXP at a cumulative dose of 15 mg/kg (5 mg/kg/3 days, intraperitoneal). CTRL: control, DRG: dorsal root ganglion, IENF: intraepidermal nerve fiber, PGP9.5: protein gene product 9.5, OXP: oxaliplatin, VEH: vehicle.
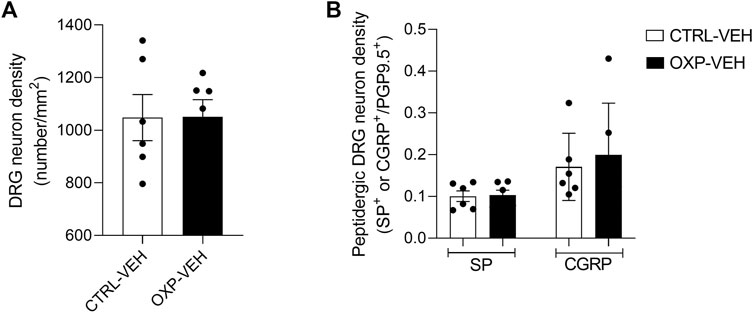
FIGURE 3. Effect of OXP on dorsal root ganglion neurons. DRG of CTRL-VEH and OXP-VEH mice were immunostained with PGP9.5 and substance P (SP) or calcitonin-gene related peptide (CGRP). (A) Quantification of PGP9.5+ total DRG neuron density. (B) The density of peptidergic neurons is expressed as SP+ or CGRP+ neurons/PGP9.5+ neurons. OXP-treated mice received OXP at a cumulative dose of 15 mg/kg (5 mg/kg/3 days, intraperitoneal). CTRL: control, DRG: dorsal root ganglion, PGP9.5: protein gene product 9.5, OXP: oxaliplatin, VEH: vehicle.
Short Course of Oxaliplatin Does Not Affect the Morphology of Sciatic Nerve Fibers
OXP did not affect the morphology nor density of myelinated nerve fibers in sciatic nerves (Figures 2B,C). There were also no noticeable changes in unmyelinated fiber morphology in OXP-VEH mice relative to CTRL-VEH mice (Figure 2C).
Short Course of Oxaliplatin Induces Significant Tactile and Cold Allodynia
There was no difference in tactile sensitivity between any groups on the reference day. OXP-VEH mice showed significant tactile allodynia compared to CTRL-VEH mice from D1 to D5 (D1: p = 0.0003, D3: p = 0.0004, D5: p = 0.0275, OXP-VEH vs. CTRL-VEH). Mechanical threshold returned to baseline 7 days after the last OXP injection (OXP-VEH vs. CTRL-VEH, p = 0.4980) (Figure 4).
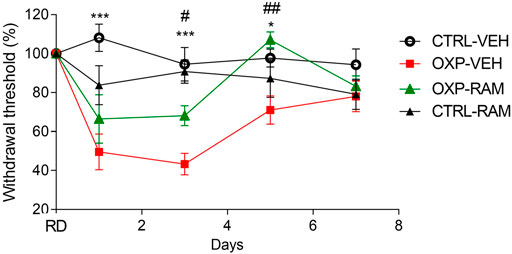
FIGURE 4. Effects of ramipril on OXP-induced mechanical allodynia. Results are compared using mixed-effects model test followed by Tukey’s multiple comparisons test (Factor treatment: F3.044, 102 = 17.79, p < 0.0001, time: F3, 36 = 15.73, p < 0.0001 and interaction: F12, 134 = 4.653, p < 0.0001). Each group represents the mean of 8–10 animals, and the error bar indicates the SEM. ***p < 0.001, *p < 0.05 OXP-VEH vs. CTRL-VEH groups. ##p < 0.01, #p < 0.05 OXP-VEH vs. OXP-RAM groups. n = 10 per group. OXP-treated mice received OXP at a cumulative dose of 15 mg/kg (5 mg/kg/3 days, intraperitoneal). RAM-treated mice received daily injection of ramipril at the dose of 3 mg/kg (intraperitoneal). OXP: oxaliplatin, RAM: ramipril, RD: reference day, VEH: vehicle.
OXP-treated mice showed cold induced allodynia on D1 (OXP-VEH vs. CTRL-VEH, p < 0.0001) and D3 (OXP-VEH vs. CTRL-VEH, p = 0.0134), as the AUC (representing the number of jumps according to temperature) of CTRL-OXP-VEH mice was higher than that of CTRL-VEH mice (Figure 5).
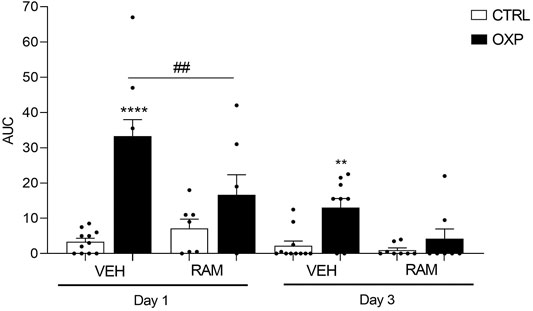
FIGURE 5. Effects of ramipril on OXP-induced cold hypersensitivity. Results are compared using mixed-effects model test followed by Tukey’s multiple comparisons test (Factor treatment: F5, 47 = 15.57, p < 0.0001, time: F1, 45 = 38.68, p < 0.0001 and interaction: F5, 45 = 4.621, p = 0.0017). Each group represents the mean of 8–10 animals, and the error bar indicates the SEM. ****p < 0.001, **p < 0.01 OXP-VEH vs. CTRL-VEH groups. ##p < 0.01 OXP-VEH vs. OXP-RAM. n = 10 per group. OXP-treated mice received OXP at a cumulative dose of 15 mg/kg (5 mg/kg/3 days, intraperitoneal). RAM-treated mice received daily injection of ramipril at the dose of 3 mg/kg (intraperitoneal). AUC: area under the curve, OXP: oxaliplatin, RAM: ramipril, VEH: vehicle.
Effect of Ramipril On Oxaliplatin-Induced Pain
Ramipril Alleviates Oxaliplatin-Induced Tactile Allodynia
Ramipril had no effect on mechanical responses of the control groups on D1 (CTRL-RAM vs. CTRL-VEH, p = 0.1527), D3 (CTRL-RAM vs. CTRL-VEH, p = 0.9318), or D5 (CTRL-RAM vs. CTRL-VEH, p = 0.6117) (Figure 3). Ramipril tended to improve tactile sensitivity in OXP-treated mice at D3 (OXP-RAM vs. OXP-VEH, p = 0.0396) and significantly restored normal tactile sensitivity at D5 (OXP-RAM vs. OXP-VEH, p = 0.0055 and vs. CTRL-VEH, p = 0.6610) (Figure 4).
Ramipril Restores Cold Sensitivity
Ramipril did not influence cold responses in the control group on D1 (CTRL-RAM vs. CTRL-VEH, p = 0.5265) and D3 (CTRL-RAM vs. CTRL-VEH, p = 0.9908) (Figure 4). Ramipril significantly improved cold sensitivity on D1 in OXP mice (OXP-RAM vs. OXP-VEH, p = 0.0014) and restored normal cold sensitivity on D3 (OXP-RAM vs. CTRL-VEH, p = 0.9953) (Figure 5).
Discussion
Our main findings were: 1) the development and characterization of a mouse OIAS model, without evidence of nerve degeneration, and 2) ramipril alleviates cold allodynia and improves mechanical sensitivity in OXP-treated mice.
Mice treated with short course of OXP did not develop abnormal motor coordination or muscular strength behaviors, suggesting that proprioceptive and motor Aα-fibers were not altered by OXP injections. This was confirmed by electron microscopy. The absence of motor deficit is consistent with observations made in other mouse strains; C57BL6J and BALB/c adult male mice (Ta et al., 2009; Andoh et al., 2011; Descoeur et al., 2011; Nassini et al., 2011; Renn et al., 2011; Zhao et al., 2012; Azevedo et al., 2013; Toyama et al., 2014; Zhao et al., 2014). The hot plate test showed no alteration of heat sensitivity, as previously shown in mouse models (Ta et al., 2009; Renn et al., 2011; Toyama et al., 2014). Immunohistochemistry analysis and sciatic nerve electron microscopic examination showed no axonal degeneration in the DRG, neuropeptide depletion, loss of IENFs, or nerve degeneration, meaning our model mimics acute neurotoxicity, without leading to long term degeneration. Previous studies on various experimental models showed that cisplatin or OXP do not induce axonal degeneration, consistent with our findings (Barajon et al., 1996; Jamieson et al., 2005). However, IENF loss has been previously described with different experimental conditions (Boyette-Davis and Dougherty, 2011; Xiao et al., 2012; Toyama et al., 2014). Nevertheless, in these studies, OXP was administered for several weeks, inducing neuropathic symptoms for a longer period than in our study. Moreover, loss of IENFs cannot explain pain in all cases, suggesting that different mechanism underpin the genesis of pain during anticancer therapy (Koskinen et al., 2011).
The relevance of our OIAS model resides in its being transient. Indeed, mice developed transient cold hypersensitivity, which consistently reproduced OXP-induced acute symptoms in humans, typically appearing with the second or third cycle of treatment during the infusion or within one or two days of OXP administration and disappearing within a few days (Staff et al., 2017). Clinically, cold-induced symptoms are the most prominent and disabling manifestations of OXP in the short term (Argyriou et al., 2008). Our OXP mice also develop transient tactile allodynia, highlighted by the von Frey test. Tactile allodynia has been previously described in patients as a symptom of OXP neurotoxicity (Binder et al., 2007) and widely described in rodent models (Hopkins et al., 2016).
Molecular basis of OIAS was not fully elucidated, although several interesting tracks have been explored in the literature. Among them, studies suggested that OXP induces a hyperexcitability of primary sensory neurons related to a dysregulation or variation of the expression of ion channels involved in mechanical and cold sensitivity (Grolleau et al., 2001; Park et al., 2009; Park et al., 2011; Kawashiri et al., 2012). More particularly, others recently reported that OIAS was associated with a downregulation of K+ channels in the DRG, following a single dose of OXP (Pereira et al., 2021). Another mechanism of OIAS is the generation of oxidative stress at the spinal level. The resulting reactive oxygen species (ROS) would induce a neuroinflammation, at the origin of hypersensitization of primary sensory neurons (Di Cesare Mannelli et al., 2012; Zhang et al., 2020; Agnes et al., 2021).
In summary, under our experimental conditions, three injections of OXP at 5 mg/kg in Swiss mice induced OIAS, characterized by transient tactile allodynia and cold hypersensitivity, associated with no impairment in motor performance or heat nociception, without overt nerve degeneration.
Ramipril, an ACE inhibitor, decreases Ang II synthesis and so AT1R/AT2R stimulation (Weber, 1999). Here we showed that ramipril improves tactile sensitivity and prevents cold sensitivity. The effect of ramipril could be mediated by the increase activity of ACE2 which converts angiotensinogen to Ang (1–7) producing antinociceptive action via Mas receptor (Forte et al., 2016; Ogata et al., 2019). Moreover, it was demonstrated that antinociceptive effect of Ang (1–7) involved K+ channels (Costa et al., 2014). Ramipril was previously shown to improve neuropathic pain in a chronic constriction-injury mouse model due to its antioxidant properties, following the decrease in Ang II levels (Kaur et al., 2015). In these study, ramipril treatment improves effects of nerve injury-induced increase in thiobarbituric acid substances (TBARS) and decrease in glutathione levels. Moreover, blockade of ACE prevents the formation of Ang II but also avoid the degradation of kinins and downregulates the bradykinin B1 receptor (Estrela et al., 2020). B1 receptor is upregulated during neuroinflammation and is involved in neuropathic pain (Ferreira et al., 2005; Cernit et al., 2020). Thus, downregulation of B1 receptor could be involved in the beneficial effect of ramipril. As oxidative stress and neuroinflammation have been shown to contribute to OIPN, they could contribute to the antinociceptive effect of ramipril.
In our study, beneficial effect of ramipril seemed to be unequal between cold and tactile pain. Tactile and cold sensitivity are transmitted by various types of fibers. As Aβ fibers participate in touch i.e. a non-painful stimulus, they are involved in the development of tactile allodynia, a non-nociceptive sensation. C and Aδ fibers are responsible for noxious and non-noxious perception of cold. Thus, the simplest explanation for such a difference in the effect of ramipril between tactile and cold allodynia is that these manifestations are linked to different fiber/DRG neuron subpopulations.
To conclude, we have shown that high dose of ramipril improves the recovery of cold sensitivity and alleviates OXP-induced tactile allodynia. Further investigation using a dose-response protocol to demonstrate whether a decrease in the dose of ramipril correlates with a decrease or not in the anti-allodynic effect in this model would be needed. As ramipril is a relatively inexpensive drug which has been demonstrated to be safe and well tolerated, our results favor clinical evaluation of the preventive therapeutic potential of ramipril in OXP-treated patients. Retrospective observational studies showed that ACEI administration in cancer patients under anticancer therapy is well tolerated (Roldan et al., 2017; Uchida et al., 2018). Moreover, the same studies showed that long lasting treatment with ACEI/ARB seems to protect sensory myelinated nerve fiber function from chemotherapy-induced neurotoxicity (Roldan et al., 2017). Thus, demonstrating the preventive effect of this drug on the development of chronic CIPN, would be the next step towards evaluation of the preventive therapeutic potential of ramipril in patients receiving OXP-based chemotherapy.
Data Availability Statement
The original contributions presented in the study are included in the article/Supplementary Material, further inquiries can be directed to the corresponding author.
Ethics Statement
The animal study was reviewed and approved by the Ethics commitee on animal experimentation n°33, Minister of Higher Education, Research and Innovation.
Author Contributions
HB: conceptualization, methodology, formal analysis, resources, investigation, writing-original draft, writing-review and editing, visualization, AD: conceptualization, formal analysis, resources, writing-original draft, writing-review and editing, visualization, funding acquisition, AB: formal analysis, FB: investigation, formal analysis, writing-review and editing, FS: supervision, DB: writing-review and editing, LM: writing-review and editing, CD: conceptualization, methodology, funding acquisition, supervision, project administration. All authors discussed the results and commented on the manuscript.
Funding
This work was supported by a grant from “Ligue Contre le Cancer 87-AAP2019” and by a grant from the region Nouvelle-Aquitaine (AAP2020). AD was supported by a grant from the CHU de Limoges (APREL (Appel à projets équipes émergentes / équipes labellisées « 2018 »), sponsored by ARS Nouvelle Aquitaine.
Conflict of Interest
The authors declare that the research was conducted in the absence of any commercial or financial relationships that could be construed as a potential conflict of interest.
Acknowledgments
The authors also thank Camille Mourgaud (University of Clermont-Ferrand, France) for technical support and Alex Edelman & associates (Malakoff, France) for improving the English of the manuscript. CD is a member of the French Neurospychopharmacology Consortium of the SFPT (“Société Française de Pharmacologie et de Thérapeutique”).
Abbreviation
CIPN, chemotherapy-induced peripheral neuropathy; OIAS, oxaliplatin-induced acute pain syndrome; OIPN, oxaliplatin-induced peripheral neuropathy; OXP, oxaliplatin; RAM, Ramipril; RAS, renin angiotensin system.
References
Agnes, J. P., Santos, V. W. d., das Neves, R. N., Gonçalves, R. M., Delgobo, M., Girardi, C. S., et al. (2021). Antioxidants Improve Oxaliplatin-Induced Peripheral Neuropathy in Tumor-Bearing Mice Model: Role of Spinal Cord Oxidative Stress and Inflammation. The J. Pain. doi:10.1016/j.jpain.2021.03.142
Alhusban, A., Al-Azayzih, A., Goc, A., Gao, F., Fagan, S. C., and Somanath, P. R. (2014). Clinically Relevant Doses of Candesartan Inhibit Growth of Prostate Tumor Xenografts In Vivo through Modulation of Tumor Angiogenesis. J. Pharmacol. Exp. Ther. 350, 635–645. doi:10.1124/jpet.114.216382
Andoh, T., Echigo, N., Kamiya, Y., Hayashi, M., Kudoh, I., and Goto, T. (2011). Effects of Erythropoietin on Intracellular Calcium Concentration of Rat Primary Cortical Neurons. Brain Res. 1387, 8–18. doi:10.1016/j.brainres.2011.02.077
Argyriou, A. A., Polychronopoulos, P., Iconomou, G., Chroni, E., and Kalofonos, H. P. (2008). A Review on Oxaliplatin-Induced Peripheral Nerve Damage. Cancer Treat. Rev. 34, 368–377. doi:10.1016/j.ctrv.2008.01.003
Azevedo, M. I., Pereira, A. F., Nogueira, R. B., Rolim, F. E., Brito, G. A., Wong, D. V. T., et al. (2013). The Antioxidant Effects of the Flavonoids Rutin and Quercetin Inhibit Oxaliplatin-Induced Chronic Painful Peripheral Neuropathy. Mol. Pain 9, 1744–8069. doi:10.1186/1744-8069-9-53
Barajon, I., Bersani, M., Quartu, M., Del Fiacco, M., Cavaletti, G., Holst, J. J., et al. (1996). Neuropeptides and Morphological Changes in Cisplatin-Induced Dorsal Root Ganglion Neuronopathy. Exp. Neurol. 138, 93–104. doi:10.1006/exnr.1996.0050
Bessaguet, F., Danigo, A., Bouchenaki, H., Duchesne, M., Magy, L., Richard, L., et al. (2018). Neuroprotective Effect of Angiotensin II Type 2 Receptor Stimulation in Vincristine-Induced Mechanical Allodynia. Pain 159, 2538–2546. doi:10.1097/j.pain.0000000000001361
Bessaguet, F., Danigo, A., Magy, L., Sturtz, F., Desmoulière, A., and Demiot, C. (2017). Candesartan Prevents Resiniferatoxin-Induced Sensory Small-Fiber Neuropathy in Mice by Promoting Angiotensin II-Mediated AT2 Receptor Stimulation. Neuropharmacology 126, 142–150. doi:10.1016/j.neuropharm.2017.08.039
Bessaguet, F., Magy, L., Desmoulière, A., and Demiot, C. (2016). The Therapeutic Potential of Renin Angiotensin Aldosterone System (RAAS) in Chronic Pain: from Preclinical Studies to Clinical Trials. Expert Rev. Neurotherapeutics 16, 331–339. doi:10.1586/14737175.2016.1150179
Binder, A., Stengel, M., Maag, R., Wasner, G., Schoch, R., Moosig, F., et al. (2007). Pain in Oxaliplatin-Induced Neuropathy - Sensitisation in the Peripheral and central Nociceptive System. Eur. J. Cancer 43, 2658–2663. doi:10.1016/j.ejca.2007.07.030
Bonin, R. P., Bories, C., and De Koninck, Y. (2014). A Simplified Up-Down Method (SUDO) for Measuring Mechanical Nociception in Rodents Using von Frey Filaments. Mol. Pain 10, 1744–8069. doi:10.1186/1744-8069-10-26
Boyette-Davis, J., and Dougherty, P. M. (2011). Protection against Oxaliplatin-Induced Mechanical Hyperalgesia and Intraepidermal Nerve Fiber Loss by Minocycline. Exp. Neurol. 229, 353–357. doi:10.1016/j.expneurol.2011.02.019
Caillaud, M., Msheik, Z., Ndong-Ntoutoume, G. M.-A., Vignaud, L., Richard, L., Favreau, F., et al. (2020). Curcumin-cyclodextrin/cellulose Nanocrystals Improve the Phenotype of Charcot-Marie-Tooth-1a Transgenic Rats through the Reduction of Oxidative Stress. Free Radic. Biol. Med. 161, 246–262. doi:10.1016/j.freeradbiomed.2020.09.019
Callizot, N., Andriambeloson, E., Glass, J., Revel, M., Ferro, P., Cirillo, R., et al. (2008). Interleukin-6 Protects against Paclitaxel, Cisplatin and Vincristine-Induced Neuropathies without Impairing Chemotherapeutic Activity. Cancer Chemother. Pharmacol. 62, 995–1007. doi:10.1007/s00280-008-0689-7
Cernit, V., Sénécal, J., Othman, R., and Couture, R. (2020). Reciprocal Regulatory Interaction between TRPV1 and Kinin B1 Receptor in a Rat Neuropathic Pain Model. Ijms 21, 821. doi:10.3390/ijms21030821
Costa, A., Galdino, G., Romero, T., Silva, G., Cortes, S., Santos, R., et al. (2014). Ang-(1-7) Activates the NO/cGMP and ATP-Sensitive K+ Channels Pathway to Induce Peripheral Antinociception in Rats. Nitric Oxide 37, 11–16. doi:10.1016/j.niox.2013.12.007
Danigo, A., Rovini, A., Bessaguet, F., Bouchenaki, H., Bernard, A., Sturtz, F., et al. (2021). The Angiotensin II Type 2 Receptor, a Target for Protection and Regeneration of the Peripheral Nervous System? Pharmaceuticals 14, 175. doi:10.3390/ph14030175
Descoeur, J., Pereira, V., Pizzoccaro, A., Francois, A., Ling, B., Maffre, V., et al. (2011). Oxaliplatin‐induced Cold Hypersensitivity Is Due to Remodelling of Ion Channel Expression in Nociceptors. EMBO Mol. Med. 3, 266–278. doi:10.1002/emmm.201100134
Di Cesare Mannelli, L., Zanardelli, M., Failli, P., and Ghelardini, C. (2012). Oxaliplatin-Induced Neuropathy: Oxidative Stress as Pathological Mechanism. Protective Effect of Silibinin. J. Pain 13, 276–284. doi:10.1016/j.jpain.2011.11.009
Estrela, G. R., Wasinski, F., Gregnani, M. F., Freitas-Lima, L. C., Arruda, A. C., Morais, R. L., et al. (2020). Angiotensin-Converting Enzyme Inhibitor Protects against Cisplatin Nephrotoxicity by Modulating Kinin B1 Receptor Expression and Aminopeptidase P Activity in Mice. Front. Mol. Biosci. 7, 96. doi:10.3389/fmolb.2020.00096
Ferreira, J., Beirith, A., Mori, M. A. S., Araújo, R. C., Bader, M., Pesquero, J. B., et al. (2005). Reduced Nerve Injury-Induced Neuropathic Pain in Kinin B1 Receptor Knock-Out Mice. J. Neurosci. 25, 2405–2412. doi:10.1523/JNEUROSCI.2466-04.2005
Forte, B. L., Slosky, L. M., Zhang, H., Arnold, M. R., Staatz, W. D., Hay, M., et al. (2016). Angiotensin-(1-7)/Mas Receptor as an Antinociceptive Agent in Cancer-Induced Bone Pain. Pain 157, 2709–2721. doi:10.1097/j.pain.0000000000000690
Gallinat, S., Yu, M., Dorst, A., Unger, T., and Herdegen, T. (1998). Sciatic Nerve Transection Evokes Lasting Up-Regulation of Angiotensin AT2 and AT1 Receptor mRNA in Adult Rat Dorsal Root Ganglia and Sciatic Nerves. Mol. Brain Res. 57, 111–122. doi:10.1016/s0169-328x(98)00079-5
Gewandter, J. S., Freeman, R., Kitt, R. A., Cavaletti, G., Gauthier, L. R., McDermott, M. P., et al. (2017). Chemotherapy-induced Peripheral Neuropathy Clinical Trials. Neurology 89, 859–869. doi:10.1212/WNL.0000000000004272
Grolleau, F., Gamelin, L., Boisdron-Celle, M., Lapied, B., Pelhate, M., and Gamelin, E. (2001). A Possible Explanation for a Neurotoxic Effect of the Anticancer Agent Oxaliplatin on Neuronal Voltage-Gated Sodium Channels. J. Neurophysiol. 85, 2293–2297. doi:10.1152/jn.2001.85.5.2293
Hopkins, H. L., Duggett, N. A., and Flatters, S. J. L. (2016). Chemotherapy-induced Painful Neuropathy. Curr. Opin. Support. Palliat. Care 10, 119–128. doi:10.1097/SPC.0000000000000204
Jamieson, S., Liu, J., Connor, B., and McKeage, M. (2005). Oxaliplatin Causes Selective Atrophy of a Subpopulation of Dorsal Root Ganglion Neurons without Inducing Cell Loss. Cancer Chemother. Pharmacol. 56, 391–399. doi:10.1007/s00280-004-0953-4
Jordan, B., Margulies, A., Cardoso, F., Cavaletti, G., Haugnes, H. S., Jahn, P., et al. (2020). Systemic Anticancer Therapy-Induced Peripheral and central Neurotoxicity: ESMO-EONS-EANO Clinical Practice Guidelines for Diagnosis, Prevention, Treatment and Follow-Up. Ann. Oncol. 31, 1306–1319. doi:10.1016/j.annonc.2020.07.003
Kaur, P., Muthuraman, A., and Kaur, J. (2015). Ameliorative Potential of Angiotensin-Converting Enzyme Inhibitor (Ramipril) on Chronic Constriction Injury of Sciatic Nerve Induced Neuropathic Pain in Mice. J. Renin Angiotensin Aldosterone Syst. 16, 103–112. doi:10.1177/1470320314556171
Kawashiri, T., Egashira, N., Kurobe, K., Tsutsumi, K., Yamashita, Y., Ushio, S., et al. (2012). L Type Ca2+ Channel Blockers Prevent Oxaliplatin-Induced Cold Hyperalgesia and TRPM8 Overexpression in Rats. Mol. Pain 8, 1744–8069. doi:10.1186/1744-8069-8-7
Kim, E., Hwang, S.-H., Kim, H.-K., Abdi, S., and Kim, H. K. (2019). Losartan, an Angiotensin II Type 1 Receptor Antagonist, Alleviates Mechanical Hyperalgesia in a Rat Model of Chemotherapy-Induced Neuropathic Pain by Inhibiting Inflammatory Cytokines in the Dorsal Root Ganglia. Mol. Neurobiol. 56, 7408–7419. doi:10.1007/s12035-019-1616-0
Koskinen, M. J., Kautio, A. L., Haanpää, M. L., Haapasalo, H. K., Kellokumpu-Lehtinen, P. L., Saarto, T., et al. (2011). Intraepidermal Nerve Fibre Density in Cancer Patients Receiving Adjuvant Chemotherapy. Anticancer Res. 31, 4413–4416.
Lauria, G., Cornblath, D. R., Johansson, O., McArthur, J. C., Mellgren, S. I., Nolano, M., et al. (2005). EFNS Guidelines on the Use of Skin Biopsy in the Diagnosis of Peripheral Neuropathy. Eur. J. Neurol. 12, 747–758. doi:10.1111/j.1468-1331.2005.01260.x
Loprinzi, C. L., Lacchetti, C., Bleeker, J., Cavaletti, G., Chauhan, C., Hertz, D. L., et al. (2020). Prevention and Management of Chemotherapy-Induced Peripheral Neuropathy in Survivors of Adult Cancers: ASCO Guideline Update. Jco 38, 3325–3348. doi:10.1200/JCO.20.01399
Lucius, R., Gallinat, S., Rosenstiel, P., Herdegen, T., Sievers, J., and Unger, T. (1998). The Angiotensin II Type 2 (AT2) Receptor Promotes Axonal Regeneration in the Optic Nerve of Adult Rats. J. Exp. Med. 188, 661–670. doi:10.1084/jem.188.4.661
Nassini, R., Gees, M., Harrison, S., De Siena, G., Materazzi, S., Moretto, N., et al. (2011). Oxaliplatin Elicits Mechanical and Cold Allodynia in Rodents via TRPA1 Receptor Stimulation. Pain 152, 1621–1631. doi:10.1016/j.pain.2011.02.051
Ogata, Y., Nemoto, W., Yamagata, R., Nakagawasai, O., Shimoyama, S., Furukawa, T., et al. (2019). Anti‐hypersensitive Effect of Angiotensin (1‐7) on Streptozotocin‐induced Diabetic Neuropathic Pain in Mice. Eur. J. Pain 23, 739–749. doi:10.1002/ejp.1341
Oltman, C. L., Davidson, E. P., Coppey, L. J., Kleinschmidt, T. L., Lund, D. D., and Yorek, M. A. (2008). Attenuation of Vascular/Neural Dysfunction in Zucker Rats Treated with Enalapril or Rosuvastatin. Obesity 16, 82–89. doi:10.1038/oby.2007.19
Pachman, D. R., Qin, R., Seisler, D. K., Smith, E. M. L., Beutler, A. S., Ta, L. E., et al. (2015). Clinical Course of Oxaliplatin-Induced Neuropathy: Results from the Randomized Phase III Trial N08CB (Alliance). Jco 33, 3416–3422. doi:10.1200/JCO.2014.58.8533
Park, S. B., Goldstein, D., Lin, C. S.-Y., Krishnan, A. V., Friedlander, M. L., and Kiernan, M. C. (2009). Acute Abnormalities of Sensory Nerve Function Associated with Oxaliplatin-Induced Neurotoxicity. Jco 27, 1243–1249. doi:10.1200/JCO.2008.19.3425
Park, S. B., Lin, C. S.-Y., Krishnan, A. V., Goldstein, D., Friedlander, M. L., and Kiernan, M. C. (2011). Dose Effects of Oxaliplatin on Persistent and Transient Na+ Conductances and the Development of Neurotoxicity. PLoS One 6, e18469. doi:10.1371/journal.pone.0018469
Pasetto, L. M., D’Andrea, M. R., Rossi, E., and Monfardini, S. (2006). Oxaliplatin-related Neurotoxicity: How and Why? Crit. Rev. Oncology/Hematology 59, 159–168. doi:10.1016/j.critrevonc.2006.01.001
Patil, J., Schwab, A., Nussberger, J., Schaffner, T., Saavedra, J. M., and Imboden, H. (2010). Intraneuronal Angiotensinergic System in Rat and Human Dorsal Root Ganglia. Regul. Peptides 162, 90–98. doi:10.1016/j.regpep.2010.03.004
Pavel, J., Oroszova, Z., Hricova, L., and Lukacova, N. (2013). Effect of Subpressor Dose of Angiotensin II on Pain-Related Behavior in Relation with Neuronal Injury and Activation of Satellite Glial Cells in the Rat Dorsal Root Ganglia. Cell. Mol. Neurobiol. 33, 681–688. doi:10.1007/s10571-013-9934-7
Percie du Sert, N., Ahluwalia, A., Alam, S., Avey, M. T., Baker, M., Browne, W. J., et al. (2020). Reporting Animal Research: Explanation and Elaboration for the ARRIVE Guidelines 2.0. Plos Biol. 18, e3000411. doi:10.1371/journal.pbio.3000411
Pereira, V., Lamoine, S., Cuménal, M., Lolignier, S., Aissouni, Y., Pizzoccaro, A., et al. (2021). Epigenetics Involvement in Oxaliplatin-Induced Potassium Channel Transcriptional Downregulation and Hypersensitivity. Mol. Neurobiol. doi:10.1007/s12035-021-02361-6
Renn, C. L., Carozzi, V. A., Rhee, P., Gallop, D., Dorsey, S. G., and Cavaletti, G. (2011). Multimodal Assessment of Painful Peripheral Neuropathy Induced by Chronic Oxaliplatin-Based Chemotherapy in Mice. Mol. Pain 7, 1744–8069. doi:10.1186/1744-8069-7-29
Rettig, R., Healy, D. P., and Printz, M. P. (1986). Cardiovascular Effects of Microinjections of Angiotensin II into the Nucleus Tractus Solitarii. Brain Res. 364, 233–240. doi:10.1016/0006-8993(86)90835-8
Roldan, C. J., Song, J., Engle, M. P., and Dougherty, P. M. (2017). Angiotensin-Converting Enzyme Inhibitors and Angiotensin Receptor Blockers Modulate the Function of Myelinated Fibers after Chemotherapy: A Quantitative Sensory Testing Study. Pain Phys. 4, 281–292. doi:10.36076/ppj.2017.292
Selvy, M., Pereira, B., Kerckhove, N., Gonneau, C., Feydel, G., Pétorin, C., et al. (2020). Long-Term Prevalence of Sensory Chemotherapy-Induced Peripheral Neuropathy for 5 Years after Adjuvant FOLFOX Chemotherapy to Treat Colorectal Cancer: A Multicenter Cross-Sectional Study. Jcm 9, 2400. doi:10.3390/jcm9082400
Sprowl, J. A., Ciarimboli, G., Lancaster, C. S., Giovinazzo, H., Gibson, A. A., Du, G., et al. (2013). Oxaliplatin-induced Neurotoxicity Is Dependent on the Organic Cation Transporter OCT2. Proc. Natl. Acad. Sci. 110, 11199–11204. doi:10.1073/pnas.1305321110
Staff, N. P., Grisold, A., Grisold, W., and Windebank, A. J. (2017). Chemotherapy-induced Peripheral Neuropathy: A Current Review. Ann. Neurol. 81, 772–781. doi:10.1002/ana.24951
Ta, L. E., Low, P. A., and Windebank, A. J. (2009). Mice with Cisplatin and Oxaliplatin-Induced Painful Neuropathy Develop Distinct Early Responses to thermal Stimuli. Mol. Pain 5, 1744–8069. doi:10.1186/1744-8069-5-9
Toyama, S., Shimoyama, N., Ishida, Y., Koyasu, T., Szeto, H. H., and Shimoyama, M. (2014). Characterization of Acute and Chronic Neuropathies Induced by Oxaliplatin in Mice and Differential Effects of a Novel Mitochondria-Targeted Antioxidant on the Neuropathies. Anesthesiology 120, 459–473. doi:10.1097/01.anes.0000435634.34709.65
Uchida, M., Kawazoe, H., Takatori, S., Namba, H., Uozumi, R., Tanaka, A., et al. (2018). Preventive Effects of Renin-Angiotensin System Inhibitors on Oxaliplatin-Induced Peripheral Neuropathy: A Retrospective Observational Study. Clin. Ther. 40, 1214–1222. doi:10.1016/j.clinthera.2018.05.011
Weber, M. (1999). Interrupting the Renin-Angiotensin System: the Role of Angiotensin-Converting Enzyme Inhibitors and Angiotensin Ii Receptor Antagonists in the Treatment of Hypertension. Am. J. Hypertens. 12, 189–194. doi:10.1016/S0895-7061(99)00105-3
Xiao, W. H., Zheng, H., and Bennett, G. J. (2012). Characterization of Oxaliplatin-Induced Chronic Painful Peripheral Neuropathy in the Rat and Comparison with the Neuropathy Induced by Paclitaxel. Neuroscience 203, 194–206. doi:10.1016/j.neuroscience.2011.12.023
Yuksel, T. N., Halici, Z., Demir, R., Cakir, M., Calikoglu, C., Ozdemir, G., et al. (2015). Investigation of the Effect of Telmisartan on Experimentally Induced Peripheral Nerve Injury in Rats. Int. J. Neurosci. 125, 464–473. doi:10.3109/00207454.2014.948115
Zhang, X., Guan, Z., Wang, X., Sun, D., Wang, D., Li, Y., et al. (2020). Curcumin Alleviates Oxaliplatin-Induced Peripheral Neuropathic Pain through Inhibiting Oxidative Stress-Mediated Activation of NF-Κb and Mitigating Inflammation. Biol. Pharm. Bull. 43, 348–355. doi:10.1248/bpb.b19-00862
Zhao, M., Isami, K., Nakamura, S., Shirakawa, H., Nakagawa, T., and Kaneko, S. (2012). Acute Cold Hypersensitivity Characteristically Induced by Oxaliplatin Is Caused by the Enhanced Responsiveness of TRPA1 in Mice. Mol. Pain 8, 1744–8069. doi:10.1186/1744-8069-8-55
Keywords: oxaliplatin, ramipril, cold allodynia, mechanical allodynia, neuropathic pain
Citation: Bouchenaki H, Danigo A, Bernard A, Bessaguet F, Richard L, Sturtz F, Balayssac D, Magy L and Demiot C (2021) Ramipril Alleviates Oxaliplatin-Induced Acute Pain Syndrome in Mice. Front. Pharmacol. 12:712442. doi: 10.3389/fphar.2021.712442
Received: 20 May 2021; Accepted: 25 June 2021;
Published: 19 July 2021.
Edited by:
Robert M. Caudle, University of Florida, United StatesReviewed by:
Takao Shimazoe, Kyushu University, JapanMariana Spetea, University of Innsbruck, Austria
Copyright © 2021 Bouchenaki, Danigo, Bernard, Bessaguet, Richard, Sturtz, Balayssac, Magy and Demiot. This is an open-access article distributed under the terms of the Creative Commons Attribution License (CC BY). The use, distribution or reproduction in other forums is permitted, provided the original author(s) and the copyright owner(s) are credited and that the original publication in this journal is cited, in accordance with accepted academic practice. No use, distribution or reproduction is permitted which does not comply with these terms.
*Correspondence: Claire Demiot, Y2xhaXJlLmRlbWlvdEB1bmlsaW0uZnI=