- 1Centre for Drug and Herbal Research Development, Faculty of Pharmacy, Universiti Kebangsaan Malaysia, Kuala Lumpur, Malaysia
- 2Program of Biomedical Science, Centre of Applied and Health Sciences, Faculty of Health Sciences, Universiti Kebangsaan Malaysia, Kuala Lumpur, Malaysia
- 3Department of Pharmacology, Faculty of Medicine, Universiti Kebangsaan Malaysia, Kuala Lumpur, Malaysia
- 4Unit of Pharmacology, Faculty of Medicine and Defence Health, Universiti Pertahanan Nasional Malaysia, Kuala Lumpur, Malaysia
Cardiovascular diseases have become a major clinical burden globally. Heart failure is one of the diseases that commonly emanates from progressive uncontrolled hypertension. This gives rise to the need for a new treatment for the disease. Sacubitril/valsartan is a new drug combination that has been approved for patients with heart failure. This review aims to detail the mechanism of action for sacubitril/valsartan in cardiac remodeling, a cellular and molecular process that occurs during the development of heart failure. Accumulating evidence has unveiled the cardioprotective effects of sacubitril/valsartan on cellular and molecular modulation in cardiac remodeling, with recent large-scale randomized clinical trials confirming its supremacy over other traditional heart failure treatments. However, its molecular mechanism of action in cardiac remodeling remains obscure. Therefore, comprehending the molecular mechanism of action of sacubitril/valsartan could help future research to study the drug’s potential therapy to reduce the severity of heart failure.
1 Introduction
Heart failure following long-standing uncontrolled hypertension and myocardial infarction (MI) remains a significant public health problem worldwide. Hypertrophic cardiomyopathy can also evolve into heart failure (Brieler et al., 2017). Despite emerging medical advancements in the therapy of cardiovascular disease, death and disability due to heart failure have raised enormously (Bhattacharya and Sil, 2018). Cardiac remodeling is generally accepted as a determinant of the clinical course of heart failure, and is now recognized as an important aspect of cardiovascular disease progression that is therefore emerging as a therapeutic target in heart failure of all etiologies (Mann, 2005).
Cardiac remodeling is a complex structural transformation marked by changes in the size and shape of myocardium. At the beginning, remodeling is an adaptive response to preserve normal heart function in circumstances such as chronic hypertension and MI (Nakamura and Sadoshima, 2018). Continuous remodeling is maladaptive, however, resulting in left ventricular dilation, hypertrophy, and dysfunction, involving molecular and cellular alterations such as cardiomyocyte hypertrophy, fibrosis, changes in cardiac extracellular matrix, and gene expression. These alterations within the myocyte are followed by cell death induced by apoptosis or necrosis, while in progressed compensated hypertrophy to dilated heart failure, the alterations result in myocyte lengthening, extracellular matrix remodeling, chamber dilation, and impaired systolic or diastolic functions (Gajarsa and Kloner, 2011).
Many pharmacological therapies have been used to treat and decrease the severity of heart failure for patients with reduced ejection fraction (HFrEF). Among them angiotensin-converting enzyme (ACE) inhibitors, β-blockers, mineralocorticoid receptor antagonists, and angiotensin receptor blockers, as well as two new classes of drugs—angiotensin receptor-neprilysin inhibitor (ARNI) and sodium-glucose co-transporter 2 (SGLT2) inhibitors (Burnett et al., 2017; Balan et al., 2021). Analysis has suggested that ARNI monotherapy is more effective than angiotensin receptor blockers or ACE inhibitors monotherapy (Burnett et al., 2017). Currently, sacubitril/valsartan (Figure 1), formerly known as LCZ696, and marketed by Novartis International AG as Entresto∗, is the only drug that belongs to the ARNI group (Hubers and Brown, 2016).
Sacubitril is a neprilysin inhibitor, while valsartan is an angiotensin II (Ang II) type 1 receptor (AT1R) blocker. Neprilysin is an enzyme involved in the breakdown of circulating natriuretic peptides that have a blood pressure-lowering property (Vilela-Martin, 2016). Therefore, the combination drug acts by simultaneously maintaining circulating natriuretic peptides and blocking the renin-angiotensin-aldosterone system (RAAS) (Cuthbert et al., 2020). Studies have revealed that inhibition of neprilysin by sacubitril alone failed to improve the prognosis of heart failure. This is likely due to its counterbalance effect (Cleland and Swedberg, 1998; Greenberg, 2020; Sutanto et al., 2021), resulting from an accumulation of vasoconstrictors Ang II and endothelin-1, which are also broken down by neprilysin (Vilela-Martin, 2016). Both vasoconstrictors could promote the development of cardiac hypertrophy (Miyauchi and Sakai, 2019; Siti et al., 2021a). Simultaneous inhibition of RAAS and neprilysin produces more effective neurohormonal modulation, preventing clinical deterioration in patients with heart failure (Choi and Shin, 2020; Książczyk and Lelonek, 2020).
Sacubitril/valsartan is the first drug with a double-acting pharmaceutical design conveying two pharmacological effects synchronously (Abumayyaleh et al., 2020). It was approved by the European Medicine Agency and the United States Food and Drug Administration in 2015 (Vilela-Martin, 2016; Chrysant and Chrysant, 2018), and was included a year later as a Class I recommendation, indicated for chronic heart failure patients with HFrEF (Kaplinsky, 2016). The drug is tolerated well and linked to a low incidence of angioedema due to a smaller increase in bradykinin in patients as compared with ACE inhibitors such as enalapril (Desai et al., 2015; McCormack, 2016; Yandrapalli et al., 2017; Myhre et al., 2019; Tanase et al., 2019). The drug is reported to be superior to enalapril in decreasing the risk of mortality in patients with heart failure (Desai et al., 2015). Moreover, omapatrilat, the first dual inhibitor of both neprilysin and ACE, produced a higher incidence of potentially life-threatening angioedema than enalapril, despite its greater efficacy in reducing blood pressure. Hence, further development of omapatrilat as an antihypertensive was terminated (Greenberg 2020; Pascual-Figal et al., 2021).
In addition to neprilysin inhibition and AT1R blockade, sacubitril/valsartan inhibits multiple targets such as signaling pathways involved in cardiac fibrosis, matrix remodeling, and apoptosis. This review provides updates on the molecular mechanisms of sacubitril/valsartan in cardiac remodeling modulation, with the goals of better understanding the drug combination’s role in the management of patients with heart failure and promoting future studies.
2 Effects of sacubitril/valsartan on renin-angiotensin-aldosterone system and the natriuretic peptide system
Neprilysin, a component of RAAS, is predominantly expressed in the kidneys, lungs, heart, and brain (Bellis et al., 2020; Nalivaeva et al., 2020). Even though the kidneys execute an important role in regulating the enzyme in systemic circulation (Pavo et al., 2020), the heart is the main origin of soluble circulating neprilysin (Arrigo et al., 2018). Surprisingly, myocardial expression of the enzyme decreased in heart failure (Pavo et al., 2020) and following repeated episodes of heart ischemia-reperfusion (Pavo et al., 2017) in animal experiments. To the best of our knowledge, the expression of the enzyme in a failing human heart has not been reported. Augmenting the natriuretic peptide system is the rationale for inhibiting circulating neprilysin in patients with HFrEF (Singh et al., 2017). As previously mentioned, sacubitril/valsartan simultaneously inhibits neprilysin and RAAS. Circulating natriuretic peptides—atrial (ANP), B-type (BNP) and C-type (CNP) natriuretic peptides (Kuwahara, 2021)—as well as Ang II and endothelin-1 levels are increased following neprilysin inhibition (Ferro et al., 1998; Khder et al., 2017; Pavo et al., 2020) by sacubitril (Figure 2). However, the detrimental efffects of Ang II on the heart and vascular system are blocked by valsartan (Kaplinsky, 2016). Sacubitril/valsartan was also reported to decrease the expresssion of hepatic endothelin-1 (Hsu et al., 2020) and its plasma level (Clements et al., 2019) in hypertensive rats. The inhibition of neprilysin was confirmed by a significant time-dependent reduction in plasma activity of soluble neprilysin as well as an elevation of ANP levels in patients with chronic heart failure after receiving sacubitril/valsartan for 30 and 90 days. BNP and N-terminal pro-BNP (NT-pro-BNP)—a prohormone of BNP with a longer half-life than BNP—levels remained stable in these patients (Nougué et al., 2019). Unlike BNP, NT-pro-BNP is not a substrate of neprilysin (Haynes et al., 2018).
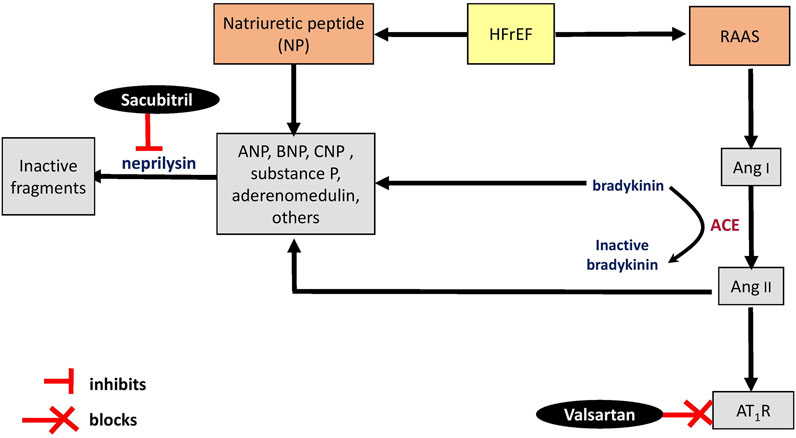
FIGURE 2. Schematic representation of mechanisms of sacubitril/valsartan on renin-angiotensin-aldosterone system and natriuretic peptide system. ACE, angiotensin converting enzyme; Ang, angiotensin; ANP, atrial natriuretic peptide; ARB, angiotensin receptor blocker; ARNI, angiotensin receptor-neprilysin inhibitor; AT1R, angiotensin type 1 receptor; BNP, B-type natriuretic peptide; CNP, C-type natriuretic peptide; HFrEF, heart failure with reduced ejection fraction; NP natriuretic peptide; RAAS, renin-angiotensin-aldosterone system; ↑, increase.
Additionally, the apelinergic system (composed of apelin and elabela as well as APJ receptors) is believed to be a promising target for the treatment of cardiovascular disease. Both apelin and elabela possess inodilator properties (Sainsily et al., 2021). However, the effects of sacubitril/valsartan on apelin and elabela have not been studied. Neprilysin cleaves apelin (McKinnie et al., 2016). Therefore, it could be hypothesized that the drug would increase the level of apelin. Apelin promotes ACE2 expression—in other words, it stimulates formation of vasodilating substrates—and antagonizes Ang II (Chatterjee et al., 2020). These properties of apelin would be beneficial for patients with heart failure.
Even though sacubitril/valsartan exerts beneficial effects in the management of cardiovascular disease, there is a concern regarding its effects on Alzheimer’s disease. Neprilysin is known to be involved in the degradation of β-amyloid peptide in the brain. Therefore, inhibition of the enzyme would promote deposition of β-amyloid peptide, a hallmark pathology in Alzheimer’s disease diagnosis (Murphy and LeVine, 2010; Nalivaeva et al., 2020). Possible effects of sacubitril/valsartan in patients with heart failure and Alzheimer’s disease should be investigated further.
3 Mechanistic roles of sacubitril/valsartan in cardiac remodeling
3.1 Effects on cardiac function
In heart failure patients, left ventricular ejection fraction (LVEF) and left ventricular volumes reflect global left ventricular systolic performance and are associated with left ventricular remodeling. Initiation of sacubitril/valsartan therapy in these patients has been shown to induce reverse remodeling of left ventricle, with a significant improvement of ventricular volume overload and dimension indices which subsequently resulting in an augmented LVEF (Liu L. W. et al., 2020; Hu et al., 2020; Karagodin et al., 2020; Mazzetti et al., 2020; Rezq et al., 2021). The improvement was observed with the reduction in left ventricular end-diastolic (LVEDV) and end-systolic (LVESV) volume, left atrial volume index, and estimated mean pulmonary capillary wedge pressure (PCWP) (Januzzi et al., 2019; Martín-Garcia et al., 2020; Masarone et al., 2020). Sacubitril/valsartan also exerts beneficial effects on right ventricular function, marked by increases in peak systolic S wave and tricuspid anular plane systolic excursion (TAPSE), as well as decreases in medium pulmonary artery pressure (mPAP) and pulmonary artery systolic pressure (PASP) (Masarone et al., 2020; Yenerçağ et al., 2021) (Table 1).
In terms of diastolic function, diastolic dysfunction alters the relationship between early diastolic filling velocity (E) and late left ventricular filling (A). E/A ratio is defined as the ratio of peak velocity blood flow from left ventricular relaxation in early diastole to peak velocity flow in late diastole caused by atrial contraction. Sacubitril/valsartan improved E/A ratio in patients with HFrEF (Romano et al., 2019; Nakou et al., 2020; Russo et al., 2020). However, the use of ratio of mitral inflow velocity to mitral annular relaxation velocity (E/E’) ratio is more sensitive than E/A ratio for measuring left ventricular diastolic function. A significant improvement in E/E’ ratio was seen with sacubitril/valsartan therapy in heart failure patients (Kang et al., 2019; Hu et al., 2020; Nakou et al., 2020). Measurement of myocardial performance index (Tei index) provides an evaluation of both systolic and diastolic function simultaneously, as does global longitudinal strain, a relatively novel measure of myocardial contractility which is superior to LVEF in predicting cardiovascular outcomes (Ashish et al., 2019). Therapy with sacubitril/valsartan was shown to improve Tei index (Gokhroo et al., 2021) and global longitudinal strain in heart failure patients (Valentim Gonçalves et al., 2019; Valentim Gonçalves et al., 2020a; Mirić et al., 2021). In addition, sacubitril/valsartan improves fractional shortening, cardiac output, load-dependent indices of left ventricular contractility (dp/dtmax), and relaxation (dp/dtmin) in animal heart failure models (Maslov et al., 2019b; Ge et al., 2020; Liu Y. et al., 2021; Raj et al., 2021) (Table 2).
Combination therapy of sacubitril/valsartan therapy with SGLT2 inhibitors—originally developed as anti-diabetic drugs—has shown additional benefits in patients with diabetic cardiomyopathy (Kim et al., 2021; Karabulut et al., 2022). The combination exhibits synergistic effects through independent mechanisms that offer greater prominent improvements in cardiac function, observed with the augmented LVEF and reduction in E/E’ ratio, as well as a decrease in the risk of cardiovascular death. Additional cardiovascular benefits observed in the patients are suggested to be conferred by SGLT2 inhibitors via their natriuretic and osmotic diuretic effects, along with an amelioration of myocardial bioenergetics (Kim et al., 2021; Lin et al., 2022). However, two other clinical trials reported no significant combinatory effects of SGLT2 inhibitors (dapagliflozin or empagliflozin) and sacubitril/valsartan in patients with heart failure, even though the effect magnitudes were numerically bigger than in those receiving SGLT2 inhibitor alone (Solomon et al., 2020; Packer et al., 2021). One possible reason could be small sample size (508 and 727 patients, respectively). Further studies are needed to achieve a conclusive finding. To the best of our knowledge, no clinical trial has been performed to investigate possible combinatory effects of other antidiabetic drugs and sacubitril/valsartan on heart failure.
Calcium levels regulate myocardial contraction in cardiomyocytes, which is significantly altered in failing hearts. Na+/K+-ATPase, Na+/Ca2+ exchanger (NCX), ryanodine receptor 2 (RyR2), and sarcoplasmic/endoplasmic reticulum calcium ATPase (SERCA) are among the calcium regulators (Salim et al., 2020). Sacubitril/valsartan increased LVEF without affecting the expressions of NCX, RyR2 or SERCA, but downregulated phosphorylated calmodulin-dependent protein kinase II (CaMKII-p) expression in a MI-heart failure rabbit model (Chang et al., 2020). Increased intracellular calcium activates CaMKII, resulting in phosphorylation of the L-type calcium channel and increases in SERCA calcium cycling. CaMKII is upregulated in heart failure (Beckendorf et al., 2018). A study by Eiringhaus et al. (2020) demonstrated that sacubitril/valsartan diminished diastolic calcium-spark frequency (limited sarcoplasmic reticulum calcium release events) and sarcoplasmic reticulum calcium leaks in isolated mouse cardiomyocytes that were exposed to catecholaminergic stress, as well as in human left ventricular cardiomyocytes isolated from explanted hearts of patients with end-stage heart failure. Similar effects were also observed in cardiomyocytes treated with sacubitril alone, but they were lacking when valsartan was used alone, suggesting the direct effects of sacubitril on improving calcium homeostasis in failing cardiomyocytes. The study also reported that sacubitril did not compromise systolic calcium release, sarcoplasmic reticulum calcium transient kinetic and load, as well as SERCA activity (Eiringhaus et al., 2020). Therefore, the findings convey that sacubitril/valsartan promotes cardiac function partly by improving myocardial calcium homeostasis.
Collectively, therapy with sacubitril/valsartan has shown beneficial effects on systolic and diastolic function in patients with heart failure and in animal studies, likely via improvement of myocardial contractility, leading to increased ejection fraction. The role of the drug on calcium handling such as Na+/K+-ATPase in other heart failure models should be studied further. Certain genes that play a role in the progression of hypertrophy—SUZ12/PRC2-MEF2A, EZH2-CaMKI, and miR-675-CaMKIId (Liu L. et al., 2020)—should also be explored. Sarcomere is the “motor” of cardiomyocyte mechanotransduction that generates force transmission. Cysteine rich protein 3 (also known as muscle LIM protein) and titin are the proteins that participate in force transmission within sarcomere (Lyon et al., 2015); sacubitril/valsartan may modulate these proteins.
3.2 Effects on cardiac structure and hypertrophy
Cardiac hypertrophy is an adaptive and compensatory mechanism (characterized by an increase in cardiomyocyte size and thickening of ventricular walls) that involves alterations in cell structure and protein synthesis (Zhang et al., 2003; Siti et al., 2020). The development of pathological cardiac hypertrophy is usually accompanied by increased expression of typical cardiac genes ANP and BNP (Tham et al., 2015; Siti et al., 2021b). Thus, the plasma level of these peptides are measured as indicators for cardiac dysfunction, including cardiac hypertrophy (Moyes and Hobbs, 2019). Other than these peptides, NT-pro-BNP and cardiac troponin T are more routinely used to assess cardiac function (Berardi et al., 2020).
The effectiveness of sacubitril/valsartan therapy in patients with HFrEF has been appraised in many studies. The drug reduced plasma levels of NT-proBNP, high-sensitivity cardiac troponin T (hs-cTnT), and soluble suppressor of tumorigenicity 2 (sST2) in the patients, high levels of which reflect ventricular wall stress and myocardial injury (Nougué et al., 2019; Murphy et al., 2021) (Table 3). NT-proBNP level and left ventricular mass are positively correlated, and this fact is useful for identifying patients with cardiac hypertrophy (Morillas et al., 2008; Kahveci et al., 2009).
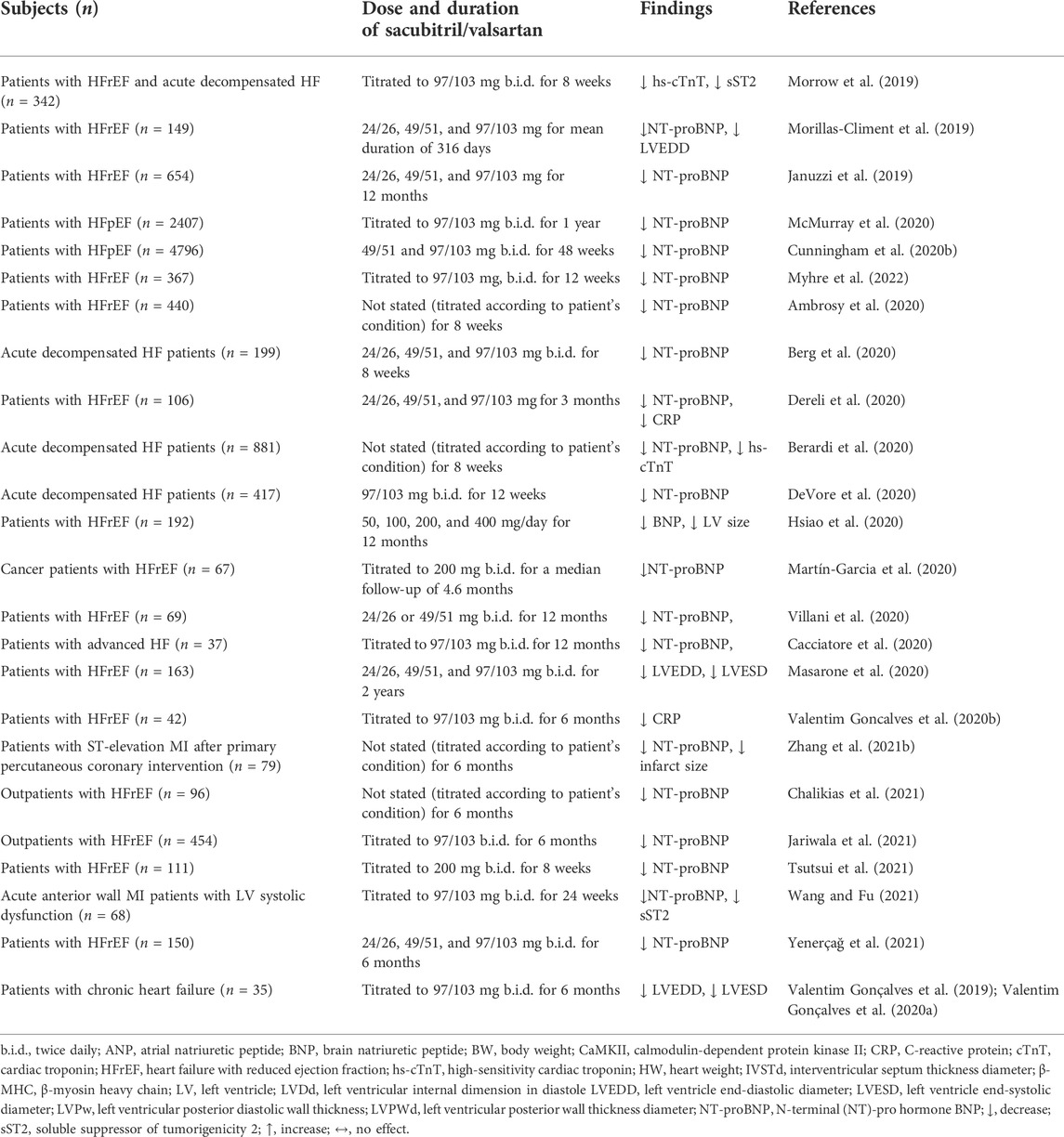
TABLE 3. The effects of sacubitril/valsartan on cardiac structure and biomarkers of cardiac hypertrophy in clinical studies.
The beneficial effects of sacubitril/valsartan were also demonstrated in animal models. Its administration has resulted in decreased heart weight to body weight ratio in spontaneous hypertensive rats and in rats with induced cardiac hypertrophy (Chang et al., 2019; Zhao et al., 2019; Nordén et al., 2021). It inhibited left ventricular hypertrophy in various models of cardiac hypertrophy, which was confirmed echocardiographically and histologically. Echocardiographic analysis revealed that sacubitril/valsartan decreased left ventricular wall thickness as shown by decreased interventricular septum thickness diameter, left ventricular posterior diastolic wall thickness, left ventricular mass, and left ventricular end-systolic diameter (Chang et al., 2019; Zhao et al., 2019; Tashiro et al., 2020), leading to improved cardiac geometry (Sung et al., 2020). Meanwhile, histological analysis showed that the increases in cardiomyocyte size induced by Ang II was significantly attenuated by the treatment (Tashiro et al., 2020). However, a detailed molecular mechanism of sacubitril/valsartan inhibition on left ventricular hypertrophy was not fully characterized. Table 4 summarizes the findings of sacubitril/valsartan on cardiac structure and biomarkers of cardiac hypertrophy in animal studies.
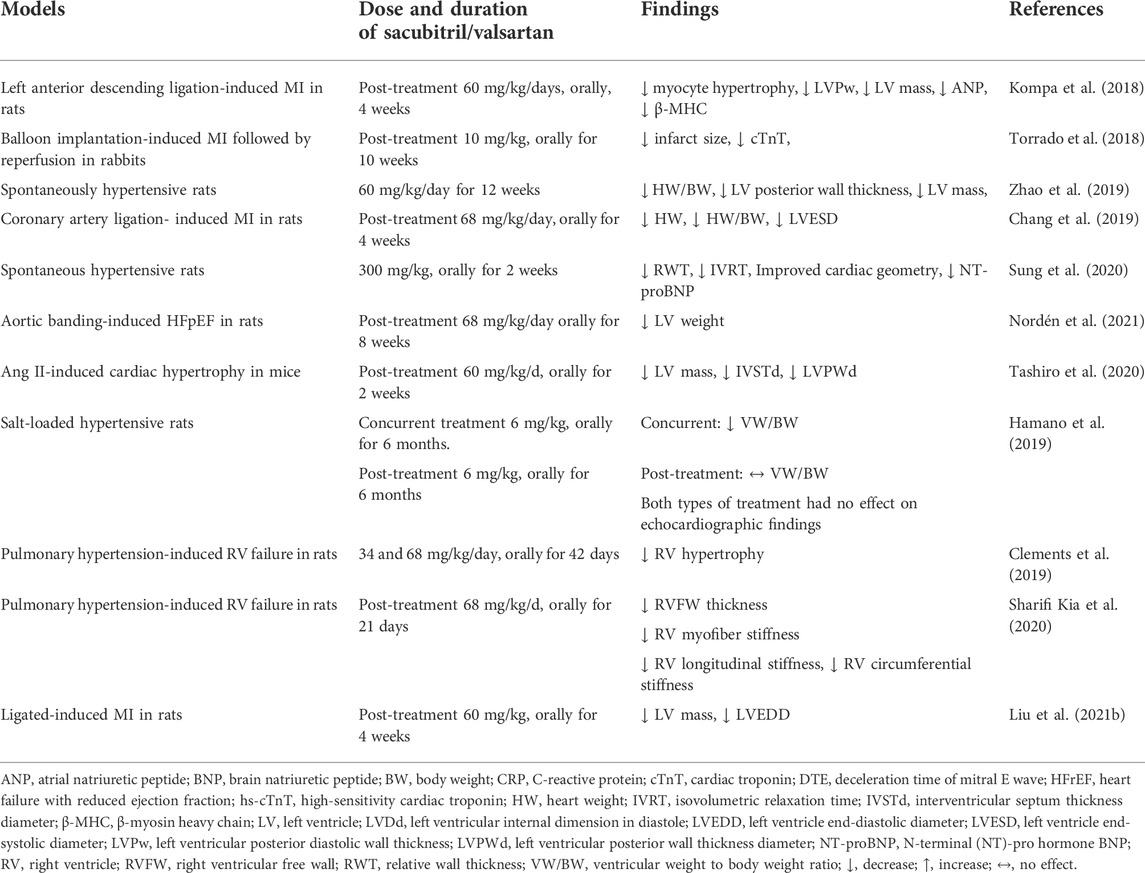
TABLE 4. The effects of sacubitril/valsartan on cardiac structure and biomarkers of cardiac hypertrophy in animal studies.
Sacubitril/valsartan administration lowers blood pressure in patients with chronic HFrEF, primarily by reducing blood volume and enhancing natriuresis (Böhm et al., 2017). However, studies have indicated that the antihypertrophic effects of sacubitril/valsartan were independent of blood pressure. Tashiro et al. (2020) exhibited that the fewest hypertrophic changes—interventricular septum thickness diameter, left ventricular posterior wall thickness diameter and cardiomyocyte cross-sectional area—took place in the sacubutril/valsartan-treated mice treated with Ang II, despite the similar blood pressure-lowering effect of sacubitril/valsartan, enalapril, and valsartan (Tashiro et al., 2020). A similar finding was also noted in a rat model of heart failure with preserved ejection fraction (HFpEF) (Nordén et al., 2021). In the study, sacubitril/valsartan had no significant effect on mean arterial pressure in the rats exposed to cardiac pressure overload, as opposed to reduced effect by valsartan. However, the left ventricular weight of the former group was significantly lower than the latter and vehicle-treated groups (Nordén et al., 2021). The findings of both studies suggest that sacubitril/valsartan provides a direct cardioprotection against hypertrophic changes.
Sacubitril/valsartan also demonstrated a potential to inhibit right ventricular hypertrophy in a rat model of pulmonary hypertension. In studies, oral sacubitril/valsartan (34 and 68 mg/kg/day) was administered for 21 (Sharifi Kia et al., 2020) or 42 days (Clements et al., 2019) in rats induced with pulmonary hypertension, resulting in a reduction of right ventricular hypertrophy with reduced right ventricular longitudinal and circumferential stiffness as well as reduced right ventricular free wall thickness. The protective effect was possibly owing to a mitigation of pulmonary artery pressure which subsequently improved right ventricular afterload, leading to regression in right ventricular hypertrophy (Clements et al., 2019). Collectively, post-treatment of sacubitril/valsartan decreases left and right ventricular hypertrophy and cardiac associated release of biomarkers. The findings are confirmative of the therapeutic effects of the drug on cardiac hypertrophy, as seen in patients with HFrEF.
Studies have further explored the possible mechanism of the protective effects of sacubitril/valsartan on cardiac hypertrophy. Its effect on extracellular signal-regulated kinase (ERK) was investigated in mice with pregnancy-associated cardiomyopathy (PAC) treated with lentiviruses stably transfected with sh-ERK for silencing ERK. This treatment resulted in reduced expression of ERK compared to its negative control group (treated with sh-NC) and was associated with a lower heart size (Wang et al., 2019a). However, ERK silencing had no effect on phosphorylated ERK (pERK). Sacubitril/valsartan did not affect ERK expression in PAC mice that were treated with sh-NC, but it did reduce pERK, whereas in the groups given sh-ERK, the drug significantly reduced the expression of both ERK and pERK. Mice that were treated with the drug had smaller hearts than the control group, regardless of whether they were treated with sh-NC or sh-ERK. However, sacubitril/valsartan further reduced heart size in the group given sh-ERK, compared to the group treated with the drug and sh-NC. Other hypertrophic markers were also inhibited (Wang et al., 2019a). Similar findings were also observed in cardiomyocytes that were transfected with sh-ERK and exposed to Ang II to induce hypertrophy before treatment with sacubitril/valsartan. In the same study, it was also noted that the drug increased the expression of ACE-2 in the Ang II-treated cells (Wang et al., 2019a). ACE2 converts Ang II into Ang 1–7, a heptapeptide which has antiremodeling and cardioprotective properties, by suppressing the synthesis of cardiac fibroblast extracellular matrix and release of hypertrophic growth factors (Iwata et al., 2011; Arendse et al., 2019). However, the possible role of the drug on the peptide level has not been reported. In summary, the findings suggest that role of ERK in the pathogenesis of cardiac hypertrophy is crucial and sacubitril/valsartan protects the heart by blocking the activation of ERK and inhibiting the Ang II receptor pathway (Figure 3).
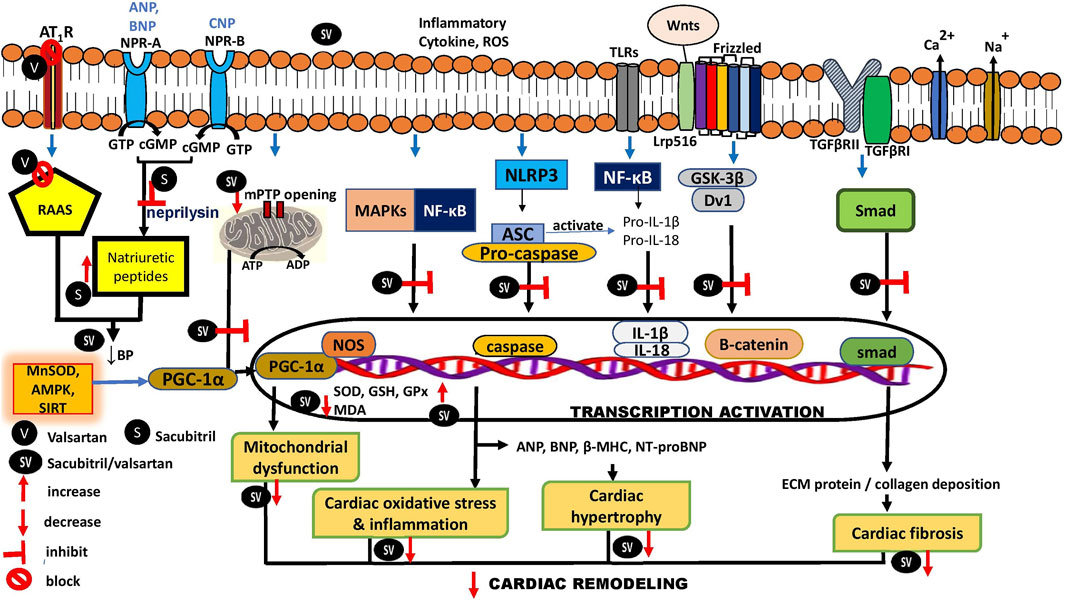
FIGURE 3. Schematic regulatory mechanisms for sacubitril/valsartan during cardiac remodeling. AMPK, AMP-activated protein kinase; ANP, atrial natriuretic peptide; ASC, apoptosis-associated speck-like protein containing a caspase; ATP, adenosine triphosphate; BNP, B-type natriuretic peptide; BP, blood pressure; cGMP, cyclic guanosine monophosphate; CNP, C-type natriuretic peptide; CTGF, connective tissue growth factor; Drp1, Dynamin‐related protein one; Dv1, signal transduction molecule disheveled; ECM, extracellular matrix; ERK, extracellular signal-regulated kinase; GPx, glutathione peroxidase; GSH, glutathione; GTP, guanosine triphosphate; IL, interleukin; JNK, c-Jun N-terminal kinases; MAPK, mitogen-activated protein kinase; MDA, malondialdehyde; MFN2, Mitofusin-2; β-MHC, β-myosin heavy chain; MMPs, matrix metalloproteinases; MnSOD, manganese suoeroxide dismutase; mPTP, mitochondrial permeability transition pore; NF-κB, nulear factor-κB; NLRP3, NOD-like receptor protein three; NOS, nitric oxide synthases; NPR-A, natriuretic peptide receptor A; NPR-B, natriuretic peptide receptor B; PGC-1α, peroxisome proliferator-activated-receptor-1α; NT-proBNP, N-terminal pro-BNP; RAAS, renin-angiotensin-aldosterone system; SIRT, mitochondria sirtuin; TGF-βR, transforming growth factor β receptor, TLRs, Toll-like receptors.
3.3 Effects on cardiac fibrosis and matrix remodeling
Cardiac fibrosis is one of the features in cardiac remodeling underlying the progression of heart failure. It occurs due to an imbalance in extracellular matrix production and degradation in the myocardium, and arises from pathological attempts to repair tissue injury in various cardiac diseases including MI and diabetic hypertrophic cardiomyopathy (Hinderer and Schenke-Layland, 2019; Siti et al., 2020). It involves increased synthesis of matrix metalloproteinases (MMP) and collagen that occurs during inflammation, resulting in fibrosis (Nattel, 2017). Fibrotic tissues become stiffer and less compliant, thus predisposed to the progression of cardiac dysfunction. Therefore, the balance between extracellular matrix synthesis and degeneration is crucial for maintaining cardiac structural integrity (Ma et al., 2018).
The effects of sacubitril/valsartan on cardiac fibrosis through the regulation of transforming growth factor-β1/small mothers against decapentaplegic (TGF-β/Smad) signaling pathways were investigated using numerous animal models and cardiac fibroblast cells (Table 5). TGF-β1 is a molecular mediator that plays a role in the development of cardiac fibrosis (Khan et al., 2014; Yue et al., 2017). It is activated following a cardiac injury (Annes et al., 2003) via Smad proteins after binding to its receptor, leading to the recruitment and activation of downstream mediators, mainly Smad2 and Smad3 (Ma et al., 2018). The effects of sacubitril/valsartan on collagen synthesis, TGF-β1, and phosphorylated Smad3 (p-Smad3) expressions were consistent in various animal models on cardiac failure such as streptozotocin-induced diabetic cardiomyopathy (Ai et al., 2021) and left anterior descending (LAD) coronary artery ligation-induced MI (Wu M. et al., 2021) rat models. Sacubitril/valsartan effectively decreased protein expression of TGF-β1 and p-Smad3 in infarcted areas and myocardial types I and III collagen in these models (Ai et al., 2021; Wu M. et al., 2021). Apart from that, the drug (10–7−10–5 M) also inhibited cell proliferation and collagen synthesis in neonatal rat myocardial fibroblast cells exposed to hypoxia and TGF-β1 to induce collagen synthesis (Wu M. et al., 2021). Histologically, oral administration of 68 mg/kg/day sacubitril/valsartan for 5 weeks in LAD coronary artery ligation-induced MI rats significantly decreased myocardial interstitial fibrosis accompanied by reduced connective tissue growth factor (CTGF) expression, a known mediator of TGF-β activity during fibrosis (Pfau et al., 2019). Clinically, patients with chronic HFpEF and HFrEF who were treated with sacubitril/valsartan had significantly lower plasma profibrotic biomarkers assessed by the levels of aldosterone, MMP-9, sST2, tissue inhibitor of metalloproteinase-1 (TIMP-1), N-terminal propeptide of collagen I (PINP), and N-terminal propeptide of collagen III (PIIINP) after 8 and 12 months. The effects of sacubitril/valsartan on the profibrotic biomarkers were superior to that of valsartan or enalapril (Zile et al., 2019; Cunningham et al., 2020a).
The antifibrotic effect of sacubitril/valsartan was also demonstrated in other cardiac fibrosis rat models induced by doxorubicin toxicity (Boutagy et al., 2020) and high-salt diet-induced HFpEF (Zhang et al., 2021a). In these models, concurrent oral administration of sacubitril/valsartan (68 mg/kg) with doxorubicin for 6 weeks attenuated MMP activity and highly contractile protein α-smooth muscle actin (α-SMA) expression (Boutagy et al., 2020), while intragastric administration of sacubitril/valsartan (68 mg/kg/day) for 4 weeks mitigated cardiac fibrosis, associated with decreases in types I and III collagen protein and mRNA expressions, as well as MMP-2 protein expression (Zhang et al., 2021a). Tissue inhibitor of metalloproteinase-2 (TIMP-2) and Smad7 expression—an inhibitory regulator of TGF-β (Ma et al., 2018)—were increased in the rats (Zhang et al., 2021a), suggestive of a strong suppressive effect of the drug on myocardial fibrosis production.
There is considerable evidence that shows that the secreted frizzled-related protein 1 (sFRP-1)/Wnt/β-catenin signaling pathway plays a role in TGF-β-mediated fibrosis in cardiac remodeling (Akhmetshina et al., 2012; McMurray et al., 2014). Wnt proteins bind to frizzled receptors to activate signal transduction molecule disheveled (Dvl), which curbs glycogen synthase kinase-3β (GSK-3β) and increases β-catenin expressions (Piersma et al., 2015). Post-treatment of oral sacubitril/valsartan (60 mg/kg) for 4 weeks alleviated myocardial fibrosis by inhibiting the Wnt/β-catenin signal transduction pathway in a mouse model of MI. A mitigation of the expression of β-catenin and Dvl-1, along with overexpression of its inhibitor, sFRP-1, was observed in the sacubitril/valsartan-treated mice (Liu J. et al., 2021). This modulation in turn attenuated cardiac fibrosis by reducing the fibrotic area, α-SMA, TGF-β, types I and III collagen (Figure 3). As expected, treatment of sacubitril/valsartan (30 μmol/L) in primary myocardial fibroblasts stimulated with Ang II produced similar results (Liu J. et al., 2021).
Taken together, the findings suggest that sacubitril/valsartan attenuates cardiac fibrosis by inhibiting the TGF-β1/Smad3 and Wnt/β-catenin signaling pathways. Sacubitril/valsartan may also modulate the phosphatidylinositol 3-kinase/protein kinase B/glycogen synthase kinase-3β (PI3K/Akt/GSK-3β) and hypoxia-induced mitogenic actor (HIMF)-IL-6 signaling pathways, and this aspect should be studied further. These pathways play a role in regulating cardiac fibrosis. Activation of the PI3K/Akt/GSK-3β pathway results in diminished cell growth and proliferation (Syamsunarno et al., 2021), while HIMF-IL6 promotes the opposite effect, leading to fibrosis (Kumar et al., 2018).
3.4 Effects on myocardial mitochondrial function and apoptosis
Bioenergetic reserve capacity (also known as spare respiratory capacity) can abruptly increase mitochondrial respiration to a maximum for synthesizing more ATP to maintain cellular functions (Sansbury et al., 2011). Impaired mitochondrial activity decreases its energy production, leading to mitochondrial dysfunction which plays an important role in the development of cardiac remodeling and heart failure (Ramaccini et al., 2021). Oral administration of sacubitril/valsartan (68 mg/kg/day) for 10 weeks increased mitochondrial maximal respiration capacity and spare respiration capacity in rats with left ventricular pressure overload, suggesting a beneficial effect of the drug on mitochondrial bioenergetics (Li et al., 2021). A similar finding was also noted in dogs with intracoronary microembolization-induced chronic heart failure following oral doses of sacubitril/valsartan (100 mg) for 3 months. Myocardial bioenergetics was improved in the dogs’ failing myocardium, likely due to improved mitochondrial function, evidenced by marked increases in mitochondrial maximal rate of ATP synthesis and membrane potential, as well as decreased opening of the permeability transition pore (mPTP) (Sabbah et al., 2020). Alterations in mitochondrial structure and function in a remodeled heart disrupt mitochondrial membrane integrity and potential. These events trigger the opening of mPTP, leading to ATP depletion (Sharov et al., 2000; Sharov et al., 2007; Sabbah et al., 2020; Yeh et al., 2021b).
In experimental heart failure, mitochondrial complex I and IV activities involved in ATP synthesis were inhibited. Post-treatment with sacubitril/valsartan (100 mg, twice daily) for 3 months restored the activities of these complexes, thus improving oxidative phosphorylation. The drug also normalized the levels of nitric oxide synthase (NOS) isoforms—endothelial NOS (eNOS) and inducible NOS—and peroxisome proliferator-activated receptor coactivator-1α (PGC-1α), proteins involved in regulation of mitochondrial biogenesis and respiration (Sabbah et al., 2020) (Figure 3). eNOS generates nitric oxide which then activates guanylate cyclase to produce cyclic guanosine monophosphate (cGMP). cGMP transmits signals to the nucleus that lead to an induction of PGC-1α gene transcription and mitochondrial biogenesis (Jornayvaz and Shulman, 2010).
Peng et al. (2020) demonstrated that post-treatment with sacubitril/valsartan (20 mg/kg/day) for 4 weeks in mice with pressure overload-induced heart failure distinctly reversed the downregulation of manganese superoxide dismutase (MnSOD), sirtuin-3 (SIRT3), and phosphorylated 5' adenosine monophosphate-activated protein kinase (p-AMPK) expression. The protective effect of sacubitril/valsartan on MnSOD and p-AMK was abolished in SIRT3 deficiency, indicating it was via a SIRT3-dependent pathway. SIRT3 has a prominent role in mitochondrial metabolism, thus it is profoundly present in tissues with high energy metabolisms, like the heart (Cao et al., 2022). The activation of PGC-1α indirectly enhances SIRT3 expression that promotes mitochondrial function, thus protecting the organelle against damage (Brandauer et al., 2015), while MnSOD is a deacetylation target of SIRT3, which also controls its expression (Koentges et al., 2016).
Similar effects of sacubitril/valsartan were also exhibited in an obesity-related metabolic heart disease model. The administration of the drug at 100 mg/kg/day for 4 months improved cardiac energetics reflected by an elevation of rate pressure product (RPP), indicative of increased cardiac energy reserve (an index of myocardial oxygen consumption), as well as a decrease in the slope of decline phosphocreatine normalized for ATP (PCr/ATP) relative to RPP in isolated beating hearts of C57BL/6J mice (Croteau et al., 2020). The slope of change in PCr/ATP relative to RPP measures energetic expense of raised contractile conduct (Croteau et al., 2020).
Apoptosis plays a major role in mitochondrial function, the elevation of which increases mitochondrial death. Sacubitril/valsartan (60 mg/kg/day for 4 weeks) administration in mice attenuated doxorubicin-induced dilated cardiomyopathy, observed by a curb on apoptosis that preserved mitochondrial function via the dynamin-related protein 1 (Drp1)-mediated pathway (Xia et al., 2017; Yeh et al., 2021a) (Figure 3). The significant reduction in Drp1 and its phosphorylated Ser-616 expression—the activated form—observed in the study was associated with the improvement in cardiac mitochondrial functional capacity. This was evident from the increased mitochondrial respiration complex I activity and ATP content (Xia et al., 2017). Drp1 plays a key role in stimulating mitochondrial disintegration and death (Wu Q. R. et al., 2021).
Sacubitril/valsartan treatment decreased pro-apoptotic markers—cleaved caspase-3, B-cell lymphoma 2 (Bcl-2), and Bcl-2 associated X protein (Bax)—in various models of cardiomyopathy (Ge et al., 2019; Sorrentino et al., 2019; Yeh et al., 2021a; Bai et al., 2021; Dindaş et al., 2021) (Table 6). A similar reduction in apoptosis was also noted in sacubitril/valsartan-pretreated (20 µM for 30 min) H9c2 cells exposed to doxorubicin (Xia et al., 2017) and phenylephrine (Peng et al., 2020) to induce cardiomyocyte hypertrophy. Moreover, it is believed that sustained phosphorylated c-Jun N-terminal kinase (JNK) and p38 mitogen-activated protein kinase (MAPK), and nuclear factor kappa-light-chain-enhancer of activated B cells (NF-κB)—a proinflammatory factor (Mustafa et al., 2018)—translocations are also involved in high-glucose-induced apoptosis in H9c2 cardiomyocytes (Ge et al., 2019). Treatment with sacubitril/valsartan in the cells mitigated apoptosis by downregulating MAPK kinases (MKK), MAPKs, NF-κB, and mitofusin 2 (Mfn2) expressions (Ge et al., 2019; Yeh et al., 2021b) (Figure 3).
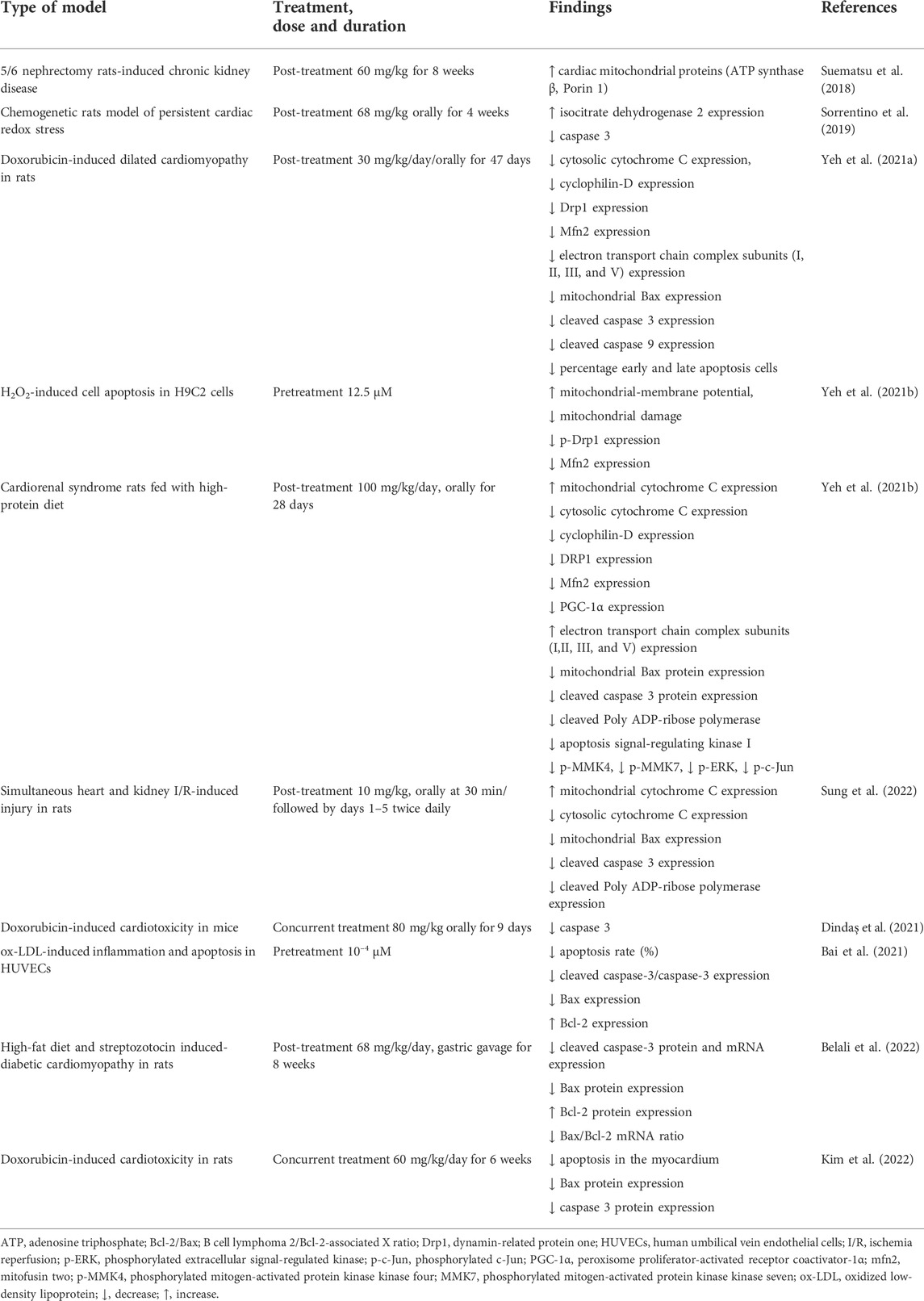
TABLE 6. The effects of sacubitril/valsartan on myocardial mitochondrial function and apoptosis in animal studies.
Collectively, these findings suggest that sacubitril/valsartan improves mitochondrial energy production, leading to increased myocardial contractile performance, possibly via a SIRT3-dependent pathway. Future studies can explore the effects of sacubitril/valsartan on nuclear respiratory factor-1 (NRF-1) and -2 (NRF-2) as well as mitochondrial transcription factor A (MTF-A; also abbreviated as TFAM). NRF-1 and NRF-2 are downstream targets for PGC-1α, while MTF-A is importantly involved in mitochondrial replication and transcription (Javadov et al., 2006). Optic atrophy 1 (OPA1), which participates in mitochondrial fusion, and fission 1 (FIS1), which participates in mitochondrial fission, could also be explored to understand the mechanistic effects of the drug on mitochondrial biogenesis. The expression of these proteins were significantly attenuated (except for FIS1, which was upregulated) in a murine model of heart failure (He et al., 2021). The effects of the drug on survivor activating factor enhancement (SAFE) signaling pathway, which participates in promoting cardiomyocyte survival (Hadebe et al., 2018) can also be investigated.
3.5 Effects on cardiac oxidative stress and inflammation
Oxidative stress and inflammation are the culprits in almost all diseases including heart failure. Sacubitril/valsartan mitigates oxidative stress and inflammation in vivo (Yang et al., 2019; Raj et al., 2021) and in vitro (Peng et al., 2020) models of heart failure as well as in patients with the disease (Acanfora et al., 2020; Bunsawat et al., 2021; Pang et al., 2021). The drug diminished production of intracellular reactive oxygen species (ROS), oxidative products such as malondialdehyde, and inflammatory factors (tumor necrosis factor-α, TNF-α; interleukins IL-6 and IL-1β) in the models. Sacubitril/valsartan restored the loss in antioxidant enzymes in the myocardium, namely superoxide dismutase, glutathione peroxidase, catalase, glutathione reductase, and glutathione S-transferase levels, as well as glutathione content in an isoprenaline-induced MI rat model, which correlated with a reduction in myocardial infarcted area (Imran et al., 2019). In a streptozotocin-induced diabetic cardiomyopathy mouse model, treatment of sacubitril/valsartan at 60 mg/kg/day for 16 weeks decreased inflammation by downregulating the expressions of NF-κB and MAPKs (JNK and p38), which in turn upregulated antioxidant expression, namely glutathione (GSH), and inhibited pro-inflammatory cytokines (IL-6, IL-1β, and TNF-α), in line with improvement in cardiac structure and function (Ge et al., 2019) (Figure 3). MAPKs play a key role in a range of fundamental cellular processes including cell growth, proliferation, death, and differentiation, which crosstalk with other pathways such as NF-κB (Yahfoufi et al., 2018). JNK and p38 play a role in the inflammatory and apoptotic response (Cargnello and Roux, 2011; Gui et al., 2019). Sacubitril/valsartan (68 mg/kg, orally, 4 weeks) also reduced 8-hydroxy guanosine (8-OHG) levels, a marker of oxidative damage, in rats with chemogenetic model of persistent cardiac redox stress that resembled experimental heart failure (Sorrentino et al., 2019).
Nucleotide-binding domain leucine-rich repeat family pyrin domain containing receptor 3 (NLRP3) inflammasome also acts as an essential mediator by inducing inflammation which could promote the profibrotic pathway (Wang et al., 2019b; Li et al., 2019). Oral administration of sacubitril/valsartan (60 mg/kg/day) for 4 weeks attenuated cardiac fibrosis, as evidenced by a reduction in the percentage of collagen volume fraction and its profibrotic factors—TGF-β, collagen I, MMP-2 and α-SMA-positive area—in mice with post-aortic debanding-induced pressure overload (Li et al., 2020). Sacubitril/valsartan inhibited cardiac inflammation and fibrosis via suppression on NF-κB and inhibition of NLRP3 inflammasome activation signaling pathways (Figure 3). The suppression of the inflammatory pathway was noted by the reduced inflammasome mediators CD45, NLRP3, and NF-κB positive-stained areas, TNF, NF-κB, caspase 1, IL-1βo-NF, and phospho-NF-κB/NF-κB expressions (Li et al., 2020).
Owing to its cardioprotective and antioxidant properties, the effects of sacubitril/valsartan on doxorubicin-induced cardiotoxicity were also investigated. Doxorubicin is a chemotherapy drug that causes an overproduction of IL-1β, IL-8, and IL-6 as well as oxidative stress in the heart leading to cardiotoxicity as an adverse effect (Quagliariello et al., 2019), with ferroptosis—accumulation of mitochondrial iron and lipid peroxides—being the underlying mechanism (Li et al., 2022). Sacubitril/valsartan was demonstrated to ameliorate doxorubicin-induced cardiotoxicity in vivo and in vitro, possibly by alleviating iron-induced mitochondrial and endoplasmic reticulum oxidative stress (Dindaş et al., 2021; Kim et al., 2022; Miyoshi et al., 2022), thereby reducing cardiac damage. It also improved LVEF in rats administered doxorubicin (Boutagy et al., 2020). Significant favorable effects of sacubitril/valsartan on cardiac function were also noted in patients with cancer therapy-related cardiac dysfunction (Sheppard and Anwar 2019; Gregorietti et al., 2020; Martín-Garcia et al., 2020; Frey et al., 2021; Xi et al., 2022).
The antihypertrophic effects of sacubitril/valsartan are frequently attributable to its capacities to reduce excessive oxidative stress and inflammation response which eventually attenuate the remodeling process. However, detailed mechanistic insights into sacubitril/valsartan’s mitigative effects on cardiac remodeling via oxidative stress and inflammation are still lacking. Modulative effects of the drug on nuclear factor erythroid 2-related factor 2 (Nrf2)/antioxidant responsive element (Nrf2/ARE) signaling pathway, as well as Kelch-like ECH-associated protein 1 (Keap1) have not been extensively studied. Keap1 forms a complex with Nrf2—a gene involved in oxidative stress regulation—to repress the transcriptional activity of the latter. After its dissociation from Keap1, Nrf2 translocates into the nucleus and establishes a complex with ARE to upregulate heme oxygenase-1 expression, which then inhibits proinflammatory genes (Syamsunarno et al., 2021). The potential role of sacubitril/valsartan in a calcineurin-nuclear factor of activated T cells (NFAT) inflammatory signaling pathway should also be of interest. In cardiac hypertrophy, calcineurin expression is elevated, while phosphorylated NFAT is downregulated (Zhou et al., 2022).
Low antioxidant status in patients is associated with the development of atrial fibrillation and cardiac remodeling (Bas et al., 2017; Gorbunova et al., 2018). Low plasma vitamin C level has been reported to increase the risk of cardiovascular disease (Berretta et al., 2020). Antioxidant supplementation comprising vitamin C (1 g), vitamin E (600 I.U.), and α-lipoic acid (0.6 g) for 30 days exerted beneficial effects on macrovascular function in patients with HFrEF (Bunsawat et al., 2020). A recent meta-analysis also revealed that vitamin C administration augmented LVEF in heart failure patients (Hemilä et al., 2022). The findings suggest that supplemental vitamin C may afford synergistic effects with sacubitril/valsartan if given together in patients with heart failure. However, oral intake of vitamin C (4 g/day) for 4 weeks posed a risk in skeletal muscle damage in patients with chronic heart failure despite improvement in vascular function (Nightingale et al., 2007), possibly due to its higher dose. Thus, studies in animals and clinical trials should be conducted to better understand the interaction between the vitamin and sacubitril/valsartan.
4 Conclusion and direction of future research
Accumulating evidence demonstrates that sacubitril/valsartan improves cardiac function and has beneficial effects on various events involved in cardiac remodeling, such as altered mitochondria function, apoptosis, oxidative stress and inflammation, fibrosis, and matrix remodeling. Thus, sacubitril/valsartan shows promising potential to be authenticated as an antihypertrophic drug particularly for heart disease. Nevertheless, future research needs to be executed to better understand its cardioprotective mechanisms. Figure 3 outlines the mechanistic sites of action of sacubitril/valsartan in cardiac remodeling. Further studies should explore the antihypertrophic effects of the drug on its possible molecular mechanisms. Insulin resistance has been reported in heart failure (Nakamura and Sadoshima, 2018). The role of insulin-like growth factor 1 (IGF1) and its modulation by sacubitril/valsartan should be investigated. IGFI is a molecule structurally similar to insulin that regulates a vast spectrum of cellular processes in the heart, including increased collagen synthesis in cardiac remodeling. Various transcription factors in cardiac remodeling such as CCAAT/enhancer binding protein-β (C/EBPβ), GATA-binding protein 4 (GATA4), and CBP/p300-interacting transactivator 4 (CITED4) could also be probed. C/EBPβ curbs proliferation and growth of cardiomyocytes; thus, its augmented expression indicates cardiomyocyte hypertrophy (Wang et al., 2022). CITED4 regulates the mammalian target of rapamycin (mTOR) and thereby protects against cardiac pathological remodeling (Lerchenmüller et al., 2020). GATA4, on the other hand, promotes cardiac hypertrophic growth (Chen et al., 2022).
Author contributions
NM drafted the manuscript. JJ, SZ, and YK supervised the work. MS, JJ, SZ, AA, and YK critically revised the manuscript.
Funding
The study was funded by the Ministry of Higher Education of Malaysia (FRGS-1-2019-SKK10-UKM-02-1).
Conflict of interest
The authors declare that the research was conducted in the absence of any commercial or financial relationships that could be construed as a potential conflict of interest.
Publisher’s note
All claims expressed in this article are solely those of the authors and do not necessarily represent those of their affiliated organizations, or those of the publisher, the editors and the reviewers. Any product that may be evaluated in this article, or claim that may be made by its manufacturer, is not guaranteed or endorsed by the publisher.
References
Abumayyaleh, M., El-Battrawy, I., Behnes, M., Borggrefe, M., and Akin, I. (2020). Current evidence of sacubitril/valsartan in the treatment of heart failure with reduced ejection fraction. Future Cardiol. 16 (4), 227–236. doi:10.2217/fca-2020-0002
Acanfora, D., Scicchitano, P., Acanfora, C., Maestri, R., Goglia, F., Incalzi, R. A., et al. (2020). Early initiation of sacubitril/valsartan in patients with chronic heart failure after acute decompensation: A case series analysis. Clin. Drug Investig. 40 (5), 493–501. doi:10.1007/s40261-020-00908-4
Ai, J., Shuai, Z., Tang, K., Li, Z., Zou, L., Liu, M., et al. (2021). Sacubitril/valsartan alleviates myocardial fibrosis in diabetic cardiomyopathy rats. Hell. J. Cardiol. 62 (5), 389–391. doi:10.1016/j.hjc.2021.04.004
Akhmetshina, A., Palumbo, K., Dees, C., Bergmann, C., Venalis, P., Zerr, P., et al. (2012). Activation of canonical Wnt signalling is required for TGF-β-mediated fibrosis. Nat. Commun. 3, 735. doi:10.1038/ncomms1734
Ambrosy, A. P., Braunwald, E., Morrow, D. A., DeVore, A. D., McCague, K., Meng, X., et al. (2020). Angiotensin receptor-neprilysin inhibition based on history of heart failure and use of renin-angiotensin system Antagonists. J. Am. Coll. Cardiol. 76 (9), 1034–1048. doi:10.1016/j.jacc.2020.06.073
Andersen, S., Axelsen, J. B., Ringgaard, S., Nyengaard, J. R., Hyldebrandt, J. A., Bogaard, H. J., et al. (2019). Effects of combined angiotensin II receptor antagonism and neprilysin inhibition in experimental pulmonary hypertension and right ventricular failure. Int. J. Cardiol. 293, 203–210. doi:10.1016/j.ijcard.2019.06.065
Annes, J. P., Munger, J. S., and Rifkin, D. B. (2003). Making sense of latent TGFbeta activation. J. Cell. Sci. 116 (2), 217–224. doi:10.1242/jcs.00229
Arendse, L. B., Danser, A., Poglitsch, M., Touyz, R. M., Burnett, J. C., Llorens-Cortes, C., et al. (2019). Novel therapeutic approaches targeting the renin-angiotensin system and associated peptides in hypertension and heart failure. Pharmacol. Rev. 71 (4), 539–570. doi:10.1124/pr.118.017129
Aroor, A. R., Mummidi, S., Lopez-Alvarenga, J. C., Das, N., Habibi, J., Jia, G., et al. (2021). Sacubitril/valsartan inhibits obesity-associated diastolic dysfunction through suppression of ventricular-vascular stiffness. Cardiovasc. Diabetol. 20 (1), 80. doi:10.1186/s12933-021-01270-1
Arrigo, M., Vodovar, N., Nougué, H., Sadoune, M., Pemberton, C. J., Ballan, P., et al. (2018). The heart regulates the endocrine response to heart failure: Cardiac contribution to circulating neprilysin. Eur. Heart J. 39 (20), 1794–1798. doi:10.1093/eurheartj/ehx679
Ashish, K., Faisaluddin, M., Bandyopadhyay, D., Hajra, A., and Herzog, E. (2019). Prognostic value of global longitudinal strain in heart failure subjects: A recent prototype. Int. J. Cardiol. Heart Vasc. 22, 48–49. doi:10.1016/j.ijcha.2018.11.009
Bai, W., Huo, T., Chen, X., Song, X., Meng, C., Dang, Y., et al. (2021). Sacubitril/valsartan inhibits ox-LDL-induced MALAT1 expression, inflammation and apoptosis by suppressing the TLR4/NF-κB signaling pathway in HUVECs. Mol. Med. Rep. 23 (6), 402. doi:10.3892/mmr.2021.12041
Balan, I., Khayo, T., Sultanova, S., and Lomakina, Y. (2021). Overview of sodium-glucose Co-transporter 2 (SGLT2) inhibitors for the treatment of non-diabetic heart failure patients. Cureus 13 (8), e17118. doi:10.7759/cureus.17118
Bas, H. A., Aksoy, F., Icli, A., Varol, E., Dogan, A., Erdogan, D., et al. (2017). The association of plasma oxidative status and inflammation with the development of atrial fibrillation in patients presenting with ST elevation myocardial infarction. Scand. J. Clin. Lab. Invest. 77 (2), 77–82. doi:10.1080/00365513.2016.1244857
Beckendorf, J., van den Hoogenhof, M., and Backs, J. (2018). Physiological and unappreciated roles of CaMKII in the heart. Basic Res. Cardiol. 113 (4), 29. doi:10.1007/s00395-018-0688-8
Belali, O. M., Ahmed, M. M., Mohany, M., Belali, T. M., Alotaibi, M. M., Al-Hoshani, A., et al. (2022). LCZ696 protects against diabetic cardiomyopathy-induced myocardial inflammation, ER stress, and apoptosis through inhibiting AGEs/NF-κB and PERK/CHOP signaling pathways. Int. J. Mol. Sci. 23 (3), 1288. doi:10.3390/ijms23031288
Bellis, A., Mauro, C., Barbato, E., Di Gioia, G., Sorriento, D., Trimarco, B., et al. (2020). The rationale of neprilysin inhibition in prevention of myocardial ischemia-reperfusion injury during ST-elevation myocardial infarction. Cells 9 (9), 2134. doi:10.3390/cells9092134
Berardi, C., Braunwald, E., Morrow, D. A., Mulder, H. S., Duffy, C. I., O'Brien, T. X., et al. (2020). Angiotensin-neprilysin inhibition in black Americans: Data from the PIONEER-HF trial. JACC. Heart Fail. 8 (10), 859–866. doi:10.1016/j.jchf.2020.06.019
Berg, D. D., Braunwald, E., DeVore, A. D., Lala, A., Pinney, S. P., Duffy, C. I., et al. (2020). Efficacy and safety of sacubitril/valsartan by dose level achieved in the PIONEER-HF trial. JACC. Heart Fail. 8 (10), 834–843. doi:10.1016/j.jchf.2020.06.008
Berretta, M., Quagliariello, V., Maurea, N., Di Francia, R., Sharifi, S., Facchini, G., et al. (2020). Multiple effects of ascorbic acid against chronic diseases: Updated evidence from preclinical and clinical studies. Antioxidants (Basel) 9 (12), 1182. doi:10.3390/antiox9121182
Bhattacharya, S., and Sil, P. C. (2018). “Promising natural cardioprotective agents in drug-and toxin-induced pathophysiology,” in Cardioprotective natural products: Promises and hopes. Editor G. Brahmachari (India: Visva-Bharati University), 47–120. doi:10.1142/9789813231160_0003
Böhm, M., Young, R., Jhund, P. S., Solomon, S. D., Gong, J., Lefkowitz, M. P., et al. (2017). Systolic blood pressure, cardiovascular outcomes and efficacy and safety of sacubitril/valsartan (LCZ696) in patients with chronic heart failure and reduced ejection fraction: Results from PARADIGM-HF. Eur. Heart J. 38 (15), 1132–1143. doi:10.1093/eurheartj/ehw570
Boutagy, N. E., Feher, A., Pfau, D., Liu, Z., Guerrera, N. M., Freeburg, L. A., et al. (2020). Dual angiotensin receptor-neprilysin inhibition with sacubitril/valsartan attenuates systolic dysfunction in experimental doxorubicin-induced cardiotoxicity. JACC. CardioOncol. 2 (5), 774–787. doi:10.1016/j.jaccao.2020.09.007
Brandauer, J., Andersen, M. A., Kellezi, H., Risis, S., Frøsig, C., Vienberg, S. G., et al. (2015). AMP-activated protein kinase controls exercise training- and AICAR-induced increases in SIRT3 and MnSOD. Front. Physiol. 6, 85. doi:10.3389/fphys.2015.00085
Brieler, J., Breeden, M. A., and Tucker, J. (2017). Cardiomyopathy: An overview. Am. Fam. Physician 96 (10), 640–646.
Bunsawat, K., Ratchford, S. M., Alpenglow, J. K., Park, S. H., Jarrett, C. L., Stehlik, J., et al. (2020). Chronic antioxidant administration restores macrovascular function in patients with heart failure with reduced ejection fraction. Exp. Physiol. 105 (8), 1384–1395. doi:10.1113/EP088686
Bunsawat, K., Ratchford, S. M., Alpenglow, J. K., Park, S. H., Jarrett, C. L., Stehlik, J., et al. (2021). Sacubitril-valsartan improves conduit vessel function and functional capacity and reduces inflammation in heart failure with reduced ejection fraction. J. Appl. Physiol. 130 (1), 256–268. doi:10.1152/japplphysiol.00454.2020
Burnett, H., Earley, A., Voors, A. A., Senni, M., McMurray, J. J., Deschaseaux, C., et al. (2017). Thirty years of evidence on the efficacy of drug treatments for chronic heart failure with reduced ejection fraction: A network meta-analysis. Circ. Heart Fail. 10 (1), e003529. doi:10.1161/CIRCHEARTFAILURE.116.003529
Cacciatore, F., Amarelli, C., Maiello, C., Pratillo, M., Tosini, P., Mattucci, I., et al. (2020). Effect of sacubitril-valsartan in reducing depression in patients with advanced heart failure. J. Affect. Disord. 272, 132–137. doi:10.1016/j.jad.2020.03.158
Cao, M., Zhao, Q., Sun, X., Qian, H., Lyu, S., Chen, R., et al. (2022). Sirtuin 3: Emerging therapeutic target for cardiovascular diseases. Free Radic. Biol. Med. 180, 63–74. doi:10.1016/j.freeradbiomed.2022.01.005
Cargnello, M., and Roux, P. P. (2011). Activation and function of the MAPKs and their substrates, the MAPK-activated protein kinases. Microbiol. Mol. Biol. Rev. 75 (1), 50–83. doi:10.1128/MMBR.00031-10
Chalikias, G., Kikas, P., Thomaidis, A., Rigopoulos, P., Pistola, A., Lantzouraki, A., et al. (2021). Effect of Sacubitril/Valsartan on Circulating Catecholamine Levels During a 6-Month Follow-Up in Heart Failure Patients. Timeo Danaos et dona ferentes? Acta Cardiol. 76 (4), 396–401. doi:10.1080/00015385.2020.1746094
Chang, P. C., Lin, S. F., Chu, Y., Wo, H. T., Lee, H. L., Huang, Y. C., et al. (2019). LCZ696 therapy reduces ventricular tachyarrhythmia inducibility in a myocardial infarction-induced heart failure rat model. Cardiovasc. Ther. 6032631. doi:10.1155/2019/6032631
Chang, P. C., Wo, H. T., Lee, H. L., Lin, S. F., Chu, Y., Wen, M. S., et al. (2020). Sacubitril/valsartan therapy ameliorates ventricular tachyarrhythmia inducibility in a rabbit myocardial infarction model. J. Card. Fail. 26 (6), 527–537. doi:10.1016/j.cardfail.2020.03.007
Chatterjee, P., Gheblawi, M., Wang, K., Vu, J., Kondaiah, P., Oudit, G. Y., et al. (2020). Interaction between the apelinergic system and ACE2 in the cardiovascular system: Therapeutic implications. Clin. Sci. 134 (17), 2319–2336. doi:10.1042/CS20200479
Chen, H., Zhou, J., Chen, H., Liang, J., Xie, C., Gu, X., et al. (2022). Bmi-1-RING1B prevents GATA4-dependent senescence-associated pathological cardiac hypertrophy by promoting autophagic degradation of GATA4. Clin. Transl. Med. 12 (4), e574. doi:10.1002/ctm2.574
Choi, H. M., and Shin, M. S. (2020). Angiotensin receptor-neprilysin inhibitor for the treatment of heart failure: A review of recent evidence. Korean J. Intern. Med. 35 (3), 498–513. doi:10.3904/kjim.2020.105
Chrysant, S. G., and Chrysant, G. S. (2018). Sacubitril/valsartan: A cardiovascular drug with pluripotential actions. Cardiovasc. Diagn. Ther. 8 (4), 543–548. doi:10.21037/cdt.2018.05.10
Cleland, J. G., and Swedberg, K. (1998). Lack of efficacy of neutral endopeptidase inhibitor ecadotril in heart failure. The international ecadotril multi-centre dose-ranging study investigators. Lancet 35 (9116), 1657–1658. doi:10.1016/s0140-6736(05)77712-6
Clements, R., Vang, A., Fernandez-Nicolas, A., Kue, N. R., Mancini, T. J., Morrison, A. R., et al. (2019). Treatment of pulmonary hypertension with angiotensin II receptor blocker and neprilysin inhibitor sacubitril/valsartan. Circ. Heart Fail. 12 (11), e005819. doi:10.1161/CIRCHEARTFAILURE.119.005819
Croteau, D., Qin, F., Chambers, J. M., Kallick, E., Luptak, I., Panagia, M., et al. (2020). Differential effects of sacubitril/valsartan on diastolic function in mice with obesity-related metabolic heart disease. JACC. Basic Transl. Sci. 5 (9), 916–927. doi:10.1016/j.jacbts.2020.07.006
Cunningham, J. W., Claggett, B. L., O'Meara, E., Prescott, M. F., Pfeffer, M. A., Shah, S. J., et al. (2020a). Effect of sacubitril/valsartan on biomarkers of extracellular matrix regulation in patients with HFpEF. J. Am. Coll. Cardiol. 76 (5), 503–514. doi:10.1016/j.jacc.2020.05.072
Cunningham, J. W., Vaduganathan, M., Claggett, B. L., Zile, M. R., Anand, I. S., Packer, M., et al. (2020b). Effects of sacubitril/valsartan on N-terminal pro-B-type natriuretic peptide in heart failure with preserved ejection fraction. JACC. Heart Fail. 8 (5), 372–381. doi:10.1016/j.jchf.2020.03.002
Cuthbert, J. J., Pellicori, P., and Clark, A. L. (2020). Cardiovascular outcomes with sacubitril-valsartan in heart failure: Emerging clinical data. Ther. Clin. Risk Manag. 16, 715–726. doi:10.2147/TCRM.S234772
Dereli, S., Kılınçel, O., Çerik, İ., and Kaya, A. (2020). Impact of sacubitril/valsartan treatment on depression and anxiety in heart failure with reduced ejection fraction. Acta Cardiol. 75 (8), 774–782. doi:10.1080/00015385.2020.1730577
Desai, A. S., McMurray, J. J., Packer, M., Swedberg, K., Rouleau, J. L., Chen, F., et al. (2015). Effect of the angiotensin-receptor-neprilysin inhibitor LCZ696 compared with enalapril on mode of death in heart failure patients. Eur. heart J. 36 (30), 1990–1997. doi:10.1093/eurheartj/ehv186
DeVore, A. D., Braunwald, E., Morrow, D. A., Duffy, C. I., Ambrosy, A. P., Chakraborty, H., et al. (2020). Initiation of angiotensin-neprilysin inhibition after acute decompensated heart failure: Secondary analysis of the open-label extension of the PIONEER-HF trial. JAMA Cardiol. 5 (2), 202–207. doi:10.1001/jamacardio.2019.4665
Dindaş, F., Güngör, H., Ekici, M., Akokay, P., Erhan, F., Doğduş, M., et al. (2021). Angiotensin receptor-neprilysin inhibition by sacubitril/valsartan attenuates doxorubicin-induced cardiotoxicity in A pretreatment mice model by interfering with oxidative stress, inflammation, and caspase 3 apoptotic pathway. Anatol. J. Cardiol. 25 (11), 821–828. doi:10.5152/AnatolJCardiol.2021.356
Eiringhaus, J., Wünsche, C. M., Tirilomis, P., Herting, J., Bork, N., Nikolaev, V. O., et al. (2020). Sacubitrilat reduces pro-arrhythmogenic sarcoplasmic reticulum Ca2+ leak in human ventricular cardiomyocytes of patients with end-stage heart failure. Esc. Heart Fail. 7 (5), 2992–3002. doi:10.1002/ehf2.12918
Ferro, C. J., Spratt, J. C., Haynes, W. G., and Webb, D. J. (1998). Inhibition of neutral endopeptidase causes vasoconstriction of human resistance vessels in vivo. Circulation 97 (23), 2323–2330. doi:10.1161/01.cir.97.23.2323
Frey, M. K., Arfsten, H., Pavo, N., Han, E., Kastl, S., Hülsmann, M., et al. (2021). Sacubitril/valsartan is well tolerated in patients with longstanding heart failure and history of cancer and improves ventricular function: Real-world data. Cardiooncology. 7 (1), 35. doi:10.1186/s40959-021-00121-y
Gajarsa, J. J., and Kloner, R. A. (2011). Left ventricular remodeling in the post-infarction heart: A review of cellular, molecular mechanisms, and therapeutic modalities. Heart fail. Rev. 16 (1), 13–21. doi:10.1007/s10741-010-9181-7
Ge, Q., Zhao, L., Liu, C., Ren, X., Yu, Y. H., Pan, C., et al. (2020). LCZ696, an angiotensin receptor-neprilysin inhibitor, improves cardiac hypertrophy and fibrosis and cardiac lymphatic remodeling in transverse aortic constriction model mice. Biomed. Res. Int. 2020, 7256862. doi:10.1155/2020/7256862
Ge, Q., Zhao, L., Ren, X. M., Ye, P., and Hu, Z. Y. (2019). LCZ696, an angiotensin receptor-neprilysin inhibitor, ameliorates diabetic cardiomyopathy by inhibiting inflammation, oxidative stress and apoptosis. Exp. Biol. Med. 244 (12), 1028–1039. doi:10.1177/1535370219861283
Gokhroo, R. K., Anushri, K., Tarik, M. T., Kailash, C., Rajesh, N., Ashish, K., et al. (2021). 1 year follow up results of "ARTIM HF TRIAL" (angiotensin receptor neprilysin inhibitor effect on TEI index & left ventricular mass in heart failure). Indian Heart J. 73 (2), 205–210. doi:10.1016/j.ihj.2021.01.010
Gorbunova, O., Panova, T., Chernysheva, E., and Popov, E. (2018). The level of protection from oxidative stress and three-year dynamics of structural and functional changes of the myocardium in chronic ischemic heart disease in men. Georgian Med. News 285, 63–69.
Greenberg, B. (2020). Angiotensin receptor-neprilysin inhibition (ARNI) in heart failure. Int. J. Heart Fail. 2 (2), 73. doi:10.36628/ijhf.2020.0002
Gregorietti, V., Fernandez, T. L., Costa, D., Chahla, E. O., and Daniele, A. J. (2020). Use of sacubitril/valsartan in patients with cardio toxicity and heart failure due to chemotherapy. Cardiooncology. 6 (1), 24. doi:10.1186/s40959-020-00078-4
Gui, J. S., Jalil, J., Jubri, Z., and Kamisah, Y. (2019). Parkia speciosa empty pod extract exerts anti-inflammatory properties by modulating NFκB and MAPK pathways in cardiomyocytes exposed to tumor necrosis factor-α. Cytotechnology 71 (1), 79–89. doi:10.1007/s10616-018-0267-8
Hadebe, N., Cour, M., and Lecour, S. (2018). The SAFE pathway for cardioprotection: Is this a promising target? Basic Res. Cardiol. 113 (2), 9. doi:10.1007/s00395-018-0670-5
Hamano, G., Yamamoto, K., Takami, Y., Takeshita, H., Shimosato, T., Moritani, T., et al. (2019). Effects of low-dose sacubitril/valsartan on different stages of cardiac hypertrophy in salt-loaded hypertensive rats. J. Cardiovasc. Pharmacol. 73 (5), 282–289. doi:10.1097/FJC.0000000000000662
Haynes, R., Judge, P. K., Staplin, N., Herrington, W. G., Storey, B. C., Bethel, A., et al. (2018). Effects of sacubitril/valsartan versus irbesartan in patients with chronic kidney disease. Circulation 138 (15), 1505–1514. doi:10.1161/CIRCULATIONAHA.118.034818
He, H., Li, C., Lu, X., Li, Y., Li, X., Sun, X., et al. (2021). RNA-seq profiling to investigate the mechanism of qishen granules on regulating mitochondrial energy metabolism of heart failure in rats. Evid. Based. Complement. Altern. Med. 2021, 5779307. doi:10.1155/2021/5779307
Hemilä, H., Chalker, E., and de Man, A. (2022). Vitamin C may improve left ventricular ejection fraction: A meta-analysis. Front. Cardiovasc. Med. 9, 789729. doi:10.3389/fcvm.2022.789729
Hinderer, S., and Schenke-Layland, K. (2019). Cardiac fibrosis - a short review of causes and therapeutic strategies. Adv. Drug Deliv. Rev. 146, 77–82. doi:10.1016/j.addr.2019.05.011
Hsiao, F. C., Wang, C. L., Chang, P. C., Lu, Y. Y., Huang, C. Y., Chu, P. H., et al. (2020). Angiotensin receptor neprilysin inhibitor for patients with heart failure and reduced ejection fraction: Real-world experience from taiwan. J. Cardiovasc. Pharmacol. Ther. 25 (2), 152–157. doi:10.1177/1074248419872958
Hsu, S. J., Huang, H. C., Chuang, C. L., Chang, C. C., Hou, M. C., Lee, F. Y., et al. (2020). Dual angiotensin receptor and neprilysin inhibitor ameliorates portal hypertension in portal hypertensive rats. Pharmaceutics 12 (4), 320. doi:10.3390/pharmaceutics12040320
Hu, J., Wu, Y., Zhou, X., Wang, X., Jiang, W., Huo, J., et al. (2020). Beneficial effects of sacubitril/valsartan at low doses in an asian real-world heart failure population. J. Cardiovasc. Pharmacol. 76 (4), 445–451. doi:10.1097/FJC.0000000000000873
Hubers, S. A., and Brown, N. J. (2016). Combined angiotensin receptor antagonism and neprilysin inhibition. Circulation 133 (11), 1115–1124. doi:10.1161/CIRCULATIONAHA.115.018622
Imran, M., Hassan, M. Q., Akhtar, M. S., Rahman, O., Akhtar, M., Najmi, A. K., et al. (2019). Sacubitril and valsartan protect from experimental myocardial infarction by ameliorating oxidative damage in wistar rats. Clin. Exp. Hypertens. 41 (1), 62–69. doi:10.1080/10641963.2018.1441862
Iwata, M., Cowling, R. T., Yeo, S. J., and Greenberg, B. (2011). Targeting the ACE2-ang-(1-7) pathway in cardiac fibroblasts to treat cardiac remodeling and heart failure. J. Mol. Cell. Cardiol. 51 (4), 542–547. doi:10.1016/j.yjmcc.2010.12.003
Januzzi, J. L., Prescott, M. F., Butler, J., Felker, G. M., Maisel, A. S., McCague, K., et al. (2019). Association of change in N-terminal pro–B-type natriuretic peptide following initiation of sacubitril-valsartan treatment with cardiac structure and function in patients with heart failure with reduced ejection fraction. JAMA 322 (11), 1085–1095. doi:10.1001/jama.2019.12821
Jariwala, P., Punjani, A., Boorugu, H., and Madhawar, D. B. (2021). A clinical experience of Indian patients with heart failure with reduced left ventricular ejection fraction using an angiotensin receptor-neprilysin inhibitor [ARNI] on an outpatient basis. Indian Heart J. 73 (2), 211–213. doi:10.1016/j.ihj.2021.01.002
Javadov, S., Purdham, D. M., Zeidan, A., and Karmazyn, M. (2006). NHE-1 inhibition improves cardiac mitochondrial function through regulation of mitochondrial biogenesis during postinfarction remodeling. Am. J. Physiol. Heart Circ. Physiol. 291 (4), H1722–H1730. doi:10.1152/ajpheart.00159.2006
Jornayvaz, F. R., and Shulman, G. I. (2010). Regulation of mitochondrial biogenesis. Essays Biochem. 47, 69–84. doi:10.1042/bse0470069
Kahveci, G., Bayrak, F., Mutlu, B., and Basaran, Y. (2009). Determinants of elevated NT-proBNP levels in patients with hypertrophic cardiomyopathy: An echocardiographic study. Heart Lung Circ. 18 (4), 266–270. doi:10.1016/j.hlc.2008.11.001
Kang, D. H., Park, S. J., Shin, S. H., Hong, G. R., Lee, S., Kim, M. S., et al. (2019). Angiotensin receptor neprilysin inhibitor for functional mitral regurgitation. Circulation 139 (11), 1354–1365. doi:10.1161/CIRCULATIONAHA.118.037077
Kaplinsky, E. (2016). Sacubitril/valsartan in heart failure: Latest evidence and place in therapy. Ther. Adv. Chronic Dis. 7 (6), 278–290. doi:10.1177/2040622316665350
Karabulut, U., Keskin, K., Karabulut, D., Yiğit, E., and Yiğit, Z. (2022). Effect of sacubitril/valsartan combined with dapagliflozin on long-term cardiac mortality in heart failure with reduced ejection fraction. Angiology 73 (4), 350–356. doi:10.1177/00033197211047329
Karagodin, I., Kalantari, S., Yu, D. B., Kim, G., Sayer, G., Addetia, K., et al. (2020). Echocardiographic evaluation of the effects of sacubitril-valsartan on vascular properties in heart failure patients. Int. J. Cardiovasc. Imaging 36 (2), 271–278. doi:10.1007/s10554-019-01708-4
Khan, S., Joyce, J., Margulies, K. B., and Tsuda, T. (2014). Enhanced bioactive myocardial transforming growth factor-β in advanced human heart failure. Circ. J. 78 (11), 2711–2718. doi:10.1253/circj.cj-14-0511
Khder, Y., Shi, V., McMurray, J., and Lefkowitz, M. P. (2017). Sacubitril/valsartan (LCZ696) in heart failure. Handb. Exp. Pharmacol. 243, 133–165. doi:10.1007/164_2016_77
Kim, B. S., Park, I. H., Lee, A. H., Kim, H. J., Lim, Y. H., Shin, J. H., et al. (2022). Sacubitril/valsartan reduces endoplasmic reticulum stress in A rat model of doxorubicin-induced cardiotoxicity. Arch. Toxicol. 96, 1065–1074. doi:10.1007/s00204-022-03241-1
Kim, H. M., Hwang, I. C., Choi, W., Yoon, Y. E., and Cho, G. Y. (2021). Combined effects of arni and Sglt2 inhibitors in diabetic patients with heart failure with reduced ejection fraction. Sci. Rep. 11 (1), 22342. doi:10.1038/s41598-021-01759-5
Koentges, C., Bode, C., and Bugger, H. (2016). SIRT3 in cardiac physiology and disease. Front. Cardiovasc. Med. 3, 38. doi:10.3389/fcvm.2016.00038
Kompa, A. R., Lu, J., Weller, T. J., Kelly, D. J., Krum, H., von Lueder, T. G., et al. (2018). Angiotensin receptor neprilysin inhibition provides superior cardioprotection compared to angiotensin converting enzyme inhibition after experimental myocardial infarction. Int. J. Cardiol. 258, 192–198. doi:10.1016/j.ijcard.2018.01.077
Książczyk, M., and Lelonek, M. (2020). Angiotensin receptor/neprilysin inhibitor-A breakthrough in chronic heart failure therapy: Summary of subanalysis on PARADIGM-HF trial findings. Heart fail. Rev. 25, 393–402. doi:10.1007/s10741-019-09879-x
Kumar, S., Wang, G., Liu, W., Ding, W., Dong, M., Zheng, N., et al. (2018). Hypoxia-induced mitogenic factor promotes cardiac hypertrophy via calcium-dependent and hypoxia-inducible factor-1α mechanisms. Hypertension 72 (2), 331–342. doi:10.1161/HYPERTENSIONAHA.118.10845
Kuwahara, K. (2021). The natriuretic peptide system in heart failure: Diagnostic and therapeutic implications. Pharmacol. Ther. 227, 107863. doi:10.1016/j.pharmthera.2021.107863
Lerchenmüller, C., Rabolli, C. P., Yeri, A., Kitchen, R., Salvador, A. M., Liu, L. X., et al. (2020). CITED4 protects against adverse remodeling in response to physiological and pathological stress. Circ. Res. 127 (5), 631–646. doi:10.1161/CIRCRESAHA.119.315881
Li, X., Braza, J., Mende, U., Choudhary, G., and Zhang, P. (2021). Cardioprotective effects of early intervention with sacubitril/valsartan on pressure overloaded rat hearts. Sci. Rep. 11 (1), 16542. doi:10.1038/s41598-021-95988-3
Li, X., Geng, J., Zhao, J., Ni, Q., Zhao, C., Zheng, Y., et al. (2019). Trimethylamine N-oxide exacerbates cardiac fibrosis via activating the NLRP3 inflammasome. Front. Physiol. 10, 866. doi:10.3389/fphys.2019.00866
Li, X., Liang, J., Qu, L., Liu, S., Qin, A., Liu, H., et al. (2022). Exploring the role of ferroptosis in the doxorubicin-induced chronic cardiotoxicity using a murine model. Chem. Biol. Interact. 363, 110008. doi:10.1016/j.cbi.2022.110008
Li, X., Zhu, Q., Wang, Q., Zhang, Q., Zheng, Y., Wang, L., et al. (2020). Protection of sacubitril/valsartan against pathological cardiac remodeling by inhibiting the NLRP3 inflammasome after relief of pressure overload in mice. Cardiovasc. Drugs Ther. 34 (5), 629–640. doi:10.1007/s10557-020-06995-x
Lin, Y., Zhang, H., Zhao, S., Chen, L., Li, J., Wang, X., et al. (2022). The efficacy and safety of the combined therapy of sodium-glucose Co-Transporter-2 inhibitors and angiotensin receptor-neprilysin inhibitor in patients with heart failure with reduced ejection fraction: A meta-analysis of the emperor-reduced and dapa-Hf sub-analysis. Front. Cardiovasc. Med. 9, 882089. doi:10.3389/fcvm.2022.882089
Liu, J., Zheng, X., Zhang, C., Zhang, C., and Bu, P. (2021b). Lcz696 alleviates myocardial fibrosis after myocardial infarction through the sFRP-1/Wnt/β-Catenin signaling pathway. Front. Pharmacol. 12, 724147. doi:10.3389/fphar.2021.724147
Liu, L. W., Wu, P. C., Chiu, M. Y., Tu, P. F., and Fang, C. C. (2020a). Sacubitril/valsartan improves left ventricular ejection fraction and reverses cardiac remodeling in Taiwanese patients with heart failure and reduced ejection fraction. Acta Cardiol. Sin. 36 (2), 125–132. doi:10.6515/ACS.202003_36(2).20190812A
Liu, L., Zhang, D., and Li, Y. (2020b). LncRNAs in cardiac hypertrophy: From basic science to clinical application. J. Cell. Mol. Med. 24 (20), 11638–11645. doi:10.1111/jcmm.15819
Liu, Y., Fan, Y., Li, J., Chen, M., Chen, A., Yang, D., et al. (2021a). Combination of LCZ696 and ACEI further improves heart failure and myocardial fibrosis after acute myocardial infarction in mice. Biomed. Pharmacother. 133, 110824. doi:10.1016/j.biopha.2020.110824
Lyon, R. C., Zanella, F., Omens, J. H., and Sheikh, F. (2015). Mechanotransduction in cardiac hypertrophy and failure. Circ. Res. 116 (8), 1462–1476. doi:10.1161/CIRCRESAHA.116.304937
Ma, Z. G., Yuan, Y. P., Wu, H. M., Zhang, X., and Tang, Q. Z. (2018). Cardiac fibrosis: New insights into the pathogenesis. Int. J. Biol. Sci. 14 (12), 1645–1657. doi:10.7150/ijbs.28103
Mann, D. L. (2005). Cardiac remodeling as therapeutic target: Treating heart failure with cardiac support devices. Heart fail. Rev. 10 (2), 93–94. doi:10.1007/s10741-005-4635-z
Martín-Garcia, A., López-Fernández, T., Mitroi, C., Chaparro-Muñoz, M., Moliner, P., Martin-Garcia, A. C., et al. (2020). Effectiveness of sacubitril-valsartan in cancer patients with heart failure. Esc. Heart Fail. 7 (2), 763–767. doi:10.1002/ehf2.12627
Masarone, D., Errigo, V., Melillo, E., Valente, F., Gravino, R., Verrengia, M., et al. (2020). Effects of sacubitril/valsartan on the right ventricular arterial coupling in patients with heart failure with reduced ejection fraction. J. Clin. Med. 9 (10), 3159. doi:10.3390/jcm9103159
Maslov, M. Y., Foianini, S., Mayer, D., Orlov, M. V., and Lovich, M. A. (2019a). Interaction between sacubitril and valsartan in preventing heart failure induced by aortic valve insufficiency in rats. J. Card. Fail. 25 (11), 921–931. doi:10.1016/j.cardfail.2019.09.008
Maslov, M. Y., Foianini, S., Mayer, D., Orlov, M. V., and Lovich, M. A. (2019b). Synergy between sacubitril and valsartan leads to hemodynamic, antifibrotic, and exercise tolerance benefits in rats with preexisting heart failure. Am. J. Physiol. Heart Circ. Physiol. 316 (2), H289–H297. doi:10.1152/ajpheart.00579.2018
Mazzetti, S., Scifo, C., Abete, R., Margonato, D., Chioffi, M., Rossi, J., et al. (2020). Short-term echocardiographic evaluation by global longitudinal strain in patients with heart failure treated with sacubitril/valsartan. Esc. Heart Fail. 7 (3), 964–972. doi:10.1002/ehf2.12656
McCormack, P. L. (2016). Sacubitril/valsartan: A review in chronic heart failure with reduced ejection fraction. Drugs 76 (3), 387–396. doi:10.1007/s40265-016-0544-9
McKinnie, S. M., Fischer, C., Tran, K. M., Wang, W., Mosquera, F., Oudit, G. Y., et al. (2016). The metalloprotease neprilysin degrades and inactivates apelin peptides. Chembiochem 17 (16), 1495–1498. doi:10.1002/cbic.201600244
McMurray, J., Jackson, A. M., Lam, C., Redfield, M. M., Anand, I. S., Ge, J., et al. (2020). Effects of sacubitril-valsartan versus valsartan in women compared with men with heart failure and preserved ejection fraction: Insights from PARAGON-HF. Circulation 141 (5), 338–351. doi:10.1161/CIRCULATIONAHA.119.044491
McMurray, J. J., Packer, M., Desai, A. S., Gong, J., Lefkowitz, M. P., Rizkala, A. R., et al. (2014). Angiotensin-neprilysin inhibition versus enalapril in heart failure. N. Engl. J. Med. 371 (11), 993–1004. doi:10.1056/NEJMoa1409077
Mirić, D., Baković, D., Eterović, D., Sorić, T., Čapkun, V., Vuković, I., et al. (2021). Left-ventricular function after 3 Months of sacubitril-valsartan in acute decompensated heart failure. J. Cardiovasc. Transl. Res. 14 (2), 290–298. doi:10.1007/s12265-020-10041-4
Miyauchi, T., and Sakai, S. (2019). Endothelin and the heart in health and diseases. Peptides 111, 77–88. doi:10.1016/j.peptides.2018.10.002
Miyoshi, T., Nakamura, K., Amioka, N., Hatipoglu, O. F., Yonezawa, T., Saito, Y., et al. (2022). Lcz696 ameliorates doxorubicin-induced cardiomyocyte toxicity in rats. Sci. Rep. 12 (1), 4930. doi:10.1038/s41598-022-09094-z
Miyoshi, T., Nakamura, K., Miura, D., Yoshida, M., Saito, Y., Akagi, S., et al. (2019). Effect of LCZ696, A dual angiotensin receptor neprilysin inhibitor, on isoproterenol-induced cardiac hypertrophy, fibrosis, and hemodynamic change in rats. Cardiol. J. 26 (5), 575–583. doi:10.5603/CJ.a2018.0048
Morillas, P., Castillo, J., Quiles, J., Nuñez, D., Guillén, S., Maceira, A., et al. (2008). Usefulness of NT-proBNP level for diagnosing left ventricular hypertrophy in hypertensive patients. A cardiac magnetic resonance study. Rev. Espanola Cardiol. 61 (9), 972–975. doi:10.1016/S1885-5857(08)60259-5
Morillas-Climent, H., Seller-Moya, J., Vicedo-López, Á., Galcerá-Jornet, E., Alania-Torres, E., Rodríguez-Pichardo., , et al. (2019). Evolution of functional Class, biochemical and echocardiographic parameters and clinical outcomes after sacubitril/valsartan initiation in daily practice. J. Comp. Eff. Res. 8 (9), 685–697. doi:10.2217/cer-2019-0014
Morrow, D. A., Velazquez, E. J., DeVore, A. D., Prescott, M. F., Duffy, C. I., Gurmu, Y., et al. (2019). Cardiovascular biomarkers in patients with acute decompensated heart failure randomized to sacubitril-valsartan or enalapril in the PIONEER-HF trial. Eur. Heart J. 40 (40), 3345–3352. doi:10.1093/eurheartj/ehz240
Moyes, A. J., and Hobbs, A. J. (2019). C-Type natriuretic peptide: A multifaceted paracrine regulator in the heart and vasculature. Int. J. Mol. Sci. 20 (9), 2281. doi:10.3390/ijms20092281
Murphy, M. P., and LeVine, H. (2010). Alzheimer's disease and the amyloid-beta peptide. J. Alzheimers Dis. 19 (1), 311–323. doi:10.3233/JAD-2010-1221
Murphy, S. P., Prescott, M. F., Maisel, A. S., Butler, J., Piña, I. L., Felker, G. M., et al. (2021). Association between angiotensin receptor-neprilysin inhibition, cardiovascular biomarkers, and cardiac remodeling in heart failure with reduced ejection fraction. Circ. Heart Fail. 14 (6), e008410. doi:10.1161/CIRCHEARTFAILURE.120.008410
Mustafa, N. H., Ugusman, A., Jalil, J., and Kamisah, Y. (2018). Anti-inflammatory property of Parkia speciosa empty pod extract in human umbilical vein endothelial cells. J. Appl. Pharm. Sci. 8 (1), 152–158. doi:10.7324/JAPS.2018.8123
Myhre, P. L., Prescott, M. F., Murphy, S. P., Fang, J. C., Mitchell, G. F., Ward, J. H., et al. (2022). Early B-type natriuretic peptide change in HFrEF patients treated with sacubitril/valsartan: A pooled analysis of EVALUATE-HF and PROVE-HF. JACC. Heart Fail. 10 (2), 119–128. doi:10.1016/j.jchf.2021.09.007
Myhre, P. L., Vaduganathan, M., Claggett, B., Packer, M., Desai, A. S., Rouleau, J. L., et al. (2019). B-Type natriuretic peptide during treatment with sacubitril/valsartan: The PARADIGM-HF trial. J. Am. Coll. Cardiol. 73 (11), 1264–1272. doi:10.1016/j.jacc.2019.01.018
Nakamura, M., and Sadoshima, J. (2018). Mechanisms of physiological and pathological cardiac hypertrophy. Nat. Rev. Cardiol. 15 (7), 387–407. doi:10.1038/s41569-018-0007-y
Nakou, E. S., Marketou, M. E., Patrianakos, A., Protonotarios, A., Vardas, P. E., Parthenakis, F. I., et al. (2020). Short-term effects of angiotensin receptor-neprilysin inhibitors on diastolic strain and tissue Doppler parameters in heart failure patients with reduced ejection fraction: A pilot trial. Hell. J. Cardiol. 61 (6), 415–418. doi:10.1016/j.hjc.2019.12.003
Nalivaeva, N. N., Zhuravin, I. A., and Turner, A. J. (2020). Neprilysin expression and functions in development, ageing and disease. Mech. Ageing Dev. 192, 111363. doi:10.1016/j.mad.2020.111363
Nattel, S. (2017). Molecular and cellular mechanisms of atrial fibrosis in atrial fibrillation. JACC. Clin. Electrophysiol. 3 (5), 425–435. doi:10.1016/j.jacep.2017.03.002
Nightingale, A. K., Crilley, J. G., Pegge, N. C., Boehm, E. A., Mumford, C., Taylor, D. J., et al. (2007). Chronic oral ascorbic acid therapy worsens skeletal muscle metabolism in patients with chronic heart failure. Eur. J. Heart Fail. 9 (3), 287–291. doi:10.1016/j.ejheart.2006.06.006
Nordén, E. S., Bendiksen, B. A., Andresen, H., Bergo, K. K., Espe, E. K., Hasic, A., et al. (2021). Sacubitril/valsartan ameliorates cardiac hypertrophy and preserves diastolic function in cardiac pressure overload. Esc. Heart Fail. 8 (2), 918–927. doi:10.1002/ehf2.13177
Nougué, H., Pezel, T., Picard, F., Sadoune, M., Arrigo, M., Beauvais, F., et al. (2019). Effects of sacubitril/valsartan on neprilysin targets and the metabolism of natriuretic peptides in chronic heart failure: A mechanistic clinical study. Eur. J. Heart Fail. 21 (5), 598–605. doi:10.1002/ejhf.1342
Packer, M., Anker, S. D., Butler, J., Filippatos, G., Ferreira, J. P., Pocock, S. J., et al. (2021). Influence of neprilysin inhibition on the efficacy and safety of empagliflozin in patients with chronic heart failure and a reduced ejection fraction: The EMPEROR-reduced trial. Eur. Heart J. 42 (6), 671–680. doi:10.1093/eurheartj/ehaa968
Pang, Z., Pan, C., Yao, Z., Ren, Y., Tian, L., Cui, J., et al. (2021). A study of the sequential treatment of acute heart failure with sacubitril/valsartan by recombinant human brain natriuretic peptide: A randomized controlled trial. Medicine 100 (16), e25621. doi:10.1097/MD.0000000000025621
Pascual-Figal, D., Bayés-Genis, A., Beltrán-Troncoso, P., Caravaca-Pérez, P., Conde-Martel, A., Crespo-Leiro, M. G., et al. (2021). Sacubitril-valsartan, clinical benefits and related mechanisms of action in heart failure with reduced ejection fraction. A Rev. Front. cardiovasc. Med. 8, 754499. doi:10.3389/fcvm.2021.754499
Pavo, I. J., Pavo, N., Kastner, N., Traxler, D., Lukovic, D., Zlabinger, K., et al. (2020). Heart failure with reduced ejection fraction is characterized by systemic NEP downregulation. JACC. Basic Transl. Sci. 5 (7), 715–726. doi:10.1016/j.jacbts.2020.05.011
Pavo, N., Lukovic, D., Zlabinger, K., Zimba, A., Lorant, D., Goliasch, G., et al. (2017). Sequential activation of different pathway networks in ischemia-affected and non-affected myocardium, inducing intrinsic remote conditioning to prevent left ventricular remodeling. Sci. Rep. 7, 43958. doi:10.1038/srep43958
Peng, S., Lu, X. F., Qi, Y. D., Li, J., Xu, J., Yuan, T. Y., et al. (2020). LCZ696 ameliorates oxidative stress and pressure overload-induced pathological cardiac remodeling by regulating the sirt3/MnSOD pathway. Oxid. Med. Cell. Longev. 2020, 9815039. doi:10.1155/2020/9815039
Pfau, D., Thorn, S. L., Zhang, J., Mikush, N., Renaud, J. M., Klein, R., et al. (2019). Angiotensin receptor neprilysin inhibitor attenuates myocardial remodeling and improves infarct perfusion in experimental heart failure. Sci. Rep. 9 (1), 5791. doi:10.1038/s41598-019-42113-0
Piersma, B., Bank, R. A., and Boersema, M. (2015). Signaling in fibrosis: TGF-β, WNT, and YAP/TAZ converge. Front. Med. 2, 59. doi:10.3389/fmed.2015.00059
Polito, M. V., Silverio, A., Rispoli, A., Vitulano, G., Auria, F., De Angelis, E., et al. (2020). Clinical and echocardiographic benefit of sacubitril/valsartan in A real-world population with HF with reduced ejection fraction. Sci. Rep. 10 (1), 6665. doi:10.1038/s41598-020-63801-2
Quagliariello, V., Coppola, C., Mita, D. G., Piscopo, G., Iaffaioli, R. V., Botti, G., et al. (2019). Low doses of bisphenol a have pro-inflammatory and pro-oxidant effects, stimulate lipid peroxidation and increase the cardiotoxicity of doxorubicin in cardiomyoblasts. Environ. Toxicol. Pharmacol. 69, 1–8. doi:10.1016/j.etap.2019.03.006
Raj, P., Sayfee, K., Parikh, M., Yu, L., Wigle, J., Netticadan, T., et al. (2021). Comparative and combinatorial effects of resveratrol and sacubitril/valsartan alongside valsartan on cardiac remodeling and dysfunction in MI-induced rats. Molecules 26 (16), 5006. doi:10.3390/molecules26165006
Ramaccini, D., Montoya-Uribe, V., Aan, F. J., Modesti, L., Potes, Y., Wieckowski, M. R., et al. (2021). Mitochondrial function and dysfunction in dilated cardiomyopathy. Front. Cell. Dev. Biol. 8, 624216. doi:10.3389/fcell.2020.624216
Rezq, A., Saad, M., and El Nozahi, M. (2021). Comparison of the efficacy and safety of sacubitril/valsartan versus ramipril in patients with ST-segment elevation myocardial infarction. Am. J. Cardiol. 143, 7–13. doi:10.1016/j.amjcard.2020.12.037
Romano, G., Vitale, G., Ajello, L., Agnese, V., Bellavia, D., Caccamo, G., et al. (2019). The effects of sacubitril/valsartan on clinical, biochemical and echocardiographic parameters in patients with heart failure with reduced ejection fraction: The "hemodynamic recovery. J. Clin. Med. 8 (12), 2165. doi:10.3390/jcm8122165
Russo, V., Bottino, R., Rago, A., Papa, A. A., Liccardo, B., Proietti, R., et al. (2020). The effect of sacubitril/valsartan on device detected arrhythmias and electrical parameters among dilated cardiomyopathy patients with reduced ejection fraction and implantable cardioverter defibrillator. J. Clin. Med. 9 (4), 1111. doi:10.3390/jcm9041111
Sabbah, H. N., Zhang, K., Gupta, R. C., Xu, J., and Singh-Gupta, V. (2020). Effects of angiotensin-neprilysin inhibition in canines with experimentally induced cardiorenal syndrome. J. Card. Fail. 26 (11), 987–997. doi:10.1016/j.cardfail.2020.08.009
Sainsily, X., Coquerel, D., Giguère, H., Dumont, L., Tran, K., Noll, C., et al. (2021). Elabela protects spontaneously hypertensive rats from hypertension and cardiorenal dysfunctions exacerbated by dietary high-salt intake. Front. Pharmacol. 12, 709467. doi:10.3389/fphar.2021.709467
Salim, S. M., Yunos, N. M., Jauri, M. H., and Kamisah, Y. (2020). Cardiotonic effects of cardiac glycosides from plants of Apocynaceae family. Chula. Med. J. 64 (4), 449–456. doi:10.14456/clmj.2020.58
Sansbury, B. E., Jones, S. P., Riggs, D. W., Darley-Usmar, V. M., and Hill, B. G. (2011). Bioenergetic function in cardiovascular cells: The importance of the reserve capacity and its biological regulation. Chem. Biol. Interact. 191 (1-3), 288–295. doi:10.1016/j.cbi.2010.12.002
Sharifi Kia, D., Benza, E., Bachman, T. N., Tushak, C., Kim, K., Simon, M. A., et al. (2020). Angiotensin receptor-neprilysin inhibition attenuates right ventricular remodeling in pulmonary hypertension. J. Am. Heart Assoc. 9 (13), e015708. doi:10.1161/JAHA.119.015708
Sharov, V. G., Todor, A., Khanal, S., Imai, M., and Sabbah, H. N. (2007). Cyclosporine A attenuates mitochondrial permeability transition and improves mitochondrial respiratory function in cardiomyocytes isolated from dogs with heart failure. J. Mol. Cell. Cardiol. 42 (1), 150–158. doi:10.1016/j.yjmcc.2006.09.013
Sharov, V. G., Todor, A. V., Silverman, N., Goldstein, S., and Sabbah, H. N. (2000). Abnormal mitochondrial respiration in failed human myocardium. J. Mol. Cell. Cardiol. 32 (12), 2361–2367. doi:10.1006/jmcc.2000.1266
Sheppard, C. E., and Anwar, M. (2019). The use of sacubitril/valsartan in anthracycline-induced cardiomyopathy: A mini case series. J. Oncol. Pharm. Pract. 25 (5), 1231–1234. doi:10.1177/1078155218783238
Singh, J., Burrell, L. M., Cherif, M., Squire, I. B., Clark, A. L., Lang, C. C., et al. (2017). Sacubitril/Valsartan: Beyond natriuretic peptides. Heart 103 (20), 1569–1577. doi:10.1136/heartjnl-2017-311295
Siti, H. N., Jalil, J., Asmadi, A. Y., and Kamisah, Y. (2021b). Parkia speciosa Hassk. Empty pod extract alleviates angiotensin II-induced cardiomyocyte hypertrophy in H9c2 cells by modulating the Ang II/ROS/NO Axis and MAPK pathway. Front. Pharmacol. 12, 741623. doi:10.3389/fphar.2021.741623
Siti, H. N., Jalil, J., Asmadi, A. Y., and Kamisah, Y. (2020). Roles of rutin in cardiac remodeling. J. Funct. Foods 64, 103606. doi:10.1016/j.jff.2019.103606
Siti, H. N., Jalil, J., Asmadi, A. Y., and Kamisah, Y. (2021a). Rutin modulates MAPK pathway differently from quercetin in angiotensin II-induced H9c2 cardiomyocyte hypertrophy. Int. J. Mol. Sci. 22 (10), 5063. doi:10.3390/ijms22105063
Solomon, S. D., Jhund, P. S., Claggett, B. L., Dewan, P., Køber, L., Kosiborod, M. N., et al. (2020). Effect of dapagliflozin in patients with HFrEF treated with sacubitril/valsartan: The DAPA-HF trial. JACC. Heart Fail. 8 (10), 811–818. doi:10.1016/j.jchf.2020.04.008
Sorrentino, A., Steinhorn, B., Troncone, L., Saravi, S., Badole, S., Eroglu, E., et al. (2019). Reversal of heart failure in a chemogenetic model of persistent cardiac redox stress. Am. J. Physiol. Heart Circ. Physiol. 317 (3), H617–H626. doi:10.1152/ajpheart.00177.2019
Suematsu, Y., Jing, W., Nunes, A., Kashyap, M. L., Khazaeli, M., Vaziri, N. D., et al. (2018). LCZ696 (Sacubitril/Valsartan), an angiotensin-receptor neprilysin inhibitor, attenuates cardiac hypertrophy, fibrosis, and vasculopathy in a rat model of chronic kidney disease. J. Card. Fail. 24 (4), 266–275. doi:10.1016/j.cardfail.2017.12.010
Sung, P. H., Chai, H. T., Yang, C. C., Chiang, J. Y., Chen, C. H., Chen, Y. L., et al. (2022). Combined levosimendan and sacubitril/valsartan markedly protected the heart and kidney against cardiorenal syndrome in rat. Biomed. Pharmacother. 148, 112745. doi:10.1016/j.biopha.2022.112745
Sung, Y. L., Lin, T. T., Syu, J. Y., Hsu, H. J., Lin, K. Y., Liu, Y. B., et al. (2020). Reverse electromechanical modelling of diastolic dysfunction in spontaneous hypertensive rat after sacubitril/valsartan therapy. Esc. Heart Fail. 7 (6), 4040–4050. doi:10.1002/ehf2.13013
Sutanto, H., Dobrev, D., and Heijman, J. (2021). Angiotensin receptor-neprilysin inhibitor (ARNI) and cardiac arrhythmias. Int. J. Mol. Sci. 22 (16), 8994. doi:10.3390/ijms22168994
Syamsunarno, M. R. A., Safitri, R., and Kamisah, Y. (2021). Protective effects of Caesalpinia sappan Linn. And its bioactive compounds on cardiovascular organs. Front. Pharmacol. 12, 725745. doi:10.3389/fphar.2021.725745
Tanase, D. M., Radu, S., Al Shurbaji, S., Baroi, G. L., Florida Costea, C., Turliuc, M. D., et al. (2019). Natriuretic peptides in heart failure with preserved left ventricular ejection fraction: From molecular evidences to clinical implications. Int. J. Mol. Sci. 20 (11), 2629. doi:10.3390/ijms20112629
Tashiro, K., Kuwano, T., Ideishi, A., Morita, H., Idemoto, Y., Goto, M., et al. (2020). Sacubitril/valsartan inhibits cardiomyocyte hypertrophy in angiotensin II-induced hypertensive mice independent of a blood pressure-lowering effect. Cardiol. Res. 11 (6), 376–385. doi:10.14740/cr1137
Tham, Y. K., Bernardo, B. C., Ooi, J. Y., Weeks, K. L., and McMullen, J. R. (2015). Pathophysiology of cardiac hypertrophy and heart failure: Signaling pathways and novel therapeutic targets. Arch. Toxicol. 89 (9), 1401–1438. doi:10.1007/s00204-015-1477-x
Torrado, J., Cain, C., Mauro, A. G., Romeo, F., Ockaili, R., Chau, V. Q., et al. (2018). Sacubitril/valsartan averts adverse post-infarction ventricular remodeling and preserves systolic function in rabbits. J. Am. Coll. Cardiol. 72 (19), 2342–2356. doi:10.1016/j.jacc.2018.07.102
Tsutsui, H., Momomura, S. I., Saito, Y., Ito, H., Yamamoto, K., Sakata, Y., et al. (2021). Efficacy and safety of sacubitril/valsartan in Japanese patients with chronic heart failure and reduced ejection fraction - results from the PARALLEL-HF study. Circ. J. 85 (5), 584–594. doi:10.1253/circj.CJ-20-0854
Valentim Gonçalves, A., Galrinho, A., Pereira-da-Silva, T., Branco, L., Rio, P., Timóteo, A. T., et al. (2020a). Myocardial work improvement after sacubitril-valsartan therapy: A new echocardiographic parameter for A new treatment. J. Cardiovasc. Med. 21 (3), 223–230. doi:10.2459/JCM.0000000000000932
Valentim Gonçalves, A., Pereira-da-Silva, T., Galrinho, A., Rio, P., Moura Branco, L., Soares, R., et al. (2019). Antiarrhythmic effect of sacubitril-valsartan: Cause or consequence of clinical improvement? J. Clin. Med. 8 (6), 869. doi:10.3390/jcm8060869
Valentim Goncalves, A., Pereira-da-Silva, T., Galrinho, A., Rio, P., Moura Branco, L., Soares, R., et al. (2020b). C-reactive protein reduction with sacubitril-valsartan treatment in heart failure patients. Am. J. Cardiovasc. Dis. 10 (3), 174–181.
Vilela-Martin, J. F. (2016). Spotlight on valsartan–sacubitril fixed-dose combination for heart failure: The evidence to date. Drug Des. devel. Ther. 10, 1627–1639. doi:10.2147/DDDT.S84782
Villani, A., Ravaro, S., Cerea, P., Caravita, S., Ciambellotti, F., Branzi, G., et al. (2020). Do the remodeling effects of sacubitril/valsartan treatment depend upon heart failure duration? J. Cardiovasc. Med. 21 (9), 682–687. doi:10.2459/JCM.0000000000001000
Vitale, G., Romano, G., Di Franco, A., Caccamo, G., Nugara, C., Ajello, L., et al. (2019). Early effects of sacubitril/valsartan on exercise tolerance in patients with heart failure with reduced ejection fraction. J. Clin. Med. 8 (2), 262. doi:10.3390/jcm8020262
Wang, H., and Fu, X. (2021). Effects of sacubitril/valsartan on ventricular remodeling in patents with left ventricular systolic dysfunction following acute anterior wall myocardial infarction. Coron. Artery Dis. 32 (5), 418–426. doi:10.1097/MCA.0000000000000932
Wang, L., Wang, P., Xu, S., Li, Z., Duan, D. D., Ye, J., et al. (2022). The cross-talk between PARylation and SUMOylation in C/EBPβ at K134 site participates in pathological cardiac hypertrophy. Int. J. Biol. Sci. 18 (2), 783–799. doi:10.7150/ijbs.65211
Wang, Y., Guo, Z., Gao, Y., Liang, P., Shan, Y., He, J., et al. (2019a). Angiotensin II receptor blocker LCZ696 attenuates cardiac remodeling through the inhibition of the ERK signaling pathway in mice with pregnancy-associated cardiomyopathy. Cell. Biosci. 9, 86. doi:10.1186/s13578-019-0348-1
Wang, Y., Li, H., Li, Y., Zhao, Y., Xiong, F., Liu, Y., et al. (2019b). Coriolus versicolor alleviates diabetic cardiomyopathy by inhibiting cardiac fibrosis and NLRP3 inflammasome activation. Phytother. Res. 33 (10), 2737–2748. doi:10.1002/ptr.6448
Wu, M., Guo, Y., Wu, Y., Xu, K., and Lin, L. (2021a). Protective effects of sacubitril/valsartan on cardiac fibrosis and function in rats with experimental myocardial infarction involves inhibition of collagen synthesis by myocardial fibroblasts through downregulating TGF-β1/smads pathway. Front. Pharmacol. 12, 696472. doi:10.3389/fphar.2021.696472
Wu, Q. R., Zheng, D. L., Liu, P. M., Yang, H., Li, L. A., Kuang, S. J., et al. (2021b). High glucose induces drp1-mediated mitochondrial fission via the Orai1 calcium channel to participate in diabetic cardiomyocyte hypertrophy. Cell. Death Dis. 12 (2), 216. doi:10.1038/s41419-021-03502-4
Xi, Q., Chen, Z., Li, T., and Wang, L. (2022). Time to switch angiotensin-converting enzyme inhibitors/angiotensin receptor blockers to sacubitril/valsartan in patients with cancer therapy-related cardiac dysfunction. J. Int. Med. Res. 50 (1), 3000605211067909. doi:10.1177/03000605211067909
Xia, Y., Chen, Z., Chen, A., Fu, M., Dong, Z., Hu, K., et al. (2017). LCZ696 improves cardiac function via alleviating drp1-mediated mitochondrial dysfunction in mice with doxorubicin-induced dilated cardiomyopathy. J. Mol. Cell. Cardiol. 108, 138–148. doi:10.1016/j.yjmcc.2017.06.003
Yahfoufi, N., Alsadi, N., Jambi, M., and Matar, C. (2018). The immunomodulatory and anti-inflammatory role of polyphenols. Nutrients 10 (11), 1618. doi:10.3390/nu10111618
Yandrapalli, S., Aronow, W. S., Mondal, P., and Chabbott, D. R. (2017). The evolution of natriuretic peptide augmentation in management of heart failure and the role of sacubitril/valsartan. Arch. Med. Sci. 13 (5), 1207–1216. doi:10.5114/aoms.2017.68813
Yang, C. C., Chen, Y. T., Chen, C. H., Li, Y. C., Shao, P. L., Huang, T. H., et al. (2019). The therapeutic impact of Entresto on protecting against cardiorenal syndrome-associated renal damage in rats on high protein diet. Biomed. Pharmacother. 116, 108954. doi:10.1016/j.biopha.2019.108954
Yeh, J. N., Sung, P. H., Chiang, J. Y., Sheu, J. J., Huang, C. R., Chu, Y. C., et al. (2021a). Early treatment with combination of SS31 and Entresto effectively preserved the heart function in doxorubicin-induced dilated cardiomyopathic rat. Biomed. Pharmacother. 141, 111886. doi:10.1016/j.biopha.2021.111886
Yeh, J. N., Yue, Y., Chu, Y. C., Huang, C. R., Yang, C. C., Chiang, J. Y., et al. (2021b). Entresto protected the cardiomyocytes and preserved heart function in cardiorenal syndrome rat fed with high-protein diet through regulating the oxidative stress and mfn2-mediated mitochondrial functional integrity. Biomed. Pharmacother. 144, 112244. doi:10.1016/j.biopha.2021.112244
Yenerçağ, M., Arslan, U., Dereli, S., Çoksevim, M., Doğduş, M., Kaya, A., et al. (2021). Effects of angiotensin receptor neprilysin inhibition on pulmonary arterial stiffness in heart failure with reduced ejection fraction. Int. J. Cardiovasc. Imaging 37 (1), 165–173. doi:10.1007/s10554-020-01973-8
Yue, Y., Meng, K., Pu, Y., and Zhang, X. (2017). Transforming growth factor beta (TGF-β) mediates cardiac fibrosis and induces diabetic cardiomyopathy. Diabetes Res. Clin. Pract. 133, 124–130. doi:10.1016/j.diabres.2017.08.018
Zhang, W., Elimban, V., Nijjar, M. S., Gupta, S. K., and Dhalla, N. S. (2003). Role of mitogen-activated protein kinase in cardiac hypertrophy and heart failure. Exp. Clin. Cardiol. 8 (4), 173–183.
Zhang, W., Liu, J., Fu, Y., Ji, H., Fang, Z., Zhou, W., et al. (2021a). Sacubitril/valsartan reduces fibrosis and alleviates high-salt diet-induced HFpEF in rats. Front. Pharmacol. 11, 600953. doi:10.3389/fphar.2020.600953
Zhang, Y., Wu, Y., Zhang, K., Ke, Z., Hu, P., Jin, D., et al. (2021b). Benefits of early administration of sacubitril/valsartan in patients with ST-elevation myocardial infarction after primary percutaneous coronary intervention. Coron. Artery Dis. 32 (5), 427–431. doi:10.1097/MCA.0000000000000955
Zhao, Y., Ma, R., Yu, X., Li, N., Zhao, X., Yu, J., et al. (2019). AHU377+Valsartan (LCZ696) modulates renin-angiotensin system (RAS) in the cardiac of female spontaneously hypertensive rats compared with valsartan. J. Cardiovasc. Pharmacol. Ther. 24 (5), 450–459. doi:10.1177/1074248419838503
Zhou, H., Xia, C., Yang, Y., Warusawitharana, H. K., Liu, X., Tu, Y., et al. (2022). The prevention role of theaflavin-3, 3'-digallate in angiotensin II induced pathological cardiac hypertrophy via CaN-NFAT signal pathway. Nutrients 14 (7), 1391. doi:10.3390/nu14071391
Keywords: sacubitril, valsartan, LCZ696, neprilysin inhibitor, fibrosis, cardiac function, cardiomyopathy, entresto
Citation: Mustafa NH, Jalil J, Zainalabidin S, Saleh MSM, Asmadi AY and Kamisah Y (2022) Molecular mechanisms of sacubitril/valsartan in cardiac remodeling. Front. Pharmacol. 13:892460. doi: 10.3389/fphar.2022.892460
Received: 09 March 2022; Accepted: 11 July 2022;
Published: 08 August 2022.
Edited by:
Fouad Antoine Zouein, American University of Beirut, LebanonReviewed by:
Mark C. Chappell, Wake Forest School of Medicine, United StatesVincenzo Quagliariello, G. Pascale National Cancer Institute Foundation (IRCCS), Italy
Copyright © 2022 Mustafa, Jalil, Zainalabidin, Saleh, Asmadi and Kamisah. This is an open-access article distributed under the terms of the Creative Commons Attribution License (CC BY). The use, distribution or reproduction in other forums is permitted, provided the original author(s) and the copyright owner(s) are credited and that the original publication in this journal is cited, in accordance with accepted academic practice. No use, distribution or reproduction is permitted which does not comply with these terms.
*Correspondence: Yusof Kamisah, a2FtaXNhaF95QHlhaG9vLmNvbQ==, a2FtaXNhaF95QHBwdWttLnVrbS5lZHUubXk=