- 1Department of Hand and Foot Surgery, Zhejiang Rongjun Hospital, Jiaxing, China
- 2Department of Neurosurgery, Zhejiang Rongjun Hospital, Jiaxing, China
Spinal cord injury (SCI) results from various pathogenic factors that destroy the normal structure and function of the spinal cord, subsequently causing sensory, motor, and autonomic nerve dysfunction. SCI is one of the most common causes of disability and death globally. It leads to severe physical and mental injury to patients and causes a substantial economic burden on families and the society. The pathological changes and underlying mechanisms within SCI involve oxidative stress, apoptosis, inflammation, etc. As a traditional therapy, acupuncture has a positive effect promoting the recovery of SCI. Acupuncture-induced neuroprotection includes several mechanisms such as reducing oxidative stress, inhibiting the inflammatory response and neuronal apoptosis, alleviating glial scar formation, promoting neural stem cell differentiation, and improving microcirculation within the injured area. Therefore, the recent studies exploring the mechanism of acupuncture therapy in SCI will help provide a theoretical basis for applying acupuncture and seeking a better treatment target and acupuncture approach for SCI patients.
Introduction
Spinal cord injury (SCI) causes structural and functional damage through direct or indirect factors, leading to motor, sensory, and autonomic nerve dysfunction (McDonald and Sadowsky, 2002). The global incidence of SCI ranges from 3.6 to 195 per 1,000,000 (Jazayeri et al., 2015). In China, the incidence of traumatic SCI was standardized to 49.8 per 1,000,000 per year based on the 2010 census, and the mean age of patients at the time of injury was 43.7 ± 17.1 years (Jiang et al., 2021). SCI is a common cause of death and disability, with severe neurological dysfunction and complications, including neuropathic pain, pressure ulcers, and urinary tract infection. In addition, it causes a substantial psychological and social burden on patients, families, and the society (Wannapakhe et al., 2015; Gedde et al., 2019; Moshi et al., 2021). Pathophysiological changes after SCI include primary and secondary injuries. Compared with the unpredictability of primary injury, the underlying mechanism and effective treatment of secondary injury is the primary focus of the current SCI research (Belegu et al., 2007; Jeong et al., 2021). SCI is a dynamic pathological process causing nerve cell and nerve fiber edema at the initial stages, followed by microcirculation disorders due to damaged blood cells (Rivlin and Tator, 1978; Tator and Fehlings, 1991). Then, the nerve cell axons degenerate or die and are gradually replaced by glial cells (O’Shea et al., 2017; Lukacova et al., 2021). Aggravation of cellular, molecular, and other factors at different stages post SCI leads to a series of pathophysiological changes, reducing the spinal cord functional recovery (Tator, 1995). Timely and efficient intervention can partially stimulate potential nerve cells and axonal regeneration and resume the function of axons and neurons. The current treatments for SCI mainly include surgery, medication, and behavioral, physical, and supportive therapies (Becker et al., 2003; Boulenguez and Vinay, 2009; Ramer et al., 2014).
Acupuncture is a substantial alternative and adjunctive therapy for SCI and is a vital component of traditional Chinese medicine. Electroacupuncture, a method based on acupuncture combined with the micro-current wave of bioelectricity, was developed by combining traditional and modern medicines. In recent years, acupuncture–electroacupuncture has been widely used in clinical practices and exerts a significant neuroprotective effect against SCI and its complications (Paola and Arnold, 2003; Shin et al., 2009; Ma et al., 2015; Fan et al., 2018; Lu et al., 2020). Compared with other therapeutic methods, acupuncture is non-toxic and has a simple operation and low cost, but its mechanism remains unclear. This article summarizes the potential mechanism of acupuncture in SCI to provide the updated theoretical basis depicting various clinical applications of acupuncture in SCI patients (Table 1).
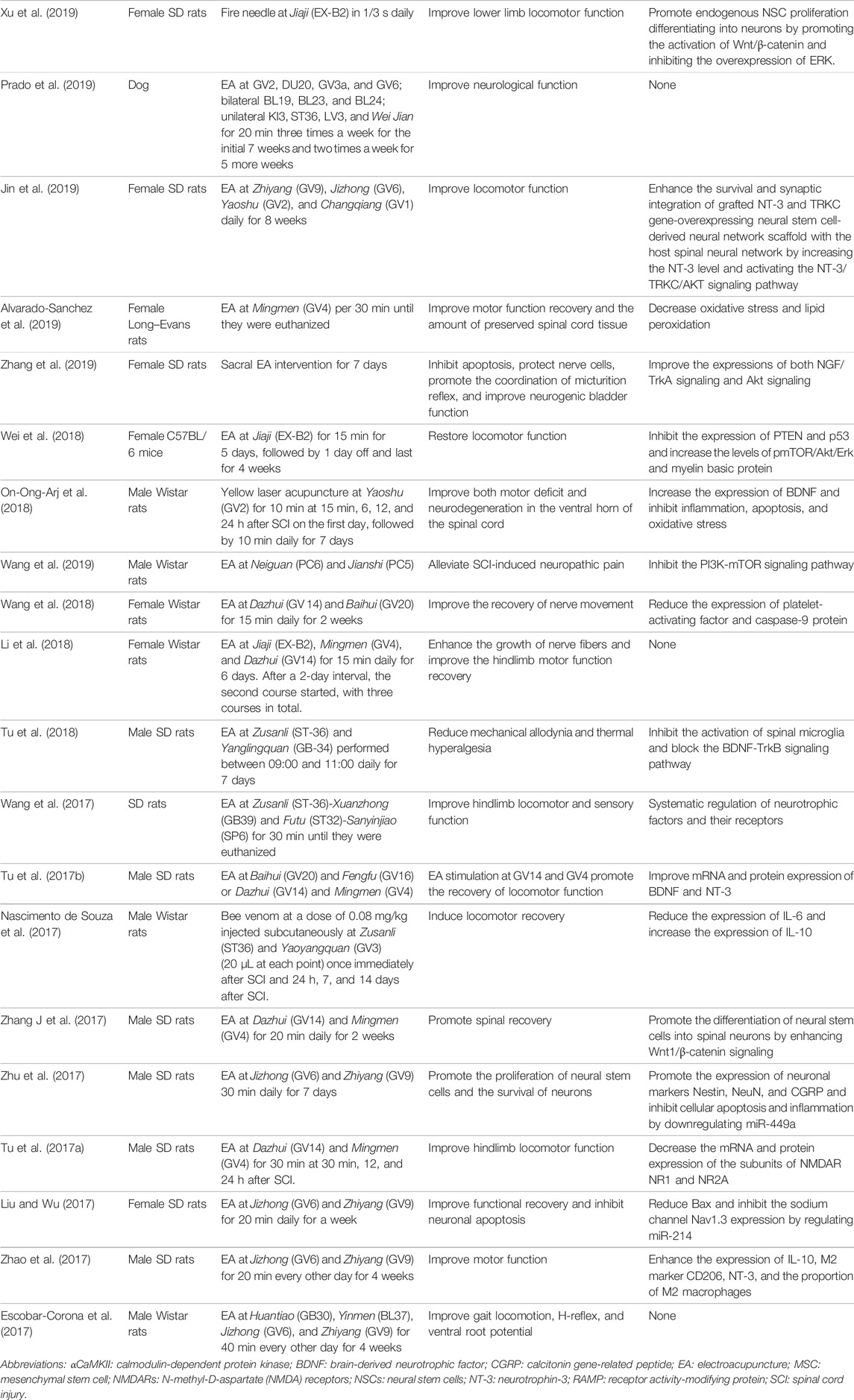
TABLE 1. Summary of preclinical studies of acupuncture therapy in spinal cord injury in recent 5 years.
Mechanism of Acupuncture Therapy in Spinal Cord Injury
Reduction of Oxidative Stress
Free radicals can be generated and released after SCI. While the degree of oxidation exceeds the ability of the antioxidant system, excessive free radicals will initiate the oxidation chain reaction (Bringans et al., 2022). Reactive oxygen species (ROS) and reactive nitrogen species (RNS) can efficiently react with intracellular macromolecules, causing cell death and tissue damage and subsequently aggravating SCI. The spinal cord contains many polyunsaturated fatty acids, thus making it sensitive to oxidative stress. The spinal cord neurons have active oxidative metabolism but low antioxidant capacity, making neurons and glial cells significantly vulnerable to oxidative stress. Hence, reactive oxygen metabolites accumulate, resulting in excessive consumption of antioxidants from tissues after SCI (Genovese and Cuzzocrea, 2008; Figueroa et al., 2013; Lim et al., 2013; Wojdasiewicz et al., 2020).
Superoxide dismutase (SOD) is an active protease scavenging free radicals and protecting cells from oxidative damage. It eliminates the oxidation products produced after SCI. The SOD level reflects the ability to clear free radicals and has a vital role in balancing oxidation and antioxidation. Malondialdehyde (MDA) is a lipid peroxidation metabolite, reflecting the degree of oxidative stress (Woźniak et al., 2016; Wu et al., 2017). The lipid peroxidation can interfere with Ca2+ transport from the cell membrane by inhibiting the Ca2+-ATPase activity, causing intracellular Ca2+ overload and enhanced ion imbalance (Rohn et al., 1993; Rohn et al., 1996). In addition, oxidative stress post SCI destroys ion homeostasis both inside and outside the membrane. Moreover, abundant Ca2+ enters and accumulates within the mitochondria, leading to mitochondrial destruction, aerobic energy metabolism dysfunction, and inhibition of ATP synthesis (Brown et al., 2006; Visavadiya et al., 2013; Scholpa and Schnellmann, 2017). Studies have revealed that acupuncture, electroacupuncture, and laser acupuncture can reduce oxidative stress after SCI (Wu et al., 2002; On-Ong-Arj et al., 2018; Alvarado-Sanchez et al., 2019). The results from a traumatic SCI model study showed that electroacupuncture at GV26 reduces radical hydroxyl concentration and increases lipid peroxidation. At the same time, stimulation of GV4 decreases oxidative stress and improves motor function recovery in the hind limbs of rats with paralysis, indicating electroacupuncture at GV4 could be a therapeutic alternative (Juarez Becerril et al., 2015). Jiang et al. found that electroacupuncture at Shuigou (DU26) and Fengfu (DU16) acupoints induce antioxidation effects by enhancing the SOD activity and decreasing the MDA level (Jiang et al., 2014). Similar to the effect of the reactive oxygen species (ROS) scavenger, acupuncture can inhibit superoxide anion production, decrease JNK/p66Shc-mediated ROS generation, upregulate the apolipoprotein E (ApoE) and nuclear factor E2-related factor 2 (Nrf2)/heme-oxygenase-1 (HO-1) signaling pathways, and reduce the ROS-induced p38MAPK and ERK activation in microglia after SCI (Choi et al., 2012; Dai et al., 2021). Notably, the inhibitory effect of electroacupuncture on p38MAPK is significantly enslaved to the acupuncture frequency (Cheng et al., 2020).
Inhibition of Neuronal Apoptosis
Apoptosis, predominantly neuronal apoptosis, is an essential pathological mechanism causing secondary spinal cord injury (Abbaszadeh et al., 2020; Shi et al., 2021). Axonal injury and neuronal apoptosis block nerve conduction pathways after SCI and aggravate secondary injuries. Therefore, inhibition of apoptosis can induce SCI recovery (Beattie, 2004). The anti-apoptotic mechanisms of acupuncture have been widely explored. Acupuncture protects the nerves and reduces apoptosis of neurons and oligodendrocytes, thus improving functional recovery after SCI (Cai and Shen, 2018). In addition, electroacupuncture can inhibit spinal cord neuronal apoptosis by increasing the Bcl-2 expression and inhibiting caspase-3 and Bax (Zhao et al., 2008; Shi et al., 2016; Liu and Wu, 2017; Zhu et al., 2017).
Poly-ADP ribose polymerase (PARP) is the most significant substrate of caspase-3, and activated PARP can cause apoptosis mediated by apoptosis-inducing factor (AIF) (Kang et al., 2004). Previous studies showed that electroacupuncture could ameliorate early brain injury after subarachnoid hemorrhage by inhibiting the PARP-1/AIF pathway (Lang et al., 2020). Moreover, electroacupuncture also reduces the PARP expression in cerebral ischemia/reperfusion and Parkinson’s disease (Sun et al., 2003; Yu et al., 2020). Furthermore, Liu et al. found that apoptosis post SCI was accompanied by cleaved PARP upregulation and electroacupuncture treatment attenuation (Liu and Wu, 2017).
BNIP3 is a member of the Bcl-2 family that induces apoptosis by promoting mitochondrial permeability transport pore opening and mitochondrial damage (Yu et al., 2018). In addition, the BNIP3 expression is elevated in rats after SCI (Yu et al., 2018), and electroacupuncture at GV20-GB7 reduced BNIP3 after intracerebral hemorrhage (Guan et al., 2021).
Heat shock protein (HSP) is an endogenous stress protein with various biological protective effects. HSP family members such as HSP 70 and HSP 72 have a protective effect on neurons after SCI (Chang et al., 2014; Xu et al., 2021a; Kim et al., 2022). Acupuncture has been demonstrated to have a neuroprotective role in cerebral ischemia by regulating HSP 70 (Xu et al., 2014; Shi et al., 2017). Gao et al. reported that HSP 90 participates in electroacupuncture-induced analgesia in chronic neuropathic pain (Gao et al., 2021). Other signaling pathways, such as PI3K/Akt/Erk, Nogo/NgR, Rho/ROCK, and mTOR, may also include the acupuncture-related beneficial effects against SCI (Renfu et al., 2014; Wei et al., 2018; Xiao et al., 2019; Li et al., 2020).
The toxic effects of excitatory amino acids play an essential role in the pathogenesis of SCI. The glutamate ion receptor activated by the N-methyl-D-aspartate (NMDA) receptor induces excessive Ca2+ influx and destroys mitochondrial function, thus stimulating the death of neurons (Xie et al., 2014; Inquimbert et al., 2018). Studies found that electroacupuncture can protect the spinal cord after SCI by reducing the expression of the NMDA receptor subunit NR1 and NR2A in the injured area (Tu et al., 2017a). It can also alleviate mechanical allodynia by inhibiting the upregulation of NR2B after chronic constrictive injury (Zhao et al., 2019).
Recent studies have observed that electroacupuncture can improve the locomotor function by regulating autophagy flux and inhibiting necroptosis after SCI (Hongna et al., 2020). Furthermore, Fang et al. depicted that pre- and post-conditioning electroacupuncture alleviates spinal cord ischemia–reperfusion injury, partly through autophagy upregulation accompanied by apoptosis inhibition (Fang et al., 2017). Moreover, studies conducted in intracerebral hemorrhage rat models show the effect of ferroptosis inhibition by acupuncture (Kong et al., 2021; Li et al., 2022). Therefore, apoptosis, autophagy, necroptosis, and ferroptosis should be clarified in future acupuncture studies on SCI.
Restrain of Inflammatory Response
After SCI, infiltrating leukocytes attracted by the innate immune response leads to an inflammatory cascade in the area of injury, and an excessive inflammatory response damages the spinal cord tissue. In addition, leukocytes, microglia, astrocytes, and macrophages release many pro-inflammatory cytokines and chemokines, including interleukin-1 (IL-1), IL-6, and tumor necrosis factor-α (TNF-α), which aggravate local inflammation and damage axons and neurons (Zhou et al., 2014a; Tang et al., 2020a; Brockie et al., 2021; Hellenbrand et al., 2021). Therefore, regulating inflammatory factors and improving neuroinflammation is of great significance for the recovery of SCI.
Neuroprotection by acupuncture is partially mediated by inhibiting inflammation and microglial activation after SCI (Choi et al., 2010; Jiang et al., 2014). However, the inflammatory response in SCI has two sides; it exerts a positive reaction against injury and aggravates secondary injury post SCI. The pro-inflammatory macrophage/microglia (M1 subsets) and anti-inflammatory macrophage/microglia (M2 subsets) are significant. Therefore, regulating the polarization of M1 and M2 macrophages/microglia can affect the inflammatory response process after SCI (Buzoianu-Anguiano et al., 2021; Ding et al., 2021; Hashemizadeh et al., 2022). Previous studies have shown that acupuncture can ameliorate SCI by regulating M1 and M2 macrophages (Zhao et al., 2017). It also reduces the release of pro-inflammatory cytokines such as IL-6, TNF-α, nitric oxide synthase, and cycloxygenase-2 (Choi et al., 2010).
The purinergic receptors P2X4 and P2X7 are overexpressed on the cell surface of spinal dorsal horn microglia involved in microglial activation, which significantly contributes to the inflammation after SCI (Deng et al., 2018; Du et al., 2019; Kobayakawa et al., 2019; Song et al., 2022). Electroacupuncture can inhibit P2X7 receptor-mediated microglial activation and attenuate neuropathic pain (Wu et al., 2021a). It can also relieve pain hypersensitivity by inhibiting P2X7 receptor-positive microglia after chronic constriction injury (Xu et al., 2016). In addition, acupuncture reduces diabetic peripheral neuralgia by downregulating the P2X4 expression in rat spinal microglia (Tang et al., 2020b).
The inflammasome is an essential component of host defense response, recognizing pathogen-associated molecular patterns and damage-associated molecular patterns. It mediates the release of pro-inflammatory factors after injury. The family of NOD-like receptors (NLRs) is a vital member of the inflammasome, with NLRP3 being the most studied inflammasome in central nervous system disorders. The ability of acupuncture to attenuate the inflammatory response through inflammasome regulation, especially NLRP3, has been explored in many neurological diseases, including autism (Zhao et al., 2022), postoperative cognitive dysfunction (Sun et al., 2021), depression (Li et al., 2021), Alzheimer’s disease (Jiang et al., 2018; Zhang et al., 2021), cerebral ischemia (Jiang et al., 2019), and vascular dementia (Du et al., 2018). Further research is needed to explore the role of the inflammasome, including NLRs, in acupuncture-induced beneficial effects against SCI.
Choi et al. demonstrated that elevated p38MAPK accelerated the microglial secretion of inflammatory mediators after SCI. Electroacupuncture can effectively downregulate the p38MAPK phosphorylation level, inhibit microglial activation, and promote nerve regeneration (Choi et al., 2010). Hu et al. demonstrated that the combination of gangliosides with electroacupuncture at Jiaojia (EX-B2) has a more substantial effect in promoting the recovery of nerve function, which could be related to the inhibition of pro-inflammatory cytokines and the Nogo-NgR signaling pathway (Hu et al., 2021).
Improvement of Microcirculation Dysfunction
SCI can cause rupture, hemorrhage, and capillary embolism, leading to microcirculation dysfunction. Improved microcirculation can reduce cellular apoptosis and promote functional recovery (Tator and Koyanagi, 1997). Reduced blood flow and intramedullary vasospasm are seen after SCI. Vasoconstriction factors such as endothelin 1 (ET-1), prostaglandin E2 (PGE2), and thromboxane A2 (TXA2) cause vasospasm aggravation and blood flow reduction. As a result, the blood–spinal cord barrier gets disrupted, leading to inflammatory cell infiltration and spinal tissue edema (Tempel and Martin, 1992; Mitsuhashi et al., 1994; McKenzie et al., 1995; Wang et al., 2007; Sinescu et al., 2010).
Clinical studies conducted in healthy adults demonstrated that acupuncture influences the tortuousness of capillary loops, the diameter of the afferent loop, and capillary refill time, thereby regulating the microcirculation (Scardina et al., 2009; Yeh et al., 2021). In animal experiments, acupuncture can also improve the blood flow within the brain after hemorrhage or ischemia. It is primarily associated with the regulation of the vascular endothelial growth factor (VEGF), angiopoietin 1 (Ang-1), Ang-2, angiotensin II type I receptor, endothelin receptor, and EphB4/EphrinB2-mediated Src/PI3K signal pathways (Tian et al., 2013; Zhou et al., 2014b; Wu et al., 2021b). In addition, a study using the intervertebral disc extrusion model revealed that electroacupuncture improves microcirculation characterized by high blood flow, micro-vessel density, and reduced vacuolation within the white matter (Jiang et al., 2015). Acupuncture can also regulate microcirculation and attenuate neurological dysfunction by suppressing the cPLA2 activity and PGE2 level (Hong et al., 2021).
Attenuation of Glial Scar Formation
Glial cells play an essential role in the physiological function inside the spinal cord microenvironment and induce excessive hyperplasia of the glial scar under pathological conditions. On the one hand, a glial scar can limit the lesion expansion and protect the surrounding tissues from injury. On the other hand, it restricts neuronal regeneration (Faulkner et al., 2004; Pekny et al., 2014; Tran et al., 2018; Gu et al., 2019). During the spinal cord recovery, astrocytes proliferate and secrete a variety of extracellular matrices to form a glial scar, hindering the neural pathway recovery. The significant molecules participating in glial scar formation are chondroitin sulfate proteoglycans (CSPGs) and keratan sulfate proteoglycans produced by astrocytes (Zhang et al., 2006; Wang et al., 2021a; Tran et al., 2021). CSPG accumulation at the injured area inhibits the axonal growth, and reducing the CSPG expression can promote axonal regeneration and remyelination (Siebert and Osterhout, 2011). Electroacupuncture can downregulate the CSPG protein expression and stimulate axonal regeneration, leading to structural and functional recovery after SCI (Ding et al., 2011). It also stimulates the differentiation of transplanted bone marrow mesenchymal stem cells (MSCs) and promotes corticospinal tract regeneration across injured sites in the caudal cord, with CSPG protein involvement (Ding et al., 2013). Numerous studies have shown that acupuncture can restrict astrogliosis and alleviate neurological dysfunction caused by diseases such as hydrocephalus (Tida et al., 2018) and cerebral ischemia (Han et al., 2010; Tao et al., 2016; Young-Wook et al., 2019).
Glial fibrillary acidic protein (GFAP) is a crucial component of astrocytes. As an important marker of glial scar formation, GFAP depicts the proliferative state of astrocytes (Brenner, 2014; Yang and Wang, 2015). In addition, GFAP secreted by astrocytes forms a physical barrier to isolate damaged tissue, provides mechanical strength, and limits axonal growth due to the physical barrier (Pekny et al., 2014). Fire needle acupuncture and electroacupuncture can decrease the GFAP expression, leading to the differentiation of neural stem cells (NSCs) and inhibition of astrocyte activation, respectively (Zhang et al., 2018; Xu et al., 2019). Liu et al. observed that electroacupuncture increases the gene and protein expression of GFAP and the platelet-derived growth factor (PDGF) after spinal cord transection, promoting locomotor function recovery (Liu et al., 2013). Interestingly, Wei et al. revealed that electroacupuncture elevates GFAP levels only at the early phase after SCI and reduces the GFAP expression later during recovery (Wei et al., 2017), indicating diverse functionalities of acupuncture in SCI. Choosing the time points and interval of acupuncture therapy exerting a better effect is an important issue that needs to be explored in future studies.
Promotion of Neural Stem Cell Proliferation and Differentiation
SCI induces damage to the segmentary neurons, axons, and glial cells at the injury site, forming a hole at the center of the spinal cord. The loss of neurons within the injured section and the disruption of the ascending sensory and descending motor tracts of axon conduction caused loss of the neurologic function. NSCs can differentiate into neurons, astrocytes, or oligodendrocytes, connect the spinal cord end, and rebuild neural pathways (Pereira et al., 2019; Vancamp et al., 2020; de Freria et al., 2021; Chen and Li, 2022). Several experimental studies have shown that acupuncture can induce the proliferation and differentiation of NSCs, thereby promoting the repair of injured nerves; however, the mechanism remains unclear (Tao et al., 2010; Zhang et al., 2013; Jiang et al., 2016; Dubrovsky et al., 2020).
Various hypotheses have been proposed to illustrate the acupuncture mechanism on NSCs. First, acupuncture could promote nerve regeneration and synaptogenesis by regulating the microenvironment of NSC transplantation and promoting SCI recovery (Tang et al., 2020c; Zhao et al., 2020; Yang et al., 2021). Second, electroacupuncture promotes the proliferation and differentiation of endogenous NSCs by regulating numerous endogenous signals. The upregulation of exosomal miR-146b, NeuroD1, the activation of the Notch pathway, and the downregulation of the PTEN expression are associated with acupuncture-induced improvement of neurological injury after ischemic stroke (Tao et al., 2014; Zhao et al., 2015; Sha et al., 2019; Zhang et al., 2020). In contrast, the potential signals of the acupuncture-induced NSC regulation in the SCI model include Wnt/β-catenin (Zhang et al., 2017a), ERK (Xu et al., 2019), miR-449a (Zhu et al., 2017), and Notch pathway (Wang et al., 2021b). Third, electroacupuncture reinforces the survival and synaptogenesis of transplanted NSC-derived neural network scaffolds as a neuronal relay bridging two severed ends of the injured spinal cord (Jin et al., 2019). Similarly, two other studies have shown that electroacupuncture facilitates the integration of the mesenchymal stem cell (MSC)–derived neural network into the transected spinal cord by elevating neurotrophin-3 (NT-3) (Ding et al., 2013; Yang et al., 2021). Moreover, pre-induction with NT-3 and retinoic acid after SCI before electroacupuncture could also promote the survival and differentiation of the grafted MSCs in gelatin sponge scaffolds (Zhang et al., 2014).
NT-3 is tightly associated with SCI recovery as the primary type of neurotrophic factor (Ding et al., 2009; Mo et al., 2016; Tu et al., 2017b). Electroacupuncture promotes the intrinsic growth ability of spinal neurons after SCI by activating the calcitonin gene-related peptide/α-calcium/calmodulin-dependent protein kinase/NT-3 pathway (Xu et al., 2021b). Additionally, electroacupuncture treatment can promote the differentiation and remyelination of MSCs and oligodendrocyte precursor cells, protect spinal motor neurons, and alleviate muscle atrophy after SCI, along with elevation of the NT-3 expression (Huang et al., 2011; Yan et al., 2011; Ding et al., 2015; Liu et al., 2015; Zhang et al., 2017b).
Summary and Prospects
SCI is characterized by high mortality and disability, with complex regeneration and repair. We explained in detail the underlying mechanisms of acupuncture therapy for SCI, including oxidative stress reduction, inflammation and apoptosis inhibition, microcirculation improvement, glial scar formation reduction, and stimulation of NSC differentiation (Figure 1). This review could provide an experimental basis for better clinical application of acupuncture in SCI. However, SCI has complex pathophysiology. Therefore, significant research should be focused on the pathogenesis of acupuncture therapy to formulate mechanism-based specific intervention strategies and help SCI patients achieve better outcomes and recovery of impaired neurological function.
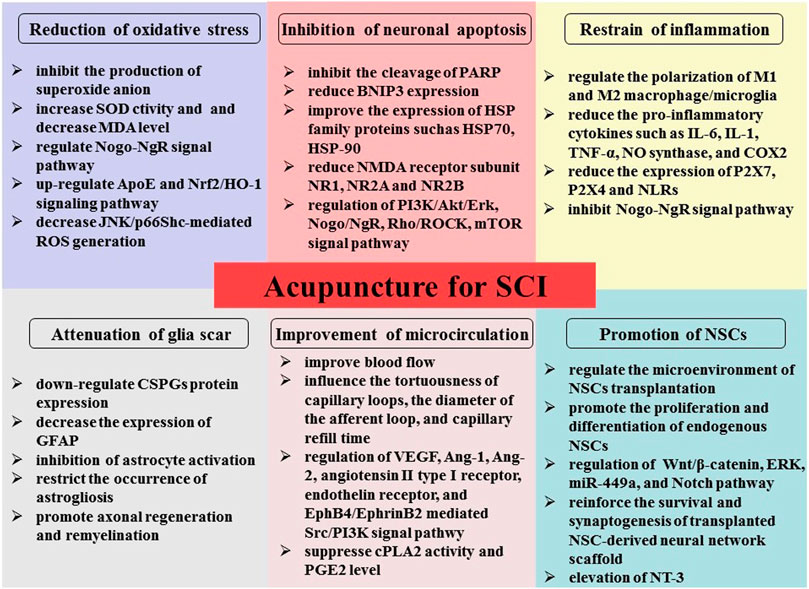
FIGURE 1. Illustration of the possible mechanism underlying acupuncture therapy in SCI, including oxidative stress reduction, inflammation and apoptosis inhibition, microcirculation improvement, reduction of glial scar formation, and stimulation of NSC differentiation and proliferation.
Although this review primarily summarizes recent preclinical studies, acupuncture clinical trials for SCI have shown positive results. Acupuncture alleviates the neurogenic bladder (Cheng et al., 1998; Honjo et al., 2000), chronic shoulder pain (Dyson-Hudson et al., 2001; Dyson-Hudson et al., 2007), neuropathic pain (Norrbrink and Lundeberg, 2011; Estores et al., 2017), and osteoporosis (Meng et al., 2014) and improves neurological (sensory and motor) functions (Wong et al., 2003). Interestingly, a study that enrolled seven healthy volunteers and three cervical SCI patients observed that the functional magnetic resonance imaging (fMRI) technique detected an activation centered at C6 and C2 cervical spinal cord levels by using acupuncture at L4 and L11, proving the existence of the meridians and points. An fMRI can be used as a harmless research and monitoring method to explore the effect of acupuncture therapy on SCI patients (Chen et al., 2007). However, most clinical trials are single-center trials with few subjects and are not conducted in a double-blinded manner.
Acupuncture can be an emerging therapy for the treatment of SCI as a simple, safe, and low-risk treatment. Although many basic studies and clinical trials have established the advantages of acupuncture in SCI, large-scale and multi-centric clinical trials are needed to authenticate the effect further. Moreover, the concept of precision medicine could further explore the best indicators in acupoint selection, stimulation frequency, starting time, and duration, for achieving individualized treatment. Thus, modern analytical techniques should be used to quantitatively analyze the variations in physiological and pathological indexes after acupuncture, which could popularize the global application of acupuncture.
Author Contributions
KJ and XC contributed substantially to the conception and design of the work and drafting and revising the manuscript for important intellectual content. YS drafted parts of the manuscript. All authors approved the final version to be published and agreed to be accountable for all aspects of the work.
Conflict of Interest
The authors declare that the research was conducted in the absence of any commercial or financial relationships that could be construed as a potential conflict of interest.
Publisher’s Note
All claims expressed in this article are solely those of the authors and do not necessarily represent those of their affiliated organizations, or those of the publisher, the editors, and the reviewers. Any product that may be evaluated in this article, or claim that may be made by its manufacturer, is not guaranteed or endorsed by the publisher.
References
Abbaszadeh, F., Fakhri, S., and Khan, H. (2020). Targeting Apoptosis and Autophagy Following Spinal Cord Injury: Therapeutic Approaches to Polyphenols and Candidate Phytochemicals. Pharmacol. Res. 160, 105069. doi:10.1016/j.phrs.2020.105069
Alvarado-Sanchez, B. G., Salgado-Ceballos, H., Torres-Castillo, S., Rodriguez-Silverio, J., Lopez-Hernandez, M. E., Quiroz-Gonzalez, S., et al. (2019). Electroacupuncture and Curcumin Promote Oxidative Balance and Motor Function Recovery in Rats Following Traumatic Spinal Cord Injury. Neurochem. Res. 44 (2), 498–506. doi:10.1007/s11064-018-02704-1
Beattie, M. S. (2004). Inflammation and Apoptosis: Linked Therapeutic Targets in Spinal Cord Injury. Trends Mol. Med. 10 (12), 580–583. doi:10.1016/j.molmed.2004.10.006
Becker, D., Sadowsky, C. L., and McDonald, J. W. (2003). Restoring Function after Spinal Cord Injury. Neurologist 9 (1), 1–15. doi:10.1097/01.nrl.0000038587.58012.05
Belegu, V., Oudega, M., Gary, D. S., and McDonald, J. W. (2007). Restoring Function after Spinal Cord Injury: Promoting Spontaneous Regeneration with Stem Cells and Activity-Based Therapies. Neurosurg. Clin. N. Am. 18 (1), 143–xi. doi:10.1016/j.nec.2006.10.012
Boulenguez, P., and Vinay, L. (2009). Strategies to Restore Motor Functions after Spinal Cord Injury. Curr. Opin. Neurobiol. 19 (6), 587–600. doi:10.1016/j.conb.2009.10.005
Brenner, M. (2014). Role of GFAP in CNS Injuries. Neurosci. Lett. 565, 7–13. doi:10.1016/j.neulet.2014.01.055
Bringans, C., Hammond, C., Hong, J., Wang, H. W., Schweder, P., Correia, J., et al. (2022). Tracking Antioxidant Status in Spinal Cord Injured Rodents: A Voltammetric Method Suited for Clinical Translation. World Neurosurg. [Epub ahead of print], S1878-8750(22)00113-9. doi:10.1016/j.wneu.2022.01.099
Brockie, S., Hong, J., and Fehlings, M. G. (2021). The Role of Microglia in Modulating Neuroinflammation after Spinal Cord Injury. Int. J. Mol. Sci. 22 (18), 706. doi:10.3390/ijms22189706
Brown, M. R., Sullivan, P. G., and Geddes, J. W. (2006). Synaptic Mitochondria Are More Susceptible to Ca2+overload Than Nonsynaptic Mitochondria. J. Biol. Chem. 281 (17), 11658–11668. doi:10.1074/jbc.M510303200
Buzoianu-Anguiano, V., Torres-Llacsa, M., and Doncel-Pérez, E. (2021). Role of Aldynoglia Cells in Neuroinflammatory and Neuroimmune Responses after Spinal Cord Injury. Cells 10 (10), 783. doi:10.3390/cells10102783
Cai, W., and Shen, W. D. (2018). Anti-Apoptotic Mechanisms of Acupuncture in Neurological Diseases: A Review. Am. J. Chin. Med. 46 (3), 515–535. doi:10.1142/S0192415X1850026X
Chang, C. K., Chou, W., Lin, H. J., Huang, Y. C., Tang, L. Y., Lin, M. T., et al. (2014). Exercise Preconditioning Protects against Spinal Cord Injury in Rats by Upregulating Neuronal and Astroglial Heat Shock Protein 72. Int. J. Mol. Sci. 15 (10), 19018–19036. doi:10.3390/ijms151019018
Chen, X., and Li, H. (2022). Neuronal Reprogramming in Treating Spinal Cord Injury. Neural Regen. Res. 17 (7), 1440–1445. doi:10.4103/1673-5374.330590
Chen, Y. X., Kong, K. M., Wang, W. D., Xie, C. H., and Wu, R. H. (2007). Functional MR Imaging of the Spinal Cord in Cervical Spinal Cord Injury Patients by Acupuncture at LI 4 (Hegu) and LI 11(Quchi). Annu. Int. Conf. IEEE Eng. Med. Biol. Soc. 2007, 3388–3391. doi:10.1109/IEMBS.2007.4353058
Cheng, M., Wu, X., Wang, F., Tan, B., and Hu, J. (2020). Electro-Acupuncture Inhibits p66Shc-Mediated Oxidative Stress to Facilitate Functional Recovery after Spinal Cord Injury. J. Mol. Neurosci. 70 (12), 2031–2040. doi:10.1007/s12031-020-01609-5
Cheng, P. T., Wong, M. K., and Chang, P. L. (1998). A Therapeutic Trial of Acupuncture in Neurogenic Bladder of Spinal Cord Injured Patients-Aa Preliminary Report. Spinal Cord 36 (7), 476–480. doi:10.1038/sj.sc.3100689
Choi, D. C., Lee, J. Y., Lim, E. J., Baik, H. H., Oh, T. H., and Yune, T. Y. (2012). Inhibition of ROS-Induced p38MAPK and ERK Activation in Microglia by Acupuncture Relieves Neuropathic Pain after Spinal Cord Injury in Rats. Exp. Neurol. 236 (2), 268–282. doi:10.1016/j.expneurol.2012.05.014
Choi, D. C., Lee, J. Y., Moon, Y. J., Kim, S. W., Oh, T. H., and Yune, T. Y. (2010). Acupuncture-mediated Inhibition of Inflammation Facilitates Significant Functional Recovery after Spinal Cord Injury. Neurobiol. Dis. 39 (3), 272–282. doi:10.1016/j.nbd.2010.04.003
Dai, N., Tang, C., Liu, H., and Huang, S. (2021). Effect of Electroacupuncture on Inhibition of Inflammatory Response and Oxidative Stress through Activating ApoE and Nrf2 in a Mouse Model of Spinal Cord Injury. Brain Behav. 11 (9), e2328. doi:10.1002/brb3.2328
de Freria, C. M., Van Niekerk, E., Blesch, A., and Lu, P. (2021). Neural Stem Cells: Promoting Axonal Regeneration and Spinal Cord Connectivity. Cells 10 (12), 296. doi:10.3390/cells10123296
Deng, Z., Li, C., Liu, C., Du, E., and Xu, C. (2018). Catestatin Is Involved in Neuropathic Pain Mediated by Purinergic Receptor P2X4 in the Spinal Microglia of Rats. Brain Res. Bull. 142, 138–146. doi:10.1016/j.brainresbull.2018.07.003
Ding, L. L., Hu, S. F., He, X. W., Zhang, P., Zhao, F. F., Liu, T. P., et al. (2020). Acupuncture Combined with Moxibustion Promote the Recovery of Spinal Cord Injury in Correlation with Shh/Gli-1 Signaling Pathway. J. Spinal Cord Med., 45(1). 1–11. doi:10.1080/10790268.2020.1766900
Ding, Y., Yan, Q., Ruan, J. W., Zhang, Y. Q., Li, W. J., Zeng, X., et al. (2011). Bone Marrow Mesenchymal Stem Cells and Electroacupuncture Downregulate the Inhibitor Molecules and Promote the Axonal Regeneration in the Transected Spinal Cord of Rats. Cel Transpl. 20 (4), 475–491. doi:10.3727/096368910X528102
Ding, Y., Yan, Q., Ruan, J. W., Zhang, Y. Q., Li, W. J., Zeng, X., et al. (2013). Electroacupuncture Promotes the Differentiation of Transplanted Bone Marrow Mesenchymal Stem Cells Overexpressing TrkC into Neuron-like Cells in Transected Spinal Cord of Rats. Cel Transpl. 22 (1), 65–86. doi:10.3727/096368912X655037
Ding, Y., Yan, Q., Ruan, J. W., Zhang, Y. Q., Li, W. J., Zhang, Y. J., et al. (2009). Electro-acupuncture Promotes Survival, Differentiation of the Bone Marrow Mesenchymal Stem Cells as Well as Functional Recovery in the Spinal Cord-Transected Rats. BMC Neurosci. 10, 35. doi:10.1186/1471-2202-10-35
Ding, Y., Zhang, D., Wang, S., Zhang, X., and Yang, J. (2021). Hematogenous Macrophages: A New Therapeutic Target for Spinal Cord Injury. Front Cel Dev Biol 9, 767888. doi:10.3389/fcell.2021.767888
Ding, Y., Zhang, R. Y., He, B., Liu, Z., Zhang, K., Ruan, J. W., et al. (2015). Combination of Electroacupuncture and Grafted Mesenchymal Stem Cells Overexpressing TrkC Improves Remyelination and Function in Demyelinated Spinal Cord of Rats. Sci. Rep. 5, 9133. doi:10.1038/srep09133
Du, S. Q., Wang, X. R., Zhu, W., Ye, Y., Yang, J. W., Ma, S. M., et al. (2018). Acupuncture Inhibits TXNIP-Associated Oxidative Stress and Inflammation to Attenuate Cognitive Impairment in Vascular Dementia Rats. CNS Neurosci. Ther. 24 (1), 39–46. doi:10.1111/cns.12773
Du, X. J., Chen, Y. X., Zheng, Z. C., Wang, N., Wang, X. Y., and Kong, F. E. (2019). Neural Stem Cell Transplantation Inhibits Glial Cell Proliferation and P2X Receptor-Mediated Neuropathic Pain in Spinal Cord Injury Rats. Neural Regen. Res. 14 (5), 876–885. doi:10.4103/1673-5374.249236
Dubrovsky, G., Ha, D., Thomas, A. L., Zhu, M., Hubacher, J., Itoh, T., et al. (2020). Electroacupuncture to Increase Neuronal Stem Cell Growth. Med. Acupunct 32 (1), 16–23. doi:10.1089/acu.2019.1381
Dyson-Hudson, T. A., Kadar, P., LaFountaine, M., Emmons, R., Kirshblum, S. C., Tulsky, D., et al. (2007). Acupuncture for Chronic Shoulder Pain in Persons with Spinal Cord Injury: a Small-Scale Clinical Trial. Arch. Phys. Med. Rehabil. 88 (10), 1276–1283. doi:10.1016/j.apmr.2007.06.014
Dyson-Hudson, T. A., Shiflett, S. C., Kirshblum, S. C., Bowen, J. E., and Druin, E. L. (2001). Acupuncture and Trager Psychophysical Integration in the Treatment of Wheelchair User's Shoulder Pain in Individuals with Spinal Cord Injury. Arch. Phys. Med. Rehabil. 82 (8), 1038–1046. doi:10.1053/apmr.2001.24888
Escobar-Corona, C., Torres-Castillo, S., Rodríguez-Torres, E. E., Segura-Alegría, B., Jiménez-Estrada, I., and Quiroz-González, S. (2017). Electroacupuncture Improves Gait Locomotion, H-Reflex and Ventral Root Potentials of Spinal Compression Injured Rats. Brain Res. Bull. 131, 7–17. doi:10.1016/j.brainresbull.2017.02.008
Estores, I., Chen, K., Jackson, B., Lao, L., and Gorman, P. H. (2017). Auricular Acupuncture for Spinal Cord Injury Related Neuropathic Pain: a Pilot Controlled Clinical Trial. J. Spinal Cord Med. 40 (4), 432–438. doi:10.1080/10790268.2016.1141489
Fan, Q., Cavus, O., Xiong, L., and Xia, Y. (2018). Spinal Cord Injury: How Could Acupuncture Help? J. Acupunct Meridian Stud. 11 (4), 124–132. doi:10.1016/j.jams.2018.05.002
Fang, B., Qin, M., Li, Y., Li, X., Tan, W., Zhang, Y., et al. (2017). Electroacupuncture Preconditioning and Postconditioning Inhibit Apoptosis and Neuroinflammation Induced by Spinal Cord Ischemia Reperfusion Injury through Enhancing Autophagy in Rats. Neurosci. Lett. 642, 136–141. doi:10.1016/j.neulet.2017.02.010
Faulkner, J. R., Herrmann, J. E., Woo, M. J., Tansey, K. E., Doan, N. B., and Sofroniew, M. V. (2004). Reactive Astrocytes Protect Tissue and Preserve Function after Spinal Cord Injury. J. Neurosci. 24 (9), 2143–2155. doi:10.1523/JNEUROSCI.3547-03.2004
Figueroa, J. D., Cordero, K., Serrano-Illan, M., Almeyda, A., Baldeosingh, K., Almaguel, F. G., et al. (2013). Metabolomics Uncovers Dietary omega-3 Fatty Acid-Derived Metabolites Implicated in Anti-nociceptive Responses after Experimental Spinal Cord Injury. Neuroscience 255, 1–18. doi:10.1016/j.neuroscience.2013.09.012
Gao, Y. H., Wang, J. Y., Han, Y. J., and Liu, J. L. (2021). Spinal Cord Toll like Receptor 4 and its Co-stimulatory Molecule Heat Shock Protein 90 May Parti-Cipate in Electroacupuncture Analgesia in Rats with Chronic Neuropathic Pain. Zhen Ci Yan Jiu 46 (9), 735–741. doi:10.13702/j.1000-0607.201103
Gedde, M. H., Lilleberg, H. S., Aßmus, J., Gilhus, N. E., and Rekand, T. (2019). Traumatic vs Non-traumatic Spinal Cord Injury: A Comparison of Primary Rehabilitation Outcomes and Complications during Hospitalization. J. Spinal Cord Med. 42 (6), 695–701. doi:10.1080/10790268.2019.1598698
Genovese, T., and Cuzzocrea, S. (2008). Role of Free Radicals and poly(ADP-Ribose)polymerase-1 in the Development of Spinal Cord Injury: New Potential Therapeutic Targets. Curr. Med. Chem. 15 (5), 477–487. doi:10.2174/092986708783503177
Gu, Y., Cheng, X., Huang, X., Yuan, Y., Qin, S., Tan, Z., et al. (2019). Conditional Ablation of Reactive Astrocytes to Dissect Their Roles in Spinal Cord Injury and Repair. Brain Behav. Immun. 80, 394–405. doi:10.1016/j.bbi.2019.04.016
Guan, R., Li, Z., Dai, X., Zou, W., Yu, X., Liu, H., et al. (2021). Electroacupuncture at GV20-GB7 R-egulates M-itophagy to P-rotect against N-eurological D-eficits F-ollowing I-ntracerebral H-emorrhage via I-nhibition of A-poptosis. Mol. Med. Rep. 24 (1), 131. doi:10.3892/mmr.2021.12131
Han, X., Huang, X., Wang, Y., and Chen, H. (2010). A Study of Astrocyte Activation in the Periinfarct Region after Cerebral Ischemia with Electroacupuncture. Brain Inj. 24 (5), 773–779. doi:10.3109/02699051003610482
Hashemizadeh, S., Pourkhodadad, S., Hosseindoost, S., Pejman, S., Kamarehei, M., Badripour, A., et al. (2022). Ac-SDKP Peptide Improves Functional Recovery Following Spinal Cord Injury in a Preclinical Model. Neuropeptides 92, 102228. doi:10.1016/j.npep.2022.102228
Hellenbrand, D. J., Quinn, C. M., Piper, Z. J., Morehouse, C. N., Fixel, J. A., and Hanna, A. S. (2021). Inflammation after Spinal Cord Injury: a Review of the Critical Timeline of Signaling Cues and Cellular Infiltration. J. Neuroinflammation 18 (1), 284. doi:10.1186/s12974-021-02337-2
Hong, E. S., Yao, H. H., Min, Y. J., Sun, J., Zhou, X., Zeng, X. B., et al. (2021). The Mechanism of Electroacupuncture for Treating Spinal Cord Injury Rats by Mediating Rho/Rho-Associated Kinase Signaling Pathway. J. Spinal Cord Med. 44 (3), 364–374. doi:10.1080/10790268.2019.1665612
Hongna, Y., Hongzhao, T., Quan, L., Delin, F., Guijun, L., Xiaolin, L., et al. (2020). Jia-Ji Electro-Acupuncture Improves Locomotor Function with Spinal Cord Injury by Regulation of Autophagy Flux and Inhibition of Necroptosis. Front. Neurosci. 14, 616864. doi:10.3389/fnins.2020.616864
Honjo, H., Naya, Y., Ukimura, O., Kojima, M., and Miki, T. (2000). Acupuncture on Clinical Symptoms and Urodynamic Measurements in Spinal-Cord-Injured Patients with Detrusor Hyperreflexia. Urol. Int. 65 (4), 190–195. doi:10.1159/000064874
Hu, H., Wang, H., and Liu, W. (2021). Effect of Ganglioside Combined with Chip Jiaji Electro-Acupuncture on Nogo-NgR Signal Pathway in SCI Rats. Saudi J. Biol. Sci. 28 (8), 4132–4136. doi:10.1016/j.sjbs.2021.02.031
Hu, R., Xu, H., Jiang, Y., Chen, Y., He, K., Wu, L., et al. (2020). EA Improves the Motor Function in Rats with Spinal Cord Injury by Inhibiting Signal Transduction of Semaphorin3A and Upregulating of the Peripheral Nerve Networks. Neural Plast. 2020, 8859672. doi:10.1155/2020/8859672
Huang, S. F., Ding, Y., Ruan, J. W., Zhang, W., Wu, J. L., He, B., et al. (2011). An Experimental Electro-Acupuncture Study in Treatment of the Rat Demyelinated Spinal Cord Injury Induced by Ethidium Bromide. Neurosci. Res. 70 (3), 294–304. doi:10.1016/j.neures.2011.03.010
Inquimbert, P., Moll, M., Latremoliere, A., Tong, C. K., Whang, J., Sheehan, G. F., et al. (2018). NMDA Receptor Activation Underlies the Loss of Spinal Dorsal Horn Neurons and the Transition to Persistent Pain after Peripheral Nerve Injury. Cell Rep 23 (9), 2678–2689. doi:10.1016/j.celrep.2018.04.107
Jazayeri, S. B., Beygi, S., Shokraneh, F., Hagen, E. M., and Rahimi-Movaghar, V. (2015). Incidence of Traumatic Spinal Cord Injury Worldwide: a Systematic Review. Eur. Spine J. 24 (5), 905–918. doi:10.1007/s00586-014-3424-6
Jeong, H. J., Yun, Y., Lee, S. J., Ha, Y., and Gwak, S. J. (2021). Biomaterials and Strategies for Repairing Spinal Cord Lesions. Neurochem. Int. 144, 104973. doi:10.1016/j.neuint.2021.104973
Jiang, B., Sun, D., Sun, H., Ru, X., Liu, H., Ge, S., et al. (2021). Prevalence, Incidence, and External Causes of Traumatic Spinal Cord Injury in China: A Nationally Representative Cross-Sectional Survey. Front. Neurol. 12, 784647. doi:10.3389/fneur.2021.784647
Jiang, D. X., Lu, Z. S., Li, G. B., Sun, S. Y., Mu, X., Lee, P., et al. (2015). Electroacupuncture Improves Microcirculation and Neuronal Morphology in the Spinal Cord of a Rat Model of Intervertebral Disc Extrusion. Neural Regen. Res. 10 (2), 237–243. doi:10.4103/1673-5374.152377
Jiang, J., Ding, N., Wang, K., and Li, Z. (2018). Electroacupuncture Could Influence the Expression of IL-1β and NLRP3 Inflammasome in Hippocampus of Alzheimer's Disease Animal Model. Evid. Based Complement. Alternat Med. 2018, 8296824. doi:10.1155/2018/8296824
Jiang, S., Chen, W., Zhang, Y., Zhang, Y., Chen, A., Dai, Q., et al. (2016). Acupuncture Induces the Proliferation and Differentiation of Endogenous Neural Stem Cells in Rats with Traumatic Brain Injury. Evid. Based Complement. Alternat Med. 2016, 2047412. doi:10.1155/2016/2047412
Jiang, S. H., Tu, W. Z., Zou, E. M., Hu, J., Wang, S., Li, J. R., et al. (2014). Neuroprotective Effects of Different Modalities of Acupuncture on Traumatic Spinal Cord Injury in Rats. Evid. Based Complement. Alternat Med. 2014, 431580. doi:10.1155/2014/431580
Jiang, T., Wu, M., Zhang, Z., Yan, C., Ma, Z., He, S., et al. (2019). Electroacupuncture Attenuated Cerebral Ischemic Injury and Neuroinflammation through α7nAChR-mediated Inhibition of NLRP3 Inflammasome in Stroke Rats. Mol. Med. 25 (1), 22. doi:10.1186/s10020-019-0091-4
Jin, H., Zhang, Y. T., Yang, Y., Wen, L. Y., Wang, J. H., Xu, H. Y., et al. (2019). Electroacupuncture Facilitates the Integration of Neural Stem Cell-Derived Neural Network with Transected Rat Spinal Cord. Stem Cel Rep. 12 (2), 274–289. doi:10.1016/j.stemcr.2018.12.015
Juarez Becerril, O., Salgado Ceballos, H., Anguiano Solis, C., Alvarado Sanchez, B., Lopez Hernandez, M. E., Diaz Ruiz, A., et al. (2015). Electro-Acupuncture at GV.4 Improves Functional Recovery in Paralyzed Rats after a Traumatic Spinal Cord Injury. Acupunct Electrother Res. 40 (4), 355.
Kang, Y. H., Yi, M. J., Kim, M. J., Park, M. T., Bae, S., Kang, C. M., et al. (2004). Caspase-independent Cell Death by Arsenic Trioxide in Human Cervical Cancer Cells: Reactive Oxygen Species-Mediated poly(ADP-Ribose) Polymerase-1 Activation Signals Apoptosis-Inducing Factor Release from Mitochondria. Cancer Res. 64 (24), 8960–8967. doi:10.1158/0008-5472.CAN-04-1830
Kim, W. K., Kim, W. H., Kweon, O. K., and Kang, B. J. (2022). Heat-Shock Proteins Can Potentiate the Therapeutic Ability of Cryopreserved Mesenchymal Stem Cells for the Treatment of Acute Spinal Cord Injury in Dogs. Stem Cel. Rev. Rep. [Epub ahead of print]. doi:10.1007/s12015-021-10316-6
Kobayakawa, K., Ohkawa, Y., Yoshizaki, S., Tamaru, T., Saito, T., Kijima, K., et al. (2019). Macrophage Centripetal Migration Drives Spontaneous Healing Process after Spinal Cord Injury. Sci. Adv. 5 (5), eaav5086. doi:10.1126/sciadv.aav5086
Kong, Y., Li, S., Zhang, M., Xu, W., Chen, Q., Zheng, L., et al. (2021). Acupuncture Ameliorates Neuronal Cell Death, Inflammation, and Ferroptosis and Downregulated miR-23a-3p after Intracerebral Hemorrhage in Rats. J. Mol. Neurosci. 71 (9), 1863–1875. doi:10.1007/s12031-020-01770-x
Lang, S., Yan, X., Wang, C., Wu, Y., Liu, X., Ma, C., et al. (2020). The Poly-ADP Ribose Polymerase-1/apoptosis-Inducing Factor Pathway May Help Mediate the Protective Effect of Electroacupuncture on Early Brain Injury after Subarachnoid Hemorrhage. Neuroreport 31 (8), 605–612. doi:10.1097/WNR.0000000000001445
Li, K., Liu, J., Song, L., Lv, W., Tian, X., Li, Z., et al. (2020). Effect of Electroacupuncture Treatment at Dazhui (GV14) and Mingmen (GV4) Modulates the PI3K/AKT/mTOR Signaling Pathway in Rats after Spinal Cord Injury. Neural Plast. 2020, 5474608. doi:10.1155/2020/5474608
Li, M. Y., Dai, X. H., Yu, X. P., Zou, W., Teng, W., Liu, P., et al. (2022). Scalp Acupuncture Protects against Neuronal Ferroptosis by Activating the P62-Keap1-Nrf2 Pathway in Rat Models of Intracranial Haemorrhage. J. Mol. Neurosci. 72 (1), 82–96. doi:10.1007/s12031-021-01890-y
Li, X., Wang, H., Li, C., Wu, J., Lu, J., Guo, J. Y., et al. (2021). Acupuncture Inhibits NLRP3 Inflammasome Activation in the Prefrontal Cortex of a Chronic Stress Rat Model of Depression. Anat. Rec. (Hoboken) 304 (11), 2470–2479. doi:10.1002/ar.24778
Li, Y. F., Li, T., Zhang, D. W., Xue, H., Chen, D., Li, C., et al. (2018). The Comprehensive Therapy of Electroacupuncture Promotes Regeneration of Nerve Fibers and Motor Function Recovery in Rats after Spinal Cord Injury. Evid. Based Complement. Alternat Med. 2018, 7568697. doi:10.1155/2018/7568697
Lim, S. N., Gladman, S. J., Dyall, S. C., Patel, U., Virani, N., Kang, J. X., et al. (2013). Transgenic Mice with High Endogenous omega-3 Fatty Acids Are Protected from Spinal Cord Injury. Neurobiol. Dis. 51, 104–112. doi:10.1016/j.nbd.2012.10.021
Liu, F., Zou, Y., Liu, S., Liu, J., and Wang, T. (2013). Electro-acupuncture Treatment Improves Neurological Function Associated with Downregulation of PDGF and Inhibition of Astrogliosis in Rats with Spinal Cord Transection. J. Mol. Neurosci. 51 (2), 629–635. doi:10.1007/s12031-013-0035-3
Liu, J., and Wu, Y. (2017). Electro-acupuncture-modulated miR-214 Prevents Neuronal Apoptosis by Targeting Bax and Inhibits Sodium Channel Nav1.3 Expression in Rats after Spinal Cord Injury. Biomed. Pharmacother. 89, 1125–1135. doi:10.1016/j.biopha.2017.02.077
Liu, Z., He, B., Zhang, R. Y., Zhang, K., Ding, Y., Ruan, J. W., et al. (2015). Electroacupuncture Promotes the Differentiation of Transplanted Bone Marrow Mesenchymal Stem Cells Preinduced with Neurotrophin-3 and Retinoic Acid into Oligodendrocyte-like Cells in Demyelinated Spinal Cord of Rats. Cel Transpl. 24 (7), 1265–1281. doi:10.3727/096368914X682099
Lu, J. Y., Ying, X. W., Chen, X. L., Tu, W. Z., Li, S. S., and Jiang, S. H. (2020). Effects of Electroacupuncture at Different Acupoints on the Histomorphology of Neurogenic Bladder and the Expression of Hyperpolarization-Activated Cyclic Nucleotide-Gated Channels in Interstitial Cells of Cajal in a Rat Model of Suprasacral Spinal Cord Injury. Ann. Palliat. Med. 9 (6), 3830–3838. doi:10.21037/apm-20-1827
Lukacova, N., Kisucka, A., Kiss Bimbova, K., Bacova, M., Ileninova, M., Kuruc, T., et al. (2021). Glial-Neuronal Interactions in Pathogenesis and Treatment of Spinal Cord Injury. Int. J. Mol. Sci. 22 (24), 13577. doi:10.3390/ijms222413577
Ma, R., Liu, X., Clark, J., Williams, G. M., and Doi, S. A. (2015). The Impact of Acupuncture on Neurological Recovery in Spinal Cord Injury: A Systematic Review and Meta-Analysis. J. Neurotrauma 32 (24), 1943–1957. doi:10.1089/neu.2014.3866
McDonald, J. W., and Sadowsky, C. (2002). Spinal-cord Injury. Lancet 359 (9304), 417–425. doi:10.1016/S0140-6736(02)07603-1
McKenzie, A. L., Hall, J. J., Aihara, N., Fukuda, K., and Noble, L. J. (1995). Immunolocalization of Endothelin in the Traumatized Spinal Cord: Relationship to Blood-Spinal Cord Barrier Breakdown. J. Neurotrauma 12 (3), 257–268. doi:10.1089/neu.1995.12.257
Meng, Q., Liu, X., Shan, Q., Yu, P., Mao, Z., Zhang, F., et al. (2014). Acupuncture for Treatment of Secondary Osteoporosis in Patients with Spinal Cord Injury: a Controlled Study. Acupunct Med. 32 (5), 381–386. doi:10.1136/acupmed-2013-010463
Mitsuhashi, T., Ikata, T., Morimoto, K., Tonai, T., and Katoh, S. (1994). Increased Production of Eicosanoids, TXA2, PGI2 and LTC4 in Experimental Spinal Cord Injuries. Paraplegia 32 (8), 524–530. doi:10.1038/sc.1994.84
Mo, Y. P., Yao, H. J., Lv, W., Song, L. Y., Song, H. T., Yuan, X. C., et al. (2016). Effects of Electroacupuncture at Governor Vessel Acupoints on Neurotrophin-3 in Rats with Experimental Spinal Cord Injury. Neural Plast. 2016, 2371875. doi:10.1155/2016/2371875
Moshi, H. I., Sundelin, G. G., Sahlen, K. G., and Sörlin, A. V. (2021). A One-Year Prospective Study on the Occurrence of Traumatic Spinal Cord Injury and Clinical Complications during Hospitalisation in North-East Tanzania. Afr. Health Sci. 21 (2), 788–794. doi:10.4314/ahs.v21i2.39
Nascimento de Souza, R., Silva, F. K., and Alves de Medeiros, M. (2017). Bee Venom Acupuncture Reduces Interleukin-6, Increases Interleukin-10, and Induces Locomotor Recovery in a Model of Spinal Cord Compression. J. Acupunct Meridian Stud. 10 (3), 204–210. doi:10.1016/j.jams.2017.04.003
Norrbrink, C., and Lundeberg, T. (2011). Acupuncture and Massage Therapy for Neuropathic Pain Following Spinal Cord Injury: an Exploratory Study. Acupunct Med. 29 (2), 108–115. doi:10.1136/aim.2010.003269
O'Shea, T. M., Burda, J. E., and Sofroniew, M. V. (2017). Cell Biology of Spinal Cord Injury and Repair. J. Clin. Invest. 127 (9), 3259–3270. doi:10.1172/JCI90608
On-Ong-Arj, P., Wattanathorn, J., Muchimapura, S., and Thukham-Mee, W. (2018). Yellow Laser Stimulation at GV2 Acupoint Mitigates Apoptosis, Oxidative Stress, Inflammation, and Motor Deficit in Spinal Cord Injury Rats. Evid. Based Complement. Alternat Med. 2018, 5407052. doi:10.1155/2018/5407052
Paola, F. A., and Arnold, M. (2003). Acupuncture and Spinal Cord Medicine. J. Spinal Cord Med. 26 (1), 12–20. doi:10.1080/10790268.2003.11753654
Pekny, M., Wilhelmsson, U., and Pekna, M. (2014). The Dual Role of Astrocyte Activation and Reactive Gliosis. Neurosci. Lett. 565, 30–38. doi:10.1016/j.neulet.2013.12.071
Pereira, I. M., Marote, A., Salgado, A. J., and Silva, N. A. (2019). Filling the Gap: Neural Stem Cells as A Promising Therapy for Spinal Cord Injury. Pharmaceuticals (Basel) 12 (2), 65. doi:10.3390/ph12020065
Prado, C., Fratini, P., de Sá Schiavo Matias, G., Monteiro, J., Dos Santos, C. J., et al. (2019). Combination of Stem Cells from Deciduous Teeth and Electroacupuncture for Therapy in Dogs with Chronic Spinal Cord Injury: A Pilot Study. Res. Vet. Sci. 123, 247–251. doi:10.1016/j.rvsc.2019.01.011
Ramer, L. M., Ramer, M. S., and Bradbury, E. J. (2014). Restoring Function after Spinal Cord Injury: towards Clinical Translation of Experimental Strategies. Lancet Neurol. 13 (12), 1241–1256. doi:10.1016/S1474-4422(14)70144-9
Renfu, Q., Rongliang, C., Mengxuan, D., Liang, Z., Jinwei, X., Zongbao, Y., et al. (2014). Anti-apoptotic Signal Transduction Mechanism of Electroacupuncture in Acute Spinal Cord Injury. Acupunct Med. 32 (6), 463–471. doi:10.1136/acupmed-2014-010526
Rivlin, A. S., and Tator, C. H. (1978). Regional Spinal Cord Blood Flow in Rats after Severe Cord Trauma. J. Neurosurg. 49 (6), 844–853. doi:10.3171/jns.1978.49.6.0844
Rohn, T. T., Hinds, T. R., and Vincenzi, F. F. (1996). Inhibition of Ca2+-Pump ATPase and the Na+/K+-pump ATPase by Iron-Generated Free Radicals. Protection by 6,7-Dimethyl-2,4-DI-1- Pyrrolidinyl-7h-Pyrrolo[2,3-D] Pyrimidine Sulfate (U-89843D), a Potent, Novel, Antioxidant/free Radical Scavenger. Biochem. Pharmacol. 51 (4), 471–476. doi:10.1016/0006-2952(95)02222-8
Rohn, T. T., Hinds, T. R., and Vincenzi, F. F. (1993). Ion Transport ATPases as Targets for Free Radical Damage. Protection by an Aminosteroid of the Ca2+ Pump ATPase and Na+/K+ Pump ATPase of Human Red Blood Cell Membranes. Biochem. Pharmacol. 46 (3), 525–534. doi:10.1016/0006-2952(93)90530-a
Scardina, G. A., Ruggieri, A., Provenzano, F., and Messina, P. (2009). The Effect of Acupuncture on Oral Microcirculation in Healthy Volunteers: an Exploratory Study. Acupunct Med. 27 (3), 114–117. doi:10.1136/aim.2009.000679
Scholpa, N. E., and Schnellmann, R. G. (2017). Mitochondrial-Based Therapeutics for the Treatment of Spinal Cord Injury: Mitochondrial Biogenesis as a Potential Pharmacological Target. J. Pharmacol. Exp. Ther. 363 (3), 303–313. doi:10.1124/jpet.117.244806
Sha, R., Han, X., Zheng, C., Peng, J., Wang, L., Chen, L., et al. (2019). The Effects of Electroacupuncture in a Rat Model of Cerebral Ischemia-Reperfusion Injury Following Middle Cerebral Artery Occlusion Involves MicroRNA-223 and the PTEN Signaling Pathway. Med. Sci. Monit. 25, 10077–10088. doi:10.12659/MSM.919611
Shi, P., Sun, L. L., Lee, Y. S., and Tu, Y. (2017). Electroacupuncture Regulates the Stress-Injury-Repair Chain of Events after Cerebral Ischemia/reperfusion Injury. Neural Regen. Res. 12 (6), 925–930. doi:10.4103/1673-5374.208574
Shi, Y., Quan, R., Li, C., Zhang, L., Du, M., Xu, J., et al. (2016). The Study of Traditional Chinese Medical Elongated-Needle Therapy Promoting Neurological Recovery Mechanism after Spinal Cord Injury in Rats. J. Ethnopharmacol 187, 28–41. doi:10.1016/j.jep.2016.04.019
Shi, Z., Yuan, S., Shi, L., Li, J., Ning, G., Kong, X., et al. (2021). Programmed Cell Death in Spinal Cord Injury Pathogenesis and Therapy. Cell Prolif 54 (3), e12992. doi:10.1111/cpr.12992
Shin, B. C., Lee, M. S., Kong, J. C., Jang, I., and Park, J. J. (2009). Acupuncture for Spinal Cord Injury Survivors in Chinese Literature: a Systematic Review. Complement. Ther. Med. 17 (5-6), 316–327. doi:10.1016/j.ctim.2009.09.001
Siebert, J. R., and Osterhout, D. J. (2011). The Inhibitory Effects of Chondroitin Sulfate Proteoglycans on Oligodendrocytes. J. Neurochem. 119 (1), 176–188. doi:10.1111/j.1471-4159.2011.07370.x
Sinescu, C., Popa, F., Grigorean, V. T., Onose, G., Sandu, A. M., Popescu, M., et al. (2010). Molecular Basis of Vascular Events Following Spinal Cord Injury. J. Med. Life 3 (3), 254
Song, J., Cao, X., Zhang, A., Fang, Z., Xu, J., and Gao, X. (2022). Posterior Tibial Nerve Stimulation Improves Neurogenic Bladder in Rats with Spinal Cord Injury through Transient Receptor potential/P2X Signaling Pathway. Neurourol Urodyn 41 (3), 756–764. doi:10.1002/nau.24885
Sun, L., Yong, Y., Wei, P., Wang, Y., Li, H., Zhou, Y., et al. (2021). Electroacupuncture Ameliorates Postoperative Cognitive Dysfunction and Associated Neuroinflammation via NLRP3 Signal Inhibition in Aged Mice. CNS Neurosci. Ther. 28(3). 399–400. doi:10.1111/cns.13784
Sun, N., Shi, J., Chen, L., Liu, X., and Guan, X. (2003). Influence of Electroacupuncture on the mRNA of Heat Shock Protein 70 and 90 in Brain after Cerebral Ischemia/reperfusion of Rats. J. Huazhong Univ. Sci. Technolog Med. Sci. 23 (2), 112–115. doi:10.1007/BF02859930
Tang, H., Guo, Y., Zhao, Y., Wang, S., Wang, J., Li, W., et al. (2020). Effects and Mechanisms of Acupuncture Combined with Mesenchymal Stem Cell Transplantation on Neural Recovery after Spinal Cord Injury: Progress and Prospects. Neural Plast. 2020, 8890655. doi:10.1155/2020/8890655
Tang, H. Y., Wang, F. J., Ma, J. L., Wang, H., Shen, G. M., and Jiang, A. J. (2020). Acupuncture Attenuates the Development of Diabetic Peripheral Neuralgia by Regulating P2X4 Expression and Inflammation in Rat Spinal Microglia. J. Physiol. Sci. 70 (1), 45. doi:10.1186/s12576-020-00769-8
Tang, R., Botchway, B. O. A., Meng, Y., Zhang, Y., Zhou, C., Jiang, J., et al. (2020). The Inhibition of Inflammatory Signaling Pathway by Secretory Leukocyte Protease Inhibitor Can Improve Spinal Cord Injury. Cell Mol Neurobiol 40 (7), 1067–1073. doi:10.1007/s10571-020-00799-1
Tao, J., Chen, B., Gao, Y., Yang, S., Huang, J., Jiang, X., et al. (2014). Electroacupuncture Enhances Hippocampal NSCs Proliferation in Cerebral Ischemia-Reperfusion Injured Rats via Activation of Notch Signaling Pathway. Int. J. Neurosci. 124 (3), 204–212. doi:10.3109/00207454.2013.840781
Tao, J., Xue, X. H., Chen, L. D., Yang, S. L., Jiang, M., Gao, Y. L., et al. (2010). Electroacupuncture Improves Neurological Deficits and Enhances Proliferation and Differentiation of Endogenous Nerve Stem Cells in Rats with Focal Cerebral Ischemia. Neurol. Res. 32 (2), 198–204. doi:10.1179/174313209X414506
Tao, J., Zheng, Y., Liu, W., Yang, S., Huang, J., Xue, X., et al. (2016). Electro-acupuncture at LI11 and ST36 Acupoints Exerts Neuroprotective Effects via Reactive Astrocyte Proliferation after Ischemia and Reperfusion Injury in Rats. Brain Res. Bull. 120, 14–24. doi:10.1016/j.brainresbull.2015.10.011
Tator, C. H., and Fehlings, M. G. (1991). Review of the Secondary Injury Theory of Acute Spinal Cord Trauma with Emphasis on Vascular Mechanisms. J. Neurosurg. 75 (1), 15–26. doi:10.3171/jns.1991.75.1.0015
Tator, C. H. (1995). Update on the Pathophysiology and Pathology of Acute Spinal Cord Injury. Brain Pathol. 5 (4), 407–413. doi:10.1111/j.1750-3639.1995.tb00619.x
Tempel, G. E., and Martin, H. F. (1992). The Beneficial Effects of a Thromboxane Receptor Antagonist on Spinal Cord Perfusion Following Experimental Cord Injury. J. Neurol. Sci. 109 (2), 162–167. doi:10.1016/0022-510x(92)90163-f
Tian, G. H., Sun, K., Huang, P., Zhou, C. M., Yao, H. J., Huo, Z. J., et al. (2013). Long-Term Stimulation with Electroacupuncture at DU20 and ST36 Rescues Hippocampal Neuron through Attenuating Cerebral Blood Flow in Spontaneously Hypertensive Rats. Evid. Based Complement. Alternat Med. 2013, 482947. doi:10.1155/2013/482947
Tida, J. A., Catalão, C. H. R., Garcia, C. A. B., Dos Santos, A. C., Salmon, C. E. G., and Lopes, L. D. S. (2018). Acupuncture at ST36 Exerts Neuroprotective Effects via Inhibition of Reactive Astrogliosis in Infantile Rats with Hydrocephalus. Acupunct Med. 36 (6), 386–393. doi:10.1136/acupmed-2017-011515
Tran, A. P., Warren, P. M., and Silver, J. (2021). New Insights into Glial Scar Formation after Spinal Cord Injury. Cell Tissue Res [Epub ahead of print]. doi:10.1007/s00441-021-03477-w
Tran, A. P., Warren, P. M., and Silver, J. (2018). The Biology of Regeneration Failure and Success after Spinal Cord Injury. Physiol. Rev. 98 (2), 881–917. doi:10.1152/physrev.00017.2017
Tu, W. Z., Chen, W. C., Xia, W., He, R., Hu, J., Jiang, M. C., et al. (2017). The Regulatory Effect of Electro-Acupuncture on the Expression of NMDA Receptors in a SCI Rat Model. Life Sci. 177, 8–14. doi:10.1016/j.lfs.2017.04.004
Tu, W. Z., Jiang, S. H., Zhang, L., Li, S. S., Gu, P. P., He, R., et al. (2017). Electro-acupuncture at Governor Vessel Improves Neurological Function in Rats with Spinal Cord Injury. Chin. J. Integr. Med. doi:10.1007/s11655-017-2968-9
Tu, W. Z., Li, S. S., Jiang, X., Qian, X. R., Yang, G. H., Gu, P. P., et al. (2018). Effect of Electro-Acupuncture on the BDNF-TrkB Pathway in the Spinal Cord of CCI Rats. Int. J. Mol. Med. 41 (6), 3307–3315. doi:10.3892/ijmm.2018.3563
Vancamp, P., Butruille, L., Demeneix, B. A., and Remaud, S. (2020). Thyroid Hormone and Neural Stem Cells: Repair Potential Following Brain and Spinal Cord Injury. Front. Neurosci. 14, 875. doi:10.3389/fnins.2020.00875
Visavadiya, N. P., McEwen, M. L., Pandya, J. D., Sullivan, P. G., Gwag, B. J., and Springer, J. E. (2013). Antioxidant Properties of Neu2000 on Mitochondrial Free Radicals and Oxidative Damage. Toxicol. Vitro 27 (2), 788–797. doi:10.1016/j.tiv.2012.12.011
Wang, G. Y., Cheng, Z. J., Yuan, P. W., Li, H. P., and He, X. J. (2021). Olfactory Ensheathing Cell Transplantation Alters the Expression of Chondroitin Sulfate Proteoglycans and Promotes Axonal Regeneration after Spinal Cord Injury. Neural Regen. Res. 16 (8), 1638–1644. doi:10.4103/1673-5374.301023
Wang, T. D., Wang, Y. H., Huang, T. S., Su, T. C., Pan, S. L., and Chen, S. Y. (2007). Circulating Levels of Markers of Inflammation and Endothelial Activation Are Increased in Men with Chronic Spinal Cord Injury. J. Formos. Med. Assoc. 106 (11), 919–928. doi:10.1016/S0929-6646(08)60062-5
Wang, X., Ju, S., Chen, S., Gao, W., Ding, J., Wang, G., et al. (2017). Effect of Electro-Acupuncture on Neuroplasticity of Spinal Cord-Transected Rats. Med. Sci. Monit. 23, 4241–4251. doi:10.12659/msm.903056
Wang, X., Wang, Q., Tian, H., Lv, W., Song, L., Li, Z., et al. (2021). Electroacupuncture in Promoting Neural Repair after Spinal Cord Injury: Inhibiting the Notch Signaling Pathway and Regulating Downstream Proteins Expression. Anat. Rec. (Hoboken) 304 (11), 2494–2505. doi:10.1002/ar.24779
Wang, Y., Zhao, Y., Ma, X., Li, J., Hou, J., and Lv, X. (2019). Beneficial Effects of Electroacupuncture on Neuropathic Pain Evoked by Spinal Cord Injury and Involvement of PI3K-mTOR Mechanisms. Biol. Res. Nurs. 21 (1), 5–13. doi:10.1177/1099800418804896
Wang, Y. L., Qi, Y. N., Wang, W., Dong, C. K., Yi, P., Yang, F., et al. (2018). Effects of Decompression Joint Governor Vessel Electro-Acupuncture on Rats with Acute Upper Cervical Spinal Cord Injury. Neural Regen. Res. 13 (7), 1241–1246. doi:10.4103/1673-5374.235062
Wannapakhe, J., Arrayawichanon, P., Saengsuwan, J., and Amatachaya, S. (2015). Medical Complications and Falls in Patients with Spinal Cord Injury during the Immediate Phase after Completing a Rehabilitation Program. J. Spinal Cord Med. 38 (1), 84–90. doi:10.1179/2045772313Y.0000000173
Wei, Z., Wang, Y., Zhao, W., and Schachner, M. (2017). Electro-Acupuncture Modulates L1 Adhesion Molecule Expression after Mouse Spinal Cord Injury. Am. J. Chin. Med. 45 (1), 37–52. doi:10.1142/S0192415X17500045
Wei, Z., Zhao, W., and Schachner, M. (2018). Electroacupuncture Restores Locomotor Functions after Mouse Spinal Cord Injury in Correlation with Reduction of PTEN and P53 Expression. Front. Mol. Neurosci. 11, 411. doi:10.3389/fnmol.2018.00411
Wojdasiewicz, P., Poniatowski, Ł. A., Turczyn, P., Frasuńska, J., Paradowska-Gorycka, A., and Tarnacka, B. (2020). Significance of Omega-3 Fatty Acids in the Prophylaxis and Treatment after Spinal Cord Injury in Rodent Models. Mediators Inflamm. 2020, 3164260. doi:10.1155/2020/3164260
Wong, A. M., Leong, C. P., Su, T. Y., Yu, S. W., Tsai, W. C., and Chen, C. P. (2003). Clinical Trial of Acupuncture for Patients with Spinal Cord Injuries. Am. J. Phys. Med. Rehabil. 82 (1), 21–27. doi:10.1097/00002060-200301000-00004
Woźniak, B., Woźniak, A., Mila-Kierzenkowska, C., and Kasprzak, H. A. (2016). Correlation of Oxidative and Antioxidative Processes in the Blood of Patients with Cervical Spinal Cord Injury. Oxid Med. Cel Longev 2016, 6094631. doi:10.1155/2016/6094631
Wu, D., Zheng, C., Wu, J., Xue, J., Huang, R., Wu, D., et al. (2017). The Pathologic Mechanisms Underlying Lumbar Distraction Spinal Cord Injury in Rabbits. Spine J. 17 (11), 1665–1673. doi:10.1016/j.spinee.2017.05.024
Wu, Q., Yue, J., Lin, L., Yu, X., Zhou, Y., Ying, X., et al. (2021). Electroacupuncture May Alleviate Neuropathic Pain via Suppressing P2X7R Expression. Mol. Pain 17, 1744806921997654. doi:10.1177/1744806921997654
Wu, Y., Hu, R., Zhong, X., Zhang, A., Pang, B., Sun, X., et al. (2021). Electric Acupuncture Treatment Promotes Angiogenesis in Rats with Middle Cerebral Artery Occlusion through EphB4/EphrinB2 Mediated Src/PI3K Signal Pathway. J. Stroke Cerebrovasc. Dis. 30 (3), 105165. doi:10.1016/j.jstrokecerebrovasdis.2020.105165
Wu, Y., Sun, Z., Li, Z., Zhao, Y., and Sun, S. (2002). Effect of Acupuncture on Free Radicals in Rats with Early Experimental Spinal Cord Injury. J. Tradit Chin. Med. 22 (1), 51
Xiao, W. P., Ding, L. L., Min, Y. J., Yang, H. Y., Yao, H. H., Sun, J., et al. (2019). Electroacupuncture Promoting Axonal Regeneration in Spinal Cord Injury Rats via Suppression of Nogo/NgR and Rho/ROCK Signaling Pathway. Neuropsychiatr. Dis. Treat. 15, 3429–3442. doi:10.2147/NDT.S216874
Xie, Y. G., Mu, H. J., Li, Z., Ma, J. H., and Wang, Y. L. (2014). Supression of Chronic central Pain by Superoxide Dismutase in Rats with Spinal Cord Injury: Inhibition of the NMDA Receptor Implicated. Exp. Ther. Med. 8 (4), 1137–1141. doi:10.3892/etm.2014.1878
Xu, B., Wang, J., Liu, S., Liu, H., Zhang, X., Shi, J., et al. (2021). HSP70 Alleviates Spinal Cord Injury by Activating the NF-kB Pathway. J. Musculoskelet. Neuronal Interact 21 (4), 542
Xu, H., Sun, H., Chen, S. H., Zhang, Y. M., Piao, Y. L., and Gao, Y. (2014). Effects of Acupuncture at Baihui (DU20) and Zusanli (ST36) on the Expression of Heat Shock Protein 70 and Tumor Necrosis Factor α in the Peripheral Serum of Cerebral Ischemia-Reperfusion-Injured Rats. Chin. J. Integr. Med. 20 (5), 369–374. doi:10.1007/s11655-014-1800-z
Xu, H., Yang, Y., Deng, Q. W., Zhang, B. B., Ruan, J. W., Jin, H., et al. (2021). Governor Vessel Electro-Acupuncture Promotes the Intrinsic Growth Ability of Spinal Neurons through Activating Calcitonin Gene-Related Peptide/α-Calcium/Calmodulin-dependent Protein Kinase/Neurotrophin-3 Pathway after Spinal Cord Injury. J. Neurotrauma 38 (6), 734–745. doi:10.1089/neu.2020.7155
Xu, J., Chen, X. M., Zheng, B. J., and Wang, X. R. (2016). Electroacupuncture Relieves Nerve Injury-Induced Pain Hypersensitivity via the Inhibition of Spinal P2X7 Receptor-Positive Microglia. Anesth. Analg 122 (3), 882–892. doi:10.1213/ANE.0000000000001097
Xu, J., Cheng, S., Jiao, Z., Zhao, Z., Cai, Z., Su, N., et al. (2019). Fire Needle Acupuncture Regulates Wnt/ERK Multiple Pathways to Promote Neural Stem Cells to Differentiate into Neurons in Rats with Spinal Cord Injury. CNS Neurol. Disord. Drug Targets 18 (3), 245–255. doi:10.2174/1871527318666190204111701
Yan, Q., Ruan, J. W., Ding, Y., Li, W. J., Li, Y., and Zeng, Y. S. (2011). Electro-acupuncture Promotes Differentiation of Mesenchymal Stem Cells, Regeneration of Nerve Fibers and Partial Functional Recovery after Spinal Cord Injury. Exp. Toxicol. Pathol. 63 (1-2), 151–156. doi:10.1016/j.etp.2009.11.002
Yang, Y., Xu, H. Y., Deng, Q. W., Wu, G. H., Zeng, X., Jin, H., et al. (2021). Electroacupuncture Facilitates the Integration of a Grafted TrkC-Modified Mesenchymal Stem Cell-Derived Neural Network into Transected Spinal Cord in Rats via Increasing Neurotrophin-3. CNS Neurosci. Ther. 27 (7), 776–791. doi:10.1111/cns.13638
Yang, Z., and Wang, K. K. (2015). Glial Fibrillary Acidic Protein: from Intermediate Filament Assembly and Gliosis to Neurobiomarker. Trends Neurosci. 38 (6), 364–374. doi:10.1016/j.tins.2015.04.003
Yeh, B. Y., Chao, Y. L., Chen, Y. S., and Yu, H. P. (2021). Effect of Acupuncture on Capillary Refill Time in Healthy Adults: A Clinical Study. Microvasc. Res. 135, 104135. doi:10.1016/j.mvr.2021.104135
Young-Wook, P., Gi Yoon, H., Min Jae, K., Seo-Yeon, L., Byung Tae, C., and Hwa Kyoung, S. (2019). Subacute Electroacupuncture at Baihui (GV 20) and Dazhui (GV 14) Promotes post-stroke Functional Recovery via Neurogenesis and Astrogliosis in a Photothrombotic Stroke Mouse Model. J. Tradit Chin. Med. 39 (6), 833–841.
Yu, D., Li, M., Nie, P., Ni, B., Zhang, Z., and Zhou, Y. (2018). Bcl-2/E1B-19KD-Interacting Protein 3/Light Chain 3 Interaction Induces Mitophagy in Spinal Cord Injury in Rats Both In Vivo and In Vitro. J. Neurotrauma 35 (18), 2183–2194. doi:10.1089/neu.2017.5280
Yu, J., Min, D., Bai, Y., Qu, L., Zou, T., and Wang, S. (2020). Electroacupuncture Alleviates Parkinson Disease and Regulates the Expression of Brain-Gut Peptides. Exp. Anim. 69 (4), 448–460. doi:10.1538/expanim.19-0153
Zhang, H., Uchimura, K., and Kadomatsu, K. (2006). Brain Keratan Sulfate and Glial Scar Formation. Ann. N. Y Acad. Sci. 1086, 81–90. doi:10.1196/annals.1377.014
Zhang, J., Li, S., and Wu, Y. (2017). Recovery of Spinal Cord Injury Following Electroacupuncture in Rats through Enhancement of Wnt/β-Catenin Signaling. Mol. Med. Rep. 16 (2), 2185–2190. doi:10.3892/mmr.2017.6801
Zhang, K., Liu, Z., Li, G., Lai, B. Q., Qin, L. N., Ding, Y., et al. (2014). Electro-acupuncture Promotes the Survival and Differentiation of Transplanted Bone Marrow Mesenchymal Stem Cells Pre-induced with Neurotrophin-3 and Retinoic Acid in Gelatin Sponge Scaffold after Rat Spinal Cord Transection. Stem Cel Rev Rep 10 (4), 612–625. doi:10.1007/s12015-014-9513-4
Zhang, M., Dai, Q., Liang, D., Li, D., Chen, S., Chen, S., et al. (2018). Involvement of Adenosine A1 Receptor in Electroacupuncture-Mediated Inhibition of Astrocyte Activation during Neuropathic Pain. Arq Neuropsiquiatr 76 (11), 736–742. doi:10.1590/0004-282X20180128
Zhang, S., Jin, T., Wang, L., Liu, W., Zhang, Y., Zheng, Y., et al. (2020). Electro-Acupuncture Promotes the Differentiation of Endogenous Neural Stem Cells via Exosomal microRNA 146b after Ischemic Stroke. Front Cel Neurosci 14, 223. doi:10.3389/fncel.2020.00223
Zhang, T., Guan, B., Tan, S., Zhu, H., Ren, D., Li, R., et al. (2021). Bushen Huoxue Acupuncture Inhibits NLRP1 Inflammasome-Mediated Neuronal Pyroptosis in SAMP8 Mouse Model of Alzheimer's Disease. Neuropsychiatr. Dis. Treat. 17, 339–346. doi:10.2147/NDT.S279304
Zhang, T., Yu, J., Huang, Z., Wang, G., and Zhang, R. (2019). Electroacupuncture Improves Neurogenic Bladder Dysfunction through Activation of NGF/TrkA Signaling in a Rat Model. J. Cel Biochem 120 (6), 9900–9905. doi:10.1002/jcb.28272
Zhang, Y. M., Zhang, Y. Q., Cheng, S. B., Chen, S. X., Chen, A. L., and Tang, C. Z. (2013). Effect of Acupuncture on Proliferation and Differentiation of Neural Stem Cells in Brain Tissues of Rats with Traumatic Brain Injury. Chin. J. Integr. Med. 19 (2), 132–136. doi:10.1007/s11655-013-1353-6
Zhang, Y. T., Jin, H., Wang, J. H., Wen, L. Y., Yang, Y., Ruan, J. W., et al. (2017). Tail Nerve Electrical Stimulation and Electro-Acupuncture Can Protect Spinal Motor Neurons and Alleviate Muscle Atrophy after Spinal Cord Transection in Rats. Neural Plast. 2017, 7351238. doi:10.1155/2017/7351238
Zhao, J., Sui, M., Lü, X., Jin, D., Zhuang, Z., and Yan, T. (2015). Electroacupuncture Promotes Neural Stem Cell Proliferation and Neurogenesis in the Dentate Gyrus of Rats Following Stroke via Upregulation of Notch1 Expression. Mol. Med. Rep. 12 (5), 6911–6917. doi:10.3892/mmr.2015.4279
Zhao, J., Wang, L., and Li, Y. (2017). Electroacupuncture Alleviates the Inflammatory Response via Effects on M1 and M2 Macrophages after Spinal Cord Injury. Acupunct Med. 35 (3), 224–230. doi:10.1136/acupmed-2016-011107
Zhao, L., Liu, J. W., Kan, B. H., Shi, H. Y., Yang, L. P., and Liu, X. Y. (2020). Acupuncture Accelerates Neural Regeneration and Synaptophysin Production after Neural Stem Cells Transplantation in Mice. World J. Stem Cell 12 (12), 1576–1590. doi:10.4252/wjsc.v12.i12.1576
Zhao, P., Fu, H., Cheng, H., Zheng, R., Yuan, D., Yang, J., et al. (2022). Acupuncture at ST36 Alleviates the Behavioral Disorder of Autistic Rats by Inhibiting TXNIP-Mediated Activation of NLRP3. J. Neuropathol. Exp. Neurol. 81 (2), 127–134. doi:10.1093/jnen/nlab132
Zhao, W., Zhao, Q., Liu, J., Xu, X. Y., Sun, W. W., Zhou, X., et al. (2008). Electro-acupuncture Reduces Neuronal Apoptosis Linked to Bax and Bcl-2 Expression in the Spinal Cords of Cats Subjected to Partial Dorsal Root Ganglionectomy. Neurochem. Res. 33 (11), 2214–2221. doi:10.1007/s11064-008-9677-x
Zhao, W. S., Jiang, Z. N., Shi, H., Xu, L. L., Yang, Y., and Wang, Y. C. (2019). Low-Frequency Electroacupuncture Alleviates Chronic Constrictive Injury-Induced Mechanical Allodynia by Inhibiting NR2B Upregulation in Ipsilateral Spinal Dorsal Horn in Rats. Chin. J. Integr. Med. 25 (6), 462–467. doi:10.1007/s11655-018-3057-4
Zhou, H. J., Tang, T., Zhong, J. H., Luo, J. K., Cui, H. J., Zhang, Q. M., et al. (2014). Electroacupuncture Improves Recovery after Hemorrhagic Brain Injury by Inducing the Expression of Angiopoietin-1 and -2 in Rats. BMC Complement. Altern. Med. 14, 127. doi:10.1186/1472-6882-14-127
Zhou, X., He, X., and Ren, Y. (2014). Function of Microglia and Macrophages in Secondary Damage after Spinal Cord Injury. Neural Regen. Res. 9 (20), 1787–1795. doi:10.4103/1673-5374.143423
Zhou, Z., Li, H., Li, H., Zhang, J., Fu, K., Cao, C., et al. (2020). Comprehensive Analysis of the Differential Expression Profile of microRNAs in Rats with Spinal Cord Injury Treated by Electroacupuncture. Mol. Med. Rep. 22 (2), 751–762. doi:10.3892/mmr.2020.11161
Keywords: acupuncture, spinal cord injury, therapy, mechanism, apoptosis, inflammation, oxidative stress, neuroprotection
Citation: Jiang K, Sun Y and Chen X (2022) Mechanism Underlying Acupuncture Therapy in Spinal Cord Injury: A Narrative Overview of Preclinical Studies. Front. Pharmacol. 13:875103. doi: 10.3389/fphar.2022.875103
Received: 13 February 2022; Accepted: 14 March 2022;
Published: 07 April 2022.
Edited by:
Anwen Shao, Zhejiang University, ChinaReviewed by:
Gaiqing Wang, Second Hospital of Shanxi Medical University, ChinaLiang Wu, Wenzhou Medical University, China
Copyright © 2022 Jiang, Sun and Chen. This is an open-access article distributed under the terms of the Creative Commons Attribution License (CC BY). The use, distribution or reproduction in other forums is permitted, provided the original author(s) and the copyright owner(s) are credited and that the original publication in this journal is cited, in accordance with accepted academic practice. No use, distribution or reproduction is permitted which does not comply with these terms.
*Correspondence: Xinle Chen, MTc4NDUxMjI5QHFxLmNvbQ==