- 1Department of Biosciences, Manipal University Jaipur, Jaipur, India
- 2School of Science, Nirwan University, Jaipur, India
Dengue has become a worldwide affliction despite incessant efforts to search for a cure for this long-lived disease. Optimistic consequences for dengue vaccine are implausible as the efficiency is tied to previous dengue virus (DENV) exposure and a very high cost is required for large-scale production of vaccine. Medicinal plants are idyllic substitutes to fight DENV infection since they constitute important components of traditional medicine and show antiviral properties, although the mechanism behind the action of bioactive compounds to obstruct viral replication is less explored and yet to be discovered. This review includes the existing traditional knowledge on how DENV infects and multiplies in the host cells, conscripting different medicinal plants that obtained bioactive compounds with anti-dengue properties, and the probable mechanism on how bioactive compounds modulate the host immune system during DENV infection. Moreover, different plant species having such bioactive compounds reported for anti-DENV efficiency should be validated scientifically via different in vitro and in vivo studies.
1 Introduction
Mosquito-borne diseases cause illness in approximately 700 million people per year globally (Genoud et al., 2018). Dengue is one of the mosquito-borne emerging viral disease endemics prominent in many urban areas of tropical countries. A considerable rise in the dengue infection incidences is noticed all over the world in recent decades. Due to its asymptomatic to mild infectious nature, actual numbers of dengue cases remain unregistered or wrongly diagnosed as other fever infections (Waggoner et al., 2016). According to the World Health Organization report, about 390 million cases of dengue virus (DENV) infections occur annually, of which nearly 96 million find medical treatment and about 20,000 individuals (mostly children and aged individuals) fail to survive the dengue infection (Bhatt et al., 2013; Saxena et al., 2017; World Health Organization 2021a). Remarkably, among 129 countries having a risk of dengue infection, Asia has almost 70% of the total infection load (Brady et al., 2012; Bhatt et al., 2013). Dengue, an arthropod-carried viral infection, is transmitted into humans by female Aedes mosquitoes (Ae. aegypti or, to a lesser extent, by Ae. albopictus and Ae. polynesiensis) (Cao-Lormeau 2009). Common symptoms in dengue infection are mild dengue fever to severe dengue hemorrhagic fever (i.e., DF and DHF), rashes, headache, vomiting, severe headache, low white blood cell count, joint and muscle pain, swollen glands, nausea, pain behind the eyes, and fever with dengue shock syndrome (DSS) (Murphy and Whitehead 2011; World Health Organization 2021a).
The natural reserve has offered various resources to mankind to combat various infectious diseases. Plants are the most frequently utilized natural sources among the local biodiversity and are linked to folk’s day-to-day needs (Robinson and Zhang 2011). Additionally, plant-based medicaments are in considerable demand because of their safer use and non-toxic nature, and because they are less harmful in comparison to synthetic treatments (Abd Kadir et al., 2013). Multiple uses, such as consumption as food, ethnomedicinal applications, cultural aspects, and sacred faith, are associated with their utilization (Svanberg and Berggren 2019; Fongnzossie et al., 2020). Traditional knowledge of plants that was commonly practiced among tribal or ethnic populations for their healthcare has now gained considerable attention from the modern populace. Nowadays, people in developing (60%–90%) as well as developed countries (23%–80%) are using ethno-medicines as the primary healthcare regimen (Borah and Prasad 2017; Sharma and Pareek 2021). Plant biodiversity used as medicaments is of utmost importance throughout human history, which has led to the accumulation of significant information in the form of scientific research and its validation (Pasa 2011). These conventional plant-based healthcare remedies led the Ayurvedic, Unani, Chinese, and Egyptian medical systems to classify various medicinal herbs (based on color, aroma, shape, flavor, and astronomic and magical attributes) (Larocca et al., 2021). Traditional use and ethnobotanical knowledge about many plant species in treating various ailments along their chemical validation through scientific research can be promising aspects in the development of contemporary medication to treat arboviral infections like dengue. Also, a plant-based antiviral product assures a more possible choice in combating dengue infection, which may be replacement of inadequate drugs with side effects (Bahuguna et al., 2019). WHO in the 1970s has effectively executed the spread of traditional knowledge via implementing Traditional Medicine Program, and consequently, following such initiative (taking into consideration the traditional knowledge and scientific research), the associated nations have been redeveloping and improving their public health systems (Larocca et al., 2021). Development of an efficient and safer anti-dengue vaccine is a challenging task, and because of its secondary infection (second time DENV serotype infection), it is linked to the serious clinical manifestations (Toman 2018; Redoni et al., 2020). Based on recent research, various plants and their derivatives have shown anti-dengue properties; among those, flavonoids are the most popular candidates, having the ability to supplement encouraging scope in the existing struggle of drug discovery (Carneiro et al., 2016). Some of the important flavonoids recently reported are discussed with various activities, such as antioxidative, anti-inflammatory, antitumoral, antiviral, and antibacterial effects (Raekiansyah et al., 2018; Deng et al., 2020b; Daneshzadeh et al., 2020; Macedo et al., 2020; Wang et al., 2020). In the present review, a brief idea of the role of these phytoconstituents has been described against dengue infections (Jayadevappa et al., 2020). In addition, the mechanism of action with future possibilities as contemporary medicaments of plant metabolites that endorses traditional knowledge is also included.
2 Epidemiology of Dengue
Dengue is a mosquito-borne viral infection, found in tropical and sub-tropical climates worldwide, mostly in urban and semi-urban areas, transmitted by Aedes aegypti and Aedes albopictus, known as the primary and secondary dengue vectors. A range of diseases has been transmitted via Ae. aegypti including yellow fever, chikungunya, zika, and, most importantly, DENV (Silva et al., 2020). DENV was first reported in the 1950s, during dengue epidemics in Thailand and Philippines. Since then, the spread of dengue has extensively widened from South Asian countries to African and South American countries. Over several decades, different countries of continental America conducted a program that aimed to eradicate Ae. aegypti. The program was proposed and commenced via the Pan American Health Organization (PAHO) in 1946 (Pinheiro and Rodrigues 1999). Venezuela, a country on the northern coast of South America, reported dengue fever outbreaks during the 1960s, when almost all South American countries had eradicated Ae. aegypti (Pinheiro and Corber 1997). Furthermore, during the 1970s, DENV-2 and DENV-3 were the main causative serotypes for dengue fever epidemics in Colombia, a country that had attained eradication of the vector during the PAHO program (Pinheiro 1997). In the 1980s, enhanced rate of dengue cases escorted the spreading of Ae. aegypti vector, and during this period, another efficient vector, the Asian mosquito, Ae. albopictus, was introduced in the region (Gubler 1989). In the last 5 decades, the American continent has been massively affected by dengue and an approximately 30-fold rise in disease level was recorded, where almost 390 million cases of dengue with 96 million medical manifestations were reported annually (Bhatt et al., 2013). In 2013, America has declared approximately 2.3 million new severe dengue cases, which was an alarming situation in viral infections (Salles et al., 2018).
Ae. albopictus was mostly found in Asia and further expanded over different countries of European and North American regions, having highly adaptive properties to survive extreme environments and therefore able to survive in cooler regions as well. The probability of an upsurge of arthropod-borne viruses is based on the abundance of vectors involved in transmission as well as the susceptibility of immune-deficient people. Hence, there is a necessity to develop an efficient way to control its population and the spread of the disease. Besides, several other causes such as increased urbanization, ecological changes, migration of people, exchange of goods, and biological contests (e.g., development of resistance for virus) stimulate the spread of disease to new regions (Chaudhry 2019).
3 Replicative Cycle and Pathogenesis of Virus
The genomic structure of DENV, approximately 10.7 kb in size, consists of ssRNA with +ve polarity (Chambers et al., 1990). Besides, the translation of viral genome was completed by three different structural proteins, i.e., Envelop (E), Capsid (C), and Pre-membrane (PrM), and seven non-structural (NS) proteins, NS1, NS2a, NS2b, NS3, NS4a, NS4b, and NS5. These proteins contribute to viral replication (Bartenschlager and Miller 2008; Xie et al., 2017). The infective form of dengue virion possesses a core of glycoprotein and ssRNA genome enclosed by icosahedral nucleocapsid (Akhtar 2019). Firstly, infected mosquito feed on host and DENV gets transmitted via injecting its saliva into the host. In the meantime, when half-length proboscis is inside the dermis, the vector releases DENV plaque-forming units (nearly 50,000) and causes infection in contiguous skin cells, i.e., Langerhans and keratinocytes (Marcial-Juárez et al., 2017; Schneider et al., 2021). Dendritic cells enter lymphatics after capturing DENV virions or antigens and transport them to local draining lymph nodes. DENV is also suggested to reach draining lymph nodes via the lymphatic flow in a cell-free manner (Yam-Puc et al., 2016). The E protein of DENV assists the binding between DENV and the cell membrane receptor. Receptor-mediated endocytosis is the principal way via which the virus is able to enter the host; a resultant sac-like structure is formed known as an endosome, depending on pH. Virus acutely penetrates and fuse with the membrane of the endosome due to the various irretrievable morphological reorganization as the endocytic vesicles become more acidic, followed by nucleocapsid opening and genetic material (RNA) release into the cytoplasm. RNA succeeds in translating into ribosomes allied to the ER (endoplasmic reticulum) by using the infected cell’s machinery; subsequently, the viral polyprotein is cleaved by cellular and viral proteases (Uno and Ross 2018). The newly synthesized RNA is wrapped in the capsid proteins (nucleocapsid), enters the host rER, and ultimately encloses the ER membrane. Further structure proteins (M and E) surround the ER-enveloped nucleocapsid forming immature virus. Subsequent processing in the Golgi apparatus results in the formation of the infectious form of the virus (Aruna 2014). Afterwards, it reaches the lymph node and further disseminates to other body organs. The virus incubation period may vary from 3 to 14 days based on the literature available (Chan and Johansson 2012). A general overview of the DENV infection cycle in humans is shown in Figure 1. After completion of the virus incubation period, the mosquito is capable of spreading the virus in the course of its life. Ae. aegypti feeds mostly on human blood and is a daytime feeder, efficiently causing infection in the early morning, and by evening, it infects multiple people during each suckling period (Weissenböck et al., 2010). The infecting efficiency of DENV for different cells including liver, blood vessels (endothelium), immune system, and retinal cells has been reported in the past (Carr et al., 2017; Begum et al., 2019).
3.1 Dengue Infection
The occurrence of dengue has intensely spread worldwide in recent decades with a majority of asymptomatic cases (World Health Organization, 2021a). The infection efficiency can vary from subclinical disease to acute flu-like symptoms. Three different categories of symptomatic dengue, namely, dengue fever (DF), dengue hemorrhagic fever (DHF), and undifferentiated fever, were classified by WHO in 1997. Due to the changing epidemiology of infection, it was difficult to fulfill the suggested WHO guidelines (for dengue), and thus, classification was re-evaluated (World Health Organization 2009). In the new classification system, DHF was further categorized up to four levels based on severity of infection, with grades III and IV as DSS. Dengue asymptomatic cases were divided into two different categories: with warning and without warning signs of severe dengue (World Health Organization 2009). Dengue is associated with several complications, which are characterized by headache, appetite loss, high fever, retro-orbital pain, vomiting, abdominal pain, diarrhea, minor bleeding, rash, fatigue, etc. Severe dengue is classified by the presence of severe plasma bleeding, organ impairment, or dengue shock conditions (Khan and Bhutta 2016). It is also designated as “break-bone fever” (Rigau-Pérez 2006; Esler 2009). The severity of infection can be strongly provoked by numerous factors, such as virus serotypes, immunity power, and genomic background of host, among others (Rico-Hesse 2010; Dussart et al., 2012). Moreover, four different serotypes of viruses (DENV-1, DENV-2, DENV-3, and DENV-4) of the Flaviviridae family were deliberated as causal forms of dengue (Guzman and Istúriz 2010). Although every serotype has an equal potential to cause infection among the four serotypes of dengue, serotype variances that only affect pathogenesis such as DENV-2 have been associated with severe infection (Halstead 1988; Vaughn et al., 2000).
Recovery from infection provides enduring immunity against that serotype, although immunity for other serotypes known as cross-immunity is temporary and partial. Consequent contagions (secondary infection) by different serotypes increase the threat of emerging severe dengue. Dengue has discrete epidemiological forms, allied with the four serotypes of the virus (Halstead 2002; Rico-Hesse 2010; Dussart et al., 2012; Kim and Hwang 2020). Different studies based on the data analysis of infection status among the Indian population have been reported (Table 1). Despite this, numerous cases have been found in different regions of India, which is still unexplored in terms of proper data illustrations.
4 Mode of Transmission
Aedes spp. mosquitoes (Ae. aegypti and Ae. albopictus) are the main source of dengue transmission. Recently, infection dispersed in almost all parts of tropical and subtropical regions and adapted to urban environments, mainly Ae. aegypti (Lambrechts et al., 2010). Among two different species, Ae. aegypti has been reported to have superior efficiency as a transmission vector; meanwhile, in urban environments, DENV is capable to endure its life cycle between humans and transmitter organisms (Lambrechts et al., 2010; Weissenböck et al., 2010). Human blood is the primary feeding source of Ae. aegypti, while Ae. albopictus (sylvatic strain) feeds on avian species as well as a variety of mammals (European Centre for Disease Prevention and Control 2018). Sylvatic strains are not that risky compared to “standard” dengue infection (Diallo et al., 2003; Vasilakis et al., 2008). In the initial stage of transmission, uninfected mosquitoes attack (susceptible to dengue) while feeding on host blood. In this process, viral particles are transferred into the mosquito midgut and start replicating and thus infecting the body cavity (hemocoel). From the hemocoel, the virus eventually makes its way to the salivary glands. Next, after completing the incubation period (approximately 2 weeks), the infected mosquito can disseminate the virus via salivary glands (Coudeville et al., 2009; Guzman et al., 2016).
5 Impact of Dengue Infection Globally and in India
Dengue has emerged as one of the most important mosquito-borne, flaviviruses diseases, deceptively intensifying as a global health issue (Zeng et al., 2021). Dengue has been designated as the topmost worldwide health risk by WHO (Kumar et al., 2017; Biswal et al., 2019). A comparison of prior approximations of entire global dengue contagions has been illustrated. Average incidences in India with maximum infected states (Karnataka, Punjab, Tamil Nadu, and Maharashtra) are shown (Alagarasu et al., 2021) (Figure 2). Moreover, dengue infection frequency of different Asian countries and their percentage contribution have been determined where India was found to comprise 9% of total dengue incidences, higher than Pakistan, Singapore, China, Thailand, Nepal, and Cambodia, while Vietnam and Philippines are the leading countries and recorded the highest number of dengue cases in 2021 (till May) (Figure 3A) (European Centre for Disease Prevention and Control 2018; World Health Organization 2021b). Also, dengue infection status recorded in the past 7 years in India can be seen where the average infection was recorded to be the maximum in 2017 (Figure 3B) (NVBDCP, 2021).
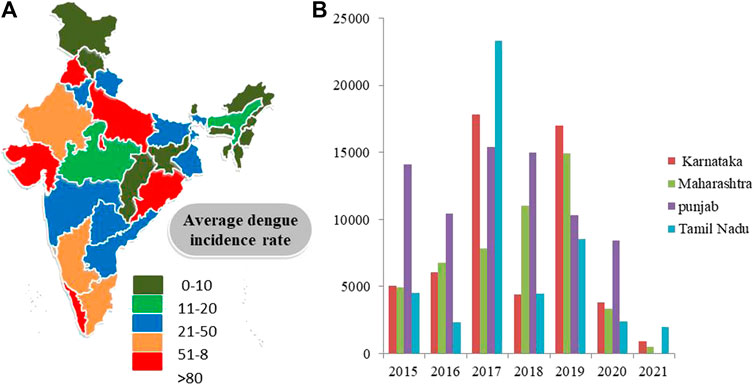
FIGURE 2. (A) Dengue cases in different regions of India 2021. (B) State-wise distribution of dengue cases (y-axis) in four states of India from 2015 to 2021 (x-axis) (Source: National Vector Borne disease Control Programme).
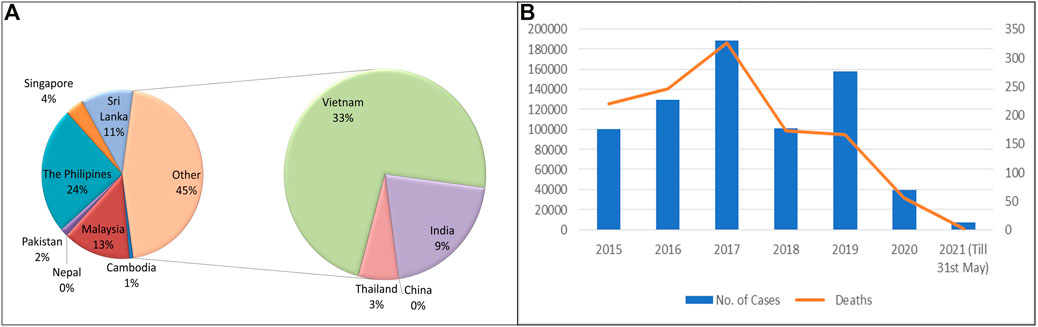
FIGURE 3. (A) Comparative analysis of dengue incidences in different Asian countries (Source: WHO and European Center for disease Prevention and Control). (B) Number of dengue cases and deaths in India (2015–2021 May). (Source: National Vector Borne disease Control Programme).
6 Plant Medicaments Used Based on Traditional Knowledge
Plants have been the main remedial source to cure a variety of ailments since ancient times. Ancestral folks empirically isolate medicinal plants for medical home remedies and pass this knowledge from generation to generation till the present day. Ethnobotany has confirmed the necessity for conserving such information in societies that utilize plants in the treatment of different types of diseases (Gu et al., 2014; Mussin and Giusiano 2020). However, it is critical to note that these practices of exploiting plant medicaments, without scientific understanding, have led to placing various plants in the IUCN Red List consisting of threatened species (Trujillo-Correa et al., 2019). The dengue treatment period is suggested to be 10–15 days, which may vary according to the infection level of severity, the regimen carried out during treatment, the conditions, and the response of the organism (Yogarajalakshmi et al., 2020). Different plant-based formulations are reported to be used in various affected parts of India against dengue and have also been approved through scientific research, although limited studies in this reference have been recorded in India (Deep et al., 2018). There are many plant species such as Curcuma longa L., Lonicera japonica Thunb., Acorus calamus L., Carica papaya L., Euphorbia hirta L., Tinospora sinensis (Lour.) Merr., and Sambucus canadensis L. used globally as ethnomedicine against dengue fever (Ichsyani et al., 2017; Lee et al., 2017; Deep et al., 2018; Yao et al., 2018; Larocca et al., 2021) (Table 2). In another report, plants from a diverse group of plant families (31 in total with 54 species) are reported to exhibit anti-dengue activity based on traditional knowledge, where major representatives reported were Lamiaceae (10.5%), Asteraceae (9.9%), Aristolochiaceae, and Loganiaceae (each 7.2%) families (Saleh and Kamisah 2021). Plants revealed by the local population to exhibit anti-dengue activity included Baccharis trimera (Less.) DC., Aristolochia surinamensis Willd., Momordica charantia L., Dysphania ambrosioides (L.) Mosyakin and Clemants, Ocimum gratissimum L., Strychnos pseudoquina A. St.-Hil., and Stachytarpheta cayennensis (Rich.) Vahl (Pongthanapisith et al., 2013; Sharma et al., 2020; Larocca et al., 2021). Although many of these plant species do not have any scientific evidence as suggested in the available literature, their ethnomedicinal potential may lead to the real success in treating arboviral diseases like dengue (Pilla et al., 2006; Mendoza et al., 2020). However, limited literature presenting scientific validation of these plants showing anti-dengue activity is available. Traditional use of C. papaya leaf juice has revealed very promising outcomes in treating dengue disease (Prakash Kala, 2012; Sarala and Paknikar 2014), which is further authorized through scientific evidence demonstrating lower cytotoxic and efficient anti-DENV effects (Saptawati et al., 2017; Sharma et al., 2019; Sarker et al., 2021). Similarly, the use of Psidium guajava L. as folkloric medication has also been cited in literature, which enhances thrombocyte count in dengue infection (Berlian et al., 2017). Traditional medicinal values being the foundation for advanced scientific research lead to the revelations of possible mechanisms behind the anti-dengue activities that suggest a promising role of phytoconstituent-associated antiviral activities via inhibition of viral cell adhesion, replication, etc. (Saptawati et al., 2017; Dewi et al., 2020). Other traditionally used plants such as Ocimum tenuiflorum L. (Tulsi), Tinospora sinensis (Lour.) Merr. (Giloy leaves and stems), Trigonella gladiata M. Bieb. (fenugreek leaves), Azadirachta indica A. Juss. (Neem leaves), Cymbopogon citratus (DC.) Stapf (Lemon grass), and Psiloxylon mauritianum (Bouton ex Hook. f) Baill. with potential ethnopharmacological uses in treating dengue still need clarification about their action mechanism technically (Parida et al., 2002; Abd Kadir et al., 2013; Ling et al., 2014; Singh et al., 2016; Deep et al., 2018; Arunabha 2019; Clain et al., 2019; Rosmalena et al., 2019; Paul et al., 2021). There are very few reports validating the antiviral activities of A. indica. According to these reports, aqueous A indica leaf extracts and Gedunin and Pongamol (neem constituents) are capable of repressing DENV-2 serotype by targeting viral replication (Parida et al., 2002) and targeting human as well as viral proteins (responsible for viral attachment) (Rao and Yeturu 2020). Quercus lusitanica Lam. and Gastrodia elata Blume have also been approved to have an antiviral response against DENV-2 replication and DENV multiplication cycle, respectively (Rahman et al., 2006; McDowell et al., 2010; Ahmad et al., 2011). Also, the polyphenol-rich extract of Aphloia theiformis (Vahl) Benn. has recently been reported against ZIKV and DENV infections, which prohibits viral entry (Clain et al., 2018).
7 Plant Extract as Mosquito Repellent and Larvicide
Ae. aegypti and Ae. albopictus, epidemiologically related arboviruses in the community health context, including Zika, dengue, and chikungunya viruses. Different types of synthetic insecticides are easily accessible in the market, associated with numerous side effects such as the development of resistance to these insecticides, having toxic effects on other organs, and ecological health problems (Rodrigues et al., 2020). However, biological control as an alternative for these vectors could be very helpful due to its eco-friendly and cost-effective nature. Govindarajan et al. (2015) reported that methanol extracts of Delonix elata (L.) Gamble leaves and seed (highest concentrations used: 5.0 mg/cm2) offered protection for over 180 and 150 min, respectively, against Ae. aegypti. Herbal oil formulation of different plant species including Eucalyptus globulus Labill., A. indica, Mentha × piperita L., Ocimum basilicum L., and rhizome of Zingiber officinale Roscoe has also been reported as repellents and bite protectors against Ae. aegypti and Ae. albopictus (Nasir et al., 2015). Terpinen-4-ol, 1,8-cineole, and β-pinene (0.4 mg/cm2) isolated from Artemisia vulgaris L. showed up to 91% inhibition of Ae. aegypti (Hwang et al., 1985). Salvia elegans Vahl possess acetate (11.4%), β-caryophyllene (6.4%), and caryophyllene oxide (13.5%), which exhibited good larvicidal activity against Ae. aegypti (Ali et al., 2015). Essential oils (EOs) extracted from the Hazomalania voyronii (Jum.) Capuron dried bark, stem, and wood are being used to attain protection against the mosquito vector Ae. aegypti (Benelli et al., 2020). As per WHO protocol, extracts were prepared using leaves of Lantana camara L., Hyptis suaveolens (L.) Poit., Nerium oleander L., and Tecoma stans (L.) Juss. ex Kunth, revealing effective larvicidal efficiency in contrast to larvae of Ae. aegypti (Hari and Mathew 2018). The chloroform bark extract of Terminalia arjuna (Roxb. ex DC.) Wight and Arn. showed maximum mortality on Ae. aegypti larvae. The LC50 and LC90 values of T. arjuna on Ae. aegypti larvae were 4.61 and 24.17 µg/ml, respectively (Tamilventhan and Jayaprakash 2019).
8 Some Phytoconstituents With Anti-Dengue Activity and Their Action Mechanism
Numerous plants and plant-based products exhibit enormous biological properties (like an antibiotic, antitumor, and antiviral), which are responsible for treating millions of individuals with serious diseases. Among those flavonoids, polysaccharides, hemicelluloses, and hydrophilic colloids are involved in the antiviral properties of plants (Table 3). Some of these biologically active compounds are based on recent research, and their promising role as contemporary medication in the near future has been discussed briefly.
8.1 Castanospermine
Castanospermine (a natural alkaloid) is a derivative of Castanospermum australae A. Cunn. and C. Fraser (black bean) (Whitby et al., 2005) that can easily be isolated through simple purification methods (Pan et al., 1993). One of the possible mechanisms through which it prevents infection is misfolding of viral proteins via obstructing the removal of glucose residues from N-linked glycans (Whitby et al., 2005). This may further lead to inhibit molecular interaction of viral and host proteins (Zhang et al., 1997; Molinari and Helenius 1999). Castanospermine has shown not only inhibitory effects on all the dengue serotypes by preventing the production of DENV but also evidence to prevent mice death when infected with DENV-2 through the intracranial route (Rathore et al., 2011). Evidence of celgosivir (6-O-butanoyl castanospermine) being an antiviral agent against DENV virus both in vitro and in vivo has also been concluded. Castanospermine exhibits glucosidase inhibitor properties that result in early-stage inhibition of glycoprotein processing. As a result, the formation of unstable complexes produces non-productive viral protein (prM and E) folding pathways and ultimately the antiviral response of Castanospermine (Courageot et al., 2000). Celgosivir is the butylated prodrug cleaved in cells to produce castanospermine, a bicyclic iminosugar (Miller et al., 2018). It causes misfolding and accumulation of NS1 (DENV non-structural protein) in the endoplasmic reticulum and also alters host protein response leading to the antiviral activity. Based on the previous research and data availability, castanospermine use in anti-dengue medication can be an important contribution in fighting dengue infection (Rathore et al., 2011).
8.2 Baicalin
Baicalin (a flavonoid) demonstrates considerable antiviral potential against DENV-2 by targeting the replication stages post-viral infection (Zandi et al., 2012). Baicalein is reported to be isolated naturally from the roots of Scutellaria baicalensis Georgi, a medicinal plant of China, and can be further converted into baicalin after intake by animals or humans (Xu et al., 2010). Based on the successful results obtained from Baicalin against dengue infection, other plant species having baicalin as a biomolecule can be explored for their anti-dengue properties. The mechanistic approach behind the anti-dengue properties has been demonstrated by researchers where possible ways of antiviral activity (96–99%) are direct inactivation of free DENV-2 particles, inhibited intracellular viral replication, and binding of DENV-2 cells to the host cell (Moghaddam et al., 2014). In molecular docking analysis, both baicalin and baicalein are reported to inhibit the DENV replication by pursuing key DENV genes and exhibit anti-DENV protease (NS2B/NS3) activity. The results of docking studies show that both baicalein and baicalin may interact with NS3–NS2B, E, and NS5 proteins, which can be responsible for their antiviral impact (Hassandarvish et al., 2016; Rasool et al., 2019). Further investigation using these phytoconstituents is still needed to obtain promising results in the form of contemporary medications and future implementation in clinical use.
8.3 Gallic Acid
Gallic acid (main phenolic plant component) exhibits various pharmacological activities (antioxidant, antiviral, antifungal, etc.) and therapeutic uses against neural disorders, dengue, cancer, asthma, and allergic rhinitis, without any cytotoxic effects (Suganthi and Ravi 2019). In a study, inhibitory response of gallic acid with no cytotoxic effect has been elucidated in in vitro conditions on DENV-2 virus along with another compound, emodin, and some plant extracts, i.e., Antennaria microphylla Rydb., Rubus scaber Weihe, Ziziphus jujuba Mill., and Commelina benghalensis L. Gallic acid was found to be active against dengue disease through prophylactic treatment rather than post-infection medication (Batool et al., 2018), while another report has suggested successful viral inhibition in both prophylactic (52.6%) and post-treatment (67.3%) approaches (Trujillo-Correa et al., 2019). More complete investigations are warranted on these plants for isolation, purification, and characterization of bioactive principles responsible for the anti-dengue activity, and to elucidate their underlying mechanisms of DENV inhibition (Batool et al., 2018). Moreover, isobutyl gallate (a derivative of gallic acid) due to low cellular toxicity and antiviral response at low concentration has been suggested as a good alternative for anti-DENV activity (by inhibiting viral replication) (Dewi et al., 2019). Considering all mentioned findings and inconsistencies about cellular toxicity and anti-dengue effects, more clarification is still needed, which can lead to formulating a successful treatment against the DENV.
8.4 Quercetin
Quercetin (flavonoid) exhibits various pharmacological responses against viruses, tumors, inflammation, etc. In accordance with previous studies available, quercetin is one of the main components of different plant extracts (E. hirta and P. guajava) exerting consistent antiviral effect against dengue infection (DENV-2) (Zandi et al., 2011; Saptawati et al., 2017; Suganthi and Ravi 2019). A possible mechanism is similar to the previously discussed metabolites that inhibit viral replication by targeting RNA polymerase enzyme. Quercetin is reported to exhibit antiviral properties via impeding viral attachment and viral replication against DENV serotypes (1–4) (Zandi et al., 2011; Sharma et al., 2018). In addition, quercetin is one of the main components present in P. guajava leaf extract demonstrating the inhibitory effect on DENV infection with no involvement in the inhibition of viral surface proteins and receptors. These findings also suggest the presence of other bioactive compounds in P. guajava leaf formulations that may exhibit anti-dengue behavior (Dewi et al., 2020). Houttuynia cordata Thunb. leaves ethyl acetate fraction (including quercetin, quercitrin, and rutin) has also been reported, which reveals the efficient anti-DENV-2 activity of bioactive components quercetin (IC50 of 176.76 μg/ml) and quercitrin (IC50 of 467.27 μg/ml). The synergistic effect of both components is also depicted in the past presenting antiviral properties with an IC50 of 176.76 μg/ml (Chiow et al., 2016). These results conclude that plant formulations and their metabolites signify a promising preventative substance in P. guajava L. leaf extract, which might prevent DENV attachment by inhibition of DENV surface protein along with the receptor inhibition (Dewi et al., 2020).
8.5 Epigallocatechin Gallate
Epigallocatechin gallate (flavonoid) and delphinidin (structurally related polyphenol) from plants and their products (wine, green tea, curcumin, and delphinidin) have proved their antiviral qualities regardless of the DENV serotypes. These compounds are suggested to exhibit virucidal effects or inhibit viral production by acting upon the viral life cycle at its attachment and entry points (Vázquez-Calvo et al., 2017; Raekiansyah et al., 2018; Clain et al., 2019). In another study, epigallocatechin gallate, a biologically active component of green tea, curcumin, and delphinidin, is reported to have the least cytotoxic effects. It is believed to prevent viral infection at early stages by direct interaction with the virion (when the virus is preincubated with epigallocatechin gallate), which leads to an approximate drop of 90% in DENV antigen present in intracellular fluid. Similarly, epigallocatechin, another bioactive compound, also has a strong repressive impact on viral growth (Raekiansyah et al., 2018). The inhibition mechanism as suggested by Raekiansyah et al. is to develop a virucidal effect through direct binding with molecules present on the DENV surface (Raekiansyah et al., 2018).
8.6 Other Bioactive Compounds
Apiofuranoside (flavanone glycosides), obtained from Faramea bahiensis Mull. Arg., is efficiently involved in the treatment of dengue via controlling viral replication as well as reducing the number of infected cells (12%) and RNA copies of DENV-2 (67%) in HepG2 cells (Nascimento et al., 2017). Flacourtoside A, a phenolic glycoside exhibited in Flacourtia indica (Burm.f.) Merr., effectively inhibited DENV replication with the 9.3 µM IC50 values (Bourjot et al., 2012). Trigocherrin A and Trigocherriolide B and C (diterpenoids) found in Trigonostemon cherrieri Veillon are also able to impede DENV replication with 12.7 µM and 3.1 and 16.0 µM IC50 values, respectively (Allard et al., 2012). Arrabidaea pulchra (Cham.) L.G. Lohmann synthesizes another important bioactive compound, verbascoside (phenyl glycoside), which is reported to display good anti-DENV-2 (IC50 = 3.4 μg/ml) properties (Brandão et al., 2013). Also, pectolinarin, a type of flavone found in Amphilophium elongatum (Vahl) L.G. Lohmann exhibited anti-DENV-2 effects with an EC50 value of 86.4 μg/ml (Simões et al., 2011).
Note that the action mechanism and the minimal cytotoxicity levels of natural compounds can contribute to the development of anti-dengue medication, which will be safer as well as more effective in use, although limited research has been done in this aspect and there are limited publications. Thus, further investigations are necessary to develop a fruitful antiviral drug (to cure DENV).
9 Immunomodulatory Response of Plant Extracts
With the onset of dengue infection, the host can experience a complex interplay of host immune factors and viral particles, which may include amplified immune cell infection due to the presence of non-deactivating antibodies and stimulation of cross-reactive autoimmunity responses (i.e., activation of T cells and autoantibodies, cytokine deregulation, complement, and coagulation systems) (Jasso-Miranda et al., 2019). Being an acute febrile infection (thrombycytopenia, arthralgia, and hemorrhagic symptoms), it may lead to the host deterioration due to the hemorrhagic attack, plasma leak, intense shock, organ collapse, and ultimately, death (Pan American Health Organization (PAHO) 2016; Jasso-Miranda et al., 2019). Dengue infection is hypothesized to be more fatal due to the antibody-dependent enhancement of infection, which is associated with the transformation of the disease. Two DENV infections in a specific sequence, the interval of two infections, and how much the human host is causative in terms of age, health, ethnicity, and genetic background (Guzman et al., 2013). Generation of cross-reactive antibodies, post-first-time dengue infection in association with the second infecting virus, results in the formation of more harmful immune compounds like non-deactivating antibodies (critical players of the pathogenic response in severe dengue conditions) (Kuczera et al., 2018). This consequently increases the infected cell count and thus increased viral output in the form of high cytokine production, which further causes vascular permeability, intense shock, and, ultimately, death (Puerta-Guardo et al., 2013). Various other immune factors like interferons (IFN-α and -γ), interleukin (IL-6, -8, and -10), and tumor necrosis factors (TNF-α), and elevated expression of cytokine signaling suppressors are linked to severe dengue infections (Bozza et al., 2008; Chen et al., 2014; Flores-Mendoza et al., 2017). Another study revealed a potential immunomodulatory response (against DENV virus) by Schwartzia brasiliensis (Choisy) Bedell ex Gir.-Cañas Choisy extract, which helps in antigen load reduction (NS1 protein, a viral load indicator) in the cells. Crude S. brasiliensis extract was most efficient against dengue activity while the dichloromethane fraction resulted in strong immunoregulatory activities. Downregulation of various immune factors like TNF-α, IFN-α, IL-6, and IL-10 was evidenced as the key reason behind the antiviral effects (Fialho et al., 2017). Significant immunoregulation and antiviral response with Uncaria tomentosa (Willd ex Schult.) DC. fractions have also been demonstrated, in which DENV-infected monocytes (human) were tested. The anti-DENV activity was associated with decreased cytokine levels such as TNF-α and modulation of IL-10 (Reis et al., 2008). Successful molecular assessment and manipulations of various immune responses and associated factors can be useful research to develop anti-dengue drugs. Traditionally, C. papaya leaf extract has been proven for its immunomodulatory activities (Pandey et al., 2016; Anjum et al., 2017; Razak et al., 2021). In dengue patients (suffering from thrombocytopenia), oral administration of papaya leaf extract has resulted in increased platelet count activity (40–48 h) (Subenthiran et al., 2013). In other similar findings with the use of C. papaya leaf extract, beneficial impacts are reported where a significant increase in WBC and platelet count is evidenced with negligible side effects (Hettige 2008; Gowda et al., 2015). Rhodiola imbricata Edgew. (a flowering plant) is also demonstrated to induce pharmacological modifications in response to the DENV infection. It induces NK cells and cytokines like interferon b (IFN), IL-8, IL-1b, IL-6, and TNF-a and upregulates phosphorylated NF-kB, eIF-2a, and PKR in DENV-infected cells (Diwaker et al., 2014). In addition, the immunomodulatory potential against DENV is stated by its ability to regulate cytokine production, phagocytic activity, and white blood cell proliferation (Razak et al., 2021).
10 Complementary and Alternative Dengue Therapeutics
Dengue vaccines have been classified into five main categories, i.e., live attenuated vaccines (CYD-TDV, TV003/TV005), DENVaxin activated virus vaccine (PIV), recombinant subunit vaccine (V180), DNA vaccine (D1ME100, TVDV), and viral vectored vaccine (TLAV Prime/PIV boost and reverse order) (Deng et al., 2020a). Dengvaxia (CYD-TDV) by Sanofi Pasteur (the first dengue vaccine) was registered in several endemic countries for individuals of the age group 9–45 years (Deep et al., 2018; Deng et al., 2020a). It consists of DENV (1–4) serotype structural genes (encoding PrM and E proteins) introduced in the attenuated yellow fever vaccine strain genome. According to the reports, it exerts 56%–61% virucidal efficacy against dengue in Asia and Latin America (Capeding et al., 2014; Biswal et al., 2019). This anti-dengue vaccine is only recommended for patients having evidence of past DENV infection due to infection severity in seronegative candidates via stimulating non-neutralizing antibody generation (Sridhar et al., 2018). This drawback is considerable in the development of other alternatives of anti-dengue drug discovery. Another tetravalent dengue medicine, TAK-003 (Takeda), consisting of live attenuated DENV-2 genetic backbone for all DENV serotypes, has been designed by experts at the Division of Vector-Borne Diseases, Centers for Disease Control and Prevention (CDC) (Huang et al., 2013). Overall vaccine efficiency is documented to be 80.9%, and the seronegative populations showed 74.9% vaccine efficacy for the new dengue vaccine (TAK-003) (Biswal et al., 2019). The vaccination priming with drugs used in combination has been reported to provide more effective immunogenic protection in animals. In addition, analysis of the immune response and the mechanism of viral elements can be a revolutionary success in vaccine development (Liu et al., 2016). Anti-dengue drugs, such as chloroquine, celgosivir, balapiravir, prednisolone, and lovastatin, have been stated to undergone medical examinations against DENV infection (Kaptein and Neyts 2016; Low et al., 2017), although no effective dengue treatment has been developed from any of these composites. Therefore, the search for more effective antiviral compounds (plant-derived or second-use medicines) is still a vital prerequisite in overcoming dengue’s lethal effects (Trujillo-Correa et al., 2019). Besides, the specific anti-dengue vaccine is not yet developed, as few to none of the potential anti-dengue candidates have been tested clinically (Razak et al., 2021).
11 Conclusion
An update about various plants with pharmacological and ethnobotanical uses against dengue treatment was presented. Besides the global status of the dengue epidemic, existing vaccination measures have been conferred in the current review. Solely, the folkloric knowledge and uses of natural resources have been promising due to the exhaustive investigation carried out on the ethnopharmacologically important plant species. Many plant species and their respective extracts and pure compounds have been shown to exhibit potential as anti-DENV medicaments. The plant bioactive metabolites exhibit antiviral response directly or through the stimulation of immunomodulatory response cascades against DENV at different infection stages, i.e., viral adsorption, intracellular replication, and proliferation. Plant metabolites also help to reduce the antigen load and manipulate various immune factors in the host. Plant-mediated immunomodulation leads to the regulated cytokine production, enhanced platelet count, and phagocytic pathway activation. However, there are several plants still used for their anti-dengue properties via traditional methods and are yet to be investigated for scientific approval. Whether the antiviral properties are a result of a single phytoconstituent or the interaction of multiple phytoconstituents present in the plant extract(s) is critical to justify. Thus, extensive research is required to assess the immunologic potentials along with the phytochemical richness of plants and further clinical probes to develop an effective medicine. Another significant aspect of medicinal plants is their insecticidal properties, which make them an eco-friendly and sustainable substitute for Aedes mosquito control. Since vaccine development is a time-consuming process, finding an alternate treatment is crucial to overcome the lethal impacts of the DENV virus. Plant natural compounds are valuable sources to accomplish the speedy discovery of anti-DENV drugs because of their safer use and positive immunomodulatory responses. Nevertheless, the most crucial task is to reveal the molecular machinery of viral components and their capability to mutate rapidly during replication. Moreover, there are some vaccines available to treat dengue infection, but with some limitations. In this scenario, research is being carried out on some medicinal plant species, i.e., Nyctanthes arbor-tristis L., Firmiana simplex (L.) W. Wight, T. sinensis, and Moringa oleifera Lam., to investigate their metabolic profiles supporting their anti-dengue properties (antilarval or anti-DENV, in the authors’ laboratory). Besides, N. arbor-tristis, M. oleifera, and T. sinensis are also reported for their larvicidal activities. Furthermore, the GC-MS profiling of F. simplex also reveals the presence of natural compounds having insecticidal properties against dengue vectors.
Besides, a lot of scientific research is dedicated towards anti-DENV drug development and further research is needed to identify phytochemicals for their antiviral efficacy, mode of action, and successful clinical implementation.
Author Contributions
MMS: Conceptualization, Validation, Supervision. MD: Writing—original draft preparation, Formal analysis, Validation, Writing—review and editing. LS: Writing—original draft preparation, Formal analysis, Validation, Writing—review and editing. AD: Data collection, Formal analysis. PD: Chemical structure.
Conflict of Interest
The authors declare that the research was conducted in the absence of any commercial or financial relationships that could be construed as a potential conflict of interest.
Publisher’s Note
All claims expressed in this article are solely those of the authors and do not necessarily represent those of their affiliated organizations, or those of the publisher, the editors and the reviewers. Any product that may be evaluated in this article, or claim that may be made by its manufacturer, is not guaranteed or endorsed by the publisher.
Acknowledgments
The authors are grateful to Manipal University Jaipur, Rajasthan, India, for supporting the present work. The entire team is thankful to Manipal University Jaipur for vital support and amenities.
References
Abd Kadir, S. L., Yaakob, H., and Mohamed Zulkifli, R. (2013). Potential Anti-dengue Medicinal Plants: A Review. J. Nat. Med. 67, 677–689. doi:10.1007/s11418-013-0767-y
Abdul Ahmad, S. A., Palanisamy, U. D., Tejo, B. A., Chew, M. F., Tham, H. W., and Syed Hassan, S. (2017). Geraniin Extracted from the Rind of Nephelium Lappaceum Binds to Dengue Virus Type-2 Envelope Protein and Inhibits Early Stage of Virus Replication. Virol. J. 14, 229. doi:10.1186/s12985-017-0895-1
Agrawal, J., and Pal, A. (2013). Nyctanthes Arbor-Tristis Linn--a Critical Ethnopharmacological Review. J. Ethnopharmacol. 146, 645–658. doi:10.1016/j.jep.2013.01.024
Ahmad, N., Fazal, H., Ayaz, M., Abbasi, B. H., Mohammad, I., and Fazal, L. (2011). Dengue Fever Treatment with Carica Papaya Leaves Extracts. Asian Pac. J. Trop. Biomed. 1, 330–333. doi:10.1016/S2221-1691(11)60055-5
Ahn, H., and Lee, G. S. (2017). Isorhamnetin and Hyperoside Derived from Water Dropwort Inhibits Inflammasome Activation. Phytomedicine 24, 77–86. doi:10.1016/j.phymed.2016.11.019
Akhtar, I. N. (2020). “Viral Genetics and Structure,” in Dengue Virus Disease: From Origin to Outbreak (Academic Press), 85–113. doi:10.1016/B978-0-12-818270-3.00006-0
Alagarasu, K., Patil, J. A., Kakade, M. B., More, A. M., Yogesh, B., Newase, P., et al. (2021). Serotype and Genotype Diversity of Dengue Viruses Circulating in India: a Multi-centre Retrospective Study Involving the Virus Research Diagnostic Laboratory Network in 2018. Int. J. Infect. Dis. 111, 242–252. doi:10.1016/j.ijid.2021.08.045
Ali, A., Tabanca, N., Demirci, B., Blythe, E. K., Ali, Z., Baser, K. H., et al. (2015). Chemical Composition and Biological Activity of Four Salvia Essential Oils and Individual Compounds against Two Species of Mosquitoes. J. Agric. Food Chem. 63, 447–456. doi:10.1021/jf504976f
Allard, P. M., Leyssen, P., Martin, M. T., Bourjot, M., Dumontet, V., Eydoux, C., et al. (2012). Antiviral Chlorinated Daphnane Diterpenoid Orthoesters from the Bark and wood of Trigonostemon Cherrieri. Phytochemistry 84, 160–168. doi:10.1016/j.phytochem.2012.07.023
Anjum, V., Arora, P., Ansari, S. H., Najmi, A. K., and Ahmad, S. (2017). Antithrombocytopenic and Immunomodulatory Potential of Metabolically Characterized Aqueous Extract of Carica Papaya Leaves. Pharm. Biol. 55, 2043–2056. doi:10.1080/13880209.2017.1346690
Arman, M., and Qader, S. A. U. (2012). Structural Analysis of Kappa-Carrageenan Isolated from Hypnea Musciformis (Red Algae) and Evaluation as an Elicitor of Plant Defense Mechanism. Carbohydr. Polym. 88, 1264–1271. doi:10.1016/j.carbpol.2012.02.003
Aruna, R. (2014). Review on Dengue Viral Replication, Assembly and Entry into the Host Cells. Int. J. Microb. Appl. Sci. 8, 1.
Arunabha, M. (2019). Trigonella Foenum-Graecum: A Review on its Traditional Uses, Phytochemistry and Pharmacology. Int. J. Adv. Scientific Res. 5 (5), e5217. doi:10.7439/ijasr
Bahuguna, V., Matura, R., and Sharma, N. (2019). Potential Use of Medicinal Plants for Dengue: a Systematic Mini Review of Scientific Evidence. J. Appl. Life Sci. 2, 10–16.
Baite, T. N., Mandal, B., and Purkait, M. K. (2021). Ultrasound Assisted Extraction of Gallic Acid from Ficus Auriculata Leaves Using green Solvent. Food Bioproducts Process. 128, 1–11. doi:10.1016/j.fbp.2021.04.008
Bartenschlager, R., and Miller, S. (2008). Molecular Aspects of Dengue Virus Replication. Future Microbiol. 3, 155–165. doi:10.2217/17460913.3.2.155
Baylor, N. W., Fu, T., Yan, Y. D., and Ruscetti, F. W. (1992). Inhibition of Human T Cell Leukemia Virus by the Plant Flavonoid Baicalin (7-glucuronic Acid, 5,6-dihydroxyflavone). J. Infect. Dis. 165, 433–437. doi:10.1093/infdis/165.3.433
Begum, F., Das, S., Mukherjee, D., Mal, S., and Ray, U. (2019). Insight into the Tropism of Dengue Virus in Humans. Viruses 11, 1. doi:10.3390/v11121136
Benelli, G., Pavela, R., Rakotosaona, R., Nzekoue, F. K., Canale, A., Nicoletti, M., et al. (2020). Insecticidal and Mosquito Repellent Efficacy of the Essential Oils from Stem Bark and wood of Hazomalania Voyronii. J. Ethnopharmacol. 248, 112333. doi:10.1016/j.jep.2019.112333
Berlian, G., Tandrasasmita, O. M., and Tjandrawinata, R. R. (2017). Trombinol, a Bioactive Fraction of Psidium Guajava , Stimulates Thrombopoietin Expression in HepG2 Cells. Asian Pac. J. Trop. Biomed. 7, 437–442. doi:10.1016/j.apjtb.2016.09.010
Bharati, P., and Sinha, R. (2012). Study the Effect of Tinospora Cardifolia (wild) Miers and Boerhaavia diffusia Linn on dengue. Int. J. Ayurvedic Herb. Med..
Bhatt, S., Gething, P. W., Brady, O. J., Messina, J. P., Farlow, A. W., Moyes, C. L., et al. (2013). The Global Distribution and burden of Dengue. Nature 496, 504–507. doi:10.1038/nature12060
Biswal, S., Reynales, H., Saez-Llorens, X., Lopez, P., Borja-Tabora, C., Kosalaraksa, P., et al. (2019). Efficacy of a Tetravalent Dengue Vaccine in Healthy Children and Adolescents. N. Engl. J. Med. 381, 2009–2019. doi:10.1056/nejmoa1903869
Borah, M. P., and Prasad, S. B. (2017). Ethnozoological Study of Animals Based Medicine Used by Traditional Healers and Indigenous Inhabitants in the Adjoining Areas of Gibbon Wildlife Sanctuary, Assam, India. J. Ethnobiol. Ethnomed. 13, 39. doi:10.1186/s13002-017-0167-6
Bourjot, M., Leyssen, P., Eydoux, C., Guillemot, J. C., Canard, B., Rasoanaivo, P., et al. (2012). Flacourtosides A-F, Phenolic Glycosides Isolated from Flacourtia Ramontchi. J. Nat. Prod. 75, 752–758. doi:10.1021/np300059n
Bozza, F. A., Cruz, O. G., Zagne, S. M., Azeredo, E. L., Nogueira, R. M., Assis, E. F., et al. (2008). Multiplex Cytokine Profile from Dengue Patients: MIP-1beta and IFN-Gamma as Predictive Factors for Severity. BMC Infect. Dis. 8, 86. doi:10.1186/1471-2334-8-86
Brady, O. J., Gething, P. W., Bhatt, S., Messina, J. P., Brownstein, J. S., Hoen, A. G., et al. (2012). Refining the Global Spatial Limits of Dengue Virus Transmission by Evidence-Based Consensus. Plos Negl. Trop. Dis. 6, e1760. doi:10.1371/journal.pntd.0001760
Brandão, G., Kroon, E., Souza, D., Filho, J., and Oliveira, A. (2013). Chemistry and Antiviral Activity of Arrabidaea Pulchra (Bignoniaceae). Molecules 18, 9919–9932. doi:10.3390/molecules18089919
Cao-Lormeau, V. M. (2009). Dengue Viruses Binding Proteins from Aedes aegypti and Aedes polynesiensis Salivary Glands. Virol. J. 6, 35. doi:10.1186/1743-422X-6-35
Capeding, M. R., Tran, N. H., Hadinegoro, S. R., Ismail, H. I., Chotpitayasunondh, T., Chua, M. N., et al. (2014). Clinical Efficacy and Safety of a Novel Tetravalent Dengue Vaccine in Healthy Children in Asia: a Phase 3, Randomised, Observer-Masked, Placebo-Controlled Trial. Lancet 384, 1358–1365. doi:10.1016/S0140-6736(14)61060-6
Carneiro, B. M., Batista, M. N., Braga, A. C. S., Nogueira, M. L., and Rahal, P. (2016). The green tea Molecule EGCG Inhibits Zika Virus Entry. Virology 496, 215–218. doi:10.1016/j.virol.2016.06.012
Carr, J. M., Ashander, L. M., Calvert, J. K., Ma, Y., Aloia, A., Bracho, G. G., et al. (2017). Molecular Responses of Human Retinal Cells to Infection with Dengue Virus. Mediators Inflamm. 2017, 3164375. doi:10.1155/2017/3164375
Chambers, T. J., Hahn, C. S., Galler, R., and Rice, C. M. (1990). Flavivirus Genome Organization, Expression, and Replication. Annu. Rev. Microbiol. 44, 649–688. doi:10.1146/annurev.mi.44.100190.003245
Chan, M., and Johansson, M. A. (2012). The Incubation Periods of Dengue Viruses. PLoS One 7, e50972. doi:10.1371/journal.pone.0050972
Chaubey, M., and Kapoor, V. P. (2001). Structure of a Galactomannan from the Seeds of Cassia Angustifolia Vahl. Carbohydr. Res. 332, 439–444. doi:10.1016/S0008-6215(01)00104-5
Chaudhry, M. R. A. (2020). “Dengue Virus Infection Outbreak: Comparison with Other Viral Infection Outbreak,” in Dengue Virus Disease: From Origin to Outbreak (IEEE), 17–35. doi:10.1016/B978-0-12-818270-3.00003-5
Chen, R. F., Yang, K. D., Lee, I. K., Liu, J. W., Huang, C. H., Lin, C. Y., et al. (2014). Augmented miR-150 Expression Associated with Depressed SOCS1 Expression Involved in Dengue Haemorrhagic Fever. J. Infect. 69, 366–374. doi:10.1016/j.jinf.2014.05.013
Chiow, K. H., Phoon, M. C., Putti, T., Tan, B. K., and Chow, V. T. (2016). Evaluation of Antiviral Activities of Houttuynia Cordata Thunb. Extract, Quercetin, Quercetrin and Cinanserin on Murine Coronavirus and Dengue Virus Infection. Asian Pac. J. Trop. Med. 9, 1–7. doi:10.1016/j.apjtm.2015.12.002
Chow, V., Batool, R., Aziz, E., Mahmood, T., and Tan, B. (2018). Inhibitory Activities of Extracts of Rumex Dentatus, Commelina Benghalensis, Ajuga Bracteosa, Ziziphus Mauritiana as Well as Their Compounds of Gallic Acid and Emodin against Dengue Virus. Asian Pac. J. Trop. Med. 11, 265. doi:10.4103/1995-7645.231466
Clain, E., Haddad, J. G., Koishi, A. C., Sinigaglia, L., Rachidi, W., Desprès, P., et al. (2019). The Polyphenol-Rich Extract from Psiloxylon Mauritianum, an Endemic Medicinal Plant from Reunion Island, Inhibits the Early Stages of Dengue and Zika Virus Infection. Int. J. Mol. Sci. 20, 1. doi:10.3390/ijms20081860
Clain, E., Sinigaglia, L., Koishi, A. C., Gorgette, O., Gadea, G., Viranaicken, W., et al. (2018). Extract from Aphloia Theiformis, an Edible Indigenous Plant from Reunion Island, Impairs Zika Virus Attachment to the Host Cell Surface. Sci. Rep. 8, 1–12. doi:10.1038/s41598-018-29183-2
Coudeville, L., Shepard, D. S., Zambrano, B., and Dayan, G. (2009). Dengue Economic burden in the Americas: Estimates from Dengue Illness. Am. J. Trop. Med. Hyg. 8, 1.
Courageot, M. P., Frenkiel, M. P., Dos Santos, C. D., Deubel, V., and Desprès, P. (2000). Alpha-glucosidase Inhibitors Reduce Dengue Virus Production by Affecting the Initial Steps of Virion Morphogenesis in the Endoplasmic Reticulum. J. Virol. 74, 564–572. doi:10.1128/jvi.74.1.564-572.2000
Daneshzadeh, M. S., Abbaspour, H., Amjad, L., and Nafchi, A. M. (2020). An Investigation on Phytochemical, Antioxidant and Antibacterial Properties of Extract from Eryngium Billardieri F. Delaroche. Food Measure 14, 708–715. doi:10.1007/s11694-019-00317-y
Deep, P., Srivastava, V., and Verma, S. (2018). Current Perspectives of Medicinal Plants Having Anti Dengue Potential. Int. J. Pharm. Sci. Rev. Res. 8, 1.
Deng, S.-Q., Yang, X., Wei, Y., Chen, J.-T., Wang, X.-J., and Peng, H.-J. (2020a). A Review on Dengue Vaccine Development. Vaccines 8, 63. doi:10.3390/vaccines8010063
Deng, Y., Li, S., Wang, M., Chen, X., Tian, L., Wang, L., et al. (2020b). Flavonoid-rich Extracts from Okra Flowers Exert Antitumor Activity in Colorectal Cancer through Induction of Mitochondrial Dysfunction-Associated Apoptosis, Senescence and Autophagy. Food Funct. 8, 1. doi:10.1039/d0fo02081h
Dewi, B. E., Angelina, M., Nuwwaaridya, F., Desti, H., and Sudiro, T. M. (2019). Antiviral Activity of Isobutyl Gallate to Dengue Virus Serotype 2 In Vitro. IOP Conf. Ser. Earth Environ. Sci. 251, 012018. doi:10.1088/1755-1315/251/1/012018
Dewi, B. E., Taufiqqurrachman, I., Desti, H., Sudiro, M., Fithriyah, , and Angelina, M. (2020). Inhibition Mechanism of Psidium Guajava Leaf to Dengue Virus Replication In Vitro. IOP Conf. Ser. Earth Environ. Sci. 462, 012034. doi:10.1088/1755-1315/462/1/012034
Diallo, M., Ba, Y., Sall, A. A., Diop, O. M., Ndione, J. A., Mondo, M., et al. (2003). Amplification of the Sylvatic Cycle of Dengue Virus Type 2, Senegal, 1999-2000: Entomologic Findings and Epidemiologic Considerations. Emerg. Infect. Dis. 9, 362–367. doi:10.3201/eid0903.020219
Diwaker, D., Mishra, K. P., Ganju, L., and Singh, S. B. (2014). Rhodiola Inhibits Dengue Virus Multiplication by Inducing Innate Immune Response Genes RIG-I, MDA5 and ISG in Human Monocytes. Arch. Virol. 159, 1975–1986. doi:10.1007/s00705-014-2028-0
Dussart, P., Baril, L., Petit, L., Beniguel, L., Quang, L. C., Ly, S., et al. (2012). Clinical and Virological Study of Dengue Cases and the Members of Their Households: The Multinational Denframe Project. Plos Negl. Trop. Dis. 6, e1482. doi:10.1371/journal.pntd.0001482
Eid, H. M., Martineau, L. C., Saleem, A., Muhammad, A., Vallerand, D., Benhaddou-Andaloussi, A., et al. (2010). Stimulation of AMP-Activated Protein Kinase and Enhancement of Basal Glucose Uptake in Muscle Cells by Quercetin and Quercetin Glycosides, Active Principles of the Antidiabetic Medicinal Plant Vaccinium Vitis-Idaea. Mol. Nutr. Food Res. 54, 991–1003. doi:10.1002/mnfr.200900218
Esler, D. (2009). Dengue - Clinical and Public Health Ramifications. Aust. Fam. Physician 38, 876–879.
European Centre for Disease Prevention and Control (2018). Aedes albopictus - {Factsheet} for Experts. Eur. Cent. Dis. Prev. Control. 1, 1.
Fialho, L. G., Da Silva, V. P., Reis, S. R. N. I., Azeredo, E. L., Kaplan, M. A. C., Figueiredo, M. R., et al. (2017). Antiviral and Immunomodulatory Effects of Norantea Brasiliensis Choisy on Dengue Virus-2. Intervirology 59, 217–227. doi:10.1159/000455855
Flores-Mendoza, L. K., Estrada-Jiménez, T., Sedeño-Monge, V., Moreno, M., Manjarrez, M. D. C., González-Ochoa, G., et al. (2017). IL-10 and Socs3 Are Predictive Biomarkers of Dengue Hemorrhagic Fever. Mediators Inflamm. 2017, 5197592. doi:10.1155/2017/5197592
Fongnzossie, E. F., Nyangono, C. F. B., Biwole, A. B., Ebai, P. N. B., Ndifongwa, N. B., Motove, J., et al. (2020). Wild Edible Plants and Mushrooms of the Bamenda Highlands in Cameroon: Ethnobotanical Assessment and Potentials for Enhancing Food Security. J. Ethnobiol. Ethnomed. 16, 12. doi:10.1186/s13002-020-00362-8
Genoud, A. P., Basistyy, R., Williams, G. M., and Thomas, B. P. (2018). Optical Remote Sensing for Monitoring Flying Mosquitoes, Gender Identification and Discussion on Species Identification. Appl. Phys. B 124, 1. doi:10.1007/s00340-018-6917-x
Govindarajan, M., Rajeswary, M., Hoti, S., Benelli, G., Benelli, G., and Amsath, A. (2015). Ovicidal Activity of Pithecellobium dulce (Family: Fabaceae) Leaf and Seed Extracts against Filariasis Vector Mosquito Culex quinquefasciatus (Diptera: Culicidae). J. Med. Herbs Ethnomed 1, 1. doi:10.5455/jmhe.2015.10.024
Gowda, A., Kumar, N., Kasture, P., and Nagabhushan, K. (2015). A Pilot Study to Evaluate the Effectiveness of Carica Papaya Leaf Extract in Increasing the Platelet Count in Cases of Dengue with Thrombocytopenia. Available at: http://medind.nic.in/ice/t15/i3/icet15i3p109.pdf.
Gu, R., Wang, Y., Long, B., Kennelly, E., Wu, S., Liu, B., et al. (2014). Prospecting for Bioactive Constituents from Traditional Medicinal Plants through Ethnobotanical Approaches. Biol. Pharm. Bull. 37, 903–915. doi:10.1248/bpb.b14-00084
Gubler, D. J. (1989). Aedes aegypti and Aedes aegypti-borne Disease Control in the 1990s: Top Down or Bottom up. Charles Franklin Craig Lecture. Am. J. Trop. Med. Hyg. 40, 571–578. doi:10.4269/ajtmh.1989.40.571
Guzman, A., and Istúriz, R. E. (2010). Update on the Global Spread of Dengue. Int. J. Antimicrob. Agents 36, S40–S42. doi:10.1016/j.ijantimicag.2010.06.018
Guzman, M. G., Alvarez, M., and Halstead, S. B. (2013). Secondary Infection as a Risk Factor for Dengue Hemorrhagic Fever/dengue Shock Syndrome: An Historical Perspective and Role of Antibody-dependent Enhancement of Infection. Arch. Virol. 158, 1445–1459. doi:10.1007/s00705-013-1645-3
Guzman, M. G., Gubler, D. J., Izquierdo, A., Martinez, E., and Halstead, S. B. (2016). Dengue Infection. Nat. Rev. Dis. Primers 2, 16055. doi:10.1038/nrdp.2016.55
Haddad, J. G., Koishi, A. C., Gaudry, A., Nunes Duarte Dos Santos, C., Viranaicken, W., Desprès, P., et al. (2019). Doratoxylon Apetalum, an Indigenous Medicinal Plant from Mascarene Islands, Is a Potent Inhibitor of Zika and Dengue Virus Infection in Human Cells. Ijms 20, 2382. doi:10.3390/ijms20102382
Hall, A., Troupin, A., Londono-Renteria, B., and Colpitts, T. M. (2017). Garlic Organosulfur Compounds Reduce Inflammation and Oxidative Stress during Dengue Virus Infection. Viruses 9, 159. doi:10.3390/v9070159
Halstead, S. B. (2002). Dengue Hemorrhagic Fever: Two Infections and Antibody Dependent Enhancement, a Brief History and Personal Memoir. Rev. Cubana Med. Trop. 54, 171–179.
Halstead, S. B. (1988). Pathogenesis of Dengue: Challenges to Molecular Biology. Science 239, 476–481. doi:10.1126/science.3277268
Han, Y., Bu, L. M., Ji, X., Liu, C. Y., and Wang, Z. H. (2005). Modulation of Multidrug Resistance by Andrographolid in a HCT-8/5-FU Multidrug-Resistant Colorectal Cancer Cell Line. Chin. J. Dig. Dis. 6, 82–86. doi:10.1111/j.1443-9573.2005.00197.x
Hari, I., and Mathew, N. (2018). Larvicidal Activity of Selected Plant Extracts and Their Combination against the Mosquito Vectors Culex quinquefasciatus and Aedes aegypti. Environ. Sci. Pollut. Res. Int. 25, 9176–9185. doi:10.1007/s11356-018-1515-3
Hassandarvish, P., Rothan, H. A., Rezaei, S., Yusof, R., Abubakar, S., and Zandi, K. (2016). In Silico study on Baicalein and Baicalin as Inhibitors of Dengue Virus Replication. RSC Adv. 6, 31235–31247. doi:10.1039/c6ra00817h
Häusler, H., Kawakami, R. P., Mlaker, E., Severn, W. B., Wrodnigg, T. M., and Stütz, A. E. (2000). Sugar Analogues with Basic Nitrogen in the Ring as Anti-infectives. J. Carbohydr. Chem. 19, 435–449. doi:10.1080/07328300008544092
Huang, C. Y., Kinney, R. M., Livengood, J. A., Bolling, B., Arguello, J. J., Luy, B. E., et al. (2013). Genetic and Phenotypic Characterization of Manufacturing Seeds for a Tetravalent Dengue Vaccine (DENVax). Plos Negl. Trop. Dis. 7, e2243. doi:10.1371/journal.pntd.0002243
Huang, N. C., Hung, W. T., Tsai, W. L., Lai, F. Y., Lin, Y. S., Huang, M. S., et al. (2017). Ficus Septica Plant Extracts for Treating Dengue Virus In Vitro. PeerJ 5, e3448. doi:10.7717/peerj.3448
Hussain, J., Ali, L., Khan, A. L., Rehman, N. U., Jabeen, F., Kim, J. S., et al. (2014). Isolation and Bioactivities of the Flavonoids Morin and Morin-3-O-β-D-Glucopyranoside from Acridocarpus Orientalis-A Wild Arabian Medicinal Plant. Molecules 19, 17763–17772. doi:10.3390/molecules191117763
Hwang, Y. S., Wu, K. H., Kumamoto, J., Axelrod, H., and Mulla, M. S. (1985). Isolation and Identification of Mosquito Repellents inArtemisia Vulgaris. J. Chem. Ecol. 11, 1297–1306. doi:10.1007/BF01024117
Ichsyani, M., Ridhanya, A., Risanti, M., Desti, H., Ceria, R., Putri, D. H., et al. (2017). Antiviral Effects of Curcuma Longa L. Against Dengue Virus In Vitro and In Vivo. IOP Conf. Ser. Earth Environ. Sci. 101, 012005. doi:10.1088/1755-1315/101/1/012005
Jasso-Miranda, C., Herrera-Camacho, I., Flores-Mendoza, L. K., Dominguez, F., Vallejo-Ruiz, V., Sanchez-Burgos, G. G., et al. (2019). Antiviral and Immunomodulatory Effects of Polyphenols on Macrophages Infected with Dengue Virus Serotypes 2 and 3 Enhanced or Not with Antibodies. Infect. Drug Resist. 12, 1833–1852. doi:10.2147/IDR.S210890
Jayadevappa, M. K., Karkera, P. R., Siddappa, R. Y., Telkar, S., and Karunakara, P. (2020). Investigation of Plant Flavonoids as Potential Dengue Protease Inhibitors. J. Herbmed Pharmacol. 9, 366–373. doi:10.34172/jhp.2020.46
Jiang, Z., Okimura, T., Yokose, T., Yamasaki, Y., Yamaguchi, K., and Oda, T. (2010). Effects of Sulfated Fucan, Ascophyllan, from the Brown Alga Ascophyllum Nodosum on Various Cell Lines: A Comparative Study on Ascophyllan and Fucoidan. J. Biosci. Bioeng. 110, 113–117. doi:10.1016/j.jbiosc.2010.01.007
Juliana, C., Lister, I. N. E., Girsang, E., Nasution, A. N., and Widowati, W. (2020). Antioxidant and Elastase Inhibitor from Black Soybean (Glycine max L.) and its Compound (Daidzein). J. Biomed. Transl. Res. 6, 11–14. doi:10.14710/jbtr.v6i1.5540
Kaptein, S. J., and Neyts, J. (2016). Towards Antiviral Therapies for Treating Dengue Virus Infections. Curr. Opin. Pharmacol. 30, 1–7. doi:10.1016/j.coph.2016.06.002
Kaushik, S., Dar, L., Kaushik, S., and Yadav, J. P. (2021). Identification and Characterization of New Potent Inhibitors of Dengue Virus NS5 Proteinase from Andrographis Paniculata Supercritical Extracts on in Animal Cell Culture and In Silico Approaches. J. Ethnopharmacol. 267, 113541. doi:10.1016/j.jep.2020.113541
Khan, A. M., and Bhutta, Z. A. (2017). “Childhood Infectious Diseases: Overview,” in International Encyclopedia of Public Health (Elsevier), 517–538. doi:10.1016/B978-0-12-803678-5.00065-5
Khanh Pham, N., Tuan Nguyen, H., and Binh Nguyen, Q. (2021). A Review on the Ethnomedicinal Uses, Phytochemistry and Pharmacology of Plant Species Belonging to Kaempferia Genus (Zingiberaceae). Pharm. Sci. Asia 48, 1–24. doi:10.29090/PSA.2021.01.19.070
Kiat, T. S., Pippen, R., Yusof, R., Ibrahim, H., Khalid, N., and Rahman, N. A. (2006). Inhibitory Activity of Cyclohexenyl Chalcone Derivatives and Flavonoids of Fingerroot, Boesenbergia Rotunda (L.), towards Dengue-2 Virus NS3 Protease. Bioorg. Med. Chem. Lett. 16, 3337–3340. doi:10.1016/j.bmcl.2005.12.075
Kim, J., and Hwang, E.-S. (2020). Multiplexed Diagnosis of Four Serotypes of Dengue Virus by Real-Time RT-PCR. Biochip J. 14, 421–428. doi:10.1007/s13206-020-4409-7
Kim, S. J., Um, J. Y., Lee, J. Y., and Lee, J. Y. (2011). Anti-inflammatory Activity of Hyperoside through the Suppression of Nuclear Factor-Κb Activation in Mouse Peritoneal Macrophages. Am. J. Chin. Med. 39, 171–181. doi:10.1142/S0192415X11008737
Kuczera, D., Assolini, J. P., Tomiotto-Pellissier, F., Pavanelli, W. R., and Silveira, G. F. (2018). Highlights for Dengue Immunopathogenesis: Antibody-dependent Enhancement, Cytokine Storm, and beyond. J. Interferon Cytokine Res. 38, 69–80. doi:10.1089/jir.2017.0037
Kumar, A., Rongpharpi, S. R., Dewan Duggal, S., Gur, R., Choudhary, S., and Khare, P. (2017). Clinical, Epidemiological and Microbiological Profile of Dengue Fever at a Tertiary Care Hospital in Delhi, India. J. Infect. Dis. Med. 02, 1. doi:10.4172/2576-1420.1000110
Lambrechts, L., Scott, T. W., and Gubler, D. J. (2010). Consequences of the Expanding Global Distribution of Aedes albopictus for Dengue Virus Transmission. Plos Negl. Trop. Dis. 4, e646. doi:10.1371/journal.pntd.0000646
Larocca, D. G., Júnior, N. G. R., Vicente, R., and Silva, I. V. (2021). Ethnobotanical Treatment of Tropical Diseases, Malaria and Dengue, Prescribed by Bioenergetico Practitioners and Profile of the Involved Population in Meridional Amazon. Revista Etnobiología. 19, 114–128.
Latgé, J. P., Debeaupuis, J. P., Moutaouakil, M., Diaquin, M., Sarfati, J., Prévost, M. C., et al. (1991). Galactomannan and the Circulating Antigens of Aspergillus fumigatus. Springer, 143–155. doi:10.1007/978-3-642-76074-7_11
Leardkamolkarn, V., Sirigulpanit, W., Phurimsak, C., Kumkate, S., Himakoun, L., and Sripanidkulchai, B. (2012). The Inhibitory Actions of Houttuynia Cordata Aqueous Extract on Dengue Virus and Dengue-Infected Cells. J. Food Biochem. 36, 86–92. doi:10.1111/j.1745-4514.2010.00514.x
Lee, Y. R., Yeh, S. F., Ruan, X. M., Zhang, H., Hsu, S. D., Huang, H. D., et al. (2017). Honeysuckle Aqueous Extract and Induced Let-7a Suppress Dengue Virus Type 2 Replication and Pathogenesis. J. Ethnopharmacol. 198, 109–121. doi:10.1016/j.jep.2016.12.049
Li, S., Zhang, Z., Cain, A., Wang, B., Long, M., and Taylor, J. (2005). Antifungal Activity of Camptothecin, Trifolin, and Hyperoside Isolated from Camptotheca Acuminata. J. Agric. Food Chem. 53, 32–37. doi:10.1021/jf0484780
Lim, S. J., Wan Aida, W. M., Schiehser, S., Rosenau, T., and Böhmdorfer, S. (2019). Structural Elucidation of Fucoidan from Cladosiphon Okamuranus (Okinawa Mozuku). Food Chem. 272, 222–226. doi:10.1016/j.foodchem.2018.08.034
Ling, A. P. K., Khoo, B. F., Seah, C. H., Foo, K. Y., Cheah, R. K., Chye, S. M., et al. (2014). “Inhibitory Activities of Methanol Extracts of Andrographis Paniculata and Ocimum Sanctum against Dengue-1 Virus,” in International Conference on Biological Environmental and Food Engineering (Bali, Indonesia: IEEE). doi:10.15242/iicbe.c814013
Liu, Y. X., Li, F. X., Liu, Z. Z., Jia, Z. R., Zhou, Y. H., Zhang, H., et al. (2016). Integrated Analysis of miRNAs and Transcriptomes in Aedes albopictus Midgut Reveals the Differential Expression Profiles of Immune-Related Genes during Dengue Virus Serotype-2 Infection. Insect Sci. 23, 377–385. doi:10.1111/1744-7917.12339
Low, J. G., Ooi, E. E., and Vasudevan, S. G. (2017). Current Status of Dengue Therapeutics Research and Development. J. Infect. Dis. 215, S96. doi:10.1093/infdis/jiw423
Macedo, T., Ribeiro, V., Oliveira, A. P., Pereira, D. M., Fernandes, F., Gomes, N. G. M., et al. (2020). Anti-inflammatory Properties of Xylopia Aethiopica Leaves: Interference with Pro-inflammatory Cytokines in THP-1-Derived Macrophages and Flavonoid Profiling. J. Ethnopharmacol. 248, 112312. doi:10.1016/j.jep.2019.112312
Marcial‐Juárez, E., Yam‐Puc, J. C., Cedillo‐Barrón, L., García‐Cordero, J., Calderón‐Amador, J., Maqueda‐Alfaro, R. A., et al. (2017). “Travelling with Dengue: from the Skin to the Nodes,” in Dengue - Immunopathology and Control Strategies (London, United Kingdom: WHO). doi:10.5772/intechopen.68338
Marudhupandi, T., and Kumar, T. T. A. (2013). Antibacterial Effect of Fucoidan from Sargassum Wightii against the Chosen Human Bacterial Pathogens. Int. Curr. Pharm. J. 2, 156–158. doi:10.3329/icpj.v2i10.16408
Mathur, V., and Mathur, N. K. (2005). Fenugreek and Other Lesser Known Legume Galactomannan-Polysaccharides: Scope for Developments. New Delhi, India: J. Sci. Ind. Res. (India).
Mbadiko, C. M., Inkoto, C. L., Gbolo, B. Z., Lengbiye, E. M., Kilembe, J. T., Matondo, A., et al. (2020). A Mini Review on the Phytochemistry, Toxicology and Antiviral Activity of Some Medically Interesting Zingiberaceae Species. Jocamr 8, 44–56. doi:10.9734/jocamr/2020/v9i430150
McDowell, M., Gonzales, S. R., Kumarapperuma, S. C., Jeselnik, M., Arterburn, J. B., and Hanley, K. A. (2010). A Novel Nucleoside Analog, 1-Beta-D-Ribofuranosyl-3-Ethynyl-[1,2,4]triazole (ETAR), Exhibits Efficacy against a Broad Range of Flaviviruses In Vitro. Antivir. Res 87, 78–80. doi:10.1016/j.antiviral.2010.04.007
Mendoza, A., Silva, M., and Castro, E. A. (2020). Etnobotánica medicinal de comunidades Ñuu Savi de la Montaña de Guerrero. México. Etnobiología 2, 1.
Miller, J. L., Tyrrell, B. E., and Zitzmann, N. (2018). Mechanisms of Antiviral Activity of Iminosugars against Dengue Virus. Adv. Exp. Med. Biol. 18, 277–301. doi:10.1007/978-981-10-8727-1_20
Minh Ly, B., Quoc Buu, N., Duy Nhut, N., Duc Thinh, P., and Thi Thanh Van, T. (2017). Studies on Fucoidan and its Production from Vietnamese Brown Seaweeds. Ajstd 22, 371. doi:10.29037/ajstd.173
Mir, M., Khurshid, R., and Aftab, R. (2012). Management of Thrombocytopenia and Flu-like Symptoms in Dengue Patients with Herbal Water of Euphorbia Hirta. J. Ayub Med. Coll. Abbottabad. 24, 6–9.
Moghaddam, E., Teoh, B. T., Sam, S. S., Lani, R., Hassandarvish, P., Chik, Z., et al. (2014). Baicalin, a Metabolite of Baicalein with Antiviral Activity against Dengue Virus. Sci. Rep. 4, 5452. doi:10.1038/srep05452
Mohd Abd Razak, M. R., Norahmad, N. A., Md Jelas, N. H., Afzan, A., Mohmad Misnan, N., Mat Ripen, A., et al. (2021). Immunomodulatory Activities of Carica Papaya L. Leaf Juice in a Non-lethal, Symptomatic Dengue Mouse Model. Pathogens 10, 501. doi:10.3390/pathogens10050501
Molinari, M., and Helenius, A. (1999). Glycoproteins Form Mixed Disulphides with Oxidoreductases during Folding in Living Cells. Nature 402, 90–93. doi:10.1038/47062
Murhekar, M. V., Kamaraj, P., Kumar, M. S., Khan, S. A., Allam, R., Barde, P., et al. (2019). Burden of Dengue Infection in India, 2017: A Cross-Sectional Population Based Serosurvey. Lancet Glob. Heal. 7 (8), e1065–e1073. doi:10.1016/S2214-109X(19)30250-5
Murphy, B. R., and Whitehead, S. S. (2011). Immune Response to Dengue Virus and Prospects for a Vaccine. Annu. Rev. Immunol. 29, 587–619. doi:10.1146/annurev-immunol-031210-101315
Mussin, J., and Giusiano, G. (2020). Ethno-Phytopharmacology: Product Validation Process Based on Traditional Knowledge of Medicinal Plants. in Agric. For. Bioindustry Biotechnol. Biodiscovery 43, 331–353. doi:10.1007/978-3-030-51358-0_17
Nascimento, A. C., Valente, L. M. M., Gomes, M., Barboza, R. S., Wolff, T., Neris, R. L. S., et al. (2017). Antiviral Activity of Faramea Bahiensis Leaves on Dengue Virus Type-2 and Characterization of a New Antiviral Flavanone Glycoside. Phytochemistry Lett. 19, 220–225. doi:10.1016/j.phytol.2017.01.013
Nasir, S., Batool, M., Qureshi, N. A., Debboun, M., Qamer, S., Nasir, I., et al. (2015). Repellency of Medicinal Plant Extracts against Dengue Vector Mosquitoes, Aedes albopictus and Ae. Aegypti (Diptera: Culicidae). Pak. J. Zool. 2, 1.
Nile, S. H., Nile, A. S., Keum, Y. S., and Sharma, K. (2017). Utilization of Quercetin and Quercetin Glycosides from Onion (Allium cepa L.) Solid Waste as an Antioxidant, Urease and Xanthine Oxidase Inhibitors. Food Chem. 235, 119–126. doi:10.1016/j.foodchem.2017.05.043
Norahmad, N. A., Mohd Abd Razak, M. R., Mohmad Misnan, N., Md Jelas, N. H., Sastu, U. R., Muhammad, A., et al. (2019). Effect of Freeze-Dried Carica Papaya Leaf Juice on Inflammatory Cytokines Production during Dengue Virus Infection in AG129 Mice. BMC Complement. Altern. Med. 19, 44. doi:10.1186/s12906-019-2438-3
Nur Fatin Nazurah, R., and Nur Hanani, Z. A. (2017). Physicochemical Characterization of Kappa-Carrageenan (Euchema Cottoni) Based Films Incorporated with Various Plant Oils. Carbohydr. Polym. 157, 1479–1487. doi:10.1016/j.carbpol.2016.11.026
NVBDCP (2021). Dengue/DHF Situation In INDIA. Available at: https://nvbdcp.gov.in/index4.php?lang=1&level=0&linkid=431&lid=3715 (Accessed June, 2021).
Ocazionez, R. E., Meneses, R., Torres, F. A., and Stashenko, E. (2010). Virucidal Activity of Colombian Lippia Essential Oils on Dengue Virus Replication In Vitro. Mem. Inst. Oswaldo Cruz 105, 304–309. doi:10.1590/S0074-02762010000300010
Ono, L., Wollinger, W., Rocco, I. M., Coimbra, T. L., Gorin, P. A., and Sierakowski, M. R. (2003). In Vitro and In Vivo Antiviral Properties of Sulfated Galactomannans against Yellow Fever Virus (BeH111 Strain) and Dengue 1 Virus (Hawaii Strain). Antivir. Res 60, 201–208. doi:10.1016/S0166-3542(03)00175-X
Padbidri, V. S., Wairagkar, N. S., Joshi, G. D., Umarani, U. B., Risbud, A. R., and Gaikwad, D. L. (2002). A Serological Survey of Arboviral Diseases Among the Human Population of the Andaman and Nicobar Islands, India. Southeast Asian J. Trop. Med. Public Health 33, 794–800.
Palanisamy, S., Vinosha, M., Marudhupandi, T., Rajasekar, P., and Prabhu, N. M. (2017). Isolation of Fucoidan from Sargassum Polycystum Brown Algae: Structural Characterization, In Vitro Antioxidant and Anticancer Activity. Int. J. Biol. Macromol. 102, 405–412. doi:10.1016/j.ijbiomac.2017.03.182
Pan American Health Organization (PAHO) (2016). Dengue. United States: Guidelines for patient care in the region of the Americas.
Pan, Y. T., Ghidoni, J., and Elbein, A. D. (1993). The Effects of Castanospermine and Swainsonine on the Activity and Synthesis of Intestinal Sucrase. Arch. Biochem. Biophys. 303, 134–144. doi:10.1006/abbi.1993.1264
Pandey, S., Cabot, P. J., Shaw, P. N., and Hewavitharana, A. K. (2016). Anti-inflammatory and Immunomodulatory Properties of Carica Papaya. J. Immunotoxicol 13, 590–602. doi:10.3109/1547691X.2016.1149528
Panya, A., Sawasdee, N., Songprakhon, P., Tragoolpua, Y., Rotarayanont, S., Choowongkomon, K., et al. (2020). A Synthetic Bioactive Peptide Derived from the Asian Medicinal Plant Acacia Catechu Binds to Dengue Virus and Inhibits Cell Entry. Viruses 12, 1. doi:10.3390/v12111267
Panya, A., Yongpitakwattana, P., Budchart, P., Sawasdee, N., Krobthong, S., Paemanee, A., et al. (2019). Novel Bioactive Peptides Demonstrating Anti-dengue Virus Activity Isolated from the Asian Medicinal Plant Acacia Catechu. Chem. Biol. Drug Des. 93, 100–109. doi:10.1111/cbdd.13400
Parida, M. M., Upadhyay, C., Pandya, G., and Jana, A. M. (2002). Inhibitory Potential of Neem (Azadirachta indica Juss) Leaves on Dengue Virus Type-2 Replication. J. Ethnopharmacol. 79, 273–278. doi:10.1016/S0378-8741(01)00395-6
Pasa, A. E. (2011). Biodiversity Study of a Smallholder-Protected forest Ecosystem in Leyte, Philippines. Biodiversity 12, 38–45. doi:10.1080/14888386.2011.573702
Paul, A., Vibhuti, A., and Raj, V. S. (2021). Evaluation of Antiviral Activity of Andrographis Paniculata and Tinospora Cordifolia Using In Silico and In Vitro Assay against DENV-2. J. Pharmacogn. Phytochem. 10, 486–496. doi:10.22271/phyto.2021.v10.i2f.13847
Pilla, M. A. C., Amorozo, M. C. d. M., and Furlan, A. (2006). Obtenção e uso das plantas medicinais no distrito de Martim Francisco, Município de Mogi-Mirim, SP, Brasil. Acta Bot. Bras. 20, 789–802. doi:10.1590/s0102-33062006000400005
Pinheiro, F. P., and Corber, S. J. (1997). Global Situation of Dengue and Dengue Haemorrhagic Fever, and its Emergence in the Americas. Geneva, Switzerland: World Heal. Stat. Q.
Pinheiro, F. (1997). Re-Emergence of Dengue and Emergence of Dengue Haemorrhagic Fever in the Americas. Geneva, Switzerland: WHO Regional Office for South-East Asia.
Pinheiro, F., and Rodrigues, S. (1999). Aedes aegypti, Dengue and Re-urbanization of Yellow Fever in Brazil and Other South American Countries Past and Present Situation and Future Presentative. Geneva, Switzerland: WHO.
Pongkitwitoon, B., Sakamoto, S., Tanaka, H., Tsuchihashi, R., Kinjo, J., Morimoto, S., et al. (2011). Development of an Enzyme-Linked Immunosorbent Assay to Determine Puerarin and its Aglycone Daidzein. J. Nat. Med. 65, 31–36. doi:10.1007/s11418-010-0448-z
Pongthanapisith, V., Ikuta, K., Puthavathana, P., and Leelamanit, W. (2013). Antiviral Protein of Momordica Charantia L. Inhibits Different Subtypes of Influenza A. Evid. Based Complement. Alternat Med. 2013, 729081. doi:10.1155/2013/729081
Powers, C. N., and Setzer, W. N. (2016). An In-Silico Investigation of Phytochemicals as Antiviral Agents against Dengue Fever. Comb. Chem. High Throughput Screen. 19, 516–536. doi:10.2174/1386207319666160506123715
Prajapati, V. D., Jani, G. K., Moradiya, N. G., Randeria, N. P., Nagar, B. J., Naikwadi, N. N., et al. (2013). Galactomannan: A Versatile Biodegradable Seed Polysaccharide. Int. J. Biol. Macromol. 60, 83–92. doi:10.1016/j.ijbiomac.2013.05.017
Prakash Kala, C. (2012). Leaf Juice of Carica Papaya L.: A Remedy of Dengue Fever. Med. Aromatic Plants 01, 1. doi:10.4172/2167-0412.1000109
Pratheeba, T., Taranath, V., Sai Gopal, D., and Natarajan, D. (2019). Antidengue Potential of Leaf Extracts of Pavetta Tomentosa and Tarenna Asiatica (Rubiaceae) against Dengue Virus and its Vector Aedes aegypti (Diptera: Culicidae). Heliyon 5, e02732. doi:10.1016/j.heliyon.2019.e02732
Puerta-Guardo, H., Raya-Sandino, A., González-Mariscal, L., Rosales, V. H., Ayala-Dávila, J., Chávez-Mungía, B., et al. (2013). The Cytokine Response of U937-Derived Macrophages Infected through Antibody-dependent Enhancement of Dengue Virus Disrupts Cell Apical-junction Complexes and Increases Vascular Permeability. J. Virol. 87, 7486–7501. doi:10.1128/jvi.00085-13
Raekiansyah, M., Buerano, C. C., Luz, M. A. D., and Morita, K. (2018). Inhibitory Effect of the green tea Molecule EGCG against Dengue Virus Infection. Arch. Virol. 163, 1649–1655. doi:10.1007/s00705-018-3769-y
Rahman, N. A., HadinurMuliawan, S., Rashid, N. N., Muhamad, M., and Yusof, R. (2006). Studies on Quercus Iusitanica Extracts on DENV-2 Replication. Dengue Bull. 1, 1.
Rao, V. B., and Yeturu, K. (2020). Possible Anti-viral Effects of Neem (Azadirachta indica) on Dengue Virus. United States: bioRxiv. doi:10.1016/s0378-8741(01)00395-6
Rasool, N., Ashraf, A., Waseem, M., Hussain, W., and Mahmood, S. (2019). Computational Exploration of Antiviral Activity of Phytochemicals against NS2B/NS3 Proteases from Dengue Virus. Turkish J. Biochem. 44, 261–277. doi:10.1515/tjb-2018-0002
Rathore, A. P., Paradkar, P. N., Watanabe, S., Tan, K. H., Sung, C., Connolly, J. E., et al. (2011). Celgosivir Treatment Misfolds Dengue Virus NS1 Protein, Induces Cellular Pro-survival Genes and Protects against Lethal challenge Mouse Model. Antivir. Res 92, 453–460. doi:10.1016/j.antiviral.2011.10.002
Redoni, M., Yacoub, S., Rivino, L., Giacobbe, D. R., Luzzati, R., and Di Bella, S. (2020). Dengue: Status of Current and Under-development Vaccines. Rev. Med. Virol. 30, e2101. doi:10.1002/rmv.2101
Rees, C. R., Costin, J. M., Fink, R. C., McMichael, M., Fontaine, K. A., Isern, S., et al. (2008). In Vitro inhibition of Dengue Virus Entry by P-Sulfoxy-Cinnamic Acid and Structurally Related Combinatorial Chemistries. Antivir. Res 80, 135–142. doi:10.1016/j.antiviral.2008.05.007
Reis, S. R., Valente, L. M., Sampaio, A. L., Siani, A. C., Gandini, M., Azeredo, E. L., et al. (2008). Immunomodulating and Antiviral Activities of Uncaria Tomentosa on Human Monocytes Infected with Dengue Virus-2. Int. Immunopharmacol. 8, 468–476. doi:10.1016/j.intimp.2007.11.010
Rico-Hesse, R. (2010). Dengue Virus Virulence and Transmission Determinants. Curr. Top. Microbiol. Immunol. 338, 45–55. doi:10.1007/978-3-642-02215-9_4
Rigau-Pérez, J. G. (2006). Severe Dengue: the Need for New Case Definitions. Lancet Infect. Dis. 3, 1. doi:10.1016/S1473-3099(06)70465-0
Robinson, M. M., and Zhang, X. (2011). Traditional Medicines. Geneva, Switzerland: global situation, issues and challenges.
Rodrigues, A., Morais, S., and Martins, V. E. (2020). Larvicidal Efficacy of Plant Extracts and Isolated Compounds from Annonaceae and Piperaceae against Aedes aegypti and Aedes albopictus. Asian Pac. J. Trop. Med. 13, 384. doi:10.4103/1995-7645.290583
Rodríguez-Barraquer, I., Solomon, S. S., Kuganantham, P., Srikrishnan, A. K., Vasudevan, C. K., Qbal, S. H., et al. (2015). The Hidden Burden of Dengue and Chikungunya in Chennai, India. PLoS Negl. Trop. Dis. 9, e0003906. doi:10.1371/journal.pntd.0003906
Rojsanga, P., Bunsupa, S., and Sithisarn, P. (2020). Flavones Contents in Extracts from Oroxylum Indicum Seeds and Plant Tissue Cultures. Molecules 25, 1. doi:10.3390/molecules25071545
Rosmalena, R., Elya, B., Dewi, B. E., Fithriyah, F., Desti, H., Angelina, M., et al. (2019). The Antiviral Effect of Indonesian Medicinal Plant Extracts against Dengue Virus In Vitro and In Silico. Pathogens 8, 1. doi:10.3390/pathogens8020085
Rothan, H. A., Zulqarnain, M., Ammar, Y. A., Tan, E. C., Rahman, N. A., and Yusof, R. (2014). Screening of Antiviral Activities in Medicinal Plants Extracts against Dengue Virus Using Dengue NS2B-NS3 Protease Assay. Trop. Biomed. 31, 286–296.
Saleh, M. S. M., and Kamisah, Y. (2021). Potential Medicinal Plants for the Treatment of Dengue Fever and Severe Acute Respiratory Syndrome-Coronavirus. Biomolecules 11, 42. doi:10.3390/biom11010042
Salles, T. S., da Encarnação Sá-Guimarães, T., De Alvarenga, E. S. L., Guimarães-Ribeiro, V., De Meneses, M. D. F., De Castro-Salles, P. F., et al. (2018). History, Epidemiology and Diagnostics of Dengue in the American and Brazilian Contexts: a Review. Parasites Vectors 11, 1. doi:10.1186/s13071-018-2830-8
Sánchez, I., Gómez-Garibay, F., Taboada, J., and Ruiz, B. H. (2000). Antiviral Effect of Flavonoids on the Dengue Virus. Phyther. Res. 1, 1. doi:10.1002/(SICI)1099-1573(200003)14:2<89:AID-PTR569>3.0.CO;2-C
Saptawati, L., Febrinasari, R. P., Yudhani, R. D., Yono, H., Faza, A. G., Luthfiani, S., et al. (2017). In Vitro study of Eight Indonesian Plants Extracts as Anti Dengue Virus. Health Sci. J. Indonesia 8, 1. doi:10.22435/hsji.v8i1.6601.12-18
Sarala, N., and Paknikar, S. (2014). Papaya Extract to Treat Dengue: A Novel Therapeutic Option? Ann. Med. Health Sci. Res. 4, 320–324. doi:10.4103/2141-9248.133452
Sarker, M. M. R., Khan, F., and Mohamed, I. N. (2021). Dengue Fever: Therapeutic Potential of Carica Papaya L. Leaves. Front. Pharmacol. 12, 1. doi:10.3389/fphar.2021.610912
Saxena, S. K., Haikerwal, A., Gadugu, S., and Bhatt, M. L. (2017). Complementary and Alternative Medicine in alliance with Conventional Medicine for Dengue Therapeutics and Prevention. Future Virol. 12, 399–402. doi:10.2217/fvl-2017-0047
Schneider, C. A., Calvo, E., and Peterson, K. E. (2021). Arboviruses: How Saliva Impacts the Journey from Vector to Host. Int. J. Mol. Sci. 22, 9173. doi:10.3390/ijms22179173
Shah, P. S., Deoshatwar, A., Karad, S., Mhaske, S., Singh, A., Bachal, R. V., et al. (2017). Seroprevalence of Dengue in a Rural and an Urbanized Village: A Pilot Study From Rural Western India. J. Vector Borne Dis. 54 (2), 172. 176.
Sharma, A., Kashyap, D., Sak, K., Tuli, H. S., and Sharma, A. K. (2018). Therapeutic Charm of Quercetin and its Derivatives: A Review of Research and Patents. Pharm. Pat. Anal. 7 (1), 15–32. doi:10.4155/ppa-2017-0030
Sharma, K., Guleria, S., and Razdan, V. K. (2020). Green Synthesis of Silver Nanoparticles Using Ocimum Gratissimum Leaf Extract: Characterization, Antimicrobial Activity and Toxicity Analysis. J. Plant Biochem. Biotechnol. 29, 213–224. doi:10.1007/s13562-019-00522-2
Sharma, N., Mishra, K. P., Chanda, S., Bhardwaj, V., Tanwar, H., Ganju, L., et al. (2019). Evaluation of Anti-dengue Activity of Carica Papaya Aqueous Leaf Extract and its Role in Platelet Augmentation. Arch. Virol. 164, 1095–1110. doi:10.1007/s00705-019-04179-z
Sharma, N., and Pareek, A. (2021). Ethnobotanical Properties of Plants Used by the Rural Community of Dausa District of rajasthan, india. Ijbi 03, 179–185. doi:10.46505/ijbi.2021.3118
Silva, N. M., Santos, N. C., and Martins, I. C. (2020). Dengue and Zika Viruses: Epidemiological History, Potential Therapies, and Promising Vaccines. TropicalMed 5, 150. doi:10.3390/tropicalmed5040150
Simões, L. R., Maciel, G. M., Brandão, G. C., Kroon, E. G., Castilho, R. O., and Oliveira, A. B. (2011). Antiviral Activity of Distictella Elongata (Vahl) Urb. (Bignoniaceae), a Potentially Useful Source of Anti-dengue Drugs from the State of Minas Gerais, Brazil. Brazil. Lett. Appl. Microbiol. 53, 602–607. doi:10.1111/j.1472-765X.2011.03146.x
Singh, K., Zeeshan, M., Ansari, V. A., Ahmad, Z., Bagga, P., and Shakya, P. (2016). Prevention and Control of Dengue by Herbal Remedies. J. Chem. Pharm. Res. 1, 1.
Skriptsova, A. V., Shevchenko, N. M., Zvyagintseva, T. N., and Imbs, T. I. (2010). Monthly Changes in the Content and Monosaccharide Composition of Fucoidan from Undaria Pinnatifida (Laminariales, Phaeophyta). J. Appl. Phycol. 22, 79–86. doi:10.1007/s10811-009-9438-5
Sood, R., Raut, R., Tyagi, P., Pareek, P. K., Barman, T. K., Singhal, S., et al. (2015). Cissampelos Pareira Linn: Natural Source of Potent Antiviral Activity against All Four Dengue Virus Serotypes. Plos Negl. Trop. Dis. 9, e0004255. doi:10.1371/journal.pntd.0004255
Sridhar, S., Luedtke, A., Langevin, E., Zhu, M., Bonaparte, M., Machabert, T., et al. (2018). Effect of Dengue Serostatus on Dengue Vaccine Safety and Efficacy. N. Engl. J. Med. 379, 327–340. doi:10.1056/NEJMoa1800820
Subenthiran, S., Choon, T. C., Cheong, K. C., Thayan, R., Teck, M. B., Muniandy, P. K., et al. (2013). Carica Papaya Leaves Juice Significantly Accelerates the Rate of Increase in Platelet Count Among Patients with Dengue Fever and Dengue Haemorrhagic Fever. Evid. Based Complement. Alternat Med. 2013, 616737. doi:10.1155/2013/616737
Suganthi, A., and Ravi, T. K. (2019). Chemical Methodologies Estimation of Anti-dengue Phytochemical Markers Gallic Acid, Rutin and Quercetin in Methanolic Extract of Euphorbia Hirta (L.) and Tawa-Tawa Capsule Formulation by Validated RP-HPLC Method. Chem. Methodol. 3, 43–54. doi:10.22034/CHEMM.2018.129381.1051
Svanberg, I., and Berggren, Å. (2019). Ant Schnapps for Health and Pleasure: The Use of Formica Rufa L. (Hymenoptera: Formicidae) to Flavour Aquavit. J. Ethnobiol. Ethnomed. 15, 68. doi:10.1186/s13002-019-0347-7
Taguchi, K., Tano, I., Kaneko, N., Matsumoto, T., and Kobayashi, T. (2020). Plant Polyphenols Morin and Quercetin rescue Nitric Oxide Production in Diabetic Mouse Aorta through Distinct Pathways. Biomed. Pharmacother. 129, 110463. doi:10.1016/j.biopha.2020.110463
Talarico, L. B., Pujol, C. A., Zibetti, R. G., Faría, P. C., Noseda, M. D., Duarte, M. E., et al. (2005). The Antiviral Activity of Sulfated Polysaccharides against Dengue Virus Is Dependent on Virus Serotype and Host Cell. Antivir. Res 66, 103–110. doi:10.1016/j.antiviral.2005.02.001
Tamilventhan, A., and Jayaprakash, A. (2019). Larvicidal Activity of Terminalia Arjuna Bark Extracts on Dengue Fever Mosquito Ades Aegypti. Rese. Jour. Pharm. Technol. 12, 87. doi:10.5958/0974-360X.2019.00017.9
Tan, Y. P., Xue, Y., Savchenko, A. I., Houston, S. D., Modhiran, N., McMillan, C. L. D., et al. (2019). Basimarols A, B, and C, Highly Oxygenated Pimarane Diterpenoids from Basilicum Polystachyon. J. Nat. Prod. 82, 2828–2834. doi:10.1021/acs.jnatprod.9b00522
Tarasuk, M., Songprakhon, P., Chimma, P., Sratongno, P., Na-Bangchang, K., and Yenchitsomanus, P. T. (2017). Alpha-mangostin Inhibits Both Dengue Virus Production and Cytokine/chemokine Expression. Virus. Res. 240, 180–189. doi:10.1016/j.virusres.2017.08.011
Toman, J. (2018). Anti-infective Properties of Epigallocatechin-3-Gallate (EGCG), a Component of green tea. J. Sci. Food Agric. 1, 1. doi:10.4137/MBI.S943
Trujillo-Correa, A. I., Quintero-Gil, D. C., Diaz-Castillo, F., Quiñones, W., Robledo, S. M., and Martinez-Gutierrez, M. (2019). In Vitro and In Silico Anti-dengue Activity of Compounds Obtained from Psidium Guajava through Bioprospecting. BMC Complement. Altern. Med. 19, 298. doi:10.1186/s12906-019-2695-1
Umesh Kanna, S., and Krishnakumar, N. (2019). Anti-dengue Medicinal Plants: A Mini Review. J. Pharmacogn. Phytochem. 1, 1. doi:10.1007/s11418-013-0767-y
Uno, N., and Ross, T. M. (2018). Dengue Virus and the Host Innate Immune Response. Emerg. Microbes Infect. 7, 167. doi:10.1038/s41426-018-0168-0
Vasilakis, N., Fokam, E. B., Hanson, C. T., Weinberg, E., Sall, A. A., Whitehead, S. S., et al. (2008). Genetic and Phenotypic Characterization of Sylvatic Dengue Virus Type 2 Strains. Virology 377, 296–307. doi:10.1016/j.virol.2008.04.044
Vaughn, D. W., Green, S., Kalayanarooj, S., Innis, B. L., Nimmannitya, S., Suntayakorn, S., et al. (2000). Dengue Viremia Titer, Antibody Response Pattern, and Virus Serotype Correlate with Disease Severity. J. Infect. Dis. 181, 2–9. doi:10.1086/315215
Vázquez-Calvo, Á., Jiménez de Oya, N., Martín-Acebes, M. A., Garcia-Moruno, E., and Saiz, J.-C. (2017). Antiviral Properties of the Natural Polyphenols Delphinidin and Epigallocatechin Gallate against the Flaviviruses West Nile Virus, Zika Virus, and Dengue Virus. Front. Microbiol. 8, 1. doi:10.3389/fmicb.2017.01314
Waggoner, J. J., Gresh, L., Vargas, M. J., Ballesteros, G., Tellez, Y., Soda, K. J., et al. (2016). Viremia and Clinical Presentation in Nicaraguan Patients Infected with Zika Virus, Chikungunya Virus, and Dengue Virus. Clin. Infect. Dis. 63, 1584–1590. doi:10.1093/cid/ciw589
Wang, M. Y., Ma, Z. L., He, C. L., and Yuan, X. Y. (2020). The Antioxidant Activities of Flavonoids in Jerusalem Artichoke (Helianthus Tuberosus L.) Leaves and Their Quantitative Analysis. Nat. Prod. Res. , 1, 1, 5.doi:doi:10.1080/14786419.2020.1839464
Warsinah, W., Baroroh, H. N., and Harwoko, H. (2020). Phytochemical Analysis and Antioxidant Activity of Brotowali (Tinospora Crispa L. Mier) Stem. Molekul 15, 73. doi:10.20884/1.jm.2020.15.2.533
Wefers, D., Tyl, C. E., and Bunzel, M. (2014). Novel Arabinan and Galactan Oligosaccharides from Dicotyledonous Plants. Front. Chem. 2, 100. doi:10.3389/fchem.2014.00100
Weissenböck, H., Hubálek, Z., Bakonyi, T., and Nowotny, N. (2010). Zoonotic Mosquito-Borne Flaviviruses: Worldwide Presence of Agents with Proven Pathogenicity and Potential Candidates of Future Emerging Diseases. Vet. Microbiol. 140, 271–280. doi:10.1016/j.vetmic.2009.08.025
Whitby, K., Pierson, T. C., Geiss, B., Lane, K., Engle, M., Zhou, Y., et al. (2005). Castanospermine, a Potent Inhibitor of Dengue Virus Infection In Vitro and In Vivo. J. Virol. 79, 8698–8706. doi:10.1128/jvi.79.14.8698-8706.2005
World Health Organization (2021a). Dengue and Severe Dengue. Available at: https://www.who.int/news-room/fact-sheets/detail/dengue-and-severe-dengueue (Accessed May 30, 2021).
World Health Organization (2009). Dengue Guidelines for Diagnosis, Treatment, Prevention and Control. Geneva, Switzerland: WHO.
World Health Organization (2021b). Dengue Situation Updates 2021. WHO. Available at: https://apps.who.int/iris/bitstream/handle/10665/341149/Dengue-20210520.pdf (Accessed May 30, 2021).
Xie, Q., Zhang, B., Yu, J., Wu, Q., Yang, F., Cao, H., et al. (2017). Structure and Function of the Non-structural Protein of Dengue Virus and its Applications in Antiviral Therapy. Curr. Top. Med. Chem. 17, 371–380. doi:10.2174/1568026616666160829155327
Xu, G., Dou, J., Zhang, L., Guo, Q., and Zhou, C. (2010). Inhibitory Effects of Baicalein on the Influenza Virus In Vivo Is Determined by Baicalin in the Serum. Biol. Pharm. Bull. 33, 238–243. doi:10.1248/bpb.33.238
Yam-Puc, J. C., Cedillo-Barrón, L., Aguilar-Medina, E. M., Ramos-Payán, R., Escobar-Gutiérrez, A., and Flores-Romo, L. (2016). The Cellular Bases of Antibody Responses during Dengue Virus Infection. Front. Immunol. 7, 1. doi:10.3389/fimmu.2016.00218
Yang, J.-Y., and Lee, H.-S. (2012). Evaluation of Antioxidant and Antibacterial Activities of Morin Isolated from mulberry Fruits (Morus alba L.). J. Korean Soc. Appl. Biol. Chem. 55, 485–489. doi:10.1007/s13765-012-2110-9
Yao, X., Ling, Y., Guo, S., Wu, W., He, S., Zhang, Q., et al. (2018). Tatanan A from the Acorus calamus L. Root Inhibited Dengue Virus Proliferation and Infections. Phytomedicine 42, 258–267. doi:10.1016/j.phymed.2018.03.018
Yogarajalakshmi, P., Venugopal Poonguzhali, T., Ganesan, R., Karthi, S., Senthil-Nathan, S., Krutmuang, P., et al. (2020). Toxicological Screening of marine Red Algae Champia Parvula (C. Agardh) against the Dengue Mosquito Vector Aedes aegypti (Linn.) and its Non-toxicity against Three Beneficial Aquatic Predators. Aquat. Toxicol. 222, 105474. doi:10.1016/j.aquatox.2020.105474
Yu, J. S., Wu, Y. H., Tseng, C. K., Lin, C. K., Hsu, Y. C., Chen, Y. H., et al. (2017). Schisandrin A Inhibits Dengue Viral Replication via Upregulating Antiviral Interferon Responses through STAT Signaling Pathway. Sci. Rep. 7, 45171. doi:10.1038/srep45171
Zandi, K., Teoh, B. T., Sam, S. S., Wong, P. F., Mustafa, M. R., and Abubakar, S. (2011). Antiviral Activity of Four Types of Bioflavonoid against Dengue Virus Type-2. Virol. J. 8, 560. doi:10.1186/1743-422X-8-560
Zandi, K., Teoh, B. T., Sam, S. S., Wong, P. F., Mustafa, M. R., and AbuBakar, S. (2012). Novel Antiviral Activity of Baicalein against Dengue Virus. BMC Complement. Altern. Med. 12, 214. doi:10.1186/1472-6882-12-214
Zeng, Z., Zhan, J., Chen, L., Chen, H., and Cheng, S. (2021). Global, Regional, and National Dengue burden from 1990 to 2017: A Systematic Analysis Based on the Global burden of Disease Study 2017. EClinicalMedicine 32, 100712. doi:10.1016/j.eclinm.2020.100712
Zhang, J. X., Braakman, I., Matlack, K. E., and Helenius, A. (1997). Quality Control in the Secretory Pathway: the Role of Calreticulin, Calnexin and BiP in the Retention of Glycoproteins with C-Terminal Truncations. Mol. Biol. Cel 8, 1943–1954. doi:10.1091/mbc.8.10.1943
Zhang, Y., Yu, X., Wang, M., Ding, Y., Guo, H., Liu, J., et al. (2021). Hyperoside from Z. Bungeanum Leaves Restores Insulin Secretion and Mitochondrial Function by Regulating Pancreatic Cellular Redox Status in Diabetic Mice. Free Radic. Biol. Med. 162, 412–422. doi:10.1016/j.freeradbiomed.2020.10.320
Zhao, Q., Chen, X. Y., and Martin, C. (2016). Scutellaria Baicalensis, the golden Herb from the Garden of Chinese Medicinal Plants. Sci. Bull. (Beijing) 61, 1391–1398. doi:10.1007/s11434-016-1136-5
Zhou, X., Zeng, L., Chen, Y., Wang, X., Liao, Y., Xiao, Y., et al. (2020). Metabolism of Gallic Acid and its Distributions in tea (Camellia Sinensis) Plants at the Tissue and Subcellular Levels. Int. J. Mol. Sci. 21, 1. doi:10.3390/ijms21165684
Keywords: dengue, traditional medicine, plant medicaments, bioactive compound, immunomodulatory response
Citation: Dhiman M, Sharma L, Dadhich A, Dhawan P and Sharma MM (2022) Traditional Knowledge to Contemporary Medication in the Treatment of Infectious Disease Dengue: A Review. Front. Pharmacol. 13:750494. doi: 10.3389/fphar.2022.750494
Received: 30 July 2021; Accepted: 05 January 2022;
Published: 14 March 2022.
Edited by:
Eliana Rodrigues, Federal University of São Paulo, BrazilReviewed by:
Marlen Martinez-Gutierrez, Cooperative University of Colombia, ColombiaPrashant Kaushik, University of Valencia, Spain
Copyright © 2022 Dhiman, Sharma, Dadhich, Dhawan and Sharma. This is an open-access article distributed under the terms of the Creative Commons Attribution License (CC BY). The use, distribution or reproduction in other forums is permitted, provided the original author(s) and the copyright owner(s) are credited and that the original publication in this journal is cited, in accordance with accepted academic practice. No use, distribution or reproduction is permitted which does not comply with these terms.
*Correspondence: M. M. Sharma, bWFkYW5tb2hhbi5zaGFybWFAamFpcHVyLm1hbmlwYWwuZWR1