- 1Department of Emergency, China-Japan Friendship Hospital, Beijing, China
- 2Shandong College of Traditional Chinese Medicine, Yantai, Shandong, China
- 3Beijing Key Lab for Immune-Mediated Inflammatory Diseases, Institute of Clinical Medical Sciences, China-Japan Friendship Hospital, Beijing, China
Diabetic kidney disease (DKD) is the major complications of type 1 and 2 diabetes, and is the predominant cause of chronic kidney disease and end-stage renal disease. The treatment of DKD normally consists of controlling blood glucose and improving kidney function. The blockade of renin-angiotensin-aldosterone system and the inhibition of sodium glucose cotransporter 2 (SGLT2) have become the first-line therapy of DKD, but such treatments have been difficult to effectively block continuous kidney function decline, eventually resulting in kidney failure and cardiovascular comorbidities. The complex mechanism of DKD highlights the importance of multiple therapeutic targets in treatment. Chinese herbal medicine (active compound, extract and formula) synergistically improves metabolism regulation, suppresses oxidative stress and inflammation, inhibits mitochondrial dysfunction, and regulates gut microbiota and related metabolism via modulating GLP-receptor, SGLT2, Sirt1/AMPK, AGE/RAGE, NF-κB, Nrf2, NLRP3, PGC-1α, and PINK1/Parkin pathways. Clinical trials prove the reliable evidences for Chinese herbal medicine against DKD, but more efforts are still needed to ensure the efficacy and safety of Chinese herbal medicine. Additionally, the ideal combined therapy of Chinese herbal medicine and conventional medicine normally yields more favorable benefits on DKD treatment, laying the foundation for novel strategies to treat DKD.
Introduction
Diabetic kidney disease (DKD), also called diabetic nephropathy (DN), is a microvascular complication of diabetes mellitus (DM) and characterized by microalbuminuria, declined glomerular filtration rate (GFR), and high risk of cardiovascular disease and stroke. DKD results in high morbidity and mortality worldwide (Koye et al., 2018). Clinically, DKD is defined as the presence of persistently increased urinary albumin (>300 mg/day) or urinary albumin-to-creatinine ratio (UACR>30 mg/g), accompanied by declined kidney function, eventually to end-stage renal disease (ESRD). According to pathological changes, DKD involves thickening of glomerular basement membrane, mesangial expansion, nodular sclerosis, and diabetic glomerulosclerosis (Thomas et al., 2015; Anders et al., 2018). DKD is the major complications of type 1 and 2 diabetes, and is the predominant cause of CKD that accounts for almost 50% of ESRD cases (Barrera-Chimal and Jaisser, 2020). Once DKD enters the dialysis stage, the economic burden of the patient and society greatly increases (Koye et al., 2018).
DKD treatment in early stage mainly focuses on the prevention of DM by the management of diet and lifestyle and glucose control. Once microalbuminuria occurred, the treatment needs to pay additional attention on alleviating and delaying albuminuria. Treatment targets hypertension, blood fat and uric acid also exhibit beneficial effect. The commonly used drugs are listed in Table 1. Although metformin, angiotensin-converting enzyme inhibitors (ACEIs), angiotensin receptor blockers (ARBs), glucagon-like peptide-1 (GLP-1) analogues and dipeptidyl peptidase-4 (DPP-4) inhibitors delay kidney function decline, the application of these drugs are limited. Recently, sodium glucose cotransporter 2 (SGLT2) inhibitor, as a novel type of hypoglycemic drugs, attaches a lot attention due to obvious advantages. SGLT2 excretes sugar directly through the kidneys, and only works when blood sugar exceeds the renal glucose threshold (Alicic et al., 2018). Functionally, SGLT2 inhibitors inhibit renal glucose reabsorption in the early proximal tubule, thereby lowering urinary glucose excretion and decreasing the glucose burden. In the diabetic nephron, compensatory upregulation and overexpression of the activity of SGLT2 glucose and sodium reabsorption in the proximal convoluted tubule results in decreased delivery of solutes to the macula densa. In the diabetic nephron with SGLT inhibition, lowering SGLT2-driven sodium-coupled glucose transport in the proximal convoluted tubule normalizes solute delivery to the macula densa, resulting in increasing solute and water reabsorption. However, adverse effects are unavoidable due to its mechanism, such as urinary tract infection and diabetic ketoacidosis. Therefore, the development of novel drugs and alternative strategies are urgently needed.
Overview of the pathophysiological mechanism and therapeutic target of diabetic kidney disease
Renin-angiotensin-aldosterone system
According to the characteristics of DKD, its pathophysiological mechanisms include hemodynamic and nonhemodynamic mechanisms (Figure 1). In the early phase of DKD, intraglomerular hypertension and single-nephron hyperfiltration are responsible for renal injury (Tonneijck et al., 2017). The improvement of preglomerular (afferent) and postglomerular (efferent) arteriolar tone has therefore exhibited beneficial effects on DKD treatment. The renin-angiotensin-aldosterone system (RAAS) controls water and salt metabolisms, but during DKD process the overactivation of RAAS facilitates efferent constriction and intraglomerular hypertension to promotes disease progression (Warren et al., 2019). The inhibition of RAAS, such as ACEIs and ARBs, has been used as the first-line therapy for DKD treatment by maintaining arteriolar tone balance and decreasing albuminuria (Chen et al., 2018a). However, dual RAAS blockade exerts side effects for DKD treatment indicating the harm of efferent arteriole (Parving et al., 2012; Fried et al., 2013). The combined therapy of mineralocorticoid receptor antagonists (MRAs) with ACEIs or ARBs significantly decreases albuminuria and protects glomerular structure (Zhou et al., 2016; Barrera-Chimal et al., 2019; Barrera-Chimal et al., 2022). Notably, finerenone, a kind of MRAs, has been attracted a lot of attention for DKD treatment. Compared with placebo, finerenone treatment decreases albuminuria and lowers the risks of DKD progression and cardiovascular events in patients with CKD and type 2 diabetes (Bakris et al., 2020; Filippatos et al., 2021; Pitt et al., 2021). Additionally, angiotensin-converting enzyme 2 (ACE2)/Ang(1-7) axis exhibits protection on DKD treatment in animal studies (Chou et al., 2013; Liu et al., 2020a), indicating a promising therapeutic target against DKD. Additionally, RAAS also participates in DKD progression via nonhemodynamic mechanisms. The upregulation of Ang II contributes to DKD progression by activating proinflammatory and profibrotic effects (Yang et al., 2016b; Chen L. et al., 2017), while recombinant ACE2 attenuates DKD progression by suppressing oxidative stress, fibrosis, and mesangial cell proliferation (Malek et al., 2021).
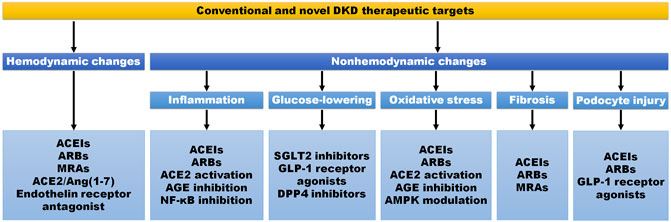
FIGURE 1. Conventional and novel DKD therapeutic targets and strategies. The therapeutic strategy of DKD includes hemodynamic and nonhemodynamic aspects. The improvement of hemodynamic changes, the glucose-lowering therapy, and the suppression of inflammation, oxidative stress, fibrosis and podocyte injury are the main therapeutic targets. ACE2, angiotensin-converting enzyme 2; ACEIs, angiotensin-converting enzyme inhibitors; AGE, advanced glycation end-product; AMPK, adenosine monophosphate-activated protein kinase; ARBs, angiotensin receptor blockers; DPP-4, dipeptidyl peptidase-4; GLP-1, glucagon-like peptide-1; MRAs, mineralocorticoid receptor antagonists; NF-κB, nuclear factor-kappa B; SGLT2, sodium glucose cotransporter 2.
Endothelin receptor
The endothelin system accounts for sodium and water metabolism. Endothelin B receptor is responsible for the natriuresis and vasodilatation at the proximal tubule, whereas endothelin A receptor activation is involved in sodium retention and vasoconstriction (Stuart et al., 2013). Endothelin receptor antagonists reduce albuminuria and prevent renal function decline by dilating the efferent arteriole. According to both short-term and long-term clinical trials, treatment with atrasentan, the selective endothelin A receptor antagonist, significantly reduces albuminuria without inducing obvious sodium retention (Kohan et al., 2015), and decreases the risk of renal events in patients with diabetes and CKD (Heerspink et al., 2019).
Sodium glucose cotransporter 2
For glucose-lowering therapies, SGLT2 inhibition and GLP-1 receptor agonists are the common choice for DKD treatment. The uptake and consumption of circulating glucose, the release of glucose by gluconeogenesis, and the reabsorption of glucose from glomerular filtrate are the main ways to maintain glucose homeostasis in the kidney (Alicic et al., 2018). SGLT2 controls tubular glucose reabsorption, and SGLT2 inhibition exhibits strong renal protection against DKD. SGLT2 inhibition improves solute delivery to the macula densa and reactivates tubuloglomerular feedback by reducing sodium and chloride reabsorption in the proximal tubule, which facilitates the reversal of afferent vasodilation and the normalization of glomerular hemodynamics. Several large clinical trials have proved the effective protection of empagliflozin and canagliflozin in patients with type 2 diabetes and CKD (Yale et al., 2013; Yale et al., 2014; Wanner et al., 2016; Cherney et al., 2017). Beyond the hemodynamic effect, reduced glucose uptake through the proximal tubular cells by SGLT2 inhibition alleviates DKD by inhibiting hyperglycemia-related tubulointerstitial injury (Anders et al., 2018; Kalantar-Zadeh et al., 2021).
Glucagon-like peptide-1 and dipeptidyl peptidase-4
GLP-1 is secreted after food ingestion and reduces postprandial glucose levels by promoting insulin secretion, inhibiting glucagon release, delaying gastric emptying, and decreasing hepatic glucose production (Müller et al., 2019). The deletion of GLP-1 in animal model results in reduced albuminuria and mesangial expansion (Fujita et al., 2014). GLP-1 is degraded by the enzyme DPP-4 in a short time indicating that DPP-4 inhibition is suitable for clinical application rather than GLP-1 (Müller et al., 2019). Two type compounds GLP-1 receptor agonists and DPP-4 inhibitors are therefor used to treat DKD. Large clinical trials have confirmed the beneficial effects of GLP-1 receptor agonists on cardiovascular, mortality, and kidney outcomes in patients with type 2 diabetes, including lixisenatide, liraglutide, semaglutide, exenatide, albiglutide, dulaglutide, and oral semaglutide (Kristensen et al., 2019; Yamada et al., 2021). GLP-1 receptor agonist, liraglutide, also coordinates lipogenic and lipolytic signals and protects renal mitochondria function against renal injury by regulating sirtuin 1 (Sirt1)/AMP-activated kinase protein (AMPK)/peroxlsome proliferator-activated receptor-γ coactlvator-1α (PGC-1α) pathways (Wang et al., 2018). The possible mechanisms of DPP-4 inhibitors for treatment of DKD is a proximally acting natriuresis that increases sodium excretion and triggers tubuloglomerular feedback (Crajoinas et al., 2011). Large clinical trials indicate that DPP-4 inhibitors, dulaglutide and albiglutide, significantly decrease the frequency of major cardiovascular events in patients with type 2 diabetes and CKD (Hernandez et al., 2018; Gerstein et al., 2019). In addition to hemodynamic mechanism, DPP-4 inhibitors reduce albuminuria, alleviate glomerular sclerosis, and suppress oxidative stress and inflammation (Cappetta et al., 2019; Marques et al., 2019).
Overview of Chinese herbal medicine in diabetic kidney disease treatment
A large number of studies have shown that Chinese herbal medicine has exhibited favorable efficacy on DKD treatment in clinics for decades, and has been the primary and additional treatment regimen. Chinese herbal medicine not only functions on abovementioned hemodynamic mechanisms also targets oxidative stress, glucose-lowering, inflammation, fibrosis, and podocyte injury to exert beneficial effects on DKD treatment (Chen et al., 2018b; Chen et al., 2019a), which attaches a lot attention. Notably, Chinese herbal medicine has been widely used to treat DKD clinically and yielded satisfactory results, which is recognized as a promisingly alternative therapy. Chinese herbal medicines are important sources for DKD treatment that prevents DKD and delays DKD progression by targeting multiple targets rather than single targets, including compounds, extracts, and Chinese herbal formulas. The present study aims to review the application of Chinese herbal medicines on DKD treatment in recent 3 years. We start from introducing DKD mechanisms and therapeutic targets, then summarize the advances on the therapeutic mechanisms and clinical application of Chinese herbal medicines on DKD treatment, and conclude by commenting on promising therapeutic candidates from Chinese herbal medicines to highlight the importance and capacity of Chinese herbal medicines on DKD treatment.
Therapeutic mechanism of Chinese herbal medicine in diabetic kidney disease treatment
Metabolism regulation
Numerous Chinese herbal medicines have exhibited beneficial efficacy on DKD treatment in clinics. Here, we describe only some of the important findings for the sake of brevity, and introduce these important findings according to their potential therapeutic targets (Figure 2; Table 2). By targeting DPP-4 and GLP-1 receptor, Abelmoschus esculentus significantly inhibits oxidative stress and renal fibrosis to improve kidney function and alleviate diabetic renal damage in streptozocin (STZ)-induced model (Peng et al., 2019). Icariin, a flavonoid extracted from Herba epimedii, activates GLP-1 receptor to alleviate tubulointerstitial fibrosis in DKD rats (Jia et al., 2021). Beyond to GLP-1 receptor, icariin alleviates inflammation by inducing NOD-like receptor thermal protein domain associated protein 3 (NLRP3) inactivation and G protein-coupled estrogen receptor (GPER) activation via Kelch-1ike ECH-associated protein l (Keap1)-nuclear factor-erythroid-2-related factor 2 (Nrf2)/heme oxygenase-1 (HO-1) axis and inhibiting toll-like receptor 4 (TLR4)/nuclear factor-kappa B (NF-κB) signal pathway (Qiao et al., 2018; Wang et al., 2020; Qi et al., 2021; Ding et al., 2022), and prevents epithelial-mesenchymal transition (EMT) of tubular epithelial cells via modulating the miR-122-5p/forkhead box p2 axis against DKD (Zang et al., 2022).
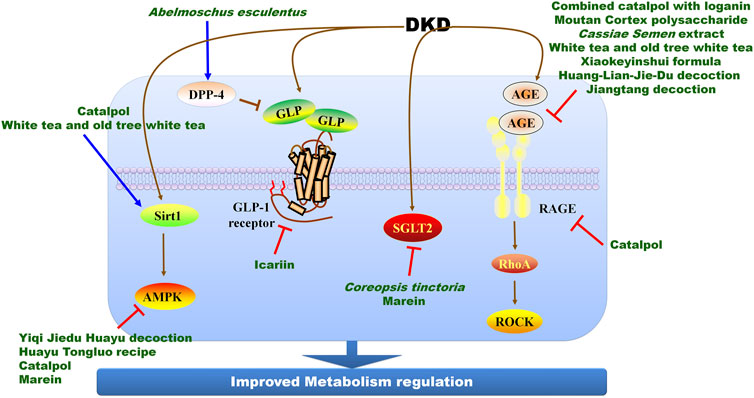
FIGURE 2. The molecular mechanisms and therapeutic targets of Chinese herbal medicine against DKD via improving metabolism regulation. AGE, advanced glycation end-product; AMPK, adenosine monophosphate-activated protein kinase; DPP-4, dipeptidyl peptidase-4; RAGE, receptors of AGE; GLP, glucagon-like peptide-1; ROCK, Rho-associated coiled-coil containing kinase; Sirt1, sirtuin 1.
Advanced glycation end-products (AGEs) are the non-enzymatic glycation products between the aldehyde group of saccharide and amino group of protein, lipid, or nucleic acid. AGEs result in the irreversible transformation of protein by glycation and facilitate DKD progression. The accumulation of AGEs and receptors of AGEs (RAGEs) accelerate glomerulus and tubule injury, and progressive proteinuria (Tang et al., 2021). AGE/AGE pathway is the pivotal therapeutic target of Chinese herbal medicine. Catalpol, an iridoid glycoside isolated from the root of Rehmannia glutinosa, significantly alleviates AGE/RAGE-induced endothelial dysfunction and inflammation in DKD mice and delays DKD progression via RAGE/RhoA/Rho-associated coiled-coil containing kinase (ROCK) pathway (Shu et al., 2021). The combined therapy of catalpol with loganin isolated from Cornus officinalis cooperatively prevents podocyte apoptosis by targeting AGE/RAGE pathway, and exerts stronger effects than used alone against DKD (Chen et al., 2020b). Catalpol also stabilizes podocyte cytoskeleton and enhances injured podocyte autophagy to prevent DKD by suppressing mammalian target of rapamycin activity and promoting transcription factor EB nuclear translocation, and inhibits oxidative stress and inflammation via AMPK/Sirt1/NF-κB pathway (Chen Y. et al., 2019; Chen et al., 2020a). The novel polysaccharide isolated from Moutan Cortex significantly reduces serum AGE and RAGE levels to prevent DKD progression in rat model (Lian et al., 2021). In addition to active compounds from Chinese herbal medicine, Cassiae Semen extract obviously controls glucose and lipid metabolism, and suppresses oxidative stress and inflammatory responses via regulating AGEs and RAGEs against DKD in STZ-induced rat model (Wang et al., 2019). White tea and old tree white tea ameliorate AGE accumulation in kidney of STZ-induced mouse model, and alleviate oxidative stress and inflammation via activating Sirt1/AMPK pathway (Xia et al., 2021). Additionally, Chinese herbal formula including Xiaokeyinshui formula, Huang-Lian-Jie-Du decoction and Jiangtang decoction exhibit renal protective effects in DKD animal models via regulating AGE/RAGE pathway (Hong et al., 2017; Zhou et al., 2020; Tang et al., 2022).
SGLT2 is an important therapeutic target of Chinese herbal medicine. Coreopsis tinctoria Nutt is widely used to treat high blood pressure and diarrhea, and SGLT2 is its potential therapeutic target. Its alcohol extract protects diabetic kidney injury in db/db mice by suppressing miR-192- and miR-200b-mediated phosphatase and tensin homolog deleted on chromosome ten (PTEN)/phosphoinositide 3-kinase (PI3K)/AKT pathway (Yu et al., 2019), while its ethyl acetate extract controls transforming growth factor-β1/Smads, AMPK, and NF-κB signaling pathways to delay DKD progression (Yao et al., 2019). Further study shows that marein and flavanomarein are the active compounds of C. tinctoria to prevent DKD progression. Marein directly inhibits SGLT2 expression and then activates AMPK/acetyl-CoA carboxylase (ACC)/PGC-1α pathway to correct hyperglycemia and dyslipidemia and diabetic kidney injury in db/db mice (Guo et al., 2020). Spleen tyrosine kinase are the potential therapeutic target of flavanomarein to ameliorate high glucose-induced extracellular matrix (ECM) against DKD (Zhang et al., 2020a). Additionally, Yiqi Jiedu Huayu decoction and Huayu Tongluo recipe suppress diabetic kidney injury in STZ-induced rat model via modulating AMPK pathway (Li et al., 2021; Xuan et al., 2021).
The inhibition of oxidative stress and inflammation
Oxidative stress and inflammation drive the development of DKD, and several novel mediators are involved in such process, including tonicity-responsive enhancer-binding protein, apoptosis signal-regulating kinase 1, serine/threonine protein kinase 25, and receptor activator of NF-κB (Chen et al., 2017a; Chen et al., 2017b; Liles et al., 2018; Chen et al., 2019b; Cansby et al., 2020; Choi et al., 2020; Ke et al., 2021; Liu et al., 2022b). Additionally, superoxide dismutase (SOD), glutathione peroxidase, malondialdehyde are considered as biomarkers of oxidative stress to evaluate the effects of Chinese herbal medicine on DKD treatment (Zhou et al., 2022). Even mechanistic studies concerning DKD progress develop a lot, mechanistic studies concerning Chinese herbal medicine against DKD is relatively hysteretic, in that most of Chinese herbal medicine alleviates oxidative stress and inflammation in DKD by modulating NF-κB and Nrf2 pathways (Figure 3; Table 2). Curcumin significantly ameliorates albumin/protein urea and increased creatinine clearance in STZ-induced DKD rats, which involves the activation of Nrf2 and the inhibition of NF-κB, NADPH oxidase and significant up-regulation of the protein kinase Cβ II (PKCβII)/p66Shc axis (AlTamimi et al., 2021). The inhibition of NLRP3 inflammasome activity and the downregulation of kidney injury molecule 1 and neutrophil gelatinase-associated lipocalin are also therapeutic targets of curcumin to suppress oxidative stress against DKD progression (Lu et al., 2017; Ghasemi et al., 2019). The combination of curcumin with antihyperglycemic agents exerts stronger effects against diabetic complications by maintaining Nrf2 pathway homeostasis (Xie et al., 2021). Fructus Arctii attenuates proteinuria in patients with diabetics, and arctigenin, a lignan extracted from F. Arctii, reduces proteinuria and podocyte injury in diabetes mouse models. Arctigenin enhances protein phosphatase 2 A (PP2A) activity to alleviate p65-mediated inflammation in vivo, and blocks endoplasmic reticulum (ER) stress signal transduction pathway to suppress apoptosis in high glucose-induced HK2 cells (Zhang et al., 2019a; Zhong et al., 2019). Specific deletion of PP2A in podocyte weakens the efficiency of arctigenin, indicating PP2A as the therapeutic target of arctigenin (Zhong et al., 2019).
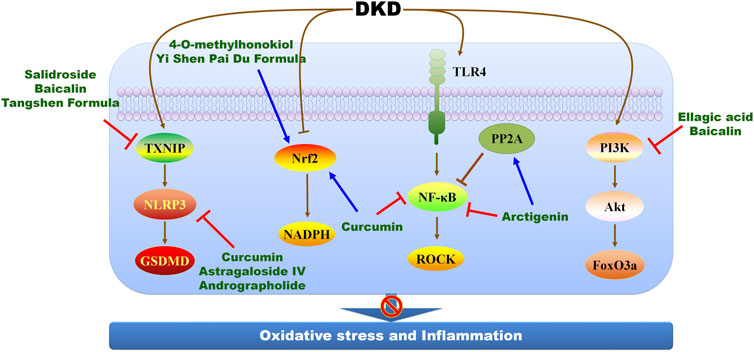
FIGURE 3. The molecular mechanisms and therapeutic targets of Chinese herbal medicine against DKD via suppressing oxidative stress and inflammation. FoxO3a, forkhead box transcription factor 3a; GSDMD, gasdermin D; NF-κB, nuclear factor-kappa B; NLRP3, NOD-like receptor thermal protein domain associated protein 3; Nrf2, nuclear factor-erythroid 2-related factor 2; PI3K, phosphoinositide 3-kinase; PP2A, protein phosphatase 2 A; ROCK, Rho-associated coiled-coil containing kinase; TLR4, toll-like receptor 4; TXNIP, thioredoxin-interacting protein.
Beyond to NF-κB and Nrf2 pathways, the suppression of mitogen-activated protein kinase (MAPK)-mediated inflammatory signaling pathway is therapeutic target of baicalin. Baicalin attenuates diabetic conditions, proteinuria, renal histopathological changes, and alleviates oxidative stress and inflammation in DKD animal model via Nrf2 and MAPK pathways (Ma et al., 2021). Ellagic acid alleviates high glucose-induced mesangial cell injury and inflammation in a concentration-dependent manner, and the underlying mechanisms involve in the activation of PI3K/Akt signaling pathway and the suppression of forkhead box transcription factor 3a (FoxO) 3a during DKD (Lin et al., 2021). Astragaloside IV alleviates podocyte injury and delays DKD progression in db/db mice via suppressing NLRP3 inflammasome-mediated inflammation (Feng et al., 2021). Yi Shen Pai Du Formula inhibits oxidative stress, inflammation, and EMT to delay DKD progression in db/db via activating Nrf2 pathway (Zhang et al., 2021a). Tangshen Formula, a Chinese formulation, exerts beneficial effects against DKD by modulating thioredoxin-interacting protein (TXNIP)-NLRP3-gasdermin D (GSDMD) axis-mediated pyroptosis in STZ-induced rat model and AGE-induced HK-2 cells (Li et al., 2020).
The modulation of mitochondrial dysfunction
PGC-1α is a prominent modulator of mitochondrial biogenesis and an attractive therapeutic target in DKD treatment (Li and Susztak, 2016; Long et al., 2016; Trembinski et al., 2020), and several Chinese herbal medicines and their active compounds exert renoprotection against DKD by modulating PGC-1α (Figure 4; Table 2). Metabolomic research proves that the abnormality on mitochondrial fuel usage and mitochondrial dysfunction occurs in patients with DKD, and berberine, the main active compounds of Rhizoma coptidis and Cortex phellodendri, modulates PGC-1α to alleviate mitochondrial injury in direct and indirect pathways. Berberine facilitates mitochondrial energy homeostasis and fatty acid oxidation by directly activating PGC-1α signaling pathway and protects glomerular podocytes via inhibiting dynamin-related protein 1-mediated mitochondrial fission and dysfunction in db/db mice model and cultured podocytes (Qin et al., 2019; Qin et al., 2020). Berberine activates AMPK pathway to upregulate PGC-1α that reduces fatty acid oxidation, lipid deposition, and protects mitochondria to mitigate diabetic renal tubulointerstitial injury (Rong et al., 2021). Additionally, berberine modulates mitochondrial reactive oxygen species (ROS) generation by stimulating the positive feedback loop of CCAAT enhancer binding protein beta (C/EBPβ)/growth arrest-specific 5 (Gas5)/miR-18a-5p (Xu et al., 2021a). The ethanol extract of Rhodiola rosea exerts beneficial protection in STZ-induced model in the early nephropathy in type 2 diabetic rats (Wang et al., 2013). Further study identifies salidroside as a major active compound of R. rosea to treat DKD. Salidroside markedly improves renal structures and reverses the downregulation of nephrin and podocin in patients with DKD. Mechanistically, salidroside treatment promotes mitochondrial DNA copy and electron transport chain proteins by enhancing Sirt1 and PGC-1α expression in STZ-induced mice (Xue et al., 2019). Salidroside also suppresses oxidative stress and ECM accumulation by modulating TXNIP-NLRP3 inflammasome pathway (Wang et al., 2017), and reduces proteinuria by attenuating caveolin-1 phosphorylation and albumin transcytosis across glomerular endothelial cells (Wu et al., 2016). Resveratrol, a potent Sirt1 agonist, attenuates podocyte damage in diabetic mice by suppressing mitochondrial oxidative stress (Zhang et al., 2019c), while 4-O-methylhonokiol, isolated from Magnolia stem bark, protects against STZ-induced DKD by activating AMPK/PGC-1α/carnitine palmitoyltransferase 1B (CPT1B)-mediated fatty acid oxidation and Nrf2/SOD2-mediated anti-oxidative stress (Ma et al., 2019). Notably, Tangshen Formula and its active compounds morroniside enhances renal cholesterol efflux to ameliorates tubular epithelial injury in db/db mice by activating PGC-1α-liver X receptor (LXR)-ATP-binding cassette transporter A1 (ABCA1) pathway (Liu et al., 2018; Gao et al., 2021a), indicating a promising candidate for DKD treatment.
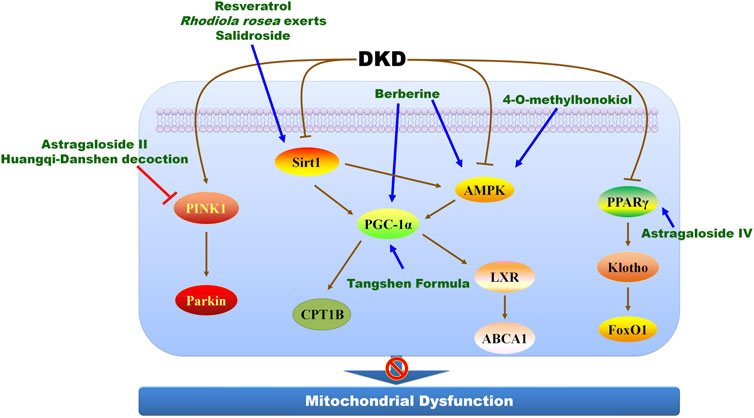
FIGURE 4. The molecular mechanisms and therapeutic targets of Chinese herbal medicine against DKD via alleviating mitochondrial dysfunction. ABCA1, ATP-binding cassette transporter A1; AMPK, adenosine monophosphate-activated protein kinase; CPT1B, carnitine palmitoyltransferase 1B; FoxO1, forkhead box transcription factor 1; LXR, liver X receptor; PGC-1α, peroxlsome proliferator-activated receptor-γ coactlvator-1α; PINK1, PTEN induced putative kinase 1; PPARγ, peroxisome proliferator-activated receptor gamma; Sirt1, sirtuin 1.
Other potential therapeutic targets are also proved, including PTEN induced putative kinase 1 (PINK1) and peroxisome proliferator-activated receptor (PPAR) (Figure 4; Table 2). Several studies show the renoprotective effects of astragaloside II and astragaloside IV, active compounds of Astragalus membranes, referring to the improvement of mitochondrial dysfunction. Astragaloside II and astragaloside IV obviously ameliorate albuminuria in DKD animal models and prevent podocyte injury from high glucose (Su et al., 2021a; Xing et al., 2021; Zang et al., 2021). Astragaloside II suppresses mitochondrial dysfunction of podocyte injury via upregulating PINK1 and Parkin, and astragaloside IV inhibits cytochrome c release and mitochondrial membrane potential to protect podocyte against DKD (Su et al., 2021a; Zang et al., 2021). Astragaloside II also mitigates podocyte apoptosis against DKD via suppressing transient receptor potential channel 6-mediated Ca2+ influx, and astragaloside IV activates PPARγ-Klotho-FoxO1 pathway to alleviate podocyte apoptosis (Xing et al., 2021; Zang et al., 2021). Ginsenoside Rb1 combines with aldose reductase to alleviate mitochondrial damage and podocyte apoptosis thereby delaying the progression of DKD (He et al., 2022). Quercetin, an active compound from Panax notoginseng, antagonizes glucose fluctuation-caused kidney injury by inhibiting aerobic glycolysis via hypoxia inducible factor-1 alpha (HIF-1α)/miR-210/ISCU/FeS pathway in glomerular mesangial cells (Xu et al., 2021b). Andrographolide isolated from Andrographis paniculate suppresses mitochondrial ROS-mediated NLRP3 inflammasome activation to ameliorate mitochondrial dysfunction during DKD (Liu et al., 2021b). Chinese herbal formula Huangqi-Danshen decoction delay DKD progress by suppressing PINK1/Parkin-mediated mitophagy (Liu et al., 2020b). Additionally, signal transducer and activator of transcription 3 is also a potential therapeutic target to modulate mitochondrial homeostasis through SDF-1α/CXCR4 pathway to ameliorate renal tubular injury in DKD (Zhang et al., 2020b).
The regulation of gut microbiota and related metabolism
Emerging evidences have confirmed the relationship between the dysfunction of gut microbiota and related metabolism and the progression of DKD indicating the importance of kidney-gut axis (Winther et al., 2020; Yang et al., 2021). According to 16S rRNA sequencing and metabolomic results, the gut microbiota structure, phenylalanine and tryptophan metabolic pathways are significantly altered in patients with DKD (Zhang et al., 2021b). Phenyl sulfate is a gut microbiota-derived metabolite, and its level increases with the progression of diabetes in rat model. Phenyl sulfate obviously contributes to albuminuria and could be used as a biomarker for DKD (Kikuchi et al., 2019). Trimethylamine N-oxide (TMAO) is a gut microbiota-derived metabolite, and serum TMAO closely relates to and mediates impaired renal function (Winther et al., 2019). As an important component of innate immunity, mitochondrial antiviral signaling protein (MAVS) maintains intestinal integrity. DKD contributes to the impairment of MAVS signaling in the kidney and intestine thus leading to the disrupted homeostasis, indicating that maintaining intestinal homeostasis may functions a novel therapeutic approach for DKD treatment (Linh et al., 2022).
Notably, gut microbiota and related metabolism are the potential therapeutic target of Chinese herbal medicine against DKD (Table 2). Punicalagin isolated from Punica granatum reshapes gut microbial ecology, reverses gut barrier dysfunction, and reduces serum lipopolysaccharide and diamine oxidase levels to delay DKD progression (Hua et al., 2022). Functioning as a prebiotic, the polysaccharide extracted from M. Cortex reconstructs gut microbiota, improves intestinal barrier function, ameliorates serum proinflammatory mediators, and upregulates short-chain fatty acid level by controlling Lactobacillus and Muribaculaceae_unclassified abundance in gut of DKD rat model (Zhang et al., 2022). Qing-Re-Xiao-Zheng formula reverses gut dysbiosis and inhibits generation of gut-derived LPS, and suppresses DKD-related inflammation by reducing TLR4 and NF-κB expression in DKD mouse model (Gao et al., 2021b). QiDiTangShen granules exert good efficacy on alleviating proteinuria in DKD mice model, and the underlying mechanisms involves the modulation of gut microbiota-bile acid axis via farnesoid X receptor (Wei et al., 2021). Shenyan Kangfu tablet, a prescription of traditional Chinese medicine, attenuates stimulated blood glucose and glycosylated hemoglobin (HbA1c) levels and alleviates renal dysfunction and inflammation in db/db mice. The underlying mechanisms refer to suppressed renal inflammatory signaling cascades and improved intestinal microbiota via elevated Firmicutes and reduced Bacteroidetes abundance (Chen et al., 2021). San-Huang-Yi-Shen capsule exhibits beneficial effects against DKD in clinics. The mechanism involves the improvement of gut microbiota by modulating arginine biosynthesis, tricarboxylic acid (TCA) cycle, tyrosine metabolism, and arginine and proline metabolism (Su et al., 2021b). In addition to above-mentioned mechanisms, Tangshen Formula attenuates diabetic renal injury and inflammation by regulating gut microbiota to reducing lipopolysaccharide and indoxyl sulfate levels (Zhao et al., 2020).
Clinical application of Chinese herbal medicine in diabetic kidney disease treatment
Even a lot of work engages to elucidate the underlying mechanisms of Chinese herbal medicine, the lack of high-quality evidences from clinical trials significantly hinders the application of Chinese herbal medicine worldwide. Here, we summarize randomized clinical trials (RCTs) of Chinese herbal medicine against DKD to highlight its beneficial efficacy, and found that Chinese herbal medicine can be used as the primary and additional treatment regimen for DKD in clinics.
Single use of Chinese herbal medicine shows beneficial efficacy on DKD treatment. A retrospective study reports the beneficial efficacy of a traditional Chinese medicine, Shenzhuo formula, on patients with DKD. The changes in estimated GFR (eGFR), creatinine clearance, serum creatinine, blood urea nitrogen, albuminuria, HbA1c, blood pressure, and lipid profile are observed. Compared with the baseline, serum creatinine significantly decreases, and estimated glomerular filtration rate (eGFR) and creatinine clearance increases after intervention at 1, 3, 6, 9, 12, and 18 months. Shenzhuo formula also reduces HbA1c, lipid levels and blood pressure (Tian et al., 2015). A multicenter, parallel-control, open-label, RCT investigates the effect of Zicuiyin decoction on DKD treatment, and the primary outcome is the change of eGFR. Zicuiyin decoction significantly increases eGFR and decreases serum creatinine to alleviate DKD via correcting gut microbiota dysbiosis (Liu et al., 2022a). Additionally, a single-blind, randomized, controlled preliminary study explores the efficacy of the acupressure at Sanyinjiao for DKD treatment, and the primary outcome measure is the UACR or logarithmic transformed UACR (log-UACR) changes. The difference in UACR and log-UACR before and after the study was higher in the Sanyinjiao group than in the sham groups, and the acupressure at Sanyinjiao for 8 weeks helps to decrease albuminuria in patients with early DKD indicated by eGFR and HbA1c (Chuang et al., 2020).
Combined therapy of Chinese herbal medicine and ARB/ACEI also exhibit favorable efficacy on DKD treatment. Huangkui capsule from traditional Chinese medicine is made from the ethanol extract of flowers in Abelmoschus manihot. A multicenter randomized double-blind parallel controlled clinical trial is designed to evaluate the effect of combined Huangkui capsule and irbesartan treatment on DKD, and the primary outcomes are changed values of albumin-to-creatinine ratio from baseline after treatment. Combined Huangkui capsule and irbesartan treatment exhibits beneficial effect on alleviating albuminuria in patients with type 2 diabetes and DKD (Zhao et al., 2022). Additionally, single Huangkui capsule therapy and combined Huangkui capsule and losartan therapy exert favorable therapeutic effect against primary glomerular disease in a prospective, multicenter randomized controlled clinical trial (Zhang et al., 2014). A multicenter double-blinded randomized placebo-controlled trial shows that accompanying by conventional ARB or ACEI treatment, Tangshen Formula treatment for continuous 24 weeks exhibits obviously beneficial efficacy compared with placebo on decreasing proteinuria and improving eGFR in DKD patients with macroalbuminuria (Li et al., 2015). In this study, primary outcomes are urinary protein level, measured by urinary albumin excretion rate (UAER) for participants with microalbuminuria, 24-h urinary protein for participants with macroalbuminuria. Except for UAER, Tangshen Formula treatment exhibits favorable effects on other primary outcomes. Further investigation indicates that urinary liver-type fatty acid binding protein is identified as the biomarker for the severity of DKD and the effects of Tangshen Formula against DKD (Yang et al., 2016a). The on-going RCT of Tangshen Formula aims to investigate its effectiveness and safety on treating type 2 DKD patients with macroalbuminuria (Yan et al., 2016). The meta-analysis of RCTs shows the satisfied efficacy of combined Tripterygium wilfordii Hook, tripterygium glycosides, Ophiocordyceps sinensis, or Jinshuibao with conventional ACEI or ARB treatment against DKD (Luo et al., 2015; Lu et al., 2018; Zhang et al., 2019b; Ren et al., 2019; Wu et al., 2020; Yang et al., 2020).
Notably, several RCT protocols have been designed to provide solid evidences for combined Chinese herbal medicine and conventional ARB or ACEI therapy against DKD. An assessor-blind, parallel, pragmatic randomized controlled clinical trial registered in Hong Kong has been engaged to evaluate the effectiveness of add-on astragalus in clinics. This trial plans to enroll 181 patients with type 2 diabetes, stage 2-3 CKD and macroalbuminuria who receive 48 weeks of add-on astragalus or standard medical care (Chan et al., 2021). A double-blind, placebo-controlled, randomized trial is designed to explore the efficacy and safety of combined Liuwei Dihuang pills with conventional metformin and ARB therapy against DKD for 4 weeks treatment and 12 weeks follow-up, and 24 h urinary protein levels from the baseline to the end of the treatment phase is the primary outcome (Liao et al., 2020). A prospective, single-center RCT aims to investigate the efficacy and safety of combined Tripterygium glycosides and ARB therapy for DKD treatment. The primary endpoint is 24 h proteinuria decreased level after treatment for 48 weeks (Lengnan et al., 2020).
Conclusion and perspectives
DKD is the leading cause of CKD and ESRD worldwide. Although the drug development of RAAS and SGLT2 inhibitors has been evolved, a large proportion of DKD patients still needs dialysis and renal transplantation. The beneficial efficacy of Chinese herbal medicine in clinical application attracts a lot attention as an alternative therapy. DKD progression is normally considered irreversible, while Chinese herbal medicine gives us hope. Reliable evidences from RCTs show that Tangshen Formula and T. wilfordii Hook extract significantly reduce proteinuria and elevate eGFR compared with ARB or ACEI (Ge et al., 2013; Li et al., 2015; Liu et al., 2021a). Chinese herbal medicine normally targets multiple and synergetic targets to alleviate DKD due to multiple active compounds, including the improvement of metabolism regulation, the inhibition of oxidative stress and inflammation, the modulation of mitochondrial dysfunction, and the regulation of gut microbiota and related metabolism. Notably, we notice that many Chinese herbal medicines synergistically target multiple key factors and pathways to ameliorate DKD, including icariin, catalpol, C. tinctoria, salidroside, and 4-O-methylhonokiol (Table 2). These promising candidates highlight the advantage that synergistically targeting multiple key factors and pathways is the important strategy to facilitate drug development for DKD treatment. These also highlight the importance and urgency to discover and identify the novel therapeutic target. Another advantage of Chinese herbal medicine is its clinical experience for thousands of years in east Asia. Chinese herbal medicine has been still used for prevent and treat DKD today, and many high-quality clinical evidences confirm the efficacy of combined Chinese herbal medicine and conventional western medicine, such as Tangshen Formula, Xiaokeyinshui formula, and T. wilfordii, which provide an alternative strategy for DKD treatment.
However, some limitations hinder the recognition and extensive use of Chinese herbal medicine beyond east Asia. One is that the lack of high-quality evidence to identify therapeutic mechanism of Chinese herbal medicine. Most research reported the modulation of Chinese herbal medicine on common mechanism rather than specific and targeted mechanism via high throughput analysis. Considerable work needs to be done to identify targeted mechanism of Chinese herbal medicine on DKD treatment. The multiple active compounds of Chinese herbal medicine contribute to the beneficial efficacy to alleviate DKD, but also results in the difficulty to control the quality of Chinese herbal medicine. The identification of active compounds and establishment of corresponding quality control system are necessary to ensure the efficacy and safety of Chinese herbal medicine. Additionally, strict and standardized toxicological research is essential to ensure the acceptable side effect. The proper dosage and duration of Chinese herbal medicine should be investigated in preclinical and clinical trial to ensure favorable efficacy. The lack of high-quality evidence from clinical medicine significantly hinders the extensive use of Chinese herbal medicine, and more efforts are needed to solve this problem. Fortunately, RCTs and mechanism studies of some Chinese herbal medicines have been completed and some is ongoing. For example, the clinical efficacy of Tangshen Formula has been investigated by RCTs, and Tangshen Formula exerts better efficacy in reducing albuminuria and elevating eGFR (Li et al., 2015). Meanwhile, mechanism studies show that Tangshen Formula synergistically targets multiple key factors or pathways to ameliorate DKD via modulating pyroptosis, enhancing renal cholesterol efflux, reshaping gut microbiota, and suppressing inflammation (Liu et al., 2018; Li et al., 2020; Zhao et al., 2020). Tangshen Formula sets up a good example that provides reliable evidences for clinical trial and therapeutic mechanism.
Given that many Chinese herbal medicines have yet to be investigated using a modern pharmacological approach, we anticipate many of them could be completed in the future. Chinese herbal medicine has its own advantages in treating DKD, including multiple therapeutic targets and rich clinical experience. New guidelines concerning Chinese herbal medicine against DKD are needed to assure safety and efficacy to amplify its application in treating DKD worldwide.
Author contributions
D-QC conceptualized and wrote the manuscript. D-QC, JW, and PL revised the manuscript and approved the submitted version.
Funding
This study was supported by the National Natural Science Foundation of China (Grant Nos. 82174296, 82104511), and the Project funded by China Postdoctoral Science Foundation (Grant Nos. 2021M693579).
Conflict of interest
The authors declare that the research was conducted in the absence of any commercial or financial relationships that could be construed as a potential conflict of interest.
Publisher’s note
All claims expressed in this article are solely those of the authors and do not necessarily represent those of their affiliated organizations, or those of the publisher, the editors and the reviewers. Any product that may be evaluated in this article, or claim that may be made by its manufacturer, is not guaranteed or endorsed by the publisher.
References
Alicic, R. Z., Johnson, E. J., and Tuttle, K. R. (2018). SGLT2 inhibition for the prevention and treatment of diabetic kidney disease: a review. Am. J. Kidney Dis. 72 (2), 267–277. doi:10.1053/j.ajkd.2018.03.022
ALTamimi, J. Z., AlFaris, N. A., Al-Farga, A. M., Alshammari, G. M., BinMowyna, M. N., and Yahya, M. A. (2021). Curcumin reverses diabetic nephropathy in streptozotocin-induced diabetes in rats by inhibition of PKCβ/p(66)Shc axis and activation of FOXO-3a. J. Nutr. Biochem. 87, 108515. doi:10.1016/j.jnutbio.2020.108515
Anders, H. J., Huber, T. B., Isermann, B., and Schiffer, M. (2018). CKD in diabetes: diabetic kidney disease versus nondiabetic kidney disease. Nat. Rev. Nephrol. 14 (6), 361–377. doi:10.1038/s41581-018-0001-y
Bakris, G. L., Agarwal, R., Anker, S. D., Pitt, B., Ruilope, L. M., Rossing, P., et al. (2020). Effect of finerenone on chronic kidney disease outcomes in type 2 diabetes. N. Engl. J. Med. 383 (23), 2219–2229. doi:10.1056/NEJMoa2025845
Barrera-Chimal, J., and Jaisser, F. (2020). Pathophysiologic mechanisms in diabetic kidney disease: A focus on current and future therapeutic targets. Diabetes Obes. Metab. 22, 16–31. doi:10.1111/dom.13969
Barrera-Chimal, J., Girerd, S., and Jaisser, F. (2019). Mineralocorticoid receptor antagonists and kidney diseases: Pathophysiological basis. Kidney Int. 96 (2), 302–319. doi:10.1016/j.kint.2019.02.030
Barrera-Chimal, J., Lima-Posada, I., Bakris, G. L., and Jaisser, F. (2022). Mineralocorticoid receptor antagonists in diabetic kidney disease - mechanistic and therapeutic effects. Nat. Rev. Nephrol. 18 (1), 56–70. doi:10.1038/s41581-021-00490-8
Cansby, E., Caputo, M., Gao, L., Kulkarni, N. M., Nerstedt, A., Ståhlman, M., et al. (2020). Depletion of protein kinase STK25 ameliorates renal lipotoxicity and protects against diabetic kidney disease. JCI Insight 5 (24), 140483. doi:10.1172/jci.insight.140483
Cappetta, D., Ciuffreda, L. P., Cozzolino, A., Esposito, G., Scavone, C., Sapio, L., et al. (2019). Dipeptidyl peptidase 4 inhibition ameliorates chronic kidney disease in a model of salt-dependent hypertension. Oxid. Med. Cell. Longev. 2019, 8912768. doi:10.1155/2019/8912768
Chan, K. W., Kwong, A. S. K., Tsui, P. N., Cheung, S. C. Y., Chan, G. C. W., Choi, W. F., et al. (2021). Efficacy, safety and response predictors of adjuvant astragalus for diabetic kidney disease (READY): Study protocol of an add-on, assessor-blind, parallel, pragmatic randomised controlled trial. BMJ Open 11 (1), e042686. doi:10.1136/bmjopen-2020-042686
Chen, D. Q., Cao, G., Chen, H., Liu, D., Su, W., Yu, X. Y., et al. (2017a). Gene and protein expressions and metabolomics exhibit activated redox signaling and wnt/β-catenin pathway are associated with metabolite dysfunction in patients with chronic kidney disease. Redox Biol. 12, 505–521. doi:10.1016/j.redox.2017.03.017
Chen, D. Q., Chen, H., Chen, L., Vaziri, N. D., Wang, M., Li, X. R., et al. (2017b). The link between phenotype and fatty acid metabolism in advanced chronic kidney disease. Nephrol. Dial. Transpl. 32 (7), 1154–1166. doi:10.1093/ndt/gfw415
Chen, L., Chen, D. Q., Wang, M., Liu, D., Chen, H., Dou, F., et al. (2017). Role of RAS/Wnt/β-catenin axis activation in the pathogenesis of podocyte injury and tubulo-interstitial nephropathy. Chem. Biol. Interact. 273, 56–72. doi:10.1016/j.cbi.2017.05.025
Chen, D. Q., Feng, Y. L., Cao, G., and Zhao, Y. Y. (2018a). Natural products as a source for antifibrosis therapy. Trends Pharmacol. Sci. 39 (11), 937–952. doi:10.1016/j.tips.2018.09.002
Chen, D. Q., Hu, H. H., Wang, Y. N., Feng, Y. L., Cao, G., and Zhao, Y. Y. (2018b). Natural products for the prevention and treatment of kidney disease. Phytomedicine 50, 50–60. doi:10.1016/j.phymed.2018.09.182
Chen, D. Q., Cao, G., Chen, H., Argyopoulos, C. P., Yu, H., Su, W., et al. (2019a). Identification of serum metabolites associating with chronic kidney disease progression and anti-fibrotic effect of 5-methoxytryptophan. Nat. Commun. 10 (1), 1476. doi:10.1038/s41467-019-09329-0
Chen, D. Q., Feng, Y. L., Chen, L., Liu, J. R., Wang, M., Vaziri, N. D., et al. (2019b). Poricoic acid A enhances melatonin inhibition of AKI-to-CKD transition by regulating Gas6/Axl-NF-κB/Nrf2 axis. Free Radic. Biol. Med. 134, 484–497. doi:10.1016/j.freeradbiomed.2019.01.046
Chen, Y., Liu, Q., Shan, Z., Mi, W., Zhao, Y., Li, M., et al. (2019). Catalpol ameliorates podocyte injury by stabilizing cytoskeleton and enhancing autophagy in diabetic nephropathy. Front. Pharmacol. 10, 1477. doi:10.3389/fphar.2019.01477
Chen, J., Yang, Y., Lv, Z., Shu, A., Du, Q., Wang, W., et al. (2020). Study on the inhibitive effect of Catalpol on diabetic nephropathy. Life Sci. 257, 118120. doi:10.1016/j.lfs.2020.118120
Chen, Y., Chen, J., Jiang, M., Fu, Y., Zhu, Y., Jiao, N., et al. (2020). Loganin and catalpol exert cooperative ameliorating effects on podocyte apoptosis upon diabetic nephropathy by targeting AGEs-RAGE signaling. Life Sci. 252, 117653. doi:10.1016/j.lfs.2020.117653
Chen, Q., Ren, D., Wu, J., Yu, H., Chen, X., Wang, J., et al. (2021). Shenyan Kangfu tablet alleviates diabetic kidney disease through attenuating inflammation and modulating the gut microbiota. J. Nat. Med. 75 (1), 84–98. doi:10.1007/s11418-020-01452-3
Cherney, D. Z. I., Zinman, B., Inzucchi, S. E., Koitka-Weber, A., Mattheus, M., von Eynatten, M., et al. (2017). Effects of empagliflozin on the urinary albumin-to-creatinine ratio in patients with type 2 diabetes and established cardiovascular disease: an exploratory analysis from the EMPA-REG OUTCOME randomised, placebo-controlled trial. Lancet. Diabetes Endocrinol. 5 (8), 610–621. doi:10.1016/s2213-8587(17)30182-1
Choi, S. Y., Lee-Kwon, W., and Kwon, H. M. (2020). The evolving role of TonEBP as an immunometabolic stress protein. Nat. Rev. Nephrol. 16 (6), 352–364. doi:10.1038/s41581-020-0261-1
Chou, C. H., Chuang, L. Y., Lu, C. Y., and Guh, J. Y. (2013). Interaction between TGF-β and ACE2-Ang-(1-7)-Mas pathway in high glucose-cultured NRK-52E cells. Mol. Cell. Endocrinol. 366 (1), 21–30. doi:10.1016/j.mce.2012.11.004
Chuang, S. M., Lee, C. C., Lo, W. Y., and Hsieh, C. L. (2020). Effect of acupressure at Sanyinjiao on albuminuria in patients with early diabetic nephropathy: A single-blind, randomized, controlled preliminary study. Explore (NY) 16 (3), 165–169. doi:10.1016/j.explore.2019.09.001
Crajoinas, R. O., Oricchio, F. T., Pessoa, T. D., Pacheco, B. P., Lessa, L. M., Malnic, G., et al. (2011). Mechanisms mediating the diuretic and natriuretic actions of the incretin hormone glucagon-like peptide-1. Am. J. Physiol. Ren. Physiol. 301 (2), F355–F363. doi:10.1152/ajprenal.00729.2010
Ding, X., Zhao, H., and Qiao, C. (2022). Icariin protects podocytes from NLRP3 activation by Sesn2-induced mitophagy through the Keap1-Nrf2/HO-1 axis in diabetic nephropathy. Phytomedicine. 99, 154005. doi:10.1016/j.phymed.2022.154005
Feng, H., Zhu, X., Tang, Y., Fu, S., Kong, B., and Liu, X. (2021). Astragaloside IV ameliorates diabetic nephropathy in db/db mice by inhibiting NLRP3 inflammasome-mediated inflammation. Int. J. Mol. Med. 48 (2), 164. doi:10.3892/ijmm.2021.4996
Filippatos, G., Anker, S. D., Agarwal, R., Pitt, B., Ruilope, L. M., Rossing, P., et al. (2021). Finerenone and cardiovascular outcomes in patients with chronic kidney disease and type 2 diabetes. Circulation 143 (6), 540–552. doi:10.1161/circulationaha.120.051898
Fried, L. F., Emanuele, N., Zhang, J. H., Brophy, M., Conner, T. A., Duckworth, W., et al. (2013). Combined angiotensin inhibition for the treatment of diabetic nephropathy. N. Engl. J. Med. 369 (20), 1892–1903. doi:10.1056/NEJMoa1303154
Fujita, H., Morii, T., Fujishima, H., Sato, T., Shimizu, T., Hosoba, M., et al. (2014). The protective roles of GLP-1R signaling in diabetic nephropathy: Possible mechanism and therapeutic potential. Kidney Int. 85 (3), 579–589. doi:10.1038/ki.2013.427
Gao, J., Liu, P., Shen, Z., Xu, K., Wu, C., Tian, F., et al. (2021). Morroniside promotes PGC-1α-mediated cholesterol efflux in sodium palmitate or high glucose-induced mouse renal tubular epithelial cells. Biomed. Res. Int. 2021, 9942152. doi:10.1155/2021/9942152
Gao, Y., Yang, R., Guo, L., Wang, Y., Liu, W. J., Ai, S., et al. (2021). Qing-Re-Xiao-Zheng formula modulates gut microbiota and inhibits inflammation in mice with diabetic kidney disease. Front. Med. 8, 719950. doi:10.3389/fmed.2021.719950
Ge, Y., Xie, H., Li, S., Jin, B., Hou, J., Zhang, H., et al. (2013). Treatment of diabetic nephropathy with tripterygium wilfordii Hook F extract: a prospective, randomized, controlled clinical trial. J. Transl. Med. 11, 134. doi:10.1186/1479-5876-11-134
Gerstein, H. C., Colhoun, H. M., Dagenais, G. R., Diaz, R., Lakshmanan, M., Pais, P., et al. (2019). Dulaglutide and cardiovascular outcomes in type 2 diabetes (REWIND): a double-blind, randomised placebo-controlled trial. Lancet 394 (10193), 121–130. doi:10.1016/s0140-6736(19)31149-3
Ghasemi, H., Einollahi, B., Kheiripour, N., Hosseini-Zijoud, S. R., and Farhadian Nezhad, M. (2019). Protective effects of curcumin on diabetic nephropathy via attenuation of kidney injury molecule 1 (KIM-1) and neutrophil gelatinase-associated lipocalin (NGAL) expression and alleviation of oxidative stress in rats with type 1 diabetes. Iran. J. Basic Med. Sci. 22 (4), 376–383. doi:10.22038/ijbms.2019.31922.7674
Guo, Y., Ran, Z., Zhang, Y., Song, Z., Wang, L., Yao, L., et al. (2020). Marein ameliorates diabetic nephropathy by inhibiting renal sodium glucose transporter 2 and activating the AMPK signaling pathway in db/db mice and high glucose-treated HK-2 cells. Biomed. Pharmacother. 131, 110684. doi:10.1016/j.biopha.2020.110684
He, J. Y., Hong, Q., Chen, B. X., Cui, S. Y., Liu, R., Cai, G. Y., et al. (2022). Ginsenoside Rb1 alleviates diabetic kidney podocyte injury by inhibiting aldose reductase activity. Acta Pharmacol. Sin. 43 (2), 342–353. doi:10.1038/s41401-021-00788-0
Heerspink, H. J. L., Parving, H. H., Andress, D. L., Bakris, G., Correa-Rotter, R., Hou, F. F., et al. (2019). Atrasentan and renal events in patients with type 2 diabetes and chronic kidney disease (SONAR): a double-blind, randomised, placebo-controlled trial. Lancet 393 (10184), 1937–1947. doi:10.1016/s0140-6736(19)30772-x
Hernandez, A. F., Green, J. B., Janmohamed, S., D'Agostino, R. B., Sr, , Granger, C. B., et al. (2018). Albiglutide and cardiovascular outcomes in patients with type 2 diabetes and cardiovascular disease (harmony outcomes): a double-blind, randomised placebo-controlled trial. Lancet 392 (10157), 1519–1529. doi:10.1016/s0140-6736(18)32261-x
Hong, J. N., Li, W. W., Wang, L. L., Guo, H., Jiang, Y., Gao, Y. J., et al. (2017). Jiangtang decoction ameliorate diabetic nephropathy through the regulation of PI3K/Akt-mediated NF-κB pathways in KK-Ay mice. Chin. Med. 12, 13. doi:10.1186/s13020-017-0134-0
Hua, Q., Han, Y., Zhao, H., Zhang, H., Yan, B., Pei, S., et al. (2022). Punicalagin alleviates renal injury via the gut-kidney axis in high-fat diet-induced diabetic mice. Food Funct. 13 (2), 867–879. doi:10.1039/d1fo03343c
Jia, Z., Wang, K., Zhang, Y., Duan, Y., Xiao, K., Liu, S., et al. (2021). Icariin ameliorates diabetic renal tubulointerstitial fibrosis by restoring autophagy via regulation of the miR-192-5p/GLP-1R pathway. Front. Pharmacol. 12, 720387. doi:10.3389/fphar.2021.720387
Kalantar-Zadeh, K., Jafar, T. H., Nitsch, D., Neuen, B. L., and Perkovic, V. (2021). Chronic kidney disease. Lancet 398 (10302), 786–802. doi:10.1016/s0140-6736(21)00519-5
Ke, G., Chen, X., Liao, R., Xu, L., Zhang, L., Zhang, H., et al. (2021). Receptor activator of NF-κB mediates podocyte injury in diabetic nephropathy. Kidney Int. 100 (2), 377–390. doi:10.1016/j.kint.2021.04.036
Kikuchi, K., Saigusa, D., Kanemitsu, Y., Matsumoto, Y., Thanai, P., Suzuki, N., et al. (2019). Gut microbiome-derived phenyl sulfate contributes to albuminuria in diabetic kidney disease. Nat. Commun. 10 (1), 1835. doi:10.1038/s41467-019-09735-4
Kohan, D. E., Lambers Heerspink, H. J., Coll, B., Andress, D., Brennan, J. J., Kitzman, D. W., et al. (2015). Predictors of atrasentan-associated fluid retention and change in albuminuria in patients with diabetic nephropathy. Clin. J. Am. Soc. Nephrol. 10 (9), 1568–1574. doi:10.2215/cjn.00570115
Koye, D. N., Magliano, D. J., Nelson, R. G., and Pavkov, M. E. (2018). The global epidemiology of diabetes and kidney disease. Adv. Chronic Kidney Dis. 25 (2), 121–132. doi:10.1053/j.ackd.2017.10.011
Kristensen, S. L., Rørth, R., Jhund, P. S., Docherty, K. F., Sattar, N., Preiss, D., et al. (2019). Cardiovascular, mortality, and kidney outcomes with GLP-1 receptor agonists in patients with type 2 diabetes: a systematic review and meta-analysis of cardiovascular outcome trials. Lancet. Diabetes Endocrinol. 7 (10), 776–785. doi:10.1016/s2213-8587(19)30249-9
Lengnan, X., Ban, Z., Haitao, W., Lili, L., Aiqun, C., Huan, W., et al. (2020). Tripterygium wilfordii Hook F treatment for stage IV diabetic nephropathy: Protocol for a prospective, randomized controlled trial. Biomed. Res. Int. 2020, 9181037. doi:10.1155/2020/9181037
Li, S. Y., and Susztak, K. (2016). The long noncoding RNA Tug1 connects metabolic changes with kidney disease in podocytes. J. Clin. Invest. 126 (11), 4072–4075. doi:10.1172/jci90828
Li, P., Chen, Y., Liu, J., Hong, J., Deng, Y., Yang, F., et al. (2015). Efficacy and safety of tangshen formula on patients with type 2 diabetic kidney disease: a multicenter double-blinded randomized placebo-controlled trial. PLoS One 10 (5), e0126027. doi:10.1371/journal.pone.0126027
Li, N., Zhao, T., Cao, Y., Zhang, H., Peng, L., Wang, Y., et al. (2020). Tangshen formula attenuates diabetic kidney injury by imparting anti-pyroptotic effects via the TXNIP-NLRP3-GSDMD Axis. Front. Pharmacol. 11, 623489. doi:10.3389/fphar.2020.623489
Li, Y., Guo, S., Yang, F., Liu, L., and Chen, Z. (2021). Huayu Tongluo recipe attenuates renal oxidative stress and inflammation through the activation of AMPK/Nrf2 signaling pathway in streptozotocin- (STZ-) induced diabetic rats. Evid. Based. Complement. Altern. Med. 2021, 5873007. doi:10.1155/2021/5873007
Lian, Y., Zhu, M., Chen, J., Yang, B., Lv, Q., Wang, L., et al. (2021). Characterization of a novel polysaccharide from Moutan Cortex and its ameliorative effect on AGEs-induced diabetic nephropathy. Int. J. Biol. Macromol. 176, 589–600. doi:10.1016/j.ijbiomac.2021.02.062
Liao, T., Zhao, K., Huang, Q., Tang, S., Chen, K., Xie, C., et al. (2020). A randomized controlled clinical trial study protocol of Liuwei Dihuang pills in the adjuvant treatment of diabetic kidney disease. Med. Baltim. 99 (31), e21137. doi:10.1097/md.0000000000021137
Liles, J. T., Corkey, B. K., Notte, G. T., Budas, G. R., Lansdon, E. B., Hinojosa-Kirschenbaum, F., et al. (2018). ASK1 contributes to fibrosis and dysfunction in models of kidney disease. J. Clin. Invest. 128 (10), 4485–4500. doi:10.1172/jci99768
Lin, W., Liu, G., Kang, X., Guo, P., Shang, Y., Du, R., et al. (2021). Ellagic acid inhibits high glucose-induced injury in rat mesangial cells via the PI3K/Akt/FOXO3a signaling pathway. Exp. Ther. Med. 22 (3), 1017. doi:10.3892/etm.2021.10449
Linh, H. T., Iwata, Y., Senda, Y., Sakai-Takemori, Y., Nakade, Y., Oshima, M., et al. (2022). Intestinal bacterial translocation contributes to diabetic kidney disease. J. Am. Soc. Nephrol. 33, 1105–1119. doi:10.1681/asn.2021060843
Liu, P., Peng, L., Zhang, H., Tang, P. M., Zhao, T., Yan, M., et al. (2018). Tangshen formula attenuates diabetic nephropathy by promoting ABCA1-mediated renal cholesterol efflux in db/db mice. Front. Physiol. 9, 343. doi:10.3389/fphys.2018.00343
Liu, Q., Lv, S., Liu, J., Liu, S., Wang, Y., and Liu, G. (2020). Mesenchymal stem cells modified with angiotensin-converting enzyme 2 are superior for amelioration of glomerular fibrosis in diabetic nephropathy. Diabetes Res. Clin. Pract. 162, 108093. doi:10.1016/j.diabres.2020.108093
Liu, X., Lu, J., Liu, S., Huang, D., Chen, M., Xiong, G., et al. (2020). Huangqi-Danshen decoction alleviates diabetic nephropathy in db/db mice by inhibiting PINK1/Parkin-mediated mitophagy. Am. J. Transl. Res. 12 (3), 989–998.
Liu, P., Zhang, J., Wang, Y., Shen, Z., Wang, C., Chen, D. Q., et al. (2021). The active compounds and therapeutic target of tripterygium wilfordii Hook. f. in attenuating proteinuria in diabetic nephropathy: A review. Front. Med. 8, 747922. doi:10.3389/fmed.2021.747922
Liu, W., Liang, L., Zhang, Q., Li, Y., Yan, S., Tang, T., et al. (2021). Effects of andrographolide on renal tubulointersticial injury and fibrosis. Evidence of its mechanism of action. Phytomedicine. 91, 153650. doi:10.1016/j.phymed.2021.153650
Liu, J., Gao, L. D., Fu, B., Yang, H. T., Zhang, L., Che, S. Q., et al. (2022). Efficacy and safety of Zicuiyin decoction on diabetic kidney disease: A multicenter, randomized controlled trial. Phytomedicine. 100, 154079. doi:10.1016/j.phymed.2022.154079
Liu, P., Zhang, J., Wang, Y., Wang, C., Qiu, X., and Chen, D. Q. (2022). Natural products against renal fibrosis via modulation of SUMOylation. Front. Pharmacol. 13, 800810. doi:10.3389/fphar.2022.800810
Long, J., Badal, S. S., Ye, Z., Wang, Y., Ayanga, B. A., Galvan, D. L., et al. (2016). Long noncoding RNA Tug1 regulates mitochondrial bioenergetics in diabetic nephropathy. J. Clin. Invest. 126 (11), 4205–4218. doi:10.1172/jci87927
Lu, M., Yin, N., Liu, W., Cui, X., Chen, S., and Wang, E. (2017). Curcumin ameliorates diabetic nephropathy by suppressing NLRP3 inflammasome signaling. Biomed. Res. Int. 2017, 1516985. doi:10.1155/2017/1516985
Lu, Q., Li, C., Chen, W., Shi, Z., Zhan, R., and He, R. (2018). Clinical efficacy of Jinshuibao capsules combined with angiotensin receptor blockers in patients with early diabetic nephropathy: A meta-analysis of randomized controlled trials. Evid. Based. Complement. Altern. Med. 2018, 6806943. doi:10.1155/2018/6806943
Luo, Y., Yang, S. K., Zhou, X., Wang, M., Tang, D., Liu, F. Y., et al. (2015). Use of Ophiocordyceps sinensis (syn. Cordyceps sinensis) combined with angiotensin-converting enzyme inhibitors (ACEI)/angiotensin receptor blockers (ARB) versus ACEI/ARB alone in the treatment of diabetic kidney disease: a meta-analysis. Ren. Fail. 37 (4), 614–634. doi:10.3109/0886022x.2015.1009820
Ma, T., Zheng, Z., Guo, H., Lian, X., Rane, M. J., Cai, L., et al. (2019). 4-O-methylhonokiol ameliorates type 2 diabetes-induced nephropathy in mice likely by activation of AMPK-mediated fatty acid oxidation and Nrf2-mediated anti-oxidative stress. Toxicol. Appl. Pharmacol. 370, 93–105. doi:10.1016/j.taap.2019.03.007
Ma, L., Wu, F., Shao, Q., Chen, G., Xu, L., and Lu, F. (2021). Baicalin alleviates oxidative stress and inflammation in diabetic nephropathy via Nrf2 and MAPK signaling pathway. Drug Des. devel. Ther. 15, 3207–3221. doi:10.2147/dddt.S319260
Malek, V., Suryavanshi, S. V., Sharma, N., Kulkarni, Y. A., Mulay, S. R., and Gaikwad, A. B. (2021). Potential of renin-angiotensin-aldosterone system modulations in diabetic kidney disease: Old players to new hope. Rev. Physiol. Biochem. Pharmacol. 179, 31–71. doi:10.1007/112_2020_50
Marques, C., Gonçalves, A., Pereira, P. M. R., Almeida, D., Martins, B., Fontes-Ribeiro, C., et al. (2019). The dipeptidyl peptidase 4 inhibitor sitagliptin improves oxidative stress and ameliorates glomerular lesions in a rat model of type 1 diabetes. Life Sci. 234, 116738. doi:10.1016/j.lfs.2019.116738
Müller, T. D., Finan, B., Bloom, S. R., D'Alessio, D., Drucker, D. J., Flatt, P. R., et al. (2019). Glucagon-like peptide 1 (GLP-1). Mol. Metab. 30, 72–130. doi:10.1016/j.molmet.2019.09.010
Parving, H. H., Brenner, B. M., McMurray, J. J., de Zeeuw, D., Haffner, S. M., Solomon, S. D., et al. (2012). Cardiorenal end points in a trial of aliskiren for type 2 diabetes. N. Engl. J. Med. 367 (23), 2204–2213. doi:10.1056/NEJMoa1208799
Peng, C. H., Lin, H. C., Lin, C. L., Wang, C. J., and Huang, C. N. (2019). Abelmoschus esculentus subfractions improved nephropathy with regulating dipeptidyl peptidase-4 and type 1 glucagon-like peptide receptor in type 2 diabetic rats. J. Food Drug Anal. 27 (1), 135–144. doi:10.1016/j.jfda.2018.07.004
Pitt, B., Filippatos, G., Agarwal, R., Anker, S. D., Bakris, G. L., Rossing, P., et al. (2021). Cardiovascular events with finerenone in kidney disease and type 2 diabetes. N. Engl. J. Med. 385 (24), 2252–2263. doi:10.1056/NEJMoa2110956
Qi, M. Y., He, Y. H., Cheng, Y., Fang, Q., Ma, R. Y., Zhou, S. J., et al. (2021). Icariin ameliorates streptozocin-induced diabetic nephropathy through suppressing the TLR4/NF-κB signal pathway. Food Funct. 12 (3), 1241–1251. doi:10.1039/d0fo02335c
Qiao, C., Ye, W., Li, S., Wang, H., and Ding, X. (2018). Icariin modulates mitochondrial function and apoptosis in high glucose-induced glomerular podocytes through G protein-coupled estrogen receptors. Mol. Cell. Endocrinol. 473, 146–155. doi:10.1016/j.mce.2018.01.014
Qin, X., Zhao, Y., Gong, J., Huang, W., Su, H., Yuan, F., et al. (2019). Berberine protects glomerular podocytes via inhibiting drp1-mediated mitochondrial fission and dysfunction. Theranostics 9 (6), 1698–1713. doi:10.7150/thno.30640
Qin, X., Jiang, M., Zhao, Y., Gong, J., Su, H., Yuan, F., et al. (2020). Berberine protects against diabetic kidney disease via promoting PGC-1α-regulated mitochondrial energy homeostasis. Br. J. Pharmacol. 177 (16), 3646–3661. doi:10.1111/bph.14935
Ren, D., Zuo, C., and Xu, G. (2019). Clinical efficacy and safety of tripterygium wilfordii Hook in the treatment of diabetic kidney disease stage IV: A meta-analysis of randomized controlled trials. Med. Baltim. 98 (11), e14604. doi:10.1097/md.0000000000014604
Rong, Q., Han, B., Li, Y., Yin, H., Li, J., and Hou, Y. (2021). Berberine reduces lipid accumulation by promoting fatty acid oxidation in renal tubular epithelial cells of the diabetic kidney. Front. Pharmacol. 12, 729384. doi:10.3389/fphar.2021.729384
Shu, A., Du, Q., Chen, J., Gao, Y., Zhu, Y., Lv, G., et al. (2021). Catalpol ameliorates endothelial dysfunction and inflammation in diabetic nephropathy via suppression of RAGE/RhoA/ROCK signaling pathway. Chem. Biol. Interact. 348, 109625. doi:10.1016/j.cbi.2021.109625
Stuart, D., Chapman, M., Rees, S., Woodward, S., and Kohan, D. E. (2013). Myocardial, smooth muscle, nephron, and collecting duct gene targeting reveals the organ sites of endothelin A receptor antagonist fluid retention. J. Pharmacol. Exp. Ther. 346 (2), 182–189. doi:10.1124/jpet.113.205286
Su, J., Gao, C., Xie, L., Fan, Y., Shen, Y., Huang, Q., et al. (2021). Astragaloside II ameliorated podocyte injury and mitochondrial dysfunction in streptozotocin-induced diabetic rats. Front. Pharmacol. 12, 638422. doi:10.3389/fphar.2021.638422
Su, X., Yu, W., Liu, A., Wang, C., Li, X., Gao, J., et al. (2021). San-huang-yi-shen capsule ameliorates diabetic nephropathy in rats through modulating the gut microbiota and overall metabolism. Front. Pharmacol. 12, 808867. doi:10.3389/fphar.2021.808867
Tang, G., Li, S., Zhang, C., Chen, H., Wang, N., and Feng, Y. (2021). Clinical efficacies, underlying mechanisms and molecular targets of Chinese medicines for diabetic nephropathy treatment and management. Acta Pharm. Sin. B 11 (9), 2749–2767. doi:10.1016/j.apsb.2020.12.020
Tang, D., He, W. J., Zhang, Z. T., Shi, J. J., Wang, X., Gu, W. T., et al. (2022). Protective effects of Huang-Lian-Jie-Du Decoction on diabetic nephropathy through regulating AGEs/RAGE/Akt/Nrf2 pathway and metabolic profiling in db/db mice. Phytomedicine. 95, 153777. doi:10.1016/j.phymed.2021.153777
Thomas, M. C., Brownlee, M., Susztak, K., Sharma, K., Jandeleit-Dahm, K. A., Zoungas, S., et al. (2015). Diabetic kidney disease. Nat. Rev. Dis. Prim. 1, 15018. doi:10.1038/nrdp.2015.18
Tian, J., Zhao, L., Zhou, Q., Liu, W., Chen, X., Lian, F., et al. (2015). Efficacy of Shenzhuo formula on diabetic kidney disease: a retrospective study. J. Tradit. Chin. Med. 35 (5), 528–536. doi:10.1016/s0254-6272(15)30135-7
Tonneijck, L., Muskiet, M. H., Smits, M. M., van Bommel, E. J., Heerspink, H. J., van Raalte, D. H., et al. (2017). Glomerular hyperfiltration in diabetes: Mechanisms, clinical significance, and treatment. J. Am. Soc. Nephrol. 28 (4), 1023–1039. doi:10.1681/asn.2016060666
Trembinski, D. J., Bink, D. I., Theodorou, K., Sommer, J., Fischer, A., van Bergen, A., et al. (2020). Aging-regulated anti-apoptotic long non-coding RNA Sarrah augments recovery from acute myocardial infarction. Nat. Commun. 11 (1), 2039. doi:10.1038/s41467-020-15995-2
Wang, Z. S., Gao, F., and Lu, F. E. (2013). Effect of ethanol extract of Rhodiola rosea on the early nephropathy in type 2 diabetic rats. J. Huazhong Univ. Sci. Technol. Med. Sci. 33 (3), 375–378. doi:10.1007/s11596-013-1127-6
Wang, S., Zhao, X., Yang, S., Chen, B., and Shi, J. (2017). Salidroside alleviates high glucose-induced oxidative stress and extracellular matrix accumulation in rat glomerular mesangial cells by the TXNIP-NLRP3 inflammasome pathway. Chem. Biol. Interact. 278, 48–53. doi:10.1016/j.cbi.2017.10.012
Wang, C., Li, L., Liu, S., Liao, G., Li, L., Chen, Y., et al. (2018). GLP-1 receptor agonist ameliorates obesity-induced chronic kidney injury via restoring renal metabolism homeostasis. PLoS One 13 (3), e0193473. doi:10.1371/journal.pone.0193473
Wang, Q., Zhou, J., Xiang, Z., Tong, Q., Pan, J., Wan, L., et al. (2019). Anti-diabetic and renoprotective effects of Cassiae Semen extract in the streptozotocin-induced diabetic rats. J. Ethnopharmacol. 239, 111904. doi:10.1016/j.jep.2019.111904
Wang, K., Zheng, X., Pan, Z., Yao, W., Gao, X., Wang, X., et al. (2020). Icariin prevents extracellular matrix accumulation and ameliorates experimental diabetic kidney disease by inhibiting oxidative stress via GPER mediated p62-dependent Keap1 degradation and Nrf2 activation. Front. Cell Dev. Biol. 8, 559. doi:10.3389/fcell.2020.00559
Wanner, C., Inzucchi, S. E., Lachin, J. M., Fitchett, D., von Eynatten, M., Mattheus, M., et al. (2016). Empagliflozin and progression of kidney disease in type 2 diabetes. N. Engl. J. Med. 375 (4), 323–334. doi:10.1056/NEJMoa1515920
Warren, A. M., Knudsen, S. T., and Cooper, M. E. (2019). Diabetic nephropathy: an insight into molecular mechanisms and emerging therapies. Expert Opin. Ther. Targets 23 (7), 579–591. doi:10.1080/14728222.2019.1624721
Wei, H., Wang, L., An, Z., Xie, H., Liu, W., Du, Q., et al. (2021). QiDiTangShen granules modulated the gut microbiome composition and improved bile acid profiles in a mouse model of diabetic nephropathy. Biomed. Pharmacother. 133, 111061. doi:10.1016/j.biopha.2020.111061
Winther, S. A., Øllgaard, J. C., Tofte, N., Tarnow, L., Wang, Z., Ahluwalia, T. S., et al. (2019). Utility of plasma concentration of trimethylamine N-oxide in predicting cardiovascular and renal complications in individuals with type 1 diabetes. Diabetes Care 42 (8), 1512–1520. doi:10.2337/dc19-0048
Winther, S. A., Henriksen, P., Vogt, J. K., Hansen, T. H., Ahonen, L., Suvitaival, T., et al. (2020). Gut microbiota profile and selected plasma metabolites in type 1 diabetes without and with stratification by albuminuria. Diabetologia 63 (12), 2713–2724. doi:10.1007/s00125-020-05260-y
Wu, D., Yang, X., Zheng, T., Xing, S., Wang, J., Chi, J., et al. (2016). A novel mechanism of action for salidroside to alleviate diabetic albuminuria: effects on albumin transcytosis across glomerular endothelial cells. Am. J. Physiol. Endocrinol. Metab. 310 (3), E225–E237. doi:10.1152/ajpendo.00391.2015
Wu, X., Huang, Y., Zhang, Y., He, C., Zhao, Y., Wang, L., et al. (2020). Efficacy of tripterygium glycosides combined with ARB on diabetic nephropathy: a meta-analysis. Biosci. Rep. 40 (11), BSR20202391. doi:10.1042/bsr20202391
Xia, X., Wang, X., Wang, H., Lin, Z., Shao, K., Xu, J., et al. (2021). Ameliorative effect of white tea from 50-year-old tree of Camellia sinensis L. (Theaceae) on kidney damage in diabetic mice via SIRT1/AMPK pathway. J. Ethnopharmacol. 272, 113919. doi:10.1016/j.jep.2021.113919
Xie, T., Chen, X., Chen, W., Huang, S., Peng, X., Tian, L., et al. (2021). Curcumin is a potential adjuvant to alleviates diabetic retinal injury via reducing oxidative stress and maintaining Nrf2 pathway homeostasis. Front. Pharmacol. 12, 796565. doi:10.3389/fphar.2021.796565
Xing, L., Fang, J., Zhu, B., Wang, L., Chen, J., Wang, Y., et al. (2021). Astragaloside IV protects against podocyte apoptosis by inhibiting oxidative stress via activating PPARγ-Klotho-FoxO1 axis in diabetic nephropathy. Life Sci. 269, 119068. doi:10.1016/j.lfs.2021.119068
Xu, J., Liu, L., Gan, L., Hu, Y., Xiang, P., Xing, Y., et al. (2021). Berberine acts on C/EBPβ/lncRNA gas5/miR-18a-5p loop to decrease the mitochondrial ROS generation in HK-2 cells. Front. Endocrinol. 12, 675834. doi:10.3389/fendo.2021.675834
Xu, W. L., Liu, S., Li, N., Ye, L. F., Zha, M., Li, C. Y., et al. (2021). Quercetin antagonizes glucose fluctuation induced renal injury by inhibiting aerobic glycolysis via HIF-1α/miR-210/ISCU/FeS pathway. Front. Med. 8, 656086. doi:10.3389/fmed.2021.656086
Xuan, C., Xi, Y. M., Zhang, Y. D., Tao, C. H., Zhang, L. Y., and Cao, W. F. (2021). Yiqi Jiedu Huayu decoction alleviates renal injury in rats with diabetic nephropathy by promoting autophagy. Front. Pharmacol. 12, 624404. doi:10.3389/fphar.2021.624404
Xue, H., Li, P., Luo, Y., Wu, C., Liu, Y., Qin, X., et al. (2019). Salidroside stimulates the Sirt1/PGC-1α axis and ameliorates diabetic nephropathy in mice. Phytomedicine 54, 240–247. doi:10.1016/j.phymed.2018.10.031
Yale, J. F., Bakris, G., Cariou, B., Yue, D., David-Neto, E., Xi, L., et al. (2013). Efficacy and safety of canagliflozin in subjects with type 2 diabetes and chronic kidney disease. Diabetes Obes. Metab. 15 (5), 463–473. doi:10.1111/dom.12090
Yale, J. F., Bakris, G., Cariou, B., Nieto, J., David-Neto, E., Yue, D., et al. (2014). Efficacy and safety of canagliflozin over 52 weeks in patients with type 2 diabetes mellitus and chronic kidney disease. Diabetes Obes. Metab. 16 (10), 1016–1027. doi:10.1111/dom.12348
Yamada, T., Wakabayashi, M., Bhalla, A., Chopra, N., Miyashita, H., Mikami, T., et al. (2021). Cardiovascular and renal outcomes with SGLT-2 inhibitors versus GLP-1 receptor agonists in patients with type 2 diabetes mellitus and chronic kidney disease: a systematic review and network meta-analysis. Cardiovasc. Diabetol. 20 (1), 14. doi:10.1186/s12933-020-01197-z
Yan, M., Wen, Y., Yang, L., Wu, X., Lu, X., Zhang, B., et al. (2016). Chinese herbal medicine tangshen formula treatment of patients with type 2 diabetic kidney disease with macroalbuminuria: Study protocol for a randomized controlled trial. Trials 17 (1), 259. doi:10.1186/s13063-016-1385-2
Yang, X., Zhang, B., Lu, X., Yan, M., Wen, Y., Zhao, T., et al. (2016). Effects of tangshen formula on urinary and plasma liver-type fatty acid binding protein levels in patients with type 2 diabetic kidney disease: Post-hoc findings from a multi-center, randomized, double-blind, placebo-controlled trial investigating the efficacy and safety of tangshen formula in patients with type 2 diabetic kidney disease. BMC Complement. Altern. Med. 16, 246. doi:10.1186/s12906-016-1228-4
Yang, X. H., Pan, Y., Zhan, X. L., Zhang, B. L., Guo, L. L., and Jin, H. M. (2016). Epigallocatechin-3-gallate attenuates renal damage by suppressing oxidative stress in diabetic db/db mice. Oxid. Med. Cell. Longev. 2016, 2968462. doi:10.1155/2016/2968462
Yang, X., Hu, C., Wang, S., and Chen, Q. (2020). Clinical efficacy and safety of Chinese herbal medicine for the treatment of patients with early diabetic nephropathy: A protocol for systematic review and meta-analysis. Med. Baltim. 99 (29), e20678. doi:10.1097/md.0000000000020678
Yang, G., Wei, J., Liu, P., Zhang, Q., Tian, Y., Hou, G., et al. (2021). Role of the gut microbiota in type 2 diabetes and related diseases. Metabolism. 117, 154712. doi:10.1016/j.metabol.2021.154712
Yao, L., Li, J., Li, L., Li, X., Zhang, R., Zhang, Y., et al. (2019). Coreopsis tinctoria Nutt ameliorates high glucose-induced renal fibrosis and inflammation via the TGF-β1/SMADS/AMPK/NF-κB pathways. BMC Complement. Altern. Med. 19 (1), 14. doi:10.1186/s12906-018-2410-7
Yu, S., Zhao, H., Yang, W., Amat, R., Peng, J., Li, Y., et al. (2019). The alcohol extract of Coreopsis tinctoria Nutt ameliorates diabetes and diabetic nephropathy in db/db mice through miR-192/miR-200b and PTEN/AKT and ZEB2/ECM pathways. Biomed. Res. Int. 2019, 5280514. doi:10.1155/2019/5280514
Zang, Y., Liu, S., Cao, A., Shan, X., Deng, W., Li, Z., et al. (2021). Astragaloside IV inhibits palmitic acid-induced apoptosis through regulation of calcium homeostasis in mice podocytes. Mol. Biol. Rep. 48 (2), 1453–1464. doi:10.1007/s11033-021-06204-4
Zang, L., Gao, F., Huang, A., Zhang, Y., Luo, Y., Chen, L., et al. (2022). Icariin inhibits epithelial mesenchymal transition of renal tubular epithelial cells via regulating the miR-122-5p/FOXP2 axis in diabetic nephropathy rats. J. Pharmacol. Sci. 148 (2), 204–213. doi:10.1016/j.jphs.2021.10.002
Zhang, L., Li, P., Xing, C. Y., Zhao, J. Y., He, Y. N., Wang, J. Q., et al. (2014). Efficacy and safety of Abelmoschus manihot for primary glomerular disease: a prospective, multicenter randomized controlled clinical trial. Am. J. Kidney Dis. 64 (1), 57–65. doi:10.1053/j.ajkd.2014.01.431
Zhang, J., Cao, P., Gui, J., Wang, X., Han, J., Wang, Y., et al. (2019). Arctigenin ameliorates renal impairment and inhibits endoplasmic reticulum stress in diabetic db/db mice. Life Sci. 223, 194–201. doi:10.1016/j.lfs.2019.03.037
Zhang, L., Yang, L., Shergis, J., Zhang, L., Zhang, A. L., Guo, X., et al. (2019). Chinese herbal medicine for diabetic kidney disease: a systematic review and meta-analysis of randomised placebo-controlled trials. BMJ Open 9 (4), e025653. doi:10.1136/bmjopen-2018-025653
Zhang, T., Chi, Y., Kang, Y., Lu, H., Niu, H., Liu, W., et al. (2019). Resveratrol ameliorates podocyte damage in diabetic mice via SIRT1/PGC-1α mediated attenuation of mitochondrial oxidative stress. J. Cell. Physiol. 234 (4), 5033–5043. doi:10.1002/jcp.27306
Zhang, N. N., Kang, J. S., Liu, S. S., Gu, S. M., Song, Z. P., Li, F. X., et al. (2020). Flavanomarein inhibits high glucose-stimulated epithelial-mesenchymal transition in HK-2 cells via targeting spleen tyrosine kinase. Sci. Rep. 10 (1), 439. doi:10.1038/s41598-019-57360-4
Zhang, Q., He, L., Dong, Y., Fei, Y., Wen, J., Li, X., et al. (2020). Sitagliptin ameliorates renal tubular injury in diabetic kidney disease via STAT3-dependent mitochondrial homeostasis through SDF-1α/CXCR4 pathway. Faseb J. 34 (6), 7500–7519. doi:10.1096/fj.201903038R
Zhang, Q., Liu, X., Sullivan, M. A., Shi, C., and Deng, B. (2021a). Protective effect of yi shen Pai Du formula against diabetic kidney injury via inhibition of oxidative stress, inflammation, and epithelial-to-mesenchymal transition in db/db mice. Oxid. Med. Cell. Longev. 2021, 7958021. doi:10.1155/2021/7958021
Zhang, Q., Zhang, Y., Zeng, L., Chen, G., Zhang, L., Liu, M., et al. (2021b). The role of gut microbiota and microbiota-related serum metabolites in the progression of diabetic kidney disease. Front. Pharmacol. 12, 757508. doi:10.3389/fphar.2021.757508
Zhang, M., Yang, L., Zhu, M., Yang, B., Yang, Y., Jia, X., et al. (2022). Moutan Cortex polysaccharide ameliorates diabetic kidney disease via modulating gut microbiota dynamically in rats. Int. J. Biol. Macromol. 206, 849–860. doi:10.1016/j.ijbiomac.2022.03.077
Zhao, T., Zhang, H., Yin, X., Zhao, H., Ma, L., Yan, M., et al. (2020). Tangshen formula modulates gut Microbiota and reduces gut-derived toxins in diabetic nephropathy rats. Biomed. Pharmacother. 129, 110325. doi:10.1016/j.biopha.2020.110325
Zhao, J., Tostivint, I., Xu, L., Huang, J., Gambotti, L., Boffa, J. J., et al. (2022). Efficacy of combined Abelmoschus manihot and irbesartan for reduction of albuminuria in patients with type 2 diabetes and diabetic kidney disease: A multicenter randomized double-blind parallel controlled clinical trial. Diabetes Care 45 (7), e113–e115. doi:10.2337/dc22-0607
Zhong, Y., Lee, K., Deng, Y., Ma, Y., Chen, Y., Li, X., et al. (2019). Arctigenin attenuates diabetic kidney disease through the activation of PP2A in podocytes. Nat. Commun. 10 (1), 4523. doi:10.1038/s41467-019-12433-w
Zhou, G., Johansson, U., Peng, X. R., Bamberg, K., and Huang, Y. (2016). An additive effect of eplerenone to ACE inhibitor on slowing the progression of diabetic nephropathy in the db/db mice. Am. J. Transl. Res. 8 (3), 1339–1354.
Zhou, J., Pan, J., Xiang, Z., Wang, Q., Tong, Q., Fang, J., et al. (2020). Xiaokeyinshui extract combination, a berberine-containing agent, exerts anti-diabetic and renal protective effects on rats in multi-target mechanisms. J. Ethnopharmacol. 262, 113098. doi:10.1016/j.jep.2020.113098
Keywords: diabetic kidney disease, Chinese herbal medicine, therapeutic mechanism, clinical application, metabolism regulation
Citation: Chen D-Q, Wu J and Li P (2022) Therapeutic mechanism and clinical application of Chinese herbal medicine against diabetic kidney disease. Front. Pharmacol. 13:1055296. doi: 10.3389/fphar.2022.1055296
Received: 27 September 2022; Accepted: 24 October 2022;
Published: 03 November 2022.
Edited by:
Divya Bhatia, Cornell University, United StatesReviewed by:
Krishna Murthy Nakuluri, The University of Iowa, United StatesPing Fu, Sichuan University, China
Copyright © 2022 Chen, Wu and Li. This is an open-access article distributed under the terms of the Creative Commons Attribution License (CC BY). The use, distribution or reproduction in other forums is permitted, provided the original author(s) and the copyright owner(s) are credited and that the original publication in this journal is cited, in accordance with accepted academic practice. No use, distribution or reproduction is permitted which does not comply with these terms.
*Correspondence: Dan-Qian Chen, Y2hlbmRhbnFpYW4yMDEzQDE2My5jb20=; Ping Li, bHA4Njc1QDE2My5jb20=