- 1Department of Clinical Laboratories Sciences, The Faculty of Applied Medical Sciences, Taif University, Taif, Saudi Arabia
- 2Centre of Biomedical Sciences Research (CBSR), Deanship of Scientific Research, Taif University, Taif, Saudi Arabia
- 3Laboratory for Nanomedicine, Division of Biological and Environmental Science and Engineering (BESE), King Abdullah University of Science and Technology (KAUST), Jeddah, Saudi Arabia
- 4Computational Bioscience Research Center (CBRC), King Abdullah University of Science and Technology, (KAUST), Jeddah, Saudi Arabia
- 5Department of Biology, College of Science, Taif University, Taif, Saudi Arabia
- 6Department of Medical Laboratory Technology, Faculty of Applied Medical Sciences in Rabigh, King Abdulaziz University, Jeddah, Saudi Arabia
- 7Department of Medical Laboratory Sciences, Faculty of Applied Medical Sciences, King Abdulaziz University, Jeddah, Saudi Arabia
- 8Center of Excellence in Genomic Medicine Research (CEGMR), King Abdulaziz University, Jeddah, Saudi Arabia
- 9King Fahd Medical Research Centre, Hematology Research Unit, King Abdulaziz University, Jeddah, Saudi Arabia
- 10Center of Innovation in Personalized Medicine (CIPM), 3D Bioprinting Unit, King Abdulaziz University (KAUST), Jeddah, Saudi Arabia
- 11Center of Innovation in Personalized Medicine (CIPM), Nanomedicine Unit, King Abdulaziz University, Jeddah, Saudi Arabia
- 12Department of Radiological Sciences, College of Applied Medical Sciences, Taif University, Taif, Saudi Arabia
- 13Department of Pharmacology and Toxicology, College of Pharmacy, University of Hail, Hail, Saudi Arabia
- 14Department of Internal Medicine, School of Medicine, Taif University, Taif, Saudi Arabia
- 15Department of Pharmacology and Toxicology, College of Pharmacy, Taif University, Taif, Saudi Arabia
- 16Addiction and Neuroscience Research Unit, Taif University, Taif, Saudi Arabia
- 17Department of Clinical Pharmacy, College of Pharmacy, Taif University, Taif, Saudi Arabia
Background: Gabapentin is widely prescribed as an off-label drug for the treatment of various diseases, including drug and alcohol addiction. Approximately 83–95% of the usage of gabapentin is off-label, accounting for more than 90% of its sales in the market, which indicates an alarming situation of drug abuse. Such misuse of gabapentin has serious negative consequences. The safety of the use of gabapentin in pregnant women has always been a serious issue, as gabapentin can cross placental barriers. The impact of gabapentin on brain development in the fetus is not sufficiently investigated, which poses difficulties in clinical decisions regarding prescriptions.
Methods: The consequences effect of prenatal gabapentin exposure on the development of ventral midbrain dopaminergic neurons were investigated using three-dimensional neuronal cell cultures. Time-mated Swiss mice were used to isolate embryos. The ventral third of the midbrain was removed and used to enrich the dopaminergic population in 3D cell cultures that were subsequently exposed to gabapentin. The effects of gabapentin on the viability, ATP release, morphogenesis and genes expression of ventral midbrain dopaminergic neurons were investigated.
Results: Gabapentin treatment at the therapeutic level interfered with the neurogenesis and morphogenesis of vmDA neurons in the fetal brain by causing changes in morphology and alterations in the expression of key developmental genes, such as Nurr1, Chl1, En1, Bdnf, Drd2, and Pitx3. The TH + total neurite length and dominant neurite length were significantly altered. We also found that gabapentin could halt the metabolic state of these neuronal cells by blocking the generation of ATP.
Conclusion: Our findings clearly indicate that gabapentin hampers the morphogenesis and development of dopaminergic neurons. This implies that the use of gabapentin could lead to serious complications in child-bearing women. Therefore, caution must be exercised in clinical decisions regarding the prescription of gabapentin in pregnant women.
Introduction
Gabapentin is most commonly prescribed to treat neuropathic pain. Gabapentin is a structural analog of the inhibitory neurotransmitter gamma-aminobutyric acid (GABA). Gabapentin does not directly act upon GABA receptors; however, it has a tendency to increase GABA and decrease the levels of glutamate neurotransmitters (Smith et al., 2016). The mechanism through which gabapentin acts is not clear; however, it has been reported that gabapentin may decrease the release of pain-related peptides and reduce opioid-induced hyperalgesia (Compton et al., 2010). Gabapentin can also bind to protein subunits of the voltage-dependent Ca++ channel complex, suggesting that it can modulate neuron signaling (Suman-Chauhan et al., 1993; Thurlow et al., 1993; Gee et al., 1996). Gabapentin was first approved for epilepsy in 1993. Later, in 2004, it was also authorized to treat postherpetic neuralgia (Mack, 2003). In 2006, UK regulatory agencies approved it as a first-line treatment for all types of neuropathic pain (Smith et al., 2016). Gabapentin is widely prescribed as an off-label drug to treat several diseases, including drug and alcohol addiction, insomnia, bipolar disorder, borderline neuropathic pain conditions, pruritic disorders, anxiety, menopausal conditions, migraines, and vertigo. Approximately 83–95% of the usage of gabapentin is off-label, which accounts for more than 90% of its sales in the market (Hamer et al., 2002; Radley et al., 2006). Pfizer was penalized a fine of $420 million for illegal marketing of gabapentin with off-label uses (Newman, 2010).
There is a growing concern about the misuse of gabapentin as it can produce a euphoria and anxiolytic effects similar to recreational drugs. The misuse of gabapentin is known to have serious consequences. There are growing concerns regarding the use of gabapentin during pregnancy because of the lack of clarity regarding the effects of gabapentin on brain development. The ability of gabapentin to cross the placental barrier has made the safety of child-bearing women a serious issue. It is still unclear whether the therapeutic dose of gabapentin impairs brain development in the fetus (Patorno et al., 2020). Considering the lack of convincing evidence concerning the impact of gabapentin on fetal brain development during pregnancy, we used embryonic ventral midbrain (VM) neurons of mice to investigate the effects of gabapentin on brain development by examining protein expression and morphological characteristics of ventral midbrain dopaminergic (vmDA) neurons, which play vital roles in controlling cognition and motor activities. In addition, vmDA neurons are involved in learning, motivation, reward association and regulation of movement. In the present study, we show for the first time the effects of gabapentin exposure on the development of vmDA neurons isolated from mouse embryos.
Materials and Methods
Separation of Primary Dopaminergic Neurons From the Ventral Midbrain of a Mouse Embryo
The experiments were approved by the King Abdulaziz University (12-CEGMR-Bioethic-2020) and Taif University (211BEC023) ethics committees, and they followed international norms for the use of animals in research. Figure 1 explains the experimental technique. Time-mated Swiss mice were used to isolate embryos. Animals were time-mated overnight, and the next morning’s sight of a vaginal plug was termed as embryonic day (E) 0.5. In ice-cold L-15 Medium (Thermo Fisher, USA), the VM of E12.5 mouse embryos was dissected. E12.5 mouse embryos have been used as it is the optimum time point for analyzing the effects of external signaling cues on developing vmDA neurons. To isolate the midbrain and cortical tissues, the telencephalon–mesencephalon boundary as well as the isthmic organizer were separated. The dopaminergic population in the culture was enriched by dissecting the ventral third of the midbrain tissue. The separated VMs were incubated for 15 min at 37 °C in a mixture of 0.05 percent trypsin (Thermo Fisher Scientific, USA) and 0.1 percent DNase (STEMCELL Technologies, USA) diluted in Ca/Mg-free Hank’s Balanced Salt Solution (HBSS) (Thermo Fisher Scientific, USA). The tissues were washed three times in HBSS before being resuspended in N2 media, which was made up of a 1:1 mixture of F12 medium and Minimum Essential Medium enhanced with 1 mM glutamine, 1 mg/ml BSA, 15 mM HEPES, 6 mg/ml glucose, 1% penicillin/streptomycin, and 1% N2 supplement. Primary neurons were cultured for three days before investigation
Neuronal Cultures
We used three-dimensional (3D) neural cell cultures to evaluate cell survival, ATP release, and morphogenesis, as well as perform real-time PCR. Freshly isolated primary embryonic mouse E12.5 vmDA neurons were sown at a density of 6 × 104 cells/well to create the 3D culture. The short peptide IIZK was then used as described in a previous publication (Alsanie et al., 2020). IIZK was prepared in 1 × Dulbecco’s phosphate-buffered saline (DPBS). The weighted IIZK peptide was resuspended in half of the needed final volume of nuclease-free sterile water. Due to the possibility of quick gelation when using DPBS, it was only used inside the cell culture well. To aid the gelation process, an appropriate volume of previously resuspended peptide in water was introduced to the culture well, followed by an equivalent volume of 2 × DPBS. To prevent cells from coming into contact with the plastic surface, a peptide base was initially added to each well. In order to achieve complete gelation, the plates were incubated for 5 min. A 3D construct was then developed on top of the peptide basal layer. The addition of peptides was followed by that of an equivalent volume of 2× DPBS containing the desired number of cells, which was then mixed briefly with the peptide. Before adding cell culture media, the plates were re-incubated for 2–3 min. The culture plates were then properly filled with N2 media. Next, the cells were incubated for 72 h. Gabapentin solution was made according to the manufacturer’s guidelines (Sigma-Aldrich, USA). Gabapentin with a final concentration of 10 µM was administered to the gabapentin treatment group immediately after seeding the cells, whereas the control groups received an identical volume of sterile 1× PBS. The 10 µM gabapentin dose was used in the current study to resemble the serum concentration levels in the therapeutic dose.
Viability and ATP Release of vmDA Neurons
After three days of culture, the change in ATP release and viability of E12.5 vmDA neuron in response to gabapentin treatment were measured by alamarBlueTM Cell Viability Reagent (Thermo Fisher, USA) according to the manufacturer’s guidelines. The fluorescence was measured using a PHERAstar FS (BMG LabTech, Germany). The CellTiter-Glo® 3D Cell Viability Assay (Promega, G9681) was used to determine the status of the metabolic activity and ATP release of the cells. The luminescent signal was read by PHERAstar FS plate reader (BMG LabTech, Germany).
Immunocytochemistry
After three days of culturing, the mouse embryonic vmDA neurons were fixed with 4% paraformaldehyde (Santa Cruz Biotechnology, sc-281692) and kept at 4°C in 1× PBS before staining. The immunocytochemistry experiment were carried out using primary antibodies against mouse neuron-specific class III beta-tubulin (TUJ1) (Promega, G7121) and tyrosine hydroxylase (TH) (Abcam, ab112). The primary antibodies TUJ1 (1:1500) and TH (1:500) diluted in blocking buffer and incubated overnight at room temperature with fixed cultures. After removing the primary antibodies, the cells were treated in blocking buffer for 1 h at room temperature. The secondary antibodies anti-mouse Alexa 488 and goat anti-rabbit IgG H&L (Alexa Fluor® 555) (Abcam, ab150078) were then added and incubated for 2 h at room temperature, diluted in blocking buffer (1:200). The cells were then rinsed and kept in 1× PBS after being treated for 5 min in 4′,6-diamidino-2-phenylindole, dihydrochloride (DAPI) (Thermo Fisher Scientific, D1306). A DMi8 inverted fluorescent microscope was used for imaging (Leica, Germany).
Morphogenesis
In stained cultures, the impacts of gabapentin on the development of VM neurons were investigated. The LAS X software (Leica, Germany) was used to assess the number of neurites, total length of neurites, length of dominant neurites, and number of branches, as detailed previously (Alsanie et al., 2017). To eliminate bias, overlapping neurites and those shorter than 20 µm were omitted. The results from the gabapentin-treated cultures were compared to the control group’s results. Following that, the data were reported as a percentage change from the control values, which were assumed to be 100%.
Real-Time PCR
The RNeasy Plus universal Mini Kit (Qiagen, Cat no. 73404) was used to isolate RNA from the VM neurons in gabapentin-treated and control cultures after three days of culturing. TissueLyser II (Qiagen) was employed as suggested in the RNeasy kit protocol to guarantee optimal cell homogenization. A negative control was RNA obtained from mouse tissues other than brain tissues. Table 1 shows the primer sequences for the designated genes. The StepOneTM Real-Time PCR System and DataAssist software (Thermo Fisher) were used to generate raw threshold cycle (CT) data for all selected genes (Table 1). The experiments were performed triplicates. The expression of each gene was measured by normalizing it to a housekeeping gene (Gapdh). The ∆∆CT method was used to calculate RNA expression. The expression levels of each gene were compared across all five groups’ samples, and p values were calculated to identify genes that were significantly expressed.
Statistical Analysis
The data were analyzed using the Student’s t test performed using GraphPad Prism v 8.1.2. All quantitative data were expressed as the mean ± SEM with the significance level set at p < 0.05.
Results
Gabapentin Disrupts the Metabolic Activity of vmDA Neurons
Neurotoxicity caused by gabapentin has been reported to occur in cases of renal failure, despite dose adjustment (Wahba and Waln, 2013). Our results showed that gabapentin did not have any impact on the viability of neurons (Figure 2A), thus confirming that it is nontoxic at therapeutic doses. However, we found a significant decrease in ATP generation in gabapentin-treated cultures. It can be thus inferred that gabapentin interferes with the mitochondrial electron transport system, causing metabolic dysfunction (Figure 2B).
Gabapentin Affects the Morphogenesis of vmDA Neurons
The impact of gabapentin on vmDA TH + neuronal morphogenesis was investigated in immune-stained cultures. We found a significant increase in the TH + total neurite length (Figure 3A) and dominant neurite length (Figure 3B) in gabapentin-treated cultures in comparison with the lengths observed in the control. However, the number of branches (Figure 3C) or neurites (Figure 3D) of TH + vmDA neurons in the gabapentin-treated cultures did not differ significantly from those in the control cultures (Figures 3E–H).
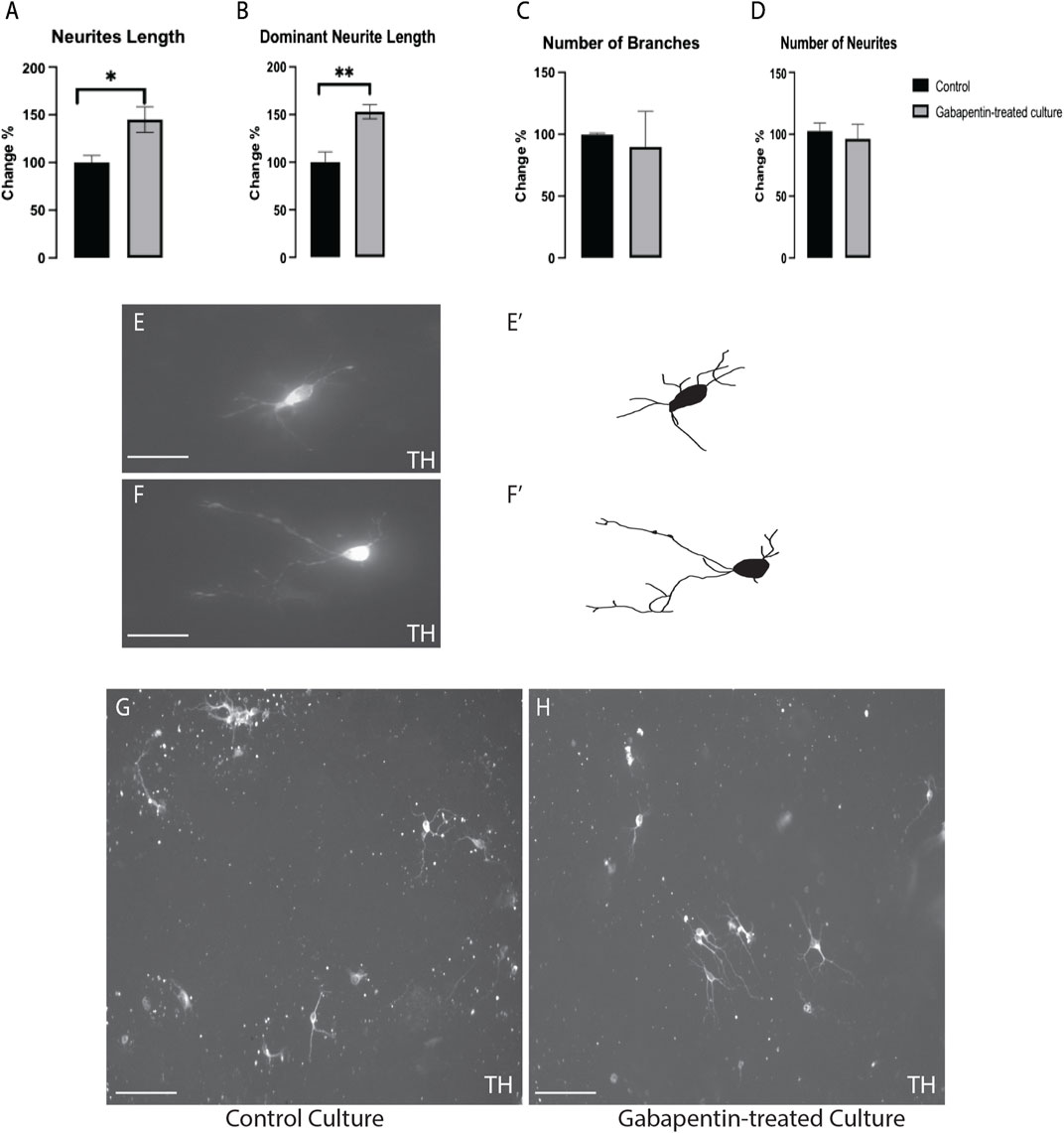
FIGURE 3. Gabapentin-induced elongation observed in total neurite length (A) and dominant neurite length (B) of TH + vmDA neurons. TH + vmDA neurons showing no significant changes in the number of branches (C) and neurites (D) of in response to gabapentin treatment. Representative images and illustration for vmDA neurons immunolabeled with TH in both groups; control (E,E’) and gabapentin-treated (F,F’) show the increase in neurites elongation in response to gabapentin exposure. (G,H) images show large field of view (20x) for both groups; control and gabapentin treated cultures, respectively. Scale bar = 50 um (E,F). Scale bar = 10 um (G,H). Data are represented as mean ± SEM, n = 4 experiments. *p < 0.05.
Gabapentin Affects the Morphogenesis of VM Non-DA Neurons
To determine if gabapentin affects the morphogenesis of all VM neurons or specifically that of vmDA neurons, we investigated the effect of gabapentin on VM non-DA (TUJ1+/TH-) neurons. We detected a significant increase in the number of branches of TUJ1+/TH- neurons (Figure 4C). However, no significant differences were observed in the total neurite length (Figure 4A), dominant neurite length (Figure 4B), or the number of neurites (Figure 4D) in TUJ1+/TH- neurons between the treatment and control cultures (Figures 4E–H). These observations reveal that the alteration of the morphogenesis of nondopaminergic and dopaminergic VM neurons was affected differently by gabapentin.
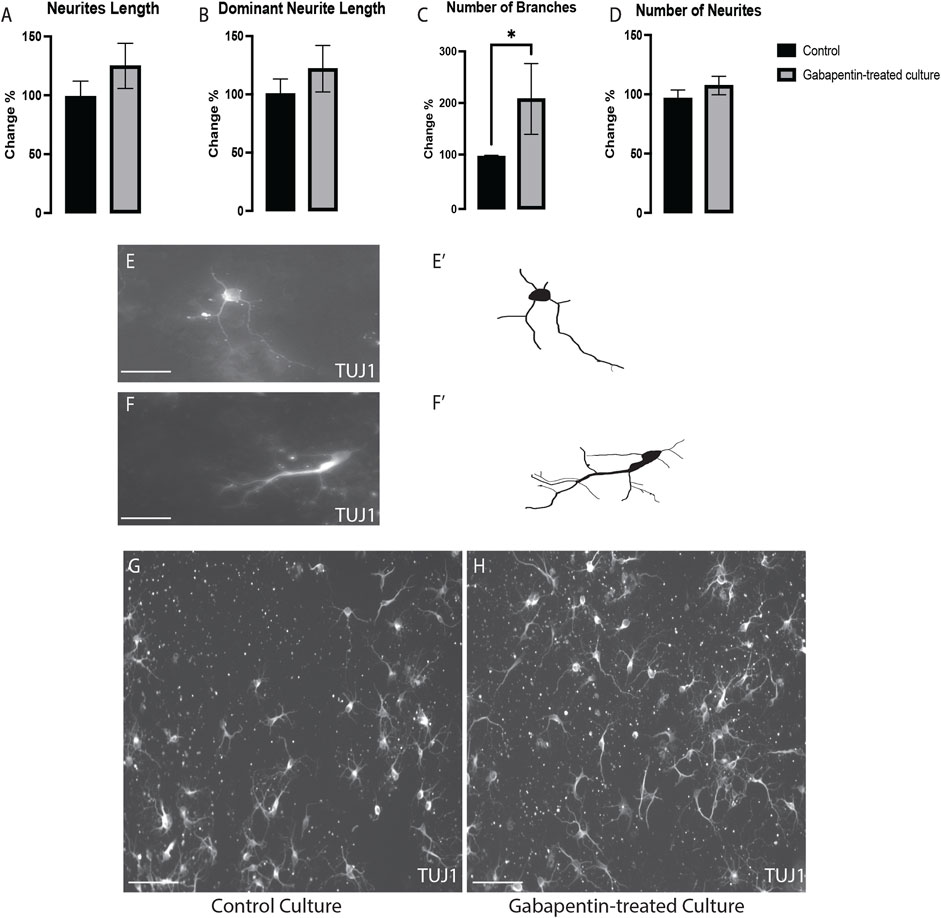
FIGURE 4. VM nondopaminergic neurons (TUJ1+/TH-) subjected to gabapentin exposure having no changes in the total neurite length (A), the dominant neurite length (B) or the number of neurites (D), while having significant increases in the number of branches (C). Representative images and illustration for VM neurons immunolabeled with TUJ1 in both groups; control (E,E’) and gabapentin-treated (F,F’) show the increase in the numbers of branches in response to gabapentin exposure. (G,H) images show large field of view (20x) for both groups; control and gabapentin treated cultures, respectively. Scale bar = 50 um (E,F). Scale bar = 10 um (G,H). Data are represented as mean ± SEM, n = 4 experiments. *p < 0.05.
Gabapentin Induces the Upregulation of Key Dopaminergic-Related Genes in vmDA Neurons
Several genes involved in neurogenesis have been discovered, such as Lmx1a/b, Mash1, and Ngn2, which play a role in early DA fate, Wnt5a/7a and Netrin1, which are involved in axonal plasticity, and Wnt5a, Pitx3, and Th, which play an important role in neuronal maturation. However, all these genes fail to encompass all activities associated with vmDA neuronal development (Burbach et al., 2003; Van den Heuvel and Pasterkamp, 2008; Fernando et al., 2014; Parish and Thompson, 2014). The fate and functional activities of mDA progenitors involve genes such as Lmx1a/b, which play an important role in neurogenesis (Doucet-Beaupré et al., 2016). Both Lmx1a and Lmx1b are closely associated and have 64% similarity in their amino acid sequence, 67 and 83% identity in the LIM domain, and 100% similarity in their homeodomain (Hobert and Westphal, 2000). The gabapentin-treated cultures in our experiments showed no significant change in Lmx1a expression compared to that in control cultures (Figure 5A). Genes such as Lmx1a, Nurr1, and Mash1 are associated with a minimal transcription factor mixture and enable vmDA neurons to be generated directly from murine and human fibroblasts without reversion to a progenitor cell stage (Caiazzo et al., 2011). Our results showed a significant upregulation in the expression of Nurr1 and En1 in the gabapentin-treated cultures (Figures 5B,C). Several studies have reported genes such as Nurr1, En1, Pitx3, Otx2, and Foxa2 to be involved in early development of vmDA neurons and are crucial for the maintenance phenotype of neurons (Doucet-Beaupr and Lvesque, 2013). Our results demonstrated a significant upregulation in the expression of Pitx3 in response to gabapentin exposure in vitro (Figure 5D). Studies have claimed that Nurr1 is activated by Lmx1a and subsequently activates Th, which is associated with vmDA neuronal differentiation (Bergman et al., 2009; Hoekstra et al., 2013). Although the expression of the maturation-related gene Pitx3 was altered in response to gabapentin exposure, the expression of Th was not significantly changed in the treated cultures in comparison with that in the control (Figure 5E). The expression profile of Pitx3 suggested that gabapentin could alter vmDA neuronal maturation. Previous studies by our group, and by others, have reported the involvement of Chl1 in vmDA development (Hoekstra et al., 2013; Alsanie et al., 2017). Our current results showed a significant upregulation in the expression of Chl1 (Figure 5F), which suggests that gabapentin may interfere with the neurogenesis and maturation of vmDA neurons. Our observations suggested that gabapentin follows the Chl1/En1/Nurr1/Pitx3 pathway for neuronal differentiation. The downstream targets of Nurr1, such as Bdnf, Dat, and Drd2, are highly important for vmDA maturation and neurogenesis (Johnson et al., 2011; Hegarty et al., 2013; Kadkhodaei et al., 2013; Torretta et al., 2020). The expression of these downstream targets was significantly altered in response to gabapentin treatment. The expression of Dat and Drd2 was significantly upregulated in gabapentin-treated cultures in comparison with that in the control (Figures 5G,H). However, Bdnf was significantly downregulated in response to gabapentin exposure (Figure 5I). These changes in the expression of the key genes might negatively affect the normal development of vmDA neurons.
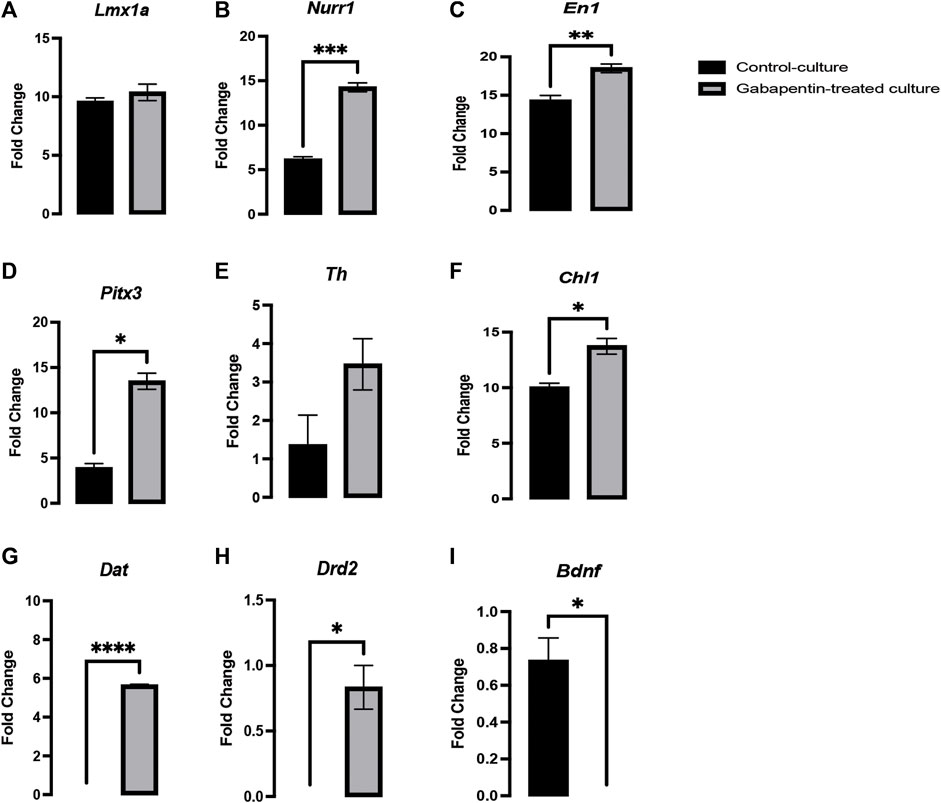
FIGURE 5. Gabapentin exposure inducing a significant upregulation in the expression of Nurr1 (B), En1 (C), Pitx3 (D), Chl1 (F), Dat (G) and Drd2 (H), significant downregulation the expression of Bdnf (I), and no significant change in the expression of Lmx1a (A) and Th (E) in vmDA neurons.
Discussion
Gabapentin is a GABA analog, which is commonly prescribed for treatment of neuropathic pain. However, it does not have an affinity for GABA receptors. Gabapentin increases GABA levels and decreases those of glutamate neurotransmitters. The neural population plays a major role in behavior, cognition, and motor activity. The fate of vmDA neurons is determined by intrinsic as well as extrinsic signaling ques. To establish a mature state and a final resting place, the neurons respond to several signals pertaining to neuronal connectivity during the cell cycle. Similar to the other neural populations, VM neurons are known to have early and late developmental stages. Our recent investigation hypothesized that gabapentinoids could affect the dopaminergic neuronal system (Althobaiti et al., 2020, 2021). In the current study, we observed that gabapentin at therapeutic doses has no impact on the survivability of VM neurons. However, our results also showed that there was a significant reduction in ATP release in VM neurons. Therefore, this study reveals that gabapentin could negatively impact the early stage of vmDA neurogenesis and morphogenesis. These results are important for the design of preclinical studies to determine the safety of gabapentin use during pregnancy.
Transcription factors such as Nurr1, Pitx3, and Lmx1a are critical for midbrain specification. We found that gabapentin could induce high expression of dopaminergic related genes, which suggested that gabapentin use might not be safe during pregnancy. Gabapentin is commonly used as a first-line treatment and is the most frequently prescribed drug in neuropathic disorders. The misuse of gabapentin is known to cause serious negative effects. Thus, several studies have been focused on investigating its safety in child-bearing women. The mechanism by which gabapentin interferes in fetal brain development is still not clear (Patorno et al., 2020). Antiepileptic drugs such as valproate have demonstrated adverse neurological effects when used alone or combined with other antiepileptics. Lamotrigine and oxcarbazepine have been associated with an increased occurrence of autism (Veroniki et al., 2017). This has made it necessary to develop optimum treatments with safe regimens (Veroniki et al., 2017). Previous studies have reported that large randomized clinical trials are required to confirm whether gabapentin does not interfere with neuronal brain development and is therefore safe to be prescribed for pregnant women (Guttuso et al., 2014). Hence, in the present study, we examined the impact of gabapentin on the development of vmDA neurons, which play an important role in rewards, motor activity and addiction. (Hegarty et al., 2013).
We treated primary mouse embryonic vmDA neurons with gabapentin to examine their survivability. We observed that gabapentin exposure had no impact on viability of vmDA neurons, confirming that gabapentin is nontoxic at therapeutic doses. Previous studies on the use of antiepileptics during pregnancy have concluded that valproic acid, lamotrigine, and carbamazepine are associated with smaller head circumference observed in the fetus (Margulis et al., 2019). Moreover, another study showed that gabapentin administration during pregnancy is associated with adverse gestational outcomes (Loudin et al., 2020). Therefore, the safety of the usage of gabapentin during pregnancy has not yet been conclusively determined (Loudin et al., 2020). Thus, antiepileptics, such as gabapentin are generally prescribed according to the risk-to-benefit ratio (Guttuso et al., 2014; Patorno et al., 2020). In the current study, we showed that gabapentin altered vmDA neuronal metabolic activity by decreasing the generation of ATP. These findings confirmed that gabapentin disrupts the activities of the electron transport chain, leading to ATP deficiency. Furthermore, we assessed the effect of gabapentin on the morphogenesis of vmDA neurons by investigating changes in the total and dominant neurite lengths following gabapentin treatment. We are reporting here that gabapentin treatment increased TH + vmDA neurites length (total and dominant neurites length). On the other hand, the numbers of neurites and branches were not significantly different between those in the treated and control groups. Contrarily, in the case of VM non-DA neurons, gabapentin significantly increased the number of branches, while the length of neurites remained the same. These findings are similar to those shown previously in our study on pregabalin, which is another drug belonging to the gabapentinoid family (Alsanie et al., 2022). However, pregabalin increased the number of neurites of VM non-DA neurons, an effect that was not detected with gabapentin treatment. Importantly, the alteration in the morphogenesis of vmDA neurons could disrupt the connectivity of these neurons with their neuronal targets. Consequently, these changes could lead to neurological disorders by abnormally changing behavior, cognition and/or motor activity.
Many genes such as Lmx1b, En1, Nurr1, Pitx3, and Lmx1a, are known to play a vital role in the development of vmDA neurons. (Yin et al., 2009; Volpicelli et al., 2012; Hoekstra et al., 2013). Previous studies have illustrated that Lmx1a and Nurr1 activate Th, which ultimately helps in vmDA neuronal differentiation and maturation (Bergman et al., 2009; Hoekstra et al., 2013). Pitx3 is regulated by Rspo2, which is activated by Lmx1a (Gyllborg et al., 2018). Considering these findings, we investigated the effects of gabapentin on the key genes associated with the development of vmDA neurons. We found that gabapentin significantly increased Nurr1, Chl1, En1, Bdnf, Drd2 and Pitx3 expression (Figure 5). Contrarily, no changes were detected in the expression profile of Lmx1a and Th. These observations suggested that gabapentin follows the Nurr1, En1, Chl1 and Pitx3 signaling pathways. Former studies have also showed that the expression of several major genes, such as Bdnf, Drd2, and Dat, is regulated by Nurr1 (Johnson et al., 2011; Hegarty et al., 2013; Kadkhodaei et al., 2013; Torretta et al., 2020). Bdnf is known to be a trophic factor for vmDA neurons and is therefore critical for their viability (Hyman et al., 1991).Drd2 and Dat are crucial genes for the development, maturation and functionality of vmDA neurons (Sung et al., 2006; Hwang et al., 2009; Hegarty et al., 2013). In the present study, we demonstrated that Drd2 and Dat are upregulated significantly by gabapentin, which may be attributed to high Nurr1 expression, which is also similar to that showed in our earlier study on pregabalin (Alsanie et al., 2022). A former study has illustrated that gabapentin exposure increases the expression of Pitx3, which in turn stimulates Dat in coordination with Nurr1 (Hwang et al., 2009). The high expression of Drd2 due to gabapentin exposure might be the reason behind the reported increase in the neurite length of TH + neurons. The elongation of dopaminergic neurons induced by DRD2 receptors has also been reported in an earlier study by Hwang and colleagues (Hwang et al., 2009). Although Bdnf is one of the downstream targets of Nurr1, our results showed that the expression of Bdnf was significantly downregulated by gabapentin treatment. Previously, we demonstrated that the expression of Bdnf was upregulated by pregabalin treatment in vmDA neuron cultures (Alsanie et al., 2022). Although gabapentin and pregabalin are both gabapentinoids, their effects on vmDA-related genes are thus shown to be different. Such alternate expression of the vital genes has been reported to be associated with several psychological, mental and neurological problems (Hansen et al., 2014; Zhang and Zhang, 2016; Tsai, 2018).
Conclusion
Our study examined the effect of gabapentin treatment on vmDA neurons, which are in charge of cognition, motion, and behavior. We used primary mouse embryonic VM neurons to investigate the impact of prenatal exposure to gabapentin on embryonic vmDA development. Our results revealed that gabapentin exposure during neurodevelopment interfered with the neurogenesis and morphogenesis of vmDA neurons. Our findings suggest that gabapentin usage in pregnant women, even at therapeutic levels, interferes with the neurogenesis and morphogenesis of vmDA neurons during fetal brain development. Furthermore, several genes involved in the influence of gabapentin on vmDA development were identified. These changes could eventually alter the functionality of vmDA neurons. These findings have important implications on the safety assessment of the therapeutic use of gabapentin during pregnancy. Future in vivo and neuronal functionality studies can be conducted to better evaluate the effects of gabapentin on vmDA neurons. In addition, neuronal cultures established from human embryonic stem cells could be used to further investigate the effects of gabapentin exposure on human neurons.
Data Availability Statement
The original contributions presented in the study are included in the article/Supplementary materials, further inquiries can be directed to the corresponding author.
Ethics Statement
The animal study was reviewed and approved by King Abdulaziz University and Taif University (1-441-133).
Author Contributions
WFA, MA, AG, ASA, SHA, CAEH, HHT, and RIF methodology, formal analysis, and data curation. SIA and WFA writing-original draft preparation. WFA, YSA, KAA, AG, YSA and YAA data writing-review and editing. WFA, KAA and YAA funding acquisition. HH, HAA and BMR conceptualization and visualization.
Funding
The present research received fund from the Deputyship for Research and Innovation, Ministry of Education, Saudi Arabia, through project number 1-441-133.
Acknowledgments
The authors are appreciatively to Dr. Christian M. Nefzgar; Institute for Molecular Bioscience, The University of Queensland, Brisbane, QLD, Australia, and to Dr. Abdulwahab Alamri; College of Pharmacy, University of Hail, Saudi Arabia for their technical support. Charlotte A. E. Hauser and Sherin Abdelrahman would like to thank kaust for their support. Also, authors extend their appreciation to the Deputyship for Research and Innovation, Ministry of Education, Saudi Arabia, for funding this work through project number 1-441-133.
Conflict of Interest
The authors declare that the research was conducted in the absence of any commercial or financial relationships that could be construed as a potential conflict of interest.
Publisher’s Note
All claims expressed in this article are solely those of the authors and do not necessarily represent those of their affiliated organizations, or those of the publisher, the editors and the reviewers. Any product that may be evaluated in this article, or claim that may be made by its manufacturer, is not guaranteed or endorsed by the publisher.
References
Alsanie, W. F., Alhomrani, M., Gaber, A., Habeeballah, H., Alkhatabi, H. A., Felimban, R. I., et al. (2022). The Effects of Prenatal Exposure to Pregabalin on the Development of Ventral Midbrain Dopaminergic Neurons. Cells 11, 852. doi:10.3390/CELLS11050852
Alsanie, W. F., Bahri, O. A., Habeeballah, H. H., Alhomrani, M., Almehmadi, M. M., Alsharif, K., et al. (2020). Generating Homogenous Cortical Preplate and Deep-Layer Neurons Using a Combination of 2D and 3D Differentiation Cultures. Sci. Rep. 10, 6272. doi:10.1038/S41598-020-62925-9
Alsanie, W. F., Penna, V., Schachner, M., Thompson, L. H., and Parish, C. L. (2017). Homophilic Binding of the Neural Cell Adhesion Molecule CHL1 Regulates Development of Ventral Midbrain Dopaminergic Pathways. Sci. Rep. 7, 9368. doi:10.1038/S41598-017-09599-Y
Althobaiti, Y. S., Alghorabi, A., Alshehri, F. S., Baothman, B., Almalki, A. H., Alsaab, H. O., et al. (2020). Gabapentin-induced Drug-seeking-like Behavior: a Potential Role for the Dopaminergic System. Sci. Rep. 10, 10445. doi:10.1038/S41598-020-67318-6
Althobaiti, Y. S., Almutairi, F. M., Alshehri, F. S., Altowairqi, E., Marghalani, A. M., Alghorabi, A. A., et al. (2021). Involvement of the Dopaminergic System in the Reward-Related Behavior of Pregabalin. Sci. Rep. 11, 1–9. doi:10.1038/s41598-021-88429-8
Bergman, O., Håkansson, A., Westberg, L., Belin, A. C., Sydow, O., Olson, L., et al. (2009). Do polymorphisms in Transcription Factors LMX1A and LMX1B Influence the Risk for Parkinson's Disease? J. Neural Transm. (Vienna) 116, 333–338. doi:10.1007/S00702-009-0187-Z
Burbach, J. P., Smits, S., and Smidt, M. P. (2003). Transcription Factors in the Development of Midbrain Dopamine Neurons. Ann. N. Y. Acad. Sci. 991, 61–68. doi:10.1111/J.1749-6632.2003.TB07463.X
Caiazzo, M., Dell'Anno, M. T., Dvoretskova, E., Lazarevic, D., Taverna, S., Leo, D., et al. (2011). Direct Generation of Functional Dopaminergic Neurons from Mouse and Human Fibroblasts. Nature 476, 224–227. doi:10.1038/NATURE10284
Compton, P., Kehoe, P., Sinha, K., Torrington, M. A., and Ling, W. (2010). Gabapentin Improves Cold-Pressor Pain Responses in Methadone-Maintained Patients. Drug Alcohol Depend. 109, 213–219. doi:10.1016/J.DRUGALCDEP.2010.01.006
Doucet-Beaupré, H., Gilbert, C., Profes, M. S., Chabrat, A., Pacelli, C., Giguère, N., et al. (2016). Lmx1a and Lmx1b Regulate Mitochondrial Functions and Survival of Adult Midbrain Dopaminergic Neurons. Proc. Natl. Acad. Sci. U. S. A. 113, E4387–E4396. doi:10.1073/PNAS.1520387113/-/DCSUPPLEMENTAL
Doucet-Beaupré, H., and Lévesque, M. (2013). The Role of Developmental Transcription Factors in Adult Midbrain Dopaminergic Neurons. OA Neurosci. 1, 3. doi:10.13172/2054-7358-1-1-947
Fernando, C. V., Kele, J., Bye, C. R., Niclis, J. C., Alsanie, W., Blakely, B. D., et al. (2014). Diverse Roles for Wnt7a in Ventral Midbrain Neurogenesis and Dopaminergic Axon Morphogenesis. Stem Cells Dev. 23, 1991–2003. doi:10.1089/SCD.2014.0166
Gee, N. S., Brown, J. P., Dissanayake, V. U., Offord, J., Thurlow, R., and Woodruff, G. N. (1996). The Novel Anticonvulsant Drug, Gabapentin (Neurontin), Binds to the Alpha2delta Subunit of a Calcium Channel. J. Biol. Chem. 271, 5768–5776. doi:10.1074/JBC.271.10.5768
Guttuso, T., Shaman, M., and Thornburg, L. L. (2014). Potential Maternal Symptomatic Benefit of Gabapentin and Review of its Safety in Pregnancy. Eur. J. Obstet. Gynecol. Reprod. Biol. 181, 280–283. doi:10.1016/J.EJOGRB.2014.08.013
Gyllborg, D., Ahmed, M., Toledo, E. M., Theofilopoulos, S., Yang, S., Ffrench-Constant, C., et al. (2018). The Matricellular Protein R-Spondin 2 Promotes Midbrain Dopaminergic Neurogenesis and Differentiation. Stem Cell Rep. 11, 651–664. doi:10.1016/j.stemcr.2018.07.014
Hamer, A. M., Haxby, D. G., McFarland, B. H., and Ketchum, K. (2002). Gabapentin Use in a Managed Medicaid Population. J. Manag. Care Pharm. 8, 266–271. doi:10.18553/JMCP.2002.8.4.266
Hansen, F. H., Skjørringe, T., Yasmeen, S., Arends, N. V., Sahai, M. A., Erreger, K., et al. (2014). Missense Dopamine Transporter Mutations Associate with Adult Parkinsonism and ADHD. J. Clin. Invest. 124, 3107–3120. doi:10.1172/JCI73778
Hegarty, S. V., Sullivan, A. M., and O'Keeffe, G. W. (2013). Midbrain Dopaminergic Neurons: A Review of the Molecular Circuitry that Regulates Their Development. Dev. Biol. 379, 123–138. doi:10.1016/J.YDBIO.2013.04.014
Hobert, O., and Westphal, H. (2000). Functions of LIM-Homeobox Genes. Trends Genet. 16, 75–83. doi:10.1016/S0168-9525(99)01883-1
Hoekstra, E. J., von Oerthel, L., van der Heide, L. P., Kouwenhoven, W. M., Veenvliet, J. V., Wever, I., et al. (2013). Lmx1a Encodes a Rostral Set of Mesodiencephalic Dopaminergic Neurons Marked by the Wnt/B-Catenin Signaling Activator R-Spondin 2. PLoS One 8, e74049. doi:10.1371/journal.pone.0074049
Hwang, D. Y., Hong, S., Jeong, J. W., Choi, S., Kim, H., Kim, J., et al. (2009). Vesicular Monoamine Transporter 2 and Dopamine Transporter Are Molecular Targets of Pitx3 in the Ventral Midbrain Dopamine Neurons. J. Neurochem. 111, 1202–1212. doi:10.1111/J.1471-4159.2009.06404.X
Hyman, C., Hofer, M., Barde, Y. A., Juhasz, M., Yancopoulos, G. D., Squinto, S. P., et al. (1991). BDNF Is a Neurotrophic Factor for Dopaminergic Neurons of the Substantia Nigra. Nature 350, 230–232. doi:10.1038/350230A0
Johnson, M. M., Michelhaugh, S. K., Bouhamdan, M., Schmidt, C. J., and Bannon, M. J. (2011). The Transcription Factor NURR1 Exerts Concentration-dependent Effects on Target Genes Mediating Distinct Biological Processes. Front. Neurosci. 5, 135. doi:10.3389/FNINS.2011.00135/ABSTRACT
Kadkhodaei, B., Alvarsson, A., Schintu, N., Ramsköld, D., Volakakis, N., Joodmardi, E., et al. (2013). Transcription Factor Nurr1 Maintains Fiber Integrity and Nuclear-Encoded Mitochondrial Gene Expression in Dopamine Neurons. Proc. Natl. Acad. Sci. U. S. A. 110, 2360–2365. doi:10.1073/PNAS.1221077110/-/DCSUPPLEMENTAL
Kim, S. Y., Kyou, C. C., Min, S. C., Myoung, H. K., Sa, Y. K., Na, Y. S., et al. (2006). The Dopamine D2 Receptor Regulates the Development of Dopaminergic Neurons via Extracellular Signal-Regulated Kinase and Nurr1 Activation. J. Neurosci. 26, 4567–4576. doi:10.1523/JNEUROSCI.5236-05.2006
Loudin, S., Haas, J., Payne, M., Linz, M. F., Meaney-Delman, D., Honein, M. A., et al. (2020). Identifying Co-exposure to Opiates and Gabapentin during Pregnancy. J. Pediatr. 217, 196–198. doi:10.1016/J.JPEDS.2019.09.029
Mack, A. (2003). Examination of the Evidence for Off-Label Use of Gabapentin. J. Manag. Care Pharm. 9, 559–568. doi:10.18553/JMCP.2003.9.6.559
Margulis, A. V., Hernandez-Diaz, S., McElrath, T., Rothman, K. J., Plana, E., Almqvist, C., et al. (2019). Relation of In-Utero Exposure to Antiepileptic Drugs to Pregnancy Duration and Size at Birth. PLoS One 14, e0214180. doi:10.1371/JOURNAL.PONE.0214180
Parish, C. L., and Thompson, L. H. (2014). Modulating Wnt Signaling to Improve Cell Replacement Therapy for Parkinson's Disease. J. Mol. Cell Biol. 6, 54–63. doi:10.1093/JMCB/MJT045
Patorno, E., Hernandez-Diaz, S., Huybrechts, K. F., Desai, R. J., Cohen, J. M., Mogun, H., et al. (2020). Gabapentin in Pregnancy and the Risk of Adverse Neonatal and Maternal Outcomes: A Population-Based Cohort Study Nested in the US Medicaid Analytic eXtract Dataset. PLoS Med. 17, e1003322. doi:10.1371/JOURNAL.PMED.1003322
Radley, D. C., Finkelstein, S. N., and Stafford, R. S. (2006). Off-label Prescribing Among Office-Based Physicians. Arch. Intern. Med. 166, 1021–1026. doi:10.1001/ARCHINTE.166.9.1021
Smith, R. V., Havens, J. R., and Walsh, S. L. (2016). Gabapentin Misuse, Abuse and Diversion: a Systematic Review. Addiction 111, 1160–1174. doi:10.1111/ADD.13324
Suman-Chauhan, N., Webdale, L., Hill, D. R., and Woodruff, G. N. (1993). Characterisation of [3H]gabapentin Binding to a Novel Site in Rat Brain: Homogenate Binding Studies. Eur. J. Pharmacol. 244, 293–301. doi:10.1016/0922-4106(93)90155-3
Thurlow, R. J., Brown, J. P., Gee, N. S., Hill, D. R., and Woodruff, G. N. (1993). [3H]gabapentin May Label a System-L-like Neutral Amino Acid Carrier in Brain. Eur. J. Pharmacol. 247, 341–345. doi:10.1016/0922-4106(93)90204-M
Torretta, S., Rampino, A., Basso, M., Pergola, G., Di Carlo, P., Shin, J. H., et al. (2020). NURR1 and ERR1 Modulate the Expression of Genes of a DRD2 Coexpression Network Enriched for Schizophrenia Risk. J. Neurosci. 40, 932–941. doi:10.1523/JNEUROSCI.0786-19.2019
Tsai, S. J. (2018). Critical Issues in BDNF Val66met Genetic Studies of Neuropsychiatric Disorders. Front. Mol. Neurosci. 11, 156. doi:10.3389/FNMOL.2018.00156/BIBTEX
Van den Heuvel, D. M., and Pasterkamp, R. J. (2008). Getting Connected in the Dopamine System. Prog. Neurobiol. 85, 75–93. doi:10.1016/J.PNEUROBIO.2008.01.003
Veroniki, A. A., Rios, P., Cogo, E., Straus, S. E., Finkelstein, Y., Kealey, R., et al. (2017). Comparative Safety of Antiepileptic Drugs for Neurological Development in Children Exposed during Pregnancy and Breast Feeding: a Systematic Review and Network Meta-Analysis. BMJ Open 7, e017248. doi:10.1136/BMJOPEN-2017-017248
Volpicelli, F., de Gregorio, R., Pulcrano, S., Perrone-Capano, C., di Porzio, U., and Bellenchi, G. C. (2012). Direct Regulation of Pitx3 Expression by Nurr1 in Culture and in Developing Mouse Midbrain. PLoS One 7, e30661. doi:10.1371/journal.pone.0030661
Wahba, M., and Waln, O. (2013). Asterixis Related to Gabapentin Intake: a Case Report and Review. Postgrad. Med. 125, 139–141. doi:10.3810/PGM.2013.09.2696
Yin, M., Liu, S., Yin, Y., Li, S., Li, Z., Wu, X., et al. (2009). Ventral Mesencephalon-Enriched Genes that Regulate the Development of Dopaminergic Neurons In Vivo. J. Neurosci. 29, 5170–5182. doi:10.1523/JNEUROSCI.5569-08.2009
Keywords: gabapentin, neurons, morphogenesis, pregnancy, development
Citation: Alsanie WF, Abdelrahman S, Alhomrani M, Gaber A, Habeeballah H, Alkhatabi HA, Felimban RI, Hauser CAE, Tayeb HH, Alamri AS, Raafat BM, Anwar S, Alswat KA, Althobaiti YS and Asiri YA (2022) Prenatal Exposure to Gabapentin Alters the Development of Ventral Midbrain Dopaminergic Neurons. Front. Pharmacol. 13:923113. doi: 10.3389/fphar.2022.923113
Received: 18 April 2022; Accepted: 22 June 2022;
Published: 22 July 2022.
Edited by:
Andleeb Khan, Jazan University, Saudi ArabiaReviewed by:
Muneeb U. Rehman, King Saud University, Saudi ArabiaSadaf Jahan, Majmaah University, Saudi Arabia
Copyright © 2022 Alsanie, Abdelrahman, Alhomrani, Gaber, Habeeballah, Alkhatabi, Felimban, Hauser, Tayeb, Alamri, Raafat, Anwar, Alswat, Althobaiti and Asiri. This is an open-access article distributed under the terms of the Creative Commons Attribution License (CC BY). The use, distribution or reproduction in other forums is permitted, provided the original author(s) and the copyright owner(s) are credited and that the original publication in this journal is cited, in accordance with accepted academic practice. No use, distribution or reproduction is permitted which does not comply with these terms.
*Correspondence: Walaa F. Alsanie, dy5hbHNhbmllQHR1LmVkdS5zYQ==