- 1Korean Medicine (KM)-Application Center, Korea Institute of Oriental Medicine (KIOM), Daegu, Republic of Korea
- 2Department of Korean Medical Science, School of Korean Medicine, Pusan National University, Yangsan, Republic of Korea
- 3Korean Convergence Medical Science Major, KIOM Campus, University of Science and Technology (UST), Daegu, Republic of Korea
Lactate dehydrogenase (LDH) is a crucial enzyme involved in energy metabolism and present in various cells throughout the body. Its diverse physiological functions encompass glycolysis, and its abnormal activity is associated with numerous diseases. Targeting LDH has emerged as a vital approach in drug discovery, leading to the identification of LDH inhibitors among natural compounds, such as polyphenols, alkaloids, and terpenoids. These compounds demonstrate therapeutic potential against LDH-related diseases, including anti-cancer effects. However, challenges concerning limited bioavailability, poor solubility, and potential toxicity must be addressed. Combining natural compounds with LDH inhibitors has led to promising outcomes in preclinical studies. This review highlights the promise of natural compounds as LDH inhibitors for treating cancer, cardiovascular, and neurodegenerative diseases.
1 Introduction
LDH is a crucial enzyme in metabolism, catalyzing the interconversion of pyruvate and lactate (Farhana and Lappin, 2022). It plays a vital role in physiological processes, including energy metabolism, glycolysis, and intracellular redox regulation (Kane, 2014). In recent years, there has been an increase recognition of the therapeutic potential of natural compounds in modulating LDH activity and expression and addressing LDH-related diseases (Gao and Chen, 2015; Li et al., 2019b; Forkasiewicz et al., 2020). The regulation of LDH can lead to various effects, including anti-oxidative stress, anti-inflammatory responses, and anti-apoptotic processes (Liu, 1995; Liu et al., 2010; Venkatesan et al., 2015). It can also affect related pathways and downstream signaling associated with the LDH (Zha et al., 2011; Miao et al., 2013; Feng et al., 2018). The involvement of LDH in several diseases, such as cancer, cardiovascular diseases, and neurodegenerative disorders, suggests that natural compounds have a broader therapeutic potential for LDH-related diseases (Liao et al., 2012; Liu et al., 2017; Morandi and Indraccolo, 2017).
Aberrant LDH activity has made the enzyme an attractive target for drug discovery (Varghese et al., 2020). Many LDH inhibitors have been discovered (Rivera et al., 2009), including natural compounds, such as polyphenols, alkaloids, and terpenoids (Gallagher et al., 2017; He et al., 2021; Ramakrishna et al., 2021). Polyphenols, abundant in plants, inhibit LDH activity by binding to its active site, decreasing lactate production (Granchi et al., 2010). Promising polyphenols for treatment of diseases related to abnormal LDH activity, such as cancer, include quercetin, kaempferol, and apigenin (Miean and Mohamed, 2001). Alkaloids, including berberine and magnoflorine, also inhibit LDH by binding to its active site, thereby preventing lactate production (Huang et al., 2009; Kooshki et al., 2022), and exert various pharmacological effects, including anti-cancer, anti-microbial, and anti-inflammatory activities (Aggarwal et al., 2011; Gurung and De, 2017; Reddy et al., 2020). Similarly, terpenoids, such as carnosic acid and artemisinin, inhibit LDH activity (Akinloye et al., 2021) by binding to the enzyme’s active site (Cameron et al., 2004; Hou et al., 2012) and show potential as anti-cancer agents, with their additional pharmacological effects including anti-inflammatory and anti-microbial activities (Salminen et al., 2008; Doughari, 2012).
However, natural compounds face challenges in terms of clinical development owing to limited bioavailability, inadequate solubility, and potential toxicity (Shishir et al., 2019; Yadav et al., 2019; Garcia-Oliveira et al., 2021). Researchers have attempted to enhance their bioavailability while minimizing toxicity using drug delivery systems (Aqil et al., 2013; Ting et al., 2014). Combining LDH inhibitors from natural compounds with synthetic compounds in therapy has shown promise in preclinical studies, suggesting that these compounds could enhance therapeutic effects (Li et al., 2013; Han et al., 2015; Cui et al., 2017).
This review explores the significance of LDH in a range of diseases, including cancer, cardiovascular diseases, and neurodegenerative diseases. It discusses the challenges in developing and using natural LDH inhibitors, their impact on downstream signaling pathways after LDH modulation, the mechanisms of their actions, and potential combination treatments with conventional medications. Natural compounds have the potential to be beneficial therapeutics for LDH-related diseases, and future research opportunities are also discussed.
2 Lactate dehydrogenase
LDH is a vital enzyme present in almost all cells, playing an essential role in energy metabolism (Le et al., 2010; Hu et al., 2016). It catalyzes the conversion of lactate to pyruvate and vice versa, depending on cellular energy demands (Gladden, 2004; Farhana and Lappin, 2022). This reaction involves the interconversion of cofactors, nicotinamide adenine dinucleotide (NAD+) and ß-nicotinamide adenine dinucleotide hydrate (NADH), which are essential for energy transfer in living organisms (Rodriguez et al., 2019). LDH operates as a proton donor, with His (193) serving as the proton donor, Arg (99) as the coenzyme, Asn(138) as the hydrogen bond donor, and Arg (106), Arg (169), and Thr (248) as substrate binding residues (Holmes and Goldberg, 2009).
LDH consists of two subunits: LDH-heart (H) and LDH-muscle (M), encoded by the genes LDHA and LDHB, respectively (Al-Jassabi, 2002). The H subunit is predominant in the brain and heart, whereas the M subunit is found in skeletal muscle tissues (Woodford et al., 2019). Two popular isoforms of LDH exist, resulting in five isotype enzymes: LDH1 (H4), LDH2 (H3M1), LDH3 (H2M2), LDH4 (H1M3), and LDH5 (M4) (Al-Jassabi, 2002). LDHA, also known as LDH5, is highly expressed in skeletal muscle and catalyzes pyruvate and NADH to lactate and NAD+, with this reaction being crucial for aerobic glycolysis metabolism in skeletal muscle (Kane, 2014). Conversely, LDHB, also known as LDH1, is abundant in the heart and brain, where it converts lactate and NAD + to pyruvate and NADH (Read et al., 2001a; Doherty and Cleveland, 2013). LDH2, LDH3, and LDH4 are found in lung tissue, bone marrow, and the pancreas (Aliberti et al., 1997; Ben et al., 2007). Each isotype enzyme exhibits intermediate activity levels from LDHA to LDHB, depending on tissue metabolic needs (Johari et al., 2018) (Figure 1).
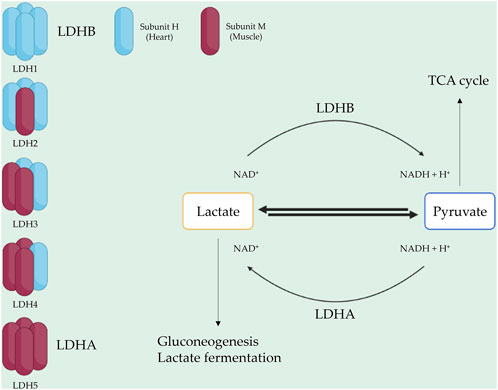
FIGURE 1. LDH composition and function. LDH is a tetrameric enzyme with five isotypes. Each isotype consists of two subunit types: H (heart) and M (muscle). LDH1 and LDH5, represented by LDHB and LDHA, play roles in converting lactate to pyruvate and pyruvate to lactate, respectively.
LDH activity is associated with various diseases, including cancer, cardiovascular diseases, and neurodegenerative disorders (Dhanasekaran and Ren, 2005; Roychoudhury et al., 2021). Dysregulated LDH activity contributes significantly to cancer development, promoting the Warburg effect (Chen et al., 2007), which involves increased glucose uptake and lactate production, even in the presence of oxygen, to meet the energy demands of rapidly proliferating cancer cells (Warburg and Minami, 1923; Dai et al., 2016b). LDHA overexpression favors pyruvate to lactate conversion, leading to tumor microenvironment acidification and aiding cancer progression and metastasis (Vander Heiden et al., 2009). Abnormal LDH activity is also observed in other diseases. For example, increased LDH activity has been reported in cardiovascular diseases, such as myocardial infarction and heart failure, reflecting cardiac tissue damage and necrosis (Ndrepepa, 2021). Neurodegenerative diseases, including Alzheimer’s disease and Parkinson’s disease, are associated with elevated LDH activity, potentially reflecting neuronal damage and inflammation (Fahrig et al., 2005; Di Domenico et al., 2017).
Given the critical role of LDH in disease development, targeting the enzyme has become an essential strategy for drug discovery. Numerous natural compounds, including polyphenols, alkaloids, and terpenoids, have shown promising results as potential LDH inhibitors for disease treatment.
3 LDH inhibitors: types, mechanisms, and therapeutic applications
3.1 Categorization of LDH inhibitors
We now explore LDH inhibitors in more depth, classifying them based on their chemical structures and modes of action into two categories: small-molecule inhibitors and RNA-based inhibitors.
3.1.1 Small-molecule inhibitors
Small-molecule inhibitors, compounds with low molecular weight, are often effective LDH inhibitors that can penetrate cell membranes and bind to the active site of the enzyme, hindering its function (Vander Heiden et al., 2010; Granchi et al., 2013). These inhibitors can be further categorized based on their chemical composition, with main subcategories including quinoline-based inhibitors with quinoline rings, benzoxazole-based inhibitors with benzoxazole rings, and benzimidazole-based inhibitors with benzimidazole rings (Holmes et al., 2006; Madapa et al., 2008; Kanwal et al., 2018). Studies have shown that these inhibitors effectively reduce LDH activity in cancerous cells and possess anti-cancer properties both in vitro and in vivo (Granchi and Minutolo, 2012; Piekuś-Słomka et al., 2019; Zhou et al., 2020). Some specific LDHA inhibitors, such as FX-11 (a benzoxazole-based inhibitor) and Compound 3a (a quinoline-based inhibitor), have shown selective inhibition of cancer cell growth. Another effective class of inhibitors is the benzimidazole anthelmintics (Miao et al., 2013; Rani and Kumar, 2016; Son et al., 2020). Depending on their chemical structure, small-molecule inhibitors may selectively target LDHA or LDHB isoforms (Fiume et al., 2014) (Figure 2).
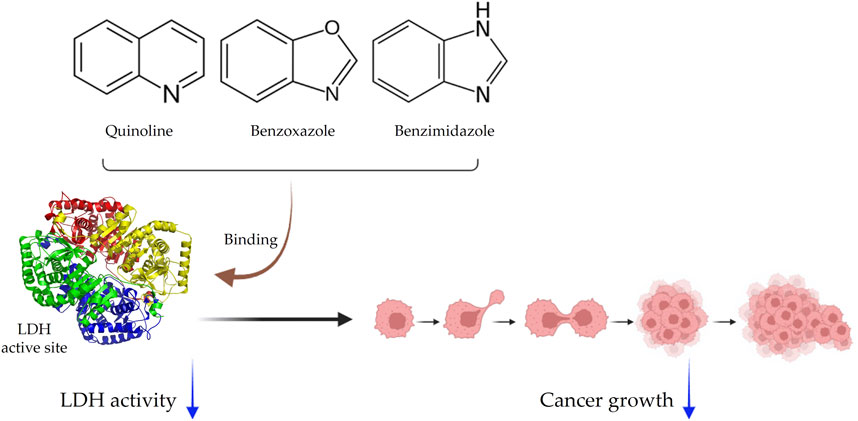
FIGURE 2. Schematic representation of small-molecule inhibitors. Inhibitors are categorized as quinolines, benzoxazoles, and benzimidazoles. They bind to the LDH active site and effectively inhibit cancer growth.
3.1.2 RNA-based inhibitors
A novel group of LDH inhibitors is RNA-based, with these inhibitors specifically hindering the expression of LDH-related enzymes (Liu et al., 2021). RNA-based inhibitors are categorized into two types based on their underlying mechanisms: RNA interference (RNAi) and antisense oligonucleotides (ASOs) (Post et al., 2019; Maruyama and Yokota, 2020).
RNAi occurs naturally, where small interfering RNAs (siRNAs) degrade mRNA, leading to gene silencing (McManus and Sharp, 2002). By pairing with complementary mRNA sequences, double-stranded RNA molecules trigger the RNA-induced silencing complex, breaking down the mRNA (Sontheimer, 2005). RNAi-based LDH inhibitors target mRNA sequences responsible for encoding LDH, resulting in its downregulation and decreased LDH activity (Yang and Zhang, 2012).
ASOs inhibit protein translation by binding to complementary mRNA sequences. ASOs are short single-stranded RNA molecules with effective inhibition capabilities (Ding and Lawrence, 2001). LDH-specific ASOs can be designed to target mRNA sequences encoding the LDH enzyme, leading to decreased expression and inhibition of its activity (Manjunath et al., 2022). RNA-based inhibitors offer advantages over small-molecule inhibitors (Blom et al., 2022). For instance, they can be designed for highly selective LDH inhibition by targeting specific mRNA sequences (Manjunath et al., 2022). Furthermore, RNA-based inhibitors can exhibit longer action time for inhibition and can be administered using viral vectors and lipid nanoparticles (Mogler and Kamrud, 2015; Bajan and Hutvagner, 2020; Aldosari et al., 2021).
3.2 Mechanisms of action
Various natural compounds can inhibit LDH activity through different mechanisms. The most common approach involves direct binding to the enzyme’s active site, leading to the inhibition of pyruvate conversion to lactate (Conners et al., 2005). Polyphenols, such as quercetin and epigallocatechin gallate (EGCG), can bind to the active site of LDH, reducing its activity levels (Gradišar et al., 2007). Additionally, some natural compounds modulate other components involved with LDH, including lactate transporters or mitochondrial enzymes. For instance, rosmarinic acid affects the lactate transporters of cancer cells (Marin-Hernandez et al., 2009; Cerella et al., 2013; Ma et al., 2018).
Another mechanism underlying inhibition of LDH by natural compounds is the regulation of LDH gene expression. Various compounds, such as curcumin, resveratrol, and quercetin, can inhibit LDH expression by reducing LDH gene transcription or promoting LDH protein degradation (Yang et al., 2018; Reyes-Farias and Carrasco-Pozo, 2019; Soni et al., 2020). In contrast to small-molecule inhibitors, RNA-based inhibitors target LDH expression by interfering with its mRNA. As discussed earlier, RNAi and ASOs are commonly used for inhibiting LDH expression, which is achieved at the mRNA level (Wood et al., 2019; Bockstahler et al., 2022).
3.2.1 Inhibition of LDH enzyme activity
As low-molecular-weight substances, small-molecule inhibitors, often referred to as drug-like molecules, can easily penetrate cells and interact with their target enzymes (Makley and Gestwicki, 2013). These inhibitors effectively block the enzyme’s catalytic activity by competing with the substrate for binding to the active site (Copeland et al., 2007). Based on their mode of action, small-molecule inhibitors can be further categorized as reversible or irreversible inhibitors (Roskoski Jr, 2016). Reversible inhibitors bind to the enzyme’s active site noncovalently and can be displaced by excess substrate, whereas irreversible inhibitors bind to the enzyme covalently, leading to permanent inactivation (Purich, 2010) Examples of small-molecule LDH inhibitors include FX11, which selectively inhibits LDHA, and gossypol, which inhibits both LDHA and LDHB (Conners et al., 2005; Granchi et al., 2011).
3.2.2 Inhibition of LDH enzyme expression
RNA-based inhibitors are employed to inhibit LDH enzyme expression, through RNAi and ASOs (Liu et al., 2021). These inhibitors induce mRNA degradation through siRNAs and inhibit mRNA translation via complementary sequences with ASOs (Ding and Lawrence, 2001; Sontheimer, 2005). RNA-based inhibitors exhibit high specificity, selectively targeting enzymes and effectively inhibiting LDH. Moreover, they can be efficiently delivered to the target using lipid nanoparticles or viral vectors (Mogler and Kamrud, 2015; Younis et al., 2021). However, some limitations, such as off-target effects and limited delivery to specific tissues or cell types, exist (Sibley et al., 2010; Lu and Thum, 2019).
3.3 Therapeutic applications
Several studies have demonstrated the potential therapeutic effects of LDH inhibitors in diseases associated with abnormal LDH activity (Granchi et al., 2010; Valvona et al., 2016). For instance, preclinical studies have shown that LDH inhibitors can suppress tumor growth both as a monotreatment and in combination with other cancer therapies, such as chemotherapy and radiation therapy (Capula et al., 2019; Qiao et al., 2021). LDH inhibitors have also been investigated as potential therapeutics in cardiovascular diseases (Ji et al., 2021), as well as for their effects on Alzheimer’s disease and Parkinson’s disease (Newington et al., 2013; Acharya et al., 2019).
3.3.1 Cancer
Cancer cells often exhibit high levels of LDH activity, which supports uncontrolled cell growth and migration, (Gallo et al., 2015), especially under hypoxic conditions (Han et al., 2021). Consequently, LDH inhibition has emerged as a promising therapeutic approach for cancer therapy (Pi et al., 2022). In preclinical studies, LDH inhibition has resulted in anti-cancer effects both under monotreatment and combination therapy with chemotherapy and radiation therapy (Abdel-Wahab et al., 2019). For example, the LDH inhibitor FX-11 has been shown to reduce tumor growth and enhance the efficacy of chemotherapy (Fantin et al., 2006). As FX-11 inhibits LDH activity, it promotes a shift toward oxidative phosphorylation and impaired cancer cell growth and survival (Le et al., 2010). LDHA inhibition by oxamate resulted in the accumulation of reactive oxygen species (ROS) and depletion of adenosine triphosphate (ATP), leading to increased sensitivity to radiotherapy in A549 and H1975 cancer cells (Yang et al., 2021).
Silibinin, a natural compound found in milk thistle, has also been proven to inhibit LDH and reduce tumor growth in various cancer cell lines (Milić et al., 2013). In a chemically induced skin cancer model in mice, silibinin reduced the expression of the tumor necrosis factor-α endogenous promoter (Zhao et al., 1999). In hepatocellular carcinoma in rats, it decreased levels of malondialdehyde (MDA)-DNA (Ramakrishnan et al., 2007). Silibinin’s potential extends to human ovarian cancer, where it inhibits tumor growth by downregulating VEGFR receptor 3 (Gallo et al., 2003). In cervical cancer, silibinin induces apoptosis through MAPK (mitogen activated protein kinase) activation, characterized by chromatin condensation and nuclear fragmentation (Huang et al., 2005). Moreover, silibinin’s pre-treatment reduces the phosphorylation of signal transducer and activator of transcription 1 (STAT1) and signal transducer and activator of transcription 3 (STAT3) induced by cytokines responsible for the proliferation of A549 human lung cancer cells in vitro. Silibinin also inhibits the AP-1 transcription factor of DNA and blocks the MAPK cascade (Chittezhath et al., 2008). In prostate cancer, it downregulates epidermal growth factor receptor (EGFR) signaling, leading to cell cycle arrest and reduced expression of tumor growth factor (TGF-α) (Singh and Agarwal, 2004). Silibinin’s effects extend to oral cancer, where it decreases cell viability by inhibiting akt phosphorylation, resulting in apoptosis (Su et al., 2013). Additionally, Silibinin shows potential in treating gastric cancer by inhibiting the growth of SGC-7901 cells, lowering p34cdc2 levels, and increasing the expression of p53 and p21 (Zhang et al., 2013b). Lastly, in colon cancer, silibinin induces dose-dependent cell cycle arrest, affects autocrine TGF-α secretion, and inhibits EGFR expression (Hogan et al., 2007). It also inhibited oxidative damage caused by lung and brain sepsis by balancing the oxidative status and modulating inflammatory mediators (Toklu et al., 2008). It induces apoptosis, suppresses angiogenesis, and decreases the expression of hypoxia-inducible factors (Sameri et al., 2021).
Similarly, the LDH inhibitor galloflavin is known to enhance efficacy in radiation therapy in preclinical studies (Kozal et al., 2021), which it achieves by sensitizing cancer cells to radiation-induced DNA damage, leading to enhanced cancer cell death (Fiume et al., 2013). Galloflavin has demonstrated its efficacy in inhibiting cell growth in endometrial cancer cell lines and primary cultures of human endometrial cancer. It achieves this by engaging with multiple signaling pathways that regulate crucial aspects such as metabolism, cell cycle progression, apoptosis, cellular stress responses, and metastasis (Han et al., 2015). Moreover, inhibition of LDH by galloflavin can exert a growth-inhibitory effect in breast cancer cells. This anti-proliferative effect may result from various mechanisms, including the downregulation of survival signaling pathways and the induction of oxidative stress states (Farabegoli et al., 2012). In Burkitt lymphoma cells, the LDH inhibitor galloflavin reduces cellular NAD levels and leads to the inhibition of sirtuin-1. As confirmed in previous studies, sirtuin-1 inhibition leads to a reduction in MYC protein levels, depriving Burkitt lymphoma cells of a crucial survival signal (Vettraino et al., 2013). Furthermore, in pancreatic cancer cells, a combination of galloflavin and metformin has been found to enhance their effectiveness in inhibiting the proliferation of cancer cells (Wendt et al., 2020).
3.3.2 Cardiovascular diseases
LDH has been implicated in the pathogenesis of various cardiovascular diseases, including cardiac failure and ischemia-reperfusion injury (Kotlyar et al., 2010). Elevated LDH levels under these conditions indicate cellular damage and impaired metabolism (Ait-Aissa et al., 2019). LDH inhibitors have exhibited protective effects against ischemia-reperfusion injury and heart failure in preclinical studies (Zhou et al., 2015). For example, in a rat model simulating myocardial ischemia-reperfusion injury, the LDH inhibitor galloflavin showed the potential to decrease infarct size and improve cardiac function (Gandhi et al., 2022). EGCG has also been shown to inhibit LDH activity, leading to reduced lactate production and cardioprotective effects (Eng et al., 2018). Additionally, S-allylcysteine treatment was shown to improve cardiac function in rats while decreasing oxidative stress and mitochondrial permeability (Aziz et al., 2021). Allicin, found in garlic, demonstrated a significant vasodilating effect on coronary arteries, leading to increased coronary blood flow in the experimental group both before ischemia and during reperfusion. This effect is attributed to a reduced concentration of LDH release (Adegbola et al., 2017). Similarly, curcumin also exhibits cardioprotective effects, as demonstrated in a rat model of acute myocardial infarction by a reduction in serum LDH levels by curcumin intake (Rahnavard et al., 2019).
3.3.3 Neurodegenerative diseases
Increased LDH activity has been detected in the brains of patients with Alzheimer’s (Bigl et al., 1999). The effects of Lycium barbarum extract on cell models of Alzheimer’s disease have been investigated, with a significant reduction in the release of LDH and a dose-dependent neuroprotective effect observed (Yu et al., 2005). This extract has also demonstrated effectiveness in treating Alzheimer’s disease by protecting against neurotoxicity caused by beta-amyloid peptides (Ho et al., 2007). EGCG has demonstrated enhanced effectiveness in neuroprotection by significantly reducing lactate dehydrogenase release in a cell model of Parkinson’s disease. Furthermore, Western blot analysis indicated that Akt might be one of the specific signaling pathways stimulated by EGCG in the context of neuroprotection (Chao et al., 2010). Overexpression and abnormal accumulation of a-synuclein are associated with Parkinson’s disease and result in increased intracellular ROS, causing mitochondrial dysfunction and oxidative damage in a Parkinson’s disease model. The use of curcumin demonstrated a reduction in LDH release, alleviating αS-induced toxicity, lowering ROS levels, and providing protection to cells against apoptosis (Wang et al., 2010).
4 Natural compounds as LDH inhibitors: Polyphenols, alkaloids, terpenoids and sulfur-containing agent
4.1 Polyphenols and cancer
Flavonoids, a group of polyphenols abundant in fruit, vegetables, and medicinal plants, function as LDH inhibitors (Huang et al., 2009). Studies have shown that the flavonoids curcumin and quercetin inhibit LDH activity and reduce lactate synthesis in cancer cells (Maurya and Vinayak, 2015; Unlu et al., 2016; Reyes-Farias and Carrasco-Pozo, 2019). Curcumin treatment reduces LDHA expression in human colorectal cancer cells, leading to decreased lactate production and cellular proliferation (Wang et al., 2015b). Quercetin, found in several foods, including apples and onions (Kopustinskiene et al., 2020), reduces LDHA activity and triggers apoptosis in cancer cells, effectively inhibiting cellular glycolysis by reducing LDHA expression, thereby suppressing lactic acid generation and glucose uptake (Jia et al., 2018). Kaempferol, found in tea and various fruit, downregulates LDHA expression in human breast cancer cells through inhibition of STAT3 activity (Narayan and Kumar, 2014). Galloflavin binds to the NADH-binding site in LDHA, inhibiting its ability to bind to single-stranded DNA and suppressing colorectal cancer growth (Fiume et al., 2013). Moreover, galloflavin has been shown to completely inhibit both LDHA and LDHB (Manerba et al., 2012). EGCG, the primary flavanol in green tea, inhibits LDHA and exhibits anti-cancer activity in pancreatic cancer cells, and it significantly slows the growth of breast cancer cells, triggering apoptosis through its action as an LDHA inhibitor (Wang et al., 2015a; Gao and Chen, 2015). Combining catechin, epicatechin, and gallocatechin with epigallocatechin enhanced the inhibitory effect on LDHA (Cheng et al., 2020). Apigenin reduces LDHA mRNA expression in HepG2 cells, a human hepatocellular carcinoma cell line (Korga et al., 2019). Although the precise mechanism underlying inhibition of LDH by flavonoids is not fully understood, it may involve direct enzyme binding, gene expression modulation, or protein stability regulation (Bader et al., 2015; Yao et al., 2022). Luteolin acts as an LDH inhibitor and has been found to bind effectively to the active pocket residues of LDH (Li et al., 2021b). Additionally, luteolin 7-O-β-d-glucoside, found in Phlomis kurdica, non-specifically inhibits both LDH-1 and LDH-5 (Bader et al., 2015).
4.2 Polyphenols and cardiovascular diseases
Flavonoids have been shown to exert beneficial effects on cardiovascular health, preventing atherosclerosis, hypertension, and myocardial infarction (Stangl et al., 2006). Quercetin treatment was found to significantly decrease infarct size and improve cardiac function in rats with myocardial infarction (Zaafan et al., 2013), with quercetin’s capacity to lower oxidative stress, inflammation, and apoptosis in the heart considered possible causes of its cardioprotective effects.
Hesperidin, found in citrus fruits, exhibits anti-hypertensive and anti-atherosclerotic properties (Mahmoud et al., 2019). It has been shown to lower blood pressure and enhance endothelial function in hypertensive rats (Morand et al., 2011), and it prevents atherosclerotic plaque formation in apolipoprotein E–knockout mice (Sugasawa et al., 2019). Catechin in tea and resveratrol in red wine also improve cardiovascular health (Gross, 2004), with the former regulating lipid metabolism and the latter reducing inflammation, oxidative stress, and platelet aggregation (Chen et al., 2016). These mechanisms contribute to improved cardiovascular disease outcomes (Gresele et al., 2011).
4.3 Polyphenols and neurodegenerative diseases
Flavonoids have been extensively studied for their potential in treating neurodegenerative diseases owing to their anti-inflammatory, anti-oxidant, and neuroprotective properties (Spagnuolo et al., 2018). In animal models of neurodegenerative diseases, including Alzheimer’s disease, Parkinson’s disease, and Huntington’s disease, flavonoids prevent neurodegeneration and cognitive decline (Solanki et al., 2015) by inhibiting oxidative stress, inflammation, and protein misfolding, and modulating the signaling pathways involved in cell survival, synaptic plasticity, and neurogenesis (Khan et al., 2020; Numakawa and Odaka, 2021). Some flavonoids may also protect against metabolic dysregulation in neurodegenerative processes by inhibiting LDHA activity in the brain (Zhang et al., 2015a). Additionally, flavonoids have been reported to alleviate brain damage caused by ischemia and reperfusion through LDH inhibition and anti-oxidant effects (Dong et al., 2013). The flavonoid oroxylin A reduces LDH expression (Sajeev et al., 2022) and shows potential for preventing and treating neurological diseases (Lu et al., 2016).
4.4 Alkaloids and cancer
Alkaloids, nitrogen-containing compounds widely distributed in the plant kingdom (Roy, 2017), have been recognized for their potential in LDH inhibition and drug discovery (Khazir et al., 2013). Berberine, an isoquinoline alkaloid found in plants, including goldenseal and barberry, possesses anti-bacterial and anti-inflammatory properties, making it a valuable component in Chinese medicine (Imanshahidi and Hosseinzadeh, 2008). Berberine is known to exhibit anti-cancer activity through inhibition of LDHA activity and reduction of lactate production in cancer cells (Tan et al., 2015). In mouse models of breast, colon, and lung cancer, berberine has demonstrated significant anti-cancer effects, inhibiting tumor growth and reducing lactate production (Sun et al., 2009; Mao et al., 2018). Moreover, berberine has shown the ability to suppress LDHA activity, inhibiting pancreatic cancer cell proliferation (Cheng et al., 2021). Papaverine, an isoquinoline-type alkaloid reported to inhibit LDHA, is currently undergoing clinical trials as a radiosensitizer aimed at reducing tumor hypoxia and enhancing the radiotherapy response in A549 non-small cell lung cancer cell (NSCLC) and EO771 breast cancer xenografts (Kapp and Whiteley, 1991; Benej et al., 2018).
4.5 Alkaloids and cardiovascular diseases
Alkaloids, such as berberine, vincamine, rutaecarpine, chelerythrine, and matrine, have been investigated for their therapeutic potential in various cardiovascular diseases (Yamamoto et al., 2001; Jia and Hu, 2010; Zhang and Yan, 2020; Zhang et al., 2021b; Cai et al., 2021). Berberine has been found to inhibit LDH activity in H9c2 cardiomyocytes, providing protection against ischemia/reperfusion injury (Zhu et al., 2020). Berberine treatment has also been shown to decrease lactate production and increase ATP production in cardiomyocytes, improving cellular energy metabolism through LDH activity inhibition (Lv et al., 2012).
Leonurine, derived from the Lamiaceae family, exhibits cardioprotective effects by decreasing LDH activity and exerting anti-oxidative activity (Liu et al., 2010). Additionally, rutaecarpine, chelerythrine, and matrine have been shown to inhibit LDH levels (Bao et al., 2011; Chen and Huang, 2012; Wu et al., 2022). Of these, rutaecarpine confers protection against myocardial cell injury by inhibiting the NADPH oxidase–ROS pathway (Tian et al., 2019).
4.6 Alkaloids and neurodegenerative diseases
In neurological diseases, LDH can serve as a marker for cell damage or death (Adan et al., 2016). Conditions such as stroke, traumatic brain injury, or neurodegenerative disorders can lead to cellular injury or necrosis (Mehta et al., 2013), causing LDH release into the extracellular space (Al Shammari et al., 2015). Elevated LDH levels in the cerebrospinal fluid or blood indicate cellular damage or loss (Fang et al., 2022). Alkaloids derived from Amaryllidaceae species have shown acetylcholinesterase (AChE) inhibitory activity, making them potential candidates for Alzheimer’s disease treatment (Marucci et al., 2021). These alkaloids protect neurons against glutamate-induced damage, reducing apoptotic nuclei and LDH release, indicating reduced cell death and damage (Cortes et al., 2015). These alkaloids may indirectly affect LDH activity through the regulation of acetylcholine levels, which impact cellular metabolism (Kim et al., 2017).
4.7 Terpenoids and cancer
Terpenoids, also known as isoprenoids, are a diverse class of chemical compounds found in a wide range of fruits, vegetables, and herbs (Thoppil and Bishayee, 2011). They exhibit numerous biological activities, including anti-cancer properties, (Yang et al., 2020), making them effective against various cancers, such as skin, breast, colon, pancreatic, and prostate cancers. Terpenoids also possess immune-modulating, anti-viral, anti-allergic, and anti-bacterial properties (Thoppil and Bishayee, 2011). Some terpenoids have shown potential for developing anti-cancer drugs as they inhibit LDH activity and reduce lactate production in cancer cells (Kooshki et al., 2022). In patients with idiopathic pulmonary fibrosis, increased levels of LDHA protein and lactate have been associated with reduced lung function (Judge et al., 2018), and gossypol, a terpenoid, has been studied for its potential to decrease the expression of hypoxia-inducible factor 1 alpha (HIF-1α) in lung fibroblast cells (Judge et al., 2017).
Artemisinins, derived from sweet wormwood (Artemisia annua), are well-known for their anti-malarial properties and are widely used for malaria treatment (Das, 2012). Dihydroartemisinin, an artemisinin derivative, exerts inhibitory effects on glycolytic metabolism in NSCLC cell lines by suppressing the glucose transporter glucose transporter 1 and impeding glucose absorption (Mi et al., 2015). This compound can also induce perturbations in lactate generation and a concomitant reduction in ATP synthesis (Guerra et al., 2018). Additional experiments have shown that dihydroartemisinin effectively reduces the expression of pyruvate kinase M2 (PKM2) in K562, HepG2, and ESCC cells (Li et al., 2019a).
Limonin, a limonoid present in tangerines, grapefruit, and oranges, exhibits diverse biological functions, including anti-inflammatory and anti-viral properties (Balestrieri et al., 2011; Yang et al., 2014; Gualdani et al., 2016). It has been reported to have anti-tumor activity against breast, liver, colon, and pancreatic cancers (Rahman et al., 2015; Murthy et al., 2021a). Limonin’s inhibitory effect on hexokinase-2 (HK-2) activity was investigated in hepatocellular carcinoma cells, where it effectively suppressed HK-2 activity, leading to decreased cell proliferation and colony formation through reduced glucose consumption and lactate production (Yao et al., 2018). Nimbolide, a limonoid derived from the neem tree (Azadirachta indica A. Juss), has demonstrated cytotoxic effects by regulating proliferation, apoptosis, migration, and invasion in various cancer cell lines (Jaiswara and Kumar, 2022).
Oleanolic acid, a natural triterpenoid, is known for its beneficial properties, including anti-inflammatory, anti-oxidant, anti-microbial, hepatoprotective, and anti-cancer activities (Liu, 1995). In endometriosis research, it inhibits LDHA activity in cell lines and induces apoptotic signaling pathways (Cho et al., 2022). Moreover, it suppresses the mTOR signaling pathway and PKM2 production in other breast and prostate cancer cell lines (Liu et al., 2014).
Ursolic acid, another triterpenoid found in various plants, including apple, basil, rosemary, and lavender (Zerin et al., 2016), exhibits various physiological functions, including antibacterial, anti-cancer, anti-diabetic, anti-inflammatory, and anti-oxidant effects (Mlala et al., 2019). For example, it has been shown to reduce LDHA expression in a breast cancer cell line (Wang et al., 2021a). Additionally, betulinic acid, astragalus saponin, and crocetin have been found to suppress LDHA activity and expression, leading to reduced glucose uptake and downregulation of the glycolysis pathway (Kim et al., 2014; Granchi et al., 2017; Guo et al., 2019; Jiao et al., 2019).
4.8 Terpenoids and cardiovascular diseases
In one study, the terpenoid ferruginol was found to reduce LDH and creatine kinase MB levels, indicators of doxorubicin-induced tissue damage (Li et al., 2021a). The study revealed that ferruginol mitigated apoptosis progression, as shown in a TUNEL assay in response to doxorubicin. Ferruginol’s cardioprotective action was demonstrated through the preservation of mitochondrial integrity, limitation of ROS-induced heart damage, and attenuation of apoptosis. These effects are likely mediated through the SIRT1 pathway, which regulates mitochondrial biogenesis and fatty acid oxidation.
Thymoquinone, known for its anti-inflammatory, anti-tumor, and analgesic properties (De Sousa, 2011; Sá et al., 2014; Sobral et al., 2014), exhibits anti-oxidant and vascular relaxant effects in experimental models of cardiovascular disease. Thymoquinone administration in mice improved superoxide dismutase activity, reduced interleukin-6 levels, and prevented cardiovascular side effects (Nemmar et al., 2011). In rats with isoproterenol-induced myocardial infarction treated with thymoquinone, dose-related decreases in plasmatic LDH, thiobarbituric acid reactive substances, and glutathione reductase were observed (Randhawa et al., 2013).
Regarding ursolic acid, prominent expression of LDH among serum marker enzymes was observed in myocardial ischemia–induced mice. Following ursolic acid treatment, significant protection against cardiac injury was evident, with a marked reduction in LDH activity (Radhiga et al., 2012).
4.9 Terpenoids and neurodegenerative diseases
Derived from Ginkgo biloba leaves, Ginkgolide B is a terpenoid diterpene lactone (Iwamoto et al., 2019) known for its anti-inflammatory and neuroprotective properties (Zhang et al., 2011). Ginkgolide B activates the Trk/Ras/MAPK signaling pathway, promoting neurite growth and secretion of brain-derived neurotrophic factors while reducing levels of ROS, LDH, caspase-3, and other proapoptotic factors.
Limonoids have been shown to enhance neuronal differentiation and neurite outgrowth in rat macrophages by activating the PKA/ERK1/2 signaling pathway (Roy and Saraf, 2006), stimulating the secretion of nerve growth factor, and attenuating LDH activity (Zhang et al., 2013a). Through activation of this pathway, limonoids promote neurite outgrowth in rat macrophages, enhancing neuronal differentiation (Gotoh et al., 1990; Yu et al., 2004).
The vibrant orange hue of carrots and sweet potatoes is due to the presence of ß-carotene (Zeb and Mehmood, 2004), a compound that serves as a precursor to vitamin A and is known for its anti-oxidant properties and potential health benefits (Thomas and Oyediran, 2008). ß-carotene plays a protective role in the brain, guarding against the harmful effects of cadmium-induced oxidative stress (Gonzalez-Burgos and Gómez-Serranillos, 2012). It enhances ATPase activity, reduces LDH activity and lipid peroxidation, and contributes to the surge of both enzymatic anti-oxidants, such as glutathione S-transferase and superoxide dismutase, and nonenzymatic anti-oxidants, including glutathione (Park et al., 2011).
Eucommia ulmoides Oliv. Bark contains geniposidic acid, one of its active ingredients (Xie et al., 2015). Geniposidic acid not only inhibits LDH but also PARP, cleaved caspase 3, MMPs, and cytochrome C, while increasing the levels of Bcl-2, Bcl-xL, and BDNF (Kwon et al., 2012). These combined effects result in an anti-apoptotic effect and suggest potential applications in the prevention or treatment of neurodegenerative diseases, such as Alzheimer’s disease (Venkatesan et al., 2015).
4.10 Sulfur-containing agents and cancer, cardiovascular disease, neurodegenerative disease
Allicin is a bioactive sulfur compound mainly stored in a precursor form in various plant parts. It is known to possess cardioprotective, anti-microbial, cholesterol-lowering, anti-inflammatory, and anti-tumor properties (Catanzaro et al., 2022). In experiments involving the combination treatment of tamoxifen and allicin on Ehrlich ascites carcinoma, both in vitro and in vivo, LDH levels were reduced, and there was a significant decrease in tumor growth (Suddek, 2014). Furthermore, in an experiment conducted on male Swiss albino mice, it was confirmed that cardiac oxidative damage was reduced when allicin and doxorubicin were administered together. This reduction in oxidative damage was attributed to a decrease in myocardial expression of activated caspase-3 and cyclooxygenase-2 (Abdel-Daim et al., 2017). In Parkinson’s disease, allicin is also known to have a protective effect against nerve damage related to Parkinson’s disease through its inherent antioxidant function and its ability to reduce LDH release (Liu et al., 2015).
Taurine, an organic compound containing sulfur in its chemical structure, possesses anti-inflammatory, anti-oxidant, and various physiological functions within the cardiovascular, kidney, endocrine, and immune systems (Kim and Cha, 2014). Treatment of the HepG2 cell line, a hepatocellular carcinoma cell line, with taurine resulted in a significant increase in apoptosis-related factors at both the gene and protein levels. Additionally, LDH activity was markedly reduced, indicating the inhibition of glycolysis and cell proliferation (Nabi et al., 2021). Furthermore, taurine has been found to prevent cardiac injury by reducing LDH activity, which is increased by cisplatin (Chowdhury et al., 2016). In neurons, taurine reduced nickel-induced LDH release and mitigated the decrease in ROS production, superoxide dismutase activity, and glutathione concentration, demonstrating its neuroprotective effect through the reduction of oxidative stress (Xu et al., 2015) (Figures 3, 4 and Table 1).
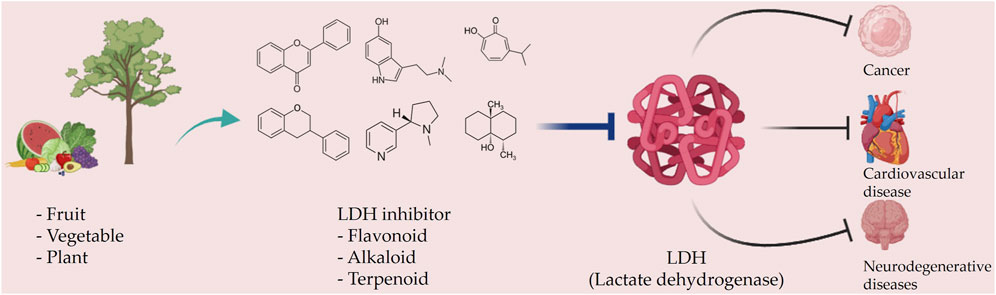
FIGURE 3. Schematic representation of natural compound LDH inhibitors. Among polyphenols, alkaloids, and terpenoids extracted from plants, certain compounds have therapeutic effects against cancer, cardiovascular diseases, and neurodegenerative diseases through inhibition of LDH.
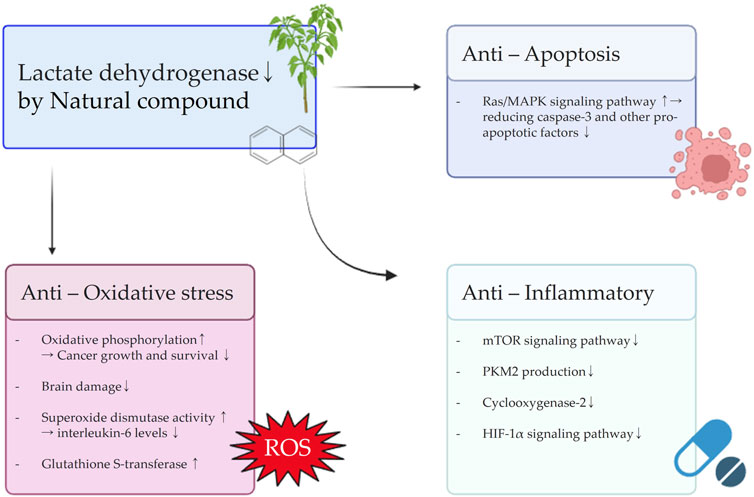
FIGURE 4. Schematic diagram illustrating the effects of natural compounds on anti-oxidative stress, anti-inflammatory, and anti-apoptosis signals resulting from the inhibition of LDH.
5 Challenges associated with using natural compounds as LDH inhibitors
Owing to their accessibility, diversity, and low toxicity, natural compounds have gained attention as potential disease treatments (David et al., 2015; Rochlani et al., 2017; Mohd Sairazi and Sirajudeen, 2020), with compounds capable of inhibiting LDH activity being of particular interest in disease therapy given its role in energy metabolism (Gallo et al., 2015; Lum et al., 2021; Zhao et al., 2021). However, using natural compounds as LDH inhibitors comes with several challenges that must be addressed before the compounds can become effective treatment options. Some of these challenges are discussed below.
5.1 Limited bioavailability
Natural compounds can be rapidly metabolized or excreted from the body, limiting their effectiveness as therapeutics (Fernandes et al., 2014). To address this issue, researchers are exploring various strategies, such as drug delivery systems, chemical modifications, and formulation approaches, to improve the bioavailability of these compounds (Prajapati et al., 2013).
5.2 Lack of specificity
Natural compounds often interact with multiple targets in the body, leading to unexpected side effects (David et al., 2015). Some natural compounds may have off-target effects, resulting in side effects, while others may lack specificity toward cancer cells, causing toxicity to normal cells (Mehta et al., 2010). Researchers are developing combination therapies to enhance the specificity of some compounds for cancer cells and minimize side effects (Koehn and Carter, 2005).
5.3 Limited understanding of the mechanism of action
The exact mechanism of action for natural compounds as LDH inhibitors remains unclear, hindering their development as therapeutic agents (Augoff et al., 2015). This lack of understanding makes it challenging to enhance their efficacy and minimize potential side effects. Researchers are employing various methods, including computational modeling, biochemical analysis, and proteomics, to elucidate the mechanisms (Lahlou, 2013).
5.4 Lack of standardized protocols
The absence of standardized protocols for screening and testing natural compounds as LDH inhibitors leads to inconsistent results. Variations in cell lines and assay conditions can contribute to discrepancies in study outcomes. To address this, many researchers are striving to establish a standardized protocol for screening and evaluating natural compounds (Cos et al., 2006).
5.5 Limited commercial interest
The nonpatentable nature of natural compounds has reduced commercial interest in their development as therapeutic agents (Li and Vederas, 2009). Additionally, the high costs associated with clinical trials and regulatory approval pose challenges for investing in natural compound–based drugs (Thomford et al., 2018). To overcome these obstacles, researchers are exploring various business models, such as open-source drug discovery, to incentivize the development of natural compounds as therapeutic agents (Sugumaran, 2012).
6 Strategies used to overcome challenges in natural compound development
Several strategies can be employed to address the challenges in developing natural compounds into viable drugs. Some of these strategies are discussed below.
6.1 Identification of bioactive compounds
To develop safe and effective drugs, it is essential to identify the major functional compounds in complex natural compounds. Techniques such as high-performance liquid chromatography, gas chromatography–mass spectrometry, and nuclear magnetic resonance, can be used for this purpose (Tsao et al., 2003; Garcia and Barbas, 2011; Wang et al., 2021b). The complexity of some natural compounds makes it challenging to pinpoint the desired therapeutic effect. Utilizing various methods can help identify the principal operational constituent within the complex blend, facilitating the formulation of effective and secure pharmaceuticals.
6.2 Optimization of bioactivity
Natural compounds often have low potency and selectivity against the target enzyme due to their low concentrations in their natural sources (Ochoa-Villarreal et al., 2016; Cazzaniga et al., 2021). Optimization of bioactivity can be achieved through structural modification, semi-synthesis, or total synthesis of natural compounds (Prachayasittikul et al., 2015). Structural modification can be applied to enhance the pharmacological properties of natural compounds (Guo, 2017). This process entails altering the compound’s stereochemistry or adding and removing functional groups. For instance, taxol, a natural compound with limited therapeutic potential owing to its low solubility in water (Dordunoo and Burt, 1996), underwent chemical modification to create a more soluble variant known as docetaxel, which has become a popular cancer medication (Hanauske et al., 1992; Fitzpatrick and Wheeler, 2003). Semi-synthesis, which involves analog production through chemical reactions, is another approach for enhancing the pharmacological properties of natural compounds (Lourenco et al., 2012). Although it is not as demanding as total synthesis, semi-synthesis remains effective in improving the compound’s bioactivity. An excellent example of semi-synthesis is the transformation of artemisinin into artesunate, resulting in a more potent variant now used in malaria treatment (White and Olliaro, 1998). Total synthesis the complete chemical synthesis of the natural compound from simple starting materials (Atanasov et al., 2021), represents the most challenging method of bioactivity optimization, but it can also be the most effective. For example, the natural compound shikonin has been completely synthesized, leading to the development of new drugs for the treatment of cancer and other diseases (Andujar et al., 2012; Wang et al., 2012).
6.3 Pharmacokinetic optimization
Pharmacokinetic properties, including solubility, stability, bioavailability, and metabolic stability, are crucial considerations in drug development (Sang et al., 2019). Some natural compounds exhibit poor pharmacokinetic properties, which can impede their development as therapeutic agents (Ma et al., 2022). To address this, prodrugs can be used, which are inert compounds that undergo metabolic transformation within the body to generate the active drug (Huttunen et al., 2011). Prodrugs can enhance the stability, solubility, and bioavailability of natural compounds, making them more effective in disease treatment. Additionally, formulation technologies, such as liposomes, nanoparticles, and cyclodextrins, can improve the solubility, stability, and release control of natural compounds, making them easier to administer and more effective (Augustin et al., 2013). Conjugation with suitable carriers, including polyethylene glycol, albumin, and dendrimers, is another approach to optimize pharmacokinetic properties, with this strategy aiming to enhance the overall efficacy of natural compounds as potential therapeutic agents (Gidwani and Vyas, 2015).
6.4 Nano-formulations and green synthesis
There are various nano-formulation types, including liposomes, hydrogels, solid lipid nanoparticles, polymeric nanomicelles, dendrimers, chitosan-based nanoparticles, metal nanoparticles, and nanocrystals, which are under investigation for their application with natural compounds (Murthy et al., 2021b). Nano-formulations can safeguard polyphenols against degradation, enhance absorption, and reduce toxicity, making them well-suited for delivering compounds (Khiev et al., 2021). These delivery systems offer advantages such as improved solubility, oral absorption, safety, and bioavailability. Researchers are currently testing the in vitro and in vivo efficacy of polyphenols like curcumin, quercetin, resveratrol, silybin, luteolin, naringenin, genistein, gossypol, ellagic acid, and hesperidin for treating various diseases (Murthy et al., 2021b).
Curcumin, a polyphenol, exhibits variations in its effects depending on the type and form of nanoparticles and has been extensively studied in various disease models, including malaria, cancer, and cerebral ischemia (Maheshwari et al., 2006; Tsai et al., 2011; Rahimi et al., 2016). The application of these nanoparticle forms has resulted in various effects, including increased solubility and circulation time, enhanced anti-tumor effects and bioavailability, improved anti-oxidative properties, and brain delivery (Liu and Chang, 2011; Liu et al., 2011; Liu et al., 2013). Camptothecin, a natural plant alkaloid, has demonstrated potent anti-tumor activity by targeting intracellular topoisomerase I (Pommier, 2006). The application of nanoformulation has been shown to enhance the efficacy of cancer treatment by addressing limiting factors such as water insolubility (Ghanbari-Movahed et al., 2021). Terpenoids have also been studied for their potential to improve the effectiveness of gastric cancer treatment by increasing anti-cancer and anti-bacterial efficacy through nanoconjugates, and by addressing shortcomings such as target delivery, stability, and half-life (Attri et al., 2023).
The green synthesis of silver nanoparticles from extracts of various plant parts has attracted widespread interest among researchers due to their unique optical and structural properties (Habeeb Rahuman et al., 2022). The green synthesis of nanoparticles is biocompatible and has potential applications in catalysts, anti-bacterial agents, energy harvesting, cancer/gene therapy, and sensing (Rana et al., 2020). Biological methods for nanoparticle synthesis are more economical, easier to implement, have a lower environmental impact, and require fewer processing steps than chemical and physical methods (Kumari et al., 2019). Plants contain polyphenols, flavonoids, alkaloids, and other biomolecules that work synergistically to inhibit oxidative damage to cellular components, leading to the reduction of metal ions into nanoparticles (Mohanpuria et al., 2008; Krishnaraj et al., 2014). Numerous studies have reported the synthesis of gold nanoparticles (AuNPs) using extracts from various plant parts. The putative biomolecules involved in the reduction of gold salts to gold nanoparticles include flavonoids, gingerol, shogaols, gingerone, paradol, catechin, proteins, aromatic amines, and aliphatic amines (Raghunandan et al., 2010; Kumar et al., 2011; Suman et al., 2014). However, the fundamental molecular mechanisms involved in nanoparticle formation are not fully understood, and further studies using natural products are needed.
6.5 Target specificity
Natural compounds often possess broad-spectrum activity against multiple targets, posing a challenge in developing specific inhibitors for the target enzyme. Target specificity is crucial in drug development, as it minimizes the potential for off-target effects and improves the therapeutic index of the drug (Mizuno et al., 2003). Several methods can be employed to enhance the specificity of natural compounds. Structure-based design uses computer modeling to develop compounds that specifically bind to target enzymes (Read et al., 2001b), with this approach being especially effective when the three-dimensional structure of the enzyme is already known (Schmidt et al., 2014). Molecular docking, employing computer algorithms, predicts compound binding to the intended enzyme (Abdolmaleki et al., 2017). To improve specificity, one strategy involves identifying compounds that favor the target enzyme while having minimal impact on off-target enzymes (Xu et al., 2021). High-throughput screening on compound libraries is another approach (Mayr and Bojanic, 2009), testing a large number of compounds to identify those that work well with the target enzyme while suppressing other enzymes (Bachovchin et al., 2009).
6.6 Toxicity
Toxicity concerns are prevalent in drug development, especially when working with natural compounds, as they have the potential to harm healthy cells (Ali Abdalla et al., 2022). One approach to reduce toxicity is through the use of prodrugs and proper carriers. Various techniques, including conjugation, can decrease toxicity by refining pharmacokinetic features, enhancing precision in targeting disease cells, and minimizing risks to healthy cells (Ma et al., 2019).
Combining natural compounds with suitable carriers has led to successful cancer treatments, such as antibody-drug conjugates, which function by attaching a toxin to an antibody that specifically targets cancer cells, delivering the toxin directly to the intended site (Dosio et al., 2011). This targeted delivery system not only reduces toxicity in healthy cells but also enhances the cancer cell specificity of natural compounds (Puthenveetil et al., 2016). An example of this progressive method involves combining a special peptide with curcumin, which specifically targets EGFR and has a more pronounced impact on suppressing breast cancer cells. The peptide–curcumin conjugate directs its attention toward EGFR-positive cancer cells, hindering their growth without significantly affecting healthy cells (Jin et al., 2017).
6.7 Intellectual property
The development of natural compounds as LDH inhibitors faces a major obstacle in the form of intellectual property challenges. Given that these compounds are found in nature, they cannot be patented, preventing companies from obtaining exclusive rights to their use (Harrison, 2014). To address this issue, one possible solution is to create modified medications with unique characteristics (Kirschning et al., 2007). These modified versions can be patented as fresh innovations, protecting the investment in drug development while still making the original natural compound available for other purposes. Additionally, exploring innovative delivery systems can enhance the potency of natural compounds and reduce their harmful effects. Overcoming the complexities of intellectual property allows companies to secure exclusive rights to the use of delivery technologies, facilitating the development of effective and commercially viable natural compound–based drugs.
6.8 Clinical trials
Clinical trials play a critical role in evaluating the safety and efficacy of drug candidates before their approval for human use (Deore et al., 2019). Extensive preclinical and clinical testing is essential for natural compounds being developed as LDH inhibitors to assess their safety and efficacy in humans. Clinical trials for LDH inhibitors present unique challenges but are vital for evaluating the safety and efficacy of these compounds in humans. Developing LDH inhibitors for cancer treatment requires specific patient populations and endpoints for clinical trials. Currently, no United States Food and Drug Administration–approved LDH inhibitors for cancer treatment exist, and their development necessitates comprehensive clinical testing to assess efficacy and safety. Some natural compounds, such as gossypol and galloflavin, have shown potential as LDH inhibitors in preclinical studies, demonstrating both LDH activity inhibition and anti-cancer properties (Cui et al., 2017; Rani and Kumar, 2017). LDHB is associated with an aggressive cancer phenotype, and there are studies aimed at identifying and clinically applying selective inhibitors for LDHB (McCleland et al., 2012; Shibata et al., 2021). In a study of clinical samples from colorectal cancer patients, a significant correlation was observed between MYC expression and the expression of multiple metabolic genes, accompanied by elevated LDHB levels, while LDHA levels remained unchanged (Satoh et al., 2017). The approach of promoting cancer cell necrosis through the inhibition of lactate transport is presently being employed in initial clinical trials as a potential cancer treatment strategy, utilizing the selective MCT-1 inhibitor known as AZD3965 (Beloueche-Babari et al., 2020).
No existing LDH inhibitors have yet shown clinically significant effects, but research is underway to discover new ones using computer-based structure-based virtual screening methods (Di Magno et al., 2022). However, the clinical advancement of these compounds faces challenges, including effectiveness, toxicity, specificity, and bioavailability. These obstacles underscore the importance of thorough preclinical and clinical testing in advancing natural compounds as LDH inhibitors.
7 Combination therapy: Natural compounds and LDH inhibitors
Natural compounds and LDH inhibitors show promise as therapeutic agents for various conditions, including cancer, inflammation, and metabolic disorders (Granchi et al., 2010; Jacobs et al., 2017). However, using these agents alone may not yield optimal treatment results in certain scenarios. Employing combination therapy, which involves multiple drugs with complementary mechanisms of action, could offer a more effective treatment option (Luo et al., 2017; Cummings et al., 2019). By combining natural compounds with LDH inhibitors, treatment efficacy can be enhanced while minimizing the risk of toxicity (Augoff et al., 2015). Natural compounds and LDH inhibitors can target different pathways relevant to disease progression, complementing each other and improving therapeutic outcomes (Gallagher et al., 2017). Additionally, using natural compounds may mitigate certain drawbacks of LDH inhibitors, such as potential toxicity and restricted specificity to targets (Fiume et al., 2014).
Several studies have investigated the combination of existing therapeutic agents and LDH inhibitors for cancer treatment (Gallo et al., 2015). For instance, in breast cancer cells, the combination of the LDH inhibitor gallic acid with the phenolic compound curcumin induces apoptosis through glutathione reduction, ROS induction, and mitochondrial dysfunction (Moghtaderi et al., 2018). In androgen-dependent prostate cancer cells (LAPC-4 and LNCaP), the combination of quercetin and arctigenin significantly inhibited the PI3K/Akt pathway, resulting in enhanced anti-proliferative effects (Wang et al., 2015c).
In glioblastoma treatment, quercetin has shown the ability to augment the effects of drugs, including temozolomide, a DNA-methylating agent. When administered together, quercetin and temozolomide induced apoptosis in T98G cells by promoting cytochrome c release and reducing mitochondrial membrane potential (∆Ψm) (Jakubowicz-Gil et al., 2013). Moreover, quercetin enhances the sensitivity of glioblastoma cells to temozolomide by inhibiting the expression of heat shock protein 27, a molecular chaperone involved in apoptosis regulation (Sang et al., 2014). Combining curcumin and quercetin provides potent protection against myocardial toxicity induced by ischemia-reperfusion injury in rats (Chakraborty et al., 2018). In glioma stem-like cells, the combination of EGCG and temozolomide exerted inhibitory effects on neurosphere formation and cell migration (Zhang et al., 2015b), affecting migration and adhesion processes (Pilorget et al., 2003).
In a rat study, the combination of resveratrol and syringic acid showed synergistic protection against cardiotoxicity by reducing nuclear factor kappa B activation and lowering tumor necrosis factor alpha levels (Shaik et al., 2020). The combined treatment of resveratrol and paclitaxel in DBTRG glioblastoma cells led to increased apoptosis marker levels, caspase 3 activity, Ca2+ fluorescence intensity, ROS levels, mitochondrial function, mitochondrial membrane depolarization, and TRPM2 current density, resulting in reduced cell viability (Øztürk et al., 2019).
The mechanism underlying the synergistic effects of combining natural compounds and LDH inhibitors in therapy is not fully understood. Nevertheless, natural compounds may enhance the anti-tumor effects of LDH inhibitors by influencing the tumor microenvironment and promoting tumor cell demise (Kooshki et al., 2022). Through LDH inhibition, natural compounds may heighten the susceptibility of tumor cells, decreasing their energy metabolism and increasing their dependence on glycolysis (Gao and Chen, 2015). Combining natural compounds and LDH inhibitors can overcome the limitations of each agent used alone by reducing their concentration in the body. This approach alleviates the toxicity of LDH inhibitors and enhances the specificity of targeting tumor cells (Akbari et al., 2022).
8 Conclusion
Natural compounds such as LDH inhibitors have shown great potential for treating various diseases, including cancer, cardiovascular diseases, and neurodegenerative diseases (Leuci et al., 2020; Mohd Sairazi and Sirajudeen, 2020). Despite notable challenges that must be addressed, preclinical studies have demonstrated the safety and effectiveness of these compounds in this inhibitory role (Rani and Kumar, 2016; 2017). Combining natural compounds with existing LDH inhibitors has also shown promise in improving therapeutic outcomes and reducing toxicity (Augoff et al., 2015). These findings suggest that developing natural compounds as LDH inhibitors could lead to new treatments for several diseases. Indeed, natural compounds could be used as monotherapy for less aggressive tumors, as adjuvants to enhance chemotherapy effectiveness, and as complementary therapy for cardiovascular diseases and neurodegenerative diseases.
However, before using natural compounds as LDH inhibitors in clinical settings, several issues need to be addressed. Improving natural compound bioavailability and pharmacokinetics is essential to ensure optimal efficacy (Nowak et al., 2019). This can be achieved through the use of delivery systems, such as nanoparticles or liposomes, or by modifying the chemical structure of natural compounds (Aqil et al., 2013; Gunasekaran et al., 2014). Additionally, more research is needed to understand the molecular mechanisms through which natural compounds act as LDH inhibitors. Identifying specific targets and pathways influenced by natural compounds could lead to more effective and targeted therapies (Dutta et al., 2019). Furthermore, thorough clinical trials are necessary to assess the safety and toxicity of natural compounds. Despite being generally considered safe, some natural compounds may have undesirable side effects or interact with other medications (Scott and Elmer, 2002; Butler, 2008). Therefore, before using natural compounds in clinical settings, their safety and toxicity must be carefully evaluated.
Despite these challenges, the potential clinical uses of natural compounds as LDH inhibitors are promising. The development of natural compounds as LDH inhibitors offers hope for patients with various diseases. They can be used as monotherapy or adjuvants in cancer treatment (Rani and Kumar, 2016; Memariani et al., 2021), as a complementary therapy in cardiovascular disease, or to halt the progression of atherosclerosis (Zhang et al., 2021a), and to reduce neuroinflammation and oxidative stress as key pathological features in neurodegenerative diseases (Chen et al., 2020). In conclusion, natural compounds have shown promise as LDH inhibitors for treating various diseases. Although challenges remain in their clinical application, further research and development could pave the way for novel treatments, offering renewed hope to patients suffering from diverse diseases.
Author contributions
JH: Conceptualization, Data curation, Investigation, Visualization, Validation, Writing–original draft, Writing–review and editing. E-JL: Conceptualization, Data curation, Investigation, Validation, Writing–review and editing, Writing–original draft. WP: Data curation, Investigation, Validation, Writing–review and editing. K-TH: Conceptualization, Data curation, Investigation, Validation, Writing–review and editing. H-SC: Conceptualization, Data curation, Funding acquisition, Project administration, Resources, Supervision, Validation, Writing–original draft, Writing–review and editing.
Funding
The author(s) declare financial support was received for the research, authorship, and/or publication of this article. This research was supported by the Korea Institute of Oriental Medicine (KIOM) grant number KSN1823231, provided by the Ministry of Science and ICT, Republic of Korea.
Conflict of interest
The authors declare that the research was conducted in the absence of any commercial or financial relationships that could be construed as a potential conflict of interest.
The author(s) declared that they were an editorial board member of Frontiers, at the time of submission. This had no impact on the peer review process and the final decision.
Publisher’s note
All claims expressed in this article are solely those of the authors and do not necessarily represent those of their affiliated organizations, or those of the publisher, the editors and the reviewers. Any product that may be evaluated in this article, or claim that may be made by its manufacturer, is not guaranteed or endorsed by the publisher.
References
Abdel-Daim, M. M., Kilany, O. E., Khalifa, H. A., and Ahmed, A. A. (2017). Allicin ameliorates doxorubicin-induced cardiotoxicity in rats via suppression of oxidative stress, inflammation and apoptosis. Cancer Chemother. Pharmacol. 80, 745–753. doi:10.1007/s00280-017-3413-7
Abdel-Wahab, A. F., Mahmoud, W., and Al-Harizy, R. M. (2019). Targeting glucose metabolism to suppress cancer progression: prospective of anti-glycolytic cancer therapy. Pharmacol. Res. 150, 104511. doi:10.1016/j.phrs.2019.104511
Abdolmaleki, A., Ghasemi, J., and Ghasemi, F. (2017). Computer aided drug design for multi-target drug design: SAR/QSAR, molecular docking and pharmacophore methods. Curr. drug targets 18, 556–575. doi:10.2174/1389450117666160101120822
Acharya, R., Chakraborty, M., and Chakraborty, J. (2019). Prospective treatment of Parkinson's disease by a siRNA–LDH nanoconjugate. MedChemComm 10, 227–233. doi:10.1039/c8md00501j
Adan, A., Kiraz, Y., and Baran, Y. (2016). Cell proliferation and cytotoxicity assays. Curr. Pharm. Biotechnol. 17, 1213–1221. doi:10.2174/1389201017666160808160513
Adegbola, P., Aderibigbe, I., Hammed, W., and Omotayo, T. (2017). Antioxidant and anti-inflammatory medicinal plants have potential role in the treatment of cardiovascular disease: a review. Am. J. Cardiovasc. Dis. 7, 19–32.
Aggarwal, B., Prasad, S., Reuter, S., Kannappan, R., R Yadav, V., Park, B., et al. (2011). Identification of novel anti-inflammatory agents from Ayurvedic medicine for prevention of chronic diseases:“reverse pharmacology” and “bedside to bench” approach. Curr. drug targets 12, 1595–1653. doi:10.2174/138945011798109464
Ait-Aissa, K., Blaszak, S. C., Beutner, G., Tsaih, S.-W., Morgan, G., Santos, J. H., et al. (2019). Mitochondrial oxidative phosphorylation defect in the heart of subjects with coronary artery disease. Sci. Rep. 9, 7623. doi:10.1038/s41598-019-43761-y
Akbari, B., Baghaei-Yazdi, N., Bahmaie, M., and Mahdavi Abhari, F. (2022). The role of plant-derived natural antioxidants in reduction of oxidative stress. BioFactors 48, 611–633. doi:10.1002/biof.1831
Akinloye, O. A., Akinloye, D. I., Lawal, M. A., Shittu, M. T., and Metibemu, D. S. (2021). Terpenoids from Azadirachta indica are potent inhibitors of Akt: validation of the anticancer potentials in hepatocellular carcinoma in male Wistar rats. J. Food Biochem. 45, e13559. doi:10.1111/jfbc.13559
Al Shammari, B., Shiomi, T., Tezera, L., Bielecka, M. K., Workman, V., Sathyamoorthy, T., et al. (2015). The extracellular matrix regulates granuloma necrosis in tuberculosis. J. Infect. Dis. 212, 463–473. doi:10.1093/infdis/jiv076
Al-Jassabi, S. (2002). Purification and kinetic properties of skeletal muscle lactate dehydrogenase from the lizard Agama stellio stellio. Biochem. Mosc. 67, 786–789. doi:10.1023/a:1016300808378
Aldosari, B. N., Alfagih, I. M., and Almurshedi, A. S. (2021). Lipid nanoparticles as delivery systems for RNA-based vaccines. Pharmaceutics 13, 206. doi:10.3390/pharmaceutics13020206
Ali Abdalla, Y. O., Subramaniam, B., Nyamathulla, S., Shamsuddin, N., Arshad, N. M., Mun, K. S., et al. (2022). Natural products for cancer therapy: a review of their mechanism of actions and toxicity in the past decade. J. Trop. Med. 2022, 5794350. doi:10.1155/2022/5794350
Aliberti, G., Pulignano, I., Proietta, M., Corvisieri, P., and De Michele, L. (1997). Lactate dehydrogenase and its isoenzymes in the marrow and peripheral blood from haematologically normal subjects. Physiological Res. 46, 435–438.
Andujar, I., Ríos, J. L., Giner, R. M., Miguel Cerda, J., and Recio, M. D. C. (2012). Beneficial effect of shikonin on experimental colitis induced by dextran sulfate sodium in BALB/c mice. Evidence-Based Complementary Altern. Med. 2012, 271606. doi:10.1155/2012/271606
Aqil, F., Munagala, R., Jeyabalan, J., and Vadhanam, M. V. (2013). Bioavailability of phytochemicals and its enhancement by drug delivery systems. Cancer Lett. 334, 133–141. doi:10.1016/j.canlet.2013.02.032
Atanasov, A. G., Zotchev, S. B., Dirsch, V. M., and Supuran, C. T. (2021). Natural products in drug discovery: advances and opportunities. Nat. Rev. Drug Discov. 20, 200–216. doi:10.1038/s41573-020-00114-z
Attri, K., Sharda, D., Chudasama, B. N., Mahajan, R., and Choudhury, D. (2023). A Review on Terpenes for Treatment of Gastric Cancer: Current Status and Nanotechnology-enabled Future. RSC Sustain. 1, 1109–1124. doi:10.1039/d2su00137c
Augoff, K., Hryniewicz-Jankowska, A., and Tabola, R. (2015). Lactate dehydrogenase 5: an old friend and a new hope in the war on cancer. Cancer Lett. 358, 1–7. doi:10.1016/j.canlet.2014.12.035
Augustin, M. A., Sanguansri, L., and Lockett, T. (2013). Nano-and micro-encapsulated systems for enhancing the delivery of resveratrol. Ann. N. Y. Acad. Sci. 1290, 107–112. doi:10.1111/nyas.12130
Aziz, N. F., Ramalingam, A., Latip, J., and Zainalabidin, S. (2021). S-allylcysteine improves ischemia/reperfusion alteration on cardiac function, antioxidant, and mitochondrial permeability. Life Sci. 269, 119080. doi:10.1016/j.lfs.2021.119080
Bachovchin, D. A., Brown, S. J., Rosen, H., and Cravatt, B. F. (2009). Identification of selective inhibitors of uncharacterized enzymes by high-throughput screening with fluorescent activity-based probes. Nat. Biotechnol. 27, 387–394. doi:10.1038/nbt.1531
Bader, A., Tuccinardi, T., Granchi, C., Martinelli, A., Macchia, M., Minutolo, F., et al. (2015). Phenylpropanoids and flavonoids from Phlomis kurdica as inhibitors of human lactate dehydrogenase. Phytochemistry 116, 262–268. doi:10.1016/j.phytochem.2015.03.007
Bajan, S., and Hutvagner, G. (2020). RNA-based therapeutics: from antisense oligonucleotides to miRNAs. Cells 9, 137. doi:10.3390/cells9010137
Balestrieri, E., Pizzimenti, F., Ferlazzo, A., Giofrè, S. V., Iannazzo, D., Piperno, A., et al. (2011). Antiviral activity of seed extract from Citrus bergamia towards human retroviruses. Bioorg. Med. Chem. 19, 2084–2089. doi:10.1016/j.bmc.2011.01.024
Bao, M.-H., Dai, W., Li, Y.-J., and Hu, C.-P. (2011). Rutaecarpine prevents hypoxia–reoxygenation-induced myocardial cell apoptosis via inhibition of NADPH oxidases. Can. J. physiology Pharmacol. 89, 177–186. doi:10.1139/Y11-006
Beloueche-Babari, M., Casals Galobart, T., Delgado-Goni, T., Wantuch, S., Parkes, H. G., Tandy, D., et al. (2020). Monocarboxylate transporter 1 blockade with AZD3965 inhibits lipid biosynthesis and increases tumour immune cell infiltration. Br. J. cancer 122, 895–903. doi:10.1038/s41416-019-0717-x
Ben, S.-Q., Ni, S.-S., Shen, H.-H., Shi, Y.-X., Huang, S.-B., Xu, J.-H., et al. (2007). The dynamic changes of LDH isoenzyme 3 and D-dimer following pulmonary thromboembolism in canine. Thrombosis Res. 120, 575–583. doi:10.1016/j.thromres.2006.12.015
Benej, M., Hong, X., Vibhute, S., Scott, S., Wu, J., Graves, E., et al. (2018). Papaverine and its derivatives radiosensitize solid tumors by inhibiting mitochondrial metabolism. Proc. Natl. Acad. Sci. 115, 10756–10761. doi:10.1073/pnas.1808945115
Bigl, M., Brückner, M., Arendt, T., Bigl, V., and Eschrich, K. (1999). Activities of key glycolytic enzymes in the brains of patients with Alzheimer's disease. J. neural Transm. 106, 499–511. doi:10.1007/s007020050174
Blom, D. J., Marais, A. D., Moodley, R., Van Der Merwe, N., Van Tonder, A., and Raal, F. J. (2022). RNA-based therapy in the management of lipid disorders: a review. Lipids Health Dis. 21, 41. doi:10.1186/s12944-022-01649-3
Bockstahler, M., Salbach, C., Müller, A. M., Kübler, A., Müller, O. J., Katus, H. A., et al. (2022). LNA oligonucleotide mediates an anti-inflammatory effect in autoimmune myocarditis via targeting lactate dehydrogenase B. Immunology 165, 158–170. doi:10.1111/imm.13421
Butler, M. S. (2008). Natural products to drugs: natural product-derived compounds in clinical trials. Nat. Product. Rep. 25, 475–516. doi:10.1039/b514294f
Cai, Y., Xin, Q., Lu, J., Miao, Y., Lin, Q., Cong, W., et al. (2021). A new therapeutic candidate for cardiovascular diseases: berberine. Front. Pharmacol. 12, 631100. doi:10.3389/fphar.2021.631100
Cameron, A., Read, J., Tranter, R., Winter, V. J., Sessions, R. B., Brady, R. L., et al. (2004). Identification and activity of a series of azole-based compounds with lactate dehydrogenase-directed anti-malarial activity. J. Biol. Chem. 279, 31429–31439. doi:10.1074/jbc.M402433200
Capula, M., Mantini, G., Funel, N., and Giovannetti, E. (2019). New avenues in pancreatic cancer: exploiting microRNAs as predictive biomarkers and new approaches to target aberrant metabolism. Expert Rev. Clin. Pharmacol. 12, 1081–1090. doi:10.1080/17512433.2019.1693256
Catanzaro, E., Canistro, D., Pellicioni, V., Vivarelli, F., and Fimognari, C. (2022). Anticancer potential of allicin: A review. Pharmacol. Res. 177, 106118. doi:10.1016/j.phrs.2022.106118
Cazzaniga, G., Mori, M., Chiarelli, L. R., Gelain, A., Meneghetti, F., and Villa, S. (2021). Natural products against key Mycobacterium tuberculosis enzymatic targets: emerging opportunities for drug discovery. Eur. J. Med. Chem. 224, 113732. doi:10.1016/j.ejmech.2021.113732
Cerella, C., Radogna, F., Dicato, M., and Diederich, M. (2013). Natural compounds as regulators of the cancer cell metabolism. Int. J. Cell Biol. 2013, 639401. doi:10.1155/2013/639401
Chakraborty, M., Ahmed, M. G., and Bhattacharjee, A. (2018). Effect of quercetin on myocardial potency of curcumin against ischemia reperfusion induced myocardial toxicity. Synergy 7, 25–29. doi:10.1016/j.synres.2018.09.001
Chao, J., Lau, W.K.-W., Huie, M. J., Ho, Y.-S., Yu, M.-S., Lai, C.S.-W., et al. (2010). A pro-drug of the green tea polyphenol (−)-epigallocatechin-3-gallate (EGCG) prevents differentiated SH-SY5Y cells from toxicity induced by 6-hydroxydopamine. Neurosci. Lett. 469, 360–364. doi:10.1016/j.neulet.2009.12.028
Chen, F., and Huang, K. (2012). Effects of the Chinese medicine matrine on experimental C. parvum infection in BALB/c mice and MDBK cells. Parasitol. Res. 111, 1827–1832. doi:10.1007/s00436-012-3030-7
Chen, S.-Y., Gao, Y., Sun, J.-Y., Meng, X.-L., Yang, D., Fan, L.-H., et al. (2020). Traditional Chinese medicine: role in reducing β-amyloid, apoptosis, autophagy, neuroinflammation, oxidative stress, and mitochondrial dysfunction of Alzheimer’s disease. Front. Pharmacol. 11, 497. doi:10.3389/fphar.2020.00497
Chen, X.-Q., Hu, T., Han, Y., Huang, W., Yuan, H.-B., Zhang, Y.-T., et al. (2016). Preventive effects of catechins on cardiovascular disease. Molecules 21, 1759. doi:10.3390/molecules21121759
Chen, Z., Lu, W., Garcia-Prieto, C., and Huang, P. (2007). The Warburg effect and its cancer therapeutic implications. J. bioenergetics Biomembr. 39, 267–274. doi:10.1007/s10863-007-9086-x
Cheng, C. S., Tan, H. Y., Wang, N., Chen, L., Meng, Z., Chen, Z., et al. (2021). Functional inhibition of lactate dehydrogenase suppresses pancreatic adenocarcinoma progression. Clin. Transl. Med. 11, e467. doi:10.1002/ctm2.467
Cheng, G., Pi, Z., Zheng, Z., Liu, S., Liu, Z., and Song, F. (2020). Magnetic nanoparticles-based lactate dehydrogenase microreactor as a drug discovery tool for rapid screening inhibitors from natural products. Talanta 209, 120554. doi:10.1016/j.talanta.2019.120554
Chittezhath, M., Deep, G., Singh, R. P., Agarwal, C., and Agarwal, R. (2008). Silibinin inhibits cytokine-induced signaling cascades and down-regulates inducible nitric oxide synthase in human lung carcinoma A549 cells. Mol. cancer Ther. 7, 1817–1826. doi:10.1158/1535-7163.MCT-08-0256
Cho, M. K., Jin, L., Han, J. H., Jin, J.-S., Cheon, S.-Y., Shin, S., et al. (2022). Water-Extracted Prunella vulgaris Alleviates Endometriosis by Reducing Aerobic Glycolysis. Front. Pharmacol. 13, 872810. doi:10.3389/fphar.2022.872810
Chowdhury, S., Sinha, K., Banerjee, S., and Sil, P. C. (2016). Taurine protects cisplatin induced cardiotoxicity by modulating inflammatory and endoplasmic reticulum stress responses. Biofactors 42, 647–664. doi:10.1002/biof.1301
Conners, R., Schambach, F., Read, J., Cameron, A., Sessions, R. B., Vivas, L., et al. (2005). Mapping the binding site for gossypol-like inhibitors of Plasmodium falciparum lactate dehydrogenase. Mol. Biochem. Parasitol. 142, 137–148. doi:10.1016/j.molbiopara.2005.03.015
Copeland, R. A., Harpel, M. R., and Tummino, P. J. (2007). Targeting enzyme inhibitors in drug discovery. Expert Opin. Ther. targets 11, 967–978. doi:10.1517/14728222.11.7.967
Cortes, N., Posada-Duque, R. A., Alvarez, R., Alzate, F., Berkov, S., Cardona-Gómez, G. P., et al. (2015). Neuroprotective activity and acetylcholinesterase inhibition of five Amaryllidaceae species: a comparative study. Life Sci. 122, 42–50. doi:10.1016/j.lfs.2014.12.011
Cos, P., Vlietinck, A. J., Berghe, D. V., and Maes, L. (2006). Anti-infective potential of natural products: how to develop a stronger in vitro proof-of-concept. J. Ethnopharmacol. 106, 290–302. doi:10.1016/j.jep.2006.04.003
Cui, Q., Wen, S., and Huang, P. (2017). Targeting cancer cell mitochondria as a therapeutic approach: recent updates. Future Med. Chem. 9, 929–949. doi:10.4155/fmc-2017-0011
Cummings, J. L., Tong, G., and Ballard, C. (2019). Treatment combinations for Alzheimer’s disease: current and future pharmacotherapy options. J. Alzheimer's Dis. 67, 779–794. doi:10.3233/JAD-180766
Dai, Q., Shestov, A. A., Lai, L., and Locasale, J. W. (2016b). A flux balance of glucose metabolism clarifies the requirements of the Warburg effect. Biophysical J. 111, 1088–1100. doi:10.1016/j.bpj.2016.07.028
Dai, Q., Yin, Q., Wei, L., Zhou, Y., Qiao, C., Guo, Y., et al. (2016a). Oroxylin A regulates glucose metabolism in response to hypoxic stress with the involvement of hypoxia-inducible factor-1 in human hepatoma HepG2 cells. Mol. Carcinog. 55, 1275–1289. doi:10.1002/mc.22369
Das, S. (2012). Artemisia annua (Qinghao): a pharmacological review. Int. J. Pharm. Sci. Res. 3, 4573–4577.
David, B., Wolfender, J.-L., and Dias, D. A. (2015). The pharmaceutical industry and natural products: historical status and new trends. Phytochem. Rev. 14, 299–315. doi:10.1007/s11101-014-9367-z
De Sousa, D. P. (2011). Analgesic-like activity of essential oils constituents. Molecules 16, 2233–2252. doi:10.3390/molecules16032233
Deore, A. B., Dhumane, J. R., Wagh, R., and Sonawane, R. (2019). The stages of drug discovery and development process. Asian J. Pharm. Res. Dev. 7, 62–67. doi:10.22270/ajprd.v7i6.616
Dhanasekaran, M., and Ren, J. (2005). The emerging role of coenzyme Q-10 in aging, neurodegeneration, cardiovascular disease, cancer and diabetes mellitus. Curr. Neurovascular Res. 2, 447–459. doi:10.2174/156720205774962656
Di Domenico, F., Tramutola, A., and Butterfield, D. A. (2017). Role of 4-hydroxy-2-nonenal (HNE) in the pathogenesis of alzheimer disease and other selected age-related neurodegenerative disorders. Free Radic. Biol. Med. 111, 253–261. doi:10.1016/j.freeradbiomed.2016.10.490
Di Magno, L., Coluccia, A., Bufano, M., Ripa, S., La Regina, G., Nalli, M., et al. (2022). Discovery of novel human lactate dehydrogenase inhibitors: structure-based virtual screening studies and biological assessment. Eur. J. Med. Chem. 240, 114605. doi:10.1016/j.ejmech.2022.114605
Ding, Y., and Lawrence, C. E. (2001). Statistical prediction of single-stranded regions in RNA secondary structure and application to predicting effective antisense target sites and beyond. Nucleic acids Res. 29, 1034–1046. doi:10.1093/nar/29.5.1034
Doherty, J. R., and Cleveland, J. L. (2013). Targeting lactate metabolism for cancer therapeutics. J. Clin. investigation 123, 3685–3692. doi:10.1172/JCI69741
Dong, S., Tong, X., Li, J., Huang, C., Hu, C., Jiao, H., et al. (2013). Total flavonoid of Litsea coreana leve exerts anti-oxidative effects and alleviates focal cerebral ischemia/reperfusion injury. Neural Regen. Res. 8, 3193–3202. doi:10.3969/j.issn.1673-5374.2013.34.003
Dordunoo, S. K., and Burt, H. M. (1996). Solubility and stability of taxol: effects of buffers and cyclodextrins. Int. J. Pharm. 133, 191–201. doi:10.1016/0378-5173(96)04443-2
Dosio, F., Brusa, P., and Cattel, L. (2011). Immunotoxins and anticancer drug conjugate assemblies: the role of the linkage between components. Toxins 3, 848–883. doi:10.3390/toxins3070848
Doughari, J. H. (2012). Phytochemicals: extraction methods, basic structures and mode of action as potential chemotherapeutic agents. Croatia: INTECH Open Access Publisher Rijeka.
Dutta, S., Mahalanobish, S., Saha, S., Ghosh, S., and Sil, P. C. (2019). Natural products: an upcoming therapeutic approach to cancer. Food Chem. Toxicol. 128, 240–255. doi:10.1016/j.fct.2019.04.012
Eng, Q. Y., Thanikachalam, P. V., and Ramamurthy, S. (2018). Molecular understanding of Epigallocatechin gallate (EGCG) in cardiovascular and metabolic diseases. J. Ethnopharmacol. 210, 296–310. doi:10.1016/j.jep.2017.08.035
Fahrig, T., Gerlach, I., and Horvath, E. (2005). A synthetic derivative of the natural product rocaglaol is a potent inhibitor of cytokine-mediated signaling and shows neuroprotective activity in vitro and in animal models of Parkinson's disease and traumatic brain injury. Mol. Pharmacol. 67, 1544–1555. doi:10.1124/mol.104.008177
Fang, Y., Wang, X., Lu, J., Shi, H., Huang, L., Shao, A., et al. (2022). Inhibition of caspase-1-mediated inflammasome activation reduced blood coagulation in cerebrospinal fluid after subarachnoid haemorrhage. EBioMedicine 76, 103843. doi:10.1016/j.ebiom.2022.103843
Fantin, V. R., St-Pierre, J., and Leder, P. (2006). Attenuation of LDH-A expression uncovers a link between glycolysis, mitochondrial physiology, and tumor maintenance. Cancer Cell 9, 425–434. doi:10.1016/j.ccr.2006.04.023
Farabegoli, F., Vettraino, M., Manerba, M., Fiume, L., Roberti, M., and Di Stefano, G. (2012). Galloflavin, a new lactate dehydrogenase inhibitor, induces the death of human breast cancer cells with different glycolytic attitude by affecting distinct signaling pathways. Eur. J. Pharm. Sci. 47, 729–738. doi:10.1016/j.ejps.2012.08.012
Farhana, A., and Lappin, S. L. (2022). Biochemistry, lactate dehydrogenase. United States: StatPearls Publishing.
Feng, Y., Xiong, Y., Qiao, T., Li, X., Jia, L., and Han, Y. (2018). Lactate dehydrogenase A: A key player in carcinogenesis and potential target in cancer therapy. Cancer Med. 7, 6124–6136. doi:10.1002/cam4.1820
Fernandes, I., Faria, A., Calhau, C., De Freitas, V., and Mateus, N. (2014). Bioavailability of anthocyanins and derivatives. J. Funct. foods 7, 54–66. doi:10.1016/j.jff.2013.05.010
Fitzpatrick, F., and Wheeler, R. (2003). The immunopharmacology of paclitaxel (Taxol®), docetaxel (Taxotere®), and related agents. Int. Immunopharmacol. 3, 1699–1714. doi:10.1016/j.intimp.2003.08.007
Fiume, L., Manerba, M., Vettraino, M., and Di Stefano, G. (2014). Inhibition of lactate dehydrogenase activity as an approach to cancer therapy. Future Med. Chem. 6, 429–445. doi:10.4155/fmc.13.206
Fiume, L., Vettraino, M., Carnicelli, D., Arfilli, V., Di Stefano, G., and Brigotti, M. (2013). Galloflavin prevents the binding of lactate dehydrogenase A to single stranded DNA and inhibits RNA synthesis in cultured cells. Biochem. biophysical Res. Commun. 430, 466–469. doi:10.1016/j.bbrc.2012.12.013
Forkasiewicz, A., Dorociak, M., Stach, K., Szelachowski, P., Tabola, R., and Augoff, K. (2020). The usefulness of lactate dehydrogenase measurements in current oncological practice. Cell. Mol. Biol. Lett. 25, 35–14. doi:10.1186/s11658-020-00228-7
Fu, X., Zhang, J., Huang, X., Mo, Z., Sang, Z., Duan, W., et al. (2021). Curcumin antagonizes glucose fluctuation-induced renal injury by inhibiting aerobic glycolysis via the mir-489/ldha pathway. Mediat. Inflamm. 2021, 6104529. doi:10.1155/2021/6104529
Gallagher, R., Motohashi, N., Vanam, A., and Gollapudi, R. (2017). Restricting anaerobic glycolysis reliance (Warburg effect): novel natural products based therapeutic strategy for cancer treatment. Archives General Intern. Med. 1, 1–10.
Gallo, D., Giacomelli, S., Ferlini, C., Raspaglio, G., Apollonio, P., Prislei, S., et al. (2003). Antitumour activity of the silybin-phosphatidylcholine complex, IdB 1016, against human ovarian cancer. Eur. J. Cancer 39, 2403–2410. doi:10.1016/s0959-8049(03)00624-5
Gallo, M., Sapio, L., Spina, A., Naviglio, D., Calogero, A., and Naviglio, S. (2015). Lactic dehydrogenase and cancer: an overview. Front. Bioscience-Landmark 20, 1234–1249. doi:10.2741/4368
Gandhi, G. R., Antony, P. J., Ceasar, S. A., Vasconcelos, A. B. S., Montalvão, M. M., Farias De Franca, M. N., et al. (2022). Health functions and related molecular mechanisms of ellagitannin-derived urolithins. Crit. Rev. Food Sci. Nutr. 2022, 1–31. doi:10.1080/10408398.2022.2106179
Gao, J.-L., and Chen, Y.-G. (2015). Natural compounds regulate glycolysis in hypoxic tumor microenvironment. BioMed Res. Int. 2015, 354143. doi:10.1155/2015/354143
Garcia, A., and Barbas, C. (2011). Gas chromatography-mass spectrometry (GC-MS)-based metabolomics. Metabolic profiling Methods Protoc. 708, 191–204. doi:10.1007/978-1-61737-985-7_11
Garcia-Oliveira, P., Otero, P., Pereira, A. G., Chamorro, F., Carpena, M., Echave, J., et al. (2021). Status and challenges of plant-anticancer compounds in cancer treatment. Pharmaceuticals 14, 157. doi:10.3390/ph14020157
Ghanbari-Movahed, M., Kaceli, T., Mondal, A., Farzaei, M. H., and Bishayee, A. (2021). Recent advances in improved anticancer efficacies of camptothecin nano-formulations: A systematic review. Biomedicines 9, 480. doi:10.3390/biomedicines9050480
Gidwani, B., and Vyas, A. (2015). A comprehensive review on cyclodextrin-based carriers for delivery of chemotherapeutic cytotoxic anticancer drugs. BioMed Res. Int. 2015, 198268. doi:10.1155/2015/198268
Gladden, L. (2004). Lactate metabolism: a new paradigm for the third millennium. J. physiology 558, 5–30. doi:10.1113/jphysiol.2003.058701
Gonzalez-Burgos, E., and Gómez-Serranillos, M. (2012). Terpene compounds in nature: a review of their potential antioxidant activity. Curr. Med. Chem. 19, 5319–5341. doi:10.2174/092986712803833335
Gotoh, Y., Nishida, E., Yamashita, T., Hoshi, M., Kawakami, M., and Sakai, H. (1990). Microtubule-associated-protein (MAP) kinase activated by nerve growth factor and epidermal growth factor in PC12 cells: identity with the mitogen-activated MAP kinase of fibroblastic cells. Eur. J. Biochem. 193, 661–669. doi:10.1111/j.1432-1033.1990.tb19384.x
Gradišar, H., Pristovšek, P., Plaper, A., and Jerala, R. (2007). Green tea catechins inhibit bacterial DNA gyrase by interaction with its ATP binding site. J. Med. Chem. 50, 264–271. doi:10.1021/jm060817o
Granchi, C., Bertini, S., Macchia, M., and Minutolo, F. (2010). Inhibitors of lactate dehydrogenase isoforms and their therapeutic potentials. Curr. Med. Chem. 17, 672–697. doi:10.2174/092986710790416263
Granchi, C., Fortunato, S., Meini, S., Rizzolio, F., Caligiuri, I., Tuccinardi, T., et al. (2017). Characterization of the saffron derivative crocetin as an inhibitor of human lactate dehydrogenase 5 in the antiglycolytic approach against cancer. J. Agric. food Chem. 65, 5639–5649. doi:10.1021/acs.jafc.7b01668
Granchi, C., and Minutolo, F. (2012). Anticancer agents that counteract tumor glycolysis. ChemMedChem 7, 1318–1350. doi:10.1002/cmdc.201200176
Granchi, C., Paterni, I., Rani, R., and Minutolo, F. (2013). Small-molecule inhibitors of human LDH5. Future Med. Chem. 5, 1967–1991. doi:10.4155/fmc.13.151
Granchi, C., Roy, S., Giacomelli, C., Macchia, M., Tuccinardi, T., Martinelli, A., et al. (2011). Discovery of N-hydroxyindole-based inhibitors of human lactate dehydrogenase isoform A (LDH-A) as starvation agents against cancer cells. J. Med. Chem. 54, 1599–1612. doi:10.1021/jm101007q
Gresele, P., Cerletti, C., Guglielmini, G., Pignatelli, P., De Gaetano, G., and Violi, F. (2011). Effects of resveratrol and other wine polyphenols on vascular function: an update. J. Nutr. Biochem. 22, 201–211. doi:10.1016/j.jnutbio.2010.07.004
Gross, M. (2004). Flavonoids and cardiovascular disease. Pharm. Biol. 42, 21–35. doi:10.3109/13880200490893483
Gualdani, R., Cavalluzzi, M. M., Lentini, G., and Habtemariam, S. (2016). The chemistry and pharmacology of citrus limonoids. Molecules 21, 1530. doi:10.3390/molecules21111530
Guerra, A. R., Duarte, M. F., and Duarte, I. F. (2018). Targeting tumor metabolism with plant-derived natural products: emerging trends in cancer therapy. J. Agric. food Chem. 66, 10663–10685. doi:10.1021/acs.jafc.8b04104
Gunasekaran, T., Haile, T., Nigusse, T., and Dhanaraju, M. D. (2014). Nanotechnology: an effective tool for enhancing bioavailability and bioactivity of phytomedicine. Asian Pac. J. Trop. Biomed. 4, S1–S7. doi:10.12980/APJTB.4.2014C980
Guo, H., Wan, B., Wang, J., Zhang, J., Yao, W., and Shen, Z. (2019). Astragalus saponins inhibit cell growth, aerobic glycolysis and attenuate the inflammatory response in a DSS-induced colitis model. Int. J. Mol. Med. 43, 1041–1048. doi:10.3892/ijmm.2018.4036
Guo, Z. (2017). The modification of natural products for medical use. Acta Pharm. Sin. B 7, 119–136. doi:10.1016/j.apsb.2016.06.003
Gurung, P., and De, P. (2017). Spectrum of biological properties of cinchona alkaloids: A brief review. J. Pharmacogn. Phytochemistry 6, 162–166.
Habeeb Rahuman, H. B., Dhandapani, R., Narayanan, S., Palanivel, V., Paramasivam, R., Subbarayalu, R., et al. (2022). Medicinal plants mediated the green synthesis of silver nanoparticles and their biomedical applications. IET nanobiotechnology 16, 115–144. doi:10.1049/nbt2.12078
Han, J. H., Kim, M., Kim, H. J., Jang, S. B., Bae, S.-J., Lee, I.-K., et al. (2021). Targeting lactate dehydrogenase A with catechin resensitizes SNU620/5FU gastric cancer cells to 5-fluorouracil. Int. J. Mol. Sci. 22, 5406. doi:10.3390/ijms22105406
Han, X., Sheng, X., Jones, H. M., Jackson, A. L., Kilgore, J., Stine, J. E., et al. (2015). Evaluation of the anti-tumor effects of lactate dehydrogenase inhibitor galloflavin in endometrial cancer cells. J. Hematol. Oncol. 8, 2–4. doi:10.1186/s13045-014-0097-x
Hanauske, A.-R., Degen, D., Hilsenbeck, S. G., Bissery, M. C., and Von Hoff, D. D. (1992). Effects of Taxotere and taxol on in vitro colony formation of freshly explanted human tumor cells. Anti-cancer drugs 3, 121–124. doi:10.1097/00001813-199204000-00008
Harrison, C. (2014). Patenting natural products just got harder. Nat. Biotechnol. 32, 403–404. doi:10.1038/nbt0514-403a
He, Y.-Q., Zhou, C.-C., Yu, L.-Y., Wang, L., Deng, J.-L., Tao, Y.-L., et al. (2021). Natural product derived phytochemicals in managing acute lung injury by multiple mechanisms. Pharmacol. Res. 163, 105224. doi:10.1016/j.phrs.2020.105224
Ho, Y.-S., Yu, M.-S., Lai, C.S.-W., So, K.-F., Yuen, W.-H., and Chang, R.C.-C. (2007). Characterizing the neuroprotective effects of alkaline extract of Lycium barbarum on β-amyloid peptide neurotoxicity. Brain Res. 1158, 123–134. doi:10.1016/j.brainres.2007.04.075
Hogan, F. S., Krishnegowda, N. K., Mikhailova, M., and Kahlenberg, M. S. (2007). Flavonoid, silibinin, inhibits proliferation and promotes cell-cycle arrest of human colon cancer. J. Surg. Res. 143, 58–65. doi:10.1016/j.jss.2007.03.080
Holmes, G. A., Rice, K., and Snyder, C. R. (2006). Ballistic fibers: a review of the thermal, ultraviolet and hydrolytic stability of the benzoxazole ring structure. J. Mater. Sci. 41, 4105–4116. doi:10.1007/s10853-005-5597-1
Holmes, R. S., and Goldberg, E. (2009). Computational analyses of mammalian lactate dehydrogenases: human, mouse, opossum and platypus LDHs. Comput. Biol. Chem. 33, 379–385. doi:10.1016/j.compbiolchem.2009.07.006
Hou, C.-W., Lin, Y.-T., Chen, Y.-L., Wang, Y.-H., Chou, J.-L., Ping, L.-Y., et al. (2012). Neuroprotective effects of carnosic acid on neuronal cells under ischemic and hypoxic stress. Nutr. Neurosci. 15, 257–263. doi:10.1179/1476830512Y.0000000021
Hu, C., Fan, L., Cen, P., Chen, E., Jiang, Z., and Li, L. (2016). Energy metabolism plays a critical role in stem cell maintenance and differentiation. Int. J. Mol. Sci. 17, 253. doi:10.3390/ijms17020253
Huang, Q., Wu, L.-J., Tashiro, S.-I., Onodera, S., Li, L.-H., and Ikejima, T. (2005). Silymarin augments human cervical cancer HeLa cell apoptosis via P38/JNK MAPK pathways in serum-free medium. J. Asian Nat. Prod. Res. 7, 701–709. doi:10.1080/1028602042000324862
Huang, W.-Y., Cai, Y.-Z., and Zhang, Y. (2009). Natural phenolic compounds from medicinal herbs and dietary plants: potential use for cancer prevention. Nutr. cancer 62, 1–20. doi:10.1080/01635580903191585
Huttunen, K. M., Raunio, H., and Rautio, J. (2011). Prodrugs—from serendipity to rational design. Pharmacol. Rev. 63, 750–771. doi:10.1124/pr.110.003459
Imanshahidi, M., and Hosseinzadeh, H. (2008). Pharmacological and therapeutic effects of Berberis vulgaris and its active constituent, berberine. Phytotherapy Res. 22, 999–1012. doi:10.1002/ptr.2399
Iwamoto, K., Kawamoto, H., Takeshita, F., Matsumura, S., Ayaki, I., Moriyama, T., et al. (2019). Mixing ginkgo biloba extract with sesame extract and turmeric oil increases bioavailability of ginkgolide A in mice brain. J. Oleo Sci. 68, 923–930. doi:10.5650/jos.ess19135
Jacobs, K., Castellano-Gonzalez, G., J Guillemin, G., and B Lovejoy, D. (2017). Major developments in the design of inhibitors along the kynurenine pathway. Curr. Med. Chem. 24, 2471–2495. doi:10.2174/0929867324666170502123114
Jaiswara, P. K., and Kumar, A. (2022). Nimbolide retards T cell lymphoma progression by altering apoptosis, glucose metabolism, pH regulation, and ROS homeostasis. Environ. Toxicol. 37, 1445–1457. doi:10.1002/tox.23497
Jakubowicz-Gil, J., Langner, E., Bądziul, D., Wertel, I., and Rzeski, W. (2013). Apoptosis induction in human glioblastoma multiforme T98G cells upon temozolomide and quercetin treatment. Tumor Biol. 34, 2367–2378. doi:10.1007/s13277-013-0785-0
Ji, N., Qi, Z., Wang, Y., Yang, X., Yan, Z., Li, M., et al. (2021). Pyroptosis: a new regulating mechanism in cardiovascular disease. J. Inflamm. Res. 14, 2647–2666. doi:10.2147/JIR.S308177
Jia, L., Huang, S., Yin, X., Zan, Y., Guo, Y., and Han, L. (2018). Quercetin suppresses the mobility of breast cancer by suppressing glycolysis through Akt-mTOR pathway mediated autophagy induction. Life Sci. 208, 123–130. doi:10.1016/j.lfs.2018.07.027
Jia, S., and Hu, C. (2010). Pharmacological effects of rutaecarpine as a cardiovascular protective agent. Molecules 15, 1873–1881. doi:10.3390/molecules15031873
Jiao, L., Wang, S., Zheng, Y., Wang, N., Yang, B., Wang, D., et al. (2019). Betulinic acid suppresses breast cancer aerobic glycolysis via caveolin-1/NF-κB/c-Myc pathway. Biochem. Pharmacol. 161, 149–162. doi:10.1016/j.bcp.2019.01.016
Jin, H., Pi, J., Zhao, Y., Jiang, J., Li, T., Zeng, X., et al. (2017). EGFR-targeting PLGA-PEG nanoparticles as a curcumin delivery system for breast cancer therapy. Nanoscale 9, 16365–16374. doi:10.1039/c7nr06898k
Johari, T. Y., Ghoneim, M. A., and Moselhy, S. S. (2018). Thyroid profile and LDH Isoenzymes as prognostic biomarkers for diabetic and/or obese subjects. Afr. Health Sci. 18, 697–706. doi:10.4314/ahs.v18i3.28
Judge, J. L., Lacy, S. H., Ku, W.-Y., Owens, K. M., Hernady, E., Thatcher, T. H., et al. (2017). The lactate dehydrogenase inhibitor gossypol inhibits radiation-induced pulmonary fibrosis. Radiat. Res. 188, 35–43. doi:10.1667/RR14620.1
Judge, J. L., Nagel, D. J., Owens, K. M., Rackow, A., Phipps, R. P., Sime, P. J., et al. (2018). Prevention and treatment of bleomycin-induced pulmonary fibrosis with the lactate dehydrogenase inhibitor gossypol. PLoS One 13, e0197936. doi:10.1371/journal.pone.0197936
Judge, J. L., Owens, K. M., Pollock, S. J., Woeller, C. F., Thatcher, T. H., Williams, J. P., et al. (2015). Ionizing radiation induces myofibroblast differentiation via lactate dehydrogenase. Am. J. Physiology-Lung Cell. Mol. Physiology 309, L879–L887. doi:10.1152/ajplung.00153.2015
Kane, D. A. (2014). Lactate oxidation at the mitochondria: a lactate-malate-aspartate shuttle at work. Front. Neurosci. 8, 366. doi:10.3389/fnins.2014.00366
Kanwal, A., Saddique, F. A., Aslam, S., Ahmad, M., Zahoor, A. F., and Mohsin, N.-U.-A. (2018). Benzimidazole ring system as a privileged template for anticancer agents. Pharm. Chem. J. 51, 1068–1077. doi:10.1007/s11094-018-1742-4
Kapp, E., and Whiteley, C. (1991). Protein ligand interactions: isoquinoline alkaloids as inhibitors for lactate and malate dehydrogenase. J. enzyme inhibition 4, 233–243. doi:10.3109/14756369109035847
Khan, H., Ullah, H., Tundis, R., Belwal, T., Devkota, H. P., Daglia, M., et al. (2020). Dietary flavonoids in the management of huntington’s disease: mechanism and clinical perspective. EFood 1, 38–52. doi:10.2991/efood.k.200203.001
Khazir, J., Mir, B. A., Mir, S. A., and Cowan, D. (2013). Natural products as lead compounds in drug discovery. Australia: Taylor and Francis.
Khiev, D., Mohamed, Z. A., Vichare, R., Paulson, R., Bhatia, S., Mohapatra, S., et al. (2021). Emerging nano-formulations and nanomedicines applications for ocular drug delivery. Nanomaterials 11, 173. doi:10.3390/nano11010173
Kim, C., and Cha, Y.-N. (2014). Taurine chloramine produced from taurine under inflammation provides anti-inflammatory and cytoprotective effects. Amino Acids 46, 89–100. doi:10.1007/s00726-013-1545-6
Kim, S. H., Kandiah, N., Hsu, J. L., Suthisisang, C., Udommongkol, C., and Dash, A. (2017). Beyond symptomatic effects: potential of donepezil as a neuroprotective agent and disease modifier in Alzheimer's disease. Br. J. Pharmacol. 174, 4224–4232. doi:10.1111/bph.14030
Kim, S. H., Lee, J. M., Kim, S. C., Park, C. B., and Lee, P. C. (2014). Proposed cytotoxic mechanisms of the saffron carotenoids crocin and crocetin on cancer cell lines. Biochem. Cell Biol. 92, 105–111. doi:10.1139/bcb-2013-0091
Kirschning, A., Taft, F., and Knobloch, T. (2007). Total synthesis approaches to natural product derivatives based on the combination of chemical synthesis and metabolic engineering. Org. Biomol. Chem. 5, 3245–3259. doi:10.1039/b709549j
Koehn, F. E., and Carter, G. T. (2005). The evolving role of natural products in drug discovery. Nat. Rev. Drug Discov. 4, 206–220. doi:10.1038/nrd1657
Kooshki, L., Mahdavi, P., Fakhri, S., Akkol, E. K., and Khan, H. (2022). Targeting lactate metabolism and glycolytic pathways in the tumor microenvironment by natural products: A promising strategy in combating cancer. BioFactors 48, 359–383. doi:10.1002/biof.1799
Kopustinskiene, D. M., Jakstas, V., Savickas, A., and Bernatoniene, J. (2020). Flavonoids as anticancer agents. Nutrients 12, 457. doi:10.3390/nu12020457
Korga, A., Ostrowska, M., Jozefczyk, A., Iwan, M., Wojcik, R., Zgorka, G., et al. (2019). Apigenin and hesperidin augment the toxic effect of doxorubicin against HepG2 cells. BMC Pharmacol. Toxicol. 20, 22–13. doi:10.1186/s40360-019-0301-2
Kotlyar, A. B., Randazzo, A., Honbo, N., Jin, Z.-Q., Karliner, J. S., and Cecchini, G. (2010). Cardioprotective activity of a novel and potent competitive inhibitor of lactate dehydrogenase. FEBS Lett. 584, 159–165. doi:10.1016/j.febslet.2009.11.022
Kozal, K., Jóźwiak, P., and Krześlak, A. (2021). Contemporary perspectives on the Warburg effect inhibition in cancer therapy. Cancer Control 28, 10732748211041243. doi:10.1177/10732748211041243
Krishnaraj, C., Muthukumaran, P., Ramachandran, R., Balakumaran, M., and Kalaichelvan, P. (2014). Acalypha indica Linn: biogenic synthesis of silver and gold nanoparticles and their cytotoxic effects against MDA-MB-231, human breast cancer cells. Biotechnol. Rep. 4, 42–49. doi:10.1016/j.btre.2014.08.002
Kumar, K. P., Paul, W., and Sharma, C. P. (2011). Green synthesis of gold nanoparticles with Zingiber officinale extract: characterization and blood compatibility. Process Biochem. 46, 2007–2013. doi:10.1016/j.procbio.2011.07.011
Kumari, S., Tyagi, M., and Jagadevan, S. (2019). Mechanistic removal of environmental contaminants using biogenic nano-materials. Int. J. Environ. Sci. Technol. 16, 7591–7606. doi:10.1007/s13762-019-02468-3
Kwon, S.-H., Kim, M.-J., Ma, S.-X., You, I.-J., Hwang, J.-Y., Oh, J.-H., et al. (2012). Eucommia ulmoides Oliv. Bark. protects against hydrogen peroxide-induced neuronal cell death in SH-SY5Y cells. J. Ethnopharmacol. 142, 337–345. doi:10.1016/j.jep.2012.04.010
Lahlou, M. (2013). The success of natural products in drug discovery. Pharmacol. Pharm. 4, 17–31. doi:10.4236/pp.2013.43A003
Le, A., Cooper, C. R., Gouw, A. M., Dinavahi, R., Maitra, A., Deck, L. M., et al. (2010). Inhibition of lactate dehydrogenase A induces oxidative stress and inhibits tumor progression. Proc. Natl. Acad. Sci. 107, 2037–2042. doi:10.1073/pnas.0914433107
Leuci, R., Brunetti, L., Poliseno, V., Laghezza, A., Loiodice, F., Tortorella, P., et al. (2020). Natural compounds for the prevention and treatment of cardiovascular and neurodegenerative diseases. Foods 10, 29. doi:10.3390/foods10010029
Li, J.W.-H., and Vederas, J. C. (2009). Drug discovery and natural products: end of an era or an endless frontier? Science 325, 161–165. doi:10.1126/science.1168243
Li, S., Huang, P., Gan, J., Ling, X., Du, X., Liao, Y., et al. (2019a). Dihydroartemisinin represses esophageal cancer glycolysis by down-regulating pyruvate kinase M2. Eur. J. Pharmacol. 854, 232–239. doi:10.1016/j.ejphar.2019.04.018
Li, S., Sun, R., and Liu, R. (2019b). Natural products in licorice for the therapy of liver diseases: Progress and future opportunities. Pharmacol. Res. 144, 210–226. doi:10.1016/j.phrs.2019.04.025
Li, W., Cao, J., Wang, X., Zhang, Y., Sun, Q., Jiang, Y., et al. (2021a). Ferruginol restores SIRT1-PGC-1α-mediated mitochondrial biogenesis and fatty acid oxidation for the treatment of dox-induced cardiotoxicity. Front. Pharmacol. 12, 773834. doi:10.3389/fphar.2021.773834
Li, W., Cui, X., and Chen, Z. (2021b). Screening of lactate dehydrogenase inhibitor from bioactive compounds in natural products by electrophoretically mediated microanalysis. J. Chromatogr. A 1656, 462554. doi:10.1016/j.chroma.2021.462554
Li, X., Lu, W., Hu, Y., Wen, S., Qian, C., Wu, W., et al. (2013). Effective inhibition of nasopharyngeal carcinoma in vitro and in vivo by targeting glycolysis with oxamate. Int. J. Oncol. 43, 1710–1718. doi:10.3892/ijo.2013.2080
Liao, Y.-T., Chen, C.-J., Li, W.-F., Hsu, L.-I., Tsai, L.-Y., Huang, Y.-L., et al. (2012). Elevated lactate dehydrogenase activity and increased cardiovascular mortality in the arsenic-endemic areas of southwestern Taiwan. Toxicol. Appl. Pharmacol. 262, 232–237. doi:10.1016/j.taap.2012.04.028
Liu, C.-H., and Chang, F.-Y. (2011). Development and characterization of eucalyptol microemulsions for topic delivery of curcumin. Chem. Pharm. Bull. 59, 172–178. doi:10.1248/cpb.59.172
Liu, C.-H., Chang, F.-Y., and Hung, D.-K. (2011). Terpene microemulsions for transdermal curcumin delivery: effects of terpenes and cosurfactants. Colloids Surfaces B Biointerfaces 82, 63–70. doi:10.1016/j.colsurfb.2010.08.018
Liu, H., Mao, P., Wang, J., Wang, T., and Xie, C.-H. (2015). Allicin protects PC12 cells against 6-OHDA-induced oxidative stress and mitochondrial dysfunction via regulating mitochondrial dynamics. Cell. Physiology Biochem. 36, 966–979. doi:10.1159/000430271
Liu, J., Chen, S., Lv, L., Song, L., Guo, S., and Huang, S. (2013). Recent progress in studying curcumin and its nano-preparations for cancer therapy. Curr. Pharm. Des. 19, 1974–1993. doi:10.2174/138161213805289327
Liu, J. (1995). Pharmacology of oleanolic acid and ursolic acid. J. Ethnopharmacol. 49, 57–68. doi:10.1016/0378-8741(95)90032-2
Liu, J., Wu, N., Ma, L., Liu, M., Liu, G., Zhang, Y., et al. (2014). Oleanolic acid suppresses aerobic glycolysis in cancer cells by switching pyruvate kinase type M isoforms. PloS one 9, e91606. doi:10.1371/journal.pone.0091606
Liu, R., Wang, X., Shen, Y., and He, A. (2021). Long non-coding RNA-based glycolysis-targeted cancer therapy: feasibility, progression and limitations. Mol. Biol. Rep. 48, 2713–2727. doi:10.1007/s11033-021-06247-7
Liu, X., Pan, L., Chen, P., and Zhu, Y. (2010). Leonurine improves ischemia-induced myocardial injury through antioxidative activity. Phytomedicine 17, 753–759. doi:10.1016/j.phymed.2010.01.018
Liu, Z., Zhou, T., Ziegler, A. C., Dimitrion, P., and Zuo, L. (2017). Oxidative stress in neurodegenerative diseases: from molecular mechanisms to clinical applications. Oxidative Med. Cell. Longev. 2017, 2525967. doi:10.1155/2017/2525967
Lourenco, A., M Ferreira, L., and S Branco, P. (2012). Molecules of natural origin, semi-synthesis and synthesis with anti-inflammatory and anticancer utilities. Curr. Pharm. Des. 18, 3979–4046. doi:10.2174/138161212802083644
Lu, D., and Thum, T. (2019). RNA-based diagnostic and therapeutic strategies for cardiovascular disease. Nat. Rev. Cardiol. 16, 661–674. doi:10.1038/s41569-019-0218-x
Lu, L., Guo, Q., and Zhao, L. (2016). Overview of oroxylin A: a promising flavonoid compound. Phytotherapy Res. 30, 1765–1774. doi:10.1002/ptr.5694
Lu, Q.-Y., Zhang, L., Yee, J. K., Go, V.-L. W., and Lee, W.-N. (2015). Metabolic consequences of LDHA inhibition by epigallocatechin gallate and oxamate in MIA PaCa-2 pancreatic cancer cells. Metabolomics 11, 71–80. doi:10.1007/s11306-014-0672-8
Lum, P. T., Sekar, M., Gan, S. H., Bonam, S. R., and Shaikh, M. F. (2021). Protective effect of natural products against Huntington’s disease: an overview of scientific evidence and understanding their mechanism of action. ACS Chem. Neurosci. 12, 391–418. doi:10.1021/acschemneuro.0c00824
Luo, D., Carter, K. A., Miranda, D., and Lovell, J. F. (2017). Chemophototherapy: an emerging treatment option for solid tumors. Adv. Sci. 4, 1600106. doi:10.1002/advs.201600106
Lv, X., Yu, X., Wang, Y., Wang, F., Li, H., Wang, Y., et al. (2012). Berberine inhibits doxorubicin-triggered cardiomyocyte apoptosis via attenuating mitochondrial dysfunction and increasing Bcl-2 expression. PLoS One 7, e47351. doi:10.1371/journal.pone.0047351
Ma, D.-L., Wu, C., Cheng, S.-S., Lee, F.-W., Han, Q.-B., and Leung, C.-H. (2019). Development of natural product-conjugated metal complexes as cancer therapies. Int. J. Mol. Sci. 20, 341. doi:10.3390/ijms20020341
Ma, Z.-J., Yan, H., Wang, Y.-J., Yang, Y., Li, X.-B., Shi, A.-C., et al. (2018). Proteomics analysis demonstrating rosmarinic acid suppresses cell growth by blocking the glycolytic pathway in human HepG2 cells. Biomed. Pharmacother. 105, 334–349. doi:10.1016/j.biopha.2018.05.129
Ma, Z., Xiang, X., Li, S., Xie, P., Gong, Q., Goh, B.-C., et al. (2022). “Targeting hypoxia-inducible factor-1, for cancer treatment: recent advances in developing small-molecule inhibitors from natural compounds,” in Seminars in cancer biology (Netherlands: Elsevier), 379–390.
Madapa, S., Tusi, Z., and Batra, S. (2008). Advances in the Syntheses of Quinoline and Quinoline-Annulated Ring Systems&#. Curr. Org. Chem. 12, 1116–1183. doi:10.2174/138527208785740300
Maheshwari, R. K., Singh, A. K., Gaddipati, J., and Srimal, R. C. (2006). Multiple biological activities of curcumin: a short review. Life Sci. 78, 2081–2087. doi:10.1016/j.lfs.2005.12.007
Mahmoud, A. M., Hernandez Bautista, R. J., Sandhu, M. A., and Hussein, O. E. (2019). Beneficial effects of citrus flavonoids on cardiovascular and metabolic health. Oxidative Med. Cell. Longev. 2019, 5484138. doi:10.1155/2019/5484138
Makley, L. N., and Gestwicki, J. E. (2013). Expanding the number of ‘Druggable’targets: non-enzymes and protein–protein interactions. Chem. Biol. drug Des. 81, 22–32. doi:10.1111/cbdd.12066
Manerba, M., Vettraino, M., Fiume, L., Di Stefano, G., Sartini, A., Giacomini, E., et al. (2012). Galloflavin (CAS 568-80-9): a novel inhibitor of lactate dehydrogenase. ChemMedChem 7, 311–317. doi:10.1002/cmdc.201100471
Manjunath, L. E., Singh, A., Som, S., and Eswarappa, S. M. (2022). Mammalian proteome expansion by stop codon readthrough. Wiley Interdiscip. Rev. RNA 14, e1739. doi:10.1002/wrna.1739
Mao, L., Chen, Q., Gong, K., Xu, X., Xie, Y., Zhang, W., et al. (2018). Berberine decelerates glucose metabolism via suppression of mTOR-dependent HIF-1α protein synthesis in colon cancer cells. Oncol. Rep. 39, 2436–2442. doi:10.3892/or.2018.6318
Marin-Hernandez, A., Gallardo-Perez, J. C., Ralph, S. J., Rodriguez-Enriquez, S., and Moreno-Sanchez, R. (2009). HIF-1alpha modulates energy metabolism in cancer cells by inducing over-expression of specific glycolytic isoforms. Mini Rev. Med. Chem. 9, 1084–1101. doi:10.2174/138955709788922610
Marucci, G., Buccioni, M., Dal Ben, D., Lambertucci, C., Volpini, R., and Amenta, F. (2021). Efficacy of acetylcholinesterase inhibitors in Alzheimer's disease. Neuropharmacology 190, 108352. doi:10.1016/j.neuropharm.2020.108352
Maruyama, R., and Yokota, T. (2020). Knocking down long noncoding RNAs using antisense oligonucleotide gapmers. Gapmers methods Protoc. 2176, 49–56. doi:10.1007/978-1-0716-0771-8_3
Maurya, A. K., and Vinayak, M. (2015). Quercetin regresses Dalton's lymphoma growth via suppression of PI3K/AKT signaling leading to upregulation of p53 and decrease in energy metabolism. Nutr. cancer 67, 354–363. doi:10.1080/01635581.2015.990574
Mayr, L. M., and Bojanic, D. (2009). Novel trends in high-throughput screening. Curr. Opin. Pharmacol. 9, 580–588. doi:10.1016/j.coph.2009.08.004
Mccleland, M. L., Adler, A. S., Shang, Y., Hunsaker, T., Truong, T., Peterson, D., et al. (2012). An integrated genomic screen identifies LDHB as an essential gene for triple-negative breast cancer. Cancer Res. 72, 5812–5823. doi:10.1158/0008-5472.CAN-12-1098
Mcmanus, M. T., and Sharp, P. A. (2002). Gene silencing in mammals by small interfering RNAs. Nat. Rev. Genet. 3, 737–747. doi:10.1038/nrg908
Mehta, A., Prabhakar, M., Kumar, P., Deshmukh, R., and Sharma, P. (2013). Excitotoxicity: bridge to various triggers in neurodegenerative disorders. Eur. J. Pharmacol. 698, 6–18. doi:10.1016/j.ejphar.2012.10.032
Mehta, R. G., Murillo, G., Naithani, R., and Peng, X. (2010). Cancer chemoprevention by natural products: how far have we come? Pharm. Res. 27, 950–961. doi:10.1007/s11095-010-0085-y
Memariani, Z., Abbas, S. Q., Ul Hassan, S. S., Ahmadi, A., and Chabra, A. (2021). Naringin and naringenin as anticancer agents and adjuvants in cancer combination therapy: efficacy and molecular mechanisms of action, a comprehensive narrative review. Pharmacol. Res. 171, 105264. doi:10.1016/j.phrs.2020.105264
Mi, Y.-J., Geng, G.-J., Zou, Z.-Z., Gao, J., Luo, X.-Y., Liu, Y., et al. (2015). Dihydroartemisinin inhibits glucose uptake and cooperates with glycolysis inhibitor to induce apoptosis in non-small cell lung carcinoma cells. PloS one 10, e0120426. doi:10.1371/journal.pone.0120426
Miao, P., Sheng, S., Sun, X., Liu, J., and Huang, G. (2013). Lactate dehydrogenase A in cancer: a promising target for diagnosis and therapy. IUBMB life 65, 904–910. doi:10.1002/iub.1216
Miean, K. H., and Mohamed, S. (2001). Flavonoid (myricetin, quercetin, kaempferol, luteolin, and apigenin) content of edible tropical plants. J. Agric. food Chem. 49, 3106–3112. doi:10.1021/jf000892m
Milić, N., Milošević, N., Suvajdžić, L., Žarkov, M., and Abenavoli, L. (2013). New therapeutic potentials of milk thistle (Silybum marianum). Nat. Product. Commun. 8, 1934578X1300801–1810. doi:10.1177/1934578x1300801236
Mittal, L., Aryal, U. K., Camarillo, I. G., Raman, V., and Sundararajan, R. (2020). Effective electrochemotherapy with curcumin in MDA-MB-231-human, triple negative breast cancer cells: A global proteomics study. Bioelectrochemistry 131, 107350. doi:10.1016/j.bioelechem.2019.107350
Mizuno, N., Niwa, T., Yotsumoto, Y., and Sugiyama, Y. (2003). Impact of drug transporter studies on drug discovery and development. Pharmacol. Rev. 55, 425–461. doi:10.1124/pr.55.3.1
Mlala, S., Oyedeji, A. O., Gondwe, M., and Oyedeji, O. O. (2019). Ursolic acid and its derivatives as bioactive agents. Molecules 24, 2751. doi:10.3390/molecules24152751
Moghtaderi, H., Sepehri, H., Delphi, L., and Attari, F. (2018). Gallic acid and curcumin induce cytotoxicity and apoptosis in human breast cancer cell MDA-MB-231. BioImpacts BI 8, 185–194. doi:10.15171/bi.2018.21
Mogler, M. A., and Kamrud, K. I. (2015). RNA-based viral vectors. Expert Rev. Vaccines 14, 283–312. doi:10.1586/14760584.2015.979798
Mohanpuria, P., Rana, N. K., and Yadav, S. K. (2008). Biosynthesis of nanoparticles: technological concepts and future applications. J. nanoparticle Res. 10, 507–517. doi:10.1007/s11051-007-9275-x
Mohd Sairazi, N. S., and Sirajudeen, K. (2020). Natural products and their bioactive compounds: neuroprotective potentials against neurodegenerative diseases. Evidence-Based Complementary Altern. Med. 2020, 6565396. doi:10.1155/2020/6565396
Morand, C., Dubray, C., Milenkovic, D., Lioger, D., Martin, J. F., Scalbert, A., et al. (2011). Hesperidin contributes to the vascular protective effects of orange juice: a randomized crossover study in healthy volunteers. Am. J. Clin. Nutr. 93, 73–80. doi:10.3945/ajcn.110.004945
Morandi, A., and Indraccolo, S. (2017). Linking metabolic reprogramming to therapy resistance in cancer. Biochimica Biophysica Acta (BBA)-Reviews Cancer 1868, 1–6. doi:10.1016/j.bbcan.2016.12.004
Murthy, K. C., Agrahari, V., and Chauhan, H. (2021b). Polyphenols against infectious diseases: Controlled release nano-formulations. Eur. J. Pharm. Biopharm. 161, 66–79. doi:10.1016/j.ejpb.2021.02.003
Murthy, K. C., Jayaprakasha, G., Safe, S., and Patil, B. S. (2021a). Citrus limonoids induce apoptosis and inhibit the proliferation of pancreatic cancer cells. Food and Funct. 12, 1111–1120. doi:10.1039/d0fo02740e
Nabi, A. A., Atta, S. A., El-Ahwany, E., Elzayat, E., and Saleh, H. (2021). Taurine upregulates miRNA-122-5p expression and suppresses the metabolizing enzymes of glycolytic pathway in hepatocellular carcinoma. Mol. Biol. Rep. 48, 5549–5559. doi:10.1007/s11033-021-06571-y
Narayan, C., and Kumar, A. (2014). Antineoplastic and immunomodulatory effect of polyphenolic components of Achyranthes aspera (PCA) extract on urethane induced lung cancer in vivo. Mol. Biol. Rep. 41, 179–191. doi:10.1007/s11033-013-2850-6
Ndrepepa, G. (2021). Aspartate aminotransferase and cardiovascular disease—a narrative review. J. Lab. Precis. Med. 6, 6. doi:10.21037/jlpm-20-93
Nemmar, A., Al-Salam, S., Zia, S., Marzouqi, F., Al-Dhaheri, A., Subramaniyan, D., et al. (2011). Contrasting actions of diesel exhaust particles on the pulmonary and cardiovascular systems and the effects of thymoquinone. Br. J. Pharmacol. 164, 1871–1882. doi:10.1111/j.1476-5381.2011.01442.x
Newington, J. T., Harris, R. A., and Cumming, R. C. (2013). Reevaluating metabolism in Alzheimer's disease from the perspective of the astrocyte-neuron lactate shuttle model. J. Neurodegener. Dis. 2013, 234572. doi:10.1155/2013/234572
Nowak, E., Livney, Y. D., Niu, Z., and Singh, H. (2019). Delivery of bioactives in food for optimal efficacy: what inspirations and insights can be gained from pharmaceutics? Trends Food Sci. Technol. 91, 557–573. doi:10.1016/j.tifs.2019.07.029
Numakawa, T., and Odaka, H. (2021). Brain-derived neurotrophic factor signaling in the pathophysiology of Alzheimer’s disease: Beneficial effects of flavonoids for neuroprotection. Int. J. Mol. Sci. 22, 5719. doi:10.3390/ijms22115719
Ochoa-Villarreal, M., Howat, S., Hong, S., Jang, M. O., Jin, Y.-W., Lee, E.-K., et al. (2016). Plant cell culture strategies for the production of natural products. BMB Rep. 49, 149–158. doi:10.5483/bmbrep.2016.49.3.264
Øztürk, Y., Günaydın, C., Yalçın, F., Nazıroğlu, M., and Braidy, N. (2019). Resveratrol enhances apoptotic and oxidant effects of paclitaxel through TRPM2 channel activation in DBTRG glioblastoma cells. Oxidative Med. Cell. Longev. 2019, 4619865. doi:10.1155/2019/4619865
Park, C. H., Tanaka, T., Kim, J. H., Cho, E. J., Park, J. C., Shibahara, N., et al. (2011). Hepato-protective effects of loganin, iridoid glycoside from Corni Fructus, against hyperglycemia-activated signaling pathway in liver of type 2 diabetic db/db mice. Toxicology 290, 14–21. doi:10.1016/j.tox.2011.08.004
Pi, M., Kuang, H., Yue, C., Yang, Q., Wu, A., Li, Y., et al. (2022). Targeting metabolism to overcome cancer drug resistance: A promising therapeutic strategy for diffuse large B cell lymphoma. Drug Resist. Updat. 61, 100822. doi:10.1016/j.drup.2022.100822
Piekuś-Słomka, N., Mikstacka, R., Ronowicz, J., and Sobiak, S. (2019). Hybrid cis-stilbene molecules: novel anticancer agents. Int. J. Mol. Sci. 20, 1300. doi:10.3390/ijms20061300
Pilorget, A., Berthet, V., Luis, J., Moghrabi, A., Annabi, B., and Béliveau, R. (2003). Medulloblastoma cell invasion is inhibited by green tea (−) epigallocatechin -3- gallate. J. Cell. Biochem. 90, 745–755. doi:10.1002/jcb.10667
Pommier, Y. (2006). Topoisomerase I inhibitors: camptothecins and beyond. Nat. Rev. Cancer 6, 789–802. doi:10.1038/nrc1977
Post, N., Yu, R., Greenlee, S., Gaus, H., Hurh, E., Matson, J., et al. (2019). Metabolism and disposition of volanesorsen, a 2′-O-(2 methoxyethyl) antisense oligonucleotide, across species. Drug Metabolism Dispos. 47, 1164–1173. doi:10.1124/dmd.119.087395
Prachayasittikul, V., Worachartcheewan, A., Shoombuatong, W., Songtawee, N., Simeon, S., Prachayasittikul, V., et al. (2015). Computer-aided drug design of bioactive natural products. Curr. Top. Med. Chem. 15, 1780–1800. doi:10.2174/1568026615666150506151101
Prajapati, V. D., Jani, G. K., Moradiya, N. G., and Randeria, N. P. (2013). Pharmaceutical applications of various natural gums, mucilages and their modified forms. Carbohydr. Polym. 92, 1685–1699. doi:10.1016/j.carbpol.2012.11.021
Purich, D. L. (2010). Enzyme kinetics: catalysis and control: a reference of theory and best-practice methods. Netherlands: Elsevier.
Puthenveetil, S., Loganzo, F., He, H., Dirico, K., Green, M., Teske, J., et al. (2016). Natural product splicing inhibitors: a new class of antibody–drug conjugate (ADC) payloads. Bioconjugate Chem. 27, 1880–1888. doi:10.1021/acs.bioconjchem.6b00291
Qiao, T., Xiong, Y., Feng, Y., Guo, W., Zhou, Y., Zhao, J., et al. (2021). Inhibition of LDH-A by oxamate enhances the efficacy of anti-PD-1 treatment in an NSCLC humanized mouse model. Front. Oncol. 11, 632364. doi:10.3389/fonc.2021.632364
Radhiga, T., Rajamanickam, C., Senthil, S., and Pugalendi, K. V. (2012). Effect of ursolic acid on cardiac marker enzymes, lipid profile and macroscopic enzyme mapping assay in isoproterenol-induced myocardial ischemic rats. Food Chem. Toxicol. 50, 3971–3977. doi:10.1016/j.fct.2012.07.067
Raghunandan, D., Bedre, M. D., Basavaraja, S., Sawle, B., Manjunath, S., and Venkataraman, A. (2010). Rapid biosynthesis of irregular shaped gold nanoparticles from macerated aqueous extracellular dried clove buds (Syzygium aromaticum) solution. Colloids Surfaces B Biointerfaces 79, 235–240. doi:10.1016/j.colsurfb.2010.04.003
Rahimi, H. R., Nedaeinia, R., Shamloo, A. S., Nikdoust, S., and Oskuee, R. K. (2016). Novel delivery system for natural products: nano-curcumin formulations. Avicenna J. phytomedicine 6, 383–398.
Rahman, A., Alam Siddiqui, S., Jakhar, R., and Chul Kang, S. (2015). Growth inhibition of various human cancer cell lines by imperatorin and limonin from Poncirus trifoliata Rafin. seeds. Anti-Cancer Agents Med. Chem. 15, 236–241. doi:10.2174/1871520614666140922122358
Rahnavard, M., Hassanpour, M., Ahmadi, M., Heidarzadeh, M., Amini, H., Javanmard, M. Z., et al. (2019). Curcumin ameliorated myocardial infarction by inhibition of cardiotoxicity in the rat model. J. Cell. Biochem. 120, 11965–11972. doi:10.1002/jcb.28480
Ramakrishna, W., Kumari, A., Rahman, N., and Mandave, P. (2021). Anticancer activities of plant secondary metabolites: rice callus suspension culture as a new paradigm. Rice Sci. 28, 13–30. doi:10.1016/j.rsci.2020.11.004
Ramakrishnan, G., Augustine, T., Jagan, S., Vinodhkumar, R., and Devaki, T. (2007). Effect of silymarin on N-nitrosodiethylamine induced hepatocarcinogenesis in rats. Exp. Oncol. 29, 39–44.
Rana, A., Yadav, K., and Jagadevan, S. (2020). A comprehensive review on green synthesis of nature-inspired metal nanoparticles: mechanism, application and toxicity. J. Clean. Prod. 272, 122880. doi:10.1016/j.jclepro.2020.122880
Randhawa, M. A., Alghamdi, M. S., and Maulik, S. K. (2013). The effect of thymoquinone, an active component of Nigella sativa, on isoproterenol induced myocardial injury. Pak J. Pharm. Sci. 26, 1215–1219.
Rani, R., and Kumar, V. (2016). Recent Update on Human Lactate Dehydrogenase Enzyme 5 (hLDH5) Inhibitors: A Promising Approach for Cancer Chemotherapy. J. Med. Chem. 59, 487–496. doi:10.1021/acs.jmedchem.5b00168
Rani, R., and Kumar, V. (2017). When will small molecule lactate dehydrogenase inhibitors realize their potential in the cancer clinic? Future Med. Chem. 9, 1113–1115. doi:10.4155/fmc-2017-0082
Read, J., Harrison, R. J., Romagnoli, B., Tanious, F. A., Gowan, S. H., Reszka, A. P., et al. (2001b). Structure-based design of selective and potent G quadruplex-mediated telomerase inhibitors. Proc. Natl. Acad. Sci. 98, 4844–4849. doi:10.1073/pnas.081560598
Read, J., Winter, V., Eszes, C., Sessions, R., and Brady, R. (2001a). Structural basis for altered activity of M-and H-isozyme forms of human lactate dehydrogenase. Proteins Struct. Funct. Bioinforma. 43, 175–185. doi:10.1002/1097-0134(20010501)43:2<175:aid-prot1029>3.0.co;2-#
Reddy, M. N., Adnan, M., Alreshidi, M. M., Saeed, M., and Patel, M. (2020). Evaluation of anticancer, antibacterial and antioxidant properties of a medicinally treasured fern tectaria coadunata with its phytoconstituents analysis by HR-LCMS. Anti-Cancer Agents Med. Chem. 20, 1845–1856. doi:10.2174/1871520620666200318101938
Reyes-Farias, M., and Carrasco-Pozo, C. (2019). The anti-cancer effect of quercetin: molecular implications in cancer metabolism. Int. J. Mol. Sci. 20, 3177. doi:10.3390/ijms20133177
Rivera, G., Bocanegra-García, V., Ordaz-Pichardo, C., Nogueda-Torres, B., and Monge, A. (2009). New therapeutic targets for drug design against Trypanosoma cruzi, advances and perspectives. Curr. Med. Chem. 16, 3286–3293. doi:10.2174/092986709788803303
Rochlani, Y., Pothineni, N. V., Kovelamudi, S., and Mehta, J. L. (2017). Metabolic syndrome: pathophysiology, management, and modulation by natural compounds. Ther. Adv. Cardiovasc. Dis. 11, 215–225. doi:10.1177/1753944717711379
Rodriguez, C., Sajjadi, S., Abrol, R., and Wen, X. (2019). A beetle antifreeze protein protects lactate dehydrogenase under freeze-thawing. Int. J. Biol. Macromol. 136, 1153–1160. doi:10.1016/j.ijbiomac.2019.06.067
Roskoski, R. (2016). Classification of small molecule protein kinase inhibitors based upon the structures of their drug-enzyme complexes. Pharmacol. Res. 103, 26–48. doi:10.1016/j.phrs.2015.10.021
Roy, A., Jamison, K. W., He, S., Engel, S. A., and He, B. (2017). Deactivation in the posterior mid-cingulate cortex reflects perceptual transitions during binocular rivalry: Evidence from simultaneous EEG-fMRI. IJPB 3, 1–11. doi:10.1016/j.neuroimage.2017.02.041
Roy, A., and Saraf, S. (2006). Limonoids: overview of significant bioactive triterpenes distributed in plants kingdom. Biol. Pharm. Bull. 29, 191–201. doi:10.1248/bpb.29.191
Roychoudhury, S., Sinha, B., Choudhury, B. P., Jha, N. K., Palit, P., Kundu, S., et al. (2021). Scavenging properties of plant-derived natural biomolecule para-coumaric acid in the prevention of oxidative stress-induced diseases. Antioxidants 10, 1205. doi:10.3390/antiox10081205
Sá, R. C. S. E., Nalone Andrade, L., Dos Reis Barreto De Oliveira, R., and De Sousa, D. P. (2014). A review on anti-inflammatory activity of phenylpropanoids found in essential oils. Molecules 19, 1459–1480. doi:10.3390/molecules19021459
Sajeev, A., Hegde, M., Girisa, S., Devanarayanan, T. N., Alqahtani, M. S., Abbas, M., et al. (2022). Oroxylin A: A Promising Flavonoid for Prevention and Treatment of Chronic Diseases. Biomolecules 12, 1185. doi:10.3390/biom12091185
Salminen, A., Lehtonen, M., Suuronen, T., Kaarniranta, K., and Huuskonen, J. (2008). Terpenoids: natural inhibitors of NF-kappaB signaling with anti-inflammatory and anticancer potential. Cell. Mol. Life Sci. 65, 2979–2999. doi:10.1007/s00018-008-8103-5
Sameri, S., Mohammadi, C., Mehrabani, M., and Najafi, R. (2021). Targeting the hallmarks of cancer: the effects of silibinin on proliferation, cell death, angiogenesis, and migration in colorectal cancer. BMC Complementary Med. Ther. 21, 160. doi:10.1186/s12906-021-03330-1
Sang, D.-P., Li, R.-J., and Lan, Q. (2014). Quercetin sensitizes human glioblastoma cells to temozolomide in vitro via inhibition of Hsp27. Acta Pharmacol. Sin. 35, 832–838. doi:10.1038/aps.2014.22
Sang, Y., Han, S., Pannecouque, C., De Clercq, E., Zhuang, C., and Chen, F. (2019). Ligand-based design of nondimethylphenyl-diarylpyrimidines with improved metabolic stability, safety, and oral pharmacokinetic profiles. J. Med. Chem. 62, 11430–11436. doi:10.1021/acs.jmedchem.9b01446
Satoh, K., Yachida, S., Sugimoto, M., Oshima, M., Nakagawa, T., Akamoto, S., et al. (2017). Global metabolic reprogramming of colorectal cancer occurs at adenoma stage and is induced by MYC. Proc. Natl. Acad. Sci. 114, E7697–E7706. doi:10.1073/pnas.1710366114
Schmidt, T., Bergner, A., and Schwede, T. (2014). Modelling three-dimensional protein structures for applications in drug design. Drug Discov. today 19, 890–897. doi:10.1016/j.drudis.2013.10.027
Scott, G. N., and Elmer, G. W. (2002). Update on natural product–drug interactions. Am. J. health-system Pharm. 59, 339–347. doi:10.1093/ajhp/59.4.339
Shaik, A. H., Manjunatha, S., Maruthi, P. E., Al Omar, S. Y., Mohammad, A., and Kodidhela, L. D. (2020). Combined cardio-protective ability of syringic acid and resveratrol against isoproterenol induced cardio-toxicity in rats via attenuating NF-kB and TNF-α pathways. Sci. Rep. 10, 3426. doi:10.1038/s41598-020-59925-0
Shibata, S., Sogabe, S., Miwa, M., Fujimoto, T., Takakura, N., Naotsuka, A., et al. (2021). Identification of the first highly selective inhibitor of human lactate dehydrogenase B. Sci. Rep. 11, 21353. doi:10.1038/s41598-021-00820-7
Shishir, M. R. I., Karim, N., Gowd, V., Zheng, X., and Chen, W. (2019). Liposomal delivery of natural product: A promising approach in health research. Trends food Sci. Technol. 85, 177–200. doi:10.1016/j.tifs.2019.01.013
Sibley, C. R., Seow, Y., and Wood, M. J. (2010). Novel RNA-based strategies for therapeutic gene silencing. Mol. Ther. 18, 466–476. doi:10.1038/mt.2009.306
Singh, R. P., and Agarwal, R. (2004). A cancer chemopreventive agent silibinin, targets mitogenic and survival signaling in prostate cancer. Mutat. Research/Fundamental Mol. Mech. Mutagen. 555, 21–32. doi:10.1016/j.mrfmmm.2004.05.017
Sobral, M. V., Xavier, A. L., Lima, T. C., and De Sousa, D. P. (2014). Antitumor activity of monoterpenes found in essential oils. Sci. World J. 2014, 953451. doi:10.1155/2014/953451
Solanki, I., Parihar, P., Mansuri, M. L., and Parihar, M. S. (2015). Flavonoid-based therapies in the early management of neurodegenerative diseases. Adv. Nutr. 6, 64–72. doi:10.3945/an.114.007500
Son, D.-S., Lee, E.-S., and Adunyah, S. E. (2020). The antitumor potentials of benzimidazole anthelmintics as repurposing drugs. Immune Netw. 20, e29. doi:10.4110/in.2020.20.e29
Soni, V. K., Shukla, D., Kumar, A., and Vishvakarma, N. K. (2020). Curcumin circumvent lactate-induced chemoresistance in hepatic cancer cells through modulation of hydroxycarboxylic acid receptor-1. Int. J. Biochem. Cell Biol. 123, 105752. doi:10.1016/j.biocel.2020.105752
Sontheimer, E. J. (2005). Assembly and function of RNA silencing complexes. Nat. Rev. Mol. Cell Biol. 6, 127–138. doi:10.1038/nrm1568
Spagnuolo, C., Moccia, S., and Russo, G. L. (2018). Anti-inflammatory effects of flavonoids in neurodegenerative disorders. Eur. J. Med. Chem. 153, 105–115. doi:10.1016/j.ejmech.2017.09.001
Stangl, V., Lorenz, M., and Stangl, K. (2006). The role of tea and tea flavonoids in cardiovascular health. Mol. Nutr. food Res. 50, 218–228. doi:10.1002/mnfr.200500118
Su, C.-H., Chen, L.-J., Liao, J. F., and Cheng, J.-T. (2013). Increase of phosphatase and tensin homolog by silymarin to inhibit human pharynx squamous cancer. J. Med. food 16, 778–784. doi:10.1089/jmf.2012.2534
Suddek, G. M. (2014). Allicin enhances chemotherapeutic response and ameliorates tamoxifen-induced liver injury in experimental animals. Pharm. Biol. 52, 1009–1014. doi:10.3109/13880209.2013.876053
Sugasawa, N., Katagi, A., Kurobe, H., Nakayama, T., Nishio, C., Takumi, H., et al. (2019). Inhibition of Atherosclerotic Plaque Development by Oral Administration of α-Glucosyl Hesperidin and Water-Dispersible Hesperetin in Apolipoprotein E Knockout Mice. J. Am. Coll. Nutr. 38, 15–22. doi:10.1080/07315724.2018.1468831
Sugumaran, G. (2012). Open Source Drug Discovery–redefining IPR through open source innovations. Curr. Sci. 2012, 1637–1639.
Suman, T., Rajasree, S. R., Ramkumar, R., Rajthilak, C., and Perumal, P. (2014). The Green synthesis of gold nanoparticles using an aqueous root extract of Morinda citrifolia L. Mol. Biomol. Spectrosc. 118, 11–16. doi:10.1016/j.saa.2013.08.066
Sun, Y., Xun, K., Wang, Y., and Chen, X. (2009). A systematic review of the anticancer properties of berberine, a natural product from Chinese herbs. Anti-cancer drugs 20, 757–769. doi:10.1097/CAD.0b013e328330d95b
Tan, W., Li, N., Tan, R., Zhong, Z., Suo, Z., Yang, X., et al. (2015). Berberine interfered with breast cancer cells metabolism, balancing energy homeostasis. Anti-Cancer Agents Med. Chem. 15, 66–78. doi:10.2174/1871520614666140910120518
Thomas, A. O., and Oyediran, O. E. (2008). Nutritional importance and micronutrient potentials of two non-conventional indigenous green leafy vegetables from Nigeria. Agric. J. 3, 362–365.
Thomford, N. E., Senthebane, D. A., Rowe, A., Munro, D., Seele, P., Maroyi, A., et al. (2018). Natural products for drug discovery in the 21st century: innovations for novel drug discovery. Int. J. Mol. Sci. 19, 1578. doi:10.3390/ijms19061578
Thoppil, R. J., and Bishayee, A. (2011). Terpenoids as potential chemopreventive and therapeutic agents in liver cancer. World J. hepatology 3, 228–249. doi:10.4254/wjh.v3.i9.228
Tian, K.-M., Li, J.-J., and Xu, S.-W. (2019). Rutaecarpine: a promising cardiovascular protective alkaloid from Evodia rutaecarpa (Wu Zhu Yu). Pharmacol. Res. 141, 541–550. doi:10.1016/j.phrs.2018.12.019
Ting, Y., Jiang, Y., Ho, C.-T., and Huang, Q. (2014). Common delivery systems for enhancing in vivo bioavailability and biological efficacy of nutraceuticals. J. Funct. Foods 7, 112–128. doi:10.1016/j.jff.2013.12.010
Toklu, H. Z., Akbay, T. T., Velioglu-Ogunc, A., Ercan, F., Gedik, N., Keyer-Uysal, M., et al. (2008). Silymarin, the antioxidant component of Silybum marianum, prevents sepsis-induced acute lung and brain injury. J. Surg. Res. 145, 214–222. doi:10.1016/j.jss.2007.03.072
Tsai, Y.-M., Chien, C.-F., Lin, L.-C., and Tsai, T.-H. (2011). Curcumin and its nano-formulation: the kinetics of tissue distribution and blood–brain barrier penetration. Int. J. Pharm. 416, 331–338. doi:10.1016/j.ijpharm.2011.06.030
Tsao, R., Yang, R., Young, J. C., and Zhu, H. (2003). Polyphenolic profiles in eight apple cultivars using high-performance liquid chromatography (HPLC). J. Agric. food Chem. 51, 6347–6353. doi:10.1021/jf0346298
Unlu, A., Nayir, E., Kalenderoglu, M. D., Kirca, O., and Ozdogan, M. (2016). Curcumin (Turmeric) and cancer. J. buon 21, 1050–1060.
Valvona, C. J., Fillmore, H. L., Nunn, P. B., and Pilkington, G. J. (2016). The regulation and function of lactate dehydrogenase a: therapeutic potential in brain tumor. Brain pathol. 26, 3–17. doi:10.1111/bpa.12299
Vander Heiden, M. G., Cantley, L. C., and Thompson, C. B. (2009). Understanding the Warburg effect: the metabolic requirements of cell proliferation. science 324, 1029–1033. doi:10.1126/science.1160809
Vander Heiden, M. G., Christofk, H. R., Schuman, E., Subtelny, A. O., Sharfi, H., Harlow, E. E., et al. (2010). Identification of small molecule inhibitors of pyruvate kinase M2. Biochem. Pharmacol. 79, 1118–1124. doi:10.1016/j.bcp.2009.12.003
Varghese, E., Samuel, S. M., Líšková, A., Samec, M., Kubatka, P., and Büsselberg, D. (2020). Targeting glucose metabolism to overcome resistance to anticancer chemotherapy in breast cancer. Cancers 12, 2252. doi:10.3390/cancers12082252
Venkatesan, R., Ji, E., and Kim, S. Y. (2015). Phytochemicals that regulate neurodegenerative disease by targeting neurotrophins: a comprehensive review. BioMed Res. Int. 2015, 814068. doi:10.1155/2015/814068
Vettraino, M., Manerba, M., Govoni, M., and Di Stefano, G. (2013). Galloflavin suppresses lactate dehydrogenase activity and causes MYC downregulation in Burkitt lymphoma cells through NAD/NADH-dependent inhibition of sirtuin-1. Anti-cancer drugs 24, 862–870. doi:10.1097/CAD.0b013e328363ae50
Wang, J., Fan, H., Chen, Q., Ma, G., Zhu, M., Zhang, X., et al. (2015b). Curcumin inhibits aerobic glycolysis and induces mitochondrial-mediated apoptosis through hexokinase II in human colorectal cancer cells in vitro. Anti-cancer drugs 26, 15–24. doi:10.1097/CAD.0000000000000132
Wang, J., Phan, T., Gordon, D., Chung, S., Henning, S. M., and Vadgama, J. V. (2015c). Arctigenin in combination with quercetin synergistically enhances the antiproliferative effect in prostate cancer cells. Mol. Nutr. food Res. 59, 250–261. doi:10.1002/mnfr.201400558
Wang, J., Wang, H., Liu, A., Fang, C., Hao, J., and Wang, Z. (2015a). Lactate dehydrogenase A negatively regulated by miRNAs promotes aerobic glycolysis and is increased in colorectal cancer. Oncotarget 6, 19456–19468. doi:10.18632/oncotarget.3318
Wang, M. S., Boddapati, S., Emadi, S., and Sierks, M. R. (2010). Curcumin reduces α-synuclein induced cytotoxicity in Parkinson's disease cell model. BMC Neurosci. 11, 1–10.
Wang, R., Yin, R., Zhou, W., Xu, D., and Li, S. (2012). Shikonin and its derivatives: a patent review. Expert Opin. Ther. Pat. 22, 977–997. doi:10.1517/13543776.2012.709237
Wang, S., Chang, X., Zhang, J., Li, J., Wang, N., Yang, B., et al. (2021a). Ursolic acid inhibits breast cancer metastasis by suppressing glycolytic metabolism via activating sp1/caveolin-1 signaling. Front. Oncol. 11, 745584. doi:10.3389/fonc.2021.745584
Wang, S., You, Y.-L., Li, F.-F., Kong, W.-R., and Wang, S.-Q. (2021b). Research progress of NMR in natural product quantification. Molecules 26, 6308. doi:10.3390/molecules26206308
Wang, Z., Wang, D., Han, S., Wang, N., Mo, F., Loo, T. Y., et al. (2013). Bioactivity-guided identification and cell signaling technology to delineate the lactate dehydrogenase A inhibition effects of Spatholobus suberectus on breast cancer. PloS one 8, e56631. doi:10.1371/journal.pone.0056631
Warburg, O., and Minami, S. (1923). Versuche an überlebendem carcinom-gewebe. Klin. Wochenschr. 2, 776–777. doi:10.1007/bf01712130
Wendt, E. H. U., Schoenrogge, M., Vollmar, B., and Zechner, D. (2020). Galloflavin plus metformin treatment impairs pancreatic cancer cells. Anticancer Res. 40, 153–160. doi:10.21873/anticanres.13936
White, N., and Olliaro, P. (1998). Artemisinin and derivatives in the treatment of uncomplicated malaria. Médecine Trop. Rev. Corps Santé Colon. 58, 54–56.
Wood, K. D., Holmes, R. P., Erbe, D., Liebow, A., Fargue, S., and Knight, J. (2019). Reduction in urinary oxalate excretion in mouse models of primary hyperoxaluria by RNA interference inhibition of liver lactate dehydrogenase activity. Biochimica Biophysica Acta (BBA)-Molecular Basis Dis. 1865, 2203–2209. doi:10.1016/j.bbadis.2019.04.017
Woodford, M. R., Chen, V. Z., Backe, S. J., Bratslavsky, G., and Mollapour, M. (2019). Structural and functional regulation of lactate dehydrogenase-A in cancer. Future Med. Chem. 12, 439–455. doi:10.4155/fmc-2019-0287
Wu, J.-S., Liu, H.-J., Han, S.-J., Mao, N.-F., and Liu, X.-F. (2022). Chelerythrine Ameliorates Acetic Acid-Induced Ulcerative Colitis via Suppression of Inflammation and Oxidation. Nat. Product. Commun. 17, 1934578X2211324. doi:10.1177/1934578x221132417
Xie, G.-P., Jiang, N., Wang, S.-N., Qi, R.-Z., Wang, L., Zhao, P.-R., et al. (2015). Eucommia ulmoides Oliv. bark aqueous extract inhibits osteoarthritis in a rat model of osteoarthritis. J. Ethnopharmacol. 162, 148–154. doi:10.1016/j.jep.2014.12.061
Xu, S., He, M., Zhong, M., Li, L., Lu, Y., Zhang, Y., et al. (2015). The neuroprotective effects of taurine against nickel by reducing oxidative stress and maintaining mitochondrial function in cortical neurons. Neurosci. Lett. 590, 52–57. doi:10.1016/j.neulet.2015.01.065
Xu, T., Zhao, H., Wang, M., Chow, A., and Fang, M. (2021). Metabolomics and in Silico docking-directed discovery of small-molecule enzyme targets. Anal. Chem. 93, 3072–3081. doi:10.1021/acs.analchem.0c03684
Yadav, D. K., Kumar, S., Teli, M. K., Yadav, R., and Chaudhary, S. (2019). Molecular targets for malarial chemotherapy: a review. Curr. Top. Med. Chem. 19, 861–873. doi:10.2174/1568026619666190603080000
Yamamoto, S., Seta, K., Morisco, C., Vatner, S. F., and Sadoshima, J. (2001). Chelerythrine rapidly induces apoptosis through generation of reactive oxygen species in cardiac myocytes. J. Mol. Cell. Cardiol. 33, 1829–1848. doi:10.1006/jmcc.2001.1446
Yang, J., Huang, J., Shen, C., Cheng, W., Yu, P., Wang, L., et al. (2018). Resveratrol treatment in different time-attenuated neuronal apoptosis after oxygen and glucose deprivation/reoxygenation via enhancing the activation of Nrf-2 signaling pathway in vitro. Cell Transplant. 27, 1789–1797. doi:10.1177/0963689718780930
Yang, W.-Q., and Zhang, Y. (2012). RNAi-mediated gene silencing in cancer therapy. Expert Opin. Biol. Ther. 12, 1495–1504. doi:10.1517/14712598.2012.712107
Yang, W., Chen, X., Li, Y., Guo, S., Wang, Z., and Yu, X. (2020). Advances in pharmacological activities of terpenoids. Nat. Product. Commun. 15, 1934578X2090355. doi:10.1177/1934578x20903555
Yang, Y., Chong, Y., Chen, M., Dai, W., Zhou, X., Ji, Y., et al. (2021). Targeting lactate dehydrogenase a improves radiotherapy efficacy in non-small cell lung cancer: from bedside to bench. J. Transl. Med. 19, 170. doi:10.1186/s12967-021-02825-2
Yang, Y., Wang, X., Zhu, Q., Gong, G., Luo, D., Jiang, A., et al. (2014). Synthesis and pharmacological evaluation of novel limonin derivatives as anti-inflammatory and analgesic agents with high water solubility. Bioorg. Med. Chem. Lett. 24, 1851–1855. doi:10.1016/j.bmcl.2014.02.003
Yao, H., Yang, F., and Li, Y. (2022). Natural products targeting human lactate dehydrogenases for cancer therapy: A mini review. Front. Chem. 10, 1013670. doi:10.3389/fchem.2022.1013670
Yao, J., Liu, J., and Zhao, W. (2018). By blocking hexokinase-2 phosphorylation, limonin suppresses tumor glycolysis and induces cell apoptosis in hepatocellular carcinoma. OncoTargets Ther. 11, 3793–3803. doi:10.2147/OTT.S165220
Younis, M. A., Khalil, I. A., Elewa, Y. H., Kon, Y., and Harashima, H. (2021). Ultra-small lipid nanoparticles encapsulating sorafenib and midkine-siRNA selectively-eradicate sorafenib-resistant hepatocellular carcinoma in vivo. J. Control. Release 331, 335–349. doi:10.1016/j.jconrel.2021.01.021
Yu, J. C., Min, Z.-D., and Ip, N. Y. (2004). Melia toosendan regulates PC12 cell differentiation via the activation of protein kinase A and extracellular signal-regulated kinases. Neurosignals 13, 248–257. doi:10.1159/000079339
Yu, M.-S., Leung, S.K.-Y., Lai, S.-W., Che, C.-M., Zee, S.-Y., So, K.-F., et al. (2005). Neuroprotective effects of anti-aging oriental medicine Lycium barbarum against β-amyloid peptide neurotoxicity. Exp. Gerontol. 40, 716–727. doi:10.1016/j.exger.2005.06.010
Zaafan, M. A., Zaki, H. F., El-Brairy, A. I., and Kenawy, S. A. (2013). Protective effects of atorvastatin and quercetin on isoprenaline-induced myocardial infarction in rats. Bull. Fac. Pharm. 51, 35–41. doi:10.1016/j.bfopcu.2013.03.001
Zeb, A., and Mehmood, S. (2004). Carotenoids contents from various sources and their potential health applications. Pak. J. Nutr. 3, 199–204. doi:10.3923/pjn.2004.199.204
Zerin, T., Lee, M., Jang, W. S., Nam, K. W., and Song, H. Y. (2016). Anti-inflammatory potential of ursolic acid in Mycobacterium tuberculosis-sensitized and Concanavalin A-stimulated cells. Mol. Med. Rep. 13, 2736–2744. doi:10.3892/mmr.2016.4840
Zha, X., Wang, F., Wang, Y., He, S., Jing, Y., Wu, X., et al. (2011). Lactate dehydrogenase B is critical for hyperactive mTOR-mediated tumorigenesis. Cancer Res. 71, 13–18. doi:10.1158/0008-5472.CAN-10-1668
Zhang, C., Tian, X., Luo, Y., and Meng, X. (2011). Ginkgolide B attenuates ethanol-induced neurotoxicity through regulating NADPH oxidases. Toxicology 287, 124–130. doi:10.1016/j.tox.2011.06.006
Zhang, C., and Yan, C. (2020). Updates of recent vinpocetine research in treating cardiovascular diseases. J. Cell. Immunol. 2, 211–219. doi:10.33696/immunology.2.045
Zhang, Q., Hu, C., Zhang, N., Wei, W.-Y., Li, L.-L., Wu, H.-M., et al. (2021b). Matrine attenuates pathological cardiac fibrosis via RPS5/p38 in mice. Acta Pharmacol. Sin. 42, 573–584. doi:10.1038/s41401-020-0473-8
Zhang, Q., Li, J.-K., Ge, R., Liang, J.-Y., Li, Q.-S., and Min, Z.-D. (2013a). Novel NGF-potentiating limonoids from the fruits of Melia toosendan. Fitoterapia 90, 192–198. doi:10.1016/j.fitote.2013.07.019
Zhang, Q., Li, Q., Ge, Y., Chen, Y., Chen, J., Dong, Y., et al. (2013b). Silibinin Triggers Apoptosis and Cell-Cycle Arrest of SGC7901 Cells. Phytotherapy Res. 27, 397–403. doi:10.1002/ptr.4733
Zhang, Q., Liu, J., Duan, H., Li, R., Peng, W., and Wu, C. (2021a). Activation of Nrf2/HO-1 signaling: An important molecular mechanism of herbal medicine in the treatment of atherosclerosis via the protection of vascular endothelial cells from oxidative stress. J. Adv. Res. 34, 43–63. doi:10.1016/j.jare.2021.06.023
Zhang, S.-F., Dong, Y.-C., Zhang, X.-F., Wu, X.-G., Cheng, J.-J., Guan, L.-H., et al. (2015a). Flavonoids from Scutellaria attenuate okadaic acid-induced neuronal damage in rats. Brain Inj. 29, 1376–1382. doi:10.3109/02699052.2015.1042053
Zhang, S.-F., Wang, S.-X., Ma, J.-W., Li, H.-Y., Ye, J.-C., Xie, S.-M., et al. (2015b). EGCG inhibits properties of glioma stem-like cells and synergizes with temozolomide through downregulation of P-glycoprotein inhibition. J. neuro-oncology 121, 41–52. doi:10.1007/s11060-014-1604-1
Zhao, C. C., Wu, X. Y., Yi, H., Chen, R., and Fan, G. (2021). The therapeutic effects and mechanisms of salidroside on cardiovascular and metabolic diseases: An updated review. Chem. Biodivers. 18, e2100033. doi:10.1002/cbdv.202100033
Zhao, J., Sharma, Y., and Agarwal, R. (1999). Significant inhibition by the flavonoid antioxidant silymarin against 12-O-tetradecanoylphorbol 13-acetate-caused modulation of antioxidant and inflammatory enzymes, and cyclooxygenase 2 and interleukin-1alpha expression in SENCAR mouse epidermis: implications in the prevention of stage I tumor promotion. Mol. Carcinog. 26, 321–333. doi:10.1002/(sici)1098-2744(199912)26:4<321:aid-mc11>3.0.co;2-9
Zhou, M., Ren, H., Han, J., Wang, W., Zheng, Q., and Wang, D. (2015). Protective effects of kaempferol against myocardial ischemia/reperfusion injury in isolated rat heart via antioxidant activity and inhibition of glycogen synthase kinase-3. Oxidative Med. Cell. Longev. 2015, 481405. doi:10.1155/2015/481405
Zhou, W., Wang, H., Yang, Y., Chen, Z.-S., Zou, C., and Zhang, J. (2020). Chloroquine against malaria, cancers and viral diseases. Drug Discov. Today 25, 2012–2022. doi:10.1016/j.drudis.2020.09.010
Zhu, N., Li, J., Li, Y., Zhang, Y., Du, Q., Hao, P., et al. (2020). Berberine Protects Against Simulated Ischemia/Reperfusion Injury-Induced H9C2 Cardiomyocytes Apoptosis In Vitro and Myocardial Ischemia/Reperfusion-Induced Apoptosis In Vivo by Regulating the Mitophagy-Mediated HIF-1α/BNIP3 Pathway. Front. Pharmacol. 11, 367. doi:10.3389/fphar.2020.00367
Keywords: lactate dehydrogenase, natural compound, LDH inhibitor, pharmacological effect, cancer, cardiovascular disease, neurodegenerative disease
Citation: Han JH, Lee E-J, Park W, Ha K-T and Chung H-S (2023) Natural compounds as lactate dehydrogenase inhibitors: potential therapeutics for lactate dehydrogenase inhibitors-related diseases. Front. Pharmacol. 14:1275000. doi: 10.3389/fphar.2023.1275000
Received: 09 August 2023; Accepted: 27 September 2023;
Published: 17 October 2023.
Edited by:
Aderbal S. Aguiar Jr, Federal University of Santa Catarina, BrazilReviewed by:
Seyed Zachariah Moradi, Kermanshah University of Medical Sciences, IranAna Cristina De Bem Alves, Federal University of Santa Catarina, Brazil
Copyright © 2023 Han, Lee, Park, Ha and Chung. This is an open-access article distributed under the terms of the Creative Commons Attribution License (CC BY). The use, distribution or reproduction in other forums is permitted, provided the original author(s) and the copyright owner(s) are credited and that the original publication in this journal is cited, in accordance with accepted academic practice. No use, distribution or reproduction is permitted which does not comply with these terms.
*Correspondence: Hwan-Suck Chung, aHNjaHVuZ0BraW9tLnJlLmty; Ki-Tae Ha, aGFnaXNAcHVzYW4uYWMua3I=