- 1Psychedelics Research Centre, National Institute of Mental Health, Prague, Czechia
- 2Third Faculty of Medicine, Charles University, Prague, Czechia
- 3Forensic Laboratory of Biologically Active Compounds, Department of Chemistry of Natural Compounds, University of Chemistry and Technology, Prague, Czechia
Introduction:N-2-methoxy-benzylated (“NBOMe”) analogues of phenethylamine are a group of new psychoactive substances (NPS) with reported strong psychedelic effects in sub-milligram doses linked to a number of severe intoxications, including fatal ones. In our present work, we provide a detailed investigation of pharmacokinetics and acute behavioural effects of 2C-B-Fly-NBOMe (2-(8-bromo-2,3,6,7-tetrahydrobenzo [1,2-b:4,5-b′]difuran-4-yl)-N-[(2-methoxybenzyl]ethan-1-amine), an analogue of popular psychedelic entactogen 2C-B (4-Bromo-2,5-dimethoxyphenethylamine).
Methods: All experiments were conducted on adult male Wistar rats. Pharmacokinetic parameters of 2C-B-Fly-NBOMe (1 mg/kg subcutaneously; s. c.) in blood serum and brain tissue were analysed over 24 h using liquid chromatography-mass spectrometry (LC/MS). For examination of behavioural parameters in open field test (OFT) and prepulse inhibition (PPI) of acoustic startle reaction (ASR), 2C-B-Fly-NBOMe (0.2, 1 and 5 mg/kg s. c.) was administered in two temporal onsets: 15 and 60 min after administration. Thermoregulatory changes were evaluated in individually and group-housed animals over 8 h following the highest dose used in behavioural experiments (5 mg/kg s. c.).
Results: Peak drug concentrations were detected 30 and 60 min after the drug application in serum (28 ng/ml) and brain tissue (171 ng/g), respectively. The parental compound was still present in the brain 8 h after administration. Locomotor activity was dose-dependently reduced by the drug in both temporal testing onsets. ASR was also strongly disrupted in both temporal onsets, drug’s effect on PPI was weaker. 2C-B-Fly-NBOMe did not cause any significant thermoregulatory changes.
Discussion: Our results suggest that 2C-B-Fly-NBOMe penetrates animal brain tissue in a relatively slow manner, induces significant inhibitory effects on motor performance, and attenuates sensorimotor gating. Its overall profile is similar to closely related analogue 2C-B and other NBOMe substances.
1 Introduction
New psychoactive substances (NPS) have become a persistent problem worldwide. According to the European Monitoring Centre for Drugs and Drug Addiction, it now consists of approximately 880 substances with 52 NPS first reported in Europe by the end of 2021 (EMCDDA, 2022). Clandestine laboratories produce legal, cheap, and easily accessible analogues (often called “bath salts’’, “legal highs” or “research chemicals”), which mimic the effects of established illicit drugs, although they are often more potent compared to parental drugs.
Currently, N-2-methoxybenzyl-substituted (NBOMe) phenethylamines are one of the most frequently appearing NPS on the illicit drugs market. They were initially developed for the research on 5-HT2A receptor-mediated functions (Elz et al., 2002), but soon after they entered the black market. Data from drug-related online forums, such as PsychonautWiki, Erowid or Bluelight, show threshold dose for psychoactive effects as low as 50 μg, recreational doses usually range between 200 and 1,000 μg. They are mostly administered buccally or sublingually via blotting papers; although nasal sprays, pills, insufflation of powder or application of suppositories are also reported to some extent (Lawn et al., 2014). Onset and duration of psychoactive effects strongly depend on the route of administration, but onset is generally fast (15 min after sublingual intake), and the effects can last for up to several hours (8–10 h after sublingual intake). Recreational users on Erowid commonly describe NBOMes as mimicking combined effects of 3,4-methylenedioxymethamphetamine (MDMA) and lysergic acid diethylamide (LSD), with intense visual hallucinations, euphoria, deep empathy, intensive perception of art and music, as well as stimulatory effects with increased heart rate and body temperature. However, their intake already caused severe intoxications including fatal ones, caused either by unintentional ingestion of NBOMe (Suzuki et al., 2014), often marketed as “legal LSD” or milder 2C drugs, or by critical variations of drug content and various impurities on blotter papers (Poklis et al., 2015; Duffau et al., 2016). Highly intoxicated individuals can suffer from anxiety, confusion, panic attacks and paranoia, they can also manifest aggressive and bizarre behaviour. In terms of adverse somatic effects, intoxications with NBOMes can cause seizures, kidney failure, toxic leukoencephalopathy, or show clinical features associated with serotonergic toxicity, such as tachycardia, hypertension, breathing difficulties, convulsions, sweating and overheating, rhabdomyolysis, acute multiorgan failure, heart arrhythmia, fibrillation, and cardiac arrest (Hill et al., 2013; Poklis et al., 2014; Stellpflug et al., 2014; Humston et al., 2017; Richeval et al., 2017). Most frequent fatal poisonings involve 25I-NBOMe, 25B-NBOMe and 25C-NBOMe (Andreasen et al., 2015; Kueppers and Cooke, 2015; Nikolaou et al., 2015; Shanks et al., 2015; Al-Imam, 2018).
It is generally acknowledged that the hallucinogenic properties of drugs are mostly exerted through the activation of cortical 5-HT2A receptors and subsequent glutamate release (Halberstadt, 2015; Schmitz et al., 2022; Wojtas et al., 2022). The addition of N-2-methoxybenzyl moiety to 2C drugs massively increases their binding affinity to 2A and 2C serotonin receptor subtypes, and moreover, binding to adrenergic α1, histaminergic H1, dopaminergic D1-3 receptors and monoamine transporters is also increased. However, affinity and potency of NBOMe analogues seem to be low at 1A and 2B serotonin receptor subtypes (Rickli et al., 2015; Eshleman et al., 2018).
2-(8-bromo-2.3,6,7-tetrahydrobenzo [1,2-b:4,5-b′]difuran-4-yl)-N-[(2-methoxybenzyl)ethan-1-amine (2C-B-Fly-NBOMe, Figure 1) represents a newly arising compound from the NBOMe series. It was first mentioned in a poster describing the development of new and highly potent 5-HT2A receptor agonists and further researched in a dissertation by Ralf Heim (Elz et al., 2002; Heim, 2003). In a positron emission tomography study in Danish Landrace pigs, 2C-B-Fly-NBOMe readily penetrated the brain tissue; it had the highest cortical uptake among the examined NBOMes. Parallel in vitro competition binding study in GF-62 cells showed the highest binding affinity of 2C-B-Fly-NBOMe to 5-HT2A receptor, although its intrinsic activity was surprisingly the lowest (Ettrup et al., 2011). In vitro analysis of its metabolites by Richter et al. (2020) found five phase I metabolites and one phase II metabolite in human hepatocellular carcinoma cells. A recent study from our team describes thirty-five phase I and nine phase II metabolites in three different systems–isolated human liver microsomes, Cunninghamella elegans mycelium and Wistar rats (Nykodemová et al., 2021).
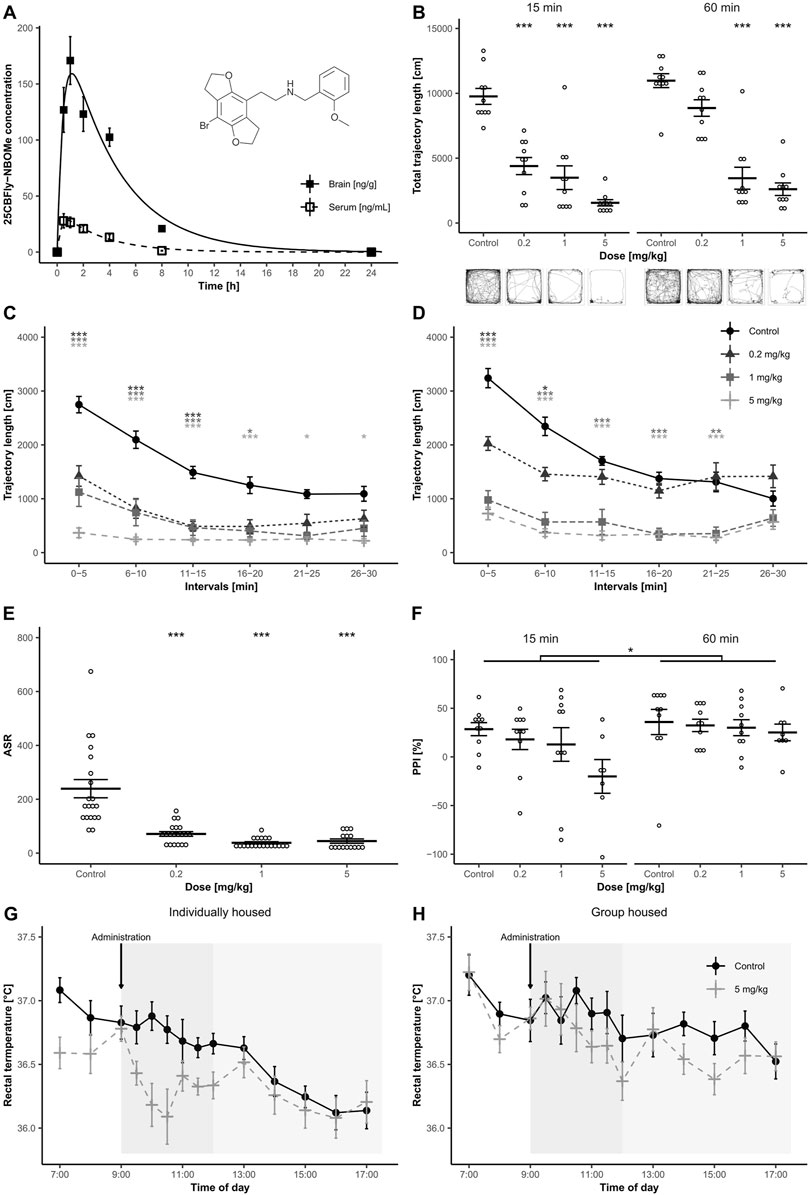
FIGURE 1. (A) Structure of 2C-B-Fly-NBOMe and graph of mean 2C-B-Fly-NBOMe concentration (1 mg/kg s. c.) in serum and brain over 24 h (n = 8 per time point). Error bars display ±1 standard error of the mean (SEM). (B) Total locomotion measured 15 and 60 min after 2C-B-Fly-NBOMe (0.2, 1 and 5 mg/kg s. c.) or ctrl administration (n = 10). Error bars display ±1 SEM, asterisks indicate significant differences from the ctrl group (*p < 0.05, **p < 0.01, ***p < 0.001). Picture inserts below demonstrate trajectory patterns induced by the drug treatment. (C,D): Means of trajectory length within 5 min blocks 15 min (C) and 60 min (D) after administration of 2C-B-Fly-NBOMe (0.2, 1 and 5 mg/kg s. c.) or ctrl. Error bars display ±1 SEM, asterisks indicate significant differences from ctrl (*p < 0.05, **p < 0.01, ***p < 0.001). (E) Mean effect of 2C-B-Fly-NBOMe (0.2, 1 and 5 mg/kg s. c.) on ASR (n = 7–10). Error bars display ±1 SEM, asterisks indicate significant differences from ctrl (*p < 0.05, **p < 0.01, ***p < 0.001). (F) Comparison of mean PPI of ASR values of 2C-B-Fly-NBOMe (0.2, 1 and 5 mg/kg s. c.) and ctrl in 15 min (A) and 60 min (B) temporal onset (n = 7–10). Error bars display ±1 SEM, asterisks indicate significant differences from the ctrl (*p < 0.05, **p < 0.01, ***p < 0.001). (G,H): The effect of 2C-B-Fly-NBOMe (5 mg/kg s. c.) on mean rectal temperature (°C) of individually housed animals (G) and 5 animals (H) per cage (n = 10). 2C-B-Fly-NBOMe or ctrl were administered at 09:00 h. Error bars display ±1 SEM.
Despite two decades have passed, there is still a critical deficit of scientific data concerning this analogue, and to the best of our knowledge, no study has focused on the changes in behavioural and physiological parameters after 2C-B-Fly-NBOMe intake up until now. So far, it is a non-controlled substance in most countries, yet data from online drug forums already suggest apparent interest in 2C-B-Fly-NBOMe, even though consequences of such ingestion are unknown and could be life-threatening, given its presumed high potency.
The aim of this study was thus to determine the pharmacokinetic profile of 2C-B-Fly-NBOMe in serum and brain over a 24 h period, and acute effects on locomotor behaviour in the open field test (OFT), sensorimotor gating in the prepulse inhibition (PPI) of acoustic startle response (ASR) and body temperature in adult male Wistar rats. As this research was part of a larger battery of experiments with NPS, we consequently used a comparable design of two temporal onsets for the behavioural experiments—15 and 60 min after the drug administration. This range is favourable for detecting a wide spectrum of acute behavioural effects. Based on our previous studies with NPS and classical serotonergic psychedelics (Páleníček et al., 2008; Páleníček et al., 2010; Páleníček et al., 2013; Tylš et al., 2016; Šíchová et al., 2022) and the literature evidence from other NBOMe analogues (Gatch et al., 2017; Halberstadt, 2017; Halberstadt and Geyer, 2017; Miliano et al., 2019), we expect that 2C-B-Fly-NBOMe is able to induce an inhibitory or biphasic effect on locomotion, and a deficit in sensorimotor gating with effects being more pronounced at 60 min after administration due to reported gradual onset of action of NBOMe substances. According to a series of case reports describing NBOMe intoxications with signs of serotonin toxicity, we also hypothesize that 2C-B-Fly-NBOMe induces hyperthermia, especially in group-housed animals–a laboratory setting mimicking crowded dance club conditions, allowing to explore possible aggregation toxicity of the investigated drug (Fantegrossi et al., 2003; Saadat et al., 2005).
2 Materials and methods
2.1 Animals
All experiments were carried out on adult male Wistar rats (Velaz, Prague, Czechia). Animals were housed in pairs in controlled conditions (12/12 h light/dark regime with light phase from 6:00 h to 18:00 h, temperature 22°C ± 2°C, humidity 30%–70%) with access to standard diet and water ad libitum. Rats were given an acclimatization period of 1 week prior to the start of each experiment, during which they were weighed twice and handled four times; the rats were approximately 9 weeks old and weighed approx. 200–250 g at the time of testing. Each experimental group consisted of 10 rats based on our previous studies with a standardized protocol for comparable data (Rohanová et al., 2008; Páleníček et al., 2013; Šíchová et al., 2022), and each subject was tested only once according to the principle of acute behavioural testing, except for the pharmacokinetic studies–to minimize the number of sacrificed animals, rats from behavioural experiments treated with saline and 1 mg/kg of 2C-B-Fly-NBOMe were used for the determination of drug concentrations in brain and serum. Experiments were conducted during the light phase of the cycle; animals were brought to the testing rooms with a controlled temperature (22°C ± 2°C) 1 h before the start of each experiment for acclimatization. All experiments respected the Guidelines of the European Union (86/609/EU) and the protocol was approved by the National Committee for the Care and Use of Laboratory Animals (Czechia) under no. 59449/2016-MZE-17214.
2.2 Drugs and chemicals
2C-B-Fly-NBOMe was synthesized, purified, and subsequently converted to 2C-B-Fly-NBOMe hydrochloride by the University of Chemistry and Technology in Prague at the Forensic Laboratory of Biologically Active Substances. The resulting hydrochloride was certified to be of 99% purity (analysed by high-performance liquid chromatography) and served as a reference standard for pharmacokinetic analyses using liquid chromatography/mass spectrometry (LC/MS). Chemicals used in a LC/MS analysis were of LC/MS grade and together with other necessary chemicals purchased from Merck (Czechia). Ultrapure water, 18.2 MΩ/cm, was produced by the Smart2Pure 12 UV system (Thermo Scientific, Germany). Homogenization beads, SiLibeads, type ZY 0.4–0.6 mm, were supplied by Ginzel (Czechia). Stock solutions of analytical standards were prepared in methanol and stored at −20°C. 2C-B-Fly-NBOMe was stored in a dry, dark place and then dissolved in deionized water and 20 μl of Tween 20 just before the experiments. The highest dose was briefly dissolved using an ultrasonic homogenizer due to poor solubility.
2.3 Dosage
Based on previously published behavioural studies with 25B-NBOMe, 25C-NBOMe and 25I-NBOMe (Halberstadt and Geyer, 2014; Elmore et al., 2018; Nakamura et al., 2018; Miliano et al., 2019) our experience with related compound 25CN-NBOMe (Šíchová et al., 2022), and reports on selectivity and potency of 2C-B-Fly-NBOMe on 5-HT2A receptors, a range of three concentrations was estimated for the experiments: 0.2, 1 and 5 mg/kg s. c. dissolved and administered in a total volume of 2 ml/kg. An equivalent volume (2 ml/kg) of deionized water and 20 μl of Tween 20 was used as negative control (ctrl).
2.4 Pharmacokinetic analysis
For the pharmacokinetic study, rats were treated with 1 mg/kg s. c. of 2C-B-Fly-NBOMe and then sacrificed by decapitation after 0.5, 1, 2, 4, 8 and 24 h (n = 8 per time point). Collected blood was left to clot in a refrigerator for 1 h, then centrifuged for 10 min at 1500 g, 10°C, and the separated serum was collected. Whole brains were excised manually using surgical instruments. All serum and brain samples were stored at −20°C until the analysis.
2.4.1 Determination of 2C-B-fly-NBOMe levels in serum and brain samples using LC/MS
Serum pre-treatment
200 μl of blood serum was diluted in a microtube with 200 μl of 1% ammonia solution and homogenized in a Bullet Blender tissue homogenizer (speed 2, time 5 min). Next, 400 μl of ethyl acetate was added by the automatic dispenser and the whole content was homogenized again (speed 3, time 5 min), additional 400 μl of ethyl acetate was added and the sample was homogenized for the last time. The homogenate was centrifuged (10 min, 25°C, 12,000 RPM), 600 μl of the top layer was transferred into a new microtube and 50 μl of 10% formic acid solution was added. After proper shaking, the solution was evaporated in the CentriVap concentrator. The residue was then dissolved in 100 μl of methanol and shaken for 10 min. Prior to the analysis, the solution was diluted 1:1 in deionized water, mixed and centrifuged.
Tissue pre-treatment
100 µL of 1% ammonium hydroxide solution was added to 200 mg of brain tissue. A mixture was homogenized with 200 mg of zirconium oxide beads in a BulletBlender for 10 min (speed 5). 400 μL of ethyl acetate was added to the mixture and homogenized (BulletBlender, speed 3, time 5 min). Additional 400 µL of ethyl acetate was added to the mixture and homogenized (BulletBlender, speed 3, time 5 min). Samples were centrifuged (10 min, 25°C, 12,000 RPM), 400 µL of supernatant was acidified and completely evaporated (Centrivap Concentrator). The dry residue was reconstituted with 400 µL of methanol. Prior to the analysis, 250 µL of the solution was diluted with 750 µL of water, mixed, and centrifuged (0.2 µm PVDF filter).
LC/MS conditions
The samples were analysed using ultra-high performance liquid chromatography-tandem mass spectrometry instrumentation (1,290 Infinity—6,460 Triple Quadrupole LC/MS Agilent Technologies with AJS electrospray ionization source). A column Kinetex Biphenyl (100 × 2.1 mm, 2.6 µm) with a pre-column was used for a chromatographic separation at 35°C with gradient elution in a system of 5 mM ammonium formate with 0.01% (v/v) formic acid (mobile phase A) and methanol with 0.01% (v/v) formic acid (mobile phase B). The gradient started at 10% B, increased from 10% to 50% B in 0.5 min and from 50% to 100% B in 1.5 min, held for 2 min at 100% B, decreased to 10% B in 0.5 min and held for 2 min at 10% B. Data were acquired in positive electrospray ionization (ESI) mode using a multiple reaction monitoring method, one quantification ion and three confirmation ions were used. 2C-B-Fly-NBOMe was quantified using an external matrix-matched calibration. The limit of quantification was 1 ng/ml (1 ng/g for brain tissue). The matrix-matched calibration was performed in duplicates; real samples were also processed in duplicates.
2.5 Behavioural experiments
2.5.1 Open field test
OFT was performed in a black square plastic arena (80 × 80 × 40 cm) in an evenly lit and sound-proof testing room. Rats were individually placed into the centre and allowed to explore the arena for 30 min while their behaviour was recorded. The experimental arena was cleaned with 70% ethanol after each subject. Arena was virtually divided into a 5 × 5 grid of identical squares; 16 squares were located next to the walls (peripheral zone) and 9 squares were in the centre (central zone). Software EthoVision XT 14.0 software (Noldus, Netherlands) was used to capture raw data used in the calculation of the following dependent variables: trajectory length (cm; corrected for deviations of <3 cm) and its temporal dynamics in 5 min intervals; thigmotaxis (∑fperipheral zones/∑fall zones, f = frequency of appearance in the zone) reflecting the probability of appearance in the peripheral zone; Tcenter reflecting time spent in the centre of the arena (∑timecentral zones).
2.5.2 Prepulse inhibition of acoustic startle response
The effect of 2C-B-Fly-NBOMe on sensorimotor gating was evaluated by a PPI of ASR test. Two identical startle chambers (SR-LAB, San Diego Instruments, California) were used, each containing a sound-proof, evenly lit and ventilated enclosure with a plexiglass stabilimeter (8.7 cm inner diameter), a piezoelectric accelerometer for detecting amplitudes of the ASR, and a high-frequency loudspeaker producing acoustic stimuli. Amplitudes of the ASR were digitized for subsequent analysis. The experimental design was based on our previous studies (Štefková et al., 2017; Šíchová et al., 2018). Two days before the experiment, the animals were habituated to the startle chambers with a background white noise (75 dB) for 5 min, followed by six presentations of pulse-alone stimuli (125 dB, 40 ms) with pseudorandomized inter-stimulus intervals. The test itself comprised a habituation period (5 min, 75 dB), followed by six pulse-alone stimuli (125 dB, 40 ms) for establishing baseline ASR; then 60 trials delivered in pseudorandomized order: (A) pulse alone: 125 dB, 40 ms; (B) prepulse-pulse: 83 or 91 dB; 20 ms prepulse; variable (30, 60, or 120 ms) inter-stimulus interval (ISI) (mean 70 ms), followed by 125 dB, 40 ms pulse; and (C) no stimulus. Finally, six pulse-alone trials (125 dB, 40 ms) were delivered.
Habituation was calculated by the percentage reduction in the ASR from the initial six baseline trials to the final six trials. PPI was calculated as [100 - (mean response for the prepulse - pulse trial/startle response for the single pulse trials) × 100].
Animals with mean ASR response lower than 10 arbitrary units were excluded from the analysis as non-responders.
2.6 Thermoregulation
For the assessment of rectal temperature changes, rats were housed individually or in groups of five per cage. They were treated with 2C-B-Fly-NBOMe (5 mg/kg) or ctrl solution s. c. at 9:00 h. During the measurements, each rat was briefly immobilized in a plexiglass tube and then returned to its home cage. In total, 14 measurements were performed: 3 hourly measurements (07:00–09:00 h) for setting the baseline temperature, and then 4 subsequent 30 min measurements (09:30–11:00 h) and 6 hourly measurements (12:00–17:00 h).
2.7 Design and statistical analysis
Statistical analyses were performed in STATISTICA (version 13.3, StatSoft, Inc.), R software (version 4.0.5) and R Studio (version 1.4.1717). Tests used a default alpha set at p = 0.05, two-tailed.
For the pharmacokinetic data, a one-compartment model with a first-order absorption equation (Wijnand, 1988) was fitted using a cubic spline interpolation:
Where C = 2C-B-Fly-NBOMe concentration, t = time, D = dose, ka = absorption rate constant, F = bioavailability, V = volume of distribution, ke = elimination rate constant.
The half-life of the drug elimination was calculated using Package PKNCA version 0.9.4 implemented in R software (Buckeridge et al., 2015).
Behavioural and thermoregulatory data were analysed using analysis of variance (ANOVA) with a factorial design. Significant main effects and inter-factor interactions were followed by pairwise comparison using Tukey post hoc test.
Locomotor activity in OFT (mean trajectory length across 5 min blocks) was evaluated using a 2 × 4 × 6 mixed factorial ANOVA with temporal testing onset (15 or 60 min) and drug treatment (2C-B-Fly-NBOMe 0.2, 1, or 5 mg/kg or ctrl) as between-subjects factors, and time blocks (6 × 5 min intervals) as a within-subjects factor. Spatial distribution of animal movement in OFT (thigmotaxis and Tcenter) and PPI parameters (habituation, ASR and % of PPI) were each analysed using a 2 × 4 factorial ANOVA with temporal testing onset (15 or 60 min) and drug treatment (2C-B-Fly-NBOMe 0.2, 1, or 5 mg/kg or ctrl) as between-subjects factors.
Data from thermoregulatory experiments were analysed using 2 × 2 × 14 mixed factorial design with drug treatment (2C-B-Fly-NBOMe at 5 mg/kg or ctrl) and home cage conditions (individually and group-housed animals) as between-subjects factors and time (14 measurements) as a within-subjects factor. The baseline temperatures of animals were compared in the same manner.
3 Results
3.1 Pharmacokinetics
Maximum mean 2C-B-Fly-NBOMe concentration was attained within 0.5 in the blood serum (28 ng/ml) and 1 h in the brain (171 ng/g) with a half-life of elimination of 1.56 h and 2.40 h, respectively. The drug was almost undetectable in the serum after 8 h, while it was still present in the brain tissue (21 ng/g; Figure 1A). More pharmacological parameters are shown in Table 1.
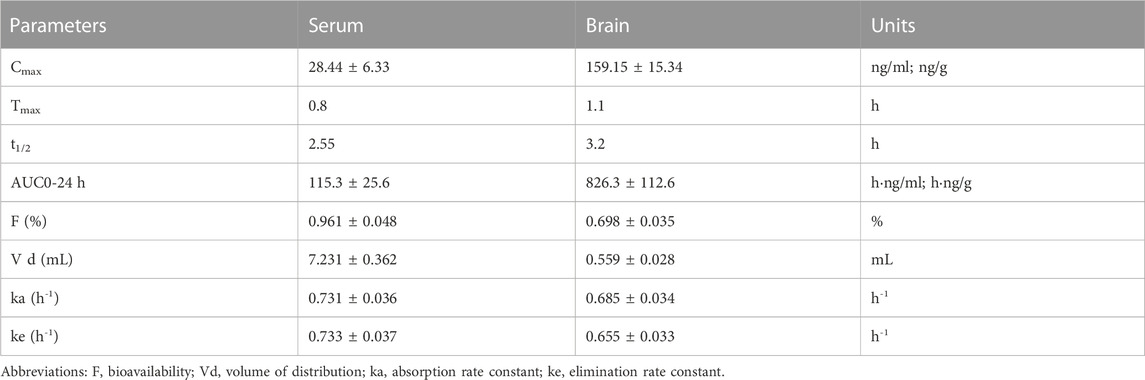
TABLE 1. Overview of pharmacokinetic parameters after 1 mg/kg s. c. 2C-B-Fly-NBOMe application. Data are presented as mean ± SEM.
3.2 Open field test
Analysis of total trajectory length revealed a main effect of drug treatment [F (3.72) = 64.91, p < 0.001], temporal onset [F (1,72) = 13.42, p < 0.001], as well as their interaction [F (3,72) = 4.55, p < 0.01]. Post hoc tests revealed significant differences in all remaining groups versus ctrl except for 0.2 mg/kg of 2C-B-Fly-NBOMe in the 60 min onset.
Further analysis of locomotion in 5 min time blocks displayed a significant main effect of time blocks [F (5,360) = 86.72, p < 0.001], and interactions of time blocks × treatment [F (15,360) = 16.01, p < 0.001], however triple interaction, treatment × testing onset × time blocks was not significant. Locomotor habituation was dose-dependently disturbed in all 2C-B-Fly-NBOMe groups in both testing onsets. Compared to ctrl, at the 15 min temporal onset, all the doses significantly decreased activity in the first three blocks (p < 0.001). In the 1 mg/kg group, the effect persisted until the fourth block (p < 0.05). The highest dose of 5 mg/kg caused significant hypolocomotion during the whole session (p < 0.05–0.001) (Figure 1C). At 60 min testing onset, all groups treated with 2C-B-Fly-NBOMe displayed reduced activity in the first two blocks (p < 0.05–0.001), which persisted to the fifth block in 1 mg/kg and 5 mg/kg groups (p < 0.01–0.001, Figure 1D). No significant differences were observed between the groups in the sixth block. For a graph of total locomotion and visualisation of trajectory patterns, see Figure 1B. Both thigmotaxis and Tcentre were not affected either by treatment, testing onset or their interaction.
3.3 PPI of ASR
Three animals from the 5 mg/kg group in 15 min onset and two animals from 5 mg/kg in 60 min onset were excluded from the analysis because of low mean ASR values. Habituation was significantly affected by drug treatment [F (3,67) = 2.79, p < 0.05], but not by temporal onset or by the interaction of these factors. ASR was significantly affected by drug treatment [F (3,67) = 25.11, p < 0.001], but not by testing onset or interaction of these two factors. Post hoc test revealed that all doses of 2C-B-Fly-NBOMe significantly diminished ASR in comparison to ctrl (p < 0.001; Figure 1E). The percentage of PPI was significantly affected by testing onset [F (1, 67) = 6.65, p < 0.05], but not by treatment or by temporal onset × treatment interaction (Figure 1F).
3.4 Body temperature
Baseline body temperatures did not significantly differ between the groups. A significant effect of the treatment on body temperature was observed [F (1,36) = 7.00, p < 0.05]. Furthermore, the home-cage condition factor was significant [F (1,36) = 14.79, p < 0.001]. The interaction of time x home-cage condition was also significant [F (13,468) = 1.98, p < 0.05], triple interaction of time x home-cage condition x time of measurement was not significant. Despite the hypothermic trend in individually housed animals during the first 90 min after s. c. application (10:00–10:30, Figure 1G), the statistical difference was not significant.
4 Discussion
2C-B-Fly-NBOMe rapidly entered the blood system; peak concentrations were detected 30 min and 1 h after the application in serum and brain, respectively. The drug was markedly present in the brain tissue for up to 8 h 2C-B-Fly-NBOMe induced strong and dose-dependent decrease of locomotor activity and marked disruption of ASR throughout the testing window (at 15 as well as 60 min testing onsets), but also a disruption of PPI in 15 min testing onset. In contrast, the animals did not demonstrate any behaviour generally acknowledged as a typical phenotype connected to 5-HT2A receptor activation by psychedelics (head-twitch response or wet dog shakes).
4.1 Pharmacokinetics
In our previous study, parental drug 2C-B (50 mg/kg s. c.) showed a similar pharmacokinetic profile, with peak concentrations attained within 30 min in blood serum and 60 min in brain, liver, and lung tissue. Presence of 2C-B was detectable for up to 5 h in all collected tissues (Rohanová et al., 2008). A recent investigation of two novel “FLY” drugs (1 mg/kg, peroral administration) by Baralla et al. (2019) showed their peak concentrations in 30 and 60 min for 1-(2,3,6,7-tetra-hydrofuro [2,3-f][1]benzofuran-4-yl)propan-2-amine (FLY) and 2-(2,3,6,7-tetrahydrofuro [2,3-f][1]benzofuran-4-yl)ethanamine (2C-FLY), respectively, in rat blood serum, with expressly distinct peak values. Our results show a markedly higher concentration of 2C-B-Fly-NBOMe in the brain tissue compared to the blood serum, suggesting that 2C-B-Fly-NBOMe readily passes through the blood-brain barrier. The long presence of 2C-B-Fly-NBOMe in the brain tissue is supportive of the prolonged behavioural and physiological effects of NBOMes in humans. Indeed, self-reports of recreational users declare peak effects of various NBOMes within 45–90 min and duration for up to 8 h (Lawn et al., 2014). Our recent study of closely related substance 25CN-NBOMe determined half-life values of 1.88 h for the blood serum and 2.28 h for the brain tissue, however, in a five-fold higher applied dose of 5 mg/kg (Šíchová et al., 2022). A higher half-life value of 2C-B-Fly-NBOMe in the brain might indicate its slower turnover and possibly a longer mechanism of action of the drug. Explanation of the concentration peak after 1 h in the brain tissue compared to the strong behavioural effects observed already 15 min after the drug administration may lay in a formation of potentially active metabolites (Stellpflug et al., 2014), after all, recent studies focused on 2C-B-Fly-NBOMe observed massive process of 2C-B-Fly-NBOMe’s metabolization (Richter et al., 2020; Nykodemová et al., 2021).
4.2 Locomotor activity
Several studies observed a biphasic effect of psychedelics such as LSD (Adams and Geyer, 1982), 2C-B (Páleníček et al., 2013) and mescaline (Páleníček et al., 2008). Generally, this effect can be described as the drug’s ability to act differently in low and high doses, as well as in different temporal onsets. These mechanisms presumably involve differential 5-HT2A/2C receptor activation and subsequent alteration of dopaminergic neurotransmission, as dopamine is the key neurotransmitter responsible for motor control (Páleníček et al., 2013). 5-HT2A receptors are most likely involved in the onset of hyperlocomotion (Ninan and Kulkarni, 1998; Halberstadt et al., 2013), whereas 5-HT2C receptors possibly mediate the inhibitory effect on locomotion (Bagdy et al., 2001; Harvey-Lewis et al., 2016). In a recent study, 25I-NBOMe induced a biphasic effect after s. c. application, but interestingly not after i. p. injection (Halberstadt, 2017). The dose of 0.1 mg/kg s. c. induced significant hyperlocomotion in 60 min temporal onset, whereas 3 mg/kg s. c. caused hypolocomotion in mice. Other examinations imply that NBOMe substances, namely 25B-NBOMe, 25C-NBOMe and 25I-NBOMe, when administered i. p., cause a dose-dependent decrease in locomotor activity (Gatch et al., 2017; Wojtas et al., 2021), which is in correlation to our observation. 25CN-NBOMe manifested a similar, yet less pronounced decrease in locomotion (Šíchová et al., 2022). It is of note that the inhibitory effect of 2C-B-Fly-NBOMe was so prominent that the animals were unable to exhibit typical thigmotactic behaviour, especially in higher doses.
4.3 PPI of ASR
Activation of 5-HT2A receptors leads to a disruption of sensorimotor gating (Sipes and Geyer, 1997), and serotonergic 5-HT1A and 5-HT2C receptors may also be of importance in this mechanism (Krebs-Thomson et al., 2006). Interestingly, a biphasic effect on PPI can be observed, depending on the dose or the temporal onset (Davis and Walters, 1977; Páleníček et al., 2013). Examination of 25I-NBOMe by Miliano et al. (2019) revealed a significant decrease of ASR in the highest dose (1 mg/kg i. p.) and dose-dependent inhibition of PPI in rats. Similarly, 25CN-NBOH also showed tendencies to disrupt PPI in rats (Halberstadt and Geyer, 2017). 25CN-NBOMe affected PPI only in the highest dose of 5 mg/kg in the 15 min onset (Šíchová et al., 2022). According to our data, 2C-B-Fly-NBOMe strongly attenuates ASR even in the lowest dose of 0.2 mg/kg, which is fully in line with our previous observations with 2C-B. Interestingly, the effect on PPI was not very prominent–rather than showing any significant effect of the drug, the results might stem from the different temporal onsets and animals’ reactions to the irritating environment.
4.4 Thermoregulatory effect
Generally, 5-HT2A receptor agonists cause elevations in body temperature, which can lead to severe hyperthermia as one of the main symptoms of serotonin syndrome (Menon and Dandiya, 1969; Murakami and Sakata, 1980; Gudelsky et al., 1986; Matuszewich and Yamamoto, 2003). According to available data from case studies of severe intoxications, NBOMe substances indeed induce hyperthermia, occasionally up to the point where active cooling of the patient’s body must be performed (Andreasen et al., 2015; Gee et al., 2016). This effect is possibly caused by their agonism on α-adrenergic receptors, leading to vasoconstriction (Tang et al., 2014). A study of 25B-NBOMe [(0.25 intraperitoneally (i. p.)] in rats suggested direct involvement of peripheral 5-HT2A receptors in hyperthermia, but only in higher ambient temperature (29°C; Nakamura et al., 2018). In another study made by Miliano et al. (2019), a medium dose of 25I-NBOMe (0.5 mg/kg i. p.) induced hyperthermia within 35–65 min in female rats, but not in males. In contrast, we observed a trend of hypothermic reaction in individually housed animals, but not in animals housed in groups. A significant hypothermic effect was observed after 25CN-NBOMe application (5 mg/kg) in individually-housed animals (Šíchová et al., 2022). Thus, there might be a different molecular mechanism of action connected to other receptors’ agonism. It is known that 5-HT1A receptors cause a decrease in body temperature (Papeschi et al., 1971; Gudelsky et al., 1986) however, according to the binding study with similar NBOMe compounds by Rickli et al. (2015), affinity to this receptor subtype is considerably diminished. Another explanation may be in the modulation of different receptors involved in thermoregulatory processes. An extreme attenuation of the movement caused by the drug could be an important factor in these results, possibly in combination with circadian rhythms, as rats’ body temperature tends to decline during the daytime (Kittrell and Satinoff, 1986). The question remains whether the results were influenced by the stress induced by repeated measurements of the animals’ temperature, although other studies from our group using the same method had shown demonstrable effects of drugs on body temperature (Páleníček et al., 2011; Štefková et al., 2017; Šíchová et al., 2022).
In conclusion, 2C-B-Fly-NBOMe is a substance with a very similar profile to 25CN-NBOMe, and to some extent other drugs from the NBOMe family, when compared with other preclinical studies. As expected, the drug readily crossed the blood-brain barrier and persisted in the system for several hours, similar to human reports. In contrast to the reports of recreational users with NBOMe compounds usually enhancing physical activity almost to the state of agitation, 2C-B-Fly-NBOMe caused strong inhibitory effects in our study. Thermoregulation also appears to be affected differently in rats compared to humans, suggesting different patterns of action in different species or a significant role of environmental factors.
Data availability statement
The raw data supporting the conclusions of this article will be made available by the authors, without undue reservation.
Ethics statement
The animal study was reviewed and approved by Hana Tejkalová, Klára Šíchová, and Tomáš Petrásek from the National Institute of Mental Health.
Author contributions
TP, MK and JH were responsible for the study concept and design. MK was responsible for the synthesis of the studied drug. KH performed and analysed the pharmacokinetic data. KS, EL, KŠ, HD, NP-L and LO-L substantially contributed to the acquisition, analysis and interpretation of behavioural data. PJ and ČV created the figures, assisted the data analysis and the interpretation of our findings. KS drafted the manuscript. TP, KŠ and MK provided critical revision of the manuscript for important intellectual content. All of the authors made a significant contribution to this study, read, revised and gave final approval for the current version of the work to be published.
Funding
This work was supported by grant from the Czech Science Foundation (project 20-25349S), Czech Health Research Council (project NU21-04-00307), Long-term conceptual development of research organization (RVO 00023752), Ministry of the interior of the Czech Republic (project VK01010212), Specific University Research, Czech Ministry of Education, Youth and Sports (project 260533/SVV/2022), programme Cooperatio, Neuroscience Charles University and private funds obtained via Foundation for Psychedelic Research (https://psyres.eu).
Conflict of interest
TP and JH declare to have shares in “Psyon s.r.o.,” and in “Společnost pro podporu neurovědního výzkumu s.r.o.” They founded “PSYRES–Psychedelic Research Foundation”. TP also reports consulting fees from GH Research and CB21-Pharma outside the submitted work. TP and JH are involved in Compass Pathways trials with psilocybin and MAPS clinical trial with MDMA outside the submitted work.
Publisher’s note
All claims expressed in this article are solely those of the authors and do not necessarily represent those of their affiliated organizations, or those of the publisher, the editors and the reviewers. Any product that may be evaluated in this article, or claim that may be made by its manufacturer, is not guaranteed or endorsed by the publisher.
References
Adams, L. M., and Geyer, M. A. (1982). LSD-induced alterations of locomotor patterns and exploration in rats. Psychopharmacol. Berl. 77, 179–185. doi:10.1007/BF00431945
Al-Imam, A. (2018). 25b-NBOMe: A case report of sudden death and insightful view of Google trends data. Iran. J. Psychiatry Behav. Sci. 12, 1–5. doi:10.5812/ijpbs.9870
Andreasen, M. F., Telving, R., Rosendal, I., Eg, M. B., Hasselstrøm, J. B., and Andersen, L. V. (2015). A fatal poisoning involving 25C-NBOMe. Forensic Sci. Int. 251, e1–e8. doi:10.1016/j.forsciint.2015.03.012
Bagdy, G., Graf, M., Anheuer, Z. E., Modos, E. A., and Kantor, S. (2001). Anxiety-like effects induced by acute fluoxetine, sertraline or m-CPP treatment are reversed by pretreatment with the 5-HT2C receptor antagonist SB-242084 but not the 5-HT1A receptor antagonist WAY-100635. Int. J. Neuropsychopharmacol. 4, 399–408. doi:10.1017/s1461145701002632
Baralla, E., Nieddu, M., Burrai, L., Varoni, M. V., Demontis, M. P., and Boatto, G. (2019). LC-MS/MS analysis of two new designer drugs (FLY serie) in rat plasma and its application to a pharmacokinetic study. Leg. Med. 38, 58–63. doi:10.1016/j.legalmed.2019.04.004
Buckeridge, E. M., Bull, A. M. J., and McGregor, A. H. (2015). Biomechanical determinants of elite rowing technique and performance. Scand. J. Med. Sci. Sports 25, e176–e183. doi:10.1111/sms.12264
Davis, M., and Walters, J. (1977). Psilocybin: Biphasic dose-response effects on the acoustic startle reflex in the rat. Pharmacol. Biochem. Behav. 6, 427–431. doi:10.1016/0091-3057(77)90180-0
Duffau, B., Camargo, C., Kogan, M., Fuentes, E., and Cassels, B. K. (2016). Analysis of 25 C NBOMe in seized blotters by HPTLC and GC-MS. J. Chromatogr. Sci. 54, 1153–1158. doi:10.1093/chromsci/bmw095
Elmore, J. S., Decker, A. M., Sulima, A., Rice, K. C., Partilla, J. S., Blough, B. E., et al. (2018). Comparative neuropharmacology of N-(2-methoxybenzyl)-2,5-dimethoxyphenethylamine (NBOMe) hallucinogens and their 2C counterparts in male rats. Neuropharmacology 142, 240–250. doi:10.1016/j.neuropharm.2018.02.033
Elz, S., Kläss, T., Heim, R., Warnke, U., and Pertz, H. (2002). Development of highly potent partial agonists and chiral antagonists as tools for the study of 5-HT2A-receptor meidated functions. Naunyn. Schmiedeb. Arch. Pharmacol. 365, R29. doi:10.1007/s00210-002-0604-4
EMCDDA (2022). European monitoring centre for drugs and drug addiction. Available at: https://www.emcdda.europa.eu/publications/edr/trends-developments/2022_en/ (last accessed 5th February 2023).
Eshleman, A. J., Wolfrum, K. M., Reed, J. F., Sunyoung, K. O., Johnson, R. A., and Janowsky, A. (2018). Neurochemical pharmacology of psychoactive substituted N-benzylphenethylamines: High potency agonists at 5-ht2a receptors. Biochem. Pharmacol. 158, 27–34. doi:10.1016/j.bcp.2018.09.024
Ettrup, A., Hansen, M., Santini, M. A., Paine, J., Gillings, N., Palner, M., et al. (2011). Radiosynthesis and in vivo evaluation of a series of substituted 11C-phenethylamines as 5-HT2A agonist PET tracers. Eur. J. Nucl. Med. Mol. Imaging 38, 681–693. doi:10.1007/s00259-010-1686-8
Fantegrossi, W. E., Godlewski, T., Karabenick, R. L., Stephens, J. M., Ullrich, T., Rice, K. C., et al. (2003). Pharmacological characterization of the effects of 3,4-methylenedioxymethamphetamine (“ecstasy”) and its enantiomers on lethality, core temperature, and locomotor activity in singly housed and crowded mice. Psychopharmacol. Berl. 166, 202–211. doi:10.1007/s00213-002-1261-5
Gatch, M. B., Dolan, S. B., and Forster, M. J. (2017). Locomotor and discriminative stimulus effects of four novel hallucinogens in rodents. Behav. Pharmacol. 28, 375–385. doi:10.1097/FBP.0000000000000309
Gee, P., Schep, L. J., Jensen, B. P., Moore, G., and Barrington, S. (2016). Case series: Toxicity from 25B-NBOMe - a cluster of N-bomb cases. Clin. Toxicol. 54, 141–146. doi:10.3109/15563650.2015.1115056
Gudelsky, G. A., Koenig, J. I., and Meltzer, H. Y. (1986). Thermoregulatory responses to serotonin (5-HT) receptor stimulation in the rat. Evidence for opposing roles of 5-HT2 and 5-HT1A receptors. Neuropharmacology 25, 1307–1313. doi:10.1016/0028-3908(86)90101-2
Halberstadt, A. L. (2015). Recent advances in the neuropsychopharmacology of serotonergic hallucinogens. Behav. Brain Res. 277, 99–120. doi:10.1016/j.bbr.2014.07.016
Halberstadt, A. L., and Geyer, M. A. (2017). Effect of hallucinogens on unconditioned behavior. Springer Berlin Heidelberg. doi:10.1007/7854
Halberstadt, A. L., and Geyer, M. A. (2014). Effects of the hallucinogen 2,5-dimethoxy-4-iodophenethylamine (2C-I) and superpotent N-benzyl derivatives on the head twitch response. Neuropharmacology 77, 200–207. doi:10.1016/j.neuropharm.2013.08.025
Halberstadt, A. L. (2017). Pharmacology and toxicology of N-benzylphenethylamine (“NBOMe”) hallucinogens. Curr. Top. Behav. Neurosci. 32, 283–311. doi:10.1007/7854_2016_64
Halberstadt, A. L., Powell, S. B., and Geyer, M. A. (2013). Role of the 5-HT₂A receptor in the locomotor hyperactivity produced by phenylalkylamine hallucinogens in mice. Neuropharmacology 70, 218–227. doi:10.1016/j.neuropharm.2013.01.014
Harvey-Lewis, C., Li, Z., Higgins, G. A., and Fletcher, P. J. (2016). The 5-HT2C receptor agonist lorcaserin reduces cocaine self-administration, reinstatement of cocaine-seeking and cocaine induced locomotor activity. Neuropharmacology 101, 237–245. doi:10.1016/j.neuropharm.2015.09.028
Heim, R. (2003). Synthesis and pharmacology of potent 5-HT2A receptor agonists which have a partial N-2-methoxybenzyl structure. Available at: https://refubium.fu-berlin.de/handle/fub188/11995 (Accessed August 4, 2019).
Hill, S. L., Doris, T. O. M., Gurung, S., Katebe, S., Lomas, A., Dunn, M., et al. (2013). Severe clinical toxicity associated with analytically confirmed recreational use of 25I – NBOMe: Case series. Clin. Toxicol. 51, 3650. doi:10.3109/15563650.2013.802795
Humston, C., Miketic, R., Moon, K., Ma, P., and Tobias, J. (2017). Toxic leukoencephalopathy in a teenager caused by the recreational ingestion of 25I-NBOMe: A case report and review of literature. J. Med. Cases 8, 174–179. doi:10.14740/jmc2811w
Kittrell, E. M. W., and Satinoff, E. (1986). Development of the circadian rhythm of body temperature in rats. Physiol. Behav. 38, 99–104. doi:10.1016/0031-9384(86)90138-1
Krebs-Thomson, K., Ruiz, E. M., Masten, V., Buell, M., and Geyer, M. A. (2006). The roles of 5-HT1A and 5-HT2 receptors in the effects of 5-MeO-DMT on locomotor activity and prepulse inhibition in rats. Psychopharmacol. Berl. 189, 319–329. doi:10.1007/s00213-006-0566-1
Kueppers, V. B., and Cooke, C. T. (2015). 25I-NBOMe related death in Australia: A case report. Forensic Sci. Int. 249, e15–e18. doi:10.1016/j.forsciint.2015.02.010
Lawn, W., Barratt, M., Williams, M., Horne, A., and Winstock, A. (2014). The NBOMe hallucinogenic drug series: Patterns of use, characteristics of users and self-reported effects in a large international sample. J. Psychopharmacol. 28 (8), 780–788. doi:10.1177/0269881114523866
Matuszewich, L., and Yamamoto, B. K. (2003). Long-lasting effects of chronic stress on DOI-induced hyperthermia in male rats. Psychopharmacol. Berl. 169, 169–175. doi:10.1007/s00213-003-1498-7
Menon, M. K., and Dandiya, P. C. (1969). Behavioural and brain neurohormonal changes produced by acute heat stress in rats: Influence of psychopharmacological agents. Eur. J. Pharmacol. 8, 284–291. doi:10.1016/0014-2999(69)90036-3
Miliano, C., Marti, M., Pintori, N., Castelli, M. P., Tirri, M., Arfè, R., et al. (2019). Neurochemical and behavioral profiling in male and female rats of the psychedelic agent 25I-NBOMe. Front. Pharmacol. 10, 1406. doi:10.3389/fphar.2019.01406
Murakami, N., and Sakata, Y. (1980). A possible role of the serotonergic system in thermoregulation in the rabbit. Neuropharmacology 19, 891–895. doi:10.1016/0028-3908(80)90089-1
Nakamura, M., Shintani-Ishida, K., and Ikegaya, H. (2018). 5-HT2A receptor agonist-induced hyperthermia is induced via vasoconstriction by peripheral 5-HT2A receptors and Brown adipose tissue thermogenesis by peripheral serotonin loss at a high ambient temperature. J. Pharmacol. Exp. Ther. 367, 356–362. doi:10.1124/jpet.118.250217
Nikolaou, P., Papoutsis, I., Stefanidou, M., Spiliopoulou, C., and Athanaselis, S. (2015). 2C-I-NBOMe, an “N-bomb” that kills with “Smiles”. Toxicological and legislative aspects. Drug Chem. Toxicol. 38, 113–119. doi:10.3109/01480545.2014.911882
Ninan, I., and Kulkarni, S. K. (1998). 5-HT(2A) receptor antagonists block MK-801-induced stereotypy and hyperlocomotion. Eur. J. Pharmacol. 358, 111–116. doi:10.1016/S0014-2999(98)00591-3
Nykodemová, J., Šuláková, A., Palivec, P., Češková, H., Rimpelová, S., Šíchová, K., et al. (2021). 2C-B-fly-NBOMe metabolites in rat urine, human liver microsomes and c. Elegans: Confirmation with synthesized analytical standards. Metabolites 11, 1–18. doi:10.3390/metabo11110775
Páleníček, T., Balíková, M., Bubeníková-Valešová, V., and Horáček, J. (2008). Mescaline effects on rat behavior and its time profile in serum and brain tissue after a single subcutaneous dose. Psychopharmacol. Berl. 196, 51–62. doi:10.1007/s00213-007-0926-5
Páleníček, T., Hliňák, Z., Bubeníková-Valešová, V., Novák, T., and Horáček, J. (2010). Sex differences in the effects of N,N-diethyllysergamide (LSD) on behavioural activity and prepulse inhibition. Prog. Neuro-Psychopharmacology Biol. Psychiatry 34, 588–596. doi:10.1016/j.pnpbp.2010.02.008
Páleníček, T., Balíková, M., Rohanová, M., Novák, T., Horáček, J., Fujáková, M., et al. (2011). Behavioral, hyperthermic and pharmacokinetic profile of para-methoxymethamphetamine (PMMA) in rats. Pharmacol. Biochem. Behav. 98, 130–139. doi:10.1016/j.pbb.2010.12.011
Páleníček, T., Fujáková, M., Brunovský, M., Horáček, J., Gorman, I., Balíková, M., et al. (2013). Behavioral, neurochemical and pharmaco-EEG profiles of the psychedelic drug 4-bromo-2,5-dimethoxyphenethylamine (2C-B) in rats. Psychopharmacol. Berl. 225, 75–93. doi:10.1007/s00213-012-2797-7
Papeschi, R., Sourkes, T. L., and Youdim, M. B. H. (1971). The effect of yohimbine on brain serotonin metabolism, motor behavior and body temperature of the rat. Eur. J. Pharmacol. 15, 318–326. doi:10.1016/0014-2999(71)90098-7
Poklis, J. L., Devers, K. G., Arbefeville, E. F., Pearson, J. M., Houston, E., and Poklis, A. (2014). Postmortem detection of 25I-NBOMe [2-(4-iodo-2,5-dimethoxyphenyl)-N-[(2-methoxyphenyl)methyl]ethanamine] in fluids and tissues determined by high performance liquid chromatography with tandem mass spectrometry from a traumatic death. Forensic Sci. Int. 234, e14–e20. doi:10.1016/j.forsciint.2013.10.015
Poklis, J. L., Raso, S. A., Alford, K. N., Poklis, A., and Peace, M. R. (2015). Analysis of 25I-NBOMe, 25B-NBOMe, 25C-NBOMe and other dimethoxyphenyl-N-[(2-Methoxyphenyl) Methyl]Ethanamine derivatives on blotter paper. J. Anal. Toxicol. 8, 617–623. doi:10.1093/jat/bkv073
Richeval, C., Boucher, A., Humbert, L., Phanithavong, M., Wiart, J. F., Moulsma, M., et al. (2017). Retrospective identification of 25I-NBOMe metabolites in an intoxication case. Toxicol. Anal. Clin. 29, 71–81. doi:10.1016/j.toxac.2017.01.001
Richter, L. H. J., Menges, J., Wagmann, L., Brandt, S. D., Stratford, A., Westphal, F., et al. (2020). In vitro toxicokinetics and analytical toxicology of three novel NBOMe derivatives: phase I and II metabolism, plasma protein binding, and detectability in standard urine screening approaches studied by means of hyphenated mass spectrometry. Forensic Toxicol. 38, 141–159. doi:10.1007/s11419-019-00498-7
Rickli, A., Luethi, D., Reinisch, J., Buchy, D., Hoener, M. C., and Liechti, M. E. (2015). Receptor interaction profiles of novel N-2-methoxybenzyl (NBOMe) derivatives of 2,5-dimethoxy-substituted phenethylamines (2C drugs). Neuropharmacology 99, 546–553. doi:10.1016/j.neuropharm.2015.08.034
Rohanová, M., Páleníček, T., and Balíková, M. (2008). Disposition of 4-bromo-2,5-dimethoxyphenethylamine (2C-B) and its metabolite 4-bromo-2-hydroxy-5-methoxyphenethylamine in rats after subcutaneous administration. Toxicol. Lett. 178, 29–36. doi:10.1016/j.toxlet.2008.01.017
Saadat, K. S., O’Shea, E., Colado, M. I., Elliott, J. M., and Green, A. R. (2005). The role of 5-HT in the impairment of thermoregulation observed in rats administered MDMA ('ecstasy’) when housed at high ambient temperature. Psychopharmacol. Berl. 179, 884–890. doi:10.1007/s00213-004-2106-1
Schmitz, G. P., Chiu, Y.-T, König, G. M., Kostenis, E., Roth, B. L., and Herman, M. A. (2022). Psychedelic compounds directly excite 5-HT2A Layer 5 Pyramidal Neurons in the Prefrontal Cortex through a 5-HT2A Gq -mediated activation mechanism. bioRxiv. doi:10.1101/2022.11.15.516655
Shanks, K. G., Sozio, T., and Behonick, G. S. (2015). Fatal intoxications with 25B-NBOMe and 25I-NBOMe in Indiana during 2014. J. Anal. Toxicol. 39, 602–606. doi:10.1093/jat/bkv058
Šíchová, K., Pinterová, N., Židková, M., Horsley, R. R., Lhotková, E., Štefková, K., et al. (2018). Mephedrone (4-Methylmethcathinone): Acute behavioral effects, hyperthermic, and pharmacokinetic profile in rats. Front. Psychiatry 8, 306–311. doi:10.3389/fpsyt.2017.00306
Šíchová, K., Syrová, K., Kofroňová, E., Pinterova-Leca, N., Vejmola, Č., Nykodemová, J., et al. (2022). Pharmacokinetics, systemic toxicity, thermoregulation and acute behavioural effects of 25CN-NBOMe. Addict. Biol. 27, 1–10. doi:10.1111/adb.13216
Sipes, T. E., and Geyer, M. A. (1997). DOI disrupts prepulse inhibition of startle in rats via 5-HT 2A receptors in the ventral pallidum. Brain Res. 761, 97–104. doi:10.1016/S0006-8993(97)00316-8
Štefková, K., Židková, M., Horsley, R. R., Pinterová, N., Šíchová, K., Uttl, L., et al. (2017). Pharmacokinetic, ambulatory, and hyperthermic effects of 3,4-methylenedioxy-n-methylcathinone (Methylone) in rats. Front. Psychiatry 8, 1–11. doi:10.3389/fpsyt.2017.00232
Stellpflug, S. J., Kealey, S. E., Hegarty, C. B., and Janis, G. C. (2014). 2-(4-Iodo-2,5-dimethoxyphenyl)-N-[(2-methoxyphenyl)methyl]ethanamine (25I-NBOMe): Clinical case with unique confirmatory testing. J. Med. Toxicol. 10, 45–50. doi:10.1007/s13181-013-0314-y
Suzuki, J., Poklis, J. L., and Poklis, A. (2014). My friend said it was good LSD”: A suicide attempt following analytically confirmed 25I-NBOMe ingestion. J. Psychoact. Drugs 46, 379–382. doi:10.1080/02791072.2014.960111
Tang, M. H. Y., Ching, C. K., Tsui, M. S. H., Chu, F. K. C., and Mak, T. W. L. (2014). Two cases of severe intoxication associated with analytically confirmed use of the novel psychoactive substances 25B-NBOMe and 25C-NBOMe. Clin. Toxicol. 52, 561–565. doi:10.3109/15563650.2014.909932
Tylš, F., Páleníček, T., Kadeřábek, L., Lipski, M., Kubešová, A., and Horáček, J. (2016). Sex differences and serotonergic mechanisms in the behavioural effects of psilocin. Behav. Pharmacol. 27, 309–320. doi:10.1097/FBP.0000000000000198
Wijnand, H. P. (1988). Pharmacokinetic model equations for the one- and two-compartment models with first-order processes in which the absorption and exponential elimination or distribution rate constants are equal. J. Pharmacokinet. Biopharm. 16, 109–128. doi:10.1007/BF01061864
Wojtas, A., Herian, M., Skawski, M., Sobocińska, M., González-Marín, A., Noworyta-Sokołowska, K., et al. (2021). Neurochemical and behavioral effects of a new hallucinogenic compound 25B-NBOMe in rats. Neurotox. Res. 39, 305–326. doi:10.1007/s12640-020-00297-8
Keywords: 2C-B-Fly-NBOMe, NBOMe series, pharmacokinetics, prepulse inhibition, thermoregulation, new psychoactive substance
Citation: Syrová K, Šíchová K, Danda H, Lhotková E, Jorratt P, Pinterová-Leca N, Vejmola Č, Olejníková-Ladislavová L, Hájková K, Kuchař M, Horáček J and Páleníček T (2023) Acute pharmacological profile of 2C-B-Fly-NBOMe in male Wistar rats—pharmacokinetics, effects on behaviour and thermoregulation. Front. Pharmacol. 14:1120419. doi: 10.3389/fphar.2023.1120419
Received: 09 December 2022; Accepted: 27 February 2023;
Published: 09 March 2023.
Edited by:
Kabirullah Lutfy, Western University of Health Sciences, United StatesReviewed by:
David Pubill, University of Barcelona, SpainCody Wenthur, University of Wisconsin-Madison, United States
Richard Glennon, Virginia Commonwealth University, United States
Copyright © 2023 Syrová, Šíchová, Danda, Lhotková, Jorratt, Pinterová-Leca, Vejmola, Olejníková-Ladislavová, Hájková, Kuchař, Horáček and Páleníček. This is an open-access article distributed under the terms of the Creative Commons Attribution License (CC BY). The use, distribution or reproduction in other forums is permitted, provided the original author(s) and the copyright owner(s) are credited and that the original publication in this journal is cited, in accordance with accepted academic practice. No use, distribution or reproduction is permitted which does not comply with these terms.
*Correspondence: Tomáš Páleníček, VG9tYXMucGFsZW5pY2VrQG51ZHouY3o=; Martin Kuchař, TWFydGluLmt1Y2hhckB2c2NodC5jeg==