- 1Center for Drug Research and Development, Guangdong Pharmaceutical University, Guangzhou, China
- 2Zhuhai People’s Hospital, Zhuhai Clinical Medical College of Jinan University, Zhuhai, China
- 3Key Specialty of Clinical Pharmacy, The First Affiliated Hospital of Guangdong Pharmaceutical University, Guangzhou, China
- 4Guangdong Key Laboratory of Pharmaceutical Bioactive Substances, Guangdong Pharmaceutical University, Guangzhou, China
NOD-like receptor protein 3 (NLRP3) inflammasome is an intracellular sensing protein complex that possesses NACHT, leucine-rich repeat, and pyrin domain, playing a crucial role in innate immunity. Activation of the NLRP3 inflammasome leads to the production of pro-inflammatory cellular contents, such as interleukin (IL)-1β and IL-18, and induction of inflammatory cell death known as pyroptosis, thereby amplifying or sustaining inflammation. While a balanced inflammatory response is beneficial for resolving damage and promoting tissue healing, excessive activation of the NLRP3 inflammasome and pyroptosis can have harmful effects. The involvement of the NLRP3 inflammasome has been observed in various cardiovascular diseases (CVD). Indeed, the NLRP3 inflammasome and its associated pyroptosis are closely linked to key cardiovascular risk factors including hyperlipidemia, diabetes, hypertension, obesity, and hyperhomocysteinemia. Exercise compared with medicine is a highly effective measure for both preventing and treating CVD. Interestingly, emerging evidence suggests that exercise improves CVD and inhibits the activity of NLRP3 inflammasome and pyroptosis. In this review, the activation mechanisms of the NLRP3 inflammasome and its pathogenic role in CVD are critically discussed. Importantly, the purpose is to emphasize the crucial role of exercise in managing CVD by suppressing NLRP3 inflammasome activity and proposes it as the foundation for developing novel treatment strategies.
1 Introduction
Cardiovascular diseases (CVD) remain a prevalent global health concern, causing a significant burden of illness and mortality, with approximately one-third of all deaths attributed to this condition (Mensah et al., 2019). The common symptoms of CVD include chest pain, shortness of breath, irregular heartbeat, fatigue, and decreased physical stamina (Tutor et al., 2023; Wong and Sattar, 2023). CVD encompass various disorders that affect the heart and blood vessels, including atherosclerosis (AS), obesity, diabetes, hyperhomocysteinemia (HHcy), myocardial infarction (MI), hypertension, heart failure (HF), and diabetic cardiomyopathy (DCM) (Konishi et al., 2022; Haidar and Horwich, 2023). The conventional risk factors that are widely recognized for CVD, such as hypertension, hypercholesteremia, diabetes mellitus, and cigarette smoking, remain acknowledged as the main factors responsible for the development and advancement of this condition (Alten et al., 2020).
NOD-like receptor protein 3 (NLRP3) inflammasome is a molecular platform that triggers caspase-1 and facilitates the secretion of interleukin (IL)-1β and IL-18 in response to cellular infection or stress (Valenzuela et al., 2023). This activation results in the cleavage of gasdermin D (GSDMD) by caspase-1, generating an N-terminal GSDMD fragment (Valenzuela et al., 2023). This fragment induces the formation of membrane pores and triggers inflammatory cell death, namely pyroptosis (Valenzuela et al., 2023). The involvement of NLRP3 inflammasome and pyroptosis has been established in cardiovascular risk factors such as hyperlipidemia, diabetes, hypertension, obesity, and HHcy (Alten et al., 2020). Targeting NLRP3 inflammasome activation and pyroptosis holds great potential for therapeutic interventions against CVD.
Extensive research has demonstrated that exercise plays a crucial role in weight management (Murray et al., 2023), blood pressure (BP) reduction (Tucker et al., 2022), blood sugar (Kar et al., 2019) and lipid regulation (Lee et al., 2020), consequently lowering the risk of CVD (Li et al., 2023). Moreover, moderate exercise improves cardiovascular system function and structure, strengthens the heart muscle, enhances cardio-pulmonary function, promotes blood circulation, and increases the heart’s tolerance and overall health (Alten et al., 2020). Furthermore, exercise exhibits a clear anti-inflammatory effect (Lee et al., 2020). It is widely acknowledged that exercise can effectively reduce chronic inflammation by inhibiting the expression of inflammatory factors while increasing the release of anti-inflammatory cytokines (Lee et al., 2020). Previous studies have indicated that exercise can decrease the activation of the NLRP3 inflammasome to substantially inhibit IL-1β, and IL-18 release (Li et al., 2023; Liu et al., 2023). This review mainly focuses on how exercise can improve CVD by influencing NLRP3 inflammasome or pyroptosis. Additionally, this review will investigate exercise as a therapeutic strategy via NLRP3 inflammasome for managing CVD and address the research questions that need to be explored in the future.1.
2 Overview of NLRP3 inflammasome
Inflammasomes are crucial components of the immune system that play a major role in initiating inflammatory responses (Chang, 2023). They are composed of sensor proteins known as pattern recognition receptors that oligomerize and form a platform for the activation of caspase-1 in response to damage-associated molecular patterns (DAMPs) or pathogen-associated molecular patterns (PAMPs) (Chang, 2023). The nucleotide-binding domain-like receptor (NLR) family all share a central nucleotide-binding domain, and most members have a C-terminal leucine-rich repeat (LRR) domain and a variable N-terminal domain (Swanson et al., 2019). While certain members such as NLRP1, NLRP3, and NLRC4 are recognized as NLRs capable of forming inflammasomes, others like NLRP6 and NLRP12 are considered potential inflammasome sensors (Swanson et al., 2019). The NLRP3 inflammasome, in particular, is essential for the host’s immune defense against various bacterial, fungal, and viral infections (Dilucca et al., 2021). However, when dysregulated, it has been implicated in the development of several inflammatory disorders, including CVD (Paerewijck and Lamkanfi, 2022).
The NLRP3 inflammasome is composed of several key components. The NLR protein in the NLRP3 inflammasome contains a conserved nucleotide-binding and oligomerization domain, C-terminal LRRs, and a pyrin domain (PYD) that facilitates multimerization (Xi et al., 2024). Upon activation of the NLRP3 inflammasome, the NLRs oligomerize through their nucleotide-binding and oligomerization domains (Fu and Wu, 2023). This leads to the recruitment of the adaptor protein apoptosis-associated speck-like protein (ASC) through PYD-PYD interactions (Fu and Wu, 2023). ASC then forms large speck-like structures and recruits pro-caspase-1 through caspase recruitment domain (CARD)-CARD interactions. Pro-caspase-1 undergoes autocatalytic cleavage, resulting in the formation of active caspase-1 p10/p20 tetramers (Ruan, 2019). These active caspase-1 tetramers mediate the maturation and secretion of IL-1β and IL-18 (Ruan, 2019; Fu and Wu, 2023). Additionally, caspase-1 can cleave GSDMD to generate GSDMD n-terminal (NT). GSDMD-NT forms plasma membrane pores, leading to the induction of pyroptosis (Lee et al., 2021; Nie et al., 2021). The canonical activation of inflammasomes is proposed to occur in two steps: priming and assembly (Bockstiegel et al., 2023). Priming involves the initial activation of toll-like receptors by agonists such as lipopolysaccharide (LPS) (Fu and Wu, 2023). This trigger signaling cascades, primarily through the nuclear factor-κB (NF-κB) pathway, which leads to the transcriptional upregulation of pro-inflammatory mediators like pro-IL-1β. Following priming, the activated inflammasome assembles in response to various PAMPs or DAMPs (Fu and Wu, 2023; Krantz et al., 2023). This assembly forms a large molecular platform that activates inflammatory caspases and processes pro-IL-1β (Huang et al., 2023). The activation of NLRP3 inflammasome requires “priming” with TLR agonists to initiate signaling cascades (primarily nuclear factor-κB (NF-κB)-dependent pathway) that ultimately promote a transcriptional response to upregulate pro-inflammatory mediators (Figure 1).
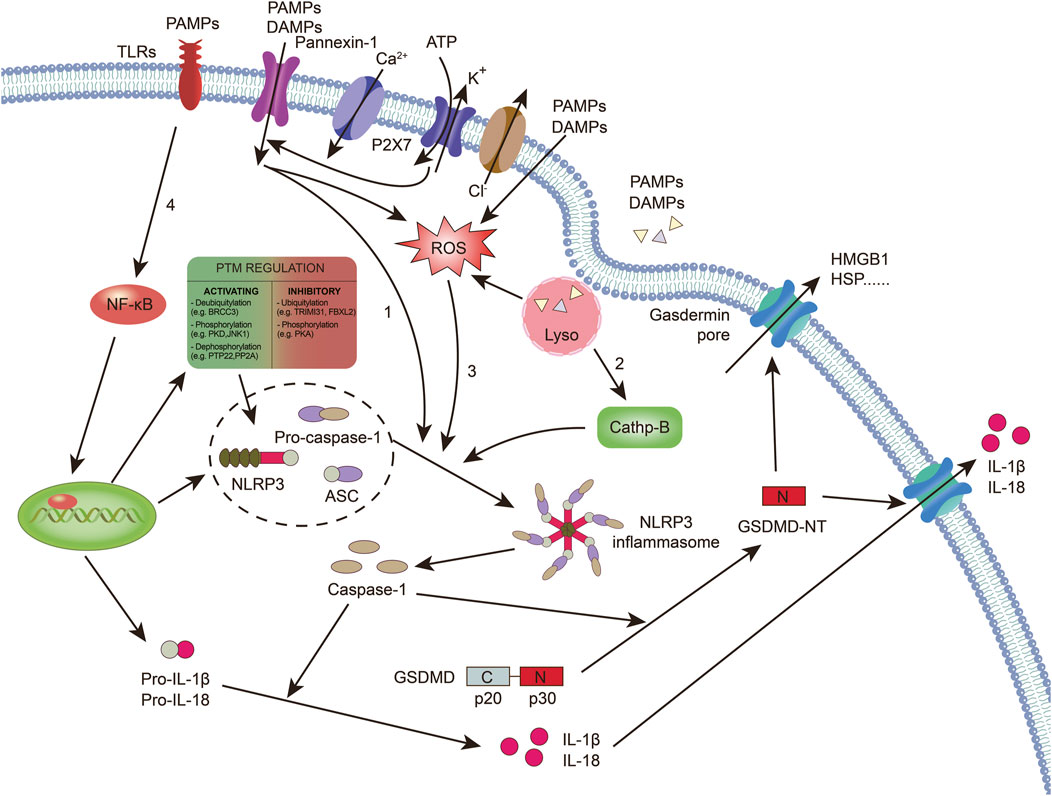
Figure 1. Caspase-1-dependent canonical pyroptotic cell death induced by NLRP3 inflammasome activation. The assembly of the NLRP3 inflammasome involves NLRP3 oligomerization and ASC recruitment, triggering the autocleavage of pro-caspase-1. This autocleavage leads to the activation of caspase-1, which converts inactive pro-IL-1β and pro-IL-18 into their bioactive and secreted forms, namely IL-1β and IL-18. Additionally, active caspase-1 cleaves GSDMD, generating GSDMD-NT, which forms pores on the plasma membrane, inducing pyroptosis. Various models have been proposed to explain the assembly of the NLRP3 inflammasome. 1): Extracellular ATP can activate the NLRP3 inflammasome through different mechanisms. This includes the activation of the P2X7 receptor leading to the opening of the pannexin-1 pore, allowing the entry of extracellular factors that directly interact with NLRP3. Alternatively, NLRP3 can sense either the efflux of K+ or the loss of membrane integrity. 2): Crystalline or particulate agonists can be phagocytosed, resulting in the release of lysosomal cathepsins B and L, which are detected by the NLRP3 inflammasome. 3): NLRP3 agonists such as DAMPs and PAMPs can trigger the production of ROS, which activates the NLRP3 inflammasome. It is important to note that these models are not mutually exclusive but rather interact with each other. 4): The activation of the NLRP3 inflammasome also requires “priming” with TLR agonists, such as LPS.. (ASC: apoptosis-associated speck-like protein; TLRs: toll-like receptors; DAMPs: damage-associated molecular patterns; GSDMD: gasdermin D; NT: n-terminal; HSP: heat shock proteins; HMGB-1: high mobility group box-1; IL: interleukin; NLRP3: NOD-like receptor protein 3; NF-κB: nuclear factor-κB; PAMPs: pathogen-associated molecular patterns; P2X7: purinergic receptor P2X, ligand-gated ion channel 7; ROS: reactive oxygen species).
K+ efflux is recognized as a crucial upstream signal for NLRP3 inflammasome activation (Zhou et al., 2020). Activation of NLRP3 also requires the mobilization of Ca2+ (Diaz-Del-Olmo et al., 2021). Mobilization of Ca2+ occurs when extracellular Ca2+ moves across channels in the plasma membrane and the Ca2+ stored in the endoplasmic reticulum is released into the cytoplasm, which can induce NLRP3 inflammasome activation (Watanabe et al., 2020; Diaz-Del-Olmo et al., 2021). In addition, Cl− was implicated in NLRP3 activation. Cl− channel blockers and elevated levels of extracellular Cl− can inhibit, whereas reduced levels of Cl− can enhance, the activation of NLRP3 (Zhou et al., 2020). Cl− efflux may be downstream of K+ efflux and affects ASC polymerization, whereas K+ efflux promotes NLRP3 oligomerization (Zhou et al., 2024).
In addition to the ion channels mentioned earlier that can activate the NLRP3 inflammasome, organelle dysfunction can also trigger inflammasome activation (Movahedpour et al., 2023). In a study, sterile lysosomal rupture caused by L-leucyl-L-leucine methyl ester is sufficient to trigger NLRP3 inflammasome activation, whereas suppression of phagosomal acidification or cathepsin B blocks NLRP3 activation (Movahedpour et al., 2023). Mitochondrial dysfunction contributes to the activation of the NLRP3 inflammasome (Kodi et al., 2024). When damaged organelles accumulate due to deficiencies in autophagic proteins, dysfunctional mitochondria release mitochondrial DNA, leading to NLRP3 inflammasome activation (Kodi et al., 2024). Additionally, mitochondrial ROS (mtROS) can initiate NLRP3 activation (Kodi et al., 2024). Inhibiting autophagy or mitophagy results in the buildup of mitochondrial ROS and subsequent NLRP3 activation (Chen et al., 2022; Lewisluján et al., 2022; Zhang et al., 2022; Lu et al., 2023).
Post-transcriptional modifications of the NLRP3 protein occur in unstimulated cells and during priming and activation to modulate its activation and function (Swanson et al., 2019). In peritoneal macrophages, tripartite motif 31 ubiquitylates NLRP3, targeting it for proteasomal degradation (Chan and Schroder, 2020). The E3 ubiquitin ligase f-box and leucine-rich repeat protein 2 are suggested to prevent NLRP3 activation by directing it to the proteasome in lung epithelial cells (Han et al., 2020). Additionally, membrane-associated ring-ch-type finger 7, another E3 ubiquitin ligase, inhibits NLRP3 downstream of dopamine-induced D1 receptor signaling (Sandall and Macdonald, 2019). Another regulatory level involves phosphorylation of NLRP3 at Ser291 (or Ser295 in humans) by protein kinase A, leading to K48- and K63-linked ubiquitination and subsequent proteasomal degradation (Ren et al., 2019). Song et al. Demonstrated that following LPS priming, NLRP3 is phosphorylated on Ser194 by c-Jun N-terminal kinase 1, promoting NLRP3 oligomerization upon activation by canonical stimuli (Spalinger et al., 2023). In monocytic cells, a study revealed that dephosphorylation of NLRP3 at Tyr861 by protein tyrosine phosphatase nonreceptor 22 induces NLRP3 activation (Spalinger et al., 2020). Whether these pathways synergize to tightly control NLRP3 activity remains elusive.
3 Mechanisms of pyroptosis
Recent advances have shed light on the molecular mechanisms of pyroptosis, a form of programmed cell death induced by the agonist of the NLRP3 inflammasome (McKee and Coll, 2020; Yuan et al., 2020; Chen et al., 2022; Zhong et al., 2023). Among these mechanisms, GSDMD has emerged as a crucial mediator of pyroptosis. GSDMD belongs to a family of proteins named GSDMs, which share a pore-forming domain (McKee and Coll, 2020). Cleavage of GSDMD by caspase-1 or caspase-4/5/11 releases GSDMD-NT, the NT domain of GSDMD (McKee and Coll, 2020). GSDMD-NT then forms these pores in the plasma membrane, leading to cell swelling and osmotic lysis (Ren et al., 2019). Other members of the GSDM family also possess pore-forming activity but are not targeted by inflammatory caspases (Chen et al., 2022). The cleavage of GSDMD occurs at a conserved residue called D276, resulting in the separation of GSDMD into two domains: GSDMD-NT (p30) and GSDMD C-terminal domain (p20) (Zhong et al., 2023). GSDMD-NT can interact with lipids in the plasma membrane and assemble into large oligomeric pores (Yuan et al., 2020). This disruption of the cell membrane integrity leads to an increase in intracellular osmotic pressure and the release of inflammatory intracellular contents, including high mobility group box-1 (HMGB-1) and heat shock protein (Yuan et al., 2020; Chan et al., 2023). This process, characterized by caspase-1-dependent cleavage of GSDMD, is known as the pyroptotic pathway (Chan et al., 2023).
4 Overview of exercise
Exercise refers to the movement of the body, typically involving the musculoskeletal system, to maintain health, improve physical fitness, promote cardiovascular health, and enhance the overall quality of life (Vella et al., 2017). It is a crucial component for maintaining both physical and mental wellbeing (Vella et al., 2017). By selecting forms of exercise that suit individual needs and goals, comprehensive health benefits can be achieved (Vella et al., 2017). The most common classification of exercise is based on the primary energy metabolism pathways, dividing it into aerobic and anaerobic exercise (Paluch et al., 2024) (Figure 2).
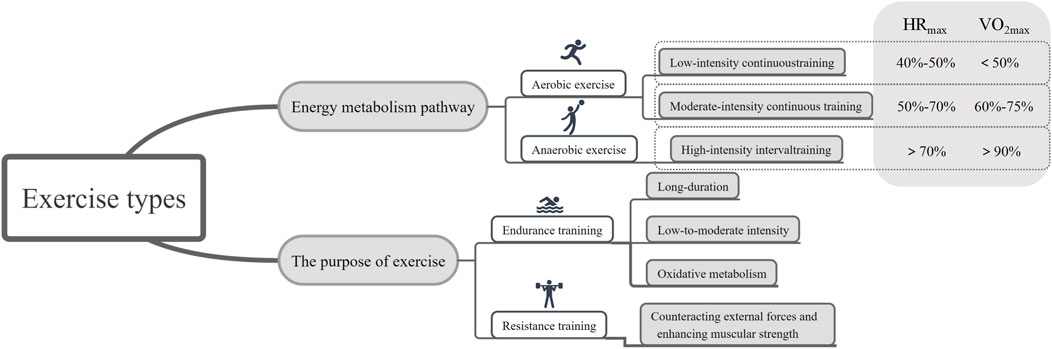
Figure 2. Regarding the specific classification of exercise and the criteria for its determination. (HRmax: maximum heart rate; VO2max: maximum volume of oxygen).
Aerobic exercise is a form of activity that generates energy through oxidative metabolism (Mueller et al., 2021). This type of exercise involves relatively low to moderate intensity over an extended period to ensure the body can supply enough oxygen to support energy production (Mueller et al., 2021). Aerobic exercise is characterized by lower intensity, safety, rhythmic, and sustained durations, with relatively minor stress on various organs, reducing the risk of exercise-related injuries (Mueller et al., 2021). Common aerobic exercise programs include low-intensity continuous training (LICT) and moderate-intensity continuous training (MICT) (Troosters et al., 2023). The intensity of exercise is typically measured using parameters such as maximum heart rate and maximum oxygen consumption (Blanks et al., 2019). For maximum heart rate (HRmax), low-intensity exercise falls within the 40%–50% range, while moderate-intensity exercise falls within the 50%–70% HRmax range (Blanks et al., 2019). Regarding the maximum volume of oxygen (VO2max), values below 60% are considered low intensity, and those between 60% and 75% are considered moderate intensity (Blanks et al., 2019).
Anaerobic exercise involves high-intensity, momentary bursts of muscle activity in an “oxygen-deprived” state (Liao et al., 2022). It is characterized by high-intensity loads and brief durations, making it challenging to sustain for extended periods, and recovery from fatigue is slower (Liao et al., 2022). Anaerobic exercise enhances muscle strength, improves adaptability, and serves as a primary source of muscle growth (Casado et al., 2023). The intensity of anaerobic exercise is relatively high, and the sustainable duration is short, resulting in high-intensity loads that can lead to muscle fatigue and soreness (Casado et al., 2023). Common anaerobic exercise programs include resistance training (RT) and high-intensity interval training (HIIT) (May et al., 2020). Recently, HIIT has gained popularity as a time-efficient exercise strategy that has been proven to improve cardiovascular risk factors in various populations (May et al., 2020). This training method employs alternating patterns of work and rest to enhance cardiorespiratory endurance, promote fat burning, and provide more efficient training effects in a shorter time (May et al., 2020). High-intensity training is identified by an HRmax exceeding 70% or VO2max exceeding 90% (Blanks et al., 2019).
Additionally, based on the primary training goals, exercise can be classified into endurance training (ET) and RT (Consitt et al., 2019). ET involves prolonged, continuous activities at relatively low loads, primarily relying on aerobic metabolism to produce energy (Rothschild and Bishop, 2020). It induces adaptations in the cardiovascular and musculoskeletal systems, supporting overall improvements in exercise capacity and performance (Miko et al., 2020). However, for older individuals who are overweight or obese, especially those with symptoms of osteoarthritis, regular ET may be uncomfortable and painful, necessitating the introduction of alternative forms of exercise (Cutrufello et al., 2020).
RT, on the other hand, offers various health benefits, supports body weight, and avoids imposing impact stress on joints (Schoenfeld et al., 2016). Therefore, RT may be an appealing exercise modality for overweight/obese older individuals. This type of training emphasizes resistance against external forces to enhance muscle strength, endurance, and mass, with each effort specifically targeting the resistance generated, designed to increase muscle strength and explosiveness (Carvalho et al., 2022).
Although most literature suggests that exercise can improve CVD, excessive endurance exercise (EEE) may have many potential adverse effects on cardiac structure and function (O'Keefe et al., 2012). Acutely, EEE can increase myocardial injury markers, lead to chamber dilation, and decrease right ventricular function (Levine, 2014). Chronically, concerns exist that this degree of EEE may lead to detrimental cardiac remodeling and fibrosis, as well as non-lethal arrhythmias, especially an increased risk of atrial fibrillation, and potentially more lethal ventricular arrhythmias (Nath et al., 2023). Recent studies also indicate that despite a more favorable overall profile of coronary heart disease risk in long-distance runners, they may have higher levels of atherosclerosis and coronary heart disease risk (Neumann et al., 2022; O'Keefe et al., 2021). However, the benefits of aerobic exercise on CVD mortality appear to be significantly diminished when running exceeds 30 miles per week or walking exceeds 46 miles per week (Schwartz et al., 2014). Even though that lack of physical activity is more prevalent than EEE in the overall population, the potential adverse effects may be more serious on a societal level for overall health and cardiovascular health (McCullough and Lavie, 2014). These studies also suggest that more is not necessarily better, and even low doses of aerobic exercise, particularly running, seem to confer benefits for long-term cardiovascular health and lifespan.
5 Mechanisms by which exercise regulates NLRP3 inflammasome activation
Exercise has long been acknowledged as an effective intervention in regulating the innate immune response (Pope and Wood, 2020). In general, LICT and MTCT have positive effects on the immune system, whereas HIIT tends to have the opposite effect (Xian et al., 2022). However, there is limited research on how exercise specifically influences the activity of the NLRP3 inflammasome.
From the perspective of material metabolism, many studies have reported that glucose and lipids can directly activate the NLRP3 inflammasome (Vandanmagsar et al., 2011; Zhang et al., 2021; Baik et al., 2023), while exercise can directly alleviate glucose and lipid metabolism, reducing the levels of blood sugar and lipids, thereby improving CVD. There is currently no literature reporting that exercise can directly regulate the activation pathway of NLRP3 inflammasome through glucose and lipid metabolism (Mardare et al., 2016; Ma et al., 2021). Therefore, it boldly speculates that the effect of exercise on NLRP3 inflammasome activation is likely to be exerted through the regulation of glucose and lipid metabolism.
From the mitochondrial standpoint, since most studies suggest that mitochondria are involved in regulating NLRP3 inflammasome activation (Chen et al., 2019; Hong et al., 2021), it is speculated that exercise adaptation of mitochondria may affect the NLRP3 inflammasome activity. Earlier studies demonstrated that MICT significantly decreased the expression of inflammatory cytokines TNF-α, IL-6, and monocyte chemoattractant protein-1 induced by metabolic disorders, coupled with an upregulation in mitochondrial proteins (Liu et al., 2017; Chen et al., 2018). Conversely, HIIT led to mitochondrial dysfunction and an augmentation in the secretion of pro-inflammatory factors (Memme et al., 2021). Moreover, additional investigations indicated that MICT facilitated mitochondrial biogenesis, bolstered antioxidant capacity, and restrained the overactivation of the NLRP3 inflammasome (Mason et al., 2020; Powers et al., 2020). Furthermore, aerobic exercise mitigated cardiac dysfunction by modulating the expression of proteins implicated in mitochondrial quality and NLRP3/caspase-1/IL-1β signaling (Salo et al., 1991). In pathological conditions, mitochondrial damage leads to increased production of mtROS (Chen et al., 2020). The elevated mtROS mediates the activation of the NLRP3 inflammasome through thioredoxin interacting protein (TXNIP) and thioredoxin (Zhang et al., 2021). These findings imply that MICT might diminish mitochondrial ROS production through the regulation of mitochondrial quality control, enhancement of mitochondrial function, and the facilitation of damaged mitochondria clearance. Consequently, this could inhibit the NLRP3 inflammasome pathway and alleviate exaggerated inflammatory responses.
From molecular mechanisms in the cell, the key factors in exercise-mediated improvement of CVD through the NLRP3 inflammasome may be related to the regulation of the NF-κB signaling pathway. Some studies have focused on the NF-κB pathway as a critical node to explore the molecular mechanisms among exercise and NLRP3 inflammasome (Wang et al., 2019; Zhou et al., 2022). For example, research has demonstrated that aerobic exercise is capable of reducing the expression of nicotinamide adenine dinucleotide phosphate oxidase 4 (NOX4), ROS, TNF-α, IL-18, NF-κB p65, and the NLRP3 inflammasome (Zhou et al., 2022). These findings suggest that exercise may ameliorate the pathological alterations in diabetes mellitus through the modulation of the NOX4/ROS/NF-κB/NLRP3 inflammasome signaling cascade (Zhou et al., 2022). In a separate study, Wang et al., 2019 observed that aerobic exercise suppresses the acetylation of forkhead box transcription factor O1 (FOXO1) in the brain tissue of diabetic rats, which in turn promotes the phosphorylation of FOXO1, thus inhibiting expression of NF-κB and NLRP3 inflammasome protein. This downregulation contributes to the inhibition of inflammatory responses, indicating that exercise may exert anti-inflammatory effects via the FOXO1/NF-κB/NLRP3 inflammasome pathway (Wang et al., 2019). Although studies have shown that exercise improves CVD through the NLRP3 inflammasome (Li et al., 2021; Zhou et al., 2022), further research is needed to elucidate the detailed molecular mechanisms by which exercise regulates the NLRP3 inflammasome.
6 Exercise, NLRP3 inflammasome, and CVD
6.1 Exercise improves AS and inhibits NLRP3 inflammasome
The pathogenesis of AS involves multiple processes, including endothelial dysfunction, low-density lipoprotein accumulation and oxidation, monocyte and lymphocyte recruitment, smooth muscle cell migration and proliferation, proinflammatory cytokine activation, and platelet adhesion (Zeng et al., 2019). Notably, the augmented release of inflammatory cytokines primarily attributed to endothelial cells, macrophages, and smooth muscle cell pyroptosis or NLRP3 inflammasome activation significantly contributes to AS formation and development (Zhaolin et al., 2019). Specifically, NLRP3 inflammasome-dependent pyroptosis triggers endothelial dysfunction, thereby acting as a catalyst for AS in these cells (Sun et al., 2017). This highlights the crucial role of NLRP3 inflammasome in promoting the release of inflammatory mediators and contributing to the pathological changes associated with AS.
Exercise can reduce the inflammatory death of local endothelial cells, slowing the development of AS plaques (Xu et al., 2019). A study found that voluntary wheel running, a natural type of aerobic exercise in the murine model could decrease the protein levels of the inflammasome components and markedly inhibit the caspase-1 activity in endothelial cells within the aorta of mice fed with a high-fat diet (HFD) (Lee et al., 2020). Recently, another study has demonstrated that exercise-induced a significant downregulation of m6A modification and methyltransferase-like 14 (Yang et al., 2023). This protein binds to the m6A sites of nuclear paraspeckle assembly transcript 1 (NEAT1) and promotes NEAT1 expression through subsequent YT521-B homology domain-containing 1, which transcriptionally promotes NLRP3 expression and endothelial pyroptosis (Yang et al., 2023). As a result, exercise effectively inhibits NLRP3 expression and endothelial pyroptosis, preventing AS plaque formation (Yang et al., 2023). In addition, it is widely recognized that fibroblast growth factor 21 (FGF21), a well-established negative risk factor for AS expressed in the aorta, exerts inhibitory effects on NLRP3 inflammasome activity, thereby attenuating aortic pyroptosis to prevent AS development (Zeng et al., 2020). Interestingly, aerobic exercise increases FGF21 sensitivity while downregulating the expression of pyroptosis-related proteins mediated by NLRP3 inflammasome in the aorta (Li et al., 2022). These findings suggest that activated FGF21 may be involved in aerobic exercise inhibiting NLRP3 inflammasome-mediated pyroptosis in the aorta. However, the current study has not investigated whether exercise improved AS via inhibiting NLRP3 inflammasome and pyroptosis in these cells, which needs further research in the future.
6.2 Exercise improves HHcy and inhibits NLRP3 inflammasome
Homocysteine (Hcy), a non-essential amino acid sulfur, is derived from methionine and is used for methylation of DNA/RNA methylation (Veeranki and Tyagi, 2013). HHcy refers to a condition where plasma Hcy levels exceed 15 μmol/L and has been associated with various diseases especially AS (Winchester et al., 2014). HHcy promotes the generation of ROS through mechanisms such as mixed disulfide formation and auto-oxidation (Veeranki and Tyagi, 2013; Winchester et al., 2014). Recent research has demonstrated that HHcy-induced activation of the NLRP3 inflammasome in macrophages contributes to vascular inflammation and AS by activating caspase-1-mediated pyroptosis (Wang et al., 2017). In addition, acid sphingomyelinase upregulation by Hcy promotes clustering of lipid rafts mediating the assembly of NADPH oxidase complex resulting in increased ROS generation followed by NLRP3 inflammasome activation leading to pyroptosis contributing towards the development of AS (Liu et al., 2022).
Although direct evidence is currently lacking regarding whether exercise specifically modulates NLRP3 inflammasome activation or pyroptosis about HHcy, it has been observed that exercise can lower Hcy levels (Liu et al., 2022). In a folate-restricted model of HHcy, exercise can reduce the increase in plasma Hcy levels by increasing betaine Hcy s-methyltransferase levels in the kidneys and promoting nonclassical remethylation to convert Hcy into methionine (Vincent et al., 2006; Neuman et al., 2013). Moreover, apart from reducing plasma Hcy levels, exercise holds the potential for mitigating Hcy-induced lipid peroxidation and ameliorating reductions in superoxide dismutase and catalase activity, both of which are implicated in the progression of AS (Neuman et al., 2013). These works suggest that exercise might also inhibit NLRP3 inflammasome activation or pyroptosis as a part of its overall impact on reducing HHcy-related complications. Given these findings, further investigation is warranted to explore whether exercise precisely influences NLRP3 inflammasome activation or pyroptosis and their role in HHcy management. Understanding this relationship could provide valuable insights into developing targeted interventions for individuals with elevated Hcy levels and associated CVD.
6.3 Exercise improves obesity and inhibits NLRP3 inflammasome
Obesity, commonly associated with chronic low-grade inflammation, is characterized by the pathological enlargement of adipose tissue (AT) primarily due to excess energy accumulation as fat (Spalding et al., 2008; Wada et al., 2017). Mice with HFD-induced obesity exhibit increased expression of caspase-1, ASC, and NLRP3. However, knocking out the Nlrp3 or Caspase-1 gene suppresses obesity-induced fat depot (Stienstra et al., 2011). Therefore, targeting the activation of NLRP3 inflammasome or pyroptosis represents a promising approach for improving obesity.
A study found that exercise decreased protein expression of inflammasome components (NLRP3 and caspase-1) in bone marrow-derived macrophages (BMDM) and AT isolated from mice with diet-induced obesity (Javaid et al., 2021). Another study investigated perform mice fed either a standard diet or an HFD and subjected to regular ET or RT and discovered that RT attenuated increased NLRP3 expression and reduced levels of IL-18 in isolated AT, while ET effectively reduced the expression of TNF-α and IL-18 in supernatant from AT, suggesting that exercise can reduce inflammasome activation in ATs and achieve systemic downregulation of inflammatory cytokines (Wada et al., 2017). Besides AT, endothelial dysfunction emerges early on in CVD associated with obesity (Stienstra et al., 2011; Wada et al., 2017). A study demonstrated that engaging in voluntary running while on an HFD led to a significant reduction in active caspase-1 levels within the endothelial cells lining the aorta when compared to sedentary mice on the same diet (Li et al., 2023). These findings indicate that voluntary running alleviates impaired blood vessel function via inhibiting NLRP3 inflammasome activation (Li et al., 2023). In addition to endothelial cells, exercise suppressed NLRP3 inflammasome activation as revealed by downregulated IL-1β and IL-18 in BMDM (Li et al., 2023). This body of research demonstrates that exercise exerts inhibitory effects on NLRP3 inflammasome activation across various cell types to ameliorate obesity.
It’s worth noting that a human study found that exercise reduces plasma IL-18 levels in obese individuals, indirectly suggesting the inhibition of the NLRP3 pathway through exercise to improve obesity (Stienstra et al., 2011). Furthermore, 8-week high-intensity and aerobic interval training (three times/week) in men and women with metabolic syndrome resulted in decreased IL-18 mRNA levels in abdominal AT and a numerical decrease in plasma IL-18 concentration (Stienstra et al., 2011; Stienstra et al., 2011; Stienstra et al., 2011; Stienstra et al., 2011). Similarly, a pilot study, conducted among thirty-seven obese individuals demonstrates that exercise intervention, primarily consisting of activities such as walking, jogging, and functional exercise circuits designed to enhance aerobic capacity and speed, leads to significant reductions in ASC mRNA expression levels when compared to the hypocaloric group without any form of exercise participation (Barrón et al., 2020). These findings indicate an inverse relationship between ASC mRNA expression and aerobic interventions. Another randomized controlled trial involving 36 obese inactive subjects further delineated the type of exercise intervention and demonstrated both HIIT and MICT significantly reduced NLRP3 gene expression in serum samples from all subjects, strongly suggesting that diversity intensity interval training can inhibit NLRP inflammasome in obese (Armannia et al., 2022).
Therefore, exercise is considered a crucial strategy for reducing inflammation and metabolic disorders associated with obesity. Further research will enhance understanding of the complex relationship between exercise and NLRP3 inflammasome, leading to more effective interventions for managing obesity-related diseases.
6.4 Exercise improves diabetes and inhibits NLRP3 inflammasome
Diabetes is a metabolic disease that poses a significant threat to human health. Type 2 diabetes, a significant risk factor for both microvascular and macrovascular diseases, accounts for 90% of diabetes cases and is a leading cause of death, particularly due to coronary heart disease (Einarson et al., 2018). Inflammatory processes play a crucial role in the development of complications associated with diabetes (Roncero-Ramos et al., 2018). Recently, NLRP3 inflammasome and pyroptosis have emerged as key contributors to insulin resistance (IR) (Roncero-Ramos et al., 2018). Peripheral blood-derived macrophages from drug-naïve patients with type 2 diabetes show increased expression of NLRP3 and ASC along with caspase-1 activation and IL-1β maturation (Lee et al., 2013). In vivo and in vitro studies have reported that high glucose stimulates IL-1β secretion in pancreatic β cells, resulting in their death through the activation of NLRP3 inflammasome (Zhou et al., 2010; Wu et al., 2019). The various modes of activating the NLRP3 inflammasome are fundamental factors influencing its complex effects on the progression of type 2 diabetes (Chen et al., 2021).
Engaging in aerobic exercise can improve IR and reduce the expression of NLRP3 and IL-1β in individuals with type 2 diabetes in aortic tissue, suggesting its positive impact on IR by inhibiting NLRP3 inflammasome (Hassanpour Soleimani et al., 2021). HMGB1 is a major pro-inflammatory cytokine released as a result of pyroptosis (Biguetti et al., 2019). This work also found that exercise can reduce the secretion of HMGB1, which is significantly increased in individuals with diabetes and contributes to disease progression (Hassanpour Soleimani et al., 2021). Previous studies have shown that exercise has the potential to lower HMGB-1 levels in circulation and tissues of diabetic patients, possibly by inhibiting NLRP3 inflammasome activation and pyroptosis (Haß et al., 2022; Haß et al., 2023). Furthermore, exercise decreased circulating levels of IL-1, which may potentially protect against IL-1-mediated destruction of β-cells (Stumvoll et al., 2005). Although there is currently no direct evidence on whether aerobic exercise specifically affects NLRP3 inflammasome activation or pyroptosis and thus decreases levels of blood glucose, these findings highlight the significant role played by exercise in regulating IR through its impact on NLRP3 inflammasome and pyroptosis.
6.5 Exercise improves DCM and inhibits NLRP3 inflammasome
DCM is a distinct cardiac phenotype observed in diabetic patients characterized by structural changes such as cardiac hypertrophy, cardiomyocyte death, and fibrosis, as well as functional abnormalities (Sun et al., 2021). The molecular mechanisms underlying DCM involve various factors including hyperglycemia, IR, fatty acids, oxidative stress, mitochondrial dysfunction, inflammation, and endothelial dysfunction (Chen et al., 2020). In particular, inflammation is believed to be present in the early stages of diabetes and plays a crucial role in promoting DCM (Luo et al., 2017). Emerging evidence has verified that NLRP3 inflammasome-mediated cardiomyocyte pyroptosis is a key participant in DCM (Xie et al., 2020). Human diabetic hearts also exhibit elevated activation of the NLRP3 inflammasome and cardiac pyroptosis compared to non-diabetic heart tissues (Zhang et al., 2015; Xie et al., 2020). Moreover, high glucose levels (35 mM) significantly induce increased protein expression of NLRP3, caspase-1, and IL-1β accompanied by noticeable cardiomyocyte pyroptosis, leading to loss of contractile units and cardiac dysfunction (Zhang et al., 2015). In contrast, silencing the Nlrp3 gene ameliorates cardiac inflammation and pyroptosis and improves cardiac function by ameliorating cardiac inflammation and pyroptosis both in vivo and in vitro experiments (Chen et al., 2020).
Exercise intervention has been shown to effectively prevent and treat DCM by modulating the NLRP3 inflammasome. For example, the expressions of NLRP3, caspase-1-p20, caspase-1p20/caspase-1, and IL-1β were increased in the myocardium of HFD-induced obese mice (Lee et al., 2018). However, treadmill exercise inhibited these parameters (Sun et al., 2021). This demonstrates that exercise training can prevent obesity-induced cardiac inflammasome formation, pyroptosis activation, and pro-inflammatory response (Sun et al., 2021). In addition, recent studies in DCM mice have demonstrated that although exercise has a limited impact on interstitial fibrosis, it can effectively reverse cardiac dysfunction by reducing the activity of NLRP3 inflammasome and inhibiting pyroptosis (Takahashi, 2019; Zhang et al., 2019). Moreover, in DCM rat models, elevated expression levels of the P2X7 receptor, NLRP3, caspase-1, and serum IL-1β were observed in the myocardium (Wang et al., 2022). However, following a 12-week treadmill running regimen in these rats, improvements were observed in terms of collagen deposition, cell disorder, as well as the expression levels of NLRP3, caspase-1, P2X7 receptor, and IL-1β within their heart (Zhang et al., 2015). Similarly, aerobic exercise also can inhibit the thioredoxin interacting protein (TXNIP)/NLRP3 inflammasome pathway and alleviate endothelial dysfunction in atherosclerotic coronary arterioles (Hong et al., 2018). These findings suggest that aerobic exercise can effectively mitigate fibrosis in the hearts subjected to an HFD and inhibit the activation of the NLRP3 inflammasome and pyroptosis in the myocardium. The effectiveness of exercise intervention on the NLRP3 inflammasome depends on the duration and intensity of exercise (Hong et al., 2018; Khakroo et al., 2019). Chronic exercise with moderate intensity significantly decreases the expression of the NLRP3 gene and levels of IL-1β, and IL-18 cytokines in serum (Khakroo et al., 2019). Conversely, chronic exercise with high intensity leads to a significant increase in gene expression of NLRP3 and levels of IL-1β, and IL-18 cytokines in serum (Khakroo et al., 2019). Therefore, personalized exercise regimens are necessary as there are currently no available guidelines; further research is needed.
6.6 Exercise improves hypertension and inhibits NLRP3 inflammasome
Hypertension is a potentially fatal yet preventable risk factor for CVD and accounts for the majority of cardiovascular mortality (Deussen and Kopaliani, 2023). Persistent inflammation plays a pivotal role in hypertension development, with activation of the inflammasome and pyroptosis being potential contributors to its onset (De et al., 2021). NLRP3 inflammasome activities have been implicated in various cell types associated with pulmonary hypertension, including pulmonary arterial smooth muscle cells, pulmonary artery endothelial cells, and systemic hypertension (Haß et al., 2023). Additionally, higher serum levels of IL-1β were observed in patients with hypertension compared to normotensive controls (Wu et al., 2022).
Exercise is commonly suggested as a lifestyle adjustment for individuals with hypertension due to various factors including inhibition of inflammation (Newman and Verdin, 2014; Chakraborty et al., 2018; Kong et al., 2021). β-Hydroxybutyrate (β-OHB) ester is primarily synthesized in the liver and transported to extrahepatic tissues, traditionally recognized as a crucial metabolic fuel during starvation periods (Newman and Verdin, 2014). Contemporary evidence indicates that ketone bodies like β-OHB can maintain physiological homeostasis by inhibiting NLRP3-inflammasome-mediated inflammation (Kong et al., 2021). Recently, a non-targeted metabolomics approach revealed nutritional intervention with β-OHB reversed the high salt-induced adverse effects including renal NLRP3-mediated inflammation, fibrosis, and hypertension (Chakraborty et al., 2018). Interestingly, exercise is associated with increased circulating levels of β-OHB (Luo et al., 2021). Therefore, exercise may raise β-OHB levels and subsequently inhibit renal NLRP3 inflammasome activation, thereby alleviating hypertension and preserving kidney function. Another in vivo study provided direct evidence of the impact of exercise training on the downregulation of NF-κB and NLRP3 pathways in the mesenteric artery of spontaneously hypertensive rats (SHR) (Luo et al., 2021), which revealed that three intensity training intensities from low to high significantly inhibited the expression of NLRP3 inflammasome component and NF-κB within the mesenteric artery and alleviate BP in SHR rat (Luo et al., 2021). In addition to the SHR rat model, Bal et al., 2022 discovered that regulated aerobic exercise also effectively reduces BP and suppresses protein expression of NLRP3, IL-1β, and caspase-1 in the heart within the deoxycorticosterone-acetate salt hypertension model. These findings from the diversity hypertension model demonstrate that exercise training effectively attenuates NLRP3 inflammasome activity, highlighting its potential as a therapeutic intervention for hypertension.
6.7 Exercise improves MI and inhibits NLRP3 inflammasome
MI, a common condition, refers to the death of heart muscle cells caused by a blockage in the coronary arteries (Wang et al., 2020). Following a heart attack, inflammatory reactions occur, characterized by the release of inflammatory cells, cytokines, and chemical hormones (Mezzaroma et al., 2011; Wang et al., 2020). These processes contribute to cardiac dysfunction, damage to the heart muscle, and remodeling (Mezzaroma et al., 2011; Wang et al., 2020). Indeed, NLRP3 inflammasome and pyroptosis-mediated inflammation have been reported to contribute to MI progression (Sandanger et al., 2013). Sandanger et al., 2013 first showed an increase in NLRP3 inflammasome activity in the left ventricle of the heart after MI. Furthermore, it appears that interfering with NLRP3 inflammasome signaling can prevent and mitigate damage caused by MI (Sandanger et al., 2013).
The research has shown that exercise training can delay the onset of ischemic reperfusion injury and MI (Thomas et al., 2016). In other words, exercise acts like ischemia preconditioning, which stimulates beneficial cellular responses. Azam Ahmadi et al., 2022 found HIIT can effectively decrease heart injury and NLRP3 expression in rats with MI. Interestingly, dynamin-related protein 1 (Drp1), an essential mitochondrial fission protein, can activate NLRP3 inflammasome through ROS generation after MI (Ahmadi et al., 2022; Jiang et al., 2022). However, the Azam Ahmadi team’s work demonstrates exercise preconditioning (EP) in a short period did not affect Drp1 (Ahmadi et al., 2022), while inhibiting NLRP3 expression. This finding indicates that EP in a short period is required to inhibit NLRP3 inflammasome independently of Drp1; the reduction of NLRP3 can occur through other mechanisms (Jiang et al., 2022).
6.8 Exercise affects HF and regulates NLRP3 inflammasome
HF means that the heart is unable to pump sufficiently to sustain blood flow to meet the needs of the body (Sacco et al., 2014). It represents the advanced stage of various CVD, including myocarditis, MI, cardiomyopathy, hypertension, atrial fibrillation, valvular heart disease, alcohol abuse, and infection (Bracey et al., 2013). Recent studies on calcineurin transgene mice have shown elevated mRNA of NLRP3 and enhanced cleavage of caspase-1 along with cardiac hypertrophy and ventricular dilatation (Bracey et al., 2013; Napodano et al., 2023). Genetic ablation of NLRP3 or administration of IL-1 receptor antagonist has been found to attenuate cardiac inflammation and systolic dysfunction (Bracey et al., 2013; Zhang et al., 2014; Napodano et al., 2023). Additionally, activation of NLRP3 inflammasome has been observed in LPS-stimulated cardiac fibroblasts and myofibroblasts, suggesting that its potential contribution to myocardial dysfunction through NLRP3 inflammasome or pyroptosis (Zhang et al., 2014; Boza et al., 2016). Although more research is needed to fully understand how the NLRP3 inflammasome or pyroptosis contributes to HF pathogenesis and progression; recent studies indicate that targeting this pathway could lead to new treatments for this debilitating condition (Lu et al., 2022; Xin et al., 2022).
Studies have shown that EP can regulate the NLRP3 inflammasome, reducing downstream inflammatory cytokines and protecting the heart (Li et al., 2020; Ma et al., 2021; Zhang et al., 2022). Li et al., 2020 found that moderate-intensity EP is more effective in protecting cardiac function and inhibiting the expression of TXNIP, NLRP3, NF-ĸB p65, and caspase-1 in the heart. As expected with moderate-intensity exercise, 8 weeks of aerobic exercise at this level inhibited protein expression related to myocardial NLRP3/caspase-1/IL-1β signaling pathways in mice with myocardial hypertrophy (Ma et al., 2021). In addition, Sandanger et al., 2013 show that the NLRP3 inflammasome is upregulated in myocardial fibroblasts post-MI, and maybe a significant contributor to infarct size development during ischemia-reperfusion. These findings highlight the potential therapeutic value of moderate-intensity exercise for cardiovascular health. However, exhaustive exercise (EE), characterized by sustained high-intensity exercise commonly practiced by athletes and soldiers alike, may lead to adverse reactions such as myocardial inflammation. It is reported that EE can increase NLRP3 expression and induce cardiac dysfunction which can be mitigated by EP at different intensities (Zhang et al., 2015). Importantly, moderate-intensity EP has cardioprotective effects on ventricular systolic and diastolic functions, indicating its superior efficacy compared to other intensities (Zhang et al., 2014).
Importantly, a clinical trial with 54 HF patients showed that exercise increased ASC methylation, decreased IL-1β and ASC mRNA levels compared to the control group in plasma, suggesting that exercise may improve HF via epigenetic regulation of ASC (Butts et al., 2018). Recently, our research team has reported for the first time that NLRP3 inflammasome-mediated pyroptosis contributes to the pathogenesis of non-ischemic dilated cardiomyopathy in cellular, murine, and human models (Zeng et al., 2020). Furthermore, numerous studies have investigated exercise intervention in patients with dilated cardiomyopathy and have demonstrated its safety and efficacy in improving exercise capacity and quality of life among these individuals (Stolen et al., 2003; Beer et al., 2008). Consequently, it is meaningful to investigate whether exercise can exert inhibitory effects on NLRP3 inflammasome activation and subsequent pyroptotic cell death in dilated cardiomyopathy. Preliminary data suggest that exercise may effectively inhibit NLRP3 inflammasome activation in dilated cardiomyopathy (unpublished data).
Although these experiments indicate that exercise improves HF by inhibiting NLRP3 inflammasome activation, there is still debate (Butts et al., 2018). For example, previous work reported that exercise training consisting of three sessions per week lasting for 60 min each, including aerobic exercises, strength exercises targeting major muscle groups, and stretching exercises have no effect on serum levels of pro-inflammatory cytokines (TNF-α, IL-1β, IL-8, and monocyte chemoattractant protein-1) of patients with severe Chagas cardiomyopathy (Rodrigues Junior et al., 2020), suggesting that exercise training may benefit patients with severe Chagas cardiomyopathy independent of its impact on inflammasome (Rodrigues Junior et al., 2020).
Most studies suggest that exercise improves HF by inhibiting the activation of NLRP3 inflammasomes. Nevertheless, a minority of research suggests that engaging in activities like EE might potentially worsen the progression of HF. It is imperative for future investigations to thoroughly examine the role played by NLRP3 inflammasome activation in exercise-induced exacerbation of HF.
7 Summary and prospects
Exercise plays an important regulatory role in the development of various CVD by influencing the activation of the NLRP3 inflammasome (Figure 3). This impact has been observed in different types of cells and tissues, both in vivo and in vitro models of CVD (Table 1). This review highlights the close relationship between aerobic exercise, NLRP3 inflammasome, and CVD. It suggests that aerobic exercise can alleviate pyroptosis and improve cardiovascular-related diseases by modulating the NLRP3 inflammatory signaling pathway. Different patterns of exercise have varying impacts on the NLRP3 inflammasome; therefore, further research is needed to determine their optimal effects on specific types of cells and their underlying molecular mechanisms involving the NLRP3 inflammasome. Currently, there is a greater emphasis on animal experiments investigating the influence of exercise on NLRP3 inflammasome or cell pyroptosis while human studies are limited with small sample sizes in this area. Conducting comprehensive research on humans would provide a scientifically sound basis for understanding how exercise regulates NLRP3 inflammasome activity and promotes overall health. Hence, there is a need for methodologically rigorous large-scale human studies to determine ideal patterns of exercise.
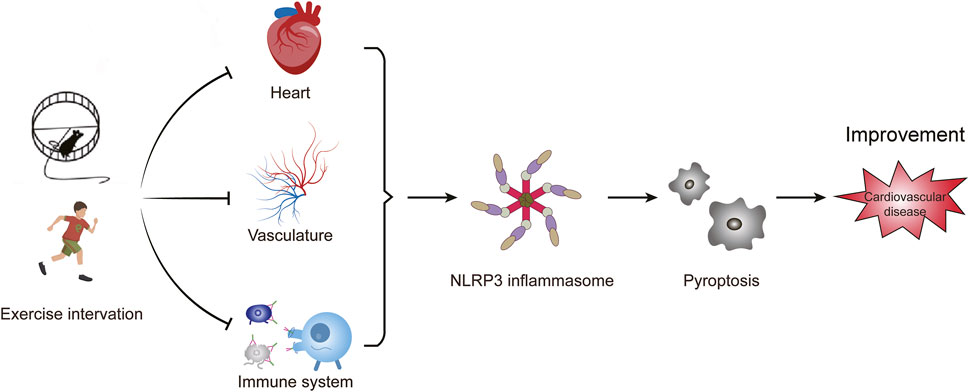
Figure 3. Exercise intervention affects cardiovascular disease by NLRP3 inflammasome-mediated pyroptosis. The activation of NLRP3 inflammasome induces pyroptosis, which promotes vascular inflammation and heart remodeling, and ultimately leads to cardiovascular diseases. Exercise affects the progression of cardiovascular diseases by inhibiting the activation of the NLRP3 inflammasome.
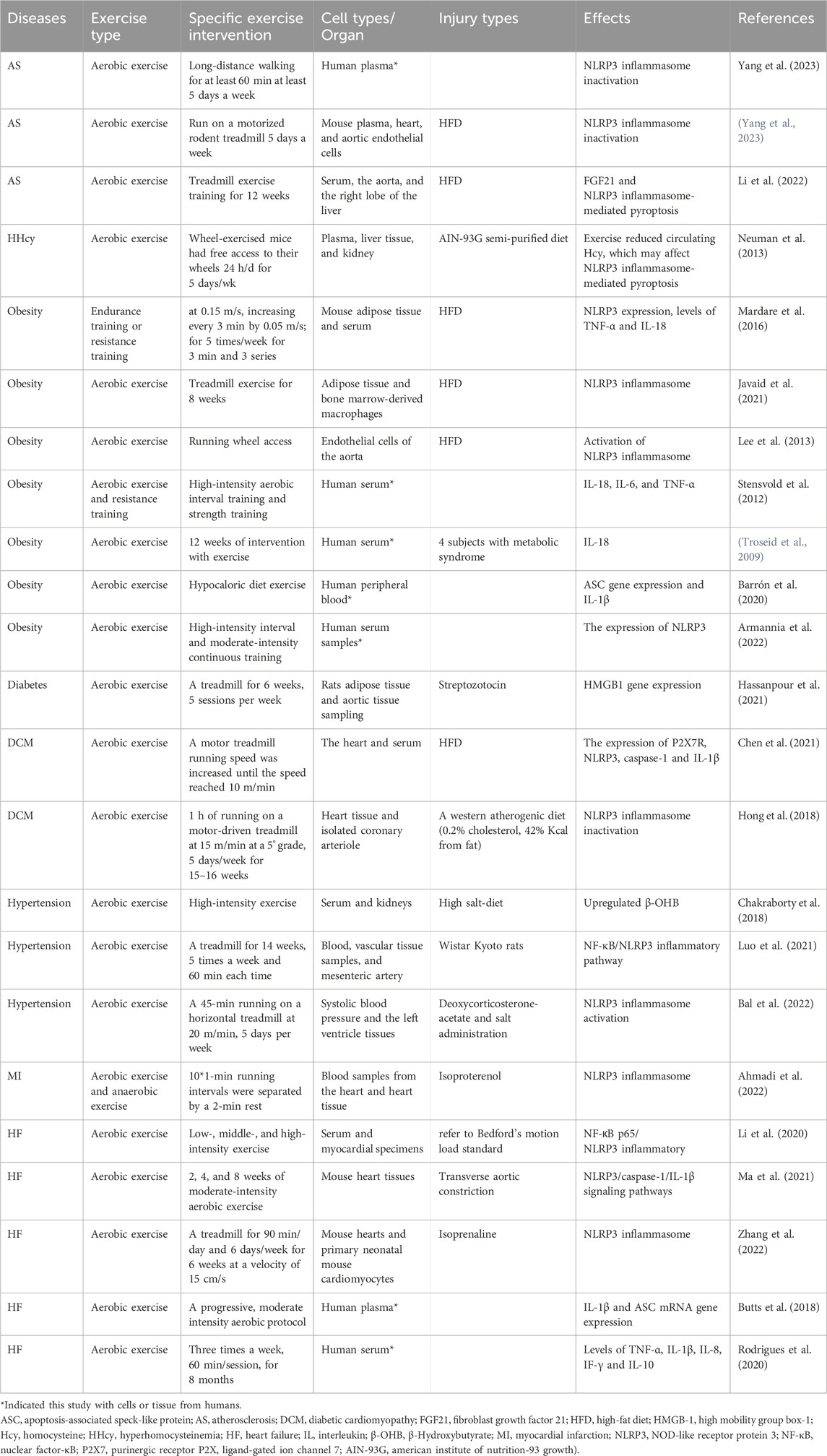
Table 1. Types of cells and tissue injury subjected to NLRP3 inflammasome by exercise intervention in various CVD.
The investigation of drugs targeting the NLRP3 inflammasome in clinical trials for CVD has been conducted. For example, the canakinumab anti-inflammatory thrombosis outcome study trial demonstrated the efficacy of IL-1-targeting therapy in preventing atherothrombotic events, indicating the potential of targeting the NLRP3 inflammasome (Ma et al., 2021; Ridker and Rane, 2021). However, the high cost of canakinumab limits its widespread use in the future. Additionally, long-term suppression of IL-1 signaling may have adverse effects such as increased susceptibility to infections and disturbances in immune homeostasis (Hettwer et al., 2022). Common cardiovascular drugs such as statins, beta-blockers, sodium-glucose cotransporter 2 inhibitors, and glucagon-like peptide 1 agonists may modulate NLRP3 inflammasome activity through different mechanisms, exerting protective effects in CVD (Terentes-Printzios et al., 2022). However, these classical medications carry certain side effects on gastrointestinal function and are prone to causing symptoms like hypotension and arrhythmias (Vaiciuleviciute et al., 2021). Interestingly, research suggests that exercise effectively modulates NLRP3 inflammasome activation, leading to improvements in AS and other CVD (Vaiciuleviciute et al., 2021; Terentes-Printzios et al., 2022). This therapeutic approach is relatively simple, feasible, and considered safe compared to using NLRP3 inhibitors and classical medications for CVD. Therefore, combining medication treatment with exercise intervention offers a potential strategy to improve the quality of life for patients.
Author contributions
PD: Data curation, Writing–original draft, Investigation. YS: Data curation, Writing–original draft, Investigation. YY: Investigation, Resources, Supervision, Writing–review and editing. CZ: Conceptualization, Funding acquisition, Investigation, Supervision, Visualization, Writing–original draft, Writing–review and editing.
Funding
The author(s) declare that financial support was received for the research, authorship, and/or publication of this article. This work was supported by the National Natural Science Foundation of China (grant number 82100379), Guangzhou Science and Technology Project (grant number 202201010148), Guangdong Provincial Medical Research Fund Project in 2021 (grant number A2021120), Zhuhai People’s Hospital Clinical Research Promotion Plan Yucai Project (2023LCTS-42) and National Key Clinical Specialty Construction Project (Clinical Pharmacy) and High-Level Clinical Key Specialty (Clinical Pharmacy) in Guangdong Province.
Conflict of interest
The authors declare that the research was conducted in the absence of any commercial or financial relationships that could be construed as a potential conflict of interest.
Publisher’s note
All claims expressed in this article are solely those of the authors and do not necessarily represent those of their affiliated organizations, or those of the publisher, the editors and the reviewers. Any product that may be evaluated in this article, or claim that may be made by its manufacturer, is not guaranteed or endorsed by the publisher.
References
Ahmadi, A., Kashef, M., Rajabi, H., and Salehpour, M. (2022). Effects of exercise preconditioning on NLRP3 and mitochondrial fission in isoproterenol-induced myocardial infarcted rats. Comp. Clin. Pathol. 32, 289–297. doi:10.1007/s00580-022-03397-3
Alten, R., Mischkewitz, M., and Nitschmann, S. (2020). Febuxostat or allopurinol in patients with gout: cardiovascular safety of febuxostat and allopurinol in patients with gout and cardiovascular morbidities (CARES). Internist (Berl). 61 (5), 530–532. doi:10.1007/s00108-020-00766-4
Armannia, F., Ghazalian, F., Shadnoush, M., Keyvani, H., and Gholami, M. (2022). Effects of high-intensity interval vs. moderate-intensity continuous training on body composition and gene expression of ACE2, NLRP3, and FNDC5 in obese adults: a randomized controlled trial Med. J. Islam Repub. Iran. 36, 161. doi:10.47176/mjiri.36.161
Baik, S. H., Ramanujan, V. K., Becker, C., Fett, S., Underhill, D. M., and Wolf, A. J. (2023). Hexokinase dissociation from mitochondria promotes oligomerization of VDAC that facilitates NLRP3 inflammasome assembly and activation. Sci. Immunol. 8 (84), eade7652. doi:10.1126/sciimmunol.ade7652
Bal, N. B., Bostanci, A., Sadi, G., Dönmez, M. O., Uludag, M. O., and Demirel, Y. E. (2022). Resveratrol and regular exercise may attenuate hypertension-induced cardiac dysfunction through modulation of cellular stress responses. Life Sci. 296, 120424. doi:10.1016/j.lfs.2022.120424
Barrón, C. E., González, B. K., Rosales, C. G., Mora, J. A., Hernández, C. I., and Martínez, L. E. (2020). Low-grade chronic inflammation is attenuated by exercise training in obese adults through down-regulation of ASC gene in peripheral blood: a pilot study. Genes. Nutr. 15, 15. doi:10.1186/s12263-020-00674-0
Beer, M., Wagner, D., Myers, J., Sandstede, J., Köstler, H., Hahn, D., et al. (2008). Effects of exercise training on myocardial energy metabolism and ventricular function assessed by quantitative phosphorus-31 magnetic resonance spectroscopy and magnetic resonance imaging in dilated cardiomyopathy. J. Am. Coll. Cardiol. 51 (19), 1883–1891. doi:10.1016/j.jacc.2007.09.075
Biguetti, C. C., Cavalla, F., Silveira, E. V., Tabanez, A. P., Francisconi, C. F., Taga, R., et al. (2019). HGMB1 and RAGE as essential components of ti osseointegration process in mice. Front. Immunol. 10, 709. doi:10.3389/fimmu.2019.00709
Blanks, A. M., Rodriguez-Miguelez, P., Looney, J., Tucker, M. A., Jeong, J., Thomas, J., et al. (2019). Whole body vibration elicits differential immune and metabolic responses in obese and normal weight individuals. Brain Behav. Immun. Health 1, 100011. doi:10.1016/j.bbih.2019.100011
Bockstiegel, J., Engelhardt, J., and Weindl, G. (2023). P2X7 receptor activation leads to NLRP3-independent IL-1β release by human macrophages. Cell. Commun. Signal 21 (1), 335. doi:10.1186/s12964-023-01356-1
Boza, P., Ayala, P., Vivar, R., Humeres, C., Cáceres, F. T., Muñoz, C., et al. (2016). Expression and function of toll-like receptor 4 and inflammasomes in cardiac fibroblasts and myofibroblasts: IL-1β synthesis, secretion, and degradation. Mol. Immunol. 74, 96–105. doi:10.1016/j.molimm.2016.05.001
Bracey, N. A., Beck, P. L., Muruve, D. A., Hirota, S. A., Guo, J., Jabagi, H., et al. (2013). The Nlrp3 inflammasome promotes myocardial dysfunction in structural cardiomyopathy through interleukin-1β. Exp. Physiol. 98, 462–472. doi:10.1113/expphysiol.2012.068338
Butts, B., Butler, J., Dunbar, S. B., Corwin, E., and Gary, R. A. (2018). Effects of exercise on ASC methylation and IL-1 cytokines in heart failure. Med. Sci. Sports Exerc 50, 1757–1766. doi:10.1249/MSS.0000000000001641
Carvalho, L., Junior, R. M., Barreira, J., Schoenfeld, B. J., Orazem, J., and Barroso, R. (2022). Muscle hypertrophy and strength gains after resistance training with different volume-matched loads: a systematic review and meta-analysis. Appl. Physiol. Nutr. Metab. 47 (4), 357–368. doi:10.1139/apnm-2021-0515
Casado, A., Foster, C., Bakken, M., and Tjelta, L. I. (2023). Does lactate-guided threshold interval training within a high-volume low-intensity approach represent the "next step" in the evolution of distance running training? Int. J. Environ. Res. Public Health 20 (5), 3782. doi:10.3390/ijerph20053782
Chakraborty, S., Galla, S., Cheng, X., Yeo, J. Y., Mell, B., Singh, V., et al. (2018). Salt-responsive metabolite, β-hydroxybutyrate, attenuates hypertension. Cell. Rep. 25, 677–689. doi:10.1016/j.celrep.2018.09.058
Chan, A. H., Burgener, S. S., Vezyrgiannis, K., Wang, X., Acklam, J., Von Pein, J. B., et al. (2023). Caspase-4 dimerisation and D289 auto-processing elicit an interleukin-1β-converting enzyme. Life Sci. Alliance 6 (10), e202301908. doi:10.26508/lsa.202301908
Chan, A. H., and Schroder, K. (2020). Inflammasome signaling and regulation of interleukin-1 family cytokines. J. Exp. Med. 217, e20190314. doi:10.1084/jem.20190314
Chang, M. X. (2023). Emerging mechanisms and functions of inflammasome complexes in teleost fish. Front. Immunol. 14, 1065181. doi:10.3389/fimmu.2023.1065181
Chen, B., Yan, Y., Yang, Y., Cao, G., Wang, X., Wang, Y., et al. (2022). A pyroptosis nanotuner for cancer therapy. Nat. Nanotechnol. 17 (7), 788–798. doi:10.1038/s41565-022-01125-0
Chen, H., Tran, D., Yang, H. C., Nylander, S., Birnbaum, Y., and Ye, Y. (2020). Dapagliflozin and ticagrelor have additive effects on the attenuation of the activation of the NLRP3 inflammasome and the progression of diabetic cardiomyopathy: an AMPK-mTOR interplay. Cardiovasc Drugs Ther. 34 (4), 443–461. doi:10.1007/s10557-020-06978-y
Chen, L., Dong, J., Liao, S., Wang, S., Wu, Z., Zuo, M., et al. (2022). Loss of Sam50 in hepatocytes induces cardiolipin-dependent mitochondrial membrane remodeling to trigger mtDNA release and liver injury. Hepatology 76 (5), 1389–1408. doi:10.1002/hep.32471
Chen, W. K., Tsai, Y. L., Shibu, M. A., Shen, C. Y., Chang-Lee, S. N., Chen, R. J., et al. (2018). Exercise training augments Sirt1-signaling and attenuates cardiac inflammation in D-galactose induced-aging rats. Aging 10, 4166–4174. doi:10.18632/aging.101714
Chen, X., Li, H., Wang, K., Liang, X., Wang, W., Hu, X., et al. (2019). Aerobic exercise ameliorates myocardial inflammation, fibrosis and apoptosis in high-fat-diet rats by inhibiting P2X7 purinergic receptors. Front. Physiol. 10, 1286. doi:10.3389/fphys.2019.01286
Chen, X., Zhang, D., Li, Y., Wang, W., Bei, W., and Guo, J. (2021). NLRP3 inflammasome and IL-1β pathway in type 2 diabetes and atherosclerosis: friend or foe? Pharmacol. Res. 173, 105885. doi:10.1016/j.phrs.2021.105885
Chen, Y., Hua, Y., Li, X., Arslan, I. M., Zhang, W., and Meng, G. (2020). Distinct types of cell death and the implication in diabetic cardiomyopathy. Front. Pharmacol. 11, 42. doi:10.3389/fphar.2020.00042
Consitt, L. A., Dudley, C., and Saxena, G. (2019). Impact of endurance and resistance training on skeletal muscle glucose metabolism in older adults. Nutrients 11 (11), 2636. doi:10.3390/nu11112636
Cutrufello, P. T., Benson, B. A., and Landram, M. J. (2020). The effect of music on anaerobic exercise performance and muscular endurance. J. Sports Med. Phys. Fit. 60 (3), 486–492. doi:10.23736/S0022-4707.19.10228-9
De, M. C., Pelegrín, P., Baroja, M. A., and Cuevas, S. (2021). Emerging role of the inflammasome and pyroptosis in hypertension. Int. J. Mol. Sci. 22, 1064. doi:10.3390/ijms22031064
Deussen, A., and Kopaliani, I. (2023). Targeting inflammation in hypertension. Curr. Opin. Nephrol. Hypertens. 32, 111–117. doi:10.1097/MNH.0000000000000862
Diaz-Del-Olmo, I., Worboys, J., Martin-Sanchez, F., Gritsenko, A., Ambrose, A. R., Tannahill, G. M., et al. (2021). Internalization of the membrane attack complex triggers NLRP3 inflammasome activation and IL-1β secretion in human macrophages. Front. Immunol. 12, 720655. doi:10.3389/fimmu.2021.720655
Dilucca, M., Ramos, S., Shkarina, K., Santos, J. C., and Broz, P. (2021). Guanylate-binding protein-dependent noncanonical inflammasome activation prevents burkholderia thailandensis-induced multinucleated giant cell formation. mBio 12 (4), e0205421. doi:10.1128/mBio.02054-21
Einarson, T. R., Acs, A., Ludwig, C., and Panton, U. H. (2018). Prevalence of cardiovascular disease in type 2 diabetes: a systematic literature review of scientific evidence from across the world in 2007-2017. Cardiovasc Diabetol. 17, 83. doi:10.1186/s12933-018-0728-6
Fu, J., and Wu, H. (2023). Structural mechanisms of NLRP3 inflammasome assembly and activation. Annu. Rev. Immunol. 41, 301–316. doi:10.1146/annurev-immunol-081022-021207
Haidar, A., and Horwich, T. (2023). Obesity, cardiorespiratory fitness, and cardiovascular disease. Curr. Cardiol. Rep. 25 (11), 1565–1571. doi:10.1007/s11886-023-01975-7
Han, X., Ni, J., Wu, Z., Wu, J., Li, B., Ye, X., et al. (2020). Myeloid-specific dopamine D2 receptor signalling controls inflammation in acute pancreatitis via inhibiting M1 macrophage. Br. J. Pharmacol. 177 (13), 2991–3008. doi:10.1111/bph.15026
Hassanpour Soleimani, S., Abbassi Daloii, A., Abdi, A., and Zilaei Bori, S. (2021). Effect of a 6-week aerobic exercise program on high-mobility group box 1 gene expression in aortic tissue of diabetic rats. Q. Horizon Med Sci. 27, 82–97. doi:10.32598/hms.27.1.2378.1
Haß, U., Heider, S., Kochlik, B., Herpich, C., Pivovarova-Ramich, O., and Norman, K. (2023). Effects of exercise and omega-3-supplemented, high-protein diet on inflammatory markers in serum, on gene expression levels in PBMC, and after ex vivo whole-Blood LPS stimulation in old adults. Int. J. Mol. Sci. 24 (2), 928. doi:10.3390/ijms24020928
Haß, U., Kochlik, B., Herpich, C., Rudloff, S., and Norman, K. (2022). Effects of an omega-3 supplemented, high-Protein diet in combination with vibration and resistance exercise on muscle power and inflammation in old adults: a pilot randomized controlled trial. Nutrients 14 (20), 4274. doi:10.3390/nu14204274
Hettwer, J., Hinterdobler, J., Miritsch, B., Deutsch, M. A., Li, X., Mauersberger, C., et al. (2022). Interleukin-1β suppression dampens inflammatory leucocyte production and uptake in atherosclerosis. Cardiovasc Res. 118 (13), 2778–2791. doi:10.1093/cvr/cvab337
Hong, J., Kim, K., Park, E., Lee, J., Markofski, M. M., Marrelli, S. P., et al. (2018). Exercise ameliorates endoplasmic reticulum stress-mediated vascular dysfunction in mesenteric arteries in atherosclerosis. Sci. Rep. 8 (1), 7938. doi:10.1038/s41598-018-26188-9
Hong, J., Park, E., Lee, J., Lee, Y., Rooney, B. V., and Park, Y. (2021). Exercise training mitigates ER stress and UCP2 deficiency-associated coronary vascular dysfunction in atherosclerosis. Sci. Rep. 11, 15449. doi:10.1038/s41598-021-94944-5
Huang, L. S., Anas, M., Xu, J., Zhou, B., Toth, P. T., Krishnan, Y., et al. (2023). Endosomal trafficking of two-pore K+ efflux channel TWIK2 to plasmalemma mediates NLRP3 inflammasome activation and inflammatory injury. Elife. 12, e83842. doi:10.7554/eLife.83842
Javaid, H. M. A., Sahar, N. E., Zhuge, D. L., and Huh, J. Y. (2021). Exercise inhibits NLRP3 inflammasome activation in obese mice via the anti-inflammatory effect of meteorin-like. Cells 10, 3480. doi:10.3390/cells10123480
Jiang, H., Chen, F., Song, D., Zhou, X., Ren, L., and Zeng, M. (2022). Dynamin-related protein 1 is involved in mitochondrial damage, defective mitophagy, and NLRP3 inflammasome activation induced by MSU crystals. Oxid. Med. Cell. Longev. 2022, 5064494. doi:10.1155/2022/5064494
Kar, S., Shahshahan, H. R., Hackfort, B. T., Yadav, S. K., Yadav, R., Kambis, T. N., et al. (2019). Exercise training promotes cardiac hydrogen sulfide biosynthesis and mitigates pyroptosis to prevent high-fat diet-induced diabetic cardiomyopathy. Antioxidants (Basel) 8, 638. doi:10.3390/antiox8120638
Khakroo, A. I., Rahmani, N. F., and Lombardi, G. (2019). The effects of acute and chronic aerobic activity on the signaling pathway of the inflammasome NLRP3 complex in young men. Med. Kaunas. 55, 105. doi:10.3390/medicina55040105
Kodi, T., Sankhe, R., Gopinathan, A., Nandakumar, K., and Kishore, A. (2024). New insights on NLRP3 inflammasome: mechanisms of activation, inhibition, and epigenetic regulation. J. Neuroimmune Pharmacol. 19 (1), 7. doi:10.1007/s11481-024-10101-5
Kong, G., Liu, J., Li, R., Lin, J., Huang, Z., Yang, Z., et al. (2021). Ketone metabolite β-hydroxybutyrate ameliorates inflammation after spinal cord injury by inhibiting the NLRP3 inflammasome. Neurochem. Res. 46, 213–229. doi:10.1007/s11064-020-03156-2
Konishi, M., Kojima, S., Uchiyama, K., Yokota, N., Tokutake, E., Wakasa, Y., et al. (2022). Effect of febuxostat on clinical outcomes in patients with hyperuricemia and cardiovascular disease. Int. J. Cardiol. 49, 127–133. doi:10.1016/j.ijcard.2021.11.076
Krantz, M., Eklund, D., Särndahl, E., and Hedbrant, A. (2023). A detailed molecular network map and model of the NLRP3 inflammasome. Front. Immunol. 14, 1233680. doi:10.3389/fimmu.2023.1233680
Lee, A. H., Shin, H. Y., Park, J. H., Koo, S. Y., Kim, S. M., and Yang, S. H. (2021). Fucoxanthin from microalgae Phaeodactylum tricornutum inhibits pro-inflammatory cytokines by regulating both NF-κB and NLRP3 inflammasome activation. Sci. Rep. 11 (1), 543. doi:10.1038/s41598-020-80748-6
Lee, H. M., Kim, J. J., Kim, H. J., Shong, M., Ku, B. J., and Jo, E. K. (2013). Upregulated NLRP3 inflammasome activation in patients with type 2 diabetes. Diabetes 62, 194–204. doi:10.2337/db12-0420
Lee, J., Hong, J., Umetani, M., Lavoy, E. C., Kim, J. H., and Park, Y. (2020). Vascular protection by exercise in obesity: inflammasome-associated mechanisms. Med. Sci. Sports Exerc 52, 2538–2545. doi:10.1249/MSS.0000000000002419
Lee, J., Lee, Y., LaVoy, E. C., Umetani, M., Hong, J., and Park, Y. (2018). Physical activity protects NLRP3 inflammasome-associated coronary vascular dysfunction in obese mice. Physiol. Rep. 6, e13738. doi:10.14814/phy2.13738
Lee, S., Ye, Q., Yang, H., Lee, S., Kim, Y., Lee, N., et al. (2024). Aiouea padiformis extract exhibits anti-inflammatory effects by inhibiting the ATPase activity of NLRP3. Sci. Rep. 14 (1), 5237. doi:10.1038/s41598-024-55651-z
Levine, B. D. (2014). Can intensive exercise harm the heart? The benefits of competitive endurance training for cardiovascular structure and function. Circulation 130 (12), 987–991. doi:10.1161/CIRCULATIONAHA.114.008142
Lewisluján, L. M., McCarty, M. F., Dinicolantonio, J. J., Gálvezruiz, J. C., Rosas-Burgos, E. C., Plascencia-Jatomea, M., et al. (2022). Nutraceuticals/drugs promoting mitophagy and mitochondrial biogenesis may combat the mitochondrial dysfunction driving progression of dry age-related macular degeneration. Nutrients 14 (9), 1985. doi:10.3390/nu14091985
Li, N., Zhang, L., Wang, X., Zhou, Y., and Gong, L. (2023). Exploring exercise-driven inhibition of pyroptosis: novel insights into treating diabetes mellitus and its complications. Front. Endocrinol. (Lausanne) 14, 1230646. doi:10.3389/fendo.2023.1230646
Li, X. H., Liu, L. Z., Chen, L., Pan, Q. N., Ouyang, Z. Y., Fan, D. J., et al. (2022). Aerobic exercise regulates FGF21 and NLRP3 inflammasome-mediated pyroptosis and inhibits atherosclerosis in mice. PLoS One 17, e0273527. doi:10.1371/journal.pone.0273527
Li, Y., Xu, P., Wang, Y., Zhang, J., Yang, M., Chang, Y., et al. (2020). Different intensity exercise preconditions affect cardiac function of exhausted rats through regulating TXNIP/TRX/NF-ĸBp65/NLRP3 inflammatory pathways. Evid. Based Complement. Altern. Med. 2020, 5809298. doi:10.1155/2020/5809298
Liao, C. C., Xu, J. W., Huang, W. C., Chang, H. C., and Tung, Y. T. (2022). Plasma proteomic changes of atherosclerosis after exercise in apoE knockout mice. Biol. (Basel). 11 (2), 253. doi:10.3390/biology11020253
Liu, D., Wang, R., Grant, A. R., Zhang, J., Gordon, P. M., Wei, Y., et al. (2017). Immune adaptation to chronic intense exercise training: new microarray evidence. BMC Genom 18, 29. doi:10.1186/s12864-016-3388-5
Liu, J., Jia, S., Yang, Y., Piao, L., Wang, Z., Jin, Z., et al. (2023). Exercise induced meteorin-like protects chondrocytes against inflammation and pyroptosis in osteoarthritis by inhibiting PI3K/Akt/NF-κB and NLRP3/caspase-1/GSDMD signaling. Biomed. Pharmacother. 158, 114118. doi:10.1016/j.biopha.2022.114118
Liu, S., Tao, J., Duan, F., Li, H., and Tan, H. (2022). HHcy induces pyroptosis and atherosclerosis via the lipid raft-mediated NOX-ROS-NLRP3 inflammasome pathway in apoE-/- mice. Cells 11, 2438. doi:10.3390/cells11152438
Lu, P., Zheng, H., Meng, H., Liu, C., Duan, L., Zhang, J., et al. (2023). Mitochondrial DNA induces nucleus pulposus cell pyroptosis via the TLR9-NF-κB-NLRP3 axis. J. Transl. Med. 21 (1), 389. doi:10.1186/s12967-023-04266-5
Lu, Y., Xiang, M., Xin, L., Zhang, Y., Wang, Y., Shen, Z., et al. (2022). Qiliqiangxin modulates the gut microbiota and NLRP3 inflammasome to protect against ventricular remodeling in heart failure. Front. Pharmacol. 13, 905424. doi:10.3389/fphar.2022.905424
Luo, B., Huang, F., Liu, Y., Liang, Y., Wei, Z., Ke, H., et al. (2017). NLRP3 inflammasome as a molecular marker in diabetic cardiomyopathy. Front. Physiol. 8, 519. doi:10.3389/fphys.2017.00519
Luo, B., Li, B., Wang, W., Liu, X., Xia, Y., Zhang, C., et al. (2014). NLRP3 gene silencing ameliorates diabetic cardiomyopathy in a type 2 diabetes rat model. PLoS One 9, e104771. doi:10.1371/journal.pone.0104771
Luo, M., Cao, C., Niebauer, J., Yan, J., Ma, X., Chang, Q., et al. (2021). Effects of different intensities of continuous training on vascular inflammation and oxidative stress in spontaneously hypertensive rats. J. Cell. Mol. Med. 25, 8522–8536. doi:10.1111/jcmm.16813
Ma, J., and Chen, X. (2021). Anti-inflammatory therapy for coronary atherosclerotic heart disease: unanswered questions behind existing successes. Front. Cardiovasc Med. 7, 631398. doi:10.3389/fcvm.2020.631398
Ma, M., Chen, W., Hua, Y., Jia, H., Song, Y., and Wang, Y. (2021). Aerobic exercise ameliorates cardiac hypertrophy by regulating mitochondrial quality control and endoplasmic reticulum stress through M2 AChR. J. Cell. Physiol. 236, 6581–6596. doi:10.1002/jcp.30342
Mardare, C., Kruger, K., Liebisch, G., Seimetz, M., Couturier, A., Ringseis, R., et al. (2016). Endurance and resistance training affect high fat diet-induced increase of ceramides, inflammasome expression, and systemic inflammation in mice. J. Diabetes. Res. 2016, 4536470. doi:10.1155/2016/4536470
Mason, S. A., Trewin, A. J., Parker, L., and Wadley, G. D. (2020). Antioxidant supplements and endurance exercise: current evidence and mechanistic insights. Redox. Biol. 35, 101471. doi:10.1016/j.redox.2020.101471
May, B. K., and Treadwell, R. E. (2020). Increasing exercise intensity: teaching high-intensity interval training to individuals with developmental disabilities using a lottery reinforcement system. Behav. Anal. Pract. 13 (4), 826–837. doi:10.1007/s40617-020-00428-9
McCullough, P. A., and Lavie, C. J. (2014). Coronary artery plaque and cardiotoxicity as a result of extreme endurance exercise. Mo Med. 111 (2), 95–98.
McKee, C. M., and Coll, R. C. (2020). NLRP3 inflammasome priming: a riddle wrapped in a mystery inside an enigma. J. Leukoc. Biol. 108, 937–952. doi:10.1002/JLB.3MR0720-513R
Memme, J. M., Erlich, A. T., Phukan, G., and Hood, D. A. (2021). Exercise and mitochondrial health. J. Physiol. 2021, 803–817. doi:10.1113/JP278853
Mensah, G. A., Roth, G. A., and Fuster, V. (2019). The global burden of cardiovascular diseases and risk factors: 2020 and beyond. J. Am. Coll. Cardiol. 74 (20), 2529–2532. doi:10.1016/j.jacc.2019.10.009
Mezzaroma, E., Toldo, S., Farkas, D., Seropian, I. M., Van Tassell, B. W., Salloum, F. N., et al. (2011). The inflammasome promotes adverse cardiac remodeling following acute myocardial infarction in the mouse. Proc. Natl. Acad. Sci. U. S. A. 108, 19725–19730. doi:10.1073/pnas.1108586108
Miko, H. C., Zillmann, N., Ring-Dimitriou, S., Dorner, T. E., Titze, S., and Bauer, R. (2020). Effects of physical activity on health. Gesundheitswesen 82 (S 03), S184-S195–S195. doi:10.1055/a-1217-0549
Movahedpour, A., Taghvaeefar, R., Asadi-Pooya, A. A., Karami, Y., Tavasolian, R., Khatami, S. H., et al. (2023). Nano-delivery systems as a promising therapeutic potential for epilepsy: current status and future perspectives. CNS Neurosci. Ther. 29 (11), 3150–3159. doi:10.1111/cns.14355
Mueller, S., Winzer, E. B., Duvinage, A., Gevaert, A. B., Edelmann, F., Haller, B., et al. (2021). Effect of high-intensity interval training, moderate continuous training, or guideline-based physical activity advice on peak oxygen consumption in patients with heart failure with preserved ejection fraction: a randomized clinical trial. Jama 325, 542–551. doi:10.1001/jama.2020.26812
Murray, K. O., Mahoney, S. A., Venkatasubramanian, R., Seals, D. R., and Clayton, Z. S. (2023). Aging, aerobic exercise, and cardiovascular health: barriers, alternative strategies and future directions. Exp. Gerontol. 173, 112105. doi:10.1016/j.exger.2023.112105
Napodano, C., Carnazzo, V., Basile, V., Pocino, K., Stefanile, A., Gallucci, S., et al. (2023). NLRP3 inflammasome involvement in heart, liver, and lung diseases-a lesson from cytokine storm syndrome. Int. J. Mol. Sci. 24 (23), 16556. doi:10.3390/ijms242316556
Nath, L. C., Elliott, A., La Gerche, A., Weir, J., Forbes, G., Thomas, G., et al. (2023). Associations between postrace atrial fibrillation and measures of performance, racing history and airway disease in horses. J. Vet. Intern Med. 37 (6), 2573–2583. doi:10.1111/jvim.16878
Neuman, J. C., Albright, K. A., and Schalinske, K. L. (2013). Exercise prevents hyperhomocysteinemia in a dietary folate-restricted mouse model. Nutr. Res. 33, 487–493. doi:10.1016/j.nutres.2013.04.008
Neumann, F. A., Jagemann, B., Makarova, N., Börschel, C. S., Aarabi, G., Gutmann, F., et al. (2022). Mediterranean diet and atrial fibrillation: lessons learned from the AFHRI case-control study. Nutrients 14 (17), 3615. doi:10.3390/nu14173615
Newman, J. C., and Verdin, E. (2014). β-hydroxybutyrate: much more than a metabolite. Diabetes Res. Clin. Pract. 106, 173–181. doi:10.1016/j.diabres.2014.08.009
Nie, Z., Chen, M., Gao, Y., Huang, D., Cao, H., Peng, Y., et al. (2021). Regulated cell death in urinary malignancies. Front. Cell. Dev. Biol. 9, 789004. doi:10.3389/fcell.2021.789004
O'Keefe, E. L., Sturgess, J. E., O'Keefe, J. H., Gupta, S., and Lavie, C. J. (2021). Prevention and treatment of atrial fibrillation via risk factor modification. Am. J. Cardiol. 160, 46–52. doi:10.1016/j.amjcard.2021.08.042
O'Keefe, J. H., Patil, H. R., Lavie, C. J., Magalski, A., Vogel, R. A., and McCullough, P. A. (2012). Potential adverse cardiovascular effects from excessive endurance exercise. Mayo Clin. Proc. 87 (6), 587–595. doi:10.1016/j.mayocp.2012.04.005
Paerewijck, O., and Lamkanfi, M. (2022). The human inflammasomes. Mol. Asp. Med. 88, 101100. doi:10.1016/j.mam.2022.101100
Paluch, A. E., Boyer, W. R., Franklin, B. A., Laddu, D., Lobelo, F., Lee, D. C., et al. (2024). Resistance exercise training in individuals with and without cardiovascular disease: 2023 update: a scientific statement from the American heart association. Circulation 149 (3), e217–e231. doi:10.1161/CIR.0000000000001189
Pope, B. S., and Wood, S. K. (2020). Advances in understanding mechanisms and therapeutic targets to treat comorbid depression and cardiovascular disease. Neurosci. Biobehav Rev. 116, 337–349. doi:10.1016/j.neubiorev.2020.06.031
Powers, S. K., Deminice, R., Ozdemir, M., Yoshihara, T., Bomkamp, M. P., and Hyatt, H. (2020). Exercise-induced oxidative stress: friend or foe? J. Sport Health Sci. 9, 415–425. doi:10.1016/j.jshs.2020.04.001
Ren, G., Zhang, X., Xiao, Y., Zhang, W., Wang, Y., Ma, W., et al. (2019). ABRO1 promotes NLRP3 inflammasome activation through regulation of NLRP3 deubiquitination. EMBO J. 38 (6), e100376. doi:10.15252/embj.2018100376
Ridker, P. M., and Rane, M. (2021). Interleukin-6 signaling and anti-interleukin-6 therapeutics in cardiovascular disease. Circ. Res. 128 (11), 1728–1746. doi:10.1161/CIRCRESAHA.121.319077
Ringseis, R., Eder, K., Mooren, F. C., and Krüger, K. (2015). Metabolic signals and innate immune activation in obesity and exercise. Exerc Immunol. Rev. 21, 58–68. doi:10.1111/jpn.12263
Rodrigues Junior, L. F., Mendes, F. S. N. S., Pinto, V. L. M., da Silva, P. S., Sperandio da Silva, G. M., Pinheiro, R. O., et al. (2020). A cardiac rehabilitation exercise program potentially inhibits progressive inflammation in patients with severe chagas cardiomyopathy: a pilot single-arm clinical trial. J. Res. Med. Sci. 25, 18. doi:10.4103/jrms.JRMS_175_18
Roncero-Ramos, I., Rangel-Zuñiga, O. A., Lopez-Moreno, J., Alcala-Diaz, J. F., Perez-Martinez, P., Jimenez-Lucena, R., et al. (2018). Mediterranean diet, glucose homeostasis, and inflammasome genetic variants: the CORDIOPREV study. Mol. Nutr. Food Res. 62 (9), e1700960. doi:10.1002/mnfr.201700960
Rothschild, J. A., and Bishop, D. J. (2020). Effects of dietary supplements on adaptations to endurance training. Sports Med. 50 (1), 25–53. doi:10.1007/s40279-019-01185-8
Ruan, J. (2019). Structural insight of gasdermin family driving pyroptotic cell death. Adv. Exp. Med. Biol. 1172, 189–205. doi:10.1007/978-981-13-9367-9_9
Sacco, S. J., Park, C. L., Suresh, D. P., and Bliss, D. (2014). Living with heart failure: psychosocial resources, meaning, gratitude and well-being. Heart Lung 43, 213–218. doi:10.1016/j.hrtlng.2014.01.012
Salo, D. C., Donovan, C. M., and Davies, K. J. (1991). HSP70 and other possible heat shock or oxidative stress proteins are induced in skeletal muscle, heart, and liver during exercise. Free Radic. Biol. Med. 11, 239–246. doi:10.1016/0891-5849(91)90119-N
Sandall, C. F., and MacDonald, J. A. (2019). Effects of phosphorylation on the NLRP3 inflammasome. Arch. Biochem. Biophys. 670, 43–57. doi:10.1016/j.abb.2019.02.020
Sandanger, Ø., Ranheim, T., Vinge, L. E., Bliksøen, M., Alfsnes, K., Finsen, A. V., et al. (2013). The NLRP3 inflammasome is up-regulated in cardiac fibroblasts and mediates myocardial ischaemia-reperfusion injury. Cardiovasc Res. 99, 164–174. doi:10.1093/cvr/cvt091
Schoenfeld, B. J., Ogborn, D., and Krieger, J. W. (2016). Effects of resistance training frequency on measures of muscle hypertrophy: a systematic review and meta-analysis. Sports Med. 46 (11), 1689–1697. doi:10.1007/s40279-016-0543-8
Schwartz, R. S., Kraus, S. M., Schwartz, J. G., Wickstrom, K. K., Peichel, G., Garberich, R. F., et al. (2014). Increased coronary artery plaque volume among male marathon runners. Mo Med. 111 (2), 89–94. doi:10.1016/j.paid.2014.03.024
Spalding, K. L., Arner, E., Westermark, P. O., Bernard, S., Buchholz, B. A., Bergmann, O., et al. (2008). Dynamics of fat cell turnover in humans. Nature 453, 783–787. doi:10.1038/nature06902
Spalinger, M. R., Kasper, S., Gottier, C., Lang, S., Atrott, K., Vavricka, S. R., et al. (2023). NLRP3 tyrosine phosphorylation is controlled by protein tyrosine phosphatase PTPN22. J. Clin. Investig. 133 (4), e169304. doi:10.1172/JCI169304
Spalinger, M. R., Schwarzfischer, M., and Scharl, M. (2020). The role of Protein tyrosine phosphatases in inflammasome activation. Int. J. Mol. Sci. 21 (15), 5481. doi:10.3390/ijms21155481
Stensvold, D., Slørdahl, S. A., and Wisløff, U. (2012). Effect of exercise training on inflammation status among people with metabolic syndrome. Metab. Syndr. Relat. Disord. 10, 267–272. doi:10.1089/met.2011.0140
Stienstra, R., van Diepen, J. A., Tack, C. J., Zaki, M. H., Perera, D., Neale, G. A., et al. (2011). Inflammasome is a central player in the induction of obesity and insulin resistance. Proc. Natl. Acad. Sci. U. S. A. 108, 15324–15329. doi:10.1073/pnas.1100255108
Stolen, K. Q., Kemppainen, J., Ukkonen, H., Kalliokoski, K. K., Luotolahti, M., Lehikoinen, P., et al. (2003). Exercise training improves biventricular oxidative metabolism and left ventricular efficiency in patients with dilated cardiomyopathy. J. Am. Coll. Cardiol. 41 (3), 460–467. doi:10.1016/s0735-1097(02)02772-9
Stumvoll, M., Goldstein, B. J., and van Haeften, T. W. (2005). Type 2 diabetes: principles of pathogenesis and therapy. Lancet 365, 1333–1346. doi:10.1016/S0140-6736(05)61032-X
Sun, H., Zhao, H., Yan, Z., Liu, X., Yin, P., and Zhang, J. (2021). Protective role and molecular mechanism of action of nesfatin-1 against high glucose-induced inflammation, oxidative stress and apoptosis in retinal epithelial cells. Exp. Ther. Med. 22, 833. doi:10.3892/etm.2021.10265
Sun, H. J., Ren, X. S., Xiong, X. Q., Chen, Y. Z., Zhao, M. X., Wang, J. J., et al. (2017). NLRP3 inflammasome activation contributes to VSMC phenotypic transformation and proliferation in hypertension. Cell. Death Dis. 8, e3074. doi:10.1038/cddis.2017.470
Sun, Y., and Ding, S. (2021). NLRP3 inflammasome in diabetic cardiomyopathy and exercise intervention. Int. J. Mol. Sci. 22, 13228. doi:10.3390/ijms222413228
Swanson, K. V., Deng, M., and Ting, J. P. (2019). The NLRP3 inflammasome: molecular activation and regulation to therapeutics. Nat. Rev. Immunol. 19 (8), 477–489. doi:10.1038/s41577-019-0165-0
Takahashi, M. (2019). Cell-specific roles of NLRP3 inflammasome in myocardial infarction. J. Cardiovasc Pharmacol. 74 (3), 188–193. doi:10.1097/FJC.0000000000000709
Terentes-Printzios, D., Ioakeimidis, N., Rokkas, K., and Vlachopoulos, C. (2022). Interactions between erectile dysfunction, cardiovascular disease and cardiovascular drugs. Nat. Rev. Cardiol. 19 (1), 59–74. doi:10.1038/s41569-021-00593-6
Thomas, K. N., Cotter, J. D., Williams, M. J., and van Rij, A. M. (2016). Repeated episodes of remote ischemic preconditioning for the prevention of myocardial injury in vascular surgery. Vasc. Endovasc. Surg. 50 (3), 140–146. doi:10.1177/1538574416639150
Troosters, T., Janssens, W., Demeyer, H., and Rabinovich, R. A. (2023). Pulmonary rehabilitation and physical interventions. Eur. Respir. Rev. 32 (168), 220222. doi:10.1183/16000617.0222-2022
Trøseid, M., Lappegård, K. T., Mollnes, T. E., Arnesen, H., and Seljeflot, I. (2009). The effect of exercise on serum levels of interleukin-18 and components of the metabolic syndrome. Metab. Syndr. Relat. Disord. 7, 579–584. doi:10.1089/met.2009.0003
Tucker, W. J., Fegers-Wustrow, I., Halle, M., Haykowsky, M. J., Chung, E. H., and Kovacic, J. C. (2022). Exercise for primary and secondary prevention of cardiovascular disease: jacc focus seminar 1/4. J. Am. Coll. Cardiol. 80, 1091–1106. doi:10.1016/j.jacc.2022.07.004
Tutor, A. W., Lavie, C. J., Kachur, S., Milani, R. V., and Ventura, H. O. (2023). Updates on obesity and the obesity paradox in cardiovascular diseases. Prog. Cardiovasc Dis. 78, 2–10. doi:10.1016/j.pcad.2022.11.013
Vaiciuleviciute, R., Bironaite, D., Uzieliene, I., Mobasheri, A., and Bernotiene, E. (2021). Cardiovascular drugs and osteoarthritis: effects of targeting ion channels. Cells 10 (10), 2572. doi:10.3390/cells10102572
Valenzuela, P. L., Ruilope, L. M., Santos-Lozano, A., Wilhelm, M., Kränkel, N., Fiuza-Luces, C., et al. (2023). Exercise benefits in cardiovascular diseases: from mechanisms to clinical implementation. Eur. Heart J. 44 (21), 1874–1889. doi:10.1093/eurheartj/ehad170
Vandanmagsar, B., Youm, Y. H., Ravussin, A., Galgani, J. E., Stadler, K., Mynatt, R. L., et al. (2011). The NLRP3 inflammasome instigates obesity-induced inflammation and insulin resistance. Nat. Med. 17, 179–188. doi:10.1038/nm.2279
Veeranki, S., and Tyagi, S. C. (2013). Defective homocysteine metabolism: potential implications for skeletal muscle malfunction. Int. J. Mol. Sci. 14, 15074–15091. doi:10.3390/ijms140715074
Vella, C. A., Taylor, K., and Drummer, D. (2017). High-intensity interval and moderate-intensity continuous training elicit similar enjoyment and adherence levels in overweight and obese adults. Eur. J. Sport Sci. 17 (9), 1203–1211. doi:10.1080/17461391.2017.1359679
Vincent, H. K., Bourguignon, C., and Vincent, K. R. (2006). Resistance training lowers exercise-induced oxidative stress and homocysteine levels in overweight and obese older adults. Obes. (Silver Spring) 14, 1921–1930. doi:10.1038/oby.2006.224
Wada, T., Ishikawa, A., Watanabe, E., Nakamura, Y., Aruga, Y., Hasegawa, H., et al. (2017). Eplerenone prevented obesity-induced inflammasome activation and glucose intolerance. J. Endocrinol. 235 (3), 179–191. doi:10.1530/JOE-17-0351
Wang, B., Zhou, R., Wang, Y., Liu, X., Shou, X., Yang, Y., et al. (2020). Effect of high-intensity interval training on cardiac structure and function in rats with acute myocardial infarct. Biomed. Pharmacother. 131, 110690. doi:10.1016/j.biopha.2020.110690
Wang, Q., Hu, J., Liu, Y., Li, J., Liu, B., Li, M., et al. (2019). Aerobic exercise improves synaptic-related proteins of diabetic rats by inhibiting FOXO1/NF-κB/NLRP3 inflammatory signaling pathway and ameliorating PI3K/Akt insulin signaling pathway. J. Mol. Neurosci. 69 (1), 28–38. doi:10.1007/s12031-019-01302-2
Wang, R., Wang, Y., Mu, N., Lou, X., Li, W., Chen, Y., et al. (2017). Activation of NLRP3 inflammasomes contributes to hyperhomocysteinemia-aggravated inflammation and atherosclerosis in apoE-deficient mice. Lab. Investig. 97, 922–934. doi:10.1038/labinvest.2017.30
Wang, T., Li, J., Li, H., Zhong, X., Wang, L., Zhao, S., et al. (2022). Aerobic exercise inhibited P2X7 purinergic receptors to improve cardiac remodeling in mice with type 2 diabetes. Front. Physiol. 13, 828020. doi:10.3389/fphys.2022.828020
Watanabe, S., Usui-Kawanishi, F., Karasawa, T., Kimura, H., Kamata, R., Komada, T., et al. (2020). Glucose regulates hypoxia-induced NLRP3 inflammasome activation in macrophages. J. Cell. Physiol. 235 (10), 7554–7566. doi:10.1002/jcp.29659
Winchester, L., Veeranki, S., Givvimani, S., and Tyagi, S. C. (2014). Exercise mitigates the adverse effects of hyperhomocysteinemia on macrophages, MMP-9, skeletal muscle, and white adipocytes. Can. J. Physiol. Pharmacol. 92, 575–582. doi:10.1139/cjpp-2014-0059
Wong, N. D., and Sattar, N. (2023). Cardiovascular risk in diabetes mellitus: epidemiology, assessment and prevention. Nat. Rev. Cardiol. 20 (10), 685–695. doi:10.1038/s41569-023-00877-z
Wu, J., Zhang, Y. F., Li, J. S., Zhu, G. L., Bi, Z. M., and Li, X. Y. (2019). The effect of high glucose-based peritoneal dialysis fluids on thioredoxin-interacting protein expression in human peritoneal mesothelial cells. Int. Immunopharmacol. 66, 198–204. doi:10.1016/j.intimp.2018.11.027
Wu, Y., Pan, B., Zhang, Z., Li, X., Leng, Y., Ji, Y., et al. (2022). Caspase-4/11-mediated pulmonary artery endothelial cell pyroptosis contributes to pulmonary arterial hypertension. Hypertension 79, 536–548. doi:10.1161/HYPERTENSIONAHA.121.17868
Xi, X., Zhang, R., Chi, Y., Zhu, Z., Sun, R., and Gong, W. (2024). TXNIP regulates NLRP3 inflammasome-induced pyroptosis related to aging via cAMP/PKA and PI3K/Akt signaling pathways. Mol. Neurobiol. doi:10.1007/s12035-024-04089-5
Xian, H., Watari, K., Sanchez-Lopez, E., Offenberger, J., Onyuru, J., Sampath, H., et al. (2022). Oxidized DNA fragments exit mitochondria via mPTP- and VDAC-dependent channels to activate NLRP3 inflammasome and interferon signaling. Immunity 55 (8), 1370–1385.e8. doi:10.1016/j.immuni.2022.06.007
Xie, Y., Huang, Y., Ling, X., Qin, H., Wang, M., and Luo, B. (2020). Chemerin/cmklr1 axis promotes inflammation and pyroptosis by activating NLRP3 inflammasome in diabetic cardiomyopathy rat. Front. Physiol. 11, 381. doi:10.3389/fphys.2020.00381
Xin, C., Zhang, J., Hao, N., Wang, J., Liu, H., Wei, H., et al. (2022). Irisin inhibits NLRP3 inflammasome activation in HG/HF incubated cardiac microvascular endothelial cells with H/R injury. Microcirculation 29 (8), e12786. doi:10.1111/micc.12786
Xu, M., Duan, Y., and Xiao, J. (2019). Exercise improves the function of endothelial cells by microrna. J. Cardiovasc Transl. Res. 12, 391–393. doi:10.1007/s12265-018-9855-4
Yang, Q., Chen, S., Wang, X., Yang, X., Chen, L., Huang, T., et al. (2023). Exercise mitigates endothelial pyroptosis and atherosclerosis by downregulating NEAT1 through N6-methyladenosine modifications. Arterioscler. Thromb. Vasc. Biol. 43, 910–926. doi:10.1161/ATVBAHA.123.319251
Yuan, B., Zhou, X. M., You, Z. Q., Xu, W. D., Fan, J. M., Chen, S. J., et al. (2020). Inhibition of AIM2 inflammasome activation alleviates GSDMD-induced pyroptosis in early brain injury after subarachnoid haemorrhage. Cell. Death Dis. 11 (1), 76. doi:10.1038/s41419-020-2248-z
Zeng, C., Duan, F., Hu, J., Luo, B., Huang, B., Lou, X., et al. (2020). NLRP3 inflammasome-mediated pyroptosis contributes to the pathogenesis of non-ischemic dilated cardiomyopathy. Redox Biol. 34, 101523. doi:10.1016/j.redox.2020.101523
Zeng, C., Wang, R., and Tan, H. (2019). Role of pyroptosis in cardiovascular diseases and its therapeutic implications. Int. J. Biol. Sci. 15, 1345–1357. doi:10.7150/ijbs.33568
Zeng, Z., Zheng, Q., Chen, J., Tan, X., Li, Q., Ding, L., et al. (2020). FGF21 mitigates atherosclerosis via inhibition of NLRP3 inflammasome-mediated vascular endothelial cells pyroptosis. Exp. Cell. Res. 393 (2), 112108. doi:10.1016/j.yexcr.2020.112108
Zhang, L., Yuan, M., Zhang, L., Wu, B., and Sun, X. (2019). Adiponectin alleviates nlrp3-inflammasome-mediated pyroptosis of aortic endothelial cells by inhibiting foxo4 in arteriosclerosis. Biochem. Biophys. Res. 514, 266–272. doi:10.1016/j.bbrc.2019.04.143
Zhang, Q., Yu, W., Lee, S., Xu, Q., Naji, A., and Le, A. D. (2015). Bisphosphonate induces osteonecrosis of the jaw in diabetic mice via NLRP3/caspase-1-dependent IL-1β mechanism. J. Bone Min. Res. 30, 2300–2312. doi:10.1002/jbmr.2577
Zhang, T., Ding, S., and Wang, R. (2021). Research progress of mitochondrial mechanism in NLRP3 inflammasome activation and exercise regulation of NLRP3 inflammasome. Int. J. Mol. Sci. 22 (19), 10866. doi:10.3390/ijms221910866
Zhang, W., Li, G., Luo, R., Lei, J., Song, Y., Wang, B., et al. (2022). Cytosolic escape of mitochondrial DNA triggers cGAS-STING-NLRP3 axis-dependent nucleus pulposus cell pyroptosis. Exp. Mol. Med. 54 (2), 129–142. doi:10.1038/s12276-022-00729-9
Zhang, W., Xu, X., Kao, R., Mele, T., Kvietys, P., Martin, C. M., et al. (2014). Cardiac fibroblasts contribute to myocardial dysfunction in mice with sepsis: the role of NLRP3 inflammasome activation. PLoS One 9, e107639. doi:10.1371/journal.pone.0107639
Zhang, X., Li, Y., Li, H., Li, W., and Li, Y. (2021). Mitochondrial ROS-induced TXNIP upregulation contributes to NLRP3 inflammasome activation in diabetic cardiomyopathy. Redox Biol. 44, 101913. doi:10.1016/j.redox.2021.101913
Zhao, N., Li, C. C., Di, B., and Xu, L. L. (2020). Recent advances in the NEK7-licensed NLRP3 inflammasome activation: mechanisms, role in diseases and related inhibitors. J. Autoimmun. 113, 102515. doi:10.1016/j.jaut.2020.102515
Zhaolin, Z., Jiaojiao, C., Peng, W., Yami, L., Tingting, Z., Jun, T., et al. (2019). OxLDL induces vascular endothelial cell pyroptosis through miR-125a-5p/TET2 pathway. J. Cell. Physiol. 234, 7475–7491. doi:10.1002/jcp.27509
Zhong, X., Zeng, H., Zhou, Z., Su, Y., Cheng, H., Hou, Y., et al. (2023). Structural mechanisms for regulation of GSDMB pore-forming activity. Nature 616 (7957), 598–605. doi:10.1038/s41586-023-05872-5
Zhou, R., Tardive, l A., Thorens, B., Choi, I., and Tschopp, J. (2010). Thioredoxin-interacting protein links oxidative stress to inflammasome activation. Nat. Immunol. 11, 136–140. doi:10.1038/ni.1831
Zhou, Y., Tong, Z., Jiang, S., Zheng, W., Zhao, J., and Zhou, X. (2020). The roles of endoplasmic reticulum in NLRP3 inflammasome activation. Cells 9 (5), 1219. doi:10.3390/cells9051219
Zhou, Z., Ying, C., Zhou, X., Shi, Y., Xu, J., Zhu, Y., et al. (2022). Aerobic exercise training alleviates renal injury in db/db mice through inhibiting Nox4-mediated NLRP3 inflammasome activation. Exp. Gerontol. 168, 111934. doi:10.1016/j.exger.2022.111934
Glossary
Keywords: cardiovascular disease, exercise, pyroptosis, NLRP3 inflammasome, intervation
Citation: Ding P, Song Y, Yang Y and Zeng C (2024) NLRP3 inflammasome and pyroptosis in cardiovascular diseases and exercise intervention. Front. Pharmacol. 15:1368835. doi: 10.3389/fphar.2024.1368835
Received: 11 January 2024; Accepted: 02 April 2024;
Published: 12 April 2024.
Edited by:
Yusof Kamisah, Faculty of Medicine Universiti Kebangaan Malaysia, MalaysiaReviewed by:
Wei Sun, University of Pittsburgh Medical Center, United StatesDezhao Lu, Zhejiang Chinese Medical University, China
Copyright © 2024 Ding, Song, Yang and Zeng. This is an open-access article distributed under the terms of the Creative Commons Attribution License (CC BY). The use, distribution or reproduction in other forums is permitted, provided the original author(s) and the copyright owner(s) are credited and that the original publication in this journal is cited, in accordance with accepted academic practice. No use, distribution or reproduction is permitted which does not comply with these terms.
*Correspondence: Cheng Zeng, emVuZ2NoZW5nQGdkcHUuZWR1LmNu
†These authors have contributed equally to this work