- 1Department of Psychiatry, School of Medicine, University of Occupational and Environmental Health, Kitakyushu, Japan
- 2Department of Psychiatry, School of Medicine, Fujita Health University, Toyoake, Japan
Background: There are complicated interactions between catecholaminergic neurons and brain-derived neurotrophic factor (BDNF) in the brain. However, no reports have addressed the relationship among 3-methoxy-4-hydroxyphenylglycol (MHPG), homovanillic acid (HVA), and BDNF in the blood.
Objective: This paper sought to investigate correlations between serum BDNF and plasma levels of MHPG and HVA in people with major depression (MD).
Materials and methods: A total of 148 patients (male/female 65/83, age 49.5 ± 12.1 years old) who satisfied criteria for MD based on the Diagnostic and Statistical Manual of Mental Disorders IV were enrolled in the present study. Plasma levels of MHPG and HVA were analyzed using high-performance liquid chromatography, and serum BDNF was measured using ELISA.
Results: No interactions were observed between plasma HVA levels (mean ± SD = 4.5 ± 1.5 ng/mL) and age, sex, HAMD scores, or serum BDNF levels (mean ± SD = 9.8 ± 2.9 ng/mL). No correlations were not also observed between plasma MHPG levels (mean ± SD = 5.9 ± 2.1 ng/mL) and age, sex, the HAMD17 scores (mean ± SD = 22.2 ± 2.9 ng/mL), or serum BDNF levels. Serum BDNF levels were negatively associated with HAMD17 scores.
Conclusion: The results suggest that there are no significant correlations between catecholamine metabolites and BDNF in the blood for MDD patients.
Introduction
Major depression (MD), which is the most prevalent and disabling form of depression, affects more than 30 million Europeans per year. In the USA, the estimated lifetime prevalence of MD is 16%. In addition to its disease burden, MD negatively impacts physical health (1). It has been speculated that catecholamines, neurotransmitters, norepinephrine, and/or dopamine play roles in MD (2), although the actions of catecholamines in the pathophysiology of MD remain incompletely understood. Norepinephrine exerts its effects by binding to α1- and β-adrenergic receptors, resulting in a stimulatory effect on cell signaling; in particular, such binding has been shown to increase intracellular phospholipase C and cyclic adenosine monophosphate (cAMP), respectively, whereas activation of the α2-adrenergic receptor suppresses intracellular cAMP and has an inhibitory influence on signaling (3). Drugs that inhibit the serotonin transporter (5-HTT) and/or the noradrenaline transporter exhibit anti-depressive efficacies. Selective serotonin reuptake inhibitors (SSRIs) and serotonin norepinephrine reuptake inhibitors (SNRIs) are first-line treatments for patients with MDD. We have demonstrated that milnacipran, an SNRI, increased plasma levels of 3-methoxy-4-hydroxyphenylglycol (MHPG), a major metabolite of norepinephrine, in the people with MD; this increase was related to milnacipran-associated clinical improvement in such patients (4). We have also reported that duloxetine, another SNRI, significantly increased plasma MHPG levels in the people with MD (5). Furthermore, in a series of prior studies, we demonstrated that the response to antidepressants was associated with plasma levels of catecholamine metabolites (6). Specifically, plasma levels of catecholamine metabolites, such as MHPG and homovanillic acid (HVA), could be used to predict response to SSRIs and SNRIs (4). The people with MD and lower plasma levels of MHPG had better response to milnacipran, whereas the people with MD and higher plasma levels of MHPG had better response to paroxetine. Given these findings, an increase in MHPG levels may play an important role in improving depressive symptoms. Furthermore, Yoon et al. (7) reported that for the people with MD, antidepressants decreased MHPG levels in cerebrospinal fluid (CSF) (7). Electroconvulsive therapy increased CSF HVA levels in the people with MD (8). In various ways, MHPG and HVA in plasma or CSF partially reflect brain status (4–8). Brain-derived neurotrophic factor (BDNF) is a neurotrophic factor that is abundant in the brain (9). BDNF is associated with neuroplasticity in the brain and plays a role in the pathophysiology of MD. According to a meta-analysis, blood levels (serum and plasma levels) of BDNF are lower in MDD patients than in healthy subjects. BDNF is synthesized and secreted in the brain, penetrates the blood–brain barrier, and is stored in platelets. The main source of BDNF in the blood is platelets, and BDNF is secreted in a calcium-dependent manner (10, 11). There exist interactions between catecholaminergic neurons and BDNF synthesis and secretion (12). We have reported that psychological stress is positively correlated with plasma MHPG and serum BDNF in healthy controls in workplaces in Japan (13, 14). There are complicated interactions between catecholaminergic neurons and BDNF in the brain. However, no studies have addressed the relationship among MHPG, HVA, and BDNF in the blood. We hypothesized that a correlation exists between plasma catecholamine metabolites and serum BDNF. In the current study, to examine this issue, we investigated correlations between serum BDNF and plasma levels of MHPG and HVA in the people with MD. The results might help shed light on catecholamine activities and BDNF in MD.
Materials and Methods
Participants
A total of 148 patients (male/female 65/83, age 49.5 ± 12.1 years old) were continuously recruited from 2004 to 2012 at Neuropsychiatry branch in University Hospital. Major depressive episodes were diagnosed using the Diagnostic and Statistical Manual of Mental Disorders IV Text Revision (15). The severity of depression was evaluated using the 17-item Hamilton Rating Scale for Depression (HAMD17) (16)-Japanese version. The evaluation was performed only once on the first day when the patients visited our branch in the hospital by Reiji Yoshimura (experienced psychiatrist over 30 years) according to the structural interview using GRID HAMD (17)-Japanese version. All patients had not taken any antidepressants at least 1 month before the evaluation. The people with MD patients whose HAMD17 scores 17 or more were enrolled in the present study. The exclusion criteria included any history of neurological disease or other physical diseases and comorbidities with other disorders, such as bipolar disorder or Axis II disorders based on the letters from former psychiatrists and/or family physicians. Blood samples and clinical evaluations were performed only once when patients first visited at our branch in the university hospital. The demographic data of the people with MD were described in Table 1. This study protocol was approved by the Ethics Committee of the University of Occupational and Environmental Health. Written informed consent was obtained from all subjects who participated in this study.
Assays of Plasma Levels of HVA and MHPG
Blood was drawn at 9:00 a.m. Plasma levels of HVA and MHPG were analyzed in duplicate, and mean value were presented each data. Plasma HVA levels were analyzed using high-performance liquid chromatography with electrochemical detection (HPLC-ECD) in accordance with a slightly modified version of the method described by Yung et al. (18) In brief, each cyano-bonded solid-phase extraction cartridge was preconditioned with methanol, followed by glass-distilled water. The plasma sample (or the standard solution) and the internal standard solution (5-hydroxyindolecarboxylic acid) were added to each cartridge. The samples were then deproteinized with acetonitrile. The samples were mixed with vortex and centrifugation, then an aliquot of supernatant was allowed to very slowly pass through the cartridge under a mild vacuum. After the cartridge was washed twice with distilled water and extracted once with ethyl acetate, the aliquot was then evaporated to dryness under N2 and dissolved in a mobile phase (phosphate buffer). Finally, a 10-µL portion of the resulting solution was injected into the HPLC-ECD apparatus. The intra- and inter-assay coefficients of variation were 6.9 and 8.8%, respectively. The recovery rate was 83.1%.
Plasma MHPG levels were also analyzed using HPLC-ECD in accordance with a previously reported method (19). Briefly, after plasma was separated by centrifugation, extraction was performed under a vacuum using Bond-Elut columns (Varian, Palo Alto, CA, USA) prepacked with C18-bonded silica in a disposable syringe with a capacity of 1 mL. The columns, which were inserted into a vacuum chamber connected to an aspirator, were prepared by washing with 1 mL methanol followed by 1 mL of water. After the addition of an internal standard (vanillyl alcohol) to the plasma, the samples were very slowly passed through the columns, followed by two rinses with distilled water to wash off residual sample and easily eluted hydrophilic compounds. The adsorbed materials were eluted with methanol into a phosphate buffer mixture. Then, a 20-µL portion of this solution was then injected into the HPLC-ECD apparatus. The intra- and inter-assay coefficients of variation were 6.2 and 8.9%, respectively. The recovery rate was 81.0%.
Assays of Serum BDNF
Blood was drawn at 9:00 a.m. Serum levels of BDNF were measured in duplicate using a Human BDNF ELISA Kit (Adipo Bioscience, Santa Clara, CA, USA), and mean value were presented each data. Protocols were performed in accordance with the manufacturer’s instructions mentioned as our previous study (20). Each well’s optical density was measured using an automated microplate reader (Emax; Molecular Devices, Sunnyvale, CA, USA).
Statistical Analysis
Student’s t-test and Pearson’s correlation coefficient were used in two factors comparison. The Shapiro–Wilk test indicated that BDNF levels were normally distributed. A generalized linear model was used to explore BDNF level associated with HVA and MHPG levels, using potentially confounding variables (age, gender, first episode or not, melancholia or not, duration of untreated illness and HAMD17 scores at baseline) as covariates, and regarded the adjusted results as the study outcomes.
All statistical tests were performed using JMP (JMP 12, SAS Japan Inc., Tokyo, Japan), and P < 0.05 was considered to be indicative of statistical significance.
Results
The demographics of the people with MD enrolled were shown in Table 1. For the examined MDD patients, plasma HVA, plasma MHPG, and serum BDNF were 4.5 ± 1.5 (mean ± SD), 5.9 ± 2.1, and 9.8 ± 2.9 ng/mL, respectively (Table 1). When we performed non-adjusted simple two correlations of two factors among serum BDNF, plasma HVA, plasma MHPG, DUI, and the HAMD17, significant correlation was only found between serum BDNF and the HAMD17 (Figure 1). Serum BDNF levels in the people with MD with melancholia (8.6 ± 2.2 ng/mL) were significantly lower than in the people with MD with non-melancholia (10.3 ± 3.1 ng/mL). Serum BDNF, plasma HVA, and plasma MHPG were not different between in MDD patients with first episode, and those in recurrent (Table 2). No associations between plasma MHPG levels and age, sex, HAMD scores, or serum BDNF levels were identified (Table 3). In addition, no associations were detected between plasma HVA levels and age, sex, HAMD scores, or serum BDNF levels (Table 3). Serum BDNF levels were negatively associated with HAMD17 scores for the people with MD analyzed by (Table 3).
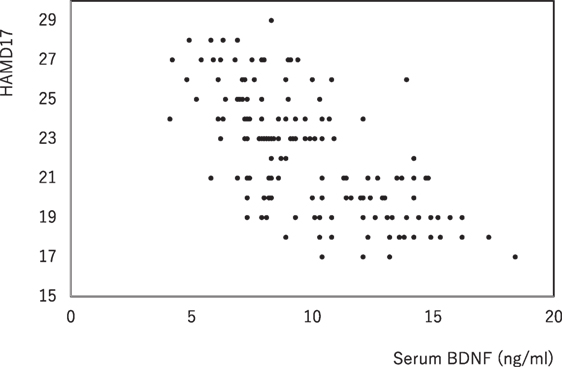
Figure 1. The changes in serum brain-derived neurotrophic factor (BDNF) and the HAMD17 scores. Data were analyzed by Pearson’s correlation coefficient (r = −0.654, p = 0.000).
Discussion
The main finding of the present study was that plasma levels of catecholamine metabolites were not associated with serum BDNF levels, even though catecholamine might influence BDNF synthesis and secretion in the brain (21) and BDNF might play an important role in the synthesis and/or secretion of catecholamine (22). We have previously reported a negative correlation between plasma MHPG and serum BDNF in healthy workers (13, 14). In this study, no correlation between serum BDNF and plasma MHPG or HVA was observed in the people with MD. Given the aforementioned findings, it is plausible that individuals’ sympathetic nerves are activated by stress, with potential imbalances when the people with major depressive state is reached. Most BDNF in serum is derived from platelets because platelets are abundant in serum. However, plasma BDNF might be derived from the brain because there are few platelets in plasma. We reported that serum BDNF levels were 14-fold higher than plasma BDNF levels, and a close relationship was found between serum and plasma BDNF levels (23). Pan et al. demonstrated that intact BDNF in the peripheral circulation crosses the BBB by a high-capacity, saturable transport system (24). The time course of central and peripheral BDNF changes might differ, it can be, however, justified to measure serum BDNF levels with a time delay to monitor brain tissue neurotrophin alterations in rats (25). From taking these findings into account, blood (serum or plasma) partially reflect the brain.
A recent meta-analysis indicated that serum levels of mature BDNF are lower in patients with MDD than in healthy controls. Serum levels of mature BDNF were found to be initially low but to increase over the course of antidepressant treatment (26). Although there was no control group in the present study, we could not compare in the MDD group and healthy subjects regarding plasma level of catecholamine metabolites or serum BDNF. Plasma HVA, plasma MHPG, and serum BDNF in healthy subjects from our pooled data in our lab (n = 218, male/female 122/96, age 34.7 ± 14.2 years old) were 5.2 ± 2.3 (mean ± SD), 4.3 ± 1.9, and 12.1 ± 3.6 ng/mL, respectively. However, we could not compare above data, because the experiments were performed different time, age and genders were not matched, and also factors influence BDNF, MHPG, HVA were heterogeneous in the both group. For example, body mass index (27), smoking (28), and personality traits also influence serum BDNF levels (29). In short, the two groups have different back ground, respectively. We have previously reported a significant correlation between HAMD17 scores and serum BDNF levels (17). In the present study, we reconfirmed this prior finding in a more robust manner. The results indicate that serum BDNF is a useful candidate biomarker for depressive severity in MDD patients. Serum BDNF was not correlated with plasma MHPG or plasma HVA in the people with MD. The results of the present study suggest that BDNF and catecholamine metabolites are not related in the periphery despite the fact that catecholaminergic neurons have important correlations with BDNF in the brain. Psychological stress generally enhances catecholaminergic activity, although there may be individual differences with respect to this reaction. One group of the people with MD might have high levels of MHPG or HVA, whereas another group of the people with MD might have low levels of MHPG or HVA; this difference may be partially determined by genetic factors such as polymorphisms. Interestingly, it has been reported that the regulation of serotonin influences both serotonin metabolites and catecholamine metabolites (30). 5-HTT is believed to affect the pathogenesis of mood disorders. Numerous genetic association studies have examined how 5-HTT functional polymorphisms relate to vulnerability to mood disorders and therapeutic responses to antidepressants. We have investigated the effects of the 5-HTT-linked polymorphic region (5-HTTLPR) and concentrations of monoamine metabolites in CSF for treatment-resistant patients with mood disorders and found a higher HVA concentration in S/S subjects than in L/L and L/S subjects and significantly higher hydroxyindole acetic acid (5-HIAA) concentrations among carriers of an S allele than among other individuals (31). Yoon et al. (7) reported that all monoamine metabolites (MHPG, HVA, and 5-HIAA) were significantly lower in moderately to severely depressed patients than in controls; they also found that antidepressants decreased levels of 5-HIAA and MHPG. In contrast, antidepressants increased HVA levels, suggesting that HVA might be useful in clinical settings (7).
Luykx et al. (32) conducted a genome-wide association study of monoamine metabolite levels in CSF for 414 human subjects from the general population. In a linear model with corrections for covariates, the authors identified one locus associated with monoamine metabolites that exhibited genome-wide significance; this locus was 20 kb from SSTR1, a gene involved in brain signal transduction and glutamate receptor signaling. Those authors performed a subsequent quantitative trait locus analysis for whole-genome expression that provided evidence that this variant controls the expression of PDE9A, a gene previously implicated in monoaminergic transmission, MD and antidepressant response; these findings will hopefully contribute to an exploration of the functional impact of the highlighted genes on monoaminergic transmission and neuropsychiatric phenotypes (32).
Based on the aforementioned findings, the transmission of catecholamine appears to be complicated and to involve many interactions. Levels of catecholamine metabolites are regulated by many genes. With respect to BDNF, we have previously reported that the BDNF Val66Met polymorphism is not correlated with serum BDNF levels (11).
Conclusion
Our hypothesis was not supported. In short, there was no strict and direct correlation between catecholamine metabolites (MHPG and HVA) and BDNF in the blood for the people with MD. The implication of this study is that plasma levels of catecholamine metabolites neither influence nor are influenced by serum BDNF levels. The present preliminary study had several serious limitations, such as the absence of a control group, a small sample, heterogeneous, and cross-sectional design. Thus, we are performing research longitudinal study with a large sample, with age- and sex-matched control group to reconfirm these preliminary results.
Highlight of the Article
• No correlation was found between plasma catecholamine metabolites (MHPG and HVA) and serum BDNF for the people with MD.
• A robust correlation was found between serum BDNF and the HAMD17.
Ethics Statement
All subjects were provided with information about the procedures. Written informed consent was obtained via forms approved by the local ethics committee of the University of Occupational and Environmental Health.
Author Contributions
RY, TK, KA, and AK conceived and designed the experiments, corrected blood samples, evaluated HAMD17 scores, measured plasma catecholamine metabolites and serum BDNF levels, analyzed the data, and wrote the first draft of the manuscript. NI read and corrected this first draft and approved the final manuscript.
Conflict of Interest Statement
RY, TK, KA, AK, and NI declare that they have no direct conflicts of interest relevant to this study. No grant support or other sources of funding were used to conduct this study or prepare this manuscript. RY has received speaker’s honoraria from Daiichi Sankyo, Dainippon Sumitomo, Eisai, Janssen, Otsuka, Meiji, MSD, and Eli Lilly. TK has received speaker’s honoraria from Daiichi Sankyo, Dainippon Sumitomo, Eisai, Janssen, Otsuka, Meiji, MSD, and Tanabe-Mitsubishi (Yoshitomi) and has received a Health Labour Sciences Research Grant and a Fujita Health University School of Medicine research grant. NI has received speaker’s honoraria from Astellas, Dainippon Sumitomo, Eli Lilly, GlaxoSmithKline, Janssen, Yoshitomi, Otsuka, Meiji, Shionogi, Novartis, and Pfizer and has received research grants from GlaxoSmithKline, Meiji, and Otsuka.
Acknowledgments
The authors thank Ms. Ishiba for her great help in preparing the samples.
References
1. Gartlehner G, Wagner G, Matyas N, Titscher V, Greimel J, Lux L, et al. Pharmacological and non-pharmacological treatments for major depressive disorder: review of systematic reviews. BMJ Open (2017) 7:e014912. doi:10.1136/bmjopen-2016-014912
2. Hamon M, Blier P. Monoamine neurocircuitry in depression and strategies for new treatments. Prog Neuropsychopharmacol Biol Psychiatry (2013) 45:54–63. doi:10.1016/j.pnpbp.2013.04.009
3. Maletic V, Eramo A, Gwin K, Offord SJ, Duffy RA. The role of norepinephrine and its α-adrenergic receptors in the pathophysiology and treatment of major depressive disorder and schizophrenia: a systematic review. Front Psychiatry (2017) 8:42. doi:10.3389/fpsyt.2017.00042
4. Shinkai K, Yoshimura R, Ueda N, Okamoto K, Nakamura J. Associations between baseline plasma MHPG (3-methoxy-4-hydroxyphenylglycol) levels and clinical responses with respect to milnacipran versus paroxetine treatment. J Clin Psychopharmacol (2004) 24:11–7. doi:10.1097/01.jcp.0000104904.75206.19
5. Atake K, Yoshimura R, Hori H, Katsuki A, Ikenouchi-Sugita A, Umene-Nakano W, et al. Duloxetine, a selective noradrenaline reuptake inhibitor, increased plasma levels of 3-methoxy-4-hydroxyphenylglycol but not homovanillic acid in patients with major depressive disorder. Clin Psychopharmacol Neurosci (2014) 12:37–40. doi:10.9758/cpn.2014.12.1.37
6. Yoshimura R, Nakamura J, Shinkai K, Ueda N. Clinical response to antidepressant treatment and 3-methoxy-4-hydroxyphenylglycol levels: mini review. Prog Neuropsychopharmacol Biol Psychiatry (2004) 28:611–6. doi:10.1016/j.pnpbp.2004.01.008
7. Yoon HS, Hattori K, Ogawa S, Sasayama D, Ota M, Teraishi T, et al. Relationships of cerebrospinal fluid monoamine metabolite levels with clinical variables in major depressive disorder. J Clin Psychiatry (2017) 78:e947–56. doi:10.4088/JCP.16m11144
8. Nikisch G, Mathé AA. CSF monoamine metabolites and neuropeptides in depressed patients before and after electroconvulsive therapy. Eur Psychiatry (2008) 23:356–9. doi:10.1016/j.eurpsy.2008.03.003
9. Schuch FB. Progress in the study of the effects of exercise on affective and anxiety disorders. Front Psychiatry (2014) 5:153. doi:10.3389/fpsyt.2014.00153
10. Yoshimura R, Mitoma M, Sugita A, Hori H, Okamoto T, Umene W, et al. Effects of paroxetine or milnacipran on serum brain-derived neurotrophic factor in depressed patients. Prog Neuropsychopharmacol Biol Psychiatry (2007) 31:1034–7. doi:10.1016/j.pnpbp.2007.03.001
11. Yoshimura R, Kishi T, Suzuki A, Umene-Nakano W, Ikenouchi-Sugita A, Hori H, et al. The brain-derived neurotrophic factor (BDNF) polymorphism Val66Met is associated with neither serum BDNF level nor response to selective serotonin reuptake inhibitors in depressed Japanese patients. Prog Neuropsychopharmacol Biol Psychiatry (2011) 35:1022–5. doi:10.1016/j.pnpbp.2011.02.009
12. Paredes D, Granholm AC, Bickford PC. Effects of NGF and BDNF on baseline glutamate and dopamine release in the hippocampal formation of the adult rat. Brain Res (2007) 1141:56–64. doi:10.1016/j.brainres.2007.01.018
13. Mitoma M, Yoshimura R, Sugita A, Umene W, Hori H, Nakano H, et al. Stress at work alters serum brain-derived neurotrophic factor (BDNF) levels and plasma 3-methoxy-4-hydroxyphenylglycol (MHPG) levels in healthy volunteers: BDNF and MHPG as possible biological markers of mental stress? Prog Neuropsychopharmacol Biol Psychiatry (2008) 32:679–85. doi:10.1016/j.pnpbp.2007.11.011
14. Okuno K, Yoshimura R, Ueda N, Ikenouchi-Sugita A, Umene-Nakano W, Hori H, et al. Relationships between stress, social adaptation, personality traits, brain-derived neurotrophic factor and 3-methoxy-4-hydroxyphenylglycol plasma concentrations in employees at a publishing company in Japan. Psychiatry Res (2011) 186:326–32. doi:10.1016/j.psychres.2010.07.023
15. American Psychiatric Association. Diagnostic and Statistical Manual of Mental Disorders (DSM-IV-TR). 4th ed. Washington, DC: American Psychiatric Association (2000).
16. Hamilton M. A rating scale for depression. J Neurol Neurosurg Psychiatry (1960) 23:56–62. doi:10.1136/jnnp.23.1.56
17. Depression Rating Scale Standardization Team (DRSST). GRID-HAMD-17, GRID-HAMD-21: Structured Interview Guide. San Diego: International Society for CNS Drug Development (2003).
18. Yung AR, Phillips LJ, Yuen HP, Francey SM, McFarlane CA, Hallgren M, et al. Psychosis prediction: 12-month follow up of a high-risk (“prodromal”) group. Schizophr Res (2003) 60:21–32. doi:10.1016/S0920-9964(02)00167-6
19. Ohnishi A, Minegishi A, Ishizaki T. Effect of β-adrenoceptor blockade on exercise-induced plasma catecholamine concentration-heart rate response relationship. J Cardiovasc Pharmacol (1987) 10:667–74. doi:10.1097/00005344-198712000-00010
20. Yoshimura R, Kishi T, Hori H, Atake K, Katsuki A, Nakano-Umene W, et al. Serum proBDNF/BDNF and response to fluvoxamine in drug-naïve first-episode major depressive disorder patients. Ann Gen Psychiatry (2014) 13:19. doi:10.1186/1744-859X-13-19
21. Parikh V, Naughton SX, Yegla B, Guzman DM. Impact of partial dopamine depletion on cognitive flexibility in BDNF heterozygous mice. Psychopharmacology (2016) 233:1361–75. doi:10.1007/s00213-016-4229-6
22. Scott AL, Zhang M, Nurse CA. Enhanced BDNF signalling following chronic hypoxia potentiates catecholamine release from cultured rat adrenal chromaffin cells. J Physiol (2015) 593:3281–99. doi:10.1113/JP270725
23. Yoshimura R, Sugita-Ikenouchi A, Hori H, Umene-Nakano W, Hayashi K, Katsuki A, et al. A close correlation between plasma and serum levels of brain-derived neurotrophic factor (BDNF) in healthy volunteers. Int J Psychiatry Clin Pract (2010) 14:220–2. doi:10.3109/13651501003748560
24. Pan W, Banks WA, Fasold MB, Bluth J, Kastin AJ. Transport of brain-derived neurotrophic factor across the blood-brain barrier. Neuropharmacology (1998) 37:1553–61. doi:10.1016/S0028-3908(98)00141-5
25. Sartorius A, Hellweg R, Litzke J, Vogt M, Dormann C, Vollmayr B, et al. Correlations and discrepancies between serum and brain tissue levels of neurotrophins after electroconvulsive treatment in rats. Pharmacopsychiatry (2009) 42:270–6. doi:10.1055/s-0029-1224162
26. Zhou C, Zhong J, Zou B, Fang L, Chen J, Deng X, et al. Meta-analyses of comparative efficacy of antidepressant medications on peripheral BDNF concentration in patients with depression. PLoS One (2017) 12:e0172270. doi:10.1371/journal.pone.0172270
27. Pillai A, Bruno D, Sarreal AS, Hernando RT, Saint-Louis LA, Nierenberg J, et al. Plasma BDNF levels vary in relation to body weight in females. PLoS One (2012) 7:e39358. doi:10.1371/journal.pone.0039358
28. Kim KW, Won YL, Ko KS, Roh JW. Smoking habits and neuropeptides: adiponectin, brain-derived neurotrophic factor, and leptin levels. Toxicol Res (2014) 30:91–7. doi:10.5487/TR.2014.30.2.091
29. Lang UE, Hellweg R, Gallinat J. BDNF serum concentrations in healthy volunteers are associated with depression-related personality traits. Neuropsychopharmacology (2004) 29:795–8. doi:10.1038/sj.npp.1300382
30. Umene-Nakano W, Yoshimura R, Ueda N, Suzuki A, Ikenouchi-Sugita A, Hori H, et al. Predictive factors for responding to sertraline treatment: views from plasma catecholamine metabolites and serotonin transporter polymorphism. J Psychopharmacol (2009) 24:1764–71. doi:10.1177/0269881109106899
31. Kishida I, Aklillu E, Kawanishi C, Bertilsson L, Ågren H. Monoamine metabolites level in CSF is related to the 5-HTT gene polymorphism in treatment-resistant depression. Neuropsychopharmacology (2007) 32:2143–51. doi:10.1038/sj.npp.1301336
Keywords: 3-methoxy-4-hydroxyphenylglycol, homovanillic acid, brain-derived neurotrophic factor, major depression, serum, plasma
Citation: Yoshimura R, Kishi T, Atake K, Katsuki A and Iwata N (2018) Serum Brain-Derived Neurotrophic Factor, and Plasma Catecholamine Metabolites in People with Major Depression: Preliminary Cross-Sectional Study. Front. Psychiatry 9:52. doi: 10.3389/fpsyt.2018.00052
Received: 09 December 2017; Accepted: 06 February 2018;
Published: 28 February 2018
Edited by:
Brisa S. Fernandes, Deakin University, AustraliaReviewed by:
Michele Fornaro, Columbia University, United StatesSerafim Carvalho, Cooperativa de Ensino Superior Politécnico e Universitário, Portugal
Copyright: © 2018 Yoshimura, Kishi, Atake, Katsuki and Iwata. This is an open-access article distributed under the terms of the Creative Commons Attribution License (CC BY). The use, distribution or reproduction in other forums is permitted, provided the original author(s) and the copyright owner are credited and that the original publication in this journal is cited, in accordance with accepted academic practice. No use, distribution or reproduction is permitted which does not comply with these terms.
*Correspondence: Reiji Yoshimura, eW9zaGk2MjFAbWVkLnVvZWgtdS5hYy5qcA==
†Co-first authors.