- 1CiberNed. Network Center for Neurodegenerative Diseases, National Spanish Health Institute Carlos III, Madrid, Spain
- 2Molecular Neurobiology Laboratory, Department of Biochemistry and Molecular Biolomedicine, Universitat de Barcelona, Barcelona, Spain
- 3School of Chemistry, Universitat de Barcelona, Barcelona, Spain
- 4Medicinal Chemistry Institute, Spanish National Research Council, Madrid, Spain
- 5Department of Biochemistry and Physiology, Faculty of Pharmacy and Food Science, Universitat de Barcelona, Barcelona, Spain
The classical terms agonists and antagonists for G protein coupled receptors (GPCRs) have often become misleading. Even the biased agonism concept does not describe all the possibilities already demonstrated for GPCRs. The cannabinoid CB2 receptor (CB2R) emerged as a promising target for a variety of diseases. Reasons for such huge potential are centered around the way drugs sit in the orthosteric and/or exosites of the receptor. On the one hand, a given drug in a specific CB2R conformation leads to a signaling cascade that differs qualitatively and/or quantitatively from that triggered by another drug. On the other hand, a given drug may lead to different signaling outputs in two different tissues (or cell contexts) in which the conformation of the receptor is affected by allosteric effects derived from interactions with other proteins or with membrane lipids. This highlights the pharmacological complexity of this receptor and the need to further unravel the binding mode of CB2R ligands in order to fine-tune signaling effects and therapeutic propositions.
Introduction
G protein-coupled receptors (GPCRs) are the target of about 40% of current drugs (Hauser et al., 2017). Although the potential of GPCRs as therapeutic targets is still considered to be high, there have been only a few recent approvals of drugs targeting these receptors. The causes are multifactorial, but perhaps the main one is the increased demands, in terms of efficacy and safety, by regulatory bodies. Functional selectivity is a property of GPCRs that has recently become relevant to overcome the issues related to the lack of success of GPCR-targeted drug candidates (Chang and Bruchas, 2014; Franco et al., 2018). For therapeutic purposes, functional selectivity of a given compound acting on the targeted receptor could achieve the desired effect(s) while minimizing side effects. A simplified version of the full potential of functional selectivity is the concept of biased agonism. Biased agonism is now considered across all platforms developing therapeutic drugs in both industry and academia. A compound selectively modulating a signaling pathway could offer a suitable therapeutic benefit compared to a another agonist that could, in parallel, induce undesired signaling events. The structural features of the cannabinoid receptors (CBRs) offer more possibilities of biased signaling as the orthosteric site is not open to the extracellular milieu. Here we aim to review the multiple therapeutic possibilities resulting from targeting the cannabinoid receptor type 2 (CB2R) orthosteric and/or non-orthosteric sites. At present, CB2R appears as more promising in drug discovery than the cannabinoid receptor type 1 (CB1R) as some of CB1R agonists have psychotropic effects and an antagonist approved for human use (for weight control) was withdrawn due to serious side effects (Christensen et al., 2007; Sam et al., 2011). In fact, ligands for CB2R seem to be generally safe and irrespective of whether they are agonists or antagonists. Safety however will not be considered in the present article.
Orthosteric and Non-Orthosteric Sites in the CB2R
Modes of Ligand Binding to the Orthosteric Site
The canonical Gα protein subunit for CB1R and CB2R is Gαi. Therefore, activation of these receptors leads to inactivation of the adenylate cyclase with the subsequent decrease in cAMP and deactivation of protein kinase A-mediated signaling. However, activation of CBRs may also lead to activation of the mitogen-activated protein kinases (MAPK) signaling cascade, regulation of ion channels, and recruitment of ß-arrestins, with subsequent regulation of Tyr kinase activity among others (Alexander et al., 2021).
Binding to GPCRs using radiolabeled compounds leads to detect one or two sites. Two sites reflect two different populations that, in the well-studied adenosine A1 GPCR, correspond to the receptor uncoupled or coupled to the G protein. Uncoupled receptors display low affinity for agonists whereas G-protein coupled receptors display high affinity. These two affinity sites for the A1 receptor can only be detected using agonists, i.e. antagonists have similar affinities for G-protein coupled and uncoupled A1 receptors (see (Casadó et al., 1990) and references therein). To our knowledge radioligand binding to the CB2R results in the detection of one single population. The two radioligands frequently used for measuring the binding to cannabinoid receptors, [3H]WIN55,212-2 and [3H]CP 55,940, are considered very potent orthosteric agonists of both CBRs, CB1R and CB2R. Competition assays using radioligands and non-labeled compounds in heterologous cells expressing CB2R showed that affinities were consistent, i.e., WIN55,212-2 competed with similar low nanomolar affinity the binding of [3H]WIN55,212-2 and of [3H]CP 55,940. In similar conditions, a naturally occurring cannabinoid, cannabigerol, competed for the binding of [3H]WIN55,212-2 or [3H]CP 55,940 with a Ki in the micromolar range (Navarro et al., 2018b; Navarro et al., 2020b). This result did not fit with the decrease in cytosolic cAMP concentration obtained by nanomolar amounts of the compound. The main difference in the experimental setup was the use of isolated membranes for radioligand binding and of living cells for cAMP level measurements. The availability of novel approaches to obtain reliable receptor binding data in living cells is fortunately increasing, indeed, these methods do not require radiolabeled compounds. On using the SNAP-tag technology in cells expressing the tagged CB2R and a validated “hot” compound (Martinez-Pinilla et al., 2016), the Ki for cannabigerol competition was 152 nM (Navarro et al., 2018b; Navarro et al., 2020b). These results show that the measured affinity of a given compound depends on the probe used for binding and allows identification of different states of the receptor or different modes to accommodate the ligand within the orthosteric center. In the case of the CB1R, differences are more extreme as, in radioligand binding assays, natural cannabinoids may compete for the binding of [3H]WIN55,212-2 but not of [3H]CP 55,940. For instance, cannabigerol binding to CB2R is similar if measured using [3H]WIN55,212-2 or [3H]CP 55,940, whereas there is no significant competition of binding to the CB1R when [3H]CP 55,940 is used. In summary, cannabigerol binds to a subcompartment of the orthosteric site of the CB1R, i.e., the orthosteric site of this receptor may be simultaneously occupied by cannabigerol and [3H]CP 55,940. These relatively recent findings add useful information to understand the variety of actions that different cannabinoids exert and also the experimental diversity between laboratories in the values of affinity and potency. This diversity may also underlie the enormous potential of cannabinoid receptors to combat a wide variety of diseases (see (Franco et al., 2020) and references therein).
Identification of Non-Orthosteric Sites
Cannabidiol, one of the main components of Cannabis Sativa L. has been instrumental to detect non-orthosteric centers in CBRs. This phytocannabinoid exerts physiological effects via a variety of receptors, located both in the cell surface and inside cells. Apart from interacting with CBRs, it may interact with serotonin and peroxisome proliferator-activated receptors (Banerjee et al., 1975; Russo et al., 2005; O’Sullivan et al., 2009; O’Sullivan and Kendall, 2010; Espejo-Porras et al., 2013; Fernández-Ruiz et al., 2013; De Gregorio et al., 2019; Franco et al., 2019b; Franco et al., 2020; Echeverry et al., 2021). At first cannabidiol was considered an orthosteric ligand able to partially activate cannabinoid receptors although with low potency (McPartland et al., 2007). Recent results in two different laboratories have shown that this compound can interact in an allosteric mode with the two CBRs (Laprairie et al., 2015; Martínez-Pinilla et al., 2017). For both receptors, CB1R and CB2R, it acts as a negative allosteric modulator (NAM) when co-administered with an orthosteric ligand. At CB2R it minimized the effects of JWH133 on the MAP kinase signaling pathway (Martínez-Pinilla et al., 2017). Thus, cannabidiol binds to an allosteric site at nanomolar concentrations while micromolar concentrations are required for significant binding to the orthosteric site. Accordingly, the in vitro results depend on the concentration while the in vivo actions at moderate doses should be mainly due to its binding to the allosteric site that has been very recently suggested to be close to the receptor entrance (Navarro et al., 2021) (See section: “Structural Insights into CB2R Binding Modes”). As would be expected from an allosteric mode of action, the binding of the compound to the allosteric site causes conformational changes in such a way that biases the effect of orthosteric agonists (Navarro et al., 2018a). A more recent report shows that structural changes in the molecule shifts negative to positive modulation (of the CB2R) thus confirming its allosteric nature (Navarro et al., 2021).
Novel approaches to achieve signaling diversity and addressing success in drug discovery are attempting the design of bitopic ligands that bind the orthosteric site and an allosteric site (Lane et al., 2013; Mohr et al., 2013; Bradley and Tobin, 2016). By combining experimental and in silico approaches an allosteric site was identified at the entrance of the orthosteric binding site of the ß-adrenergic GPCRs (González et al., 2011). This site has been termed the -extracellular- vestibule (Dror et al., 2011) or entrance (Wang et al., 2013), also metastable (Fronik et al., 2017) or secondary (González et al., 2011) binding site. Exosite is also used to describe such non-orthosteric sites when they are located at the lipidic-receptor interface (Masureel et al., 2018). Bitopic ligands designed according to these findings improve subfamily selectivity (Medina et al., 2014; Masureel et al., 2018); they also offer signaling bias and better off-rates (Valant et al., 2012; Lane et al., 2013). Knowing that unlike GPCRs for polar compounds, CBRs do not have the orthosteric center accessible from the extracellular milieu, we designed bitopic ligands able to enter into the CB2R orthosteric site but also able to interact with amino acids located at the receptor transmembrane portals (Morales et al., 2020). Signaling assays in the CB2R wild-type and specific mutants led us to discover the first CB2R bitopic ligands. These compounds, which consist of two chromenopyrazole moieties linked by methylene spacers of different lengths, can bind to the orthosteric site and to an exosite. Bitopic ligands showed to be CB2R selective and, as depicted in Figure 1, may likely extend from the orthosteric site, the vestibule and an “allosteric exosite” able to accommodate the same moiety that sits in the orthosteric site.
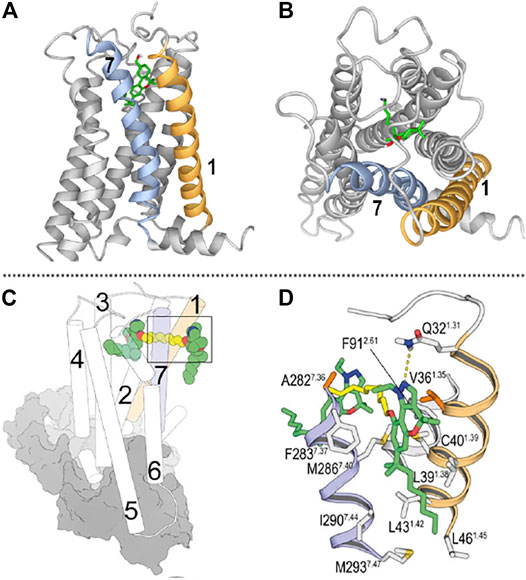
FIGURE 1. (A) Lateral view of the CB2R/AM12033 complex from the entrance portal formed by transmembrane helices (TMs) 1 and 7 (shown in orange and blue, respectively). (B) View from the outside of the cell of the CB2R in complex with the agonist AM12033 (PDB-ID 6KPF); ligand access from extracellular is blocked by the N-terminus and the EC loops. (C) General view of the binding mode of a CB2R bitopic ligand [molecule 22 in (Morales et al., 2020)] into the orthosteric site and the vestibule of the CB2R-Gi complex (depicted as cylinders for CB2R and grey surfaces for Gi). (D) Detailed view of the binding mode of ligand 22 into the receptor vestibule obtained during the MD simulations. TMs 1 and 7 are shown in orange and blue, respectively; and the pharmacophore units and spacer of bitopic ligands are shown in green and yellow tubes, respectively. (C,D) have been reproduced from our previously reported article (Morales et al., 2020); permitted reproduction under the terms and conditions of the Creative Commons Attribution (CC BY) license (https://creativecommons.org/licenses/by/4.0/).
Structural Insights Into CB2R Binding Modes
As previously mentioned, in recent years, the CB2R has been resolved in its active (Hua et al., 2020; Xing et al., 2020) and inactive (Li et al., 2019) states, enlightening the structural knowledge of crucial domains for G protein activation as well as ligand binding. Not only CB2R but also CB1R and other class A lipid GPCRs have structural features that determine the lipophilic nature of their ligands (Hua et al., 2016; Hua et al., 2017; Krishna Kumar et al., 2019; Shao et al., 2019). On the one hand, the extracellular loops and the N-terminus of these receptors are generally structured over the orthosteric binding pocket occluding ligand entrance from the extracellular milieu. Moreover, transmembrane openings have been detected in these receptors acting as portals for lateral access of ligands to the binding crevice. Therefore, hydrophobic ligands such as phytocannabinoids need to diffuse through the lipid membrane to target binding sites. Figure 1A,B shows these features in the recently released structure of CB2R in complex with Gαi.
Class A GPCR allosteric sites are widely distributed in different receptor domains including intracellular, intrahelical or exosites. For instance, the CB1R has been resolved bound to the NAM ORG27569 and the agonist CP55940 (Shao et al., 2019). This crystal structure revealed the ability of ORG27569 to target an extrahelical exosite within the inner leaflet of the lipid bilayer. Even though few CB2R allosteric modulators have been reported and none resolved in complex with the receptor, molecular dynamic and mutagenic studies have recently shown the potential allosteric site of CBD in CB2R (Navarro et al., 2021). This report shows that CBD can bind to an allosteric cavity close to the receptor entrance in a transmembrane portal defined by transmembrane helices 1 and 7. As aforementioned, concomitant binding at orthosteric and allosteric/exosites has been shown at CB2R with chromenopyrazole bitopic ligands (Morales et al., 2020). Site-directed mutagenesis and molecular dynamic studies determined key interacting residues at transmembrane helices 1 and 7 which define the entry portal for these ligands (Figures 1C,D).
The CB2R structural understanding gained in the past few years will likely accelerate the rational drug design of CB2R modulators with optimal activity to address specific physiopathological conditions.
Bidirectional Information Exchange Between Ligand and CB2Rs
On the one hand, functional selectivity can result from different agonists that activate different populations of receptors, but also from agonists that produce different conformational changes in the receptor that allow different qualitative and/or quantitative signaling outputs. On the other hand, a given agonist can give rise to different signaling outputs depending on the conformation of the receptor’s orthosteric site, which can vary depending on the cell type and the fate of the cell (Fuxe et al., 1998; Urban et al., 2007; Kenakin and Miller, 2010; Rajagopal et al., 2011; Fuxe et al., 2014; Ladarre et al., 2014; Navarro et al., 2020a; Franco et al., 2021).
By definition, allosterism produces conformational changes that alter the binding of agonists to the orthosteric site and, consequently, also modify (qualitatively or quantitatively) signal transduction. Important to highlight is that allosterism is bidirectional, i.e. an orthosteric compound binding to a receptor leads to conformational changes that likely alter the affinity of the binding of the allosteric compound to the receptor (Christopoulos and Kenakin, 2002; May and Christopoulos, 2003; Smith et al., 2011). In practice this means that if an allosteric compound is suspected on the basis of changes in affinity of radiolabeled compound to the orthosteric site, the orthosteric compound should modify the affinity of the binding of the allosteric compound to the allosteric site. In the field of GPCR, this requirement has made difficult the identification of allosteric compounds, as there are few to none radiolabeled compounds designed to measure binding to allosteric sites. In the case of CB2R, the discovery of bitopic ligands together with the structure of the receptor leaves no doubt about the possibility of regulating the functionality of the receptor by “touching” allosteric/exosites.
Different Macromolecular Environments of the CB2R Impact agonist Binding and Effect
Can a given compound be more efficacious at targeting a cell that expresses CB2R in a particular conformation? and/or can a CB2R in a particular cell type be more likely to respond to the challenge of a given compound?
The pharmacology of cannabinoid receptors is complex. As discussed above, binding data can depend on the radioligand used as the probe, and the effects of a given compound on a given receptor are not always consistent across laboratories. At present we have enough data to realize that there are many possibilities for CB2R-mediated responses that may turn into novel and powerful possibilities for drug discovery.
The complex pharmacology of the CB2R has likely delayed the identification of CB2R-containing macromolecular complexes, whose occurrence has been demonstrated in natural sources (i.e. not only in heterologous expression systems). Such interactions modify binding and/or function. Current data suggest that the receptor environment modifies the conformation and, accordingly, the binding and effects of orthosteric and non-orthosteric ligands. Interaction of the CB2R with other GPCRs may be searched in http://www.gpcr-hetnet.com/ (using the gene name: CNR2) (Borroto-Escuela et al., 2014). Figure 2 shows the STRING analysis of the interactions of the receptor which indicates mandatory interactions with G proteins, and interactions with the CB1R and with other GPCRs. In www.gpcr-hetnet.com and in Figure 2 interactions of CB2R with further GPCRs are not yet included (they have not yet been incorporated into the STRING database). Also missing are the recently described interactions of the CB2R with glutamate N-Methyl-D-Asp (NMDA) ionotropic receptors (Rivas-Santisteban et al., 2021). From a therapeutic perspective, the fact that CB2R may interact with other receptors that are also targeted by cannabinoids, for instance with GPR18 and GPR55, is of high interest (Balenga et al., 2014; Reyes-Resina et al., 2018; Martínez-Pinilla et al., 2019; Martínez-Pinilla et al., 2020; Rivas-Santisteban et al., 2021).
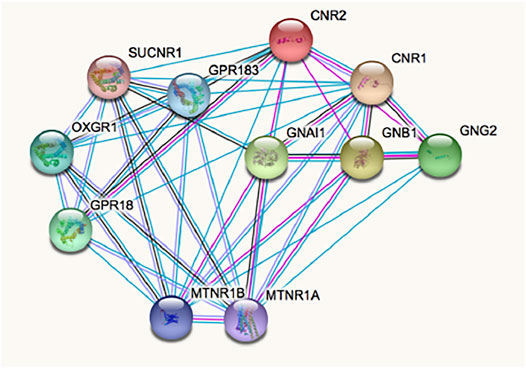
FIGURE 2. Interactions involving the CB2R according to STRING database for functional protein association networks. Abbreviations/gene products are: CNR2, CB2R; CNR1, CB1R; MTNR1A/1B, Melatonin GPCRs 1A/1B; OXGR1, Alpha-ketoglutarate receptor (a GPCR); SUCNR1, Succinate receptor 1 (a GPCR); GPR18 and GPR183 are orphan GPCRs; GNAl1, Guanine nucleotide-binding protein G(i) subunit alpha-1; GNB1, Guanine nucleotide-binding protein G(i) subunit ß-1; GNG2, Guanine nucleotide-binding protein Gi/Gs/Go subunit gamma-2.
So far, no major change has been detected concerning the nature of the G protein coupling of CB2R in a macromolecular environment as it occurs for D1 and D2 dopamine receptors. Whereas the D1 is coupled to Gαs and D2 to Gαi, the macromolecular complex formed when the two receptors are co-expressed in the same neuron couples to Gαq (Rashid et al., 2007; Hasbi et al., 2009; George et al., 2014; Perreault et al., 2015). Notwithstanding, conformational changes that affect the binding and signaling outputs produced by a given agonist have been shown in the interactions with the Gαi-coupled CB1R (Callén et al., 2012; Sierra et al., 2015; Angelats et al., 2018), the Gαs-coupled adenosine A2A receptor (Franco et al., 2019a), and the ionotropic NMDA receptor (Rivas-Santisteban et al., 2021).
In one of the first studies of biased agonism in GPCR heteromers (CB1R/CB2R), Navarro and co-workers showed that the allosteric effect of CBD was particularly noteworthy for the endocannabinoid anandamide but also that the effect tested using different agonists was smaller in the heteromer (Navarro et al., 2018a). These results confirmed that CBD acts as an allosteric modulator (for both receptors) also suggesting that the formation of the heteromer leads to conformational changes that make it less sensitive to the action of this phytocannabinoid. There are several examples of conformational changes induced by receptor-receptor interactions, i.e. by heteromer expression (Franco et al., 2007; Ferré et al., 2009; Franco et al., 2016). In the case of the CB2R, indirect evidence is provided by potentiation of receptor-mediated signaling when forming heteromers with the adenosine A2A receptor (Franco et al., 2019a).
Can Ligands Affect Conformation via Regulation of the CB2R Context?
The binding of orthosteric and non-orthosteric ligands alters the conformation of the receptor, but can ligands alter the environment? The answer to this question will take time as there is little background on the regulation of, for instance, heteromer formation.
Defining the target in the right context and delineating contextual changes due to ligand-induced regulation of the structure of the CB2R-contaning macromolecule, may further improve the rational design of therapeutic drugs (orthosteric and non-orthosteric) targeting the CB2R.
Author Contributions
RF, PM, NJ, GN and IR-R have scanned the literature and selected the cited articles. RF, PM and IR-R prepared the first draft. RF, PM, NJ, GN and IR-R agreed on the order and titles of the sections. PM prepared Figure 1. IR-R prepared Figure 2. All authors contributed to prepare an improved version. All authors edited the manuscript and have read and approved the final version of the review.
Funding
This work was partially supported by AARFD-17-503612 grant of the US Alzheimer’s Association and by grants SAF 2017-84117-R, RTI 2018-094204-B-I00, RTI 2018-095544-B-I00 and PID 2020-113430RB-I00 funded by Spanish MCIN/AEI/10.13039/501100011033 and, as appropriate, by “ERDF A way of making Europe”, by the “European Union” or by the “European Union Next Generation EU/PRTR”. PM is a postdoctoral fellow supported by the Juan de la Cierva Programme-MICIU (IJC 2019-042182-I). The research group of the University of Barcelona is considered of excellence (grup consolidat #2017 SGR 1497) by the Regional Catalonian Government, which does not provide any specific funding for reagents or for payment of services or Open Access fees.
Conflict of Interest
The authors declare that the research was conducted in the absence of any commercial or financial relationships that could be construed as a potential conflict of interest.
Publisher’s Note
All claims expressed in this article are solely those of the authors and do not necessarily represent those of their affiliated organizations, or those of the publisher, the editors and the reviewers. Any product that may be evaluated in this article, or claim that may be made by its manufacturer, is not guaranteed or endorsed by the publisher.
References
Alexander, S. P., Christopoulos, A., Davenport, A. P., Kelly, E., Mathie, A., Peters, J. A., et al. (2021). The Concise Guide to Pharmacology 2021/22: G Protein-Coupled Receptors. Br. J. Pharmacol. 178, S27–S156. doi:10.1111/BPH.15538
Angelats, E., Requesens, M., Aguinaga, D., Kreutz, M. R., Franco, R., and Navarro, G. (2018). Neuronal Calcium and cAMP Cross-Talk Mediated by Cannabinoid CB1 Receptor and EF-Hand Calcium Sensor Interactions. Front. Cell Dev. Biol. 6, 67–69. doi:10.3389/fcell.2018.00067
Balenga, N. A., Martínez-Pinilla, E., Kargl, J., Schröder, R., Peinhaupt, M., Platzer, W., et al. (2014). Heteromerization of GPR55 and Cannabinoid CB2 Receptors Modulates Signalling. Br. J. Pharmacol. 171, 5387–5406. doi:10.1111/bph.12850
Banerjee, S. P., Snyder, S. H., and Mechoulam, R. (1975). Cannabinoids: Influence on Neurotransmitter Uptake in Rat Brain Synaptosomes. J. Pharmacol. Exp. Ther. 194, 74–81. Available at: http://www.ncbi.nlm.nih.gov/pubmed/168349 (Accessed August 15, 2019).
Borroto-Escuela, D. O., Brito, I., Romero-Fernandez, W., Di Palma, M., Oflijan, J., Skieterska, K., et al. (2014). The G Protein-Coupled Receptor Heterodimer Network (GPCR-HetNet) and its Hub Components. Int. J. Mol. Sci. 15, 8570–8590. doi:10.3390/ijms15058570
Bradley, S. J., and Tobin, A. B. (2016). Design of Next-Generation G Protein-Coupled Receptor Drugs: Linking Novel Pharmacology and In Vivo Animal Models. Annu. Rev. Pharmacol. Toxicol. 56, 535–559. doi:10.1146/annurev-pharmtox-011613-140012
Callén, L., Moreno, E., Barroso-Chinea, P., Moreno-Delgado, D., Cortés, A., Mallol, J., et al. (2012). Cannabinoid Receptors CB1 and CB2 Form Functional Heteromers in Brain. J. Biol. Chem. 287, 20851–20865. doi:10.1074/jbc.M111.335273
Casadó, V., Cantí, C., Mallol, J., Canela, E. I., Lluis, C., and Franco, R. (1990). Solubilization of A1 Adenosine Receptor from Pig Brain: Characterization and Evidence of the Role of the Cell Membrane on the Coexistence of High‐ and Low‐Affinity States. J. Neurosci. Res. 26, 461–473. doi:10.1002/jnr.490260409
Chang, S. D., and Bruchas, M. R. (2014). Functional Selectivity at GPCRs: New Opportunities in Psychiatric Drug Discovery. Neuropsychopharmacology 39, 248–249. doi:10.1038/npp.2013.205
Christensen, R., Kristensen, P. K., Bartels, E. M., Bliddal, H., and Astrup, A. (2007). Efficacy and Safety of the Weight-Loss Drug Rimonabant: A Meta-Analysis of Randomised Trials. Lancet 370, 1706–1713. doi:10.1016/S0140-6736(07)61721-8
Christopoulos, A., and Kenakin, T. (2002). G Protein-Coupled Receptor Allosterism and Complexing. Pharmacol. Rev. 54, 323–374. doi:10.1124/PR.54.2.323
De Gregorio, D., McLaughlin, R. J., Posa, L., Ochoa-Sanchez, R., Enns, J., Lopez-Canul, M., et al. (2019). Cannabidiol Modulates Serotonergic Transmission and Reverses Both Allodynia and Anxiety-Like Behavior in a Model of Neuropathic Pain. Pain 160, 136–150. doi:10.1097/j.pain.0000000000001386
Dror, R. O., Pan, A. C., Arlow, D. H., Borhani, D. W., Maragakis, P., Shan, Y., et al. (2011). Pathway and Mechanism of Drug Binding to G-Protein-Coupled Receptors. Proc. Natl. Acad. Sci. U. S. A. 108, 13118–13123. doi:10.1073/pnas.1104614108
Echeverry, C., Prunell, G., Narbondo, C., de Medina, V. S., Nadal, X., Reyes-Parada, M., et al. (2021). A Comparative In Vitro Study of the Neuroprotective Effect Induced by Cannabidiol, Cannabigerol, and Their Respective Acid Forms: Relevance of the 5-HT 1A Receptors. Neurotox. Res. 39, 335–348. doi:10.1007/S12640-020-00277-Y
Espejo-Porras, F., Fernández-Ruiz, J., Pertwee, R. G., Mechoulam, R., and García, C. (2013). Motor Effects of the Non-Psychotropic Phytocannabinoid Cannabidiol that are Mediated by 5-HT1A Receptors. Neuropharmacology 75, 155–163. doi:10.1016/j.neuropharm.2013.07.024
Fernández-Ruiz, J., Sagredo, O., Pazos, M. R., García, C., Pertwee, R., Mechoulam, R., et al. (2013). Cannabidiol for Neurodegenerative Disorders: Important New Clinical Applications for This Phytocannabinoid? Br. J. Clin. Pharmacol. 75, 323–333. doi:10.1111/j.1365-2125.2012.04341.x
Ferré, S., Baler, R., Bouvier, M., Caron, M. G., Devi, L. A., Durroux, T., et al. (2009). Building a New Conceptual Framework for Receptor Heteromers. Nat. Chem. Biol. 5, 131–134. doi:10.1038/nchembio0309-131
Franco, R., Casadó, V., Cortés, A., Ferrada, C., Mallol, J., Woods, A., et al. (2007). Basic Concepts in G-Protein-Coupled Receptor Homo- and Heterodimerization. Scientific World J. 7, 48–57. doi:10.1100/tsw.2007.197
Franco, R., Martínez-Pinilla, E., Lanciego, J. L., and Navarro, G. (2016). Basic Pharmacological and Structural Evidence for Class A G-Protein-Coupled Receptor Heteromerization. Front. Pharmacol. 7, 1–10. doi:10.3389/fphar.2016.00076
Franco, R., Aguinaga, D., Jiménez, J., Lillo, J., Martínez-Pinilla, E., and Navarro, G. (2018). Biased Receptor Functionality Versus Biased Agonism in G-Protein-Coupled Receptors. Biomol. Concepts 9, 143–154. doi:10.1515/bmc-2018-0013
Franco, R., Reyes-Resina, I., Aguinaga, D., Lillo, A., Jiménez, J., Raïch, I., et al. (2019a). Potentiation of Cannabinoid Signaling in Microglia by Adenosine A2A Receptor Antagonists. Glia 67, 2410–2423. doi:10.1002/glia.23694
Franco, R., Villa, M., Morales, P., Reyes-Resina, I., Gutiérrez-Rodríguez, A., Jiménez, J., et al. (2019b). Increased Expression of Cannabinoid CB2 and Serotonin 5-HT1A Heteroreceptor Complexes in a Model of Newborn Hypoxic-Ischemic Brain Damage. Neuropharmacology 152, 58–66. doi:10.1016/j.neuropharm.2019.02.004
Franco, R., Rivas-Santisteban, R., Reyes-Resina, I., Casanovas, M., Pérez-Olives, C., Ferreiro-Vera, C., et al. (2020). Pharmacological Potential of Varinic-, Minor-, and Acidic Phytocannabinoids. Pharmacol. Res. 158, 104801. doi:10.1016/j.phrs.2020.104801
Franco, R., Rivas-Santisteban, R., Reyes-Resina, I., and Navarro, G. (2021). The Old and New Visions of Biased Agonism Through the Prism of Adenosine Receptor Signaling and Receptor/Receptor and Receptor/Protein Interactions. Front. Pharmacol. 11, 628601. doi:10.3389/fphar.2020.628601
Fronik, P., Gaiser, B. I., and Sejer Pedersen, D. (2017). Bitopic Ligands and Metastable Binding Sites: Opportunities for G Protein-Coupled Receptor (GPCR) Medicinal Chemistry. J. Med. Chem. 60, 4126–4134. doi:10.1021/acs.jmedchem.6b01601
Fuxe, K., Ferré, S., Zoli, M., and Agnati, L. F. (1998). Integrated Events in central Dopamine Transmission as Analyzed at Multiple Levels. Evidence for Intramembrane Adenosine A2A/Dopamine D2 and Adenosine A1/Dopamine D1 Receptor Interactions in the Basal Ganglia. Brain Res. Brain Res. Rev. 26, 258–273. doi:10.1016/S0165-0173(97)00049-0
Fuxe, K., Tarakanov, A., Romero Fernandez, W., Ferraro, L., Tanganelli, S., Filip, M., et al. (2014). Diversity and Bias through Receptor-Receptor Interactions in GPCR Heteroreceptor Complexes. Focus on Examples from Dopamine D2 Receptor Heteromerization. Front. Endocrinol. 5, 71–11. doi:10.3389/fendo.2014.00071
George, S. R., Kern, A., Smith, R. G., and Franco, R. (2014). Dopamine Receptor Heteromeric Complexes and Their Emerging Functions. Prog. Brain Res. 211, 183–200. doi:10.1016/B978-0-444-63425-2.00008-8
González, A., Perez-Acle, T., Pardo, L., and Deupi, X. (2011). Molecular Basis of Ligand Dissociation in β-Adrenergic Receptors. PLoS One 6, e23815. doi:10.1371/journal.pone.0023815
Hasbi, A., Fan, T., Alijaniaram, M., Nguyen, T., Perreault, M. L., O'Dowd, B. F., et al. (2009). Calcium Signaling Cascade Links Dopamine D1-D2 Receptor Heteromer to Striatal BDNF Production and Neuronal Growth. Proc. Natl. Acad. Sci. U. S. A. 106, 21377–21382. doi:10.1073/pnas.0903676106
Hauser, A. S., Attwood, M. M., Rask-Andersen, M., Schiöth, H. B., and Gloriam, D. E. (2017). Trends in GPCR Drug Discovery: New Agents, Targets and Indications. Nat. Rev. Drug Discov. 16, 829–842. doi:10.1038/nrd.2017.178
Hua, T., Vemuri, K., Pu, M., Qu, L., Han, G. W., Wu, Y., et al. (2016). Crystal Structure of the Human Cannabinoid Receptor CB1. Cell 167, 750–762.e14. doi:10.1016/j.cell.2016.10.004
Hua, T., Vemuri, K., Nikas, S. P., Laprairie, R. B., Wu, Y., Qu, L., et al. (2017). Crystal Structures of Agonist-Bound Human Cannabinoid Receptor CB1. Nature 547, 468–471. doi:10.1038/nature23272
Hua, T., Li, X., Wu, L., Iliopoulos-Tsoutsouvas, C., Wang, Y., Wu, M., et al. (2020). Activation and Signaling Mechanism Revealed by Cannabinoid Receptor-Gi Complex Structures. Cell 180, 655–665. doi:10.1016/j.cell.2020.01.008
Kenakin, T., and Miller, L. J. (2010). Seven Transmembrane Receptors as Shapeshifting Proteins: the Impact of Allosteric Modulation and Functional Selectivity on New Drug Discovery. Pharmacol. Rev. 62, 265–304. doi:10.1124/pr.108.000992
Krishna Kumar, K., Shalev-Benami, M., Robertson, M. J., Hu, H., Banister, S. D., Hollingsworth, S. A., et al. (2019). Structure of a Signaling Cannabinoid Receptor 1-G Protein Complex. Cell 176, 448–458.e12. doi:10.1016/j.cell.2018.11.040
Ladarre, D., Roland, A. B., Biedzinski, S., Ricobaraza, A., and Lenkei, Z. (2014). Polarized Cellular Patterns of Endocannabinoid Production and Detection Shape Cannabinoid Signaling in Neurons. Front. Cell. Neurosci. 8, 426. doi:10.3389/fncel.2014.00426
Lane, J. R., Sexton, P. M., and Christopoulos, A. (2013). Bridging the Gap: Bitopic Ligands of G-Protein-Coupled Receptors. Trends Pharmacol. Sci. 34, 59–66. doi:10.1016/j.tips.2012.10.003
Laprairie, R. B., Bagher, A. M., Kelly, M. E., and Denovan-Wright, E. M. (2015). Cannabidiol Is a Negative Allosteric Modulator of the Cannabinoid CB1 Receptor. Br. J. Pharmacol. 172, 4790–4805. doi:10.1111/bph.13250
Li, X., Hua, T., Vemuri, K., Ho, J. H., Wu, Y., Wu, L., et al. (2019). Crystal Structure of the Human Cannabinoid Receptor CB2. Cell 176, 459–467.e13. doi:10.1016/j.cell.2018.12.011
Martínez-Pinilla, E., Varani, K., Reyes-Resina, I., Angelats, E., Vincenzi, F., Ferreiro-Vera, C., et al. (2017). Binding and Signaling Studies Disclose a Potential Allosteric Site for Cannabidiol in Cannabinoid CB2 Receptors. Front. Pharmacol. 8, 744. doi:10.3389/fphar.2017.00744
Martínez-Pinilla, E., Aguinaga, D., Navarro, G., Rico, A. J., Oyarzábal, J., Sánchez-Arias, J. A., et al. (2019). Targeting CB1 and GPR55 Endocannabinoid Receptors as a Potential Neuroprotective Approach for Parkinson's Disease. Mol. Neurobiol. 56, 5900–5910. doi:10.1007/s12035-019-1495-4
Martínez-Pinilla, E., Rico, A. J., Rivas-Santisteban, R., Lillo, J., Roda, E., Navarro, G., et al. (2020). Expression of GPR55 and Either Cannabinoid CB1 or CB2 Heteroreceptor Complexes in the Caudate, Putamen, and Accumbens Nuclei of Control, Parkinsonian, and Dyskinetic Non-human Primates. Brain Struct. Funct. 225, 2153–2164. doi:10.1007/s00429-020-02116-4
Martínez-Pinilla, E., Rabal, O., Reyes-Resina, I., Zamarbide, M., Navarro, G., Sánchez-Arias, J. A., et al. (2016). Two Affinity Sites of the Cannabinoid Subtype 2 Receptor Identified by a Novel Homogeneous Binding Assay. J. Pharmacol. Exp. Ther. 358, 580–587. doi:10.1124/jpet.116.234948
Masureel, M., Zou, Y., Picard, L. P., van der Westhuizen, E., Mahoney, J. P., Rodrigues, J. P. G. L. M., et al. (2018). Structural Insights into Binding Specificity, Efficacy and Bias of a β2AR Partial Agonist. Nat. Chem. Biol. 14, 1059–1066. doi:10.1038/s41589-018-0145-x
May, L. T., and Christopoulos, A. (2003). Allosteric Modulators of G-Protein-Coupled Receptors. Curr. Opin. Pharmacol. 3, 551–556. doi:10.1016/S1471-4892(03)00107-3
McPartland, J. M., Glass, M., and Pertwee, R. G. (2007). Meta-Analysis of Cannabinoid Ligand Binding Affinity and Receptor Distribution: Interspecies Differences. Br. J. Pharmacol. 152, 583–593. doi:10.1038/sj.bjp.0707399
Medina, R. A., Vázquez-Villa, H., Gómez-Tamayo, J. C., Benhamú, B., Martín-Fontecha, M., de la Fuente, T., et al. (2014). The Extracellular Entrance Provides Selectivity to Serotonin 5-HT7 Receptor Antagonists with Antidepressant-like Behavior In Vivo. J. Med. Chem. 57, 6879–6884. doi:10.1021/jm500880c
Mohr, K., Schmitz, J., Schrage, R., Tränkle, C., and Holzgrabe, U. (2013). Molecular Alliance-From Orthosteric and Allosteric Ligands to Dualsteric/Bitopic Agonists at G Protein Coupled Receptors. Angew. Chem. Int. Ed. Engl. 52, 508–516. doi:10.1002/anie.201205315
Morales, P., Navarro, G., Gómez-Autet, M., Redondo, L., Fernández-Ruiz, J., Pérez-Benito, L., et al. (2020). Discovery of Homobivalent Bitopic Ligands of the Cannabinoid CB2 Receptor*. Chemistry 26, 15839–15842. doi:10.1002/chem.202003389
Navarro, G., Reyes-Resina, I., Rivas-Santisteban, R., Sánchez de Medina, V., Morales, P., Casano, S., et al. (2018a). Cannabidiol Skews Biased Agonism at Cannabinoid CB1 and CB2 Receptors with Smaller Effect in CB1-CB2 Heteroreceptor Complexes. Biochem. Pharmacol. 157, 148–158. doi:10.1016/j.bcp.2018.08.046
Navarro, G., Varani, K., Reyes-Resina, I., Sánchez de Medina, V., Rivas-Santisteban, R., Sánchez-Carnerero Callado, C., et al. (2018b). Cannabigerol Action at Cannabinoid CB1 and CB2 Receptors and at CB1-CB2 Heteroreceptor Complexes. Front. Pharmacol. 9, 632. doi:10.3389/fphar.2018.00632
Navarro, G., Gonzalez, A., Campanacci, S., Rivas-Santisteban, R., Reyes-Resina, I., Casajuana-Martin, N., et al. (2020a). Experimental and Computational Analysis of Biased Agonism on Full-Length and a C-Terminally Truncated Adenosine A2A Receptor. Comput. Struct. Biotechnol. J. 18, 2723–2732. doi:10.1016/j.csbj.2020.09.028
Navarro, G., Varani, K., Lillo, A., Vincenzi, F., Rivas-Santisteban, R., Raïch, I., et al. (2020b). Pharmacological Data of Cannabidiol- and Cannabigerol-Type Phytocannabinoids Acting on Cannabinoid CB1, CB2 and CB1/CB2 Heteromer Receptors. Pharmacol. Res. 159, 104940. doi:10.1016/j.phrs.2020.104940
Navarro, G., Gonzalez, A., Sánchez-Morales, A., Casajuana-Martin, N., Gómez-Ventura, M., Cordomí, A., et al. (2021). Design of Negative and Positive Allosteric Modulators of the Cannabinoid CB2 Receptor Derived from the Natural Product Cannabidiol. J. Med. Chem. 64, 9354–9364. doi:10.1021/ACS.JMEDCHEM.1C00561
O’Sullivan, S. E., and Kendall, D. A. (2010). Cannabinoid Activation of Peroxisome Proliferator-Activated Receptors: Potential for Modulation of Inflammatory Disease. Immunobiology 215, 611–616. doi:10.1016/J.IMBIO.2009.09.007
O’Sullivan, S. E., Sun, Y., Bennett, A. J., Randall, M. D., and Kendall, D. A. (2009). Time-Dependent Vascular Actions of Cannabidiol in the Rat Aorta. Eur. J. Pharmacol. 612, 61–68. doi:10.1016/J.EJPHAR.2009.03.010
Perreault, M. L., Shen, M. Y., Fan, T., and George, S. R. (2015). Regulation of C-Fos Expression by the Dopamine D1-D2 Receptor Heteromer. Neuroscience 285, 194–203. doi:10.1016/j.neuroscience.2014.11.017
Rajagopal, S., Ahn, S., Rominger, D. H., Gowen-MacDonald, W., Lam, C. M., Dewire, S. M., et al. (2011). Quantifying Ligand Bias at Seven-Transmembrane Receptors. Mol. Pharmacol. 80, 367–377. doi:10.1124/mol.111.072801
Rashid, A. J., So, C. H., Kong, M. M., Furtak, T., El-Ghundi, M., Cheng, R., et al. (2007). D1-D2 Dopamine Receptor Heterooligomers with Unique Pharmacology Are Coupled to Rapid Activation of Gq/11 in the Striatum. Proc. Natl. Acad. Sci. U. S. A. 104, 654–659. doi:10.1073/pnas.0604049104
Reyes-Resina, I., Navarro, G., Aguinaga, D., Canela, E. I., Schoeder, C. T., Załuski, M., et al. (2018). Molecular and Functional Interaction between GPR18 and Cannabinoid CB2 G-Protein-Coupled Receptors. Relevance in Neurodegenerative Diseases. Biochem. Pharmacol. 157, 169–179. doi:10.1016/j.bcp.2018.06.001
Rivas-Santisteban, R., Lillo, A., Lillo, J., Rebassa, J. B., Contestí, J. S., Saura, C. A., et al. (2021). N-Methyl-D-aspartate (NMDA) and Cannabinoid CB2 Receptors Form Functional Complexes in Cells of the central Nervous System: Insights into the Therapeutic Potential of Neuronal and Microglial NMDA Receptors. Alzheimers. Res. Ther. 13 (1), 184. doi:10.1186/S13195-021-00920-6
Russo, E. B., Burnett, A., Hall, B., and Parker, K. K. (2005). Agonistic Properties of Cannabidiol at 5-HT1a Receptors. Neurochem. Res. 30, 1037–1043. doi:10.1007/s11064-005-6978-1
Sam, A. H., Salem, V., and Ghatei, M. A. (2011). Rimonabant: From RIO to Ban. J. Obes. 2011, 432607. doi:10.1155/2011/432607
Shao, Z., Yan, W., Chapman, K., Ramesh, K., Ferrell, A. J., Yin, J., et al. (2019). Structure of an Allosteric Modulator Bound to the CB1 Cannabinoid Receptor. Nat. Chem. Biol. 15, 1199–1205. doi:10.1038/S41589-019-0387-2
Sierra, S., Luquin, N., Rico, A. J., Gómez-Bautista, V., Roda, E., Dopeso-Reyes, I. G., et al. (2015). Detection of Cannabinoid Receptors CB1 and CB2 within Basal Ganglia Output Neurons in Macaques: Changes Following Experimental Parkinsonism. Brain Struct. Funct. 220, 2721–2738. doi:10.1007/s00429-014-0823-8
Smith, N. J., Bennett, K. A., and Milligan, G. (2011). When Simple Agonism is Not Enough: Emerging Modalities of GPCR Ligands. Mol. Cell. Endocrinol. 331, 241–247. doi:10.1016/J.MCE.2010.07.009
Urban, J. D., Clarke, W. P., von Zastrow, M., Nichols, D. E., Kobilka, B., Weinstein, H., et al. (2007). Functional Selectivity and Classical Concepts of Quantitative Pharmacology. J. Pharmacol. Exp. Ther. 320, 1–13. doi:10.1124/jpet.106.104463
Valant, C., Robert Lane, J., Sexton, P. M., and Christopoulos, A. (2012). The Best of Both Worlds? Bitopic Orthosteric/Allosteric Ligands of G Protein-Coupled Receptors. Annu. Rev. Pharmacol. Toxicol. 52, 153–178. doi:10.1146/annurev-pharmtox-010611-134514
Wang, C., Jiang, Y., Ma, J., Wu, H., Wacker, D., Katritch, V., et al. (2013). Structural Basis for Molecular Recognition at Serotonin Receptors. Science 340, 610–614. doi:10.1126/science.1232807
Keywords: biased agonism, heteromer, health benefits, therapy, functional selectivity, cannabinoid receptor, CB2, allosterism
Citation: Franco R, Morales P, Navarro G, Jagerovic N and Reyes-Resina I (2022) The Binding Mode to Orthosteric Sites and/or Exosites Underlies the Therapeutic Potential of Drugs Targeting Cannabinoid CB2 Receptors. Front. Pharmacol. 13:852631. doi: 10.3389/fphar.2022.852631
Received: 11 January 2022; Accepted: 20 January 2022;
Published: 16 February 2022.
Edited by:
Reem Smoum, Hebrew University of Jerusalem, IsraelReviewed by:
Carlos Ferrerio-Vera, Phytoplant Research S.L., SpainCopyright © 2022 Franco, Morales, Navarro, Jagerovic and Reyes-Resina. This is an open-access article distributed under the terms of the Creative Commons Attribution License (CC BY). The use, distribution or reproduction in other forums is permitted, provided the original author(s) and the copyright owner(s) are credited and that the original publication in this journal is cited, in accordance with accepted academic practice. No use, distribution or reproduction is permitted which does not comply with these terms.
*Correspondence: Rafael Franco, rfranco123@gmail.com
†These authors have contributed equally to this work