- 1School of Health Science and Technology, University of Petroleum and Energy Studies, Bidholi, Uttarakhand, India
- 2Chitkara College of Pharmacy, Chitkara University, Rajpura, Punjab, India
- 3Institutes for Systems Genetics, Frontiers Science Center for Disease-Related Molecular Network, West China Hospital, Sichuan University, Chengdu, Sichuan, China
- 4School of Pharmaceutical Sciences, Lovely Professional University, Phagwara, Punjab, India
- 5GHG Khalsa College of Pharmacy, Gurusar Sadhar, Ludhiana, Punjab, India
- 6Pharmacy Practice Research Unit, Clinical Pharmacy Department, College of Pharmacy, Jazan University, Jazan, Saudi Arabia
- 7Department of Pharmaceutical Chemistry and Pharmacognosy, College of Pharmacy, Jazan University, Jazan, Saudi Arabia
- 8Department of Pharmacy, Faculty of Medicine and Pharmacy, University of Oradea, Oradea, Romania
- 9Doctoral School of Biomedical Sciences, University of Oradea, Oradea, Romania
Next to Alzheimer’s disease, Parkinson’s disease constitutes the second most widespread neurological disorder, primarily affecting the older population. Its symptoms are noticeable with advancing age including tremors, postural imbalance, and slow movements, and over time, these symptoms get aggravated, progressing to osteoporosis, osteopenia, and risk of fractures. These symptoms correlate to low bone density and hence weakened bones; thus, vitamin D proves to be an intricate component of the pathogenesis of the disease. Moreover, lower serum concentrations of vitamin D have been found in diseased subjects. Supplementation with vitamin D can retard the aggravation of non-motor as well as motor symptoms of Parkinson’s disease that include cognitive improvement along with the decline in risk of fractures. Also, vitamin D is extremely crucial for brain functioning, targeting dopaminergic neurons, and almost the entire functioning of the brain is affected. However, further exploration is required to determine the toxic dose of vitamin D in Parkinson’s subjects. This “sunshine vitamin” surely can be a ray of sunshine for neurologically diseased subjects.
Introduction
The most widespread neurodegenerative disorder other than Alzheimer’s disease is “shaking palsy” or Parkinson’s disease (PD), affecting about one/two people per thousand population. The incidence of developing the disorder increases as age increases, particularly affecting those above the age of 60 years (Tysnes and Storstein, 2017). The recognition of the disorder by the term “shaking palsy” is absolutely precise owing to its characteristic features that include tremors, slow movements, rigidity, and an imbalanced posture. Various sources of evidence suggest that PD patients have an abnormal vitamin D endocrine system, comprising a decline in the vitamin D level and less bone density, along with elevated bone-turnover indicators—urinary N-terminal telo-peptide and alkaline phosphatase of the bone (Abou-Raya et al., 2009). These parameters combined with postural imbalances contribute to the occurrence of fractures in diseased subjects, particularly hip fractures among geriatric females (Sato et al., 2001).
Vitamin D, a lipid-soluble steroid, the site of synthesis being the skin, is generated primarily via exposure to sunlight and also via dietary components (Cesari et al., 2011). Activation of the vitamin occurs via hydroxylation which occurs twice inside our body. The foremost hydroxylation site is the liver, converting vitamin D to 25-hydroxy vitamin D, and the other one is the kidneys, where 25-hydroxy vitamin D is transformed into calcitriol/1,25-dihydroxy vitamin D, which is the active form of vitamin D, and the effects are seen when it communicates with the receptor for vitamin D (Cesari et al., 2011). This “sunshine vitamin” proves to be extremely essential for the therapy of rheumatoid arthritis, asthma (Rappaport et al., 1934; Scheuring et al., 2007), cancer, and neurodegenerative disorders, such as PD (Bouillon, 2018). There have been numerous studies correlating vitamin D levels and PD; for instance, the patients with PD usually suffer from osteoporosis (more commonly observed in women than in men) (Invernizzi et al., 2009), leading to elevated chances of hip fractures owing to lower bone density along with low calcium (Sato et al., 2001). Also, vitamin D levels are notably lower in PD subjects than those in healthy subjects (Peterson et al., 2013a; Rimmelzwaan et al., 2016), and the level of 25-hydroxy vitamin D exhibits a gradual decline as the motor signs of PD augment (Van den Bos et al., 2013).
There occur a plethora of mechanisms that correlate neurodegenerative disorders with the “sunshine vitamin.” The vitamin plays a vital role in neuronal protection via nerve growth regulation, as well as via neurotrophic factors (Garcion et al., 2002). In fact, 1,25-dihydroxy vitamin D3 can synthesize neurotrophic factors (derived from cell lines of glial cells) as well as neurotrophin 3 (Wion et al., 1991; Brown et al., 2003), resulting in neuroprotection as observed in rats (Riaz et al., 1999; Wang et al., 2000). The toxic effects of reactive oxygen species (ROS) are also affected by vitamin D. Our immune system produces inducible nitric oxide synthase inside nerve cells along with other cells of the central nervous system (Garcion et al., 1998). Higher concentrations of nitric oxide exert a damaging effect on the nerve cells. The interesting component here is that the presence of vitamin D has an inhibitory effect on inducible nitric oxide synthase (Garcion et al., 1998). Also, in the nerve cells of the hippocampus, vitamin D downregulates the calcium ion channels (voltage sensitive) exhibiting protective effects on the neurons (Li et al., 2011). Yet another crucial factor is the reduction of oxidative stress, leading to nerve cell protection by the vitamin and causing a decline in cell death (Ibi et al., 2001). The intricate relationship between vitamin D and PD was initially identified in 1997 by Sato et al. (1997). The substantia nigra of the brain contains the receptor for vitamin D and the prominent vitamin D-activating enzyme, 1α-hydroxylase, that accounts for the fact that the deficiency of the vitamin progresses to the dysfunctional substantia nigra, the primary affected part in PD (Shen et al., 2004).
Here, we review the metabolism of vitamin D, declining concentrations of vitamin D in PD subjects, important functions of the vitamin in the brain, and its association to clinical manifestations of PD coupled with the fundamentals for administering vitamin D3 to PD subjects, utilizing the method of reviewing the referenced reviews and research papers, searching the material through the usage of appropriate keywords, and stating the facts accordingly. Moreover, complete utilization of vitamin D in PD therapy could be carried out by overcoming certain limitations mentioned in the conclusion for which adequate data stand unavailable (Higgins et al., 2019).
Vitamin D: Biotransformation and receptor interaction
The fundamental source of Vitamin D, also called “sunshine vitamin,” is sunlight. Apart from this, the vitamin can be obtained via diet after which the liver enzyme, 25-hydroxylase, transforms it into 25-hydroxy vitamin D, and this constitutes the vitamin in its circulatory form. Moreover, 25-hydroxy vitamin D serves as a biological marker to detect serum levels of the vitamin in people with PD, but this circulatory form is not the active form and thus necessitates its transformation via 25-hydroxy vitamin D-one-alpha hydroxylase enzyme to 1,25-dihydroxy vitamin D3 (process occurs inside the kidney), which is the activated form of the vitamin. This active form, when present at extremely elevated levels, can be further metabolized to calcitroic acid via the metabolic enzyme 25-hydroxy vitamin D 24 hydroxylase (Lv et al., 2020).
1,25-Dihydroxy vitamin D3 exhibits its actions through an interaction with the receptor for the vitamin, majorly confined to the nucleus since it is a nuclear receptor (DeLuca, 2004). When a ligand is attached to the receptor, the receptor exhibits an interaction with the retinoid X-receptor, resulting in the formation of a hetero-dimer. The hetero-dimer and vitamin D response elements interact, thus promoting latter’s expression (Holick, 2007). This biotransformation has been indicated in Figure 1.
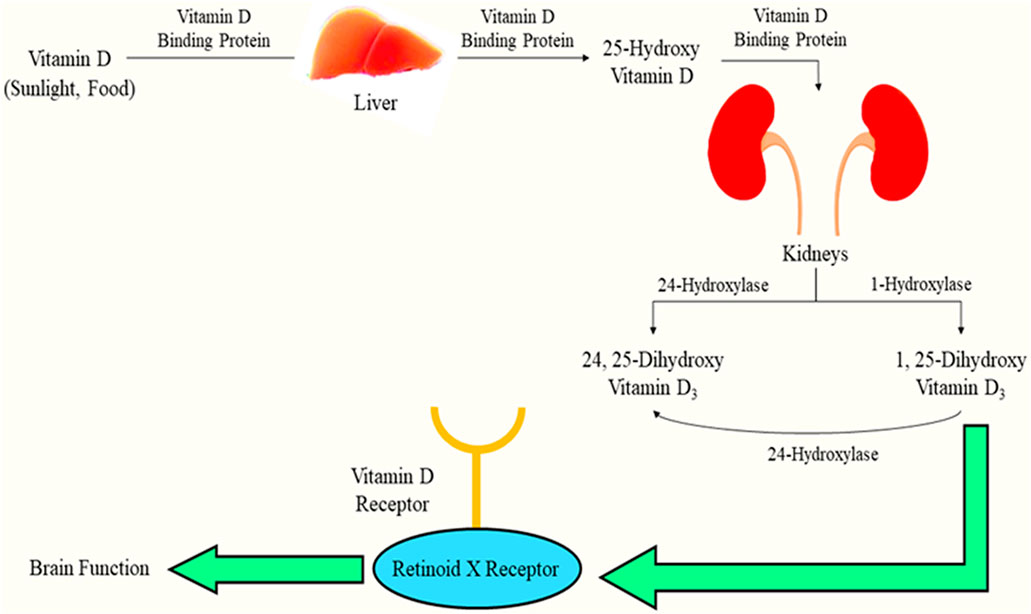
FIGURE 1. Biotransformation of vitamin D and interaction with its receptor facilitating brain function.
Vitamin D and PD in parallel: Low vitamin D levels in PD subjects
Vitamin D deficiency has become extremely common throughout the world, especially in the aged population (Dawson-Hughes et al., 2010), and recent research claims that the deficiency of the vitamin is found to be closely associated with neurodegenerative disorders such as PD (Ding et al., 2013). Probably, deficiency of vitamin D does not occur as a result of neurodegenerative diseases, and this is evident from the fact that in the case of Alzheimer’s disease, vitamin D levels are not significantly low, whereas vitamin D levels are found to be considerably low in PD (Evatt et al., 2008). The concentration of 1,25-dihydroxy vitamin D3 does not decrease in PD subjects, but the concentration of 25-hydroxy vitamin D falls significantly in serum to as low as 20 ng per milliliter (according to prospective cohort studies) (Suzuki et al., 2012). The probable cause for decreased 25-hydroxy vitamin D is that its levels in serum/blood are about a thousand times greater than 1,25-dihydroxy vitamin D3 (Suzuki et al., 2012).
There were several studies to explore the possible causes of the deficit in vitamin concentration in diseased subjects. A study came up with one of the reasons as decreased motility of PD patients and the longer course of the disease that ultimately reduces the probability of getting exposed to sunlight, leading to a decline in the synthesis of the vitamin (Suzuki et al., 2012; Peterson, 2014; Zhou et al., 2019). However, another study came up with the fact that even in PD patients who had adequate sun exposure, vitamin D levels were low owing to a decline in gastrointestinal functioning (Mueller et al., 2021). Such lowering of vitamin D levels due to a decline in gastrointestinal function was overruled by another study because 25-hydroxy vitamin D was higher in plasma and hypovitaminosis is much more common in patients with “early PD” (Evatt et al., 2011).
Vitamin D and brain functioning: An intricate association
Enzymes involved in the biological synthesis of the sunshine vitamin (25-hydroxylase, etc.) as well as the receptor for vitamin D are presented in the embryonic brain and adult brain (Eyles et al., 2005; de Abreu et al., 2009). The receptor for the vitamin is present in abundance in the amygdala, thalamus, cortex, and hippocampus (Stumpf et al., 1982) with 1α-hydroxylase being present in abundance in the substantia nigra. Vitamin D facilitates the regulation of multiplication, differentiation, and the viability of the cells via which it is synthesized, i.e., microglia and nerve cells (de Abreu et al., 2009). This vitamin also plays a crucial role in providing synaptic plasticity as well as in ameliorating brain function (Almeras et al., 2007), and this synaptic plasticity is provided by the vitamin owing to its role in the regulation of protein expression including connexin 43, growth-associated protein 43, and drebrin; transportation of kinesin, creatine kinase B, and dynactin; and maintaining the cytoskeletal structure comprising neuro-filament, tubulin, and microtubule-associated protein 2 (Almeras et al., 2007; de Abreu et al., 2009). A preclinical study showed that lower vitamin D levels at the maternal stage cause alterations in the brain such as reduced cortical thickness and anatomically increased lateral ventricle in the newborn (Eyles et al., 2003; Grecksch et al., 2009).
Vitamin D seems to be engaged in neuroprotection since the lowered level of 25-hydroxy vitamin D causes dopaminergic nerve cell death, leading to PD, and also the functions mentioned previously exhibit its crucial role in brain functioning (Suzuki et al., 2012), and there are numerous mechanisms in support of this fact. Various mechanisms state that the production of parvalbumin (calcium ion-binding protein), as well as the release of neurotrophin, is stimulated via 1,25-dihydroxy vitamin D3; gamma-glutamyl transpeptidase (necessary for the regulation of calcium ion balance and anti-oxidant effect) is upregulated, while the L-type voltage-sensitive calcium ion channel is downregulated (necessary for neurotransmission); and calcitriol does not permit the formation of inducible nitric oxide synthase, tumor necrosis factor α, and macrophage colony-stimulating factor (Brewer et al., 2001; de Sire et al., 2022). Moreover, when calcitriol is lower in the body, there occurs a rise in inflammation which is indicated by higher levels of C-reactive protein (Alfieri et al., 2017). Not only this, there are certain growth factors that the vitamin synthesizes such as ciliary neurotrophic factor and brain-derived neurotrophic factor, among others, that prevent the brain from aging and degenerating (de Sire et al., 2022). The vitamin has a critical function of maintaining calcium ion concentration in the neurons as well as in the glial cells. If this calcium ion concentration is not maintained, it will probably cause excitotoxicity in the cytoplasm due to a spike in the calcium ion concentration; hence, the injury due to excitotoxicity is avoided (Brewer et al., 2001). By reducing the production of inducible nitric oxide synthase and nuclear factor kappa-light-chain-enhancer of activated B cells (NF-kB), as well as incrementing the activity of gamma-glutamyl transpeptidase, the oxidative stress is significantly reduced (van Etten and Mathieu, 2005). Nonetheless, significantly lower vitamin D levels can actually progress to impaired functioning of the neurons of the sympathetic nervous system due to the involvement of the vitamin in the functioning of the renin–angiotensin–aldosterone system/RAAS (Ometto et al., 2016).
Vitamin D plays a major role in the functioning of the cerebrum to transmit signals in the nervous system in order to facilitate locomotor, emotional, and rewarding behavior along with intelligence. All of this is dopamine level-dependent and under the influence of vitamin D (Trinko et al., 2016). The site of dopamine production in the substantia nigra and into the tegmental area (ventral) further projects into the striatum (dorsal part) and the prefrontal cortex. The dorsal striatum participates in motor activities, whereas the tegmental area (ventral), as well as the prefrontal cortex, participates in reward behavior. When the receptor for vitamin D is overexpressed in the striatum (as seen in preclinical studies), an elevation in motivation as well as reward behaviors occurs (Burne et al., 2006; Zhang et al., 2018). Vitamin D affects locomotion; thus, the mice that do not have the receptor for vitamin D showing dysfunction in motor performance (vinh quôc Luong and Thi Hoàng Nguyên, 2012). The vitamin also plays a role in the regulation of emotions and mood owing to its presence in the hippocampus, cortex, and amygdala (i.e., limbic system) (Bertone-Johnson, 2009).
Vitamin D and its receptor facilitate the proper functioning of the neurons as mentioned previously, and to further strengthen the neuronal circuit, vitamin D also influences the generation of serotonin (Patrick and Ames, 2015; Penckofer et al., 2017). The intricate association of vitamin D with brain functioning is summarized in Figure 2.
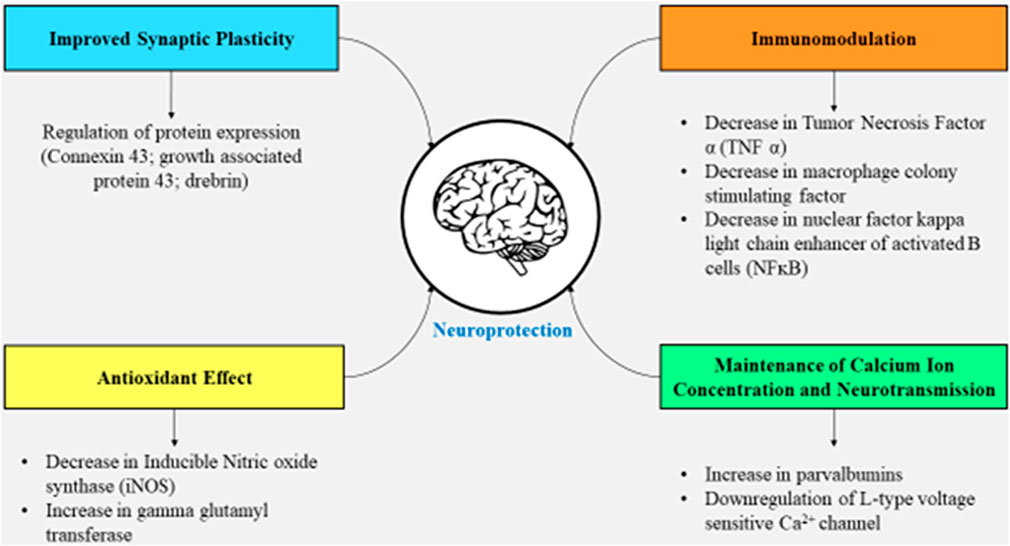
FIGURE 2. Effects of vitamin D on the brain facilitating synaptic plasticity, immunomodulation, neurotransmission, and a decline in oxidative stress.
Vitamin D: A neuroprotective agent
The re-growth of neurons, as well as the protection of dopaminergic nerve endings, is facilitated via glial cell-derived neurotrophic factors (GDNFs), and hence, the GDNFs have the potential for neuro-restoration as a part of treatment for PD (Weissmiller and Wu, 2012; Lv et al., 2020). The receptor, known as GDNF family receptor alpha 1 (GFRA1), is the binding site for GDNFs, further associating with receptor-tyrosine protein kinase Ret/C-Ret (a proto-oncogene receptor). The complexation favors signaling within the dopaminergic nerve cells (Pertile et al., 2018). Although GDNF has the potential for neuro-restoration, its inability to cross the blood–brain barrier (BBB) necessitates the need to be injected into the central nervous system, leading to numerous adverse effects (Lv et al., 2020). Thus, to overcome this limitation, administering 1,25-dihydroxy vitamin D3 is the solution, and as being lipid-soluble, it can penetrate the BBB (DeLuca et al., 2013), indicating the vital role of this hormone in the therapy of PD. 1, 25-Dihydroxy vitamin D3 further binds to the receptor for vitamin D upregulating the transcription of genes stimulated via GDNF, as well as C-Ret, both of which can decrease the generation of GDNF family receptor alpha-1 (GFRA1) (Pertile et al., 2018). The functional specificity, as well as maturity of dopaminergic nerve cells, in mice is affected (Cui et al., 2010) due to the decreased levels of calcitriol that lower the relative abundance of nuclear receptor-related 1 protein (Nurr1), p57kip2, and GDNF (Eyles et al., 2003; McGrath et al., 2004). C-Ret that activates tyrosine kinase enzyme necessary for mechanisms, such as phosphor-inositol 3 kinase (PI3K), phospholipase 3 pathway, and mitogen-activated protein kinase, is also expressed when nuclear receptor-related 1 protein exhibits its presence (Sariola and Saarma, 2003; García-Martínez et al., 2006). The activation of these mechanisms is crucial for the dopaminergic neurons to survive and acquire functional specifications.
Apart from augmenting the presence of the GDNF gene and activating the mechanisms, neuroprotection is also offered by 1, 25-dihydroxy vitamin D3 by exerting anti-oxidant effects since GDNF has the capacity to scavenge free radicals via augmentation of catalase and glutathione peroxidase enzymes in the striatum nigra (Chao and Lee, 1999). The active form of the vitamin exhibits its anti-oxidant potential via genomic and non-genomic mechanisms. When inflammation strikes in, there occurs in situ generation of calcitriol by microglia enhancing the activity of gamma-glutamyl transferase. The gamma-glutamyl transferase enzyme further promotes glutathione in the cells that exert an anti-oxidant effect (scavenges hydrogen peroxide) (Garcion et al., 1999). The nuclear factor-erythroid 2-related factor 2 (Nrf2) attaches to anti-oxidant response elements (AREs) inside the nucleus that augments the presence of anti-oxidant genes along with fos proto-oncogene (Fos) and JUN (Berridge, 2015). Another crucial benefit of administering 1, 25-dihydroxy vitamin D3 is that cell membranes are protected from damage due to oxidative stress due to the inhibition of peroxidation of lipids (Wiseman, 1993).
The active form of the vitamin also possesses anti-inflammatory properties by decreasing the inflammatory activities and augmenting the anti-inflammatory activities (Calvello et al., 2017). According to a cell culture study, the increased levels of calcium ions within the cell induce clumping of alpha-synuclein (Rcom-H’cheo-Gauthier et al., 2017). In order to maintain decreased calcium ion levels in the cytosol, the presence of L-type calcium ion channels has to be reduced, augmenting the levels of calcium-ATPase, Bcl2 (apoptosis regulator), sodium–calcium exchanger 1(NCX1), and calbindin-D28k (buffering protein) (Berridge, 2015; Phillipson, 2017). A factor that contributes to the increased oxidative stress is increased levels of metal ions such as zinc and manganese, and the level of these ions is maintained by 1,25-dihydroxy vitamin D3 by increasing their transportation via activation of SLC30A10 that augments the presence of the transporter ZnT10 (zinc transporter) (da Silva et al., 2016). This will reduce oxidative stress and damage to mitochondria as well as cell membranes. The illustration of 1,25-dihydroxy vitamin D3 as a neuroprotective agent is depicted in Figure 3.
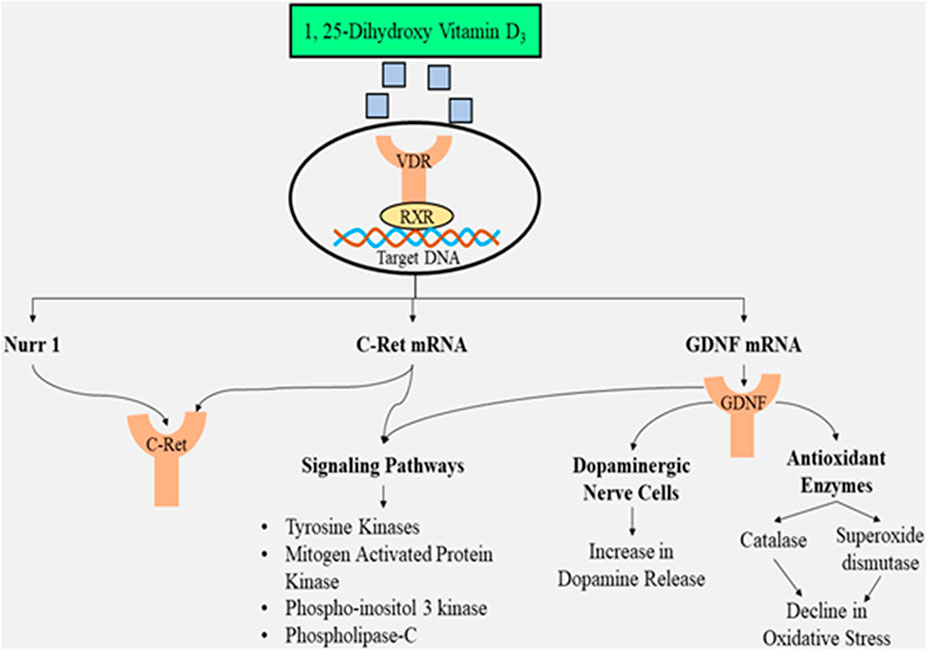
FIGURE 3. Illustration of 1,25-dihydroxy vitamin D3 as a neuroprotective agent preventing dopaminergic neuronal loss and suppressing oxidative stress along with triggering multiple signaling pathways.
The impact of calcitriol on various genes is summarized in Table 1.
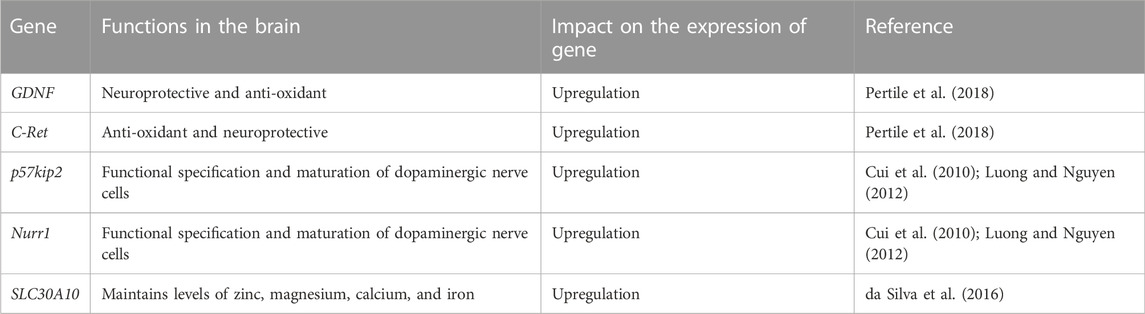
TABLE 1. Influence of 1,25-dihydroxy vitamin D3 on the expression (upregulation/downregulation) of genes.
Clinical findings in PD patients: Vitamin D therapy in PD subjects as a valuable approach
Clinical manifestations that occur in PD patients directly indicate the need for vitamin D supplementation, and this section throws light on some of these.
People who suffer from PD have increased chances of developing osteopenia along with osteoporosis (Invernizzi et al., 2009). Moreover, patients with PD can develop secondary osteoporosis (Bezza et al., 2008). According to one research conducted in Korea, out of the total 35,663 patients participated in the study, about 6,542 patients developed osteoporosis and developed fractures 6 months post-development of PD (Park et al., 2019). Patients with PD have more chances of loss of bone density, falls, and hip fractures (Lyell et al., 2015); thus, timely therapy for osteoporosis should begin, especially for elderly women (Park et al., 2019). Due to these reasons, another study came up with the fact that the administration of 700–1,000 IU (international units) of vitamin D can possibly lower the chances of falling by 19 percent without the need for calcium intake (Bischoff-Ferrari et al., 2009). Further research has established a negative correlation between the intensity of disease developed (as measured by the Unified Parkinson’s Disease Rating Scale) and the concentration of 25-hydroxy vitamin D in plasma (Suzuki et al., 2012; Chitsaz et al., 2013; Peterson, 2014; Zhou et al., 2019). Hence, treatment via vitamin D3 can retard aggravating PD symptoms (Peterson et al., 2013b). Also, a relationship exists between lower vitamin D levels and a decrease in intelligence and memory, progressing to the risk of dementia (Feart et al., 2017). This comes with the fact that was proved by yet another study that elevated levels of vitamin D improve memory and behavior (Peterson et al., 2013a). Vitamin D has an impact on memory because it affects amyloid beta protein (Hooshmand et al., 2014) that accumulates not only in Alzheimer’s but also in PD, progressing to decreased memory (Jellinger et al., 2008). According to a double-blind trial, therapy with vitamin D lowers the concentration of amyloid precursor protein as well as amyloid precursor protein mRNA (Jia et al., 2019). The lack of vitamin D alters the plasticity of synapses, thus causing decreased memory (Mayne and Burne, 2019).
Thus, in a nutshell, vitamin D3 is definitely a critical component of the pathophysiology encompassing PD and hence needs to be incorporated into PD therapy.
The major studies on the administration of the vitamin in people who suffer from PD are listed in Table 2.
Vitamin D significantly improves posture in patients with PD when administered daily, especially in the not-so-old PD patients (Hiller et al., 2018). There have been other research studies that mention a positive impact of administering 25-hydroxy vitamin D on geriatric PD patients (Muir and Montero-Odasso, 2011). However, whether the administration of vitamin D supplement has a specificity for delaying motor symptoms in PD patients or acts non-specifically to improve posture and balance is still a debatable issue (Torres-Oviedo and Ting, 2007; Asaka et al., 2011). An important thing to mention is that vitamin D as a supplement acts depending on the age of PD patients (Hiller et al., 2018). Although several drugs are available for PD and more drugs can possibly be discovered by the technique of “elicitation via abiotic stress” (Hassan et al., 2021), vitamin D can be used as a supplement along with these drugs to prevent aggravation of PD symptoms. Moreover, vitamin D3 can also be obtained from herbs as herbal drugs, which display numerous pharmacological activities (Hassan et al., 2022).
Table 3 represents the literature summary table depicting relationship between PD and vitamin D.
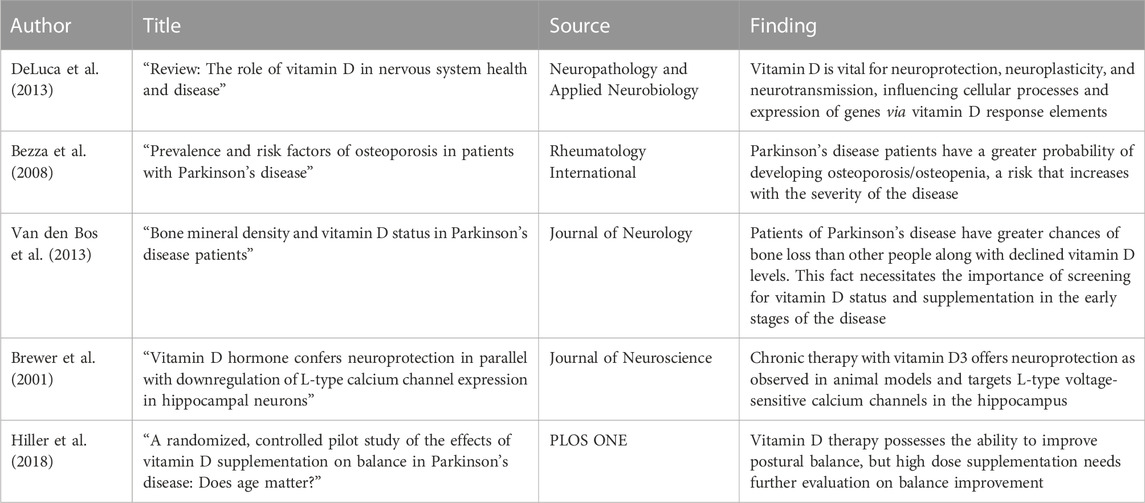
TABLE 3. Literature summary table: highlighting key points that indicate the co-relation between PD and vitamin D.
Conclusion and future perspectives
Vitamin D levels are low in patients suffering from PD, and boosting vitamin D levels indicates the possibility of improving mood, cognition, and behavior in PD patients along with preventing the aggravation of PD symptoms. The review convinces us to accept the fact that improving vitamin D levels reduces the incidence of fractures, especially hip fractures, and recovers bone density as well. At the same time, certain limitations that need to be taken into consideration for further research include the necessity to establish the effectiveness of vitamin D3 as a supplement in PD, and determining the correlation between vitamin D3 and PD will be crucial because vitamin D can act as a biomarker for PD. Also, deficiency of the vitamin has become frequent across the globe, and supplements of vitamin D are easily accessible and affordable. Thus, vitamin D can act as a potential candidate that can be used as a supplement in PD; however, another limitation to be taken into account is its toxicity profile, especially in PD subjects, and at exactly what stage of the disease is the supplementation useful.
Author contributions
All authors listed have made a substantial, direct, and intellectual contribution to the work and approved it for publication.
Funding
Funding for the publication of this paper was provided by the University of Oradea, Oradea, Romania, through an internal project.
Conflict of interest
Author RS was employed by the company iGlobal Research and Publishing Foundation.
The remaining authors declare that the research was conducted in the absence of any commercial or financial relationships that could be construed as a potential conflict of interest.
Publisher’s note
All claims expressed in this article are solely those of the authors and do not necessarily represent those of their affiliated organizations, or those of the publisher, the editors, and the reviewers. Any product that may be evaluated in this article, or claim that may be made by its manufacturer, is not guaranteed or endorsed by the publisher.
References
Abou-Raya, S., Helmii, M., and Abou-Raya, A. (2009). Bone and mineral metabolism in older adults with Parkinson's disease. Age Ageing 38 (6), 675–680. doi:10.1093/ageing/afp137
Alfieri, D. F., Lehmann, M. F., Oliveira, S. R., Flauzino, T., Delongui, F., de Araújo, M. C. M., et al. (2017). Vitamin D deficiency is associated with acute ischemic stroke, C-reactive protein, and short-term outcome. Metab. Brain Dis. 32 (2), 493–502. doi:10.1007/s11011-016-9939-2
Almeras, L., Eyles, D., Benech, P., Laffite, D., Villard, C., Patatian, A., et al. (2007). Developmental vitamin D deficiency alters brain protein expression in the adult rat: implications for neuropsychiatric disorders. Proteomics 7 (5), 769–780. doi:10.1002/pmic.200600392
Asaka, T., Yahata, K., Mani, H., and Wang, Y. (2011). Modulations of muscle modes in automatic postural responses induced by external surface translations. J. Mot. Behav. 43 (2), 165–172. doi:10.1080/00222895.2011.552079
Berridge, M. J. (2015). Vitamin D cell signalling in health and disease. Biochem. Biophys. Res. Commun. 460 (1), 53–71. doi:10.1016/j.bbrc.2015.01.008
Bertone-Johnson, E. R. (2009). Vitamin D and the occurrence of depression: causal association or circumstantial evidence? Nutr. Rev. 67 (8), 481–492. doi:10.1111/j.1753-4887.2009.00220.x
Bezza, A., Ouzzif, Z., Naji, H., Achemlal, L., Mounach, A., Nouijai, M., et al. (2008). Prevalence and risk factors of osteoporosis in patients with Parkinson’s disease. Rheumatol. Int. 28 (12), 1205–1209. doi:10.1007/s00296-008-0632-6
Bischoff-Ferrari, H. A., Dawson-Hughes, B., Staehelin, H. B., Orav, J. E., Stuck, A. E., Theiler, R., et al. (2009). Fall prevention with supplemental and active forms of vitamin D: a meta-analysis of randomised controlled trials. Bmj 339, b3692. doi:10.1136/bmj.b3692
Bouillon, R. (2018). Extra-skeletal effects of vitamin D. Front. Horm. Res. 50, 72–88. doi:10.1159/000486072
Brewer, L. D., Thibault, V., Chen, K. C., Langub, M. C., Landfield, P. W., and Porter, N. M. (2001). Vitamin D hormone confers neuroprotection in parallel with downregulation of L-type calcium channel expression in hippocampal neurons. J. Neurosci. 21 (1), 98–108. doi:10.1523/JNEUROSCI.21-01-00098.2001
Brown, J., Bianco, J. I., McGrath, J. J., and Eyles, D. W. (2003). 1, 25-dihydroxyvitamin D3 induces nerve growth factor, promotes neurite outgrowth and inhibits mitosis in embryonic rat hippocampal neurons. Neurosci. Lett. 343 (2), 139–143. doi:10.1016/s0304-3940(03)00303-3
Burne, T. H., Johnston, A. N., McGrath, J. J., and Mackay-Sim, A. (2006). Swimming behaviour and post-swimming activity in Vitamin D receptor knockout mice. Brain Res. Bull. 69 (1), 74–78. doi:10.1016/j.brainresbull.2005.10.014
Calvello, R., Cianciulli, A., Nicolardi, G., De Nuccio, F., Giannotti, L., Salvatore, R., et al. (2017). Vitamin D treatment attenuates neuroinflammation and dopaminergic neurodegeneration in an animal model of Parkinson’s disease, shifting M1 to M2 microglia responses. J. Neuroimmune Pharmacol. 12 (2), 327–339. doi:10.1007/s11481-016-9720-7
Cesari, M., Incalzi, R. A., Zamboni, V., and Pahor, M. (2011). Vitamin D hormone: a multitude of actions potentially influencing the physical function decline in older persons. Geriatr. Gerontol. Int. 11 (2), 133–142. doi:10.1111/j.1447-0594.2010.00668.x
Chao, C. C., and Lee, E. H. (1999). Neuroprotective mechanism of glial cell line-derived neurotrophic factor on dopamine neurons: role of antioxidation. Neuropharmacology 38 (6), 913–916. doi:10.1016/s0028-3908(99)00030-1
Chitsaz, A., Maracy, M., Basiri, K., Izadi Boroujeni, M., Tanhaei, A. P., Rahimi, M., et al. (2013). 25-hydroxyvitamin d and severity of Parkinson’s disease. Int. J. Endocrinol. 2013, 689149. doi:10.1155/2013/689149
Cui, X., Pelekanos, M., Burne, T. H., McGrath, J. J., and Eyles, D. W. (2010). Maternal vitamin D deficiency alters the expression of genes involved in dopamine specification in the developing rat mesencephalon. Neurosci. Lett. 486 (3), 220–223. doi:10.1016/j.neulet.2010.09.057
da Silva, T. C., Hiller, C., Gai, Z., and Kullak-Ublick, G. A. (2016). Vitamin D3 transactivates the zinc and manganese transporter SLC30A10 via the Vitamin D receptor. J. Steroid Biochem. Mol. Biol. 163, 77–87. doi:10.1016/j.jsbmb.2016.04.006
Dawson-Hughes, B., Mithal, A., Bonjour, J. P., Boonen, S., Burckhardt, P., Fuleihan, G. H., et al. (2010). IOF position statement: vitamin D recommendations for older adults. Osteoporos. Int. 21 (7), 1151–1154. doi:10.1007/s00198-010-1285-3
de Abreu, D. F., Eyles, D., and Feron, F. (2009). Vitamin D, a neuro-immunomodulator: implications for neurodegenerative and autoimmune diseases. Psychoneuroendocrinology 34, S265–S277. doi:10.1016/j.psyneuen.2009.05.023
de Sire, A., Gallelli, L., Marotta, N., Lippi, L., Fusco, N., Calafiore, D., et al. (2022). Vitamin D deficiency in women with breast cancer: A correlation with osteoporosis? A machine learning approach with multiple factor analysis. Nutrients 14 (8), 1586. doi:10.3390/nu14081586
DeLuca, G. C., Kimball, S. M., Kolasinski, J., Ramagopalan, S. V., and Ebers, G. C. (2013). Review: the role of vitamin D in nervous system health and disease. Neuropathol. Appl. Neurobiol. 39 (5), 458–484. doi:10.1111/nan.12020
DeLuca, H. F. (2004). Overview of general physiologic features and functions of vitamin D. Am. J. Clin. Nutr. 80 (6), 1689S–1696S. doi:10.1093/ajcn/80.6.1689S
Ding, H., Dhima, K., Lockhart, K. C., Locascio, J. J., Hoesing, A. N., Duong, K., et al. (2013). Unrecognized vitamin D3 deficiency is common in Parkinson disease: harvard biomarker study. Neurology 81 (17), 1531–1537. doi:10.1212/WNL.0b013e3182a95818
Evatt, M. L., DeLong, M. R., Khazai, N., Rosen, A., Triche, S., and Tangpricha, V. (2008). Prevalence of vitamin D insufficiency in patients with Parkinson disease and Alzheimer disease. Arch. Neurol. 65 (10), 1348–1352. doi:10.1001/archneur.65.10.1348
Evatt, M. L., DeLong, M. R., Kumari, M., Auinger, P., McDermott, M. P., and Tangpricha, V.Parkinson Study Group DATATOP Investigators (2011). High prevalence of hypovitaminosis D status in patients with early Parkinson disease. Arch. Neurol. 68 (3), 314–319. doi:10.1001/archneurol.2011.30
Eyles, D., Brown, J., Mackay-Sim, A., McGrath, J., and Feron, F. (2003). Vitamin D3 and brain development. Neuroscience 118 (3), 641–653. doi:10.1016/s0306-4522(03)00040-x
Eyles, D. W., Smith, S., Kinobe, R., Hewison, M., and McGrath, J. J. (2005). Distribution of the vitamin D receptor and 1α-hydroxylase in human brain. J. Chem. Neuroanat. 29 (1), 21–30. doi:10.1016/j.jchemneu.2004.08.006
Feart, C., Helmer, C., Merle, B., Herrmann, F. R., Annweiler, C., Dartigues, J. F., et al. (2017). Associations of lower vitamin D concentrations with cognitive decline and long-term risk of dementia and Alzheimer's disease in older adults. Alzheimers Dement. 13 (11), 1207–1216. doi:10.1016/j.jalz.2017.03.003
García-Martínez, J. M., Pérez-Navarro, E., Gavaldà, N., and Alberch, J. (2006). Glial cell line-derived neurotrophic factor promotes the arborization of cultured striatal neurons through the p42/p44 mitogen-activated protein kinase pathway. J. Neurosci. Res. 83 (1), 68–79. doi:10.1002/jnr.20713
Garcion, E., Sindji, L., Montero-Menei, C., Andre, C., Brachet, P., and Darcy, F. (1998). Expression of inducible nitric oxide synthase during rat brain inflammation: regulation by 1, 25-dihydroxyvitamin D3. Glia 22 (3), 282–294. doi:10.1002/(sici)1098-1136(199803)22:3<282::aid-glia7>3.0.co;2-7
Garcion, E., Sindji, L., Leblondel, G., Brachet, P., and Darcy, F. (1999). 1, 25-Dihydroxyvitamin D3 regulates the synthesis of γ-glutamyl transpeptidase and glutathione levels in rat primary astrocytes. J. Neurochem. 73 (2), 859–866. doi:10.1046/j.1471-4159.1999.0730859.x
Garcion, E., Wion-Barbot, N., Montero-Menei, C. N., Berger, F., and Wion, D. (2002). New clues about vitamin D functions in the nervous system. Trends Endocrinol. Metab. 13 (3), 100–105. doi:10.1016/s1043-2760(01)00547-1
Grecksch, G., Rüthrich, H., Höllt, V., and Becker, A. (2009). Transient prenatal vitamin D deficiency is associated with changes of synaptic plasticity in the dentate gyrus in adult rats. Psychoneuroendocrinology 34, S258–S264. doi:10.1016/j.psyneuen.2009.07.004
Hassan, S. S. U., Muhammad, I., Abbas, S. Q., Hassan, M., Majid, M., Jin, H. Z., et al. (2021). Stress driven discovery of natural products from actinobacteria with anti-oxidant and cytotoxic activities including docking and admet properties. Int. J. Mol. Sci. 22 (21), 11432. doi:10.3390/ijms222111432
Hassan, S. S. U., Zhang, W. D., Jin, H. Z., Basha, S. H., and Priya, S. S. (2022). In-silico anti-inflammatory potential of guaiane dimers from Xylopia vielana targeting COX-2. J. Biomol. Struct. Dyn. 40 (1), 484–498. doi:10.1080/07391102.2020.1815579
J. P. Higgins, J. Thomas, J. Chandler, M. Cumpston, T. Li, M. J. Pageet al. (Editors) (2019). Cochrane handbook for systematic reviews of interventions (US: John Wiley & Sons).
Hiller, A. L., Murchison, C. F., Lobb, B. M., O’Connor, S., O’Connor, M., and Quinn, J. F. (2018). A randomized, controlled pilot study of the effects of vitamin D supplementation on balance in Parkinson's disease: Does age matter? PloS one 13 (9), e0203637. doi:10.1371/journal.pone.0203637
Holick, M. F. (2007). Vitamin D deficiency. N. Engl. J. Med. 357 (3), 266–281. doi:10.1056/NEJMra070553
Hooshmand, B., Lökk, J., Solomon, A., Mangialasche, F., Miralbell, J., Spulber, G., et al. (2014). Vitamin D in relation to cognitive impairment, cerebrospinal fluid biomarkers, and brain volumes. J. Gerontol. A Biol. Sci. Med. Sci. 69 (9), 1132–1138. doi:10.1093/gerona/glu022
Ibi, M., Sawada, H., Nakanishi, M., Kume, T., Katsuki, H., Kaneko, S., et al. (2001). Protective effects of 1 alpha, 25-(OH)(2)D(3) against the neurotoxicity of glutamate and reactive oxygen species in mesencephalic culture. Neuropharmacology 40 (6), 761–771. doi:10.1016/s0028-3908(01)00009-0
Invernizzi, M., Carda, S., Viscontini, G. S., and Cisari, C. (2009). Osteoporosis in Parkinson's disease. Park. Relat. Disord. 15 (5), 339–346. doi:10.1016/j.parkreldis.2009.02.009
Jellinger, K. A., Graeber, M. B., and Pearce, R. K. (2008). Striatal beta-amyloid deposition in Parkinson disease with dementia. J. Neuropathol. Exp. Neurol. 67 (5), 484. doi:10.1097/NEN.0b013e3181713cb1
Jia, J., Hu, J., Huo, X., Miao, R., Zhang, Y., and Ma, F. (2019). Effects of vitamin D supplementation on cognitive function and blood aβ-related biomarkers in older adults with Alzheimer’s disease: a randomised, double-blind, placebo-controlled trial. J. Neurol. Neurosurg. Psychiatry 90 (12), 1347–1352. doi:10.1136/jnnp-2018-320199
Li, Z., Chalazonitis, A., Huang, Y. Y., Mann, J. J., Margolis, K. G., Yang, Q. M., et al. (2011). Essential roles of enteric neuronal serotonin in gastrointestinal motility and the development/survival of enteric dopaminergic neurons. J. Neurosci. 31 (24), 8998–9009. doi:10.1523/JNEUROSCI.6684-10.2011
Luong, V. Q. K., and Nguyen, L. T. H. (2012). Vitamin D and Parkinson's disease. J. Neurosci. Res. 90 (12), 2227–2236. doi:10.1002/jnr.23115
Lv, L., Tan, X., Peng, X., Bai, R., Xiao, Q., Zou, T., et al. (2020). The relationships of vitamin D, vitamin D receptor gene polymorphisms, and vitamin D supplementation with Parkinson’s disease. Transl. Neurodegener. 9 (1), 34–13. doi:10.1186/s40035-020-00213-2
Lyell, V., Henderson, E., Devine, M., and Gregson, C. (2015). Assessment and management of fracture risk in patients with Parkinson's disease. Age Ageing 44 (1), 34–41. doi:10.1093/ageing/afu122
Mayne, P. E., and Burne, T. H. (2019). Vitamin D in synaptic plasticity, cognitive function, and neuropsychiatric illness. Trends Neurosci. 42 (4), 293–306. doi:10.1016/j.tins.2019.01.003
McGrath, J. J., Féron, F. P., Burne, T. H., Mackay-Sim, A., and Eyles, D. W. (2004). Vitamin D3—implications for brain development. J. Steroid Biochem. Mol. Biol. 89, 557–560. doi:10.1016/j.jsbmb.2004.03.070
Mueller, S. J., Teckentrup, V., Rebollo, I., Hallschmid, M., and Kroemer, N. B. (2021). Vagus nerve stimulation increases stomach-brain coupling via a vagal afferent pathway. bioRxiv.
Muir, S. W., and Montero-Odasso, M. (2011). Effect of vitamin D supplementation on muscle strength, gait and balance in older adults: a systematic review and meta-analysis. J. Am. Geriatr. Soc. 59 (12), 2291–2300. doi:10.1111/j.1532-5415.2011.03733.x
Ometto, F., Stubbs, B., Annweiler, C., Duval, G. T., Jang, W., Kim, H. T., et al. (2016). Hypovitaminosis D and orthostatic hypotension: a systematic review and meta-analysis. J. Hypertens. 34 (6), 1036–1043. doi:10.1097/HJH.0000000000000907
Park, S. B., Chung, C. K., Lee, J. Y., Lee, J. Y., and Kim, J. (2019). Risk factors for vertebral, hip, and femoral fractures among patients with Parkinson's disease: a 5-year follow-up in Korea. J. Am. Med. Dir. Assoc. 20 (5), 617–623. doi:10.1016/j.jamda.2018.08.014
Patrick, R. P., and Ames, B. N. (2015). Vitamin D and the omega-3 fatty acids control serotonin synthesis and action, part 2: relevance for ADHD, bipolar disorder, schizophrenia, and impulsive behavior. FASEB J. 29 (6), 2207–2222. doi:10.1096/fj.14-268342
Penckofer, S., Byrn, M., Adams, W., Emanuele, M. A., Mumby, P., Kouba, J., et al. (2017). Vitamin D supplementation improves mood in women with type 2 diabetes. J. Diabetes Res. 2017, 8232863. doi:10.1155/2017/8232863
Pertile, R. A., Cui, X., Hammond, L., and Eyles, D. W. (2018). Vitamin D regulation of GDNF/Ret signaling in dopaminergic neurons. FASEB J. 32 (2), 819–828. doi:10.1096/fj.201700713R
Peterson, A. L., Mancini, M., and Horak, F. B. (2013a). The relationship between balance control and vitamin D in Parkinson's disease—a pilot study. Mov. Disord. 28 (8), 1133–1137. doi:10.1002/mds.25405
Peterson, A. L., Murchison, C., Zabetian, C., Leverenz, J. B., Watson, G., Montine, T., et al. (2013b). Memory, mood, and vitamin D in persons with Parkinson's disease. J. Park. Dis. 3 (4), 547–555. doi:10.3233/JPD-130206
Peterson, A. L. (2014). A review of vitamin D and Parkinson's disease. Maturitas 78 (1), 40–44. doi:10.1016/j.maturitas.2014.02.012
Phillipson, O. T. (2017). Alpha-synuclein, epigenetics, mitochondria, metabolism, calcium traffic, & circadian dysfunction in Parkinson’s disease. An integrated strategy for management. Ageing Res. Rev. 40, 149–167. doi:10.1016/j.arr.2017.09.006
Rappaport, B. Z., Reed, C. I., Hathaway, M. L., and Struck, H. C. (1934). The treatment of hay fever and asthma with viosterol of high potency. J. Allergy 5 (6), 541–553. doi:10.1016/s0021-8707(34)90130-1
Rcom-H'cheo-Gauthier, A. N., Meedeniya, A. C., and Pountney, D. L. (2017). Calcipotriol inhibits α-synuclein aggregation in SH-SY 5Y neuroblastoma cells by a Calbindin-D28k-dependent mechanism. J. Neurochem. 141 (2), 263–274. doi:10.1111/jnc.13971
Riaz, S., Malcangio, M., Miller, M., and Tomlinson, D. R. (1999). A vitamin D3 derivative (CB1093) induces nerve growth factor and prevents neurotrophic deficits in streptozotocin-diabetic rats. Diabetologia 42 (11), 1308–1313. doi:10.1007/s001250051443
Rimmelzwaan, L. M., van Schoor, N. M., Lips, P., Berendse, H. W., and Eekhoff, E. M. (2016). Systematic review of the relationship between vitamin D and Parkinson’s disease. J. Park. Dis. 6 (1), 29–37. doi:10.3233/JPD-150615
Sariola, H., and Saarma, M. (2003). Novel functions and signalling pathways for GDNF. J. Cell Sci. 116 (19), 3855–3862. doi:10.1242/jcs.00786
Sato, Y., Kikuyama, M., and Oizumi, K. (1997). High prevalence of vitamin D deficiency and reduced bone mass in Parkinson's disease. Neurology 49 (5), 1273–1278. doi:10.1212/wnl.49.5.1273
Sato, Y., Kaji, M., Tsuru, T., and Oizumi, K. (2001). Risk factors for hip fracture among elderly patients with Parkinson’s disease. J. Neurol. Sci. 182 (2), 89–93. doi:10.1016/s0022-510x(00)00458-5
Scheuring, S., Boudier, T., and Sturgis, J. N. (2007). From high-resolution AFM topographs to atomic models of supramolecular assemblies. J. Struct. Biol. 159 (2), 268–276. doi:10.1016/j.jsb.2007.01.021
Shen, X., Zhang, K., and Kaufman, R. J. (2004). The unfolded protein response—a stress signaling pathway of the endoplasmic reticulum. J. Chem. Neuroanat. 28 (1-2), 79–92. doi:10.1016/j.jchemneu.2004.02.006
Stumpf, W. E., Sar, M., Clark, S. A., and DeLuca, H. F. (1982). Brain target sites for 1, 25-dihydroxyvitamin D3. Science 215 (4538), 1403–1405. doi:10.1126/science.6977846
Suzuki, M., Yoshioka, M., Hashimoto, M., Murakami, M., Kawasaki, K., Noya, M., et al. (2012). 25-hydroxyvitamin D, vitamin D receptor gene polymorphisms, and severity of Parkinson's disease. Mov. Disord. 27 (2), 264–271. doi:10.1002/mds.24016
Torres-Oviedo, G., and Ting, L. H. (2007). Muscle synergies characterizing human postural responses. J. Neurophysiol. 98 (4), 2144–2156. doi:10.1152/jn.01360.2006
Trinko, J. R., Land, B. B., Solecki, W. B., Wickham, R. J., Tellez, L. A., Maldonado-Aviles, J., et al. (2016). Vitamin D3: a role in dopamine circuit regulation, diet-induced obesity, and drug consumption. Eneuro 3 (3), ENEURO.0122, 15.2016. doi:10.1523/ENEURO.0122-15.2016
Tysnes, O. B., and Storstein, A. (2017). Epidemiology of Parkinson’s disease. J. Neural Transm. 124 (8), 901–905. doi:10.1007/s00702-017-1686-y
Van den Bos, F., Speelman, A. D., Van Nimwegen, M., Van Der Schouw, Y. T., Backx, F. J. G., Bloem, B. R., et al. (2013). Bone mineral density and vitamin D status in Parkinson’s disease patients. J. Neurol. 260 (3), 754–760. doi:10.1007/s00415-012-6697-x
van Etten, E., and Mathieu, C. (2005). Immunoregulation by 1, 25-dihydroxyvitamin D3: basic concepts. J. Steroid Biochem. Mol. Biol. 97 (1-2), 93–101. doi:10.1016/j.jsbmb.2005.06.002
vinh quôc Luong, K., and Thi Hoàng Nguyên, L. (2012). Vitamin D and Parkinson's disease. J. Neurosci. Res. 90 (12), 2227–2236. doi:10.1002/jnr.23115
Wang, Y., Chiang, Y. H., Su, T. P., Hayashi, T., Morales, M., Hoffer, B. J., et al. (2000). Vitamin D3 attenuates cortical infarction induced by middle cerebral arterial ligation in rats. Neuropharmacology 39 (5), 873–880. doi:10.1016/s0028-3908(99)00255-5
Weissmiller, A. M., and Wu, C. (2012). Current advances in using neurotrophic factors to treat neurodegenerative disorders. Transl. Neurodegener. 1 (1), 14–19. doi:10.1186/2047-9158-1-14
Wion, D., MacGrogan, D., Neveu, I., Jehan, F., Houlgatte, R., and Brachet, P. (1991). 1, 25-Dihydroxyvitamin D3 is a potent inducer of nerve growth factor synthesis. J. Neurosci. Res. 28 (1), 110–114. doi:10.1002/jnr.490280111
Wiseman, H. (1993). Vitamin D is a membrane antioxidant Ability to inhibit iron-dependent lipid peroxidation in liposomes compared to cholesterol, ergosterol and tamoxifen and relevance to anticancer action. FEBS Lett. 326 (1-3), 285–288. doi:10.1016/0014-5793(93)81809-e
Zhang, P., Rhodes, J. S., Garland, T., Perez, S. D., Southey, B. R., and Rodriguez-Zas, S. L. (2018). Brain region-dependent gene networks associated with selective breeding for increased voluntary wheel-running behavior. PloS one 13 (8), e0201773. doi:10.1371/journal.pone.0201773
Keywords: vitamin D, Parkinson’s disease, neuroprotection, supplement, neurological disorder
Citation: Behl T, Arora A, Singla RK, Sehgal A, Makeen HA, Albratty M, Meraya AM, Najmi A and Bungau SG (2022) Understanding the role of "sunshine vitamin D" in Parkinson’s disease: A review. Front. Pharmacol. 13:993033. doi: 10.3389/fphar.2022.993033
Received: 13 July 2022; Accepted: 01 December 2022;
Published: 19 December 2022.
Edited by:
Philippe De Deurwaerdere, Université de Bordeaux, FranceReviewed by:
Manar Fayiz Atoum, Hashemite University, JordanMd Zamshed Alam Begh, Daffodil International University, Bangladesh
Copyright © 2022 Behl, Arora, Singla, Sehgal, Makeen, Albratty, Meraya, Najmi and Bungau. This is an open-access article distributed under the terms of the Creative Commons Attribution License (CC BY). The use, distribution or reproduction in other forums is permitted, provided the original author(s) and the copyright owner(s) are credited and that the original publication in this journal is cited, in accordance with accepted academic practice. No use, distribution or reproduction is permitted which does not comply with these terms.
*Correspondence: Tapan Behl, dGFwYW5iZWhsMzFAZ21haWwuY29t; Rajeev K. Singla, cmFqZWV2c2luZ2xhMjZAZ21haWwuY29t, cmFqZWV2a3VtYXJAc2N1LmVkdS5jbg==; Simona Gabriela Bungau, c2ltb25hYnVuZ2F1QGdtYWlsLmNvbQ==