- 1Department of Biochemistry and Pharmacology, School of Biomedical Sciences, Faculty of Medicine, Dentistry and Health Sciences, The University of Melbourne, Parkville, VIC, Australia
- 2Department of Ophthalmology, Maimonides Biomedical Research Institute of Cordoba (IMIBIC), Reina Sofia University Hospital and University of Cordoba, Cordoba, Spain
- 3Department of Optometry and Vision Sciences, The University of Melbourne, Parkville, VIC, Australia
Highly effective cystic fibrosis (CF) transmembrane conductance regulator (CFTR) modulator therapies (HEMT), including elexacaftor-tezacaftor-ivacaftor, correct the underlying molecular defect causing CF. HEMT decreases general symptom burden by improving clinical metrics and quality of life for most people with CF (PwCF) with eligible CFTR variants. This has resulted in more pregnancies in women living with CF. All HEMT are known to be able pass through the placenta and into breast milk in mothers who continue on this therapy while pregnant and breast feeding. Toxicity studies of HEMT in young rats demonstrated infant cataracts, and case reports have reported the presence of congenital cataracts in early life exposure to HEMT. This article reviews the evidence for how HEMT influences the dynamic and interdependent processes of healthy and abnormal lens development in the context of HEMT exposure during pregnancy and breastfeeding, and raises questions that remain unanswered.
Introduction
Since the approval of the highly effective modulator therapies (HEMT) targeting the cystic fibrosis transmembrane conductance regulator (CFTR) protein, the use of HEMT have been crucial alongside the supplementary symptomatic treatments for cystic fibrosis (CF) (Bell et al., 2020a). HEMT are small molecules designed to target the specific underlying gene defect, with the ability to modulate CFTR protein synthesis, trafficking, and functioning, ultimately restoring the CFTR channels residing on various epithelial cell surfaces. From the early first-generation modulator ivacaftor as a monotherapy to the progression of ivacaftor combination therapies including ivacaftor-lumacaftor and ivacaftor-tezacaftor, HEMT have displayed safety and efficacy in preclinical and clinical trials (Cai et al., 2011). In recent years, triple combination of elexacaftor-tezacaftor and ivacaftor (ETI), as well as the ongoing phase II trials involving vanzacaftor-tezacaftor-deutivacaftor combination have been the main focus to increase treatment options for CF patients (Ghelani and Schneider-Futschik, 2020).
Along with the progression in drug development, some adverse events have been noticed through preclinical and clinical trials involving HEMT. The use of ivacaftor in juvenile rodents and young patients is associated with non-congenital cataracts and this has been observed in several ivacaftor—combination therapies (FDA, 2021). Due to the ongoing lens development in humans from birth to adulthood, the influence of chemicals including drugs is hypothesised as a potential explanation for the ocular defects observed in clinical studies (Van Cruchten et al., 2017).
As a result of the novel ETI therapy, more women with CF (wwCF) are reaching childbearing age, doubling the number of pregnancies from 2019 to 2021 (Figure 1) (Foundation, 2022). Since pregnant patients and babies are typically excluded from clinical trials, all safety data to date stems from case reports/series. Recently, clinical case series provided evidence of an increased risk of developing congenital cataracts in newborns after in utero exposure to ETI (Jain et al., 2022). The embryonic and fetal stages are particularly vulnerable to exogenous agents, making drug use during these stages highly likely to influence ocular development (Sachdeva et al., 2009; Li D and Schneider-Futschik, 2023).
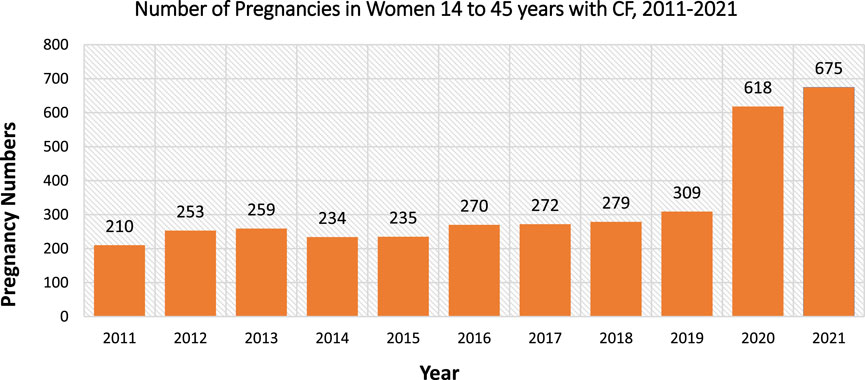
FIGURE 1. United States cystic fibrosis registry data on the number of pregnancies per year in women with cystic fibrosis at 14–45 years from 2011 to 2021. Data obtained from Cystic Fibrosis Foundation Patient Registry 2021 Annual Data Report (Foundation, 2022).
Cystic fibrosis
CF is an autosomal recessive condition resulting from variations in the CFTR gene, which encodes for the CFTR ion channel that conducts chloride and bicarbonate transport (Kerem et al., 1989; Rang et al., 2020). Since CFTR is abundant on epithelial cells, CF symptoms can manifest in various organs e.g., lung, liver, and pancreas (Rang et al., 2020; Li D and Schneider-Futschik, 2023). Therefore, despite lung symptoms being the primary cause of mortality, CF is considered a multi-organ disease (Bell et al., 2020a).
The CFTR gene has over 2,000 possible variants that can be categorised into 6 classes (Rang et al., 2020). These classes provide the basis for “mutation-specific therapies” which aim to use the same therapeutic strategy for mutations categorized in the same class (Amaral and Kunzelmann, 2007). This propelled the discovery of highly effective modulator therapies (HEMT) through high throughput screening of compounds tested on specific CFTR mutation expressing cell lines (Lopes-Pacheco, 2019). Currently, HEMT can be categorised into either correctors or potentiators which both act to modulate the CFTR protein directly to target the underlying gene defect in CF. Correctors can rescue the defect trafficking of CFTR onto the cell surface and can be used in combination with potentiators that maintains the opening time and opening probability of CFTR channels (Ramsey et al., 2011; Bell et al., 2020a; Ghelani and Schneider-Futschik, 2020).
HEMT
Ivacaftor is the first CFTR potentiator approved for patients ≥3 months old with at least one gating variation e.g., G551D class III, which is present in around 4% of the CF patient population (Ghelani and Schneider-Futschik, 2020). In 2023, after the completion of a phase 3 ARRIVAL clinical trial that evaluated ivacaftor use in infants under 24 months old, the United States Food and Drug Administration expanded the use of ivacaftor for infants up to 1 month old that are carrying at least one mutation responsive to ivacaftor (FDA, 2023a). The ARRIVAL study revealed that the pharmacokinetics of ivacaftor in children aged 12–24 months were similar in distribution to older children and adults, and the adverse events were unlikely to be associated with ivacaftor use (Rosenfeld et al., 2018; Davies et al., 2021). In addition, the observed clinical efficacy through biomarkers of CFTR function overall supports the use of ivacaftor to slow or prevent CF progression in newborns and young patients.
Since ivacaftor is limited to mostly class III and rarer mutations, correctors that target the F508del mutation which would be available for 90% of CF patients were developed to benefit the wider population (Cai et al., 2011). Lumacaftor-ivacaftor combination as a corrector-potentiator combination acts to first rescue the defective processing of CFTR protein in the endoplasmic reticulum and then enhance the gating activity of CFTR proteins with F508del mutations (Van Goor et al., 2011). In vitro studies highlighted the combination effect of lumacaftor-ivacaftor inducing greater CFTR-mediated chloride transport than lumacaftor alone, and this similar observation of higher clinical benefit compared to ivacaftor alone was replicated in studies on homozygous F508del patients (Van Goor et al., 2011; Wainwright et al., 2015). Based on the successful phase 3 study in 2021, FDA has approved the use of lumacaftor-ivacaftor for children over 1 year old with the homozygous F508del mutation (FDA, 2022a; Rayment et al., 2022). Similarly, tezacaftor-ivacaftor is a synergistic corrector-potentiator combination therapy that is available for children aged 6 years old who have at least one mutation responsive to therapy (FDA, 2022b). Compared to the lumacaftor-ivacaftor combination, tezacaftor-ivacaftor does not interact with CYP3A4 enzymes which reduces potential drug interaction and metabolising issues but maintains its clinical efficacy (Donaldson et al., 2018; Schneider, 2018).
In 2019 (US) and 2021 (Australia), the first triple combination therapy involving elexacaftor-tezacaftor-ivacaftor was approved for patients aged over 12 years old. Elexacaftor is a next-generation modulator that is proposed to act as both corrector and potentiator (Shaughnessy et al., 2021). In phase 3 studies, clinical end points of FEV1 were superior to the tezacaftor-ivacaftor combination with fewer adverse events (Heijerman et al., 2019; Middleton et al., 2019). Following on, clinical trials have confirmed the safety profile, efficacy and pharmacokinetics of ETI in patients aged 2–5 years old (Rayment et al., 2022; FDA, 2023b; Goralski et al., 2023). To date, the FDA has announced the availability of ETI for children from 2 years old with an F508del allele or any mutation responsive to therapy.
Apart from the approved modulators, there is ongoing development involving a novel triple combination of vanzacaftor-tezacaftor-deutivacaftor that has undergone phase 2 clinical trials in patients over 18 years. It is aimed to achieve once daily dosing for patients who have at least one copy of the F508del mutation and exceed the clinical benefit of ETI which is currently the benchmark therapy for F508del mutations (Uluer et al., 2023). Undoubtedly, if it displays superior efficacy and tolerable safety profiles, clinical trials will proceed to young patients since it is hoped that HEMT treatment options can extend to younger age groups to control manifestations of CF symptoms early.
Cystic fibrosis and pregnancy
Symptoms of CF extend to the reproductive system (Kaplan et al., 1968). Since the 1970 s, wwCF were described having lower fertility rates compared with healthy women (Kopito et al., 1973; Shteinberg et al., 2019). Dehydrated cervical mucus and disrupted pH balance can disrupt sperm viability for successful fertilisation (Kopito et al., 1973; Chan et al., 2002; Wang et al., 2003; Hughan et al., 2019). Furthermore, CFTR has been identified in the hypothalamus of both rats and humans where the regulation of homeostatic reproductive functions such as gonadotrophin-releasing hormone production may consequently be disrupted in CF patients (Mulberg et al., 1998; Hughan et al., 2019; Qiu et al., 2020).
Apart from indirectly enhancing health status, CFTR modulators may also directly restore fertility by correcting CFTR channel located in the cervix to decrease cervical mucus tenacity (Jones and Walshaw, 2015). Jones et al. support the hypothesis by displaying restored fertility in women despite no significant changes in the overall health status (Jones and Walshaw, 2015). Additionally, hysteroscopic images of the vagina highlights the clearance of mucus plugs after starting ivacaftor, further emphasising that restoration of CFTR localised in the reproductive system can improve fertility (Jain and Taylor-Cousar, 2021). The approval of ETI consequently escalated the number of pregnancies due to the high drug efficacy and mild adverse events with wide population suitability (Figure 1) (Middleton et al., 2019). Specifically, ETI have been proposed to have the potential to increase conception, even in patients with a history of subfertility (O'Connor et al., 2021).
Safety of HEMT in pregnancy
It is well established in preclinical and clinical studies that all components of HEMT will pass through the placenta which raises concern for the effect it may have on fetal development (Trimble et al., 2018; FDA, 2021; Qiu et al., 2021; Collins et al., 2022; Jain et al., 2022). As more pregnancies are reported under ETI treatment, this paragraph focuses on ETI.
In animal reproductive models, the use of ETI during the organogenesis period at normal doses is safe, and at maternally toxic doses, ETI does not cause significant developmental defects (FDA, 2021). Similarly, in clinical cases reported, first-generation CFTR modulatory drugs are well tolerated, and emerging case reports of ETI use indicates that most maternal and fetal complications are unrelated to its use (Table 1) (Nash et al., 2020; Taylor-Cousar, 2020). Moreover, a withdrawal effect initially proposed by Trimble et al. is observed after stopping ETI treatment in which the patient’s pulmonary function deteriorates significantly (Trimble and Donaldson, 2018). The impact of the withdrawal effect has prompted many wwCF to re-initiate therapy (Trimble and Donaldson, 2018; Nash et al., 2020; Taylor-Cousar and Jain, 2021). Conversely, despite some promising and increasing use of ETI reported, the government’s drug for pregnancy categorisation systems have indicated that ultimately, the lack of sufficient clinical studies of ETI use during pregnancy makes it difficult to establish certainty in its safety. Therefore, observations of complications from individual case reports should be prioritized for evaluation.
HEMT and the development of cataracts
Non-congenital cataract formation with ivacaftor treatment has been reported in preclinical rat studies and in children receiving both ivacaftor monotherapy and ivacaftor combined with other CFTR modulators like lumacaftor. (Talamo Guevara and McColley, 2017). However, the exact pathophysiology or critical periods of exposure of ivacaftor-associated cataracts remains uncertain. (Kramer and Clancy, 2016).
Juvenile rats that were dosed from post-natal days 7–35 developed cataracts even at doses 0.1 times the maximum recommended human dose (FDA, 2023a). Since this was only observed in the juvenile age group, it potentiates that ivacaftor may modulate early ocular developmental milestones which are subsequently observed in clinical studies. A number of studies associated with the manufacturer of HEMT found that 4.17% of CF patients between 2 and 6 years old developed cortical cataracts within 84 weeks of starting ivacaftor and 0.57% of CF patients 12 years and older developed subcapsular cataracts within 96 weeks of starting treatment. (R/0106 K.-E. H. C,). Similarly, 1.72% of CF patients aged between 6 and 11 developed unspecified forms of cataracts within 24 weeks of starting lumacaftor combined with ivacaftor, and 2 out of 130 participants aged 6 years or older undertaking combination of ivacaftor-tezacaftor experienced cataract (Incorporated, 2017a; European Medicines Agency, 2020). Although in the recent clinical trials involving young patients under 1 year old, ivacaftor did not cause cataract development, 1 case of lenticular opacity was observed in children from 2 to 5 years old under ETI combination regime (Rosenfeld et al., 2018; Davies et al., 2021; Goralski et al., 2023). These concerning findings prompted the FDA to suggest the conductance of ophthalmologic examination before and following ivacaftor monotherapy or combination modulator treatments in clinical settings. Given the anticipated rise in the number of younger patients who will be on modulator therapy in the future, this is an urgent research priority as the development of cataracts and risk of blindness will be devastating for these children and should not be accepted as trading one life-changing disease with another.
A recent clinical series in which 3 out of 23 infants were diagnosed with congenital cataracts after being born to mothers who were taking ETI highlights a potential risk of ETI use during pregnancy (Table 2) (Jain et al., 2022). In all cases of cataracts, mothers carrying at least one copy of the F508del mutation had been on ETI before their conception and continued treatment throughout pregnancy. There were no known risk factors and no family history of cataracts, suggesting that ETI may be the main contributor to this defect. Previous cases of non-congenital cataracts of unknown pathophysiology associated with ivacaftor use in juvenile animals and pediatric patients have already raised concern about the possible ocular influences ivacaftor may have (Table 2). Despite subsequent recommendations to assess for cataracts after in utero exposure to ivacaftor-containing therapies not revealing any clinical cases of cataracts in infants, there are limited cases studies and formal ophthalmic tests conducted to draw a definite conclusion (Nash et al., 2020). Currently, the three cases of cataracts are < 3 mm which is classified as visually insignificant thus they do not require surgical intervention at this stage. However, this study brings awareness to the potential adverse effect of ETI-related cataract development in newborns since no animal toxicity study has been conducted using ETI as the three-drug combination therapy concomitantly. Since recent reports of babies whose mothers were taking ETI during pregnancy are becoming increasingly common, there is the need to further validate the safety of ETI during these critical developmental periods.
Lens development
Eye development, regulated by the transcriptional factor Pax6, begins with the protrusion of optic vesicles from the diencephalon by gestational day (GD) 25 (Chow and Lang, 2001). The optic vesicle contacts the surface ectoderm layer and invaginates into the midsagittal plane, resulting in the ectodermal cells forming the lens and the optic vesicle forming the retina (Santana and Waiswo, 2011). Simultaneously, the basement membrane of the surface ectodermal cells are positioned as the outer surface and become the lens capsule to encapsulate the detached lens vesicle by gestational week (GW) 6 (Danysh and Duncan, 2009; O'Rahilly, 1975). This transparent membrane thickens via deposition of matrix from lens cells to function as a key anchor for ciliary zonules that control lens accommodation, to regulate the passage of metabolic components into the avascular lens and act as a barrier against infectious agents (Danysh and Duncan, 2009). Lens capsule formation is an important developmental stage, as prior to this stage, the lens is vulnerable to infectious agents. In a study on in utero exposure to rubella virus after the human lens capsule has been formed at GW6, Karkinen-Jääskeläinen et al. observed normal eye morphogenesis, which contrasted to the lens fibre degeneration that occurred when the exposure to rubella was at GW4-5, before lens capsule formation was complete (Karkinen-Jääskeläinen et al., 1975). Thus, this highlights that a well-developed lens capsule may mitigate damaging factors during embryogenesis.
Primary lens fibre cells differentiate from lens epithelial cells and elongate to form the embryonic nucleus within the lens vesicle by the end of GW7 (Figure 2) (Van Cruchten et al., 2017). Subsequently, differentiated secondary lens fibres form concentric layers upon the original fibre mass and meet to form Y-shaped sutures visible at the end of the 3rd gestational month (O'Rahilly, 1975). Critically, to maintain lens transparency, central lens fibre cells will accumulate crystallin proteins and undergo constitutive de-nucleation and degradation of organelles. This process continues within the structurally compact lens and in the context of uniform proliferation of secondary fibre cells throughout life (McAvoy et al., 1999).
Despite the adult lens being completely avascular, during development, it is supplied by a dense network of transient vessels that surround the lens capsule. To support lens growth by providing nutrients and oxygen, the anterior pupillary membrane and tunica vasculosa lentis from the hyaloid vasculature envelop the anterior and posterior hemispheres of the lens respectively by GW9 (Wang et al., 2019). The importance of hyaloid vasculature is supported by observations of smaller lenses and nuclear cataracts in mouse studies where the hyaloid capillaries were not formed in fetal life (Garcia et al., 2009). However, normal regression of fetal vasculatures involving hyalocytes and macrophages is equally crucial since the persistence of capillaries after birth will lead to congenital ocular anomalies such as persistent pupillary membranes (Zhu et al., 2000; Wang et al., 2019).
Rat eye development
To gain preclinical insight from animal toxicity studies on eye development, it is essential to identify the differences between rat models and humans. Structurally, rat eyes are a quarter the size of humans’, but the ratio of their lens thickness to axial length is around four-fold greater than humans’ (Shibuya et al., 2015). Zonular fibres that originate from the ciliary muscle and attach to the lens capsule are present in both species (Van Cruchten et al., 2017). Yet due to the poor development of this ciliary musculature in and the apparent lack of accommodation needs in rat eyes, the functional significance of the zonular fibres to modulate lens shape is less clear in rat eyes (Parker and Picut, 2016). Despite these differences, the embryogenesis process in rats is similar to humans, where the invagination of the ventral forebrain from GD11 begins the process of optic vesicle formation (Parker and Picut, 2016). However, in the short 21–23 days of gestational period, rat eye morphogenesis is significantly less developed at birth as seen in the eyelids, iris, and hyaloid vasculatures (Table 3).
Childhood cataract
Cataracts are opacifications within the lens that can be classified based on their location and time of onset (Bell et al., 2020b). Childhood cataracts of both congenital and juvenile onset have a rarer incidence of 1.8–3.6/10,000 per year compared to age-related cataracts (Sheeladevi et al., 2016). The aetiology of childhood cataracts is diverse and, in some cases, the cause is unknown (Lam et al., 2015; Sheeladevi et al., 2016). While hereditary genetic conditions account for a majority of congenital bilateral cataracts, environmental factors including metabolic disorders and trauma can also lead to cataracts at birth (Bell et al., 2020b). Additionally, intrauterine influences including maternal infections, radiation exposure and maternal drug use may also increase the risks (Churchill and Graw, 2011).
Drug-induced cataract
Eye malformations can occur during the critical embryonic stage due to in utero exposure to drugs (Figure 3). (Strömland et al., 1991). Ethanol is a known teratogenic substance shown to disrupt the early induction of the eye primordium via altering gene expression (Miles, 1995). Cook et al. suggest that major teratogenic effects are only observed in the mouse fetal eye when ethanol is administered before GD8 Once the fetal eye is exposed, the altered eye morphogenesis is irreversible (Cook et al., 1987). In past aetiology studies, maternal ingestion of abortifacients, anti-epileptic, anti-diabetic drugs, and corticosteroids are proposed to be associated with congenital cataracts (Angra, 1987; Strömland et al., 1991). Despite the specific mechanism of action being unknown, apart from genetic modification, other possible pathological mechanisms induced by drug exposure include lens osmotic dysregulation, oxidative stress, and metabolic disturbances (Jobling and Augusteyn, 2002). Research by Jobling et al. hypothesises that steroid exposure causes cataract by altering growth factor expression to signal lens epithelial cells to migrate and aggregate at the posterior pole of lens (Jobling and Augusteyn, 2002). Whilst in exposure to anti-depressant drug (TP0446131), the observations of lens fibre degeneration were proposed to be due to TP0446131-related disturbance of cholesterol biosynthesis that was essential for lens fibre saturation (Iwasaki et al., 2020). Ultimately, various mechanisms can disrupt the micro-environment of the lens biochemistry, leading to abnormal light absorption or light scattering of the eye that can progresses to vision-impairing cataract (Bell et al., 2020b).
Conclusion
Emerging data from case reports and case series (paired with some animal reproduction data) of the use of HEMT during pregnancy provides encouragement about drug safety during pregnancy and breastfeeding. However, due to reports of acute deterioration in health following cessation of HEMT risks to the mother’s health due to discontinuation of HEMT must be weighed carefully against the unknown risks to the fetus. The development of non-congenital cataracts in juvenile rats and published case reports in paediatric patients highlight the need for infant ophthalmologic exams. Thus, a better understanding of the potential risks of HEMT during early life exposure is urgently needed.
Author contributions
YZ: Conceptualization, Writing–review and editing. DL: Writing–review and editing. FR-O: Funding acquisition, Validation, Writing–review and editing. HC: Conceptualization, Funding acquisition, Supervision, Writing–review and editing. ES-F: Conceptualization, Funding acquisition, Supervision, Writing–review and editing.
Funding
The author(s) declare financial support was received for the research, authorship, and/or publication of this article. ES-F is supported by the NHMRC (Grant ID: APP1157287) and Cystic Fibrosis Australia. FR-O is supported by the Plan Andaluz de Investigación, Desarrollo e Innovación (PAIDI 2020) Fellowship supported by Consejería de Economía, Conocimiento, Empresas y Universidad, Junta de Andalucía co-funded by Fondo Social Europeo de Andalucía 2014–2020.
Conflict of interest
The authors declare that the research was conducted in the absence of any commercial or financial relationships that could be construed as a potential conflict of interest.
Publisher’s note
All claims expressed in this article are solely those of the authors and do not necessarily represent those of their affiliated organizations, or those of the publisher, the editors and the reviewers. Any product that may be evaluated in this article, or claim that may be made by its manufacturer, is not guaranteed or endorsed by the publisher.
Abbreviations
CF, Cystic fibrosis; CFTR, Cystic fibrosis transmembrane conductance regulator; HEMT, Highly effective modulator treatment; FEV1, Forced expiratory volume in 1 s; wwCF, Women with CF; ETI, Elexacaftor tezacaftor ivacaftor.
References
Amaral, M. D., and Kunzelmann, K. (2007). Molecular targeting of CFTR as a therapeutic approach to cystic fibrosis. Trends Pharmacol. Sci. 28, 334–341. doi:10.1016/j.tips.2007.05.004
Angra, S. K. (1987). Etiology and management of congenital cataract. Indian J. Pediatr. 54, 673–677. doi:10.1007/BF02751275
Balmpouzis, Z., Faure van Rossum, A., Baud, D., Panchaud, A., Mitropoulou, G., Mazza Stalder, J., et al. (2022). Successful pregnancy in a cystic fibrosis patient with a severe impairment of lung function receiving Elexacaftor-Tezacaftor-Ivacaftor. Respir. Med. Case Rep. 40, 101776. doi:10.1016/j.rmcr.2022.101776
Bell, S. C., Mall, M. A., Gutierrez, H., Macek, M., Madge, S., Davies, J. C., et al. (2020a). The future of cystic fibrosis care: A global perspective. Lancet Respir. Med. 8, 65–124. doi:10.1016/S2213-2600(19)30337-6
Bell, S. J., Oluonye, N., Harding, P., and Moosajee, M. (2020b). Congenital cataract: A guide to genetic and clinical management. Ther. Adv. Rare Dis. 1, 2633004020938061. doi:10.1177/2633004020938061
Braekevelt, C. R., and Hollenberg, M. J. (1970). The development of the retina of the albino rat. Am. J. Anat. 127, 281–301. doi:10.1002/aja.1001270305
Burn, S. C., Looby, A. A., Johnson, K., Cross, S. N., and Jones, C. (2022). Trikafta in pregnancy: A clinical case series. Am. J. Obstetrics Gynecol. 226, S393–S394. doi:10.1016/j.ajog.2021.11.658
Cai, Z. W., Liu, J., Li, H. Y., and Sheppard, D. N. (2011). Targeting F508del-CFTR to develop rational new therapies for cystic fibrosis. Acta Pharmacol. Sin. 32, 693–701. doi:10.1038/aps.2011.71
Chamagne, M., Farabet, C., Grenet, D., and Ayoubi, J. M. (2022). Comparison of two pregnancies with and without elexacaftor-tezacaftor-ivacaftor in a woman with cystic fibrosis. Respir. Med. Res. 83, 100953. doi:10.1016/j.resmer.2022.100953
Chan, L. N., Tsang, L. L., Rowlands, D. K., Rochelle, L. G., Boucher, R. C., Liu, C. Q., et al. (2002). Distribution and regulation of ENaC subunit and CFTR mRNA expression in murine female reproductive tract. J. Membr. Biol. 185, 165–176. doi:10.1007/s00232-001-0117-y
Chow, R. L., and Lang, R. A. (2001). Early eye development in vertebrates. Annu. Rev. Cell Dev. Biol. 17, 255–296. doi:10.1146/annurev.cellbio.17.1.255
Churchill, A., and Graw, J. (2011). Clinical and experimental advances in congenital and paediatric cataracts. Philos. Trans. R. Soc. Lond B Biol. Sci. 366, 1234–1249. doi:10.1098/rstb.2010.0227
Cimino, G., Sorrenti, S., Murciano, M., Galoppi, P., Ascenzioni, F., Botta, B., et al. (2023). “Sapienza university working group on cystic fibrosis in, P,” in Use of elexacaftor/tezacaftor/ivacaftor combination in pregnancy (Rome, Italy: Sapienza University).
Collins, B., Fortner, C., Cotey, A., Esther, C. R. J., and Trimble, A. (2022). Drug exposure to infants born to mothers taking Elexacaftor, Tezacaftor, and Ivacaftor. J. Cyst. Fibros. 21, 725–727. doi:10.1016/j.jcf.2021.12.004
Cook, C. S., Nowotny, A. Z., and Sulik, K. K. (1987). Fetal alcohol syndrome: Eye malformations in a mouse model. Archives Ophthalmol. 105, 1576–1581. doi:10.1001/archopht.1987.01060110122045
Danysh, B. P., and Duncan, M. K. (2009). The lens capsule. Exp. Eye Res. 88, 151–164. doi:10.1016/j.exer.2008.08.002
Davies, J. C., Wainwright, C. E., Sawicki, G. S., Higgins, M. N., Campbell, D., Harris, C., et al. (2021). Ivacaftor in infants aged 4 to <12 Months with cystic fibrosis and a gating mutation. Results of a two-Part Phase 3 clinical trial. Am. J. Respir. Crit. Care Med. 203, 585–593. doi:10.1164/rccm.202008-3177OC
Donaldson, S. H., Pilewski, J. M., Griese, M., Cooke, J., Viswanathan, L., Tullis, E., et al. (2018). Tezacaftor/Ivacaftor in Subjects with Cystic Fibrosis and F508del/F508del-CFTR or F508del/G551D-CFTR. Am. J. Respir. Crit. Care Med. 197, 214–224. doi:10.1164/rccm.201704-0717OC
FDA (2021). Trikafta (elexacaftor/tezacaftor/ivacaftor). Bethesda, Maryland: Prescribing Information, United States Food and Drug Administration.
Fortner, C. N., Seguin, J. M., and Kay, D. M. (2021). Normal pancreatic function and false-negative CF newborn screen in a child born to a mother taking CFTR modulator therapy during pregnancy. J. Cyst. Fibros. 20, 835–836. doi:10.1016/j.jcf.2021.03.018
Foundation, C. F. (2022). Cystic fibrosis foundation patient registry 2021 annual data report. Bethesda, Maryland: SciELO Brasil.
Garcia, C. M., Shui, Y. B., Kamath, M., DeVillar, J., Johnson, R. S., Gerber, H. P., et al. (2009). The function of VEGF-A in lens development: Formation of the hyaloid capillary network and protection against transient nuclear cataracts. Exp. Eye Res. 88, 270–276. doi:10.1016/j.exer.2008.07.017
Ghelani, D. P., and Schneider-Futschik, E. K. (2020). Emerging cystic fibrosis transmembrane conductance regulator modulators as new drugs for cystic fibrosis: A portrait of in vitro Pharmacology and clinical translation. ACS Pharmacol. Transl. Sci. 3, 4–10. doi:10.1021/acsptsci.9b00060
Gómez-Montes, E., Salcedo Lobato, E., Galindo, A., García Alcázar, D., Villalain, C., Moral-Pumarega, M. T., et al. (2023). Prenatal cystic fibrosis transmembrane conductance regulator modulator therapy: A promising way to change the impact of cystic fibrosis. Fetal Diagnosis Ther. 20, 1. doi:10.1159/000530261
Goralski, J. L., Hoppe, J. E., Mall, M. A., McColley, S. A., McKone, E., Ramsey, B., et al. (2023). Phase 3 Open-Label Clinical Trial of Elexacaftor/Tezacaftor/Ivacaftor in Children Aged 2 Through 5 Years with Cystic Fibrosis and at Least One F508del Allele. Am. J. Respir. Crit. Care Med. 10. doi:10.1164/rccm.202301-0084OC
Heijerman, H. G. M., McKone, E. F., Downey, D. G., Van Braeckel, E., Rowe, S. M., Tullis, E., et al. (2019). Efficacy and safety of the elexacaftor plus tezacaftor plus ivacaftor combination regimen in people with cystic fibrosis homozygous for the F508del mutation: A double-blind, randomised, phase 3 trial. Lancet 394, 1940–1948. doi:10.1016/S0140-6736(19)32597-8
Hughan, K. S., Daley, T., Rayas, M. S., Kelly, A., and Roe, A. (2019). Female reproductive health in cystic fibrosis. J. Cyst. Fibros. 18 (2), S95–S104. doi:10.1016/j.jcf.2019.08.024
Incorporated, V. P. (2017a). A phase 3 rollover study of lumacaftor in combination with ivacaftor in subjects 12 Years and older with cystic fibrosis. ClinicalTrials.gov. 10.
Incorporated, V. P. (2017b). Roll-over study of ivacaftor in cystic fibrosis pediatric subjects with a CF transmembrane conductance regulator gene gating mutation. Clinicaltrials.gov. 14.
Incorporated, V. P. (2017c). Study of lumacaftor in combination with ivacaftor in subjects 6 through 11 Years of age with cystic fibrosis, homozygous for the F508del-CFTR mutation. ClinicalTrials.gov. 80.
Iwasaki, H., Wakamatsu, M., Sugihara, K., Kamio, K., Tsuji, S., Morita, J., et al. (2020). Drug-induced lenticular opacity and accumulation of cholesterol-related substances in the lens cortex of dogs. J. Toxicol. Sci. 45, 201–218. doi:10.2131/jts.45.201
Jain, R., and Taylor-Cousar, J. L. (2021). Fertility, pregnancy and lactation considerations for women with CF in the CFTR modulator era. J. Pers. Med. 11, 418. doi:10.3390/jpm11050418
Jain, R., Wolf, A., Molad, M., Taylor-Cousar, J., Esther, C. R., and Shteinberg, M. (2022). Congenital bilateral cataracts in newborns exposed to elexacaftor-tezacaftor-ivacaftor in utero and while breast feeding. J. Cyst. Fibros. 21, 1074–1076. doi:10.1016/j.jcf.2022.10.004
Jobling, A. I., and Augusteyn, R. C. (2002). What causes steroid cataracts? A review of steroid-induced posterior subcapsular cataracts. Clin. Exp. Optom. 85, 61–75. doi:10.1111/j.1444-0938.2002.tb03011.x
Jones, G. H., and Walshaw, M. J. (2015). Potential impact on fertility of new systemic therapies for cystic fibrosis. Paediatr. Respir. Rev. 16 (1), 25–27. doi:10.1016/j.prrv.2015.07.013
Kaplan, E., Shwachman, H., Perlmutter, A. D., Rule, A., Khaw, K.-T., and Holsclaw, D. S. (1968). Reproductive failure in males with cystic fibrosis. N. Engl. J. Med. 279, 65–69. doi:10.1056/NEJM196807112790203
Karkinen-Jääskeläinen, M., Saxén, L., Vaheri, A., and Leinikki, P. (1975). Rubella cataract in vitro: Sensitive period of the developing human lens. J. Exp. Med. 141, 1238–1248. doi:10.1084/jem.141.6.1238
Kendle, A. M., Roekner, J. T., Santillana, E. C., Kis, L. E., and Cain, M. A. (2021). Cystic fibrosis transmembrane conductance regulator modulators during pregnancy: A case series. Cureus 13, e17427. doi:10.7759/cureus.17427
Kerem, B., Rommens, J. M., Buchanan, J. A., Markiewicz, D., Cox, T. K., Chakravarti, A., et al. (1989). Identification of the cystic fibrosis gene: Genetic analysis. Science 245, 1073–1080. doi:10.1126/science.2570460
Kopito, L. E., Kosasky, H. J., and Shwachman, H. (1973). Water and electrolytes in cervical mucus from patients with cystic fibrosis. Fertil. Steril. 24, 512–516. doi:10.1016/s0015-0282(16)39790-4
Kramer, E. L., and Clancy, J. P. (2016). CFTR modulator therapies in pediatric cystic fibrosis: Focus on ivacaftor. Expert Opin. Orphan Drugs 4, 1033–1042. doi:10.1080/21678707.2016.1226800
Lam, D., Rao, S. K., Ratra, V., Liu, Y., Mitchell, P., King, J., et al. (2015). Cataract. Nat. Rev. Dis. Prim. 1, 15014. doi:10.1038/nrdp.2015.14
Li D, H. M., and Schneider-Futschik, E. K. (2023). Utero mapping and development role of CFTR in lung and gastrointestinal tract of cystic fibrosis patients. ACS Pharmacol. Transl. Sci. doi:10.1021/acsptsci.2c00233
Lopes-Pacheco, M. (2019). CFTR modulators: The changing face of cystic fibrosis in the era of precision medicine. Front. Pharmacol. 10, 1662. doi:10.3389/fphar.2019.01662
McAvoy, J. W., Chamberlain, C. G., de Iongh, R. U., Hales, A. M., and Lovicu, F. J. (1999). Lens development. Eye (Lond) 13, 425–437. doi:10.1038/eye.1999.117
Middleton, P. G., Mall, M. A., Drevinek, P., Lands, L. C., McKone, E. F., Polineni, D., et al. (2019). Elexacaftor-Tezacaftor-Ivacaftor for Cystic Fibrosis with a Single Phe508del Allele. N. Engl. J. Med. 381, 1809–1819. doi:10.1056/NEJMoa1908639
Mulberg, A. E., Weyler, R. T., Altschuler, S. M., and Hyde, T. M. (1998). Cystic fibrosis transmembrane conductance regulator expression in human hypothalamus. Neuroreport 9, 141–144. doi:10.1097/00001756-199801050-00028
Nash, E. F., Middleton, P. G., and Taylor-Cousar, J. L. (2020). Outcomes of pregnancy in women with cystic fibrosis (CF) taking CFTR modulators - an international survey. J. Cyst. Fibros. 19, 521–526. doi:10.1016/j.jcf.2020.02.018
O'Connor, K. E., Goodwin, D. L., NeSmith, A., Garcia, B., Mingora, C., Ladores, S. L., et al. (2021). Elexacafator/tezacaftor/ivacaftor resolves subfertility in females with CF: A two center case series. J. Cyst. Fibros. 20, 399–401. doi:10.1016/j.jcf.2020.12.011
O'Rahilly, R. (1975). The prenatal development of the human eye. Exp. Eye Res. 21, 93–112. doi:10.1016/0014-4835(75)90075-5
Parker, G. A., and Picut, C. A. (2016). Atlas of Histology of the juvenile rat sara tenney. Cambridge, MA, USA: Academic Press.
Parmigiani, C., and McAvoy, J. (1984). Localisation of laminin and fibronectin during rat lens morphogenesis. Differentiation 28, 53–61. doi:10.1111/j.1432-0436.1984.tb00266.x
Qiu, F., Habgood, M. D., Huang, Y., Dziegielewska, K. M., Toll, S., and Schneider-Futschik, E. K. (2021). Entry of cystic fibrosis transmembrane conductance potentiator ivacaftor into the developing brain and lung. J. Cyst. Fibros. 20, 857–864. doi:10.1016/j.jcf.2021.06.005
Qiu, F., Habgood, M., and Schneider-Futschik, E. K. (2020). The balance between the safety of mother, fetus, and newborn undergoing cystic fibrosis transmembrane conductance regulator treatments during pregnancy. ACS Pharmacol. Transl. Sci. 3, 835–843. doi:10.1021/acsptsci.0c00098
Ramsey, B. W., Davies, J., McElvaney, N. G., Tullis, E., Bell, S. C., Dřevínek, P., et al. (2011). A CFTR potentiator in patients with cystic fibrosis and the G551D mutation. N. Engl. J. Med. 365, 1663–1672. doi:10.1056/NEJMoa1105185
Rang, C., Keating, D., Wilson, J., and Kotsimbos, T. (2020). Re-Imagining cystic fibrosis care: Next generation thinking. Eur. Respir. J. 55, 1902443. doi:10.1183/13993003.02443-2019
Rayment, J. H., Asfour, F., Rosenfeld, M., Higgins, M., Liu, L., Mascia, M., et al. (2022). A phase 3, open-label study of lumacaftor/ivacaftor in children 1 to less than 2 Years of age with cystic fibrosis homozygous for F508del-CFTR. Am. J. Respir. Crit. Care Med. 206, 1239–1247. doi:10.1164/rccm.202204-0734OC
Rosenfeld, M., Wainwright, C. E., Higgins, M., Wang, L. T., McKee, C., Campbell, D., et al. (2018). Ivacaftor treatment of cystic fibrosis in children aged 12 to <24 months and with a CFTR gating mutation (ARRIVAL): A phase 3 single-arm study. Lancet Respir. Med. 6, 545–553. doi:10.1016/S2213-2600(18)30202-9
Sachdeva, P., Patel, B. G., and Patel, B. K. (2009). Drug use in pregnancy; a point to ponder. Indian J. Pharm. Sci. 71, 1–7. doi:10.4103/0250-474X.51941
Santana, A., and Waiswo, M. (2011). The genetic and molecular basis of congenital cataract. Arq. Bras. Oftalmol. 74, 136–142. doi:10.1590/s0004-27492011000200016
Schneider, E. K. (2018). Cytochrome P450 3A4 induction: Lumacaftor versus ivacaftor potentially resulting in significantly reduced plasma concentration of ivacaftor. Drug Metab. Lett. 12, 71–74. doi:10.2174/1872312812666180328105259
Shaughnessy, C. A., Zeitlin, P. L., and Bratcher, P. E. (2021). Elexacaftor is a CFTR potentiator and acts synergistically with ivacaftor during acute and chronic treatment. Sci. Rep. 11, 19810. doi:10.1038/s41598-021-99184-1
Sheeladevi, S., Lawrenson, J. G., Fielder, A. R., and Suttle, C. M. (2016). Global prevalence of childhood cataract: A systematic review. Eye (Lond) 30, 1160–1169. doi:10.1038/eye.2016.156
Shibuya, K., Tomohiro, M., Sasaki, S., and Otake, S. (2015). Characteristics of structures and lesions of the eye in laboratory animals used in toxicity studies. J. Toxicol. Pathol. 28, 181–188. doi:10.1293/tox.2015-0037
Shteinberg, M., Lulu, A. B., Downey, D. G., Blumenfeld, Z., Rousset-Jablonski, C., Perceval, M., et al. (2019). Failure to conceive in women with CF is associated with pancreatic insufficiency and advancing age. J. Cyst. Fibros. 18, 525–529. doi:10.1016/j.jcf.2018.10.009
Sionidou, M., Vogiatzoglou, A., Hatzi- Mitrova, M., Papadaki, E., and Manika, K. (2023). Pregnancy in cystic fibrosis in the era of CFTR modulators: A case series. Pneumon 36, 1–4. doi:10.18332/pne/157586
Strömland, K., Miller, M., and Cook, C. (1991). Ocular teratology. Surv. Ophthalmol. 35, 429–446. doi:10.1016/0039-6257(91)90107-q
Szentpetery, S., Foil, K., Hendrix, S., Gray, S., Mingora, C., Head, B., et al. (2022). A case report of CFTR modulator administration via carrier mother to treat meconium ileus in a F508del homozygous fetus. J. Cyst. Fibros. 21, 721–724. doi:10.1016/j.jcf.2022.04.005
Talamo Guevara, M., and McColley, S. A. (2017). The safety of lumacaftor and ivacaftor for the treatment of cystic fibrosis. Expert Opin. Drug Saf. 16, 1305–1311. doi:10.1080/14740338.2017.1372419
Taylor-Cousar, J. L. (2020). CFTR modulators: Impact on fertility, pregnancy, and lactation in women with cystic fibrosis. J. Clin. Med. 9, 2706. doi:10.3390/jcm9092706
Taylor-Cousar, J. L., and Jain, R. (2021). Maternal and fetal outcomes following elexacaftor-tezacaftor-ivacaftor use during pregnancy and lactation. J. Cyst. Fibros. 20, 402–406. doi:10.1016/j.jcf.2021.03.006
Trimble, A., McKinzie, C., Terrell, M., Stringer, E., and Esther, C. R. (2018). Measured fetal and neonatal exposure to Lumacaftor and Ivacaftor during pregnancy and while breastfeeding. J. Cyst. Fibros. 17, 779–782. doi:10.1016/j.jcf.2018.05.009
Trimble, A. T., and Donaldson, S. H. (2018). Ivacaftor withdrawal syndrome in cystic fibrosis patients with the G551D mutation. J. Cyst. Fibros. 17, e13–e16. doi:10.1016/j.jcf.2017.09.006
Uluer, A. Z., MacGregor, G., Azevedo, P., Indihar, V., Keating, C., Mall, M. A., et al. (2023). Safety and efficacy of vanzacaftor-tezacaftor-deutivacaftor in adults with cystic fibrosis: Randomised, double-blind, controlled, phase 2 trials. Lancet Respir. Med. 11, 550–562. doi:10.1016/S2213-2600(22)00504-5
Van Cruchten, S., Vrolyk, V., Perron Lepage, M. F., Baudon, M., Voute, H., Schoofs, S., et al. (2017). Pre- and postnatal development of the eye: A species comparison. Birth Defects Res. 109, 1540–1567. doi:10.1002/bdr2.1100
Van Goor, F., Hadida, S., Grootenhuis, P. D., Burton, B., Stack, J. H., Straley, K. S., et al. (2011). Correction of the F508del-CFTR protein processing defect in vitro by the investigational drug VX-809. Proc. Natl. Acad. Sci. U. S. A. 108, 18843–18848. doi:10.1073/pnas.1105787108
Vrolyk, V., Haruna, J., and Benoit-Biancamano, M. O. (2018). Neonatal and juvenile ocular development in sprague-dawley rats: A histomorphological and immunohistochemical study. Vet. Pathol. 55, 310–330. doi:10.1177/0300985817738098
Wainwright, C. E., Elborn, J. S., Ramsey, B. W., Marigowda, G., Huang, X., Cipolli, M., et al. (2015). Lumacaftor-Ivacaftor in Patients with Cystic Fibrosis Homozygous for Phe508del CFTR. N. Engl. J. Med. 373, 1783–1784. doi:10.1056/NEJMc1510466
Wang, X. F., Zhou, C. X., Shi, Q. X., Yuan, Y. Y., Yu, M. K., Ajonuma, L. C., et al. (2003). Involvement of CFTR in uterine bicarbonate secretion and the fertilizing capacity of sperm. Nat. Cell Biol. 5, 902–906. doi:10.1038/ncb1047
Wang, Z., Liu, C. H., Huang, S., and Chen, J. (2019). Assessment and characterization of hyaloid vessels in mice. J. Vis. Exp. doi:10.3791/59222
Keywords: cystic fibrosis, ocular development, CFTR modulator, catarat, eye malformations
Citation: Zhu Y, Li D, Reyes-Ortega F, Chinnery HR and Schneider-Futschik EK (2023) Ocular development after highly effective modulator treatment early in life. Front. Pharmacol. 14:1265138. doi: 10.3389/fphar.2023.1265138
Received: 22 July 2023; Accepted: 06 September 2023;
Published: 19 September 2023.
Edited by:
Miquéias Lopes-Pacheco, University of Lisbon, PortugalReviewed by:
Kelvin D. MacDonald, Oregon Health and Science University, United StatesPeter R. Corridon, Khalifa University, United Arab Emirates
Copyright © 2023 Zhu, Li, Reyes-Ortega, Chinnery and Schneider-Futschik. This is an open-access article distributed under the terms of the Creative Commons Attribution License (CC BY). The use, distribution or reproduction in other forums is permitted, provided the original author(s) and the copyright owner(s) are credited and that the original publication in this journal is cited, in accordance with accepted academic practice. No use, distribution or reproduction is permitted which does not comply with these terms.
*Correspondence: Felisa Reyes-Ortega, felisa.reyes@imibic.org; Holly R. Chinnery, holly.chinnery@unimelb.edu.au; Elena K. Schneider-Futschik, elena.schneider@unimelb.edu.au