- 1Chinese Medicine Centre, Western Sydney University, Sydney, NSW, Australia
- 2School of Health Sciences, Western Sydney University, Sydney, NSW, Australia
- 3College of Chinese Medicine, College of Integrated Chinese and Western Medicine, Nanjing University of Chinese Medicine, Nanjing, China
- 4College of the First Clinical Medical, Nanjing University of Chinese Medicine, Nanjing, China
- 5NICM Health Research Institute, Western Sydney University, Westmead, NSW, Australia
Introduction: Tamoxifen (TAM) is the most commonly used hormone therapeutic drug for the treatment of estrogen receptor-positive (ER+) breast cancer. 30%–70% of clinical breast cancer patients use natural products, which may increase the likelihood of drug interactions.
Objective: To evaluate the evidence for the interactions between natural products and TAM in breast cancer.
Methods: Electronic databases, including PubMed, CINAHL Plus (via EbscoHost), European PMC, Medline, and Google Scholar, were searched for relevant publications. The search terms include complementary and alternative medicine, natural products, plant products, herbs, interactions, tamoxifen, breast cancer, and their combinations.
Results: Various in vitro and in vivo studies demonstrated that the combined use of natural products with TAM produced synergistic anti-cancer effects, including improved inhibition of tumor cell growth and TAM sensitivity and reduced side effects or toxicity of TAM. In contrast, some natural products, including Angelica sinensis (Oliv.) Diels [Apiaceae], Paeonia lactiflora Pall., Rehmannia glutinosa (Gaertn.) DC., Astragalus mongholicus Bunge, and Glycyrrhiza glabra L. [Fabaceae], showed estrogen-like activity, which may reduce the anti-cancer effect of TAM. Some natural products, including morin, silybin, epigallocatechin gallate (EGCG), myricetin, baicalein, curcumin, kaempferol, or quercetin, were found to increase the bioavailability of TAM and its metabolites in vivo. However, three are limited clinical studies on the combination of natural products and TAM.
Conclusion: There is evidence for potential interactions of various natural products with TAM in pre-clinical studies, although the relevant clinical evidence is still lacking. Further studies are warranted to evaluate the potential interactions of natural products with TAM in clinical settings.
1 Introduction
Breast cancer is one of the more frequently diagnosed cancers and the leading cause of cancer deaths in women worldwide (Jemal et al., 2011). The latest cancer statistics in 2019 show that breast cancer accounts for around 30% of all the new cancer diagnoses in women in the United States, of which approximately 1 in 6.5 (15.5%) will die of cancer (Siegel et al., 2019). According to the presence of immunohistochemistry markers, such as estrogen receptor (ER), progesterone receptor (PR), or human epidermal growth factor 2 (HER2) molecular markers, breast cancer can be classified into three main subtypes: hormone receptor-positive (ER+, PR+, HER2−), HER2 positive (ER−, PR−, HER2+), and triple-negative breast cancer TNBC (ER−, PR−, HER2−). Among them, estrogen receptor-positive (ER+) breast cancer accounts for approximately 60%–75% of all breast cancer patients. Adjuvant endocrine therapy is an established effective systemic treatment for most ER+ breast cancers and has become the most widely employed therapy to treat hormone receptor-positive breast cancers.
Tamoxifen (TAM) is a selective estrogen receptor modulator, acting as an estrogen antagonist in the breast while acting as an estrogen agonist in the uterus. TAM binds to the estrogen receptor to form a nuclear complex that blocks the estrogen receptor transcriptional activity, thereby reducing DNA synthesis and inhibiting the effect of estrogen (Ali et al., 2016). TAM demonstrated clinically significant effects of reducing breast cancer recurrence rate by 40%–50% and could reduce the mortality and temporary remission rate after treatment and may reduce mortality and increase remission rate (Karn et al., 2010). However, on top of the concern for survival and growth of TAM-tolerant cells, there are adverse events (AE) associated with TAM therapy, including hot flashes, increased risk of endometrial cancer, and, in rare instances, liver abnormalities (Viedma-Rodríguez et al., 2014).
Recently there has been an increase in studies on the anti-cancer properties of natural products (Wu et al., 2017; Lichota and Gwozdzinski, 2018) and increasing use of various natural products by cancer patients (Chen XW et al., 2011). Of all the cancer patients, breast cancer patients, in particular, are more likely to turn to complementary and alternative medicine (CAM) treatment (Wanchai et al., 2010). An Australian study found that 55% of patients using biological-based CAM and 80% of patients using nonbiological-based CAM did not discuss their use of CAM with their oncologist (Kremser et al., 2008). Many studies report that herbal or dietary supplements can affect various molecular targets and signaling pathways, leading to the possible use of breast cancer combination therapy (Chen et al., 2011a; Mansour et al., 2012; Oleaga et al., 2012; Pawlik et al., 2016). However, there is still a lack of evidence-based information or guidance for clinicians and consumers on the interaction between herbs and drugs used to treat and prevent cancer (Sparreboom et al., 2004).
This review aimed to evaluate the evidence available from the literature on the combined use of natural or plant products and TAM in breast cancer studies and identify the current gaps and possible future studies for improving treatment strategies for breast cancer.
2 Literature Search
Electronic databases, including PubMed, CINAHL Plus (via EbscoHost), European PMC, Medline, and Google Scholar, were searched for relevant publications. The search terms include “complementary and alternative medicine,” “natural product*,” “plant product*,” “herb*,” “interaction*,” “tamoxifen,” “breast cancer,” and “endoxifen” and their combinations. The search results include publications from their inceptions to 2021 on the single herbs or a combination of herbs or natural products except for unpublished studies or abstracts that do not have sufficient data for analysis. The exclusion criteria are as follows: articles that did not analyze herb and TAM in combination or separately or comment on herb-TAM interactions; articles that included a cocktail of endocrine agents; non-English publications (not including articles with English abstracts, tables, or figures); and contribution articles such as letters, editorials, commentaries, or letters to the editor.
3 Results
A total of 100 relevant articles were identified among 1,518 entries found in the literature search, including 24 pharmacokinetic studies on herbal medicines and 76 on pharmacodynamics studies. A summary of the findings is shown in Tables 1–6, with Table 1 on pharmacokinetics interactions, Table 2, 3 on synergetic anti-effects, Table 4 on sensitization, Table 5 on side effects or toxicity of combined use, and Table 6 on antagonizing effects.
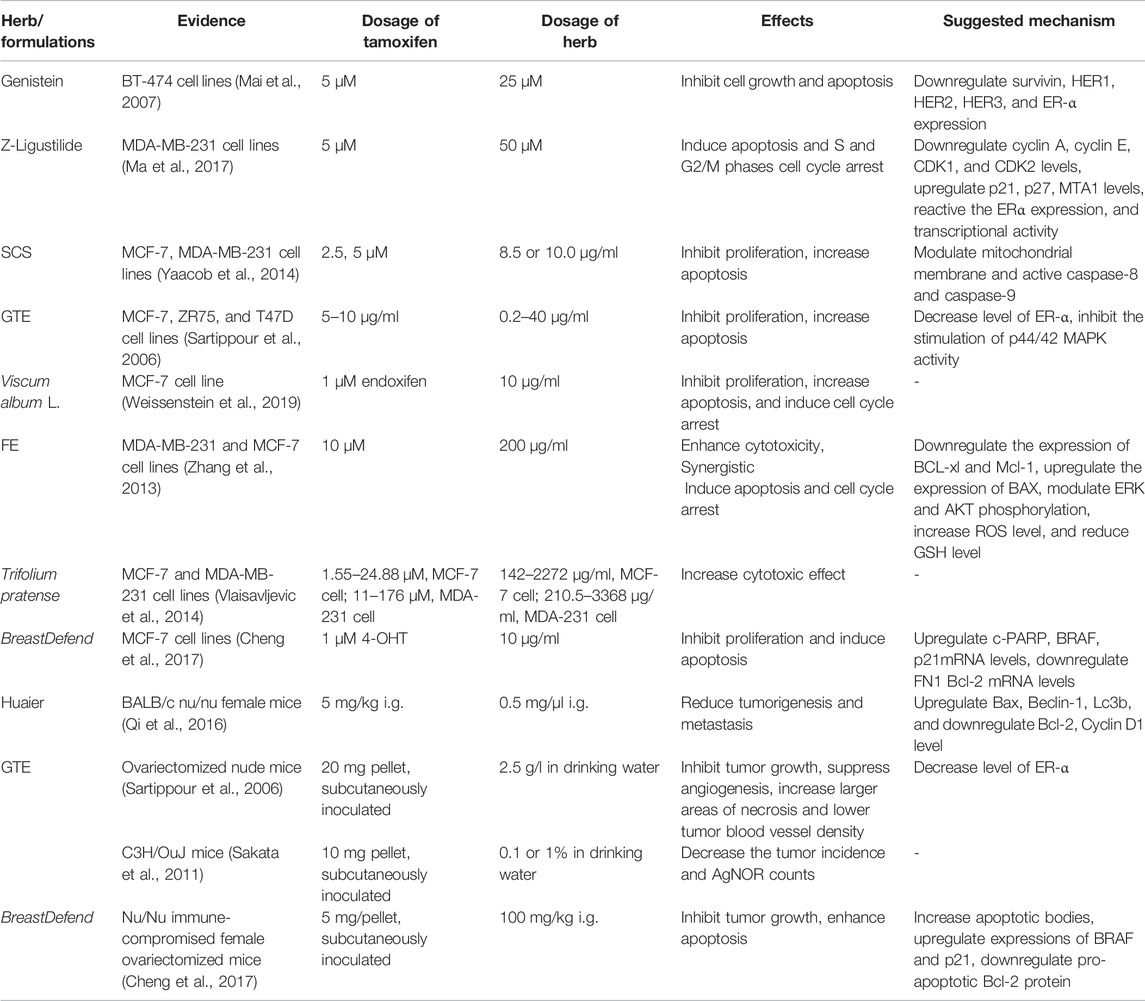
TABLE 2. Reported synergetic effects of a combination of inhibiting tumor growth, inhibiting proliferation, or inducing apoptosis.
TAM has a relatively lower affinity for the estrogen receptor (Brauch et al., 2009; Kim et al., 2021). After a single oral administration, the maximum plasma concentration of the parent drug and demethylated metabolites can be reached within a few hours. Within the therapeutic margin, it is highly bound to serum proteins, which will then undergo extensive liver metabolism. Metabolism of TAM takes place mainly through two main pathways, 4-hydroxylation and N-demethylation, from which TAM is converted to primary metabolites 4-hydroxy-tamoxifen and N-desmethyl-tamoxifen (Klein et al., 2013).
Both primary metabolites are further converted to secondary metabolite endoxifen, catalyzed by CYP3A4/5 and CYP2D6 (Desta et al., 2004). Endoxifen competes with estrogen and binds to ER with nearly 100 times higher affinity than TAM (Brauch et al., 2009). It exhibits estrogenic activity by binding to ligand-regulated transcription factors ERα/ERβ. The resulting nuclear complex affects the transcription of estrogen-responsive genes, which is responsible for the production of various growth-promoting signals (Riggs and Hartmann, 2003).
3.1 Pharmacokinetic Interactions
Several in vitro and in vivo studies on the bioavailability and metabolism of TAM and its metabolite 4-hydroxy-tamoxifen found that morin (Shin et al., 2008), silybin (Kim et al., 2010), Epigallocatechin gallate (EGCG) (Shin and hoi, 2009), myricetin (Li et al., 2011b), baicalein (Li et al., 2011a), curcumin (Cho et al., 2012), kaempferol (Piao et al., 2008), and quercetin (Shin et al., 2006) significantly changed the pharmacokinetics of oral TAM, resulting in reduced systemic clearance (CL/F); increased the area under the plasma concentration-time curve (AUC0-∞), in the peak plasma concentration (Cmax); and increased absolute and relative bioavailability, which may be the result of reducing first-pass metabolism in the intestine and liver.
Among these, morin and kaempferol had no significant effect on the formation of 4-hydroxy-tamoxifen (Piao et al., 2008; Shin et al., 2008). However, silybin, EGCG, myricetin, baicalein, curcumin, and quercetin significantly changed AUC0-∞ and the metabolite-maternal ratio (MR) of 4- hydroxy-tamoxifen, indicating that it can significantly increase the bioavailability of TAM and affect the formation of 4-hydroxy-tamoxifen (Shin et al., 2006; Shin and Choi, 2009; Kim et al., 2010; Li et al., 2011a; Li et al., 2011b; Cho et al., 2012). These findings highlight that natural or plant products can interfere with the pharmacokinetics of TAM, as seen in Table 1. If clinical studies further confirm the results of the above pharmacokinetic studies, an adjustment of clinical TAM doses may be needed when co-administrated with these products to avoid potential drug–herb interactions.
On the contrary, studies found no interactions between certain natural products and TAM. For example, Ginkgo biloba L. extract is an effective herb widely used in diseases including breast cancer (Jacobs and Browner, 2000). A prospective open-label cross-over on the effect of clinical Ginkgo biloba L. extract co-administration on the pharmacokinetics of TAM found that there was no significant difference in drug concentration and toxicity before and after 3-week Ginkgo biloba L. extract treatment according to the LC-MS/MS results of 20 women with early breast cancer who took TAM received Ginkgo biloba L. extract (EGb 761) (120 mg twice daily), indicating that co-administration of Ginkgo biloba L. extract would not significantly affect the pharmacokinetics of TAM (Vardy et al., 2013).
Fucoidan is a polysaccharide compound naturally occurring in brown algae. In vivo and in vitro studies have shown that fucoidan can prevent and treat breast cancer, promyelocytic leukemia, colon cancer, breast cancer, liver cancer, and melanoma (Atashrazm et al., 2015). An open-label non-crossover study of patients with active malignant tumors taking letrozole or TAM (n = 10 per group) found that the results of HPLC-CAD (high-performance liquid chromatography with aerosol detector) of patients who took oral fucoidan in the form of fucoidan extract for 3 weeks (500 mg twice daily) showed no significant difference in letrozole, TAM concentration, and the steady-state plasma concentration of TAM metabolites and no adverse effects of fucoidan. These results indicate that, at research, fucoidan does not interact with letrozole and TAM (Tocaciu et al., 2018).
Another study investigating the effects of combined treatment of MCF-7 with a fermented mistletoe preparation (VAE) and TAM reported that the combination of clinically relevant doses of VAE with TAM metabolite endoxifen did not show significant inhibition of TAM metabolism catalyzed by CYP3A4/5 and CYP2D6, indicating mistletoe (Viscum album L.) preparations can be combined with TAM without the risk of HDI in vitro (Weissenstein et al., 2019).
3.2 Pharmacodynamic Interactions
The key mechanisms of action of anti-cancer drugs involve apoptosis, autophagy, and cell cycle arrest (Chen and Karantza-Wadsworth, 2009; Wang et el., 2011; Kon et al., 2013). In the normal state, apoptosis is a highly specific and carefully controlled programmed cell death. Under pathological conditions, especially in cancer, cells lose their ability to induce apoptosis and proliferate uncontrollably. In the catalytic activation of the apoptotic cascade, caspase family proteases and BCL-2 family proteins such as Bcl-2-associated X (BAX) and B-cell lymphoma 2 (Bcl-2) play an important role (Pistritto et al., 2016).
Programmed cell death (PCD) is not limited to apoptosis (type I) PCD but also autophagic (type II) PCD (Bursch et al., 2000). Regulating autophagy can also improve anti-cancer treatment (Moretti et al., 2007). It is an important process in programmed cell death and the phagocytosis and degradation of non-essential or abnormal organelles and proteins (Denton et al., 2012). In the process of autophagy, there is an increase in the expression of autophagy genes Beclin 1, PI3KC3, and LC3-II (Kang et al., 2010; Liu et al., 2010), while p62 can be degraded by autophagy (Bjørkøy et al., 2005). As deregulation of the cell cycle is one of the main characteristics of cancer cells, cell cycle arrest may be an effective strategy to control the abnormal proliferation of cancer cells (Novák and Tyson, 2003).
Genome replication and cell division are regulated by cell cycle progression (Malumbres and Barbacid, 2009). The control and coordination of cell cycle progression involve cyclin-dependent kinases (CDK) and cyclins, including p53, p21, p16, and cdc25 (Malumbres and Barbacid, 2009). Current evidence, summarized in Tables 2, 3, reports that natural or plant products and TAM can target the physiological characteristics of cancer cells, inhibit cell proliferation, and exert anti-cancer effects via participation in the aforementioned cellular mechanisms such as apoptosis, autophagy, and cell cycle. There is also evidence of the combined use of some natural or plant products combined with TAM, in which the natural or plant products can significantly increase the sensitivity of drug-resistant cells to TAM in vitro (Table 4).
3.2.1 Inhibiting Tumour Cell Growth and Increasing Apoptosis
3.2.1.1 In Vitro Studies
The plant flavonoid hesperidin (HES) is a natural product with obvious anti-tumor effects and is widely present in citrus fruits, piperine (PIP), and bee venom (BV) extracted from black pepper (Piper nigrum L.) and long pepper (Piper longum L.) (Park et al., 2011; Palit et al., 2015; Deng et al., 2016). A recent study has shown that the combined use of HES, PIP, and BV with TAM showed an enhanced anti-proliferative effect on MCF7 and T47D and can significantly increase cell apoptosis (Khamis et al., 2018). Furthermore, this study reports that the mRNA level of the pro-apoptotic factor BAX is upregulated, whereas the mRNA level of the anti-apoptotic protein Bcl-2 is downregulated (Khamis et al., 2018). It can reduce the expression of breast cancer-related receptors EGFR and ERα and induce the G2/M phase arrest (Khamis et al., 2018). Tangerine, a flavonoid present in oranges, inhibits the growth of MCF-7/6 breast cancer cells in a manner similar to TAM in vitro, activates cell adhesion, and prevents invasion (Bracke et al., 1999). The combined use of tangerine with TAM exhibited a synergistic inhibitory effect on the growth of MCF-7/6 cells (Bracke et al., 1999).
Isoflavone genistein is one of the most important phytoestrogens. When combined with TAM, it can further inhibit the proliferation of MCF-7, MDA-231, and MDA-435 cells (Tanos et al., 2002). Another study showed that the combination of genistein and TAM could further inhibit the proliferation of BT-474 cells, increase apoptosis, arrest the cell cycle in the G1 phase, and be involved in the synergistic downregulation of the expression of survivin (Mai et al., 2007). Persin is a novel phytotoxin, which, combined with 4-hydroxytamoxifen on MCF-7 and T-47D cells, increases the sensitivity of cells to TAM, increases cell apoptosis, reduces ERαmRNA expression, and increases B-cell lymphoma 2 interacting mediator of cell death (BIM) mRNA expression (Roberts et al., 2007). Canola oil (CAN) has a low content of saturated fatty acids and a high content of unsaturated fatty acids. When combined with TAM, it exhibits a significant synergistic inhibitory effect on MCF-7 and T-47D cell growth (Cho et al., 2010).
Z-Ligustilide, a phthalate compound, is the primary active ingredient of the Chinese herbal medicine Angelica sinensis (Oliv.) Diels. When used in combination with TAM, it has been found to increase the growth inhibitory effect in ERα-breast cancer cells MDA-MB-231 and induce cell apoptosis and cell cycle arrest in S and G2/M phases (Ma et al., 2017). A combination of Z-ligustilide with TAM is also found to activate the expression and transcriptional activity of ERα of MDA-MB-231 cells and reduce the expression of metastasis-associated protein 1 (MTA1) (Yaacob et al., 2015).
When an active subcomponent (SCS) in the leaves of the traditional medicinal plant flowering shrub (Strobilanthes crispa (L.) Blume) in Malaysia and Indonesia used in combination with TAM under low doses of antiestrogens, it has been reported to synergistically induce cell cycle arrest in MCF-7 and MDA-MB-23 in the G1 phase, via mechanisms involving the regulation of cyclin D1, p21 CDK inhibitor and p53 tumor suppressor protein, modulation of mitochondrial membrane potential, and activation of caspase-8 and caspase-9 (Yaacob et al., 2014). The study also reports that the anti-cancer effect of SRS involves the downregulation of ERα protein; however, it is independent of ER-mediated mechanism in MDA-MB-231 cells (Yaacob et al., 2014).
In addition to water, green tea (Camellia sinensis (L.) Kuntze [Theaceae]) is the most widely consumed beverage in the world; current studies have reported that drinking green tea can improve the prognosis of breast cancer (Nagata et al., 1998; Fujiki et al., 1999). The combination of green tea extract (GTE) and TAM can synergistically inhibit the proliferation of MCF-7 cells, with the underlying mechanism relating to the inhibition of MAPK signal transduction (Sartippour et al., 2006). The combination of the main active ingredient of green tea epigallocatechin-3-gallate (EGCG) and TAM also exhibited significant inhibition of MCF-7 cell proliferation (Sakata et al., 2011).
Mistletoe (Viscum album L.) derived medicinal preparations are widely used and registered as medicines in many European countries. Clinical studies have reported significant anti-cancer effects of Viscum album L. extracts (VAE) in breast cancer patients (Kienle et al., 2009; Tröger et al., 2014). A clinical study on the combined use of mistletoe with the active metabolite of TAM (E/Z) endoxifen reports that clinically relevant doses of VAE did not affect the growth inhibition and cytotoxicity effect of (E/Z) endoxifen on tumor cells and could significantly induce cell cycle in the G0/G1 phase (Weissenstein et al., 2019).
Fucoidan extract (FE) in combination with TAM has been reported with significant induction of cell growth inhibition, apoptosis, and cell cycle modification in MDA-MB-231 and MCF-7 cells (Zhang et al., 2013). The study also found significant downregulation in expression levels of anti-apoptotic proteins Bcl-xL and Mcl-1; significant reduction in the phosphorylation of ERK and Akt in MDA-MB-231 cells but an increased ERK phosphorylation in MCF-7 cells; additionally, a synergistic increase in intracellular ROS level of breast cancer cells and reduction in the level of intracellular glutathione (Zhang et al., 2013).
Huaier, also known as Trametes robiniophila Murr., has been used as a traditional Chinese medicine in China for about 1,600 years, with its extracts documented for anti-tumor effects, including inhibition of cell proliferation, anti-metastasis, interference with tumor angiogenesis, induction of autophagic cell death, and tumor-specific immune regulation (Pan et al., 2019). In MCF-7 and T47D cells, the Huaier extract combined with TAM can reduce cell viability and synergistically induce cell autophagy, apoptosis, and G0/G1 cell cycle arrest via the inhibition of the AKT/mTOR signaling pathway (Qi et al., 2016).
Red clover, Trifolium pratense L., is a member of the legume family, used as a health food for humans, and has been extracted for various isoflavone preparations used in nutritional supplements (Vlaisavljevic et al., 2014). The combination of hydroethanolic extract of red clover and TAM can significantly reduce the survival of MCF-7 and MDA-MB-231 cells. The CI values across different dose combinations on the two cell lines were less than 1, implying that all combinations exhibited a synergistic effect (Khazaei and Pazhouhi, 2019). At the same time, the DRI value of TAM > 1 indicates that the TAM dose can be reduced under a given therapeutic effect (Khazaei and Pazhouhi, 2019).
HPC 03 herbal formula contains extracts of Angelica gigas Nakai, Ligusticum officinale (Makino) Kitag, and Cinnamomum cassia (L.) J. Presl. Combined with TAM, they can inhibit the proliferation of MCF-7 cells and reduce the mRNA expression of estrogen-responsive genes: Psen2, PGR, and CTSD (Chang et al., 2018). BreastDefend® (BD) is a dietary supplement formula that includes Ganoderma lucidum (Leyss ex Fr.) Karst., Curcuma longa L., and quercetin. Combined with 4-hydroxytamoxifen (4-OHT), it exhibits significant inhibition of cell proliferation and induction of MCF-7 cell apoptosis (Cheng et al., 2017). The study evaluated the effect of 4-OHT and BD combinations on gene expression involved with apoptosis and TAM resistance. At the translation level, increased expression levels of BRAF and p21 mRNA and suppressed expression levels of FN1 and Bcl-2 in MCF-7 cells were detected (Cheng et al., 2017). Sanhuang Decoction (SHD) comprises Astragalus mongholicus Bunge, Rheum palmatum L. [Polygonaceae], and Curcuma longa L. in a ratio of 3:1:1 (Xu et al., 2016). The combination of SHD and TAM exhibited additive effects on MCF-7 cell growth inhibition and apoptosis induction (Xu et al., 2016). It can also inhibit the growth of breast cancer cells by downregulating the Aurora A expression and enhancing the chemosensitivity to other anti-tumor drugs (Xu et al., 2016).
3.2.1.2 In Vivo Studies
Ginkgo leaf extract (GbE) is an effective herbal medicine widely used as a CAM for diseases including breast cancer (Jacobs and Browner, 2000). In a study adopting 7,12-dimethyl-benz(a)anthracene- (DMBA-) induced breast cancer animal model, the combined use of GbE and TAM was demonstrated to a significant reduction in the tumor area, increase in the tumor necrosis area, and reduction in the number of proliferating cell nuclear antigen- (PCNA-) positive cells (Dias et al., 2013). The combined use of Huaier extract and TAM in vivo can enhance the inhibitory effects of TAM on the growth of subcutaneous tumors of MCF-7 breast cancer cells and synergistically induce autophagy and apoptosis in ER+ breast cancer cells via suppression of the AKT/mTOR pathway (Qi et al., 2016).
In a study involving an MCF-7 cell xenograft mouse model, it was reported that the combination of green tea extract (GTE) and TAM significantly inhibit the growth and angiogenesis of tumor tissues (Sartippour et al., 2006). The study also reports that the combination of significant reduction expression levels of ER-α, ∼50% inhibition of transcription of ERE-CAT reporter gene, and an increase in tumor cell apoptosis and larger areas of tumor cell necrosis (Sartippour et al., 2006). Another study on the combined GTE and TAM reports a significant reduction in the number of hyperplastic alveolar nodules (HAN) and the number of argyrophilic nucleolar organization areas (AgNOR) (Sakata et al., 2011).
BD alone can inhibit the growth and infiltration of highly metastatic triple-negative human breast cancer cells in vitro and in vivo (Jiang et al., 2011; Jiang et al., 2012). In a study that adopted the MCF-7 cell xenograft model, it was reported that TAM and BD co-treatment could significantly enhance cell apoptosis, suppress tumor growth, and increase the expression of TAM resistance proteins BRAF and p21 (Cheng et al., 2017). Among all CAM products used by breast cancer patients, flaxseed (FS) is the third most commonly used (Boon et al., 2007). FS has two major anti-cancer components, secoisolariciresinol diglucoside (SDG) lignan and flaxseed oil (FO). When used in combination with TAM, it can effectively reduce the growth of MCF-7 breast tumors in ovariectomized (OVX) athymic mice at low circulating estrogen levels (Saggar et al., 2010). The underlying mechanism includes ER and growth factor-signaling pathways and involves proteins such as phosphorylated mitogen-activated protein kinase, PAKT, BCL2, and angiogenesis and vascular endothelial growth factor (Saggar et al., 2010).
3.2.2 Increasing Sensitivity to TAM and Reducing Resistance
TAM is an endocrine drug that is most commonly used to block the effects of estrogen at all stages of breast cancer, especially in postmenopausal patients. While the ERα status has been used to identify breast cancer patients who may respond to TAM, there are still 30%–50% of treated Erα-positive breast cancer cells that show resistance to TAM (Early Breast Cancer Trialists' Collaborative Group, 1998). Therefore, in the experimental studies, many TAM-resistant cell lines were derived. At present, the mechanism of TAM resistance mainly includes the alteration or loss of ER expression; the activation or inactivation of various signal pathways involved in cellular processes, such as survival, proliferation, stress response, cell cycle, apoptosis inhibition regulated by the BCL-2 family, autophagy, and changes in mRNA expression (Rondón-Lagos et al., 2016).
A study involving the TAM-resistant cells named MCF-7TR5 (TAM resistant to 5 μM) reported that Z-ligustilide can enhance the inhibitory effect of TAM on the cell viability in MCF-7TR5 and sensitized MCF-7TR5 cells to TAM via promoting caspase-independent cell death (Qi et al., 2017). The study also reported that the combined use of Z-ligustilide with TAM can also induce DNA damage and apoptosis by restoring Nur77 expression and proteins degraded by autophagy in MCF-7TR5 (Qi et al., 2017).
Curcumin is a bioactive compound of the Chinese medicine turmeric that exhibits effective anti-cancer activity. Curcumin and its analogs also exhibit multi-target biological effects and have been used to enhance targeted therapy to assist breast cancer chemotherapy treatment (Nagaraju et al., 2012). A study involving the TAM-resistant cell lines named MCF-7/LCC2 and MCF-7/LCC9 reported that the combined use of curcumin and TAM resulted in synergistic growth inhibition, an increase in the sensitivity of MCF-7/LCC2 and MCF-7/LCC9 cells to TAM, and a reduction in IC50 of TAM (Jiang et al., 2013).
Antrodia cinnamomea (AC) is a medical mushroom that could have a novel role in breast cancer management (Lin et al., 2018). In a study involving TAM-resistant cell line MCF-7 tam-R, while 10–6 M TAM has no proliferation inhibitory effect on MCF-7 tam-R, the combined treatment of 10–6 M TAM with ethanol extract of AC was able to increase the effect of TAM to inhibit the proliferation of MCF-7 tam-R cells (Lin et al., 2018).
Evn-50 is an ethyl acetate extract of Vitex negundo L. When used in combination with TAM to treat TAM-resistant MCF-7/TAM-R cells, it significantly reduces cell viability, inhibits cell growth, induces apoptosis, and reverses TAM resistance of MCF-7/TAM-R cells (Hu et al., 2012). The study reports a decrease in phosphorylated AKT and p-MAPK44/42 levels, while total AKT and MAPK44/42 expressions were stable. This highlights that the underlying mechanism may be related to the downregulation of AKT and MAPK signaling pathways (Hu et al., 2012).
The combined use of persin and 4-OHT on TAM-R cells showed a synergistic induction of drug-resistant cell apoptosis pre-dominantly mediated by the CHOP-dependent endoplasmic reticulum stress (ERS) signaling cascades (McCloy et al., 2013). Jaeumkanghwa soup (JEKHT) is a compound composed of 12 traditional herbs (Jung et al., 2010). In antiestrogen-resistant LCC9 human breast cancer cells, the combination of JEKHT with 4-OHT can increase the sensitivity of the cells to TAM (De Oliveira Andrade et al., 2019). All the above evidence shows that natural or plant products can increase the sensitivity of drug-resistant cell lines to TAM.
On the contrary, some studies showed no significant interactions between TAM and certain natural products. In ovariectomized athymic mice with established MCF-7 that had only TAM implanted, increased biomechanical bone strength and bone mineral content (BMC) and density (BMD) in the femur and lumbar spine were reported (Chen et al., 2011b). In the mice group receiving a combination of SDG and FO diet with TAM therapy, no effects on the BMC and BMD of the femur or vertebrae were reported, indicating that the combined use of FS and TAM exhibited no obvious effect on bone health (Chen et al., 2011b).
Jiawei Xiaoyao San (JWXYS) is a very common Chinese herbal formula used by the Chinese for thousands of years (Chen et al., 2014). In MCF-7 cells, a study reports no statistically significant difference between TAM only and combined JWXYS with TAM groups in terms of cell number, cell cycle progression, and cell proliferation signal proteins such as AKT, ERK, P38, p27 (Kip1), and light chain (LC3) I, II (Chen et al., 2014). In the MCF-7 xenograft mouse model, the study found no significant change in tumor weight and the protein expression levels of AKT and ERK when compared to the TAM-only group, indicating no obvious interaction between JWXYS and TAM in vivo (Chen et al., 2014).
A study utilizing uterotrophic assay in 21-day immature female SD rats reports that the combined use of EGb 761 and TAM showed no significant effect on absolute or relative uterine weight, luminal epithelial cell height (LECH), and luminal circumference (LCO), indicating that EGB761 has no agonism nor antagonism on utero (Moon et al., 2018).
3.2.3 Reducing the Side Effects of TAM
Although the use of TAM as an adjuvant endocrine therapy has effectively reduced the recurrence of ER+ breast patients and improved their survival rate, more and more studies have reported a series of side effects related to TAM that include hot flashes, night sweats, vaginal dryness, sleep, and emotional problems (Lorizio et al., 2012). Occasionally, more severe adverse events may occur, including endometrial cancer (Fisher et al., 1994; Kloos et al., 2002) and abnormal liver function (Yang et al., 2013). However, some natural or plant products can reduce the side effects of TAM, as shown in Table 5.
3.2.3.1 Hot Flashes
A clinical observation study assessing the effect of Actaea racemosa L. [Ranunculaceae] (CR BNO 1055) and TAM combination on the number and intensity of hot flashes found that the number and severity of hot flashes decreased in patients after 12 months of combined use (Hernández Muñoz & Pluchino, 2003). Only 24.4% of the patients in the group receiving combined therapy reported severe hot flashes, while 73.9% of the patients in the control group reported severe hot flashes, showing that the combination of TAM and CR BNO 1055 for 12 months can provide a satisfactory reduction of the number and severity of hot flashes (Hernández Muñoz & Pluchino, 2003). A prospective observational study on the combined use of black cohosh (Actaea racemosa L.) and TAM in the treatment of breast cancer observed improvement in symptoms of hot flashes, sweating, sleep problems, and anxiety after 6 months of treatment; 90% of the study participants reported “very good/good” tolerability to black cohosh extract (Rostock et al., 2011).
3.2.3.2 Endometrial Disease
In retrospective study of all breast cancer patients who received TAM treatment in the Taiwan Health Insurance Research Database between 1 January 1998 and 31 December 2008, it was found that among breast cancer survivors aged 20–79, the risk of endometrial cancer in patients treated with Angelica sinensis (Oliv.) Diels is lower when compared with patients who have never used Angelica sinensis (Oliv.) Diels (HR: 0.61, 95% CI: 0.44–0.84) (Wu et al., 2014). When compared with people who have never used Chinese herbal medicine, breast cancer survivors who take Chinese herbal medicines containing coumestrol, genistein, or daidzein concurrently with TAM therapy have no higher risk ratio for the subsequent development of endometrial cancer (Hu et al., 2015). More than half of the female breast cancer patients receiving TAM have used at least a Chinese herbal product (CHP). Two of the most commonly used CHPs are Jiawei Xiaoyao San and Shujing Huoxue Decoction (Tsai et al., 2014). Compared with non-CHP users, the risk ratio for the development of endometrial cancer among CHP users was 0.50 (95% CI = 0.38–0.64) (Tsai et al., 2014). The above results indicate that the combination of natural or plant products with TAM may reduce the risk of endometrial cancer after breast cancer.
JEKHT is a compound consisting of 12 traditional herbs (Jung et al., 2010). In a study adopting DMBA-induced ER+ breast cancer rat model, it was reported that the combined treatment of JEKHT with TAM can reduce risk of recurrence and developing TAM resistance, prevent the development of precancerous endometrial lesions, and prevent the immunosuppressive effects of TAM (De Oliveira Andrade et al., 2019). When compared with the TAM-only group, the combination of JEKHT and TAM significantly downregulated Il-6, Foxp3/T regulatory cell (Treg) markers, and Tgfβ1 that activate Treg (De Oliveira Andrade et al., 2019).
3.2.3.3 Liver Damage
In TAM-intoxicated rats, a study found significantly increased levels of serum glutamic-oxaloacetic transaminase (sGOT), serum glutamic-pyruvic transaminase (sGPT), alkaline phosphatase (ALP), triglycerides (TG), cholesterol, urea, uric acid, bilirubin, and creatinine. The study proposed that the change in parameters may be related to extensive liver damage during TAM treatment (Rahate and Rajasekaran, 2015). Desmostachya bipinnata (L.) Stapf (PFDB) is used in Indian traditional medicine and is commonly called “sacrificial grass” in English (Rahate and Rajasekaran, 2015). The study reported that rats pre-treated with PFDB were effectively protected from TAM-induced hepatotoxicity. Reduced levels of the liver function test parameters, as discussed above, in serum were detected in these rats. Degree of liver cell necrosis was observed in these rats in histopathological studies (Rahate and Rajasekaran, 2015). The protective effect is dose-dependent (Rahate and Rajasekaran, 2015).
GTE also show hepatoprotective effect against TAM-induced liver injury in rats. A study where GTE was administered to TAM-intoxicated rats reported significant increase in antioxidant enzymes, reduction of glutathione concomitant, and significant decrease in the levels of thiobarbituric acid reactive substance (TBARS) and liver transaminase (El-Beshbishy, 2005a).
Rats treated with TAM showed elevated aminotransaminase, carbonyl groups (CGs), and 8-hydroxydeoxyguanosine (8OHdG) (Codoñer-Franch et al., 2013). When dried apple snack enriched with mandarin juice was used in combination with TAM, restoration of oxidative damage to protein and DNA in liver were observed with a significant reduction in plasma and liver markers of oxidative stress recorded, exhibiting a protective effect on the oxidative stress induced by TAM in rats (Codoñer-Franch et al., 2013).
Dimethyl-4.4'-dimethoxy-5,6,5',6'-dimethylenedioxybiphenyl-2.2'-dicarboxylate (DDB), a structural analog of Schizandrin B and Schizandrin C, is one of many compounds isolated from Chinese herbal medicine Schisandra chinensis (Turcz.) Baill. (Kim et al., 1999). Oxidative stress status of TAM-intoxicated liver injury in rats is determined by the change in levels of antioxidant enzymes (glutathione-S-transferase, glutathione peroxidase, and catalase), glutathione, TBARS, liver transaminase, sGPT, and sGOT (El-Beshbishy, 2005a). DDB is found to alleviate the oxidative stress status of TAM-intoxicated liver injury in rats, with the study reporting a significant increase in antioxidant enzymes, a significant decrease in the activity of sGPT and sGOT, reduction of glutathione concomitant, and a significant reduction of TBARS and liver transaminases (El-Beshbishy, 2005b).
4 Natural Products With Estrogen-Like Effects
There is growing evidence on the potential benefits of complementary and alternative medicine usage in treatment of cancer (Horneber et al., 2012). Prevalence rate of CAM usage among breast cancer survivor was reported to fall within 60-70%; with herbal preparations, vitamin and diet supplements being the more common CAM products used (Matthews et al., 2007).
While some natural products have a better effect on alleviating the adverse reactions during TAM treatment, there are some natural or plant products that have estrogen-like effects and mutual adverse effects when combined with TAM (Table 6).
4.1 Reduce Anti-Cancer Effect
In vivo Xanthorrhizol is a sesquiterpene compound isolated from turmeric and has been reported to have antimicrobial, antibacterial, anti-metastatic, and anti-inflammatory effects (Oon et al., 2015). In a study adopting TAM-treated MCF-7-implanted athymic nude mouse model, significantly increased tumor volume, larger tumor size, and increased protein expression of P38 and P27 (Kip1) were found in the group receiving combined treatment of Xanthorrhizol and TAM compared to the group receiving TAM only (Noomhorm et al., 2014).
Si-Wu-Tang (SWT) is a Chinese herbal medicine composition consisting of Angelica sinensis (Oliv.) Diels, Paeonia lactiflora Pall., Ligusticum striatum DC., and Rehmannia glutinosa (Gaertn.) DC. (Chen et al., 2013). In a study using an MCF-7-implanted female athymic nude mouse model, it is found that the combination of SWT and TAM can reverse the anti-proliferative effect of TAM on tumor weight and tumor volume and increase the expression of estrogen receptor α and N-cadherin (Chen et al., 2013). The combination of SWT and TAM also increased protein expressions of ERK, AKT, P38, and p27 (Kip1), which could indicate the phytoestrogenic effect of SWT that could contribute to adverse effects when SWT interacts with TAM in the body (Chen et al., 2013)
Baihe Zhimu Tang (BZ) is a traditional Chinese medicine formula that consists of Lilium brownii var. viridulum Baker and Anemarrhena asphodeloides Bunge, often used to treat depression (Li et al., 2019). In the mouse xenograft model of human breast cancer MCF-7 cells, BZ reduced the effectiveness of TAM in the treatment of breast cancer and the concentration of endoxifen and 4-OH-tamoxifen in tumor-bearing mice (Li et al., 2019). The BZ formula and its major component, mangiferin, also significantly inhibited the activity of CYP450 enzymes in rat liver microsomes (Li et al., 2019).
In female nude mice inoculated subcutaneously with MCF-7/6 human breast adenocarcinoma cells, the combination of tangeretin and TAM counteracted the tumor growth inhibition effect of TAM (Bracke et al., 1999). This evidence reports adverse effects that occur between the interaction of certain herbal or plant products with TAM that doctors and patients should pay attention to during treatment of ER+ breast cancer.
5 Conclusion
This review has covered the potential interactions of TAM with 33 commonly used natural or plant products and 5 commonly used herbal formulas. With further in-depth research on breast cancer treatment drugs, much evidence reports that the combination of natural or plant products with conventional breast cancer treatment drug TAM contributes to the increased anti-tumor effect, inhibition of tumor cell proliferation, sensitizing breast cancer cells to TAM, and minimizing adverse effect of drugs. However, there are also studies that report no effect or adverse effects when certain natural or plant products are combined with TAM.
While there are significant discoveries and advancements in the study of drug combinations, there are still limitations in the experimental design of some studies. Firstly, while most in vitro studies adopted single cell line models, in vitro tumor environments tend to be vastly different, limiting the relevance of the research evidence. Secondly, studies on the mechanism of synergistic anti-tumor effect need to be further elucidated, particularly on contributions by different signaling pathways. A possible approach is to compare and analyze the structure-activity relationships of phytochemicals, TAM, and estradiol with chemical structures diagram retrieved from National Center for Biotechnology Information (2022). Table 7 includes some of the phytochemicals that have been investigated in the selected reviewed studies. Studies have shown that just the monitoring of protein expression levels is insufficient in understanding transcription activity in vitro (Gordân et al., 2009).
Additionally, there are still issues relating to herbal formulations and their composition that is often unaddressed, such as the clarification of herbal formula composition and quality control of herbal products. Moreover, in studies that investigate herbal formulations or plant extracts, there is increased complexity in the determination of which combinations of compounds are producing the observed additive, synergistic, or antagonistic effects.
Natural or plant products have been used in clinical practice for thousands of years, with numerous modern-day studies reporting the anti-cancer effects of such herbal medicines and compounds. While continuous efforts in scientific and laboratory investigations of individual herbal and herbal compositions in breast cancer treatment have given rise to various evidence from the derivation of major anti-cancer components and especially evaluation of effects from combination with conventional breast cancer treatment drugs, it is necessary to conduct further research and review them systematically, in particular, clinical studies to verify the herb–drug interactions. In this regard, cooperation between medical doctors, traditional Chinese medicine practitioners, and allied health practitioners that use herbal products to monitor and evaluate the use of natural or plant products in patients receiving TAM endocrine treatment for breast cancer is important to ensure the effectiveness of the treatments and the safety of patients. Such efforts, including further research, are critical for the integration of the scientific evidence into clinical practice to guide the use of natural or plant products and anti-cancer drugs such as TAM for better care of breast cancer patients.
Author Contributions
CY and FZ wrote the draft manuscript. FZ, CY, ZY, and XZ searched the related literature. CL guided the writing and critically revised the manuscript. All authors have read and approved the manuscript.
Conflict of Interest
The authors declare that the research was conducted in the absence of any commercial or financial relationships that could be construed as a potential conflict of interest.
Publisher’s Note
All claims expressed in this article are solely those of the authors and do not necessarily represent those of their affiliated organizations or those of the publisher, the editors, and the reviewers. Any product that may be evaluated in this article, or claim that may be made by its manufacturer, is not guaranteed or endorsed by the publisher.
References
Ali, S., Rasool, M., Chaoudhry, H., N Pushparaj, P., Jha, P., Hafiz, A., et al. (2016). Molecular Mechanisms and Mode of Tamoxifen Resistance in Breast Cancer. Bioinformation 12 (3), 135–139. doi:10.6026/97320630012135
Atashrazm, F., Lowenthal, R. M., Woods, G. M., Holloway, A. F., and Dickinson, J. L. (2015). Fucoidan and Cancer: A Multifunctional Molecule with Anti-Tumor Potential. Mar. Drugs 13 (4), 2327–2346. doi:10.3390/md13042327
Bjørkøy, G., Lamark, T., Brech, A., Outzen, H., Perander, M., Overvatn, A., et al. (2005). p62/SQSTM1 Forms Protein Aggregates Degraded by Autophagy and Has a Protective Effect on Huntingtin-Induced Cell Death. J. Cel. Biol. 171 (4), 603–614. doi:10.1083/jcb.200507002
Boon, H. S., Olatunde, F., and Zick, S. M. (2007). Trends in Complementary/Alternative Medicine Use by Breast Cancer Survivors: Comparing Survey Data from 1998 and 2005. BMC Womens Health 7, 4. doi:10.1186/1472-6874-7-4
Bracke, M. E., Depypere, H. T., Boterberg, T., Van Marck, V. L., Vennekens, K. M., Vanluchene, E., et al. (1999). Influence of Tangeretin on Tamoxifen's Therapeutic Benefit in Mammary Cancer. J. Natl. Cancer Inst. 91 (4), 354–359. doi:10.1093/jnci/91.4.354
Brauch, H., Mürdter, T. E., Eichelbaum, M., and Schwab, M. (2009). Pharmacogenomics of Tamoxifen Therapy. Clin. Chem. 55 (10), 1770–1782. doi:10.1373/clinchem.2008.121756
Bursch, W., Ellinger, A., Gerner, C., Fröhwein, U., and Schulte-Hermann, R. (2000). Programmed Cell Death (PCD). Apoptosis, Autophagic PCD, or Others? Ann. N. Y Acad. Sci. 926, 1–12. doi:10.1111/j.1749-6632.2000.tb05594.x
Chang, B. Y., Kim, D. S., Kim, H. S., and Kim, S. Y. (2018). Evaluation of Estrogenic Potential by Herbal Formula, HPC 03 for In Vitro and In Vivo. Reproduction 155 (2), 105–115. doi:10.1530/REP-17-0530
Chen, J., Saggar, J. K., Corey, P., and Thompson, L. U. (2011a). Flaxseed Cotyledon Fraction Reduces Tumour Growth and Sensitises Tamoxifen Treatment of Human Breast Cancer Xenograft (MCF-7) in Athymic Mice. Br. J. Nutr. 105 (3), 339–347. doi:10.1017/S0007114510003557
Chen, J., Saggar, J. K., Ward, W. E., and Thompson, L. U. (2011b). Effects of Flaxseed Lignan and Oil on Bone Health of Breast-Tumor-Bearing Mice Treated with or without Tamoxifen. J. Toxicol. Environ. Health A. 74 (12), 757–768. doi:10.1080/15287394.2011.567950
Chen, J. L., Chang, C. J., Wang, J. Y., Wen, C. S., Tseng, L. M., Chang, W. C., et al. (2014). In Vitro and In Vivo Effects of Jia-Wei-Xiao-Yao-San in Human Breast Cancer MCF-7 Cells Treated with Tamoxifen. Integr. Cancer Ther. 13 (3), 226–239. doi:10.1177/1534735414520970
Chen, J. L., Wang, J. Y., Tsai, Y. F., Lin, Y. H., Tseng, L. M., Chang, W. C., et al. (2013). In Vivo and In Vitro Demonstration of Herb-Drug Interference in Human Breast Cancer Cells Treated with Tamoxifen and Trastuzumab. Menopause 20 (6), 646–654. doi:10.1097/gme.0b013e31827b2240
Chen, N., and Karantza-Wadsworth, V. (2009). Role and Regulation of Autophagy in Cancer. Biochim. Biophys. Acta 1793 (9), 1516–1523. doi:10.1016/j.bbamcr.2008.12.013
Chen, X. W., Sneed, K. B., and Zhou, S. F. (2011). Pharmacokinetic Profiles of Anticancer Herbal Medicines in Humans and the Clinical Implications. Curr. Med. Chem. 18 (21), 3190–3210. doi:10.2174/092986711796391624
Cheng, S., Castillo, V., Welty, M., Alvarado, M., Eliaz, I., Temm, C. J., et al. (2017). BreastDefend Enhances Effect of Tamoxifen in Estrogen Receptor-Positive Human Breast Cancer In Vitro and In Vivo. BMC Complement. Altern. Med. 17 (1), 115. doi:10.1186/s12906-017-1621-7
Cho, K., Mabasa, L., Fowler, A. W., Walsh, D. M., and Park, C. S. (2010). Canola Oil Inhibits Breast Cancer Cell Growth in Cultures and In Vivo and Acts Synergistically with Chemotherapeutic Drugs. Lipids 45 (9), 777–784. doi:10.1007/s11745-010-3462-8
Cho, Y. A., Lee, W., and Choi, J. S. (2012). Effects of Curcumin on the Pharmacokinetics of Tamoxifen and its Active Metabolite, 4-Hydroxytamoxifen, in Rats: Possible Role of CYP3A4 and P-Glycoprotein Inhibition by Curcumin. Pharmazie 67 (2), 124–130.
Codoñer-Franch, P., Betoret, E., López-Jaén, A. B., Betoret, N., Fito, P., and Valls-Bellés, V. (2013). Dried Apple Enriched with Mandarin Juice Counteracts Tamoxifen-Induced Oxidative Stress in Rats. Int. J. Food Sci. Nutr. 64 (7), 815–821. doi:10.3109/09637486.2013.798267
De Oliveira Andrade, F., Yu, W., Zhang, X., Carney, E., Hu, R., Clarke, R., et al. (2019). Effects of Jaeumkanghwa-Tang on Tamoxifen Responsiveness in Preclinical ER+ Breast Cancer Model. Endocr. Relat. Cancer 26 (3), 339–353. doi:10.1530/ERC-18-0393
Deng, Y., Sriwiriyajan, S., Tedasen, A., Hiransai, P., and Graidist, P. (2016). Anti-Cancer Effects of Piper Nigrum via Inducing Multiple Molecular Signaling In Vivo and In Vitro. J. Ethnopharmacol. 188, 87–95. doi:10.1016/j.jep.2016.04.047
Denton, D., Nicolson, S., and Kumar, S. (2012). Cell Death by Autophagy: Facts and Apparent Artefacts. Cell Death Differ. 19 (1), 87–95. doi:10.1038/cdd.2011.146
Desta, Z., Ward, B. A., Soukhova, N. V., and Flockhart, D. A. (2004). Comprehensive Evaluation of Tamoxifen Sequential Biotransformation by the Human Cytochrome P450 System In Vitro: Prominent Roles for CYP3A and CYP2D6. J. Pharmacol. Exp. Ther. 310 (3), 1062–1075. doi:10.1124/jpet.104.065607
Dias, M. C., Furtado, K. S., Rodrigues, M. A., and Barbisan, L. F. (2013). Effects of Ginkgo Biloba on Chemically-Induced Mammary Tumors in Rats Receiving Tamoxifen. BMC Complement. Altern. Med. 13, 93. doi:10.1186/1472-6882-13-93
Early Breast Cancer Trialists' Collaborative Group (1998). Tamoxifen for Early Breast Cancer: An Overview of the Randomised Trials. Early Breast Cancer Trialists' Collaborative Group. Lancet 351 (9114), 1451–1467.
El-Beshbishy, H. A. (2005a). Hepatoprotective Effect of Green Tea (Camellia Sinensis) Extract Against Tamoxifen-Induced Liver Injury in Rats. J. Biochem. Mol. Biol. 38 (5), 563–570. doi:10.5483/bmbrep.2005.38.5.563
El-Beshbishy, H. A. (2005b). The Effect of Dimethyl Dimethoxy Biphenyl Dicarboxylate (DDB) Against Tamoxifen-Induced Liver Injury in Rats: DDB Use Is Curative or Protective. J. Biochem. Mol. Biol. 38 (3), 300–306. doi:10.5483/bmbrep.2005.38.3.300
Fisher, B., Costantino, J. P., Redmond, C. K., Fisher, E. R., Wickerham, D. L., and Cronin, W. M. (1994). Endometrial Cancer in Tamoxifen-Treated Breast Cancer Patients: Findings from the National Surgical Adjuvant Breast and Bowel Project (NSABP) B-14. J. Natl. Cancer Inst. 86 (7), 527–537. doi:10.1093/jnci/86.7.527
Fujiki, H., Suganuma, M., Okabe, S., Sueoka, E., Suga, K., Imai, K., et al. (1999). Mechanistic Findings of Green Tea as Cancer Preventive for Humans. Proc. Soc. Exp. Biol. Medsociety Exp. Biol. Med. (N.Y, N.Y.) 220 (4), 225–228. doi:10.1046/j.1525-1373.1999.d01-38.x
Gordân, R., Hartemink, A. J., and Bulyk, M. L. (2009). Distinguishing Direct versus Indirect Transcription Factor-DNA Interactions. Genome Res. 19 (11), 2090–2100. doi:10.1101/gr.094144.109
Hernández Muñoz, G., and Pluchino, S. (2003). Cimicifuga Racemosa for the Treatment of Hot Flushes in Women Surviving Breast Cancer. Maturitas 44 (Suppl. 1), S59–S65. doi:10.1016/s0378-5122(02)00349-3
Horneber, M., Bueschel, G., Dennert, G., Less, D., Ritter, E., and Zwahlen, M. (2012). How Many Cancer Patients Use Complementary and Alternative Medicine: A Systematic Review and Metaanalysis. Integr. Cancer Ther. 11 (3), 187–203. doi:10.1177/1534735411423920
Hu, H. Y., Zhou, J., Wan, F., Dong, L. F., Zhang, F., Wang, Y. K., et al. (2012). Zhejiang da xue xue bao. Yi xue ban = Journal of Zhejiang University. Med. Sci. 498–505.
Hu, Y. C., Wu, C. T., Lai, J. N., and Tsai, Y. T. (2015). Detection of a Negative Correlation Between Prescription of Chinese Herbal Products Containing Coumestrol, Genistein or Daidzein and Risk of Subsequent Endometrial Cancer Among Tamoxifen-Treated Female Breast Cancer Survivors in Taiwan Between 1998 and 2008: A Population-Based Study. J. Ethnopharmacol. 169, 356–362. doi:10.1016/j.jep.2015.04.028
Jacobs, B. P., and Browner, W. S. (2000). Ginkgo Biloba: A Living Fossil. Am. J. Med. 108 (4), 341–342. doi:10.1016/s0002-9343(00)00290-4
Jemal, A., Bray, F., Center, M. M., Ferlay, J., Ward, E., and Forman, D. (2011). Global Cancer Statistics. CA Cancer J. Clin. 61 (2), 69–90. doi:10.3322/caac.20107
Jiang, J., Thyagarajan-Sahu, A., Loganathan, J., Eliaz, I., Terry, C., Sandusky, G. E., et al. (2012). BreastDefend™ Prevents Breast-To-Lung Cancer Metastases in an Orthotopic Animal Model of Triple-Negative Human Breast Cancer. Oncol. Rep. 28 (4), 1139–1145. doi:10.3892/or.2012.1936
Jiang, J., Wojnowski, R., Jedinak, A., and Sliva, D. (2011). Suppression of Proliferation and Invasive Behavior of Human Metastatic Breast Cancer Cells by Dietary Supplement BreastDefend. Integr. Cancer Ther. 10 (2), 192–200. doi:10.1177/1534735410386953
Jiang, M., Huang, O., Zhang, X., Xie, Z., Shen, A., Liu, H., et al. (2013). Curcumin Induces Cell Death and Restores Tamoxifen Sensitivity in the Antiestrogen-Resistant Breast Cancer Cell Lines MCF-7/LCC2 and MCF-7/LCC9. Molecules 18 (1), 701–720. doi:10.3390/molecules18010701
Jung, D., Ha, H., Lee, H. Y., Lee, J.-A., Lee, J.-K., Huang, D.-S., et al. (2010). Stimulation of the Immune Response by Yin-Tonifying Formula. J. Korean Oriental Med. 32 (5), 112–123.
Kang, R., Tang, D., Schapiro, N. E., Livesey, K. M., Farkas, A., Loughran, P., et al. (2010). The Receptor for Advanced Glycation End Products (RAGE) Sustains Autophagy and Limits Apoptosis, Promoting Pancreatic Tumor Cell Survival. Cel. Death Differ. 17 (4), 666–676. doi:10.1038/cdd.2009.149
Karn, A., Jha, A. K., Shrestha, S., Acharya, B., Poudel, S., and Bhandari, R. B. (2010). Tamoxifen for Breast Cancer. JNMA J. Nepal Med. Assoc. 49 (177), 62–67. doi:10.31729/jnma.140
Khamis, A. A. A., Ali, E. M. M., El-Moneim, M. A. A., Abd-Alhaseeb, M. M., El-Magd, M. A., and Salim, E. I. (2018). Hesperidin, Piperine and Bee Venom Synergistically Potentiate the Anticancer Effect of Tamoxifen Against Breast Cancer Cells. Biomed. Pharmacother. 105, 1335–1343. doi:10.1016/j.biopha.2018.06.105
Khazaei, M., and Pazhouhi, M. (2019). Antiproliferative Effect of Trifolium Pratens L. Extract in Human Breast Cancer Cells. Nutr. Cancer 71 (1), 128–140. doi:10.1080/01635581.2018.1521443
Kienle, G. S., Glockmann, A., Schink, M., and Kiene, H. (2009). Viscum Album L. Extracts in Breast and Gynaecological Cancers: A Systematic Review of Clinical and Preclinical Research. J. Exp. Clin. Cancer Res. 28 (1), 79. doi:10.1186/1756-9966-28-79
Kim, C. S., Choi, S. J., Park, C. Y., Li, C., and Choi, J. S. (2010). Effects of Silybinin on the Pharmacokinetics of Tamoxifen and its Active Metabolite, 4-Hydroxytamoxifen in Rats. Anticancer Res. 30 (1), 79–85.
Kim, J. J., Liang, W., Kang, C. C., Pegram, M. D., and Herr, A. E. (2021). Single-Cell Immunoblotting Resolves Estrogen Receptor-α Isoforms in Breast Cancer. PloS One 16 (7), e0254783. doi:10.1371/journal.pone.0254783
Kim, S. N., Kim, S. Y., Yim, H. K., Lee, W. Y., Ham, K. S., Kim, S. K., et al. (1999). Effect of Dimethyl-4,4'-Dimethoxy-5,6,5',6'-Dimethylenedioxybiphenyl-2,2'- Dicarboxylate (DDB) on Chemical-Induced Liver Injury. Biol. Pharm. Bull. 22 (1), 93–95. doi:10.1248/bpb.22.93
Klein, D. J., Thorn, C. F., Desta, Z., Flockhart, D. A., Altman, R. B., and Klein, T. E. (2013). PharmGKB Summary: Tamoxifen Pathway, Pharmacokinetics. Pharmacogenet. Genomics 23 (11), 643–647. doi:10.1097/FPC.0b013e3283656bc1
Kloos, I., Delaloge, S., Pautier, P., Di Palma, M., Goupil, A., Duvillard, P., et al. (2002). Tamoxifen-Related Uterine Carcinosarcomas Occur Under/After Prolonged Treatment: Report of Five Cases and Review of the Literature. Int. J. Gynecol. Cancer 12 (5), 496–500. doi:10.1046/j.1525-1438.2002.01134.x
Kon, A., Yuan, B., Hanazawa, T., Kikuchi, H., Sato, M., Furutani, R., et al. (2013). Contribution of Membrane Progesterone Receptor α to the Induction of Progesterone-Mediated Apoptosis Associated with Mitochondrial Membrane Disruption and Caspase Cascade Activation in Jurkat Cell Lines. Oncol. Rep. 30 (4), 1965–1970. doi:10.3892/or.2013.2657
Kremser, T., Evans, A., Moore, A., Luxford, K., Begbie, S., Bensoussan, A., et al. (2008). Use of Complementary Therapies by Australian Women with Breast Cancer. Breast 17 (4), 387–394. doi:10.1016/j.breast.2007.12.006
Li, C., Kim, M., Choi, H., and Choi, J. (2011a). Effects of Baicalein on the Pharmacokinetics of Tamoxifen and its Main Metabolite, 4-Hydroxytamoxifen, in Rats: Possible Role of Cytochrome P450 3A4 and P-Glycoprotein Inhibition by Baicalein. Arch. Pharm. Res. 34 (11), 1965–1972. doi:10.1007/s12272-011-1117-9
Li, C., Lim, S. C., Kim, J., and Choi, J. S. (2011b). Effects of Myricetin, an Anticancer Compound, on the Bioavailability and Pharmacokinetics of Tamoxifen and its Main Metabolite, 4-Hydroxytamoxifen, in Rats. Eur. J. Drug Metab. Pharmacokinet. 36 (3), 175–182. doi:10.1007/s13318-011-0036-y
Li, H., Wu, C., Liu, Y., Zhang, S., and Gao, X. (2019). Baihe Zhimu Formula Attenuates the Efficacy of Tamoxifen Against Breast Cancer in Mice Through Modulation of CYP450 Enzymes. BMC Complement. Altern. Med. 19 (1), 240. doi:10.1186/s12906-019-2651-0
Lichota, A., and Gwozdzinski, K. (2018). Anticancer Activity of Natural Compounds from Plant and Marine Environment. Int. J. Mol. Sci. 19 (11), 3533. doi:10.3390/ijms19113533
Lin, Y. S., Lin, Y. Y., Yang, Y. H., Lin, C. L., Kuan, F. C., Lu, C. N., et al. (2018). Antrodia Cinnamomea Extract Inhibits the Proliferation of Tamoxifen-Resistant Breast Cancer Cells through Apoptosis and skp2/microRNAs Pathway. BMC Complement. Altern. Med. 18 (1), 152. doi:10.1186/s12906-018-2204-y
Liu, Y. L., Yang, P. M., Shun, C. T., Wu, M. S., Weng, J. R., and Chen, C. C. (2010). Autophagy Potentiates the Anti-Cancer Effects of the Histone Deacetylase Inhibitors in Hepatocellular Carcinoma. Autophagy 6 (8), 1057–1065. doi:10.4161/auto.6.8.13365
Lorizio, W., Wu, A. H., Beattie, M. S., Rugo, H., Tchu, S., Kerlikowske, K., et al. (2012). Clinical and Biomarker Predictors of Side Effects from Tamoxifen. Breast Cancer Res. Treat. 132 (3), 1107–1118. doi:10.1007/s10549-011-1893-4
Ma, H., Li, L., Dou, G., Wang, C., Li, J., He, H., et al. (2017). Z-Ligustilide Restores Tamoxifen Sensitivity of ERa Negative Breast Cancer Cells by Reversing MTA1/IFI16/HDACs Complex Mediated Epigenetic Repression of ERa. Oncotarget 8 (17), 29328–29345. doi:10.18632/oncotarget.16440
Mai, Z., Blackburn, G. L., and Zhou, J. R. (2007). Genistein Sensitizes Inhibitory Effect of Tamoxifen on the Growth of Estrogen Receptor-Positive and HER2-Overexpressing Human Breast Cancer Cells. Mol. Carcinog. 46 (7), 534–542. doi:10.1002/mc.20300
Malumbres, M., and Barbacid, M. (2009). Cell Cycle, CDKs and Cancer: A Changing Paradigm. Nat. Rev. Cancer 9 (3), 153–166. doi:10.1038/nrc2602
Mansour, A., Daba, A., Baddour, N., El-Saadani, M., and Aleem, E. (2012). Schizophyllan Inhibits the Development of Mammary and Hepatic Carcinomas Induced by 7,12 Dimethylbenz(α)anthracene and Decreases Cell Proliferation: Comparison with Tamoxifen. J. Cancer Res. Clin. Oncol. 138 (9), 1579–1596. doi:10.1007/s00432-012-1224-0
Matthews, A. K., Sellergren, S. A., Huo, D., List, M., and Fleming, G. (2007). Complementary and Alternative Medicine Use Among Breast Cancer Survivors. J. Altern. Complement. Med. 13 (5), 555–562. doi:10.1089/acm.2007.03-9040
McCloy, R. A., Shelley, E. J., Roberts, C. G., Boslem, E., Biden, T. J., Nicholson, R. I., et al. (2013). Role of Endoplasmic Reticulum Stress Induction by the Plant Toxin, Persin, in Overcoming Resistance to the Apoptotic Effects of Tamoxifen in Human Breast Cancer Cells. Br. J. Cancer 109 (12), 3034–3041. doi:10.1038/bjc.2013.693
Miller, K. D., Nogueira, L., Mariotto, A. B., Rowland, J. H., Yabroff, K. R., Alfano, C. M., et al. (2019). Cancer Treatment and Survivorship Statistics, 2019. CA Cancer J. Clin. 69 (1), 363–385. doi:10.3322/caac.21565
Moon, B., Kim, W., Park, C. H., and Oh, S. M. (2018). Ginkgo Biloba Extract (EGb761) Did Not Express Estrogenic Activity in an Immature Rat Uterotrophic Assay. Environ. Health Toxicol. 33 (3), e2018016. doi:10.5620/eht.e2018016
Moretti, L., Yang, E. S., Kim, K. W., and Lu, B. (2007). Autophagy Signaling in Cancer and its Potential as Novel Target to Improve Anticancer Therapy. Drug Resist. Updat. 10 (4-5), 135–143. doi:10.1016/j.drup.2007.05.001
Nagaraju, G. P., Aliya, S., Zafar, S. F., Basha, R., Diaz, R., and El-Rayes, B. F. (2012). The Impact of Curcumin on Breast Cancer. Integr. Biol. (Camb) 4 (9), 996–1007. doi:10.1039/c2ib20088k
Nagata, C., Kabuto, M., and Shimizu, H. (1998). Association of Coffee, Green Tea, and Caffeine Intakes with Serum Concentrations of Estradiol and Sex Hormone-Binding Globulin in Premenopausal Japanese Women. Nutr. Cancer 30 (1), 21–24. doi:10.1080/01635589809514635
National Center for Biotechnology Information (2022). PubChem. Available at: https://pubchem.ncbi.nlm.nih.gov/ (Accessed March 21, 2022).
Noomhorm, N., Chang, C. J., Wen, C. S., Wang, J. Y., Chen, J. L., Tseng, L. M., et al. (2014). In Vitro and In Vivo Effects of Xanthorrhizol on Human Breast Cancer MCF-7 Cells Treated with Tamoxifen. J. Pharmacol. Sci. 125 (4), 375–385. doi:10.1254/jphs.14024fp
Novák, B., and Tyson, J. J. (2003). Modelling the Controls of the Eukaryotic Cell Cycle. Biochem. Soc. Trans. 31 (Pt 6), 1526–1529. doi:10.1042/bst0311526
Oleaga, C., García, M., Solé, A., Ciudad, C. J., Izquierdo-Pulido, M., and Noé, V. (2012). CYP1A1 Is Overexpressed Upon Incubation of Breast Cancer Cells with a Polyphenolic Cocoa Extract. Eur. J. Nutr. 51 (4), 465–476. doi:10.1007/s00394-011-0231-2
Oon, S. F., Nallappan, M., Tee, T. T., Shohaimi, S., Kassim, N. K., Sa'ariwijaya, M. S., et al. (2015). Xanthorrhizol: A Review of its Pharmacological Activities and Anticancer Properties. Cancer Cel. Int. 15, 100. doi:10.1186/s12935-015-0255-4
Palit, S., Kar, S., Sharma, G., and Das, P. K. (2015). Hesperetin Induces Apoptosis in Breast Carcinoma by Triggering Accumulation of ROS and Activation of ASK1/JNK Pathway. J. Cel. Physiol. 230 (8), 1729–1739. doi:10.1002/jcp.24818
Pan, J., Yang, C., Jiang, Z., and Huang, J. (2019). Trametes Robiniophila Murr: A Traditional Chinese Medicine with Potent Anti-Tumor Effects. Cancer Manag. Res. 11, 1541–1549. doi:10.2147/CMAR.S193174
Park, M. H., Choi, M. S., Kwak, D. H., Oh, K. W., Yoon, D. Y., Han, S. B., et al. (2011). Anti-Cancer Effect of Bee Venom in Prostate Cancer Cells Through Activation of Caspase Pathway via Inactivation of NF-Κb. Prostate 71 (8), 801–812. doi:10.1002/pros.21296
Pawlik, A., Słomińska-Wojewódzka, M., and Herman-Antosiewicz, A. (2016). Sensitization of Estrogen Receptor-Positive Breast Cancer Cell Lines to 4-Hydroxytamoxifen by Isothiocyanates Present in Cruciferous Plants. Eur. J. Nutr. 55 (3), 1165–1180. doi:10.1007/s00394-015-0930-1
Piao, Y., Shin, S. C., and Choi, J. S. (2008). Effects of Oral Kaempferol on the Pharmacokinetics of Tamoxifen and One of its Metabolites, 4-Hydroxytamoxifen, After Oral Administration of Tamoxifen to Rats. Biopharm. Drug Dispos 29 (4), 245–249. doi:10.1002/bdd.593
Pistritto, G., Trisciuoglio, D., Ceci, C., Garufi, A., and D'Orazi, G. (2016). Apoptosis as Anticancer Mechanism: Function and Dysfunction of its Modulators and Targeted Therapeutic Strategies. Aging (Albany NY) 8 (4), 603–619. doi:10.18632/aging.100934
Qi, H., Jiang, Z., Wang, C., Yang, Y., Li, L., He, H., et al. (2017). Sensitization of Tamoxifen-Resistant Breast Cancer Cells by Z-Ligustilide through Inhibiting Autophagy and Accumulating DNA Damages. Oncotarget 8 (17), 29300–29317. doi:10.18632/oncotarget.16832
Qi, W., Sun, M., Kong, X., Li, Y., Wang, X., Lv, S., et al. (2016). Huaier Extract Synergizes with Tamoxifen to Induce Autophagy and Apoptosis in ER-Positive Breast Cancer Cells. Oncotarget 7 (18), 26003–26015. doi:10.18632/oncotarget.8303
Rahate, K. P., and Rajasekaran, A. (2015). Hepatoprotection by Active Fractions from Desmostachya Bipinnata Stapf (L.) against Tamoxifen-Induced Hepatotoxicity. Indian J. Pharmacol. 47 (3), 311–315. doi:10.4103/0253-7613.157130
Riggs, B. L., and Hartmann, L. C. (2003). Selective Estrogen-Receptor Modulators -- Mechanisms of Action and Application to Clinical Practice. N. Engl. J. Med. 348 (7), 618–629. doi:10.1056/NEJMra022219
Roberts, C. G., Gurisik, E., Biden, T. J., Sutherland, R. L., and Butt, A. J. (2007). Synergistic Cytotoxicity between Tamoxifen and the Plant Toxin Persin in Human Breast Cancer Cells Is Dependent on Bim Expression and Mediated by Modulation of Ceramide Metabolism. Mol. Cancer Ther. 6 (10), 2777–2785. doi:10.1158/1535-7163.MCT-07-0374
Rondón-Lagos, M., Villegas, V. E., Rangel, N., Sánchez, M. C., and Zaphiropoulos, P. G. (2016). Tamoxifen Resistance: Emerging Molecular Targets. Int. J. Mol. Sci. 17 (8), 1357. doi:10.3390/ijms17081357
Rostock, M., Fischer, J., Mumm, A., Stammwitz, U., Saller, R., and Bartsch, H. H. (2011). Black Cohosh (Cimicifuga Racemosa) in Tamoxifen-Treated Breast Cancer Patients with Climacteric Complaints - a Prospective Observational Study. Gynecol. Endocrinol. 27 (10), 844–848. doi:10.3109/09513590.2010.538097
Saggar, J. K., Chen, J., Corey, P., and Thompson, L. U. (2010). Dietary Flaxseed Lignan or Oil Combined with Tamoxifen Treatment Affects MCF-7 Tumor Growth Through Estrogen Receptor- and Growth Factor-Signaling Pathways. Mol. Nutr. Food Res. 54 (3), 415–425. doi:10.1002/mnfr.200900068
Sakata, M., Ikeda, T., Imoto, S., Jinno, H., and Kitagawa, Y. (2011). Prevention of Mammary Carcinogenesis in C3H/OuJ Mice by Green Tea and Tamoxifen. Asian Pac. J. Cancer Prev. 12 (2), 567–571.
Sartippour, M. R., Pietras, R., Marquez-Garban, D. C., Chen, H. W., Heber, D., Henning, S. M., et al. (2006). The Combination of Green Tea and Tamoxifen Is Effective Against Breast Cancer. Carcinogenesis 27 (12), 2424–2433. doi:10.1093/carcin/bgl066
Shin, S. C., and Choi, J. S. (2009). Effects of Epigallocatechin Gallate on the Oral Bioavailability and Pharmacokinetics of Tamoxifen and its Main Metabolite, 4-Hydroxytamoxifen, in Rats. Anticancer Drugs 20 (7), 584–588. doi:10.1097/CAD.0b013e32832d6834
Shin, S. C., Choi, J. S., and Li, X. (2006). Enhanced Bioavailability of Tamoxifen After Oral Administration of Tamoxifen with Quercetin in Rats. Int. J. Pharm. 313 (1-2), 144–149. doi:10.1016/j.ijpharm.2006.01.028
Shin, S. C., Piao, Y. J., and Choi, J. S. (2008). Effects of Morin on the Bioavailability of Tamoxifen and its Main Metabolite, 4-Hydroxytamoxifen, in Rats. In Vivo 22 (3), 391–395.
Sparreboom, A., Cox, M. C., Acharya, M. R., and Figg, W. D. (2004). Herbal Remedies in the United States: Potential Adverse Interactions with Anticancer Agents. J. Clin. Oncol. 22 (12), 2489–2503. doi:10.1200/JCO.2004.08.182
Tanos, V., Brzezinski, A., Drize, O., Strauss, N., and Peretz, T. (2002). Synergistic Inhibitory Effects of Genistein and Tamoxifen on Human Dysplastic and Malignant Epithelial Breast Cells In Vitro. Eur. J. Obstet. Gynecol. Reprod. Biol. 102 (2), 188–194. doi:10.1016/s0301-2115(01)00582-6
Tocaciu, S., Oliver, L. J., Lowenthal, R. M., Peterson, G. M., Patel, R., Shastri, M., et al. (2018). The Effect of Undaria Pinnatifida Fucoidan on the Pharmacokinetics of Letrozole and Tamoxifen in Patients with Breast Cancer. Integr. Cancer Ther. 17 (1), 99–105. doi:10.1177/1534735416684014
Tröger, W., Zdrale, Z., Tišma, N., and Matijašević, M. (2014). Additional Therapy with a Mistletoe Product During Adjuvant Chemotherapy of Breast Cancer Patients Improves Quality of Life: An Open Randomized Clinical Pilot Trial. Evid Based. Complement. Altern. Med.. London 2014. 430518. doi:10.1155/2014/430518
Tsai, Y. T., Lai, J. N., and Wu, C. T. (2014). The Use of Chinese Herbal Products and its Influence on Tamoxifen Induced Endometrial Cancer Risk Among Female Breast Cancer Patients: A Population-Based Study. J. Ethnopharmacol. 155 (2), 1256–1262. doi:10.1016/j.jep.2014.07.008
Vardy, J., Dhillon, H. M., Clarke, S. J., Olesen, I., Leslie, F., Warby, A., et al. (2013). Investigation of Herb-Drug Interactions with Ginkgo Biloba in Women Receiving Hormonal Treatment for Early Breast Cancer. SpringerPlus 2 (1), 126. doi:10.1186/2193-1801-2-126
Viedma-Rodríguez, R., Baiza-Gutman, L., Salamanca-Gómez, F., Diaz-Zaragoza, M., Martínez-Hernández, G., Ruiz Esparza-Garrido, R., et al. (2014). Mechanisms Associated with Resistance to Tamoxifen in Estrogen Receptor-Positive Breast Cancer (Review). Oncol. Rep. 32 (1), 3–15. doi:10.3892/or.2014.3190
Vlaisavljevic, S., Kaurinovic, B., Popovic, M., Djurendic-Brenesel, M., Vasiljevic, B., Cvetkovic, D., et al. (2014). Trifolium Pratense L. As a Potential Natural Antioxidant. Molecules 19 (1), 713–725. doi:10.3390/molecules19010713
Wanchai, A., Armer, J. M., and Stewart, B. R. (2010). Complementary and Alternative Medicine Use Among Women with Breast Cancer: A Systematic Review. Clin. J. Oncol. Nurs. 14 (4), E45–E55. doi:10.1188/10.CJON.E45-E55
Wang, X., Gao, P., Long, M., Lin, F., Wei, J. X., Ren, J. H., et al. (2011). Essential Role of Cell Cycle Regulatory Genes P21 and P27 Expression in Inhibition of Breast Cancer Cells by Arsenic Trioxide. Med. Oncol. 28 (4), 1225–1254. doi:10.1007/s12032-010-9552-x
Weissenstein, U., Kunz, M., Oufir, M., Wang, J. T., Hamburger, M., Urech, K., et al. (2019). Absence of Herb-Drug Interactions of Mistletoe with the Tamoxifen Metabolite (E/Z)-Endoxifen and Cytochrome P450 3A4/5 and 2D6 In Vitro. BMC Complement. Altern. Med. 19 (1), 23. doi:10.1186/s12906-019-2439-2
Wu, C. T., Lai, J. N., and Tsai, Y. T. (2014). The Prescription Pattern of Chinese Herbal Products that Contain Dang-Qui and Risk of Endometrial Cancer Among Tamoxifen-Treated Female Breast Cancer Survivors in Taiwan: A Population-Based Study. PloS One 9 (12), e113887. doi:10.1371/journal.pone.0113887
Wu, N., Xie, Y., and Yang, B. B. (2017). Anti-Cancer Drugs for Cardioprotection. Cell Cycle (Georgetown, Tex.) 16 (2), 155–156. doi:10.1080/15384101.2016.1242536,
Xu, Y., Chen, X., Chen, X., Bian, W., Yao, C., Zhang, X., et al. (2016). San Huang Decoction Downregulates Aurora Kinase A to Inhibit Breast Cancer Cell Growth and Enhance Chemosenstivity to Anti-Tumor Drugs. Pathol. Res. Pract. 212 (8), 696–703. doi:10.1016/j.prp.2016.05.004
Yaacob, N. S., Kamal, N. N., and Norazmi, M. N. (2014). Synergistic Anticancer Effects of a Bioactive Subfraction of Strobilanthes Crispus and Tamoxifen on MCF-7 and MDA-MB-231 Human Breast Cancer Cell Lines. BMC Complement. Altern. Med. 14, 252. doi:10.1186/1472-6882-14-252
Yaacob, N. S., Nik Mohamed Kamal, N. N., Wong, K. K., and Norazmi, M. N. (2015). Cell Cycle Modulation of MCF-7 and MDA-MB-231 by a Sub- Fraction of Strobilanthes Crispus and its Combination with Tamoxifen. Asian Pac. J. Cancer Prev. 16 (18), 8135–8140. doi:10.7314/apjcp.2015.16.18.8135
Yang, G., Nowsheen, S., Aziz, K., and Georgakilas, A. G. (2013). Toxicity and Adverse Effects of Tamoxifen and Other Anti-Estrogen Drugs. Pharmacol. Ther. 139 (3), 392–404. doi:10.1016/j.pharmthera.2013.05.005
Keywords: breast cancer, natural products, herbal medicines, tamoxifen, pharmacodynamic, pharmacokinetic, interactions, mechanisms
Citation: Yen C, Zhao F, Yu Z, Zhu X and Li CG (2022) Interactions Between Natural Products and Tamoxifen in Breast Cancer: A Comprehensive Literature Review. Front. Pharmacol. 13:847113. doi: 10.3389/fphar.2022.847113
Received: 01 January 2022; Accepted: 14 April 2022;
Published: 02 June 2022.
Edited by:
Rosa Ventura-Martinez, Universidad Nacional Autónoma de México, MexicoReviewed by:
Paramita Basu, University of Pittsburgh, United StatesPatricia Garcia-Lopez, National Institute of Cancerology (INCAN), Mexico
Mariano Martinez-Vazquez, Universidad Nacional Autónoma de México, Mexico
Copyright © 2022 Yen, Zhao, Yu, Zhu and Li. This is an open-access article distributed under the terms of the Creative Commons Attribution License (CC BY). The use, distribution or reproduction in other forums is permitted, provided the original author(s) and the copyright owner(s) are credited and that the original publication in this journal is cited, in accordance with accepted academic practice. No use, distribution or reproduction is permitted which does not comply with these terms.
*Correspondence: Chun Guang Li, Yy5saUB3ZXN0ZXJuc3lkbmV5LmVkdS5hdQ==
†These authors have contributed equally to this work