- 1School of Life Sciences and Medicine, Shandong University of Technology, Zibo, China
- 2Shandong Qingyujiangxing Biotechnology Co., Ltd., Zibo, China
- 3Key Laboratory of Novel Food Resources Processing, Ministry of Agriculture and Rural Affairs/Key Laboratory of Agro-Products Processing Technology of Shandong Province/Institute of Agro-Food Science and Technology, Shandong Academy of Agricultural Sciences, Jinan, China
Hyperuricemia is the result of increased production and/or underexcretion of uric acid. Hyperuricemia has been epidemiologically associated with multiple comorbidities, including metabolic syndrome, gout with long-term systemic inflammation, chronic kidney disease, urolithiasis, cardiovascular disease, hypertension, rheumatoid arthritis, dyslipidemia, diabetes/insulin resistance and increased oxidative stress. Dysregulation of xanthine oxidoreductase (XOD), the enzyme that catalyzes uric acid biosynthesis primarily in the liver, and urate transporters that reabsorb urate in the renal proximal tubules (URAT1, GLUT9, OAT4 and OAT10) and secrete urate (ABCG2, OAT1, OAT3, NPT1, and NPT4) in the renal tubules and intestine, is a major cause of hyperuricemia, along with variations in the genes encoding these proteins. The first-line therapeutic drugs used to lower serum uric acid levels include XOD inhibitors that limit uric acid biosynthesis and uricosurics that decrease urate reabsorption in the renal proximal tubules and increase urate excretion into the urine and intestine via urate transporters. However, long-term use of high doses of these drugs induces acute kidney disease, chronic kidney disease and liver toxicity. Therefore, there is an urgent need for new nephroprotective drugs with improved safety profiles and tolerance. The current systematic review summarizes the characteristics of major urate transporters, the mechanisms underlying the pathogenesis of hyperuricemia, and the regulation of uric acid biosynthesis and transport. Most importantly, this review highlights the potential mechanisms of action of some naturally occurring bioactive compounds with antihyperuricemic and nephroprotective potential isolated from various medicinal plants.
Introduction
Uric acid (2,6,8-trioxypurine, C5H4N4O3) is a heterocyclic organic compound with a molecular weight of 168.11 Da. It is the end-product of purine (endogenous and exogenous) metabolism in humans and great apes because of loss-of-function mutations during primate evolution in the gene encoding the uricase enzyme, which oxidizes uric acid to produce more soluble allantoin (Wu et al., 1992; So and Thorens, 2010). Due to uricase inactivation, the serum urate level is 7- to 8-fold higher in humans (≈240–360 μM) than in other mammals (≈30–50 μM in mice) (So and Thorens, 2010). Considering the pKa value of uric acid (5.75) that exists as soluble urate at physiological pH (7.4), more uric acid than urate is present in the urine (pH 5–6), and a pH less than 7.4 in the tissue microenvironment favors urate crystal formation. In humans, the serum urate concentration is approximately 10 times higher than that of ascorbic acid, showing that urate is a potent radical scavenger in plasma. Perhaps 7- to 8-fold higher serum urate levels might have selectively protected the neurons of hominids during evolution from the detrimental effects of reactive oxygen species (ROS) and allowed them to become the most intelligent species on Earth. In addition, elevated serum urate levels have been shown to reduce the risk of neurodegenerative diseases, specifically Alzheimer’s disease (Ye et al., 2016), Parkinson’s disease (Schwarzschild et al., 2008; Ascherio et al., 2009), and multiple sclerosis (Liu et al., 2012). Recently, integral membrane protein 2B (ITM2B) has been shown to be a potential regulatory link between urate homeostasis and neurodegenerative disorders (Mandal and Mount, 2019). Elevated serum urate levels may have given hominids a selective advantage during evolution. Taken together, these results suggest a potential role of soluble urate in maintaining memory and intelligence.
Approximately two-thirds of serum uric acid in humans is produced endogenously, while the remaining third comes from dietary purines (Schlesinger 2005). Under physiological concentrations, uric acid in its soluble form as urate acts as a protective powerful antioxidant, demonstrating the ability to scavenge ROS such as superoxide, hydroxyl radicals, and singlet oxygen (Ames et al., 1981; Davies et al., 1986), which is closely comparable to the scavenging ability of vitamin C (Ames et al., 1981). However, under conditions of poor solubility and a high uric acid concentration in crude urine, urate crystal deposition occurs in the renal tubular lumens and ureters, which contributes to obstructive nephropathy (Moe et al., 2002). The urate crystals adhere to the surfaces of renal epithelial cells and induce an acute inflammatory response (Koka et al., 2000). In addition to inducing kidney stone formation, such effects can reduce the glomerular filtration rate (Spencer et al., 1976). Urate can also act as a pro-oxidant inside cells, as it can induce the activity of NADPH oxidases, resulting in mitochondrial alterations and endothelial dysfunction (Sánchez-Lozada et al., 2012).
Hyperuricemia is a common finding in patients with metabolic syndrome. Clinically, the prevalence of hyperuricemia is much higher than that of hypouricemia. Hyperuricemia in humans is defined by the serum urate level >7.0 mg/dl among men and >5.7 mg/dl among women. Statistically, the morbidity due to hyperuricemia and gout is higher in men than in women, probably because of the influence of sex hormones. With increasing age after menopause, the discrepancy in prevalence is reduced between men and women (Chen-Xu et al., 2019). The prevalence of hyperuricemia increased from 10.5% to 16.6% in Caucasian or Australian representative populations from 2011 to 2020 (Pathmanathan et al., 2021). In Ireland, the prevalence increased from 19.7% to 25.0% among men and from 20.5% to 24.1% among women between 2006 and 2014 (Kumar A U et al., 2018). The pooled prevalence of hyperuricemia was 19.4% in men and 7.9% in women from 2000 to 2014 and was high in mainland China (Liu et al., 2015). Long-term hyperuricemia can induce renal mitochondrial dysfunction associated with oxidative stress in the renal cortex as well as tubular damage (Cristóbal-García et al., 2015). Moreover, it can lead to urate crystal formation that causes gout (a type of inflammatory arthritis), leading to joint damage, loss of motion and an acute inflammatory response (Koka et al., 2000). Hyperuricemia is the causative risk factor for gout (Choi et al., 2005) and increases the risks for chronic kidney disease (CKD) (Johnson et al., 2018), cardiovascular disease (Feig et al., 2008; Gaffo et al., 2009), hypertension (Feig and Johnson, 2003), insulin resistance (Facchini et al., 1991; Yoo et al., 2005), and diabetic kidney disease (Kim et al., 2012; Qu et al., 2022). Currently, it is recognized that hyperuricemia alone is not sufficient to cause gout and that other factors also play roles in urate crystal formation. It is also acknowledged that lowering serum urate levels is an effective strategy to prevent gout attacks (Shoji et al., 2004).
The normal serum urate level in humans is the result of balance among biosynthesis of uric acid primarily in the liver, reabsorption of urate in renal proximal tubules and secretion in the renal tubules and intestine (Mandal and Mount, 2015). Dysregulation of xanthine oxidoreductase (XOD), the enzyme that catalyzes the endogenous production of uric acid primarily in the liver, and urate transporters that reabsorb urate in renal proximal tubules and secrete urate in renal tubules and the intestine, is the major cause of hyperuricemia, along with variability in the genes encoding these proteins. Notably, most urate is filtered freely in the kidney, with approximately 90% of the urate from glomerular filtrate being reabsorbed via urate transporters in the proximal tubules (Alexandru and Moe, 2012). Approximately 70% of the total serum urate in the human body is excreted via the kidney, and the rest is excreted via intestinal and biliary secretion (Schlesinger 2005). Ultimately, after urate reabsorption, only 3–10% of the filtered urate is eliminated in the urine (Taniguchi and Kamatani, 2008). Abnormalities in urate metabolism and decreased urate metabolite in the kidney are major inducers of hyperuricemia and gout development (Terkeltaub 2003; Liu et al., 2015). Genome-wide association studies have also found associations between polymorphisms in urate transporters and the risk for hyperuricemia/gout (Köttgen et al., 2013; Phipps-Green et al., 2016; Tin et al., 2019). In this review, advances in urate excretion and possible therapeutic herbal extracts are discussed to provide novel insights regarding the development and treatment of hyperuricemia.
Relationship between hyperuricemia and CKD
Long-term hyperuricemia is considered an independent risk factor for the occurrence and progression of CKD (Jalal et al., 2013; Su et al., 2020). The prevalence of hyperuricemia and CKD has been steadily increasing (Levey et al., 2007; Jalal et al., 2013). Patients with long-term hyperuricemia have poor quality of life and high mortality rates. Because of advances in research, CKD can now be detected using simple laboratory tests, and there are treatments to prevent or delay abnormal kidney function, slow the progression of kidney disease, and reduce the risk of CKD. However, the debate regarding whether hyperuricemia plays a causal role in the progression of CKD or is simply a marker of renal dysfunction continues. The causal relationship between hyperuricemia and CKD remains controversial, and the pathophysiological mechanisms of hyperuricemia-induced renal injury are not entirely clear. Serum urate is often elevated in subjects with CKD but is not always associated with the development and progression of CKD. The causal role of hyperuricemia in the progression of CKD has not been fully established. Currently, there is no clear cutoff serum urate level associated with the risk for kidney damage, and there is not sufficient evidence to recommend the widespread use of uric acid-lowering therapy to prevent or slow the progression of CKD. Elevated urate levels in PO-induced (PO, potassium oxonate; a uricase inhibitor) hyperuricemic rats have been shown to cause intrarenal oxidative stress, increased NOX-4 and angiotensin II expression, increased juxtaglomerular renin and decreased nitric oxide bioavailability, renal vasoconstriction, glomerular hypertrophy, glomerulosclerosis and afferent arteriolopathy (Mazzali et al., 2001; Kang et al., 2002; Nakagawa et al., 2003; Sánchez-Lozada et al., 2005; Sánchez-Lozada et al., 2008). Furthermore, treatment with allopurinol partially prevents cortical vasoconstriction and fully prevents arteriolopathy and glomerular hypertension (Nakagawa et al., 2003; Sánchez-Lozada et al., 2005).
Excessive serum urate causes hyperuricemic nephropathy, which is characterized by inflammatory infiltration of macrophages, neutrophils and lymphocytes and tubulointerstitial fibrosis (Lai and Zhou, 2013; Pan et al., 2021a). Recent findings suggest that asymptomatic hyperuricemia has no effect on CKD progression unless urate crystallizes in the kidney (Sellmayr et al., 2020). However, when experimental animals with CKD are made hyperuricemic, renal disease progresses rapidly despite an absence of crystals in the kidney. This experimental results link hyperuricemia with the progression of CKD as in most mammals, uric acid levels are relatively low (compared to those in humans) because of the presence of liver uricase, which degrades uric acid to 5-hydroxyisourate and eventually to soluble allantoin. All humans are essentially “uricase knockouts,” exhibiting elevations in serum urate levels that can be treated. Reducing urate levels with losartan may slow renal disease (Miao et al., 2011) and reduce cardiovascular events (Smink et al., 2012). The renoprotective drug febuxostat (a xanthine oxidase inhibitor) does not alleviate the decline in kidney function in patients with stage 3 CKD and asymptomatic hyperuricemia (Kimura et al., 2018). Evidence obtained from basic research suggests that hyperuricemia plays a pathogenic role in the development of CKD and cardiovascular disease by inducing inflammation, endothelial dysfunction, proliferation of vascular smooth muscle cells, and activation of the renin–angiotensin system (Saito et al., 1978; Mazzali et al., 2001; Perlstein et al., 2004). In human first trimester uterine trophoblast cell lines, monosodium urate (MSU) crystals have been shown to induce inflammatory cytokine production in response to activation of the NOD-like receptor superfamily pyrin domain containing 3 (NLRP3) inflammasome (Mulla et al., 2011; Mulla et al., 2013). CKD is most often associated with obesity and metabolic syndrome (Copur et al., 2022), and there is insufficient evidence to suggest that uric acid-lowering therapy can prevent CKD progression.
Relationship between hyperuricemia and insulin resistance
Serum urate is elevated in metabolic syndrome and diabetes (Choi et al., 2007; Choi and Ford, 2007; Copur et al., 2022) as a consequence of insulin resistance and insulin-mediated reductions in urinary urate excretion (Quiñones Galvan et al., 1995). There is a positive relationship between serum insulin and elevated serum urate levels in healthy individuals and people with diabetes (Mandal et al., 2021). Insulin resistance also leads to impaired urate excretion at a low urinary pH, contributing to the formation of urate stones (Spatola et al., 2017). Genetic variation in insulin signaling pathways is also associated with variations in serum urate levels (Köttgen et al., 2013; Phipps-Green et al., 2016; Tin et al., 2019). These genetic data are consistent with a role of insulin in controlling serum urate levels (Mandal et al., 2021). In an oocyte expression system and transfected cells overexpressing individual urate transporters, insulin was recently suggested to activate both “reabsorptive” urate transporters (GLUT9 and OAT10) and “secretory” urate transporters (OAT1, OAT3, NPT1, and ABCG2) via phosphoinositide 3-kinase/protein kinase (PI3K/AKT) and mitogen-activated protein kinase/extracellular signal-regulated kinase signaling pathways (Mandal et al., 2021).
Various urate transporters involved in renal excretion
Well-characterized urate transporters that are involved in reabsorption of urate from the glomerular filtrate in the human renal proximal tubules include human urate transporter 1 (URAT1, encoded by the SLC22A12 gene), organic anion transporters (OAT10/ORCTL3, encoded by the SLC22A13 gene, and OAT4, encoded by the SLC22A11 gene), and glucose transporter member 9 (GLUT9, encoded by the SLC2A9 gene). The urate transporters that are involved in secretion of urate into the urine from serum include OAT1 (encoded by the SLC22A6 gene), OAT2 (encoded by the SLC22A7 gene), OAT3 (encoded by the SLC22A8 gene), ATP-binding cassette, subfamily G, member 2 (ABCG2, encoded by the ABCG2 gene), ABCC4 (encoded by the ABCC4 gene), sodium-dependent phosphate transporters types (NPT1, encoded by the SLC17A1 gene) and NPT4 (encoded by the SLC17A3 gene) (Mandal and Mount, 2015; Mandal et al., 2017). Urate reabsorption in the proximal tubule involves the coordinated activity of several transporters. Sodium-dependent reabsorption of organic monocarboxylates by the apical Na+-dependent monocarboxylate transporters SMCT1 (encoded by the SLC5A8 gene) and SMCT2 (encoded by the SLC5A12 gene) (Coady et al., 2004; Srinivas et al., 2005; Mandal et al., 2017) increases the intracellular concentrations of monocarboxylate anions that can then be exchanged with luminal urate via the urate–anion exchangers URAT1 and OAT10.
URAT1 (SLC22A12)
URAT1 is expressed in the apical membrane of the proximal tubules in the kidney and has been regarded as the dominant apical urate/anion exchanger in humans mediating urate reabsorption (Figure 1). URAT1 transports urate in exchange for intracellular nicotinate and pyrazinoate, but not lactate (Mandal et al., 2017). In an oocyte expression system, the urate/anion exchange activity of URAT1 has been found to be independent of Na+ ions, but complete removal of Cl− ions in the extracellular medium increases the urate uptake activity of URAT1 3- to 4-fold. URAT1 is also transported in exchange for pyrazinoate. Uricosuric drugs such as benzbromarone, probenecid, tranilast (Mandal et al., 2017), lesinurad (Miner et al., 2016b; Zhao et al., 2020), fenofibrate (Uetake et al., 2010), and losartan, an angiotensin II receptor antagonist (Nakashima et al., 1992), are potent inhibitors of URAT1.
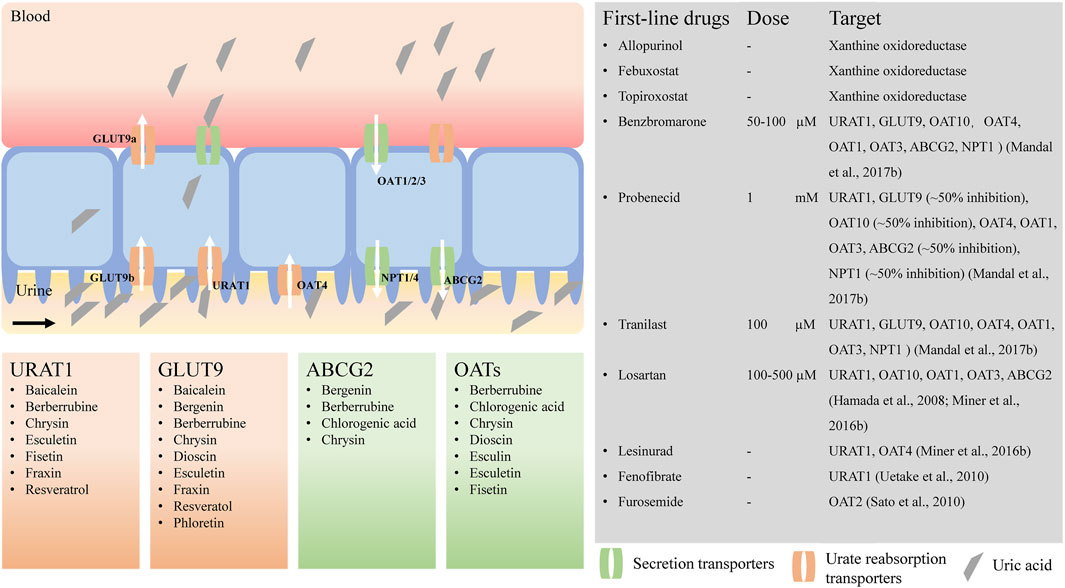
FIGURE 1. Urate transporters and potential natural products for hyperuricemia treatment. URAT1, urate transporter 1; GLUT9, glucose transporter member 9; ABCG2, ATP-binding cassette transporter, subfamily G, member 2; OATs, organic anion transporters; NPTs, sodium-dependent phosphate cotransporter types. The activities of urate transporters were inhibited by benzbromarone (Mandal et al., 2017), probenecid (Mandal et al., 2017), tranilast (Mandal et al., 2017), losartan (Hamada et al., 2008; Miner et al., 2016b), lesinurad (Miner et al., 2016b), fenofibrate (Uetake et al., 2010), furosemide (Sato et al., 2010), respectively.
Loss-of-function mutations in the SLC22A12 gene are associated with hypouricemia (Dinour et al., 2011). Hypouricemia has been previously shown to be associated with common variants associated with several single nucleotide polymorphisms (SNPs), including rs12800450 (G65W), rs121907896 (R90H), rs121907892 (W258X), rs139316841 (W277R), rs749900943 (R349W), rs147647315 (R434H), rs201423508 (T450I) and rs747742344 (E458K) (Ichida et al., 2004; Tin et al., 2011; Sakiyama et al., 2016). The identification of missense mutations (T217M, E298D, G269A, R406C, G412A, G444R, G490A, A1145T, and T1289C) in patients with hypouricemia validates URAT1 as the primary reabsorption urate transporter (Enomoto et al., 2002; Ichida et al., 2004; Dinour et al., 2011). In an SLC22A12-knockout mouse model, fractional urate excretion was significantly greater than that in wild-type mice, confirming the role of URAT1 in urate reabsorption (Hosoyamada et al., 2010). Based on this information, regulation of the URAT1 expression level and its activity is an effective strategy for maintaining a suitable serum urate concentration. Benzbromarone is a potent uricosuric agent that has been used in the treatment of gout for over 30 years. It functions by increasing urate excretion in human kidney proximal tubules through effective inhibition of the dominant apical (luminal) urate exchanger URAT1 at low doses (Enomoto et al., 2002; Mandal et al., 2017). This blockade reduces urate reabsorption, increasing urate elimination via the urine (Enomoto et al., 2002). Benzbromarone was withdrawn from the market by Sanofi-Synthelabo in 2003 after reports of severe hepatotoxicity (Jansen et al., 2004). Aggravation of hepatic steatosis in obese individuals and gastrointestinal problems associated with benzbromarone treatment limit the clinical use of this drug but warrant further affirmation in vivo (Heel et al., 1977; Sun et al., 2018). Recently, many studies have indicated that SNPs in the SLC12A22 gene are strongly associated with hyperuricemia and gout. In an analysis of the Korean Cancer Prevention Study-II (KCPS-II) cohort, sequencing of URAT1 in 68 male Korean subjects revealed that the pattern most strongly associated with hyperuricemia included a common variant consisting of rs7929627 (IVS7-103A/G, noncoding variants), rs75786299 (IVS3+11A/G, noncoding variants) and rs3825017 (N82N, coding variant). Moreover, rs11602903 (788A/T, promoter variants) and rs121907892 (W258X, coding variant) were negatively correlated with hyperuricemia (Cho et al., 2015). In addition, other SNPs, including rs3825017 (N82N), rs3825016 (C/T), and rs11231825(H142H), are linked with the risk of hyperuricemia and gout (Li et al., 2014; Pavelcova et al., 2020).
GLUT9 (SLC2A9)
GLUT9 is a voltage-driven high-capacity urate transporter that is mainly expressed in the proximal tubules of the human kidney, the liver, and the intestine (Figure 1) (Anzai et al., 2008; Döring et al., 2008; Vitart et al., 2008; Wallace et al., 2008). It is the sole transporter that transports reabsorbed urate from the proximal tubular epithelium to the blood. Loss-of-function mutations in GLUT9 have been identified in familial hypouricemia, and SNPs are associated with reduced serum urate, indicating that GLUT9 is a major determinant of serum urate levels (Mandal and Mount, 2015). GLUT9 exists in two isoforms, GLUT9a and GLUT9b, which differ in their amino-terminal cytoplasmic domains; GLUT9a is located in the basolateral membrane, and GLUT9b is located in the apical membrane of the proximal tubules in human kidney (Augustin et al., 2004; Kimura et al., 2014). However, in mice, Glut9a is expressed in the proximal convoluted and straight tubules, and Glut9b is expressed in distal convoluted tubules and connecting tubules (Bibert et al., 2009). Thus, mouse Glut9 in the kidney differs from human GLUT9. Human GLUT9a is 540 amino acids in length and is encoded by 12 exons, whereas GLUT9b is 511 amino acids in length and is encoded by 13 exons of the splice variants of RNA of the SLC2A9 gene. In both humans and mice, GLUT9b is expressed only in the liver and kidney, whereas GLUT9a is present in many more tissues, such as the liver, kidney, intestine, leukocytes, and chondrocytes (Augustin et al., 2004; Keembiyehetty et al., 2006; Kimura et al., 2014).
Although GLUT9 was initially reported to mediate glucose/fructose transport (Augustin et al., 2004; Doblado and Moley, 2009), it was later confirmed to be a high-capacity robust urate transporter without any detectable glucose/fructose transport activity (Caulfield et al., 2008; Vitart et al., 2008; Mandal et al., 2017; Mandal et al., 2021). Furthermore, SLC2A9-knockout mice exhibit increased serum urate levels with impaired enterocyte urate transport kinetics. SLC2A9 deficiency in mice can induce the occurrence of early-onset metabolic syndrome, suggesting a role of SLC2A9 in regulating enterocyte urate clearance (DeBosch et al., 2014). Importantly, uricosuric drugs such as benzbromarone, probenecid, and tranilast (Mandal et al., 2017), but not losartan or lesinurad, are potent inhibitors of GLUT9 (Miner et al., 2016a). In a oocyte expression system, urate uptake activity was found to be almost completely inhibited by 100 µM benzbromarone (Mandal et al., 2017). The uptake of urate mediated by GLUT9 is inhibited by benzbromarone (100 µM) and losartan (1 mM) by approximately 90 and 50%, respectively (Bibert et al., 2009). Moreover, electrophysiological measurements suggest that urate transport by mouse GLUT9 is electrogenic and voltage-dependent but independent of Na+ and Cl− transmembrane gradients (Bibert et al., 2009). However, in the oocyte expression system, complete removal of Cl− ions in the extracellular medium increased the urate uptake activity of GLUT9 3- to 4-fold. Similarly, complete replacement of Na+ ions by K+ ions in the extracellular medium increased the urate uptake activity of human GLUT9 4- to 5-fold (Mandal et al., 2017).
ABCG2 (ABCG2)
ABCG2 protein, first identified as a multidrug resistance protein (Doyle et al., 1998) that transports a wide range of structurally and functionally diverse chemotherapeutics, has been characterized as a high-capacity urate secretion transporter (Matsuo et al., 2009; Woodward et al., 2009; Nakayama et al., 2011). ABCG2 is located on chromosome 4q, as identified by genome-wide association studies related to hyperuricemia and gout (Nakayama et al., 2011). It is expressed in the apical membrane of the human kidney proximal tubules (Figure 1) (Huls et al., 2008; Woodward et al., 2009), the intestine and the liver (Nakayama et al., 2011).
In white, African and Asian populations, the SNP rs2231142 in exon 5 of the ABCG2 gene, which generally causes the Gln141Lys amino acid substitution, is the strongest link between ABCG2 and gout/hyperuricemia (Dehghan et al., 2008). Functional studies have shown that the Q141K substitution causes an approximately 53% reduction in ABCG2-mediated urate export activity compared with that of the wild-type protein (Woodward et al., 2009). Approximately one-third of uric acid is excreted from the intestines in humans (Matsuo et al., 2014). Thus, ABCG2 plays a crucial role as an essential renal and intestinal urate exporter, as its dysfunction is associated with abnormal serum uric acid levels and gout/hyperuricemia risk. In an oocyte expression system, benzbromarone and probenecid were shown to inhibit ABCG2 urate export activity (Mandal et al., 2017; Fujita et al., 2019), but lesinurad was not (Miner et al., 2016a).
OATs (SLC22A)
OATs, encoded by SLC22 family of genes, are expressed in the barrier epithelia of the major excretory organs of the body, the kidney and the liver (Figure 1). These transporters play important roles in renal drug elimination, as they interact with endogenous metabolic end products such as urate and a multitude of widely used drugs, including antibiotics, antihypertensives, antivirals, anti-inflammatory drugs, diuretics and uricosurics (Rizwan and Burckhardt, 2007). OATs also play important roles in both the renal secretion and reabsorption of urate. Five of the characterized OATs are expressed in the renal proximal tubules: OAT1 (encoded by the SLC22A6 gene), OAT2 (encoded by the SLC22A7 gene) and OAT3 (encoded by the SLC22A8 gene) are located in the basolateral membrane, whereas OAT4 (encoded by the SLC22A11 gene) and OAT10/ORCTL3 (encoded by the SLC22A13 gene) are located in the apical membrane of the renal proximal tubules (Eraly et al., 2008). The basolateral urate transporters OAT1, OAT2 and OAT3 function in urate secretion (Eraly et al., 2008), transporting urate from blood into proximal tubular cells for secretion at the apical membrane (Mandal et al., 2017). OAT1 and OAT3 are Na+-independent and exchange urate with divalent anions such as α-ketoglutarate (Aslamkhan et al., 2003; Bakhiya et al., 2003; Sweet et al., 2003), indicating that basolateral entry of urate from the blood into the proximal tubular epithelium is driven by intracellular α-KG during urate secretion. In an oocyte expression system, the urate transport activity of murine OAT3 was found to be cis-inhibited by extracellular salicylate, nicotinate or pyrazinoate anion (Mandal et al., 2017). OAT2 is chloride-dependent and has more restricted substrate specificity than OAT1 and OAT3 (Sato et al., 2010). The urate transport activity of OAT2 is cis-inhibited by the antiuricosuric agents pyrazinecarboxylic acid and nicotinate (Sato et al., 2010), and OAT3 is cis-inhibited by pyrazinecarboxylic acid, nicotinate and salicylate (Mandal et al., 2017). Human OAT4 transports urate in exchange for divalent organic dicarboxylate ions (Hagos et al., 2007; Mandal et al., 2017). OAT10 is a urate transporter and high-affinity urate/nicotinate exchanger dependent on Cl− ions (Bahn et al., 2008; Mandal et al., 2017). OAT10 and URAT1 share functional similarities, as both of them transport urate and nicotinate and as the urate transport activity of both is trans-stimulated by intracellular nicotinate or pyrazine carboxylate (Mandal et al., 2017). Oat1-and Oat3-null mice exhibit decreased secretion of urate rather than reabsorption (Eraly et al., 2008). Benzbromarone, probenecid and tranilast were shown to inhibit the urate transport activity of OAT10, OAT4, OAT1, and OAT3 in an oocyte expression system (Mandal et al., 2017). Benzbromarone, probenecid, losartan, telmisartan, hydrochlorothiazide, and furosemide have been shown to inhibit the urate transport activity of OAT2 (Sato et al., 2010). In addition, lesinurad inhibits OAT4 but does not inhibit OAT1 or OAT3 in the clinical setting (Sato et al., 2010).
NPTs (SLC17A)
NPT1 and NPT4, encoded by SLC17A1 and SLC17A3 genes, respectively, are located at the apical membrane of the proximal tubule and mediate net tubular urate secretion (Figure 1). Genome-wide association studies have identified a region in chromosome 6p23-p21.3, where SLC17A1 and SLC17A3 are located, that is associated with serum urate concentrations (Kolz et al., 2009). NPT1 is the first identified member of the SLC17A phosphate transporter family. Human NPT1 transports organic anions such as urate, p-aminohippurate and acetylsalicylate (aspirin), and salicylate in a voltage-driven and Cl−-dependent manner (Reimer and Edwards, 2004; Iharada et al., 2010). The identification of a common gain-of-function variant, rs1165196 (T806C), in Japanese patients with significantly decreased risk of renal underexcretion gout enhanced understanding of the physiological role of NPT1 as a renal urate exporter (Chiba et al., 2015). Benzbromarone, probenecid and tranilast have been shown to inhibit the urate transport activity of NPT1 in an oocyte expression system (Mandal et al., 2017). NPT4 is also a voltage-dependent organic anion transporter, similar to porcine OAT1/3, with lower affinity urate transport (Jutabha et al., 2011). Two thiazide drugs, chlorothiazide-2 and trichlormethiazide-3, and two loop diuretics, bumetanide-4 and furosemide-5, have been shown to inhibit the urate transport activity of NPT4 in an oocyte expression system (Jutabha et al., 2011), which suggests the involvement of NPT4 in diuretic-induced hyperuricemia. Two SNPs, rs1165205 within intron 1 of SLC17A3 and rs116205 identified in the SLC17A3 gene, correlate well with the changes in serum urate concentration (Riches et al., 2009). The in vivo role of NPT4 is supported by the presence of missense mutations (N68H and F304S) in SLC17A3 in underexcretion-type hyperuricemia patients (Jutabha et al., 2010).
Management of hyperuricemia by herbal extracts and natural bioactive compounds
The field of traditional Chinese medicine (TCM) is valued for its holistic view of the human body. TCM provides a massive amount of information on natural products and disease phenotypes observable as clinical symptoms that are crucial for clinical diagnosis and treatment (Table 1). Various aqueous or ethanolic herbal extracts used in TCM have been demonstrated to be beneficial in various disease conditions. The 70% ethanol extract (comprising polyphenols and flavonoids) of Eurycoma longifolia, a tropical medicinal plant, has been reported to significantly reduce serum urate levels by downregulating the protein expression levels of Urat1 and Glut9 in rats with PO-induced hyperuricemia and an adenine-/PO-induced hyperuricemia mouse model (Bao et al., 2019). The macroporous resin extract of Dendrobium officinale leaves has been reported to reduce serum urate levels in rats with fructose- and PO-induced hyperuricemia by inhibiting XOD activity and regulating the expression of Abcg2, Urat1, and Glut9 (Wang et al., 2022). Sunflower calathide aqueous extract has been shown to reduce serum urate levels to a degree comparable to that mediated by allopurinol and benzbromarone in rats with yeast extract-induced hyperuricemia with renal injury (Dai et al., 2021). Sunflower calathide aqueous extract has also been shown to downregulate the cytokines COX-2, PGE2, NO, and IFN-γ in lipopolysaccharide (LPS)-treated RAW264.7 cells (Dai et al., 2021). Ethanol extract of the bark of Liriodendron chinense (Hemsl.) Sarg has been shown to reduce serum urate in adenine and PO-induced hyperuricemia mice with nephropathy by suppressing the activation of nuclear factor-kappa B (NF-κB) and the Janus kinase/signal transducer and activator of transcription 3 (JAK/STAT3) signaling pathway, reducing inflammatory factor infiltration and urate accumulation in the kidney (Pan et al., 2021a). A polysaccharide (molecular weight, 46.56 kDa) obtained from the green algae Enteromorpha prolifera, comprising rhamnose, glucuronic acid, galactose, arabinose, and xylose at a molar ratio of 20.45:12.74:10.99:5.84:1.95, has been shown to significantly reduce serum urate, serum XOD and hepatic XOD; upregulate the mRNA and protein expression of urate secretion transporters Abcg2, Oat1, and Npt1; and downregulate the urate reabsorption transporter, maintaining the stability of the intestinal flora in mice with hyperuricemia (Li X. et al., 2021). Fufang Zhenzhu Tiaozhi capsule prevents renal injury, inflammation and fibrosis in mice with hypoxanthine- and PO-induced hyperuricemia by promoting urate excretion and inhibiting the PI3K/AKT/NF-κB signaling pathway (Li et al., 2022). The Third National Health and Nutrition Examination Survey (1988–1994), using data from 14,758 men and women aged ≥20 years, showed that coffee consumption is associated with reduced serum urate levels (Choi and Curhan, 2007). Dioscin, a spirostane glycoside with anti-inflammatory and antiallergic properties found in the rhizome of Dioscorea spongiosa, has been shown to significantly reduce serum urate by downregulating Glut9 and upregulating Oat1 in rats with PO-induced hyperuricemia alone and in mice with adenine-/PO-induced hyperuricemia (Tao et al., 2018; Zhang et al., 2018; Li J. et al., 2021). Theobromine, a natural dimethylxanthine present in high amounts in cocoa, may be clinically useful in the treatment of nephrolithiasis, as it inhibits nucleation and urate crystal growth (Grases et al., 2014). Curcumin, a hydrophobic polyphenol extracted from the rhizome of Curcuma longa, has been shown to significantly reduce serum urate in PO-induced hyperuricemia rats modulating the gut microbiota, fortifying the intestinal barrier, attenuating metabolic endotoxemia, and consequently protecting renal function (Xu et al., 2021).
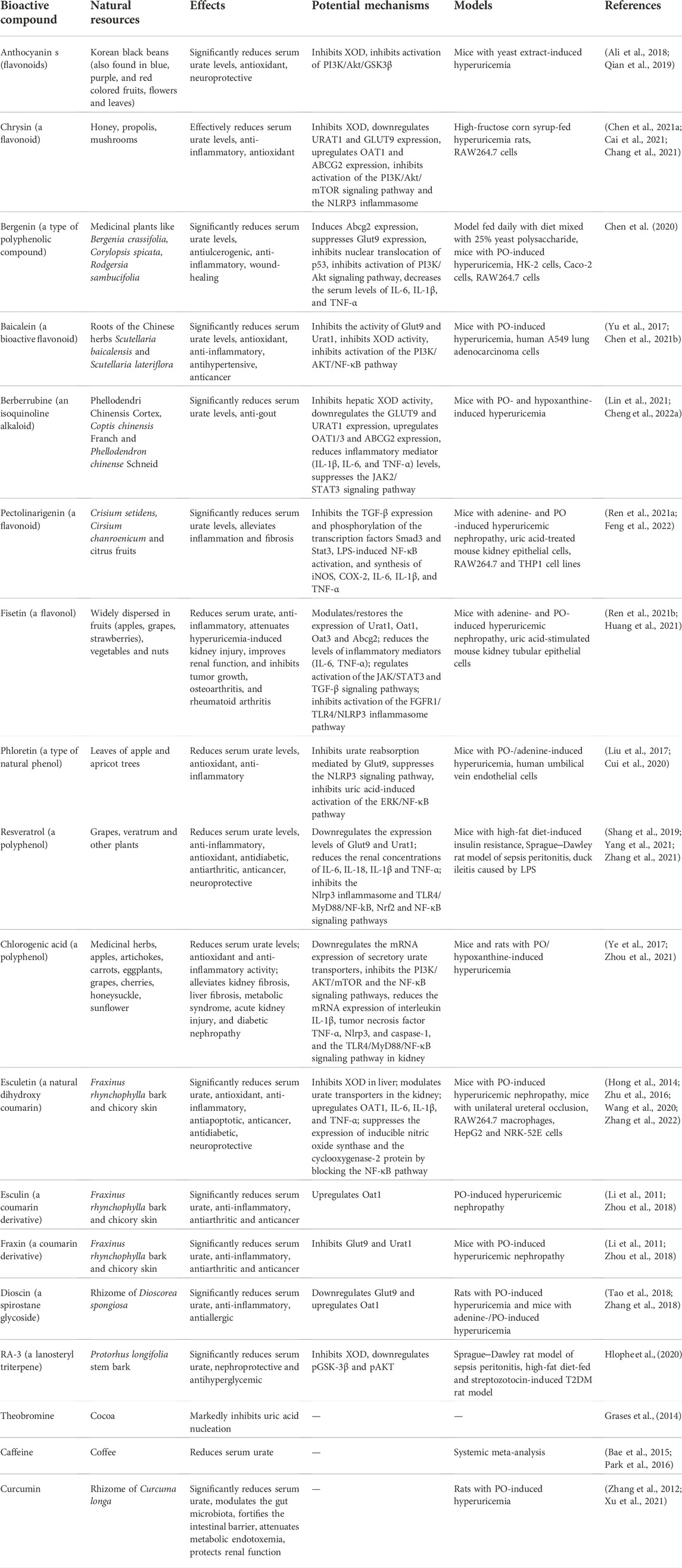
TABLE 1. Summary of the effects and potential mechanisms of candidates for hyperuricemia treatment. PO, potassium oxonate; LPS, lipopolysaccharide; URAT1, human urate transporter 1; GLUT9, glucose transporter member 9; ABCG2, ATP-binding cassette transporter, subfamily G, member 2; OAT, organic anion transporter; NPT, sodium-dependent phosphate cotransporter type; NLRP3, NOD-like receptor family pyrin domain-containing 3; IL-6, interleukin-6; IL-1β, interleukin-1β; NF-κB, nuclear factor-kappa B; TGF-β, transforming growth factor-beta; PI3K/AKT, phosphoinositide 3-kinase/protein kinase; JAK/STAT3, Janus kinase/signal transducer and activator of transcription 3, TLR4, Toll-like receptor 4; XOD, xanthine oxidoreductase.
These results suggest that TCM herbal extracts have potential benefits in the management of hyperuricemia and related kidney impairment. Due to the complex ingredient structures and compositions of TCM herbal extracts, it is difficult to comprehensively elucidate the mechanisms of the pharmacological effects of the extracts. Identification and exploration of natural bioactive compounds from TCMs might be promising directions for hyperuricemia management.
The PI3K/Akt signaling pathway, a potential target for the treatment of hyperuricemia
Generally, MSU deposition causes acute gout flares and recurrent gout attacks that destroy joints. Macrophages are thought to initiate and drive MSU-induced inflammation. MSU-induced acute gouty arthritis in mice has been shown to cause activation of the PI3K/Akt pathway (Cao et al., 2021). In addition, insulin stimulation of urate uptake in human proximal tubular cells (PTC-05) and HEK293T cells is effectively abrogated by inhibitors of protein tyrosine kinase and PI3K (Mandal et al., 2021). In an oocyte expression system, insulin was shown to stimulate the urate transport activity of GLUT9a, GLUT9b, OAT10, OAT3, OAT1, NPT1, and ABCG2, which are directly activated by insulin signaling through the PI3K/Akt signaling pathway (Mandal et al., 2021). Anthocyanins, a group of natural flavonoids found in Korean black beans (also found in blue-, purple-, and red-colored fruits, flowers, and leaves) that regulate the PI3K/Akt/GSK3β pathways (Ali et al., 2018), have been reported to significantly reduce serum urate levels and XOD activity in the serum and livers of mice with yeast extract-induced hyperuricemia (Qian et al., 2019). Future studies are warranted to investigate whether anthocyanins regulate the expression of urate transporters via the PI3K/Akt signaling pathway. Chrysin, a flavonoid compound naturally found in honey, propolis and mushrooms that exerts anti-inflammatory and antioxidant effects, has been shown to effectively reduce serum urate levels by inhibiting the activity of XOD in the liver of high-fructose corn syrup-fed hyperuricemia rats by downregulating the protein expression of Urat1 and Glut9 and upregulating the protein expression of Oat1 and Abcg2 (Chang et al., 2021). The anti-inflammatory effect of chrysin is mediated by the PI3K/Akt/mTOR signaling pathway in RAW264.7 cells (Cai et al., 2021). Bergenin, a type of polyphenol compound with antiulcerogenic, anti-inflammatory, and wound-healing properties that induces ABCG2 expression and suppresses SLC2A9 expression by inhibiting the nuclear translocation of p53 in HK-2 cells, reduces serum urate levels in mice with yeast polysaccharide-induced hyperuricemia by promoting renal and gut urate excretion (Chen et al., 2020).
The JAK/STAT3 signaling pathway, a potential target for the treatment of hyperuricemia
Kidney fibrosis is a histologic hallmark of CKD that is possibly caused by hyperuricemia. Pharmacological inhibition of the activation of the JAK/STAT3 pathway has been shown to reduce serum urate levels and delay the progression of kidney fibrosis and CKD in adenine- and PO-induced hyperuricemia mouse models (Pan et al., 2021b). Berberrubine is an isoquinoline alkaloid that is the primary metabolite of berberine, the main component of Phellodendri Chinensis Cortex, which is found in Coptis chinensis Franch and Phellodendron chinense Schneid (Cheng H. et al., 2022). Berberrubine possesses antihyperuricemic and antigout effects and significantly decreases the serum urate levels in mice with PO- and hypoxanthine-induced hyperuricemia (Lin et al., 2021). Berberrubine has also been shown to reduce hepatic XOD activity, downregulate the expression of Glut9 and Urat1 and upregulate the expression of Oat1/3 and Abcg2 at both the protein and mRNA levels in mice with hyperuricemia as well as to suppress activation of the JAK/STAT3 signaling pathway (Lin et al., 2021). The natural flavonoid pectolinarigenin has been reported to significantly reduce serum urate levels in mice with adenine- and PO-induced hyperuricemic nephropathy (Ren et al., 2021a). Pectolinarigenin also inhibits the expression of transforming growth factor-beta (TGF-β)1 as well as the phosphorylation of the transcription factors Smad3 and Stat3, suggesting that suppression of inflammation and fibrosis by pectolinarigenin occurs through inhibition of Smad3 and Stat3 signaling pathway activation in mice with hyperuricemic nephropathy (Ren et al., 2021a). Fisetin (3,3′,4′,7-tetrahydroxyflavone), a naturally occurring flavonol, reduces serum urate by modulating the expression of kidney urate transporters, including Urat1, Oat1/3, and Abcg2, via the STAT3 and TGF-β signaling pathways in mice with PO- and adenine-induced hyperuricemia (Ren et al., 2021b). Fisetin treatment also reduces the levels of proinflammatory mediators, including tumor necrosis factor-alpha (TNF-α), interleukin 6 (IL-6) and monocyte chemoattractant protein-1; attenuates kidney fibrosis; and restores the expression of alpha-smooth muscle actin, collagen I and fibronectin via modulation of the STAT3 and TGF-β signaling pathways (Ren et al., 2021b). In a cell-based urate transport assay, fisetin was shown to be a strong URAT1 inhibitor with a half-maximal inhibitory concentration of 7.5 µM (Toyoda et al., 2022). Curcumin has been shown to reduce fructose-induced hyperuricemia and renal endothelial dysfunction by inhibiting activation of NO-mediated JAK/STAT signaling in rats (Zhang et al., 2012).
NLRP3 and the NF-κB/TLR4 signaling pathway, potential targets for the treatment of hyperuricemia
The inflammasome is a by multiprotein cytoplasmic complex that plays important roles in host defense and inflammatory responses through activating caspase-1 and promoting secretion of the proinflammatory cytokines interleukin 1beta (IL-1β) and IL-18 (Franchi et al., 2009). Nod-like receptor protein members, including NLRP1, NLRP3 and NLRC4, and the adaptor ASC (apoptosis-related specific protein) constitute the inflammasome (Franchi et al., 2009). The NLRP3 inflammasome, an intracellular sensor, plays an important role in innate immunity and is therefore the most investigated inflammasome (Franchi et al., 2009). For activation of the NLRP3 inflammasome, activation of NF-κB is required to upregulate the expression of NLRP3, pro-IL-1β, and caspase 1, which is accomplished via stimulation of Toll-like receptors (TLRs) (Toma et al., 2010; Qiao et al., 2012). MSU, identified as a danger signal formed after the release of uric acid from dying cells (Shi et al., 2003), has been shown to trigger the cellular inflammatory response through the NLRP3 inflammasome, resulting in the production of the active inflammatory cytokines IL-1β and IL-18 (Martinon et al., 2006). MSU-induced inflammation and oxidative stress proceed through the NF-κB/NLRP3 and Nrf2 pathways, leading to increased production of the inflammatory cytokines IL-1β, IL-6, IL-18, and TNF-α (Alberts et al., 2019; Cheng J.-J. et al., 2022). The bacterial cell wall component crude LPS can also activate the NRLP3 inflammasome (Martinon et al., 2006).
In this review, we have shown the mechanisms by which some natural bioactive compounds suppress activation of the NLRP3 inflammasome in mice with hyperuricemia (Table 1). In a PO-induced hyperuricemia mouse model, reduction of serum urate by treatment with the XOD inhibitor N-(9,10-anthraquinone-2-yl-carbonyl), downregulates Glut9 protein expression and upregulates Oat1 and Oat3 protein expression, resulting in decrease in the levels of TNF-α, IL-6, and other inflammatory factors in the serum and kidney of mice and inhibition of NLRP3 pathway-mediated inflammation (Gao et al., 2022). Chlorogenic acid, a polyphenolic compound found in medicinal herbs with antioxidant and anti-inflammatory activity, has been shown to inhibit XOD and downregulate the mRNA expression of secretory uric acid transporters in a hypoxanthine- and PO-induced hyperuricemia mouse model (Zhou et al., 2021). In addition, chlorogenic acid reduces the mRNA expression of IL-1β, TNF-α, Nlrp3, and caspase-1. It also inhibits activation of the TLR 4/MyD88/NF-κB signaling pathway in the kidney and reduces the mRNA expression of ileal IL-1β and IL-6, resulting in inflammation relief in the above hyperuricemic mice and in LPS-induced acute kidney injury (Ye et al., 2017; Zhou et al., 2021). Berberrubine has been shown to significantly reduce serum urate levels and the levels of inflammatory mediators (IL-1β, IL-6, and TNF-α) in mice with PO- and hypoxanthine-induced hyperuricemia (Lin et al., 2021). Pectolinarigenin, in addition to reducing serum urate in mice with hyperuricemic nephropathy (Ren et al., 2021a), inhibits LPS-induced NF-κB activation by interfering with the degradation of IκB-α and the synthesis of inducible nitric oxide synthase, cyclooxygenase-2, IL-6, IL-1β, and TNF-α in RAW264.7 and THP1 cell lines (Feng et al., 2022). Chrysin improves vascular permeability and alleviates the inflammatory response in lung tissue by suppressing the IRE1α/TXNIP/NLRP3 signaling pathway, thereby alleviating LPS-induced acute lung injury in mice (Chen M. et al., 2021). Bergenin reduced the levels of serum urate, IL-6, IL-1β, and TNF-α in hyperuricemic mice and promotes a polarization shift from the M1 to the M2 phenotype in RAW264.7 cells (Chen et al., 2020). Esculetin is a natural dihydroxy coumarin found in Fraxinus rhynchophylla bark and chicory skin that has been shown to reduce serum urate by increasing renal urate excretion, inhibiting XOD expression and activity in the liver, and modulating urate transporters in the kidney (Hong et al., 2014; Wang et al., 2020). Esculetin has been shown to attenuate elevations in the levels of proinflammatory cytokines, including IL-6, IL-1β, and TNF-α, and serum urate and suppress inducible nitric oxide synthase and cyclooxygenase-2 protein expression by blocking the NF-κB pathway and suppressing the generation of proinflammatory mediators, including nitric oxide and prostaglandin E2, in LPS-induced RAW264.7 macrophages and mice (Hong et al., 2014; Zhu et al., 2016). Huang et al. reported that fisetin, a naturally occurring flavonol, inhibits inflammatory responses in experimental periodontitis in rats and LPS-induced human gingival fibroblasts through the FGFR1/TLR4/NLRP3 inflammasome pathway, which mitigates kidney injury to restore the normal expression of Urat1 (Huang et al., 2021). Phloretin, a dihydrochalcone, is a type of natural phenol found in the leaves of apple and apricot trees with antioxidant and anti-inflammatory properties. Phloretin has been shown to effectively attenuate urate-induced renal injury by inhibiting Nlrp3 and urate reabsorption mediated by Glut9 and promoting urinary urate excretion in mice with PO-/adenine-induced hyperuricemia (Cui et al., 2020). In human umbilical vein endothelial cells, phloretin also significantly attenuates proinflammatory factor expression and reduces GLUT9-mediated urate uptake by inhibiting activation of the ERK/NF-κB pathway (Liu et al., 2017). Resveratrol, a polyphenolic, non-flavonoid plant-derived antitoxin, has been shown to reduce serum urate levels by downregulating the expression levels of Glut9 and Urat1 and to improve kidney inflammation, possibly via the TLR4 AND NLRP3 signaling pathways, in mice with high-fat diet-induced insulin resistance (Zhang et al., 2021). In a classic Sprague‒Dawley rat model of sepsis peritonitis, resveratrol significantly decreases LPS-induced expression of NF-κB, TNF-α, IL6, IL-1β, and TLR4 and increases the expression of p-PI3K, p-AKT, and p-mTOR in the myocardium (Shang et al., 2019). Thus, resveratrol has the potential to protect the myocardium in sepsis by activating the PI3K/AKT/mTOR signaling pathway and inhibiting the NF-κB signaling pathway and related inflammatory factors (Shang et al., 2019). Resveratrol also reduces LPS-induced inflammation by reducing the levels of inflammatory cytokines (IL-6, IL-18 and TNF-α) in duck ileitis (Yang et al., 2021). Bergenin, a C-glucoside of 4-O-methyl gallic acid isolated from several medicinal plants, has been shown to reduce serum urate levels in a hyperuricemia-induced mouse model by elevating Abcg2 expression in both the kidney and intestine and by suppressing Slc2a9 expression in the kidney (Chen et al., 2020). Bergenin also reduces the serum levels of IL-6, IL-1β, and TNF-α in hyperuricemic mice (Chen et al., 2020). Baicalein, a bioactive flavonoid exhibiting antioxidant, anti-inflammatory, antihypertensive and anticancer properties, has been reported to significantly reduce serum urate and enhance renal urate excretion in mice with PO-induced hyperuricemia by specifically inhibiting the [14C]-urate uptake activities of Glut9 and Urat1 in a noncompetitive manner, with a half-maximal inhibitory concentration values of 30.17 ± 8.68 and 31.56 ± 1.37 μM, respectively (Chen Y. et al., 2021). Baicalein also downregulates Glut9 and Urat1 expression in the kidney and suppressed XOD activity in the serum and liver (Chen Y. et al., 2021). Cisplatin resistance is one of the major obstacles in the treatment of non-small cell lung cancer. Combining baicalein with cisplatin has been shown to significantly attenuate cisplatin resistance in human A549 lung adenocarcinoma cells through inhibition of the PI3K/AKT/NF-κB pathway (Yu et al., 2017).
Present-day first-line medications for the management of hyperuricemia/gout
It is not clear whether elevated uric acid is the primary cause of kidney disease or the oxidant (H2O2) produced as byproduct by the activation of XOD in addition to uric acid. Are uric acid-lowering agents more beneficial than angiotensin-converting enzyme inhibitors in subjects with CKD? At present, the first-line medications for hyperuricemia/gout are XOD inhibitors, such as allopurinol (a purine analog and competitive xanthine oxidase inhibitor), or the more renoprotective febuxostat/topiroxostat (a nonpurine analog), which inhibits uric acid production (Figure 1). Although allopurinol can successfully control serum urate levels in hyperuricemia/gout patients, long-term use of a high dose of allopurinol to promote the dissolution of urate crystals has been linked to an increased risk of liver, kidney and cardiovascular diseases (Arellano and Sacristán, J. A. 1993; Yang et al., 2015). Febuxostat is used only in the subset of CKD patients with impaired renal function due to its hepatic elimination. There is a risk that all xanthine oxidase inhibitors can increase urinary xanthine levels, which can be nephrotoxic. Uricosurics lower uric acid levels by promoting urate excretion through interactions with urate transporters. Uricosuric diuretics such as probenecid and benzbromarone increase urine output, but high-dose or long-term use of diuretics increases the risk of hepatotoxicity and renal failure (Stamp and Chapman, 2014). Probenecid is ineffective in patients with renal impairment (Stamp et al., 2005). Benzbromarone is effective in allopurinol-intolerant patients with renal failure, solid organ transplant or tophaceous/polyarticular gout. In an oocyte expression system, benzbromarone has been found to inhibit ABCG2, the major transporter for urate excretion in the kidney and intestine, along with OAT1, OAT3 and NPT1 (Mandal et al., 2017). Notably, benzbromarone was withdrawn from the market by Sanofi-Synthelabo in 2003, after reports of serious hepatotoxicity. Fenofibrate may reduce serum urate but it is associated with the decline in renal function (Stamp et al., 2005). In transplant recipients, there is a risk of adverse effects and potentially severe interactions between hypouricemic and immunosuppressive drugs (Stamp et al., 2005).
Conclusion
Hyperuricemia is associated with multiple comorbidities, including metabolic syndrome, CKD, urolithiasis, cardiovascular disease, hypertension, dyslipidemia and diabetes, creating a demand for new antihyperuricemic and nephroprotective drugs. Long-term use of high doses of first-line medications for hyperuricemia likely induces acute kidney disease, CKD, liver toxicity, and adverse effects in transplant recipients. Therefore, there is an urgent need for new nephroprotective drugs with improved safety profiles and tolerance. To avoid oxidative stress and excessive inflammatory responses, new therapeutic drugs with strong antioxidant and anti-inflammatory activities would be preferred. There is substantial evidence of naturally occurring bioactive compounds from various medicinal plants with antihyperuricemic and nephroprotective potential that can protect the kidney from various insults and maintain their integrity and functions. The current review has covered some of the compounds that influence the expression and activity of urate transporters, including URAT1, GLUT9, ABCG2, NPTs, and OATs, and their regulatory pathways, such as the PI3K/AKT, JAK/STAT3, NLRP3, and NF-κB pathways.
Author contributions
SX and SW directed and guided this review. YB wrote and edited the whole article. XM, LS, XX, CT, and DL reviewed and made some comments on the article. All authors read and approved the final article.
Funding
Subproject of Major Science and Technology Innovation Project of Shandong Province (2019JZZY020612), Zhangdian School-City Integrated Development Project (2021PT0001), National Natural Science Foundation of China (81903878), Natural Science Foundation of Shandong Province (ZR202112010171, ZR2022MH306).
Conflict of interest
SX and SW were employed by the Shandong Qingyujiangxing Biotechnology Co., Ltd.
The authors declare that the research was conducted in the absence of any commercial or financial relationships that could be construed as a potential conflict of interest.
Publisher’s note
All claims expressed in this article are solely those of the authors and do not necessarily represent those of their affiliated organizations, or those of the publisher, the editors and the reviewers. Any product that may be evaluated in this article, or claim that may be made by its manufacturer, is not guaranteed or endorsed by the publisher.
Abbreviations
ABCG2, ATP-binding cassette transporter, subfamily G, member 2; CKD, chronic kidney disease; GLUT9, glucose transporter member 9; IL-1β, interleukin-1β; IL-6, interleukin-6; JAK/STAT3, Janus kinase/signal transducer and activator of transcription 3, MSU, monosodium urate; NF-κB, nuclear factor-kappa B; NLRP3, NOD-like receptor family pyrin domain-containing 3; NPT, sodium-dependent phosphate cotransporter type; OAT, organic anion transporter; PI3K/AKT, phosphoinositide 3-kinase/protein kinase; PO, potassium oxonate; ROS, reactive oxygen species; LPS, lipopolysaccharide; SNPs, single nucleotide polymorphisms; TCMs, traditional Chinese medicines, TGF-β, transforming growth factor-beta; TNF-α, tumor necrosis factor-alpha; TLR4, Toll-like receptor 4; URAT1, human urate transporter 1; XOD, xanthine oxidoreductase.
References
Alberts, B. M., Barber, J. S., Sacre, S. M., Davies, K. A., Ghezzi, P., and Mullen, L. M. (2019). Precipitation of soluble uric acid is necessary for in vitro activation of the NLRP3 inflammasome in primary human monocytes. J. Rheumatol. 46, 1141–1150. doi:10.3899/jrheum.180855
Alexandru, B. I., and Moe, O. W. (2012). Renal transport of uric acid: Evolving concepts and uncertainties. Adv. Chronic Kidney Dis. 19, 358–371. doi:10.1053/j.ackd.2012.07.009
Ali, T., Kim, T., Rehman, S., Sohail, K. M., AminUl, F., Khan, M., et al. (2018). Natural dietary supplementation of anthocyanins via PI3K/Akt/Nrf2/HO-1 pathways mitigate oxidative stress, neurodegeneration, and memory impairment in a mouse model of alzheimer's disease. Mol. Neurobiol. 55, 6076–6093. doi:10.1007/s12035-017-0798-6
Ames, B. N., Cathcart, R., Schwiers, E., and Hochstein, P. (1981). Uric acid provides an antioxidant defense in humans against oxidant- and radical-caused aging and cancer: A hypothesis. Proc. Natl. Acad. Sci. U. S. A. 78, 6858–6862. doi:10.1073/pnas.78.11.6858
Anzai, N., Ichida, K., Jutabha, P., Kimura, T., Babu, E., JinJi, C., et al. (2008). Plasma urate level is directly regulated by a voltage-driven urate efflux transporter URATv1 (SLC2A9) in humans. J. Biol. Chem. 283, 26834–26838. doi:10.1074/jbc.C800156200
Arellano, F., and Sacristán, J. A. (1993). Allopurinol hypersensitivity syndrome: A review. Ann. Pharmacother. 27, 337–343. doi:10.1177/106002809302700317
Ascherio, A., LeWitt, P. A., Xu, K., Eberly, S., Watts, A., Matson, W. R., et al. (2009). Urate as a predictor of the rate of clinical decline in Parkinson disease. Arch. Neurol. 66, 1460–1468. doi:10.1001/archneurol.2009.247
Aslamkhan, A., Han, Y.-H., Walden, R., Sweet, D. H., and Pritchard, J. B. (2003). Stoichiometry of organic anion/dicarboxylate exchange in membrane vesicles from rat renal cortex and hOAT1-expressing cells. Am. J. Physiol. Ren. Physiol. 285, F775–F783. Renal physiology. doi:10.1152/ajprenal.00140.2003
Augustin, R., Carayannopoulos, M. O., Dowd, L. O., Phay, J. E., Moley, J. F., and Moley, K. H. (2004). Identification and characterization of human glucose transporter-like protein-9 (GLUT9): Alternative splicing alters trafficking. J. Biol. Chem. 279, 16229–16236. doi:10.1074/jbc.M312226200
Bae, J., Park, P. S., Chun, B.-Y., Choi, B. Y., Kim, M. K., Shin, M.-H., et al. (2015). The effect of coffee, tea, and caffeine consumption on serum uric acid and the risk of hyperuricemia in Korean Multi-Rural Communities Cohort. Rheumatol. Int. 35, 327–336. doi:10.1007/s00296-014-3061-8
Bahn, A., Hagos, Y., Reuter, S., Balen, D., Brzica, H., Krick, W., et al. (2008). Identification of a new urate and high affinity nicotinate transporter, hOAT10 (SLC22A13). J. Biol. Chem. 283, 16332–16341. doi:10.1074/jbc.M800737200
Bakhiya, A., Bahn, A., Burckhardt, G., and Wolff, N. (2003). Human organic anion transporter 3 (hOAT3) can operate as an exchanger and mediate secretory urate flux. Cell. Physiol. biochem. 13, 249–256. doi:10.1159/000074539
Bao, R., Liu, M., Wang, D., Wen, S., Yu, H., Zhong, Y., et al. (2019). Effect of eurycoma longifolia stem extract on uric acid excretion in hyperuricemia mice. Front. Pharmacol. 10, 1464. doi:10.3389/fphar.2019.01464
Bibert, S., Hess, S., Kharoubi, F. D., Thorens, B., Geering, K., Horisberger, J.-D., et al. (2009). Mouse GLUT9: Evidences for a urate uniporter. Am. J. Physiol. Ren. Physiol. 297, F612–F619. Renal physiology. doi:10.1152/ajprenal.00139.2009
Cai, S., Li, Q., Zhou, H., Xu, Y., Song, J., Gan, C., et al. (2021). Mechanism of PI3K/AKT/mTOR signaling pathway for mediating anti-inflammatory and anti-oxidant effects of chrysin: A protein microarray-based study. Nan Fang. Yi Ke Da Xue Xue Bao 41, 1554–1561. doi:10.12122/j.issn.1673-4254.2021.10.15
Cao, L., Zhao, T., Xue, Y., Xue, L., Chen, Y., Quan, F., et al. (2021). The anti-inflammatory and uric acid lowering effects of Si-Miao-San on gout. Front. Immunol. 12, 777522. doi:10.3389/fimmu.2021.777522
Caulfield, M. J., Munroe, P. B., O'Neill, D., Witkowska, K., Charchar, F. J., Doblado, M., et al. (2008). SLC2A9 is a high-capacity urate transporter in humans. PLoS Med. 5, e197. doi:10.1371/journal.pmed.0050197
Chang, Y.-H., Chiang, Y.-F., Chen, H.-Y., Huang, Y.-J., Wang, K.-L., Hong, Y.-H., et al. (2021). Anti-inflammatory and anti-hyperuricemic effects of chrysin on a high fructose corn syrup-induced hyperuricemia rat model via the amelioration of urate transporters and inhibition of NLRP3 inflammasome signaling pathway. Antioxidants (Basel, Switz. 10, 564. doi:10.3390/antiox10040564
Chen, M., Li, J., Liu, X., Song, Z., Han, S., Shi, R., et al. (2021a). Chrysin prevents lipopolysaccharide-induced acute lung injury in mice by suppressing the IRE1α/TXNIP/NLRP3 pathway. Pulm. Pharmacol. Ther. 68, 102018. doi:10.1016/j.pupt.2021.102018
Chen, M., Ye, C., Zhu, J., Zhang, P., Jiang, Y., Lu, X., et al. (2020). Bergenin as a novel urate-lowering therapeutic strategy for hyperuricemia. Front. Cell Dev. Biol. 8, 703. doi:10.3389/fcell.2020.00703
Chen, Y., Zhao, Z., Li, Y., Yang, Y., Li, L., Jiang, Y., et al. (2021b). Baicalein alleviates hyperuricemia by promoting uric acid excretion and inhibiting xanthine oxidase. Phytomedicine 80, 153374. doi:10.1016/j.phymed.2020.153374
Chen-Xu, M., Yokose, C., Rai, S. K., Pillinger, M. H., and Choi, H. K. (2019). Contemporary prevalence of gout and hyperuricemia in the United States and decadal trends: The national health and nutrition examination survey, 2007-2016. Arthritis Rheumatol. 71, 991–999. doi:10.1002/art.40807
Cheng, H., Liu, J., Tan, Y., Feng, W., and Peng, C. (2022a). Interactions between gut microbiota and berberine, a necessary procedure to understand the mechanisms of berberine. J. Pharm. Anal. 12, 541–555. doi:10.1016/j.jpha.2021.10.003
Cheng, J.-J., Ma, X.-D., Ai, G.-X., Yu, Q.-X., Chen, X.-Y., Yan, F., et al. (2022b). Palmatine protects against MSU-induced gouty arthritis via regulating the NF-κB/NLRP3 and Nrf2 pathways. Drug Des. devel. Ther. 16, 2119–2132. doi:10.2147/DDDT.S356307
Chiba, T., Matsuo, H., Kawamura, Y., Nagamori, S., Nishiyama, T., Wei, L., et al. (2015). NPT1/SLC17A1 is a renal urate exporter in humans and its common gain-of-function variant decreases the risk of renal underexcretion gout. Arthritis Rheumatol. 67, 281–287. doi:10.1002/art.38884
Cho, S., Kim, S., Chung, J.-Y., and Jee, S. H. (2015). Discovery of URAT1 SNPs and association between serum uric acid levels and URAT1. BMJ open 5, e009360. doi:10.1136/bmjopen-2015-009360
Choi, H. K., and Curhan, G. (2007). Coffee, tea, and caffeine consumption and serum uric acid level: The third national health and nutrition examination survey. Arthritis Rheum. 57, 816–821. doi:10.1002/art.22762
Choi, H. K., Ford, E. S., Li, C., and Curhan, G. (2007). Prevalence of the metabolic syndrome in patients with gout: The third national health and nutrition examination survey. Arthritis Rheum. 57, 109–115. doi:10.1002/art.22466
Choi, H. K., and Ford, E. S. (2007). Prevalence of the metabolic syndrome in individuals with hyperuricemia. Am. J. Med. 120, 442–447. doi:10.1016/j.amjmed.2006.06.040
Choi, H. K., Mount, D. B., and Reginato, A. M. (2005). Pathogenesis of gout. Ann. Intern. Med. 143, 499–516. doi:10.7326/0003-4819-143-7-200510040-00009
Coady, M. J., Chang, M.-H., Charron, F. M., Plata, C., Wallendorff, B., Sah, J., et al. (2004). The human tumour suppressor gene SLC5A8 expresses a Na+-monocarboxylate cotransporter. J. Physiol. 557, 719–731. doi:10.1113/jphysiol.2004.063859
Copur, S., Demiray, A., and Kanbay, M. (2022). Uric acid in metabolic syndrome: Does uric acid have a definitive role? Eur. J. Intern. Med. 103, 4–12. doi:10.1016/j.ejim.2022.04.022
Cristóbal-García, M., García-Arroyo, F. E., Tapia, E., Osorio, H., Arellano-Buendía, A. S., Madero, M., et al. (2015). Renal oxidative stress induced by long-term hyperuricemia alters mitochondrial function and maintains systemic hypertension. Oxid. Med. Cell. Longev. 2015, 535686. doi:10.1155/2015/535686
Cui, D., Liu, S., Tang, M., Lu, Y., Zhao, M., Mao, R., et al. (2020). Phloretin ameliorates hyperuricemia-induced chronic renal dysfunction through inhibiting NLRP3 inflammasome and uric acid reabsorption. Phytomedicine 66, 153111. doi:10.1016/j.phymed.2019.153111
Dai, H., Lv, S., Qiao, Z., Wang, K., Zhou, X., Bao, C., et al. (2021). The active components of sunflower (helianthus annuus L.) calathide and the effects on urate nephropathy based on COX-2/PGE2 signaling pathway and the urate transporter URAT1, ABCG2, and GLUT9. Front. Nutr. 8, 769555. doi:10.3389/fnut.2021.769555
Davies, K. J., Sevanian, A., Muakkassah-Kelly, S. F., and Hochstein, P. (1986). Uric acid-iron ion complexes. A new aspect of the antioxidant functions of uric acid. Biochem. J. 235, 747–754. doi:10.1042/bj2350747
DeBosch, B. J., Kluth, O., Fujiwara, H., Schürmann, A., and Moley, K. (2014). Early-onset metabolic syndrome in mice lacking the intestinal uric acid transporter SLC2A9. Nat. Commun. 5, 4642. doi:10.1038/ncomms5642
Dehghan, A., Köttgen, A., Yang, Q., Hwang, S.-J., Linda, Kao W., Rivadeneira, F., et al. (2008). Association of three genetic loci with uric acid concentration and risk of gout: A genome-wide association study. Lancet (London, Engl. 372, 1953–1961. doi:10.1016/S0140-6736(08)61343-4
Dinour, D., Bahn, A., Ganon, L., Ron, R., Geifman-Holtzman, O., Knecht, A., et al. (2011). URAT1 mutations cause renal hypouricemia type 1 in Iraqi Jews. Nephrol. Dial. Transpl. 26, 2175–2181. doi:10.1093/ndt/gfq722
Doblado, M., and Moley, K. H. (2009). Facilitative glucose transporter 9, a unique hexose and urate transporter. Am. J. Physiol. Endocrinol. Metab. 297, E831–E835. doi:10.1152/ajpendo.00296.2009
Döring, A., Gieger, C., Mehta, D., Gohlke, H., Prokisch, H., Coassin, S., et al. (2008). SLC2A9 influences uric acid concentrations with pronounced sex-specific effects. Nat. Genet. 40, 430–436. doi:10.1038/ng.107
Doyle, L. A., Yang, W., Abruzzo, L. V., Krogmann, T., Gao, Y., Rishi, A. K., et al. (1998). A multidrug resistance transporter from human MCF-7 breast cancer cells. Proc. Natl. Acad. Sci. U. S. A. 95, 15665–15670. doi:10.1073/pnas.95.26.15665
Enomoto, A., Kimura, H., Chairoungdua, A., Shigeta, Y., Jutabha, P., Ho, Cha S., et al. (2002). Molecular identification of a renal urate anion exchanger that regulates blood urate levels. Nature 417, 447–452. doi:10.1038/nature742
Eraly, S. A., Vallon, V., Rieg, T., Gangoiti, J. A., Wikoff, W. R., Siuzdak, G., et al. (2008). Multiple organic anion transporters contribute to net renal excretion of uric acid. Physiol. Genomics 33, 180–192. doi:10.1152/physiolgenomics.00207.2007
Facchini, F., Chen, Y. D., Hollenbeck, C. B., and Reaven, G. M. (1991). Relationship between resistance to insulin-mediated glucose uptake, urinary uric acid clearance, and plasma uric acid concentration. JAMA 266, 3008–3011. doi:10.1001/jama.1991.03470210076036
Feig, D. I., and Johnson, R. J. (2003). Hyperuricemia in childhood primary hypertension. Hypertension 42, 247–252. doi:10.1161/01.HYP.0000085858.66548.59
Feig, D. I., Kang, D.-H., and Johnson, R. J. (2008). Uric acid and cardiovascular risk. N. Engl. J. Med. 359, 1811–1821. doi:10.1056/NEJMra0800885
Feng, Y., Bhandari, R., Li, C., Shu, P., and ShaikhIbrahim., I. (2022). Pectolinarigenin suppresses LPS-induced inflammatory response in macrophages and attenuates DSS-induced colitis by modulating the NF-κB/Nrf2 signaling pathway. Inflammation. doi:10.1007/s10753-022-01710-4
Franchi, L., Eigenbrod, T., Muñoz-Planillo, R., and Nuñez, G. (2009). The inflammasome: A caspase-1-activation platform that regulates immune responses and disease pathogenesis. Nat. Immunol. 10, 241–247. doi:10.1038/ni.1703
Fujita, K., Yamada, H., Iijima, M., and Ichida, K. (2019). Electrochemical analysis of uric acid excretion to the intestinal lumen: Effect of serum uric acid-lowering drugs and 5/6 nephrectomy on intestinal uric acid levels. PloS one 14, e0226918. doi:10.1371/journal.pone.0226918
Gaffo, A. L., Lawrence, E. N., and Saag, K. G. (2009). Gout. Hyperuricemia and cardiovascular disease: How strong is the evidence for a causal link? Arthritis Res. Ther. 11, 240. doi:10.1186/ar2761
Gao, T., Xu, J., Xiao, Y., Li, J., Hu, W., Su, X., et al. (2022). Therapeutic effects and mechanisms of N-(9, 10-anthraquinone-2-ylcarbonyl) xanthine oxidase inhibitors on hyperuricemia. Front. Pharmacol. 13, 950699. doi:10.3389/fphar.2022.950699
Grases, F., Rodriguez, A., and Costa-Bauza, A. (2014). Theobromine inhibits uric acid crystallization. A potential application in the treatment of uric acid nephrolithiasis. PloS one 9, e111184. doi:10.1371/journal.pone.0111184
Hagos, Y., Stein, D., Ugele, B., Burckhardt, G., and Bahn, A. (2007). Human renal organic anion transporter 4 operates as an asymmetric urate transporter. J. Am. Soc. Nephrol. 18, 430–439. doi:10.1681/ASN.2006040415
Hamada, T., Ichida, K., Hosoyamada, M., Mizuta, E., Yanagihara, K., Sonoyama, K., et al. (2008). Uricosuric action of losartan via the inhibition of urate transporter 1 (URAT 1) in hypertensive patients. Am. J. Hypertens. 21, 1157–1162. doi:10.1038/ajh.2008.245
Heel, R. C., Brogden, R. N., Speight, T. M., and Avery, G. S. (1977). Benzbromarone: A review of its pharmacological properties and therapeutic use in gout and hyperuricaemia. Drugs 14, 349–366. doi:10.2165/00003495-197714050-00002
Hlophe, N. B., Opoku, A. R., Osunsanmi, F. O., Djarova-Daniels, T. G., Lawal, O. A., and Mosa, R. A. (2020). A lanosteryl triterpene (RA-3) exhibits antihyperuricemic and nephroprotective effects in rats. Mol. Basel, Switz. 25, E4010. doi:10.3390/molecules25174010
Hong, S. H., Jeong, H.-K., HanHo, M., Park, C., and Choi, Y. H. (2014). Esculetin suppresses lipopolysaccharide-induced inflammatory mediators and cytokines by inhibiting nuclear factor-κB translocation in RAW 264.7 macrophages. Mol. Med. Rep. 10, 3241–3246. doi:10.3892/mmr.2014.2613
Hosoyamada, M., Takiue, Y., Morisaki, H., Cheng, J., Ikawa, M., Okabe, M., et al. (2010). Establishment and analysis of SLC22A12 (URAT1) knockout mouse. Nucleosides Nucleotides Nucleic Acids 29, 314–320. doi:10.1080/15257771003738634
Huang, X., Shen, H., Liu, Y., Qiu, S., and Guo, Y. (2021). Fisetin attenuates periodontitis through FGFR1/TLR4/NLRP3 inflammasome pathway. Int. Immunopharmacol. 95, 107505. doi:10.1016/j.intimp.2021.107505
Huls, M., Brown, C. D. A., Windass, A. S., Sayer, R., van den Heuvel, J. J. M. W., Heemskerk, S., et al. (2008). The breast cancer resistance protein transporter ABCG2 is expressed in the human kidney proximal tubule apical membrane. Kidney Int. 73, 220–225. doi:10.1038/sj.ki.5002645
Ichida, K., Hosoyamada, M., Hisatome, I., Enomoto, A., Hikita, M., Endou, H., et al. (2004). Clinical and molecular analysis of patients with renal hypouricemia in Japan-influence of URAT1 gene on urinary urate excretion. J. Am. Soc. Nephrol. 15, 164–173. doi:10.1097/01.asn.0000105320.04395.d0
Iharada, M., Miyaji, T., Fujimoto, T., Hiasa, M., Anzai, N., Omote, H., et al. (2010). Type 1 sodium-dependent phosphate transporter (SLC17A1 Protein) is a Cl(-)-dependent urate exporter. J. Biol. Chem. 285, 26107–26113. doi:10.1074/jbc.M110.122721
Jalal, D. I., Chonchol, M., Chen, W., and Targher, G. (2013). Uric acid as a target of therapy in CKD. Am. J. Kidney Dis. 61, 134–146. doi:10.1053/j.ajkd.2012.07.021
Jansen, T. L. Th A., Reinders, M. K., van Roon, E. N., and Brouwers, J. R. B. J. (2004). Benzbromarone withdrawn from the European market: Another case of "absence of evidence is evidence of absence. Clin. Exp. Rheumatol. 22, 651.
Johnson, R. J., Bakris, G. L., Borghi, C., Chonchol, M. B., Feldman, D., Lanaspa, M. A., et al. (2018). Hyperuricemia, acute and chronic kidney disease, hypertension, and cardiovascular disease: Report of a scientific workshop organized by the national kidney foundation. Am. J. Kidney Dis. 71, 851–865. doi:10.1053/j.ajkd.2017.12.009
Jutabha, P., Anzai, N., Kitamura, K., Taniguchi, A., Kaneko, S., Yan, K., et al. (2010). Human sodium phosphate transporter 4 (hNPT4/SLC17A3) as a common renal secretory pathway for drugs and urate. J. Biol. Chem. 285, 35123–35132. doi:10.1074/jbc.M110.121301
Jutabha, P., Anzai, N., Wempe, M. F., Wakui, S., Endou, H., and Sakurai, H. (2011). Apical voltage-driven urate efflux transporter NPT4 in renal proximal tubule. Nucleosides Nucleotides Nucleic Acids 30, 1302–1311. doi:10.1080/15257770.2011.616564
Kang, D.-H., Nakagawa, T., Feng, L., Watanabe, S., Han, L., Mazzali, M., et al. (2002). A role for uric acid in the progression of renal disease. J. Am. Soc. Nephrol. 13, 2888–2897. doi:10.1097/01.asn.0000034910.58454.fd
Keembiyehetty, C., Augustin, R., Carayannopoulos, M. O., Steer, S., Manolescu, A., Cheeseman, C. I., et al. (2006). Mouse glucose transporter 9 splice variants are expressed in adult liver and kidney and are up-regulated in diabetes. Mol. Endocrinol. 20, 686–697. doi:10.1210/me.2005-0010
Kim, S.-M., Choi, Y.-W., Seok, H.-Y., Jeong, K.-H., Lee, S.-H., Lee, T.-W., et al. (2012). Reducing serum uric acid attenuates TGF-β1-induced profibrogenic progression in type 2 diabetic nephropathy. Nephron. Exp. Nephrol. 121, e109–e121. doi:10.1159/000343567
Kimura, K., Hosoya, T., Uchida, S., Inaba, M., Makino, H., Maruyama, S., et al. (2018). Febuxostat therapy for patients with stage 3 CKD and asymptomatic hyperuricemia: A randomized trial. Am. J. Kidney Dis. 72, 798–810. doi:10.1053/j.ajkd.2018.06.028
Kimura, T., Takahashi, M., Yan, K., and Sakurai, H. (2014). Expression of SLC2A9 isoforms in the kidney and their localization in polarized epithelial cells. PloS one 9, e84996. doi:10.1371/journal.pone.0084996
Koka, R. M., Huang, E., and Lieske, J. C. (2000). Adhesion of uric acid crystals to the surface of renal epithelial cells. Am. J. Physiol. Ren. Physiol. 278, F989–F998. Renal physiology. doi:10.1152/ajprenal.2000.278.6.F989
Kolz, M., Johnson, T., Sanna, S., Teumer, A., Vitart, V., Perola, M., et al. (2009). Meta-analysis of 28, 141 individuals identifies common variants within five new loci that influence uric acid concentrations. PLoS Genet. 5, e1000504. doi:10.1371/journal.pgen.1000504
Köttgen, A., Albrecht, E., Teumer, A., Vitart, V., Krumsiek, J., Hundertmark, C., et al. (2013). Genome-wide association analyses identify 18 new loci associated with serum urate concentrations. Nat. Genet. 45, 145–154. doi:10.1038/ng.2500
Kumar, A. U. A., Browne, L. D., Li, X., Adeeb, F., Perez-Ruiz, F., Fraser, A. D., et al. (2018). Temporal trends in hyperuricaemia in the Irish health system from 2006-2014: A cohort study. PloS one 13, e0198197. doi:10.1371/journal.pone.0198197
Lai, S., and Zhou, X. (2013). Inflammatory cells in tissues of gout patients and their correlations with comorbidities. Open Rheumatol. J. 7, 26–31. doi:10.2174/1874312901307010026
Levey, A. S., Atkins, R., Coresh, J., Cohen, E. P., Collins, A. J., Eckardt, K.-U., et al. (2007). Chronic kidney disease as a global public health problem: Approaches and initiatives - a position statement from kidney disease improving global outcomes. Kidney Int. 72, 247–259. doi:10.1038/sj.ki.5002343
Li, C., Yu, Q., Han, L., Wang, C., Chu, N., and Liu, S. (2014). The hURAT1 rs559946 polymorphism and the incidence of gout in Han Chinese men. Scand. J. Rheumatol. 43, 35–42. doi:10.3109/03009742.2013.808375
Li, J.-M., Zhang, X., Wang, X., Xie, Y.-C., and Kong, L.-D. (2011). Protective effects of cortex fraxini coumarines against oxonate-induced hyperuricemia and renal dysfunction in mice. Eur. J. Pharmacol. 666, 196–204. doi:10.1016/j.ejphar.2011.05.021
Li, J., Wang, T., Liu, P., Yang, F., Wang, X., Zheng, W., et al. (2021a). Hesperetin ameliorates hepatic oxidative stress and inflammation via the PI3K/AKT-Nrf2-ARE pathway in oleic acid-induced HepG2 cells and a rat model of high-fat diet-induced NAFLD. Food Funct. 12, 3898–3918. doi:10.1039/d0fo02736g
Li, M.-H., Guan, J., Chen, Z., Mo, J.-X., Wu, K.-R., Hu, X.-G., et al. (2022). Fufang Zhenzhu Tiaozhi capsule ameliorates hyperuricemic nephropathy by inhibition of PI3K/AKT/NF-κB pathway. J. Ethnopharmacol. 298, 115644. doi:10.1016/j.jep.2022.115644
Li, X., Gao, X., Zhang, H., Liu, Y., SarkerRahman, M. M., Wu, Y., et al. (2021b). The anti-hyperuricemic effects of green alga Enteromorpha prolifera polysaccharide via regulation of the uric acid transporters in vivo. Food Chem. Toxicol. 158, 112630. doi:10.1016/j.fct.2021.112630
Lin, G., Yu, Q., Xu, L., Huang, Z., Mai, L., Jiang, L., et al. (2021). Berberrubine attenuates potassium oxonate- and hypoxanthine-induced hyperuricemia by regulating urate transporters and JAK2/STAT3 signaling pathway. Eur. J. Pharmacol. 912, 174592. doi:10.1016/j.ejphar.2021.174592
Liu, B., Shen, Y., Xiao, K., Tang, Y., Cen, L., and Wei, J. (2012). Serum uric acid levels in patients with multiple sclerosis: A meta-analysis. Neurol. Res. 34, 163–171. doi:10.1179/1743132811Y.0000000074
Liu, R., Han, C., Wu, D., Xia, X., Gu, J., Guan, H., et al. (2015). Prevalence of hyperuricemia and gout in mainland China from 2000 to 2014: A systematic review and meta-analysis. Biomed. Res. Int. 2015, 762820. doi:10.1155/2015/762820
Liu, S., Yuan, Y., Zhou, Y., Zhao, M., Chen, Y., Cheng, J., et al. (2017). Phloretin attenuates hyperuricemia-induced endothelial dysfunction through co-inhibiting inflammation and GLUT9-mediated uric acid uptake. J. Cell. Mol. Med. 21, 2553–2562. doi:10.1111/jcmm.13176
Mandal, A. K., Leask, M. P., Estiverne, C., Choi, H. K., Merriman, T. R., and Mount, D. B. (2021). Genetic and physiological effects of insulin on human urate homeostasis. Front. Physiol. 12, 713710. doi:10.3389/fphys.2021.713710
Mandal, A. K., Mercado, A., Foster, A., Zandi-Nejad, K., and Mount, D. B. (2017). Uricosuric targets of tranilast. Pharmacol. Res. Perspect. 5, e00291. doi:10.1002/prp2.291
Mandal, A. K., and Mount, D. B. (2019). Interaction between ITM2B and GLUT9 links urate transport to neurodegenerative disorders. Front. Physiol. 10, 1323. doi:10.3389/fphys.2019.01323
Mandal, A. K., and Mount, D. B. (2015). The molecular physiology of uric acid homeostasis. Annu. Rev. Physiol. 77, 323–345. doi:10.1146/annurev-physiol-021113-170343
Martinon, F., Pétrilli, V., Mayor, A., Tardivel, A., and Tschopp, J. (2006). Gout-associated uric acid crystals activate the NALP3 inflammasome. Nature 440, 237–241. doi:10.1038/nature04516
Matsuo, H., Takada, T., Ichida, K., Nakamura, T., Nakayama, A., Ikebuchi, Y., et al. (2009). Common defects of ABCG2, a high-capacity urate exporter, cause gout: A function-based genetic analysis in a Japanese population. Sci. Transl. Med. 1, 5ra11. doi:10.1126/scitranslmed.3000237
Matsuo, H., Takada, T., Nakayama, A., Shimizu, T., Sakiyama, M., Shimizu, S., et al. (2014). ABCG2 dysfunction increases the risk of renal overload hyperuricemia. Nucleosides Nucleotides Nucleic Acids 33, 266–274. doi:10.1080/15257770.2013.866679
Mazzali, M., Hughes, J., Kim, Y. G., Jefferson, J. A., Kang, D. H., Gordon, K. L., et al. (2001). Elevated uric acid increases blood pressure in the rat by a novel crystal-independent mechanism. Hypertension 38, 1101–1106. doi:10.1161/hy1101.092839
Miao, Y., Ottenbros, S. A., Laverman, G. D., Brenner, B. M., Cooper, M. E., Parving, H.-H., et al. (2011). Effect of a reduction in uric acid on renal outcomes during losartan treatment: A post hoc analysis of the reduction of endpoints in non-insulin-dependent diabetes mellitus with the angiotensin II antagonist losartan trial. Hypertension 58, 2–7. doi:10.1161/HYPERTENSIONAHA.111.171488
Miner, J. N., Tan, P. K., Hyndman, D., Liu, S., Iverson, C., Nanavati, P., et al. (2016a). Erratum to: Lesinurad, a novel, oral compound for gout, acts to decrease serum uric acid through inhibition of urate transporters in the kidney. Arthritis Res. Ther. 18, 236. doi:10.1186/s13075-016-1150-7
Miner, J. N., Tan, P. K., Hyndman, D., Liu, S., Iverson, C., Nanavati, P., et al. (2016b). Lesinurad, a novel, oral compound for gout, acts to decrease serum uric acid through inhibition of urate transporters in the kidney. Arthritis Res. Ther. 18, 214. doi:10.1186/s13075-016-1107-x
Moe, O. W., Abate, N., and Sakhaee, K. (2002). Pathophysiology of uric acid nephrolithiasis. Endocrinol. Metab. Clin. North Am. 31, 895–914. doi:10.1016/s0889-8529(02)00032-4
Mulla, M. J., Myrtolli, K., Potter, J., Boeras, C., Kavathas, P. B., Sfakianaki, A. K., et al. (2011). Uric acid induces trophoblast IL-1β production via the inflammasome: Implications for the pathogenesis of preeclampsia. Am. J. Reprod. Immunol. 65, 542–548. (New York, N.Y, 1989). doi:10.1111/j.1600-0897.2010.00960.x
Mulla, M. J., Salmon, J. E., Chamley, L. W., Brosens, J. J., Boeras, C. M., Kavathas, P. B., et al. (2013). A role for uric acid and the Nalp3 inflammasome in antiphospholipid antibody-induced IL-1β production by human first trimester trophoblast. PloS one 8, e65237. doi:10.1371/journal.pone.0065237
Nakagawa, T., Mazzali, M., Kang, D.-H., Kanellis, J., Watanabe, S., Sanchez-Lozada, L. G., et al. (2003). Hyperuricemia causes glomerular hypertrophy in the rat. Am. J. Nephrol. 23, 2–7. doi:10.1159/000066303
Nakashima, M., Uematsu, T., Kosuge, K., and Kanamaru, M. (1992). Pilot study of the uricosuric effect of DuP-753, a new angiotensin II receptor antagonist, in healthy subjects. Eur. J. Clin. Pharmacol. 42, 333–335. doi:10.1007/BF00266358
Nakayama, A., Matsuo, H., Takada, T., Ichida, K., Nakamura, T., Ikebuchi, Y., et al. (2011). ABCG2 is a high-capacity urate transporter and its genetic impairment increases serum uric acid levels in humans. Nucleosides Nucleotides Nucleic Acids 30, 1091–1097. doi:10.1080/15257770.2011.633953
Pan, J., Shi, M., Guo, F., Ma, L., and Fu, P. (2021b). Pharmacologic inhibiting STAT3 delays the progression of kidney fibrosis in hyperuricemia-induced chronic kidney disease. Life Sci. 285, 119946. doi:10.1016/j.lfs.2021.119946
Pan, J., Zhang, C., Shi, M., Guo, F., Liu, J., Li, L., et al. (2021a). Ethanol extract of Liriodendron chinense (Hemsl.) Sarg barks attenuates hyperuricemic nephropathy by inhibiting renal fibrosis and inflammation in mice. J. Ethnopharmacol. 264, 113278. doi:10.1016/j.jep.2020.113278
Park, K. Y., Kim, H. J., Ahn, H. S., Hee, K. S., Ji, P. E., Yim, S.-Y., et al. (2016). Effects of coffee consumption on serum uric acid: Systematic review and meta-analysis. Semin. Arthritis Rheum. 45, 580–586. doi:10.1016/j.semarthrit.2016.01.003
Pathmanathan, K., Robinson, P. C., Hill, C. L., and Keen, H. I. (2021). The prevalence of gout and hyperuricaemia in Australia: An updated systematic review. Semin. Arthritis Rheum. 51, 121–128. doi:10.1016/j.semarthrit.2020.12.001
Pavelcova, K., Bohata, J., Pavlikova, M., Bubenikova, E., Pavelka, K., and Stiburkova, B. (2020). Evaluation of the influence of genetic variants of SLC2A9 (GLUT9) and SLC22A12 (URAT1) on the development of hyperuricemia and gout. J. Clin. Med. 9, E2510. doi:10.3390/jcm9082510
Perlstein, T. S., Gumieniak, O., Hopkins, P. N., Murphey, L. J., Brown, N. J., Williams, G. H., et al. (2004). Uric acid and the state of the intrarenal renin-angiotensin system in humans. Kidney Int. 66, 1465–1470. doi:10.1111/j.1523-1755.2004.00909.x
Phipps-Green, A. J., Merriman, M. E., Topless, R., Altaf, S., Montgomery, G. W., Franklin, C., et al. (2016). Twenty-eight loci that influence serum urate levels: Analysis of association with gout. Ann. Rheum. Dis. 75, 124–130. doi:10.1136/annrheumdis-2014-205877
Qian, X., Wang, X., Luo, J., Liu, Y., Pang, J., Zhang, H., et al. (2019). Hypouricemic and nephroprotective roles of anthocyanins in hyperuricemic mice. Food Funct. 10, 867–878. doi:10.1039/c8fo02124d
Qiao, Y., Wang, P., Qi, J., Zhang, L., and Gao, C. (2012). TLR-induced NF-κB activation regulates NLRP3 expression in murine macrophages. FEBS Lett. 586, 1022–1026. doi:10.1016/j.febslet.2012.02.045
Qu, Y.-L., Dong, Z.-Y., Cheng, H.-M., Liu, Q., Wang, Q., Yang, H.-T., et al. (2022). Evaluation of renal impairment in patients with diabetic kidney disease by integrated Chinese and western medicine. Chin. J. Integr. Med. doi:10.1007/s11655-022-3520-0
Quiñones Galvan, A., Natali, A., Baldi, S., Frascerra, S., Sanna, G., Ciociaro, D., et al. (1995). Effect of insulin on uric acid excretion in humans. Am. J. Physiol. 268, E1–E5. doi:10.1152/ajpendo.1995.268.1.E1
Reimer, R. J., and Edwards, R. H. (2004). Organic anion transport is the primary function of the SLC17/type I phosphate transporter family. Pflugers Arch. 447, 629–635. doi:10.1007/s00424-003-1087-y
Ren, Q., Tao, S., Guo, F., Wang, B., Yang, L., Ma, L., et al. (2021b). Natural flavonol fisetin attenuated hyperuricemic nephropathy via inhibiting IL-6/JAK2/STAT3 and TGF-β/SMAD3 signaling. Phytomedicine. 87, 153552. doi:10.1016/j.phymed.2021.153552
Ren, Q., Wang, B., Guo, F., Huang, R., Tan, Z., Ma, L., et al. (2021a). Natural flavonoid pectolinarigenin alleviated hyperuricemic nephropathy via suppressing tgfβ/SMAD3 and JAK2/STAT3 signaling pathways. Front. Pharmacol. 12, 792139. doi:10.3389/fphar.2021.792139
Riches, P. L., Wright, A. F., and Ralston, S. H. (2009). Recent insights into the pathogenesis of hyperuricaemia and gout. Hum. Mol. Genet. 18, R177–R184. doi:10.1093/hmg/ddp369
Rizwan, A. N., and Burckhardt, G. (2007). Organic anion transporters of the SLC22 family: Biopharmaceutical, physiological, and pathological roles. Pharm. Res. 24, 450–470. doi:10.1007/s11095-006-9181-4
Saito, I., Saruta, T., Kondo, K., Nakamura, R., Oguro, T., Yamagami, K., et al. (1978). Serum uric acid and the renin-angiotensin system in hypertension. J. Am. Geriatr. Soc. 26, 241–247. doi:10.1111/j.1532-5415.1978.tb02396.x
Sakiyama, M., Matsuo, H., Shimizu, S., Nakashima, H., Nakamura, T., Nakayama, A., et al. (2016). The effects of URAT1/SLC22A12 nonfunctional variants, R90H and W258X, on serum uric acid levels and gout/hyperuricemia progression. Sci. Rep. 6, 20148. doi:10.1038/srep20148
Sánchez-Lozada, L. G., Soto, V., Tapia, E., Avila-Casado, C., Sautin, Y. Y., Nakagawa, T., et al. (2008). Role of oxidative stress in the renal abnormalities induced by experimental hyperuricemia. Am. J. Physiol. Ren. Physiol. 295, F1134–F1141. Renal physiology. doi:10.1152/ajprenal.00104.2008
Sánchez-Lozada, L. G., Tapia, E., Santamaría, J., Avila-Casado, C., Soto, V., Nepomuceno, T., et al. (2005). Mild hyperuricemia induces vasoconstriction and maintains glomerular hypertension in normal and remnant kidney rats. Kidney Int. 67, 237–247. doi:10.1111/j.1523-1755.2005.00074.x
Sánchez-LozadaGabriela, L., Lanaspa, M. A., Cristóbal-García, M., García-Arroyo, F., Soto, V., Cruz-Robles, D., et al. (2012). Uric acid-induced endothelial dysfunction is associated with mitochondrial alterations and decreased intracellular ATP concentrations. Nephron. Exp. Nephrol. 121, e71–e78. doi:10.1159/000345509
Sato, M., Mamada, H., Anzai, N., Shirasaka, Y., Nakanishi, T., and Tamai, I. (2010). Renal secretion of uric acid by organic anion transporter 2 (OAT2/SLC22A7) in human. Biol. Pharm. Bull. 33, 498–503. doi:10.1248/bpb.33.498
Schlesinger, N. (2005). Dietary factors and hyperuricaemia. Curr. Pharm. Des. 11, 4133–4138. doi:10.2174/138161205774913273
Schwarzschild, M. A., Schwid, S. R., Marek, K., Watts, A., Lang, A. E., Oakes, D., et al. (2008). Serum urate as a predictor of clinical and radiographic progression in Parkinson disease. Arch. Neurol. 65, 716–723. doi:10.1001/archneur.2008.65.6.nct70003
Sellmayr, M., Hernandez PetzscheRoman, M. Ma Q., Krüger, N., Liapis, H., Brink, A., Lenz, B., et al. (2020). Only hyperuricemia with crystalluria, but not asymptomatic hyperuricemia, drives progression of chronic kidney disease. J. Am. Soc. Nephrol. 31, 2773–2792. doi:10.1681/ASN.2020040523
Shang, X., Lin, K., Yu, R., Zhu, P., Zhang, Y., Wang, L., et al. (2019). Resveratrol protects the myocardium in sepsis by activating the phosphatidylinositol 3-kinases (PI3K)/AKT/mammalian target of rapamycin (mTOR) pathway and inhibiting the nuclear factor-κb (NF-κB) signaling pathway. Med. Sci. Monit. 25, 9290–9298. doi:10.12659/MSM.918369
Shi, Y., Evans, J. E., and Rock, K. L. (2003). Molecular identification of a danger signal that alerts the immune system to dying cells. Nature 425, 516–521. doi:10.1038/nature01991
Shoji, A., Yamanaka, H., and Kamatani, N. (2004). A retrospective study of the relationship between serum urate level and recurrent attacks of gouty arthritis: Evidence for reduction of recurrent gouty arthritis with antihyperuricemic therapy. Arthritis Rheum. 51, 321–325. doi:10.1002/art.20405
Smink, P. A., Bakker, S. J. L., Laverman, G. D., Berl, T., Cooper, M. E., Zeeuw, D. D., et al. (2012). An initial reduction in serum uric acid during angiotensin receptor blocker treatment is associated with cardiovascular protection: A post-hoc analysis of the RENAAL and IDNT trials. J. Hypertens. 30, 1022–1028. doi:10.1097/HJH.0b013e32835200f9
So, A., and Thorens, B. (2010). Uric acid transport and disease. J. Clin. Invest. 120, 1791–1799. doi:10.1172/JCI42344
Spatola, L., Angelini, C., Badalamenti, S., Maringhini, S., and Gambaro, G. (2017). Kidney stones diseases and glycaemic statuses: Focus on the latest clinical evidences. Urolithiasis 45, 457–460. doi:10.1007/s00240-016-0956-8
Spencer, H. W., Yarger, W. E., and Robinson, R. R. (1976). Alterations of renal function during dietary-induced hyperuricemia in the rat. Kidney Int. 9, 489–500. doi:10.1038/ki.1976.63
Srinivas, S. R., Gopal, E., Zhuang, L., Itagaki, S., Martin, P. M., Fei, Y.-J., et al. (2005). Cloning and functional identification of slc5a12 as a sodium-coupled low-affinity transporter for monocarboxylates (SMCT2). Biochem. J. 392, 655–664. doi:10.1042/BJ20050927
Stamp, L. K., and Chapman, P. T. (2014). Urate-lowering therapy: Current options and future prospects for elderly patients with gout. Drugs Aging 31, 777–786. doi:10.1007/s40266-014-0214-0
Stamp, L., Searle, M., O'Donnell, J., and Chapman, P. (2005). Gout in solid organ transplantation: A challenging clinical problem. Drugs 65, 2593–2611. doi:10.2165/00003495-200565180-00004
Su, H.-Y., Yang, C., Liang, D., and Liu, H.-F. (2020). Research advances in the mechanisms of hyperuricemia-induced renal injury. Biomed. Res. Int. 2020, 5817348. doi:10.1155/2020/5817348
Sun, P., Zhu, J.-J., Wang, T., Huang, Q., Zhou, Y.-R., Yu, B.-W., et al. (2018). Benzbromarone aggravates hepatic steatosis in obese individuals. Biochim. Biophys. Acta. Mol. Basis Dis. 1864, 2067–2077. –2077. doi:10.1016/j.bbadis.2018.03.009
Sweet, D. H., Chan, L. M. S., Walden, R., Yang, X.-P., Miller, D. S., and Pritchard, J. B. (2003). Organic anion transporter 3 (Slc22a8) is a dicarboxylate exchanger indirectly coupled to the Na+ gradient. Am. J. Physiol. Ren. Physiol. 284, F763–F769. doi:10.1152/ajprenal.00405.2002
Taniguchi, A., and Kamatani, N. (2008). Control of renal uric acid excretion and gout. Curr. Opin. Rheumatol. 20, 192–197. doi:10.1097/BOR.0b013e3282f33f87
Tao, X., Yin, L., Xu, L., and Peng, J. (2018). Dioscin: A diverse acting natural compound with therapeutic potential in metabolic diseases, cancer, inflammation and infections. Pharmacol. Res. 137, 259–269. doi:10.1016/j.phrs.2018.09.022
Terkeltaub, R. A. (2003). Clinical practice. Gout. N. Engl. J. Med. 349, 1647–1655. doi:10.1056/NEJMcp030733
Tin, A., Marten, J., Halperin Kuhns, V. L., Li, Y., Wuttke, M., Kirsten, H., et al. (2019). Target genes, variants, tissues and transcriptional pathways influencing human serum urate levels. Nat. Genet. 51, 1459–1474. doi:10.1038/s41588-019-0504-x
Tin, A., Woodward, O. M., Hong Linda, K. W., Liu, C.-T., Lu, X., Nalls, M. A., et al. (2011). Genome-wide association study for serum urate concentrations and gout among African Americans identifies genomic risk loci and a novel URAT1 loss-of-function allele. Hum. Mol. Genet. 20, 4056–4068. doi:10.1093/hmg/ddr307
Toma, C., Higa, N., Koizumi, Y., Nakasone, N., Ogura, Y., McCoy, A. J., et al. (2010). Pathogenic Vibrio activate NLRP3 inflammasome via cytotoxins and TLR/nucleotide-binding oligomerization domain-mediated NF-kappa B signalingJ. Immunol.184Baltimore, Md, 5287–5297. doi:10.4049/jimmunol.0903536
Toyoda, Y., Takada, T., Saito, H., Hirata, H., Ota-Kontani, A., Tsuchiya, Y., et al. (2022). Soy isoflavone genistein inhibits an axillary osmidrosis risk factor ABCC11: In vitro screening and fractional approach for ABCC11-inhibitory activities in plant extracts and dietary flavonoids. Nutrients 14, E2452. doi:10.3390/nu12082452
Uetake, D., Ohno, I., Ichida, K., Yamaguchi, Y., Saikawa, H., Endou, H., et al. (2010). Effect of fenofibrate on uric acid metabolism and urate transporter 1. Intern. Med. 49, 89–94. doi:10.2169/internalmedicine.49.2597
Vitart, V., Rudan, I., Hayward, C., Gray, N. K., Floyd, J., Palmer, C. N. A., et al. (2008). SLC2A9 is a newly identified urate transporter influencing serum urate concentration, urate excretion and gout. Nat. Genet. 40, 437–442. doi:10.1038/ng.106
Wallace, C., Newhouse, S. J., Braund, P., Zhang, F., Tobin, M., Falchi, M., et al. (2008). Genome-wide association study identifies genes for biomarkers of cardiovascular disease: Serum urate and dyslipidemia. Am. J. Hum. Genet. 82, 139–149. doi:10.1016/j.ajhg.2007.11.001
Wang, Y.-Z., Zhou, C., Zhu, L.-J., He, X.-L.-S., Li, L.-Z., Zheng, X., et al. (2022). Effects of macroporous resin extract of Dendrobium officinale leaves in rats with hyperuricemia induced by fructose and potassium oxonate. Comb. Chem. High. Throughput Screen. 25, 1294–1303. doi:10.2174/1386207324666210528114345
Wang, Y., Kong, W., Wang, L., Zhang, T., Huang, B., Meng, J., et al. (2020). Multiple-purpose connectivity map analysis reveals the benefits of esculetin to hyperuricemia and renal fibrosis. Int. J. Mol. Sci. 21, E7695. doi:10.3390/ijms21207695
Woodward, O. M., Köttgen, A., Coresh, J., Boerwinkle, E., Guggino, W. B., and Köttgen, M. (2009). Identification of a urate transporter, ABCG2, with a common functional polymorphism causing gout. Proc. Natl. Acad. Sci. U. S. A. 106, 10338–10342. doi:10.1073/pnas.0901249106
Wu, X. W., Muzny, D. M., Lee, C. C., and Caskey, C. T. (1992). Two independent mutational events in the loss of urate oxidase during hominoid evolution. J. Mol. Evol. 34, 78–84. doi:10.1007/BF00163854
Xu, X., Wang, H., Guo, D., Man, X., Liu, J., Li, J., et al. (2021). Curcumin modulates gut microbiota and improves renal function in rats with uric acid nephropathy. Ren. Fail. 43, 1063–1075. doi:10.1080/0886022X.2021.1944875
Yang, C.-Y., Chen, C.-H., Deng, S.-T., Huang, C.-S., Lin, Y.-J., Chen, Y.-J., et al. (2015). Allopurinol use and risk of fatal hypersensitivity reactions: A nationwide population-based study in taiwan. JAMA Intern. Med. 175, 1550–1557. doi:10.1001/jamainternmed.2015.3536
Yang, H., Wang, Y., Jin, S., Pang, Q., Shan, A., and Feng, X. (2021). Dietary resveratrol alleviated lipopolysaccharide-induced ileitis through Nrf2 and NF-κB signalling pathways in ducks (Anas platyrhynchos). J. Anim. Physiol. Anim. Nutr. 106 (6), 1306–1320. doi:10.1111/jpn.13657
Ye, B. S., Lee, W. W., Ham, J. H., Lee, J. J., Lee, P. H., Sohn, Y. H., et al. (2016). Does serum uric acid act as a modulator of cerebrospinal fluid Alzheimer's disease biomarker related cognitive decline? Eur. J. Neurol. 23, 948–957. doi:10.1111/ene.12969
Ye, H.-Y., Jin, J., Jin, L.-W., Chen, Y., Zhou, Z.-H., and Li, Z.-Y. (2017). Chlorogenic acid attenuates lipopolysaccharide-induced acute kidney injury by inhibiting TLR4/NF-κB signal pathway. Inflammation 40, 523–529. doi:10.1007/s10753-016-0498-9
Yoo, T. W., Sung, K. C., Shin, H. S., Kim, B. J., Kim, B. S., Kang, J. H., et al. (2005). Relationship between serum uric acid concentration and insulin resistance and metabolic syndrome. Circ. J. 69, 928–933. doi:10.1253/circj.69.928
Yu, M., Qi, B., Xiaoxiang, W., Xu, J., and Liu, X. (2017). Baicalein increases cisplatin sensitivity of A549 lung adenocarcinoma cells via PI3K/Akt/NF-κB pathway. Biomed. Pharmacother. = Biomedecine Pharmacother. 90, 677–685. doi:10.1016/j.biopha.2017.04.001
Zhang, D.-M., Li, Y.-C., De, X., Ding, X.-Q., and Kong, L.-D. (2012). Protection of curcumin against fructose-induced hyperuricaemia and renal endothelial dysfunction involves NO-mediated JAK-STAT signalling in rats. Food Chem. 134, 2184–2193. doi:10.1016/j.foodchem.2012.04.026
Zhang, L., Xie, Q., and Li, X. (2022). Esculetin: A review of its pharmacology and pharmacokinetics. Phytother. Res. 36, 279–298. doi:10.1002/ptr.7311
Zhang, X., Nie, Q., Zhang, Z., Zhao, J., Zhang, F., Wang, C., et al. (2021). Resveratrol affects the expression of uric acid transporter by improving inflammation. Mol. Med. Rep. 24, 564. doi:10.3892/mmr.2021.12203
Zhang, Y., Jin, L., Liu, J., Wang, W., Yu, H., Li, J., et al. (2018). Effect and mechanism of dioscin from Dioscorea spongiosa on uric acid excretion in animal model of hyperuricemia. J. Ethnopharmacol. 214, 29–36. doi:10.1016/j.jep.2017.12.004
Zhao, T., Meng, Q., Sun, Z., Chen, Y., Ai, W., Zhao, Z., et al. (2020). Novel human urate transporter 1 inhibitors as hypouricemic drug candidates with favorable druggability. J. Med. Chem. 63, 10829–10854. doi:10.1021/acs.jmedchem.0c00223
Zhou, X., Zhang, B., Zhao, X., Lin, Y., Wang, J., Wang, X., et al. (2021). Chlorogenic acid supplementation ameliorates hyperuricemia, relieves renal inflammation, and modulates intestinal homeostasis. Food Funct. 12, 5637–5649. doi:10.1039/d0fo03199b
Zhou, Y., Zhang, X., Li, C., Yuan, X., Han, L., Li, Z., et al. (2018). Research on the pharmacodynamics and mechanism of Fraxini Cortex on hyperuricemia based on the regulation of URAT1 and GLUT9. Biomed. Pharmacother. = Biomedecine Pharmacother. 106, 434–442. doi:10.1016/j.biopha.2018.06.163
Keywords: hyperuricemia, urate transporters, natural products, chronic kidney disease, renal urate extraction
Citation: Yang B, Xin M, Liang S, Xu X, Cai T, Dong L, Wang C, Wang M, Cui Y, Song X, Sun J and Sun W (2022) New insight into the management of renal excretion and hyperuricemia: Potential therapeutic strategies with natural bioactive compounds. Front. Pharmacol. 13:1026246. doi: 10.3389/fphar.2022.1026246
Received: 23 August 2022; Accepted: 26 October 2022;
Published: 22 November 2022.
Edited by:
Dan Tang, Guangdong Pharmaceutical University, ChinaReviewed by:
Asim Kumar Mandal, Brigham and Women’s Hospital, United StatesPing Fu, Sichuan University, China
Copyright © 2022 Yang, Xin, Liang, Xu, Cai, Dong, Wang, Wang, Cui, Song, Sun and Sun. This is an open-access article distributed under the terms of the Creative Commons Attribution License (CC BY). The use, distribution or reproduction in other forums is permitted, provided the original author(s) and the copyright owner(s) are credited and that the original publication in this journal is cited, in accordance with accepted academic practice. No use, distribution or reproduction is permitted which does not comply with these terms.
*Correspondence: Wenlong Sun, NTEyNjQ5MTEzQHFxLmNvbQ==; Jinyue Sun, bW9vbl9zNzMxQGhvdG1haWwuY29t; Xinhua Song, ODkyNDQyNTcyQHFxLmNvbQ==