- 1Personalized Drug Therapy Key Laboratory of Sichuan Province, Department of Pharmacy, Sichuan Academy of Medical Science and Sichuan Provincial People’s Hospital, University of Electronic Science and Technology of China, Chengdu, China
- 2The State Key Laboratory of Southwestern Chinese Medicine Resources, Department of Pharmacy, Chengdu University of Traditional Chinese Medicine, Chengdu, China
- 3School of Chinese Medicine, Li Ka Shing Faculty of Medicine, The University of Hong Kong, Hong Kong SAR, China
PI3K/Akt, an essential signaling pathway widely present in cells, has been shown to be relevant to neurological disorders. As an important class of natural products, terpenoids exist in large numbers and have diverse backbones, so they have a great chance to be identified as neuroprotective agents. In this review, we described and summarized recent research for a range of terpenoid natural products associated with the PI3K/Akt pathway by classifying their basic chemical structures of the terpenes, identified by electronic searches on PubMed, Web of Science for research, and Google Scholar websites. Only articles published in English were included. Our discussion here concerned 16 natural terpenoids and their mechanisms of action, the associated diseases, and the methods of experimentation used. We also reviewed the discovery of their chemical structures and their derivatives, and some compounds have been concluded for their structure–activity relationships (SAR). As a result, terpenoids are excellent candidates for research as natural neuroprotective agents, and our content will provide a stepping stone for further research into these natural products. It may be possible for more terpenoids to serve as neuroprotective agents in the future.
Introduction
The brain is a complex and fragile system closely related to our studies and life. By altering its structure and function, the brain can store information, but it is also susceptible to damage, invasion by pathogens, and neurodegeneration (Heneka et al., 2018). Neurodegenerative disease (NDD) is caused by degeneration and subsequent loss of neurons because nerve cells cannot regenerate or replace. Gradually, the patient’s cognition and peripheral and central nervous system functions will decrease (Fakhri et al., 2021). It is widely recognized that neurodegenerative diseases include Alzheimer’s disease (AD), Parkinson’s disease (PD), Huntington’s disease (HD), and diseases affecting the central nervous system (CNS) (Gitler et al., 2017; Ahmed et al., 2018; Li et al., 2021). In recent years, with an aging population worldwide, neurological diseases have become a major health concern and economic burden, posing a severe threat to human health. Some researchers have even predicted that the number of patients with AD will exceed 100 million by the year 2050 (Prince et al., 2013; Pohl and Kong Thoo Lin, 2018). However, as of today, these nerve diseases cannot be radically cured, instead relying solely on applicable drugs to alleviate their symptoms.
In ancient times, plants were used as medicine, and natural products of all kinds were used as medicine, including plants, animals, microbes, and marine organisms (Shi et al., 2010b; Chen et al., 2021b). Natural products exhibit a high level of biological activity due to their unique chemical diversity, which is crucial to the development of new lead compounds and chemical molecular scaffolds. In the process of discovering new drugs, natural products have offered abundant chemical structures and new pharmacological mechanisms. With their significant curative properties and small side effects, natural products have become one of the major sources of drug discovery in our time. In the past few years, the FDA has approved more than 60% of natural medicines (Galm et al., 2007; Rodrigues et al., 2016; Yuan et al., 2016). Among these natural medicines is galantamine, which has been approved by the FDA as an anti-AD medicine isolated from the bulbs and flowers of snowdrop Galanthus woronowii (Amaryllidaceae) (Yoo and Park, 2012). A number of synthetic drugs can also be prescribed to treat nervous system issues, including idebenone, diazepam, doxepin, and memantine hydrochloride, but these drugs all have unwanted side effects. Thus, developing natural neuroprotective agents with high efficacy and low toxicity may increase the therapeutic prospects for neurological disorders (Chen et al., 2021c).
Terpenoids, among the largest natural product families, possess complex chemical structures and play a significant role in the drug discovery of NDD. Based on the number of units of the isoprene unit (C5), terpenes can be classified as hemi- (C5), mono- (C10), sesqui- (C15), di- (C20), sester- (C25), tri- (C30), tetra- (C40), or polyterpenes (n > 8). In addition, many related modification products and derivatives have also been characterized. Moreover, terpenoids have been reported to have a number of benefits, including potential anti-inflammatory, anti-carcinogenic, and neuroprotective effects, which may lead to improved human health (Kiyama, 2017).
In the nervous system, the PI3K/Akt pathway is an important signaling pathway that contributes to neuronal growth, proliferation, survival, and function at all stages of development (Coelho and Leevers, 2000; Huang et al., 2005). In the downstream of PI3K, Akt is a key target in mediating anti-cell apoptosis and regulating cellular metabolism (Manning and Cantley, 2007). As shown in Figure 1, PI3K can be activated and promote the phosphorylation of Akt, which regulates the phosphorylation of various downstream proteins and ultimately affects the activity of several cellular physiological processes. It should be noted that PTEN (phosphatase and tensin homolog deleted on chromosome 10) can downregulate the PI3K/Akt signaling pathway (Chen et al., 2021a). Then, the activation of Akt leads to the nuclear translocation of Nrf2 and p-CREB, which promotes cell survival and prevents apoptosis. The transcription factor Nrf2 (nuclear factor-erythroid 2-related factor 2) regulates the transcription of antioxidant genes such as HO-1 (heme oxygenase-1) and inhibits the oxidative stress response (Li et al., 2016). High activity of Nrf2 also has the ability to downregulate the NF-κB pathway, which is associated with anti-inflammatory and antioxidant activities. Also, CREB (cAMP-response element-binding protein) is a transcription factor that promotes the expression of neuroprotective proteins (Belgacem and Borodinsky, 2017). It is well known that the Wnt/β-catenin signaling pathway is vital in regulating brain development, growth, and differentiation (Kurimoto et al., 2015). Its downstream effector, GSK-3β (glycogen synthase kinase-3 beta), is activated by Akt, which inhibits the β-catenin expression and reduces neuronal activity. Moreover, AKT can promote mTOR (mammalian target of rapamycin) expression, which regulates cellular nutrition and energy supply and stimulates protein synthesis (Ling et al., 2019). Akt can also inhibit the expression of the pro-apoptotic protein Bax (BCL2-associated X) and increase cell survival, as well as increase the expression of the anti-apoptotic protein Bcl-2 (B-cell lymphoma 2) and reduce apoptosis in cells. In general, PI3K and Akt are known to activate several substrates and downstream effectors involved in metabolism, cell survival and apoptosis, differentiation, and proliferation (Hannan et al., 2020).
We classified terpenoids according to their chemical structure and searched numerous academic websites for articles on terpenoid natural products related to the neuroprotective pathway PI3K/Akt. We reviewed their neuroprotective activities via the PI3K/Akt pathway, some of these terpenoid derivatives, and their structure–activity relationship studies. Hopefully, our study will inspire researchers and promote the development of terpenoid neuroprotective agents.
Monoterpenes
Monoterpenes are isoprene dimers with the composition C10H16. Catalpol and geniposide described in the following sections are both iridoids that belong to special monoterpenoids. Usually, they are linked with sugars to form glycosides, which are collectively referred to as iridoid glycosides.
Catalpol and its derivatives
Catalpol is a monoterpene containing an aldehyde acetal, which belongs to the iridoids. Having been isolated from plants in 1888, it has been extensively studied for its anti-inflammatory, antioxidant, and other biological properties (Claassen, 1888; Villasenor and Chemistry, 2007). The primary active ingredient in Rehmanniae Radix is catalpol, which prevents multiple central nervous system diseases (Jiang et al., 2015). Catalpol has been found to provide antioxidant and neuroprotective effects on mouse models of depression, improving their depressive behavior by upregulating the PI3K/Akt/Nrf2/HO-1 signaling pathway, indicating that this pathway could be used as a potential target and biomarker for the treatment of depression with catalpol (Wang et al., 2021a; Wu et al., 2021). In addition, some studies have reported that catalpol activated the PI3K/Akt/mTOR pathway, decreasing the expression of miR-124 and increasing the expression of downstream protein S6, thus enhancing in vivo axon growth and neuronal survival in stroke models (Wang et al., 2019; Zhu et al., 2019).
A number of catalpol derivatives are usually derived from plants. Different substituents at different positions will produce significant functional differences when the main skeletons of compounds are the same. The catalpol derivatives mentioned following have been listed in terms of their chemical structures (Figure 2). In another study, researchers summarized the natural derivatives of catalpol discovered between 1888 and 2018, categorizing them into three categories: 6-O-acyl catalpols, 6-O-glycosyl catalpols, and other derivatives (Zhang et al., 2019a). A wide range of pharmacological activities was observed in these derivatives, but only the picroside mentioned was neuroprotective. The PI3K/Akt pathway was only mentioned by these researchers in the section of cardiovascular protection. As small-molecule drugs, catalpol and its derivatives have obvious disadvantages, such as their hydrophilicity and rapid metabolism, which inhibit their penetration of the blood–brain barrier (Ginter et al., 2014). Therefore, chemical modification of its derivatives must result in improved bioavailability. Liu et al. (2020) used computer-aided drug design (CADD) to dock catalpol crotonates with glutathione peroxidase (GSH-Px) which has neuroprotective properties. By using microwave-assisted synthesis (MWAS) as a synthesis method, they were successful in designing and synthesizing catalpol hexacrotonate (CC-6). The kinetic simulation study of CC-6 indicated that it was a successful combination with GSH-Px, and in vitro experiments also showed that it was neuroprotective. In order to determine if it is effective in treating diabetic encephalopathy, more studies need to be conducted in vivo.
Geniposide and its derivatives
As a bioactive iridoid glycoside, geniposide has been isolated from a wide range of plant species, including the Rubiaceae family. As early as 1969, Inouye and Saito were the first to isolate the compound from the gardenia fruit (Inouye et al., 1969). There has been research showing that geniposide activated Akt and then increased PI3K and GSK-3β expression, thus improving pathological symptoms in epileptic rats (Wei et al., 2018). Moreover, Wang et al. (2021b) have confirmed that geniposide could inhibit hippocampal neurons’ apoptosis via the aforementioned pathway, resulting in antidepressant properties in the brain. It is well known that neuropathic pain (NP) results from a lesion or disease of the somatosensory system, including peripheral fibers and central nerves (Colloca et al., 2017). As a neurodegenerative disorder, NP is thought to arise from degeneration of inhibitory interneurons in the dorsal horn of the spinal cord, following peripheral nerve injury (Apkarian and Scholz, 2006). Geniposide could inhibit the EGFR/PI3K/Akt signaling pathway, thereby alleviating the pain symptoms in sciatic nerve injury in the chronic constriction injury (CCI) model of NP, which provided a basis for further clinical trials to treat NP (Zhang et al., 2022).
Approximately 90 species of geniposide derivatives exist in nature with various pharmacological properties, including neuroprotective properties. Geniposide can be hydrolyzed into genipin by β-glucosidase in vivo. The primary metabolite of genipin and geniposide is genipin sulfate, which is highly toxic in the body (Hou et al., 2008). During the early years, a series of 1-alkyloxygenipins were designed and synthesized based on CADD studies of genipin’s structure and electronic properties. These researchers changed the hydroxyl group of genipin at position 1 into an alkoxy group, thereby obtaining (1R)-isopropyloxygenipin, which had better stability and was physiologically similar to genipin. By converting the hydroxyl group of genipin to the alkoxy group at position 1, they obtained (1R)-isopropyloxygenipin, which exhibits similar physiological properties and has enhanced physiological stability compared to genipin (Figure 3) (Suzuki et al., 2010). Cai et al. (2020) synthesized and designed an acetylated derivative of geniposide, penta-acetyl geniposide [(Ac) 5 GP], which improved the lipophilicity and pharmacological properties of geniposide. In chronic unpredictable mild stress (CUMS) depression rats, (Ac) 5 GP might be effective.
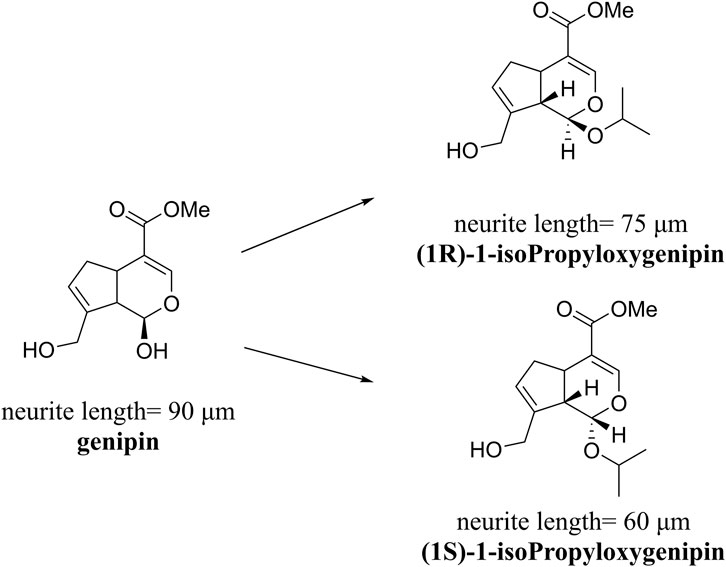
FIGURE 3. Neurite lengths in PC12 cells were measured after 2 days of treatment with compounds (20 mM). Relative active: genipin ≈ 1-isopropyloxygenipin (Suzuki et al., 2010).
Gardenamide A and its derivatives
Gardenamide A (GA) is an analog of genipin isolated from Rothmannia urcelliformis, which can inhibit cell apoptosis through the PI3K/Akt pathway (Wang et al., 2015b). Novel GA derivatives have been reported in recent years. Some researchers developed this series of derivatives based on the piperazine scaffold (Figure 4), which has shown promise in the development of drugs for neurodegenerative diseases. In the end, four derivative compounds were found to be potent multifunctional neuroprotective agents (Zhang et al., 2019b). We presented a table containing the chemical structures of the four derivatives and the results of their evaluation against each of oxygen–glucose deprivation (OGD)-, hydrogen peroxide (H2O2)-, and amyloid-β1-42 (Aβ1-42)-induced experimental models and compared with GA (Table 1). The SAR analysis revealed two conclusions: 1) substitution of a 2-isopropyl group on GA resulted in higher cell survival than the substitution of a 2-methyl group in GA; 2) compounds with an electron-withdrawing group on the benzene ring showed better neuroprotective activity.
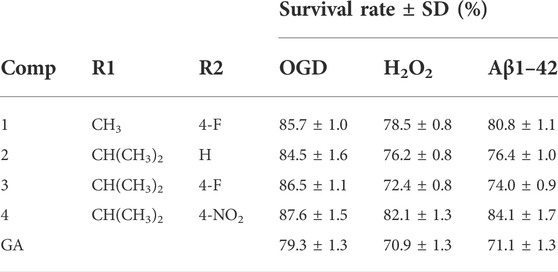
TABLE 1. Chemical structures of the four derivatives and comparison of cell viability of GA with four derivatives at 10 μM (Zhang et al., 2019b).
Sesquiterpenoids
Among terpenoids, sesquiterpenes are composed of three isoprene units, which can be roughly divided into acyclic and cyclic types according to their chemical structures. The natural products described in the following section are all classified as cyclic sesquiterpenes.
Bilobalide and its derivatives
The Ginkgo biloba (EGb) tree is the oldest species of trees in existence. It has been used as a traditional Chinese medicine for thousands of years and is currently being used worldwide (Nakanishi and chemistry, 2005). The “Encyclopedia of Chinese Herbal Medicines” recorded that it could be used to treat strokes and myocardial infarctions (Chevallier, 2016). In 1970, highly concentrated and stable substances were first extracted from Ginkgo biloba leaves by Dr. Willmar Schwabe Pharmaceuticals (Karlsruhe, Germany), who developed a standard extraction method for Ginkgo biloba leaves and determined the chemical structure of this extract (Le Bars, 2003). The standardized extract of Ginkgo biloba (EGb) usually contains 6% terpene trilactones (TTLs), and bilobalide (BB) is the only sesquiterpene trilactone found in TTLs (van Beek and Montoro, 2009). Several studies have suggested that the neuroprotective properties of the Ginkgo biloba extract might be attributable to the presence of BB (Defeudis, 2002; Huang et al., 2003; Jiang et al., 2014). By antagonizing the GABA receptor, BB could increase the levels of glutamic acid decarboxylase (GAD) in the brain, reducing the transmission of GABA in the brain and resulting in an anticonvulsant effect (Sasaki et al., 1999). Furthermore, it could inhibit the release of choline caused by activating phospholipase A2, which reduced the death of neurons (Weichel et al., 1999; Huang et al., 2003; Strømgaard and Nakanishi, 2004).
In recent years, a study has revealed that BB’s neuroprotective properties are mediated by the PI3K/Akt pathway. BB has been shown to exert anti-apoptotic effects by activating this pathway involved in neuronal apoptosis in an in vitro experiment model (Shi et al., 2010a). In addition, in vivo studies verified that BB supports neuronal cell survival in patients suffering from ischemic stroke (Zheng et al., 2018). Therefore, the development of BB as a treatment for neurodegenerative diseases is worth continuing.
Due to the limitations to molecular scaffold reconstruction in medicinal chemistry, BB’s SAR research has not been completed. Recently, some researchers have conducted SAR studies on BB to clarify the relationship between the modification of lactone structure and its biological activity. Four derivatives of BB were synthesized following structural modifications: BB-diether, diAc-isoBB, diBrBn-isoBB, and diBn-BB-aldehyde (Figure 5). In vitro, the biological identification found that BB-diether without a lactone group neither protected Aβ (1–40) peptides nor promoted the growth of nerve axons, but other compounds showed positive effects, which proved the importance of lactone groups. Furthermore, both diAc-isoBB and diBrBn-isoBB possessed better activity than BB, indicating that it is beneficial to replace the hydroxyl groups in BB (Usuki et al., 2020).
Artesunate
Artesunate (ART), a derivative of artemisinin with numerous biological functions, possesses a good permeability to the blood–brain barrier and is usually given intravenously to patients in its water-soluble ionic form (Leicach and Chludil, 2014). A study has shown that ART effectively ameliorated the insufficient endogenous neural stem/progenitor cell (NSPC) proliferation caused by ischemia. By stimulating the PI3K/Akt signaling pathway, ART could increase the phosphorylation level of FOXO-3a, downregulate p27kip1, and inhibit the transcription of FOXO-3a, thus recovering brain function (Zhang et al., 2020). According to a SAR study, the endoperoxide bridge was essential for the activity of ART, but the lactone group was not, and reducing lactone carbonyl to dihydroartemisinin (DART) could improve its solubility (Schmidt, 2006).
Methyl lucidone
Methyl lucidone (MLC) is extracted from the fruit of Lindera erythrocarpa Makino (Lauraceae) distributed in Korea, Taiwan, Japan, and China (Ichino et al., 1988). By activating the PI3K/Akt pathway, MLC could promote phosphorylation of Nrf2 and HO-1 transcription and then play an antioxidant role, which might have therapeutic potential for neurological disorders (Park et al., 2019).
Diterpenoids
A diterpene consists of four isoprene units and contains 20 carbon atoms. The following is a description of the diterpene ginkgolides.
Ginkgolides and their derivatives
In general, diterpene ginkgolides are known as ginkgolides A, B, C, J, and M, which are rare natural compounds with t-Bu groups. Ginkgolides are also bioactive components of the EGb extract, just like bilobalide. The five main components of ginkgolides differ only in the number and position of their hydroxy groups, and all have six five-membered rings: a spiro [4.4]-nonane carbocyclic ring, three lactones, and a tetrahydrofuran ring (Figure 6). There is no doubt that they are special due to their tert-butyl structure (Strømgaard and Nakanishi, 2004).
The diterpene ginkgolide meglumine injection (DGMI) is often used to treat ischemic stroke in the clinic, whose main component is ginkgolide B (GB). Studies have reported that DGMI significantly activated the PI3K/Akt signaling pathway in vitro and in vivo to promote cell survival and inhibit apoptosis, which might be one mechanism for its efficacy in stroke treatment (Jin et al., 2017; Zhang et al., 2018; Liu et al., 2019). According to a study in vitro, ginkgolide K (GK) had a superior therapeutic potential than GB since GK was easier to upregulate PI3K and p-Akt expression, affecting downstream pathways, thereby contributing to anti-inflammatory and antioxidant effects (Yu et al., 2018b). Furthermore, GK can activate diverse signaling pathways in order to serve a neuroprotective function. For example, some researchers suspected that GK activated the PI3K/Akt pathway to treat PD by upregulating BDNF, but this hypothesis remains unsubstantiated (Yu et al., 2018a).
The results of an early SAR study on GB indicated that it could exert anti-inflammatory and anticoagulant effects by antagonizing the platelet-activating factor receptor (PAFR) (Strømgaard and Nakanishi, 2004). In addition to inhibiting the PAFR, ginkgolides have also been proven to be potent and selective inhibitors of the inhibitory glycine receptor (GlyR), which is a critical target for brain function (Kondratskaya and Krishtal, 2002; Jaracz et al., 2004a). Some researchers selectively derivatized the hydroxyl groups of ginkgolide C (GC) to produce various ether, ester, and carbamoyl derivatives (Jaracz et al., 2004b). The derivatives with only one benzyl substituent at 10-OH had better activity among them, but even the best compound 12 was far less potent than the parent compound GC, so the hydroxyl group was very important to the inhibitory activity of GlyR.
In addition, a study reported the SAR between ginkgolides and different subtypes of GlyR. Due to the previous discovery that lactone structures C and F inhibited GlyR, researchers modified these structures chemically and synthesized four series of derivatives: 1) both lactones were complete and derived from different positions; 2) lactone C had been opened or removed or modified but not the remaining lactones; 3) different lactones had been reduced in the chemical structure; and 4) to derive or oxidize 7-positions. They studied the biological functions of these derivatives in vitro and found only six derivatives were effective, confirming that both lactones were essential for inhibiting GlyR. Meanwhile, ginkgolide M (GM) was the most active of all ginkgolide lactones for inhibiting GlyR in a fluorescence-based membrane potential assay. The structural peculiarity of GM was the lack of 3-OH, suggesting that 3-OH was not favorable for GlyR activity. Following this, a 3D pharmacophore model was generated for use in virtual screening. All hit compounds failed to exhibit any activity in pharmacological testing due to their less rigid structures than ginkgolides, indicating that rigid structures were necessary for blocking GlyR (Jensen et al., 2007). 10-O-(N,N-dimethylaminoethyl)-ginkgolide B methane-sulfonate (XQ-1H) (Figure 6) is a derivative of GB (Deng et al., 2009). An in vivo study has found that XQ-1H promoted angiogenesis by activating the PI3K/Akt signaling pathway, which is a potential stroke treatment (Fei et al., 2021).
Triterpenes
Triterpenes are a class of terpenoids constructed from six isoprene units. In general, triterpene saponins are composed of glycosides, sugars, and uronic acids, among which Panax notoginseng saponin is a well-studied natural neuroprotectant related to the PI3K/Akt signaling pathway. We mainly discussed asiatic acid and asiaticoside, two triterpenoids that have been extracted from the Centella asiatica extract, which are rich in triterpenoids. The pharmacological mechanisms of other reviewed triterpenoids are also related to the PI3K/Akt pathway, but there are only a few medicinal chemistry studies available.
Alisol A 24-acetate
Alisol A 24-acetate (24A) is a protostane-type tetracyclic triterpenoid compound found in the well-known traditional Chinese medicinal herb Alisma orientale (Sam.) Juz. (Chen et al., 2020b). Lu et al. (2021) explored the possible neuroprotective mechanism of 24A, finding that 24A activated the PI3K/Akt signaling pathway to promote the expression of downstream anti-apoptosis proteins, making it an effective treatment for cerebral ischemia-reperfusion injuries. Unfortunately, the chemical properties of 24A are unstable, and it can be converted into 23-acetate by acetylation (Makabel et al., 2008). Currently, there is no new research on improving its structure.
Polygalasaponin F
Polygalasaponin F (PGSF), an oleanane-type triterpenoid saponin, is the main terpene in Polygala japonica Houtt. Zhang et al. (1995a) and Zhang et al. (1995b) isolated it for the first time in 1995 from Polygala japonica. It has been reported that PGSF might play an anti-apoptotic role by mediating the PI3K/Akt pathway, and further animal and clinical trials should be conducted to verify the pharmacological effect of PGSF to treat cerebral ischemia (Xie et al., 2020). A study has found that the NR2A-containing NMDAR/PI3K/Akt signaling pathway might be one of the neuroprotective mechanisms by which PGSF inhibits the excitotoxicity of cells in a concentration-dependent manner (Sun et al., 2022).
The development of isolation and structure identification technology has promoted the discovery of active ingredients in Polygala japonica Houtt over the past few decades. Although the modification of PGSF has not been reported yet, research studies about separating more triterpene saponins from Polygala japonica Houtt have never stopped.
Panax notoginseng saponins
Panax notoginseng saponins (PNS) are extracted from the root or rhizome of the herb Panax notoginseng (Bruk.) F. H. Chen (Araliaceae). There are five main components of PNS: notoginsenoside R1, ginsenoside Rg1, ginsenoside Re, ginsenoside Rb1, and ginsenoside Rd. PNS can treat vascular and cardiovascular diseases such as ischemic stroke because of its anti-hypoxia, anti-aging, and immune-boosting functions (Yang et al., 2014; Duan et al., 2017). Wu et al. (2020a) demonstrated that the neuroprotective property of PNS was mediated by the EGFR/PI3K/Akt signaling pathway, which was one of the most representative neuroprotective pathways. EGFR was transactivated after an ischemic stroke in an organism and then stimulated downstream signal cascades to protect and promote the growth of cells. An in vivo study by Yang et al. (2018) also confirmed that PNS promoted the growth of neurites and plays a neuroprotective role by activating the EGFR/PI3K/Akt pathway. The well-known Xueshuantong for injection (lyophilized) (血栓通注射液, XST) has an excellent curative effect against vascular and cardiovascular diseases, and its main component is PNS.
A study has shown that PNS activated the PI3K/Akt/mTOR pathway in both in vivo and in vitro experiment models (Pan et al., 2021). By upregulating mTOR, this pathway inhibited autophagy and promoted neuronal survival. In addition, some researchers have studied the therapeutic effect of notoginsenoside R1 (NGR1) on hypoxic-ischemic brain damage (HIBD). They found that NGR1 targeted the endoplasmic reticulum and regulated the PI3K-Akt-mTOR/JNK signaling pathway. As a result of the interaction between the endoplasmic reticulum and PI3K, Akt/mTOR was activated, thus inhibiting JNK downstream of the endoplasmic reticulum. Ultimately, NGR1 could inhibit neuronal apoptosis and promote neuronal survival, which has a significant neuroprotective effect on newborns and contributes to long-term neurological recovery (Tu et al., 2018). Vinaginsenoside R4 (VGN4) is a minor component of Panax notoginseng and Panax japonicus (Zhu et al., 2016). In an in vivo PD model, VGN4 activated the PI3K/Akt/GSK-3β signaling pathway. Then, the NF-κB pathway was triggered to resist an organism’s oxidative stress reaction and control cell apoptosis and proliferation (Luo et al., 2020). Thus, VGN4 has the potential to treat PD and can be further studied later.
Notoginseng saponins are all dammarane-type saponins, classified as PPDs and PPTs. A first step in the biosynthesis process (Figure 7) involves cyclizing 2,3-oxidosqualene to dammarenediol-II. It is then hydroxylated to produce protopanaxadiol (PPD),β,12β,20-trihydroxydammar-24-ene. On the one hand, O-glycosylation of PPD results in the biosynthesis of various PPD-type saponins, such as ginsenosides Rb1 and Rd. On the other hand, PPD is hydroxylated into protopanaxatriol (PPT) and 3β,6α,12β,20-tetrahydroxydammar-24-ene. Additionally, PPT is biosynthesized through O-glycosylation into PPT-type saponins, such as ginsenoside Rg1 and ginsenoside Re (Shin et al., 2015). In terms of chemical structure, both PPD and PPT types contain hydroxyl groups at C-3, C-12, and C-20 positions, but PPD has one less hydroxyl group at position 6 than PPT. Furthermore, sugar units are linked to them in different positions such that PPD is either at C-3 or C-20, but PPT is either at C-6 or C-20 (Wu et al., 2019b). It is known that Panax ginseng processing involves oxidation, hydrolysis, and/or dehydration, which can produce a series of artificial compounds that are biologically active. Some researchers have summarized the structural characteristics of over 100 ginsenosides found in the processing (Shin et al., 2015).
In a SAR study, different saponin chemical backbones have been shown to affect autophagy, which plays a role in neurodegenerative disease pathogenesis. Saponins of the PPT type inhibit autophagy, while saponins of the PPD type and their transformed types promote autophagy (Wu et al., 2019b). Stereoisomers significantly influence PNS’s pharmacological activity in particular. In the processing of raw Panax notoginseng, the C-20 position of the saponin is deglycosylated to form optical saponin epimers, and 20(S)-Rg3 is more neuroprotective than 20(R)-Rg3 (Peng et al., 2018).
Echinocystic acid and its derivatives
Echinocystic acid (EA) is an oleanane pentacyclic triterpenoid with wide pharmacological activity, extracted from plants of Gleditsia sinensis Lam. In an in vivo study, Chen et al. (2020a) reported that EA served as a neuroprotective agent by activating the PI3K/Akt pathway. It is possible that EA can be used in clinical trials in order to treat intracerebral hemorrhage (ICH).
Due to EA’s complex structure, stable ring system, and limited active sites, few reports have been published regarding its chemical modification. The chemical modification of EA is mainly concentrated in 3-hydroxyl, 16-hydroxyl, C12–C13 double bonds, and 28-carboxyl groups, but their pharmacological effects are anti-inflammation and anti-hepatitis C virus (HCV) (Georgatza et al., 2016; Yu et al., 2016). By coupling EA to cyclodextrin (CD), it is possible to increase EA’s water solubility without causing adverse effects (Jiao et al., 2019). Moreover, Wang et al. (2015a) expanded and opened the rings A and/or C of EA to synthesize a series of derivatives of EA. As a result, this modification could decrease their bioactivity, suggesting that a rigid skeleton is essential for their bioactivity.
In order to improve bioavailability, some researchers wished to obtain related compounds through microbial transformation, followed by activity detection and modifications to the structure. Using the aforementioned method, Feng et al. (2010) have reported that 3-β-OH and 16-α-OH of EA could be distinguished for the first time, providing new insights into the structural modification of EA. As compared to traditional chemical methods, microbial transformations have greater advantages in oxidizing unactivated C–H bonds, and we can activate saturated C–H bonds of EA to expand its structural diversity and develop its pharmaceutical properties (Xu et al., 2017). Some studies have found that a new method of biotransformation could provide EA derivatives with cleavage and 7-member-lactone of ring A and derivatives with single or double hydroxylations, proving that this method could overcome the shortcomings of traditional chemical modification methods (Fu et al., 2018; Fu et al., 2019).
Asiatic acid and asiaticoside
A plant belonging to the family Apiaceae, Centella asiatica (L.) Urban, is inexpensive and easy to obtain as a medicinal remedy. Historically, ayurvedic practitioners have used it as a neuroprotective agent, and its major components are asiatic acid (AA) and asiaticoside (AS). Early evidence has suggested that AS could be used to treat PD and dementia (Xu et al., 2012; Lin et al., 2013; Chen et al., 2014). In an in vitro PD model, AS inhibited the decrease in PI3K expression induced by rotenone, eliminating ROT-induced abnormal neuronal dynamics at the synapse, thus affecting PI3K/Akt and thus activating the cytoprotective pathway to treat PD (Gopi et al., 2017). Both in vivo and in vitro studies have demonstrated that AA activated the PI3K/Akt pathway, which inhibited the activity of GSK-3β and reduced neuronal apoptosis (Nataraj et al., 2017; Cheng et al., 2018). It is known that diabetic encephalopathy is a complication of diabetes that causes cognitive impairment. The molecular mechanism of Centella asiatica for treating this disease has been reported in recent years. A study of AS has demonstrated that the compound upregulated the expression of synaptic proteins through the PI3K/Akt/NF-κB pathway in vitro, helping to restore synaptic connectivity (Yin et al., 2015). In recent years, some researchers have conducted in vivo experiments with an aqueous extract of the C. asiatica leaf aqueous extract in the diabetes rat model and found that PI3K and Akt levels increased in the rat brain, prolonging the survival time of cells (Giribabu et al., 2020).
In a study on the chemical composition of Centella asiatica extracts, Wu et al. (2020b) identified six new compounds, three pairs of oleanolic and ursolic pentacyclic triterpenoid isomers. Among these compounds, compound III was similar to asiaticoside B in chemical structure and was named 11-oxo-asiaticoside B (Figure 8). Compound III inhibited oxidative stress response, reduced apoptosis, and played a neuroprotective role through the PI3K signaling pathway. In the future, compound III should be further developed in order to explore its therapeutic potential.
So far, many derivatives have been synthesized by chemical modification of the structure of asiaticoside, usually on C-2, C-3, and C-23 hydroxyl groups, a C-12 olefin group, and a C-28 carboxylic acid group, which generally have less toxicity, better bioavailability, and efficacy (Lv et al., 2018; Nagoor Meeran et al., 2018). These derivatives can treat cancer, diabetes, and other diseases. In the future, we will also need to work on developing such derivatives for neurological disorders.
Pachymic acid
Pachymic acid (PA) is a natural triterpene of Poria cocos origin and a derivative of the lanostane skeleton (Zheng and Yang, 2008; Ríos, 2011). PA inhibited neuronal apoptosis by downregulating the expression of caspase-3, following activation of the PI3K/Akt pathway to reduce brain damage (Pang et al., 2020). In the future, PA may be used to treat hemic cerebrovascular diseases at an early stage. Furthermore, Zhu et al. (2021) have described the PA’s synthetic pathway and the genes involved in the synthesis. PA and ganoderic acid are homologous derivatives with highly similar carbon skeletons, so they looked for inspiration in the synthesis of G. lucidum and discovered that WcSOAT gene plays a role in the biosynthesis of PA in Wolfiporia cocos.
Stellettin B
A marine organism-derived compound, stellettin B (SB), is an isomalabaricane triterpenoid found in the sponge Jaspis stellifera. SB has been shown to reverse the downregulation of the PI3K/Akt signaling pathway caused by 6-OHDA in vitro and to reverse ataxia in a zebrafish model of PD in vivo (Feng et al., 2019). Therefore, SB is expected to become a leading anti-apoptotic and antioxidant compound for the treatment of PD. Interestingly, a study using a homogenous time-resolved fluorescence (HTRF) assay suggested that SB might target a signal protein upstream of the Akt pathway rather than PI3K (Wu et al., 2019a). The SAR analysis of SB revealed that the lactone rings with α,β-unsaturated carbonyl groups were essential for its efficacy (Song et al., 2018).
Tetraterpenes
A tetraterpene is a class of terpenoids composed of eight isoprene units. In general, tetraterpenoids refer to carotenoids.
The tetraterpene pigments carotenoids, including lycopene and astaxanthin, are found in photosynthetic bacteria, some species of archaea and fungi, algae, plants, and animals. Over the past decade, a number of studies have demonstrated the potential to act as neuroprotective agents for carotenoids.
Lycopene
Lycopene is a carotenoid of wide biological activity, which is mainly found in tomatoes and other red fruits, and has a stronger antioxidant effect than beta-carotene. There is no doubt that its neuroprotective properties are well known. Huang et al. (2019) found that lycopene reversed the neurotoxicity induced by tert-butyl hydroperoxide (t-BHP) in an in vitro AD model and elucidated that its neuroprotective mechanism involved the PI3K/Akt pathway. Recently, researchers have developed a rapid and efficient HPLC-DAD-MS2 method to isolate and characterize some carotenoids and their geometrical isomers from tomato products, including some derivatives of lycopene first discovered such as di-hydroxy cyclolycopene adduct and di-methoxy lycopene (Daood et al., 2022).
Astaxanthin
Astaxanthin (AST) is a marine carotenoid commonly found in crustaceans (shrimp and crab) (Zarneshan et al., 2020). Both in vitro and in vivo studies have demonstrated that AST was neuroprotective. AST exerts anti-apoptotic and neuroprotective effects by upregulating the phosphorylation of PI3K and Akt, which can treat neurological diseases such as PD, AD, and ischemic stroke (Wang et al., 2018; Deng et al., 2019). Adonixanthin and adonirubin are intermediates in the synthesis of AST, and like AST, they can improve brain damage associated with ICH by inhibiting lipid peroxidation and quenching singlet oxygen. The antioxidant and anti-inflammatory effects of adonixanthin are attributed to its characteristic structure, in which polyene (conjugated double bond) is the key to turning singlet oxygen into stable triplet oxygen. Additionally, there are differences in the ionone rings between adonixanthin and adonirubin compared to AST, resulting in structural polarity, which contributes to a stronger neuroprotective effect (Maoka et al., 2013; Kishimoto et al., 2016; Iwata et al., 2018).
Discussion and prospects
So far, in many studies, terpenoids have been found to exert neuroprotective effects by modulating the PI3K/Akt pathway (Table 2), of which triterpenoids account for the majority.
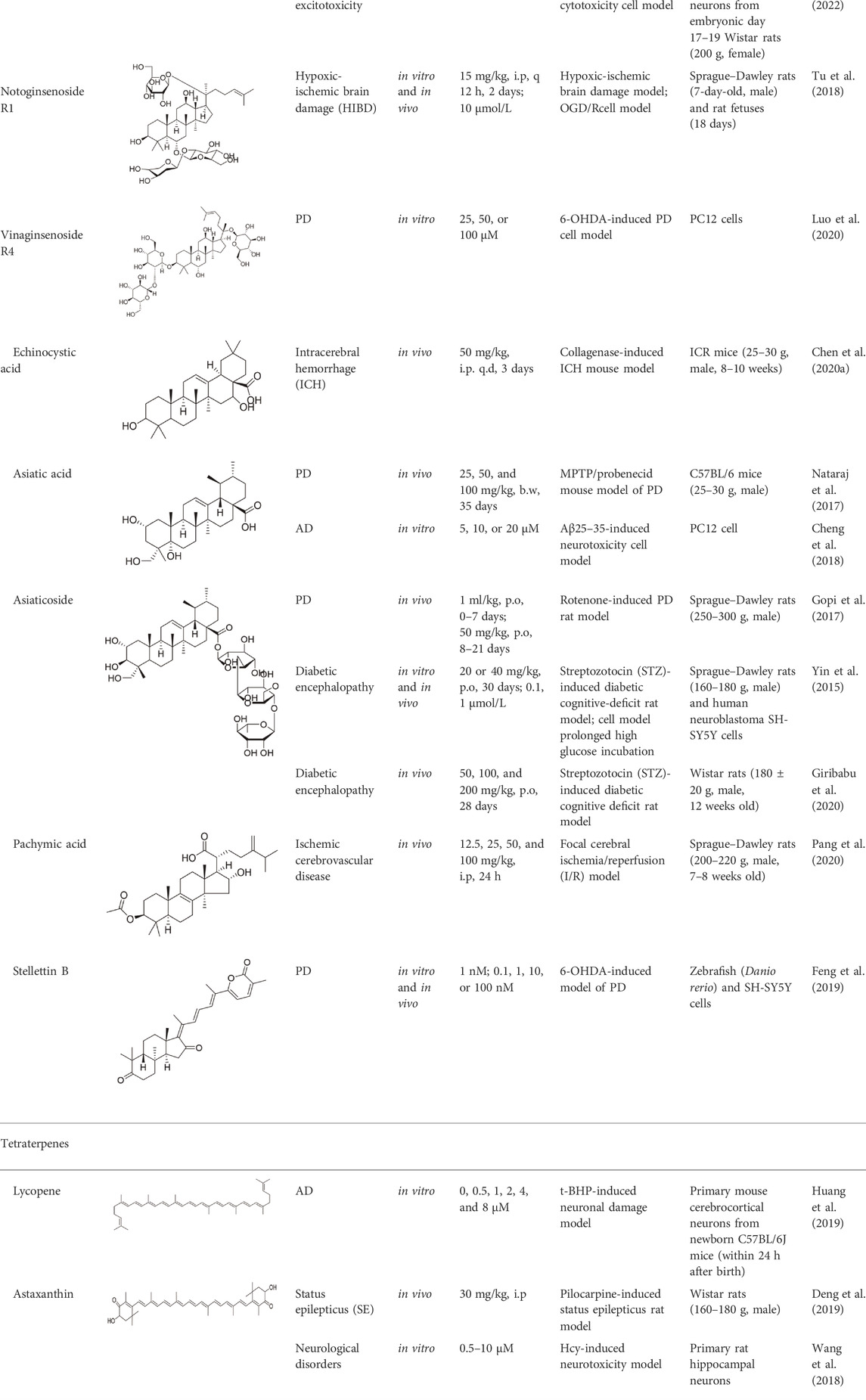
TABLE 2. Experimental evidence supporting the neuroprotective role of terpenoids in various neurological disorders.
In terms of pharmacology, the neuroprotective effects of some terpenoid natural products have not been fully proven, so it is crucial to establish appropriate experimental models to determine the efficacy of natural products, so those good drug candidates may be identified. In terms of chemical structures, CADD can be used to study chemical structures systematically, such as the studies of catalpol, geniposide, and ginkgolide derivatives previously discussed. The SAR analyses of catalpol and ginkgolides are based on computer simulations of binding to the relevant receptors. To make chemical modifications of ginkgolides, researchers have even established pharmacophore models. CADD can instruct us to make chemical modifications based on the structures of known compounds, improving the properties of compounds, such as enhancing bioavailability, reducing toxicity, and changing solubility. Derivatives of artesunate, methyl lucidone, polygalasaponin F, pachymic acid, and stellettin B are still less studied.
It is sometimes difficult to develop new drugs using traditional chemical modification methods. Researchers may be able to gain some new insights from EA’s use of novel microbial transformations in order to obtain new compounds. In addition, new natural products can be discovered more efficiently by developing advanced separation and characterization technologies.
Thus, we generally have three ideas for developing new neuroprotective terpenoid natural products: 1) exploring the neuroprotective properties of existing natural terpenoids; 2) extracting and isolating new terpenoids from natural herbs that extracted terpenoids with neuroprotective effects; and 3) modifying known terpenoid natural neuroprotective agents chemically to develop small-molecule new terpenoid drugs.
In conclusion, future research should employ comprehensive in vivo and in vitro experiments to illustrate the key role of PI3K/Akt pathways in neuroprotection for natural products, followed by scientific clinical trials. Developing derivatives of natural products will also promote the discovery of new neuroprotective drugs.
Author contributions
BX and LB contributed to the conception of this review and preparation of the manuscript, tables, and figures; LC revised tables, figures and reviewed manuscript; JS, YF, and RT contributed to the conception, supervision, and revision of the manuscript. All authors have approved the final article and are included in the disclosure.
Funding
This work is funded by the National Natural Science Foundation of China (No. 82073311), the Key Research and Development Program of Science and Technology Department of Sichuan Province (No. 2019YFS0514), the Natural Science Foundation of Sichuan Province (No. 2022JDTD0025), the screening and evaluation of anti-hepatic fibrosis varieties in traditional Chinese medicine formula granules (No. 2022HX006), and the research on vacuum freeze-drying technology of chuanxiong based on the intelligent control algorithm neural network model (No. 2021HX026).
Conflict of interest
The authors declare that the research was conducted in the absence of any commercial or financial relationships that could be construed as a potential conflict of interest.
Publisher’s note
All claims expressed in this article are solely those of the authors and do not necessarily represent those of their affiliated organizations, or those of the publisher, the editors, and the reviewers. Any product that may be evaluated in this article, or claim that may be made by its manufacturer, is not guaranteed or endorsed by the publisher.
References
Ahmed, R. M., Ke, Y. D., Vucic, S., Ittner, L. M., Seeley, W., Hodges, J. R., et al. (2018). Physiological changes in neurodegeneration—mechanistic insights and clinical utility. Nat. Rev. Neurol. 14, 259–271. doi:10.1038/nrneurol.2018.23
Apkarian, A. V., and Scholz, J. (2006). Shared mechanisms between chronic pain and neurodegenerative disease. Drug Discov. Today Dis. Mech. 3, 319–326. doi:10.1016/j.ddmec.2006.09.006
Belgacem, Y. H., and Borodinsky, L. (2017). Creb at the crossroads of activity-dependent regulation of nervous system development and function. Adv. Exp. Med. Biol. 1015, 19–39. doi:10.1007/978-3-319-62817-2
Cai, L., Mu, Y.-R., Liu, M.-M., Tang, W.-J., and Li, R. (2020). Antidepressant-like effects of penta-acetyl geniposide in chronic unpredictable mild stress-induced depression rat model: Involvement of inhibiting neuroinflammation in prefrontal cortex and regulating hypothalamic-pituitaryadrenal Axis. Int. Immunopharmacol. 80, 106182. doi:10.1016/j.intimp.2019.106182
Chen, B., Zhao, Y., Li, W., Hang, J., Yin, M., and Yu, H. (2020a). Echinocystic acid provides A neuroprotective effect via the pi3k/akt pathway in intracerebral haemorrhage mice. Ann. Transl. Med. 8, 6. doi:10.21037/atm.2019.12.35
Chen, J.-X., Li, H.-Y., Li, T.-T., Fu, W.-C., Du, X., Liu, C.-H., et al. (2020b). Alisol A-24-acetate promotes glucose uptake via activation of ampk in C2c12 myotubes. BMC Complement. Med. Ther. 20, 22–29. doi:10.1186/s12906-019-2802-3
Chen, J., Zhang, C., Li, S., Li, Z., Lai, X., and Xia, Q. (2021a). Exosomes derived from nerve stem cells loaded with Fty720 promote the recovery after spinal cord injury in rats by pten/akt signal pathway. J. Immunol. Res. 2021, 8100298. doi:10.1155/2021/8100298
Chen, S., Yin, Z.-J., Jiang, C., Ma, Z.-Q., Fu, Q., Qu, R., et al. (2014). Asiaticoside attenuates memory impairment induced by transient cerebral ischemia–reperfusion in mice through anti-inflammatory mechanism. Pharmacol. Biochem. Behav. 122, 7–15. doi:10.1016/j.pbb.2014.03.004
Chen, X., Drew, J., Berney, W., and Lei, W. J. C. (2021b). Neuroprotective natural products for Alzheimer's disease. Cells 10, 1309. doi:10.3390/cells10061309
Chen, Y.-Y., Liu, Q.-P., An, P., Jia, M., Luan, X., Tang, J.-Y., et al. (2021c). Ginsenoside Rd: A promising natural neuroprotective agent. Phytomedicine 95, 153883. doi:10.1016/j.phymed.2021.153883
Cheng, W., Chen, W., Wang, P., and Chu, J. (2018). Asiatic acid protects differentiated PC12 cells from Aβ25-35-induced apoptosis and tau hyperphosphorylation via regulating PI3K/Akt/GSK-3β signaling. Life Sci. 208, 96–101. doi:10.1016/j.lfs.2018.07.016
Chevallier, A. (2016). Encyclopedia of herbal medicine: 550 herbs and remedies for common ailments. Penguin: DK Pub.
Coelho, C. M., and Leevers, S. J (2000). Do growth and cell division rates determine cell. J. Cell Sci. 113, 2927–2934. doi:10.1242/jcs.113.17.2927
Colloca, L., Ludman, T., Bouhassira, D., Baron, R., Dickenson, A. H., Yarnitsky, D., et al. (2017). Neuropathic pain. Nat. Rev. Dis. Prim. 3, 1–19. doi:10.1038/nrdp.2017.2
Daood, H. G., Ráth, S., Palotás, G., Halász, G., Hamow, K., and Helyes, L. (2022). Efficient HPLC separation on a core-C30 column with MS2 characterization of isomers, derivatives and unusual carotenoids from tomato products. J. Chromatogr. Sci. 60, 336–347. doi:10.1093/chromsci/bmab085
Defeudis, F. V. (2002). Bilobalide and neuroprotection. Pharmacol. Res. 46, 565–568. doi:10.1016/s1043-6618(02)00233-5
Deng, X., Wang, M., Hu, S., Feng, Y., Shao, Y., Xie, Y., et al. (2019). The neuroprotective effect of astaxanthin on pilocarpine-induced status epilepticus in rats. Front. Cell. Neurosci. 13, 123. doi:10.3389/fncel.2019.00123
Deng, Y., Fang, W., Li, Y., Cen, J., Fang, F., Lv, P., et al. (2009). Blood-brain barrier breakdown by paf and protection by xq-1h due to antagonism of paf effects. Eur. J. Pharmacol. 616, 43–47. doi:10.1016/j.ejphar.2009.06.017
Duan, L., Xiong, X., Hu, J., Liu, Y., Li, J., and Wang, J. (2017). Panax notoginseng saponins for treating coronary artery disease: A functional and mechanistic overview. Front. Pharmacol. 8, 702. doi:10.3389/fphar.2017.00702
Fakhri, S., Iranpanah, A., Gravandi, M. M., Moradi, S. Z., Ranjbari, M., Majnooni, M. B., et al. (2021). Natural products attenuate pi3k/akt/mtor signaling pathway: A promising strategy in regulating neurodegeneration. Phytomedicine. 91, 153664. doi:10.1016/j.phymed.2021.153664
Fei, Y., Zhao, B., Zhu, J., Fang, W., and Li, Y. (2021). XQ-1H promotes cerebral angiogenesis via activating PI3K/Akt/GSK3β/β-catenin/VEGF signal in mice exposed to cerebral ischemic injury./Β-Catenin/Vegf Signal. Life Sci. 272, 119234. doi:10.1016/j.lfs.2021.119234
Feng, C.-W., Chen, N.-F., Wen, Z.-H., Yang, W.-Y., Kuo, H.-M., Sung, P.-J., et al. (2019). Vitro and in vivo neuroprotective effects of stellettin B through anti-apoptosis and the Nrf2/Ho-1 pathway. Mar. Drugs 17, 315. doi:10.3390/md17060315
Feng, X., Zou, Z., Fu, S., Sun, L., Su, Z., and Sun, D.-A. (2010). Microbial oxidation and glucosidation of echinocystic acid by Nocardia corallina by. J. Mol. Catal. B Enzym. 66, 219–223. doi:10.1016/j.molcatb.2010.05.012
Fu, S.-B., Feng, X., Meng, Q.-F., Cai, Q., and Sun, D.-A. (2019). Two new echinocystic acid derivatives catalyzed by filamentous fungus Gliocladium roseum CGMCC 3.3657. Nat. Prod. Res. 33, 1842–1848. doi:10.1080/14786419.2018.1477148
Fu, S., Feng, X., and Sun, D.-A. (2018). Microbe-mediate transformation of echinocystic acid by whole cells of filamentous fungus Cunninghamella blakesleana CGMCC 3.910. Mol. Biol. Rep. 45, 2795–2800. doi:10.1007/s11033-018-4357-7
Galm, U., and Shen, B. (2007). Natural product drug discovery: The times have never been better. Chem. Biol. 1, 1098–1104. doi:10.1016/j.chembiol.2007.10.004
Georgatza, D., Gorgogietas, V. A., Kylindri, P., Charalambous, M. C., Papadopoulou, K. K., Hayes, J. M., et al. (2016). The triterpene echinocystic acid and its 3-O-glucoside derivative are revealed as potent and selective glucocorticoid receptor agonists. Int. J. Biochem. Cell Biol. 79, 277–287. doi:10.1016/j.biocel.2016.08.028
Ginter, E., Simko, V., and Panakova, V. (2014). Antioxidants in health and disease. Bratisl. Lek. Listy 115, 603–606. doi:10.4149/bll_2014_116
Giribabu, N., Karim, K., Kilari, E. K., Nelli, S. R., and Salleh, N. J. I. (2020). Oral administration of Centella asiatica (L.) urb leave aqueous extract ameliorates cerebral oxidative stress, inflammation, and apoptosis in male rats with type-2 diabetes. Inflammopharmacology 28, 1599–1622. doi:10.1007/s10787-020-00733-3
Gitler, A. D., Dhillon, P., and Shorter, J. (2017). Neurodegenerative disease: Models, mechanisms, and A new hope. Dis. Model. Mech. 10, 499–502. doi:10.1242/dmm.030205
Gopi, M., and Janardhanam, V. A (2017). Asiaticoside: Attenuation of rotenone induced oxidative burden in A rat model of hemiparkinsonism by maintaining the phosphoinositide-mediated synaptic integrity. Pharmacol. Biochem. Behav. 155, 1–15. doi:10.1016/j.pbb.2017.02.005
Hannan, M. A., Dash, R., Haque, M. N., Mohibbullah, M., Sohag, A. A. M., Rahman, M. A., et al. (2020). Neuroprotective potentials of marine algae and their bioactive metabolites: Pharmacological insights and therapeutic advances. Mar. Drugs 18, 347. doi:10.3390/md18070347
Heneka, M. T., Mcmanus, R. M., and Latz, E. J. (2018). Inflammasome signalling in brain function and neurodegenerative disease. Nat. Rev. Neurosci. 19, 610–621. doi:10.1038/s41583-018-0055-7
Hou, Y., Tsai, S., Lai, P., Chen, Y., Chao, P. J. F., and Toxicology, C. (2008). Metabolism and pharmacokinetics of genipin and geniposide in rats. Food Chem. Toxicol. 46, 2764–2769. doi:10.1016/j.fct.2008.04.033
Huang, C., Wen, C., Yang, M., Gan, D., Fan, C., Li, A., et al. (2019). Lycopene protects against T-bhp-induced neuronal oxidative damage and apoptosis via activation of the pi3k/akt pathway. Mol. Biol. Rep. 46, 3387–3397. doi:10.1007/s11033-019-04801-y
Huang, S. H., Duke, R. K., Chebib, M., Sasaki, K., Wada, K., and Johnston, G. A. (2003). Bilobalide, a sesquiterpene trilactone from Ginkgo biloba, is an antagonist at recombinant alpha1beta2gamma2L GABA(A) receptors. Eur. J. Pharmacol. 464, 1–8. doi:10.1016/s0014-2999(03)01344-x
Huang, T.-J., Verkhratsky, A., Fernyhough, P. J. M., and Neuroscience, C. (2005). Insulin enhances mitochondrial inner membrane potential and increases atp levels through phosphoinositide 3-kinase in adult sensory neurons. Mol. Cell. Neurosci. 28, 42–54. doi:10.1016/j.mcn.2004.08.009
Ichino, K., Tanaka, H., Ito, K., Tanaka, T., and Mizuno, M. (1988). Two new dihydrochalcones from Lindera erythrocarpa. J. Nat. Prod. 51, 915–917.
Inouye, H., Saito, S., Taguchi, H., and Endo, T. J. T. L. (1969). Two new iridoid glycosides from gardenia jasminoides: Gasdenoside and geniposide. Tetrahedron Lett.
Iwata, S., Imai, T., Shimazawa, M., Ishibashi, T., Hayashi, M., Hara, H., et al. (2018). Protective effects of the astaxanthin derivative, adonixanthin, on brain hemorrhagic injury. Brain Res. 1698, 130–138. doi:10.1016/j.brainres.2018.08.009
Jaracz, S., Nakanishi, K., Jensen, A. A., and Strømgaard, K. (2004a). Ginkgolides and Glycine receptors: A structure–activity relationship study. Chemistry 10, 1507–1518. doi:10.1002/chem.200305473
Jaracz, S., Nakanishi, K., Jensen, A. A., and Strømgaard, K. J. C. A. E. J. (2004b). Ginkgolides and glycine receptors: A structure-activity relationship study. Chemistry 10, 1507–1518. doi:10.1002/chem.200305473
Jensen, A. A., Begum, N., Vogensen, S. B., Knapp, K. M., Gundertofte, K., Dzyuba, S. V., et al. 2007. Probing the pharmacophore of ginkgolides as Glycine receptor antagonists, J. Med. Chem. 50, 1610–1617. doi:10.1021/jm070003n
Jiang, B., Shen, R., Bi, J., Tian, X., Hinchliffe, T., and Xia, Y. (2015). Catalpol: A potential therapeutic for neurodegenerative diseases. Curr. Med. Chem. 22, 1278–1291. doi:10.2174/0929867322666150114151720
Jiang, M., Li, J., Peng, Q., Liu, Y., Liu, W., Luo, C., et al. (2014). Neuroprotective effects of bilobalide on cerebral ischemia and reperfusion injury are associated with inhibition of pro-inflammatory mediator production and down-regulation of jnk1/2 and P38 mapk activation. J. Neuroinflammation 11, 167–217. doi:10.1186/s12974-014-0167-6
Jiao, P., Wang, S., Liang, S., Li, M., Gao, Q., Ji, D., et al. (2019). Facile preparation and characterization of novel oleanane-type triterpene functionalized Β-cyclodextrin conjugates. Chin. Chem. Lett. 30, 690–693. doi:10.1016/j.cclet.2018.10.009
Jin, Z., Xu, Z., Liu, Q., Zhou, J., Cao, L., Ding, G., et al. (2017). Diterpene ginkgolides meglumine injection inhibits oxidative stress induced by oxygen-glucose deprivation by activating Akt/Nrf2 pathway in SH-SY5Y cells. Chin. J. Pharmacol. Toxicol., 65–72. doi:10.3867/j.issn.1000-3002.2017.01.008
Kishimoto, Y., Yoshida, H., and Kondo, K. (2016). Potential anti-atherosclerotic properties of astaxanthin. Mar. Drugs 14, 35. doi:10.3390/md14020035
Kiyama, R. (2017). Estrogenic terpenes and terpenoids: Pathways, functions and applications. Eur. J. Pharmacol. 815, 405–415. doi:10.1016/j.ejphar.2017.09.049
Kondratskaya, E. L., and Krishtal, O. A. (2002). Effects of Ginkgo biloba extract constituents on glycine-activated strychnine-sensitive receptors in hippocampal pyramidal neurons of the rat. Neurophysiology 34, 155–157. doi:10.1023/a:1020774410527
Kurimoto, S., Jung, J., Tapadia, M., Lengfeld, J., Agalliu, D., Waterman, M., et al. (2015). Activation of the Wnt/β-catenin signaling cascade after traumatic nerve injury. Neuroscience 294, 101–108. doi:10.1016/j.neuroscience.2015.02.049
Le Bars, P. J. P. (2003). Magnitude of effect and special approach to Ginkgo biloba extract egb 761® in cognitive disorders. Pharmacopsychiatry 36, 44–49. doi:10.1055/s-2003-40458
Leicach, S. R., and Chludil, H. D. 2014. Plant secondary metabolites: Structure–activity relationships in human health prevention and treatment of common diseases, Stud. Nat. Prod. Chem. 42, 267–304. doi:10.1016/B978-0-444-63281-4.00009-4
Li, B., Yang, Y., Wang, Y., Zhang, J., Ding, J., Liu, X., et al. (2021). Acetylation of NDUFV1 induced by a newly synthesized HDAC6 inhibitor HGC rescues dopaminergic neuron loss in Parkinson models. iScience 24, 102302. doi:10.1016/j.isci.2021.102302
Li, Z., Ma, Q.-Q., Yan, Y., Xu, F.-D., Zhang, X.-Y., Zhou, W.-Q., et al. (2016). Edaravone attenuates hippocampal damage in an infant mouse model of pneumococcal meningitis by reducing Hmgb1 and inos expression via the Nrf2/Ho-1 pathway. Acta Pharmacol. Sin. 37, 1298–1306. doi:10.1038/aps.2016.71
Lin, X., Huang, R., Zhang, S., Wei, L., Zhuo, L., Wu, X., et al. (2013). Beneficial effects of asiaticoside on cognitive deficits in senescence-accelerated mice. Fitoterapia 87, 69–77. doi:10.1016/j.fitote.2013.03.023
Ling, Y., Li, Y., Zhu, R., Qian, J., Liu, J., Gao, W., et al. (2019). Hydroxamic acid derivatives of β-carboline/hydroxycinnamic acid hybrids inducing apoptosis and autophagy through the PI3K/Akt/mTOR pathways. J. Nat. Prod. 82, 1442–1450. doi:10.1021/acs.jnatprod.8b00843
Liu, Q., Jin, Z., Xu, Z., Yang, H., Li, L., Li, G., et al. (2019). Antioxidant effects of ginkgolides and bilobalide against cerebral ischemia injury by activating the akt/nrf2 pathway in vitro and in vivo. Cell Stress Chaperones 24, 441–452. doi:10.1007/s12192-019-00977-1
Liu, S., Cheng, X., Li, X., Kong, Y., Jiang, S., Dong, C., et al. (2020). Design, microwave synthesis, and molecular docking studies of catalpol crotonates as potential neuroprotective agent of diabetic encephalopathy. Sci. Rep. 10, 20415–20510. doi:10.1038/s41598-020-77399-y
Lu, T., Li, H., Zhou, Y., Wei, W., Ding, L., Zhan, Z., et al. (2021). Neuroprotective effects of alisol A 24-acetate on cerebral ischemia-reperfusion injury by regulating pi3k/akt pathway. J. Neuroinflammation 19, 37. doi:10.1186/s12974-022-02392-3
Luo, Y., Jiang, Y., He, Y., Shen, T., Ji, L., Li, F., et al. (2020). Vina-ginsenoside R4 from panax ginseng leaves alleviates 6-ohda-induced neurotoxicity in Pc12 cells via the pi3k/akt/gsk-3β signaling pathway. J. Agric. Food Chem. 68, 15239–15248. doi:10.1021/acs.jafc.0c06474
Lv, J., Sharma, A., Zhang, T., Wu, Y., and Ding, X. (2018). Pharmacological review on asiatic acid and its derivatives: A potential compound. SLAS Technol. 23, 111–127. doi:10.1177/2472630317751840
Makabel, B., Zhao, Y., Wang, B., Bai, Y., Zhang, Q., Wu, L., et al. (2008). Stability and structure studies on alisol A 24-acetate. Chem. Pharm. Bull.56, 41–45. doi:10.1248/cpb.56.41
Manning, B. D., and Cantley, L. C. J. C. (2007). AKT/PKB signaling: Navigating downstream. Cell 129, 1261–1274. doi:10.1016/j.cell.2007.06.009
Maoka, T., Yasui, H., Ohmori, A., Tokuda, H., Suzuki, N., Osawa, A., et al. (2013). Anti-oxidative, anti-tumor-promoting, and anti-carcinogenic activities of adonirubin and adonixanthin. J. Oleo Sci. 62, 181–186. doi:10.5650/jos.62.181
Nagoor Meeran, M. F., Goyal, S. N., Suchal, K., Sharma, C., Patil, C. R., and Ojha, S. K. (2018). Pharmacological properties, molecular mechanisms, and pharmaceutical development of asiatic acid: A pentacyclic triterpenoid of therapeutic promise. Front. Pharmacol. 9, 892. doi:10.3389/fphar.2018.00892
Nakanishi, K. J. B., and Chemistry, M. (2005). Terpene trilactones from gingko biloba: From ancient times to the 21st century. Bioorg. Med. Chem. 13, 4987–5000. doi:10.1016/j.bmc.2005.06.014
Nataraj, J., Manivasagam, T., Justin Thenmozhi, A., and Essa, M. M. (2017). Neurotrophic effect of asiatic acid, A triterpene of Centella asiatica against chronic 1-methyl 4-phenyl 1, 2, 3, 6-tetrahydropyridine hydrochloride/probenecid mouse model of Parkinson’s disease: The role of mapk, pi3k-akt-gsk3β and mtor signalling pathways. Neurochem. Res. 42, 1354–1365. doi:10.1007/s11064-017-2183-2
Pan, Y.-W., Wu, D.-P., Liang, H.-F., Tang, G.-Y., Fan, C.-L., Shi, L., et al. (2021). Total saponins of panax notoginseng activate akt/mtor pathway and exhibit neuroprotection in vitro and in vivo against ischemic damage. Chin. J. Integr. Med. 28, 410–418. doi:10.1007/s11655-021-3454-y
Pang, Y., Zhu, S., and Pei, H. (2020). Pachymic acid protects against cerebral ischemia/reperfusion injury by the pi3k/akt signaling pathway. Metab. Brain Dis. 35, 673–680. doi:10.1007/s11011-020-00540-3
Park, J.-Y., Amarsanaa, K., Cui, Y., Lee, J.-H., Wu, J., Yang, Y.-S., et al. (2019). Methyl lucidone exhibits neuroprotective effects on glutamate-induced oxidative stress in ht-22 cells via nrf-2/Ho-1 signaling. Appl. Biol. Chem. 62, 67–69. doi:10.1186/s13765-019-0474-9
Peng, M., Yi, Y. X., Zhang, T., Ding, Y., and Le, J. (2018). Stereoisomers of saponins in panax notoginseng (sanqi): A review. Front. Pharmacol. 9, 188. doi:10.3389/fphar.2018.00188
Pohl, F., and Kong Thoo Lin, P. (2018). The potential use of plant natural products and plant extracts with antioxidant properties for the prevention/treatment of neurodegenerative diseases: In vitro, in vivo and clinical trials. Molecules 23, 3283. doi:10.3390/molecules23123283
Prince, M., Prina, M., and Guerchet, M. (2013). World alzheimer report 2013: Journey of caring: An analysis of long-term care for dementia. London, UK: Alzheimer’s Disease International.
Ríos, J.-L. (2011). Chemical constituents and pharmacological properties ofPoria cocos. Planta Med. 77, 681–691. doi:10.1055/s-0030-1270823
Rodrigues, T., Reker, D., Schneider, P., and Schneider, G. J. N. C. (2016). Counting on natural products for drug design. Nat. Chem. 8, 531–541. doi:10.1038/nchem.2479
Sasaki, K., Hatta, S., Haga, M., and Ohshika, H. (1999). Effects of bilobalide on Γ-aminobutyric acid levels and glutamic acid decarboxylase in mouse brain. Eur. J. Pharmacol. 367, 165–173. doi:10.1016/s0014-2999(98)00968-6
Schmidt, T. J. (2006). Structure-activity relationships in Studies in Natural Products Chemistry. Editor R. Atta Ur. Elsevier 33, 309–392. doi:10.1016/S1572-5995(06)80030-X
Shi, C., Wu, F., Yew, D. T., Xu, J., and Zhu, Y. J. A. (2010a). Bilobalide prevents apoptosis through activation of the PI3K/Akt pathway in SH-SY5Y cells. Apoptosis 15, 715–727. doi:10.1007/s10495-010-0492-x
Shi, Q., Li, L., Huo, C., Zhang, M., Wang, Y., and Drugs, H. (2010b). Study on natural medicinal chemistry and new drug development. Chin. Traditional Herb. Drugs 41, 1583–1589.
Shin, B.-K., Kwon, S. W., and Park, J. H. (2015). Chemical diversity of ginseng saponins from Panax ginseng. J. Ginseng Res. 39, 287–298. doi:10.1016/j.jgr.2014.12.005
Song, X., Xiong, Y., Qi, X., Tang, W., Dai, J., Gu, Q., et al. (2018). Molecular targets of active anticancer compounds derived from marine sources. Mar. Drugs 16, 175. doi:10.3390/md16050175
Strømgaard, K., and Nakanishi, K. (2004). Chemistry and biology of terpene trilactones from Ginkgo biloba. Angew. Chem. Int. Ed. Engl. 43, 1640–1658. doi:10.1002/anie.200300601
Sun, C., Cao, X.-C., Liu, Z.-Y., Ma, C.-L., and Li, B.-M. (2022). Polygalasaponin F protects hippocampal neurons against glutamate-induced cytotoxicity. Neural Regen. Res. 17, 178–184. doi:10.4103/1673-5374.314321
Suzuki, H., Yamazaki, M., Chiba, K., Uemori, Y., Sawanishi, H. J. C., and Bulletin, P. (2010). Neuritogenic activities of 1-alkyloxygenipins. Chem. Pharm. Bull. 58, 168–171. doi:10.1248/cpb.58.168
Tu, L., Wang, Y., Chen, D., Xiang, P., Shen, J., Li, Y., et al. (2018). Protective effects of notoginsenoside R1 via regulation of the pi3k-akt-mtor/jnk pathway in neonatal cerebral hypoxic–ischemic brain injury. Neurochem. Res. 43, 1210–1226. doi:10.1007/s11064-018-2538-3
Usuki, T., Yoshimoto, Y., Sato, M., Takenaka, T., Takezawa, R., Yoshida, Y., et al. (2020). Bilobalide and PC12 cells: A structure activity relationship study. Bioorg. Med. Chem. 28, 115251. doi:10.1016/j.bmc.2019.115251
Van Beek, T. A., and Montoro, P. (2009). Chemical analysis and quality control of Ginkgo biloba leaves, extracts, and phytopharmaceuticals. J. Chromatogr. A 1216, 2002–2032. doi:10.1016/j.chroma.2009.01.013
Villasenor, I. M. J. A.-I., and Chemistry, A.-A. (2007). Bioactivities of iridoids. Antiinflamm. Antiallergy. Agents Med. Chem. 6, 307–314. doi:10.2174/187152307783220040
Wang, H., Yu, F., Peng, Y., Wang, Q., Han, X., Xu, R., et al. (2015a). Synthesis and biological evaluation of ring A and/or C expansion and opening echinocystic acid derivatives for anti-hcv entry inhibitors. Eur. J. Med. Chem. 102, 594–599. doi:10.1016/j.ejmech.2015.08.034
Wang, J., Chen, R., Liu, C., Wu, X., and Zhang, Y. (2021a). Antidepressant mechanism of catalpol: Involvement of the PI3K/Akt/Nrf2/HO-1 signaling pathway in rat hippocampus. Eur. J. Pharmacol. 909, 174396. doi:10.1016/j.ejphar.2021.174396
Wang, J., Wan, D., Wan, G., Wang, J., Zhang, J., and Zhu, H. (2019). Catalpol induces cell activity to promote axonal regeneration via the pi3k/akt/mtor pathway in vivo and in vitro stroke model. Ann. Transl. Med. 7, 756. doi:10.21037/atm.2019.11.101
Wang, M., Yang, L., Chen, Z., Dai, L., Xi, C., Wu, X., et al. (2021b). Geniposide ameliorates chronic unpredictable mild stress induced depression-like behavior through inhibition of ceramide-PP2A signaling via the PI3K/Akt/GSK3β axis. Psychopharmacology 238, 2789–2800. doi:10.1007/s00213-021-05895-8
Wang, R., Yang, J., Peng, L., Zhao, J., Mu, N., Huang, J., et al. (2015b). Gardenamide A attenuated cell apoptosis induced by serum deprivation insult via the erk1/2 and pi3k/akt signaling pathways. Neuroscience 286, 242–250. doi:10.1016/j.neuroscience.2014.11.056
Wang, X.-J., Chen, W., Fu, X.-T., Ma, J.-K., Wang, M.-H., Hou, Y.-J., et al. (2018). Reversal of homocysteine-induced neurotoxicity in rat hippocampal neurons by astaxanthin: Evidences for mitochondrial dysfunction and signaling crosstalk. Cell Death Discov. 4, 50–11. doi:10.1038/s41420-018-0114-x
Wei, H., Duan, G., He, J., Meng, Q., Liu, Y., Chen, W., et al. (2018). Geniposide attenuates epilepsy symptoms in A mouse model through the pi3k/akt/gsk-3β signaling pathway. Exp. Ther. Med. 15, 1136–1142. doi:10.3892/etm.2017.5512
Weichel, O., Hilgert, M., Chatterjee, S. S., Lehr, M., and Klein, J. (1999). Bilobalide, a constituent of Ginkgo biloba, inhibits NMDA-induced phospholipase A2 activation and phospholipid breakdown in rat hippocampus. Naunyn. Schmiedeb. Arch. Pharmacol. 360, 609–615. doi:10.1007/s002109900131
Wu, Q., Nay, B., Yang, M., Ni, Y., Wang, H., Yao, L., et al. (2019a). Marine sponges of the genus stelletta as promising drug sources: Chemical and biological aspects. Acta Pharm. Sin. B 9, 237–257. doi:10.1016/j.apsb.2018.10.003
Wu, S., Yang, T., Cen, K., Zou, Y., Shi, X., Zhou, D., et al. (2020a). Vitro evaluation of the neuroprotective effect of panax notoginseng saponins by activating the egfr/pi3k/akt pathway. Evid. Based Complement. Altern. Med. 2020, 1403572. doi:10.1155/2020/1403572
Wu, T., Kwaku, O. R., Li, H.-Z., Yang, C.-R., Ge, L.-J., and Xu, M. (2019b). Sense ginsenosides from ginsengs: Structure-activity relationship in autophagy. Nat. Product. Commun. 14, 1934578X1985822. doi:10.1177/1934578X19858223
Wu, X., Wang, J., Song, L., Guan, Y., Cao, C., Cui, Y., et al. (2021). Catalpol weakens depressive-like behavior in mice with streptozotocin-induced hyperglycemia via pi3k/akt/nrf2/Ho-1 signaling pathway. Neuroscience 473, 102–118. doi:10.1016/j.neuroscience.2021.07.029
Wu, Z.-W., Li, W.-B., Zhou, J., Liu, X., Wang, L., Chen, B., et al. (2020b). Oleanane-and ursane-type triterpene saponins from Centella asiatica exhibit neuroprotective effects. J. Agric. Food Chem. 68, 6977–6986. doi:10.1021/acs.jafc.0c01476
Xie, W., Wulin, H., Shao, G., Wei, L., Qi, R., Ma, B., et al. (2020). Polygalasaponin F inhibits neuronal apoptosis induced by oxygen‐glucose deprivation and reoxygenation through the pi3k/akt pathway. Basic Clin. Pharmacol. Toxicol. 127, 196–204. doi:10.1111/bcpt.13408
Xu, C.-L., Wang, Q.-Z., Sun, L.-M., Li, X.-M., Deng, J.-M., Li, L.-F., et al. (2012). Asiaticoside: Attenuation of neurotoxicity induced by mptp in A rat model of parkinsonism via maintaining redox balance and up-regulating the ratio of bcl-2/bax. Pharmacol. Biochem. Behav. 100, 413–418. doi:10.1016/j.pbb.2011.09.014
Xu, S.-H., Wang, W.-W., Zhang, C., Liu, X.-F., Yu, B.-Y., and Zhang, J. (2017). Site-selective oxidation of unactivated C–H sp bonds of oleanane triterpenes by Streptomyces griseus ATCC 13273. Tetrahedron 73, 3086–3092. doi:10.1016/j.tet.2017.04.036
Yang, T., Guo, Q., Shi, X., Wu, S., Li, Y., Sun, Y., et al. (2018). Panax notoginseng saponins promotes cerebral recovery from ischemic injury by downregulating lingo-1 and activating the egfr/pi3k/akt signaling pathways in vivo. J. Traditional Chin. Med. Sci. 5, 151–160. doi:10.1016/j.jtcms.2018.03.009
Yang, X., Xiong, X., Wang, H., Wang, J. J. E.-B. C., and Medicine, A. (2014). Protective effects of panax notoginseng saponins on cardiovascular diseases: A comprehensive overview of experimental studies. Evid. Based Complement. Altern. Med. 2014, 204840. doi:10.1155/2014/204840
Yin, Z., Yu, H., Chen, S., Ma, C., Ma, X., Xu, L., et al. (2015). Asiaticoside attenuates diabetes-induced cognition deficits by regulating pi3k/akt/nf-?b pathway. Behav. Brain Res. 292, 288–299. doi:10.1016/j.bbr.2015.06.024
Yoo, K.-Y., and Park, S.-Y. J. M. (2012). Terpenoids as potential anti-Alzheimer's disease therapeutics. Molecules 17, 3524–3538. doi:10.3390/molecules17033524
Yu, F., Wang, Q., Wang, H., Si, L.-L., Liu, J.-X., Han, X., et al. (2016). Synthesis and biological evaluation of echinocystic acid derivatives as hcv entry inhibitors. Eur. J. Med. Chem. 102, 594–599. doi:10.1016/j.ejmech.2015.08.034
Yu, W. B., Cao, L., Zhao, Y. Y., Xiao, W., and Xiao, B. G. (2018b). Comparing the role of Ginkgolide B and Ginkgolide K on cultured astrocytes exposed to oxygen‑glucose deprivation. Mol. Med. Rep. 18, 4417–4427. doi:10.3892/mmr.2018.9450
Yu, W., Chen, S., Cao, L., Tang, J., Xiao, W., and Xiao, B. (2018a). Ginkgolide K promotes the clearance of A53T mutation alpha-synuclein in SH-SY5Y cells. Cell Biol. Toxicol. 34, 291–303. doi:10.1007/s10565-017-9419-4
Yuan, H., Ma, Q., Ye, L., and Piao, G. J. M. (2016). The traditional medicine and modern medicine from natural products. Molecules 21, 559. doi:10.3390/molecules21050559
Zarneshan, S. N., Fakhri, S., Farzaei, M. H., Khan, H., Saso, L. J. F., and Toxicology, C. (2020). Astaxanthin targets PI3K/Akt signaling pathway toward potential therapeutic applications. Food Chem. Toxicol. 145, 111714. doi:10.1016/j.fct.2020.111714
Zhang, D.-D., Chen, Q.-Q., and Li, Y. (2022). Geniposide alleviates neuropathic pain in cci rats by inhibiting the egfr/pi3k/akt pathway and Ca2+ channels. Neurotox. Res. 40, 1057–1069. doi:10.1007/s12640-022-00531-5
Zhang, D., Miyase, T., Kuroyanagi, M., Umehara, K., Ueno, A. J. C., and Bulletin, P. (1995b). Studies on the constituents of Polygala japonica HOUTT. II. Structures of polygalasaponins XI-XIX. Chem. Pharm. Bull. 43, 966–970. doi:10.1248/cpb.43.966
Zhang, D., Miyase, T., Kuroyanagi, M., Umehara, K., Ueno, A. J. C., and Bulletin, P. (1995a). Studies on the constituents of Polygala japonica Houtt. I. Structures of polygalasaponins I-X. Chem. Pharm. Bull. 43, 115–120. doi:10.1248/cpb.43.115
Zhang, K., Yang, Y., Ge, H., Wang, J., Chen, X., Lei, X., et al. (2020). Artesunate promotes the proliferation of neural stem/progenitor cells and alleviates ischemia-reperfusion injury through pi3k/akt/foxo-3a/P27kip1 signaling pathway. Aging 12, 8029–8048. doi:10.18632/aging.103121
Zhang, L.-Q., Chen, K.-X., and Li, Y.-M. (2019a). Bioactivities of natural catalpol derivatives. Curr. Med. Chem. 26, 6149–6173. doi:10.2174/0929867326666190620103813
Zhang, W., Song, J.-K., Yan, R., Li, L., Xiao, Z.-Y., Zhou, W.-X., et al. (2018). Diterpene ginkgolides protect against cerebral ischemia/reperfusion damage in rats by activating Nrf2 and creb through pi3k/akt signaling. Acta Pharmacol. Sin. 39, 1259–1272. doi:10.1038/aps.2017.149
Zhang, Z., Wang, Y., Zhang, Y., Li, J., Huang, W., and Wang, L. J. M. (2019b). The synthesis and biological evaluation of novel gardenamide A derivatives as multifunctional neuroprotective agents. Medchemcomm 10, 1180–1186. doi:10.1039/c9md00211a
Zheng, Y., Wu, Z., Yi, F., Orange, M., Yao, M., Yang, B., et al. (2018). By activating akt/enos bilobalide B inhibits autophagy and promotes angiogenesis following focal cerebral ischemia reperfusion. Cell. Physiol. biochem. 47, 604–616. doi:10.1159/000490016
Zheng, Y., and Yang, X.-W. (2008). Two new lanostane triterpenoids from Poria cocos. J. Asian Nat. Prod. Res. 10, 289–292. doi:10.1080/10286020801892250
Zhu, H., Wang, J., Shao, Y., and Wan, D. (2019). Catalpol may improve axonal growth via regulating mir-124 regulated pi3k/akt/mtor pathway in neurons after ischemia. Ann. Transl. Med. 7, 306. doi:10.21037/atm.2019.06.25
Zhu, T., Li, F., Chen, B., Deng, Y., Wang, M., and Li, L. J. C. J. A. E. B. (2016). Studies on the saponins from the leaves of Panax ginseng. Chin. J. Appl. Environ. Biol. 22, 70–74. doi:10.3724/SP.J.1145.2015.06008
Keywords: natural products, neuroprotection, PI3K/Akt pathway, derivatives, terpenoids
Citation: Xu B, Bai L, Chen L, Tong R, Feng Y and Shi J (2022) Terpenoid natural products exert neuroprotection via the PI3K/Akt pathway. Front. Pharmacol. 13:1036506. doi: 10.3389/fphar.2022.1036506
Received: 04 September 2022; Accepted: 26 September 2022;
Published: 13 October 2022.
Edited by:
Wei Peng, Chengdu University of Traditional Chinese Medicine, ChinaCopyright © 2022 Xu, Bai, Chen, Tong, Feng and Shi. This is an open-access article distributed under the terms of the Creative Commons Attribution License (CC BY). The use, distribution or reproduction in other forums is permitted, provided the original author(s) and the copyright owner(s) are credited and that the original publication in this journal is cited, in accordance with accepted academic practice. No use, distribution or reproduction is permitted which does not comply with these terms.
*Correspondence: Rongsheng Tong, dG9uZ3JzQDEyNi5jb20=; Yibin Feng, eWZlbmdAaGt1Lmhr; Jianyou Shi, c2hpamlhbnlvdWRlQDEyNi5jb20=
†These authors share first authorship