- 1Molecular and Medicine Research Center, Khomein University of Medical Sciences, Khomein, Iran
- 2Department of Medical Parasitology and Mycology, Arak University of Medical Sciences, Arak, Iran
- 3Microbial Biotechnology Research Center, Iran University of Medical Sciences, Tehran, Iran
- 4Department of Microbiology, School of Medicine, Iran University of Medical Sciences, Tehran, Iran
- 5Department of Laboratory Sciences, School of Paramedical Sciences, Sabzevar University of Medical Sciences, Sabzevar, Iran
- 6Cellular and Molecular Research Center, Sabzevar University of Medical Sciences, Sabzevar, Iran
- 7Student Research Committee, Khomein University of Medical Sciences, Khomein, Iran
- 8Department of Microbiology, School of Medicine, Hamadan University of Medical Sciences, Hamadan, Iran
The biofilm communities of Candida are resistant to various antifungal treatments. The ability of Candida to form biofilms on abiotic and biotic surfaces is considered one of the most important virulence factors of these fungi. Extracellular DNA and exopolysaccharides can lower the antifungal penetration to the deeper layers of the biofilms, which is a serious concern supported by the emergence of azole-resistant isolates and Candida strains with decreased antifungal susceptibility. Since the biofilms’ resistance to common antifungal drugs has become more widespread in recent years, more investigations should be performed to develop novel, inexpensive, non-toxic, and effective treatment approaches for controlling biofilm-associated infections. Scientists have used various natural compounds for inhibiting and degrading Candida biofilms. Curcumin, cinnamaldehyde, eugenol, carvacrol, thymol, terpinen-4-ol, linalool, geraniol, cineole, saponin, camphor, borneol, camphene, carnosol, citronellol, coumarin, epigallocatechin gallate, eucalyptol, limonene, menthol, piperine, saponin, α-terpineol, β–pinene, and citral are the major natural compounds that have been used widely for the inhibition and destruction of Candida biofilms. These compounds suppress not only fungal adhesion and biofilm formation but also destroy mature biofilm communities of Candida. Additionally, these natural compounds interact with various cellular processes of Candida, such as ABC-transported mediated drug transport, cell cycle progression, mitochondrial activity, and ergosterol, chitin, and glucan biosynthesis. The use of various drug delivery platforms can enhance the antibiofilm efficacy of natural compounds. Therefore, these drug delivery platforms should be considered as potential candidates for coating catheters and other medical material surfaces. A future goal will be to develop natural compounds as antibiofilm agents that can be used to treat infections by multi-drug-resistant Candida biofilms. Since exact interactions of natural compounds and biofilm structures have not been elucidated, further in vitro toxicology and animal experiments are required. In this article, we have discussed various aspects of natural compound usage for inhibition and destruction of Candida biofilms, along with the methods and procedures that have been used for improving the efficacy of these compounds.
Introduction
The incidence of fungal infections, and therapeutic resistance of fungi, is increasing worldwide, intensifying the need for new and more effective antifungal strategies (El-Baz et al., 2021). Candidiasis, caused by various Candida species, is an important and life-threatening infection through which Candida can enter the bloodstream and host organs during invasive infections (Achkar and Fries, 2010). Worldwide, these fungi rank fifth in causing hospital-acquired infections and are responsible for 50% of catheter-related deaths. Candida albicans is the most commonly isolated strain from clinical samples (Brown et al., 2012). Other Candida species such as Candida tropicalis, Candida parapsilosis, Candida glabrata, and Candida krusei can also cause invasive infections with a high mortality rate in humans (Pappas et al., 2018).
The ability of Candida cells to form biofilms and produce hydrolytic enzymes, such as phospholipases and hemolysins, plays an important role in the pathogenesis and drug resistance (Mba and Nweze, 2020). Biofilms are easily formed on the objects that enter the body, such as intravascular and urinary catheters, artificial valves, intrauterine devices, contact lenses, and other foreign objects, or on host surfaces. Biofilm formation begins with the attachment of Candida to the surface of a medical device or host (Doke et al., 2014). After the attachment, the yeast is transformed from the planktonic to hyphal state, and the biofilm formation and invasion begin (Sudbery et al., 2004; Finkel and Mitchell, 2011). The extracellular matrix of a biofilm consists of exopolymeric materials that have a protective role against various toxic compounds, such as antifungals, and which also protect the fungal cells from the host immune system (Gupta et al., 2018).
Increased use of catheters and medical implant devices along with biofilm formation, in addition to negatively affecting their performance, leads to persistent infections with the emergence of widespread antifungal resistance (Ramage et al., 2006). Furthermore, many antifungal drugs are currently associated with limitations such as increased resistance, narrow antifungal spectrum, toxicity, and high treatment costs for patients (Lone and Ahmad, 2020). Thus, due to the emergence of multidrug-resistant Candida and need for treating its resistant forms, such as biofilms, the application of natural products as new and effective therapies has gained a special place in clinical treatments (Ahmad et al., 2015). Studies have shown that plant products, including essential oils (EOs), extracts, and pure compounds, have significant effects on the resistant and biofilm-forming species of Candida (Ahmad et al., 2015). The natural compounds obtained from different parts of a plant, such as roots, leaves, and fruits, which have medicinal properties, show different medicinal effects once changes are applied to them (Rangel et al., 2018).
Recent studies have indicated that various natural compounds such as curcumin, cinnamaldehyde, eugenol, and thymol not only inhibit biofilm formation but also eliminate mature biofilm structures (Doke et al., 2014; Rangel et al., 2018). Additionally, the combination treatment with antifungal drugs and different natural compounds can be an effective solution to treat common diseases such as candidiasis due to its increased potency and effectiveness, lower drug toxicity, lower dosages, and reduced likelihood of developing resistant strains (Shinde et al., 2013).
Accordingly, this review concentrates on the interactions of different natural compounds with biofilm communities of Candida and various drug delivery platforms used for enhancing the therapeutic efficacy of natural compounds. The review also focuses on the synergistic antimicrobial activity of these compounds with antifungal drugs to facilitate their widespread use in clinical practice.
Curcumin
Curcumin is one of the main phytochemical ingredients of Curcuma longa (Zingiberaceae family). Curcumin usually shows no to low cytotoxicity at active dose range and oral doses as high as 8–12 g per day. This compound has shown antioxidant, antiviral, antimalarial, anticancer, and antibiofilm characteristics in studies and show promising synergistic effects in combination with other drugs (Hewlings and Kalman, 2017; Shariati et al., 2019). Because of this, scientists are interested in employing this compound for degrading Candida biofilms. For instance, a recently published study reported that curcumin at high concentrations (0.1–2 mg/ml) effectively suppressed the planktonic and biofilm community of Candida (Narayanan et al., 2020). In this section, we have reviewed studies that have applied curcumin for inhibition and degradation of Candida biofilm in different stages.
As mentioned previously, fungal adhesion is an important stage in biofilm formation. Thus, if a compound disrupts microbial adhesion to different surfaces, it could be considered a potential antibiofilm agent. In this regard, Shahzad et al. (2014) reported that curcumin showed superior antibiofilm activity, remarkably suppressing initial C. albicans adhesion following pre-coating with this compound. This repressive effect diminished with the delay in curcumin exposure after the inoculation; however, curcumin could still suppress the biofilm formation by 50% when added 4 h after inoculation. The authors proposed that the antibiofilm activity of curcumin may be attributed to two α,β-unsaturated carbonyl groups that connect two phenol groups; additionally, the presence of two unsaturated double bonds in the central connecting chain could further boost its antibiofilm activity. Notably, curcumin reduced the expression of Agglutinin-like sequence 3 (ALS3), an adhesion-associated gene, and the hyphal protein HWP1 (Shahzad et al., 2014). The Als adhesive proteins are one of the most extensively studied virulence characteristics of C. albicans, and the elimination of Als3 results in a significant reduction in fungal adhesion (Hoyer and Cota, 2016). These findings are in line with a recently published study that reported that curcumin (50 µg/ml) inhibited C. albicans adhesion, This activity of curcumin could be further boosted by the pre-treatment of yeast cells with the compound to obtain a remarkably greater inhibition of adhesion. Additionally, curcumin affected various immature morphological forms of C. albicans, such as germlings and yeast. Transcriptional analyses corroborated these findings and indicated that treatment with curcumin suppressed the expression of key adhesion-related genes ALS3 and ALS1, while the expression of aggregation-related genes AAF1 and ALS5 increased (Alalwan et al., 2017). Notably, Shariati et al. (2019) reported that the human alveolar epithelial cells were well-spread, and there was no distinct change in morphology after 24 h of incubation with 50–200 µg/ml of curcumin compared to control cells. Therefore, curcumin could be used for the destruction of Candida biofilms without cytotoxic effects on human cells; however, more confirmatory studies are required in this area.
These data show that curcumin has an anti-adhesive activity against C. albicans and the compound interferes with the expression of adhesion- and biofilm-associated genes. It has also been reported that the treatment of C. albicans SC5314 strain biofilm with curcumin led to a decrease in the biofilm mass and proteinase and phospholipase activity of the biofilm community. However, proteinase gene expression was not downregulated after the curcumin treatment. C. albicans secretes proteinases and phospholipases for purposes such as host invasion, tissue degradation, and hypha formation. Thus, curcumin not only decreased the biomass of C. albicans biofilm but also suppressed critical pathogenic factors of this fungus (Chen et al., 2018).
The satisfactory performance of curcumin against Candida biofilms has led to the use of this compound for inhibiting drug-resistant strains. In this regard, Dong et al. (2021) used curcumin to restore the effectiveness of fluconazole against fluconazole-resistant Candida. Curcumin alone or in combination with fluconazole suppressed biofilm formation and yeast-to-hypha morphological transition in fluconazole-resistant C. albicans. Furthermore, this compound combined with fluconazole disrupted membrane permeability and reduced intracellular ATPase production. The synergism of curcumin and fluconazole has introduced this compound as a new therapeutic agent against drug-resistant Candida strains (Dong et al., 2021). However, there is limited data on the curcumin and antifungals interactions; thus, further studies are required.
In a biofilm community, microorganisms with different antibiotic resistance properties interact with each other; thus, treating an infection of a multi-species biofilm community is more difficult than that of a mono-species biofilm. In this regard, recent studies have evaluated the inhibitory effect of curcumin against mixed-species biofilms. In one such study, the authors assessed the inhibitory effect of curcumin against the dual-species biofilms formed by Streptococcus mutans and C. albicans. Curcumin suppressed C. albicans and S. mutans in both mono- and dual-species biofilms. Notably, S. mutans was more sensitive to treatment in the dual-species biofilms than in mono-species biofilms, while C. albicans was less sensitive to curcumin in dual-species biofilms. Curcumin downregulated the expression of quorum sensing-related genes and glucosyltransferase in S. mutans and ALS1 and ALS3 family in C. albicans in dual-species biofilms. Accordingly, curcumin, due to its inhibitory effect on various biofilm-associated molecular pathways of C. albicans and S. mutans, could be used for controlling caries-related dual-species plaque biofilms (Li et al., 2019).
In yet another investigation, curcumin exhibited antibiofilm activity against a mixed community of Acinetobacter baumannii and C. albicans (Raorane et al., 2019). In this mixed biofilm community, Hyr1p of C. albicans binds to the outer membrane protein of A. baumannii, FhaB; it has been reported that HYR1 knockdown remarkably decreased C. albicans hyphae binding to A. baumannii (Darwish Alipour Astaneh et al., 2017; Uppuluri et al., 2018). However, curcumin interactions and mentioned genes were not reported in this study (Raorane et al., 2019). Finally, the results of the study by Tan et al. (2019) showed that 2-aminobenzimidazole in combination with curcumin effectively degraded C. albicans and Staphylococcus aureus mixed biofilm on silicone surface. Collectively, curcumin showed good inhibitory effects against mixed bacterial and fungal biofilm. Hence, this compound could be used for mixed biofilm-associated infections such as oral disorders and infections of implants.
Nonetheless, like other natural compounds, curcumin has limited usage in the clinical setting due to its various drawbacks, including low oral bioavailability, water insolubility, rapid metabolism and degradation, poor absorption from the gut, and low blood plasma levels (Shariati et al., 2019). To address these issues, researchers have been interested in using various drug delivery platforms to enhance curcumin-antibiofilm efficacy. For instance, Rajasekar et al. (2021) used the curcumin-sophorolipid nanocomplex for the degradation of C. albicans biofilm. Sophorolipids, which are biologically-derived surfactants, are practical candidates for the delivery of hydrophobic molecules. The synthesized curcumin-sophorolipids remarkably suppressed fungal attachment, subsequent biofilm formation, filamentation, and maturation. Additionally, this compound downregulated different pathogenic genes such as EFG1, ALS1, SAP8, and EAP1 (Rajasekar et al., 2021). In another investigation, the authors used graphene oxide functionalized with curcumin and polyethylene glycol (PEG) for the eradication of C. albicans biofilm. The results showed that this compound decreased fungal proliferation, adhesion, and biofilm formation. Thus, the authors suggested that combining curcumin and PEG molecules on the graphene oxide surface results in a strong antibiofilm effect, which can also suppress the local growth of C. albicans cells not adhering to the surface (Palmieri et al., 2018). In line with these findings, the results of a recently published study demonstrated that curcumin-loaded chitosan nanoparticles (NPs) had acceptable antibiofilm effects against S. aureus and C. albicans mixed-species and mono-species biofilms. Microscopic analysis revealed that the synthesized compound reduced biofilm thickness and killed the microbial cells embedded in the biofilm on silicone surfaces. Thus, curcumin-loaded chitosan NPs could be used as an effective agent to eliminate infections caused by Candida biofilms, particularly those associated with mixed-species biofilms (Ma et al., 2020).
As is evident from the above studies, various drug delivery platforms could be used to enhance the antibiofilm efficacy of curcumin. However, data in this area are very limited, and more in-depth investigations should be carried out before the clinical usage of curcumin-based drug delivery platforms.
Finally, recent studies have employed the combination of photodynamic therapy and curcumin for Candida biofilm elimination. This type of treatment is in its initial stages, with the studies described in Table 1.
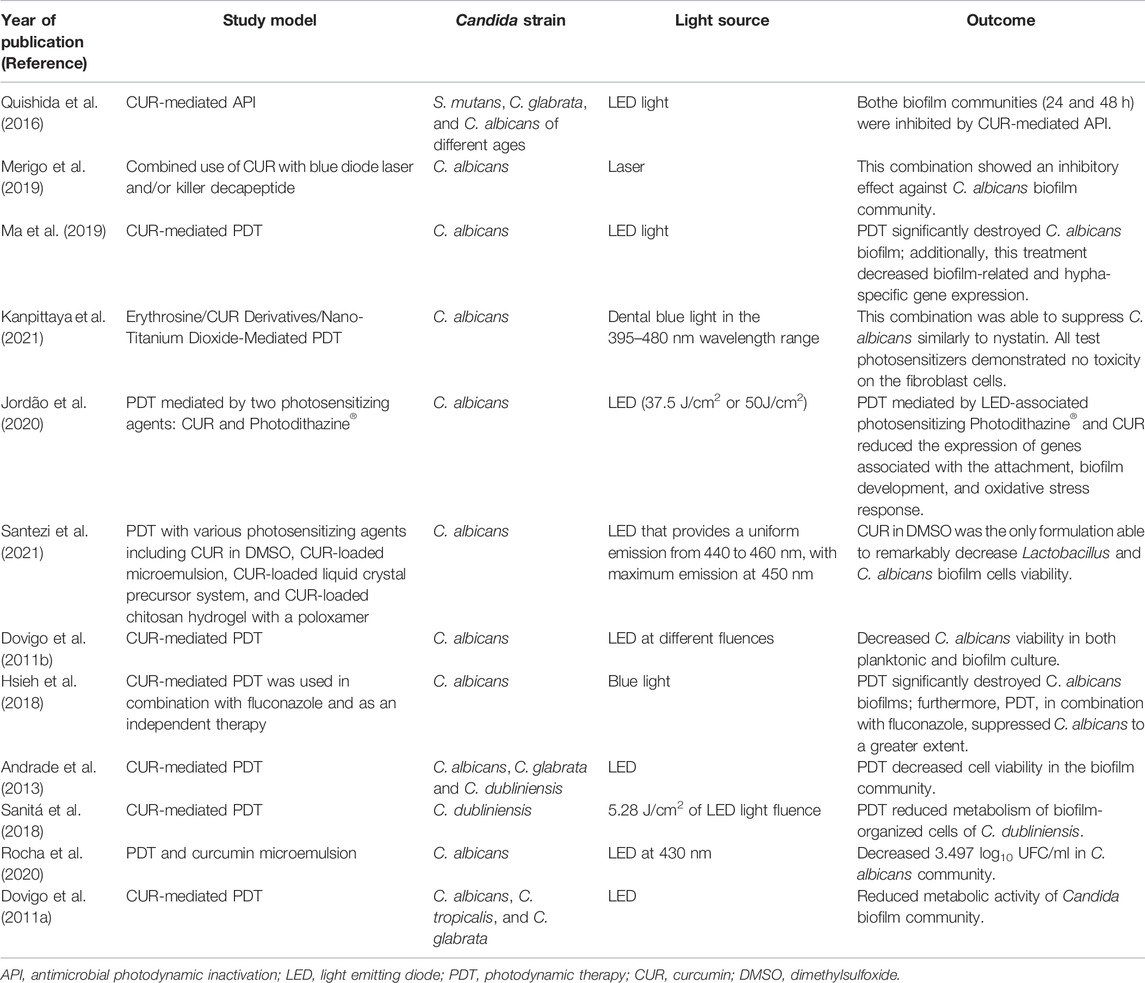
TABLE 1. Studies that have applied a combination of photodynamic therapy and curcumin for the elimination of Candida biofilm.
Thymol
Thymol, 2-isopropyl-5-methyl phenol, is an isomeric phenolic monoterpene with a strong odor and good solubility in alcohol and other organic solvents but is poorly water-soluble. Thymol is abundantly found in certain plants and has been used in traditional medicine for many years because of its different pharmacological properties, such as anti-rheumatic, antioxidant, antiseptic, anticancer, antiviral, antibacterial, and antifungal (Salehi et al., 2018; Schnitzler, 2019; Kowalczyk et al., 2020; Gholami-Ahangaran et al., 2022).
Scientists have evaluated the antibiofilm potential of this compound and reported that thymol could inhibit biofilm formation and has the capacity to reduce viable cells as well as eliminate mature biofilm community of various Candida (Figure 1) (Braga et al., 2008; Dalleau et al., 2008; De Vasconcelos et al., 2014; Shu et al., 2016; Chatrath et al., 2019). Further, gas chromatography-mass spectrometry analysis in recent studies has indicated thymol as the main ingredient of plant EOs, including that of Carum copticum, Thymus vulgaris, Satureja hortensis L, Carum copticum L, and Trachyspermum ammi. Notably, these EOs showed antibiofilm activity against Candida biofilms (Khan et al., 2014; Sharifzadeh et al., 2016; Arabi Monfared et al., 2020; Hejazi et al., 2021). Furthermore, C. copticum and T. vulgaris EOs could cause a >70% decrease in the hydrophobicity of the cell surface, and reduce hemolysin, and proteinase production (Khan et al., 2014).
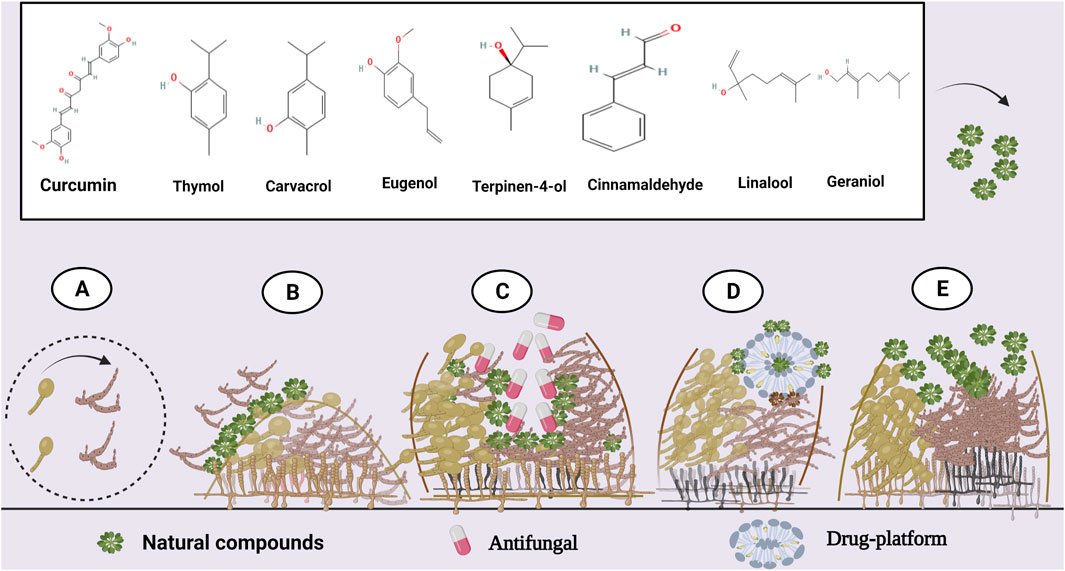
FIGURE 1. Antibiofilm activity of various natural compounds against Candida. (A) Inhibition of yeast-to-hypha morphological transition and (B) initial Candida adhesion. (C) Synergistic combination with antifungals. (D) Drug delivery platforms could enhance natural compounds' antibiofilm efficacy. (E) Destruction of an established biofilm. Created with BioRender.com
Additionally, another study evaluated the antibiofilm effect of thymol against the mixed biofilm of S. mutans and C. albicans. Thymol significantly reduced the biofilm formation and virulence of both S. mutans and C. albicans, including acidogenicity (acid production from the dietary carbohydrates), acidurity (able to survive under lethal pH condition), and yeast-to-hyphal transition, in single- and mixed-species biofilm communities. Notably, these microorganisms did not show resistance against thymol, which could be associated with the fact that this compound showed regulatory effects on many genes/transcriptional regulators of both organisms (Priya et al., 2021b). The synergistic interaction between S. mutans and C. albicans within the carious biofilm leads to enhanced virulence of both pathogens. Recent investigations have demonstrated that the presence of C. albicans supports the extensive colonization of S. mutans in the dental biofilm (Waltimo et al., 2000; Priya et al., 2021b). Thus, thymol, due to its ability to inhibit fungal filamentation and morphogenesis, and impair S. mutans acidogenic and aciduric ability, could be a practical candidate for the prevention and treatment of early childhood caries; however, more confirmatory investigations are required.
Combined use of thymol with other natural compounds and antifungals also showed promising results in inhibiting the Candida biofilm community. Priya et al. (2021a) evaluated the synergistic effect of thymol and piperine, the major bioactive component of pepper, against biofilms formed by some clinical isolates of C. albicans through checkerboard assay. Both of these compounds at a concentration of 32 μg/ml inhibited the biofilm community of C. albicans, whereas the combined use of piperine and thymol indicated synergistic effects at four different combinations of concentrations (piperine and thymol at 8 and 8, 8 and 4, 8 and 2, and 4 and 8 μg/ml). The combination therapy led to the reduction of different virulence factors of C. albicans, including those related to the attachment, morphological transformation, and hyphal extension. In addition, hyphal elongation was controlled by limited exposure to synergistic combinations. The molecular assessment also confirmed these findings and showed that the expression of tup1 and nrg1, the negative transcriptional regulators of filamentous growth, was upregulated under the influence of thymol and piperine. On the other hand, the expression of ume6, a positive regulator of the filamentation morphology, was remarkably downregulated. Collectively, these findings suggest that thymol and piperine could interact synergistically in exerting antihyphal and antibiofilm functions against C. albicans (Priya et al., 2021a). Along similar lines, another study evaluated the interaction of thymol and carvacrol against mono- and mixed-species growth of Staphylococcus epidermidis and C. albicans. This combination exhibited anti-adhesion, antibiofilm, and antihyphal actions. It also eliminated mono- and mixed-species highly tolerant persister cells and reduced the risk of resistance expansion (Swetha et al., 2020). Notably, a recently published study showed that the treatment of HEK 293 (human embryonic kidney) cells with 250 μg/ml thymol led to a loss of nearly 100% viability (Al-Ani and Heaselgrave, 2022). Therefore, it seems that the antibiofilm effect of thymol at a concentration of 32 μg/ml could be achieved without damaging the eukaryotic cells, although more studies are needed.
Another study reported synergistic effects of thymol and antifungal drugs against the drug-resistant (itraconazole and fluconazole) strains of C. albicans and C. tropicalis. Thymol reduced biofilm formation by these fungi either by preventing the attachment or interfering with the subsequent biofilm maturation. The microscopic evaluation also revealed deformed structures and disaggregation of C. albicans biofilm community cells and reduced hyphae formation of C. tropicalis biofilm cells at sub-minimum inhibitory concentrations (MICs) of thymol. Notably, thymol had a significant effect on the structure of the preformed biofilm of both C. tropicalis and C. albicans. Thymol also demonstrated synergy with fluconazole against both the planktonic and biofilm community of C. tropicalis and C. albicans. Nevertheless, synergy with amphotericin B (AMB) was evident only in the planktonic Candida cells (Jafri and Ahmad, 2020).
These results support the findings of Pemmaraju et al. (2013), who reported synergistic effects for thymol and fluconazole in the reduction of C. albicans biofilm formation. The adherence assay indicated 30% viability of fungal cells after 2 h of treatment with 0.05% (v/v) fluconazole and thymol. The authors proposed that improved antifungal action of fluconazole in the presence of thymol could be related to the alternations in the membrane permeability and fluidity and inhibition of ergosterol biosynthesis by thymol, which could lead to cytoplasm membrane disruption, cell contents leakage and cell wall degradation. The microscopic analysis confirmed this proposition and revealed alterations in the number of cells and structural design of C. albicans biofilm. Thymol with fluconazole showed the highest synergistic effect on biofilm inhibition compared to the other natural compounds, including eugenol and menthol (Pemmaraju et al., 2013).
Thus, synergistic combinations of thymol with other natural compounds such as piperine and carvacrol, as well as antifungals, could be a promising treatment for C. albicans biofilm-associated infections. Further, these combinations could possibly be used in the formulation of dentifrices that are particularly effective for oral candidiasis treatment. However, the mode of synergistic action of thymol with other natural compounds and antifungals is not clear at present; thus, these encouraging findings will need to be confirmed by in vivo and translational investigations before clinical usage can be realized.
Carvacrol
Carvacrol, a phenolic monoterpenoid, is known as one of the main ingredients of EOs of various aromatic plants, such as oregano (Origanum vulgare), pepperwort (Lepidium flavum), and thyme (Thymus vulgaris). Carvacrol has been used as a food preservative, additive, and flavoring, as well as a fragrance in cosmetic products. Recent studies have revealed various biological activities of carvacrol, including antioxidant, anticancer, and antimicrobial activities (Sharifi-Rad et al., 2018; Javed et al., 2021). Because of its various properties, such as the presence of a free hydroxyl group and phenol moiety and hydrophobicity, carvacrol exhibited better antimicrobial activity than other volatile compounds (Sharifi-Rad et al., 2018). Various investigations have used carvacrol for inhibiting and degrading Candida biofilms (Dalleau et al., 2008).
Miranda-Cadena et al. (2021b) used various natural compounds, including carvacrol and cinnamaldehyde, against the planktonic and sessile cells of Candida. The authors evaluated the reduction in the biofilm metabolic activity and biomass during the adhesion as well as mature biofilm phases. Carvacrol remarkably reduced both the metabolic activity and biomass of the mature biofilm and even degraded the biofilms of azole-resistant strains. The microscopic observations also showed a loss of the cell viability of Candida biofilm community after carvacrol treatment. Cinnamaldehyde showed a better function in preventing biofilm formation, while carvacrol more effectively degraded mature Candida biofilm (Miranda-Cadena et al., 2021b). Thus, the combined use of carvacrol and cinnamaldehyde could be a promising approach for suppressing Candida biofilm-associated infections due to its interference with various biofilm formation stages. These results support the findings of Touil et al. (2020), who reported that using a combination of terpenoids (farnesol, carvacrol, and cuminaldehyde) could inhibit biofilm formation and kill drug-resistant C. albicans. Carvacrol significantly suppressed the biofilm formation of C. albicans (80% at 2,000 μg/ml). Additionally, carvacrol at a concentration of 2,000 μg/ml reduced the cells of C. albicans by more than 75% during the biofilm development with the co-isolated bacteria. Hence, carvacrol had destructive effects on the interactions between the mixed-species biofilms and suppressed the biofilm development. Notably, the combined use of carvacrol and farnesol revealed synergistic suppressive effects not only on C. albicans hyphae and yeast, but also on biofilms formed from single and mixed-species (Touil et al., 2020).
Gomes et al. (2022) reported that at a concentration of 100 μg/ml, carvacrol has a moderately toxic effect on L929 (mouse fibroblast) cells. Therefore, high concentrations of carvacrol can have a detrimental effect on eukaryotic cells, which should be evaluated in future studies.
In addition to cinnamaldehyde and farnesol, the combination of thymol and carvacrol also showed promising results for C. albicans biofilm inhibition. The carvacrol and thymol combination showed anti-adhesion, antibiofilm, and anti-hyphal activities. Further, this combination eliminated the highly tolerant persister cells of mono- and mixed-species of both S. epidermidis and C. albicans biofilms and was associated with less risk of resistance development (Swetha et al., 2020).
The combinations of carvacrol and antifungals also showed promising results for degrading C. albicans biofilm. Doke et al. (2014) reported that the mature biofilm of C. albicans was highly resistant to fluconazole but susceptible to natural compounds such as carvacrol. Sensitization of fungal cells by carvacrol at sub-inhibitory concentrations led to the inhibition of biofilm formation and destruction of mature biofilm of C. albicans at low fluconazole concentrations (0.032 mg/ml). On the other hand, thymol and eugenol combinations with fluconazole did not show useful interaction against C. albicans mature biofilms (Doke et al., 2014). Thus, carvacrol has the potential for treating drug-resistant C. albicans. It could additionally be used in combination with other natural compounds and antifungals for better antibiofilm action. Nonetheless, the efficacy of combination therapies with carvacrol and other natural compounds needs to be demonstrated in animal model studies and translational investigations before its use in clinical practice.
A recently published study reported carvacrol as the main ingredient of Origanum majorana L. EO. This EO exhibited antifungal and antibiofilm activities against C. albicans. In addition to biofilm inhibition, this EO also suppressed germ tube formation, one of the main virulence factors of C. albicans, and modulated cell surface hydrophobicity. Hence, carvacrol prevented biofilm formation, degraded mature C. albicans biofilm, and suppressed various virulence factors of this fungus. Accordingly, carvacrol has the potential to inhibit C. albicans pathogenesis and could be considered in patients with the risk of C. albicans infection or in the with common antifungals was without sufficient success (Kaskatepe et al., 2022). However, for this purpose, further in vivo and clinical studies are needed to evaluate the carvacrol efficiency in clinical setting and further enhance its activity against Candida biofilm.
To this end, various drug delivery platforms have been used for enhancing carvacrol antibiofilm efficacy. Vitali et al. (2021) used an ionic gelation procedure to synthesize chitosan NPs loaded with carvacrol. Afterward, the antibiofilm activity of carvacrol-loaded NPs was evaluated against Candida biofilms. The results showed that the antibiofilm activity of synthesized NPs was significantly dependent on the Candida species; accordingly, C. krusei and C. tropicalis showed the most susceptibility to the treatment. The authors suggested that the structures of biofilms in the studied species could affect the response to carvacrol-loaded NPs (Vitali et al., 2021). Studies indicate that the mature biofilm structure of C. albicans has a heterogeneous structure, composed of hyphae and blastospores surrounded by extracellular polysaccharides. On the other hand, C. glabrata biofilm community consists of yeast cells multilayer, intimately packed or arranged in cell clusters. Furthermore, C. tropicalis biofilm consists of a network of yeast, pseudohyphae, and hyphal yeast, with intense hyphal budding (Chandra et al., 2001; Bizerra et al., 2008; Silva et al., 2009; Vitali et al., 2021). Thus, it can be concluded that the antibiofilm effect of carvacrol and drug delivery platforms based on this compound should be evaluated against various Candida species. Since different Candida species have different biofilm structures, the antibiofilm efficacy of carvacrol could be different across various species.
In yet another investigation, the authors incorporated carvacrol into electrospun membranes of poly(lactic acid) (PLA) for the elimination of C. albicans and S. aureus mixed biofilm structure. Carvacrol showed good compatibility with PLA, improved the extensibility and flexibility of the matrix, and acted as a plasticizer. The gradual release of carvacrol from PLA membranes led to antimicrobial activity for up to 144 h and a reduction of biofilm formation by 88–95% for C. albicans and 92–96% for S. aureus in single and mixed cultures. Furthermore, the findings indicated a huge reduction in biomass and cell count, as well as metabolic activity of 24- and 48-h biofilms formed after the treatment (Scaffaro et al., 2018). These results highlight the potential of nanobiotechnology such as electrospun nanofibrous membranes as a practical delivery platform for carvacrol, an ecological substitution in developing new antibiofilm approaches, and a a promising agent for managing Candida biofilm-associated infections.
Eugenol
Eugenol or 2-methoxy-4-[2-propenyl] phenol, a phenolic aromatic compound mainly derived from Cinnamomum and clove EO, is a natural microbiocidal compound belonging to the phenylpropanoid class of organic compounds and has been used for a long time as an analgesic in dentistry (Hassan et al., 2018; Wijesinghe et al., 2021). Eugenol can be synthesized by guaiacol allylation with allyl chloride or produced through a biotransformation process that involves microorganisms such as Bacillus cereus, Corynebacterium spp., and Escherichia coli (Abrahão et al., 2013). Eugenol also has anesthetic, neuroprotective, antidiabetic, insecticidal, analgesic, anti-inflammatory, and antifungal properties, which makes it a versatile natural ingredient that helps prevent and cure various disorders (Kozam, 1977; Reddy and Lokesh, 1994; Hassan et al., 2018; Nisar et al., 2021). Eugenol exhibits significant antifungal activity against various fungal species, including the dermatophytes Aspergillus and Candida, primarily due to damage to the fungal cell envelope and inhibitory action against biofilm communities and various virulence factors (Chami et al., 2005; Hassan et al., 2018). As a result, scientists are interested in using eugenol to suppress Candida biofilm communities and various cellular pathways of these fungi.
He et al. (2007) reported that eugenol reduced the adhesion capacity of cells and inhibited biofilm formation in C. albicans. Furthermore, the treatment of C. albicans cells with eugenol produced scant biofilms with suppressed filamentous growth (He et al., 2007). Notably, C. albicans is a dimorphic fungus that can switch from the yeast phase to the filamentous phase. The filamentous phenotype of this fungus is essential for pathogenicity and could have an important role in producing the spatially organized architecture seen in mature, highly structured C. albicans biofilms (López-Ribot, 2005). These data suggest that eugenol inhibited biofilm formation in C. albicans, affected the fungal cells’ morphogenesis, and weakened their invasive capacity (He et al., 2007).
Furthermore, molecular docking analysis indicated that eugenol impacts the C. albicans Als3 protein (El-Baz et al., 2021). The binding capacity of eugenol to Als3 was higher than that of other natural compounds such as cinnamaldehyde (Hoyer and Cota, 2016). Thus, the interaction of eugenol and Als3 may be the underlying mechanism of inhibition of C. albicans adhesion and biofilm formation by this compound (El-Baz et al., 2021).
Additionally, as a significant constituent of Cinnamomum, eugenol has demonstrated promising antibiofilm effects against Candida. In a study conducted in 2018, the authors used the Cinnamomum zeylanicum EO to inhibit Candida biofilm. The results showed that C. zeylanicum EO (500 μg/ml) significantly inhibited the formation of Candida biofilm and reduced mono- (C. tropicalis) and multi-species biofilm communities formed by these fungi. On the other hand, this EO at 1,000 μg/ml concentrations did not reduce human red blood cell viability. Notably, phytochemical evaluations pointed out eugenol as the main component (68.96%) of the EO extracted from C. zeylanicum Blume leaves (Rangel et al., 2018). A recent study also reported eugenol as the main compound (77.22%) of Cinnamomum verum EO. This EO suppressed initial adhesion, germ tube formation, and biofilm progression of Candida. Furthermore, the microscopic assessments showed decreased hyphal formation, cell wall damage, and cell shrinkage after treatment with this EO. Nevertheless, no lethal effect of C. verum EO was observed using the Galleria mellonella experimental model at the various concentrations tested (Wijesinghe et al., 2020).
These observations support the findings by Wijesinghe et al. (2021), who reported eugenol as the main compound of C. verum EO. This EO also suppressed established biofilm and hyphal production in Candida by causing cell wall injuries and cellular shrinkage. On the other hand, C. verum EO did not exhibit any effect on HaCaT (aneuploid immortal keratinocyte cell line from adult human skin) cells (Wijesinghe et al., 2021). Hence, Cinnamomum EO contains eugenol as its major chemical component, which can suppress the biofilm community and cause cell wall damage in Candia. Various studies indicate low cytotoxicity of this compound in different human cell lines.
Eugenol can be used to treat Candida biofilm-associated oral disorders. In a study conducted by Jafri et al. (2019), eugenol was used to inhibit single and mixed biofilms of S. mutans and C. albicans resistant to AMB, itraconazole, and ketoconazole. The interaction of these two significant oral pathogens may result in recalcitrant and resistant infections in the oral cavity, thereby increasing the complexity of managing them. Microscopic analysis revealed that eugenol treatment leads to cell shape alterations, reduced cell aggregation, and disordered single and mixed biofilms. At sub-MIC of 100 μg/ml, eugenol significantly suppressed single and mixed biofilms formed by the drug-resistant strains of the two oral pathogens (Jafri et al., 2019). Another investigation also reported inhibitory effects of eugenol against the planktonic and biofilm community of C. tropicalis and Candida dubliniensis (dose-dependent and fluconazole-resistant strains) isolated from the oral cavity of HIV-positive patients. No metabolic activity was detected in the biofilms after 24 h of treatment with eugenol (500 μg/ml); moreover, this compound markedly decreased the biofilm cells on denture material surfaces. It is noteworthy that eugenol significantly inhibited the adhesion of Candida to the polystyrene and HEp-2 cells; thus, the authors proposed that eugenol may have an additional beneficial effect in the treatment of local candidiasis (De Paula et al., 2014).
Previous studies have investigated the combination therapy of eugenol and fluconazole against Candida biofilms. A study conducted in 2014 described a synergistic effect of fluconazole and eugenol against a planktonic community of C. albicans. The established biofilm of this fungus was highly resistant to fluconazole, while sensitization of fungal cells by eugenol (at sub-inhibitory concentrations) led to the inhibition of biofilm formation at low fluconazole concentrations. The authors hypothesized that eugenol destabilizes the cytoplasmic membrane and causes specific signal intervention. Hence, this compound caused sensitization of C. albicans biofilms, boosting fluconazole penetration and leading to the inhibition of biofilm formation (Doke et al., 2014).
Additionally, Khan et al. reported that preformed C. albicans biofilms showed ≥1,024 x increased resistance to fluconazole but no increased tolerance to eugenol. This compound inhibited the biofilm formation in C. albicans and exhibited a synergistic interaction with fluconazole against biofilms formed by the test strains. The microscopic evaluation showed the effect of eugenol on the cell membrane integrity, as evidenced by the shrinkage of the cell surface in the biofilm cells. Therefore, the authors proposed that when eugenol, which possesses cidal activity against the biofilm cells, is combined with fluconazole, the drug’s fungistatic nature is converted to fungicidal (Khan and Ahmad, 2012).
In line with these observations, another investigation performed in 2020 in India reported that eugenol suppressed S. mutans and C. albicans mixed biofilms. The sessile MIC of fluconazole was increased up to 1,000-fold over planktonic MIC. Notably, eugenol was highly synergistic with fluconazole against C. albicans single and mixed biofilms. The microscopic analysis also confirmed these findings and showed distorted cell structure, decreased matrix production, and elimination of single and mixed biofilm cells of S. mutans and C. albicans in the samples treated with eugenol. Therefore, eugenol may disrupt cell membrane integrity and boost the drug entry into the microbial cell. This phenomenon increases the antimicrobial drug availability in the deeper layers of biofilm, consequently improving treatment efficacy (Jafri et al., 2020).
The results reported in the above studies indicate that besides having fungicidal activity, eugenol could potentially inhibit the adhesion capacity of planktonic cells of Candida. The compound suppressed biofilm formation and destroyed the established biofilm of these fungi formed on various surfaces. Thus, eugenol is a natural product with potential for non-toxic therapeutic application in the treatment of candidiasis by interfering with the most important virulence factors of Candida species, such as germ tube formation, adhesion to various host surfaces, and biofilm formation. However, the exact mechanism of antifungal and antibiofilm effects of eugenol against Candida is not clearly understood; hence, further studies are required.
Terpinen-4-ol
Terpinen-4-ol is a monoterpene and the main bioactive ingredient of tea tree oil (TTO). It is found in many aromatic plants such as mandarins, Japanese cedar, black pepper, oregano, oranges, and New Zealand lemonwood tree (Pino et al., 2003; Shapira et al., 2016). Monoterpenes are major plant-derived secondary metabolites extensively found in natural products, such as vegetables, herbs, and fruits. They are known to be related with the plant defense activity (Gershenzon and Dudareva, 2007; Shapira et al., 2016). Previous studies have reported antibacterial and antifungal properties of terpinen-4-ol (Dryden et al., 2004; Mertas et al., 2015). Thus, scientists have been interested in applying this compound for the inhibition and degradation of Candida biofilm.
Francisconi et al. (2020a) reported that TTO and its main component terpinen-4-ol suppressed the growth of C. albicans. The application of TTO and terpinen-4-ol for 60 s (rinse simulation) at the concentrations of 17.92 mg/ml and 8.86 mg/ml, respectively, disrupted the biofilm formation of C. albicans. Thus, terpinen-4-ol could suppress biofilm formation at a lower concentration than the TTO. Furthermore, the microscopic analysis showed that this compound penetrated the cytoplasmic membrane, caused C. albicans cell membrane disruption, and interfered with the pathogen cell’s integrity and physiology. The authors suggested that the lipophilicity of TTO and terpinen-4-ol could be related to the mentioned antifungal characteristics (Francisconi et al., 2020a). In another study, the authors used TTO and terpinen-4-ol for inhibiting the biofilm community of C. albicans. TTO and terpinen-4-ol killed the planktonic C. albicans isolates; also, terpinen-4-ol showed potent activity against the biofilm structure of this fungus. Notably, terpinen-4-ol was not cytotoxic at 0.5 × MIC50 (the concentration able to suppress C. albicans growth) against OKF6-TERT2 epithelial cells. Accordingly, the authors concluded that the use of terpinen-4-ol, the main ingredient of TTO, has advantages over the complete EO in terms of product consistency as well as safety and may be useful for preventing and treating various C. albicans biofilm-associated infections, especially established oropharyngeal candidiasis (Ramage et al., 2012).
Another study has shown that the combined use of the terpinen-4-ol and antifungals like nystatin exhibited synergistic effects in inhibiting Candida biofilms. Nystatin binds to the steroids within the cell membranes of susceptible fungi, interferes with the cell membrane permeability, and causes the cytoplasmic content to leak from the cell. Nonetheless, this antifungal drug has some adverse effects in humans, such as an unpleasant taste and gastrointestinal disorders, and fungal resistance to nystatin has been reported (Francisconi et al., 2020a). Hence, the synergistic effects of nystatin with various natural compounds could help reduce the nystatin dosage and its unwanted side effects, prevent the development of resistance to this antifungal drug and boost its clinical application.
In a recent study, the authors assessed the antifungal activity of terpinen-4-ol in combination with nystatin against C. albicans and C. tropicalis mixed-species biofilm. The results indicated that terpinen-4-ol and nystatin at 4.53 mg/ml and 0.008 mg/ml, respectively, were able to suppress biofilm growth. A synergistic antifungal effect was observed with the drug combination, and a reduction of nystatin inhibitory concentration up to eight times in the mono-species biofilm of C. albicans, and up to two times in mixed-species biofilm was achieved. Further, terpinen-4-ol limited the adhesion of C. tropicalis to oral keratinocytes, while this compound did not show inhibitory effects on the fungal enzymatic activity (Tonon et al., 2018). Terpinen-4-ol, like other monoterpenes, has a hydrophobic nature and a capacity for binding to different lipophilic structures of fungi, such as the plasma membrane. Accordingly, this compound enhanced non-specific cell permeability and caused the loss of essential electrolytes required for cell survival (Carson et al., 2006). Thus, it seems that terpinen-4-ol disturbs the Candida cell membrane permeability and increases the penetration of nystatin to the deeper layers of biofilms.
In another interesting study, Francisconi et al. (2020b) developed a liquid crystalline system (LCS) incorporated with nystatin and terpinen-4-ol to assess its antibiofilm and synergistic/modulatory function against C. albicans. The LCS containing nystatin and terpinen-4-ol significantly suppressed the C. albicans growth and biofilm formation at a lower concentration of each compound, displaying a synergistic action. The mucoadhesion analyses indicated that the addition of artificial saliva to the LCS improved its mucoadhesive and viscosity properties. Thus, the authors suggested LCS incorporated with nystatin and terpinen-4-ol as a promising antibiofilm agent for preventing and treating the C. albicans infection; however, more confirmatory studies are required (Francisconi et al., 2020b). Thus, nystatin combination with terpinen-4-ol could lower the nystatin quantity used in antifungal treatments, thereby diminishing unwanted side effects.
In addition to the above drug platform for terpinen-4-ol delivery, the researchers have developed other platforms for boosting the antibiofilm efficacy of this compound against Candida biofilms and overcoming high volatilization and nonwettability characteristics of terpinen-4-ol, which limits its clinical usage,. In one of such studies, the authors used PEG-stabilized lipid NPs loaded with terpinen-4-ol for C. albicans biofilm elimination. The results showed that this platform could suppress the biofilm community of this fungus. Various analyses confirmed that terpinen-4-ol-loaded lipid NP’s antibiofilm activity results from its capacity to destroy the cell membrane structure of C. albicans and block the respiratory chain by inhibiting succinate dehydrogenase attached to the inner mitochondrial membrane of cells (Sun et al., 2012). In line with these results, Souza et al. (2017) also used nanostructured lipid carriers (synthesized by a proprietary method) for the delivery of TTO and degradation of Candida biofilms. The chromatographic profile indicated that the TTO oil was in accordance with ISO 4730, with 41.9% terpinen-4-ol as a major constituent. The synthesized TTO NPs significantly reduced the biofilm formation of various Candida species including C. albicans, C. glabrata, C. parapisilosis, C. tropicalis, and Candida membranafaciens. Furthermore, the TTO NPs lowered the levels of the biofilm protein and exopolysaccharides (Souza et al., 2017).
The above studies suggest that the use of nanobiotechnology for delivery of terpinen-4-ol could enhance its antibiofilm activity, making it a suitable alternative against Candida biofilm-associated infections; however, there are very limited data in this area, and more studies are required. Collectively, terpinen-4-ol could be used for the treatment of Candida infections, especially for use in prophylactic oral hygiene products such as mouth rinses and denture cleansers.
Cinnamaldehyde
Recent investigations have focused on the cinnamaldehyde’s ability to inhibit Candida biofilm formation, thereby limiting the extension of decreasing or resistant antifungal selective pressure. Cinnamaldehyde, one of the main Cinnamomum ingredients constituting about 65% of it, could have an antimicrobial activity due to its acrolein group (α,β-unsaturated carbonyl moiety). Cinnamaldehyde does not suffer from antibiotic resistance despite its strong effect on pathogenic infections (Bae et al., 1992). In recent years, in addition to its antimicrobial effect, scientists have been interested in using cinnamon and its derivative components, especially cinnamaldehyde, to inhibit microbial biofilms (Kosari et al., 2020). This section primarily focuses on the role of cinnamaldehyde in the suppression and elimination of Candida biofilms to facilitate its possible widespread use in clinical practice.
Choonharuangdej et al. (2021) used Cinnamomum oil to inhibit preformed C. albicans biofilm on dental devices made from heat Polymerized Polymethyl Methacrylate (PMMA) resin. PMMA is associated with the development of mild to severe candidiasis in patients who wear it. Cinnamomum oil eliminated 99% of the pre-established Candida biofilm on this resin. Additionally, coating the PMMA samples with this oil for 24 h also reduced the formation of C. albicans biofilm by almost 70.0% (Choonharuangdej et al., 2021). In addition, the results of a recently published study illustrated intense antifungal activity for cinnamaldehyde against Candida species isolated from patients with oral candidiasis. Moreover, this compound decreased the metabolic activity and biomass of mature biofilms (Miranda-Cadena et al., 2021a). Notably, the reduction of biofilm biomass may have an essential role in handling resistant infections because biofilms are a source for dispersal of cells, resulting in the formation of new biofilms and enhancement of virulence and adhesion (Uppuluri et al., 2010; Nobile and Johnson, 2015).
It should be noted that the results of a study published in 2021 showed that Cinnamomum verum EO at concentrations of 1,000–2,000 µg/ml has no toxic effects on normal human keratinocyte cell line (Wijesinghe et al., 2021).
In addition to the research mentioned above, some studies examined the molecular interactions of cinnamaldehyde with Candida biofilms and obtained interesting results. A study conducted by El-Baz et al. (2021) reported that C. verum EO had an inhibitory effect against the biofilms formed by C. albicans strains isolated from different clinical samples. This EO also suppressed the hemolysin and phospholipase activity of this fungus. Microscopic images showed a decreased biofilm formation due to the suppressed adhesion. Molecular docking showed that cinnamaldehyde, as the main component of C. verum EO, has an impact on Als3 (El-Baz et al., 2021). Thus, the interaction of cinnamaldehyde and Als3 holds promise for using this compound to inhibit C. albicans adhesion and biofilm formation (El-Baz et al., 2021).
Another study discovered that cinnamaldehyde inhibited biofilm formation and affected Candida cellular development, as detected by specific features such as the absence of chlamydoconidia and the expression of rare pseudo-hyphae. Molecular docking analysis revealed that cinnamaldehyde presented negative ligand-receptor interaction energy at the studied targets with the most affinity for squalene epoxidase and thymidylate synthase. Therefore, the authors hypothesized that cinnamaldehyde could restrict the formation of biofilms in Candida by affecting important targets present in the fungal cell and nucleus; however, further docking studies are required for the precise identification of the proteins targeted by cinnamaldehyde (Da Nóbrega Alves et al., 2020). Furthermore, Gupta et al. discovered that cinnamaldehyde destroyed the biofilms formed by C. glabrata clinical isolates on biomaterials such as urinary catheters and contact lenses. Cinnamaldehyde enhanced cell lysis, ROS production, and plasma membrane ergosterol content; It also suppressed the phospholipase, catalase, and proteinase activity of C. glabrata cells. Detailed molecular analysis revealed that cinnamaldehyde downregulated the expression of FKS1, AUS1, KRE1, and CDR1 genes related to the 1,3-β-glucan synthase, sterol importer, GPI-anchored protein, and multi-drug transporter, respectively. As a result, the authors hypothesized that interactions between cinnamaldehyde and ergosterol, result in the formation of pores that alter the permeability and integrity of the cell membrane and ultimately result in intracellular content leakage and cell lysis (Gupta et al., 2018). According to the above-mentioned studies, the interaction of cinnamaldehyde with different cellular pathways of Candida could inhibit biofilm formation and suppress various virulence phenotypes of this fungus. However, the data on this subject are scarce, necessitating additional research.
Due to the poor solubility of cinnamaldehyde in aqueous solutions and its instability and volatility, the typical dosage of oral rinses enables only short exposure to the compound and may not be suitable to treat active infections. Different drug platforms have been considered in recent years to overcome this limitation and increase the efficiency of cinnamaldehyde against Candida biofilms. Mishra et al. (2021a) addressed the above issue by incorporating cinnamaldehyde into electrospun gellan (GA)/polyvinyl alcohol (PVA) nanofibers. These nanofibers eliminated 50.45% and 89.29% of C. albicans and C. glabrata biofilm, respectively. The authors proposed that the unique characteristics of C. albican’s biofilm, such as a multi-layered structure consisting of blastosphores, hyphae, and pseudohyphae, could be related to the lower antibiofilm activity of the nanofibers against it. On the other hand, C. glabrata biofilm, consisting of a monolayer of cells with psuedohyphal and yeast morphology and a discontinuous matrix is more susceptible to the treatment (Mishra et al., 2021a). Another investigation evaluated the antibiofilm effect of cinnamaldehyde encapsulated in multilamellar liposomes (CM-ML) against C. albicans clinical isolates. CM-ML containing cinnamaldehyde showed improved fungicidal effects than cinnamaldehyde alone, possibly due to sustained release of cinnamaldehyde in the encapsulated form (Khan et al., 2017).
Finally, in a recent study, cinnamaldehyde was loaded onto poly (DL-lactide-co-glycolide) (PLGA) NPs (CA-PLGA NPs) and their antibiofilm activity against C. albicans was assessed. The results showed that the amount of active ingredient loaded in CA-PLGA NPs was much lower than the free cinnamaldehyde, and a strong antifungal effect was obtained even at this rate. Notably, the post-biofilm application of CA-PLGA NPs was more effective than the pre-biofilm application (Gursu et al., 2021). Therefore, the use of drug delivery systems for increasing the effectiveness of cinnamaldehyde may allow the use of lower doses to eliminate Candida biofilms. Different drug platforms can also mitigate the cytotoxicity caused by cinnamaldehyde. In this regard, future studies should focus more on encapsulating cinnamaldehyde in different carriers to enhance Candida biofilm inhibition.
Linalool
Linalool, an unsaturated monoterpene alcohol with a pleasant odor, is produced by over 200 plant species belonging to different families, such as Rutaceae (citrus), Lauraceae (cinnamon, laurels, rosewood), and Lamiaceae (mints) (Kamatou and Viljoen, 2008; Elsharif et al., 2015). Linalool is known as a chemical intermediate in the biosynthesis of vitamin E. Additionally, this compound is one of the main ingredients of various EOs with different biological activities such as antiplasmodial, anti-inflammatory, antinociceptive, antihyperalgesic, and antibacterial (Peana et al., 2006; Van Zyl et al., 2006; Kamatou and Viljoen, 2008). Since linalool’s discovery, this compound has been extensively used in various clinical and industrial investigations. Previous studies have introduced linalool as a promising antibiofilm agent against Candida biofilm, and this section reviews these studies.
In one of these studies, the authors reported linalool as the main component (29%) of Iranian S. macrosiphon EO. This EO at concentrations of 4–8 μg/ml showed antibiofilm activity against various species of Candida (Motamedi et al., 2019). Another study also showed that linalool-rich EO from Croton cajucara inhibited the growth of various oral microorganisms such as C. albicans. In this study, purified linalool also inhibited the C. albicans biofilm community. The electron microscopy indicated that linalool treatment led to abnormal germination and cell size reduction in the fungus. Germ tube production is an essential virulence factor for C. albicans invasion; the inhibition of this virulence factor could be a possible treatment option for Candida infections (Alviano et al., 2005). Souza et al. (2016) evaluated the cytotoxicity effects of linalool on normal human lung fibroblasts, and the compound’s IC50 value (50% inhibition of cell line) was 1,253.00 ± 2.83 μg/ml.
Other studies evaluated the cellular interactions of linalool and Candida biofilms. A recent study indicated that linalool significantly suppressed C. albicans biofilm formation without affecting the planktonic cell growth. This compound, combined with α-longipinene (the main ingredient of cascarilla bark oil) and helichrysum oil, synergistically reduced biofilm formation. Further, linalool potentially inhibited C. albicans hypha formation. Notably, the biofilm community C. albicans is composed of hyphal, psuedohyphal, and yeast elements, and the transition from the yeast-to-hyphal stage could be an important virulence factor (Manoharan et al., 2017a). Abnormal biofilm communities developed in strains with defects in hyphae formation; the biofilm structure was thin, densely packed with yeast cells, and easily detached from the substratum (Nobile and Mitchell, 2006). Accordingly, the authors proposed that linalool-mediated inhibition of C. albicans biofilm formation could result from its interference with the production and induction of filaments. Additionally, using linalool with other natural compounds as a combinational therapy could be more useful in fungal biofilm elimination (Manoharan et al., 2017a). These results support the findings of Hsu et al. (2013), who reported that linalool eliminated the preformed biofilm structure of C. albicans by >80% at 2 × MIC. Further, this compound suppressed germ tube and biofilm formation in this fungus. The molecular analysis indicated that linalool downregulated the expression of various pathogenic genes in C. albicans such as ALS3 and HWP1 (adhesion-related genes), CPH1 and CYR1 (encoding components of the Mitogen-activated protein kinase and CAMP-Protein kinase A hyphal formation regulatory pathways, respectively) as well as EED1, HGC1, and UME6 (long-term hyphae maintenance-associated genes) (Hsu et al., 2013).
These findings indicate that linalool not only suppresses C. albicans attachment and biofilm formation but also exerts an inhibitory effect on the pathways that regulate hyphal transformation and the mechanisms related to hyphal maintenance. The above studies highlight the antibiofilm activities of linalool for treating Candida biofilm-associated infections, such as those associated with implant medical devices, and the potential application of this compound in treating human and animal infections. However, more studies are required for a better understanding of linalool’s interactions with Candida biofilms.
Geraniol
Geraniol, trans-3,7-dimethyl-2,6-octadien-1-ol, is an acyclic monoterpene alcohol detected in various EOs such as those of geranium, rose, and lemongrass. Because of its distinctive floral aroma and sweetness, this compound is one of the main commercial fragrance compounds that has been used in 58% of perfumes, 26% of cosmetic creams, and 40% of deodorants (Chacón et al., 2019). Recent studies have suggested additional commercial uses for geraniol as an anti-inflammatory, pain-relieving, and anticancer compound (Peralta-Yahya and Keasling, 2010; De Cássia Da Silveira E Sá et al., 2013; Chacón et al., 2019). The antimicrobial activity of this compound against the planktonic communities of various Candida species has also been reported. Thus, researchers have been interested in employing geraniol for inhibiting and degrading the biofilm structures of these fungi (Leite et al., 2015; Gupta et al., 2021a).
A recent study reported that geraniol not only suppressed both planktonic and sessile growth of various C. glabrata strains, but also degraded the mature biofilm structure of these fungi. Microscopic and molecular analysis indicated that geraniol lowered the eDNA and carbohydrate content and hydrolytic enzyme activity in C. glabrata extracellular matrix. This compound targeted β-glucan and chitin in the cell wall, reduced the cell membrane ergosterol content, altered the activity of mitochondria, increased Ca2+ uptake, and blocked ABC drug efflux pumps. Furthermore, the real-time polymerase chain reaction analysis indicated that the expression of ERG4 and CDR1, which is related to ergosterol biosynthesis and multi-drug efflux transport, respectively, was downregulated by geraniol (Gupta et al., 2021a). These results align with the results obtained by Singh et al. (2016), who evaluated the interactions of geraniol with C. albicans cellular pathways. The findings revealed that geraniol suppressed both the biofilm formation and virulence attributes of hyphal morphogenesis in C. albicans. Further, this compound caused depletion of ergosterol levels, alteration of plasma membrane ATPase activity, iron genotoxicity, homeostasis dysregulation, and mitochondrial dysfunction (Singh et al., 2016).
In addition to the above Candida species, geraniol (at 500 μg/ml) remarkably reduced the number of viable biofilm cells and inhibited biofilm formation in a clinical strain of C. tropicalis after exposure for 48 h. The microscopic examination after treatment with this compound revealed a complex architecture with the upper layer of filamentous cells and basal layer of yeast cells. Additionally, geraniol at concentrations that were active against C. tropicalis, did not show cytotoxic effects on normal human lung fibroblasts (Souza et al., 2016). The above findings indicate that geraniol has negative effects on various Candida cellular pathways such as ABC transporter-mediated drug transport, ergosterol, chitin, and glucan biosynthesis and on cell cycle progression and mitochondrial activity (Figure 2). Thus, geraniol should be considered as a new antifungal agent against various Candida species infections.
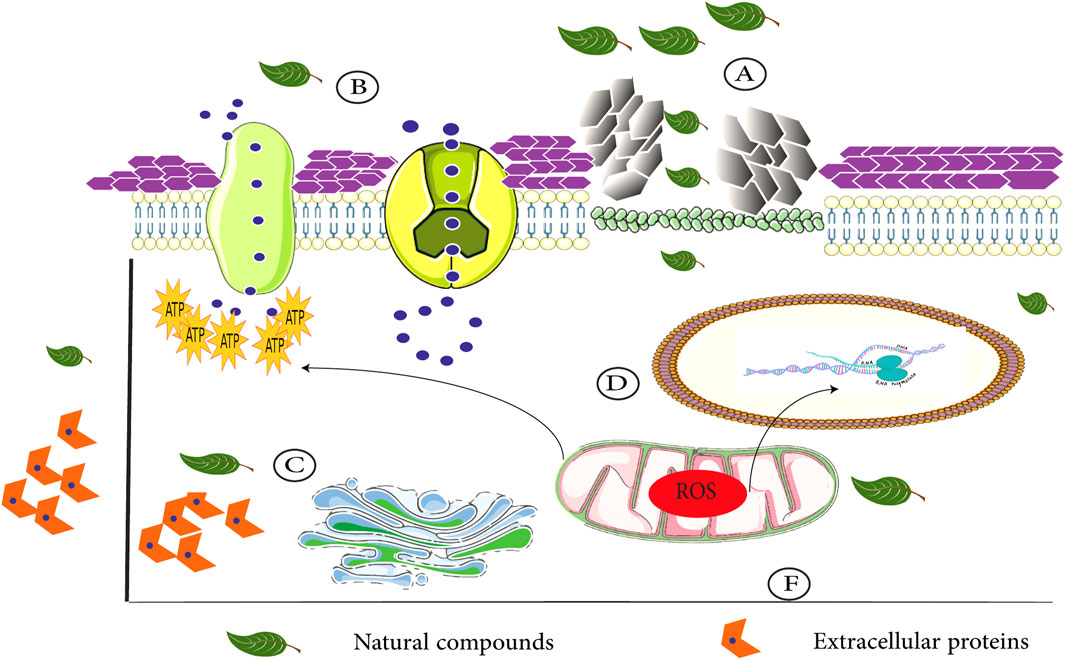
FIGURE 2. Different interactions of natural compounds with Candida cellular pathways. (A) Ergosterol biosynthesis inhibition (cytoplasm membrane disruption) and cell wall degradation. (B) Negative effects on various Candida cellular pathways such as ABC drug transport: additionally, modulated efflux pump activity. (C) Blockage of proteinase and phospholipase activity and intracellular ATPase production. (D) Downregulation of the expression of various adhesion and biofilm-associated genes. (F) Natural compounds lead to mitochondrial dysfunction.
The combination of geraniol and fluconazole also showed promising outcomes for the inhibition of C. albicans biofilm. The results of a study published in 2018 demonstrated that geraniol decreased biofilm biomass, impaired fungal adherence to the epithelial cells, and was synergistic with fluconazole against C. albicans. The authors proposed that geraniol modulated efflux pump activity by binding to the active site of CaCdr1p (drug efflux pump). Hence, modulation of efflux pump activity by geraniol and fluconazole synergism represents a promising approach for combinatorial treatment of candidiasis (Singh et al., 2018). Geraniol (at a MIC of 152 μg/ml) showed a good inhibitory effect against both fluconazole-sensitive and resistant C. albicans strains. The use of fluconazole with geraniol boosted the antifungal function of the combination, mainly against the resistant strain. Geraniol caused cell wall thickening and irregularities in the membrane structure, reduced ergosterol biosynthesis, and inhibited biofilm formation in C. albicans (Cardoso et al., 2016). Collectively, geraniol could boost the fluconazole activity by inhibiting C. albicans efflux pump activity and degrading the external structure of this fungi. The ability of geraniol to sensitize Candida cells to fluconazole opens up new options for combination therapy; however, more studies in animal models and clinical trials are needed before clinical application of this combination becomes a reality. Its noteworthy that other studies have used natural compounds for inhibition and destruction of Candida biofilm are presented in Table 2.
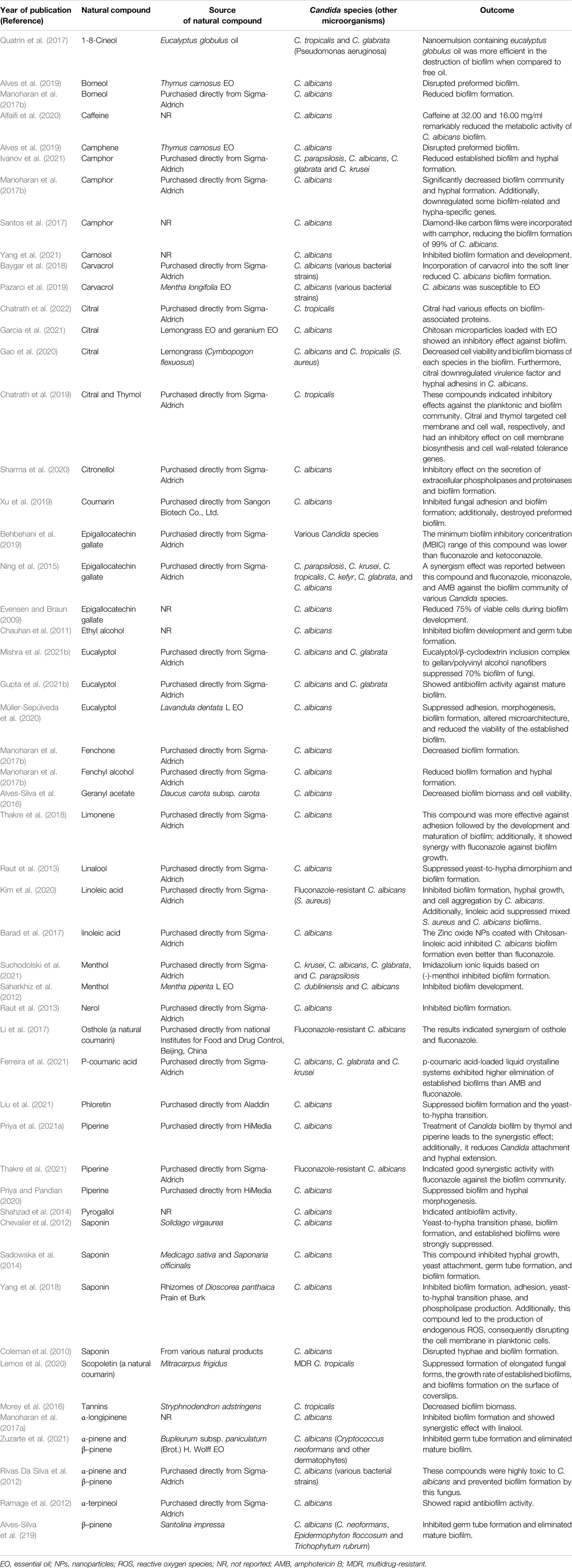
TABLE 2. The studies that have employed various natural compounds for inhibition and degradation of the Candida biofilm. Natural compounds that are the main ingredient of their-associated plants are reported here.
Conclusion
This article discussed various natural compounds with the potential for inhibiting and degrading Candida biofilm. These compounds have shown negative effects on different cellular mechanisms of Candida and could boost the efficacy of antifungals against biofilm communities. Thus, natural compounds should be considered for the treatment of Candida biofilm-associated infections, especially for established oropharyngeal candidiasis, since local treatment is easier in these infections. Disadvantages such as low oral bioavailability, water insolubility, and rapid metabolism and degradation limit the clinical application of many natural compounds. The use of various drug delivery platforms could be useful in such cases and should be evaluated in future studies. Most studies have evaluated the antibiofilm efficacy of natural compounds only in C. albicans, and that also in vitro. As such, future studies should evaluate the antibiofilm activity of natural compounds against other clinical important clinically important species such as Candida auris. Furthermore, before major clinical usage of natural compounds, more animal studies and clinical trials are required to confirm in vitro findings.
Author Contributions
AS and MD conceived and designed the study. AS and FS contributed in comprehensive research. ZC, AS, and MD wrote the paper. SR and MH participated in manuscript editing. Notably, all authors have read and approved the manuscript.
Conflict of Interest
The authors declare that the research was conducted in the absence of any commercial or financial relationships that could be construed as a potential conflict of interest.
Publisher’s Note
All claims expressed in this article are solely those of the authors and do not necessarily represent those of their affiliated organizations, or those of the publisher, the editors and the reviewers. Any product that may be evaluated in this article, or claim that may be made by its manufacturer, is not guaranteed or endorsed by the publisher.
Abbreviations
EOs, essential oils; ALS, agglutinin‐like sequence; PEG, polyethylene glycol; NPs, nanoparticles; GC-GC/MS, gas chromatography-mass spectrometry; MICs, minimum inhibitory concentrations; PLA, poly (lactic acid); AMB, amphotericin B; PMMA, polymerized Polymethyl Methacrylate; TTO, tea tree oil; LCS, liquid crystalline system; GA, electrospun gellan; PVA, polyvinyl alcohol; CM-ML, cinnamaldehyde in a multilamellar liposome; PLGA, poly (DL-lactide-co-glycolide).
References
Abrahão, M. R., Molina, G., and Pastore, G. M. (2013). Endophytes: Recent Developments in Biotechnology and the Potential for Flavor Production. Food Res. Int. 52, 367–372. doi:10.1016/j.foodres.2013.03.007
Achkar, J. M., and Fries, B. C. (2010). Candida Infections of the Genitourinary Tract. Clin. Microbiol. Rev. 23, 253–273. doi:10.1128/CMR.00076-09
Ahmad, A., Wani, M. Y., Khan, A., Manzoor, N., and Molepo, J. (2015). Synergistic Interactions of Eugenol-Tosylate and its Congeners with Fluconazole against Candida Albicans. Plos one 10, e0145053. doi:10.1371/journal.pone.0145053
Al-Ani, E., and Heaselgrave, W. (2022). The Investigation of Thymol Formulations Containing Poloxamer 407 and Hydroxypropyl Methylcellulose to Inhibit Candida Biofilm Formation and Demonstrate Improved Bio-Compatibility. Pharm. (Basel) 15, 71. doi:10.3390/ph15010071
Alalwan, H., Rajendran, R., Lappin, D. F., Combet, E., Shahzad, M., Robertson, D., et al. (2017). The Anti-adhesive Effect of Curcumin on Candida Albicans Biofilms on Denture Materials. Front. Microbiol. 8, 659. doi:10.3389/fmicb.2017.00659
Alfaifi, A. A., Lin, W. S., Aldhaian, B. A., Levon, J. A., and Gregory, R. L. (2020). Impact of Caffeine on Metabolic Activity and Biofilm Formation of Candida Albicans on Acrylic Denture Resin in the Presence of Nicotine. J. Prosthet. Dent. 123, 875–879. doi:10.1016/j.prosdent.2019.09.007
Alves, M., Gonçalves, M. J., Zuzarte, M., Alves-Silva, J. M., Cavaleiro, C., Cruz, M. T., et al. (2019). Unveiling the Antifungal Potential of Two Iberian Thyme Essential Oils: Effect on C. Albicans Germ Tube and Preformed Biofilms. Front. Pharmacol. 10, 446. doi:10.3389/fphar.2019.00446
Alves-Silva, J. M., Zuzarte, M., Gonçalves, M. J., Cavaleiro, C., Cruz, M. T., Cardoso, S. M., et al. (2016). New Claims for Wild Carrot (Daucus Carota Subsp. Carota) Essential Oil. Evid. Based Complement. Altern. Med. 2016, 9045196. doi:10.1155/2016/9045196
Alves-Silva, J. M., Zuzarte, M., Gonçalves, M. J., Cruz, M. T., Cavaleiro, C., and Salgueiro, L. (2019). Unveiling the Bioactive Potential of the Essential Oil of a Portuguese Endemism, Santolina Impressa. J. Ethnopharmacol. 244, 112120. doi:10.1016/j.jep.2019.112120
Alviano, W. S., Mendonça-Filho, R. R., Alviano, D. S., Bizzo, H. R., Souto-Padrón, T., Rodrigues, M. L., et al. (2005). Antimicrobial Activity of Croton Cajucara Benth Linalool-Rich Essential Oil on Artificial Biofilms and Planktonic Microorganisms. Oral Microbiol. Immunol. 20, 101–105. doi:10.1111/j.1399-302X.2004.00201.x
Andrade, M. C., Ribeiro, A. P., Dovigo, L. N., Brunetti, I. L., Giampaolo, E. T., Bagnato, V. S., et al. (2013). Effect of Different Pre-irradiation Times on Curcumin-Mediated Photodynamic Therapy against Planktonic Cultures and Biofilms of Candida Spp. Arch. Oral Biol. 58, 200–210. doi:10.1016/j.archoralbio.2012.10.011
Arabi Monfared, A., Ayatollahi Mousavi, S. A., Zomorodian, K., Mehrabani, D., Iraji, A., and Moein, M. R. (2020). Trachyspermum Ammi Aromatic Water: A Traditional Drink with Considerable Anti-Candida Activity. Curr. Med. Mycol. 6, 1–8. doi:10.18502/cmm.6.3.3979
Bae, K.-H., Ji, J.-M., and Park, K.-L. (1992). The Antibacterial Component from Cinnamomi Cortex against a Cariogenic bacteriumStreptococcus Mutans OMZ 176. Arch. Pharm. Res. 15, 239–241. doi:10.1007/bf02974062
Barad, S., Roudbary, M., Omran, A. N., and Daryasari, M. P. (2017). Preparation and Characterization of ZnO Nanoparticles Coated by Chitosan-Linoleic Acid; Fungal Growth and Biofilm Assay. Bratisl. Lek. Listy 118, 169–174. doi:10.4149/BLL_2017_034
Baygar, T., Ugur, A., Sarac, N., Balci, U., and Ergun, G. (2018). Functional Denture Soft Liner with Antimicrobial and Antibiofilm Properties. J. Dent. Sci. 13, 213–219. doi:10.1016/j.jds.2017.10.002
Behbehani, J. M., Irshad, M., Shreaz, S., and Karched, M. (2019). Synergistic Effects of Tea Polyphenol Epigallocatechin 3-O-Gallate and Azole Drugs against Oral Candida Isolates. J. Mycol. Med. 29, 158–167. doi:10.1016/j.mycmed.2019.01.011
Bizerra, F. C., Nakamura, C. V., De Poersch, C., Estivalet Svidzinski, T. I., Borsato Quesada, R. M., Goldenberg, S., et al. (2008). Characteristics of Biofilm Formation by Candida tropicalis and Antifungal Resistance. FEMS Yeast Res. 8, 442–450. doi:10.1111/j.1567-1364.2007.00347.x
Braga, P. C., Culici, M., Alfieri, M., and Dal Sasso, M. (2008). Thymol Inhibits Candida Albicans Biofilm Formation and Mature Biofilm. Int. J. Antimicrob. Agents 31, 472–477. doi:10.1016/j.ijantimicag.2007.12.013
Brown, G. D., Denning, D. W., Gow, N. A., Levitz, S. M., Netea, M. G., and White, T. C. (2012). Hidden Killers: Human Fungal Infections. Sci. Transl. Med. 4, 165rv13–165rv113. doi:10.1126/scitranslmed.3004404
Cardoso, N. N., Alviano, C. S., Blank, A. F., Romanos, M. T., Fonseca, B. B., Rozental, S., et al. (2016). Synergism Effect of the Essential Oil from Ocimum Basilicum Var. Maria Bonita and its Major Components with Fluconazole and its Influence on Ergosterol Biosynthesis. Evid. Based Complement. Altern. Med. 2016, 5647182. doi:10.1155/2016/5647182
Carson, C. F., Hammer, K. A., and Riley, T. V. (2006). Melaleuca Alternifolia (Tea Tree) Oil: a Review of Antimicrobial and Other Medicinal Properties. Clin. Microbiol. Rev. 19, 50–62. doi:10.1128/CMR.19.1.50-62.2006
Chacón, M. G., Marriott, A., Kendrick, E. G., Styles, M. Q., and Leak, D. J. (2019). Esterification of Geraniol as a Strategy for Increasing Product Titre and Specificity in Engineered Escherichia coli. Microb. Cell Fact. 18, 105. doi:10.1186/s12934-019-1130-0
Chami, N., Bennis, S., Chami, F., Aboussekhra, A., and Remmal, A. (2005). Study of Anticandidal Activity of Carvacrol and Eugenol In Vitro and In Vivo. Oral Microbiol. Immunol. 20, 106–111. doi:10.1111/j.1399-302X.2004.00202.x
Chandra, J., Kuhn, D. M., Mukherjee, P. K., Hoyer, L. L., Mccormick, T., and Ghannoum, M. A. (2001). Biofilm Formation by the Fungal Pathogen Candida Albicans: Development, Architecture, and Drug Resistance. J. Bacteriol. 183, 5385–5394. doi:10.1128/jb.183.18.5385-5394.2001
Chatrath, A., Kumar, M., and Prasad, R. (2022). Comparative Proteomics and Variations in Extracellular Matrix of Candida tropicalis Biofilm in Response to Citral. Protoplasma 259, 263–275. doi:10.1007/s00709-021-01658-6
Chatrath, A., Gangwar, R., Kumari, P., and Prasad, R. (2019). In Vitro Anti-Biofilm Activities of Citral and Thymol against Candida Tropicalis. J. Fungi (Basel) 5, 13. doi:10.3390/jof5010013
Chauhan, N. M., Raut, J. S., and Karuppayil, S. M. (2011). A Morphogenetic Regulatory Role for Ethyl Alcohol in Candida Albicans. Mycoses 54, e697–703. doi:10.1111/j.1439-0507.2010.02002.x
Chen, E., Benso, B., Seleem, D., Ferreira, L. E. N., Pasetto, S., Pardi, V., et al. (2018). Fungal-Host Interaction: Curcumin Modulates Proteolytic Enzyme Activity of Candida Albicans and Inflammatory Host Response In Vitro. Int. J. Dent. 2018, 2393146. doi:10.1155/2018/2393146
Chevalier, M., Medioni, E., and Prêcheur, I. (2012). Inhibition of Candida Albicans Yeast-Hyphal Transition and Biofilm Formation by Solidago Virgaurea Water Extracts. J. Med. Microbiol. 61, 1016–1022. doi:10.1099/jmm.0.041699-0
Choonharuangdej, S., Srithavaj, T., and Thummawanit, S. (2021). Fungicidal and Inhibitory Efficacy of Cinnamon and Lemongrass Essential Oils on Candida Albicans Biofilm Established on Acrylic Resin: An In Vitro Study. J. Prosthet. Dent. 125, 707. e706. doi:10.1016/j.prosdent.2020.12.017
Coleman, J. J., Okoli, I., Tegos, G. P., Holson, E. B., Wagner, F. F., Hamblin, M. R., et al. (2010). Characterization of Plant-Derived Saponin Natural Products against Candida Albicans. ACS Chem. Biol. 5, 321–332. doi:10.1021/cb900243b
da Nóbrega Alves, D., Monteiro, A. F. M., Andrade, P. N., Lazarini, J. G., Abílio, G. M. F., Guerra, F. Q. S., et al. (2020). Docking Prediction, Antifungal Activity, Anti-biofilm Effects on Candida spp., and Toxicity against Human Cells of Cinnamaldehyde. Molecules 25, 5969. doi:10.3390/molecules25245969
Dalleau, S., Cateau, E., Bergès, T., Berjeaud, J. M., and Imbert, C. (2008). In Vitro activity of Terpenes against Candida Biofilms. Int. J. Antimicrob. Agents 31, 572–576. doi:10.1016/j.ijantimicag.2008.01.028
Darwish Alipour Astaneh, S., Rasooli, I., and Mousavi Gargari, S. L. (2017). Filamentous Hemagglutinin Adhesin FhaB Limits A.Baumannii Biofilm Formation. Front. Biosci. (Elite Ed. 9, 266–275. doi:10.2741/e801
De Cássia Da Silveira E Sá, R., Andrade, L. N., and De Sousa, D. P. (2013). A Review on Anti-inflammatory Activity of Monoterpenes. Molecules 18, 1227–1254.
De Paula, S. B., Bartelli, T. F., Di Raimo, V., Santos, J. P., Morey, A. T., Bosini, M. A., et al. (2014). Effect of Eugenol on Cell Surface Hydrophobicity, Adhesion, and Biofilm of Candida tropicalis and Candida Dubliniensis Isolated from Oral Cavity of HIV-Infected Patients. Evidence-Based Complementary Altern. Med. 2014 (7), 505204. doi:10.1155/2014/505204
De Vasconcelos, L. C., Sampaio, F. C., Albuquerque, Ade. J., and Vasconcelos, L. C. (2014). Cell Viability of Candida Albicans against the Antifungal Activity of Thymol. Braz Dent. J. 25, 277–281. doi:10.1590/0103-6440201300052
Doke, S. K., Raut, J. S., Dhawale, S., and Karuppayil, S. M. (2014). Sensitization of Candida Albicans Biofilms to Fluconazole by Terpenoids of Plant Origin. J. Gen. Appl. Microbiol. 60, 163–168. doi:10.2323/jgam.60.163
Dong, H. H., Wang, Y. H., Peng, X. M., Zhou, H. Y., Zhao, F., Jiang, Y. Y., et al. (2021). Synergistic Antifungal Effects of Curcumin Derivatives as Fungal Biofilm Inhibitors with Fluconazole. Chem. Biol. Drug Des. 97, 1079–1088. doi:10.1111/cbdd.13827
Dovigo, L. N., Pavarina, A. C., Carmello, J. C., Machado, A. L., Brunetti, I. L., and Bagnato, V. S. (2011a). Susceptibility of Clinical Isolates of Candida to Photodynamic Effects of Curcumin. Lasers Surg. Med. 43, 927–934. doi:10.1002/lsm.21110
Dovigo, L. N., Pavarina, A. C., Ribeiro, A. P., Brunetti, I. L., Costa, C. A., Jacomassi, D. P., et al. (2011b). Investigation of the Photodynamic Effects of Curcumin against Candida Albicans. Photochem Photobiol. 87, 895–903. doi:10.1111/j.1751-1097.2011.00937.x
Dryden, M. S., Dailly, S., and Crouch, M. (2004). A Randomized, Controlled Trial of Tea Tree Topical Preparations versus a Standard Topical Regimen for the Clearance of MRSA Colonization. J. Hosp. Infect. 56, 283–286. doi:10.1016/j.jhin.2004.01.008
El-Baz, A. M., Mosbah, R. A., Goda, R. M., Mansour, B., Sultana, T., Dahms, T. E. S., et al. (2021). Back to Nature: Combating candida Albicans Biofilm, Phospholipase and Hemolysin Using Plant Essential Oils. Antibiot. (Basel) 10, 81. doi:10.3390/antibiotics10010081
Elsharif, S. A., Banerjee, A., and Buettner, A. (2015). Structure-odor Relationships of Linalool, Linalyl Acetate and Their Corresponding Oxygenated Derivatives. Front. Chem. 3, 57. doi:10.3389/fchem.2015.00057
Evensen, N. A., and Braun, P. C. (2009). The Effects of Tea Polyphenols on Candida Albicans: Inhibition of Biofilm Formation and Proteasome Inactivation. Can. J. Microbiol. 55, 1033–1039. doi:10.1139/w09-058
Ferreira, P. S., Victorelli, F. D., Rodero, C. F., Fortunato, G. C., Araújo, V. H. S., Fonseca-Santos, B., et al. (2021). p-Coumaric Acid Loaded into Liquid Crystalline Systems as a Novel Strategy to the Treatment of Vulvovaginal Candidiasis. Int. J. Pharm. 603, 120658. doi:10.1016/j.ijpharm.2021.120658
Finkel, J. S., and Mitchell, A. P. (2011). Genetic Control of Candida Albicans Biofilm Development. Nat. Rev. Microbiol. 9, 109–118. doi:10.1038/nrmicro2475
Francisconi, R. S., Huacho, P. M. M., Tonon, C. C., Bordini, E. A. F., Correia, M. F., Sardi, J. C. O., et al. (2020a). Antibiofilm Efficacy of Tea Tree Oil and of its Main Component Terpinen-4-Ol against Candida Albicans. Braz Oral Res. 34, e050. doi:10.1590/1807-3107bor-2020.vol34.0050
Francisconi, R. S., Maquera-Huacho, P. M., Tonon, C. C., Calixto, G. M. F., de Cássia Orlandi Sardi, J., Chorilli, M., et al. (2020b). Terpinen-4-ol and Nystatin Co-loaded Precursor of Liquid Crystalline System for Topical Treatment of Oral Candidiasis. Sci. Rep. 10, 12984. doi:10.1038/s41598-020-70085-z
Gao, S., Liu, G., Li, J., Chen, J., Li, L., Li, Z., et al. (2020). Antimicrobial Activity of Lemongrass Essential Oil (Cymbopogon Flexuosus) and its Active Component Citral against Dual-Species Biofilms of Staphylococcus aureus and Candida Species. Front. Cell. Infect. Microbiol. 10, 603858. doi:10.3389/fcimb.2020.603858
Garcia, L. G. S., Da Rocha, M. G., Lima, L. R., Cunha, A. P., De Oliveira, J. S., De Andrade, A. R. C., et al. (2021). Essential Oils Encapsulated in Chitosan Microparticles against Candida Albicans Biofilms. Int. J. Biol. Macromol. 166, 621–632. doi:10.1016/j.ijbiomac.2020.10.220
Gershenzon, J., and Dudareva, N. (2007). The Function of Terpene Natural Products in the Natural World. Nat. Chem. Biol. 3, 408–414. doi:10.1038/nchembio.2007.5
Gholami-Ahangaran, M., Ahmadi-Dastgerdi, A., Azizi, S., Basiratpour, A., Zokaei, M., and Derakhshan, M. (2022). Thymol and Carvacrol Supplementation in Poultry Health and Performance. Vet. Med. Sci. 8, 267–288. doi:10.1002/vms3.663
Gomes, D. S., Negrão-Corrêa, D. A., Miranda, G. S., Rodrigues, J. G. M., Guedes, T. J. F. L., De Lucca Junior, W., et al. (2022). Lippia Alba and Lippia Gracilis Essential Oils Affect the Viability and Oviposition of Schistosoma Mansoni. Acta Trop. 231, 106434. doi:10.1016/j.actatropica.2022.106434
Gupta, P., Gupta, H., and Poluri, K. M. (2021a). Geraniol Eradicates Candida Glabrata Biofilm by Targeting Multiple Cellular Pathways. Appl. Microbiol. Biotechnol. 105, 5589–5605. doi:10.1007/s00253-021-11397-6
Gupta, P., Gupta, S., Sharma, M., Kumar, N., Pruthi, V., and Poluri, K. M. (2018). Effectiveness of Phytoactive Molecules on Transcriptional Expression, Biofilm Matrix, and Cell Wall Components of Candida Glabrata and its Clinical Isolates. ACS omega 3, 12201–12214. doi:10.1021/acsomega.8b01856
Gupta, P., Pruthi, V., and Poluri, K. M. (2021b). Mechanistic Insights into Candida Biofilm Eradication Potential of Eucalyptol. J. Appl. Microbiol. 131, 105–123. doi:10.1111/jam.14940
Gursu, B. Y., Dag, İ., and Dikmen, G. (2021). Antifungal and Antibiofilm Efficacy of Cinnamaldehyde-Loaded poly(DL-Lactide-Co-Glycolide) (PLGA) Nanoparticles against Candida Albicans. Int. Microbiol. 25 (2), 245–258. doi:10.1007/s10123-021-00210-z
Hassan, H. A., Geniady, M. M., Abdelwahab, S. F., Abd-Elghany, M. I., Sarhan, H. A., Abdelghany, A. A., et al. (2018). Topical Eugenol Successfully Treats Experimental Candida Albicans-Induced Keratitis. Ophthalmic Res. 60, 69–79. doi:10.1159/000488907
He, M., Du, M., Fan, M., and Bian, Z. (2007). In Vitro activity of Eugenol against Candida Albicans Biofilms. Mycopathologia 163, 137–143. doi:10.1007/s11046-007-0097-2
Hejazi, M., Zareshahrabadi, Z., Ashayeri, S., Saharkhiz, M. J., Iraji, A., Alishahi, M., et al. (2021). Characterization and Physical and Biological Properties of Tissue Conditioner Incorporated with Carum Copticum L. Biomed. Res. Int. 2021, 5577760. doi:10.1155/2021/5577760
Hewlings, S. J., and Kalman, D. S. (2017). Curcumin: A Review of its Effects on Human Health. Foods 6, 92. doi:10.3390/foods6100092
Hoyer, L. L., and Cota, E. (2016). Candida Albicans Agglutinin-like Sequence (Als) Family Vignettes: a Review of Als Protein Structure and Function. Front. Microbiol. 7, 280. doi:10.3389/fmicb.2016.00280
Hsieh, Y. H., Zhang, J. H., Chuang, W. C., Yu, K. H., Huang, X. B., Lee, Y. C., et al. (2018). An In Vitro Study on the Effect of Combined Treatment with Photodynamic and Chemical Therapies on Candida Albicans. Int. J. Mol. Sci. 19, 337. doi:10.3390/ijms19020337
Hsu, C. C., Lai, W. L., Chuang, K. C., Lee, M. H., and Tsai, Y. C. (2013). The Inhibitory Activity of Linalool against the Filamentous Growth and Biofilm Formation in Candida Albicans. Med. Mycol. 51, 473–482. doi:10.3109/13693786.2012.743051
Ivanov, M., Kannan, A., Stojković, D. S., Glamočlija, J., Calhelha, R. C., Ferreira, I., et al. (2021). Camphor and Eucalyptol-Anticandidal Spectrum, Antivirulence Effect, Efflux Pumps Interference and Cytotoxicity. Int. J. Mol. Sci. 22, 483. doi:10.3390/ijms22020483
Jafri, H., and Ahmad, I. (2020). Thymus Vulgaris Essential Oil and Thymol Inhibit Biofilms and Interact Synergistically with Antifungal Drugs against Drug-Resistant Strains of Candida Albicans and Candida tropicalis. J. Mycol. Med. 30, 100911. doi:10.1016/j.mycmed.2019.100911
Jafri, H., Banerjee, G., Khan, M. S. A., Ahmad, I., Abulreesh, H. H., and Althubiani, A. S. (2020). Synergistic Interaction of Eugenol and Antimicrobial Drugs in Eradication of Single and Mixed Biofilms of Candida Albicans and Streptococcus Mutans. Amb. Express 10, 185–189. doi:10.1186/s13568-020-01123-2
Jafri, H., Khan, M. S. A., and Ahmad, I. (2019). In Vitro efficacy of Eugenol in Inhibiting Single and Mixed-Biofilms of Drug-Resistant Strains of Candida Albicans and Streptococcus Mutans. Phytomedicine 54, 206–213. doi:10.1016/j.phymed.2018.10.005
Javed, H., Meeran, M. F. N., Jha, N. K., and Ojha, S. (2020). Carvacrol, a Plant Metabolite Targeting Viral Protease (Mpro) and ACE2 in Host Cells Can Be a Possible Candidate for COVID-19. Front. Plant Sci. 11, 601335. doi:10.3389/fpls.2020.601335
Jordão, C. C., Viana De Sousa, T., Inêz Klein, M., Mendonça Dias, L., Pavarina, A. C., and Carmello, J. C. (2020). Antimicrobial Photodynamic Therapy Reduces Gene Expression of Candida Albicans in Biofilms. Photodiagnosis Photodyn. Ther. 31, 101825.
Kamatou, G. P., and Viljoen, A. M. (2008). Linalool–A Review of a Biologically Active Compound of Commercial Importance. Nat. Product. Commun. 3, 1. doi:10.1177/1934578x0800300727
Kanpittaya, K., Teerakapong, A., Morales, N. P., Hormdee, D., Priprem, A., Weera-Archakul, W., et al. (2021). Inhibitory Effects of Erythrosine/Curcumin Derivatives/Nano-Titanium Dioxide-Mediated Photodynamic Therapy on Candida Albicans. Molecules 26, 2405. doi:10.3390/molecules26092405
Kaskatepe, B., Aslan Erdem, S., Ozturk, S., Safi Oz, Z., Subasi, E., Koyuncu, M., et al. (2022). Antifungal and Anti-virulent Activity of Origanum Majorana L. Essential Oil on Candida Albicans and In Vivo Toxicity in the Galleria Mellonella Larval Model. Molecules 27, 663. doi:10.3390/molecules27030663
Khan, M. S., and Ahmad, I. (2012). Antibiofilm Activity of Certain Phytocompounds and Their Synergy with Fluconazole against Candida Albicans Biofilms. J. Antimicrob. Chemother. 67, 618–621. doi:10.1093/jac/dkr512
Khan, M. S., Ahmad, I., Cameotra, S. S., and Botha, F. (2014). Sub-MICs of Carum Copticum and Thymus Vulgaris Influence Virulence Factors and Biofilm Formation in Candida Spp. BMC Complement. Altern. Med. 14, 337. doi:10.1186/1472-6882-14-337
Khan, S. N., Khan, S., Iqbal, J., Khan, R., and Khan, A. U. (2017). Enhanced Killing and Antibiofilm Activity of Encapsulated Cinnamaldehyde against Candida Albicans. Front. Microbiol. 8, 1641. doi:10.3389/fmicb.2017.01641
Kim, Y. G., Lee, J. H., Park, J. G., and Lee, J. (2020). Inhibition of Candida Albicans and Staphylococcus aureus Biofilms by Centipede Oil and Linoleic Acid. Biofouling 36, 126–137. doi:10.1080/08927014.2020.1730333
Kosari, F., Taheri, M., Moradi, A., Hakimi Alni, R., and Alikhani, M. Y. (2020). Evaluation of Cinnamon Extract Effects on clbB Gene Expression and Biofilm Formation in Escherichia coli Strains Isolated from Colon Cancer Patients. BMC cancer 20, 267–268. doi:10.1186/s12885-020-06736-1
Kowalczyk, A., Przychodna, M., Sopata, S., Bodalska, A., and Fecka, I. (2020). Thymol and Thyme Essential Oil-New Insights into Selected Therapeutic Applications. Molecules 25, 4125. doi:10.3390/molecules25184125
Kozam, G. (1977). The Effect of Eugenol on Nerve Transmission. Oral Surg. Oral Med. Oral Pathol. 44, 799–805. doi:10.1016/0030-4220(77)90390-5
Leite, M. C., De Brito Bezerra, A. P., De Sousa, J. P., and De Oliveira Lima, E. (2015). Investigating the Antifungal Activity and Mechanism(s) of Geraniol against Candida Albicans Strains. Med. Mycol. 53, 275–284. doi:10.1093/mmy/myu078
Lemos, A. S. O., Florêncio, J. R., Pinto, N. C. C., Campos, L. M., Silva, T. P., Grazul, R. M., et al. (2020). Antifungal Activity of the Natural Coumarin Scopoletin against Planktonic Cells and Biofilms from a Multidrug-Resistant Candida tropicalis Strain. Front. Microbiol. 11, 1525. doi:10.3389/fmicb.2020.01525
Li, D. D., Chai, D., Huang, X. W., Guan, S. X., Du, J., Zhang, H. Y., et al. (2017). Potent In Vitro Synergism of Fluconazole and Osthole against Fluconazole-Resistant Candida Albicans. Antimicrob. Agents Chemother. 61, e00436–17. doi:10.1128/AAC.00436-17
Li, X., Yin, L., Ramage, G., Li, B., Tao, Y., Zhi, Q., et al. (2019). Assessing the Impact of Curcumin on Dual-Species Biofilms Formed by Streptococcus Mutans and Candida Albicans. Microbiologyopen 8, e937. doi:10.1002/mbo3.937
Liu, N., Zhang, N., Zhang, S., Zhang, L., and Liu, Q. (2021). Phloretin Inhibited the Pathogenicity and Virulence Factors against Candida Albicans. Bioengineered 12, 2420–2431. doi:10.1080/21655979.2021.1933824
Lone, S. A., and Ahmad, A. (2020). Inhibitory Effect of Novel Eugenol Tosylate Congeners on Pathogenicity of Candida Albicans. BMC Complement. Med. Ther. 20, 131–214. doi:10.1186/s12906-020-02929-0
López-Ribot, J. L. (2005). Candida Albicans Biofilms: More Than Filamentation. Curr. Biol. 15, R453–R455. doi:10.1016/j.cub.2005.06.020
Ma, J., Shi, H., Sun, H., Li, J., and Bai, Y. (2019). Antifungal Effect of Photodynamic Therapy Mediated by Curcumin on Candida Albicans Biofilms In Vitro. Photodiagnosis Photodyn. Ther. 27, 280–287. doi:10.1016/j.pdpdt.2019.06.015
Ma, S., Moser, D., Han, F., Leonhard, M., Schneider-Stickler, B., and Tan, Y. (2020). Preparation and Antibiofilm Studies of Curcumin Loaded Chitosan Nanoparticles against Polymicrobial Biofilms of Candida Albicans and Staphylococcus aureus. Carbohydr. Polym. 241, 116254. doi:10.1016/j.carbpol.2020.116254
Manoharan, R. K., Lee, J. H., Kim, Y. G., Kim, S. I., and Lee, J. (2017a). Inhibitory Effects of the Essential Oils α-longipinene and Linalool on Biofilm Formation and Hyphal Growth of Candida Albicans. Biofouling 33, 143–155. doi:10.1080/08927014.2017.1280731
Manoharan, R. K., Lee, J. H., and Lee, J. (2017b). Antibiofilm and Antihyphal Activities of Cedar Leaf Essential Oil, Camphor, and Fenchone Derivatives against Candida Albicans. Front. Microbiol. 8, 1476. doi:10.3389/fmicb.2017.01476
Mba, I. E., and Nweze, E. I. (2020). Mechanism of Candida Pathogenesis: Revisiting the Vital Drivers. Eur. J. Clin. Microbiol. Infect. Dis. 39, 1797–1819. doi:10.1007/s10096-020-03912-w
Merigo, E., Chevalier, M., Conti, S., Ciociola, T., Fornaini, C., Manfredi, M., et al. (2019). Antimicrobial Effect on Candida Albicans Biofilm by Application of Different Wavelengths and Dyes and the Synthetic Killer Decapeptide KP. Laser Ther. 28, 180–186. doi:10.5978/islsm.28_19-OR-14
Mertas, A., Garbusińska, A., Szliszka, E., Jureczko, A., Kowalska, M., and Król, W. (2015). The Influence of Tea Tree Oil (Melaleuca Alternifolia) on Fluconazole Activity against Fluconazole-Resistant Candida Albicans Strains. Biomed. Res. Int. 2015, 590470. doi:10.1155/2015/590470
Miranda-Cadena, K., Marcos-Arias, C., Mateo, E., Aguirre-Urizar, J. M., Quindós, G., and Eraso, E. (2021b). In Vitro activities of Carvacrol, Cinnamaldehyde and Thymol against Candida Biofilms. Biomed. Pharmacother. 143, 112218. doi:10.1016/j.biopha.2021.112218
Miranda-Cadena, K., Marcos-Arias, C., Mateo, E., Aguirre-Urizar, J. M., Quindós, G., and Eraso, E. (2021a). In Vitro activities of Carvacrol, Cinnamaldehyde and Thymol against Candida Biofilms. Biomed. Pharmacother. 143, 112218. doi:10.1016/j.biopha.2021.112218
Mishra, P., Gupta, P., Srivastava, A. K., Poluri, K. M., and Prasad, R. (2021b). Eucalyptol/β-cyclodextrin Inclusion Complex Loaded Gellan/PVA Nanofibers as Antifungal Drug Delivery System. Int. J. Pharm. 609, 121163. doi:10.1016/j.ijpharm.2021.121163
Mishra, P., Gupta, P., and Pruthi, V. (2021a). Cinnamaldehyde Incorporated Gellan/PVA Electrospun Nanofibers for Eradicating Candida Biofilm. Mater. Sci. Eng. C 119, 111450. doi:10.1016/j.msec.2020.111450
Morey, A. T., De Souza, F. C., Santos, J. P., Pereira, C. A., Cardoso, J. D., De Almeida, R. S., et al. (2016). Antifungal Activity of Condensed Tannins from Stryphnodendron Adstringens: Effect on Candida tropicalis Growth and Adhesion Properties. Curr. Pharm. Biotechnol. 17, 365–375. doi:10.2174/1389201017666151223123712
Motamedi, M., Saharkhiz, M. J., Pakshir, K., Amini Akbarabadi, S., Alikhani Khordshami, M., Asadian, F., et al. (2019). Chemical Compositions and Antifungal Activities of Satureja Macrosiphon against Candida and Aspergillus Species. Curr. Med. Mycol. 5, 20–25. doi:10.18502/cmm.5.4.2162
Müller-Sepúlveda, A., Chevecich, C. C., Jara, J. A., Belmar, C., Sandoval, P., Meyer, R. S., et al. (2020). Chemical Characterization of Lavandula Dentata Essential Oil Cultivated in Chile and its Antibiofilm Effect against Candida Albicans. Planta Med. 86, 1225–1234. doi:10.1055/a-1201-3375
Narayanan, V. S., Muddaiah, S., Shashidara, R., Sudheendra, U. S., Deepthi, N. C., and Samaranayake, L. (2020). Variable Antifungal Activity of Curcumin against Planktonic and Biofilm Phase of Different candida Species. Indian J. Dent. Res. 31, 145–148. doi:10.4103/ijdr.IJDR_521_17
Ning, Y., Ling, J., and Wu, C. D. (2015). Synergistic Effects of Tea Catechin Epigallocatechin Gallate and Antimycotics against Oral Candida Species. Arch. Oral Biol. 60, 1565–1570. doi:10.1016/j.archoralbio.2015.07.001
Nisar, M. F., Khadim, M., Rafiq, M., Chen, J., Yang, Y., and Wan, C. C. (2021). Pharmacological Properties and Health Benefits of Eugenol: A Comprehensive Review. Oxid. Med. Cell Longev. 2021, 2497354. doi:10.1155/2021/2497354
Nobile, C. J., and Johnson, A. D. (2015). Candida Albicans Biofilms and Human Disease. Annu. Rev. Microbiol. 69, 71–92. doi:10.1146/annurev-micro-091014-104330
Nobile, C. J., and Mitchell, A. P. (2006). Genetics and Genomics of Candida Albicans Biofilm Formation. Cell Microbiol. 8, 1382–1391. doi:10.1111/j.1462-5822.2006.00761.x
Palmieri, V., Bugli, F., Cacaci, M., Perini, G., Maio, F., Delogu, G., et al. (2018). Graphene Oxide Coatings Prevent Candida Albicans Biofilm Formation with a Controlled Release of Curcumin-Loaded Nanocomposites. Nanomedicine (Lond) 13, 2867–2879. doi:10.2217/nnm-2018-0183
Pappas, P. G., Lionakis, M. S., Arendrup, M. C., Ostrosky-Zeichner, L., and Kullberg, B. J. (2018). Invasive Candidiasis. Nat. Rev. Dis. Prim. 4, 18026. doi:10.1038/nrdp.2018.26
Pazarci, O., Tutar, U., and Kilinc, S. (2019). Investigation of the Antibiofilm Effects of Mentha Longifolia Essential Oil on Titanium and Stainless Steel Orthopedic Implant Surfaces. Eurasian J. Med. 51, 128–132. doi:10.5152/eurasianjmed.2019.18432
Peana, A. T., Rubattu, P., Piga, G. G., Fumagalli, S., Boatto, G., Pippia, P., et al. (2006). Involvement of Adenosine A1 and A2A Receptors in (-)-Linalool-Induced Antinociception. Life Sci. 78, 2471–2474. doi:10.1016/j.lfs.2005.10.025
Pemmaraju, S. C., Pruthi, P. A., Prasad, R., and Pruthi, V. (2013). Candida Albicans Biofilm Inhibition by Synergistic Action of Terpenes and Fluconazole. Indian J. Exp. Biol. 51, 1032–1037.
Peralta-Yahya, P. P., and Keasling, J. D. (2010). Advanced Biofuel Production in Microbes. Biotechnol. J. 5, 147–162. doi:10.1002/biot.200900220
Pino, J. A., Marbot, R., and Fuentes, V. (2003). Characterization of Volatiles in Bullock's Heart (Annona Reticulata L.) Fruit Cultivars from Cuba. J. Agric. Food Chem. 51, 3836–3839. doi:10.1021/jf020733y
Priya, A., Nivetha, S., and Pandian, S. K. (2021a). Synergistic Interaction of Piperine and Thymol on Attenuation of the Biofilm Formation, Hyphal Morphogenesis and Phenotypic Switching in Candida Albicans. Front. Cell Infect. Microbiol. 11, 780545. doi:10.3389/fcimb.2021.780545
Priya, A., and Pandian, S. K. (2020). Piperine Impedes Biofilm Formation and Hyphal Morphogenesis of Candida Albicans. Front. Microbiol. 11, 756. doi:10.3389/fmicb.2020.00756
Priya, A., Selvaraj, A., Divya, D., Karthik Raja, R., and Pandian, S. K. (2021b). In Vitro and In Vivo Anti-infective Potential of Thymol against Early Childhood Caries Causing Dual Species Candida Albicans and Streptococcus Mutans. Front. Pharmacol. 12, 760768. doi:10.3389/fphar.2021.760768
Quatrin, P. M., Verdi, C. M., De Souza, M. E., De Godoi, S. N., Klein, B., Gundel, A., et al. (2017). Antimicrobial and Antibiofilm Activities of Nanoemulsions Containing Eucalyptus Globulus Oil against Pseudomonas aeruginosa and Candida Spp. Microb. Pathog. 112, 230–242. doi:10.1016/j.micpath.2017.09.062
Quishida, C. C., De Oliveira Mima, E. G., Jorge, J. H., Vergani, C. E., Bagnato, V. S., and Pavarina, A. C. (2016). Photodynamic Inactivation of a Multispecies Biofilm Using Curcumin and LED Light. Lasers Med. Sci. 31, 997–1009. doi:10.1007/s10103-016-1942-7
Rajasekar, V., Darne, P., Prabhune, A., Kao, R. Y. T., Solomon, A. P., Ramage, G., et al. (2021). A Curcumin-Sophorolipid Nanocomplex Inhibits Candida Albicans Filamentation and Biofilm Development. Colloids Surf. B Biointerfaces 200, 111617. doi:10.1016/j.colsurfb.2021.111617
Ramage, G., Martínez, J. P., and López-Ribot, J. L. (2006). Candida Biofilms on Implanted Biomaterials: a Clinically Significant Problem. FEMS Yeast Res. 6, 979–986. doi:10.1111/j.1567-1364.2006.00117.x
Ramage, G., Milligan, S., Lappin, D. F., Sherry, L., Sweeney, P., Williams, C., et al. (2012). Antifungal, Cytotoxic, and Immunomodulatory Properties of Tea Tree Oil and its Derivative Components: Potential Role in Management of Oral Candidosis in Cancer Patients. Front. Microbiol. 3, 220. doi:10.3389/fmicb.2012.00220
Rangel, M. D. L., Aquino, S. G. D., Lima, J. M. D., Castellano, L. R., and Castro, R. D. D. (2018). In Vitro effect of Cinnamomum Zeylanicum Blume Essential Oil on Candida Spp. Involved in Oral Infections. Evidence-Based Complementary Altern. Med. 2018, 1–13. doi:10.1155/2018/4045013
Raorane, C. J., Lee, J. H., Kim, Y. G., Rajasekharan, S. K., García-Contreras, R., and Lee, J. (2019). Antibiofilm and Antivirulence Efficacies of Flavonoids and Curcumin against Acinetobacter Baumannii. Front. Microbiol. 10, 990. doi:10.3389/fmicb.2019.00990
Raut, J. S., Shinde, R. B., Chauhan, N. M., and Karuppayil, S. M. (2013). Terpenoids of Plant Origin Inhibit Morphogenesis, Adhesion, and Biofilm Formation by Candida Albicans. Biofouling 29, 87–96. doi:10.1080/08927014.2012.749398
Reddy, A. C., and Lokesh, B. R. (1994). Studies on Anti-inflammatory Activity of Spice Principles and Dietary N-3 Polyunsaturated Fatty Acids on Carrageenan-Induced Inflammation in Rats. Ann. Nutr. Metab. 38, 349–358. doi:10.1159/000177833
Rivas Da Silva, A. C., Lopes, P. M., Barros De Azevedo, M. M., Costa, D. C., Alviano, C. S., and Alviano, D. S. (2012). Biological Activities of α-pinene and β-pinene Enantiomers. Molecules 17, 6305–6316. doi:10.3390/molecules17066305
Rocha, M. P., Ruela, A. L. M., Rosa, L. P., Santos, G. P. O., and Rosa, F. C. S. (2020). Antimicrobial Photodynamic Therapy in Dentistry Using an Oil-In-Water Microemulsion with Curcumin as a Mouthwash. Photodiagnosis Photodyn. Ther. 32, 101962. doi:10.1016/j.pdpdt.2020.101962
Sadowska, B., Budzyńska, A., Więckowska-Szakiel, M., Paszkiewicz, M., Stochmal, A., Moniuszko-Szajwaj, B., et al. (2014). New Pharmacological Properties of Medicago Sativa and Saponaria officinalis Saponin-Rich Fractions Addressed to Candida Albicans. J. Med. Microbiol. 63, 1076–1086. doi:10.1099/jmm.0.075291-0
Saharkhiz, M. J., Motamedi, M., Zomorodian, K., Pakshir, K., Miri, R., and Hemyari, K. (2012). Chemical Composition, Antifungal and Antibiofilm Activities of the Essential Oil of Mentha Piperita L. ISRN Pharm. 2012, 718645. doi:10.5402/2012/718645
Salehi, B., Mishra, A. P., Shukla, I., Sharifi-Rad, M., Contreras, M. D. M., Segura-Carretero, A., et al. (2018). Thymol, Thyme, and Other Plant Sources: Health and Potential Uses. Phytother. Res. 32, 1688–1706. doi:10.1002/ptr.6109
Sanitá, P. V., Pavarina, A. C., Dovigo, L. N., Ribeiro, A. P. D., Andrade, M. C., and Mima, E. G. O. (2018). Curcumin-mediated Anti-microbial Photodynamic Therapy against Candida Dubliniensis Biofilms. Lasers Med. Sci. 33, 709–717. doi:10.1007/s10103-017-2382-8
Santezi, C., Reina, B. D., De Annunzio, S. R., Calixto, G., Chorilli, M., and Dovigo, L. N. (2021). Photodynamic Potential of Curcumin in Bioadhesive Formulations: Optical Characteristics and Antimicrobial Effect against Biofilms. Photodiagnosis Photodyn. Ther. 35, 102416. doi:10.1016/j.pdpdt.2021.102416
Santos, T. B., Vieira, A. A., Paula, L. O., Santos, E. D., Radi, P. A., Khouri, S., et al. (2017). Flexible Camphor Diamond-like Carbon Coating on Polyurethane to Prevent Candida Albicans Biofilm Growth. J. Mech. Behav. Biomed. Mater 68, 239–246. doi:10.1016/j.jmbbm.2017.02.013
Scaffaro, R., Lopresti, F., D'arrigo, M., Marino, A., and Nostro, A. (2018). Efficacy of Poly(lactic Acid)/carvacrol Electrospun Membranes against Staphylococcus aureus and Candida Albicans in Single and Mixed Cultures. Appl. Microbiol. Biotechnol. 102, 4171–4181. doi:10.1007/s00253-018-8879-7
Schnitzler, P. (2019). Essential Oils for the Treatment of Herpes Simplex Virus Infections. Chemotherapy 64, 1–7. doi:10.1159/000501062
Shahzad, M., Sherry, L., Rajendran, R., Edwards, C. A., Combet, E., and Ramage, G. (2014). Utilising Polyphenols for the Clinical Management of Candida Albicans Biofilms. Int. J. Antimicrob. Agents 44, 269–273. doi:10.1016/j.ijantimicag.2014.05.017
Shapira, S., Pleban, S., Kazanov, D., Tirosh, P., and Arber, N. (2016). Terpinen-4-ol: A Novel and Promising Therapeutic Agent for Human Gastrointestinal Cancers. PLoS One 11, e0156540. doi:10.1371/journal.pone.0156540
Shariati, A., Asadian, E., Fallah, F., Azimi, T., Hashemi, A., Yasbolaghi Sharahi, J., et al. (2019). Evaluation of Nano-Curcumin Effects on Expression Levels of Virulence Genes and Biofilm Production of Multidrug-Resistant Pseudomonas aeruginosa Isolated from Burn Wound Infection in Tehran, Iran. Infect. Drug Resist 12, 2223–2235. doi:10.2147/IDR.S213200
Sharifi-Rad, M., Varoni, E. M., Iriti, M., Martorell, M., Setzer, W. N., Del Mar Contreras, M., et al. (2018). Carvacrol and Human Health: A Comprehensive Review. Phytother. Res. 32, 1675–1687. doi:10.1002/ptr.6103
Sharifzadeh, A., Khosravi, A. R., and Ahmadian, S. (2016). Chemical Composition and Antifungal Activity of Satureja Hortensis L. Essentiall Oil against Planktonic and Biofilm Growth of Candida Albicans Isolates from Buccal Lesions of HIV(+) Individuals. Microb. Pathog. 96, 1–9. doi:10.1016/j.micpath.2016.04.014
Sharma, Y., Rastogi, S. K., Perwez, A., Rizvi, M. A., and Manzoor, N. (2020). β-Citronellol Alters Cell Surface Properties of Candida Albicans to Influence Pathogenicity Related Traits. Med. Mycol. 58, 93–106. doi:10.1093/mmy/myz009
Shinde, R. B., Raut, J. S., Chauhan, N. M., and Karuppayil, S. M. (2013). Chloroquine Sensitizes Biofilms of Candida Albicans to Antifungal Azoles. Braz J. Infect. Dis. 17, 395–400. doi:10.1016/j.bjid.2012.11.002
Shu, C., Sun, L., and Zhang, W. (2016). Thymol Has Antifungal Activity against Candida Albicans during Infection and Maintains the Innate Immune Response Required for Function of the P38 MAPK Signaling Pathway in Caenorhabditis elegans. Immunol. Res. 64, 1013–1024. doi:10.1007/s12026-016-8785-y
Silva, S., Henriques, M., Martins, A., Oliveira, R., Williams, D., and Azeredo, J. (2009). Biofilms of Non-Candida Albicans Candida Species: Quantification, Structure and Matrix Composition. Med. Mycol. 47, 681–689. doi:10.3109/13693780802549594
Singh, S., Fatima, Z., Ahmad, K., and Hameed, S. (2018). Fungicidal Action of Geraniol against Candida Albicans Is Potentiated by Abrogated CaCdr1p Drug Efflux and Fluconazole Synergism. PLoS One 13, e0203079. doi:10.1371/journal.pone.0203079
Singh, S., Fatima, Z., and Hameed, S. (2016). Insights into the Mode of Action of Anticandidal Herbal Monoterpenoid Geraniol Reveal Disruption of Multiple MDR Mechanisms and Virulence Attributes in Candida Albicans. Arch. Microbiol. 198, 459–472. doi:10.1007/s00203-016-1205-9
Souza, C. M., Pereira Junior, S. A., Moraes, Tda. S., Damasceno, J. L., Amorim Mendes, S., Dias, H. J., et al. (2016). Antifungal Activity of Plant-Derived Essential Oils on Candida tropicalis Planktonic and Biofilms Cells. Med. Mycol. 54, 515–523. doi:10.1093/mmy/myw003
Souza, M. E., Lopes, L. Q., Bonez, P. C., Gündel, A., Martinez, D. S., Sagrillo, M. R., et al. (2017). Melaleuca Alternifolia Nanoparticles against Candida Species Biofilms. Microb. Pathog. 104, 125–132. doi:10.1016/j.micpath.2017.01.023
Suchodolski, J., Feder-Kubis, J., and Krasowska, A. (2021). Antiadhesive Properties of Imidazolium Ionic Liquids Based on (-)-Menthol against Candida Spp. Int. J. Mol. Sci. 22, 7543. doi:10.3390/ijms22147543
Sudbery, P., Gow, N., and Berman, J. (2004). The Distinct Morphogenic States of Candida Albicans. Trends Microbiol. 12, 317–324. doi:10.1016/j.tim.2004.05.008
Sun, L. M., Zhang, C. L., and Li, P. (2012). Characterization, Antibiofilm, and Mechanism of Action of Novel PEG-Stabilized Lipid Nanoparticles Loaded with Terpinen-4-Ol. J. Agric. Food Chem. 60, 6150–6156. doi:10.1021/jf3010405
Swetha, T. K., Vikraman, A., Nithya, C., Hari Prasath, N., and Pandian, S. K. (2020). Synergistic Antimicrobial Combination of Carvacrol and Thymol Impairs Single and Mixed-Species Biofilms of Candida Albicans and Staphylococcus Epidermidis. Biofouling 36, 1256–1271. doi:10.1080/08927014.2020.1869949
Tan, Y., Leonhard, M., Moser, D., Ma, S., and Schneider-Stickler, B. (2019). Antibiofilm Efficacy of Curcumin in Combination with 2-aminobenzimidazole against Single- and Mixed-Species Biofilms of Candida Albicans and Staphylococcus aureus. Colloids Surf. B Biointerfaces 174, 28–34. doi:10.1016/j.colsurfb.2018.10.079
Thakre, A., Jadhav, V., Kazi, R., Shelar, A., Patil, R., Kharat, K., et al. (2021). Oxidative Stress Induced by Piperine Leads to Apoptosis in Candida Albicans. Med. Mycol. 59, 366–378. doi:10.1093/mmy/myaa058
Thakre, A., Zore, G., Kodgire, S., Kazi, R., Mulange, S., Patil, R., et al. (2018). Limonene Inhibits Candida Albicans Growth by Inducing Apoptosis. Med. Mycol. 56, 565–578. doi:10.1093/mmy/myx074
Tonon, C. C., Francisconi, R. S., Bordini, E. A. F., Huacho, P. M. M., Sardi, J. C. O., and Spolidorio, D. M. P. (2018). Interactions between Terpinen-4-Ol and Nystatin on Biofilm of Candida Albicans and Candida tropicalis. Braz Dent. J. 29, 359–367. doi:10.1590/0103-6440201802073
Touil, H. F. Z., Boucherit, K., Boucherit-Otmani, Z., Kohder, G., Madkour, M., Soliman, S. S. M., et al. (20202020). Optimum Inhibition of Amphotericin-B-Resistant Candida Albicans Strain in Single- and Mixed-Species Biofilms by Candida and Non-Candida Terpenoids. Biomolecules 10, 342. doi:10.3390/biom10020342
Uppuluri, P., Chaturvedi, A. K., Srinivasan, A., Banerjee, M., Ramasubramaniam, A. K., Köhler, J. R., et al. (2010). Dispersion as an Important Step in the Candida Albicans Biofilm Developmental Cycle. PLoS Pathog. 6, e1000828. doi:10.1371/journal.ppat.1000828
Uppuluri, P., Lin, L., Alqarihi, A., Luo, G., Youssef, E. G., Alkhazraji, S., et al. (2018). The Hyr1 Protein from the Fungus Candida Albicans Is a Cross Kingdom Immunotherapeutic Target for Acinetobacter Bacterial Infection. PLoS Pathog. 14, e1007056. doi:10.1371/journal.ppat.1007056
Van Zyl, R. L., Seatlholo, S. T., Van Vuuren, S. F., and Viljoen, A. M. (2006). The Biological Activities of 20 Nature Identical Essential Oil Constituents. J. Essent. Oil Res. 18, 129–133. doi:10.1080/10412905.2006.12067134
Vitali, A., Stringaro, A., Colone, M., Muntiu, A., and Angiolella, L. (2021). Antifungal Carvacrol Loaded Chitosan Nanoparticles. Antibiot. (Basel) 11, 11. doi:10.3390/antibiotics11010011
Waltimo, T. M., Ørstavik, D., Sirén, E. K., and Haapasalo, M. P. (2000). In Vitro yeast Infection of Human Dentin. J. Endod. 26, 207–209. doi:10.1097/00004770-200004000-00002
Wijesinghe, G. K., De Oliveira, T. R., Maia, F. C., De Feiria, S. B., Barbosa, J. P., Joia, F., et al. (2021). Efficacy of True Cinnamon (Cinnamomum Verum) Leaf Essential Oil as a Therapeutic Alternative for Candida Biofilm Infections. Iran. J. Basic Med. Sci. 24, 787–795. doi:10.22038/ijbms.2021.53981.12138
Wijesinghe, G. K., Maia, F. C., De Oliveira, T. R., De Feiria, S. N. B., Joia, F., Barbosa, J. P., et al. (2020). Effect of Cinnamomum Verum Leaf Essential Oil on Virulence Factors of Candida Species and Determination of the In-Vivo Toxicity with Galleria Mellonella Model. Memórias do Inst. Oswaldo Cruz 115, e200349. doi:10.1590/0074-02760200349
Xu, K., Wang, J. L., Chu, M. P., and Jia, C. (2019). Activity of Coumarin against Candida Albicans Biofilms. J. Mycol. Med. 29, 28–34. doi:10.1016/j.mycmed.2018.12.003
Yang, L., Sui, Y., Zhong, L., Ma, T., Ma, Z., and Liu, X. (2022). Carnosol Inhibits the Growth and Biofilm of Candida Albicans. J. Mycol. Med. 32, 101234. doi:10.1016/j.mycmed.2021.101234
Yang, L., Liu, X., Zhuang, X., Feng, X., Zhong, L., and Ma, T. (2018). Antifungal Effects of Saponin Extract from Rhizomes of Dioscorea panthaica Prain et Burk against Candida albicans. Evid. Based Complement. Altern. Med. 2018, 6095307. doi:10.1155/2018/6095307
Keywords: natural compounds, Candida biofilm, new treatment, essential oil, antibiobilm
Citation: Shariati A, Didehdar M, Razavi S, Heidary M, Soroush F and Chegini Z (2022) Natural Compounds: A Hopeful Promise as an Antibiofilm Agent Against Candida Species. Front. Pharmacol. 13:917787. doi: 10.3389/fphar.2022.917787
Received: 11 April 2022; Accepted: 30 May 2022;
Published: 11 July 2022.
Edited by:
Mustofa Mustofa, Gadjah Mada University, IndonesiaReviewed by:
Viviane de Cássia Oliveira, University of São Paulo, BrazilDr. Adnan Amin, Gomal University, Pakistan
Copyright © 2022 Shariati, Didehdar, Razavi, Heidary, Soroush and Chegini. This is an open-access article distributed under the terms of the Creative Commons Attribution License (CC BY). The use, distribution or reproduction in other forums is permitted, provided the original author(s) and the copyright owner(s) are credited and that the original publication in this journal is cited, in accordance with accepted academic practice. No use, distribution or reproduction is permitted which does not comply with these terms.
*Correspondence: Aref Shariati, YXJlZnNoYXJpYXRpMDExMUBzYm11LmFjLmly; Zahra Chegini, cGFyaXNhLmNoZWdpbmk3MkBnbWFpbC5jb20=