- College of Biological and Chemical Engineering, Panzhihua University, Panzhihua, China
Cerebral ischemia with high mortality and morbidity still requires the effectiveness of medical treatments. A growing number of investigations have shown strong links between inflammation and cerebral ischemia. Natural medicine’s treatment methods of cerebral ischemic illness have amassed a wealth of treatment experience and theoretical knowledge. This review summarized recent progress on the disease inflammatory pathways as well as 26 representative natural products that have been routinely utilized to treat cerebral ischemic injury. These natural products have exerted anti-inflammatory effects in cerebral ischemia based on their inflammatory mechanisms, including their inflammatory gene expression patterns and their related different cell types, and the roles of inflammatory mediators in ischemic injury. Overall, the combination of the potential therapeutic interventions of natural products with the inflammatory mechanisms will make them be applicable for cerebral ischemic patients in the future.
Introduction
Stroke is a brain disease that causes numerous deaths and disabilities (Yuan et al., 2021), and it is the 3rd in disease mortality rate worldwide (Lo et al., 2003). Stroke is subdivided into ischemia and hemorrhage. Ischemic strokes account for approximately 87% of all deaths and were the focus of major drug trials (Rosenzweig et al., 2007). While ischemia is a pathologic condition in which blood flow is reduced to the point where normal cellular activity is disrupted (Adams et al., 2005; Sacco et al., 2006; Shang et al., 2013). The complex pathophysiology of cerebral ischemia primarily includes inflammatory pathways except ionic imbalances, neuroprotection, apoptosis, oxidative damage and angiogenesis (Deb et al., 2010; Dodd et al., 2021). There is growing evidences that some inflammatory mechanisms play a key role during the setting or acute phase of cerebral ischemia owing to subarachnoid hemorrhage, brain injury or cardiac arrest.
Inflammation, a dynamic tissue response mechanism to resist the invasion of pathogens, evolves over the course of evolution (Headland and Norling, 2015). The role of inflammatory mechanisms is crucial in stroke-related brain injury and tissue damage after ischemia (Przykaza, 2021). The understanding inflammatory of response mechanisms will help workers to choose appropriate intervention measures of blocking the cerebral ischemic injury cascade, and reducing neuronal death, and more efficiently preventing cerebral ischemia and treat disease in the theoretical and clinical applications. As a requirement of novel drug development for cerebral ischemia, there is a focus on the anti-inflammatory mechanisms and effects of natural products. Therefore, understanding and treatments of cerebral ischemic disease will be esential for scientists and clinicians to chooose the suitable anti-inflammatory measures and agents. Some specific natural products discovered from natural medical resources with the potentials in possessing anti-inflammatory effects on cerebral ischemia will increase the opportunity in laboratory and clinical trials.
Methodology
Using exhaustive searches in the PubMed, China National Knowledge Internet (CNKI), Springer and Elsevier SDOL database, the present review gathered data of the research and trials from 2002 until February 2022 for 2 decades by adopting the following keywords and MeSH (medical subject heading) terms according to the Preferred Reporting Items for Systematic Reviews (Bayat et al., 2021). This review mainly summarized the inflammatory mechanisms and some representative natural products that have been commonly used in experimental research for the treatment of cerebral ischemia according to the inflammatory mechanism of ischemia.
Inflammatory Mechanisms of Ischaemia
Inflammation acts as a critical role in the pathological progression of cerebral ischemia. Inflammation progresses in response to various stimuli are produced after ischemia. These progresses require activation and undergoes the rapid upregulation of multifarious genes, invasion of leukocyte (monocytes and neutrophils), and stimulation the activity of microglia, astrocytes, and endothelial cells following ischemia with the duration from hours to days (Huang et al., 2006; Zaleska et al., 2009), including many different inflammatory mediators and extracellular receptors. To avoid the evolution of acute resolving into persistent chronic inflammatory symptoms and further tissue damages, the inflammatory reaction should be actively resolved. Taken all together, the following discussions provided insights into the contributions of key inflammatory gene expression, different cell types, and inflammatory mediators to ischemic injury (Figure 1).
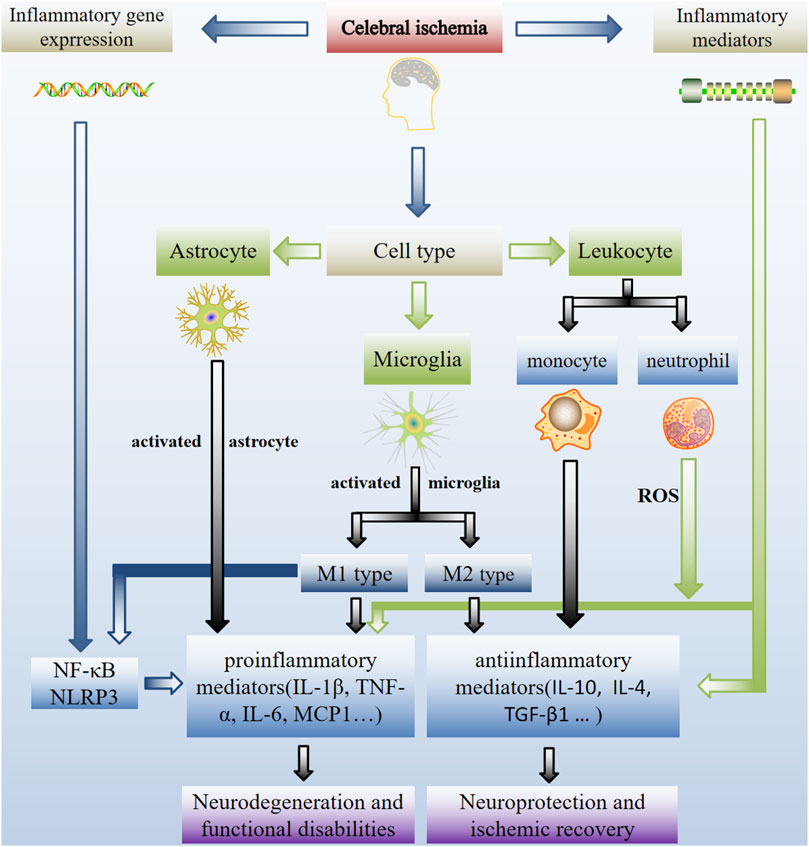
FIGURE 1. Schematic representation of inflammatory mechanisms in cerebral ischemia. Inflammation is crucial to the pathogenesis of ischemic stroke. Critical inflammatory and anti-inflammatory events involved in inflammatory gene expression, different cell types and inflammatory mediators contribute to ischemic injury. Inflammation inhibition is a possible treatment option for ischemic stroke-related neuro-inflammatory damage. Activation of local microglia and infiltrating macrophages from the compromised BBB occurs during cerebral ischemia. Microglia activation has two-way role (a double-edged sword). Stimulation the activity of microglia migrate to injured neurons and then switche to the M1 or M2 phenotype, which is implicated in nerve injury and repair. As a result, after an ischemic stroke, controlling the M1/m2 phenotype of microglia is critical for brain healing.
Inflammatory Gene Expression
Ischemic injury in the brain parenchyma sets off an inflammatory cascade that exacerbates tissue damage. Each of these events may be affected by heredity, which makes it difficult to prove the classical pattern of heredity. Within minutes of occlusion, pro-inflammatory genes (heat shock proteins, transcription factors, adhesion molecules, chemokines and cytokines) that produce mediators of inflammation are upregulated (Raghay et al., 2020). Among these transcription factors, NF-κB (nuclear factor) regulated the expression of TNF-α (tumor necrosis factor α), NOS (nitric oxide synthase), IL-1β (interleukin-1β), IL-6 (interleukin-6), COX-2 (cyclooxygenase-2) and MCP1 (monocyte chemoattractant protein 1) in vitro (Deb et al., 2010). This has been demonstrated by reduced ischemic damage in mutant mice that targeted disruption of these genes (Adibhatla and Hatcher, 2008; Tănăsescu et al., 2008). Intercellular adhesion molecule-1 (ICAM-1) was found to appear in the postischemic no-reflow regions during cerebral ischemia. And it was also a mediator of leukocyte-endothelial cell adhesion (Sun et al., 2019). Matrix metalloproteinase (MMP) levels, especially MMP-9, have been found that associated with infarct growth, bleeding, transformation events, and neurological dysfunction (Montaner et al., 2003; Deb et al., 2010).
Cell Types in Inflammation
The aggregation of inflammatory cells and mediators during cerebral ischemia is a characteristic of inflammation. After vascular occlusion, ischemic injury causes inflammatory cascade and further aggravates tissue injury (Amantea et al., 2014; Soliman et al., 2021). Reactive microglia, leukocytes (inflammatory cells) and macrophages have found to be recruited into the ischemic brain, and then resulting in inflammatory injury and the generation of inflammatory mediators by these cells, neurons and astrocytes.
Microglia
Microglia play critical roles in brain inflammation following stroke, especially in the penumbral region of damage (Subedi and Gaire, 2021). Promoting the polarization of microglia and macrophages from the pro-inflammatory M1 to the anti-inflammatory M2 phenotype has been demonstrated to be a possible treatment for ischemic stroke (Li et al., 2021). M1 is generally considered to be neurotoxic, while M2 exhibits neuroprotective effects. Microglia are in a resting phase. And they account for 5–20% of glial cells (Deb et al., 2010). During ischemia, these cells get the significantly morphological and metabolic changes, as well as rapid and extensive genetic upregulations. These cells are the principal central nervous system (CNS) source of cytokines including transforming growth factor-β (TGF-β), TNF-α and IL-1β (Zarruk et al., 2018). Microglial cells have been indicated that can also produce pro-inflammatory cytokines and neuroprotective factors, such as TGF-β1, metallothionein-2 and erythropoietin. Because of the mixed nature of destructive and protective factors from microglial and astrocytes, the overall role of glia may differ at different time points following stroke insult, with protective or regenerative activities occurring days to weeks after the onset of ischemia. Several studies imply that activated microglia induce to injury (Wang et al., 2007). However, whether microglia cause damage following cerebral ischemia is not clear, and their anti-inflammatory potential is considered to be an significant neuroprotective factor after ischemic injury (Jin et al., 2017; Fukumoto et al., 2019; Gaire, 2021).
Leukocytes
With increasing evidences, leukocytes are related to the pathogenesis of ischemic stroke. An increase in peripheral leukocyte counts are involved in the earliest inflammatory reactions in stroke. Neutrophils are the first leukocytes to respond, and their level is related to the infarct volume. From minutes to hours after reperfusion, neutrophil adhesion and migration have been observed in cerebral venules. After ischemia, the recruited cell population were switched from polymorphonuclear cells to mononuclear leukocytes and lymphocytes, and leukocyte recruitment continued days to weeks (Yilmaz et al., 2006; Gavins et al., 2007). Leukocyte-endothelial cell adhesion was prevented using adhesion molecule antibodies against stroke (Ishikawa et al., 2004). Therefore, leukocytes accumulate in the tissue after ischemia before tissue injury, and specific targeting of leukocytes provides substantive protection for ischemic injury.
Astrocytes
In the human CNS, astrocytes are the main glial cell type and generally exceed the number of neurons. Astrocyte response is one of the earliest and the most significant changes in the CNS after ischemic injury. Astrocytes express different inflammatory mediators in inflammatory responses that may influence the outcome of ischemic injury (Falsig et al., 2006; Denes et al., 2008; Sofroniew, 2020).
Inflammatory Mediators
Inflammatory mediators of cell adhesion to cerebral ischemia are primarily divided into cytokines and chemokines. Cytokines are a flock of small glycoproteins (∼25kd) from different sources. As a kind of cytokines, chemokines have chemotactic properties, which can stimulate cells to migrate to the source of chemokines. Chemokines polypeptides have been found to play the role in inflammation, immunological activation, cell differentiation, and cell death.
Cytokines
Cytokines associated with inflammation in stroke, such as IL-1, TNF-α, IL-6, TGF-β and IL-10, are released and serve as intercellular messengers. Messengers mediate inflammatory and immunological responses. IL-6, IL-10 and TGF-β may have neuroprotective effect. IL-1 and TNF-α aggravate brain injury.
IL-1: IL-1 has two isoforms (IL-1α and IL-1β) and an endogenous inhibitor, IL-1 receptor antagonist (IL-1ra). Its pro-inflammatory properties were neutralized by IL-1ra administration (Emsley et al., 2005), and ischemic injury in IL-1ra deficient mice increased significantly (Pinteaux et al., 2006).
IL-10: an anti-inflammatory cytokine. Lower IL-10 levels are related to one of increased risks in stroke. Guizhi Fuling Capsule significantly upregulate the level of IL-10 mRNA and protein expression and its receptor in rats with focal cerebral ischemia and reperfusion (I/R) (Sun et al., 2015).
IL-6: a pro-inflammatory cytokine with unclear function in stroke. The clinical focus of IL-6 has revealed that IL-6 serum concentrations was an excellent independent predictor value for in-hospital mortality. (Rallidis et al., 2006).
TNF-α: TNF-α is a cellular signal protein involved in systemic inflammation and one of the cytokines involved in acute phase response. Activated macrophages primarily produce TNF-α. Its expression occurs peri-infarct areas in the ischemic core, adjacent distal areas in brain.
TGF-β: TGF-β may helpful for the rehabilitation therapy of ischemic stroke. Microglia secrete TGF-β1 that is reported to protect cultured neurons from ischemia-like injury (Lu et al., 2005). Its expression suggests a neuroprotective function in the penumbra during the recovery phase of cerebral injury.
Chemokines
Chemokines play roles in cellular communication and inflammatory cell recruitment in host defense. Cytokines stimulate the production and release of chemokines C, CC, CXC, and CX3C [location based on the cysteine residues (C)] and MCP-1 (monocyte chemoattractant protein-1) in cerebral ischemia.
CX3C: CX3C expression was limited to surviving neurons around infarction and some endothelial cells during ischemia, and the CX3CR1 expression (CX3C receptor) was found only on microglia. Those suggest that fractalkine is involved in neuron-microglia signal transduction (Tarozzo et al., 2002).
MCP-1: MCP-1 overexpression significantly exacerbated the ischemic injury. This phenomenon is related to the activation of inflammatory cells. MCP-1 increased the cell permeability in vitro, and it generated changes in tight junction proteins, which suggest that it helps to ‘open’ the blood-brain barrier (BBB) (Stamatovic et al., 2005).
The Anti-Inflammatory Effects of Natural Products
Historically, as folk medicines, natural products have been revealed the vast potentials for drug discoveries and the managements of various human diseases (Mu et al., 2020; Newman and Cragg, 2020; Atanasov et al., 2021). To date, the natural products used for anti-inflammation have been widely investigated and reported (Azab et al., 2016). These natural products with anti-inflammation effects are extensively distributed in different classes, e.g., flavonoids, terpenoids, saponins, phenylpropanoidss, anthraquinones, alkaloids, and phenols, etc., (Wang and Zeng, 2019) (Figure 2). These natural products exert significant anti-inflammatory effects via acting on different drug targets and cell signaling pathways.
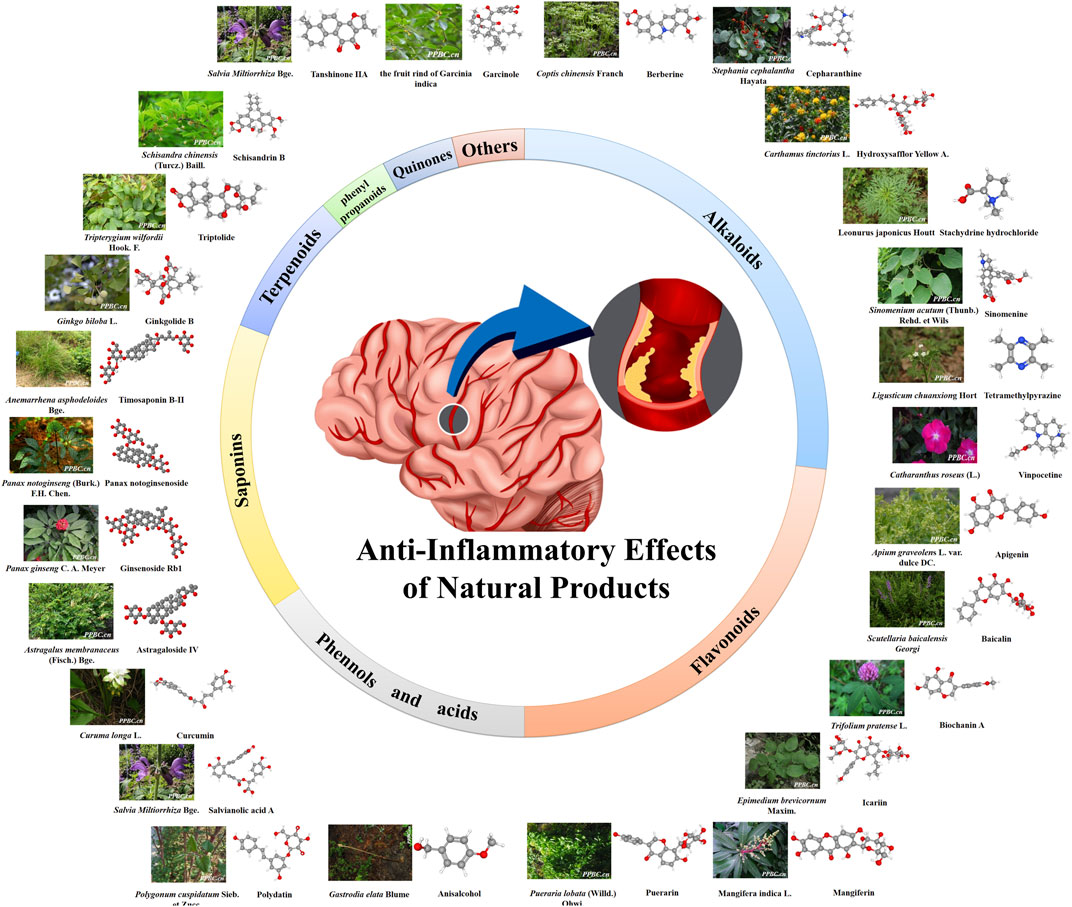
FIGURE 2. Anti-inflammatory effect of natural products. The color figures of medicine are viewed in www.iplant.cn. The club model comes from PubChem and Guidechem.
Anti-Inflammatory Effects of Flavonoids in Cerebral Ischemia
Flavonoids are low molecular weight phenolic compounds including A-, B- and C- rings. Most flavonoids that discovered so far have displayed a remarkable array of biochemical and pharmacological actions including anti-inflammatory effects (Romano et al., 2013). Several mechanisms have been proposed to explain the anti-inflammatory effects of flavonoids in vivo, including reducing the production of pro-inflammatory molecules and regulating the function of inflammatory cells (Serafini et al., 2010). The natural accuring flavonoids, such as apigenin, baicalin, biochanin A, icariin, mangiferin, and puerarin the flavonoid examples have exhibited anti-inflammatory effects of flavonoids (Table 1).
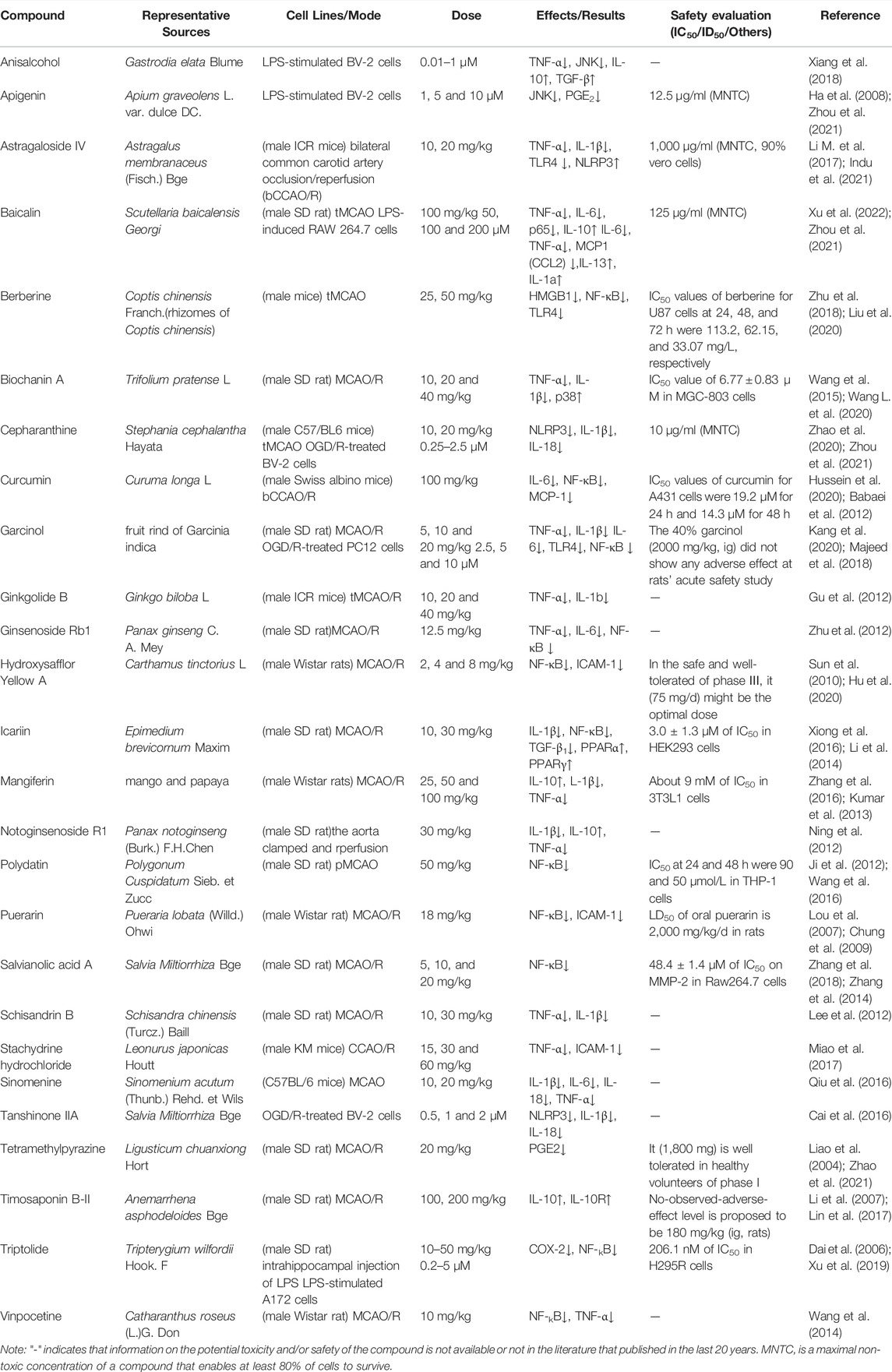
TABLE 1. Twenty-six active compounds from natural medicine with anti-inflammatory effects in cerebral ischemia.
Anti-Inflammatory Effects of Alkaloids in Cerebral Ischemia
Alkaloids are nitrogen-containing organic compounds with an alkali like properties. They that are widely distributed in plants with diverse anti-inflammatory activities provide various potentials for the design and discovery of new anti-inflammatory drugs (Bai et al., 2021). Some alkaloids have strong anti-inflammatory activities and play important roles in the treatment of vascular disease (Alasvand et al., 2019). Anti-inflammatory alkaloids can be mainly divided into the following categories: isoquinoline alkaloids, indole alkaloids, pyridine alkaloids, terpenoid alkaloids, organic amine alkaloids, etc (Li S. et al., 2020). Several studies support the significance of the anti-inflammatory activity as an underlying mechanism for most of the pharmacological activities of the alkaloid (Tian et al., 2019; Geetha and Ramachandran, 2021). Alkaloids, such as berberine, cepharanthine, hydroxysafflor yellow A, sinomenine, tetramethylpyrazine, and vinpocetine, with anti-inflammatory effects in cerebral ischemia treatment, were summaried in Table 1.
Anti-Inflammatory Effects of Saponins in Cerebral Ischemia
Saponins are glycosides that release sugar(s) and an aglycone (sapogenin) after acid hydrolysis. The aglycone of saponins can be triterpenoid or steroidal in nature. Steroidal saponins and saponins have been found to have diverse activities of inflammatory cytokines on a variety of inflammatory models (Passos et al., 2022). Studies support the anti-inflammatory activity as an underlying mechanism of the saponin in cerebral ischemia treatment (Sun et al., 2020; Yang F. et al., 2020; Xue et al., 2021). The anti-inflammatory sapnins, such as astragaloside IV, Ginsenoside Rb1, notoginsenoside R1, and Timosaponin B-II were summaried in Table 1.
Anti-Inflammatory Effects of Terpenoids in Cerebral Ischemia
Terpenoids are secondary metabolites widely distributed in nature with diverse structures, and they represent a promising chemical group with potential beneficial effects in neurological diseases in view of the pleiotropic effects on cell death and survival, which consolidate their therapeutic value (Gonzalez-Cofrade et al., 2019; Proshkina et al., 2020). Several studies support the therapeutic potential compounds known to be effective among various inflammatory diseases (Downer, 2020; Khoshnazar et al., 2020; Kim et al., 2020). Terpenoids, combined with the studied mechanism and commonly used drugs, may be new strategy for further anti-inflammatory treatment. The anti-inflammatory effects of terpenoids including ginkgolide B and triptolide are reviewed in cerebral ischemic treatment (Table 1).
Anti-Inflammatory Effects of Phenols and Acids in Cerebral Ischemia
(Poly) Phenols are natural substances with variable phenolic structures, and they are generally known to possess potent anti-inflammatory properties. It has been found that phenols can affect the polarization of M1/M2 via TLR-4/NF-κB pathway and exert anti-inflammatory properties to treat ischemic stroke (Li et al., 2022). The anti-inflammatory effects of phenols and acids including anisalcohol, curcumin, polydatin and salvianolic acid A are reviewed in cerebral ischemic treatment (Table 1).
Anti-Inflammatory Effects of Quinones, Phenylpropanoids and Others in Cerebral Ischemia
In addition to the types of compounds mentioned above, phenylpropanoids, quinones and others are a large group of organic compounds in the nature (Table 1). Anti-inflammatory effects of them are found and reported (Gui et al., 2020; Yang et al., 2021).
Major Anti-Inflammatory Natural Products From Natural Medicines
Natural products have been long used as folk drugs all over the world although the resources and applications vary among different regions. In China, many natural medicines have been accustomed to treat stroke for years. The direct experience gained from human subjects provides a huge resource for new drug candidates and treatment methods for stroke. Therefore, this review primarily discussed several representative natural products (compounds) with anti-inflammatory effects in cerebral ischemia treatment.
Anisalcohol is a phenolic compound that was originally isolated from Gastrodia elata Blume (Chen H. L. et al., 2020). Anisalcohol has ability to significantly decrease TNF-α and increase IL-10 and TGF-β in lipopolysaccharide (LPS)-stimulated BV-2 cells (a murine microglial cell line). Anisalcohol has also found to inhibit c-Jun N-terminal kinase (JNK) phosphorylation and suppress NF-κB activation. Therefore, anisalcohol reduces the generation of inflammatory mediators and cytokines by suppressing NF-κB and mitogen-activated protein kinase (MAPK) activation (Xiang et al., 2018).
Apigenin is one of the active ingredients in the leaves of Apium graveolens L. var. dulce DC. Apigenin decreased prostaglandin E2 (PGE2) levels by inhibiting COX-2 expression and mediating inflammation in LPS-stimulated BV-2 cells. They found that LPS induced p38 MAPK and apigenin inhibited the phosphorylation of JNK in BV-2 microglia, suggesting that it played an anti-inflammatory role through p38 MAPK and JNK. However, apigenin had no significant effect on extracellular signal-regulated kinase activation (Ha et al., 2008).
Astragaloside IV is an ingredient isolated from the dried roots of Astragalus membranaceus (Fisch.) Bge (Zhang et al., 2020). As shown by Morris water maze test, bilateral common carotid artery occlusion in mice can lead to serious memory impairment. Oral administration of astragaloside IV (10 and 20 mg/kg, once daily, beginning 7 days before surgery and continued for 7 days after surgery) could reduce Toll-like receptor-4 (TLR4) expression and its downstream adaptor proteins, including tumor necrosis factor receptor associated factor-6 (TRAF6), so NF-κB phosphorylation was inhibited as a result (Li M. et al., 2017). These findings show that astragaloside IV protects against transient cerebral I/R by suppressing the TLR4 signaling pathway, in part through its anti-inflammatory properties.
Baicalin is an important flavonoid that is extracted from the root of Scutellaria baicalensis Georgi (Chen H. et al., 2020; Li Y. et al., 2020). The report was found that baicalin inhibited the secretion of NO, IL-6, TNF-α, and CCL22 (C-C motif chemokine ligand 22) in macrophages, promoted the secretion of IL-13, IFNG (interferon gamma), and IL-1a in vitro (Xu et al., 2022). It inhibited CCL2 expression, reduced the phosphorylation levels of p65 and IκBα protein, and downregulated the level of CCR2 in vivo. Meanwhile, it discovered that baicalin had a neuroprotective effect, most likely via blocking NF-κB p65 activation, which improved neurological functioning and reduced the extent of cerebral infarction (Xue et al., 2010). The anti-inflammatory and anti-apoptotic benefits of baicalin were validated by Tu et al. (Tu et al., 2009), and the molecular mechanism may be related to the downregulated levels of iNOS, COX-2, and cleaved caspase-3 protein, as well as the enhanced enzymatic activity of myeloperoxidase (MPO). Current studies indicate that baicalin protects from ischemic injury by inhibiting of NF-κB–mediated inflammation.
Berberine is a non-basic and quaternary benzylisoquinoline alkaloid in berberine-containing plants (the berberidaceae family) worldwide, and it is an active ingredient isolated from the dried roots of Coptidis rhizoma (rhizomes of Coptis chinensis) in China (Neag et al., 2018). In I/R injury, berberine dramatically lowered the levels of pro-inflammatory cytokines, which was prevented by a SIRT1 inhibitor (Yu et al., 2016). It found that pretreatment with berberine dose-dependently inhibited high-mobility group box 1 (HMGB1) and NF-κB translocation from the nucleus to the cytoplasm in cerebral I/R injury and decreased Toll-like receptor 4 (TLR4) expression in ischemic cortical tissue (Zhu et al., 2018). According to new findings, berberine’s anti-inflammatory benefits against the injury are aided by reducing the activation of the HMGB1/TLR4/NF-κB signaling pathway. In clinical studies, berberine administration lowered serum levels of IL-6 and macrophage migration inhibitory factor, as well as plasma levels of C-reactive protein, TNF-α, and IL-6 (Li et al., 2016; Liu et al., 2019). Berberine appears to reduce I/R injury by reducing excessive inflammatory responses in patients according to recent research. Berberine could be employed as an alternate therapeutic technique for I/R injury management.
Biochanin A is an O-methylated natural isoflavonoid isolated from Trifolium pratense L. (red clover). In a rat model, the anti-inflammatory properties of biochanin A against I/R damage were examined. TNF-α and IL-1β expression, as well as p38 phosphorylation, were assessed using RT-PCR or WB. As a result, TNF-α and IL-1β levels were markedly elevated following I/R injury, and biochanin A therapy significantly reduced these inflammatory processes. Meawhile, the increase in p-p38 levels in I/R brain tissue was reduced by biochanin A (Wang et al., 2015). These results suggest that biochanin A protects against focal cerebral I/R in rats via inhibition of p38-mediated inflammatory responses.
Cepharanthine is a bibenzylisoquinoline alkaloid isolated from Stephania cephalantha Hayata (Huang H. et al., 2014). Cepharanthine reduced microglia activation in transient middle cerebral artery occlusion (tMCAO) mouse models and in hypoxia, glucose deprivation/reoxygenation microglia models (OGD/R). Cepharanthine reduced microglial activation. Following tMCAO, cepharanthine reduced elevation of NLR family pyrin domain containing 3 (NLRP3) immunoreactivity in Ibal-labeled microglia as well as total Iba1 and NLRP3 expression in the brain, according to immunofluorescence labeling. Cepharanthine reduced the overproduction of the M1 microglia-regulated pro-inflammatory cytokines IL-1 and IL-18 generated by tMCAO and OGD/R (Zhao et al., 2020). Cepharanthine reduced cerebral I/R injury by reducing microglial activation and inflammation generated by the NLRP3 inflammasome.
Curcumin is an effective ingredient from the dried roots of Curuma longa L. Curcumin (100 mg/kg) decreased the degree of neutrophilic granulocyte infiltration in cerebral tissues and inhibited TNF-α expression in a cerebral I/R injury model (Lei et al., 2010). Treatment with curcumin (IP) decreased IL-6, NF-κB and MCP-1 levels after I/R injury (Hussein et al., 2020). These findings suggest that curcumin has a neuroprotective role by inhibiting the inflammatory reaction.
Garcinol is a benzophenone compound isolated from the fruit rind of Garcinia indica (Balasubramanyam et al., 2004). In MCAO/R-induced animals and OGD/R-treated cells of models, garcinol reduced model-induced inflammation, including inhibiting the production of IL-6, IL-1β, and TNF-α, and it also inhibited TLR4 and NF-κB (p65) expression. The data indicate that garcinol protects against cerebral I/R injury. And garcinol attenuates inflammation by suppression of the TLR4/NF-ĸB signaling pathway (Kang et al., 2020).
Ginkgolide B is a compound extracted from the dried leaves of Ginkgo biloba L. The tMCAO model of mice was made by an intraluminal filament technique. Ginkgolide B (10, 20 and 40 mg/kg in 2 h after MCAO, iv) inhibited I/R-induced NF-κB, microglial activation and the production of pro-inflammatory cytokines (Gu et al., 2012). Taken together, the information indicates that ginkgolide B has an anti-inflammatory function by inhibition of NF-κB pathway.
Ginsenoside Rb1 (Rb1) is one of the primary active compounds of Panax ginseng C. A. Mey. Male Sprague-Dawley (SD) rats were given Rb1 (12.5 mg/kg/d) intranasally for 7 days before being subjected to transient blockage of the right middle cerebral artery and reperfusion. The rats were slaughtered 6 hours, 12 hours, 24 hours, and 72 hours following reperfusion, and brain tissues were taken for testing. Key inflammatory aspects of CNS, such as inflammatory cells, pro-inflammatory cytokines, and transcription factors, were used to assess Rb1’s neuroprotection. Rb1 inhibited microglia activity in the penumbra from 24 to 72 h after reperfusion, as well as microglia conversion to phagocytic microglia. In the Rb1 group, the mRNA and protein level of TNF-α was lower 12 h after reperfusion. From 6 to 72 h, Rb1 partially reduced NF-κB pathway activation (Zhu et al., 2012). These findings suggest that local inflammation suppression during cerebral ischemia may be one mechanism contributing to Rb1’s neuroprotective benefits.
Hydroxysafflor Yellow A is a component of flavonoids extracted from the flower of the safflower plant Carthamus tinctorius L. Hydroxysafflor Yellow A (2, 4, 8 mg/kg, respectively, iv) prevented brain injury in a focal cerebral I/R model by inhibiting thrombin generation. Hydroxysafflor yellow A treatment suppressed NF-κB p65 nuclear translation and p65 binding activity, as well as ICAM-1 mRNA and protein levels and neutrophil infiltration. Hydroxysafflor yellow A enhanced the number of CA1 pyramidal cells in the hippocampus CA1 and lowered plasma angiotensin II levels, all of which improved neurological deficit scores (Sun et al., 2010). Another study revealed the neuroprotective effect of hydroxysafflor yellow A on GSK-3β/NF-κB-mediated inflammatory pathways (Yang X. et al., 2020). These findings demonstrated that hydroxysafflor yellow A had an anti-inflammatory effect by reducing angiotensin II content or regulating NF-κB.
Icariin is a natural compound of flavonoids extracted from Epimedium brevicornum Maxim. Pretreatment with icariin (10, 30 mg/kg) decreased NF-κB activation, IL-1β and TGF-β1 protein levels in the cerebral I/R model of rats. Icarin also increased the levels of peroxisome proliferator-activated receptor (PPARα and PPARγ) protein in this study. These results suggest that icariin protects rats from ischemic stroke by inhibiting inflammatory responses mediated by NF-κB, PPARα and PPARγ (Xiong et al., 2016).
Mangiferin is a natural compund found in papaya and mango (the Anacardiaceae and Gentianaceae families). Mangiferin (25, 50, 100 mg/kg, respectively, iv) increased IL-10 levels and reduced IL-1β and TNF-α levels in the rat brain tissues with cerebral I/R injury. These results demonstrate that mangiferin has a significant protective ability that may be its effect to inhibit the overproduction of inflammatory cytokines (Zhang et al., 2016).
Notoginsenoside R1 is an active component extracted from the dried roots of Panax notoginseng (Burk.) F.H. Chen. After administration notoginsenoside R1 for 3 days, bilateral CCA were occluded with artery clip for 20 min followed by reperfusion for 24 h in C57BL/6 mice, notoginsenoside R1 reduced both TNF-α and ICAM-1 mRNA (Huang et al., 2015). Panax notoginsenoside (30 mg/kg, including notoginsenoside R1) was injected intraperitoneally approximately 30 min before aortic clamping and reperfusion, and it decreased the expression of IL-1β, IL-10, and TNF-α levels in this acute spinal cord I/R injury model, as shown by immunohistochemistry and Western blotting (Ning et al., 2012). These results indicated that panax notoginsenoside effectively decreased inflammation in damaged spinal cord tissues, but it did not completely ameliorate the symptoms.
Polydatin is a stilbene chemical isolated from Polygonum cuspidatum Sieb. et Zuccdried.'s roots (Ji et al., 2012; Tang and Tan, 2019). Polydatin (50 mg/kg) decreased the number of cells positive for NF-κB in an SD rat model of permanent MCAO (pMCAO) compared to the blank control group 24 and 72 h after pMCAO (Ji et al., 2012). These findings indicate that polydatin inhibits the inflammation in response to exert its neuroprotective effects after pMCAO.
Puerarin is one of the main active ingredients from the dried roots of Pueraria lobata (Willd.) Ohwi. (Wei et al., 2014). A cerebral I/R injury model was created using MCAO in rats. Puerarin (18 mg/kg) was administered just before occlusion and immediately after reperfusion, and it decreased ICAM-1 protein level and the nuclear translocation of the NF-κB p65 subunit (Lou et al., 2007). These effects may be due to its inhibition of the neutrophil-mediated inflammatory response.
Salvianolic acid A is one of the main active components isolated from Salvia Miltiorrhiza Bge. MCAO operation was used to create a focal cerebral I/R model for 1.5 h of ischemia, followed by reperfusion after 24 h. Salvianolic acid A (5, 10, and 20 mg/kg, respectively, i. v.) administration kept off cerebral NF-κB p65 activation and released the inflammatory response (Chien et al., 2016; Zhang et al., 2018; Yang et al., 2022). These findings suggest that salvianolic acid A attenuates I/R-induced rat brain injury by protecting the BBB via anti-inflammation.
Schisandrin B is a compound isolated from the dried fruits of Schisandra chinensis (Turcz.) Baill. Schisandrin B (10, 30 mg/kg, i. p.) was administered twice 30 min before the onset of ischemia and 2 h after reperfusion. Schisandrin B therapy suppressed the TNF-α and IL-1β protein expression in ischemic hemispheres (Lee et al., 2012). These findings show that schisandrin B therapy protects rats from neurodegeneration by suppressing inflammation.
Stachydrine hydrochloride is an alkaloid isolated from Leonurus japonicus Houtt. Stachydrine hydrochloride (15, 30 and 60 mg/kg) was administered in a repetitive cerebral ischemia reperfusion mouse model, and it reduced the levels of TNF-α, ICAM-1, and MPO. These results suggest that stachydrine hydrochloride inhibits inflammatory reactions after ischemia (Miao et al., 2017).
Sinomenine is an isoquinoline-type alkaloid originally extracted from the Sinomenium acutum (Thunb.) Rehd. et Wils and S. acutum (Thunb.) Rehd. et Wils var. cinereum Rehd. et Wils. (Zhao et al., 2015). Sinomenine inhibited pro-inflammatory factors including IL-1β, TNF-α, IL-6 and IL-18 to protect BBB integrity and improve neurological functions (Qiu et al., 2016). It reduced the activation of microglia and astrocytes, and it also decreased inflammasome-related molecules (NLRP3, ASC, and caspase-1) and proinflammatory cytokines in ischemic brains in vivo and cultured microglia in vitro (Yang et al., 2016). Therefore, sinomenine inhibits inflammatory reactions in ischemic stroke treatment.
Tanshinone IIA is an active ingredients isolated from Salvia Miltiorrhiza Bge. (Subedi and Gaire, 2021). Tanshinone IIA has an anti-inflammatory impact in N2a cells, where it increases cell viability and reduces inflammation via the PI3K/Akt/mTOR signaling pathway (Wang J. et al., 2020). Another study in OGD/R-exposed microglia found that Salvia Miltiorrhiza extract containing tanshinone IIA significantly reduced inflammatory responses, as shown by lower expression of NLRP3, caspase-1, IL-1, and IL-18 (Cai et al., 2016). Tanshinone IIA also had anti-inflammatory effects in OGD/R-activated astrocytes by reducing proliferation, GFAP staining, HIF-1 expression, and SDF-1, ERK, and Akt signaling (Huang X. et al., 2014). It enhanced cell viability while lowering NO production and NF-κB signaling (Dong et al., 2009). These studies suggest that tanshinone IIA prevents inflammatory responses after ischemia.
Tetramethylpyrazine is an active compound from Ligusticum chuanxiong Hort . The MCAO model was produced in rats by occluding the right middle cerebral artery for 90 min and then reperfusing for 3 days, using tetramethylpyrazine (20 mg/kg) administered intraperitoneally 60 min before the occlusion. Tetramethylpyrazine significantly reduced inflammatory cell activation and pro-inflammatory mediator synthesis in the brain after ischemia and reperfusion. In cultured glial cells, it reduced inflammation generated by lipopolysaccharide and interferon, as well as the formation of prostaglandin E2 (PGE2) (Liao et al., 2004). These findings show that one of tetramethylpyrazine’s neuroprotective properties to prevent brain injury is its anti-inflammatory capability.
Timosaponin B-II is an active ingredient isolated from the dried roots of Anemarrhena asphodeloides Bge. Timosaponin B-II treatment significantly increased IL-10 and IL-10R mRNA expression in an SD rat model of focal cerebral I/R produced by transient MCAO (2 h) (Li et al., 2007). The results demonstrated that the anti-dementia effect of timosaponin B-II is at least partially due to its anti-inflammatory properties.
Triptolide is a biologically active natural chemical derived from the Tripterygium wilfordii Hook plant. F. Triptolide (0.2–5 mg/L) suppressed the astroglial response and NF-κB/DNA binding activity in vitro, while triptolide (10–50 mg/kg) lowered LPS-induced COX-2 expression in vivo and in vitro (Dai et al., 2006). These findings imply that triptolide protects against neuroinflammation by suppressing COX-2 production, at least in part through suppression of the NF-κB signaling pathway.
Vinpocetine is one of the major active ingredients in Catharanthus roseus (L.) Don. Vinpocetine (10 mg/kg) was intraperitoneally injected in a murine model of transient MCAO (2 h) and then reperfusion. Vinpocetine decreased the expression of NF-κB and TNF-α at 24 h and 3 days and inhibited the inflammatory response after cerebral I/R (Jeon et al., 2010; Wang et al., 2014).
Strcture-Activity Relationship Between Inflammation and Natural Products
Structure activity relationship (SAR) has been demonstrated as a useful tool for investigating the bioactivities of various classes of compounds (Li X. et al., 2017). The SAR of biological activity is mainly related to the type (or basic parent nucleus) of compounds and the position of main functional groups (Xie et al., 2021; Zhou et al., 2021). Flavonoids are based on 2-phenylchromone as the skeleton. The position of C-5 and 7 on the ring A of flavonoids that coupled with hydroxyl groups has found to affect the cell secretion process, mitosis and cell interactions with strong anti-inflammatory effects (Xie et al., 2021). Forthermore, C5-OH, C7-OH, C2 = C3 and C4 = O functional groups that present in the flavonoids has also been indicated to performa greater anti-inflammatory effect. C3-OH or glycosylation group at the ring A has greatly effection in the decrease the anti-inflammatory (Zhang et al., 2019). For alkaloids and other types of natural compounds mentioned above, due to the number or complex structure of compounds, this review did not draw a suitable SAR.
Conclusion
Even though cerebral ischemia as a serious issue with global health concern, the lack of effective drug options still limit its treatment (Gaire, 2021). The present study provides a wealth of information to elucidate the important role of inflammation in ischemic brain injury. Natural medicines with anti-inflammatory effects in cerebral ischemia have great potential for treatment based on the above mentioned 26 active ingredients in experimental studies (Table 1). This review fully demonstrated that compounds of natural medicine have protective effects against cerebral ischemia via anti-inflammatory mechanisms.
Inflammation is a critical mediator of cerebral ischemic injury, and it is increasingly regarded as the important factor to the pathological processes in cerebral ischemia. Experimental anti-inflammatory strategies to diminish cerebral ischemic injury have certainly been useful. In-depth study of the inflammatory response of cerebral ischemic injury offers a novel method for the prevention and treatment. However, whether inflammation is harmful or useful may depend on the severity of the ischemia and the stage of ischemia in which inflammatory responses contribute, and detailed mechanisms of inflammation-induced injury or recovery are far from complete. In the laboratory, a lot of time and money has gone into studying the mechanisms, but it hasn't translated into effective treatments in the clinic. Compound preparation is difficult to prepare for the international market because internationality only admits the curative effect of monomers, which is worthy of attention in the future.
The use of natural medicine compounds to study the anti-inflammatory effect of cerebral ischemia should strengthen the recent research achievements of physiology, pathology, immunology, molecular biology, neurobiology, neuroscience and omics methods. Using the research method of Western-style drugs and recognizing each step of the inflammatory response leads to the possibility of developing a new compound from natural medicines that interferes with a specific inflammatory mechanism of ischemic injury and creating a new situation that prevents and treats cerebral ischemic injury using compounds of natural medicines for anti-inflammatory effects. Review of the inflammatory mechanism and anti-inflammatory effect of the above mentioned 26 compounds revealed great new progress and breakthroughs in the future, thanks to a strong scientific research capability to protect cerebral ischemia with natural products based on the inflammatory theory.
Author Contributions
YS and JT designed and revised this review article. YS wrote the manuscript. ZZ, XL and JT amended the text.
Funding
This work was supported by the Department of Science and Technology of Sichuan Province (2021YFN0101).
Conflict of Interest
The authors declare that the research was conducted in the absence of any commercial or financial relationships that could be construed as a potential conflict of interest.
Publisher’s Note
All claims expressed in this article are solely those of the authors and do not necessarily represent those of their affiliated organizations, or those of the publisher, the editors and the reviewers. Any product that may be evaluated in this article, or claim that may be made by its manufacturer, is not guaranteed or endorsed by the publisher.
References
Adams, H., Adams, R., Del Zoppo, G., and Goldstein, L. B. (2005). Guidelines for the Early Management of Patients with Ischemic Stroke: 2005 Guidelines Update a Scientific Statement from the Stroke Council of the American Heart Association/American Stroke Association. Stroke 36, 916–923. doi:10.1161/01.STR.0000163257.66207.2d
Adibhatla, R. M., and Hatcher, J. F. (2008). Tissue Plasminogen Activator (tPA) and Matrix Metalloproteinases in the Pathogenesis of Stroke: Therapeutic Strategies. CNS Neurol. Disord. Drug Targets 7, 243–253. doi:10.2174/187152708784936608
Alasvand, M., Assadollahi, V., Ambra, R., Hedayati, E., Kooti, W., and Peluso, I. (2019). Antiangiogenic Effect of Alkaloids. Oxid. Med. Cell Longev. 2019, 9475908. doi:10.1155/2019/9475908
Amantea, D., Tassorelli, C., Petrelli, F., Certo, M., Bezzi, P., Micieli, G., et al. (2014). Understanding the Multifaceted Role of Inflammatory Mediators in Ischemic Stroke. Curr. Med. Chem. 21, 2098–2117. doi:10.2174/0929867321666131227162634
Atanasov, A. G., Zotchev, S. B., Dirsch, V. M., International Natural Product Sciences Taskforce, , and Supuran, C. T. (2021). Natural Products in Drug Discovery: Advances and Opportunities. Nat. Rev. Drug Discov. 20, 200–216. doi:10.1038/s41573-020-00114-z
Azab, A., Nassar, A., and Azab, A. N. (2016). Anti-Inflammatory Activity of Natural Products. Molecules 21 (10), 1321. doi:10.3390/molecules21101321
Babaei, E., Sadeghizadeh, M., Hassan, Z. M., Feizi, M. A., Najafi, F., and Hashemi, S. M. (2012). Dendrosomal Curcumin Significantly Suppresses Cancer Cell Proliferation In Vitro and In Vivo. Int. Immunopharmacol. 12, 226–234. doi:10.1016/j.intimp.2011.11.015
Bai, R., Yao, C., Zhong, Z., Ge, J., Bai, Z., Ye, X., et al. (2021). Discovery of Natural Anti-inflammatory Alkaloids: Potential Leads for the Drug Discovery for the Treatment of Inflammation. Eur. J. Med. Chem. 213, 113165. doi:10.1016/j.ejmech.2021.113165
Balasubramanyam, K., Altaf, M., Varier, R. A., Swaminathan, V., Ravindran, A., Sadhale, P. P., et al. (2004). Polyisoprenylated Benzophenone, Garcinol, a Natural Histone Acetyltransferase Inhibitor, Represses Chromatin Transcription and Alters Global Gene Expression. J. Biol. Chem. 279, 33716–33726. doi:10.1074/jbc.M402839200
Bayat, P., Farshchi, M., Yousefian, M., Mahmoudi, M., and Yazdian-Robati, R. (2021). Flavonoids, the Compounds with Anti-Inflammatory and Immunomodulatory Properties, as Promising Tools in Multiple Sclerosis (MS) Therapy: A Systematic Review of Preclinical Evidence. Int. Immunopharmacol. 95, 107562. doi:10.1016/j.intimp.2021.107562
Cai, L., Yi, X. B., Yuan, L. B., and Gong, G. (2016). The Protective Effect of Tanshinone IIA on Oxygen-Glucose Deprivation and Reperfusion Injury of MicrogliaThrough the NLRP3 Inflammatory Signaling Pathway. J. Sichuan Univ. Med. Sci. Ed. 47, 660–664. doi:10.13464/j.scuxbyxb.2016.05.006
Chen, H., He, Y., Chen, S., Qi, S., and Shen, J. (2020a). Therapeutic Targets of Oxidative/Nitrosative Stress and Neuroinflammation in Ischemic Stroke: Applications for Natural Product Efficacy with Omics and Systemic Biology. Pharmacol. Res. 158, 104877. doi:10.1016/j.phrs.2020.104877
Chen, H. L., Jia, W. J., Li, H. E., Han, H., Li, F., Zhang, X. L., et al. (2020b). Scutellarin Exerts Anti-Inflammatory Effects in Activated Microglia/Brain Macrophage in Cerebral Ischemia and in Activated BV-2 Microglia through Regulation of MAPKs Signaling Pathway. Neuromolecular Med. 22, 264–277. doi:10.1007/s12017-019-08582-2
Chien, M. Y., Chuang, C. H., Chern, C. M., Liou, K. T., Liu, D. Z., Hou, Y. C., et al. (2016). Salvianolic Acid A Alleviates Ischemic Brain Injury through the Inhibition of Inflammation and Apoptosis and the Promotion of Neurogenesis in Mice. Free Radic. Biol. Med. 99, 508–519. doi:10.1016/j.freeradbiomed.2016.09.006
Chung, H. J., Chung, M. J., Houng, S.-J., Jeun, J., Kweon, D.-K., Choi, C. H., et al. (2009). Toxicological Evaluation of the Isoflavone Puerarin and its Glycosides. Eur. Food Res. Technol. 230, 145–153. doi:10.1007/s00217-009-1156-3
Dai, Y. Q., Jin, D. Z., Zhu, X. Z., and Lei, D. L. (2006). Triptolide Inhibits COX-2 Expression via NF-kB Pathway in Astrocytes. Neurosci. Res. 55, 154–160. doi:10.1016/j.neures.2006.02.013
Deb, P., Sharma, S., and Hassan, K. M. (2010). Pathophysiologic Mechanisms of Acute Ischemic Stroke: An Overview with Emphasis on Therapeutic Significance beyond Thrombolysis. Pathophysiology 17, 197–218. doi:10.1016/j.pathophys.2009.12.001
Dénes, A., Ferenczi, S., Halász, J., Környei, Z., and Kovács, K. J. (2008). Role of CX3CR1 (Fractalkine Receptor) in Brain Damage and Inflammation Induced by Focal Cerebral Ischemia in Mouse. J. Cereb. Blood Flow. Metab. 28, 1707–1721. doi:10.1038/jcbfm.2008.64
Dodd, W. S., Laurent, D., Dumont, A. S., Hasan, D. M., Jabbour, P. M., Starke, R. M., et al. (2021). Pathophysiology of Delayed Cerebral Ischemia after Subarachnoid Hemorrhage: A Review. J. Am. Heart Assoc. 10, e021845. doi:10.1161/JAHA.121.021845
Dong, K., Xu, W., Yang, J., Qiao, H., and Wu, L. (2009). Neuroprotective Effects of Tanshinone IIA on Permanent Focal Cerebral Ischemia in Mice. Phytother. Res. 23, 608–613. doi:10.1002/ptr.2615
Downer, E. J. (2020). Anti-Inflammatory Potential of Terpenes Present in Cannabis Sativa L. ACS Chem. Neurosci. 11, 659–662. doi:10.1021/acschemneuro.0c00075
Emsley, H. C., Smith, C. J., Georgiou, R. F., Vail, A., Hopkins, S. J., Rothwell, N. J., et al. (2005). A Randomised Phase II Study of Interleukin-1 Receptor Antagonist in Acute Stroke Patients. J. Neurol. Neurosurg. Psychiatry 76, 1366–1372. doi:10.1136/jnnp.2004.054882
Falsig, J., Pörzgen, P., Lund, S., Schrattenholz, A., and Leist, M. (2006). The Inflammatory Transcriptome of Reactive Murine Astrocytes and Implications for Their Innate Immune Function. J. Neurochem. 96, 893–907. doi:10.1111/j.1471-4159.2005.03622.x
Fukumoto, Y., Tanaka, K. F., Parajuli, B., Shibata, K., Yoshioka, H., Kanemaru, K., et al. (2019). Neuroprotective Effects of Microglial P2Y1 Receptors against Ischemic Neuronal Injury. J. Cereb. Blood Flow. Metab. 39, 2144–2156. doi:10.1177/0271678X18805317
Gaire, B. P. (2021). Microglia as the Critical Regulators of Neuroprotection and Functional Recovery in Cerebral Ischemia. Cell Mol. Neurobiol. doi:10.1007/s10571-021-01145-9 https://link.springer.com/article/10.1007/s10571-021-01145-9
Gavins, F., Yilmaz, G., and Granger, D. N. (2007). The Evolving Paradigm for Blood Cell-Endothelial Cell Interactions in the Cerebral Microcirculation. Microcirculation 14, 667–681. doi:10.1080/10739680701404903
Geetha, R. G., and Ramachandran, S. (2021). Recent Advances in the Anti-Inflammatory Activity of Plant-Derived Alkaloid Rhynchophylline in Neurological and Cardiovascular Diseases. Pharmaceutics 13 (8), 1170. doi:10.3390/pharmaceutics13081170
González-Cofrade, L., de Las Heras, B., Apaza Ticona, L., and Palomino, O. M. (2019). Molecular Targets Involved in the Neuroprotection Mediated by Terpenoids. Planta Med. 85, 1304–1315. doi:10.1055/a-0953-6738
Gu, J. H., Ge, J. B., Li, M., Wu, F., Zhang, W., and Qin, Z. H. (2012). Inhibition of NF-κB Activation is Associated with Anti-inflammatory and Anti-Apoptotic Effects of Ginkgolide B in a Mouse Model of Cerebral Ischemia/Reperfusion Injury. Eur. J. Pharm. Sci. 47, 652–660. doi:10.1016/j.ejps.2012.07.016
Gui, Y. H., Liu, L., Wu, W., Zhang, Y., Jia, Z. L., Shi, Y. P., et al. (2020). Discovery of Nitrogenous Sesquiterpene Quinone Derivatives from Sponge Dysidea Septosa with Anti-Inflammatory Activity In Vivo Zebrafish Model. Bioorg Chem. 94, 103435. doi:10.1016/j.bioorg.2019.103435
Ha, S. K., Lee, P., Park, J. A., Oh, H. R., Lee, S. Y., Park, J. H., et al. (2008). Apigenin Inhibits the Production of NO and PGE2 in Microglia and Inhibits Neuronal Cell Death in a Middle Cerebral Artery Occlusion-Induced Focal Ischemia Mice Model. Neurochem. Int. 52, 878–886. doi:10.1016/j.neuint.2007.10.005
Headland, S. E., and Norling, L. V. (2015). The Resolution of Inflammation: Principles and Challenges. Semin. Immunol. 27, 149–160. doi:10.1016/j.smim.2015.03.014
Hu, M. Z., Zhou, Z. Y., Zhou, Z. Y., Lu, H., Gao, M., Liu, L. M., et al. (2020). Effect and Safety of Hydroxysafflor Yellow A for Injection in Patients with Acute Ischemic Stroke of Blood Stasis Syndrome: A Phase II, Multicenter, Randomized, Double-Blind, Multiple-Dose, Active-Controlled Clinical Trial. Chin. J. Integr. Med. 26, 420–427. doi:10.1007/s11655-020-3094-7
Huang, H., Hu, G., Wang, C., Xu, H., Chen, X., and Qian, A. (2014a). Cepharanthine, an Alkaloid from Stephania Cepharantha Hayata, Inhibits the Inflammatory Response in the RAW264.7 Cell and Mouse Models. Inflammation 37, 235–246. doi:10.1007/s10753-013-9734-8
Huang, J., Upadhyay, U. M., and Tamargo, R. J. (2006). Inflammation in Stroke and Focal Cerebral Ischemia. Surg. Neurol. 66, 232–245. doi:10.1016/j.surneu.2005.12.028
Huang, X., Li, Y., Li, J., Feng, Y., and Xu, X. (2014b). Tanshinone IIA Dampens the Cell Proliferation Induced by Ischemic Insult in Rat Astrocytes via Blocking the Activation of HIF-1α/SDF-1 Signaling. Life Sci. 112, 59–67. doi:10.1016/j.lfs.2014.07.020
Huang, X. P., Ding, H., Lu, J. D., Tang, Y. H., Deng, B. X., and Deng, C. Q. (2015). Effects of the Combination of the Main Active Components of Astragalus and Panax Notoginseng on Inflammation and Apoptosis of Nerve Cell after Cerebral Ischemia-Reperfusion. Am. J. Chin. Med. 43, 1419–1438. doi:10.1142/S0192415X15500809
Hussein, Y. A., Al-sarraf, A. M., and Alfalluji, W. L. (2020). Modulation of Oxidative Stress, Inflammatory and Apoptotic Response by Curcumin against Cerebral Ischemia Reperfusion Injury in a Mouse Model. Interdiscip. Neurosurg. 21, 100741. doi:10.1016/j.inat.2020.100741
Indu, P., Arunagirinathan, N., Rameshkumar, M. R., Sangeetha, K., Divyadarshini, A., and Rajarajan, S. (2021). Antiviral Activity of Astragaloside II, Astragaloside III and Astragaloside IV Compounds against Dengue Virus: Computational Docking and In Vitro Studies. Microb. Pathog. 152, 104563. doi:10.1016/j.micpath.2020.104563
Ishikawa, M., Zhang, J. H., Nanda, A., and Granger, D. N. (2004). Inflammatory Responses to Ischemia and Reperfusion in the Cerebral Microcirculation. Front. Biosci. 9, 1339–1347. doi:10.2741/1330
Jeon, K. I., Xu, X., Aizawa, T., Lim, J. H., Jono, H., Kwon, D. S., et al. (2010). Vinpocetine Inhibits NF-kappaB-Dependent Inflammation via an IKK-dependent but PDE-independent Mechanism. Proc. Natl. Acad. Sci. U. S. A. 107, 9795–9800. doi:10.1073/pnas.0914414107
Ji, H., Zhang, X., Du, Y., Liu, H., Li, S., and Li, L. (2012). Polydatin Modulates Inflammation by Decreasing NF-κB Activation and Oxidative Stress by Increasing Gli1, Ptch1, SOD1 Expression and Ameliorates Blood-Brain Barrier Permeability for its Neuroprotective Effect in pMCAO Rat Brain. Brain Res. Bull. 87, 50–59. doi:10.1016/j.brainresbull.2011.09.021
Jin, W. N., Shi, S. X., Li, Z., Li, M., Wood, K., Gonzales, R. J., et al. (2017). Depletion of Microglia Exacerbates Postischemic Inflammation and Brain Injury. J. Cereb. Blood Flow. Metab. 37, 2224–2236. doi:10.1177/0271678X17694185
Kang, Y., Sun, Y., Li, T., and Ren, Z. (2020). Garcinol Protects against Cerebral Ischemia-Reperfusion Injury In Vivo and In Vitro by Inhibiting Inflammation and Oxidative Stress. Mol. Cell Probes 54, 101672. doi:10.1016/j.mcp.2020.101672
Khoshnazar, M., Parvardeh, S., and Bigdeli, M. R. (2020). Alpha-Pinene Exerts Neuroprotective Effects via Anti-Inflammatory and Anti-Apoptotic Mechanisms in a Rat Model of Focal Cerebral Ischemia-Reperfusion. J. Stroke Cerebrovasc. Dis. 29, 104977. doi:10.1016/j.jstrokecerebrovasdis.2020.104977
Kim, T., Song, B., Cho, K. S., and Lee, I. S. (2020). Therapeutic Potential of Volatile Terpenes and Terpenoids from Forests for Inflammatory Diseases. Int. J. Mol. Sci. 21 (6), 2187. doi:10.3390/ijms21062187
Kumar, B. D., Krishnakumar, K., Jaganathan, S. K., and Mandal, M. (2013). Effect of Mangiferin and Mahanimbine on Glucose Utilization in 3T3-L1 Cells. Pharmacogn. Mag. 9, 72–75. doi:10.4103/0973-1296.108145
Lee, T. H., Jung, C. H., and Lee, D. H. (2012). Neuroprotective Effects of Schisandrin B against Transient Focal Cerebral Ischemia in Sprague-Dawley Rats. Food Chem. Toxicol. 50, 4239–4245. doi:10.1016/j.fct.2012.08.047
Lei, J. R., Jun, Q., Zhang, J., Huang, K. M., Fu, R., and Zhou, Z. M. (2010). Effects of Curcumin on Inflammatory Reaction and Blood-Brain Barrierpermeability in Rats Following Cerebral Ischemic Injury. Chin. Pharmacol. Bull. 26, 120–123.
Li, L., Gan, H., Jin, H., Fang, Y., Yang, Y., Zhang, J., et al. (2021). Astragaloside IV Promotes Microglia/Macrophages M2 Polarization and Enhances Neurogenesis and Angiogenesis through PPARγ Pathway after Cerebral Ischemia/reperfusion Injury in Rats. Int. Immunopharmacol. 92, 107335. doi:10.1016/j.intimp.2020.107335
Li, M., Li, H., Fang, F., Deng, X., and Ma, S. (2017a). Astragaloside IV Attenuates Cognitive Impairments Induced by Transient Cerebral Ischemia and Reperfusion in Mice via Anti-Inflammatory Mechanisms. Neurosci. Lett. 639, 114–119. doi:10.1016/j.neulet.2016.12.046
Li, R., Zhou, Y., Zhang, S., Li, J., Zheng, Y., and Fan, X. (2022). The Natural (Poly)phenols as Modulators of Microglia Polarization via TLR4/NF-κB Pathway Exert Anti-Inflammatory Activity in Ischemic Stroke. Eur. J. Pharmacol. 914, 174660. doi:10.1016/j.ejphar.2021.174660
Li, S., Liu, X., Chen, X., and Bi, L. (2020a). Research Progress on Anti-Inflammatory Effects and Mechanisms of Alkaloids from Chinese Medical Herbs. Evid. Based Complement. Altern. Med. 2020, 1303524. doi:10.1155/2020/1303524
Li, T. J., Qiu, Y., Yang, P. Y., Rui, Y. C., and Chen, W. S. (2007). Timosaponin B-II Improves Memory and Learning Dysfunction Induced by Cerebral Ischemia in Rats. Neurosci. Lett. 421, 147–151. doi:10.1016/j.neulet.2007.04.082
Li, X., Li, N., Sui, Z., Bi, K., and Li, Z. (2017b). An Investigation on the Quantitative Structure-Activity Relationships of the Anti-Inflammatory Activity of Diterpenoid Alkaloids. Molecules 22 (3), 363. doi:10.3390/molecules22030363
Li, Y., Song, K., Zhang, H., Yuan, M., An, N., Wei, Y., et al. (2020b). Anti-Inflammatory and Immunomodulatory Effects of Baicalin in Cerebrovascular and Neurological Disorders. Brain Res. Bull. 164, 314–324. doi:10.1016/j.brainresbull.2020.08.016
Li, Y., Wang, P., Chai, M. J., Yang, F., Li, H. S., Zhao, J., et al. (2016). Effects of Berberine on Serum Inflammatory Factors and Carotid Atherosclerotic Plaques in Patients with Acute Cerebral Ischemic Stroke. China J. Chin. Materia Medica 41, 4066–4071. doi:10.4268/cjcmm20162128
Li, Z., Cheung, F. S., Zheng, J., Chan, T., Zhu, L., and Zhou, F. (2014). Interaction of the Bioactive Flavonol, Icariin, with the Essential Human Solute Carrier Transporters. J. Biochem. Mol. Toxicol. 28, 91–97. doi:10.1002/jbt.21540
Liao, S. L., Kao, T. K., Chen, W. Y., Lin, Y. S., Chen, S. Y., Raung, S. L., et al. (2004). Tetramethylpyrazine Reduces Ischemic Brain Injury in Rats. Neurosci. Lett. 372, 40–45. doi:10.1016/j.neulet.2004.09.013
Lin, N., Liu, B., Zhang, J., Long, Y., Dong, G., Jin, H., et al. (2017). Acute Toxicity, 28-day Repeated-Dose Toxicity and Toxicokinetic Study of Timosaponin BII in Rats. Regul. Toxicol. Pharmacol. 90, 244–257. doi:10.1016/j.yrtph.2017.09.021
Liu, D. Q., Chen, S. P., Sun, J., Wang, X. M., Chen, N., Zhou, Y. Q., et al. (2019). Berberine Protects against Ischemia-Reperfusion Injury: A Review of Evidence from Animal Models and Clinical Studies. Pharmacol. Res. 148, 104385. doi:10.1016/j.phrs.2019.104385
Liu, Z., Chen, Y., Gao, H., Xu, W., Zhang, C., Lai, J., et al. (2020). Berberine Inhibits Cell Proliferation by Interfering with Wild-Type and Mutant P53 in Human Glioma Cells. Onco Targets Ther. 13, 12151–12162. doi:10.2147/OTT.S279002
Lo, E. H., Dalkara, T., and Moskowitz, M. A. (2003). Mechanisms, Challenges and Opportunities in Stroke. Nat. Rev. Neurosci. 4, 399–415. doi:10.1038/nrn1106
Lou, H. Y., Wei, X. b., Wang, R. X., and Zhang, X. M. (2007). Inhibitory Effects of Puerarin on Inflammation Following Focal Brain Ischemia-Reperfusion Injury in Rats. Chin. J. Pathophysiol. 23, 366–369.
Lu, Y. Z., Lin, C. H., Cheng, F. C., and Hsueh, C. M. (2005). Molecular Mechanisms Responsible for Microglia-Derived Protection of Sprague-Dawley Rat Brain Cells during In Vitro Ischemia. Neurosci. Lett. 373, 159–164. doi:10.1016/j.neulet.2004.10.004
Majeed, M., Bani, S., Bhat, B., Pandey, A., Mundkur, L., and Neupane, P. (2018). Safety Profile of 40% Garcinol from garcinia Indica in Experimental Rodents. Toxicol. Rep. 5, 750–758. doi:10.1016/j.toxrep.2018.06.009
Miao, M., Wang, T., Lou, X., MingBai, M., Xi, P., Liu, B., et al. (2017). The Influence of Stachydrine Hydrochloride on the Reperfusion Model of Mice with Repetitive Cerebral Ischemia. Saudi J. Biol. Sci. 24, 658–663. doi:10.1016/j.sjbs.2017.01.039
Montaner, J., Molina, C. A., Monasterio, J., Abilleira, S., Arenillas, J. F., Ribó, M., et al. (2003). Matrix Metalloproteinase-9 Pretreatment Level Predicts Intracranial Hemorrhagic Complications after Thrombolysis in Human Stroke. Circulation 107, 598–603. doi:10.1161/01.cir.0000046451.38849.90
Mu, J. K., Li, Y. Q., Shi, T. T., Yu, L. P., Yang, Y. Q., Gu, W., et al. (2020). Remedying the Mitochondria to Cure Human Diseases by Natural Products. Oxid. Med. Cell Longev. 2020, 5232614. doi:10.1155/2020/5232614
Neag, M. A., Mocan, A., Echeverría, J., Pop, R. M., Bocsan, C. I., Crişan, G., et al. (2018). Berberine: Botanical Occurrence, Traditional Uses, Extraction Methods, and Relevance in Cardiovascular, Metabolic, Hepatic, and Renal Disorders. Front. Pharmacol. 9, 557. doi:10.3389/fphar.2018.00557
Newman, D. J., and Cragg, G. M. (2020). Natural Products as Sources of New Drugs over the Nearly Four Decades from 01/1981 to 09/2019. J. Nat. Prod. 83, 770–803. doi:10.1021/acs.jnatprod.9b01285
Ning, N., Dang, X., Bai, C., Zhang, C., and Wang, K. (2012). Panax Notoginsenoside Produces Neuroprotective Effects in Rat Model of Acute Spinal Cord Ischemia-Reperfusion Injury. J. Ethnopharmacol. 139, 504–512. doi:10.1016/j.jep.2011.11.040
Passos, F. R. S., Araújo-Filho, H. G., Monteiro, B. S., Shanmugam, S., Araújo, A. A. D. S., Almeida, J. R. G. D. S., et al. (2022). Anti-Inflammatory and Modulatory Effects of Steroidal Saponins and Sapogenins on Cytokines: A Review of Pre-clinical Research. Phytomedicine 96, 153842. doi:10.1016/j.phymed.2021.153842
Pinteaux, E., Rothwell, N. J., and Boutin, H. (2006). Neuroprotective Actions of Endogenous Interleukin-1 Receptor Antagonist (IL-1ra) Are Mediated by Glia. Glia 53, 551–556. doi:10.1002/glia.20308
Proshkina, E., Plyusnin, S., Babak, T., Lashmanova, E., Maganova, F., Koval, L., et al. (2020). Terpenoids as Potential Geroprotectors. Antioxidants (Basel) 9, 529. doi:10.3390/antiox9060529
Przykaza, Ł. (2021). Understanding the Connection between Common Stroke Comorbidities, Their Associated Inflammation, and the Course of the Cerebral Ischemia/Reperfusion Cascade. Front. Immunol. 12, 782569. doi:10.3389/fimmu.2021.782569
Qiu, J., Yan, Z., Tao, K., Li, Y., Li, Y., Li, J., et al. (2016). Sinomenine Activates Astrocytic Dopamine D2 Receptors and Alleviates Neuroinflammatory Injury via the CRYAB/STAT3 Pathway after Ischemic Stroke in Mice. J. Neuroinflammation 13, 263–276. doi:10.1186/s12974-016-0739-8
Raghay, K., Akki, R., Bensaid, D., and Errami, M. (2020). Ghrelin as an Anti-inflammatory and Protective Agent in Ischemia/Reperfusion Injury. Peptides 124, 170226. doi:10.1016/j.peptides.2019.170226
Rallidis, L. S., Vikelis, M., Panagiotakos, D. B., Rizos, I., Zolindaki, M. G., Kaliva, K., et al. (2006). Inflammatory Markers and In-Hospital Mortality in Acute Ischaemic Stroke. Atherosclerosis 189, 193–197. doi:10.1016/j.atherosclerosis.2005.11.032
Romano, B., Pagano, E., Montanaro, V., Fortunato, A. L., Milic, N., and Borrelli, F. (2013). Novel Insights into the Pharmacology of Flavonoids. Phytother. Res. 27, 1588–1596. doi:10.1002/ptr.5023
Rosenzweig, H. L., Minami, M., Lessov, N. S., Coste, S. C., Stevens, S. L., Henshall, D. C., et al. (2007). Endotoxin Preconditioning Protects against the Cytotoxic Effects of TNFalpha after Stroke: A Novel Role for TNFalpha in LPS-Ischemic Tolerance. J. Cereb. Blood Flow. Metab. 27, 1663–1674. doi:10.1038/sj.jcbfm.9600464
Sacco, R. L., Adams, R., Albers, G., Alberts, M. J., Benavente, O., Furie, K., et al. (2006). Guidelines for Prevention of Stroke in Patients with Ischemic Stroke or Transient Ischemic Attack: A Statement for Healthcare Professionals from the American Heart Association/American Stroke Association Council on Stroke: Co-Sponsored by the Council on Cardiovascular Radiology and Intervention: The American Academy of Neurology Affirms the Value of This Guideline. Stroke 37, 577–617. doi:10.1161/01.STR.0000199147.30016.74
Serafini, M., Peluso, I., and Raguzzini, A. (2010). Flavonoids as Anti-Inflammatory Agents. Proc. Nutr. Soc. 69, 273–278. doi:10.1017/S002966511000162X
Shang, Y. H., Tian, J. F., Hou, M., and Xu, X. Y. (2013). Progress on the Protective Effect of Compounds from Natural Medicines on Cerebral Ischemia. Chin. J. Nat. Med. 11, 588–595. doi:10.1016/S1875-5364(13)60068-0
Sofroniew, M. V. (2020). Astrocyte Reactivity: Subtypes, States, and Functions in CNS Innate Immunity. Trends Immunol. 41, 758–770. doi:10.1016/j.it.2020.07.004
Soliman, A. M., Das, S., and Mahakkanukrauh, P. (2021). Inflammatory Molecular Mediators and Pathways Involved in Vascular Aging and Stroke: A Comprehensive Review. Curr. Med. Chem. 28, 1–21. doi:10.2174/0929867328666210901122359
Stamatovic, S. M., Shakui, P., Keep, R. F., Moore, B. B., Kunkel, S. L., Van Rooijen, N., et al. (2005). Monocyte Chemoattractant Protein-1 Regulation of Blood-Brain Barrier Permeability. J. Cereb. Blood Flow. Metab. 25, 593–606. doi:10.1038/sj.jcbfm.9600055
Subedi, L., and Gaire, B. P. (2021). Tanshinone IIA: A Phytochemical as a Promising Drug Candidate for Neurodegenerative Diseases. Pharmacol. Res. 169, 105661. doi:10.1016/j.phrs.2021.105661
Sun, K., Fan, J., and Han, J. (2015). Ameliorating Effects of Traditional Chinese Medicine Preparation, Chinese Materia Medica and Active Compounds on Ischemia/Reperfusion-Induced Cerebral Microcirculatory Disturbances and Neuron Damage. Acta Pharm. Sin. B 5, 8–24. doi:10.1016/j.apsb.2014.11.002
Sun, P., Bu, F., Min, J. W., Munshi, Y., Howe, M. D., Liu, L., et al. (2019). Inhibition of Calcium/Calmodulin-Dependent Protein Kinase Kinase (CaMKK) Exacerbates Impairment of Endothelial Cell and Blood-Brain Barrier after Stroke. Eur. J. Neurosci. 49, 27–39. doi:10.1111/ejn.14223
Sun, T., Wang, P., Deng, T., Tao, X., Li, B., and Xu, Y. (2020). Effect of Panax Notoginseng Saponins on Focal Cerebral Ischemia-Reperfusion in Rat Models: A Meta-Analysis. Front. Pharmacol. 11, 572304. doi:10.3389/fphar.2020.572304
Sun, X., Wei, X., Qu, S., Zhao, Y., and Zhang, X. (2010). Hydroxysafflor Yellow A Suppresses Thrombin Generation and Inflammatory Responses Following Focal Cerebral Ischemia-Reperfusion in Rats. Bioorg Med. Chem. Lett. 20, 4120–4124. doi:10.1016/j.bmcl.2010.05.076
Tănăsescu, R., Nicolau, A., Ticmeanu, M., Luca, D., Caraiola, S., Cojocaru, I. M., et al. (2008). An Immunological Approach to Cerebral Ischemia (I). Immune Cells and Adhesion Molecules. Rom. J. Intern Med. 46, 3–8. doi:10.1890/03-0369
Tang, K. S., and Tan, J. S. (2019). The Protective Mechanisms of Polydatin in Cerebral Ischemia. Eur. J. Pharmacol. 842, 133–138. doi:10.1016/j.ejphar.2018.10.039
Tarozzo, G., Campanella, M., Ghiani, M., Bulfone, A., and Beltramo, M. (2002). Expression of Fractalkine and its Receptor, CX3CR1, in Response to Ischaemia-Reperfusion Brain Injury in the Rat. Eur. J. Neurosci. 15, 1663–1668. doi:10.1046/j.1460-9568.2002.02007.x
Tian, K. M., Li, J. J., and Xu, S. W. (2019). Rutaecarpine: A Promising Cardiovascular Protective Alkaloid from Evodia Rutaecarpa (Wu Zhu Yu). Pharmacol. Res. 141, 541–550. doi:10.1016/j.phrs.2018.12.019
Tu, X. K., Yang, W. Z., Shi, S. S., Wang, C. H., and Chen, C. M. (2009). Neuroprotective Effect of Baicalin in a Rat Model of Permanent Focal Cerebral Ischemia. Neurochem. Res. 34, 1626–1634. doi:10.1007/s11064-009-9953-4
Wang, C., Luo, Y., Lu, J., Wang, Y., and Sheng, G. (2016). Polydatin Induces Apoptosis and Inhibits Growth of Acute Monocytic Leukemia Cells. J. Biochem. Mol. Toxicol. 30, 200–205. doi:10.1002/jbt.21779
Wang, H., Zhang, K., Zhao, L., Tang, J., Gao, L., and Wei, Z. (2014). Anti-Inflammatory Effects of Vinpocetine on the Functional Expression of Nuclear Factor-Kappa B and Tumor Necrosis Factor-Alpha in a Rat Model of Cerebral Ischemia-Reperfusion Injury. Neurosci. Lett. 566, 247–251. doi:10.1016/j.neulet.2014.02.045
Wang, J., Tong, H., Wang, X., Wang, X., and Wang, Y. (2020a). Tanshinone IIA Alleviates the Damage of Neurocytes by Targeting GLUT1 in Ischaemia Reperfusion Model (In Vivo and In Vitro Experiments). Folia Neuropathol. 58, 176–193. doi:10.5114/fn.2020.96983
Wang, L., Li, L., Han, Q., Wang, X., Zhao, D., and Liu, J. (2020b). Identification and Biological Evaluation of Natural Product Biochanin A. Bioorg Chem. 97, 103674. doi:10.1016/j.bioorg.2020.103674
Wang, Q., Tang, X. N., and Yenari, M. A. (2007). The Inflammatory Response in Stroke. J. Neuroimmunol. 184, 53–68. doi:10.1016/j.jneuroim.2006.11.014
Wang, W., Tang, L., Li, Y., and Wang, Y. (2015). Biochanin A Protects against Focal Cerebral Ischemia/Reperfusion in Rats via Inhibition of P38-Mediated Inflammatory Responses. J. Neurol. Sci. 348, 121–125. doi:10.1016/j.jns.2014.11.018
Wang, Y. H., and Zeng, K. W. (2019). Natural Products as a Crucial Source of Antiinflammatorydrugs Recent Trends and Advancements. Tradit. Med. Res. 4, 257–268. doi:10.53388/tmr20190831133
Wei, S. Y., Chen, Y., and Xu, X. Y. (2014). Progress on the Pharmacological Research of Puerarin: A Review. Chin. J. Nat. Med. 12, 407–414. doi:10.1016/s1875-5364(14)60064-9
Xiang, B., Xiao, C., Shen, T., and Li, X. (2018). Anti-Inflammatory Effects of Anisalcohol on Lipopolysaccharide-Stimulated BV2 Microglia via Selective Modulation of Microglia Polarization and Down-Regulation of NF-κB P65 and JNK Activation. Mol. Immunol. 95, 39–46. doi:10.1016/j.molimm.2018.01.011
Xie, Q., Li, H., Lu, D., Yuan, J., Ma, R., Li, J., et al. (2021). Neuroprotective Effect for Cerebral Ischemia by Natural Products: A Review. Front. Pharmacol. 12, 607412. doi:10.3389/fphar.2021.607412
Xiong, D., Deng, Y., Huang, B., Yin, C., Liu, B., Shi, J., et al. (2016). Icariin Attenuates Cerebral Ischemia-Reperfusion Injury through Inhibition of Inflammatory Response Mediated by NF-κB, PPARα and PPARγ in Rats. Int. Immunopharmacol. 30, 157–162. doi:10.1016/j.intimp.2015.11.035
Xu, L. Y., Wu, W., Cheng, R., Sun, L. X., Jiang, Z. Z., Zhang, L. Y., et al. (2019). Toxic Effects of Triptolide on Adrenal Steroidogenesis in H295R Cells and Female Rats. J. Biochem. Mol. Toxicol. 33, e22394. doi:10.1002/jbt.22394
Xu, T., Wang, X., Ma, C., Ji, J., Xu, W., Shao, Q., et al. (2022). Identification of Potential Regulating Effect of Baicalin on NFκB/CCL2/CCR2 Signaling Pathway in Rats with Cerebral Ischemia by Antibody-Based Array and Bioinformatics Analysis. J. Ethnopharmacol. 284, 114773. doi:10.1016/j.jep.2021.114773
Xue, X., Qu, X. J., Yang, Y., Sheng, X. H., Cheng, F., Jiang, E. N., et al. (2010). Baicalin Attenuates Focal Cerebral Ischemic Reperfusion Injury through Inhibition of Nuclear Factor κB P65 Activation. Biochem. Biophys. Res. Commun. 403, 398–404. doi:10.1016/j.bbrc.2010.11.042
Xue, Z., Cao, Z., Jin, M., Zhang, X., Wang, X., Dou, J., et al. (2021). New Steroid Saponins from Dioscorea Zingiberensis Yam and Their Medicinal Use Against I/R via Anti-Inflammatory Effect. Food Funct. 12, 8314–8325. doi:10.1039/d1fo01301g
Yang, F., Ma, Q., Matsabisa, M. G., Chabalala, H., Braga, F. C., and Tang, M. (2020a). Panax Notoginseng for Cerebral Ischemia: A Systematic Review. Am. J. Chin. Med. 48, 1331–1351. doi:10.1142/S0192415X20500652
Yang, L., Liu, R., Fang, Y., and He, J. (2021). Anti-Inflammatory Effect of Phenylpropanoids from Dendropanax Dentiger in TNF-α-Induced MH7A Cells via Inhibition of NF-κB, Akt and JNK Signaling Pathways. Int. Immunopharmacol. 94, 107463. doi:10.1016/j.intimp.2021.107463
Yang, S., Ning, F., Li, J., Guo, D., Zhang, L., Cui, R., et al. (2016). Therapeutic Effect Analysis of Sinomenine on Rat Cerebral Ischemia-Reperfusion Injury. J. Stroke Cerebrovasc. Dis. 25, 1263–1269. doi:10.1016/j.jstrokecerebrovasdis.2016.02.023
Yang, X., Chen, L., Li, Y., Gao, F., Yan, Z., Zhang, P., et al. (2020b). Protective Effect of Hydroxysafflor Yellow A on Cerebral Ischemia Reperfusion-Injury by Regulating GSK3β-Mediated Pathways. Neurosci. Lett. 736, 135258. doi:10.1016/j.neulet.2020.135258
Yang, Y., Song, J., Liu, N., Wei, G., Liu, S., Zhang, S., et al. (2022). Salvianolic Acid A Relieves Cognitive Disorder after Chronic Cerebral Ischemia: Involvement of Drd2/Cryab/NF-κB Pathway. Pharmacol. Res. 175, 105989. doi:10.1016/j.phrs.2021.105989
Yilmaz, G., Arumugam, T. V., Stokes, K. Y., and Granger, D. N. (2006). Role of T Lymphocytes and Interferon-Gamma in Ischemic Stroke. Circulation 113, 2105–2112. doi:10.1161/CIRCULATIONAHA.105.593046
Yu, L., Li, Q., Yu, B., Yang, Y., Jin, Z., Duan, W., et al. (2016). Berberine Attenuates Myocardial Ischemia/Reperfusion Injury by Reducing Oxidative Stress and Inflammation Response: Role of Silent Information Regulator 1. Oxid. Med. Cell Longev. 2016, 1689602. doi:10.1155/2016/1689602
Yuan, Q., Yuan, Y., Zheng, Y., Sheng, R., Liu, L., Xie, F., et al. (2021). Anti-Cerebral Ischemia Reperfusion Injury of Polysaccharides: A Review of the Mechanisms. Biomed. Pharmacother. 137, 111303. doi:10.1016/j.biopha.2021.111303
Zaleska, M. M., Mercado, M. L., Chavez, J., Feuerstein, G. Z., Pangalos, M. N., and Wood, A. (2009). The Development of Stroke Therapeutics: Promising Mechanisms and Translational Challenges. Neuropharmacology 56, 329–341. doi:10.1016/j.neuropharm.2008.10.006
Zarruk, J. G., Greenhalgh, A. D., and David, S. (2018). Microglia and Macrophages Differ in Their Inflammatory Profile after Permanent Brain Ischemia. Exp. Neurol. 301, 120–132. doi:10.1016/j.expneurol.2017.08.011
Zhang, P., Mak, J. C., Man, R. Y., and Leung, S. W. (2019). Flavonoids Reduces Lipopolysaccharide-Induced Release of Inflammatory Mediators in Human Bronchial Epithelial Cells: Structure-Activity Relationship. Eur. J. Pharmacol. 865, 172731. doi:10.1016/j.ejphar.2019.172731
Zhang, T., Xu, J., Li, D., Chen, J., Shen, X., Xu, F., et al. (2014). Salvianolic Acid A, a Matrix Metalloproteinase-9 Inhibitor of Salvia Miltiorrhiza, Attenuates Aortic Aneurysm Formation in Apolipoprotein E-Deficient Mice. Phytomedicine 21, 1137–1145. doi:10.1016/j.phymed.2014.05.003
Zhang, W., Song, J. K., Zhang, X., Zhou, Q. M., He, G. R., Xu, X. N., et al. (2018). Salvianolic Acid A Attenuates Ischemia Reperfusion Induced Rat Brain Damage by Protecting the Blood Brain Barrier through MMP-9 Inhibition and Anti-inflammation. Chin. J. Nat. Med. 16, 184–193. doi:10.1016/s1875-5364(18)30046-3
Zhang, Y., Tao, C., Xuan, C., Jiang, J., and Cao, W. (2020). Transcriptomic Analysis Reveals the Protection of Astragaloside IV against Diabetic Nephropathy by Modulating Inflammation. Oxid. Med. Cell Longev. 2020, 9542165. doi:10.1155/2020/9542165
Zhang, Y., Chen, W. A., Huang, S. S., and Wang, H. M. (2016). Protective Effects of Mangiferin on Cerebral Ischemia-Reperfusion Injury and its Mechanisms. Eur. J. Pharmacol. 771, 145–151. doi:10.1016/j.ejphar.2015.12.003
Zhao, C., Lv, Y., Cui, H., Zhu, Y., Wei, M., Xia, Y., et al. (2021). Phase I Safety, Tolerability, and Pharmacokinetic Studies of Tetramethylpyrazine Nitrone in Healthy Chinese Volunteers. Drug Dev. Res. 82, 97–107. doi:10.1002/ddr.21733
Zhao, J., Piao, X., Wu, Y., Liang, S., Han, F., Liang, Q., et al. (2020). Cepharanthine Attenuates Cerebral Ischemia/Reperfusion Injury by Reducing NLRP3 Inflammasome-Induced Inflammation and Oxidative Stress via Inhibiting 12/15-LOX Signaling. Biomed. Pharmacother. 127, 110151. doi:10.1016/j.biopha.2020.110151
Zhao, Z., Xiao, J., Wang, J., Dong, W., Peng, Z., and An, D. (2015). Anti-Inflammatory Effects of Novel Sinomenine Derivatives. Int. Immunopharmacol. 29, 354–360. doi:10.1016/j.intimp.2015.10.030
Zhou, J., Wen, B., Xie, H., Zhang, C., Bai, Y., Cao, H., et al. (2021). Advances in the Preparation and Assessment of the Biological Activities of Chitosan Oligosaccharides with Different Structural Characteristics. Food Funct. 12, 926–951. doi:10.1039/d0fo02768e
Zhu, J., Jiang, Y., Wu, L., Lu, T., Xu, G., and Liu, X. (2012). Suppression of Local Inflammation Contributes to the Neuroprotective Effect of Ginsenoside Rb1 in Rats with Cerebral Ischemia. Neuroscience 202, 342–351. doi:10.1016/j.neuroscience.2011.11.070
Keywords: anti-inflammatory effect, inflammatory mechanism, compound, cerebral ischemia, inflammation, natural product
Citation: Shang Y, Zhang Z, Tian J and Li X (2022) Anti-Inflammatory Effects of Natural Products on Cerebral Ischemia. Front. Pharmacol. 13:914630. doi: 10.3389/fphar.2022.914630
Received: 07 April 2022; Accepted: 30 May 2022;
Published: 20 June 2022.
Edited by:
Jack Arbiser, Emory University, United StatesReviewed by:
Sivareddy Challa, University of Illinois, United StatesEmiliano Barreto, Federal University of Alagoas, Brazil
Copyright © 2022 Shang, Zhang, Tian and Li. This is an open-access article distributed under the terms of the Creative Commons Attribution License (CC BY). The use, distribution or reproduction in other forums is permitted, provided the original author(s) and the copyright owner(s) are credited and that the original publication in this journal is cited, in accordance with accepted academic practice. No use, distribution or reproduction is permitted which does not comply with these terms.
*Correspondence: Jinfeng Tian, dGpmYXN5aEAxNjMuY29t