- 1Department of Rehabilitation Sciences, Faculty of Health and Social Sciences, Hong Kong Polytechnic University, Kowloon, Hong Kong SAR, China
- 2Department of Pharmacology and Pharmacy, The University of Hong Kong, Pokfulam, Hong Kong SAR, China
- 3State Key Laboratory of Quality Research in Chinese Medicine and Institute of Chinese Medical Sciences, University of Macau, Taipa Macao SAR, China
Angiogenesis, the formation of new capillaries from pre-existing vascular networks, plays an important role in many physiological and pathological processes. The use of pro-angiogenic agents has been proposed as an attractive approach for promoting wound healing and treating vascular insufficiency-related problems, such as ischemic heart disease and stroke, which are the leading causes of death worldwide. Traditional herbal medicine has a long history; however, there is still a need for more in-depth studies and evidence-based confirmation from controlled and validated trials. Many in vitro and in vivo studies have reported that herbal medicines and their bioactive ingredients exert pro-angiogenic activity. The most frequently studied pro-angiogenic phytochemicals include ginsenosides from Panax notoginseng, astragalosides and calycosin from Radix Astragali, salvianolic acid B from Salvia miltiorrhiza, paeoniflorin from Radix Paeoniae, ilexsaponin A1 from Ilex pubescens, ferulic acid from Angelica sinensis, and puerarin from Radix puerariae. This review summarizes the progress in research on these phytochemicals, particularly those related to pro-angiogenic mechanisms and applications in ischemic diseases, tissue repair, and wound healing. In addition, an outline of their limitations and challenges during drug development is presented.
Introduction
Angiogenesis is the formation of new blood vessels. It plays a critical role in regulating a wide range of physiological activities such as embryonic development, menstrual cycle, wound healing, tissue repair after surgery, and traumatic injury (Carmeliet, 2003). Angiogenesis is tightly regulated by several stimulators and inhibitors. An imbalance between these stimulators and inhibitors is implicated in diseases including cancer, cardio-cerebral diseases, age-related macular degeneration, arthritis, psoriasis, obesity, and even asthma. Therefore, the manipulation of angiogenesis is considered an attractive and promising approach for curing these pathological conditions.
Process of Angiogenesis
Angiogenesis is categorized into two major types: sprouting and intussusceptive angiogenesis (Kurz, 2000). In sprouting angiogenesis, the processes of endothelial cell migration and proliferation, sprout fusion, and lumen formation are precisely coordinated (Franco et al., 2009). When endothelial cells are stimulated by pro-angiogenic factors, they produce proteases that break down the surrounding basement membrane, allowing them to move out of the pre-existing blood vessel lumen. The endothelial cells proliferate extensively in the surrounding matrix. Thus, solid sprouts are formed. After recruiting mural cells (i.e., pericytes and smooth muscle cells), the sprouts are ultimately transformed into mature blood vessels (Carmeliet, 2000; Duran et al., 2017).
In intussusceptive angiogenesis, blood vessels are divided into two new types (Burri et al., 2004). This process starts with the projection of opposing capillary walls inside the lumen of the original blood vessel, and inter-endothelial contact is built up. Afterward, growth factors and cells such as fibroblasts and pericytes enter. A pillar is formed between the two new vessels. This pillar eventually disappears and the two independent blood vessels split (Burri et al., 2004).
Sprouting and intussusceptive angiogenesis are functionally distinct. Sprouting angiogenesis promotes the formation of blood vessels in previously unvascularized tissues, whereas intussusceptive angiogenesis allows for the rapid-recovery adaption of existing microvascular networks (Heil et al., 2006). Sprouting and intussusceptive angiogenesis can occur concurrently during the development of certain organs or tissues, such as the lung, heart, and yolk sac (Kurz, 2000). It has been suggested that sprouting angiogenesis is more common during brain development, whereas intussusceptive angiogenesis is predominant in the lungs (Kurz, 2000; Burri and Djonov, 2002). Interestingly, increasing evidence has shown that intussusceptive angiogenesis is more important than sprouting angiogenesis in embryonic development. This is because there are inadequate resources to produce large numbers of endothelial cells during embryonic development. Intussusceptive angiogenesis can rapidly and massively increase the number of blood vessels without a corresponding increase in endothelial cells (Carmeliet, 2003; Djonov et al., 2003).
Regulation of Angiogenesis
Endogenous pro-angiogenic and anti-angiogenic factors are listed in Table 1. VEGF is the most representative pro-angiogenic factor, inducing endothelial cell survival, proliferation, migration, invasion, lumen formation, and blood vessel maturation (Cébe-Suarez et al., 2006). Hypoxia-inducible factor (HIF)-1 is a major driver of angiogenesis. HIF-1 comprises an oxygen-regulated α-subunit and ubiquitously expressed ß-subunit. Under hypoxia, the hydroxylation of HIF-1α is inhibited. HIF-1α, therefore, accumulates and translocates to the nucleus, where it forms a dimer with HIF-1β and binds to the VEGF promoter. Thus, the expression of VEGF mRNA and the subsequent release of VEGF are increased.
Mechanistically, VEGF regulates the secretion and activation of enzymes and factors [e.g. matrix metallopeptidases (MMP) and plasminogen activators] involved in the degradation of the extracellular matrix, a critical step in angiogenesis (Carmeliet, 2000). Moreover, VEGF protects newly formed blood vessels against apoptosis by promoting the anti-apoptotic factors B-cell lymphoma (Bcl)-2 and survivin (Karaman et al., 2018). Basic information on VEGF family members, such as splice variants, sources, receptors, and functions, is given in Table 2.
VEGF receptors (VEGFR) comprise extracellular domains with seven immunoglobulin-like subdomains, a single transmembrane spanning domain, and an intracellular tyrosine kinase domain (Hoeben et al., 2004). The VEGFR family comprises three members. Among these, VEGFR-2 is the most important receptor involved in VEGF-induced angiogenesis (Holmes et al., 2007; Wang et al., 2020). On stimulation with VEGFR-2, the subsequent dimerization of two receptor monomers causes phosphorylation of tyrosine residues in the intracellular domain, which induces the activation of a series of signaling pathways, leading to the formation of new blood vessels (Peach et al., 2018; Fallah et al., 2019; Wang et al., 2020) (Figure 1). The downstream signaling pathways of VEGFR-2 include the protein kinase C (PKC)/proto-oncogene serine/threonine-protein kinase (Raf)/mitogen-activated protein kinase (MEK)/extracellular signal-regulated kinase (ERK) signaling pathway, which is related to endothelial cell proliferation; the SH2 domain containing adapter protein B (Shb)/focal adhesion kinase (FAK) and cell division control protein 42 homolog (Cdc42)/p38 MAPK signaling pathways, which are responsible for endothelial cell migration; inhibition of the Bcl-2 associated death (BAD) promoter and caspase-9 expression by the PI3K/protein kinase B (Akt)-dependent pathway, which is associated with endothelial cell survival; and PKC/endothelial nitric oxide synthase (eNOS), which catalyzes the production of nitric oxide, increasing vascular permeability.
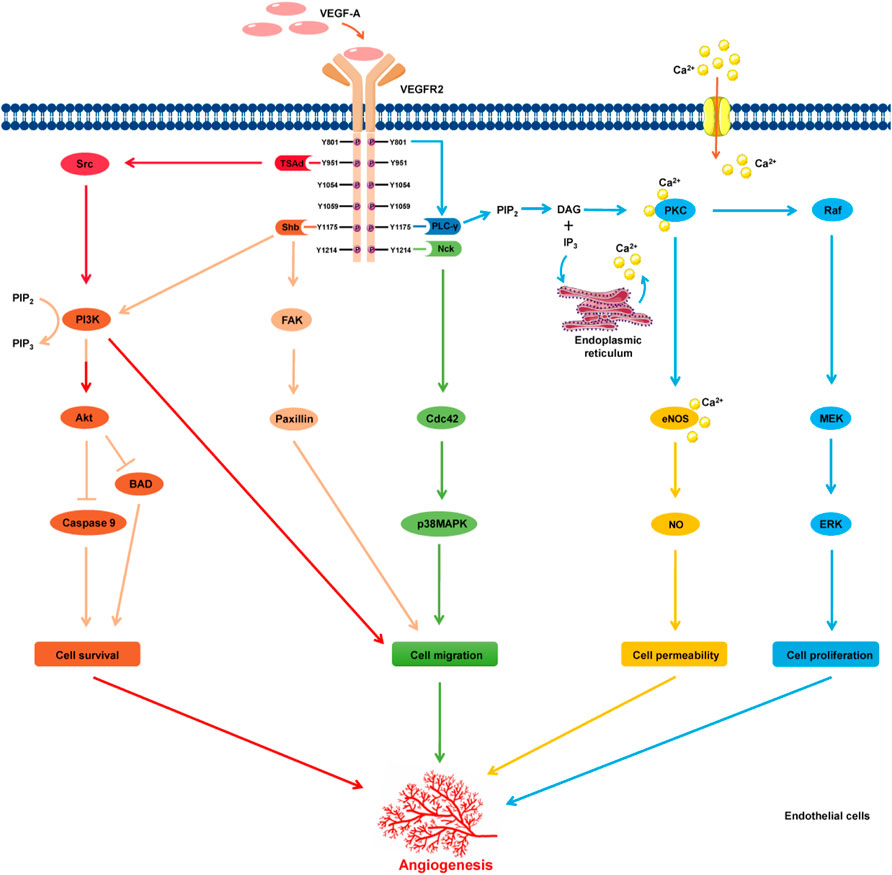
FIGURE 1. Schematic illustration of VEGFR-2 signaling pathways. Stimulation of VEGFR-2 by VEGF-A induces dimerization and autophosphorylation of specific intracellular tyrosine residues. Subsequently, the activation of the downstream signal transduction pathways induces the proliferation, migration, and survival of endothelial cells, and increases vascular permeability.
Relationship Between Angiogenesis and Diseases
Increased rates of angiogenesis are associated with the pathogenesis of diseases such as cancer (Weis and Cheresh, 2011), psoriasis (Weidemann et al., 2013), rheumatoid arthritis (Koch and Distler, 2007), diabetic retinopathy (Behl and Kotwani, 2015), and age-related macular degeneration (Ng and Adamis, 2005). VEGF is implicated in the pathology of these conditions. Anti-angiogenic therapy has been used for the treatment of cancer and macular degeneration (Carmeliet, 2005; Ng and Adamis, 2005; Liu et al., 2006; Copur, 2008; Wilhelm et al., 2008; Escudier et al., 2009; Capdevila et al., 2012; Al-Ostoot et al., 2021). In addition to VEGF, deficiency of leucine-rich α-2-glycoprotein 1 (LRG1), a novel pro-angiogenic factor, also leads to several pathophysiological processes such as retinopathy and diabetic wounds. The mechanism involves the TGF-β accessory receptor, endoglin, apoptosis-activating factor-1, and HIF-1α stability (Wang et al., 2013a; Gao et al., 2020; Jemmerson et al., 2021). Angiopoietins and vasohibin-2 are two additional angiogenic factors involved in pathogenesis. It has been reported that the imbalance of the angiopoietin-1 and angiopoietin-2 ratio and deficiency of vasohibin-2 play a role in the pathogenesis of glomerular diseases (Zhang et al., 2020).
In contrast, insufficient angiogenesis leads to diseases such as ischemic heart failure and stroke, neurodegeneration, osteoporosis, respiratory distress, preeclampsia, endometriosis, and ovarian hyperstimulation syndrome (Carmeliet, 2003). Theoretically, pro-angiogenic agents can improve blood perfusion, transport survival factors to ischemic tissues, mobilize regenerative stem cell populations, and ultimately restore tissue functions (Majewska and Gendaszewska-Darmach, 2011; Mitsos et al., 2012). Pro-angiogenic therapy has been suggested as a promising approach for wound healing and tissue repair. A clinical trial has been conducted on patients with coronary artery disease, and their conditions can be improved after the injection of human VEGF (Sarkar et al., 2001). In addition, improved vascularization of regenerated tissue is necessary after tissue implantation and subsequent major operations. The incorporation of VEGF and FGF may increase the cure and surgical success rates in critically ill patients (Nomi et al., 2002).
Herbal Medicine and Phytochemicals as Novel Pro-Angiogenic Agents and Their Potential Implications
Native pro-angiogenic agents inside the body, such as VEGF and FGF, are not ideal options for pro-angiogenic therapy because their therapeutic efficacy is often transient. This is related to the development of drug resistance and degradation over time. Small-molecule drugs may be a better alternative, for many reasons. First, they are more stable than proteins under a wider range of conditions. Second, they are less expensive in terms of production costs. Third, they may act on multiple targets, reducing the risk of drug resistance. Many small-molecule drugs used clinically are derived either directly or indirectly from plants. According to the National Administration of Traditional Chinese Medicine, the advantages of traditional Chinese medicine are particularly prominent in treating 95 types of diseases, including diseases closely related to angiogenesis, such as ischemic diseases and diabetic ulcers (National Administration of Traditional Chinese Medicine, 2019). Therefore, many studies have attempted to explore the potential pro-angiogenic effects of herbal medicines and their active ingredients. The following examples of herbal medicines were chosen because they are better studied and their pro-angiogenic effects are more widely reported. They have been used for a long time; therefore, their efficacy and safety are well recognized. The chemical structures, pharmacology, and potential applications of phytochemicals in these herbal medicines are given in Figure 2 and Table 3.
Panax notoginseng
The root of Panax notoginseng (Burkill) F.H. Chen, also known as San Qi in Chinese, is a traditional herbal medicine used in Asia to treat blood stasis and promote blood circulation (Yu et al., 2007; Cheung et al., 2011). Moreover, P. notoginseng has been proposed for the treatment of cardiovascular diseases, inflammatory diseases, and traumatic injuries. The basic active ingredients in P. notoginseng are saponins (Liu et al., 2014); more than 60 saponins have been identified in this plant (Wang et al., 2013b), of which ginsenoside Rg1, ginsenoside Rb1, and notoginsenoside R1 are the major P. notoginseng saponins (PNS). These compounds possess promising angiogenic effects in vitro and in vivo at multiple sites of action (Hong et al., 2009; Zheng et al., 2013a). Interestingly, the saponins extracted from the flowers of P. notoginseng exhibit similar pro-angiogenic properties, although the types of saponins may be different from those in the roots (Yang et al., 2016a).
It has been reported that PNS can rescue myocardial infarct size and cardiac function in left anterior descending coronary artery ligation-operated mice, an in vivo model of acute myocardial infarction. PNS inhibits platelet aggregation and enhances angiogenesis in response to myocardial infarction injury (Wang et al., 2021a). In vitro studies have shown that PNS promotes tube formation in endothelial cells through adenosine monophosphate kinase- and eNOS-dependent signaling pathways (Wang et al., 2017). Moreover, notoginsenoside R1 stimulates the proliferation, migration, and tube formation of endothelial cells. It also exerts pro-angiogenic effects in zebrafish models. Its pro-angiogenic action is mediated through the activation of the VEGF-KDR/Flk-1 and PI3K-Akt-eNOS signaling pathways (Yang et al., 2016b). Another saponin, notoginsenoside Ft1, increases the release of VEGF via the HIF-1α-dependent pathway. In addition, notoginsenoside Ft1 induces the activation of PI3K/Akt and Raf/MEK/ERK signaling pathways, which are crucial in the process of angiogenesis (Shen et al., 2012).
The active ingredients of Panax notoginseng have promising effects on wound healing. In streptozotocin-induced diabetic rats, ginsenoside Rg1 promoted angiogenesis during wound healing. This is probably mediated through the reduction of miR-23a levels, which removes its inhibitory regulation on interferon regulatory factor-1. Subsequently, interferon regulatory factor-1 activates inducible nitric oxide synthase (iNOS). The increased production of NO increases VEGF expression, proliferation, anti-apoptotic efficacy, and the migration ability of endothelial cells (Cai et al., 2019).
Interestingly, the PNS acts not only on endothelial cells but also on fibroblasts, another important cell type involved in angiogenesis and wound healing. The PNS promotes the proliferation and migration of fibroblasts and increases the expression of collagen and fibronectin. Further mechanistic studies indicate that the PNS plays a role in the phosphorylation of PI3K, Akt, and ERK-dependent pathways (Yu et al., 2015). Ginsenoside Rb1 improved the wound healing rate in a rat model of second-degree burn injury, probably due to the upregulation of PDGF-BB, PDGFR-β, and FGF-2 expression (Zhang et al., 2021). In human dermal fibroblasts, notoginsenoside Ft1 increases cell proliferation and collagen production via the PI3K/Akt/mechanistic target of the rapamycin (mTOR) signaling pathway. Notoginsenoside Ft1 shortens the wound closure time in an excisional wound-splinting model established in db/db diabetic mice by promoting fibroblast proliferation and attenuating the inflammatory response (Zhang et al., 2016).
Although the pro-angiogenic effects of PNS and their potential applications have been widely proposed, clinical data are relatively rare. Interestingly, a clinical study reported that a combination of granulocyte colony-stimulating factor (G-CSF)-mobilized peripheral blood mononuclear cell (PBMNC) transplantation and PNS is useful for the treatment of unreconstructable critical limb ischemia (Wang et al., 2014). The combination resulted in a greater reduction in the ulcer area and severity of rest pain than PBMNC transplantation alone. Improvements in parameters such as ankle-brachial index, transcutaneous oxygen pressure, and walking distance were significantly improved after combining PBMNC and PNS.
Radix Astragali
Radix Astragali, the dried root of Astragalus mongholicus Bunge, is also known in Chinese as Huang Qi. It is one of the most popular traditional herbal medicines used to reinforce vital energy. It possesses cardiotonic, hepatoprotective, hypotensive, immunostimulatory, anti-aging, anti-oxidative, antidiabetic, and anti-inflammatory activities. The pro-angiogenic potential of Radix Astragali is reflected in a work showing that Radix Astragali extract can stimulate the proliferation, migration, and tube formation of endothelial cells. The mechanism of action involves the upregulation of VEGF and stimulation of the PI3K-Akt-eNOS pathway (Zhang et al., 2009).
Astragaloside IV (AS-IV) is one of the active ingredients responsible for the pro-angiogenic effects of Radix Astragali. AS-IV can reproduce the effects of Radix Astragali extract on endothelial cells, and it has been shown to rescue blood vessel loss in zebrafish models (Zhang et al., 2012). In terms of the mechanism of action, AS-IV increases HIF-1α through the PI3K/Akt pathway in endothelial cells, and the increase in HIF-1α stimulates downstream VEGF signaling pathways and regulates angiogenesis in a hypoxic environment (Zhang et al., 2011). In addition, AS-IV stimulates endothelial cells to produce small ubiquitin-related modifier 1, which stabilizes the HIF-1α/VEGF pathway and improves angiogenesis (Wang et al., 2021b; Wang et al., 2021c).
The pro-angiogenic effect of AS-IV may be useful in the treatment of myocardial infarction. In rat models of myocardial infarction established by ligation of the left anterior descending artery, AS-IV significantly improved the morphology of the myocardium and the number of new blood vessels. The cardioprotective effect of AS-IV is mediated by its pro-angiogenic effect through the protein kinase D1-histone deacetylase 5-VEGF pathway (Yang et al., 2019). Apart from its cardioprotective effect, AS-IV promotes cerebral protection against ischemic brain injury via its pro-angiogenic action. The specific mechanism involves the activation of the HIF/VEGF/Notch signaling pathway via miRNA-210 (Liang et al., 2020). Interestingly, in addition to the pro-angiogenic effect, AS-IV improves ischemic brain tissue repair by promoting neurogenesis and neurological functional recovery, which is partially mediated by transforming microglia/macrophages from the M1 to M2 phenotype in a PPARγ-dependent manner (Li et al., 2021a).
AS-IV has been reported to be effective in skin wound repair, leading to significant improvements in wound closure, collagen synthesis, and skin tensile strength recovery (Chen et al., 2012; Zhang et al., 2012). When cultured human umbilical cord blood-derived endothelial progenitor cells are treated with AS-IV cells and then combined with human blood plasma gel, the application of this gel can repair full-thickness skin defects in a mouse model (Huang et al., 2021). In addition to its action on endothelial cells, AS-IV and cycloastragenol-6-O-beta-D-glucoside (the major metabolite of astragalosides) increase the proliferation and migration of skin cells via activation of the epidermal growth factor (EGFR)/ERK signaling pathway, resulting in the improvement of wound healing (Lee et al., 2018). Moreover, AS-IV mediates keratinocyte proliferation and migration by downregulating ß-catenin (Li et al., 2012). AS-IV also augments skin re-epithelialization and enhances matrix synthesis. Finally, AS-IV induces the development of polarized alternatively activated macrophages, which may reduce inflammation and facilitate wound healing (Luo et al., 2016).
It is interesting to note that AS-IV accelerates bone regeneration by simultaneously enhancing osteogenesis and preosteoclast-induced angiogenesis, partially through Akt/glycogen synthase kinase-3β/β-catenin signaling. Therefore, AS-IV has been proposed as a potential therapeutic agent for patients undergoing distraction osteogenesis treatment (Wang et al., 2021d).
Apart from AS-IV, other saponins in Radix Astragali, such as cycloastragenol, cyclocephaloside I, and cyclocanthoside E increase fibroblast proliferation and migration. These effects were observed to be stronger with cycloastragenol which also possesses a more remarkable wound-healing effect compared with astragaloside IV, with a more regularly organized dermis and more newly formed blood vessels (Sevimli-Gür et al., 2011). Furthermore, calycosin, a major isoflavonoid isolated from Radix Astragali, possesses pro-angiogenic effects. Calycosin induces angiogenesis in cultured endothelial cells and zebrafish models by upregulating VEGF, VEGFR-1, and VEGFR-2 mRNA levels. In addition to the VEGF-dependent pathway, calycosin also modulates the FGF and EGFR signaling pathways (Li et al., 2011). Interestingly, calycosin is similar to other selective estrogen receptor modulators, such as raloxifene and tamoxifen, in the sense that it has selective potency and affinity for estrogen receptors α and ß. Through its interaction with estrogen receptors, the subsequent activation of the MAPK-dependent pathway leads to a pro-angiogenic effect (Tang et al., 2010).
Salvia miltiorrhiza
Salvia miltiorrhiza Bunge, also known as Dan Shen in Chinese, is a widely used Chinese herbal medicine, particularly for the treatment of cardiovascular and trauma-related diseases. The crude extract of S. miltiorrhiza enhances the growth and differentiation of endothelial cells, probably through the upregulation of MMP-2, VEGF, and VEGFR-2 gene expression (Lay et al., 2003). In a mouse model of left anterior descending coronary artery ligation, S. miltiorrhiza treatment partially rescued the angiogenesis defect and heart failure with an increase in the mRNA and protein expression levels of HIF-1α and VEGF-A (Ai et al., 2015). In a rat model, S. miltiorrhiza decreased the amount of necrosis in burn wounds by increasing tissue perfusion and neovascularization. This is promising because burn healing is a complicated process and most treatments are ineffective (Irmak et al., 2018). Salvianolic acid tanshinone IIA is the major active ingredient of S. miltiorrhiza.
Salvianolic acids increase the number of endothelial progenitor cells and promote endothelial progenitor cell migration, adhesion, and vasculogenesis (Li et al., 2010). Danshensu [(R)-3- (3, 4-Dihydroxyphenyl)-2-hydroxypropanoic acid] has the basic chemical structure of various salvianolic acids. Salvianolic acid A is formed by one danshensu molecule and two caffeic acid molecules. Salvianolic acid B is formed by three danshensu molecules and one caffeic acid molecule. Sodium danshensu significantly increased the newly formed arteries and the diameter of the collateral arteries, leading to enhanced local cerebral blood flow recovery after a stroke. In addition to its effect on angiogenesis, sodium danshensu improves stroke recovery by stimulating neurogenesis in the post-ischemic brain. The mechanism of action involves the increased expression of VEGF, stromal-derived factor-1, brain-derived neurotrophic factor (BDNF), and eNOS in the peri-infarct region (Wei et al., 2018). Salvianolic acid A reduced the infarct size and increased the capillary density in the infarct region in rat myocardial infarction models. This effect is attributed to the increased formation of VEGF, VEGFR-2, and MMP-9, and the promotion of the number and migration capacity of endothelial progenitor cells (Li et al., 2014).
The pro-angiogenic effects and potential applications of salvianolic acid B have been widely studied. Salvianolic acid B enhances the growth and differentiation of endothelial cells (Lay et al., 2003). It also ameliorates left ventricular dysfunction in the hearts of streptozotocin-induced diabetic mice (Li et al., 2020a). Salvianolic acid B promotes angiogenesis in the heart by increasing the expression of VEGFR-2 and VEGF-A. Moreover, it reduces hyperglycemia-induced insulin-like growth factor-binding protein-3 expression, induces ERK and Akt activities, and activates VEGFR-2/VEGFA signaling in endothelial cells (Li et al., 2020a).
Although direct evidence is still unavailable, many studies have indicated that salvianolic acid B is a promising candidate for accelerating wound healing. In addition to its action on angiogenesis, salvianolic acid B inhibits the activities of human MMP-1, MMP-2, and MMP-9. The effects of salvianolic acid B on MMPs are one of the mechanisms accounting for the effects of Salvia miltiorrhiza on chronic wounds because MMPs are a family of endopeptidases that can degrade extracellular matrix components. This proteolytic property is important during wound healing to remove debris and facilitate cell migration (Liang et al., 2009). Moreover, salvianolic acid B enhances proliferation and collagen production in human skin cells (Chen et al., 2014), which may accelerate wound healing. Interestingly, salvianolic acid B has been shown to accelerate early-stage bone fracture healing. In a rat tibial fracture model, X-ray imaging and histological data showed that the salvianolic acid B treatment group had better callus growth and improved bone remodeling. Increased alkaline phosphatase activity is also involved in the healing process (He and Shen, 2014).
Tanshinone IIA elicits a significant cardioprotective effect in rats with myocardial infarction by improving heart function, reducing infarct size, and increasing the survival rate. Tanshinone IIA promotes angiogenesis and upregulates VEGF expression in myocardial infarction by enhancing HIF-1α mRNA expression (Xu et al., 2009). In addition to tanshinone IIA, 10 new imidazole-tanshinones and one oxazole-tanshinone have been isolated, and their angiogenesis activities were determined in a zebrafish model. Interestingly, one of the imidazole-tanshinones exhibited potent vascular protective and restorative activity with an EC50 value of 0.026 µM, which is very potent. The mechanism underlying its pro-angiogenesis effect involves the VEGF/FGF-Src-MAPK and PI3K-P38 MAPK signaling pathways (Zhang et al., 2014). Cryptotanshinone is a diterpene quinone compound found in Salvia miltiorrhiza. In an excisional wound-splinting model in diabetic mice, cryptotanshinone accelerated wound closure. A significant increase in re-epithelialization and granulation tissue formation was observed. Mechanistically, cryptotanshinone suppressed leukocyte infiltration and chemokine C-X-C motif ligand-1 and -2 expressions. In addition, cryptotanshinone increases the blood vessel density, expression levels of VEGF and Ang-1, and activation of eNOS. In addition, extracellular matrix remodeling is enhanced by cryptotanshinone by promoting fibroblast transformation and inhibiting MMP-2 and MMP-9. These findings indicate that the wound-healing effect of cryptotanshinone is mediated by a combined modulatory effect on angiogenesis, inflammation, and extracellular matrix remodeling (Song et al., 2020). Finally, a mouse model showed that while cryptotanshinone accelerated wound healing, the scar margins were narrow and there was less collagen deposition in the regenerated tissue. This indicates that cryptotanshinone can prevent and reduce scarring, which may be an extra advantage (Li et al., 2016).
Angelica sinensis
Angelica sinensis (Oliv.) Diels, also known as Dong Quai in Chinese, has been traditionally used to treat blood-related ailments including menstrual cramps, blood deficiencies, uterine disorders, and cardiovascular and cerebrovascular ischemia. The in vitro and in vivo pro-angiogenic effects of A. sinensis have been demonstrated. A. sinensis extract stimulates the proliferation, migration, and tube formation of endothelial cells. The extract has been shown to promote the formation of subintestinal vessels in zebrafish models. The pro-angiogenic effects probably involve the increased expression of VEGF, p38, and JNK 1/2 phosphorylation (Lam et al., 2008). Moreover, the A. sinensis extract effectively attenuated cerebral infarcts and improved neurological deficits in animal models. A. sinensis extract pretreatment provides neuroprotective effects against astrocyte-mediated cerebral infarction by activating pro-angiogenic and anti-apoptotic signaling pathways. The pro-angiogenic and anti-apoptotic effects of the A. sinensis extract can be attributed to the activation of the p38 MAPK/HIF-1/VEGF-A signaling pathway and p38 MAPK/HIF-1/VEGF-A/p-BAD-related regulation of the cytochrome c/caspase-3 signaling pathway, respectively, in the cortical ischemic penumbra after reperfusion (Cheng et al., 2017). The active ingredient responsible for the pro-angiogenic effect of A. sinensis has not yet been confirmed. Sodium ferulate and n-butylidenephthalide are the two major components of A. sinensis. Bone marrow-derived mesenchymal stem/stromal cells (BM-MSCs) have been demonstrated to enhance angiogenesis and the proliferation of reactive astrocytes, which subsequently leads to the amelioration of neurological injury. Interestingly, a combination of sodium ferulate and n-butylidenephthalide with BM-MSCs further enhances the expression of astrocyte-derived VEGF and BDNF, which contribute to angiogenesis after cerebral ischemia, and the underlying mechanism is associated with the activation of the astrocytic Akt/mTOR signaling pathway (Zhang et al., 2017).
A low molecular weight fraction of Angelica sinensis possesses strong wound-healing activity in a diabetic mouse wound-healing model and a human/severe combined immunodeficiency mouse chimera wound-healing model. In both models, this fraction of A. sinensis was compared favorably with becaplermin, which is a human PDGF approved by the Food and Drug Administration (FDA) for wound-healing treatment. Mechanistically, this fraction strongly stimulates endothelial cell proliferation and tri-dimensional endothelial cell network formation, which are important in the process of angiogenesis. This fraction stimulates the proliferation of human dermal fibroblasts and type I collagen secretion (Zhao et al., 2006). The mechanism of the wound-healing effects of the A. sinensis extract and its active water-soluble component, ferulic acid, has been studied using proteomic and biochemical analyses. The treatment of fibroblasts with A. sinensis extract modulated the upregulation of proteins associated with cell growth, metabolism, calcium ion regulation, and anti-apoptosis. In contrast, ferulic acid has the unique ability to modulate the upregulation of proteins associated with mobility, anti-oxidative functions, and calcium ion regulation in fibroblasts (Hsiao et al., 2012).
Radix Puerariae
Radix puerariae lobatae is the dried root of Pueraria lobata (Wild.) Ohwi, also known as Ge Gen in China. It is a traditional herbal medicine used for the treatment of a wide range of diseases, including the common cold, influenza, shoulder stiffness, diarrhea, deafness, and cardiovascular disease. The major active ingredient in Radix puerariae is puerarin, which is useful in treating patients with coronary artery disease. Puerarin induces angiogenesis in the non-ischemic and ischemic myocardium, which is one of the mechanisms for reducing the infarct area in animal models. The pro-angiogenic effect of puerarin is due to the induction of gene expression and activation of VEGF, HIF-1α, eNOS synthase, and Akt (Zhang et al., 2006). Interestingly, the cardioprotective effect of puerarin is mediated by the alleviation of oxidative stress and the activation of the PI3K/Akt pathway, which limits endothelial cell migration (Zhang et al., 2008; Li et al., 2020b). Moreover, PKCε and miR-21 contribute to the cardioprotective effects of puerarin by inhibiting apoptosis and oxidative stress in cardiomyocytes (Tang et al., 2014; Xu et al., 2019). Apart from cardioprotection, puerarin prevents stroke by improving microcirculation, which results from an increase in cerebral blood perfusion both by arteriole relaxation and p42/44 MAPK-mediated angiogenesis (Wu et al., 2014).
Radix Paeoniae
Radix Paeoniae Alba is the dried root of Paeonia lactiflora Pall., also named Bai Shao in Chinese, and is used as an herbal medicine for the treatment of various ailments such as liver disease, inflammation, and emotion-related diseases. It is also used to activate blood circulation and remove blood stasis. Paeoniflorin is an active ingredient in Radix Paeoniae. The pro-angiogenic effects of paeoniflorin have been demonstrated in endothelial cells and a zebrafish vascular insufficiency model. Paeoniflorin enhances the proliferation, migration, and tube formation of endothelial cells. Paeoniflorin rescues VEGF tyrosine kinase inhibitor II-induced blood vessel loss in a zebrafish model (Xin et al., 2018). Interestingly, molecular docking studies have revealed that paeoniflorin has an excellent binding ability to PI3K and Akt. A Western blot analysis showed that paeoniflorin suppressed the phosphorylation of PI3K and Akt. This provides clues to the mechanism of the pro-angiogenic action of paeoniflorin (Dong et al., 2020).
The therapeutic application of paeoniflorin in ischemic diseases, particularly those related to the brain, has been proposed. In a rat model of ischemic stroke, paeoniflorin reduced cerebral infarction, alleviated pathological injury, induced the secretion of pro-angiogenic factors, and increased cerebral vascular density. The angiogenic action of paeoniflorin is mediated through the upregulation of VEGF/VEGFR-2 expression (Liu et al., 2021). Moreover, paeoniflorin significantly attenuates cerebral infarction and the severity of intimal hyperplasia in animal models of ischemia/reperfusion injury. Paeoniflorin acts not only on endothelial cells but also on vascular smooth muscle cells. It reduces PDGF-stimulated vascular smooth muscle cell proliferation and migration, probably by modulating the Ras/MAPK/ERK signaling pathway (Chen et al., 2013).
Paeoniflorin improves wound healing in rat models of diabetic foot ulcers. In vitro experiments confirmed that paeoniflorin alleviates oxidative stress, increases proliferation and migration, decreases apoptosis, and upregulates VEGF and TGF-β1 expression in endothelial cells (Sun et al., 2020). In addition to its effect on angiogenesis, paeoniflorin accelerates wound healing through collagen deposition (Dong et al., 2020). Furthermore, paeoniflorin exerts anti-inflammatory effects by downregulating interleukin-1β, interleukin-18, and tumor necrosis factor-alpha. Paeoniflorin also decreases expression levels of the chemokine receptor and nuclear factor-kappa B and increases the expression levels of the inhibitor of nuclear factor kappa B. These findings suggest that paeoniflorin significantly attenuates wound inflammation, which may lead to improved wound healing (Sun et al., 2021).
Ilex pubescens
The roots of Ilex pubescens Hook. & Arn., also known as Mao Dong Qing in Chinese, has been used as a traditional herbal medicine for the treatment of stroke, coronary arterial disease, and peripheral vascular diseases. It has been reported that the ethanolic extract of I. pubescens promotes cerebral ischemic tolerance and exerts neuroprotective effects. In rats subjected to middle cerebral artery occlusion/reperfusion injury, the ethanolic extract of I. pubescens significantly reduces the cerebral infarct volume and increases neurological deficit scores. The underlying mechanism involves the toll-like receptor 4 signaling pathway through the inhibition of the production of proteins or cytokines downstream of the myeloid differentiation factor 88-dependent pathway and the activation of the toll/interleukin-1 receptor domain-containing adapter inducing interferon-β-dependent anti-inflammatory pathways (Fang et al., 2020). Ilexsaponin A1 is a major bioactive ingredient in Ilex pubescens. The pro-angiogenic effect of ilexsaponin A1 is reflected in its ability to promote proliferation, migration, invasion, and tube formation of endothelial cells and rescue blood vessel loss in zebrafish models. The mechanism of action probably involves the activation of the Akt/mTOR-, MAPK/ERK-, and FAK-dependent signaling pathways (Li et al., 2017).
The flavonoids in Ilex pubescens possess pro-angiogenic effects, which may account for its cerebrovascular protective effect. In a rat model of cerebral ischemia with blood stasis, total flavonoids of I. pubescens improved blood circulation, energy metabolism, endogenous anti-oxidative capability, and anti-apoptotic effects, thereby relieving nerve cell injury (Miao et al., 2017). Flavonoids in I. pubescens not only reduce damage to brain nerve cells but also significantly reduce the content of nitric oxide in brain homogenates and increase ATP synthetase enzyme activity. Thus, cerebral ischemia-reperfusion injury is improved (Yan et al., 2017). In addition, the neuroprotective effect of flavonoids from I. pubescens is linked to the decreased production of pro-inflammatory cytokines such as interleukin-1β and tumor necrosis factor-α and increased production of anti-inflammatory cytokines such as interleukin-10 and neurotrophic factors (Fang et al., 2017).
Limitation of Natural Products and Future Directions
Although the pharmacological actions of phytochemicals have been widely reported, in-depth studies on their pharmacokinetic and pharmacodynamic properties should be conducted. Drug delivery is a critical factor for the success of drug development. In the treatment of ischemic heart or brain diseases, either injections or oral dosage forms are usually used. For the oral route of administration, drug dissolution and absorption rates are affected mainly by the chemical properties of the drugs. Some phytochemicals, such as astragaloside IV, calycosin, tanshinone IIA, and puerarin, are poorly water-soluble, which may lead to slow dissolution and low bioavailability. The use of different salt forms or derivatives of compounds is an easy approach to enhancing drug absorption. For instance, it has been reported that sodium tanshinone IIA sulfonate, a water-soluble derivative of tanshinone IIA, shows a better bioavailability than tanshinone II does (Shang et al., 2012). Similar to tanshinone II, sodium tanshinone IIA sulfonate has a good pro-angiogenic effect, as it can improve perfusion recovery and increase capillary densities in the ischemic hind limbs of diabetic mice (Chen et al., 2019). In addition to drug absorption, the intraluminal metabolic activity occurring in the intestine due to bacterial flora and the first-pass effect due to hepatic metabolism may affect the amount of drugs reaching the systemic circulation. Therefore, it would be useful to develop a derivative that is more resistant to metabolism. In addition, it is critical to study whether these metabolites are bioactive.
For the topical treatment of wound healing, the drug should adhere to the skin for an adequate amount of time, and the drug release rate should be well controlled. It has been reported that ginsenoside Rg1 incorporated into collagen/chitosan–gelatin microsphere scaffolds is advantageous for wound healing and tissue regeneration (Zheng et al., 2013b). Insufficient skin-surface penetration by drugs is another challenge. These problems can be overcome by particle size manipulation through the application of nanotechnology and by encapsulating drugs into nanoformulations. Another advantage of encapsulation is that it allows sustained controlled-release delivery, which is useful for the lengthy wound-healing process (Kant et al., 2021).
Regarding the pharmacodynamics of pro-angiogenic compounds, a more comprehensive understanding of their mechanisms of action is required. For instance, the wound-healing process is related not only to blood vessel formation but also to the proliferation of skin cells and anti-inflammation. Therefore, phytochemicals with bioactivity toward multiple targets are valuable. Furthermore, the structure-activity and dose-effect relationships of phytochemicals should be further explored. Many pro-angiogenic phytochemicals are glycosides. It is important to know whether the number and type of glycosides affect the pro-angiogenic activity. Glycosides may be removed by glycosidases released by the gut flora, so it is necessary to know if aglycones possess pro-angiogenic activities. In addition, the structural modification of phytochemicals may change the binding affinity to their corresponding receptors, thereby affecting the efficiency of the drugs. Finally, the dose-response relationship should be studied because it is plausible that phytochemicals may have different pharmacological effects at different concentrations. Salvianolic acid B is one example; its effects on osteoblast bone formation, angiogenesis, and adipogenesis have been studied in a mouse model of glucocorticoid-induced osteoporosis (Cui et al., 2012). A low dose of salvianolic acid B prevents glucocorticoid-induced cancellous bone loss and increases adipogenesis by improving local microcirculation through capillary dilation. Higher doses of salvianolic acid B not only prevent glucocorticoid-induced osteopenia but also increase cancellous bone mass and thickness, which is associated with an increase in marrow bone morphogenetic protein expression, inhibition of adipogenesis, and a further increase in microvessel diameters. Information on the dose-response relationship can provide important information for dose selection in clinical studies.
Accumulating evidence from cell and animal models has demonstrated that herbal medicines and phytochemicals have promising pro-angiogenic effects. However, more rigorously designed randomized controlled clinical trials should be conducted to verify their effectiveness and to clarify our understanding of their therapeutic potential. Relatively few randomized controlled trials have been conducted on herbal medicines for several reasons. First, the registration of certain herbal products in certain countries including China, Japan, Korea, and India does not require data from clinical trials if their formulations have been used for a long time. This is because they are considered safe and effective. Second, prescriptions containing herbal medicines are usually used for treatment. Clinical trials involving the use of a single herb are rare. Therefore, clinical data for specific herbs are usually unavailable. Third, quality control of herbal products is difficult, which makes the governmental authority or regulatory body unwilling to issue certificates for conducting clinical trials.
Future studies are required to design a dosing regimen, establish a delivery route, and demonstrate the safety of use. Regarding the phytochemicals mentioned in this review, ginsenosides, Panax notoginseng saponin, and astragaloside are safe and tolerated in humans (Xu et al., 2013; Xu et al., 2015; Fan et al., 2020). Clinical data regarding the safety of calycosin and ilexsaponin A1 are not available; however, in long-term clinical use, total glucosides of peony have produced no severe adverse reaction (Zhou et al., 2020). When patients use a combination of salvianolic acids and basic Western medicine therapy to treat acute cerebral infarction, salvianolic acids increase the occurrence of adverse events, such as headache, dizziness, hemorrhage of the digestive tract or skin, and mucosa, liver, or kidney injury. However, these adverse effects can be easily controlled or eliminated after the termination of salvianolic acid treatment (Xin et al., 2020). The use of tanshinones in pregnancy should be pursued with caution because they increase the drug permeability of the placental barrier (Zhou et al., 2019). Ferulic acid is a low-toxicity phytochemical, but it can aggravate renal cancer in rats with diabetes mellitus and renal cancer, and it causes renal damage after long-term treatment of chronic kidney disease (Li et al., 2021b). In addition, a meta-analysis of randomized controlled trials concluded that puerarin injection causes mild adverse reactions including nausea, vomiting, gastrointestinal discomfort, facial flushing, dizziness, and allergy (Zheng et al., 2017). In addition, some potential problems should be considered when pro-angiogenic therapy is applied. For instance, it is not known whether accelerated plaque angiogenesis leads to plaque rupture. Moreover, it remains unclear whether the use of pro-angiogenic agents increases the risk of angiogenesis-borne diseases, such as tumor formation and metastasis. Therefore, further studies are required.
Conclusion
This study focuses on the potential use of herbal medicine and bioactive ingredients for the treatment of vascular insufficiency-related diseases such as ischemic cardiovascular and cerebrovascular disorders, and wound healing and tissue repair (Figure 3). The most frequently studied pro-angiogenic phytochemicals include ginsenosides from Panax notoginseng, astragalosides, calycosin from Radix Astragali, salvianolic acid B from Salvia miltiorrhiza, paeoniflorin from Radix Paeoniae, ilexsaponin A1 from Ilex pubescens, ferulic acid from Angelica sinensis, and puerarin from Radix puerariae. Their pro-angiogenic effects are promising and have been demonstrated in various cell and animal models. The major mechanisms of pro-angiogenic action are mostly related to VEGF-dependent signaling pathways. However, further pharmacokinetic and pharmacodynamic studies are warranted. In addition, there is still a need for more evidence-based confirmation through controlled and validated clinical trials. Traditional herbal medicine provides a fruitful resource for pro-angiogenic phytochemicals with different targets in the angiogenesis process. With the rapid development of various chromatographic separation technologies and different in vitro, ex vivo, and in vivo screening platforms, many pro-angiogenic compounds will be discovered. The application of medicinal chemistry and advancements in drug delivery technology has also accelerated the improvement of pharmacodynamic and pharmacokinetic properties of drug candidates. Therefore, the prospect of developing pro-angiogenic agents from herbal medicines and phytochemicals is promising.
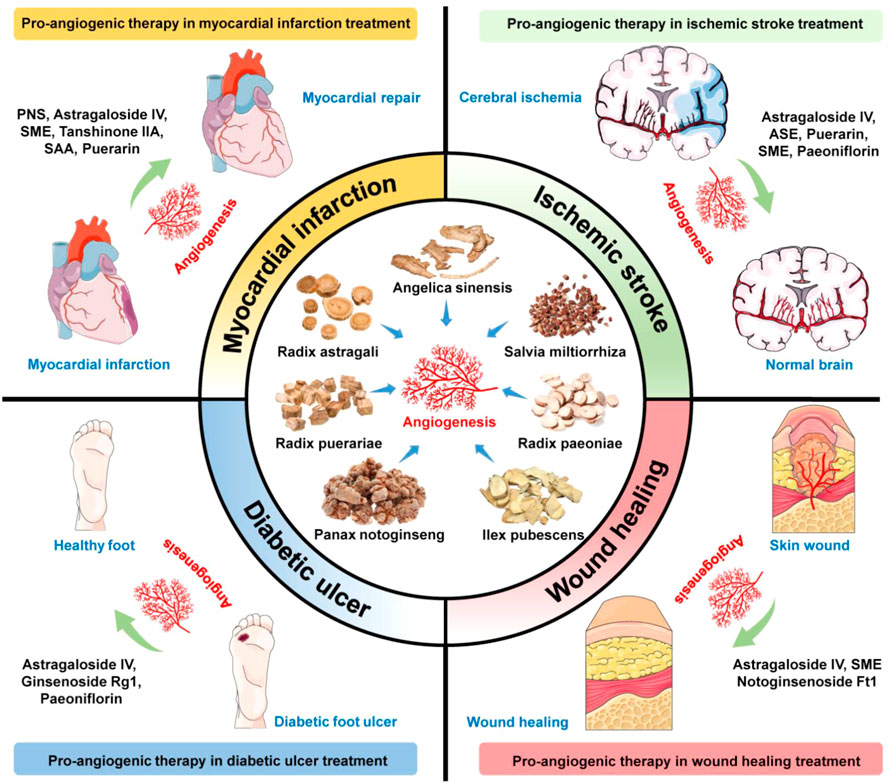
FIGURE 3. Summarized findings of pro-angiogenic phytochemicals for ischemic diseases, tissue repairing, and wound healing. Bioactive compounds or crude extracts of Panax notoginseng, Radix Astragali, Salvia miltiorrhiza, Radix Paeoniae, Ilex pubescens, Angelica sinensis, and Radix puerariae with pro-angiogenic activities show potential applications in treating ischemic stroke, myocardial infarction, diabetic ulcer, and wound healing. PNS, Panax notoginseng saponins; SME, Salvia miltiorrhiza extract; SAA, Salvianolic acid A; ASE, Angelica sinensis extract.
Author Contributions
JL and RL drafted the manuscript. SL and GL reviewed the manuscript. XW, CZ, PS and PR aided in the design of the illustrations. All the authors read and approved the final manuscript.
Conflict of Interest
The authors declare that the research was conducted in the absence of any commercial or financial relationships that could be construed as a potential conflict of interest.
Publisher’s Note
All claims expressed in this article are solely those of the authors and do not necessarily represent those of their affiliated organizations, or those of the publisher, the editors, and the reviewers. Any product that may be evaluated in this article, or claim that may be made by its manufacturer, is not guaranteed or endorsed by the publisher.
References
Ai, F., Chen, M., Li, W., Yang, Y., Xu, G., Gui, F., et al. (2015). Danshen Improves Damaged Cardiac Angiogenesis and Cardiac Function Induced by Myocardial Infarction by Modulating HIF1α/VEGFA Signaling Pathway. Int. J. Clin. Exp. Med. 8 (10), 18311–18318.
Al-Ostoot, F. H., Salah, S., Khamees, H. A., and Khanum, S. A. (2021). Tumor Angiogenesis: Current Challenges and Therapeutic Opportunities. Cancer Treat. Res. Commun. 28, 100422. doi:10.1016/j.ctarc.2021.100422
Behl, T., and Kotwani, A. (2015). Exploring the Various Aspects of the Pathological Role of Vascular Endothelial Growth Factor (VEGF) in Diabetic Retinopathy. Pharmacol. Res. 99, 137–148. doi:10.1016/j.phrs.2015.05.013
Burri, P. H., and Djonov, V. (2002). Intussusceptive Angiogenesis-Tthe Alternative to Capillary Sprouting. Mol. Asp. Med. 23 (6S), S1–S27. doi:10.1016/s0098-2997(02)00096-1
Burri, P. H., Hlushchuk, R., and Djonov, V. (2004). Intussusceptive Angiogenesis: Its Emergence, its Characteristics, and its Significance. Dev. Dyn. 231 (3), 474–488. doi:10.1002/dvdy.20184
Cai, H. A., Huang, L., Zheng, L. J., Fu, K., Wang, J., Hu, F. D., et al. (2019). Ginsenoside (Rg-1) Promoted the Wound Closure of Diabetic Foot Ulcer through iNOS Elevation via miR-23a/IRF-1 axis. Life Sci. 233, 116525. doi:10.1016/j.lfs.2019.05.081
Capdevila, J., Iglesias, L., Halperin, I., Segura, A., Martínez-Trufero, J., Vaz, M. Á., et al. (2012). Sorafenib in Metastatic Thyroid Cancer. Endocr. Relat. Cancer 19 (2), 209–216. doi:10.1530/ERC-11-0351
Carmeliet, P. (2003). Angiogenesis in Health and Disease. Nat. Med. 9 (6), 653–660. doi:10.1038/nm0603-653
Carmeliet, P. (2000). Mechanisms of Angiogenesis and Arteriogenesis. Nat. Med. 6 (4), 389–395. doi:10.1038/74651
Carmeliet, P. (2005). VEGF as a Key Mediator of Angiogenesis in Cancer. Oncology 69 Suppl 3, 4–10. doi:10.1159/000088478
Cébe-Suarez, S., Zehnder-Fjällman, A., and Ballmer-Hofer, K. (2006). The Role of VEGF Receptors in Angiogenesis; Complex Partnerships. Cell. Mol. Life Sci. 63 (5), 601–615. doi:10.1007/s00018-005-5426-3
Chen, L., He, W., Peng, B., Yuan, M., Wang, N., Wang, J., et al. (2019). Sodium Tanshinone IIA Sulfonate Improves Post-ischemic Angiogenesis in Hyperglycemia. Biochem. Biophys. Res. Commun. 520 (3), 580–585. doi:10.1016/j.bbrc.2019.09.106
Chen, X., Peng, L. H., Li, N., Li, Q. M., Li, P., Fung, K. P., et al. (2012). The Healing and Anti-scar Effects of Astragaloside IV on the Wound Repair In Vitro and In Vivo. J. Ethnopharmacol. 139 (3), 721–727. doi:10.1016/j.jep.2011.11.035
Chen, Y. F., Wu, K. J., and Wood, W. G. (2013). Paeonia Lactiflora Extract Attenuating Cerebral Ischemia and Arterial Intimal Hyperplasia Is Mediated by Paeoniflorin via Modulation of VSMC Migration and Ras/MEK/ERK Signaling Pathway. Evid. Based Complement. Altern. Med. 2013, 482428. doi:10.1155/2013/482428
Chen, Y. S., Lee, S. M., Lin, Y. J., Chiang, S. H., and Lin, C. C. (2014). Effects of Danshensu and Salvianolic Acid B from Salvia Miltiorrhiza Bunge (Lamiaceae) on Cell Proliferation and Collagen and Melanin Production. Molecules 19 (2), 2029–2041. doi:10.3390/molecules19022029
Cheng, C. Y., Ho, T. Y., Hsiang, C. Y., Tang, N. Y., Hsieh, C. L., Kao, S. T., et al. (2017). Angelica Sinensis Exerts Angiogenic and Anti-apoptotic Effects against Cerebral Ischemia-Reperfusion Injury by Activating p38MAPK/HIF-1[Formula: See Text]/VEGF-A Signaling in Rats. Am. J. Chin. Med. 45 (8), 1683–1708. doi:10.1142/S0192415X17500914
Cheung, L. W., Leung, K. W., Wong, C. K., Wong, R. N., and Wong, A. S. (2011). Ginsenoside-Rg1 Induces Angiogenesis via Non-genomic Crosstalk of Glucocorticoid Receptor and Fibroblast Growth Factor Receptor-1. Cardiovasc Res. 89, 419–425. doi:10.1093/cvr/cvq300
Copur, M. S. (2008). Sorafenib in Advanced Hepatocellular Carcinoma. N. Eng. J. Med. 359 (23), 2498.
Cui, L., Li, T., Liu, Y., Zhou, L., Li, P., Xu, B., et al. (2012). Salvianolic Acid B Prevents Bone Loss in Prednisone-Treated Rats through Stimulation of Osteogenesis and Bone Marrow Angiogenesis. PLoS One 7 (4), e34647. doi:10.1371/journal.pone.0034647
Djonov, V., Baum, O., and Burri, P. H. (2003). Vascular Remodeling by Intussusceptive Angiogenesis. Cell. Tissue Res. 314 (1), 107–117. doi:10.1007/s00441-003-0784-3
Dong, X., He, Z., Xiang, G., Cai, L., Xu, Z., Mao, C., et al. (2020). Paeoniflorin Promotes Angiogenesis and Tissue Regeneration in a Full-Thickness Cutaneous Wound Model through the PI3K/AKT Pathway. J. Cell. Physiol. 235 (12), 9933–9945. doi:10.1002/jcp.29808
Duran, C. L., Howell, D. W., Dave, J. M., Smith, R. L., Torrie, M. E., Essner, J. J., et al. (2017). Molecular Regulation of Sprouting Angiogenesis. Compr. Physiol. 8 (1), 153–235. doi:10.1002/cphy.c160048
Escudier, B., Eisen, T., Stadler, W. M., Szczylik, C., Oudard, S., Staehler, M., et al. (2009). Sorafenib for Treatment of Renal Cell Carcinoma: Final Efficacy and Safety Results of the Phase III Treatment Approaches in Renal Cancer Global Evaluation Trial. J. Clin. Oncol. 27 (20), 3312–3318. doi:10.1200/JCO.2008.19.5511
Fallah, A., Sadeghinia, A., Kahroba, H., Samadi, A., Heidari, H. R., Bradaran, B., et al. (2019). Therapeutic Targeting of Angiogenesis Molecular Pathways in Angiogenesis-dependent Diseases. Biomed. Pharmacother. 110, 775–785. doi:10.1016/j.biopha.2018.12.022
Fan, S., Zhang, Z., Su, H., Xu, P., Qi, H., Zhao, D., et al. (2020). Panax Ginseng Clinical Trials: Current Status and Future Perspectives. Biomed. Pharmacother. 132, 110832. doi:10.1016/j.biopha.2020.110832
Fang, X., Li, Y., Qiao, J., Guo, Y., and Miao, M. (2017). Neuroprotective Effect of Total Flavonoids from Ilex Pubescens against Focal Cerebral Ischemia/reperfusion Injury in Rats. Mol. Med. Rep. 16 (5), 7439–7449. doi:10.3892/mmr.2017.7540
Fang, X., Li, Y., Zheng, Y., Wang, Y., Feng, S., and Miao, M. (2020). Ethanol Extracts from Ilex Pubescens Promotes Cerebral Ischemic Tolerance via Modulation of TLR4-MyD88/TRIF Signaling Pathway in Rats. J. Ethnopharmacol. 256, 112680. doi:10.1016/j.jep.2020.112680
Franco, C. A., Liebner, S., and Gerhardt, H. (2009). Vascular Morphogenesis: a Wnt for Every Vessel? Curr. Opin. Genet. Dev. 19 (5), 476–483. doi:10.1016/j.gde.2009.09.004
Gao, Y., Xie, Z., Ho, C., Wang, J., Li, Q., Zhang, Y., et al. (2020). LRG1 Promotes Keratinocyte Migration and Wound Repair through Regulation of HIF-1α Stability. J. Invest. Dermatol 140 (2), 455–e8. doi:10.1016/j.jid.2019.06.143
He, X., and Shen, Q. (2014). Salvianolic Acid B Promotes Bone Formation by Increasing Activity of Alkaline Phosphatase in a Rat Tibia Fracture Model: a Pilot Study. BMC Complement. Altern. Med. 14, 493. doi:10.1186/1472-6882-14-493
Heil, M., Eitenmüller, I., Schmitz-Rixen, T., and Schaper, W. (2006). Arteriogenesis versus Angiogenesis: Similarities and Differences. J. Cell. Mol. Med. 10 (1), 45–55. doi:10.1111/j.1582-4934.2006.tb00290.x
Hoeben, A., Landuyt, B., Highley, M. S., Wildiers, H., Van Oosterom, A. T., and De Bruijn, E. A. (2004). Vascular Endothelial Growth Factor and Angiogenesis. Pharmacol. Rev. 56 (4), 549–580. doi:10.1124/pr.56.4.3
Holmes, K., Roberts, O. L., Thomas, A. M., and Cross, M. J. (2007). Vascular Endothelial Growth Factor Receptor-2: Structure, Function, Intracellular Signalling and Therapeutic Inhibition. Cell. Signal 19 (10), 2003–2012. doi:10.1016/j.cellsig.2007.05.013
Hong, S. J., Wan, J. B., Zhang, Y., Hu, G., Lin, H. C., Seto, S. W., et al. (2009). Angiogenic Effect of Saponin Extract from Panax Notoginseng on HUVECs In Vitro and Zebrafish In Vivo. Phytother. Res. 23 (5), 677–686. doi:10.1002/ptr.2705
Hsiao, C. Y., Hung, C. Y., Tsai, T. H., and Chak, K. F. (2012). A Study of the Wound Healing Mechanism of a Traditional Chinese Medicine, Angelica Sinensis, Using a Proteomic Approach. Evid. Based Complement. Altern. Med. 2012, 467531. doi:10.1155/2012/467531
Huang, N. C., Dai, L. G., Kang, L. Y., Huang, N. C., Fu, K. Y., Hsieh, P. S., et al. (2021). Beneficial Effects of Astragaloside IV-Treated and 3-Dimensional-Cultured Endothelial Progenitor Cells on Angiogenesis and Wound Healing. Ann. Plast. Surg. 86 (2S Suppl. 1), S3–S12. doi:10.1097/SAP.0000000000002655
Irmak, F., Kurt Yazar, S., Şirvan, S. S., Serin, M., Özağarı, A., and Karasoy Yeşilada, A. (2018). Beneficial Effects of Salvia Miltiorrhiza in the Healing of Burn Wounds: an Experimental Study in Rats. J. Plast. Surg. Hand Surg. 52 (4), 229–233. doi:10.1080/2000656X.2018.1461631
Jemmerson, R., Staskus, K., Higgins, L., Conklin, K., and Kelekar, A. (2021). Intracellular Leucine-Rich Alpha-2-Glycoprotein-1 Competes with Apaf-1 for Binding Cytochrome C in Protecting MCF-7 Breast Cancer Cells from Apoptosis. Apoptosis 26 (1–2), 71–82. doi:10.1007/s10495-020-01647-9
Kant, V., Kumari, P., Jitendra, D. K., Ahuja, M., and Kumar, V. (2021). Nanomaterials of Natural Bioactive Compounds for Wound Healing: Novel Drug Delivery Approach. Curr. Drug Deliv. 18 (10), 1406–1425. doi:10.2174/1567201818666210729103712
Karaman, S., Leppänen, V. M., and Alitalo, K. (2018). Vascular Endothelial Growth Factor Signaling in Development and Disease. Development 145 (14), dev151019. doi:10.1242/dev.151019
Koch, A. E., and Distler, O. (2007). Vasculopathy and Disordered Angiogenesis in Selected Rheumatic Diseases: Rheumatoid Arthritis and Systemic Sclerosis. Arthritis Res. Ther. 9 Suppl 2 (Suppl. 2), S3. doi:10.1186/ar2187
Kurz, H. (2000). Physiology of Angiogenesis. J. Neurooncol 50 (1-2), 17–35. doi:10.1023/a:1006485716743
Lam, H. W., Lin, H. C., Lao, S. C., Gao, J. L., Hong, S. J., Leong, C. W., et al. (2008). The Angiogenic Effects of Angelica Sinensis Extract on HUVEC In Vitro and Zebrafish In Vivo. J. Cell. Biochem. 103 (1), 195–211. doi:10.1002/jcb.21403
Lay, I. S., Chiu, J. H., Shiao, M. S., Lui, W. Y., and Wu, C. W. (2003). Crude Extract of Salvia Miltiorrhiza and Salvianolic Acid B Enhance In Vitro Angiogenesis in Murine SVR Endothelial Cell Line. Planta Med. 69 (1), 26–32. doi:10.1055/s-2003-37034
Lee, S. Y., Chang, W. L., Li, Z. X., Kirkby, N. S., Tsai, W. C., Huang, S. F., et al. (2018). Astragaloside VI and Cycloastragenol-6-O-Beta-D-Glucoside Promote Wound Healing In Vitro and In Vivo. Phytomedicine 38, 183–191. doi:10.1016/j.phymed.2017.12.003
Li, C. L., Liu, B., Wang, Z. Y., Xie, F., Qiao, W., Cheng, J., et al. (2020). Salvianolic Acid B Improves Myocardial Function in Diabetic Cardiomyopathy by Suppressing IGFBP3. J. Mol. Cell. Cardiol. 139, 98–112. doi:10.1016/j.yjmcc.2020.01.009
Li, D., Rui, Y. X., Guo, S. D., Luan, F., Liu, R., and Zeng, N. (2021). Ferulic Acid: A Review of its Pharmacology, Pharmacokinetics and Derivatives. Life Sci. 284, 119921. doi:10.1016/j.lfs.2021.119921
Li, F. L., Li, X., Wang, Y. F., Xiao, X. L., Xu, R., Chen, J., et al. (2012). Astragaloside IV Downregulates β-Catenin in Rat Keratinocytes to Counter LiCl-Induced Inhibition of Proliferation and Migration. Evid. Based Complement. Altern. Med. 2012, 956107. doi:10.1155/2012/956107
Li, J., Zhang, J., Zou, L., Lee, S. M., Yang, C., Seto, S. W., et al. (2017). Pro-angiogenic Effects of Ilexsaponin A1 on Human Umbilical Vein Endothelial Cells In Vitro and Zebrafish In Vivo. Phytomedicine 36, 229–237. doi:10.1016/j.phymed.2017.10.006
Li, L., Gan, H., Jin, H., Fang, Y., Yang, Y., Zhang, J., et al. (2021). Astragaloside IV Promotes Microglia/macrophages M2 Polarization and Enhances Neurogenesis and Angiogenesis through PPARγ Pathway after Cerebral Ischemia/reperfusion Injury in Rats. Int. Immunopharmacol. 92, 107335. doi:10.1016/j.intimp.2020.107335
Li, S., Lou, S., Lei, B. U., Chan, T. F., Kwan, Y. W., Chan, S. W., et al. (2011). Transcriptional Profiling of Angiogenesis Activities of Calycosin in Zebrafish. Mol. Biosyst. 7 (11), 3112–3121. doi:10.1039/c1mb05206c
Li, X., Sun, S., Chen, D., Yuan, T., Chen, Y., Wang, D., et al. (2020). Puerarin Attenuates the Endothelial-Mesenchymal Transition Induced by Oxidative Stress in Human Coronary Artery Endothelial Cells through PI3K/AKT Pathway. Eur. J. Pharmacol. 886, 173472. doi:10.1016/j.ejphar.2020.173472
Li, Y., Shi, S., Gao, J., Han, S., Wu, X., Jia, Y., et al. (2016). Cryptotanshinone Downregulates the Profibrotic Activities of Hypertrophic Scar Fibroblasts and Accelerates Wound Healing: A Potential Therapy for the Reduction of Skin Scarring. Biomed. Pharmacother. 80, 80–86. doi:10.1016/j.biopha.2016.03.006
Li, Y. J., Duan, C. L., and Liu, J. X. (2014). Salvianolic Acid A Promotes the Acceleration of Neovascularization in the Ischemic Rat Myocardium and the Functions of Endothelial Progenitor Cells. J. Ethnopharmacol. 151 (1), 218–227. doi:10.1016/j.jep.2013.10.019
Li, Y. J., Duan, C. L., Liu, J. X., and Xu, Y. G. (2010). Pro-angiogenic Actions of Salvianolic Acids on In Vitro Cultured Endothelial Progenitor Cells and Chick Embryo Chorioallantoic Membrane Model. J. Ethnopharmacol. 131 (3), 562–566. doi:10.1016/j.jep.2010.07.040
Liang, C., Ni, G. X., Shi, X. L., Jia, L., and Wang, Y. L. (2020). Astragaloside IV Regulates the HIF/VEGF/Notch Signaling Pathway through miRNA-210 to Promote Angiogenesis after Ischemic Stroke. Restor. Neurol. Neurosci. 38 (3), 271–282. doi:10.3233/RNN-201001
Liang, Y. H., Li, P., Huang, Q. F., Zhao, J. X., Liu, X., and Dai, M. K. (2009). Salvianolic Acid B In Vitro Inhibited Matrix Metalloproteinases-1, -2, and -9 Activities. Zhong Xi Yi Jie He Xue Bao 7 (2), 145–150. doi:10.3736/jcim20090210
Liu, J., Wang, Y., Qiu, L., Yu, Y., and Wang, C. (2014). Saponins of Panax Notoginseng: Chemistry, Cellular Targets and Therapeutic Opportunities in Cardiovascular Diseases. Expert Opin. Investig. Drugs 23, 523–539. doi:10.1517/13543784.2014.892582
Liu, L., Cao, Y., Chen, C., Zhang, X., McNabola, A., Wilkie, D., et al. (2006). Sorafenib Blocks the RAF/MEK/ERK Pathway, Inhibits Tumor Angiogenesis, and Induces Tumor Cell Apoptosis in Hepatocellular Carcinoma Model PLC/PRF/5. Cancer Res. 66 (24), 11851–11858. doi:10.1158/0008-5472.CAN-06-1377
Liu, R., Zheng, Y., Han, T., Lan, J., He, L., and Shi, J. (2021). Angiogenic Actions of Paeoniflorin on Endothelial Progenitor Cells and in Ischemic Stroke Rat Model. Am. J. Chin. Med. 49 (4), 863–881. doi:10.1142/S0192415X21500415
Luo, X., Huang, P., Yuan, B., Liu, T., Lan, F., Lu, X., et al. (2016). Astragaloside IV Enhances Diabetic Wound Healing Involving Upregulation of Alternatively Activated Macrophages. Int. Immunopharmacol. 35, 22–28. doi:10.1016/j.intimp.2016.03.020
Majewska, I., and Gendaszewska-Darmach, E. (2011). Proangiogenic Activity of Plant Extracts in Accelerating Wound Healing - a New Face of Old Phytomedicines. Acta Biochim. Pol. 58 (4), 449–460. doi:10.18388/abp.2011_2210
Miao, M., Cao, L., Xu, K., Xin, W., and Zheng, Y. (2017). Intervention Action of Total Flavonoids from Root of Ilex Pubescens in Cerebral Ischemic Tolerance with Blood Stasis. Saudi J. Biol. Sci. 24 (3), 729–736. doi:10.1016/j.sjbs.2017.01.049
Mitsos, S., Katsanos, K., Koletsis, E., Kagadis, G. C., Anastasiou, N., Diamantopoulos, A., et al. (2012). Therapeutic Angiogenesis for Myocardial Ischemia Revisited: Basic Biological Concepts and Focus on Latest Clinical Trials. Angiogenesis 15 (1), 1–22. doi:10.1007/s10456-011-9240-2
National Administration of Traditional Chinese Medicine. Available at: www.satcm.gov.cn/hudongjiaoliu/guanfangweixin/2019-01-25/8909.html.
Ng, E. W., and Adamis, A. P. (2005). Targeting Angiogenesis, the Underlying Disorder in Neovascular Age-Related Macular Degeneration. Can. J. Ophthalmol. 40 (3), 352–368. doi:10.1016/S0008-4182(05)80078-X
Nomi, M., Atala, A., Coppi, P. D., and Soker, S. (2002). Principals of Neovascularization for Tissue Engineering. Mol. Asp. Med. 23 (6), 463–483. doi:10.1016/s0098-2997(02)00008-0
Peach, C. J., Mignone, V. W., Arruda, M. A., Alcobia, D. C., Hill, S. J., Kilpatrick, L. E., et al. (2018). Molecular Pharmacology of VEGF-A Isoforms: Binding and Signalling at VEGFR2. Int. J. Mol. Sci. 19 (4), 1264. doi:10.3390/ijms19041264
Sarkar, N., Rück, A., Källner, G., Y-Hassan, S., Blomberg, P., Islam, K. B., et al. (2001). Effects of Intramyocardial Injection of phVEGF-A165 as Sole Therapy in Patients with Refractory Coronary Artery Disease--12Mmonth Follow-Up: Angiogenic Gene Therapy. J. Intern Med. 250 (5), 373–381. doi:10.1046/j.1365-2796.2001.00905.x
Sevimli-Gür, C., Onbaşılar, I., Atilla, P., Genç, R., Cakar, N., Deliloğlu-Gürhan, I., et al. (2011). In Vitro growth Stimulatory and In Vivo Wound Healing Studies on Cycloartane-type Saponins of Astragalus Genus. J. Ethnopharmacol. 134 (3), 844–850. doi:10.1016/j.jep.2011.01.030
Shang, Q., Xu, H., and Huang, L. (2012). Tanshinone IIA: A Promising Natural Cardioprotective Agent. Evid. Based Complement. Altern. Med. 2012, 716459. doi:10.1155/2012/716459
Shen, K., Ji, L., Gong, C., Ma, Y., Yang, L., Fan, Y., et al. (2012). Notoginsenoside Ft1 Promotes Angiogenesis via HIF-1α Mediated VEGF Secretion and the Regulation of PI3K/AKT and Raf/MEK/ERK Signaling Pathways. Biochem. Pharmacol. 84 (6), 784–792. doi:10.1016/j.bcp.2012.05.024
Song, M., Chen, L., Zhang, L., Li, C., Coffie, J. W., Fang, Z., et al. (2020). Cryptotanshinone Enhances Wound Healing in Type 2 Diabetes with Modulatory Effects on Inflammation, Angiogenesis and Extracellular Matrix Remodelling. Pharm. Biol. 58 (1), 845–853. doi:10.1080/13880209.2020.1803369
Sun, X., Wang, X., Zhao, Z., Chen, J., Li, C., and Zhao, G. (2020). Paeoniflorin Accelerates Foot Wound Healing in Diabetic Rats Though Activating the Nrf2 Pathway. Acta histochem. 122 (8), 151649. doi:10.1016/j.acthis.2020.151649
Sun, X., Wang, X., Zhao, Z., Chen, J., Li, C., and Zhao, G. (2021). Paeoniflorin Inhibited Nod-like Receptor Protein-3 Inflammasome and NF-Κb-Mediated Inflammatory Reactions in Diabetic Foot Ulcer by Inhibiting the Chemokine Receptor CXCR2. Drug Dev. Res. 82 (3), 404–411. doi:10.1002/ddr.21763
Tang, J. Y., Li, S., Li, Z. H., Zhang, Z. J., Hu, G., Cheang, L. C., et al. (2010). Calycosin Promotes Angiogenesis Involving Estrogen Receptor and Mitogen-Activated Protein Kinase (MAPK) Signaling Pathway in Zebrafish and HUVEC. PLoS One 5 (7), e11822. doi:10.1371/journal.pone.0011822
Tang, L., Liu, D., Yi, X., Xu, T., Liu, Y., Luo, Y., et al. (2014). The Protective Effects of Puerarin in Cardiomyocytes from Anoxia/reoxygenation Injury Are Mediated by PKCε. Cell. Biochem. Funct. 32 (4), 378–386. doi:10.1002/cbf.3026
Wang, B., Zhang, C., Chu, D., Ma, X., Yu, T., Liu, X., et al. (2021). Astragaloside IV Improves Angiogenesis under Hypoxic Conditions by Enhancing Hypoxia-inducible F-actor-1α SUMOylation. Mol. Med. Rep. 23 (4), 244. doi:10.3892/mmr.2021.11883
Wang, B. S., Ma, X. F., Zhang, C. Y., Li, Y. X., Liu, X. Z., and Hu, C. Q. (2021). Astragaloside IV Improves Angiogenesis and Promotes Wound Healing in Diabetic Rats via the Activation of the SUMOylation Pathway. Biomed. Environ. Sci. 34 (2), 124–129. doi:10.3967/bes2021.018
Wang, D., Jie, Q., Liu, B., Li, Y., Dai, L., Luo, J., et al. (2017). Saponin Extract from Panax notoginseng Promotesangiogenesis through AMPK- and eNOS-dependent P-athways in HUVECs. Mol. Med. Rep. 16 (4), 5211–5218. doi:10.3892/mmr.2017.7280
Wang, D., Koh, H. L., Hong, Y., Zhu, H. T., Xu, M., Zhang, Y. J., et al. (2013). Chemical and Morphological Variations of Panax Notoginseng and Their Relationship. Phytochemistry 93, 88–95. doi:10.1016/j.phytochem.2013.03.007
Wang, D., Lv, L., Xu, Y., Jiang, K., Chen, F., Qian, J., et al. (2021). Cardioprotection of Panax Notoginseng Saponins against Acute Myocardial Infarction and Heart Failure through Inducing Autophagy. Biomed. Pharmacother. 136, 111287. doi:10.1016/j.biopha.2021.111287
Wang, F., Qian, H., Kong, L., Wang, W., Wang, X., Xu, Z., et al. (2021). Accelerated Bone Regeneration by Astragaloside IV through Stimulating the Coupling of Osteogenesis and Angiogenesis. Int. J. Biol. Sci. 17 (7), 1821–1836. doi:10.7150/ijbs.57681
Wang, X., Abraham, S., McKenzie, J. A. G., Jeffs, N., Swire, M., Tripathi, V. B., et al. (2013). LRG1 Promotes Angiogenesis by Modulating Endothelial TGF-β Signalling. Nature 499 (7458), 306–311. doi:10.1038/nature12345
Wang, X., Bove, A. M., Simone, G., and Ma, B. (2020). Molecular Bases of VEGFR-2-Mediated Physiological Function and Pathological Role. Front. Cell. Dev. Biol. 8, 599281. doi:10.3389/fcell.2020.599281
Wang, X., Jiang, L., Wang, X., Yin, F., Li, G., Feng, X., et al. (2014). Combination of Autologous Transplantation of G-CSF-Mobilized Peripheral Blood Mononuclear Cells and Panax Notoginseng Saponins in the Treatment of Unreconstructable Critical Limb Ischemia. Ann. Vasc. Surg. 28 (6), 1501–1512. doi:10.1016/j.avsg.2014.03.002
Wei, Z. Z., Chen, D., Liu, L. P., Gu, X., Zhong, W., Zhang, Y. B., et al. (2018). Enhanced Neurogenesis and Collaterogenesis by Sodium Danshensu Treatment after Focal Cerebral Ischemia in Mice. Cell. Transpl. 27 (4), 622–636. doi:10.1177/0963689718771889
Weidemann, A. K., Crawshaw, A. A., Byrne, E., and Young, H. S. (2013). Vascular Endothelial Growth Factor Inhibitors: Investigational Therapies for the Treatment of Psoriasis. Clin. Cosmet. Investig. Dermatol 6, 233–244. doi:10.2147/CCID.S35312
Weis, S. M., and Cheresh, D. A. (2011). Tumor Angiogenesis: Molecular Pathways and Therapeutic Targets. Nat. Med. 17 (11), 1359–1370. doi:10.1038/nm.2537
Wilhelm, S. M., Adnane, L., Newell, P., Villanueva, A., Llovet, J. M., and Lynch, M. (2008). Preclinical Overview of Sorafenib, a Multikinase Inhibitor that Targets Both Raf and VEGF and PDGF Receptor Tyrosine Kinase Signaling. Mol. Cancer Ther. 7 (10), 3129–3140. doi:10.1158/1535-7163.MCT-08-0013
Wu, X. D., Wang, C., Zhang, Z. Y., Fu, Y., Liu, F. Y., and Liu, X. H. (2014). Puerarin Attenuates Cerebral Damage by Improving Cerebral Microcirculation in Spontaneously Hypertensive Rats. Evid. Based Complement. Altern. Med. 2014, 408501. doi:10.1155/2014/408501
Xin, M., Hao, Y., Huang, G., Wang, X., Liang, Z., Miao, J., et al. (2020). The Efficacy and Safety of Salvianolic Acids on Acute Cerebral Infarction Treatment: A Protocol for Systematic Review and Meta Analysis. Med. Baltim. 99 (23), e20059. doi:10.1097/MD.0000000000020059
Xin, Q. Q., Yang, B. R., Zhou, H. F., Wang, Y., Yi, B. W., Cong, W. H., et al. (2018). Paeoniflorin Promotes Angiogenesis in A Vascular Insufficiency Model of Zebrafish In Vivo and in Human Umbilical Vein Endothelial Cells In Vitro. Chin. J. Integr. Med. 24 (7), 494–501. doi:10.1007/s11655-016-2262-2
Xu, D., Huang, P., Yu, Z., Xing, D. H., Ouyang, S., and Xing, G. (2015). Efficacy and Safety of Panax Notoginseng Saponin Therapy for Acute Intracerebral Hemorrhage, Meta-Analysis, and Mini Review of Potential Mechanisms of Action. Front. Neurol. 5, 274. doi:10.3389/fneur.2014.00274
Xu, H. X., Pan, W., Qian, J. F., Liu, F., Dong, H. Q., and Liu, Q. J. (2019). MicroRNA-21 C-ontributes to the P-uerarin-induced C-ardioprotection via S-uppression of A-poptosis and O-xidative S-tress in a C-ell M-odel of I-schemia/reperfusion I-njury. Mol. Med. Rep. 20 (1), 719–727. doi:10.3892/mmr.2019.10266
Xu, M., Yin, J., Xie, L., Zhang, J., Zou, C., Zou, J., et al. (2013). Pharmacokinetics and Tolerance of Toal Astragalosides after Intravenous Infusion of Astragalosides Injection in Healthy Chinese Volunteers. Phytomedicine 20 (12), 1105–1111. doi:10.1016/j.phymed.2013.05.004
Xu, W., Yang, J., and Wu, L. M. (2009). Cardioprotective Effects of Tanshinone IIA on Myocardial Ischemia Injury in Rats. Pharmazie 64 (5), 332–336. doi:10.1691/ph.2009.8771
Yan, X., Li, H., Bai, M., and Miao, M. (2017). Effect of Total Flavonoids of Radix Ilicis Pubescentis on Cerebral Ischemia Reperfusion Model. Saudi J. Biol. Sci. 24 (3), 595–602. doi:10.1016/j.sjbs.2017.01.031
Yang, B. R., Cheung, K. K., Zhou, X., Xie, R. F., Cheng, P. P., Wu, S., et al. (2016). Amelioration of Acute Myocardial Infarction by Saponins from Flower Buds of Panax Notoginseng via Pro-angiogenesis and Anti-apoptosis. J. Ethnopharmacol. 181, 50–58. doi:10.1016/j.jep.2016.01.022
Yang, B. R., Hong, S. J., Lee, S. M., Cong, W. H., Wan, J. B., Zhang, Z. R., et al. (2016). Pro-angiogenic Activity of Notoginsenoside R1 in Human Umbilical Vein Endothelial Cells In Vitro and in a Chemical-Induced Blood Vessel Loss Model of Zebrafish In Vivo. Chin. J. Integr. Med. 22 (6), 420–429. doi:10.1007/s11655-014-1954-8
Yang, L., Liu, N., Zhao, W., Li, X., Han, L., Zhang, Z., et al. (2019). Angiogenic Function of Astragaloside IV in Rats with Myocardial Infarction Occurs via the PKD1-HDAC5-VEGF Pathway. Exp. Ther. Med. 17 (4), 2511–2518. doi:10.3892/etm.2019.7273
Yu, L., Xie, J., Xin, N., and Wang, Z. (2015). Panax Notoginseng Saponins Promote Wound Repair of Anterior Cruciate Ligament through Phosphorylation of PI3K, AKT and ERK. Int. J. Clin. Exp. Pathol. 8 (1), 441–449.
Yu, L. C., Chen, S. C., Chang, W. C., Huang, Y. C., Lin, K. M., Lai, P. H., et al. (2007). Stability of Angiogenic Agents, Ginsenoside Rg1 and Re, Isolated from Panax Ginseng: In Vitro and In Vivo Studies. Int. J. Pharm. 328, 168–176. doi:10.1016/j.ijpharm.2006.08.009
Zhang, A., Fang, H., Chen, J., He, L., and Chen, Y. (2020). Role of VEGF-A and LRG1 in Abnormal Angiogenesis Associated with Diabetic Nephropathy. Front. Physiol. 11, 1064. doi:10.3389/fphys.2020.01064
Zhang, E., Gao, B., Yang, L., Wu, X., and Wang, Z. (2016). Notoginsenoside Ft1 Promotes Fibroblast Proliferation via PI3K/Akt/mTOR Signaling Pathway and Benefits Wound Healing in Genetically Diabetic Mice. J. Pharmacol. Exp. Ther. 356 (2), 324–332. doi:10.1124/jpet.115.229369
Zhang, L., Hu, Q., Jin, H., Yang, Y., Yang, Y., Yang, R., et al. (2021). Effects of Ginsenoside Rb1 on Second-Degree Burn Wound Healing and FGF-2/PDGF-BB/PDGFR-β Pathway Modulation. Chin. Med. 16 (1), 45. doi:10.1186/s13020-021-00455-w
Zhang, L., Liu, Q., Lu, L., Zhao, X., Gao, X., and Wang, Y. (2011). Astragaloside IV Stimulates Angiogenesis and Increases Hypoxia-Inducible Factor-1α Accumulation via Phosphatidylinositol 3-kinase/Akt Pathway. J. Pharmacol. Exp. Ther. 338 (2), 485–491. doi:10.1124/jpet.111.180992
Zhang, Q., Chen, Z. W., Zhao, Y. H., Liu, B. W., Liu, N. W., Ke, C. C., et al. (2017). Bone Marrow Stromal Cells Combined with Sodium Ferulate and N-Butylidenephthalide Promote the Effect of Therapeutic Angiogenesis via Advancing Astrocyte-Derived Trophic Factors after Ischemic Stroke. Cell. Transpl. 26 (2), 229–242. doi:10.3727/096368916X693536
Zhang, S., Chen, S., Shen, Y., Yang, D., Liu, X., Sun-Chi, A. C., et al. (2006). Puerarin Induces Angiogenesis in Myocardium of Rat with Myocardial Infarction. Biol. Pharm. Bull. 29 (5), 945–950. doi:10.1248/bpb.29.945
Zhang, S. Y., Chen, G., Wei, P. F., Huang, X. S., Dai, Y., Shen, Y. J., et al. (2008). The Effect of Puerarin on Serum Nitric Oxide Concentration and Myocardial eNOS Expression in Rats with Myocardial Infarction. J. Asian Nat. Prod. Res. 10 (3–4), 373–381. doi:10.1080/10286020801892250
Zhang, Y., Hu, G., Li, S., Li, Z. H., Lam, C. O., Hong, S. J., et al. (2012). Pro-angiogenic Activity of Astragaloside IV in HUVECs In Vitro and Zebrafish In Vivo. Mol. Med. Rep. 5 (3), 805–811. doi:10.3892/mmr.2011.716
Zhang, Y., Hu, G., Lin, H. C., Hong, S. J., Deng, Y. H., Tang, J. Y., et al. (2009). Radix Astragali Extract Promotes Angiogenesis Involving Vascular Endothelial Growth Factor Receptor-Related Phosphatidylinositol 3-kinase/Akt-dependent Pathway in Human Endothelial Cells. Phytother. Res. 23 (9), 1205–1213. doi:10.1002/ptr.2479
Zhang, Z. R., Li, J. H., Li, S., Liu, A. L., Hoi, P. M., Tian, H. Y., et al. (2014). In Vivo angiogenesis Screening and Mechanism of Action of Novel Tanshinone Derivatives Produced by One-Pot Combinatorial Modification of Natural Tanshinone Mixture from Salvia Miltiorrhiza. PLoS One 9 (7), e100416. doi:10.1371/journal.pone.0100416
Zhao, H., Mortezaei, R., Wang, Y., Sheng, X., Aria, F., and Bojanowski, K. (2006). SBD.4 Stimulates Regenerative Processes In Vitro, and Wound Healing in Genetically Diabetic Mice and in Human Skin/severe-Combined Immunodeficiency Mouse Chimera. Wound Repair Regen. 14 (5), 593–601. doi:10.1111/j.1743-6109.2006.00166.x
Zheng, H., Liu, C., Ou, Y., Zhang, Y., and Fu, X. (2013). Total Saponins of Panax Notoginseng Enhance VEGF and Relative Receptors Signals and Promote Angiogenesis Derived from Rat Bone Marrow Mesenchymal Stem Cells. J. Ethnopharmacol. 147, 595–602. doi:10.1016/j.jep.2013.03.043
Zheng, Q. H., Li, X. L., Mei, Z. G., Xiong, L., Mei, Q. X., Wang, J. F., et al. (2017). Efficacy and Safety of Puerarin Injection in Curing Acute Ischemic Stroke: A Meta-Analysis of Randomized Controlled Trials. Med. Baltim. 96, e5803. doi:10.1097/MD.0000000000005803
Zheng, Y., Feng, Z., You, C., Jin, Y., Hu, X., Wang, X., et al. (2013). In Vitro evaluation of Panax Notoginseng Rg1 Released from Collagen/chitosan-Gelatin Microsphere Scaffolds for Angiogenesis. Biomed. Eng. Online 12, 134. doi:10.1186/1475-925X-12-134
Zhou, Y. X., Gong, X. H., Zhang, H., and Peng, C. (2020). A Review on the Pharmacokinetics of Paeoniflorin and its Anti-inflammatory and Immunomodulatory Effects. Biomed. Pharmacother. 130, 110505. doi:10.1016/j.biopha.2020.110505
Zhou, Z. Y., Zhao, W. R., Zhang, J., Chen, X. L., and Tang, J. Y. (2019). Sodium Tanshinone IIA Sulfonate: A Review of Pharmacological Activity and Pharmacokinetics. Biomed. Pharmacother. 118, 109362. doi:10.1016/j.biopha.2019.109362
Glossary
Akt protein kinase B
AMPK 5′ adenosine monophosphate-activated protein kinase
Ang-1 angiopoietin-1
BAD B-cell lymphoma-2 associated death
Bcl B-cell lymphoma
BDNF brain-derived neurotrophic factor
BM-MSCs bone marrow-derived mesenchymal stem/stromal cells
BMSCs bone marrow mesenchymal stem cells
CAM chick embryo chorioallantoic membrane
Cdc42 cell division control protein 42 homolog
DAG diacylglycerol
DO distraction osteogenesis
EGF epidermal growth factor
EGFR epidermal growth factor receptors
eNOS endothelial nitric oxide
EPCs human endothelial progenitor cells
ER estrogen receptor
ERK extracellular signal-regulated kinases
FAK focal adhesion kinase
FGF fibroblast growth factor
G-CSF granulocyte colony-stimulating factor
GSK-3β glycogen synthase kinase-3β
HDAC5 histone deacetylase 5
HIF hypoxia-inducible factor
HIF-1α hypoxia-inducible factor-1α
HUVECs human umbilical vein endothelial cells
IP3 inositol 1,4,5-trisphosphate
IRF-1 interferon Regulatory Factor 1
JNK c-Jun N-terminal kinase
LAD left anterior descending
MCAO middle cerebral artery occlusion
MAPK mitogen-activated protein kinase
MECs microvascular endothelial cells
MMP matrix metalloproteinase
mTOR mechanistic target of rapamycin
N/A not applicable
Nck non-catalytic region of the tyrosine kinase adapter protein
NO nitric oxide
PBMNCs peripheral blood mononuclear cells
PDGF platelet-derived growth factor
PI3K phosphoinositide 3-kinase
PIP2 phosphatidylinositol 4,5-bisphosphate
PKC protein kinase C
PKD1 protein kinase D1
PLC phospholipase C
PNS Panax notoginseng saponins
PPARγ proliferator-activated receptor γ
Raf proto-oncogene serine/threonine-protein kinase
Shb SH2 domain containing adapter protein B
SUMOylation small ubiquitin-related modifier-mediated modification
TGF transforming growth factor
VEGF vascular endothelial growth factor
VEGFRs vascular endothelial growth factor receptors
Keywords: herbal medicine, phytochemicals, pro-angiogenic, wound healing, ischemic diseases
Citation: Li J, Li R, Wu X, Zheng C, Shiu PH-T, Rangsinth P, Lee SM-Y and Leung GP-H (2022) An Update on the Potential Application of Herbal Medicine in Promoting Angiogenesis. Front. Pharmacol. 13:928817. doi: 10.3389/fphar.2022.928817
Received: 26 April 2022; Accepted: 20 June 2022;
Published: 19 July 2022.
Edited by:
Ramoji Kosuru, Versiti Blood Research Institute, United StatesReviewed by:
Le-Le Zhang, Chengdu University, ChinaBin-Nan Wu, Kaohsiung Medical University, Taiwan
Hongwei Gao, Guangxi University of Chinese Medicine, China
Amro M. Soliman, University of Alberta, Canada
Yifan Zhang, Shanghai Jiao Tong University, China
Copyright © 2022 Li, Li, Wu, Zheng, Shiu, Rangsinth, Lee and Leung. This is an open-access article distributed under the terms of the Creative Commons Attribution License (CC BY). The use, distribution or reproduction in other forums is permitted, provided the original author(s) and the copyright owner(s) are credited and that the original publication in this journal is cited, in accordance with accepted academic practice. No use, distribution or reproduction is permitted which does not comply with these terms.
*Correspondence: George Pak-Heng Leung, Z3BobGV1bmdAaGt1Lmhr