- 1Department of Respiratory Medicine, Hospital of Chengdu University of Traditional Chinese Medicine, Chengdu, China
- 2College of Pharmacy, Chengdu University of Traditional Chinese Medicine, Chengdu, China
- 3State Key Laboratory of Southwestern Chinese Medicine Resources, Innovative Institute of Chinese Medicine and Pharmacy, Chengdu University of Traditional Chinese Medicine, Chengdu, China
Cancer is the leading cause of death and one of the greatest barriers to increased life expectancy worldwide. Currently, chemotherapy with synthetic drugs remains one of the predominant ways for cancer treatment, which may lead to drug resistance and normal organ damage. Increasing researches have suggested that apoptosis, a type of programmed cell death, is a promising way for cancer therapy. Furthermore, natural products are important sources for finding new drugs with high availability, low cost and low toxicity. As a well-known isoquinoline alkaloid, accumulating evidence has revealed that berberine (BBR) exerts potential pro-apoptotic effects on multiple cancers, including breast, lung, liver, gastric, colorectal, pancreatic, and ovarian cancers. The related potential signal pathways are AMP-activated protein kinase, mitogen-activated protein kinase, and protein kinase B pathways. In this review, we provide a timely and comprehensive summary of the detailed molecular mechanisms of BBR in treating three types of cancer (breast, lung and liver cancer) by inducing apoptosis. Furthermore, we also discuss the existing challenges and strategies to improve BBR’s bioavailability. Hopefully, this review provides valuable information for the comprehension of BBR in treating three types of cancer and highlight the pro-apoptotic effects of BBR, which would be beneficial for the further development of this natural compound as an effective clinical drug for treating cancers.
Introduction
Cancer is one of the leading causes of death worldwide with estimated 19.3 million new cases and approximately 10 million deaths in 2020 (gco.iarc.fr; Sung et al., 2021). According to the Global Cancer Observatory, lung cancer is the second most diagnosed cancer and the leading cause of cancer mortality, and it is also the leading cause of death in men. In 2020, 2.2 million new lung cancer cases and 1.8 million deaths were predicted, accounting for 18% of all cancer-related deaths. Among women, lung cancer is the principal cause of cancer-related death in many countries, such as most cities in Eastern Europe, West Asia, East Asia (China), and Southeast Asia (gco.iarc.fr). The incidence rate is generally higher in young women than in young men, which cannot be explained by smoking (Fidler-Benaoudia et al., 2020). Breast cancer is the most commonly diagnosed cancer and the most common cause of death in women. In 2020, approximately 2.3 million women were newly diagnosed with breast cancer and 684,996 women with breast cancer died (gco.iarc.fr). Although the incidence and mortality of breast cancer in high-income countries have been declining, those in low- and middle-income countries have been increasing (Lortet-Tieulent et al., 2020). Globally, the incidence of breast cancer is still on the rise, and there has been a 3.1% annual rate of increase in global breast cancer incidences beginning with 641,000 cases in 1980 to over 1.6 million in 2010 (Bray et al., 2015). Liver cancer was the sixth most commonly diagnosed cancer in the world in 2020 and the third leading cause of cancer-related deaths, with approximately 906,000 new cases and 830,000 deaths. The morbidity and mortality rate of liver cancer in men is two to three times that of women worldwide (gco.iarc.fr). Liver cancer progresses rapidly unless diagnosed early, and the patient’s odds are very low (Gravitz, 2014). Currently, the common treatment methods for cancer include radiotherapy, chemotherapy, and surgery; as research progresses, targeted therapy (Mollaei et al., 2019; Thakkar et al., 2020; Song et al., 2021), immunotherapy (Anderson et al., 2021; Myers and Miller, 2021) and metabolic therapy (Park et al., 2020) have also been used to treat various types of cancer. However, despite the advances that have been made in therapeutic approaches, these treatments may lead to chemotherapy resistance (Zhang et al., 2019d), cancer development (Zhao et al., 2020) and normal organ damage (Zhang et al., 2020b). Owing to socio-economic development, population aging, and lifestyle changes, the incidence of cancer continues to rise worldwide, necessitating the search for safe and effective therapeutic drugs (Sung et al., 2021).
Various natural products have been proven to have anticancer effects and the potential to be effective in cancer treatment (Peng et al., 2015; Wu et al., 2015; Luo et al., 2019a). For example, curcumin can inhibit cancer development and proliferation (Giordano and Tommonaro, 2019), and baicalin promotes apoptosis in pancreatic cancer (Huang et al., 2019b). Natural products are believed to be safer than chemical products (Hou et al., 2021; Xu et al., 2021), as they kill cancer cells without affecting normal cells (El Khalki et al., 2020). Owing to their high availability, low cost, and low toxicity, the application of natural products in cancer therapy has been recommended (Anwanwan et al., 2020). Berberine (BBR) is a well-known isoquinoline alkaloid with multiple biological effects that has been used in medicine for many years (Habtemariam, 2020). It can be easily obtained from medicinal plants and can be synthesized (Kong et al., 2004; Huang et al., 2011). BBR has attracted attention because of its low cytotoxicity and wide variety of pharmacological effects, including antiviral, hypoglycemic, anti-inflammatory, hypotensive, hypolipidemic, and anticancer activities (Feng et al., 2019; Wang et al., 2019; Samadi et al., 2020; Song et al., 2020; Warowicka et al., 2020).
Recently, BBR, a well-known natural alkaloid from the Coptis chinensis Franch. has shown good potential anticancer effects via the inhibition of cell proliferation, metastasis and invasiveness through apoptosis. The involved mechanisms were related to the regulation of the AMP-activated protein kinase (AMPK), mitogen-activated protein kinase (MAPK), and protein kinase B (AKT) pathways (Figure 1). In this review, we offer a comprehensive summary of the mechanisms of BBR in treating breast, lung and liver cancer, providing a valuable reference for future research.
Physicochemical Properties of BBR
BBR (C20H18NO4), with a molecular weight of 336.36 g/mol, is a quaternary amine isoquinoline alkaloid. It is yellow, odorless, and has a very bitter taste. BBR has a wide range of sources, such as Coptis chinensis Franch., Phellodendron chinense C. K. Schneid., Berberis vulgaris L., Hydrastis canadensis L., Arcangelisia flava (L.) Merr., Berberis aquifolium Pursh, and Berberis aristata DC. (Peng et al., 2004; Wang et al., 2004) (Table 1). Berberis vulgaris L. is the most widely distributed natural source of BBR, containing approximately 2.44% BBR (Ahmad et al., 2019) (Figure 2). BBR is slightly soluble in water, ethanol, or methanol, readily soluble in hot water and hot ethanol, and hardly soluble in organic solvents such as benzene, chloroform and acetone. The salt form is relatively soluble, especially in the sulfate and phosphate forms (Battu et al., 2010; Wang et al., 2017c; Guo and Kang, 2018). Methanol, ethanol, and aqueous or acidified methanol and ethanol are the most commonly used extraction solvents (Teng and Choi, 2014). BBR is sensitive to decomposition under high-temperature and light conditions, and it should be kept at a proper temperature and away from light (Neag et al., 2018).
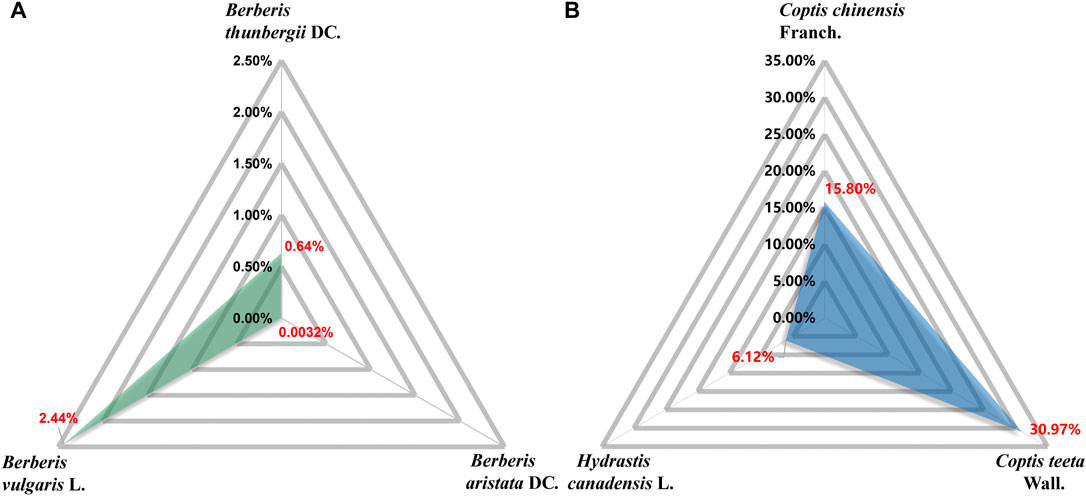
FIGURE 2. Content of BBR in corresponding plant parts. Berberis vulgaris L. dry root: 2.44% (Ahmad et al., 2019). Berberis thunbergii DC.: 0.64% (Och et al., 2017). Berberis aristata DC. stem bark: 0.0032% (Khan et al., 2020). Hydrastis canadensis L. root: 6.12% (Ettefagh et al., 2011). Coptis chinensis Franch. water extracts: 15.80% (Roh et al., 2020). Coptis teeta Wall. rhizome: 30.97% (Goswami et al., 2019).
The ADMET Properties of BBR
BBR has poor intestinal absorption and oral bioavailability in humans and rats. Its absolute oral bioavailability is only 0.37 ± 0.11% (Feng et al., 2020). In humans, after oral administrations of 400 mg BBR, the mean maximum plasma concentration is approximately 0.4 ng/ml (Hua et al., 2007). The most ionized form of BBR under physiological conditions (pKa = ∼15) results in lower oral bioavailability (Spinozzi et al., 2014). P-glycoprotein (P-gp) expressed in the apical membrane of intestinal mucosal cells secretes xenobiotics into the lumen, thereby limiting the net intestinal absorption of BBR (Maeng et al., 2002). In addition, extensive intestinal first-pass elimination leads to BBR remaining in the gastrointestinal lumen, which is finally excreted in the stool (Liu et al., 2010; Wang et al., 2017c). Moreover, after absorption through the intestine, BBR is widely distributed in organs, predominantly in liver (Liu et al., 2010; Tan et al., 2013), thereby making the BBR content of the organs much higher than the plasma concentration (Wang et al., 2017b). In vivo, BBR undergoes extensive metabolism and simultaneously exists with its metabolites (Wang et al., 2017b) (Figure 3).
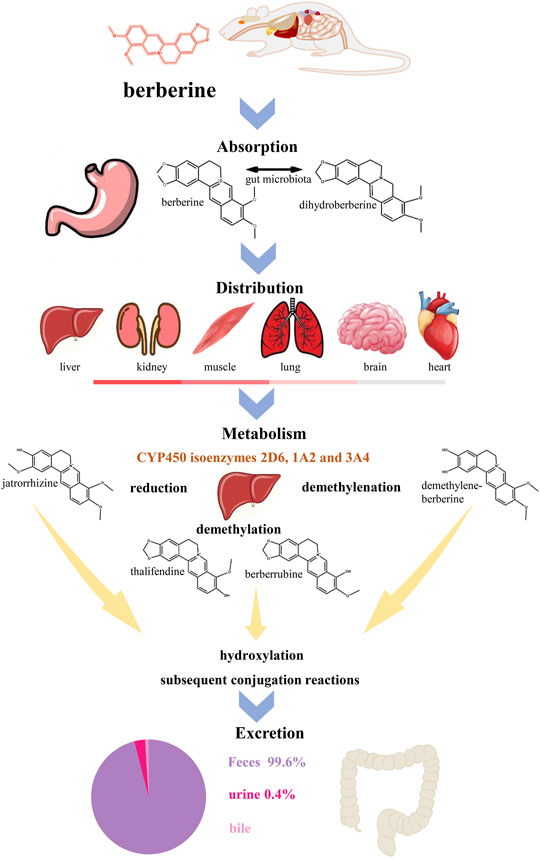
FIGURE 3. The ADME process of BBR in rats. BBR has a poor oral bioavailability which is below 1% (Habtemariam, 2020). After oral administration in rats, BBR is absorptive by gastrointestinal system, involving the reciprocal transformation between BBR and dihydroberberine (Feng et al., 2015). BBR has wildly distribution in the liver, kidney, muscle, lung, brain and heart (decreasing order) (Tan et al., 2013). In liver, BBR metabolizes in five ways, reduction, demethylation, demethylenation, hydroxylation and subsequent conjugation reactions (Wang et al., 2017b). Cytochrome P450 (CYP450) isoenzymes 2D6, 1A2 and 3A4 (Li et al., 2011) play an important role in metabolism and jatrorrhizine, thalifendine, berberrubine and demethylene-berberine are the main products of phase I metabolism. Meanwhile, the four metabolites can be detected in feces, urine and bile (Ma et al., 2013). The main way of metabolism of BBR and its metabolites is through feces.
The acute toxicity of BBR depends on the method of administration, and basic acute toxicity studies have illustrated that the median lethal dose (LD50) of BBR from intravenous and intraperitoneal injection is 9.0386 and 57.6103 mg/kg, respectively, in mice. Interestingly, no LD50 was found after oral administration, possibly because of its poor oral bioavailability (Kheir et al., 2010). Previous studies have revealed that BBR is cardiotoxic, leading to total amplitude and beating rate inhibition at 10 μM in cardiomyocytes (Zhang et al., 2018). Animal experiments have suggested that BBR exerts toxic and teratogenic effects in developing zebrafish embryos at 400 mg/L for a-48 h administration, as evidenced by pericardial edema, damaged heart morphogenesis, vascular defects, and death (Martini et al., 2020). BBR treatment during vertebrate development leads to the impairment of cardiovascular system morphogenesis and functionality, suggesting it should be used with caution during pregnancy and lactation. In addition, BBR shows a risk of potential neurotoxicity and immunotoxicity (Kysenius et al., 2014; Mahmoudi et al., 2016), and high doses of BBR (10 mg/kg) can suppress both cellular and humoral immune functions in treated hosts. BBR at 5 mg/kg appeared to affect only delayed-type hypersensitivity responses and lymphoproliferation (Mahmoudi et al., 2016). Moreover, several studies have shown that the concomitant use of BBR with macrolides or 5-aminosalicylic acid may induce cardiac or splenic toxicity, respectively (Zhi et al., 2015; Li et al., 2016). However, the above-mentioned data are derived from animal experiments, and recent clinical trials have shown that BBR is well tolerated in the human body with only some gastrointestinal side effects (Zhang et al., 2020c; Zhao et al., 2021b). Cardioprotective effects have also been confirmed (Qing et al., 2018; Mercurio et al., 2020; Zhao et al., 2021a). These differences may be related to administration time, concentration, and species. Overall, current research proves that BBR is safe for humans, but the rare embryo- and immune-related toxicity suggests its potential risk for pregnant women and children. In addition, the interaction between BBR and drugs requires further research (Table 2).
Mechanisms of BBR Anticancer Effects
BBR is shown to exert pro-apoptotic effects in multiple cancers (Table 3). In the following section, we provide a comprehensive summary of the anticancer mechanisms.
Breast Cancer
Numerous experiments have shown that BBR is effective for treating breast cancer through diverse mechanisms, including the inhibition of cancer cell proliferation, metastasis; regulation of metabolism, the immune system, and cancer-related pathways; and the promotion of DNA damage (Figure 4).
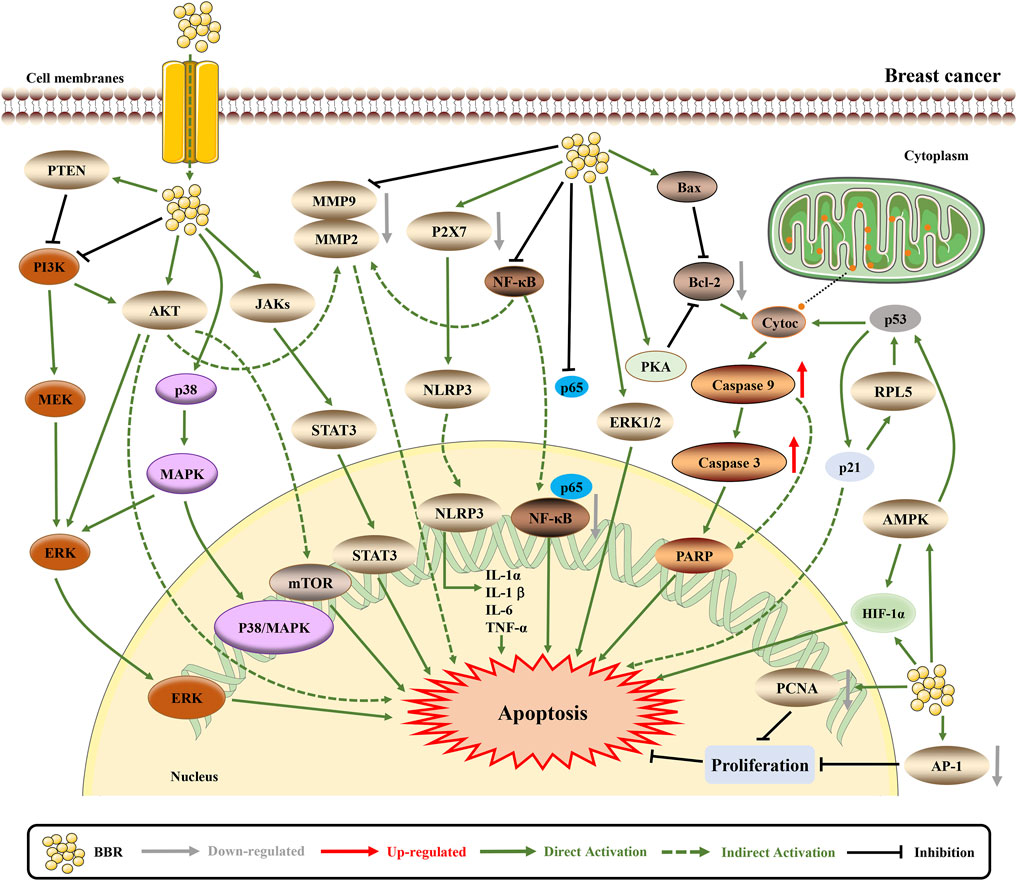
FIGURE 4. The pro-apoptotic mechanisms of BBR in breast cancer. BBR exerts anti-cancer effects by influencing breast cancer related pathways. BBR induces apoptosis through downregulating MTDH (Sun et al., 2019). BBR actives the mitochondrial apoptotic pathway and upregulates AMPK to promote apoptosis (Pan et al., 2017; Zhao et al., 2017; Ma et al., 2020). Furthermore, BBR downregulates AKT/ERK pathway to induce cell cycle arrest and apoptosis (Lin et al., 2019). BBR inhibits NLRP3 and NF-κB inflammasome pathway to suppress inflammation and EMT, thus inducing apoptosis (Karnam et al., 2017; Yao et al., 2019; Zhao and Zhang, 2020). The mechanism of BBR inhibiting cell proliferation, invasion and growth may be induced by EGFR/ERK and EGFR/AKT signaling pathways (Kim et al., 2018c; Jabbarzadeh Kaboli et al., 2019). BBR affects certain protein expression and structure via VASP, and downregulates AP-1 and PCNA to inhibit cell proliferation and migration, which also decreases resistance to apoptosis (Su et al., 2016; Karnam et al., 2017; Jeong et al., 2018).
BBR Inhibits Cell Proliferation to Induce Apoptosis
Proliferation and apoptosis are considered opposing cellular phenomena, and the occurrence of tumors is believed to be related to blocked apoptosis and abnormal proliferation. According to the literature, higher doses of BBR (100 μM) are predominantly distributed in the nucleolus, which causes a nucleolar stress response to upregulate p53 and induce cell proliferation inhibition and cell death (Sakaguchi et al., 2020). In addition, BBR downregulates metadherin (MTDH) to exert an antiproliferative effect (Sun et al., 2019). In triple-negative breast cancer (TNBC) cell lines, BBR inhibits the proliferation of TNBC cells with IC50 values ranging from 0.19 to 16.7 µM including different pathways, such as AKT/extracellular regulated protein kinases (ERK) pathways and p38. Moreover, the cell cycle kinase complex cyclin A/cyclin-dependent kinase (CDK) 1, and cyclin D/CDK4 are suppressed (Lin et al., 2019; El Khalki et al., 2020). Furthermore, recent studies have indicated that, with cell proliferation inhibition, BBR induces apoptotic cell death through the activation of caspase-9/cytochrome c; the cleavage of poly ADP-ribose polymerase (PARP), caspase-7, and caspase-8 proteins, and upregulation of pro-apoptotic B-cell lymphoma-2 (Bcl-2) proteins via p53 (Zhao et al., 2017; Carneiro and El-Deiry, 2020; El Khalki et al., 2020).
BBR Inhibits Cell Invasion and Metastasis to Potentially Induce Apoptosis
Metastasis is a characteristic of tumor invasiveness. Several studies have shown that BBR inhibits colony formation and migration by decreasing the phosphorylation of c-Jun and c-Fos (Zhao et al., 2017; Yao et al., 2019; Zhao and Zhang, 2020). Recent studies have indicated that BBR downregulates ephrin-B2 and transforming growth factor beta 1 (TGF-β1) to decrease the expression levels of matrix metalloproteinase (MMP)2 and MMP9, thereby inhibiting cell growth and migration (Ma et al., 2017; Kim et al., 2018a). MMPs are known to be involved in all steps of tumor progression. For example, MMP11 inhibits apoptosis and promotes cancer development. As an MMP inhibitor, BBR may be able to regulate MMPs and tissue inhibitors of MMPs to induce apoptosis (Cabral-Pacheco et al., 2020). In addition, BBR suppresses the toll-like receptor 9 (TLR9)-myeloid differentiation factor 88 (MyD88)–nuclear factor kappa-B (NF-κB) pathway and partly reverses doxorubicin (Dox)-exacerbated breast cancer metastasis (Zheng et al., 2021). NF-κB is a transcription factor that reduces apoptosis and has been shown to be involved in cell apoptosis in the phosphoinositide-3-kinase (PI3K)/AKT/NF-κB/MMP9 signaling pathway (Yang et al., 2019). The overexpression of vasodilator-stimulated phosphoprotein (VASP) and fibronectin (FN) is related to the poor prognosis of breast cancer, BBR not only binds to VASP to induce changes in its secondary structure but also decreases the expression of FN to inhibit cell proliferation and migration (Su et al., 2016; Jeong et al., 2018). In particular, serine phosphorylation of VASP regulates colon cancer cell survival and apoptosis, while FN protects lung cancer cells against docetaxel-induced apoptosis (Ali et al., 2015; Qin et al., 2016). Ultimately, the inhibition of cell invasion and metastasis may be a potential target for BBR to induce apoptosis.
BBR Regulates Metabolism to Synergistically Induce Apoptosis
Metabolic disorders are characterized by several pathological states such as chronic inflammation, dyslipidemia and insulin resistance, which are related to the incidence of breast cancer. Therefore, targeting the metabolism to treat cancer is a feasible approach. BBR has the ability to regulate metabolism or metabolic functions to affect the outcome of breast cancer (Cazzaniga and Bonanni, 2018). Previous studies have shown that BBR interferes with breast cancer cell metabolism and induces apoptosis via a mitochondrial-dependent pathway (Patil et al., 2010; Tan et al., 2015a; Tan et al., 2015b). As mentioned earlier, BBR targets p53, which regulates multiple metabolic pathways, including glucose, lipid, mitochondrial, serine, nucleotide metabolism and oxidative phosphorylation, thereby synergistically inducing apoptosis (Liu et al., 2019a). Although its specific mechanism remains unclear, the use of BBR in breast cancer patients has been recommended (Cazzaniga and Bonanni, 2018).
BBR Regulates the Immune System to Active Apoptosis Pathway
Cancer immunosurveillance usually challenges cells that have undergone tumor transformation. Only the most immune-evasive or highly mutagenic tumor cells can escape immune surveillance and produce clinically relevant tumors. Therefore, cancer cells in established tumors can resist antitumor immunity. Thus, overcoming the immune-evasive phenotype has become a new strategy for effective cancer therapies (Garg and Agostinis, 2017). Physiologically, the immune system can activate the mitochondrial apoptosis pathway and the Fas death receptor apoptosis pathway without causing inflammation or tissue destruction (Nagata and Tanaka, 2017). Recently, BBR and exercise have been shown to slow the progression of breast cancer in 4T1 tumor-bearing rats. Synergistic therapy regulates intestinal microbial metabolites to improve the immune system by activating the mitochondrial apoptosis pathway and the Fas death receptor apoptosis pathway, thereby exerting anticancer effects (Ma et al., 2020). Collectively, these results provide evidence that BBR regulates immunity during cancer treatment.
BBR Promotes DNA Damage to Induce Apoptosis
DNA fragmentation is a hallmark of apoptosis, and BBR can induce DNA fragmentation in MDA-MB-231 cells. Further studies have shown that BBR markedly downregulates the levels of X-ray cross-complementing protein 1 and excision repair cross-complementing group 1, thereby inhibiting cell DNA repair and sensitizing MDA-MB-231 cells to chemotherapeutic drugs (Gao et al., 2019). Another study showed (El Khalki et al., 2020) that after 120 h of BBR treatment, both BT-20 and HCC70 showed significant H2A.X variant histone (H2AX) phosphorylation, which is a sign of DNA damage. Finally, DNA damage promotes p53 expression, leading to the activation of apoptosis (Hafner et al., 2019).
BBR Suppresses Inflammation to Induce Apoptosis
Infection and inflammation account for approximately a quarter of the causes of cancers, and tumors can be described as wounds that never heal and are infiltrated by numerous inflammatory and immune cells. Tumor-related chronic inflammation is generally believed to be a sign of cancer that promotes the progression to metastatic stage, and plays an important role in tumor development and treatment (Suarez-Carmona et al., 2017; Murata, 2018). After BBR treatment, P2X7-mediated NLR family pyrin domain containing 3 (NLRP3) inflammasome activation was inhibited, as evidenced by the suppressed expressing of NLRP3, caspase-1, and interleukin 1 beta (IL-1β) in MDA-MB-231 cells (Yao et al., 2019). BBR can also inhibit the secretion of tumor necrosis factor alpha (TNF-α) and interleukin-6 (IL-6) by increasing the expression of the nuclear factor of kappa light polypeptide gene enhancer in B-cells inhibitor, alpha (IκBα), thus inhibiting p65 protein and NF-κB (Zhao and Zhang, 2020). Similarly, in breast cancer-bearing rats treated with BBR (50 mg/kg), the increased levels of lipid peroxide (malonaldehyde), pro-inflammatory cytokines (IL-1β, IL-6 and TNF-α), enzymatic antioxidants (superoxide dismutase and catalase), non-enzymatic antioxidants (glutathione and vitamin C), and transcription factor NF-κB were significantly decreased by the administration of BBR. Furthermore, NF-κB and proliferating cell nuclear antigen (PCNA) in breast cancer were also downregulated (Karnam et al., 2017). Finally, with NF-κB downregulation, apoptosis was induced in breast cancer cells.
BBR Regulates Cancer-Related Pathways to Induce Apoptosis
AMPK Pathway
In breast cancer, AMPK phosphorylation induces multiple cellular responses, including cell growth inhibition, cell cycle arrest and apoptosis (Ponnusamy et al., 2020b). Salt-inducible kinase 3 (SIK3) belongs to the AMPK-related kinase family. The combination of emodin and BBR attenuates SIK3, leading to cell cycle arrest, decreased cell growth and increased apoptosis in breast cancer cells (Ponnusamy et al., 2020a). Interestingly, low-dose BBR enhances Dox sensitivity to drug-resistant breast cancer through the AMPK- hypoxia-inducible factor-1α (HIF-1α) -P-gp pathway. High-dose BBR directly induces apoptosis by activating the AMPK-p53 pathway. Therefore, BBR is both a chemotherapy sensitizer and chemotherapy drug (Pan et al., 2017).
MAPK Pathway
Overwhelming evidence indicates that the aberrant activation of the MAPK pathway contributes to breast cancer growth, invasion, metastasis, and reduced apoptosis. For example, MAPK promotes breast cancer cell invasion and metastasis via interleukin 32 (Wen et al., 2019). MAPK promotes breast cancer cell growth via linc-RoR (Peng et al., 2017). Downregulated MAPK has been shown to induce apoptosis and inhibit the proliferation of breast cancer cells (Zhu et al., 2020). The epidermal growth factor receptor (EGFR) is a surface marker of breast cancer. Dysregulation of EGFR/MAPK is common in this disease. BBR downregulates EGFR/MAPK to induce apoptosis (Jabbarzadeh Kaboli et al., 2019). BBR also reduces the expression of interleukin-8 (IL-8) by downregulating the EGFR/mitogen-activated protein kinase (MEK)/ERK pathway to induce cell growth and inhibit invasion (Kim et al., 2018c).
AKT Pathway
Aberrant AKT expression is associated with increased invasion, metastasis and drug resistance (Shariati and Meric-Bernstam, 2019). Several lines of evidence have suggested that BBR induces cell cycle arrest and death by suppressing AKT (Tak et al., 2019; Ponnusamy et al., 2020a). BBR modulates the phosphatase and tensin homolog (PTEN)/AKT/mammalian target of rapamycin (mTOR) signaling pathway to reverse Dox resistance and induce apoptosis (Wang et al., 2020).
BBR Reverses Drug Resistance to Induce Apoptosis
Lapatinib is a new type of human epidermal growth factor receptor 2 (HER2)/EGFR tyrosine kinase inhibitor used for the treatment of HER2-positive breast cancer. However, acquired drug resistance is inevitable. Studies have shown that BBR can increase the level of reactive oxygen species (ROS) to induce apoptosis in lapatinib-resistant cells and reverse the drug resistance of breast cancer cells (Zhang et al., 2016). In addition, BBR and Dox synergistically enhanced the inhibitory effect on cancer cells by reducing P-gp and multidrug resistance protein 1 expression with the best BBR/Dox ratio of = 2:1 (Qian et al., 2021).
Lung Cancer
As documented in the literature, BBR exhibits anti-lung cancer activity via multiple molecular mechanisms (Figure 5). BBR can significantly inhibit the growth of lung cancer cells by reducing the protein expression of transcription factors Sp1 and 3-phosphoinositide dependent protein kinase 1 (PDPK1), both of which are related to cancer growth and progression (Zheng et al., 2018a). Furthermore, BBR induces apoptosis by modulating cell cyclins, inflammation, and the PI3K/AKT pathway. However, it was also observed that the proliferation inhibitory effect of BBR was transient, and no significant inhibitory effect was observed in the second-generation cells (Fu et al., 2013; Kumar et al., 2020). Another study showed that the tissue factor (TF) is a direct inhibitory target of miR-19a in non-small cell lung cancer (NSCLC) cells and BBR induces apoptosis through the miR-19a/TF/MAPK axis (Chen et al., 2019). In addition, BBR can cause mitochondrial dysfunction. By activating the ROS/AMPK pathway, BBR inhibits lipogenesis and cell proliferation (Fan et al., 2018). The combination of BBR and UVA can significantly increase cytotoxicity, and the phototoxicity of BBR is mediated by the production of ROS, mitochondrial membrane permeabilization and caspase-9/caspase-3 activation (Bhattacharyya et al., 2017). BBR also mediates epigenetic reprogramming by inhibiting histone deacetylase (HDAC) to downregulate oncogenes such as TNF-α, cyclooxygenase (COX)-2, MMP2, and MMP9 and cancer suppressor genes (p21 and p53) mRNA and protein upregulation. Besides, BBR regulates Bcl-2/Bcl-2 associated X protein (Bax) family proteins and triggers the caspase cascade apoptotic pathway (Kalaiarasi et al., 2016).
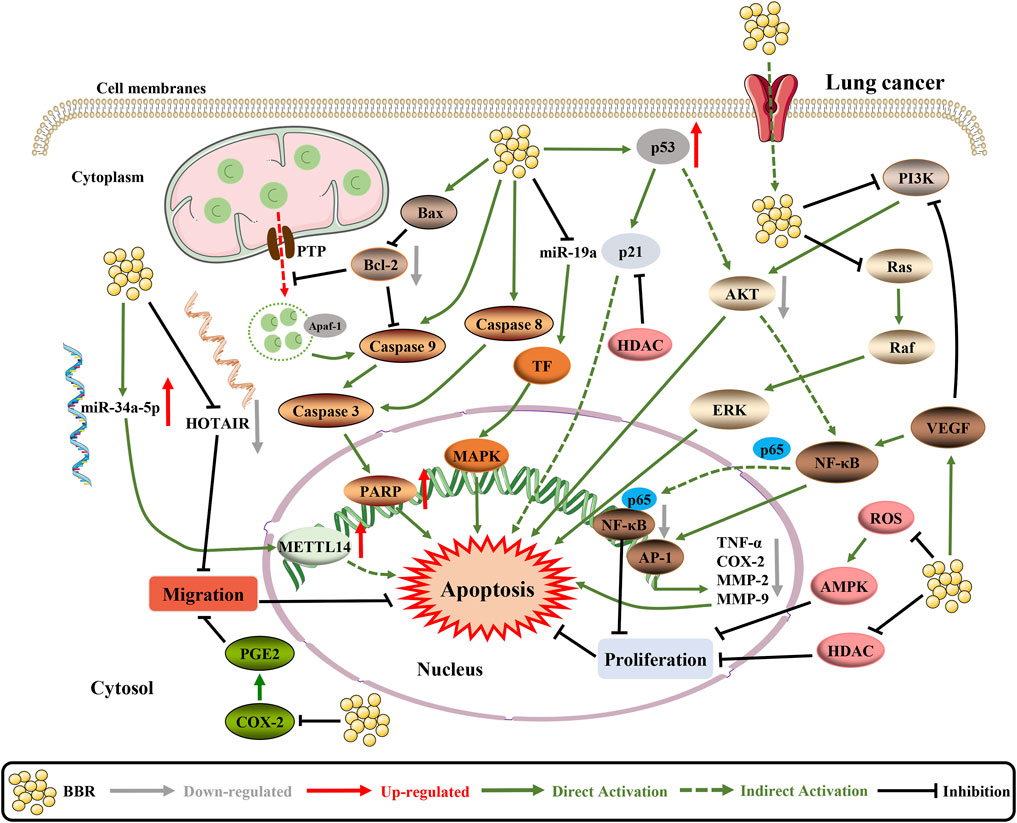
FIGURE 5. The pro-apoptotic mechanisms of BBR in lung cancer. BBR increases the activity of the Bcl-2/Bax signaling pathway and inhibits the VEGF/NF-κB/AP-1 signaling pathway to enhance cell apoptosis (Li et al., 2018b). BBR upregulates tumor suppressor genes (p21 and p53) mRNA and protein by inhibiting HDAC (Kalaiarasi et al., 2016). On the top of this, BBR can regulate the expression and interaction of HOTAIR and miR-34a-5p in human lung cancer cells, which significantly inhibits EMT and induces apoptosis (Zheng et al., 2020). In addition, BBR inhibits the cell proliferation by targeting PI3K/AKT and ROS/AMPK signaling pathways to activate apoptosis (Fu et al., 2013; Bhattacharyya et al., 2017).
Epithelial-mesenchymal transition (EMT) is a process through which epithelial cells acquire mesenchymal features. EMT is associated with cancer initiation, invasion, metastasis, and resistance to therapy (Nieto et al., 2016). BBR and gefitinib can regulate the expression and interaction of HOX transcript antisense intergenic RNA (HOTAIR) and miR-34a-5p in human lung cancer cells to inhibit EMT. HOTAIR is an important oncogenic lncRNA, that is involved in tumorigenesis and invasion, and miR-34a-5p is a tumor suppressor. HOTAIR is significantly elevated in NSCLC cells and promotes migration and invasion, whereas depletion of HOTAIR reduces the migration and invasion ability of NSCLC cells (Zheng et al., 2020).
In vitro studies have shown that continuous Dox treatment causes transformation of HL-60 cells to N2 neutrophils, thereby inducing chemotherapy resistance. The combined treatment of Dox and 2 µM BBR caused HL-60 cells to differentiate into N1 neutrophils and stimulates immune clearance in HL-60 cells. In addition, BBR can also downregulate the expression of Dox-derived neutrophils CD133 and CD309 to prevent chemotherapy sensitivity and immune rejection (Zhang et al., 2019d).
Liver Cancer
Through continued research, numerous anti-liver cancer activities of BBR are being recognized (Figure 6).
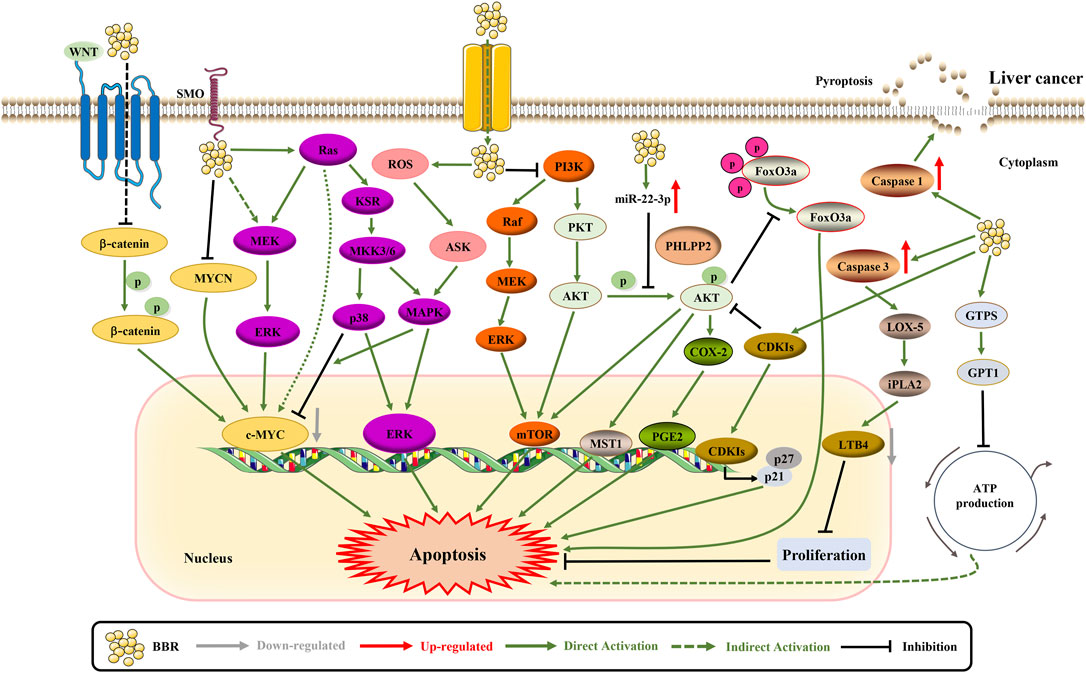
FIGURE 6. The pro-apoptotic mechanisms of BBR in liver cancer. BBR promotes apoptosis by suppressing the expression of SLC1A5 and c-MYC (Zhang et al., 2019c; Tai et al., 2020). BBR increases the expression of CDKIs p21Cip1 and p27Kip1 via regulating the AKT/FoxO3a signaling pathway (Guo et al., 2020). Likewise, BBR induces apoptosis through iPLA2/LOX-5/LTB4 pathway and PHLPP2-AKT-MST1 axis (Saxena et al., 2018; Zhao et al., 2020). Besides, BBR inhibits cell proliferation through ERK1/2 and GPT1 axis (Chuang et al., 2017; Guo et al., 2020). BBR can regulate adenylate cyclase signaling in association with MKK3/6 stabilization to exert necroptosis (Sengupta et al., 2017). BBR upregulates miR-22-3p to inhibit cell proliferation (Chen et al., 2016). Furthermore, BBR simultaneously inhibits NF-κB p65 and β-catenin translation to promote apoptosis (Li et al., 2017). The apoptosis promotion of BBR may also be related to the inhibition of PI3K/AKT, p38 MAPK/ERK-COX2 and HIF-1α (Luo et al., 2019b; Song et al., 2019b; Qi and Liu, 2021).
BBR Inhibits Cell Proliferation and Growth to Induce Apoptosis
Cancer cells undergo specific metabolic reprogramming to sustain their proliferation. In Hep3B and BEL-7404 cells, BBR treatment decreases the rate of glutamine uptake by inhibiting solute carrier family 1 member 5 (SLC1A5) to in turn inhibit cell proliferation (Zhang et al., 2019c). In addition, BBR treatment inhibits chemotherapy-exacerbated hepatocellular carcinoma (HCC) cell population through the caspase-3-calcium-independent phospholipase A2 (iPLA2) -COX-2 pathway (Zhang et al., 2019b). BBR treatment can also inhibit cell proliferation by targeting Sp1 to upregulate miR-22-3p and suppress NF-κB p65 to induce apoptosis (Li et al., 2017). The pleckstrin homology domain leucine-rich repeat protein phosphatase 2 (PHLPP2), a tumor suppressor gene, can upregulate PHLPP2 from the PHLPP2-AKT-mammalian sterile 20-like kinase 1 (MST1) axis, exerting anti-proliferative effects (Saxena et al., 2018). A recent study revealed that BBR treatment enhanced ROS production and increased apoptosis (Tai et al., 2020). BBR treatment inhibits the growth of HCC cells by inhibiting glutamic-pyruvic transaminase 1 (GPT1) (Guo et al., 2020). Similarly, BBR treatment inhibits HCC cell proliferation by activating ERK1/2 phosphorylation (Chuang et al., 2017). The combination of S-allyl-cysteine and BBR can regulate adenylate cyclase signaling in association with stabilization of mitogen-activated protein kinase 3/6 (MKK3/6) to inhibit necroptosis and proliferation (Sengupta et al., 2017). BBR also regulates miR-22-3p to inhibit HCC development and progression (Chen et al., 2016). Overall, these results suggest that BBR induces apoptosis by inhibiting cell proliferation and growth.
BBR Inhibits Cell Invasion and Metastasis to Potentially Induce Apoptosis
Cyclin D1 is overexpressed in HCC and is associated with invasiveness. Studies have shown that BBR can inhibit cyclin D1 expression in liver cancer cells (Wang et al., 2016). Apoptotic cancer cells induced by chemotherapeutics can also change the tumor microenvironment by activating the lipoxygenase (LOX) pathway, and release inflammatory factors such as leukotriene B4 (LTB4) to stimulate the adhesion and migration of a small number of surviving cells. BBR can reverse FN adhesion and migration in HepG2 cells by inhibiting the iPLA2/LOX-5/LTB4 pathway (Zhao et al., 2020).
BBR Regulates Cancer-Related Pathways to Induce Apoptosis
First, BBR promotes the expression of cyclin-dependent kinase inhibitors (CDKIs) p21Cip1 and p27Kip1 by regulating the AKT/Forkhead box O3a (FoxO3a)/S-phase kinase-associated protein 2 (Skp2) axis and further induces HCC cell cycle arrest (Li et al., 2018a). Second, BBR antagonizes the β-catenin pathway to induce apoptosis, thereby reducing the survival rate of HCC cells (Vishnoi et al., 2021). However, BBR combined with HMQ1611 has also been shown to inhibit the WNT/β-catenin pathway and inhibit the proliferation and metastasis of HCC (Dai et al., 2019). Third, BBR can inhibit the PI3K/AKT pathway to inhibit cell growth, migration and invasion, and induce apoptosis (Song et al., 2019b). In Western countries, liver cancer is increasingly caused by nonalcoholic fatty liver disease/nonalcoholic steatohepatitis (NAFLD/NASH). Interestingly, BBR might alleviate NASH-HCC via the p38MAPK and ERK/COX-2 pathways (Luo et al., 2019b).
BBR Induces Cell Pyroptosis
Pyroptosis is a caspase-1-dependent programmed cell death. However, the role of pyroptosis in HCC remains unclear. Pyrolysis in HCC tissues and cells is suppressed. Administration of BBR inhibited the viability, migration and invasion of HepG2 cells by inducing cell pyrolysis, which was attenuated by the caspase-1 inhibitor Ac-YVAD-CMK. In summary, pyroptosis is involved in the pathogenesis of HCC and may be a new target for HCC treatment (Chu et al., 2016).
BBR Promotes Chemosensitivity and Radiosensitivity to Induce Apoptosis
Regorafenib resistance is an important barrier to the treatment of advanced liver cancer. BBR enhances the cytotoxicity of regorafenib in liver cancer cells. The combination of these two factors significantly inhibits the proliferation of HCC cells and induces apoptosis (Wang et al., 2021). Similarly, BBR can induce cell apoptosis and inhibit proliferation by resisting sorafenib resistance (Huang et al., 2018). 10-hydroxycamptothecin (10-HCPT) is an effective topoisomerase I inhibitor used in the treatment of liver cancer. The high expression of HIF-1α in liver cancer tissues is related to 10-HCPT resistance, which is considered a potential cancer target for natural products (Li et al., 2020). The upregulation of HIF-1α has been confirmed to exert anti-apoptotic effects (Tang et al., 2021). Conversely, BBR can inhibit the expression of HIF-1α as a synergistic treatment for liver cancer (Qi and Liu, 2021). Radiotherapy, which can be enhanced by BBR, is a common treatment for liver cancer. For instance, BBR enhances radiation-induced oxidative stress and apoptosis in Huh7 and HepG2 cells by suppressing the nuclear factor erythroid 2–related factor 2 (Nrf2) signaling pathway (You et al., 2019).
Anticancer Effect of BBR Against Other Cancers
There is growing evidence that BBR exhibits other anticancer activities. Nasopharyngeal carcinoma (NPC) is a malignancy derived from the epithelial cells of the nasopharynx cavity, and is closely associated with Epstein-Barr virus infection, BBR decreases the expression of EBNA1 to exhibit an antitumor effect against NPC (Wang et al., 2017a). BBR represses human gastric cancer cell growth by inducing cytostatic autophagy via the inhibition of MAPK/mTOR/p70 ribosomal S6 protein kinase and AKT (Zhang et al., 2020a). Moreover, BBR can regulate metabolism and exert anticancer effects. Lipid metabolism is a significant component of energy homeostasis, and BBR may partially inhibit colon cancer growth by targeting the SREBP cleavage-activating protein/sterol regulatory element-binding protein-1 pathway driving lipogenesis (Liu et al., 2020b). BBR also inhibits overactive glucose metabolism in colon cancer cells by suppressing mTOR-dependent HIF-1α protein synthesis (Mao et al., 2018). Furthermore, BBR promotes the degradation of sonic hedgehog mRNA in colorectal cancer cells, interrupting the paracrine hedgehog signaling pathway activity thus suppressing colorectal cancer growth (Shen et al., 2021). Additionally, BBR regulates the TGF-β pathway and reverses EMT in normal colonic epithelial cells (Huang et al., 2019a). In gynecology, BBR has been shown to suppress the growth and metastasis of endometrial cancer cells via miR-101/COX-2, and the combination therapy of BBR and cisplatin markedly enhances ovarian cancer cell death by inducing apoptosis and necroptosis, which may improve the anticancer effect of chemotherapy drugs (Liu et al., 2019b). Collectively, these results indicate that BBR has a wide range of anticancer effects.
Anticancer Effect of BBR Derivatives
BBR derivatives have better bioavailability, specific targeting, and stronger anticancer effects than those of BBR. The BBR derivative 8-cetylBBR (HBBR) is more effective for targeting lungs, and its anticancer properties are also significantly stronger than those of BBR. Animal experiments have proven that the oral administration of HBBR at a dose of 10 mg/kg significantly inhibited tumor growth and was more effective than the 120 mg/kg dose of BBR treatment (Xiao et al., 2018). Esters, amides, and sulfonates of BBR have also been developed for small-molecule cancer immunotherapy, such as 2,3-methylenedioxy-9-((2,2,3,3-tetramethylcyclopropane-1-carbonyl) oxy)-10-methoxyprotoberberine chloride and 2,3-methylenedioxy-9-(2-(adamantan-1-yl) acetoxy)-10-methoxyberberine chloride (Wang et al., 2018). BBR derivatives with a long alkyl chain branched by hydroxyl and methoxycarbonyl groups at position 9 showed 3.6-fold higher intracellular concentrations and 60-fold increased anti-proliferation activity against A549 cells compared with BBR (Liu et al., 2020c). 9-O-gernylberberrubine bromide and 9-O-farnesylberberrubine bromide showed greater growth inhibition, cell cycle regulation, and migration reduction (Chang et al., 2021). Combined with nanotechnology, 9-O-octadecyl substituted BBR derivative induce mitochondrial apoptosis (Song et al., 2019a). 13-dichlorophenylalkyl BBR semisynthetic derivatives, especially 13-[3-(2,4-dichlorophenyl)propyl]-9,10-dimethoxy-5,6-dihy-drobenzo[g]-1,3benzodioxolo[5,6-a]quinolizinium chloride induced apoptosis in breast cancer cells, while, 13-[2-(4-chlorophenyl)ethyl]berberine iodide (NAX014) reduced HER2 overexpressing breast cancer cell migration, and intragastric administration of 20 mg/kg NAX014 in HER2/neu transgenic mice delayed the onset of mammary tumors with no negative effects on health and survival (Pierpaoli et al., 2018; Pierpaoli et al., 2021).13-Ethylberberine induces apoptosis through the mitochondria-related apoptotic pathway in radiotherapy-resistant breast cancer cells (Jin et al., 2019). 9-/13-lipophilic substituted BBR derivatives, such as 9-O-dodecyl-BBR, 13-dodecyl-BBR, and 13-O-dodecyl-BBR, show significant photocytotoxic effects on HepG2 cells and induce remarkable cancer cell apoptosis (Lin et al., 2020).
Collectively, although BBR derivatives have higher anticancer efficacy, their specific mechanisms need to be further studied, and preclinical evidence is scarce.
Strategies to Improve the Bioavailability of BBR
The poor bioavailability of BBR limits its clinical application in cancer treatment. At present, apart from BBR derivatives, several other effective methods have been adopted. Structural modification of BBR not only improves its bioavailability but also enhances its anticancer effects. As mentioned earlier, BBR is sensitive to temperature and is widely distributed in organs after absorption. Evidence indicates that cationic or lipophilic substituted BBR derivatives increase their potential to penetrate the phospholipid bilayer (Popiołek et al., 2019; Lin et al., 2020). Demonstrating good stability at 4°C and 25°C, BBR liposomes showed a sustained-release behavior and displayed significantly increased retention in rat blood circulation, as evidenced by the significantly increased half-life (t1/2) and area under the curve (AUC) compared with BBR. In addition, BBR liposomes selectively increased the concentration in the liver, lungs and tumors, while reducing their distribution in the heart and kidney (Wang et al., 2017d). Similarly, the forms of esters, amides, and sulfonates of BBR have also been developed (Wang et al., 2018). In particular, BBR hydrochloride and BBR sulfate have higher solubilities than BBR (Feng et al., 2020). In addition, BBR hydrochloride induced cell proliferation and apoptosis of A549 cells by increasing the activity of the Bcl-2/Bax signaling pathway and inhibiting the Janus kinase 2 (Jak2)/vascular endothelial growth factor (VEGF)/NF-κB/transcription factor AP-1 (AP-1) signaling pathway (Li et al., 2018b). Second, co-administration can significantly improve the bioavailability and anticancer effects of BBR, such as theophylline (Hashemi-Niasari et al., 2018), cinnamaldehyde (Meng et al., 2017), icotinib (Chen et al., 2021), evodiamine (Du et al., 2017), HMQ1611 (Dai et al., 2019), s-allyl-cysteine (Sengupta et al., 2017), resveratrol (D’arcy et al., 2021), and Dox (Zhang et al., 2020b). All these factors may have a synergistic effect with BBR. Furthermore, the advent of nanotechnology has led to considerable improvements in enhancing the targeting and efficacy of BBR. For example, nanofabrication methods generate BBR-loaded liposomes with uniform size, high entrapment efficiency, and extended drug release time (Duong et al., 2021). Collagen gold nanoparticulate nanocarriers conjugated with BBR showed greater cell uptake capacity and pro-apoptotic effect on breast cancer cells (Chiu et al., 2021). Similarly, BBR-loaded Janus gold mesoporous silica nanocarriers were used in chemo/radio/photothermal therapy for the treatment of liver cancer and had a protective effect on normal tissues (Li et al., 2019b). The fluorescence of BBR-loaded polylactic acid nanoparticles (NPs) taken up by HCT116 colon carcinoma cells was approximately 2-fold higher than that of BBR (Ghaffarzadegan et al., 2020). Nano-co-delivery can further improve these effects. Nanoliposomes loaded with P-gp inhibitors and BBR chloride significantly increased BBR chloride content in cells (Vanti et al., 2021). Nano-co-delivery of BBR and Dox using poly lactic-co-glycolic acid NPs resulted in an almost 14-fold increase in half-life and an increased plasma concentration in rats (Khan et al., 2019), while BBR and zinc oxide-based NPs provided safe chemo-photothermal therapy for lung cancers (Kim et al., 2018b). Dual-functionalized spray-dried casein micelles combined with BBR and diosmin showed superior cytotoxicity and higher cellular uptake in HepG2 cells (Abdelmoneem et al., 2018). Targeting the lung delivery of layer-by-layer lipid NPs for the dual delivery of BBR and rapamycin (RAP) enhanced the sensitivity of lung cancer cells to RAP and the toxicity of drugs to cancer cells (Kabary et al., 2018). With BBR mitochondrial targeting, a paclitaxel-ss-BBR conjugate (PTX-ss-BBR NPs) and camptothecin-ss-BBR were prepared, which had stronger anti-A549 cell proliferation and pro-apoptotic effects (Cheng et al., 2020; Cheng and Ji, 2020). In addition, BBR derivatives and Dox nanomedicines target mitochondria-enhanced apoptosis (Lin et al., 2021). Dendrimer-encapsulated BBR improved pharmacokinetic parameters, such as AUC and half-life (t1/2) of BBR (Gupta et al., 2017). The gram-scale production of carrier-free fluorescent BBR microrods exhibited good optical properties and, pH-responsive drug-release behavior (Zheng et al., 2018b).
Therefore, the enhancement of BBR bioavailability primarily includes derivatizations, modifications, co-administration, and nanotechnology.
Conclusion and Future Perspectives
Lung cancer, breast cancer, and liver cancer are common types of cancers globally with a rising incidence rate. Conventional cancer therapies may lead to drug resistance and serious toxicities. Thus, novel therapeutic approaches are urgently needed. Natural products are a valuable source of new drugs, and BBR is a known isoquinoline alkaloid with outstanding activities. It can be extracted from various medicinal plants, such as C. chinensis, P. chinense, and B. vulgaris. In recent decades, accumulated studies have shown that BBR has considerable anticancer effects. As mentioned above, BBR can significantly inhibit the proliferation, metastasis, and invasiveness of cancer cells to induce apoptosis, the related signal pathways are AMPK, MAPK, and AKT pathways. This article reviews the chemical properties, ADMET, therapeutic effects and mechanisms of BBR in treating three types of cancers (breast, lung and liver cancer). Which are expected to serve as a reference for follow-up research.
However, there are some issues require further clarification in future research before clinical usage of this natural compound. Firstly, due to multi-target characteristics, the anticancer mechanisms of BBR have not yet been fully elucidated, and more verification is still needed, for example, study shows BBR regulates immunity during cancer treatment, but its targets are not fully clarified, more research is needed in the future. By means of network pharmacology, research has demonstrated that traditional medicines show a synergistic effect through multiple targets and pathways (Yuan et al., 2017). Therefore, we suggest to search for possible targets of BBR and to find drugs or natural products that have a synergistic effect with BBR through network pharmacology.
Secondly, most of the current existing researches focus on the cellular level, there is still a lack of corresponding clinical data to evaluate anticancer efficacy and corresponding dose in humans, which should be studied further.
Thirdly, the pharmacokinetics of BBR in humans have not been fully elucidated. The metabolites of BBR in the body also need to be further studied. A previous study identified 96 metabolites in rats, with most metabolites excreted in urine (Wang et al., 2017b). However, how metabolites interact with BBR requires further research to better understand their biological activities. Therefore, we recommend more in vivo studies to understand the metabolism of BBR.
Fourthly, low bioavailability of BBR seriously limits its clinical application, fortunately, structural modification of BBR has a significant effect on improving efficacy, selectivity, and safety. Some BBR derivatives, such as position 9 or 13 of the alkaloid skeleton, have better bioavailability and anticancer effects, which have been predicted to improve clinical efficacy and safety of BBR (Zhang et al., 2019a). Besides, nanotechnology have significantly enhanced the bioavailability of BBR, also, nanotechnology enables BBR to reach specific sites and exert pharmacological effects. Moreover, nanocarriers can enhance the anticancer effects of BBR through co-delivery. Therefore, a combination of structural modification, BBR derivatives, and nanotechnology is expected to be a comprising way to improve bioavailability of BBR.
Fifthly, regarding safety, as described herein, toxicity of BBR depends on the method of administration and mild gastrointestinal reactions may occur in some patients after oral administration (Imenshahidi and Hosseinzadeh, 2019). Paradoxically, BBR has shown adverse effects and even toxicity under specific circumstances, such as liver toxicity, cardiotoxicity and immunotoxicity. But BBR has been shown to possess cardioprotective, hepatoprotective effects and immunomodulatory capacities in human body. Such contradictory results may be related to mode of administration, dose of BBR and administration time. Hence, we suggest comprehensive strategies to investigate the balance between toxicity and therapeutic efficacy of BBR. Especially, long-term clinical trials are needed to better determine the human safety of BBR.
Sixthly, recently, clinical research on BBR has increased, primarily focusing on metabolic diseases, such as nonalcoholic liver disease, diabetes, and hyperlipidemia (Li et al., 2019a; Zhang et al., 2020c; Di Bisceglie et al., 2020; Zhao et al., 2021b; Harrison et al., 2021), and digestive system diseases, such as ulcerative colitis (Xu et al., 2020) and colorectal adenoma (Chen et al., 2020). BBR ursodeoxycholate and BBR chloride are involved in this process. They provide reliable evidence for the safety and effectiveness of the clinical use of BBR, but a lack of high-quality clinical research on cancers remains. Furthermore, most research is limited to Chinese studies, which may not be appliance for other groups of people. So large sample and multi-center studies are recommended.
Lastly, multidrug resistance has become a challenge in anticancer therapy and BBR has been valued as a chemosensitizer, chemoprotector and radiotherapy protector. Therefore, the sensitization effect of BBR on radiotherapy and chemotherapy warrants further study.
In conclusion, many studies have revealed anticancer effects of BBR through apoptosis, and more in vivo and in vitro studies are needed to further confirm anticancer effects of BBR. Hopefully, with further research, the therapeutic effect of BBR on cancers will likely be clinically accepted and applied.
Author Contributions
YZ and KL searched the literature, wrote the first draft of the manuscript. NX and YL conducted ADMET analysis. YC and YY draught mechanism diagrams. YN processed the radar graph and the table in the manuscript. CZ and JS contributed to the manuscript revision. All authors read and approved the submitted version.
Funding
Financial support was provided by the Sichuan Science and technology program, Grant/Award Number: 2020JDRC0114, 2020YFH0164; Special subject of scientific research on traditional Chinese Medicine of Sichuan Administration of Traditional Chinese Medicine, Grant/Award Number: 2021MS093; “100 Talent Plan” Project of Hospital of Chengdu University of Traditional Chinese Medicine, Grant/Award Number: Hospital office (2021) 42.
Conflict of Interest
The authors declare that the research was conducted in the absence of any commercial or financial relationships that could be construed as a potential conflict of interest.
Publisher’s Note
All claims expressed in this article are solely those of the authors and do not necessarily represent those of their affiliated organizations, or those of the publisher, the editors and the reviewers. Any product that may be evaluated in this article, or claim that may be made by its manufacturer, is not guaranteed or endorsed by the publisher.
References
Abdelmoneem, M. A., Mahmoud, M., Zaky, A., Helmy, M. W., Sallam, M., Fang, J. Y., et al. (2018). Dual-Targeted Casein Micelles as Green Nanomedicine for Synergistic Phytotherapy of Hepatocellular Carcinoma. J. Control. Release 287, 78–93. doi:10.1016/j.jconrel.2018.08.026
Ahmad, S., Hussain, A., Hussain, A., Abdullah, I., Ali, M. S., Froeyen, M., et al. (2019). Quantification of Berberine in Berberis Vulgaris L. Root Extract and its Curative and Prophylactic Role in Cisplatin-Induced In Vivo Toxicity and In Vitro Cytotoxicity. Antioxidants (Basel) 8, 185. doi:10.3390/antiox8060185
Ali, M., Rogers, L. K., and Pitari, G. M. (2015). Serine Phosphorylation of Vasodilator-Stimulated Phosphoprotein (Vasp) Regulates Colon Cancer Cell Survival and Apoptosis. Life Sci. 123, 1–8. doi:10.1016/j.lfs.2014.12.018
American Chemical Society, (2022). American Chemical Society. [Online]. Available: https://www.acs.org/content/acs/en/molecule-of-the-week/archive/b/berberine.html (Accessed November 21, 2021).
Anderson, N. R., Minutolo, N. G., Gill, S., and Klichinsky, M. (2021). Macrophage-Based Approaches for Cancer Immunotherapy. Cancer Res. 81, 1201–1208. doi:10.1158/0008-5472.Can-20-2990
Andola, H. C., Gaira, K. S., Rawal, R. S., Rawat, M. S., and Bhatt, I. D. (2010). Habitat-Dependent Variations in Berberine Content of Berberis Asiatica Roxb. Ex. Dc. In Kumaon, Western Himalaya. Chem. Biodivers. 7, 415–420. doi:10.1002/cbdv.200900041
Anwanwan, D., Singh, S. K., Singh, S., Saikam, V., and Singh, R. (2020). Challenges in Liver Cancer and Possible Treatment Approaches. Biochim. Biophys. Acta Rev. Cancer 1873 (1), 188314. doi:10.1016/j.bbcan.2019.188314
Battu, S. K., Repka, M. A., Maddineni, S., Chittiboyina, A. G., Avery, M. A., and Majumdar, S. (2010). Physicochemical Characterization of Berberine Chloride: A Perspective in the Development of a Solution Dosage Form for Oral Delivery. AAPS PharmSciTech 11, 1466–1475. doi:10.1208/s12249-010-9520-y
Bhattacharyya, R., Saha, B., Tyagi, M., Bandyopadhyay, S. K., Patro, B. S., and Chattopadhyay, S. (2017). Differential Modes of Photosensitisation in Cancer Cells by Berberine and Coralyne. Free Radic. Res. 51, 723–738. doi:10.1080/10715762.2017.1368506
Bray, F., Ferlay, J., Laversanne, M., Brewster, D. H., Gombe Mbalawa, C., Kohler, B., et al. (2015). Cancer Incidence in Five Continents: Inclusion Criteria, Highlights from Volume X and the Global Status of Cancer Registration. Int. J. Cancer 137, 2060–2071. doi:10.1002/ijc.29670
Cabral-Pacheco, G. A., Garza-Veloz, I., Castruita-De La Rosa, C., Ramirez-Acuña, J. M., Perez-Romero, B. A., Guerrero-Rodriguez, J. F., et al. (2020). The Roles of Matrix Metalloproteinases and Their Inhibitors in Human Diseases. Ijms 21 (24), 9739. doi:10.3390/ijms21249739
Campisi, A., Acquaviva, R., Bonfanti, R., Raciti, G., Amodeo, A., Mastrojeni, S., et al. (2014). Antioxidant Properties of Berberis Aetnensis C. Presl (Berberidaceae) Roots Extract and Protective Effects on Astroglial Cell Cultures. ScientificWorldJournal 2014, 315473. doi:10.1155/2014/315473
Carneiro, B. A., and El-Deiry, W. S. (2020). Targeting Apoptosis in Cancer Therapy. Nat. Rev. Clin. Oncol. 17, 395–417. doi:10.1038/s41571-020-0341-y
Cazzaniga, M., and Bonanni, B. (2018). Relationship between Metabolic Disorders and Breast Cancer Incidence and Outcomes. Is There a Preventive and Therapeutic Role for Berberine? Anticancer Res. 38, 4393–4402. doi:10.21873/anticanres.12741
Chan, E. (1993). Displacement of Bilirubin from Albumin by Berberine. Biol. Neonate. 63, 201–208. doi:10.1159/000243932
Chang, J. M., Wu, J. Y., Chen, S. H., Chao, W. Y., Chuang, H. H., Kam, K. H., et al. (2021). 9-O-Terpenyl-Substituted Berberrubine Derivatives Suppress Tumor Migration and Increase Anti-human Non-small-cell Lung Cancer Activity. Int. J. Mol. Sci. 22, 9864. doi:10.3390/ijms22189864
Chen, J., Wu, F. X., Luo, H. L., Liu, J. J., Luo, T., Bai, T., et al. (2016). Berberine Upregulates Mir-22-3p to Suppress Hepatocellular Carcinoma Cell Proliferation by Targeting Sp1. Am. J. Transl. Res. 8, 4932–4941.
Chen, P., Dai, C. H., Shi, Z. H., Wang, Y., Wu, J. N., Chen, K., et al. (2021). Synergistic Inhibitory Effect of Berberine and Icotinib on Non-small Cell Lung Cancer Cells via Inducing Autophagic Cell Death and Apoptosis. Apoptosis 26, 639–656. doi:10.1007/s10495-021-01694-w
Chen, Q. Q., Shi, J. M., Ding, Z., Xia, Q., Zheng, T. S., Ren, Y. B., et al. (2019). Berberine Induces Apoptosis in Non-small-cell Lung Cancer Cells by Upregulating Mir-19a Targeting Tissue Factor. Cancer Manag. Res. 11, 9005–9015. doi:10.2147/cmar.S207677
Chen, Y. X., Gao, Q. Y., Zou, T. H., Wang, B. M., Liu, S. D., Sheng, J. Q., et al. (2020). Berberine versus Placebo for the Prevention of Recurrence of Colorectal Adenoma: A Multicentre, Double-Blinded, Randomised Controlled Study. Lancet Gastroenterol. Hepatol. 5, 267–275. doi:10.1016/s2468-1253(19)30409-1
Cheng, Y., and Ji, Y. (2020). Mitochondria-Targeting Nanomedicine Self-Assembled from Gsh-Responsive Paclitaxel-Ss-Berberine Conjugate for Synergetic Cancer Treatment with Enhanced Cytotoxicity. J. Control. Release 318, 38–49. doi:10.1016/j.jconrel.2019.12.011
Cheng, Y., Ji, Y., and Tong, J. (2020). Triple Stimuli-Responsive Supramolecular Nanoassembly with Mitochondrial Targetability for Chemophotothermal Therapy. J. Control. Release 327, 35–49. doi:10.1016/j.jconrel.2020.08.006
Chi, C. W., Chang, Y. F., Chao, T. W., Chiang, S. H., P'eng, F. K., Lui, W. Y., et al. (1994). Flowcytometric Analysis of the Effect of Berberine on the Expression of Glucocorticoid Receptors in Human Hepatoma Hepg2 Cells. Life Sci. 54, 2099–2107. doi:10.1016/0024-3205(94)00719-5
Chiu, C. F., Fu, R. H., Hsu, S. H., Yu, Y. A., Yang, S. F., Tsao, T. C., et al. (2021). Delivery Capacity and Anticancer Ability of the Berberine-Loaded Gold Nanoparticles to Promote the Apoptosis Effect in Breast Cancer. Cancers (Basel) 13, 5317. doi:10.3390/cancers13215317
Chu, Q., Jiang, Y., Zhang, W., Xu, C., Du, W., Tuguzbaeva, G., et al. (2016). Pyroptosis Is Involved in the Pathogenesis of Human Hepatocellular Carcinoma. Oncotarget 7, 84658–84665. doi:10.18632/oncotarget.12384
Chuang, T. Y., Wu, H. L., Min, J., Diamond, M., Azziz, R., and Chen, Y. H. (2017). Berberine Regulates the Protein Expression of Multiple Tumorigenesis-Related Genes in Hepatocellular Carcinoma Cell Lines. Cancer Cel Int 17, 59. doi:10.1186/s12935-017-0429-3
D'arcy, M. S., Pike, C. V. S., and Coussons, P. J. (2021). A Novel Combined Resveratrol/Berberine Phytochemotheraputic Using the Hepg2 Cell Line as a Model for the Treatment of Hepatocarcinoma. Cell Biol Int 45, 2499–2509. doi:10.1002/cbin.11695
Dai, B., Ma, Y., Yang, T., Fan, M., Yu, R., Su, Q., et al. (2019). Synergistic Effect of Berberine and Hmq1611 Impairs Cell Proliferation and Migration by Regulating Wnt Signaling Pathway in Hepatocellular Carcinoma. Phytother. Res. 33, 745–755. doi:10.1002/ptr.6267
Di Bisceglie, A. M., Watts, G. F., Lavin, P., Yu, M., Bai, R., and Liu, L. (2020). Pharmacokinetics and Pharmacodynamics of Htd1801 (Berberine Ursodeoxycholate, Budca) in Patients with Hyperlipidemia. Lipids Health Dis. 19, 239. doi:10.1186/s12944-020-01406-4
Du, J., Sun, Y., Lu, Y. Y., Lau, E., Zhao, M., Zhou, Q. M., et al. (2017). Berberine and Evodiamine Act Synergistically against Human Breast Cancer Mcf-7 Cells by Inducing Cell Cycle Arrest and Apoptosis. Anticancer Res. 37, 6141–6151. doi:10.21873/anticanres.12063
Duong, T. T., Isomäki, A., Paaver, U., Laidmäe, I., Tõnisoo, A., Yen, T. T. H., et al. (2021). Nanoformulation and Evaluation of Oral Berberine-Loaded Liposomes. Molecules 26 (9), 2591. doi:10.3390/molecules26092591
El Khalki, L., Maire, V., Dubois, T., and Zyad, A. (2020). Berberine Impairs the Survival of Triple Negative Breast Cancer Cells: Cellular and Molecular Analyses. Molecules 25 (3), 506. doi:10.3390/molecules25030506
Ettefagh, K. A., Burns, J. T., Junio, H. A., Kaatz, G. W., and Cech, N. B. (2011). Goldenseal (Hydrastis Canadensis L.) Extracts Synergistically Enhance the Antibacterial Activity of Berberine via Efflux Pump Inhibition. Planta Med. 77, 835–840. doi:10.1055/s-0030-1250606
Fan, X. X., Leung, E. L., Xie, Y., Liu, Z. Q., Zheng, Y. F., Yao, X. J., et al. (2018). Suppression of Lipogenesis via Reactive Oxygen Species-Ampk Signaling for Treating Malignant and Proliferative Diseases. Antioxid. Redox Signal. 28, 339–357. doi:10.1089/ars.2017.7090
Feng, R., Shou, J. W., Zhao, Z. X., He, C. Y., Ma, C., Huang, M., et al. (2015). Transforming Berberine into its Intestine-Absorbable Form by the Gut Microbiota. Sci. Rep. 5, 12155. doi:10.1038/srep12155
Feng, X., Sureda, A., Jafari, S., Memariani, Z., Tewari, D., Annunziata, G., et al. (2019). Berberine in Cardiovascular and Metabolic Diseases: From Mechanisms to Therapeutics. Theranostics 9, 1923–1951. doi:10.7150/thno.30787
Feng, X., Wang, K., Cao, S., Ding, L., and Qiu, F. (2020). Pharmacokinetics and Excretion of Berberine and its Nine Metabolites in Rats. Front. Pharmacol. 11, 594852. doi:10.3389/fphar.2020.594852
Fernández-Poyatos, M. D. P., Ruiz-Medina, A., Zengin, G., and Llorent-Martínez, E. J. (2019). Phenolic Characterization, Antioxidant Activity, and Enzyme Inhibitory Properties of Berberis Thunbergii Dc. Leaves: A Valuable Source of Phenolic Acids. Molecules 24 (22), 4171. doi:10.3390/molecules24224171
Fidler-Benaoudia, M. M., Torre, L. A., Bray, F., Ferlay, J., and Jemal, A. (2020). Lung Cancer Incidence in Young Women vs. Young Men: A Systematic Analysis in 40 Countries. Int. J. Cancer 147, 811–819. doi:10.1002/ijc.32809
Fu, L., Chen, W., Guo, W., Wang, J., Tian, Y., Shi, D., et al. (2013). Berberine Targets AP-2/hTERT, NF-Κb/cox-2, HIF-1α/VEGF and Cytochrome-c/Caspase Signaling to Suppress Human Cancer Cell Growth. PLoS One 8, e69240. doi:10.1371/journal.pone.0069240
Gao, X., Wang, J., Li, M., Wang, J., Lv, J., Zhang, L., et al. (2019). Berberine Attenuates Xrcc1-Mediated Base Excision Repair and Sensitizes Breast Cancer Cells to the Chemotherapeutic Drugs. J. Cel. Mol. Med. 23, 6797–6804. doi:10.1111/jcmm.14560
Garg, A. D., and Agostinis, P. (2017). Cell Death and Immunity in Cancer: From Danger Signals to Mimicry of Pathogen Defense Responses. Immunol. Rev. 280, 126–148. doi:10.1111/imr.12574
Ghaffarzadegan, R., Khoee, S., and Rezazadeh, S. (2020). Fabrication, Characterization and Optimization of Berberine-Loaded Pla Nanoparticles Using Coaxial Electrospray for Sustained Drug Release. DARU 28, 237–252. doi:10.1007/s40199-020-00335-y
Giordano, A., and Tommonaro, G. (2019). Curcumin and Cancer. Nutrients 11, 2376. doi:10.3390/nu11102376
Global Cancer Observatory, (2021). Global Cancer Observatory. [Online]. Available: https://gco.iarc.fr/(Accessed November 21, 2021).
Goswami, A. K., Gogoi, N., Shakya, A., and Sharma, H. K. (2019). Development and Validation of High-Performance Thin-Layer Chromatographic Method for Quantification of Berberine in Rhizomes of Coptis Teeta Wall, an Endangered Species Collected from Arunachal Pradesh, India. J. Chromatogr. Sci. 57, 411–417. doi:10.1093/chromsci/bmz009
Gu, Y., Qian, D., Duan, J. A., Wang, Z., Guo, J., Tang, Y., et al. (2010). Simultaneous Determination of Seven Main Alkaloids of Chelidonium Majus L. By Ultra-performance Lc with Photodiode-Array Detection. J. Sep. Sci. 33, 1004–1009. doi:10.1002/jssc.200900690
Guo, L., and Kang, W. Y. (2018). Chemistry of Chinese Materia Medica. Beijing: China Medical Science Press.
Guo, W., Tan, H. Y., Li, S., Wang, N., and Feng, Y. (2020). Glutamic-Pyruvic Transaminase 1 Facilitates Alternative Fuels for Hepatocellular Carcinoma Growth-A Small Molecule Inhibitor, Berberine. Cancers (Basel) 12, 1854. doi:10.3390/cancers12071854
Gupta, L., Sharma, A. K., Gothwal, A., Khan, M. S., Khinchi, M. P., Qayum, A., et al. (2017). Dendrimer Encapsulated and Conjugated Delivery of Berberine: A Novel Approach Mitigating Toxicity and Improving In Vivo Pharmacokinetics. Int. J. Pharm. 528, 88–99. doi:10.1016/j.ijpharm.2017.04.073
Habtemariam, S. (2020). Berberine Pharmacology and the Gut Microbiota: A Hidden Therapeutic Link. Pharmacol. Res. 155, 104722. doi:10.1016/j.phrs.2020.104722
Hafner, A., Bulyk, M. L., Jambhekar, A., and Lahav, G. (2019). The Multiple Mechanisms that Regulate P53 Activity and Cell Fate. Nat. Rev. Mol. Cel Biol. 20, 199–210. doi:10.1038/s41580-019-0110-x
Harrison, S. A., Gunn, N., Neff, G. W., Kohli, A., Liu, L., Flyer, A., et al. (2021). A Phase 2, Proof of Concept, Randomised Controlled Trial of Berberine Ursodeoxycholate in Patients with Presumed Non-alcoholic Steatohepatitis and Type 2 Diabetes. Nat. Commun. 12, 5503. doi:10.1038/s41467-021-25701-5
Hashemi-Niasari, F., Rabbani-Chadegani, A., Razmi, M., and Fallah, S. (2018). Synergy of Theophylline Reduces Necrotic Effect of Berberine, Induces Cell Cycle Arrest and Parp, Hmgb1, Bcl-2 Family Mediated Apoptosis in Mda-Mb-231 Breast Cancer Cells. Biomed. Pharmacother. 106, 858–867. doi:10.1016/j.biopha.2018.07.019
Hou, Y., Wang, X., Zhang, Y., Wang, S., and Meng, X. (2021). Highland Mate: Edible and Functional Foods in Traditional Medicine for the Prevention and Treatment of Hypoxia-Related Symptoms. Curr. Opin. Pharmacol. 60, 306–314. doi:10.1016/j.coph.2021.07.018
Hua, W., Ding, L., Chen, Y., Gong, B., He, J., and Xu, G. (2007). Determination of Berberine in Human Plasma by Liquid Chromatography-Electrospray Ionization-Mass Spectrometry. J. Pharm. Biomed. Anal. 44, 931–937. doi:10.1016/j.jpba.2007.03.022
Huang, C., Tao, L., Wang, X. L., and Pang, Z. (2019a). Berberine Reversed the Epithelial-Mesenchymal Transition of normal Colonic Epithelial Cells Induced by SW480 Cells through Regulating the Important Components in the TGF-β Pathway. J. Cel. Physiol 234, 11679–11691. doi:10.1002/jcp.27835
Huang, Q., Zhang, J., Peng, J., Zhang, Y., Wang, L., Wu, J., et al. (2019b). Effect of Baicalin on Proliferation and Apoptosis in Pancreatic Cancer Cells. Am. J. Transl. Res. 11, 5645–5654.
Huang, Y., Jiang, Z., Wang, J., Yin, G., Jiang, K., Tu, J., et al. (2019c). Quality Evaluation of Mahonia Bealei (Fort.) Carr. Using Supercritical Fluid Chromatography with Chemical Pattern Recognition. Molecules 24 (20), 3684. doi:10.3390/molecules24203684
Huang, Y., Wang, K., Gu, C., Yu, G., Zhao, D., Mai, W., et al. (2018). Berberine, a Natural Plant Alkaloid, Synergistically Sensitizes Human Liver Cancer Cells to Sorafenib. Oncol. Rep. 40, 1525–1532. doi:10.3892/or.2018.6552
Huang, Z. J., Zeng, Y., Lan, P., Sun, P. H., and Chen, W. M. (2011). Advances in Structural Modifications and Biological Activities of Berberine: An Active Compound in Traditional Chinese Medicine. Mini Rev. Med. Chem. 11, 1122–1129. doi:10.2174/138955711797655362
Imanshahidi, M., and Hosseinzadeh, H. (2008). Pharmacological and Therapeutic Effects of Berberis Vulgaris and its Active Constituent, Berberine. Phytother Res. 22, 999–1012. doi:10.1002/ptr.2399
Imenshahidi, M., and Hosseinzadeh, H. (2019). Berberine and Barberry (Berberis Vulgaris): A Clinical Review. Phytother. Res. 33, 504–523. doi:10.1002/ptr.6252
Inbaraj, J. J., Kukielczak, B. M., Bilski, P., Sandvik, S. L., and Chignell, C. F. (2001). Photochemistry and Photocytotoxicity of Alkaloids from Goldenseal (Hydrastis Canadensis L.) 1. Berberine. Chem. Res. Toxicol. 14, 1529–1534. doi:10.1021/tx0155247
Jabbarzadeh Kaboli, P., Leong, M. P., Ismail, P., and Ling, K. H. (2019). Antitumor Effects of Berberine against Egfr, Erk1/2, P38 and Akt in Mda-Mb231 and Mcf-7 Breast Cancer Cells Using Molecular Modelling and In Vitro Study. Pharmacol. Rep. 71, 13–23. doi:10.1016/j.pharep.2018.07.005
Jeong, Y., You, D., Kang, H. G., Yu, J., Kim, S. W., Nam, S. J., et al. (2018). Berberine Suppresses Fibronectin Expression through Inhibition of C-Jun Phosphorylation in Breast Cancer Cells. J. Breast Cancer 21, 21–27. doi:10.4048/jbc.2018.21.1.21
Jin, H., Ko, Y. S., Park, S. W., Chang, K. C., and Kim, H. J. (2019). 13-Ethylberberine Induces Apoptosis through the Mitochondria-Related Apoptotic Pathway in Radiotherapy-Resistant Breast Cancer Cells. Molecules 24 (13), 2448. doi:10.3390/molecules24132448
Kabary, D. M., Helmy, M. W., Elkhodairy, K. A., Fang, J. Y., and Elzoghby, A. O. (2018). Hyaluronate/Lactoferrin Layer-By-Layer-Coated Lipid Nanocarriers for Targeted Co-delivery of Rapamycin and Berberine to Lung Carcinoma. Colloids Surf. B Biointerfaces 169, 183–194. doi:10.1016/j.colsurfb.2018.05.008
Kalaiarasi, A., Anusha, C., Sankar, R., Rajasekaran, S., John Marshal, J., Muthusamy, K., et al. (2016). Plant Isoquinoline Alkaloid Berberine Exhibits Chromatin Remodeling by Modulation of Histone Deacetylase to Induce Growth Arrest and Apoptosis in the A549 Cell Line. J. Agric. Food Chem. 64, 9542–9550. doi:10.1021/acs.jafc.6b04453
Karnam, K. C., Ellutla, M., Bodduluru, L. N., Kasala, E. R., Uppulapu, S. K., Kalyankumarraju, M., et al. (2017). Preventive Effect of Berberine against Dmba-Induced Breast Cancer in Female Sprague Dawley Rats. Biomed. Pharmacother. 92, 207–214. doi:10.1016/j.biopha.2017.05.069
Khan, I., Joshi, G., Nakhate, K. T., AjazuddinKumar, R., Kumar, R., and Gupta, U. (2019). Nano-Co-Delivery of Berberine and Anticancer Drug Using Plga Nanoparticles: Exploration of Better Anticancer Activity and In Vivo Kinetics. Pharm. Res. 36, 149. doi:10.1007/s11095-019-2677-5
Khan, M. I., Rahman, M. A., Khalid, M., Khushtar, M., and Mujahid, M. (2020). Quality Control Standardization and Evaluation of Antimicrobial Potential of Daruhaldi (Berberis Aristata Dc) Stem Bark. J. Diet. Suppl. 17, 97–109. doi:10.1080/19390211.2018.1484405
Kheir, M. M., Wang, Y., Hua, L., Hu, J., Li, L., Lei, F., et al. (2010). Acute Toxicity of Berberine and its Correlation with the Blood Concentration in Mice. Food Chem. Toxicol. 48, 1105–1110. doi:10.1016/j.fct.2010.01.033
Kim, J. B., Lee, K. M., Ko, E., Han, W., Lee, J. E., Shin, I., et al. (2008). Berberine Inhibits Growth of the Breast Cancer Cell Lines Mcf-7 and Mda-Mb-231. Planta Med. 74, 39–42. doi:10.1055/s-2007-993779
Kim, S., Lee, J., You, D., Jeong, Y., Jeon, M., Yu, J., et al. (2018a). Berberine Suppresses Cell Motility through Downregulation of TGF-Β1 in Triple Negative Breast Cancer Cells. Cell Physiol. Biochem. 45, 795–807. doi:10.1159/000487171
Kim, S., Lee, S. Y., and Cho, H. J. (2018b). Berberine and Zinc Oxide-Based Nanoparticles for the Chemo-Photothermal Therapy of Lung Adenocarcinoma. Biochem. Biophys. Res. Commun. 501, 765–770. doi:10.1016/j.bbrc.2018.05.063
Kim, S., You, D., Jeong, Y., Yu, J., Kim, S. W., Nam, S. J., et al. (2018c). Berberine Down-Regulates Il-8 Expression through Inhibition of the Egfr/Mek/Erk Pathway in Triple-Negative Breast Cancer Cells. Phytomedicine 50, 43–49. doi:10.1016/j.phymed.2018.08.004
Kong, W., Wei, J., Abidi, P., Lin, M., Inaba, S., Li, C., et al. (2004). Berberine Is a Novel Cholesterol-Lowering Drug Working through a Unique Mechanism Distinct from Statins. Nat. Med. 10, 1344–1351. doi:10.1038/nm1135
Kumar, R., Awasthi, M., Sharma, A., Padwad, Y., and Sharma, R. (2020). Berberine Induces Dose-dependent Quiescence and Apoptosis in A549 Cancer Cells by Modulating Cell Cyclins and Inflammation Independent of Mtor Pathway. Life Sci. 244, 117346. doi:10.1016/j.lfs.2020.117346
Kysenius, K., Brunello, C. A., and Huttunen, H. J. (2014). Mitochondria and Nmda Receptor-dependent Toxicity of Berberine Sensitizes Neurons to Glutamate and Rotenone Injury. PLoS One 9, e107129. doi:10.1371/journal.pone.0107129
Lee, H. Y., He, X., and Ahn, J. (2010). Enhancement of Antimicrobial and Antimutagenic Activities of Korean Barberry (Berberis Koreana Palib.) by the Combined Process of High-Pressure Extraction with Probiotic Fermentation. J. Sci. Food Agric. 90, 2399–2404. doi:10.1002/jsfa.4098
Li, F., Dong, X., Lin, P., and Jiang, J. (2018a). Regulation of Akt/Foxo3a/Skp2 Axis Is Critically Involved in Berberine-Induced Cell Cycle Arrest in Hepatocellular Carcinoma Cells. Int. J. Mol. Sci. 19, 327. doi:10.3390/ijms19020327
Li, G., Zhao, M., Qiu, F., Sun, Y., and Zhao, L. (2019a). Pharmacokinetic Interactions and Tolerability of Berberine Chloride with Simvastatin and Fenofibrate: An Open-Label, Randomized, Parallel Study in Healthy Chinese Subjects. Drug Des. Devel Ther. 13, 129–139. doi:10.2147/dddt.S185487
Li, J., Liu, F., Jiang, S., Liu, J., Chen, X., Zhang, S., et al. (2018b). Berberine Hydrochloride Inhibits Cell Proliferation and Promotes Apoptosis of Non-small Cell Lung Cancer via the Suppression of the Mmp2 and Bcl-2/Bax Signaling Pathways. Oncol. Lett. 15, 7409–7414. doi:10.3892/ol.2018.8249
Li, M., Zhang, M., Zhang, Z. L., Liu, N., Han, X. Y., Liu, Q. C., et al. (2017). Induction of Apoptosis by Berberine in Hepatocellular Carcinoma HepG2 Cells via Downregulation of NF-Κb. Oncol. Res. 25, 233–239. doi:10.3727/096504016x14742891049073
Li, R. L., He, L. Y., Zhang, Q., Liu, J., Lu, F., Duan, H. X., et al. (2020). HIF-1α Is a Potential Molecular Target for Herbal Medicine to Treat Diseases. Drug Des. Devel. Ther. 14, 4915–4949. doi:10.2147/dddt.S274980
Li, X.-D., Wang, Z., Wang, X.-R., Shao, D., Zhang, X., Li, L., et al. (2019b). Berberine-Loaded Janus Gold Mesoporous Silica Nanocarriers for Chemo/Radio/Photothermal Therapy of Liver Cancer and Radiation-Induced Injury Inhibition. Ijn 14, 3967–3982. doi:10.2147/ijn.S206044
Li, Y., Ren, G., Wang, Y. X., Kong, W. J., Yang, P., Wang, Y. M., et al. (2011). Bioactivities of Berberine Metabolites after Transformation through Cyp450 Isoenzymes. J. Transl. Med. 9, 62. doi:10.1186/1479-5876-9-62
Li, Y. H., Zhang, M., Fu, H. B., Xiao, H. T., and Bian, Z. X. (2016). Pre-Clinical Toxicity of a Combination of Berberine and 5-Aminosalicylic Acid in Mice. Food Chem. Toxicol. 97, 150–158. doi:10.1016/j.fct.2016.08.031
Lin, C., Yang, X., Li, H., Zou, Y., Mohammad, I. S., Rong, H., et al. (2021). Self-Assembled Nanomedicine Combining a Berberine Derivative and Doxorubicin for Enhanced Antitumor and Antimetastatic Efficacy via Mitochondrial Pathways. Nanoscale 13, 6605–6623. doi:10.1039/d1nr00032b
Lin, H. J., Ho, J. H., Tsai, L. C., Yang, F. Y., Yang, L. L., Kuo, C. D., et al. (2020). Synthesis and In Vitro Photocytotoxicity of 9-/13-Lipophilic Substituted Berberine Derivatives as Potential Anticancer Agents. Molecules 25, 677. doi:10.3390/molecules25030677
Lin, Y. S., Chiu, Y. C., Tsai, Y. H., Tsai, Y. F., Wang, J. Y., Tseng, L. M., et al. (2019). Different Mechanisms Involved in the Berberine-Induced Antiproliferation Effects in Triple-Negative Breast Cancer Cell Lines. J. Cel Biochem. 120, 13531–13544. doi:10.1002/jcb.28628
Liu, J., Zhang, C., Hu, W., and Feng, Z. (2019a). Tumor Suppressor P53 and Metabolism. J. Mol. Cel Biol. 11, 284–292. doi:10.1093/jmcb/mjy070
Liu, L., Fan, J., Ai, G., Liu, J., Luo, N., Li, C., et al. (2019b). Berberine in Combination with Cisplatin Induces Necroptosis and Apoptosis in Ovarian Cancer Cells. Biol. Res. 52, 37. doi:10.1186/s40659-019-0243-6
Liu, L., Wang, Z. B., Song, Y., Yang, J., Wu, L. J., Yang, B. Y., et al. (2016). Simultaneous Determination of Eight Alkaloids in Rat Plasma by Uhplc-Ms/Ms after Oral Administration of Coptis Deltoidea C. Y. Cheng Et Hsiao and Coptis Chinensis Franch. Molecules 21, 913. doi:10.3390/molecules21070913
Liu, T. H., Zhang, X. M., Tian, S. Z., Chen, L. G., and Yuan, J. L. (2020a). Bioinformatics Analysis of Endophytic Bacteria Related to Berberine in the Chinese Medicinal Plant Coptis Teeta Wall. 3 Biotech. 10, 96. doi:10.1007/s13205-020-2084-y
Liu, Y., Hua, W., Li, Y., Xian, X., Zhao, Z., Liu, C., et al. (2020b). Berberine Suppresses Colon Cancer Cell Proliferation by Inhibiting the Scap/Srebp-1 Signaling Pathway-Mediated Lipogenesis. Biochem. Pharmacol. 174, 113776. doi:10.1016/j.bcp.2019.113776
Liu, Y., Zhu, K. X., Cao, L., Xie, Z. F., Gu, M., Lü, W., et al. (2020c). Berberine Derivatives with a Long Alkyl Chain Branched by Hydroxyl Group and Methoxycarbonyl Group at 9-Position Show Improved Anti-proliferation Activity and Membrane Permeability in A549 Cells. Acta Pharmacol. Sin 41, 813–824. doi:10.1038/s41401-019-0346-1
Liu, Y. T., Hao, H. P., Xie, H. G., Lai, L., Wang, Q., Liu, C. X., et al. (2010). Extensive Intestinal First-Pass Elimination and Predominant Hepatic Distribution of Berberine Explain its Low Plasma Levels in Rats. Drug Metab. Dispos. 38, 1779–1784. doi:10.1124/dmd.110.033936
Lortet-Tieulent, J., Georges, D., Bray, F., and Vaccarella, S. (2020). Profiling Global Cancer Incidence and Mortality by Socioeconomic Development. Int. J. Cancer 147, 3029–3036. doi:10.1002/ijc.33114
Luo, H., Vong, C. T., Chen, H., Gao, Y., Lyu, P., Qiu, L., et al. (2019a). Naturally Occurring Anti-cancer Compounds: Shining from Chinese Herbal Medicine. Chin. Med. 14, 48. doi:10.1186/s13020-019-0270-9
Luo, Y., Tian, G., Zhuang, Z., Chen, J., You, N., Zhuo, L., et al. (2019b). Berberine Prevents Non-alcoholic Steatohepatitis-Derived Hepatocellular Carcinoma by Inhibiting Inflammation and Angiogenesis in Mice. Am. J. Transl. Res. 11, 2668–2682.
Ma, J. Y., Feng, R., Tan, X. S., Ma, C., Shou, J. W., Fu, J., et al. (2013). Excretion of Berberine and its Metabolites in Oral Administration in Rats. J. Pharm. Sci. 102, 4181–4192. doi:10.1002/jps.23718
Ma, W., Zhu, M., Zhang, D., Yang, L., Yang, T., Li, X., et al. (2017). Berberine Inhibits the Proliferation and Migration of Breast Cancer Zr-75-30 Cells by Targeting Ephrin-B2. Phytomedicine 25, 45–51. doi:10.1016/j.phymed.2016.12.013
Ma, W., Zhang, Y., Yu, M., Wang, B., Xu, S., Zhang, J., et al. (2020). In‐Vitro and In‐Vivo Anti-breast Cancer Activity of Synergistic Effect of Berberine and Exercise through Promoting the Apoptosis and Immunomodulatory Effects. Int.Immunopharmacology 87, 106787. doi:10.1016/j.intimp.2020.106787
Maeng, H. J., Yoo, H. J., Kim, I. W., Song, I. S., Chung, S. J., and Shim, C. K. (2002). P-Glycoprotein-Mediated Transport of Berberine across Caco-2 Cell Monolayers. J. Pharm. Sci. 91, 2614–2621. doi:10.1002/jps.10268
Mahmoudi, M., Zamani Taghizadeh Rabe, S., Balali-Mood, M., Karimi, G., Memar, B., Rahnama, M., et al. (2016). Immunotoxicity Induced in Mice by Subacute Exposure to Berberine. J. Immunotoxicol. 13, 255–262. doi:10.3109/1547691x.2015.1058306
Mandal, S. K., Maji, A. K., Mishra, S. K., Ishfaq, P. M., Devkota, H. P., Silva, A. S., et al. (2020). Goldenseal (Hydrastis Canadensis L.) and its Active Constituents: A Critical Review of Their Efficacy and Toxicological Issues. Pharmacol. Res. 160, 105085. doi:10.1016/j.phrs.2020.105085
Mao, L., Chen, Q., Gong, K., Xu, X., Xie, Y., Zhang, W., et al. (2018). Berberine Decelerates Glucose Metabolism via Suppression of mTOR-dependent HIF-1α Protein Synthesis in colon Cancer Cells. Oncol. Rep. 39, 2436–2442. doi:10.3892/or.2018.6318
Martini, D., Pucci, C., Gabellini, C., Pellegrino, M., and Andreazzoli, M. (2020). Exposure to the Natural Alkaloid Berberine Affects Cardiovascular System Morphogenesis and Functionality during Zebrafish Development. Sci. Rep. 10, 17358. doi:10.1038/s41598-020-73661-5
Meng, M., Geng, S., Du, Z., Yao, J., Zheng, Y., Li, Z., et al. (2017). Berberine and Cinnamaldehyde Together Prevent Lung Carcinogenesis. Oncotarget 8, 76385–76397. doi:10.18632/oncotarget.20059
Mercurio, V., Pucci, G., Bosso, G., Fazio, V., Battista, F., Iannuzzi, A., et al. (2020). A Nutraceutical Combination Reduces Left Ventricular Mass in Subjects with Metabolic Syndrome and Left Ventricular Hypertrophy: A Multicenter, Randomized, Double-Blind, Placebo-Controlled Trial. Clin. Nutr. 39, 1379–1384. doi:10.1016/j.clnu.2019.06.026
Min, Y. D., Yang, M. C., Lee, K. H., Kim, K. R., Choi, S. U., and Lee, K. R. (2006). Protoberberine Alkaloids and Their Reversal Activity of P-Gp Expressed Multidrug Resistance (Mdr) from the Rhizome of Coptis Japonica Makino. Arch. Pharm. Res. 29, 757–761. doi:10.1007/bf02974076
Mollaei, H., Safaralizadeh, R., and Rostami, Z. (2019). Microrna Replacement Therapy in Cancer. J. Cel Physiol 234, 12369–12384. doi:10.1002/jcp.28058
Murata, M. (2018). Inflammation and Cancer. Environ. Health Prev. Med. 23, 50. doi:10.1186/s12199-018-0740-1
Myers, J. A., and Miller, J. S. (2021). Exploring the Nk Cell Platform for Cancer Immunotherapy. Nat. Rev. Clin. Oncol. 18, 85–100. doi:10.1038/s41571-020-0426-7
Nagata, S., and Tanaka, M. (2017). Programmed Cell Death and the Immune System. Nat. Rev. Immunol. 17, 333–340. doi:10.1038/nri.2016.153
Neag, M. A., Mocan, A., Echeverría, J., Pop, R. M., Bocsan, C. I., Crişan, G., et al. (2018). Berberine: Botanical Occurrence, Traditional Uses, Extraction Methods, and Relevance in Cardiovascular, Metabolic, Hepatic, and Renal Disorders. Front. Pharmacol. 9, 557. doi:10.3389/fphar.2018.00557
Nieto, M. A., Huang, R. Y., Jackson, R. A., and Thiery, J. P. (2016). Emt: 2016. Cell 166, 21–45. doi:10.1016/j.cell.2016.06.028
Ning, N., Wang, Y. Z., Zou, Z. Y., Zhang, D. Z., Wang, D. Z., and Li, X. G. (2015). Pharmacological and Safety Evaluation of Fibrous Root of Rhizoma Coptidis. Environ. Toxicol. Pharmacol. 39, 53–69. doi:10.1016/j.etap.2014.11.006
Och, A., Szewczyk, K., Pecio, Ł., Stochmal, A., Załuski, D., and Bogucka-Kocka, A. (2017). Uplc-Ms/Ms Profile of Alkaloids with Cytotoxic Properties of Selected Medicinal Plants of the Berberidaceae and Papaveraceae Families. Oxid Med. Cel Longev 2017, 9369872. doi:10.1155/2017/9369872
Pan, Y., Zhang, F., Zhao, Y., Shao, D., Zheng, X., Chen, Y., et al. (2017). Berberine Enhances Chemosensitivity and Induces Apoptosis through Dose-Orchestrated Ampk Signaling in Breast Cancer. J. Cancer 8, 1679–1689. doi:10.7150/jca.19106
Park, J. H., Pyun, W. Y., and Park, H. W. (2020). Cancer Metabolism: Phenotype, Signaling and Therapeutic Targets. Cells 9, 2308. doi:10.3390/cells9102308
Patil, J. B., Kim, J., and Jayaprakasha, G. K. (2010). Berberine Induces Apoptosis in Breast Cancer Cells (Mcf-7) through Mitochondrial-dependent Pathway. Eur. J. Pharmacol. 645, 70–78. doi:10.1016/j.ejphar.2010.07.037
Peng, P. L., Hsieh, Y. S., Wang, C. J., Hsu, J. L., and Chou, F. P. (2006). Inhibitory Effect of Berberine on the Invasion of Human Lung Cancer Cells via Decreased Productions of Urokinase-Plasminogen Activator and Matrix Metalloproteinase-2. Toxico.l Appl. Pharmacol. 214, 8–15. doi:10.1016/j.taap.2005.11.010
Peng, W., Hu, C., Shu, Z., Han, T., Qin, L., and Zheng, C. (2015). Antitumor Activity of Tatariside F Isolated from Roots of Fagopyrum Tataricum (L.) Gaertn against H22 Hepatocellular Carcinoma via Up-Regulation of P53. Phytomedicine 22, 730–736. doi:10.1016/j.phymed.2015.05.003
Peng, W. H., Wu, C. R., Chen, C. S., Chen, C. F., Leu, Z. C., and Hsieh, M. T. (2004). Anxiolytic Effect of Berberine on Exploratory Activity of the Mouse in Two Experimental Anxiety Models: Interaction with Drugs Acting at 5-Ht Receptors. Life Sci. 75, 2451–2462. doi:10.1016/j.lfs.2004.04.032
Peng, W. X., Huang, J. G., Yang, L., Gong, A. H., and Mo, Y. Y. (2017). Linc-Ror Promotes Mapk/Erk Signaling and Confers Estrogen-independent Growth of Breast Cancer. Mol. Cancer 16, 161. doi:10.1186/s12943-017-0727-3
Philogène, B. J., Arnason, J. T., Towers, G. H., Abramowski, Z., Campos, F., Champagne, D., et al. (1984). Berberine: A Naturally Occurring Phototoxic Alkaloid. J. Chem. Ecol. 10, 115–123. doi:10.1007/bf00987648
Pierpaoli, E., Fiorillo, G., Lombardi, P., Salvatore, C., Geroni, C., Piacenza, F., et al. (2018). Antitumor Activity of Nax060: A Novel Semisynthetic Berberine Derivative in Breast Cancer Cells. Biofactors 44, 443–452. doi:10.1002/biof.1440
Pierpaoli, E., Piacenza, F., Fiorillo, G., Lombardi, P., Orlando, F., Salvatore, C., et al. (2021). Antimetastatic and Antitumor Activities of Orally Administered Nax014 Compound in a Murine Model of Her2-Positive Breast Cancer. Int. J. Mol. Sci. 22, 2653. doi:10.3390/ijms22052653
Ponnusamy, L., Kothandan, G., and Manoharan, R. (2020a). Berberine and Emodin Abrogates Breast Cancer Growth and Facilitates Apoptosis through Inactivation of Sik3-Induced Mtor and Akt Signaling Pathway. Biochim. Biophys. Acta Mol. Basis Dis. 1866, 165897. doi:10.1016/j.bbadis.2020.165897
Ponnusamy, L., Natarajan, S. R., Thangaraj, K., and Manoharan, R. (2020b). Therapeutic Aspects of Ampk in Breast Cancer: Progress, Challenges, and Future Directions. Biochim. Biophys. Acta Rev. Cancer 1874, 188379. doi:10.1016/j.bbcan.2020.188379
Popiołek, I., Niziołek, A., Kamiński, K., Kwolek, U., Nowakowska, M., and Szczubiałka, K. (2019). Cellular Delivery and Enhanced Anticancer Activity of Berberine Complexed with a Cationic Derivative of γ-cyclodextrin. Bioorg. Med. Chem. 27, 1414–1420. doi:10.1016/j.bmc.2019.02.042
Qi, Y., and Liu, G. (2021). Berberine-10-hydroxy Camptothecine-Loaded Lipid Microsphere for the Synergistic Treatment of Liver Cancer by Inhibiting Topoisomerase and HIF-1α. Drug Deliv. 28, 171–182. doi:10.1080/10717544.2020.1870020
Qian, K., Tang, C.-y., Chen, L.-y., Zheng, S., Zhao, Y., Ma, L.-s., et al. (2021). Berberine Reverses Breast Cancer Multidrug Resistance Based on Fluorescence Pharmacokinetics In Vitro and In Vivo. ACS Omega 6, 10645–10654. doi:10.1021/acsomega.0c06288
Qin, S., Zhang, B., Xiao, G., Sun, X., Li, G., Huang, G., et al. (2016). Fibronectin Protects Lung Cancer Cells against Docetaxel-Induced Apoptosis by Promoting Src and Caspase-8 Phosphorylation. Tumour Biol. 37, 13509–13520. doi:10.1007/s13277-016-5206-8
Qing, Y., Dong, X., Hongli, L., and Yanhui, L. (2018). Berberine Promoted Myocardial Protection of Postoperative Patients through Regulating Myocardial Autophagy. Biomed. Pharmacother. 105, 1050–1053. doi:10.1016/j.biopha.2018.06.088
Roh, K. B., Ryu, D. H., Cho, E., Weon, J. B., Park, D., Kweon, D. H., et al. (2020). Coptis Chinensis Franch Directly Inhibits Proteolytic Activation of Kallikrein 5 and Cathelicidin Associated with Rosacea in Epidermal Keratinocytes. Molecules 25, 5556. doi:10.3390/molecules25235556
Sakaguchi, M., Kitaguchi, D., Morinami, S., Kurashiki, Y., Hashida, H., Miyata, S., et al. (2020). Berberine-Induced Nucleolar Stress Response in a Human Breast Cancer Cell Line. Biochem. Biophys. Res. Commun. 528, 227–233. doi:10.1016/j.bbrc.2020.05.020
Samadi, P., Sarvarian, P., Gholipour, E., Asenjan, K. S., Aghebati-Maleki, L., Motavalli, R., et al. (2020). Berberine: A Novel Therapeutic Strategy for Cancer. IUBMB life 72, 2065–2079. doi:10.1002/iub.2350
Saxena, S., Shukla, S., and Kakkar, P. (2018). Berberine Induced Modulation of Phlpp2-Akt-Mst1 Kinase Signaling Is Coupled with Mitochondrial Impairment and Hepatoma Cell Death. Toxicol. Appl. Pharmacol. 347, 92–103. doi:10.1016/j.taap.2018.03.033
Sengupta, D., Chowdhury, K. D., Chatterjee, S., Sarkar, A., Paul, S., Sur, P. K., et al. (2017). Modulation of Adenylate Cyclase Signaling in Association with Mkk3/6 Stabilization under Combination of Sac and Berberine to Reduce Hepg2 Cell Survivability. Apoptosis 22, 1362–1379. doi:10.1007/s10495-017-1407-x
Shariati, M., and Meric-Bernstam, F. (2019). Targeting Akt for Cancer Therapy. Expert Opin. Investig. Drugs 28, 977–988. doi:10.1080/13543784.2019.1676726
Shen, Z. Q., Wang, J., Tan, W. F., and Huang, T. M. (2021). Berberine Inhibits Colorectal Tumor Growth by Suppressing Shh Secretion. Acta Pharmacol. Sin. 42, 1190–1194. doi:10.1038/s41401-020-00514-2
Slobodníková, L., Kost'álová, D., Labudová, D., Kotulová, D., and Kettmann, V. (2004). Antimicrobial Activity of Mahonia Aquifolium Crude Extract and its Major Isolated Alkaloids. Phytother. Res. 18, 674–676. doi:10.1002/ptr.1517
Song, D., Hao, J., and Fan, D. (2020). Biological Properties and Clinical Applications of Berberine. Front. Med. 14, 564–582. doi:10.1007/s11684-019-0724-6
Song, J., Lin, C., Yang, X., Xie, Y., Hu, P., Li, H., et al. (2019a). Mitochondrial Targeting Nanodrugs Self-Assembled from 9-O-Octadecyl Substituted Berberine Derivative for Cancer Treatment by Inducing Mitochondrial Apoptosis Pathways. J. Control. Release 294, 27–42. doi:10.1016/j.jconrel.2018.11.014
Song, L., Luo, Y., Wang, X., Almutairi, M. M., Pan, H., Li, W., et al. (2019b). Exploring the Active Mechanism of Berberine against Hcc by Systematic Pharmacology and Experimental Validation. Mol. Med. Rep. 20, 4654–4664. doi:10.3892/mmr.2019.10698
Song, X., Liu, C., Wang, N., Huang, H., He, S., Gong, C., et al. (2021). Delivery of Crispr/Cas Systems for Cancer Gene Therapy and Immunotherapy. Adv. Drug Deliv. Rev. 168, 158–180. doi:10.1016/j.addr.2020.04.010
Spinozzi, S., Colliva, C., Camborata, C., Roberti, M., Ianni, C., Neri, F., et al. (2014). Berberine and its Metabolites: Relationship between Physicochemical Properties and Plasma Levels after Administration to Human Subjects. J. Nat. Prod. 77, 766–772. doi:10.1021/np400607k
Su, K., Hu, P., Wang, X., Kuang, C., Xiang, Q., Yang, F., et al. (2016). Tumor Suppressor Berberine Binds Vasp to Inhibit Cell Migration in Basal-like Breast Cancer. Oncotarget 7, 45849–45862. doi:10.18632/oncotarget.9968
Suarez-Carmona, M., Lesage, J., Cataldo, D., and Gilles, C. (2017). Emt and Inflammation: Inseparable Actors of Cancer Progression. Mol. Oncol. 11, 805–823. doi:10.1002/1878-0261.12095
Sun, Y., Wang, W., and Tong, Y. (2019). Berberine Inhibits Proliferative Ability of Breast Cancer Cells by Reducing Metadherin. Med. Sci. Monit. 25, 9058–9066. doi:10.12659/msm.914486
Sung, H., Ferlay, J., Siegel, R. L., Laversanne, M., Soerjomataram, I., Jemal, A., et al. (2021). Global Cancer Statistics 2020: Globocan Estimates of Incidence and Mortality Worldwide for 36 Cancers in 185 Countries. CA Cancer J. Clin. 71, 209–249. doi:10.3322/caac.21660
Tai, C. J., Jassey, A., Liu, C. H., Tai, C. J., Richardson, C. D., Wong, S. H., et al. (2020). Targeting Autophagy Augments Bbr-Mediated Cell Death in Human Hepatoma Cells Harboring Hepatitis C Virus Rna. Cells 9, 908. doi:10.3390/cells9040908
Tak, J., Sabarwal, A., Shyanti, R. K., and Singh, R. P. (2019). Berberine Enhances Posttranslational Protein Stability of P21/Cip1 in Breast Cancer Cells via Down-Regulation of Akt. Mol. Cel. Biochem. 458, 49–59. doi:10.1007/s11010-019-03529-4
Tan, W., Li, N., Tan, R., Zhong, Z., Suo, Z., Yang, X., et al. (2015a). Berberine Interfered with Breast Cancer Cells Metabolism, Balancing Energy Homeostasis. Anticancer Agents Med. Chem. 15, 66–78. doi:10.2174/1871520614666140910120518
Tan, W., Zhong, Z., Wang, S., Suo, Z., Yang, X., Hu, X., et al. (2015b). Berberine Regulated Lipid Metabolism in the Presence of C75, Compound C, and Tofa in Breast Cancer Cell Line Mcf-7. Evid. Based Complement. Alternat Med. 2015, 396035. doi:10.1155/2015/396035
Tan, X. S., Ma, J. Y., Feng, R., Ma, C., Chen, W. J., Sun, Y. P., et al. (2013). Tissue Distribution of Berberine and its Metabolites after Oral Administration in Rats. PLoS One 8, e77969. doi:10.1371/journal.pone.0077969
Tang, Y., Hou, Y., Zeng, Y., Hu, Y., Zhang, Y., Wang, X., et al. (2021). Salidroside Attenuates CoCl2-Simulated Hypoxia Injury in PC12 Cells Partly by Mitochondrial protection. Eur. J. Pharmacol. 912, 174617. doi:10.1016/j.ejphar.2021.174617
Teng, H., and Choi, Y. H. (2014). Optimization of Ultrasonic-Assisted Extraction of Bioactive Alkaloid Compounds from Rhizoma Coptidis (Coptis Chinensis Franch.) Using Response Surface Methodology. Food Chem. 142, 299–305. doi:10.1016/j.foodchem.2013.06.136
Thakkar, S., Sharma, D., Kalia, K., and Tekade, R. K. (2020). Tumor Microenvironment Targeted Nanotherapeutics for Cancer Therapy and Diagnosis: A Review. Acta Biomater. 101, 43–68. doi:10.1016/j.actbio.2019.09.009
Vanti, G., Coronnello, M., Bani, D., Mannini, A., Bergonzi, M. C., and Bilia, A. R. (2021). Co-Delivery of Berberine Chloride and Tariquidar in Nanoliposomes Enhanced Intracellular Berberine Chloride in a Doxorubicin-Resistant K562 Cell Line Due to P-Gp Overexpression. Pharmaceutics 13, 306. doi:10.3390/pharmaceutics13030306
Vishnoi, K., Ke, R., Saini, K. S., Viswakarma, N., Nair, R. S., Das, S., et al. (2021). Berberine Represses β-Catenin Translation Involving 4E-BPs in Hepatocellular Carcinoma Cells. Mol. Pharmacol. 99, 1–16. doi:10.1124/molpharm.120.000029
Wang, C., Wang, H., Zhang, Y., Guo, W., Long, C., Wang, J., et al. (2017a). Berberine Inhibits the Proliferation of Human Nasopharyngeal Carcinoma Cells via an Epstein-Barr Virus Nuclear Antigen 1-Dependent Mechanism. Oncol. Rep. 37, 2109–2120. doi:10.3892/or.2017.5489
Wang, F., Zhao, G., Cheng, L., Zhou, H. Y., Fu, L. Y., and Yao, W. X. (2004). Effects of Berberine on Potassium Currents in Acutely Isolated Ca1 Pyramidal Neurons of Rat Hippocampus. Brain Res. 999, 91–97. doi:10.1016/j.brainres.2003.11.036
Wang, J., Wang, L., Lou, G. H., Zeng, H. R., Hu, J., Huang, Q. W., et al. (2019). Coptidis Rhizoma: A Comprehensive Review of its Traditional Uses, Botany, Phytochemistry, Pharmacology and Toxicology. Pharm. Biol. 57, 193–225. doi:10.1080/13880209.2019.1577466
Wang, K., Chai, L., Feng, X., Liu, Z., Liu, H., Ding, L., et al. (2017b). Metabolites Identification of Berberine in Rats Using Ultra-high Performance Liquid Chromatography/Quadrupole Time-Of-Flight Mass Spectrometry. J. Pharm. Biomed. Anal. 139, 73–86. doi:10.1016/j.jpba.2017.02.038
Wang, K., Feng, X., Chai, L., Cao, S., and Qiu, F. (2017c). The Metabolism of Berberine and its Contribution to the Pharmacological Effects. Drug Metab. Rev. 49, 139–157. doi:10.1080/03602532.2017.1306544
Wang, K., Yu, G., Lin, J., Wang, Z., Lu, Q., Gu, C., et al. (2021). Berberine Sensitizes Human Hepatoma Cells to Regorafenib via Modulating Expression of Circular Rnas. Front. Pharmacol. 12, 632201. doi:10.3389/fphar.2021.632201
Wang, N., Feng, Y., Zhu, M., Tsang, C. M., Man, K., Tong, Y., et al. (2010). Berberine Induces Autophagic Cell Death and Mitochondrial Apoptosis in Liver Cancer Cells: The Cellular Mechanism. J. Cel. Biochem. 111, 1426–1436. doi:10.1002/jcb.22869
Wang, N., Wang, X., Tan, H. Y., Li, S., Tsang, C. M., Tsao, S. W., et al. (2016). Berberine Suppresses Cyclin D1 Expression through Proteasomal Degradation in Human Hepatoma Cells. Int. J. Mol. Sci. 17, 1899. doi:10.3390/ijms17111899
Wang, W., Li, Q., Liu, Y., and Chen, B. (2015a). Ionic Liquid-Aqueous Solution Ultrasonic-Assisted Extraction of Three Kinds of Alkaloids from Phellodendron Amurense Rupr and Optimize Conditions Use Response Surface. Ultrason. Sonochem. 24, 13–18. doi:10.1016/j.ultsonch.2014.10.009
Wang, W., Ma, X., Guo, X., Zhao, M., Tu, P., and Jiang, Y. (2015b). A Series of Strategies for Solving the Shortage of Reference Standards for Multi-Components Determination of Traditional Chinese Medicine, Mahoniae Caulis as a Case. J. Chromatogr. A. 1412, 100–111. doi:10.1016/j.chroma.2015.08.015
Wang, X., Wang, Q., Liu, Z., and Zheng, X. (2017d). Preparation, Pharmacokinetics and Tumour-Suppressive Activity of Berberine Liposomes. J. Pharm. Pharmacol. 69, 625–632. doi:10.1111/jphp.12692
Wang, Y., Liu, Y., Du, X., Ma, H., and Yao, J. (2020). Berberine Reverses Doxorubicin Resistance by Inhibiting Autophagy through the Pten/Akt/Mtor Signaling Pathway in Breast Cancer. Onco Targets Ther. 13, 1909–1919. doi:10.2147/ott.S241632
Wang, Y. X., Pang, W. Q., Zeng, Q. X., Deng, Z. S., Fan, T. Y., Jiang, J. D., et al. (2018). Synthesis and Biological Evaluation of New Berberine Derivatives as Cancer Immunotherapy Agents through Targeting Ido1. Eur. J. Med. Chem. 143, 1858–1868. doi:10.1016/j.ejmech.2017.10.078
Warowicka, A., Nawrot, R., and Goździcka-Józefiak, A. (2020). Antiviral Activity of Berberine. Arch. Virol. 165, 1935–1945. doi:10.1007/s00705-020-04706-3
Wen, S., Hou, Y., Fu, L., Xi, L., Yang, D., Zhao, M., et al. (2019). Cancer-associated Fibroblast (CAF)-derived IL32 Promotes Breast Cancer Cell Invasion and Metastasis via Integrin β3-p38 MAPK Signalling. Cancer Lett. 442, 320–332. doi:10.1016/j.canlet.2018.10.015
Wu, L. S., Jia, M., Chen, L., Zhu, B., Dong, H. X., Si, J. P., et al. (2015). Cytotoxic and Antifungal Constituents Isolated from the Metabolites of Endophytic Fungus Do14 from Dendrobium Officinale. Molecules 21, E14. doi:10.3390/molecules21010014
Xiao, Y., Tian, C., Huang, T., Han, B., Wang, M., Ma, H., et al. (2018). 8-Cetylberberine Inhibits Growth of Lung Cancer In Vitro and In Vivo. Life Sci. 192, 259–269. doi:10.1016/j.lfs.2017.11.012
Xu, L., Zhang, Y., Xue, X., Liu, J., Li, Z. S., Yang, G. Y., et al. (2020). A Phase I Trial of Berberine in Chinese with Ulcerative Colitis. Cancer Prev. Res. (Phila) 13, 117–126. doi:10.1158/1940-6207.Capr-19-0258
Xu, T., Wu, X., Liu, J., Sun, J., Wang, X., Fan, G., et al. (2021). The Regulatory Roles of Dietary Fibers on Host Health via Gut Microbiota-Derived Short Chain Fatty Acids. Curr. Opin. Pharmacol. 62, 36–42. doi:10.1016/j.coph.2021.11.001
Yang, H. L., Thiyagarajan, V., Shen, P. C., Mathew, D. C., Lin, K. Y., Liao, J. W., et al. (2019). Anti-Emt Properties of Coq0 Attributed to Pi3k/Akt/Nfkb/Mmp-9 Signaling Pathway through Ros-Mediated Apoptosis. J. Exp. Clin. Cancer Res. 38, 186. doi:10.1186/s13046-019-1196-x
Yao, M., Fan, X., Yuan, B., Takagi, N., Liu, S., Han, X., et al. (2019). Berberine Inhibits Nlrp3 Inflammasome Pathway in Human Triple-Negative Breast Cancer Mda-Mb-231 Cell. BMC Complement. Altern. Med. 19, 216. doi:10.1186/s12906-019-2615-4
You, X., Cao, X., and Lin, Y. (2019). Berberine Enhances the Radiosensitivity of Hepatoma Cells by Nrf2 Pathway. Front. Biosci. (Landmark Ed. 24, 1190–1202. doi:10.2741/4775
Yuan, H., Ma, Q., Cui, H., Liu, G., Zhao, X., Li, W., et al. (2017). How Can Synergism of Traditional Medicines Benefit from Network Pharmacology? Molecules 22, 1135. doi:10.3390/molecules22071135
Zhang, C., Sheng, J., Li, G., Zhao, L., Wang, Y., Yang, W., et al. (2019a). Effects of Berberine and its Derivatives on Cancer: A Systems Pharmacology Review. Front. Pharmacol. 10, 1461. doi:10.3389/fphar.2019.01461
Zhang, F., Jia, Y., Zheng, X., Shao, D., Zhao, Y., Wang, Z., et al. (2019b). Janus Nanocarrier-Based Co-delivery of Doxorubicin and Berberine Weakens Chemotherapy-Exacerbated Hepatocellular Carcinoma Recurrence. Acta Biomater. 100, 352–364. doi:10.1016/j.actbio.2019.09.034
Zhang, M. Y., Yu, Y. Y., Wang, S. F., Zhang, Q., Wu, H. W., Wei, J. Y., et al. (2018). Cardiotoxicity Evaluation of Nine Alkaloids from Rhizoma Coptis. Hum. Exp. Toxicol. 37, 185–195. doi:10.1177/0960327117695633
Zhang, P., Wang, Q., Lin, Z., Yang, P., Dou, K., and Zhang, R. (2019c). Berberine Inhibits Growth of Liver Cancer Cells by Suppressing Glutamine Uptake. Onco Targets Ther. 12, 11751–11763. doi:10.2147/ott.S235667
Zhang, Q., Wang, X., Cao, S., Sun, Y., He, X., Jiang, B., et al. (2020a). Berberine Represses Human Gastric Cancer Cell Growth In Vitro and In Vivo by Inducing Cytostatic Autophagy via Inhibition of Mapk/Mtor/P70s6k and Akt Signaling Pathways. Biomed. Pharmacother. 128, 110245. doi:10.1016/j.biopha.2020.110245
Zhang, R., Qiao, H., Chen, S., Chen, X., Dou, K., Wei, L., et al. (2016). Berberine Reverses Lapatinib Resistance of Her2-Positive Breast Cancer Cells by Increasing the Level of Ros. Cancer Biol. Ther. 17, 925–934. doi:10.1080/15384047.2016.1210728
Zhang, R., Zhang, Y., Zhang, Y., Wang, X., Gao, X., Liu, Y., et al. (2020b). Ratiometric Delivery of Doxorubicin and Berberine by Liposome Enables superior Therapeutic index Than DoxilⓇ. Asian J. Pharm. Sci. 15, 385–396. doi:10.1016/j.ajps.2019.04.007
Zhang, S., Zhou, L., Zhang, M., Wang, Y., Wang, M., Du, J., et al. (2019d). Berberine Maintains the Neutrophil N1 Phenotype to Reverse Cancer Cell Resistance to Doxorubicin. Front. Pharmacol. 10, 1658. doi:10.3389/fphar.2019.01658
Zhang, Y., Gu, Y., Ren, H., Wang, S., Zhong, H., Zhao, X., et al. (2020c). Gut Microbiome-Related Effects of Berberine and Probiotics on Type 2 Diabetes (The Premote Study). Nat. Commun. 11, 5015. doi:10.1038/s41467-020-18414-8
Zhao, J. V., Yeung, W. F., Chan, Y. H., Vackova, D., Leung, J. Y. Y., Ip, D. K. M., et al. (2021a). Effect of Berberine on Cardiovascular Disease Risk Factors: A Mechanistic Randomized Controlled Trial. Nutrients 13, 2550. doi:10.3390/nu13082550
Zhao, L., and Zhang, C. (2020). Berberine Inhibits Mda-Mb-231 Cells by Attenuating Their Inflammatory Responses. Biomed. Res. Int. 2020, 3617514. doi:10.1155/2020/3617514
Zhao, M. M., Lu, J., Li, S., Wang, H., Cao, X., Li, Q., et al. (2021b). Berberine Is an Insulin Secretagogue Targeting the Kcnh6 Potassium Channel. Nat. Commun. 12, 5616. doi:10.1038/s41467-021-25952-2
Zhao, Y., He, K., Zheng, H., Sun, M., Shi, T., Zheng, X., et al. (2020). Berberine Inhibits the Apoptosis-Induced Metastasis by Suppressing the Ipla2/Lox-5/Ltb4 Pathway in Hepatocellular Carcinoma. Onco Targets Ther. 13, 5223–5230. doi:10.2147/ott.S243357
Zhao, Y., Jing, Z., Lv, J., Zhang, Z., Lin, J., Cao, X., et al. (2017). Berberine Activates Caspase-9/Cytochrome C-Mediated Apoptosis to Suppress Triple-Negative Breast Cancer Cells In Vitro and In Vivo. Biomed. Pharmacother. 95, 18–24. doi:10.1016/j.biopha.2017.08.045
Zheng, F., Li, J., Ma, C., Tang, X., Tang, Q., Wu, J., et al. (2020). Novel Regulation of Mir-34a-5p and Hotair by the Combination of Berberine and Gefitinib Leading to Inhibition of Emt in Human Lung Cancer. J. Cel. Mol. Med. 24, 5578–5592. doi:10.1111/jcmm.15214
Zheng, F., Wu, J., Tang, Q., Xiao, Q., Wu, W., and Hann, S. S. (2018a). The Enhancement of Combination of Berberine and Metformin in Inhibition of Dnmt1 Gene Expression through Interplay of Sp1 and Pdpk1. J. Cel. Mol. Med. 22, 600–612. doi:10.1111/jcmm.13347
Zheng, X., Zhang, F., Shao, D., Zhang, Z., Cui, L., Zhang, J., et al. (2018b). Gram-Scale Production of Carrier-free Fluorescent Berberine Microrods for Selective Liver Cancer Therapy. Biofactors 44, 496–502. doi:10.1002/biof.1450
Zheng, X., Zhao, Y., Jia, Y., Shao, D., Zhang, F., Sun, M., et al. (2021). Biomimetic Co-assembled Nanodrug of Doxorubicin and Berberine Suppresses Chemotherapy-Exacerbated Breast Cancer Metastasis. Biomaterials 271, 120716. doi:10.1016/j.biomaterials.2021.120716
Zhi, D., Feng, P. F., Sun, J. L., Guo, F., Zhang, R., Zhao, X., et al. (2015). The Enhancement of Cardiac Toxicity by Concomitant Administration of Berberine and Macrolides. Eur. J. Pharm. Sci. 76, 149–155. doi:10.1016/j.ejps.2015.05.009
Zhou, H., and Gu, Y. (1995). Determination of Berberine in Phellodendron Chinense Schneid and its Processed Products by Tlc (Thin Layer Chromatography) Densitometry. Zhongguo Zhong Yao Za Zhi 20, 405447–447. doi:10.3321/j.issn:1001-5302.1995.07.019
Zhu, X., Qiu, J., Zhang, T., Yang, Y., Guo, S., Li, T., et al. (2020). Microrna-188-5p Promotes Apoptosis and Inhibits Cell Proliferation of Breast Cancer Cells via the Mapk Signaling Pathway by Targeting Rap2c. J. Cel. Physiol. 235, 2389–2402. doi:10.1002/jcp.29144
Keywords: berberine, bioavailability, ADMET, anticancer effects, apoptosis induction
Citation: Zhu Y, Xie N, Chai Y, Nie Y, Liu K, Liu Y, Yang Y, Su J and Zhang C (2022) Apoptosis Induction, a Sharp Edge of Berberine to Exert Anti-Cancer Effects, Focus on Breast, Lung, and Liver Cancer. Front. Pharmacol. 13:803717. doi: 10.3389/fphar.2022.803717
Received: 28 October 2021; Accepted: 10 January 2022;
Published: 27 January 2022.
Edited by:
Hong Zhang, Shanghai University of Traditional Chinese Medicine, ChinaReviewed by:
Dhanasekhar Reddy, Central University of Kerala, IndiaLei Gao, Southern Medical University, China
Xiaoping Pan, Zhejiang Chinese Medical University, China
Copyright © 2022 Zhu, Xie, Chai, Nie, Liu, Liu, Yang, Su and Zhang. This is an open-access article distributed under the terms of the Creative Commons Attribution License (CC BY). The use, distribution or reproduction in other forums is permitted, provided the original author(s) and the copyright owner(s) are credited and that the original publication in this journal is cited, in accordance with accepted academic practice. No use, distribution or reproduction is permitted which does not comply with these terms.
*Correspondence: Chuantao Zhang, zhangchuantao@cdutcm.edu.cn; Jinsong Su, 1508113999@qq.com
†These authors have contributed equally to this work and share first authorship