- Department of Clinical Laboratory Science, College of Applied Medical Sciences-Qurayyat, Jouf University, Al Jouf, Saudi Arabia
Withania somnifera (L.) Dunal (Solanaceae) (also known as Ashwagandha) is a botanical drug that has been used for centuries to treat many chronic diseases like high blood pressure, arthritis, diabetes, Alzheimer’s disease, and depression. As many botanical drugs, w. Somnifera possesses anti-inflammatory, antioxidant, anticarinogenic, anti-diabetic, and anti-asthmatic properties. W. somnifera is often compared to the ginseng plant due to its ability to reduce stress, improve cognitive functions (e.g., memory), and promote a healthy immune system. It promotes immunomodulatory effects whose function is to balance the humoral and cellular responses of the adaptive immune system. The therapeutic effect of w. Somnifera is attributed to active ingredients like alkaloids, steroidal lactones (such as withanolides, withaferins), and steroidal saponins. Although w. Somnifera is safe and highly recommended for treating various diseases, the current knowledge and understanding of its operational mechanisms are limited. One of the proposed mechanisms states that w. Somnifera promotes cellular-mediated immunity or initiates chemical interactions that contribute to therapeutic effects. Withania somnifera has been shown to play a significant role in immunological diseases by modulating several cytokines, increasing T-cell proliferation and enhancing macrophages functions. In this review, we will discuss the latest therapeutic effects of w. Somnifera on a number of diseases through modulating immunological markers and which specific components of w. Somnifera induce these therapeutic activities. We will also focus on the chemical properties in w. Somnifera components and their immunomodulatory role in type 2 allergic diseases where type 2 inflammation is highly imbalanced.
1 Introduction
The immune system deals with a spectrum of foreign antigens derived from microbes (e.g., bacteria, viruses) while maintaining normal responses to self-antigens from host tissues. Normally, invaders of the immune system are eliminated by the innate immune organs and cells, however, when innate immunity fails, adaptive immunity is initiated. Components of the innate immune system include skin, tears, low stomach pH, and body temperature while the adaptive immune system is mainly composed of T and B lymphocytes. When the innate immune system fails to clear microbes, the adaptive immune system responds in two main ways (cell-mediated and humoral) based on the type of organism. Viral and intracellular bacteria require cell-mediated immunity. In the case of microbial infection by viruses, the innate natural killer cells (NK cells), the adaptive T helper 1 (Th1) and cytotoxic CD8+ cells are responsible for clearing virally infected cells (Sun and Lanier, 2011). NK cells differ from CD8 cells in many ways such as antigen recognition, specificity, and memory, however, but the final aim for both types of cells is similar. On the other hand, parasitic and extracellular infections require humoral immunity which involves T helper 2 (Th2), B lymphocytes, and antibodies. Initially, when bacteria are introduced into the body, neutrophils are the first innate immune responders (Kobayashi et al., 2018). Next, antigen-presenting cells (APCs) such as dendritic cells engulf the microbe (i.e., bacteria) in a process called phagocytosis and migrate to the secondary lymphoid organs such as lymph nodes to present a small part of the microbe (antigenic peptide) to adaptive Th2 cells (Kim et al., 2006). Once Th2 is activated by the antigen peptide, it will provide the activation signal to specific B lymphocytes (that encountered the same antigen probably in a different part of the body), thus resulting in the conversion of B lymphocytes into plasma cells (known antibody producer cell) (Zhao et al., 2021). There are five types of antibodies (called immunoglobulins): IgM, IgA, IgG, IgE, and IgD (Schroeder and Cavacini, 2010). The production of antibodies is mainly based on the type of microbe, for instance, viral infections result in the production of IgG while parasites lead to the production of IgE antibodies which play important role in allergic diseases (Bell, 1996). Normally, microbial infections are eliminated and few memory CD4 and CD8 cells reside for the elimination of subsequent infections by the same microbe at later times.
Immunogenic components of microbes provoke beneficial immune responses like those seen in viral and parasitic infections; these responses are utilized for therapeutic purposes. For instance, viruses and bacteria contain immunogenic double-stranded RNA (dsRNA) structures and unmethylated Cytosine-phosphate-Guanine (CpG) motifs, respectively (Hartmann, 2017). DsRNA and CpG DNA are called adjuvants and stimulate immune responses through innate receptors like toll-like receptors (TLRs) three and nine which result in the production of type I interferon (IFN) and CD4+ and CD8+ activation (Coffman et al., 2010; She et al., 2019). Many adjuvants are used for purposes like enhancing vaccine responses or antitumor responses such as TLR2 and TLR4 ligands (Urban-Wojciuk et al., 2019). Alternatively, several studies showed that botanical medicines can be used as immunomodulators or adjuvants (Kumar et al., 2012; Shi et al., 2021). The use of natural botanical products as immunomodulators is much safer compared to conventional adjuvants because there have been fewer side effects reported when consuming them and most of these botanical products have been consumed by people for thousands of years.
Withania somnifera L.) Dunal (Solanaceae) or w. Somnifera is a botanical medicine that has been used for centuries for various purposes such as dietary supplementations and treating various diseases. The clinical use of w. Somnifera as an immunomodulator or adjuvant in mice and humans has yielded very favorable results (e.g., anticancerogenic, arthritis treatment) (Agarwal et al., 1999; Khan et al., 2009). Adjuvants or immunomodulators work by initiating signaling pathways mainly through immune receptors found on innate cells such as dendritic cells. TLRs are essential innate immune receptors that respond to a variety of natural and synthesized agonists (Kawasaki and Kawai, 2014). Similar to microbial components, w. Somnifera has been shown to modulate TLRs by inhibiting influenza A virus-induced stimulation of TLR2/4 and mRNA expression of TLR2 and TLR4 (Kashyap et al., 2020). W. somnifera has also been used to treat allergic diseases where type 2 inflammation is dominating (Agarwal et al., 1999).
1.1 Role of w. somnifera in type 2 inflammation
The immune system responds to a verity of foreign particles in many ways while maintaining tolerance to self or harmless particles. Generally, harmless particles do not provoke significant immune responses, however, some particles (called allergens) stimulate unwanted immune responses such as skin rash, excessive mucus production, constriction of respiratory smooth muscles, diarrhea, and others (Galli et al., 2008). The main immunoglobulin responsible for many of the allergic manifestations is IgE antibody (Galli et al., 2008). IgE antibody act as a receptor on mast cells and basophils to equip these cells for future allergen exposure (Stone et al., 2010). Upon second exposure, allergens bind to the receptor on these cells (i.e., IgE) and enhance type 2 allergic inflammation (Galli et al., 2008). Type 2 allergic inflammation is characterized by Th2 and B cell activation which eventually leads to the production of IgE antibodies, an increase in eosinophils, high numbers of group 2 innate lymphoid cells (ILC2), and elevated Th2 cytokines (IL-4, IL-5, IL-13) (Galli et al., 2008).
Excessive type 2 inflammation is the driving factor for several allergic diseases including asthma, eczema, dermatitis, and several others. The incidents of allergic diseases are rapidly increasing in developed countries. More than 300 million patients worldwide are living with asthma and it is expected that the cases would reach 400 million by 2025 (Serebrisky and Wiznia, 2019). The sudden increase in allergic diseases in recent years was attributed to the reduced exposure to microbes “hygiene hypothesis”, and increased exposures to indoor allergens or environmental factors (Okada et al., 2010). It is now clear that both innate and adaptive immune responses significantly contribute to allergic responses seen in patients (Maeda et al., 2019). Initially, it was thought that the adaptive T helper (Th2) cell is the main player in type 2 inflammation, but recent studies show that innate immune cells such as ILC2 cells are important for the establishment of allergic diseases (Halim et al., 2014). Stimulating innate cells through immunomodulators or adjuvants using active concentration of 1.0 mg/kg or as low as 0.001 mg/kg intratracheally was shown to skew Th2 immune response to Th1 response resulting in attenuated allergic immunity, reduced histamine, and decreased eosinophil levels in allergic mice models (Duechs et al., 2011). One of the strategies proposed to reduce type 2 inflammation seen in asthma is the use of the w. Somnifera (Speers et al., 2021). In a recent study, it was shown that w. Somnifera reduces the levels of type 2 cytokines (e.g., IL-4, IL-13) and type 2 inflammation markers such as TNF-α and IgE induced by OVA suggesting that w. Somnifera has immunomodulatory properties that could be utilized to attenuate type 2 inflammation (Saggam et al., 2021). One of the current immunotherapy strategies to reduce type 2 inflammation using adjuvants is by skewing Th2 immune response into Th1 immune response. It would be interesting to know the immunotherapeutic effect of w. Somnifera in various allergic diseases such as atopic dermatitis and hay fever.
1.2 W. somnifera and other immunomodulators
Immunomodulators can refer to substances that modify immune responses by stimulating, suppressing, or modulating the components of the innate and adaptive immune system. Adjuvants confer immunomodulatory effects when combined with vaccines by enhancing stronger immune responses mediated by T and B cells. Adjuvants, such as alum, which has been used since ∼1930 enhance immunity through stimulating Th2 immune responses via dendritic cells and priming CD4+ and CD8+ cells (McKee et al., 2009). The use of adjuvants has then broadened to skewing immune responses from Th2 to Th1 such as the case in allergic diseases (Kirtland et al., 2020). Ligands of TLR3, TLR4, and TLR9 have all been used as adjuvants to skew type 2 immune responses towards Th1 responses or Regulatory T cells (Tregs) responses (Sel et al., 2007; Gunawardana and Durham, 2018). Several types of immunomodulators including adjuvants are currently under clinical trials for their use in immunotherapy in various medical conditions like cancers such as Poly (I: C), synthetic dsRNA (Sultan et al., 2020). In many cases however, the use of these adjuvants has complicated and systemic side effects making them unfavorable to use especially in serious diseases like autoimmune diseases (Saxena et al., 2019). Therefore, more studies are now exploring the use of natural plant immunomodulators as a substitute to synthetic adjuvants.
There are many fungi or natural botanical drugs that modulate the immune system in ways akin to synthetic adjuvants. For instance, Ganoderma lucidum stimulates the activation of immune cells such as Т cells, macrophages, and natural killer cells (Habijanic et al., 2015). Ganoderma lucidum also induces antitumor and inflammatory activities by secretion of interferons, interleukins, and tumor necrosis factor cytokines (Habijanic et al., 2015; Jin et al., 2016). Also, Curcuma longa L. induces a number of immunomodulatory properties including anti-inflammatory, antiseptic, antitumor, and antioxidative properties and most importantly antiallergic properties through inhibition of histamine production (Kurup and Barrios, 2008).
Moreover, Lentinula edodes (Shiitake) mushroom is also shown to enhance the immune system functions through increased γδ-T and NKT cells, elevated IL-4, IL-10, TNF-α, and IL-1α levels, and reduced macrophage inflammatory protein-1α/chemokine C-C ligand 3 (MIP-1α/CCL3) and inflammatory protein C-reactive protein (CRP) (Dai et al., 2015).
The immunomodulatory activities of w. Somnifera have been tested in several studies (Figure 1). Withania somnifera root powder was shown to inhibit several inflammatory mediators such as excessive complement activation, T-lymphocytes proliferation and humoral antibody response (Rasool and Varalakshmi, 2006). The role of w. Somnifera in attenuating Arthritis, which is an autoimmune disease characterized by increased inflammatory markers such as IL-6, IL-10 and TNF-α, has also been evaluated (Shrivastava et al., 2015). Administration of 300 mg/kg of w. Somnifera orally is sufficient to reduce pro inflammatory cytokines seen in arthritis such as TNF-α, IL-1β, and IL-6 in vivo (Khan et al., 2019). The immunomodulatory effect of w. Somnifera is due to presence of chemical substances such as steroids (withanolides), flavonoids and lactones (Rasool and Varalakshmi, 2006).
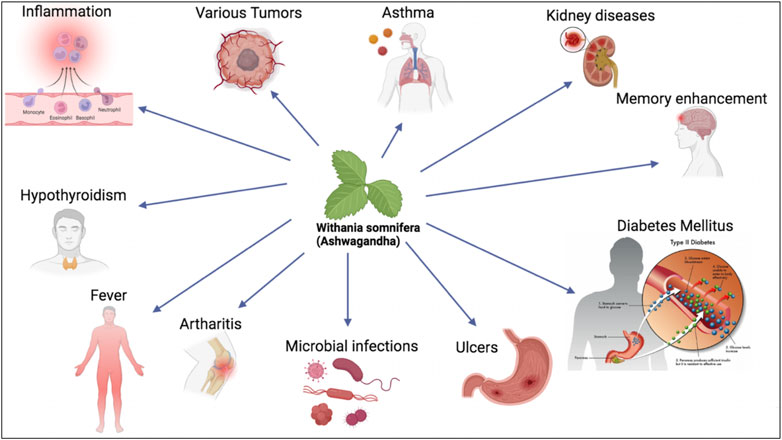
FIGURE 1. W. somnifera is used as botanical drug to treat various chronic diseases including asthma, kidney diseases, diabetes mellitus and others.
1.3 Chemical properties of w. somnifera compared to other immunomodulators
The steroidal lactones of group C28 known as withanolides are found in nature. Its structure consists of three cyclohexane rings, one cyclopentane ring, and four cycloalkane ring configurations (Vanden Berghe et al., 2012). Withaferin A is highly reactive due to its unsaturated lactone ring, epoxide in the B ring, and ketone-containing unsaturated A ring. For the most part, ring A’s double bond and epoxide ring are what cause cytotoxicity. The ergostane skeleton undergoes an oxidation reaction with withaferin A and associated steroidal metabolites, resulting in the formation of a six-membered delta lactone unit on the 22nd and 26th carbons. These analogs are being researched for their potential to treat inflammatory, neoplastic, autoimmune, and neurological diseases.
It has been suggested that the anti-inflammatory actions of withaferin A are due to its ability to inhibit NF-κB, AP1, and alpha-2 macroglobulin (Saleem et al., 2020). There are many withanolides that selectively inhibit COX-2 in response to inflammation (Ichikawa et al., 2006). Withaferin A’s anti-tumor capabilities were first tested in PC-3 human prostate cancer cell line xenografts in nude mice. Androgen receptor (AR)-dependent cytotoxicity was shown. In an in vivo pancreatic model, it suppresses tumor growth through ATP-independently inhibiting the heat shock protein 90 (HSP90) (Yu et al., 2010). In investigations using cancer cell culture, it exhibits growth-inhibitory behavior, demonstrating its cytotoxic and apoptotic capabilities. There is an increase in Mcl-1 expression in vitro models of breast cancer apoptosis. By covalently switching the cysteine residue in the mainly preserved alpha-helical coiled 2B domain, withaferin A can bind to the intermediate filament protein and vimentin (Bargagna-Mohan et al., 2007). Withaferin A can lead to apoptosis by causing vimentin accumulation and F-actin aggregation. In both in vitro and in vivo experiments, WA was applied to mouse sarcoma 180 (S-180) solid and ascites tumor cells. When the substance was observed using an electron microscope, it was discovered that the cells’ spindle microtubules were affected (Shohat et al., 1976). At a non-toxic concentration of about 2 μM, WA revealed a sensitizer enhancement ratio of 1.5 for in vitro cell death of V79 Chinese hamster cells (Devi, 1996).
Through the combination of withaferin A and the steroid lactone withanolide E, withaferin A specifically suppresses the immune system in human B-cell and T-cell as well as mice thymocytes (Shohat et al., 1978). These chemicals prevent typical B-cells and T-cells from forming the E rosette and the EAC rosette at very low concentrations. Withaferin A has specific effects on antigen recognition and the ability of both B-cells and T-cells to proliferate (Mohan et al., 2004). According to a recent study, withaferin plays a part in inhibiting Zap70 kinase activity, which is essential for human T-cell function in both sickness and health (Fazil et al., 2021).
Through the induction of Par-4, withaferin A reduces cancer cells’ capacity for invasion and their ability to move around the body (Rah et al., 2012). Withaferin stops the spread of cancer cells by preventing them from invading because the ability of cancer cells to infiltrate the extracellular matrix (ECM) is a critical determinant in the progression of cancer. Withaferin derivatives reduce the capacity of cancer cells to form colonies in a dose-dependent manner (Rah et al., 2012). Directed growth, adhesion, and migration are three biological functions that depend on actin. Withaferin A can alter the organization of the cytoskeleton by forming a covalent binding with the flexible adaptor protein annexin II and by boosting the activity of the basal F-actin cross-linking mechanism (Falsey et al., 2006).
It effectively inhibits angiogenesis (Yu et al., 2010). Chymotrypsin is inhibited by withaferin A, which has anti-angiogenic and anti-tumor properties, whilst protein kinase C is inhibited, which induces apoptosis (Sen et al., 2007). There have also been reports of withaferin A activating caspase-3.
Since withaferin A influences the immune system, it may explain why it is used as a general tonic to increase energy and ward against illness. The immunomodulatory and central nervous system (antistress, memory, and learning) effects of glycowithanolides and a combination of sitoindosides IX and X isolated from withaferin A were investigated in Swiss mice (15–25 g, 5–6 months old) and Wistar breeds albino rats (120–150 g and 250–300 g) (Nascimento et al., 2016). Both medicines significantly recruited and activated peritoneal macrophages in addition to phagocytosis and elevated lysosomal enzyme activity. Both medications significantly improved learning and memory retention in both young and old animals and drastically reduced stress in albino mice and rats (50–200 mg/kg orally). Three animal myelosuppression models using cyclophosphamide, azathioprine, or prednisolone were used to assess the root extract of withaferin A for immunomodulatory activities (Ziauddin et al., 1996). Withaferin A medication led to statistically significant increases (p 0.05) in hemoglobin concentration, red blood cell count, white blood cell count, platelet count, and body weight as compared to untreated control mice.
Additionally, the impact of withaferin A on the functions of macrophages obtained from mice given the carcinogen ochratoxin A was investigated (OTA) (Dhuley, 1997). Mice subjected to OTA therapy for weeks saw a considerable decline in the macrophages’ chemotactic activity. Production of TNF-α and IL-1 was also significantly decreased.
Plants and their byproducts are important sources for the creation of novel medications with special features for a range of medical uses. The widespread tendency of synthetic medications has recently given way to the use of botanical drugs (Ekor, 2014). Chemical species called free radicals and reactive oxygen species (ROS) develop in cells because of chemical reactions and metabolic activities. Free radicals can start the oxidation of biomolecules, resulting in cell damage and numerous illnesses in humans (Sharma et al., 2018). It goes without saying that antioxidant phytochemicals act as prophylactic metabolites by shielding cells from the harmful effects of free radicals and other oxidants (He et al., 2017). Alkaloids, terpenoids, polysaccharides, tannins, steroids, phenols, and flavonoids are only a few examples of the bioactive metabolites that medicinal plants produce in the form of distinctive active antioxidants that have certain biological actions against specific human diseases. The most significant adaptogen among medicinal plants is w. Somnifera, a treasured botanical drug known as “Rasayana” in Indian Ayurvedic medicine and a nerve tonic (Singh et al., 2011). Ashwagandha nolides are listed as active markers, along with 12 alkaloids, 40 withanolides, and sitoindosides (VII, VIII, IX, and X), according to chemical characterization of roots (Kulkarni and Dhir, 2008). Ashwagandha has been used as a vermifuge, diuretic, aphrodisiac, narcotic, astringent, and thermogenic supplement (Aggarwal et al., 2011). A tall, deciduous vine known as Tinospora sinensis (Malabar Gulbel) is known for having two novel lignan glucosides called tinoposide A and tinoposide B in its stems. When combined with other botanical drugs, these metabolites are believed to help treat muscle stiffness, lessen pain, and promote mental calmness (Haque et al., 2017). Historically, ulcerative sores, hemorrhoids, and chronic rheumatism have all been treated with the sap of the stem and leaves. The energizing fruit Phyllanthus emblica, often known as Indian gooseberry, gives the body its lost vigor and energy back. Amla is a very healthy fruit that is high in tannins, vitamin C, alkaloids, flavonoids including gallic acid, emblicanin A and B, phyllatidine, and phyllatine (Nguse et al., 2022). In addition to acting as an antiplatelet agent, vasodilator, and antiatherogenic, they have antiastringent, antidyspeptic, anticolitis, hemorrhoidal, hematuria, hepatoprotective, anti-aging, and gastroprotective effects (Hashem-Dabaghian et al., 2018). Since ancient times, Bacopa monneri, also known as “Medhya Rasayana,” or nootropic, has been used as a brain tonic to improve memory in seizure disorders and has been effective against neurological disorders. It contains bacosides A and B, brahmin, which is the main alkaloid, herpestine, and nicotine (Mannan et al., 2015). Ocimum basilicum has a group of 20 monoterpenes, triterpenes, steroids, phenols, flavonoids, and sesquiterpenes that have antihyperglycemic and hepatoprotective properties, earning it the nickname “Royal Herb” in French (Sestili et al., 2018). Its roots are classified as an anti-diabetic, galactogogue, nourishing tonic with ten different groups of steroids, such as shatavaroside, alkaloids, such as asparagine A, and specific flavonoids, and are traditionally used to treat “Vata,” hypertension, and heart disease. The miraculous botanical drug known as asparagus racemosus, sometimes known as the “Queen of Herbs,” helps to maintain cellular vitality (Gautam et al., 2004). Shatavari increases immunity, enhances mental abilities, helps in avoiding aging and longevity difficulties connected to stress, and promotes reproductive health (Pandey et al., 2018). The female reproductive system is nourished and supported by the uterine tonics; shatavari root tonic is typically administered to women (Pandey et al., 2018). The botanical drugs mentioned above are frequently mentioned in ancient Ayurvedic texts as a revitalizing “Rasayana” with a variety of health advantages. The potential for the presence of phytocomponents that function as cytoprotectors, anti-cancer agents, anti-inflammatory agents, immunomodulators, and immunological adjuvants led to the selection of there particular plant sections. The alleged conventional botanical drug is used in numerous recipes. The pharmacognostic and therapeutic uses of botanical drugs including ashwagandha have been demonstrated in both in vitro and in vivo models. Although there are several reports and articles on the health advantages of traditional botanical antioxidants, comprehensive combinatorial studies are still needed to increase the availability of chemical components and strengthen the body’s immune defenses.
1.4 Potential use for w. somnifera as pharmacologic agent
Withanolides are the primary biological components of w. Somnifera (steroidal lactones with ergostane skeleton). The withanolides have a six-membered lactone ring and a C28 steroidal nucleus with a C9 side chain. Withanolides are highly oxygenated phytochemicals, and structural differences between distinct classes of withanolides are caused by oxidation at diverse skeleton locations (Logie and Vanden Berghe, 2020). In addition, numerous classes of secondary bioactive metabolites of the plant with broad-spectrum therapeutic potential were identified and described, including withanosides, glycowithanolides, sitoindosides, alkaloids, saponins, amino acids, phenolic metabolites, flavonoids, and many others (Mishra et al., 2000; Dhar et al., 2015).
Two of the main components of ashwagandha are withaferin-A and withanolide-D, which are regarded to be largely in charge of the botanical drug’s pharmacological activity (Kashyap et al., 2022). W. somnifera has been the subject of in-depth toxicological investigation, and findings from several clinical research initiatives have demonstrated that the plant is safe at a variety of realistic doses. Therefore, it is reasonable to believe that the doses at which its preparations are advised on humans are similarly quite safe. To date, there have not yet been any documented botanical drug-other drugs or botanical drug-botanical drug interactions involving w. Somnifera in the literature. The adverse effects and long-term safety of w. Somnifera are currently unknown. The effects of somnifera could include diarrhea, vomiting, and nausea. According to a small body of research on humans, w. Somnifera can cause sleepiness, potentially fatal respiratory depression, low blood pressure, and irregular heartbeats (Kataria et al., 2011). The findings of studies on the chemistry and pharmacology of numerous types of w. Somnifera extracts derived from different portions of the plant clearly suggest that these extracts, when combined with other plants, could be used to treat and prevent a variety of illnesses and chronic diseases. The primary emphasis of this chapter is on recent and historical advancements in the chemistry and pharmacology of w. Somnifera.
1.5 Role of W. Somnifera in COVID-19 infection
The beneficial and inhibitory role of w. Somnifera has been tested in COVID-19 infection. It was shown that w. Somnifera disrupts the interaction between the SARS-COV-2 spike protein domain and the angiotensin-converting enzyme 2 (ACE2) receptor (Saggam et al., 2021). ACE2 receptor is considered the main receptor of entry for SARS-COV-2 into cells and is expressed in many tissues including the heart, kidneys, and lung tissues (Beyerstedt et al., 2021). In addition, certain w. Somnifera constituents are believed to suppress the main protease of SARS-CoV-2 which is very important for viral replication (Tripathi et al., 2021; Chakraborty et al., 2022; Shree et al., 2022). Withanoside V is one of the natural constituents of w. Somnifera that inhibit the main protease of SARS-CoV-2 (Tripathi et al., 2021). Moreover, several independent studies showed that w. Somnifera is very effective in treating COVID-19 infection probably through its ability to induce Th1 immune responses and inhibit inflammatory responses (via inhibiting NF-κB). W. Somnifera contains many other phytoconstituents such as withanolide A and B, withaferin A, withanone, and withanolides that reduce the replication and transcription of COVID-19 virus (Brahmbhatt, 2020). W. Somnifera induces very minimal side effects while still maintaining its therapeutic efficacy making it one of the best natural therapeutic candidates for COVID-19 infection.
2 Discussion
Withania somnifera is a potent immune stimulator and its efficacy has been determined in multiple diseases. Withania somnifera induces anti-tumor, anti-stress, anti-inflammatory and immunomodulatory effects that lead to favorable and less harmful immune responses (Figure 2, Figure 3). For instance, the therapeutic effects of w. Somnifera on robust Th2 immune responses and airway inflammation is very promising. When administered in mice, w. Somnifera upregulates Th1 immune responses accompanied by an increase in CD4 and CD8 T cells, thus, balancing the Th2-Th1 immune responses (Bani et al., 2006). In asthma mice models, pre-administration of 20–80 mg/kg of withaferin A (isolated from w. somnifera) was effective in inhibiting airway inflammation through downregulating the expression of pro-inflammatory cytokines in the lungs (Zhao et al., 2019). Evidently, w. Somnifera works as an immunomodulator that mitigates the airway overresponsiveness seen in mice asthmatic models suggesting that botanical drugs, like known adjuvants, can also modulate diseases in a favorable manner (Zhao et al., 2019). Although several other botanical drugs (besides w. somnifera) modulate pathophysiological diseases through immune responses, the use of natural w. Somnifera as an adjuvant is highly encouraged. It is possible that w. Somnifera stimulates immune responses via signaling through immune receptors (e.g., TLR3, 9) found on innate cells like dendritic cells. Activating these receptors eventually results in desired immune responses.
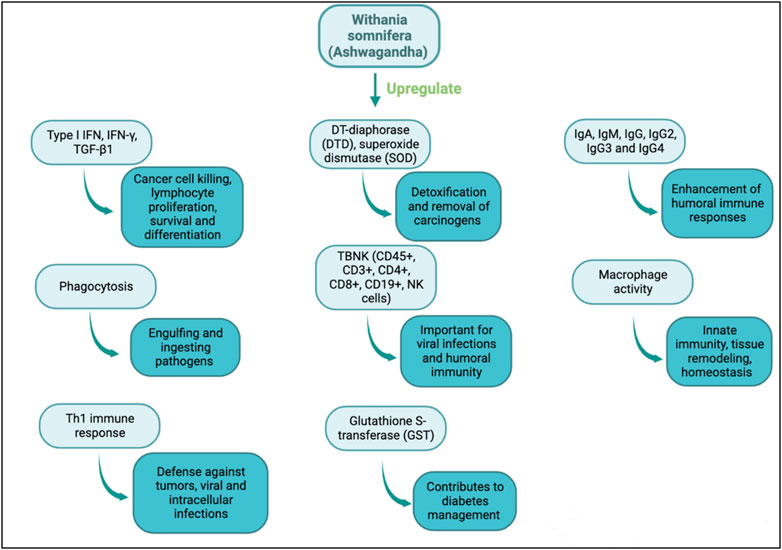
FIGURE 2. Immunomodulatory actions of w. Somnifera through upregulating immune mediators, cytokines, cells and enzymes.
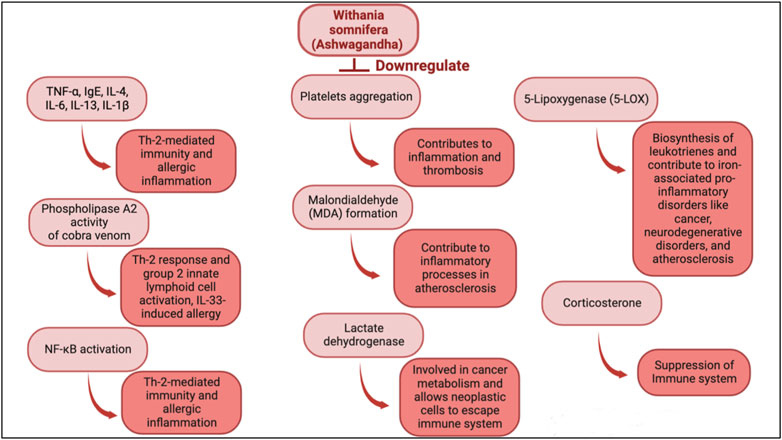
FIGURE 3. Immunomodulatory actions of w. Somnifera through downregulating immune mediators, cytokines, cells and enzymes.
There are two main types for adjuvants. Adjuvants that promote Th1 immune responses (cell-mediated) and adjuvants that promote Th2 immune responses (humoral) (Silva et al., 2004). In prophylactic studies, Th1 adjuvants (e.g., dsRNA) stimulate cells through innate sensors (e.g., TLR3) which shape the adaptive immunity (Chattopadhyay and Sen, 2014). In this case, Th1 adjuvants promote upregulation of interferon stimulatory gene (ISGs) response and activation of immune cells necessary for viral elimination (Chattopadhyay and Sen, 2014). Moreover, Th1 adjuvants suppress Th2 responses and can therefore be utilized to alleviate diseases where Th2 responses are hyperactive (commonly seen in allergic diseases) (Oriss et al., 1997).
3 Conclusion, future directions, and limitations for using W. Somnifera as an immunomodulator
Earlier studies on w. Somnifera have showed its effectiveness in treating several diseases (e.g., bacterial and viral), and allergic conditions like asthma (Alam et al., 2012). It would be intriguing to test the specific mechanisms employed by w. Somnifera to reduce the severity of diseases and allergic inflammation. Most of the studies investigating the therapeutic role of w. Somnifera (Table 1), do not fully provide complete signaling pathways starting with the initial stimulation of cells until a certain effector function of immune components is executed. It is likely that w. Somnifera active components (e.g., withaferin A) treat chronic diseases through stimulation of immune receptors found on innate cells (e.g., dendritic cells and macrophages). Such stimulation of these cells results in activation of downstream adaptor proteins (e.g., TRIFF or MAVS) which ultimately results in stimulation of transcription factors (e.g., IRF3) and upregulation of beneficial immune responses like Th1 immune response. Recent studies focus more on the therapeutic effects of w. Somnifera in many diseases including immunological diseases. In this review, we described some of the signaling mechanisms induced by w. Somnifera to reduce allergic conditions and diseases. For instance, in allergic conditions (where type 2 immune response is high), w. Somnifera provokes the production of type I IFN which suppresses type 2 immune responses. Compared to using current synthetic adjuvants which sometimes cause cytotoxicity to the host and unwanted immune responses, using a medicinal botanical drug such as w. Somnifera provides a safer and highly effective substitute. Defining the full strategies employed by w. Somnifera when used as a therapeutic agent, would empower and promote its use in many clinical trials investigating solutions for cancer, inflammatory and metabolic disorders. Further research is highly encouraged to determine the potential of w. Somnifera as an immunomodulator in the future.
Like any botanical drugs and pharmaceutical drugs, there have been some adverse effects reported after w. Somnifera consumption. In a clinical trial, seven adult males reported feeling sleepiness, mild abdominal and joint pain after consuming ashwagandha capsule (Chauhan et al., 2022). Withania somnifera is still not approved by the U.S. Food and Drug Administration (FDA) and has not been used legally to treat diseases on large scale. All performed clinical trials have not been able to fully address its efficacy, mechanism, and long-term effects. In addition, there is no consensus on the adequate doses and the treatment duration for w. Somnifera which indicates more clinical trial research is needed. Moreover, studies investigating the potential interactions of w. Somnifera with other drugs are very limited.
Overall, although w. Somnifera induces many beneficial outcomes and reduces the severity of several diseases, there are some gaps regarding its therapeutic use as a botanical drug. Clinical studies focusing on defining the mechanisms, appropriate doses, time duration, and long-term adverse effects of w. Somnifera should provide knowledge that fills these gaps.
Author contributions
HHA: Manuscript design, manuscript writing, illustrations. EE: Manuscript writing. HHA and EE: manuscript revision.
Acknowledgments
The authors acknowledge (Department of Clinical Laboratory Science, College of Applied Medical Sciences-Qurayyat, Jouf University, Al Jouf, Saudi Arabia) for supporting this work.
Conflict of interest
The authors declare that the research was conducted in the absence of any commercial or financial relationships that could be construed as a potential conflict of interest.
Publisher’s note
All claims expressed in this article are solely those of the authors and do not necessarily represent those of their affiliated organizations, or those of the publisher, the editors and the reviewers. Any product that may be evaluated in this article, or claim that may be made by its manufacturer, is not guaranteed or endorsed by the publisher.
References
Agarwal, R., Diwanay, S., Patki, P., and Patwardhan, B. (1999). Studies on immunomodulatory activity of Withania somnifera (Ashwagandha) extracts in experimental immune inflammation. J. Ethnopharmacol. 67 (1), 27–35. doi:10.1016/s0378-8741(99)00065-3
Aggarwal, B. B., Prasad, S., Reuter, S., Kannappan, R., Yadev, V. R., Park, B., et al. (2011). Identification of novel anti-inflammatory agents from ayurvedic medicine for prevention of chronic diseases: "reverse pharmacology" and "bedside to bench" approach. Curr. Drug Targets 12 (11), 1595–1653. doi:10.2174/138945011798109464
Alam, N., Hossain, M., Mottalib, M. A., Sulaiman, S. A., Gan, S. H., and Khalil, M. I. (2012). Methanolic extracts of Withania somnifera leaves, fruits and roots possess antioxidant properties and antibacterial activities. BMC Complement. Altern. Med. 12, 175. doi:10.1186/1472-6882-12-175
Bani, S., Gautam, M., Sheikh, F. A., Khan, B., Satti, N. K., Suri, K. A., et al. (2006). Selective Th1 up-regulating activity of Withania somnifera aqueous extract in an experimental system using flow cytometry. J. Ethnopharmacol. 107 (1), 107–115. doi:10.1016/j.jep.2006.02.016
Bargagna-Mohan, P., Hamza, A., Kim, Y. e., Khuan Abby Ho, Y., Mor-Vaknin, N., Wendschlag, N., et al. (2007). The tumor inhibitor and antiangiogenic agent withaferin A targets the intermediate filament protein vimentin. Chem. Biol. 14 (6), 623–634. doi:10.1016/j.chembiol.2007.04.010
Bell, R. G. (1996). IgE, allergies and helminth parasites: A new perspective on an old conundrum. Immunol. Cell Biol. 74 (4), 337–345. doi:10.1038/icb.1996.60
Beyerstedt, S., Casaro, E. B., and Rangel, E. B. (2021). COVID-19: Angiotensin-converting enzyme 2 (ACE2) expression and tissue susceptibility to SARS-CoV-2 infection. Eur. J. Clin. Microbiol. Infect. Dis. 40 (5), 905–919. doi:10.1007/s10096-020-04138-6
Brahmbhatt, R. V. (2020). Herbal medicines in management and prevention of COVID-19. J. Pharmacogn. Phytochemistry 9 (3), 1221–1223. doi:10.1016/j.ccmp.2022.100021
Chakraborty, S., Mallick, D., Goswami, M., Guengerich, F. P., Chakrabarty, A., and Chowdhury, G. (2022). The natural products withaferin A and withanone from the medicinal Herb withania somnifera are covalent inhibitors of the SARS-CoV-2 main protease. J. Nat. Prod. 85 (10), 2340–2350. doi:10.1021/acs.jnatprod.2c00521
Chattopadhyay, S., and Sen, G. C. (2014). dsRNA-activation of TLR3 and RLR signaling: gene induction-dependent and independent effects. J. Interferon Cytokine Res. 34 (6), 427–436. doi:10.1089/jir.2014.0034
Chauhan, S., Srivastava, M. K., and Pathak, A. K. (2022). Effect of standardized root extract of ashwagandha (withania somnifera) on well-being and sexual performance in adult males: A randomized controlled trial. Health Sci. Rep. 5 (4), e741. doi:10.1002/hsr2.741
Coffman, R. L., Sher, A., and Seder, R. A. (2010). Vaccine adjuvants: Putting innate immunity to work. Immunity 33 (4), 492–503. doi:10.1016/j.immuni.2010.10.002
Dai, X., Stanilka, J. M., Rowe, C. A., Esteves, E. A., Nieves, C., Spaiser, S. J., et al. (2015). Consuming Lentinula edodes (shiitake) mushrooms daily improves human immunity: A randomized dietary intervention in healthy young adults. J. Am. Coll. Nutr. 34 (6), 478–487. doi:10.1080/07315724.2014.950391
Devi, P. U. (1996). Withania somnifera dunal (ashwagandha): Potential plant source of a promising drug for cancer chemotherapy and radiosensitization. Indian J. Exp. Biol. 34 (10), 927–932.
Dhar, N., Razdan, S., Rana, S., Bhat, W. W., Vishwakarma, R., and Lattoo, S. K. (2015). A decade of molecular understanding of withanolide biosynthesis and in vitro studies in withania somnifera (L.) dunal: Prospects and perspectives for pathway engineering. Front. Plant Sci. 6, 1031. doi:10.3389/fpls.2015.01031
Dhuley, J. N. (1997). Effect of some Indian herbs on macrophage functions in ochratoxin A treated mice. J. Ethnopharmacol. 58 (1), 15–20. doi:10.1016/s0378-8741(97)00072-x
Duechs, M. J., Hahn, C., Benediktus, E., Werner-Klein, M., Braun, A., Hoymann, H. G., et al. (2011). TLR agonist mediated suppression of allergic responses is associated with increased innate inflammation in the airways. Pulm. Pharmacol. Ther. 24 (2), 203–214. doi:10.1016/j.pupt.2010.12.009
Ekor, M. (2014). The growing use of herbal medicines: Issues relating to adverse reactions and challenges in monitoring safety. Front. Pharmacol. 4, 177. doi:10.3389/fphar.2013.00177
Falsey, R. R., Marron, M. T., Gunaherath, G. M. K. B., Shirahatti, N., Mahadevan, D., Gunatilaka, A. A. L., et al. (2006). Actin microfilament aggregation induced by withaferin A is mediated by annexin II. Nat. Chem. Biol. 2 (1), 33–38. doi:10.1038/nchembio755
Fazil, M., Chirumamilla, C. S., Perez-Novo, C., Wong, B. H. S., Kumar, S., Sze, S. K., et al. (2021). The steroidal lactone withaferin A impedes T-cell motility by inhibiting the kinase ZAP70 and subsequent kinome signaling. J. Biol. Chem. 297 (6), 101377. doi:10.1016/j.jbc.2021.101377
Galli, S. J., Tsai, M., and Piliponsky, A. M. (2008). The development of allergic inflammation. Nature 454 (7203), 445–454. doi:10.1038/nature07204
Gautam, M., Diwanay, S., Gairola, S., Shinde, Y., Patki, P., and Patwardhan, B. (2004). Immunoadjuvant potential of Asparagus racemosus aqueous extract in experimental system. J. Ethnopharmacol. 91 (2-3), 251–255. doi:10.1016/j.jep.2003.12.023
Gunawardana, N. C., and Durham, S. R. (2018). New approaches to allergen immunotherapy. Ann. Allergy Asthma Immunol. 121 (3), 293–305. doi:10.1016/j.anai.2018.07.014
Habijanic, J., Berovic, M., Boh, B., Plankl, M., and Wraber, B. (2015). Submerged cultivation of Ganoderma lucidum and the effects of its polysaccharides on the production of human cytokines TNF-alpha, IL-12, IFN-gamma, IL-2, IL-4, IL-10 and IL-17. N. Biotechnol. 32 (1), 85–95. doi:10.1016/j.nbt.2014.07.007
Halim, T. Y., Steer, C. A., Matha, L., Gold, M. J., Martinez-Gonzalez, I., McNagny, K. M., et al. (2014). Group 2 innate lymphoid cells are critical for the initiation of adaptive T helper 2 cell-mediated allergic lung inflammation. Immunity 40 (3), 425–435. doi:10.1016/j.immuni.2014.01.011
Haque, M. A., Jantan, I., and Abbas Bukhari, S. N. (2017). Tinospora species: An overview of their modulating effects on the immune system. J. Ethnopharmacol. 207, 67–85. doi:10.1016/j.jep.2017.06.013
Hartmann, G. (2017). Nucleic acid immunity. Adv. Immunol. 133, 121–169. doi:10.1016/bs.ai.2016.11.001
Hashem-Dabaghian, F., Ziaee, M., Ghaffari, S., Nabati, F., and Kianbakht, S. (2018). A systematic review on the cardiovascular pharmacology of Emblica officinalis Gaertn. J. Cardiovasc Thorac. Res. 10 (3), 118–128. doi:10.15171/jcvtr.2018.20
He, L., He, T., Farrar, S., Ji, L., Liu, T., and Ma, X. (2017). Antioxidants maintain cellular redox homeostasis by elimination of reactive oxygen species. Cell Physiol. Biochem. 44 (2), 532–553. doi:10.1159/000485089
Ichikawa, H., Takada, Y., Shishodia, S., Jayaprakasam, B., Nair, M. G., and Aggarwal, B. B. (2006). Withanolides potentiate apoptosis, inhibit invasion, and abolish osteoclastogenesis through suppression of nuclear factor-kappaB (NF-kappaB) activation and NF-kappaB-regulated gene expression. Mol. Cancer Ther. 5 (6), 1434–1445. doi:10.1158/1535-7163.MCT-06-0096
Jin, X., Ruiz Beguerie, J., Sze, D. M. Y., and Chan, G. C. F. (2016). Ganoderma lucidum (Reishi mushroom) for cancer treatment. Cochrane Database Syst. Rev. 4 (4), CD007731. doi:10.1002/14651858.CD007731.pub3
Kashyap, V. K., Dhasmana, A., Yallapu, M. M., Chauhan, S. C., and Jaggi, M. (2020). Withania somnifera as a potential future drug molecule for COVID-19. Future Drug Discov. 2 (4), FDD50. doi:10.4155/fdd-2020-0024
Kashyap, V. K., Peasah-Darkwah, G., Dhasmana, A., Jaggi, M., Yallapu, M. M., and Chauhan, S. C. (2022). Withania somnifera: Progress towards a pharmaceutical agent for immunomodulation and cancer therapeutics. Pharmaceutics 14 (3), 611. doi:10.3390/pharmaceutics14030611
Kataria, H., Shah, N., Kaul, S. C., Wadhwa, R., and Kaur, G. (2011). Water extract of ashwagandha leaves limits proliferation and migration, and induces differentiation in glioma cells. Evid. Based Complement. Altern. Med. 2011, 267614. doi:10.1093/ecam/nep188
Kawasaki, T., and Kawai, T. (2014). Toll-like receptor signaling pathways. Front. Immunol. 5, 461. doi:10.3389/fimmu.2014.00461
Khan, M. A., Ahmed, R. S., Chandra, N., and Arora, V. K. (2019). In vivo, extract from withania somnifera root ameliorates arthritis via regulation of key immune mediators of inflammation in experimental model of arthritis. Antiinflamm. Antiallergy Agents Med. Chem. 18 (1), 55–70. doi:10.2174/1871523017666181116092934
Khan, S., Malik, F., Suri, K. A., and Singh, J. (2009). Molecular insight into the immune up-regulatory properties of the leaf extract of Ashwagandha and identification of Th1 immunostimulatory chemical entity. Vaccine 27 (43), 6080–6087. doi:10.1016/j.vaccine.2009.07.011
Kim, R., Emi, M., and Tanabe, K. (2006). Functional roles of immature dendritic cells in impaired immunity of solid tumour and their targeted strategies for provoking tumour immunity. Clin. Exp. Immunol. 146 (2), 189–196. doi:10.1111/j.1365-2249.2006.03215.x
Kirtland, M. E., Tsitoura, D. C., Durham, S. R., and Shamji, M. H. (2020). Toll-like receptor agonists as adjuvants for allergen immunotherapy. Front. Immunol. 11, 599083. doi:10.3389/fimmu.2020.599083
Kobayashi, S. D., Malachowa, N., and DeLeo, F. R. (2018). Neutrophils and bacterial immune evasion. J. Innate Immun. 10 (5-6), 432–441. doi:10.1159/000487756
Kulkarni, S. K., and Dhir, A. (2008). Withania somnifera: An Indian ginseng. Prog. Neuropsychopharmacol. Biol. Psychiatry 32 (5), 1093–1105. doi:10.1016/j.pnpbp.2007.09.011
Kumar, D., Arya, V., Kaur, R., Bhat, Z. A., Gupta, V. K., and Kumar, V. (2012). A review of immunomodulators in the Indian traditional health care system. J. Microbiol. Immunol. Infect. 45 (3), 165–184. doi:10.1016/j.jmii.2011.09.030
Kurup, V. P., and Barrios, C. S. (2008). Immunomodulatory effects of curcumin in allergy. Mol. Nutr. Food Res. 52 (9), 1031–1039. doi:10.1002/mnfr.200700293
Logie, E., and Vanden Berghe, W. (2020). Tackling chronic inflammation with withanolide phytochemicals-A withaferin a perspective. Antioxidants (Basel) 9 (11), 1107. doi:10.3390/antiox9111107
Maeda, K., Caldez, M. J., and Akira, S. (2019). Innate immunity in allergy. Allergy 74 (9), 1660–1674. doi:10.1111/all.13788
Mannan, A., Abir, A. B., and Rahman, R. (2015). Antidepressant-like effects of methanolic extract of Bacopa monniera in mice. BMC Complement. Altern. Med. 15, 337. doi:10.1186/s12906-015-0866-2
McKee, A. S., Munks, M. W., MacLeod, M. K. L., Fleenor, C. J., Van Rooijen, N., Kappler, J. W., et al. (2009). Alum induces innate immune responses through macrophage and mast cell sensors, but these sensors are not required for alum to act as an adjuvant for specific immunity. J. Immunol. 183 (7), 4403–4414. doi:10.4049/jimmunol.0900164
Mishra, L. C., Singh, B. B., and Dagenais, S. (2000). Scientific basis for the therapeutic use of withania somnifera (ashwagandha): A review. Altern. Med. Rev. 5 (4), 334–346.
Mohan, R., Hammers, H. J., Bargagna-Mohan, P., Zhan, X. H., Herbstritt, C. J., Ruiz, A., et al. (2004). Withaferin A is a potent inhibitor of angiogenesis. Angiogenesis 7 (2), 115–122. doi:10.1007/s10456-004-1026-3
Nascimento, D. K., Souza, I. A. D., Oliveira, A. F. M. D., Barbosa, M. O., Santana, M. A. N., Pereira, D. F., et al. (2016). Phytochemical screening and acute toxicity of aqueous extract of leaves of conocarpus erectus linnaeus in Swiss albino mice. Acad Bras Cienc 88 (3), 1431–1437. doi:10.1590/0001-3765201620150391
Nguse, M., Yang, Y., Fu, Z., Xu, J., Ma, L., and Bu, D. (2022). Phyllanthus emblica (amla) fruit powder as a supplement to improve preweaning dairy calves' health: Effect on antioxidant capacity, immune response, and gut bacterial diversity. Biol. (Basel) 11 (12), 1753. doi:10.3390/biology11121753
Okada, H., Kuhn, C., Feillet, H., and Bach, J. F. (2010). The 'hygiene hypothesis' for autoimmune and allergic diseases: An update. Clin. Exp. Immunol. 160 (1), 1–9. doi:10.1111/j.1365-2249.2010.04139.x
Oriss, T. B., McCarthy, S. A., Morel, B. F., Campana, M. A., and Morel, P. A. (1997). Crossregulation between T helper cell (Th)1 and Th2: Inhibition of Th2 proliferation by IFN-gamma involves interference with IL-1. J. Immunol. 158 (8), 3666–3672. doi:10.4049/jimmunol.158.8.3666
Pandey, A. K., Gupta, A., Tiwari, M., Prasad, S., Pandey, A. N., Yadav, P. K., et al. (2018). Impact of stress on female reproductive health disorders: Possible beneficial effects of shatavari (Asparagus racemosus). Biomed. Pharmacother. 103, 46–49. doi:10.1016/j.biopha.2018.04.003
Rah, B., Amin, H., Yousuf, K., Khan, S., Jamwal, G., Mukherjee, D., et al. (2012). A novel MMP-2 inhibitor 3-azidowithaferin A (3-azidoWA) abrogates cancer cell invasion and angiogenesis by modulating extracellular Par-4. PLoS One 7 (9), e44039. doi:10.1371/journal.pone.0044039
Rasool, M., and Varalakshmi, P. (2006). Immunomodulatory role of Withania somnifera root powder on experimental induced inflammation: An in vivo and in vitro study. Vasc. Pharmacol. 44 (6), 406–410. doi:10.1016/j.vph.2006.01.015
Saggam, A., Limgaokar, K., Borse, S., Chavan-Gautam, P., Dixit, S., Tillu, G., et al. (2021). Withania somnifera (L.) dunal: Opportunity for clinical repurposing in COVID-19 management. Front. Pharmacol. 12, 623795. doi:10.3389/fphar.2021.623795
Saleem, S., Muhammad, G., Hussain, M. A., Altaf, M., and Bukhari, S. N. A. (2020). Withania somnifera L.: Insights into the phytochemical profile, therapeutic potential, clinical trials, and future prospective. Iran. J. Basic Med. Sci. 23 (12), 1501–1526. doi:10.22038/IJBMS.2020.44254.10378
Saxena, M., Sabado, R. L., La Mar, M., Mohri, H., Salazar, A. M., Dong, H., et al. (2019). Poly-ICLC, a TLR3 agonist, induces transient innate immune responses in patients with treated HIV-infection: A randomized double-blinded placebo controlled trial. Front. Immunol. 10, 725. doi:10.3389/fimmu.2019.00725
Schroeder, H. W., and Cavacini, L. (2010). Structure and function of immunoglobulins. J. Allergy Clin. Immunol. 125, S41–S52. doi:10.1016/j.jaci.2009.09.046
Sel, S., Wegmann, M., Sel, S., Bauer, S., Garn, H., Alber, G., et al. (2007). Immunomodulatory effects of viral TLR ligands on experimental asthma depend on the additive effects of IL-12 and IL-10. J. Immunol. 178 (12), 7805–7813. doi:10.4049/jimmunol.178.12.7805
Sen, N., Banerjee, B., Das, B. B., Ganguly, A., Sen, T., Pramanik, S., et al. (2007). Apoptosis is induced in leishmanial cells by a novel protein kinase inhibitor withaferin A and is facilitated by apoptotic topoisomerase I-DNA complex. Cell Death Differ. 14 (2), 358–367. doi:10.1038/sj.cdd.4402002
Serebrisky, D., and Wiznia, A. (2019). Pediatric asthma: A global epidemic. Ann. Glob. Health 85 (1), 6. doi:10.5334/aogh.2416
Sestili, P., Ismail, T., Calcabrini, C., Guescini, M., Catanzaro, E., Turrini, E., et al. (2018). The potential effects of ocimum basilicum on health: A review of pharmacological and toxicological studies. Expert Opin. Drug Metab. Toxicol. 14 (7), 679–692. doi:10.1080/17425255.2018.1484450
Sharma, G. N., Gupta, G., and Sharma, P. (2018). A comprehensive review of free radicals, antioxidants, and their relationship with human ailments. Crit. Rev. Eukaryot. Gene Expr. 28 (2), 139–154. doi:10.1615/CritRevEukaryotGeneExpr.2018022258
She, L., Alanazi, H. H., Yan, L., Zou, Y., Sun, Y., Dube, P. H., et al. (2019). Immune sensing of aeroallergen-associated double-stranded RNA triggers an IFN response and modulates type 2 lung inflammation. J. Immunol. 203 (9), 2520–2531. doi:10.4049/jimmunol.1900720
Shi, J., Weng, J. H., and Mitchison, T. J. (2021). Immunomodulatory drug discovery from herbal medicines: Insights from organ-specific activity and xenobiotic defenses. Elife 10, e73673. doi:10.7554/eLife.73673
Shohat, B., Kirson, I., and Lavie, D. (1978). Immunosuppressive activity of two plant steroidal lactones withaferin A and withanolide E. Immunosuppr. activity two plant steroidal Lact. withaferin A withanolide E. Biomed. 28 (1), 18–24.
Shohat, B., Shaltiel, A., Ben-Bassat, M., and Joshua, H. (1976). The effect of withaferin A, a natural steroidal lactone, on the fine structure of S-180 tumor cells. Cancer Lett. 2 (2), 71–77. doi:10.1016/s0304-3835(76)80014-6
Shree, P., Mishra, P., Selvaraj, C., Singh, S. K., Chaube, R., Garg, N., et al. (2022). Targeting COVID-19 (SARS-CoV-2) main protease through active phytochemicals of ayurvedic medicinal plants - withania somnifera (Ashwagandha), Tinospora cordifolia (Giloy) and Ocimum sanctum (Tulsi) - a molecular docking study. J. Biomol. Struct. Dyn. 40 (1), 190–203. doi:10.1080/07391102.2020.1810778
Shrivastava, A. K., Singh, H. V., Raizada, A., Singh, S. K., Pandey, A., Singh, N., et al. (2015). Inflammatory markers in patients with rheumatoid arthritis. Allergol. Immunopathol. Madr. 43 (1), 81–87. doi:10.1016/j.aller.2013.11.003
Silva, D. G., Cooper, P. D., and Petrovsky, N. (2004). Inulin-derived adjuvants efficiently promote both Th1 and Th2 immune responses. Immunol. Cell Biol. 82 (6), 611–616. doi:10.1111/j.1440-1711.2004.01290.x
Singh, N., Bhalla, M., de Jager, P., and Gilca, M. (2011). An overview on ashwagandha: A Rasayana (rejuvenator) of ayurveda. Afr. J. Tradit. Complement. Altern. Med. 8, 208–213. doi:10.4314/ajtcam.v8i5S.9
Speers, A. B., Cabey, K. A., Soumyanath, A., and Wright, K. M. (2021). Effects of withania somnifera (ashwagandha) on stress and the stress- related neuropsychiatric disorders anxiety, depression, and insomnia. Curr. Neuropharmacol. 19 (9), 1468–1495. doi:10.2174/1570159X19666210712151556
Stone, K. D., Prussin, C., and Metcalfe, D. D. (2010). IgE, mast cells, basophils, and eosinophils. J. Allergy Clin. Immunol. 125, S73–S80. doi:10.1016/j.jaci.2009.11.017
Sultan, H., Wu, J., Fesenkova, V. I., Fan, A. E., Addis, D., Salazar, A. M., et al. (2020). Poly-IC enhances the effectiveness of cancer immunotherapy by promoting T cell tumor infiltration. J. Immunother. Cancer 8 (2), e001224. doi:10.1136/jitc-2020-001224
Sun, J. C., and Lanier, L. L. (2011). NK cell development, homeostasis and function: Parallels with CD8⁺ T cells. Nat. Rev. Immunol. 11 (10), 645–657. doi:10.1038/nri3044
Tripathi, M. K., Singh, P., Sharma, S., Singh, T. P., Ethayathulla, A. S., and Kaur, P. (2021). Identification of bioactive molecule from Withania somnifera (Ashwagandha) as SARS-CoV-2 main protease inhibitor. J. Biomol. Struct. Dyn. 39 (15), 5668–5681. doi:10.1080/07391102.2020.1790425
Urban-Wojciuk, Z., Khan, M. M., Oyler, B. L., Fahraeus, R., Marek-Trzonkowska, N., Nita-Lazar, A., et al. (2019). The role of TLRs in anti-cancer immunity and tumor rejection. Front. Immunol. 10, 2388. doi:10.3389/fimmu.2019.02388
Vanden Berghe, W., Sabbe, L., Kaileh, M., Haegeman, G., and Heyninck, K. (2012). Molecular insight in the multifunctional activities of Withaferin A. Biochem. Pharmacol. 84 (10), 1282–1291. doi:10.1016/j.bcp.2012.08.027
Yu, Y., Hamza, A., Zhang, T., Gu, M., Zou, P., Newman, B., et al. (2010). Withaferin A targets heat shock protein 90 in pancreatic cancer cells. Biochem. Pharmacol. 79 (4), 542–551. doi:10.1016/j.bcp.2009.09.017
Zhao, H. M., Gao, Z. W., Xie, S. X., Han, X., and Sun, Q. S. (2019). Withaferin A attenuates ovalbumin induced airway inflammation. Front. Biosci. (Landmark Ed. 24 (3), 576–596. doi:10.2741/4737
Zhao, H., Wu, L., Yan, G., Chen, Y., Zhou, M., Wu, Y., et al. (2021). Inflammation and tumor progression: Signaling pathways and targeted intervention. Signal Transduct. Target Ther. 6 (1), 263. doi:10.1038/s41392-021-00658-5
Keywords: immunomodulation, immunostimulatory effects, withania somnifera, innate immunity, immune receptors, adaptive immunity
Citation: Alanazi HH and Elfaki E (2023) The immunomodulatory role of withania somnifera (L.) dunal in inflammatory diseases. Front. Pharmacol. 14:1084757. doi: 10.3389/fphar.2023.1084757
Received: 30 October 2022; Accepted: 13 February 2023;
Published: 22 February 2023.
Edited by:
Shahnawaz Ali Bhat, Aligarh Muslim University, IndiaReviewed by:
Vineet Kumar, Regional Centre for Biotechnology (RCB), IndiaJitendra Kanshana, University of Pittsburgh, United States
Copyright © 2023 Alanazi and Elfaki. This is an open-access article distributed under the terms of the Creative Commons Attribution License (CC BY). The use, distribution or reproduction in other forums is permitted, provided the original author(s) and the copyright owner(s) are credited and that the original publication in this journal is cited, in accordance with accepted academic practice. No use, distribution or reproduction is permitted which does not comply with these terms.
*Correspondence: Hamad H. Alanazi, SGhhbGFuYXppQGp1LmVkdS5zYQ==