- 1State Key Laboratory of Characteristic Chinese Medicine Resources in Southwest China, Chengdu University of Traditional Chinese Medicine, Chengdu, China
- 2School of Pharmacy, Chengdu University of Traditional Chinese Medicine, Chengdu, China
Jatrorrhizine, an isoquinoline alkaloid, is a bioactive metabolite in common medicinal plants, such as Berberis vernae Schneid., Tinospora sagittata (Oliv.) Gagnep. and Coptis chinensis Franch. These plants have been used for centuries in traditional medicine for their wide-ranging pharmacological properties. This review emphasizes the latest and comprehensive information on the sources, pharmacology, pharmacokinetics and toxicity of jatrorrhizine. Studies on this alkaloid were collected from scientific internet databases, including the Web of Science, PubMed, ScienceDirect, Google Scholar, Elsevier, Springer, Wiley Online Library and Europe PMC and CNKI, using a combination of keywords involving “jatrorrhizine”, “sources”, “pharmacology,” “pharmacokinetics,” and “toxicology”. Jatrorrhizine exhibits anti-diabetic, antimicrobial, antiprotozoal, anticancer, anti-obesity and hypolipidemic properties, along with central nervous system activities and other beneficial activity. Studies of jatrorrhizine have laid the foundation for its application to the treatment of various diseases, but some issues still exist. Further investigations might emphasize 1) specific curative mechanisms of jatrorrhizine and clinical utility, 2) application prospect in the treatment of metabolic disorders, 3) comprehensive investigations of the toxicity mechanisms and 4) interactions of jatrorrhizine with other pharmaceuticals and development of derivatives.
Introduction
Plants are sources of metabolites with varied biological activities, clinical effectiveness. The therapeutical benefits and safety of plant-derived metabolites have been proven in long-standing traditional medicinal practices across the world (Gurib-Fakim, 2006; Porras et al., 2020). The bioactive metabolites and related derivatives are increasingly used for the production of new drugs and may have broad clinical applications.
Alkaloids are important natural products derived mostly from amino acids. These chemicals display substantial physiological and pharmacological activities. Jatrorrhizine is a well-known isoquinoline alkaloid of the protoberberine type. Its molecular structure is 2,9,10-trimethoxy-5,6-dihydroisoquinolino[2,1-b]isoquinolin-7-ium-3-ol (molecular formula: C20H20NO4, Figure 1). Jatrorrhizine is a major bioactive metabolite with wide distribution across plant families. Several species have been used medicinally for centuries, such as Berberis vernae C.K.Schneid. (Li et al., 2020), Mahonia bealei (Fortune) Carrière (He and Mu, 2015), Tinospora sagittata (Oliv.) Gagnep. (Zhang et al., 2006), Coptis chinensis Franch. (Chen et al., 2017) and Corydalis yanhusuo (Y.H.Chou and Chun C. Hsu) W.T.Wang ex Z.Y.Su and C.Y.Wu (Xiao et al., 2011). One of the active constituents in herbal formulae, such as Zoujinwan, Jiaotai Pills and San-Huang decoction, is considered to be jatrorrhizine (Yan et al., 2012; Sun et al., 2018; Su et al., 2020). Modern pharmacological studies demonstrate that this alkaloid exhibits anti-diabetic, antimicrobial (Ali et al., 2013), antiprotozoal (Malebo et al., 2013), anticancer (Sun et al., 2019), anti-obesity and hypolipidemic properties (Yang et al., 2016). Central nervous system activities are also reported (Luo et al., 2011; Xiao et al., 2011; Bacq et al., 2012).
Jatrorrhizine has attracted the attention of researchers due to its wide distribution across a variety of plant species and its potential for clinical use certain diseases. We summarise and discuss, in this review, the latest and comprehensive information on the plant sources, synthesis, pharmacological effects, pharmacokinetics and toxicity of jatrorrhizine. This information will be beneficial for future examination of therapeutic potential of this active metabolite and subsequent development of clinical applications.
Sources
Plant Sources of Jatrorrhizine
Medicinal plants are major sources of jatrorrhizine. This metabolite is isolated from various plant families, such as Annonaceae, Berberidaceae, Menispermaceae, Papaveraceae, Ranunculaceae and Rutaceae (Table 1). Many species in these families are used in folk medicinal plants and Chinese herbal medicine. Several Annickia species from the Annonaceae family, are multi-purpose medicinal plants used widely for the treatment of malaria and other ailments across tropical Africa. Protoberberine alkaloids (including jatrorrhizine and palmatine) are the major anti-protozoal agents in these plants (Malebo et al., 2013; Olivier et al., 2015; Odoh et al., 2018). Numerous species of the Berberis, Mahonia, Tinospora, Corydalis, Coptis, Thalictrum and Phellodendron genera are commonly used medicinal plants and important sources of jatrorrhizine (Alamzeb et al., 2015; Bajpai et al., 2016; Du et al., 2018; Feng et al., 2018; Abdykerimova et al., 2020; Sharma et al., 2020). In China, the stems of Mahonia bealei (Fortune) Carrière and Mahonia fortunei (Lindl.) Fedde (named Mahoniae Caulis), rhizomes of Coptis chinensis Franch., Coptis deltoidea C.Y.Cheng and P.K.Hsiao and Coptis teeta Wall. (named Coptidis Rhizoma) and barks from Phellodendron amurense Rupr. (named Phellodendri amurensis Cortex) and Phellodendron chinense C.K.Schneid. (named Phellodendri Chinensis Cortex) are known for antipyretic and analgesic properties. These traditional medicines have been widely used to treat abdominal pain and diarrhea, inflammatory disorders and gastrointestinal diseases (Ryuk et al., 2012; He and Mu, 2015; Meng et al., 2018). However, the wild resources of the three Coptis species are almost endangered (Chen et al., 2017). Jatrorrhizine is also found in the Rutaceae stem barks of several Zanthoxylum species. The anti-cancer activity of these plants might be attributed to quaternary alkaloids (Tian et al., 2017).
Synthesis of Jatrorrhizine
Access to natural products with complex structures is a major challenge because of slow growth and limited production (Romanowski and Eustáquio, 2020). Chemical synthesis has thus become an effective way to obtain some plant metabolites. Total synthesis of jatrorrhizine has been achieved through an efficient syntheses strategy in four steps (Figure 2). The alkaloid was synthesized from phenethylamine and 2,2-dimethoxyacetaldehyde using the Pictet–Spengler reaction to provide tetrahydroisoquinoline. This intermediate then reductively aminated with 2,3-dimethoxybenzaldehyde to afford the tertiary amine. Friedel–Crafts cyclization and subsequent oxidation deliver isomerically pure jatrorrhizine. This synthesis of jatrorrhizine displayed a 20% overall yield (Mori-Quiroz et al., 2018).
Microbial biosynthesis might become a fast and efficient way to obtain natural products. Identification and characterization of the biosynthetic pathway of jatrorrhizine is a prerequisite for its heterologous expression and production. Isoquinoline alkaloids are an important group of specialized plant metabolites. Biosynthesis proceeds by common early steps to form (S)-reticuline (Figure 3). This pivotal intermediate is the branch-point intermediate in the biosynthesis of many isoquinoline alkaloids (He et al., 2018). Sequentially, (S)-scoulerine is formed from (S)-reticuline by berberine bridge enzyme. Pyne et al. (2020) reported a yeast platform for high-level synthesis of tetrahydroisoquinoline alkaloids, and the production of the central intermediate (S)-reticuline increased to 4.6 g/L. However, the subsequent pathway leading to production jatrorrhizine remains unknown. Hagel and Facchini proposed that 3-O-demethylation of (S)-scoulerine combined with 2-O- and 9-O-methylation might lead to jatrorrhizine (Hagel and Facchini, 2010); enzymes that might catalyse these reactions have not been identified to date. Hence, more research needed to clarify the biosynthetic pathway of jatrorrhizine.
Pharmacological Activities of Jatrorrhizine
Anti-Obesity and Hypolipidemic Activity
Obesity is a challenging health problem worldwide. Plants and their active phytochemical constituents are used as natural anti-obesity agents and dietary supplements for weight loss. Jatrorrhizine increased the expression of hepatic low-density lipoprotein receptor (LDLR) in Hep G2 cells in vitro and produced a significant reduction in cellular lipid accumulation (Zhou et al., 2014). Jatrorrhizine (46.7 mg/kg×day) was administered to high-fat and high-cholesterol (HFHC)-induced hyperlipidemic hamsters. This treatment reduced the serum total cholesterol (TC) and total triglyceride (TG), decreased the low-density lipoprotein cholesterol (LDL-C) levels, reduced protein levels of 3-hydroxy-3-methyl glutaryl coenzyme A reductase (HMGCR) and significantly increased the expression of cholesterol 7α-hydroxylase (CYP7A1) and LDLR, as well as elevated fecal excretion of cholesterol and TBA (He et al., 2016). In addition, jatrorrhizine decreased body weights of C57BL/6 mice on a HFHC diet and increased HDL-C levels (Yang et al., 2016). The anti-obesity and hypolipidemic effect of jatrorrhizine may thus be related to regulating the expression of LDLR, CYP7A1 and HMGCR, increasing lipid metabolism, and promoting excretion of TBA. All of these effects would lead to increase metabolism and excretion of cholesterol.
Jatrorrhizine ameliorated the pathophysiological changes observed in the livers of hyperlipidemic mice (e.g., swelling of hepatocytes, lipid accumulation, and so on) and caused in a significant decrease in serum aspartate transaminase (AST) and alanine aminotransferase (ALT) levels. Jatrorrhizine also downregulated the hepatic sterol regulatory element binding transcription factor 1c (SREBP-1c) and fatty acid synthase (FAS) levels and upregulated peroxisome proliferator activated receptor-α (PPAR-α) and carnitine palmitoyl transferase 1A (CPT1A) expression. Hence, jatrorrhizine may counter hyperlipidemia through inhibition of fatty acid synthesis and activation of fatty acid β oxidation (Yang et al., 2016). Obesity is a complex disorder that significantly increases the risk of multiple metabolic disorders, such cardiovascular disease and diabetes (Karri et al., 2019). The clinical use of jatrorrhizine might helpful in the management of obesity and associated disorders.
Anti-Diabetic Activity
Type 2 diabetes mellitus (T2DM), an expanding global health problem, is characterized by insulin resistance and impaired insulin secretion (DeFronzo et al., 2015). Some botanical drugs containing jatrorrhizine, such as Coptidis Rhizoma, are widely used in traditional Chinese medicine for treating diabetes (Ma et al., 2016; Meng et al., 2018).
The potential of jatrorrhizine as a hypoglycaemic agent was manifest by inhibition of α-glucosidase and aldose reductase (AR) (Patel MB. and Mishra S., 2012; Patel M. B. and Mishra S. M., 2012). Jatrorrhizine displayed anti-diabetic activity in vitro (RINm5F cells and HepG2 cells) and in vivo (glucose-loaded rats and hyperlipidemic mice) via promoting insulin secretion, improving glucose tolerance and insulin sensitivity and inhibiting hepatic gluconeogenesis, thus improve postprandial hyperglycemia (Patel and Mishra, 2011; Chen et al., 2012; Yang et al., 2016; Li et al., 2020).
Jatrorrhizine protected rats with induced diabetes mellitus and restored vascular endothelial dysfunction through upregulating the Akt/AMPK/eNOS signaling pathway and reducing IL-1β and tumor necrosis factor α (TNF-α) in blood vessels (Wang, et al., 2017). The alkaloid regulated glucose uptake and utilization and reduced insulin resistance through upregulating the expression of insulin receptor substrate 2 (IRS2), phosphoinositide-3-kinase regulatory subunit 1 (PI3KR1), phosphorylated protein kinase B (p-AKT), phospho-AMP-activated protein kinase (p-AMPK) and glucose transporter 4/1/2 (GLUT4/1/2) (Zhu et al., 2018). Jatrorrhizine, a primary active component of Coptidis Rhizoma, displayed potent inhibition of gut microbiota modulation and reduction of blood glucose in db/db mice (Lyu et al., 2021).
Jatrorrhizine is thus considered to be an active ingredient with multiple manners that reduce hypoglycaemia (Table 2). However, a comparative study of jatrorrhizine and existing anti-diabetic drugs is not available. Systematic clinical research and molecular studies of jatrorrhizine are still needed to elucidate definite mechanism of action. It is reported that other alkaloids in Coptidis Rhizoma also exhibit anti-diabetic effects, such as berberine, coptisine and palmatine (Lyu et al., 2021). The synergy between this natural metabolite with other alkaloids in Coptidis Rhizoma is of special interest, including interacts with berberine, coptisine and palmatine.
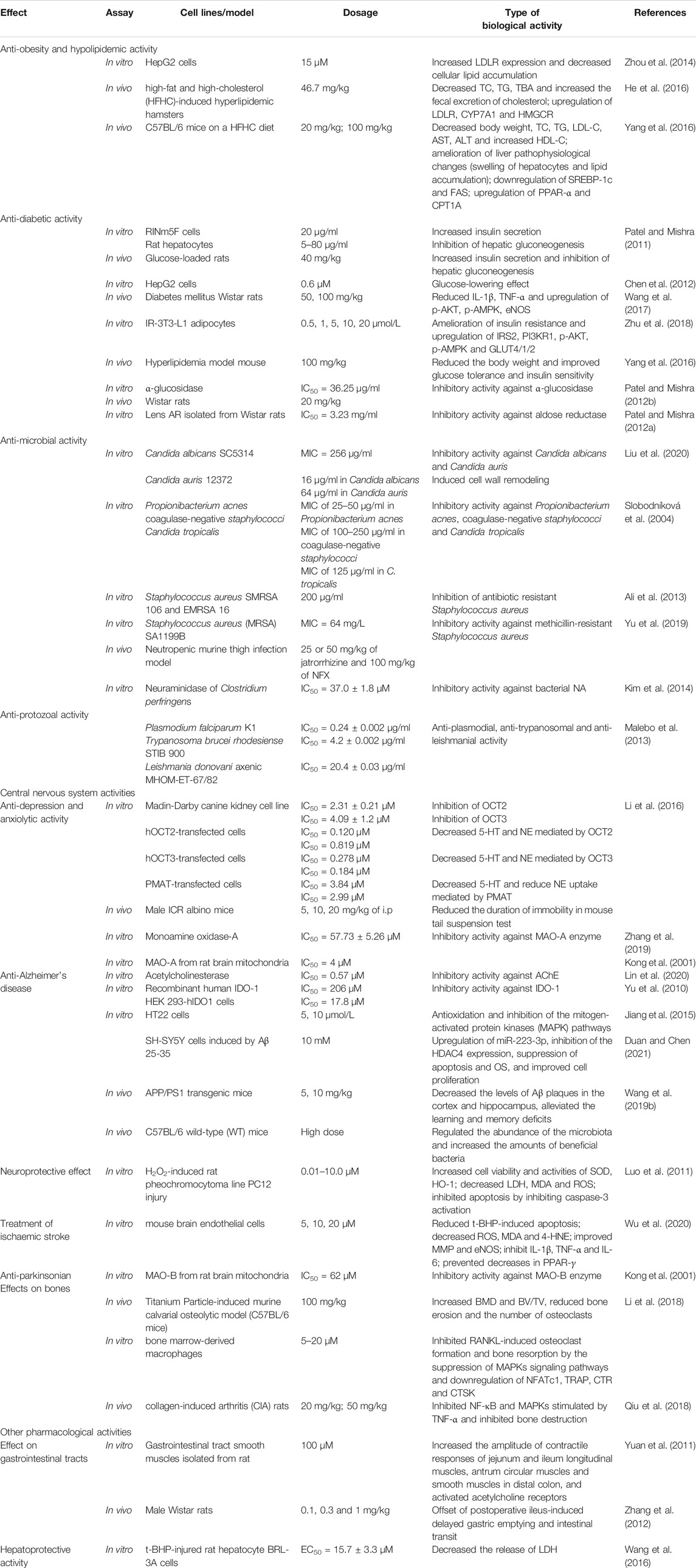
TABLE 2. Anti-diabetic, antimicrobial, antiprotozoal, and central nervous system activities and mechanisms of jatrorrhizine in in vitro and in vivo assays.
Anti-Microbial and Anti-protozoal Activity
Jatrorrhizine, in plants such as Mahonia aquifolium (Pursh) Nutt., Berberis brevissima Jafri and Coptis chinensis Franch. (Slobodníková et al., 2004; Ali et al., 2013; Tseng et al., 2021), is a notable among natural products for its varied anti-microbial properties. This metabolite strongly inhibited the growth of some bacteria, such as Candida albicans SC5314 (MIC = 256 μg/ml), Candida auris 12372 (MIC = 256 μg/ml), Candida tropicalis (MIC = 125 μg/ml), Propionibacterium acnes (MIC between 25 and 50 μg/ml), coagulase-negative staphylococci (MIC between 100 and 250 μg/ml) and Staphylococcus aureus (200 μg/ml) (Slobodníková et al., 2004; Ali et al., 2013; Liu et al., 2020). This alkaloid induced cell wall remodeling at 16 μg/ml in Candida albicans and 64 μg/ml in Candida auris (Liu et al., 2020). The mechanism underlying the antimycotic effect was inhibition of drug efflux and expression of the NorA multi-drug efflux pump (Yu et al., 2019). Further, a combination of jatrorrhizine (25 or 50 mg/kg) and norfloxacin (NFX, 100 mg/kg) significantly decreased bacterial count in a murine thigh infection model, suggesting in vivo synergistic bactericidal activity. Moreover, the combination of five berberine alkaloids (berberine: coptisine: jatrorrhizine: palmatine: epiberberine = 0.702 : 0.863: 1: 0.491: 0.526) exhibited broad-spectrum antibacterial activity, and this activity was verified in vivo using cyclophosphamide-immunocompromised mouse model and in vitro against Escherichia coli, Staphylococcus aureus, Staphylococcus dysenteriae, and Staphylococcus pneumonia. Hence, jatrorrhizine may act synergistically with other alkaloids (Luo et al., 2013). Moreover, jatrorrhizine showed a synergistic effect with colistin antibacterial activity against carbapenem-resistant Klebsiella pneumoniae, exhibiting one-to two-fold reductions of colistin MIC (Tseng et al., 2021).
Neuraminidase (NA) is a novel target for the development of therapeutic agents to treat bacterial or viral infections (Kim et al., 2014; Luo et al., 2020). As documented in literature, jatrorrhizine showed inhibitory activity on bacterial NA with an IC50 value of 37.0 ± 1.8 μΜ and suppressed viral NA activity against rvH1N1 and H5N1 with IC50 values of 66.2 ± 4.2 μΜ and 76.3 ± 2.1 μΜ (Kim et al., 2014). Molecular modelling and docking studies indicated that jatrorrhizine might be a potent agent against transmembrane protease serine 2 (TMPRSS2) enzyme for treating SARS-CoV-2 (Pooja et al., 2021). It bound to human immunodeficiency virus-1 (HIV-1) as an effective inhibitor of HIV (Namthabad and Mamidala, 2014). Therefore, jatrorrhizine is a promising therapeutic agent and a natural metabolite of the combination therapy for microbial diseases.
Annickia affinis (Exell) Versteegh and Sosef and Annickia chlorantha (Oliv.) Setten and Maas containing jatrorrhizine are used for the treatment of malaria across tropical Africa (Olivier et al., 2015; Odoh et al., 2018). In vitro antiprotozoal studies on jatrorrhizine have shown its anti-plasmodial activity against multi-drug resistant strains of Plasmodium falciparum K1 (IC50 = 0.24 ± 0.002 μg/ml), anti-trypanosomal activity against the Trypanosoma brucei rhodesiense STIB 900 (IC50 = 4.2 ± 0.002 μg/ml) and anti-leishmanial activity against Leishmania donovani axenic MHOM-ET-67/82 strain (IC50 = 20.4 ± 0.03 μg/ml) (Malebo et al., 2013).
In vivo validation of naturally occurring anti-microbials and the development of effective alternatives to anti-biotics is crucial in the current era of microorganism resistance. Several studies report anti-microbial and anti-protozoal activity of jatrorrhizine in vitro, but clinical efficacy, therapeutic doses, safety and mechanisms remain largely unknown.
Effects on the Central Nervous System
Anti-depressant Activity
Jatrorrhizine demonstrated anti-depressant activity via several targets in anti-depressant therapeutics. It showed strong inhibitory activity against monoamine oxidase A (MAO-A) (IC50 = 4 μM). This inhibitory activity was greater than that of berberine (IC50 = 126 μM), which lacks the phenolic hydroxyl of jatrorrhizine (Kong et al., 2001; Zhang et al., 2019). Furthermore, several studies have shown that organic cation transporters (OCTs) play roles in anti-anxiety and anti-depressant processes (Bacq et al., 2012) and plasma membrane monoamine transporter (PMAT) is a novel anti-depressant target. Jatrorrhizine was proved to be a high-affinity substrate for OCTs and a potent inhibitor of OCT2 (IC50 = 2.31 ± 0.21 μM) and OCT3 (IC50 = 4.09 ± 1.2 μM) (Li et al., 2016). Moreover, jatrorrhizine strongly reduced serotonin (5-HT) and norepinephrine (NE) uptake mediated by hOCT2, hOCT3, and hPMAT in vitro. Meanwhile, jatrorrhizine reduced 5-HT and NE uptake at 50 μM in mouse synaptosomes, and reduced the duration of immobility and reversed the effect of stress in tail suspension tests, consistent with an anti-depressant effect. However, more in vivo experiments are needed to verify and clarify the complex anti-depressant activity of jatrorrhizine.
Anti-Alzheimer’s Disease
The Alzheimer’s disease (AD) is currently attributed to extracellular aggregates of amyloid β (Aβ) plaques and intracellular neurofibrillary tangles in cortical and limbic areas of the human brain (Tiwari et al., 2019). Defects in acetylcholine and cholinergic neurotransmission can be observed along with the accumulation of β-amyloid. The use of acetylcholinesterase (AChE) inhibitors, which activate central cholinergic function, is a treatment strategy for AD. Jatrorrhizine demonstrated inhibitory activity against AChE with IC50 values of 0.57 μM (Lin et al., 2020), 106.1 μM (Zhao et al., 2016) and 2.08 μM (Xiao et al., 2011), respectively. The differences in these values might be explained by the different sources and concentrations of the enzyme and substrate used for testing. Further, a jatrorrhizine derivative with -NH2 linked at the 3-position (IC50 = 0.301 μM) exhibited the greater AChE inhibitory activity than jatrorrhizine (IC50 = 0.872 μM) (Jiang et al., 2017). Hence, structural modification of jatrorrhizine may be effective for modulating its activity.
Indoleamine 2, 3-dioxygenase 1 (IDO-1) is a rate-limiting enzyme in the kynurenine pathway of tryptophan metabolism. The accumulation of a downstream neurotoxic metabolite via overexpression or over activation of IDO1 is involved in neurodegenerative disease (Zhang et al., 2017; Röhrig et al., 2019). Jatrorrhizine was able to irreversibly inhibit IDO1, and had IC50 values of 206 μM (recombinant human IDO-1) and 17.8 μM (in HEK 293-hIDO1 cells) (Yu et al., 2010).
In vivo treatment of APP/PS1 transgenic mice with 5 mg/kg or 10 mg/kg jatrorrhizine reduced levels of Aβ plaques in the cortex and hippocampus, and alleviated the learning and memory deficits (Wang S. et al., 2019). Learning and memory impairment in AD is related to dysfunction in gut microbiota (Vogt et al., 2017). Microbial colonies of APP/PS1 mice showed altered composition compared to C57BL/6 wild-type (WT) mice. High dose jatrorrhizine treatment modulated microbiota populations and enriched the numbers of beneficial bacteria, such as Faecalibaculum, Lactobacillus acidophilus and Bifidobacterium (Wang S. et al., 2019). Thus, jatrorrhizine might affect the learning and memory capabilities by regulating the intestinal flora.
Neuroprotective effects of jatrorrhizine are mainly attributed to its anti-oxidant property and anti-apoptosis activity. The alkaloid alleviates alleviated oxidative damage and suppresses neuronal apoptosis. Jatrorrhizine exhibited neuroprotective activity on okadaic acid (OA)-induced cytotoxicity and apoptosis in HT22 cells. This effect ascribed to increase cell viability, enhance anti-oxidant status (SOD and GSH) and maintenance of mitochondrial membrane potential (MMP). Reduced lactate dehydrogenase (LDH) release, lipid peroxidation (MDA) levels and reactive oxygen species (ROS) were also observed. Other responses included downregulation of expression of phosphorylated extracellular signal-regulated kinases 1/2 (p-ERK1/2), phosphorylated c-Jun N-terminal kinases (p-JNK) and phosphorylated p38 (p-p38), along with upregulation of B cell lymphoma 2 (Bcl-2), reduction in cleaved caspase-3 and BCL-2-associated X protein (Bax) levels, and inhibition of NF-κB p65 subunit activation (Jiang et al., 2015). A possible mechanism was inhibition of mitogen-activated protein kinase (MAPK) pathways. Similarly, jatrorrhizine was effective in mitigating hydrogen peroxide (H2O2)-induced rat pheochromocytoma PC12 injury via reducing oxidative stress and inhibiting apoptosis (Luo et al., 2011). Furthermore, treatment with jatrorrhizine (10 mM) alleviated Aβ 25-35-induced nerve cell injury through upregulating miR-223-3p and inhibiting histone deacetylase 4 (HDAC4) expression. The alkaloid also suppressed apoptosis and oxidative stress (OS) and improved SH-SY5Y cell proliferation (Duan and Chen, 2021).
Other Effects on the Central Nervous System
Jatrorrhizine might have therapeutic potential for ischaemic stroke associated with endothelial dysfunction. The alkaloid produced protective effect in mouse brain endothelial cells (MBECs) treated with tert-butyl hydroperoxide (t-BHP) via reducing cell apoptosis, inhibiting oxidative damage and ameliorating mitochondrial dysfunction. Jatrorrhizine also prevented the expression of IL-1β, TNF-α and IL-6, and upregulated endothelial nitric oxide synthase (eNOS) and prevented decreases in PPAR-γ protein expression in MBECs (Wu et al., 2020). Jatrorrhizine also non-competitively inhibited MAO-B from rat brain mitochondria with an IC50 value of 62 mM (Kong et al., 2001). This activity was intended to be helpful for the prevention and adjunct treatment of Parkinson’ disease.
Anti-Cancer Activity
Globally, cancer is one of the major diseases that cause a large number of deaths, and the incidence of cancer is increasing in recent years (Sharma et al., 2019). Inhibition of apoptosis, unlimited proliferation of cancer cells, invasion of normal organs and destruction of normal tissues are the main reasons that cancer threatens human health (Rosell and Karachaliou, 2015; Roy and Saikia, 2016). Over the past decades, development of anti-tumor agents from natural products has been one of the fresh approaches for therapeutic candidate discovery. Jatrorrhizine exhibited anti-cancer activity in various cancer cells (in vitro) and a few in vivo models (Table 3). Its multidirectional mechanisms involve in inhibiting cancer cell proliferation and tumor growth, preventing metastasis, while the important mechanism is promoting apoptosis of cancer cells (Figure 4).
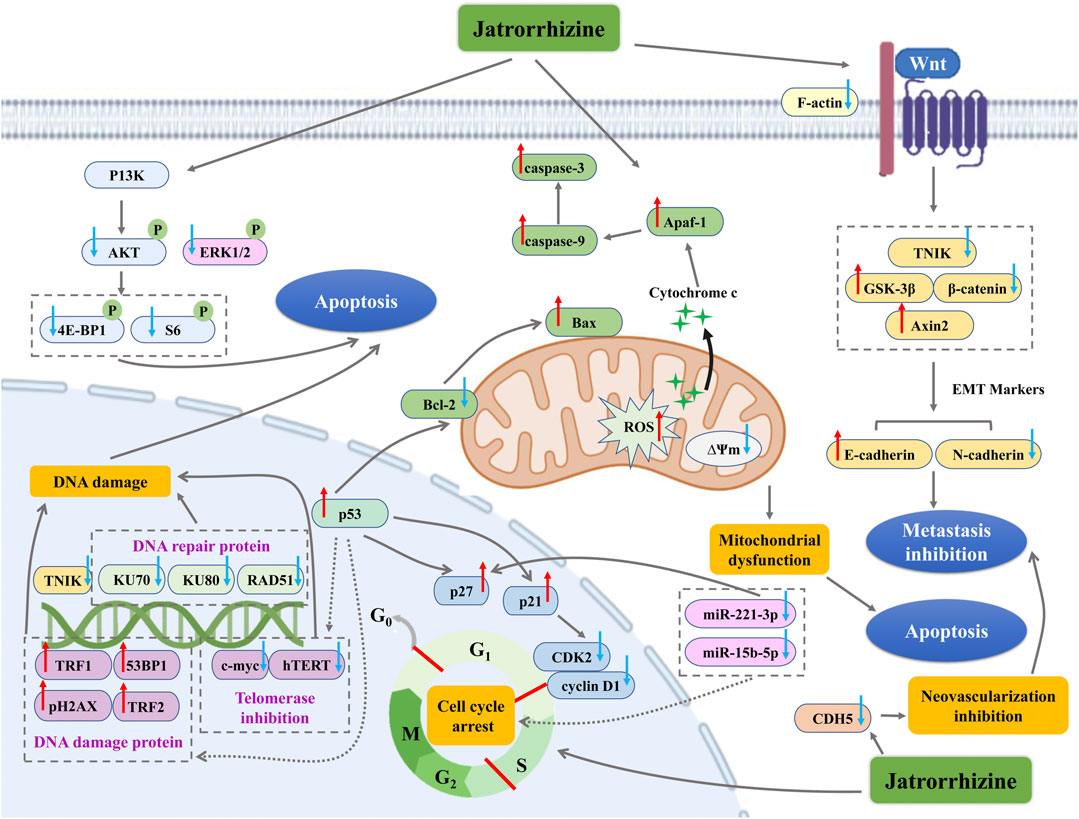
FIGURE 4. The antitumor mechanism of jatrorrhizine. ↑ with red color indicate increase/promotion, ↓with blue color indicate inhibition/reduction.
Inhibition of Cell Proliferation
The rapid and unlimited proliferation of cancer cells is attributed to the loss or gene mutations of critical checkpoint controlling cycling of cell phase (Andrade-Tomaz et al., 2020). As an important process in cancer development, cell cycle modulation is a well-established therapeutic schedule. Jatrorrhizine (5–15 μM) affected human colorectal carcinoma HCT-116 and HT-29 cells proliferation by blocking cell cycle in S phase and it (5 mg/kg) could prolong the survival of nude mice xenografted HCT-116 cells (Wang P. et al., 2019). In C8161 human metastatic melanoma cells, jatrorrhizine (160 mmol/L) inhibited cell proliferation through inducing cell cycle arrest in the G0/G1 phases and upregulating expression of cyclin-dependent kinase (CDK) inhibitors (p21and p27) and the tumor suppressor p53 (Liu et al., 2013). The derivative, Pt(II) complexes with jatrorrhizine blocked cell cycle at G1 phase in human bladder T-24 tumor cells, which was associated with inhibiting the levels of cyclin D1 and CDK2 (Qin et al., 2019a). microRNAs play a prominent role in modulation of cell proliferation by directly targeting cell cycle regulators, such as cyclin, c-myc, p27 and p57 (Yu et al., 2010). Jatrorrhizine (16.0 µM) inhibited cell viabilities of HepG2 and HCCLM3 liver cancer cells by down-regulating miR-221-3p and miR-15b-5p expressions (Deng and Wan, 2021).
Inhibition of Cancer Cell Metastasis
Abnormal vascularization and epithelial-mesenchymal transition (EMT) are essential for metastatic spread of cancer cells (Dewaguet et al., 2021). In BALB/C nude mice xenografted metastatic melanoma C8161 cell, jatrorrhizine (50 μg) reduced neovascularization of tumor, probably due to its suppression of CDH5 expression, which encodes the vascular endothelial cadherin (Liu et al., 2013). Traf2 and Nck interacting serine protein kinase (TNIK) has been considered as an important activator of Wnt signaling pathway to promote tumor progression and invasion (Yang et al., 2021). MDA-MB-231 human breast cancer cells targeted knockout of TNIK validated that the disruption of TNIK restrained the key proteins expression of Wnt/β-catenin signalling and EMT (Sun et al., 2019). Interestingly, jatrorrhizine exhibited good binding affinity and interaction with TNIK. The alkaloid effectively downregulated TNIK, p-TNIK, β-catenin, F-actin and N-cadherin expression levels, and upregulated GSK-3β and E-cadherin in in vitro (MDA-MB-231 cells and MCF-7 cells) and in vivo models (Orthotopic 4T1 tumour bearing mouse) (Sun et al., 2019). Moreover, jatrorrhizine (5 mg/kg) also reduced tumor volume and weight, and inhibited lung metastasis in nude mice xenografted HCT-116 colorectal carcinoma cells via suppressing Wnt signaling pathway and the process of EMT (Wang P. et al., 2019). Hence, jatrorrhizine is expected to be an anticancer drug targeting TNIK and EMT.
Promotion of Apoptosis
Apoptosis is considered as a major barrier for the development and progression of cancer (Boudreau et al., 2019). Jatrorrhizine (10–30 μM) triggered mitochondrial dysfunction and apoptosis in MDA-MB-231 breast cancer cells. The relevant mechanism was related to disruption of ∆Ψm, upregulation of the pro-apoptotic protein Bax, and downregulation of the anti-apoptotic protein Bcl-2, as well as decrease of apoptosis-related proteins including Procaspase-3, Procaspase-8, Procas-pase-9 and PARP (Sun et al., 2019). Similarly, jatrorrhizine showed disruptive effect on ∆Ψm and nuclear morphological changes in human colorectal carcinoma HCT-116 and HT-29 cells, indicating mitochondrial dysfunction and early apoptosis (Wang P. et al., 2019). Besides, jatrorrhizine-Platinum(II) complex promoted DNA damage of thyroid cancer SW1736 and BHP7-13 cells via increasing pH2AX protein (DNA damage protein) and decreasing DNA repair protein KU70, KU80 and RAD51, while activated apoptosis by upregulating ROS and cleaved caspase-3, and downregulating PI3K/AKT/mTOR pathway (pS6, p-ERK1/2, p-4E-BP1, and p-AKT levels) (Lu et al., 2020). In mice bearing SW1736 tumor xenografts, this derivate suppressed tumor growth and tumor tissues expression of pH2AX, which confirmed its anti-cancer activity in vivo (Lu et al., 2020). The complexes of jatrorrhizine with platinum (Pt1 and Pt2) induced apoptosis in HeLa cancer cells, which was manifested in that these derivates targeted p53 and telomerase and further caused DNA damage via suppression of c-myc and human telomerase reverse transcriptase (hTERT), and activation of 53BP1, pH2AX, TRF1, and TRF2 (Qin et al., 2019b). Consistent with that in HeLa cancer cells, a novel Pt(II) complex as well modulated telomerase related-proteins and DNA damage. It also successfully achieved the induction of apoptosis to decrease ∆Ψm and Bcl-2, increase the release of ROS and cytochrome c, and up-regulate caspase-9, caspase-3 and apoptotic protease activating factor 1 (Apaf-1) (Qin et al., 2019a).
Several studies have illustrated that jatrorrhizine and its derivates exert anti-cancer effect with low systemic toxicity in in vivo models, which inhibited tumor growth and metastasis and prolong the survival time for mice bearing tumor xenografts (Liu et al., 2013; Qin et al., 2019a; Wang P. et al., 2019; Sun et al., 2019). Additionally, the complexes of jatrorrhizine with platinum had good effects in the induction of cisplatin-resistant cancer SK-OV-3 cells and could reduce the side effects of anti-tumor drugs such as cisplatin (Qin et al., 2019a; Lu et al., 2020).
On the whole, jatrorrhizine and its derivatives may be a logical agent for tumor therapy. However, in the retrieved studies, the experiments also lacked the information on the selectivity index. More positive controls (anti-cancer drugs for clinical use) are required to further confirm the anticancer effects of jatrorrhizine. We observed that jatrorrhizine inhibited different types of cancer, but in vivo models of different cancer stages including tumorigenesis, development and metastasis were not fully considered. More importantly, elaborative consideration needs to be taken into the validation criteria of the models used. In addition, its clinical efficacy, specific targets and long-term drug safety during cancer treatment are critical issues to be addressed.
Effects on Bones
Jatrorrhizine inhibited osteolysis in titanium particle-induced murine calvarial osteolytic (C57BL/6 mice). Treatment with the alkaloid (100 mg/kg) significantly increased bone mineral density (BMD) as well as bone volume/tissue volume (BV/TV), and reduced bone erosion and the number of osteoclasts (Li et al., 2018). In bone marrow-derived macrophages, jatrorrhizine inhibited receptor activator of nuclear factor κ-B ligand (RANKL)-induced osteoclast formation and bone resorption. Mechanism analysis revealed that these effects were mediated via suppression of MAPK (p38 and ERK) signaling and downregulation of nuclear factor of activated T-cells cytoplasmic 1 (NFATc1) and NFATc1-associated osteoclastic genes including tartrate-resistant acid phosphatase (TRAP), calcitonin receptor (CTR) and cathepsin K (CTSK) (Li et al., 2018). Also, jatrorrhizine suppressed the activation of NF-κB and MAPK stimulated by TNF-α, thereby inhibiting inflammatory responses and bone destruction in collagen-induced arthritis (CIA) rats (Qiu et al., 2018). Bone protection and anti-inflammatory effects suggest that jatrorrhizine may be beneficial in reducing infection after orthopaedic titanium implantation. Overall, this natural product may be useful agents for treatment of bone disorders.
Other Pharmacological Activities of Jatrorrhizine
Effects on Gastrointestinal Tracts
Jatrorrhizine at concentrations from 1.0 to 300 μM increased the amplitude of spontaneous contractions of gastrointestinal tract smooth muscles isolated from rats in a concentration-dependent manner. Jatrorrhizine (100 μM) markedly increased contractile responses of jejunum and ileum longitudinal muscles, antrum circular muscles and smooth muscles in the distal colon. These effects were mediated by activation of acetylcholine receptors (probably M3 receptors) and associated with calcium agonistic effects, including enhancing Ca2+ influx through L-type Ca2+ channel and Ca2+ release via IP3 and ryanodine pathways (Yuan et al., 2011). Moreover, in vivo experiments in rats demonstrated that jatrorrhizine (0.1, 0.3 and 1 mg/kg) offset postoperative ileus-induced delayed gastric emptying and intestinal transit in a dose-dependent manner (Zhang et al., 2012). Hence, jatrorrhizine may be useful for treatment of functional disorders of the gastrointestinal tract.
Hepatoprotective Activity
Jatrorrhizine is one of the constituents in three traditional Chinese medicine formulae with hepatoprotective activity for treating jaundice, namely Zhi-Zi-Da-Huang-Tang, Yin-Chen-Hao-Tang and Da-Huang-Xiao-Shi-Tang. The alkaloid decreased the release of LDH (EC50 = 15.7 ± 3.3 μM) in a study on t-BHP-injured rat hepatocyte BRL-3A cells. LDH release is an indicator of liver damage and reduced release is evidence of a hepatoprotective effect against oxidative damage (Wang et al., 2016).
Pharmacokinetics of Jatrorrhizine
Pharmacokinetic evaluation of drugs provides increasingly important information for clinical research. The pharmacokinetic profile of jatrorrhizine after oral or intravenous administration was assessed in rats and rabbits using liquid chromatography-tandem mass spectrometry (LC-MS/MS), LC-MS/MS combined with brain micro-dialysis, ultra-high performance liquid chromatography-mass spectrometry (UPLC-MS/MS), UPLC-orbitrap mass spectrometry and liquid chromatography quadrupole time-of-flight mass spectrometry (LC-qTOF-MS) (Deng et al., 2008; Liu et al., 2011; Shi et al., 2012; He W. et al., 2014; Zhang et al., 2018). The pharmacokinetic parameters of these studies are shown in Table 4.
Cui et al. (2015) reported permeability and absorption of jatrorrhizine in rats after oral administration. An apparent permeability coefficient of jatrorrhizine (0.23–0.36 × 10−6 cm s−1) indicated limited ability to cross cell membranes. P-glycoprotein (P-gp) efflux had a significant effect on the absorption of this compound, which may explain its poor bioavailability. Intestinal perfusion experiments confirmed absorption into the rat jejunum (8.98 ± 2.43%) and ileum (7.54 ± 1.45%) (Cui et al., 2015). Jiaotai Pill extracts containing jatrorrhizine (15.52 mg/kg) was administered orally to rats and the pharmacokinetic parameters in rat brain were assessed using LC-MS/MS. The half-life of terminal elimination phase (T1/2), AUC (0-t), AUC (0-∞), and Cmax in insomnic rats were increased compared with normal controls (Zhang et al., 2018). Thus, the absorption and bioavailability of jatrorrhizine may increase under pathological conditions. A study on plasma pharmacokinetics reported similar results (He W. et al., 2014). Rat plasma jatrorrhizine concentrations showed a biphasic decline, dose-independent clearance (CL) and T1/2, and dose dependent AUC (0-∞) after intravenous administration (0.1 mg/kg to 3 mg/kg). Large distribution volumes (Vd) indicated that jatrorrhizine might be distributed across tissues (Shi et al., 2012). Also, three peaks were observed in both individual and mean plasma-concentration curves of jatrorrhizine in rats after oral gavage with Coptis–Evodia powder (1.086 g/kg). This finding may be explained by distribution, re-absorption and enterohepatic circulation (Deng et al., 2008). In addition, absorption and elimination of jatrorrhizine are also influenced by compounds and other herbs coexisting with it, such as Astragali Radix, Lonicerae Japonicae Flos and Fructus Evodiae (Yan et al., 2012; Sun et al., 2018; Zhao et al., 2018). It is required to explore and confirm the compound-compound and compound-herb interaction mechanism in the further research.
Biotransformation of jatrorrhizine was similar among liver microsomes from rats, rhesus monkeys and humans. C20H20O7N was the major metabolite in these species (Li et al., 2015). Seventeen metabolites in rat urine, thirteen metabolites in rat faeces (including eight phase I metabolites and five phase II metabolites) and eleven metabolites in rat plasma (including six phase I metabolites and five phase II metabolites) were detected after oral administration of jatrorrhizine (34 mg/kg) to healthy rats. Further, seventeen and nine metabolites were identified after incubating jatrorrhizine with rat intestinal flora and liver microsomes, respectively (Zhang et al., 2008). Also, jatrorrhizine metabolism exhibited high consistency between human and zebrafish (Li et al., 2015). Additionally, seven phase I metabolites of jatrorrhizine after demethylation, dehydrogenation and dihydroxylation, and eleven phase II metabolites including glucuronide and methyl conjugates were detected in rat urine (Han et al., 2006). Cytochrome P450 and UDP-glucuronosyltransferase enzymes are responsible for the metabolism of jatrorrhizine in human liver microsomes, including CYP1A2 and multiple UGT1A isoforms (UGT1A1, UGT1A3, UGT1A7, UGT1A8, UGT1A9 and UGT1A10) (Zhou et al., 2013). CYP3A1/2 and CYP2D2 were also involved in demethylation of jatrorrhizine and UGT1A1 and UGT 1A3 were associated with glucuronidation in rat liver microsomes (Shi et al., 2012).
Toxicity of Jatrorrhizine
Toxicity and safety are critical for the assessment of clinical applications of natural products (Li et al., 2021). Jatrorrhizine is a bioactive metabolite in some commonly used medicinal plants and has been used for centuries in traditional medicine. However, analyses of the composition of traditional medicines and modern pharmacology research indicate that some natural products may produce adverse effects or even overt toxicity under certain conditions despite their beneficial pharmacological properties. Jatrorrhizine exhibited anti-cancer activity on SW480 cells (human colon cancer) and HepG2 cells (hepatocellular carcinoma) as discussed above. However, it also showed cytotoxicity against these cancer cells the at the concentrations of 200 μg/ml and 100 μM, respectively (Chen et al., 2012; Singh et al., 2016). Slight cytotoxicity was also reported in normal MCF10A normal breast eptithelial cells in vitro (100 μM) (Sun et al., 2019). However, jatrorrhizine did not cause cytotoxicity to MCF-7 cells at the concentration below 10 µM (Lo et al., 2017). Additionally, Sun et al. (2019) reported that gross necropsy did not show signs of toxicity in the jatrorrhizine-treated (2.5 and 5 mg/kg) 4T1tumour-bearing mice (Sun et al., 2019). Also, there was no significant changes in body weight and serum ALT and AST levels in jatrorrhizine (25 and 100 mg/kg)-treated Ti particle-induced mice, as compared with the untreated and control groups (Li et al., 2018). The alkaloid was also non-cytotoxic to rheumatoid arthritis-derived fibroblast-like synoviocyte MH7A cells, and no damage to liver function was observed in CIA rats at administered doses of 20 and 50 mg/kg (Qiu et al., 2018).
An acute LD50 value for jatrorrhizine was about 5,500 mg/kg in Kunming mice, and significantly higher than that of the related alkaloid, berberine (763 mg/kg). No influences on body weight and organ weight were observed in rats in a sub-chronic study, and no abnormalities in urinalysis and haematological parameters, gross necropsy or histology were reported after administration of jatrorrhizine (70.05 mg/kg day) over 90 days (Wu et al., 2014). Few indications of adverse effects of jatrorrhizine are available, but we should explore mechanisms for any toxicity observed in particular clinical circumstances.
Conclusion and Future Perspectives
Jatrorrhizine is encountered in a variety of traditional medicinal plants. This bioactive metabolite possesses numerous pharmacological properties via modulation of multiple signaling pathways and targets (Figure 5), such as AR, NA, MAO-A, OCT, PMAT, AChE, IDO-1, TNIK, and Wnt/β-catenin, MAPK, PI3K/AKT/mTOR, NF-κB, PPAR, and insulin signaling pathway. This review summarizes molecular mechanisms for possible use in the treatment of diseases, discusses pharmacokinetic parameters and evaluates toxicity and safety. However, some issues still need clarification in the future studies.
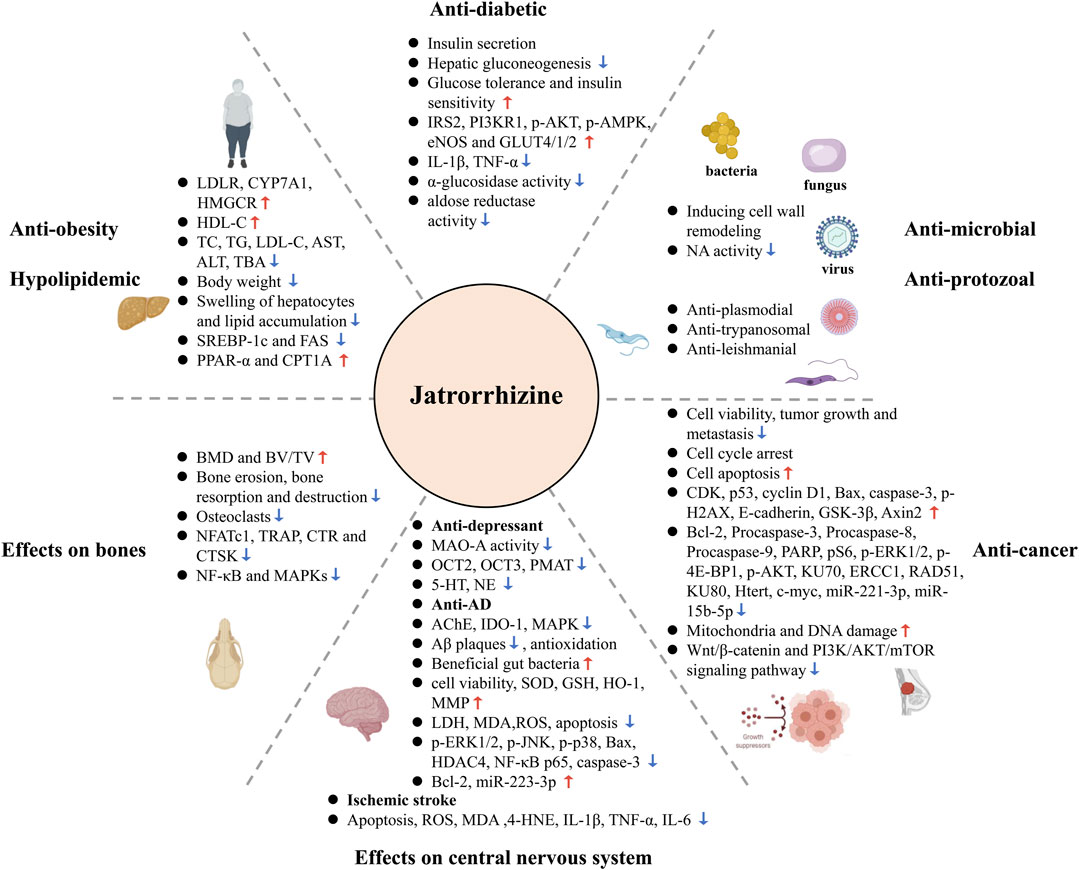
FIGURE 5. Summary of the therapeutic potential of jatrorrhizine through multiple pathways and multiple targets.
Curative Mechanisms of Jatrorrhizine and Clinical Validity Confirmation
We recognise a potential for jatrorrhizine to be a therapeutic ingredient in medications, reflecting its impacts on multiple pathways and targets. However, its specific mechanisms of action against various diseases are not fully understood. Clarification the molecular targets of jatrorrhizine is conducive to a more scientific understanding and development of this natural metabolite. Currently, this natural metabolite is rarely alone used clinically to treat specific diseases even though it is the main active substance in some medicinal materials and extracts and has a role in the treatment of diabetes, gastrointestinal diseases, and Alzheimer’s disease in traditional medicine. Proper positive controls are necessary for future work to ensure the reproducibility and data quality for comparisons of therapeutic effects on various diseases and different disease stages. Sufficient evidence is available to support the detailed assessment of curative mechanisms of jatrorrhizine, structure-activity studies and clinical trials in humans. Such work should be approached systematically to fully explore the clinical utility of the alkaloid.
Jatrorrhizine is widely reported to exhibit pro-apoptotic effects in multiple cancer cells. However, it displays a protective role in AD and ischaemic stroke via reducing apoptosis. Mechanisms underlying these disparate effects require elucidation to understand actions on apoptosis in neuroprotection and cancer therapy, and subsequently improve targeting and specificity of jatrorrhizine through structural modification and dosage form optimization (e.g., as nanoparticles and liposome).
Application Prospect of Jatrorrhizine in the Treatment of Metabolic Disorders
Long-term metabolic disorders and hyperglycemia remain a challenge in medical practice. These conditions cause a series of complications, such as cardiovascular disease, retinopathy, neuropathy and nephropathy. Current therapeutics for metabolic diseases require a multi-drug regimen. However, major problems of this therapeutic method are poor patient compliance, side effects and drug-drug interactions (Lillich et al., 2021). Multi-target ligands and drugs have been proposed as promising approaches to developing therapies for complex diseases (González-Álvarez et al., 2021). Jatrorrhizine is a multi-purpose natural metabolite that affects multiple targets. The alkaloid effectively modulates glucose and lipid metabolism and exhibits anti-inflammatory, anti-oxidant and anti-cancer effects. It is also a safe and controllable natural product. Therefore, the use of jatrorrhizine, alone or as a supplement to other nutraceuticals, is a potential strategy to address multiple pathways and targets to delay metabolic disorders and affect the long-term treatment of related complications.
Comprehensive Investigations of Toxicity Mechanisms
We found that jatrorrhizine exerts cytotoxic effects under specific circumstances in vitro. High dose and long-term administration may lead to cytotoxicity in a few cancer cell lines, such as colon cancer and hepatocellular carcinoma cells and normal breast epithelial cells. However, there was no studies that report target-organ toxicity of jatrorrhizine in different disease models. The existing in vivo studies indicated that jatrorrhizine is non-cytotoxic and has no influence on liver function or other tissues. Therefore, further comprehensive investigation for mechanisms of toxicity is needed and further exploration of whether jatrorrhizine has target organ toxicity under special circumstances in vivo is of significance. Such studies will serve as a basis to further evaluate the safety of jatrorrhizine in the treatment of different diseases and for chronic administration.
Interaction Mechanism of Jatrorrhizine With Other Compounds and Development of Derivatives
Jatrorrhizine displays low permeability and poor bioavailability. Interestingly, jatrorrhizine may interact with other constituents and thereby alter its absorption and elimination. Additionally, different salt forms of quaternary ammonium compounds show varying physicochemical properties. Thus, salts of jatrorrhizine might exhibit different pharmacokinetic properties in vivo (Neef et al., 1984; Cui et al., 2019). Berberine, an alkaloid similar in chemical structure to jatrorrhizine, displays better bioavailability of its organic acid salts (fumarate, malate, succinate and citrate) than inorganic acid salts (hydrochloride) (Cui et al., 2019). The hydrochloride salt is commonly used in clinical practice and pharmacological research. However, almost no reports on the pharmacokinetics of other salts and comparative studies of different salt forms are available for jatrorrhizine. Therefore, the investigation of interactions between jatrorrhizine and other compounds and the effects of different salt forms on pharmacokinetics is crucial. The improvement of the bioavailability of jatrorrhizine and development of jatrorrhizine derivatives with high bioavailability and low toxicity also needs to be explored.
Author Contributions
FZ and YM designed this work of review. FZ and YC collected the literatures related to jatrorrhizine. FZ, YC, JC, YL, and HL analyzed literatures and summarized results. FZ wrote the manuscript. HL, YM revised the manuscript.
Funding
This work was supported by Sichuan Science and Technology Support Program (2020YFN0152), and National Wild Plant Germplasm Resource Center for South China Botanic Garden, CAS (ZWGX 1905).
Conflict of Interest
The authors declare that the research was conducted in the absence of any commercial or financial relationships that could be construed as a potential conflict of interest.
Publisher’s Note
All claims expressed in this article are solely those of the authors and do not necessarily represent those of their affiliated organizations, or those of the publisher, the editors, and the reviewers. Any product that may be evaluated in this article, or claim that may be made by its manufacturer, is not guaranteed or endorsed by the publisher.
Abbreviations
3OHase, tyrosine/tyramine 3-hydroxylase; 4-HNE, 4-hydroxynonenal; 4HPPDC, 4-hydroxyphenylpuruvate decarboxylase; 4′OMT, 3′-hydroxy-N-methyl-(S)-coclaurine 4′-O-methyltransferase; 53BP1, p53-binding protein 1; 5-HT, serotonin; 6OMT, (S)-norcoclaurine 6-O-methyltransferase; Aβ, amyloid β; AChE, acetylcholinesterase; Apaf-1, apoptotic protease activating factor 1; ALT, alanine aminotransferase; AR, aldose reductase; AST, aspartate transaminase; Bax, BCL-2-associated X protein; BBE, berberine bridge enzyme; Bcl-2, B cell lymphoma 2; BMD, bone mineral density; BV/TV, bone volume/tissue volume; CDK, cyclin-dependent kinase; CNMT, (S)-coclaurine N-methyltransferase; CPT1A, carnitine palmitoyltransferase 1A; CODM, codeine-O-demethylase; CTR, calcitonin receptor; CTSK, cathepsin K; CYP7A1, cholesterol 7α-hydroxylase; eNOS, endothelial nitric oxide synthase; EMT, epithelial-mesenchymal transition; ERCC1, excision repair cross-complementation group 1; FAS, fatty acid synthase; GLUT4/1/2, glucose transporter 4/1/2; GSH, glutathione; GSK-3β, glycogen synthase kinase-3β; HDAC4, histone deacetylase 4; HDL-C, high-density lipoprotein cholesterol; HMGCR, 3-hydroxy-3-methyl glutaryl coenzyme A reductase; HO-1, heme oxygenase-1; hTERT, human telomerase reverse transcriptase; IDO-1, indoleamine 2, 3-dioxygenase 1; IL-1β, interleukin-1β; IRS2, insulin receptor substrate 2; LDH, lactate dehydrogenase; LDL-C, low-density lipoprotein cholesterol; LDLR, low density lipoprotein receptor; MAO, monoamine oxidase; MAPK, mitogen-activated protein kinases; MDA, lipid peroxidation; MIC, minimum inhibitory concentration; MMP (∆Ψm), mitochondrial membrane potential; NA, neuraminidase; NCS, (S)-norcoclaurine synthase; NE, norepinephrine; NFATc1, T-cells cytoplasmic 1; NF-κB, nuclear factor kappa-B; NMCH, (S)-N-methylcoclaurine-3′-hydroxylase; OCT, organic cation transporter; OMT, O-methyltransferase; p-4E-BP1, phosphorylated 4E-binding protein 1; PARP, ADP-ribose polymerase; p-AKT, phosphorylated protein kinase B; p-AMPK, phospho-AMP-activated protein kinase; p-ERK1/2, phosphorylated extracellular signal-regulated kinases 1/2; pH2AX, phosphorylated H2A histone X; PI3KR1, phosphoinositide-3-kinase regulatory subunit 1; p-JNK, phosphorylated c-Jun N-terminal kinases; PMAT, plasma membrane monoamine transporter; PPAR, peroxisome proliferator activated receptor; p-p38, phosphorylated p38; ROS, oxygen species; SOMT, (S)-scoulerine 9-O-methyltransferase; SREBP-1c, sterol regulatory element binding transcription factor 1c; STOX, (S)-tetrahydroprotoberberine oxidase; T2DM, type 2 diabetes mellitus; TBA, total bile acids; TC, total cholesterol; TRF1, TTAGGG repeat binding factor 1; TG, total triglyceride; TNF-α, tumor necrosis factor α TRAP, tartrate-resistant acid phosphatase; TYDC, tyrosine decarboxylase; TyrAT, tyrosine aminotransferase.
References
Abdykerimova, S., Sakipova, Z., Nakonieczna, S., Koch, W., Biernasiuk, A., Grabarska, A., et al. (2020). Superior Antioxidant Capacity of Berberis Iliensis-HPLC-Q-TOF-MS Based Phytochemical Studies and Spectrophotometric Determinations. Antioxidants (Basel) 9 (6), 504. doi:10.3390/antiox9060504
Achenbach, H., and Hemrich, H. (1991). Clerodane-Type Diterpenes and Other Constituents of Penianthus Zenkeri. Phytochemistry 30 (6), 1957–1962. doi:10.1016/0031-9422(91)85047-4
Alamzeb, M., Khan, M. R., Mamoon-Ur-Rashid, , Ali, S., and Khan, A. A. (2015). A New Isoquinoline Alkaloid with Anti-Microbial Properties from Berberis Jaeschkeana Schneid. Var. Jaeschkeana. Nat. Prod. Res. 29 (8), 692–697. doi:10.1080/14786419.2014.981187
Al-Howiriny, T. A., Zemaitis, M. A., Lin, F. T., Beal, J. L., and Schiff, P. L. (2002). Alkaloids of Thalictrum Angustifolium. J. Herb. Pharmacother. 2 (2), 1–10. doi:10.1080/j157v02n02_01
Ali, S., Igoli, J., Clements, C., Alamzeb, M., Shah, S. Q., Ferro, V. A., et al. (2013). Anti-diabetic and Antimicrobial Activities of Fractions and Compounds Isolated from Berberis Brevissima Jafri and Berberis Parkeriana Schneid. Bangl. J. Pharmacol. 8 (3), 336–342. doi:10.3329/bjp.v8i3.13888
Andrade-Tomaz, M., de Souza, I., Rocha, C. R. R., and Gomes, L. R. (2020). The Role of Chaperone-Mediated Autophagy in Cell Cycle Control and its Implications in Cancer. Cells 9 (9), 2140. doi:10.3390/cells9092140
Bacq, A., Balasse, L., Biala, G., Guiard, B., Gardier, A. M., Schinkel, A., et al. (2012). Organic Cation Transporter 2 Controls Brain Norepinephrine and Serotonin Clearance and Antidepressant Response. Mol. Psychiatry 17 (9), 926–939. doi:10.1038/mp.2011.87
Bajpai, V., Singh, A., Chandra, P., Negi, M. P., Kumar, N., and Kumar, B. (2016). Analysis of Phytochemical Variations in Dioecious Tinospora Cordifolia Stems Using HPLC/QTOF MS/MS and UPLC/QqQLIT -MS/MS. Phytochem. Anal. 27 (2), 92–99. doi:10.1002/pca.2601
Basera, I. A., Girme, A., Bhatt, V. P., and Shah, M. B. (2021). A Validated High-Performance Thin-Layer Chromatography Method for the Simultaneous Estimation of Berberine, Berbamine, Palmatine, Magnoflorine and Jatrorrhizine from Berberis Aristata. Jpc-j. Planar Chromat. 34, 147–155. doi:10.1007/s00764-021-00094-9
Boudreau, M. W., Peh, J., and Hergenrother, P. J. (2019). Procaspase-3 Overexpression in Cancer: a Paradoxical Observation with Therapeutic Potential. ACS Chem. Biol. 14 (11), 2335–2348. doi:10.1021/acschembio.9b00338
Chao, J., Lu, T. C., Liao, J. W., Huang, T. H., Lee, M. S., Cheng, H. Y., et al. (2009). Analgesic and Anti-inflammatory Activities of Ethanol Root Extract of Mahonia Oiwakensis in Mice. J. Ethnopharmacol. 125 (2), 297–303. doi:10.1016/j.jep.2009.06.024
Chen, H. Y., Ye, X. L., Cui, X. L., He, K., Jin, Y. N., Chen, Z., et al. (2012). Cytotoxicity and Antihyperglycemic Effect of Minor Constituents from Rhizoma Coptis in HepG2 Cells. Fitoterapia 83 (1), 67–73. doi:10.1016/j.fitote.2011.09.014
Chen, H., Fan, G., and He, Y. (2017). Species Evolution and Quality Evaluation of Four Coptis Herbal Medicinal Materials in Southwest China. 3 Biotech. 7 (1), 62. doi:10.1007/s13205-017-0679-8
Constantine, G. H., Vitek, M. R., Sheth, K., Catalfomo, P., and Sciuchetti, L. A. (1966). Chromatographic Study of the Alkaloids of Aquilegia Formosa. J. Pharm. Sci. 55 (9), 982–984. doi:10.1002/jps.2600550926
Cui, H. M., Zhang, Q. Y., Wang, J. L., Chen, J. L., Zhang, Y. L., and Tong, X. L. (2015). Poor Permeability and Absorption Affect the Activity of Four Alkaloids from Coptis. Mol. Med. Rep. 12 (5), 7160–7168. doi:10.3892/mmr.2015.4288
Cui, H., Hu, Y., Li, J., Yuan, K., and Guo, Y. (2019). Preparation and Evaluation of Antidiabetic Agents of Berberine Organic Acid Salts for Enhancing the Bioavailability. Molecules 24 (1), 103. doi:10.3390/molecules24010103
Da-Cunha, E. V., Fechinei, I. M., Guedes, D. N., Barbosa-Filho, J. M., and Da Silva, M. S. (2005). Protoberberine Alkaloids. Alkaloids Chem. Biol. 62, 1–75. doi:10.1016/s1099-4831(05)62001-9
Dary, C., Bun, S. S., Herbette, G., Mabrouki, F., Bun, H., Kim, S., et al. (2017). Chemical Profiling of the Tuber of Stephania Cambodica Gagnep. (Menispermaceae) and Analytical Control by UHPLC-DAD. Nat. Prod. Res. 31 (7), 802–809. doi:10.1080/14786419.2016.1247077
DeFronzo, R. A., Ferrannini, E., Groop, L., Henry, R. R., Herman, W. H., Holst, J. J., et al. (2015). Type 2 Diabetes Mellitus. Nat. Rev. Dis. Primers 1 (1), 15019–15022. doi:10.1038/nrdp.2015.19
Deng, B., and Wan, M. (2021). Jatrorrhizine Inhibits Liver Cancer Cell Growth by Targeting the Expressions of miR-221-3p and miR-15b-5p. Trop. J. Pharm. Res. 20 (8), 1573–1578. doi:10.4314/tjpr.v20i8.4
Deng, Y., Liao, Q., Li, S., Bi, K., Pan, B., and Xie, Z. (2008). Simultaneous Determination of Berberine, Palmatine and Jatrorrhizine by Liquid Chromatography-Tandem Mass Spectrometry in Rat Plasma and its Application in a Pharmacokinetic Study after Oral Administration of Coptis-Evodia Herb Couple. J. Chromatogr. B Analyt Technol. Biomed. Life Sci. 863 (2), 195–205. doi:10.1016/j.jchromb.2007.12.028
Desgrouas, C., Taudon, N., Bun, S. S., Baghdikian, B., Bory, S., Parzy, D., et al. (2014). Ethnobotany, Phytochemistry and Pharmacology of Stephania Rotunda Lour. J. Ethnopharmacol. 154 (3), 537–563. doi:10.1016/j.jep.2014.04.024
Dewaguet, J., Copin, M. C., Duhamel, A., Faivre, J. B., Deken, V., Sedlmair, M., et al. (2021). Dual-Energy CT Perfusion of Invasive Tumor Front in Non-Small Cell Lung Cancers. Radiology, 210600. doi:10.1148/radiol.2021210600
Du, W., Jin, L., Li, L., Wang, W., Zeng, S., Jiang, H., et al. (2018). Development and Validation of a HPLC-ESI-MS/MS Method for Simultaneous Quantification of Fourteen Alkaloids in Mouse Plasma after Oral Administration of the Extract of Corydalis Yanhusuo Tuber: Application to Pharmacokinetic Study. Molecules 23 (4), 714. doi:10.3390/molecules23040714
Duan, W., and Chen, X. (2021). Jatrorrhizine Can Improve Nerve Cell Injury Induced by Aβ 25-35, Acting through miR-223-3p/HDAC4 axis. Am. J. Transl. Res. 13 (5), 4644–4655.
Fechine, I. M., Lima, M. A., Navarro, V. R., Da Cunha, E. V. L., Silva, M. S., Barbosa-Filho, J. M., et al. (2002). Alcalóides de Duguetia trunciflora Maas (Annonaceae). Rev. Bras. Farmacogn. 12, 17–19. doi:10.1590/s0102-695x2002000300009
Feng, T., Du, H., Chen, H., Xiao, Q., He, Y., and Fan, G. (2018). Comparative Analysis of Genetic and Chemical Differences between Four Berberis Herbs Based on Molecular Phylogenetic and HPLC Methods. Biol. Pharm. Bull. 41 (12), 1870–1873. doi:10.1248/bpb.b18-00327
Furuya, T., Yoshikawa, T., and Kiyohara, H. (1983). Alkaloid Production in Cultured Cells of Dioscoreophyllum Cumminsii. Phytochemistry 22 (7), 1671–1673. doi:10.1016/0031-9422(83)80108-3
González-Álvarez, H., Bravo-Jiménez, A., Martínez-Arellanes, M., Gamboa-Osorio, G. O., Chávez-Gutiérrez, E., González-Hernández, L. A., et al. (2021). In Silico-Based Design and In Vivo Evaluation of an Anthranilic Acid Derivative as a Multitarget Drug in a Diet-Induced Metabolic Syndrome Model. Pharmaceuticals 14 (9), 914. doi:10.3390/ph14090914
Gurib-Fakim, A. (2006). Medicinal Plants: Traditions of Yesterday and Drugs of Tomorrow. Mol. Aspects Med. 27 (1), 1–93. doi:10.1016/j.mam.2005.07.008
Hagel, J. M., and Facchini, P. J. (2010). Dioxygenases Catalyze the O-Demethylation Steps of Morphine Biosynthesis in Opium Poppy. Nat. Chem. Biol. 6 (4), 273–275. doi:10.1038/nchembio.317
Han, F. M., Zhu, M. M., Chen, H. X., and Chen, Y. (2006). Liquid Chromatography-Tandem Electrospray Ionization Ion Trap Mass Spectrometric Assay for the Metabolites of Jatrorrhizine in Rat Urine. Yao Xue Xue Bao 41 (9), 846–851. doi:10.16438/j.0513-4870.2006.09.009
He, J. M., and Mu, Q. (2015). The Medicinal Uses of the Genus Mahonia in Traditional Chinese Medicine: An Ethnopharmacological, Phytochemical and Pharmacological Review. J. Ethnopharmacol. 175, 668–683. doi:10.1016/j.jep.2015.09.013
He, W., Liu, G., Cai, H., Sun, X., Hou, W., Zhang, P., et al. (2014a). Integrated Pharmacokinetics of Five Protoberberine-type Alkaloids in normal and Insomnic Rats after Single and Multiple Oral Administration of Jiao-Tai-Wan. J. Ethnopharmacol. 154 (3), 635–644. doi:10.1016/j.jep.2014.04.040
He, Y., Hou, P., Fan, G., Arain, S., and Peng, C. (2014b). Comprehensive Analyses of Molecular Phylogeny and Main Alkaloids for Coptis (Ranunculaceae) Species Identification. Biochem. Syst. Ecol. 56, 88–94. doi:10.1016/j.bse.2014.05.002
He, K., Kou, S., Zou, Z., Hu, Y., Feng, M., Han, B., et al. (2016). Hypolipidemic Effects of Alkaloids from Rhizoma Coptidis in Diet-Induced Hyperlipidemic Hamsters. Planta Med. 82 (8), 690–697. doi:10.1055/s-0035-1568261
He, S. M., Liang, Y. L., Cong, K., Chen, G., Zhao, X., Zhao, Q. M., et al. (2018). Identification and Characterization of Genes Involved in Benzylisoquinoline Alkaloid Biosynthesis in Coptis Species. Front. Plant Sci. 9, 731. doi:10.3389/fpls.2018.00731
Hussain, R. A., Kim, J., Beecher, C. W., and Douglas Kinghorn, A. (1989). Unambiguous Carbon-13 NMR Assignments of Some Biologically Active Photoberberine Alkaloids. Heterocycles 29 (12), 2257–2260.
Ikuta, A., Syōno, K., and Furuya, T. (1975). Alkaloids in Plants Regenerated from Coptis Callus Cultures. Phytochemistry 14 (5-6), 1209–1210. doi:10.1016/s0031-9422(00)98596-0
Iwasa, K., Takahashi, T., Nishiyama, Y., Moriyasu, M., Sugiura, M., Takeuchi, A., et al. (2008). Online Structural Elucidation of Alkaloids and Other Constituents in Crude Extracts and Cultured Cells of Nandina Domestica by Combination of LC-MS/MS, LC-NMR, and LC-CD Analyses. J. Nat. Prod. 71 (8), 1376–1385. doi:10.1021/np8001496
Jiang, W., Duan, W. B., Li, S., Shen, X. Y., Zhou, Y., Luo, T., et al. (2015). Jatrorrhizine Protects against Okadaic Acid Induced Oxidative Toxicity through Inhibiting the Mitogen-Activated Protein Kinases Pathways in HT22 Hippocampal Neurons. CNS Neurol. Disord. Drug Targets 14 (10), 1334–1342. doi:10.2174/1871527314666150821104455
Jiang, X., Zhang, N., Xiong, N., Liu, Y., Cao, J., and Qiu, S. (2017). Synthesis and Biological Evaluation of Novel Jatrorrhizine Derivatives with Amino Groups Linked at the 3-position as Inhibitors of Acetylcholinesterase. J. Chem-ny 2017, 3261520. doi:10.1155/2017/3261520
Karri, S., Sharma, S., Hatware, K., and Patil, K. (2019). Natural Anti-obesity Agents and Their Therapeutic Role in Management of Obesity: A Future Trend Perspective. Biomed. Pharmacother. 110, 224–238. doi:10.1016/j.biopha.2018.11.076
Kim, J. H., Ryu, Y. B., Lee, W. S., and Kim, Y. H. (2014). Neuraminidase Inhibitory Activities of Quaternary Isoquinoline Alkaloids from Corydalis Turtschaninovii Rhizome. Bioorg. Med. Chem. 22 (21), 6047–6052. doi:10.1016/j.bmc.2014.09.004
Kong, L. D., Cheng, C. H., and Tan, R. X. (2001). Monoamine Oxidase Inhibitors from Rhizoma of Coptis Chinensis. Planta Med. 67 (1), 74–76. doi:10.1055/s-2001-10874
Kukula-Koch, W. (2017). The Elevation of LC-ESI-Q-TOF-MS Response in the Analysis of Isoquinoline Alkaloids from Some Papaveraceae and Berberidaceae Representatives. J. Anal. Methods Chem. 2017, 8384107. doi:10.1155/2017/8384107
Le, P. M., McCooeye, M., and Windust, A. (2014). Application of UPLC-QTOF-MS in MS(E) Mode for the Rapid and Precise Identification of Alkaloids in Goldenseal (Hydrastis canadensis). Anal. Bioanal. Chem. 406 (6), 1739–1749. doi:10.1007/s00216-013-7558-x
Li, Y., Wang, H., Si, N., Ren, W., Han, L., Xin, S., et al. (2015). Metabolic Profiling Analysis of Berberine, Palmatine, Jatrorrhizine, Coptisine and Epiberberine in Zebrafish by Ultra-High Performance Liquid Chromatography Coupled with LTQ Orbitrap Mass Spectrometer. Xenobiotica 45 (4), 302–311. doi:10.3109/00498254.2014.979270
Li, L., Sun, S., Weng, Y., Song, F., Zhou, S., Bai, M., et al. (2016). Interaction of Six Protoberberine Alkaloids with Human Organic Cation Transporters 1, 2 and 3. Xenobiotica 46 (2), 175–183. doi:10.3109/00498254.2015.1056283
Li, H., Wang, J., Sun, Q., Chen, G., Sun, S., Ma, X., et al. (2018). Jatrorrhizine Hydrochloride Suppresses RANKL-Induced Osteoclastogenesis and Protects against Wear Particle-Induced Osteolysis. Int. J. Mol. Sci. 19 (11), 3698. doi:10.3390/ijms19113698
Li, Q., Zhao, C., Zhang, Y., Du, H., Xu, T., Xu, X., et al. (2020). 1H NMR-Based Metabolomics Coupled with Molecular Docking Reveal the Anti-Diabetic Effects and Potential Active Components of Berberis Vernae on Type 2 Diabetic Rats. Front. Pharmacol. 11, 932. doi:10.3389/fphar.2020.00932
Li, X., Zhang, C., Ma, W., Xie, X., and Huang, Q. (2021). Oridonin: A Review of its Pharmacology, Pharmacokinetics and Toxicity. Front. Pharmacol. 12, 1642. doi:10.3389/fphar.2021.645824
Lillich, F. F., Imig, J. D., and Proschak, E. (2021). Multi-Target Approaches in Metabolic Syndrome. Front. Pharmacol. 11, 554961. doi:10.3389/fphar.2020.554961
Lin, Y., Guo, H. C., Kuang, Y., Shang, Z. P., Li, B., Chen, K., et al. (2020). AChE Inhibitory Alkaloids from Coptis Chinensis. Fitoterapia 141, 104464. doi:10.1016/j.fitote.2019.104464
Liu, F., Li, Z., Shi, X., and Zhong, M. (2011). Determination of Berberine, Palmatine and Jatrorrhizine in Rabbit Plasma by Liquid Chromatography-Electrospray Ionization-Mass Spectrometry. J. Pharm. Biomed. Anal. 56 (5), 1006–1015. doi:10.1016/j.jpba.2011.08.001
Liu, R., Cao, Z., Pan, Y., Zhang, G., Yang, P., Guo, P., et al. (2013). Jatrorrhizine Hydrochloride Inhibits the Proliferation and Neovascularization of C8161 Metastatic Melanoma Cells. Anticancer Drugs 24 (7), 667–676. doi:10.1097/CAD.0b013e328361ab28
Liu, J., Li, Q., Wang, C., Shao, J., Wang, T., Wu, D., et al. (2020). Antifungal Evaluation of Traditional Herbal Monomers and Their Potential for Inducing Cell wall Remodeling in Candida Albicans and Candida Auris. Biofouling 36 (3), 319–331. doi:10.1080/08927014.2020.1759559
Lo, S. N., Wang, C. W., Chen, Y. S., Huang, C. C., Wu, T. S., Li, L. A., et al. (2017). Berberine Activates Aryl Hydrocarbon Receptor but Suppresses CYP1A1 Induction through miR-21-3p Stimulation in MCF-7 Breast Cancer Cells. Molecules 22 (11), 1847. doi:10.3390/molecules22111847
Lou, Z. C., Gao, C. Y., Lin, F. T., Lin, M. C., Zhang, J., Slatkin, D. J., et al. (1987). Quaternary Alkaloids of Thalictrum Glandulosissimum. Planta Med. 53 (11), 498–499. doi:10.1055/s-2006-962784
Lu, K., Wei, W., Hu, J., Wen, D., Ma, B., Liu, W., et al. (2020). Apoptosis Activation in Thyroid Cancer Cells by Jatrorrhizine-Platinum(II) Complex via Downregulation of PI3K/AKT/Mammalian Target of Rapamycin (mTOR) Pathway. Med. Sci. Monit. 26, e922518-1–e922518-10. doi:10.12659/MSM.922518
Luo, T., Zhang, H., Zhang, W. W., Huang, J. T., Song, E. L., Chen, S. G., et al. (2011). Neuroprotective Effect of Jatrorrhizine on Hydrogen Peroxide-Induced Cell Injury and its Potential Mechanisms in PC12 Cells. Neurosci. Lett. 498 (3), 227–231. doi:10.1016/j.neulet.2011.05.017
Luo, J., Yan, D., Yang, M., Dong, X., and Xiao, X. (2013). Multicomponent Therapeutics of Berberine Alkaloids. Evid. Based Complement. Alternat Med. 2013, 545898. doi:10.1155/2013/545898
Luo, S., Guo, L., Sheng, C., Zhao, Y., Chen, L., Li, C., et al. (2020). Rapid Identification and Isolation of Neuraminidase Inhibitors from Mockstrawberry (Duchesnea Indica Andr.) Based on Ligand Fishing Combined with HR-ESI-Q-TOF-MS. Acta Pharm. Sin. B 10 (10), 1846–1855. doi:10.1016/j.apsb.2020.04.001
Lyu, Y., Lin, L., Xie, Y., Li, D., Xiao, M., Zhang, Y., et al. (2021). Blood-Glucose-Lowering Effect of Coptidis Rhizoma Extracts from Different Origins via Gut Microbiota Modulation in Db/db Mice. Front. Pharmacol. 12, 684358. doi:10.3389/fphar.2021.684358
Ma, H., Hu, Y., Zou, Z., Feng, M., Ye, X., and Li, X. (2016). Antihyperglycemia and Antihyperlipidemia Effect of Protoberberine Alkaloids from Rhizoma Coptidis in HepG2 Cell and Diabetic KK-Ay Mice. Drug Dev. Res. 77 (4), 163–170. doi:10.1002/ddr.21302
Malebo, H. M., Wenzler, T., Cal, M., Swaleh, S. M., Omolo, M. O., Hassanali, A., et al. (2013). Anti-Protozoal Activity of Aporphine and Protoberberine Alkaloids from Annickia Kummeriae (Engl. & Diels) Setten & Maas (Annonaceae). BMC Complement. Altern. Med. 13 (1), 48–10. doi:10.1186/1472-6882-13-48
Mao, Z., Di, X., Zhang, J., Wang, X., Liu, Y., and Di, X. (2017). Rapid and Cost-Effective Method for the Simultaneous Quantification of Seven Alkaloids in Corydalis Decumbens by Microwave-Assisted Extraction and Capillary Electrophoresis. J. Sep. Sci. 40 (14), 3008–3014. doi:10.1002/jssc.201700051
Meng, F. C., Wu, Z. F., Yin, Z. Q., Lin, L. G., Wang, R., and Zhang, Q. W. (2018). Coptidis Rhizoma and its Main Bioactive Components: Recent Advances in Chemical Investigation, Quality Evaluation and Pharmacological Activity. Chin. Med. 13 (1), 13–18. doi:10.1186/s13020-018-0171-3
Mori-Quiroz, L. M., Hedrick, S. L., De Los Santos, A. R., and Clift, M. D. (2018). A Unified Strategy for the Syntheses of the Isoquinolinium Alkaloids Berberine, Coptisine, and Jatrorrhizine. Org. Lett. 20 (14), 4281–4284. doi:10.1021/acs.orglett.8b01702
Namthabad, S., and Mamidala, E. (2014). Molecular Docking of HIV-1 Protease Using Alkaloids from Tinospora Cordifolia. Int. J. Res. Appl. 1 (1), 12–16.
Neef, C., Oosting, R., and Meijer, D. K. (1984). Structure-Pharmacokinetics Relationship of Quaternary Ammonium Compounds. Elimination and Distribution Characteristics. Naunyn Schmiedebergs Arch. Pharmacol. 328 (2), 103–110. doi:10.1007/BF00512058
Nishiyama, Y., Moriyasu, M., Ichimaru, M., Iwasa, K., Kato, A., Mathenge, S. G., et al. (2004). Quaternary Isoquinoline Alkaloids from Xylopia Parviflora. Phytochemistry 65 (7), 939–944. doi:10.1016/j.phytochem.2003.12.010
Odoh, U. E., Uzor, P. F., Eze, C. L., Akunne, T. C., Onyegbulam, C. M., and Osadebe, P. O. (2018). Medicinal Plants Used by the People of Nsukka Local Government Area, South-Eastern Nigeria for the Treatment of Malaria: An Ethnobotanical Survey. J. Ethnopharmacol. 218, 1–15. doi:10.1016/j.jep.2018.02.034
Olivier, D. K., Van Vuuren, S. F., and Moteetee, A. N. (2015). Annickia Affinis and A. Chlorantha (Enantia Chlorantha)--A Review of Two Closely Related Medicinal Plants from Tropical Africa. J. Ethnopharmacol. 176, 438–462. doi:10.1016/j.jep.2015.10.021
Patel, M. B., and Mishra, S. (2011). Hypoglycemic Activity of Alkaloidal Fraction of Tinospora Cordifolia. Phytomedicine 18 (12), 1045–1052. doi:10.1016/j.phymed.2011.05.006
Patel, M. B., and Mishra, S. (2012a). Isoquinoline Alkaloids from Tinospora Cordifolia Inhibit Rat Lens Aldose Reductase. Phytother. Res. 26 (9), 1342–1347. doi:10.1002/ptr.3721
Patel, M. B., and Mishra, S. M. (2012b). Magnoflorine from Tinospora Cordifolia Stem Inhibits α-glucosidase and Is Antiglycemic in Rats. J. Funct. Foods 4 (1), 79–86. doi:10.1016/j.jff.2011.08.002
Pérez, E. G., and Cassels, B. K. (2010). Alkaloids from the Genus Duguetia. Alkaloids: Chem. Biol. 68, 83–156. doi:10.1016/s1099-4831(10)06803-3
Peng, C. Y., Liu, J. Q., Zhang, R., and Shu, J. C. (2014). A New Alkaloid from the Fruit of Nandina Domestica Thunb. Nat. Prod. Res. 28 (15), 1159–1164. doi:10.1080/14786419.2014.921166
M, P., Reddy, G. J., Hema, K., Dodoala, S., and Koganti, B. (2021). Unravelling High-Affinity Binding Compounds towards Transmembrane Protease Serine 2 Enzyme in Treating SARS-CoV-2 Infection Using Molecular Modelling and Docking Studies. Eur. J. Pharmacol. 890, 173688. doi:10.1016/j.ejphar.2020.173688
Porras, G., Chassagne, F., Lyles, J. T., Marquez, L., Dettweiler, M., Salam, A. M., et al. (2020). Ethnobotany and the Role of Plant Natural Products in Antibiotic Drug Discovery. Chem. Rev. 121 (6), 3495–3560. doi:10.1021/acs.chemrev.0c00922
Pyne, M. E., Kevvai, K., Grewal, P. S., Narcross, L., Choi, B., Bourgeois, L., et al. (2020). A Yeast Platform for High-Level Synthesis of Tetrahydroisoquinoline Alkaloids. Nat. Commun. 11 (1), 3337. doi:10.1038/s41467-020-17172-x
Qin, R., and Jiang, H. (2011). Determination the Content of Jatrorrhizine in Thalictrum of Changbai Mountain by the Method of Thin-Layer Chromatography. North. Hortic. 14, 175–176.
Qin, Q. P., Wang, Z. F., Huang, X. L., Tan, M. X., Luo, Z. H., Wang, S. L., et al. (2019a). Two Telomerase-Targeting Pt(ii) Complexes of Jatrorrhizine and Berberine Derivatives Induce Apoptosis in Human Bladder Tumor Cells. Dalton Trans. 48 (40), 15247–15254. doi:10.1039/c9dt02381j
Qin, Q. P., Zou, B. Q., Wang, Z. F., Huang, X. L., Zhang, Y., Tan, M. X., et al. (2019b). High In vitro and In vivo Antitumor Activities of Luminecent Platinum(II) Complexes with Jatrorrhizine Derivatives. Eur. J. Med. Chem. 183, 111727. doi:10.1016/j.ejmech.2019.111727
Qiu, H., Sun, S., Ma, X., Cui, C., Chen, G., Liu, Z., et al. (2018). Jatrorrhizine Hydrochloride Suppresses Proliferation, Migration, and Secretion of Synoviocytes In Vitro and Ameliorates Rat Models of Rheumatoid Arthritis In Vivo. Int. J. Mol. Sci. 19 (5), 1514. doi:10.3390/ijms19051514
Rao, G. X., Zhang, S., Wang, H. M., Li, Z. M., Gao, S., and Xu, G. L. (2009). Antifungal Alkaloids from the Fresh rattan Stem of Fibraurea Recisa Pierre. J. Ethnopharmacol. 123 (1), 1–5. doi:10.1016/j.jep.2009.02.046
Röhrig, U. F., Reynaud, A., Majjigapu, S. R., Vogel, P., Pojer, F., and Zoete, V. (2019). Inhibition Mechanisms of Indoleamine 2,3-Dioxygenase 1 (Ido1). J. Med. Chem. 62 (19), 8784–8795. doi:10.1021/acs.jmedchem.9b00942
Romanowski, S., and Eustáquio, A. S. (2020). Synthetic Biology for Natural Product Drug Production and Engineering. Curr. Opin. Chem. Biol. 58, 137–145. doi:10.1016/j.cbpa.2020.09.006
Rosell, R., and Karachaliou, N. (2015). Relationship between Gene Mutation and Lung Cancer Metastasis. Cancer Metastasis Rev. 34 (2), 243–248. doi:10.1007/s10555-015-9557-1
Roy, P. S., and Saikia, B. J. (2016). Cancer and Cure: A Critical Analysis. Indian J. Cancer 53 (3), 441–442. doi:10.4103/0019-509X.200658
Ryuk, J. A., Zheng, M. S., Lee, M. Y., Seo, C. S., Li, Y., Lee, S. H., et al. (2012). Discrimination of Phellodendron Amurense and P. Chinense Based on DNA Analysis and the Simultaneous Analysis of Alkaloids. Arch. Pharm. Res. 35 (6), 1045–1054. doi:10.1007/s12272-012-0612-y
Sharma, P., Mehta, M., Dhanjal, D. S., Kaur, S., Gupta, G., Singh, H., et al. (2019). Emerging Trends in the Novel Drug Delivery Approaches for the Treatment of Lung Cancer. Chem. Biol. Interact 309, 108720. doi:10.1016/j.cbi.2019.06.033
Sharma, N., Kumar, V., Chopra, M. P., Sourirajan, A., Dev, K., and El-Shazly, M. (2020). Thalictrum Foliolosum: A Lesser Unexplored Medicinal Herb from the Himalayan Region as a Source of Valuable Benzyl Isoquinoline Alkaloids. J. Ethnopharmacol. 255, 112736. doi:10.1016/j.jep.2020.112736
Shi, R., Zhou, H., Ma, B., Ma, Y., Wu, D., Wang, X., et al. (2012). Pharmacokinetics and Metabolism of Jatrorrhizine, a Gastric Prokinetic Drug Candidate. Biopharm. Drug Dispos. 33 (3), 135–145. doi:10.1002/bdd.1779
Singh, S., Verma, M., Malhotra, M., Prakash, S., and Singh, T. D. (2016). Cytotoxicity of Alkaloids Isolated from Argemone Mexicana on SW480 Human colon Cancer Cell Line. Pharm. Biol. 54 (4), 740–745. doi:10.3109/13880209.2015.1073334
Singh, A., Bajpai, V., Kumar, S., Singh Rawat, A. K., and Kumar, B. (2017). Analysis of Isoquinoline Alkaloids from Mahonia Leschenaultia and Mahonia Napaulensis Roots Using UHPLC-Orbitrap-MSn and UHPLC-QqQLIT-MS/MS. J. Pharm. Anal. 7 (2), 77–86. doi:10.1016/j.jpha.2016.10.002
Slavík, J., and Slavíková, L. (1989). Alkaloids from Corydalis Nobilis (L.) Pers. And C. intermedia (L.) Mérat. Collect. Czech. Chem. Commun. 54 (7), 2009–2020.
Slobodníková, L., KoSt'álová, D., Labudová, D., Kotulová, D., and Kettmann, V. (2004). Antimicrobial Activity of Mahonia Aquifolium Crude Extract and its Major Isolated Alkaloids. Phytother. Res. 18 (8), 674–676. doi:10.1002/ptr.1517
Su, C. R., Ueng, Y. F., Dung, N. X., Vijaya Bhaskar Reddy, M., and Wu, T. S. (2007). Cytochrome P3A4 Inhibitors and Other Constituents of Fibraurea Tinctoria. J. Nat. Prod. 70 (12), 1930–1933. doi:10.1021/np0704248
Su, H., Zhang, C., Zou, X., Lu, F., Zeng, Y., Guan, H., et al. (2020). Jiao-tai-wan Inhibits Inflammation of the Gut-Brain-Axis and Attenuates Cognitive Impairment in Insomnic Rats. J. Ethnopharmacol 250, 112478. doi:10.1016/j.jep.2019.112478
Sun, L., Ding, F., You, G., Liu, H., Wang, M., Ren, X., et al. (2018). Development and Validation of an Uplc-Ms/ms Method for Pharmacokinetic Comparison of Five Alkaloids from Jinqi Jiangtang Tablets and its Monarch Drug Coptidis Rhizoma. Pharmaceutics 10 (1), 4. doi:10.3390/pharmaceutics10010004
Sun, Y., Gao, X., Wu, P., Wink, M., Li, J., Dian, L., et al. (2019). Jatrorrhizine Inhibits Mammary Carcinoma Cells by Targeting TNIK Mediated Wnt/β-Catenin Signalling and Epithelial-Mesenchymal Transition (EMT). Phytomedicine 63, 153015. doi:10.1016/j.phymed.2019.153015
Tian, Y., Zhang, C., and Guo, M. (2017). Comparative Study on Alkaloids and Their Anti-Proliferative Activities from Three Zanthoxylum Species. BMC Complement. Altern. Med. 17 (1), 460. doi:10.1186/s12906-017-1966-y
Tiwari, S., Atluri, V., Kaushik, A., Yndart, A., and Nair, M. (2019). Alzheimer's Disease: Pathogenesis, Diagnostics, and Therapeutics. Int. J. Nanomedicine 14, 5541–5554. doi:10.2147/IJN.S200490
Tseng, C., Sun, M., Kao, T., Li, T., and Lin, C. (2021). Role of Coptis Chinensis in Antibiotic Susceptibility of Carbapenem-Resistant Klebsiella pneumoniae. J. Microbiol. Immunol. Infect. 7, 1–10. doi:10.1016/j.jmii.2021.07.003
Vogt, N. M., Kerby, R. L., Dill-McFarland, K. A., Harding, S. J., Merluzzi, A. P., Johnson, S. C., et al. (2017). Gut Microbiome Alterations in Alzheimer's Disease. Sci. Rep. 7 (1), 13537. doi:10.1038/s41598-017-13601-y
Wang, S., Li, X., Niu, Y., Liu, Y., Zhu, Y., Lu, X., et al. (2016). Identification and Screening of Chemical Constituents with Hepatoprotective Effects from Three Traditional Chinese Medicines for Treating Jaundice. J. Sep. Sci. 39 (19), 3690–3699. doi:10.1002/jssc.201600437
Wang, Y., Zhang, H., and Zhang, X. (2017). Effects of Jatrorrhizine on Akt/AMPK/eNOS Signaling Pathways in Blood Vessel of Diabetes Rats. Her. Med. 36 (10), 1107–1111.
Wang, P., Gao, X. Y., Yang, S. Q., Sun, Z. X., Dian, L. L., Qasim, M., et al. (2019a). Jatrorrhizine Inhibits Colorectal Carcinoma Proliferation and Metastasis through Wnt/β-Catenin Signaling Pathway and Epithelial-Mesenchymal Transition. Drug Des. Devel Ther. 13, 2235–2247. doi:10.2147/DDDT.S207315
Wang, S., Jiang, W., Ouyang, T., Shen, X. Y., Wang, F., Qu, Y. H., et al. (2019b). Jatrorrhizine Balances the Gut Microbiota and Reverses Learning and Memory Deficits in APP/PS1 Transgenic Mice. Sci. Rep. 9 (1), 19575. doi:10.1038/s41598-019-56149-9
Wen, L. N., and Xie, M. X. (2017). Spectroscopic Investigation of the Interaction between G-Quadruplex of KRAS Promoter Sequence and Three Isoquinoline Alkaloids. Spectrochim Acta A. Mol. Biomol. Spectrosc. 171, 287–296. doi:10.1016/j.saa.2016.08.013
Wu, H., He, K., Wang, Y., Xue, D., Ning, N., Zou, Z., et al. (2014). The Antihypercholesterolemic Effect of Jatrorrhizine Isolated from Rhizoma Coptidis. Phytomedicine 21 (11), 1373–1381. doi:10.1016/j.phymed.2014.05.002
Wu, G., Mu, T., Zhang, L., and Chen, X. (2020). Jatrorrhizine Hydrochloride Alleviates Tert-Butyl Hydroperoxide-Induced Endothelial Cell Injury through its Anti-inflammatory Activity and PPAR-γ Activation. Cel Mol Biol (Noisy-le-grand) 66 (2), 125–129. doi:10.14715/cmb/2020.66.2.20
Xiang, Q., Hashi, Y., and Chen, Z. (2016). Simultaneous Detection of Eight Active Components in Radix Tinosporae by Ultra High Performance Liquid Chromatography Coupled with Electrospray Tandem Mass Spectrometry. J. Sep. Sci. 39 (11), 2036–2042. doi:10.1002/jssc.201600042
Xiao, H. T., Peng, J., Liang, Y., Yang, J., Bai, X., Hao, X. Y., et al. (2011). Acetylcholinesterase Inhibitors from Corydalis Yanhusuo. Nat. Prod. Res. 25 (15), 1418–1422. doi:10.1080/14786410802496911
Yan, R., Wang, Y., Liu, Y., and Di, X. (2012). Comparative Pharmacokinetics of Berberine, Palmatine and Jatrorrhizine in Rat Plasma after Oral Administration of Rhizoma Coptidis and Zuojinwan Using Liquid Chromatography-Tandem Mass Spectrometry. Iran. J. Pharm. Res. 11 (3), 949–957.
Yang, W., She, L., Yu, K., Yan, S., Zhang, X., Tian, X., et al. (2016). Jatrorrhizine Hydrochloride Attenuates Hyperlipidemia in a High-Fat Diet-Induced Obesity Mouse Model. Mol. Med. Rep. 14 (4), 3277–3284. doi:10.3892/mmr.2016.5634
Yang, Y.-F., Yu, B., Zhang, X.-X., and Zhu, Y.-H. (2021). Identification of TNIK as a Novel Potential Drug Target in Thyroid Cancer Based on Protein Druggability Prediction. Medicine 100 (16), e25541. doi:10.1097/md.0000000000025541
Yu, C. J., Zheng, M. F., Kuang, C. X., Huang, W. D., and Yang, Q. (2010). Oren-gedoku-to and its Constituents with Therapeutic Potential in Alzheimer's Disease Inhibit Indoleamine 2, 3-dioxygenase Activity In Vitro. J. Alzheimers Dis. 22 (1), 257–266. doi:10.3233/JAD-2010-100684
Yu, H., Wang, Y., Wang, X., Guo, J., Wang, H., Zhang, H., et al. (2019). Jatrorrhizine Suppresses the Antimicrobial Resistance of Methicillin-Resistant Staphylococcus A. Exp. Ther. Med. 18 (5), 3715–3722. doi:10.3892/etm.2019.8034
Yuan, S., Fang, J., Tu Qing, M., Tu, Z., Nie, G., and Chen, L. (2010). Chemical Constituents and Antimicrobial Effect of Tinospora Sagittata (oliv.) Gagnep. Chin. Pharm. J. 10, 48–52.
Yuan, J., Zhou, J., Hu, Z., Ji, G., Xie, J., and Wu, D. (2011). The Effects of Jatrorrhizine on Contractile Responses of Rat Ileum. Eur. J. Pharmacol. 663 (1-3), 74–79. doi:10.1016/j.ejphar.2011.05.002
Zhang, L.-B., and Rao, G.-X. (2009). Aporphine, Protoberberine and Morphine Alkaloids from the Tubers of Stephania Yunnanensis. Biochem. Syst. Ecol. 37 (5), 622–625. doi:10.1016/j.bse.2009.08.005
Zhang, Y., Shi, Q., Shi, P., Zhang, W., and Cheng, Y. (2006). Characterization of Isoquinoline Alkaloids, Diterpenoids and Steroids in the Chinese Herb Jin-Guo-Lan (Tinospora Sagittata and Tinospora Capillipes) by High-Performance Liquid Chromatography/electrospray Ionization with Multistage Mass Spectrometry. Rapid Commun. Mass. Spectrom. 20 (15), 2328–2342. doi:10.1002/rcm.2593
Zhang, Y., Wu, W., Han, F., and Chen, Y. (2008). LC/MS/MS for Identification of In Vivo and In Vitro Metabolites of Jatrorrhizine. Biomed. Chromatogr. 22 (12), 1360–1367. doi:10.1002/bmc.1066
Zhang, B., Cao, A., Zhou, J., Hu, Z., and Wu, D. (2012). Effect of Jatrorrhizine on Delayed Gastrointestinal Transit in Rat Postoperative Ileus. J. Pharm. Pharmacol. 64 (3), 413–419. doi:10.1111/j.2042-7158.2011.01407.x
Zhang, S., Liu, X., and Yang, Q. (2017). The Study of Indoleamine 2, 3-dioxygenase 1 and its Inhibitors. Fudan Univ. J. Med. Sci. 44 (1), 1–7.
Zhang, X.-j., Chen, J.-h., Luo, L., He, W., Liu, G.-h., Gong, J., et al. (2018). Comparative Brain Pharmacokinetic Study of Jiaotai Pills in Normal and Insomnic Rats Using Brain Microdialysis Combinated with LC-MS/MS. Chin. Herbal Medicines 10 (2), 206–214. doi:10.1016/j.chmed.2018.03.006
Zhang, Y., Wang, Q., Liu, R., Zhou, H., Crommen, J., Moaddel, R., et al. (2019). Rapid Screening and Identification of Monoamine Oxidase-A Inhibitors from Corydalis Rhizome Using Enzyme-Immobilized Magnetic Beads Based Method. J. Chromatogr. A. 1592, 1–8. doi:10.1016/j.chroma.2019.01.062
Zhao, H., Zhou, S., Zhang, M., Feng, J., Wang, S., Wang, D., et al. (2016). An In Vitro AChE Inhibition Assay Combined with UF-HPLC-ESI-Q-TOF/MS Approach for Screening and Characterizing of AChE Inhibitors from Roots of Coptis Chinensis Franch. J. Pharm. Biomed. Anal. 120, 235–240. doi:10.1016/j.jpba.2015.12.025
Zhao, X., Wang, Y., Zheng, L., Sun, C., Wang, C., Cong, H., et al. (2018). Comparative Pharmacokinetics Study of Five Alkaloids in Rat Plasma and Related Compound-Herb Interactions Mechanism after Oral Administration of Shuanghua Baihe Tablets. Nat. Prod. Res. 32 (17), 2031–2036. doi:10.1080/14786419.2017.1365075
Zhou, H., Shi, R., Ma, B., Ma, Y., Wang, C., Wu, D., et al. (2013). CYP450 1A2 and Multiple UGT1A Isoforms Are Responsible for Jatrorrhizine Metabolism in Human Liver Microsomes. Biopharm. Drug Dispos. 34 (3), 176–185. doi:10.1002/bdd.1835
Zhou, Y., Cao, S., Wang, Y., Xu, P., Yan, J., Bin, W., et al. (2014). Berberine Metabolites Could Induce Low Density Lipoprotein Receptor Up-Regulation to Exert Lipid-Lowering Effects in Human Hepatoma Cells. Fitoterapia 92, 230–237. doi:10.1016/j.fitote.2013.11.010
Keywords: jatrorrhizine, natural products, pharmacological properties, toxicology, pharmacokinetics
Citation: Zhong F, Chen Y, Chen J, Liao H, Li Y and Ma Y (2022) Jatrorrhizine: A Review of Sources, Pharmacology, Pharmacokinetics and Toxicity. Front. Pharmacol. 12:783127. doi: 10.3389/fphar.2021.783127
Received: 29 September 2021; Accepted: 14 December 2021;
Published: 13 January 2022.
Edited by:
Michael Heinrich, UCL School of Pharmacy, United KingdomReviewed by:
Guozheng Huang, Anhui University of Technology, ChinaJuan Carlos Sepúlveda-Arias, Technological University of Pereira, Colombia
Copyright © 2022 Zhong, Chen, Chen, Liao, Li and Ma. This is an open-access article distributed under the terms of the Creative Commons Attribution License (CC BY). The use, distribution or reproduction in other forums is permitted, provided the original author(s) and the copyright owner(s) are credited and that the original publication in this journal is cited, in accordance with accepted academic practice. No use, distribution or reproduction is permitted which does not comply with these terms.
*Correspondence: Yuntong Ma, Mayuntong@cdutcm.edu.cn