- 1Infectious Diseases Unit, Western Health, Melbourne, VIC, Australia
- 2The Center for Global Health and Diseases, Case Western Reserve University, Cleveland, OH, United States
- 3Victorian Infectious Diseases Service, Royal Melbourne Hospital, Melbourne, VIC, Australia
- 4Peter Doherty Institute of Infection and Immunity, Melbourne, VIC, Australia
Primaquine, an 8-aminoquinoline, is the only medication approved by the World Health Organization to treat the hypnozoite stage of Plasmodium vivax and P. ovale malaria. Relapse, triggered by activation of dormant hypnozoites in the liver, can occur weeks to years after primary infection, and provides the predominant source of transmission in endemic settings. Hence, primaquine is essential for individual treatment and P. vivax elimination efforts. However, primaquine use is limited by the risk of life-threatening acute hemolytic anemia in glucose-6-phosphate dehydrogenase (G6PD) deficient individuals. More recently, studies have demonstrated decreased efficacy of primaquine due to cytochrome P450 2D6 (CYP2D6) polymorphisms conferring an impaired metabolizer phenotype. Failure of standard primaquine therapy has occurred in individuals with decreased or absent CYP2D6 activity. Both G6PD and CYP2D6 are highly polymorphic genes, with considerable geographic and interethnic variability, adding complexity to primaquine use. Innovative strategies are required to overcome the dual challenge of G6PD deficiency and impaired primaquine metabolism. Further understanding of the pharmacogenetics of primaquine is key to utilizing its full potential. Accurate CYP2D6 genotype-phenotype translation may optimize primaquine dosing strategies for impaired metabolizers and expand its use in a safe, efficacious manner. At an individual level the current challenges with G6PD diagnostics and CYP2D6 testing limit clinical implementation of pharmacogenetics. However, further characterisation of the overlap and spectrum of G6PD and CYP2D6 activity may optimize primaquine use at a population level and facilitate region-specific dosing strategies for mass drug administration. This precision public health approach merits further investigation for P. vivax elimination.
Introduction
Plasmodium vivax and P. ovale are unique human malaria species in their ability to develop into hypnozoites, a liver stage that can remain dormant until relapse occurs weeks to years later (Krotoski 1985). Previously considered a benign disease there is now clear evidence that P. vivax can cause severe malaria (Baird 2013). Relapses can result in significant morbidity, and provide the predominant source for ongoing transmission in endemic settings, with up to 85% of P. vivax blood stage infections occurring due to reactivation of dormant hypnozoites (Ross et al., 2016; Commons et al., 2020). This poses a considerable challenge for global elimination efforts. Clearance of hypnozoites requires an 8-aminoquinoline (8AQ), such as primaquine (PQ) or tafenoquine (TQ), to achieve radical cure. However, use of 8AQ derivatives is limited by the risk of life-threatening acute haemolytic anemia (AHA) in glucose-6-phosphate dehydrogenase deficient (G6PDd) individuals. Additionally, because this risk is difficult to quantify in pregnancy, lactating women and infants, 8AQs are contraindicated in these groups. This safety concern has hampered widespread use, both at the individual level and as an elimination tool through mass drug administration (MDA).
Recently an additional issue with PQ efficacy has been identified, when multiple cases of P. vivax relapse were reported in patients treated with standard courses of PQ (Bennett et al., 2013, Ingram et al., 2014). The lack of PQ efficacy has been associated with cytochrome P450 2D6 (CYP2D6) polymorphisms conferring impaired metabolizer phenotypes of drug substrates of this hepatic detoxification enzyme (Baird et al., 2018b). There is significant geographic and interethnic variability in CYP2D6 metabolizer phenotypes, with high proportions of impaired metabolizers in P. vivax endemic areas, which may have considerable implications for the role of PQ for P. vivax elimination (Baird et al., 2018a).
Hence, despite 8AQs being in clinical use for more than 60 years, malaria-endemic countries remain unable to utilize their full potential. With no alternative hypnozoiticidal agents nearing licensure, innovative solutions are required to target the hypnozoite reservoir.
Primaquine – Safety and Efficacy Issues
Safety – Glucose-6-Phosphate Dehydrogenase Deficiency
Primaquine has been the mainstay of hypnozoiticidal therapy since it was first licenced as an anti-malarial in 1952. The description of AHA in G6PDd individuals taking PQ in 1956 was one of the earliest described pharmacogenetic associations (Dern et al., 1954; Alving et al., 1956). The X-linked defect of G6PDd has a highly polymorphic genotype, with 217 mutations identified (Gómez-Manzo et al., 2016). Enzyme activity varies depending on the severity of the variant and the gender of the patient (Luzzatto and Seneca 2014). In malaria-endemic countries the estimated frequency of deficiency alleles is 8% (Howes et al., 2012). However, there is considerable geographic and interethnic variability in G6PDd, with prevalence of G6PDd up to 32.5% in some regions (Figure 1) (Howes et al., 2012). In P. vivax endemic countries 14.3% of the population are estimated to be ineligible for PQ based on G6PDd and contraindications of pregnancy, lactation and age <6 months (Baird et al., 2018a). Currently, WHO recommends G6PD testing prior to PQ administration; however in most malaria-endemic countries PQ is withheld due to inability to test G6PD activity (Recht et al., 2018; World Health Organization 2021). Regulatory authorities have recently approved single dose TQ for P. vivax radical cure. However, due its long terminal elimination half-life (12–16 days) and the risk of AHA, higher G6PD activity (70%) is required, significantly limiting its use (Lacerda et al., 2019; Chu and Hwang 2021). Thus, PQ remains the only hypnozoiticidal agent recommended by WHO for radical cure of P. vivax (World Health Organization 2021).
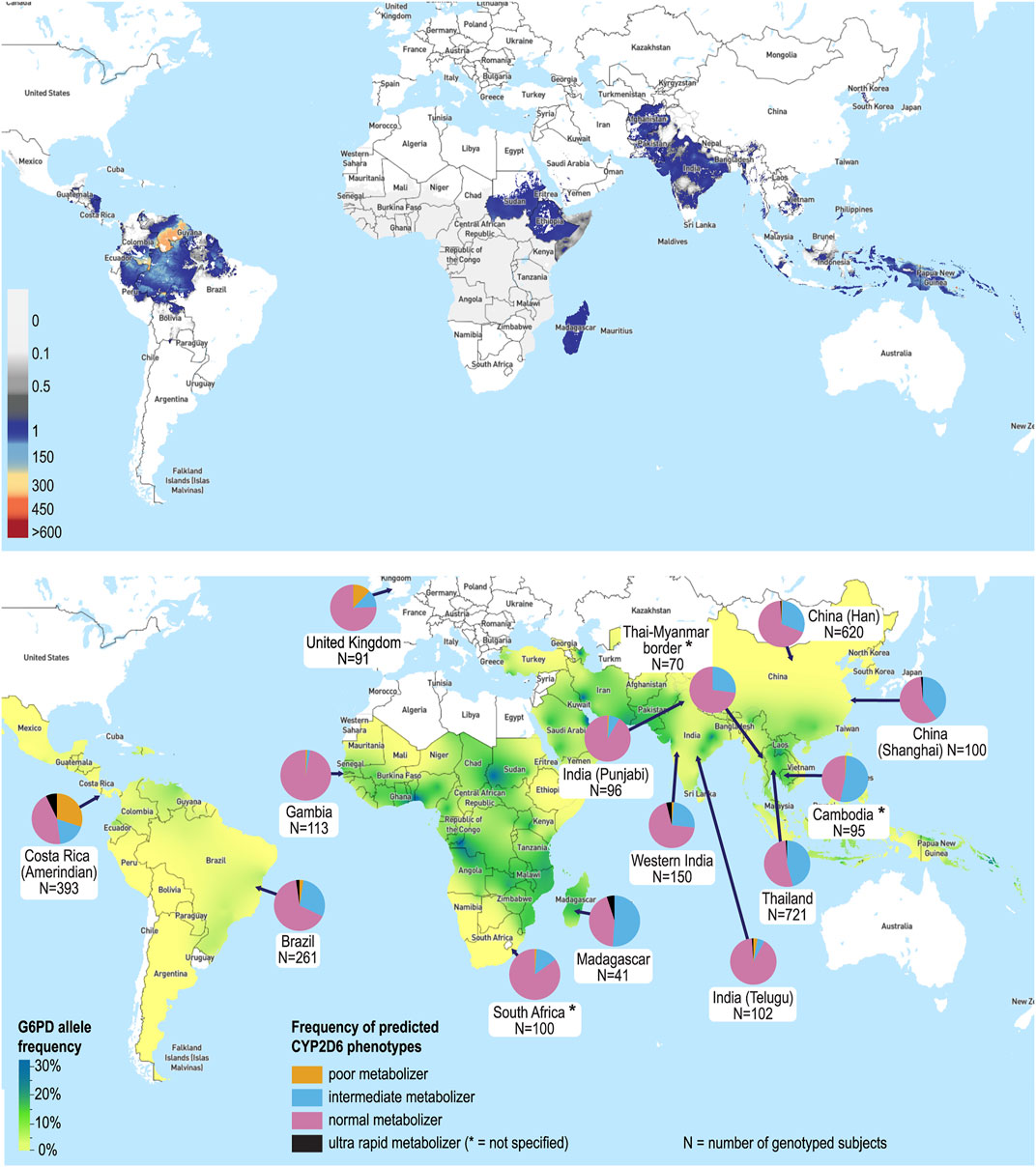
FIGURE 1. The global distribution of Plasmodium vivax, G6PDd and CYP2D6 metabolizer status. (A) Predicted incidence of P. vivax in 2017 [incidence in cases per 1,000 people per year are shown on a spectrum of white (zero incidence) to dark grey (1 case per 1,000) and then blue to red (>1 case per 1,000 to >600 cases per 1,000)] (Battle et al., 2019). (B) Map of the median predicted allele frequency of G6PDd (Howes et al., 2012), and CYP2D6 metabolizer status by country and/or ethnicity (selected sample represented) (Dodgen et al., 2016; Leitão et al., 2020; Spring et al., 2020; Koopmans et al., 2021; Mehlotra et al., 2021). Maps used with permission from the Malaria Atlas Project (https://malariaatlas.org).
Efficacy – CYP2D6 Polymorphisms
Primaquine is a pro-drug that requires metabolic transformation to metabolites active against hypnozoites. Primaquine’s mechanism of action is complex and still to be definitively defined. However, the hydroxylation pathway has been demonstrated in mouse and human studies to be CYP2D6 dependent (Pybus et al., 2012; Pybus et al., 2013; Potter et al., 2015; Popovici et al., 2021). Clinical evidence for CYP2D6 mediated metabolism, and its role in PQ efficacy comes from initial observations by Bennett et al., in 2013, where PQ treatment failures occurred in two subjects with CYP2D6 genotypes conferring impaired metabolism (Bennett et al., 2013). Further evidence of clinical failures associated with impaired CYP2D6 activity has been demonstrated in studies from Papua New Guinea, Indonesia, Brazil and China (Ingram et al., 2014; Silvino et al., 2016; Baird et al., 2018b; Brasil et al., 2018; Silvino et al., 2020; Huang et al., 2021). Over 20% of the population in P. vivax endemic areas are estimated to carry CYP2D6 alleles conferring impaired enzyme function, and are therefore at risk for PQ treatment failure (Baird et al., 2018a).
As outlined in the accompanying perspective piece by Olvany et al., the activity score (AS) metric, based on genotype, is used to predict the phenotype translation (poor (PM), intermediate (IM), normal (NM) and ultra-rapid metabolizers (UM)). Heterogeneity in the genotype-phenotype relationship has been observed, with significant inter- and intra-individual phenotypic variation (Gaedigk et al., 2018). In addition to single nucleotide polymorphisms, small insertions and deletions, copy number variations and the non-functional CYP2D7 pseudogene make it challenging to accurately assign phenotypes using genotyping approaches (Del Tredici et al., 2018; Nofziger et al., 2020). Additional genetic modifiers are hypothesized to play a role in phenotypic variability, and greater understanding of these modifiers is required to accurately determine metabolizer status (Gaedigk et al., 2018).
Regional variations in CYP2D6 enzyme activity are well characterized (Figure 1). While populations of European heritage have the highest frequency of PMs, common reduced function variants (e.g., *10, *17 and *41) result in a higher frequency of IMs in East Asian, African and Middle Eastern populations (Sistonen et al., 2007; LLerena et al., 2014). The allele conferring reduced function, CYP2D6*10, predominates in Southeast Asia (frequency up to 40%), the region with the highest P. vivax burden, with 1.4 billion people at risk (Gething et al., 2012; LLerena et al., 2014). Population admixture adds further complexity, as extrapolation by ethnicity is hampered by differences in biogeographical ancestry, unique allele combinations and frequency patterns (Suarez-Kurtz et al., 2014; Nofziger et al., 2020). From a clinical perspective pharmacogenetic knowledge of interethnic variability and admixture can influence choice of drugs for national formularies and dosing guidelines (Roederer and McLeod 2010). For example, in Brazil warfarin dosing algorithms based on CYP2C9 and VKORC1 genes were unreliable in regions with different population admixture (Botton et al., 2011; Suarez-Kurtz 2011).
Drug-drug and food-drug interactions can also influence CYP2D6 metabolic activity, with concomitant CYP2D6 inhibitors causing phenoconversion (Sasaki et al., 2017; Gaedigk et al., 2018; Nofziger et al., 2020). Similarly, physiologic and environmental factors may affect the CYP2D6 phenotype and AS (Gaedigk et al., 2018). Further characterization of these interactions, the need for adjustment to phenotype prediction or potential dosing algorithms will be required to ensure accurate application of PQ pharmacogenetics in clinical practice.
Clinical Implications
G6PDd and CYP2D6 Pharmacogenetics in Clinical Practice
Rapid progress in pharmacogenetic research has led to increased recognition of clinically actionable gene-drug pairs, with more than 50 drugs identified by the international Clinical Pharmacogenetics Implementation Consortium (CPIC) and the Dutch Pharmacogenetics Working Group (DPWG), and development of pharmacogenetic labelling (Swen et al., 2011; Yoon et al., 2020). However, there has been slow translation of pharmacogenetic testing and guided prescribing into clinical practice.
This is particularly true for malaria–despite the known association of G6PDd with PQ for over 60 years qualitative point-of-care (POC) G6PD diagnostics have only recently become available, and use remains limited in many areas (Thriemer et al., 2017). The first generation of these tests report enzyme activity >30% as “normal” and hence are not suitable for determining eligibility for TQ (LaRue et al., 2014). Additionally, qualitative tests cannot identify female heterozygotes with intermediate activity, and they therefore remain at risk of clinically significant hemolysis (Chu et al., 2017). Promisingly, quantitative POC G6PD diagnostics have recently been developed (e.g. SD Biosensor Standard™ G6PD), allowing identification of individuals with intermediate activity (Alam et al., 2018; Pal et al., 2019). While these represent significant progress, issues of accessibility, usability and cost remain (Thriemer et al., 2017).
Pharmacogenetic testing for CYP2D6 diplotypes has the potential to play a significant role in patient management prior to use of PQ, particularly for IM where alternative dosing strategies may be required. However, limitations for clinical CYP2D6 testing include laboratory expertise required, prolonged turnaround time, cost, low number of alleles included in commercial testing (particularly for those in less well studied populations), accuracy issues due to short-read sequencing and incomplete CYP2D6 genotype databases (Haga 2016; Hippman and Nislow 2019). These limitations preclude the use of pharmacogenetic testing in P. vivax endemic areas. In practice POC testing would be required for clinical use. However, due to the complexity of the CYP2D6 gene locus, this is not yet possible. Importantly, POC CYP2D6 testing would need to include common variations in regions where the test is deployed, given the geographic and ethnic variability in CYP2D6 diplotypes (Haga 2016).
Implementation of clinical pharmacogenetic testing requires accurate prediction of phenotype and corresponding dosing guidelines. Prior discrepancies in the categorization of AS and metabolizer status between CPIC and DPWG guidelines have now been resolved, with recent standardization of CYP2D6 genotype-phenotype translation (Caudle et al., 2020). Uptake of this consensus translation system by clinical laboratories and therapeutic guidelines will ensure consistent pharmacogenetic implementation. Activity scores can be used in high-resource settings to make therapeutic recommendations, such as for codeine (Crews et al., 2014). However, further refinement of the CYP2D6 genotype-phenotype relationship is required to make such AS recommendations for PQ (Gaedigk et al., 2018).
Primaquine Dosing
Optimizing PQ dosing will be essential in G6PDd and impaired PQ metabolizers, as the total dose of PQ administered, influences efficacy for radical cure, while AHA occurs in a dose-dependent manner, with decreased dosing frequency used as a strategy to mitigate this risk in populations with milder variants (John et al., 2012). In individuals with G6PDd of the African A− variant weekly dosing of PQ over 8 weeks (0.75 mg base/kg/week) has been shown to be a safe alternative to daily dosing for 14 days (0.25–0.5 mg base/kg/day), allowing for controlled hemolysis (Alving et al., 1960). However, the safety of such PQ dosing depends on the G6PDd variant in question, with safety of this regimen less clear in patients with more severe G6PDd variants (e.g. Mediterranean, Mahidol, Viangchan). In Pakistan, no hematological adverse effects were noted in a G6PDd patient (likely Mediterranean variant) included in a randomized trial of weekly PQ (Leslie et al., 2008). However, in Cambodia, where the Viangchan variant predominates, significant drops in hemoglobin were noted with weekly dosing, including a patient that required blood transfusion (Kheng et al., 2015). Drug-drug interactions may have compounded hemolysis in this patient (Kheng et al., 2015), highlighting the clinical complexity, including the need to consider concomitant medications, both from a safety and efficacy perspective.
P. vivax relapses after PQ therapy are most commonly associated with CYP2D6 diplotypes with an AS < 0.5; however relapses have also occurred in individuals with diplotypes with an AS = 1.5, a NM phenotype (Baird et al., 2018b). Therefore, a greater understanding of the role of genetic variation on PQ metabolism is required, and no definitive AS threshold for radical cure has been established. Potential dosing strategies, as outlined by Olvany et al., will need to balance an increased PQ dose for efficacy with the potential risk of AHA. In a recent randomized control trial high dose short-course PQ (1 mg base/kg/day for 7 days) was non-inferior and well tolerated in G6PD normal individuals (Taylor et al., 2019), highlighting an approach worth testing in clinical trials to assess greater PQ efficacy in IM.
Public Health Implications
Mass Drug Administration for P. vivax Elimination
Given the significant challenges integrating G6PDd and CYP2D6 phenotypes into individual case management, a population approach, utilizing pharmacogenetics in MDA merits consideration. Historically, PQ use in MDA has been an effective strategy for P. vivax control in eradication settings (Newby et al., 2015). In the majority of settings PQ was administered in the absence of G6PD testing (where known G6PDd prevalence varied between 1 and 39%) (Newby et al., 2015). However, close monitoring was undertaken and adverse effects were rare (Newby et al., 2015). In analysis of daily PQ use in these MDA programs the incidence of significant hemolysis was estimated at 1.8 cases per million exposed (Recht et al., 2014). The MDA strategy led to suppression of transmission in Papua New Guinea, China, Afghanistan, Azerbaijan, Tajikistan and Democratic People’s Republic of Korea, and sustained interruption of transmission on Aneityum island, Vanuatu (Kaneko et al., 2000; Hsiang et al., 2013; Kondrashin et al., 2014; Newby et al., 2015). Currently the WHO does not recommend MDA for P. vivax (World Health Organization 2020), in large part due to the recommendation for G6PD testing prior to PQ administration. Some experts believe that PQ for radical cure can be administered in certain populations without G6PD testing, depending on the balance of population-risk of hemolysis versus the benefits of radical cure (Thriemer et al., 2017). In appropriately selected regions PQ for radical cure is administered without G6PD testing. In southern Papua (G6PDd prevalence 3%) the beneficial effects of PQ, such as lower risk of P. vivax related severe anemia, hospital admission or representation, outweighed the risks (Thriemer et al., 2020). However, in the Brazilian Amazon two deaths secondary to PQ-induced AHA have been reported (Lacerda et al., 2012). In this region G6PDd prevalence is also 3% (predominantly the mild A− variant) (Santana et al., 2009). This highlights the risk of rare but life-threatening adverse effects when PQ administration is based on population data.
Without the ability to test all individuals for G6PDd the acceptable risk-benefit balance in PQ MDA remains unresolved. Although treatment of P. vivax infection confers direct benefit to the individual, when used in MDA, some participants may not be hypnozoite carriers, and therefore at risk of harm with no possible clinical benefit (Jamrozik et al., 2015). Further, if population coverage is poor then risks of adverse events secondary to PQ may outweigh the overall benefits of an MDA program aiming for elimination (Cheah and White 2016).
Achieving success with MDA depends on the therapeutic efficacy of the drug administered and ensuring 80–90% population coverage (Newby et al., 2015; Tanner et al., 2015). With expanding knowledge of the effect of CYP2D6 polymorphisms on PQ efficacy this must be factored into MDA planning. Baird et al. have estimated that 38.8% of the population living in P. vivax endemic areas would be excluded from receiving standard PQ regimens based on G6PDd and impaired PQ metabolism (Baird et al., 2018a). Hence, with current PQ dosing regimens it may not be possible to reach the population threshold for interruption of transmission. Utilizing population knowledge of G6PDd and CYP2D6 genotypes may facilitate dosing strategies that reduce the proportion of individuals currently deemed “ineligible” for radical cure and allow coverage thresholds for MDA to be reached.
The Role of Pharmacogenomics in MDA - Challenges and Potential Solutions
Population-scale sequencing projects, such as the 1,000 Genome Project, provide a global overview of genetic diversity and interethnic variability (Abecasis et al., 2012). However, genomic databases continue to significantly under-represent developing countries and ethnically diverse populations (Jarvis et al., 2019; Sivadas and Scaria 2019). National and regional population screening programs are gathering pace (e.g. H3 Africa, African Genome Variation Project, SEAPharm) and will contribute to closing this gap (Gurdasani et al., 2015; Mulder et al., 2018; Chumnumwat et al., 2019). These projects may lead to higher resolution mapping of G6PDd and CYP2D6 polymorphisms. The unique overlay and spectrum of G6PDd and impaired PQ metabolism will influence population-dosing algorithms for safe and efficacious use of PQ in specific regions. One significant challenge is the highly polymorphic nature of both the CYP2D6 and G6PD genes. The combined complexity may make integrating pharmacogenetic knowledge at a population level challenging.
Modeling suggests that ascending dose regimens in mild-moderate G6PDd may be effective and well tolerated, with optimal regimens allowing for slow hemolysis and minimal drops in hemoglobin without the need for G6PD testing (Watson et al., 2017). Further modeling to incorporate increased dosing for impaired PQ metabolizers into ascending dose regimens may facilitate strategies to ensure both safety and efficacy in MDA. Projected population coverage, taking into account regional pharmacogenetic-guided dosing regimens, will inform regional feasibility of MDA, and whether an acceptable risk-benefit threshold is met. Although these are complex challenges to navigate there is the potential for reducing P. vivax burden through region-specific MDA strategies, aligned with the WHO “High burden to high impact approach”, a country led, targeted strategy, as opposed to the current dogma of one-size fits all (World Health Organization 2019).
Future Directions
Currently the use of pharmacogenetics for P. vivax radical cure is beyond reach due to insufficient understanding of the role of CYP2D6 in PQ metabolism and efficacy. A number of key knowledge gaps (Table 1) must be addressed; including the clinical and public health implications of CYP2D6 and G6PD pharmacogenetics, to ensure translation into clinical practice is pragmatic for P. vivax endemic areas.
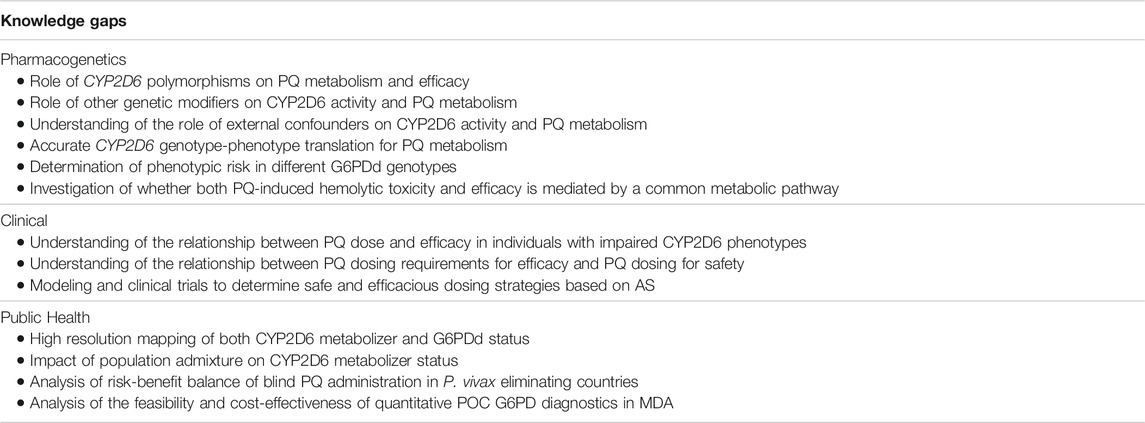
TABLE 1. Key knowledge gaps in CYP2D6 and G6PDd pharmacogenetics for PQ use in P. vivax radical cure.
Firstly, will tailored dosing strategies be effective? Modeling is the initial step, but ultimately clinical trials are required to assess dosing regimens based on population G6PDd and PQ metabolizer status. If evidence supports tailored dosing strategies to account for population G6PDd, will national malaria control programs support MDA of PQ without testing? Region-specific dosing will not completely mitigate the risk of PQ toxicity; the level of acceptable risk-benefit balance and ethical issues surrounding use of PQ in MDA require further debate. In populations where the risk outweighs the benefit, quantitative G6PD testing prior to PQ administration may facilitate higher dosing to ensure safety and efficacy.
Altered PQ dosing regimens may be more complex, with potential for poor adherence, risk of incorrect administration and interactions with concomitant drugs or foods that increase the risk of AHA or decrease the efficacy of PQ due to CYP2D6 inhibition. Will this complexity be too high for MDA operational feasibility? If blind administration is approved rigorous pharmacovigilance will be required.
Will it be possible to reach the 80–90% MDA coverage required for successful elimination of P. vivax? In addition to the well-described operational challenges in rolling out MDA, from a pharmacogenetic perspective, population admixture will need to be taken into account. Will it be possible to achieve safe and efficacious population dosing guided by pharmacogenetic data?
Population pharmacogenetics integrated in national public health policy to guide safe and efficacious PQ dosing for radical cure has the potential to enable MDA for P. vivax elimination, and build towards individualized case management as progress is made with POC pharmacogenetic testing. This is a challenging prospect, and one that ultimately may not be feasible. However, it deserves further investigation given the limited drugs available for P. vivax radical cure and the underutilization of PQ to date.
Data Availability Statement
The original contributions presented in the study are included in the article/Supplementary Material, further inquiries can be directed to the corresponding author.
Author Contributions
AS wrote the first draft. PZ and JM designed the research and secured the funding for the overall study. All authors approved the final version.
Funding
This study was supported by grants from the National Institutes of Health, United States (1R01AI148469 to PZ).
Conflict of Interest
The authors declare that the research was conducted in the absence of any commercial or financial relationships that could be construed as a potential conflict of interest.
Publisher’s Note
All claims expressed in this article are solely those of the authors and do not necessarily represent those of their affiliated organizations, or those of the publisher, the editors and the reviewers. Any product that may be evaluated in this article, or claim that may be made by its manufacturer, is not guaranteed or endorsed by the publisher.
Acknowledgments
We thank Katharine Trenholme for preparation of Figure 1.
References
Abecasis, G. R., Abecasis, G. R., Auton, A., Brooks, L. D., DePristo, M. A., Durbin, R. M., et al. (2012). An Integrated Map of Genetic Variation from 1,092 Human Genomes. Nature 491 (7422), 56–65. doi:10.1038/nature11632
Alam, M. S., Kibria, M. G., Jahan, N., Thriemer, K., Hossain, M. S., Douglas, N. M., et al. (2018). Field Evaluation of Quantitative point of Care Diagnostics to Measure Glucose-6-Phosphate Dehydrogenase Activity. PloS one 13 (11), e0206331. doi:10.1371/journal.pone.0206331
Alving, A. S., Carson, P. E., Flanagan, C. L., and Ickes, C. E. (1956). Enzymatic Deficiency in Primaquine-Sensitive Erythrocytes. Science 124 (3220), 484–485. doi:10.1126/science.124.3220.484-a
Alving, A. S., Johnson, C. F., Tarlov, A. R., Brewer, G. J., Kellermeyer, R. W., and Carson, P. E. (1960). Mitigation of the Haemolytic Effect of Primaquine and Enhancement of its Action against Exoerythrocytic Forms of the Chesson Strain of Piasmodium Vivax by Intermittent Regimens of Drug Administration: a Preliminary Report. Bull. World Health Organ. 22 (6), 621–631.
Baird, J. K., Battle, K. E., and Howes, R. E. (2018a). Primaquine Ineligibility in Anti-relapse Therapy of Plasmodium Vivax Malaria: the Problem of G6PD Deficiency and Cytochrome P-450 2D6 Polymorphisms. Malar. J. 17 (1), 42. doi:10.1186/s12936-018-2190-z
Baird, J. K. (2013). Evidence and Implications of Mortality Associated with Acute Plasmodium Vivax Malaria. Clin. Microbiol. Rev. 26 (1), 36–57. doi:10.1128/cmr.00074-12
Baird, J. K., Louisa, M., Noviyanti, R., Ekawati, L., Elyazar, I., Subekti, D., et al. (2018b). Association of Impaired Cytochrome P450 2D6 Activity Genotype and Phenotype with Therapeutic Efficacy of Primaquine Treatment for Latent Plasmodium Vivax Malaria. JAMA Netw. Open 1 (4), e181449. doi:10.1001/jamanetworkopen.2018.1449
Battle, K. E., Lucas, T. C. D., Nguyen, M., Howes, R. E., Nandi, A. K., Twohig, K. A., et al. (2019). Mapping the Global Endemicity and Clinical burden of Plasmodium Vivax, 2000-17: a Spatial and Temporal Modelling Study. Lancet 394 (10195), 332–343. doi:10.1016/s0140-6736(19)31096-7
Bennett, J. W., Pybus, B. S., Yadava, A., Tosh, D., Sousa, J. C., McCarthy, W. F., et al. (2013). Primaquine Failure and Cytochrome P-450 2D6 in Plasmodium Vivax Malaria. N. Engl. J. Med. 369 (14), 1381–1382. doi:10.1056/NEJMc1301936
Botton, M. R., Bandinelli, E., Rohde, L. E., Amon, L. C., and Hutz, M. H. (2011). Influence of Genetic, Biological and Pharmacological Factors on Warfarin Dose in a Southern Brazilian Population of European Ancestry. Br. J. Clin. Pharmacol. 72 (3), 442–450. doi:10.1111/j.1365-2125.2011.03942.x
Brasil, F., Santoro, A. B., Almeida, A. C. G., Kühn, A., Ramasawmy, R., Lacerda, M. V. G., et al. (2018). CYP2D6 Activity and the Risk of Recurrence of Plasmodium Vivax Malaria in the Brazilian Amazon: a Prospective Cohort Study. Malar. J. 17 (1), 57. doi:10.1186/s12936-017-2139-7
Caudle, K. E., Sangkuhl, K., Whirl-Carrillo, M., Swen, J. J., Haidar, C. E., Klein, T. E., et al. (2020). Standardizing CYP2D6 Genotype to Phenotype Translation: Consensus Recommendations from the Clinical Pharmacogenetics Implementation Consortium and Dutch Pharmacogenetics Working Group. Clin. Transl Sci. 13 (1), 116–124. doi:10.1111/cts.12692
Cheah, P. Y., and White, N. J. (2016). Antimalarial Mass Drug Administration: Ethical Considerations. Int. Health 8 (4), 235–238. doi:10.1093/inthealth/ihw027
Chu, C. S., Bancone, G., Moore, K. A., Win, H. H., Thitipanawan, N., Po, C., et al. (2017). Haemolysis in G6PD Heterozygous Females Treated with Primaquine for Plasmodium Vivax Malaria: A Nested Cohort in a Trial of Radical Curative Regimens. Plos Med. 14 (2), e1002224. doi:10.1371/journal.pmed.1002224
Chu, C. S., and Hwang., J. (2021). Tafenoquine: a Toxicity Overview. Expert Opin. Drug Saf. 20 (3), 349–362. doi:10.1080/14740338.2021.1859476
Chumnumwat, S., Lu, Z. H., Sukasem, C., Winther, M. D., Capule, F. R., Abdul Hamid, Aaat., et al. (2019). Southeast Asian Pharmacogenomics Research Network (SEAPharm): Current Status and Perspectives. Public Health Genomics 22 (3-4), 132–139. doi:10.1159/000502916
Commons, R. J., Simpson, J. A., Watson, J., White, N. J., and Price, R. N. (2020). Estimating the Proportion of Plasmodium Vivax Recurrences Caused by Relapse: A Systematic Review and Meta-Analysis. Am. J. Trop. Med. Hyg. 103 (3), 1094–1099. doi:10.4269/ajtmh.20-0186
Crews, K. R., Gaedigk, A., Dunnenberger, H. M., Leeder, J. S., Klein, T. E., Caudle, K. E., et al. (2014). Clinical Pharmacogenetics Implementation Consortium Guidelines for Cytochrome P450 2D6 Genotype and Codeine Therapy: 2014 Update. Clin. Pharmacol. Ther. 95 (4), 376–382. doi:10.1038/clpt.2013.254
Del Tredici, A. L., Malhotra, A., Dedek, M., Espin, F., Roach, D., Zhu, G. D., et al. (2018). Frequency of CYP2D6 Alleles Including Structural Variants in the United States. Front. Pharmacol. 9, 305. doi:10.3389/fphar.2018.00305
Dern, R. J., Beutler, E., and Alving, A. S. (1954). The Hemolytic Effect of Primaquine. II. The Natural Course of the Hemolytic Anemia and the Mechanism of its Self-Limited Character. J. Lab. Clin. Med. 44 (2), 171–176.
Dodgen, T. M., Labuschagne, C. J., van Schalkwyk, A., Steffens, F. E., Gaedigk, A., Cromarty, A. D., et al. (2016). Pharmacogenetic Comparison of CYP2D6 Predictive and Measured Phenotypes in a South African Cohort. Pharmacogenomics J. 16 (6), 566–572. doi:10.1038/tpj.2015.76
Gaedigk, A., Dinh, J. C., Jeong, H., Prasad, B., and Leeder, J. S. (2018). Ten Years' Experience with the CYP2D6 Activity Score: A Perspective on Future Investigations to Improve Clinical Predictions for Precision Therapeutics. J. Pers Med. 8 (2), 15. doi:10.3390/jpm8020015
Gething, P. W., Elyazar, I. R., Moyes, C. L., Smith, D. L., Battle, K. E., Guerra, C. A., et al. (2012). A Long Neglected World Malaria Map: Plasmodium Vivax Endemicity in 2010. Plos Negl. Trop. Dis. 6 (9), e1814. doi:10.1371/journal.pntd.0001814
Gómez-Manzo, S., Marcial-Quino, J., Vanoye-Carlo, A. D., Serrano-PosadaCastillo-Rodríguez, H., Ortega-Cuellar, D., González-Valdez, A., et al. (2016). Glucose-6-Phosphate Dehydrogenase: Update and Analysis of New Mutations Around the World. Int. J. Mol. Sci. 17 (12), 2069. doi:10.3390/ijms17122069
Gurdasani, D., Carstensen, T., Tekola-Ayele, F., Pagani, L., Tachmazidou, I., Hatzikotoulas, K., et al. (2015). The African Genome Variation Project Shapes Medical Genetics in Africa. Nature 517 (7534), 327–332. doi:10.1038/nature13997
Haga, S. B. (2016). Challenges of Development and Implementation of point of Care Pharmacogenetic Testing. Expert Rev. Mol. Diagn. 16 (9), 949–960. doi:10.1080/14737159.2016.1211934
Hippman, C., and Nislow, C. (2019). Pharmacogenomic Testing: Clinical Evidence and Implementation Challenges. J. Pers Med. 9 (3), e30040. doi:10.3390/jpm9030040
Howes, R. E., Piel, F. B., Patil, A. P., Nyangiri, O. A., Gething, P. W., Dewi, M., et al. (2012). G6PD Deficiency Prevalence and Estimates of Affected Populations in Malaria Endemic Countries: a Geostatistical Model-Based Map. Plos Med. 9 (11), e1001339. doi:10.1371/journal.pmed.1001339
Hsiang, M. S., Hwang, J., Tao, A. R., Liu, Y., BennettShanks, A., Shanks, G. D., et al. (2013). Mass Drug Administration for the Control and Elimination of Plasmodium Vivax Malaria: an Ecological Study from Jiangsu Province, China. Malar. J. 12 (1), 383. doi:10.1186/1475-2875-12-383
Huang, H., Dong, Y., Xu, Y., Deng, Y., Zhang, C., Liu, S., et al. (2021). The Association of CYP2D6 Gene Polymorphisms in the Full-Length Coding Region with Higher Recurrence Rate of Vivax Malaria in Yunnan Province, China. Malar. J. 20 (1), 160. doi:10.1186/s12936-021-03685-3
Ingram, R. J. H., Joan, H., Crenna-Darusallam, C., Soebianto, S., Noviyanti, R., and Baird, J. K. (2014). Chelzie Crenna-Darusallam, Saraswati Soebianto, Rintis Noviyanti, and J. Kevin BairdThe Clinical and Public Health Problem of Relapse Despite Primaquine Therapy: Case Review of Repeated Relapses of Plasmodium Vivax Acquired in Papua New Guinea. Malar. J. 13, 488. doi:10.1186/1475-2875-13-488
Jamrozik, E., de la Fuente-Núñez, V., Reis, A., Ringwald, P., Selgelid, M. J., and Selgelid, (2015). Ethical Aspects of Malaria Control and Research. Malar. J. 14 (1), 518. doi:10.1186/s12936-015-1042-3
Jarvis, J. P., Peter, A. P., and Shaman, J. A. (2019). Consequences of CYP2D6 Copy-Number Variation for Pharmacogenomics in Psychiatry. Front. Psychiatry 10 (432), 432. doi:10.3389/fpsyt.2019.00432
John, G. K., Douglas, N. M., von Seidlein, L., Nosten, F., Baird, J. K., White, N. J., et al. (2012). Primaquine Radical Cure of Plasmodium Vivax: a Critical Review of the Literature. Malar. J. 11, 280. doi:10.1186/1475-2875-11-280
Kaneko, A., Taleo, G., Kalkoa, M., Yamar, S., Kobayakawa, T., and Björkman, A. (2000). Malaria Eradication on Islands. Lancet 356 (9241), 1560–1564. doi:10.1016/s0140-6736(00)03127-5
Kheng, S., Muth, S., Taylor, W. R. J., Tops, N., Kosal, K., Sothea, K., et al. (2015). Narann Tops, Khem Kosal, Khon Sothea, Phum Souy, Saorin Kim, Chuor Meng Char, Chan Vanna, Po Ly, Pascal Ringwald, Virak Khieu, Alexandra Kerleguer, Pety Tor, John K. Baird, Steven Bjorge, Didier Menard, and Eva ChristophelTolerability and Safety of Weekly Primaquine against Relapse of Plasmodium Vivax in Cambodians with Glucose-6-Phosphate Dehydrogenase Deficiency. BMC Med. 13 (1), 203. doi:10.1186/s12916-015-0441-1
Kondrashin, A., Baranova, A. M., Ashley, E. A., Recht, J., White, N. J., and Sergiev, V. P. (2014). Mass Primaquine Treatment to Eliminate Vivax Malaria: Lessons from the Past. Malar. J. 13, 51. doi:10.1186/1475-2875-13-51
Koopmans, A. B., Braakman, M. H., Vinkers, D. J., Hoek, H. W., and van Harten, P. N. (2021). Meta-analysis of Probability Estimates of Worldwide Variation of CYP2D6 and CYP2C19. Transl Psychiatry 11 (1), 141. doi:10.1038/s41398-020-01129-1
Krotoski, W. A. (1985). Discovery of the Hypnozoite and a New Theory of Malarial Relapse. Trans. R. Soc. Trop. Med. Hyg. 79 (1), 1–11. doi:10.1016/0035-9203(85)90221-4
Lacerda, M. V., Fragoso, S. C., Alecrim, M. G., Alexandre, M. A., Magalhães, B. M., Siqueira, A. M., et al. (2012). Postmortem Characterization of Patients with Clinical Diagnosis of Plasmodium Vivax Malaria: to what Extent Does This Parasite Kill. Clin. Infect. Dis. 55 (8), e67–74. doi:10.1093/cid/cis615
Lacerda, M. V. G., Llanos-Cuentas, A., Krudsood, S., Lon, C., Saunders, D. L., Mohammed, R., et al. (2019). Single-Dose Tafenoquine to Prevent Relapse of Plasmodium Vivax Malaria. N. Engl. J. Med. 380 (3), 215–228. doi:10.1056/NEJMoa1710775
LaRue, N., Kahn, M., Murray, M., Leader, B. T., Bansil, P., McGray, S., et al. (2014). Comparison of Quantitative and Qualitative Tests for Glucose-6-Phosphate Dehydrogenase Deficiency. Am. J. Trop. Med. Hyg. 91 (4), 854–861. doi:10.4269/ajtmh.14-0194
Leitão, L. P. C., SouzaSouzaRodrigues, T. P, Rodrigues, J. C. G., Fernandes, M. R., Santos, S., and Santos, N. P. C. (2020). The Metabolization Profile of the CYP2D6 Gene in Amerindian Populations: A Review. Genes (Basel) 11 (3), 262. doi:10.3390/genes11030262
Leslie, T., Mayan, I., Mohammed, N., Erasmus, P., Kolaczinski, J., Whitty, C. J., et al. (2008). A Randomised Trial of an Eight-Week, once Weekly Primaquine Regimen to Prevent Relapse of Plasmodium Vivax in Northwest Frontier Province, Pakistan. PloS one 3 (8), e2861. doi:10.1371/journal.pone.0002861
LLerena, A., Naranjo, M. E., Rodrigues-Soares, F., Penas-LLedó, E. M., Fariñas, H., and Tarazona-Santos, E. (2014). Interethnic Variability of CYP2D6 Alleles and of Predicted and Measured Metabolic Phenotypes across World Populations. Expert Opin. Drug Metab. Toxicol. 10 (11), 1569–1583. doi:10.1517/17425255.2014.964204
Luzzatto, L., and Seneca, E. (2014). G6PD Deficiency: a Classic Example of Pharmacogenetics with On-Going Clinical Implications. Br. J. Haematol. 164 (4), 469–480. doi:10.1111/bjh.12665
Mehlotra, R. K., Gaedigk, A., Howes, R. E., Rakotomanga, T. A., Ratsimbasoa, A. C., and Zimmerman, P. A. (2021). CYP2D6 Genetic Variation and its Implication for Vivax Malaria Treatment in Madagascar. Front. Pharmacol. 12, 654054. doi:10.3389/fphar.2021.654054
Mulder, N., Abimiku, A., Adebamowo, S. N., de Vries, J., Matimba, A., Olowoyo, P., et al. (2018). H3Africa: Current Perspectives. Pharmgenomics Pers Med. 11, 59–66. doi:10.2147/pgpm.S141546
Newby, G., Hwang, J., Koita, K., Chen, I., Greenwood, B., von Seidlein, L., et al. (2015). Review of Mass Drug Administration for Malaria and its Operational Challenges. Am. J. Trop. Med. Hyg. 93 (1), 125–134. doi:10.4269/ajtmh.14-0254
Nofziger, C., Turner, A. J., Sangkuhl, K., Whirl-Carrillo, M., Agúndez, J. A. G., Black, J. L., et al. (2020). PharmVar GeneFocus: CYP2D6. Clin. Pharmacol. Ther. 107 (1), 154–170. doi:10.1002/cpt.1643
Pal, S., Bansil, P., Bancone, G., Hrutkay, S., Kahn, M., Gornsawun, G., et al. (2019). Evaluation of a Novel Quantitative Test for Glucose-6-Phosphate Dehydrogenase Deficiency: Bringing Quantitative Testing for Glucose-6-Phosphate Dehydrogenase Deficiency Closer to the Patient. Am. J. Trop. Med. Hyg. 100 (1), 213–221. doi:10.4269/ajtmh.18-0612
Popovici, J., Tebben, K., Witkowski, B., and Serre, D. (2021). Primaquine for Plasmodium Vivax Radical Cure: What We Do Not Know and Why it Matters. Int. J. Parasitol. Drugs Drug Resist. 15, 36–42. doi:10.1016/j.ijpddr.2020.12.004
Potter, B. M., Xie, L. H., Vuong, C., Zhang, J., Zhang, P., Duan, D., et al. (2015). Differential CYP 2D6 Metabolism Alters Primaquine Pharmacokinetics. Antimicrob. Agents Chemother. 59 (4), 2380–2387. doi:10.1128/aac.00015-15
Pybus, B. S., Marcsisin, S. R., Jin, X., Deye, G., Sousa, J. C., Li, Q., et al. (2013). The Metabolism of Primaquine to its Active Metabolite Is Dependent on CYP 2D6. Malar. J. 12, 212. doi:10.1186/1475-2875-12-212
Pybus, B. S., Sousa, J. C., Jin, X., Ferguson, J. A., Christian, R. E., Barnhart, R., et al. (2012). CYP450 Phenotyping and Accurate Mass Identification of Metabolites of the 8-aminoquinoline, Anti-malarial Drug Primaquine. Malar. J. 11, 259. doi:10.1186/1475-2875-11-259
Recht, J., Ashley, E. A., and White, N. J. (2018). Use of Primaquine and Glucose-6-Phosphate Dehydrogenase Deficiency Testing: Divergent Policies and Practices in Malaria Endemic Countries. Plos Negl. Trop. Dis. 12 (4), e0006230. doi:10.1371/journal.pntd.0006230
Recht, J., Ashley, E. A., and White, N. (2014). Safety of 8-aminoquinoline Antimalarial Medicines. Geneva, Switzerland: World Health Organization.
Roederer, M. W., and McLeod, H. L. (2010). Applying the Genome to National Drug Formulary Policy in the Developing World. Pharmacogenomics 11 (5), 633–636. doi:10.2217/pgs.10.55
Ross, A., Koepfli, C., Schoepflin, S., Timinao, L., Siba, P., Smith, T., et al. (2016). The Incidence and Differential Seasonal Patterns of Plasmodium Vivax Primary Infections and Relapses in a Cohort of Children in Papua New Guinea. Plos Negl. Trop. Dis. 10 (5), e0004582. doi:10.1371/journal.pntd.0004582
Santana, M. S., de Lacerda, M. V., Barbosa, Md., Alecrim, W. D., and Alecrim, Md. (2009). Glucose-6-phosphate Dehydrogenase Deficiency in an Endemic Area for Malaria in Manaus: a Cross-Sectional Survey in the Brazilian Amazon. PLoS One 4 (4), e5259. doi:10.1371/journal.pone.0005259
Sasaki, T., Sato, Y., Kumagai, T., Yoshinari, K., and Nagata, K. (2017). Effect of Health Foods on Cytochrome P450-Mediated Drug Metabolism. J. Pharm. Health Care Sci. 3, 14. doi:10.1186/s40780-017-0083-x
Silvino, A. C., Costa, G. L., Araújo, F. C., Ascher, D. B., Pires, D. E., Fontes, C. J., et al. (2016). Variation in Human Cytochrome P-450 Drug-Metabolism Genes: A Gateway to the Understanding of Plasmodium Vivax Relapses. PLoS One 11 (7), e0160172. doi:10.1371/journal.pone.0160172
Silvino, A. C. R., Kano, F. S., Costa, M. A., Fontes, C. J. F., Soares, I. S., de Brito, C. F. A., et al. (2020). Novel Insights into Plasmodium Vivax Therapeutic Failure: CYP2D6 Activity and Time of Exposure to Malaria Modulate the Risk of Recurrence. Antimicrob. Agents Chemother. 64 (5), e02056–19. doi:10.1128/AAC.02056-19
Sistonen, J., Sajantila, A., Lao, O., Corander, J., Barbujani, G., and Fuselli, S. (2007). CYP2D6 Worldwide Genetic Variation Shows High Frequency of Altered Activity Variants and No continental Structure. Pharmacogenet Genomics 17 (2), 93–101. doi:10.1097/01.fpc.0000239974.69464.f2
Sivadas, A., and Scaria, V. (2019). Population-scale Genomics-Enabling Precision Public Health. Adv. Genet. 103, 119–161. doi:10.1016/bs.adgen.2018.09.001
Spring, M. D., Chann, S., Kuntawunginn, W., Kheang Heng, T., Nou, S., Arsanok, M., et al. (2020). Prevalence of CYP2D6 Genotypes and Predicted Phenotypes in a Cohort of Cambodians at High Risk for Infections with Plasmodium Vivax. Am. J. Trop. Med. Hyg. 103 (2), 756–759. doi:10.4269/ajtmh.20-0061
Suarez-Kurtz, G., Paula, D. P., and Struchiner, C. J. (2014). Pharmacogenomic Implications of Population Admixture: Brazil as a Model Case. Pharmacogenomics 15 (2), 209–219. doi:10.2217/pgs.13.238
Suarez-Kurtz, G. (2011). Population Diversity and the Performance of Warfarin Dosing Algorithms. Br. J. Clin. Pharmacol. 72 (3), 451–453. doi:10.1111/j.1365-2125.2011.04018.x
Swen, J. J., Nijenhuis, M., de Boer, A., Grandia, L., Maitland-van der Zee, A. H., Mulder, H., et al. (2011). Pharmacogenetics: from Bench to Byte-Aan Update of Guidelines. Clin. Pharmacol. Ther. 89 (5), 662–673. doi:10.1038/clpt.2011.34
Tanner, M., Greenwood, B., Whitty, C. J., Ansah, E. K., Price, R. N., Dondorp, A. M., et al. (2015). Malaria Eradication and Elimination: Views on How to Translate a Vision into Reality. BMC Med. 13, 167. doi:10.1186/s12916-015-0384-6
Taylor, W. R. J., Thriemer, K., von Seidlein, L., Yuentrakul, P., Assawariyathipat, T., Assefa, A., et al. (2019). Short-course Primaquine for the Radical Cure of Plasmodium Vivax Malaria: a Multicentre, Randomised, Placebo-Controlled Non-inferiority Trial. Lancet 394 (10202), 929–938. doi:10.1016/s0140-6736(19)31285-1
Thriemer, K., Ley, B., Bobogare, A., Dysoley, L., Alam, M. S., Pasaribu, A. P., et al. (2017). Challenges for Achieving Safe and Effective Radical Cure of Plasmodium Vivax: a Round Table Discussion of the APMEN Vivax Working Group. Malar. J. 16 (1), 141. doi:10.1186/s12936-017-1784-1
Thriemer, K., Poespoprodjo, J. R., Kenangalem, E., Douglas, N. M., Sugiarto, P., Anstey, N. M., et al. (2020). The Risk of Adverse Clinical Outcomes Following Treatment of Plasmodium Vivax Malaria with and without Primaquine in Papua, Indonesia. Plos Negl. Trop. Dis. 14 (11), e0008838. doi:10.1371/journal.pntd.0008838
Watson, J., Taylor, W. R., Menard, D., Kheng, S., and White, N. J. (2017). Modelling Primaquine-Induced Haemolysis in G6PD Deficiency. Elife 6, 23061. doi:10.7554/eLife.23061
World Health Organization (Editor) (2019). High Burden to High Impact: A Targeted Malaria Response (Geneva, Switzerland: World Health Organization).
World Health Organization (Editor) (2021). WHO Guidelines for Malaria (Geneva: World Health Organization).
World Health Organization (2020). WHO Technical Brief for Countries Preparing Malaria Funding Requests for the Global Fund (2020–2022). Geneva: World Health Organization.
Keywords: Plasmodium vivax, primaquine, CYP2D6, G6PD (glucose-6-phosphate dehydrogenase), pharmacogenetics, elimination, mass drug administration (MDA)
Citation: Stewart AGA, Zimmerman PA and McCarthy JS (2021) Genetic Variation of G6PD and CYP2D6: Clinical Implications on the Use of Primaquine for Elimination of Plasmodium vivax. Front. Pharmacol. 12:784909. doi: 10.3389/fphar.2021.784909
Received: 28 September 2021; Accepted: 05 November 2021;
Published: 26 November 2021.
Edited by:
Marcelo Rizzatti Luizon, Federal University of Minas Gerais, BrazilReviewed by:
Eng Wee Chua, Universiti Kebangsaan Malaysia, MalaysiaNathalie K. Zgheib, American University of Beirut, Lebanon
Copyright © 2021 Stewart, Zimmerman and McCarthy. This is an open-access article distributed under the terms of the Creative Commons Attribution License (CC BY). The use, distribution or reproduction in other forums is permitted, provided the original author(s) and the copyright owner(s) are credited and that the original publication in this journal is cited, in accordance with accepted academic practice. No use, distribution or reproduction is permitted which does not comply with these terms.
*Correspondence: Alexandra G. A. Stewart, YWxleGFuZHJhLnN0ZXdhcnQwM0BnbWFpbC5jb20=