- Department of Cardiovascular Medicine, The Second Xiangya Hospital, Central South University, Changsha, Hunan, China
Background: Pulmonary arterial hypertension (PAH) is a malignant pulmonary vascular disease that negatively impacts quality of life, exercise capacity, and mortality. This study sought to investigate the relationship between serum uric acid (UA) level and the disease severity and treatment response of patients with PAH and congenital heart disease (PAH-CHD).
Methods: This study included 225 CHD patients and 40 healthy subjects. Serum UA was measured in all patients, and UA levels and haemodynamic parameters were re-evaluated in 20 patients who had received PAH-specific drug treatment for at least 7 ± 1 month.
Results: Serum UA levels were significantly higher in PAH-CHD patients than in CHD patients with a normal pulmonary artery pressure and normal subjects (347.7 ± 105.7 μmol/L vs. 278.3 ± 84.6 μmol/L; 347.7 ± 105.7 μmol/L vs. 255.7 ± 44.5 μmol/L, p < 0.05). UA levels in the intermediate and high risk groups were significantly higher than those in the low-risk group (365.6 ± 107.8 μmol/L vs. 311.2 ± 82.8 μmol/L; 451.6 ± 117.6 μmol/L vs. 311.2 ± 82.8 μmol/L, p < 0.05). Serum UA levels positively correlated with mean pulmonary arterial pressure, WHO functional class, pulmonary vascular resistance, and NT-proBNP (r = 0.343, 0.357, 0.406, 0.398; p < 0.001), and negatively with mixed venous oxygen saturation (SvO2) and arterial oxygen saturation (SaO2) (r = −0.293, −0.329; p < 0.001). UA significantly decreased from 352.7 ± 97.5 to 294.4 ± 56.8 μmol/L (p = 0.001) after PAH-specific drug treatment for at least 6 months, along with significant decreases in mean pulmonary arterial pressure and pulmonary vascular resistance and increases in cardiac index and mixed SvO2.
Conclusion: Serum UA can be used as a practical and economic biomarker for risk stratification and the evaluation of PAH-specific drug treatment effects for patients with PAH-CHD.
1 Introduction
Pulmonary hypertension (PH) is a malignant pulmonary vascular disease that is characterized by a progressive increase in pulmonary vascular resistance (PVR) that can eventually lead to right heart failure and death (Galiè et al., 2015). PH is currently sub-classified into five categories. PAH associated with congenital heart disease (PAH-CHD) is the first category of PH, and is primarily characterized by pulmonary arteriole remodeling (Galiè et al., 2015; Simonneau et al., 2019). Nearly 50% of PAH patients have idiopathic, heritable, or drug-induced PAH in Western countries (Simonneau et al., 2019). However, CHD is the most common cause of PAH in China (Jiang and Jing, 2013).
Most CHD patients are diagnosed late for many reasons, at which time the pulmonary vessels have already undergone irreversible remodeling and in some cases Eisenmenger syndrome (ES) is established. ES is not correctable surgically (Dimopoulos et al., 2014). While PAH-specific drugs improve survival (Galiè et al., 2015; Arnott et al., 2018), many patients continually deteriorate. In a Danish nationwide study, Schwartz found that the 1-, 5-, and 10-year mortality rates of PAH-CHD patients were 24%, 44%, and 52%, respectively (Schwartz et al., 2018). Early diagnosis, accurate and convenient disease severity assessment, and the ability to predict changes due to the disease are key prognostic factors for PAH-CHD patients.
The utility of serological biomarkers in PAH has been a recent subject of significant research. Brain natriuretic peptide (BNP), N-terminal pro BNP (NT-proBNP), troponin T (TNT), endothelin-1 (ET-1) and C-reactive protein (CRP) have all been associated with the development of PAH. However, only the measurement of BNP and NT-proBNP are recommended by clinical guidelines and widely used in practice (Nagaya et al., 2000; Leuchte et al., 2007; Foris et al., 2013; Galiè et al., 2015; Pezzuto et al., 2015).
Serum uric acid (UA) is the final product of purine metabolism and is a marker of low cardiac output (CO) and/or tissue hypoxia (Foris et al., 2013; Galiè et al., 2015; Pezzuto et al., 2015). UA plays an important role in the disease evaluation and prognosis of PAH patients with idiopathic PAH and that associated with connective tissue disease (Nagaya et al., 1999; Castillo-Martínez et al., 2016). However, the clinical significance of UA in patients with PAH-CHD has been poorly studied, and quantitative risk stratification of PAH using UA levels has never been attempted. This study aimed to analyze the role of UA in assessing the disease status, risk stratification, and treatment response of PAH-CHD patients to provide the basis for its clinical use.
2 Patients and methods
2.1 Study population
This study was performed at the Department of Cardiovascular Medicine of the Second Xiangya Hospital, Central South University, China. Two-hundred consecutive CHD patients diagnosed with PAH (PAH-CHD group) from July 2017 to June 2019 were enrolled. The inclusion criteria were: 1) a diagnosis of PAH, defined as an mPAP ≥25 mmHg, pulmonary artery wedge pressure (PAWP) ≤15 mmHg, and PVR ≥3 Wood; 2) all measurements were performed via right heart catheterization (RHC). The exclusion criteria were: 1) any other cause of PH, including connective tissue disease, left heart disease, lung disease and/or hypoxia, and chronic thromboembolic disease; 2) patients with gout, liver disease, kidney disease, hypertension, coronary heart disease, an age <18 years old, missing data, a history of CHD surgery, or prior use of PAH-specific drugs. Twenty-five CHD patients with normal pulmonary arterial pressures (non-PAH-CHD group) and 40 healthy adults (normal group) were used as controls. The inclusion criteria for the healthy adult group were age older than 18 years old and no history of hypertension, obesity, diabetes, coronary heart disease, stroke, or other cardiovascular disease. The study protocol was approved by the ethics committee of The Second Xiangya Hospital of Central South University, and all participants provided informed consent before enrollment. The flow chart for this study was described in Figure 1.
2.2 Clinical and laboratory data
Baseline clinical information, including gender, age, body mass index (BMI), systolic blood pressure (SBP), diastolic blood pressure (DBP), arterial oxygen saturation (SaO2), heart rate, WHO functional class, and previous medical history were collected. Blood samples were drawn from the unilateral cubital vein after an overnight fast and prior to RHC. Medications, including diuretics, cardiac inotropes, and targeted drugs, were initiated based on an adequate risk status assessment. This protocol was implemented to reduce the influence of medication regimen on baseline serum UA level to the greatest extent possible. A complete blood count was analyzed using a SYSMEX Blood Analyzer XN10 (Kobe, Japan). Serum UA levels were measured using the uricase-peroxidase method. Other biochemical parameters, including creatinine (Cr), alanine aminotransferase (ALT), aspartate aminotransferase (AST), direct bilirubin (DBIL), total bilirubin (TBIL), D-Dimer, and NT-proBNP, were analyzed using a Hitachi Automatic Biochemical Analyzer 7,600-020 (Tokyo, Japan) in the laboratory at The Second Xiangya Hospital of Central South University. The reference range for UA was 142.0–416.0 μmol/L.
2.3 Echocardiography
An experienced cardiologist performed transthoracic echocardiography (TTE) on all patients using a commercially available device (Vivid 7, Vingmed, GE, United States of America). Using left ventricular (LV) long axis, LV short axis, and apical 4-chamber views, we evaluated and recorded the right atrial end-systolic diameter (RAS), right ventricular end-diastolic diameter (RVD), internal diameter of the pulmonary artery (PA), aortic inner diameter (AO), and left ventricular ejection fraction (LVEF). Systolic tricuspid regurgitation velocity (TRV) was calculated using continuous-wave Doppler echocardiography, and the peak TRV was used to estimate the peak tricuspid regurgitation pressure gradient (PTR) using a simplified Bernoulli equation. Systolic PA pressure (sPAP) was estimated by adding PTR to the estimated right atrial pressure based on the size and change in diameter of the inferior vena cava during respiration.
2.4 Right heart catheterization
With the exception of healthy subjects, all patients underwent RHC via their right femoral vein at the Interventional Catheterization Centre of The Second Xiangya Hospital of Central South University. We used a Siemens Axiom Artis X-ray device (SIEMENS Co., Munich, Germany). Baseline haemodynamic parameters, including systolic pulmonary arterial pressure (SPAP), diastolic pulmonary arterial pressure (DPAP), mPAP, mean right atrial pressure (mRAP), PAWP, and oximetry samples of various heart chambers, were measured using a Swan-Ganz catheter. Cardiac index (CI) was calculated as CO based on Fick’s principle divided by body surface area. PVR was calculated using the following formula: (mPAP-PAWP)/CO. We used Wood units as the units for PVR. ES was defined as Qp/Qs < 1.0 and PVR >10 Wood. All studied patients were diagnosed with PAH-CHD via RHC according to the following standard criteria: an mPAP≥25 mmHg and PVR>3 Wood units at rest in the presence of a normal PAWP (≤15 mmHg).
2.5 Risk assessment
The pre-treatment risk assessment for the 200 PAH-CHD patients was based on the comprehensive risk stratification recommended by the European Heart Journal in 2018 (Kylhammar et al., 2018). This risk assessment strategy includes eight variables: World Health Organization functional class (FC), 6-min walking distance (6MWD), CI, right atrial pressure, NT-proBNP, mixed venous oxygen saturation (SvO2), right atrial area, and the presence of a pericardial effusion. All of the aforementioned variables were a part of the risk assessment instrument proposed by the ESC/ERS 2015 guidelines (Galiè et al., 2015). Each variable was categorized as low, intermediate, or high risk based on pre-specified values and given one, two, or three points, respectively. We then divided the sum of all grades by the number of available variables for each patient, resulting in a mean grade. The risk group for each patient was defined by the mean grade rounded off to the nearest integer. PAH-CHD patients were categorized into three risk groups: low (PAH-CHD-L group), intermediate (PAH-CHD-M group), or high (PAH-CHD-H group).
2.6 Treatment and follow-up
Surgical indications at our center were a pulmonary-to-systemic flow ratio (Qp/Qs) ≥1.5 and a PVR ≤ 3Wood. The 138 PAH-CHD patients who were not candidates for transcatheter closure or surgery received individualized treatments. In addition to conventional treatment, including oxygen, diuretics, or digitalis, patients with a positive acute pulmonary vasodilator test received a calcium channel blocker. Patients with a negative acute pulmonary vasodilator test received at least one disease-specific drug, such as an endothelin receptor antagonist (ERA), phosphodiesterase type 5 inhibitor (PDE-5i), or prostacyclin analogue (PGI2). We followed all PAH-CHD patients who received PAH-specific drug therapies. Twenty PAH-CHD patients underwent repeated RHC after an average follow-up period of 7 ± 1 month. Additional information, including biochemical indicators and echocardiographic parameters, were also collected for these patients.
2.7 Statistical analysis
Statistical analyses were performed using the Statistical Package for Social Science version 22.0 for Windows (SPSS Inc., Chicago, IL, United States). Quantitative data (Clinical features, biochemical indicators, echocardiography parameters, and haemodynamic variables) were described as means and standard deviations (normal or approximately normal distributions) or medians and interquartile ranges (IQR) (non-normal distributions). Normally distributed data were compared using a one-way analysis of variance for repeated measurement data, and non-normal distributions were compared using Friedman’s M test. Qualitative data and ranked data (WHO FC) were described as a number and percentage. Ranked data were compared using Friedman’s M test, and qualitative data were compared using Cochran’s Q test. Correlation coefficients between two variables were calculated using Pearson’s correlation. A receiver operating characteristic (ROC) curve was used to confirm the serum UA level that provided the best diagnostic significance for intermediate to high-risk PAH-CHD patients. A paired t-test was performed to compare baseline and post-treatment echocardiographic, haemodynamic, and serological features. Two-sided p-values less than 0.05 were considered statistically significant in all analyses.
3 Results
3.1 Baseline characteristics
This study included 200 PAH-CHD patients (mean age 37.7 ± 14.1 years; range 15–72 years; 145 female). The PAH-CHD-L group had 94 patients (47.0%), the PAH-CHD-M group 88 (44.0%), and the PAH-CHD-H group 18 (9.0%). All PAH-CHD patients received standard therapy with diuretics. Compared with the nPAH-CHD group and the control group, the body weight and BMI of the PAH-CHD group were lower (p < 0.05). There was no statistically significant difference in BMI between the low, medium, and high-risk patients in the PAH-CHD group (p > 0.05). There were no significant differences in baseline ALT, AST, total bile acid (TBA), blood Urea Nitrogen (BUN), Cr, international standardized ratio (INR), and D-Dimer levels between groups. Airect bilirubin (DBIL), NT-proBNP, and platelet levels were higher in the PAH-CHD group than in the normal control and nPAH-CHD groups (p < 0.05). Subgroup analysis showed that NT-proBNP and platelet levels in the PAH-CHD-M and PAH-CHD-H groups were significantly higher than in the PAH-CHD-L group (p < 0.05), but there was no statistical difference between the two groups (p > 0.05). The red blood cell distribution width (RDW) in the PAH-CHD group was equivalent to that of the non-PAH-CHD and control groups (p > 0.05). However, our subgroup analysis showed that the RDW level of the PAH-CHD-H group was higher than that of the PAH-CHD-M group (p < 0.05), but equivalent to the PAH-CHD-L group (Table 1).
3.2 Hemodynamic data
The mean RAS, RVD, PA, and TRV of the PAH-CHD (including all subgroups) and nPAH-CHD groups were significantly higher than those of the control group (p < 0.05). The mean PA and TRV levels in the PAH-CHD group (including all subgroups) were both higher than those of the nPAH-CHD group (p < 0.05) (Table 1).
Compared with the nPAH-CHD group, the PAH-CHD group (including all subgroups) had significantly increased pulmonary artery systolic pressure (SPAP), pulmonary artery diastolic pressure (DPAP), pulmonary artery mean pressure (mPAP), right ventricular mean pressure (mRVP), and total pulmonary resistance (PVR) (p < 0.05). The levels of SPAP and mPAP in the PAH-CHD-H group were significantly higher than those of the PAH-CHD-M and PAH-CHD-L groups (p < 0.05), but there was no statistically significant difference in SPAP between the PAH-CHD-M and PAH-CHD-L groups (p > 0.05). The mean DPAP and mRVP of the PAH-CHD-H and PAH-CHD-M groups were higher than those of the PAH-CHD-L group (p < 0.05). However, there was no statistically significant difference in DPAP and mRVP between the PAH-CHD-H and the PAH-CHD-M group (p > 0.05). SvO2 was significantly lower in the PAH-CHD group (including all subgroups), and decreased with increased PAH risk stratification level (p < 0.05) (Table 1).
3.3 UA levels and ROC curve analysis
Baseline UA levels were significantly higher in PAH-CHD patients than in CHD patients with normal pulmonary pressures or normal control subjects (Table 1). Patients in the intermediate- and high-risk groups had significantly higher UA levels than low-risk patients (Figure 2). A total of 40 patients presented with hyperuricemia (UA > 416.0 μmol/L), including 2 non-PAH-CHD patients, 10 PAH-CHD-L patients, 20 PAH-CHD-M patients, and 8 PAH-CHD-H patients, accounting for 8.0%, 10.6%, 22.7%, and 44.4% of each group. As no significant difference in UA levels was observed between the PAH-CHD-L and non-PAH-CHD groups, we performed ROC analysis and identified a cutoff serum UA level of 330.9 μmol/L to achieve the maximum Youden index {sensitivity [65.1%]-[1-specificity (71.4%)]}. The area under the curve (AUC) was 0.706 (95% CI 0.638-0.773) for predicting intermediate-high risk PAH (Figure 3).
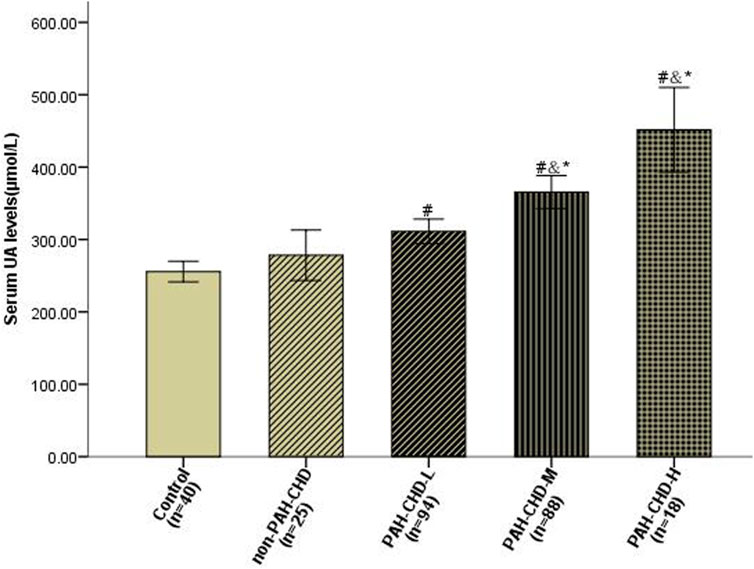
FIGURE 2. Serum uric acid levels in PAH-CHD patients compared with those in non-PAH-CHD group and normal subjects. #P < 0.05 versus normal control subjects; &P < 0.05 versus non-PAH-CHD group; *P < 0.05 versus PAH-CHD-L group.
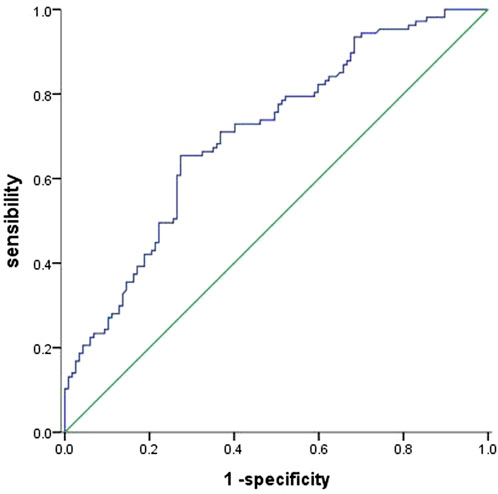
FIGURE 3. ROC curve evaluating the diagnostic value of serum UA levels for predicting PAH-CHD of intermediate and high risk. An area under the curve (AUC) of 0.706 (95% CI 0.638 −0.773) was obtained with a cut-off point of 330.9 μmol/L.
3.4 Correlations between serum UA levels and haemodynamic features and risk assessment variables
Table 2 presents correlations between serum UA levels and different variables. Serum UA levels were positively correlated with mPAP, SPAP, WHO functional class, NT-proBNP, and PVR, and a negatively correlated with SvO2, and SaO2 (Figure 4) the influence of serum UA levels on clinical indicators.
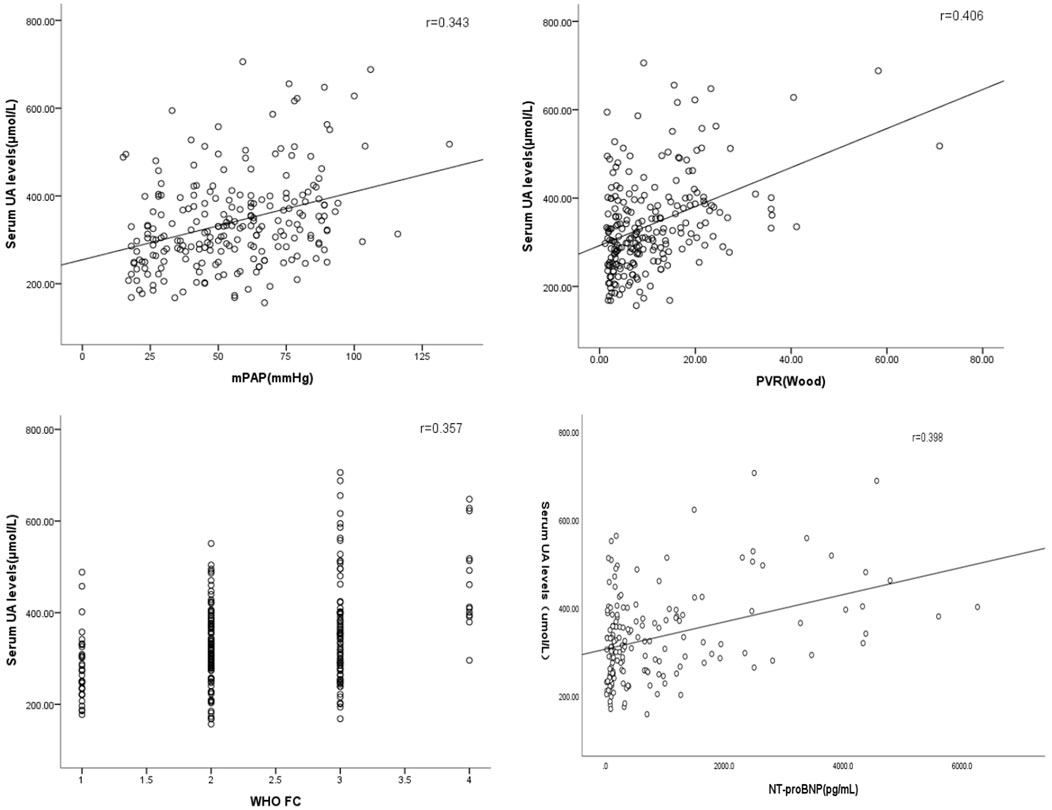
FIGURE 4. Correlations between serum UA levels, haemodynamic features, and other risk assessment variables.
The optimal cutoff value for UA diagnosis for medium to high-risk PAH-CHD patients was calculated using ROC curve analysis. This study divided PAH-CHD patients into UA > 330.9 μmol and UA ≤ 330.9 μmol groups. Differences in patient characteristics, biochemical indicators, imaging measurements, and hemodynamic parameters were compared between the two groups. Cardiac function grading, NT-proBNP, RAS, RVD, mean PA, and PVR were significantly increased in the higher UA level group than the UA ≤ 330.9 μmol group, while SaO2, SvO2 and right CI were significantly decreased in the higher UA level group (p < 0.05). These results indicate that high levels of UA can indicate more severe PAH and a worse prognosis in PAH-CHD patients (Table 3).
3.5 Changes in echocardiographic, haemodynamic, and serologic parameters following vasodilator treatment
All PAH-CHD patients were treated with one or two targeted drugs. Twenty patients followed up for an average of 7 ± 1 month and underwent repeat RHC. During that follow-up period, 10 of the 20 patients received an ERA combined with a PDE-5i, 5 received an ERA with PGI2, 1 received a PDE-5i with PGI2, 1 received PGI2 monotherapy, and the remaining three received ERA monotherapy. After at least 6 months of therapy, serum UA level and other clinical variables were re-examined. Changes in serum UA levels, echocardiographic data, and haemodynamic parameters are presented in Table 4. Haemodynamic and echocardiographic parameters, such as RAS, RVD, sPAP, mPAP, and PVR were significantly decreased, while CI and mixed SvO2 significantly improved after vasodilator treatment. Serum UA levels significantly decreased after vasodilator treatment, from 352.7 ± 97.5 μmol/L to 294.4 ± 56.8 μmol/L (p = 0.001). However, no significant decrease in mRAP was observed after treatment.
4 Discussion
To the best of our knowledge, most of the current research on the role of serum UA levels in PAH has focused on patients with connective tissue diseases and IPAH. The present study found that serum UA levels were significantly elevated in intermediate and high-risk PAH-CHD patients, suggesting that UA is predictive of disease severity. We also found that serum UA levels positively correlated with mPAP, SPAP, WHO functional class, and PVR, and negatively correlated with CI, mixed SvO2, and SaO2. Serum UA levels also significantly decreased following treatment with PAH-specific drugs. These results suggest that serum UA levels have the potential to serve as an indicator of disease severity and treatment response in PAH-CHD patients.
4.1 Increased serum UA levels in PAH-CHD patients
UA levels are be affected by a diverse range of factors, such as gender, age, race, and diet. Hyperuricemia is common in PAH (Nagaya et al., 1999; Voelkel et al., 2000; Dimitroulas et al., 2011). Nagaya et al. (1999) found that increased serum UA levels in idiopathic PAH patients are negatively correlated with CO, and constitute an independent risk factor for long-term mortality. Dimitroulas et al. (2011) reported that systemic sclerosis patients with PAH had higher serum UA levels than those without PAH, and that serum UA levels correlated with 6-min walking and other functional capacity tests. A study conducted on patients with primary and secondary PAH found that serum UA levels were higher in patients with severe PAH (Voelkel et al., 2000).
The results of our research are in agreement with these studies. We found that serum UA levels were significantly higher in patients with PAH-CHD than in non-PAH-CHD patients or healthy subjects. We also noted that hyperuricemia in the present study was mainly present in high-risk PAH-CHD patients.
Although the exact mechanism as to why PAH patients have elevated serum UA levels is unclear, tissue ischaemia and/or hypoxia may play an important role. CHD patients with ES have hypoxic exacerbations due to right-to-left shunting, which may lead to increased serum UA levels (Fathallah and Krasuki, 2018). We hypothesized that the differences in serum UA levels observed within the PAH-CHD group may be due to the higher incidence of ES in the intermediate and high-risk groups. SaO2 levels were lowest in PAH-CHD-H patients, and UA levels were negatively correlated with SaO2, SvO2, and CI, suggesting that uric acid overproduction reflects damage to oxidative metabolism.
Reduced CI and renal perfusion may also be responsible for the elevated serum UA levels. A study by Ross et al. (1986) found that elevated UA levels in ES patients were due to inappropriately low uric acid excretion and enhanced urate reabsorption. Bendayan et al. (2003) reported that hyperuricemia in patients with worsening PAH is caused by impaired renal excretion of UA, which was associated with decreased CO and renal perfusion pressure (Prince et al., 2012). Although serum UA levels were negatively correlated with right CI in the present work, no statistically significant association was found with either serum Cr or LVEF in any of the five groups. These results suggest that patients with normal intrarenal dynamics can appropriately manage elevated UA levels.
Hoeper et al. (1999) found a strong association between serum UA levels and mRAP. This was not the case in our study, although intermediate-risk PAH-CHD patients higher mRAP than CHD patients with normal pulmonary artery pressures. Interestingly, no increases in mRAP were observed in high-risk PAH-CHD patients, which may be explained by right-to-left shunting reducing right atrial pressure.
Diuretic therapy is known to increase serum UA levels by stimulating urate reabsorption in the proximal tubule. All PAH patients received diuretics in our study. However, their baseline renal function was normal, so no significant differences were found between groups. We therefore believe that diuretic use cannot explain the elevated UA level observed in PAH-CHD patients.
4.2 Is UA a pathogenic factor in PAH?
While the degree to which elevated UA levels contribute to the development of PAH is unknown, several mechanisms may be proposed to this regard. First, persistent hyperuricemia may result in endothelial dysfunction, which in turn may lead to PAH progression through the promotion of oxidative stress. Besides being a key enzyme for UA production, xanthine oxidoreductase (XOR) is closely related to vascular oxidative stress, plausibly through the generation of reactive oxygen species (ROS) (Berry and Hare, 2004). ROS produced by increased XOR activity may exceeds cellular antioxidant capacity, inducing oxidative stress and endothelial dysfunction (Cai and Harrison, 2000). Second, disruption of NO signaling pathways by UA may be related to the pathobiology of PAH. Pulmonary vascular endothelial cells can induce pulmonary vessel vasodilation by synthesizing NO through the L-arginine-eNOS pathway, a complex process that is catalyzed by eNOS with arginine as a substrate (Zharikov et al., 2008). Third, elevated UA levels stimulate the release of a variety of inflammatory mediators and induce smooth muscle cell proliferation, thereby promoting the development and progression of pulmonary vascular disease (Rao et al., 1991; Kanellis et al., 2003; Kang et al., 2005). The present study lacked a long follow-up time or the use of cardiovascular events as endpoints, but it did find that serum UA levels significantly correlated with prognostic parameters. UA levels may therefore indirectly reflect hemodynamic status, thereby assessing the severity of PAH-CHD.
All 20 patients who received PAH-specific therapy for at least 6 months demonstrated significant improvements in their WHO functional class, echocardiographic parameters, and haemodynamic variables. Serum UA levels decreased and mixed SvO2 and right heart CI increased. Oya et al. found that serum UA levels vary in ES patients, with reduced PVR after treatment with PGI2 (Oya et al., 2000). These results suggest that serum UA levels may serve as a useful indicator of disease progression and treatment efficacy in PAH-CHD patients.
4.3 Targeted therapies in PAH-CHD patients
PAH-CHD is a heterogeneous patient population with various phenotypes of pulmonary vascular disease that range from increased pulmonary blood flow to Eisenmenger physiology with shunt reversal due to supra-systemic pulmonary pressures and right-to-left shunting. There is now additional evidence that PAH-targeted therapies are of benefit to patients with PAH-CHD, and that these therapies are commonly used in this patient population (Rosenkranz et al., 2015; Hidayati et al., 2020; Kaemmerer et al., 2021). This study followed 20 PAH-CHD patients who were re-catherized after at least 6 months of targeted drug treatment. We found that right heart size was significantly improved and hemodynamic indicators, including PVR and PAP, were significantly decreased. This suggests that targeted drug treatment can improve the symptoms and reduce the incidence of cardiovascular events in patients with PAH-CHD. We look forward to a further confirming the role of targeted drugs in congenital heart disease with PH with a larger scale clinical study.
4.4 Clinical implications
Serum UA levels are simple to measure in a non-invasive and inexpensive manner. Several additional biochemical markers, including BNP, TNT, ET-1, and CRP, have been proposed, (Nagaya et al., 1999; Leuchte et al., 2007; Castillo-Martínez et al., 2016; Kylhammar et al., 2018), but serum UA may be superior in that it performs as a predictor of the disease severity and mortality of patients with PAH-CHD over long-term follow-up. The present work also found that serum UA levels decreased following PAH-specific drug therapy. Based on these findings, we suggest that serum UA levels be repeatedly measured to evaluate the treatment response of PAH-CHD patients in both the outpatient and inpatient settings.
4.5 Limitations
The findings of the present work have to be interpreted in the context of its limitations. First, this is a single center study. Second, although this study had a relatively large sample size compared with related studies in the literature, it was still small for stratified analysis. A larger multi-center prospective study is necessary to further refine our findings. Third, only a few patients underwent repeated RHC after treatment with PAH-specific drugs. The current data can only partially reflect the relationship between decreased UA levels and improved haemodynamic indicators. Fourth, this study lacked a long follow-up time and the use of cardiovascular events as endpoints. We also failed to confirm that whether serum UA levels could act as an independent risk factor for CHD patients with PAH.
4.6 Conclusion
In conclusion, we associated serum UA levels with clinical and haemodynamic severity in PAH-CHD patients. Serum UA levels may be a practical biomarker for assessing risk stratification in patients with PAH, and for evaluating treatment response to PAH-specific drugs. Further studies with larger sample sizes are necessary to confirm the results of this study.
Data availability statement
The raw data supporting the conclusion of this article will be made available by the authors, without undue reservation.
Ethics statement
The studies involving humans were approved by The Second Xiangya Hospital of Central South University. The studies were conducted in accordance with the local legislation and institutional requirements. The participants provided their written informed consent to participate in this study.
Author contributions
JL and YL did the literature search, data collection, study design, analysis of data and manuscript preparation. JC, HQ, and WC designed the study, analysed the data and reviewed the manuscript. XL, YC, and YT designed the study and prepared and reviewed the manuscript. JL analysed the data. All authors contributed to the article and approved the submitted version.
Funding
This work was supported by National Natural Science Foundation of China (Project 81870233 and 81600249), Natural Science Foundation of Hunan Province (2022JJ30823), Hunan Provincial Health Commission Foundation of China (Project 202103010961), Natural Science Foundation of Changsha city (Project kq2202392) and China International Medical Foundation (2022-N-01-23).
Acknowledgments
The authors express their gratitude to all the participants for their cooperation.
Conflict of interest
The authors declare that the research was conducted in the absence of any commercial or financial relationships that could be construed as a potential conflict of interest.
Publisher’s note
All claims expressed in this article are solely those of the authors and do not necessarily represent those of their affiliated organizations, or those of the publisher, the editors and the reviewers. Any product that may be evaluated in this article, or claim that may be made by its manufacturer, is not guaranteed or endorsed by the publisher.
References
Arnott, C., strange, G., Bullockn, A., Kirby, A. C., Donnell, C., Radford, D. J., et al. (2018). Pulmonary vasodilator therapy is associated with greater survival in Eisenmenger syndrome. Heart 104, 732–737. doi:10.1136/heartjnl-2017-311876
Bendayan, D., Shitrit, D., Ygla, M., Huerta, M., Fink, G., and Kramer, M. R. (2003). Hyperuricemia as a prognostic factor in pulmonary arterial hypertension. Respir. Med. 97 (2), 130–133. doi:10.1053/rmed.2003.1440
Berry, C. E., and Hare, J. M. (2004). Xanthine oxidoreductase and cardiovascular disease: molecular mechanisms and pathophysiological implications. J. Physiol. 555 (3), 589–606. doi:10.1113/jphysiol.2003.055913
Cai, H., and Harrison, D. G. (2000). Endothelial dysfunction in cardiovascular diseases: the role of oxidant stress. Circ. Res. 87 (10), 840–844. doi:10.1161/01.res.87.10.840
Castillo-Martínez, D., Marroquín-Fabián, E., Lozada-Navarro, A. C., Mora-Ramirez, M., Juárez, M., Sánchez-Muñoz, F., et al. (2016). Levels of uric acid may predict the future development of pulmonary hypertension in systemic lupus erythematosus: a seven-year follow-up study. Lupus 25 (1), 61–66. doi:10.1177/0961203315600539
Dimitroulas, T., Giannakoulas, G., Dimitroula, H., Sfetsios, T., Parcharidou, D., Karvounis, H., et al. (2011). Significance of serum uric acid in pulmonary hypertension due to systemic sclerosis: a pilot study. Rheumatol. Int. 31 (2), 263–267. doi:10.1007/s00296-010-1557-4
Dimopoulos, K., Wort, S. J., and Michael, A. (2014). Pulmonary hypertension related to congenital heart disease: a call for action. Euro Heart J. 35 (11), 691–700. doi:10.1093/eurheartj/eht437
Fathallah, M., and Krasuki, R. A. (2018). A multifaceted approach to pulmonary hypertension in adults with congenital heart disease. Prog. Cardiovasc Dis. 61 (3-4), 320–327. doi:10.1016/j.pcad.2018.07.017
Foris, V., Kovacs, G., Tscherner, M., Olschewski, A., and Olschewski, H. (2013). Biomarkers in pulmonary hypertension: what do we know? Chest 144 (1), 274–328 3. doi:10.1378/chest.12-1246
Galiè, N., Humbert, M., Vachiery, J. L., Gibbs, S., Lang, I., Torbicki, A., et al. (2015). 2015 ESC/ERS guidelines for the diagnosis and treatment of pulmonary hypertension: the joint task force for the diagnosis and treatment of pulmonary hypertension of the European society of cardiology (ESC) and the European respiratory society (ERS): endorsed by: association for European paediatric and congenital cardiology (AEPC), international society for heart and lung transplantation (ISHLT). Eur. Respir. J. 46 (4), 903–975. doi:10.1183/13993003.01032-2015
Hidayati, F., Gharini, P. P. R., Hartopo, A. B., Anggrahini, D. W., and Dinarti, L. K. (2020). The effect of oral sildenafil therapy on health-related quality of life in adults with pulmonary arterial hypertension related to uncorrected secundum atrial septal defect: a quasi experimental study. Health Qual. Life Outcomes 18 (1), 278. doi:10.1186/s12955-020-01498-7
Hoeper, M. M., Hohlfeld, J. M., and Fabel, H. (1999). Hyperuricaemia in patients with right or left heart failure. Eur. Respir. J. 13, 682–685. doi:10.1183/09031936.99.13368299
Jiang, X., and Jing, Z. C. (2013). Epidemiology of pulmonary arterial hypertension. Curr. Hypertens. Rep. 15 (6), 638–649. doi:10.1007/s11906-013-0397-5
Kaemmerer, A. S., Gorenflo, M., Huscher, D., Pittrow, D., Ewert, P., Pausch, C., et al. (2021). Medical treatment of pulmonary hypertension in adults with congenital heart disease: updated and extended results from the international COMPERA-CHD registry. Cardiovasc Diagn Ther. 11 (6), 1255–1268. doi:10.21037/cdt-21-351
Kanellis, J., Watanabe, S., Li, J. H., Kang, D. H., Li, P., Nakagawa, T., et al. (2003). Uric acid stimulates monocyte chemoattractant protein-1 production in vascular smooth muscle cells via mitogen-activated protein kinase and cyclooxygenase-2. Hypertension 41 (6), 1287–1293. doi:10.1161/01.HYP.0000072820.07472.3B
Kang, D. H., Park, S. K., Lee, I. K., and Johnson, R. J. (2005). Uric acid-induced C-reactive protein expression: implication on cell proliferation and nitric oxide production of human vascular cells. J. Am. Soc. Nephrol. 16 (12), 3553–3562. doi:10.1681/ASN.2005050572
Kylhammar, D., Kjellström, B., Hjalmarsson, C., Jansson, K., Nisell, M., Söderberg, S., et al. (2018). A comprehensive risk stratification at early follow-up determines prognosis in pulmonary arterial hypertension. Euro Heart J. 39 (47), 4175–4181. doi:10.1093/eurheartj/ehx257
Leuchte, H. H., Nounou, M. E., Tuerpe, J. C., Hartmann, B., Baumgartner, R. A., Vogeser, M., et al. (2007). N-terminal pro-brain natriuretic peptide and renal insufficiency as predictors of mortality in pulmonary hypertension. Chest 131 (2), 402–409. doi:10.1378/chest.06-1758
Nagaya, N., Nishikimi, T., Uematsu, M., Satoh, T., Kyotani, S., Sakamaki, F., et al. (2000). Plasma brain natriuretic peptide as a prognostic indicator in patients with primary pulmonary hypertension. Circ 102 (8), 865–870. doi:10.1161/01.cir.102.8.865
Nagaya, N., Uematsu, M., Satoh, T., Kyotani, S., Sakamaki, F., Nakanishi, N., et al. (1999). Serum uric acid levels correlate with the severity and the mortality of primary pulmonary hypertension. Am. J. Respir. Crit. Care Med. 160 (2), 487–492. doi:10.1164/ajrccm.160.2.9812078
Oya, H., Nagaya, N., Satoh, T., Sakamaki, F., Kyotani, S., Fujita, M., et al. (2000). Haemodynamic correlates and prognostic significance of serum uric acid in adult patients with Eisenmenger syndrome. Heart 84 (1), 53–58. doi:10.1136/heart.84.1.53
Pezzuto, B., Badagliacca, R., Poscia, R., Ghio, S., D'Alto, M., Vitulo, P., et al. (2015). Circulating biomarkers in pulmonary arterial hypertension: update and future direction. J. Heart Lung Transpl. 34 (3), 282–305. doi:10.1016/j.healun.2014.12.005
Prince, L. C., Wort, S. J., Perros, F., Dorfmüller, P., Huertas, A., Montani, D., et al. (2012). Inflammation in pulmonary arterial hypertension. Chest 141 (1), 210–221. doi:10.1378/chest.11-0793
Rao, G. N., Corson, M. A., and Berk, B. C. (1991). Uric acid stimulates vascular smooth muscle cell proliferation by increasing platelet derived growth factor A-chain expression. J. Biol. Chem. 266 (13), 8604–8608. doi:10.1016/s0021-9258(18)93017-6
Rosenkranz, S., Ghofrani, H. A., Beghetti, M., Ivy, D., Frey, R., Fritsch, A., et al. (2015). Riociguat for pulmonary arterial hypertension associated with congenital heart disease. Heart 101 (22), 1792–1799. doi:10.1136/heartjnl-2015-307832
Ross, E. A., PerloV, J. K., Danovitch, G. M., Child, J. S., and Canobbio, M. M. (1986). Renal function and urate metabolism in late survivors with cyanotic congenital heart disease. Circ 73 (3), 396–400. doi:10.1161/01.cir.73.3.396
Schwartz, S. S., Madsen, N., Laursen, H. B., Hirsch, R., and Olsen, M. S. (2018). Incidence and mortality of adults with pulmonary hypertension and congenital heart disease. Am. J. Cardiol. 121 (12), 1610–1616. doi:10.1016/j.amjcard.2018.02.051
Simonneau, G., Montani, D., Celermajer, D. S., Denton, C. P., Gatzoulis, M. A., Krowka, M., et al. (2019). Haemodynamic definitions and updated clinical classification of pulmonary Hypertension. Eur. Respir. J. 53 (1), 1801913. doi:10.1183/13993003.01913-2018
Voelkel, M. A., Wynne, K. M., Badesch, D. B., Groves, B. M., and Voelkel, N. F. (2000). Hyperuricemia in severe pulmonary hypertension. Chest 117 (1), 19–24. doi:10.1378/chest.117.1.19
Keywords: uric acid, congenital heart disease, pulmonary arterial hypertension, eisenmenger syndrome, risk stratification
Citation: Luo J, Li Y, Chen J, Qiu H, Chen W, Luo X, Chen Y, Tan Y and Li J (2023) Evaluating the role of serum uric acid in the risk stratification and therapeutic response of patients with pulmonary arterial hypertension associated with congenital heart disease (PAH-CHD). Front. Pharmacol. 14:1238581. doi: 10.3389/fphar.2023.1238581
Received: 12 June 2023; Accepted: 09 August 2023;
Published: 28 August 2023.
Edited by:
Xianwei Wang, Xinxiang Medical University, ChinaReviewed by:
Tao You, Gansu Provincial Hospital, ChinaXuan Zheng, Wuhan University, China
Siyi He, General Hospital of Western Theater Command, China
Copyright © 2023 Luo, Li, Chen, Qiu, Chen, Luo, Chen, Tan and Li. This is an open-access article distributed under the terms of the Creative Commons Attribution License (CC BY). The use, distribution or reproduction in other forums is permitted, provided the original author(s) and the copyright owner(s) are credited and that the original publication in this journal is cited, in accordance with accepted academic practice. No use, distribution or reproduction is permitted which does not comply with these terms.
*Correspondence: Jiang Li, TGlqaWFuZ2NzQGNzdS5lZHUuY24=