- 1Laboratory of Metabolic Abnormalities and Vascular Aging, Liyuan Hospital Affiliated to Huazhong University of Science and Technology, Department of Integrated Chinese and Western Medicine, City Wuhan, Hubei Province, China
- 2Hubei University of Chinese Medicine, City Wuhan, Hubei Province, China
- 3Liyuan Hospital Affiliated to Huazhong University of Science and Technology, Geriatric Department, City Wuhan, Hubei Province, China
A metabolic bone disorder called osteoporosis is characterized by decreased bone mass and compromised microarchitecture. This condition can deteriorate bones and raise the risk of fractures. The two main causes of osteoporosis are an increase in osteoclast activity or quantity and a decrease in osteoblast viability. Numerous mechanisms, including estrogen shortage, aging, chemical agents, and decreased mechanical loads, have been linked to osteoporosis. Inflammation and oxidative stress have recently been linked to osteoporosis, according to an increasing number of studies. The two primary medications used to treat osteoporosis at the moment are bisphosphonates and selective estrogen receptor modulators (SERMs). These medications work well for osteoporosis brought on by aging and estrogen deprivation, however, they do not target inflammation and oxidative stress-induced osteoporosis. In addition, these drugs have some limitations that are attributed to various side effects that have not been overcome. Traditional Chinese medicine (TCM) has been applied in osteoporosis for many years and has a high safety profile. Therefore, in this review, literature related to botanical drugs that have an effect on inflammation and oxidative stress-induced osteoporosis was searched for. Moreover, the pharmacologically active ingredients of these herbs and the pathways were discussed and may contribute to the discovery of more safe and effective drugs for the treatment of osteoporosis.
1 Introduction
Osteoporosis is a chronic metabolic disease that is connected with estrogen deficiency, aging, chemical agents, decreased mechanical load (Nai-Dan et al., 2016), inflammatory (BoCun-Yu, 2022), oxidative stress (Jeff et al., 2021), and other factors. The prevalence of osteoporosis is widespread and it affects approximately 75 million people and an estimated 10 million women worldwide (Kerri et al., 2011). Hip fractures due to osteoporosis are expected to increase to 6.3 million by the year 2050 (Hong-Tao et al., 2006). Osteoporosis is characterized by decreased bone mass and damaged bone structure, which predisposes one to variations of fractures. Statistically, one in three older women is affected in this way (SalilaDorothy and Ethel, 2014). Clinically approved drugs for the treatment and prevention of osteoporosis primarily include bisphosphonates, selective estrogen receptor modulators (SERMs), and so on. However, gastrointestinal reaction, renal toxicity, and other side effects may be induced due to the poor bioavailability and low permeability of bisphosphonates (Suman et al., 2021). SERMs may increase the incidence rate of breast and endometrial cancer (Rossouw et al., 2002). Furthermore, given that these drugs are commonly used for estrogen deficiency, aging, and certain hormone-induced osteoporosis, studies on the specific effect on inflammation and oxidative stress-induced osteoporosis are relatively. scarce Therefore, potential drugs with fewer side effects and more specific treatments should be actively sought to make up for the shortcomings of existing drug therapies.
Traditional Chinese medicine (TCM) has been broadly used in treating multifarious diseases on account of its tiny adverse reaction. Many Chinese medicines have been shown to cure osteoporosis caused by estrogen deficiency, aging, glucocorticoid, and decreased mechanical load (Emmanuel et al., 2014; Yinbo et al., 2015; LinBeibei et al., 2019; Joon-Ho et al., 2022). Moreover, more Chinese botanical drugs have been proved to be applicable in treating osteoporosis on account of their anti-inflammatory and antioxidant effects (Xiaobin et al., 2020; Mao et al., 2021; Cheng et al., 2022). In order to create greater efficacy and reduce side effects, various botanical drugs are mixed and boiled into decoctions according to the prescription theory rather than a single TCM (Zhen G. et al., 2013). Therefore, it is difficult to figure out which component of the medicine is responsible for the treatment, which also limits the popularity of TCM worldwide. However, with the development of network pharmacology, the active ingredients of drugs and their target genes can be excavated by public website information. In additional, bioinformatics analysis can be employed to reveal the pathways through which botanical drugs may exert their anti-osteoporosis effects.
This review summarizes Chinese botanical drugs that can be utilized to treat osteoporosis through their anti-inflammatory and antioxidant properties and provides an in-depth analysis of their active ingredients. Finally, combined with network pharmacology and bioinformatics indications, the pathways that may be involved in the anti-osteoporosis effects were estimated. This may help to better explore its mechanisms and develop potential therapeutic agents.
2 Oxidative stress and inflammation induced osteoporosis
Oxidative stress is a pathological process in which cells produce excessive reactive oxygen species (ROS) that cannot be neutralized by tissues and antioxidant systems, causing damage to cellular molecules such as DNA, proteins, and lipids (Tarique et al., 2016). Inflammation is a defense mechanism when the body is infected by microorganisms or viruses, and exposed to allergens or any other foreign sources (Palanisamy et al., 2016). Several studies have linked inflammation and oxidative stress to the progress of cancer, mental illness, and cardiovascular disease (Simone et al., 2010; Daniel et al., 2017; Noemí et al., 2017; Bee and Mohd, 2019). Oxidative stress mediates the occurrence of diseases through two main mechanisms. One is lipid peroxidation mediated by hydroxyl radical (•OH) and peroxynitrite (ONOO−). The second is that the activity of antioxidant enzymes such as glutathione, thioredoxin, and NADPH is inhibited (Henry and Hongqiao, 2021). The pathogenesis of inflammation mainly includes interleukin, tumor necrosis factor, and other inflammatory factors that change the cell environment; the interaction between inflammatory factors and oxidation reaction damages DNA and affects the homeostasis of endoplasmic reticulum, mitochondria, and other organelles; and activation of inflammasome (Prof and Prof, 2001; Ji-Chun et al., 2016; Myles et al., 2016; Gregory et al., 2021; Paul N Cori and Russell, 2022).
Recently, increasing research has demonstrated that inflammation and oxidative stress are highly associated with osteoarticular disease, especially osteoporosis (Jeff et al., 2021; BoCun-Yu, 2022). The increase of inflammatory factors such as interleukin-1 (IL-1), interleukin-6 (IL-6), and tumor necrosis factor-α (TNF-α) during the inflammatory response can inhibit the activity and formation of osteoblasts by suppressing the expression of runt-related transcription factor 2 (RUNX2, a regulator of osteoblast differentiation) and stimulate the release of RANKL to promote the activity and formation of osteoclasts. Excessive RANKL binding with RANK will lead to the recruitment of TNF receptor-associated factor 6 (TRAF6) (Yunpeng et al., 2013; Izawa et al., 2014). As a result, the downstream pathway including nuclear factor kappa-light-chain-enhancer of activated B Cells (NF-κB), c-hJun N-terminal kinase (JNK), ERK (extracellular regulated protein kinases)and p38 will be activated and the expression of osteoclast transcription factors such as proto-oncogene c-fos and nuclear factor of activated T Cells, cytoplasmic, calcineurin-dependent 1 (NFATc1) will be further increased (Lin KM. et al., 2019; Janhavi et al., 2022). The increased expression of transcription factors induces the expression of triiodothyronine receptor auxiliary protein (TRAP), cathepsin K, and matrix metalloprotein (MMP9), which are specific to osteoclasts (Hiroyuki et al., 2016). The overexpression of the Wnt family such as Wnt10a and Wnt10b greatly restrains lipogenic differentiation and promotes osteogenic differentiation of mesenchymal stem cells (MSC) through a canonical β-catenin-dependent pathway (Cawthorn et al., 2012). However, TNF-α has the ability to relieve the osteoblastogenic effects of canonical Wnt signaling. Activation of NF-Kb is attributed to the upregulation of smurf1 and smurf2, thus promoting the degradation of β-catenin (Jia et al., 2013). In addition, inflammatory cytokines also activate the NF-κB pathway by stimulating IκB kinases (IKKs) phosphorylation and allowing NF-κB and IKKs complexes to disintegrate into the nucleus (Jeff et al., 2021). Excessive ROS produced by oxidative stress can promote bone loss and contribute to osteoporosis (Teresa et al., 2023). ROS can enhance the permeability of the mitochondrial membrane and release cytochrome C (Cytc) and apoptosis-inducing factors (AIF) inside (Rui et al., 2020; Bianca et al., 2021). These factors, when combined with apoptotic protein kinase activator-1 (APKA-1), activate the caspase-9 and caspase-3 pathways in the cytoplasm, resulting in osteoblast apoptosis (Keda et al., 2022). In order to consume excessive ROS produced by oxidative stress, cells will activate various pathways resulting in inflammation, which in turn will activate NF-κB and mitogen-activated protein kinase (MAPK) pathways which include JNK, ERK, and P38 contributing to increased osteoclast activity and generation (Kerri et al., 2011; Dinesh et al., 2017; Zhikun et al., 2018). Nuclear factor E2-related factor 2 (Nrf2) is a transcription factor for a variety of cell protective enzymes such as heme oxygenase-1 (HO-1), NADPH: quinone oxidoreductase 1 (NQO-1), γ-glutamylcysteine synthetase (GCS), and glucose 6-phosphate dehydrogenase (G6PD) (Agnieszka et al., 2016). Oxidative stress reduces the nuclear localization of Nrf2 and thus reduces the production of cell protective enzymes. As a consequence, oxidative stress is aggravated; in contrast, low expression of Nrf2 directly causes increased osteoclast generation (Teresa et al., 2023). The mechanism of osteoporosis induced by oxidative stress and inflammation is shown in Figure 1.
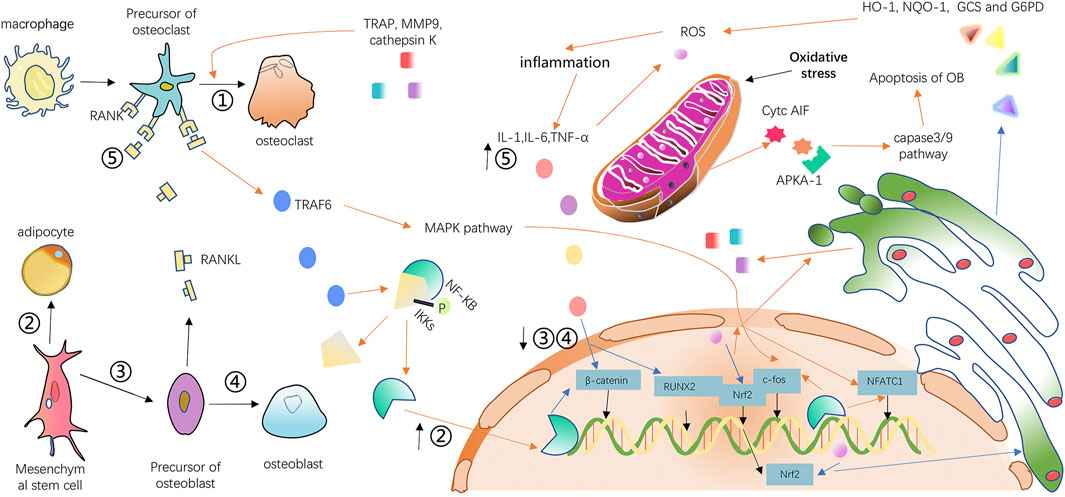
FIGURE 1. Mechanism diagram of osteoporosis mediated by inflammation and oxidative stress. The orange arrows indicate that paths have been enhanced, and the blue arrows represent that paths process has been weakened. The upward black arrow next to the number indicates that the path has been reinforced, while the downward arrow represents that the path corresponding to the number has been weakened.
3 Single TCM with anti-inflammatory and antioxidant effects in osteoporosis
TCM mainly includes animal medicine, plant medicine, and shell medicine. The primary parts of animal drugs were the whole scale, viscera production, horn, or the whole animal body. The medicinal parts of plant medicine include the heels, stem, leaf, seed, and flower. Shell drugs are generally in the form of ground powder decoctions. Since the use of animal drugs is against the humanitarian spirit and shell drugs need to be highly processed, plant drugs have become predominant in TCM, the form of which is decocted in water In the course of thousands of years of application of TCM, TCM has been used in accordance with TCM theories such as Yin and Yang theory, five elements theory (Yan Zou, from 324 BC to 250 BC), Qi, blood and body fluid theory (“Emperor Neijing”, from Qin dynasty to Han Dynasty), eight principles of dialectics (Zhongjing Zhang, Han dynasty, “Treatise on Febrile Disease”) and so on, thus deviating from modern pharmacology knowledge to a certain extent. In recent years, with the extensive application of TCM and the continuous development of TCM pharmacology, there have been specialized websites for systematic pharmacological analysis and induction of TCM ingredients (https://old.tcmsp-e.com/tcmsp.php). Among them, many TCM have been found to have therapeutic effects on a variety of diseases due to their anti-inflammatory and antioxidant activities. Such as Alzheimer, cancer, stroke, liver diseases, and osteoporosis (Jia et al., 2016; Kathy et al., 2016; Yangyang H. et al., 2020; Hansen et al., 2020; Ting et al., 2021; Yi and Meng, 2022). Here we summarize the botanical drugs that can be utilized to treat osteoporosis (Table 1). The pharmacokinetics of the active ingredients of Chinese medicines is shown in Table 2.
3.1 Epimedium brevicornu maxim
Epimedium brevicornu Maxim belongs to the Berberidaceae family. A commonly used part for medicine is the dried aerial. It contains abundant nutrients and phytochemicals, such as protein, essential elements, alkaloids, terpenoids, polysaccharides, lignans, and flavonoids (Xiao-Hua et al., 2020). Icariin (ICA) is a flvonoid glucoside active component isolated from Epimedium pubescens. Lipopolysaccharide (LPS) can induce an inflammatory response causing bone resorption and further osteoporosis. A research study elucidated that Icariine restored LPS-induced osteoporosis by suppressing miR-34c level, and further inhibited the pathways of JNK, p38, and NF-kB (Jian et al., 2016). Wear particles can generate chronic inflammation and osteolysis. Therefore, they were thought to be associated with osteoporosis. ICA could treat lesions caused by wear particles by suppressing the expression of inflammatory cytokines TNF-α and IL-1β and the RANK-RANKL signaling pathway (Jingfu et al., 2014). LPS recruits inflammatory cells, produces prostaglandins such as Prostaglandin E2 (PGE2), and synthesizes cytokines IL-6 and TNF-α to mediate inflammatory responses (Shamima et al., 2007). PGE2 and hypoxia-inducible factor-1α (HIF-1α) have the ability to stimulate osteoclast differentiation. ICA suppresses osteoclasts differentiation and inflammatory bone loss by restraining the activation of the MAPK pathway and the expression of PGGE2 and HIF-1α (Tsai-Pei et al., 2011). Inducible nitric oxide synthase (iNOS) is responsible for manufacturing nitric oxide (NO). Excessive NO can cause diversified inflammatory diseases such as inflammatory bone loss (Sunil, 2008; Monia et al., 2009). Ikarisoside A is a natural flavonoid that has antioxidant properties and is in the root of Epimedium brevicornu Maxim Ikarisoside A suppresses the expression of LPS-induced inflammatory mediators NO, TNF-α, and IL-1β as well as iNOS in macrophages via inhibiting p38 kinase and NF-κB signaling (Hwa et al., 2008). Osteoprotegerin (OPG) is a natural antagonist of RANKL. The formation of osteoclast and bone resorption is promoted when the expression of OPG is restrained (Sri and Gallagher, 2014). ICA suppresses the formation and activation of osteoclast which was induced by Ti particles, a wear particle, by decreasing the RANKL/OPG ratio (Hongguo et al., 2015). Excess iron ions are associated with osteoporosis because they facilitate the formation of ROS and are followed by impaired mitochondrial function. Therefore, iron overload has been confirmed as a risk factor for osteoporosis (Viktória, 2017). ICA reverses excess iron ions-induced bone loss by decreasing the production of ROS and ameliorating the impairment of mitochondria (Xingzhi et al., 2019). Iron overload leads to retardation and autophagy of osteoblast progenitors by inhibiting the Phosphatidylinositol 3-kinase (PI3K)/Akt/mammalian target of rapamycin (mTOR) signaling pathway (Wan-Jing et al., 2018). ICA could alleviate mitochondrial dysfunction and ROS production caused by iron overload in MSCs via initiating PI3K/AKT/mTOR and inhibiting MAPK pathways (Xudong et al., 2019), which cause MSCs to differentiate into osteoblasts unceasingly.
3.2 Salvia miltiorrhiza bunge
Part of the roots of Salvia miltiorrhiza Bunge [Lamiaceae] has been used in the treatment of cardiovascular disease as a medicine (Chun-Yan et al., 2015). Recently, various studies uncovered that it could be applied to treat inflammatory bone disease, especially osteoporosis. Some researchers have demonstrated that a high-fat-sucrose (HFS) diet will increase ROS and lipid peroxidation which inevitably causes oxidative stress injury (Yanjun et al., 2015). Salvianolic acid B and danshensu have been identified as major active ingredients in Salvia miltiorrhiza Bunge aqueous extract (SMA). A study proved that SMA could act against lipid peroxidation and ROS level in tissues of HFS-fed ovariectomized rats As a consequence, it ameliorates bone loss and promotes preosteoblast cell proliferation and differentiation (Dong et al., 2018). The plasma malondialdehyde (MDA) level represents the plasma lipid peroxidation level, which indirectly reflects plasma oxidative stress (Draper and Hadley, 1990). Ethanol extract of Salvia miltiorrhiza Bunge, the primary components of which are tanshinone IIA and cryptotanshinone, inhibit osteoclast proliferation and maturation via reducing the production of NO and MDA to ameliorate impairment of oxidative stress (Yan et al., 2011). In addition, cryptotanshinone could significantly reduce bone loss by restraining the expression of osteoclast differentiation regulators including cathepsin K, NFATc1, and c-fos (Wenjiu et al., 2022). Dexamethasone (Dex) can lead to glucocorticoid-induced osteoporosis (GIO) by inducing oxidative stress (Fabien et al., 2009; Maria et al., 2011). Tanshinol {D (+)β-3,4-dihydroxyphenyl lactic acid} is one of the water-soluble components of Salvia miltiorrhiza Bunge. Relevant evidence has uncovered that tanshinol could act against oxidative stress and has an inhibitory effect on osteoblastic differentiation induced by Dex via suppressing excessive ROS generation (Shiying et al., 2016). Prednisone is a glucocorticoid like dexamethasone that can induce osteoporosis. It was reported that salvianolic acid B could increase bone mass and prevent prednisone-induced osteoporosis by depressing adipogenesis and stimulating angiogenesis as well as osteogenesis (Liao et al., 2012). Glutathione (GSH) is an antioxidant.
3.3 Pueraria montana var. lobata
Pueraria montana var. lobata, the dried root of Pueraria montana var. lobata from the family of Fabaceae, is a perennial winding botanical drug that grows naturally in Southeast Asia. Modern pharmacology has proved that Pueraria montana var. lobata has anti-depression, anti-oxidation, prevention of osteonecrosis, lowering of blood lipids, and other pharmacological effects (Zhen Z. et al., 2013). Cadmium exposure contributes to oxidative injury by reducing the levels of antioxidant glutathione (GSH), and intercellular ROS in osteoblast-like Saos-2 cells (Spenser et al., 2009). Simultaneously, cadmium exposure ameliorates bone density and destructs the microstructural of trabecular bone (Mayra et al., 2021). Puerarin is an isoflavonoid metabolite isolated from the botanical drug puerariae. Previous research demonstrated that puerarin can alleviate oxidative damage from cadmium exposure by regulating autophagy (Xishuai et al., 2022). BMP families can not only facilitate the differentiation of MSCs into osteoblasts and chondrocytes but also promote the differentiation of osteoblast progenitor cells into osteoblasts (Jay et al., 2002). A research study indicated that puerarin promotes the proliferation of osteoblasts probably by initiating bone morphogenetic protein (BMP) and inhibiting the NO pathway (Shiow-Yunn et al., 2012). Cyclooxygenase-2 (COX-2), a variety of inflammatory mediators, mediates inflammatory disease by regulating the oxygenated metabolites of arachidonic acid. Isoorientin is an active component extracted from the tubers of Pueraria tuberosa. It alleviates macrophage inflammatory and oxidative stress damage adequately via decreasing inflammatory mediators such as iNOS and COX-2, inflammatory factors such as TNF-α and IL-1β, and nuclear translocation of NF-KB (Kotha et al., 2017).
3.4 Gynochthodes officinalis
Gynochthodes officinalis is a plant belonging to the genus Morinda of the family Rubiaceae. The dried roots are commonly used in medicine. Modern pharmacology has illustrated that Morinda officinalis possesses anti-depression, antioxidant, pro-fertility, and other pharmacological effects (Meng-Yun et al., 2022). The BMP/Smads signaling pathway is highly associated with bone formation and has been identified to affect the differentiation of osteoblasts (Valerie et al., 2016). Morinada officinalis polysaccharide (MOP) is an active ingredient deriving from Morinada officinalis. MOP, consisting mainly of galactose, galacturonic acid, and arabinose, could inhibit osteoclast differentiation, facilitate osteoblast maturation and proliferation, and elevate the antioxidant capacity to withstand bone injury caused by tetramethylthiuram disulfide through the BMP/Smads pathway (Chaodong et al., 2022). Interleukin-6 (IL-6), an interleukin, is both a pro-inflammatory and an anti-inflammatory cytokine. MOP improves bone quality and postpones osteoporosis development by converting IL-6 into an anti-inflammatory cytokine (Zhu et al., 2008). Peroxisome proliferator-activated receptor γ (PPARγ) and its coactivator-1α (PGC-1α) are connected with osteoporosis caused by oxidative stress (Bo et al., 2018; Jian et al., 2018). There is evidence that MOP could inhibit oxidative stress in ovariectomized rats through the hindrance of the PGC-1α/PPARγ pathway to increase the level of reducing agents superoxide dismutase (SOD) and GSH (Kai et al., 2022). This further elucidates that MOP could reduce oxidative stress in ovariectomized rats. Bajijiasu, a variety of dimeric fructose, is extracted from the root of Gynochthodes officinalis. It has been demonstrated that bajijiasu could mitigate oxidative stress induced by RANKL by inhibiting NF-κB and NFATc1 activation. As a result, it is able to attenuate bone resorption and osteoclast formation (Guoju et al., 2017).
3.5 Corethrodendron multijugum
Corethrodendron multijugum is a Fabaceae plant widely used to strengthen the body’s natural defenses. Recently, many studies have shown that Corethrodendron multijugum has anti-inflammatory activity and can regulate the growth balance between osteoclasts and osteoblasts (Young et al., 2005; Li-Jie et al., 2011). The p38 pathway regulates the transcription of pro-inflammatory cytokines. Flavonoids isolated from the roots of Corethrodendron multijugum are capable of inhibiting the production of pro-inflammatory cytokines such as IL-6, IL-12, and TNF-α by suppressing the p38 and MAPK pathways. This restrains the toxic effect of pro-inflammatory cytokines on bone marrow-derived dendritic cells (Wei et al., 2014). As we have described before, iron overload is associated with the development of osteoporosis. Astragaloside IV, a tetracyclic triterpenoid saponin extracted from Corethrodendron multijugum, is suggested to block the differentiation of MSCs into adipocytes and bone loss under the condition of iron overload; it also simultaneously stimulates the differentiation of MSCs into osteoblasts (Hui et al., 2021). Aqueous extracted Corethrodendron multijugum (the main component is astragaloside IV) has been proven to suppress the generation of NO and transcription of iNOS mRNA in LPS co-cultured RAW 264.7 macrophages (Young et al., 2005). In summary, we believe that astragalus can treat osteoporosis through its anti-inflammatory and antioxidant properties.
3.6 Eclipta prostrata
The dried Eclipta prostrata [Asteraceae] is a perennial botanical drug that is frequently used medicinally to treat musculoskeletal disorders (Zhicai et al., 2022). There is evidence that the total flavonoids of Eclipta prostrata (TFRD) augment the levels of antioxidant SOD and GSH and reduce the generation of ROS. Therefore, we can conclude that TFRD (including naringin, naringenin, and neoeriocitrin) has an antioxidant property. PI3K/AKT is able to promote the transcription of the osteoblast-related gene RUNX2. This article also demonstrated that TFRD can facilitate osteoblast maturation by inhibiting the phosphorylation of the PI3K/AKT pathway. As a result, TFRD actively enhanced antioxidant capacity, increased bone density, and reduced bone loss (Panyun et al., 2021). In a prednisone-induced oxidative stress osteoporosis model, the production of excess oxygen radicals resulted in decreased protein concentration and increased carbyl content in mice, which was reversed by the 75% alcohol concentration of the Eclipta prostrata extract (the main component is Naringin) (Xinyu et al., 2012). Naringin has also been reported to prevent glucocorticoid-induced inflammatory bone loss by inhibiting the levels of the inflammatory factor TNF-α (Chengli et al., 2018). This suggests that the 75% ethanol extraction of Eclipta prostrata has the ability to inhibit oxidative stress induced by glucocorticoids.
3.7 Curculigo orchioides gaertn
Curculigo orchioides Gaertn is a tropical and subtropical Hypoxidaceae plant. Dried Curculigo orchioides Gaertn was used in TCM to treat knee arthritis, diarrhea, and leg fatigue (Zhicai et al., 2022). According to a recent study, Curculigo orchioides Gaertn seems to have antioxidant effects as a treatment for osteoporosis. Curculigoside (CCG) is the dominant bioactive metabolite in Curculigo orchioides Gaertn. CCG can not only improve the differentiation and proliferation of osteoblasts but also inhibit osteoclastogenesis by reducing dexamethasone-induced production of ROS and pro-inflammatory cytokines such as interleukin-1β (IL-1β) and TNF-α (Fang-Bing et al., 2015). Amyloid beta peptide (Aβ) deposition causes oxidative damage, contributing to osteoporosis (Carolin et al., 2014). CCG mitigated bone loss following Aβ deposition-induced oxidative damage by reinforcing SOD activity and reducing the generation of pro-inflammatory cytokines IL-6 and TNF-α (Lu et al., 2015). Forkhead Box Protein O1 (FoxO1) enhanced the activity of the antioxidant enzyme SOD after transfer from the cytoplasm to the nucleus. However, the phosphorylation of serine/threonine kinase (AKT) and the overactivation of insulin-like growth factor receptor (IGFR) results in the degradation of FoxO1. CCG counteracts iron overload-induced bone loss principally by inhibiting the IGFR/Akt pathway to increase FoxO1 expression in the nucleus (Quanlong et al., 2019). Orcinol glucoside (OG) is a phenolic glycoside isolated from Curculigo orchioides Gaertn. Nrf2 is associated with the regulation of oxidative stress at the cellular and tissue levels by negatively regulating ROS (Hiroyuki et al., 2016). Keap1 negatively regulates Nrf2 expression by inhibiting the nuclear translocation of Nrf2, which leads to decreased production of antioxidants (including HO-1, GCS, NQO1, and G6PD) associated with Nrf2. Activation of autophagy is connected with osteoclastogenesis. However, this autophagy process can be negatively regulated by the redox-sensitive factor mTOR to restrain osteoclast generation (Xishuai et al., 2019). It has been reported that OG can suppress osteoclast formation, eliminate ROS, up-regulate antioxidant enzyme levels, and inhibit autophagy by activating the Nrf2/Keap1 pathway and mTOR pathway (Wan et al., 2022). In addition, there is a study that demonstrated that CCG can reduce ROS production and osteoclastogenesis by activating the Nrf2 pathway and restraining the NF-KB pathway (Mengqin et al., 2021). Moreover, CCG can reverse the inhibition of hydrogen peroxide in the activity of osteoblasts, the number of mineralized nodules, and the promotion of reactive oxygen generation so as to enhance the activity of osteoblasts and ameliorate oxidative damage by the MAPK and NF-kB pathways (Ying et al., 2012). In conclusion, with a variety of anti-inflammatory and antioxidant active ingredients, Curculigo orchioides Gaertn can be applied to prevent and treat osteoporosis.
3.8 Ligustrum lucidum
Ligustrum lucidum (FLL) is a plant of the Oleaceae family, the medical part of which is its ripe, dry fruit, which has been applied to treat diseases associated with aging. In the case of excessive ROS produced by oxidative stress, the deficiency of sirt6 expression will promote and inhibit the formation of osteoclasts and osteoblasts respectively by regulating the NF-kB signaling pathway, thus resulting in osteoporosis (Lin L. et al., 2019). Ligustrum lucidum aqueous extraction (including nuezhenide and salidroside) reverses the adverse effects of oxidative stress. Furthermore, nuezhenide and salidroside inhibit bone loss caused by oxidative damage by enhancing the activity of the antioxidant enzyme superoxide dismutase (SOD) (Yi et al., 2021). NADPH oxidase 4 (Nox4) is an enzyme that controls the oxidative stress response. Upregulated Nox4 may augment oxidative stress and lead to mitochondrial dysfunction (Junya et al., 2010; Carolin et al., 2014). There is evidence that FLL aqueous extraction improves bone mass in ovariectomized mice through the regulation of the Nox4/ROS/NF-kB pathway (Lili et al., 2017). Specifically, FLL aqueous extraction suppresses the expression of Nox4, thus obliterating excessive ROS, and relieving the mitochondrial dysfunction caused by excessive ROS. Bone mass is then increased by regulating the NF-kB pathway. Salidroside (SAL) is an active component derived from FLL. Terttert-butyl hydroperoxide (t-BHP) can induce oxidative stress in osteoblast cells by causing galactose and fatty acid metabolism disorders (Yi-Fei et al., 2022). When galactose metabolism is impaired, it is converted to galactosyl by aldose reductase (AR). Excessive galactosyl in cells leads to the accumulation of free radicals (Dandan et al., 2020), and superfluous AR contributes to the formation of advanced glycosylation end products (AGEs) (Yan-Ying et al., 2013). Subsequently, the accumulation of AGEs increases ROS production, causing mitochondrial dysfunction and cellular inflammation (Ramin and Sorayya, 2018). Furthermore, the disruption of fatty acid metabolism caused by t-BHP can produce oxygen free radicals and lipid peroxides, thus causing oxidative stress damage to osteoblasts. Interestingly, SAL can enhance antioxidant enzyme activity and reduce oxidative stress damage caused by galactose and fatty acid metabolism disturbance by activating Nrf2 (Yi-Fei et al., 2022). As a result, osteoblast cells were protected from oxidative damage caused by t-BHP.
In addition to the botanical drugs mentioned above that have therapeutic and preventive effects on inflammation and oxidative stress-induced osteoporosis, there are also many botanical drugs that have not been mentioned. For example, the Chinese botanical drug medicine Psoralea corylifolia can effectively inhibit lipopolysaccharide-induced excess NO production by macrophages and also reduce bone loss by decreasing ROS production (Hisashi et al., 2009; Yinglong et al., 2023). In addition, the water-soluble extract of the Chinese botanical drug medicine Eucommiae Cortex has also been reported to downregulate lipopolysaccharide-induced iNOS, COX-2, and TNF-α in a dose-dependent manner, thus potentially becoming a safe drug for the treatment of inflammatory osteoporosis (Wonil et al., 2017). The chemical structure formula of the active ingredients of Chinese medicine is shown in Figure 2.
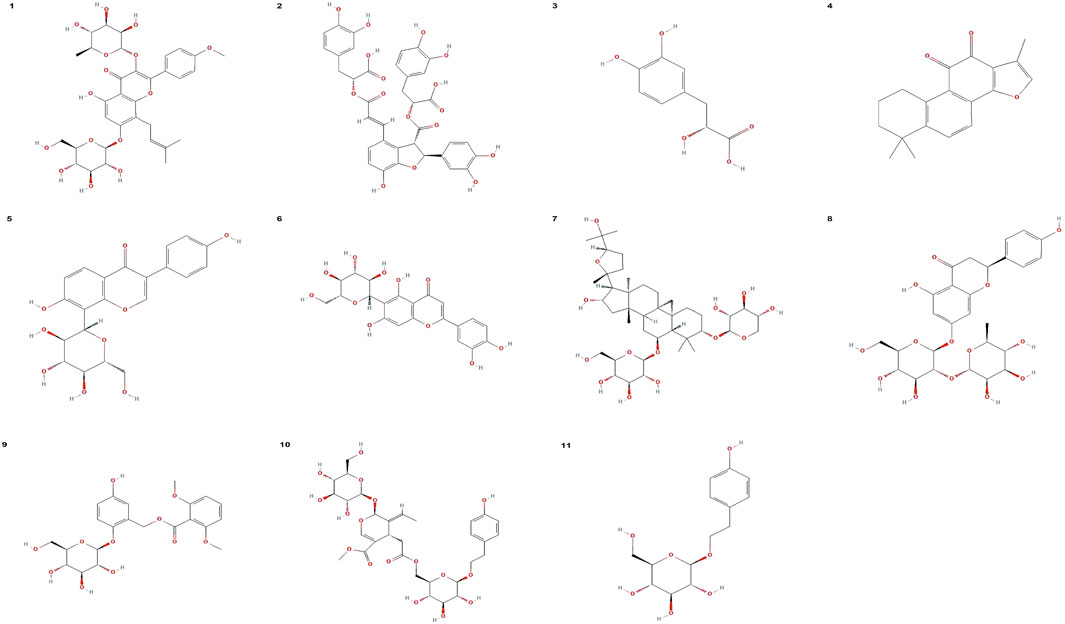
FIGURE 2. Chemical structure formula of effecttive ingredients of Chinese medicine. (1) Chemical structure formula of icariin. (2) Chemical structure formula of salvianolic acid B. (3) Chemical structure formula of danshensu. (4) Chemical structure formula of Tanshinone IIA. (5) Chemical structure formula of puerarin. (6) Chemical structure formula of isoorientin. (7) Chemical structure formula of astragaloside IV. (8) Chemical structure formula of Naringin. (9) Chemical structure formula of curculigoside. (10) Chemical structure formula of nuezhenide. (11) Chemical structure formula of salidroside. All chemical structure formulas were downloaded from PubChem (https://pubchem.ncbi.nlm.nih.gov/).
4 Chinese herbal compound preparation with anti-inflammatory and antioxidant effects in osteoporosis
Although the composition of TCM compound preparations is complicated and the pharmacological active ingredients are difficult to elucidate, they are still widely used as therapeutic drugs due to the advantages of long-term use and few side effects. This review aims to discuss and summarize the anti-inflammatory and antioxidant properties of TCM preparations for the treatment of osteoporosis (Table 3).
As previously mentioned, TNF-α, as an inflammatory factor, can inhibit the differentiation of MSCs into osteoblasts and affect the development and maturation of osteoblasts by inhibiting osteogenic factors such as Runx2. Duhuo JiSheng decoction was first recorded in “Qianjin Yaofang” of Simiao Sun, consisting of 15 traditional Chinese medicines such as Achyranthes bidentata Blume [Amaranthaceae], Cinnamomum verum [Lauraceae], Asarum heterotropoides F. Schmidt [Aristolochiaceae], Gentiana macrophylla Pall [Gentianaceae], Taxillus chinensis Danser [Loranthaceae], Angelica biserrata [Apiaceae], Eucommia ulmoides Oliv [Eucommiaceae], Smilax glabra Roxb [Smilacaceae], Saposhnikovia divaricata Schischk [Apiaceae], Conioselinum anthriscoides [Apiaceae], Panax ginseng C.A.Mey [Araliaceae], Glycyrrhiza glabra L. [Fabaceae], Angelica sinensis Diels [Apiaceae], Paeonia lactiflora Pall [Paeoniaceae], and Rehmannia glutinosa [Orobanchaceae] (Jinyu et al., 2020). It was reported that Duhuo JiSheng decoction could significantly decrease inflammatory cytokine TNF-α in ovariectomized rats to prevent bone loss (Xudong et al., 2022). Er-xian decoction, which includes Phellodendron chinense C.K.Schneid [Rutaceae], Epimedium brevicornu Maxim, Angelica sinensis Diels [Apiaceae], Anemarrhena asphodeloides Bunge [Asparagaceae], Curculigo orchioides Gaertn [Hypoxidaceae], Gynochthodes officinalis [Rubiaceae], is often used for menopause symptomsand is now being applied more and more widely in the treatment of osteoporosis. It has been demonstrated that Er-xian decoction could inhibit the oxidative stress induced by hydrogen peroxide by increasing the content of antioxidant enzymes SOD and GSH, as well as decreasing the levels of nitric oxide and MDA, thereby increasing bone density (Nani et al., 2019). Moreover, Er-xian decoction seems to reduce oxidative stress damage of bone tissue by regulating fatty acid metabolism (Yujie et al., 2023). FoxO1 was discovered to prompt osteoblast proliferation due to its ability to resist oxidative stress (Marie-Therese et al., 2010). Liuwei Dihuang pills were initially recorded in “Children’s Medicine Prescription Strightt Formula” of Yi Qian, consisting of six traditional Chinese medicines such as Dioscorea oppositifolia L [Dioscoreaceae], Cornus officinalis [Cornaceae], Rehmannia glutinosa [Orobanchaceae], Smilax glabra Roxb [Smilacaceae], Alisma plantago-aquatica subsp. orientale [Alismataceae], and Paeonia × suffruticosa Andrews [Paeoniaceae]. Based on the network pharmacological analysis of the target pathway of Liuwei Dihuang pills, it may inhibit the inflammatory response and oxidative stress by acting on the pro-inflammatory cytokines IL-1, IL-6, interleukin-17 (IL-17), and the FoxO1 signaling pathway, thereby treating postmenopausal osteoporosis (Qingchan et al., 2022). Qing E pills, which are composed of Eucommia ulmoides Oliv [Eucommiaceae], Cullen corylifolium [Fabaceae], Juglans regia L [Juglandaceae], and Allium sativum L [Amaryllidaceae], originated from the Song Dynasty book of “Tai Ping Hui Min He Ji Ju Fang” (Nai-Dan et al., 2016). The colonization of the intestinal tract by Firmicutes is related to the intestinal inflammatory response, which mediates the specific expression of pro-inflammatory cytokines TNF-α and Th17. Ultimately, this inflammation will lead to the activation of osteoclasts and induce osteoporosis (Thea and Emilio, 2013; Kyoung-Woon et al., 2015). Qing E pills decrease the level of Firmicutes in the intestinal tract and reverse adverse effects on bone mass (Hui et al., 2022). Siwu decoction was first written about by Daoren Lin during the Tang dynasty in the book “Xianshou Lishang Xuduan Mifang”. Siwu decoction, which is comprised of Angelica sinensis [Apiaceae], Rehmannia glutinosa [Orobanchaceae], Conioselinum anthriscoides ‘Chuanxiong’ [Apiaceae], and Paeonia lactiflora Pall [Paeoniaceae], has been used to treat chronic inflammatory diseases. We can infer that it inhibits osteoporosis due to its anti-inflammatory properties (Chi-Ming et al., 2013). Zuo Gui Wan was first recorded in the “Jingyue Quanshu”, consisting of Cornus officinalis [Cornaceae], Achyranthes bidentata Blume [Amaranthaceae], Lycium barbarum L [Solanaceae], Cuscuta australis [Convolvulaceae], deer horn gum, Turtle horn gum, Dioscorea oppositifolia L [Dioscoreaceae], and Rehmannia glutinosa [Orobanchaceae]. Aging MSCs secrete inflammatory cytokines and proteases that deprive their potential to differentiate into osteoblasts. Fortunately, Zuo Gui Wan can delay the senescence of MSCs to prevent osteoporosis by inhibiting the Wnt/β-catenin signaling pathway (Xiangping et al., 2022). In addition, Zuo Gui Wan alleviates Dex-Induced spinal osteoporosis (Gengyang et al., 2022). It is clear that these TCM preparations play an anti-osteoporosis role through their anti-inflammatory and antioxidant effects.
In recent years, with the continuous development of pharmacology, in addition to the compatibility of traditional fixed TCM, newly developed TCM-compatible preparations have been to applied in the treatment of osteoporosis. New Chinese medicine formulations including BuShenHuoXue capsules, Jianpi Qingchang Bushen decoction, Yishen Bugu Ye, Yunnan Baiyao, and Zhuanggu Zhitong capsules reduce inflammatory bone loss by directly regulating the level of pro-inflammatory factors or indirectly influencing the level of autophagy, thereby regulating the expression of inflammatory factors (Yangyang L. et al., 2020; Jia-Cheng et al., 2020; Xiaobin et al., 2020; Ya-Li et al., 2022; Zhitao et al., 2022). Moreover, Gushudan, Fufang Lurong Jiangu capsules, and Yishen Zhuanggu decoction rely on their antioxidant effect to relieve cells suffering from oxidative stress and thus prevent the occurrence of osteoporosis (Shuo et al., 2020; Wenqi et al., 2020; Bole et al., 2022).
5 Pathway analysis of pharmacological effects of network pharmacological on traditional Chinese Medicine
There are numerous active ingredients in TCM. Reasonable screening of active ingredients that can play a therapeutic role and actively search for targeted pathways of drug therapy are conducive to uncovering the mystery of TCM and accurately understanding the whole pharmacological process of TCM. Network pharmacology, which combines computer technology and systems biology, can identify drug targets and active ingredients, and then find disease-related targets and pathways through biological information analysis. Finally, the main pathway of drug treatment of disease will be explored after the intersection of drug targets and disease targets through bioinformatics analysis.
KEGG enrichment analysis (http://www.kegg.jp/) is an integrated database resource for the biological interpretation of genome sequences and other high-throughput data (Minoru et al., 2016). KEGG enrichment analysis showed that an extract of Salvia miltiorrhiza Bunge could significantly regulate the TNF pathway, then inhibit the levels of IL-6, IL-12, and TNF-α, and reduce the inflammatory damage of bone marrow macrophages (Tingting et al., 2021). In addition, the NF-kB pathway has also been verified to be involved in the progression of danshensu against macrophage inflammatory damage (Tingting et al., 2020). Some studies have demonstrated that Morindae Officinalis Radix plays an anti-osteoporosis role by regulating the MAPK pathway to affect the inflammatory response and nitric oxide biosynthesis through a combination of network pharmacology and bioinformation analysis (Shao-Qi et al., 2020). Ecliptae herba may influence the expression of PPARγ by acting on the cancer pathway and the P13K-Akt signaling pathway, thereby associated with protein targets that influence inflammatory responses. The result was the inhibition of glucocorticoid-induced osteoporosis (Fangqing et al., 2022). According to the results of KEGG and gene ontology enrichment analysis, Fructus ligustri lucidi may affect the biological process of the inflammatory response by regulating the P13K-Akt pathway, thus stimulating the osteogenic differentiation of MSCs (Yuanhang et al., 2022). Although there is no direct evidence that Siwu decoction plays an anti-osteoporosis role through a specific pathway, network pharmacological analysis suggests that it can regulate the metabolism of arachidonic acid and thus participate in the inflammatory process. Therefore, it can be inferred that Siwu decoction has certain anti-inflammatory properties (Hua-Li et al., 2021). Moreover, Bushenhuoxue capsules might be connected with reducing the inflammatory response and delaying the biological process of osteoporosis by controlling osteoclast differentiation, phagosome, leukocyte transendothelial migration, and other pathways (Jia-Cheng et al., 2020). Biological analysis indicated that Zhuanggu Zhitong capsules could participate in the metabolic process of ROS and the response to steroid hormones, as well as alleviate bone loss through Akt and apoptosis signaling pathways (Jiangtao et al., 2021). This indicates that Zhuanggu Zhitong capsules could be used to treat oxidative stress-induced osteoporosis depending on the antioxidant activity.
Although there are many studies that have confirmed the targeting pathways and biological processes as well as the active ingredients of these TCMs with anti-inflammatory and antioxidant activities through network pharmacology, there are still many TCMs, especially TCM compound preparations, which have not been fully explored in network pharmacology and basic research. This makes it difficult to elucidate the mechanism of action of TCM and makes it difficult to standardize the doses and ratios of drugs used; therefore, a clear investigation of the mechanism through network pharmacology and basic experiments is now a top priority.
6 Discussion
TCM is a vast resource waiting to be exploited. TCM and its formulations contain abundant anti-inflammatory and antioxidant active ingredients, including flvonoid glucoside, flavonoids, isoflavonoids, saponin, and iridoid glycoside. Icariin from Epimedii herba regulates bone metabolism by suppressing the release of inflammatory cytokines and ameliorating the accumulation of oxidative products. Morinada officinalis polysaccharide from Morindae officinalis Radix has been reported to increase bone mineral density and alleviate bone absorption, attributed to its anti-inflammation and anti-oxidation properties. Curculigoside from Curculiginis rhizome is an antioxidant that alleviates oxidative stress damage in the bone. Although there are a few reports on this subject, the scientific quality of these articles varies as some of them describe the drug preparation methods and pharmacological monitoring methods in detail but some of the reports directly purchased from ready-to-use herbal medicines from reagent dealers and there is no clear mention of the drug composition dose, extraction methods, and active ingredient detection methods This deserves attention in future ethnomedical studies with a view to obtaining more qualitative scientific reports. In addition, a review of the research process of the literature covered in this article showed that a few articles did not explore the drug dose concentrations, only one drug concentration was involved, and negative controls were also lacking. These active ingredients play a role through the multiple targets and multiple pathways. Moreover, with a low price and few adverse reactions, TCM can not only restore the bone microstructure and increase the physiological quality of the bone but also alleviate secondary symptoms such as back pain, and waist and leg weakness (Zhu et al., 2012). However, since the use of TCM and formulations was employed under the guidance of TCM theory, there has not been a unified mode of action for general reference in pharmacology so far, which suggests that more research is urgently needed to fully understand the natural mechanism of drug action and optimize the matching of the drugs.
In conclusion, TCM contains many anti-inflammatory and antioxidant active substances, and its value in the treatment of osteoporosis is beyond doubt. However, since the action mechanism of TCM is multi-targeted and multi-pathway, more pharmacological knowledge is needed to confirm the effectiveness and accuracy of the mechanism of TCM. In brief, it is urgent to develop more effective and safer forms of TCM with clear mechanisms of action. This review elucidates the active ingredients and pathways of action of various herbal medicines, which are relatively wide-ranging but have certain limitations. This review is based on the summary of basic experiments and network pharmacology and lacks a collection of clinical information and clinical verification of the usefulness of the drugs. In addition, the mechanism of action of the active ingredient of the drug on osteoporosis was elucidated in detail, the interaction between the active ingredient of the drug and the active ingredient was not clearly taken into account. Another major limitation is that the efficacy and/or safety of many herbal products relies heavily on low-quality studies that may be limited by the inadequacy or inconsistency of the methods used and the risk of bias in the included studies, thus failing to draw definitive efficacy conclusions. Therefore, more high-quality studies are needed in the field of herbal medicine to clearly determine the efficacy and safety of herbal products.
Author contributions
All the authors were involved in the manuscript writing process. QL and CT contributed equally to this work and share first authorship. These authors contributed equally to this work and share senior authorship. HL provided scientific ideas for the study and reviewed the manuscript. QL and CT wrote the original draft. XL provided help with project administration and funding acquisition. DL contributed to the background investigation. All authors contributed to the article and approved the submitted version.
Funding
The authors acknowledge funding from the Vice President and Director of the Geriatrics Department, Liyuan Hospital Affiliated with Huazhong University of Science and Technology. This project was supported by the Key Technologies Research and Development Program “Technology for Proactive Health and Aging” (2020YFC2006500).
Conflict of interest
The authors declare that the research was conducted in the absence of any commercial or financial relationships that could be construed as a potential conflict of interest.
Publisher’s note
All claims expressed in this article are solely those of the authors and do not necessarily represent those of their affiliated organizations, or those of the publisher, the editors and the reviewers. Any product that may be evaluated in this article, or claim that may be made by its manufacturer, is not guaranteed or endorsed by the publisher.
References
Agnieszka, L., Milena, D., Elzbieta, P., Alicja, J., and Jozef, D. (2016). Role of Nrf2/HO-1 system in development, oxidative stress response and diseases: An evolutionarily conserved mechanism. Cell Mol. Life Sci. 73 (17), 3221–3247. doi:10.1007/s00018-016-2223-0
Bee, L. T., and Mohd, E. N. (2019). Effect of high-fat diets on oxidative stress, cellular inflammatory response and cognitive function. Nutrients 11 (11), 2579. doi:10.3390/nu11112579
Bianca, S., Ana, C. R., Ângela, Z., Alexandre, U. A., Thabata, F., Kaleb, P. S., et al. (2021). S-adenosylmethionine induces mitochondrial dysfunction, permeability transition pore opening and redox imbalance in subcellular preparations of rat liver. J. Bioenerg. Biomembr. 53 (5), 525–539. doi:10.1007/s10863-021-09914-3
Bo, Y., Lihong, H., Yunsong, L., Peng, D., John, S., Jiong, L., et al. (2018). PGC-1α controls skeletal stem cell fate and bone-fat balance in osteoporosis and skeletal aging by inducing TAZ. Cell Stem Cell 23 (2), 193–209.e5. doi:10.1016/j.stem.2018.06.009
BoCun-Yu, Y. W. (2022). Osteoporosis and periodontal diseases - an update on their association and mechanistic links. Periodontol 89 (1), 99–113. doi:10.1111/prd.12422
Bole, L., Changqing, J., and Xinyu, Z. (2022). Combined therapy of yishen Zhuanggu decoction and caltrate D600 alleviates postmenopausal osteoporosis by targeting FoxO3a and activating the Wnt/β-catenin pathway. Evid. Based Complement. Altern. Med. 2022, 7732508. doi:10.1155/2022/7732508
Carolin, C., Guido, K., Rosalia, C., Rosanna, D. P., Angela, K., Francesca, L., et al. (2014). Osteoporosis and alzheimer pathology: Role of cellular stress response and hormetic redox signaling in aging and bone remodeling. Front. Pharmacol. 5, 120. doi:10.3389/fphar.2014.00120
Cawthorn, W. P., Bree, A. J., Yao, Y., Du, B., Hemati, N., Martinez-Santibañez, G., et al. (2012). Wnt6, Wnt10a and Wnt10b inhibit adipogenesis and stimulate osteoblastogenesis through a β-catenin-dependent mechanism. Bone 50, 477–489. doi:10.1016/j.bone.2011.08.010
Chaodong, Z., Tingting, X., Luxi, L., Aftab, S., Xishuai, T., Ke, Y., et al. (2022). Morinda officinalis polysaccharides ameliorates bone growth by attenuating oxidative stress and regulating the gut microbiota in thiram-induced tibial dyschondroplasia chickens. compounds 12 (10), 958. doi:10.3390/metabo12100958
Cheng, W., Shu, D., Lihong, G., Ke, F., and Cheng, M. (2022). A review of pharmacology, toxicity and pharmacokinetics of 2,3,5,4'-tetrahydroxystilbene-2-O-β-D-Glucoside. Front. Pharmacol. 12, 791214. doi:10.3389/fphar.2021.791214
Chengli, L., Jun, Z., Fang, L., Xingtao, G., and Gang, L. (2018). Naringin protects against bone loss in steroid-treated inflammatory bowel disease in a rat model. Arch. Biochem. Biophys. 650, 22–29. doi:10.1016/j.abb.2018.05.011
Chi-Ming, W., Po-Chun, C., Te-Mao, L., Yi-Chin, F., and Chih-Hsin, T. (2013). Si-Wu-tang extract stimulates bone formation through PI3K/Akt/NF-κB signaling pathways in osteoblasts. BMC Complement. Altern. Med. 13, 277. doi:10.1186/1472-6882-13-277
Chun-Yan, S., Qian-Liang, M., Khalid, R., Ting, H., and Lu-Ping, Q. (2015). Salvia miltiorrhiza: Traditional medicinal uses, chemistry, and pharmacology. Chin. J. Nat. Med. 13 (3), 163–182. doi:10.1016/S1875-5364(15)30002-9
Dandan, X., Liqin, J., Yao, L., and Zhenwei, L. (2020). Antioxidant activity of selenium-enriched Chrysomyia megacephala (Fabricius) larvae powder and its impact on intestinal microflora in D-galactose induced aging mice. BMC Complement. Med. Ther. 20 (1), 264. doi:10.1186/s12906-020-03058-4
Daniel, L., Firdaus, S. D., Christina, M. H., and Felipe, A. J. (2017). Oxidative stress, inflammation and treatment response in major depression. Psychoneuroendocrinology 76, 197–205. doi:10.1016/j.psyneuen.2016.11.031
Dinesh, T., Pradip, C., and Chaudhari, P. (2017). Carnosic acid attenuates RANKL-induced oxidative stress and osteoclastogenesis via induction of Nrf2 and suppression of NF-κB and MAPK signalling. J. Mol. Med. Berl. 95 (10), 1065–1076. doi:10.1007/s00109-017-1553-1
Dong, X. L., Yu, W. X., Li, C. M., He, S., Zhou, L. P., Poon, C. W., et al. (2018). Danshen (Salvia miltiorrhiza) protects ovariectomized rats fed with high-saturated fat-sucrose diet from bone loss. Osteoporos. Int. 29 (1), 223–235. doi:10.1007/s00198-017-4254-2
Draper, H. H., and Hadley, M. (1990). Malondialdehyde determination as index of lipid peroxidation. Methods Enzymol. 186, 421–431. doi:10.1016/0076-6879(90)86135-i
Du, Y., Zhang, Q., Chen, G. G., Wei, P., and Tu, C. Y. (2005). Pharmacokinetics of Astragaloside IV in rats by liquid chromatography coupled with tandem mass spectrometry. Eur. J. Drug Metab. Pharmacokinet. 30 (4), 269–273. doi:10.1007/BF03190631
Emmanuel, M., Fei, X., Man-Sau, W., and Yan, Z. (2014). Chinese herbal medicine for bone health. Pharm. Biol. 52 (9), 1223–1228. doi:10.3109/13880209.2014.884606
Fabien, W., Laurent, L., Véronique, C., Jérôme, G., and Yohann, W. (2009). Oxidative stress in bone remodelling and disease. Trends Mol. Med. 15 (10), 468–477. doi:10.1016/j.molmed.2009.08.004
Fang-Bing, Z., Jian-Yue, W., Ying-Liang, Z., Ren-Fu, Q., Zhen-Shuang, Y., Lin-Ru, Z., et al. (2015). Curculigoside regulates proliferation, differentiation, and pro-inflammatory cytokines levels in dexamethasone-induced rat calvarial osteoblasts. Int. J. Clin. Exp. Med. 8 (8), 12337–12346.
Fangqing, Z., Qiuyue, L., Jiashuo, W., Haonan, R., Chuanrui, S., Jia, Z., et al. (2022). Total flavonoids of drynariae rhizoma improve glucocorticoid-induced osteoporosis of rats: UHPLC-MS-based qualitative analysis, network pharmacology strategy and pharmacodynamic validation. Front. Endocrinol. (Lausanne) 13, 920931. doi:10.3389/fendo.2022.920931
Gengyang, S., Qi, S., Zhida, Z., Wenhua, Z., Honglin, C., Ibrayinjan, M., et al. (2022). Zuo-gui-wan aqueous extract ameliorates glucocorticoid-induced spinal osteoporosis of rats by regulating let-7f and autophagy. Front. Endocrinol. (Lausanne) 13, 878963. doi:10.3389/fendo.2022.878963
Gregory, H. J., Courtney, M. V., Omar, F. P., and Rodrigo, M. (2021). Inflammatory signaling mechanisms in bipolar disorder. J. Biomed. Sci. 28 (1), 45. doi:10.1186/s12929-021-00742-6
Guoju, H., Lin, Z., Xuguang, S., Wei, H., Haibin, W., Qiushi, W., et al. (2017). Bajijiasu abrogates osteoclast differentiation via the suppression of RANKL signaling pathways through NF-κB and NFAT. Int. J. Mol. Sci. 18 (1), 203. doi:10.3390/ijms18010203
Hansen, C., Yacong, H., Shuang, C., Suhua, Q., and Jiangang, S. (2020). Therapeutic targets of oxidative/nitrosative stress and neuroinflammation in ischemic stroke: Applications for natural product efficacy with omics and systemic biology. Pharmacol. Res. 158, 104877. doi:10.1016/j.phrs.2020.104877
Henry, J. F., and Hongqiao, Z. (2021). Targeting oxidative stress in disease: Promise and limitations of antioxidant therapy. Nat. Rev. Drug Discov. 20 (9), 689–709. doi:10.1038/s41573-021-00233-1
Hiroyuki, K., Fumiaki, S., Itohiya, K., Yuuki, Y., Sari, F., Yutaka, M., et al. (2016). Molecular regulatory mechanisms of osteoclastogenesis through cytoprotective enzymes. Redox Biol. 8, 186–191. doi:10.1016/j.redox.2016.01.006
Hisashi, M., Sachie, K., Sachiko, S., Shin, A., Seikou, N., and Masayuki, Y. (2009). Bioactive constituents from Chinese natural medicines. XXXIII. Inhibitors from the seeds of Psoralea corylifolia on production of nitric oxide in lipopolysaccharide-activated macrophages. Biol. Pharm. Bull. 32 (1), 147–149. doi:10.1248/bpb.32.147
Hong-Tao, X., Lu-Ping, Q., Hai-Liang, X., Qiao-Yan, Z., Yi-min, L., and Nancy, E. L. (2006). Epidemiology, etiology, and diagnosis of osteoporosis. Am. J. Obstet. Gynecol. 194 (2), S3–S11. doi:10.1016/j.ajog.2005.08.047
Hongguo, S., Ji, S., Mingjun, W., Jingfu, C., Yijun, W., Shijun, Z., et al. (2015). Icariin protects against titanium particle-induced osteolysis and inflammatory response in a mouse calvarial model. Biomaterials 60, 92–99. doi:10.1016/j.biomaterials.2015.04.048
Hua-Li, Z., Qian-Ru, Z., Cen, C., Feng-Qing, Y., Hua, Y., and Yuan-Jia, (2021). Molecular evidence of herbal formula: A network-based analysis of Si-Wu decoction. HuPhytochem Anal. 32 (2), 198–205. doi:10.1002/pca.2965
Hui, J., Jianyang, D., Huan, R., Guofu, Y., Wenbo, W., and Jianyang, D. (2021). Astragaloside IV protects against iron loading-induced abnormal differentiation of bone marrow mesenchymal stem cells (BMSCs). FEBS Open Bio 11 (4), 1223–1236. doi:10.1002/2211-5463.13082
Hui, X., Zhengying, H., Mengyu, G., Shangyang, L., Yaqian, Z., Zebin, W., et al. (2022). Gut microbiota and metabonomics used to explore the mechanism of Qing'e Pills in alleviating osteoporosis. Pharm. Biol. 60 (1), 785–800. doi:10.1080/13880209.2022.2056208
Hwa, J. C., Jae-Soon, E., Young-Ran, P., Dae Keun, K., Rihua, L., Woo, S. M., et al. (2008). Ikarisoside A inhibits inducible nitric oxide synthase in lipopolysaccharide-stimulated RAW 264.7 cells via p38 kinase and nuclear factor-kappaB signaling pathways. Eur. J. Pharmacol. 601 (1-3), 171–178. doi:10.1016/j.ejphar.2008.09.032
Izawa, A., Ishihara, Y., Mizutani, H., Kobayashi, S., and Goto, H. (2014). Inflammatory bone loss in experimental periodontitis induced by Aggregatibacter actinomycetemcomitans in interleukin-1 receptor antagonist knockout mice. Infect. Immun. 82 (5), 1904–1913. Epub 2014 Feb 24. doi:10.1128/IAI.01618-13
Janhavi, J. D., Mary, J. D. S., Hannah, L. V., Nicole, C. A. S., and Connie, J. R. (2022). The role of prunes in modulating inflammatory pathways to improve bone health in postmenopausal women. Adv. Nutr. 13 (5), 1476–1492. doi:10.1093/advances/nmab162
Jay, R. L., Aaron, D., and Thomas, A. E. (2002). The role of growth factors in the repair of bone. Biology and clinical applications. J. Bone Jt. Surg. Am. 84 (6), 1032–1044. doi:10.2106/00004623-200206000-00022
Jeff, S. K., Joey, P. J., and DuWayne, A. C. (2021). Oxidative stress and osteoporosis. J. Bone Jt. Surg. Am. 103 (15), 1451–1461. doi:10.2106/JBJS.20.00989
Ji-Chun, Z., Wei, Y., and Kenji, H. (2016). Brain-derived neurotrophic factor (BDNF)-TrkB signaling in inflammation-related depression and potential therapeutic targets. Curr. Neuropharmacol. 14 (7), 721–731. doi:10.2174/1570159x14666160119094646
Jia, C., Fei, L., Min, L., Benjamin, W., Kang, T., Janette, N. Z., et al. (2013). NF-κB inhibits osteogenic differentiation of mesenchymal stem cells by promoting β-catenin degradation. Proc. Natl. Acad. Sci. U. S. A. 110 (23), 9469–9474. doi:10.1073/pnas.1300532110
Jia, L., Lingyu, H., Junhua, W., Jiquan, Z., and Jiansheng, S. (2016). Linarin promotes osteogenic differentiation by activating the BMP-2/RUNX2 pathway via protein kinase A signaling. Int. J. Mol. Med. 37 (4), 901–910. doi:10.3892/ijmm.2016.2490
Jia-Cheng, L., Xue-Zhen, L., Di, L., Bo-Zhao, Y., Jin-Bao, L., and Gang, L. (2020). Study on the molecular mechanism of BuShenHuoXue capsule in treatment of steroid-induced osteonecrosis of the femoral head. Ann. Transl. Med. 8 (24), 1680. doi:10.21037/atm-20-7040
Jian, L., Danqing, L., Xuying, S., Yuting, W., Qiangbing, X., and Anmin, C. (2016). Icariine restores LPS-induced bone loss by downregulating miR-34c level. Inflammation 39 (5), 1764–1770. doi:10.1007/s10753-016-0411-6
Jian, W., Gang, W., Li, G., Guanwen, S., Bin, S., Huhe, B., et al. (2018). Isopsoralen regulates PPAR-γ/WNT to inhibit oxidative stress in osteoporosis. Mol. Med. Rep. 17 (1), 1125–1131. doi:10.3892/mmr.2017.7954
Jiangtao, M., Maolin, Y., Ying, L., Shuang, C., Hong, H., Xiaohang, L., et al. (2021). Zhuanggu Zhitong Capsule alleviates osteosarcopenia in rats by up-regulating PI3K/Akt/Bcl2 signaling pathway. Biomed. Pharmacother. 142, 111939. doi:10.1016/j.biopha.2021.111939
Jingfu, C., Mo, Z., Shijun, Z., Guixian, W., Yaozeng, X., and Dechun, G. (2014). Inhibitory effect of icariin on Ti-induced inflammatory osteoclastogenesis. J. Surg. Res. 192 (2), 447–453. doi:10.1016/j.jss.2014.05.038
Jinyu, L., Wei, W., Guiyu, F., Jian, D., Shengqian, K., Zhe, L., et al. (2020). Efficacy and safety of Duhuo jisheng decoction for postmenopausal osteoporosis: A systematic review and meta-analysis. Evid. Based Complement. Altern. Med. 2020, 6957825. doi:10.1155/2020/6957825
Joon-Ho, L., Yuan-Ji, W., Zhong-Yan, Z., Yu-Ming, H., and Cheng-Long, W. (2022). Efficacy of the herbal pair, Radix Achyranthis Bidentatae and Eucommiae Cortex, in preventing glucocorticoid-induced osteoporosis in the zebrafish model. J. Integr. Med. 20 (1), 83–90. doi:10.1016/j.joim.2021.11.003
Junya, K., Tetsuro, A., Shouji, M., Peiyong, Z., Michael, D. S., and Junichi, S. (2010). NADPH oxidase 4 (Nox4) is a major source of oxidative stress in the failing heart. Proc. Natl. Acad. Sci. U. S. A. 107 (35), 15565–15570. doi:10.1073/pnas.1002178107
Kai, R., Pingbo, C., Yi, L., Yaowu, Z., Zhan, W., Fengli, W., et al. (2022). Morinda officinalis polysaccharide attenuates osteoporosis in rats underwent bilateral ovariectomy by suppressing the PGC-1α/PPARγ pathway. J. Orthop. Surg. Hong. Kong) 30 (3), 10225536221130824. doi:10.1177/10225536221130824
Kanaze, F. I., Bounartzi, M. I., Georgarakis, M., and Niopas, I. (2007). Pharmacokinetics of the citrus flavanone aglycones hesperetin and naringenin after single oral administration in human subjects. Eur. J. Clin. Nutr. 61 (4), 472–477. doi:10.1038/sj.ejcn.1602543
Kathy, K. A., Quan-Bin, H., and Joshua, K. K. (2016). Astragalus membranaceus: A review of its protection against inflammation and gastrointestinal cancers. Am. J. Chin. Med. 44 (1), 1–22. doi:10.1142/S0192415X16500014
Keda, Y., Fangming, C., Yuchuan, X., Lin, T., and Yue, Z. (2022). Three classes of antioxidant defense systems and the development of postmenopausal osteoporosis. Front. Physiol. 13, 840293. doi:10.3389/fphys.2022.840293
Kerri, Ds, Kristi, R., and Rachel, N. (2011). Osteoporosis update. J. Midwifery Womens Health 56 (6), 615–627. doi:10.1111/j.1542-2011.2011.00135.x
Kotha, A., Gorla, V. R., Rajaram, A., Nagendra, S. Y., Gangappa, D., Anand, S., et al. (2017). Evaluation of anti-inflammatory properties of isoorientin isolated from tubers of Pueraria tuberosa. Oxid. Med. Cell Longev. 2017, 5498054. doi:10.1155/2017/5498054
Kyoung-Woon, K., Hae-Rim, K., Bo-Mi, K., Mi-La, C., and Sang-Heon, L. (2015). Th17 cytokines regulate osteoclastogenesis in rheumatoid arthritis. Am. J. Pathol. 185 (11), 3011–3024. doi:10.1016/j.ajpath.2015.07.017
Li-Jie, Z., Hui-Kang, L., Ping-Chun, H., Li-Ming, Y. K., I-Jung, L., Tian-Shung, W., et al. (2011). New isoflavonoid glycosides and related constituents from astragali radix (Astragalus membranaceus) and their inhibitory activity on nitric oxide production. J. Agric. Food Chem. 59 (4), 1131–1137. doi:10.1021/jf103610j
Liao, C., Ting, L., Yuyu, L., Le, Z., Pinghua, L., Bilian, X., et al. (2012). Salvianolic acid B prevents bone loss in prednisone-treated rats through stimulation of osteogenesis and bone marrow angiogenesis. PLoS One 7 (4), e34647. doi:10.1371/journal.pone.0034647
Lili, W., Rufeng, M., Yubo, G., Jing, S., Haixia, L., Ruyuan, Z., et al. (2017). Antioxidant effect of Fructus ligustri lucidi aqueous extract in ovariectomized rats is mediated through nox4-ROS-NF-κb pathway. Front. Pharmacol. 8, 266. doi:10.3389/fphar.2017.00266
Lin, K. M., Lu, C. L., Hung, K. C., Wu, P. C., Pan, C. F., Wu, C. J., et al. (2019a). The paradoxical role of uric acid in osteoporosis. Nutrients 11 (9), 2111. doi:10.3390/nu11092111
Lin, L., Beibei, C., Ruyuan, Z., Rui, L. Y. T., Chenyue, L., Qiangqiang, J., et al. (2019b). Fructus Ligustri Lucidi preserves bone quality through the regulation of gut microbiota diversity, oxidative stress, TMAO and Sirt6 levels in aging mice. Aging (Albany NY) 11 (21), 9348–9368. doi:10.18632/aging.102376
LinBeibei, L. C., Ruyuan, Z., Rui, L., and Yimiao, T. (2019). Fructus Ligustri Lucidi preserves bone quality through the regulation of gut microbiota diversity, oxidative stress, TMAO and Sirt6 levels in aging mice. Aging (Albany NY) 11 (21), 9348–9368. doi:10.18632/aging.102376
Lu, Z., Sha, L., Yin, W., Qiaoyan, Z., Wenjuan, Z., Zejian, W., et al. (2015). Effects of curculigoside on memory impairment and bone loss via anti-oxidative character in APP/PS1 mutated transgenic mice. PLoS One 10 (7), e0133289. doi:10.1371/journal.pone.0133289
Mao, D., Huijuan, C., Jiaying, L., Jiawen, S., Long, X., and Xiaofang, L. (2021). Calycosin: A review of its pharmacological effects and application prospects. Expert Rev. Anti Infect. Ther. 19 (7), 911–925. doi:10.1080/14787210.2021.1863145
Maria, A., Li, H., Elena, A., Robert, S. W., and Stavros, C. M. (2011). Glucocorticoids and tumor necrosis factor α increase oxidative stress and suppress Wnt protein signaling in osteoblasts. J. Biol. Chem. 286 (52), 44326–44335. doi:10.1074/jbc.M111.283481
Marie-Therese, R., Aruna, K., Lili, X., Yoshihiro, Y., Ji-Hye, P., Ronald, A. D., et al. (2010). FoxO1 is a positive regulator of bone formation by favoring protein synthesis and resistance to oxidative stress in osteoblasts. Cell Metab. 11 (2), 147–160. doi:10.1016/j.cmet.2010.01.001
Mayra, A. G., Héctor, F. T., Juan, M. J., Joel, H., Marcela, A., and Sergio, M. (2021). Cadmium exposure negatively affects the microarchitecture of trabecular bone and decreases the density of a subset of sympathetic nerve fibers innervating the developing rat femur. Biometals 34 (1), 87–96. doi:10.1007/s10534-020-00265-x
Meng-Yun, L., Zhen-Hua, L., Zi-En, C., Yi-Ran, Q., Qiu-Yi, L., and Ping, D. (2022). Quality markers for processed products of Morinda officinalis how based on the "oligosaccharides-spectrum-effect. J. Pharm. Biomed. Anal. 208, 114403. doi:10.1016/j.jpba.2021.114403
Mengqin, L., Sha, L., Qi, Z., Yingqi, F., Yanwei, Y., Lulin, Z., et al. (2021). Curculigoside attenuates oxidative stress and osteoclastogenesis via modulating Nrf2/NF-κB signaling pathway in RAW264.7 cells. J. Ethnopharmacol. 275, 114129. doi:10.1016/j.jep.2021.114129
Minoru, K., Yoko, S., Masayuki, K., Miho, F., and Mao, T. (2016). KEGG as a reference resource for gene and protein annotation. Nucleic Acids Res. 44 (1), D457–D462. doi:10.1093/nar/gkv1070
Monia, O., Oriana, T., Arianna, V., Monica, M., and Eleonora, S. (2009). Nitric oxide production during the osteogenic differentiation of human periodontal ligament mesenchymal stem cells. Acta histochem. 111 (1), 15–24. doi:10.1016/j.acthis.2008.02.005
Myles, R. M., Juliet, M. T., and Peter, J. C. (2016). The contribution of neuroinflammation to amyloid toxicity in Alzheimer's disease. J. Neurochem. 136 (3), 457–474. doi:10.1111/jnc.13411
Na, G., Zhiwei, H., Xiaoxu, F., Jian, Z., Dehui, Z., Tao, X., et al. (2012). Simultaneous determination of salidroside and its aglycone metabolite p-tyrosol in rat plasma by liquid chromatography-tandem mass spectrometry. Molecules 17 (4), 4733–4754. doi:10.3390/molecules17044733
Nai-Dan, Z., Ting, H., Bao-Kang, H., Khalid, R., Yi-Ping, J., Xu, H. T., et al. (2016). Traditional Chinese medicine formulas for the treatment of osteoporosis: Implication for antiosteoporotic drug discovery. J. Ethnopharmacol. 189, 61–80. doi:10.1016/j.jep.2016.05.025
Nani, W., Pingcui, X., Xuping, W., Weixuan, Y., Zhongming, Y., Renjie, W., et al. (2019). Integrated pathological cell fishing and network pharmacology approach to investigate main active components of Er-Xian decotion for treating osteoporosis. J. Ethnopharmacol. 241, 111977. doi:10.1016/j.jep.2019.111977
Ning, S., Xuran, Z., Lin, Y., Zhiyong, Z., Peipei, J., Xiaoxu, Z., et al. (2014). Pharmacokinetic and excretion study of three secoiridoid glycosides and three flavonoid glycosides in rat by LC-MS/MS after oral administration of the Swertia pseudochinensis extract. J. Chromatogr. B Anal. Technol. Biomed. Life Sci. 967, 75–83. doi:10.1016/j.jchromb.2014.07.010
Noemí, G., Cecilia, Z., and Leopoldo, A. (2017). Oxidative stress and inflammation in cardiovascular disease. Oxid. Med. Cell Longev. 2017, 5853238. doi:10.1155/2017/5853238
Palanisamy, A., Masoumeh, T. F., Woan, S. T., Sivapragasam, G., and Sharida, F. (2016). Role of antioxidants and natural products in inflammation. Oxid. Med. Cell Longev. 2016, 5276130. doi:10.1155/2016/5276130
Panyun, M., Yimei, H., Xu, M., Jingru, S., Zhendong, Z., and Lingyuan, H. (2021). Total flavonoids of Rhizoma Drynariae combined with calcium attenuate osteoporosis by reducing reactive oxygen species generation. Exp. Ther. Med. 21 (6), 618. doi:10.3892/etm.2021.10050
Paul N Cori, R., and Russell, C. (2022). Cardio-Rheumatology: Prevention of cardiovascular disease in inflammatory disorders. Med. Clin. North Am. 106 (2), 349–363. doi:10.1016/j.mcna.2021.11.010
Peng, L., Yue, X., Zhifeng, X., Zhe, M., Bing, Z., Hui, P., et al. (2019). Pharmacokinetics of salvianolic acid B, rosmarinic acid and Danshensu in rat after pulmonary administration of Salvia miltiorrhiza polyphenolic acid solution. Biomed. Chromatogr. 33 (8), e4561. doi:10.1002/bmc.4561
Prof, F. B., and Prof, A. M. (2001). Inflammation and cancer: Back to virchow? Lancet 357 (9255), 539–545. doi:10.1016/S0140-6736(00)04046-0
Qingchan, W., Ping, H., Chenjie, X., and Danqing, F. (2022). Network pharmacology-based strategy to investigate pharmacological mechanism of Liuwei Dihuang Pill against postmenopausal osteoporosis. Med. Baltim. 101 (47), e31387. doi:10.1097/MD.0000000000031387
Quanlong, Z., Lu, Z., Yi, S., Yuqiong, H., Gang, C., Ming, Y., et al. (2019). Curculigoside protects against excess-iron-induced bone loss by attenuating akt-FoxO1-dependent oxidative damage to mice and osteoblastic mc3t3-E1 cells. Oxid. Med. Cell Longev. 2019, 9281481. doi:10.1155/2019/9281481
Ramin, G., and Sorayya, K. (2018). Carnosine and advanced glycation end products: A systematic review. Amino Acids 50 (9), 1177–1186. doi:10.1007/s00726-018-2592-9
Ran, Y., Qingwen, W., Huajin, Z., Zao, Q., Jianjun, L., and Lingbo, Q. (2011). Determination of puerarin in biological samples and its application to a pharmacokinetic study by flow-injection chemiluminescence. Luminescence 26 (5), 368–373. doi:10.1002/bio.1281
Rossouw, J. E., Anderson, G. L., Prentice, R. L., LaCroix, A. Z., Kooperberg, C., Stefanick, M. L., et al. (2002). Risks and benefits of estrogen plus progestin in healthy postmenopausal women: Principal results from the women's health initiative randomized controlled trial. JAMA 288 (3), 321–333. doi:10.1001/jama.288.3.321
Rui, Z., Lin, T., Shui, Q., Lin, S., and Yihao, T. (2020). Melatonin rescues glucocorticoid-induced inhibition of osteoblast differentiation in MC3T3-E1 cells via the PI3K/AKT and BMP/Smad signalling pathways. Life Sci. 257, 118044. doi:10.1016/j.lfs.2020.118044
SalilaDorothy, K. A. F., and Ethel, S. S. (2014). Osteoporosis-associated fracture and diabetes. Endocrinol. Metab. Clin. North Am. 43 (1), 233–243. doi:10.1016/j.ecl.2013.09.004
Shamima, I., Ferdaus, H., Gantsetseg, T., Jargalsaikhan, D., and Naoki, K. (2007). Bacterial lipopolysaccharide induces osteoclast formation in RAW 264.7 macrophage cells. Biochem. Biophys. Res. Commun. 360 (2), 346–351. doi:10.1016/j.bbrc.2007.06.023
Shao-Qi, F., Zi-Yuan, W., Zheng-Meng, J., Zhi-Ming, B., and E-Hu, L. (2020). Integration of zebrafish model and network pharmacology to explore possible action mechanisms of Morinda officinalis for treating osteoporosis. Chem. Biodivers. 17 (5), e2000056. doi:10.1002/cbdv.202000056
Shiow-Yunn, S., Chia-Chung, T., Jui-Sheng, S., Ming-Hong, C., Man-Hai, L., and Man-Ger, S. (2012). Stimulatory effect of puerarin on bone formation through co-activation of nitric oxide and bone morphogenetic protein-2/mitogen-activated protein kinases pathways in mice. Chin. Med. J. Engl. 125 (20), 3646–3653.
Shiying, L., Yajun, Y., Jingfeng, C., Zhiguo, Z., Hongxin, H., Jingjing, Z., et al. (2016). Tanshinol stimulates bone formation and attenuates dexamethasone-induced inhibition of osteogenesis in larval zebrafish. J. Orthop. Transl. 4, 35–45. Published online 2015 Sep 2. doi:10.1016/j.jot.2015.07.002
Shuo, L., Xuemei, Y., Chang, M., Jing, Z., and Zhili, X. (2020). 1H-NMR-based urinary metabolomic analysis for the preventive effects of gushudan on glucocorticoid-induced osteoporosis rats. Anal. Biochem. 610, 113992. doi:10.1016/j.ab.2020.113992
Simone, R., Subash, C. G., Madan, M. C., and Bharat, B. A. (2010). Oxidative stress, inflammation, and cancer: How are they linked? Free Radic. Biol. Med. 49 (11), 1603–1616. doi:10.1016/j.freeradbiomed.2010.09.006
Spenser, S. S., Jackeline, R. R., Kate, S. A., Wendy, A. H., Lindsey, M. H., and Sara, J. H. (2009). Cadmium-induced decrease in RUNX2 mRNA expression and recovery by the antioxidant N-acetylcysteine (NAC) in the human osteoblast-like cell line, Saos-2. Toxicol Vitro 23 (1), 60–66. doi:10.1016/j.tiv.2008.10.011
Sri, H. T., and Gallagher, J. C. (2014). Prevention and treatment of postmenopausal osteoporosis. J. Steroid Biochem. Mol. Biol. 142, 155–170. doi:10.1016/j.jsbmb.2013.09.008
Suman, G., Prashant, K., Afsana, S., Farhan, J. A., Ritu, T., and Sushama, T. (2021). Formulation development and in vitro-in vivo assessment of protransfersomal gel of anti-resorptive drug in osteoporosis treatment. Int. J. Pharm. 608, 121060. doi:10.1016/j.ijpharm.2021.121060
Sunil, J. W. (2008). Nitric oxide: Novel therapy for osteoporosis. Expert Opin. Pharmacother. 9 (17), 3025–3044. doi:10.1517/14656560802197162
Tarique, H., Bie, T., Yulong, Y., Francois, B., Myrlene, C. B. T., and Najma, R. (2016). Oxidative stress and inflammation: What polyphenols can do for us? Oxid. Med. Cell Longev. 2016, 7432797. doi:10.1155/2016/7432797
Teresa, I., Cecilia, R., Gaia, P., Simone, D., Irene, F., Francesca, M., et al. (2023). Oxidative stress and inflammation in osteoporosis: Molecular mechanisms involved and the relationship with microRNAs. Int. J. Mol. Sci. 24 (4), 3772. doi:10.3390/ijms24043772
Thea, M., and Emilio, J. (2013). The interaction between gut microbiota and age-related changes in immune function and inflammation. Immun. Ageing 10 (1), 31. doi:10.1186/1742-4933-10-31
Ting, Z., Lei, W., Yicheng, F., Guibo, S., and Xiaobo, S. (2021). Classical active ingredients and extracts of Chinese herbal medicines: Pharmacokinetics, pharmacodynamics, and molecular mechanisms for ischemic stroke. Oxid. Med. Cell Longev. 2021, 8868941. doi:10.1155/2021/8868941
Ting-ting, Y., Hong-tao, X., Liang, Z., Lei, L., Yong-jing, H., and Nan-dan, Z. (2015). Pharmacokinetic and tissue distribution profile of curculigoside after oral and intravenously injection administration in rats by liquid chromatography-mass spectrometry. Fitoterapia 101, 64–72. doi:10.1016/j.fitote.2014.12.012
Tingting, Y., Difeifei, X., Yufei, L., Shuqing, G., Luquan, Z., et al. (2020). Inhibition of nuclear factor kappa B as a mechanism of Danshensu during Toll-like receptor 2-triggered inflammation in macrophages. Int. Immunopharmacol. 83, 106419. doi:10.1016/j.intimp.2020.106419
Tingting, Y., Yufei, L., Difeifei, X., Shuqing, G., Luquan, Z., Bailing, L., et al. (2021). Combination of Danshen and ligustrazine has dual anti-inflammatory effect on macrophages and endothelial cells. J. Ethnopharmacol. 266, 113425. doi:10.1016/j.jep.2020.113425
Tsai-Pei, H., Shiow-Yunn, S., Jui-Sheng, S., and Ming-Hong, C. (2011). Icariin inhibits osteoclast differentiation and bone resorption by suppression of MAPKs/NF-κB regulated HIF-1α and PGE(2) synthesis. Phytomedicine 18 (2-3), 176–185. doi:10.1016/j.phymed.2010.04.003
Valerie, S. S., Laura, W. G., and Vicki, R. (2016). BMP signalling in skeletal development, disease and repair. Nat. Rev. Endocrinol. 12 (4), 203–221. doi:10.1038/nrendo.2016.12
Viktória, J. (2017). Clinical impact and cellular mechanisms of iron overload-associated bone loss. Front. Pharmacol. 8, 77. doi:10.3389/fphar.2017.00077
Wan, G., Mengqin, L., Qi, Z., Quanlong, Z., Yang, W., Qiming, Z., et al. (2022). Orcinol glucoside improves senile osteoporosis through attenuating oxidative stress and autophagy of osteoclast via activating nrf2/keap1 and mTOR signaling pathway. Oxid. Med. Cell Longev. 2022, 5410377. doi:10.1155/2022/5410377
Wan-Jing, C., Yi, F., Shu-Shu, L., Liang-Wei, H., Tao, Z., Wu, Z., et al. (2018). Iron overload induces G1 phase arrest and autophagy in murine preosteoblast cells. J. Cell Physiol. 233 (9), 6779–6789. doi:10.1002/jcp.26405
Wei, L., Ya Nan, S., Xi, T. Y., Seo, Y. Y., Sohyun, K., Young, M. L., et al. (2014). Flavonoids from Astragalus membranaceus and their inhibitory effects on LPS-stimulated pro-inflammatory cytokine production in bone marrow-derived dendritic cells. Arch. Pharm. Res. 37 (2), 186–192. doi:10.1007/s12272-013-0174-7
Wenjiu, Y., Jing, H., Shuo, G., Jun, Z., Tengbo, Y., and Jinfeng, M. (2022). Cryptotanshinone suppressed postmenopausal osteoporosis by preventing RANKL-mediated osteoclastogenesis against kidney injury. Evid. Based Complement. Altern. Med. 2022, 2821984. Published online 2022 Jan 29. doi:10.1155/2022/2821984
Wenqi, J., Xiaoqian, Z., Fan, Y., Xiaohao, X., Xuenan, C., Zongjian, L., et al. (2020). Cytoprotective effect of Fufang Lurong Jiangu capsule against hydrogen peroxide-induced oxidative stress in bone marrow stromal cell-derived osteoblasts through the Nrf2/HO-1 signaling pathway. Biomed. Pharmacother. 121, 109676. doi:10.1016/j.biopha.2019.109676
Wonil, K., Joon-Shik, S., Jinho, L., Ha, I. H., Hee, L., Sang, K. L., et al. (2017). Anti-inflammatory effect of Cortex Eucommiae via modulation of the toll-like receptor 4 pathway in lipopolysaccharide-stimulated RAW 264.7 macrophages. J. Ethnopharmacol. 209, 255–263. doi:10.1016/j.jep.2017.08.001
Xiangping, K., Long, C., Shuchen, Y., Zhangbin, G., Haiyan, H., Xueli, Z., et al. (2022). Zuogui Wan slowed senescence of bone marrow mesenchymal stem cells by suppressing Wnt/β-catenin signaling. J. Ethnopharmacol. 294, 115323. doi:10.1016/j.jep.2022.115323
Xiao-Hua, Y., Lu, L., Yao-Bi, X., Xue-Xue, Z., and Jie-Hua, T. (2020). Flavonoids from Epimedium pubescens: Extraction and mechanism, antioxidant capacity and effects on CAT and GSH-px of Drosophila melanogaster. PeerJ 8, e8361. doi:10.7717/peerj.8361
Xiaobin, R., Yanping, Z., Liangkun, X., Mingzhu, Z., Lihui, G., and Hongbing, H. (2020). Yunnan Baiyao diminishes lipopolysaccharide-induced inflammation in osteoclasts. J. Food Biochem. 44 (6), e13182. doi:10.1111/jfbc.13182
Xingzhi, J., Ting, D., Kun, C., Jiachao, G., Wei, X., Xudong, Y., et al. (2019). Icariin protects against iron overload-induced bone loss via suppressing oxidative stress. J. Cell Physiol. 234 (7), 10123–10137. doi:10.1002/jcp.27678
Xinyu, L., Shangshang, Z., Xiumei, L., Shuning, Z., Famei, L., and Zhili, X. (2012). Metabonomic study on the anti-osteoporosis effect of Rhizoma Drynariae and its action mechanism using ultra-performance liquid chromatography-tandem mass spectrometry. J. Ethnopharmacol. 139 (1), 311–317. doi:10.1016/j.jep.2011.11.017
Xishuai, T., Gengsheng, Y., Qingyang, L., Xueqing, Z., Jianchun, B., Zongping, L., et al. (2022). Puerarin alleviates cadmium-induced oxidative damage to bone by reducing autophagy in rats. Environ. Toxicol. 37 (4), 720–729. doi:10.1002/tox.23437
Xishuai, T., Jianhong, G., Ruilong, S., Dong, W., Ziqiang, S., Chen, S., et al. (2019). Osteoprotegerin inhibit osteoclast differentiation and bone resorption by enhancing autophagy via AMPK/mTOR/p70S6K signaling pathway in vitro. J. Cell Biochem. 120 (2), 1630–1642. doi:10.1002/jcb.27468
Xudong, H., Zhou, Z., Yingyi, Z., Guoshuai, F., Baihe, N., Meichen, L., et al. (2022). Network pharmacological study on mechanism of the therapeutic effect of modified Duhuo jisheng decoction in osteoporosis. Front. Endocrinol. (Lausanne) 13, 860649. doi:10.3389/fendo.2022.860649
Xudong, Y., Xingzhi, J., Jiachao, G., Kai, S., Yi, D., Yong, Z., et al. (2019). Icariin protects bone marrow mesenchymal stem cells against iron overload induced dysfunction through mitochondrial fusion and fission, PI3K/AKT/mTOR and MAPK pathways. Front. Pharmacol. 10, 163. doi:10.3389/fphar.2019.00163
Ya-Li, Z., Qian, C., Lie, Z., Zi-Wei, Z., Yu-Jun, C., Yan-Cheng, D., et al. (2022). Jianpi Qingchang Bushen decoction improves inflammatory response and metabolic bone disorder in inflammatory bowel disease-induced bone loss. World J. Gastroenterol. 28 (13), 1315–1328. doi:10.3748/wjg.v28.i13.1315
Yan, C., Bidur, B., Anu, M., Geum-Hwa, L., Bo, L., Do-Sung, K., et al. (2011). Characterization of Salvia miltiorrhiza ethanol extract as an anti-osteoporotic agent. BMC Complement. Altern. Med. 11, 120. doi:10.1186/1472-6882-11-120
Yan-Ying, L., Bhushan, V. N., Peter, T-H. W., and Jin-Song, B. (2013). Hydrogen sulfide protects SH-SY5Y neuronal cells against d-galactose induced cell injury by suppression of advanced glycation end products formation and oxidative stress. Neurochem. Int. 62 (5), 603–609. doi:10.1016/j.neuint.2012.12.010
Yangyang, H., Xiaoyu, W., Zihan, Y., Xiaofei, F., Binxin, C., Tianming, Z., et al. (2020a). Scoparone as a therapeutic drug in liver diseases: Pharmacology, pharmacokinetics and molecular mechanisms of action. Pharmacol. Res. 160, 105170. doi:10.1016/j.phrs.2020.105170
Yangyang, L., Yongfeng, Z., Weiqi, M., Yutong, L., Tao, H., Di, W., et al. (2020b). The antiosteoporosis effects of yishen Bugu Ye based on its regulation on the differentiation of osteoblast and osteoclast. Biomed. Res. Int. 2020, 9467683. doi:10.1155/2020/9467683
Yanjun, L., Jie, X., Yongli, G., Yong, X., Jingfeng, W., and Changhu, X. (2015). Ameliorative effect of vanadyl(IV)-ascorbate complex on high-fat high-sucrose diet-induced hyperglycemia, insulin resistance, and oxidative stress in mice. J. Trace Elem. Med. Biol. 32, 155–161. doi:10.1016/j.jtemb.2015.07.007
Yi, W., Yusheng, H., Zeguang, Z., Lina, X., Ye, C., Tongtong, L., et al. (2021). Protective effects of water extract of Fructus ligustri lucidi against oxidative stress-related osteoporosis in vivo and in vitro. Vet. Sci. 8 (9), 198. doi:10.3390/vetsci8090198
Yi, Z., and Meng, Z. (2022). Neuroprotective effects of Morinda officinalis How.: Anti-inflammatory and antioxidant roles in Alzheimer's disease. Front. Aging Neurosci. 14, 963041. doi:10.3389/fnagi.2022.963041
Yi-Fei, W., Yue-Yue, C., Xue-Meng, Z., Meng-Ting, G., Qiu-Lan, Z., Xin, L., et al. (2022). Salidroside protects against osteoporosis in ovariectomized rats by inhibiting oxidative stress and promoting osteogenesis via Nrf2 activation. Phytomedicine 99, 154020. doi:10.1016/j.phymed.2022.154020
Yinbo, N., Chenrui, L., Yalei, P., Yuhua, L., and Xianghe, K. (2015). Treatment of Radix Dipsaci extract prevents long bone loss induced by modeled microgravity in hindlimb unloading rats. Pharm. Biol. 53 (1), 110–116. doi:10.3109/13880209.2014.911920
Ying, W., Lu, Z., Yin, W., Jinlong, X., Yan, N., Yuanhui, G., et al. (2012). Curculigoside isolated from Curculigo orchioides prevents hydrogen peroxide-induced dysfunction and oxidative damage in calvarial osteoblasts. Acta Biochim. Biophys. Sin. (Shanghai) 44 (5), 431–441. doi:10.1093/abbs/gms014
Yinglong, X., Dezhi, S., Xixi, L., Hui, P., Yuangang, S., Jiamin, L., et al. (2023). Corylifol A protects against ovariectomized-induced bone loss and attenuates RANKL-induced osteoclastogenesis via ROS reduction, ERK inhibition, and NFATc1 activation. Free Radic. Biol. Med. 196, 121–132. doi:10.1016/j.freeradbiomed.2023.01.017
Young, S. L., Ok, K. H., Chan, W. P., Chae, H. Y., Tae, W. J., Wang, K. Y., et al. (2005). Pro-inflammatory cytokine gene expression and nitric oxide regulation of aqueous extracted Astragali radix in RAW 264.7 macrophage cells. J. Ethnopharmacol. 100 (3), 289–294. doi:10.1016/j.jep.2005.03.009
Yuanhang, K., Xinnan, M., Xin, Z., Leilei, W., Dechun, C., Bo, S., et al. (2022). The potential mechanism of Fructus Ligustri Lucidi promoting osteogenetic differentiation of bone marrow mesenchymal stem cells based on network pharmacology, molecular docking and experimental identification. Bioengineered 13 (4), 10640–10653. doi:10.1080/21655979.2022.2065753
Yujie, M., Jing, H., Changheng, S., Pei, L., Yin, C., Yuhan, W., et al. (2023). Er-Xian decoction attenuates ovariectomy-induced osteoporosis by modulating fatty acid metabolism and IGF1/PI3K/AKT signaling pathway. J. Ethnopharmacol. 301, 115835. doi:10.1016/j.jep.2022.115835
Yunpeng, J., Tanghong, J., Weiming, G., Paul, H., and Shang-You, Y. (2013). Effects of Ti, PMMA, UHMWPE, and Co-Cr wear particles on differentiation and functions of bone marrow stromal cells. J. Biomed. Mater Res. A 101 (10), 2817–2825. doi:10.1002/jbm.a.34595
Zeng-Jun, G., Yu, Z., Xing, T., Hui, L., and Qi-Shi, S. (2008). Pharmacokinetic interaction between tanshinones and polyphenolic extracts of salvia miltinorrhiza BUNGE after intravenous administration in rats. Biol. Pharm. Bull. 31 (8), 1469–1474. doi:10.1248/bpb.31.1469
Zhangyang, B., Wei, Z., and Xiaoyan, Y. (2022). Anti-inflammatory and immunoregulatory effects of icariin and icaritin. Biomed. Pharmacother. 151, 113180. doi:10.1016/j.biopha.2022.113180
Zhao, F. Q., Zheng, N. X., Sato, H., Adachi, I., and Horikoshi, I. (1997). Pharmacokinetics of a Chinese traditional medicine, danshensu (3,4-dihydroxyphenyllactic acid), in rabbits using high-performance liquid chromatography. Biol. Pharm. Bull. 20 (3), 285–287. doi:10.1248/bpb.20.285
Zhen, G., Yong, L., Halmurat, U., Jing, J., and Dan, X. (2013a). Study of osteoporosis treatment principles used historically by ancient physicians in Chinese Medicine. Chin. J. Integr. Med. 19 (11), 862–868. doi:10.1007/s11655-013-1328-z
Zhen, Z., Tai-Ning, L., and Zhong, Z. (2013b). Radix puerariae: An overview of its chemistry, pharmacology, pharmacokinetics, and clinical use. J. Clin. Pharmacol. 53 (8), 787–811. doi:10.1002/jcph.96
Zhicai, P., Ronghua, X., and Qinjian, Y. (2022). Role of traditional Chinese medicine in bone regeneration and osteoporosis. Front. Bioeng. Biotechnol. 10, 911326. doi:10.3389/fbioe.2022.911326
Zhikun, L., Chao, C., Xiaodong, Z., Yifan, L., Ronghua, Y., and Wei, X. (2018). Glycyrrhizin suppresses RANKL-induced osteoclastogenesis and oxidative stress through inhibiting NF-κB and MAPK and activating AMPK/Nrf2. Calcif. Tissue Int. 103 (3), 324–337. doi:10.1007/s00223-018-0425-1
Zhitao, M., Chengjun, H., Min, X., Jiangtao, M., Lei, W., Jiachun, H., et al. (2022). Zhuanggu Zhitong Capsule alleviates postmenopausal osteoporosis in ovariectomized rats by regulating autophagy through AMPK/mTOR signaling pathway. Ann. Transl. Med. 10 (16), 900. doi:10.21037/atm-22-3724
Zhu, H. M., Qin, L., Garnero, P., Genant, H. K., Zhang, G., Dai, K., et al. (2012). The first multicenter and randomized clinical trial of herbal Fufang for treatment of postmenopausal osteoporosis. Osteoporos. Int. 23 (4), 1317–1327. doi:10.1007/s00198-011-1577-2
Zhu, M. Y., Wang, C., Zhang, H., Pei, X., and Fen, J. (2008). Protective effect of polysaccharides from morinda officinalis on bone loss in ovariectomized rats. Int. J. Biol. Macromol. 43 (3), 276–278. doi:10.1016/j.ijbiomac.2008.06.008
Glossary
Keywords: inflammatory, oxidative stress, osteoporosis, traditional Chinese medicine, network pharmacology
Citation: Li Q, Tian C, Liu X, Li D and Liu H (2023) Anti-inflammatory and antioxidant traditional Chinese Medicine in treatment and prevention of osteoporosis. Front. Pharmacol. 14:1203767. doi: 10.3389/fphar.2023.1203767
Received: 21 April 2023; Accepted: 13 June 2023;
Published: 27 June 2023.
Edited by:
Wei Peng, Chengdu University of Traditional Chinese Medicine, ChinaReviewed by:
Yajun Yang, Guangdong Medical University, ChinaPeng Zhang, Guangzhou University of Chinese Medicine, China
Copyright © 2023 Li, Tian, Liu, Li and Liu. This is an open-access article distributed under the terms of the Creative Commons Attribution License (CC BY). The use, distribution or reproduction in other forums is permitted, provided the original author(s) and the copyright owner(s) are credited and that the original publication in this journal is cited, in accordance with accepted academic practice. No use, distribution or reproduction is permitted which does not comply with these terms.
*Correspondence: Hao Liu, NzI5NDgxOTM3QHFxLmNvbQ==
†These authors have contributed equally to this work and share first authorship