- 1Department of Nephrology, The First Affiliated Hospital of Zhengzhou University, Zhengzhou, Henan, China
- 2Institute of Integrated Traditional Chinese and Western Medicine, Tongji Hospital, Tongji Medical College, Huazhong University of Science and Technology, Wuhan, Hubei, China
- 3Nephrology Department of Integrated Traditional Chinese and Western Medicine, The First Affiliated Hospital of Zhengzhou University, Zhengzhou, Henan, China
The prevalence of metabolic syndrome (MetS) is drastically growing worldwide, resulting in MetS-associated kidney disease. According to traditional theories, preventing blood pressure, lipid, glycose, and obesity and improving insulin resistance (IR), a couple of medications are required for MetS. It not only lowers patients’ compliance but also elevates adverse reactions. Accordingly, we attempted to seek answers from complementary and alternative medicine. Ultimately, berberine (BBR) was chosen due to its efficacy and safety on MetS through multi-pathways and multi-targets. The effects and mechanisms of BBR on obesity, IR, diabetic nephropathy, hypertension, hyperlipidemia, and hyperuricemia were elaborated. In addition, the overall properties of BBR and interventions for various kidney diseases were also collected. However, more clinical trials are expected to further identify the beneficial effects of BBR.
1 Introduction
With socioeconomic advances and altered lifestyles, metabolic syndrome (MetS) has gained increasing attention and was first defined by a Swedish physician in the 1920s (Studien, 1923). Although the diagnostic criteria of MetS proposed by various agencies, such as the National Cholesterol Education Program’s Adult Treatment Panel III (NCEP-ATP III), the International Diabetes Federation (IDF), the World Health Organization, and the Chinese Diabetes Society (CDS), differ, and abdominal obesity and insulin resistance (IR) are the common denominators. MetS is characterized by IR, obesity, hyperglycemia, hyperlipidemia, and hypertension. It is initiated by abdominal obesity and centered on IR. In other words, visceral obesity leads to IR, which leads to abnormal components of MetS.
MetS is approximately three times more common than diabetes, and the estimated global prevalence is approximately one-quarter of the world population (Saklayen, 2018). Currently, a growing number of studies have reported that obesity (Xu et al., 2021), hypertension (Sun et al., 2020), diabetes, and hyperlipidemia (Qian et al., 2021) can induce a wide range of kidney diseases. Therefore, MetS is prone to cause kidney injury. To our knowledge, MetS-associated kidney injury was first recorded by a Chinese physician in 2002 (Yun-Kai et al., 2002). Interventions for MetS-associated kidney disease include weight loss, lowering hypertension, glucose control, lifestyle improvement, lipid adjustment, Chinese herbal medicines, probiotics, and stem cell therapy (Lin et al., 2022). These therapies are aimed at different components of MetS, resulting in the prescription of many medications for patients with MetS. Hence, exploring novel therapeutic strategies is necessary. Inspired by the holistic concept of Traditional Chinese Medicine, natural-derived medicine was further investigated.
After retrieving numerous studies, berberine (BBR) is highlighted due to its wide spectrum of pharmacological activities and intervention mechanisms. The earliest information on the medical use of Rhizoma coptidis containing BBR dates back to A.D. 200 (Feng et al., 2019). BBR is a plant isoquinoline alkaloid widely applied in Chinese and Ayurvedic medicine and is extracted from various plants of the Berberidaceae, Ranunculaceae, and Papaveraceae families, such as Berberis aristata (5% in roots and 4.2% in stem bark), Berberis petiolaris (0.43%), Berberis vulgaris (1.24%), Berberis aquifolium, Berberis thunbergii, Berberis asiatica, Coptis teeta (rhizome 8%–9%), Hydrastis canadensis, Coptis chinensis, Phellodendron amurense, and Caulis mahoniae (Singh and Mahajan, 2013). Recently, most oral BBR has been found in its synthetic form of chloride or sulfate. BBR is a yellow powder that is bitter and slightly soluble in water and ethanol (Kumar et al., 2015). The chemical structure and metabolites of BBR are shown in Figure 1.
Initially, BBR was applied to treat diarrhea and gastrointestinal infection (Yu et al., 2020). Afterward, numerous studies demonstrated its weight loss (Park et al., 2020), antihypertensive (Ma et al., 2017), glucose-lowering (Di et al., 2021), lipid-lowering (Pirillo and Catapano, 2015), uric acid-lowering (Li X. et al., 2021), anti-inflammatory (Oshima et al., 2018; Lu et al., 2022), antifibrotic (Ahmedy et al., 2022), antiproliferative (Bonon et al., 2013), antiapoptotic (Hu et al., 2012), antiaging (Xu Z. et al., 2017), antioxidant (Shou et al., 2022), antibacterial (Liao et al., 2020), anticancer (Liu et al., 2022), immunomodulatory (Huang et al., 2021), gut microbiome adjustment (Wang H. et al., 2022), neuroprotective (Domitrović et al., 2013), cardioprotective (Cai et al., 2021), and neuroprotective (Shou et al., 2022) effects (Figure 2). According to these pharmacological properties, BBR is promising in treating various diseases, such as MetS (Och et al., 2022), hyperuricemia (Li X. et al., 2021), acute kidney injury (Shen et al., 2020), rheumatoid arthritis (Huang et al., 2021), cardiovascular disease (Feng et al., 2019), gastric cancer (Liu et al., 2022), neuropsychiatric disorder (Hao et al., 2022), and polycystic ovary syndrome (Zhang et al., 2021).
Regarding nephroprotective effects, a wide range of kidney diseases could be treated by BBR, such as MetS-associated, membranous nephropathy (Sha et al., 2018), kidney transplant (Wu et al., 2005), ischemia-reperfusion injury (Zheng et al., 2018), kidney fibrosis (Shao et al., 2021), medication- or toxin-induced injury (Othman et al., 2014; Ibrahim Fouad and Ahmed, 2021), autosomal dominant polycystic kidney disease (Bonon et al., 2013), kidney stones (Bashir and Gilani, 2011), kidney aging (El-Horany et al., 2020), and Wilms’ tumor (Liu and Liu, 2013) (Figure 3).
In contrast to chemical drugs, which act on special targets and treat unique diseases, herb-derived BBR is multipotent with multiple targets, consistent with the holistic concept of Traditional Chinese Medicine. Consequently, the potential therapeutic action and mechanism of BBR in MetS-associated kidney injury requires summarizing knowledge and research trends (Figure 4).
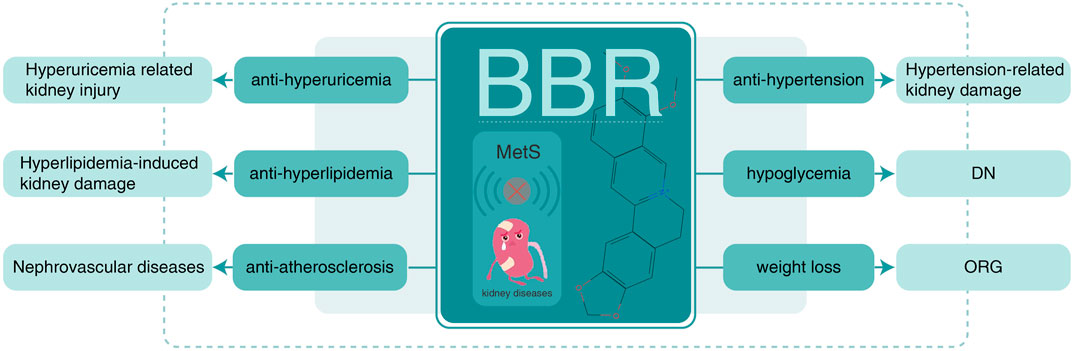
FIGURE 4. BBR on MetS-associated kidney disease. BBR, berberine; MetS, metabolic syndrome; ORG, obesity-related glomerulopathy; DN, diabetic nephropathy.
1.1 BBR and obesity
Obesity prevalence is progressively growing as living conditions rise and physical labor decreases. Obesity is most likely caused by a complex mix of changes in the dietary environment; physical activity; and socioeconomic, environmental, and genetic variables. Since 1980, the global prevalence of being overweight and obese has more than doubled, with over a third of the world’s population categorized as overweight or obese. Obesity rates have risen across all ages and sexes, regardless of geographical location, race, or financial level (Chooi et al., 2019).
Obesity-related glomerulopathy (ORG) is becoming increasingly common with the global obesity epidemic. Glomerular hypertrophy and focal segmental glomerulosclerosis (FSGS), particularly the perihilar form, are pathologic characteristics of ORG, and the degree of foot process effacement in ORG is generally less than that in primary FSGS. Obesity-induced increases in glomerular filtration rate, renal plasma flow, filtration fraction, and tubular sodium reabsorption cause the glomerulus to expand. Although most patients have stable or slowly progressing proteinuria, up to one-third suffer progressive renal failure and end-stage renal disease (D'Agati et al., 2016). Insights from Mendelian randomization and human kidney transcriptomics suggest that a putatively causal association of obesity with renal health is primarily independent of blood pressure and type 2 diabetes and reveal the signatures of obesity on the transcriptome of the human kidney (Xu et al., 2021).
BBR, as a botanical compound, is widely employed in ameliorating obesity, although no available research has been mentioned regarding its usage in ORG. To date, many previous studies have demonstrated that BBR effectively inhibits the development of obesity via modulation of the gut microbiota, intestinal permeability, gene regulation, and hepatic gluconeogenesis (Ilyas et al., 2020). The detailed potential mechanisms of BBR against obesity are summarized in Table 1 and are as follows.
First, the modulation of gut microbiota: the imbalance of intestinal flora is an important cause of obesity. BBR could reduce the exogenous antigen load in the host and elevate short-chain fatty acid levels in the intestine (Zhang et al., 2012), modulate gut microbiota, restore the gut barrier, and improve gut peptide levels (Xu J. H. et al., 2017).
Second, anti-inflammatory effects: long-term chronic inflammation exists in obesity. BBR treatment notably decreases the phosphorylation state of JNK1 in both hepatoma H4IIE cells and mouse primary hepatocytes, suggesting that improving diet-induced obesity is largely attributable to BBR’s suppression of inflammation (Guo et al., 2016). Furthermore, BBR significantly increases the CD206 + M2 adipose tissue macrophage (ATM) population while significantly reducing tumor necrosis factor-α (TNF-α), C-C motif chemokine ligand 2 (CCL2), CCL4, CCL5, and C-X-C motif chemokine receptor 4 (CXCR4) (Noh et al., 2022).
Third, mitochondrial energy metabolism is regulated. BBR significantly increases fasting-induced adipose factor (Fiaf, a key protein negatively regulated by intestinal microbes) expression in intestinal and visceral adipose tissues. BBR considerably increases AMP-activated protein kinase (AMPK) mRNA, peroxisome proliferator-activated receptor gamma (PPARG) coactivator 1-alpha (PGC1α), uncoupling protein 2 (UCP2), carnitine palmitoyl transferase 1-alpha (CPT1α), and Hadhb related mitochondrial energy metabolism (Xie et al., 2011).
Fourth, the central obesity-related pathway is regulated. By regulating the central obesity-related pathway, microinjections of BBR in the hypothalamus area of rats lower food intake and glucose rise and prevent obesity (Park et al., 2020).
Finally, there are other mechanisms. Because BBR lowers mouse weight gain and food intake with the downregulation of PPAR and upregulation of GATA binding protein 3 (GATA-3), transcription factors function in high-fat diet (HFD)-induced mice (Hu and Davies, 2010). Additionally, through the bitter-taste receptor (TAS2R) signaling pathway, BBR increases the production of glucagon-like peptide-1 (GLP-1) in vivo and ex vivo, encourages tuft cell proliferation, and secretes IL-25 in obesity (Sun et al., 2022).
1.2 BBR and IR
IR is generally secondary to obesity or diabetes. Although it does not directly affect the kidney, its complications, such as MetS, obesity, hypertension, and diabetes, could result in a series of kidney impairments. In the case of these potential kidney injuries, it is imperative to intervene in IR from the origin. BBR is suggested to play a prominent role in attenuating IR via different signaling pathways in the cell, animal, and clinical trials involving multiple molecules, proteins, and multiple tissues. The detailed potential mechanisms of BBR against IR are summarized in Table 2 and are as follows.
First, the AMPK activity is activated. BBR appears to reduce IR in H9c2 cardiomyocytes, at least in part, by stimulating AMPK activity, as shown by BBR’s considerably enhanced AMPK activity (Chang et al., 2013). The anti-inflammatory benefits of BBR are achieved by activating AMPK-dependent autophagy in ATMs, hence reducing IR (Zhou et al., 2017). Furthermore, BBR increases the protein expression of phospho-AMP-activated protein kinase (p-AMPK) and protects against IR caused by fructose (Li et al., 2020).
Second, insulin sensitivity and insulin receptor (InsR) are increased. Preliminary findings show that suppressing fat storage and altering the adipokine composition improve insulin sensitivity in human preadipocytes and patients with MetS. BBR increases InsR mRNA and protein expression in human liver cells. Meanwhile, BBR boosts insulin sensitivity, InsR, and protein kinase C (PKC) activity in type 2 diabetes mellitus rat liver (Kong et al., 2009). BBR may reduce IR in rats with non-alcoholic fatty liver disease (NAFLD) by increasing IRS-2 mRNA and protein levels (Xing et al., 2011). In HFD-induced IR rats, BBR may ameliorate the development of IR by differentially preventing alterations of IR, IRS-1, and glucagon in β-cells, α-cells, and hepatocytes (Gu et al., 2012).
Third, BBR has anti-inflammatory effects. Inflammation also participates in the pathological process of IR. According to a meta-analysis of randomized controlled trials (RCTs) on Chinese subjects with MetS and associated illnesses, high-sensitivity C-reactive protein (hs-CRP) was strongly connected with IR (r = 0.9929, p < 0.05) (Cao and Su, 2019). BBR efficiently suppressed IL-6 and TNF-α production in palmitate (PA)-stimulated hepatocytes, indicating that BBR may increase insulin sensitivity in conjunction with its anti-inflammatory effects (Lou et al., 2011). BBR inhibited PA-induced NLRP3 inflammasome activation and caspase 1 and interleukin-1 (IL-1) release in HFD-induced IR (Zhou et al., 2017). Additionally, BBR alleviates IR in HepG2 cells via a cholinergic anti-inflammatory mechanism (Li F. et al., 2016).
Fourth, the gut microbiota is regulated. BBR improved IR in HFD-fed mice, which was connected not only with gut microbiota changes in branched-chain amino acid (BCAA) production but also with BCAA catabolism in liver and adipose tissues (Yue et al., 2019). The prevention of IR by BBR in HFD-fed rats is at least partially mediated by structural modulation of the gut microbiota, which may help alleviate inflammation by reducing the exogenous antigen load in the host and elevating short-chain fatty acid levels in the intestine (Zhang et al., 2012). BBR reversed dysbacteriosis and inhibited Toll-like receptor 4 (TLR4)/TNF-α activation, resulting in increased InsR and InsR substrate-1 expression in the liver, suggesting that BBR may reduce IR, at least in part by modulating the gut microbiota (Liu et al., 2018).
Fifth, hypoxia-inducible factor (HIF) is inhibited and could alleviate IR by inhibiting fatty acid oxidation (FAO)-mediated activation of the NLRP3 inflammasome (Li X. et al., 2021). In HFD-fed mice, BBR reduced hypoxia-inducible factor 2α (HIF-2α)-induced ceramide production and attenuated ceramide-induced IR (Xia et al., 2022). In IR adipocyte models, BBR improves insulin sensitivity, and the beneficial effects of BBR are possibly realized through the inhibition of HIF3α methylation (Wang et al., 2019).
Finally, other mechanisms are involved, such as reducing PPARG and fatty acid transferase (FAT)/CD36 (Chen et al., 2009), inhibition of cyclophilin protein (Dong et al., 2021), M1 macrophage activation (Ye et al., 2016), the leukotriene B4 (LTB4)–BLT1 axis (Gong et al., 2021), I-kappa-B kinase-β (IKκβ) (Yi et al., 2008), mitogen-activated protein (MAP) kinase kinase kinase (MEKK) 1, MAP kinase (MEK) 1/2, ERK1/2 (Li et al., 2022), nuclear transcription factor-kappa B (NF-κB) p65 (Yi et al., 2007), increased liver X receptor (LXR) α, PPARα, and sterol regulatory element-binding protein (SREBP) (Liu et al., 2010), enhancing mitochondrial architecture via sirtuin 1 (SIRT1)/optic atrophy 1 (Opa1) (Xu et al., 2022), regulating microRNA-146b/SIRT1 (Sui et al., 2021), and bone morphogenetic protein 4 (BMP4) transcription (Li G. S. et al., 2016).
1.3 BBR and diabetic nephropathy
Hyperglycemia in MetS is composed of either prediabetes or diabetes. The odds ratios (ORs) for isolated microalbuminuria and impaired fasting glucose were 1.58 (1.10–2.25), suggesting that prediabetes, combining impaired fasting glucose and impaired glucose tolerance, may be detrimental to the kidney. Additionally, among individuals with prediabetes, the OR for isolated decreased kidney function was 2.57 (1.31–5.06) (Markus et al., 2018). Without immediate prevention, prediabetes is susceptible to progression into diabetes. Fortunately, BBR slows the progression of prediabetes to diabetes in diabetic fatty rats by enhancing the intestinal secretion of glucagon-like peptide-2 and improving the gut microbiota (Wang et al., 2021).
A meta-analysis of observational studies showed that the overall pooled prevalence of diabetic nephropathy (DN) was 21.8% in China (Zhang X. X. et al., 2020). DN is responsible for 30%–50% of all end-stage renal disease causes. The increased filtration and total renal size of early-stage diabetes are correlated with elevations in glomerular and tubular size. The mesangial compartment of the glomerulus grows as matrix formation rises, likely as the mesangial cell population increases (Ibrahim and Hostetter, 1997).
BBR is frequently used in the prevention and control of DN due to its blood glucose lowering, anti-inflammation, anti-fibrosis, and flora adjustment effects. The comprehensive potential mechanisms of BBR against DN are summarized in Table 3 and are as follows.
First, the NF-κB pathway is inhibited. BBR decreased renal impairment in the streptozotocin (STZ)-induced DN rat model, as shown by lowering fasting blood glucose, kidney weight to body weight ratio, 24-h proteinuria, urea nitrogen (BUN), and creatinine (Cr) levels. BBR suppressed the TLR4/NF-κB pathway and decreased the systemic and renal cortex inflammatory responses in STZ-induced DN rats and high glucose (HG)-induced podocytes (Zhu L. et al., 2018). Another study revealed that under diabetic conditions, BBR lowers fibronectin (FN) expression by acting on the sphingosine 1-phosphate (S1P) 2 receptor in the mesangium, which might be related to its inhibitory action on NF-κB activation (Huang et al., 2012). A current study shows that BBR can prevent type 2 diabetes by suppressing NF-κB-driven renal inflammation and the transforming growth factor (TGF)/Smad3 signaling pathway (Sun et al., 2015).
Second, mesangial proliferation was reduced. Glomerular mesangial cell proliferation is one of the main pathological changes in DN. A current study found that Huang-Gui solid dispersion (HGSD), a novel BBR formulation, prevented DN by reducing renal mesangial matrix growth and activating autophagy, which might be linked to AMPK phosphorylation activation (Zhang M. et al., 2020). Furthermore, BBR can prevent the progression of DN, perhaps by blocking the PI3K/Akt/AS160/glucose transporter 1 (GLUT1) signaling pathway, which regulates HG-induced aberrant glomerular mesangial cell (GMC) proliferation and the cell cycle (Ni et al., 2022).
Third, BBR has anti-inflammatory effects. In the DN hamster kidney, NLRP3–Caspase-1–Gasdermin D (GSDMD) signaling was increased. BBR can diminish oxidative stress damage by modulating antioxidative Nrf2 and then NLRP3–Caspase-1–GSDMD signaling to prevent pyroptosis and antagonize DN inflammation-induced damage (Ding et al., 2021). BBR inhibited HG-induced epithelial-to-mesenchymal transition (EMT) and renal interstitial fibrosis by inhibiting the NLRP3 inflammasome, implying that BBR might be utilized as a new medication to treat tubulointerstitial fibrosis in DN (Ma et al., 2022).
Fourth, BBR has anti-fibrotic effects. Glomerular mesangial cell proliferation is one of the main pathological changes in DN. Consistently, BBR inhibits renal tubular EMT and renal interstitial fibrosis through Notch/snail pathway regulation (Yang et al., 2017). Through the Smad signaling pathway, BBR can maintain the dynamic equilibrium of TGF-β1/SnoN expression in renal tissues, hence mitigating renal dysfunction (Liu et al., 2012).
Finally, there are other mechanisms. The renoprotective effects of BBR on DN may be connected with alterations in the extracellular matrix via control of the matrix metalloproteinase (MMP)/tissue inhibitor of metalloproteinase (TIMP) pathway in the rat kidney (Ni et al., 2015). BBR (100, 200 mg/kg) alleviated DN histopathology, which may be related to the control of β-arrestin expression, as well as intercellular cell adhesion molecule-1 (ICAM-1) and vascular cell adhesion molecule-1 (VCAM-1) levels in the rat kidney (Tang et al., 2016).
1.4 BBR and hypertension nephropathy
In MetS, high blood pressure is defined as prehypertension or hypertension according to varying diagnosis criteria. A meta-analysis revealed that prehypertension showed an increased risk of chronic kidney disease (CKD) (pooled RR = 1.28) compared with the optimal BP values. Therefore, prehypertension is a potential cause of CKD (Li Y. et al., 2016). Meanwhile, hypertension and malignant hypertension can induce hypertensive kidney damage with different kidney pathological features. Secondary hypertension is commonly induced by parenchymal and renovascular diseases. Thus, hypertension and renal injury interact, ultimately cultivating a vicious cycle. Anti-hypertension is especially crucial for blood pressure-dependent kidney damage, regardless of which reason induces hypertension.
Abnormal hemodynamics, activation of the renin–angiotensin–aldosterone system (RAAS), oxidative stress, inflammation, genetic factors, and metabolic disorders contribute to hypertensive kidney damage (Fang-Ming et al., 2015). The pathological features of benign hypertension nephropathy are characterized by a thickened interlobular artery intima, stratified elastic layer, and arteriole hyalatosis, with/without media smooth muscle cell proliferation. Pathological manifestations of malignant hypertension kidney injury are malignant lesions of the arteries, including fibrinous necrosis of the arteries (acute lesions), “scallion-skin-like” changes in intimal hyperplasia of the arteries (chronic lesions), fibrinous necrosis of glomerular vascular loops, and benign lesions of the arteries (Shao-Shan et al., 2015).
BBR exerts certain beneficial effects on hypertension nephropathy. A randomized, double-blind, placebo-controlled clinical trial indicated a significant decrease in systolic blood pressure (SBP) (123 ± 7 vs. 115 ± 9 mmHg, p < 0.01), which was observed after BBR administration compared with placebo (Perez-Rubio et al., 2013). The anti-hypertension mechanisms of BBR are summarized in Table 4 and are as follows.
First, endothelial function is improved. Endothelial dysfunction is an important determinant risk factor for the development of hypertension and its complications. Hence, improving endothelial function has major clinical importance. In spontaneously hypertensive rats (SHRs) with carotid arteries, BBR lowers endothelium-dependent contractions (EDCs), most likely by activating AMPK, which then prevents endoplasmic reticulum stress and scavenges reactive oxygen species (ROS), causing cyclooxygenase-2 (COX-2) to be downregulated (Liu et al., 2015). Endothelial dysfunction and arterial stiffness are linked to abnormal alterations in circulating microparticles (MPs) and endothelial progenitor cells (EPCs) in SHRs. BBR enhanced endothelial function by preserving superior endothelium-dependent vasodilation and retained arterial elasticity by reducing aortic pulse wave velocity (aPWV) and increasing the content of arterial media elastin fiber (Zhang G. et al., 2020).
Second, there is vasodilation. By activating the large conductance calcium-activated K+ 504 channels (BKCa) in vascular smooth muscle cells (VSMCs), BBR decreased blood pressure and enhanced vasodilation in diabetic rats, suggesting that BBR could provide a combined treatment for regulating hyperglycemia and blood pressure in diabetes (Ma et al., 2017). The angiotensin-converting enzyme inhibitory impact, direct release of nitric oxide/cyclic guanosine monophosphate (NO/cGMP), and α1-adrenoreceptor antagonistic activity of BBR all contribute to its vasodilatory effect, which may explain the drop in blood pressure (Derosa et al., 2012).
Third, BBR affects antioxidants. In 2K1C renovascular hypertensive rats, chronic infusion of BBR decreased mean arterial pressure (MAP), paraventricular nucleus Fra-like activity, and plasma levels of norepinephrine (NE), as well as NADPH oxidase 2 (NOX2), NOX4, ERK1/2, and inducible nitric oxide synthase (iNOS). This finding suggests that BBR attenuates hypertension and sympathoexcitation by inhibiting the ROS/ERK1/2/iNOS pathway (Tian et al., 2019).
Finally, the gut microbiota is regulated. BBR administration dramatically reduced vascular dysfunction and pathological remodeling in Ang II-induced hypertensive mice, suggesting that the protective effect of BBR in hypertension may be attributable (at least in part) to the reduction in trimethylamine (TMA)/trimethylamine-N-oxide (TMAO) formation through gut microbiota regulation (Wang Z. et al., 2022).
However, a systematic review involving 614 participants revealed that the data from randomized trials are insufficient to prove the efficacy and safety of BBR in the treatment of hypertension as its evidence is restricted, of low quality, and ultimately inconclusive (Suadoni and Atherton, 2021).
1.5 BBR and hyperlipidemia
Hyperlipidemia is frequently secondary to nephrotic syndrome, chronic renal failure, and postrenal transplant conditions. Because these disorders appear to enhance the risk of coronary heart disease, lowering blood lipids is particularly important (Grundy, 1990). Moreover, feeding cholesterol to experimental animals induced the initiation and progression of glomerular injury, and treatment of hyperlipidemic animals with lipid-lowering drugs slowed the development of glomerulosclerosis (Kamanna et al., 1998).
BBR, a natural lipid-lowering agent, is salutary in animal and clinical experiments. Scholars showed that BBR improved adipose tissue remodeling by activating Sirtuin 3 (SIRT3), which could contribute to the anti-hyperlipidemic effect (Li 2022). After 8 weeks of treatment with BBR, cholesterol was significantly decreased in a rat model of MetS (Li et al., 2015). Patients who received BBR also showed statistically significant improvements in total cholesterol (MD, −0.58; 95% CI, −0.74 to −0.41) and low-density lipoprotein (LDL) (MD, 0.52; 95% CI, −0.68 to −0.35) in antipsychotic-associated weight gain and MetS in patients with schizophrenia (Chan et al., 2022). In hyperlipidemic patients with chronic hepatitis or liver cirrhosis, BBR significantly lowered blood cholesterol, triglycerides, and LDL-cholesterol (LDL-c) (Zhao et al., 2008). The exhaustive potential hypolipidemic mechanisms of BBR are shown in Table 5 and are as follows.
First, BBR inhibits lipid synthesis in human hepatocytes through the direct and indirect activation of AMPK in peripheral tissues (notably the liver and muscles) (Brusq et al., 2006; Kim et al., 2009; Wang et al., 2016). BBR can protect the lean body mass from excessive lipid accumulation by promoting mitochondrial biogenesis and improving FAO in an AMPK/PGC-1α-dependent manner (Yao et al., 2020). BBR moderates lipid metabolism through AMPk-p38 mitogen-activated protein kinase (MAPK)-GLUT4 (Zhang et al., 2011). In addition, AMPKα1 is essential for BBR to improve glucose and lipid metabolism in HepG2 cells (Ren et al., 2020). However, prolonged activation of AMPK increases CD36 expression in hepatocytes, resulting in fatty acid uptake linked to hepatocellular lipid accumulation and fatty liver (Choi et al., 2017).
Second, BBR and its metabolites exhibit lipid-lowering effects by upregulating LDL receptor (LDLR) expression (Pisciotta et al., 2012; Zhou et al., 2014; Zhu X. et al., 2018). A study demonstrated that fatty liver could be improved by BBR administration by reversing the abnormal expression of microsomal triglyceride transfer protein (MTTP) and LDLR and inhibiting lipid synthesis (Chen et al., 2021).
Third, BBR could significantly modify the structure and composition of gut microbiota (Yang et al., 2022), and the changes in gut microbiota and metabolites are correlated with BBR improving lipid metabolism disturbances (Fang et al., 2022). Other studies demonstrated that BBR significantly inhibited the 7α-dehydroxylation conversion of cholic acid to deoxycholic acid, and the hypocholesterolemic effect of orally administered BBR was involved in its effect on modulating the turnover of bile acids and the farnesoid X receptor signaling pathway (Gu et al., 2015; Sun et al., 2017).
Fourth, BBR exhibits a dual effect on maintaining lipid homeostasis through hepatocyte nuclear factor (HNF)-4α-regulated miR-122 expression (Wei et al., 2016). BBR promotes lipid metabolism by activating the expression of miR-192, downregulating steroid regulatory element binding transcription factor 1 (SREBF1) and PPARG, increasing PPARG phosphorylation, and reducing JNK phosphorylation (Dai J. et al., 2021a; Dai J. G. et al., 2021b).
Finally, BBR alleviates lipid deposition by improving the HG-induced reduction in FAO (Rong et al., 2021). BBR can reduce steatosis by increasing ATP-binding cassette transporter A1 (ABCA1) protein levels through PKCδ to reduce the phosphorylation of serine residues in ABCA1 (Liang and Wang, 2018). Moreover, BBR represses complex I in the gut and liver and consequently inhibits lipid metabolism, leading to alleviation of obesity and fatty liver (Yu et al., 2021).
1.6 BBR and hyperuricemia-related kidney disease
Hyperuricemia does not belong to the scope of MetS. However, hyperuricemia is induced by abnormal metabolism and is closely linked to MetS. Hence, hyperuricemia-related kidney disease is discussed under this condition. The mechanisms of hyperuricemia-related kidney disease involve RAAS activation, direct damage to uric acid (UA) crystals, and inflammation. The pathological feature of hyperuricemia-related kidney disease is characterized by tubule-interstitial injury. BBR exerts antihyperuricemic and nephroprotective effects in hyperuricemic kidney disease (Li X. et al., 2021). The mechanisms of BBR in hyperuricemia and related kidney disease are listed below.
First, BBR lowers UA by inhibiting UA biosynthesis and promoting UA excretion. BBR suppresses the expression of xanthine oxidase (XOD), urate transporter 1 (URAT1), and glucose transporter 9 (GLUT9) to lower UA (Shan et al., 2022). In addition, BBR effectively reduced serum UA levels in hyperuricemic rats by increasing urine uric acid levels and urate fractional excretion.
Second, BBR has anti-inflammatory effects. Inflammation is involved in hyperuricemia-related kidney injury. BBR drastically reduced the levels of UA, BUN, and Cr in a mouse model of hyperuricemia created by potassium oxonate and hypoxanthine, as well as kidney injury. BBR inhibits the activation of the NLRP3 inflammasome and decreases TNF-α (Shan et al., 2022), IL-1β (Liu et al., 2016), and IL-18 levels, as well as NLRP3, ASC, caspase-1, and URAT1 expression (Li Q. et al., 2021).
Finally, the gut microbiota is regulated. Subsequently, 16S rRNA sequencing data showed that BBR enriched the abundance of Coprococcus, Bacteroides, Akkermansia, and Prevotella in potassium oxonate-induced hyperuricemia, clarifying that BBR ameliorates hyperuricemia by modulating the gut microbiota (Shan et al., 2022).
2 Discussion
MetS-associated kidney diseases can be induced by different components of MetS, such as obesity-related ORG, hypertension-related hypertension nephropathy, hyperglycemia-related DN, hyperlipidemia and hyperlipidemia-associated kidney injury, and hyperuricemia and hyperuricemia-associated kidney disease. One or several components of MetS can contribute to kidney damage alone or together with other elements. Hence, the clinical manifestation and pathological features of MetS-associated kidney disease are different according to the component(s) involved. In addition, the pathological features are not always consistent with clinical manifestations. Therefore, the specific component may not cause this component-associated kidney disease. In this situation, renal biopsy is a unique way to diagnose MetS-associated kidney disease. Due to the complexity of MetS, its therapy is renal pathology-oriented.
Regarding renal pathology, MetS can lead to glomerulopathy (associated with obesity and disorders of glucose and lipid metabolism), lesions of the small arteries of the kidney (associated with hypertension and diabetes), and tubule-interstitial lesions (associated with hyperuricemia and secondary to renal arterioles and glomerulus lesions) (Yi-Pu, 2006). Thus, renal biopsy is crucial for the precise diagnosis of MetS-associated kidney diseases. The treatment plan varies depending on different kidney lesions.
In this review, we summarized the effects of BBR on different components of MetS and its kidney injury. More attention was given to improving IR, lipid-lowering, and glucose-lowering than to controlling obesity, hypertension, and hyperuricemia. On the one hand, the levels of insulin, lipids, and glucose are easy to determine and show efficacy in the short term. On the other hand, the effects of losing weight and lowering blood pressure are difficult to realize unless IR, lipids, and glucose are well-controlled. The antihyperuricemic effect of BBR is rarely reported, possibly because it is beyond the scope of MetS. Anti-inflammation and modulation of gut microbiota are common mechanisms of BBR, which participate in intervening in nearly all components of MetS. The diagram of BBR on metabolic pathways of IR in liver and muscle cells, hypertension and hyperuricemia in endothelial cells, and hyperlipidemia in the tubular epithelial cell is shown in Figure 5.
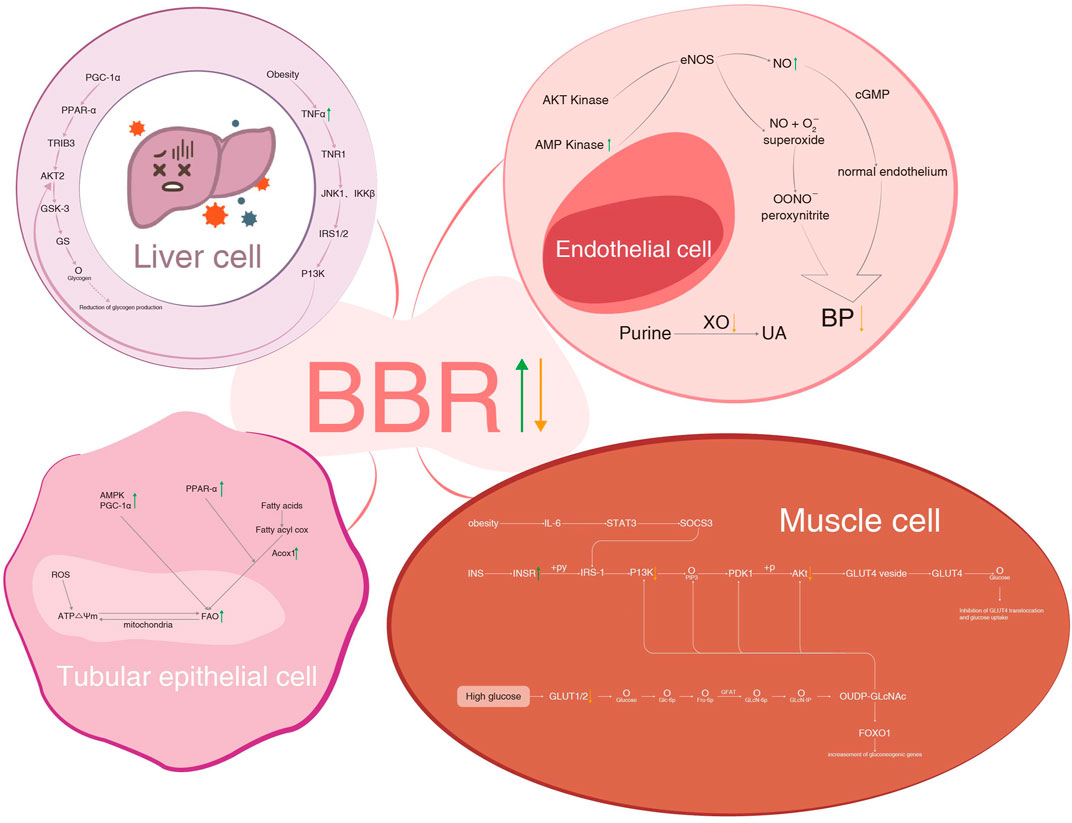
FIGURE 5. BBR on metabolic pathways of IR in liver cell and muscle cells, hypertension and hyperuricemia in endothelial cells, and hyperlipidemia in tubular epithelial cells. BBR, berberine; IR, insulin resistance; PGC-1α, peroxisome proliferator-activated receptor-γ coactivator 1α; PPAR-α, peroxisome proliferator-activated receptor α; GLUT4, glucose transporter 4; TR1B3, tribbles homologue 3; AKT2, RAC serine/threonine-protein kinase; GSK-3, glycogen synthase kinase-3; GS, glycogen synthase; TNF-α, tumor necrosis factor-α; TNR1, tumor necrosis factor receptor 1; JNK1, c-Jun-N-terminal kinase 1; IKKβ, inhibitor of nuclear factor kappa-B kinase subunit beta; IRS1/2, insulin receptor substrate 1/2; P13K, phosphatidylinositol-3-kinase; AMP, adenosine 5'-monophosphate activated protein; cGMP, cyclic guanosine monophosphate; ROS, reactive oxygen species; Acox1, acyl-coenzyme a oxidase 1; IL-6, interleukin-6; STAT3, signal transducer and activator of transcription 3; SOCS3, suppressor of cytokine signaling 3; INS, insulin; INSR, insulin receptor; +py, tyrosine phosphorylation; PDK1, phosphoinositide-dependent kinase-1; GLUT4 vesicle, glucose transporter 4 vesicle; GFAT, glutamine fructose phosphate transaminase; FOXO1, forkhead box O1.
Meanwhile, several limitations and recommendations for these included studies should be noted. First, different modeling methods are applied in different components; even for the same component, varying modeling methods are used. In addition, the same modeling method is employed in different components. For example, obesity, diabetes, and IR models could be established by HFD. Therefore, the efficacy and severity of different modeling methods are difficult to estimate, as are the efficacy and safety of BBR. Second, few studies on MetS-associated kidney disease have been reported due to a lack of awareness among clinicians. We found that MetS-associated kidney disease is replaced by isolated component-induced kidney damage, such as DN, ORG, and hypertension nephropathy. Third, renal biopsy is not performed in most studies. Although clinical renal lesions are presented, including proteinuria and abnormal BUN or Cr, the gold standard for kidney injury tends to be ignored. Based on the renal biopsy, the final pathological diagnosis of diabetic patients is possibly non-diabetic nephropathy. If these diabetic patients are treated according to DN, kidney diseases may be delayed or aggravated. Fourth, altered purity, doses, duration, and administration routes of BBR dependent on different design protocols are responsible for the variance of effects. Fifth, the bioavailability of BBR is rather low after it is absorbed by the gastrointestinal tract. After a single oral dose of 400 mg of BBR, the highest concentration (Cmax) of BBR in human plasma is 0.4 ng/ml (Hua et al., 2007). BBR is commonly applied at high doses to increase Cmax, which may induce adverse gastrointestinal effects. Consequently, novel formulations and derivatives or analogs of BBR may enhance bioavailability. Sixth, most recent studies concerning BBR are derived from cells or animals, and the definitive efficacy and safety of BBR urgently need to be further confirmed in large-scale, high-quality, multicenter RCTs. Finally, most of the research is from China, so race bias may be an issue.
BBR is believed to be omnipotent for different organs and tissues’ illnesses, which makes us concerned if its efficacy is exaggerated to some extent. However, BBR properties are based on its beneficial effects on dysmetabolism, so it is acceptable in some way. Nonetheless, it may be long before BBR is available for patients with dysmetabolic diseases, including MetS and related kidney injuries.
3 Conclusion
Overall, this review provides an important account of the impact of BBR on MetS and its nephroprotective effects. The “one-drug with multiple mechanisms and multiple targets” property of BBR enables its applications in obesity, IR, hypertension, hyperglycemia, hyperlipidemia, and hyperuricemia. Among these abundant mechanisms of BBR, anti-inflammation and regulation of gut microbiota seem to be the common mechanisms. Due to its low cost, easy obtainability, efficacy, and safety, BBR is promising in MetS-associated kidney disease, especially in developing countries.
Author contributions
Y-FL selected the dissertation topic, searched and analyzed the literature, and drafted the manuscript. H-HW searched the literature and drafted the manuscript. Y-HG, S-HT, and HuW contributed to the manuscript revision. Y-FL contributed to figure design and editing.
Funding
This work was supported by the National Natural Science Foundation of China (82174154).
Acknowledgments
We thank Ya-Ru Liu (Beijing Hanyi International Culture Communication Co., Ltd) for her contribution to figure design and editing.
Conflict of interest
The authors declare that the research was conducted in the absence of any commercial or financial relationships that could be construed as a potential conflict of interest.
Publisher’s note
All claims expressed in this article are solely those of the authors and do not necessarily represent those of their affiliated organizations or those of the publisher, the editors, and the reviewers. Any product that may be evaluated in this article, or claim that may be made by its manufacturer, is not guaranteed or endorsed by the publisher.
References
Ahmedy, O. A., El-Tanbouly, D. M., Al-Mokaddem, A. K., and El-Said, Y. A. M. (2022). Insights into the role of P2X7R/DUSP6/ERK1/2 and SIRT2/MDM2 signaling in the nephroprotective effect of berberine against cisplatin-induced renal fibrosis in rats. Life Sci. 309, 121040. doi:10.1016/j.lfs.2022.121040
Bashir, S., and Gilani, A. H. (2011). Antiurolithic effect of berberine is mediated through multiple pathways. Eur. J. Pharmacol. 651 (1-3), 168–175. doi:10.1016/j.ejphar.2010.10.076
Bonon, A., Mangolini, A., Pinton, P., Del Senno, L., and Aguiari, G. (2013). Berberine slows cell growth in autosomal dominant polycystic kidney disease cells. Biochem. Biophys. Res. Commun. 441 (3), 668–674. doi:10.1016/j.bbrc.2013.10.076
Brusq, J. M., Ancellin, N., Grondin, P., Guillard, R., Martin, S., Saintillan, Y., et al. (2006). Inhibition of lipid synthesis through activation of AMP kinase: An additional mechanism for the hypolipidemic effects of berberine. J. Lipid Res. 47 (6), 1281–1288. doi:10.1194/jlr.M600020-JLR200
Cai, Y., Xin, Q., Lu, J., Miao, Y., Lin, Q., Cong, W., et al. (2021). A new therapeutic candidate for cardiovascular diseases: Berberine. Front. Pharmacol. 12, 631100. doi:10.3389/fphar.2021.631100
Cao, C., and Su, M. (2019). Effects of berberine on glucose-lipid metabolism, inflammatory factors and insulin resistance in patients with metabolic syndrome. Exp. Ther. Med. 17 (4), 3009–3014. doi:10.3892/etm.2019.7295
Chan, M., Qin, Z., Man, S. C., Lam, M., Lai, W. H., Ng, R. M. K., et al. (2022). Adjunctive berberine reduces antipsychotic-associated weight gain and metabolic syndrome in patients with schizophrenia: A randomized controlled trial. Psychiatry Clin. Neurosci. 76 (3), 77–85. doi:10.1111/pcn.13323
Chang, W., Zhang, M., Li, J., Meng, Z., Wei, S., Du, H., et al. (2013). Berberine improves insulin resistance in cardiomyocytes via activation of 5'-adenosine monophosphate-activated protein kinase. Metabolism 62 (8), 1159–1167. doi:10.1016/j.metabol.2013.02.007
Chen, P., Li, Y., and Xiao, L. (2021). Berberine ameliorates nonalcoholic fatty liver disease by decreasing the liver lipid content via reversing the abnormal expression of MTTP and LDLR. Exp. Ther. Med. 22 (4), 1109. doi:10.3892/etm.2021.10543
Chen, Y., Li, Y., Wang, Y., Wen, Y., and Sun, C. (2009). Berberine improves free-fatty-acid-induced insulin resistance in L6 myotubes through inhibiting peroxisome proliferator-activated receptor gamma and fatty acid transferase expressions. Metabolism 58 (12), 1694–1702. doi:10.1016/j.metabol.2009.06.009
Choi, Y. J., Lee, K. Y., Jung, S. H., Kim, H. S., Shim, G., Kim, M. G., et al. (2017). Activation of AMPK by berberine induces hepatic lipid accumulation by upregulation of fatty acid translocase CD36 in mice. Toxicol. Appl. Pharmacol. 316, 74–82. doi:10.1016/j.taap.2016.12.019
Chooi, Y. C., Ding, C., and Magkos, F. (2019). The epidemiology of obesity. Metabolism 92, 6–10. doi:10.1016/j.metabol.2018.09.005
D'Agati, V. D., Chagnac, A., de Vries, A. P., Levi, M., Porrini, E., Herman-Edelstein, M., et al. (2016). Obesity-related glomerulopathy: Clinical and pathologic characteristics and pathogenesis. Nat. Rev. Nephrol. 12 (8), 453–471. doi:10.1038/nrneph.2016.75
Dai, J. G., Huang, X. M., Zhang, C., and Gao, J. M. (2021). Mechanisms of lipid metabolism promoted by berberine via peroxisome proliferator-activated receptor gamma during in vitro maturation of porcine oocytes. Anim. Sci. J. 92 (1), e13582. doi:10.1111/asj.13582
Dai, J., Huang, X., Zhang, C., Luo, X., Cao, S., Wang, J., et al. (2021). Berberine regulates lipid metabolism via miR-192 in porcine oocytes matured in vitro. Veterinary Med. Sci 7 (3), 950–959. doi:10.1002/vms3.393
Derosa, G., Maffioli, P., and Cicero, A. F. (2012). Berberine on metabolic and cardiovascular risk factors: An analysis from preclinical evidences to clinical trials. Expert Opin. Biol. Ther. 12 (8), 1113–1124. doi:10.1517/14712598.2012.704014
Di, S., Han, L., An, X., Kong, R., Gao, Z., Yang, Y., et al. (2021). In silico network pharmacology and in vivo analysis of berberine-related mechanisms against type 2 diabetes mellitus and its complications. J. Ethnopharmacol. 276, 114180. doi:10.1016/j.jep.2021.114180
Ding, B., Geng, S., Hou, X., Ma, X., Xu, H., Yang, F., et al. (2021). Berberine reduces renal cell pyroptosis in golden hamsters with diabetic nephropathy through the nrf2-NLRP3-caspase-1-GSDMD pathway. Evid. Based Complement. Altern. Med. 2021, 5545193. doi:10.1155/2021/5545193
Domitrović, R., Cvijanović, O., Pernjak-Pugel, E., Škoda, M., Mikelić, L., and Crnčević-Orlić, Z. (2013). Berberine exerts nephroprotective effect against cisplatin-induced kidney damage through inhibition of oxidative/nitrosative stress, inflammation, autophagy and apoptosis. Food Chem. Toxicol. 62, 397–406. doi:10.1016/j.fct.2013.09.003
Dong, Z. H., Lin, H. Y., Chen, F. L., Che, X. Q., Bi, W. K., Shi, S. L., et al. (2021). Berberine improves intralipid-induced insulin resistance in murine. Acta Pharmacol. Sin. 42 (5), 735–743. doi:10.1038/s41401-020-0493-4
El-Horany, H. E., Gaballah, H. H., and Helal, D. S. (2020). Berberine ameliorates renal injury in a rat model of D-galactose-induced aging through a PTEN/Akt-dependent mechanism. Arch. Physiol. Biochem. 126 (2), 157–165. doi:10.1080/13813455.2018.1499117
Fang, X., Wu, H., Wang, X., Lian, F., Li, M., Miao, R., et al. (2022). Modulation of gut microbiota and metabolites by berberine in treating mice with disturbances in glucose and lipid metabolism. Front. Pharmacol. 13, 870407. doi:10.3389/fphar.2022.870407
Fang-Ming, Z., Yan-Xiang, G., and Jin-Gang, Z. (2015). Advances in the pathogenesis of hypertensive renal damage. J. China-Japan Friendsh. Hosp. 29 (06), 364–366.
Feng, X., Sureda, A., Jafari, S., Memariani, Z., Tewari, D., Annunziata, G., et al. (2019). Berberine in cardiovascular and metabolic diseases: From mechanisms to therapeutics. Theranostics 9 (7), 1923–1951. doi:10.7150/thno.30787
Gong, M., Duan, H., Wu, F., Ren, Y., Gong, J., Xu, L., et al. (2021). Berberine alleviates insulin resistance and inflammation via inhibiting the LTB4-BLT1 Axis. Front. Pharmacol. 12, 722360. doi:10.3389/fphar.2021.722360
Grundy, S. M. (1990). Management of hyperlipidemia of kidney disease. Kidney Int. 37 (3), 847–853. doi:10.1038/ki.1990.57
Gu, J. J., Gao, F. Y., and Zhao, T. Y. (2012). A preliminary investigation of the mechanisms underlying the effect of berberine in preventing high-fat diet-induced insulin resistance in rats. J. Physiol. Pharmacol. 63 (5), 505–513.
Gu, S., Cao, B., Sun, R., Tang, Y., Paletta, J. L., Wu, X., et al. (2015). A metabolomic and pharmacokinetic study on the mechanism underlying the lipid-lowering effect of orally administered berberine. Mol. Biosyst. 11 (2), 463–474. doi:10.1039/c4mb00500g
Guo, T., Woo, S. L., Guo, X., Li, H., Zheng, J., Botchlett, R., et al. (2016). Berberine ameliorates hepatic steatosis and suppresses liver and adipose tissue inflammation in mice with diet-induced obesity. Sci. Rep. 6, 22612. doi:10.1038/srep22612
Hao, Y., Li, J., Yue, S., Wang, S., Hu, S., and Li, B. (2022). Neuroprotective effect and possible mechanisms of berberine in diabetes-related cognitive impairment: A systematic review and meta-analysis of animal studies. Front. Pharmacol. 13, 917375. doi:10.3389/fphar.2022.917375
Hu, J., Chai, Y., Wang, Y., Kheir, M. M., Li, H., Yuan, Z., et al. (2012). PI3K p55γ promoter activity enhancement is involved in the anti-apoptotic effect of berberine against cerebral ischemia-reperfusion. Eur. J. Pharmacol. 674 (2-3), 132–142. doi:10.1016/j.ejphar.2011.11.014
Hu, Y., and Davies, G. E. (2010). Berberine inhibits adipogenesis in high-fat diet-induced obesity mice. Fitoterapia 81 (5), 358–366. doi:10.1016/j.fitote.2009.10.010
Hua, W., Ding, L., Chen, Y., Gong, B., He, J., and Xu, G. (2007). Determination of berberine in human plasma by liquid chromatography-electrospray ionization-mass spectrometry. J. Pharm. Biomed. Anal. 44 (4), 931–937. doi:10.1016/j.jpba.2007.03.022
Huang, D. N., Wu, F. F., Zhang, A. H., Sun, H., and Wang, X. J. (2021). Efficacy of berberine in treatment of rheumatoid arthritis: From multiple targets to therapeutic potential. Pharmacol. Res. 169, 105667. doi:10.1016/j.phrs.2021.105667
Huang, K., Liu, W., Lan, T., Xie, X., Peng, J., Huang, J., et al. (2012). Berberine reduces fibronectin expression by suppressing the S1P-S1P2 receptor pathway in experimental diabetic nephropathy models. PLoS One 7 (8), e43874. doi:10.1371/journal.pone.0043874
Ibrahim Fouad, G., and Ahmed, K. A. (2021). The protective impact of berberine against doxorubicin-induced nephrotoxicity in rats. Tissue Cell 73, 101612. doi:10.1016/j.tice.2021.101612
Ibrahim, H. N., and Hostetter, T. H. (1997). Diabetic nephropathy. J. Am. Soc. Nephrol. 8 (3), 487–493. doi:10.1681/asn.V83487
Ilyas, Z., Perna, S., Al-Thawadi, S., Alalwan, T. A., Riva, A., Petrangolini, G., et al. (2020). The effect of berberine on weight loss in order to prevent obesity: A systematic review. Biomed. Pharmacother. 127, 110137. doi:10.1016/j.biopha.2020.110137
Kamanna, V. S., Roh, D. D., and Kirschenbaum, M. A. (1998). Hyperlipidemia and kidney disease: Concepts derived from histopathology and cell biology of the glomerulus. Histol. Histopathol. 13 (1), 169–179. doi:10.14670/hh-13.169
Karihaloo, A. (2012). Anti-fibrosis therapy and diabetic nephropathy. Curr. Diab. Rep. 12 (4), 414–422. doi:10.1007/s11892-012-0290-7
Kim, W. S., Lee, Y. S., Cha, S. H., Jeong, H. W., Choe, S. S., Lee, M. R., et al. (2009). Berberine improves lipid dysregulation in obesity by controlling central and peripheral AMPK activity. Am. J. Physiol. Endocrinol. Metab. 296 (4), E812–E819. doi:10.1152/ajpendo.90710.2008
Kong, W. J., Zhang, H., Song, D. Q., Xue, R., Zhao, W., Wei, J., et al. (2009). Berberine reduces insulin resistance through protein kinase C-dependent up-regulation of insulin receptor expression. Metabolism 58 (1), 109–119. doi:10.1016/j.metabol.2008.08.013
Kumar, A., EkavaliChopra, K., Chopra, M., Mukherjee, R., Pottabathini, D. K., and Dhull, D. K. (2015). Current knowledge and pharmacological profile of berberine: An update. Eur. J. Pharmacol. 761, 288–297. doi:10.1016/j.ejphar.2015.05.068
Li, D. (2022). Berberine remodels adipose tissue to attenuate metabolic disorders by activating sirtuin 3. Acta Pharmacol. Sin. 43 (5), 1285–1298. doi:10.1038/s41401-021-00736-y
Li, F., Zhao, Y. B., Wang, D. K., Zou, X., Fang, K., and Wang, K. F. (2016). Berberine relieves insulin resistance via the cholinergic anti-inflammatory pathway in HepG2 cells. J. Huazhong Univ. Sci. Technol. Med. Sci. 36 (1), 64–69. doi:10.1007/s11596-016-1543-5
Li, G. S., Liu, X. H., Li, X. Y., Gao, Z. N., Huang, L., and Liu, Y. L. (2016). Regulations of berberine on gene expression of BMP4 transcriptional pathways to improve visceral white adipose tissues insulin resistance in type 2 diabetic hamsters. Zhongguo Zhong Yao Za Zhi 41 (3), 514–520. doi:10.4268/cjcmm20160326
Li, Q., Huang, Z., Liu, D., Zheng, J., Xie, J., Chen, J., et al. (2021). Effect of berberine on hyperuricemia and kidney injury: A network pharmacology analysis and experimental validation in a mouse model. Drug Des. Devel Ther. 15, 3241–3254. doi:10.2147/dddt.s317776
Li, X. X., Li, C. B., Xiao, J., Gao, H. Q., Wang, H. W., Zhang, X. Y., et al. (2015). Berberine attenuates vascular remodeling and inflammation in a rat model of metabolic syndrome. Biol. Pharm. Bull. 38 (6), 862–868. doi:10.1248/bpb.b14-00828
Li, X., Zhang, X., Xia, J., Zhang, L., Chen, B., Lian, G., et al. (2021). Macrophage HIF-2α suppresses NLRP3 inflammasome activation and alleviates insulin resistance. Cell Rep. 36 (8), 109607. doi:10.1016/j.celrep.2021.109607
Li, Y., Chen, X., Chen, Y., Yu, D., Jiang, R., Kou, X., et al. (2022). Berberine improves TNF-α-induced hepatic insulin resistance by targeting MEKK1/MEK pathway. Inflammation 45 (5), 2016–2026. doi:10.1007/s10753-022-01671-8
Li, Y., Wang, B., Shen, J., Bai, M., and Xu, E. (2020). Berberine attenuates fructose-induced insulin resistance by stimulating the hepatic LKB1/AMPK/PGC1α pathway in mice. Pharm. Biol. 58 (1), 385–392. doi:10.1080/13880209.2020.1756349
Li, Y., Xia, P., Xu, L., Wang, Y., and Chen, L. (2016). A meta-analysis on prehypertension and chronic kidney disease. PLoS One 11 (6), e0156575. doi:10.1371/journal.pone.0156575
Liang, H., and Wang, Y. (2018). Berberine alleviates hepatic lipid accumulation by increasing ABCA1 through the protein kinase C δ pathway. Biochem. Biophys. Res. Commun. 498 (3), 473–480. doi:10.1016/j.bbrc.2018.03.003
Liao, Z., Xie, Y., Zhou, B., Zou, B., Xiao, D., Liu, W., et al. (2020). Berberine ameliorates colonic damage accompanied with the modulation of dysfunctional bacteria and functions in ulcerative colitis rats. Appl. Microbiol. Biotechnol. 104 (4), 1737–1749. doi:10.1007/s00253-019-10307-1
Lin, L., Tan, W., Pan, X., Tian, E., Wu, Z., and Yang, J. (2022). Metabolic syndrome-related kidney injury: A review and update. Front. Endocrinol. (Lausanne) 13, 904001. doi:10.3389/fendo.2022.904001
Liu, D., Zhang, Y., Liu, Y., Hou, L., Li, S., Tian, H., et al. (2018). Berberine modulates gut microbiota and reduces insulin resistance via the TLR4 signaling pathway. Exp. Clin. Endocrinol. Diabetes 126 (8), 513–520. doi:10.1055/s-0043-125066
Liu, L., Liu, J., Huang, Z., Yu, X., Zhang, X., Dou, D., et al. (2015). Berberine improves endothelial function by inhibiting endoplasmic reticulum stress in the carotid arteries of spontaneously hypertensive rats. Biochem. Biophys. Res. Commun. 458 (4), 796–801. doi:10.1016/j.bbrc.2015.02.028
Liu, Q., Tang, J., Chen, S., Hu, S., Shen, C., Xiang, J., et al. (2022). Berberine for gastric cancer prevention and treatment: Multi-step actions on the Correa's cascade underlie its therapeutic effects. Pharmacol. Res. 184, 106440. doi:10.1016/j.phrs.2022.106440
Liu, S., Yu, N., Zhang, X. L., Chen, X. Q., and Tang, L. Q. (2012). Regulatory effect of berberine on unbalanced expressions of renal tissue TGF-beta1/SnoN and smad signaling pathway in rats with early diabetic nephropathy. Zhongguo Zhong Yao Za Zhi 37 (23), 3604–3610.
Liu, X., Li, G., Zhu, H., Huang, L., Liu, Y., Ma, C., et al. (2010). Beneficial effect of berberine on hepatic insulin resistance in diabetic hamsters possibly involves in SREBPs, LXRα and PPARα transcriptional programs. Endocr. J. 57 (10), 881–893. doi:10.1507/endocrj.k10e-043
Liu, Y.-F., Wen, C.-Y.-Z., Chen, Z., Wang, Y., Huang, Y., and Tu, S.-H. (2016). Effects of berberine on NLRP3 and IL-1β expressions in monocytic THP-1 cells with monosodium urate crystals-induced inflammation. Biomed. Res. Int. 2016, 2503703–2503707. doi:10.1155/2016/2503703
Liu, Y., and Liu, S. (2013). Berberine inhibits Wilms' tumor cell progression through upregulation of Wilms' tumor gene on the X chromosome. Mol. Med. Rep. 8 (5), 1537–1541. doi:10.3892/mmr.2013.1665
Lou, T., Zhang, Z., Xi, Z., Liu, K., Li, L., Liu, B., et al. (2011). Berberine inhibits inflammatory response and ameliorates insulin resistance in hepatocytes. Inflammation 34 (6), 659–667. doi:10.1007/s10753-010-9276-2
Lu, Y., Zhang, X., He, J., Dai, Z., Shi, P., Lu, Y., et al. (2022). The effects of berberine on inflammatory markers in Chinese patients with metabolic syndrome and related disorders: A meta‑analysis of randomized controlled trials. Inflammopharmacology 30 (3), 1063–1077. doi:10.1007/s10787-022-00976-2
Ma, Y. G., Liang, L., Zhang, Y. B., Wang, B. F., Bai, Y. G., Dai, Z. J., et al. (2017). Berberine reduced blood pressure and improved vasodilation in diabetic rats. J. Mol. Endocrinol. 59 (3), 191–204. doi:10.1530/JME-17-0014
Ma, Z., Zhu, L., Wang, S., Guo, X., Sun, B., Wang, Q., et al. (2022). Berberine protects diabetic nephropathy by suppressing epithelial-to-mesenchymal transition involving the inactivation of the NLRP3 inflammasome. Ren. Fail 44 (1), 923–932. doi:10.1080/0886022X.2022.2079525
Markus, M. R. P., Ittermann, T., Baumeister, S. E., Huth, C., Thorand, B., Herder, C., et al. (2018). Prediabetes is associated with microalbuminuria, reduced kidney function and chronic kidney disease in the general population: The KORA (Cooperative Health Research in the Augsburg Region) F4-Study. Nutr. Metab. Cardiovasc Dis. 28 (3), 234–242. doi:10.1016/j.numecd.2017.12.005
Ni, W.-J., Ding, H.-H., Zhou, H., Qiu, Y.-Y., and Tang, L.-Q. (2015). Renoprotective effects of berberine through regulation of the MMPs/TIMPs system in streptozocin-induced diabetic nephropathy in rats. Eur. J. Pharmacol. 764, 448–456. doi:10.1016/j.ejphar.2015.07.040
Ni, W. J., Guan, X. M., Zeng, J., Zhou, H., Meng, X. M., and Tang, L. Q. (2022). Berberine regulates mesangial cell proliferation and cell cycle to attenuate diabetic nephropathy through the PI3K/Akt/AS160/GLUT1 signalling pathway. J. Cell Mol. Med. 26 (4), 1144–1155. doi:10.1111/jcmm.17167
Noh, J. W., Jun, M. S., Yang, H. K., and Lee, B. C. (2022). Cellular and molecular mechanisms and effects of berberine on obesity-induced inflammation. Biomedicines 10 (7). doi:10.3390/biomedicines10071739
Och, A., Och, M., Nowak, R., Podgórska, D., and Podgórski, R. (2022). Berberine, a herbal metabolite in the metabolic syndrome: The risk factors, course, and consequences of the disease. Molecules 27 (4). doi:10.3390/molecules27041351
Oshima, N., Shimizu, T., Narukawa, Y., Hada, N., and Kiuchi, F. (2018). Quantitative analysis of the anti-inflammatory activity of orengedokuto II: Berberine is responsible for the inhibition of NO production. J. Nat. Med. 72 (3), 706–714. doi:10.1007/s11418-018-1209-7
Othman, M. S., Safwat, G., Aboulkhair, M., and Abdel Moneim, A. E. (2014). The potential effect of berberine in mercury-induced hepatorenal toxicity in albino rats. Food Chem. Toxicol. 69, 175–181. doi:10.1016/j.fct.2014.04.012
Park, H. J., Jung, E., and Shim, I. (2020). Berberine for appetite suppressant and prevention of obesity. Biomed. Res. Int. 2020, 3891806. doi:10.1155/2020/3891806
Pérez-Rubio, K. G., González-Ortiz, M., Martínez-Abundis, E., Robles-Cervantes, J. A., and Espinel-Bermúdez, M. C. (2013). Effect of berberine administration on metabolic syndrome, insulin sensitivity, and insulin secretion. Metab. Syndr. Relat. Disord. 11 (5), 366–369. doi:10.1089/met.2012.0183
Pirillo, A., and Catapano, A. L. (2015). Berberine, a plant alkaloid with lipid- and glucose-lowering properties: From in vitro evidence to clinical studies. Atherosclerosis 243 (2), 449–461. doi:10.1016/j.atherosclerosis.2015.09.032
Pisciotta, L., Bellocchio, A., and Bertolini, S. (2012). Nutraceutical pill containing berberine versus ezetimibe on plasma lipid pattern in hypercholesterolemic subjects and its additive effect in patients with familial hypercholesterolemia on stable cholesterol-lowering treatment. Lipids Health Dis. 11, 123. doi:10.1186/1476-511x-11-123
Qian, C., Yang, Q., Guo, L., Zhu, H., You, X., Liu, H., et al. (2021). Exercise reduces hyperlipidemia-induced kidney damage in apolipoprotein E-deficient mice. Exp. Ther. Med. 21 (2), 153. doi:10.3892/etm.2020.9585
Ren, G., Guo, J. H., Qian, Y. Z., Kong, W. J., and Jiang, J. D. (2020). Berberine improves glucose and lipid metabolism in HepG2 cells through AMPKα1 activation. Front. Pharmacol. 11, 647. doi:10.3389/fphar.2020.00647
Rong, Q., Han, B., Li, Y., Yin, H., Li, J., and Hou, Y. (2021). Berberine reduces lipid accumulation by promoting fatty acid oxidation in renal tubular epithelial cells of the diabetic kidney. Front. Pharmacol. 12, 729384. doi:10.3389/fphar.2021.729384
Saklayen, M. G. (2018). The global epidemic of the metabolic syndrome. Curr. Hypertens. Rep. 20 (2), 12. doi:10.1007/s11906-018-0812-z
Sha, W., Shen, L., Zhou, L., Xu, D., Yang, J., and Lu, G. (2018). Silencing of CXCL12 performs a protective effect on C5b-9-induced injury in podocytes. Int. Urol. Nephrol. 50 (8), 1535–1544. doi:10.1007/s11255-018-1799-8
Shan, B., Wu, M., Chen, T., Tang, W., Li, P., and Chen, J. (2022). Berberine attenuates hyperuricemia by regulating urate transporters and gut microbiota. Am. J. Chin. Med. 50, 2199–2221. doi:10.1142/S0192415X22500951
Shao, M., Ye, C., Bayliss, G., and Zhuang, S. (2021). New insights into the effects of individual Chinese herbal medicines on chronic kidney disease. Front. Pharmacol. 12, 774414. doi:10.3389/fphar.2021.774414
Shao-Shan, L., Wei-Bo, L., Dan-Dan, L., Hao, C., Feng, X., Hui-Ping, C., et al. (2015). Clinico-pathological characteristics and outcome of patients with biopsy-proven hypertensive nephrosclerosis. J. Nephrol. Dialy. Transplant. 24 (01), 32–37. doi:10.1186/s12882-016-0254-2
Shen, J., Wang, W., Shao, X., Wu, J., Li, S., Che, X., et al. (2020). Integrated analysis of m6A methylome in cisplatin-induced acute kidney injury and berberine alleviation in mouse. Front. Genet. 11, 584460. doi:10.3389/fgene.2020.584460
Shou, J. W., Li, X. X., Tang, Y. S., Lim-Ho Kong, B., Wu, H. Y., Xiao, M. J., et al. (2022). Novel mechanistic insight on the neuroprotective effect of berberine: The role of PPARδ for antioxidant action. Free Radic. Biol. Med. 181, 62–71. doi:10.1016/j.freeradbiomed.2022.01.022
Singh, I. P., and Mahajan, S. (2013). Berberine and its derivatives: A patent review (2009 - 2012). Expert Opin. Ther. Pat. 23 (2), 215–231. doi:10.1517/13543776.2013.746314
Studien, K. E. (1923). Hypertonie-hyperglykamie-hyperurikamiessyndrome. Zentralblatt fur Inn. Med. 44, 105–127.
Suadoni, M. T., and Atherton, I. (2021). Berberine for the treatment of hypertension: A systematic review. Complement. Ther. Clin. Pract. 42, 101287. doi:10.1016/j.ctcp.2020.101287
Sui, M., Jiang, X., Sun, H., Liu, C., and Fan, Y. (2021). Berberine ameliorates hepatic insulin resistance by regulating microRNA-146b/SIRT1 pathway. Diabetes Metab. Syndr. Obes. 14, 2525–2537. doi:10.2147/dmso.S313068
Sun, D., Wang, J., Shao, W., Wang, J., Yao, L., Li, Z., et al. (2020). Pathogenesis and damage targets of hypertensive kidney injury. J. Transl. Int. Med. 8 (4), 205–209. doi:10.2478/jtim-2020-0033
Sun, R., Yang, N., Kong, B., Cao, B., Feng, D., Yu, X., et al. (2017). Orally administered berberine modulates hepatic lipid metabolism by altering microbial bile acid metabolism and the intestinal FXR signaling pathway. Mol. Pharmacol. 91 (2), 110–122. doi:10.1124/mol.116.106617
Sun, S. F., Zhao, T. T., Zhang, H. J., Huang, X. R., Zhang, W. K., Zhang, L., et al. (2015). Renoprotective effect of berberine on type 2 diabetic nephropathy in rats. Clin. Exp. Pharmacol. Physiol. 42 (6), 662–670. doi:10.1111/1440-1681.12402
Sun, S., Yang, Y., Xiong, R., Ni, Y., Ma, X., Hou, M., et al. (2022). Oral berberine ameliorates high-fat diet-induced obesity by activating TAS2Rs in tuft and endocrine cells in the gut. Life Sci. 311, 121141. doi:10.1016/j.lfs.2022.121141
Tang, L. Q., Ni, W. J., Cai, M., Ding, H. H., Liu, S., and Zhang, S. T. (2016). Renoprotective effects of berberine and its potential effect on the expression of β-arrestins and intercellular adhesion molecule-1 and vascular cell adhesion molecule-1 in streptozocin-diabetic nephropathy rats. J. Diabetes 8 (5), 693–700. doi:10.1111/1753-0407.12349
Tian, H., Kang, Y. M., Gao, H. L., Shi, X. L., Fu, L. Y., Li, Y., et al. (2019). Chronic infusion of berberine into the hypothalamic paraventricular nucleus attenuates hypertension and sympathoexcitation via the ROS/Erk1/2/iNOS pathway. Phytomedicine 52, 216–224. doi:10.1016/j.phymed.2018.09.206
Wang, C., Jiang, J. D., Wu, W., and Kong, W. J. (2016). The compound of mangiferin-berberine salt has potent activities in modulating lipid and glucose metabolisms in HepG2 cells. Biomed. Res. Int. 2016, 8753436. doi:10.1155/2016/8753436
Wang, H., Zhang, H., Gao, Z., Zhang, Q., and Gu, C. (2022). The mechanism of berberine alleviating metabolic disorder based on gut microbiome. Front. Cell Infect. Microbiol. 12, 854885. doi:10.3389/fcimb.2022.854885
Wang, Y., Gong, W., Lv, S., Qu, H., and He, Y. (2019). Berberine improves insulin resistance in adipocyte models by regulating the methylation of hypoxia-inducible factor-3α. Biosci. Rep. 39 (10). doi:10.1042/bsr20192059
Wang, Y., Liu, H., Zheng, M., Yang, Y., Ren, H., Kong, Y., et al. (2021). Berberine slows the progression of prediabetes to diabetes in zucker diabetic fatty rats by enhancing intestinal secretion of glucagon-like peptide-2 and improving the gut microbiota. Front. Endocrinol. (Lausanne) 12, 609134. doi:10.3389/fendo.2021.609134
Wang, Z., Wu, F., Zhou, Q., Qiu, Y., Zhang, J., Tu, Q., et al. (2022). Berberine improves vascular dysfunction by inhibiting trimethylamine-N-oxide via regulating the gut microbiota in angiotensin II-induced hypertensive mice. Front. Microbiol. 13, 814855. doi:10.3389/fmicb.2022.814855
Wei, S., Zhang, M., Yu, Y., Lan, X., Yao, F., Yan, X., et al. (2016). Berberine attenuates development of the hepatic gluconeogenesis and lipid metabolism disorder in type 2 diabetic mice and in palmitate-incubated HepG2 cells through suppression of the HNF-4α miR122 pathway. PLoS One 11 (3), e0152097. doi:10.1371/journal.pone.0152097
Wu, X., Li, Q., Xin, H., Yu, A., and Zhong, M. (2005). Effects of berberine on the blood concentration of cyclosporin A in renal transplanted recipients: Clinical and pharmacokinetic study. Eur. J. Clin. Pharmacol. 61 (8), 567–572. doi:10.1007/s00228-005-0952-3
Xia, Q. S., Wu, F., Wu, W. B., Dong, H., Huang, Z. Y., Xu, L., et al. (2022). Berberine reduces hepatic ceramide levels to improve insulin resistance in HFD-fed mice by inhibiting HIF-2α. Biomed. Pharmacother. 150, 112955. doi:10.1016/j.biopha.2022.112955
Xie, W., Gu, D., Li, J., Cui, K., and Zhang, Y. (2011). Effects and action mechanisms of berberine and Rhizoma coptidis on gut microbes and obesity in high-fat diet-fed C57BL/6J mice. PLoS One 6 (9), e24520. doi:10.1371/journal.pone.0024520
Xing, L. J., Zhang, L., Liu, T., Hua, Y. Q., Zheng, P. Y., and Ji, G. (2011). Berberine reducing insulin resistance by up-regulating IRS-2 mRNA expression in nonalcoholic fatty liver disease (NAFLD) rat liver. Eur. J. Pharmacol. 668 (3), 467–471. doi:10.1016/j.ejphar.2011.07.036
Xu, J. H., Liu, X. Z., Pan, W., and Zou, D. J. (2017). Berberine protects against diet-induced obesity through regulating metabolic endotoxemia and gut hormone levels. Mol. Med. Rep. 15 (5), 2765–2787. doi:10.3892/mmr.2017.6321
Xu, J., Zhang, Y., Yu, Z., Guan, Y., Lv, Y., Zhang, M., et al. (2022). Berberine mitigates hepatic insulin resistance by enhancing mitochondrial architecture via the SIRT1/Opa1 signalling pathway. Abbs 54, 1464–1475. doi:10.3724/abbs.2022146
Xu, X., Eales, J. M., Jiang, X., Sanderson, E., Drzal, M., Saluja, S., et al. (2021). Contributions of obesity to kidney health and disease: Insights from mendelian randomization and the human kidney transcriptomics. Cardiovasc Res. 118, 3151–3161. doi:10.1093/cvr/cvab357
Xu, Z., Feng, W., Shen, Q., Yu, N., Yu, K., Wang, S., et al. (2017). Rhizoma coptidis and berberine as a natural drug to combat aging and aging-related diseases via anti-oxidation and AMPK activation. Aging Dis. 8 (6), 760–777. doi:10.14336/AD.2016.0620
Yang, G., Zhao, Z., Zhang, X., Wu, A., Huang, Y., Miao, Y., et al. (2017). Effect of berberine on the renal tubular epithelial-to-mesenchymal transition by inhibition of the Notch/snail pathway in diabetic nephropathy model KKAy mice. Drug Des. Devel Ther. 11, 1065–1079. doi:10.2147/dddt.S124971
Yang, S., Cao, S., Li, C., Zhang, J., Liu, C., Qiu, F., et al. (2022). Berberrubine, a main metabolite of berberine, alleviates non-alcoholic fatty liver disease via modulating glucose and lipid metabolism and restoring gut microbiota. Front. Pharmacol. 13, 913378. doi:10.3389/fphar.2022.913378
Yao, S., Yuan, Y., Zhang, H., Meng, X., Jin, L., Yang, J., et al. (2020). Berberine attenuates the abnormal ectopic lipid deposition in skeletal muscle. Free Radic. Biol. Med. 159, 66–75. doi:10.1016/j.freeradbiomed.2020.07.028
Ye, L., Liang, S., Guo, C., Yu, X., Zhao, J., Zhang, H., et al. (2016). Inhibition of M1 macrophage activation in adipose tissue by berberine improves insulin resistance. Life Sci. 166, 82–91. doi:10.1016/j.lfs.2016.09.025
Yi, P., Lu, F. E., and Chen, G. (2007). Molecular mechanism of berberine in improving insulin resistance induced by free fatty acid through inhibiting nuclear trascription factor-kappaB p65 in 3T3-L1 adipocytes. Zhongguo Zhong Xi Yi Jie He Za Zhi 27 (12), 1099–1104.
Yi, P., Lu, F. E., Xu, L. J., Chen, G., Dong, H., and Wang, K. F. (2008). Berberine reverses free-fatty-acid-induced insulin resistance in 3T3-L1 adipocytes through targeting IKKbeta. World J. Gastroenterol. 14 (6), 876–883. doi:10.3748/wjg.14.876
Yu, M., Alimujiang, M., Hu, L., Liu, F., Bao, Y., and Yin, J. (2021). Berberine alleviates lipid metabolism disorders via inhibition of mitochondrial complex I in gut and liver. Int. J. Biol. Sci. 17 (7), 1693–1707. doi:10.7150/ijbs.54604
Yu, M., Jin, X., Liang, C., Bu, F., Pan, D., He, Q., et al. (2020). Berberine for diarrhea in children and adults: A systematic review and meta-analysis. Ther. Adv. Gastroenterol. 13, 1756284820961299. doi:10.1177/1756284820961299
Yue, S. J., Liu, J., Wang, A. T., Meng, X. T., Yang, Z. R., Peng, C., et al. (2019). Berberine alleviates insulin resistance by reducing peripheral branched-chain amino acids. Am. J. Physiol. Endocrinol. Metab. 316 (1), E73–e85. doi:10.1152/ajpendo.00256.2018
Yun-Kai, B., Hui, L., Hua, X., and Yan-Feng, L. (2002). Observation on renal lesion due to metabolic syndrome. Acad. J. Kunming Med. Coll. 23 (1), 69–71. doi:10.3969/j.issn.1003-4706.2002.01.019
Zhang, G., Lin, X., Shao, Y., Su, C., Tao, J., and Liu, X. (2020). Berberine reduces endothelial injury and arterial stiffness in spontaneously hypertensive rats. Clin. Exp. Hypertens. 42 (3), 257–265. doi:10.1080/10641963.2019.1632339
Zhang, M., Zhang, Y., Xiao, D., Zhang, J., Wang, X., Guan, F., et al. (2020). Highly bioavailable berberine formulation ameliorates diabetic nephropathy through the inhibition of glomerular mesangial matrix expansion and the activation of autophagy. Eur. J. Pharmacol. 873, 172955. doi:10.1016/j.ejphar.2020.172955
Zhang, Q., Xiao, X., Feng, K., Wang, T., Li, W., Yuan, T., et al. (2011). Berberine moderates glucose and lipid metabolism through multipathway mechanism. Evidence-Based Complementary Altern. Med. 2011, 1–10. doi:10.1155/2011/924851
Zhang, S. W., Zhou, J., Gober, H. J., Leung, W. T., and Wang, L. (2021). Effect and mechanism of berberine against polycystic ovary syndrome. Biomed. Pharmacother. 138, 111468. doi:10.1016/j.biopha.2021.111468
Zhang, X. X., Kong, J., and Yun, K. (2020). Prevalence of diabetic nephropathy among patients with type 2 diabetes mellitus in China: A meta-analysis of observational studies. J. Diabetes Res. 2020, 2315607. doi:10.1155/2020/2315607
Zhang, X., Zhao, Y., Zhang, M., Pang, X., Xu, J., Kang, C., et al. (2012). Structural changes of gut microbiota during berberine-mediated prevention of obesity and insulin resistance in high-fat diet-fed rats. PLoS One 7 (8), e42529. doi:10.1371/journal.pone.0042529
Zhao, W., Xue, R., Zhou, Z. X., Kong, W. J., and Jiang, J. D. (2008). Reduction of blood lipid by berberine in hyperlipidemic patients with chronic hepatitis or liver cirrhosis. Biomed. Pharmacother. 62 (10), 730–731. doi:10.1016/j.biopha.2008.01.007
Zheng, H., Lan, J., Li, J., and Lv, L. (2018). Therapeutic effect of berberine on renal ischemia-reperfusion injury in rats and its effect on Bax and Bcl-2. Exp. Ther. Med. 16 (3), 2008–2012. doi:10.3892/etm.2018.6408
Zhou, H., Feng, L., Xu, F., Sun, Y., Ma, Y., Zhang, X., et al. (2017). Berberine inhibits palmitate-induced NLRP3 inflammasome activation by triggering autophagy in macrophages: A new mechanism linking berberine to insulin resistance improvement. Biomed. Pharmacother. 89, 864–874. doi:10.1016/j.biopha.2017.03.003
Zhou, Y., Cao, S., Wang, Y., Xu, P., Yan, J., Bin, W., et al. (2014). Berberine metabolites could induce low density lipoprotein receptor up-regulation to exert lipid-lowering effects in human hepatoma cells. Fitoterapia 92, 230–237. doi:10.1016/j.fitote.2013.11.010
Zhu, L., Han, J., Yuan, R., Xue, L., and Pang, W. (2018). Berberine ameliorates diabetic nephropathy by inhibiting TLR4/NF-κB pathway. Biol. Res. 51 (1), 9. doi:10.1186/s40659-018-0157-8
Keywords: berberine, metabolic syndrome, obesity, diabetic nephropathy, insulin resistance, hyperlipidemia, kidney disease
Citation: Liu Y-F, Wang H-H, Geng Y-H, Han L, Tu S-H and Wang H (2023) Advances of berberine against metabolic syndrome-associated kidney disease: Regarding effect and mechanism. Front. Pharmacol. 14:1112088. doi: 10.3389/fphar.2023.1112088
Received: 30 November 2022; Accepted: 17 January 2023;
Published: 06 February 2023.
Edited by:
Weidong Xie, Tsinghua University, ChinaReviewed by:
Dan Li, Chengdu University of Traditional Chinese Medicine, ChinaQingsheng Liu, Hangzhou Hospital of Traditional Chinese Medicine, China
Copyright © 2023 Liu, Wang, Geng, Han, Tu and Wang. This is an open-access article distributed under the terms of the Creative Commons Attribution License (CC BY). The use, distribution or reproduction in other forums is permitted, provided the original author(s) and the copyright owner(s) are credited and that the original publication in this journal is cited, in accordance with accepted academic practice. No use, distribution or reproduction is permitted which does not comply with these terms.
*Correspondence: Ya-Fei Liu, eWFmZWlsaXV0amhAZ21haWwuY29t