- 1School of Pharmacy, Hangzhou Normal University, Hangzhou, China
- 2Department of Medical Oncology, The Affiliated Hospital of Hangzhou Normal University, Hangzhou Normal University, Hangzhou, China
Tuft cells are solitary chemosensory epithelial cells with microvilli at the top, which are found in hollow organs such as the gastrointestinal tract, pancreas, and lungs. Recently, an increasing number of studies have revealed the chemotactic abilities and immune function of the tuft cells, and numerous efforts have been devoted to uncovering the role of tuft cells in tumors. Notably, accumulating evidence has shown that the specific genes (POU2F3, DCLK1) expressed in tuft cells are involved in vital processes related with carcinogenesis and cancer development. However, the interaction between the tuft cells and cancer remains to be further elucidated. Here, based on an introduction of biological functions and specific markers of the tuft cells, we have summarized the functional roles and potential therapeutic implications of tuft cells in cancers, including pancreatic cancer, lung cancer, gastric cancer, colon cancer, and liver cancer, which is in the hope of inspiring the future research in validating tuft cells as novel strategies for cancer therapies.
Introduction
Tuft cells are unusual epithelial cells that were firstly observed in an apical brush border of the rat trachea by Rhodin et al. 60 years ago (Rhodin and Dalhamn, 1956). And Jarvi et al. also reported the discovery of cells with similar morphological features in the mouse stomach in the same year (Jarvi and Keyrilainen, 1956). Later, the presence of tuft cells were detected in the human trachea (Rhodin, 1959). Over the past decades, transmission electron microscopy, scanning electron microscopy, and histochemical techniques have contributed to characterizing the morphological criterion for identifying these unusual epithelial cells, and “tuft” was finally used to describe the cellular morphology in detail (Isomaki, 1973; Luciano and Reale, 1979). In some circumstances, tuft cells have been referred to as “brush-like” “vesicular”, “peculiar”, “fibrillovesicular”, or “caveolated” cells, which all displayed the similar ultrastructures with a microvillus tuft (Reid et al., 2005; Hoover et al., 2017; Hendel et al., 2022). In this paper, we will refer to these cells collectively as tuft cells since they appear to be rather closely associated across tissues.
Generally, tuft cells are located in epithelium that are predominantly present at mucosal surfaces of vertebrates derived from endoderm. In the respiratory tract, tuft cells are distributed in the respiratory and olfactory epithelium of the nose, trachea, and proximal airway (O'Leary et al., 2022; Ualiyeva et al., 2020; Zaragosi et al., 2020). In the gastrointestinal tract, tuft cells are present in the stomach, small and large intestines, as well as the pancreatic bile tract (Hoover et al., 2017; O'Leary et al., 2019). Moreover, tuft cells are found in the columnar epithelium of the urethra, ear canal, conjunctival nasal cavity, the gallbladder bile ducts, and thymus (O'Leary et al., 2019; Bornstein et al., 2018; Miller et al., 2018; Minton, 2022).
Over the past decade, plenty of studies have shown the relationship between tuft cells and the biological processes closely related with cancer, which suggested the potential role of tuft cells in clinical applications of cancer treatment (Yamada et al., 2021; Yamada et al., 2022). Meanwhile, evidence from specific genes of tuft cells has also confirmed that the involvement of tuft cells in cancer. Doublecortin-like kinase1 (DCLK1), a characteristic gene of tuft cells, was associated with progression of gastric cancer, pancreatic cancer, colon cancer, and renal cancer (Weygant et al., 2016; Ge et al., 2018), and involved in the initiation, growth, metastasis, epithelial-mesenchymal transition (EMT), and stemness of the tumor (Westphalen et al., 2017; Sureban et al., 2011; Ito et al., 2016). Interleukin-25 (IL-25), one of the most important effector factors secreted by tuft cells, also plays an irreplaceable function in the immune processes (Hayakawa and Wang, 2018).
Physiological functions of tuft cells
The entire gastrointestinal tract (the esophagus, stomach, and intestines) serves as a physical barrier and the largest immune organ against the outside environment, and is responsible for nutrient absorption and immune surveillance (Takiishi et al., 2017). Tuft cells are particularly useful in regulation of immune responses, especially for recognizing helminth and protist infection (Hendel et al., 2022). What’s more, as remote chemosensory epithelial cells, tuft cells facilitate the transmission of chemical signals via producing effector molecules such as IL-25, cysteinyl leukotrienes, DCLK1, Tas2r, etc. (Luo et al., 2019; Cao et al., 2020; Ualiyeva et al., 2021).
As mentioned above, the presence of tuft cells was found in the lung, pancreas, stomach, intestine, and trachea. Significantly, the studies of tuft cells that focus on the intestine are relatively elaborated. Evidence from intestinal epithelial cell lines suggested that tuft cells originated from leucine-rich-repeat-containing G-protein-coupled receptor 5 (Lgr5+) stem cells were the source of tuft cells (van Es et al., 2019; McKernan and Egan, 2015). By using a knock-in mice model, the in vivo lineage tracing experiments demonstrated that Lgr5 served as a stem cell marker of tuft cells in the small intestine and colon (Barker et al., 2007). And hairy/enhancer of split 1 (HES1) or atonal homologue 1 (ATOH1) determines the cell fate into absorptive or secretory cell (Gerbe et al., 2011). In addition, POU2F3 is also crucial. The mechanism of M-cells formation deserves further exploration (Figure 1).
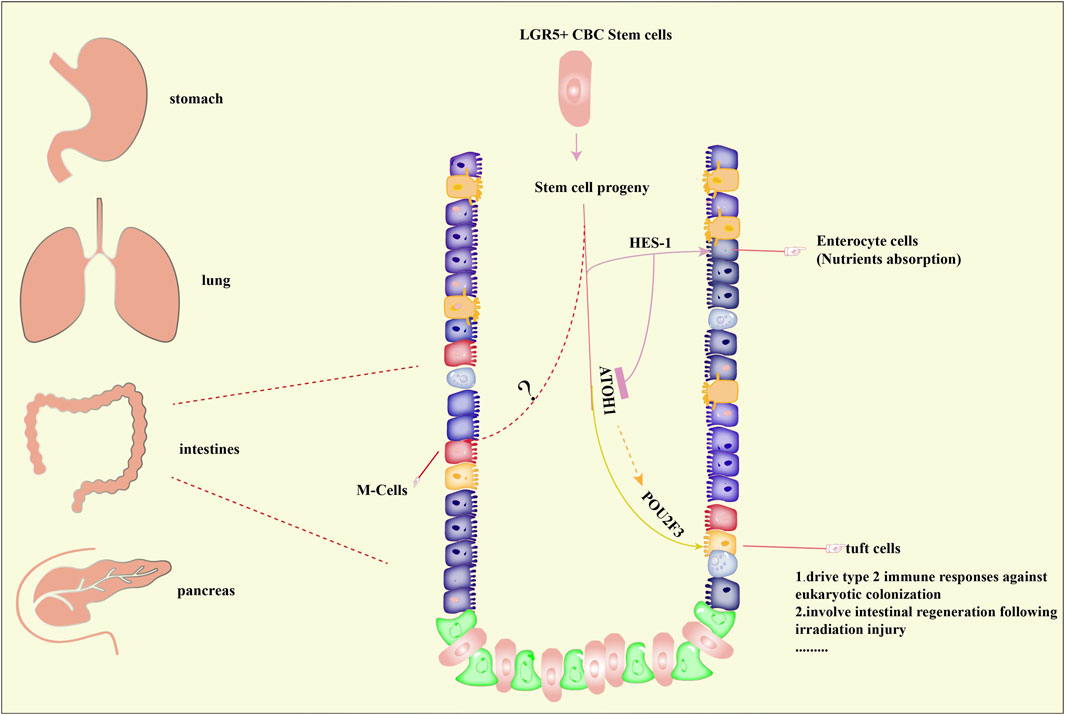
FIGURE 1. Distribution and formation of tuft cells. Tuft cells have been reported to be present in organs including the stomach, lung, intestine, pancreas. The formation of tuft cells in intestines has been predominantly studied and well elucidated. Briefly, stem cell progenitors originated from Lgr5+ crypt base columnar (CBC) stem cells can differentiate into tuft cells under the control of atonal homologue 1 (ATOH1) and POU Class 2 Homeobox 3 (POU2F3), both of which are master regulators of tuft cells. On the other hand, hairy/enhancer of split 1 (HES1) directs the formation of enterocyte cells, which is important for nutrients absorption. However, the underlying mechanisms of M-cells formation remains unclear. Importantly, tuft cells are associated with type 2 immune responses and regeneration of intestines.
Intestinal helminths constitute a diverse group of pathogens which lead to infection of intestines in over one billion people world wide (Bąska and Norbury, 2022). To eliminate gastro-intestinal helminths, an integrated response orchestrated by epithelial, neural, and immune cells are used to expel the parasites, increased goblet cell mucus production and muscle contractions result in helminths expulsion from the gut and release outside the body, which is known as “weep and sweep” (Hildersley et al., 2021). Upon infection with helminths or protists, epithelial tuft cells release IL-25 to activate ILC2, which initiates type 2 immune response (von Moltke et al., 2016). Firstly, succinate receptor 1 (SUCNR1; also known as G protein-coupled receptor), a specific marker of tuft cells in small intestine and trachea (Nadjsombati et al., 2018), binds with succinate secreted by pathogens and leads to depolymerization of guanine nucleotide-binding protein G(t) subunit α3 (GNAT3) when the organism was infected with pathogens such as helminths (Schneider et al., 2019; Hoffman et al., 2021). Subunit βγ from GNAT3 activates downstream phospholipase Cβ2 (PLCβ2) to regulate cleavage of phosphatidylinositol-4,5-bisphosphate PtdIns (4,5) P2 (PtdIns (4,5) P2) into inositol-1,4,5- trisphosphate (InsP3) and diacylglycerol (DAG) (Rössler et al., 1998; Schneider et al., 2019). Then, InsP3 binds with its receptor (InsP3Rs) on the endoplasmic reticulum and causes the release of intracellular calcium (Ca2+) (Simon et al., 2006). Increased Ca2+ in the cytoplasm activates transient receptor potential cation channel subfamily M member 5 (TRPM5) inside tuft cells and leads to the flow of Na+ into tuft cells, which depolarizes the tuft cells (Liu and Liman, 2003), and elevated Ca2+ leads to choline acetyltransferase (CHAT)-mediated release of acetylcholine (ACh) (Fu et al., 2018; Hollenhorst et al., 2020). Importantly, ACh generated by tuft cells is involved in a variety of pathophysiological processes including epithelial homeostasis (Middelhoff et al., 2020; Westphalen et al., 2014), airway remodeling (Pieper et al., 2007), respiratory reflexes (Krasteva et al., 2011), inflammation (Ualiyeva et al., 2020), carcinogenesis (Huang et al., 2018), and ATP production (Dando and Roper, 2012). In addition, activated TRPM5 also promotes the secretion of IL-25 in tuft cells, which is an essential cytokine involved in type 2 immune responses (Nadjsombati et al., 2018; Harris, 2016). Epithelial tuft cells are the only source of IL-25, when IL-25 is released outside of tuft cells, it binds to receptors of innate lymphoid cells (ILC2) to promote the secretion of immune factors including IL-5, IL-9, and IL-13, then IL-13 promotes the formation of tuft cells and subsequently increases IL-25, which is regarded as a response circuit for type 2 immune responses in tuft cells (von Moltke et al., 2016; Howitt et al., 2016) (Figure 2). The researchers further identified and characterized a tuft-cell deficient mice line. The analysis found that POU2F3, DCLK1, and SOX9 were all absent, demonstrating the absence of tuft cells. The authors revealed the critical function of tuft-cell in initiating mucosal type 2 responses after infecting worms with IL-25 secretion. In the absence of tuft cells, IL-25 and IL-13 expression remained low, and type 2 mucosal reactions and helminth expulsion were delayed. In addition, tuft cells are required upstream of IL-4/IL-13. These cytokines drive tuft cells proliferation and thus amplify the feedforward loop to coordinate rapid and effective anthelmintic immunity (Gerbe et al., 2016; Schneider et al., 2018).
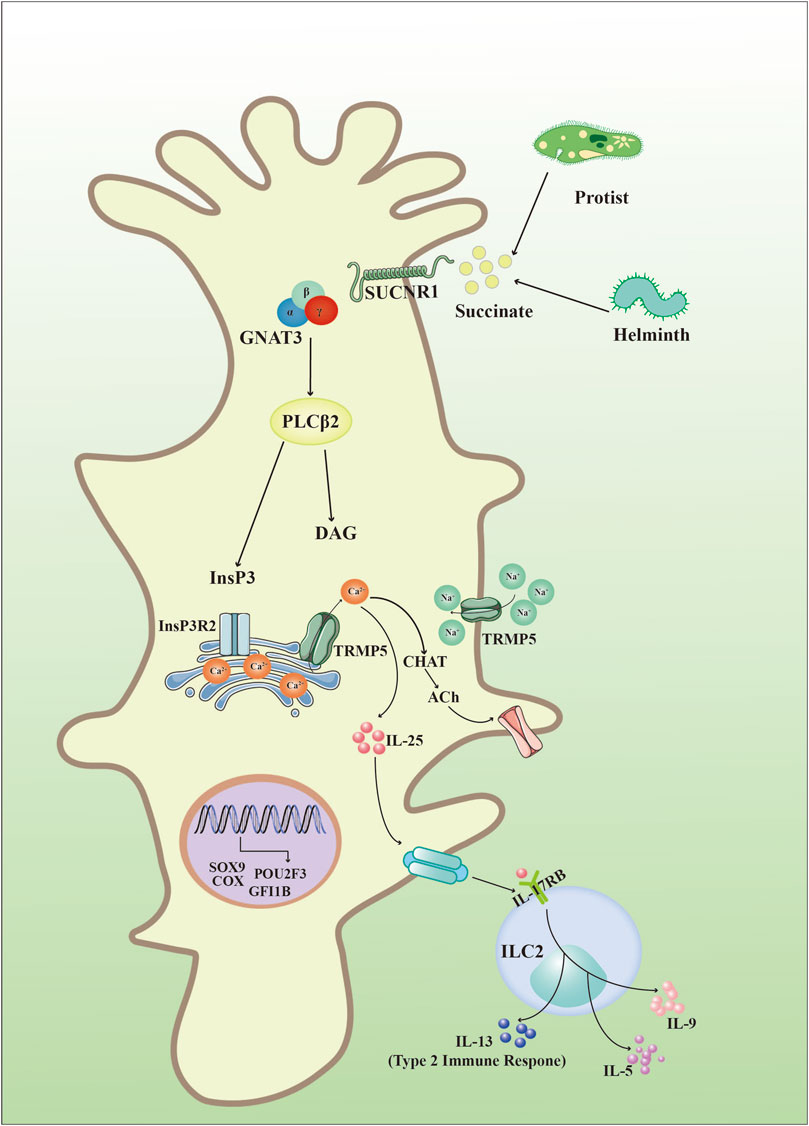
FIGURE 2. Intestinal tuft cell-specific signaling. Invasive organisms including helminths and protozoa produce succinate to bind with succinate receptor 1 (SUCNR1), which further interacts with guanine nucleotide-binding protein G(t) subunit α3 (GNAT3) and activates phospholipase Cβ2 (PLCβ2) to produce inositol-1,4,5- trisphosphate (InsP3) and diacylglycerol (DAG). InsP3 then binds to its receptors (InsP3Rs) in the endoplasmic reticulum and causes the release of Ca2+ into the cytoplasm. Subsequently, increased Ca2+ in the cytoplasm activates transient receptor potential cation channel subfamily M member 5 (TRPM5) and results in: (1) choline acetyltransferase (CHAT)-mediated release of acetylcholine (ACh); (2) secretion of IL-25 to bind with interleukin 17 receptor B (IL-17RB) in group 2 innate lymphoid cells (ILC2s) and synthesize cytokines such as IL-5, IL-9, and IL-13.
By producing cytokines such as IL-25 and ACh in various tissues, tuft cells show impacts on many physiological and pathological processes, which extends the understanding of the applications of tuft cells in diseases.
Markers of tuft cells
It has been reported that tuft cells constitute the fifth cell type in the intestinal epithelium, thus, it is important to define tuft cells with specific characteristics. Although morphological features help to distinguish tuft cells from other chemosensory epithelial cells, the unique molecular characteristics of tuft cells are essential for the definition of tuft cells and the validation of their functions (Table 1).
Pou class 2 homeobox 3 (POU2F3), also known as OCT-11 and Skn-1a (Huang et al., 2018), is constitutively expressed in all tuft cells and a specific master regulator for differentiation of tuft cells (Ohmoto et al., 2013), and POU2F3 deficiency results in the entire absence of tuft cells in mice (Gerbe et al., 2016). Although the underlying transcriptional mechanisms of POU2F3 in regulating formation of tuft cells remains poorly understood, it has been confirmed the binding of POU2F3 to POU2AF2 (also known as C11orf53 or OCA-T1) and POU2AF3 (also known as COLCA2 or OCA-T2) is important in the tuft cell lineage (Szczepanski et al., 2022; Wu et al., 2022). By using a Balb/cJ (Balb) mice strain with fewer intestinal tuft cells than C57BL/6J (B6) mice, it was identified that POU2AF2 isoform usage was a novel regulator of tuft cells differentiation and tuned innate type 2 immunity in the small intestine (Nadjsombati et al., 2022). In addition, POU2AF2 and POU2AF3 were identified as novel vulnerabilities in tuft cell-like SCLC and POU2AF3 functioned as a co-activator for POU2F3 to drive the transcriptional program critical for tuft cell-like SCLC (Zhou et al., 2022). It was found that 11q23.1 trans-eQTL (expression Quantitative Trait Loci) targets comprised a POU2AF2-related network, which might be specific for tuft cells and associated with abundance of tuft cells (Harris et al., 2022). Furthermore, by using a POU2F3 deficient mice strain Yamashita et al. demonstrated that POU2F3 as a master regulator for the generation of TRPM5-expressing chemosensory cells, like intestinal tuft cells, and was required for generation of tuft cells (Yamashita et al., 2017). ATOH1, also known as Math1, is another transcription factor that dominates the differentiation of tuft cells (Gerbe et al., 2011). It has been found that ATOH1 determined the fate of early differentiation of epithelial stem cells, which distinguishes tuft cells from other cell types of the intestinal epithelium including cup cells, discoid cells, and intestinal endocrine cells (Gerbe et al., 2011; Gracz et al., 2018). Notably, tuft cells were not present in ATOH1 knockout mice and differentiation of all secretory cells was impaired without ATOH1 (Shroyer et al., 2007). Thus, POU2F3 and ATOH1 are specific transcription factors in defining tuft cells, but more studies are needed to further illustrate the transcriptional program in regulating formation of tuft cells. SOX9, a member of the SOX gene family that regulates the development, cell-fate determination, and differentiation of multiple organs (Pritchett et al., 2011), has been confirmed as a marker of tuft cells (McKinley et al., 2017) and played a key role in tumor development and progression, such as glioma and colorectal cancer (Lü et al., 2008; Swartling et al., 2009). Studies have found that the expression of SOX9 was positively associated with transcription factors POU2F3 and TRPM5 (Yuan et al., 2021).
Besides those markers mentioned above, several other proteins involved in the regulatory function of the tuft cells have also been used to identify tuft cells. For example, DCLK1, a serine/threonine protein kinase associated with microtubules, is the most commonly used and prevalent marker in tuft cells (Westphalen et al., 2017; Yi et al., 2019). Arachidonate 5-lipoxygenase (ALOX5), an important enzyme for the synthesis of leukotrienes from arachidonic acid, is also observed extensively expressed in tuft cells (McGinty et al., 2020). Both cyclooxygenases 1 (COX1) and cyclooxygenases 2 (COX2) are key enzymes needed for the production of prostaglandin H2 (PGH2), and studies have confirmed that tuft cells were the only epithelial cells in the uninflamed intestine that expressed COX1 and COX2 (Sauvé, 2019; Zhang, 2021). Thus, the expression of COX1 and COX2 indicates the presence of tuft cells in the intestinal epithelium. Advillin, a member of the villin/gelsolin superfamily, is a sensory neuron-specific actin-binding protein (Marks et al., 1998) and has been identified as a specific marker of tuft cells in the epithelium of the gastrointestinal and biliary tract (Ruppert et al., 2020). Studies have shown that advillin binds to F-actin and regulates the migration of cancer cells (Xie et al., 2020). In addition, advillin is responsible for the depolymerization of gelsolin and villin (Khurana, 2006). Although the function of advillin has not been fully studied, it was reported to be involved in the inflammatory response to helminths and bacterial microbiota cells and participated in the transmission of sensory signals to the enteric nervous system as well as type 2 immune responses (Esmaeilniakooshkghazi et al., 2020). In addition, proteins including SUCNR1, TRPM5, CHAT, and IL-25 that are involved in intestinal tuft cell-specific signaling are also regarded as critical markers of tuft cells.
Accumulative advances have gradually uncovered the significance of tuft cells in human diseases. Although increasing cell markers are being discovered and their association with diseases has been identified, much more efforts should be devoted to elucidating the underlying mechanisms and the potential of tuft cells in diseases including cancer.
The potential roles and underlying mechanisms of tuft cells in cancer
Over the past decade, increasing evidence from genes specifically expressed in tuft cells has proven the significance of tuft cells in the development and progression of cancers including pancreatic cancer, lung cancer, and colon cancer. Here, we will summarize the functions and underlying mechanisms of tuft cells in those tumors.
Pancreatic cancer
It has been reported that two-thirds of neuroendocrine carcinoma (NEC) were found present in the digestive system, and the pancreas cancer is among the most common ones (Yachida et al., 2022). Pancreatic cancer is one of the prevalent malignancies of the digestive tract and is regarded as the king of carcinoma (Luo et al., 2020). Tuft cells have been found in both human and mouse pancreas, and play a significant role in pancreatic cancer (Schütz et al., 2019; DelGiorno et al., 2020a).
By using mouse models, a massive increase of tuft cells was found during chronic pancreatitis and acinar-to-ductal metaplasia (ADM) in response to pancreatic injury, and tuft cells were transformed from alveolar cells following complex changes, which was similar to the process that a reservist (alveolar cells) leaped from a civilian to a warrior (tuft cells) to fight against inflammatory factors (DelGiorno et al., 2020b). In a KC mice model of pancreatic ductal adenocarcinoma (PDAC), increased in tuft cells and elevated prostaglandin D2 (PGD2) levels were found in KC mice in comparison with that in wild-type mice. In addition, deficiency in the differentiation of tuft cells by silencing POU2F3 resulted in decreased tuft cells and inhibition of the progression of PDAC in KC mice (DelGiorno et al., 2020a). However, in LSL-KRASG12D/+ and Ptf1aCre/+ mice models of pancreatic tumorigenesis, researchers found that tuft cells were most abundant in ADM and became less abundant during disease progression, with 11.2% of mPanIN-1 epithelium, 8.7% of mPanIN-2, and 2.9% of mPanIN-3, while tuft cells were absent in invasive disease. In addition, they also found that abnormality of pancreatic tuft cells was common in KRASG12D-induced pancreatic disease and is accompanied by an epithelial expression with SOX17, which promoted KRASG12D-induced tumorigenesis in mice (Delgiorno et al., 2014). It was also found that overexpression of IL-17RB was associated with metastasis and poor clinical outcome of pancreatic cancer, and impairing IL-17B-IL-17RB signaling blocked metastasis of pancreatic cancer (Wu et al., 2015).
Lung cancer
Tuft cells are scattered as isolated cells in the epithelium of the airways and are generally absent in the alveoli except in cases of post-viral remodeling (Rane et al., 2019). In 2018, a small cell lung cancer (SCLC) derived from tuft cells was identified, and a tuft cell-like gene expression profile was found in this type of SCLC, which was characterized by exclusively expression of POU2F3 (Huang et al., 2018). Tuft cell-like tumors with co-expression of POU2F3, GFI1B, and KIT were identified among pulmonary squamous cell carcinomas, adenocarcinomas, and large cell neuroendocrine carcinoma, and new molecular subsets were delineated by their tuft cell-like signatures among the lung cancer histotypes (Yamada et al., 2021).
Pathologically, POU2F3-positive tumors were defined as one type of true small cell lung cancer with specific morphological characteristics and extremely high proliferation rates (Baine et al., 2020). Based on the critical role of POU2F3 in lung cancer, it was uncovered that binding between POU2F3 and its coactivators (OCA-T1, OCA-T2) was essential in tuft-cell-like small-cell lung cancer, and POU2F3–OCA-T complex was the master regulator for identity of tuft cells and a potential target in treating tuft-cell-like small-cell lung cancer (Wu et al., 2022). Thus, the therapeutic strategy that aims at blocking POU2F3 function or ablating the tuft cell lineage is expected to have a wide therapeutic scope for patients with tuft cell-like lung cancers.
Considering the extremely poor prognosis of tuft-cell-like small-cell lung cancer, it is urgent to improve the early diagnosis and treatment options for those patients. Specific markers of tuft cells such as POU2F3 may be an Achilles’ heel for tuft cell-like lung cancer patients, but much more is needed to further clarify the relationship of tuft cells with lung cancer.
Gastric cancer
Emerging evidence has been suggesting the oncogenic function of tuft cells in gastric cancer. High expression of POU2F3 was found in three neuroendocrine carcinoma of the gastrointestinal systemincluding colon, esophageal and gastric cancers (Yachida et al., 2022). Inhibition of the tuft cell-ILC2 axis by genetic ablation of tuft cells, ILC2s or antibody-mediated neutralization of IL-13 or IL-25 attenuated gastric tumor development in mice (O’Keefe et al., 2022). DCLK1-expressing tuft cells are one of the two main sources of ACh within the gastric mucosa (Hayakawa et al., 2017). Furthermore, dual immunofluorescence analysis of cholinergic marker CHAT and tuft cells specific markers such as advillin identified that tuft cells were the unique source for epithelial biosynthesis of ACh in the human alimentary tract (Schütz et al., 2019). Notably, it was found that ACh secreted by DCLK1+ tuft cells and nerves within the gastric mucosa stimulated nerve growth factor (NGF) expression in gastric epithelium to promote gastric carcinogenesis, and ablation of DCLK1+ cells inhibited epithelial proliferation and tumorigenesis (Hayakawa et al., 2017). Thus, this feed-forward ACh-NGF axis derived by tuft cells was recommended as a compelling target for the prevention and treatment of gastric carcinoma. In conclusion, tuft cells play an important role in gastrointestinal tumors.
Colon cancer
In the small intestine, tuft cells are rarely present under homeostasis, but infection with various related pathogens will promote the differentiation of Lgr5 stem cells into tuft cells, and ultimately increase the number of tuft cells and goblet cell fraction to promote pathogen clearance (von Moltke et al., 2016). In the case of tissue damage, intestinal progenitor cells would proliferate quickly and differentiate into tuft cells to promote type 2 immunity to eliminate inflammation and prevent the progression of ulcerative colitis (Sunaga et al., 2022). In addition, berberine, the main active component of an ancient Chinese herb Coptis Chinensis French, was found to regulate the repairment of damaged intestinal tract by promoting the IL-25-ILC2-IL-13 immune pathway in tuft cells (Xiong et al., 2021). Regarding that ulcerative colitis is usually considered to be a precancerous lesion of colon cancer (Kvorjak et al., 2020), it is believed that tuft cells may be involved in the initiation of colon cancer.
By using a lineage-tracing assay, it was found that DCLK1 marked tumor stem cells that continuously produce tumor progeny rather than normal stem cells in the intestine, and specific ablation of DCLK1-positive tumor stem cells lead to significant regression of polyps without significant damage to the normal intestine (Nakanishi et al., 2013). It has been reported that DCLK1 played an important role in promoting intestinal tumorigenesis, and depletion of DCLK1 reduced the stemness and inhibited progression of tumors, thus suggesting its role in regulating pro-survival signals and tumor cell pluripotency (Chandrakesan et al., 2017). Thus, tuft cells may serve as a novel choice in the treatment of colon cancer by targeting DCLK1-positive cancer stem cells. Similarly, IL-17RB expression was also found in human colorectal cancer stem cells instead of normal stem cells by genealogical tracking, and long-term ablation of IL-17RB resulted in the inhibition of cancer stem cells growth in vivo, which all validated the implication of IL-17RB+ cancer stem cells as clinical targets for cancer therapy (Goto et al., 2019). Moreover, overexpression of SOX9 has been shown to be involved in human colorectal carcinogenesis by in vitro and in vivo studies (Reineking et al., 2022).
Liver cancer
The presence of tuft cells in the liver has not been confirmed yet. However, it was found hyperplastic epithelial tuft cells in the colon induced by gut microbiota dysbiosis might partly be the source of IL-25 and increased IL-25 promoted the progression of hepatocellular carcinoma (HCC) via alternative activation of macrophages in the tumor microenvironment (Li et al., 2019). Thus, it is hypothesized that colonic epithelial tuft cells could promote HCC progression by secretion of effector factors.
In summary, we have summarized the role of tuft cells in tumors and indicated the potential possibilities of tuft cells as a novel target for tumor treatment, and strategies targeting specific molecules including DCLK1 and POU2F3 can become good choices for cancers with tuft cell signatures. Therapeutic approaches targeting specific markers of tuft cells by kinase inhibitors, monoclonal antibodies, and chimeric antigen receptor T cells (CAR-T) are preferred (Cao et al., 2020). However, much more remains to be explored in deepening our understanding of tuft cells.
Heterogeneity of tuft cells
As rare chemosensory epithelial cells and critical orchestrators in mediating type-2 immunity (Gerbe et al., 2012), tuft cells have been revealed to be heterogeneous in their lineage and functions. Recent advances from single cell sequencing confirmed diversity of individual tuft cells (Haber et al., 2017). By using single-cell survey of small intestinal epithelial cells, tuft cells were clustered into four subsets including two representing progenitors (early and late) and two representing mature cells (tuft-1 and tuft-2) (Haber et al., 2017). In response to helminth infections, protozoan colonization, and bacterial ecological dysregulation in the small intestine, tuft cells initiate type 2 immune responses characterized by tissue remodeling. In the airway, tuft cells sense external noxious stimuli through their own functions and make a series of corresponding responses (Billipp et al., 2021). When infected with protist or when mice are treated with succinate to mimic protist infection, no tuft cell hyperplasia is observed in the gallbladder, colon, and cecum (von Moltke et al., 2016; Nadjsombati et al., 2018). And it has been reported that tuft cells were increased in the small intestine and DCLK1+ tuft cells were largely absent in the colon in the ATOH1 ablation mice model (Herring et al., 2018), which suggested that ATOH1 might be required for colonic tuft cells differentiation. Also, the proliferation of intestinal tuft cells is influenced by the luminal environment, and when invaded by microbiota, colonic tuft cells of germ-free mice are temporarily increased (McKinley et al., 2017). A20 (Tnfaip3) deficient mice (Tnfaip3loxP/loxP; IL5RFP-Cre) only have tuft cell hyperplasia in the small intestine but not the colon, cecum, and stomach (Schneider et al., 2018). In addition, tuft cells in the thymus promoted the canonical taste transduction pathway and secreted IL-25, which was similar with that in the intestine. On the other hand, thymic tuft cells were unique in their ability to present antigens (Miller et al., 2018). Thymic tuft cells also express a variety of typical taste receptors that have been described in airway tuft cells but seem to be largely absent in intestinal tuft cells (Krasteva et al., 2011; Nadjsombati et al., 2018), which suggested that tuft cells from different tissues have distinct cellular properties, which potentially can be traced back to distinct gene expression programs (Haber et al., 2017; Bornstein et al., 2018; Plasschaert et al., 2018; Shanahan et al., 2021). Even within the same tissue, there are different types of tuft cells. For example, in the intestine, tuft cells have been found to have two subtypes, which are termed tuft-1 and tuft-2. Tuft-2 cells are associated with immunity, while tuft-1 cells are associated with neuronal development (Haber et al., 2017). Tuft cells are also heterogeneous in injury-inducing lung models, Barr et al. have found transcriptional heterogeneity in this lung tuft cell, and the locus from 'basal- > tuft' to 'tuft-2' and 'tuft-1' clusters was identified. Although the tuft cells in the injured lung were consistent with intestinal tuft cells in their POU2F3 dependence, lung tuft cells were not dependent on type 2 immune signaling, unlike the intestinal (Barr et al., 2022). Different from the intestinal and the injury-inducing lung, in the airway, tuft cells are divided into three clusters, immature tuft, tuft-1, and tuft-2 cells. Tuft-1 expresses genes related to taste transduction, and tuft-2 is associated with immunity (Montoro et al., 2018).
Discussion
Increasing studies have been focused on tuft cells, and the impacts of tuft cells are gradually being reported. As a relatively rare epithelial cell population, tuft cells exhibit a specific expression profile, and are involved in the processes of cell repair, inflammation, and parasite-induced type 2 immunity.
In recent years, the role of tufts cells in tumors has been increasingly reported and their role in tumors is gaining attention. In this review, based on the introduction of the physiological functions and specific markers of tuft cells, we have summarized the roles and underlying mechanisms of tuft cells in cancer, in hope of inspiring more research in this field and providing clues for scientists with interests in tuft cells. Here, we demonstrated that tuft cells were increased in pancreatic ductal adenocarcinoma, small cell lung cancer, and colon cancer, and ablation of tuft cells by silencing specific markers inhibited the progression of pancreatic ductal adenocarcinoma and colon cancer, which all indicated the tumor-promoter role of tuft cells in cancer. These unique gene markers of tuft cells also help to explore the association of tuft cells with diseases including cancer. For example, DCLK1 was found up-regulated in renal clear cell cancer and colorectal cancer (Weygant et al., 2015; Gao et al., 2016; Mohammadi et al., 2018). As a potential effector of KRAS, DCLK1 contributes functionally to the pathogenesis of pancreatic cancer, and marks quiescent pancreatic progenitor cells of pancreatic origin (Westphalen et al., 2016). In addition, DCLK1-IN-1, a highly selective inhibitor with good uptake and bioavailability properties that targets DCLK1, has been proven to inhibit tumorigenesis in DCLK1+ organs (Ferguson et al., 2020). However, the role of DCLK1 in tuft cells remains to be further elucidated. Thus, we hypothesized that the functions of tuft cells might vary in cancers depending on the tumor types and stages. Considering the critical role of tuft cells in type 2 immunity, it is also inferred that tuft cells could be potential targets in uncovering novel immunotherapy strategies. Tuft cells have shown great potential in the development of antitumor drugs, but more evidence is still needed to illustrate the functions and underlying mechanisms, which will hold the promise of clinical application in tumors by targeting tuft cells.
However, the limited number of tuft cells as chemoreceptors in tissues is one of the obstacles to expanding their implications in cancer treatment, and it remains to be clarified whether cooperation with other epithelial cells is required to fulfill the functions of tuft cells. And it is of great importance to reveal more specific genes in tuft cells to facilitate studies in this field. With a comprehensive understanding of tuft cells, we hope drugs targeting tuft cells will benefit patients with tuft cell-like cancers.
Author contributions
XS and TD guided and designed the research; LL and MM contributed materials information gathering and data analysis. LL and MM wrote the manuscript with contributions from the other authors.
Funding
This work was financially funded by the grants the Science and Technology Development Fund, Macau SAR (No. 0098/2021/A2, to XS), Zhejiang Provincial Natural Science Foundation of China (No. LQ20H160013, TD).
Acknowledgments
The authors are grateful for comments from teachers.
Conflict of interest
The authors declare that the research was conducted in the absence of any commercial or financial relationships that could be construed as a potential conflict of interest.
Publisher’s note
All claims expressed in this article are solely those of the authors and do not necessarily represent those of their affiliated organizations, or those of the publisher, the editors and the reviewers. Any product that may be evaluated in this article, or claim that may be made by its manufacturer, is not guaranteed or endorsed by the publisher.
References
Adelizzi, R. A. (1999). COX-1 and COX-2 in health and disease. J. Am. Osteopath Assoc. 99, S7–s12. doi:10.7556/jaoa.1999.99.11.S7
Baine, M. K., Hsieh, M. S., Lai, W. V., Egger, J. V., Jungbluth, A. A., Daneshbod, Y., et al. (2020). SCLC subtypes defined by ASCL1, NEUROD1, POU2F3, and YAP1: A comprehensive immunohistochemical and histopathologic characterization. J. Thorac. Oncol. 15 (12), 1823–1835. doi:10.1016/j.jtho.2020.09.009
Banerjee, A., Herring, C. A., Chen, B., Kim, H., Simmons, A. J., Southard-Smith, A. N., et al. (2020). Succinate produced by intestinal microbes promotes specification of tuft cells to suppress ileal inflammation. Gastroenterology 159 (6), 2101–2115. doi:10.1053/j.gastro.2020.08.029
Barker, N., van Es, J. H., Kuipers, J., Kujala, P., van den Born, M., Cozijnsen, M., et al. (2007). Identification of stem cells in small intestine and colon by marker gene Lgr5. Nature 449 (7165), 1003–1007. doi:10.1038/nature06196
Barr, J., Gentile, M. E., Lee, S., Kotas, M. E., Fernanda de Mello Costa, M., Holcomb, N. P., et al. (2022). Injury-induced pulmonary tuft cells are heterogenous, arise independent of key Type 2 cytokines, and are dispensable for dysplastic repair. Elife 11, e78074. doi:10.7554/eLife.78074
Bąska, P., and Norbury, L. J. (2022). The role of the intestinal epithelium in the "weep and sweep" response during gastro-intestinal helminth infections. Animals. 12, 175. doi:10.3390/ani12020175
Billipp, T. E., Nadjsombati, M. S., and von Moltke, J. (2021). Tuning tuft cells: New ligands and effector functions reveal tissue-specific function. Curr. Opin. Immunol. 68, 98–106. doi:10.1016/j.coi.2020.09.006
Bjerknes, M., Khandanpour, C., Moroy, T., Fujiyama, T., Hoshino, M., Klisch, T. J., et al. (2012). Origin of the brush cell lineage in the mouse intestinal epithelium. Dev. Biol. 362 (2), 194–218. doi:10.1016/j.ydbio.2011.12.009
Bornstein, C., Nevo, S., Giladi, A., Kadouri, N., Pouzolles, M., Gerbe, F., et al. (2018). Single-cell mapping of the thymic stroma identifies IL-25-producing tuft epithelial cells. Nature 559 (7715), 622–626. doi:10.1038/s41586-018-0346-1
Cao, Z., Weygant, N., Chandrakesan, P., Houchen, C. W., Peng, J., and Qu, D. (2020). Tuft and cancer stem cell marker DCLK1: A new target to enhance anti-tumor immunity in the tumor microenvironment. Cancers (Basel) 12 (12), E3801. doi:10.3390/cancers12123801
Chandrakesan, P., Yao, J., Qu, D., May, R., Weygant, N., Ge, Y., et al. (2017). Dclk1, a tumor stem cell marker, regulates pro-survival signaling and self-renewal of intestinal tumor cells. Mol. Cancer 16 (1), 30. doi:10.1186/s12943-017-0594-y
Dando, R., and Roper, S. D. (2012). Acetylcholine is released from taste cells, enhancing taste signalling. J. Physiol. 590, 3009–3017. doi:10.1113/jphysiol.2012.232009
DelGiorno, K. E., Chung, C. Y., Vavinskaya, V., Maurer, H. C., Novak, S. W., Lytle, N. K., et al. (2020). Tuft cells inhibit pancreatic tumorigenesis in mice by producing prostaglandin D(2). Gastroenterology 159 (5), 1866–1881. doi:10.1053/j.gastro.2020.07.037
Delgiorno, K. E., Hall, J. C., Takeuchi, K. K., Pan, F. C., Halbrook, C. J., Washington, M. K., et al. (2014). Identification and manipulation of biliary metaplasia in pancreatic tumors. Gastroenterology 146 (1), 233–244. doi:10.1053/j.gastro.2013.08.053
DelGiorno, K. E., Naeem, R. F., Fang, L., Chung, C. Y., Ramos, C., Luhtala, N., et al. (2020). Tuft cell formation reflects epithelial plasticity in pancreatic injury: Implications for modeling human pancreatitis. Front. Physiol. 11, 88. doi:10.3389/fphys.2020.00088
Esmaeilniakooshkghazi, A., George, S. P., Biswas, R., and Khurana, S. (2020). Mouse intestinal tuft cells express advillin but not villin. Sci. Rep. 10 (1), 8877. doi:10.1038/s41598-020-65469-0
Ferguson, F. M., Nabet, B., Raghavan, S., Liu, Y., Leggett, A. L., Kuljanin, M., et al. (2020). Discovery of a selective inhibitor of doublecortin like kinase 1. Nat. Chem. Biol. 16 (6), 635–643. doi:10.1038/s41589-020-0506-0
Fu, Z., Ogura, T., Luo, W., and Lin, W. (2018). ATP and odor mixture activate TRPM5-expressing microvillous cells and potentially induce acetylcholine release to enhance supporting cell endocytosis in mouse main olfactory epithelium. Front. Cell. Neurosci. 12, 71. doi:10.3389/fncel.2018.00071
Gao, T., Wang, M., Xu, L., Wen, T., Liu, J., and An, G. (2016). DCLK1 is up-regulated and associated with metastasis and prognosis in colorectal cancer. J. Cancer Res. Clin. Oncol. 142 (10), 2131–2140. doi:10.1007/s00432-016-2218-0
Ge, Y., Weygant, N., Qu, D., May, R., Berry, W. L., Yao, J., et al. (2018). Alternative splice variants of DCLK1 mark cancer stem cells, promote self-renewal and drug-resistance, and can be targeted to inhibit tumorigenesis in kidney cancer. Int. J. Cancer 143 (5), 1162–1175. doi:10.1002/ijc.31400
Gerbe, F., Brulin, B., Makrini, L., Legraverend, C., and Jay, P. (2009). DCAMKL-1 expression identifies Tuft cells rather than stem cells in the adult mouse intestinal epithelium. Gastroenterology 137, 2179–2180. doi:10.1053/j.gastro.2009.06.072
Gerbe, F., Legraverend, C., and Jay, P. (2012). The intestinal epithelium tuft cells: Specification and function. Cell. Mol. Life Sci. 69, 2907–2917. doi:10.1007/s00018-012-0984-7
Gerbe, F., Sidot, E., Smyth, D. J., Ohmoto, M., Matsumoto, I., Dardalhon, V., et al. (2016). Intestinal epithelial tuft cells initiate type 2 mucosal immunity to helminth parasites. Nature 529 (7585), 226–230. doi:10.1038/nature16527
Gerbe, F., van Es, J. H., Makrini, L., Brulin, B., Mellitzer, G., Robine, S., et al. (2011). Distinct ATOH1 and Neurog3 requirements define tuft cells as a new secretory cell type in the intestinal epithelium. J. Cell Biol. 192 (5), 767–780. doi:10.1083/jcb.201010127
Goto, N., Fukuda, A., Yamaga, Y., Yoshikawa, T., Maruno, T., Maekawa, H., et al. (2019). Lineage tracing and targeting of IL17RB(+) tuft cell-like human colorectal cancer stem cells. Proc. Natl. Acad. Sci. U. S. A. 116 (26), 12996–13005. doi:10.1073/pnas.1900251116
Gracz, A. D., Samsa, L. A., Fordham, M. J., Trotier, D. C., Zwarycz, B., Lo, Y. H., et al. (2018). Sox4 promotes atoh1-independent intestinal secretory differentiation toward tuft and enteroendocrine fates. Gastroenterology 155 (5), 1508–1523. doi:10.1053/j.gastro.2018.07.023
Grimm, D., Bauer, J., Wise, P., Kruger, M., Simonsen, U., Wehland, M., et al. (2020). The role of SOX family members in solid tumours and metastasis. Semin. Cancer Biol. 67, 122–153. doi:10.1016/j.semcancer.2019.03.004
Gzil, A., Szylberg, L., Jaworski, D., Dominiak, J., Zarebska, I., and Grzanka, D. (2019). The essential role of DCLK1 in pathogenesis, diagnostic procedures and prognostic stratification of colorectal cancer. Anticancer Res. 39 (6), 2689–2697. doi:10.21873/anticanres.13394
Haber, A. L., Biton, M., Rogel, N., Herbst, R. H., Shekhar, K., Smillie, C., et al. (2017). A single-cell survey of the small intestinal epithelium. Nature 551 (7680), 333–339. doi:10.1038/nature24489
Harris, B. T., Rajasekaran, V., Blackmur, J. P., O'Callaghan, A., Donnelly, K., Timofeeva, M., et al. (2022). Transcriptional dynamics of colorectal cancer risk associated variation at 11q23.1 correlate with tuft cell abundance and marker expression in silico. Sci. Rep. 12 (1), 13609. doi:10.1038/s41598-022-17887-5
Harris, N. (2016). IMMUNOLOGY. The enigmatic tuft cell in immunity. Science 351 (6279), 1264–1265. doi:10.1126/science.aaf5215
Hayakawa, Y., Sakitani, K., Konishi, M., Asfaha, S., Niikura, R., Tomita, H., et al. (2017). Nerve growth factor promotes gastric tumorigenesis through aberrant cholinergic signaling. Cancer Cell 31 (1), 21–34. doi:10.1016/j.ccell.2016.11.005
Hayakawa, Y., and Wang, T. C. (2018). The tuft cell-ILC2 circuit integrates intestinal defense and homeostasis. Cell 174 (2), 251–253. doi:10.1016/j.cell.2018.06.037
Hendel, S. K., Kellermann, L., Hausmann, A., Bindslev, N., Jensen, K. B., and Nielsen, O. H. (2022). Tuft cells and their role in intestinal diseases. Front. Immunol. 13, 822867. doi:10.3389/fimmu.2022.822867
Herring, C. A., Banerjee, A., McKinley, E. T., Simmons, A. J., Ping, J., Roland, J. T., et al. (2018). Unsupervised trajectory analysis of single-cell RNA-seq and imaging data reveals alternative tuft cell origins in the gut. Cell Syst. 6 (1), 37–51. doi:10.1016/j.cels.2017.10.012
Hildersley, K. A., McNeilly, T. N., Gillan, V., Otto, T. D., Löser, S., Gerbe, F., et al. (2021). Tuft cells increase following ovine intestinal parasite infections and define evolutionarily conserved and divergent responses. Front. Immunol. 12, 781108. doi:10.3389/fimmu.2021.781108
Hoffman, M. T., Kemp, S. B., Salas-Escabillas, D. J., Zhang, Y., Steele, N. G., The, S., et al. (2021). The gustatory sensory G-protein GNAT3 suppresses pancreatic cancer progression in mice. Cell. Mol. Gastroenterol. Hepatol. 11 (2), 349–369. doi:10.1016/j.jcmgh.2020.08.011
Hollenhorst, M. I., Jurastow, I., Nandigama, R., Appenzeller, S., Li, L., Vogel, J., et al. (2020). Tracheal brush cells release acetylcholine in response to bitter tastants for paracrine and autocrine signaling. Faseb J. 34, 316–332. doi:10.1096/fj.201901314RR
Hoover, B., Baena, V., Kaelberer, M. M., Getaneh, F., Chinchilla, S., and Bohorquez, D. V. (2017). The intestinal tuft cell nanostructure in 3D. Sci. Rep. 7 (1), 1652. doi:10.1038/s41598-017-01520-x
Howitt, M. R., Lavoie, S., Michaud, M., Blum, A. M., Tran, S. V., Weinstock, J. V., et al. (2016). Tuft cells, taste-chemosensory cells, orchestrate parasite type 2 immunity in the gut. Science 351 (6279), 1329–1333. doi:10.1126/science.aaf1648
Huang, X., Oshima, T., Akiba, Y., Yoshimoto, T., Chen, J., Taki, M., et al. (2022). Duodenal cholinergic tuft cell number is increased in functional dyspepsia. Neurogastroenterol. Motil. 34, e14378. doi:10.1111/nmo.14378
Huang, Y. H., Klingbeil, O., He, X. Y., Wu, X. S., Arun, G., Lu, B., et al. (2018). POU2F3 is a master regulator of a tuft cell-like variant of small cell lung cancer. Genes Dev. 32 (13-14), 915–928. doi:10.1101/gad.314815.118
Isomaki, A. M. (1973). A new cell type (tuft cell) in the gastrointestinal mucosa of the rat. A transmission and scanning electron microscopic study. Acta Pathol. Microbiol. Scand. A, 1–35.
Ito, H., Tanaka, S., Akiyama, Y., Shimada, S., Adikrisna, R., Matsumura, S., et al. (2016). Dominant expression of DCLK1 in human pancreatic cancer stem cells accelerates tumor invasion and metastasis. PLoS One 11, e0146564. doi:10.1371/journal.pone.0146564
Jarvi, O., and Keyrilainen, O. (1956). On the cellular structures of the epithelial invasions in the glandular stomach of mice caused by intramural application of 20-methylcholantren. Acta Pathol. Microbiol. Scand. 39, 72–73. doi:10.1111/j.1600-0463.1956.tb06739.x
Kaske, S., Krasteva, G., Konig, P., Kummer, W., Hofmann, T., Gudermann, T., et al. (2007). TRPM5, a taste-signaling transient receptor potential ion-channel, is a ubiquitous signaling component in chemosensory cells. BMC Neurosci. 8, 49. doi:10.1186/1471-2202-8-49
Khurana, S. (2006). Structure and function of villin. Adv. Mol. Cell Biol. 27, 89–117. doi:10.1016/S1569-2558(06)37005-1
Krasteva, G., Canning, B. J., Hartmann, P., Veres, T. Z., Papadakis, T., Muhlfeld, C., et al. (2011). Cholinergic chemosensory cells in the trachea regulate breathing. Proc. Natl. Acad. Sci. U. S. A. 108 (23), 9478–9483. doi:10.1073/pnas.1019418108
Kvorjak, M., Ahmed, Y., Miller, M. L., Sriram, R., Coronnello, C., Hashash, J. G., et al. (2020). Cross-talk between colon cells and macrophages increases ST6GALNAC1 and MUC1-sTn expression in ulcerative colitis and colitis-associated colon cancer. Cancer Immunol. Res. 8 (2), 167–178. doi:10.1158/2326-6066.CIR-19-0514
Lei, W., Ren, W., Ohmoto, M., Urban, J. F., Matsumoto, I., Margolskee, R. F., et al. (2018). Activation of intestinal tuft cell-expressed Sucnr1 triggers type 2 immunity in the mouse small intestine. Proc. Natl. Acad. Sci. U. S. A. 115 (21), 5552–5557. doi:10.1073/pnas.1720758115
Li, Q., Ma, L., Shen, S., Guo, Y., Cao, Q., Cai, X., et al. (2019). Intestinal dysbacteriosis-induced IL-25 promotes development of HCC via alternative activation of macrophages in tumor microenvironment. J. Exp. Clin. Cancer Res. 38 (1), 303. doi:10.1186/s13046-019-1271-3
Liu, D., and Liman, E. R. (2003). Intracellular Ca2+ and the phospholipid PIP2 regulate the taste transduction ion channel TRPM5. Proc. Natl. Acad. Sci. U. S. A. 100 (25), 15160–15165. doi:10.1073/pnas.2334159100
Lü, B., Fang, Y., Xu, J., Wang, L., Xu, F., Xu, E., et al. (2008). Analysis of SOX9 expression in colorectal cancer. Am. J. Clin. Pathol. 130, 897–904. doi:10.1309/ajcpw1w8gjbqgcni
Luciano, L., and Reale, E. (1979). A new morphological aspect of the brush cells of the mouse gallbladder epithelium. Cell Tissue Res. 201 (1), 37–44. doi:10.1007/BF00238045
Luo, W., Tao, J., Zheng, L., and Zhang, T. (2020). Current epidemiology of pancreatic cancer: Challenges and opportunities. Chin. J. Cancer Res. 32 (6), 705–719. doi:10.21147/j.issn.1000-9604.2020.06.04
Luo, X. C., Chen, Z. H., Xue, J. B., Zhao, D. X., Lu, C., Li, Y. H., et al. (2019). Infection by the parasitic helminth Trichinella spiralis activates a Tas2r-mediated signaling pathway in intestinal tuft cells. Proc. Natl. Acad. Sci. U. S. A. 116 (12), 5564–5569. doi:10.1073/pnas.1812901116
Marks, P. W., AraiM., , Bandura, J. L., and Kwiatkowski, D. J. (1998). Advillin (p92): A new member of the gelsolin/villin family of actin regulatory proteins. J. Cell Sci. 111, 2129–2136. doi:10.1242/jcs.111.15.2129
McGinty, J. W., Ting, H. A., Billipp, T. E., Nadjsombati, M. S., Khan, D. M., Barrett, N. A., et al. (2020). Tuft-cell-derived leukotrienes drive rapid anti-helminth immunity in the small intestine but are dispensable for anti-protist immunity. Immunity 52 (3), 528–541. doi:10.1016/j.immuni.2020.02.005
McKernan, D. P., and Egan, L. J. (2015). The intestinal epithelial cell cycle: Uncovering its 'cryptic' nature. Curr. Opin. Gastroenterol. 31, 124–129. doi:10.1097/mog.0000000000000154
McKinley, E. T., Sui, Y., Al-Kofahi, Y., Millis, B. A., Tyska, M. J., Roland, J. T., et al. (2017). Optimized multiplex immunofluorescence single-cell analysis reveals tuft cell heterogeneity. JCI Insight 2 (11), 93487. doi:10.1172/jci.insight.93487
Middelhoff, M., Nienhüser, H., Valenti, G., Maurer, H. C., Hayakawa, Y., Takahashi, R., et al. (2020). Prox1-positive cells monitor and sustain the murine intestinal epithelial cholinergic niche. Nat. Commun. 11, 111. doi:10.1038/s41467-019-13850-7
Miller, C. N., Proekt, I., von Moltke, J., Wells, K. L., Rajpurkar, A. R., Wang, H., et al. (2018). Thymic tuft cells promote an IL-4-enriched medulla and shape thymocyte development. Nature 559 (7715), 627–631. doi:10.1038/s41586-018-0345-2
Minton, K. (2022). Bile duct tuft cells regulate immune defences. Nat. Rev. Immunol. 22 (5), 273. doi:10.1038/s41577-022-00712-5
Mohammadi, Y., Tavangar, S. M., Saidijam, M., Amini, R., Etemadi, K., Karimi Dermani, F., et al. (2018). DCLK1 plays an important role in colorectal cancer tumorgenesis through the regulation of miR-200c. Biomed. Pharmacother. 103, 301–307. doi:10.1016/j.biopha.2018.04.042
Montoro, D. T., Haber, A. L., Biton, M., Vinarsky, V., Lin, B., Birket, S. E., et al. (2018). A revised airway epithelial hierarchy includes CFTR-expressing ionocytes. Nature 560 (7718), 319–324. doi:10.1038/s41586-018-0393-7
Nadjsombati, M. S., Niepoth, N., Webeck, L. M., Kennedy, E. A., Jones, D. L., Baldridge, M. T., et al. (2022). Genetic mapping reveals Pou2af2-dependent tuning of tuft cell differentiation and intestinal type 2 immunity. bioRxiv [Preprint]. Available at: https://www.biorxiv.org/content/10.1101/2022.10.19.512785v1.
Nadjsombati, M. S., McGinty, J. W., Lyons-Cohen, M. R., Jaffe, J. B., DiPeso, L., Schneider, C., et al. (2018). Detection of succinate by intestinal tuft cells triggers a type 2 innate immune circuit. Immunity 49 (1), 33–41. doi:10.1016/j.immuni.2018.06.016
Nakanishi, Y., Seno, H., Fukuoka, A., Ueo, T., Yamaga, Y., Maruno, T., et al. (2013). Dclk1 distinguishes between tumor and normal stem cells in the intestine. Nat. Genet. 45 (1), 98–103. doi:10.1038/ng.2481
Nevo, S., Kadouri, N., and Abramson, J. (2019). Tuft cells: From the mucosa to the thymus. Immunol. Lett. 210, 1–9. doi:10.1016/j.imlet.2019.02.003
O'Leary, C. E., Ma, Z., Culpepper, T., Novak, S. W., and DelGiorno, K. E. (2022). New insights into tuft cell formation: Implications for structure-function relationships. Curr. Opin. Cell Biol. 76, 102082. doi:10.1016/j.ceb.2022.102082
O'Leary, C. E., Schneider, C., and Locksley, R. M. (2019). Tuft cells-systemically dispersed sensory epithelia integrating immune and neural circuitry. Annu. Rev. Immunol. 37, 47–72. doi:10.1146/annurev-immunol-042718-041505
Ohmoto, M., Yamaguchi, T., Yamashita, J., Bachmanov, A. A., Hirota, J., and Matsumoto, I. (2013). Pou2f3/Skn-1a is necessary for the generation or differentiation of solitary chemosensory cells in the anterior nasal cavity. Biosci. Biotechnol. Biochem. 77 (10), 2154–2156. doi:10.1271/bbb.130454
O’Keefe, R. N., Carli, A. L. E., Baloyan, D., Asfahar-Sterle, S., Eissmann, M. F., Poh, A. R., et al. (2022). Inhibition of the tuft cell/ILC2 axis reduces gastric tumor development in mice. bioRxiv [Preprint]. Available at: https://www.biorxiv.org/content/10.1101/2022.02.16.480779v2.
Panneck, A. R., Rafiq, A., Schutz, B., Soultanova, A., Deckmann, K., Chubanov, V., et al. (2014). Cholinergic epithelial cell with chemosensory traits in murine thymic medulla. Cell Tissue Res. 358 (3), 737–748. doi:10.1007/s00441-014-2002-x
Pieper, M. P., Chaudhary, N. I., and Park, J. E. (2007). Acetylcholine-induced proliferation of fibroblasts and myofibroblasts in vitro is inhibited by tiotropium bromide. Life Sci. 80, 2270–2273. doi:10.1016/j.lfs.2007.02.034
Plasschaert, L. W., Zilionis, R., Choo-Wing, R., Savova, V., Knehr, J., Roma, G., et al. (2018). A single-cell atlas of the airway epithelium reveals the CFTR-rich pulmonary ionocyte. Nature 560 (7718), 377–381. doi:10.1038/s41586-018-0394-6
Pritchett, J., Athwal, V., Roberts, N., Hanley, N. A., and Hanley, K. P. (2011). Understanding the role of SOX9 in acquired diseases: Lessons from development. Trends Mol. Med. 17 (3), 166–174. doi:10.1016/j.molmed.2010.12.001
Rane, C. K., Jackson, S. R., Pastore, C. F., Zhao, G., Weiner, A. I., Patel, N. N., et al. (2019). Development of solitary chemosensory cells in the distal lung after severe influenza injury. Am. J. Physiol. Lung Cell. Mol. Physiol. 316 (6), L1141–L1149. doi:10.1152/ajplung.00032.2019
Reid, L., Meyrick, B., Antony, V. B., Chang, L. Y., Crapo, J. D., and Reynolds, H. Y. (2005). The mysterious pulmonary brush cell: A cell in search of a function. Am. J. Respir. Crit. Care Med. 172 (1), 136–139. doi:10.1164/rccm.200502-203WS
Reineking, W., Schauerte, I. E., Junginger, J., and Hewicker-Trautwein, M. (2022). Sox9, Hopx, and survivin and tuft cell marker DCLK1 expression in normal canine intestine and in intestinal adenoma and adenocarcinoma. Vet. Pathol. 59 (3), 415–426. doi:10.1177/03009858221079666
Rhodin, J., and Dalhamn, T. (1956). Electron microscopy of the tracheal ciliated mucosa in rat. Z. Zellforsch. Mikrosk. Anat. 44 (4), 345–412. doi:10.1007/BF00345847
Rhodin, J. (1959). LXVII ultrastructure of the tracheal ciliated mucosa in rat and man. Ann. Otol. Rhinol. Laryngol. 68 (4), 964–974. doi:10.1177/000348945906800402
Rössler, P., Kroner, C., Freitag, J., Noè, J., and Breer, H. (1998). Identification of a phospholipase C beta subtype in rat taste cells. Eur. J. Cell Biol. 77, 253–261. doi:10.1016/s0171-9335(98)80114-3
Ruppert, A. L., Keshavarz, M., Winterberg, S., Oberwinkler, J., Kummer, W., and Schutz, B. (2020). Advillin is a tuft cell marker in the mouse alimentary tract. J. Mol. Histol. 51 (4), 421–435. doi:10.1007/s10735-020-09893-6
Saqui-Salces, M., Keeley, T. M., Grosse, A. S., Qiao, X. T., El-Zaatari, M., Gumucio, D. L., et al. (2011). Gastric tuft cells express DCLK1 and are expanded in hyperplasia. Histochem. Cell Biol. 136 (2), 191–204. doi:10.1007/s00418-011-0831-1
Sauvé, J. P. (2019). COX-expressing tuft cells initiate Crohn’s disease-like intestinal inflammation in SHIP-/- mice. Colombia: UCB.
Schneider, C., O'Leary, C. E., and Locksley, R. M. (2019). Regulation of immune responses by tuft cells. Nat. Rev. Immunol. 19 (9), 584–593. doi:10.1038/s41577-019-0176-x
Schneider, C., O'Leary, C. E., von Moltke, J., Liang, H. E., Ang, Q. Y., Turnbaugh, P. J., et al. (2018). A metabolite-triggered tuft cell-ILC2 circuit drives small intestinal remodeling. Cell 174 (2), 271–284. doi:10.1016/j.cell.2018.05.014
Schutz, B., Jurastow, I., Bader, S., Ringer, C., von Engelhardt, J., Chubanov, V., et al. (2015). Chemical coding and chemosensory properties of cholinergic brush cells in the mouse gastrointestinal and biliary tract. Front. Physiol. 6, 87. doi:10.3389/fphys.2015.00087
Schütz, B., Ruppert, A. L., Strobel, O., Lazarus, M., Urade, Y., Buchler, M. W., et al. (2019). Distribution pattern and molecular signature of cholinergic tuft cells in human gastro-intestinal and pancreatic-biliary tract. Sci. Rep. 9 (1), 17466. doi:10.1038/s41598-019-53997-3
Shanahan, M. T., Kanke, M., Oyesola, O. O., Hung, Y. H., Koch-Laskowski, K., Singh, A. P., et al. (2021). Multiomic analysis defines the first microRNA atlas across all small intestinal epithelial lineages and reveals novel markers of almost all major cell types. Am. J. Physiol. Gastrointest. Liver Physiol. 321 (6), G668–g681. doi:10.1152/ajpgi.00222.2021
Shroyer, N. F., Helmrath, M. A., Wang, V. Y. C., Antalffy, B., Henning, S. J., and Zoghbi, H. Y. (2007). Intestine-specific ablation of mouse atonal homolog 1 (Math1) reveals a role in cellular homeostasis. Gastroenterology 132 (7), 2478–2488. doi:10.1053/j.gastro.2007.03.047
Simon, S. A., de Araujo, I. E., Gutierrez, R., and Nicolelis, M. A. L. (2006). The neural mechanisms of gustation: A distributed processing code. Nat. Rev. Neurosci. 7 (11), 890–901. doi:10.1038/nrn2006
Sunaga, S., Tsunoda, J., Teratani, T., Mikami, Y., and Kanai, T. (2022). Heterogeneity of ILC2s in the intestine; homeostasis and pathology. Front. Immunol. 13, 867351. doi:10.3389/fimmu.2022.867351
Sureban, S. M., May, R., Lightfoot, S. A., Hoskins, A. B., Lerner, M., Brackett, D. J., et al. (2011). DCAMKL-1 regulates epithelial-mesenchymal transition in human pancreatic cells through a miR-200a-dependent mechanism. Cancer Res. 71, 2328–2338. doi:10.1158/0008-5472.Can-10-2738
Swartling, F. J., Ferletta, M., Kastemar, M., Weiss, W. A., and Westermark, B. (2009). Cyclic GMP-dependent protein kinase II inhibits cell proliferation, Sox9 expression and Akt phosphorylation in human glioma cell lines. Oncogene 28, 3121–3131. doi:10.1038/onc.2009.168
Szczepanski, A. P., Tsuboyama, N., Watanabe, J., Hashizume, R., Zhao, Z., and Wang, L. (2022). POU2AF2/C11orf53 functions as a co-activator of POU2F3 by maintaining chromatin accessibility and enhancer activity. Sci. Adv. 8, eabq2403. doi:10.1126/sciadv.abq2403
Takiishi, T., Fenero, C. I. M., and Câmara, N. O. S. (2017). Intestinal barrier and gut microbiota: Shaping our immune responses throughout life. Tissue Barriers 5 (4), e1373208. doi:10.1080/21688370.2017.1373208
Ting, H. A., and von Moltke, J. (2019). The immune function of tuft cells at gut mucosal surfaces and beyond. J. Immunol. 202 (5), 1321–1329. doi:10.4049/jimmunol.1801069
Ualiyeva, S., Hallen, N., Kanaoka, Y., Ledderose, C., Matsumoto, I., Junger, W. G., et al. (2020). Airway brush cells generate cysteinyl leukotrienes through the ATP sensor P2Y2. Sci. Immunol. 5 (43), eaax7224. doi:10.1126/sciimmunol.aax7224
Ualiyeva, S., Lemire, E., Aviles, E. C., Wong, C., Boyd, A. A., Lai, J., et al. (2021). Tuft cell-produced cysteinyl leukotrienes and IL-25 synergistically initiate lung type 2 inflammation. Sci. Immunol. 6 (66), eabj0474. doi:10.1126/sciimmunol.abj0474
van Es, J. H., Wiebrands, K., Lopez-Iglesias, C., van de Wetering, M., Zeinstra, L., van den Born, M., et al. (2019). Enteroendocrine and tuft cells support Lgr5 stem cells on Paneth cell depletion. Proc. Natl. Acad. Sci. U. S. A. 116 (52), 26599–26605. doi:10.1073/pnas.1801888117
von Moltke, J., Ji, M., Liang, H. E., and Locksley, R. M. (2016). Tuft-cell-derived IL-25 regulates an intestinal ILC2-epithelial response circuit. Nature 529 (7585), 221–225. doi:10.1038/nature16161
Wang, D., and Dubois, R. N. (2010). Eicosanoids and cancer. Nat. Rev. Cancer 10, 181–193. doi:10.1038/nrc2809
Westphalen, C. B., Asfaha, S., Hayakawa, Y., Takemoto, Y., Lukin, D. J., Nuber, A. H., et al. (2014). Long-lived intestinal tuft cells serve as colon cancer-initiating cells. J. Clin. Invest. 124, 1283–1295. doi:10.1172/jci73434
Westphalen, C. B., Quante, M., and Wang, T. C. (2017). Functional implication of Dclk1 and Dclk1-expressing cells in cancer. Small GTPases 8 (3), 164–171. doi:10.1080/21541248.2016.1208792
Westphalen, C. B., Takemoto, Y., Tanaka, T., Macchini, M., Jiang, Z., Renz, B. W., et al. (2016). Dclk1 defines quiescent pancreatic progenitors that promote injury-induced regeneration and tumorigenesis. Cell Stem Cell 18 (4), 441–455. doi:10.1016/j.stem.2016.03.016
Weygant, N., Ge, Y., Qu, D., Kaddis, J. S., Berry, W. L., May, R., et al. (2016). Survival of patients with gastrointestinal cancers can Be predicted by a surrogate microRNA signature for cancer stem-like cells marked by DCLK1 kinase. Cancer Res. 76 (14), 4090–4099. doi:10.1158/0008-5472.CAN-16-0029
Weygant, N., Qu, D., May, R., Tierney, R. M., Berry, W. L., Zhao, L., et al. (2015). DCLK1 is a broadly dysregulated target against epithelial-mesenchymal transition, focal adhesion, and stemness in clear cell renal carcinoma. Oncotarget 6 (4), 2193–2205. doi:10.18632/oncotarget.3059
Wu, H. H., Hwang-Verslues, W. W., Lee, W. H., Huang, C. K., Wei, P. C., Chen, C. L., et al. (2015). Targeting IL-17B-IL-17RB signaling with an anti-IL-17RB antibody blocks pancreatic cancer metastasis by silencing multiple chemokines. J. Exp. Med. 212 (3), 333–349. doi:10.1084/jem.20141702
Wu, X. S., He, X. Y., Ipsaro, J. J., Huang, Y. H., Preall, J. B., Ng, D., et al. (2022). OCA-T1 and OCA-T2 are coactivators of POU2F3 in the tuft cell lineage. Nature 607 (7917), 169–175. doi:10.1038/s41586-022-04842-7
Xie, Z., Janczyk, P., Zhang, Y., Liu, A., Shi, X., Singh, S., et al. (2020). A cytoskeleton regulator AVIL drives tumorigenesis in glioblastoma. Nat. Commun. 11, 3457. doi:10.1038/s41467-020-17279-1
Xiong, X., Cheng, Z., Wu, F., Hu, M., Liu, Z., Dong, R., et al. (2021). Berberine in the treatment of ulcerative colitis: A possible pathway through tuft cells. Biomed. Pharmacother. 134, 111129. doi:10.1016/j.biopha.2020.111129
Yachida, S., Totoki, Y., Noe, M., Nakatani, Y., Horie, M., Kawasaki, K., et al. (2022). Comprehensive genomic profiling of neuroendocrine carcinomas of the gastrointestinal system. Cancer Discov. 12 (3), 692–711. doi:10.1158/2159-8290.CD-21-0669
Yamada, Y., Bohnenberger, H., Kriegsmann, M., Kriegsmann, K., Sinn, P., Goto, N., et al. (2022). Tuft cell-like carcinomas: Novel cancer subsets present in multiple organs sharing a unique gene expression signature. Br. J. Cancer 127, 1876–1885. doi:10.1038/s41416-022-01957-6
Yamada, Y., Simon-Keller, K., Belharazem-Vitacolonnna, D., Bohnenberger, H., Kriegsmann, M., Kriegsmann, K., et al. (2021). A tuft cell-like signature is highly prevalent in thymic squamous cell carcinoma and delineates new molecular subsets among the major lung cancer histotypes. J. Thorac. Oncol. 16 (6), 1003–1016. doi:10.1016/j.jtho.2021.02.008
Yamashita, J., Ohmoto, M., Yamaguchi, T., Matsumoto, I., and Hirota, J. (2017). Skn-1a/Pou2f3 functions as a master regulator to generate Trpm5-expressing chemosensory cells in mice. PLoS One 12 (12), e0189340. doi:10.1371/journal.pone.0189340
Yi, J., Bergstrom, K., Fu, J., Shan, X., McDaniel, J. M., McGee, S., et al. (2019). Dclk1 in tuft cells promotes inflammation-driven epithelial restitution and mitigates chronic colitis. Cell Death Differ. 26 (9), 1656–1669. doi:10.1038/s41418-018-0237-x
Yuan, X., Huang, L., Luo, W., Zhao, Y., Nashan, B., Yu, F., et al. (2021). Diagnostic and prognostic significances of SOX9 in thymic epithelial tumor. Front. Oncol. 11, 708735. doi:10.3389/fonc.2021.708735
Zaragosi, L. E., Deprez, M., and Barbry, P. (2020). Using single-cell RNA sequencing to unravel cell lineage relationships in the respiratory tract. Biochem. Soc. Trans. 48 (1), 327–336. doi:10.1042/BST20191010
Keywords: immune function, tuft cells, cancer therapy, POU2F3, DCLK1
Citation: Li L, Ma M, Duan T and Sui X (2022) The critical roles and therapeutic implications of tuft cells in cancer. Front. Pharmacol. 13:1047188. doi: 10.3389/fphar.2022.1047188
Received: 17 September 2022; Accepted: 21 November 2022;
Published: 09 December 2022.
Edited by:
Lianming Liao, Fujian Medical University, ChinaReviewed by:
Xue-Yan He, Cold Spring Harbor Laboratory, United StatesYosuke Yamada, Kyoto University, Japan
Copyright © 2022 Li, Ma, Duan and Sui. This is an open-access article distributed under the terms of the Creative Commons Attribution License (CC BY). The use, distribution or reproduction in other forums is permitted, provided the original author(s) and the copyright owner(s) are credited and that the original publication in this journal is cited, in accordance with accepted academic practice. No use, distribution or reproduction is permitted which does not comply with these terms.
*Correspondence: Ting Duan, ZHVhbnRpbmdAaHpudS5lZHUuY24=; Xinbing Sui, aHp6anVAaHpudS5lZHUuY24=
†These authors have contributed equally to this work