- 1Xiangya School of Pharmaceutical Sciences, Central South University, Changsha, China
- 2Hunan Provincial Key Laboratory of Cardiovascular Research, Xiangya School of Pharmaceutical Sciences, Central South University, Changsha, China
- 3Department of Pharmacy, Xiangya Hospital, Central South University, Changsha, China
- 4National Clinical Research Center for Geriatric Disorders, Xiangya Hospital, Central South University, Changsha, China
Radiotherapy (RT), as one of the main methods of clinical tumor treatment, has been applied to the treatment of most solid tumors. However, the effect of RT is compromised by the radiation resistance of tumor hypoxic environment and non-specific damage caused by high-dose radiation. Bismuth chalcogenides (Bi2X3, X = S, Se) based nanodrugs have attracted widespread attention as highly efficient radiosensitizers due to their high photoelectric effect and excellent biocompatibility. More importantly, specially designed nanocomposites can effectively alleviate the radiation resistance of tumor tissues. Here, for the first time, we systematically summarize the latest progresses of Bi2X3 nanodrugs to enhance RT by alleviating the hypoxic tumor microenvironment. These emerging Bi2X3 nanodrugs mainly include three aspects, which are Bi2X3 nanocomposites with high-efficient O2 supply, non-O2-dependent Bi2X3 nanocomposites RT enhancers, and Bi2X3 nanocomposites-based photothermal-enhanced radiosensitizers. These Bi2X3 nanodrugs can effectively overcome the RT resistance of tumor hypoxic microenvironment, and have extremely high therapeutic effects and clinical application prospects. Finally, we put forward the challenges and prospects of Bi2X3 nanomaterials in the field of RT.
Introduction
Radiotherapy (RT) has many advantages for cancer treatment compared with surgery or chemotherapy, like non-invasive, excellent targeting, and low cost (Begg et al., 2011). Currently, half of new cancers are treated with RT (Bentzen, 2006). RT adopts ionizing radiation (usually X-ray) to irradiate the tumor site through direct and indirect action to induce cancer cell death. Ionizing radiation can directly destroy DNA or protein by breaking the bonds in these molecules. More importantly, high-energy ionizing radiation can easily ionize and split H2O to produce many reactive oxygen species (ROS) in tumor tissues (Eq. 1) (Le Caër, 2011). These ROS further cause the death of cancer cells by damaging DNA and proteins (Wu et al., 2019; Yao et al., 2021a). This indirect effect is the main tumor-killing effect of RT because the water content is the highest (generally 65%) in tumor tissues. However, there are two bottlenecks which greatly limit the effectiveness of RT. Firstly, a larger dose of X-rays is usually required to kill tumor cells because cancer tissues absorb X-rays very weakly, which also cause damage to normal tissues, especially the immune system (De Martino et al., 2021). Secondly, the hypoxic tumor microenvironment (TME) greatly reduces the effect of RT. O2 is a very important RT sensitizer and is easy to accept a free electron to form superoxide radicals (O2−·), which is then further converted into other highly oxidative active ROS (e.g. hydrogen peroxide and hydroxyl radicals) (Zhao et al., 2022; Zhu et al., 2022). Compared with normal cells, cancer cells are 3-times more resistant to RT-induced killing in a tumor hypoxia environment (Evans et al., 1997).
Drugs containing high atomic number elements can be very effective in enhancing RT because they have a much higher X-ray absorption capacity than human tissues. Currently, many kinds of elements with high atomic number have been researched for radiosensitization, such as Au, Ta, W, Yb, Hf, and Bi (Tang et al., 2019; Xie et al., 2019; Zang et al., 2019; Peng et al., 2020; Liu et al., 2021; Xue et al., 2021). For example, NBTXR3 based on HfO2 has been approved by the FDA to enter Phase Ⅲ clinical studies, and demonstrated excellent RT effect for advanced soft-tissue sarcoma (Bonvalot et al., 2019). However, most of the high-Z elements are heavy metal elements with high toxicity, and their application in the field of biomedicine has been greatly restricted.
Bismuth, as an element with high atomic number (Z = 83), has surprising biocompatibility and been active in the biomedical field for hundreds of years. A variety of bismuth-based compounds have been widely used to treat diseases such as gastrohelcoma and bacterial infections (Peterson et al., 1996; Nomiya et al., 2004). Bismuth chalcogenides (Bi2X3, X = S, Se) based nanodrugs have been favored in tumor RT due to their many unique characteristics: 1) low toxicity and high biological safety in vivo; 2) low cost and easy synthesis; 3) strong X-ray absorption (The X-ray attenuation coefficient of Bi element is 5.74 > Au = 5.16 > Pt = 4.99 > Ta = 4.3 cm2 g−1 at 100 keV). After Bi2X3-based nanodrugs specifically enrich in the tumor area by passively or actively targeting effect, the tumor can be effectively killed at a lower X-ray dose, and the damage to other normal tissues can also be greatly reduced (Zhang et al., 2014; Song et al., 2017; Alejo-Martinez et al., 2019). Nevertheless, the RT effect of these nanodrugs is still greatly reduced by the hypoxic tumor microenvironment. Currently, many emerging Bi2X3 nanodrugs are developed to further improve the efficiency of RT, and have demonstrated very impressive tumor-killing effects. Here, a systematic review is provided to summarize the breakthrough progresses of Bi2X3 nanodrugs for overcoming the limitations of the tumor hypoxia microenvironment in the field of RT. Currently, three strategies have been developed to improve the RT efficiency of Bi2X3 nanodrugs (Figure 1; Table 1). Firstly, elaborately designed Bi2X3-based nanocomposites increase the supply of O2 to relieve the hypoxic state of the TME; the second strategy is non-O2 dependent RT: Bi2X3-based nanocomposites with distinctive heterojunction structure to promote the production of non-O2 dependent radicals; the third is photothermal-enhanced RT: local high temperature of the tumor site can not only relieve the hypoxic tumor microenvironment, but also increase the yield and speed of ROS production in RT. Finally, we discussed the challenges and prospects of bismuth chalcogenides nanocomposites in the field of cancer RT.
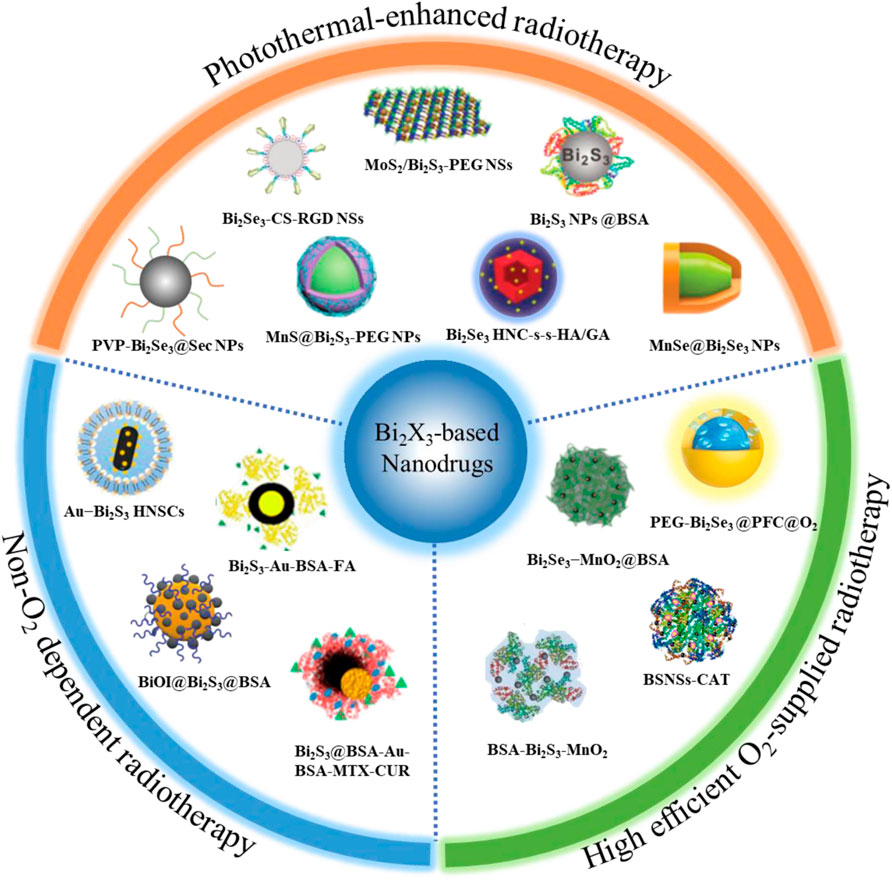
FIGURE 1. Overview of radiosensitization strategies based on Bi2X3 nanodrugs, which mainly including three aspects, the first is high efficient O2-supplied Bi2X3-based nanodrugs: such as perfluorocarbon-loaded hollow Bi2Se3 nanoparticles (PEG-Bi2Se3@PFC@O2 NPs), bismuth sulfide−albumin composite nanospheres followed by catalase conjugation (BSNSs-CAT), bovine serum albumin-coated Bi2S3 and MnO2 nanocomposites (BSA-Bi2S3-MnO2), bovine serum albumin-coated Bi2Se3 and MnO2 nanocomposites (Bi2Se3-MnO2@BSA); the second is Non-O2 dependent Bi2X3-based nanodrugs: such as Schottky-type heterostructure of Au-Bi2S3 (Au-Bi2S3 HNSCs), co-drug (MTX and CUR) loaded Bi2S3@BSA-Au semiconductor-metal heterojunction nanoparticles (Bi2S3@BSA-Au-BSA-MTX-CUR), folic acid (FA) functionalized and BSA-modified Bi2S3-Au heterodimers (Bi2S3-Au-BSA-FA); the third is photothermal-enhanced Bi2X3-based nanodrugs, such as PEGylated 2D MoS2/Bi2S3 composite nanosheets (MoS2/Bi2S3-PEG NSs), BSA-stabilized Bi2S3 Nanoparticles (Bi2S3 NPs@BSA), poly(vinylpyrollidone)-and selenocysteine-modified Bi2Se3 nanoparticles (PVP-Bi2Se3@Sec NPs), HA-functionalized gambogic acid (GA) loaded Bi2Se3 hollow nanocube (HNC-s-s-HA/GA) and MnSe@Bi2Se3 core–shell nanoparticles (MnSe@Bi2Se3 NPs).
High-Efficient O2-Supplied Radiotherapy
Many well-designed Bi2X3-based nanocomposites have shown great potential in improving tumor hypoxia and RT efficiency. Bi2X3-based nanocomposites with ideal structure and morphology can be prepared through specific synthesis strategies due to their unique and flexible physical and chemical properties, such as hollow structure (Song et al., 2016; Zhang et al., 2020), mesoporous structure (Sun et al., 2019; Yang et al., 2021), core-shell structure (Li et al., 2017; Li et al., 2018). For example, Song et al. (2016) prepared PEGylated hollow Bi2Se3 nanoparticles (PEG-Bi2Se3 NPs) through cation exchange reaction based on the Kirkendall effect. Perfluorocarbon, a highly efficient oxygen loading solvent, was then filled into the hollow structure of PEG-Bi2Se3 NPs (PEG-Bi2Se3@PFC@O2). The oxygen carrying capacity of PEG-Bi2Se3@PFC@O2 was significantly higher than that of the hollow PEG-Bi2Se3 NPs, up to 96.9 ± 9.4 μmol/g of PEG-Bi2Se3. Moreover, the O2 retention time exceeded 1 h, and the gradual release of O2 effectively improved the hypoxic microenvironment in the tumor site. At the same X-ray dose, the anti-tumor effect of PEG-Bi2Se3@PFC@O2 was significantly better than that of PEG-Bi2Se3 and RT group. Another effective strategy to improve tumor hypoxia is to convert the high concentration H2O2 into O2 in the tumor microenvironment (Zhang et al., 2018; Zhang et al., 2019; Yuzhu Yao et al., 2021). For example, Zhang et al. (2018) developed a Bi2S3-albumin composite nanospheres combined with catalase (abbreviated as BSNSs-CAT) for cancer treatment. CAT at BSNSs-CAT efficiently catalyzed the conversion of H2O2 into O2 after BSNSs-CAT accumulated in tumor tissues through enhanced penetration and retention effect (EPR effect). The percentage of O2 saturation concentration treated with BSNSs-CAT increased significantly from 52.5% to about 59.2% in the tumor site. BSNSs-CAT + RT had the best tumor growth inhibition effect thanks to the strong reflective absorption of Bi and the improvement of the hypoxic microenvironment, followed by BSNSs + RT, then RT group. However, CAT, as a natural enzyme, is easily degraded and inactivated by proteases in vivo. Some catalase-mimick nanozymes can catalyze H2O2 to produce H2O and O2 (Dai et al., 2021). Very recently, Yuzhu Yao et al. (2021) developed a nanocomposite of Bi2Se3, MnO2 and bovine serum albumin (Bi2Se3-MnO2@BSA) for RT. MnO2 showed high-efficiency catalase-like properties and excellent stability in vivo. Moreover, the CAT activity of Bi2Se3-MnO2@BSA was 2.46 times higher than that of MnO2@BSA, because the Mn atoms of Bi2Se3-MnO2@BSA was in an electron-rich state and easier to provide electrons for H2O2. The Bi2Se3-MnO2@BSA + RT group showed a stronger tumor-killing effect compared to the MnO2@BSA + RT group and the RT group in the in vivo treatments.
Non-O2 Dependent Radiotherapy
Non-O2 dependent RT has great advantages in RT, because it can directly avoid the RT resistance from the hypoxic microenvironment. As we all know, Bi chalcogenide compounds, as a narrow band gap semiconductor, can theoretically be excited by X-rays to generate free electrons and holes in the conduction band (CB) and valence band (VB), respectively (Meng et al., 2016; Waiskopf et al., 2016). These electron-hole pairs further react with H2O or H2O2 to generate highly cytotoxic hydroxyl radicals (·OH) to induce cancer cells apoptosis by intense oxidative damages. However, the generation of ·OH is significantly suppressed in Bi chalcogenide nanomaterials due to the rapid recombination of electron-hole pairs (Zhang et al., 2012). The heterojunction structure of Bi2X3 nanocomposites can separate electrons and holes to greatly reduce the recombination of electron and hole pairs (Wang et al., 2019; Abhari et al., 2020; Nosrati et al., 2022). For example, Wang et al. (2019) designed Au-Bi2S3 nanocomposites with Schottky-type heterostructures (Au-Bi2S3 HNSCs) for non-O2 dependent RT. Au-Bi2S3 HNSCs were prepared by in-situ growth of gold nanocrystals on Bi2S3 nanorods. The Schottky barrier was a low interface voltage region on the metal-semiconductor boundary. Semiconductor Bi2S3 generated low-energy electron-hole pairs under X-ray irradiation in Au-Bi2S3 HNSCs, and then electrons and holes were effectively separated because the electrons were easily transferred to gold via Schottky barrier. The current response of Au-Bi2S3 HNSCs was 1.5-times higher than pure Bi2S3 and the ·OH production was 1.6-times than that of Au and Bi2S3 mixture under X-ray irradiation. More importantly, the RT effect of Au-Bi2S3 HNSCs was significantly better than that of the pure Bi2S3 group or the Au and Bi2S3 mixture group both in the in vitro and in vivo experiments. In addition, Bi2S3-Au Schottky-type heterostructures can be adopted as a multifunctional drug delivery platform to combine chemotherapy and RT. This combination therapy has shown great potential in improving the efficiency of RT and minimizing the systemic toxicity of chemotherapeutic drugs (Nadar et al., 2021). Very recently, Nosrati et al. (2022) developed a methotrexate and curcumin co-loaded BSA-encapsulated Bi2S3-Au nanocomposite (Bi2S3@BSA-Au-BSA-MTX-CUR) for the combined treatment of chemotherapy and RT. In Bi2S3@BSA-Au-BSA-MTX-CUR, Bi2S3@BSA-Au heterojunctions enhance the generation of ·OH to increase the RT efficiency, while MTX efficiently promoted cellular uptake and interfere the biosynthesis of DNA of cancer cells. Interestingly, the combined treatment of chemotherapy and RT achieved a significant anti-cancer effect in vivo only under a single dose Bi2S3@BSA-Au-BSA-MTX-CUR injection and one-time X-ray irradiation, and the tumors was completely eradicated after 20 days of treatment.
Photothermal-Enhanced Radiotherapy
In recent years, photothermal therapy (PTT), as a specific emerging cancer therapy, has been extensively researched in the field of tumor treatment (Liu et al., 2019; Danewalia and Singh, 2021). Many transition metal nanomaterials have been researched for PTT, such as MoS2-based nanomaterials (Jianling Wang et al., 2021), CoS2 nanomaterials (Wang et al., 2020a), copper-based nanomaterials (Ai et al., 2021; Wang et al., 2021b; Li et al., 2021), titanium-based nanomaterials (Wang et al., 2020b; Wang et al., 2021c), covalent organic frameworks (COFs) (Yao et al., 2021b), etc. Compared with above PTT agents, Bi2X3-based nanomaterials have been proven to be a kind of more excellent photosensitizers due to the strong near-infrared absorption performance and high photothermal conversion efficiency of Bi2X3 (Xie et al., 2016; Cheng et al., 2018). Local high temperature can directly increase the oxygen content of the tumor microenvironment by increasing blood flow in the tumor. Moreover, high temperature induced by PTT can facilitate the generation of O2-dependent ROS for RT by inhibiting the expression of hypoxia-inducible factor (HIF-1α) to increase oxygen concentration in tumor site. In addition, photothermal effects also interfere with DNA repair by reducing the expression of DNA repair related proteins (DNA repair enzymes, PARP, Rad 51), and downregulating angiogenic factors to inhibit tumor metastasis (Oei et al., 2015; Cheng et al., 2017). Therefore, the combination of photothermal therapy and RT is an effective radiosensitization strategy. For example, Wang et al. (2016) prepared ultra-small BSA-coated Bi2S3 nanodots (BSA-Bi2S3 NPs) for photothermal-enhanced RT. BSA-Bi2S3 NPs had the excellent X-ray and photothermal response properties (the photothermal conversion efficiency was as high as 51%). Moreover, The BSA-Bi2S3 NPs with ultra-small size (about only 6 nm) were more conducive to being taken up by tumor cells. Compared with the RT sensitization group (Bi2S3+X-ray) or the PTT group (Bi2S3+NIR), the 4T1-tumor bearing mice treated with radio-photothermal combination therapy group (Bi2S3+X-ray + NIR) achieved complete tumor eradication, and the survival rate of mice reached 100% over 40 days after treatment. In addition, it is also extremely important to protect adjacent normal tissues from radiation damage during RT. Recently, Du et al. (2017) reported a Bi2Se3 nanoparticles modified with polyvinylpyrrolidone and selenocysteine (PVP-Bi2Se3@Sec NPs) for photothermal-enhanced RT. The photothermal effect of Bi2Se3 NPs effectively improved tumor hypoxia microenvironment to enhance the radiosensitivity of cancer cells. Moreover, the PVP-Bi2Se3@Sec NPs were degraded in vivo, and part of the Se released from the NPs to enhance the body’s immune function. Compared with RT, the PVP-Bi2Se3@Sec NPs group effectively protected the immune system, and the key cytokines level (like interleukin 6 and 2) were restored in the blood.
The efficiency of RT can be further increased by improving the photothermal conversion efficiency of the Bi2X3-based nanocomposites. Fox example, Fei Gao et al. (2020) developed heterogeneous Bi2S3-MoS2 nanoparticles (BMNPs) for photothermal enhanced RT. BMNPs had a higher photothermal conversion efficiency than Bi2S3 nanoparticles (BNPs) (35.8 vs 28.1%). The BMNPs reduced the quasi-threshold X-ray dose from 1.39 to 0.92 Gy, and the sensitivity enhancement ratio increased by 17.9%. The effect of NIR + RT + BMNP group was much better than that of RT group and RT + BMNPs group in the treatment of triple-negative breast cancer. The survival rate of mice in the NIR + RT + BMNP group was as high as 100% at 28 days after treatment, while the RT group and RT + BMNPs group had only 0 and 20%, respectively. When the temperature of the tumor area rises, the tumor cells resisting heating-caused damage by up-regulating the expression of heat shock proteins (HSPs) (Ge Gao et al., 2020). Therefore, the photothermal enhanced RT can be further increased by inhibiting the activity of HSPs. Moreover, avoiding thermal damage and inflammation of adjacent normal tissues caused by hyperthermia also needs to be considered. Recently, Song et al. reported a hyaluronic acid (HA) modification and gambogic acid (GA) loaded hollow Bi2Se3 nanotube (HNC-ss-HA/GA) for low-temperature radio-photothermal combination therapy. HA ligands promoted the accumulation of HNC-ss-HA/GA in tumors due to its specifical affinity with CD44 receptor in cancer cells. Glutathione, one of the most important antioxidants in cells, is known to be overexpressed in cancer cells (Ding et al., 2021). Interestingly, the disulfide bond between HNC and HA can be rapidly cleaved by glutathione to release GA. GA, as an effective inhibitor of HSPs, which could enhance the heat sensitivity of cancer cells (Su et al., 2021), thereby improve the efficacy of photothermal-enhanced RT. The combined therapy group (HNC-s-s-HA/GA + NIR + X-ray) demonstrated the strongest suppress tumor growth effect in vivo compared to other monotherapy groups (HNC-s-s-HA/GA + NIR and HNC-s-s-HA/GA + X-ray).
Summary and Outlook
In summary, this review summarizes the latest research progress of Bi2X3-based nanodrugs for RT. Bi2X3-based nanodrugs have great clinical application prospects in the field of RT because of their super-high RT effect and biocompatibility. Nevertheless, there are still many challenges to overcome in achieving clinical translation of these treatment strategies. Firstly, the excellent RT effects of these Bi2X3-based nanodrugs are all achieved in mice models. However, the huge species difference between human and mice makes these nanodrugs face a big bottleneck for clinical translation. For example, mice tumor models generally take about 15 days, while human cancers often take months or even years. Therefore, the tumor microenvironment of human may be very different from that of mice models, which may lead to unsatisfactory clinical effects of Bi2X3-based nanodrugs. Therefore, from the perspective of clinical application, it is necessary to verify the radiosensitizing effect of Bi2X3-based nanodrugs in humanized animal models, such as the monkey models. Secondly, metabolic pathway of Bi2X3-based nanodrugs needs further study in vivo. As we all know, as a heavy metal element, excessive Bi may cause some side effects such as renal toxicity, brain toxicity and neurological decline, which can be attributed to the tendency of Bi to bind to sulfhydryl groups in many important enzymes in the human body, resulting in the denaturation of enzymes and destroys its functionality. At present, most of the metabolism and toxicity of Bi2X3-based nanodrugs have only been done for about a month, and the longer-term toxicity and metabolic mechanisms still need to be further explored. Therefore, exploring biodegradable and clearable Bi2X3-based nanodrugs is of great significance for thier clinical translation (Wang et al., 2021a). Fortunately, there is rare Bi element in the human body itself. Therefore, the distribution, metabolism, and excretion process of Bi2X3-based nanodrugs can be easily tracked by the content and valence of Bi in vivo. Thirdly, the large-scale and controllable preparation of Bi2X3-based nanodrugs need to be further optimized. In commercial preparation, it is necessary to maintain precise control of the size, morphology, charge, and composition of nanomaterials to ensure uniformity and strict quality control. Therefore, exploring a simpler, faster, more precise and controllable synthesis process is vital for the clinical translation and commercial production of Bi2X3-based nanodrugs in the field of RT. Nevertheless, Bi2X3-based nanodrugs still have great clinical application prospects of RT. As mentioned earlier, NBTXR3 based on HfO2 have shown excellent effects in clinical phase III. In theory, Bi2X3-based nanodrugs have stronger biocompatibility and radiosensitization effect than HfO2 nanoparticles. We believe that Bi2X3-based nanodrugs will achieve true clinical RT treatment with the joint efforts of scientists from multiple disciplines such as chemistry, medicine, and biology in the near future.
Author Contributions
All authors listed have made a substantial, direct, and intellectual contribution to the work and approved it for publication.
Funding
This work was supported by the National Natural Science Foundation of China (Nos. 21974134 and 81974508), the Hunan Science Fund for Distinguished Young Scholar of China (No. 2021JJ10067), Innovation-Driven Project of Central South University (No. 202045005), Hunan Provincial Natural Science Foundation of China (No. 2021JJ31066), Changsha Science and Technology Project (No. kq2001048), Key Research Project of Ningxia Hui Autonomous Region in 2021 of China (Major Project) (No. 2021BEG01001).
Conflict of Interest
The authors declare that the research was conducted in the absence of any commercial or financial relationships that could be construed as a potential conflict of interest.
Publisher’s Note
All claims expressed in this article are solely those of the authors and do not necessarily represent those of their affiliated organizations, or those of the publisher, the editors and the reviewers. Any product that may be evaluated in this article, or claim that may be made by its manufacturer, is not guaranteed or endorsed by the publisher.
References
Abhari, F., Charmi, J., Rezaeejam, H., Karimimoghaddam, Z., Nosrati, H., Danafar, H., et al. (2020). Folic Acid Modified Bismuth Sulfide and Gold Heterodimers for Enhancing Radiosensitization of Mice Tumors to X-ray Radiation. ACS Sustain. Chem. Eng. 8 (13), 5260–5269. doi:10.1021/acssuschemeng.0c00182
Ai, K., Huang, J., Xiao, Z., Yang, Y., Bai, Y., and Peng, J. (2021). Localized Surface Plasmon Resonance Properties and Biomedical Applications of Copper Selenide Nanomaterials. Mater. Today Chem. 20, 100402. doi:10.1016/j.mtchem.2020.100402
Alejo-Martinez, H., Sevilla-Moreno, A. C., Ondo-Mendéz, A., Quintero, J. H., and Páez, C. J. (2019). Comparison of Bi 2 S 3 and Ta 2 O 5 as Alternative Materials to Gold in Nanoparticles Used as Agents to Increase the Dose in Radiotherapy. J. Phys. Conf. Ser. 1247 (1), 012050. doi:10.1088/1742-6596/1247/1/012050
Begg, A. C., Stewart, F. A., and Vens, C. (2011). Strategies to Improve Radiotherapy with Targeted Drugs. Nat. Rev. Cancer 11 (4), 239–253. doi:10.1038/nrc3007
Bentzen, S. M. (2006). Preventing or Reducing Late Side Effects of Radiation Therapy: Radiobiology Meets Molecular Pathology. Nat. Rev. Cancer 6 (9), 702–713. doi:10.1038/nrc1950
Bonvalot, S., Rutkowski, P. L., Thariat, J., Carrère, S., Ducassou, A., Sunyach, M. P., et al. (2019). NBTXR3, a First-In-Class Radioenhancer Hafnium Oxide Nanoparticle, Plus Radiotherapy versus Radiotherapy Alone in Patients with Locally Advanced Soft-Tissue Sarcoma (Act.In.Sarc): a Multicentre, Phase 2-3, Randomised, Controlled Trial. Lancet Oncol. 20 (8), 1148–1159. doi:10.1016/S1470-2045(19)30326-2
Cheng, L., Shen, S., Shi, S., Yi, Y., Wang, X., Song, G., et al. (2016). FeSe2-Decorated Bi2Se3 Nanosheets Fabricated via Cation Exchange for Chelator-free 64Cu-Labeling and Multimodal Image-Guided Photothermal-Radiation Therapy. Adv. Funct. Mater. 26 (13), 2185–2197. doi:10.1002/adfm.201504810
Cheng, X., Yong, Y., Dai, Y., Song, X., Yang, G., Pan, Y., et al. (2017). Enhanced Radiotherapy Using Bismuth Sulfide Nanoagents Combined with Photo-thermal Treatment. Theranostics 7 (17), 4087–4098. doi:10.7150/thno.20548
Cheng, Y., Chang, Y., Feng, Y., Jian, H., Tang, Z., and Zhang, H. (2018). Deep-Level Defect Enhanced Photothermal Performance of Bismuth Sulfide-Gold Heterojunction Nanorods for Photothermal Therapy of Cancer Guided by Computed Tomography Imaging. Angew. Chem. Int. Ed. Engl. 57 (1), 246–251. doi:10.1002/anie.201710399
Dai, Y., Ding, Y., and Li, L. (2021). Nanozymes for Regulation of Reactive Oxygen Species and Disease Therapy. Chin. Chem. Lett. 32 (9), 2715–2728. doi:10.1016/j.cclet.2021.03.036
Danewalia, S. S., and Singh, K. (2021). Bioactive Glasses and Glass-Ceramics for Hyperthermia Treatment of Cancer: State-Of-Art, Challenges, and Future Perspectives. Mater. Today Bio 10, 100100. doi:10.1016/j.mtbio.2021.100100
De Martino, M., Daviaud, C., and Vanpouille-Box, C. (2021). Radiotherapy: An Immune Response Modifier for Immuno-Oncology. Semin. Immunol. 52, 101474. doi:10.1016/j.smim.2021.101474
Ding, Y., Dai, Y., Wu, M., and Li, L. (2021). Glutathione-mediated Nanomedicines for Cancer Diagnosis and Therapy. Chem. Eng. J. 426, 128880. doi:10.1016/j.cej.2021.128880
Du, J., Gu, Z., Yan, L., Yong, Y., Yi, X., Zhang, X., et al. (2017). Poly(Vinylpyrollidone)- and Selenocysteine-Modified Bi2 Se3 Nanoparticles Enhance Radiotherapy Efficacy in Tumors and Promote Radioprotection in Normal Tissues. Adv. Mater. 29 (34), 1701268. doi:10.1002/adma.201701268
Evans, S. M., Jenkins, W. T., Shapiro, M., and Koch, C. J. (1997). “Evaluation of the Concept of “Hypoxic Fraction” as a Descriptor of Tumor Oxygenation Status,” in Oxygen Transport to Tissue XVIII. Editors E. M. Nemoto, J. C. LaManna, C. Cooper, D. Delpy, K. Groebe, T. K. Huntet al. (Boston, MA: Springer US), 215–225. doi:10.1007/978-1-4615-5865-1_26
Gao, F., Wang, D., Zhang, T., Ghosal, A., Guo, Z., Miao, Y., et al. (2020). Facile Synthesis of Bi2S3-MoS2 Heterogeneous Nanoagent as Dual Functional Radiosensitizer for Triple Negative Breast Cancer Theranostics. Chem. Eng. J. 395, 125032. doi:10.1016/j.cej.2020.125032
Gao, G., Jiang, Y. W., Guo, Y., Jia, H. R., Cheng, X., Deng, Y., et al. (2020). Enzyme‐Mediated Tumor Starvation and Phototherapy Enhance Mild‐Temperature Photothermal Therapy. Adv. Funct. Mater. 30 (16), 1909391. doi:10.1002/adfm.201909391
Le Caër, S. (2011). Water Radiolysis: Influence of Oxide Surfaces on H2 Production under Ionizing Radiation. Water 3 (1), 235–253. doi:10.3390/w3010235
Li, Y., Sun, Y., Cao, T., Su, Q., Li, Z., Huang, M., et al. (2017). A Cation-Exchange Controlled Core-Shell MnS@Bi2S3 Theranostic Platform for Multimodal Imaging Guided Radiation Therapy with Hyperthermia Boost. Nanoscale 9 (38), 14364–14375. doi:10.1039/c7nr02384g
Li, L., Lu, Y., Jiang, C., Zhu, Y., Yang, X., Hu, X., et al. (2018). Actively Targeted Deep Tissue Imaging and Photothermal-Chemo Therapy of Breast Cancer by Antibody-Functionalized Drug-Loaded X-Ray-Responsive Bismuth Sulfide@Mesoporous Silica Core-Shell Nanoparticles. Adv. Funct. Mater. 28 (5), 1704623. doi:10.1002/adfm.201704623
Li, X., Yuan, H. J., Tian, X. M., Tang, J., Liu, L. F., and Liu, F. Y. (2021). Biocompatible Copper Sulfide-Based Nanocomposites for Artery Interventional Chemo-Photothermal Therapy of Orthotropic Hepatocellular Carcinoma. Mater. Today Bio 12, 100128. doi:10.1016/j.mtbio.2021.100128
Liu, Y., Bhattarai, P., Dai, Z., and Chen, X. (2019). Photothermal Therapy and Photoacoustic Imaging via Nanotheranostics in Fighting Cancer. Chem. Soc. Rev. 48 (7), 2053–2108. doi:10.1039/c8cs00618k
Liu, J., Zhang, J., Song, K., Du, J., Wang, X., Liu, J., et al. (2021). Tumor Microenvironment Modulation Platform Based on Composite Biodegradable Bismuth-Manganese Radiosensitizer for Inhibiting Radioresistant Hypoxic Tumors. Small 17 (34), 2101015. doi:10.1002/smll.202101015
Mao, F., Wen, L., Sun, C., Zhang, S., Wang, G., Zeng, J., et al. (2016). Ultrasmall Biocompatible Bi2Se3 Nanodots for Multimodal Imaging-Guided Synergistic Radiophotothermal Therapy against Cancer. ACS Nano 10 (12), 11145–11155. doi:10.1021/acsnano.6b06067
Meng, X., Liu, L., Ouyang, S., Xu, H., Wang, D., Zhao, N., et al. (2016). Nanometals for Solar-To-Chemical Energy Conversion: From Semiconductor-Based Photocatalysis to Plasmon-Mediated Photocatalysis and Photo-Thermocatalysis. Adv. Mater. 28 (32), 6781–6803. doi:10.1002/adma.201600305
Nadar, R. A., Franssen, G. M., Van Dijk, N. W. M., Codee-van der Schilden, K., de Weijert, M., Oosterwijk, E., et al. (2021). Bone Tumor-Targeted Delivery of Theranostic 195mPt-Bisphosphonate Complexes Promotes Killing of Metastatic Tumor Cells. Mater. Today Bio 9, 100088. doi:10.1016/j.mtbio.2020.100088
Nomiya, K., Sekino, K., Ishikawa, M., Honda, A., Yokoyama, M., Chikaraishi Kasuga, N., et al. (2004). Syntheses, crystal Structures and Antimicrobial Activities of Monomeric 8-coordinate, and Dimeric and Monomeric 7-coordinate Bismuth(III) Complexes with Tridentate and Pentadentate Thiosemicarbazones and Pentadentate Semicarbazone Ligands. J. Inorg. Biochem. 98 (4), 601–615. doi:10.1016/j.jinorgbio.2004.01.011
Nosrati, H., Attari, E., Abhari, F., Barsbay, M., Ghaffarlou, M., Mousazadeh, N., et al. (2022). Complete Ablation of Tumors Using Synchronous Chemoradiation with Bimetallic Theranostic Nanoparticles. Bioactive Mater. 7, 74–84. doi:10.1016/j.bioactmat.2021.05.015
Oei, A. L., Vriend, L. E., Crezee, J., Franken, N. A., and Krawczyk, P. M. (2015). Effects of Hyperthermia on DNA Repair Pathways: One Treatment to Inhibit Them All. Radiat. Oncol. 10 (1), 165. doi:10.1186/s13014-015-0462-0
Peng, C., Liang, Y., Chen, Y., Qian, X., Luo, W., Chen, S., et al. (2020). Hollow Mesoporous Tantalum Oxide Based Nanospheres for Triple Sensitization of Radiotherapy. ACS Appl. Mater. Inter. 12 (5), 5520–5530. doi:10.1021/acsami.9b20053
Peterson, W. L., Ciociola, A. A., Sykes, D. L., McSorley, D. J., and Webb, D. D. (1996). Ranitidine Bismuth Citrate Plus Clarithromycin Is Effective for Healing Duodenal Ulcers, Eradicating H. pylori and Reducing Ulcer Recurrence. RBC H. pylori Study Group. Aliment. Pharmacol. Ther. 10 (3), 251–261. doi:10.1111/j.0953-0673.1996.00251.x
Song, G., Liang, C., Gong, H., Li, M., Zheng, X., Cheng, L., et al. (2015). Core-Shell MnSe@Bi2 Se3 Fabricated via a Cation Exchange Method as Novel Nanotheranostics for Multimodal Imaging and Synergistic Thermoradiotherapy. Adv. Mater. 27 (40), 6110–6117. doi:10.1002/adma.201503006
Song, G., Liang, C., Yi, X., Zhao, Q., Cheng, L., Yang, K., et al. (2016). Perfluorocarbon-Loaded Hollow Bi2Se3 Nanoparticles for Timely Supply of Oxygen under Near-Infrared Light to Enhance the Radiotherapy of Cancer. Adv. Mater. 28 (14), 2716–2723. doi:10.1002/adma.201504617
Song, Z., Chang, Y., Xie, H., Yu, X.-F., Chu, P. K., and Chen, T. (2017). Decorated Ultrathin Bismuth Selenide Nanosheets as Targeted Theranostic Agents for In Vivo Imaging Guided Cancer Radiation Therapy. NPG Asia Mater. 9 (10), e439. doi:10.1038/am.2017.167
Song, Y., Wang, Y., Zhu, Y., Cheng, Y., Wang, Y., Wang, S., et al. (2019). Biomodal Tumor-Targeted and Redox-Responsive Bi2 Se3 Hollow Nanocubes for MSOT/CT Imaging Guided Synergistic Low-Temperature Photothermal Radiotherapy. Adv. Healthc. Mater. 8 (16), e1900250. doi:10.1002/adhm.201900250
Su, X., Cao, Y., Liu, Y., Ouyang, B., Ning, B., Wang, Y., et al. (2021). Localized Disruption of Redox Homeostasis Boosting Ferroptosis of Tumor by Hydrogel Delivery System. Mater. Today Bio 12, 100154. doi:10.1016/j.mtbio.2021.100154
Sun, L., Hou, M., Zhang, L., Qian, D., Yang, Q., Xu, Z., et al. (2019). PEGylated mesoporous Bi2S3 nanostars loaded with chlorin e6 and doxorubicin for fluorescence/CT imaging-guided multimodal therapy of cancer. Nanomedicine 17, 1–12. doi:10.1016/j.nano.2018.12.013
Tang, W., Dong, Z., Zhang, R., Yi, X., Yang, K., Jin, M., et al. (2019). Multifunctional Two-Dimensional Core-Shell MXene@Gold Nanocomposites for Enhanced Photo-Radio Combined Therapy in the Second Biological Window. ACS Nano 13 (1), 284–294. doi:10.1021/acsnano.8b05982
Wang, J., Sui, L., Huang, J., Miao, L., Nie, Y., Wang, K., et al. (2021). MoS2-based Nanocomposites for Cancer Diagnosis and Therapy. Bioact Mater. 6 (11), 4209–4242. doi:10.1016/j.bioactmat.2021.04.021
Waiskopf, N., Ben-Shahar, Y., Galchenko, M., Carmel, I., Moshitzky, G., Soreq, H., et al. (2016). Photocatalytic Reactive Oxygen Species Formation by Semiconductor-Metal Hybrid Nanoparticles. Toward Light-Induced Modulation of Biological Processes. Nano Lett. 16 (7), 4266–4273. doi:10.1021/acs.nanolett.6b01298
Wang, S., Li, X., Chen, Y., Cai, X., Yao, H., Gao, W., et al. (2015). A Facile One-Pot Synthesis of a Two-Dimensional MoS2/Bi2S3 Composite Theranostic Nanosystem for Multi-Modality Tumor Imaging and Therapy. Adv. Mater. 27 (17), 2775–2782. doi:10.1002/adma.201500870
Wang, Y., Wu, Y., Liu, Y., Shen, J., Lv, L., Li, L., et al. (2016). BSA-mediated Synthesis of Bismuth Sulfide Nanotheranostic Agents for Tumor Multimodal Imaging and Thermoradiotherapy. Adv. Funct. Mater. 26 (29), 5335–5344. doi:10.1002/adfm.201601341
Wang, X., Zhang, C., Du, J., Dong, X., Jian, S., Yan, L., et al. (2019). Enhanced Generation of Non-oxygen Dependent Free Radicals by Schottky-type Heterostructures of Au-Bi2S3 Nanoparticles via X-ray-Induced Catalytic Reaction for Radiosensitization. ACS Nano 13 (5), 5947–5958. doi:10.1021/acsnano.9b01818
Wang, X., Zhong, X., Zha, Z., He, G., Miao, Z., Lei, H., et al. (2020). Biodegradable CoS2 Nanoclusters for Photothermal-Enhanced Chemodynamic Therapy. Appl. Mater. Today 18, 100464. doi:10.1016/j.apmt.2019.100464
Wang, X., Wang, X., Zhong, X., Li, G., Yang, Z., Gong, Y., et al. (2020). V-TiO2 Nanospindles with Regulating Tumor Microenvironment Performance for Enhanced Sonodynamic Cancer Therapy. Appl. Phys. Rev. 7 (4), 041411. doi:10.1063/5.0027606
Wang, X., Zhong, X., Li, J., Liu, Z., and Cheng, L. (2021). Inorganic Nanomaterials with Rapid Clearance for Biomedical Applications. Chem. Soc. Rev. 50 (15), 8669–8742. doi:10.1039/d0cs00461h
Wang, X., Shi, Q., Zha, Z., Zhu, D., Zheng, L., Shi, L., et al. (2021). Copper Single-Atom Catalysts with Photothermal Performance and Enhanced Nanozyme Activity for Bacteria-Infected Wound Therapy. Bioact Mater. 6 (12), 4389–4401. doi:10.1016/j.bioactmat.2021.04.024
Wang, X., Zhong, X., and Cheng, L. (2021). Titanium-based Nanomaterials for Cancer Theranostics. Coord. Chem. Rev. 430, 213662. doi:10.1016/j.ccr.2020.213662
Wu, M., Ding, Y., and Li, L. (2019). Recent Progress in the Augmentation of Reactive Species with Nanoplatforms for Cancer Therapy. Nanoscale 11 (42), 19658–19683. doi:10.1039/c9nr06651a
Xie, H., Li, Z., Sun, Z., Shao, J., Yu, X. F., Guo, Z., et al. (2016). Metabolizable Ultrathin Bi2 Se3 Nanosheets in Imaging-Guided Photothermal Therapy. Small 12 (30), 4136–4145. doi:10.1002/smll.201601050
Xie, J., Gong, L., Zhu, S., Yong, Y., Gu, Z., and Zhao, Y. (2019). Emerging Strategies of Nanomaterial-Mediated Tumor Radiosensitization. Adv. Mater. 31 (3), e1802244. doi:10.1002/adma.201802244
Xue, J., Duosiken, D., Zhong, S., Cao, J. J., Hu, L. Y., Sun, K., et al. (2021). The Dependence of Radio-Sensitization Efficiency on Mitochondrial Targeting with NaGdF4:Yb,Er Nanoparticles. Acta Biomater. 131, 508–518. doi:10.1016/j.actbio.2021.06.041
Yang, C., Chang, M., Yuan, M., Jiang, F., Ding, B., Zhao, Y., et al. (2021). NIR‐Triggered Multi‐Mode Antitumor Therapy Based on Bi 2 Se 3/Au Heterostructure with Enhanced Efficacy. Small 17 (28), 2100961. doi:10.1002/smll.202100961
Yao, S., Zhao, X., Wan, X., Wang, X., Huang, T., Zhang, J., et al. (2021). π-π Conjugation Promoted Nanocatalysis for Cancer Therapy Based on a Covalent Organic Framework. Mater. Horiz 8 (12), 3457–3467. doi:10.1039/d1mh01273h
Yao, S., Liu, Z., and Li, L. (2021). Recent Progress in Nanoscale Covalent Organic Frameworks for Cancer Diagnosis and Therapy. Nanomicro Lett. 13 (1), 176. doi:10.1007/s40820-021-00696-2
Yao, Y., Li, P., He, J., Wang, D., Hu, J., and Yang, X. (2021). Albumin-Templated Bi2Se3-MnO2 Nanocomposites with Promoted Catalase-like Activity for Enhanced Radiotherapy of Cancer. ACS Appl. Mater. Inter. 13 (24), 28650–28661. doi:10.1021/acsami.1c05669
Zang, Y., Gong, L., Mei, L., Gu, Z., and Wang, Q. (2019). Bi2WO6 Semiconductor Nanoplates for Tumor Radiosensitization through High- Z Effects and Radiocatalysis. ACS Appl. Mater. Inter. 11 (21), 18942–18952. doi:10.1021/acsami.9b03636
Zhang, Z., Wang, W., Wang, L., and Sun, S. (2012). Enhancement of Visible-Light Photocatalysis by Coupling with Narrow-Band-Gap Semiconductor: A Case Study on Bi2S3/Bi2WO6. ACS Appl. Mater. Inter. 4 (2), 593–597. doi:10.1021/am2017199
Zhang, X.-D., Chen, J., Min, Y., Park, G. B., Shen, X., Song, S.-S., et al. (2014). Metabolizable Bi2Se3Nanoplates: Biodistribution, Toxicity, and Uses for Cancer Radiation Therapy and Imaging. Adv. Funct. Mater. 24 (12), 1718–1729. doi:10.1002/adfm.201302312
Zhang, Q., Chen, J., Ma, M., Wang, H., and Chen, H. (2018). A Bioenvironment-Responsive Versatile Nanoplatform Enabling Rapid Clearance and Effective Tumor Homing for Oxygen-Enhanced Radiotherapy. Chem. Mater. 30 (15), 5412–5421. doi:10.1021/acs.chemmater.8b02251
Zhang, L., Chen, Q., Zou, X., Chen, J., Hu, L., Dong, Z., et al. (2019). Intelligent Protein-Coated Bismuth Sulfide and Manganese Oxide Nanocomposites Obtained by Biomineralization for Multimodal Imaging-Guided Enhanced Tumor Therapy. J. Mater. Chem. B 7 (34), 5170–5181. doi:10.1039/c9tb00991d
Zhang, C., Li, D., Pei, P., Wang, W., Chen, B., Chu, Z., et al. (2020). Rod-based Urchin-like Hollow Microspheres of Bi2S3: Facile Synthesis, Photo-Controlled Drug Release for Photoacoustic Imaging and Chemo-Photothermal Therapy of Tumor Ablation. Biomaterials 237, 119835. doi:10.1016/j.biomaterials.2020.119835
Zhao, T., Wu, W., Sui, L., Huang, Q., Nan, Y., Liu, J., et al. (2022). Reactive Oxygen Species-Based Nanomaterials for the Treatment of Myocardial Ischemia Reperfusion Injuries. Bioactive Mater. 7, 47–72. doi:10.1016/j.bioactmat.2021.06.006
Keywords: bismuth chalcogenides, nanomaterials, cancer radiotherapy, radiosensitizers, tumor hypoxia microenvironment
Citation: Huang J, Huang Q, Liu M, Chen Q and Ai K (2022) Emerging Bismuth Chalcogenides Based Nanodrugs for Cancer Radiotherapy. Front. Pharmacol. 13:844037. doi: 10.3389/fphar.2022.844037
Received: 27 December 2021; Accepted: 28 January 2022;
Published: 18 February 2022.
Edited by:
Christian Celia, University of Studies G. d’Annunzio Chieti and Pescara, ItalyCopyright © 2022 Huang, Huang, Liu, Chen and Ai. This is an open-access article distributed under the terms of the Creative Commons Attribution License (CC BY). The use, distribution or reproduction in other forums is permitted, provided the original author(s) and the copyright owner(s) are credited and that the original publication in this journal is cited, in accordance with accepted academic practice. No use, distribution or reproduction is permitted which does not comply with these terms.
*Correspondence: Kelong Ai, YWlrZWxvbmdAY3N1LmVkdS5jbg==
†These authors have contributed equally to this work