- 1College of Life Sciences, Northwest A & F University, Xi’an, China
- 2College of Life Sciences and Medicine, Zhejiang Sci-Tech University, Hangzhou, China
Diabetes mellitus is a fast-growing disease with a major influence on people’s quality of life. Oral hypoglycemic drugs and insulin are currently the main effective drugs in the treatment of diabetes, but chronic consumption of these drugs has certain side effects. Polysaccharides, saponins, flavonoids, and phenolics are the primary secondary metabolites isolated from the rhizomes of Polygonatum sibiricum Redouté [Asparagaceae], Polygonatum kingianum Collett & Hemsl [Asparagaceae], or Polygonatum cyrtonema Hua [Asparagaceae], which have attracted much more attention owing to their unique therapeutic role in the treatment and prevention of diabetes. However, the research on the mechanism of these three Polygonatum spp. in diabetes has not been reviewed. This review provides a summary of the research progress of three Polygonatum spp. on diabetes and its complications, reveals the potential antidiabetic mechanism of three Polygonatum spp., and discusses the effect of different processed products of three Polygonatum spp. in treating diabetes, for the sake of a thorough understanding of its effects on the prevention and treatment of diabetes and diabetes complications.
Introduction
Diabetes mellitus (DM) is a comprehensive endocrine and metabolic disease characterized by glucose metabolism disorders, mainly resulting from insulin resistance or insufficient insulin secretion (Xiao et al., 2019). According to the American Diabetes Association, it is divided into four major types: type 1 diabetes (T1DM), type 2 diabetes (T2DM), gestational diabetes (GDM), and diabetes from other causes. T2DM has the highest incidence among these diseases (Skyler and Oddo, 2010). The key factors that cause T2DM are pancreas β-cell failure, insulin resistance, and its complex interrelationships (Thorens, 2011). More importantly, long-term hyperglycemia may cause malfunction and long-term damage in a variety of tissues and organs, particularly the eyes, nerves, kidneys, heart, and blood vessels (Pham et al., 2019; Sloan, 2019).
In recent years, DM has become one of the primary diseases endangering modern people, and the number of patients has been increasing year by year. Almost all patients require oral hypoglycemic agents or injecting insulin. Diabetes is difficult to control in a maintainable long-term lifestyle (Tahrani et al., 2011; Herman et al., 2018). Current oral hypoglycemic agents include the earlier developed metformin and sulfonamides, as well as some novel hypoglycemic agents targeting the pancreas or liver, such as sodium-dependent glucose transporter 2 (SGLT2) inhibitors, dipeptidyl peptidase-4 (DPP-4) inhibitors, and glucagon-like peptide 1 (GLP-1) receptor agonists, which can enhance insulin activity, exert insulin-like effects, or alleviate glucose metabolism disorders (Tahrani et al., 2016). However, the clinical application of insulin often causes hypoglycemia, insulin resistance, lipoatrophy, and other side effects. Although the newly developed drugs have had a lower risk of hypoglycemia in recent years, they are more expensive than other early-developed drugs such as sulfonylureas, and long-term safety has yet to be determined. Therefore, it is essential to screen the natural products with antidiabetic activity and investigate their material basis, pharmacodynamics, and mechanism of action to provide new ideas for developing high efficiency and low toxicity antidiabetic drugs.
Traditional Chinese medicines (TCMs) are gaining popularity as a result of their success in the treatment and prevention of diabetes, such as Panax ginseng C. A. Mey. [Araliaceae], Lycium barbarum L. [Solanaceae], Coptis chinensis Franch. [Ranunculaceae], Abelmoschus esculentus (L.) Moench [Malvaceae], Angelica sinensis (Oliv.) Diels [Apiaceae], and Andrographis paniculata (Burm.f.) Nees [Acanthaceae] (Xu et al., 2012; Sun et al., 2014; Zhao et al., 2014; Wang et al., 2016a; Cui et al., 2016; Liao et al., 2019). Polygonatum is a common genus from the Asparagaceae family widely distributed in China and has been used as medicine or food for more than 2,000 years because it invigorates the spleen, moisturizes the lungs, and invigorates qi. The plant part used is the dry rhizome of Polygonatum sibiricum Redouté (P.sibiricum), Polygonatum kingianum Collett & Hemsl (P. kingianum), or Polygonatum cyrtonema Hua (P. cyrtonema), introduced in the 2020 edition of the Pharmacopoeia of the People’s Republic of China (Commission, 2020). The underlying pharmacological applications of Polygonatum are gaining popularity in clinical diseases, such as fatty liver disease, Alzheimer’s disease, diabetes mellitus, and cancer (Yu et al., 2021). Such biological activities are closely related to the secondary metabolites of Polygonatum, including polysaccharides, saponins, flavonoids, phenolics, alkaloids, anthraquinones, lignans, and a variety of beneficial amino acids (Jiang et al., 2017). Recently, Polygonatum spp. have become widely used TCMs in improving diabetes.
In this work, we comprehensively analyzed the antidiabetic-related research work on three Polygonatum spp. The purpose of this work is to review the secondary metabolites of Polygonatum and their antidiabetic mechanism, investigate the effects of different processed products of Polygonatum on treatment, and lay the foundation for the clinical application and product development of Polygonatum.
Data Collection
According to published reports from 2011 to 2021, “Polygonatum” or “Rhizoma polygonti” combined with “diabetes” or “anti-diabetic,” “secondary metabolites,” and “processed products” were used as search keywords. The data were collected by various online databases, including PUBMED, Web of Science, Science Direct, SpringerLink, Wiley Online Library, Wanfang, and China Knowledge Network. About 189 papers were found by reading abstracts to exclude repetitive and irrelevant papers. The data were further extracted from the above studies: P. sibiricum, P. kingianum, and P. cyrtonema were used according to the 2020 edition of the Pharmacopoeia of the People’s Republic of China; test design with the control group and functional verification; and dose use strictly in line with the standard (rat dose = human dose g * 0.018/0.02 kg). Eventually, we found that 47 articles met the screening standard and brought into this paper by critically reviewing and analyzing the data, aiming to identify secondary metabolites and processed products of Polygonatum involved in the antidiabetic mechanism.
Secondary Metabolites of Polygonatum
Currently, the secondary metabolites of Polygonatum have been reported to include polysaccharides, saponins (steroidal saponins and triterpenoids), flavonoids, phenols, alkaloids, lignans, phytosterols, and volatile oils, of which the first four ones are the major ingredients and have been studied most frequently. Additionally, polysaccharides and saponins were the highest in P. cyrtonema, and flavonoids and other phenolics were the highest in P. sibiricum (Table 1).
Polysaccharides
Polysaccharide is not only an active important component of Polygonatum but also an important evaluation index of its quality. It has been reported that polysaccharide is composed of many monosaccharides including fructose (Fru), glucose (Glc), mannose (Man), galactose (Gal), arabinose (Ara), and rhamnose (Rha), as well as a handful of glucuronic acid (GlcA) and xylose (Xyl). The molecular weights of polysaccharides from Polygonatum plants are estimated to be approximately 2,734∼3.6 × 105 Da (Zhao et al., 2018). Two new polysaccharides (PSP50-2-1 and PSP50-2-2) were isolated and purified from the rhizome of P. sibiricum, both of which were homogeneous polysaccharides by the analysis of the specific optical rotation. Meanwhile, the result of monosaccharide composition indicated that PSP50-2-1 and PSP50-2-2 were made up of Glc, Gal, and Fru (Liu et al., 2021), with the molecular weight of 7.7 and 7.0 kDa, respectively. More importantly, Wang et al. found that four polysaccharides isolated from P. sibiricum (PSP1, PSP2, PSP3, and PSP4) were made up of Gal, Rha, Man, Glu, and Xyl in different proportions, and the immune activity of polysaccharides was closely related to that of Rha residues, with the molecular weight of 4.415, 2.236, 7.743, and 6.467 kDa, respectively (Wang et al., 2020). Zhao et al. found that polysaccharides isolated from P. sibiricum, P. kingianum, and P. cyrtonema were mainly made up of Fru and pectins, with a molecular weight of more than 4.1 × 105 Da (Zhao et al., 2020).
Saponins
Although saponins are another main active component of Polygonatum, their content is relatively low. According to the different structures of saponins in Polygonatum, saponins were divided into steroidal saponins and triterpenoid saponins. Zhao et al. summarized 162 saponins from 18 species of Polygonatum genus, among which 70 steroidal saponins and 12 triterpenoid saponins were isolated from P. sibiricum, P. kingianum, and P. cyrtonema (Zhao et al., 2018). Subsequently, some studies provided novel findings of five novel steroidal saponins isolated from P. sibiricum, 3-O-β-d-glucopyranosyl(1→2)-β-d-glucopyranosyl(1→4)-β-d-fucopyranosyl-(25R)-spirost-5-en-3β,17α-diol, 3-O-β-d-glucopyranosyl(1→4)-β-d-fucopyranosyl-(25R/S)-spirost-5-en-3β,12β-diol, 3-O-β-d-glucopyranosyl(1→4)-β-d-fucopyranosyl-(25R)-spirost-5-en-3β,17α-diol, 3-O-β-d-glucopyranosyl(1→4)-β-d-fucopyranosyl-(25S)-spirost-5-en-3β,17α-diol, and kingianoside Z (Zhang et al., 2017; Tang et al., 2019). Two new steroidal saponins were isolated from P. kingianum, named polygokingiaside A and polygokingiaside B, respectively (Ha et al., 2021). A novel steroidal saponin was isolated from P. cyrtonema, named Huangjingsterol B (Huang et al., 2020). On the other hand, no new triterpenoid saponins were found in Polygonatum plants because triterpenoid saponins are found principally in the Magnoliopsida class, and steroidal saponins are distributed widely in the Liliopsida class (Faizal and Geelen, 2013).
Phenolics
Phenolics include flavonoids, phenolics, and lignins. Flavonoids are ubiquitous in natural plants and have a broad spectrum of biological activities. Until now, 34 flavonoids have been isolated from P. sibiricum, P. kingianum, and P. cyrtonema, which can be divided into six types in accordance with the structure of the parent nucleus: homoisoflavones, isoflavones, flavones, chalcones, dihydroflavones, and rosandalanes (Table 2). Among them, homoisoflavones are the most abundant in Polygonatum, such as 4′,5,7-trihydroxy-6-methyl-8-methoxy-homoisoflavanon, disporopsin, and polygonatone H.
Phenolics in plants are secondary metabolites synthesized during the normal development of plants. Relatively rare studies have been conducted on the structural properties of the phenolics and lignans from Polygonatum. Wang et al. identified two known compounds (narcissoside and nicotiflorin) from P. sibiricum by 1D/2D NMR and MS data (Wang et al., 2016b). Zhai and Wang isolated syringaresinol-di-O-β-d-glucoside from P. sibiricum (Zhai and Wang, 2018). Chen et al. isolated a benzofuran-type lignan (polygonneolignanoside A) from P. sibiricum (Chen et al., 2020).
Other Secondary Metabolites
The contents of alkaloids, phytosterols, and volatile compounds in Polygonatum were extremely low, and their structures were less studied. Polygonatine A and Polygonatine B isolated from P. sibiricum were identified as alkaloids (Sun et al., 2005). Four phytosterol compounds have already been identified in P. sibiricum and P. kingianum, including β-sitosterol, carotenoside, palmitate-3β sitosterol, ester and (22S)-cholest-5-ene-1β,3β,16β,22-tetrol 1-O-α-l-rhamnopyranosyl 16-O-β-d-glucopyranoside (Li et al., 2008; Ahn et al., 2011). Volatile compounds were found in the rhizomes of P. cyrtonema, which accounted for 95.97% of the total volatile oils (Yu et al., 2008).
Potential Antidiabetic Mechanism of Polygonatum on Diabetes
Studies have shown that certain active ingredients of traditional Chinese herbal medicines have apparent effects of lowering blood sugar and blood lipids, such as polysaccharides, saponins, flavonoids, phenols, and alkaloids (Xu et al., 2018; Xu et al., 2019; Deng et al., 2020; Hou et al., 2020; Zhuang et al., 2020). Polygonatum is rich in these substances and hence is a Chinese herbal medicine with great medicinal value. The number of research papers on secondary metabolites and biological activities of Polygonatum is increasing in recent decades.
To date, there are three models to study the antidiabetic mechanism of secondary metabolites from Polygonatum: cells, diabetic animal models, and humans (Figure 1). For example, in in vitro studies, in which the IR-3T3-L1 adipocytes and IR-HepG2 cells were cultured, it was found that Polygonatum could increase glucose intake by alleviating oxidative stress and inflammation (Cai et al., 2019; Luo et al., 2020). In animal models of diabetes, for the sake of identifying the metabolic impact of the Polygonatum rhizome extract, high-fat diet (HFD)-, streptozotocin (STZ)-, or alloxan-induced rats were administered Polygonatum orally at a certain dose for a period. It is suggested that Polygonatum could decrease high blood glucose by analyzing various factors related to metabolic syndrome (Pang et al., 2018; Gu et al., 2020; Li et al., 2020). In addition, Polygonatum also improves homeostasis model assessment of insulin sensitivity (HOMA-IS) and homeostasis model assessment of insulin resistance (HOMA-IR) of patients with diabetes in clinical studies (Ping, 2021).
In Vitro Models
The nuclear factor erythroid 2-related factor 2/heme oxygenase-1 (Nrf2/HO-1) signaling pathway is closely related to pancreatic β-cell injury, obesity, glucose metabolism disorders, and insulin resistance. Polysaccharides of P. sibiricum (PSP) (50, 100, and 250 μg/ml) can alleviate IR and proliferation of IR-3T3-L1 adipocytes by activating Nrf2/HO-1 signaling pathway in IR-3T3-L1 adipocytes, they promoted the expression of Nrf2 and HO-1 and lessened the expression levels of inflammatory cytokines [interleukin-1β (IL-1β), interleukin-6 (IL-6), and tumor necrosis factor-α (TNF-α)], and subsequently enhanced glucose intake by stimulating the expression of transporter subtype-4 (GLUT4). When the Nrf2 gene was silenced, the expressions of inflammatory cytokines, HO-1, GLUT4, and glucose intake were elevated, thereby reversing the therapeutic effect of PSP in IR-3T3-L1 adipocytes (Cai et al., 2019).
In IR-HepG2 cells, polysaccharides from P. kingianum (PKP) enhanced the levels of glucose utilization efficiency at doses of 6.25, 12.5, and 25 mg/L (Li et al., 2020); saponins from P. sibiricum (PSS) could significantly inhibit insulin resistance in a dose-dependent manner in HepG2 cells, but it was noteworthy that when the concentration of PSS was above 500 μg/ml, it affected cell viability. Moreover, PSS also could markedly attenuated the activities of α-glucosidase and α-amylase in vitro (Luo et al., 2020).
Animal Models
Polysaccharides of P. cyrtonema (PCP) (450–900 mg/kg) significantly improved the survival rate of STZ-induced T1DM female rats by inhibiting weight loss, suppressing inflammatory cytokine expression in the liver, and increasing insulin receptor substrate (IRS) expression, thereby improving the hepatic immune response (Wang et al., 2019b). More importantly, both low (120 mg/kg) and high (480 mg/kg) doses of PKP improved diabetic symptoms by increasing short-chain fatty acid (SCFA) levels, modulating gut microbiota composition, and reducing inflammation in HFD rats (Gu et al., 2020).
Saponins from P. kingianum (TSPK) also have antidiabetic effects. STZ-induced diabetic rats were given TSPK for 8 weeks at 0.025 and 0.1 g/kg, TSPK could alleviate hyperlipidemia and hyperglycemia in diabetic rats, and the genome-wide expression indicated that expression of GLUT4 was significantly upregulated. In contrast, the expression of G6P was downregulated in the insulin signal pathway (Lu et al., 2016). The structure and number of gut microbiota of rats treated with TSPK were significantly changed, so TSPK may prevent T2DM by regulating gut microbiota and the secretion of SCFAs (Yan et al., 2017). Furthermore, PSS can activate hexokinase and then converts glucose to glucose-6-phosphatase (G6P), which promotes glycogen synthesis and ultimately reduces insulin resistance. Interestingly, the number of bacteria changed in the dung of the T2DM rats treated with PSS (1, 1.5, and 2 g/kg), with the result that the number of probiotics increased and the number of harmful bacteria decreased (Luo et al., 2020).
Shu et al. found that total flavonoids of P. sibiricum (TFP) have significant hypoglycemic effects on both T1DM and T2DM. Compared to those of the control group, the hypoglycemic effects of 100 and 200 mg/kg of TFP were similar to those of 20 mg/kg of acarbose in STZ-induced T1DM rats. In HFD- and alloxan-induced T2DM rats, 200 mg/kg of TFP had a similar hypoglycemic effect to 15 mg/kg of gliclazide. After 9 days of treatment with 100 and 200 mg/kg of TFP, the fasting blood glucose (FBG) of rats decreased in a dose-dependent manner. Besides, TFP significantly inhibited α-amylase activity in a dose-dependent manner in vitro (Shu et al., 2012). Overall, TFP may have multiple beneficial effects on lessening hyperglycemia induced by alloxan, STZ, and HFD in diabetic rats, respectively.
However, there is another class of phenolic compound (syringaresinol-di-O-β-d-glucoside (SOG)) isolated from P. sibiricum that exerts an antidiabetic effect. Treatment with SOG (25, 50, and 75 mg/kg) facilitated insulin secretion and reduced the levels of lipid metabolism and oxidative stress in the STZ-induced diabetic rats, as well as downregulated the expression of nitrotyrosine (NT) and TGF-β1 in kidneys (Zhai and Wang, 2018). Thus, SOG showed a significant antidiabetic effect by suppressing oxidative stress.
In summary, polysaccharides, saponins, flavonoids, and other phenolics of Polygonatum have a prominent role in lowering blood sugar and blood lipids in DM (Table 3). The minimum dose of Polygonatum secondary metabolites is 25 mg/kg, and the maximum dose is 2 g/kg.
Clinical Application
To date, clinical studies verified that a few Chinese patent medicines containing Polygonatum have a beneficial effect on diabetes, such as Tangwei capsules, Jinlida granules, Tangmaikang granules, Jiangtangjia tablets, and Qizhi Jiangtang capsules (Tables 4, 5). Jinlida granules could significantly decrease the level of hemoglobin A1c (HbA1c) and fasting plasma glucose (FPG) in the 2-h postprandial blood glucose (2hPG) in the individuals who received Jinlida granules (9 g) compared to the control groups (Lian et al., 2019). Jiangtang Tongmai capsules (1.05 g) combined with glibenclamide can reduce the blood glucose level, improve HOMA-IS and HOMA-IR of patients with T2DM, and reduce the severity of clinical symptoms of T2DM (Ping, 2021). HbA1c and HOMA-IR were significantly decreased after treatment with Jiangtangshu tablets (1.5 g) combined with repaglinide, while GLP-1 and fasting serum insulin (FINS) levels were significantly increased (Li and Li, 2019). After treatment with Qizhi Jiangtang capsules (2.5 g), NO serum content was increased, and endothelin-1 (ET-1), thromboxane B2 (TXB2), blood urea nitrogen (BUN), serum creatinine (SCr) contents were lower than those in the control group, which eventually improved renal microcirculation and dysfunction (Si and Xue, 2021). In conclusion, these Chinese patent medicines could effectively control blood glucose and inhibit insulin resistance without significant adverse effects and could be used as an adjuvant drug for the treatment of T2DM and its complications.
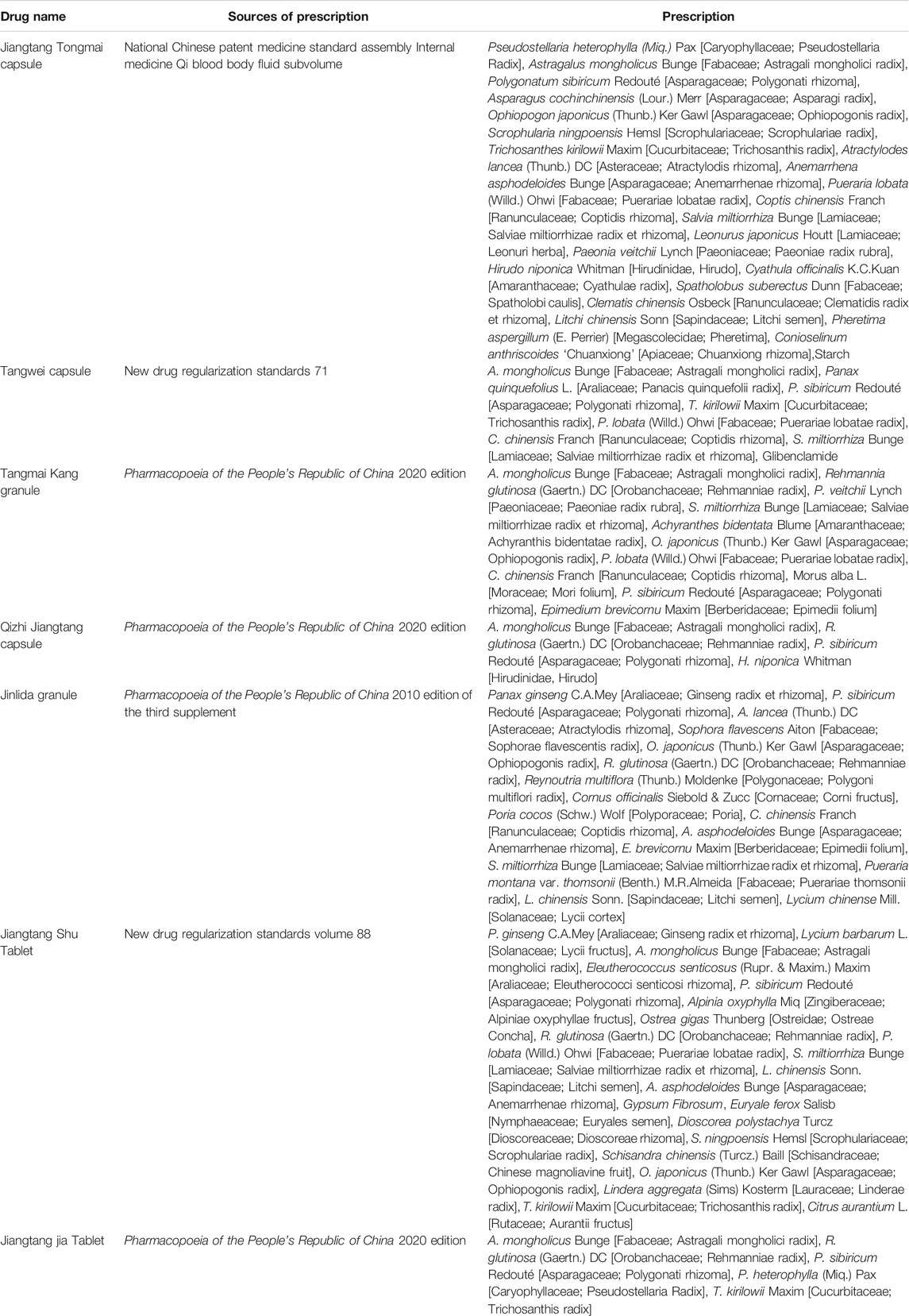
TABLE 5. Chinese patent medicine prescription containing Polygonatum with hypoglycemic effect (data from db.yaozh.com).
Potential Antidiabetic Mechanism of Polygonatum on Diabetes Complications
Diabetes can also lead to complications of other diseases, such as acute kidney injury (AKI), diabetic retinopathy (DR), and diabetic nephropathy (DN). The p38 MAPK is the most critical and common signaling pathway in protecting against inflammatory kidney injury (Ahmed and Mohamed, 2018). Gentamicin (GM) can stimulate the secretion of inflammatory cytokines via activation of p38 mitogen-activated protein kinase (MAPK)/activation transcription factor 2 (p38 MAPK/ATF2) pathway, triggering a set of inflammatory cascade reactions that result in kidney injury. However, PSP could markedly decrease the expression levels of neutrophil gelatinase-associated lipocalin (NGAL) and kidney injury molecule-1 (KIM-1), preventing the p38 MAPK/ATF2 pathway to suppress the secretion of inflammatory cytokines in the kidney (Han et al., 2020). As a result, PSP has a potential pharmacotherapy on GM-induced AKI rats.
VEGF is a crucial angiogenic growth factor that facilitates the migration, proliferation, and angiogenesis of vascular endothelial cells (Hu et al., 2015). Moreover, some growth factors can promote retinal cell proliferation, such as transforming growth factor-β (TGF-β), which can contribute to cell proliferation and differentiation and suppress DNA synthesis of vascular endothelial cells (Sharma et al., 2015). Epidermal growth factor (EGF) works on the proliferation of retinal capillary endothelial (Sugimoto et al., 2013). However, the treatment of PSP notably reduced the expression of VEGF, TGF-β, and EGF in the DR retina (Wang et al., 2019c). In STZ-induced DR rats, the expression of apoptotic protein B-cell lymphoma-2 factor (Bcl-2) was enhanced, while the expression of Bcl2-associated X protein (Bax) and p38 was reduced in PSP-treated rats. p38 MAPK is pivotal in the regulation of apoptosis. In addition, PSP can also reduce the activity of the superoxide dismutase (SOD) enzyme and increase the content of malondialdehyde (MDA), thus reducing oxidative stress of DM rats (Wang et al., 2017).
Wnt/β-catenin pathway (Wnt) signaling is involved in pancreas development and islet function (Liu and Habener, 2008; Wang et al., 2015; Palsgaard et al., 2016) and plays a vital role in modulating GLP-1 through regulating the transcription of the proglucagon gene in T2DM (Welzel et al., 2009). Zou et al. proved that Shen’an granules could regulate urinary protein, renal function, and dyslipidemia in DN rats, and such effects are achieved by suppressing the activation of the Wnt/β-catenin signaling pathway (Zou et al., 2016). Furthermore, the hypoglycemic effect of PSS on T2DM was also related to the Wnt/β-catenin signaling pathway. There is evidence that the expression of Wnt4 and β-catenin in the DN model group has been notably enhanced compared with that of the control group. In contrast, the expression of Wnt4 and β-catenin in the high-dose and low-dose PSS groups notably decreased (Jing, 2019). Therefore, PSS can suppress the process of tubulointerstitial fibrosis by blocking the activation of the Wnt/β-catenin signaling pathway and finally plays a vital role in kidney protection.
In brief, the studies of molecular mechanisms suggest that Polygonatum influences the development of diabetic complications by regulating MAPK, adenosine monophosphate-activated protein kinase (AMPK), and Wnt/β-catenin signaling pathway (Figure 2).
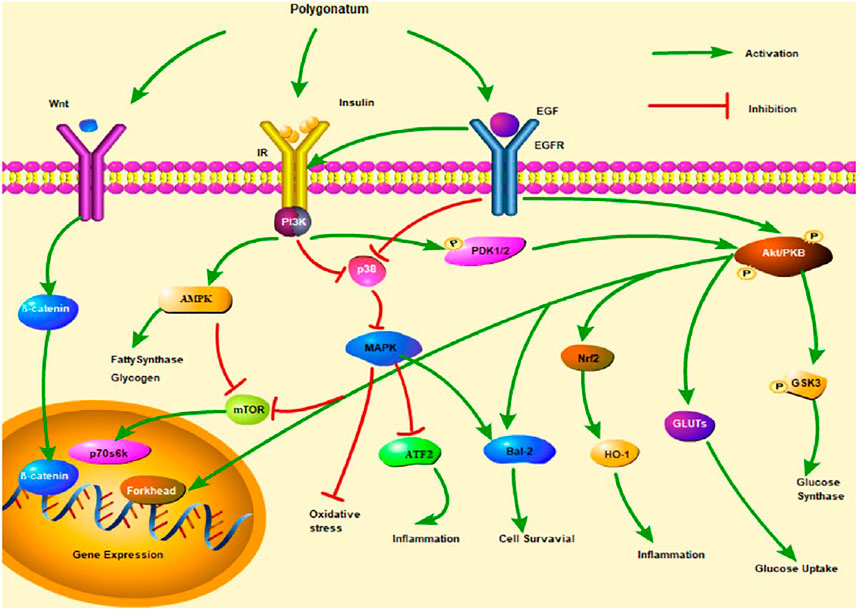
FIGURE 2. Insulin signaling pathways of three Polygonatum spp. Note. mTOR, mammalian target of rapamycin; PDK1/2, 3-phosphoinositide-dependent protein kinase-1/2; GSK3, glycogen synthase kinase 3β.
Effects of Different Processed Products of Polygonatum on Diabetes
TCMs need to be processed to have a better therapeutic effect, unlike Western medicine. Processed TCMs have an apparent therapeutic effect, low toxicity, and convenience for storability. Moreover, different processing methods of the same drug show different efficacy.
An effort was made to compare the effect of hypoglycemic and hypolipidemic among ninefold-processed P. kingianum and four products of P. kingianum processed with different auxiliary materials (wine, black beans, Rehmannia glutinosa (Gaertn.) DC [Orobanchaceae] and L. barbarum L. [Solanaceae]). All five processed P. kingianum products were administered in high-glucose rats and high-fat rats at doses of 1.95 and 1.35 mg/g, respectively. The results confirmed that the hypoglycemic effect of ninefold-processed P. kingianum and P. kingianum processed with R. glutinosa (Gaertn.) DC. had markedly enhanced effects, while L. barbarum L. processed P. kingianum shows no significant effect. Additionally, ninefold-processed P. kingianum has the best hypolipidemic effect, and L. barbarum L. processed P. kingianum has the lowest effect through detecting the content of four factors related to lipid metabolism [total cholesterol (TC), triglyceride (TG), high-density lipoprotein cholesterol (HDL-C), and low-density lipoprotein cholesterol (LDL-C)] (Zhang, 2019). Other studies revealed that water extracts from each processed product of P. sibiricum (10 g/kg) were given by intragastric administration for 6 weeks; fourfold processing of P. sibiricum can better improve Qi and Yin deficiency syndrome by increasing the body weight and tail diameter of rats and regulating the glucose and lipid metabolism, compared to ninefold-processed P. sibiricum (Ma et al., 2019).
Li et al. found that fermented P. sibiricum (FPS) could lower insulin, FBG, and lipid metabolism than P. sibiricum. FPS showed greater efficacy than P. sibiricum in decreasing insulin resistance by increasing the p-AKT/AKT ratio, and FPS had a hypolipidemic effect on liver and fat in STZ-induced diabetic rats by improving lipolysis and inhibiting adipogenesis (Li et al., 2021).
Future Prospects
The beneficial effects of antidiabetes may be related to the metabolites of natural products in the human body. For instance, conjugated (glucuronidated and sulfated) metabolites of hydroxytyrosol and oleuropein are detected in plasma and urine following oleuropein consumption at a single dose of 76.6 mg per person. The concentration of oleuropein metabolites was significantly increased compared with oleuropein (149 vs. 3.55 ng/ml) in plasma (Bock et al., 2013). However, there are no studies on the beneficial effects of chemical components of Polygonatum against diabetes, and this may be related to metabolites in humans, and the specific mechanism needs to be further studied. Beyond that, it also is worth further exploring whether different active components of Polygonatum work alone or in a particular proportion with better curative effect against diabetes.
Conclusion
Diabetes mellitus, known as thirst dissipation in ancient China, was characterized by polydipsia, polyuria, polyphagia, emaciation, fatigue, and frequent urination. Now, it is common knowledge that DM is a group of metabolic diseases characterized by hyperglycemia, which is a chronic disease that cannot be cured by pharmaceutical means, but treatments can alleviate the development and symptoms of diabetes. With the increasing number of diabetic patients, natural products of Polygonatum (polysaccharides, saponins, flavonoids, and phenols) have attracted wide attention on account of their efficacy in lowering blood sugar and blood lipids. However, there are more studies on the hypoglycemic effect of polysaccharides and saponins than that of flavonoids and phenols. However, flavonoids and other phenolics are worthy of being studied. In addition, this review also summarizes the three insulin signaling pathways—p38MAPK, AMPK, and Wnt/β-catenin signaling pathways—that might be involved in the treatment of diabetes with Polygonatum, whereas these signaling pathways could result in a variety of biological activities to change, such as glucose uptake and glycogen synthesis, cell survival, oxidative stress, inflammation, and lipid metabolism. Consequently, the mechanism of action and targets of Polygonatum have been studied from the perspective of its unique chemical components, which is crucial to lay the foundation for clinical research.
Preclinical and clinical studies have shown that Polygonum has a positive therapeutic effect on diabetes. However, there is still a lack of research on Polygonum intake in humans. It is worth noting that the minimum effective dose of Polygonum must be determined in clinical studies due to individual differences.
Overall, the antidiabetic efficacy of Polygonum is well-known, and the antidiabetic benefits of bioactive components, especially polysaccharides and saponins, have widely been reported. Meanwhile, the combination use of Polygonatum and other clinical hypoglycemic drugs could enhance the therapeutic effect of hypoglycemic drugs, giving Polygonatum a broader application prospect in the treatment of diabetes and its complications.
Author Contributions
SL and Q-JJ: conceptualization, writing-original draft. Y-QP, TF, and SH: collected the literatures. JD and Z-SL: writing-review and editing.
Funding
The present study was supported by the Key R&D program project from Zhejiang Province (2017C02034), Basic Public Welfare Research Plan of Zhejiang Province (LGN21C020008), Characteristic Industrial Chain Project of Shaanxi Provincial Science and Technology Department (2019TSLSF02-02), and Key Program of Shandong Province, China (S190002030001).
Conflict of Interest
The authors declare that the research was conducted in the absence of any commercial or financial relationships that could be construed as a potential conflict of interest.
Publisher’s Note
All claims expressed in this article are solely those of the authors and do not necessarily represent those of their affiliated organizations, or those of the publisher, the editors, and the reviewers. Any product that may be evaluated in this article, or claim that may be made by its manufacturer, is not guaranteed or endorsed by the publisher.
References
Ahmed, H. I., and Mohamed, E. A. (2018). Candesartan and Epigallocatechin-3-Gallate Ameliorate Gentamicin-Induced Renal Damage in Rats through P38-MAPK and NF-Κb Pathways. J. Biochem. Mol. Toxicol. 33 (3), e22254. doi:10.1002/jbt.22254
Ahn, M. J., Cho, H., Lee, M. K., Bae, J. Y., and Kim, J. (2011). A Bisdesmosidic Cholestane Glycoside from the Rhizomes of Polygonatum Sibiricum. Nat. Prod. Sci. 17, 183–188. Available at: https://www.researchgate.net/publication/286580335_A_bisdesmosidic_cholestane_glycoside_from_the_rhizomes_of_Polygonatum_sibiricum
Cai, J., Zhu, Y., Zuo, Y., Tong, Q., Zhang, Z., Yang, L., et al. (2019). Polygonatum Sibiricum Polysaccharide Alleviates Inflammatory Cytokines and Promotes Glucose Uptake in High-glucose- and H-igh-insulin-induced 3T3-L1 A-dipocytes by P-romoting Nrf2 E-xpression. Mol. Med. Rep. 20 (4), 3951–3958. doi:10.3892/mmr.2019.10626
Chen, H., Zhu, Y., Kong, J. B., Sun, Y. J., Gao, L., Bian, H., et al. (2020). A New Benzofuran Lignan from Rhizomes of Polygonatum Sibiricum. Chin. Tradit. Herb. Drugs 51 (01), 21–25. doi:10.7501/j.issn.0253-2670.2020.01.004
Chen, X., and Zhang, H. (2019). Clinical Analysis of Tangwei Capsule Combined with Alogliptin in the Treatment of Type 2 Diabetes Mellitus. World J. Diabetes 2 2 (22), 56–57. doi:10.16658/j.cnki.1672-4062.2019.22.056
Commission, C. P. (2020). Pharmacopoeia of the People’s Republic of China. Beijing: China Medical Science Press, 319–320.
Cui, L., Liu, M., Chang, X., and Sun, K. (2016). The Inhibiting Effect of the Coptis Chinensis Polysaccharide on the Type II Diabetic Mice. Biomed. Pharmacother. 81, 111–119. doi:10.1016/j.biopha.2016.03.038
de Bock, M., Thorstensen, E. B., Derraik, J. G., Henderson, H. V., Hofman, P. L., and Cutfield, W. S. (2013). Human Absorption and Metabolism of Oleuropein and Hydroxytyrosol Ingested as Olive (Olea Europaea L.) Leaf Extract. Mol. Nutr. Food Res. 57 (11), 2079–2085. doi:10.1002/mnfr.201200795
Deng, N., Zheng, B., Li, T., Hu, X., and Liu, R. H. (2020). Phenolic Profiles, Antioxidant, Antiproliferative, and Hypoglycemic Activities of Ehretia Macrophyla Wall. (EMW) Fruit. J. Food Sci. 85 (7), 2177–2185. doi:10.1111/1750-3841.15185
Faizal, A., and Geelen, D. (2013). Saponins and Their Role in Biological Processes in Plants. Phytochem. Rev. 12 (4), 877–893. doi:10.1007/s11101-013-9322-4
Fan, H. J., Sun, X. F., Yin, L., Yang, X., and Dong, Q. J. (2021). Clinical Study on Jinlida Granules Combined with Nateglinide in Treatment of Type 2 Diabetes. Drugs Clinic 36 (02), 269–273. doi:10.7501/j.issn.1674-5515.2021.02.012
Fan, M. (2012). Effect of Jiangtangjia Tablet and Metformin Hydrochloride Tablet in Combined Treatment of Diabetic Nephropathy (DN). Sichuan J. Physiol. Sci. 34 (03), 112–114. doi:10.3969/j.issn.1671-3885.2012.03.007
Gan, L.-S., Chen, J.-J., Shi, M.-F., and Zhou, C.-X. (2013). A New Homoisoflavanone from the Rhizomes of Polygonatum Cyrtonema. Nat. Product. Commun. 8 (5), 1934578X1300800. doi:10.1177/1934578x1300800513
Gao, Y., Qi, C. L., Zhang, L., Yue, X. M., and Wang, H. (2015). Study on the Chemical Constituents of Fresh Polygonatum Sibiricum. Pharm. Clin. Res. 23 (04), 365–367. doi:10.13664/j.cnki.pcr.2015.04.009
Gu, W., Wang, Y., Zeng, L., Dong, J., Bi, Q., Yang, X., et al. (2020). Polysaccharides from Polygonatum Kingianum Improve Glucose and Lipid Metabolism in Rats Fed a High Fat Diet. Biomed. Pharmacother. 125, 109910. doi:10.1016/j.biopha.2020.109910
Ha, T. T. T., Huy, T. Q., Chinh, P. T., and Van Kiem, P. (2021). Polygokingiasides A and B: Two New Spirostane Glycosides from the Roots of Polygonatum Kingianum with Nitric Oxide Inhibitory Activity. Nat. Product. Commun. 16 (1), 1934578X2098563. doi:10.1177/1934578X20985634
Han, C., Sun, T., Liu, Y., Fan, G., Zhang, W., and Liu, C. (2020). Protective Effect of Polygonatum Sibiricum Polysaccharides on Gentamicin-Induced Acute Kidney Injury in Rats via Inhibiting P38 MAPK/ATF2 Pathway. Int. J. Biol. Macromol. 151, 595–601. doi:10.1016/j.ijbiomac.2020.02.049
Herman, W. H., Pan, Q., Edelstein, S. L., Mather, K. J., Perreault, L., Barrett-Connor, E., et al. (2018). Erratum. Impact of Lifestyle and Metformin Interventions on the Risk of Progression to Diabetes and Regression to Normal Glucose Regulation in Overweight or Obese People with Impaired Glucose Regulation. Diabetes Care 2017;40:1668-1677. Diabetes Care 41 (12), 913–1677. doi:10.2337/dc18-er04
Hou, B.-Y., Zhao, Y.-R., Ma, P., Xu, C.-Y., He, P., Yang, X.-Y., et al. (2020). Hypoglycemic Activity of Puerarin through Modulation of Oxidative Stress and Mitochondrial Function via AMPK. Chin. J. Nat. Medicines 18 (11), 818–826. doi:10.1016/s1875-5364(20)60022-x
Hu, J., Li, T., Du, S., Chen, Y., Wang, S., Xiong, F., et al. (2015). The MAPK Signaling Pathway Mediates the GPR91-dependent Release of VEGF from RGC-5 Cells. Int. J. Mol. Med. 36 (1), 130–138. doi:10.3892/ijmm.2015.2195
Huang, L., Wu, Y., Yin, H., Yang, X., Yuan, M., and Ning, H. (2020). Two New Compounds from the Stewed Polygonatum Cyrtonema Hua and Their Protective Effect against Aβ25-35 Induced Cytotoxicity and Oxidative Stress Damage. Nat. Prod. Res. 39 (05), 1–8. doi:10.1080/14786419.2020.1753735
Jiang, C. X., Zhang, T. J., Chen, C. Q., Xiao, X. K., and Liu, L. C. X. (2017). Research Progress in Polygonati Rhizoma and Predictive Analysis on Q-Marker. Chin. Tradit. Herb. Drugs 48 (1), 1–16. doi:10.7501/j.issn.0253-2670.2017.01.001
Jiao, J., Chen, L. M., Sun, Z. R., Liu, F., and Liang, Z. S. (2016). Comparison and Analysis of the Major Chemical Constituents of Polygonati Rhizoma from Various Production Areas. J. Chin. Med. Mater. 39 (03), 519–522. doi:10.13863/j.issn1001-4454.2016.03.013
Jing, P. (2019). Protective Effects of Polygonatum Sibiricum Saponin on Kidney and Wnt/β-Catenin Signaling Pathway in Diabetic Nephropathy Rats. Chin. Tradit. Pat. Med. 41 (10), 2518–2521. doi:10.3969/j.issn.1001-1528.2019.10.048
Li, B., Wang, M.-x., Wang, Y. F., Zhang, B. G., and Luo, S. D. (2008). Diffusion-driven Instability and Hopf Bifurcation in Brusselator System. Appl. Math. Mech.-Engl. Ed. 29, 825–832. doi:10.3321/j.issn:0253-2670.2008.06.00910.1007/s10483-008-0614-y
Li, C., Li, J., Shang, Y., Wang, Y., Gao, J., Xue, N., et al. (2021). Hypoglycemic and Hypolipidemic Activity of Polygonatum Sibiricum Fermented with Lactobacillus Brevis YM 1301 in Diabetic C57BL/6 Mice. J. Med. Food 24 (7), 720–731. doi:10.1089/jmf.2021.K.0034
Li, R., Tao, A., Yang, R., Fan, M., Zhang, X., Du, Z., et al. (2020). Structural characterization, hypoglycemic effects and antidiabetic mechanism of a novel polysaccharides from Polygonatum kingianum Coll. et Hemsl. Biomed. Pharmacother. 131, 110687. doi:10.1016/j.biopha.2020.110687
Li, W., and Li, J. (2019). Clinical Study on Jiangtangshu Tablets Combined with Repaglinide in Treatment of Type 2 Diabetes Mellitus. Drugs Clinic 34 (12), 203–206. doi:10.7501/j.issn.1674-5515.2019.12.043
Lian, F., Jin, D., Bao, Q., Zhao, Y., and Tong, X. (2019). Effectiveness of Traditional Chinese Medicine Jinlida Granules as an Add-On Therapy for Type 2 Diabetes: A System Review and Meta-Analysis of Randomized Controlled Trials. J. Diabetes 11 (7), 540–551. doi:10.1111/1753-0407.12877
Liao, Z., Zhang, J., Wang, J., Yan, T., Xu, F., Wu, B., et al. (2019). The Anti-nephritic Activity of a Polysaccharide from Okra (Abelmoschus Esculentus (L.) Moench) via Modulation of AMPK-Sirt1-PGC-1α Signaling axis Mediated Anti-oxidative in Type 2 Diabetes Model Mice. Int. J. Biol. Macromol. 140, 568–576. doi:10.1016/j.ijbiomac.2019.08.149
Liu, J., Li, T., Chen, H., Yu, Q., and Yan, C. (2021). Structural Characterization and Osteogenic Activity In Vitro of Novel Polysaccharides from the Rhizome of Polygonatum Sibiricum. Food Funct. 12, 6626–6636. doi:10.1039/D1FO00938A
Liu, Z., and Habener, J. F. (2008). Glucagon-like Peptide-1 Activation of TCF7L2-dependent Wnt Signaling Enhances Pancreatic Beta Cell Proliferation. J. Biol. Chem. 283 (13), 8723–8735. doi:10.1074/jbc.M706105200
Lu, J. M., Wang, Y. F., Yan, H. L., Lin, P., Gu, W., and Yu, J. (2016). Antidiabetic Effect of Total Saponins from Polygonatum Kingianum in Streptozotocin-Induced Daibetic Rats. J. Ethnopharmacol 179, 291–300. doi:10.1016/j.jep.2015.12.057
Luo, J., Chai, Y., Zhao, M., Guo, Q., and Bao, Y. (2020). Hypoglycemic Effects and Modulation of Gut Microbiota of Diabetic Mice by Saponin from Polygonatum Sibiricum. Food Funct. 11 (5), 4327–4338. doi:10.1039/D0FO00428F
Ma, M., Dong, Y. J., Lei, S. S., Wang, X. Q., Lv, G. Y., and Chen, S. H. (2019). Pharmacodynamic Effects of Different Processed Products of Rhizoma Polygonati on Rat Model of Qi and Yin Deficiency. Shanghai J. Tradit. Chin. Med. 53 (10), 83–89. doi:10.16305/j.1007-1334.2019.10.020
Palsgaard, J., Emanuelli, B., Winnay, J. N., Sumara, G., Karsenty, G., and Kahn, C. R. (2016). Cross-talk between Insulin and Wnt Signaling in Preadipocytes: Role of Wnt Co-receptor Low Density Lipoprotein Receptor-Related Protein-5 (LRP5). J. Biol. Chem. 287 (32), 12016–12026. doi:10.1074/jbc.A111.33704810.1074/jbc.M111.337048
Pang, H. X., Cui, J., Fan, G. Q., Wei, Z., and Ma, N. (2018). Extraction Optimization of Saponin from Polygonatum Sibiricum by Design-Expert and Preliminary Study on its Hypoglycemic Effect. China Pharmacist 21 (09), 1531–1534+1546. doi:10.3969/j.issn.1008-049X.2018.09.006
Pham, V. M., Matsumura, S., Katano, T., Funatsu, N., and Ito, S. (2019). Diabetic Neuropathy Research: from Mouse Models to Targets for Treatment. Neural Regen. Res. 14 (11), 1870–1879. doi:10.4103/1673-5374.259603
Ping, T. (2021). Effect of Adjuvant Therapy of Jiangtang Tongmai Capsule on HOMA-IS and HOMA-IR Levels in Patients with Type 2 Diabetes Mellitus. Pract. Clini J. Integr. Tradit. Chin. West. Med. 21 (06), 129–130. doi:10.13638/j.issn.1671-4040.2021.06.064
Schinner, S., Hermann, B., Kraus-Tiefenbacher, U., and Wenz, F. (2009). Wnt-signalling and the Metabolic Syndrome. Horm. Metab. Res. 41 (02), 159–163. doi:10.1055/s-0028-1119408
Sharma, S., Purohit, S., Sharma, A., Hopkins, D., Steed, L., Bode, B., et al. (2015). Elevated Serum Levels of Soluble TNF Receptors and Adhesion Molecules Are Associated with Diabetic Retinopathy in Patients with Type-1 Diabetes. Mediators Inflamm. 2015, 279393. doi:10.1155/2014/27939310.1155/2015/279393
Shu, X. S., Lv, J. H., Chen, D. M., and Chen, Y. H. (2012). Anti-diabetic Effects of Total Flavonoids from Polygonatum Sibiricum Red in Induced Diabetic Mice and Induced Diabetic Rats. Herald J. Biochem. Bioinformatic 2 (1), 014–019. Available at: https://xueshu.baidu.com/usercenter/paper/show?paperid=d5262888104cacc226615e415305d3caandsite=xueshu_se
Si, L., and Xue, Y. (2021). Effect of Qizhi Jiangtang Capsule on Microcirculation and Renal Function in Diabetic Nephropathy Patients. Chronic Pathematology J. 22 (04), 588–589+592. doi:10.16440/j.cnki.1674-8166.2021.04.033
Skyler, J. S., and Oddo, C. (2010). Diabetes Trends in the USA. Diabetes Metab. Res. Rev. 18 (S3), S21–S26. doi:10.1002/dmrr.289
Sloan, L. A. (2019). Review of Glucagon-like Peptide-1 Receptor Agonists for the Treatment of Type 2 Diabetes Mellitus in Patients with Chronic Kidney Disease and Their Renal Effects. J. Diabetes 11 (12), 938–948. doi:10.1111/1753-0407.12969
Sugimoto, M., Cutler, A., Shen, B., Moss, S. E., Iyengar, S. K., Klein, R., et al. (2013). Inhibition of EGF Signaling Protects the Diabetic Retina from Insulin-Induced Vascular Leakage. Am. J. Pathol. 183 (3), 987–995. doi:10.1016/j.ajpath.2013.05.017
Sun, C., Chen, Y., Li, X., Tai, G., Fan, Y., and Zhou, Y. (2014). Anti-hyperglycemic and Anti-oxidative Activities of Ginseng Polysaccharides in STZ-Induced Diabetic Mice. Food Funct. 5 (5), 845–848. doi:10.1039/c3fo60326a
Sun, L. R., Li, X., and Wang, S. X. (2005). Two New Alkaloids from the Rhizome of Polygonatum Sibiricum. J. Asian Nat. Prod. Resj. Asian Nat. Prod. Res. 7 (2), 127–130. doi:10.1080/10286020310001625157
Tahrani, A. A., Bailey, C. J., Del Prato, S., and Barnett, A. H. (2011). Management of Type 2 Diabetes: New and Future Developments in Treatment. Lancet 378 (9786), 182–197. doi:10.1016/S0140-6736(11)60207-9
Tahrani, A. A., Barnett, A. H., and Bailey, C. J. (2016). Pharmacology and Therapeutic Implications of Current Drugs for Type 2 Diabetes Mellitus. Nat. Rev. Endocrinol. 12 (10), 566–592. doi:10.1038/nrendo.2016.86
Tang, C., Yu, Y. M., Qi, Q. L., Wu, X. D., Wang, J., and Tang, S. A. (2019). Steroidal Saponins from the Rhizome of Polygonatum Sibiricum. J. Asian Nat. Prod. Res. 21 (3), 197–206. doi:10.1080/10286020.2018.1478815
Thorens, B. (2011). Of Fat, β Cells, and Diabetes. Cell Metab 14 (4), 439–440. doi:10.1016/j.cmet.2011.09.006
Wang, J., Lu, C. S., Liu, D. Y., Xu, Y. T., Zhu, Y., and Wu, H. H. (2016b). Constituents from Polygonatum Sibiricum and Their Inhibitions on the Formation of Advanced Glycosylation End Products. J. Asian Nat. Prod. Res. 18 (7), 697–704. doi:10.1080/10286020.2015.1135905
Wang, J., Zhao, J., Zhang, J., Luo, X., Gao, K., Zhang, M., et al. (2015). Association of Canonical Wnt/β-Catenin Pathway and Type 2 Diabetes: Genetic Epidemiological Study in Han Chinese. Nutrients 7 (6), 4763–4777. doi:10.3390/nu7064763
Wang, K., Tang, Z., Zheng, Z., Cao, P., Shui, W., Li, Q., et al. (2016a). Protective Effects of Angelica Sinensis Polysaccharide against Hyperglycemia and Liver Injury in Multiple Low-Dose Streptozotocin-Induced Type 2 Diabetic BALB/c Mice. Food Funct. 7 (12), 4889–4897. doi:10.1039/c6fo01196a
Wang, Q. L., Tong, X. H., Li, X. D., Han, R. C., Peng, D. Y., and Yu, N. J. (2019b). Hypoglycemic Effects of Polysaccharide of Polygonatum Cyrtonema Hua on Type1 Diabetic Mice Induced by STZ. J. Yunnan Univ. Tradit. Chin. Med. 42 (01), 1–7. doi:10.19288/j.cnki.issn.1000-2723.2019.01.001
Wang, W., Dabu, X., He, J., Yang, H., Yang, S., Chen, J., et al. (2019a). Polygonatone H, a New Homoisoflavanone with Cytotoxicity from Polygonatum Cyrtonema Hua. Nat. Prod. Res. 33 (12), 1727–1733. doi:10.1080/14786419.2018.1434645
Wang, Y., Lan, C., Liao, X., Chen, D., Song, W., and Zhang, Q. (2019c). Polygonatum Sibiricum Polysaccharide Potentially Attenuates Diabetic Retinal Injury in a Diabetic Rat Model. J. Diabetes Investig. 10 (4), 915–924. doi:10.1111/jdi.12976
Wang, Y., Liu, N., Xue, X., Li, Q., Sun, D., and Zhao, Z. (2020). Purification, Structural Characterization and In Vivo Immunoregulatory Activity of a Novel Polysaccharide from Polygonatum Sibiricum. Int. J. Biol. Macromol. 160, 688–694. doi:10.1016/j.ijbiomac.2020.05.245
Wang, Y., Qin, S., Pen, G., Chen, D., Han, C., Miao, C., et al. (2017). Original Research: Potential Ocular protection and Dynamic Observation of Polygonatum Sibiricum Polysaccharide against Streptozocin-Induced Diabetic Rats' Model. Exp. Biol. Med. (Maywood) 242 (1), 92–101. doi:10.1177/1535370216663866
Xiao, Y., Zheng, L., Zou, X., Wang, J., Zhong, J., and Zhong, T. (2019). Extracellular Vesicles in Type 2 Diabetes Mellitus: Key Roles in Pathogenesis, Complications, and Therapy. J. Extracell. Vesicles. 8 (1), 1625677. doi:10.1080/20013078.2019.1625677
Xu, J., Li, Z., Cao, M., Zhang, H., Sun, J., Zhao, J., et al. (2012). Synergetic Effect of Andrographis Paniculata Polysaccharide on Diabetic Nephropathy with Andrographolide. Int. J. Biol. Macromol. 51 (5), 738–742. doi:10.1016/j.ijbiomac.2012.06.035
Xu, J., Wang, S., Feng, T., Chen, Y., and Yang, G. (2018). Hypoglycemic and Hypolipidemic Effects of Total Saponins from Stauntonia Chinensis in Diabetic Db/db Mice. J. Cell. Mol. Med. 22 (12), 6026–6038. doi:10.1111/jcmm.13876
Xu, Y., Zhang, X., Yan, X. H., Zhang, J. L., Wang, L. Y., Xue, H., et al. (2019). Characterization, Hypolipidemic and Antioxidant Activities of Degraded Polysaccharides from Ganoderma Lucidum. Int. J. Biol. Macromol. 135, 706–716. doi:10.1016/j.ijbiomac.2019.05.166
Yan, H., Lu, J., Wang, Y., Gu, W., Yang, X., and Yu, J. (2017). Intake of Total Saponins and Polysaccharides from Polygonatum Kingianum Affects the Gut Microbiota in Diabetic Rats. Phytomedicine 26, 45–54. doi:10.1016/j.phymed.2017.01.007
Yong, J., Wang, Y. N., and Yong, X. W. (2019). Clinical Study of Tangmakang Granule Combined with Glimepiride in the Treatment of Type 2 Diabetes Mellitus. Drugs Clinic 34 (07), 2156–2159. doi:10.7501/j.issn.1674-5515.2019.07.049
Yu, C., Yunmei, Z., Dan, L., and Cheng, P. (2021). Research Progress on Modern Pharmacological Effect of Polygonati Rhizoma. J. Chin. Med. Mater. 44 (01), 240–244. doi:10.13863/j.issn1001-4454.2021.01.046
Yu, H., Zhang, X., Deng, M., Ye, H., and Jiang, J. (2008). Study on Constituents and Biological Activity of Volatile Oil from Tubers of Polygonatum Cyrtonema Hua. Chin. J. Exp. Tradit Med. Form (05), 4–6. doi:10.3969/j.issn.1005-9903.2008.05.002
Yu, Y. M., Ma, X. Y., Zhang, T. J., and Tang, C. (2016). Rapid Characterization on Components of Polygonatum Sibiricum Based on HPLC-MS Technology. Lishizhen Med. Mate. Med. Res. 27 (04), 794–796. doi:10.3969/j.issn.1008-0805.2016.04.009
Zhai, L., and Wang, X. (2018). Syringaresinol-di-O-β-D-glucoside, a P-henolic C-ompound from Polygonatum sibiricum, Exhibits an Antidiabetic and Antioxidative Effect on a Streptozotocin-induced M-ouse M-odel of D-iabetes. Mol. Med. Rep. 18 (6), 5511–5519. doi:10.3892/mmr.2018.9580
Zhang, H. (2019). A Preliminary Study on the Effect Difference of Processed Products of Rhizoma Polygonti. Strait Pharm. J. 31 (06), 36–39. doi:10.3969/j.issn.1006-3765.2019.06.014
Zhang, H. Y., Hu, W. C., Ma, G. X., Zhu, N. L., Sun, X. B., Wu, H. F., et al. (2017). A New Steroidal Saponin from Polygonatum Sibiricum. J. Asian Nat. Prod. Res. 20, 586–592. doi:10.1080/10286020.2017.1351436
Zhao, P., Li, X., Wang, Y., Yan, L., GuoHuangGao, L. L. Q. W., Huang, L., et al. (2020). Characterisation and Saccharide Mapping of Polysaccharides from Four Common Polygonatum Spp. Carbohydr. Polym. 233, 115836. doi:10.1016/j.carbpol.2020.115836
Zhao, P., Zhao, C., Li, X., Gao, Q., Huang, L., Xiao, P., et al. (2018). The Genus Polygonatum: A Review of Ethnopharmacology, Phytochemistry and Pharmacology. J. Ethnopharmacol 214, 274–291. doi:10.1016/j.jep.2017.12.006
Zhao, R., Qiu, B., Li, Q., Zhang, T., Zhao, H., Chen, Z., et al. (2014). LBP-4a Improves Insulin Resistance via Translocation and Activation of GLUT4 in OLETF Rats. Food Funct. 5 (4), 811–820. doi:10.1039/c3fo60602c
Zhuang, Z., Wang, Z. H., Huang, Y. Y., Zheng, Q., and Pan, X. D. (2020). Protective Effect and Possible Mechanisms of Ligustrazine Isolated from Ligusticum Wallichii on Nephropathy in Rats with Diabetes: A Preclinical Systematic Review and Meta-Analysis. J. Ethnopharmacol. 252, 112568. doi:10.1016/j.jep.2020.112568
Keywords: Polygonatum, antidiabetic mechanism, hypoglycemic, hypolipidemic, diabetes
Citation: Liu S, Jia Q-, Peng Y-, Feng T-, Hu S-, Dong J- and Liang Z- (2022) Advances in Mechanism Research on Polygonatum in Prevention and Treatment of Diabetes. Front. Pharmacol. 13:758501. doi: 10.3389/fphar.2022.758501
Received: 14 August 2021; Accepted: 03 January 2022;
Published: 08 February 2022.
Edited by:
John Thor Arnason, University of Ottawa, CanadaReviewed by:
Acharaporn Duangjai, University of Phayao, ThailandKe Han, Harbin University of Commerce, China
Xuanbin Wang, Hubei University of Medicine, China
Copyright © 2022 Liu, Jia, Peng, Feng, Hu, Dong and Liang. This is an open-access article distributed under the terms of the Creative Commons Attribution License (CC BY). The use, distribution or reproduction in other forums is permitted, provided the original author(s) and the copyright owner(s) are credited and that the original publication in this journal is cited, in accordance with accepted academic practice. No use, distribution or reproduction is permitted which does not comply with these terms.
*Correspondence: Juan-e Dong, ZGplMDA5QDEyNi5jb20=; Zong-Suo Liang, bGlhbmd6c0B6c3R1LmVkdS5jbg==
†These authors have contributed equally to this work